- 1Infectious Diseases Research Center (IDRC), Arak University of Medical Sciences, Arak, Iran
- 2Department of Genetics, Faculty of Advanced Science and Technology, Tehran Medical Sciences, Islamic Azad University, Tehran, Iran
- 3Department of Microbiology, School of Medicine, Hamadan University of Medical Sciences, Hamadan, Iran
- 4Department of Microbiology, School of Medicine, Iran University of Medical Sciences, Tehran, Iran
- 5Molecular and Medicine Research Center, Khomein University of Medical Sciences, Khomein, Iran
Pseudomonas aeruginosa biofilm is a community of bacteria that adhere to live or non-living surfaces and are encapsulated by an extracellular polymeric substance. Unlike individual planktonic cells, biofilms possess a notable inherent resistance to sanitizers and antibiotics. Overcoming this resistance is a substantial barrier in the medical and food industries. Hence, while antibiotics are ineffective in eradicating P. aeruginosa biofilm, scientists have explored alternate strategies, including the utilization of natural compounds as a novel treatment option. To this end, curcumin, carvacrol, thymol, eugenol, cinnamaldehyde, coumarin, catechin, terpinene-4-ol, linalool, pinene, linoleic acid, saponin, and geraniol are the major natural compounds extensively utilized for the management of the P. aeruginosa biofilm community. Noteworthy, the exact interaction of natural compounds and the biofilm of this bacterium is not elucidated yet; however, the interference with the quorum sensing system and the inhibition of autoinducer production in P. aeruginosa are the main possible mechanisms. Noteworthy, the use of different drug platforms can overcome some drawbacks of natural compounds, such as insolubility in water, limited oral bioavailability, fast metabolism, and degradation. Additionally, drug platforms can deliver different antibiofilm agents simultaneously, which enhances the antibiofilm potential of natural compounds. This article explores many facets of utilizing natural compounds to inhibit and eradicate P. aeruginosa biofilms. It also examines the techniques and protocols employed to enhance the effectiveness of these compounds.
1 Introduction
Most bacteria in nature, including those that cause hospital-acquired illnesses, exist in the form of biofilms, which are communities of microorganisms attached to surfaces (Persat et al., 2015). Biofilm communities are surrounded by a strong matrix consisting of proteins, extracellular DNA, and polysaccharides. This matrix makes them less vulnerable to antimicrobial drugs and the immune system (Walsh et al., 2019a). The concept of bacterial biofilm was initially introduced in 1987. It refers to a population of microorganisms that can attach to surfaces and produce an exopolysaccharide and extracellular matrix (Chegini et al., 2020). Biofilms readily develop on foreign objects introduced into the body, such as intravascular and urinary catheters, prosthetic valves, intrauterine devices, contact lenses, and other foreign objects, as well as on surfaces within the body (Shariati et al., 2022). Biofilms exhibit significantly higher resistance to antibiotics, ranging from 10 to 1,000 times, compared to individual planktonic cells. This enhanced resistance is mostly attributed to the limited ability of antibiotics to penetrate the intricate polysaccharide matrix, known as glycocalyx, present in biofilms (Spoering and Lewis, 2001).
Pseudomonas aeruginosa is a Gram-negative and rod-shaped bacterium with a pronounced tendency to form biofilms. It is also an opportunistic pathogen that exhibits multi-drug resistance (MDR) (Walsh et al., 2019a). Infection with this bacterium results in diseases characterized by a significant death rate in individuals suffering from cystic fibrosis, cancer, severe burns, and immunocompromised patients (Bahramian et al., 2019). This bacterium exhibits the ability to persist on water, various surfaces, and medical devices through the utilization of its potent adhesive elements, including flagella, pili, and biofilms. P. aeruginosa is highly prevalent in both natural and man-made settings, including lakes, hospitals, and domestic sink drains (Remold et al., 2011). The rising prevalence of this bacteria in generating diverse diseases and its contribution to the rise of antibiotic resistance has led to a significant global issue of treatment failure. P. aeruginosa exhibits substantial inherent resistance to many antibiotics, such as beta-lactams, fluoroquinolones, and aminoglycosides, leading to notable rates of morbidity and mortality (Breidenstein et al., 2011).
Therefore, the presence of biofilms and the intrinsic and acquired antibiotic resistance mechanisms of P. aeruginosa have led to a rise in the occurrence of MDR strains in recent years, with very few medications that are completely effective in halting the growth of this bacterium. Moreover, numerous antibacterial medications are now linked to constraints such as heightened resistance, limited antibacterial range, toxicity, and elevated treatment expenses for patients (Lone and Ahmad, 2020). To this end, scientists are seeking novel methods to impede the growth of P. aeruginosa biofilms (Lou et al., 2015). The utilization of natural compounds as novel and efficacious medicines has acquired a prominent position in clinical therapies (Al-khazraji et al., 2022; Alarkwazi et al., 2022; Hossain et al., 2022; Salman, 2022). Research has demonstrated that plant-derived substances, such as essential oils (EOs), extracts, and pure chemicals, have notable impacts on bacteria that are resistant to treatment and capable of producing biofilms (Ahmad et al., 2015).
The natural compounds derived from various plant components, including roots, leaves, and fruits, provide therapeutic attributes that exhibit distinct medicinal effects upon modification (Rangel et al., 2018). Plant extracts have been examined for their inherent antioxidant, antibacterial, anti-inflammatory, and antiseptic properties, as well as their role as precursors in the production of medicinal compounds (Radulović et al., 2013). In this regard, this review article will examine various aspects of the interaction between natural compounds with the quorum sensing (QS) system and the biofilm community of P. aeruginosa. Additionally, it will explore different drug delivery platforms that can enhance the therapeutic effectiveness of natural compounds and promote their extensive utilization in clinical practice.
2 Quorum sensing in P. aeruginosa
QS is a bacterial communication system that is dependent on cell density. It allows P. aeruginosa to coordinate with different determinants of virulence factors, which helps the bacteria colonize the host and develop resistance to antibiotics. QS is a system that operates on the principle of signal-response. In this system, the levels of secreted signal molecules, known as autoinducers (AIs), rise as the population density increases. These AIs are then recognized by transcriptional regulators that control specific genes (Davies et al., 1998). P. aeruginosa mainly possesses two QS systems: lasI/lasR and rhlI/rhlR. In these systems, lasI and rhlI (signal synthase genes) synthesize AIs like N-(3-oxododecanoyl)- L-homoserine lactone (OdDHL) and N-butyryl-L-Homoserine lactone (BHL), respectively. These AIs are then specifically recognized by intracellular receptors, LasR and RhlR. P. aeruginosa possesses an additional QS system known as Pqs, which specifically detects the Pseudomonas quinolone signal (PQS, 2-heptyl-3-hydroxy-4(1H)-quinolone). This system interacts with the transcriptional regulator PqsR (MvfR). Upon stimulation by their endogenous signals, these receptors will form homodimers and function as transcription factors to control the expression of the specified gene(s). Under normal circumstances, the las system is commonly considered the primary controller of the QS systems in P. aeruginosa and triggers the activation of both the Rhl and Pqs circuits (Lee and Zhang, 2015).
The DNA-binding transcription regulator LasR activates the production of the rhl and pqs, QS circuits, as well as virulence factors including lasA (staphylolytic), lasB (elastase), toxA (exotoxin A), aprA (alkaline protease), and hcnABC (hydrogen cyanide synthase). Therefore, under normal circumstances, the las system has traditionally been considered the primary controller of the QS systems in P. aeruginosa. Within this bacterium, the second QS pathway, known as the Rhl system, triggers the activation of the rhlI gene (responsible for signal synthesis), resulting in the production of BHL, which is recognized by the RhlR receptor. The BHL-RhlR complex serves as the transcriptional activator for various genes, including rpoS (a sigma factor associated with stationary phase), pqs, rhlAB (genes involved in rhamnolipid synthesis), lecA (a type I lectin), and lecB (a type II lectin) (Kim et al., 2015; Rathinam et al., 2017).
P. aeruginosa employs N-acyl homoserine lactone (AHL) and non-AHL-mediated cell density-dependent complicated QS signaling to control its virulence. To achieve this objective, AHL-driven QS consists of two hierarchical subsystems known as LasI/R and RhlI/R (Gambello and Iglewski, 1991; Pearson et al., 1995). The LasI/R and RhlI/R systems employ 3-oxo-C12-HSL and C4-HSL, respectively, to control the expression of approximately 10% of the genes in the P. aeruginosa genome. These genes control important virulence factors, including elastase, protease, hydrogen cyanide, pyocyanin, lipase, pyoverdin, rhamnolipid, swarming, and biofilm formation (Schuster et al., 2003; Wagner et al., 2003). Furthermore, P. aeruginosa employs two intercellular signal molecules, namely, 2-heptyl-3-hydroxy-4-quinolone (PQS) and 2-(2-hydroxyphenyl)-thiazole-4-carbaldehyde (IQS), which are not AHL-based, to control its fundamental pathogenicity (Tang et al., 1996; Pesci et al., 1999; Smith and Iglewski, 2003; Lee et al., 2013; Sethupathy et al., 2016).
Therefore, as mentioned, given the substantial role of the QS mechanism in microbial pathogenicity, inhibiting QS serves as an alternate strategy for managing nosocomial infections associated with biofilms. Anti-QS drugs, both synthetic and natural, have demonstrated efficacy in combating P. aeruginosa infections by reducing the thickness of biofilms and diminishing pathogenicity (Kumar et al., 2009). It is noteworthy to mention that QS suppression primarily happens through two methods: signal degradation and signal mimicking. These mechanisms lead to the inhibition of genetic control systems and the disruption of downstream virulence and biofilm genes (Ni et al., 2009; Rathinam et al., 2017). QS/biofilm inhibitors have been observed to impose limited or no selection pressure on infections to develop resistance. This is because they specifically target the production of virulence factors and the creation of biofilms without significantly affecting the growth of the pathogens. Therefore, it is crucial to identify and assess novel non-toxic substances that can effectively combat biofilm infections by inhibiting bacterial communication and biofilm formation. Various natural compounds have a considerable impact on different aspects of QS in P. aeruginosa. In the following part, we will explore the interactions between natural compounds and the QS system in this bacterium.
3 Curcumin
Curcumin is a curcuminoid compound that is present in Curcuma longa, a medicinal plant from the ginger family. Curcumin is widely regarded as a remarkable phytochemical due to its anti-Alzheimer, anticancer, apoptotic, anti-inflammatory, and antioxidant activities. In addition, curcumin is renowned for its antibacterial and antifungal characteristics, making it a popular ingredient in traditional medicine in India, China, and other Southeast-Asian nations. Numerous reports indicated that this remarkable phytochemical can also function as QS inhibitors, as demonstrated in studies with Candida albicans and Aeromonas hydrophila (Neyestani et al., 2019; Tanhay Mangoudehi et al., 2020). To this end, scientists are interested in employing this compound for degrading P. aeruginosa biofilm.
Sethupathy et al. (2016) found that curcumin reduces the activity of QS and the production of biofilms by disrupting the balance of iron levels and the response to oxidative stress in P. aeruginosa. This study found that curcumin modulates proteins responsible for the detoxification of reactive oxygen species (ROS), iron uptake, the tricarboxylic acid cycle, and the synthesis of pyoverdin and pyocyanin. Consequently, the authors propose that curcumin regulates the response to oxidative stress and the acquisition of iron in P. aeruginosa PAO1 strain by reducing the activity of antioxidant enzymes such as catalase, superoxide dismutases (SOD), peroxidase, ferroxidase, etc. This inhibition leads to the prevention of biofilm formation and the controlled production of protease, elastase, and pyocyanin through QS. Another study has identified curcumin as a QS-antagonist, which can effectively inhibit the generation of extracellular polymeric substances (EPS), biofilm, pyocyanin, and rhamnolipid, along with improving the susceptibility to antibiotics (Shukla et al., 2021). Therefore, the inhibition of QS by curcumin could inhibit the virulence factor and biofilm formation of P. aeruginosa.
To this end, the inhibitory effect of curcumin on QS and inhibition of the P. aeruginosa biofilm community were reported in other studies (Rudrappa and Bais, 2008; Packiavathy et al., 2014; Bali et al., 2019; Shukla et al., 2020; Fernandes et al., 2023). For instance, a recently published study reported that curcumin has inhibitory effects on the LuxS/AI-2 and LasI/LasR QS systems, resulting in a reduction of 33%–77% and 21%, respectively. Significantly, curcumin (at a concentration of 200 μg/mL) resulted in a 21% decrease in the generation of 3-oxo-C12-HSL by P. aeruginosa PA14 strain (Fernandes et al., 2023). Additionally, Rudrappa and Bais (2008) found that curcumin decreased the overall production of AHLs in PAO1 bacteria, and its supplementation resulted in a decrease in bacterial pathogenicity. Similarly, the introduction of external AHL along with curcumin reversed the harmful effects of the bacteria, resulting in the restoration of greater levels of bacterial populations. These observations suggest that curcumin may affect the QS responses in PAO1 by inhibiting the synthesis of AHL or preventing the reception of QS signals.
Noteworthy, curcumin exhibits a significant structural resemblance to AHLs. Curcumin has aromatic ring structures that consist of phenols and are linked by two α, β-unsaturated carbonyl groups. The phenolic component of curcumin imitates the lactone component of AHL. The ketone moiety present in curcumin is structurally identical to the one observed in AHLs (Packiavathy et al., 2012; Packiavathy et al., 2014). To this end, the results of an in silico investigation showed that curcumin can form bonds with both LasR and LuxR through distinct combinations of hydrogen bonding and hydrophobic interactions. These interactions can result in the deactivation of both proteins, enabling this plant-derived organic AHL antagonist to be categorized as a QS inhibitor (Shukla et al., 2020). In line with these results, another in silico study also found that the LasR protein has the highest binding affinities for curcumin (Bajire et al., 2023).
Therefore, it could be concluded that the spontaneous interaction between curcumin and AHL receptors can modify their structure, preventing them from activating a group of genes that are responsible for curcumin’s role as an inhibitor of QS (Shukla et al., 2021). Recently published study showed that the process of forming biofilms in various species is controlled by QS regulation. As previously stated, curcumin hindered the development of biofilm by disrupting the signal molecule-dependent QS mechanism. Considering the prevalence of signal-mediated QS systems, it has been discovered that disrupting the QS system could potentially hinder the generation of signal-mediated biofilms and the resulting biofilm-related illnesses. Therefore, curcumin can be utilized as an anti-pathogenic substance, specifically targeting P. aeruginosa, due to its ability to inhibit QS. Nevertheless, curcumin, like other natural chemicals, has restricted applicability in clinical settings due to several disadvantages, including insolubility in water, limited oral bioavailability, fast metabolism, and degradation, as well as inadequate absorption from the gut and low blood plasma levels (Shariati et al., 2019).
In this regard, researchers have been interested in using nano-curcumin and various drug delivery platforms for enhancing curcumin-antibiofilm efficacy. Notably, the higher solubility and longer half-life of nano-curcumin compared to curcumin and its higher resistance to enzymatic hydrolysis have been reported in recently published studies. Nano-curcumin exhibits more tissue reabsorption and a prolonged half-life compared to curcumin. Consequently, this molecule has been extensively studied for its potential impact on biofilm formation. The bioavailability and solubility of nano-curcumin are superior to those of curcumin. This improved water solubility can be attributed to the greater surface area of nano-curcumin, which facilitates dissolution (Shariati et al., 2019; Sharifian et al., 2020; Rahimi et al., 2023). To this end, different methods, such as the wet-milling technique and the ultrasonic homogenizer sonication method, were used for the production of nano-curcumin, and it was reported that the synthesized nanoparticles could significantly inhibit P. aeruginosa biofilm (Shariati et al., 2019; Sharifian et al., 2020; Rahimi et al., 2023). Additionally, the combined usage of nano-curcumin and other antibacterial agents, such as natural compounds and other nanoparticles, also showed a synergistic effect against P. aeruginosa biofilm (Loo et al., 2016; Rahimi et al., 2023). Xue et al. (2020) also fabricated nano-curcumin using solution-enhanced dispersion by supercritical CO2, and the results showed better anti-P. aeruginosa activity than the original curcumin. Therefore, nano-curcumin, in comparison to the original curcumin, not only has an improved dissolution rate and bioavailability, but also has improved antibacterial activity against the P. aeruginosa biofilm community.
In addition to nano-curcumin, different drug platforms were also used for the enhancement of the antibiofilm activity of curcumin. In one study that was published in 2021, curcumin was loaded onto a bioactive bone substitute (hydroxyapatite, HA) using a single-step precipitation process. The release of curcumin from HA was limited, indicating the presence of robust hydrogen bonding and van der Waals contacts between curcumin molecules and calcium ions of HA, which impeded the release of curcumin. Although the curcumin release was modest, the curcumin-functionalized HA successfully prevented bacterial cell attachment and the subsequent stages of biofilm generation in P. aeruginosa. The findings indicated that the quantity of curcumin released from HA was adequate to hinder the formation of biofilm and regulate the count of viable planktonic cells concurrently (Lee et al., 2021). Furthermore, other studies also used curcumin-based drug platforms for inhibition of P. aeruginosa biofilm (Table 1). Nanocarriers and drug platforms enhance the solubility and transportation of curcumin in bacterial suspensions, hence intensifying its antibacterial efficacy. Moreover, the utilization of a drug platform can result in a prolonged and controlled release of curcumin, reducing the required dosage of this chemical and improving its therapeutic efficacy.
It should be noted that when we use a drug platform, in addition to curcumin, other compounds can also be used along with curcumin. For instance, Sadeghi Mohammadi et al. (2022) used gentamicin- and curcumin-loaded lipid-polymer hybrid nanoparticles for inhibition of the P. aeruginosa biofilm community. The findings demonstrated that synthesized nanoparticles have excellent antibacterial activity compared to pure curcumin at the same concentration, due to the increased solubility of curcumin with nanoparticles. Additionally, simultaneous usage of curcumin and gentamycin enhanced antibacterial activity. The hybrid nanoparticles exhibit a notable increase in both anti-inflammatory and antibacterial effectiveness when hydrophilic gentamicin and hydrophobic curcumin are used as the drug molecules for loading. Altogether, stimuli-responsive nano-systems can enhance the delivery of drugs to specific targets with spatial and temporal control. This has the potential to be beneficial for treating biofilm-related infections using topical therapy. Curcumin should be regarded as a potential co-delivery system for dual medicines with both hydrophilic and hydrophobic properties. This approach can boost antibacterial activities and effectively cure chronic and persistent illnesses associated with biofilm formation.
Finally, it is noteworthy to mention that curcumin was used in photodynamic therapy (PDT) for the reduction of bacterial biofilm. PDT is an effective method for combating antibiotic-resistant bacteria without the need for intrusive procedures. PDT utilizes non-toxic dyes and innocuous visible light to generate ROS, which specifically induces the death of microbial cells. Curcumin was employed as a photosensitizer in recently conducted research, targeting both the planktonic and biofilm forms of P. aeruginosa (Abdulrahman et al., 2020; Mirzahosseinipour et al., 2020; Zangirolami et al., 2020; Ghasemi et al., 2021; Silva et al., 2023). The use of curcumin, then PDT, reduced the number of viable bacteria (Ghasemi et al., 2021). Noteworthy, curcumin, acting as a photosensitizer, can penetrate the bacterial cell. Subsequently, when exposed to light of a specific wavelength, the photosensitizer molecules become activated and transfer their energy to the nearby molecular oxygen, leading to the production of ROS. ROS can interact with crucial molecular constituents of cells, such as DNA, membrane components, lipids, and proteins, resulting in a retardation of growth and ultimately the demise of the cell (Sánchez-López et al., 2020; Ghasemi et al., 2021). Abdulrahman et al. (2020) also reported that curcumin, when exposed to 405 nm laser light, decreased the synthesis of EPS by P. aeruginosa biofilm, therefore inhibiting biofilm formation. Furthermore, following curcumin-mediated PDT, it was observed that the genes associated with QS were downregulated. Additionally, a higher intensity of light resulted in a significant decrease in gene expression. On the other hand, Silva et al. (2023) documented that PDT serves as an antibacterial substitute; however, the arrangement of the biofilm poses challenges for photodynamic penetration. In addition, P. aeruginosa is a Gram-negative bacterium that impedes the interactions between the cell wall and molecules. In this particular scenario, the application of sonodynamic therapy utilizing ultrasound waves can mechanically break the biofilm. When combined with curcumin as a sonosensitizer, this process results in the generation of ROS. However, it is important to note that the level of ROS produced is insufficient for complete elimination of the microbes. Therefore, the authors reported that sonophotodynamic inactivation, the combination of photodynamic and sonodynamic, using curcumin could be a much more efficient approach for biofilm inactivation. However, this is a preliminary study, and more confirmatory studies are needed in this field.
Collectively, the recently published studies introduce curcumin as a sensitizer that can function as a photo and sonosensitizer, leading to highly effective inactivation of the P. aeruginosa biofilm. Curcumin-induced antimicrobial-PDT effectively hinders the production of biofilms by generating ROS. The regulation of biofilm formation may involve the reduction of the QS system in P. aeruginosa, leading to the elimination of EPS. Therefore, curcumin-mediated PDT has the potential to be a viable therapeutic method for controlling P. aeruginosa biofilm-induced infections. Further research should be conducted to explore the effectiveness of this treatment in managing biofilm communities of drug-resistant bacteria.
4 Cinnamaldehyde
Cinnamaldehyde, a primary constituent of cinnamomum, accounts for approximately 65% of its composition. Its antimicrobial activity may be attributed to the presence of an acrolein group (α, β-unsaturated carbonyl moiety). Despite its potent impact on pathogen infections, cinnamaldehyde is not susceptible to typical antibiotic resistance mechanisms (Bae et al., 1992). Recently, scientists have become interested in utilizing cinnamomum and its derivative components, particularly cinnamaldehyde, not only for their antibacterial properties but also for their ability to hinder microbial biofilm formation. Cinnamaldehyde effectively eradicated P. aeruginosa biofilms after exhibiting its antibacterial action through several routes (Firmino et al., 2018; Ferro et al., 2019; Kosari et al., 2020).
The principal mechanism by which cinnamaldehyde inhibits the formation of P. aeruginosa biofilm is through the reduction of QS. Recent reviews indicate that the sub-inhibitory concentration of cinnamaldehyde, without having any bactericidal effect, resulted in the suppression of both the las and rhl systems by inhibiting the activity of the regulatory proteins LasR and RhlR. This effect not only reduced the production of extracellular virulence agents such as elastase, pyocyanin, and protease but also repressed the expression of the rhamnolipid gene and impeded biofilm formation in P. aeruginosa PAO1. This study did not identify the precise mechanism by which cinnamaldehyde inhibits QS; however, the authors put forward the hypothesis that this chemical functions as a QS antagonist (Ahmed et al., 2019).
To this end, the synergistic effect of cinnamaldehyde and conventional antibiotics was investigated to eradicate P. aeruginosa biofilm by inhibiting QS. A recent study found that cinnamaldehyde suppressed the expression of lasB, rhlA, and pqsA, indicating its ability to inhibit QS. The concurrent application of cinnamaldehyde and tobramycin demonstrated potent inhibitory effects on QS. In addition, the utilization of combination therapy showed an additional impact of cinnamaldehyde when administered in conjunction with tobramycin and colistin, resulting in the inhibition of PAO1 biofilm growth and the dispersion of preexisting biofilm. This effect was more pronounced compared to the use of each treatment individually (Topa et al., 2020). In line with these findings, Brackman et al. used a P. aeruginosa mutant incapable of QS (due to a disruption of both rhlI and lasI, this strain cannot produce AHL signaling molecules). In comparison to the wild type, this mutant exhibited a significantly higher susceptibility to tobramycin, thereby confirming the involvement of a functional QS system in resistance. Furthermore, although cinnamaldehyde caused the wild-type strain to become more vulnerable to tobramycin, this effect was not detected in the QS mutant strain. This suggests that the cinnamaldehyde impact is dependent on the presence of a functional QS system. Therefore, these data indicate that the combination of tobramycin and cinnamaldehyde could enhance the effectiveness of a treatment by improving the ability of tobramycin to combat infections caused by biofilms (Brackman et al., 2011).
Additionally, the combination of cinnamaldehyde and gentamycin demonstrated a synergistic effect and showed potential action against QS. This combination therapy demonstrated the ability to inhibit the generation of AHL and reduce the expression of important QS genes in P. aeruginosa PAO1. The molecular docking analysis demonstrated robust binding interactions between the QS receptors and cinnamaldehyde, confirming its significant potential as a QS modulator. This dual treatment effectively eliminated pre-existing biofilms and suppressed the creation of new biofilms by disrupting the production of EPS (Chadha et al., 2022). Noteworthy, the authors suggested that the synergistic interaction between the drugs can be related to their unique mechanisms of action. Cinnamaldehyde causes membrane rupture and oxidative damage, while gentamycin inhibits bacterial protein production. This renders the bacterial cell more vulnerable as the medications can simultaneously target two separate cellular areas. In addition, cinnamaldehyde efficiently inhibits the QS circuits in P. aeruginosa by reducing the expression of QS genes and preventing the production of AHL molecules (Shaw and Wuest, 2020; Chadha et al., 2022). The mentioned function of cinnamaldehyde was attributed to its potent interaction with the QS receptors of P. aeruginosa. As a result, this reduces the activity of the virulence factors and the creation of biofilms associated with the QS system, consequently decreases the pathogenesis of the bacterium. Furthermore, the addition of gentamycin increases the effectiveness of inhibiting QS and enhances the ability of cinnamaldehyde to reduce virulence (Chadha et al., 2022).
In another study, Kart et al. (2021a) reported that the combined use of cinnamaldehyde and ciprofloxacin demonstrated a greater decrease in the minimum biofilm eradication concentration compared to the use of ciprofloxacin alone. The scientists found that cinnamaldehyde hindered the process of QS and the creation of alginate. As a result, it prevented the formation of biofilms by PAO1 and enhanced the effectiveness of ciprofloxacin in combating biofilms. As a result of these findings, there is a possibility that cinnamaldehyde could enhance the effectiveness of antibiotic treatment in combination therapy by reducing QS and thereby increasing the vulnerability of bacterial biofilms to antibiotics. Nevertheless, this hypothesis has not been experimentally verified. Collectively, cinnamaldehyde is a powerful phytochemical that has been shown to effectively combat the virulence of P. aeruginosa. It has the potential to be used alongside conventional antibiotics to develop new intervention methods. However, further comprehensive investigations are necessary to assess its therapeutic effectiveness using in vivo models in order to utilize its potential for combating virulent infections in future clinical applications.
Furthermore, a recent study has revealed that cinnamaldehyde not only inhibits QS but also inhibits the activity of Cyclic di-guanosine monophosphate (c-di-GMP) (Topa et al., 2018). C-di-GMP is recognized as a crucial intracellular signal and secondary messenger that regulates virulence, cell cycle progression, movement, and various other activities, including the life cycle of biofilms in multiple bacterial species (Ryan et al., 2006). To this end, Topa et al. (2018) reported that cinnamaldehyde decreased c-di-GMP expression by 66.2% after 5 h in comparison to the untreated control. To this end, cinnamaldehyde interfered with the transmembrane potential, pre-existing biofilms, and swarming motility of PAO1. The authors proposed that the carbon atoms of cinnamaldehyde could potentially form bonds with nitrogen-containing components, such as proteins, in the cytoplasmic membrane. This interaction could lead to changes in the structure of the proteins and result in a loss of membrane integrity. Nevertheless, there is a scarcity of information regarding the connection between cinnamaldehyde and intracellular c-di-GMP levels. Consequently, the precise chemical mechanism via which cinnamaldehyde influences variations in c-di-GMP levels is still unknown. Therefore, although the specific mechanism by which cinnamaldehyde counteracts the QS system is not yet understood, it seems to function as both a QS and c-di-GMP antagonist. Cinnamaldehyde not only eradicates P. aeruginosa biofilm but also eliminates the bacterium’s virulence factors via blocking QS-related factors and c-di-GMP. This occurrence would enhance the effectiveness of the host’s innate immunity and other antibiofilm agents. The administration of cinnamaldehyde to boost antibiofilm agents has promising potential for future research. However, there is limited understanding of the molecular-level effects of such synergy. Therefore, further investigation is necessary to validate the aforementioned discoveries.
5 Carvacrol
Carvacrol, a phenolic monoterpenoid, is a prominent component found in EOs derived from fragrant herbs like pepperwort (Lepidium flavum), oregano (Origanum vulgare), and thyme (Thymus vulgaris). Carvacrol is utilized as a food preservative, additive, and flavoring, as well as a fragrance in cosmetic items. Carvacrol has been found to exhibit several biological properties, such as antioxidant, anticancer, and antibacterial activity, according to recent studies (Sharifi-Rad et al., 2018; Javed et al., 2021). Carvacrol has been demonstrated to disrupt the cytoplasmic membrane by enhancing its fluidity, resulting in the release of ions, a reduction in the pH gradient across the membrane, and the suppression of Adenosine triphosphate (ATP) synthesis in bacteria (Ultee et al., 2002; Sharifi-Rad et al., 2018). Additionally, the results of recently published studies demonstrated that carvacrol could significantly inhibit the biofilm community of P. aeruginosa (Ceylan and Ugur, 2015; Walsh et al., 2019b; Pazarci et al., 2019; Tapia-Rodriguez et al., 2019; Walczak et al., 2021). For instance, the findings of a study indicated that carvacrol inhibited the formation of biofilms by up to 74%–88% for P. aeruginosa and decreased the enzyme activity of the biofilm by up to 40%–100% (Walczak et al., 2021). A biofilm eradication assay in another study also showed the potential of carvacrol for inhibition of Staphylococcus epidermidis and P. aeruginosa (Walsh et al., 2019b). In addition, Pazarci et al. identified carvacrol as the primary constituent of Mentha longifolia EO. This EO showed efficacy against P. aeruginosa and fungal biofilms that develop on the surface of steel and titanium implants. Hence, the authors suggested that the use of M. longifolia EO could be advantageous in treating infections associated with implant biofilms (Pazarci et al., 2019).
Noteworthy, the precise mechanism of how carvacrol interacts with bacterial biofilm has not been fully understood. However, a docking analysis conducted in a study revealed that this molecule does interact with the QS system in bacteria. The study revealed that when exposed to carvacrol, P. aeruginosa exhibited a 60% decrease in the production of AHLs. Importantly, this reduction in AHLs did not have any impact on the viability of the cells, suggesting a decrease in the activity of the LasI synthase enzyme. Carvacrol decreased the expression of the lasR gene while leaving the lasI gene unaffected. Furthermore, computational docking analysis revealed that carvacrol interacts with certain amino acids located in the active site pocket of LasI and within the binding pocket of LasR. Carvacrol action led to a decrease in AHLs, perhaps impacting LasI activity, resulting in the suppression of the sequence of signals, pathways, and virulence-related gene expression in P. aeruginosa (Tapia-Rodriguez et al., 2019). Therefore, the aforementioned research offers pertinent data regarding the impact of carvacrol on P. aeruginosa biofilm by disrupting the QS system. These discoveries can aid in the advancement of natural anti-QS compounds, which can impact the biofilm community and pathogenicity of P. aeruginosa.
Noteworthy, researchers also explored the synergistic effects of carvacrol in combination with other antibacterial agents for the management of P. aeruginosa biofilm. A study published in 2023 investigated the effectiveness of two proteolytic enzymes, pepsin and trypsin, in breaking down P. aeruginosa biofilms. The study also explored the combination of these enzymes with carvacrol. Pepsin and trypsin, both mammalian digestive enzymes, rely on certain amino acids in the polypeptide chain to break down proteins through hydrolysis. The P. aeruginosa biofilm population experienced a greater reduction when treated with a combination of pepsin or trypsin along with carvacrol compared to treatment with carvacrol alone. The reduction of biofilms was much greater when sequential enzyme treatments were followed by carvacrol treatment, as opposed to employing a single enzyme followed by carvacrol. The enhanced efficiency is also apparent in the analysis of epifluorescence microscopy. It was observed that the combined treatment involving enzymes, either individually or in sequence, followed by carvacrol, demonstrated a synergistic effect. This effect resulted from the enzymes’ ability to disperse biofilms and carvacrol antimicrobial activity. As a result, the biofilms exhibited a greater reduction in terms of both biomass and viability (Mechmechani et al., 2023). It is important to mention that these two enzymes, by potentially interacting with proteins, such as outer membrane proteins in P. aeruginosa, can lead to structural defects in the biofilms and weaken their protective properties. This interaction may enhance the entry of carvacrol and decrease the ability of the cells to survive. Therefore, the aforementioned discoveries indicate that proteins can serve as viable targets for elimination, enabling the successful infiltration of antibiofilm agents like carvacrol to deactivate P. aeruginosa cells within the biofilm (Mechmechani et al., 2023).
Gobin et al. (2022) also reported that the combination of gallic acid and carvacrol exhibits a potent and synergistic effect in eliminating both mono- and dual-species mature biofilms of Staphylococcus aureus and P. aeruginosa. The antibiofilm efficacy of gallic acid may be attributed to alterations in the characteristics of the plasma membrane. Indeed, besides modifying bacterial adhesion, these alterations can result in the release of intracellular constituents. Evidence has demonstrated that gallic acid stimulates the outward flow of potassium ions (K+) from P. aeruginosa. The release of intracellular components, such as ions, can ultimately lead to cell death through various mechanisms, including changes in gene expression, disruption of cellular ion balance, and interference with cell signaling. Moreover, gallic acid possesses the ability to form complexes with Ca2+ and Fe2+, two crucial metals required for bacterial growth and certain enzyme functions (Borges et al., 2013; Sarjit et al., 2015; Gobin et al., 2022). Therefore, gallic acid has the potential to modify the activity of membrane proteins and bacterial iron uptake mechanisms, such as siderophore. Gallic acid possesses an anti-adherence property that can disrupt biofilm, enhancing the diffusion and effectiveness of carvacrol, which in turn permeabilize the bacterial membrane and causes bacterial demise. Hence, the amalgamation of these molecules not only produces an additive effect but also enhances the activity of active molecules, resulting in the synergistic eradication properties of the combination (Gobin et al., 2022).
Another investigation also revealed that the concurrent use of carvacrol and nerol can effectively dismantle the preexisting biofilm structure and enhance the elimination of bacteria after subsequent water rinsing. Nerol is an acyclic monoterpenoid that is the cis-isomer of geraniol. It possesses a main alcohol functional group and has a more pleasant rose odor compared to geraniol (Kasthuri et al., 2022). The exceptional chemical composition of carvacrol and nerol is responsible for their extensive antibacterial efficacy across a wide range of bacteria. Carvacrol has a secondary alcohol known as phenolic alcohol, which is a–OH functional group in the benzene ring. Acyclic monoterpenoid nerol contains the functional group–OH at an acyclic ring structure called primary alcohol (Elsharif and Buettner, 2018). To this end, efficient broad-spectrum antibacterial action is achieved by combining the bactericidal effect of both carvacrol and nerol, which are monocyclic phenols and acyclic primary alcohols, respectively. Furthermore, there is speculation that the combined action of carvacrol and nerol, namely, their–OH groups, effectively depolarizes the cell membrane by dissipating pH and protons (H+ and K+) gradients across it (Kasthuri et al., 2022). Thus, as mentioned, the combined treatment using carvacrol and other antibacterial treatments, including natural products and enzymes, shows potential as a viable strategy for eliminating P. aeruginosa biofilm infections. Implementing carvacrol-based combination therapy would additionally decrease the use of chemical agents, energy expenditures, and water usage required for biofilm management.
Finally, it is noteworthy to mention that different drug platforms and techniques were used for the enhancement of carvacrol activity against bacterial biofilm. Mechmechani et al. (2022b) used the spray drying method, one of the most common microencapsulation techniques, to develop microcapsules containing carvacrol.
The results showed that the minimum inhibitory concentration (MIC) of carvacrol against P. aeruginosa, when encapsulated, was four times lower compared to carvacrol in its free form. Additionally, encapsulated carvacrol was able to reduce biofilm below the detection limit for this bacterium after 15 min of treatment (Mechmechani et al., 2022b). In line with these results, in another study, spray drying method was also used to produce novel microencapsulated (ME) proteases, pepsin and trypsin, and carvacrol. The findings of this study revealed that the sequential treatment in the order ME-trypsin, ME-pepsin, and ME-carvacrol resulted in more efficient biofilm removal with a maximum reduction of 5 log CFU/mL for P. aeruginosa (Mechmechani et al., 2022a). Notably, the pharmaceutical and food industries have extensively employed ME techniques to regulate the release of active compounds, enhance formulation stability, and conceal odors. Moreover, encapsulation aims to safeguard the active chemicals against external elements like light, water, oxygen, pH, etc., thereby preserving their antibacterial efficacy for an extended duration. This approach can hinder the interactions between antimicrobials and the biofilm EPS matrix, resulting in the rejection or retention of the biocide. This prevents the biocide from interacting with microbial cells, allowing for effective disinfection of the deep layers of the biofilm (Dias et al., 2015; Yan et al., 2022). Therefore, the results have demonstrated a favorable potential for utilizing ME carvacrol as a substitute for traditional sanitization approaches in combating biofilms in medical settings. However, before using these encapsulated products as anti-biofilm agents, they must undergo approval and registration by regulatory agencies.
Furthermore, in other studies, the researchers also loaded carvacrol on different materials, including chitosan polymers, antimicrobial polymers, and carvacrol-loaded polylactic acid (PLA) scaffolds. All of the mentioned platforms showed enhanced antibiofilm activity in comparison to the individual compounds. The increased effectiveness of the described drug platforms in preventing the formation of biofilms can be related to the specific transport of carvacrol to the biofilm. This transport is facilitated by the electrostatic contact between the positively charged delivery vehicles, in the form of polymeric micelles, and the negatively charged bacteria. Moreover, chitosan nanoparticles have the potential to enhance the infiltration of carvacrol into the biofilm population of P. aeruginosa (Namivandi-Zangeneh et al., 2020; Bernal-Mercado et al., 2022; Farto-Vaamonde et al., 2022). Therefore, the successful performance of drug platforms in enhancing the antibiofilm activity of carvacrol demonstrates the potential application of these platforms as both therapeutic agents and delivery vehicles for hydrophobic compounds or drugs. These platforms can be used to specifically deliver cargo molecules to the P. aeruginosa biofilm.
6 Eugenol
Eugenol, also known as 2-methoxy-4-[2-propenyl] phenol, is a phenolic aromatic compound primarily obtained from EO found in nutmeg, clove, basil, cinnamon, and bay leaf. It belongs to a unique group of microbiocidal phenylpropanoids and has been extensively utilized as a dental analgesic for a considerable duration (Hassan et al., 2018; Wijesinghe et al., 2021a). Eugenol can be created via guaiacol allylation using allyl chloride or produced through a biotransformation process using microorganisms such as Escherichia coli, Corynebacterium spp., and Bacillus cereus (Abrahão et al., 2013). Eugenol has been documented to exhibit several pharmacological activities, including anti-cancer, anti-inflammatory, analgesic, anesthetic, antioxidant, and antibacterial effects (Kozam, 1977; Reddy and Lokesh, 1994; Hassan et al., 2018; Nisar et al., 2021). Noteworthy, eugenol is a lipophilic molecule and has the potential to effectively pass through the phospholipid bilayer of the cell membrane. This causes changes in the membrane’s fluidity and permeability, ultimately leading to its disruption. Additionally, published data indicates that eugenol can enhance the protein leakage of cell membranes in both Gram-positive and Gram-negative bacteria. The compromised integrity of the cell walls and subsequent cellular leakages ultimately result in the demise of the microbial cell (Marchese et al., 2017; Wijesinghe et al., 2021b).
In addition, recently published studies reported that eugenol could inhibit the biofilm community of P. aeruginosa and the inhibitory effect of eugenol on the QS system of this bacterium is one of the most important possible mechanisms for inhibition of biofilm (Zhou et al., 2013; Rathinam et al., 2017; Rathinam and Viswanathan, 2018; Wijesinghe et al., 2021b; Lahiri et al., 2021; Shariff et al., 2022; Wang et al., 2023). Wang et al. (2023) reported that eugenol can reduce the amount of the QS signaling molecule 3-oxo-C12-homoseine lactone outside of cells by influencing the activity of the efflux pump MexAB-OprM. This indirectly disrupted the bacterial QS mechanism, thereby impeding the production of biofilms. The result of another study also showed that eugenol had a significant impact on the biofilm structure by causing a decrease in the protein and carbohydrate levels of the EPS. In addition, eugenol has the potential to impact the production of QS proteins, specifically LasA and LasB, as well as virulence factors like pyocyanin and rhamnolipid, which significantly impede the formation of biofilm (Lahiri et al., 2021).
In line with these findings, another investigation also reported that eugenol effectively decreased biofilm formation on urinary catheters and inhibited the production of virulence factors such as extracellular polysaccharides, rhamnolipid, elastase, protease, pyoverdin, and pyocyanin. Furthermore, computational docking analysis demonstrated a consistent molecular interaction between eugenol and LasR and RhlR receptors, indicating a strong binding affinity between eugenol and the QS receptors, as compared to the corresponding naturally released signal molecules (autoinducers). An examination of reporter strains has verified that eugenol competitively binds to a QS receptor (LasR), potentially inhibiting QS and resulting in substantial suppression of genes related to QS and virulence factors (Rathinam et al., 2017). Molecular modeling studies published in 2016 also demonstrated that eugenol, the main constituent of clove bud oil, effectively attaches to the QS receptor through hydrophobic interactions and hydrogen bonding with Arg61 and Tyr41, which are important amino acid residues of the LasR receptor (Jayalekshmi et al., 2016). Moreover, it was reported in another experiment that the inhibitory effect of eugenol on QS is likely due to its ability to decrease the ability of LasR transcription factors to detect their specific signal molecules, resulting in a decrease in the expression of quorum-controlled virulence factor genes and signal synthase genes (Rathinam and Viswanathan, 2018).
Finally, Zhou et al. (2013) data showed that eugenol inhibits the las system by reducing both the elastase activity of PAO1 and the transcriptional activation of lasB in E. coli. Moreover, eugenol demonstrated a decrease in both the pyocyanin production of PAO1 and the transcriptional activation of pqsA in E. coli. This suggests that eugenol acts as an inhibitor of the pqs system. By inhibiting the las and pqs systems, which control the expression of multiple genes linked to virulence, the use of eugenol would greatly reduce the virulence of P. aeruginosa. Altogether, according to the docking study and competitive test, it is hypothesized that the anti-QS actions are initiated by eugenol binding to the LasR receptor due to its demonstrated binding stability. Eugenol’s binding to the LasR signal receptor results in the inhibition of its role as a transcriptional activator. Moreover, eugenol most likely inhibited QS by reducing the activity of QS systems such as las, rhl, and pqs. Eugenol not only reduces the production of virulence components such as proteases, elastase, rhamnolipid, and pyocyanin in the biofilm community of P. aeruginosa but also significantly decreases their levels. Thus, it may be inferred that this molecule can impede the operation of various components of QS systems, namely, las, rhl, and pqs, at both the transcriptional and post-translational stages (Wang et al., 2023).
Prior research has indicated that eugenol is susceptible to degradation when exposed to oxygen, light, high temperatures, and humidity. Moreover, this compound exhibits a high degree of volatility, limited solubility in water, and inherent instability. Consequently, the aforementioned drawbacks have the potential to diminish the effectiveness, biological potency, and stability of eugenol (Karmakar et al., 2012; Shao et al., 2018). Therefore, given the drawbacks of eugenol in terms of its solubility, instability, and volatilization, employing various drug delivery methods could serve as a viable solution to address these issues and offer a stable, secure, and efficient antibiofilm agent for clinical application. To achieve this objective, Rathinam et al. applied sol-gel thin films containing eugenol onto urinary catheters and assessed the antibiofilm properties of the eugenol-coated portions against P. aeruginosa. The application of eugenol-coated silicone segments resulted in a decrease in the number of bacteria associated with the biofilm and effectively prevented bacterial attachment to the surface, even when a conditioning film was present. The study observed a notable decrease in the activity of genes related to virulence and biofilm formation, which confirms that silicone segments coated with eugenol have qualities that can block the expression of these genes and prevent biofilm formation (Rathinam et al., 2021). Noteworthy, silica sol-gels exhibit exceptional drug release characteristics, which may be extensively modified by manipulating the nanostructure network of the sol-gel. This modification results in a desirable therapeutic effect. Sol-gels are synthesized as thin films, coatings, and hydrogels for use in the antimicrobial and catalytic fields. They are also used for encapsulating enzymes, proteins, growth factors, and antibacterial agents (Dehghanghadikolaei et al., 2018; Rathinam et al., 2021). Therefore, sol-gel-coated silicone implants with prolonged release of eugenol could be a promising agent for managing P. aeruginosa biofilm; however, more confirmatory results are needed.
Another study also showed that eugenol and its nanoemulsion demonstrated that eugenol and its nanoemulsion exhibited 36% and 63% inhibition, respectively, of biofilm formation by P. aeruginosa at a dosage of 0.2 mg/mL. The eugenol nanoemulsion had a notable impact on the production of biofilm by this bacterium, in contrast to pure eugenol. In addition, eugenol and its nanoemulsion demonstrated the ability to decrease the activity of genes responsible for producing 3-oxo-C12-HSL and C4-HSL AHL. The impact was more prominent in the eugenol nanoemulsion compared to the pure eugenol for both synthase genes. Overall, due to its ability to hinder all the QS-mediated virulence factors and biofilm development of P. aeruginosa, the eugenol nanoemulsion shows promise as a new antibacterial and antibiofilm agent that targets QS, making it effective in controlling this bacterium (Lou et al., 2019).
Finally, the recently published study assessed the molecular docking of eugenol-conjugated silver nanoparticles (AgNPs) on QS regulator proteins (LasR, LasI, and MvfR). AgNPs coupled with eugenol exhibited significant binding affinities with proteins related to QS. The findings revealed that the synthesized nanoparticle can deactivate the transcriptional regulator LasR and suppress AHL synthesis. Moreover, the synthesized nanoparticle exhibits positive interactions with MvfR, potentially reducing the activity of the QS signaling system in P. aeruginosa. In this regard, eugenol-conjugated AgNPs may be regarded as a highly promising option for combating the production of P. aeruginosa biofilms (Shah et al., 2019). Therefore, as mentioned, the use of eugenol-based nanoplatforms could not only enhance the eugenol antibiofilm activity, but also suppress the QS system in P. aeruginosa (Figure 1). To this end, the use of these drug platforms should be considered in future studies where pure eugenol is not effective against the biofilm community.
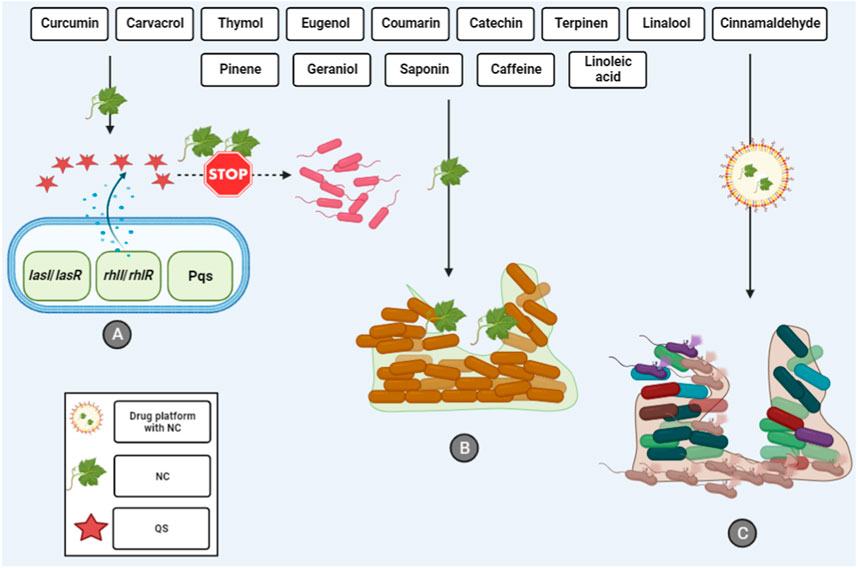
Figure 1. Interactions between natural compounds and P. aeruginosa biofilm. (A) Inhibition of QS system that inhibits the first step of biofilm formation. (B) Natural compounds can destroy the biofilm community and (C) The usage of different drug platforms can enhance the antibiofilm activity of natural compounds. NC, natural compounds; QS, quorum sensing.
7 Catechin
Catechins are bioactive compounds that are classified as polyphenolic phytochemicals. Catechins are present in a diverse range of foods and herbs, such as tea, apples, persimmons, grapes, and berries. There is a growing body of research that links the use of foods high in catechins to the prevention and treatment of chronic diseases in humans. Moreover, catechins have antioxidant properties, the ability to decrease tumor growth, and antibacterial effects (Fan et al., 2017). Noteworthy, irreversible damage to the microbial cytoplasmic membrane has been reported as the antimicrobial mechanism of catechins (Hirasawa et al., 2006). To this end, the antibiofilm activity of these compounds has also been considered by researchers.
Mombeshora et al., 2021 identified catechins as the predominant phytochemical present in Triumfetta welwitschii, a traditional African medicinal plant. This chemical effectively decreased both the amount of capsular polysaccharide in biofilms generated by P. aeruginosa and the amount of extracellular DNA in these bacterial biofilms. Noteworthy, the exact antibiofilm mechanism of catechin has not been elucidated yet; however, a recently published study indicated that catechin can disrupt QS in P. aeruginosa. In this concept, the study’s findings demonstrated that catechin effectively decreased the formation of biofilm, elastase, pyocyanin, and rhamnolipid, while having no impact on growth. The in silico analysis of this work demonstrated that catechin-7-xyloside can interact with the QS regulator LasR (Zhong et al., 2020). Another study also investigated the inhibitory effect of 19 different natural compounds on the QS system of P. aeruginosa. The results showed a high potency of catechin to interfere with LasR; therefore, the authors suggested catechin as a potent QS antagonist (Chaieb et al., 2022). In line with these results, the co-administration of catechin and gallic acid markedly reduced the expression of lasI and lasR in P. aeruginosa. The docking study revealed that catechin was successfully positioned in the ligand-binding domain of LasR. Catechin formed hydrogen bonds with Thr-115 and Ser-129 amino acid residues, which are known to play a role in the interaction with the autoinducer ligand. This supports the notion that the combination of catechin and gallic acid has a strong potential to inhibit the LasR and disrupt both QS and biofilm formation in P. aeruginosa (Abdel Bar et al., 2022).
Finally, Vandeputte et al. (2010) found that catechin had a notable inhibitory impact on the synthesis of pyocyanin and elastase, as well as on the formation of biofilm. Additionally, catechin affected the expression of the genes lasB and rhlA, as well as the important QS regulatory genes lasI, lasR, rhlI, and rhlR. Utilizing RhlR and LasR-based biosensors, it was observed that catechin might potentially disrupt the detection of the QS signal N-butanoyl-L-homoserine lactone by RhlR. As a result, this interference leads to a decrease in the production of QS factors. Therefore, as mentioned, the las system is the main QS regulator of the biofilm and the interaction of catechin with this system can significantly inhibit the biofilm community of P. aeruginosa. To this end, catechin may be proposed as an anti-QS agent, able to reduce the biofilm, virulence, and pathogenicity of P. aeruginosa.
8 Thymol
Thymol, also known as 2-isopropyl-5-methyl phenol, is a kind of phenolic monoterpene that has a powerful smell and can dissolve well in alcohol and other organic solvents but does not dissolve well in water (Shariati et al., 2022). Thymol is plentifully present in specific plants and has been utilized in traditional medicine for an extended period due to its diverse pharmacological characteristics (Salehi et al., 2018). Thymol has been proven to possess antibacterial and anti-inflammatory properties against microbes. This compound has been found to possess potent antibacterial activities, which make it effective in treating respiratory infections, oral cavity infections, and intestinal disorders (Jo et al., 2022). Thymol has been observed to display antibacterial properties against both planktonic and biofilm communities of Gram-negative and Gram-positive bacteria such as E. coli, S. aureus, Salmonella spp., and P. aeruginosa (Flores et al., 2019).
According to Walczak et al. (2021), when dealing with the biofilm formed on surfaces made of polypropylene, polyvinyl chloride, polyethylene, and stainless steel, commonly used in medical technologies and food production, thymol reduced the amount of P. aeruginosa biofilm by 70%–77% after 3 days of exposure. In addition, following a 10-day exposure to thymol, the production of biofilm was significantly decreased by 80%–100%. In another study that was published in 2022, the antibiofilm efficacy of thymol against biofilms generated by ciprofloxacin-resistant P. aeruginosa (CRPA) was examined on tympanostomy tubes. Noteworthy, the placement of these devices frequently results in ear infections, with otorrhea being the predominant consequence in youngsters. The authors of this study observed significant reductions in the viability and adhesion of CRPA in response to thymol therapy, which depended on the concentration of thymol. Thymol exposure also hindered the development of CRPA biofilms. In addition, thymol was found to improve the elimination of fully matured biofilms created by CRPA and also contributed to a decrease in the rates of CRPA breakdown. Moreover, thymol was found to effectively eliminate CRPA biofilms that had developed on the surface of tympanostomy tubes. The data suggest that thymol is a powerful inhibitor of CRPA biofilms, making it a promising therapeutic drug for treating biofilm-related post-tympanostomy tube otorrhea caused by CRPA infection. It is noteworthy to mention that the authors propose that thymol antibiofilm activity may be due to its ability to disrupt the surface adherence of antibiotic-resistant strains and damage the structure of the cell membrane (Walczak et al., 2021).
In line with these results, the recently published study also demonstrated that thymol, with a concentration ranging from 52.33% to 62.46%, was the primary constituent in the thyme EOs. The strongest antibacterial and anti-biofilm activity was shown in thyme oil obtained through distillation of fresh plant material gathered at the onset of the flowering period. This oil demonstrated efficacy against many microorganisms, including P. aeruginosa (Bakó et al., 2023). Finally, a further study on the anti-biofilm properties of thymol found that this powerful plant compound is mostly present in log (30.28%) and stationary phase (20.89%) extracts. The log phase extracts exhibited the lowest MIC (25 mg/mL) in comparison to other developmental phases. The extracts obtained from the stationary phase demonstrated the most effective biofilm dispersal activity against P. aeruginosa, with a rate of 80%. The log phase extracts exhibited the most potent biofilm inhibitory effect against P. aeruginosa (66%). Overall, leaf extracts of Plectranthus amboinicus in the log phase demonstrated a multifaceted mechanism of action. They exhibited antibacterial and antibiofilm actions while also lowering the motility and hydrophobicity of P. aeruginosa, which are crucial components in the development of this pathogen (Sawant et al., 2022). Therefore, as mentioned, thymol has the potential to inhibit bacterial biofilm. Thymol’s antibacterial effectiveness is linked to its ability to disturb both the outer and inner membranes. This disruption has been observed through the following indicators: a decrease in membrane potential and the release of potassium ions, ATP, and carboxyfluorescein (Gómez-Sequeda et al., 2020). In addition, thymol can inhibit extracellular polysaccharides present in the biofilm matrix (Valliammai et al., 2020). Thymol also inhibits cdrA which contributes to P. aeruginosa biofilm formation and stabilization (Jo et al., 2022). Noteworthy, CdrA serves as the cargo for the two-partner secretion system, which is encoded by the cdrAB operon. Inside the matrix, the bound CdrA-Psl creates strong and resistant bacterial aggregates that strengthen the structure of the biofilm (Cendra and Torrents, 2021). CdrA can bind to unidentified EPS molecules, which aids in the development of biofilms and enhances their stability (Reichhardt et al., 2018). To this end, the interactions of thymol and cdrA could inhibit the P. aeruginosa biofilm; however, data in this field is limited, and more confirmatory studies are needed.
Finally, the results of recently published study showed that thymol, derived from the root exudates of Sedum alfredii, has the ability to hinder the production of protease and elastase in P. aeruginosa. This is achieved by reducing the expression of lasB, without causing any notable impact on the primary las system. Thymol shows promise as a potential natural inhibitor of QS (Zhu et al., 2021). Additionally, thymol showed inhibitory effects against the QS system in other microorganisms; however, data about the interactions of this compound with the P. aeruginosa QS system is limited. Thus, the inhibitory effect of thymol on the QS system of P. aeruginosa is one possible antibiofilm effect of this natural compound; however, more confirmatory studies are required on this issue (Cáceres et al., 2020; Scandorieiro et al., 2023).
Although thymol has beneficial antimicrobial effects, it is limited by poor solubility in water, easy degradation, inadequate distribution on target sites, and chemical and biological instability caused by its volatile nature. These limitations restrict the applicability of thymol as an antimicrobial agent (Piri-Gharaghie et al., 2022). In this regard, as mentioned earlier, researchers are looking for more effective ways, such as new methods of drug delivery with this compound. To this end, Velázquez-Carriles et al. developed a novel nanohybrid structure consisting of zinc-layered hydroxide salt (ZnLHS) and thymol to inhibit bacterial growth. The synthesized pharmacological platform effectively suppressed P. aeruginosa biofilm by over 90%. Noteworthy, common disinfectants induce oxidation of the cell membrane before the formation of biofilm; therefore, the authors suggested that the hydroxide salt, owing to its anionic character, can impede the synthesis of this polysaccharide (Köse and Yapar, 2017). Furthermore, scientific evidence has demonstrated that thymol effectively inhibits the expression of genes linked with biofilm development. Consequently, the combined use of these substances may enhance the rate at which biofilm formation is inhibited (Sreelatha et al., 2021). Collectively, the hydroxide salt possesses an anionic character that facilitates oxidation, whereas thymol effectively inhibits the growth of bacterial biofilm. The greater degree of inhibition found with thymol–(ZnLHS) in comparison to thymol alone can be due to the latter’s hydrophilic characteristics. The hydrophilic nature of this substance, when it is stabilized in a colloidal dispersion, can enhance its ability to spread across the polysaccharide matrix, which also has a polar nature. Unlike thymol, ZnLHS possesses a hydrophobic property that allows it to specifically engage with the bacterial membrane. Therefore, the nanohybrid improves inhibition by capitalizing on a synergistic effect (Velázquez-Carriles et al., 2022).
Furthermore, one potential approach to addressing the constraints of thymol is to encapsulate it in nanocarriers (Fathi-Achachelouei et al., 2019). Nanocarriers, due to their small size and physicochemical characteristics, enhance the stability of the compound and can transport significant quantities of medicine (Heidari et al., 2018). In this regard, Piri-Gharaghie et al. also fabricated thymol-loaded chitosan nanogels. These nanogels can be used as a drug delivery system due to their low cytotoxicity, biodegradability, and biocompatibility (Pourebrahim et al., 2021). The antibacterial activity test showed that the thymol-loaded chitosan nanogel (thymol -CsNG) decreased the MIC by 4–6 times compared to free thymol. Thus, the authors propose that the increased antibacterial properties of thymol-CsNG can be attributed to the amalgamation of chitosan with the bacterial cell membrane and the specific discharge of thymol into the bacterial cell. Furthermore, thymol-CsNG may have an anti-biofilm effect by decreasing the number of microorganisms, leading to a subsequent reduction in biofilm development. One of the reasons is that the antibiotic can more effectively enter the biofilm structure because thymol-CsNG binds to the biofilm matrix, resulting in a decrease in viable counts and a reduction in the formation of biofilms. Furthermore, the thymol-CsNG compound effectively suppressed the expression of ompA and pgaB genes in bacterial strains. This suppression is likely a result of thymol interactions with transcription factors, leading to their inactivation and subsequent inhibition of biofilm gene transcription. Consequently, the expression of biofilm genes is diminished. Based on the findings, it can be inferred that thymol-CsNG has the potential to be a good candidate for improving antibacterial and anti-biofilm properties (Pourebrahim et al., 2021; Piri-Gharaghie et al., 2022).
In addition to the methods mentioned for how to use thymol, multiple research studies have examined the combined impact of thymol and blue light (BL) and how they work together. BL with a wavelength range of 400–495 nm is an emerging antibacterial method, especially effective against skin infections. Recent research has confirmed the effectiveness of BL in eliminating bacteria, regardless of their resistance profiles (Zhang et al., 2014). The precise process by which BL operates remains ambiguous. Nevertheless, there is a widespread consensus that BL triggers the activation of internal protoporphyrin-like derivatives and initiates a cascade of ROS generation. The hazardous ROS interacts with many constituents of bacteria and ultimately causes the cell to rupture. The broad-spectrum and rapid effects of ROS reduce the likelihood of bacterial resistance to different antibiotics such as beta-lactam antibiotics (Zhang et al., 2014). Similarly, Lu et al. (2021) established an antibacterial synergy between BL and thymol. This study investigates a combinatory approach to eliminate MDR P. aeruginosa, targeting both planktonic cells and established biofilms. The strategy successfully reduces bacterial populations by up to 7.5 log in less than 30 min. When used in combination, BL and thymol effectively sterilized acute infected or biofilm-associated wounds and successfully inhibited the spread of infection throughout the body in mice. BL and thymol induced oxidative bursts specifically in bacteria due to the presence of proporphyrin-like chemicals that are more numerous in bacteria than in mammalian cells. These compounds convert thymol, which is normally innocuous, into blue-laser sensitizers called thymoquinone and thymohydroquinone. These agents, when exposed to light, increased the generation of ROS and triggered a series of harmful effects in bacteria without causing any harm to the human tissue. The work reveals a hitherto unrecognized characteristic of thymol as a pro-photosensitizer, similar to a prodrug, which is specifically active in bacteria (Lu et al., 2021).
Again, in 2022, the researchers of the previous study investigated another synergistic effect. The study showed that the combination of BL and oregano EO effectively decreased P. aeruginosa planktonic cells and mature biofilms by more than 7log10. In contrast, the treatment with only BL or oregano EO did not result in a substantial reduction of bacteria. The findings indicate that thymol and carvacrol are the main constituents accountable for the bactericidal properties of oregano EO. The fundamental reason for the bactericidal effect of oregano EO is its ability to enhance the generation of ROS triggered by BL. This activation stimulates the endogenous tetrapyrrole macrocycles in bacteria, leading to their destruction. Importantly, this phototoxic action is limited to the bacteria and does not harm the surrounding tissues. An examination of the ingredients of oregano EO, together with microbiological tests, revealed that carvacrol and its isomer thymol are the primary phytochemicals that work together with BL to achieve synergistic killing. This research provides compelling evidence for the use of carvacrol and thymol as helpful benchmarks in evaluating and establishing the antibacterial effectiveness of different oregano EO products (Lu et al., 2022a). Therefore, as mentioned, in addition to the use of a thymol-based drug platform, the combined use of BL and thymol could be a promising antibiofilm approach for managing P. aeruginosa infections; however, more confirmatory studies are needed in this field.
9 Conclusion
The biofilm community of P. aeruginosa is one of the most important reasons for antibiotic resistance in this bacterium. As reported by recently published studies, natural compounds can inhibit biofilm formation and enhance the antibiofilm efficacy of conventional antibiotics and other antibacterial agents. The exact interactions between natural compounds and P. aeruginosa biofilm have not been elucidated yet; however, inhibition and interference with the QS system are the main possible mechanisms that have been reported until now. Additionally, the use of different nanostructures and drug platforms could overcome the disadvantages of natural compounds such as rapid metabolism and degradation, water insolubility, and low oral bioavailability. Hence, it is vital to contemplate the utilization of drug platforms in forthcoming investigations that prioritize the assessment of the antibiofilm efficacy of natural compounds. It is important to note that there is a lack of animal studies and clinical studies on this subject. Therefore, future research should focus on evaluating the effectiveness of natural compounds in preventing biofilm formation through these types of investigations. Additionally, data about some of the natural compound interactions with the biofilm community is limited. To this end, focusing on these natural compounds can lead to finding new ways to manage the P. aeruginosa biofilm community.
Author contributions
AS: Conceptualization, Investigation, Writing–original draft, Writing–review and editing. MN: Investigation, Writing–original draft. MA: Data curation, Investigation, Writing–original draft. AK: Formal Analysis, Methodology, Writing–original draft. AF: Supervision, Writing–original draft. ZC: Conceptualization, Investigation, Writing–original draft, Writing–review and editing.
Acknowledgments
We greatly appreciate the input from the BioRender team (BioRender.com) for their collaboration with us in figure design.
Conflict of interest
The authors declare that the research was conducted in the absence of any commercial or financial relationships that could be construed as a potential conflict of interest.
The authors declared that they were an editorial board member of Frontiers, at the time of submission. This had no impact on the peer review process and the final decision.
Publisher’s note
All claims expressed in this article are solely those of the authors and do not necessarily represent those of their affiliated organizations, or those of the publisher, the editors and the reviewers. Any product that may be evaluated in this article, or claim that may be made by its manufacturer, is not guaranteed or endorsed by the publisher.
References
Abdel Bar, F. M., Alossaimi, M. A., Elekhnawy, E., Alzeer, M. a.A., Abo Kamer, A., Moglad, E., et al. (2022). Anti-quorum sensing and anti-biofilm activity of Pelargonium × hortorum root extract against Pseudomonas aeruginosa: combinatorial effect of catechin and gallic acid. Molecules 27 (22), 7841. doi:10.3390/molecules27227841
Abdulrahman, H., Misba, L., Ahmad, S., and Khan, A. U. (2020). Curcumin induced photodynamic therapy mediated suppression of quorum sensing pathway of Pseudomonas aeruginosa: an approach to inhibit biofilm in vitro. Photodiagnosis Photodyn. Ther. 30, 101645. doi:10.1016/j.pdpdt.2019.101645
Abrahão, M. R., Molina, G., and Pastore, G. M. (2013). Endophytes: recent developments in biotechnology and the potential for flavor production. Food Res. Int. 52 (1), 367–372. doi:10.1016/j.foodres.2013.03.007
Ahmad, A., Wani, M. Y., Khan, A., Manzoor, N., and Molepo, J. (2015). Synergistic interactions of eugenol-tosylate and its congeners with fluconazole against Candida albicans. Plos one 10 (12), e0145053. doi:10.1371/journal.pone.0145053
Ahmad, W., Ansari, M. A., Yusuf, M., Amir, M., Wahab, S., Alam, P., et al. (2022). Antibacterial, anticandidal, and antibiofilm potential of fenchone: in vitro, molecular docking and in silico/ADMET study. Study 11 (18), 2395. doi:10.3390/plants11182395
Ahmed, B., Syed, A., Ali, K., Elgorban, A. M., Khan, A., Lee, J., et al. (2021). Synthesis of gallotannin capped iron oxide nanoparticles and their broad spectrum biological applications. RSC Adv. 11 (17), 9880–9893. doi:10.1039/d1ra00220a
Ahmed, S., Rudden, M., Smyth, T. J., Dooley, J. S. G., Marchant, R., and Banat, I. M. (2019). Natural quorum sensing inhibitors effectively downregulate gene expression of Pseudomonas aeruginosa virulence factors. Appl. Microbiol. Biotechnol. 103 (8), 3521–3535. doi:10.1007/s00253-019-09618-0
Alarkwazi, R. K., Abdulreda, G. R., and Ali, A. S. (2022). Secondary metabolism compounds study of essential oils for the Mentha spicata L. And ocimum basilicum L. J. Biomed. Biochem. 1 (1), 27–33. doi:10.57238/jbb.2022.18906
Al-Khazraji, S. M., Hossain, M. H., and Hassoon, A. S. (2022). Estimation of some bioactive substances and antibacterial activity of zingiber officinale (ginger) extract. J. Biomed. Biochem. 1 (2), 29–33. doi:10.57238/jbb.2022.5544.1017
Bae, K.-H., Ji, J.-M., and Park, K.-L. (1992). The antibacterial component from Cinnamomi Cortex against a cariogenic bacterium Streptococcus mutans OMZ 176. Archives Pharmacal Res. 15 (3), 239–241. doi:10.1007/bf02974062
Bahari, S., Zeighami, H., Mirshahabi, H., Roudashti, S., and Haghi, F. (2017). Inhibition of Pseudomonas aeruginosa quorum sensing by subinhibitory concentrations of curcumin with gentamicin and azithromycin. J. Glob. Antimicrob. Resist 10, 21–28. doi:10.1016/j.jgar.2017.03.006
Bahramian, A., Khoshnood, S., Shariati, A., Doustdar, F., Chirani, A. S., and Heidary, M. (2019). Molecular characterization of the pilS2 gene and its association with the frequency of Pseudomonas aeruginosa plasmid pKLC102 and PAPI-1 pathogenicity island. Infect. Drug Resist. 12, 221–227. doi:10.2147/IDR.S188527
Bajire, S. K., Ghate, S. D., Shetty, S., Banerjee, S., Rao, R. S. P., Shetty, V., et al. (2023). Unveiling the role of hub proteins in controlling quorum sensing regulated virulence through analogues in Pseudomonas aeruginosa PAO1: a functional protein-protein network biology approach. Biochem. Biophys. Res. Commun. 660, 13–20. doi:10.1016/j.bbrc.2023.03.079
Bakó, C., Balázs, V. L., Kerekes, E., Kocsis, B., Nagy, D. U., Szabó, P., et al. (2023). Flowering phenophases influence the antibacterial and anti-biofilm effects of Thymus vulgaris L. essential oil. BMC Complement. Med. Ther. 23 (1), 168. doi:10.1186/s12906-023-03966-1
Bali, E. B., Türkmen, K. E., Erdönmez, D., and Sağlam, N. (2019). Comparative study of inhibitory potential of dietary phytochemicals against quorum sensing activity of and biofilm formation by chromobacterium violaceum 12472, and swimming and swarming behaviour of Pseudomonas aeruginosa PAO1. Food Technol. Biotechnol. 57 (2), 212–221. doi:10.17113/ftb.57.02.19.5823
Bernal-Mercado, A. T., Juarez, J., Valdez, M. A., Ayala-Zavala, J. F., Del-Toro-Sánchez, C. L., and Encinas-Basurto, D. (2022). Hydrophobic chitosan nanoparticles loaded with carvacrol against Pseudomonas aeruginosa biofilms. Molecules 27 (3), 699. doi:10.3390/molecules27030699
Borges, A., Ferreira, C., Saavedra, M. J., and Simões, M. (2013). Antibacterial activity and mode of action of ferulic and gallic acids against pathogenic bacteria. Microb. Drug Resist 19 (4), 256–265. doi:10.1089/mdr.2012.0244
Bose, S. K., Chauhan, M., Dhingra, N., Chhibber, S., and Harjai, K. (2020a). Terpinen-4-ol attenuates quorum sensing regulated virulence factors and biofilm formation in Pseudomonas aeruginosa. Future Microbiol. 15, 127–142. doi:10.2217/fmb-2019-0204
Bose, S. K., Nirbhavane, P., Batra, M., Chhibber, S., and Harjai, K. (2020b). Nanolipoidal α-terpineol modulates quorum sensing regulated virulence and biofilm formation in Pseudomonas aeruginosa. Nanomedicine Lond, 15(18), 1743–1760. doi:10.2217/nnm-2020-0134
Brackman, G., Cos, P., Maes, L., Nelis, H. J., and Coenye, T. (2011). Quorum sensing inhibitors increase the susceptibility of bacterial biofilms to antibiotics in vitro and in vivo. Antimicrob. Agents Chemother. 55 (6), 2655–2661. doi:10.1128/aac.00045-11
Breidenstein, E. B., De La Fuente-Núñez, C., and Hancock, R. E. (2011). Pseudomonas aeruginosa: all roads lead to resistance. Trends Microbiol. 19 (8), 419–426. doi:10.1016/j.tim.2011.04.005
Cáceres, M., Hidalgo, W., Stashenko, E., Torres, R., and Ortiz, C. (2020). Essential oils of aromatic plants with antibacterial, anti-biofilm and anti-quorum sensing activities against pathogenic bacteria. Antibiot. Basel 9 (4), 147. doi:10.3390/antibiotics9040147
Cendra, M. D. M., and Torrents, E. (2021). Pseudomonas aeruginosa biofilms and their partners in crime. Biotechnol. Adv. 49, 107734. doi:10.1016/j.biotechadv.2021.107734
Ceylan, O., and Ugur, A. (2015). Chemical composition and anti-biofilm activity of Thymus sipyleus BOISS. subsp. sipyleus BOISS. var. davisianus RONNIGER essential oil. Arch. Pharm. Res. 38 (6), 957–965. doi:10.1007/s12272-014-0516-0
Chadha, J., Singh, J., Chhibber, S., and Harjai, K. (2022). Gentamicin augments the quorum quenching potential of cinnamaldehyde in vitro and protects Caenorhabditis elegans from Pseudomonas aeruginosa infection. Front. Cell Infect. Microbiol. 12, 899566. doi:10.3389/fcimb.2022.899566
Chadha, J., Singh, J., and Harjai, K. (2023). α-Terpineol synergizes with gentamicin to rescue Caenorhabditis elegans from Pseudomonas aeruginosa infection by attenuating quorum sensing-regulated virulence. Life Sci. 313, 121267. doi:10.1016/j.lfs.2022.121267
Chaieb, K., Kouidhi, B., Hosawi, S. B., Baothman, O. a.S., Zamzami, M. A., and Altayeb, H. N. (2022). Computational screening of natural compounds as putative quorum sensing inhibitors targeting drug resistance bacteria: molecular docking and molecular dynamics simulations. Comput. Biol. Med. 145, 105517. doi:10.1016/j.compbiomed.2022.105517
Chakraborty, P., Dastidar, D. G., Paul, P., Dutta, S., Basu, D., Sharma, S. R., et al. (2020). Inhibition of biofilm formation of Pseudomonas aeruginosa by caffeine: a potential approach for sustainable management of biofilm. Arch. Microbiol. 202 (3), 623–635. doi:10.1007/s00203-019-01775-0
Chegini, Z., Khoshbayan, A., Taati Moghadam, M., Farahani, I., Jazireian, P., and Shariati, A. (2020). Bacteriophage therapy against Pseudomonas aeruginosa biofilms: a review. Ann. Clin. Microbiol. Antimicrob. 19, 45–17. doi:10.1186/s12941-020-00389-5
Chipenzi, T., Baloyi, G., Mudondo, T., Sithole, S., Fru Chi, G., and Mukanganyama, S. (2020). An evaluation of the antibacterial properties of tormentic acid congener and extracts from callistemon viminalis on selected ESKAPE pathogens and effects on biofilm formation. Adv. Pharmacol. Pharm. Sci. 2020, 8848606. doi:10.1155/2020/8848606
Danaraj, J., Mariasingarayan, Y., Ayyappan, S., and Karuppiah, V. (2020). Seagrass Halodule pinifolia active constituent 4-methoxybenzioic acid (4-MBA) inhibits quorum sensing mediated virulence production of Pseudomonas aeruginosa. Microb. Pathog. 147, 104392. doi:10.1016/j.micpath.2020.104392
Das, S., Paul, P., Dastidar, D. G., Chakraborty, P., Chatterjee, S., Sarkar, S., et al. (2023). Piperine exhibits potential antibiofilm activity against Pseudomonas aeruginosa by accumulating reactive oxygen species, affecting cell surface hydrophobicity and quorum sensing. Affect. Cell Surf. Hydrophobicity Quorum Sens. 195 (5), 3229–3256. doi:10.1007/s12010-022-04280-1
Davies, D. G., Parsek, M. R., Pearson, J. P., Iglewski, B. H., Costerton, J. W., and Greenberg, E. P. (1998). The involvement of cell-to-cell signals in the development of a bacterial biofilm. Science 280 (5361), 295–298. doi:10.1126/science.280.5361.295
Dehghanghadikolaei, A., Ansary, J., and Ghoreishi, R. (2018). Sol-gel process applications: a mini-review. Proc. Nat. Res. Soc. 2 (1), 02008–02029. doi:10.11605/j.pnrs.201802008
Dias, M. I., Ferreira, I. C., and Barreiro, M. F. (2015). Microencapsulation of bioactives for food applications. Food Funct. 6 (4), 1035–1052. doi:10.1039/c4fo01175a
Di Salle, A., Viscusi, G., Di Cristo, F., Valentino, A., Gorrasi, G., Lamberti, E., et al. (2021). Antimicrobial and antibiofilm activity of curcumin-loaded electrospun nanofibers for the prevention of the biofilm-associated infections. Molecules 26 (16), 4866. doi:10.3390/molecules26164866
Elsharif, S. A., and Buettner, A. (2018). Structure-odor relationship study on geraniol, nerol, and their synthesized oxygenated derivatives. J. Agric. Food Chem. 66 (10), 2324–2333. doi:10.1021/acs.jafc.6b04534
Fan, F. Y., Sang, L. X., and Jiang, M. (2017). Catechins and their therapeutic benefits to inflammatory bowel disease. Molecules 22 (3), 484. doi:10.3390/molecules22030484
Farto-Vaamonde, X., Diaz-Gomez, L., Parga, A., Otero, A., Concheiro, A., and Alvarez-Lorenzo, C. (2022). Perimeter and carvacrol-loading regulate angiogenesis and biofilm growth in 3D printed PLA scaffolds. J. Control Release 352, 776–792. doi:10.1016/j.jconrel.2022.10.060
Fathi-Achachelouei, M., Knopf-Marques, H., Ribeiro Da Silva, C. E., Barthès, J., Bat, E., Tezcaner, A., et al. (2019). Use of nanoparticles in tissue engineering and regenerative medicine. Front. Bioeng. Biotechnol. 7, 113. doi:10.3389/fbioe.2019.00113
Fernandes, S., Borges, A., Gomes, I. B., Sousa, S. F., and Simões, M. (2023). Curcumin and 10-undecenoic acid as natural quorum sensing inhibitors of LuxS/AI-2 of Bacillus subtilis and LasI/LasR of Pseudomonas aeruginosa. Food Res. Int. 165, 112519. doi:10.1016/j.foodres.2023.112519
Ferro, T. a.F., Souza, E. B., Suarez, M. a.M., Rodrigues, J. F. S., Pereira, D. M. S., Mendes, S. J. F., et al. (2019). Topical application of cinnamaldehyde promotes faster healing of skin wounds infected with Pseudomonas aeruginosa. Molecules 24 (8), 1627. doi:10.3390/molecules24081627
Firmino, D. F., Cavalcante, T. T. A., Gomes, G. A., Firmino, N. C. S., Rosa, L. D., De Carvalho, M. G., et al. (2018). Antibacterial and antibiofilm activities of cinnamomum sp. Essential oil and cinnamaldehyde: antimicrobial activities. ScientificWorldJournal 2018, 7405736. doi:10.1155/2018/7405736
Flores, P. I. G., Valenzuela, R. B., Ruiz, L. D., López, C. M., and Cháirez, F. E. (2019). Antibacterial activity of five terpenoid compounds: carvacrol, limonene, linalool, α-terpinene and thymol. Trop. subtropical Agroecosyst. 22 (2). doi:10.56369/tsaes.2838
Gambello, M. J., and Iglewski, B. H. (1991). Cloning and characterization of the Pseudomonas aeruginosa lasR gene, a transcriptional activator of elastase expression. J. Bacteriol. 173 (9), 3000–3009. doi:10.1128/jb.173.9.3000-3009.1991
Gambino, E., Maione, A., Guida, M., Albarano, L., Carraturo, F., Galdiero, E., et al. (2022). Evaluation of the pathogenic-mixed biofilm formation of Pseudomonas aeruginosa/Staphylococcus aureus and treatment with limonene on three different materials by a dynamic model. Model 19 (6), 3741. doi:10.3390/ijerph19063741
Ghannay, S., Aouadi, K., Kadri, A., and Snoussi, M. (2022a). GC-MS profiling, vibriocidal, antioxidant, antibiofilm, and anti-quorum sensing properties of Carum carvi L. Essential oil: in vitro and in silico approaches. Essent. Oil Vitro Silico Approaches 11 (8), 1072. doi:10.3390/plants11081072
Ghannay, S., Aouadi, K., Kadri, A., and Snoussi, M. (2022b). In vitro and in silico screening of anti-Vibrio spp., antibiofilm, antioxidant and anti-quorum sensing activities of Cuminum cyminum L. Volatile oil. Plants Basel 11 (17), 2236. doi:10.3390/plants11172236
Ghasemi, M., Khorsandi, K., and Kianmehr, Z. (2021). Photodynamic inactivation with curcumin and silver nanoparticles hinders Pseudomonas aeruginosa planktonic and biofilm formation: evaluation of glutathione peroxidase activity and ROS production. World J. Microbiol. Biotechnol. 37 (9), 149. doi:10.1007/s11274-021-03104-4
Gholami, M., Zeighami, H., Bikas, R., Heidari, A., Rafiee, F., and Haghi, F. (2020). Inhibitory activity of metal-curcumin complexes on quorum sensing related virulence factors of Pseudomonas aeruginosa PAO1. Amb. Express 10 (1), 111. doi:10.1186/s13568-020-01045-z
Gobin, M., Proust, R., Lack, S., Duciel, L., Des Courtils, C., Pauthe, E., et al. (2022). A combination of the natural molecules gallic acid and carvacrol eradicates P. aeruginosa and S. aureus mature biofilms. Int. J. Mol. Sci. 23 (13), 7118. doi:10.3390/ijms23137118
Gómez-Sequeda, N., Cáceres, M., Stashenko, E. E., Hidalgo, W., and Ortiz, C. (2020). Antimicrobial and antibiofilm activities of essential oils against Escherichia coli O157:H7 and methicillin-resistant Staphylococcus aureus (MRSA). Antibiot. (Basel) 9 (11), 730. doi:10.3390/antibiotics9110730
Gutiérrez-Barranquero, J. A., Reen, F. J., Mccarthy, R. R., and O'gara, F. (2015). Deciphering the role of coumarin as a novel quorum sensing inhibitor suppressing virulence phenotypes in bacterial pathogens. Appl. Microbiol. Biotechnol. 99 (7), 3303–3316. doi:10.1007/s00253-015-6436-1
Häkkinen, S. T., Soković, M., Nohynek, L., Ćirić, A., Ivanov, M., Stojković, D., et al. (2021). Chicory extracts and sesquiterpene lactones show potent activity against bacterial and fungal pathogens. Pathogens 14 (9), 941. doi:10.3390/ph14090941
Hassan, H. A., Geniady, M. M., Abdelwahab, S. F., Abd-Elghany, M. I., Sarhan, H. A., Abdelghany, A. A., et al. (2018). Topical eugenol successfully treats experimental Candida albicans-induced keratitis. Ophthalmic Res. 60 (2), 69–79. doi:10.1159/000488907
He, R., Zhang, Z., Xu, L., Chen, W., Zhang, M., Zhong, Q., et al. (2022). Antibacterial mechanism of linalool emulsion against Pseudomonas aeruginosa and its application to cold fresh beef. World J. Microbiol. Biotechnol. 38 (4), 56. doi:10.1007/s11274-022-03233-4
Heidari, Z., Salehzadeh, A., Sadat Shandiz, S. A., and Tajdoost, S. (2018). Anti-cancer and anti-oxidant properties of ethanolic leaf extract of Thymus vulgaris and its bio-functionalized silver nanoparticles. 3 Biotech. 8 (3), 177. doi:10.1007/s13205-018-1199-x
Hirasawa, M., Takada, K., and Otake, S. (2006). Inhibition of acid production in dental plaque bacteria by green tea catechins. Caries Res. 40 (3), 265–270. doi:10.1159/000092236
Hossain, M. H., Al-Khazraji, S. M., and Hassoon, A. S. (2022). Estimation of some Essential oils and study Antibacterial activity of Rosmarinus officinalis Extract. J. Biomed. Biochem. 1 (3), 34–38. doi:10.57238/jbb.2022.20108
Husain, F. M., Ahmad, I., Khan, M. S., Ahmad, E., Tahseen, Q., Khan, M. S., et al. (2015a). Sub-MICs of Mentha piperita essential oil and menthol inhibits AHL mediated quorum sensing and biofilm of Gram-negative bacteria. Front. Microbiol. 6, 420. doi:10.3389/fmicb.2015.00420
Husain, F. M., Ahmad, I., Khan, M. S., and Al-Shabib, N. A. (2015b). Trigonella foenum-graceum (seed) extract interferes with quorum sensing regulated traits and biofilm formation in the strains of Pseudomonas aeruginosa and Aeromonas hydrophila. Evid. Based Complement. Altern. Med. 2015, 879540. doi:10.1155/2015/879540
Ivanov, M., Novović, K., Malešević, M., Dinić, M., Stojković, D., Jovčić, B., et al. (2022). Polyphenols as inhibitors of antibiotic resistant bacteria-mechanisms underlying rutin interference with bacterial virulence. Pharm. Basel. 15 (3), 385. doi:10.3390/ph15030385
Jagani, S., Chelikani, R., and Kim, D. S. (2009). Effects of phenol and natural phenolic compounds on biofilm formation by Pseudomonas aeruginosa. Biofouling 25 (4), 321–324. doi:10.1080/08927010802660854
Javed, H., Meeran, M. F. N., Jha, N. K., and Ojha, S. (2021). Carvacrol, a plant metabolite targeting viral protease (mpro) and ACE2 in host cells can Be a possible candidate for COVID-19. Front. Plant Sci. 11, 601335. doi:10.3389/fpls.2020.601335
Jayalekshmi, H., Omanakuttan, A., Pandurangan, N., Vargis, S. V., Maneesh, M., Nair, G. B., et al. (2016). Clove bud oil reduces kynurenine and inhibits pqs A gene expression in P. aeruginosa. Appl. Microbiol. Biotechnol. 100 (8), 3681–3692. doi:10.1007/s00253-016-7313-2
Jo, E. R., Oh, J., and Cho, S. I. (2022). Inhibitory effect of thymol on tympanostomy tube biofilms of methicillin-resistant Staphylococcus aureus and ciprofloxacin-resistant Pseudomonas aeruginosa. Microorganisms 10 (9), 1867. doi:10.3390/microorganisms10091867
Kačániová, M., Vukovic, N. L., Čmiková, N., Galovičová, L., Schwarzová, M., Šimora, V., et al. (2023). Salvia sclarea essential oil chemical composition and biological activities. Int. J. Mol. Sci. 24 (6), 5179. doi:10.3390/ijms24065179
Karmakar, S., Choudhury, M., Das, A. S., Maiti, A., Majumdar, S., and Mitra, C. (2012). Clove (Syzygium aromaticum Linn) extract rich in eugenol and eugenol derivatives shows bone-preserving efficacy. Nat. Prod. Res. 26 (6), 500–509. doi:10.1080/14786419.2010.511216
Kart, D., Reçber, T., Nemutlu, E., and Sagiroglu, M. (2021a). Sub-inhibitory concentrations of ciprofloxacin alone and combinations with plant-derived compounds against P. aeruginosa biofilms and their effects on the metabolomic profile of P. aeruginosa biofilms. Antibiotics 10 (4), 414. doi:10.3390/antibiotics10040414
Kart, D., Reçber, T., Nemutlu, E., and Sagiroglu, M. (2021b). Sub-inhibitory concentrations of ciprofloxacin alone and combinations with plant-derived compounds against P. aeruginosa biofilms and their effects on the metabolomic profile of P. aeruginosa biofilms. Antibiot. Basel 10 (4), 414. doi:10.3390/antibiotics10040414
Kasthuri, T., Swetha, T. K., Bhaskar, J. P., and Pandian, S. K. (2022). Rapid-killing efficacy substantiates the antiseptic property of the synergistic combination of carvacrol and nerol against nosocomial pathogens. Arch. Microbiol. 204 (9), 590. doi:10.1007/s00203-022-03197-x
Khaleghi, M., Haghi, F., Gholami, M., Hourfar, H., Shahi, F., Mir Mousavi Zekoloujeh, A., et al. (2023). A fabricated hydrogel of hyaluronic acid/curcumin shows super-activity to heal the bacterial infected wound. Amb. Express 13 (1), 29. doi:10.1186/s13568-023-01533-y
Khan, F., Park, S. K., Bamunuarachchi, N. I., Oh, D., and Kim, Y. M. (2021). Caffeine-loaded gold nanoparticles: antibiofilm and anti-persister activities against pathogenic bacteria. Appl. Microbiol. Biotechnol. 105 (9), 3717–3731. doi:10.1007/s00253-021-11300-3
Kim, H. S., Cha, E., Ham, S. Y., Park, J. H., Nam, S., Kwon, H., et al. (2021). Linoleic acid inhibits Pseudomonas aeruginosa biofilm formation by activating diffusible signal factor-mediated quorum sensing. Biotechnol. Bioeng. 118 (1), 82–93. doi:10.1002/bit.27552
Kim, H. S., Lee, S. H., Byun, Y., and Park, H. D. (2015). 6-Gingerol reduces Pseudomonas aeruginosa biofilm formation and virulence via quorum sensing inhibition. Sci. Rep. 5, 8656. doi:10.1038/srep08656
Kosari, F., Taheri, M., Moradi, A., Alni, R. H., and Alikhani, M. Y. (2020). Evaluation of cinnamon extract effects on clbB gene expression and biofilm formation in Escherichia coli strains isolated from colon cancer patients. BMC cancer 20 (1), 267–268. doi:10.1186/s12885-020-06736-1
Köse, H., and Yapar, N. (2017). The comparison of various disinfectants? efficacy on Staphylococcus aureus and Pseudomonas aeruginosa biofilm layers. Turk J. Med. Sci. 47 (4), 1287–1294. doi:10.3906/sag-1605-88
Kouki, H., Polito, F., De Martino, L., Mabrouk, Y., Hamrouni, L., Amri, I., et al. (2022). Chemistry and bioactivities of six Tunisian Eucalyptus species. Pharm. Basel. 15 (10), 1265. doi:10.3390/ph15101265
Kozam, G. (1977). The effect of eugenol on nerve transmission. Oral Surg. Oral Med. Oral Pathol. 44 (5), 799–805. doi:10.1016/0030-4220(77)90390-5
Kumar, R., Chhibber, S., and Harjai, K. (2009). Quorum sensing is necessary for the virulence of Pseudomonas aeruginosa during urinary tract infection. Kidney Int. 76 (3), 286–292. doi:10.1038/ki.2009.183
Kumar Bose, S., Sharma, K., Chhibber, S., and Harjai, K. (2021). Therapeutic potential of nanolipoidal α-terpineol in combating keratitis induced by Pseudomonas aeruginosa in the murine model. Int. J. Pharm. 594, 120175. doi:10.1016/j.ijpharm.2020.120175
Lahiri, D., Nag, M., Dutta, B., Dey, S., Mukherjee, D., Joshi, S. J., et al. (2021). Antibiofilm and anti-quorum sensing activities of eugenol and linalool from Ocimum tenuiflorum against Pseudomonas aeruginosa biofilm. J. Appl. Microbiol. 131 (6), 2821–2837. doi:10.1111/jam.15171
Lakshmanan, D., Harikrishnan, A., Vishnupriya, S., and Jeevaratnam, K. (2019). Swarming inhibitory potential of cinnamtannin B1 from cinnamomum tamala T. Nees and eberm on Pseudomonas aeruginosa. ACS Omega 4 (16), 16994–16998. doi:10.1021/acsomega.9b02471
Lee, J., Wu, J., Deng, Y., Wang, J., Wang, C., Wang, J., et al. (2013). A cell-cell communication signal integrates quorum sensing and stress response. Nat. Chem. Biol. 9 (5), 339–343. doi:10.1038/nchembio.1225
Lee, J., and Zhang, L. (2015). The hierarchy quorum sensing network in Pseudomonas aeruginosa. Protein Cell 6 (1), 26–41. doi:10.1007/s13238-014-0100-x
Lee, W. H., Rohanizadeh, R., and Loo, C. Y. (2021). In situ functionalizing calcium phosphate biomaterials with curcumin for the prevention of bacterial biofilm infections. Colloids Surf. B Biointerfaces 206, 111938. doi:10.1016/j.colsurfb.2021.111938
Li, W. R., Ma, Y. K., Shi, Q. S., Xie, X. B., Sun, T. L., Peng, H., et al. (2018). Diallyl disulfide from garlic oil inhibits Pseudomonas aeruginosa virulence factors by inactivating key quorum sensing genes. Appl. Microbiol. Biotechnol. 102 (17), 7555–7564. doi:10.1007/s00253-018-9175-2
Li, W. R., Zeng, T. H., Zhang, Z. Q., Shi, Q. S., and Xie, X. B. (2023). Geraniol attenuates virulence factors by inhibiting quorum sensing of Pseudomonas aeruginosa. Front. Microbiol. 14, 1190619. doi:10.3389/fmicb.2023.1190619
Liu, L., Xiao, X., Li, K., Li, X., Yu, K., Liao, X., et al. (2020). Prevention of bacterial colonization based on self-assembled metal-phenolic nanocoating from rare-earth ions and catechin. ACS Appl. Mat. Interfaces 12 (19), 22237–22245. doi:10.1021/acsami.0c06459
Lone, S. A., and Ahmad, A. (2020). Inhibitory effect of novel Eugenol Tosylate Congeners on pathogenicity of Candida albicans. BMC complementary Med. Ther. 20 (1), 131. doi:10.1186/s12906-020-02929-0
Loo, C. Y., Rohanizadeh, R., Young, P. M., Traini, D., Cavaliere, R., Whitchurch, C. B., et al. (2016). Combination of silver nanoparticles and curcumin nanoparticles for enhanced anti-biofilm activities. J. Agric. Food Chem. 64 (12), 2513–2522. doi:10.1021/acs.jafc.5b04559
Lou, Z., Letsididi, K. S., Yu, F., Pei, Z., Wang, H., and Letsididi, R. (2019). Inhibitive effect of eugenol and its nanoemulsion on quorum sensing-mediated virulence factors and biofilm formation by Pseudomonas aeruginosa. J. Food Prot. 82 (3), 379–389. doi:10.4315/0362-028x.jfp-18-196
Lou, Z., Tang, Y., Song, X., and Wang, H. (2015). Metabolomics-based screening of biofilm-inhibitory compounds against Pseudomonas aeruginosa from burdock leaf. Molecules 20 (9), 16266–16277. doi:10.3390/molecules200916266
Lu, M., Li, Y., and Wu, M. X. (2021). Bacteria-specific pro-photosensitizer kills multidrug-resistant Staphylococcus aureus and Pseudomonas aeruginosa. Commun. Biol. 4 (1), 408. doi:10.1038/s42003-021-01956-y
Lu, M., Wong, K. I., Li, X., Wang, F., Wei, L., Wang, S., et al. (2022a). Oregano oil and harmless blue light to synergistically inactivate multidrug-resistant pseudomonas aeruginosa. Front. Microbiol. 13, 810746. doi:10.3389/fmicb.2022.810746
Lu, M., Wong, K. I., Li, X., Wang, F., Wei, L., Wang, S., et al. (2022b). Oregano oil and harmless blue light to synergistically inactivate multidrug-resistant Pseudomonas aeruginosa. Front. Microbiol. 13, 810746. doi:10.3389/fmicb.2022.810746
Luciardi, M. C., Blázquez, M. A., Alberto, M. R., Cartagena, E., and Arena, M. E. (2020). Grapefruit essential oils inhibit quorum sensing of Pseudomonas aeruginosa. Food Sci. Technol. Int. 26(3), 231–241. doi:10.1177/1082013219883465
Maisetta, G., Batoni, G., Caboni, P., Esin, S., Rinaldi, A. C., and Zucca, P. (2019). Tannin profile, antioxidant properties, and antimicrobial activity of extracts from two Mediterranean species of parasitic plant Cytinus. BMC Complement. Altern. Med. 19 (1), 82. doi:10.1186/s12906-019-2487-7
Marchese, A., Barbieri, R., Coppo, E., Orhan, I. E., Daglia, M., Nabavi, S. F., et al. (2017). Antimicrobial activity of eugenol and essential oils containing eugenol: a mechanistic viewpoint. Crit. Rev. Microbiol. 43 (6), 668–689. doi:10.1080/1040841x.2017.1295225
Mechmechani, S., Gharsallaoui, A., El Omari, K., Fadel, A., Hamze, M., and Chihib, N. E. (2022a). Hurdle technology based on the use of microencapsulated pepsin, trypsin and carvacrol to eradicate Pseudomonas aeruginosa and Enterococcus faecalis biofilms. Biofouling 38 (9), 903–915. doi:10.1080/08927014.2022.2151361
Mechmechani, S., Gharsallaoui, A., Fadel, A., El Omari, K., Khelissa, S., Hamze, M., et al. (2022b). Microencapsulation of carvacrol as an efficient tool to fight Pseudomonas aeruginosa and Enterococcus faecalis biofilms. PLoS One 17 (7), e0270200. doi:10.1371/journal.pone.0270200
Mechmechani, S., Gharsallaoui, A., Karam, L., El Omari, K., Fadel, A., Hamze, M., et al. (2023). Pepsin and trypsin treatment combined with carvacrol: an efficient strategy to fight Pseudomonas aeruginosa and Enterococcus faecalis biofilms. Microorganisms 11 (1), 143. doi:10.3390/microorganisms11010143
Mirzahosseinipour, M., Khorsandi, K., Hosseinzadeh, R., Ghazaeian, M., and Shahidi, F. K. (2020). Antimicrobial photodynamic and wound healing activity of curcumin encapsulated in silica nanoparticles. Photodiagnosis Photodyn. Ther. 29, 101639. doi:10.1016/j.pdpdt.2019.101639
Mombeshora, M., Chi, G. F., and Mukanganyama, S. (2021). Antibiofilm activity of extract and a compound isolated from Triumfetta welwitschii against Pseudomonas aeruginosa. Biochem. Res. Int. 2021, 9946183. doi:10.1155/2021/9946183
Namivandi-Zangeneh, R., Yang, Y., Xu, S., Wong, E. H. H., and Boyer, C. (2020). Antibiofilm platform based on the combination of antimicrobial polymers and essential oils. Biomacromolecules 21 (1), 262–272. doi:10.1021/acs.biomac.9b01278
Neyestani, Z., Ebrahimi, S. A., Ghazaghi, A., Jalili, A., Sahebkar, A., and Rahimi, H. R. (2019). Review of anti-bacterial activities of curcumin against Pseudomonas aeruginosa. Crit. Rev. Eukaryot. Gene Expr. 29 (5), 377–385. doi:10.1615/CritRevEukaryotGeneExpr.2019029088
Ni, N., Li, M., Wang, J., and Wang, B. (2009). Inhibitors and antagonists of bacterial quorum sensing. Med. Res. Rev. 29 (1), 65–124. doi:10.1002/med.20145
Nisar, M. F., Khadim, M., Rafiq, M., Chen, J., Yang, Y., and Wan, C. C. (2021). Pharmacological properties and health benefits of eugenol: a comprehensive review. Oxid. Med. Cell Longev. 2021, 2497354. doi:10.1155/2021/2497354
Niu, C., and Gilbert, E. S. (2004). Colorimetric method for identifying plant essential oil components that affect biofilm formation and structure. Appl. Environ. Microbiol. 70 (12), 6951–6956. doi:10.1128/aem.70.12.6951-6956.2004
Norizan, S. N., Yin, W. F., and Chan, K. G. (2013). Caffeine as a potential quorum sensing inhibitor. Sensors (Basel) 13 (4), 5117–5129. doi:10.3390/s130405117
Noumi, E., Merghni, A., M, M. A., Haddad, O., Akmadar, G., De Martino, L., et al. (2018). Chromobacterium violaceum and Pseudomonas aeruginosa PAO1: models for evaluating anti-quorum sensing activity of melaleuca alternifolia essential oil and its main component terpinen-4-ol. Molecules 23, 2672. doi:10.3390/molecules23102672
O'may, C., Ciobanu, A., Lam, H., and Tufenkji, N. (2012). Tannin derived materials can block swarming motility and enhance biofilm formation in Pseudomonas aeruginosa. Biofouling 28 (10), 1063–1076. doi:10.1080/08927014.2012.725130
Packiavathy, I. A., Priya, S., Pandian, S. K., and Ravi, A. V. (2014). Inhibition of biofilm development of uropathogens by curcumin - an anti-quorum sensing agent from Curcuma longa. Food Chem. 148, 453–460. doi:10.1016/j.foodchem.2012.08.002
Packiavathy, I. a.S. V., Agilandeswari, P., Musthafa, K. S., Pandian, S. K., and Ravi, A. V. (2012). Antibiofilm and quorum sensing inhibitory potential of Cuminum cyminum and its secondary metabolite methyl eugenol against Gram negative bacterial pathogens. Food Res. Int. 45 (1), 85–92. doi:10.1016/j.foodres.2011.10.022
Pazarci, O., Tutar, U., and Kilinc, S. (2019). Investigation of the antibiofilm effects of Mentha longifolia essential oil on titanium and stainless steel orthopedic implant surfaces. Eurasian J. Med. 51 (2), 128–132. doi:10.5152/eurasianjmed.2019.18432
Pearson, J. P., Passador, L., Iglewski, B. H., and Greenberg, E. P. (1995). A second N-acylhomoserine lactone signal produced by Pseudomonas aeruginosa. Proc. Natl. Acad. Sci. U. S. A. 92 (5), 1490–1494. doi:10.1073/pnas.92.5.1490
Pejčić, M., Stojanović-Radić, Z., Genčić, M., Dimitrijević, M., and Radulović, N. (2020). Anti-virulence potential of basil and sage essential oils: inhibition of biofilm formation, motility and pyocyanin production of Pseudomonas aeruginosa isolates. Food Chem. Toxicol. 141, 111431. doi:10.1016/j.fct.2020.111431
Pejin, B., Ciric, A., Glamoclija, J., Nikolic, M., and Sokovic, M. (2015). In vitro anti-quorum sensing activity of phytol. Nat. Prod. Res. 29 (4), 374–377. doi:10.1080/14786419.2014.945088
Persat, A., Nadell, C. D., Kim, M. K., Ingremeau, F., Siryaporn, A., Drescher, K., et al. (2015). The mechanical world of bacteria. Cell 161 (5), 988–997. doi:10.1016/j.cell.2015.05.005
Pesci, E. C., Milbank, J. B., Pearson, J. P., Mcknight, S., Kende, A. S., Greenberg, E. P., et al. (1999). Quinolone signaling in the cell-to-cell communication system of Pseudomonas aeruginosa. Proc. Natl. Acad. Sci. U. S. A. 96 (20), 11229–11234. doi:10.1073/pnas.96.20.11229
Piri-Gharaghie, T., Beiranvand, S., Riahi, A., Shirin, N. J., Badmasti, F., Mirzaie, A., et al. (2022). Fabrication and characterization of thymol-loaded chitosan nanogels: improved antibacterial and anti-biofilm activities with negligible cytotoxicity. Chem. Biodivers. 19 (3), e202100426. doi:10.1002/cbdv.202100426
Pourebrahim, M., Nejabatdoust, A., Mirmiran, S. D., Daemi, H. B., Meftahpour, H., and Salehzadeh, A. (2021). Aminoglycosides–loaded glucose-conjugated chitosan nanoparticles for in vitro antimicrobial and antibiofilm screening on Klebsiella pneumonia. BioNanoScience 11 (3), 901–914. doi:10.1007/s12668-021-00878-7
Prateeksha, , Rao, C. V., Das, A. K., Barik, S. K., and Singh, B. N. (2019). ZnO/Curcumin nanocomposites for enhanced inhibition of Pseudomonas aeruginosa virulence via LasR-RhlR quorum sensing systems. Mol. Pharm. 16 (8), 3399–3413. doi:10.1021/acs.molpharmaceut.9b00179
Qais, F. A., Khan, M. S., Ahmad, I., Husain, F. M., Khan, R. A., Hassan, I., et al. (2021). Coumarin exhibits broad-spectrum antibiofilm and antiquorum sensing activity against gram-negative bacteria: in vitro and in silico investigation. Vitro Silico Investigation 6 (29), 18823–18835. doi:10.1021/acsomega.1c02046
Quatrin, P. M., Verdi, C. M., De Souza, M. E., De Godoi, S. N., Klein, B., Gundel, A., et al. (2017). Antimicrobial and antibiofilm activities of nanoemulsions containing Eucalyptus globulus oil against Pseudomonas aeruginosa and Candida spp. Microb. Pathog. 112, 230–242. doi:10.1016/j.micpath.2017.09.062
Radulović, N. S., Blagojević, P. D., Stojanović-Radić, Z. Z., and Stojanović, N. M. (2013). Antimicrobial plant metabolites: structural diversity and mechanism of action. Curr. Med. Chem. 20 (7), 932–952. doi:10.2174/092986713805219136
Rahimi, M., Piroozmand, A., Shayestehpour, M., Salamat, S., Peik Falak, F., Shakerimoghaddam, A., et al. (2023). Effect of curcumin nanoparticles and alcoholic extract of Falcaria vulgaris on the growth rate, biofilm, and gene expression in Pseudomonas aeruginosa isolated from burn wound infection. Mol. Biol. Rep. 50 (8), 6681–6690. doi:10.1007/s11033-023-08559-2
Ramasamy, M., Lee, J. H., and Lee, J. (2017a). Development of gold nanoparticles coated with silica containing the antibiofilm drug cinnamaldehyde and their effects on pathogenic bacteria. Int. J. Nanomedicine 12, 2813–2828. doi:10.2147/ijn.s132784
Ramasamy, M., Lee, J. H., and Lee, J. (2017b). Direct one-pot synthesis of cinnamaldehyde immobilized on gold nanoparticles and their antibiofilm properties. Colloids Surf. B Biointerfaces 160, 639–648. doi:10.1016/j.colsurfb.2017.10.018
Ramesh, G., Kaviyil, J. E., Paul, W., Sasi, R., and Joseph, R. (2022). Gallium-curcumin nanoparticle conjugates as an antibacterial agent against Pseudomonas aeruginosa: synthesis and characterization. ACS Omega 7 (8), 6795–6809. doi:10.1021/acsomega.1c06398
Rangel, M. L., De Aquino, S. G., De Lima, J. M., Castellano, L. R., and De Castro, R. D. (2018). In vitro effect of cinnamomum zeylanicum blume essential oil on Candida spp. involved in oral infections. Evid. Based Complement. Altern. Med. 2018, 4045013. doi:10.1155/2018/4045013
Rathinam, P., Murari, B. M., and Viswanathan, P. (2021). Biofilm inhibition and antifouling evaluation of sol-gel coated silicone implants with prolonged release of eugenol against Pseudomonas aeruginosa. Biofouling 37 (5), 521–537. doi:10.1080/08927014.2021.1933960
Rathinam, P., Vijay Kumar, H. S., and Viswanathan, P. (2017). Eugenol exhibits anti-virulence properties by competitively binding to quorum sensing receptors. Biofouling 33 (8), 624–639. doi:10.1080/08927014.2017.1350655
Rathinam, P., and Viswanathan, P. (2018). Anti-virulence potential of eugenol-rich fraction of Syzygium aromaticum against multidrug resistant uropathogens isolated from catheterized patients. Avicenna J. Phytomed 8 (5), 416–431.
Reddy, P., and Lokesh, B. R. (1994). Studies on anti-inflammatory activity of spice principles and dietary n-3 polyunsaturated fatty acids on carrageenan-lnduced inflammation in rats. Ann. Nutr. Metabolism 38 (6), 349–358. doi:10.1159/000177833
Reichhardt, C., Wong, C., Passos Da Silva, D., Wozniak, D. J., and Parsek, M. R. (2018). CdrA interactions within the Pseudomonas aeruginosa biofilm matrix safeguard it from proteolysis and promote cellular packing. mBio 9 (5), e01376-18. doi:10.1128/mBio.01376-18
Remold, S. K., Brown, C. K., Farris, J. E., Hundley, T. C., Perpich, J. A., and Purdy, M. E. (2011). Differential habitat use and niche partitioning by Pseudomonas species in human homes. Microb. Ecol. 62, 505–517. doi:10.1007/s00248-011-9844-5
Rosi-Marshall, E. J., Kincaid, D. W., Bechtold, H. A., Royer, T. V., Rojas, M., and Kelly, J. J. (2013). Pharmaceuticals suppress algal growth and microbial respiration and alter bacterial communities in stream biofilms. Ecol. Appl. 23 (3), 583–593. doi:10.1890/12-0491.1
Roudashti, S., Zeighami, H., Mirshahabi, H., Bahari, S., Soltani, A., and Haghi, F. (2017). Synergistic activity of sub-inhibitory concentrations of curcumin with ceftazidime and ciprofloxacin against Pseudomonas aeruginosa quorum sensing related genes and virulence traits. World J. Microbiol. Biotechnol. 33 (3), 50. doi:10.1007/s11274-016-2195-0
Rudrappa, T., and Bais, H. P. (2008). Curcumin, a known phenolic from Curcuma longa, attenuates the virulence of Pseudomonas aeruginosa PAO1 in whole plant and animal pathogenicity models. J. Agric. Food Chem. 56 (6), 1955–1962. doi:10.1021/jf072591j
Ryan, R. P., Fouhy, Y., Lucey, J. F., Crossman, L. C., Spiro, S., He, Y.-W., et al. (2006). Cell–cell signaling in Xanthomonas campestris involves an HD-GYP domain protein that functions in cyclic di-GMP turnover. Proc. Natl. Acad. Sci. 103 (17), 6712–6717. doi:10.1073/pnas.0600345103
Sadeghi Mohammadi, S., Vaezi, Z., and Naderi-Manesh, H. (2022). Improvement of anti-biofilm activities via co-delivery of curcumin and gentamicin in lipid-polymer hybrid nanoparticle. J. Biomater. Sci. Polym. Ed. 33 (2), 174–196. doi:10.1080/09205063.2021.1982159
Salehi, B., Mishra, A. P., Shukla, I., Sharifi-Rad, M., Contreras, M. D. M., Segura-Carretero, A., et al. (2018). Thymol, thyme, and other plant sources: health and potential uses. Phytother. Res. 32 (9), 1688–1706. doi:10.1002/ptr.6109
Salman, A. S. (2022). Antibacterial effect of onion‘s infusion and garlic‘s infusion on Escherichia coli isolated from urine samples. science 10, 11. doi:10.57238/jbb.2022.20107
Sánchez-López, E., Gomes, D., Esteruelas, G., Bonilla, L., Lopez-Machado, A. L., Galindo, R., et al. (2020). Metal-based nanoparticles as antimicrobial agents: an overview. Nanomater. Basel 10 (2), 292. doi:10.3390/nano10020292
Sarjit, A., Wang, Y., and Dykes, G. A. (2015). Antimicrobial activity of gallic acid against thermophilic Campylobacter is strain specific and associated with a loss of calcium ions. Food Microbiol. 46, 227–233. doi:10.1016/j.fm.2014.08.002
Sawant, S., Baldwin, T. C., Khan, H., and Rahman, A. (2022). Evaluation of the effect of leaf development in Plectranthus amboinicus L. On antimicrobial activity and virulence factors of Pseudomonas aeruginosa PAO1 and Staphylococcus aureus NCTC8325. Curr. Microbiol. 80 (1), 24. doi:10.1007/s00284-022-03126-7
Scandorieiro, S., Teixeira, F., Nogueira, M. C. L., Panagio, L. A., De Oliveira, A. G., Durán, N., et al. (2023). Antibiofilm effect of biogenic silver nanoparticles combined with oregano derivatives against carbapenem-resistant Klebsiella pneumoniae. Antibiot. Basel 12 (4), 756. doi:10.3390/antibiotics12040756
Schuster, M., Lostroh, C. P., Ogi, T., and Greenberg, E. P. (2003). Identification, timing, and signal specificity of Pseudomonas aeruginosa quorum-controlled genes: a transcriptome analysis. J. Bacteriol. 185 (7), 2066–2079. doi:10.1128/jb.185.7.2066-2079.2003
Sethupathy, S., Prasath, K. G., Ananthi, S., Mahalingam, S., Balan, S. Y., and Pandian, S. K. (2016). Proteomic analysis reveals modulation of iron homeostasis and oxidative stress response in Pseudomonas aeruginosa PAO1 by curcumin inhibiting quorum sensing regulated virulence factors and biofilm production. J. Proteomics 145, 112–126. doi:10.1016/j.jprot.2016.04.019
Shafiei, M., Abdi-Ali, A., Shahcheraghi, F., Vali, H., Shahbani Zahiri, H., and Akbari Noghabi, K. (2017). Analysis of Pseudomonas aeruginosa PAO1 biofilm protein profile after exposure to n-butanolic cyclamen coum extract alone and in combination with ciprofloxacin. Appl. Biochem. Biotechnol. 182 (4), 1444–1457. doi:10.1007/s12010-017-2409-4
Shah, S., Gaikwad, S., Nagar, S., Kulshrestha, S., Vaidya, V., Nawani, N., et al. (2019). Biofilm inhibition and anti-quorum sensing activity of phytosynthesized silver nanoparticles against the nosocomial pathogen Pseudomonas aeruginosa. Biofouling 35 (1), 34–49. doi:10.1080/08927014.2018.1563686
Shao, Y., Wu, C., Wu, T., Li, Y., Chen, S., Yuan, C., et al. (2018). Eugenol-chitosan nanoemulsions by ultrasound-mediated emulsification: formulation, characterization and antimicrobial activity. Carbohydr. Polym. 193, 144–152. doi:10.1016/j.carbpol.2018.03.101
Shariati, A., Asadian, E., Fallah, F., Azimi, T., Hashemi, A., Yasbolaghi Sharahi, J., et al. (2019). Evaluation of Nano-curcumin effects on expression levels of virulence genes and biofilm production of multidrug-resistant Pseudomonas aeruginosa isolated from burn wound infection in Tehran, Iran. Infect. Drug Resist 12, 2223–2235. doi:10.2147/idr.s213200
Shariati, A., Didehdar, M., Razavi, S., Heidary, M., Soroush, F., and Chegini, Z. (2022). Natural compounds: a hopeful promise as an antibiofilm agent against Candida species. Front. Pharmacol. 13, 917787. doi:10.3389/fphar.2022.917787
Shariff, M., Chatterjee, M., Morris, S. D., Paul, V., Vasudevan, A. K., Mohan, C. G., et al. (2022). Enhanced inhibition of Pseudomonas aeruginosa virulence factor production and biofilm development by sublethal concentrations of eugenol and phenyllactic acid. Lett. Appl. Microbiol. 75 (5), 1336–1345. doi:10.1111/lam.13803
Sharifian, P., Yaslianifard, S., Fallah, P., Aynesazi, S., Bakhtiyari, M., and Mohammadzadeh, M. (2020). Investigating the effect of nano-curcumin on the expression of biofilm regulatory genes of Pseudomonas aeruginosa. Infect. Drug Resist 13, 2477–2484. doi:10.2147/idr.s263387
Sharifi-Rad, M., Varoni, E. M., Iriti, M., Martorell, M., Setzer, W. N., Del Mar Contreras, M., et al. (2018). Carvacrol and human health: a comprehensive review. Phytother. Res. 32 (9), 1675–1687. doi:10.1002/ptr.6103
Shaw, E., and Wuest, W. M. (2020). Virulence attenuating combination therapy: a potential multi-target synergy approach to treat Pseudomonas aeruginosa infections in cystic fibrosis patients. RSC Med. Chem. 11 (3), 358–369. doi:10.1039/c9md00566h
Shukla, A., Parmar, P., Rao, P., Goswami, D., and Saraf, M. (2020). Twin peaks: presenting the antagonistic molecular interplay of curcumin with LasR and LuxR quorum sensing pathways. Curr. Microbiol. 77 (8), 1800–1810. doi:10.1007/s00284-020-01997-2
Shukla, A., Shukla, G., Parmar, P., Patel, B., Goswami, D., and Saraf, M. (2021). Exemplifying the next generation of antibiotic susceptibility intensifiers of phytochemicals by LasR-mediated quorum sensing inhibition. Sci. Rep. 11 (1), 22421. doi:10.1038/s41598-021-01845-8
Silva, E. C. I., Pratavieira, S., Salvador Bagnato, V., and Alves, F. (2023). Sonophotodynamic inactivation of Pseudomonas aeruginosa biofilm mediated by curcumin. Biofouling 39 (6), 606–616. doi:10.1080/08927014.2023.2241385
Smith, R. S., and Iglewski, B. H. (2003). Pseudomonas aeruginosa quorum sensing as a potential antimicrobial target. J. Clin. Invest. 112 (10), 1460–1465. doi:10.1172/jci20364
Spoering, A. L., and Lewis, K. (2001). Biofilms and planktonic cells of Pseudomonas aeruginosa have similar resistance to killing by antimicrobials. J. Bacteriol. 183 (23), 6746–6751. doi:10.1128/JB.183.23.6746-6751.2001
Sreelatha, S., Kumar, N., Yin, T. S., and Rajani, S. (2021). Evaluating the antibacterial activity and mode of action of thymol-loaded chitosan nanoparticles against plant bacterial pathogen xanthomonas campestris pv. campestris. Front. Microbiol. 12, 792737. doi:10.3389/fmicb.2021.792737
S'thebe, N. W., Aribisala, J. O., and Sabiu, S. (2023). Cheminformatics bioprospection of sunflower seeds' oils against quorum sensing system of Pseudomonas aeruginosa. Antibiot. Basel. 12 (3), 504. doi:10.3390/antibiotics12030504
Taganna, J. C., Quanico, J. P., Perono, R. M., Amor, E. C., and Rivera, W. L. (2011). Tannin-rich fraction from Terminalia catappa inhibits quorum sensing (QS) in Chromobacterium violaceum and the QS-controlled biofilm maturation and LasA staphylolytic activity in Pseudomonas aeruginosa. J. Ethnopharmacol. 134 (3), 865–871. doi:10.1016/j.jep.2011.01.028
Tang, H. B., Dimango, E., Bryan, R., Gambello, M., Iglewski, B. H., Goldberg, J. B., et al. (1996). Contribution of specific Pseudomonas aeruginosa virulence factors to pathogenesis of pneumonia in a neonatal mouse model of infection. Infect. Immun. 64 (1), 37–43. doi:10.1128/iai.64.1.37-43.1996
Tanhay Mangoudehi, H., Zamani, H., Shahangian, S. S., and Mirzanejad, L. (2020). Effect of curcumin on the expression of ahyI/R quorum sensing genes and some associated phenotypes in pathogenic Aeromonas hydrophila fish isolates. World J. Microbiol. Biotechnol. 36 (5), 70. doi:10.1007/s11274-020-02846-x
Tapia-Rodriguez, M. R., Bernal-Mercado, A. T., Gutierrez-Pacheco, M. M., Vazquez-Armenta, F. J., Hernandez-Mendoza, A., Gonzalez-Aguilar, G. A., et al. (2019). Virulence of Pseudomonas aeruginosa exposed to carvacrol: alterations of the quorum sensing at enzymatic and gene levels. J. Cell Commun. Signal 13 (4), 531–537. doi:10.1007/s12079-019-00516-8
Targhi, A. A., Moammeri, A., Jamshidifar, E., Abbaspour, K., Sadeghi, S., Lamakani, L., et al. (2021). Synergistic effect of curcumin-Cu and curcumin-Ag nanoparticle loaded niosome: enhanced antibacterial and anti-biofilm activities. Bioorg Chem. 115, 105116. doi:10.1016/j.bioorg.2021.105116
Topa, S. H., Palombo, E. A., Kingshott, P., and Blackall, L. L. (2020). Activity of Cinnamaldehyde on quorum Sensing and biofilm susceptibility to antibiotics in Pseudomonas aeruginosa. Microorganisms 8 (3), 455. doi:10.3390/microorganisms8030455
Topa, S. H., Subramoni, S., Palombo, E. A., Kingshott, P., Rice, S. A., and Blackall, L. L. (2018). Cinnamaldehyde disrupts biofilm formation and swarming motility of Pseudomonas aeruginosa. Microbiology 164 (9), 1087–1097. doi:10.1099/mic.0.000692
Ultee, A., Bennik, M. H., and Moezelaar, R. (2002). The phenolic hydroxyl group of carvacrol is essential for action against the food-borne pathogen Bacillus cereus. Appl. Environ. Microbiol. 68 (4), 1561–1568. doi:10.1128/aem.68.4.1561-1568.2002
Valliammai, A., Selvaraj, A., Yuvashree, U., Aravindraja, C., and Karutha Pandian, S. (2020). sarA-dependent antibiofilm activity of thymol enhances the antibacterial efficacy of rifampicin against Staphylococcus aureus. Front. Microbiol. 11, 1744. doi:10.3389/fmicb.2020.01744
Vandeputte, O. M., Kiendrebeogo, M., Rajaonson, S., Diallo, B., Mol, A., El Jaziri, M., et al. (2010). Identification of catechin as one of the flavonoids from Combretum albiflorum bark extract that reduces the production of quorum-sensing-controlled virulence factors in Pseudomonas aeruginosa PAO1. Appl. Environ. Microbiol. 76 (1), 243–253. doi:10.1128/aem.01059-09
Velázquez-Carriles, C., Macías-Rodríguez, M. E., Ramírez-Alvarado, O., Corona-González, R. I., Macías-Lamas, A., García-Vera, I., et al. (2022). Nanohybrid of thymol and 2D simonkolleite enhances inhibition of bacterial growth, biofilm formation, and free radicals. Molecules 27 (19), 6161. doi:10.3390/molecules27196161
Wagner, V. E., Bushnell, D., Passador, L., Brooks, A. I., and Iglewski, B. H. (2003). Microarray analysis of Pseudomonas aeruginosa quorum-sensing regulons: effects of growth phase and environment. J. Bacteriol. 185 (7), 2080–2095. doi:10.1128/jb.185.7.2080-2095.2003
Walczak, M., Michalska-Sionkowska, M., Olkiewicz, D., Tarnawska, P., and Warżyńska, O. (2021). Potential of carvacrol and thymol in reducing biofilm formation on technical surfaces. Molecules 26 (9), 2723. doi:10.3390/molecules26092723
Walsh, D. J., Livinghouse, T., Goeres, D. M., Mettler, M., and Stewart, P. S. (2019a). Antimicrobial activity of naturally occurring phenols and derivatives against biofilm and planktonic bacteria. Front. Chem. 7, 653. doi:10.3389/fchem.2019.00653
Walsh, D. J., Livinghouse, T., Goeres, D. M., Mettler, M., and Stewart, P. S. (2019b). Antimicrobial activity of naturally occurring phenols and derivatives against biofilm and planktonic bacteria. Front. Chem. 7, 653. doi:10.3389/fchem.2019.00653
Wang, W. Q., Feng, X. C., Shi, H. T., Wang, Y. M., Jiang, C. Y., Xiao, Z. J., et al. (2023). Biofilm inhibition based on controlling the transmembrane transport and extracellular accumulation of quorum sensing signals. Environ. Res. 221, 115218. doi:10.1016/j.envres.2023.115218
Wijesinghe, G. K., De Oliveira, T. R., Maia, F. C., De Feiria, S. B., Barbosa, J. P., Joia, F., et al. (2021a). Efficacy of true cinnamon (Cinnamomum verum) leaf essential oil as a therapeutic alternative for Candida biofilm infections. Iran. J. Basic Med. Sci. 24 (6), 787–795. doi:10.22038/ijbms.2021.53981.12138
Wijesinghe, G. K., Feiria, S. B., Maia, F. C., Oliveira, T. R., Joia, F., Barbosa, J. P., et al. (2021b). In-vitro antibacterial and antibiofilm activity of cinnamomum verum leaf oil against Pseudomonas aeruginosa, Staphylococcus aureus and Klebsiella pneumoniae. Acad Bras Cienc 93 (1), e20201507. doi:10.1590/0001-3765202120201507
Wira Septama, A., Arvia Chiara, M., Turnip, G., Nur Tasfiyati, A., Triana Dewi, R., Anggrainy Sianipar, E., et al. (2023). Essential oil of zingiber cassumunar roxb. And zingiber officinale rosc.: a comparative study on chemical constituents, antibacterial activity, biofilm formation, and inhibition of Pseudomonas aeruginosa quorum sensing system. Chem. Biodivers. 20 (6), e202201205. doi:10.1002/cbdv.202201205
Xue, B., Huang, J., Zhang, H., Li, B., Xu, M., Zhang, Y., et al. (2020). Micronized curcumin fabricated by supercritical CO(2) to improve antibacterial activity against Pseudomonas aeruginosa. Artif. Cells Nanomed Biotechnol. 48 (1), 1135–1143. doi:10.1080/21691401.2020.1815755
Yan, C., Kim, S. R., Ruiz, D. R., and Farmer, J. R. (2022). Microencapsulation for food applications: a review. ACS Appl. Bio Mater 5 (12), 5497–5512. doi:10.1021/acsabm.2c00673
Zangirolami, A. C., Dias, L. D., Blanco, K. C., Vinagreiro, C. S., Inada, N. M., Arnaut, L. G., et al. (2020). Avoiding ventilator-associated pneumonia: curcumin-functionalized endotracheal tube and photodynamic action. Proc. Natl. Acad. Sci. U. S. A. 117 (37), 22967–22973. doi:10.1073/pnas.2006759117
Zhang, Y., Sass, A., Van Acker, H., Wille, J., Verhasselt, B., Van Nieuwerburgh, F., et al. (2018). Coumarin reduces virulence and biofilm formation in Pseudomonas aeruginosa by affecting quorum sensing, type III secretion and C-di-GMP levels. Front. Microbiol. 9, 1952. doi:10.3389/fmicb.2018.01952
Zhang, Y., Zhu, Y., Gupta, A., Huang, Y., Murray, C. K., Vrahas, M. S., et al. (2014). Antimicrobial blue light therapy for multidrug-resistant Acinetobacter baumannii infection in a mouse burn model: implications for prophylaxis and treatment of combat-related wound infections. J. Infect. Dis. 209 (12), 1963–1971. doi:10.1093/infdis/jit842
Zhong, L., Ravichandran, V., Zhang, N., Wang, H., Bian, X., Zhang, Y., et al. (2020). Attenuation of Pseudomonas aeruginosa quorum sensing by natural products: virtual screening, evaluation and biomolecular interactions. Int. J. Mol. Sci. 21 (6), 2190. doi:10.3390/ijms21062190
Zhou, L., Zheng, H., Tang, Y., Yu, W., and Gong, Q. (2013). Eugenol inhibits quorum sensing at sub-inhibitory concentrations. Biotechnol. Lett. 35 (4), 631–637. doi:10.1007/s10529-012-1126-x
Keywords: natural compounds, P. aeruginosa, quorum sensing, biofilm, curcumin
Citation: Shariati A, Noei M, Askarinia M, Khoshbayan A, Farahani A and Chegini Z (2024) Inhibitory effect of natural compounds on quorum sensing system in Pseudomonas aeruginosa: a helpful promise for managing biofilm community. Front. Pharmacol. 15:1350391. doi: 10.3389/fphar.2024.1350391
Received: 05 December 2023; Accepted: 19 March 2024;
Published: 02 April 2024.
Edited by:
Rizwan Shahid, Alberta Health Services, CanadaReviewed by:
Carlos R. Pungitore, National Scientific and Technical Research Council (CONICET), ArgentinaViviane de Cássia Oliveira, University of São Paulo, Brazil
Copyright © 2024 Shariati, Noei, Askarinia, Khoshbayan, Farahani and Chegini. This is an open-access article distributed under the terms of the Creative Commons Attribution License (CC BY). The use, distribution or reproduction in other forums is permitted, provided the original author(s) and the copyright owner(s) are credited and that the original publication in this journal is cited, in accordance with accepted academic practice. No use, distribution or reproduction is permitted which does not comply with these terms.
*Correspondence: Aref Shariati, arefshariati0111@gmail.com; Zahra Chegini, parisa.chegini72@gmail.com