- 1School of Basic Medicine, Hebei University of Chinese Medicine, Shijiazhuang, China
- 2Central Laboratory, Hebei Academy of Chinese Medicine Sciences, Shijiazhuang, China
- 3Turbidity and Toxicity Laboratory, Hebei Key Laboratory of Turbidity, Shijiazhuang, China
Introduction: Acori Tatarinowii Rhizoma (ATR) is a well-known traditional Chinese medicine that is used for treating neuropathic diseases. However, there is little information about the safety of ATR.
Methods: The present study evaluated the acute and subacute oral toxicity of a water extract of ATR in Institute of Cancer Research (ICR) mice. In acute trials, a single administration of extract at a dose 5,000 mg/kg body weight led to no clinical signs of toxicity or mortality, indicating that the lethal dose (LD50) exceeded 5,000 mg/kg. A subacute toxicity test was done using daily doses of 1,250, 2,500, and 5,000 mg/kg of the ATR extract for 28 days, which did not show any adverse clinical symptoms or mortality. However, the male renal organ index and urea level in mice given 5,000 mg/kg was obviously abnormal, which was consistent with pathological results and suggested that this dose might cause kidney injury.
Results: Doses of ATR lower than 2,500 mg/kg could be regarded as safe, although the potential cumulative effects of long-term use of high doses of ATR need to be considered.
Discussion: The study highlights the function of ATR in reducing blood lipids and provides a new idea for its widespread clinical use in the future.
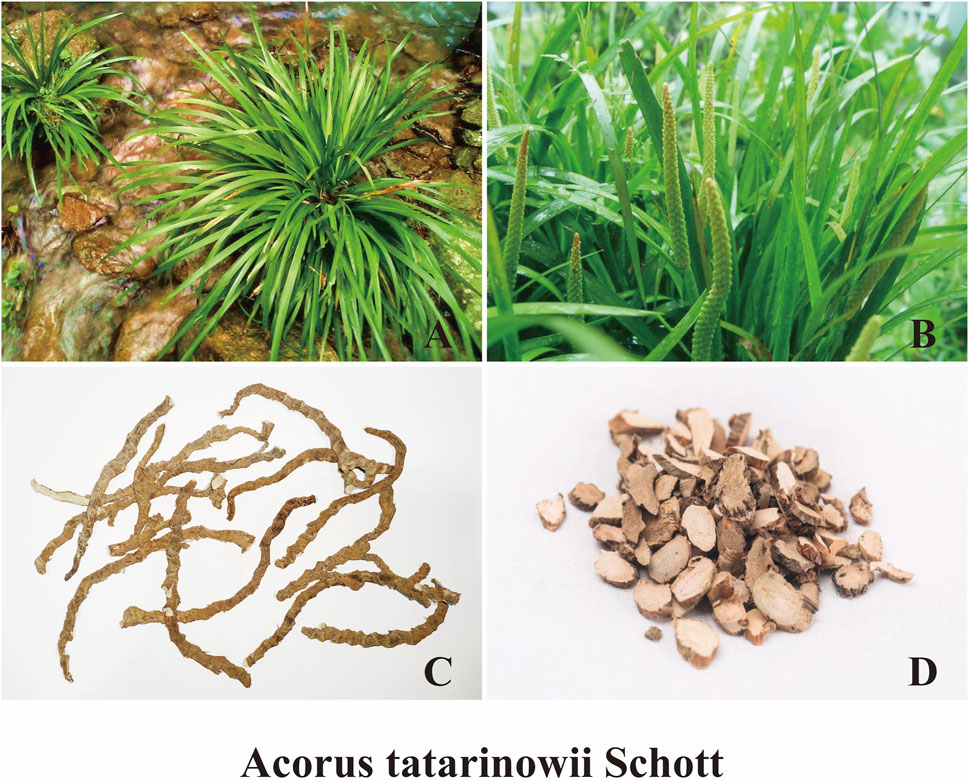
GRAPHICAL ABSTRACT | Representative image of Acori Tatarinowii Rhizoma. (A) Whole plant, (B) rhizomes, (C) dry rhizomes, (D) Chinese herb pieces.
1 Introduction
Acori Tatarinowii Rhizoma (ATR) is an important medicinal plant in traditional Chinese medicine, which is one of the most frequently used drug for treating brain diseases (Zhang et al., 2024), and over the past few decades, it has received continuous attention from medical and pharmaceutical researchers. According to reports, over 160 different structural types compounds have been identified in ATR. The involatile components include flavonoids, quinones, alkaloids, triterpenoid, saponins, phenylpropanoids, and organic acids, and the volatile components include α-asarone, β-asarone, methylisoeugenol, β-caryophyllene. Amino acids, sugars (Zhang et al., 2015), and trace elements have also been identified.
ATR has been shown to improve Alzheimer’s disease (AD) (Saki et al., 2020; Wang et al., 2021; Chen et al., 2022; Xiong et al., 2022) and shows outstanding performance as an antioxidant (Zhang et al., 2015) and anti-fatigue treatment (Zhu et al., 2014; Zhu et al., 2020). Significantly, ATR exhibits strong neuroprotective effects and reduces brain-nerve damage by improving cerebral blood circulation (Dong et al., 2014; Zhang et al., 2021). The key pharmacological components of Acori Tatarinowii Rhizoma extract are α-asarone and β-asarone. Modern pharmacological studies show that these active ingredients are widely used in the treatment of neurological diseases, such as depression, anxiety, epilepsy, and convulsions (Nandakumar et al., 2013; Koga et al., 2015; Liu et al., 2015; Tian et al., 2017; Zhang et al., 2019; Zhao et al., 2022; Xie et al., 2023), especially, α-asaronol promoted oligodendrocyte precursor cell differentiation and alleviated dysmyelination through the sensitization of PPARγ-GLT-1 signaling (Ge et al., 2022).
ATR is widely used as a natural medicinal plant and has broad development prospects, but related research has mainly focused on its clinical efficacy and pharmacological mechanisms (Li et al., 2020; Lu et al., 2023). There is little public safety information about its overall safety and side effects. The adverse reactions of natural medicinal plants have been attracting increasing attention in recent years (Traesel et al., 2016; You et al., 2020; Zhai et al., 2022). Therefore, in this study, a safety validation experiment was designed to explore the acute and subacute toxicity of ATR water extracts. The research provides a scientific basis for the clinical application of ATR and protecting public health and safety.
2 Materials and methods
2.1 Preparation of ATR water extract
ATR (batch number: 221001) was purchased from Anguo Yikang Pharmaceutical Co., Ltd. (Hebei, China) and satisfied the quality standards of the Chinese Pharmacopoeia (2020), as shown in graphical abstract. The sample was identified by Professor Xiao-gang Wang from the Pharmacy Department of Hebei Academy of Chinese Medicine Sciences (Hebei, China). ATR water extracts were prepared according to the following standard procedures.
A 1-kg sample of crude powder and 10 L of distilled water (ultra-pure water system, model OS007XXM1, ELGA, United Kingdom) were put into a multifunctional extraction tank (Shanghai Chaohong Instrument Equipment Co., Ltd., China), heated at 60°C, reflowed for 90 min, and filtered with 200-mesh screens. During the second extraction, 10 L of distilled water were added, extraction was done at 60°C for 60 min, and the extract filtered with 200-mesh screens. The extraction solution was concentrated to a relative density of 1.05–1.20 (60°C) using a rotary evaporator (Shanghai Yarong Biochemical Instrument Co., Ltd., China) at a vacuum of 80°C (pressure value 0.08 Mpa).
Next, the concentrate was spray-dried at 70°C, the spray powder was collected, and finally, a water extract powder of ATR was obtained. The brown powder was passed through a 50-mesh sieve and kept in a cool and dry environment (25°C, 35%–50% humidity) until use in experiments. ATR suspensions were freshly prepared daily with purified water before administration.
2.2 Experimental animals and housing conditions
Male and female Institute of Cancer Research (ICR) mice (6–8 weeks old and weighing 20–25 g) were provided by Beijing Vital River Laboratory Animal Technology Co., Ltd., Beijing, China, with Chinese animal use license SCXK (Beijing) 2021-0006. Before the experiment, the mice were allowed to adapt in cages for 7 days (22°C ± 2°C, 40%–70% humidity, light/dark reverse cycle for 12 h each). The mice were separated by sex and given free access to pure water and standard food. The animal experiments were conducted in accordance with the Good Laboratory Practice (GLP) principles of the National Medical Administration of China (NMPA) in the Animal Laboratory of Hebei Academy of Chinese Medicine Sciences and were approved by the Animal Care and Use Ethics Committee of Hebei University of Chinese Medicine (Ethics No. DWLL202302019).
2.3 Acute toxicity study
Acute oral toxicity studies were done according to the recommendations of protocol 423 of the OECD guidelines (OECD, 2001; Yang et al., 2019; Maimaiti et al., 2021; Ozan et al., 2023; Ru et al., 2023). The experiment was weighted and separated based on weight differences, and the 40 mice were divided into the following 4 groups of 10 each (5 males and 5 females). The groups received either no ATR water extract (0 mg/kg; control group), a low dose (1,250 mg/kg), a medium dose (2,500 mg/kg), or a high-dose (5,000 mg/kg). A volume of 20 mL/kg was administered by gavage.
At 16 h before the experiment, the animals were fasted. On the first day of administration, the mortality rate and general behavioural changes of all animals were regularly observed at 30 min, 1, 2, 4, 8, and 24 h. Starting from the second day, the mortality rate and abnormal clinical symptoms of the treated mice were observed every day for 14 consecutive days, as shown in Table 1. The median lethal dose (LD50) was determined using the acute toxicity method.
Body weight, food/water consumption, and corresponding changes were recorded daily. The mice received a known amount of food/water, and the remaining was measured 24 h later to determine the daily food/water intake as the difference. The mortality and abnormalities of the mice were also recorded. At the end of administration, mice were anesthetized with inhaled isoflurane, and then blood samples were taken. The animals were dissected, and organ weights were recorded.
2.4 Subacute toxicity study
Subacute toxicity experiments were performed for 28 days according to protocol 407 of the OECD guidelines (OECD, 2008; Yang et al., 2019; Maimaiti et al., 2021; Hisamuddin et al., 2023; Ozan et al., 2023; Ru et al., 2023). The experiments used 40 mice that were divided into 4 groups based on the same principle as the previous experiment with (10 mice in each group, 5 females and 5 males; control group (pure water), low-dose group (1,250 mg/kg), medium-dose group (2,500 mg/kg), and high-dose group (5,000 mg/kg)). All animals were gavaged at a weight of 20 mL/kg once a day for 28 consecutive days. The observations are shown in Table 1. Mice were anesthetized by inhalation using 4% isoflurane (batch number 20220803, Ruihua Life Technology Co., Ltd., Shenzhen, China) in the induction anesthesia room of the multi-channel small animal anesthesia device (R550, Ruihua Life Technology Co., Ltd., Shenzhen, China).
An anticoagulant vessel was used to collect about 0.5 mL of blood, which was immediately used in a fully automated blood cell analyser (Mindray, model BC6800 plus, Shenzhen, China). The following blood parameters were detected: white blood cell count (WBC), red blood cell count (RBC), haemoglobin (HGB), haematocrit (HCT), mean red blood cell volume (MCV), mean red blood cell haemoglobin (MCH), mean red blood cell haemoglobin concentration (MCHC), mean haemoglobin concentration (CHCM), red blood cell distribution width (RDW), red blood cell albumin distribution width (HDW), platelet count (PLT), mean platelet volume (MPV), neutrophils (NEU%), lymphocytes (LYM%), monocytes (MON%), eosinophils (EOS%), and basophils (BAS%). About 1.8 mL blood was collected and centrifuged at 3,000 rpm for 10 min to obtain serum for the detection of the following activity of the enzymes (Rayto Life Technologies, Chemray 800, Shenzhen, China): serum alanine aminotransferase (ALT), aspartate aminotransferase (AST), alkaline phosphatase (ALP), creatinine (CRE), total bilirubin (TBIL), glutamyltranspeptidase (GGT), urea, total protein (TP), albumin (ALB), glucose (GLU), total cholesterol (CHOL), triglycerides (TG), and the concentrations of sodium (Na+), chlorine (Cl−), and potassium (K+). The brains, hearts, livers, spleens, kidneys, lungs, testicles (males), uteruses and ovaries (females), thymuses, and bladders were weighed, and an organ index was calculated (absolute organ weight/body weight). All tissues and organs were stored in 10% neutral buffer formalin for histopathological examination.
2.5 Statistical analysis
The statistical analysis was conducted using the Windows version of GraphPad Prism 5.0 and plotted using Adobe Illustrator. The data were represented by the mean and standard deviation (SD). When the variance was homogeneous, the intergroup differences were evaluated using a one-way analysis of variance (ANOVA) and Dunnett’s test. When the variance was uneven, a Kruskal–Wallis nonparametric test was used. A difference was considered statistically significant at p < 0.05.
3 Results
3.1 α- and β-asarone quantification
Figure 1 shows the chromatograms of α- and β-asarone quantification from 0.1 g of ATR extract and the peak maxima. The content of α- and β-asarone is approximately 6.63% and 14.68% in 0.1 g of ATR extract, respectively. The respective elution times of α- and β-asarone were recorded at 23.473 min and 19.206 min.
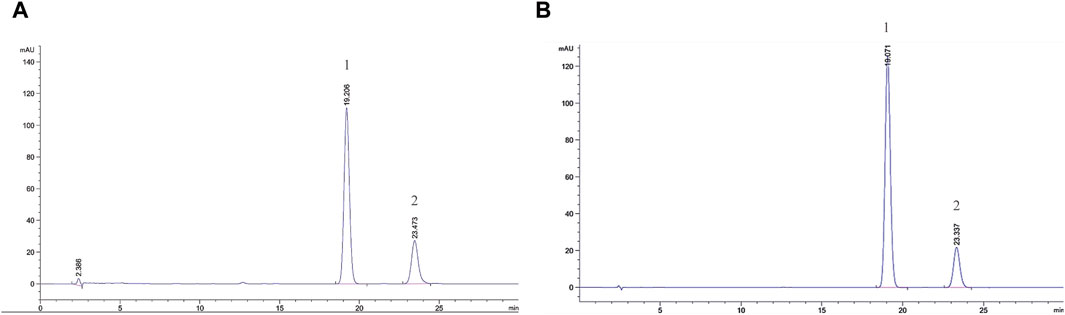
FIGURE 1. Comprehensive peak characterization trace showing Acori Tatarinowii Rhizoma and α- and β-asarone from HPLC analyses. (A) Sample map of water extract of Acori Tatarinowii Rhizoma, (B) Map of standard substance. 1: β-asarone. 2: α-asarone.
3.2 Acute oral toxicity in mice
3.2.1 General behaviours
During the 14 days of observation, no animal deaths were observed (Table 2). Compared with the control group, there was no significant difference in weight and food/water consumption between each group (p > 0.05). The weight of the treatment groups was slightly lower than in the control group, as shown in Figure 1. All mice curled up for 1–5 min after gavage and then started normal activity. On the first day, two high-dose male mice showed less activity, while one medium-dose male mouse was insensitive to noise stimulation. On the third day, one low-dose and one high-dose female mouse had loose stool. These clinical signs all appeared briefly, and the mice recovered within 48 h, as shown in Table 2. The mice showed no other abnormalities.
After dissection, there was no abdominal bleeding, and significant changes in organ colour and volume were observed. The organ coefficient values of the hearts, livers, spleens, lungs, kidneys, and brains were all within the normal ranges (Table 3). Therefore, we assumed that the LD50 of ATR exceeds the high dose based on these observations.
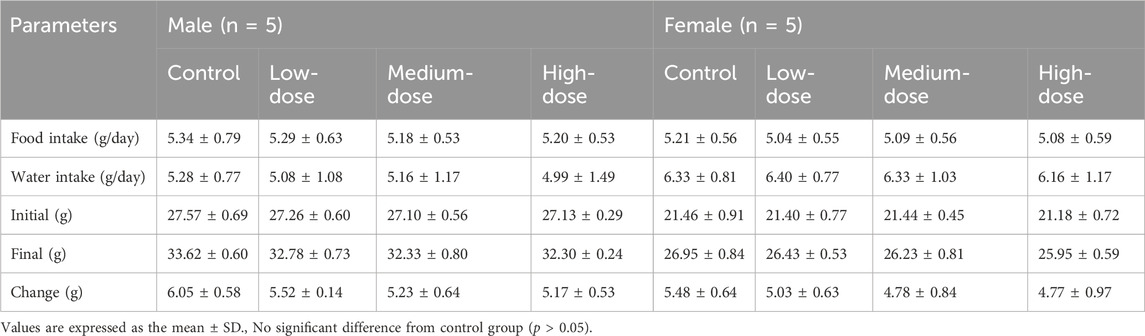
TABLE 3. Body weight, food/water consumption in acute toxicity study with ATR water extract in mice.
3.2.2 Weight, consumption, post-mortem examination, and organ index
The body weight and food/water consumption are shown in Figure 2 and Table 3. There were no significant changes in all groups (p > 0.05). The autopsy revealed no significant associated changes. There was no statistical significance for organ coefficients in each group (p > 0.05), as shown in Table 4.
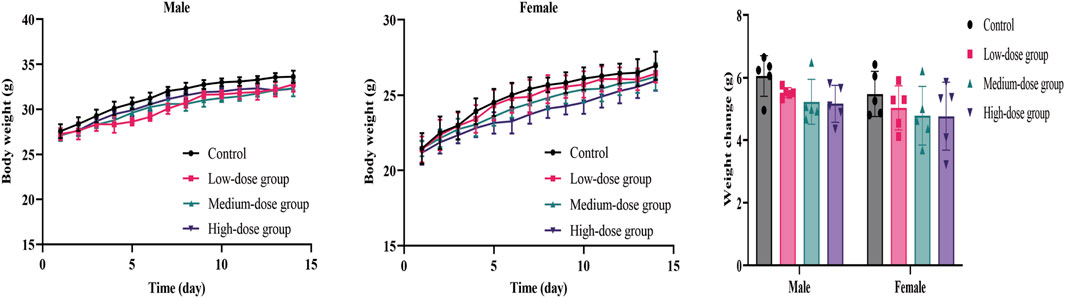
FIGURE 2. Mean body weight and weight changes of mice during the 14-day acute toxicity study. Error bars represent ±standard deviation.
3.3 Subacute oral toxicity in mice
3.3.1 General behaviours
There were no animal deaths or abnormal clinical symptoms associated with ATR. There were no obvious abnormalities in fur colour, behaviour, eating, drinking, breathing, or defecation in all mice. On the 10th day, depilation and dishevelled hair were observed in two low-dose male mice, but there was no alopecia areata, skin ulcers, or swelling. The condition was found to be related to a fight between male animals after observation. On day 17, slight wheezing and reduced activity were recorded in one medium-dose female mouse, which was possibly due to a manipulation error during gastric perfusion and returned to normal within 48 h. No other abnormal behavioural changes were seen in all groups, as shown in Table 5.
3.3.2 Body weight and food/water consumption
The results showed that weight-grain rates of male mice treated with ATR slowed down. The weight-gain rates of male mice in the low-dose, medium-dose and high-dose groups were 38.5%, 36.5%, and 35.7%, respectively, which were lower than the control group (41.0%), but there was no significant difference. However, the body weight of female mice in medium-dose group and high-dose group was significantly different from that in the control group (p < 0.05). These mice showed increases of 31.2%% and 24.6%, respectively, which were lower than that in the control group (47.9%). There was no difference in the amount of food/water consumed by either group during administration. Therefore, ATR water extract did not damage the total food intake of the animals. The body weight and food/water intake are shown in Figure 3 and Table 6.
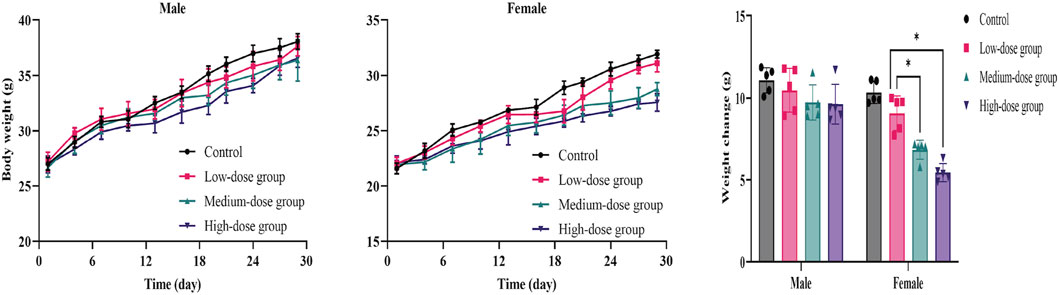
FIGURE 3. Mean body weight and weight changes of mice during the 28-day in subacute toxicity study. Error bars represent ±standard deviation.
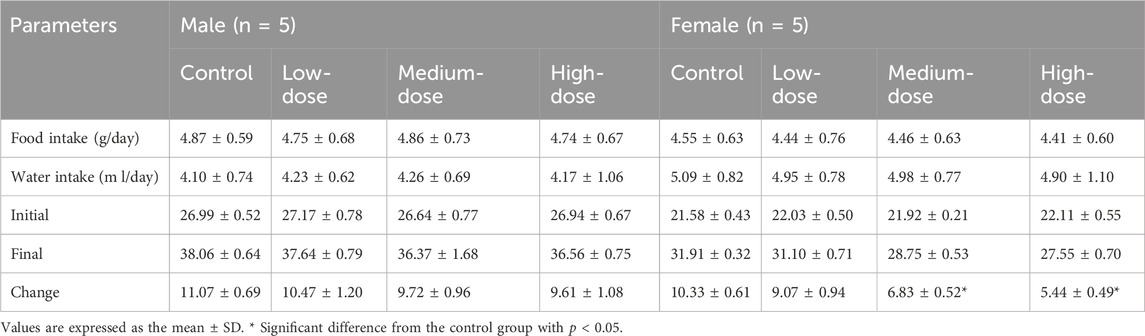
TABLE 6. Body weight, food/water consumption in subacute toxicity study with ATR water extract in mice.
3.3.3 Post-mortem examination, organ index, and histopathology
After 28 days of administration, no significant abnormalities were found during the autopsy. The results showed that the thymus organ index was significantly higher in the medium-dose group and the high-dose groups of female mice than in the control group (p < 0.05), while no abnormality was observed in the male groups. However, this may have not been due to drug effects because there were abnormalities in the histopathological examination of thymus tissues in corresponding groups. Compared with the control group, the renal organ index of the male high-dose group had a statistically significant difference (p < 0.05), while the corresponding female mice had no change. The coefficient changes of other organs were not statistically significant (p > 0.05), as shown in Table 7.
Representative pathological images of major organs in mice are shown in Figure 4. There were slightly thickened alveolar walls, a small increase in neutrophils, and occasional slight dilation of alveolar cavities in the lungs of each group, suggesting mild pneumonia. There was only mild cellular hydrodegeneration in the liver and kidneys, but there was no significant inflammation in each group and no significant difference between the administration groups and the control group. Therefore, we considered that the pathological change was spontaneous and not related to drug damage.
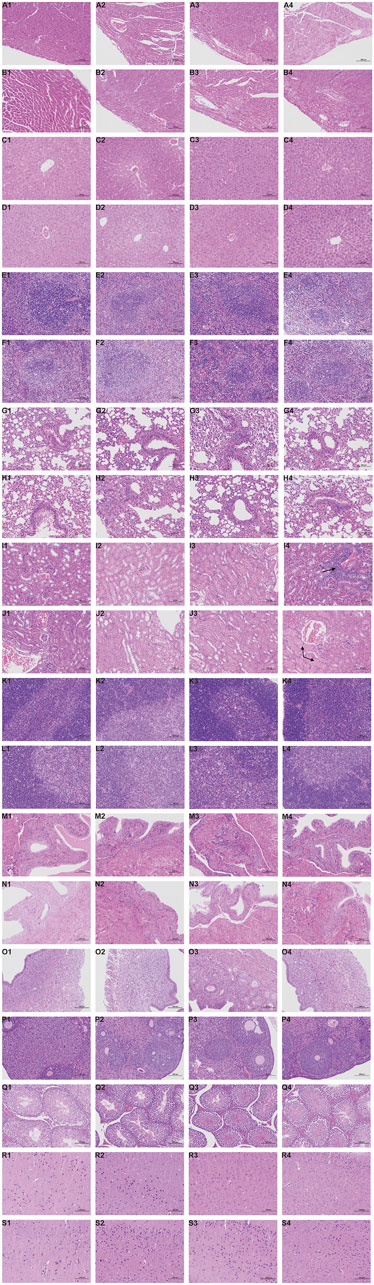
FIGURE 4. Histopathological results during the 28-day in subacute toxicity study. Stained with H&E, 200 ×, Scale Bars: 100 μm. Heart: A1 (Control, male); A2 (Low dose of ATR, male); A3 (Medium dose of ATR, male); A4 (High dose of ATR, male). Heart: B1 (Control, female); B2 (Low dose of ATR, female); B3 (Medium dose of ATR, female); B4 (High dose of ATR, female). Liver: C1 (Control, male); C2 (Low dose of ATR, male); C3 (Medium dose of ATR, male); C4 (High dose of ATR, male). Liver: D1 (Control, female); D2 (Low dose of ATR, female); D3 (Medium dose of ATR, female); D4 (High dose of ATR, female). Spleen: E1 (Control, male); E2 (Low dose of ATR, male); E3 (Medium dose of ATR, male); E4 (High dose of ATR, male). Spleen: F1 (Control, female); F2 (Low dose of ATR, female); F3 (Medium dose of ATR, female); F4 (High dose of ATR, female). Lung: G1 (Control, male); G2 (Low dose of ATR, male); G3 (Medium dose of ATR, male); G4 (High dose of ATR, male). Lung: H1 (Control, female); H2 (Low dose of ATR, female); H3 (Medium dose of ATR, female); H4 (High dose of ATR, female). Kidney: I1 (Control, male); I2 (Low dose of ATR, male); I3 (Medium dose of ATR, male); I4 (High dose of ATR, male). Kidney: J1 (Control, female); J2 (Low dose of ATR, female); J3 (Medium dose of ATR, female); J4 (High dose of ATR, female). Thymus: K1 (Control, male); K2 (Low dose of ATR, male); K3 (Medium dose of ATR, male); K4 (High dose of ATR, male). Thymus: L1 (Control, female); L2 (Low dose of ATR, female); L3 (Medium dose of ATR, female); L4 (High dose of ATR, female). Bladder: M1 (Control, male); M2 (Low dose of ATR, male); M3 (Medium dose of ATR, male); M4 (High dose of ATR, male). Bladder: N1 (Control, female); N2 (Low dose of ATR, female); N3 (Medium dose of ATR, female); N4 (High dose of ATR, female). Testis: Q1 (Control, male); Q2 (Low dose of ATR, male); Q3 (Medium dose of ATR, male); Q4 (High dose of ATR, male). Uterus: O1 (Control, female); O2 (Low dose of ATR, female); O3 (Medium dose of ATR, female); O4 (High dose of ATR, female). Ovary: P1 (Control, female); P2 (Low dose of ATR, female); P3 (Medium dose of ATR, female); P4 (High dose of ATR, female). Brain: R1 (Control, male); R2 (Low dose of ATR, male); R3 (Medium dose of ATR, male); R4 (High dose of ATR, male). Brain: S1 (Control, female); S2 (Low dose of ATR, female); S3 (Medium dose of ATR, female); S4 (High dose of ATR, female).
However, there was vacuolar degeneration of renal tubular epithelial cells and occasional minor glomerular capillary congestion in the female high-dose group. Similarly, in the male high-dose group, focal lymphocyte infiltration was observed in the renal cortex. In addition, compared with the control group, the renal organ index of the male high-dose group also showed significant differences, suggesting that the high dose may cause minor damage to the kidneys of mice.
3.3.4 Hematology
The blood routine results of the mice from after the administration are shown in Table 8. Compared with the control group, the female low-dose group showed a significant decrease in HGB and MCH, although there was no dose-response relationship. Therefore, we assume that there was no toxicological significance. NEU (%) was significantly increased in both sexes of mice in the high-dose group compared to the control group, but the results were within the normal range for mice (52%–72%). PLT and MPV in the male group decreased with a dose-response relationship, but the difference was not statistically significant (p > 0.05). Therefore, we assume that the result had no toxicological significance.
3.3.5 Serum biochemistry
The results of serum biochemistry are shown in Table 9. Compared with the control group, TG levels in female medium-dose and high-dose groups were significantly decreased (p < 0.05). The same happened in the male high-dose group (p < 0.05). Other data showed a downward trend in TC in the treated group of mice, although the result was not statistically significant. Furthermore, all values were within the normal range. Therefore, these changes were not considered to be adverse reactions associated with the test substance.
However, there was a statistically significant difference in urea between the male high-dose group and the control group, although the experimental values of the mice were within the normal range (Ozan et al., 2023). Compared to the control group, the Na+ concentration in the female medium-dose group was significantly decreased (p < 0.05), while the Cl− concentration in the male high-dose group was decreased (p < 0.05). The experimental values of both were within the normal range for mice (Ru et al., 2023) and lacked a dose correlation. In addition, electrolytes in living organisms are dynamically changing and not static, so we considered this result to have no significant biological meaning and to be unrelated to toxicity. Other detection indexes were normal.
4 Discussion
The safety assessment of natural medicinal plants is a concern, especially for avoiding their adverse effects on health (Bernstein et al., 2021). The toxic potential of active ingredients present in natural medicinal plants has been extensively studied (Hudson et al., 2018; Roytman et al., 2018; Zárybnický et al., 2018). These studies involve safe doses and times, toxicological evaluations, and clinical application recommendations to reduce or avoid related adverse reactions. For hundreds of years, ATR has been used to treat diseases, but so far, evaluations of its toxicity have not been reported. Acute and subacute toxicity tests have been used to evaluate the toxicity or side effects of many natural extracts. Due to the lack of comprehensive information on its safety in the scientific literature, this study evaluated the safety of ATR extracts.
Mice are always one of the main mammals used in preclinical research, including pharmacological mechanism research and safety evaluations. Currently, international guidelines for chemicals and drugs require mice as models for safety assessment (Yang et al., 2019). Mice are small, low in cost, easy to obtain and study, and suitable for studying all stages of life, which makes them an ideal model organism for various experiments.
In the acute toxicity study, no deaths or adverse clinical symptoms were observed in each dose group, and no significant changes in body weight were observed. Therefore, according to the method of acute toxicity classification (Chushak et al., 2021), it can be considered that the plant extract is non-toxic. However, it is worth noting that long-term use of drugs may lead to accumulation in the body, gradually affecting tissues and organs. Therefore, as a continuation of acute toxicity studies, subacute toxicity tests were conducted using three doses of ATR extract.
Changes in weight in humans and animals directly reflect their overall health status, and there are many factors that affect it, such as genes, diet, and exercise. During the subacute toxicity experiment, although there was no difference in food intake among the groups, the weight of the female medium-dose group and female high-dose group decreased significantly. When acute poisoning occurs, animals may have symptoms such as drowsiness, anorexia, diarrhoea, and elevated liver enzyme levels (Amaral et al., 2022). In our study, no relevant symptoms were found, thus ruling out signs of toxicity.
Literature has shown that components of ATR raise HDL and lower triglyceride and total cholesterol levels, thereby lowering blood lipid levels, and it even showed comparable performance to simvastatin (Rodríguez-Páez et al., 2003; Argüelles et al., 2010; Mendieta et al., 2014; Thakare et al., 2016). In addition, other studies found am ethnic medicinal plant from the same genus, Acorus calamus L., has therapeutic effects on diabetes and cardiovascular complications through a mechanism of insulin sensitization (Parab et al., 2002; Wu et al., 2009). Therefore, combined with the results of weight and biochemical tests which TG and TC showed a downward trend in this study, we speculated that ATR reduced blood lipids and changed metabolism, ultimately leading to weight loss, which was consistent with the results of previous studies (Lee et al., 2010). Significantly, the result is the same as the theory in traditional Chinese medicine.
The blood system is sensitive to toxic chemicals and is an important indicator for monitoring physiological changes in both humans and animals. Biochemical parameters are crucial for studying organs and tissues (Devi et al., 2022), especially in the kidneys and liver since one is used for excreting waste, and the other is used for uptake metabolism. In order to evaluate the toxicity of any new compound, it is necessary to understand the status of these two important organs, which can be validated through biochemical evaluation (Sureshkumar et al., 2018). Serum levels of three enzymes (ALT, AST, and ALP) are commonly used in studies as clinical biochemical markers related to liver injury (Yun et al., 2018). Our results showed no significant differences in their serum levels between all groups.
Histopathological examination provides a comprehensive assessment of the degree of injury and the specific organs affected. In addition, renal function can be evaluated by changes in urea, and changes in parameters indicate impaired renal function (Ezeja et al., 2014). The abnormal changes in ureal evels in our biochemical analysis support the pathological abnormalities in renal tissue at high doses. Accordingly, we assume that high-dose ATR extracts may have potential toxicity, which could lead to nephrotoxic side effects. However, the specific mechanism of the toxic effects and the chemical composition that may cause toxicity need further investigation.
However, the blood biochemistry results were not completely consistent with the pathological results. The pathological results showed that all groups had mild pneumonia, but there were no abnormal numbers of white blood cells, although they were generally high. This may have been due to the antibacterial and anti-inflammatory effects of ATR, which reduces white blood cells to some extent (Joshi, 2016). Therefore, in the pathological manifestations of pneumonia, the overall white blood cell count did not exceed the normal range. Blood tests are dynamic and variable results, unlike pathological results, which are long lasting and difficult to eliminate. In summary, the overall analysis of all ATR water-extract doses showed no significant damage to the important organs and reproductive organs of the treated animals, indicating that the low dose and medium dose are safe, while the high dose may cause minor kidney damage.
The no-observed-adverse-effect level (NOAEL) value was determined to be the medium-dose per body weight of ATR water extract. When converting the NOAEL of ATR water extract to the equivalent human dose (HED), it is calculated based on the body surface area and body weight (Nair et al., 2018). The HED value was calculated to be 191.65 mg/kg, which is equivalent to a dose of 11,499 mg for a 60-kg person, which is much higher than the clinical dosage for ATR prescribed by the Chinese Pharmacopoeia Committee (Committee of National Pharmacopoeia, 2020). These studies provide important information on the safety of oral ATR water extracts and support the sustained development and use of ATR water extracts as natural medicinal plants.
5 Conclusion
In conclusion, our research findings indicate that a single high-dose exposure to ATR water extract does not lead to acute toxicity in mice, but prolonged exposure to the high dose may lead to histopathological changes, especially in the kidneys. The results showed that the LD50 value of ATR water extract was greater than the high dose, and the NOAEL value was 2,500 mg/kg/day. However, in order to accurately determine NOAEL values, it is necessary to apply repeated subchronic doses for at least 90 days, as shown in Figure 5.
A surprising discovery was that ATR reduced serum lipid levels and altered metabolism. This could provide new ideas for the prevention of cardiovascular disease and the development of weight-loss drugs. As far as we know, this study is the first to report the safety assessment of ATR water extract and provides valuable preliminary data for the toxicity profile of ATR.
Data availability statement
The original contributions presented in the study are included in the article/Supplementary Materials, further inquiries can be directed to the corresponding authors.
Ethics statement
The animal study was approved by Animal Care and Use Ethics Committee of Hebei University of Chinese Medicine Hebei University of Chinese Medicine. The study was conducted in accordance with the local legislation and institutional requirements.
Author contributions
LP: Writing–review and editing, Supervision, Project administration, Funding acquisition. JL: Writing–original draft, Software, Methodology, Funding acquisition, Conceptualization. XP: Writing–review and editing, Writing–original draft, Funding acquisition, Conceptualization. S-JS: Writing–review and editing, Software, Methodology. JY: Writing–original draft, Software, Formal Analysis. YL: Writing–review and editing, Supervision, Funding acquisition, Formal Analysis.
Funding
The author(s) declare that financial support was received for the research, authorship, and/or publication of this article. This research was supported by the State Administration of Traditional Chinese Medicine of the People’s Republic of China Funding Project: LP National Prestigious Chinese Physicians Inheritance Studio [State TCM Human Education Letter (2022) No. 75]. LP is the recipient of the funding awards. Hebei Provincial Administration of Traditional Chinese Medicine Funding Project (Nos. 2022112 to XP; Nos. 2022113 to JL), and Hebei Province Graduate Innovation Funding Project (Nos. XCXZZBS2023011 to JL). Hebei Provincial Administration of Traditional Chinese Medicine Funding Project (Nos.2024075 to YL).
Conflict of interest
The authors declare that the research was conducted in the absence of any commercial or financial relationships that could be construed as a potential conflict of interest.
Publisher’s note
All claims expressed in this article are solely those of the authors and do not necessarily represent those of their affiliated organizations, or those of the publisher, the editors and the reviewers. Any product that may be evaluated in this article, or claim that may be made by its manufacturer, is not guaranteed or endorsed by the publisher.
References
Argüelles, N., Sánchez-Sandoval, E., Mendieta, A., Villa-Tanaca, L., Garduño-Siciliano, L., Jiménez, F., et al. (2010). Design, synthesis, and docking of highly hypolipidemic agents: Schizosaccharomyces pombe as a new model for evaluating alpha-asarone-based HMG-CoA reductase inhibitors. Bioorg Med. Chem. 18, 4238–4248. doi:10.1016/j.bmc.2010.04.096
Bernstein, N., Akram, M., Yaniv-Bachrach, Z., and Daniyal, M. (2021). Is it safe to consume traditional medicinal plants during pregnancy? Phytother. Res. 35, 1908–1924. doi:10.1002/ptr.6935
Bodnar, M. J., Ratuski, A. S., and Weary, D. M. (2023). Mouse isoflurane anesthesia using the drop method. Lab. Anim. 57 (6), 623–630. doi:10.1177/00236772231169550
Chen, J., Hao, W., Zhang, C., Cui, M., Sun, Y., Zhang, Y., et al. (2022). Explore the therapeutic composition and mechanism of schisandra chinensis-acorus tatarinowii schott on Alzheimer's disease by using an integrated approach on chemical profile, network Pharmacology, and UPLC-QTOF/MS-Based metabolomics analysis. Oxid. Med. Cell Longev. 2022, 6362617. doi:10.1155/2022/6362617
Cheng, Z., Shu, H., Zhang, S., Luo, B., Gu, R., Zhang, R., et al. (2020). From folk taxonomy to species confirmation of acorus (acoraceae): evidences based on phylogenetic and metabolomic analyses. Front. Plant Sci. 11, 965. doi:10.3389/fpls.2020.00965
Chushak, Y., Gearhart, J. M., and Ott, D. (2021). In silico assessment of acute oral toxicity for mixtures. Chem. Res. Toxicol. 34, 345–354. doi:10.1021/acs.chemrestox.0c00256
Devi, T. B., Jena, S., Patra, B., Singh, K. D., Chawla, S., Raina, V., et al. (2022). Acute and sub-acute toxicity evaluation of dihydro-p-coumaric acid isolated from leaves of Tithoni adiversifolia Hemsl. A. Gray in BALB/c mice. Front. Pharmacol. 13, 1055765. doi:10.3389/fphar.2022.1055765
do Amaral, L. A., Farias Pereira Subtil Cavalcante, A. C., da Silva Fleming de Almeida, T., Marques Romeiro Santos, M., Candeloro Portugal, L., Suzuki Dos Santos, B., et al. (2022). Acute and subacute (28 days) oral toxicity studies of tucum almond oil (Bactris Setosa Mart.) in mice. Drug Chem. Toxicol. 45, 1754–1760. doi:10.1080/01480545.2021.1871737
Dong, H., Gao, Z., Rong, H., Jin, M., and Zhang, X. (2014). β-asarone reverses chronic unpredictable mild stress-induced depression-like behavior and promotes hippocampal neurogenesis in rats. Molecules 19, 5634–5649. doi:10.3390/molecules19055634
Ezeja, M. I., Anaga, A. O., and Asuzu, I. U. (2014). Acute and sub-chronic toxicity profile of methanol leaf extract of Gouania longipetala in rats. J. Ethnopharmacol. 151, 1155–1164. doi:10.1016/j.jep.2013.12.034
Ge, Y. H., Zhen, F., Liu, Z. Q., Feng, Z. W., Wang, G., Zhang, C., et al. (2022). Alpha-asaronol alleviates dysmyelination by enhancing glutamate transport through the activation of PPARγ-GLT-1 signaling in hypoxia-ischemia neonatal rats. Front. Pharmacol. 13, 766744. doi:10.3389/fphar.2022.766744
Hisamuddin, A. S. B., Naomi, R., Bin Manan, K. A., Bahari, H., Yazid, M. D., Othman, F., et al. (2023). Phytochemical component and toxicological evaluation of purple sweet potato leaf extract in male Sprague-Dawley rats. Front. Pharmacol. 14, 1132087. doi:10.3389/fphar.2023.1132087
Hohlbaum, K., Bert, B., Dietze, S., Palme, R., Fink, H., and Thöne-Reineke, C. (2017). Severity classification of repeated isoflurane anesthesia in C57BL/6JRj mice-Assessing the degree of distress. PLoS One 12 (6), e0179588. doi:10.1371/journal.pone.0179588
Hudson, A., Lopez, E., Almalki, A. J., Roe, A. L., and Calderón, A. I. (2018). A review of the toxicity of compounds found in herbal dietary supplements. Planta Med. 84, 613–626. doi:10.1055/a-0605-3786
Joshi, R. K. (2016). Acorus calamus Linn.: phytoconstituents and bactericidal property. World J. Microbiol. Biotechnol. 32, 164. doi:10.1007/s11274-016-2124-2
Koga, K., Descalzi, G., Chen, T., Ko, H. G., Lu, J. S., Li, S., et al. (2015). Coexistence of two forms of LTP in ACC provides a synaptic mechanism for the interactions between anxiety and chronic pain. Neuron 85, 377–389. doi:10.1016/j.neuron.2014.12.021
Lee, H., Kang, R., and Yoon, Y. (2010). SH21B, an anti-obesity herbal composition, inhibits fat accumulation in 3T3-L1 adipocytes and high fat diet-induced obese mice through the modulation of the adipogenesis pathway. J. Ethnopharmacol. 127, 709–717. doi:10.1016/j.jep.2009.12.002
Li, Y., Zhang, X. L., Huang, Y. R., Zheng, Y. Y., Zheng, G. Q., and Zhang, L. P. (2020). Extracts or active components from acorus gramineus aiton for cognitive function impairment: preclinical evidence and possible mechanisms. Oxid. Med. Cell Longev. 2020, 6752876. doi:10.1155/2020/6752876
Liu, H., Song, Z., Liao, D. G., Zhang, T. Y., Liu, F., Zhuang, K., et al. (2015). Anticonvulsant and sedative effects of eudesmin isolated from Acorus tatarinowii on mice and rats. Phytother. Res. 29, 996–1003. doi:10.1002/ptr.5337
Lu, H., Luo, M., Chen, R., Luo, Y., Xi, A., Wang, K., et al. (2023). Efficacy and safety of traditional Chinese medicine for the treatment of epilepsy: a updated meta-analysis of randomized controlled trials. Epilepsy Res. 189, 107075. doi:10.1016/j.eplepsyres.2022.107075
Maimaiti, A., Jing-Jing, L., and Shi, L. (2021). Investigating the acute and sub-acute toxicity of medicinal Cuscuta chinensis Lam plant. J. Ethnopharmacol. 273, 114005. doi:10.1016/j.jep.2021.114005
Mendieta, A., Jiménez, F., Garduño-Siciliano, L., Mojica-Villegas, A., Rosales-Acosta, B., Villa-Tanaca, L., et al. (2014). Synthesis and highly potent hypolipidemic activity of alpha-asarone- and fibrate-based 2-acyl and 2-alkyl phenols as HMG-CoA reductase inhibitors. Bioorg Med. Chem. 22, 5871–5882. doi:10.1016/j.bmc.2014.09.022
Nair, A., Morsy, M. A., and Jacob, S. (2018). Dose translation between laboratory animals and human in preclinical and clinical phases of drug development. Drug Dev. Res. 79, 373–382. doi:10.1002/ddr.21461
Nandakumar, S., Menon, S., and Shailajan, S. (2013). A rapid HPLC-ESI-MS/MS method for determination of β-asarone, a potential anti-epileptic agent, in plasma after oral administration of Acorus calamus extract to rats. Biomed. Chromatogr. 27, 318–326. doi:10.1002/bmc.2794
Oecd, (2001). Test No. 423: acute oral toxicity–acute toxic class method. OECD guidelines for testing of chemicals, adopted 22nd march 1996, and revised method adopted 17th december 2001. Paris: OECD.
Oecd, (2008). Test No. 407: repeated dose 28-day oral toxicity in rodents, OECD guidelines for the testing of chemicals, section 4. Paris: OECD.
Ozan, G., Cumbul, A., Sumer, E., Aydin, A., and Ekinci, F. Y. (2023). Safety assessment of European cranberrybush (Viburnum opulus L.) fruit juice: acute and subacute oral toxicity. Food Chem. Toxicol. 181, 114082. doi:10.1016/j.fct.2023.114082
Parab, R. S., and Mengi, S. A. (2002). Hypolipidemic activity of Acorus calamus L. in rats. Fitoterapia 73, 451–455. doi:10.1016/s0367-326x(02)00174-0
Rodríguez-Páez, L., Juárez-Sanchez, M., Antúnez-Solís, J., Baeza, I., and Wong, C. (2003). Alpha-asarone inhibits HMG-CoA reductase, lowers serum LDL-cholesterol levels and reduces biliary CSI in hypercholesterolemic rats. Phytomedicine 10, 397–404. doi:10.1078/0944-7113-00274
Roytman, M. M., Poerzgen, P., and Navarro, V. (2018). Botanicals and hepatotoxicity. Clin. Pharmacol. Ther. 104, 458–469. doi:10.1002/cpt.1097
Ru, L., Liu, R., Xing, H., Yuan, Y., Yuan, Z., Xu, Z., et al. (2023). Acute and subacute oral toxicity assessment of Gancao Xiexin decoction in Sprague-Dawley rats. Front. Pharmacol. 13, 1078665. doi:10.3389/fphar.2022.1078665
Saki, G., Eidi, A., Mortazavi, P., Panahi, N., and Vahdati, A. (2020). Effect of β-asarone in normal and β-amyloid-induced Alzheimeric rats. Arch. Med. Sci. 16, 699–706. doi:10.5114/aoms.2020.94659
Sureshkumar, D., Begum, S., Johannah, N. M., Maliakel, B., and Krishnakumar, I. M. (2018). Toxicological evaluation of a saponin-rich standardized extract of fenugreek seeds (FenuSMART®): acute, sub-chronic and genotoxicity studies. Toxicol. Rep. 5, 1060–1068. doi:10.1016/j.toxrep.2018.10.008
Thakare, M. M., and Surana, S. J. (2016). β-Asarone modulate adipokines and attenuates high fat diet-induced metabolic abnormalities in Wistar rats. Pharmacol. Res. 103, 227–235. doi:10.1016/j.phrs.2015.12.003
Tian, J., Tian, Z., Qin, S. L., Zhao, P. Y., Jiang, X., and Tian, Z. (2017). Anxiolytic-like effects of α-asarone in a mouse model of chronic pain. Metab. Brain Dis. 32, 2119–2129. doi:10.1007/s11011-017-0108-z
Traesel, G. K., Menegati, S. E., Dos Santos, A. C., Carvalho Souza, R. I., Villas Boas, G. R., Justi, P. N., et al. (2016). Oral acute and subchronic toxicity studies of the oil extracted from pequi (Caryocar brasiliense, Camb.) pulp in rats. Food Chem. Toxicol. 97, 224–231. doi:10.1016/j.fct.2016.09.018
Wang, M., Tang, H. P., Wang, S., Hu, W. J., Li, J. Y., Yu, A. Q., et al. (2023). Acorus tatarinowii schott: a review of its botany, traditional uses, phytochemistry, and Pharmacology. Molecules 28, 4525. doi:10.3390/molecules28114525
Wang, N., Wang, H., Pan, Q., Kang, J., Liang, Z., and Zhang, R. (2021). The combination of β-asarone and icariin inhibits amyloid-β and reverses cognitive deficits by promoting mitophagy in models of alzheimer’ s disease. Oxid. Med. Cell Longev. 2021, 7158444. doi:10.1155/2021/7158444
Wu, H. S., Zhu, D. F., Zhou, C. X., Feng, C. R., Lou, Y. J., Yang, B., et al. (2009). Insulin sensitizing activity of ethyl acetate fraction of Acorus calamus L. in vitro and in vivo. J. Ethnopharmacol. 123, 288–292. doi:10.1016/j.jep.2009.03.004
Xie, Y., Yang, Y., Yuan, X., Liu, Y., and He, X. (2023). Herb pair of Polygala tenuifolia Willd and Acorus tatarinowii Schott decoction attenuates seizures and alleviates anxiety in mice: evidence for modulating inflammation, alleviating oxidative stress and mediating GABA pathway. Pak J. Pharm. Sci. 36, 565–577.
Xiong, W., Zhao, X., Xu, Q., Wei, G., Zhang, L., Fan, Y., et al. (2022). Qisheng Wan formula ameliorates cognitive impairment of Alzheimer’s disease rat via inflammation inhibition and intestinal microbiota regulation. J. Ethnopharmacol. 282, 114598. doi:10.1016/j.jep.2021.114598
Yang, M., Wu, Z., Wang, Y., Kai, G., Singor Njateng, G. S., Cai, S., et al. (2019). Acute and subacute toxicity evaluation of ethanol extract from aerial parts of Epigynum auritum in mice. Food Chem. Toxicol. 131, 110534. doi:10.1016/j.fct.2019.05.042
You, L., Yang, C., Du, Y., Wang, W., Sun, M., Liu, J., et al. (2020). A systematic review of the Pharmacology, Toxicology and pharmacokinetics of matrine. Front. Pharmacol. 11, 01067. doi:10.3389/fphar.2020.01067
Yun, J. W., Kwon, E., Kim, Y. S., Kim, S. H., You, J. R., Kim, H. C., et al. (2018). Assessment of acute, 14-day, and 13-week repeated oral dose toxicity of Tiglium seed extract in rats. BMC Complement. Altern. Med. 18, 251. doi:10.1186/s12906-018-2315-5
Zárybnický, T., Boušová, I., Ambrož, M., and Skálová, L. (2018). Hepatotoxicity of monoterpenes and sesquiterpenes. Arch. Toxicol. 92, 1–13. doi:10.1007/s00204-017-2062-2
Zhai, J., Dong, X., Yan, F., Guo, H., and Yang, J. (2022). Oleandrin: a systematic review of its natural sources, structural properties, detection methods, pharmacokinetics and Toxicology. Front. Pharmacol. 13, 822726. doi:10.3389/fphar.2022.822726
Zhang, F. H., Wang, Z. M., Liu, Y. T., Huang, J. S., Liang, S., Wu, H. H., et al. (2019). Bioactivities of serotonin transporter mediate antidepressant effects of Acorus tatarinowii Schott. J. Ethnopharmacol. 241, 111967. doi:10.1016/j.jep.2019.111967
Zhang, W., Song, D., Xu, D., Wang, T., Chen, L., and Duan, J. (2015). Characterization of polysaccharides with antioxidant and immunological activities from Rhizoma Acori Tatarinowii. Carbohydr. Polym. 133, 154–162. doi:10.1016/j.carbpol.2015.07.018
Zhang, Y., Long, Y., Yu, S., Li, D., Yang, M., Guan, Y., et al. (2021). Natural volatile oils derived from herbal medicines: a promising therapy way for treating depressive disorder. Pharmacol. Res. 164, 105376. doi:10.1016/j.phrs.2020.105376
Zhang, Y., Tian, J., Ni, J., Wei, M., Li, T., and Shi, J. (2024). Polygala tenuifolia and Acorus tatarinowii in the treatment of Alzheimer's disease: a systematic review and meta-analysis. Front. Pharmacol. 12 (14), 1268000. doi:10.3389/fphar.2023.1268000
Zhao, X., Liang, L., Xu, R., Cheng, P., Jia, P., Bai, Y., et al. (2022). Revealing the antiepileptic effect of α-asaronol on pentylenetetrazole-induced seizure rats using NMR-based metabolomics. ACS Omega 7, 6322–6334. doi:10.1021/acsomega.1c06922
Zhu, M., Zhu, H., Tan, N., Zeng, G., Zeng, Z., Chu, H., et al. (2014). The effects of Acorus tatarinowii Schott on 5-HT concentrations, TPH2 and 5-HT1B expression in the dorsal raphe of exercised rats. J. Ethnopharmacol. 158, 431–436. doi:10.1016/j.jep.2014.10.026
Keywords: Acori Tatarinowii Rhizoma, acute toxicity, subacute toxicity, hematology, serum biochemistry, histopathology, general behavior
Citation: Liu J, Ping X, Sun S-j, Yang J, Lu Y and Pei L (2024) Safety assessment of Acori Tatarinowii Rhizoma: acute and subacute oral toxicity. Front. Pharmacol. 15:1377876. doi: 10.3389/fphar.2024.1377876
Received: 28 January 2024; Accepted: 06 March 2024;
Published: 19 March 2024.
Edited by:
Jong-Choon Kim, Chonnam National University, Republic of KoreaCopyright © 2024 Liu, Ping, Sun, Yang, Lu and Pei. This is an open-access article distributed under the terms of the Creative Commons Attribution License (CC BY). The use, distribution or reproduction in other forums is permitted, provided the original author(s) and the copyright owner(s) are credited and that the original publication in this journal is cited, in accordance with accepted academic practice. No use, distribution or reproduction is permitted which does not comply with these terms.
*Correspondence: Ye Lu, 314749164@qq.com; Lin Pei, peilin13831190309@126.com
†These authors have contributed equally to this work and share first authorship