- 1Department of Child and Adolescent Psychiatry and Psychotherapy, Central Institute of Mental Health, Medical Faculty Mannheim, University of Heidelberg, Mannheim, Germany
- 2Department of Child and Adolescent Psychiatry and Psychotherapy, University Hospital of Psychiatry Zurich, University of Zurich, Zurich, Switzerland
- 3Neuroscience Center Zurich, University of Zurich and ETH Zurich, Zurich, Switzerland
- 4Donders Center for Brain, Cognition and Behavior, Radboud University Nijmegen, Nijmegen, Netherlands
- 5Department for Cognitive Neuroscience, Radboud University Medical Center Nijmegen, Nijmegen, Netherlands
Introduction: Growing evidence suggests that adverse experiences have long-term effects on executive functioning and underlying neural circuits. Previous work has identified functional abnormalities during inhibitory control in frontal brain regions in individuals exposed to adversities. However, these findings were mostly limited to specific adversity types such as maltreatment and prenatal substance abuse.
Methods: We used data from a longitudinal birth cohort study (n = 121, 70 females) to investigate the association between adversities and brain responses during inhibitory control. At the age of 33 years, all participants completed a stop-signal task during fMRI and an Adult Self-Report scale. We collected seven prenatal and postnatal adversity measures across development and performed a principal component analysis to capture common variations across those adversities, which resulted in a three-factor solution. Multiple regression analysis was performed to identify links between adversities and brain responses during inhibitory control using the identified adversity factors to show the common effect and single adversity measures to show the specific contribution of each adversity. To find neural correlates of current psychopathology during inhibitory control, we performed additional regression analyses using Adult Self-Report subscales.
Results: The first adversity factor reflecting prenatal maternal smoking and postnatal psychosocial adversities was related to higher activation during inhibitory control in bilateral inferior frontal gyri, insula, anterior cingulate cortex, and middle temporal gyri. Similar results were found for the specific contribution of the adversities linked to the first adversity factor. In contrast, we did not identify any significant association between brain responses during inhibitory control and the second adversity factor reflecting prenatal maternal stress and obstetric risk or the third adversity factor reflecting lower maternal sensitivity. Higher current depressive symptoms were associated with higher activation in the bilateral insula and anterior cingulate cortex during inhibitory control.
Conclusion: Our findings extended previous work and showed that early adverse experiences have a long-term effect on the neural circuitry of inhibitory control in adulthood. Furthermore, the overlap between neural correlates of adversity and depressive symptomatology suggests that adverse experiences might increase vulnerability via neural alterations, which needs to be investigated by future longitudinal research.
1 Introduction
Executive functions (EF) are essential cognitive skills for adaptation, social functioning, and goal-directed behavior (1). Deficits in EF have been documented in several psychiatric disorders, indicating that EF impairments may be a transdiagnostic correlate for psychopathology (2, 3). Previous findings showed that exposure to adverse childhood experiences is associated with both EF difficulties (4, 5) and poor mental health outcomes (6, 7), which lead to a developmental model suggesting that childhood adversities and other sources of stress may disrupt the neural systems supporting EF and thereby increase the risk of developing psychopathology (1). However, due to scarcity of longitudinal neuroimaging research, temporal dynamics of these relations have not been elucidated yet.
Inhibitory control is a core component of EF and a fundamental aspect of self-regulation, which requires suppressing a behavior or emotion when responding is no longer necessary or inappropriate (8). At the neural level, successful behavioral inhibition requires the involvement of several frontal regions, such as the inferior frontal gyrus, and the pre-supplementary motor area (9–13), whereas unsuccessful inhibition leads to enhanced activity in the dorsal anterior cingulate cortex (10, 13, 14), more likely reflecting error processing (15). Several studies found that individuals exposed to adverse childhood experiences showed altered frontal activation in these regions during inhibitory control tasks (16–22). However, the direction of the alterations changed based on the specific experimental paradigm used. Higher adversity was associated with higher activation in frontal regions in studies using the stop-signal task (16–19), whereas the reverse pattern was identified in the studies using the Go/No Go task (20–22). Although both Go/No Go and stop-signal tasks were commonly used in the context of response inhibition and require suppression of a dominant response, they involve distinct mechanisms, namely action restraint and action cancellation, respectively (23), which might explain conflicting directional associations found in previous literature.
Similar neural alterations, as found for adversities, were also identified in the context of psychopathology. Previous studies have reported abnormal prefrontal cortex activation during inhibitory control in several clinical conditions, including depression (24, 25), attention deficit and hyperactivity disorder (26), posttraumatic stress disorder (27), and eating disorder (28), indicating that altered frontal activation during inhibitory control can be a neural vulnerability correlate of psychopathology. However, the direction of the alteration was not consistent across the studies.
Although previous studies provided evidence for the relationship between childhood adversities and neural correlates of inhibitory control, there are several gaps in the literature that need to be addressed. First, the previous studies examined the effect of a single adversity measure on neural inhibitory network. However, it is plausible that different adversities could have common effects in addition to distinct associations with neural systems. Moreover, different adversities tend to occur together (29). Focusing on a single adversity measure may not only reflect the effect of specific adversity but also the effect of co-occurring adversities. Therefore, investigating shared effects as well as specific effects of diverse experiences will bring new insights. Second, most of the studies limited their findings to adolescent samples, except two previous studies conducted in adults using the Go/No Go paradigm (20, 22). Therefore, complimentary research is necessary to show if the long-term effect of adversities on neural inhibitory network is identifiable in other inhibitory control contexts. Third, most of the previous studies utilized liberal thresholds for reporting neuroimaging results (16, 17, 19, 21, 22), and some additionally investigated small sample sizes (16, 17, 19, 21).
The current study aimed to address these gaps by investigating the specific and cumulative effects of several lifespan adversities on neural responses during inhibitory control using the stop-signal task in a cohort of adults followed since birth. We collected several risk measures across development, which included prenatal factors such as maternal stress and maternal smoking, perinatal factors such as obstetric adversity, and postnatal factors such as low maternal care, family adversity, stressful life events, and self-reported childhood trauma. We hypothesized that adversities across development would be associated with increased functional activation in several frontal regions, including the inferior frontal gyrus (IFG), pre-supplementary motor area (pre-SMA), and dorsal anterior cingulate cortex (dACC) during inhibitory control. Furthermore, altered frontal activation would be associated with lower inhibition success and higher psychopathology symptoms in adulthood.
2 Methods
2.1 Participants
The current study was conducted within the framework of Mannheim Study of Children at Risk. The initial sample included 384 infants recruited from two obstetric and six children’s hospitals in the Rhine-Neckar region of Germany between 1986 and 1988. Participants were followed from their birth up to around the age of 33 years (age range: 31.7–34.5 years) across 11 assessment waves. At the last assessment wave (T11), 256 participants (67%) agreed to participate in the study and completed several psychological measurements. fMRI data for the stop-signal task was available for 170 participants. We used an extensive quality check procedure covering fMRI data quality (e.g., head motion, signal loss etc.), and task performance metrics based on a consensus guide for the stop-signal task (30) (see Supplementary material S1 for a detailed description of exclusion criteria). Four participants were excluded due to low fMRI data quality. An additional 45 participants were excluded due to poor task performance during go trials (correct go <80%), having inhibition success lower than 25% or greater than 75%, and having greater mean reaction time for unsuccessful stop trials than go trials. The final sample included 121 participants (Table 1). Of 121 participants, 16 participants fulfilled the criteria for a current psychopathology including major depressive disorder (n = 5), anxiety disorder (n = 7), alcohol and substance abuse (n = 3), and schizophrenia (n = 1).
2.2 Psychological measurements
2.2.1 Adversity measurements
The Mannheim Study of Children Risk included several adversity measures across the development, which were previously associated with abnormal brain development and functioning (16, 20, 31–33). For the prenatal period, we included maternal stress (34) and maternal smoking (20), which were measured using a standardized interview during the 3-month assessment at T1. Obstetric adversity (35) included obstetric complications as a measure of perinatal risk. Postnatal measures included several psychosocial measures such as maternal stimulation (36) during infancy (3-month assessment), family adversity (37) from birth to up to 11 years (T5), stressful life events (38) from birth to up to around 33 years (T11), and self-reported childhood trauma at T9 (23 years) using the Childhood Trauma Questionnaire (CTQ) (39). Detailed descriptions for each adversity measure can be found in Table 2. Similar to our previous study (41), we applied a principal component analysis in IBM SPSS (version 27) using the above-mentioned adversity measures to reduce the dimensionality and account for correlative nature of the adversity measures (Supplementary Table S1). We identified three components with an eigenvalue >1, which in total explained 66.8% of the variance in the data (see details in Results).
2.2.2 Psychopathology
We used the Adult Self-Report (42) to assess current symptoms of psychopathology. The Adult Self-Report includes 126 items rated on a 3-point Likert scale (0 = “not true”, 1 = “somewhat or sometimes true”, 2 = “very true or often true”) assessing mental health problems, adaptive functioning, and substance use. As measures of psychopathology, we used the total scores of six DSM-oriented subscales, including depression, anxiety, avoidant personality, somatic problems, attention deficit and hyperactivity disorder (ADHD) and antisocial personality scales.
2.3 Experimental paradigm
We used the stop-signal task (10) to assess inhibitory control during fMRI (Supplementary Figure S1). The task contained 160 trials (6.37 min), which consisted of two types of trials (go trials and stop trials). Each trial began with a fixation cross, which was followed by an arrow pointing to the left or right (go-signal). In the majority of trials (75%), participants were required to respond as quickly and accurately as possible by pressing the left or right button according to the previously shown arrow. Infrequently (25%), an arrow pointing upward (stop-signal) followed the go signal. During the stop trials, participants were asked to inhibit their response, which resulted in either successful or unsuccessful inhibition. The delay between go-signal and stop-signal started at 250 ms and increased by 50 ms if participants successfully inhibited their response (max 900 ms) or decreased by 50 ms if they failed (min 50 ms). This procedure enabled an approximately equal number of successful and unsuccessful stop trials. The inhibition success (successful stop trials / all stop trials) was on average 58.1% (SD = 8.9) in the current sample.
2.4 Data acquisition and preprocessing
The functional and structural images were acquired on a Siemens Magnetom Prisma Fit (Siemens, Erlangen, Germany) 3 T MRI scanner with a standard 32-channel head coil. During the stop-signal task, 186 volumes were obtained using a gradient echo-planar sequence sensitive to blood oxygen level-dependent (BOLD) contrast (36 slices, TE = 35 ms, TR = 2,100 ms, voxel size = 3 × 3 × 3 mm). More information on the scanning parameters can be found in the Supplementary material S4 (Supplementary Tables S2, S3).
Functional data was preprocessed using SPM 12.1 The first six volumes were discarded to allow for equilibration of the magnetic field. The preprocessing steps included slice timing correction of volumes to the middle slice, realignment to the first volume using a rigid body linear transformation, structural and functional image co-registration, segmentation, normalization to the Montreal Neurological Institute template, and smoothing using a kernel with a full-width half-maximum of 8 mm.
2.5 Generalized linear modeling
2.5.1 First-level generalized linear modeling
Experimental conditions (correct go trials, successful stop trials, and unsuccessful stop trials) were convolved with a canonical hemodynamic response function using SPM 12. To quantify head motion, we calculated framewise displacement based on six motion parameters (43). If a participant had scans with framewise displacement greater than 0.5, we then censored those scans by creating a dummy-coded regressor (44). Six motion parameters, the regressor representing the censored scans, and time series from white matter and cerebrospinal fluid were entered into the first-level analysis as nuisance covariates to correct for motion and physiological noise. Having performed the first-level analysis, we created two widely used t-contrasts (10, 18, 45) in the literature: Successful stop trials > correct go trials and successful stop trials > unsuccessful stop trials.
2.5.2 Second-level generalized linear modeling
All second-level analyses were conducted using SPM 12. We first performed a one-sample t-test to identify brain regions showing the main task effect. Results were thresholded at p < 0.05 (whole-brain family-wise error (FWE) corrected, cluster size >10).
We then conducted a series of multiple regression analyses to examine the association between three adversity factors and brain responses during inhibitory control on a whole-brain level. Sex and current psychopathology were included as covariates of no interest in all analyses. The same regression analysis was performed for each adversity measure separately.
In addition, we conducted regression analyses to explore brain-behavior relationship using task performance and psychopathology measures. We calculated several task performance metrics including the percentage of successful stop trials (i.e., number of correct stop trials / all stop trials) as a measure of inhibition success and stop-signal reaction time (SSRT) (46) as a measure of inhibition speed (47). Previous literature suggests that lower SSRT is related to higher inhibitory control (47). Unexpectedly, lower SSRT here was associated with higher commission errors during go trials (rs = −0.27, p < 0.01), indicating that the higher the inhibition speed, the higher the commission error. This could be the case because healthy adults can develop a strategy (e.g., waiting longer) to increase inhibition success. Given the high correlation between the two metrics (r = 0.62, p < 0.001) and conflicting results regarding the SSRT, we opted to use inhibition success as a measure of task performance since inhibition success might be a more meaningful measure than inhibition speed in real-life settings. The results for the SSRT are presented in the Supplementary material S10.
To identify if there is an overlap between neural correlates of adversity and specific psychopathology, we first performed regression analyses using the above-mentioned six DSM-oriented Adult Self-Report subscales and then identified the regions showing both adversity and psychopathology effects by intersecting SPM whole-brain association maps.
All results were thresholded at a whole-brain level using p < 0.001 as a cluster-forming threshold, and the clusters with p < 0.05 corrected for FWE are reported in the results.
2.6 Statistical analyses
All statistical analyses were performed in IBM SPSS version 27. The analyses encompassed demographics for sample characteristics and correlation analyses to examine association the association between adversities and psychopathology. P was set to 0.05 (two-tailed). Due to non-normally distributed data for psychopathology measures (n = 6), we conducted a Spearman’s correlation test and applied Bonferroni correction to correct for multiple testing problem (p < 0.05 /6 = 0.008).
2.7 Post-hoc analyses
We identified adversity related alterations only during successful versus unsuccessful stop trials. However, this differential contrast did not reveal whether the activation difference was arisen due to more activation or less deactivation in one condition compared to other. Therefore, we further performed one sample t-tests using contrast images for successful stop trials (versus baseline) and unsuccessful stop trials (versus baseline). We then intersected the task and adversity effect maps obtained from second-level SPM analysis to identify regions showing shared effect.
Moreover, having identified the relationship between the first adversity factor and psychopathology measures, we conducted mediation analysis to see if neural responses mediates this relationship. For this purpose, we extracted mean activation from the clusters significantly related to adversity using the MarsBar toolbox.2 Mediation analysis was performed using the PROCESS toolbox (48) implemented in IBM SPSS version 27. In total, we tested 30 mediation models (five clusters x six psychopathology scales) using the model 4 from the PROCESS toolbox. To approximate the rigor of multiple comparisons correction, we set our confidence intervals to 99% and increased the number of bootstrap samples to 10,000. Each mediation model included mean activation from a cluster associated with adversity as a mediator (M) to explain the impact of the first adversity factor (X) on psychopathology symptoms (Y). In the current study, we used the sum scores of two psychosocial adversity measures that were assessed at multiple time points across development, namely family adversity (presence of 11 adverse family factors up to 11 years) and stressful life events (sum scores of the life events at each assessment wave up to 33 years). However, this approach does not allow to disentangle timing effects of adversities on neural systems. Given that the literature suggests that adverse experiences may exert more detrimental effects during specific developmental windows than the others (49), we performed regression analyses using the time-specific measures for family adversity (n = 5) and stressful life events (n = 11) (p < 0.001 at whole-brain, p < 0.05 FWE corrected at cluster level) in a further exploratory sensitivity analysis. All analyses were controlled for sex and current psychopathology. Due to the high number of tests and correlative nature, the findings should be considered preliminary.
3 Results
3.1 Behavioral results
The principal component analysis identified three adversity factors (Supplementary Table S4). The first adversity factor was strongly informed by stressful life events, family adversity, maternal smoking, and childhood trauma questionnaire. The second adversity factor was strongly related to obstetric adversity and maternal stress. The third adversity factor mostly reflected maternal stimulation. Similar to our previous work with a larger sample (50), we found that the first adversity factor was associated with higher scores in all psychopathology measures except for somatic problems (all p < 0.05, Bonferroni-corrected, Supplementary material S6). We did not identify any association between psychopathology measures and the second and third adversity factors, although the latter association was significant in the larger sample (50).
3.2 Task effect
We performed a one-sample t-test to identify neural correlates of inhibitory control. The results are shown in Figure 1. During successful stop versus go trials, we found increased activation in several brain regions, including bilateral angular gyrus, middle temporal gyrus, cerebellum, precuneus, occipital regions, motor regions (precentral gyrus, postcentral gyrus, supplementary motor area), posterior insula, IFG, anterior cingulate cortex, orbitofrontal cortex, middle frontal gyrus, hippocampus, right amygdala, and left parahippocampal gyrus (p < 0.05, whole-brain FWE corrected; Supplementary Table S5). We additionally identified decreased activation in the bilateral anterior insula extending to the posterior IFG, bilateral putamen, and left midbrain (p < 0.05, whole-brain FWE corrected; Supplementary Table S5).
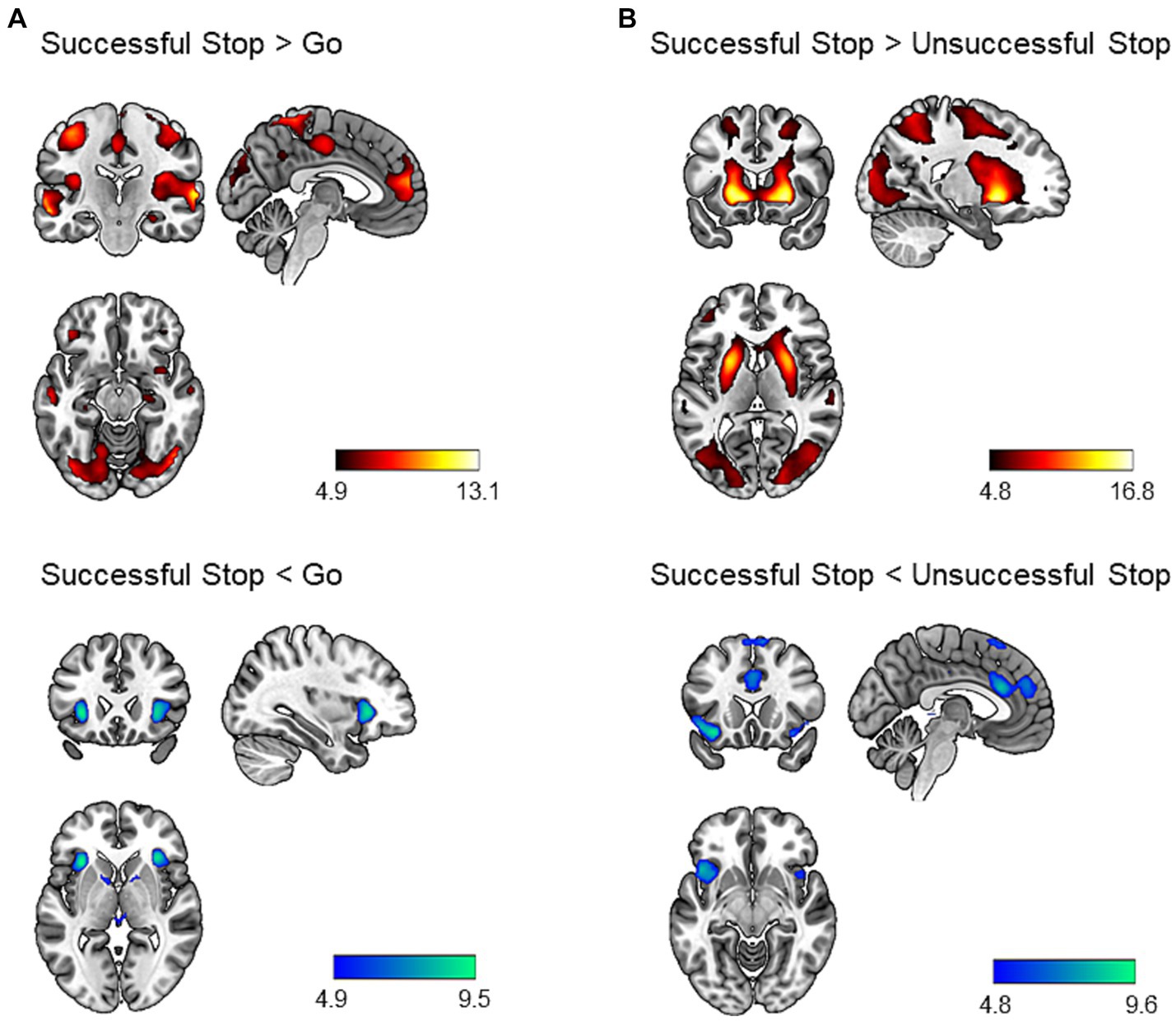
Figure 1. Brain regions showing task effect during the stop signal task (p < 0.05, whole-brain FWE corrected). Successful stop versus go trials (A) and successful stop versus unsuccessful stop trials (B) contrasts were chosen to identify neural correlates of inhibitory control. The hot colors represent increased activation, whereas the cold colors represent decreased activation for the contrast of interest. Results were mapped on the brain surface using MRIcroGL (https://www.nitrc.org/projects/mricrogl).
Similarly, during successful versus unsuccessful stop trials, we identified increased activation in a large cluster including bilateral occipital regions, striatum, frontal regions (middle, superior, and inferior frontal gyrus), motor regions (precentral gyrus, postcentral gyrus, SMA), middle temporal gyrus, superior temporal gyrus, precuneus, angular gyrus, amygdala, hippocampus, and left cerebellum (p < 0.05, whole-brain FWE corrected; Supplementary Table S5). In addition, we found decreased activation bilaterally in the anterior insula, orbitofrontal cortex, superior medial prefrontal cortex, dACC, and pre-SMA (p < 0.05, whole-brain FWE corrected; Supplementary Table S5).
3.3 Adversity effect
3.3.1 Adversity factors
We identified five clusters showing positive correlation with the first adversity factor representing postnatal psychosocial adversities and prenatal maternal smoking (all p < 0.05, cluster-level FWE corrected; Figure 2). These clusters included left middle temporal gyrus (MTG) (t = 5.25, k = 95, p < 0.001), right MTG (t = 4.34, k = 64, p = 0.04), left insula extending to left IFG and left orbitofrontal cortex (t = 5.10, k = 259, p < 0.001), right insula extending to right IFG and right superior temporal gyrus (STG) (t = 4.78, k = 306, p < 0.001), and superior medial prefrontal cortex (mPFC) extending to dACC and middle cingulum (t = 5.19, k = 277, p < 0.001). Higher scores in the first adversity factor were associated higher activation in these regions during the successful versus unsuccessful stop trials.
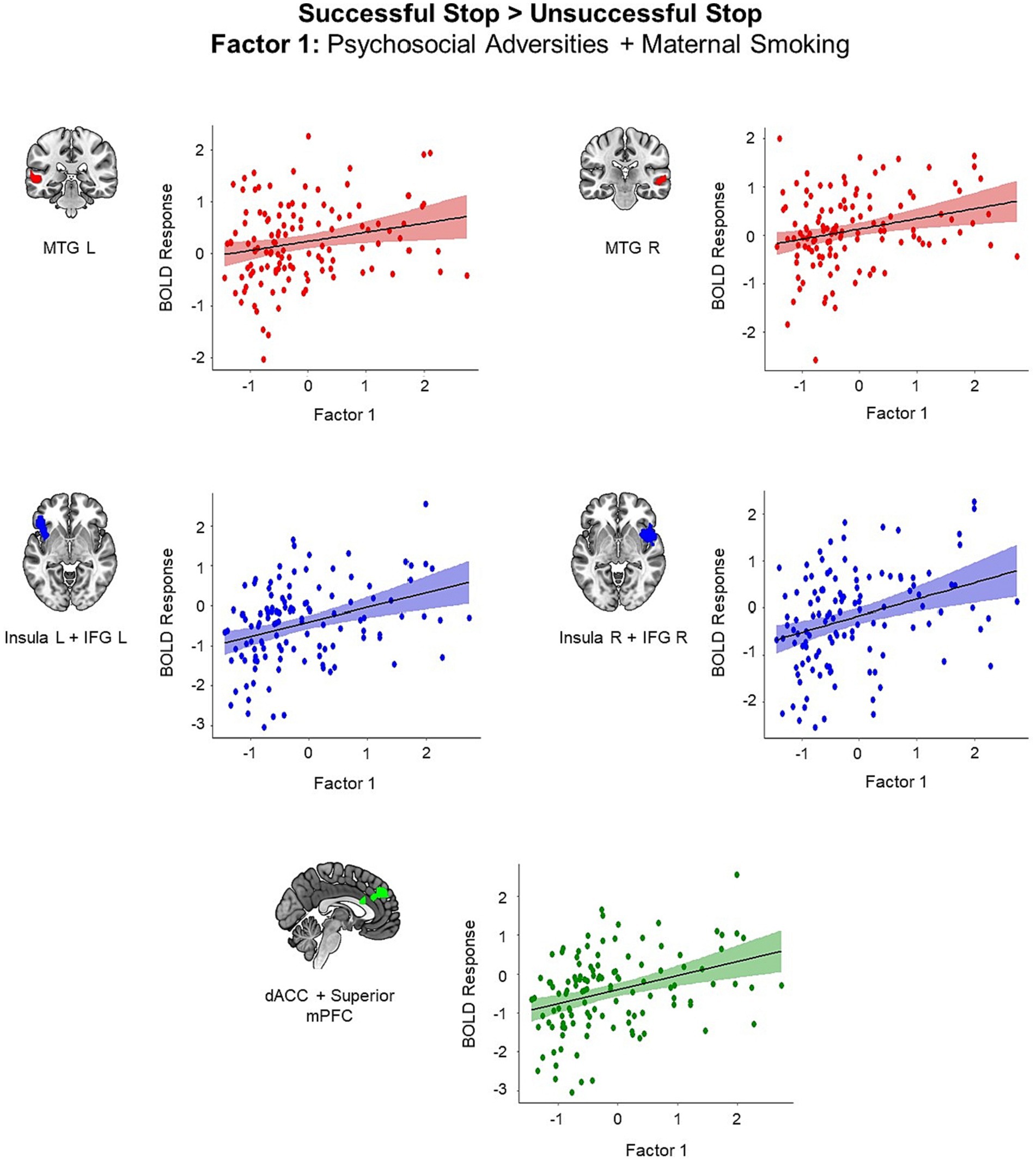
Figure 2. Brain regions showing positive associations with the first adversity factor during the successful versus unsuccessful stop trials (all p < 0.05, cluster-level FWE corrected). Scatter plots show the association between the scores in the first adversity factor and the mean BOLD response in the identified clusters for visualization purposes. dACC, dorsal anterior cingulate cortex; IFG, inferior frontal gyrus; mPFC, medial prefrontal cortex, MTG, middle temporal gyrus.
We did not identify any cluster exhibiting correlation with the second and third adversity factors. Additionally, we did not identify any adversity-related alteration in brain activation for the successful stop versus go trials contrast.
3.3.2 Specific adversity measures
We found similar results for the adversity measures constituting the first adversity factor, namely stressful life events, family adversity, prenatal maternal smoking and self-reported childhood trauma. All identified clusters showed increased activation during successful versus unsuccessful stop trials in individuals with higher adversity scores (all p < 0.05, cluster-level corrected, Figure 3). We identified four clusters that showed positive associations with stressful life events, including the left MTG (t = 5.13, k = 92, p = 0.01), right IFG extending to right orbitofrontal cortex, right insula, and right STG (t = 4.69, k = 113, p = 0.004), superior mPFC extending to left pre-SMA, middle cingulum, and left dACC (t = 4.40, k = 209, p < 0.001), and right insula (t = 4.22, k = 64, p = 0.04). Higher family adversity was linked to higher activation in the left insula extending to left IFG, left orbitofrontal cortex, and left STG (t = 5.20, k = 174, p < 0.001), right insula extending to right STG (t = 5.71, k = 179, p < 0.001), and midbrain (t = 4.20, k = 91, p = 0.01). Maternal smoking was related to higher activation in the left pre-SMA extending to left middle cingulum (t = 4.39, k = 88, p = 0.01), and right middle cingulum extending to right dACC (t = 4.34, k = 89, p = 0.01). Higher scores in the childhood trauma questionnaire were associated with higher activation in right MTG and STG (t = 4.45, k = 152, p < 0.001).
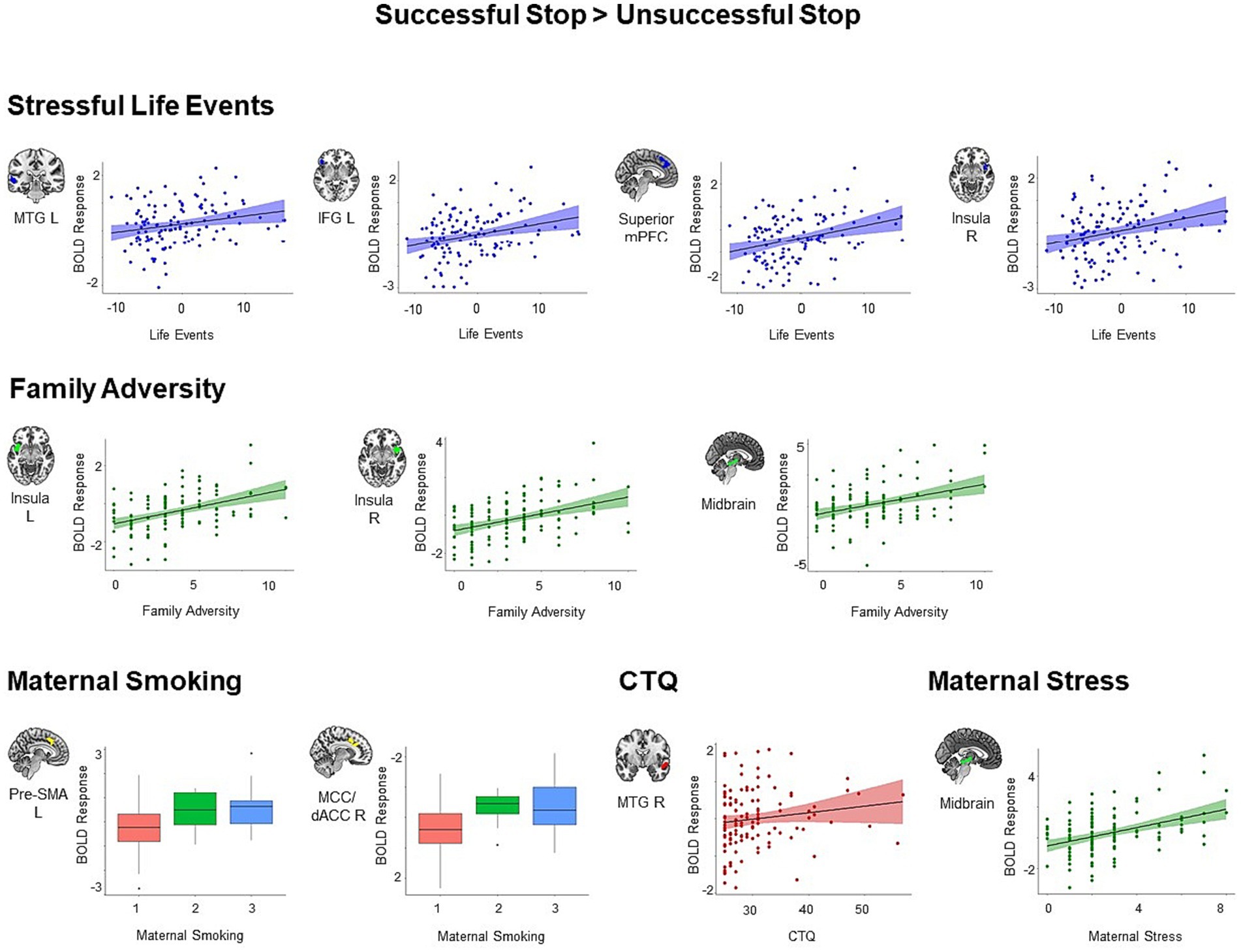
Figure 3. Positive associations between specific adversity measures and brain responses during inhibitory control (p < 0.05, cluster-level FWE corrected). Scatter plots show the association between the scores in the specific adversity measures and mean BOLD response in the identified clusters for visualization purposes. CTQ, Childhood Trauma Questionnaire; dACC, dorsal anterior cingulate cortex; IFG, inferior frontal gyrus; MCC, middle cingulate cortex; MTG, middle temporal gyrus; pre-SMA, pre-supplementary motor area.
Moreover, maternal stress loaded to the second adversity factor was associated with higher activation in the midbrain (t = 4.14, k = 119, p = 0.003). We did not identify any significant cluster for lower maternal stimulation and obstetric adversity during successful versus unsuccessful stop trials.
Post-hoc analysis on the overlap between adversity and task effects (successful stop versus baseline, unsuccessful stop versus baseline, and successful stop versus unsuccessful stop) was reported in the Supplementary material S8.
Furthermore, our exploratory sensitivity analyses on the timing effect of adversities revealed that family adversity between the ages of 2 years and 11 years was related to higher activation in insula (all p < 0.05, FWE-corrected at cluster level; Supplementary Table S8 and Supplementary Figure S9). We found higher activation in MTG at T2, left insula\IFG at T5, and dACC at T7 and T9 during successful stop trials in response to stressful life events (all p < 0.05, FWE-corrected at cluster level; Supplementary Table S9 and Supplementary Figure S9).
3.4 Brain-behavior association
To explain the meaning of adversity related alterations, we conducted regression analyses with behavioral measures, a task performance measure (i.e., inhibition success) and psychopathology measures, using the successful versus unsuccessful stop trials contrast. Among the significant results, only the regions showing adversity effect were visualized in Figure 4 (p < 0.05, cluster-level FWE corrected).
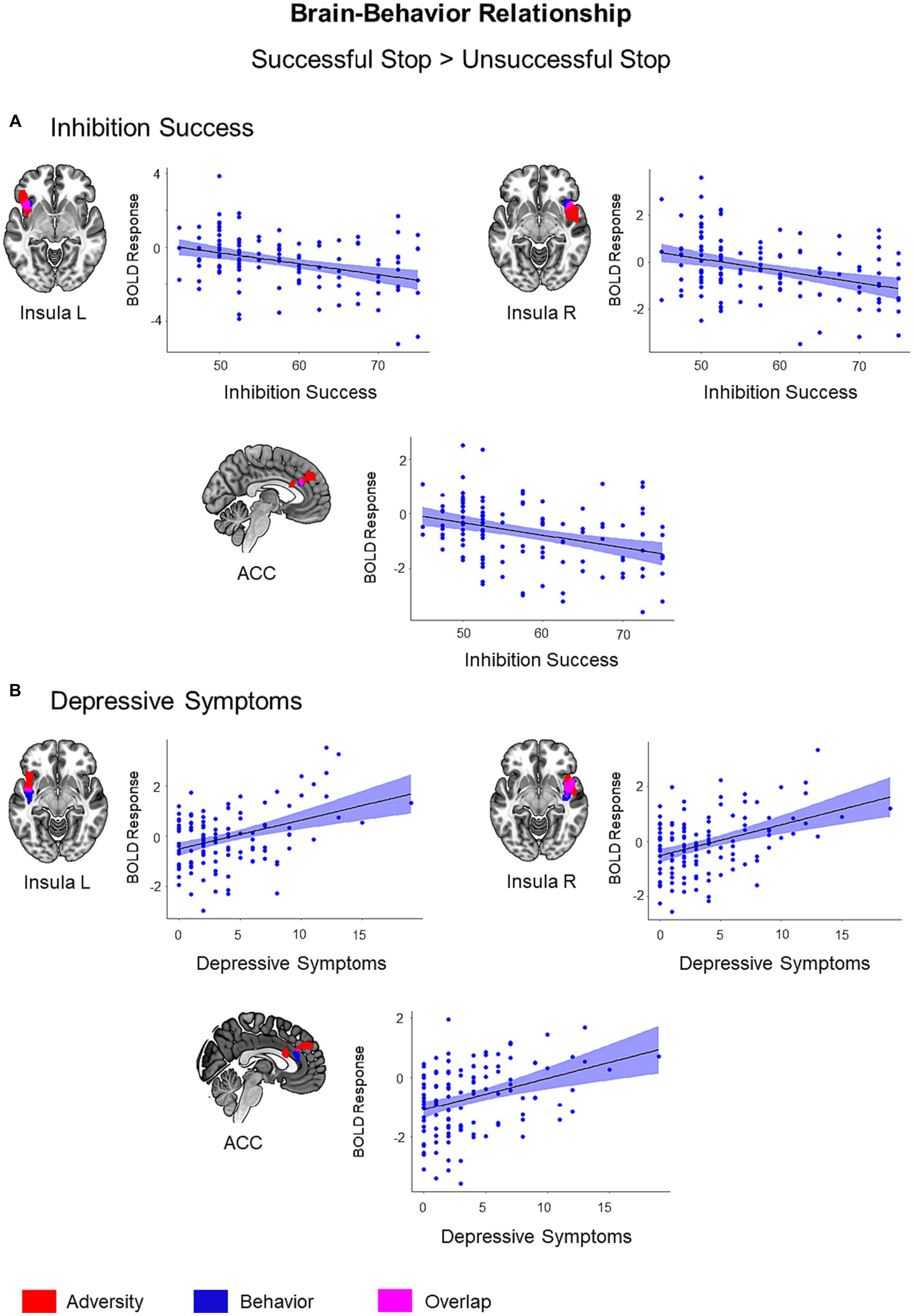
Figure 4. Brain-Behavior Relationship. Brain regions showing associations with inhibition success (A) and depressive symptoms (B) during successful versus unsuccessful stop trials were visualized in blue color on the brain surface (p < 0.05, cluster-level FWE corrected). Inhibition success showed a negative association with BOLD response, while depressive symptoms showed a positive association with BOLD response during successful versus unsuccessful stop trials. Adversity effect and overlap between adversity and behavior were visualized with red and pink color, respectively. Scatter plots show correlations between behavioral scores (inhibition success and depressive symptoms) and mean BOLD response extracted from the clusters associated with respective behavior (blue).
3.4.1 Inhibition success
Inhibitory control success was associated with lower activation in the left insula (t = 4.38, k = 69, p = 0.03), right insula (t = 4.38, k = 70, p = 0.03), and ACC (t = 4.13, k = 63, p = 0.04) during the successful versus unsuccessful stop trials. These regions also exhibited an overlap with the adversity effect (i.e., the first adversity factor) (Figure 4). Bilateral insula activation also showed an overlap with stressful life events and family adversity (Supplementary Figure S5). However, the overlap between adversity and lower inhibition success was more visible in the left insula. In addition, inhibitory control success was linked to higher activation in the left inferior occipital gyrus (t = 4.74, k = 425, p < 0.001), right inferior occipital gyrus (t = 4.43, k = 270, p < 0.001), right pre- and postcentral gyrus (t = 4.61, k = 444, p < 0.001), and precuneus/posterior cingulate cortex (t = 4.54, k = 272, p < 0.001).
3.4.2 Psychopathology
Out of six psychopathology measures, only depressive symptoms were linked to higher activation in the right insula (t = 4.96, k = 260, p < 0.001), left insula (t = 4.55, k = 70, p = 0.03), and ACC (t = 3.87, k = 72, p = 0.03) during the successful versus unsuccessful stop trials. These regions also exhibited an overlap with the adversity effect (Figure 4). The effect of depressive symptoms overlapped with stressful life events and family adversity in bilateral insula (Supplementary Figure S6) and with inhibition success in the right insula and ACC (Supplementary Figure S7).
Furthermore, the mediation analysis revealed that right insula activation partially mediated the relationship between the first adversity factor and depressive symptoms (interaction effect (a×b) = 0.33, CI = [0.02 0.76], Figure 5). No other brain region mediated the relationship between the first adversity factor and depressive symptoms or other psychopathology symptoms.
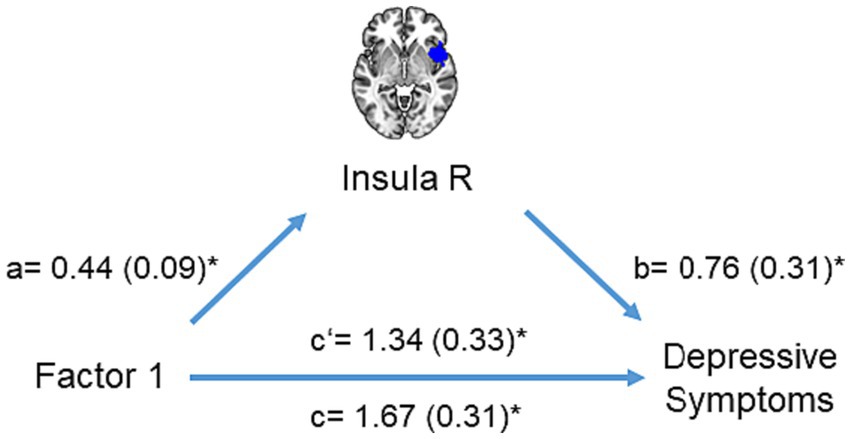
Figure 5. Mediation analysis. The mediation model included right insula activation as a mediator (M) to explain the impact of the first adversity factor (X) on depressive symptoms (Y). Significant paths are shown with asterisk.
4 Discussion
We here investigated the long-term effect of lifespan adversities on neural inhibitory network during the stop-signal task. Our results showed that lifespan adversities such as prenatal and postnatal psychosocial measures were associated with increased activation in several brain regions including IFG, dACC, insula, and MTG during successful versus unsuccessful stop trials in adults. Furthermore, increased activation in the insula and dACC was related to lower inhibition success and higher depressive symptoms. Taken together, our study contributes to the existing literature on adversity and inhibitory control by providing evidence for brain-behavior associations that were not explicitly demonstrated in previous studies. (16, 18, 19). Specifically, our findings regarding the stop-signal task and adversity-related neural alterations offer new insights into the neural mechanisms involved. This adds a novel dimension to our understanding of how adversity impacts brain function, particularly in the context of inhibitory control tasks.
The first adversity factor informed by postnatal psychosocial adversities and prenatal maternal smoking was associated with higher insula and dACC activation during successful compared to unsuccessful stop trials. In other words, individuals with higher adversity exhibited lower activation in these regions during the failed inhibition (i.e., unsuccessful versus successful stop trials). This effect was also partially overlapped with the task effect, where we found higher insula and dACC activation during failed inhibition across the participants (Supplementary material S8). Moreover, we found adversity-related neural alterations in both regions for stressful life events, only in insula for family adversity, and only in dACC for maternal smoking. Our exploratory analysis indicated an increased sensitivity of the insula for adversity during childhood and ACC during young adulthood. Taken together, these results indicate that insula and dACC activation were lower during failed inhibition in individuals with higher adversity with potentially different sensitive windows.
Insula together with dACC is a part of salience network and involves in error monitoring process (15, 51, 52). Previous studies utilizing directional connectivity methods reported a feedforward connectivity from the anterior insula to dACC following an error (51, 52), suggesting that the anterior insula might be involved in detecting saliency and signaling dACC that more attention is required to optimize a behavior after an error. Thus, reduced neural activation in the insula and dACC during failed inhibition might be related to reduced allocation of attention to errors in individuals with higher lifespan adversity, which in turn might lead to lower post-error behavioral adjustment. Indeed, we found that lower bilateral insula and dACC activation during unsuccessful versus successful trials were related to lower inhibitory control.
Furthermore, lower activation in the insula and dACC during failed inhibition was associated with higher depressive symptoms. Depression-related alterations also overlapped with the adversity effect. Several studies showed that depressed patients have difficulties in error monitoring (53), exhibit altered neural responses in dACC during error monitoring (24, 25), and abnormal resting-state salience network connectivity (54). Taken together, these results suggest that neural alterations in insula and dACC during error monitoring can be a potential vulnerability correlate for depressive symptoms and can be identified in non-clinical risk groups. Interestingly, although executive dysfunctions are assumed to be an important developmental pathway from early life stress to psychopathology in general (1) and previous studies have also identified error processing abnormalities in other clinical samples such as ADHD (26, 55), we only found an association with depressive symptoms. This might be related to our sample characteristics such as having lower variance in other psychopathology scales and a higher prevalence of depression in adulthood. Future research should also include clinical groups with adversity exposure to make inferences about the vulnerability aspect.
Higher scores in the first adversity factor as well as stressful life events and family adversity were related to higher IFG activation during successful versus unsuccessful stop trials. This result is compatible with a previous study showing higher IFG activation during inhibitory control in adolescents with early caregiver deprivation (16). Several studies found that IFG plays an important role in behavioral inhibition (12, 56). In line with the literature (9, 10, 14), we found that IFG was more active during successful stop trials compared to both go trials and unsuccessful stop trials across the participants. However, the adversity effect as well as inhibition success did not overlap with increased IFG activation (Supplementary material S8). Therefore, our results do not indicate that higher IFG activation in individuals with higher adversity is linked to higher inhibitory control. Moreover, enhanced IFG activation is not only found in inhibitory control tasks but also in several attentionally demanding tasks (57, 58). In addition, IFG activation is found to be modulated by task difficulty during the stop-signal task (59), suggesting higher IFG activation with more difficult stop trials. Indeed, a previous study showed that adolescents with prenatal alcohol exposure exhibited greater activation in several frontal regions with higher task difficulty (19). Taken together, higher IFG activation in individuals with higher lifespan adversity might be related to a compensatory recruitment due to higher attentional demand or task difficulty.
Additionally, we found that higher scores in the first adversity factor were related to higher activation in bilateral MTG during successful versus unsuccessful stop trials. This effect was also present for stressful life events and CTQ. MTG was more active during successful inhibition across the participants (Supplementary material S8), which is in line with other studies reporting increased activation in temporal regions during response inhibition (11, 60). However, none of the previous studies reported abnormal MTG activity related to adverse experiences during inhibitory control tasks, although altered MTG activation in relation to adversities was identified in other cognitive tasks such as sustained attention (61), working memory task (62, 63), and affective Stroop task (64). MTG is considered to be a part of the default-mode network and is associated with several cognitive functions including language processing, semantic memory and reasoning (65). However, due to a lack of behavioral associations and limited knowledge of its role in inhibitory control, it is difficult to explain why MTG activation is altered during inhibitory control in individuals with higher adversity.
In terms of specific adversity effects, most neural alterations were observed for stressful life events and family adversity. For maternal smoking, we identified an additional neural alteration in pre-SMA which was not identified in the common adversity factor. Higher maternal smoking was also related to higher dACC activation. With this finding, we replicated our previous work showing altered ACC activation in young adults exposed to prenatal maternal smoking during flanker/no-go task (20). Interestingly, higher total CTQ scores were only linked to higher MTG activation. We did not identify another region showing altered activation in relation to self-reported childhood trauma. However, these results must be interpreted with caution since the sample had low trauma exposure. Lastly, although the literature underscores the importance of parental behavior on cognitive development (66), we did not find any abnormal activation in individuals with lower maternal stimulation during infancy. However, our maternal sensitivity variable measures the socioemotional component of mother-infant interactions. Providing a cognitively rich environment (e.g., books and activities) can have different consequences on cognitive development than simply being emotionally available for the child. Therefore, the effect of cognitively stimulating home environment on executive functioning and brain responses should be further investigated by future studies.
To further address the reliability of our results, we investigated adversity-related neural alterations within the inhibitory control network using a longitudinal design that enables examination of long-term effects of adversities on adult brain functioning, a relatively large sample size, and a stringent methodological framework. The latter included comprehensive exclusion criteria for task performance and conservative thresholding, which collectively may enhance the generalizability and reliability of our findings. However, this study includes several limitations and needs to be interpreted with caution. First, although longitudinal studies offer valuable insights into how adverse experiences affect brain functions later in development, they do not provide enough evidence to make causal inferences. We here measured brain responses to inhibitory control only at the age of 33 years, whereas the adversity measures were collected across development. Thus, longitudinal neuroimaging studies are necessary and can offer a better understanding in terms of causality. Second, our exploratory analysis on the timing effect of psychosocial adversities should be interpreted with caution. Family adversity measures family characteristics that are tend to consistent across development, and it was not assessed beyond childhood. Except for stressful life events, our analysis did not include another adversity measure covering adolescence and adulthood periods. Third, our reliance on the Adult Self-Report for psychological assessments introduces potential biases inherent in self-report methods. These include recall bias, where participants may not accurately remember past events or feelings, recency bias, which might lead to overemphasis on recent experiences, and response bias, affecting the authenticity of the responses. We acknowledge these as critical limitations in interpreting our findings, given the retrospective nature of the data collected at each assessment wave. Fourth, we implemented principal component analysis to identify adversity factors that take into account the correlative nature of different adversity measures. Although it is helpful to model linear relations, non-linear relationships between variables could exist and be worth investigating. Therefore, future studies can implement machine learning approaches for clustering adversities to offer a better understanding of complex interactions between adversities.
In conclusion, our results indicated that higher psychosocial adversities and prenatal maternal smoking were linked to altered responses during successful versus unsuccessful stop trials in several brain regions that are important for successful response inhibition and error monitoring such as IFG, insula, and dACC. Lower insula and dACC activation during failed inhibition (i.e., unsuccessful versus successful stop trials) was further associated with lower inhibition success and higher depressive symptomology. Taken together, these results suggest that lifespan adversities are related to neural changes potentially heightening the risk of developing psychopathology. However, this aspect needs to be further examined by future studies using repeated prospective assessments of adversity and neural responses together.
Data availability statement
The data analyzed in this study is subject to the following licenses/restrictions: Data available on request due to privacy/ethical restrictions. Requests to access these datasets should be directed to nathalie.holz@zi-mannheim.de.
Ethics statement
The studies involving humans were approved by the local ethics committee of the medical faculty of the University of Heidelberg. The studies were conducted in accordance with the local legislation and institutional requirements. The participants provided their written informed consent to participate in this study.
Author contributions
SS: Formal Analysis, Visualization, Writing – original draft, Writing – review & editing. PMA: Writing – review & editing. MM: Data curation, Writing – review & editing. AK: Writing – review & editing. DB: Conceptualization, Writing – review & editing. TB: Conceptualization, Funding acquisition, Resources, Supervision, Writing – review & editing. NEH: Conceptualization, Funding acquisition, Investigation, Supervision, Writing – review & editing.
Funding
The author(s) declare financial support was received for the research, authorship, and/or publication of this article. NEH gratefully acknowledges grant support from the German Research Foundation (grant numbers DFG HO 5674/2-1, GRK2350/1) and in the framework of the Radboud Excellence Fellowship. TB gratefully acknowledges grant support by the German Federal Ministry of Education and Research (01EE1408E ESCAlife; FKZ 01GL1741[X] ADOPT; 01EE1406C Verbund AERIAL; 01EE1409C Verbund ASD-Net; 01GL1747C STAR; 01GL1745B IMAC-Mind), by the German Research Foundation (TRR 265/1), by the Innovative Medicines Initiative Joint Undertaking (IMI JU FP7 115300 EU-AIMS; grant 777394 EU-AIMS-2-TRIALS) and the European Union – H2020 (Eat2beNICE, grant 728018; PRIME, grant 847879).
Acknowledgments
The authors would like to thank the participants for their continued participation in the Mannheim Study of Children at Risk.
Conflict of interest
DB served as an unpaid scientific consultant for an EU-funded neurofeedback trial, which is unrelated to the present work. TB served in an advisory or consultancy role for eye level, Infectopharm, Lundbeck, Medice, Neurim Pharmaceuticals, Oberberg GmbH, Roche, and Takeda. He received conference support or speaker’s fee by Janssen, Medice and Takeda. He received royalities from Hogrefe, Kohlhammer, CIP Medien, Oxford University Press.
The remaining authors declare that the research was conducted in the absence of any commercial or financial relationships that could be construed as a potential conflict of interest.
Publisher’s note
All claims expressed in this article are solely those of the authors and do not necessarily represent those of their affiliated organizations, or those of the publisher, the editors and the reviewers. Any product that may be evaluated in this article, or claim that may be made by its manufacturer, is not guaranteed or endorsed by the publisher.
Supplementary material
The Supplementary material for this article can be found online at: https://www.frontiersin.org/articles/10.3389/fpsyt.2024.1298695/full#supplementary-material
Footnotes
References
1. Zelazo, PD . Executive function and psychopathology: a neurodevelopmental perspective. Annu Rev Clin Psychol. (2020) 16:431–54. doi: 10.1146/annurev-clinpsy-072319-024242
2. Snyder, HR, Miyake, A, and Hankin, BL. Advancing understanding of executive function impairments and psychopathology: bridging the gap between clinical and cognitive approaches. Front Psychol. (2015) 6:328. doi: 10.3389/fpsyg.2015.00328
3. Snyder, HR, Friedman, NP, and Hankin, BL. Transdiagnostic mechanisms of psychopathology in youth: executive functions, dependent stress, and rumination. Cognit Ther Res. (2019) 43:834–51. doi: 10.1007/s10608-019-10016-z
4. Lund, JI, Toombs, E, Radford, A, Boles, K, and Mushquash, C. Adverse childhood experiences and executive function difficulties in children: a systematic review. Child Abuse Negl. (2020) 106:104485. doi: 10.1016/j.chiabu.2020.104485
5. Lund, JI, Boles, K, Radford, A, Toombs, E, and Mushquash, CJ. A systematic review of childhood adversity and executive functions outcomes among adults. Arch Clin Neuropsychol. (2022) 37:1118–32. doi: 10.1093/arclin/acac013
6. McKay, MT, Cannon, M, Chambers, D, Conroy, RM, Coughlan, H, Dodd, P, et al. Childhood trauma and adult mental disorder: a systematic review and meta-analysis of longitudinal cohort studies. Acta Psychiatr Scand. (2021) 143:189–205. doi: 10.1111/acps.13268
7. Hughes, K, Bellis, MA, Hardcastle, KA, Sethi, D, Butchart, A, Mikton, C, et al. The effect of multiple adverse childhood experiences on health: a systematic review and meta-analysis. Lancet Public Health. (2017) 2:e356–66. doi: 10.1016/S2468-2667(17)30118-4
8. Diamond, A . Executive functions. Annu Rev Psychol. (2013) 64:135–68. doi: 10.1146/annurev-psych-113011-143750
9. Aron, AR, and Poldrack, RA. Cortical and subcortical contributions to stop signal response inhibition: role of the subthalamic nucleus. J Neurosci. (2006) 26:2424–33. doi: 10.1523/JNEUROSCI.4682-05.2006
10. Rubia, K, Smith, AB, Brammer, MJ, and Taylor, E. Right inferior prefrontal cortex mediates response inhibition while mesial prefrontal cortex is responsible for error detection. Neuroimage. (2003) 20:351–8. doi: 10.1016/S1053-8119(03)00275-1
11. Steele, VR, Aharoni, E, Munro, GE, Calhoun, VD, Nyalakanti, P, Stevens, MC, et al. A large scale (N=102) functional neuroimaging study of response inhibition in a go/NoGo task. Behav Brain Res. (2013) 256:529–36. doi: 10.1016/j.bbr.2013.06.001
12. Cai, W, Cannistraci, CJ, Gore, JC, and Leung, HC. Sensorimotor-independent prefrontal activity during response inhibition. Hum Brain Mapp. (2014) 35:2119–36. doi: 10.1002/hbm.22315
13. Korucuoglu, O, Harms, MP, Astafiev, SV, Golosheykin, S, Kennedy, JT, Barch, DM, et al. Test-retest reliability of neural correlates of response inhibition and error monitoring: an fMRI study of a stop-signal task. Front Neurosci. (2021) 15:1–18. doi: 10.3389/fnins.2021.624911
14. Duann, JR, Ide, JS, Luo, X, and Li, CSR. Functional connectivity delineates distinct roles of the inferior frontal cortex and presupplementary motor area in stop signal inhibition. J Neurosci. (2009) 29:10171–9. doi: 10.1523/JNEUROSCI.1300-09.2009
15. Dali, G, Brosnan, M, Tiego, J, Johnson, BP, Fornito, A, Bellgrove, MA, et al. Examining the neural correlates of error awareness in a large fMRI study. Cereb Cortex. (2023) 33:458–68. doi: 10.1093/cercor/bhac077
16. Mueller, SC, Maheu, FS, Dozier, M, Peloso, E, Mandell, D, Leibenluft, E, et al. Early-life stress is associated with impairment in cognitive control in adolescence: an fMRI study. Neuropsychologia. (2010) 48:3037–44. doi: 10.1016/j.pscychresns.2011.02.003
17. Lim, L, Hart, H, Mehta, MA, Simmons, A, Mirza, K, and Rubia, K. Neural correlates of error processing in young people with a history of severe childhood abuse: an fMRI study. Am J Psychiatry. (2015) 172:892–900. doi: 10.1176/appi.ajp.2015.14081042
18. Lees, B, Aguinaldo, L, Squeglia, LM, Infante, MA, Wade, NE, Hernandez Mejia, M, et al. Parental family history of alcohol use disorder and neural correlates of response inhibition in children from the adolescent brain cognitive development (ABCD) study. Alcohol Clin Exp Res. (2020) 44:1234–44. doi: 10.1111/acer.14343
19. Ware, AL, Infante, MA, O’Brien, JW, Tapert, SF, Jones, KL, Riley, EP, et al. An fMRI study of behavioral response inhibition in adolescents with and without histories of heavy prenatal alcohol exposure. Behav Brain Res. (2015) 278:137–46. doi: 10.1016/j.bbr.2014.09.037
20. Holz, NE, Boecker, R, Baumeister, S, Hohm, E, Zohsel, K, Buchmann, AF, et al. Effect of prenatal exposure to tobacco smoke on inhibitory control neuroimaging results from a 25-year prospective study. JAMA Psychiatry. (2014) 71:786–96. doi: 10.1001/jamapsychiatry.2014.343
21. Bruce, J, Fisher, PA, Graham, AM, Moore, WE III, Peake, SJ, and Mannering, AA. Patterns of brain activation in Foster children and nonmaltreated children during an inhibitory control task. Dev Psychopathol. (2013) 25:931–41. doi: 10.1017/S095457941300028X
22. Demers, LA, Hunt, RH, Cicchetti, D, Cohen-Gilbert, JE, Rogosch, FA, Toth, SL, et al. Impact of childhood maltreatment and resilience on behavioral and neural patterns of inhibitory control during emotional distraction. Dev Psychopathol. (2022) 34:1260–71. doi: 10.1017/S0954579421000055
23. Raud, L, Westerhausen, R, Dooley, N, and Huster, RJ. Differences in unity: the go/no-go and stop signal tasks rely on different mechanisms. NeuroImage. (2020) 210:116582. doi: 10.1016/j.neuroimage.2020.116582
24. Malejko, K, Hafner, S, Plener, PL, Bonenberger, M, Groen, G, Abler, B, et al. Neural signature of error processing in major depression. Eur Arch Psychiatry Clin Neurosci. (2021) 271:1359–68. doi: 10.1007/s00406-021-01238-y
25. Nixon, NL, Liddle, PF, Worwood, G, Liotti, M, and Nixon, E. Prefrontal cortex function in remitted major depressive disorder. Psychol Med. (2013) 43:1219–30. doi: 10.1017/S0033291712002164
26. Massat, I, Slama, H, Villemonteix, T, Mary, A, Baijot, S, Albajara Sáenz, A, et al. Hyperactivity in motor response inhibition networks in unmedicated children with attention deficit-hyperactivity disorder. World J Biol Psychiatry. (2018) 19:101–11. doi: 10.1080/15622975.2016.1237040
27. Aupperle, RL, Stillman, AN, Simmons, AN, Flagan, T, Allard, CB, Thorp, SR, et al. Intimate partner violence PTSD and neural correlates of inhibition. J Trauma Stress. (2016) 29:33–40. doi: 10.1002/jts.22068
28. Bartholdy, S, O’Daly, OG, Campbell, IC, Banaschewski, T, Barker, G, Bokde, ALW, et al. Neural correlates of failed inhibitory control as an early marker of disordered eating in adolescents. Biol Psychiatry. (2019) 85:956–65. doi: 10.1016/j.biopsych.2019.01.027
29. Holz, NE, Berhe, O, Sacu, S, Schwarz, E, Tesarz, J, Heim, CM, et al. Early social adversity, altered brain functional connectivity, and mental health. Biol Psychiatry. (2023) 93:430–41. doi: 10.1016/j.biopsych.2022.10.019
30. Verbruggen, F, Aron, AR, Band, GPH, Beste, C, Bissett, PG, Brockett, AT, et al. A consensus guide to capturing the ability to inhibit actions and impulsive behaviors in the stop-signal task. elife. (2019) 8:1–26. doi: 10.7554/eLife.46323
31. Philip, NS, Sweet, LH, Tyrka, AR, Price, LH, Bloom, RF, and Carpenter, LL. Decreased default network connectivity is associated with early life stress in medication-free healthy adults. Eur Neuropsychopharmacol. (2013) 23:24–32. doi: 10.1016/j.euroneuro.2012.10.008
32. Hart, H, Lim, L, Mehta, MA, Curtis, C, Xu, X, Breen, G, et al. Altered functional connectivity of fronto-cingulo-striatal circuits during error monitoring in adolescents with a history of childhood abuse. Front Hum Neurosci. (2018) 12:1–14. doi: 10.3389/fnhum.2018.00007
33. Entringer, S, Buss, C, and Wadhwa, PD. Prenatal stress, development, health and disease risk: a psychobiological perspective – 2015 Curt Richter award winner. Psychoneuroendocrinology. (2015) 62:366–75. doi: 10.1016/j.psyneuen.2015.08.019
34. Zohsel, K, Buchmann, AF, Blomeyer, D, Hohm, E, Schmidt, MH, Esser, G, et al. Mothers’ prenatal stress and their children’s antisocial outcomes – a moderating role for the dopamine D4 receptor (DRD4) gene. J Child Psychol Psychiatry Allied Discip. (2014) 55:69–76. doi: 10.1111/jcpp.12138
35. Laucht, M, Esser, G, Baving, L, Gerhold, M, Hoesch, I, Ihle, W, et al. Behavioral sequelae of perinatal insults and early family adversity at 8 years of age. J Am Acad Child Adolesc Psychiatry. (2000) 39:1229–37. doi: 10.1097/00004583-200010000-00009
36. Holz, NE, Boecker-Schlier, R, Jennen-Steinmetz, C, Hohm, E, Buchmann, AF, Blomeyer, D, et al. Early maternal care may counteract familial liability for psychopathology in the reward circuitry. Soc Cogn Affect Neurosci. (2018) 13:1191–201. doi: 10.1093/scan/nsy087
37. Holz, NE, Boecker-Schlier, R, Buchmann, AF, Blomeyer, D, Jennen-Steinmetz, C, Baumeister, S, et al. Ventral striatum and amygdala activity as convergence sites for early adversity and conduct disorder. Soc Cogn Affect Neurosci. (2017) 12:261–72. doi: 10.1093/scan/nsw120
38. Maier-Diewald, W, Wittchen, H-U, Hecht, H, and Werner-Eilert, K. Die Münchner Ereignisliste (MEL) – Anwendungsmanual. München: Max-Plank-Institut für Psychiatrie (1983).
39. Bernstein, DP, Fink, L, Handelsman, L, Foote, J, Lovejoy, M, Wenzel, K, Sapareto, E, Ruggiero, J, et al. Initial reliability and validity of a new retrospective measure of child abuse and neglect. Am J Psychiatry. (1994) 151:1132–6. doi: 10.1176/ajp.151.8.1132
40. Wingenfeld, K, Hill, A, Gast, U, Beblo, T, Höpp, H, Schlosser, N, et al. Die deutsche Version des Childhood Trauma Questionnaire (CTQ): Erste Befunde zu den psychometrischen Kennwerten. Psychother Psychosom Med Psychol. (2010) 60:442–50. doi: 10.1055/s-0030-1247564
41. Holz, NE, Zabihi, M, Kia, SM, Monninger, M, Aggensteiner, PM, Siehl, S, et al. A stable and replicable neural signature of lifespan adversity in the adult brain. Nat Neurosci. (2023) 26:1603–12. doi: 10.1038/s41593-023-01410-8
42. Achenbach, TM, and Rescorla, LA. Manual for the ASEBA adult forms & profiles. Burlington, VT, USA: Research Center for Children, Youth, & Families (2003).
43. Power, JD, Mitra, A, Laumann TOSnyder, AZ, Schlaggar, BL, and Petersen, SE. Methods to detect, characterize, and remove motion artifact in resting state fMRI. NeuroImage. (2014) 84:320–41. doi: 10.1016/j.neuroimage.2013.08.048
44. Cisler, JM, Privratsky, A, Smitherman, S, Herringa, RJ, and Kilts, CD. Large-scale brain organization during facial emotion processing as a function of early life trauma among adolescent girls. Neuroimage Clin. (2018) 17:778–85. doi: 10.1016/j.nicl.2017.12.001
45. Li, LM, Violante, IR, Leech, R, Hampshire, A, Opitz, A, McArthur, D, et al. Cognitive enhancement with salience network electrical stimulation is influenced by network structural connectivity. NeuroImage. (2019) 185:425–33. doi: 10.1016/j.neuroimage.2018.10.069
46. Logan, GD, Van Zandt, T, Verbruggen, F, and Wagenmakers, EJ. On the ability to inhibit thought and action: general and special theories of an act of control. Psychol Rev. (2014) 121:66–95. doi: 10.1037/a0035230
47. Mennes, M, Potler, NV, Kelly, C, Di Martino, A, Castellanos, FX, and Milham, MP. Resting state functional connectivity correlates of inhibitory control in children with attention-deficit/hyperactivity disorder. Front Psych. (2012) 2:1–17. doi: 10.3389/fpsyt.2011.00083
48. Hayes, AF . PROCESS: A versatile computational tool for observed variable mediation, moderation, and conditional process modeling. (2012). 1–39 p. Available at: http://www.afhayes.com/%0Apublic/process2012.pdf.
49. Weiss, MJ, and Wagner, SH. What explains the negative consequences of adverse childhood experiences on adult health? Insights from cognitive and neuroscience research. Am J Prev Med. (1998) 14:356–60. doi: 10.1016/s0749-3797(98)00011-7
50. Sacu, S, Dubois, M, Aggensteiner, PM, Monninger, M, Brandeis, D, Banaschewski, T, et al. Early life adversities affect expected value signaling in the adult brain. bioRxiv. (2023):1–35. doi: 10.1101/2023.06.19.545539
51. Bastin, J, Deman, P, David, O, Gueguen, M, Benis, D, Minotti, L, et al. Direct recordings from human anterior insula reveal its leading role within the error-monitoring network. Cereb Cortex. (2017) 27:bhv352–bhv1557. doi: 10.1093/cercor/bhv352
52. Ham, T, Leff, A, de Boissezon, X, Joffe, A, and Sharp, DJ. Cognitive control and the salience network: an investigation of error processing and effective connectivity. J Neurosci. (2013) 33:7091–8. doi: 10.1523/JNEUROSCI.4692-12.2013
53. Schroder, HS, Moran, TP, Infantolino, ZP, and Moser, JS. The relationship between depressive symptoms and error monitoring during response switching. Cogn Affect Behav Neurosci. (2013) 13:790–802. doi: 10.3758/s13415-013-0184-4
54. Manoliu, A, Meng, C, Brandl, F, Doll, A, Tahmasian, M, Scherr, M, et al. Insular dysfunction within the salience network is associated with severity of symptoms and aberrant inter-network connectivity in major depressive disorder. Front Hum Neurosci. (2014) 7:1–17. doi: 10.3389/fnhum.2013.00930
55. Albrecht, B, Brandeis, D, Uebel, H, Heinrich, H, Ueli, C, Hasselhorn, M, et al. Action monitoring in boys with ADHD, their nonaffected siblings and normal controls: evidence for an endophenotype. Biol Psychiatry. (2008) 64:615–25. doi: 10.1016/j.biopsych.2007.12.016.Action
56. Zandbelt, BB, Bloemendaal, M, Hoogendam, JM, Kahn, RS, and Vink, M. Transcranial magnetic stimulation and functional MRI reveal cortical and subcortical interactions during stop-signal response inhibition. J Cogn Neurosci. (2013) 25:157–74. doi: 10.1162/jocn_a_00309
57. Erika-Florence, M, Leech, R, and Hampshire, A. A functional network perspective on response inhibition and attentional control. Nat Commun. (2014) 5:4073. doi: 10.1038/ncomms5073
58. Hampshire, A, Chamberlain, SR, Monti, MM, Duncan, J, and Owen, AM. The role of the right inferior frontal gyrus: inhibition and attentional control. NeuroImage. (2010) 50:1313–9. doi: 10.1016/j.neuroimage.2009.12.109
59. Hughes, ME, Johnston, PJ, Fulham, WR, Budd, TW, and Michie, PT. Stop-signal task difficulty and the right inferior frontal gyrus. Behav Brain Res. (2013) 256:205–13. doi: 10.1016/j.bbr.2013.08.026
60. Congdon, E, Mumford, JA, Cohen, JR, Galvan, A, Aron, AR, Xue, G, et al. Engagement of large-scale networks is related to individual differences in inhibitory control. NeuroImage. (2010) 53:653–63. doi: 10.1016/j.neuroimage.2010.06.062
61. Lim, L, Hart, H, Mehta, MA, Simmons, A, Mirza, K, and Rubia, K. Neurofunctional abnormalities during sustained attention in severe childhood abuse. PLoS One. (2016) 11:1–20. doi: 10.1371/journal.pone.0165547
62. Philip, NS, Sweet, LH, Tyrka, AR, Carpenter, SL, Albright, SE, Price, LH, et al. Exposure to childhood trauma is associated with altered n-back activation and performance in healthy adults: implications for a commonly used working memory task. Brain Imaging Behav. (2016) 10:124–35. doi: 10.1007/s11682-015-9373-9
63. Philip, NS, Sweet, LH, Tyrka, AR, Price, LH, Carpenter, LL, Kuras, YI, et al. Early life stress is associated with greater default network deactivation during working memory in healthy controls: a preliminary report. Brain Imaging Behav. (2013) 7:204–12. doi: 10.1007/s11682-012-9216-x
64. Blair, KS, Aloi, J, Crum, K, Meffert, H, White, SF, Taylor, BK, et al. Association of different types of childhood maltreatment with emotional responding and response control among youths. JAMA Netw Open. (2019) 2:1–22. doi: 10.1001/jamanetworkopen.2019.4604
65. Xu, J, Wang, J, Fan, L, Li, H, Zhang, W, Hu, Q, et al. Tractography-based parcellation of the human middle temporal gyrus. Sci Rep. (2015) 5:18883. doi: 10.1038/srep18883
Keywords: adverse experiences, early life stress, stop-signal task, fMRI, inhibitory control
Citation: Sacu S, Aggensteiner P-M, Monninger M, Kaiser A, Brandeis D, Banaschewski T and Holz NE (2024) Lifespan adversities affect neural correlates of behavioral inhibition in adults. Front. Psychiatry. 15:1298695. doi: 10.3389/fpsyt.2024.1298695
Edited by:
Charlotte Lotta Borg Skoglund, Uppsala University, SwedenReviewed by:
Lisa Wagels, University Hospital RWTH Aachen, GermanyDarren William Roddy, Trinity College Dublin, Ireland
Copyright © 2024 Sacu, Aggensteiner, Monninger, Kaiser, Brandeis, Banaschewski and Holz. This is an open-access article distributed under the terms of the Creative Commons Attribution License (CC BY). The use, distribution or reproduction in other forums is permitted, provided the original author(s) and the copyright owner(s) are credited and that the original publication in this journal is cited, in accordance with accepted academic practice. No use, distribution or reproduction is permitted which does not comply with these terms.
*Correspondence: Nathalie E. Holz, nathalie.holz@zi-mannheim.de
†These authors have contributed equally to this work