- Hubei Key Laboratory of Cognitive and Affective Disorders, Wuhan Institute of Biomedical Sciences, School of Medicine, Jianghan University, Wuhan, China
Prevalent neurological disorders such as Alzheimer’s disease, Parkinson’s disease, and stroke are increasingly becoming a global burden as society ages. It is well-known that degeneration and loss of neurons are the fundamental underlying processes, but there are still no effective therapies for these neurological diseases. In recent years, plenty of studies have focused on the pharmacology and feasibility of natural products as new strategies for the development of drugs that target neurological disorders. Antrodia camphorata has become one of the most promising candidates, and the crude extracts and some active metabolites of it have been reported to play various pharmacological activities to alleviate neurological symptoms at cellular and molecular levels. This review highlights the current evidence of Antrodia camphorata against neurological disorders, including safety evaluation, metabolism, blood-brain barrier penetration, neuroprotective activities, and the potential on regulating the gut-microbiome-brain axis. Furthermore, potential strategies to resolve problematic issues identified in previous studies are also discussed. We aim to provide an overview for the ongoing development and utilization of Antrodia camphorata in cerebral neuropathology.
1 Introduction
Antrodia camphorata (M. Zang & C.H. Su) Sheng H. Wu, Ryvarden & T.T. Chang (AC) also called Antrodia cinnamomea or Taiwanofungus camphoratus or Ganoderma camphoratum, locally known as Niu-Chang-Chih in (Su et al., 2022), is a valuable edible mushroom, with a large potential for biological and medicinal health benefits including anti-cancer, anti-inflammatory, anti-oxidative, hepatoprotective, and neuroprotective properties (Zhang et al., 2022). Naturally, the growth of AC is extremely slow and parasitic on the inner wall of a unique and native tree in Taiwan called Cinnamomum kanehirai Hayata on the mountain ranges between 450 to 1200 m higher (Li et al., 2022; Su et al., 2022). AC was first identified in 1990, and was recognized and used as highly beneficial Chinese folk medicine (Chen et al., 2023). As a fungus, AC belongs to the phylum Basidiomycota, the Fomitopsidaceae family, and the Antrodia genus (Li et al., 2023). The appearance of fruiting bodies is generally red-orange, but in certain regions of Taiwan, rarely yellow and white variants also occur. Metabolomic Profiling indicated that red AC possesses relatively higher contents of triterpenoids and diverse metabolites than yellow AC and white AC (Su et al., 2023).
Ever since the spread of AC to the mainland, products like camphor mushroom drop pills and camphor mushroom oral liquid have become popular in the form of healthcare products (Cheng et al., 2005). Wild-grown AC is rare and valuable, but demands have increased in recent years. Therefore, research has been carried out on artificial cultivation including solid state (cutting wood, agar plate medium) culture and liquid culture (submerged fermentation) (Zhang et al., 2019). Although cultured fungus may possess bioactivities similar to those of the naturally occurring fungus, there are several differences in the constituents of ingredients and the content of bioactive metabolites (Du et al., 2012; Tung et al., 2014).
Currently, more than 200 metabolites have been extracted and identified from AC. Many investigations have revealed their pharmacological activities and mechanisms, and some of them are generally recognized by the U.S. Food and Drug Administration (FDA) as potential drugs for clinical trials (Angamuthu et al., 2019). Each stage of the fungal life cycle creates metabolites usually differently. Mycelium, an exponential phase of AC, and polysaccharide is usually produced in this stage (Zhang et al., 2018). It is not easy to artificially cultivate fruiting bodies. The chemical metabolites of the fruiting body are different from the mycelium, and in general, the metabolites of the mycelium are also found in the fruiting body; more secondary metabolites are produced during this mature phase (Chang et al., 2006).
The physiologically active substances in AC can be mainly divided into triterpenoids, polysaccharides, derivatives of ubiquinone, and derivatives of maleic acid and succinic acid (Liu et al., 2023). Triterpenoids are one of the main metabolites of AC, and the content of triterpenoids is approximately 63% in the fruiting body (Geethangili and Tzeng, 2011). Antcins, a typical class of triterpenoids, have high medicinal activity (Kuang et al., 2021). AC polysaccharides are mainly composed of a variety of monosaccharides linked by glycosidic bonds (Lee et al., 2002) and exhibit excellent biological activity of anticancer and anti-inflammatory properties (Yang et al., 2022). Ubiquinones are a class of lipophilic quinones and antroquinonol, probably the most valuable derivatives of ubiquinone in AC, has strong biological activity (Zhang et al., 2017). Maleic acid and succinic acid derivatives also are characteristic active metabolites of AC, mainly antrodin and antrocinnamomin, which are mainly found in the mycelium stage (Nakamura et al., 2004).
Neurological diseases such as Alzheimer’s disease (AD), Parkinson’s disease (PD), and stroke are becoming serious public health issues, and currently there is still no effective cure or prevention strategies. AD is the most common cause of dementia in older individuals with the pathological hallmarks of amyloid plaques composed of amyloid-β (Aβ) and neurofibrillary tangles consist of phosphorylated tau protein (Kalampokini et al., 2019; Marino et al., 2020). Currently, approved drugs to treat AD are mainly effective in improving the symptoms (Alzheimer’s Association, 2023; Yan et al., 2020). PD is the second-most common neurodegenerative disease, the pathological characteristics include the loss of dopaminergic neurons in the substantia nigra and the increase of Lewy body, which is caused by aggregation of α-synuclein in neurons (Beitz, 2014; Breijyeh et al., 2020). Current treatment of PD is limited to symptomatic relief (So et al., 2024). Stroke is one of the primary causes of disability and death worldwide, and ischemic stroke is the most common type (Zhao et al., 2022). Intravascular thrombosis is the primary pathogenic cause of ischemic stroke to result in brain damage, including cerebral tissue lesions and deficiencies in the neurons (Das et al., 2023). Therefore, preventative and improve of neural injuries strategies caused by stroke are of great clinical value.
Although various clinical manifestations are present in neurological disorders, common signaling pathways are shared by AD, PD, and ischemic stroke, including neuronal cell death, inflammation, and oxidative stress (Sun et al., 2022). Figure 1 shows the common signaling pathways in neurological disorders. Hence, research on the inhibition of the common pathway will be useful for the development of new drugs against all neurological disorders. AC has been reported to have excellent neuroprotective effects. In this focused review, we summarize the most recent findings on the neuroprotective properties of the extracts and bioactive metabolites of AC.
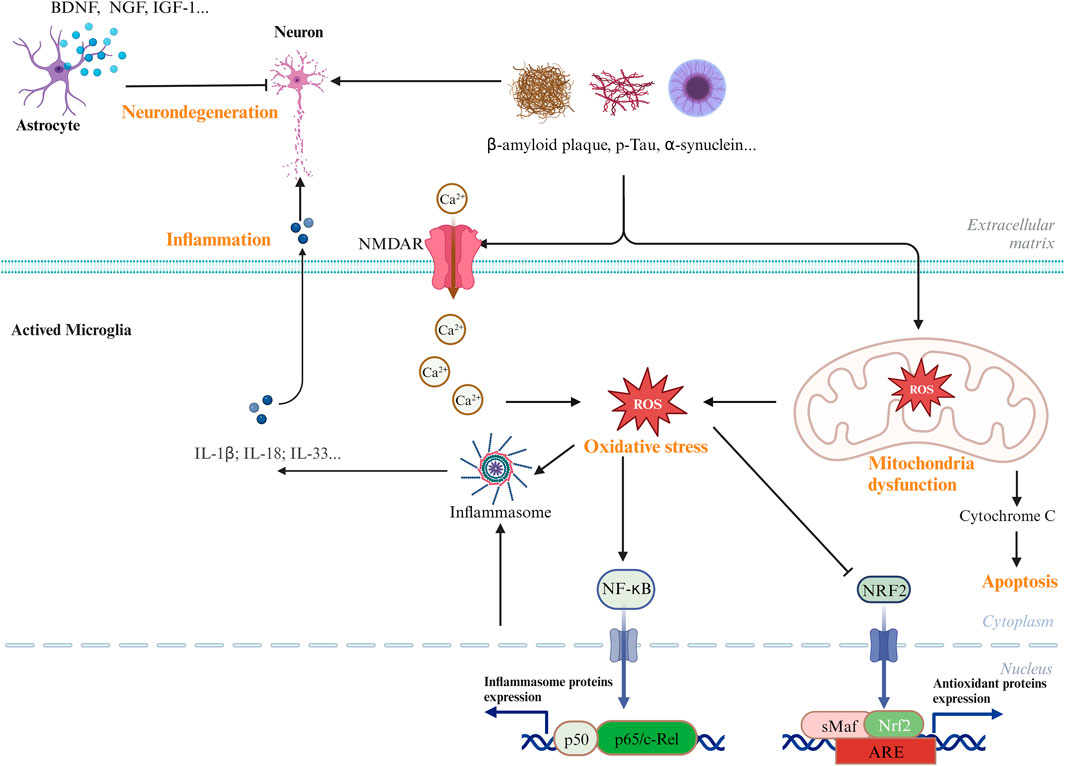
Figure 1. Common and major pathways in the CNS diseases. Biological processes, including oxidative stress, neuroinflammatory, and mitochondrial dysfunctions, have been involved in the development and pathogenesis of CNS diseases.
2 Safety evaluation of AC
Traditionally, AC has been used as a health food, and the historical use of this mushroom as a food supplement supports its safety. Previous studies on genotoxicity, teratotoxicity, and oral toxicity have showed the no-observed-adverse-effect-level (NOAEL) of AC in Sprague–Dawley (SD) rats (Lin et al., 2015). A recent study showed that fruiting body powders of dish-cultured AC did not cause mortality and clinical symptoms of toxicity (Liu et al., 2022). The freeze-dried mycelium of AC can be considered as a novel food by the European Commission, and the European Food Safety Authority (EFSA) Panel on Nutrition, Novel Foods, and Food Allergens has reported no adverse effects at the proposed use level (Turck et al., 2022). In addition to showing an acceptable safety and tolerability profile at doses of up to 2,988 mg/day with no appreciable side effects, a phase I clinical study in healthy adults also raised the possibility that LEAC-102 (a novel botanical drug extracted from AC, major compounds are antcin B, antcin K, and antcin H) may have novel immunomodulatory activities (Liao et al., 2023). Taken together, these findings indicate that crude extracts of AC and its products have no adverse effects on humans within a certain dose range.
The toxicology of metabolites from AC also has been studied. To assess the safety of antroquinonol, a phase I clinic trial was carried out using 50–600 mg daily for 1 month in patients with metastatic non-small-cell lung cancer. Antroquinonol exhibited a mild toxicity profile at all dose levels. Of the five patients with high does at 600 mg, three were evaluable for treatment response, and two achieved stable disease, which was generally considered safe and tolerable without dose-limiting toxicities. Then, the recommended dose for phase II clinic trial is at least 600 mg daily for non-small-cell lung cancer (Lee et al., 2015).
β-glucan (∼65% pure) from AC was analyzed in the subchronic toxicity and mutagenicity study in CD (SD) IGS rats (∼6 weeks old, 12/sex/group), and a daily dose of 2 g/kg for 3 months of oral treatment did not show any adverse effects and genotoxicity (Chen et al., 2018). Therefore, AC and some of its bioactive metabolites showed no obvious toxicity within a reasonable dose range.
3 Metabolism and blood-brain barrier penetration of AC
As a dietary supplement and adjuvant therapeutic agent, AC is widely used, and the biological activities of different extracts of AC have been thoroughly investigated. Understanding the biological effects and safety of botanical drugs highly depends on preclinical research regarding the metabolism and pharmacokinetics.
To investigate the metabolism of AC in vivo, several reports performed mice experiments. A previous study performed oral administration of the ethanol extract of AC in SD rat, only antrodin B and C were detected in plasma (Liu et al., 2010). The metabolism and pharmacokinetics after oral administration of AC in male SD rats were studied (Qiao et al., 2015), and a total of 18 triterpenoids and 8 metabolites were detected in rat plasma after oral administration. Antcins K and H were the major exposure metabolites of AC. While the lanostanes were retained in the plasma at a low concentration for a considerable amount of time, the ergostanes were typically quickly absorbed and removed. Actin H was found in the tumor tissue of ICR mice with xenogra S180 tumor model after oral administration of AC extract (Li et al., 2018). In a Caco-2 cell monolayer model, most ergostanes exhibited high permeability (Wang et al., 2015). In detail, antcin H and antcin B could easily pass through the Caco-2 cell layer; antcins A, B, C, H, and K were absorbed through passive transcellular diffusion; and the permeability of lanostanes was poor, including dehydrosulphurenic acid, 15α-acetyldehydrosulphurenic acid, dehydroeburicoic acid, and eburicoic acid. Components could be absorbed in plasma is the basis for its entry into the brain regions by passing through the blood-brain barrier (BBB) or through the nose-to-brain pathway. The above-mentioned ergostanes in AC may exhibit biological activity for the treatment of neurologic diseases.
Using a SD rat model, the pharmacokinetic properties of ergosterol were investigated (Zhao et al., 2011). After a single oral administration of ergosterol (100 mg/kg) to SD rats, two metabolites (ERG1 and ERG2) were identified in the plasma, urine, and fecal samples. The side-chain was oxidized with β-d-glucopyranoside structure. The peak concentration (Cmax) time was 8.00 ± 1.18 h. Approximately 62.5% of the administered ergosterol was excreted from the feces, while 3.2% was eliminated from the urine. It remains unclear whether ergosterol can transport and metabolism in the brain.
The pharmacokinetics of antroquinonol by administration were evaluated in patients with metastatic non-small-cell lung cancer in a clinical study (Liao et al., 2023). The mean elimination half-life ranged from 1.30 to 4.33 h, regardless of the treatment dose. Four metabolites of antroquinonol were identified by NMR spectroscopic analysis from the male Wistar rats’ urine following oral treatment (Chen et al., 2014). These results suggest that antroquinonol has good bioavailability, but the biological activity of four metabolites of antroquinonol was not determined.
The ability of drugs to cross the blood-brain barrier is critical for their neuroprotective function. After oral intake of antroquinonol in mice, no adverse effects were observed, and antroquinonol could penetrate through the blood-brain barrier (Chang et al., 2012). And adenosine from AC can enter brain and the brain efflux index was shown to be capable of reaching 90.1%±1.5% (Isakovic et al., 2004). More reports showed that the extracts and some active metabolites from AC have neuroprotective function, but the ability of them to cross the blood-brain barrier were not be detected. Further investigations need to be performed to clarify the usable of the candidates for neurological diseases.
4 Neuroprotection potential of AC
As a rich source of biologically active metabolites, AC exerts excellent effects on a variety of physiological processes and produces various bioactivities. Many other utilizable activities of AC await discovery. Hence, AC has enormous potential for the development of new drugs. The most recent findings on the therapeutic benefits, underlying mechanisms, and active metabolites of AC in the management and avoidance of neurological disorders are thoroughly reviewed in this article.
4.1 Neuroprotective activities of AC extracts
There is increasing evidence that AC is an encouraging candidate for various neurological disorders (Wang et al., 2019). Here, we have summarized the effects of AC in chronic neurodegenerative diseases including AD, PD, and other acute neurodegenerative diseases, mainly stroke.
4.1.1 Alzheimer’s disease
Neuroinflammation, the production of free radicals in the brain, amyloidogenic processing, and the ensuing Aβ cascade-triggered neuronal dysfunction and death are the pathological hallmarks of AD and other forms of dementia, which are the potential targets for AD treatment (Simpson and Oliver, 2020). Pharmacological therapy for neurodegenerative diseases provides only temporary symptomatic relief; hence, more effective drugs need to be developed (Passeri et al., 2022). Natural products with diverse structure and excellent activity are the main sources of new drugs.
The accumulation of Aβ in the brain may directly contribute to the degeneration of neurons during the pathogenesis of AD. Several isoforms of Aβ peptide have been isolated in vivo including Aβ1–38, Aβ1–39, Aβ1–40, Aβ1–42, and Aβ1–43. Aβ1–40 is the predominant sequence isolated from cerebrospinal fluid, while Aβ1–42 is the predominant component of senile plaques in parenchyma (Miyashita et al., 2009). Meanwhile, Aβ1-40, which is more abundantly produced by the cells than Aβ1-42, is commonly colocalized with Aβ1-42 in the plaque. Likewise, Aβ25-35 fragment can also induce aggregation and toxicity, similar to Aβ1-42. Aβ peptide is with important applications in the establishment of AD cell model. Methanol extract (10–50 μg/mL) from the wild fruiting body could suppress the inflammation induced by Aβ25-35 in EOC13.31 microglia at a dose-dependent manner, indicating that AC might be useful for the prevention of inflammation in the neurodegenerative brain (Liu et al., 2007). A study was conducted to evaluate the effect of AC fruiting body and mycelium on alleviating neurotoxicity induced by Aβ1-40 in the PC12 cell model and AD animal model (Wang et al., 2012). The results showed that AC can improve the memory and learning abilities by inhibiting several AD risk factors, including reactive oxygen species (ROS), p-tau, and BACE expression, as well as Aβ1-40 accumulation, suggesting that the fruiting body has stronger anti-oxidant and anti-inflammatory abilities to inhibit Aβ1-40-induced neurotoxicity than the mycelium. Another study also reported that AC ethanol extract (ACEE) protected PC12 cells activated by Aβ25-35 via increasing the BcL-2/Bax ratio to resist apoptosis, reducing ROS, and modulating adenosine A1 receptor (ADORA1) to enhance neuroprotective bioactivity and adenosine A2 receptor (ADORA2) to inhibit neurodegeneration. It also prevented the formation of Aβ25-35 fibrils and the production of tumor necrosis factor-alpha (TNF-α), ROS, malondialdehyde (MDA), and NO. Binding the function and the composition determination, it is demonstrated that the high content of triterpenoids, phenolics, and adenosine in ACEE was responsible for the rescue of these detrimental effects (Chang et al., 2012).
4.1.2 Parkinson’s disease
Typically, PD is mainly caused by intra-cytoplasmic α-synuclein aggregation in the dopaminergic neurons of the substantia nigra compacta, leading to a decrease in the release of dopamine in the striatum. Several hypotheses of the dopaminergic cell death include mitochondrial dysfunction, iron accumulation, and inflammation (Morris et al., 2024). The preference for natural products is attributed to their efficiency and comparably fewer side effects.
1-Methyl-4-phenyl-1,2,3,6-tetrahydropyridine (MPTP) is one of the most used neurotoxins in PD animal models. Recently, an in vivo study in a MPTP induced PD mouse model demonstrated the protective role of AC extract administration significantly reduce α-synuclein-positive neuron numbers, and protect the brain from MPTP-induced loss of TH+ neurons, neuroinflammation, and oxidative stress to prevent dopaminergic cell death and glial activation (Lanza et al., 2023). 6-Hydroxydopamine (6-OHDA) is the most sought-after neurotoxin to identify oxidative stress-induced PD model in vitro and in vivo because it generates ROS and mimics the neuropathological and biochemical features, which can cause neuronal degradation and apoptosis in the dopaminergic neurons (Hernandez-Baltazar et al., 2017). Among solid-state-cultured mycelium of AC extracts, 11 metabolites belonging to quinone, phenolic acid derivatives, ubiquinone derivatives, alkaloids, and triterpenoid were identified to exhibit potent protective effects against 6-OHDA-induced toxicity to decrease dopaminergic neuronal loss in PC12 cells. The underlying mechanism likely involved the restoration of morphological changes in the nuclei, the reduction of ROS production and caspase 3 activity (Zou et al., 2022).
4.1.3 Stroke
The most prevalent type of stroke is ischemic stroke, which causes degeneration and death of neurons. The pathology of ischemic stroke is extremely complex, oxidative stress and inflammation are the two major players (Chamorro et al., 2021). Neuroprotection is a promising strategy for stroke treatment. Natural products are reported to have excellent antioxidant and anti-inflammatory activities (Chen et al., 2020).
The activation of JNK and p38 and concurrent inhibition of ERK are critical for induction of apoptosis in both neuronal and non-neuronal cells (Xia et al., 1995). It has been reported that AC could effectively prevent serum-deprived apoptosis of PC12 cells by increasing phosphorylated ERK and decreasing phosphorylated JNK and p38, and by regulating a PKA/CREB-dependent pathway (Huang et al., 2005; Lu et al., 2008). Oral treatment of AC extract provided neuroprotection in rats with thromboembolic stroke by reducing infarct volume, improves neurological outcome (Lee et al., 2014). Furthermore, downregulation of iNOS/HO-1/Bax/caspase-3 and inhibition of hydroxyl radical formation were the main molecular pathways behind the AC neuroprotective activity against cerebral ischemia in rats (Yang et al., 2015). Ethyl acetate crude extract of AC (EtOAc-AC) showed protective effects both in acute ischemic stroke (AIS) injured mice and oxygen-glucose deprivation (OGD) induced Neuro 2A cells by inhibiting inflammation and apoptosis (Wang et al., 2019). Cobalt chloride (CoCl2) acts as hypoxia mimetic agent that increases the ROS production, resulting to the increase of inflammatory mediators such as TNF-α, interleukin 1 beta (IL-1β), inducible nitric oxide synthase (iNOS), and cyclooxygenase-2 (COX-2) (Zhong et al., 2014). In a recent study, the authors found that AC alcohol extracts (AC-AE) reduced cell damage against CoCl2-induced hypoxic toxicity in both C6 neuronal and C6 glial cells, and significantly reduced the stroke infarct size and decreased the level of proinflammatory iNOS and COX-2, and increased anti-inflammatory Nrf2 and HO-1 content in experimental rats, suggesting that AC-AE possesses protective effects in the ischemic stroke model (Kong et al., 2021).
Evidence from the above studies support that the medical benefits of AC can alleviate neuronal disorders. This great potential on the neuroprotective drug development of AC has further encouraged researchers to identify its functional metabolites.
4.2 Neuroprotective activities of isolated metabolites
The protective role of AC on neuronal disorders has been demonstrated using in vitro and in vivo experiments. Common pathways including anti-apoptosis, anti-oxidant, and anti-inflammatory play crucial roles in the degeneration and loss of axons and neurons (Moujalled et al., 2021). The effects of metabolites isolated from AC on neurological disorders are reviewed below.
4.2.1 Anti-apoptotic activities
Extensive neuronal loss was observed in neuronal disorders, and the apoptosis marker activated caspase-3 has been observed in AD, PD, and stroke, which suggested that apoptosis plays crucial roles in the pathological processes (Robertson et al., 2000). A common feature of most neurological diseases is the degeneration of neurons, thus, drugs that inhibit neuronal apoptosis could thus be candidates for therapy of neurodegenerative disorders (D'Mello and Chin, 2005). ADORA2 encodes the G protein-coupled adenosine receptor known as adenosine receptor subtype A2A, which can promote neuronal polarization and axon formation (Alçada-Morais et al., 2021). It is reported that adenosine (ADO) from AC could protect PC12 cells from serum deprivation by activation of the ADORA2 (Lu et al., 2006). Further research showed that adenosine suppressed JNK and p38 activities through a protein kinase A (PKA) pathway in serum-deprived PC12 cells (Lu et al., 2008).
4.2.2 Antioxidant activities
Increasing ROS is demonstrated to be susceptible to neuronal damage and functional deficits, which results in neurological disorders including AD, PD, and ischemic stroke (Teleanu et al., 2022). Brain tissue is characterized by high levels of oxygen consumption and high metabolic demand, but relatively low levels of antioxidant enzymes (HO-1, SOD, CAT, and GPx) and non-enzymatic antioxidants (vitamin A, C, and E) (Halliwell, 1992). Therefore, supplementation of antioxidants represents an effective strategy of prevention and treatment to restore the functionality and survival of neuronal cells against oxidative stress.
Current evidence has shown that AC is a potent scavenger of direct oxygen free radicals that protects cells from oxidative damage. Polysaccharides isolated from AC (ACP) have been found to comprehensively improve the neuroethology of PD mice, including autonomic activity, coordination, motility ability, and cognitive ability. Investigation of the mechanisms showed that ACP can enhance the expression levels of dopamine and dihydroxyphenylacetic acid in the striatum, and significantly decrease the expression of NLRP3 inflammasome and downstream inflammatory factors in a dose-dependent manner (Han et al., 2019). NLRP3 inflammasome can be activated not only by a variety of exogenous pathogens, but also by certain endogenous signals and metabolites. ROS is an upstream signal for NLRP3 activation (Hernandes et al., 2014). Further study demonstrated that ACP intervention can lead to significantly decreased ROS-NLRP3 activation, reduce intracellular ROS, and the apoptotic rate at the cellular and in vivo levels (Han et al., 2020).
A recent study in amyloid precursor protein (APP) transgenic mice revealed that antroquinonol, a ubiquinone derivative isolated from AC, might significantly improve memory acquisition, alleviate Aβ plaque pathology, and inflammation, mainly by decreasing histone deacetylase 2 (HDAC2) and increasing Nrf2 levels (Chang et al., 2005). As the catalytic subunit of deacetylase repressor complexes, the increase of HDAC2 level and activity in AD have been linked to the worsening of neuronal and synaptic function (Gonzalez-Zuninga et al., 2014). Further antroquinonol administration studies showed that anxiety-related behavior and cognitive abilities in 3XTgAD mice could be significantly improved; furthermore, inflammatory markers, AD biomarkers, and oxidative stress markers showed a significant decrease (Francesca et al., 2022). The results are consistent with previously study in the APP transgenic mice, which confirmed the antioxidant capacity of antroquinonol in AD model.
4.2.3 Anti-inflammatory activities
The prominent role inflammation plays in various age-related diseases such as AD, PD, and central nervous system (CNS) injury (Khadka et al., 2020). Glial cells and immune cells are associated with the instigation of neuroinflammation. Plenty of potential inflammatory targets for intervention have been proposed (DiSabato et al., 2016).
Several intracellular signaling and transcription factors mediated by the activation of microglia in turn activate the inflammatory pathway in cerebral haemorrhage. The US patent US20180353520 has claimed that the active metabolites dehydroeburicoic acid, dehydrosulphurenic acid, and 4,7-dimethoxy-5-methyl-1,3-benzodioxole from AC can be used for the treatment of stroke (Wu et al., 2018). Their anti-inflammatory bioactivities have been demonstrated by several studies (Shen et al., 2004; Deng et al., 2013; Shie et al., 2016). Oxidative stress is reported to play an important role in the activation of the inflammatory cascade (Fan et al., 2023). Antcin C is a well-known metabolite of AC, which exerts its hepatoprotective and antioxidative activities by modulating the Nrf2 pathway. It is also reported that antcin C treatment in rats with cerebral injury could reduce the oxidative stress parameters and inflammatory cytokine levels via inhibiting the TLR-4 pathway (Ling et al., 2020).
Ergosta-7,9 (11),22-trien-3β-ol (Ergostatrien-3β-ol; EK100), a triterpenoid metabolite abundant in both the fruiting bodies and (Chao et al., 2021), also showed promising medicinal uses in neurological disorders. EK100 and antrodin C effectively improved the autonomous behavior and social ability and alleviated amyloid plaque burden in APP/PS1 mice (Tsay et al., 2021). EK100 from AC improved AD symptoms in a Drosophila model with Aβ42 overexpression by preventing the activation of microglial (Liu et al., 2021). EK100 showed a significant anti-inflammatory effect against LPS-induced NO production in BV2 cells, with IC50 values of 18 ± 2 μM, which was almost as potent as the NF-κB inhibitor. In the AIS mouse model, EK100 treatment reduced ischemic brain injury by decreasing the expression of p65 and caspase 3. EK100 also promoted endogenous neurogenesis through GSK-3 inhibition and β-catenin activation by activating PI3K/Akt signaling, suggesting EK100 may have other targets in addition to anti-inflammatory activity and it may act as potent brain protective agent (Wang et al., 2019). In another study, EK100 treatment showed neuroprotective effects on ipsilateral injuries in a mouse model of collagenase-induced intracerebral hemorrhage (ICH) via inhibiting COX-2 and MMP-9; EK100 treatment also exerted significant anti-inflammatory function by downregulating JNK activation in BV-2 cells and ICH mice (Hsueh et al., 2021; Huang et al., 2021). The above reports demonstrated that EK100 isolated from AC showed obvious anti-inflammatory effects through interfering with several pathways and anti-neuropathy in vivo and in vitro.
Our previous study showed that ergosterol isolated from AC, an isomer of EK100, could suppress the activation of BV2 treated with LPS by inhibiting the NF-κB, MAPK, and AKT signaling pathways (Sun et al., 2023). Further investigation in LPS-treated ICR mice found that ergosterol exhibited anti-neuroinflammatory activity and maintained the synaptic proteins. Another group also reported that ergosterol isolated from Auricularia polytricha or Cordyceps militaris attenuated neuroinflammation in BV2 cells (Nallathamby et al., 2015; Sillapachaiyaporn et al., 2022a), and exhibited neuroprotective activity in TNF-α-induced HT-22 cells via regulating the expression of N-methyl-D-aspartate receptors (NMDARs) and antioxidant enzyme, and cell survival pathways (Sillapachaiyaporn et al., 2022b). Therefore, ergosterol exerts its neuroprotective effect mainly through its anti-neuroinflammatory property via multiple pathways.
4.2.4 Stimulation of neurite regrowth and NGF synthesis
Neurite regrowth promoters were found to be possible therapies for neurological disorders. These neuritogenic substances have the ability to stimulate the growth of neurites in neuronal cells and are expected to be effective in the treatment of nerve injuries (More et al., 2012). Nerve growth factor (NGF), brain derived neurotrophic factor (BDNF), neurotrophin 3 (NT-3), and glia-derived neurotrophic factor (GDNF) are identified as important factors called neurotrophins for the survival and differentiation of neurons as well as neuronal maintenance (Numakawa and Kajihara, 2023). Neurotrophic factors are one of the key mediators of neural plasticity and functional recovery. They have great potential as therapeutic drugs against neurodegenerative diseases.
Some lanostane triterpenoids such as tumulosic acid, polyporenic acid C, 16α-hydroxyeburicoic acid, dehydrotumulosic acid, and pachymic acid are also widely found in medicinal mushrooms other than AC and can induce neurite outgrowth in human astrocytoma 1321N1 and PC12 cells, which might be due to inducing the expression of neurotrophic factors, NGF, and BDNF (Hassan et al., 2022). Further investigation demonstrated that these metabolisms identified as neurotrophins show weak cytotoxic effects on mammalian cells, but they are lacking certain structural features. It is worthwhile to further study these fungi, including their secondary metabolisms, the function structural and so on, which might reveal the interesting chemotaxonomic relationships as well as hitherto unprecedented biological activities of the constituents from AC.
4.2.5 Inhibition of beta-amyloid toxicity
Aβ peptide is formed by a cleavage process of APP via beta-cleavage by the secretases beta (BACE1) and gamma-cleavage of gamma secretases. Oligomeric and fibrillar beta-amyloid are both toxic to neurons. Aβ-dependent neuronal death can cause changes in cell membrane fluidity and integrity, leading to ion leakage, disruption of cellular calcium balance, decreased membrane potential, ultimately promoting apoptosis and synaptic loss (Silva et al., 2023).
Five metabolites—19-hydroxylabda-8 (17)-en-16, 15- olide; 3b,19-dihydroxylabda-8 (17), 11E-dien-16,15-olide; 13-epi-3b, 19-dihydroxylabda-8 (17), 11E-dien-16, 15- olide; 19-hydroxylabda-8 (17), 13-dien-16,15-olide, and 14-deoxy-11, 12-didehydroandrographolide—were obtained from the fruiting bodies of AC, and showed neuronal protection against Aβ25–35 damage (Chen et al., 2006). The above-mentioned studies have been demonstrated to be the basic substance of AC for neuroprotection.
Metabolites derived from AC possess potential neuroprotective properties via its various bioactivities. We have summarized the effects of some active metabolites from the AC on CNS diseases in Table 1.
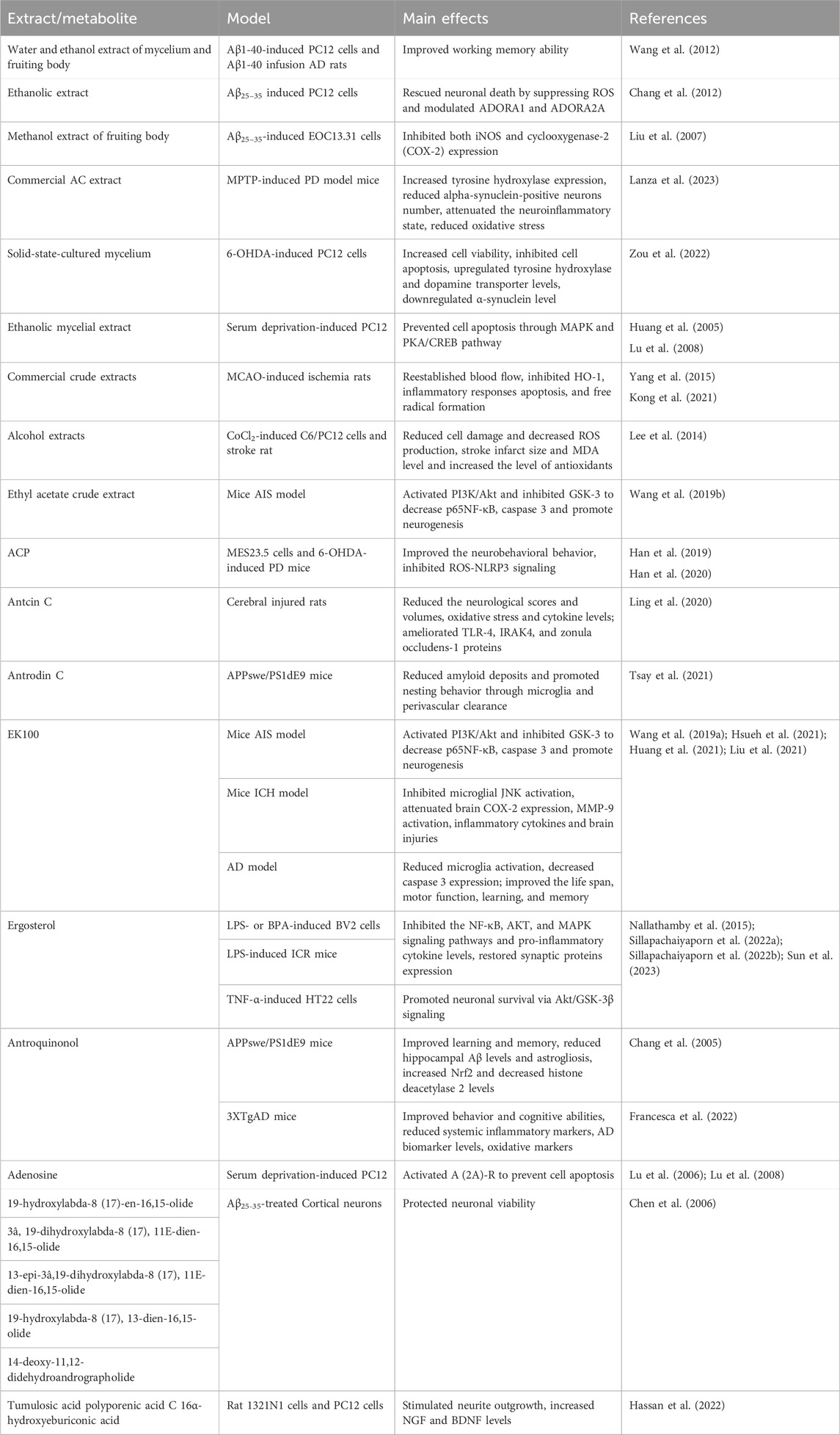
Table 1. Summary of the main in vitro and preclinical studies on Antrodia camphorata and some active metabolites in the CNS diseases.
5 Potential with the gut-microbiome-brain axis of AC
The gut microbiome plays a key role in human health, such as overall homeostasis maintenance, immune system moderation, and central nervous system regulation. Increasing clinical and preclinical evidence points to the existence of the microbiota-gut-brain axis that forms a bidirectional network between the CNS and the gut (Singh et al., 2016; Sharon et al., 2019). Microbial imbalance is particularly linked to various neurological disorders including AD (Cattaneo et al., 2017), PD (Forsyth et al., 2011), and stroke (Sorboni et al., 2022). Plenty of research has attempted to define the molecular cross-talk between the host and microbiome to provide novel perspectives on promising therapeutic approaches for the management of neurological disorders (Mou et al., 2022).
A previous study in the leptin-induced Caco-2 cells model found that AC had a positive effect on intestinal microflora by repairing intestinal-barrier damage and enhancing the integrity of the intestinal barrier (Tsai et al., 2020). Another study in high-fat diet (HFD)-fed mice demonstrated that AC showed protective effects, maintained the intestinal barrier integrity, reduced the Firmicutes/Bacteroidetes ratio and increased Akkermansia muciniphila level (Chang et al., 2018). It is also reported that solid-state cultured AC can reduce hyperglycemia and tend to alleviate metabolic disorder in HFD-induced obese mice, mainly reduce the relative abundance of Firmicutes-to-Bacteroidetes ratio and elevate the relative abundance of Akkermansia spp. (Wang et al., 2020). The arrangement of the enterocyte was not disrupted by AC extract treatment, but intactness and denseness of hepatic tissue was elevated by regulating redox and cytoskeleton-related proteins and increasing the abundance of Akkermansia spp. in the gut microbiota of C57BL/6 mice (Tsai et al., 2021). Akkermansia spp. has reported to improve the situation of AD and PD (He et al., 2022). The above studies demonstrated that AC has neuroprotective potential in the gut microbiome by increasing probiotic bacteria Akkermansia spp.
In addition to crude extracts, the functions of AC metabolites on the microbiota-gut-brain axis have also been studied. In LPS-stressed slow-growing broiler breeds model, dietary supplement with 100–400 mg/kg ACP showed beneficial effects on liver damage and the bacterial microbiota species richness and diversity (Ye et al., 2022). Additionally, ACP inhibited the rise of Proteobacteria in LPS-induced group, while restored the beneficial cecal microbiota (typically Lactobacillus, Faecalibacterium, and Christensenellaceae R-7 group). Thus, ACP enhanced the species richness, and diversity indices might be related to the anti-inflammatory effect. Intragastric administration of exopolysaccharides from AC in lincomycin hydrochloride (LIH)-induced mice greatly reduced serum inflammatory cytokine levels and alleviated immune organs damage, while also regulating the microbial environment via enhancing the relative abundance of beneficial microbiota in the intestine (typically Lactobacillus, Roseburia, Ligilactobacillus, and Lachnospiraceae_NK4A136_group), and reducing the relative abundances of harmful microbes such as Enterococcus and Shigella (Lu et al., 2022), which were increased in AD subjects (Hou et al., 2021). Antrodin A from AC can alleviate the alcohol-induced metabolic disorders via regulating the composition of intestinal microbiota by decreasing Clostridium sensu stricto 1, Lachnospiraceae_NK4A136_group, Prevotellaceae_NK3B31_group, and Prevotellaceae_UCG-001, and increasing the relative abundance of Lactobacillus and Dubosiella (Yi et al., 2021). Another study demonstrated that the ethyl acetate layer of AC mycelium extract, especially antrodin A, and antroquinonol decreased the abundance of intestinal Helicobacteraceae and increased the relative abundance of Lachnospiraceae and Ruminococcaceae to prevent alcohol-induced oxidative stress and inflammation in mice (Yi et al., 2020). The decrease of Ruminococcaceae could act as a predictive marker for the rapidly progressive mild cognitive impairment (Yang et al., 2023). According to these studies, AC metabolites may have the potential to modulate the brain-gut axis.
The composition of the gut microbiota is important for host homeostatic functions. AC and its active metabolites showed prebiotic effects on the gut microbiome to affect the state of the brain and nervous system. AC might show its neuroprotective activity on the gut-microbiota-brain axis. We summarized the effects of AC in the gut microbiome in Table 2. Figure 2 presents the neuroprotective effects of bioactive molecules contained within AC by working on nervous system and gut-microbiota.
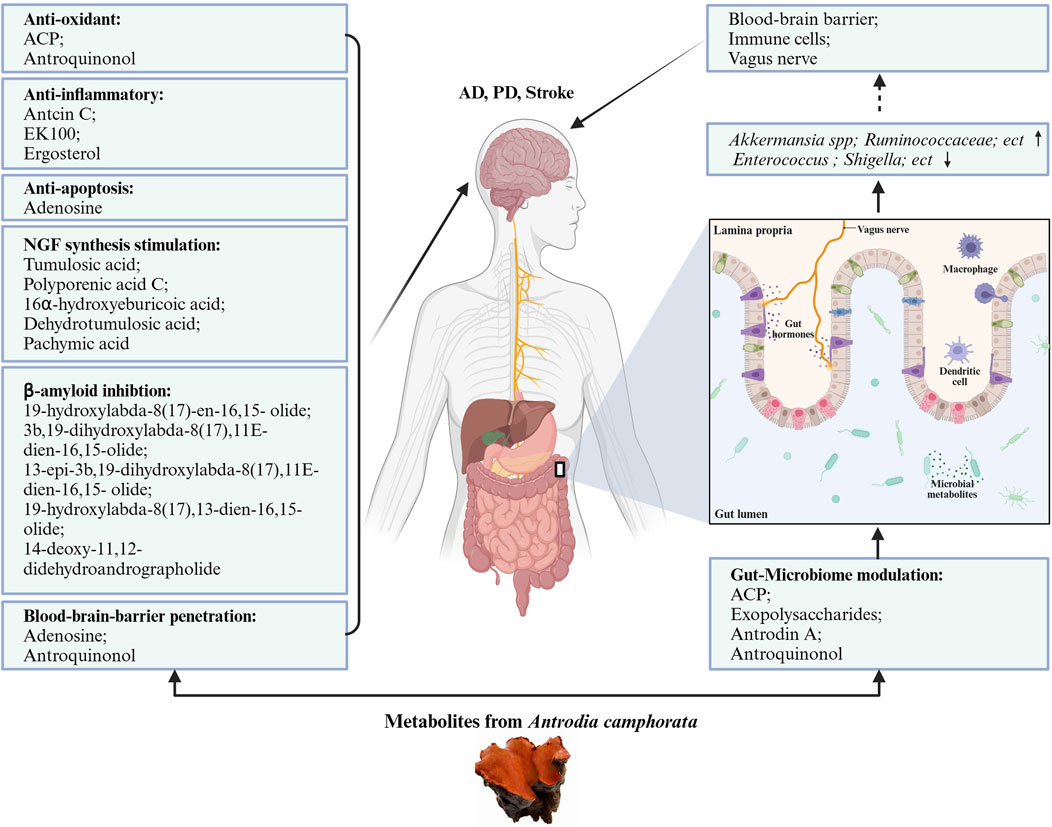
Figure 2. Neuroprotective potentials of Antrodia camphorata. The bioactive metabolites from AC play neuroprotective function directly by anti-oxidant, anti-inflammatory, anti-apoptotic, stimulation NGF synthesis, and beta-amyloid inhibition pattern, also indirectly by gut-microbiome modulation.
6 Future perspectives
AC is a valuable and medicinal fungus known widely and has tremendous medicinal properties and nutritional value for brain health. It possesses several health-endorsing properties such as anti-oxidant, anti-apoptosis, and anti-inflammatory and probably improves the gut microbiome in neurologic disorders. In general, the isolated metabolites from AC have not been deeply and systematically studied in neuroscience. There are still metabolites that have not been identified; hence, AC has great potential. For metabolites that have entered clinical evaluation, production should be increased by applying different strategies. Furthermore, in-depth pharmacokinetic studies and modification of AC metabolites are also lacking. The following aspects of AC research could be improved:
6.1 Increase the production of the bioactive metabolites in AC
Genomic and transcriptomic analyzes will help to develop strategies to increase the production of useful metabolites (Lu et al., 2014). For tissue-specific metabolites, secondary metabolites biosynthesis pathway genes were enriched, including 14-α-demethylase (CYP51F1) for the conversion of lanosterol to ergosteroidal triterpenes in fruiting bodies, coenzyme Q (COQ) for the synthesis of antroquinonol in mycelia, and polyketide synthase for the synthesis of antrocamphin in fruiting bodies. It was reported that talc enhanced the yield of the bioactive secondary metabolite antioxidant antrodin C in the submerged fermentation of AC by increasing the permeability and fluidity of the cell membrane, upregulating the key genes and then improving the biosynthesis process (Fan J. H. et al., 2023). Another study showed that oxidative stressors supplementation (such as hydrogen peroxide) can increase the yield of antrodin C in submerged fermentation of AC (Hu et al., 2020). For instance, biosynthesis is a strategy to achieve industrial-scale production for the identification of novel metabolites and improvement of the yield of previously known valuable metabolites.
6.2 Specific investigation of metabolite pharmacology and pharmacokinetics
As an emerging medicinal fungus, AC has a variety of important biological activities and pharmacological functions, a variety of bioactive metabolites from its fruiting bodies and mycelia that have been isolated and purified, but the underlying mechanism such as the pharmacokinetics and pharmacodynamics remain unclear, which are important for further biochemical research and clinical trials. The establishment of an intracerebral pharmacokinetic research model will truly reflect the process of treatment and action of drugs after entering the brain tissue, ensuring the effective concentration of brain-targeted drugs in the brain and preventing the damage of non-brain-targeted drugs to neurological function, thereby greatly improving the effectiveness and safety. Most pharmacological studies were derived from in vitro laboratory investigations; hence, further preclinical studies are necessitated for a full evaluation of these valuable metabolites from AC in vivo experiments to promote clinical applications and complete the clinical validation for potential therapeutic benefits.
6.3 Strategies for bioavailability improvement of the metabolites from AC
Structure function studies are important for the design of more effective drugs, and many pharmacological actions and its molecular mechanisms are defined. The structures of terpenoids isolated from AC are closely related to the anti-inflammatory activity. The substituents on C15 are crucial to the anti-inflammatory properties of lanostane-type metabolites (Yang et al., 2022).
Chemical modification is necessary to enhance the structural diversity and druggability of natural products. It has been demonstrated that side-chain esterification increased the antitumor activity of Antrodia ergosteroids (Li et al., 2020). Furthermore, a number of investigations have shown that amide derivatives of triterpenoids containing nitrogen heterocycles or anilines at the side chain exhibit higher cytotoxicity than the original metabolites (Li et al., 2021). Ergosterol lacks aqueous solubility, but a study found that solubility and bioavailability can be improved by using nanostructured lipid carriers (NLCs). Nanoparticles drug delivery system can also improve the bioavailability of ergosterol following oral administration (Zhang et al., 2016). AC modified the nanocarrier β-cyclodextrin (BCD) inclusion complex could potentially unlock its full potential and attempt to make it suitable for improving skeletal muscle health and possible to develop evidence-based drug (Menon et al., 2022). The blood-brain barrier penetration of potential drugs is a critical issue, and delivery of these neuroprotective metabolites into the brain requires a tremendous effort, for which nanomedicine has emerged as a promising approach.
Author contributions
WL: Writing–original draft, Writing–review and editing. PW: Writing–review and editing. JQ: Funding acquisition, Writing–review and editing. YL: Writing–review and editing. QP: Writing–review and editing. ZZ: Funding acquisition, Writing–review and editing. XS: Supervision, Writing–review and editing. YX: Conceptualization, Writing–review and editing. BS: Conceptualization, Writing–review and editing.
Funding
The author(s) declare financial support was received for the research, authorship, and/or publication of this article. This work was supported by the Research Fund of Jianghan University (grants number: 08190006; 2022SXZX28) and Wuhan Municipal Education Bureau (CXY202204).
Acknowledgments
The authors acknowledge Biorender that is used to create schematic Figures 1, 2.
Conflict of interest
The authors declare that the research was conducted in the absence of any commercial or financial relationships that could be construed as a potential conflict of interest.
Publisher’s note
All claims expressed in this article are solely those of the authors and do not necessarily represent those of their affiliated organizations, or those of the publisher, the editors and the reviewers. Any product that may be evaluated in this article, or claim that may be made by its manufacturer, is not guaranteed or endorsed by the publisher.
References
Alçada-Morais, S., Gonçalves, N., Moreno-Juan, V., Andres, B., Ferreira, S., Marques, J. M., et al. (2021). Adenosine A2A receptors contribute to the radial migration of cortical projection neurons through the regulation of neuronal polarization and axon formation. Cereb. Cortex 31 (12), 5652–5663. doi:10.1093/cercor/bhab188
Alzheimer’s Association (2023). Alzheimer's disease facts and figures. Alzheimers Dement. 194, 1598–1695. doi:10.1002/alz.13016
Angamuthu, V., Shanmugavadivu, M., Nagarajan, G., and Velmurugan, B. K. (2019). Pharmacological activities of antroquinonol- Mini review. Chem. Biol. Interact. 297, 8–15. doi:10.1016/j.cbi.2018.10.009
Beitz, J. M. (2014). Parkinson's disease: a review. Front. Biosci. Sch. Ed. 6 (1), 65–74. doi:10.2741/s415
Breijyeh, Z., and Karaman, R. (2020). Comprehensive review on Alzheimer's disease: causes and treatment. Molecules 25 (24), 5789. doi:10.3390/molecules25245789
Cattaneo, A., Cattane, N., Galluzzi, S., Provasi, S., Lopizzo, N., Festari, C., et al. (2017). Association of brain amyloidosis with pro-inflammatory gut bacterial taxa and peripheral inflammation markers in cognitively impaired elderly. Neurobiol. Aging 49, 60–68. doi:10.1016/j.neurobiolaging.2016.08.019
Chamorro, Á., Lo, E. H., Renú, A., van Leyen, K., and Lyden, P. D. (2021). The future of neuroprotection in stroke. J. Neurol. Neurosurg. Psychiatry 92 (2), 129–135. doi:10.1136/jnnp-2020-324283
Chang, C. H., Wang, H. E., Liaw, P. Y., Peng, C. C., and Peng, R. Y. (2012). Antrodia cinnamomea exhibits a potent neuroprotective effect in the PC12 Cell-Aβ25-35 model - pharmacologically through adenosine receptors and mitochondrial pathway. Planta Med. 78 (17), 1813–1823. doi:10.1055/s-0032-1315397
Chang, C. J., Lu, C. C., Lin, C. S., Martel, J., Ko, Y. F., Ojcius, D. M., et al. (2018). Antrodia cinnamomea reduces obesity and modulates the gut microbiota in high-fat diet-fed mice. Int. J. Obes. (Lond). 42 (2), 231–243. doi:10.1038/ijo.2017.149
Chang, C. Y., Lee, C. L., and Pan, T. M. (2006). Statistical optimization of medium components for the production of Antrodia cinnamomea AC0623 in submerged cultures. Appl. Microbiol. Biotechnol. 72 (4), 654–661. doi:10.1007/s00253-006-0325-6
Chao, T. Y., Hsieh, C. C., Hsu, S. M., Wan, C. H., Lian, G. T., Tseng, Y. H., et al. (2021). Ergostatrien-3β-ol (EK100) from Antrodia camphorata attenuates oxidative stress, inflammation, and liver Injury in vitro and in vivo. Prev. Nutr. Food Sci. 26 (1), 58–66. doi:10.3746/pnf.2021.26.1.58
Chen, C. C., Shiao, Y. J., Lin, R. D., Shao, Y. Y., Lai, M. N., Lin, C. C., et al. (2006). Neuroprotective diterpenes from the fruiting body of Antrodia camphorata. J. Nat. Prod. 69 (4), 689–691. doi:10.1021/np0581263
Chen, C. K., Kang, J. J., Wen, W. C., Chiang, H. F., and Lee, S. S. (2014). Metabolites of antroquinonol found in rat urine following oral administration. J. Nat. Prod. 77 (4), 1061–1064. doi:10.1021/np400670a
Chen, H., He, Y., Chen, S., Qi, S., and Shen, J. (2020). Therapeutic targets of oxidative/nitrosative stress and neuroinflammation in ischemic stroke: applications for natural product efficacy with omics and systemic biology. Pharmacol. Res. 158, 104877. doi:10.1016/j.phrs.2020.104877
Chen, J. J., Zhang, Z., Wang, Y., Yuan, X. L., Wang, J., Yang, Y. M., et al. (2023). Transcriptome analysis of Antrodia cinnamomea mycelia from different wood substrates. Mycobiology 51 (1), 49–59. doi:10.1080/12298093.2023.2175434
Chen, S. N., Chang, C. S., Chen, S., and Soni, M. (2018). Subchronic toxicity and genotoxicity studies of Antrodia mushroom β-glucan preparation. Regul. Toxicol. Pharmacol. 92, 429–438. doi:10.1016/j.yrtph.2017.12.022
Cheng, J. J., Yang, C. J., Cheng, C. H., Wang, Y. T., Huang, N. K., and Lu, M. K. (2005). Characterization and functional study of Antrodia camphorata lipopolysaccharide. J. Agric. Food Chem. 53 (2), 469–474. doi:10.1021/jf049281a
Das, T. K., Ganesh, B. P., and Fatima-Shad, K. (2023). Common signaling pathways involved in Alzheimer's disease and stroke: two faces of the same coin. J. Alzheimers Dis. Rep. 7 (1), 381–398. doi:10.3233/ADR-220108
Deng, J. S., Huang, S. S., Lin, T. H., Lee, M. M., Kuo, C. C., Sung, P. J., et al. (2013). Analgesic and anti-inflammatory bioactivities of eburicoic acid and dehydroeburicoic acid isolated from Antrodia camphorata on the inflammatory mediator expression in mice. J. Agric. Food Chem. 61 (21), 5064–5071. doi:10.1021/jf303820k
DiSabato, D. J., Quan, N., and Godbout, J. P. (2016). Neuroinflammation: the devil is in the details. Journal of neurochemistry 139 (2), 136–153. doi:10.1111/jnc.13607
D'Mello, S. R., and Chin, P. C. (2005). Treating neurodegenerative conditions through the understanding of neuronal apoptosis. Curr. Drug Targets CNS Neurol. Disord. 4 (1), 3–23. doi:10.2174/1568007053005118
Du, Y. C., Wu, T. Y., Chang, F. R., Lin, W. Y., Hsu, Y. M., Cheng, F. T., et al. (2012). Chemical profiling of the cytotoxic triterpenoid-concentrating fraction and characterization of ergostane stereo-isomer ingredients from Antrodia camphorata. J. Pharm. Biomed. Anal. 58, 182–192. doi:10.1016/j.jpba.2011.09.007
Fan, H., Bai, Q., Yang, Y., Shi, X., Du, G., Yan, J., et al. (2023a). The key roles of reactive oxygen species in microglial inflammatory activation: regulation by endogenous antioxidant system and exogenous sulfur-containing compounds. Eur. J. Pharmacol. 956, 175966. doi:10.1016/j.ejphar.2023.175966
Fan, J. H., Lai, K. S., Huang, Y. Y., Chen, H. Y., Xiong, L. Q., Guo, H. K., et al. (2023b). Efficient production of Antrodin C by microparticle-enhanced cultivation of medicinal mushroom Antrodia cinnamomea. J. Biosci. Bioeng. 135 (3), 232–237. doi:10.1016/j.jbiosc.2022.12.013
Forsyth, C. B., Shannon, K. M., Kordower, J. H., Voigt, R. M., Shaikh, M., Jaglin, J. A., et al. (2011). Increased intestinal permeability correlates with sigmoid mucosa alpha-synuclein staining and endotoxin exposure markers in early Parkinson's disease. PloS one 6 (12), e28032. doi:10.1371/journal.pone.0028032
Francesca, F., Caitlin, A., Sarah, L., and Robyn, G. L. (2022). Antroquinonol administration in animal preclinical studies for Alzheimer's disease (AD): a new avenue for modifying progression of AD pathophysiology. Brain Behav. Immun. Health 21, 100435. doi:10.1016/j.bbih.2022.100435
Geethangili, M., and Tzeng, Y. M. (2011). Review of pharmacological effects of Antrodia camphorata and its bioactive compounds. Evid. Based Complement. Altern. Med. 2011, 212641. doi:10.1093/ecam/nep108
Gonzalez-Zuñiga, M., Contreras, P. S., Estrada, L. D., Chamorro, D., Villagra, A., Zanlungo, S., et al. (2014). c-Abl stabilizes HDAC2 levels by tyrosine phosphorylation repressing neuronal gene expression in Alzheimer's disease. Mol. Cell 56 (1), 163–173. doi:10.1016/j.molcel.2014.08.013
Halliwell, B. (1992). Reactive oxygen species and the central nervous system. J. Neurochem. 59, 1609–1623. doi:10.1111/j.1471-4159.1992.tb10990.x
Han, C., Guo, L., Yang, Y., Li, W., Sheng, Y., Wang, J., et al. (2019). Study on Antrodia camphorata polysaccharide in alleviating the neuroethology of PD mice by decreasing the expression of NLRP3 inflammasome. Phytother. Res. 33 (9), 2288–2297. doi:10.1002/ptr.6388
Han, C., Shen, H., Yang, Y., Sheng, Y., Wang, J., Li, W., et al. (2020). Antrodia camphorata polysaccharide resists 6-OHDA-induced dopaminergic neuronal damage by inhibiting ROS-NLRP3 activation. Brain Behav. 10 (11), e01824. doi:10.1002/brb3.1824
Hassan, K., Matio Kemkuignou, B., Kirchenwitz, M., Wittstein, K., Rascher-Albaghdadi, M., Chepkirui, C., et al. (2022). Neurotrophic and immunomodulatory lanostane triterpenoids from wood-inhabiting Basidiomycota. Int. J. Mol. Sci. 23 (21), 13593. doi:10.3390/ijms232113593
He, X., Yan, C., Zhao, S., Zhao, Y., Huang, R., and Li, Y. (2022). The preventive effects of probiotic Akkermansia muciniphila on D-galactose/AlCl3 mediated Alzheimer's disease-like rats. Exp. Gerontol. 170, 111959. doi:10.1016/j.exger.2022.111959
Hernandes, M. S., D'Avila, J. C., Trevelin, S. C., Reis, P. A., Kinjo, E. R., Lopes, L. R., et al. (2014). The role of Nox2-derived ROS in the development of cognitive impairment after sepsis. J. Neuroinflammation 11, 36. doi:10.1186/1742-2094-11-36
Hernandez-Baltazar, D., Zavala-Flores, L. M., and Villanueva-Olivo, A. (2017). The 6-hydroxydopamine model and parkinsonian pathophysiology: novel findings in an older model. Neurologia 32 (8), 533–539. doi:10.1016/j.nrl.2015.06.011
Hou, M., Xu, G., Ran, M., Luo, W., and Wang, H. (2021). APOE-ε4 carrier status and gut microbiota dysbiosis in patients with Alzheimer disease. Front. Neurosci. 15, 619051. doi:10.3389/fnins.2021.619051
Hsueh, P. J., Wang, M. H., Hsiao, C. J., Chen, C. K., Lin, F. L., Huang, S. H., et al. (2021). Ergosta-7,9(11),22-trien-3β-ol alleviates intracerebral hemorrhage-induced brain injury and BV-2 microglial activation. Molecules 26 (10), 2970. doi:10.3390/molecules26102970
Hu, P. F., Huang, J., Chen, L., Ding, Z., Liu, L., Molnár, I., et al. (2020). Oxidative stress induction is a rational strategy to enhance the productivity of Antrodia cinnamomea fermentations for the antioxidant secondary metabolite Antrodin C. J. Agric. Food Chem. 68 (13), 3995–4004. doi:10.1021/acs.jafc.9b07965
Huang, N. K., Cheng, J. J., Lai, W. L., and Lu, M. K. (2005). Antrodia camphorata prevents rat pheochromocytoma cells from serum deprivation-induced apoptosis. FEMS Microbiol. Lett. 244 (1), 213–219. doi:10.1016/j.femsle.2005.01.048
Huang, Y. P., Chen, D. R., Lin, W. J., Lin, Y. H., Chen, J. Y., Kuo, Y. H., et al. (2021). Ergosta-7,9(11),22-trien-3β-ol attenuates inflammatory responses via inhibiting MAPK/AP-1 induced IL-6/JAK/STAT pathways and activating Nrf2/HO-1 signaling in LPS-stimulated macrophage-like cells. Antioxidants 10 (9), 1430. doi:10.3390/antiox10091430
Isakovic, A. J., Abbott, N. J., and Redzic, Z. B. (2004). Brain to blood efflux transport of adenosine: blood-brain barrier studies in the rat. J. Neurochem. 90 (2), 272–286. doi:10.1111/j.1471-4159.2004.02439.x
Kalampokini, S., Becker, A., Fassbender, K., Lyros, E., and Unger, M. M. (2019). Nonpharmacological modulation of chronic inflammation in Parkinson's disease: role of diet interventions. Park. Dis. 2019, 7535472. doi:10.1155/2019/7535472
Khadka, B., Lee, J. Y., Park, D. H., Kim, K. T., and Bae, J. S. (2020). The role of natural compounds and their nanocarriers in the treatment of CNS inflammation. Biomolecules 10 (10), 1401. doi:10.3390/biom10101401
Kong, Z. L., Hsu, Y. T., Johnson, A., Tsai, T. H., Miao, S., He, J. L., et al. (2021). Protective effects of Antrodia camphorata extract against hypoxic cell injury and ischemic stroke brain damage. Phytother. Res. 35 (3), 1609–1620. doi:10.1002/ptr.6928
Kuang, Y., Li, B., Wang, Z., Qiao, X., and Ye, M. (2021). Terpenoids from the medicinal mushroom Antrodia camphorata: chemistry and medicinal potential. Nat. Prod. Rep. 38 (1), 83–102. doi:10.1039/d0np00023j
Lanza, M., Cucinotta, L., Casili, G., Filippone, A., Basilotta, R., Capra, A. P., et al. (2023). The transcription factor Nrf2 mediates the effects of Antrodia camphorata extract on neuropathological changes in a mouse model of Parkinson's disease. Int. J. Mol. Sci. 24 (11), 9250. doi:10.3390/ijms24119250
Lee, I. H., Huang, R. L., Chen, C. T., Chen, H. C., Hsu, W. C., and Lu, M. K. (2002). Antrodia camphorata polysaccharides exhibit anti-hepatitis B virus effects. FEMS Microbiol. Lett. 209 (1), 63–67. doi:10.1111/j.1574-6968.2002.tb11110.x
Lee, Y. C., Ho, C. L., Kao, W. Y., and Chen, Y. M. (2015). A phase I multicenter study of antroquinonol in patients with metastatic non-small-cell lung cancer who have received at least two prior systemic treatment regimens, including one platinum-based chemotherapy regimen. Mol. Clin. Oncol. 3 (6), 1375–1380. doi:10.3892/mco.2015.642
Lee, Y. M., Chang, C. Y., Yen, T. L., Geraldine, P., Lan, C. C., Sheu, J. R., et al. (2014). Extract of Antrodia camphorata exerts neuroprotection against embolic stroke in rats without causing the risk of hemorrhagic incidence. Sci. World J. 2014, 686109. doi:10.1155/2014/686109
Li, B., Kuang, Y., He, J. B., Tang, R., Xu, L. L., Leung, C. H., et al. (2020). Antcamphorols A-K, cytotoxic and ROS scavenging triterpenoids from Antrodia camphorata. J. Nat. Prod. 83 (1), 45–54. doi:10.1021/acs.jnatprod.9b00580
Li, B., Kuang, Y., Yi, Y., Qiao, X., Liang, L., and Ye, M. (2021). Chemical modifications of ergostane-type triterpenoids from Antrodia camphorata and their cytotoxic activities. Bioorg Med. Chem. Lett. 43, 128066. doi:10.1016/j.bmcl.2021.128066
Li, H., Dai, J., Shi, Y., Zhu, X., Jia, L., and Yang, Z. (2023). Molecular regulatory mechanism of the iron-ion-promoted asexual sporulation of Antrodia cinnamomea in submerged fermentation revealed by comparative transcriptomics. J. Fungi (Basel) 9 (2), 235. doi:10.3390/jof9020235
Li, H. X., Wang, J. J., Lu, C. L., Gao, Y. J., Gao, L., and Yang, Z. Q. (2022). Review of bioactivity, isolation, and identification of active compounds from Antrodia cinnamomea. Bioeng. (Basel) 9 (10), 494. doi:10.3390/bioengineering9100494
Li, Z. W., Ji, S., Li, B., Wang, S., Tzeng, Y. M., Qiao, X., et al. (2018). Metabolites identification and quantification of antcin H in mice tumors after oral administration of the anticancer mushroom Antrodia camphorata. TMR Mod. Herb. Med. 1, 40. doi:10.53388/tmrmhm2017a15
Liao, Y. T., Huang, K. W., Chen, W. J., and Lai, T. H. (2023). A botanical drug extracted from Antrodia cinnamomea: a first-in-human phase I study in healthy volunteers. J. Am. Nutr. Assoc. 42 (3), 274–284. doi:10.1080/07315724.2022.2032868
Lin, C. C., Kumar, K. J. S., Liao, J. W., Kuo, Y. H., and Wang, S. Y. (2015). Genotoxic, teratotoxic and oral toxic assessments of Antrodia cinnamomea health food product (Leader Deluxe Antrodia cinnamomea®). Toxicol. Rep. 2, 1409–1417. doi:10.1016/j.toxrep.2015.10.007
Ling, W., Cui, Y., Gao, J., Li, R., Jiang, X., Tian, Y., et al. (2020). Antcin C ameliorates neuronal inflammation due to cerebral haemorrhage by inhibiting the TLR-4 pathway. Folia Neuropathol. 58, 317–323. doi:10.5114/fn.2020.102434
Liu, D. Z., Liang, H. J., Chen, C. H., Su, C. H., Lee, T. H., Huang, C. T., et al. (2007). Comparative anti-inflammatory characterization of wild fruiting body, liquid-state fermentation, and solid-state culture of Taiwanofungus camphoratus in microglia and the mechanism of its action. J. Ethnopharmacol. 113, 45–53. doi:10.1016/j.jep.2007.03.037
Liu, H. P., Kuo, Y. H., Cheng, J., Chang, L. Z., Chang, M. S., Su, L. W., et al. (2021). Ergosta-7,9(11),22-trien-3β-ol rescues AD deficits by modulating microglia activation but not oxidative stress. Molecules 26, 5338. doi:10.3390/molecules26175338
Liu, S. C., Wu, T. Y., Hsu, T. H., Lai, M. N., Wu, Y. C., and Ng, L. T. (2022). Chemical composition and chronic toxicity of disc-cultured Antrodia cinnamomea fruiting bodies. Toxics 10, 587. doi:10.3390/toxics10100587
Liu, X., Yu, S., Zhang, Y., Zhang, W., Zhong, H., Lu, X., et al. (2023). A review on the protective effect of active components in Antrodia camphorata against alcoholic liver injury. J. Ethnopharmacol. 300, 115740. doi:10.1016/j.jep.2022.115740
Liu, Y., Di, X., Liu, X., Shen, W., and Leung, K. S. (2010). Development of a LC-MS/MS method for the determination of antrodin B and antrodin C from Antrodia camphorata extract in rat plasma for pharmacokinetic study. J. Pharm. Biomed. Anal. 53, 781–784. doi:10.1016/j.jpba.2010.05.017
Lu, C. L., Lee, B. H., Ren, Y. L., Ji, D., Rao, S. Q., Li, H. X., et al. (2022). Effects of exopolysaccharides from Antrodia cinnamomea on inflammation and intestinal microbiota disturbance induced by antibiotics in mice. Food Biosci. 50, 102116. doi:10.1016/j.fbio.2022.102116
Lu, M. K., Cheng, J. J., Lai, W. L., Lin, Y. J., and Huang, N. K. (2008). Fermented Antrodia cinnamomea extract protects rat PC12 cells from serum deprivation-induced apoptosis: the role of the MAPK family. J. Agric. Food Chem. 56, 865–874. doi:10.1021/jf072828b
Lu, M. K., Cheng, J. J., Lai, W. L., Lin, Y. R., and Huang, N. K. (2006). Adenosine as an active component of Antrodia cinnamomea that prevents rat PC12 cells from serum deprivation-induced apoptosis through the activation of adenosine A(2A) receptors. Life Sci. 79, 252–258. doi:10.1016/j.lfs.2005.12.042
Lu, M. Y., Fan, W. L., Wang, W. F., Chen, T., Tang, Y. C., Chu, F. H., et al. (2014). Genomic and transcriptomic analyses of the medicinal fungus Antrodia cinnamomea for its metabolite biosynthesis and sexual development. Proc. Natl. Acad. Sci. U. S. A. 111, E4743–E4752. doi:10.1073/pnas.1417570111
Marino, B. L. B., de Souza, L. R., Sousa, K. P. A., Ferreira, J. V., Padilha, E. C., da Silva, C. H. T. P., et al. (2020). Parkinson's disease: a review from pathophysiology to treatment. Mini Rev. Med. Chem. 20, 754–767. doi:10.2174/1389557519666191104110908
Menon, M. P., Chien, Y. H., Thomas, J., Yu, Y. H., Chang, C. T., and Hua, K. F. (2022). Nano modification of Antrodia cinnamomea exhibits anti-inflammatory action and improves the migratory potential of myogenic progenitors. Cells 11, 2512. doi:10.3390/cells11162512
Miyashita, N., Straub, J. E., and Thirumalai, D. (2009). Structures of beta-amyloid peptide 1-40, 1-42, and 1-55-the 672-726 fragment of APP-in a membrane environment with implications for interactions with gamma-secretase. J. Am. Chem. Soc. 131 (49), 17843–17852. doi:10.1021/ja905457d
More, S. V., Koppula, S., Kim, I. S., Kumar, H., Kim, B. W., and Choi, D. K. (2012). The role of bioactive compounds on the promotion of neurite outgrowth. Molecules 17 (6), 6728–6753. doi:10.3390/molecules17066728
Morris, H. R., Spillantini, M. G., Sue, C. M., and Williams-Gray, C. H. (2024). The pathogenesis of Parkinson's disease. Lancet 403 (10423), 293–304. doi:10.1016/S0140-6736(23)01478-2
Mou, Y., Du, Y., Zhou, L., Yue, J., Hu, X., Liu, Y., et al. (2022). Gut microbiota interact with the brain through systemic chronic inflammation: implications on neuroinflammation, neurodegeneration, and aging. Front. Immunol. 13, 796288. doi:10.3389/fimmu.2022.796288
Moujalled, D., Strasser, A., and Liddell, J. R. (2021). Molecular mechanisms of cell death in neurological diseases. Cell Death Differ. 28 (7), 2029–2044. doi:10.1038/s41418-021-00814-y
Nakamura, N., Hirakawa, A., Gao, J. J., Kakuda, H., Shiro, M., Komatsu, Y., et al. (2004). Five new maleic and succinic acid derivatives from the mycelium of Antrodia camphorata and their cytotoxic effects on LLC tumor cell line. J. Nat. Prod. 67, 46–48. doi:10.1021/np030293k
Nallathamby, N., Guan-Serm, L., Vidyadaran, S., Abd Malek, S. N., Raman, J., and Sabaratnam, V. (2015). Ergosterol of Cordyceps militaris attenuates LPS induced inflammation in BV2 microglia cells. Nat. Prod. Commun. 10, 1934578X1501000–886. doi:10.1177/1934578x1501000623
Numakawa, T., and Kajihara, R. (2023). Neurotrophins and other growth factors in the pathogenesis of Alzheimer's disease. Life (Basel) 13, 647. doi:10.3390/life13030647
Passeri, E., Elkhoury, K., Morsink, M., Broersen, K., Linder, M., Tamayol, A., et al. (2022). Alzheimer's disease: treatment strategies and their limitations. Int. J. Mol. Sci. 23 (22), 13954. doi:10.3390/ijms232213954
Qiao, X., Wang, Q., Ji, S., Huang, Y., Liu, K. D., Zhang, Z. X., et al. (2015). Metabolites identification and multi-component pharmacokinetics of ergostane and lanostane triterpenoids in the anticancer mushroom Antrodia cinnamomea. J. Pharm. Biomed. Anal. 111, 266–276. doi:10.1016/j.jpba.2015.04.010
Robertson, G. S., Crocker, S. J., Nicholson, D. W., and Schulz, J. B. (2000). Neuroprotection by the inhibition of apoptosis. Brain Pathol. 10, 283–292. doi:10.1111/j.1750-3639.2000.tb00262.x
Sharon, G., Cruz, N. J., Kang, D. W., Gandal, M. J., Wang, B., Kim, Y. M., et al. (2019). Human gut microbiota from autism spectrum disorder promote behavioral symptoms in mice. Cell 177, 1600–1618. doi:10.1016/j.cell.2019.05.004
Shen, Y. C., Chou, C. J., Wang, Y. H., Chen, C. F., Chou, Y. C., and Lu, M. K. (2004). Anti-inflammatory activity of the extracts from mycelia of Antrodia camphorata cultured with water-soluble fractions from five different Cinnamomum species. FEMS Microbiol. Lett. 231, 137–143. doi:10.1016/S0378-1097(03)00953-4
Shie, P. H., Wang, S. Y., Lay, H. L., and Huang, G. J. (2016). 4,7-Dimethoxy-5-methyl-1,3-benzodioxole from Antrodia camphorata inhibits LPS-induced inflammation via suppression of NF-κB and induction HO-1 in RAW264.7 cells. Int. Immunopharmacol. 31, 186–194. doi:10.1016/j.intimp.2015.12.030
Sillapachaiyaporn, C., Chuchawankul, S., Nilkhet, S., Moungkote, N., Sarachana, T., Ung, A. T., et al. (2022a). Ergosterol isolated from cloud ear mushroom (Auricularia polytricha) attenuates bisphenol A-induced BV2 microglial cell inflammation. Food Res. Int. 157, 111433. doi:10.1016/j.foodres.2022.111433
Sillapachaiyaporn, C., Mongkolpobsin, K., Chuchawankul, S., Tencomnao, T., and Baek, S. J. (2022b). Neuroprotective effects of ergosterol against TNF-α-induced HT-22 hippocampal cell injury. Biomed. Pharmacother. 154, 113596. doi:10.1016/j.biopha.2022.113596
Silva, A. M., Preto, M., Grosso, C., Vieira, M., Delerue-Matos, C., Vasconcelos, V., et al. (2023). Tracing the path between mushrooms and Alzheimer's disease-a literature review. Molecules 28, 5614. doi:10.3390/molecules28145614
Simpson, D. S. A., and Oliver, P. L. (2020). ROS generation in microglia: understanding oxidative stress and inflammation in neurodegenerative disease. Antioxidants (Basel) 9 (8), 743. doi:10.3390/antiox9080743
Singh, V., Roth, S., Llovera, G., Sadler, R., Garzetti, D., Stecher, B., et al. (2016). Microbiota dysbiosis controls the neuroinflammatory response after stroke. J. Neurosci. 36, 7428–7440. doi:10.1523/JNEUROSCI.1114-16.2016
So, Y. J., Lee, J. U., Yang, G. S., Yang, G., Kim, S. W., Lee, J. H., et al. (2024). The potentiality of natural products and herbal medicine as novel medications for Parkinson's disease: a promising therapeutic approach. Int. J. Mol. Sci. 25 (2), 1071. doi:10.3390/ijms25021071
Sorboni, S. G., Moghaddam, H. S., Jafarzadeh-Esfehani, R., and Soleimanpour, S. (2022). A comprehensive review on the role of the gut microbiome in human neurological disorders. Clin. Microbiol. Rev. 35, e0033820. doi:10.1128/CMR.00338-20
Su, C. H. (2002). Health guardian angel: Antrodia camphorata. 1st edition. Taipei, Taiwan: EKS Book Publishing.
Su, C. H., Hsieh, Y. C., Chng, J. Y., Lai, M. N., and Ng, L. T. (2023). Metabolomic profiling of different Antrodia cinnamomea phenotypes. J. Fungi (Basel) 9, 97. doi:10.3390/jof9010097
Su, R. C., Leu, J. G., Chen, Y. H., Chen, C. Y., Yang, Y. F., Yen, C. C., et al. (2022). Topical application of Antrodia cinnamomea ointment in diabetic wound healing. Life (Basel) 12 (4), 507. doi:10.3390/life12040507
Sun, P., Li, W., Guo, J., Peng, Q., Ye, X., Hu, S., et al. (2023). Ergosterol isolated from Antrodia camphorata suppresses LPS-induced neuroinflammatory responses in microglia cells and ICR mice. Molecules 28, 2406. doi:10.3390/molecules28052406
Sun, Y., Yang, Y., Liu, S., Yang, S., Chen, C., Lin, M., et al. (2022). New therapeutic approaches to and mechanisms of ginsenoside Rg1 against neurological diseases. Cells 11, 2529. doi:10.3390/cells11162529
Teleanu, D. M., Niculescu, A. G., Lungu, I. I., Radu, C. I., Vladâcenco, O., Roza, E., et al. (2022). An overview of oxidative stress, neuroinflammation, and neurodegenerative diseases. Int. J. Mol. Sci. 23, 5938. doi:10.3390/ijms23115938
Tsai, Y. T., Ruan, J. W., Chang, C. S., Ko, M. L., Chou, H. C., Lin, C. C., et al. (2020). Antrodia cinnamomea confers obesity resistance and restores intestinal barrier integrity in leptin-deficient obese mice. Nutrients 12, 726. doi:10.3390/nu12030726
Tsai, Y. T., Ruan, J. W., Chang, C. S., Ko, M. L., Chou, H. C., Lin, C. C., et al. (2021). Proteomic and microbial assessments on the effect of Antrodia cinnamomea in C57BL/6 mice. Arch. Biochem. Biophys. 713, 109058. doi:10.1016/j.abb.2021.109058
Tsay, H. J., Liu, H. K., Kuo, Y. H., Chiu, C. S., Liang, C. C., Chung, C. W., et al. (2021). EK100 and antrodin C improve brain amyloid pathology in APP/PS1 transgenic mice by promoting microglial and perivascular clearance pathways. Int. J. Mol. Sci. 22, 10413. doi:10.3390/ijms221910413
Tung, Y. T., Tsai, T. C., Kuo, Y. H., Yen, C. C., Sun, J. Y., Chang, W. H., et al. (2014). Comparison of solid-state-cultured and wood-cultured Antrodia camphorata in anti-inflammatory effects using NF-κB/luciferase inducible transgenic mice. Phytomedicine 21, 1708–1716. doi:10.1016/j.phymed.2014.08.011
Turck, D., Bohn, T., Castenmiller, J., De Henauw, S., Hirsch-Ernst, K. I., Maciuk, A., et al. (2022). Safety of freeze-dried mycelia of Antrodia camphorata as a novel food pursuant to regulation (EU) 2015/2283. EFSA J. 20, e07380. doi:10.2903/j.efsa.2022.7380
Wang, C., Zhang, W., Wong, J. H., Ng, T., and Ye, X. (2019a). Diversity of potentially exploitable pharmacological activities of the highly prized edible medicinal fungus Antrodia camphorata. Appl. Microbiol. Biotechnol. 103 (19), 7843–7867. doi:10.1007/s00253-019-10016-9
Wang, L. C., Wang, S. E., Wang, J. J., Tsai, T. Y., Lin, C. H., Pan, T. M., et al. (2012). In vitro and in vivo comparisons of the effects of the fruiting body and mycelium of Antrodia camphorata against amyloid β-protein-induced neurotoxicity and memory impairment. Appl. Microbiol. Biotechnol. 94, 1505–1519. doi:10.1007/s00253-012-3941-3
Wang, Q., Qiao, X., Qian, Y., Li, Z. W., Tzeng, Y. M., Zhou, D. M., et al. (2015). Intestinal absorption of ergostane and lanostane triterpenoids from Antrodia cinnamomea using Caco-2 cell monolayer model. Nat. Prod. Bioprospect 5, 237–246. doi:10.1007/s13659-015-0072-4
Wang, Y. H., Chern, C. M., Liou, K. T., Kuo, Y. H., and Shen, Y. C. (2019b). Ergostatrien-7,9(11),22-trien-3β-ol from Antrodia camphorata ameliorates ischemic stroke brain injury via downregulation of p65NF-κ-B and caspase 3, and activation of Akt/GSK3/catenin-associated neurogenesis. Food Funct. 10, 4725–4738. doi:10.1039/c9fo00908f
Wang, Y. Y., Hsieh, Y. H., Kumar, K. J. S., Hsieh, H. W., Lin, C. C., and Wang, S. Y. (2020). The regulatory effects of a formulation of Cinnamomum osmophloeum Kaneh and Taiwanofungus camphoratus on metabolic syndrome and the gut microbiome. Plants (Basel) 9, 383. doi:10.3390/plants9030383
Wu, Y., Lo, J., Shih, Y., and Liang, H. J. (2018). Method for treating stroke or reducing nerve injury. US20180353520. U.S. Patent.
Xia, Z., Dickens, M., Raingeaud, J., Davis, R. J., and Greenberg, M. E. (1995). Opposing effects of ERK and JNK-p38 MAP kinases on apoptosis. Science 270 (5240), 1326–1331. doi:10.1126/science.270.5240.1326
Yan, Y., Yang, H., Xie, Y., Ding, Y., Kong, D., and Yu, H. (2020). Research progress on Alzheimer's disease and resveratrol. Neurochem. Res. 45, 989–1006. doi:10.1007/s11064-020-03007-0
Yang, J., Wang, L., Liu, H., Xu, H., Liu, F., Song, H., et al. (2023). Dysregulation of Ruminococcaceae and Megamonas could be predictive markers for rapid progression of mild cognitive impairment. Microb. Pathog. 183, 106272. doi:10.1016/j.micpath.2023.106272
Yang, P. S., Lin, P. Y., Chang, C. C., Yu, M. C., Yen, T. L., Lan, C. C., et al. (2015). Antrodia camphorata potentiates neuroprotection against cerebral ischemia in rats via downregulation of iNOS/HO-1/Bax and activated caspase-3 and inhibition of hydroxyl radical formation. Evid. Based Complement. Altern. Med. 2015, 232789. doi:10.1155/2015/232789
Yang, X., Wang, X., Lin, J., Lim, S., Cao, Y., Chen, S., et al. (2022a). Structure and anti-inflammatory activity relationship of ergostanes and lanostanes in Antrodia cinnamomea. Foods 11, 1831. doi:10.3390/foods11131831
Yang, Y., Han, C., Sheng, Y., Wang, J., Li, W., Zhou, X., et al. (2022b). Antrodia camphorata polysaccharide improves inflammatory response in liver injury via the ROS/TLR4/NF-κB signal. J. Cell Mol. Med. 26, 2706–2716. doi:10.1111/jcmm.17283
Ye, J., Zhang, C., Fan, Q., Lin, X., Wang, Y., Azzam, M., et al. (2022). Antrodia cinnamomea polysaccharide improves liver antioxidant, anti-inflammatory capacity, and cecal flora structure of slow-growing broiler breeds challenged with lipopolysaccharide. Front. Vet. Sci. 9, 994782. doi:10.3389/fvets.2022.994782
Yi, Z., Liu, X., Liang, L., Wang, G., Xiong, Z., Zhang, H., et al. (2021). Antrodin A from Antrodia camphorata modulates the gut microbiome and liver metabolome in mice exposed to acute alcohol intake. Food Funct. 12, 2925–2937. doi:10.1039/d0fo03345f
Yi, Z. W., Xia, Y. J., Liu, X. F., Wang, G. Q., Xiong, Z. Q., and Ai, L. Z. (2020). Antrodin A from mycelium of Antrodia camphorata alleviates acute alcoholic liver injury and modulates intestinal flora dysbiosis in mice. J. Ethnopharmacol. 254, 112681. doi:10.1016/j.jep.2020.112681
Zhang, B. B., Guan, Y. Y., Hu, P. F., Chen, L., Xu, G. R., Liu, L., et al. (2019). Production of bioactive metabolites by submerged fermentation of the medicinal mushroom Antrodia cinnamomea: recent advances and future development. Crit. Rev. Biotechnol. 39, 541–554. doi:10.1080/07388551.2019.1577798
Zhang, B. B., Hu, P. F., Huang, J., Hu, Y. D., Chen, L., and Xu, G. R. (2017). Current advances on the structure, bioactivity, synthesis, and metabolic regulation of novel ubiquinone derivatives in the edible and medicinal mushroom Antrodia cinnamomea. J. Agric. Food Chem. 65, 10395–10405. doi:10.1021/acs.jafc.7b04206
Zhang, H. Y., Firempong, C. K., Wang, Y. W., Xu, W. Q., Wang, M. M., Cao, X., et al. (2016). Ergosterol-loaded poly(lactide-co-glycolide) nanoparticles with enhanced in vitro antitumor activity and oral bioavailability. Acta Pharmacol. 37, 834–844. doi:10.1038/aps.2016.37
Zhang, Y., Lv, P., Ma, J., Chen, N., Guo, H., Chen, Y., et al. (2022). Antrodia cinnamomea exerts an anti-hepatoma effect by targeting PI3K/AKT-mediated cell cycle progression in vitro and in vivo. Acta Pharm. Sin. B 12 (2), 890–906. doi:10.1016/j.apsb.2021.07.010
Zhang, Y., Wang, Z., Li, D., Zang, W., Zhu, H., Wu, P., et al. (2018). A polysaccharide from Antrodia cinnamomea mycelia exerts antitumor activity through blocking of TOP1/TDP1-mediated DNA repair pathway. Int. J. Biol. Macromol. 120, 1551–1560. doi:10.1016/j.ijbiomac.2018.09.162
Zhao, Y., Zhang, X., Chen, X., and Wei, Y. (2022). Neuronal injuries in cerebral infarction and ischemic stroke: from mechanisms to treatment (Review). Int. J. Mol. Med. 49, 15. doi:10.3892/ijmm.2021.5070
Zhao, Y. Y., Cheng, X. L., Liu, R., Ho, C. C., Wei, F., Yan, S. H., et al. (2011). Pharmacokinetics of ergosterol in rats using rapid resolution liquid chromatography-atmospheric pressure chemical ionization multi-stage tandem mass spectrometry and rapid resolution liquid chromatography/tandem mass spectrometry. J. Chromatogr. B Anal. Technol. Biomed. Life Sci. 879, 1945–1953. doi:10.1016/j.jchromb.2011.05.025
Zhong, X., Lin, R., Li, Z., Mao, J., and Chen, L. (2014). Effects of Salidroside on cobalt chloride-induced hypoxia damage and mTOR signaling repression in PC12 cells. Biol. Pharm. Bull. 37 (7), 1199–1206. doi:10.1248/bpb.b14-00100
Zou, X. G., Xu, M. T., Dong, X. L., Ying, Y. M., Guan, R. F., Wu, W. C., et al. (2022). Solid-state-cultured mycelium of Antrodia camphorata exerts potential neuroprotective activities against 6-hydroxydopamine-induced toxicity in PC12 cells. J. Food Biochem. 46, e14208. doi:10.1111/jfbc.14208
Glossary
Keywords: Antrodia camphorata, neuroprotective activities, CNS disease, secondary metabolite, gut-microbiome-brain axis
Citation: Li W, Wan P, Qiao J, Liu Y, Peng Q, Zhang Z, Shu X, Xia Y and Sun B (2024) Current and further outlook on the protective potential of Antrodia camphorata against neurological disorders. Front. Pharmacol. 15:1372110. doi: 10.3389/fphar.2024.1372110
Received: 17 January 2024; Accepted: 02 April 2024;
Published: 17 April 2024.
Edited by:
Qi Liang, Air Force Medical University, ChinaReviewed by:
Jyh-Fei Liao, National Yang-Ming University, TaiwanYuhao Xie, St. John’s University, United States
Copyright © 2024 Li, Wan, Qiao, Liu, Peng, Zhang, Shu, Xia and Sun. This is an open-access article distributed under the terms of the Creative Commons Attribution License (CC BY). The use, distribution or reproduction in other forums is permitted, provided the original author(s) and the copyright owner(s) are credited and that the original publication in this journal is cited, in accordance with accepted academic practice. No use, distribution or reproduction is permitted which does not comply with these terms.
*Correspondence: Binlian Sun, binlian17@jhun.edu.cn; Yiyuan Xia, XYY@jhun.edu.cn
†These authors have contributed equally to this work