- 1Department of Public Health, Pharmacology, and Toxicology, University of Nairobi, Nairobi, Kenya
- 2Department of Medical Laboratory Science, Jomo Kenyatta University of Agriculture and Technology, Nairobi, Kenya
- 3Department of Veterinary Pathology, Microbiology, and Parasitology, University of Nairobi, Nairobi, Kenya
- 4Department of Chemistry, School of Physical and Biological Sciences, University of Nairobi, Nairobi, Kenya
- 5Department of Pharmacology and Toxicology, Moi University, Eldoret, Kenya
Background: The present study investigated the efficacy of Conyza bonariensis, Commiphora africana, Senna obtusifolia, Warburgia ugandensis, Vernonia glabra, and Zanthoxylum usambarense against Bitis arietans venom (BAV), Naja ashei venom (NAV), and Naja subfulva venom (NSV).
Methods: 40 extracts and fractions were prepared using n-hexane, dichloromethane, ethyl acetate, and methanol. In vitro efficacy against snake venom phospholipase A2 (svPLA2) was determined in 96-well microtiter and agarose-egg yolk coagulation assays. in vivo efficacy against venom-induced cytotoxicity was determined using Artemia salina. Two commercial antivenoms were used for comparison.
Results: The 96-well microtiter assay revealed poor svPLA2 inhibition of BAV by antivenom (range: 20.76% ± 13.29% to 51.29% ± 3.26%) but strong inhibition (>90%) by dichloromethane and hexane fractions of C. africana, hexane and ethyl acetate extracts and fraction of W. ugandensis, dichloromethane fraction of V. glabra, and the methanol extract of S. obtusifolia. The methanol extract and fraction of C. africana, and the hexane extract of Z. usambarense strongly inhibited (>90%) svPLA2 activity in NAV. The hexane and ethyl acetate fractions of V. glabra and the dichloromethane, ethyl acetate, and methanol extracts of C. africana strongly inhibited (>90%) svPLA2 in NSV. The agarose egg yolk coagulation assay showed significant inhibition of BAV by the dichloromethane fraction of C. africana (EC50 = 3.51 ± 2.58 μg/mL), significant inhibition of NAV by the methanol fraction of C. africana (EC50 = 7.35 ± 1.800 μg/mL), and significant inhibition of NSV by the hexane extract of V. glabra (EC50 = 7.94 ± 1.50 μg/mL). All antivenoms were non-cytotoxic in A. salina but the methanol extract of C. africana and the hexane extracts of V. glabra and Z. usambarense were cytotoxic. The dichloromethane fraction of C. africana significantly neutralized BAV-induced cytotoxicity, the methanol fraction and extract of C. africana neutralized NAV-induced cytotoxicity, while the ethyl acetate extract of V. glabra significantly neutralized NSV-induced cytotoxicity. Glycosides, flavonoids, phenolics, and tannins were identified in the non-cytotoxic extracts/fractions.
Conclusion: These findings validate the local use of C. africana and V. glabra in snakebite but not C. bonariensis, S. obtusifolia, W. ugandensis, and Z. usambarense. Further work is needed to isolate pure compounds from the effective plants and identify their mechanisms of action.
Introduction
An estimated 5 million people are bitten by snakes every year, about half of whom experience clinical illness, and up to 140,000 die from complications related to envenomation (Chippaux, 1998; Kasturiratne et al., 2008). Snakebites are prevalent among low-income individuals residing in rural, tropical areas with limited access to healthcare (Oliveira et al., 2023). Consequently, local people frequently rely on folk medicine, which includes the use of medicinal plants. Several such plants, including C. bonariensis, C. africana, S. obtusifolia, Warburgia ugandensis, Vernonia glabra, and Zanthoxylum usambarense have gained notoriety among the Luo people in Kisumu, Kenya, due to their putative anti-snake venom properties (Owuor et al., 2005; Owuor and Kisangau, 2006). These plants are known by the locals as “yadh asere” (C. bonariensis), “arupiny” (C. africana), “olusia” (V. glabra), “sogo” (W. ugandensis), and “roko” (Z. usambarense). They have widespread ethnomedicinal use locally including in snakebite and share phylogenetic relationships with plants previously reported as anti-snake bite remedies, e.g., Senna siamea, Conyza sumatrensis, and Zanthoxylum chalybeum (Owuor and Kisangau, 2006). Treatments include the use of cut, suck, and bind techniques, followed by the application of plant leaf and root poultices secured with bark or cloth strips (Owuor et al., 2005). However, there is a general concern about the efficacy and safety of alternative remedies in managing diseases (Puzari et al., 2022). Rigorous scientific scrutiny of these remedies is essential to determine the validity of the ethnomedicinal claims and to ensure the development of safe and efficacious interventions for snakebite victims (Puzari et al., 2022).
B. arietans, N. ashei, and N. subfulva are snakes of medical importance in sub-Saharan Africa (Calvete et al., 2007; Currier, 2012; Tasoulis and Isbister, 2017; Onyango, 2018; Okumu et al., 2020; Dyba et al., 2021) (Figure 1). Antivenom is the mainstay of treatment for envenomation by these snakes but is expensive, has limited availability, and does not sufficiently neutralize some key venom toxins, e.g., cytotoxins which cause dermonecrosis in snake bite victims. Medicinal plants are used to plug this gap, but they lack scientific validity. This study employed a combination of in vitro and in vivo methods to evaluate the antivenom properties of C. bonariensis, C. africana, S. obtusifolia, W. ugandensis, V. glabra, and Z. usambarense against B. arietans, N. ashei, and N. subfulva venoms.
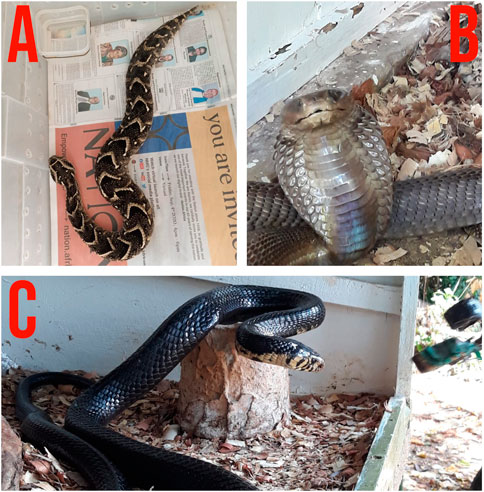
Figure 1. Photos of Bitis arietans (A), Naja ashei (B), and Naja subfulva (C). Photos by Mitchel Okumu.
Materials and methods
Collection and identification of medicinal plants
Plant materials were collected in November 2016 in Kisumu County. The East African Herbarium in Nairobi, Kenya identified and verified the plant specimens, as shown in (Supplementary Figure S1) (Supplementary Section). REF NMK/BOT/CTX/1/2/1. The selection of the plants was based on five factors: 1) their extensive local ethnopharmacological use in treating snakebites; 2) their evolutionary link to other plants used for the same purpose; 3) the findings of an Owuor and Kisangau survey on the use and practice of herbal medicine (Owuor and Kisangau, 2006), 4) the lack of published research outlining the plants’ bioactive ingredients, and 5) their availability for evaluation. An overview of the plants used in this study is as shown in (Figure 2).
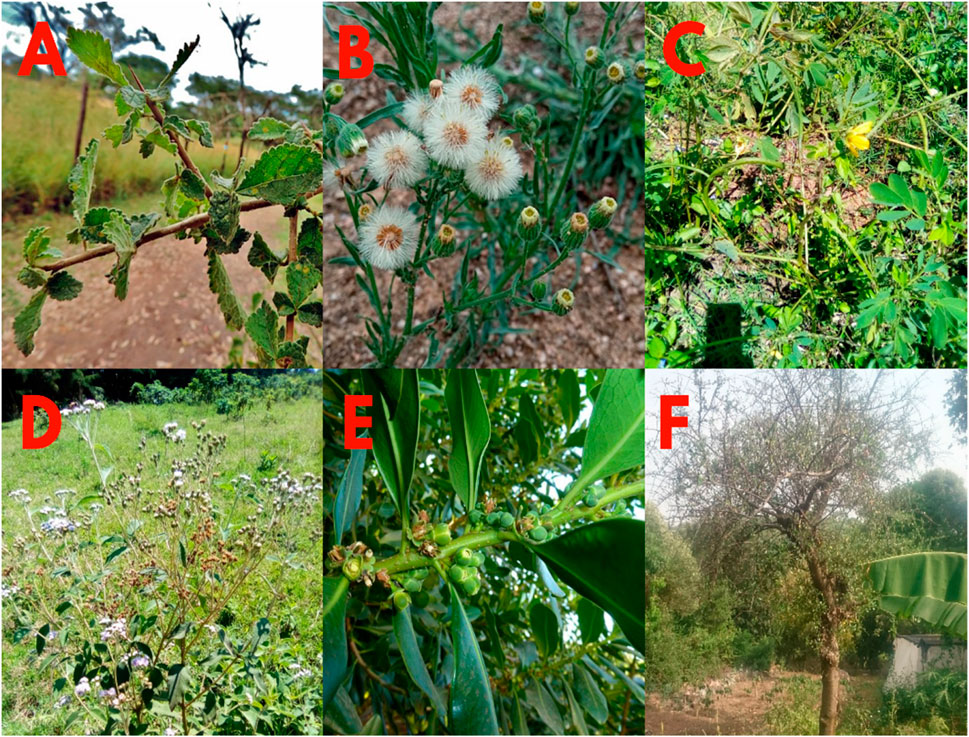
Figure 2. Photos of Commiphora africana (A. Rich) Engl (A), Conyza bonariensis (L.)., Cronquist (B), Senna obtusifolia (L.) Irwin and Barneby (C), Vernonia glabra (Streetz) (D), Warburgia ugandensis (Sprague) (E), and Zanthoxylum usambarense (Engl.) Kokwaro (F) used in this study.
Preparation of plant material
After being cleaned to get rid of any dust that stuck to them, the plant materials were shade-dried and then ground into a powder using an electric mill (Retsch Grindomax, Germany).
Chemical and reagents
n-hexane, dichloromethane, ethyl acetate, and methanol were purchased from Loba Chemie (India). Phosphate buffered saline (PBS) tablets, Calcium chloride, Fuchsin acid (Carbol Fuchsin), gallic acid, catechin, agarose, and rutin were bought from Sigma Aldrich (USA). Sodium carbonate, Folin-phenol reagent, Folin-Denis reagent, aluminum chloride, sodium hydroxide pellets, lead acetate, Sodium hydrogen phosphate, and picric acid were bought from FINAR (India). The antivenoms used in this study were manufactured in India and Mexico.
Soxhlet extraction of the medicinal plants
Powdered plant materials were sequentially extracted by Soxhlet extraction using n-hexane, dichloromethane, ethyl acetate, and methanol and concentrated under reduced pressure at 40°C on a rotary evaporator (Stuart, Cole-Parmer-UK) (Janardhan et al., 2014). The percentage yield of the extracts was calculated as %w/w.
Extraction of medicinal plants using a modified maceration technique
Powdered plant materials were separately mixed with methanol, macerated for 72 h, and concentrated at 40°C under reduced pressure on a rotary evaporator (Stuart, Cole-Parmer-UK). The methanol extracts were separated into four parts, distributed in de-ionized water, partitioned sequentially with n-hexane, dichloromethane, ethyl acetate, and concentrated under reduced pressure at 40°C on a rotary evaporator (Stuart, Cole-Parmer-UK) (Alsayari et al., 2018). The percentage yield of the extracts was calculated as %w/w.
Ethics
The biosafety, animal care, and use committee of the University of Nairobi was consulted before the authors handled any experimental animal, as shown in Supplementary Figure S2 (Supplementary section) (REF BAUEC/2019/220).
Snake venom
Nine specimens of the large brown spitting cobras (N. ashei), Eastern Forest cobras (N. subfulva) and puff adders (B. arietans) were collected in the wild and identified by a herpetologist at Bioken snake farm, Kenya. Venom was collected from these snakes using the beaker method, snap frozen, lyophilized (Labconco, USA), and kept as a powder at −20°C until it was reconstituted in phosphate buffered saline.
Determination of the in vitro anti-snake venom phospholipase A2 activity of the prepared extracts
The 96-well microtiter plate assay
The methods of Iwanaga and Suzuki (Iwanaga and Suzuki, 1979) and Molander and colleagues (Molander et al., 2014) were used. 10 μL of a 10 μg/mL concentration of each of the venoms (in 0.1 M phosphate buffered saline) and 20 µL of a 100 μg/mL concentration of each of the prepared extracts were micro pipetted (Finnpipette, Thermo Fisher Scientific, USA) into 96-well microtiter plates (Costar®3590, USA) before 200 µL of a 1.1% egg yolk suspension in 0.1 M PBS adjusted to pH 8.1 and 0.2 mM CaCl2 was added to each well, and the absorbance of the mixtures was taken at 620 nm on a multi plate reader (Thermo Fisher Multiskan, USA). The plates were incubated (Memmert, Germany) at 37°C for 20 min and the absorbance measured again at 620 nm. svPLA2 activity was measured as the decrease in turbidity of the egg yolk suspension from 0 to 20 min. The inhibition of svPLA2 activity by the extract was expressed as percentage inhibition of enzymatic activity taking the absorbance of a well to which no venom was added as 100%. Extracts were tested in triplicate and antivenom was used as a positive reference.
The agarose-egg yolk coagulation assay
Extracts with >90% inhibition of the svPLA2 activity in the aforementioned assay were further evaluated in the agarose egg yolk coagulation assay described by Habermann and Hardt (Habermann and Hardt, 1972) as follows.
1. Group I (Venom only group): 10 µL of graded (0.5 μg/mL to 10.0 μg/mL) dilutions of venom only.
2. Group II (Venom + extract/fraction mixture group): Pre-incubated mixture of 10 μL of venom (0.5 μg/mL to 10.0 μg/mL) + 20 µL of a 100 μg/mL concentration of each of the extracts/fractions.
3. Group III (Venom + antivenom): Pre-incubated mixture of 10 μL of venom (0.5 μg/mL to 10.0 μg/mL) + 20 µL of a 100 μg/mL concentration of each of the antivenoms.
These mixtures were micro pipetted into 0.5 mm wells on an agarose-egg yolk medium and incubated (Memmert, Germany) at 50°C for 24 h. 10% Carbol Fuchsin was used to visualize the enzymatic halos in each group and the diameter of the enzymatic halos was measured using a digital vernier calliper (Rolson, United Kingdom) and expressed as the minimum phospholipase concentration (MPC) i.e., the least dose of venom which is responsible for an enzymatic halo of 10 mm in the case of BAV and 15 mm in the case of NAV and NSV.
Cytotoxicity of the venoms, extracts, and antivenoms in Artemia salina
The in vivo toxicities of the extracts, venoms, and antivenoms were evaluated in Artemia salina according to the method described by Meyer et al. (1982) with modifications as described by Nguta et al. (2014). This was replicated in 5 different sample tubes for each venom, extract, or antivenom concentration. Physiological buffer saline (1 mL) was used as the negative control and vincristine sulphate was used as the positive control.
Neutralization of Artemia salina venom-induced cytotoxicity by the extracts and antivenom
The WHO pre-incubation neutralization protocol was used and adjusted to A. salina (WHO, 2016). Varying doses of the extracts or antivenom (50 μg/mL, 100 μg/mL, 200 μg/mL, 400 μg/mL, and 800 μg/mL) were incubated (Memmert, Germany) with a 2LC50 dose of each of the venoms at 37°C for 30 min. The resulting mixtures were added to vials containing A. salina and the survivors were counted after 24, 48, and 72 h of exposure. The median effective concentration of the extracts was defined as the minimum amount of extract (in µL) required to neutralize 1 mg of venom.
Initial screening of the extracts for phytochemicals
Standard methods were used for preliminary phytochemical screening of the extracts and fractions (Kokate et al., 2006; Evans, 2009; Kumar et al., 2013). The presence of alkaloids (dragendorrf’s test), anthraquinones, carboxylic acids, cardiac glycosides (keller-killiani test), flavonoids (alkaline reagent test), phenolics (Ferric chloride test), phytosterols, resins, saponins (foam test), tannins (Ferric chloride test), and terpenoids (Salkowski test) were investigated.
Quantitative phytochemical composition
Total phenolics, flavonoids, glycosides, and tannins were estimated using a UV-VIS spectrophotometer (Spectronic 21-D, USA). Analytical grade gallic acid, catechin, and rutin were used as standards.
Determination of total phenolic content (TPC)
The method of Harnafi et al. was used (Harnafi et al., 2008). The extracts/fractions were mixed with 7.5% w/v Na2CO3 solution and 2.5 mL of Folin-Ciocalteau reagent (FINAR, India), and the absorbance was read at 765 nm on a UV-VIS spectrophotometer (Spectronic 21-D, USA) and a gallic acid standard curve was generated. The assay was performed in triplicate and the results were expressed as milligrams of Gallic acid equivalents per Gram of the dry plant material (mg.GAE.g-1).
Determination of total flavonoid content (TFC)
The method of Atanassova et al. was used (Atanassova et al., 2011). The extract/fractions were mixed with distilled water, 5% w/v sodium nitrite (NaNO2), 10% w/v aluminum chloride (AlCl3), and 1 M sodium hydroxide (NaOH), and the absorbance was read on a UV-VIS spectrophotometer (Spectronic 21-D, USA) at 510 nm. The flavonoid content was determined from a catechin standard curve. The assay was performed in triplicate and the results were calculated as milligrams of Catechin equivalents per Gram of the dry plant material (mg. CE. g-1).
Tannin content
The method of Amadi et al. was used (Amadi et al., 2004). The extracts/fractions were boiled gently for 1 h and mixed with 2.5 mL of Folin-Denis reagent, 5 mL of saturated Na2CO3 solution, and 25 mL of distilled water. The mixture was left to stand for 30 min in a water bath (Memmert, Germany) at 25°C and the absorbance was read on a UV-VIS spectrophotometer (Spectronic 21-D, USA) at 700 nm. The tannin content was determined from a tannic acid standard curve. The assay was performed in triplicate and the results were calculated as below:
Where C is concentration of tannic acid read off the graph.
Cardiac glycoside content
The method described by Muhamad and Abubakar (Muhammad and Abubakar, 2016) was used. The extracts/fractions were mixed with distilled water, 12.5% lead acetate, 47% w/v Na2HPO4, and Baljet reagent (95 mL of 1% picric acid+5 mL of 10% NaOH). A blank titration was carried out using 10 mL distilled water and 10 mL Baljet reagent (95 mL of 1% picric acid+5 mL of 10% NaOH). This mixture was allowed to stand for 1 hour and the absorbance was read on a UV-VIS spectrophotometer (Spectronic 21-D, USA) at 495 nm. The percentage (%) of total glycosides present in extracts/fractions was calculated as % of total glycosides= (A×100)/77 g %. Where A = absorbance of samples.
Data analysis
The effect of each of the extracts/fractions/antivenoms on the minimum phospholipase concentration of venom (s) was compared using one way-ANOVA and Dunnet’s multiple comparison test. The lethality of venoms, extracts, fractions, and antivenoms in A. salina and their capacity to neutralize venom-induced cytotoxicity in the same model was analyzed using probit regression analysis. Results on the phytochemical composition of the extracts/fractions were summarized in a table. p < 0.05 was considered significant.
Results
The percentage yield of extracts
The percentage yield of the hexane root extract of C. africana prepared by the Soxhlet method was the lowest (0.23%), while the percentage yield of the dichloromethane leaf extract of V. glabra prepared by the maceration method was the highest (54.65%), as observed in (Supplementary Table S1).
Information on the snakes whose venom was used in the study
Most of the snakes used in this study were sourced from the Watamu area in Kenya. (Supplementary Table S2) in vitro microtiter well svPLA2 neutralization assay.
The microtiter well assay revealed poor (<90%) anti-svPLA2 inhibition of BAV by the tested antivenoms (range: 20.76% ± 13.29% to 51.29% ± 3.26%) but potent (>90%) anti-svPLA2 inhibition of the venom by dichloromethane and hexane fractions of C. africana stem bark, hexane and ethyl acetate extracts and fraction of W. ugandensis leaves, dichloromethane fraction of V. glabra leaves, and the methanol extract of S. obtusifolia leaves.
>90% anti-svPLA2 inhibition was observed against NAV with the methanol extract and fraction of C. africana stem bark, the methanol extract from the C. africana bark, and the hexane extract of Z. usambarense leaves.
>90% anti-svPLA2 inhibition was noted against NSV with hexane and ethyl acetate fractions of V. glabra leaves and dichloromethane, ethyl acetate, and methanol extracts of C. africana bark (Table 1).
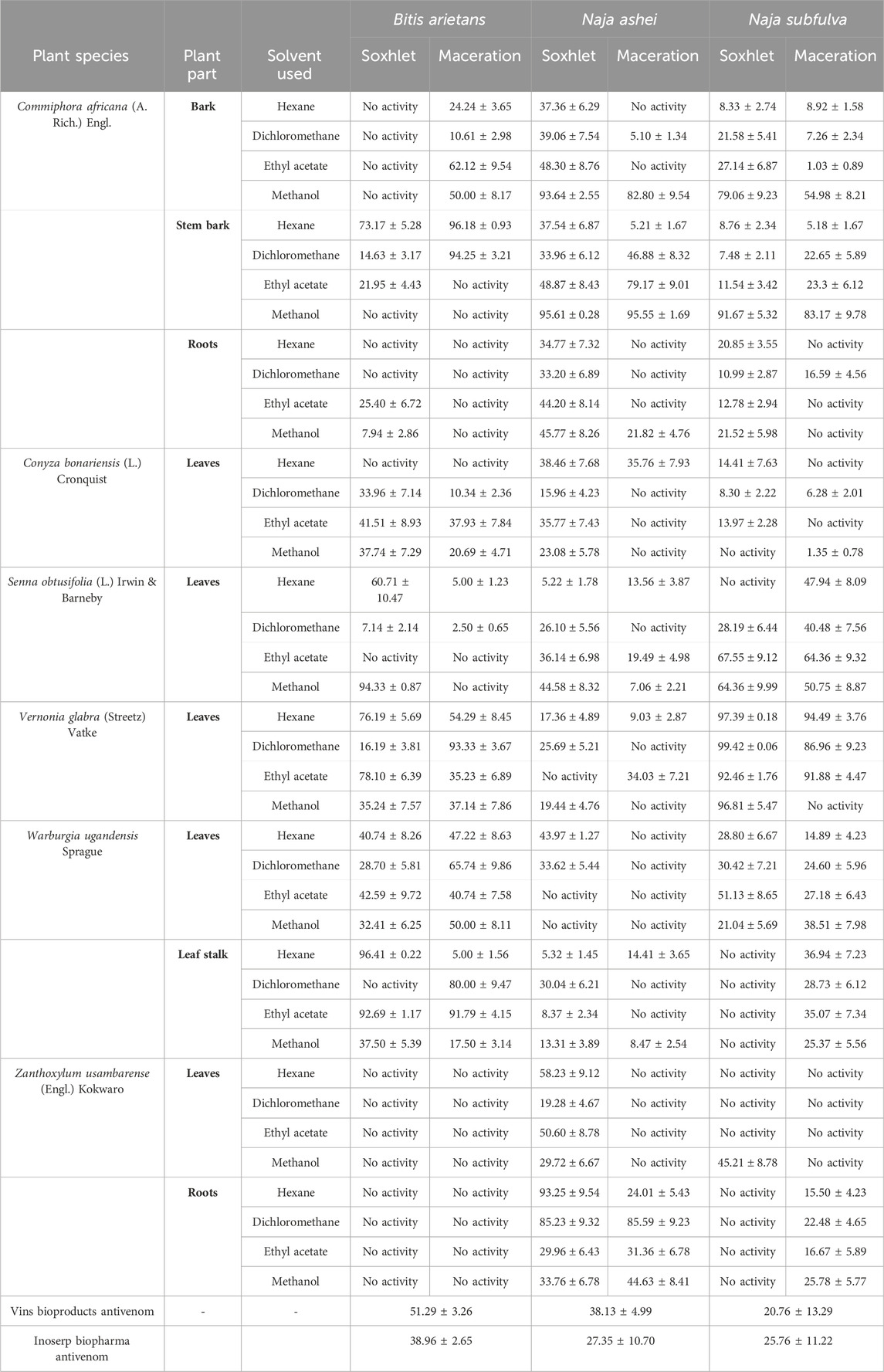
Table 1. The in vitro neutralization capacity of antivenom, extracts, and fractions of Commiphora africana, Conyza bonariensis, Senna obtusifolia, Vernonia glabra, Warburgia ugandensis, and Zanthoxylum usambarense against snake venom phospholipase A2.
In vitro agarose-egg yolk svPLA2 neutralization assay
BAV had a minimum phospholipase concentration (MPC) of 1.102 ± 0.423 μg/mL. When separately incubated with various extracts, fractions, and antivenom, the MPC of the venom ranged from 1.908 ± 0.498 μg/mL to 9.016 ± 0.756 μg/mL. However, the only test substances that significantly inhibited B. arietans venom were Vins bioproducts antivenom, MPC = 9.016 ± 0.756 μg/mL (p < 0.0001) and the dichloromethane fraction of C. africana stem bark, MPC = 3.506 ± 2.560 μg/mL (p = 0.0007) (Figure 3).
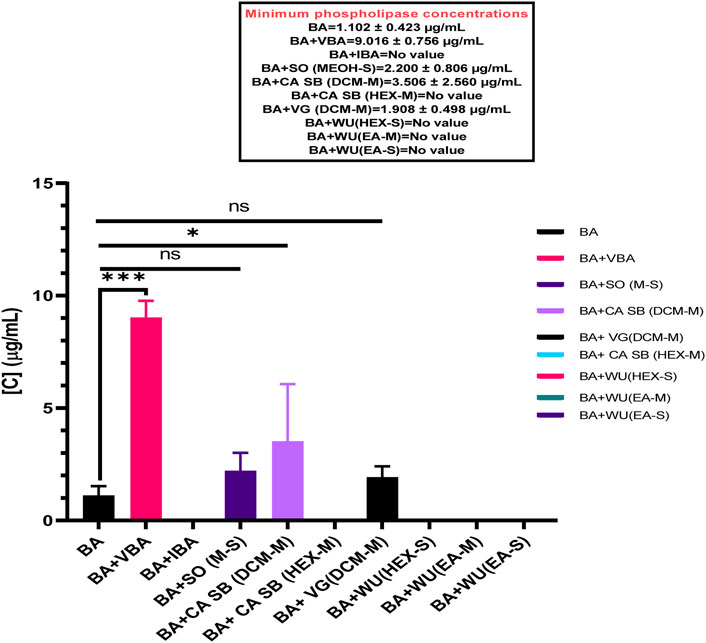
Figure 3. The effects of extracts, fractions, and antivenoms on the minimum phospholipase concentration of Bitis arietans venom. BA: Bitis arietans, VG (DCM-M): Dichloromethane fraction of Vernonia glabra leaves, CA SB (HEX-M): Hexane fraction of Commiphora africana stem bark, CA SB (DCM-M): Dichloromethane fraction of Commiphora africana stem bark. SO (MEOH-S): Methanol extract of Senna obtusifolia leaves, WU (HEX-S): Hexane extract of Warburgia ugandensis leaf stalk, WU (EA-M): Ethyl acetate fraction of Warburgia ugandensis leaf stalk, VBA: Vins bioproducts antivenom, IBA: Inoserp biopharma antivenom.
NAV had an MPC of 1.156 ± 0.148 μg/mL. When separately incubated with various extracts, fractions, and antivenom, the MPC of the venom ranged from 3.586 ± 1.196 μg/mL to 7.348 ± 1.800 μg/mL. All the tested extracts, fractions, and antivenom significantly inhibited the phospholipase A2 activity of N. ashei including the methanol extract of C. africana bark, MPC = 3.586 ± 1.196 μg/mL (p = 0.0343), the hexane extract of Z. usambarense roots, MPC = 3.701 ± 2.344 μg/mL (p = 0.0248), Inoserp biopharma antivenom, MPC = 3.791 ± 1.259 μg/mL (p = 0.0191), the methanol extract of C. africana stem bark, MPC = 4.223 ± 0.289 μg/mL (p = 0.0051), Vins bioproducts antivenom, MPC = 6.332 ± 1.883 μg/mL (p < 0.001), and the methanol fraction of C. africana stem bark, MPC = 7.348 ± 1.800 μg/mL (p < 0.0001). (Figure 4).
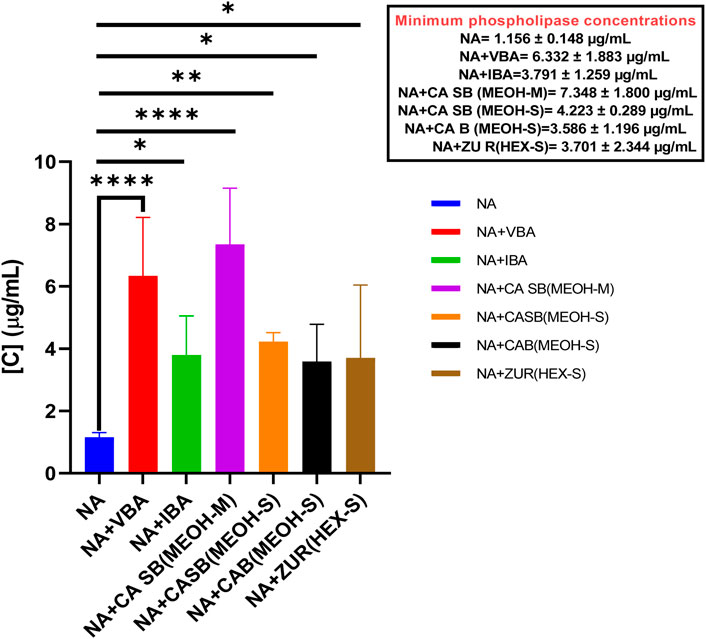
Figure 4. The effects of extracts, fractions, and antivenoms on the minimum phospholipase concentration of Naja ashei venom. NA: Naja ashei, CA B (MEOH-S): Methanol extract of Commiphora africana bark, ZU R (HEX-S): Hexane extract of Zanthoxylum usambarense root, CA SB (MEOH-S): Methanol extract of Commiphora africana stem bark, CA SB (MEOH-M): Methanol extract of Commiphora africana stem bark, VBA: Vins bioproducts antivenom, IBA: Inoserp biopharma antivenom.
NSV venom had an MPC of 1.006 ± 0.249 μg/mL. When separately incubated with various extracts, fractions, and antivenom, the MPC of the venom ranged from 1.210 ± 0.103 μg/mL to 7.936 ± 1.497 μg/mL. However, the only test substances that significantly inhibited Naja subfulva venom were Vins bioproducts antivenom, MPC = 4.563 ± 3.433 μg/mL (p = 0.0049), the ethyl acetate extract of V. glabra leaves, MPC = 6.578 ± 2.374 μg/mL, the hexane extract of V. glabra leaves, MPC = 7.936 ± 1.497 μg/mL (p < 0.0001), and the methanol extract of C. africana stem bark, MPC = 5.192 ± 0.25 μg/mL (p = 0.0022) (Figure 5).
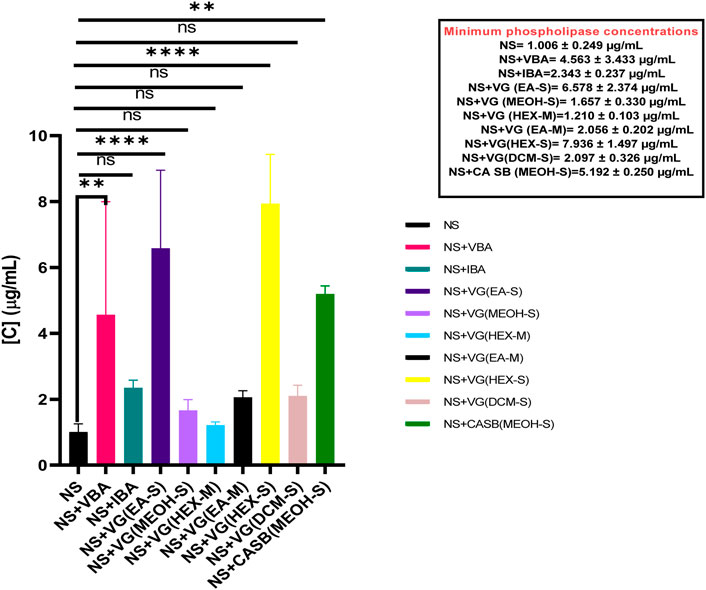
Figure 5. The effects of extracts, fractions, and antivenoms on the minimum phospholipase concentration of Naja subfulva venom in the snake venom phospholipase A2 agarose-egg yolk assay. NS: Naja subfulva, VG (DCM-S): Dichloromethane extract of Vernonia glabra leaves, VG (HEX-S): Hexane extract of Vernonia glabra leaves, VG (EA-M), Ethyl acetate fraction of Vernonia glabra, VG (HEX-M): Hexane fraction of Vernonia glabra, VG (MEOH-S): Methanol extract of Vernonia glabra leaves, VG (EA-S): Ethyl acetate extract of Vernonia glabra leaves, CA SB (MEOH-S): Methanol extract of Commiphora africana stem bark, VBA: Vins bioproducts antivenom, IA: Inoserp antivenom.
Cytotoxicity of the extracts, fractions, and antivenom in Artemia salina
The methanol extract of C. africana stem bark, the hexane extracts of V. glabra leaves and Z. usambarense leaf stalk were cytotoxic to A. salina with LC50 values of 611.72 (251.06-3437.50) µg/mL, 0.04 μg/mL, and 31.54 (22.50-44.03) µg/mL respectively whereas the methanol stem bark fraction of C. africana, Vins bioproducts antivenom, and Inoserp antivenom were the least cytotoxic (Table 2).
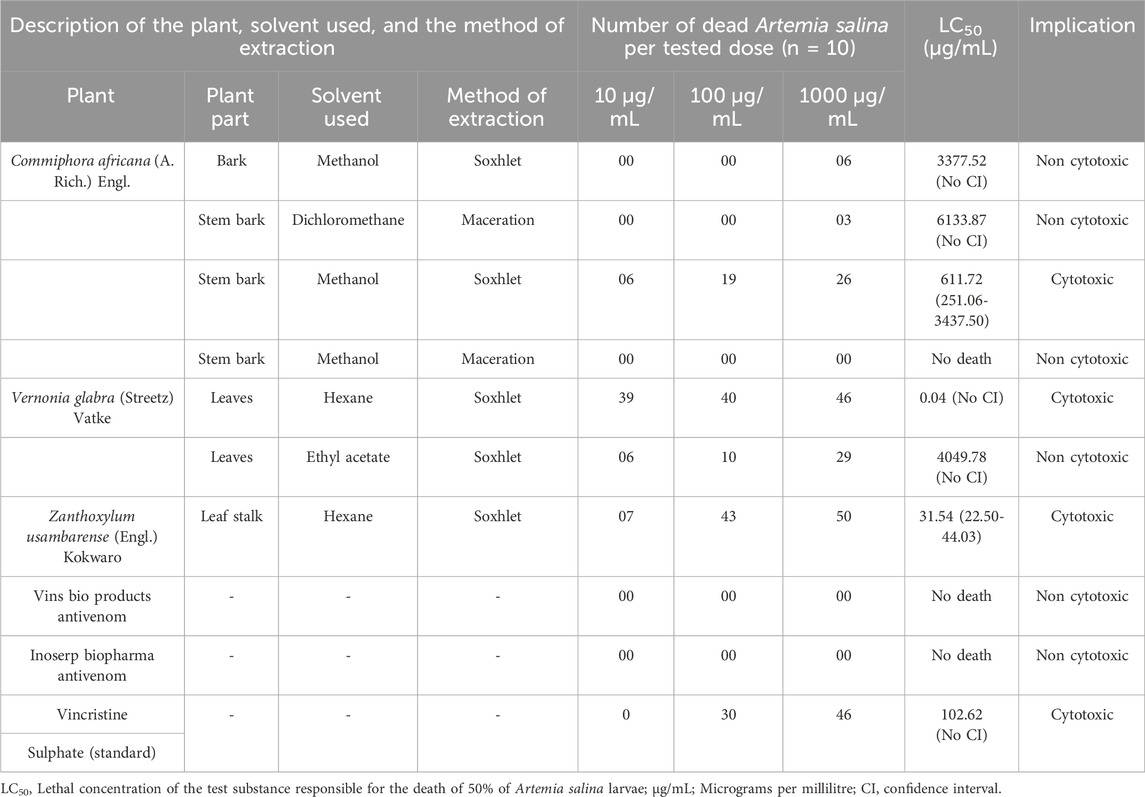
Table 2. The cytotoxicity of antivenom, extracts, and fractions of Commiphora africana, Vernonia glabra, and Zanthoxylum usambarense in Artemia salina.
Qualitative phytochemical composition of extracts and fractions
Flavonoids, phenolics, glycosides, and tannins were found to be present in the dichloromethane and methanol fractions of C. africana stem bark, the methanol extract of C. africana bark, and the ethyl acetate extract of V. glabra leaves. However, alkaloids, carboxylic acids, phytosterols, and terpenoids were absent in the extracts and fractions (Table 3).
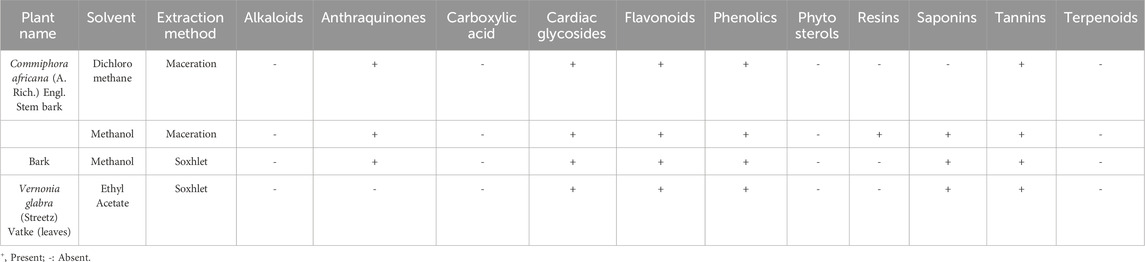
Table 3. Qualitative phytochemical composition of the extracts and fractions of Commiphora africana and Vernonia glabra.
Quantitative phytochemical composition of the non-cytotoxic extracts and fractions
The ethyl acetate extract of V. glabra leaves had the highest glycoside (0.003%), total flavonoid (2.990 mg/g catechin equivalents), and tannic acid content (0.010%) while the methanol extract of C. africana stem bark had the highest phenolic content (2.180 mg/g gallic acid equivalents) (Table 4).
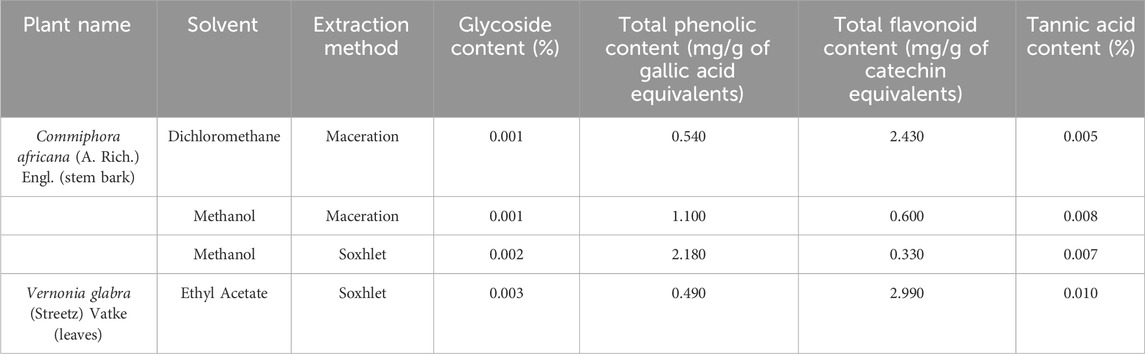
Table 4. Quantitative phytochemical composition of the extracts and fractions of Commiphora africana and Vernonia glabra.
Neutralization of venom-induced cytotoxicity by extracts, fractions, and antivenom
The dichloromethane fraction of C. africana stem bark had an effective concentration of 336.12 ± 59.97 μg/mL against BAV-induced cytotoxicity in A. salina. The methanol extract of C. africana bark was the most effective against NAV-induced cytotoxicity in A. salina with an EC50 of 221.37 ± 30.33 μg/mL. The ethyl acetate extract of V. glabra leaves had an effective concentration of 329.39 ± 15.92 against NSV-induced cytotoxicity in A. salina. However, the test antivenoms were ineffective in neutralizing BAV, NAV and NSV-induced cytotoxicity in A. salina (Table 5).
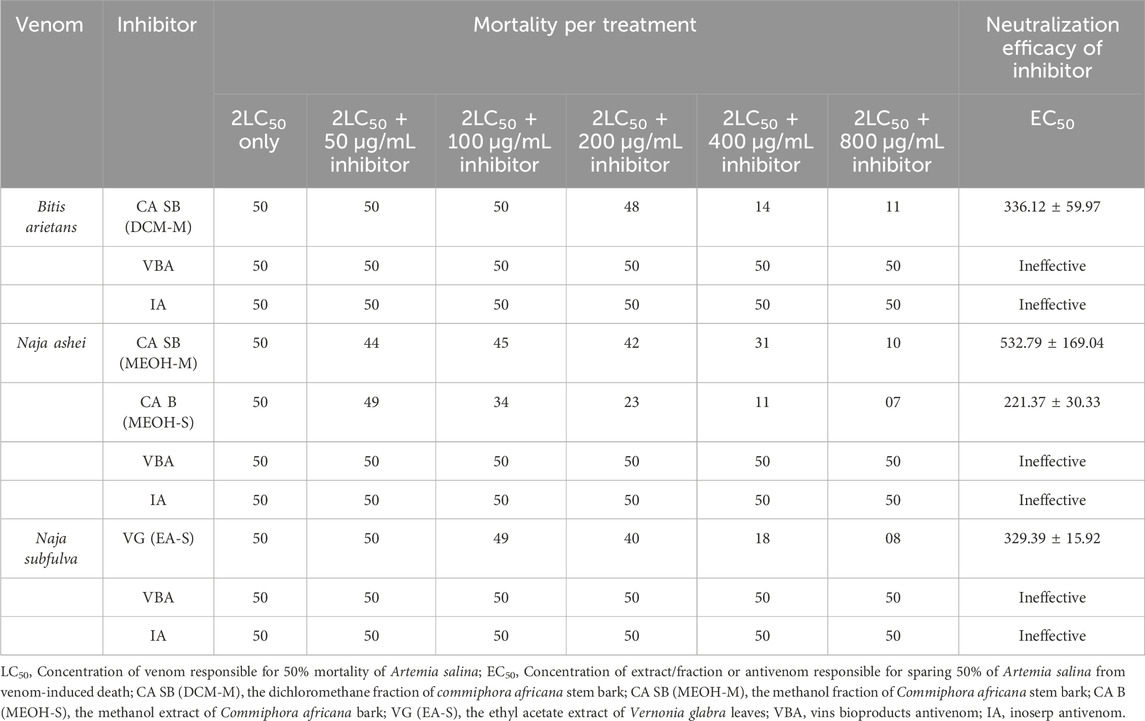
Table 5. Neutralization of snake venom-induced cytotoxicity in Artemia salina by antivenom, extracts, and fractions of Commiphora africana and Vernonia glabra.
Discussion
Snake venom phospholipases A2 (svPLA2) are enzymes which hydrolyze phospholipids and induce several pharmacological effects including edema, modulation of platelet aggregation, neurotoxicity, and myotoxicity (Six and Dennis, 2000; Kini, 2003; Pereanez et al., 2011). The present study observed that extracts and fractions of C. africana, S. obtusifolia, V. glabra, and W. ugandensis effectively neutralized svPLA2s in BAV, NAV, and NSV. A similar study by Molander and colleagues evaluated the neutralization capacity of 226 extracts from 94 different plant species where it was reported that 11 water extracts and 28 ethanol extracts showed more than 90% inhibition against svPLA2 in Bitis arietans and Naja nigricollis venoms (Molander et al., 2014). These plants included Lanea acida, Spondias mombin, and Capparis tometosa (Molander et al., 2014).
Phytochemical analysis revealed that the extracts were rich in phenolics, tannins, saponins, and cardiac glycosides. Previous authors have demonstrated that phenolics, tannins, and saponins have antivenom properties (da Silva et al., 2007; Sia et al., 2011; de Moura et al., 2016; Salama et al., 2018; Liu et al., 2024). These antivenom properties were observed when Saxifraga stolonifera, Rosmarinus officinalis, Plathymenia reticulata, Mimosa pudica, and Pentaclethra macroloba were tested against venom from Bothrops atrox, Cerastes, and Naja kaouthia (da Silva et al., 2007; Sia et al., 2011; de Moura et al., 2016; Salama et al., 2018; Liu et al., 2024).
Cytotoxicity studies in A. salina revealed that some extracts of V. glabra leaves, W. ugandensis leaf stalk, and C. africana stem bark were cytotoxic to A. salina. Previous studies by Wanna, Karani, Anywar, Mwangi and their colleagues have shown that V. glabra was cytotoxic in A. salina (LC50 = 658 μg/mL) (Wanna et al., 2023), W. ugandensis was non-cytotoxic in Vero cells (CC50 of >250 μg/mL) (Karani et al., 2013) but cytotoxic to human glioblastoma cells (IC50 = 7.6 μg/mL) (Anywar et al., 2022) and C. africana was cytotoxic to Vero cells (CC50 > 20 μg/mL) (Mwangi et al., 2020). The compounds responsible for the toxicity of V. glabra and Z. usambarense have not been studied in detail but a study by Wairagu and colleagues established that cedrol, 9-octadecanoic acid-ethyl-ester, octadecadien-1-ol, citronellyl formate, n-hexadecenoic acid, and 1,2-dihydro-6-methoxy-naphthalene isolated from the dichloromethane crude fraction of C. africana resin were toxic to bedbugs (Cimex lectularius) (Wairagu et al., 2022). Moreover, E-resveratol 3-O-rutinoside isolated from the methanol fraction of C. africana stem bark was highly cytotoxic to breast (MCF-7), liver (HepG2), lung (A549), and prostate (PC3) cancer cell lines (Segun et al., 2019). In the case of W. ugandensis, compounds such as polygodial, warbuganal, ugandensolide, and mukaadial have been identified to be toxic against the maize weevil (Sitophilus zeamais Motchulsky) and the larger grain borer (Prostephanus truncates Horn) while compounds such as muzigadial have been found to be highly toxic to brine shrimp (A. salina) and in vitro trypanocidal activity against both drug-resistant and drug-sensitive trypanosome strains (Olila and Opuda-Asibo, 2001; Opiyo, 2020).
The A. salina model has been used to evaluate the cytotoxicity of medicinal plants (Nguta et al., 2011; Mwangi et al., 2015), environmental contaminants (Barahona and Sanchez-Fortun, 1999; Sanchez-Fortun and Barahona, 2009), and venom (Damotharan et al., 2015; Okumu et al., 2021). The present work was a continuation of our previous work where we investigated the capacity of two antivenoms to neutralize NAV-induced cytotoxicity in A. salina (Okumu et al., 2020). Moreover, we showed in another study that the A. salina model was a good surrogate for dermonecrosis in mice (Okumu et al., 2021). The present study established that some extracts and fractions of C. africana were effective in prolonging the survival of A. salina exposed to NAV. Isa and colleagues in a previous research reported that the crude methanol extract and fraction of C. africana dose-dependently neutralized N. nigricollis envenomation in mice (Isa et al., 2022). Abdullahi et al. reported the anti-snake venom properties of a C. africana related plant, i.e., Commiphora pedunculata against N. nigricollis venom (Abdullahi et al., 2017). While this study has highlighted the capacity of the prepared extracts to neutralize key effects of medically important sub-Saharan snakes, it did not evaluate the capacity of the extracts/fractions to neutralize other key toxins in the studied snake venoms including protease, hyaluronidase, and neurotoxins (3FTx’s). Moreover, further work is needed to understand the identity of the compounds responsible for the observed extract/fraction induced cytotoxicity in A. salina.
Conclusion
These findings validate the local use of C. africana and V. glabra in snakebite envenomation and provide a basis for further work aimed at isolating pure compounds from these plants and identifying their mechanism of action. However, C. bonariensis, S. obtusifolia, W. ugandensis, and Z. usambarense use in snakebite is limited by poor efficacy and cytotoxicity.
Data availability statement
The raw data supporting the conclusion of this article will be made available by the authors, without undue reservation.
Ethics statement
The animal study was approved by The Biosafety, Animal Use and Ethics Committee of the University of Nairobi. The study was conducted in accordance with the local legislation and institutional requirements.
Author contributions
MO: Conceptualization, Data curation, Formal Analysis, Funding acquisition, Investigation, Methodology, Project administration, Resources, Software, Visualization, Writing–original draft, Writing–review and editing. JM: Investigation, Project administration, Resources, Supervision, Validation, Writing–original draft, Writing–review and editing. JG: Conceptualization, Data curation, Investigation, Methodology, Project administration, Resources, Supervision, Writing–original draft, Writing–review and editing. PM: Data curation, Investigation, Project administration, Resources, Supervision, Writing–original draft, Writing–review and editing. VM: Data curation, Investigation, Project administration, Resources, Supervision, Writing–original draft, Writing–review and editing. FO: Conceptualization, Investigation, Methodology, Project administration, Resources, Writing–original draft, Writing–review and editing.
Funding
The author(s) declare that financial support was received for the research, authorship, and/or publication of this article. This study received financial support from the National Research Fund (Kenya). The grant was issued to Mitchel Otieno Okumu (1st author). REF NRF/Ph.D./02/158.
Acknowledgments
All authors are grateful for the support of the technical staff at the Department of Public Health, Pharmacology and Toxicology, University of Nairobi.
Conflict of interest
The authors declare that the research was conducted in the absence of any commercial or financial relationships that could be construed as a potential conflict of interest.
Publisher’s note
All claims expressed in this article are solely those of the authors and do not necessarily represent those of their affiliated organizations, or those of the publisher, the editors and the reviewers. Any product that may be evaluated in this article, or claim that may be made by its manufacturer, is not guaranteed or endorsed by the publisher.
Supplementary material
The Supplementary Material for this article can be found online at: https://www.frontiersin.org/articles/10.3389/fphar.2024.1369768/full#supplementary-material
Abbreviations
CaCl2, Calcium Chloride; CHCl3, Chloroform; ELISA, Enzyme Linked Immunosorbent Assay; EC50, Effective concentration of extract/antivenom that spares 50% of A. salina from death; FECl3, Ferric Chloride; H2SO4, Sulphuric acid, HCl, Hydrochloric acid; LC50, Lethal concentration that kills 50% of A. salina, Na2CO3, Sodium Carbonate, UV-VIS, Ultraviolet and visible, mg. GAE.g-1, milligrams of gallic acid equivalents per Gram, mg. CE. g-1, milligrams of catechin equivalents per Gram; MPC, Minimum phospholipase concentration; µL, Microliter; µg/mL, Microgram per millilitre; mM, millimoles; NaOH: sodium hydroxide; Na2WO4, Sodium tungstate, H3PO4, Phosphoric acid; SvPLA2, Snake venom phospholipase A2; WHO, World Health Organization.
References
Abdullahi, H. L., Muhammed, H. S., Tajuddeen, N., Hamza, S. A., and Ibrahim, M. A. (2017). Effect of methanolicstem-bark extract of Commiphora pedunculata on plasma recalcification time of Najani gricollis venom treated plasma. Bayero J. Pure Appl. Sci. 10, 233–237. doi:10.4314/bajopas.v10i2.38
Alsayari, A., Kopel, L., Ahmed, M. S., Soliman, H. S. M., Annadurai, S., and Halaweish, F. T. (2018). Isolation of anticancer constituents from Cucumis prophetarum var. prophetarum through bioassay-guided fractionation. BMC Complement. Altern. Med. 18, 274. doi:10.1186/s12906-018-2295-5
Amadi, B. A., Agomuo, E. N., and Ibegbulem, C. O. (2004). Proximate analysis. Res. Methods Biochem. Supreme Publ. Owerri, Niger., 105–115.
Anywar, G. U., Kakudidi, E., Oryem-Origa, H., Schubert, A., and Jassoy, C. (2022). Cytotoxicity of medicinal plant species used by traditional healers in treating people suffering from HIV/AIDS in Uganda. Front. Toxicol. 4, 832780. doi:10.3389/ftox.2022.832780
Atanassova, M., Georgieva, S., and Ivancheva, K. (2011). Total phenolic and total flavonoid contents, antioxidant capacity and biological contaminants in medicinal herbs. J. Univ. Chem. Technol. Metall. 46.
Barahona, M. V., and Sanchez-Fortun, S. (1999). Toxicity of carbamates to the brine shrimp Artemia salina and the effect of atropine, BW284c51, iso-OMPA and 2-PAM on carbaryl toxicity. Environ. Pollut. 104, 469–476. doi:10.1016/s0269-7491(98)00152-3
Calvete, J. J., Escolano, J. J., and Sanz, L. (2007). Snake venomics of Bitis species reveals large intragenus venom toxin composition variation: application to taxonomy of congeneric taxa. J. Proteome Res. 6, 2732–2745. doi:10.1021/pr0701714
Chippaux, J. P. (1998). Snake-bites: appraisal of the global situation. Bull. World Health Organ. 76, 515–524. doi:10.1086/505866
Currier, R. (2012). Investigating venom synthesis: exploring the composition, variation and gene expression dynamics of Bitis arietans venom. The University of Liverpool Repository. Available at: https://livrepository.liverpool.ac.uk.
Damotharan, P., Veeruraj, A., Arumugam, M., and Balasubramanian, T. (2015). Isolation and characterization of biologically active venom protein from sea snake Enhydrina schistosa. J. Biochem. Mol. Toxicol. 29, 140–147. doi:10.1002/jbt.21678
da Silva, J. O., Fernandes, R. S., Ticli, F. K., Oliveira, C. Z., Mazzi, M. V., Franco, J. J., et al. (2007). Triterpenoid saponins, new metalloprotease snake venom inhibitors isolated from Pentaclethra macroloba. Toxicon 50, 283–291. doi:10.1016/j.toxicon.2007.03.024
de Moura, V. M., da Silva, W. C. R., Raposo, J. D. A., Freitas-de-Sousa, L. A., Dos-Santos, M. C., de Oliveira, R. B., et al. (2016). The inhibitory potential of the condensed-tannin-rich fraction of Plathymenia reticulata Benth.(Fabaceae) against Bothrops atrox envenomation. J. Ethnopharmacol. 183, 136–142. doi:10.1016/j.jep.2016.02.047
Dyba, B., Rudolphi-Szydło, E., Barbasz, A., Czyżowska, A., Hus, K. K., Petrilla, V., et al. (2021). Effects of 3FTx protein fraction from Naja ashei venom on the model and native membranes: recognition and implications for the mechanisms of toxicity. Molecules 26, 2164. doi:10.3390/molecules26082164
Habermann, E., and Hardt, K. L. (1972). A sensitive and specific plate test for the quantitation of phospholipases. Anal. Biochem. 50, 163–173. doi:10.1016/0003-2697(72)90495-2
Harnafi, H., Caid, H. S., el Houda Bouanani, N., Aziz, M., and Amrani, S. (2008). Hypolipemic activity of polyphenol-rich extracts from Ocimum basilicum in Triton WR-1339-induced hyperlipidemic mice. Food Chem. 108, 205–212. doi:10.1016/j.foodchem.2007.10.062
Isa, H. I., Saleh, A., Shittu, M., Suleiman, M. M., and Ambali, S. F. (2022). Prolongation of survival duration by extract and fraction of Commiphora africana in mice injected with LD 99 of Naja nigricollis crude venom. Savannah Vet. J. 5. doi:10.36759/svj.2021.155
Janardhan, B., Shrikanth, V. M., Mirajkar, K. K., and More, S. S. (2014). In vitro screening and evaluation of antivenom phytochemicals from Azima tetracantha Lam. leaves against Bungarus caeruleus and Vipera russelli. J. Venom. Anim. Toxins Incl. Trop. Dis. 20, 12. doi:10.1186/1678-9199-20-12
Karani, L. W., Tolo, F. M., Karanja, S. M., and Khayeka, C. (2013). Safety of Prunus africana and Warburgia ugandensis in asthma treatment. South Afr. J. Bot. 88, 183–190. doi:10.1016/j.sajb.2013.07.007
Kasturiratne, A., Wickremasinghe, A. R., De Silva, N., Gunawardena, N. K., Pathmeswaran, A., Premaratna, R., et al. (2008). The global burden of snakebite: a literature analysis and modelling based on regional estimates of envenoming and deaths. PLoS Med. 5, e218–e1604. doi:10.1371/journal.pmed.0050218
Kini, R. M. (2003). Excitement ahead: structure, function and mechanism of snake venom phospholipase A2 enzymes. Toxicon 42, 827–840. doi:10.1016/j.toxicon.2003.11.002
Kokate, C. K., Purohit, A. P., and Gokhale, S. B. (2006). Pharmacognosy. Nirali Prakashan 35, 133–525.
Kumar, R. S., Venkateshwar, C., Samuel, G., and Rao, S. G. (2013). Phytochemical screening of some compounds from plant leaf extracts of holoptelea integrifolia (planch.) and celestrus emarginata (grah.) used by gondu tribes at adilabad district, andhrapradesh, India. R. Suman Kumar1, C. Venkateshwar 2, G. Samuel3, S. Gangadhar Rao4. Int. J. Eng. Sci. Invent. 2, 65–70.
Liu, Z.-T., Zhang, Y., Fang, R., Simmonds, M., Zhang, X.-J., Zhang, T.-T., et al. (2024). Evaluation of Saxifraga stolonifera phenolic extracts as a potential antivenom against Deinagkistrodon acutus venom: in vitro and in vivo studies. J. Ethnopharmacol. 318, 116964. doi:10.1016/j.jep.2023.116964
Molander, M., Nielsen, L., Søgaard, S., Staerk, D., Rønsted, N., Diallo, D., et al. (2014). Hyaluronidase, phospholipase A 2 and protease inhibitory activity of plants used in traditional treatment of snakebite-induced tissue necrosis in Mali, DR Congo and South Africa. J. Ethnopharmacol. 157, 171–180. doi:10.1016/j.jep.2014.09.027
Muhammad, S. A., and Abubakar, S. M. (2016). Qualitative and quantitative determination of phytochemicals in aqueous extract of chrysophyllumalbidum seed kernel. Biosci. Biotechnol. Res. Asia 13, 1201–1206. doi:10.13005/bbra/2153
Mwangi, C., Nimbeshaho, F., Abdulai, Y., Chacha, M. R., Ndirangu, E. G., Ajaiyeoba, E. O., et al. (2020). Antimycobacterial activity, cytotoxicity and phytochemical screening of organic extracts of Commiphora africana stem bark from Kenya. MUT Library. Available at repository.mut.ac.ke:8080/xmlui/handle/123456789/4411.
Mwangi, G. G., Wagacha, J. M., Nguta, J. M., and Mbaria, J. M. (2015). Brine shrimp cytotoxicity and antimalarial activity of plants traditionally used in treatment of malaria in Msambweni district. Pharm. Biol. 53, 588–593. doi:10.3109/13880209.2014.935861
Nguta, J. M., Mbaria, J. M., Gathumbi, P. K., Kabasa, J. D., and Kiama, S. G. (2011). Biological screeing of Kenya medicinal plants using Artemia salina L. (Artemiidae). Pharmacologyonline 2, 458–478.
Okumu, M. O., Mbaria, J. M., Gikunju, J. K., Mbuthia, P. G., Madadi, V. O., and Ochola, F. O. (2020). Enzymatic activity and brine shrimp lethality of venom from the large brown spitting cobra (Naja ashei) and its neutralization by antivenom. BMC Res. Notes 13, 325–327. doi:10.1186/s13104-020-05167-2
Okumu, M. O., Mbaria, J. M., Gikunju, J. K., Mbuthia, P. G., Madadi, V. O., Ochola, F. O., et al. (2021). Artemia salina as an animal model for the preliminary evaluation of snake venom-induced toxicity. Toxicon X 12, 100082. doi:10.1016/j.toxcx.2021.100082
Olila, D., Opuda-Asibo, J., and Olwa-Odyek, (2001). Bioassay-guided studies on the cytotoxic and in vitro trypanocidal activities of a sesquiterpene (Muzigadial) derived from a Ugandan medicinal plant (Warburgia ugandensis). Afr. Health Sci. 1, 12–15.
Oliveira, I. S., Pucca, M. B., Cerni, F. A., Vieira, S., Sachett, J., Seabra de Farias, A., et al. (2023). Snakebite envenoming in Brazilian children: clinical aspects, management and outcomes. J. Trop. Pediatr. 69, fmad010. doi:10.1093/tropej/fmad010
Onyango, O. K. (2018). Isolation and characterization of snake venom proteins and peptides from members of viperidae and Elapidae snake families from kilifi county. Jomo Kenyatta University of Agriculture and Technology. Available at: ir.jkuat.ac.ke/handle/123456789/4764.
Opiyo, S. A. (2020). Evaluation of Warburgia ugandensis extracts and compounds for crop protection against Prostephanus truncates. MUT Library. Available at repository.mut.ac.ke:8080/xmlui/handle/123456789/4453.
Owuor, B. O., and Kisangau, D. P. (2006). Kenyan medicinal plants used as antivenin: a comparison of plant usage. J. Ethnobiol. Ethnomed. 2, 7. doi:10.1186/1746-4269-2-7
Owuor, B. O., Mulemi, B. A., and Kokwaro, J. O. (2005). Indigenous snake bite remedies of the Luo of western Kenya. J. Ethnobiol. 25, 129–141. doi:10.2993/0278-0771_2005_25_129_isbrot_2.0.co_2
Pereanez, J. A., Nunez, V., Patino, A. C., Londono, M., and Quintana, J. C. (2011). Inhibitory effects of plant phenolic compounds on enzymatic and cytotoxic activities induced by a snake venom phospholipase A2. Vitae 18, 295–304. doi:10.17533/udea.vitae.10653
Puzari, U., Fernandes, P. A., and Mukherjee, A. K. (2022). Pharmacological re-assessment of traditional medicinal plants-derived inhibitors as antidotes against snakebite envenoming: a critical review. J. Ethnopharmacol. 292, 115208. doi:10.1016/j.jep.2022.115208
Salama, W. H., Abdel-Aty, A. M., and Fahmy, A. S. (2018). Rosemary leaves extract: anti-snake action against Egyptian Cerastes cerastes venom. J. Tradit. Complement. Med. 8, 465–475. doi:10.1016/j.jtcme.2017.10.001
Sanchez-Fortun, S., and Barahona, M. V. (2009). Toxicity and characterization of cholinesterase-inhibition induced by diisopropyl fluorophosphate in Artemia salina larvae. Ecotoxicol. Environ. Saf. 72, 775–780. doi:10.1016/j.ecoenv.2007.11.004
Segun, P. A., Ogbole, O. O., Ismail, F. M. D., Nahar, L., Evans, A. R., Ajaiyeoba, E. O., et al. (2019). Resveratrol derivatives from Commiphora africana (A. Rich.) Endl. display cytotoxicity and selectivity against several human cancer cell lines. Phyther. Res. 33, 159–166. doi:10.1002/ptr.6209
Sia, F. Y., Vejayan, J., Jamuna, A., and Ambu, S. (2011). Efficacy of tannins from Mimosa pudica and tannic acid in neutralizing cobra (Naja kaouthia) venom. J. Venom. Anim. Toxins Incl. Trop. Dis. 17, 42–48. doi:10.1590/s1678-91992011000100006
Six, D. A., and Dennis, E. A. (2000). The expanding superfamily of phospholipase A2 enzymes: classification and characterization. Biochim. Biophys. Acta (BBA)-Molecular Cell Biol. Lipids 1488, 1–19. doi:10.1016/s1388-1981(00)00105-0
Tasoulis, T., and Isbister, G. K. (2017). A review and database of snake venom proteomes. Toxins (Basel). 9, 290. doi:10.3390/toxins9090290
Wairagu, N. W., Wachira, B. M., Githiomi, J. K., Oduor, N., and Ng’ang’a, M. M. (2022). Repellency and toxicity of selected fractions, identified compounds and blends of Commiphora africana resin against bedbugs. Nat. Prod. Commun. 17, 1934578X2211068. doi:10.1177/1934578x221106898
Wanna, D. Y., Mzula, A., and Mwega, E. D. (2023). Antibacterial potential and safety level of Euphorbia tirucalli and Vernonia glabra commonly used by residents in iringa, Tanzania. J. Med. plants By-product 12, 283–292. doi:10.22034/JMPB.2023.361209.1525
WHO (2016). Post ecbs version expert committee on biological standardization Geneva, 17 to 21 october 2016 WHO guidelines for the production, control and regulation of snake antivenom immunoglobulins. World Health Organization. Available at: https://www.who.int/publications/m/item/snake-antivenon-immunoglobulins-annex-5-trs-no-1004, 1–138.
Keywords: Bitis arietans, medicinal plants, Naja ashei, preclinical efficacy evaluation, Naja subfulva, snake venom, Artemia salina bioassay
Citation: Okumu M, Mbaria J, Gikunju J, Mbuthia P, Madadi V and Ochola F (2024) Exploring nature’s antidote: unveiling the inhibitory potential of selected medicinal plants from Kisumu, Kenya against venom from some snakes of medical significance in sub-Saharan Africa. Front. Pharmacol. 15:1369768. doi: 10.3389/fphar.2024.1369768
Received: 12 January 2024; Accepted: 28 March 2024;
Published: 12 April 2024.
Edited by:
Hellen Oketch-Rabah, United States Pharmacopeial Convention, United StatesReviewed by:
Tushar Dhanani, Florida Agricultural and Mechanical University, United StatesBhargab Kalita, Amrita Vishwa Vidyapeetham (kochi campus), India
Mirtha Navarro-Hoyos, University of Costa Rica (UCR), Costa Rica
Copyright © 2024 Okumu, Mbaria, Gikunju, Mbuthia, Madadi and Ochola. This is an open-access article distributed under the terms of the Creative Commons Attribution License (CC BY). The use, distribution or reproduction in other forums is permitted, provided the original author(s) and the copyright owner(s) are credited and that the original publication in this journal is cited, in accordance with accepted academic practice. No use, distribution or reproduction is permitted which does not comply with these terms.
*Correspondence: Mitchel Okumu, mytchan88@gmail.com