- 1Carthage University, Field Crop Laboratory, National Institute for Agricultural Research of Tunisia (INRAT), Menzah 1, Tunisia
- 2Laboratory of Biotechnology and Biomonitoring of the Environment and Oasis Ecosystems, Faculty of Sciences of Gafsa, University of Gafsa, Gafsa, Tunisia
- 3AgroBioSciences Program, College for Agriculture and Environmental Science, Mohammed VI Polytechnic University, Ben Guerir, Morocco
In this current study, we explored the preventive capacity of the aqueous extract of Orobanche foetida (OF), a root holoparasite, against CCl4 prompt hepatotoxicity in rats. LC-MS/MS profiling revealed the existence of 32 compounds belonging to organic acids, benzoic acid derivatives, and hydroxycinnamic acids along with their glycosides and derivatives as well as several flavonoids. In vitro, OF demonstrated substantial antioxidant potential at DPPH and ABTS assays. Results showed that the pretreatment with OF for 6 weeks at the doses (25 mg/kg bw) and (50 mg/kg bw) countered the CCl4-induced liver injury by restoring liver injuries indicators (ALT, AST, LDH, ALP, GGT and bilirubin), normalizing lipid profile (TC, TG, LDL-C, and HDL-C), as well as, impeding DNA fragmentation. Furthermore, OF blocked the hepatic oxidative stress spurred by CCl4 administration through boosting antioxidant enzymes (GSH, CAT, and SOD) responsible of diminishing lipid peroxidation. exhibited an anti-inflammatory effect by downregulating TNF-α and IL-6 levels. OF suppressive effect on proinflammatory cytokines is further exerted by its capacity to modulate the expression of the NF-κB gene. In silico investigation revealed that among the 32 identified compounds, vanillic acid glucoside and dihydroxybenzoic acid glucoside have strong and stable bindings with the active sites of three key inflammatory proteins (PARP-1, TNF-α, IL-6), which could highlight the antioxidant and anti-inflammatory capacity of. Overall, this research provides a preliminary pharmacological support for the medicinal applications of Orobanche foetida for addressing inflammatory and hepato-pathological conditions.
1 Introduction
Parasitic plants are a very distinct group, notorious for their capacity to penetrate the living tissues of another plant (the host) from which they obtain some or all the nutrients needed for their development throughout their life cycle. This group, comprising over 4,750 species from 26 families, makes up 1.6% of the angiosperm population (Nickrent, 2020). These species, often overlooked and dismissed as mere parasites, have played versatile roles in human societies and have been used throughout the world as food, medicine, and in cultural customs (Kuijt, 1969; Büssing, 2000; Těšitel et al., 2021). More importantly, they possess a noteworthy arsenal of bioactive molecules such as alkaloids, flavonoids, terpenoids, and phenolic compounds that may have potential therapeutic applications in the treatment of various diseases (Ben Attia et al., 2020; Gawenda-Kempczyńska et al., 2022; Suaza-Gaviria et al., 2023). As a matter of fact, the two families Orobanchaceae and Loranthaceae are the most recurrently reported and studied for their therapeutic properties among the parasitic plants’ families (Těšitel et al., 2021).
The genus Orobanche, the largest of the Orobanchaceae family with over 170 species distributed throughout the world, includes mostly holoparasitic plant species that depends entirely on the host for their growth and survival due to the lack of chlorophyll (Konarska and Chmielewski, 2020). Orobanche foetida, very distinct by its vibrant red color and fetid smell, is a root parasite known to be one of the most devastating species in the Mediterranean region (Abbes et al., 2010). In Tunisia, this species poses a growing challenge for various leguminous plant fields (Amri et al., 2019; Amri et al., 2021). Its presence and large spreading into farmers’ fields has been reported as an emerging issue affecting these crops (Nefzi et al., 2016; Trabelsi et al., 2016; Abbes et al., 2020).
Despite their parasitic and damaging nature, several Orobanche species were used for centuries in traditional and folk medicine among several ancient civilizations (Shi et al., 2020). Numerous studies have been conducted on them in the effort of foregrounding their vast spectrum of bioactivities and discovering the wealth of their phytochemical compounds. Within this framework, the biological activities of O. crenata phytochemical compounds, including anti-inflammatory and antioxidant activities (Abbes et al., 2014; Nada and El-Chaghaby, 2015; Genovese et al., 2020), anticancer activity (Ben Attia et al., 2020; Hegazy et al., 2020) and hepatoprotective activity (Abo-Qotb et al., 2022), have been studied in depth. Meanwhile, the screening for bioactive compounds with interesting therapeutic traits from O. foetida is little laid out. A limited number of studies suggest that O. foetida might be a reservoir of antioxidant and antibacterial properties and it could be deployed in human nutrition as well as in various commercial and pharmaceutical products (Abbes et al., 2014; Ben Attia et al., 2020).
The aims of our study were to elaborate a preliminary phytochemical analysis of O. foetida and to study how well the aqueous extracts of O. foetida (OF) could suppress an induced hepatotoxicity. For this matter, our study offered conclusive evidence regarding the potential free radical scavenging capabilities of compounds, along with their antioxidant properties and their ability to protect DNA and hepatic tissues. Moreover, molecular docking techniques were employed to examine the nature of binds formed between several phenolic compounds and some key proteins engaged in the induced hepatotoxicity.
2 Material and methods
2.1 Chemicals
Chemicals used in conducting mass spectrometry assays were designated as MS-grade, implying a higher purity level suitable for such analyses, while the remaining reagents were classified under analytical reagent grade. LCgrade acetic acid and acetonitrile were obtained from Fluka (Switzerland) and Thermo Fisher (Waltham, MA, USA), respectively. Methanol, employed for the dissolution of samples, was purchased from Panreac (Barcelona, Spain). Double-deionized water was produced using a Milli-Q system (Millipore, Bedford, MA, USA). Standard compounds were supplied by Sigma–Aldrich (St. Louis, MO, USA). Additionally, both CCl4 and kits for biochemical assays were obtained from the same supplier, Sigma–Aldrich (St. Louis, MO, USA).
2.2 Plant sampling and extraction
In May 2021, O. foetida growing on faba bean (Vicia faba L.) were gathered from the research station of Beja in Tunisia. The newly cut entire plants were dried under shade in a drying chamber at ambient temperature and active ventilation. The Khurm et al. (2023) method was modified slightly to extract the dry powder from the entire plant (9 g) using 0.25L of water in a Soxhlet system (Buchi, France) during 6 hours. The extract, after being filtered and concentrated using a rotary evaporator at a temperature fixed at 38°C, was stored at 4°C for further analysis.
2.3 Phytocontents
The procedure of Mahdi et al. (2023) with slight adjustments was used to assess both total phenolic content (TPC) and total flavonoids content (TFC). To determine the TPC, The Folin-Ciocalteu colorimetric test was employed. The results have been reported as gallic acid equivalent per g dry weight (mg GAE/g DW). The aluminum chloride (AlCl3) method was employed to estimate the TFC. The results were presented as mg quercetin equivalents per g dry weight (mg QE/g DW). To estimate the Total tannin contents (TTC), we used the Yao et al. (2023) procedure. The results were expressed as milligrams of catechin equivalent per Gram dry weight (mg CATE/g DW).
2.4 LC-MS analysis
HPLC-PDA-MS/MS system, which consists of a Shimadzu Japan system (Tokyo, Japan) coupled with an MS 8050 mass spectrometer equipped with an electrospray ionization (ESI) source, was used to assess OF phytochemical composition, as previously described by Tawfeek et al. (2023). The fragmentation process was carried out using C18 reversed-phase column (Zorbax Eclipse XDBC18, rapid resolution, 4.6 × 150 mm, 3.5 µm, Agilent, Santa Clara, CA, USA). A gradient of water and acetonitrile (ACN) with 0.1% formic acid each was applied, ranging from 5% to 30% ACN over a 60-minuteperiod, at a flow rate of 1 mL/min. Sample injection was automated using the SIL-40C xs autosampler, and the instrument was operated using LC solution software from Shimadzu, Japan. The MS was performed in the negative mode.
2.5 Evaluation of in vitro activities
2.5.1 DPPH assay
The Mufti et al. (2022) approach was used to test the extract’s capacity to scavenge DPPH. Briefly, 125 µL of DPPH solution was added to 500 µL of extract at gradient concentrations and 375 µL of deionized water. The control tube contained all reagents except the plant extract. The absorbance was measured at 517 nm. The following formula was used to compute the inhibitory activity:
where: Ac: Absorbance of the control. As: Absorbance of the sample.
2.5.2 ABTS assay
In accordance with the procedure outlined by Hamed et al. (2020), this test was carried out. Firstly, ABTS (7 mM) was incubated with K2S2O8 (2.45 mM) in the dark at 37°C for 12–16 h to obtain ABTS + radical. The solution mixture was provided with distilled water to achieve an absorbance of around 0.70 ± 0.02 at 734 nm. 10 μL of either ascorbic acid (the positive control) or OF samples with different concentrations were blended with 200 µL of a diluted ABTS + solution. The absorbance was measured at 734 nm after 6 min incubation period. The equation used to measure scavenging activity is:
with (A) the measured absorbance and (A0) the reagent blank reading.
2.6 Hepatoprotective activity assays
2.6.1 Animals and treatments
The proposed experiment was carried out on peer male Wistar rats, weighed around 250 g at the beginning of the experience. The rats were purchased from the Central Pharmacy, Tunisia, and housed in the laboratory cages (Faculty of Sciences, Gafsa, Tunisia) under controlled conditions (Temperature: 23°C ± 2°C, Relative Humidity: 55% ± 5%, and 12 h light/dark cycle, fed on a standard chow diet and water ad libitum).
2.6.2 Acute toxicity study
Over a 24-h period, and in order to ascertain the safety of O. foetida extract, we monitored five groups administered with different doses (5, 10, 25, and 50 mg/kg body weight) and compared their response to non-treated control group.
2.6.3 Experimental design
Following a fortnight period of acclimation and observation, rats were randomly allocated into six main groups (n = 6), in order to ascertain the pretreatment with OF consequences on CCl4-intoxicated rats.
Group I (C): Rats received corn oil and fed regular diet ad libitum for 6 weeks.
Group II (OF1): Rats were administered OF (25 mg/kg b. w) dissolved in corn oil for 6 weeks.
Group III (OF2): Rats were administered OF (50 mg/kg b. w.) dissolved in corn oil for 6 weeks.
Group (CCl4) IV: Rats were injected with 2 mL/kg b. w. dose of carbon tetrachloride (CCl4) intraperitoneally (IP) dissolved in corn oil (Taamalli et al., 2020).
Group V (OF1 + CCl4): Rats were pre-treated with 25 mg/kg b. w of orally administered at a dose for 6 weeks, and simultaneous treatment with CCl4 dissolved in corn oil.
Group VI (OF2 + CCl4): Rats received 50 mg/kg b. w of for 6 weeks, and simultaneously they were intraperitoneally injected with CCl4 dissolved in corn oil.
The CCl4 was administered by gastric gavage twice per week over 6 weeks. A daily pretreatment with OF1 or OF2 was fulfilled 7 days prior to the CCl4 exposure and then daily for the duration of the research assay by mean of gastric gavage.
The animals were cervically decapitated following 6 weeks. Plasma was isolated from blood samples via centrifugation for 15 min at 2000 g. For biochemical and molecular research, the plasma and portions of the liver were kept at −20°C. The remaining organs portions were fixed in 10% formalin for the histological analysis.
2.6.4 Lipidic profile
Following the manufacturer guidelines, kits purchased from BIOMAGHREB (Tunisia) were employed to discern the lipidic profile by quantifying plasmatic levels of LDL-C, total cholesterol (TC), triglyceride (TG), and HDL-C by means of spectrophotometric methods.
2.6.5 Biochemical analysis of liver function enzyme
The activities of aspartate aminotransferase (AST), alanine aminotransferase (ALT), glutamyl transferase (GGT), lactate dehydrogenase (LDH), alkaline phosphatase (ALP) and bilirubin were evaluated spectrophotometrically in the plasma samples, according to the manufacturer’s guidelines. The levels of IL-6, and TNF-α in the plasmatic homogenates were measured using the ELISA assay which was carried out using the diagnostic kits from BIOMAGHREB (Tunisia).
2.6.6 Oxidative stress indicators
Superoxide dismutase (SOD) activity was estimated accordingly to Marklund and Marklund (1974), where the results were presented as units (U) per mg of protein. Catalase (CAT) activity was determined based on the method established by Aebi (1984), and its activity was presented as micromoles of H2O2 broken down per minute per mg of protein. Finally, the activity of glutathione peroxidase (GPx) was measured following the approach reported by Flohé and Günzler (1984), and results were indicated as nanomoles of GSH oxidized per minute per mg of protein.
2.6.7 NF-ƙB gene expression by semi-quantitative RT-PCR
Liver tissue total RNA samples from both the control and experimental groups were isolated using the iScript™ RTqPCR Sample Preparation Reagent (170–8,898, Bio-Rad) according to the manufacturer’s guidelines. The concentration and purity of the RNA were assessed by determining the absorbance A260/A280 ratios with a NanoPhotometer™ (Implen, GmBH) as described by A. Feriani et al. (2020). Subsequently, 2 µg of RNA was reverse-transcribed using superscript reverse transcriptase (Invitrogen, France) with oligo (dT) 12–18 as a primer in a total volume of 20 µL. The real-time cycler conditions included an initial denaturation step at 70°C for 10 min, followed by 26 cycles of denaturation at 94°C for 5 min, annealing at 58°C, and extension at 72°C for 1 min. For NF-ƙB gene amplification, the primers sequences used were as follows (Forward 5′-GCCGTGGAGTACGACAACATC-3′ and Reverse 5′-TTTGAGAAGAGCTG CCAGCC-3′).
2.6.8 DNA fragmentation
Hepatic tissues’ DNAs from different groups were isolated using the method phenol–chloroform–isoamyl alcohol (25:24:1). Qualitative assessment of genomic DNA damage was performed by electrophoresis of genomic DNA samples on ethidium bromide-stained 0.8% agarose gels according to a previously described method by Feriani et al. (2020). The unaltered and fragmented DNA fractions were visualized using UV light.
2.6.9 Histopathological examinations
The paraffin-embedded fixed hepatic tissues were sliced into 4–6 µm thick pieces for various histopathological colorations. Hematoxylin-Eosin (H-E) staining was applied to elaborate morphological structure. Different images of the liver from each experimental group were captured at a ×200 magnification via a light microscope.
2.6.10 Molecular docking procedure
Molecular docking procedure were performed according to Horchani et al. (2022). Initially, the SDF files of the identified compounds were downloaded from the website https://pubchem.ncbi.nlm.nih.gov/. The ACD (3D Viewer) freeware was used to optimize the correct geometry of these compounds. The three-dimensional (3D) structures of PARP-1 (PDB ID: 7KK6), TNF-α (PDBID: 7JRA), and interleukin 6 (PDB ID: 1ALU) were obtained from the RCSB Protein Data Bank. SPDBV4.10 was used to manipulate and optimize these structures. OpenBabel 2.4.1 software was used to perform format conversion. The AutoDockTools 1.5.6 package was used to generate docking input files and results. Finally, Biovia Discovery Studio 2019 and Pymol (Edu-Pymol version) were used to visualize, analyze interactions and also used to demonstrate two-dimensional (2D) structures.
2.7 Statistical methods
The statistical analysis was conducted using Prism 7.01 (GraphPad, San Diego, CA, USA). All data were subjected to one-way analysis of variance (ANOVA) procedures at a significance level of p < 0.05. The outcomes were presented as the mean ± SD, and comparisons between treatment means were highlighted using the Tukey post hoc test.
3 Results
3.1 Phytochemical characterization
Using HPLC-PDAMS/MS, the identification of peaks in the sample and standards was accomplished by analyzing their MS, MS2 fragments, and retention time. The phytoconstituents analysis revealed the presence of 32 compounds, mainly consisting of phenolic acids and flavonoids (Table 1; Figure 1).
The identified compounds included various derivatives of benzoic acid, including mono- and di-hydroxy forms, and the corresponding glycosides. In addition, several hydroxy cinnamic acids and their glycosides were identified. The extracts showed the presence of several flavonoids, namely, kaempferol, diosmetin, quercetin, isorhamnetin, and myricetin, and their respective mono- and di-glycosides. Besides, two organic acids, malic acid and quinic acid, were identified.
The Phytocontents dosage and the in vitro antioxidant activity results are presented in Table 2. They demonstrate that OF possessed notable polyphenols and flavonoids levels, and it displayed a remarkable antioxidant activity when compared to the standard antioxidant ascorbic acid.
3.2 Lipid profile studies
Table 3 shows that the rats receiving CCl4 showed a dramatic elevation in TC, TG, and LDL-C levels by 129%, 104% and 1,308%, respectively, and a decrease in HDL-C plasmatic level by 57% when compared to control group rats. However, compared to the CCl4 Group alone, the pretreatment by OF1 or OF2 restored all the lipid profile indicators. Since the amounts of TC, TG, and LDL-C dropped by 33% 30%, and 48.5%, respectively, for rats treated with OF1 and 25% 40%, and 35% for those treated with OF2. Meanwhile, the assessed level of HDL-C increased by 48% for rats pretreated with OF1 and 61% for rats receiving OF2.
3.3 Lipid peroxidation analysis
Figure 2 illustrates the amounts of TBARS found in the livers of all experimental animals. Data confirmed that, in contrast to the untreated group, the TBARS’ concentration in the CCl4-treated rats soared by 292%. The level of this marker in the liver was recovered after being pre-treated by the two doses OF1 and OF2 prior to the CCl4 injection. Given that, when compared to the CCl4 group only, the amount of TBARS was reduced to 64% and 69% for (OF1 + CCl4) and (OF2+CCl4), respectively.
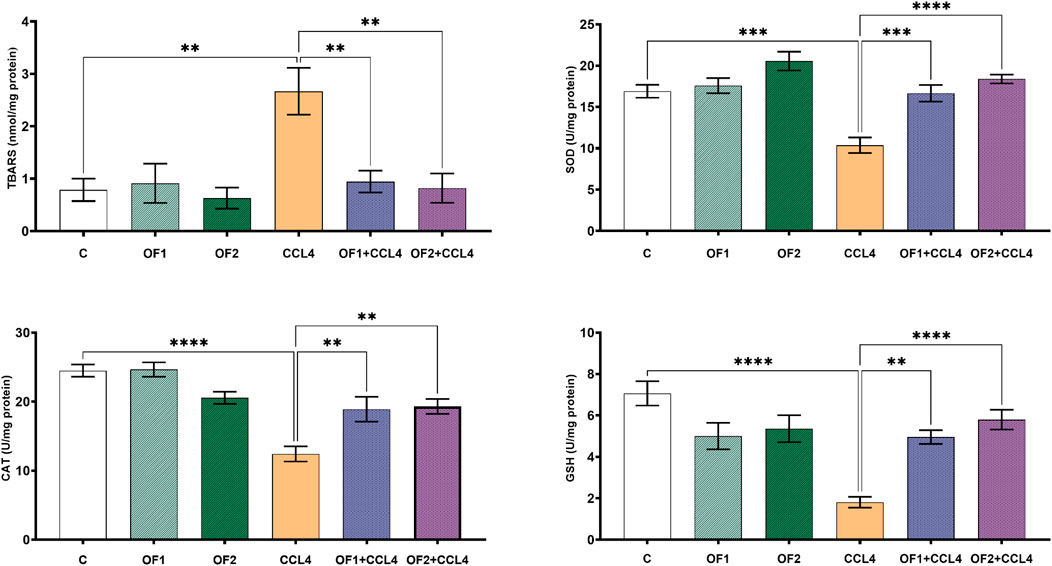
FIGURE 2. Variation in level of lipids peroxidation and antioxidant biomarkers. Values are given as mean ± sd for groups of six rats **p < 0.01; ***p < 0.001 and ****p < 0.0001: control vs. CCl4, CCl4 vs. OF1 + CCl4 and CCl4 vs. OF2 + CCl4.
3.4 Effects on enzymatic antioxidant levels in the liver
To assess the hepatopreventive activity of, we evaluated its impact on some hepatic oxidative stress markers (SOD, GSH and CAT) after CCl4 administration (Figure 2). Aligned with the previous in vitro findings, the in vivo test results confirmed that the administration of at both doses (OF1 and OF2) mitigates CCl4-prompt oxidative stress. Compared to the control group, the hepatotoxicity brought on by CCl4 dramatically lowered the activities of SOD, GSH, and CAT in the hepatic tissues by 38% 74%, and 49%, respectively. In comparison to CCl4-treated rats alone, pretreatment with the two doses of followed by the injection of CCl4 scientifically restored the activities of these enzymatic antioxidants. SOD, GSH, and CAT levels were significantly enhanced by 60%,173%, and 52% respectively, in the (OF1 + CCl4) group. And analogously, in the (OF2 + CCl4) group, they were increased by 77%, 63% and 55%, respectively.
3.5 Effect on the liver injury biomarkers
Table 4 illustrates the outcome of the hepatic injury biomarkers assessment in six groups. The CCl4-treated animals had a significant increase in LDH, GGT, ALT, AST, ALP, and Bilirubin activities by 159%, 127%, 214%, 162%, 81% and 303%, respectively, as opposed to the control ones. Yet, compared to CCl4-treated groups, the pretreatment of animals with OF1 or OF2 mitigated the impact of CCl4 and decreased the level of the marker enzymes LDH, GGT, ALT, AST, ALP, and Bilirubin by 40%, 27%, 34%, 34%, 16% and 20%, respectively, for (OF1 + CCl4) group and 38%, 46%, 49%, 30%, 27%, and 43%, respectively, for (OF2 + CCl4) group.
3.6 Effects on cytokines levels and NF-κB gene expression
TNF-α and IL-6 are two key pro-inflammatory cytokines. Their evaluation is crucial to confirm hepatic inflammation response (Figure 3). In this study, CCl4 is the culprit for a significant rise in both IL-6 and TNF-α around 103% and 202%, respectively. The pre-administration OF1 or OF2 provoked a noteworthy decrease in the elevated TNF-a and IL-6 (p < 0.05) compared to the CCl4 group. These IL-6 and TNF-α reductions were 8% and 15%, respectively, for the (OF1 + CCl4) group and 22% and 26% for the (OF2 + CCl4) group.
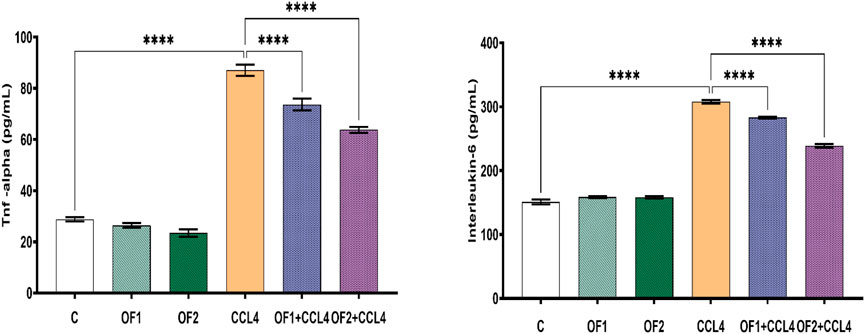
FIGURE 3. Effect of the pretreatment the aqueous extract of Orobanche foetida on IL-6 and TNF-a levels. Values are presented as mean ± sd for groups of six rats ****p < 0.0001: control vs. CCl4, CCl4 vs. OF1 + CCl4 and CCl4 vs. OF2 + CCl4.
Figure 4 depicts the hepatic NF-κB mRNA expression levels in both the control and treated groups. Analysis through semi-quantitative real-time PCR revealed a substantial upregulation of NF-κB mRNA in response to CCl4 compared to the control group. Furthermore, the findings indicated that pretreatment with OF1 and OF2 significantly alleviated the expression of NF-κB mRNA compared to the CCl4 group. Nevertheless, the mRNA expressions in the extract-treated groups remained unaltered when compared to the control group.
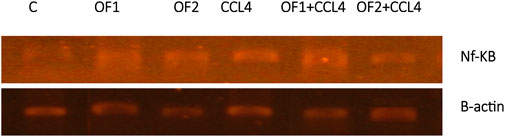
FIGURE 4. Hepatic gene expression of the nuclear factor kappa B (NF-κB) in control and experimental groups.
3.7 Histopathological findings
Figure 5 depicts the results of the histological examination using H-E of the control and the treated groups. The control group and the OF1 and OF2 treated groups’ hepatic tissue had a normal structure, with polyhedral hepatocytes arranged around the central vein and spaced by normal sinusoids. The CCl4-treated group exhibited dilated sinusoids, excessive congestion of the centrilobular vein, and infiltration of inflammatory cells. The liver injuries that CCl4 induced in the rats were greatly reduced by the pre-treatment with OF at both doses.
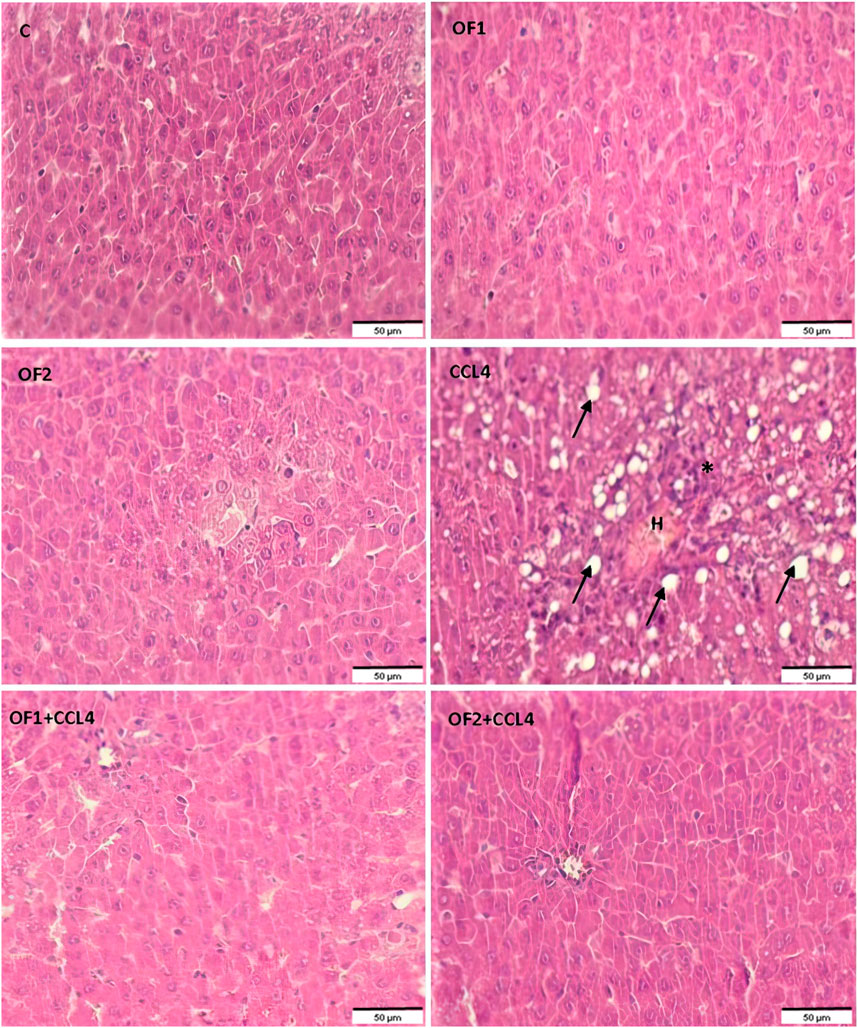
FIGURE 5. Histological sections of liver of control and experimental treated rats. Liver tissue stained with Hematoxylin and Eosin (G ×200). Asterix: infammatory cell infiltration; arrow: foci of lipid, H: Hemorrhage.
3.8 Evaluation of DNA ladder fragmentation in liver tissue
The variations in the genomic DNA extracted from the liver tissues of the control and experimental groups are shown in Figure 6. An intact band of DNA was visible on an agarose gel electrophoresis of genomic DNA purified from the hepatic tissues of control (lane 1), OF1, and OF2-treated rats (lane 2) and (lane 3), respectively. DNA laddering revealed substantial differences between the CCl4-intoxicated group (lane 4) and the control group. Finally, the CCl4-induced genotoxicity was reduced by the pre-treatment with OF by the two doses, as evidenced by decreased genomic DNA fragmentation (lane 5) and (lane 6).
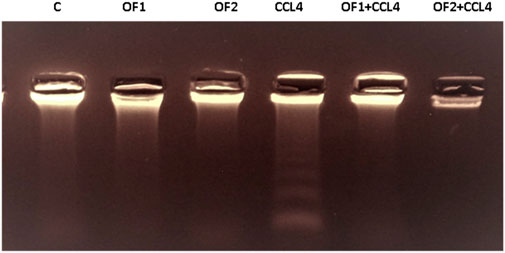
FIGURE 6. Agarose gel electrophoresis analysis of hepatic DNA from control (Lane 1), OF1 (Lane 2), OF2 (Lane 3); CCl4 (Lane 4), OF1 + CCl4 (Lane 5) and OF2 + CCl4 (Lane 6) treated group.
3.9 Molecular docking inhibition of PARP-1, TNF-α, and IL-6
Out of the 32 compounds identified from the extract, several were excluded due to their molecular weights surpassing 500 Da (Table 1). The remaining compounds underwent molecular docking with PARP-1, TNF-α, and IL-6. The 13 assessed compounds exhibited noteworthy binding energy, as evidenced in (Table 5). Results showed that 12 compounds for both PARP-1 and TNF-α and 10 compounds for IL-6 exhibited free energies lower to −5 kcal/mol. Table 6 summarizes the docking analysis’ outcomes. Besides their favorable binding affinities, several compounds, namely, quinic acid, dihydroxybenzoic acid glucoside, coumaroyl glucose, vanillic acid glucoside, and caffeic acid pentosyl glucuronide, have displayed a multitude of H-bonds surpassing the number of H-bonds formed between the reference drug (Silymarin) and the three enzymes. It is noteworthy that all the compounds have displayed a significant number of H-bonds even with at least one enzyme. Table 6 presents the amino acid residues that could ignite the inhibition of one of three enzymes. This is achieved by highlighting and tracing the specific residues involved in the binding interactions with the reference drug silymarin across all the compounds. Silymarin establishes H-bond with specific amino acid residues within the active sites of the three enzymes, PARP-1, TNF-α, and IL-6: These residues are GLY863, SER864, ASP766, ASP770, ILE879 for PARP-1; PHE140, ASN168 for TNF-α and GLN175 and MET67 for IL-6. We can note that these key residues are recurrently present in several OF compound interactions. In our investigation, we also identified and highlighted some key residues involved in other types of interactions other than H-bond interactions. Vanillic acid glucoside and dihydroxybenzoic acid glucoside, besides not exhibiting any violation of Lipinski’s rules, displayed good energy scores with the three enzymes and formed a significant number of H-bond with several key amino acid residues (Figures 7, 8).
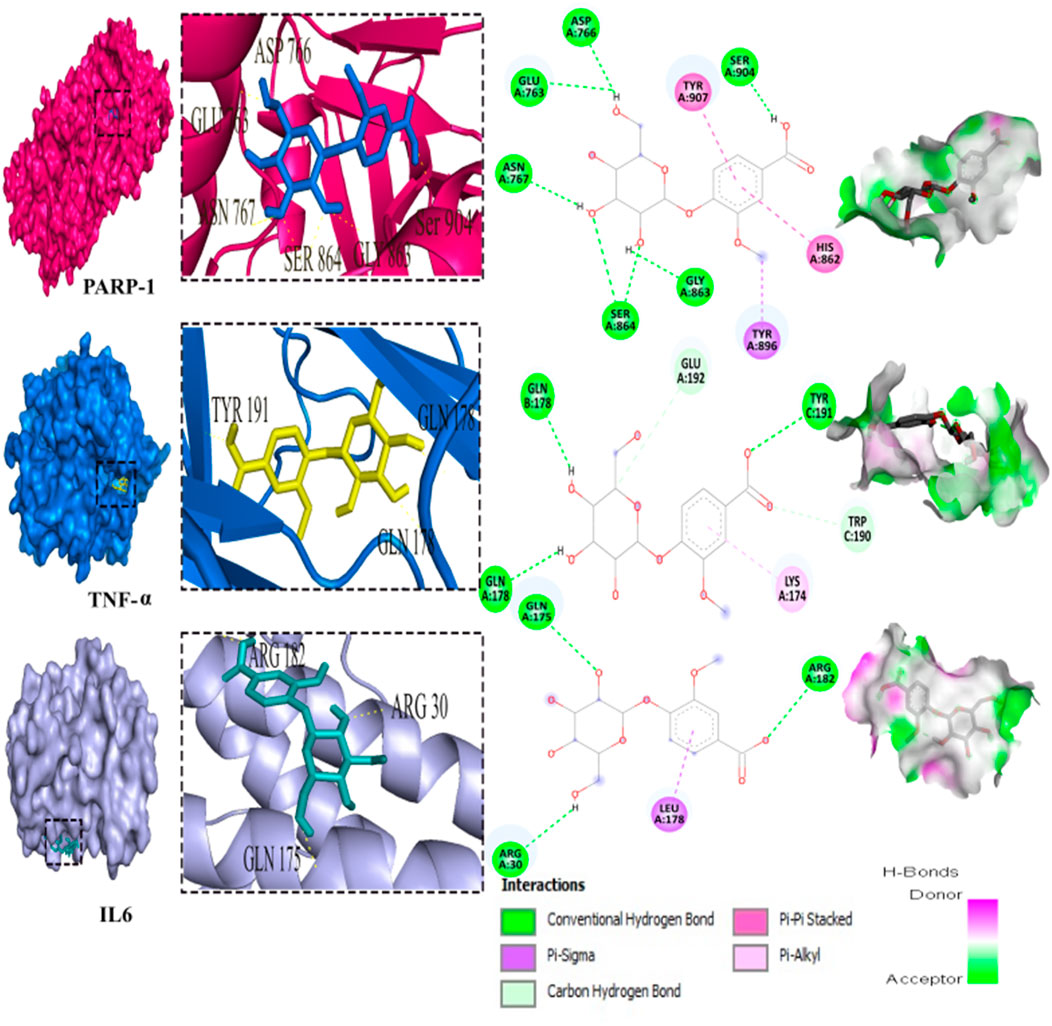
FIGURE 7. 3D representation and 2D diagram of interactions of Vanillic acid glucoside with the best docking scores, bounded to the pocket region of PARP-1, TNF-α and IL-6 with illustration of hydrogen bond.
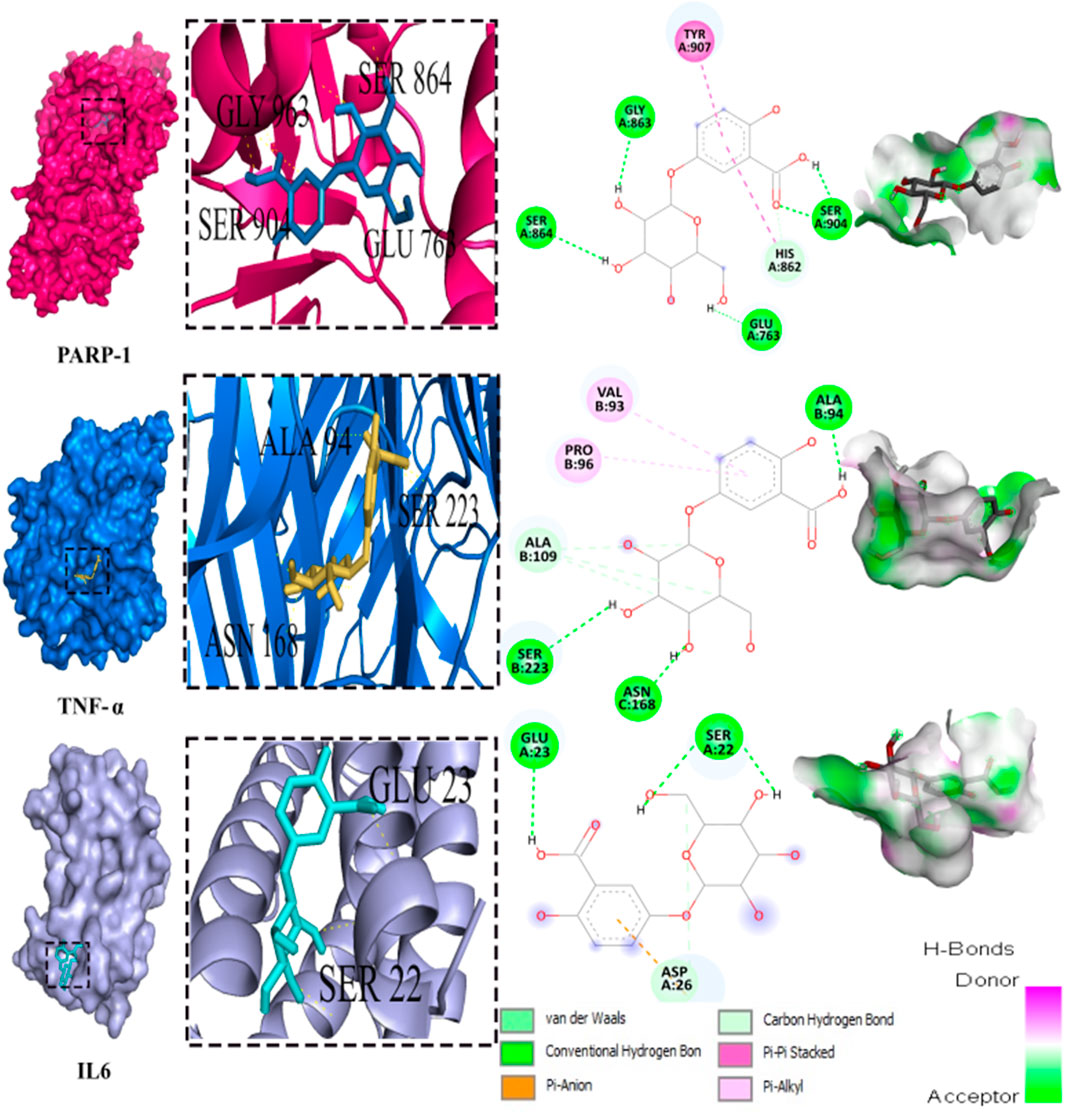
FIGURE 8. 3D representation and 2D diagram of interactions of Dihydroxybenzoic acid glucoside with the best docking scores, bounded to the pocket region of PARP-1, TNF-α and IL-6 with illustration of hydrogen bond.
4 Discussion
This study is one of the few studies that documented and investigated the phytochemical composition of O. foetida and its potential use in diseases protective effect. Phenolic compounds are known to be the primary bio-antioxidants present in plants (Kumar and Goel, 2019). In our preliminary analysis, the approximate levels of TPC, TFC and TTC were found to be around 23.13 mg GAE/g DW, 19.31 mg QE/g DW, and 1.08 mg CATE/g DW, respectively. These findings are consistent with the research conducted by Abbes et al. (2014). That further supports our decision to utilize an aqueous extract rather than a methanolic extract, as both extracts demonstrated similar chemical profiles.
The use of chromatographic analysis (LC/MS-MS) enabled the identification of 32 compounds. It is important to note that several peaks observed in the chromatogram remain unidentified, and their identification could potentially provide valuable insights into the phytochemical characterization of this species, particularly if they turn out to be phenylethanoid glycosides. As previous research on phytochemicals from parasitic plants has placed significant emphasis on these phenylethanoid glycosides due to their occurrence exclusively in parasitic plants and their notable antioxidant properties (Kartbaeva et al., 2017; Jedrejek and et al., 2020).
Liver injury is a multifactorial pathology that can arise from several etiological factors, including viral infection, drug overdose, alcohol abuse, and toxicity from exposure to various xenobiotics. Carbon tetrachloride (CCl4) is a chlorinated hydrocarbon utilized in several industries. Prolonged or excessive exposure to this substance can induce hepatotoxicity and liver damage. CCl4 is metabolized by a group of enzymes known as the cytochrome P450 giving trichloromethyl radical (CCl3∗) (Dua et al., 2023). This CCl3∗ radical interacts with reactive oxygen species (ROS), leading to the generation of another radical known as trichloromethyl peroxyl radical (OOCCl3∗). The presence of the two radicals CCl3∗ and OOCCl3∗ contributes to an increase in oxidative stress, triggering various pathways including lipid peroxidation (Erdaş et al., 2021), disruption of the glutathione pathway (Bikheet et al., 2022) and the production of pro-inflammatory cytokines, specifically TNF-α (Devi et al., 2021) and IL-6 (Wali et al., 2021). These cumulative effects ultimately contribute to liver damage. Therefore, in experimental models of hepatotoxicity, carbon tetrachloride (CCl4)-induced injury is a well-established model that closely mimics the physiopathology of human liver injury (Okoro et al., 2022).
Antioxidants are infamous for playing a critical role in minimizing cellular damage and subsequently apoptosis (Ali et al., 2020; Feriani et al., 2021). we examined the antioxidant potential of using two complementary assays, including assessments of its scavenging activity against DPPH and ABTS. Through these evaluations, we sought to gain a deeper understanding of’s antioxidant properties and its ability to mitigate the damaging effects of oxidative stress on cells. Results confirmed OF’s significant ability to scavenge free radicals, as opposed to ascorbic acid, owing to its bio-antioxidant molecules’ richness, as previously reported by (Genovese et al., 2021).
On the other hand, according to the results of our current study, OF contains secondary metabolites such as tannins, flavonoids, and phenols, as indicated by our phytochemical analyses. It is worth noting that several of these compounds have previously been demonstrated to exhibit antioxidant and hepatoprotective activities (Tir et al., 2019; Feriani et al., 2020; Saadaoui et al., 2023). Hence, the intent was to investigate the potential antioxidant and hepatoprotective effects OF’s phenolic phytochemicals. A possible explanation for these effects is the presence of quinic acid reported to possess antioxidant properties, which may be responsible for the observed reduction in oxidative damage to liver tissue in experimental animals (Lekmine et al., 2020). Furthermore, both the antioxidant and hepatoprotective effects of the extract can also be attributed to the flavonoids present in the extract, especially quercetin. quercetin a well-documented anti-inflammatory and antioxidant flavonoid (Başaran et al., 2022).
In vivo experiments showed that the biomarkers (AST, ALT, LDH, ALP, Bilirubin, and GGT) amounts were significantly increased after CCl4 treatment, aligned with the previous results of Tlili et al. (2018). As reported by Wali et al. (2021), these biomarkers suggest that liver injury may be due to altered membrane permeability and/or hepatocyte necrosis. Our current study confirmed this hypothesis and demonstrated extensive damage to liver tissue, including necrotic cells, centrilobular venous occlusion, leukocyte infiltration, and Kupffer cell proliferation. These results are aligned with previous studies on O. crenata that generated similar findings (Abo-Qotb et al., 2022). The fact that administrating OF significantly decreased high AST, ALT, LDH, ALP, Bilirubin and GGT levels further supports the idea that this extract may be able to shield the liver from the harm that CCl4-induced exposure can cause. The capacity of to preserve the integrity of liver cell membranes and enhance liver function may be the origin of this hepatopreventive action. The outcomes of this study revealed a noteworthy reductions of hepatic injury ant pro-oxidative indicators following the pre-treatment with OF prior the exposure to CCl4. In addition, taking into account the adverse effects in CCl4 group, there was a considerable decrease in antioxidant enzymes consumption. In fact, Hepatocytes have evolved complex defense systems based on different antioxidant enzymes, including CAT, GPx, GST, and GSH, to counteract CCl4 damages (Kostic et al., 2022). The excessive production of reactive oxygen species (ROS) might overwhelm antioxidant defense mechanisms in pathological situations like acute CCl4 toxicity and result in cellular damage. By triggering the release of proinflammatory cytokines like tumor necrosis factor (TNF-α), interleukin-1 (IL-1), interleukin-6 (IL-6), and nitric oxide (NO), such damage might further accentuate inflammatory responses (Yu et al., 2021). The obtained results showed that pretreatment with OF extract was capable of reducing the oxidative stress state brought on by CCl4. To achieve this effect, the OF had to enhance the activities of crucial endogenous antioxidant (SOD, CAT, and GSH) and counters protein oxidation and lipid peroxidation, according to previous studies (Ahmed et al., 2011). According to these results, OF has strong antioxidant properties that may help protect the liver from oxidative stress’s harmful effects. Moreover, caffeic acid present in OF has been previously reported for its ability to increase the endogenous antioxidant enzymes, especially GSH, as well as its ability to decrease the lipid peroxidation in hepatic tissues (Yang et al., 2013).
The study’s findings demonstrated that CCl4 exposure led to significant alterations in the lipid profile. However, the alteration of lipid markers brought on by CCl4 in the plasma of rats was mitigated by the pre-administration with OF. The inhibitory effects on pancreatic lipase, the most significant enzyme involved in fat digestion, may be the cause of’ hypolipidemic impact. Moreover, the inhibition of HMG-CoA reductase, a crucial enzyme in cholesterol biosynthesis, may be the cause of the observed hypocholesterolemia. This impact has previously been documented in research by (Allagui et al., 2015; Chang et al., 2020). The rise in HDL-C levels due to OF administration is linked to SR-BI receptor’ suppression, which is a widely known HDL receptor that stimulates HDL production, as noted in previous studies (Cariello et al., 2021). Additionally, the decrease in circulating LDL-C levels after OF pre-treatment may be attributed to hepatic LDL-R gene’ safeguarding, resulting in active elimination and uptake of LDL fractions from circulation and ultimately leading to reduced LDL-C in the blood, as documented by Feriani et al. (2020).
The progression of hepatic tissue injury is known to be considerably enhanced by oxidative stress-induced hepatocyte apoptosis, according to the literature (Ghosh et al., 2023). Results demonstrating that CCl4 treatment dramatically enhances DNA damage and apoptosis, are consistent with this concept. These findings are aligned with those published by (Maalej et al., 2017; Khan et al., 2019), which showed that administering CCl4 causes DNA damage by producing ROS. The current study demonstrates that administering OF within liver tissue decreases DNA fragmentation, suggesting that the pre-treatment with OF reduces oxidative stress-induced apoptosis. This may correlate with the presence of quercetin pentosyl-glucoside, a flavonoid proven to have antioxidant and DNA-protective properties (Ansar et al., 2016).
The histological results showcase that pre-treatment with OF significantly mitigates the occurrence of centrilobular necrosis in animals that had been subjected to the acute toxic effects of CCl4, as well as the inflammatory infiltrate. These results suggest that OF might mitigate the liver damage brought on by CCl4, possibly through its bioactive components.
The potential hepatopreventive effect of may be attributed to its richness in phenolic compounds, especially flavonoids. Flavonoid-rich plants are well documented to exhibit hepatoprotective effects by inhibiting key enzymes involved in inflammatory processes, such as PARP-1, TNF-α, and IL-6 (Iqbal et al., 2022; Huang and kraus, 2023). Taking account of the pivotal implication of inflammation in hepatic injury prompted by CCl4, ROS are not only the source of liver damage, they initiated inflammation by releasing proinflammatory cytokines from activated macrophages (Lin et al., 2012). CCl4 activates Kupffer cells to release TNF-α which stimulates the release of IL-1β, and IL-6 that eventually cause hepatic necrosis and alter plasmatic biomarkers (Iqbal et al., 2022; Lu et al., 2015). Besides oxidative stress triggers the expression of NF-κB expediting the synthesis of proinflammatory cytokines. Thereby treatment with OF significantly impedes NF-κB expression, contributing to the inhibition of the inflammatory cascade and ultimately reducing hepatic fibrosis. Previous studies have underscored the capability of bioactive compounds to alleviate the production of pro-inflammatory cytokines by inhibiting the expression of profibrotic mediators such as NF-κB. (Eltahir et al., 2020; Feriani et al., 2020).
As for PARP-1, this enzyme is mainly known for its involvement in DNA damage repair and the regulation of the expression of inflammatory factors. Still, the overactivation of PARP-1, occurring by exposure to CCl4 is culprit for the pathophysiological mechanism of hepatic fibrosis. Since it can trigger the activation of hepatic stellate cells HSC that are responsible for liver fibrosis (Huang and kraus, 2023).
Using molecular docking simulations, this work displays the interaction between several phytocompounds found in OF with the binding sites of the three enzymes (PARP-1, TNF-α, and IL-6). The interactions were analyzed to predict their binding mode and to explain their hepatopreventive activity. Ten identified compounds of the extract (Dihydroxybenzoic acid glucoside, vanillic acid glucoside, caffeic acid pentosyl glucuronide, dihydroxybenzoic acid, caffeic acid, coumaroyl quinic acid, caffeoyl glucose, sinapoyl glucose, isorhamnetin glucoside) subjected to molecular docking fit perfectly to the three proteins’ active sites and showed good binding energy scores ranged from −5.0 to −10.4 Kcal/Mol analogously to the reference drug Silymarin. These findings may explain the OF potential hepatopreventive effect highlighted by the previous assays’ outcomes. By comparing the interactions between all the molecular docking studies compounds in the OF and the three enzymes (PARP-1, TNF-alpha, and IL-6) with the interactions of the reference drug silymarin with the previously mentioned proteins, we can note that some specific residues could play a significant role in their inhibition. The residues GLY863, SER864, HIS862, ASP766, and ASP770 for PARP-1, the residues GLN175, ARG182, and LEU178 for IL-6, and the residue ASN168 for TNF-α, were found to be highly involved and represented among several OF compounds. These findings can prove that these residues must be engaged in the inhibition of these inflammation-associated proteins. Subsequently, the interactions between these specific residues and several OF compounds, especially via H-bond, foreground the promising anti-inflammatory and hepatopreventive effect of the extract.
Both vanillic acid glucoside and dihydroxybenzoic acid glucoside stand out as two promising candidates for this hepatopreventive effect through their good binding score, their multiple hydrogen bonds, and their alignment with some key amino residues. Numerous studies have already outlined diverse protective effects of vanillic acid and its derivatives against cancer, diabetes, obesity, neurodegenerative, cardiovascular, and hepatic diseases (Anbalagan et al., 2017; Taqvi et al., 2021; Kaur et al., 2022; Hu et al., 2022) suggested that the supplementation of Vanillic acid glucoside can alleviate oxidative stress and reduce the level of pro-inflammatory cytokines (IL-1β, IL-2, IL-6, and TNF-α).
Every scientific investigation is bounded by certain limitations, and our study is no exception. Firstly, our decision to use only the entire plant of O. foetida may have obscured the potential differences and richness in phenolic compounds across different plant parts (stem, flowers, and seeds …) as reported in previous studies. (Khanam et al., 2015; Bitwell et al., 2023). Secondly, Expanding the sample size (n = 6) in our in vivo study could enhance the statistical power and address any ambiguity regarding parameter variations. Concerning the histopathological studies, employing additional staining techniques like Sirius red and Masson’s trichrome staining could foreground other anomalies, such as the distribution of total collagen (Peugnet-Gonzále et al., 2023). Besides, in molecular docking studies, by following the Lipinski rule of five, we excluded some powerful bioactive compounds like quercetin, kaempferol, and myricetin due to their high molecular weight. Finally, regarding the clinical relevance to human health, our study, though carried out on an animal model, is a promising preliminary investigation that could ignite considerations for humans uses. To start, we need to address the conundrum of the extrapolation of our results to human uses by recognizing and exploring the interspecific variations specially in hepatoprotection involved pathways and implementing future clinical trials to validate the observed effects in human subjects, bridging the gap between preclinical research and the therapeutic applications of O. foetida.
5 Conclusion
In this study the authors explored the potential antioxidant and hepatopreventive activities of O. foetida phytochemical composition via LCMS/MS. Results exhibited a noteworthy level of interesting molecules with potent hepatopreventive and antioxidant activities. In vitro and in vivo assays have revealed the remarkable antioxidant properties of, as well as, its capacity to mitigate CCI4-induced hepatotoxicity by counteracting oxidative stress, DNA fragmentation, and hepatic tissue alterations. Molecular docking analysis underscored the strong and stable binding pattern between two OF compounds: Vanillic acid glucoside and Dihydroxybenzoic acid glucoside with PARP-1, TNF-α, and IL-6, three enzymes infamous for their role in hepatotoxicity and inflammatory response.
Further advanced work is yet needed to isolate the interesting bioactive molecules and to fully grasp the pathways and the mechanisms of action involved in their antioxidant and hepatopreventive activities, for the purpose of developing a new natural, non-hazardous pharmaceutical drug.
Data availability statement
The original contributions presented in the study are included in the article/Supplementary material, further inquiries can be directed to the corresponding authors.
Ethics statement
The animal study was reviewed and approved by the University of Gafsa, Tunisia (Ethical approval number FSG-AE-20-23). The study was conducted in accordance with the local legislation and institutional requirements.
Author contributions
AB: Conceptualization, Data curation, Formal Analysis, Methodology, Software, Validation, Writing–original draft. AM: Data curation, Formal Analysis, Methodology, Writing–review and editing. AF: Conceptualization, Data curation, Formal Analysis, Methodology, Software, Supervision, Validation, Writing–review and editing. HB: Formal Analysis, Methodology, Writing–review and editing. AB: Data curation, Formal Analysis, Methodology, Writing–review and editing. MK: Funding acquisition, Project administration, Validation, Visualization, Writing–review and editing. MS: Methodology, Validation, Writing–review and editing. MA: Funding acquisition, Methodology, Project administration, Supervision, Validation, Visualization, Writing–review and editing. ZA: Conceptualization, Funding acquisition, Investigation, Methodology, Project administration, Resources, Supervision, Validation, Visualization, Writing–review and editing.
Funding
The author(s) declare that no financial support was received for the research, authorship, and/or publication of this article.
Acknowledgments
The authors wish to thank The Ministry of Higher Education and Scientific Research and the project Prima Section 2 (ZeroParasitic) for their financial support and the technical staff of the laboratories for their kind help.
Conflict of interest
The authors declare that the research was conducted in the absence of any commercial or financial relationships that could be construed as a potential conflict of interest.
The author(s) declared that they were an editorial board member of Frontiers, at the time of submission. This had no impact on the peer review process and the final decision.
References
Abbes, Z., Bouallegue, A., Trabelsi, I., Trabelsi, N., Taamalli, A., Amri, M., et al. (2020). Investigation of some biochemical mechanisms involved in the resistance of faba bean (Vicia faba L.) varieties to Orobanche spp. Plant Prot. Sci. 56 (4), 317–328. doi:10.17221/103/2019-pps
Abbes, Z., El Abed, N., Amri, M., Kharrat, M., and Ben Hadj Ahmed, S. (2014). Antioxidant and antibacterial activities of the parasitic plants Orobanche foetida and Orobanche crenata collected on faba bean in Tunisia. J. Anim. Plant Sci. 24, 310–314.
Abbes, Z., Kharrat, M., Shaaban, K., and Bayaa, B. (2010). Comportement de différentes accessions améliorées de féverole (Vicia faba L.) vis-à-vis d'Orobanche crenata Forsk. et Orobanche foetida Poir. Cah. Agric. 19 (3), 194–199. doi:10.1684/agr.2010.0396
Abo-Qotb, S. M., Hassanein, A. M., Desoukey, S. Y., Wanas, A. S., Tawfik, H. M., and Orabi, M. A. (2022). In vivo anti-inflammatory and hepatoprotective activities of Orobanche crenata (Forssk.) aerial parts in relation to its phytomolecules. Nat. Prod. Res. 36 (4), 1067–1072. doi:10.1080/14786419.2020.1845167
Aebi, H. (1984). Catalase in vitro. Method. Enzymol. 105, 121–126. doi:10.1016/s0076-6879(84)05016-3
Ahmed, A. F., Mahmoud, M. F., Ouf, M. A., and Abd El-Fathaah, E. (2011). Aminoguanidine potentiates the hepatoprotective effect of silymarin in CCL4 treated rats. Ann. Hepatol. 10 (2), 207–215. doi:10.1016/S1665-2681(19)31570-4
Ali, B. M., Boothapandi, M., and Nasar, A. S. (2020). Nitric oxide, DPPH and hydrogen peroxide radical scavenging activity of TEMPO terminated polyurethane dendrimers: data supporting antioxidant activity of radical dendrimers. Data Brief. 28, 104972. doi:10.1016/j.dib.2019.104972
Allagui, M. S., Hachani, R., Saidi, S., Feriani, A., Murat, J. C., Kacem, K., et al. (2015). Pleiotropic protective roles of melatonin against aluminium-induced toxicity in rats. Gen. Physiol. Biophys. 34 (4), 415–424. doi:10.4149/gpb_2015028
Amri, M., Abbes, Z., Trabelsi, I., Ghanem, M. E., Mentag, R., and Kharrat, M. (2021). Chlorophyll content and fluorescence as physiological parameters for monitoring Orobanche foetida Poir. infection in faba bean. Plos One 16 (5), e0241527. doi:10.1371/journal.pone.0241527
Amri, M., Trabelsi, I., Abbes, Z., and Kharrat, M. (2019). Release of a new faba bean variety “Chourouk” resistant to the parasitic plants Orobanche foetida and O. crenata in Tunisia. Int. J. Agric. Biol. 21, 499–505. doi:10.17957/IJAB/15.0921
Anbalagan, V., Raju, K., and ShAnMugAM, M. (2017). Assessment of lipid peroxidation and antioxidant status in vanillic acid treated 7, 12-dimethylbenz [a] anthracene induced hamster buccal pouch carcinogenesis. J. Clin. Diagn. Res. 11 (3), BF01–BF04. doi:10.7860/JCDR/2017/23537.9369
Ansar, S., Siddiqi, N. J., Zargar, S., Ganaie, M. A., and Abudawood, M. (2016). Hepatoprotective effect of Quercetin supplementation against Acrylamide-induced DNA damage in wistar rats. Bmc. Complem. Altern. Med. 16, 327–335. doi:10.1186/s12906-016-1322-7
Başaran, E., Öztürk, A. A., Şenel, B., Demirel, M., and Sarica, Ş. (2022). Quercetin, rutin and quercetin-rutin incorporated hydroxypropyl β-cyclodextrin inclusion complexes. Eur. J. Pharm. Sc. 172, 106153. doi:10.1016/j.ejps.2022.106153
Ben Attia, I., Zucca, P., Marincola, F. C., Nieddu, M., Piras, A., Rosa, A., et al. (2020). Evaluation of the antioxidant and cytotoxic activities on cancer cell line of extracts of parasitic plants harvested in Tunisia. Pol. J. Food. Nutr. Sci. 70 (3), 253–263. doi:10.31883/pjfns/122040
Bikheet, M. M., Mahmoud, M. E., Yassien, E. E., and Hassan, H. M. (2022). Effect of lactic acid bacteria isolated from some fermented dairy products on carbon tetrachloride-induced hepatotoxicity and nephrotoxicity of albino rats. Environ. Sci. Pollut. Res. Int. 29 (8), 11790–11800. doi:10.1007/s11356-021-16524-4
Bitwell, C., Sen, S. I., Luke, C., and Kakoma, M. K. (2023). UHPLC-MS/MS phytochemical screening, polyphenolic content and antioxidant potential of Diplorhynchus condylocarpon (Müll. Arg.) Pichon (Apocynaceae), a medicinal plant. Sci. Afr. 20, e01712. doi:10.1016/j.sciaf.2023.e01712
Büssing, A. (2000). “Introduction: history of mistletoe uses,” in Mistletoe (Amsterdam: CRC Press), 17–22.
Cariello, M., Salvia, R., Härdfeldt, J., Piglionica, M., Rutigliano, D., Caldarola, P., et al. (2021). Intracoronary monocyte expression pattern and HDL subfractions after non-ST elevation myocardial infarction. Biochimica Biophysica Acta Mol. Basis. Dis. 1867 (6), 166116. doi:10.1016/j.bbadis.2021.166116
Chang, T. T., Yang, H. Y., Chen, C., and Chen, J. W. (2020). CCL4 inhibition in atherosclerosis: effects on plaque stability, endothelial cell adhesiveness, and macrophages activation. Int. J. Mol. Sci. 21 (18), 6567. doi:10.3390/ijms21186567
Devi, A. B., Rahigude, A. B., Parab, P. B., Engineer, A. S., Dhakephalkar, P. K., and Apte, K. G. (2021). A study to evaluate the hepatoprotective activity of prebiotics, probiotics, and synbiotic in CCl4 induced hepatotoxicity in rats. J. Appl. Pharm. Sci. 11 (3), 141–153. doi:10.7324/japs.2021.110317-1
Dua, T. K., Ashraf, G. J., Palai, S., Baishya, T., Nandi, G., Sahu, R., et al. (2023). The protective role of probiotics in the mitigation of carbon tetrachloride (CCl4) induced hepatotoxicity. Food. Chem. Adv. 2, 100205. doi:10.1016/j.focha.2023.100205
Eltahir, H. M., Fawzy, M. A., Mohamed, E. M., Alrehany, M. A., Shehata, A. M., and Abouzied, M. M. (2020). Antioxidant, anti-inflammatory and anti-fibrotic effects of Boswellia serrate gum resin in CCl4-induced hepatotoxicity. Exp. Ther. Med. 19 (2), 1313–1321. doi:10.3892/etm.2019.8353
Erdaş, M., Akyüz, F., Can, B., Özkoç, M., Öz, S., and Dönmez, D. B. (2021). In vivo effects of Viscum album and probiotics against carbon tetrachloride-induced liver injury. J. Bioenerg. Biomembr. 53, 139–148. doi:10.1007/s10863-021-09883-7
Feriani, A., Tir, M., Gomez-Caravaca, A. M., del Mar Contreras, M., Taamalli, A., Segura-Carretero, A., et al. (2020). Zygophyllum album leaves extract prevented hepatic fibrosis in rats, by reducing liver injury and suppressing oxidative stress, inflammation, apoptosis and the TGF-β1/Smads signaling pathways. Exploring of bioactive compounds using HPLC–DAD–ESI–QTOF-MS/MS. Inflammopharmacology 28, 1735–1750. doi:10.1007/s10787-020-00700-y
Feriani, A., Tir, M., Hachani, R., Allagui, M. S., Tlili, N., Nahdi, S., et al. (2021). Permethrin induced arterial retention of native and oxidized LDL in rats by promoting inflammation, oxidative stress and affecting LDL receptors, and collagen genes. Ecotox. Environ. Safe. 207, 111269. doi:10.1016/j.ecoenv.2020.111269
Flohé, L., and Günzler, W. (1984). Assays of glutathione peroxidase. Methods. Enzymol. 105, 114–121. doi:10.1016/s0076-6879(84)05015-1
Gawenda-Kempczyńska, D., Olech, M., Balcerek, M., Nowak, R., Załuski, T., and Załuski, D. (2022). Phenolic acids as chemotaxonomic markers able to differentiate the Euphrasia species. Phytochemistry 203, 113342. doi:10.1016/j.phytochem.2022.113342
Genovese, C., Acquaviva, R., Ronsisvalle, S., Tempera, G., Antonio Malfa, G., D’Angeli, F., et al. (2020). In vitro evaluation of biological activities of Orobanche crenata Forssk. leaves extract. Nat. Prod. Res. 34 (22), 3234–3238. doi:10.1080/14786419.2018.1552697
Genovese, C., D’Angeli, F., Attanasio, F., Caserta, G., Scarpaci, K. S., and Nicolosi, D. (2021). Phytochemical composition and biological activities of Orobanche crenata Forssk.: a review. Nat. Prod. Res. 35 (22), 4579–4595. doi:10.1080/14786419.2020.1739042
Ghosh, P., Sasaki, K., Pulido Ruiz, I. A., King, K. E., Weinman, S. A., and Wozniak, A. L. (2023). Inflammatory macrophage to hepatocyte signals can be prevented by extracellular vesicle reprogramming. J. Cell. Sci. 136 (9), jcs260691. doi:10.1242/jcs.260691
Hamed, M., Bougatef, H., Karoud, W., Krichen, F., Haddar, A., Bougatef, A., et al. (2020). Polysaccharides extracted from pistachio external hull: characterization, antioxidant activity and potential application on meat as preservative. Ind. Crop. Prod. 148, 112315. doi:10.1016/j.indcrop.2020.112315
Hegazy, M. G., Imam, A. M., and Abdelghany, B. E. (2020). Evaluation of cytotoxic and anticancer effect of Orobanche crenata methanolic extract on cancer cell lines. Tumor. Biol. 42 (5), 1010428320918685. doi:10.1177/1010428320918685
Horchani, M., Heise, N. V., Csuk, R., Ben Jannet, H., Harrath, A. H., and Romdhane, A. (2022). Synthesis and in silico docking study towards M-pro of novel heterocyclic compounds derived from pyrazolopyrimidinone as putative SARS-CoV-2 inhibitors. Molecules 27 (16), 5303. doi:10.3390/molecules27165303
Hu, R., Wu, S., Li, B., Tan, J., Yan, J., Wang, Y., et al. (2022). Dietary ferulic acid and vanillic acid on inflammation, gut barrier function and growth performance in lipopolysaccharide-challenged piglets. Anim. Nutr. 8, 144–152. doi:10.1016/j.aninu.2021.06.009
Huang, D., and Kraus, W. L. (2022). The expanding universe of PARP1-mediated molecular and therapeutic mechanisms. Mol. Cell. 82, 2315–2334. doi:10.1016/j.molcel.2022.02.021
Iqbal, N., Zubair, H. M., Almutairi, M. H., Abbas, M., Akhtar, M. F., Aleya, L., et al. (2022). Hepatoprotective effect of Cordia rothii extract against CCl4-induced oxidative stress via Nrf2–NFκB pathways. Biomed. Pharmacother. 156, 113840. doi:10.1016/j.biopha.2022.113840
Jedrejek, D., Pawelec, S., Piwowarczyk, R., Pecio, Ł., and Stochmal, A. (2020). Identification and occurrence of phenylethanoid and iridoid glycosides in six Polish broomrapes (Orobanche spp. and Phelipanche spp., Orobanchaceae). Phytochemistry 170, 112189. doi:10.1016/j.phytochem.2019.112189
Kartbaeva, E. B., Donald, G. R., Sakipova, Z. B., Ibragimova, L. N., Bekbolatova, E. N., Ternynko, I. I., et al. (2017). Antinociceptive activity of Cistanche salsa stolons, growing in the Republic of Kazakhstan. Rev. Bras. Farmacogn. 27, 587–591. doi:10.1016/j.bjp.2017.05.013
Kaur, J., Gulati, M., Singh, S. K., Kuppusamy, G., Kapoor, B., Mishra, V., et al. (2022). Discovering multifaceted role of vanillic acid beyond flavours: nutraceutical and therapeutic potential. Trends. Food. Sci. Tech. 122, 187–200. doi:10.1016/j.tifs.2022.02.023
Khan, A., Shal, B., Naveed, M., Shah, F. A., Atiq, A., Khan, N. U., et al. (2019). Matrine ameliorates anxiety and depression-like behaviour by targeting hyperammonemia-induced neuroinflammation and oxidative stress in CCl4 model of liver injury. Neurotoxicology 72, 38–50. doi:10.1016/j.neuro.2019.02.002
Khanam, Z., Wen, C. S., and Bhat, I. U. H. (2015). Phytochemical screening and antimicrobial activity of root and stem extracts of wild Eurycoma longifolia Jack (Tongkat Ali). J. King Saud. Univ. Sci. 27 (1), 23–30. doi:10.1016/j.jksus.2014.04.006
Khurm, M., Guo, Y., Wu, Q., Zhang, X., Ghori, M. U., Rasool, M. F., et al. (2023). Conocarpus lancifolius (combretaceae): pharmacological effects, LC-ESI-MS/MS profiling and in silico attributes. Metabolites 13 (7), 794. doi:10.3390/metabo13070794
Konarska, A., and Chmielewski, P. (2020). Taxonomic traits in the microstructure of flowers of parasitic Orobanche picridis with particular emphasis on secretory structures. Protoplasma 257 (1), 299–317. doi:10.1007/s00709-019-01438-3
Kostic, T., Popović, D., Perisic, Z., Stanojevic, D., Dakic, S., Saric, S., et al. (2022). The hepatoprotective effect of aminoguanidine in acute liver injury caused by CCl4 in rats. Biomed. Pharmacother. 156, 113918. doi:10.1016/j.biopha.2022.113918
Kuijt, J. (1969). “The biology of parasitic flowering plants,” in The biology of parasitic flowering plants (Berkeley: University of California Press). U.C. Berkeley.
Kumar, N., and Goel, N. (2019). Phenolic acids: natural versatile molecules with promising therapeutic applications. Biotechnol. Rep. 24, e00370. doi:10.1016/j.btre.2019.e00370
Lekmine, S., Boussekine, S., Kadi, K., Martín-García, A. I., Kheddouma, A., Nagaz, K., et al. (2020). A comparative study on chemical profile and biological activities of aerial parts (stems, flowers, leaves, pods and seeds) of Astragalus gombiformis. Biocatal. Agr. Biotech. 27, 101668. doi:10.1016/j.bcab.2020.101668
Lin, X., Huang, R., Zhang, S., Zheng, L., Wei, L., He, M., et al. (2012). Methyl helicterate protects against CCl4-induced liver injury in rats by inhibiting oxidative stress, NF-κB activation, Fas/FasL pathway and cytochrome P4502E1 level. Food. Chem. Toxicol. 50 (10), 3413–3420. doi:10.1016/j.fct.2012.07.053
Lu, D. H., Guo, X. Y., Qin, S. Y., Luo, W., Huang, X. L., Chen, M., et al. (2015). Interleukin-22 ameliorates liver fibrogenesis by attenuating hepatic stellate cell activation and downregulating the levels of inflammatory cytokines. World. J. Gastroentero. 21 (5), 1531–1545. doi:10.3748/wjg.v21.i5.1531
Maalej, A., Mahmoudi, A., Bouallagui, Z., Fki, I., Marrekchi, R., and Sayadi, S. (2017). Olive phenolic compounds attenuate deltamethrin-induced liver and kidney toxicity through regulating oxidative stress, inflammation and apoptosis. Food. Chem. Toxicol. 106, 455–465. doi:10.1016/j.fct.2017.06.010
Mahdi, I., Fahsi, N., Annaz, H., Drissi, B., Barakate, M., Mahmoud, M. F., et al. (2023). Thymus satureioides coss.: mineral composition, nutritional value, phytochemical profiling, and dermatological properties. Molecules 28 (12), 4636. doi:10.3390/molecules28124636
Marklund, S., and Marklund, G. (1974). Involvement of the superoxide anion radical in the autoxidation of pyrogallol and a convenient assay for superoxide dismutase. Eur. J. Biochem. 47 (3), 469–474. doi:10.1111/j.1432-1033.1974.tb03714.x
Mufti, A., Tir, M., Zarei, A., Contreras, M. D. M., Gómez-Cruz, I., Feriani, A., et al. (2022). Phytochemical profiling of Ephedra alata subsp. alenda seeds by high-performance liquid chromatography—electrospray ionization—quadrupole-time-of-flight-mass spectrometry (HPLC-ESI-QTOF-MS), molecular docking, and antioxidant, anti-diabetic, and acetylcholinesterase inhibition. Anal. Lett. 55 (15), 2450–2466. doi:10.1080/00032719.2022.2059082
Nada, S. A., and El-Chaghaby, G. A. (2015). Nutritional evaluation, phytoconstituents analysis and biological activity of the parasitic plant Orobanche crenata. J. Chem. Biol. Sci. Sect. A 5, 171–180.
Nefzi, F., Trabelsi, I., Amri, M., Triki, E., Kharrat, M., and Abbes, Z. (2016). Response of some chickpea (Cicer arietinum L.) genotypes to Orobanche foetida Poir. Parasit. Chil. J. Agr. Res. 76 (2), 170–178. doi:10.4067/s0718-58392016000200006
Nickrent, D. L. (2020). Parasitic angiosperms: how often and how many? Taxon 69 (1), 5–27. doi:10.1002/tax.12195
Okoro, I. O., Okoro, E. O., Isoje, F. E., and Oyubu, G. (2022). Protective effects of Alstonia congensis Methanolic extract against CCl4 induced liver damage in Wistar rats. Sci. Afr. 17, e01315. doi:10.1016/j.sciaf.2022.e01315
Peugnet-González, I., Martínez-Hernández, S. L., Ávila-Blanco, M. E., Hernández-Marín, D. A., Macias-Pérez, J. R., Aldaba-Muruato, L. R., et al. (2023). Hepatoprotective and antifibrotic activity of watercress extract in a model of CCl4-induced liver fibrosis in Wistar rats. J. Funct. Foods 109, 105760. doi:10.1016/j.jff.2023.105760
Saadaoui, N., Mathlouthi, A., Zaiter, A., El-Bok, S., Mokni, M., Harbi, M., et al. (2023). Phytochemical profiling, antioxidant potential and protective effect of leaves extract of tunisian Vitis vinifera autochthonous accessions against acute CCl4-injured hepatotoxicity in mice. Heliyon 9 (5), e16377. doi:10.1016/j.heliyon.2023.e16377
Shi, R., Zhang, C., Gong, X., Yang, M., Ji, M., Jiang, L., et al. (2020). The genus Orobanche as food and medicine: an ethnopharmacological review. J. Ethnopharmacol. 263, 113154. doi:10.1016/j.jep.2020.113154
Suaza-Gaviria, V., Mesa Vanegas, A. M., and Fonegra, Z. M. (2023). Pharmacological potential of parasitic Angiosperms against microorganisms. Bol. Latinoam. Caribe Plant. Med. Aromat. 22 (2), 180–193. doi:10.37360/blacpma.23.22.2.13
Taamalli, A., Feriani, A., Lozano-Sanchez, J., Ghazouani, L., El Mufti, A., Allagui, M. S., et al. (2020). Potential hepatoprotective activity of super critical carbon dioxide olive leaf extracts against CCl4-induced liver damage. Foods 9 (6), 804. doi:10.3390/foods9060804
Taqvi, S., Bhat, E. A., Sajjad, N., Sabir, J. S., Qureshi, A., Rather, I. A., et al. (2021). Protective effect of vanillic acid in hydrogen peroxide-induced oxidative stress in D. Mel-2 cell line. Saudi. J. Biol. Sci. 28 (3), 1795–1800. doi:10.1016/j.sjbs.2020.12.023
Tawfeek, N., Fikry, E., Mahdi, I., Ochieng, M. A., Bakrim, W. B., Taarji, N., et al. (2023). Cupressus arizonica greene: phytochemical profile and cosmeceutical and dermatological properties of its leaf extracts. Molecules 28 (3), 1036. doi:10.3390/molecules28031036
Těšitel, J., Li, A. R., Knotková, K., McLellan, R., Bandaranayake, P. C., and Watson, D. M. (2021). The bright side of parasitic plants: what are they good for? Plant. Physiol. 185 (4), 1309–1324. doi:10.1093/plphys/kiaa069
Tir, M., Feriani, A., Labidi, A., Mufti, A., Saadaoui, E., Nasri, N., et al. (2019). Protective effects of phytochemicals of Capparis spinosa seeds with cisplatin and CCl4 toxicity in mice. Food. Biosci. 28, 42–48. doi:10.1016/j.fbio.2019.01.002
Tlili, N., Tir, M., Feriani, A., Yahia, Y., Allagui, M. S., Saadaoui, E., et al. (2018). Potential health advantages of Periploca laevigata: preliminary phytochemical analysis and evaluation of in vitro antioxidant capacity and assessment of hepatoprotective, anti-inflammatory and analgesic effects. J. Funct. Foods 48, 234–242. doi:10.1016/j.jff.2018.06.028
Trabelsi, I., Abbes, Z., Amri, M., and Kharrat, M. (2016). Study of some resistance mechanisms to Orobanche spp. Infestation in faba bean (Vicia faba L.) breeding lines in Tunisia. Plant. Prod. Sci. 19, 562–573. doi:10.1080/1343943x.2016.1221734
Wali, A. F., Ali, S., Rashid, S., Alsaffar, R. M., Arafah, A., Qamar, W., et al. (2021). Attenuation of oxidative damage-associated hepatotoxicity by piperine in CCl4-induced liver fibrosis. J. King Saud. Univ. Sci. 33 (8), 101629. doi:10.1016/j.jksus.2021.101629
Yang, W. S., Jeong, D., Yi, Y. S., Park, J. G., Seo, H., Moh, S. H., et al. (2013). IRAK1/4-targeted anti-inflammatory action of caffeic acid. Mediat. Inflamm. 2013, 518183. doi:10.1155/2013/518183
Yao, K. A., Tiho, T., Silué, N., Assidjo, N. E., and Koné, K. Y. (2023). Total polyphenols, total flavonoids, condensed tannins, and antioxidant activity of Borassus aethiopum (arecaceae) ripe fruits’ peels, and peel-pulps, dried at different temperatures. Asian J. Chem. Sci. 13 (2), 20–33. doi:10.9734/ajocs/2023/v13i2236
Yu, H. H., Qiu, Y. X., Li, B., Peng, C. Y., Zeng, R., and Wang, W. (2021). Kadsura heteroclita stem ethanol extract protects against carbon tetrachloride-induced liver injury in mice via suppression of oxidative stress, inflammation, and apoptosis. J. Ethnopharmacol. 267, 113496. doi:10.1016/j.jep.2020.113496
Keywords: Orobanche foetida, phenolic compounds, hepatopreventive, antioxidant, molecular docking
Citation: Bedoui A, Mufti A, Feriani A, Baccari H, Bouallegue A, Kharrat M, Sobeh M, Amri M and Abbes Z (2024) Unlocking the hepatoprotective potential of the parasitic plant Orobanche foetida Poir. aqueous extract against CCl4-induced liver injury in rat. Front. Pharmacol. 14:1320062. doi: 10.3389/fphar.2023.1320062
Received: 11 October 2023; Accepted: 11 December 2023;
Published: 04 January 2024.
Edited by:
Sílvio Terra Stefanello, Westfälischen Wilhelms-Universität Münster, GermanyReviewed by:
Milton Prabu, Annamalai University, IndiaCadmiel Moldovan, Luliu Hațieganu University of Medicine and Pharmacy, Romania
Copyright © 2024 Bedoui, Mufti, Feriani, Baccari, Bouallegue, Kharrat, Sobeh, Amri and Abbes. This is an open-access article distributed under the terms of the Creative Commons Attribution License (CC BY). The use, distribution or reproduction in other forums is permitted, provided the original author(s) and the copyright owner(s) are credited and that the original publication in this journal is cited, in accordance with accepted academic practice. No use, distribution or reproduction is permitted which does not comply with these terms.
*Correspondence: Zouhaier Abbes, zouhaier.abbes@isste.ucar.tn; Moez Amri, moez.amri@um6p.ma; Mansour Sobeh, mansour.sobeh@um6p.ma