- 1Undergraduate Program in Physiology and Neuroscience, Dayton, OH, United States
- 2Department of Neuroscience, Cell Biology and Physiology, Boonshoft School of Medicine and College of Science and Mathematics, Wright State University, Dayton, OH, United States
Tetraethylammonium (TEA), a quaternary ammonium compound, is a well-known blocker of potassium channels belonging to various subfamilies, such as KV1-3, KCa1, 2 and prokaryotic KcsA. In many cases, TEA acts from the extracellular side by open pore blockade. TEA can also block transient receptor potential (TRP) cation channels, such as TRPM7, in a voltage-dependent manner. In human T lymphocytes, intracellular (cytosolic) TEA and its analog TMA (tetramethylammonium) inhibit TRPM7 channel currents in the outward but not inward direction. By contrast, intracellular Mg2+, protons and polyamines inhibit both outward and inward current components equally. Likewise, the majority of available pharmacological tools inhibit TRPM7 channels in a voltage-independent manner. Since TRPM7 is a steeply outwardly rectifying conductance, voltage-dependent blockers can be useful for studying the cellular functions of this channel. TRPM7 protein is endogenously expressed in diverse cell lines, including HEK, HeLa, CHO, RBL and Jurkat. Using patch-clamp electrophysiology, we found that incubating HEK293 and Jurkat T cells overnight in the presence of 20 mM TEA-Cl, resulted in the nearly complete blockade of whole-cell TRPM7 outward current, measured at break-in. By contrast, the inward current was unchanged in TEA-loaded cells. The blockade was fully reversible after washout of intracellular solution in whole-cell but not in perforated-patch recording configurations. Overnight incubation with 20 mM TMA-Cl resulted in a more modest blockade of the outward TRPM7 current. Internal 129 mM TMA and TEA eliminated most of the outward current. TEA uptake in transfected HEK293 cells led to blockade of recombinant murine TRPM7 and the Mg2+ and pH insensitive Ser1107Arg variant. Unexpectedly, Tris-HCl, a widely used pH buffer, could similarly be loaded into Jurkat and HEK cells, and preferentially blocked outward TRPM7 currents. 20 mM and 129 mM Tris in the internal solution blocked TRPM7 current in outward but not inward direction. Voltage-dependent channel blockade by TEA, TMA and Tris loading will be useful for studying the properties and functions of TRPM7-mediated ion transport in intact cells.
Materials and methods
Cell culture
Human embryonic kidney HEK293 and Jurkat Clone E61 leukemic T cells were purchased from ATCC (Manassas, VA) and maintained in RPMI 1640 (Gibco, Thermo-Fisher Scientific, Waltham, MA) supplemented with 10% fetal bovine serum (FBS) (Biowest USA, Brandenton, FL) and penicillin/streptomycin (Gibco) in a CO2 incubator at 37°C (Forma Scientific, Marietta, OH) (Chokshi et al., 2012b). Jurkat T cells were passaged 2 or 3 times a week by diluting the cell suspension in fresh culture medium. HEK293 cells were passaged twice a week using trypsin-EDTA (Life Technologies, Grand Island, NY) and Cellstripper reagent (Mediatech, Manassas, VA). The normal RPMI medium contains 0.42 mM [Ca2+] and 0.4 mM [Mg2+] (Mellott et al., 2020). For preparing the low magnesium medium, custom-made divalent metal free (DVF) RPMI (Gibco) was supplemented with 10% FBS, treated with Chelex 100 sodium resin beads (Sigma-Aldrich, St. Louis, MO) and passed through a sterile 0.22 µm polyethersulfone filter (Thermo-Fisher). 0.42 mM CaCl2, 10 µM HEDTA, 10 µM MgCl2 were added to Chelex-treated DVF RPMI, yielding approximately 8 µM free Mg2+. In a few experiments, 40 µM HEDTA was used with 0.42 mM CaCl2 and 10 µM MgCl2 (Mellott et al., 2020). The high potassium medium (see Figure 5) was prepared by adding 25 mM KCl to RPMI medium, yielding 30.3 mM final K+ concentration.
Heterologous expression of murine wildtype TRPM7 and S1107R variant
HEK293 cells were chemically transfected with pEGFP-mTRPM7 and pEGFP-mTRPM7 S1107R plasmids using polyethyleneimine (PEI) (Polysciences, Warrington, PA) or LT1 (Mirus Bio, Madison, WI) by mixing with DNA and Dulbecco’s phosphate-buffered saline (DPBS) and adding to cells in 6-well polystyrene plates (Corning Incorporated, Kennebunk, ME) (Zhelay et al., 2018). Plasmid DNA was purified using PureYield plasmid midiprep kit (Promega, Madison, WI).
Patch clamp electrophysiology
Endogenous and over-expressed TRPM7 channel currents were recorded in whole-cell and perforated patch configurations in HEK293 and Jurkat T cells as previously described in detail (Kozak et al., 2002; Chokshi et al., 2012b; Mellott et al., 2020). Briefly, on the day of experiment cells were transferred to a glass-bottom plexiglass recording chamber grounded with a silver-silver chloride electrode connected to EPC10 amplifier headstage (HEKA Elektronik, Lambrecht, Germany). Patch pipettes were manufactured from borosilicate glass capillaries (Harvard Apparatus, Holliston, MA) using a horizontal P-1000 puller (Sutter Instrument, Novato, CA). The pipette tips were fire-polished on a microforge (MF-830, Narishige, Tokyo, Japan), yielding resistances of ∼2–3 MOhm. TRPM7 currents were recorded by applying −100 to 100 mV command voltage ramps every 1.5 s (Chokshi et al., 2012b). The voltage was held at 0 mV between ramps. The standard “0.4 Ca/0.4 Mg” bath (external) solution contained (in mM): 0.4 CaCl2, 0.4 MgCl2, 140 sodium aspartate, 4.5 KCl, 3 CsCl, 10 HEPES sodium, pH 7.3. In some experiments the external solution contained 2 mM CaCl2 and no Mg2+ (see Figure 6). “DVF” external solution contained (in mM) 6 mM HEDTA, 140 aspartic acid, 10 HEPES acid, pH 7.3 with CsOH. The whole-cell internal solution contained (in mM) 112 glutamic acid, 10 HEDTA, 8 NaCl, 10 HEPES acid, pH 7.3 with CsOH. In experiments with TEA-Cl and Tris-HCl addition, the internal solution contained (in mM) 100 glutamic acid, 10 HEDTA, 8 NaCl, 10 HEPES acid, 133 CsOH, pH 7.3. For 129 mM internal TEA, TMA and Tris-HCl experiments the pipette solution contained: 129 mM TEA, TMA or Tris-HCl, 5 mM CsF, 1 mM EGTA, 10 mM HEPES, pH 7.3. Osmolalities of all solutions were measured with a freezing point osmometer (Osmette III, Precision Systems, Natick, MA) and adjusted with D-mannitol as needed. Aliquots of 1 M TEA-Cl, 1 M TMA-Cl, 1 M Tris-HCl prepared in deionized water were added to cell cultures at specified final concentrations for 1–3 days. Perforated-patch recordings were performed as previously described (Kozak et al., 2005; Zhelay et al., 2018; Mellott et al., 2020). A frozen aliquot of amphotericin B (Sigma) stock solution in DMSO (6 mg/10 µL) was thawed and diluted in the recording solution which contained 50 mM Cs2SO4, 50 mM CsCl, 7 mM MgCl2, 1 mM CaCl2, 10 mM HEPES, pH 7.3. Pipette tips were briefly immersed in antibiotic-free recording solution before backfilling with antibiotic-containing solution. After gigaohm seal formation, successful perforation was assessed as a gradual reduction in access resistance occurring over ∼10–20 min. Recordings were discarded if the access resistance exceeded 20 MOhm or a spontaneous break-in occurred. All patch clamp recordings were performed at room temperature in the absence of TEA, TMA and Tris in the bath solution. In HEK293 cells transfected with TRPM7 constructs, the recordings were performed 2–3 days after transfection, and successfully transfected cells were identified by GFP fluorescence on an inverted Nikon microscope equipped with a fluorescent illumination system (89 North, Williston, VT).
Data analysis
In order to quantify the extent of block in TEA and Tris loaded cells in the Ca2+-containing bath solution, TRPM7 current magnitude measured at break-in (I0) was divided by maximum current magnitude after washout (Imax) measured at 98.67 mV. For block of the monovalent TRPM7 current recorded in DVF, the break-in current magnitude at 98.67 mV was divided by current magnitude at −100 mV (R = I0 (+98.67)/I0 (−100), followed by division by the ratio of maximum current magnitudes (Imax) at these voltages (r = Imax (+98.67)/Imax (−100). R/r ratio accounts for differences in the degree of monovalent TRPM7 current preactivation (basal current magnitude) due to cellular Mg2+ depletion (Mellott et al., 2020). In some recordings the washout trace was normalized to the amplitude of the break-in trace measured at −100 mV as specified in figure legends. Two sample Student’s t-test was used for statistical comparisons of means. Patchmaster (HEKA), Microsoft Excel and Origin (v. 2017, 9.0, Originlab, Northampton, MA) were used for data analysis and graphing.
Chemicals
DPBS was purchased from HyClone (Logan, UT). Aspartic acid, CsOH, Cs2SO4, CsCl and D-mannitol were from Sigma. Tetraethylammonium chloride (TEA-Cl), 1.0 M CaCl2 and 1.0 M MgCl2 were from Fluka Honeywell (Muskegon, MI). Glutamic acid, HEDTA, HEPES sodium, tetramethylammonium chloride (TMA-Cl) and NaCl were from Thermo Fisher. HEPES acid and sodium aspartate were from Alfa Aesar (Ward Hill, MA). KCl was from Fisher Scientific. Tris(hydroxymethyl)aminomethane hydrochloride (Tris-HCl) was from Promega (Madison, WI).
Introduction
TRPM7 (transient receptor potential melastatin 7) channels belong to the superfamily of TRP cation channels, and are highly expressed in cells of the immune system: lymphocytes, macrophages, mast cells and neutrophils (Nadolni and Zierler, 2018; Hoeger and Zierler, 2022; Liang et al., 2022; Alavi et al., 2024). TRPM7 is expressed in widely used human cell lines such as Jurkat, HEK293, HeLa, K562, MCF7 (Numata et al., 2007; Dhennin-Duthille et al., 2011; Chokshi et al., 2012b; Takahashi et al., 2018). This protein is unique in that its C-terminus includes a functional serine/threonine protein kinase domain (Yamaguchi et al., 2001; Ryazanova et al., 2004; Matsushita et al., 2005; Visser et al., 2014). TRPM7 channels are sensitive to cytosolic Mg2+, pH and other divalent metal cations (Kozak et al., 2002; Kozak and Cahalan, 2003; Chokshi et al., 2012b; c). Intracellular magnesium nucleotides such as MgATP and MgGTP were also reported to inhibit TRPM7 channels (Demeuse et al., 2006).
Pharmacological tools often used in TRPM7 research include channel inhibitors SKF-96365, 2-APB, spermine, philanthotoxin, NS8593, FTY-720, quinine, salicylate, lanthanides and others (Kozak et al., 2002; Kerschbaum et al., 2003; Gwanyanya et al., 2004; Wykes et al., 2007; Chokshi et al., 2012a; Chubanov et al., 2012; Qin et al., 2013; Mellott et al., 2020; Chokshi et al., 2021). Several TRPM7 channel blockers (2-APB, naproxen, ibuprofen, salicylate, acetylsalicylate) inhibit TRPM7 channels through a cytosolic acidification mechanism (Chokshi et al., 2012a; Chokshi et al., 2021). Unfortunately, TRPM7 inhibitors are not specific and target other channels and receptors (Cheng et al., 2022). The majority inhibit TRPM7 in a voltage-independent manner. By contrast, external polyamines (spermine, spermidine, putrescine, philanthotoxin), tetraethylammonium (TEA), tetramethylammonium (TMA) and La3+ are voltage-dependent blockers (Kozak et al., 2005). External La3+, at concentrations not exceeding 1 mM, blocks only the inward TRPM7 current (Kozak and Cahalan, 2001; Mellott et al., 2020). Polyamines block the inward monovalent TRPM7 current at micromolar concentrations (Kozak et al., 2002; Kerschbaum et al., 2003), whereas intracellular TEA and TMA block the outward monovalent current at millimolar concentrations (Kozak et al., 2005). At concentrations of 200 µM and higher, external polyamines also block the outward TRPM7 current (Kerschbaum et al., 2003).
The tools available for specifically eliminating the outward TRPM7 current have been limited, since TEA and TMA blockade occurs when added to the internal recording solution. In the present study, we report that in intact HEK293 and Jurkat T cells, TEA, TMA blocked outward TRPM7 current by overnight incubation with these compounds. Additionally, we discovered that intracellular Tris-HCl (tris(hydroxymethyl)aminomethane), is a voltage-dependent blocker of TRPM7 channels that can enter HEK293 and Jurkat cytosol from the outside. TEA was an effective blocker of recombinant murine TRPM7 and its constitutively active variant S1107R (Zhelay et al., 2018). Thus, TEA, TMA, Tris accumulate in cell cytosol and inhibit the outward TRPM7 current in a concentration-dependent manner, without affecting the inward current. We propose that cellular uptake of these compounds can be useful for dissecting the functions of TRPM7 current components.
Results
Blockade of native TRPM7 channels in cells loaded with TEA and TMA
Previously, we showed that 20 mM TEA or 20 mM TMA in the internal solution blocks whole-cell monovalent TRPM7 current in a voltage-dependent manner. Both TEA, and to a lesser degree TMA, reduced the magnitude of the outward but not inward current in human T lymphocytes (Kozak et al., 2005). In order to evaluate if these compounds could also be useful for blocking outward TRPM7 currents in intact cells, we incubated Jurkat T cells overnight in Mg2+- deficient RPMI medium, in the presence of TEA. Maintaining Jurkat T cells in 8 µM [Mg2+]/0.42 mM [Ca2+] medium for 1–3 days results in cytosolic Mg2+ depletion and almost complete activation of TRPM7 current (Mellott et al., 2020). In cells maintained under normal Mg2+ conditions ([Mg](RPMI) = 0.4 mM), the majority of TRPM7 channels are inhibited, and full current activation requires 5–10 min of recording with Mg2+-free internal solution (Chokshi et al., 2012b; Mellott et al., 2020).
Activation of TRPM7 current in intact cells by prior Mg2+ depletion, allows the study of TEA blockade separately from Mg2+ inhibition. Additionally, it separates internal Mg2+ washout from TEA washout. Figure 1A shows whole-cell current-voltage (I-V) relations obtained after overnight incubation with 20 mM TEA, at break-in (red trace) and after several minutes of recording (black). The break-in (t = 0) trace represents the current present in an intact cell in external 0.4 Ca/0.4 Mg, before cytosol exchange with the pipette solution. The I-V was modified by a preferential reduction of current at positive membrane potentials. TEA blockade was fully reversible after exchange with TEA-free internal solution (Figure 1A).
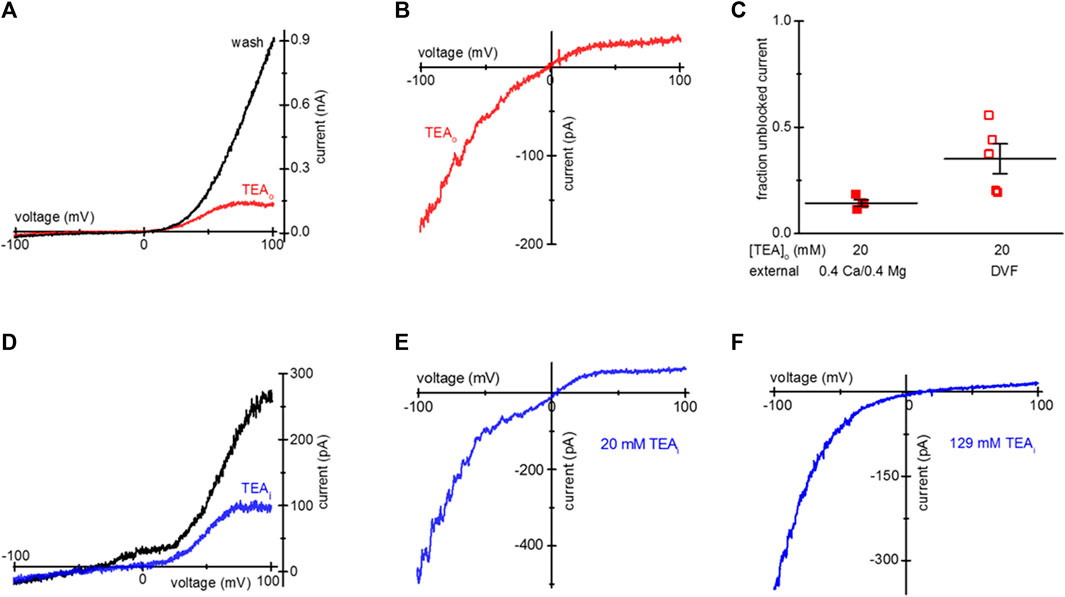
Figure 1. Blockade of TRPM7 current in TEA-loaded Mg2+- depleted Jurkat T cells. (A) TRPM7 I-V relations at break-in (red trace) and after 20 mM TEA-Cl washout (black) recorded in 0.4 Ca/0.4 Mg external solution. (B) Monovalent TRPM7 current I-V in a 20 mM TEA-Cl loaded cell at break-in. (C) Summary of TRPM7 current blockade by uptake of 20 mM TEAo, measured in 0.4 Ca/0.4 Mg (filled squares) and DVF (empty squares) external solutions. (D) Blockade of TRPM7 current by 20 mM TEA added to the internal solution. I-V relations at break-in (black trace) and after blockade (blue) with 20 mM TEA. (E,F) Blockade of monovalent TRPM7 current by internal 20 mM and 129 mM TEA. Jurkat T cells were incubated in low Mg2+ RPMI (A–D) supplemented with TEA-Cl (A–C) for 1–2 days. In (D–F) the cells were not exposed to external TEA.
Upon removal of external divalent cations (e.g., Ca2+ and Mg2+), TRPM7 current becomes semi-linear due to removal of tonic inward current blockade (Kozak et al., 2002). Thus, it is possible to study the effect of the blockers in the full voltage range by recording TRPM7 monovalent currents. We, therefore, recorded monovalent TRPM7 currents in external DVF. As expected, monovalent current was blocked in the outward direction, giving rise to inward rectification (Figure 1B). The extent of 20 mM TEA block in 0.4 Ca/0.4 Mg and DVF is summarized in Figure 1C. For comparison, inclusion of 20 mM TEA in the pipette resulted in blockade of outward TRPM7 currents both in 0.4 Ca/0.4 Mg and in DVF (Figures 1D, E). 129 mM internal TEA eliminated almost all monovalent outward current without affecting the inward current (Figure 1F). These experiments demonstrated that after prolonged incubation, TEA enters intact Jurkat cells, blocks TRPM7 channels from the cytosolic side, and the blockade is reversible.
HEK293 cells endogenously express TRPM7 channels, and are commonly used for heterologous expression of TRPM7 (Chokshi et al., 2012c; Zhelay et al., 2018). We, therefore, tested if TEA uptake can occur in HEK293 cells. We incubated HEK293 cells overnight in low Mg2+ RPMI and 1–20 mM TEA. Similar to Jurkat T cells, this treatment resulted in cytosolic Mg2+ depletion and activation of TRPM7 channels concomitant with TEA uptake and voltage-dependent blockade. Figures 2A–C show TEA blockade of endogenous TRPM7 channels in HEK293 cells. Figure 2A shows I-V relations obtained in 0.4 Ca/0.4 Mg at break-in and after TEA washout. Figures 2B, C show monovalent TRPM7 current blocked in cells incubated with 10 mM and 20 mM TEA, respectively. The blockade occurred only in the outward direction. The summary of TRPM7 current blockade by various concentrations of TEA in HEK293 cells is presented in Figure 2D.
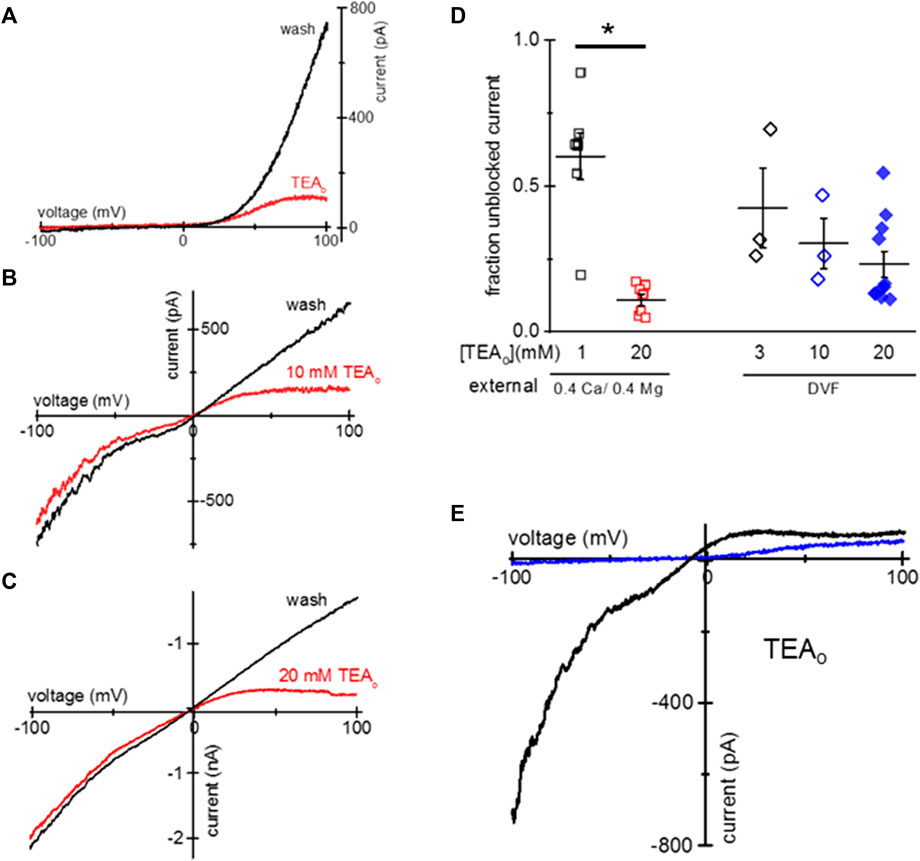
Figure 2. Blockade of TRPM7 current in TEA-loaded Mg2+- depleted HEK293 cells. (A) TRPM7 I-V relations at break-in (red trace) and after 20 mM TEA washout (black) in 0.4 Ca/0.4 Mg solution. (B,C) Monovalent TRPM7 I-V in 10 mM and 20 mM TEA loaded cells at break-in and after washout. (D) Summary of TRPM7 current blockade by uptake of indicated concentrations of TEAo for 0.4 Ca/0.4 Mg and DVF external solutions. (E) Perforated patch recording of TRPM7 current in 0.4 mM Ca/0.4 mM Mg (blue) and DVF (black) in a cell loaded with 20 mM TEA. No washout of TEA block was observed during perforated-patch recording. HEK cells were incubated in low Mg2+ RPMI supplemented with TEA for 1–2 days (A–E). Asterisk denotes Student’s two sample test p < 0.05.
We performed similar experiments using amphotericin perforated-patch recording, which prevents the exchange of divalent ions between the cytosol and the pipette solution (Rae et al., 1991; Mellott et al., 2020). Figure 2E shows the almost complete blockade of outward TRPM7 currents recorded in 0.4 Ca/0.4 Mg and DVF in the same cell. Unlike with conventional whole-cell recording, the blockade persisted during prolonged recording (up to 9 min, data not shown) suggesting that cytosolic TEA cannot diffuse out of the cell through amphotericin channels.
Similar to TEA, tetramethylammonium (TMA), a closely related quaternary ammonium cation blocks TRPM7 channels at positive membrane potentials (Kozak et al., 2005), we therefore tested if it is taken up by Jurkat and HEK293 cells. Overnight incubation with low Mg2+ and 20 mM TMA-Cl resulted in monovalent TRPM7 current blockade in the outward direction (Figures 3A, B). The extent of current blockade measured at 98.67 mV was similar in both human cell lines (Figure 3C). Like TEA (Figure 1), 129 mM TMA in the internal solution blocked most of the outward monovalent current in Jurkat cells (Figure 3D). In conclusion, TMA can be loaded into Jurkat and HEK293, resulting in native TRPM7 channel blockade in intact cells.
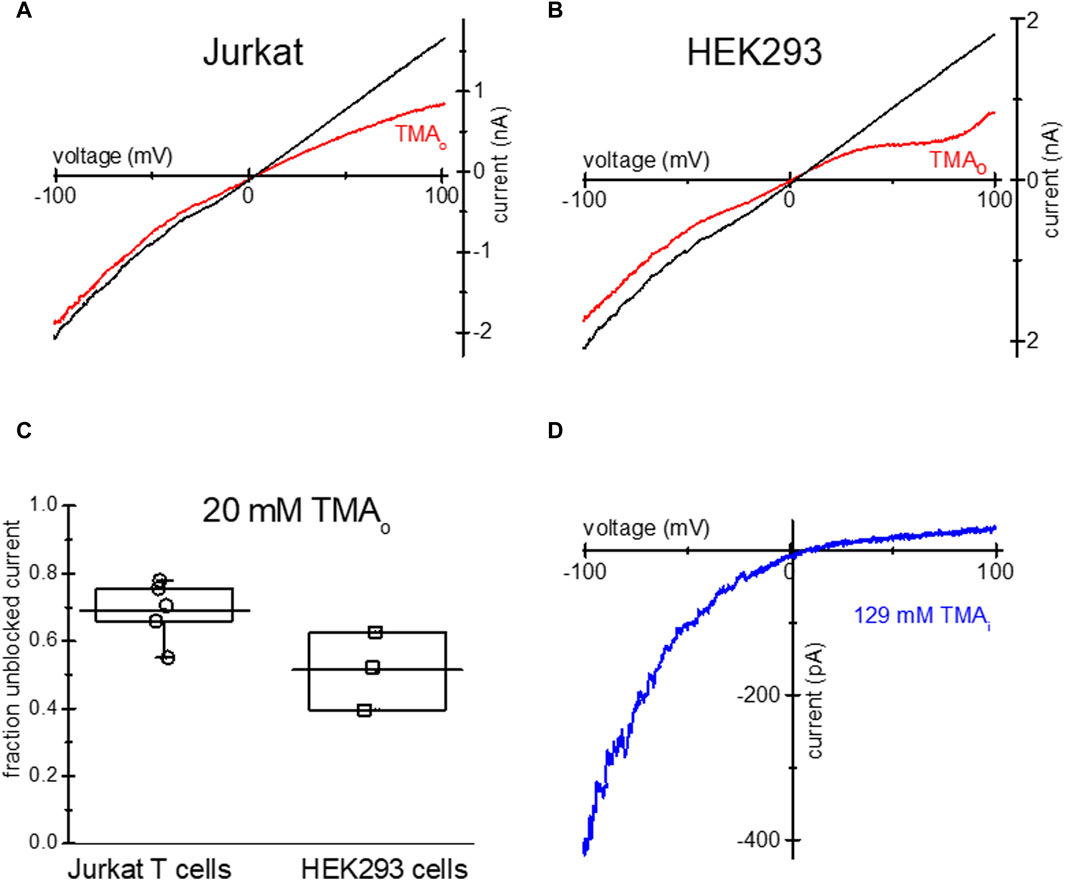
Figure 3. Blockade of TRPM7 monovalent current in TMA-loaded Mg2+- depleted Jurkat and HEK293 cells. TRPM7 I-V relations at break in (red) and after 20 mM TMA washout (black) in Jurkat T cell (A) and HEK293 cell (B) in DVF external solution. (C) Summary of TMA uptake experiments, showing the fraction of unblocked monovalent current in both cell lines. (D) Blockade of monovalent TRPM7 current by internal 129 mM TMA in a Jurkat T cell. In (A,B) the washout trace magnitude was normalized to break-in current magnitude measured at −100 mV.
Blockade of heterologously expressed TRPM7 channels by TEA uptake
We tested if TEA loading can be used for inhibition of heterologously expressed TRPM7 channels. HEK293 were transfected with murine TRPM7 cDNA, and TEA was added to normal RPMI medium 1 day after transfection. Unlike the native channels, heterologously expressed TRPM7 shows a substantial basal activity at break-in, without prior Mg2+ depletion (Matsushita et al., 2005; Zhelay et al., 2018). Figure 4A shows WT wildtype (WT) TRPM7 channel current blockade in TEA-loaded HEK cells in 0.4 Ca/0.4 Mg. The red trace shows TRPM7 I-V at break-in, and the black trace shows full washout of cytosolic TEA. In Figure 4B, TRPM7 I-Vs were collected immediately after break-in (red), following TEA washout in 0.4 Ca/0.4 Mg (black) and DVF (wine). Inclusion of 20 mM TEA in the internal solution resulted in voltage-dependent block of WT TRPM7 channels at break-in (red), after achieving maximal magnitude (black) and upon switching to DVF (blue) (Figure 4C). As was for endogenous currents (Figures 1, 2), TEA preferentially blocked outward WT TRPM7 currents.
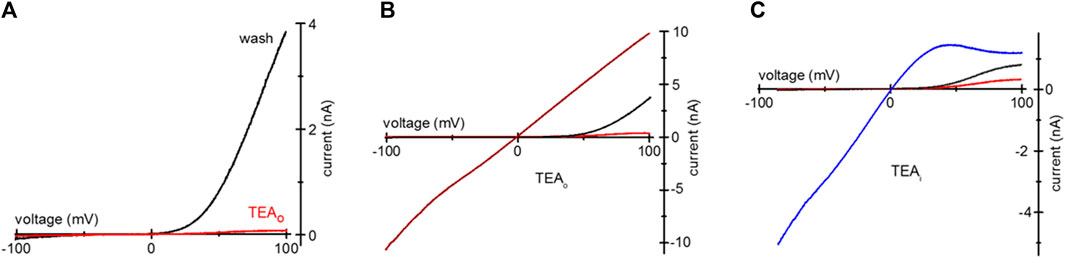
Figure 4. TEA blockade of overexpressed mTRPM7 channels. (A) TRPM7 channel current after uptake of 20 mM TEA in a HEK293 cell overexpressing wildtype murine TRPM7. Red and black I-Vs were recorded at break-in and after TEA washout, respectively. (B) mTRPM7 currents in a cell loaded with 20 mM TEA recorded at break-in (red), after washout (black) and after removal of divalent cations (wine). (C) Blockade of mTRPM7 currents with internal 20 mM TEA. The red and black traces represent break-in and maximal currents. The blue trace represents monovalent current in the same cell, recorded after current had reached maximum in the 0.4 Ca/0.4 Mg solution. In (C) the cell was not exposed to external TEA and the command voltage ramps spanned −85 mV–100 mV range.
S1107R variant forms ion channels that have I-V relations identical to WT TRPM7, but are largely insensitive to cytosolic Mg2+, pH and polyamines (Zhelay et al., 2018). In essence, under physiological ionic conditions S1107R and other substitutions of S1107 make the channel constitutively active (Hofmann et al., 2014; Zhelay et al., 2018). We tested if TEA uptake is effective in blocking S1107R. Figure 5A shows that in HEK cells loaded with 20 mM TEA, outward S1107R current was blocked similar to WT. Figure 5B shows I-V relations recorded at break-in and after maximal current was achieved in a S1107R expressing HEK cell not exposed to TEA.
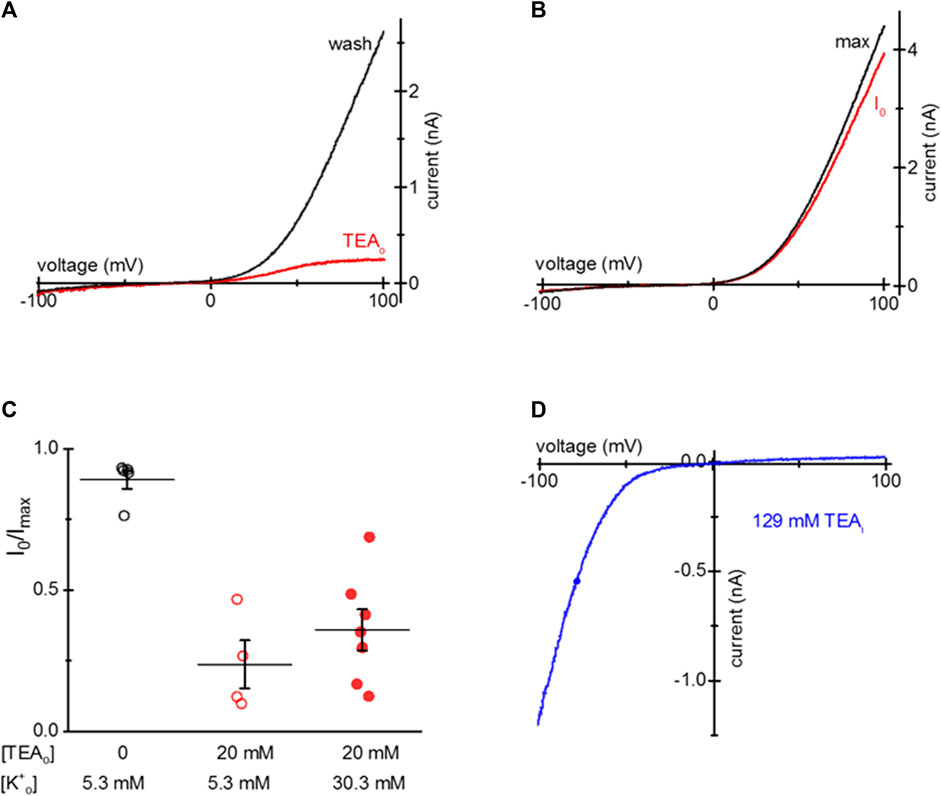
Figure 5. TEA blockade of mTRPM7 S1107R channels. (A) TRPM7 S1107R channel current at break-in (red) and after washout (black) in a HEK293 cell after 20 mM TEA uptake. (B) S1107R current at break-in and after several minutes of recording in a control HEK293 cell not exposed to TEA. (C) Summary of S1107R TRPM7 current block by TEA for HEK cells grown in normal (5.3 mM) and elevated (30.3 mM) [K+] containing RPMI in the presence of 20 mM TEA for 1–2 days. The external solution was 0.4 Ca/0.4 Mg. (D) Blockade of monovalent S1107R TRPM7 current by internal 129 mM TEA in a HEK293 cell not exposed to external TEA.
Our next goal was to test if TEA entry into HEK cells depends on the membrane potential since TEA is a cation. To this end, we supplemented low Mg2+ RPMI with 30.3 mM KCl and compared the extent of TEA loading in cells grown in normal (5 mM) and elevated [K+]. Figure 5C shows that the extent of TEA blockade was somewhat reduced by depolarizing HEK293 cells but the difference did not reach statistical significance. Inclusion of 129 mM TEA in the pipette blocked most of the outward S1107R current, similar to WT TRPM7 (Figure 5D). These results suggest that TEA block does not depend on Mg2+ sensitivity of TRPM7 channels and occurs through a different mechanism, which probably involves pore blockade.
Blockade of TRPM7 channels by Tris uptake
Tris is a positively charged compound widely used in biochemistry as a pH buffer. Tris-buffered Tyrode’s is used in cell physiology and immunology [e.g., (Okazaki et al., 1975; Hess et al., 1982)]. Similar to TEA, overnight incubation of HEK293 and Jurkat T cells with 20 mM Tris-HCl resulted in the voltage-dependent blockade of endogenous TRPM7 channels (Figure 6). Panels A and B show TRPM7 current block by 20 mM Tris uptake in Mg2+-depleted Jurkat T cells in 0.4 Ca/0.4 Mg and DVF. 20 mM Tris blocked TRPM7 channels in HEK293 cells as well (Figures 6E, F). 20 mM Tris blocked in the outward direction, albeit to a lesser degree than 20 mM TEA: approximately half of outward current was blocked (Figure 6G). Blockade was reversed by diffusion into the patch pipette over several minutes (Figures 6A, B, E, F). Inclusion of 20 and 129 mM Tris in the pipette solution blocked the outward but TRPM7 current in a concentration-dependent manner without affecting the inward current (Figures 6C, D). These experiments demonstrate that Tris is a previously unknown voltage-dependent blocker of TRPM7 channels, and Jurkat and HEK cells can accumulate it after prolonged incubation.
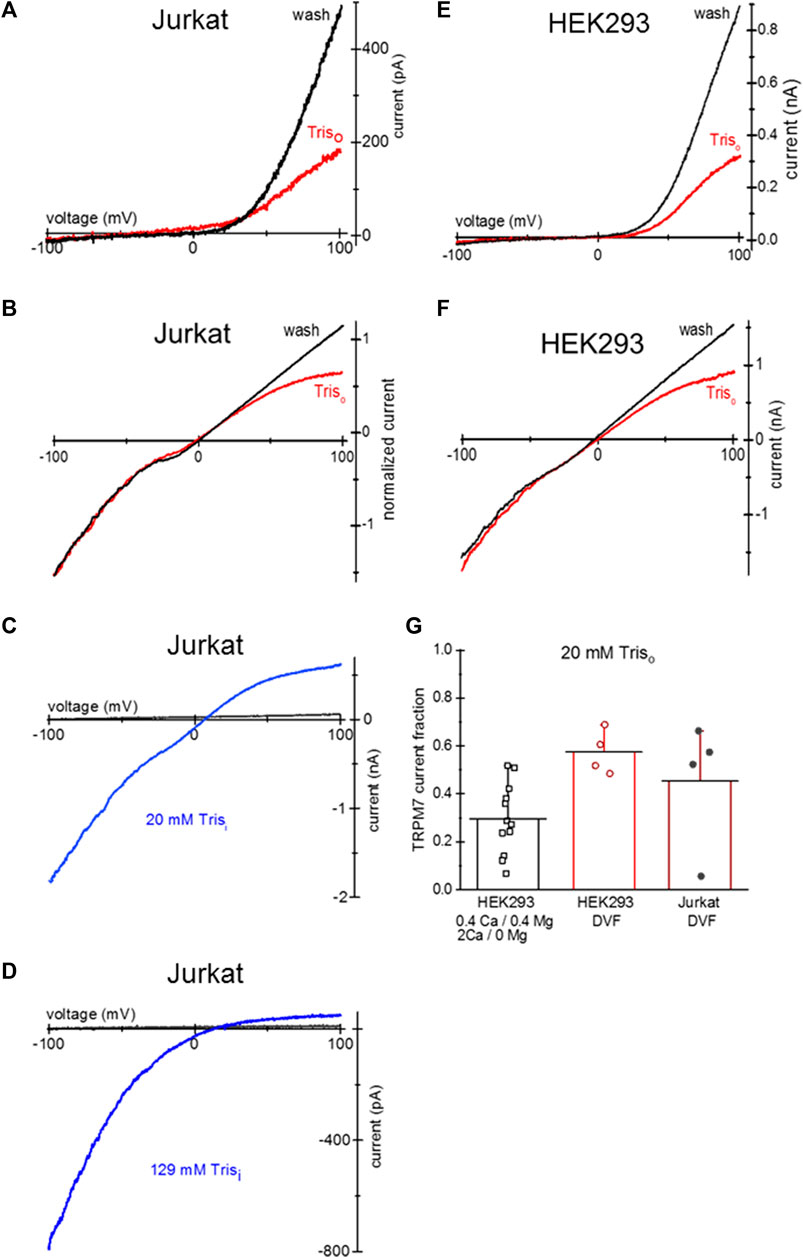
Figure 6. Tris uptake and blockade of TRPM7 channels. (A,B) TRPM7 channel current at break-in (red trace) and after washout (black) in a Jurkat T cell loaded with 20 mM Tris-HCl. The external solutions in (A,B) were 0.4 Ca/0.4 Mg and DVF, respectively. (C) TRPM7 channel monovalent current block by 20 mM Tris added to pipette in a Jurkat T cell. (D) TRPM7 channel monovalent current block by internal 129 mM Tris in a Jurkat T cell. In (C,D) black traces represent break-in and blue traces maximal current after intracellular Mg2+ depletion, respectively. (E,F) TRPM7 channel current at break-in (red) and after washout (black) in a HEK293 cell loaded with 20 mM Tris-HCl. The external solutions in (E,F) were 0.4 Ca/0.4 Mg and DVF, respectively. (G) Summary of the effects of external 20 mM Tris uptake on native TRPM7 in HEK293 cells. Extent of outward current block in 0.4 Ca/0.4 Mg and DVF was calculated as described in Methods. Cells in (A,B,E,F) were grown in low Mg2+ RPMI for 1–2 days. In (B) the current was normalized to −100 mV amplitude.
Discussion
Here we report a simple experimental approach for preferentially blocking outward TRPM7 currents in intact cells by loading with TEA, TMA and Tris. In the presence of external divalent cations, TRPM7 has a characteristic steeply outwardly rectifying I-V relation, reversing near 0 mV. At hyperpolarized membrane potentials, the native TRPM7 channels give rise to a small 10–20 pA inward (negative) current. In the absence of external divalent cations, the inward current is substantially larger and whole-cell I-V is semi-linear with unchanged reversal potential (Kozak et al., 2002; Chokshi et al., 2021). Thus, the ratio of current magnitudes measured at +98 mV and −98 mV, which describes the degree of rectification, is greater than 30 in 0.4 mM Ca/0.4 mM Mg, whereas in DVF it is ∼0.9 (unpublished data). The steep outward rectification arises from preferential block of the inward monovalent current by external Ca2+ and Mg2+ (Kozak et al., 2002; Kerschbaum et al., 2003). The slight inward rectification of monovalent current is due to smaller single-channel conductance at positive membrane potentials (Chokshi et al., 2012c). Previously, we showed that internal TEA and TMA blocked the outward TRPM7 in human T lymphocytes in DVF solution (Kozak et al., 2005). However, we are not aware of any tools that allow specifically blocking the outward current in intact cells. Here we report that overnight incubation with TEA and TMA block outward TRPM7 current recorded in 0.4 Ca/0.4 Mg bath solution, which mimics RPMI cell culture medium (Figures 1, 2). Blockade by TEA and TMA was fully reversible in whole cell recording. In perforated patch recording, which closely mimics the situation in intact cells, TRPM7 current was blocked in TEA-loaded cells (Figure 2E). In RBL cells, internal 25 mM TEA blocked the monovalent TRPM7 current at positive but not negative membrane potentials (Gwanyanya et al., 2004). TEA blockade of TRPM7 current is reminiscent of voltage dependent blockade by Mg2+ and spermine resulting in strong inward rectification of Kir potassium channels such as Kir2.1 and Kir4.1 (Oliver et al., 2000). Interestingly, for TRPM7 channels intracellular Mg2+ and spermine are also inhibitory, but without voltage dependence (Kozak et al., 2002; Kozak et al., 2005; Zhelay et al., 2018).
The majority of studies of TRPM7 cellular function have focused on the inward component of the current, even though it is substantially smaller than the outward component (e.g., Figures 1A, 2A). One of the reasons has been the voltage independence of available inhibitors, which block both inward and outward current components. It has been argued that the small inward TRPM7 current is primarily carried by divalent metal cations Ca2+, Mg2+, Ba2+, Sr2+ and others (Monteilh-Zoller et al., 2003; Li et al., 2007; Schmitz et al., 2014; Chubanov et al., 2018; Takahashi et al., 2018). Specifically, TRPM7 channels are thought to mediate cellular Mg2+ entry (Monteilh-Zoller et al., 2003; He et al., 2005; Ryazanova et al., 2010; Komiya and Runnels, 2015). We found recently, however, that elimination of inward current by La3+ block did not prevent Mg2+ uptake, whereas inhibition of both inward and outward currents by NS8593 abolished Mg2+ entry in the majority of treated Jurkat T cells (Mellott et al., 2020). This suggests that, paradoxically, the outward TRPM7 current may play a role in Mg2+ transport into the cell. It should be noted, that a portion of Jurkat T cells exhibited an additional pathway for Mg2+ entry even when all TRPM7 channels were blocked.
We report here that intracellular Tris is a voltage-dependent blocker of TRPM7 channels. 20 mM and 129 mM Tris-HCl in the internal solution resulted in a concentration-dependent block of outward TRPM7 current (Figure 6). Similar to TEA and TMA, overnight incubation with 20 mM Tris resulted in its uptake and blockade of outward current in intact cells (Figure 6). Tris did not reduce TRPM7 current magnitude in the inward direction.
Intracellular TEA and TMA block or modify several K+ channels including voltage-gated, Ca2+-activated K+ channels (Hermann and Gorman, 1981; Villarroel et al., 1988; Im and Quandt, 1992; Gonzalez-Perez et al., 2008). Intracellular TEA blocks Kv1.3 channels which are highly expressed in T cells (Rauer and Grissmer, 1996; Prutting and Grissmer, 2011), thus, uptake of these cations will be expected to block these K+ channels also. ATP-gated P2X channels are expressed in T cells and other immune cells (Bohmwald et al., 2021; Vinnenberg et al., 2021) and their properties would be altered upon Tris uptake since this cation is somewhat permeant (Evans et al., 1996).
We did not investigate the identity of pathways for TEA, TMA and Tris entry into Jurkat and HEK293 cells. Maintaining cells in elevated K+ (30 mM), which would be expected to depolarize the cells, did not significantly reduce TEA cation loading (Figure 5C). Of note, high extracellular K+, reaching 40–50 mM occurs in tumor environment and has a suppressive effect on T lymphocyte function, serving as an “ionic checkpoint” mediating immunosuppression and enabling malignant cells to escape immune attack (Ong et al., 2019). It has been reported that organic cation transporter proteins OCT1 and 2 can serve as pathways for TEA entry in other cell types (Wright et al., 2004; Pelis et al., 2007; Kimura et al., 2009). It would be instructive to determine if HEK293 and Jurkat T cells express the human homologs of OCT proteins.
Future investigation will address several questions of interest: is TEA, TMA, Tris uptake temperature sensitive or can it also occur at room temperature? Does the uptake of these cations depend on TRPM7 channel activity? Based on our findings, entry of TEA is not Mg2+ dependent and can occur both in reduced (8 µM) (Figure 1) and normal (0.4 mM) external Mg2+ (Figure 4). This makes it unlikely that TRPM7 participates in the uptake of these cations. TEA and TMA uptake may occur through other channels. For example, aquaporin 1 (AQP1) water channels also conduct cations K+, Cs+, Na+ and TEA, likely through the central pore of the AQP1 tetramer (Boassa et al., 2006; Yu et al., 2006). Pilot data showed that incubating cells in presence of TEA, TMA and Tris for up to 2 days, was not visibly toxic, and can therefore be used for long-term experiments aiming to dissect the physiological roles of TRPM7 channels.
Data availability statement
The raw data supporting the conclusion of this article will be made available by the authors, without undue reservation.
Ethics statement
Ethical approval was not required for the studies on humans in accordance with the local legislation and institutional requirements because only commercially available established cell lines were used. Ethical approval was not required for the studies on animals in accordance with the local legislation and institutional requirements because only commercially available established cell lines were used.
Author contributions
KH: Investigation, Writing–original draft, Data curation. JK: Investigation, Writing–original draft, Conceptualization, Funding acquisition, Methodology, Supervision, Writing–review and editing.
Funding
The author(s) declare that financial support was received for the research, authorship, and/or publication of this article. This work was funded by grants 1R01AI114804 and 1R21AI144337 from the National Institute of Allergy and Infectious Diseases and by funds from The Neuroscience Institute and Boonshoft School of Medicine (Wright State University) (to JK).
Acknowledgments
We thank Jananie Rockwood for help with cell culture and molecular biology, Masayuki Matsushita (University of the Ryukyus) for providing the pEGFP-mTRPM7 plasmid and Vera Moiseenkova-Bell (University of Pennsylvania) for sharing the PEI transfection protocol.
Conflict of interest
The authors declare that the research was conducted in the absence of any commercial or financial relationships that could be construed as a potential conflict of interest.
Publisher’s note
All claims expressed in this article are solely those of the authors and do not necessarily represent those of their affiliated organizations, or those of the publisher, the editors and the reviewers. Any product that may be evaluated in this article, or claim that may be made by its manufacturer, is not guaranteed or endorsed by the publisher.
References
Alavi, M. S., Soheili, V., and Roohbakhsh, A. (2024). The role of transient receptor potential (TRP) channels in phagocytosis: a comprehensive review. Eur. J. Pharmacol. 964, 176302. doi:10.1016/j.ejphar.2023.176302
Boassa, D., Stamer, W. D., and Yool, A. J. (2006). Ion channel function of aquaporin-1 natively expressed in choroid plexus. J. Neurosci. 26, 7811–7819. doi:10.1523/JNEUROSCI.0525-06.2006
Bohmwald, K., Galvez, N. M. S., Andrade, C. A., Mora, V. P., Munoz, J. T., Gonzalez, P. A., et al. (2021). Modulation of adaptive immunity and viral infections by ion channels. Front. Physiol. 12, 736681. doi:10.3389/fphys.2021.736681
Cheng, X. Y., Li, S. F., Chen, Y., Zhao, Y. J., Hu, W., Lu, C., et al. (2022). Transient receptor potential melastatin 7 and their modulators. Eur. J. Pharmacol. 931, 175180. doi:10.1016/j.ejphar.2022.175180
Chokshi, R., Bennett, O., Zhelay, T., and Kozak, J. A. (2021). NSAIDs naproxen, ibuprofen, salicylate, and aspirin inhibit TRPM7 channels by cytosolic acidification. Front. Physiol. 12, 727549. doi:10.3389/fphys.2021.727549
Chokshi, R., Fruasaha, P., and Kozak, J. A. (2012a). 2-Aminoethyl diphenyl borinate (2-APB) inhibits TRPM7 channels through an intracellular acidification mechanism. Channels (Austin) 6, 362–369. doi:10.4161/chan.21628
Chokshi, R., Matsushita, M., and Kozak, J. A. (2012b). Detailed examination of Mg2+ and pH sensitivity of human TRPM7 channels. Am. J. Physiol. Cell Physiol. 302, C1004–C1011. doi:10.1152/ajpcell.00422.2011
Chokshi, R., Matsushita, M., and Kozak, J. A. (2012c). Sensitivity of TRPM7 channels to Mg2+ characterized in cell-free patches of Jurkat T lymphocytes. Am. J. Physiol. Cell Physiol. 302, C1642–C1651. doi:10.1152/ajpcell.00037.2012
Chubanov, V., Mederos Y Schnitzler, M., Meissner, M., Schafer, S., Abstiens, K., Hofmann, T., et al. (2012). Natural and synthetic modulators of SK (K(Ca)2) potassium channels inhibit magnesium-dependent activity of the kinase-coupled cation channel TRPM7. Br. J. Pharmacol. 166, 1357–1376. doi:10.1111/j.1476-5381.2012.01855.x
Chubanov, V., Mittermeier, L., and Gudermann, T. (2018). Role of kinase-coupled TRP channels in mineral homeostasis. Pharmacol. Ther. 184, 159–176. doi:10.1016/j.pharmthera.2017.11.003
Demeuse, P., Penner, R., and Fleig, A. (2006). TRPM7 channel is regulated by magnesium nucleotides via its kinase domain. J. Gen. Physiol. 127, 421–434. doi:10.1085/jgp.200509410
Dhennin-Duthille, I., Gautier, M., Faouzi, M., Guilbert, A., Brevet, M., Vaudry, D., et al. (2011). High expression of transient receptor potential channels in human breast cancer epithelial cells and tissues: correlation with pathological parameters. Cell Physiol. Biochem. 28, 813–822. doi:10.1159/000335795
Evans, R. J., Lewis, C., Virginio, C., Lundstrom, K., Buell, G., Surprenant, A., et al. (1996). Ionic permeability of, and divalent cation effects on, two ATP-gated cation channels (P2X receptors) expressed in mammalian cells. J. Physiol. 497 (Pt 2), 413–422. doi:10.1113/jphysiol.1996.sp021777
Gonzalez-Perez, V., Neely, A., Tapia, C., Gonzalez-Gutierrez, G., Contreras, G., Orio, P., et al. (2008). Slow inactivation in Shaker K channels is delayed by intracellular tetraethylammonium. J. Gen. Physiol. 132, 633–650. doi:10.1085/jgp.200810057
Gwanyanya, A., Amuzescu, B., Zakharov, S. I., Macianskiene, R., Sipido, K. R., Bolotina, V. M., et al. (2004). Magnesium-inhibited, TRPM6/7-like channel in cardiac myocytes: permeation of divalent cations and pH-mediated regulation. J. Physiol. 559, 761–776. doi:10.1113/jphysiol.2004.067637
He, Y., Yao, G., Savoia, C., and Touyz, R. M. (2005). Transient receptor potential melastatin 7 ion channels regulate magnesium homeostasis in vascular smooth muscle cells: role of angiotensin II. Circ. Res. 96, 207–215. doi:10.1161/01.RES.0000152967.88472.3e
Hermann, A., and Gorman, A. L. (1981). Effects of tetraethylammonium on potassium currents in a molluscan neurons. J. Gen. Physiol. 78, 87–110. doi:10.1085/jgp.78.1.87
Hess, P., Metzger, P., and Weingart, R. (1982). Free magnesium in sheep, ferret and frog striated muscle at rest measured with ion-selective micro-electrodes. J. Physiol. 333, 173–188. doi:10.1113/jphysiol.1982.sp014447
Hoeger, B., and Zierler, S. (2022). Engulf and digest - TRPM7 as key regulator of macrophage phagosome maturation. Cell Calcium 106, 102636. doi:10.1016/j.ceca.2022.102636
Hofmann, T., Schafer, S., Linseisen, M., Sytik, L., Gudermann, T., and Chubanov, V. (2014). Activation of TRPM7 channels by small molecules under physiological conditions. Pflügers Archiv Eur. J. Physiology 466, 2177–2189. doi:10.1007/s00424-014-1488-0
Im, W. B., and Quandt, F. N. (1992). Mechanism of asymmetric block of K channels by tetraalkylammonium ions in mouse neuroblastoma cells. J. Membr. Biol. 130, 115–124. doi:10.1007/BF00231890
Kerschbaum, H. H., Kozak, J. A., and Cahalan, M. D. (2003). Polyvalent cations as permeant probes of MIC and TRPM7 pores. Biophys. J. 84, 2293–2305. doi:10.1016/S0006-3495(03)75035-8
Kimura, N., Masuda, S., Katsura, T., and Inui, K. (2009). Transport of guanidine compounds by human organic cation transporters, hOCT1 and hOCT2. Biochem. Pharmacol. 77, 1429–1436. doi:10.1016/j.bcp.2009.01.010
Komiya, Y., and Runnels, L. W. (2015). TRPM channels and magnesium in early embryonic development. Int. J. Dev. Biol. 59, 281–288. doi:10.1387/ijdb.150196lr
Kozak, J. A., and Cahalan, M. D. (2001). Pharmacological properties of the 40 pS monovalent current in T cells and RBL cells. Soc. Neurosci. Abstr. 27.
Kozak, J. A., and Cahalan, M. D. (2003). MIC channels are inhibited by internal divalent cations but not ATP. Biophys. J. 84, 922–927. doi:10.1016/S0006-3495(03)74909-1
Kozak, J. A., Kerschbaum, H. H., and Cahalan, M. D. (2002). Distinct properties of CRAC and MIC channels in RBL cells. J. Gen. Physiol. 120, 221–235. doi:10.1085/jgp.20028601
Kozak, J. A., Matsushita, M., Nairn, A. C., and Cahalan, M. D. (2005). Charge screening by internal pH and polyvalent cations as a mechanism for activation, inhibition, and rundown of TRPM7/MIC channels. J. Gen. Physiol. 126, 499–514. doi:10.1085/jgp.200509324
Li, M., Du, J., Jiang, J., Ratzan, W., Su, L. T., Runnels, L. W., et al. (2007). Molecular determinants of Mg2+ and Ca2+ permeability and pH sensitivity in TRPM6 and TRPM7. J. Biol. Chem. 282, 25817–25830. doi:10.1074/jbc.M608972200
Liang, H. Y., Chen, Y., Wei, X., Ma, G. G., Ding, J., Lu, C., et al. (2022). Immunomodulatory functions of TRPM7 and its implications in autoimmune diseases. Immunology 165, 3–21. doi:10.1111/imm.13420
Matsushita, M., Kozak, J. A., Shimizu, Y., Mclachlin, D. T., Yamaguchi, H., Wei, F. Y., et al. (2005). Channel function is dissociated from the intrinsic kinase activity and autophosphorylation of TRPM7/ChaK1. J. Biol. Chem. 280, 20793–20803. doi:10.1074/jbc.M413671200
Mellott, A., Rockwood, J., Zhelay, T., Luu, C. T., Kaitsuka, T., and Kozak, J. A. (2020). TRPM7 channel activity in Jurkat T lymphocytes during magnesium depletion and loading: implications for divalent metal entry and cytotoxicity. Pflügers Archiv Eur. J. Physiology 472, 1589–1606. doi:10.1007/s00424-020-02457-3
Monteilh-Zoller, M. K., Hermosura, M. C., Nadler, M. J., Scharenberg, A. M., Penner, R., and Fleig, A. (2003). TRPM7 provides an ion channel mechanism for cellular entry of trace metal ions. J. Gen. Physiol. 121, 49–60. doi:10.1085/jgp.20028740
Nadolni, W., and Zierler, S. (2018). The Channel-kinase TRPM7 as novel regulator of immune system homeostasis. Cells 7, 109. doi:10.3390/cells7080109
Numata, T., Shimizu, T., and Okada, Y. (2007). TRPM7 is a stretch- and swelling-activated cation channel involved in volume regulation in human epithelial cells. Am. J. Physiol. Cell Physiol. 292, C460–C467. doi:10.1152/ajpcell.00367.2006
Okazaki, T., Ilea, V., Okazaki, A., Reisman, R. E., and Arbesman, C. E. (1975). The effect of Tris-buffered media and Tyrode physiologic saline solution on the antigenic release of histamine from human leukocytes. J. Allergy Clin. Immunol. 56, 27–32. doi:10.1016/0091-6749(75)90031-7
Oliver, D., Baukrowitz, T., and Fakler, B. (2000). Polyamines as gating molecules of inward-rectifier K+ channels. Eur. J. Biochem. 267, 5824–5829. doi:10.1046/j.1432-1327.2000.01669.x
Ong, S. T., Ng, A. S., Ng, X. R., Zhuang, Z., Wong, B. H. S., Prasannan, P., et al. (2019). Extracellular K(+) dampens T cell functions: implications for immune suppression in the tumor microenvironment. Bioelectricity 1, 169–179. doi:10.1089/bioe.2019.0016
Pelis, R. M., Hartman, R. C., Wright, S. H., Wunz, T. M., and Groves, C. E. (2007). Influence of estrogen and xenoestrogens on basolateral uptake of tetraethylammonium by opossum kidney cells in culture. J. Pharmacol. Exp. Ther. 323, 555–561. doi:10.1124/jpet.107.126748
Prutting, S., and Grissmer, S. (2011). A novel current pathway parallel to the central pore in a mutant voltage-gated potassium channel. J. Biol. Chem. 286, 20031–20042. doi:10.1074/jbc.M110.185405
Qin, X., Yue, Z., Sun, B., Yang, W., Xie, J., Ni, E., et al. (2013). Sphingosine and FTY720 are potent inhibitors of the transient receptor potential melastatin 7 (TRPM7) channels. Br. J. Pharmacol. 168, 1294–1312. doi:10.1111/bph.12012
Rae, J., Cooper, K., Gates, P., and Watsky, M. (1991). Low access resistance perforated patch recordings using amphotericin B. J. Neurosci. Methods 37, 15–26. doi:10.1016/0165-0270(91)90017-t
Rauer, H., and Grissmer, S. (1996). Evidence for an internal phenylalkylamine action on the voltage-gated potassium channel Kv1.3. Mol. Pharmacol. 50, 1625–1634.
Ryazanova, L. V., Dorovkov, M. V., Ansari, A., and Ryazanov, A. G. (2004). Characterization of the protein kinase activity of TRPM7/ChaK1, a protein kinase fused to the transient receptor potential ion channel. J. Biol. Chem. 279, 3708–3716. doi:10.1074/jbc.M308820200
Ryazanova, L. V., Rondon, L. J., Zierler, S., Hu, Z., Galli, J., Yamaguchi, T. P., et al. (2010). TRPM7 is essential for Mg(2+) homeostasis in mammals. Nat. Commun. 1, 109. doi:10.1038/ncomms1108
Schmitz, C., Brandao, K., and Perraud, A. L. (2014). The channel-kinase TRPM7, revealing the untold story of Mg(2+) in cellular signaling. Magnes. Res. 27, 9–15. doi:10.1684/mrh.2014.0357
Takahashi, K., Umebayashi, C., Numata, T., Honda, A., Ichikawa, J., Hu, Y., et al. (2018). TRPM7-mediated spontaneous Ca(2+) entry regulates the proliferation and differentiation of human leukemia cell line K562. Physiol. Rep. 6, e13796. doi:10.14814/phy2.13796
Villarroel, A., Alvarez, O., Oberhauser, A., and Latorre, R. (1988). Probing a Ca2+-activated K+ channel with quaternary ammonium ions. Pflügers Archiv Eur. J. Physiology 413, 118–126. doi:10.1007/BF00582521
Vinnenberg, L., Bock, S., Hundehege, P., Ruck, T., and Meuth, S. G. (2021). Impact of diverse ion channels on regulatory T cell functions. Cell Physiol. Biochem. 55, 145–156. doi:10.33594/000000375
Visser, D., Middelbeek, J., Van Leeuwen, F. N., and Jalink, K. (2014). Function and regulation of the channel-kinase TRPM7 in health and disease. Eur. J. Cell Biol. 93, 455–465. doi:10.1016/j.ejcb.2014.07.001
Wright, S. H., Evans, K. K., Zhang, X., Cherrington, N. J., Sitar, D. S., and Dantzler, W. H. (2004). Functional map of TEA transport activity in isolated rabbit renal proximal tubules. Am. J. Physiol. Ren. Physiol. 287, F442–F451. doi:10.1152/ajprenal.00115.2004
Wykes, R. C., Lee, M., Duffy, S. M., Yang, W., Seward, E. P., and Bradding, P. (2007). Functional transient receptor potential melastatin 7 channels are critical for human mast cell survival. J. Immunol. 179, 4045–4052. doi:10.4049/jimmunol.179.6.4045
Yamaguchi, H., Matsushita, M., Nairn, A. C., and Kuriyan, J. (2001). Crystal structure of the atypical protein kinase domain of a TRP channel with phosphotransferase activity. Mol. Cell 7, 1047–1057. doi:10.1016/s1097-2765(01)00256-8
Yu, J., Yool, A. J., Schulten, K., and Tajkhorshid, E. (2006). Mechanism of gating and ion conductivity of a possible tetrameric pore in aquaporin-1. Structure 14, 1411–1423. doi:10.1016/j.str.2006.07.006
Keywords: lymphocyte, channel-kinase, HEK293, tetramethylammonium ion, tetraethylammonium ion, Tris hydrochloride, Jurkat T cell, magnesium
Citation: Holderby KG and Kozak JA (2024) Use of tetraethylammonium (TEA) and Tris loading for blocking TRPM7 channels in intact cells. Front. Pharmacol. 15:1341799. doi: 10.3389/fphar.2024.1341799
Received: 22 November 2023; Accepted: 26 March 2024;
Published: 10 April 2024.
Edited by:
Heike Wulff, University of California, Davis, United StatesReviewed by:
Vaibhavkumar S. Gawali, Frontage Laboratories, Inc., United StatesThomas Gudermann, Ludwig Maximilian University of Munich, Germany
Copyright © 2024 Holderby and Kozak. This is an open-access article distributed under the terms of the Creative Commons Attribution License (CC BY). The use, distribution or reproduction in other forums is permitted, provided the original author(s) and the copyright owner(s) are credited and that the original publication in this journal is cited, in accordance with accepted academic practice. No use, distribution or reproduction is permitted which does not comply with these terms.
*Correspondence: J. Ashot Kozak, juliusz.kozak@wright.edu