- 1Research Center for Child Mental Development, Kanazawa University, Kanazawa, Japan
- 2United Graduate School of Child Development, Osaka University, Osaka, Japan
- 3Department of Neuropsychiatry, Kansai Medical University, Osaka, Japan
- 4Department of Occupational Therapy, Faculty of Rehabilitation Kansai Medical University, Osaka, Japan
- 5Department of Neuropsychiatry, Kansai Medical University Medical Center, Osaka, Japan
- 6Occupational Therapy Major, Graduate School of Rehabilitation Science, Osaka Metropolitan University, Habikino, Japan
- 7Department of Biofunctional Imaging, Preeminent Medical Photonics Education & Research Center, Hamamatsu University School of Medicine, Hamamatsu, Japan
- 8Department of Psychiatry and Neurobiology, Graduate School of Medical Science, Kanazawa University, Kanazawa, Japan
Introduction
The coronavirus pandemic had considerable effects on people's emotions, such as fear of infection, anxiety, and depressive mood (1, 2). From the perspective of preventing the spread of the virus, it would be ideal for everyone to avoid all occasions of interpersonal contact. To solve this problem, there may be a need to establish a telemedical system for the treatment of mental diseases (3).
At present, there is an increasing use of online diagnosis with digital transformation (DX) technologies, typically via telemedicine (4, 5). DX involves using information technology to transform existing mechanisms in order to provide new services (6). One advantage of medical care using DX is the ability to take action anywhere and anytime, which reduces labor costs and other expenses for both patients and healthcare providers.
Recent advancements in psychiatric treatment include non-invasive brain stimulation (NIBS) techniques, such as transcranial magnetic stimulation and transcranial electrical stimulation (tES) (7, 8). Several studies have examined the efficacy of NIBS techniques in treating psychiatric diseases (9). For example, transcranial direct current stimulation (tDCS) is a NIBS technique wherein simple equipment is used to pass a weak direct current between electrodes placed on the scalp. Due to the high level of safety with tDCS (10), there has been a sharp increase in high-quality research involving tDCS on cognition and emotion regulation (11, 12), and treatment with tDCS devices is considered suitable for use in telemedicine. It is ideal for home-based medical care because treatment is provided entirely by electronic devices, and it is easy to manage changes in stimulation parameters (13). Consequently, several ideas for telemedicine using tDCS have been proposed (14–23). Recently, a telemedicine depression trial was conducted with tDCS (24). Given the popularity of the research and the need for more telemedicine options highlighted by the COVID-19 pandemic, we sought to discuss the future of tDCS-telemedicine systems compatible with DX.
Overview of Neurophysiology
Coronavirus infections not only threaten human life but also leave serious sequelae after recovery. Several reports have shown the significant decrease of cognitive function in groups of former patients who had apparently recovered from infection (25, 26), signifying negative effects on the working memory (WM) system that supports higher cognitive function (27). These dysfunctions are governed by the prefrontal cortex (28, 29). When studying brain interventions, it is important to concurrently evaluate how tDCS stimulation changes brain activity.
For future applications of telemedicine at home, electroencephalography (EEG) is likely to be the leading neuroimaging technique that can be used. Thus, we provide an overview of studies that targeted the dorsolateral prefrontal cortex (DLPFC) and investigated the effects of tDCS via EEG and magnetoencephalography (MEG), which can offer practically equivalent indices to EEG.
Cognitive Function
Numerous studies have examined DLPFC stimulation for improving cognitive function, but the results are mixed. While some reports have indicated significant improvement effects, others have not shown any effects or indicated reduced cognitive performance (30–32). However, even when no behavioral changes were observed, it is possible that brain activity patterns had changed. Therefore, researchers need a process using neurophysiological evidence to verify whether these changes have triggered the expected behavior and, thereby, determine the efficacy of tDCS treatment.
Regarding brain activity, which is closely related to cognitive functions measured by EEG, oscillation analysis can extract the activity within a specific frequency bandwidth (33, 34). The effect of tDCS depends on the state of the participant (35). For example, research comparing patients with mild cognitive impairment and healthy participants suggests that the effects of tDCS for elevating cognitive functions cannot be measured in fully functioning participants (36). Conversely, tDCS performed with four patients who had experienced brain damage resulted in improved WM, raising our hopes for its application in the rehabilitation sector (37).
One meta-analysis targeted patients with a disease but did not evaluate their brain function. They reported that, although tDCS was shown to improve mood disorders in some patients and cognitive function in patients with schizophrenia, these results were not seen in patients with Alzheimer's dementia or MCI (38). Another meta-analysis showed that tDCS promoted cognitive function in patients with schizophrenia (39). Regarding the lack of effects of elevated cognitive function in a meta-analysis of tDCS targeting healthy participants (40), it is possible that the effects of tDCS differ between healthy participants and patients with mood disorders (41). Within the population of patients whose cognitive function has temporarily declined, a group exists in which tDCS might be effective.
Mood
Through our overview, we found that the analytical methods used when simultaneously evaluating the effects of tDCS on emotions and neurophysiology are rich in variety. When the cathode was placed above the left DLPFC and the anode was placed on the contralateral supraorbital area, the subjective magnitude of pain measured by the visual analog scale slightly decreased by active stimulation, despite no changes observed in visually inspected EEG (42). Maeoka et al. (43) examined whether pain-related unpleasant emotions induced by unpleasant photos were decreased by tDCS. A decrease in alpha power and an increase in beta power were observed after stimulation in the active-stimulation group.
In another study, researchers attempting to stimulate the ventromedial prefrontal cortex placed cathodes underneath the chin of participants and asked them to look at some happy faces and some fearful faces. According to their results, happy faces were associated with greater neural reactivity arousal (44). When the same regions were stimulated with tDCS, looking at pleasant photographs increased the strength of the magnetic field presumed to be generated by the medial prefrontal cortex (45).
Palm et al. (46) examined a patient with depression and evaluated the effects of tDCS with anodes placed above the left DLPFC, employing a quantitative EEG analysis method called low-resolution brain electromagnetic tomography (LORETA) (47). The results showed a decrease in current densities in the frontal region. LORETA has examined the predicted changes in anxiety owing to tDCS and described its possibilities (48). The use of EEGs also has diverse hidden possibilities. Additionally, the components of event-related potential N170, P3, and N400 are being investigated (49–51). Given all of this new evidence and the continuing progress in methods of analysis, we anticipate further growth and development in the field.
Neurophysiological Indices
Various neurophysiological indices are being used to track cognition and emotion, as evaluations based on neurophysiological indices using only stimulation sites are insufficient. To evaluate the state of the brain of each participant, it is necessary to consider what is happening inside each brain region. This is because, as a theory suggests, there are possible differences between healthy individuals and patients with depression in terms of the sites and balance of brain activation (52). Understanding the neurophysiological basis of tDCS on neural oscillations in the local activation and inter-regional neural networks and neurotransmitters will be the key to evaluating the state of the individual brain (Figure 1A).
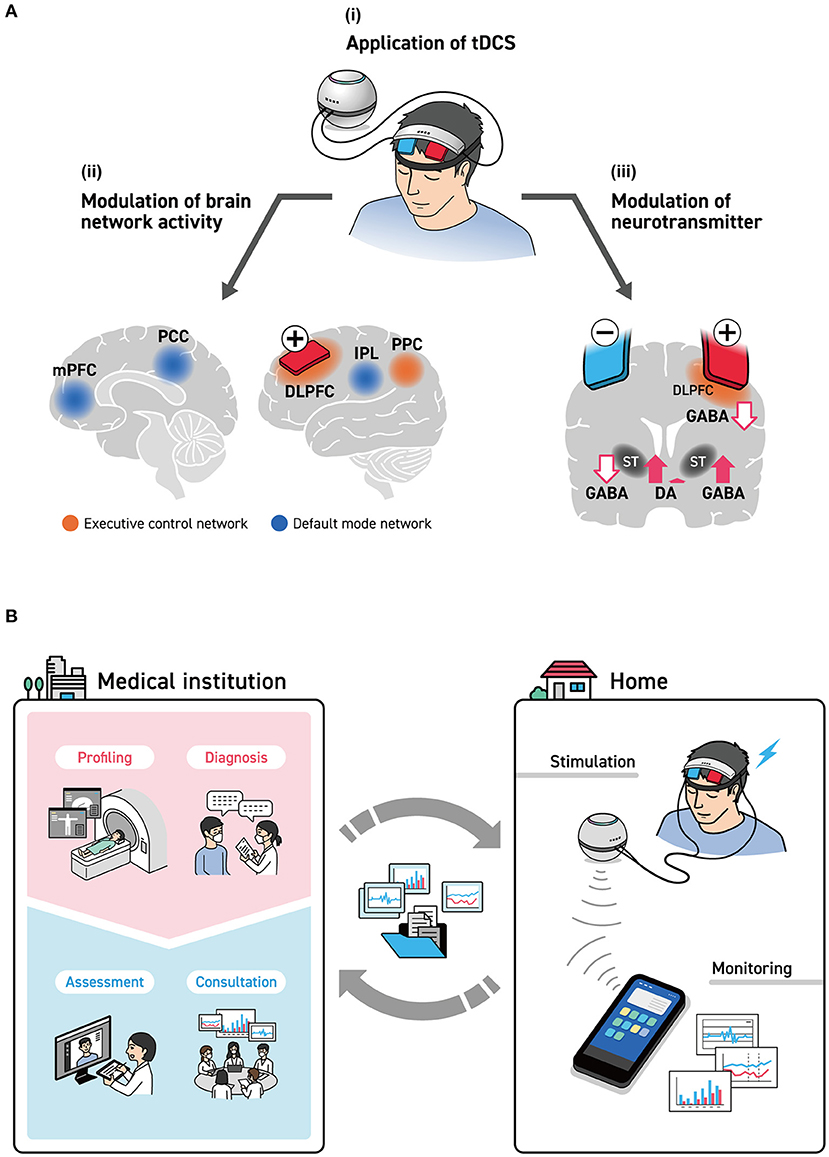
Figure 1. (A) brain stimulation by tDCS. (i) With the intent of stimulating the left DLPFC to improve cognitive function and mood, an anode was installed on F3 as defined under the International Standard ten-twenty electrode system. Many studies, however, have installed cathodes on either the F4 of the right hemisphere or the forehead. (ii) The spread of the effects of tDCS to the cerebral cortex, as estimated by the EEG/MEG studies are seen with the executive control network (DLPFC, SFC, and PPC) and the default mode network (mPFC, PCC, and IPL). (iii) The influence of tDCS stimulation of the left DLPFC on neurotransmitters. This brings about the release of dopamine in the right striatum and a reduction in GABA concentration, while it brings about an increase in GABA concentration in the left striatum. DLPFC, dorsolateral prefrontal cortex; IPL, inferior parietal lobule; mPFC, medial prefrontal cortex; PCC, posterior cingulate cortex; PPC, posterior parietal cortex; SFC, superior frontal cortex; ST, striatum. (B) tDCS-based telemedicine system, including medical institutions. In hospitals, meticulous examinations and brain measurements are done by physicians and specialists, who formulate stimulation parameters. Here, the structural data of the head were obtained by CT and MRI, and more detailed data on brain functions were obtained by fMRI, MEG, and PET to determine the optimal positions on the scalp for providing tDCS as well as parameters that are suited to individual patients. Brain condition before and after stimulation was measured by simple EEG, and the effects of tDCS were monitored as needed. These data are delivered to medical specialists via smart devices and are used to confirm the effects of treatment and formulate the next treatment plan.
The influence of NIBS on the brain function network has been previously reported (53). For example, stimulation applied to the DLPFC affects not only the executive control network (ECN), which is important for the WM, but also the default mode network (DMN), which is inversely correlated with ECN (54). An earlier study on the simultaneous measurement of fMRI and EEG found an inverse correlation with EEG activity between the frontoparietal network, which is a type of ECN, and the alpha band (55). Other studies focused on the relationship between DMN and EEG activity in the alpha and beta bands, as well as on the relationship between DMN and a combination of alpha and theta bands (56). The relationship between brain stimulation and RSNs is likely to attract growing research attention.
The effects of tDCS are often explained on the basis of electrophysiological mechanisms such as induced depolarization and hyperpolarization of the membrane potential (57). However, more studies have looked at the manifestation of its effects from a different perspective, such as those that support the remote effect hypothesis of tDCS, claiming that it affects the neurotransmitter system in the deep brain. In a study related to dopamine using PET, researchers performed tDCS by placing anodes on the left DLPFC and cathodes on the right DLPFC; they found that dopamine was released from the right ventral striatum and that attention and execution functions were reinforced (58). The effect of tDCS on the GABA system, which plays an important role in the modulation of the basal ganglia, was shown in a study using magnetic resonance spectroscopy (59). Here, GABA levels had decreased, unlike the release of dopamine from the right striatum seen with tDCS, and GABA concentration had conversely increased in the left striatum. tDCS appears to have an effect not only on the brain's surfaces but also on the neurotransmitter systems in the basal ganglia found in the deep brain.
Discussion
Using DX technology may make it possible to integrate the necessary information into smart devices to create efficient systems to provide mental healthcare that minimizes the burden on both the medical institution and the patient. A technique that is suited for evaluating whether stimulation has reached the patient's brain is EEG, which, in addition to being safe and convenient, has a long-known history related to numerous neuromarkers. Also, as wireless battery-operated EEG devices are already commercially available, it should not be too difficult to produce integrated devices.
tDCS-Based Telemedicine System Including Medical Institutions
Researchers have noted individual differences in the state of the brain, including functional and biological changes caused by the presence of disease (60), and differences in the histological structures of the brain, including the skull (61). It may be considered that tDCS is moving in the direction of individual optimization, as seen in recent trends in depression treatment (62). Herein, we clarified the roles of tDCS in telemedicine, especially for home-based care; simultaneously, we discussed the need to build an integrated system that will allow specialists at medical institutions to verify its effects (Figure 1B).
The key here is to create a system that enables profiling and diagnoses at medical institutions rather than requiring participants at home to complete the process on their own. The emergence of the effects of treatment appears to be influenced by the difference in the activity of existing functional networks and neurotransmitters. Therefore, measures to obtain detailed structural images of the head via MRI, ascertain the activity status via brain function imaging methods (63), and enhance the provision of effect predictions will probably be effective in the near future. The efficacy of tDCS will be enhanced by using these predictions as a starting point and then optimizing various parameters, such as the positions of the tDCS electrodes, electrical current levels, and stimulation time.
Information on the situation and effects should be communicated to the hospital via mobile devices. Although the electrical current used in tDCS is weak, cases of adverse reactions—such as burns and skin discomfort—have been reported (64). However, these problems can be resolved along with the further development of the equipment with monitoring. The information such as on/off-line effect and with/without stress is also important (65, 66).
Digital technologies must be used to send large data safety. Although this capacity may not be achievable until the equipment and Internet device technologies are more evolved, these goals could be reached in the near future, given the rate of innovation in the Internet of Things.
The relationship between the social system and industry is important to the future development of tDCS usage. It is necessary to prepare the social infrastructure, including the human resources necessary to run the medical system and develop training (24, 67). Quality control of devices is essential to ensure the safety of users in case of failure, and technology must be developed to enhance data transmission security (68).
After implementation, the day's stimulation status and the brain activity data are sent to medical institutions for assessment; then, based on the data, therapeutic policies are decided following consultation within a team. Current video conferencing systems allow physicians and patients to communicate whenever necessary, and physicians can check on a patient's current status, discuss consultations, and adjust stimulation parameters without the patient having to visit a medical institution. However, if treatment policies were determined by multiple specialists rather than the personal judgment of one individual, be it a patient or healthcare provider, treatment appropriateness and safety would be better guaranteed.
Conclusions
To guarantee safety and efficacy, researchers must make gains in technology, uniting tES and neurophysiology. Additionally, the establishment of a system that allows for medical institutions to provide comprehensive evaluations and consultations with expert follow-ups is vital for tES used in telemedicine. This concept cannot be realized by the healthcare industry alone, and cooperation with industries and social systems will be necessary. Now that awareness of the productivity and importance of telemedicine has grown because of the coronavirus pandemic, these perspectives toward the future have become realistic and comprise the visions for the future that we can pursue.
Author Contributions
TI and KN conceptualized and wrote the draft. TI, KN, and YO reviewed the work. All authors contributed to the article and approved the submitted version.
Funding
All authors received funding from the Osaka University Centre of Innovation (COI) program of the Japan Science and Technology Agency (JST, No. JPMJCE1310). KN received Grant-in-Aid for Young Scientists (B) (KAKEN 26860950) and MY received a Grant-in-Aid for Scientific Research (C) (KAKEN 19K08056). This work was partially supported by The Collaborative Research Network for Asian Children with Developmental Disorders (CRNACDD).
Conflict of Interest
The authors declare that the research was conducted in the absence of any commercial or financial relationships that could be construed as a potential conflict of interest.
Publisher's Note
All claims expressed in this article are solely those of the authors and do not necessarily represent those of their affiliated organizations, or those of the publisher, the editors and the reviewers. Any product that may be evaluated in this article, or claim that may be made by its manufacturer, is not guaranteed or endorsed by the publisher.
Acknowledgments
We thank Prof. Ogura for advice regarding this manuscript. We also would like to thank Editage (www.editage.com) for English language editing.
References
1. Ettman CK, Abdalla SM, Cohen GH, Sampson L, Vivier PM, Galea S. Prevalence of depression symptoms in US adults before and during the COVID-19 pandemic. JAMA Netw Open. (2020) 3:e2019686. doi: 10.1001/jamanetworkopen.2020.19686
2. Bueno-Notivol J, Gracia-Garcia P, Olaya B, Lasheras I, Lopez-Anton R, Santabarbara J. Prevalence of depression during the COVID-19 outbreak: a meta-analysis of community-based studies. Int J Clin Health Psychol. (2021) 21:100196. doi: 10.1016/j.ijchp.2020.07.007
3. O'Brien M, McNicholas F. The use of telepsychiatry during COVID-19 and beyond. Ir J Psychol Med. (2020) 37:250–5. doi: 10.1017/ipm.2020.54
4. Brem A, Viardot E, Nylund PA. Implications of the coronavirus (COVID-19) outbreak for innovation: which technologies will improve our lives? Technol Forecast Soc Change. (2021) 163:120451. doi: 10.1016/j.techfore.2020.120451
5. Kinoshita S, Cortright K, Crawford A, Mizuno Y, Yoshida K, Hilty D, et al. Changes in telepsychiatry regulations during the COVID-19 pandemic: 17 countries and regions' approaches to an evolving healthcare landscape. Psychol Med. (2020) 1−8. doi: 10.1017/S0033291720004584
6. Vial G. Understanding digital transformation: a review and a research agenda. J Strateg Inf Syst. (2019) 28:118–44. doi: 10.1016/j.jsis.2019.01.003
7. Zugliani MM, Fidry M, Steffen RE, Lan K, Brietzke E, Milev R, et al. Clinical effectiveness of non-tms neurostimulation in depression: clinical trials from 2010 to 2020. Prog Neuropsychopharmacol Biol Psychiatry. (2021) 110:110287. doi: 10.1016/j.pnpbp.2021.110287
8. Brunoni AR, Chaimani A, Moffa AH, Razza LB, Gattaz WF, Daskalakis ZJ, et al. Repetitive transcranial magnetic stimulation for the acute treatment of major depressive episodes: a systematic review with network meta-analysis. JAMA Psychiatry. (2017) 74:143–52. doi: 10.1001/jamapsychiatry.2016.3644
9. Fregni F, El-Hagrassy MM, Pacheco-Barrios K, Carvalho S, Leite J, Simis M, et al. Evidence-based guidelines and secondary meta-analysis for the use of transcranial direct current stimulation in neurological and psychiatric disorders. Int J Neuropsychopharmacol. (2021) 24:256–313. doi: 10.1093/ijnp/pyaa051
10. Antal A, Alekseichuk I, Bikson M, Brockmoller J, Brunoni AR, Chen R, et al. Low intensity transcranial electric stimulation: safety, ethical, legal regulatory and application guidelines. Clin Neurophysiol. (2017) 128:1774–809. doi: 10.1016/j.clinph.2017.06.001
11. Brunoni AR, Moffa AH, Sampaio-Junior B, Borrione L, Moreno ML, Fernandes RA, et al. Trial of electrical direct-current therapy versus escitalopram for depression. N Engl J Med. (2017) 376:2523–33. doi: 10.1056/NEJMoa1612999
12. Choi KM, Scott DT, Lim S-L. The modulating effects of brain stimulation on emotion regulation and decision-making. Neuropsychiatr Electrophysiol. (2016) 2:1–24. doi: 10.1186/s40810-016-0018-z
13. Castelo-Branco L, Fregni F. Home-based transcranial direct current stimulation (tDCS) to prevent and treat symptoms related to stress: a potential tool to remediate the behavioral consequences of the COVID-19 isolation measures? Front Integr Neurosci. (2020) 14:46. doi: 10.3389/fnint.2020.00046
14. Agarwal S, Pawlak N, Cucca A, Sharma K, Dobbs B, Shaw M, et al. Remotely-supervised transcranial direct current stimulation paired with cognitive training in parkinson's disease: an open-label study. J Clin Neurosci. (2018) 57:51–7. doi: 10.1016/j.jocn.2018.08.037
15. Charvet L, Shaw M, Dobbs B, Frontario A, Sherman K, Bikson M, et al. Remotely supervised transcranial direct current stimulation increases the benefit of at-home cognitive training in multiple sclerosis. Neuromodulation. (2018) 21:383–9. doi: 10.1111/ner.12583
16. Charvet LE, Dobbs B, Shaw MT, Bikson M, Datta A, Krupp LB. Remotely supervised transcranial direct current stimulation for the treatment of fatigue in multiple sclerosis: results from a randomized, sham-controlled trial. Mult Scler. (2018) 24:1760–9. doi: 10.1177/1352458517732842
17. Charvet LE, Kasschau M, Datta A, Knotkova H, Stevens MC, Alonzo A, et al. Remotely-supervised transcranial direct current stimulation (tdcs) for clinical trials: guidelines for technology and protocols. Front Syst Neurosci. (2015) 9:26. doi: 10.3389/fnsys.2015.00026
18. Dobbs B, Pawlak N, Biagioni M, Agarwal S, Shaw M, Pilloni G, et al. Generalizing remotely supervised transcranial direct current stimulation (tDCS): feasibility and benefit in parkinson's disease. J Neuroeng Rehabil. (2018) 15:114. doi: 10.1186/s12984-018-0457-9
19. Kasschau M, Reisner J, Sherman K, Bikson M, Datta A, Charvet LE. Transcranial direct current stimulation is feasible for remotely supervised home delivery in multiple sclerosis. Neuromodulation. (2016) 19:824–31. doi: 10.1111/ner.12430
20. Kasschau M, Sherman K, Haider L, Frontario A, Shaw M, Datta A, et al. A protocol for the use of remotely-supervised transcranial direct current stimulation (tDCS) in multiple sclerosis (MS). J Vis Exp. (2015) 106:e53542. doi: 10.3791/53542
21. Riggs A, Patel V, Paneri B, Portenoy RK, Bikson M, Knotkova H. At-home transcranial direct current stimulation (tDCS) with telehealth support for symptom control in chronically-ill patients with multiple symptoms. Front Behav Neurosci. (2018) 12:93. doi: 10.3389/fnbeh.2018.00093
22. Shaw MT, Kasschau M, Dobbs B, Pawlak N, Pau W, Sherman K, et al. Remotely supervised transcranial direct current stimulation: an update on safety and tolerability. J Vis Exp. (2017) 128:56211. doi: 10.3791/56211
23. Im JJ, Jeong H, Bikson M, Woods AJ, Unal G, Oh JK, et al. Effects of 6-month at-home transcranial direct current stimulation on cognition and cerebral glucose metabolism in Alzheimer's disease. Brain Stimul. (2019) 12:1222–8. doi: 10.1016/j.brs.2019.06.003
24. Cappon D, den Boer T, Jordan C, Yu W, Lo A, LaGanke N, et al. Safety and feasibility of tele-supervised home-based transcranial direct current stimulation for major depressive disorder. Front Aging Neurosci. (2021) 13:765370. doi: 10.3389/fnagi.2021.765370
25. Hampshire A, Trender W, Chamberlain SR, Jolly AE, Grant JE, Patrick F, et al. Cognitive deficits in people who have recovered from COVID-19. EClinicalMedicine. (2021) 39:101044. doi: 10.1016/j.eclinm.2021.101044
26. Zhou H, Lu S, Chen J, Wei N, Wang D, Lyu H, et al. The landscape of cognitive function in recovered COVID-19 patients. J Psychiatr Res. (2020) 129:98–102. doi: 10.1016/j.jpsychires.2020.06.022
27. Graham EL, Clark JR, Orban ZS, Lim PH, Szymanski AL, Taylor C, et al. Persistent neurologic symptoms and cognitive dysfunction in non-hospitalized COVID-19 “long haulers”. Ann Clin Transl Neurol. (2021) 8:1073–85. doi: 10.1002/acn3.51350
28. D'Argembeau A, Xue G, Lu ZL, Van der Linden M, Bechara A. Neural correlates of envisioning emotional events in the near and far future. Neuroimage. (2008) 40:398–407. doi: 10.1016/j.neuroimage.2007.11.025
29. Frith C, Dolan R. The role of the prefrontal cortex in higher cognitive functions. Brain Res Cogn Brain Res. (1996) 5:175–81. doi: 10.1016/S0926-6410(96)00054-7
30. Polizzotto NR, Ramakrishnan N, Cho RY. Is it possible to improve working memory with prefrontal tDCS? bridging currents to working memory models. Front Psychol. (2020) 11:939. doi: 10.3389/fpsyg.2020.00939
31. Galli G, Vadillo MA, Sirota M, Feurra M, Medvedeva A. A systematic review and meta-analysis of the effects of transcranial direct current stimulation (tDCS) on episodic memory. Brain Stimul. (2019) 12:231–41. doi: 10.1016/j.brs.2018.11.008
32. Mancuso LE, Ilieva IP, Hamilton RH, Farah MJ. Does transcranial direct current stimulation improve healthy working memory? : a meta-analytic review. J Cogn Neurosci. (2016) 28:1063–89. doi: 10.1162/jocn_a_00956
33. Ishii R, Shinosaki K, Ukai S, Inouye T, Ishihara T, Yoshimine T, et al. Medial prefrontal cortex generates frontal midline theta rhythm. Neuroreport. (1999) 10:675–9. doi: 10.1097/00001756-199903170-00003
34. Roux F, Uhlhaas PJ. Working memory and neural oscillations: alpha-gamma versus theta-gamma codes for distinct WM information? Trends Cogn Sci. (2014) 18:16–25. doi: 10.1016/j.tics.2013.10.010
35. Splittgerber M, Salvador R, Brauer H, Breitling-Ziegler C, Prehn-Kristensen A, Krauel K, et al. Individual baseline performance and electrode montage impact on the effects of anodal tDCS over the left dorsolateral prefrontal cortex. Front Hum Neurosci. (2020) 14:349. doi: 10.3389/fnhum.2020.00349
36. Emonson MRL, Fitzgerald PB, Rogasch NC, Hoy KE. Neurobiological effects of transcranial direct current stimulation in younger adults, older adults and mild cognitive impairment. Neuropsychologia. (2019) 125:51–61. doi: 10.1016/j.neuropsychologia.2019.01.003
37. O'Neil-Pirozzi TM, Doruk D, Thomson JM, Fregni F. Immediate memory and electrophysiologic effects of prefrontal cortex transcranial direct current stimulation on neurotypical individuals and individuals with chronic traumatic brain injury: a pilot study. Int J Neurosci. (2017) 127:592–600. doi: 10.1080/00207454.2016.1216415
38. Ciullo V, Spalletta G, Caltagirone C, Banaj N, Vecchio D, Piras F, et al. Transcranial direct current stimulation and cognition in neuropsychiatric disorders: systematic review of the evidence and future directions. Neuroscientist. (2021) 27:285–309. doi: 10.1177/1073858420936167
39. Narita Z, Stickley A, DeVylder J, Yokoi Y, Inagawa T, Yamada Y, et al. Effect of multi-session prefrontal transcranial direct current stimulation on cognition in schizophrenia: a systematic review and meta-analysis. Schizophr Res. (2020) 216:367–73. doi: 10.1016/j.schres.2019.11.011
40. Horvath JC, Forte JD, Carter O. Quantitative review finds no evidence of cognitive effects in healthy populations from single-session transcranial direct current stimulation (tDCS). Brain Stimul. (2015) 8:535–50. doi: 10.1016/j.brs.2015.01.400
41. Koshikawa Y, Nishida K, Yamane T, Yoshimura M, Onohara A, Ueda S, et al. Disentangling cognitive inflexibility in major depressive disorder: a transcranial direct current stimulation study. Psychiatry Clin Neurosci. (2022). doi: 10.1111/pcn.13364. [Epub ahead of print].
42. Tadini L, El-Nazer R, Brunoni AR, Williams J, Carvas M, Boggio P, et al. Cognitive, mood, and electroencephalographic effects of noninvasive cortical stimulation with weak electrical currents. J ECT. (2011) 27:134–40. doi: 10.1097/YCT.0b013e3181e631a8
43. Maeoka H, Matsuo A, Hiyamizu M, Morioka S, Ando H. Influence of transcranial direct current stimulation of the dorsolateral prefrontal cortex on pain related emotions: a study using electroencephalographic power spectrum analysis. Neurosci Lett. (2012) 512:12–6. doi: 10.1016/j.neulet.2012.01.037
44. Winker C, Rehbein MA, Sabatinelli D, Dohn M, Maitzen J, Wolters CH, et al. Noninvasive stimulation of the ventromedial prefrontal cortex modulates emotional face processing. Neuroimage. (2018) 175:388–401. doi: 10.1016/j.neuroimage.2018.03.067
45. Junghofer M, Winker C, Rehbein MA, Sabatinelli D. Noninvasive stimulation of the ventromedial prefrontal cortex enhances pleasant scene processing. Cereb Cortex. (2017) 27:3449–56. doi: 10.1093/cercor/bhx073
46. Palm U, Kumpf U, Behler N, Wulf L, Kirsch B, Worsching J, et al. Home use, remotely supervised, and remotely controlled transcranial direct current stimulation: a systematic review of the available evidence. Neuromodulation. (2018) 21:323–33. doi: 10.1111/ner.12686
47. Pascual-Marqui RD, Michel CM, Lehmann D. Low resolution electromagnetic tomography: a new method for localizing electrical activity in the brain. Int J Psychophysiol. (1994) 18:49–65. doi: 10.1016/0167-8760(84)90014-X
48. Nishida K, Koshikawa Y, Morishima Y, Yoshimura M, Katsura K, Ueda S, et al. Pre-stimulus brain activity is associated with state-anxiety changes during single-session transcranial direct current stimulation. Front Hum Neurosci. (2019) 13:266. doi: 10.3389/fnhum.2019.00266
49. Kuehne M, Schmidt K, Heinze HJ, Zaehle T. Modulation of emotional conflict processing by high-definition transcranial direct current stimulation (HD-tDCS). Front Behav Neurosci. (2019) 13:224. doi: 10.3389/fnbeh.2019.00224
50. Lafontaine MP, Theoret H, Gosselin F, Lippe S. Transcranial direct current stimulation of the dorsolateral prefrontal cortex modulates repetition suppression to unfamiliar faces: an erp study. PLoS ONE. (2013) 8:e81721. doi: 10.1371/journal.pone.0081721
51. Shahsavar Y, Ghoshuni M, Talaei A. Quantifying clinical improvements in patients with depression under the treatment of transcranial direct current stimulation using event related potentials. Australas Phys Eng Sci Med. (2018) 41:973–83. doi: 10.1007/s13246-018-0696-x
52. Roiser JP, Sahakian BJ. Hot and cold cognition in depression. CNS Spectr. (2013) 18:139–49. doi: 10.1017/S1092852913000072
53. Fox MD, Buckner RL, Liu H, Chakravarty MM, Lozano AM, Pascual-Leone A. Resting-state networks link invasive and noninvasive brain stimulation across diverse psychiatric and neurological diseases. Proc Natl Acad Sci U S A. (2014) 111:E4367–75. doi: 10.1073/pnas.1405003111
54. Fox MD, Snyder AZ, Vincent JL, Corbetta M, Van Essen DC, Raichle ME. The human brain is intrinsically organized into dynamic, anticorrelated functional networks. Proc Natl Acad Sci U S A. (2005) 102:9673–8. doi: 10.1073/pnas.0504136102
55. Laufs H, Kleinschmidt A, Beyerle A, Eger E, Salek-Haddadi A, Preibisch C, et al. Eeg-correlated fMRI of human alpha activity. Neuroimage. (2003) 19:1463–76. doi: 10.1016/S1053-8119(03)00286-6
56. Chang C, Liu Z, Chen MC, Liu X, Duyn JH. Eeg correlates of time-varying bold functional connectivity. Neuroimage. (2013) 72:227–36. doi: 10.1016/j.neuroimage.2013.01.049
57. Nitsche MA, Nitsche MS, Klein CC, Tergau F, Rothwell JC, Paulus W. Level of action of cathodal DC polarisation induced inhibition of the human motor cortex. Clin Neurophysiol. (2003) 114:600–4. doi: 10.1016/S1388-2457(02)00412-1
58. Fukai M, Bunai T, Hirosawa T, Kikuchi M, Ito S, Minabe Y, et al. Endogenous dopamine release under transcranial direct-current stimulation governs enhanced attention: a study with positron emission tomography. Transl Psychiatry. (2019) 9:115. doi: 10.1038/s41398-019-0443-4
59. Bunai T, Hirosawa T, Kikuchi M, Fukai M, Yokokura M, Ito S, et al. tDCS-induced modulation of GABA concentration and dopamine release in the human brain: a combination study of magnetic resonance spectroscopy and positron emission tomography. Brain Stimul. (2021) 14:154–60. doi: 10.1016/j.brs.2020.12.010
60. Hsu TY, Juan CH, Tseng P. Individual differences and state-dependent responses in transcranial direct current stimulation. Front Hum Neurosci. (2016) 10:643. doi: 10.3389/fnhum.2016.00643
61. Kim JH, Kim DW, Chang WH, Kim YH, Kim K, Im CH. Inconsistent outcomes of transcranial direct current stimulation may originate from anatomical differences among individuals: electric field simulation using individual MRI data. Neurosci Lett. (2014) 564:6–10. doi: 10.1016/j.neulet.2014.01.054
62. Williams LM. Precision psychiatry: a neural circuit taxonomy for depression and anxiety. Lancet Psychiatry. (2016) 3:472–80. doi: 10.1016/S2215-0366(15)00579-9
63. Boes AD, Prasad S, Liu H, Liu Q, Pascual-Leone A, Caviness VS, et al. Network localization of neurological symptoms from focal brain lesions. Brain. (2015) 138:3061–75. doi: 10.1093/brain/awv228
64. Matsumoto H, Ugawa Y. Adverse events of tDCS and tACS: a review. Clin Neurophysiol Pract. (2017) 2:19–25. doi: 10.1016/j.cnp.2016.12.003
65. Friehs MA, Frings C. Offline beats online: transcranial direct current stimulation timing influences on working memory. Neuroreport. (2019) 30:795–9. doi: 10.1097/WNR.0000000000001272
66. Friehs MA, Frings C. Evidence against combined effects of stress and brain stimulation on working memory. Open Psychol. (2020) 2:40–56. doi: 10.1515/psych-2020-0004
67. Charvet LE, Shaw MT, Bikson M, Woods AJ, Knotkova H. Supervised transcranial direct current stimulation (tDCS) at home: a guide for clinical research and practice. Brain Stimul. (2020) 13:686–93. doi: 10.1016/j.brs.2020.02.011
68. Rossi S, Antal A, Bestmann S, Bikson M, Brewer C, Brockmoller J, et al. Safety and recommendations for Tms Use in healthy subjects and patient populations, with updates on training, ethical and regulatory issues: expert guidelines. Clin Neurophysiol. (2021) 132:269–306. doi: 10.1016/j.clinph.2020.10.003
Keywords: digital transformation (DX), telemedicine, transcranial direct current stimulation (tDCS), home-use device, cognitive function, emotion regulation, mood disorders, electroencephalography (EEG)
Citation: Ikeda T, Nishida K, Yoshimura M, Ishii R, Tsukuda B, Bunai T, Ouchi Y and Kikuchi M (2022) Toward the Development of tES- Based Telemedicine System: Insights From the Digital Transformation and Neurophysiological Evidence. Front. Psychiatry 13:782144. doi: 10.3389/fpsyt.2022.782144
Received: 23 September 2021; Accepted: 31 May 2022;
Published: 11 July 2022.
Edited by:
Hiroyuki Toda, National Defense Medical College, JapanReviewed by:
Kyung Mook Choi, Suinjae Brain Science, South KoreaMaximilian Achim Friehs, Max Planck Institute for Human Cognitive and Brain Sciences, Germany
Copyright © 2022 Ikeda, Nishida, Yoshimura, Ishii, Tsukuda, Bunai, Ouchi and Kikuchi. This is an open-access article distributed under the terms of the Creative Commons Attribution License (CC BY). The use, distribution or reproduction in other forums is permitted, provided the original author(s) and the copyright owner(s) are credited and that the original publication in this journal is cited, in accordance with accepted academic practice. No use, distribution or reproduction is permitted which does not comply with these terms.
*Correspondence: Keiichiro Nishida, nishidak@takii.kmu.ac.jp