- 1Department of Obstetrics and Gynecology, Medical University of Graz, Graz, Austria
- 2Gynecology Research Unit, Hannover Medical School, Hannover, Germany
Preeclampsia (PE) is a complex pregnancy-related disorder characterized by hypertension, followed by organ dysfunction and uteroplacental abnormalities. It remains a major cause of maternal and neonatal morbidity and mortality worldwide. Although the pathophysiology of PE has not been fully elucidated, a two-stage model has been proposed. In this model, a poorly perfused placenta releases various factors into the maternal circulation during the first stage, including pro-inflammatory cytokines, anti-angiogenic factors, and damage-associated molecular patterns into the maternal circulation. In the second stage, these factors lead to a systemic vascular dysfunction with consecutive clinical maternal and/or fetal manifestations. Despite advances in feto-maternal management, effective prophylactic and therapeutic options for PE are still lacking. Since termination of pregnancy is the only curative therapy, regardless of gestational age, new treatment/prophylactic options are urgently needed. Hydroxychloroquine (HCQ) is mainly used to treat malaria as well as certain autoimmune conditions such as systemic lupus and rheumatoid arthritis. The exact mechanism of action of HCQ is not fully understood, but several mechanisms of action have been proposed based on its pharmacological properties. Interestingly, many of them might counteract the proposed processes involved in the development of PE. Therefore, based on a literature review, we aimed to investigate the interrelated biological processes of HCQ and PE and to identify potential molecular targets in these processes.
1 Introduction
Preeclampsia (PE) is a hypertensive disorder, occurring solely during pregnancy and is characterized by high blood pressure, proteinuria and/or other organ manifestations such as liver or kidneys (Brown et al., 2018; Author Anonymous, 2019). The exact aetiology of PE is still unknown. However, abnormal placentation in early pregnancy is thought to cause significant physiologic impairment in the mother later on (Zhou et al., 1997). Due to vascular sclerosis and atypical arteriole remodelling in the placenta, there is a gradual onset of placental ischemia (Rana et al., 2019). Consequently, the emergence of anti-angiogenic and pro-inflammatory factors leads to an imbalance that exacerbates competition for binding sites with important angiogenic and growth factors. As a consequence, impaired vessel formation and insufficient vascular adaptation, particularly affecting various organ systems, including cardiovascular, renal, and hepatic systems are subsequent outcomes (Author Anonymous, 2019). One of the main problems in pregnancies complicated by PE, especially at early gestation is the lack of effective, especially causative therapeutic options. Delivery, sometimes unfortunately immediately after diagnosis, is still the main therapy. If untreated, PE might lead to severe maternal complications such as eclampsia, liver rupture, pulmonary oedema, apoplectic insult or renal insufficiency (Jena et al., 2020). The conventional treatment of PE consists of magnesium sulphate for seizure prevention and fetal neuroprotection, as well as antihypertensive agents, either orally or intravenous and delivery (Author Anonymous, 2019). However, preterm delivery due to PE with its consequences is still a huge problem worldwide and prolongation of pregnancy after especially maternal stabilization should therefore be of highest priority.
Several attempts such as supplements and medications have unfortunately not reached its expectations for prevention of PE. Only low-dose aspirin was found to have a modest benefit in reducing the rate of PE. This benefit might only be achieved if low-dose aspirin was started before 16 weeks of gestation (Author Anonymous, 2019). PE is associated with serious and especially long-term maternal and neonatal complications: for the child due to (iatrogenic) preterm delivery as well as fetal growth restriction (FGR) and for the mother an increased risk of cardiovascular morbidity and mortality. (Freeman et al., 2004; Vitoratos et al., 2010; Drost et al., 2012; Groenhof et al., 2017; Cho et al., 2019; Fox et al., 2019). According to the literature, 30% of women with PE develop arterial hypertension and 25% a metabolic syndrome (Hermes et al., 2013; Veerbeek et al., 2015). PE also has an overall negative effect on offspring’s cardiovascular and neurological health (Lu and Hu, 2019). A few studies also have shown the connection between PE and postpartum depression (Mbarak et al., 2019; Caropreso et al., 2020). Poel et al. revealed in their work from 2009, that 1/5 of all women with PE require psychological treatment for several years (Poel et al., 2009). Therefore, the need for new effective medication for PE is high.
Hydroxychloroquine (HCQ) is an antimalarial drug that has been used to treat autoimmune diseases like rheumatoid arthritis (RA) (Kerschbaumer et al., 2020) and systemic lupus erythematosus (SLE) (Fanouriakis et al., 2019). While its exact mechanism of action is not fully understood, it has been shown that HCQ has multiple effects on the systemic and cellular processes. Among them, anti-inflammatory, immunomodulatory and vascular effects could be beneficial for the treatment of PE (Plantone and Koudriavtseva, 2018; Petri et al., 2021). HCQ’s anti-inflammatory effects lie in its capability to reduce the production and release of inflammatory mediators. Since PE is associated with increased systemic inflammation, HCQ-induced reduction of inflammation could potentially have a beneficial effect. Moreover, HCQ immunomodulatory properties are reflected by the inhibition of certain immune cells and suppression of immune responses (Plantone and Koudriavtseva, 2018). PE is thought to involve immune system dysregulation, and modulating immune responses could potentially help in managing the condition. In addition, HCQ has been shown to improve vascular function and reduce endothelial damage (Petri et al., 2021; Dong et al., 2022), which are common aspects of PE. Therefore, it could be beneficial for the management of PE if HCQ could improve these impairments.
This review aims to elucidate the immunological interactions between HCQ and PE focusing on identifying potential molecular targets within these intersecting domains. Based on a literature search we identified well-described mechanisms of action of HCQ in various conditions. Subsequently, we sought to compare these mechanisms with the processes implicated in the development of PE, aiming to identify the most promising targets for future research.
2 Pathophysiology of preeclampsia (PE) and its medical approach
PE is a pregnancy-related syndrome (Myatt and Roberts, 2015) that globally affects 4.6% of all pregnancies (Abalos et al., 2013). It is characterized by new onset of hypertension (systolic >140 mmHg and diastolic >90 mmHg) after 20 weeks of gestation, accompanied by proteinuria, dysfunction of maternal organs, haematological abnormalities, and uteroplacental dysfunction (Brown et al., 2018; Author Anonymous, 2019). With over 70,000 maternal deaths and 500,000 fetal deaths every year, PE is one of the leading causes of maternal mortality and morbidity worldwide (Rana et al., 2019). Based on the time of clinical onset of PE symptoms, PE can be classified as early-onset preeclampsia (EO-PE) occurring before 34 weeks of gestation, or late-onset preeclampsia (LO-PE) occurring after 34 weeks of gestation (Tranquilli et al., 2013). However, more than just the difference in time of occurrence divides these two subtypes of PE. EO-PE is less common but is considered the more severe form based on clinical and laboratory outcomes (Wojtowicz et al., 2019). Contrary, LO-PE is usually characterized exclusively by maternal manifestations and is mild to moderate in severity (Lisonkova and Joseph, 2013; Wojtowicz et al., 2019).
Although the underlying mechanisms of PE remain still unclear, several systemic processes have been proposed. Redman postulated a two-stage model of the disease (Redman, 1991) which has been updated in the past years and has been revised by Staff (Staff, 2019). The latest revision of this model takes into account maternal factors and differences between EO-PE and LO-PE (Staff, 2019). In the newest revision of Redman´s model, stage 1 is characterized by placental dysfunction, while stage 2 represents the clinical manifestation in the mother, both mediated by syncytiotrophoblast stress (Staff, 2019). The pathophysiological process of EO-PE is thought to initiate abnormal trophoblast invasion early in pregnancy, leading to inadequate remodelling of spiral arteries and poor placental perfusion with consecutive oxidative stress and dysfunction of the syncytiotrophoblast. Huppertz et al. have shown that in PE syncytiotrophoblast shedding occurs rather in a necrotic than in an apoptotic manner (Huppertz et al., 2003). As a result, syncytiotrophoblasts release trophoblast debris containing damage-associated molecular patterns (DAMPs), pro-inflammatory cytokines and anti-angiogenic factors.
In contrast, LO-PE occurs in term or post-term pregnancies and is characterized by the decline in placental function resulting in increased compression of the intervillous space and hypoxia, which in turn leads to various clinical manifestations of placental dysfunction such as PE, fetal growth restriction, and even stillbirth (Staff, 2019). Both pathways are known to be due to syncytiotrophoblast stress and have similar maternal consequences (Staff, 2019). However, they are triggered by different causes at different times during pregnancy.
Currently, there is still no effective medication for curing PE, and the only curative therapy for PE is the termination of pregnancy. However, according to clinical practice guidelines, the decision for immediate delivery depends on gestational age and maternal and fetal status (Brown et al., 2018). In cases of LO-PE, delivery is advised (Brown et al., 2018; Author Anonymous, 2019). However, for those with PE onset occurring before 34th weeks of gestation (EO-PE), a strategy of expectant management is typically recommended (Brown et al., 2018; Author Anonymous, 2019). Different medications like low-dose aspirin (Phipps et al., 2019), statins (Smith and Costantine, 2022) and calcium (Burton et al., 2019) have been proposed for prevention or even stabilization of PE. However, they are just partially effective in reducing the risk of PE or prolongation of pregnancy.
Only low-dose aspirin was found to have a modest benefit in reducing the rate of PE; this benefit might be achieved if low-dose aspirin was started before 16 weeks of gestation. However, this does not apply to LO-PE. The molecular mechanism of low-dose aspirin in PE is nicely reviewed by Walsh and Straus (Walsh and Strauss, 2021). By blocking its main target cyclooxygenase enzymes, low-dose aspirin specifically reduces the production of thromboxane in maternal platelets without impacting the synthesis of prostacyclin. Furthermore, it seems to similarly target and reduce thromboxane production in the placenta, along with mitigating oxidative stress within the placenta (Gimenez-Bastida et al., 2019; Walsh and Strauss, 2021). However, it has been postulated that the use of aspirin during pregnancy might be associated with increased postpartum bleeding and postpartum hematoma (Hastie et al., 2021; Jiang et al., 2023). Therefore, “Lifestyle modification”, such as optimizing pre-pregnancy weight might be a promising approach (Author Anynomus, 2018; Roberge et al., 2018).
Other candidates are statins, specifically pravastatin. Although preclinical studies (Brownfoot et al., 2015; Brownfoot et al., 2016) and clinical studies with women at high risk of PE (Costantine et al., 2016) showed that pravastatin increases Placental Growing Factor (PlGF) while decreasing Soluble Vascular Endothelial Growth Factor Receptor 1 (sFlt-1) and soluble endoglin (sENG), clinical trials which included EO-PE patients did not observe the same (Ahmed et al., 2020).
High-dose calcium reduces the risk of PE in women destined for the development of PE, while low-dose calcium did not have a clear effect (Hofmeyr et al., 2018). The need for new treatment options is therefore high. Considering the diverse underlying mechanisms and clinical presentations of PE, it is crucial to acknowledge that a single drug cannot universally address all cases. The heterogeneity of this condition necessitates tailored approaches for effective treatment.
2.1 Innate immune system dysregulation in PE
As stated before, in EO-PE necrotic syncytiotrophoblasts release a mixture of molecules, including DAMPs, into the maternal circulation (Staff, 2019). DAMPs are endogenous molecules released from damaged or stressed cells in the body, such as DNA and RNA fragments, ATP, uric acid, heat shock proteins, etc (Piccinini and Midwood, 2010). Similar to pathogen-associated molecular patterns, they can be recognized by pattern recognition receptors on immune cells, such as Toll-like receptors (TLRs). Depending on the type of TLR it can be either present on the cellular membrane or intracellularly inside of endosome.
The main function of endosomal TLRs is to recognize free amino acids derived from bacteria and viruses or necrotic cells. TLR3 bind double-strand RNA (dsRNA) and signals through a MyD88-independent pathway. This way it activates interferon regulatory factor 3 (IRF-3) and increases interferons production in cells (Piccinini and Midwood, 2010). However, in the later phase, it can also activate the nuclear factor kappa-light-chain-enhancer of activated B cells (NF-κB) transcriptional factors and increase pro-inflammatory cytokines (Piccinini and Midwood, 2010). In contrast, TLR7/8 and TLR9 recognise single-strand RNA (ssRNA) and hypomethylated 2′-deoxyribo (cytidine–phosphate–guanosine) (CpG)-rich DNA motifs, respectively (Piccinini and Midwood, 2010). This signal goes through the MyD88-dependent pathway leading to the release of NF-κB translocating to the nucleus, where it triggers the transcription of pro-inflammatory genes, such as cytokines and chemokines (Piccinini and Midwood, 2010).
In the context of PE, TLR3, 7/8 and 9 are found to be increased in PE placentas (Pineda et al., 2011; Chatterjee et al., 2012; He et al., 2018; Ozeki et al., 2019; Gierman et al., 2021). Moreover, the expression of TLR9 co-localised with TLR3 in placental compartments: the trophoblast (syncytium) covering the entire cellular area and in the stromal vascular endothelium (Pineda et al., 2011). In addition to its increased presence in the placenta, TLR9 and 3 expression is also increased in peripheral blood mononuclear cells (PBMCs) in maternal circulation (Liao et al., 2009; Panda et al., 2012).
Regarding ligands of endosomal TLRs, their amount and composition are investigated in the context of PE, too. The presence of cell-free nucleic acids in maternal circulation is not limited to cell-free fetal DNA (cffDNA) but also includes mitochondrial DNA, messenger RNA, microRNA and long non-coding RNA species (Hahn et al., 2014). However, cffDNA has been the most studied one.
Compared to maternal DNA, cffDNA is hypo-methylated (Schroeder et al., 2013) making it recognizable by TLR9. In healthy pregnancies, the concentration of cffDNA in maternal circulation increases with gestational age due to apoptosis of syncytium (Halperin et al., 2000). As previously described, during PE shedding of syncytium is more in a necrotic manner, which is due to placental oxidative stress (Huppertz et al., 2003). Consistent with this, the increase of cffDNA in the maternal circulation is also associated with oxidative stress in the placenta (Tjoa et al., 2006). Therefore, numerous studies have likely detected higher cffDNA levels in pregnancies with PE compared with those without complications (Amash et al., 2012; Yu et al., 2013; Dong and Yin, 2014; Ma et al., 2019; Ozeki et al., 2019) suggesting a possible link between PE and elevated cffDNA levels. In addition, cffDNA levels also correlate with disease severity (Zhong et al., 2001).
Among the different types of cell-free RNA, composition is more important than quantity. Transcriptomic analyses show two different RNA profiles in normotensive and PE patients (Moufarrej et al., 2022; Zhou et al., 2023). However, it would be very plausible that dsRNA and ssRNA are increased in the maternal circulation and can activate TLRs in surrounding cells. In addition, it is likely that high concentrations of nucleic acids in the maternal circulation act as a danger signal to the mother (Scharfe-Nugent et al., 2012).
The connection between endosomal TLRs activation by their ligands and PE pathophysiology is supported by multiple in vitro and in vivo experiments (Nakada et al., 2009; Tinsley et al., 2009; Chatterjee et al., 2012; Scharfe-Nugent et al., 2012; Goulopoulou et al., 2016; Conka et al., 2017; He et al., 2018; Ozeki et al., 2019). Scharfe-Nugent et al. showed that, PBMCs from pregnant women incubated with cffDNA lead to activation of the pro-inflammatory transcriptional factor NF-κB and the production of interleukin (IL)-6 (Scharfe-Nugent et al., 2012). Moreover, Nakada and colleagues observed that after stimulation of HTR-8/SVneo, trophoblast cell line model, with TLR3 specific ligand there is an activation of both NF-κB and IRF3 pathways, leading to elevated inflammatory mediators’ expression and - more interestingly - PE-related anti-angiogenic factor sFlt-1 (Nakada et al., 2009). In addition, it has been shown that activation of TLR9 with cffDNA causes syncytial inflammation (Scharfe-Nugent et al., 2012), promoting both IL-6 and IL-8 secretion by human umbilical vein endothelial cells (HUVECs) (Ozeki et al., 2019) and a sFlt-1 increase while decreasing vascular endothelial growth factor A (VEGFA) in mouse placenta and HTR-8/SVneo trophoblast cell line (He et al., 2018). In vivo experiments in rodents have shown, with one exception (Conka et al., 2017), that cffDNA as well as TLR3 and TLR7/8 ligands can trigger fetal loss and induce PE-like conditions (Tinsley et al., 2009; Chatterjee et al., 2012; Scharfe-Nugent et al., 2012; Goulopoulou et al., 2016; He et al., 2018).
The identified findings emphasize the significance of nucleic acids (especially cffDNA) in the development of PE, indicating their potential role as diagnostic markers for early detection of PE (Sekizawa et al., 2003; Karapetian et al., 2021; Moufarrej et al., 2022; Zhou et al., 2023). Moreover, these findings suggest that endosomal TLRs activation could serve as a potential target for the treatment of PE (Pineda et al., 2011; Panda et al., 2012; Scharfe-Nugent et al., 2012).
Another well-characterized TLR is TLR4, which is responsible for the recognition of cell wall components from Gram-negative bacteria (Asea et al., 2002). From the DAMPs, it is responsible for the recognition of heat shock proteins (HSP) (Banerjee et al., 2021), High-Mobility Group Box 1 protein (HMGB1) and uric acid. TLR4 expression was found to be higher in PE (Chen et al., 2015), especially in EO-PE (Kim et al., 2005; Xie et al., 2010; Pineda et al., 2011; Zhang and Yang, 2012; Nizyaeva et al., 2019; Zhao et al., 2019; Romao-Veiga et al., 2020). An increased expression in both first and third-trimester placenta (Zhang and Yang, 2012), interstitial trophoblasts (Kim et al., 2005), syncytiotrophoblast and endothelial cells (Nizyaeva et al., 2019; Zhao et al., 2019) was detectable where it co-localises with TLR2 (Pineda et al., 2011). In addition to placental expression, baseline expression of TLR4 was found to be significantly elevated in monocytes (Chen et al., 2015; Romao-Veiga et al., 2020) and neutrophils (Xie et al., 2010).
Among the DAMPs, HSPs have varying roles in PE. For instance, cell-free HSP70s are increased in the sera of PE patients (Fukushima et al., 2005; Molvarec et al., 2006; Hromadnikova et al., 2016), particularly in EO-PE cases. (Jirecek et al., 2002; Peracoli et al., 2013; Alvarez-Cabrera et al., 2018; Romao-Veiga et al., 2020). They are believed to contribute to the systemic inflammatory response in PE (Asea et al., 2002; Piccinini and Midwood, 2010; Romao-Veiga et al., 2022). Contrary, HSP70 has been found to be increased in the placental tissue (Padmini et al., 2012) but here it has a rather protective function. Moreover, data regarding HSP90 and HSP60 in PE is more diverse. While HSP90 was found to be increased in the sera of PE patients during the third trimester (Guzel et al., 2019), its concentration in endothelial cells isolated from PE placentas showed contrary results (Gu et al., 2006; Padmini et al., 2012). Some studies showed increased levels of HSP60 in PE patients’ sera (Alvarez-Cabrera et al., 2018), while others did not observe any difference (Peracoli et al., 2013).
HMGB1 is a molecule with a dual role. It acts as a transcription factor, when inside cells, while upon release from immune or necrotic cells, it works like a danger signal (Nadeau-Vallee et al., 2016). HMGB1 has been found to be increased in the placenta and maternal circulation in cases of PE (Wang et al., 2011; Chen et al., 2016; Hu et al., 2018). Its presence in syncytiotrophoblasts is particularly associated with severe and EO-PE, (Chen et al., 2016), being released into the maternal circulation following hypoxia-induced necrosis (Hu et al., 2018). The release of HMGB1 from hypoxic trophoblast has been linked to increased permeability (Jiang et al., 2014) and secretion of pro-thrombotic microparticles in HUVECs (Hu et al., 2018) through experiments done on primary human villous trophoblasts, primary mouse trophoblasts and JEG-3 trophoblast cell line. Although, HMGB1 can be recognized by multiple receptors on various cell types (Tang et al., 2011), in HUVECs, HMGB1 induced endothelial permeability through the TLR4– caveolin-1 pathway (Jiang et al., 2014). This pathway may play a role in the clinical features of PE, including general oedema and proteinuria. Furthermore, it has been implicated that HMGB1 could cause increasing reactive oxygen species (ROS) levels as well as IL-6, IL-8 and monocyte chemoattractant protein-1 secretion from Sw.71 human trophoblast cell line (Shirasuna et al., 2016). Additionally, HMGB1 derived from necrotic cells serves as a crucial activator of dendritic cells (DCs) (Tang et al., 2011).
3 Hydroxychloroquine (HCQ)
HCQ is a less toxic hydroxylated analogue of an anti-malaria drug chloroquine (Gies et al., 2020). Nowadays, HCQ is widely used in the prevention and treatment of different autoimmune diseases, such as SLE (Fanouriakis et al., 2019), RA (Kerschbaumer et al., 2020), antiphospholipid syndrome (APS) (Tektonidou et al., 2019), and Sjögren’s syndrome (Brito-Zeron et al., 2014; Demarchi et al., 2017; Wang et al., 2017). HCQ has been shown to cross the placental barrier (Costedoat-Chalumeau et al., 2002), and its safety during pregnancy has been already confirmed in a few studies (Abarientos et al., 2011; Kaplan et al., 2016; Clowse et al., 2022). HCQ is associated with higher gestational age at delivery, suggesting its positive effect on the prolongation of pregnancy (Sciascia et al., 2016; Kroese et al., 2017; Gerde et al., 2021) and an increase in live births in patients with APS (Mekinian et al., 2015; Sciascia et al., 2016; Gerde et al., 2021). Some retrospective studies and meta-analyses associated HCQ with a lower risk of PE in SLE (Do et al., 2020; Hu et al., 2023; Phillips et al., 2023) and in APS patients (Sciascia et al., 2016; Latino et al., 2020) who continued to take it during pregnancy.
The most well-known role of HCQ is the alkalization of lysosomes (Derendorf, 2020). In its chemical structure, HCQ has a basic side chain that makes it a weak base. HCQ easily passes through phospholipid membranes and enters the cell and cell compartments. Because of its basic chain, it accumulates in a lysosomal compartment (Gies et al., 2020). There, it alkylates lysosomal pH, which affects several cellular processes (Ponticelli and Moroni, 2017) such as activation of TLRs, antigen processing, major histocompatibility complex class II and autophagy (Mauthe et al., 2018).
Overall, HCQ´s mechanism of action involves inhibiting TLR-mediated cell activation, reducing cytokine production, inhibiting antigen presentation, and modulation of T- and B-cell activation and differentiation. Since PE is a multifactorial disease, there are multiple sources and triggers of cytokine storms. Some of the most important triggers are DAMPs and their receptors on different cells are the first connection between HCQ and PE.
3.1 HCQ effect on toll-like receptors and PE
There are two proposed mechanisms for how HCQ inhibits the activation of TLR3, 7, 8 and 9 (Abarientos et al., 2011). The first is the alkalization of lysosomes. An increase in pH prevents proteolytic cleavage of TLR which is required for TLR3, 7 and 9 activation (Ewald et al., 2008; Ewald et al., 2011). The second mechanism involves direct binding to DNA and RNA via electrostatic forces, hydrogen bonds, and van der Waals forces. Thus, HCQ prevents the binding of nucleic acids to TLR receptors present in the endosome (Kuznik et al., 2011). Regardless of the mechanism of action, HCQ prevents the activation of endosomal TLRs in both cases. Therefore, there is no activation of downstream molecules and no increase in inflammation.
Considering the HCQ mechanisms of action, we might expect HCQ to block either the binding of DAMPs specific for endosomal TLRs or the activation of endosomal TLRs in PE (Figure 1). This may lead downstream to a decrease of IL-6 and IL-8 production by PBMCs, endothelial cells and trophoblast (Scharfe-Nugent et al., 2012; Ozeki et al., 2019). Further, it is plausible that HCQ could also decrease sFLT-1 and increase VEGFA in maternal circulation (He et al., 2018), which would improve endothelial function and vascularization. However, in a study on term placental explants, HCQ did not significantly decrease the secretion of pro-inflammatory or transcription of angiogenic molecules, induced by TLR7 and TLR9 activation (Scott et al., 2019). The exception was IL-10 secretion, where HCQ increased basal secretion by term placenta explants as well as reversed the negative effect of TLR7 agonist on term placenta explants IL-10 production (Scott et al., 2019). HCQ could most likely improve migration and invasion in early placentation through a TLR9-dependent manner, as this concept was tested in trophoblast cell lines and placental explants (He et al., 2018; Scott et al., 2019; Leon-Martinez et al., 2023).
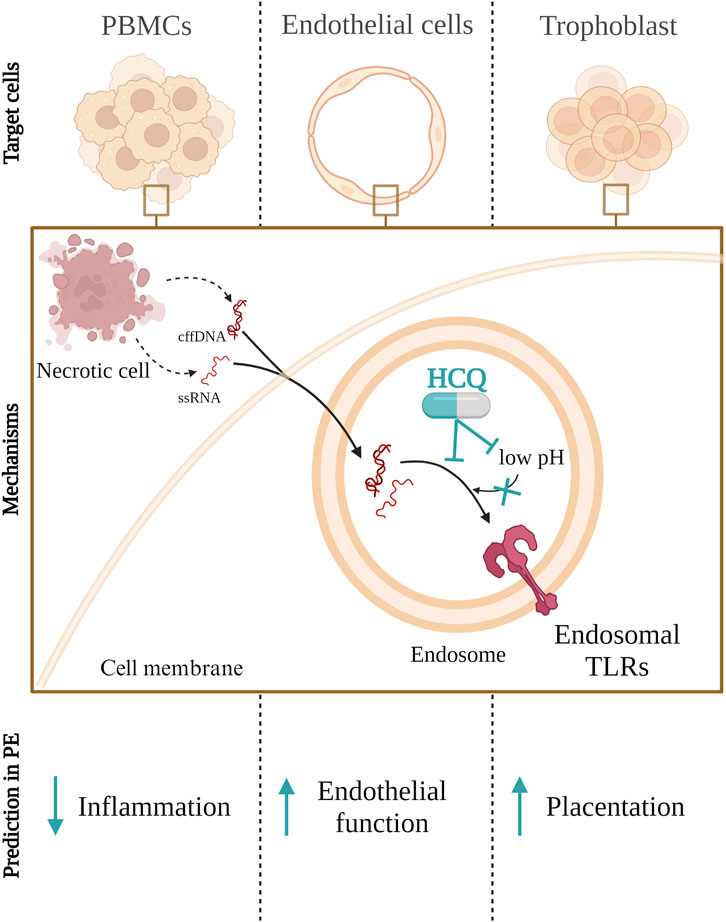
FIGURE 1. Hydroxychloroquine (HCQ) inhibits the activation of Toll like receptor (TLR) 3, 7, 8, and 9 through two mechanisms: by increasing lysosomal pH, preventing proteolytic cleavage required for TLR activation, and/or by directly binding to DNA and RNA, thus preventing their interaction with TLR receptors in endosomes. This inhibition of endosomal TLR activation in peripheral blood mononuclear cells (PMBCs), endothelial or trophoblasts cells could lead to a reduction in inflammation as well as improve endothelial function and placentation in peeclamptic patients. cffDNA–cell free fetal DNA; ssRNA–single strand RNA.
In contrast to intracellular TLR receptors, HCQ does not affect the activation of TLR4 but reduces the total mRNA and protein amount of TLR4 in cells and it may affect cytokine production (Molvarec et al., 2006; Romao-Veiga et al., 2020). The mechanism of action, in this case, is different from that for endosomal TLRs. HCQ’s impact on TLR4 and HMGB1 modulation has shown promising results (Hu J. et al., 2022; Marchetti et al., 2014; Yang et al., 2013; van den Borne et al., 1997). Studies conducted on microglia and BeWo cells have indicated that HCQ administration inhibits inflammatory responses and affects the expression of TLR4, potentially inhibiting the TLR4/NF-κB signalling pathway (Yang et al., 2013; Marchetti et al., 2014; Hu J. et al., 2022). Additionally, it has been demonstrated that HCQ effectively inhibits the release and pro-inflammatory function of HMGB1 in several in vitro cell types and in animal models of endotoxemia and sepsis (Yang et al., 2013).
Moreover, chloroquine (CQ), the precursor for HCQ, is found to decrease the production of Tumor necrosis factor-alpha (TNF-α), IL-1 beta (β) and IL-6 from lipopolysaccharide (LPS)-stimulated human monocytes (Jang et al., 2006). Here it was described that CQ blocks the conversion of cell-associated TNF-α precursor to mature soluble protein, whereas it reduces the levels of IL-1β and IL-6 mRNA, at least in part, by decreasing their stability and by a pH-dependent mechanism. Moreover, LPS-induced IL-6 and TNF-α production were inhibited by both CQ and HCQ in PBMC (van den Borne et al., 1997). Contrary, other authors reported that HCQ was not able to prevent TNF-α secretion triggered by TLR4 activation (Sacre et al., 2012; Alves et al., 2017).
The mechanism shown in Figure 2 described in other conditions could also apply to PE. Therefore, HCQ could lead to improvement of PE symptoms by decreasing the total amount of TLR4 might be expected. That would consequentially lead to reduce the secretion of pro-inflammatory cytokines by monocytes and neutrophils. In addition, HCQ could decrease the release of HMGB1 from multiple cells in PE, hence TLR4 activation would not occur. Therefore, HCQ could also improve endothelial dysfunction and thrombosis as well as reduce activation of DCs.
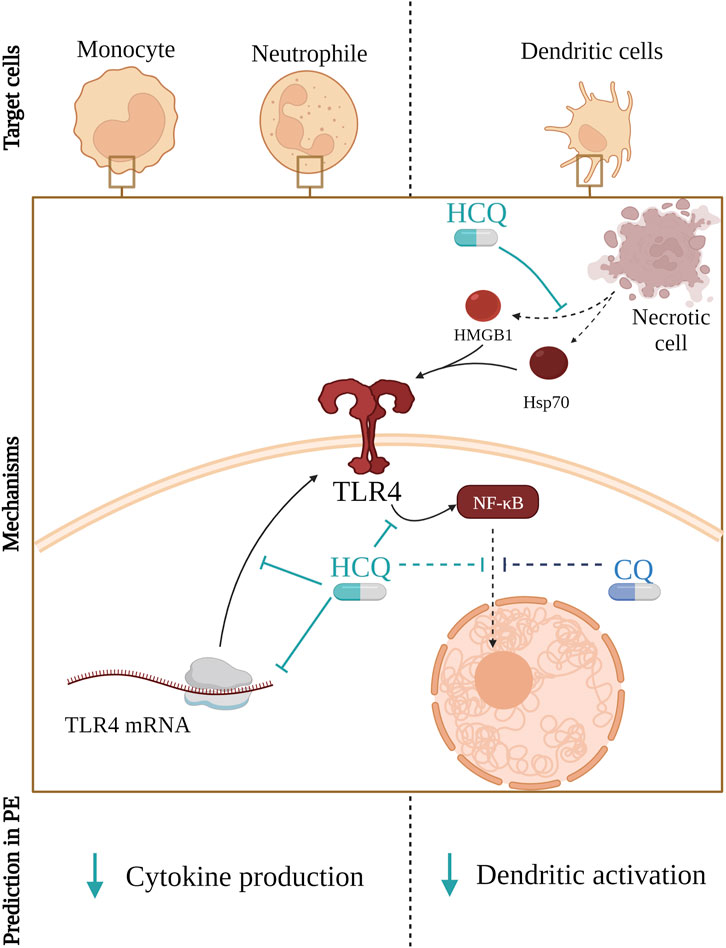
FIGURE 2. Hydroxychloroquine (HCQ) reduces the amount of available TLR4 on the cell membrane by affecting its mRNA transcription and translation. Furthermore, it blocks signal transduction from TLR4 to NF-κB and, same as Chloroquine (CQ), it prevents NF-κB transport from cytoplasm to the nucleus. Consequentially, that leads to reduced secretion of pro-inflammatory cytokines by monocytes and neutrophils. Furthermore, HCQ mitigates the release of HMGB1 from various cells, thereby preventing TLR4 activation potentially improving endothelial dysfunction and reducing the risk of thrombosis.
3.2 Targeting dysregulated complement in PE with HCQ
When overlapping the information, it is evident that both PE and certain autoimmune conditions, where HCQ is effective, involve the dysregulation of the complement system. The ability of HCQ to inhibit complement activation suggests its potential utility in managing conditions like PE, especially considering its protective effects in related pregnancy complications. However, when we focus on just research that shows the exact components of complement that are targets of HCQ and compare them to components of complement that are different in PE, the overlap is missing.
In the realm of PE, excessive activation of the complement system is widely recognized as a contributing factor to its development, a point underscored by multiple researchers (Alrahmani and Willrich, 2018; Burwick and Feinberg, 2022). In a study that involved 701 pregnant women, Lynch et al. found that elevated levels of the complement activation product Bb in early pregnancy were linked to an elevated risk of PE (Lynch et al., 2008). In contrast, Derzsy et al. did not observe any difference in circulating levels of Bb between healthy and PE women (Derzsy et al., 2010). Multiple authors found an increased presence of C4d in PE placental tissue (Buurma et al., 2012; Yonekura Collier et al., 2019). Moreover, they also found a strong association between these two molecules with and well-known anti-angiogenic factor, sFlt-1 (Yonekura Collier et al., 2019). In serum from pregnant women C4d, C3a, and SC5b9 were higher (Derzsy et al., 2010; Denny et al., 2013; Guseh et al., 2015; Burwick et al., 2018; He et al., 2020).
Conversely, HCQ’s role in inhibiting complement system activation, particularly in pregnancy-related complications and autoimmune diseases, has been explored but lacks specificity in the context of PE. A study by Bertolaccini et al. found that HCQ prevents placental ischemia and abnormal foetal brain development in mice models for antiphospholipid syndrome by inhibiting complement activation (Bertolaccini et al., 2016). In these mice, C3a and C5a levels strongly decreased after HCQ treatment (Bertolaccini et al., 2016). However, we do not know if the reason for that is decreased antiphospholipid antibody binding or if HCQ additionally affects C3 and C convertase. In a study done on syncytiotrophoblast, HCQ impaired complement-dependent antigen-antibody reactions and showed potential protection in APS (Wu et al., 2011; Mekinian et al., 2015). Therefore, the HCQ effect on complement is connected to APS antibodies (Wu et al., 2011). In addition, it has been observed that HCQ therapy in Japanese SLE patients even increases C3 and C4 (Ikeda et al., 2019). However, it was not clarified how. It could be through the inactivation of convertases or by an increase in the production of these molecules. If it is further, that would not be favourable for PE patients. In summary, while HCQ shows promise in modulating the complement system, its specific effects on the complement components involved in PE require further investigation. Direct studies focusing on HCQ’s application in PE are essential to determine its effectiveness and safety in this particular context.
4 Adaptive immune system in PE and HCQ immunomodulatory properties
The activation of innate immunity cells and antigen-presenting cells detected in PE results in the production of inflammatory cytokines and activation of cells of adaptive immunity, leading to increased inflammation. Miller et al. have provided a comprehensive review of available literature about the role of each immune cell type response in healthy and PE pregnancies (Miller et al., 2022) focusing on T cell subtypes in placental and decidua (Miller et al., 2022). Thus, our focus is on subtypes of T helper cells in maternal circulation, their contribution to cytokine storm and potential interaction with HCQ.
4.1 Cytokine effects on Th cell polarization in PE
CD4+ T lymphocytes orchestrate adaptive humoral and cell-mediated immune responses (Honey and Rudensky, 2003). Their activation depends on three steps: recognition of antigenic fragments on MHC class II molecules on APC cells, co-stimulating by APC cells and inflammatory cytokines produced by the APC or other cells at the site of T cell activation. This leads to the proliferation and differentiation of activated CD4+ T cells (Magombedze et al., 2013). Depending on the particular cytokine milieu, naïve CD4+ T lymphocytes can differentiate into five major subsets: T helper (Th) 1, Th2, Th17, T regulatory (Treg), and follicular Th cells (Magombedze et al., 2013; Zhu and Zhu, 2020). Multiple studies have shown that the balance between Th1, Th2, Th17 and Treg lymphocytes in the circulation of PE patients is disturbed (Saito et al., 1999; Santner-Nanan et al., 2009; Darmochwal-Kolarz et al., 2012; Ribeiro et al., 2017; Zhang et al., 2017; Salazar Garcia et al., 2018; Eghbal-Fard et al., 2019; Romao-Veiga et al., 2022). Salazar Gracia and colleagues reported that elevated Th17/Treg cell ratios in peripheral blood happen in early pregnancy and it associated with PE, suggesting a potential predictive marker (Salazar Garcia et al., 2018). Others have shown that the severity of PE is related to the change in Th17/Treg ratio (Ribeiro et al., 2017; Zhang et al., 2017). Multiple authors proposed that an imbalance between Treg cell and Th17 cell differentiation may explain PE pathogenesis (Santner-Nanan et al., 2009; Saito, 2010; Salazar Garcia et al., 2018; Eghbal-Fard et al., 2019). He et al. postulated the hypothesis that sENG produced by endothelial cells and syncytiotrophoblasts may disturb Treg cell differentiation (He et al., 2018). Therefore, drugs with immunomodulatory properties which would be able to turn over Th balance in favour of Treg and Th2 would be good candidate for PE treatment.
Cytokines are important for the differentiation of Th cells. However, each Th cell type is also secreting a specific cytokine profile that promotes or blocks differentiation of other Th lymphocytes, leading to a complex feedback loop and an overall cytokine storm. Th1 and Th17 are considered more pro-inflammatory subtypes because they are major producers of TNF-α and INF-γ or IL-17 as well as IL-21 and IL-22, respectively. Contrary, Th2 secreting IL-4, IL-5, IL-6 and IL-13 and Treg secreting TGF-β and IL-10 are considered more anti-inflammatory (Figueiredo and Schumacher, 2016). High levels of pro-inflammatory cytokines are characteristic of early implantation and parturition (Mor et al., 2011; Rinaldi et al., 2017). However, their persistent state leads to pregnancy-related complications (Saini et al., 2011). In women with PE, the cytokine profiles in peripheral blood are mainly Th1 and Th17 dominant (Arriaga-Pizano et al., 2005).
TNF-α is a multifunctional Th1 cytokine and one of the most important inflammatory cytokines. While some studies did not report a difference in TNF-α concentration between maternal sera/plasma from normal and PE pregnancies (Valencia-Ortega et al., 2019), multiple other studies revealed significantly higher TNF-α levels (Serin et al., 2002; Pinheiro et al., 2015; Salazar Garcia et al., 2018; Aggarwal et al., 2019). In addition, Ribeiro et al. showed that this increase is more prominent in EO-PE than in LO-PE (Ribeiro et al., 2017). The increase of systemic TNF-α levels during pregnancy, besides PE, is associated with miscarriages, late fetal losses, and preterm birth (Dai et al., 2022). It also inhibits trophoblast-derived JEG-3 cell line integration into endothelial cellular networks in vitro co-culture with uterine-derived endothelial cells (Xu et al., 2011). Furthermore, it inhibited EVT invasion (Bauer et al., 2004; Renaud et al., 2005; Otun et al., 2011), implicating possible effects on first-trimester trophoblast invasion and remodelling of the spiral artery. In addition, it has been shown that the challenge of first-trimester placenta explants with TNF-α would drastically increase the shed of syncytiotrophoblasts (Chen et al., 2010) suggesting a TNF-α role in PE origin. PMBCs from PE patients also produce a higher amount of TNF-α compared to PMBCs from healthy pregnant women (Romao-Veiga et al., 2022). Thus, TNF-α acts as one major mediator in the pathogenesis of severe PE (Alijotas-Reig et al., 2017).
The second Th1-produced cytokine is IFN-γ (Figueiredo and Schumacher, 2016). Its circulating (Arriaga-Pizano et al., 2005; Yang et al., 2014; Pinheiro et al., 2015; Deng et al., 2020) and placenta tissue (Sheibak et al., 2020; Liu et al., 2021) levels are increased in PE patients. It has been proposed that IFN-γ could be responsible for the shallow invasion of extravillous trophoblasts since IFN-γ reduced the invasion of the HTR-8/SVneo trophoblast cell line (Liu et al., 2021; Nurzadeh et al., 2023).
Similar to TNF-α, data on IL-6 concentration in the maternal circulation are inconsistent. Various studies demonstrated increased (Takacs et al., 2003; Xiao et al., 2012; Pinheiro et al., 2015; Li et al., 2016; Aggarwal et al., 2019; Ma et al., 2019; Valencia-Ortega et al., 2019; Gencheva et al., 2022; Kadife et al., 2022) rather than unchanged (Borekci et al., 2007; Salazar Garcia et al., 2018) IL-6 concentration in maternal sera and plasma. Xiao et al. found a connection between IL-6 and the severity of PE (Xiao et al., 2012). However, Gencheva et al. did not observe the same connection (Gencheva et al., 2022). In addition, Takacs et al. show that HUVECs incubated with plasma from PE patients secrete more IL-6 (Takacs et al., 2003).
Data regarding IL-1β in PE patients is also varied. While some authors did not observe any difference between maternal sera concentration of IL-1β (Valencia-Ortega et al., 2019; Deng et al., 2020), others found significantly increased concentrations in both maternal sera and in placentas of women with PE (Amash et al., 2012; Dong and Yin, 2014; Ma et al., 2019).
While IL-6 and IL-1β are important for Th17 differentiation, IL-17 is produced by them and plays a well-established role in driving various inflammatory pathways. It is also increased in sera from women with PE (Saito, 2010; Szarka et al., 2010; Molvarec et al., 2015; Eghbal-Fard et al., 2019; El Shahaway et al., 2019; Deng et al., 2020). Its dynamic role involves recruiting and activating neutrophils, which stands as a distinctive characteristic of the inflammatory response observed in PE (El Shahaway et al., 2019). In a pregnant rodent treated with TLR3 and TLR7 protein amount of IL-17 was elevated.
Contrary to that, IL-10 was found to be decreased in both placenta and maternal serum in PE (Borekci et al., 2007; Aggarwal et al., 2019; Valencia-Ortega et al., 2019), as well as IL-4 (Arriaga-Pizano et al., 2005; Aggarwal et al., 2019). Therefore, a disturbed TNF-α/IL-10 ratio in maternal circulation is associated with PE (Salazar Garcia et al., 2018).
The study by Vitoratos et al. showed that TNF-α and IL-6 remain elevated in PE women, resulting in persistent inflammatory stress up to 12–14 weeks postpartum, even after all other signs of PE have resolved (Vitoratos et al., 2010). Furthermore, Freeman et al. observed that even after 20 years of the index pregnancy, women who had PE still exhibited a significantly higher IL-6/IL-10 ratio (Freeman et al., 2004). Hence, PE is associated with not only short-term but also long-term changes in inflammatory status (Freeman et al., 2004; Vitoratos et al., 2010).
4.2 Immunomodulatory effect of HCQ
Although HCQ cannot directly protect cells from cytokine storms (Gajić et al., 2023), it could indirectly decrease their overall production them. We have already discussed in previous chapters how HCQ could influence cytokine production by TLRs modulation. However, that effect goes even deeper when we consider the importance of cytokine surroundings for the maturation of the adaptive immune system and its role in PE. There are two different ways in which HCQ´s immunomodulatory properties can improve PE: First, HCQ would directly inhibit TLRs activation and MHC II assembling in DCs and other antigen-presenting cells, and their cytokine production. In that way, HCQ could also affect the ratio between the Th subgroup as presented in Figure 3A. The other way is by directly affecting T and B lymphocytes.
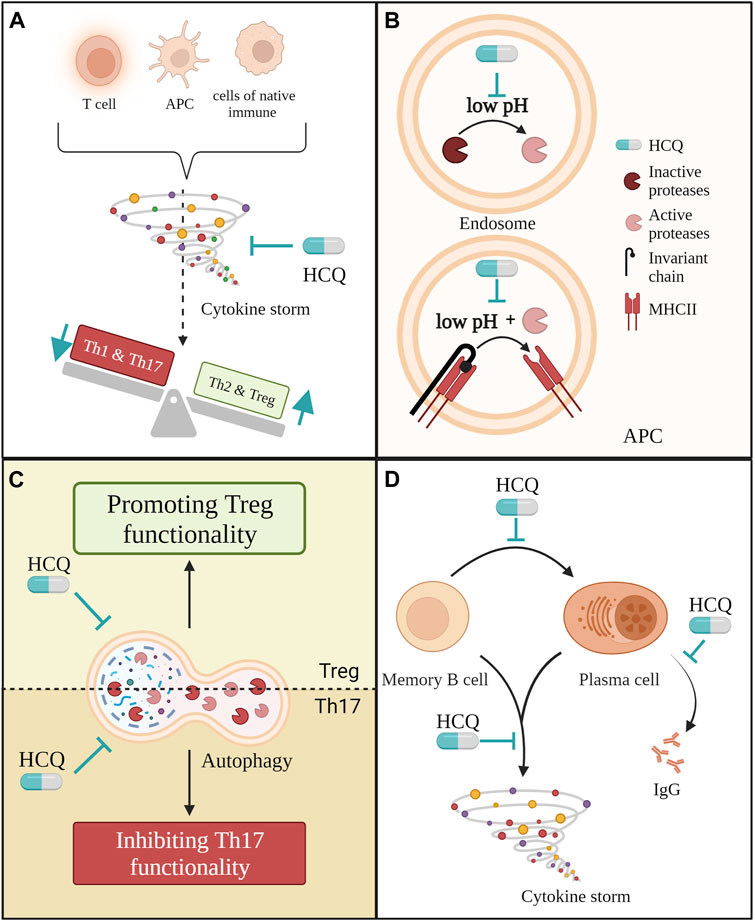
FIGURE 3. Several ways in which hydroxychloroquine (HCQ) may have an immunomodulatory effect on adaptive immune cells in PE. (A) HCQ could reduce cytokine production from different cell types in PE such are antigen-presenting cells, T cells and cells from native immune system like monocites, macrophages, natural killer cells and neutrophils. This way, it would reduce cytokine storm and indirectly improve the ratio between pro-inflammatory and anti-inflammatory T helper cells; (B) Through its direct effect on pH in endosomes HCQ could prevent the expression of MHC II molecules. Low pH is obligatory for the activation of proteases that cleave of invariant chain from MHC II as well as for the process of cleaving itself; (C) By affecting autophagy in Th17 and Treg cells mitigated HCQ could improve Th17/Treg ratio; (D) Throughout multiple different ways HCQ could reduce the pro-inflammatory effect of B cells in PE.
It is a known fact that HCQ can affect the production of cytokines by different cells and consequently reduce the concentration of specific cytokines in circulation (Scharfe-Nugent et al., 2012; Scott et al., 2019; van den Borne et al., 1997; Alves et al., 2017; Piconi et al., 2011; Silva et al., 2013; Ghasemnejad-Berenji et al., 2018; Torigoe et al., 2018; Sperber et al., 1993; Ulander et al., 2021; Hjorton et al., 2018; Meng et al., 2022).
The effect of HCQ on Th1-produced cytokines was observed in SLE and HIV patients who were taking HCQ and had lower IL-6 plasma concentrations (Piconi et al., 2011). After incubation with increasing dosage of HCQ, PMBCs from SLE and RA patients reduced secretion of IL-6, IL-17 and IL-22, which are cytokines connected to Th17 cells (Silva et al., 2013). Therefore, by decreasing IL-6 production, HCQ could improve the Th17/Treg ratio (van den Borne et al., 1997; Silva et al., 2013; Sperber et al., 1993; Ulander et al., 2021) because IL-6 together with IL-1β promotes the differentiation of naive T cells into Th17 cells and even the conversion of Treg cells into Th17 cells. Since Th17 cells induce chronic inflammation, resulting in endothelial dysfunction (Saito, 2010), the HCQ effect on the Th17/Treg ratio would also have a positive effect on endothelial dysfunction. HCQ could also improve the TNF-α/IL-10 ratio in PE patients, as it has been shown in patients with repeated implantation failure (Ghasemnejad-Berenji et al., 2018). Similar to PE, these patients had high TNF-α and low IL-10 concentrations in blood circulation. However, after treatment with HCQ, the TNF-α/IL-10 ratio improved (Ghasemnejad-Berenji et al., 2018). In addition, HCQ reduces basal production of IFNs (van den Borne et al., 1997) and TLR3 and TLR9 activated (Alves et al., 2017; Hjorton et al., 2018) in PBMC from SLE patients. Moreover, it reduces the presence of endometrium in women treated with HCQ for repeated implantation failure (Ghasemnejad-Berenji et al., 2018).
Besides HCQ, CQ was also able to reduce the secretion of TNF-α, IL-6, IFN-γ and IL-1β in human primary PBMCs, monocytes/macrophages and two different monocyte cell lines stimulated with LPS or fetal DNA (Scharfe-Nugent et al., 2012; van den Borne et al., 1997; Jang et al., 2006). It has been suggested that CQ inhibited TNF-α synthesis at a post-translational step (Jang et al., 2006) while inhibiting IL-1β and IL-6 synthesis on their mRNA levels (Jang et al., 2006). In this setup, the HCQ inhibitory effect on the secretion of TNF-α, IL-6 and IL-1β was confirmed in monocytes/macrophages (Jang et al., 2006). Hypothetically HCQ should have same the effect as CQ, but this needs to be tested in an experimental setup, favourably in the context of PE. Although CQ and HCQ lead to a decrease in cytokine secretion in all Th subtypes, Th2 cytokine secretion appears to be less sensitive to their inhibitory effects compared to Th1 and Th17 cells (Schmidt et al., 2017), which would be suitable for PE treatment.
DCs are the primary and most potent antigen-presenting cells, playing an essential role in the presentation of antigens to CD8 + and CD4 + T lymphocytes and their polarization.
We have already mentioned that HCQ interferes with lysosomal acidification and TLR activation (Ponticelli and Moroni, 2017). DCs can be activated through various cell surface receptors and cytosolic sensors, but their dominant mode of activation for endogenous or exogenous nucleic acids and HMGB1 is recognized by TLR4, TLR7 and TLR9. Following activation, DCs upregulate the expression of major histocompatibility complex (MHC II) molecules, costimulatory molecules and cytokines, leading to the priming of T cells for subsequent immune responses. In SLE and RA patients, HCQ affects TLR7 and TLR9 activation and decreases the percentage of DCs as well as their production of IFN-α (Sacre et al., 2012; Alves et al., 2017; Hjorton et al., 2018; Zeidi et al., 2019; Meng et al., 2022), IL-6 (Piconi et al., 2011; Hjorton et al., 2018; Meng et al., 2022) and TNF-α (Sacre et al., 2012; Hjorton et al., 2018; Han et al., 2020).
Besides TLR activation, endosomal low pH is necessary for the activation and functioning of proteases. These enzymes are crucial for antigen presentation because they cleave the invariant chain from the MHC II molecule leaving it available for antigen. As shown in Figure 3B, by alkalisation of the lysosome, HCQ can partially inhibit the activation of proteases as well as block the cleaving of the invariant chain from MHC II. As a result, antigen presentation by antigen-presenting cells, such as DCs, is inhibited (Honey and Rudensky, 2003; Al-Bari, 2015). In both cases, by blocking the activation of DCs and by blocking MHC II assembling, HCQ would indirectly affect the Th cells ratio in PE.
Besides the indirect effect on antigen-presenting cells and cytokine storm, HCQ could also directly affect T and B lymphocytes. Another mechanism through which HCQ could affect Th17/Treg ratio is by inhibiting autophagy in both cell populations. In experiments with rodents and PBMC isolated from SLE and HIV patients, HCQ attenuated Th17 differentiation, proliferation, and production of IL-17 while promoting the immunosuppressive effect of Tregs (Piconi et al., 2011; An et al., 2017; Yang et al., 2018; Kowatsch et al., 2023). Due to that, An et al. suggested that HCQ might have different effects of autophagy on diverse T-cell subsets (An et al., 2017). This difference in effect on autophagy in Th17 and Treg is presented in Figure 3C. While HCQ reduces IL-17 secretion by inhibiting autophagy in Th17 cells, thereby also reversing Th17 cell over-activity, it leads to blockage of autophagy-induced degradation of Foxp3 in Treg cells, thereby promoting Treg cell functionality (An et al., 2017).
Besides priming T cells, HCQ also affects their proliferation. Both CQ and HCQ have been found to suppress T-cell proliferation (Piconi et al., 2011; Kim et al., 2021; Hu Y. et al., 2022). The suggested mechanism behind it is that HCQ causes an increase in O2− (superoxide) production during T-cell receptor stimulation, which leads to the inhibition of T-cell proliferation (Kim et al., 2021).
Further, HCQ also affects B lymphocytes as shown in Figure 3D. HCQ leads to a decrease of TNF-α, IL-6 and IL-1β secretion by all subsets of B cells (Torigoe et al., 2018). Notably, the percentage of memory B cells and plasma cell precursors is significantly increased in PE women (Liao et al., 2009). Furthermore, the percentages of plasma cells generated upon in vitro stimulation with CpG were significantly higher in the PE group than in the control group (Liao et al., 2009). These cells present another potential target for HCQ since HCQ effectively suppresses the class-switch of memory B cells to plasma cells and also decreases the production of IgG (Torigoe et al., 2018; Kowatsch et al., 2023), both of which are increased in PE (Liao et al., 2009).
5 Vascularity in PE and HCQ
In a typical placental implantation process, the extravillous trophoblast migrates into the maternal uterine vasculature, resulting in significant alterations to the maternal spiral arteries, which become high-capacity, high-flow vessels (Rana et al., 2019). However, in pregnancies with subsequent PE, there are abnormalities in extravillous trophoblast behaviour that contribute to shallow placental implantation, leading to incomplete spiral artery transformation and placental ischemia (Rana et al., 2019). This will affect the vasculature of both mother and foetus, leading to high blood pressure and organ failure in mothers and potential fetal growth restriction (Thilaganathan and Kalafat, 2019; Opichka et al., 2021).
5.1 Angiogenic factors and endothelial dysfunction in PE
PE is a complex disorder characterized by an imbalance of pro- and anti-angiogenic factors, which directly influence endothelial function. Angiogenesis and the importance of nitric oxide (NO) for health and PE have been reviewed in detail in the following papers (Sutton et al., 2020; Opichka et al., 2021). Here we will focus on the most important molecules and processes to explain the potential effect of HCQ on this part of PE (Sutton et al., 2020; Opichka et al., 2021).
The equilibrium between circulating pro-angiogenic and anti-angiogenic factors has been implicated as a mechanism underlying endothelial and NO dysfunction in PE (Opichka et al., 2021). The main players in this interplay are VEGFA, PlGF, sFLT1, sENG and endothelin 1 (ET1). In healthy pregnancies, the pro-angiogenic factors, VEGFA and PlGF, bind to VEGF1 and VEGF2 receptors, stimulating angiogenesis, vascular permeability, and cell migration (Geva et al., 2002; Chau et al., 2017). These factors also lead to robust activation of endothelial nitric oxide synthase (eNOS) (Papapetropoulos et al., 1997; Grummer et al., 2009), increasing the availability of NO and promoting healthy vascular function. However, in PE, both VEGFA and PlGF are significantly decreased, contributing to the endothelial dysfunction observed in this condition (Molvarec et al., 2011; Molvarec et al., 2015; Chau et al., 2017; He et al., 2018).
The soluble form of Vascular Endothelial Growing Factor Receptor 1 (VEGFR1), also known as sFLT-1, is found to be increased in PE (Molvarec et al., 2011; Molvarec et al., 2015; He et al., 2018; Ozeki et al., 2019). This soluble receptor acts as a scavenger for VEGFA, further reducing its bioavailability in maternal serum. It has been shown that sFlt-1 secretion is increased in HTR-8/SVneo trophoblast cell line, pregnant mice and term placenta explants when challenged with either TLR3, TLR4 or TLR9 agonists (Nakada et al., 2009; He et al., 2018; Scott et al., 2019). The sFlt-1/PlGF ratio is found to be disturbed in PE, its utility as a predictive and even diagnostic biomarker has been described by various authors (Molvarec et al., 2011; Verlohren et al., 2012; Molvarec et al., 2015; Herraiz et al., 2018). Besides in patients, the difference in sFlt-1/PlGF was also observed in the secretion profile of villus tissue explants from term placentas incubated with TLR9 agonist. In addition to VEGF-related factors, sENG has been found to be elevated in PE (Ozeki et al., 2019) and is also considered a potential diagnostic tool. In addition, when challenged with PE sera JEG-3 and Sw.71 cells, a model for placental trophoblast, increased secretion of ENG (Aoki et al., 2012; Ozeki et al., 2019). Another potent vasoconstrictor that incites reactive oxygen species production and inflammatory signalling in endothelial cells is ET1 (Kowalczyk et al., 2015).
Endothelial dysfunction is one key feature of PE (Chambers et al., 2001; Echeverria et al., 2020), manifesting a barrier disruption and impaired vasodilatory capacity. Studies have demonstrated that NO availability is diminished in women with PE (Sutton et al., 2020). Oxidative stress and elevated levels of ROS are commonly observed in PE and likely contribute to impaired NO signalling and vascular dysfunction (McElwain et al., 2020). Furthermore, this leads to overall vasocontraction and high blood pressure. Therefore, affecting any of these molecules would be beneficial for PE treatment.
5.1.1 HCQ impact role on angiogenic factors and endothelial dysfunction
Data concerning the effect of HCQ on angiogenic molecules and endothelial dysfunction appears to be quite heterogeneous. Concerning angiogenic molecules, HCQ seems to reduce sFLT-1 secretion from cytotrophoblast cells and slightly increase PlGF, but it does not seem to affect ENG or the secretion of these molecules from placental explants (Scott et al., 2019; Rahman et al., 2020; Kadife et al., 2022). However, HCQ reversed the effect of TLR9 agonist on third-trimester placenta explants secretion, leading to a reduction in sFlt-1 production and improvement of sFlt1/PlGF ratio (Scott et al., 2019).
In a mouse model for SLE and APS, HCQ reduces ROS levels, increases NO bioavailability and helps to prevent endothelial dysfunction. This is achieved either through the blocking of NADPH oxidase (NOX) present in endosomes or by modulation of eNOS, respectively (Gomez-Guzman et al., 2014; Miranda et al., 2019). The same mechanism presented in Figure 4 would be also beneficial for PE patients. Consistent with this, HCQ demonstrated a reduction in clot formation and thrombin generation in adult endothelial cells, APS mouse model and patients with primary APS (Nuri et al., 2017; Miranda et al., 2019; Kravvariti et al., 2020). Since thrombin generation is elevated in PE (de Boer et al., 1989; Xin et al., 2012) use of HCQ would be beneficial in this case, too. In the context of PE, HCQ has been shown to reduce PE serum-triggered production of ET-1 and NOX in HUVECs (Rahman et al., 2016). However, conflicting results have been obtained on the same type of cells for TNF-α-triggered ET-1 secretion (Rahman et al., 2016; Kadife et al., 2022).
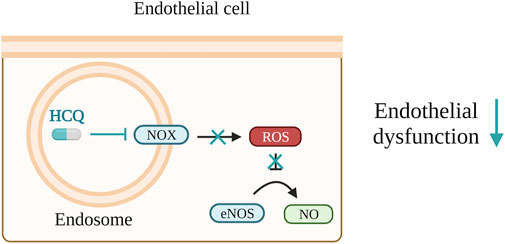
FIGURE 4. Hydroxychloroquine (HCQ) lowers reactive oxygen species (ROS) levels, enhances nitrogen monoxide (NO) bioavailability, and contributes to the prevention of endothelial dysfunction. These effects are accomplished by either inhibiting the activity of NADPH oxidase (NOX) within endosomes or by regulating endothelial nitric oxide synthase (eNOS).
Furthermore, HCQ seems to have opposing effects on fetal endothelial cells under different conditions. For instance, in high-glucose conditions, HCQ decreased ROS production in endothelial cells but also diminished NO production and angiogenesis (Rezabakhsh et al., 2017). Conversely, when exposed to a mixture of pro-inflammatory cytokines, HCQ did not affect angiogenesis in fetal arterial endothelial cells (Gajić et al., 2023), while in the presence of just TNF-α, HCQ improved angiogenesis in HUVECs (Rahman et al., 2016).
The high variability of the HCQ effect on angiogenic factors and endothelial dysfunction is due to differences in tested conditions. Thus, these special effects of HCQ need to be confirmed in PE or PE-like experimental conditions.
5.2 HCQ and regulators of leukocyte rolling and adhesion
An alternative mechanism, by which HCQ might affect PE is its effect on the regulation of leukocyte rolling and adhesion. The most important molecules in this process are ICAM-1, VCAM-1 and selectins (McEver and Zhu, 2010). When endothelial cells undergo inflammatory activation, they increase the expression of selectins, VCAM-1, and ICAM-1. In turn, they promote monocytes adherence and transfer to sites of inflammation.
Circulating soluble forms of all three molecules (ICAM-1, VCAM-1 and Selectin E and P) were found to be increased in PE (Austgulen et al., 1997; Kim et al., 2004; Molvarec et al., 2011; Dogan et al., 2014; Docheva et al., 2019; Valencia-Ortega et al., 2019; Kornacki et al., 2020). Furthermore, sVCAM was specifically more prominent in severe and EO-PE (Kim et al., 2004; Dogan et al., 2014). At the same time, multiple authors even found a correlation between sVCAM-1 and sICAM-1 with the systolic/diastolic blood pressure values, and renal and liver function parameters in PE patients (Szarka et al., 2010; Dogan et al., 2014).
It has been shown that HCQ induces a decline of VCAM-1 in endothelial cells (Le et al., 2014; Li et al., 2018; Hu Y. et al., 2022; Kadife et al., 2022; Gajić et al., 2023). It has been suggested that this inhibition goes through the activation of ERK5 (Le et al., 2014; Cheng et al., 2018). Alongside the reduction of VCAM-1, multiple studies have reported a decrease in ICAM-1 (Li et al., 2018; Sciascia et al., 2020; Hu Y. et al., 2022). Furthermore, transcription of Selectin P was negatively correlated with HCQ dose in platelets from SLE patients and this confirmed HCQ (Cornwell et al., 2021). Furthermore, there is an association between high HCQ blood levels and reduced thrombotic events in SLE patients (Petri et al., 2021).
Besides the effect on gene and protein levels, HCQ was also able to decrease the serum concentrations of sICAM, sVCAM and sSELE in insulin resistance-induced endothelial dysfunction in rats (Abdel-Hamid and Firgany, 2016). Further, HCQ improves altered endothelium-dependent relaxation in the context of APS (Miranda et al., 2019). Therefore, HCQ could potentially decrease the concentration of circulating VCAM-1, ICAM-1 and selectins in PE patients, which potentially could affect their high blood pressure. Moreover, HCQ could improve endothelial-dependent relaxation and decrease thrombosis in PE patients. Besides the effect on the vasculature, by reducing VCAM-1 on endothelial cells, HCQ could also decrease monocyte penetration to tissue and inflammatory reactions in general.
6 Conclusion
While there is a substantial body of literature discussing the potential utility of HCQ as a treatment or prophylaxis for PE, the current state of research falls short in terms of rigorous experimental and clinical investigation in the context of PE. The majority of available studies draw upon HCQ data derived from patients with conditions such as SLE and RA, leaving a noticeable gap in dedicated research on PE. Furthermore, some clinical studies reporting a reduced incidence of PE in pregnant women who used HCQ are essentially meta-analyses of trials conducted with SLE patients.
Given that the effectiveness of HCQ appears to be influenced by the specific disease or condition for which it is being tested, it is imperative to explore its impact under PE-specific conditions. In our review, we endeavoured to elucidate the mechanisms of HCQ action and identify areas of convergence with the pathophysiology of PE. More precisely, we aimed to highlight distinct molecular targets, cell types, and potential outcome measures that could guide future experimental investigations.
Author contributions
MG: Data curation, Formal Analysis, Investigation, Methodology, Validation, Visualization, Writing–original draft. BS-H: Writing–review and editing, Formal Analysis. KM-P: Conceptualization, Funding acquisition, Supervision, Writing–review and editing.
Funding
The author(s) declare financial support was received for the research, authorship, and/or publication of this article. This research was funded by the Austrian Science Fund (FWF) (DOC 31-B26) and the Medical University of Graz through the Ph.D. Program Inflammatory Disorders in Pregnancy (DP-iDP).
Conflict of interest
The authors declare that the research was conducted in the absence of any commercial or financial relationships that could be construed as a potential conflict of interest.
Publisher’s note
All claims expressed in this article are solely those of the authors and do not necessarily represent those of their affiliated organizations, or those of the publisher, the editors and the reviewers. Any product that may be evaluated in this article, or claim that may be made by its manufacturer, is not guaranteed or endorsed by the publisher.
References
Abalos, E., Cuesta, C., Grosso, A. L., Chou, D., and Say, L. (2013). Global and regional estimates of preeclampsia and eclampsia: a systematic review. Eur. J. Obstet. Gynecol. Reprod. Biol. 170 (1), 1–7. doi:10.1016/j.ejogrb.2013.05.005
Abarientos, C., Sperber, K., Shapiro, D. L., Aronow, W. S., Chao, C. P., and Ash, J. Y. (2011). Hydroxychloroquine in systemic lupus erythematosus and rheumatoid arthritis and its safety in pregnancy. Expert Opin. Drug Saf. 10 (5), 705–714. doi:10.1517/14740338.2011.566555
Abdel-Hamid, A. A. M., and Firgany, A. E. L. (2016). Favorable outcomes of hydroxychloroquine in insulin resistance may be accomplished by adjustment of the endothelial dysfunction as well as the skewed balance of adipokines. Acta histochem. 118 (6), 560–573. doi:10.1016/j.acthis.2016.06.002
Aggarwal, R., Jain, A. K., Mittal, P., Kohli, M., Jawanjal, P., and Rath, G. (2019). Association of pro- and anti-inflammatory cytokines in preeclampsia. J. Clin. Lab. Anal. 33 (4), e22834. doi:10.1002/jcla.22834
Ahmed, A., Williams, D. J., Cheed, V., Middleton, L. J., Ahmad, S., Wang, K., et al. (2020). Pravastatin for early-onset pre-eclampsia: a randomised, blinded, placebo-controlled trial. BJOG 127 (4), 478–488. doi:10.1111/1471-0528.16013
Al-Bari, M. A. (2015). Chloroquine analogues in drug discovery: new directions of uses, mechanisms of actions and toxic manifestations from malaria to multifarious diseases. J. Antimicrob. Chemother. 70 (6), 1608–1621. doi:10.1093/jac/dkv018
Alijotas-Reig, J., Esteve-Valverde, E., Ferrer-Oliveras, R., Llurba, E., and Gris, J. M. (2017). Tumor necrosis factor-alpha and pregnancy: focus on biologics. An updated and comprehensive review. Clin. Rev. Allergy Immunol. 53 (1), 40–53. doi:10.1007/s12016-016-8596-x
Alrahmani, L., and Willrich, M. A. V. (2018). The complement alternative pathway and preeclampsia. Curr. Hypertens. Rep. 20 (5), 40. doi:10.1007/s11906-018-0836-4
Alvarez-Cabrera, M. C., Barrientos-Galeana, E., Barrera-Garcia, A., Osorio-Caballero, M., Acevedo, J. F., Flores-Herrera, O., et al. (2018). Secretion of heat shock -60, -70 kD protein, IL-1β and TNFα levels in serum of a term normal pregnancy and patients with pre-eclampsia development. J. Cell Mol. Med. 22 (11), 5748–5752. doi:10.1111/jcmm.13824
Alves, P., Bashir, M. M., Wysocka, M., Zeidi, M., Feng, R., and Werth, V. P. (2017). Quinacrine suppresses tumor necrosis factor-α and IFN-α in dermatomyositis and Cutaneous lupus erythematosus. J. Investig. Dermatol Symp. Proc. 18 (2), S57–S63. doi:10.1016/j.jisp.2016.11.001
Amash, A., Holcberg, G., Sapir, O., and Huleihel, M. (2012). Placental secretion of interleukin-1 and interleukin-1 receptor antagonist in preeclampsia: effect of magnesium sulfate. J. Interferon Cytokine Res. 32 (9), 432–441. doi:10.1089/jir.2012.0013
An, N., Chen, Y., Wang, C., Yang, C., Wu, Z. H., Xue, J., et al. (2017). Chloroquine autophagic inhibition rebalances Th17/treg-mediated immunity and ameliorates systemic lupus erythematosus. Cell Physiol. Biochem. 44 (1), 412–422. doi:10.1159/000484955
Aoki, Y., Yamamoto, T., Fumihisa, C., Nakamura, A., Asanuma, A., and Suzuki, M. (2012). Effect on the production of soluble endoglin from human choriocarcinoma cells by preeclampsia sera. Am. J. Reprod. Immunol. 67 (5), 413–420. doi:10.1111/j.1600-0897.2011.01086.x
Arriaga-Pizano, L., Jimenez-Zamudio, L., Vadillo-Ortega, F., Martinez-Flores, A., Herrerias-Canedo, T., and Hernandez-Guerrero, C. (2005). The predominant Th1 cytokine profile in maternal plasma of preeclamptic women is not reflected in the choriodecidual and fetal compartments. J. Soc. Gynecol. Investig. 12 (5), 335–342. doi:10.1016/j.jsgi.2005.02.005
Asea, A., Rehli, M., Kabingu, E., Boch, J. A., Bare, O., Auron, P. E., et al. (2002). Novel signal transduction pathway utilized by extracellular HSP70: role of toll-like receptor (TLR) 2 and TLR4. J. Biol. Chem. 277 (17), 15028–15034. doi:10.1074/jbc.M200497200
Austgulen, R., Lien, E., Vince, G., and Redman, C. W. (1997). Increased maternal plasma levels of soluble adhesion molecules (ICAM-1, VCAM-1, E-selectin) in preeclampsia. Eur. J. Obstet. Gynecol. Reprod. Biol. 71 (1), 53–58. doi:10.1016/s0301-2115(96)02647-4
Author Anonymous (2019). Gestational hypertension and preeclampsia: ACOG practice bulletin, number 222. Obstet. Gynecol. 133 (1), 1. doi:10.1097/AOG.0000000000003891
Author Anynomus (2018). ACOG committee opinion No. 743: low-dose aspirin use during pregnancy. Obstet. Gynecol. 132 (1), e44–e52. doi:10.1097/AOG.0000000000002708
Banerjee, S., Huang, Z., Wang, Z., Nakashima, A., Saito, S., Sharma, S., et al. (2021). Etiological value of sterile inflammation in preeclampsia: is it a non-infectious pregnancy complication? Front. Cell Infect. Microbiol. 11, 694298. doi:10.3389/fcimb.2021.694298
Bauer, S., Pollheimer, J., Hartmann, J., Husslein, P., Aplin, J. D., and Knofler, M. (2004). Tumor necrosis factor-alpha inhibits trophoblast migration through elevation of plasminogen activator inhibitor-1 in first-trimester villous explant cultures. J. Clin. Endocrinol. Metab. 89 (2), 812–822. doi:10.1210/jc.2003-031351
Bertolaccini, M. L., Contento, G., Lennen, R., Sanna, G., Blower, P. J., Ma, M. T., et al. (2016). Complement inhibition by hydroxychloroquine prevents placental and fetal brain abnormalities in antiphospholipid syndrome. J. Autoimmun. 75, 30–38. doi:10.1016/j.jaut.2016.04.008
Borekci, B., Aksoy, H., Al, R. A., Demircan, B., and Kadanali, S. (2007). Maternal serum interleukin-10, interleukin-2 and interleukin-6 in pre-eclampsia and eclampsia. Am. J. Reprod. Immunol. 58 (1), 56–64. doi:10.1111/j.1600-0897.2007.00491.x
Brito-Zeron, P., and Ramos-Casals, M. (2014). Advances in the understanding and treatment of systemic complications in Sjogren's syndrome. Curr. Opin. Rheumatol. 26 (5), 520–527. doi:10.1097/BOR.0000000000000096
Brown, M. A., Magee, L. A., Kenny, L. C., Karumanchi, S. A., McCarthy, F. P., Saito, S., et al. (2018). The hypertensive disorders of pregnancy: ISSHP classification, diagnosis and management recommendations for international practice. Pregnancy Hypertens. 13, 291–310. doi:10.1016/j.preghy.2018.05.004
Brownfoot, F. C., Tong, S., Hannan, N. J., Binder, N. K., Walker, S. P., Cannon, P., et al. (2015). Effects of pravastatin on human placenta, endothelium, and women with severe preeclampsia. Hypertension 66 (3), 687–697. ; discussion 445. doi:10.1161/HYPERTENSIONAHA.115.05445
Brownfoot, F. C., Tong, S., Hannan, N. J., Hastie, R., Cannon, P., and Kaitu'u-Lino, T. J. (2016). Effects of simvastatin, rosuvastatin and pravastatin on soluble fms-like tyrosine kinase 1 (sFlt-1) and soluble endoglin (sENG) secretion from human umbilical vein endothelial cells, primary trophoblast cells and placenta. BMC Pregnancy Childbirth 16, 117. doi:10.1186/s12884-016-0902-3
Burton, G. J., Redman, C. W., Roberts, J. M., and Moffett, A. (2019). Pre-eclampsia: pathophysiology and clinical implications. BMJ BMJ Publ. Group 366, l2381. doi:10.1136/bmj.l2381
Burwick, R. M., and Feinberg, B. B. (2022). Complement activation and regulation in preeclampsia and hemolysis, elevated liver enzymes, and low platelet count syndrome. Am. J. Obstet. Gynecol. 226 (2S), S1059–S1070. doi:10.1016/j.ajog.2020.09.038
Burwick, R. M., Velasquez, J. A., Valencia, C. M., Gutierrez-Marin, J., Edna-Estrada, F., Silva, J. L., et al. (2018). Terminal complement activation in preeclampsia. Obstet. Gynecol. 132 (6), 1477–1485. doi:10.1097/AOG.0000000000002980
Buurma, A., Cohen, D., Veraar, K., Schonkeren, D., Claas, F. H., Bruijn, J. A., et al. (2012). Preeclampsia is characterized by placental complement dysregulation. Hypertension 60 (5), 1332–1337. doi:10.1161/HYPERTENSIONAHA.112.194324
Caropreso, L., de Azevedo Cardoso, T., Eltayebani, M., and Frey, B. N. (2020). Preeclampsia as a risk factor for postpartum depression and psychosis: a systematic review and meta-analysis. Arch. Womens Ment. Health 23 (4), 493–505. doi:10.1007/s00737-019-01010-1
Chambers, J. C., Fusi, L., Malik, I. S., Haskard, D. O., De Swiet, M., and Kooner, J. S. (2001). Association of maternal endothelial dysfunction with preeclampsia. JAMA 285 (12), 1607–1612. doi:10.1001/jama.285.12.1607
Chatterjee, P., Weaver, L. E., Doersch, K. M., Kopriva, S. E., Chiasson, V. L., Allen, S. J., et al. (2012). Placental Toll-like receptor 3 and Toll-like receptor 7/8 activation contributes to preeclampsia in humans and mice. PLoS One 7 (7), e41884. doi:10.1371/journal.pone.0041884
Chau, K., Hennessy, A., and Makris, A. (2017). Placental growth factor and pre-eclampsia. J. Hum. Hypertens. 31 (12), 782–786. doi:10.1038/jhh.2017.61
Chen, L. M., Liu, B., Zhao, H. B., Stone, P., Chen, Q., and Chamley, L. (2010). IL-6, TNFalpha and TGFbeta promote nonapoptotic trophoblast deportation and subsequently causes endothelial cell activation. Placenta 31 (1), 75–80. doi:10.1016/j.placenta.2009.11.005
Chen, Q., Yin, Y. X., Wei, J., Tong, M., Shen, F., Zhao, M., et al. (2016). Increased expression of high mobility group box 1 (HMGB1) in the cytoplasm of placental syncytiotrophoblast from preeclamptic placentae. Cytokine 85, 30–36. doi:10.1016/j.cyto.2016.06.001
Chen, W., Qian, L., Wu, F., Li, M., and Wang, H. (2015). Significance of toll-like receptor 4 signaling in peripheral blood monocytes of pre-eclamptic patients. Hypertens. Pregnancy 34 (4), 486–494. doi:10.3109/10641955.2015.1077860
Cheng, F., Desai, R. J., Handy, D. E., Wang, R., Schneeweiss, S., Barabasi, A. L., et al. (2018). Network-based approach to prediction and population-based validation of in silico drug repurposing. Nat. Commun. 9 (1), 2691. doi:10.1038/s41467-018-05116-5
Cho, G. J., Jung, U. S., Sim, J. Y., Lee, Y. J., Bae, N. Y., Choi, H. J., et al. (2019). Is preeclampsia itself a risk factor for the development of metabolic syndrome after delivery? Obstet. Gynecol. Sci. 62 (4), 233–241. doi:10.5468/ogs.2019.62.4.233
Clowse, M. E. B., Eudy, A. M., Balevic, S., Sanders-Schmidler, G., Kosinski, A., Fischer-Betz, R., et al. (2022). Hydroxychloroquine in the pregnancies of women with lupus: a meta-analysis of individual participant data. Lupus Sci. Med. 9 (1), e000651. doi:10.1136/lupus-2021-000651
Conka, J., Konecna, B., Laukova, L., Vlkova, B., and Celec, P. (2017). Fetal DNA does not induce preeclampsia-like symptoms when delivered in late pregnancy in the mouse. Placenta 52, 100–105. doi:10.1016/j.placenta.2017.02.008
Cornwell, M. G., Luttrell-Williams, E. S., Golpanian, M., El Bannoudi, H., Myndzar, K., Izmirly, P., et al. (2021). Hydroxychloroquine is associated with lower platelet activity and improved vascular health in systemic lupus erythematosus. Lupus Sci. Med. 8 (1), e000475. doi:10.1136/lupus-2021-000475
Costantine, M. M., Cleary, K., Hebert, M. F., Ahmed, M. S., Brown, L. M., Ren, Z., et al. (2016). Safety and pharmacokinetics of pravastatin used for the prevention of preeclampsia in high-risk pregnant women: a pilot randomized controlled trial. Am. J. Obstet. Gynecol. 214 (6), 720 e1–e17. doi:10.1016/j.ajog.2015.12.038
Costedoat-Chalumeau, N., Amoura, Z., Aymard, G., Le, T. H., Wechsler, B., Vauthier, D., et al. (2002). Evidence of transplacental passage of hydroxychloroquine in humans. Arthritis Rheum. 46 (4), 1123–1124. doi:10.1002/art.10150
Dai, F. F., Hu, M., Zhang, Y. W., Zhu, R. H., Chen, L. P., Li, Z. D., et al. (2022). TNF-α/anti-TNF-α drugs and its effect on pregnancy outcomes. Expert Rev. Mol. Med. 24, e26. doi:10.1017/erm.2022.18
Darmochwal-Kolarz, D., Kludka-Sternik, M., Tabarkiewicz, J., Kolarz, B., Rolinski, J., Leszczynska-Gorzelak, B., et al. (2012). The predominance of Th17 lymphocytes and decreased number and function of Treg cells in preeclampsia. J. Reprod. Immunol. 93 (2), 75–81. doi:10.1016/j.jri.2012.01.006
de Boer, K., ten Cate, J. W., Sturk, A., Borm, J. J., and Treffers, P. E. (1989). Enhanced thrombin generation in normal and hypertensive pregnancy. Am. J. Obstet. Gynecol. 160 (1), 95–100. doi:10.1016/0002-9378(89)90096-3
Demarchi, J., Papasidero, S., Medina, M. A., Klajn, D., Chaparro Del Moral, R., Rillo, O., et al. (2017). Primary Sjogren's syndrome: extraglandular manifestations and hydroxychloroquine therapy. Clin. Rheumatol. 36 (11), 2455–2460. doi:10.1007/s10067-017-3822-3
Deng, Z., Zhang, L., Tang, Q., Xu, Y., Liu, S., and Li, H. (2020). Circulating levels of IFN-gamma, IL-1, IL-17 and IL-22 in pre-eclampsia: a systematic review and meta-analysis. Eur. J. Obstet. Gynecol. Reprod. Biol. 248, 211–221. doi:10.1016/j.ejogrb.2020.03.039
Denny, K. J., Coulthard, L. G., Finnell, R. H., Callaway, L. K., Taylor, S. M., and Woodruff, T. M. (2013). Elevated complement factor C5a in maternal and umbilical cord plasma in preeclampsia. J. Reprod. Immunol. 97 (2), 211–216. doi:10.1016/j.jri.2012.11.006
Derendorf, H. (2020). Excessive lysosomal ion-trapping of hydroxychloroquine and azithromycin. Int. J. Antimicrob. Agents 55 (6), 106007. doi:10.1016/j.ijantimicag.2020.106007
Derzsy, Z., Prohaszka, Z., Rigo, J., Fust, G., and Molvarec, A. (2010). Activation of the complement system in normal pregnancy and preeclampsia. Mol. Immunol. 47 (7-8), 1500–1506. doi:10.1016/j.molimm.2010.01.021
Do, S. C., Rizk, N. M., Druzin, M. L., and Simard, J. F. (2020). Does hydroxychloroquine protect against preeclampsia and preterm delivery in systemic lupus erythematosus pregnancies? Am. J. Perinatol. 37 (9), 873–880. doi:10.1055/s-0039-3402752
Docheva, N., Romero, R., Chaemsaithong, P., Tarca, A. L., Bhatti, G., Pacora, P., et al. (2019). The profiles of soluble adhesion molecules in the "great obstetrical syndromes". J. Matern. Fetal Neonatal Med. 32 (13), 2113–2136. doi:10.1080/14767058.2018.1427058
Dogan, E., Demir, S. C., and Gulec, U. K. (2014). Maternal soluble vascular cytoplasmic adhesion molecule-1 and fibronectin levels in early- and late-onset preeclamptic pregnancies. Clin. Exp. Obstet. Gynecol. 41 (6), 681–684. doi:10.12891/ceog17532014
Dong, W., and Yin, L. (2014). Expression of lipoxin A4, TNFα and IL-1β in maternal peripheral blood, umbilical cord blood and placenta, and their significance in pre-eclampsia. Hypertens. Pregnancy 33 (4), 449–456. doi:10.3109/10641955.2014.931419
Dong, Y., Lu, Y., Xia, Y., and Wang, X. (2022). Effect of hydroxychloroquine on antiphospholipid antibodies-inhibited endometrial angiogenesis. J. Matern. Fetal Neonatal Med. 35 (25), 7084–7092. doi:10.1080/14767058.2021.1943656
Drost, J. T., Arpaci, G., Ottervanger, J. P., de Boer, M. J., van Eyck, J., van der Schouw, Y. T., et al. (2012). Cardiovascular risk factors in women 10 years post early preeclampsia: the Preeclampsia Risk EValuation in FEMales study (PREVFEM). Eur. J. Prev. Cardiol. 19 (5), 1138–1144. doi:10.1177/1741826711421079
Echeverria, C., Eltit, F., Santibanez, J. F., Gatica, S., Cabello-Verrugio, C., and Simon, F. (2020). Endothelial dysfunction in pregnancy metabolic disorders. Biochim. Biophys. Acta Mol. Basis Dis. 1866 (2), 165414. doi:10.1016/j.bbadis.2019.02.009
Eghbal-Fard, S., Yousefi, M., Heydarlou, H., Ahmadi, M., Taghavi, S., Movasaghpour, A., et al. (2019). The imbalance of Th17/Treg axis involved in the pathogenesis of preeclampsia. J. Cell Physiol. 234 (4), 5106–5116. doi:10.1002/jcp.27315
El Shahaway, A. A., Abd Elhady, R. R., Abdelrhman, A. A., and Yahia, S. (2019). Role of maternal serum interleukin 17 in preeclampsia: diagnosis and prognosis. J. Inflamm. Res. 12, 175–180. doi:10.2147/JIR.S206800
Ewald, S. E., Engel, A., Lee, J., Wang, M., Bogyo, M., and Barton, G. M. (2011). Nucleic acid recognition by Toll-like receptors is coupled to stepwise processing by cathepsins and asparagine endopeptidase. J. Exp. Med. 208 (4), 643–651. doi:10.1084/jem.20100682
Ewald, S. E., Lee, B. L., Lau, L., Wickliffe, K. E., Shi, G. P., Chapman, H. A., et al. (2008). The ectodomain of Toll-like receptor 9 is cleaved to generate a functional receptor. Nature 456 (7222), 658–662. doi:10.1038/nature07405
Fanouriakis, A., Kostopoulou, M., Alunno, A., Aringer, M., Bajema, I., Boletis, J. N., et al. (2019). 2019 update of the EULAR recommendations for the management of systemic lupus erythematosus. Ann. Rheum. Dis. 78(6):736–745. doi:10.1136/annrheumdis-2019-215089
Figueiredo, A. S., and Schumacher, A. (2016). The T helper type 17/regulatory T cell paradigm in pregnancy. Immunology 148 (1), 13–21. doi:10.1111/imm.12595
Fox, R., Kitt, J., Leeson, P., Aye, C. Y. L., and Lewandowski, A. J. (2019). Preeclampsia: risk factors, diagnosis, management, and the cardiovascular impact on the offspring. J. Clin. Med. 8 (10), 1625. doi:10.3390/jcm8101625
Freeman, D. J., McManus, F., Brown, E. A., Cherry, L., Norrie, J., Ramsay, J. E., et al. (2004). Short- and long-term changes in plasma inflammatory markers associated with preeclampsia. Hypertension 44 (5), 708–714. doi:10.1161/01.HYP.0000143849.67254.ca
Fukushima, A., Kawahara, H., Isurugi, C., Syoji, T., Oyama, R., Sugiyama, T., et al. (2005). Changes in serum levels of heat shock protein 70 in preterm delivery and pre-eclampsia. J. Obstet. Gynaecol. Res. 31 (1), 72–77. doi:10.1111/j.1447-0756.2005.00244.x
Gajić, M., Schröder-Heurich, B., Horvat Mercnik, M., Cervar-Zivkovic, M., Wadsack, C., von Versen-Höynck, F., et al. (2023). The impact of hydroxychloroquine on primary feto-placental endothelial cells from healthy and early-onset preeclamptic placentas. Int. J. Mol. Sci. 24 (13), 10934. doi:10.3390/ijms241310934
Gencheva, D., Nikolov, F., Uchikova, E., Mihaylov, R., Pencheva, B., and Vasileva, M. (2022). Interleukin-6 and its correlations with maternal characteristics and echocardiographic parameters in pre-eclampsia, gestational hypertension and normotensive pregnancy. Cardiovasc J. Afr. 33 (2), 65–73. doi:10.5830/CVJA-2021-040
Gerde, M., Ibarra, E., Mac Kenzie, R., Fernandez Suarez, C., Heer, C., Alvarez, R., et al. (2021). The impact of hydroxychloroquine on obstetric outcomes in refractory obstetric antiphospholipid syndrome. Thromb. Res. 206, 104–110. doi:10.1016/j.thromres.2021.08.004
Geva, E., Ginzinger, D. G., Zaloudek, C. J., Moore, D. H., Byrne, A., and Jaffe, R. B. (2002). Human placental vascular development: vasculogenic and angiogenic (branching and nonbranching) transformation is regulated by vascular endothelial growth factor-A, angiopoietin-1, and angiopoietin-2. J. Clin. Endocrinol. Metab. 87 (9), 4213–4224. doi:10.1210/jc.2002-020195
Ghasemnejad-Berenji, H., Ghaffari Novin, M., Hajshafiha, M., Nazarian, H., Hashemi, S. M., Ilkhanizadeh, B., et al. (2018). Immunomodulatory effects of hydroxychloroquine on Th1/Th2 balance in women with repeated implantation failure. Biomed. Pharmacother. 107, 1277–1285. doi:10.1016/j.biopha.2018.08.027
Gierman, L. M., Silva, G. B., Pervaiz, Z., Rakner, J. J., Mundal, S. B., Thaning, A. J., et al. (2021). TLR3 expression by maternal and fetal cells at the maternal-fetal interface in normal and preeclamptic pregnancies. J. Leukoc. Biol. 109 (1), 173–183. doi:10.1002/JLB.3MA0620-728RR
Gies, V., Bekaddour, N., Dieudonne, Y., Guffroy, A., Frenger, Q., Gros, F., et al. (2020). Beyond anti-viral effects of chloroquine/hydroxychloroquine. Front. Immunol. 11, 1409. doi:10.3389/fimmu.2020.01409
Gimenez-Bastida, J. A., Boeglin, W. E., Boutaud, O., Malkowski, M. G., and Schneider, C. (2019). Residual cyclooxygenase activity of aspirin-acetylated COX-2 forms 15 R-prostaglandins that inhibit platelet aggregation. FASEB J. 33 (1), 1033–1041. doi:10.1096/fj.201801018R
Gomez-Guzman, M., Jimenez, R., Romero, M., Sanchez, M., Zarzuelo, M. J., Gomez-Morales, M., et al. (2014). Chronic hydroxychloroquine improves endothelial dysfunction and protects kidney in a mouse model of systemic lupus erythematosus. Hypertension 64 (2), 330–337. doi:10.1161/HYPERTENSIONAHA.114.03587
Goulopoulou, S., Wenceslau, C. F., McCarthy, C. G., Matsumoto, T., and Webb, R. C. (2016). Exposure to stimulatory CpG oligonucleotides during gestation induces maternal hypertension and excess vasoconstriction in pregnant rats. Am. J. Physiol. Heart Circ. Physiol. 310 (8), H1015–H1025. doi:10.1152/ajpheart.00834.2015
Groenhof, T. K. J., van Rijn, B. B., Franx, A., Roeters van Lennep, J. E., Bots, M. L., and Lely, A. T. (2017). Preventing cardiovascular disease after hypertensive disorders of pregnancy: searching for the how and when. Eur. J. Prev. Cardiol. 24 (16), 1735–1745. doi:10.1177/2047487317730472
Grummer, M. A., Sullivan, J. A., Magness, R. R., and Bird, I. M. (2009). Vascular endothelial growth factor acts through novel, pregnancy-enhanced receptor signalling pathways to stimulate endothelial nitric oxide synthase activity in uterine artery endothelial cells. Biochem. J. 417 (2), 501–511. doi:10.1042/BJ20081013
Gu, Y., Lewis, D. F., Zhang, Y., Groome, L. J., and Wang, Y. (2006). Increased superoxide generation and decreased stress protein Hsp90 expression in human umbilical cord vein endothelial cells (HUVECs) from pregnancies complicated by preeclampsia. Hypertens. Pregnancy 25 (3), 169–182. doi:10.1080/10641950600912950
Guseh, S. H., Feinberg, B. B., Dawood, H. Y., Yamamoto, H. S., Fichorova, R. N., and Burwick, R. M. (2015). Urinary excretion of C5b-9 is associated with the anti-angiogenic state in severe preeclampsia. Am. J. Reprod. Immunol. 73 (5), 437–444. doi:10.1111/aji.12349
Guzel, C., van den Berg, C. B., Duvekot, J. J., Stingl, C., van den Bosch, T. P. P., van der Weiden, M., et al. (2019). Quantification of calcyclin and heat shock protein 90 in sera from women with and without preeclampsia by mass spectrometry. Proteomics Clin. Appl. 13 (3), e1800181. doi:10.1002/prca.201800181
Hahn, S., Giaglis, S., Buser, A., Hoesli, I., Lapaire, O., and Hasler, P. (2014). Cell-free nucleic acids in (maternal) blood: any relevance to (reproductive) immunologists? J. Reprod. Immunol. 104-105, 26–31. doi:10.1016/j.jri.2014.03.007
Halperin, R., Peller, S., Rotschild, M., Bukovsky, I., and Schneider, D. (2000). Placental apoptosis in normal and abnormal pregnancies. Gynecol. Obstet. Invest. 50 (2), 84–87. doi:10.1159/000010287
Han, J., Li, X., Luo, X., He, J., Huang, X., Zhou, Q., et al. (2020). The mechanisms of hydroxychloroquine in rheumatoid arthritis treatment: inhibition of dendritic cell functions via Toll like receptor 9 signaling. Biomed. Pharmacother. 132, 110848. doi:10.1016/j.biopha.2020.110848
Hastie, R., Tong, S., Wikstrom, A. K., Sandstrom, A., Hesselman, S., and Bergman, L. (2021). Aspirin use during pregnancy and the risk of bleeding complications: a Swedish population-based cohort study. Am. J. Obstet. Gynecol. 224 (1), 95 e1–e12. doi:10.1016/j.ajog.2020.07.023
He, B., Yang, X., Li, Y., Huang, D., Xu, X., Yang, W., et al. (2018). TLR9 (Toll-Like receptor 9) agonist suppresses angiogenesis by differentially regulating VEGFA (vascular endothelial growth factor A) and sFLT1 (soluble vascular endothelial growth factor receptor 1) in preeclampsia. Hypertension 71 (4), 671–680. doi:10.1161/HYPERTENSIONAHA.117.10510
He, Y. D., Xu, B. N., Wang, M. L., Wang, Y. Q., Yu, F., Chen, Q., et al. (2020). Dysregulation of complement system during pregnancy in patients with preeclampsia: a prospective study. Mol. Immunol. 122, 69–79. doi:10.1016/j.molimm.2020.03.021
Hermes, W., Koopmans, C. M., van Pampus, M. G., Franx, A., Bloemenkamp, K. W., van der Post, J., et al. (2013). Induction of labour or expectant monitoring in hypertensive pregnancy disorders at term: do women's postpartum cardiovascular risk factors differ between the two strategies? Eur. J. Obstet. Gynecol. Reprod. Biol. 171 (1), 30–34. doi:10.1016/j.ejogrb.2013.08.006
Herraiz, I., Llurba, E., Verlohren, S., and Galindo, A.Spanish Group for the Study of Angiogenic Markers in Preeclampsia (2018). Update on the diagnosis and prognosis of preeclampsia with the aid of the sFlt-1/PlGF ratio in singleton pregnancies. Fetal Diagn Ther. 43 (2), 81–89. doi:10.1159/000477903
Hjorton, K., Hagberg, N., Israelsson, E., Jinton, L., Berggren, O., Sandling, J. K., et al. (2018). Cytokine production by activated plasmacytoid dendritic cells and natural killer cells is suppressed by an IRAK4 inhibitor. Arthritis Res. Ther. 20 (1), 238. doi:10.1186/s13075-018-1702-0
Hofmeyr, G. J., Lawrie, T. A., Atallah, Á. N., and Torloni, M. R. (2018). Calcium supplementation during pregnancy for preventing hypertensive disorders and related problems. Cochrane database Syst. Rev. 10 (10), CD001059–CD. doi:10.1002/14651858.CD001059.pub3
Honey, K., and Rudensky, A. Y. (2003). Lysosomal cysteine proteases regulate antigen presentation. Nat. Rev. Immunol. 3 (6), 472–482. doi:10.1038/nri1110
Hromadnikova, I., Dvorakova, L., Kotlabova, K., Kestlerova, A., Hympanova, L., Novotna, V., et al. (2016). Circulating heat shock protein mRNA profile in gestational hypertension, pre-eclampsia and foetal growth restriction. Indian J. Med. Res. 144 (2), 229–237. doi:10.4103/0971-5916.195037
Hu, J., Wang, X., Chen, X., Fang, Y., Chen, K., Peng, W., et al. (2022a). Hydroxychloroquine attenuates neuroinflammation following traumatic brain injury by regulating the TLR4/NF-κB signaling pathway. J. Neuroinflammation 19 (1), 71. doi:10.1186/s12974-022-02430-0
Hu, Y., Li, Z., Chen, G., Li, Z., Huang, J., Huang, H., et al. (2022b). Hydroxychloroquine alleviates EAU by inhibiting uveitogenic T cells and ameliorating retinal vascular endothelial cells dysfunction. Front. Immunol. 13, 859260. doi:10.3389/fimmu.2022.859260
Hu, Y., Yan, R., Zhang, C., Zhou, Z., Liu, M., Wang, C., et al. (2018). High-Mobility group box 1 from hypoxic trophoblasts promotes endothelial microparticle production and thrombophilia in preeclampsia. Arterioscler. Thromb. Vasc. Biol. 38 (6), 1381–1391. doi:10.1161/ATVBAHA.118.310940
Hu, Z., Gao, R., Huang, W., Wang, H., Qin, L., and Kim, Y. (2023). Effect of hydroxychloroquine on lupus activity, preeclampsia and intrauterine growth restriction in pregnant women with systemic lupus erythematosus and/or antiphospholipid syndrome: a systematic review and meta-analysis. J. Clin. Med. 12 (2), 3296. doi:10.3390/jcm12093296
Huppertz, B., Kingdom, J., Caniggia, I., Desoye, G., Black, S., Korr, H., et al. (2003). Hypoxia favours necrotic versus apoptotic shedding of placental syncytiotrophoblast into the maternal circulation. Placenta 24 (2-3), 181–190. doi:10.1053/plac.2002.0903
Ikeda, Y., Tamaki, H., and Okada, M. (2019). 91 the effect of hydroxychloroquine on complement status in patients with systemic lupus erythematosus; Analysis of Japanese real-world patients with SLE in a large single center over twelve-month period. Lupus Sci. Med. 6 (Suppl. 1), A68. doi:10.1136/lupus-2019-lsm.91
Jang, C. H., Choi, J. H., Byun, M. S., and Jue, D. M. (2006). Chloroquine inhibits production of TNF-alpha, IL-1beta and IL-6 from lipopolysaccharide-stimulated human monocytes/macrophages by different modes. Rheumatol. Oxf. 45 (6), 703–710. doi:10.1093/rheumatology/kei282
Jena, M. K., Sharma, N. R., Petitt, M., Maulik, D., and Nayak, N. R. (2020). Pathogenesis of preeclampsia and therapeutic approaches targeting the placenta. Biomolecules 10 (6), 953. doi:10.3390/biom10060953
Jiang, R., Cai, J., Zhu, Z., Chen, D., Wang, J., Wang, Q., et al. (2014). Hypoxic trophoblast HMGB1 induces endothelial cell hyperpermeability via the TRL-4/caveolin-1 pathway. J. Immunol. 193 (10), 5000–5012. doi:10.4049/jimmunol.1303445
Jiang, Y., Chen, Z., Chen, Y., Wei, L., Gao, P., Zhang, J., et al. (2023). Low-dose asprin use during pregnancy may be a potential risk for postpartum hemorrhage and increased blood loss: a systematic review and meta-analysis. Am. J. Obstet. Gynecol. MFM 5 (4), 100878. doi:10.1016/j.ajogmf.2023.100878
Jirecek, S., Hohlagschwandtner, M., Tempfer, C., Knofler, M., Husslein, P., and Zeisler, H. (2002). Serum levels of heat shock protein 70 in patients with preeclampsia: a pilot-study. Wien Klin. Wochenschr 114 (15-16), 730–732.
Kadife, E., Hannan, N., Harper, A., Binder, N., Beard, S., and Brownfoot, F. C. (2022). Hydroxychloroquine reduces soluble Flt-1 secretion from human cytotrophoblast, but does not mitigate markers of endothelial dysfunction in vitro. PLoS One 17 (11), e0271560. doi:10.1371/journal.pone.0271560
Kaplan, Y. C., Ozsarfati, J., Nickel, C., and Koren, G. (2016). Reproductive outcomes following hydroxychloroquine use for autoimmune diseases: a systematic review and meta-analysis. Br. J. Clin. Pharmacol. 81 (5), 835–848. doi:10.1111/bcp.12872
Karapetian, A. O., Baev, O. R., Sadekova, A. A., Krasnyi, A. E. M., and Sukhikh, G. T. (2021). Cell-free foetal DNA as a useful marker for preeclampsia prediction. Reprod. Sci. 28 (5), 1563–1569. doi:10.1007/s43032-021-00466-w
Kerschbaumer, A., Sepriano, A., Smolen, J. S., van der Heijde, D., Dougados, M., van Vollenhoven, R., et al. (2020). Efficacy of pharmacological treatment in rheumatoid arthritis: a systematic literature research informing the 2019 update of the EULAR recommendations for management of rheumatoid arthritis. Ann. Rheum. Dis. 79 (6), 744–759. doi:10.1136/annrheumdis-2019-216656
Kim, M. L., Hardy, M. Y., Edgington-Mitchell, L. E., Ramarathinam, S. H., Chung, S. Z., Russell, A. K., et al. (2021). Hydroxychloroquine inhibits the mitochondrial antioxidant system in activated T cells. iScience 24 (12), 103509. doi:10.1016/j.isci.2021.103509
Kim, S. Y., Ryu, H. M., Yang, J. H., Kim, M. Y., Ahn, H. K., Lim, H. J., et al. (2004). Maternal serum levels of VCAM-1, ICAM-1 and E-selectin in preeclampsia. J. Korean Med. Sci. 19 (5), 688–692. doi:10.3346/jkms.2004.19.5.688
Kim, Y. M., Romero, R., Oh, S. Y., Kim, C. J., Kilburn, B. A., Armant, D. R., et al. (2005). Toll-like receptor 4: a potential link between "danger signals," the innate immune system, and preeclampsia? Am. J. Obstet. Gynecol. 193 (3 Pt 2), 921–927. doi:10.1016/j.ajog.2005.07.076
Kornacki, J., Wirstlein, P., and Wender-Ozegowska, E. (2020). Markers of endothelial injury and dysfunction in early- and late-onset preeclampsia. Life (Basel) 10 (10), 239. doi:10.3390/life10100239
Kowalczyk, A., Kleniewska, P., Kolodziejczyk, M., Skibska, B., and Goraca, A. (2015). The role of endothelin-1 and endothelin receptor antagonists in inflammatory response and sepsis. Arch. Immunol. Ther. Exp. Warsz. 63 (1), 41–52. doi:10.1007/s00005-014-0310-1
Kowatsch, M. M., Lajoie, J., Mwangi, L., Omollo, K., Oyugi, J., Hollett, N., et al. (2023). Hydroxychloroquine reduces T cells activation recall antigen responses. PLoS One 18 (8), e0287738. doi:10.1371/journal.pone.0287738
Kravvariti, E., Koutsogianni, A., Samoli, E., Sfikakis, P. P., and Tektonidou, M. G. (2020). The effect of hydroxychloroquine on thrombosis prevention and antiphospholipid antibody levels in primary antiphospholipid syndrome: a pilot open label randomized prospective study. Autoimmun. Rev. 19 (4), 102491. doi:10.1016/j.autrev.2020.102491
Kroese, S. J., de Hair, M. J. H., Limper, M., Lely, A. T., van Laar, J. M., Derksen, R., et al. (2017). Hydroxychloroquine use in lupus patients during pregnancy is associated with longer pregnancy duration in preterm births. J. Immunol. Res. 2017, 2810202. doi:10.1155/2017/2810202
Kuznik, A., Bencina, M., Svajger, U., Jeras, M., Rozman, B., and Jerala, R. (2011). Mechanism of endosomal TLR inhibition by antimalarial drugs and imidazoquinolines. J. Immunol. 186 (8), 4794–4804. doi:10.4049/jimmunol.1000702
Latino, J. O., Udry, S., Aranda, F., Wingeyer, S. P., Romero, D. S. F., Belizna, C., et al. (2020). Risk factors for early severe preeclampsia in obstetric antiphospholipid syndrome with conventional treatment. The impact of hydroxychloroquine. Lupus 29 (13), 1736–1742. doi:10.1177/0961203320952850
Le, N. T., Takei, Y., Izawa-Ishizawa, Y., Heo, K. S., Lee, H., Smrcka, A. V., et al. (2014). Identification of activators of ERK5 transcriptional activity by high-throughput screening and the role of endothelial ERK5 in vasoprotective effects induced by statins and antimalarial agents. J. Immunol. 193 (7), 3803–3815. doi:10.4049/jimmunol.1400571
Leon-Martinez, D., Lynn, T., and Abrahams, V. M. (2023). Cell-free fetal DNA impairs trophoblast migration in a TLR9-dependent manner and can be reversed by hydroxychloroquine. J. Reprod. Immunol. 157, 103945. doi:10.1016/j.jri.2023.103945
Li, R., Lin, H., Ye, Y., Xiao, Y., Xu, S., Wang, J., et al. (2018). Attenuation of antimalarial agent hydroxychloroquine on TNF-α-induced endothelial inflammation. Int. Immunopharmacol. 63, 261–269. doi:10.1016/j.intimp.2018.08.008
Li, Y., Wang, Y., Ding, X., Duan, B., Li, L., and Wang, X. (2016). Serum levels of TNF-α and IL-6 are associated with pregnancy-induced hypertension. Reprod. Sci. 23 (10), 1402–1408. doi:10.1177/1933719116641760
Liao, A. H., Liu, L. P., Ding, W. P., and Zhang, L. (2009). Functional changes of human peripheral B-lymphocytes in pre-eclampsia. Am. J. Reprod. Immunol. 61 (5), 313–321. doi:10.1111/j.1600-0897.2009.00697.x
Lisonkova, S., and Joseph, K. S. (2013). Incidence of preeclampsia: risk factors and outcomes associated with early-versus late-onset disease. Am. J. Obstet. Gynecol. 209 (6), 544 e1–e544. doi:10.1016/j.ajog.2013.08.019
Liu, H., Wang, W., and Liu, C. (2021). Increased expression of IFN-gamma in preeclampsia impairs human trophoblast invasion via a SOCS1/JAK/STAT1 feedback loop. Exp. Ther. Med. 21 (2), 112. doi:10.3892/etm.2020.9544
Lu, H. Q., and Hu, R. (2019). Lasting effects of intrauterine exposure to preeclampsia on offspring and the underlying mechanism. AJP Rep. 9 (3), e275–e291. doi:10.1055/s-0039-1695004
Lynch, A. M., Murphy, J. R., Byers, T., Gibbs, R. S., Neville, M. C., Giclas, P. C., et al. (2008). Alternative complement pathway activation fragment Bb in early pregnancy as a predictor of preeclampsia. Am. J. Obstet. Gynecol. 198 (4), 385 e1–e9. doi:10.1016/j.ajog.2007.10.793
Ma, Y., Ye, Y., Zhang, J., Ruan, C. C., and Gao, P. J. (2019). Immune imbalance is associated with the development of preeclampsia. Med. Baltim. 98 (14), e15080. doi:10.1097/MD.0000000000015080
Magombedze, G., Reddy, P. B., Eda, S., and Ganusov, V. V. (2013). Cellular and population plasticity of helper CD4(+) T cell responses. Front. Physiol. 4, 206. doi:10.3389/fphys.2013.00206
Marchetti, T., Ruffatti, A., Wuillemin, C., de Moerloose, P., and Cohen, M. (2014). Hydroxychloroquine restores trophoblast fusion affected by antiphospholipid antibodies. J. Thromb. Haemost. 12 (6), 910–920. doi:10.1111/jth.12570
Mauthe, M., Orhon, I., Rocchi, C., Zhou, X., Luhr, M., Hijlkema, K. J., et al. (2018). Chloroquine inhibits autophagic flux by decreasing autophagosome-lysosome fusion. Autophagy 14 (8), 1435–1455. doi:10.1080/15548627.2018.1474314
Mbarak, B., Kilewo, C., Kuganda, S., and Sunguya, B. F. (2019). Postpartum depression among women with pre-eclampsia and eclampsia in Tanzania; a call for integrative intervention. BMC Pregnancy Childbirth 19 (1), 270. doi:10.1186/s12884-019-2395-3
McElwain, C. J., Tuboly, E., McCarthy, F. P., and McCarthy, C. M. (2020). Mechanisms of endothelial dysfunction in pre-eclampsia and gestational diabetes mellitus: windows into future cardiometabolic health? Front. Endocrinol. (Lausanne) 11, 655. doi:10.3389/fendo.2020.00655
McEver, R. P., and Zhu, C. (2010). Rolling cell adhesion. Annu. Rev. Cell Dev. Biol. 26, 363–396. doi:10.1146/annurev.cellbio.042308.113238
Mekinian, A., Lazzaroni, M. G., Kuzenko, A., Alijotas-Reig, J., Ruffatti, A., Levy, P., et al. (2015). The efficacy of hydroxychloroquine for obstetrical outcome in anti-phospholipid syndrome: data from a European multicenter retrospective study. Autoimmun. Rev. 14 (6), 498–502. doi:10.1016/j.autrev.2015.01.012
Meng, Y., Ma, J., Yao, C., Ye, Z., Ding, H., Liu, C., et al. (2022). The NCF1 variant p.R90H aggravates autoimmunity by facilitating the activation of plasmacytoid dendritic cells. J. Clin. Invest. 132 (16), e153619. doi:10.1172/JCI153619
Miller, D., Motomura, K., Galaz, J., Gershater, M., Lee, E. D., Romero, R., et al. (2022). Cellular immune responses in the pathophysiology of preeclampsia. J. Leukoc. Biol. 111 (1), 237–260. doi:10.1002/JLB.5RU1120-787RR
Miranda, S., Billoir, P., Damian, L., Thiebaut, P. A., Schapman, D., Le Besnerais, M., et al. (2019). Hydroxychloroquine reverses the prothrombotic state in a mouse model of antiphospholipid syndrome: role of reduced inflammation and endothelial dysfunction. PLoS One 14 (3), e0212614. doi:10.1371/journal.pone.0212614
Molvarec, A., Czegle, I., Szijarto, J., and Rigo, J. (2015). Increased circulating interleukin-17 levels in preeclampsia. J. Reprod. Immunol. 112, 53–57. doi:10.1016/j.jri.2015.05.007
Molvarec, A., Prohaszka, Z., Nagy, B., Szalay, J., Fust, G., Karadi, I., et al. (2006). Association of elevated serum heat-shock protein 70 concentration with transient hypertension of pregnancy, preeclampsia and superimposed preeclampsia: a case-control study. J. Hum. Hypertens. 20 (10), 780–786. doi:10.1038/sj.jhh.1002060
Molvarec, A., Szarka, A., Walentin, S., Beko, G., Karadi, I., Prohaszka, Z., et al. (2011). Serum leptin levels in relation to circulating cytokines, chemokines, adhesion molecules and angiogenic factors in normal pregnancy and preeclampsia. Reprod. Biol. Endocrinol. 9, 124. doi:10.1186/1477-7827-9-124
Mor, G., Cardenas, I., Abrahams, V., and Guller, S. (2011). Inflammation and pregnancy: the role of the immune system at the implantation site. Ann. N. Y. Acad. Sci. 1221 (1), 80–87. doi:10.1111/j.1749-6632.2010.05938.x
Moufarrej, M. N., Vorperian, S. K., Wong, R. J., Campos, A. A., Quaintance, C. C., Sit, R. V., et al. (2022). Early prediction of preeclampsia in pregnancy with cell-free RNA. Nature 602 (7898), 689–694. doi:10.1038/s41586-022-04410-z
Myatt, L., and Roberts, J. M. (2015). Preeclampsia: syndrome or disease? Curr. Hypertens. Rep. 17 (11), 83. doi:10.1007/s11906-015-0595-4
Nadeau-Vallee, M., Obari, D., Palacios, J., Brien, M. E., Duval, C., Chemtob, S., et al. (2016). Sterile inflammation and pregnancy complications: a review. Reproduction 152 (6), R277–R92. doi:10.1530/REP-16-0453
Nakada, E., Walley, K. R., Nakada, T., Hu, Y., von Dadelszen, P., and Boyd, J. H. (2009). Toll-like receptor-3 stimulation upregulates sFLT-1 production by trophoblast cells. Placenta 30 (9), 774–779. doi:10.1016/j.placenta.2009.07.001
Nizyaeva, N. V., Kulikova, G. V., Nagovitsyna, M. N., and Shchegolev, A. I. (2019). Peculiarities of the expression of TLR4 and inhibitor of TLR-cascade tollip in the placenta in earlyand late-onset preeclampsia. Bull. Exp. Biol. Med. 166 (4), 507–511. doi:10.1007/s10517-019-04383-6
Nuri, E., Taraborelli, M., Andreoli, L., Tonello, M., Gerosa, M., Calligaro, A., et al. (2017). Long-term use of hydroxychloroquine reduces antiphospholipid antibodies levels in patients with primary antiphospholipid syndrome. Immunol. Res. 65 (1), 17–24. doi:10.1007/s12026-016-8812-z
Nurzadeh, M., Ghalandarpoor-Attar, S. M., Ghalandarpoor-Attar, S. N., and Rabiei, M. (2023). The role of interferon (IFN)-gamma in extravillous trophoblast cell (EVT) invasion and preeclampsia progression. Reprod. Sci. 30 (5), 1462–1469. doi:10.1007/s43032-022-01110-x
Opichka, M. A., Rappelt, M. W., Gutterman, D. D., Grobe, J. L., and McIntosh, J. J. (2021). Vascular dysfunction in preeclampsia. Cells 10 (11), 3055. doi:10.3390/cells10113055
Otun, H. A., Lash, G. E., Innes, B. A., Bulmer, J. N., Naruse, K., Hannon, T., et al. (2011). Effect of tumour necrosis factor-α in combination with interferon-γ on first trimester extravillous trophoblast invasion. J. Reprod. Immunol. 88 (1), 1–11. doi:10.1016/j.jri.2010.10.003
Ozeki, A., Tani, K., Takahashi, H., Suzuki, H., Nagayama, S., Hirashima, C., et al. (2019). Preeclamptic patient-derived circulating cell-free DNA activates the production of inflammatory cytokines via toll-like receptor 9 signalling in the human placenta. J. Hypertens. 37 (12), 2452–2460. doi:10.1097/HJH.0000000000002208
Padmini, E., Uthra, V., and Lavanya, S. (2012). Effect of HSP70 and 90 in modulation of JNK, ERK expression in preeclamptic placental endothelial cell. Cell Biochem. Biophys. 64 (3), 187–195. doi:10.1007/s12013-012-9371-0
Panda, B., Panda, A., Ueda, I., Abrahams, V. M., Norwitz, E. R., Stanic, A. K., et al. (2012). Dendritic cells in the circulation of women with preeclampsia demonstrate a pro-inflammatory bias secondary to dysregulation of TLR receptors. J. Reprod. Immunol. 94 (2), 210–215. doi:10.1016/j.jri.2012.01.008
Papapetropoulos, A., Garcia-Cardena, G., Madri, J. A., and Sessa, W. C. (1997). Nitric oxide production contributes to the angiogenic properties of vascular endothelial growth factor in human endothelial cells. J. Clin. Invest. 100 (12), 3131–3139. doi:10.1172/JCI119868
Peracoli, J. C., Bannwart-Castro, C. F., Romao, M., Weel, I. C., Ribeiro, V. R., Borges, V. T., et al. (2013). High levels of heat shock protein 70 are associated with pro-inflammatory cytokines and may differentiate early-from late-onset preeclampsia. J. Reprod. Immunol. 100 (2), 129–134. doi:10.1016/j.jri.2013.08.003
Petri, M., Konig, M. F., Li, J., and Goldman, D. W. (2021). Association of higher hydroxychloroquine blood levels with reduced thrombosis risk in systemic lupus erythematosus. Arthritis Rheumatol. 73 (6), 997–1004. doi:10.1002/art.41621
Phillips, J., Nathan, E., and Graham, D. (2023). Preeclampsia in women with lupus - influence of aspirin and hydroxychloroquine on pregnancy outcome. Pregnancy Hypertens. 31, 14–16. doi:10.1016/j.preghy.2022.11.006
Phipps, E. A., Thadhani, R., Benzing, T., and Karumanchi, S. A. (2019). Pre-eclampsia: pathogenesis, novel diagnostics and therapies. Nat. Rev. Nephrol. Nat. Publ. Group 15, 275–289. doi:10.1038/s41581-019-0119-6
Piccinini, A. M., and Midwood, K. S. (2010). DAMPening inflammation by modulating TLR signalling. Mediat. Inflamm. 2010, 672395. doi:10.1155/2010/672395
Piconi, S., Parisotto, S., Rizzardini, G., Passerini, S., Terzi, R., Argenteri, B., et al. (2011). Hydroxychloroquine drastically reduces immune activation in HIV-infected, antiretroviral therapy-treated immunologic nonresponders. Blood 118 (12), 3263–3272. doi:10.1182/blood-2011-01-329060
Pineda, A., Verdin-Teran, S. L., Camacho, A., and Moreno-Fierros, L. (2011). Expression of toll-like receptor TLR-2, TLR-3, TLR-4 and TLR-9 is increased in placentas from patients with preeclampsia. Arch. Med. Res. 42 (5), 382–391. doi:10.1016/j.arcmed.2011.08.003
Pinheiro, M. B., Gomes, K. B., Ronda, C. R., Guimaraes, G. G., Freitas, L. G., Teixeira-Carvalho, A., et al. (2015). Severe preeclampsia: association of genes polymorphisms and maternal cytokines production in Brazilian population. Cytokine 71 (2), 232–237. doi:10.1016/j.cyto.2014.10.021
Plantone, D., and Koudriavtseva, T. (2018). Current and future use of chloroquine and hydroxychloroquine in infectious, immune, neoplastic, and neurological diseases: a mini-review. Clin. Drug Investig. 38 (8), 653–671. doi:10.1007/s40261-018-0656-y
Poel, Y. H., Swinkels, P., and de Vries, J. I. (2009). Psychological treatment of women with psychological complaints after pre-eclampsia. J. Psychosom. Obstet. Gynaecol. 30 (1), 65–72. doi:10.1080/01674820802545990
Ponticelli, C., and Moroni, G. (2017). Hydroxychloroquine in systemic lupus erythematosus (SLE). Expert Opin. Drug Saf. 16 (3), 411–419. doi:10.1080/14740338.2017.1269168
Rahman, R., Murthi, P., Singh, H., Gurusinghe, S., Mockler, J. C., Lim, R., et al. (2016). The effects of hydroxychloroquine on endothelial dysfunction. Pregnancy Hypertens. 6 (4), 259–262. doi:10.1016/j.preghy.2016.09.001
Rahman, R. A., Murthi, P., Singh, H., Gurungsinghe, S., Leaw, B., Mockler, J. C., et al. (2020). Hydroxychloroquine mitigates the production of 8-isoprostane and improves vascular dysfunction: implications for treating preeclampsia. Int. J. Mol. Sci. 21 (7), 2504. doi:10.3390/ijms21072504
Rana, S., Lemoine, E., Granger, J. P., and Karumanchi, S. A. (2019). Preeclampsia: pathophysiology, challenges, and perspectives. Circ. Res. 124 (7), 1094–1112. doi:10.1161/CIRCRESAHA.118.313276
Redman, C. W. (1991). Current topic: pre-eclampsia and the placenta. Placenta 12 (4), 301–308. doi:10.1016/0143-4004(91)90339-h
Renaud, S. J., Postovit, L. M., Macdonald-Goodfellow, S. K., McDonald, G. T., Caldwell, J. D., and Graham, C. H. (2005). Activated macrophages inhibit human cytotrophoblast invasiveness in vitro. Biol. Reprod. 73 (2), 237–243. doi:10.1095/biolreprod.104.038000
Rezabakhsh, A., Montazersaheb, S., Nabat, E., Hassanpour, M., Montaseri, A., Malekinejad, H., et al. (2017). Effect of hydroxychloroquine on oxidative/nitrosative status and angiogenesis in endothelial cells under high glucose condition. Bioimpacts 7 (4), 219–226. doi:10.15171/bi.2017.26
Ribeiro, V. R., Romao-Veiga, M., Romagnoli, G. G., Matias, M. L., Nunes, P. R., Borges, V. T. M., et al. (2017). Association between cytokine profile and transcription factors produced by T-cell subsets in early- and late-onset pre-eclampsia. Immunology 152 (1), 163–173. doi:10.1111/imm.12757
Rinaldi, S. F., Makieva, S., Saunders, P. T., Rossi, A. G., and Norman, J. E. (2017). Immune cell and transcriptomic analysis of the human decidua in term and preterm parturition. Mol. Hum. Reprod. 23 (10), 708–724. doi:10.1093/molehr/gax038
Roberge, S., Bujold, E., and Nicolaides, K. H. (2018). Aspirin for the prevention of preterm and term preeclampsia: systematic review and metaanalysis. Am. J. Obstetrics Gynecol. Mosby Inc. 218, 287–293. doi:10.1016/j.ajog.2017.11.561
Romao-Veiga, M., Bannwart-Castro, C. F., Borges, V. T. M., Golim, M. A., Peracoli, J. C., and Peracoli, M. T. S. (2020). Increased TLR4 pathway activation and cytokine imbalance led to lipopolysaccharide tolerance in monocytes from preeclamptic women. Pregnancy Hypertens. 21, 159–165. doi:10.1016/j.preghy.2020.06.002
Romao-Veiga, M., Ribeiro, V. R., Matias, M. L., Nunes, P. R., Romagnoli, G. G., Peracoli, J. C., et al. (2022). DAMPs are able to skew CD4(+) T cell subsets and increase the inflammatory profile in pregnant women with preeclampsia. J. Reprod. Immunol. 149, 103470. doi:10.1016/j.jri.2021.103470
Sacre, K., Criswell, L. A., and McCune, J. M. (2012). Hydroxychloroquine is associated with impaired interferon-alpha and tumor necrosis factor-alpha production by plasmacytoid dendritic cells in systemic lupus erythematosus. Arthritis Res. Ther. 14 (3), R155. doi:10.1186/ar3895
Saini, V., Arora, S., Yadav, A., and Bhattacharjee, J. (2011). Cytokines in recurrent pregnancy loss. Clin. Chim. Acta 412 (9-10), 702–708. doi:10.1016/j.cca.2011.01.002
Saito, S. (2010). Th17 cells and regulatory T cells: new light on pathophysiology of preeclampsia. Immunol. Cell Biol. 88 (6), 615–617. doi:10.1038/icb.2010.68
Saito, S., Sakai, M., Sasaki, Y., Tanebe, K., Tsuda, H., and Michimata, T. (1999). Quantitative analysis of peripheral blood Th0, Th1, Th2 and the Th1:Th2 cell ratio during normal human pregnancy and preeclampsia. Clin. Exp. Immunol. 117 (3), 550–555. doi:10.1046/j.1365-2249.1999.00997.x
Salazar Garcia, M. D., Mobley, Y., Henson, J., Davies, M., Skariah, A., Dambaeva, S., et al. (2018). Early pregnancy immune biomarkers in peripheral blood may predict preeclampsia. J. Reprod. Immunol. 125, 25–31. doi:10.1016/j.jri.2017.10.048
Santner-Nanan, B., Peek, M. J., Khanam, R., Richarts, L., Zhu, E., Fazekas de St Groth, B., et al. (2009). Systemic increase in the ratio between Foxp3+ and IL-17-producing CD4+ T cells in healthy pregnancy but not in preeclampsia. J. Immunol. 183 (11), 7023–7030. doi:10.4049/jimmunol.0901154
Scharfe-Nugent, A., Corr, S. C., Carpenter, S. B., Keogh, L., Doyle, B., Martin, C., et al. (2012). TLR9 provokes inflammation in response to fetal DNA: mechanism for fetal loss in preterm birth and preeclampsia. J. Immunol. 188 (11), 5706–5712. doi:10.4049/jimmunol.1103454
Schmidt, R. L., Jutz, S., Goldhahn, K., Witzeneder, N., Gerner, M. C., Trapin, D., et al. (2017). Chloroquine inhibits human CD4(+) T-cell activation by AP-1 signaling modulation. Sci. Rep. 7, 42191. doi:10.1038/srep42191
Schroeder, D. I., Blair, J. D., Lott, P., Yu, H. O., Hong, D., Crary, F., et al. (2013). The human placenta methylome. Proc. Natl. Acad. Sci. U. S. A. 110 (15), 6037–6042. doi:10.1073/pnas.1215145110
Sciascia, S., Hunt, B. J., Talavera-Garcia, E., Lliso, G., Khamashta, M. A., and Cuadrado, M. J. (2016). The impact of hydroxychloroquine treatment on pregnancy outcome in women with antiphospholipid antibodies. Am. J. Obstet. Gynecol. 214 (2), 273 e1–e273. doi:10.1016/j.ajog.2015.09.078
Sciascia, S., Schreiber, K., Radin, M., Roccatello, D., Kramer, B. K., and Stach, K. (2020). Hydroxychloroquine reduces IL-6 and pro-thrombotic status. Autoimmun. Rev. 19 (7), 102555. doi:10.1016/j.autrev.2020.102555
Scott, R. E., Greenwood, S. L., Hayes, D. J. L., Baker, B. C., Jones, R. L., and Heazell, A. E. P. (2019). Effects of hydroxychloroquine on the human placenta-Findings from in vitro experimental data and a systematic review. Reprod. Toxicol. 87, 50–59. doi:10.1016/j.reprotox.2019.05.056
Sekizawa, A., Jimbo, M., Saito, H., Iwasaki, M., Matsuoka, R., Okai, T., et al. (2003). Cell-free fetal DNA in the plasma of pregnant women with severe fetal growth restriction. Am. J. Obstet. Gynecol. 188 (2), 480–484. doi:10.1067/mob.2003.27
Serin, I. S., Ozcelik, B., Basbug, M., Kilic, H., Okur, D., Erez, R., et al. (2002). Predictive value of tumor necrosis factor alpha (TNF-alpha) in preeclampsia. Eur. J. Obstet. Gynecol. Reprod. Biol. 100 (2), 143–145. doi:10.1016/s0301-2115(01)00484-5
Sheibak, N., Mahmoudzadeh-Sagheb, H., Moudi, B., and Heidari, Z. (2020). Elevated immunoexpression of interferon-gamma in placenta tissue samples from pregnancies complicated with preeclampsia compared to the placenta previa. Pregnancy Hypertens. 22, 175–180. doi:10.1016/j.preghy.2020.08.003
Shirasuna, K., Seno, K., Ohtsu, A., Shiratsuki, S., Ohkuchi, A., Suzuki, H., et al. (2016). AGEs and HMGB1 increase inflammatory cytokine production from human placental cells, resulting in an enhancement of monocyte migration. Am. J. Reprod. Immunol. 75 (5), 557–568. doi:10.1111/aji.12506
Silva, J. C., Mariz, H. A., Rocha, L. F., Oliveira, P. S., Dantas, A. T., Duarte, A. L., et al. (2013). Hydroxychloroquine decreases Th17-related cytokines in systemic lupus erythematosus and rheumatoid arthritis patients. Clin. (Sao Paulo. 68 (6), 766–771. doi:10.6061/clinics/2013(06)07
Smith, D. D., and Costantine, M. M. (2022). The role of statins in the prevention of preeclampsia. Am. J. Obstet. Gynecol. 226 (2S), S1171–S1181. doi:10.1016/j.ajog.2020.08.040
Sperber, K., Quraishi, H., Kalb, T. H., Panja, A., Stecher, V., and Mayer, L. (1993). Selective regulation of cytokine secretion by hydroxychloroquine: inhibition of interleukin 1 alpha (IL-1-alpha) and IL-6 in human monocytes and T cells. J. Rheumatol. 20 (5), 803–808.
Staff, A. C. (2019). The two-stage placental model of preeclampsia: an update. J. Reprod. Immunol. 134-135, 1–10. doi:10.1016/j.jri.2019.07.004
Sutton, E. F., Gemmel, M., and Powers, R. W. (2020). Nitric oxide signaling in pregnancy and preeclampsia. Nitric Oxide 95, 55–62. doi:10.1016/j.niox.2019.11.006
Szarka, A., Rigo, J., Lazar, L., Beko, G., and Molvarec, A. (2010). Circulating cytokines, chemokines and adhesion molecules in normal pregnancy and preeclampsia determined by multiplex suspension array. BMC Immunol. 11, 59. doi:10.1186/1471-2172-11-59
Takacs, P., Green, K. L., Nikaeo, A., and Kauma, S. W. (2003). Increased vascular endothelial cell production of interleukin-6 in severe preeclampsia. Am. J. Obstet. Gynecol. 188 (3), 740–744. doi:10.1067/mob.2003.134
Tang, D., Kang, R., Zeh, H. J., and Lotze, M. T. (2011). High-mobility group box 1, oxidative stress, and disease. Antioxid. Redox Signal 14 (7), 1315–1335. doi:10.1089/ars.2010.3356
Tektonidou, M. G., Andreoli, L., Limper, M., Amoura, Z., Cervera, R., Costedoat-Chalumeau, N., et al. (2019). EULAR recommendations for the management of antiphospholipid syndrome in adults. Ann. Rheum. Dis. 78 (10), 1296–1304. doi:10.1136/annrheumdis-2019-215213
Thilaganathan, B., and Kalafat, E. (2019). Cardiovascular system in preeclampsia and beyond. Hypertension 73 (3), 522–531. doi:10.1161/HYPERTENSIONAHA.118.11191
Tinsley, J. H., Chiasson, V. L., Mahajan, A., Young, K. J., and Mitchell, B. M. (2009). Toll-like receptor 3 activation during pregnancy elicits preeclampsia-like symptoms in rats. Am. J. Hypertens. 22 (12), 1314–1319. doi:10.1038/ajh.2009.185
Tjoa, M. L., Cindrova-Davies, T., Spasic-Boskovic, O., Bianchi, D. W., and Burton, G. J. (2006). Trophoblastic oxidative stress and the release of cell-free feto-placental DNA. Am. J. Pathol. 169 (2), 400–404. doi:10.2353/ajpath.2006.060161
Torigoe, M., Sakata, K., Ishii, A., Iwata, S., Nakayamada, S., and Tanaka, Y. (2018). Hydroxychloroquine efficiently suppresses inflammatory responses of human class-switched memory B cells via Toll-like receptor 9 inhibition. Clin. Immunol. 195, 1–7. doi:10.1016/j.clim.2018.07.003
Tranquilli, A. L., Brown, M. A., Zeeman, G. G., Dekker, G., and Sibai, B. M. (2013). The definition of severe and early-onset preeclampsia. Statements from the international society for the study of hypertension in pregnancy (ISSHP). Pregnancy Hypertens. 3 (1), 44–47. doi:10.1016/j.preghy.2012.11.001
Ulander, L., Tolppanen, H., Hartman, O., Rissanen, T. T., Paakkanen, R., Kuusisto, J., et al. (2021). Hydroxychloroquine reduces interleukin-6 levels after myocardial infarction: the randomized, double-blind, placebo-controlled OXI pilot trial. Int. J. Cardiol. 337, 21–27. doi:10.1016/j.ijcard.2021.04.062
Valencia-Ortega, J., Zarate, A., Saucedo, R., Hernandez-Valencia, M., Cruz, J. G., and Puello, E. (2019). Placental proinflammatory state and maternal endothelial dysfunction in preeclampsia. Gynecol. Obstet. Invest. 84 (1), 12–19. doi:10.1159/000491087
van den Borne, B. E., Dijkmans, B. A., de Rooij, H. H., le Cessie, S., and Verweij, C. L. (1997). Chloroquine and hydroxychloroquine equally affect tumor necrosis factor-alpha, interleukin 6, and interferon-gamma production by peripheral blood mononuclear cells. J. Rheumatol. 24 (1), 55–60.
Veerbeek, J. H., Hermes, W., Breimer, A. Y., van Rijn, B. B., Koenen, S. V., Mol, B. W., et al. (2015). Cardiovascular disease risk factors after early-onset preeclampsia, late-onset preeclampsia, and pregnancy-induced hypertension. Hypertension 65 (3), 600–606. doi:10.1161/HYPERTENSIONAHA.114.04850
Verlohren, S., Herraiz, I., Lapaire, O., Schlembach, D., Moertl, M., Zeisler, H., et al. (2012). The sFlt-1/PlGF ratio in different types of hypertensive pregnancy disorders and its prognostic potential in preeclamptic patients. Am. J. Obstet. Gynecol. 206 (1), 58 e1–e8. doi:10.1016/j.ajog.2011.07.037
Vitoratos, N., Economou, E., Iavazzo, C., Panoulis, K., and Creatsas, G. (2010). Maternal serum levels of TNF-alpha and IL-6 long after delivery in preeclamptic and normotensive pregnant women. Mediat. Inflamm. 2010, 908649. doi:10.1155/2010/908649
Walsh, S. W., and Strauss, J. F. (2021). The road to low-dose aspirin therapy for the prevention of preeclampsia began with the placenta. Int. J. Mol. Sci. 22 (13), 6985. doi:10.3390/ijms22136985
Wang, B., Koga, K., Osuga, Y., Hirata, T., Saito, A., Yoshino, O., et al. (2011). High mobility group box 1 (HMGB1) levels in the placenta and in serum in preeclampsia. Am. J. Reprod. Immunol. 66 (2), 143–148. doi:10.1111/j.1600-0897.2010.00975.x
Wang, S. Q., Zhang, L. W., Wei, P., and Hua, H. (2017). Is hydroxychloroquine effective in treating primary Sjogren's syndrome: a systematic review and meta-analysis. BMC Musculoskelet. Disord. 18 (1), 186. doi:10.1186/s12891-017-1543-z
Wojtowicz, A., Zembala-Szczerba, M., Babczyk, D., Kolodziejczyk-Pietruszka, M., Lewaczynska, O., and Huras, H. (2019). Early- and late-onset preeclampsia: a comprehensive cohort study of laboratory and clinical findings according to the new ISHHP criteria. Int. J. Hypertens. 2019, 4108271. doi:10.1155/2019/4108271
Wu, X. X., Guller, S., and Rand, J. H. (2011). Hydroxychloroquine reduces binding of antiphospholipid antibodies to syncytiotrophoblasts and restores annexin A5 expression. Am. J. Obstet. Gynecol. 205 (6), e7–e14. doi:10.1016/j.ajog.2011.06.064
Xiao, J. P., Yin, Y. X., Gao, Y. F., Lau, S., Shen, F., Zhao, M., et al. (2012). The increased maternal serum levels of IL-6 are associated with the severity and onset of preeclampsia. Cytokine 60 (3), 856–860. doi:10.1016/j.cyto.2012.07.039
Xie, F., Hu, Y., Turvey, S. E., Magee, L. A., Brunham, R. M., Choi, K. C., et al. (2010). Toll-like receptors 2 and 4 and the cryopyrin inflammasome in normal pregnancy and pre-eclampsia. BJOG 117 (1), 99–108. doi:10.1111/j.1471-0528.2009.02428.x
Xin, H., Zhang, Y., Wang, H., and Sun, S. (2012). Alterations of profibrinolytic receptor annexin A2 in pre-eclampsia: a possible role in placental thrombin formation. Thromb. Res. 129 (5), 563–567. doi:10.1016/j.thromres.2011.07.039
Xu, B., Nakhla, S., Makris, A., and Hennessy, A. (2011). TNF-α inhibits trophoblast integration into endothelial cellular networks. Placenta 32 (3), 241–246. doi:10.1016/j.placenta.2010.12.005
Yang, J., Yang, X., Yang, J., and Li, M. (2018). Hydroxychloroquine inhibits the differentiation of Th17 cells in systemic lupus erythematosus. J. Rheumatol. 45 (6), 818–826. doi:10.3899/jrheum.170737
Yang, M., Cao, L., Xie, M., Yu, Y., Kang, R., Yang, L., et al. (2013). Chloroquine inhibits HMGB1 inflammatory signaling and protects mice from lethal sepsis. Biochem. Pharmacol. 86 (3), 410–418. doi:10.1016/j.bcp.2013.05.013
Yang, Y., Su, X., Xu, W., and Zhou, R. (2014). Interleukin-18 and interferon gamma levels in preeclampsia: a systematic review and meta-analysis. Am. J. Reprod. Immunol. 72 (5), 504–514. doi:10.1111/aji.12298
Yonekura Collier, A. R., Zsengeller, Z., Pernicone, E., Salahuddin, S., Khankin, E. V., and Karumanchi, S. A. (2019). Placental sFLT1 is associated with complement activation and syncytiotrophoblast damage in preeclampsia. Hypertens. Pregnancy 38 (3), 193–199. doi:10.1080/10641955.2019.1640725
Yu, H., Shen, Y., Ge, Q., He, Y., Qiao, D., Ren, M., et al. (2013). Quantification of maternal serum cell-free fetal DNA in early-onset preeclampsia. Int. J. Mol. Sci. 14 (4), 7571–7582. doi:10.3390/ijms14047571
Zeidi, M., Kim, H. J., and Werth, V. P. (2019). Increased myeloid dendritic cells and TNF-α expression predicts poor response to hydroxychloroquine in cutaneous lupus erythematosus. J. Invest. Dermatol 139 (2), 324–332. doi:10.1016/j.jid.2018.07.041
Zhang, L., and Yang, H. (2012). Expression and localization of TLR4 and its negative regulator Tollip in the placenta of early-onset and late-onset preeclampsia. Hypertens. Pregnancy 31 (2), 218–227. doi:10.3109/10641955.2011.642434
Zhang, Z., Liu, H., Shi, Y., Xu, N., Wang, Y., Li, A., et al. (2017). Increased circulating Th22 cells correlated with Th17 cells in patients with severe preeclampsia. Hypertens. Pregnancy 36 (1), 100–107. doi:10.1080/10641955.2016.1239737
Zhao, L., Ma, R., Zhang, L., Yuan, X., Wu, J., He, L., et al. (2019). Inhibition of HIF-1a-mediated TLR4 activation decreases apoptosis and promotes angiogenesis of placental microvascular endothelial cells during severe pre-eclampsia pathogenesis. Placenta 83, 8–16. doi:10.1016/j.placenta.2019.06.375
Zhong, X. Y., Laivuori, H., Livingston, J. C., Ylikorkala, O., Sibai, B. M., Holzgreve, W., et al. (2001). Elevation of both maternal and fetal extracellular circulating deoxyribonucleic acid concentrations in the plasma of pregnant women with preeclampsia. Am. J. Obstet. Gynecol. 184 (3), 414–419. doi:10.1067/mob.2001.109594
Zhou, S., Li, J., Yang, W., Xue, P., Yin, Y., Wang, Y., et al. (2023). Noninvasive preeclampsia prediction using plasma cell-free RNA signatures. Am. J. Obstet. Gynecol. 229, 553.e1–553.e16. doi:10.1016/j.ajog.2023.05.015
Zhou, Y., Damsky, C. H., and Fisher, S. J. (1997). Preeclampsia is associated with the failure of human cytotrophoblasts to mimic a vascular adhesion phenotype. One cause of defective endovascular invasion in this syndrome? J. Clin. Invest. 99 (9), 2152–2164. doi:10.1172/JCI119388
Keywords: preeclampsia, Hydroxychloroquine, immunity, pathophysiology, hypertension
Citation: Gajić M, Schröder-Heurich B and Mayer-Pickel K (2024) Deciphering the immunological interactions: targeting preeclampsia with Hydroxychloroquine’s biological mechanisms. Front. Pharmacol. 15:1298928. doi: 10.3389/fphar.2024.1298928
Received: 22 September 2023; Accepted: 23 January 2024;
Published: 05 February 2024.
Edited by:
Ariela Hoxha, University Hospital of Padua, ItalyReviewed by:
Valentina Canti, San Raffaele Hospital (IRCCS), ItalyJosé Martin Murrieta-Coxca, University Hospital Jena, Germany
Copyright © 2024 Gajić, Schröder-Heurich and Mayer-Pickel. This is an open-access article distributed under the terms of the Creative Commons Attribution License (CC BY). The use, distribution or reproduction in other forums is permitted, provided the original author(s) and the copyright owner(s) are credited and that the original publication in this journal is cited, in accordance with accepted academic practice. No use, distribution or reproduction is permitted which does not comply with these terms.
*Correspondence: Karoline Mayer-Pickel, karoline.pickel@medunigraz.at