- 1Hospital of Chengdu University of Traditional Chinese Medicine, Chengdu, China
- 2Hubei University of Chinese Medicine, Wuhan, China
Introduction: The objective of this study is to systematically evaluate the effect of ligustrazine on animal models of ischemic stroke and investigate its mechanism of action.
Materials and Methods: The intervention of ligustrazine in ischemic diseases research on stroke model animals was searched in the Chinese National Knowledge Infrastructure (CNKI), Wanfang Database (Wanfang), VIP Database (VIP), Chinese Biomedical Literature Database (CBM), Cochrane Library, PubMed, Web of Science, and Embase databases. The quality of the included literature was evaluated using the Cochrane risk of bias tool. The evaluation included measures such as neurological deficit score (NDS), percentage of cerebral infarction volume, brain water content, inflammation-related factors, oxidative stress-related indicators, apoptosis indicators (caspase-3), and blood-brain barrier (BBB) permeability (Claudin-5).
Results: A total of 32 studies were included in the analysis. The results indicated that ligustrazine significantly improved the neurological function scores of ischemic stroke animals compared to the control group (SMD = −1.84, 95% CI −2.14 to −1.55, P < 0.00001). It also reduced the percentage of cerebral infarction (SMD = −2.97, 95% CI −3.58 to −2.36, P < 0.00001) and brain water content (SMD = −2.37, 95% CI −3.63 to −1.12, P = 0.0002). In addition, ligustrazine can significantly improve various inflammatory factors such as TNF-α (SMD = −7.53, 95% CI −11.34 to −3.72, P = 0.0001), IL-1β (SMD = −2.65, 95% CI −3.87 to −1.44, P < 0.0001), and IL-6 (SMD = −5.55, 95% CI −9.32 to −1.78, P = 0.004). It also positively affects oxidative stress-related indicators including SOD (SMD = 4.60, 95% CI 2.10 to 7.10, P = 0.0003), NOS (SMD = −1.52, 95% CI −2.98 to −0.06, P = 0.04), MDA (SMD = −5.31, 95% CI −8.48 to −2.14, P = 0.001), and NO (SMD = −5.33, 95% CI −8.82 to −1.84, P = 0.003). Furthermore, it shows positive effects on the apoptosis indicator caspase-3 (SMD = −5.21, 95% CI −7.47 to −2.94, P < 0.00001) and the expression level of the sex-related protein Claudin-5, which influences BBB permeability (SMD = 7.38, 95% CI 3.95 to 10.82, P < 0.0001).
Conclusion: Ligustrazine has been shown to have a protective effect in animal models of cerebral ischemic injury. Its mechanism of action is believed to be associated with the reduction of inflammation and oxidative stress, the inhibition of apoptosis, and the repair of BBB permeability. However, further high-quality animal experiments are required to validate these findings.
1 Background
Stroke is the second leading cause of death and the third leading cause of disability in the world (Lozano et al., 2012; Murray et al., 2012). In 2016, there were 13.7 million new stroke events worldwide, with 87% of them being ischemic strokes. During the same year, 2.7 million people died from stroke. Cerebral ischemic stroke (CIS) imposes a significant health and economic burden on the world, particularly on low- and middle-income countries (Collaborators, 2019; Saini et al., 2021). CIS is typically caused by embolism or thrombotic artery occlusion, resulting in reduced cerebral blood flow and various forms of brain damage, including tissue and structural damage, as well as neuronal death and defects (Xing and Bai, 2020; Zhao et al., 2022). Recombinant tissue plasminogen activator (tPA) is currently the only CIS therapy approved by the U.S. Food and Drug Administration. However, this therapy has a limited time window of 4.5 h and carries the potential risk of hemorrhagic transformation, resulting in only 10% of stroke patients benefiting from it (Bansal et al., 2013; Tao et al., 2020). Recent advancements in the research of stroke neuroprotective drugs have identified promising candidates such as human urinary kininogenase (HUK), statins, edaravone, 3K3A-activating protein C (APC), and vinpocetine. These drugs target multiple pathophysiological pathways involved in stroke-related brain injury, with a primary focus on inflammation and oxidative stress. (Paul and Candelario-Jalil, 2021). While these studies provide optimism for CIS treatment, further research is needed to fully address the complexities of this condition. Moreover, there is an urgent need to enhance the current treatment’s effectiveness in addressing motor dysfunction and neurological damage caused by CIS. Therefore, it is crucial to explore new complementary and alternative therapies to overcome the limitations of existing treatments in stroke management.
Ligustrazine, an alkaloid monomer derived from the dried rhizome of Ligusticum striatum DC [Apiaceae] (Figure 1). Ligusticum striatum DC was first documented in Shennong’s Materia Medica, a compilation of Chinese herbal medicine information dating back to 2800 BC (Li et al., 2021). According to traditional Chinese medicine, CIS is attributed to wind, fire, phlegm, blood stasis, and deficiency, with L. striatum DC to improve clinical symptoms of CIS by enhancing qi and blood circulation, activating blood flow, and resolving blood stasis. Modern scientific studies have shown that L. striatum DC can be effective in stroke treatment, with its mechanism of action linked to the regulation of the TNF/IL-17 signaling pathway (Zhang et al., 2023). Ligustrazine, an active compound in L. striatum DC, plays a key role in these therapeutic effects. Preclinical studies have demonstrated that ligustrazine has the potential to mitigate CIS injury through various mechanisms, including alleviating inflammatory response, resisting apoptosis, protecting the BBB, combating oxidative stress, inhibiting calcium overload and glutamate excitotoxicity, and enhancing synaptic plasticity (Figure 2). Specifically, ligustrazine has been shown to regulate inflammation-related factors by inhibiting the immunoreactivity of ED-1, DNA fragmentation, caspase-3 activation, and Cyt c release. Additionally, it inhibits the activation of the JAK/STAT signaling pathway and matrix metalloproteinase-9 (MMP-9) expression to reduce BBB permeability. Ligustrazine also reduces the concentration of ROS and malondialdehyde (MDA) while upregulating the activity of superoxide to relieve oxidative stress. Furthermore, it intervenes in the course of ischemia-reperfusion by reducing Ca2+ overload and trough amino acid excitotoxicity, as well as improving synaptic ultrastructure to enhance synaptic plasticity (Liu et al., 2022).
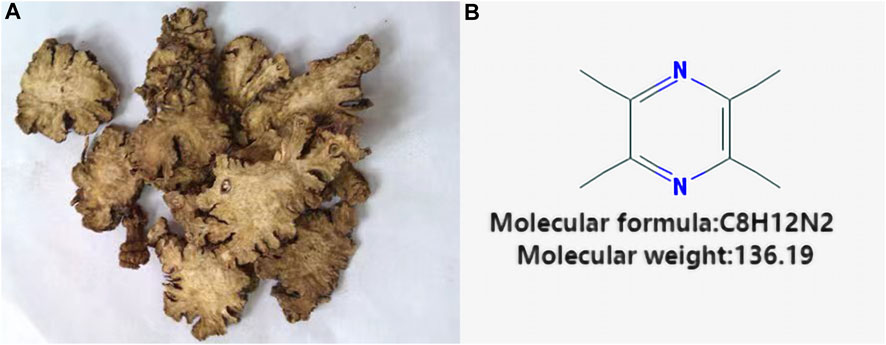
FIGURE 1. Vegetal products of Ligusticum striatum DC and chemical structure of Ligustrazine. (A) Vegetal products of Ligusticum striatum DC. (B) Chemical structure of Ligustrazine.
While numerous studies have delved into the neuroprotective effects and potential mechanisms of ligustrazine in CIS models, most have primarily focused on specific pathways or a limited set of efficacy indicators. A comprehensive and quantitative analysis of the various mechanisms of action of ligustrazine in CIS has yet to be reported. Although some studies have conducted systematic reviews and meta-analyses of clinical trials on ligustrazine for treating CIS (Shao et al., 2021), it remains essential to quantify and evaluate its mechanism of action through preclinical meta-analysis. This study aims to systematically evaluate and quantify the intervention effect of ligustrazine on CIS across multiple mechanisms and efficacy indicators. By synthesizing existing data, the study seeks to uncover the therapeutic potential and multifaceted action mechanism of ligustrazine in CIS, offering insights for future clinical research.
2 Materials and methods
2.1 Search strategy
Two researchers conducted independent searches on several databases including CNKI, Wanfang, VIP, CBM, Cochrane Library, PubMed, Web of Science, and Embase. The studies focused on the intervention of ligustrazine in animal models of CIS. The search period ranged from the establishment of the databases to January 2024. Additionally, the researchers traced the references of included articles and visited the International Clinical Trials Registry of the National Institutes of Health and the Chinese Clinical Trials Registry to supplement the acquisition of relevant literature. Any disagreements were resolved by involving a third researcher. The search terms used were: “brain infarction” or “brain ischemia” or “cerebral ischemia reperfusion injury” or “cerebral thrombosis” or “cerebral embolism” or “ischemic stroke” or “middle cerebral artery occlusion” or “MCAO” and “tetramethylpyrazine” or “ligustrazine".
2.2 Inclusion criteria and outcome indicators
2.2.1 Outcome indicators
1) Primary outcome measures: Neurological deficit score (NDS).
2) Secondary outcome measures:
a. Percentage of infarct volume
b. Brain water content
c. Inflammation related factors, including tumor necrosis factor-α (TNF-α), interleukin -1β (IL-1β) and interleukin −6 (IL-6).
d. Oxidative stress-related indicators, including Superoxide Dismutase (SOD), Nitric Oxide Synthase (NOS), MDA and Nitric Oxide (NO).
e. Apoptosis marker caspase-3.
f. BBB -related protein Claudin-5.
2.2.2 Inclusion criteria
1)Study Type: This study focuses on animal experiments conducted on rats or mice using permanent CIS or ischemic stroke/reperfusion models. 2) Interventional measures: experimental group was treated with ligustrazine without restriction, control group was treated with placebo only, placebo included saline, PBS, etc., 3) Language: There are no language restrictions for this study.
2.2.3 Exclusion criteria
1) Animal experiments in permanent CIS or ischemic stroke/reperfusion models other than rats or mice. 2) Drug combination therapy. 3) Review, conference, case report, dissertation, clinical trial research. 4) Duplicated studies are excluded according to time. 5) Failure to reach the result index or to extract effective indicators. 6) Clinical studies or in vitro experiments.
2.3 Literature screening and data extraction
Two reviewers independently screened the literature, extracted data, and cross-checked. In the event of disagreements, a third party was consulted for assistance in making a judgment. If any data were missing, the authors were contacted to provide additional information. During the literature screening process, the title and abstract were initially read, and after excluding obviously irrelevant literature, the full text was further examined to determine its inclusion. The data extraction process mainly involved capturing the basic characteristics of the study, such as the study name, publication date, animal species, animal weight, and animal model. It also included specific details such as intervention measures, control measures, intervention time, drug dosage, and other relevant information used in the study. Key elements for evaluating the risk of bias were also considered, along with the outcome indicators involved in the study. For studies reporting data only in the form of images, we used the GetData Graph Digitizer software to extract the data from the images.
2.4 Quality evaluation of the included studies
In this study, two researchers conducted a systematic review of the included studies using the Cochrane risk of bias assessment tool (Cumpston et al., 2019). The evaluation focused on several key aspects, including selection bias (whether the random sequence generation was adequately described and whether allocation concealment was implemented), implementation bias (whether the subjects and experimenters were blinded), measurement bias (whether the outcome assessors were blinded), follow-up bias (whether the outcome data was complete), reporting bias, and other potential biases.
2.5 Statistical methods
Statistical analysis of the data was conducted using Review Manager 5.4 software, and sensitivity analysis was performed using Stata16. This study utilizes relative risk (RR) and 95% confidence interval (CI) as indicators for dichotomous variables, while Standardized mean difference (SMD) and 95% CI are used for continuous variables. Heterogeneity between studies was analyzed using the χ2 test. If I2 ≤ 50%, it indicates small heterogeneity between studies, and the fixed effects model is applied. On the other hand, if I2 ≥ 50%, it suggests large heterogeneity between studies, and a random effects model is utilized. Subgroup analysis or sensitivity analysis is employed when necessary. The presence of publication bias was assessed by analyzing outcome indicators from studies with a minimum of 10. A statistically significant difference is considered when p < 0.05.
2.6 Publication bias
Publication bias was assessed using a funnel plot and calculated with STATA 16 using Begg’s test.
3 Results
3.1 Literature screening process and results
A total of 3,398 relevant documents were retrieved. After applying the inclusion and exclusion criteria, 32 studies (Liao et al., 2004; Kao et al., 2006; Chang et al., 2007; Li et al., 2008; Qi et al., 2008; Ren et al., 2008; Zhang et al., 2008; Jia et al., 2009; Zhu et al., 2009; Hu et al., 2010a; Hu et al., 2010b; Chen et al., 2010; Fang et al., 2010; Ma and Chen, 2010; Xiao et al., 2010; Yang and Ren, 2011; Zhang et al., 2011; Kao et al., 2013; Li et al., 2013; Han et al., 2014; Tian et al., 2014; Tan et al., 2015; Liu, 2016; Li et al., 2017; Gong et al., 2019; Zhu et al., 2020; Ge et al., 2021; Li et al., 2022; Liang et al., 2022; Chang et al., 2023; Feng et al., 2023; Gao et al., 2023) were included. The detailed literature search and screening process is illustrated in Figure 3.
3.2 Basic characteristics of included literature
A total of 32 studies were included in this study. Among these, three studies used Wistar rats (Chang et al., 2007; Li et al., 2008; Zhang et al., 2011) weighing 250–320 g, two studies used Kunming mice (Hu et al., 2010a; Hu et al., 2010b) weighing 30–40 g, and one study used C57BL/6 mice (Li et al., 2022), but the weight of the mice was not reported. The remaining 26 studies utilized SD rats weighing 220–350 g. Five studies employed the MCAO model, while 27 studies used the MCAO/reperfusion model. Intraperitoneal injection was used in 29 studies, tail vein injection in two studies (Ma and Chen, 2010; Zhu et al., 2020), and oral gavage in one study (Qi et al., 2008). The basic characteristics of the 32 included documents are presented in Table 1, and information on the preparations used in the study can be found in supplementary documents.
3.3 Risk of bias
A total of 12 studies did not provide a description of random random-sequence generation, indicating a high risk of selection bias. None of the studies mentioned allocation concealment, resulting in unclear judgments for both performance bias and detection bias. Since the animal’s baseline characteristics and data have been fully reported, both attrition bias and reporting bias are considered to have low risks. The authors of four studies (Hu et al., 2010a; Hu et al., 2010b; Ma and Chen, 2010; Li et al., 2013) had published similar papers during the same period, indicating a high risk. However, due to multiple factors influencing the risk of bias, the remaining 28 studies were judged to have an unclear risk. The specific bias situation is illustrated in Figure 4.
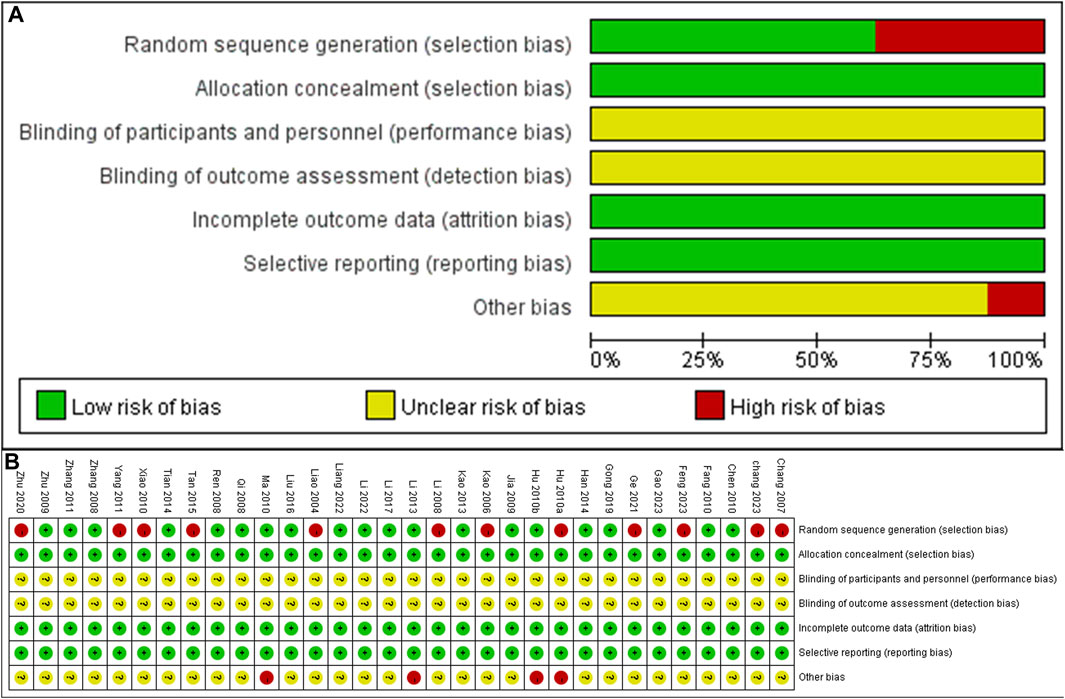
FIGURE 4. Risk of bias summary and Risk of bias graph (A) Risk of bias graph (B) Risk of bias summary.
3.4 Analysis results
3.4.1 NDS
A total of 18 studies (Li et al., 2008; Qi et al., 2008; Ren et al., 2008; Jia et al., 2009; Zhu et al., 2009; Fang et al., 2010; Yang and Ren, 2011; Zhang et al., 2011; Kao et al., 2013; Han et al., 2014; Tian et al., 2014; Tan et al., 2015; Liu, 2016; Li et al., 2017; Gong et al., 2019; Li et al., 2022; Liang et al., 2022; Gao et al., 2023) reported NDS. The heterogeneity test showed a significance of p = 0.02, with an I2 value of 44%. Therefore, a fixed effects model was adopted. The results indicated that ligustrazine could reduce the NDS after CIS (SMD = −1.84, 95% CI -2.14 to −1.55, p < 0.00001, Figure 5A). To enhance the reliability of the results, a subgroup analysis was conducted based on the animal modeling method and animal species, which demonstrated the robustness of the findings (Figures 5B, C).
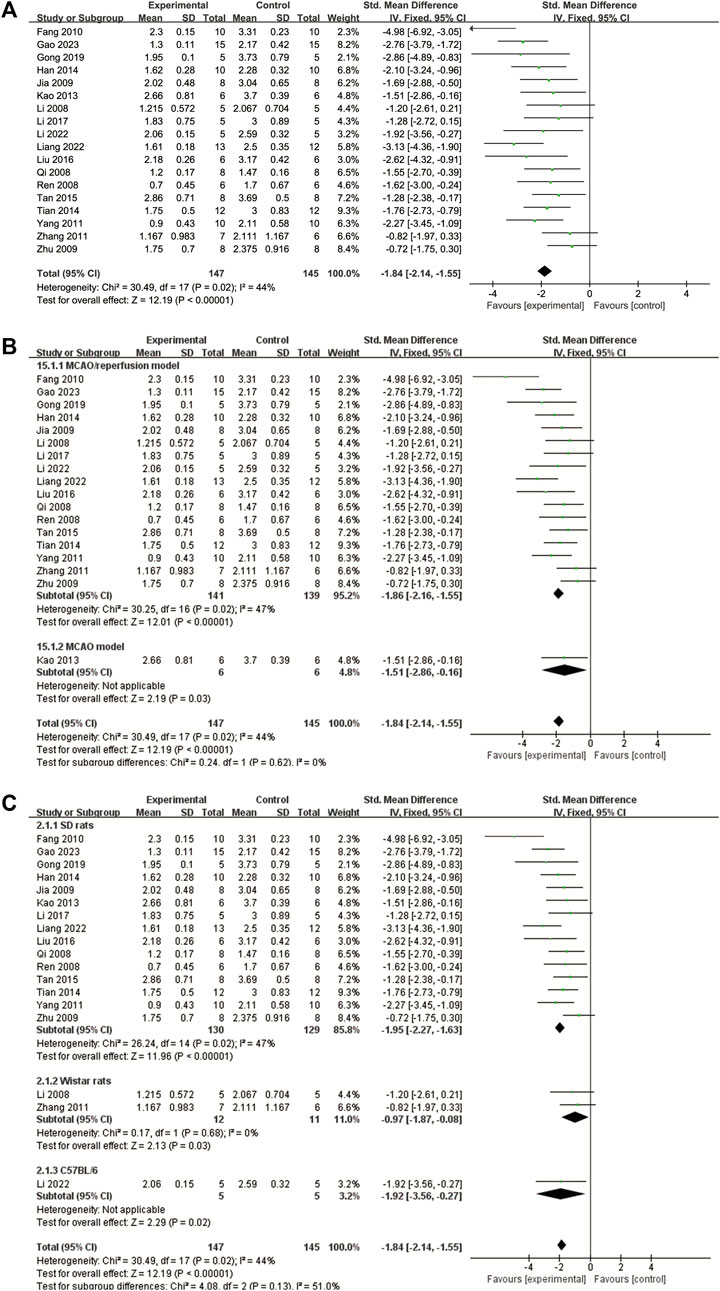
FIGURE 5. The forest plot of NDS. (A) The forest plot of NDS. (B) The forest plot of subgroup analysis based on the animal modeling method. (C) The forest plot of subgroup analysis based on the animal species.
3.4.2 Percentage of infarct volume
A total of nine studies (Kao et al., 2006; Fang et al., 2010; Kao et al., 2013; Tan et al., 2015; Liu, 2016; Li et al., 2017; Gong et al., 2019; Li et al., 2022; Chang et al., 2023) reported the percentage of infarct volume. The heterogeneity test showed p = 0.09 and I2 = 41%. Therefore, a fixed effects model was adopted. The results demonstrated that ligustrazine significantly reduced the percentage of cerebral infarct volume in CIS (SMD = −2.97, 95% CI -3.58 to −2.36, p < 0.00001, Figure 6A). To enhance the reliability of the findings, subgroup analysis was conducted based on the animal modeling method and animal species, which confirmed the robustness of the results (Figures 6B, C).
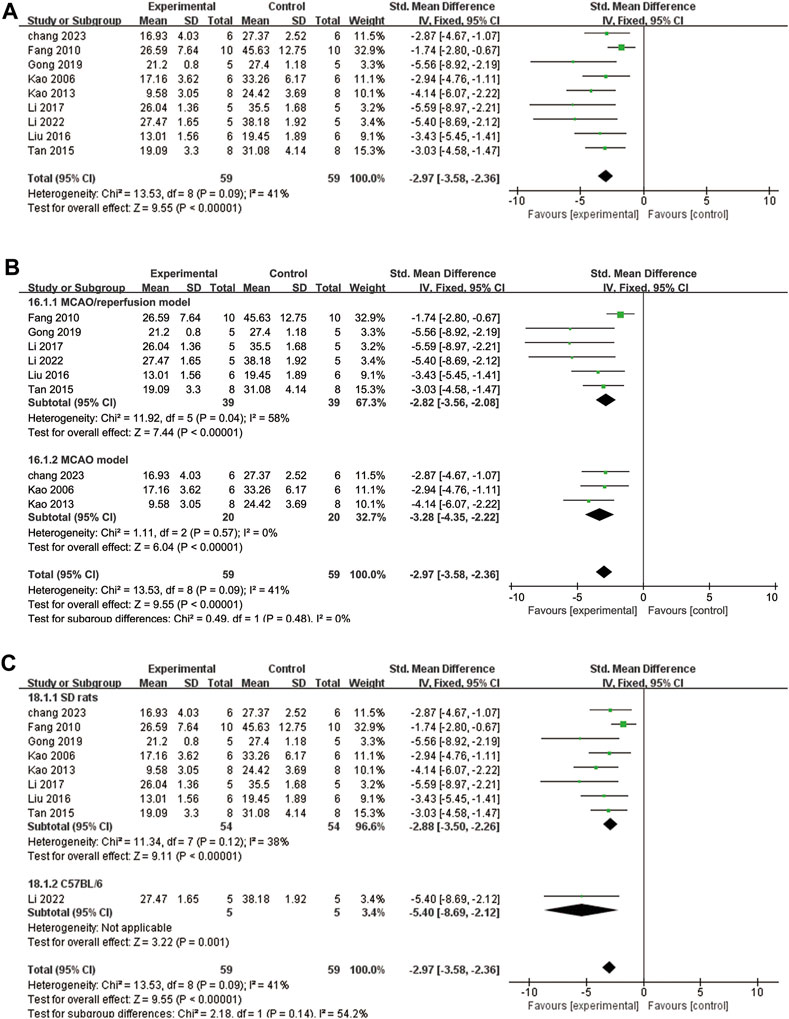
FIGURE 6. The forest plot of percentage of infarct volume. (A) The forest plot of percentage of infarct volume. (B) The forest plot of subgroup analysis based on the animal modeling method. (C) The forest plot of subgroup analysis based on the animal species.
3.4.3 Brain water content
A total of six studies (Liao et al., 2004; Xiao et al., 2010; Tan et al., 2015; Li et al., 2017; Gong et al., 2019; Zhu et al., 2020) examined brain water content. To account for high heterogeneity (p = 0.003, I2 = 73%), a random effects model was applied. The findings indicate that ligustrazine can reduce brain water content in patients with CIS (SMD = −2.37, 95% CI -3.63 to −1.12, p = 0.0002, Figure 7A). To enhance the reliability of the results and identify the source of heterogeneity, we performed a subgroup analysis based on the animal modeling method. The results demonstrated the robustness of the findings (Figure 7B). However, the animal modeling method did not contribute to the observed heterogeneity according to the subgroup analysis (Figure 7B).
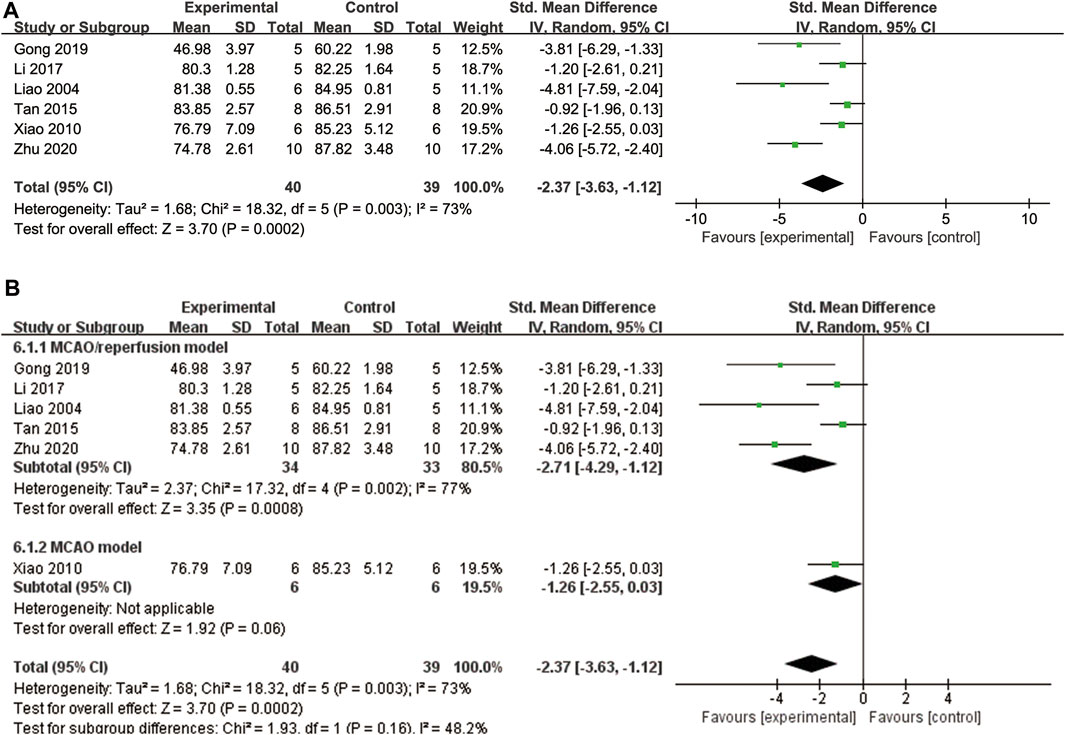
FIGURE 7. The forest plot of percentage of brain water content. (A) The forest plot of percentage of brain water content. (B) The forest plot of subgroup analysis based on the animal modeling method.
3.4.4 Inflammation related factors
A total of four studies (Chang et al., 2007; Li et al., 2017; Zhu et al., 2020; Ge et al., 2021) reported TNF-α levels. Due to high heterogeneity (p = 0.002, I2 = 79%), a random effects model was used. The findings indicated that ligustrazine was effective in reducing TNF-α indicators in animals with CIS (SMD = −7.53, 95% CI -11.34 to −3.72, p = 0.0001, Figure 8A).
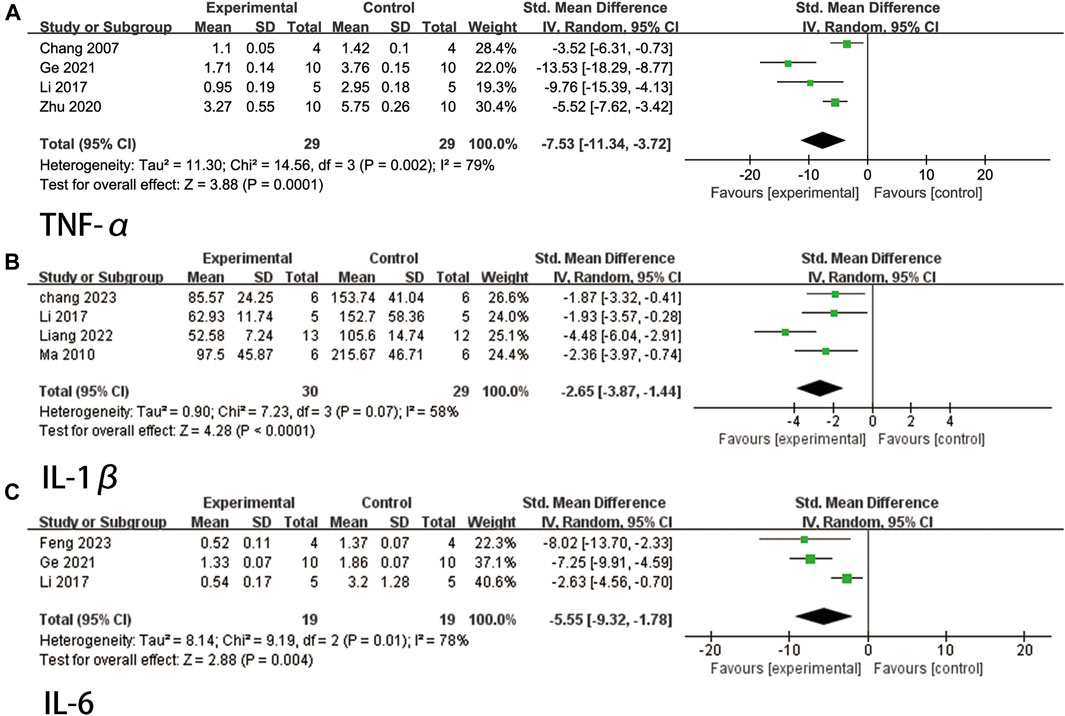
FIGURE 8. The forest plot of percentage of Inflammation related factors. (A) The forest plot of percentage of TNF-α. (B) The forest plot of percentage of IL-1β. (C) The forest plot of percentage of IL-6.
A total of four studies (Ma and Chen, 2010; Li et al., 2017; Liang et al., 2022; Chang et al., 2023) reported IL-1β levels. The heterogeneity test indicated a p-value of 0.07 and an I2 value of 58%. Therefore, a random effects model was employed. The findings demonstrated that ligustrazine had a significant impact in reducing IL-1β indicators in animals with CIS (SMD = −2.65, 95% CI -3.87 to −1.44, p < 0.0001, Figure 8B).
A total of three studies (Li et al., 2017; Ge et al., 2021; Feng et al., 2023) reported IL-6 levels. Due to high heterogeneity (p = 0.01, I2 = 78%), a random effects model was used. The findings indicated that ligustrazine could effectively reduce IL-6 indicators in animals with CIS (SMD = −5.55, 95% CI -9.32 to −1.78, p = 0.004, Figure 8C).
3.4.5 Oxidative stress-related indicators
Five studies (Zhang et al., 2008; Chen et al., 2010; Zhang et al., 2011; Liu, 2016; Ge et al., 2021) reported the measurement of SOD, and due to significant heterogeneity (p < 0.00001, I2 = 87%), a random effects model was employed. The findings demonstrated that ligustrazine had the ability to decrease SOD levels in animals with CIS (SMD = 4.60, 95% CI 2.10 to 7.10, p = 0.0003, Figure 9A).
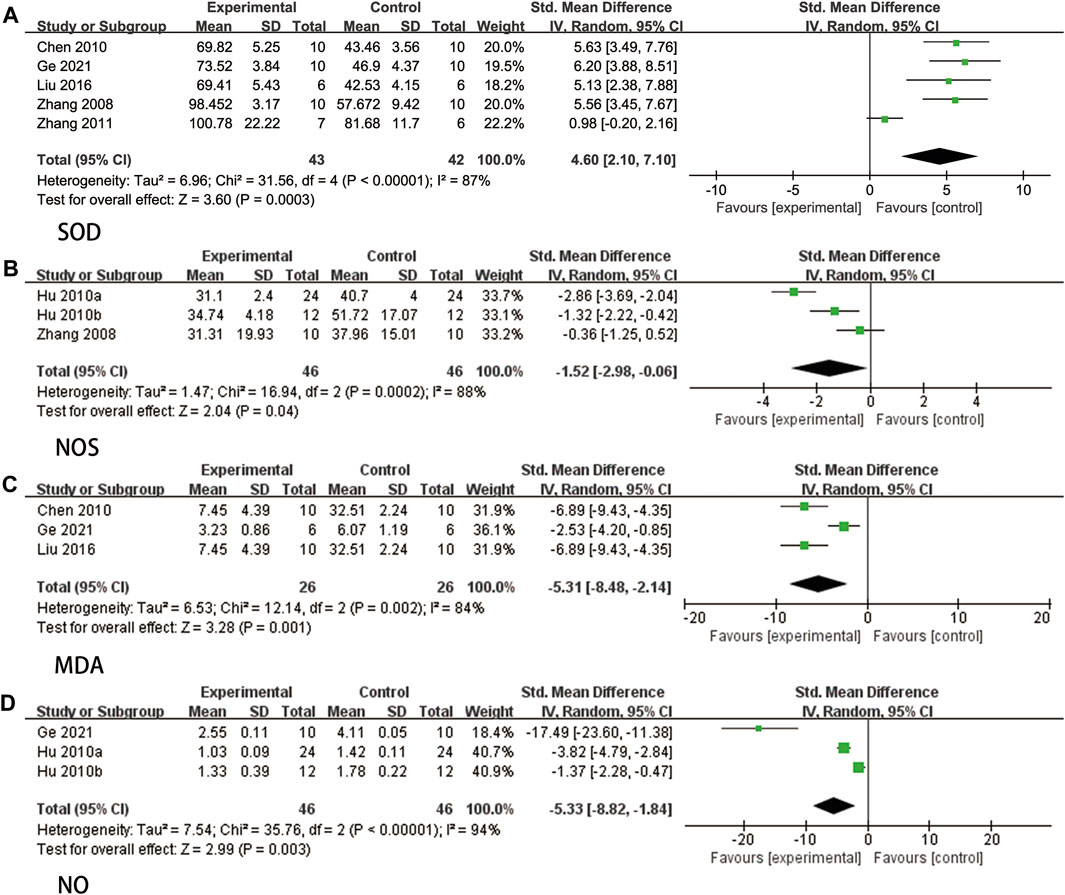
FIGURE 9. The forest plot of percentage of Oxidative stress-related indicators. (A) The forest plot of percentage of SOD. (B) The forest plot of percentage of NOS. (C) The forest plot of percentage of MDA. (D) The forest plot of percentage of NO.
Three studies (Zhang et al., 2008; Hu et al., 2010a; Hu et al., 2010b) reported NOS. Due to high heterogeneity (p = 0.0002, I2 = 88%), a random effects model was used. The results indicated that ligustrazine could reduce NOS indicators in animals with CIS (SMD = −1.52, 95% CI -2.98 to −0.06, p = 0.04, Figure 9B).
Three studies (Chen et al., 2010; Liu, 2016; Ge et al., 2021) reported MDA levels, and due to significant heterogeneity (p = 0.002, I2 = 84%), a random effects model was employed. The findings indicated that ligustrazine demonstrated a reduction in MDA indicators among animals with CIS (SMD = −5.31, 95% CI -8.48 to −2.14, p = 0.001, Figure 9C).
Three studies (Hu et al., 2010a; Hu et al., 2010b; Ge et al., 2021) reported NO, and due to high heterogeneity (p < 0.00001, I2 = 94%), a random effects model was employed. The findings indicated that ligustrazine had the potential to decrease NO indicators in animals with CIS (SMD = −5.33, 95% CI -8.82 to −1.84, p = 0.003, Figure 9D).
3.4.6 Caspase-3
Two studies (Chang et al., 2007; Li et al., 2022) reported caspase-3 levels, and the heterogeneity test showed no significant variation (p = 0.67, I2 = 0%). Therefore, a fixed effects model was used. The results indicated that ligustrazine had a significant effect in reducing caspase-3 levels in animals with CIS (SMD = −5.21, 95% CI -7.47 to −2.94, p < 0.00001, Figure 10).
3.4.7 Claudin-5
Three studies (Li et al., 2013; Tan et al., 2015; Gong et al., 2019) examined Claudin-5, and after conducting a heterogeneity test (p = 0.007, I2 = 80%), a random effects model was applied. The findings revealed that ligustrazine had the potential to decrease the Claudin-5 index in animals with CIS (SMD = 7.38, 95% CI 3.95 to 10.82, p < 0.0001, Figure 11).
3.5 Sensitivity analysis
3.5.1 Brain water content
Due to the significant heterogeneity observed in this study, a sensitivity analysis was conducted. After excluding the study conducted by Zhu 2020 (Zhu et al., 2020), the heterogeneity decreased to 61%. These findings continue to support the notion that ligustrazine can effectively reduce the water content of brain tissue in animals with CIS. Further literature analysis revealed that only Zhu 2020 administered the treatment via tail vein injection, whereas the remaining studies utilized intraperitoneal injection. This observed heterogeneity is likely attributed to the differences in the method of administration.
3.5.2 Inflammation related factors
TNF-α exhibits significant heterogeneity, prompting a sensitive analysis. Upon excluding Ge 2021 (Ge et al., 2021), the heterogeneity decreased to 50%. A comprehensive literature analysis identified a total of four studies contributing to this indicator. Notably, Ge 2021 utilized a dosage of 50 mg/kg, while the remaining three studies employed dosages of 20, 20, and 10 mg/kg respectively. Hence, it is speculated that the heterogeneity is attributable to the varying dosages of Ge 2021, resulting in divergent medication effects. As for IL-6, which also displays substantial heterogeneity, a heterogeneity analysis was conducted. After excluding Li 2017 (Li et al., 2017), the heterogeneity decreased to 0%. Literature analysis suggests that this heterogeneity may arise from differences in administration time and two other studies.
3.5.3 Oxidative stress-related indicators
SOD exhibited high heterogeneity, thus a sensitivity analysis was conducted. After excluding Zhang 2011 (Zhang et al., 2011), the heterogeneity decreased to 0%. Literature analysis revealed that this study employed Wistar rats, while the other four studies utilized SD rats. Hence, the heterogeneity of SOD indicators may be attributed to the different rat species.
NOS also demonstrated high heterogeneity, thus a sensitivity analysis was conducted. After excluding Hu 2020a (Hu et al., 2010a), the heterogeneity decreased to 55%. Since only three studies were included, it is speculated that the heterogeneity is linked to the limited number of experimental animals. However, the research results still support the notion that ligustrazine can regulate oxidative stress through NOS indicators, thereby protecting animals with CIS.
MDA displayed high heterogeneity, prompting a sensitivity analysis, which resulted in a heterogeneity decrease to 0% after excluding Ge 2021 (Ge et al., 2021). Literature analysis suggested that the heterogeneity may be due to varying dosages.
The heterogeneity of NO is also high, thus a sensitivity analysis was performed. Despite excluding studies one by one, the heterogeneity remained high. It is speculated that this is due to the small number of experimental animals. Nevertheless, the research results still support the idea that ligustrazine can regulate oxidative stress through NO indicators, effectively protecting animals with CIS.
3.5.4 Claudin-5
Claudin-5 exhibits high heterogeneity, thus necessitating a sensitivity analysis. Upon excluding the study conducted by Li 2013 (Li et al., 2013), the heterogeneity decreased to 0%. Further examination of the literature revealed that this reduction could be attributed to the limited sample size of experimental animals. Nevertheless, the research findings continue to support the notion that Ligustrazine can safeguard animals with CIS by modulating oxidative stress through Claudin-5 indicators.
3.6 Publication bias
Given that the NDS had more than 10 articles among the outcome indicators, publication bias was evaluated. The findings indicated that there was no significant publication bias in the NDS (z = −1.63, p = 0.103, Figure 12); however, it is important to exercise caution when interpreting these results.
4 Discussion
CIS is a clinical syndrome caused by cerebral vascular disease, resulting in a disruption of cerebral blood supply, tissue necrosis due to brain tissue hypoxia and glucose deficiency, and neurological impairment (Chen et al., 2021). Current guidelines recommend thrombolysis and endovascular therapy (Kapil et al., 2017). However, thrombolytic treatment can result in rapid reoxygenation during reperfusion, leading to the generation of reactive oxygen species and subsequent oxidative stress reaction and inflammatory storm, which can be detrimental to the brain. Moreover, due to strict time constraints and various contraindications, many patients are unable to benefit from this treatment. As a result, complementary and alternative therapies are gaining increasing attention from clinical practitioners. Ligustrazine, a new calcium channel antagonist, has shown promise in the treatment of ischemic cerebrovascular disease and coronary atherosclerotic disease (Zhao et al., 2016).
Over the past 2 decades, there has been a growing body of evidence demonstrating the positive effects of ligustrazine on CIS in both in vivo and in vitro studies (Zhu et al., 2021). The mechanism of action of ligustrazine involves several beneficial processes, such as activating free radical scavenging (Yang et al., 2017; Zhang et al., 2018a), reducing BBB destruction (Tan et al., 2015; Gong et al., 2019), inhibiting inflammation (Zhou et al., 2019; Chang et al., 2023), maintaining mitochondrial function (Zhang et al., 2018b), preventing apoptosis (Chang et al., 2007), and promoting oligodendrogenesis and gliogenesis (Zhang et al., 2018a). To date, no studies have conducted a systematic review and meta-analysis on the mechanism of action of ligustrazine. In this study, we aimed to provide a comprehensive evaluation of various studies on the treatment of CIS using ligustrazine. Ultimately, we included 32 studies to elucidate the effectiveness and mechanism of action of this treatment. Studies have demonstrated that following an ischemic stroke, there is a likelihood of significant brain edema and the occurrence of large-area infarcts. Additionally, neurons may undergo extensive necrosis and apoptosis, leading to severe damage to the cell structure (Jurcau and Simion, 2021; Fu et al., 2023). This study conducted a statistical analysis of the included studies and found that ligustrazine exhibits a significant improvement in cerebral edema, reduction in cerebral infarction area, and amelioration of neurological damage. Furthermore, the statistical analysis of outcome indicators in the included studies revealed that ligustrazine can effectively enhance inflammatory factor-related indicators (TNF-α, IL-1β, and IL-6), oxidative stress-related indicators (SOD, NOS, MDA, and NO), apoptosis indicator caspase-3, and BBB permeability-related protein Claudin-5.
Inflammation plays a crucial role in the pathophysiology of ischemic stroke. Specifically, inflammatory cytokines IL -1, IL -6, and TNF have been identified as key regulators of the immune response following ischemic stroke (Lambertsen et al., 2019; Clausen et al., 2020). In the hyperacute window after ischemia, resident microglia are recruited from the site of cellular injury due to the action of various cytokines such as TNF-α, IL-6, and IL-1β. This localized inflammation then triggers systemic inflammation, resulting in the breakdown of the BBB, brain edema, and neuronal death (Jin et al., 2010; Naik et al., 2023). The findings of this study demonstrate that ligustrazine can effectively modulate TNF - α, IL-6, and IL-1β levels in animals with ischemic stroke, thereby improving the damage caused by the stroke.
During ischemia reperfusion, the level of NO initially increased, then decreased, and then increased again. In pathological conditions, there was a significant increase in inducible NOS (INOS), which led to excessive production of NO. This excessive NO production caused damage to nerve tissue, resulting in a neurotoxic effect (Fan et al., 2022) Cerebral ischemia reperfusion injury leads to the production of a large amount of oxygen free radicals, which in turn cause peroxidation reactions and changes in the basic characteristics of cell membranes. This process results in the production of MDA in large quantities. SOD, as a main enzyme responsible for scavenging oxygen free radicals, plays a crucial role in protecting cells against oxidative damage (Korenić et al., 2014; Deng et al., 2022). In this study, mice in the ligustrazine intervention group showed a significant decrease in oxidative stress index levels of NO, NOS, and MDA, as well as a significant increase in the antioxidant index SOD level. These findings suggest that ligustrazine effectively regulates the oxidative balance in brain tissue injury, thereby reducing damage caused by oxygen free radicals and protecting nerve cells.
Apoptosis, a process of programmed cell death, is activated during cerebral ischemia (Love, 2003). Caspase-3, a member of the cysteine protease family, plays a crucial role in mediating apoptosis (Uzdensky, 2019). The destruction of the BBB is a significant pathophysiological process in acute ischemic stroke, which can result in destructive malignant brain edema and hemorrhagic transformation (Qiu et al., 2021). Previous research has demonstrated that ligustrazine has the ability to penetrate the BBB and distribute across various brain regions (Tsai and Liang, 2001). However, its biological half-life when taken orally is limited to 0.5–2 h. Utilizing ligustrazine - loaded liposomes (Xia et al., 2016), transdermal administration drugs (Qi et al., 2002), intranasal administration (Feng et al., 2009), and intraocular administration (Mao et al., 2019) can enhance the efficiency of ligustrazine in crossing the BBB and reaching the brain. Moreover, ligustrazine exhibits protective effects against CIS damage by reducing BBB permeability (Tan et al., 2015; Jin et al., 2021), possibly through the inhibition of the JAK/STAT signaling pathway (Gong et al., 2019) and the reduction of MMP-9 levels (Jin et al., 2021). Claudin-5, a specific protein found in the tight junctions of brain microvascular endothelial cells, has been identified as an inducer of BBB formation (Ueno, 2007). The analysis conducted in this study reveals that ligustrazine can reduce the expression of Caspase-3 and increase Claudin-5 levels. These findings suggest that ligustrazine may protect against cerebral ischemic injury by reducing apoptosis and repairing the BBB.
We conducted a meta-analysis of animal studies investigating the effects of ligustrazine intervention in CIS for the first time. We summarized and classified the indicators included in the study, finding that the mechanism of ligustrazine is closely related to inflammatory response, oxidative stress, apoptosis, and BBB permeability. This provides valuable insights for future studies in this area. However, there are several limitations in our study that should be acknowledged. Firstly, most of the included articles did not mention blinding of participants and results, which increases the risk of bias. Secondly, the secondary outcome indicators in our study showed heterogeneity. Although we identified the source of heterogeneity through sensitivity analysis, the limited data available may have led to inaccurate results. Thirdly, some indicators had small sample sizes, which increases the risk of bias. Fourthly, the safety of ligustrazine could not be evaluated in our study as most included studies did not record adverse reactions in animal models. Lastly, the extraction of outcome measures from images using GetData Graph Digitizer software may introduce inaccuracies. These limitations should be considered when interpreting the findings of our study.
A meta-analysis of clinical trials examining the combination of ligustrazine injection with western medicine for acute cerebral infarction showed better efficacy when compared to using western medicine alone (Shao et al., 2021). These findings align with the results of our study. While ligustrazine may cause damage to the gastrointestinal, peripheral nervous, or central nervous systems, most adverse effects are self-limiting and the compound is generally considered safe (Lin et al., 2022). However, due to the limited depth of research on the toxicology and side effects of ligustrazine, it is crucial to strictly adhere to the recommended indications and consider the patient’s drug allergy history. Researchers should prioritize investigating the toxicology and side effects of ligustrazine in patients with CIS to enhance its therapeutic potential for human use.
5 Conclusion
Our study suggests that ligustrazine has a protective effect on animal models of CIS. The mechanism behind this effect may be attributed to the reduction of inflammation and oxidative stress, inhibition of apoptosis, and repair of BBB permeability. However, discrepancies between animal models of CIS and the physiological and pathological processes in humans pose challenges in translating preclinical findings to clinical applications. Future preclinical investigations on ligustrazine for CIS treatment should adhere rigorously to predefined protocols to mitigate bias in subsequent studies.
Data availability statement
The original contributions presented in the study are included in the article/Supplementary material, further inquiries can be directed to the corresponding author.
Author contributions
ZmW: Writing–review and editing, Writing–original draft, Visualization, Validation, Supervision, Software, Resources, Project administration, Methodology, Investigation, Funding acquisition, Formal Analysis, Data curation, Conceptualization. ZhW: Conceptualization, Data curation, Formal Analysis, Funding acquisition, Investigation, Methodology, Project administration, Resources, Software, Supervision, Validation, Visualization, Writing–original draft, Writing–review and editing. YM: Writing–review and editing, Writing–original draft. AH: Writing–review and editing, Writing–original draft. HC: Writing–review and editing, Writing–original draft. SZ: Writing–original draft, Writing–review and editing. ML: Writing–review and editing, Writing–original draft. SG: Writing–review and editing, Writing–original draft. YiL: Writing–review and editing, Writing–original draft. YuL: Writing–review and editing, Writing–original draft, Visualization, Validation, Supervision, Software, Resources, Project administration, Methodology, Investigation, Funding acquisition, Formal Analysis, Data curation, Conceptualization.
Funding
The author(s) declare that no financial support was received for the research, authorship, and/or publication of this article.
Conflict of interest
The authors declare that the research was conducted in the absence of any commercial or financial relationships that could be construed as a potential conflict of interest.
Publisher’s note
All claims expressed in this article are solely those of the authors and do not necessarily represent those of their affiliated organizations, or those of the publisher, the editors and the reviewers. Any product that may be evaluated in this article, or claim that may be made by its manufacturer, is not guaranteed or endorsed by the publisher.
Abbreviations
APC, 3K3A-activating protein C; BBB, blood-brain barrier; CBM, Chinese Biomedical Literature Database; CI, confidence interval; CIS, cerebral ischemic stroke; CNKI, China National Knowledge Infrastructure; HPLC, high-performance liquid chromatography; HUK, human urinary kininogenase; IL, interleukin; INOS, inducible NOS; MCAO, middle cerebral artery occlusion; MDA, malondialdehyde; MMP-9, matrix metalloproteinase-9; NDS, neurological deficit score; NO, nitric oxide; NOS, nitric oxide synthase; RR, relative risk; SMD, standardized mean difference; SOD, superoxide dismutase; TNF, tumor necrosis facto; tPA, tissue plasminogen activator; VIP, VIP Database.
References
Bansal, S., Sangha, K. S., and Khatri, P. (2013). Drug treatment of acute ischemic stroke. Am. J. Cardiovasc. Drugs. 13 (1), 57–69. doi:10.1007/s40256-013-0007-6
Chang, C. Y., Wu, C. C., Pan, P. H., Wang, Y. Y., Lin, S. Y., Liao, S. L., et al. (2023). Tetramethylpyrazine alleviates mitochondrial abnormality in models of cerebral ischemia and oxygen/glucose deprivation Reoxygenation. Exp. Neurol. 367, 114468. doi:10.1016/j.expneurol.2023.114468
Chang, Y., Hsiao, G., Chen, S. H., Chen, Y. C., Lin, J. H., Lin, K. H., et al. (2007). Tetramethylpyrazine suppresses HIF-1alpha, TNF-alpha, and activated caspase-3 expression in middle cerebral artery occlusion-induced brain ischemia in rats. Acta Pharmacol. Sin. 28 (3), 327–333. doi:10.1111/j.1745-7254.2007.00514.x
Chen, D. S., Guo, H. L., Li, L., and Fan, J. M. (2010). Effects of tetramethylpyrazine on local cerebral ischemia-reperfusion injury in rats. J. Shanxi Med. Univ. 41 (09), 780–783.
Chen, L., Wang, W., Zhang, S., Liu, H., Yuan, X., Yang, X., et al. (2021). Value of Barthel, PLAN and NIHSS scores for predicting the death of patients with acute ischemic stroke during their 5-year follow-up. J. Clin. Neurosci. 90, 94–98. doi:10.1016/j.jocn.2021.05.049
Clausen, B. H., Wirenfeldt, M., Høgedal, S. S., Frich, L. H., Nielsen, H. H., Schrøder, H. D., et al. (2020). Characterization of the TNF and IL-1 systems in human brain and blood after ischemic stroke. Acta Neuropathol. Commun. 8 (1), 81. doi:10.1186/s40478-020-00957-y
Collaborators, G. S. (2019). Global, regional, and national burden of stroke, 1990-2016: a systematic analysis for the Global Burden of Disease Study 2016. Lancet Neurol. 18 (5), 439–458. doi:10.1016/S1474-4422(19)30034-1
Cumpston, M., Li, T., Page, M. J., Chandler, J., Welch, V. A., Higgins, J. P., et al. (2019). Updated guidance for trusted systematic reviews: a new edition of the Cochrane Handbook for Systematic Reviews of Interventions. Cochrane Database Syst. Rev. 10 (10), ED000142. doi:10.1002/14651858.ED000142
Deng, Z., Li, J., Tang, X., Li, D., Wang, Y., Wu, S., et al. (2022). Leonurine reduces oxidative stress and provides neuroprotection against ischemic injury via modulating oxidative and NO/NOS pathway. Int. J. Mol. Sci. 23 (17), 10188. doi:10.3390/ijms231710188
Fan, X., Chen, H., Xu, C., Wang, Y., Yin, P., Li, M., et al. (2022). Inhibiting sphingosine 1-phosphate receptor subtype 3 attenuates brain damage during ischemia-reperfusion injury by regulating nNOS/NO and oxidative stress. Front. Neurosci. 16, 838621. doi:10.3389/fnins.2022.838621
Fang, Z. Y., Xiong, L., Huang, J., Yang, T. Z., Ma, Y. J., and You, C. J. (2010). Effects of tetramethylpyrazine on neurological function and neuroelectrophysiology in rats with cerebral ischemia-reperfusion. Shandong Med. 50 (02), 19–20.
Feng, J., Li, F., Zhao, Y., Feng, Y., and Abe, Y. (2009). Brain pharmacokinetics of tetramethylpyrazine after intranasal and intravenous administration in awake rats. Int. J. Pharm. 375 (1-2), 55–60. doi:10.1016/j.ijpharm.2009.03.034
Feng, X., Li, M., Lin, Z., Lu, Y., Zhuang, Y., Lei, J., et al. (2023). Tetramethylpyrazine promotes axonal remodeling and modulates microglial polarization via JAK2-STAT1/3 and GSK3-NFκB pathways in ischemic stroke. Neurochem. Int. 170, 105607. doi:10.1016/j.neuint.2023.105607
Fu, L., Zhang, L. M., Guan, L. N., Song, Y. C., Zhang, D. X., Kang, L. Q., et al. (2023). Advanced MRI to assess hippocampal injury after incomplete cerebral ischemia-reperfusion in rats. J. Neuroimaging. 33 (5), 742–751. doi:10.1111/jon.13134
Gao, S. H., Yang, Y. C., Zhang, X. L., and Hou, M. M. (2023). Protective effect of tetramethylpyrazine on cerebral ischemia-reperfusion injury in rats. Clin. Med. 43 (07), 104–106. doi:10.19528/j.issn.1003-3548.2023.07.033
Ge, L., Cao, H. L., Zhang, J., Xu, M., Zhu, X. F., and Chen, Y. F. (2021). Effects of tetramethylpyrazine on oxidative stress, Ca∼(2+)-ATPase activity and inflammatory factors after cerebral ischemia reperfusion injury in rats. Inter J. lab. Med. 42 (05), 517–520.
Gong, P., Zhang, Z., Zou, Y., Tian, Q., Han, S., Xu, Z., et al. (2019). Tetramethylpyrazine attenuates blood-brain barrier disruption in ischemia/reperfusion injury through the JAK/STAT signaling pathway. Eur. J. Pharmacol. 854, 289–297. doi:10.1016/j.ejphar.2019.04.028
Han, J., Wan, H. T., Yang, J. H., Zhang, Y. Y., Ge, L. J., and Bie, X. D. (2014). Effect of ligustrazine on levels of amino acid neurotransmitters in rat striatum after cerebral ischemia-reperfusion injury. J. Asian Nat. Prod. Res. 16 (11), 1060–1067. doi:10.1080/10286020.2014.935347
Hu, Y. H., Zhou, A. M., Tian, Y., and Zhu, Y. B. (2010a). Effects of tetramethylpyrazine on bax, bcl-2, NO and NOS after cerebral ischemia reperfusion in mice. J. Difficult Dis. 9 (09), 648–727.
Hu, Y. H., Zhou, A. M., Tian, Y., and Zhu, Y. B. (2010b). Effect and mechanism of tetramethylpyrazine on the formation of cerebral ischemic tolerance. Chin. J. Basic Med. 16 (08), 671–674. doi:10.19945/j.cnki.issn.1006-3250.2010.08.016
Jia, J., Zhang, X., Hu, Y. S., Wu, Y., Wang, Q. Z., Li, N. N., et al. (2009). Protective effect of tetraethyl pyrazine against focal cerebral ischemia/reperfusion injury in rats: therapeutic time window and its mechanism. Thromb. Res. 123 (5), 727–730. doi:10.1016/j.thromres.2008.11.004
Jin, R., Yang, G., and Li, G. (2010). Inflammatory mechanisms in ischemic stroke: role of inflammatory cells. J. Leukoc. Biol. 87 (5), 779–789. doi:10.1189/jlb.1109766
Jin, Z., Liang, J., and Kolattukudy, P. E. (2021). Tetramethylpyrazine preserves the integrity of blood-brain barrier associated with upregulation of MCPIP1 in a murine model of focal ischemic stroke. Front. Pharmacol. 12, 710358. doi:10.3389/fphar.2021.710358
Jurcau, A., and Simion, A. (2021). Neuroinflammation in cerebral ischemia and ischemia/reperfusion injuries: from pathophysiology to therapeutic strategies. Int. J. Mol. Sci. 23 (1), 14. doi:10.3390/ijms23010014
Kao, T. K., Chang, C. Y., Ou, Y. C., Chen, W. Y., Kuan, Y. H., Pan, H. C., et al. (2013). Tetramethylpyrazine reduces cellular inflammatory response following permanent focal cerebral ischemia in rats. Exp. Neurol. 247, 188–201. doi:10.1016/j.expneurol.2013.04.010
Kao, T. K., Ou, Y. C., Kuo, J. S., Chen, W. Y., Liao, S. L., Wu, C. W., et al. (2006). Neuroprotection by tetramethylpyrazine against ischemic brain injury in rats. Neurochem. Int. 48 (3), 166–176. doi:10.1016/j.neuint.2005.10.008
Kapil, N., Datta, Y. H., Alakbarova, N., Bershad, E., Selim, M., Liebeskind, D. S., et al. (2017). Antiplatelet and anticoagulant therapies for prevention of ischemic stroke. Clin. Appl. Thromb.-Hemost. 23 (4), 301–318. doi:10.1177/1076029616660762
Korenić, A., Boltze, J., Deten, A., Peters, M., Andjus, P., and Radenović, L. (2014). Astrocytic mitochondrial membrane hyperpolarization following extended oxygen and glucose deprivation. PLoS One 9 (2), e90697. doi:10.1371/journal.pone.0090697
Lambertsen, K. L., Finsen, B., and Clausen, B. H. (2019). Post-stroke inflammation-target or tool for therapy? Acta Neuropathol. 137 (5), 693–714. doi:10.1007/s00401-018-1930-z
Li, D., Long, Y., Yu, S., Shi, A., Wan, J., Wen, J., et al. (2021). Research advances in cardio-cerebrovascular diseases of Ligusticum chuanxiong hort. Front. Pharmacol. 12, 832673. doi:10.3389/fphar.2021.832673
Li, J., Ma, L., and Pan, Y. (2008). Protective effect of ligustrazine on local cerebral ischemia-reperfusion injury in rats. New drug Clin. Pharmacol. tradi Chin. Med. (06), 445–446. doi:10.19378/j.issn.1003-9783.2008.06.009
Li, L., Zhang, D., Yao, W., Wu, Z., Cheng, J., Ji, Y., et al. (2022). Ligustrazine exerts neuroprotective effects via circ_0008146/miR-709/Cx3cr1 axis to inhibit cell apoptosis and inflammation after cerebral ischemia/reperfusion injury. Brain Res. Bull. 190, 244–255. doi:10.1016/j.brainresbull.2022.10.011
Li, P. F., Shen, M. H., Wu, J. P., and Zhang, C. B. (2017). Mechanism of ligustrazine in inhibiting cerebral ischemia-reperfusion injury in rats. Chin. J. Gerontol. 37 (07), 1561–1565.
Li, Y. F., Liu, K., Ni, Y. Q., Yang, Z. H., and Fu, W. S. (2013). Protective effects of tetramethylpyrazine on blood-brain barrier and the expression of claudin-5 after cerebral ischemia-reperfusion in rats. J. Chin. Med. 42 (06), 511–514.
Liang, B., Zhao, K., Ma, X. D., and Li, J. (2022). Experimental study on anti-apoptosis effect and mechanism of ligustrazine on neurons in rats with cerebral ischemia/reperfusion. Chin. J. Tradi Chin. Med. 40 (06), 205–289. doi:10.13193/j.issn.1673-7717.2022.06.047
Liao, S. L., Kao, T. K., Chen, W. Y., Lin, Y. S., Chen, S. Y., Raung, S. L., et al. (2004). Tetramethylpyrazine reduces ischemic brain injury in rats. Neurosci. Lett. 372 (1-2), 40–45. doi:10.1016/j.neulet.2004.09.013
Lin, J., Wang, Q., Zhou, S., Xu, S., and Yao, K. (2022). Tetramethylpyrazine: a review on its mechanisms and functions. Biomed. Pharmacother. 150, 113005. doi:10.1016/j.biopha.2022.113005
Liu, J. F. (2016). Protective effect of ligustrazine on local cerebral ischemia-reperfusion injury in rats. J. Math. Med. 29 (08), 1189–1191.
Liu, Y., Yang, G., Cui, W., Zhang, Y., and Liang, X. (2022). Regulatory mechanisms of tetramethylpyrazine on central nervous system diseases: a review. Front. Pharmacol. 13, 948600. doi:10.3389/fphar.2022.948600
Love, S. (2003). Apoptosis and brain ischaemia. Prog. Neuro-Psychopharmacol. Biol. Psychiatry. 27 (2), 267–282. doi:10.1016/S0278-5846(03)00022-8
Lozano, R., Naghavi, M., Foreman, K., Lim, S., Shibuya, K., Aboyans, V., et al. (2012). Global and regional mortality from 235 causes of death for 20 age groups in 1990 and 2010: a systematic analysis for the Global Burden of Disease Study 2010. Lancet 380 (9859), 2095–2128. doi:10.1016/S0140-6736(12)61728-0
Ma, Y. C., and Chen, Y. (2010). Effects of tetramethylpyrazine on the size of cerebral infarction and the expression of ED1, IL-1β and TNF-α after cerebral ischemia reperfusion in rats. Chin. J. Tradi Chin. Med. Inf. 17 (05), 33–35.
Mao, D., Li, F., Ma, Q., Dai, M., Zhang, H., Bai, L., et al. (2019). Intraocular administration of tetramethylpyrazine hydrochloride to rats: a direct delivery pathway for brain targeting? Drug Deliv. 26 (1), 841–848. doi:10.1080/10717544.2019.1650849
Murray, C. J., Vos, T., Lozano, R., Naghavi, M., Flaxman, A. D., Michaud, C., et al. (2012). Disability-adjusted life years (DALYs) for 291 diseases and injuries in 21 regions, 1990-2010: a systematic analysis for the Global Burden of Disease Study 2010. Lancet 380 (9859), 2197–2223. doi:10.1016/S0140-6736(12)61689-4
Naik, A., Adeleye, O., Koester, S. W., Winkler, E. A., Hartke, J. N., Karahalios, K., et al. (2023). Cerebrospinal fluid biomarkers for diagnosis and the prognostication of acute ischemic stroke: a systematic review. Int. J. Mol. Sci. 24 (13), 10902. doi:10.3390/ijms241310902
Paul, S., and Candelario-Jalil, E. (2021). Emerging neuroprotective strategies for the treatment of ischemic stroke: an overview of clinical and preclinical studies. Exp. Neurol. 335, 113518. doi:10.1016/j.expneurol.2020.113518
Qi, C. F., Liu, Y., Zhang, J. S., Tian, Y. M., Chen, X. L., and Zhang, J. F. (2008). Neuroprotective effect of ligustrazine on cerebral ischemia-reperfusion injury in rats. J. Mod. Integr. Tradi Chin. Med. (25), 3908–3964.
Qi, X., Ackermann, C., Sun, D., Liu, R., Sheng, M., and Hou, H. (2002). The prediction of plasma and brain levels of 2,3,5,6-tetramethylpyrazine following transdermal application. AAPS PharmSci 4 (4), E46. doi:10.1208/ps040446
Qiu, Y. M., Zhang, C. L., Chen, A. Q., Wang, H. L., Zhou, Y. F., Li, Y. N., et al. (2021). Immune cells in the BBB disruption after acute ischemic stroke: targets for immune therapy? Front. Immunol. 12, 678744. doi:10.3389/fimmu.2021.678744
Ren, Z. C., Guo, J. X., Yang, Y. C., and Liang, Y. G. (2008). Expression of cyclooxygenase-2 in cerebral cortex after cerebral ischemia and reperfusion and effect of tetramethylpyrazine. J. Anat. 31 (06), 831–847.
Saini, V., Guada, L., and Yavagal, D. R. (2021). Global epidemiology of stroke and access to acute ischemic stroke interventions. Neurology 97, S6–S16. 20 Suppl 2. doi:10.1212/WNL.0000000000012781
Shao, H., He, X., Zhang, L., Du, S., Yi, X., Cui, X., et al. (2021). Efficacy of ligustrazine injection as adjunctive therapy in treating acute cerebral infarction: a systematic review and meta-analysis. Front. Pharmacol. 12, 761722. doi:10.3389/fphar.2021.761722
Tan, F., Fu, W., Cheng, N., Meng, D. I., and Gu, Y. (2015). Ligustrazine reduces blood-brain barrier permeability in a rat model of focal cerebral ischemia and reperfusion. Exp. Ther. Med. 9 (5), 1757–1762. doi:10.3892/etm.2015.2365
Tao, T., Liu, M., Chen, M., Luo, Y., Wang, C., Xu, T., et al. (2020). Natural medicine in neuroprotection for ischemic stroke: challenges and prospective. Pharmacol. Ther. 216, 107695. doi:10.1016/j.pharmthera.2020.107695
Tian, Y., Li, Y. P., and Han, R. D. (2014). Effects of tetramethylpyrazine on apoptosis and caspase 12 expression after cerebral ischemia reperfusion in rats. J. Clin. Exper Med. 13 (01), 16–19.
Tsai, T. H., and Liang, C. (2001). Pharmacokinetics of tetramethylpyrazine in rat blood and brain using microdialysis. Int. J. Pharm. 216 (1-2), 61–66. doi:10.1016/s0378-5173(01)00572-5
Ueno, M. (2007). Molecular anatomy of the brain endothelial barrier: an overview of the distributional features. Curr. Med. Chem. 14 (11), 1199–1206. doi:10.2174/092986707780597943
Uzdensky, A. B. (2019). Apoptosis regulation in the penumbra after ischemic stroke: expression of pro- and antiapoptotic proteins. Apoptosis 24 (9-10), 687–702. doi:10.1007/s10495-019-01556-6
Xia, H., Cheng, Z., Cheng, Y., and Xu, Y. (2016). Investigating the passage of tetramethylpyrazine-loaded liposomes across blood-brain barrier models in vitro and ex vivo. Mat. Sci. Eng. c-mater. Biol. Appl. 69, 1010–1017. doi:10.1016/j.msec.2016.08.001
Xiao, X., Liu, Y., Qi, C., Qiu, F., Chen, X., Zhang, J., et al. (2010). Neuroprotection and enhanced neurogenesis by tetramethylpyrazine in adult rat brain after focal ischemia. Neurol. Res. 32 (5), 547–555. doi:10.1179/174313209X414533
Xing, Y., and Bai, Y. (2020). A review of exercise-induced neuroplasticity in ischemic stroke: pathology and mechanisms. Mol. Neurobiol. 57 (10), 4218–4231. doi:10.1007/s12035-020-02021-1
Yang, G., Qian, C., Wang, N., Lin, C., Wang, Y., Wang, G., et al. (2017). Tetramethylpyrazine protects against oxygen-glucose deprivation-induced brain microvascular endothelial cells injury via rho/rho-kinase signaling pathway. Cell. Mol. Neurobiol. 37 (4), 619–633. doi:10.1007/s10571-016-0398-4
Yang, Y. C., and Ren, Z. C. (2011). Effect of tetramethylpyrazine on COX-2 expression in cerebral cortex of rats after cerebral ischemia reperfusion. J. North China Coal Med. 13 (02), 144–145. doi:10.19539/j.cnki.2095-2694.2011.02.002
Zhang, C. B., Ding, X., and Zhan, Q. (2008). Protective effect of ligustrazine hydrochloride on cerebral ischemia reperfusion (I/R) injury in rats. Jiangsu Tradit. Chin. Med. (04), 77–79.
Zhang, G., Zhang, T., Li, N., Wu, L., Gu, J., Li, C., et al. (2018a). Tetramethylpyrazine nitrone activates the BDNF/Akt/CREB pathway to promote post-ischaemic neuroregeneration and recovery of neurological functions in rats. Br. J. Pharmacol. 175 (3), 517–531. doi:10.1111/bph.14102
Zhang, G., Zhang, T., Wu, L., Zhou, X., Gu, J., Li, C., et al. (2018b). Neuroprotective effect and mechanism of action of tetramethylpyrazine nitrone for ischemic stroke therapy. Neuromolecular Med. 20 (1), 97–111. doi:10.1007/s12017-018-8478-x
Zhang, J., Cai, C., Luo, Y., Wu, Y., and Qiu, F. (2023). The diagnostic model of Ligusticum chuanxiong hort. Against cerebral stroke using network Pharmacology and transcriptomics analyses. J. Integr. Neurosci. 22 (6), 149. doi:10.31083/j.jin2206149
Zhang, J. Q., Zhao, X. Q., Ji, X. M., Shi, W. J., Wang, P., Liu, Z. Q., et al. (2011). Effects of tetramethylpyrazine on cerebral ischemia/reperfusion injury in rats. Clin. Misdiagnosis 24 (02), 41–43.
Zhao, Y., Liu, Y., and Chen, K. (2016). Mechanisms and clinical application of tetramethylpyrazine (an interesting natural compound isolated from Ligusticum wallichii): current status and perspective. Oxidative Med. Cell. Longev. 2016, 2124638. doi:10.1155/2016/2124638
Zhao, Y., Zhang, X., Chen, X., and Wei, Y. (2022). Neuronal injuries in cerebral infarction and ischemic stroke: from mechanisms to treatment (Review). Int. J. Mol. Med. 49 (2), 15. doi:10.3892/ijmm.2021.5070
Zhou, P., Du, S., Zhou, L., Sun, Z., Zhuo, L. H., He, G., et al. (2019). Tetramethylpyrazine-2'O-sodium ferulate provides neuroprotection against neuroinflammation and brain injury in MCAO/R rats by suppressing TLR-4/NF-κB signaling pathway. Pharmacol. Biochem. Behav. 176, 33–42. doi:10.1016/j.pbb.2018.08.010
Zhu, B. K., Wang, X. X., and Zhao, H. (2020). Ligustrazine alleviates cerebral ischemia reperfusion injury in rats by activating VEGF-mediated PI 3K/AKT signaling pathway. HeBei Med. 26 (06), 912–917.
Zhu, T., Wang, L., Feng, Y., Sun, G., and Sun, X. (2021). Classical active ingredients and extracts of Chinese herbal medicines: pharmacokinetics, pharmacodynamics, and molecular mechanisms for ischemic stroke. Oxidative Med. Cell. Longev. 2021, 8868941. doi:10.1155/2021/8868941
Keywords: ligustrazine, ischemic stroke, inflammation, oxidative stress, apoptosis, meta-analysis
Citation: Wang Z, Wu Z, Miao Y, Hao A, Chen H, Zhao S, Luo M, Guo S, Liu Y and Lu Y (2024) The protective effects of ligustrazine on ischemic stroke: a systematic review and meta-analysis of preclinical evidence and possible mechanisms. Front. Pharmacol. 15:1373663. doi: 10.3389/fphar.2024.1373663
Received: 20 January 2024; Accepted: 29 February 2024;
Published: 13 March 2024.
Edited by:
Rajeev K. Singla, Sichuan University, ChinaReviewed by:
Irina Ielciu, University of Medicine and Pharmacy Iuliu Hatieganu, RomaniaDongyue Wang, Sichuan University, China
Xuesong Bai, Capital Medical University, China
Copyright © 2024 Wang, Wu, Miao, Hao, Chen, Zhao, Luo, Guo, Liu and Lu. This is an open-access article distributed under the terms of the Creative Commons Attribution License (CC BY). The use, distribution or reproduction in other forums is permitted, provided the original author(s) and the copyright owner(s) are credited and that the original publication in this journal is cited, in accordance with accepted academic practice. No use, distribution or reproduction is permitted which does not comply with these terms.
*Correspondence: Yun Lu, 0550@cdutcm.edu.cn
†These authors have contributed equally to this work and share first authorship