- 1Department of Medical Biochemistry and Molecular Biology, School of Medicine, Jinan University, Guangzhou, Guangdong, China
- 2School of Materials Science and Engineering, Sun Yat-sen University, Guangzhou, China
Upconversion nanoparticles (UCNPs) have experienced significant advancements, finding applications in diverse fields over the past decade. The growing demand for UCNP-based nanoplatforms with multifunctionality to address complex scenarios has led to the emergence of the microemulsion confined self-assembly method, which allows for the integration of different UCNPs or UCNPs with additional functional materials within a single entity, resulting in a nanoplatform that possesses a wide range of properties suitable for specific applications. This comprehensive review aimed to summarize recent developments in the design of UCNP assemblies using the microemulsion confined self-assembly method, which focused on exploring their applications in critical areas such as color encoding, bioimaging, and programmable therapeutics. Furthermore, the review acknowledged the existing limitations associated with the microemulsion confined self-assembly method and provided an in-depth discussion of potential solutions to overcome these challenges, aiming to foster further progress and innovation in the design and application of UCNP assemblies.
1 Introduction
The utilization of near-infrared (NIR) light-excitable upconversion nanoparticles (UCNPs) has garnered significant attention in recent times due to their distinctive capability as on-demand energy transducers to convert low-energy NIR light to high-energy ultraviolet (UV)/visible light (Chen et al., 2015a; Idris et al., 2015; Li et al., 2015; Zhou et al., 2015; Zhu et al., 2017b; Zheng et al., 2019; Chen and Wang, 2020a; Chen and Wang, 2020b; Cheng et al., 2020; Dong et al., 2020; Nonat and Charbonnière, 2020), thereby enabling a range of applications such as molecular detection (Zheng et al., 2015; Chen et al., 2016; Shikha et al., 2017; Gu and Zhang, 2018; Gao et al., 2019), drug delivery (Yan et al., 2012; Li et al., 2013; Liu et al., 2013; Yang et al., 2013; Bansal and Zhang, 2014; Zhao et al., 2014; Yang et al., 2015; Wang et al., 2016), optogenetics (Wu et al., 2016; Chen et al., 2018; Liang Zou, 2020), and photodynamic therapy (PDT) (Wang et al., 2011; Idris et al., 2012; Cui et al., 2013; Liang et al., 2016; Bansal et al., 2018; Qiu et al., 2018; Liu et al., 2019). However, the limited extinction coefficients, quantum efficiency, and fixed energy levels of UCNPs hindered their broader applications in specific multiplexed scenarios. To circumvent these limitations, researchers have focused on designing and synthesizing UCNPs with increasingly complex structures, involving multi-step and time-consuming procedures (Chen et al., 2015a; Wang et al., 2015a; Chen et al., 2015b; Wang et al., 2016; Ju et al., 2018; Leng et al., 2018; Fan et al., 2019). To resolve these problems, UCNP hybrid assemblies containing various UCNPs or UCNPs and other functional counterparts were developed to achieve desired properties tailored to specific applications, such as cell imaging (Li et al., 2013; Li and Lu, 2015; Xue et al., 2019), drug delivery (Cai et al., 2017; Zhang et al., 2019; Zhang et al., 2020a; Zhang et al., 2020b; Zhang et al., 2021a; Zhang and Zhang, 2021), molecular detection (Wang and Li, 2006; Liu et al., 2018), and programmed therapy (Zhang et al., 2019; Zhang et al., 2020a). The UCNP assembly not only preserves the inherent upconverting characteristics of UCNPs but also possesses additional variable functional properties, including environmental responsiveness, magnetic targeting, and catalytic activity, which assist in enhancing performance and overcoming the limitations of traditional UCNPs. Moreover, the composition and assembly approach of individual components could be finely tuned to obtain desired properties and adapt to specific environmental conditions as needed.
Several synthesis methods have been developed for UCNP assemblies, including interface-based approaches (Ye et al., 2010; Ren et al., 2012; Liu et al., 2016), microemulsion confined self-assembly (Bai et al., 2007; Wang et al., 2013a; Wang et al., 2013b), and polymer/DNA-meditated methods (Li et al., 2013; Chen et al., 2019; Zhang et al., 2020b). Among these methods, due to its superior versatility and efficiency, microemulsion confined self-assembly has demonstrated exceptional potential for fabricating highly useful UCNP-based nanoplatforms, particularly in emerging fields such as bioimaging, controllable molecular delivery, and programmable therapeutics. A comprehensive overview of recent developments and achievements in UCNP assemblies reported in the past years would facilitate the effective utilization of UCNP-based materials. Accordingly, Section 2 provides a concise introduction to the microemulsion confined self-assembly of UCNP-based multifunctional materials. Additionally, Section 3 discusses related applications, as well as the challenges and perspectives of UCNP assemblies are summarized in Section 4.
2 Advancements in microemulsion-based fabrication of UCNP-based multifunctional materials
Microemulsion is a process in which the continuous phase, comprising either an organic or aqueous medium, undergoes fragmentation, leading to the dispersion of oil or water droplets within an aqueous or oil solution containing surfactants (Bai et al., 2007; Lu and Yin, 2012; Wang et al., 2013a; Lee et al., 2015; Boles et al., 2016; Xiao et al., 2017; Liu et al., 2022; Zhang et al., 2022; Zhou et al., 2023). In a typical oil-in-water (O/W) microemulsion system, for instance, emulsification is achieved by subjecting the oil phase to mechanical stirring or sonication in the presence of surfactants. Subsequently, the low-temperature boiling oil phase is eliminated through evaporation, causing the materials within the oil droplets to aggregate and form larger assemblies exhibiting a 3D spherical morphology (Figure 1A).
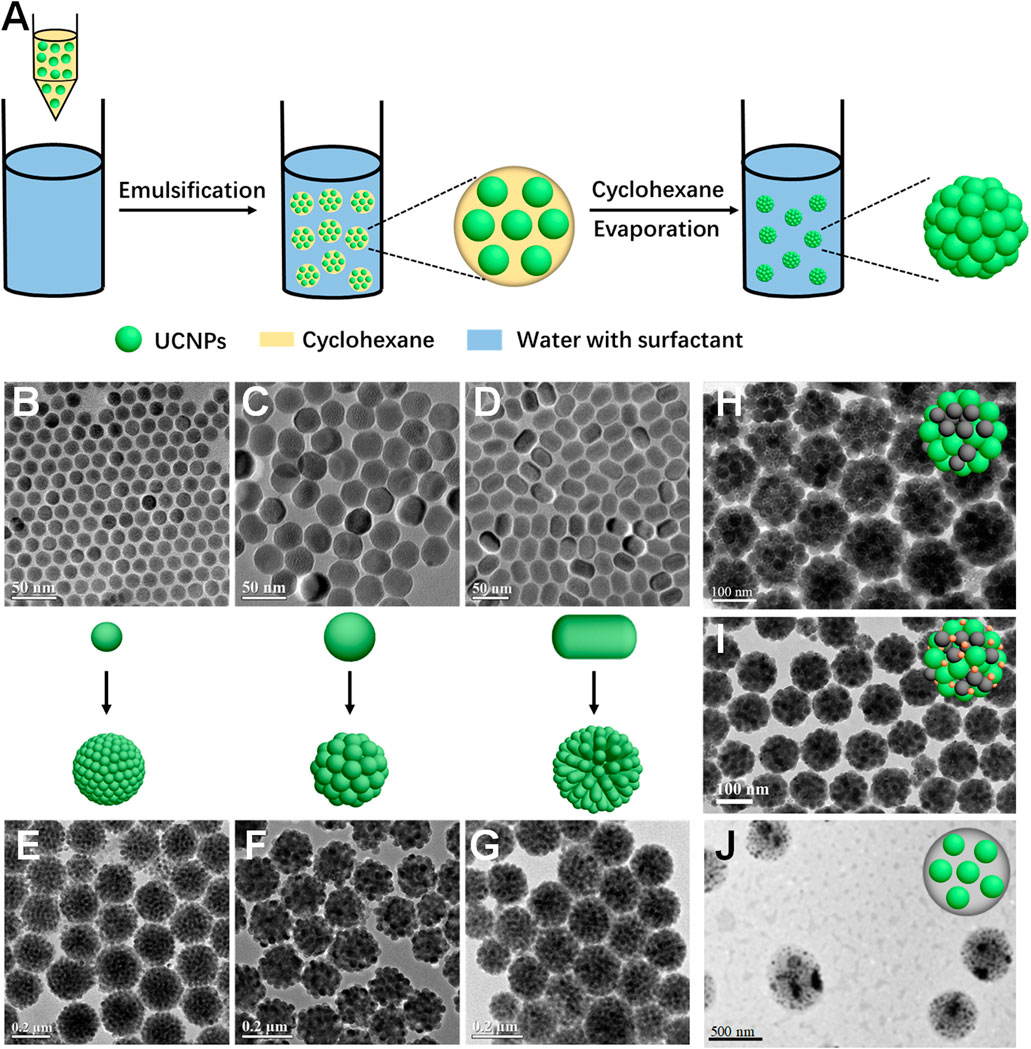
FIGURE 1. (A), Schematics showing the microemulsion approach for the fabrication of the UCNP cluster. TEM image of UCNPs with different sizes and shapes (B–D) and their corresponding cluster (E–G). (B, C), spherical, (D), rod-like. Reproduced from (Zhang et al., 2020a), copyright right 2020, American Chemical Society. TEM images of UCNPs-based hybrid nanomaterials with other functional materials. (H), Fe3O4, reproduced form (Liu et al., 2023), copyright right 2023, John Wiley and Sons. (I), Fe3O4/Au, reproduced form (Liang et al., 2023), copyright right 2023, Springer Nature. (J), PLGA. Reproduced from (Zhao et al., 2017), copyright 2017, Springer Nature.
2.1 Assemblies of UCNPs in various types
Taking advantage of the microemulsion confined self-assembly method, Zhang’s group assembled various UCNPs into UCNP clusters, demonstrating the broad applicability of this approach. Initially, a set of spherical UCNPs (diameter is about 17 and 34 nm) and rod-shaped UCNPs (length about 34 nm) were selected to form spherical UCNP clusters (Figures 1B–G) (Zhang et al., 2020a). The size, composition, and surface charge of these 3D colloidal spheres can be precisely controlled by manipulating experiment parameters. This approach paves the way for the preparation of a 3D UCNP assembly derived from a single UCNP.
2.2 Assemblies of UCNPs and other inorganic nanoparticles
After a thorough exploration of the principles behind the microemulsion confined self-assembly method, Zhang’s group recognized the potential for other functional inorganic materials, possessing similar surface properties as OA-capped UCNPs, to form their own assemblies or hybrid assemblies. Therefore, Fe3O4 nanoparticles were selected and combined with UCNPs to construct multifunctional superparticles (MFSPs), exhibiting both upconversion and magnetic targeting capabilities, which significantly highlighted the versatility of the microemulsion confined self-assembly method (Figure 1H) (Liu et al., 2023). The synthesized MFSPs, comprising two distinct types of functional nanoparticles, demonstrated remarkable performance in terms of green-colored upconversion luminescence upon excitation with a 980 nm laser. Additionally, under the influence of a magnetic field, the MFSPs exhibited controlled and directed movement. The simultaneous manifestation of both functionalities in all MFSPs provided strong evidence for the successful combination of UCNPs and Fe3O4 nanoparticles within a single MFSP. Expanding on this concept, Zhang and his collaborators aimed to enhance the versatility of the microemulsion approach by incorporating Au nanoparticles into the UCNPs/Fe3O4 MFSPs system (Figure 1I) (Liang et al., 2023), which further demonstrated that tailored nanoplatforms with enhanced functionalities can be achieved according to the specific requirements of practical applications.
2.3 Assemblies of UCNPs with organic materials
The microemulsion method offers a versatile approach not only for constructing UCNP-inorganic nanoparticle assemblies but also for combining UCNPs with other materials such as organic small molecules and polymers to create UCNP-based inorganic/organic hybrid nanosystems. Dai’s group reported a facile and straightforward synthesis of ZnPc/NaGdF4:Yb, Er clusters, utilizing an amphiphilic copolymer, PMAO-PEG (poly (maleic anhydride-alt-1-octadecen-poly (ethylene glycol)) as an additive in the organic phase to form a microemulsion (Wang et al., 2013b). After complete evaporation of the organic solvent, the UCNPs, ZnPc, and PMAO-PEG aggregated together, forming a 3D hybrid cluster. The hydrophilic PEG molecules extended to the outside of the cluster, ensuring its stability, dispersivity and biocompatibility for in vitro and in vivo applications. In 2017, Wang et al. introduced pH-dependent multifunctional nanocapsules based on pH-simultaneous PLGA and UCNPs (NaYF4:Yb,Er@NaGdF4) for drug delivery of an antitumor drug (DOX) (Figure 1I) (Zhao et al., 2017).
3 Advancements in applications of UCNP-based multifunctional materials
In recent years, UCNP assemblies with diverse functionalities have found application in various practical scenarios, such as dual-modality cell imaging, controllable drug delivery, and programmable therapeutics, etc. Summarizing the achievements in these applications over the past few years can provide valuable insights into the self-assembly of UCNPs and facilitate the exploration of more useful and desired applications of UCNP-based nanoplatforms through microemulsion-based fabrication methods.
3.1 Color encoding
Traditional methods for tuning the color emissions of UCNPs typically involve complex and time-consuming fabrication processes, which require multiple synthesis steps and precise control over the composition and thickness of each layer. However, by utilizing the microemulsion system, UCNPs with different emission characteristics can be easily incorporated into a single hybrid cluster, allowing for color encoding without the need for complex structural modifications. For instance, by mixing UCNPs with blue and green emission in different intensity ratios and exciting them with 980 nm excitation, diverse UCNP cluster solutions with varying blue-to-green fluorescence ratios at 450 nm and 550 nm were successfully created (Figure 2A) (Zhang et al., 2020a).
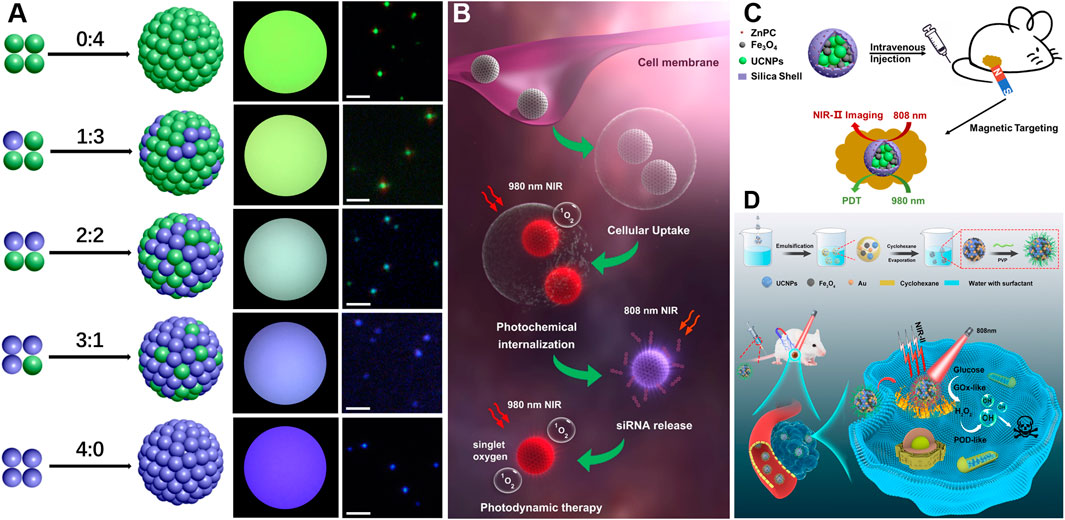
FIGURE 2. (A), Scheme shows color encoding by mixing different blue-to-green fluorescence intensity ratios. (B), OUCNPs utilized for programmable therapeutics including endosomal escape, siRNA release, and PDT. Reproduced with permission from (Zhang et al., 2019), copyright 2019, Springer Nature. (C), Schematic illustration of the multifunctional UCNPs/Fe3O4 superparticles for magnetic targeting PDT and real-time NIR-Ⅱ imaging. Reproduced from (Liu et al., 2023), copyright right 2020, John Wiley and Sons. (D), Schematic illustration of the microemulsion-based fabrication process of UCNPs/Au/Fe3O4 hybrid nanoplatforms for magnetically targeted and photothermal enhanced catalytic therapy under the guidance of NIR-II imaging for efficient tumor therapy. Reproduced from (Liang et al., 2023), copyright right 2023, Springer Nature.
Besides, recent studies have focused on the development of UCNPs with orthogonal emissive emissions (OUCNPs) to meet the complex requirements of various applications, due to their superior temporal separation and precise molecular photoactivations. However, the synthesis of OUCNPs requires multi-core-shell structures, where different lanthanide activator/emitter cations are doped in isolated shells, and an inert shell is desired to prevent energy transfer between the shells. The behavior of these multi-core-shell OUCNPs is often limited in quantum yield and luminescence stability. Zhang’s group addressed this challenge by modularly assembling two types of UCNPs, namely, spherical UCNPs A (NaYF4:60%Yb,20%Gd,2%Er@NaLuF4:25%Y) and dumbbell-like UCNPs B (NaYF4:30Yb,0.5%Tm@NaYF4:10%Yb@NaNdF4:10%Yb), which emits red light upon excitation at 980 nm and UV/blue light when exposed to 808 nm irradiation, respectively (Zhang et al., 2019; Zhang et al., 2020a). This approach focused on synthesizing individual UCNPs with a simple structure containing a single activator and then assembling them while maintaining their individual luminescence properties. Just by changing the laser power ratio of 980 nm–808 nm, different colors from blue to orange could be achieved easily.
3.2 Bioimaging
UCNPs have emerged as promising candidates for bioimaging applications, due to their remarkable optical properties, including large anti-Stokes shift, long lifetime emission, and narrow band emission (Wang et al., 2010; Zhou et al., 2012; Park et al., 2015; Wolfbeis, 2015; Wang et al., 2019). Moreover, the microemulsion method allows for the combination of UCNPs with other materials possessing different properties, resulting in hybrid systems with enhanced functionalities for bioimaging. One promising candidate among these functional counterparts is persistent luminescence (PL), which exhibits the ability to emit light for several minutes or even hours after the excitation source has been removed (Zhou et al., 2023). Li’s group utilized the microemulsion confined method to construct UCNP/PL hybrid cluster which involved the combination of UCNPs (NaYbF4:Tm@NaYF4) with PL nanoparticles (Zn1.1Ga1.8Ge0.1O4:0.5%Cr) in a hybrid nanocluster (Xue et al., 2019). In this hybrid system, the UV light generated by a 980 nm laser served as a secondary excitation source for the PL nanoparticles, resulting in afterglow emission at 700 nm. This NIR-to-NIR bioimaging hybrid cluster exhibited remarkable reactivation capabilities even covered by a 10 mm layer of pork, showcasing its high tissue penetration ability in the NIR range for bioimaging in vivo.
3.3 Programmable therapeutics
Traditional UCNPs with fixed emissions pose limitations in performing multiple tasks, while utilizing UCNPs with orthogonal emissive emissions (OUCNPs) makes it possible to independently control the photoactivation of different functions by simply adjusting the external excitation light. This capability allows for precise temporal control and targeting of multiple specific biological processes (Boyer et al., 2010; Wang et al., 2014; Wang et al., 2015b; Huang et al., 2018; Zheng et al., 2018; Zhang et al., 2021b; Zhang and Zhang, 2021). So far, the synthesis of OUCNPs is complicated and tedious because different lanthanides need to be incorporated into the different shells (Boyer et al., 2010; Lai et al., 2014; Wang et al., 2015b; Li et al., 2016; Dong et al., 2017; Mei et al., 2019; Lei et al., 2020). To address these bottleneck problems, Zhang et al. utilized the microemulsion method to assemble two distinct UCNPs with different emissions into OUCNP clusters (Zhang et al., 2020a). These UCNPs can be individually activated by irradiation with the 980 and 808 nm light, resulting in red and UV/blue emission, respectively, which were applied for cell imaging and drug delivery in a controllable manner. Furthermore, the same group achieved enhanced PDT using OUCNP clusters through programmed photoactivation of multiple therapeutic processes, including endosomal escape through photochemical internalization for enhancing cellular uptake, gene knockdown of superoxide dismutase-1 to increase sensitivity to reactive oxygen species, and PDT to ensure a higher therapeutic efficacy (Figure 2B) (Zhang et al., 2019).
In addition to the controlled photoactivation of OUCNP clusters through external excitation light, hybrid nanoplatforms combining UCNPs with other materials could be programmed to suit various practical applications. Zhang’s research group utilized the microemulsion based method to assemble UCNPs and Fe3O4 nanoparticles into multifunctional UCNP/Fe3O4 superparticles with highly integrated functionalities including magnetic targeting, PDT and real-time NIR-Ⅱ imaging, which allows for the guidance of PDT using 980 nm and 808 nm excitations (Figure 2C) (Liu et al., 2023). In order to further enhance the efficacy of tumor theragnostic, Zhang’s group incorporated Au nanoparticles into the superparticles, which act as catalysts for the conversion of glucose to gluconic acid and H2O2. Importantly, the generated H2O2 serves as a reactant source for the production of ⋅OH due to the nanoenzyme-like peroxidase activity of Fe3O4 nanoparticles. Furthermore, under 808 nm irradiation, the Au nanoparticles exhibit enhanced catalytic properties due to their photothermal conversion capability (Liang et al., 2023). This multifunctional nanoplatform demonstrates a synergistic effect of different nanoparticles for cancer treatment. By utilizing the microemulsion confined modular assembly technology, the construction of such multifunctional intelligent systems brings us closer to the realization of effective cancer diagnosis and therapy integration.
4 Summary and outlook
Over the past few years, the synthesis of UCNPs have made tremendous progress and high-quality UCNPs greatly expand their application potential in various fields, including imaging, detection, delivery, PDT, PTT, and programmable control of therapeutic processes. Although advancements have been made, UCNPs still suffer form certain challenges, such as low quantum efficiency and extinction coefficients, and fixed emissions, which limit their boarder practical applications. To address these limitations and draw inspiration from the strengths of other materials, researchers have focused on constructing UCNP nanoplatforms that incorporate different types of UCNPs or other functional counterparts. By leveraging the advantages of diverse materials, UCNP-based hybrid nanoplatforms can exhibit improved performance and overcome the inherent limitations of UCNPs alone. However, synthesizing UCNP-based hybrid nanoplatforms with different functionalities is a challenging and time-consuming process. A possible solution to this issue is the modular assembly of UCNPs with other materials through a microemulsion confined method. This approach allows for the synthesis of individual materials with simple structures, which can then be bonded together to create a complex and versatile nanoplatform that meets the requirements of various applications. By simplifying the synthesis process, this method offers a promising and efficient way to create hybrid nanoplatforms with diverse properties. Moreover, the exploration of alternative approaches for efficient and convenient synthesizing UCNP-based multifunctional materials is expected to become a key focus in future research and developments.
This review provides an overview of the recent advancements and applications of the microemulsion confined method in fabricating functional materials based on UCNPs. Despite the significant progress made in the past decade, several limitations need to be addressed before these materials can be effectively translated into practical applications. One significant factor to consider is the size of UCNP clusters, as it has a substantial impact on their interaction with biological systems (Hoshyar et al., 2016; Dolai et al., 2021), such as cellular uptake (Arnida et al., 2010; Dasgupta et al., 2014), tumor penetration (Perrault et al., 2009; Zhang et al., 2009; Huang et al., 2012), and tissue biodistribution (De Jong et al., 2008; Sonavane et al., 2008; Hirn et al., 2011; Duadi et al., 2013). Therefore, it is crucial to study the interactions between nanoparticles and cells and tissues to determine the optimal size for UCNP clusters. Previous lectures have indicated that nanoparticles should have a diameter larger than 10 nm to prevent kidney filtration (de Barros et al., 2012; Zuckerman et al., 2012). However, if the diameter exceeds 200 nm, the nanoparticles can activate the complement system, leading to their removal from the bloodstream and accumulation in the liver and spleen (Faraji and Wipf, 2009; Kulkarni and Feng, 2013). Furthermore, the colloidal properties of nanoparticles necessitate their size to be less than 200 nm, as larger nanoparticles tend to settle out due to the gravitational forces (Karagoz et al., 2014). Additionally, nanoparticles with a size of 100–200 nm are more efficiently internalized by cancer cells (Zhu et al., 2017a). Therefore, most of the UCNP clusters described in this review have been designed to be around 100–200 nm to fulfill these size-related requirements for applications in vitro and in vivo. Achieving precise control over the microemulsion confined self-assembly while obtaining desired properties in the final nanostructures remains a major challenge. Another challenge arises from the inherent dependence on high mechanical forces such as sonication agitation, or homogenization during the process of the microemulsion-based assembly method. Therefore, a wide range of cluster sizes is often obtained, making it difficult to guarantee excellent reproducibility of the products across different batches. This poses a significant hurdle in the practical utilization of microemulsion for large-scale production of monodisperse structures at the sub-micrometer scale. In summary, the microemulsion-based method for the fabrication of UCNP-based assemblies with different functional materials has experienced rapid development in recent years. However, there are still numerous areas and directions that require further explorations to address the challenges mentioned above and unlock the full potential of microemulsion-based fabrication techniques.
Author contributions
YZ: Conceptualization, Funding acquisition, Writing–original draft, Writing–review and editing. QM: Conceptualization, Writing–original draft, Writing–review and editing, Supervision. ZZ: Conceptualization, Supervision, Writing–original draft, Writing–review and editing, Funding acquisition, Software.
Funding
The author(s) declare financial support was received for the research, authorship, and/or publication of this article. We gratefully acknowledge the financial support from the Natural Science Foundation of China (No. 52103276), Guangdong Basic and Applied Basic Research Foundation (No. 2022A1515010947 and 2022A1515111012), Science and Technology Projects of Guangzhou (No. 201804010173), Science and Technology Plan of Haizhu District (No. 2022-51), Open Project Program of Jiangsu Key Laboratory of Environmentally Friendly Polymeric Materials (PML2204), Fundamental Research Funds for the Central Universities, Sun Yat-Sen University (No. 23qnpy03), and China Postdoctoral Science Foundation (2023M731314).
Conflict of interest
The authors declare that the research was conducted in the absence of any commercial or financial relationships that could be construed as a potential conflict of interest.
Publisher’s note
All claims expressed in this article are solely those of the authors and do not necessarily represent those of their affiliated organizations, or those of the publisher, the editors and the reviewers. Any product that may be evaluated in this article, or claim that may be made by its manufacturer, is not guaranteed or endorsed by the publisher.
References
Arnida, , Malugin, A., and Ghandehari, H. (2010). Cellular uptake and toxicity of gold nanoparticles in prostate cancer cells: a comparative study of rods and spheres. J. Appl. Toxicol. 30 (3), 212–217. doi:10.1002/jat.1486
Bai, F., Wang, D., Huo, Z., Chen, W., Liu, L., Liang, X., et al. (2007). A versatile bottom-up assembly approach to colloidal spheres from nanocrystals. Angew. Chem. Int. Ed. 46 (35), 6650–6653. doi:10.1002/anie.200701355
Bansal, A., Yang, F., Xi, T., Zhang, Y., and Ho, J. S. (2018). In vivo wireless photonic photodynamic therapy. Proc. Natl. Acad. Sci. 115 (7), 1469–1474. doi:10.1073/pnas.1717552115
Bansal, A., and Zhang, Y. (2014). Photocontrolled nanoparticle delivery systems for biomedical applications. Acc. Chem. Res. 47 (10), 3052–3060. doi:10.1021/ar500217w
Boles, M. A., Engel, M., and Talapin, D. V. (2016). Self-assembly of colloidal nanocrystals: from intricate structures to functional materials. Chem. Rev. 116 (18), 11220–11289. doi:10.1021/acs.chemrev.6b00196
Boyer, J. C., Carling, C. J., Gates, B. D., and Branda, N. R. (2010). Two-way photoswitching using one type of near-infrared light, upconverting nanoparticles, and changing only the light intensity. J. Am. Chem. Soc. 132 (44), 15766–15772. doi:10.1021/ja107184z
Cai, H., Shen, T., Kirillov, A. M., Zhang, Y., Shan, C., Li, X., et al. (2017). Self-assembled upconversion nanoparticle clusters for nir-controlled drug release and synergistic therapy after conjugation with gold nanoparticles. Inorg. Chem. 56 (9), 5295–5304. doi:10.1021/acs.inorgchem.7b00380
Chen, B., and Wang, F. (2020a). Combating concentration quenching in upconversion nanoparticles. Acc. Chem. Res. 53 (2), 358–367. doi:10.1021/acs.accounts.9b00453
Chen, B., and Wang, F. (2020b). Emerging Frontiers of upconversion nanoparticles. Trends Chem. 2 (5), 427–439. doi:10.1016/j.trechm.2020.01.008
Chen, C., Li, C., and Shi, Z. (2016). Current advances in lanthanide-doped upconversion nanostructures for detection and bioapplication. Adv. Sci. 3 (10), 1600029. doi:10.1002/advs.201600029
Chen, G., Ågren, H., Ohulchanskyy, T. Y., and Prasad, P. N. (2015a). Light upconverting core–shell nanostructures: nanophotonic control for emerging applications. Chem. Soc. Rev. 44 (6), 1680–1713. doi:10.1039/C4CS00170B
Chen, S., Weitemier, A. Z., Zeng, X., He, L., Wang, X., Tao, Y., et al. (2018). Near-infrared deep brain stimulation via upconversion nanoparticle–mediated optogenetics. Science 359 (6376), 679–684. doi:10.1126/science.aaq1144
Chen, X., Peng, D., Ju, Q., and Wang, F. (2015b). Photon upconversion in core–shell nanoparticles. Chem. Soc. Rev. 44 (6), 1318–1330. doi:10.1039/C4CS00151F
Chen, Y., Ren, J., Tian, D., Li, Y., Jiang, H., and Zhu, J. (2019). Polymer–upconverting nanoparticle hybrid micelles for enhanced synergistic chemo–photodynamic therapy: effects of emission–absorption spectral match. Biomacromolecules 20 (10), 4044–4052. doi:10.1021/acs.biomac.9b01211
Cheng, X., Tu, D., Zheng, W., and Chen, X. (2020). Energy transfer designing in lanthanide-doped upconversion nanoparticles. Chem. Commun. 56 (96), 15118–15132. doi:10.1039/D0CC05878E
Cui, S., Yin, D., Chen, Y., Di, Y., Chen, H., Ma, Y., et al. (2013). In vivo targeted deep-tissue photodynamic therapy based on near-infrared light triggered upconversion nanoconstruct. ACS Nano 7 (1), 676–688. doi:10.1021/nn304872n
Dasgupta, S., Auth, T., and Gompper, G. (2014). Shape and orientation matter for the cellular uptake of nonspherical particles. Nano Lett. 14 (2), 687–693. doi:10.1021/nl403949h
de Barros, A. B., Tsourkas, A., Saboury, B., Cardoso, V. N., and Alavi, A. (2012). Emerging role of radiolabeled nanoparticles as an effective diagnostic technique. EJNMMI Res. 2 (1), 39. doi:10.1186/2191-219X-2-39
De Jong, W. H., Hagens, W. I., Krystek, P., Burger, M. C., Sips, A. J. A. M., and Geertsma, R. E. (2008). Particle size-dependent organ distribution of gold nanoparticles after intravenous administration. Biomaterials 29 (12), 1912–1919. doi:10.1016/j.biomaterials.2007.12.037
Dolai, J., Mandal, K., and Jana, N. R. (2021). Nanoparticle size effects in biomedical applications. ACS Appl. Nano Mat. 4 (7), 6471–6496. doi:10.1021/acsanm.1c00987
Dong, H., Sun, L. D., Feng, W., Gu, Y., Li, F., and Yan, C. H. (2017). Versatile spectral and lifetime multiplexing nanoplatform with excitation orthogonalized upconversion luminescence. ACS Nano 11 (3), 3289–3297. doi:10.1021/acsnano.7b00559
Dong, H., Sun, L. D., and Yan, C. H. (2020). Upconversion emission studies of single particles. Nano Today 35, 100956. doi:10.1016/j.nantod.2020.100956
Duadi, H., Fixler, D., and Popovtzer, R. (2013). Dependence of light scattering profile in tissue on blood vessel diameter and distribution: a computer simulation study. J. Biomed. Opt. 18 (11), 111408. doi:10.1117/1.jbo.18.11.111408
Fan, Y., Liu, L., and Zhang, F. (2019). Exploiting lanthanide-doped upconversion nanoparticles with core/shell structures. Nano Today 25, 68–84. doi:10.1016/j.nantod.2019.02.009
Faraji, A. H., and Wipf, P. (2009). Nanoparticles in cellular drug delivery. Biorg. Med. Chem. 17 (8), 2950–2962. doi:10.1016/j.bmc.2009.02.043
Gao, M., Wu, R., Mei, Q., Zhang, C., Ling, X., Deng, S., et al. (2019). Upconversional nanoprobes with highly efficient energy transfer for ultrasensitive detection of alkaline phosphatase. ACS Sensors 4 (11), 2864–2868. doi:10.1021/acssensors.9b00858
Gu, B., and Zhang, Q. (2018). Recent advances on functionalized upconversion nanoparticles for detection of small molecules and ions in biosystems. Adv. Sci. 5 (3), 1700609. doi:10.1002/advs.201700609
Hirn, S., Semmler-Behnke, M., Schleh, C., Wenk, A., Lipka, J., Schäffler, M., et al. (2011). Particle size-dependent and surface charge-dependent biodistribution of gold nanoparticles after intravenous administration. Eur. J. Pharm. Biopharm. 77 (3), 407–416. doi:10.1016/j.ejpb.2010.12.029
Hoshyar, N., Gray, S., Han, H., and Bao, G. (2016). The effect of nanoparticle size on in vivo pharmacokinetics and cellular interaction. Nanomedicine 11 (6), 673–692. doi:10.2217/nnm.16.5
Huang, B., Wu, Q., Peng, X., Yao, L., Peng, D., and Zhan, Q. (2018). One-scan fluorescence emission difference nanoscopy developed with excitation orthogonalized upconversion nanoparticles. Nanoscale 10 (45), 21025–21030. doi:10.1039/c8nr07017b
Huang, K., Ma, H., Liu, J., Huo, S., Kumar, A., Wei, T., et al. (2012). Size-dependent localization and penetration of ultrasmall gold nanoparticles in cancer cells, multicellular spheroids, and tumors in vivo. ACS Nano 6 (5), 4483–4493. doi:10.1021/nn301282m
Idris, N. M., Gnanasammandhan, M. K., Zhang, J., Ho, P. C., Mahendran, R., and Zhang, Y. (2012). In vivo photodynamic therapy using upconversion nanoparticles as remote-controlled nanotransducers. Nat. Med. 18 (10), 1580–1585. doi:10.1038/nm.2933
Idris, N. M., Jayakumar, M. K. G., Bansal, A., and Zhang, Y. (2015). Upconversion nanoparticles as versatile light nanotransducers for photoactivation applications. Chem. Soc. Rev. 44 (6), 1449–1478. doi:10.1039/C4CS00158C
Ju, D., Song, F., Khan, A., Song, F., Zhou, A., Gao, X., et al. (2018). Simultaneous dual-mode emission and tunable multicolor in the time domain from lanthanide-doped core-shell microcrystals. Nanomaterials 8 (12), 1023. doi:10.3390/nano8121023
Karagoz, B., Esser, L., Duong, H. T., Basuki, J. S., Boyer, C., and Davis, T. P. (2014). Polymerization-induced self-assembly (pisa) – control over the morphology of nanoparticles for drug delivery applications. Polym. Chem. 5 (2), 350–355. doi:10.1039/C3PY01306E
Kulkarni, S. A., and Feng, S.-S. (2013). Effects of particle size and surface modification on cellular uptake and biodistribution of polymeric nanoparticles for drug delivery. Pharm. Res. 30 (10), 2512–2522. doi:10.1007/s11095-012-0958-3
Lai, J., Zhang, Y., Pasquale, N., and Lee, K. B. (2014). An upconversion nanoparticle with orthogonal emissions using dual nir excitations for controlled two-way photoswitching. Angew. Chem. Int. Ed. 53 (52), 14419–14423. doi:10.1002/anie.201408219
Lee, N., Yoo, D., Ling, D., Cho, M. H., Hyeon, T., and Cheon, J. (2015). Iron oxide based nanoparticles for multimodal imaging and magnetoresponsive therapy. Chem. Rev. 115 (19), 10637–10689. doi:10.1021/acs.chemrev.5b00112
Lei, Z., Ling, X., Mei, Q., Fu, S., Zhang, J., and Zhang, Y. (2020). An excitation navigating energy migration of lanthanide ions in upconversion nanoparticles. Adv. Mat. 32 (9), 1906225. doi:10.1002/adma.201906225
Leng, Z., Li, L., Zhang, D., and Li, G. (2018). Tunable green/red dual-mode luminescence via energy management in core-multishell nanoparticles. Mat. Des. 152, 119–128. doi:10.1016/j.matdes.2018.04.054
Li, L.-L., and Lu, Y. (2015). Regiospecific hetero-assembly of DNA-functionalized plasmonic upconversion superstructures. J. Am. Chem. Soc. 137 (16), 5272–5275. doi:10.1021/jacs.5b01092
Li, L.-L., Wu, P., Hwang, K., and Lu, Y. (2013). An exceptionally simple strategy for DNA-functionalized up-conversion nanoparticles as biocompatible agents for nanoassembly, DNA delivery, and imaging. J. Am. Chem. Soc. 135 (7), 2411–2414. doi:10.1021/ja310432u
Li, X., Guo, Z., Zhao, T., Lu, Y., Zhou, L., Zhao, D., et al. (2016). Filtration shell mediated power density independent orthogonal excitations–emissions upconversion luminescence. Angew. Chem. Int. Ed. 55 (7), 2464–2469. doi:10.1002/anie.201510609
Li, X., Zhang, F., and Zhao, D. (2015). Lab on upconversion nanoparticles: optical properties and applications engineering via designed nanostructure. Chem. Soc. Rev. 44 (6), 1346–1378. doi:10.1039/C4CS00163J
Liang, L., Care, A., Zhang, R., Lu, Y., Packer, N. H., Sunna, A., et al. (2016). Facile assembly of functional upconversion nanoparticles for targeted cancer imaging and photodynamic therapy. ACS Appl. Mat. Inter. 8 (19), 11945–11953. doi:10.1021/acsami.6b00713
Liang, Y., Liu, Y., Lei, P., Zhang, Z., and Zhang, H. (2023). Tumor microenvironment-responsive modular integrated nanocomposites for magnetically targeted and photothermal enhanced catalytic therapy. Nano Res. 16 (7), 9826–9834. doi:10.1007/s12274-023-5706-y
Liang Zou, H. T., and Tian, H. (2020). Upconversion nanoparticles-mediated optogenetics for minimally invasive neural interface. Acta phys.-chim. Sin. 36 (12), 2003042–2003050. doi:10.3866/PKU.WHXB202003042
Liu, J., Bu, W., Pan, L., and Shi, J. (2013). Nir-triggered anticancer drug delivery by upconverting nanoparticles with integrated azobenzene-modified mesoporous silica. Angew. Chem. Int. Ed. 52 (16), 4375–4379. doi:10.1002/anie.201300183
Liu, X., Liu, M., Chen, J., Li, Z., and Yuan, Q. (2018). Rational design and biomedical applications of DNA-functionalized upconversion nanoparticles. Chin. Chem. Lett. 29 (9), 1321–1332. doi:10.1016/j.cclet.2018.03.004
Liu, X., Ni, Y., Zhu, C., Fang, L., Kou, J., Lu, C., et al. (2016). Controllable self-assembly of Naref4upconversion nanoparticles and their distinctive fluorescence properties. Nanotechnology 27 (29), 295605. doi:10.1088/0957-4484/27/29/295605
Liu, Y., Ke, F., Li, Y., Shi, Y., Zhang, Z., and Chen, Y. (2022). Emulsion confined block copolymer self-assembly: recent progress and prospect. Nano Res. 16 (1), 564–582. doi:10.1007/s12274-022-4850-0
Liu, Y., Liang, Y., Lei, P., Zhang, Z., and Chen, Y. (2023). Multifunctional superparticles for magnetically targeted nir-ii imaging and photodynamic therapy. Adv. Sci. 10 (2), 2203669. doi:10.1002/advs.202203669
Liu, Y., Meng, X., and Bu, W. (2019). Upconversion-based photodynamic cancer therapy. Coord. Chem. Rev. 379, 82–98. doi:10.1016/j.ccr.2017.09.006
Lu, Z., and Yin, Y. (2012). Colloidal nanoparticle clusters: functional materials by design. Chem. Soc. Rev. 41 (21), 6874–6887. doi:10.1039/C2CS35197H
Mei, Q., Bansal, A., Jayakumar, M. K. G., Zhang, Z., Zhang, J., Huang, H., et al. (2019). Manipulating energy migration within single lanthanide activator for switchable upconversion emissions towards bidirectional photoactivation. Nat. Commun. 10, 4416. doi:10.1038/s41467-019-12374-4
Nonat, A. M., and Charbonnière, L. J. (2020). Upconversion of light with molecular and supramolecular lanthanide complexes. Coord. Chem. Rev. 409, 213192. doi:10.1016/j.ccr.2020.213192
Park, Y. I., Lee, K. T., Suh, Y. D., and Hyeon, T. (2015). Upconverting nanoparticles: a versatile platform for wide-field two-photon microscopy and multi-modal in vivo imaging. Chem. Soc. Rev. 44 (6), 1302–1317. doi:10.1039/C4CS00173G
Perrault, S. D., Walkey, C., Jennings, T., Fischer, H. C., and Chan, W. C. W. (2009). Mediating tumor targeting efficiency of nanoparticles through design. Nano Lett. 9 (5), 1909–1915. doi:10.1021/nl900031y
Qiu, H., Tan, M., Ohulchanskyy, Y. T., Lovell, F. J., and Chen, G. (2018). Recent progress in upconversion photodynamic therapy. Nanomaterials 8 (5), 344. doi:10.3390/nano8050344
Ren, Y., Chen, M., Hu, L., Fang, X., and Wu, L. (2012). Oil/water interfacial self-assembly for the organization of hydrophobic Nayf4:Yb, Er nanoplatelets into closely-packed fluorescent nanofilms. J. Mat. Chem. 22 (3), 944–950. doi:10.1039/C1JM13381K
Shikha, S., Zheng, X., and Zhang, Y. (2017). Upconversion nanoparticles-encoded hydrogel microbeads-based multiplexed protein detection. Nano-Micro Lett. 10 (2), 31. doi:10.1007/s40820-017-0184-y
Sonavane, G., Tomoda, K., and Makino, K. (2008). Biodistribution of colloidal gold nanoparticles after intravenous administration: effect of particle size. Colloids Surf. B 66 (2), 274–280. doi:10.1016/j.colsurfb.2008.07.004
Wang, C., Tao, H., Cheng, L., and Liu, Z. (2011). Near-infrared light induced in vivo photodynamic therapy of cancer based on upconversion nanoparticles. Biomaterials 32 (26), 6145–6154. doi:10.1016/j.biomaterials.2011.05.007
Wang, F., Banerjee, D., Liu, Y., Chen, X., and Liu, X. (2010). Upconversion nanoparticles in biological labeling, imaging, and therapy. Analyst 135 (8), 1839–1854. doi:10.1039/C0AN00144A
Wang, H., Han, R.-l., Yang, L.-m., Shi, J.-h., Liu, Z.-j., Hu, Y., et al. (2016). Design and synthesis of core–shell–shell upconversion nanoparticles for nir-induced drug release, photodynamic therapy, and cell imaging. ACS Appl. Mat. Inter. 8 (7), 4416–4423. doi:10.1021/acsami.5b11197
Wang, K., Qincheng, W., Zhang, Y., Qiao, R., Li, S., and Li, Z. (2015a). Synthesis of Nd3+/Yb3+ sensitized upconversion core–shell nanocrystals with optimized hosts and doping concentrations. RSC Adv. 5 (77), 62899–62904. doi:10.1039/C5RA09873D
Wang, L., Dong, H., Li, Y., Liu, R., Wang, Y.-F., Bisoyi, H. K., et al. (2015b). Luminescence-driven reversible handedness inversion of self-organized helical superstructures enabled by a novel near-infrared light nanotransducer. Adv. Mat. 27 (12), 2065–2069. doi:10.1002/adma.201405690
Wang, L., Dong, H., Li, Y., Xue, C., Sun, L.-D., Yan, C.-H., et al. (2014). Reversible near-infrared light directed reflection in a self-organized helical superstructure loaded with upconversion nanoparticles. J. Am. Chem. Soc. 136 (12), 4480–4483. doi:10.1021/ja500933h
Wang, L., and Li, Y. (2006). Green upconversion nanocrystals for DNA detection. Chem. Commun. 28 (24), 2557–2559. doi:10.1039/B604871D
Wang, T., LaMontagne, D., Lynch, J., Zhuang, J., and Cao, Y. C. (2013a). Colloidal superparticles from nanoparticle assembly. Chem. Soc. Rev. 42 (7), 2804–2823. doi:10.1039/C2CS35318K
Wang, X., Zhang, Q., Zhao, J., and Dai, J. (2013b). One-step self-assembly of znpc/nagdf4:Yb,Er nanoclusters for simultaneous fluorescence imaging and photodynamic effects on cancer cells. J. Mat. Chem. B 1 (36), 4637–4643. doi:10.1039/C3TB20533A
Wang, Y., Song, S., Zhang, S., and Zhang, H. (2019). Stimuli-responsive nanotheranostics based on lanthanide-doped upconversion nanoparticles for cancer imaging and therapy: current advances and future challenges. Nano Today 25, 38–67. doi:10.1016/j.nantod.2019.02.007
Wolfbeis, O. S. (2015). An overview of nanoparticles commonly used in fluorescent bioimaging. Chem. Soc. Rev. 44 (14), 4743–4768. doi:10.1039/C4CS00392F
Wu, X., Zhang, Y., Takle, K., Bilsel, O., Li, Z., Lee, H., et al. (2016). Dye-sensitized core/active shell upconversion nanoparticles for optogenetics and bioimaging applications. ACS Nano 10 (1), 1060–1066. doi:10.1021/acsnano.5b06383
Xiao, M., Hu, Z., Wang, Z., Li, Y., Tormo, A. D., Le Thomas, N., et al. (2017). Bioinspired bright noniridescent photonic melanin supraballs. Sci. Adv. 3 (9), e1701151. doi:10.1126/sciadv.1701151
Xue, W., Di, Z., Zhao, Y., Zhang, A., and Li, L. (2019). DNA-mediated coordinative assembly of upconversion hetero-nanostructures for targeted dual-modality imaging of cancer cells. Chin. Chem. Lett. 30 (4), 899–902. doi:10.1016/j.cclet.2019.03.022
Yan, B., Boyer, J.-C., Habault, D., Branda, N. R., and Zhao, Y. (2012). Near infrared light triggered release of biomacromolecules from hydrogels loaded with upconversion nanoparticles. J. Am. Chem. Soc. 134 (40), 16558–16561. doi:10.1021/ja308876j
Yang, D., Ma, P. a., Hou, Z., Cheng, Z., Li, C., and Lin, J. (2015). Current advances in lanthanide ion (Ln3+)-Based upconversion nanomaterials for drug delivery. Chem. Soc. Rev. 44 (6), 1416–1448. doi:10.1039/C4CS00155A
Yang, Y., Liu, F., Liu, X., and Xing, B. (2013). Nir light controlled photorelease of sirna and its targeted intracellular delivery based on upconversion nanoparticles. Nanoscale 5 (1), 231–238. doi:10.1039/C2NR32835F
Ye, X., Collins, J. E., Kang, Y., Chen, J., Chen, D. T. N., Yodh, A. G., et al. (2010). Morphologically controlled synthesis of colloidal upconversion nanophosphors and their shape-directed self-assembly. Proc. Natl. Acad. Sci. 107 (52), 22430–22435. doi:10.1073/pnas.1008958107
Zhang, G., Yang, Z., Lu, W., Zhang, R., Huang, Q., Tian, M., et al. (2009). Influence of anchoring ligands and particle size on the colloidal stability and in vivo biodistribution of polyethylene glycol-coated gold nanoparticles in tumor-xenografted mice. Biomaterials 30 (10), 1928–1936. doi:10.1016/j.biomaterials.2008.12.038
Zhang, Y., Zhu, X., and Zhang, Y. (2021a). Exploring heterostructured upconversion nanoparticles: from rational engineering to diverse applications. ACS Nano 15 (3), 3709–3735. doi:10.1021/acsnano.0c09231
Zhang, Z., Chen, Y., and Zhang, Y. (2022). Self-assembly of upconversion nanoparticles based materials and their emerging applications. Small 18 (9), 2103241. doi:10.1002/smll.202103241
Zhang, Z., Jayakumar, M. K. G., Shikha, S., Zhang, Y., Zheng, X., and Zhang, Y. (2020a). Modularly assembled upconversion nanoparticles for orthogonally controlled cell imaging and drug delivery. ACS Appl. Mat. Inter. 12 (11), 12549–12556. doi:10.1021/acsami.0c00672
Zhang, Z., Jayakumar, M. K. G., Zheng, X., Shikha, S., Zhang, Y., Bansal, A., et al. (2019). Upconversion superballs for programmable photoactivation of therapeutics. Nat. Commun. 10 (1), 4586. doi:10.1038/s41467-019-12506-w
Zhang, Z., Liu, Y., and Chen, Y. (2021b). Recent progress in utilizing upconversion nanoparticles with switchable emission for programmed therapy. Adv. Ther. 5 (1), 2100172. doi:10.1002/adtp.202100172
Zhang, Z., Rahmat, J. N., Mahendran, R., and Zhang, Y. (2020b). Controllable assembly of upconversion nanoparticles enhanced tumor cell penetration and killing efficiency. Adv. Sci. 7 (24), 2001831. doi:10.1002/advs.202001831
Zhang, Z., and Zhang, Y. (2021). Orthogonal emissive upconversion nanoparticles: material design and applications. Small 17 (11), 2004552. doi:10.1002/smll.202004552
Zhao, J., Yang, H., Li, J., Wang, Y., and Wang, X. (2017). Fabrication of ph-responsive plga(ucnps/dox) nanocapsules with upconversion luminescence for drug delivery. Sci. Rep. 7 (1), 18014. doi:10.1038/s41598-017-16948-4
Zhao, L., Peng, J., Huang, Q., Li, C., Chen, M., Sun, Y., et al. (2014). Near-infrared photoregulated drug release in living tumor tissue via yolk-shell upconversion nanocages. Adv. Funct. Mat. 24 (3), 363–371. doi:10.1002/adfm.201302133
Zheng, K., Han, S., Zeng, X., Wu, Y., Song, S., Zhang, H., et al. (2018). Rewritable optical memory through high-registry orthogonal upconversion. Adv. Mat. 30 (30), 1801726. doi:10.1002/adma.201801726
Zheng, K., Loh, K. Y., Wang, Y., Chen, Q., Fan, J., Jung, T., et al. (2019). Recent advances in upconversion nanocrystals: expanding the kaleidoscopic toolbox for emerging applications. Nano Today 29, 100797. doi:10.1016/j.nantod.2019.100797
Zheng, W., Huang, P., Tu, D., Ma, E., Zhu, H., and Chen, X. (2015). Lanthanide-doped upconversion nano-bioprobes: electronic structures, optical properties, and biodetection. Chem. Soc. Rev. 44 (6), 1379–1415. doi:10.1039/C4CS00178H
Zhou, H., Sheng, J., Wang, W., Liu, W., Cao, Y., Zhou, X., et al. (2023). Microemulsion-based fabrication of organic nanocrystals for persistent phosphorescence bioimaging. ACS Appl. Nano Mat. 6 (8), 6693–6699. doi:10.1021/acsanm.3c00429
Zhou, J., Liu, Q., Feng, W., Sun, Y., and Li, F. (2015). Upconversion luminescent materials: advances and applications. Chem. Rev. 115 (1), 395–465. doi:10.1021/cr400478f
Zhou, J., Liu, Z., and Li, F. (2012). Upconversion nanophosphors for small-animal imaging. Chem. Soc. Rev. 41 (3), 1323–1349. doi:10.1039/C1CS15187H
Zhu, K., Deng, Z., Liu, G., Hu, J., and Liu, S. (2017a). Photoregulated cross-linking of superparamagnetic iron oxide nanoparticle (spion) loaded hybrid nanovectors with synergistic drug release and magnetic resonance (mr) imaging enhancement. Macromolecules 50 (3), 1113–1125. doi:10.1021/acs.macromol.6b02162
Zhu, X., Su, Q., Feng, W., and Li, F. (2017b). Anti-Stokes shift luminescent materials for bio-applications. Chem. Soc. Rev. 46 (4), 1025–1039. doi:10.1039/C6CS00415F
Keywords: upconversion, microemulsion, self-assembly, bioimaging, molecular delivery, programmed therapeutics
Citation: Zhang Y, Mei Q and Zhang Z (2024) Advancements in microemulsion-based fabrication of upconversion-mediated multifunctional materials. Front. Photonics 5:1363223. doi: 10.3389/fphot.2024.1363223
Received: 30 December 2023; Accepted: 18 January 2024;
Published: 02 February 2024.
Edited by:
Renren Deng, Zhejiang University, ChinaReviewed by:
Kezhi Zheng, South China Normal University, ChinaCopyright © 2024 Zhang, Mei and Zhang. This is an open-access article distributed under the terms of the Creative Commons Attribution License (CC BY). The use, distribution or reproduction in other forums is permitted, provided the original author(s) and the copyright owner(s) are credited and that the original publication in this journal is cited, in accordance with accepted academic practice. No use, distribution or reproduction is permitted which does not comply with these terms.
*Correspondence: Qingsong Mei, qsmei@jnu.edu.cn; Zhen Zhang, zhangzh379@mail.sysu.edu.cn