- 1MBC Group, Edmonton, AB, Canada
- 2Department of Chemistry, Faculty of Science, University of Alberta, Edmonton, AB, Canada
Fire debris analysis is focused on the recovery and identification of ignitable liquids to provide context for fire investigation. Investigators use a variety of methods to select suspicious debris for analysis, with ignitable liquid detection canines being one of the most popular. When properly trained and certified, ignitable liquid detection canines offer continuous sampling with high sensitivity and the ability to discriminate between irrelevant and suspicious odours to rapidly locate debris which may contain ignitable liquid residues. However, canine indications are presumptive as they cannot be sufficiently scrutinised by the legal process without confirmatory laboratory analysis. Standard debris analysis methods detect very small amounts of ignitable liquid residue (∼1-0.1 μL) without maximising sensitivity which minimises the risk from false positives and from detection of background petroleum which is ubiquitous in our environment. For canine-selected debris, the goal of the laboratory analysis should be to provide data to confirm or refute the validity of the canine indication. For such confirmatory analysis to be useful, analytical sensitivity should approximate the sensitivity of the canine. The sensitivity of fire debris analysis is most influenced by the selection of the extraction device and tuning of extraction conditions. Non-destructive extractions are preferred for forensic analyses, and solid phase microextraction (SPME) offers an excellent option. However, the original SPME fibres are fragile and tend to skew the chromatographic profile which can lead to high costs and a risk of ignitable liquid misclassification. Herein, we present an optimised SPME extraction method suited to confirmatory analysis of canine-selected exhibits. The method is non-destructive and non-exhaustive, is easily applied to cans of debris, and yields chromatographic profiles equivalent to those obtained by the gold-standard passive headspace sampling (PHS) methods based on activated carbon. Fibre selection, debris temperature, fibre temperature, and extraction time were optimised to yield chromatographic profiles with maximum comparability to reference samples collected as neat liquids or standard PHS extracts. The optimised method is applied to samples recovered from another study which estimated the threshold of the canine’s sensitivity, with the laboratory result compared to the canine result for each sample.
1 Introduction
Fire debris analysis is an important aspect of fire investigation, where investigators collect material from selected areas of a fire scene to determine whether an ignitable liquid (IL) may have been used to set or promote growth of the fire. The most frequently encountered ILs in fire debris are petroleum products, which may be either foreign or native to the scene (Bertsch and Zhang, 1990; Cherry, 1996; Lentini et al., 2000; Lentini, 2001; Hetzel and Moss, 2005; Stauffer et al., 2008). These ILs often show significant depletion due to consumption by the fire and post-fire losses from weathering or degradation (Mann and Gresham, 1990; Kirkbride et al., 1992; Chalmers et al., 2001; Ferrino-McAllister et al., 2006; Zorzetti et al., 2011; Turner and Goodpaster, 2012; Hutches, 2013; Turner et al., 2017). Fire debris is selected at the scene using investigative techniques (DeHaan, 2006; National Fire Protection Association, 2017) ranging from inspection of visual indicators left by the fire to the use of in-situ screening techniques such as photoionisation detectors and ignitable liquid detection canines (ILDCs). ILDCs rely on highly sensitive olfaction to detect and localise foreign odours to indicate the possible presence of ignitable liquids within the scene (DeHaan, 1994; Kurz et al., 1994; Tindall and Lothridge, 1995; National Fire Protection Association, 2017). ILDCs have been in use since at least 1986 and are especially popular because of their ability to perform a quick and sensitive screening of the fire scene with greater selectivity than other screening tools like the photoionisation detector (Almirall and Furton, 2004). With proper training and certification, ILDCs can be highly reliable, but legal scrutiny of forensic examinations means their indications must be considered presumptive and require laboratory confirmation. For this reason, the sensitivity of ILDCs must be understood so the laboratory can apply a method with an equivalent sensitivity. There have been many published attempts to characterise the lower limit of ILDC sensitivity towards the complex mixtures of volatiles found in petroleum products (DeHaan, 1994; Kurz et al., 1994; Tindall and Lothridge, 1995; Kurz et al., 1996), but the canine sensitivity has consistently exceeded the minimum volumes tested. However, a first estimate was recently reported for the minimum volume of gasoline required for ILDC detection under conditions approximating a fire scene (Abel et al., 2020). The reported sensitivity of canines to 50 pL of 75% evaporated regular gasoline greatly exceeds the range of sensitivities offered by the most widely applied ASTM methods for fire debris analysis (Bertsch and Ren, 1999; ASTM International, 2013; ASTM International, 2015a; ASTM International, 2016; Martín-Alberca et al., 2016). The most popular methodologies are estimated to provide chromatograms sufficient to identify and properly classify ignitable liquids from amounts in the range of 1–0.1 μL, depending on the ignitable liquid composition and the extraction technique applied (Thatcher, 1986; DeHaan, 1994; Kurz et al., 1994; Tindall and Lothridge, 1995; Kurz et al., 1996; Armstrong et al., 2004; Abel et al., 2018).
The long history of ILDC assistance in fire investigations, and the mismatch between laboratory and canine sensitivity has led to a dangerous situation where some canine handlers have taken their canine indications to court without proper laboratory confirmation due to frustration over negative laboratory findings for exhibits eliciting strong canine indications (Chasteen et al., 1995; Katz and Midkiff, 1998; Abel et al., 2020). An appropriate solution to this issue is for the laboratory to apply an extraction method with canine-like sensitivity for confirmatory analysis of exhibits selected by ILDCs, while retaining the widely accepted standard sensitivity for all other analyses. In this way, false negatives can be avoided for the canine-selected exhibits, while the risk of false positives can still be controlled for routine casework. The use of highly sensitive laboratory extraction for exhibits selected by ILDC is justified, in spite of the prevalence of petroleum in the background of our environment (Lentini et al., 2000; DeHaan, 2002), because properly trained and certified canines provide additional selectivity by discriminating between background petroleum common to the scene and materials with potentially foreign ignitable liquids.
To achieve the high sensitivity required for confirmatory analysis of ILDC indications while avoiding spoliation of evidence, an ideal extraction technique would be either non-exhaustive or non-destructive, easily repeatable, and would not interfere with the integrity of other secondary extractions should they be necessary. This would ensure that in situations where the canine has located a high abundance ignitable liquid, and a high sensitivity extraction has yielded badly skewed chromatographic profiles or overloading of the analytical system, any secondary extraction of routine sensitivity would still provide the necessary result.
Solid phase microextraction (SPME) is an attractive technique for highly sensitive extraction, and has been repeatedly explored for application to fire debris samples (Furton et al., 1995; Almirall et al., 1996; Bertsch and Ren, 1999; Ahmad and Voon, 2001; Borusiewicz, 2002; Ahmad and Selvaraju, 2007; Yoshida et al., 2008; Fettig et al., 2014; ASTM International, 2015b; Martín-Alberca et al., 2016; Grafit et al., 2018; Lashgari et al., 2019). It offers the advantage of a non-exhaustive extraction where an insignificant mass of volatile content is removed from the headspace volume. This leaves the exhibit effectively unaltered and fit for subsequent extractions by other techniques when the first extraction is overloaded. However, under the consensus standards published by ASTM for fire debris analysis, SPME is treated primarily as a screening technique and the standard asserts that SPME is to be used “in conjunction with other extraction techniques” described by other ASTM standards (ASTM International, 2015b). Secondary confirmation by other ASTM extraction methods is typically much less sensitive than SPME and may fail to produce any of the chromatographic features of trace ignitable liquids observed with the more sensitive SPME screening extraction. This can result in ambiguous situations where a forensic exhibit has been found positive by one technique and later found negative by another more recognised technique due to the characteristics of the extraction rather than the content of the exhibit.
The original SPME fibre format also has a tendency to produce chromatographic profiles with different relative peak recoveries and chromatographic profiles relative to other standard fire debris extraction techniques (Furton et al., 1995; Bertsch and Ren, 1999; Ren and Bertsch, 1999; Yoshida et al., 2008; Fettig et al., 2014). Laboratories are required by the ASTM E1618 standard to maintain ignitable liquid and substrate databases for comparison to forensic exhibits (ASTM International, 2014). Most forensic laboratories acquire their ignitable liquid databases from neat liquids and their substrate databases from passive headspace extracts using activated carbon strips (PHS via ACS). Standard SPME extracts may compare poorly to these databases and it is often impractical to re-acquire entire databases under new conditions. This limits broader adoption of SPME and has lead many laboratories to adhere to less sensitive methods for canine-selected exhibits. Other high sensitivity extraction methods are available for fire debris (e.g. purge and trap for thermal desorption analysis (ASTM International, 2013) but due to the exhaustive and often destructive nature of these alternatives, they have also had limited adoption within the forensic community. Any high sensitivity extraction is prone to overloading or skewing of the characteristic peak-to-peak ratios within critical compound groupings which are relied upon for the proper identification and classification of ignitable liquid residues, meaning a non-exhaustive and/or non-destructive extraction technique is effectively required for confirmatory analysis of canine-selected exhibits.
In spite of some challenges, SPME extraction represents the best intersection of desired characteristics: minimally invasive, highly sensitive, non-exhaustive, short extraction times (under kinetic conditions), etc. (Steffen and Pawliszyn, 1996; Pawliszyn, 2000). Several experimental factors influence the character of an SPME extract and may be optimised to produce a representative chromatographic profile to ensure proper classification (Furton et al., 1995; Almirall et al., 1996; Furton et al., 1996; Steffen and Pawliszyn, 1996; Ren and Bertsch, 1999; Almirall et al., 2000; Pawliszyn, 2000). Extraction time must be long enough to yield good sensitivity, but short enough to avoid skewing of chromatographic profiles towards heavier components (Pawliszyn, 2000). The chemistry of the fibre coating must have an affinity for hydrocarbons to ensure efficient extraction of petroleum products. The temperature of the headspace and the fibre will also influence the extraction speed and the representativeness of the extract. Finally, the delicate nature of the SPME fibre must be considered during insertion and exposure in the debris container, especially if extracts are to be compared (e.g. comparison of debris and packaging controls to exhibits).
The original SPME fibre format was commercialised by Supelco® (now Millipore Sigma) and is available in a variety of fibre chemistries and configurations, with the advantage of direct compatibility with existing GC inlets. However, a variety of newer SPME formats have been described including the “SPME Arrow,” a commercial option introduced by Restek which has gained significant attention (Kremser et al., 2016a; Kremser et al., 2016b; Eckert et al., 2018). Although the Arrow fibres require a larger GC inlet path due to their increased diameter, they offer larger coating volumes for increased sensitivity, and are continuously metal-supported and metal-tipped. In the original SPME fibre configuration the coating is adhered to a fused silica support which is susceptible to delamination of the coating or various modes of breakage of the support, while the increased robustness of the Arrow fibres’ metal support and tip offers greater robustness during insertions and exposures. Because lower fibre temperatures shift the extraction equilibria towards the sorptive coating, the thermally conductive metal substrate may also improve recovery of the most volatile components during heated headspace extractions by conducting heat away from the coating towards those fibre components kept outside of the headspace environment. External cooling of the fibre housing may also further improve analyte recovery (Haddadi and Pawliszyn, 2009; Menezes and de Lourdes Cardeal, 2011; Menezes et al., 2013).
In this study, two standard SPME fibres (i.e. the original SPME format) described as suitable for fire debris extraction (Lloyd and Edmiston, 2003; Yoshida et al., 2008; ASTM International, 2015b), and an SPME Arrow fibre were evaluated for highly sensitive extraction of fire debris for confirmatory analysis of canine-selected exhibits. A variety of extraction conditions were evaluated to provide: 1) an optimised method with canine-like sensitivity towards petroleum based ignitable liquids (ASTM International, 2014; ASTM International, 2016), and 2) extracts comparable to pre-existing reference databases. SPME extraction parameters selected for optimisation were fibre type, extraction time, debris temperature, and fibre temperature. SPME extract representativeness was evaluated by comparison to analyses of neat liquid samples and standard extracts using PHS via ACS to ensure comparability to pre-existing ignitable liquid and substrate databases. The resulting extraction method provides canine-like sensitivity for confirmatory analysis of ILDC indications, which is demonstrated through its successful application to samples selected by ILDCs which were preserved following another study (Abel et al., 2020).
2 Materials and Methods
2.1 SPME Fibres
Two standard SPME fibre configurations were selected for initial extraction optimisation based on their recommendation within the fire debris literature (Furton et al., 1995; Ren and Bertsch, 1999; Almirall et al., 2000; Borusiewicz, 2002; Harris and Wheeler, 2003; Cornett and Wermeling, 2005; Bodle and Hardy, 2007; Yoshida et al., 2008; Heitmann et al., 2009; Monfreda and Gregori, 2011; Tankiewicz et al., 2013; ASTM International, 2015b). 100 µm polydimethylsiloxane (PDMS) and 50/30 µm divinylbenzene-Carboxen-polydimethylsiloxane (DVB-CAR-PDMS) fibres were obtained from Chromatographic Specialties (Brockville, ON) and conditioned according to the manufacturer’s criteria by heating under helium flow in a standalone GC inlet assembly (5.0 grade; Praxair®, Edmonton, AB). An SPME Arrow fibre with the 120 µm “Carbon Wide Range”-polydimethylsiloxane (CAR-WR-PDMS) configuration designed for sampling hydrocarbons across a wide carbon number range was later obtained from Restek (Pittsburgh, PA, United States) for additional testing under the extraction conditions determined from the standard SPME fibres. Restek’s GC inlet conversion kit and specialised inlet liners were also installed into the GC instrumentation to accommodate the larger diameter of the SPME Arrow fibres relative to the standard SPME format.
2.2 Ignitable Liquid Standards
One-quart unlined steel paint cans were purchased from Uline (Edmonton, AB) and each loaded with a single Kimwipe disposable tissue (VWR International, Edmonton, AB) to provide a wicking substrate. A regular gasoline (87 octane) and a Diesel were purchased from an Edmonton area 7-Eleven® service station. Prior to use these gasoline and Diesel samples were confirmed to be in good condition, both showing good clarity, no sediments or residues, and routine composition shown by cursory GC analyses of neat aliquots. Aliquots of both ignitable liquids were transferred into GC vials, placed into a heated 24-well evaporator (Cole-Parmer, Montreal, QC, Canada), and evaporated with high purity nitrogen gas (5.0 grade; Praxair®, Edmonton, AB) until approximately 75% of the original gasoline volume and approximately 25% of the original Diesel volume were lost.
Three additional ignitable liquids were used in another study assessing ILDC sensitivity (Abel et al., 2020), consisting of Ronsonol® brand lighter fluid purchased from an Edmonton area Canadian Tire® retail store, another regular gasoline (87 octane) and another Diesel both purchased from an Edmonton area Shell service station. Aliquots of the lighter fluid and gasoline were evaporated under a high purity nitrogen stream (5.0 grade; Praxair®, Edmonton, AB) until approximately 75% of the original volume had been lost. An aliquot of the Diesel was similarly treated until approximately 50% of the original volume had been lost. These three ignitable liquids represent light (lighter fluid), medium (evaporated gasoline), and heavy (Diesel) petroleum products and cover the range of volatility typically found for ignitable liquids recovered from fire scenes.
The oxygenates class of ignitable liquids was not assessed due to the ASTM E1618 requirement for an oxygenate to be an order of magnitude greater in abundance than other matrix peaks. In highly sensitive confirmatory analysis of canine indications, it is unlikely that the matrix peaks would be substantially less abundant than a trace oxygenate requiring high sensitivity recovery.
Aliquots of the evaporated ignitable liquids were delivered into cans by manual pipetting using calibrated glass pipettes (Drummond Scientific, Broomall, PA, United States) with liquids deposited directly onto the Kimwipe substrate. For the initial SPME extraction optimisation, two sets of samples were prepared from the gasoline and Diesel obtained from 7-Eleven® to produce standard headspace extracts via ACS from volumes of 0.1, 1, 20, and 50 μL of gasoline, and from 0.5, 5, 20, and 50 μL of Diesel. Diesel is a more complex fuel with a larger number of components at lower individual concentrations per unit volume than gasoline; consequently, the two lowest abundance Diesel samples were prepared with larger volumes. Two duplicate samples, one with 0.1 μL gasoline and the other with 0.5 μL Diesel were prepared for SPME extraction. Samples at 1 μL and greater were pipetted directly as neat liquids onto the Kimwipes®, while the lower abundance samples (i.e. 0.1 and 0.5 μL) could not be accurately pipetted as neat ignitable liquids and were instead applied by pipetting appropriate volumes of 50 μL mL−1 ignitable liquid diluted in GC-MS grade SupraSolv® dichloromethane (EMD Millipore, Burlington, MA, United States). After pipetting diluted ignitable liquids, the cans were left open briefly (∼2 min) to allow evaporation of the dichloromethane.
2.3 Preliminary SPME Method Development
A series of preliminary tests were performed using the standard SPME fibres. The extraction time was assessed with durations of 60, 120, 300, 600, and 1,500 s at a maximum variation of ±1 s, to identify the interval offering the best compromise between maximising sensitivity and providing chromatographic similarity to the neat ignitable liquid. The two SPME coating chemistries (PDMS and DVB-CAR-PDMS) were evaluated at each of the above extraction intervals for their comparative ability to provide chromatograms similar to the neat ignitable liquid. The headspace temperature for these initial tests was first maintained at the ambient room temperature of approximately 24°C. After fabrication of a heated sleeve to encircle individual cans, 60, 80, and 90°C were also evaluated.
2.3.1 Sampling of Canine-Searched Cans and Real Debris
In the prior study (Abel et al., 2020) evaluating the canine sensitivity for locating petroleum products, lighter fluid, gasoline, and Diesel were presented to the canines at varying concentrations. The target cans from the wheel searches in that study were recovered and preserved, and were subsequently extracted and analysed using the optimised SPME extraction method. Samples of debris were also collected from a controlled structure fire deliberately accelerated with ignitable liquid. Those samples were homogenised and split equally into two cans at the time of their collection at the fire scene. One can from each sample pair was subjected to extraction by ACS and the other subjected to serial extractions using the optimised method with the DVB-CAR-PDMS fibre and then the CAR-WR-PDMS Arrow fibre. The chromatographic profiles from each fibre platform were compared to the ACS extracts. In this case, the presence or absence of ignitable liquid was not considered. Instead, the total headspace chromatographic profiles were compared visually as an assessment of the overall representativeness of each fibre platform.
2.4 ACS Sample Extractions
Sample cans were loaded with an ACS strip (Albrayco, Cromwell, CT, United States) pierced by a dichloromethane-cleaned safety pin and magnetically suspended from the interior surface of the can lid by a rare earth magnet (Lee Valley, Edmonton, AB) placed on the lid exterior. Cans were sealed and placed in a 60°C Cenco laboratory oven (Fairfax, VA, United States) overnight for passive headspace extraction (ASTM International, 2016). The cans were then removed and allowed to cool to room temperature. Once cool, the ACS strips were recovered into GC autosampler vials and eluted with 600 μL of high purity CS2 (>99.9%, low benzene, Sigma Aldrich, Saint Louis, MO, United States). 50 μL aliquots of each extract were transferred into separate low-volume GC autosampler vials equipped with glass inserts (Chromatographic Specialties, Brockville, ON) for subsequent analysis.
2.5 SPME Sample Extractions
Sample cans were stored at room temperature prior to extraction if their contents could be rapidly heated (i.e. cans of low thermal mass such as those containing Kimwipes® or small slate tiles). Cans filled with debris were pre-heated to 60°C overnight (no less than 12 h) in a Cenco laboratory oven (Fairfax, VA, United States) to ensure full warming of the substrate and equilibration of the headspace. During SPME extraction cans were transferred into a thick-walled aluminum sleeve heated on a VWR Professional Series ceramic hot plate with digital temperature control (VWR International, Edmonton, AB). Temperature was maintained at the setpoint by feedback from a K-type thermocouple inserted into a bore hole in the side of the aluminum sleeve (Figure 1). Immediately prior to performing the first SPME extraction of each can, a section of the lid was wiped clean with a Kimwipe dampened with purified water, then punctured with a dichloromethane-cleaned nail with a diameter similar to the SPME fibre sheath, followed by insertion of the SPME fibre through the puncture hole. The fibre was subsequently exposed for a precise amount of time (maximum variation ±1 s), then withdrawn into its protective housing and transferred directly to the heated inlet of the analytical system for desorption. Once the SPME fibre was removed, the punctured hole was immediately re-sealed using a pre-prepared rectangular piece of masking tape with a smaller square of clean aluminum foil placed on the underside. This step limits exposure of the sample headspace to the adhesive which could introduce interferents or sorb low-abundance analyte. The tape-and-foil seals were re-opened and re-sealed for access during subsequent SPME extractions.
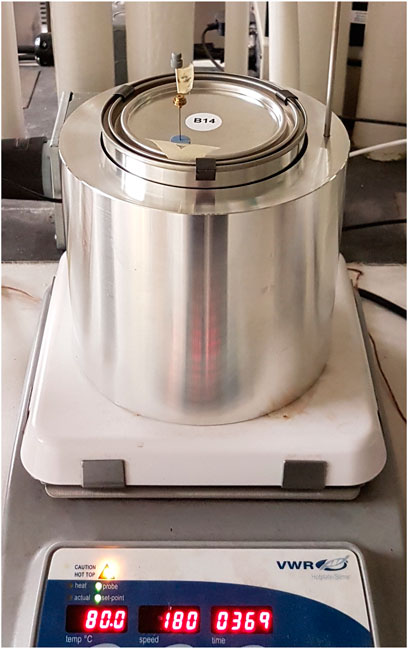
FIGURE 1. Can Sleeve and Hotplate for Heated Headspace SPME Sampling. An aluminum sleeve offers a high thermal mass and effective heat transfer to uniformly heat the can exterior. The metal rod extending from the right of the aluminum sleeve is a K-type thermocouple providing feedback to the hotplate temperature control. The SPME fibre is inserted through a puncture hole (adjacent to the ‘B14’ sticker label). The tan coloured triangle near the fibre is the piece of masking tape with clean aluminum foil on the adhesive side.
2.6 Instrumental Analysis
All GC×GC-MS analyses were performed using a Pegasus 4D GC×GC-TOF (LECO, St. Joseph, MI, United States) composed of an Agilent 7890A gas chromatograph with a secondary oven and a quad-jet dual-stage modulator using liquid nitrogen as coolant. Fibre desorption occurred in the GC inlet operated in splitless mode at 250°C for a duration of 3 min to ensure complete desorption, at which time the fibre was retracted and removed, and the split vent opened. The GC×GC column configuration consisted of a first-dimension column of 30 m × 0.25 mm; 0.25 µm thick 5% phenyl phase (actual length ∼26 m), and a second-dimension poly(ethylene glycol) wax phase column of 1.7 m × 0.25 mm; 0.25 µm film thickness. The instrument was operated using helium carrier gas (5.0 grade; Praxair®, Edmonton, AB) under speed-optimised flow conditions (2.0 mL min−1 based on column geometry) (Klee and Blumberg, 2002), and a modulation period of 1.3 s. The primary oven program started with an initial temperature of 40°C held for 3 min, followed by ramping to a final temperature of 255°C under optimum heating rate conditions (10°C tM−1; tM = column void time in min) (Blumberg and Klee, 2000) with the final temperature held for 2.5 min. The second-dimension oven was operated at an offset of +15°C relative to the primary oven up to the column maximum of 260°C. The mass spectrometer was set to a potential of 70 eV in electron impact mode, tuned according to the manufacturer’s criteria prior to use. The detector voltage was set to an offset of +200 V relative to the tune voltage, and acquisition was between 25 and 500 m/z at a rate of 200 spectra per second. Liquid samples were injected by an Agilent 7683A autosampler using a volume of 1 μL with the inlet operated in split mode at a 1:80 ratio, otherwise using identical instrument conditions.
2.7 Interpretation of Chromatographic Results
Interpretation of all chromatographic data for ignitable liquid identification proceeded as described in our prior work (Abel et al., 2018; Abel and Harynuk, 2020) and in accordance with the general interpretation and classification rules laid out in ASTM E1618, including comparison with standards of known ignitable liquids (in this case, the neat ignitable liquids) and a reference collection of pyrolysed substrates. Additional description of the interpretive approach and basis for identification of ignitable liquids from each examined class is provided in the Supplementary Material. Chromatographic figures were generated as single EICs from a total sum of ions from each class of petroleum components (i.e. alkane, cycloalkane, aromatic, indane, and naphthalene) to reduce the visual contribution of matrix components from other chemical classes.
3 Results
3.1 Optimisation of the SPME Extraction Method
The best compromise between sensitivity and a chromatographic profile consistent with ACS extracts and neat ignitable liquids was found at a 5-min extraction time (i.e. 300 ± 1 s). The SPME Arrow CAR-PDMS fibre provided more representative extraction than the standard SPME format, yielding chromatographic profiles with a higher degree of similarity to the ACS extracts due to more uniform recovery of components across the entire volatility range. The SPME Arrow fibre was also significantly more robust against damage during exposure compared to the standard SPME format (Figure 2). Pre-heating of debris at 60°C ensured a uniform internal temperature such that ignitable liquid residues would not be sequestered in cold portions of the debris, and an elevated extraction temperature of 80°C provided the most representative peak profiles which was consistent with prior literature (Furton et al., 1995; Bertsch and Ren, 1999; Ren and Bertsch, 1999; Yoshida et al., 2008). The 80°C temperature also limited the significant distension of the cans encountered at 90°C, and was found to give good recovery of both light and heavy distillate peaks (for the SPME Arrow fibre) in contrast to other prior studies (Furton et al., 1995; Yoshida et al., 2008; Fettig et al., 2014). Finally, external cooling of the fibre provided only a marginal improvement in the recovery of the lighter volatiles and did not significantly improve comparability to the neat ignitable liquids or ACS extracts, especially relative to the much greater improvement observed for the SPME Arrow fibre over to the standard DVB-CAR-PDMS fibre.
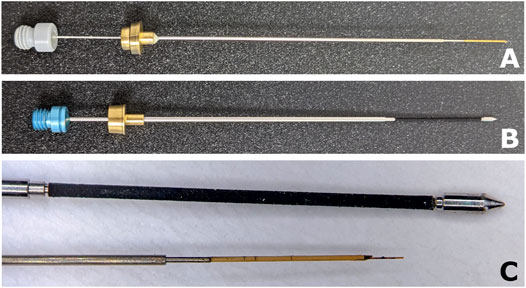
FIGURE 2. Standard SPME Fibre and SPME Arrow Fibre Comparison. (A) The standard SPME format. The thickest length is the fibre sheath, followed by the metal pin inside the sheath (right), bearing the off-white/yellowish coating over fused silica. The fibre in (A) is damaged from delamination of the coating after light contact with a Kimwipe. The SPME Arrow format in (B) shows the wider gauge and more rugged construction. At the far right of the fibre is the “Arrow” tip which protects the coating from collisions during exposure into debris. The relative scale of the fibres is visible in (C) showing the much greater coating volume on the Arrow fibre (further expansions provided in Figure 7). The standard SPME fibre in (C) incurred the damage shown within 5 debris extractions while the SPME Arrow fibre remains unaltered after more than 100 debris extractions.
3.2 Comparison of SPME Arrow Debris Extract Profiles to PHS via ACS
The following optimised method was developed from the SPME optimisation study. The selected Arrow fibre became commercially available after the optimisation study was complete, so the optimised SPME method was used with the SPME Arrow fibre. The SPME Arrow fibre provided improved sensitivity and representativeness using the optimised method, so no additional method development was pursued.
1) warming the debris to 60°C overnight prior to extraction,
2) holding the can in the 80°C aluminum sleeve for at least 20 min,
3) wiping part of the lid with a water-dampened Kimwipe,
4) puncturing the wiped spot with a clean nail,
5) immediate insertion and 300 ± 1 s extraction with the SPME Arrow fibre,
6) rapid retraction of the fibre and immediate sealing with aluminum foil under tape,
7) immediate desorption in the GC inlet at 250°C for 3 min.
The sample pairs of the three types of debris collected from a controlled structure fire (burned carpet, charred drywall, and burned carpet underlay) were sampled by the optimised SPME extraction method and the standard PHS via ACS extraction. In spite of the obvious presence of ignitable liquid peak profiles, no specific assessment of ignitable liquid content was made for these samples. Instead, the comparison shown in Figure 3 demonstrates that the SPME Arrow extracts provide the same overall qualitative chromatographic character as the more familiar ACS extracts for both ignitable liquid and matrix content. Consequently, it was decided that no further optimisation of conditions was needed for the Arrow fibre. The chromatograms shown in Figure 3 demonstrate that apart from overloading of some peaks in the SPME extracts, the overall range of extracted volatiles and the elution region with maximal peak abundance is consistent between both techniques. Both techniques yield equivalent and representative chromatographic results for equivalent samples, and comparison to both ignitable liquid and substrate databases should yield the same interpretive findings for ACS and SPME Arrow extracts.
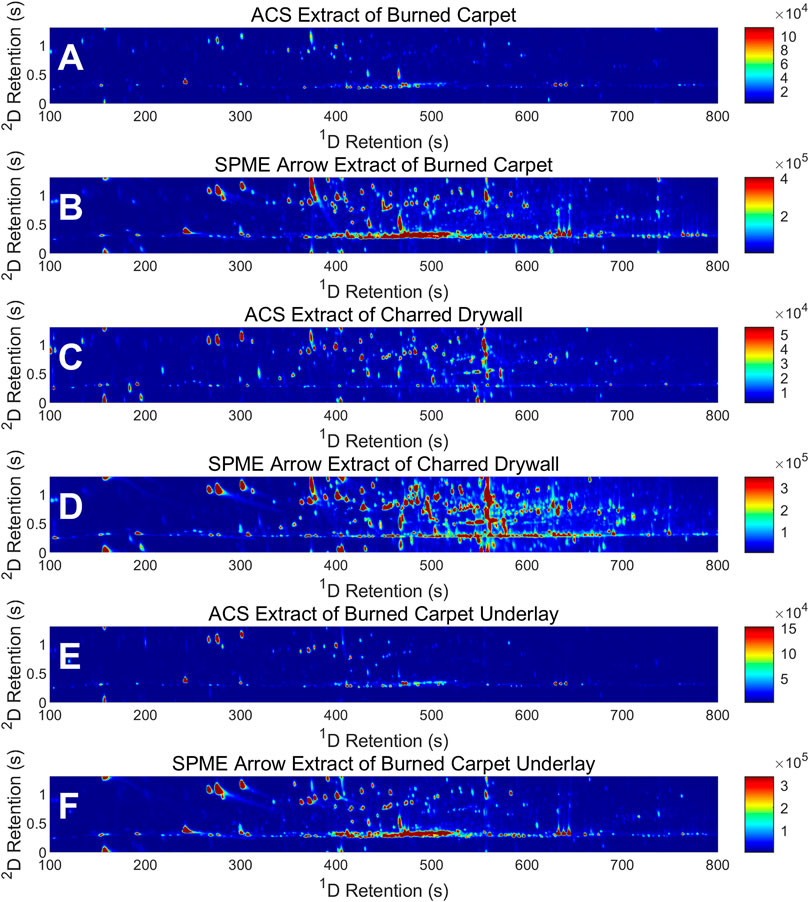
FIGURE 3. Comparison of ACS and SPME Arrow Extraction of Real Fire Debris. (A,C,E) show the ACS extracts of burned carpet, charred drywall, and burned carpet underlay. (B,D,F) Show similar chromatographic profiles yielded by the SPME Arrow extracts of the same materials. Note that these plots have been scaled to 10% of the maximum peak abundance for each chromatogram to facilitate visual comparison.
3.3 Confirmatory Analysis of Recovered Canine-Searched Cans
The results of confirmatory analysis for the recovered sample cans from the wheel search in the prior canine study (Abel et al., 2020) using the optimised SPME extraction method are summarised in Table 1 (for the first ILDC team) and Table 2 (for the second ILDC team). One modification to the optimised extraction method was made for these samples due to their precious nature, their low substrate mass and resultant high headspace volume, and the extremely low amounts of ignitable liquid which may remain after their exposure and manipulation during the canine searches. To avoid spoliation of the samples by exacerbating the loss of the most volatile compounds, the cans containing lighter fluid were only heated to 30°C, the cans containing gasoline were heated to 60°C, and the cans containing Diesel were heated to 90°C.
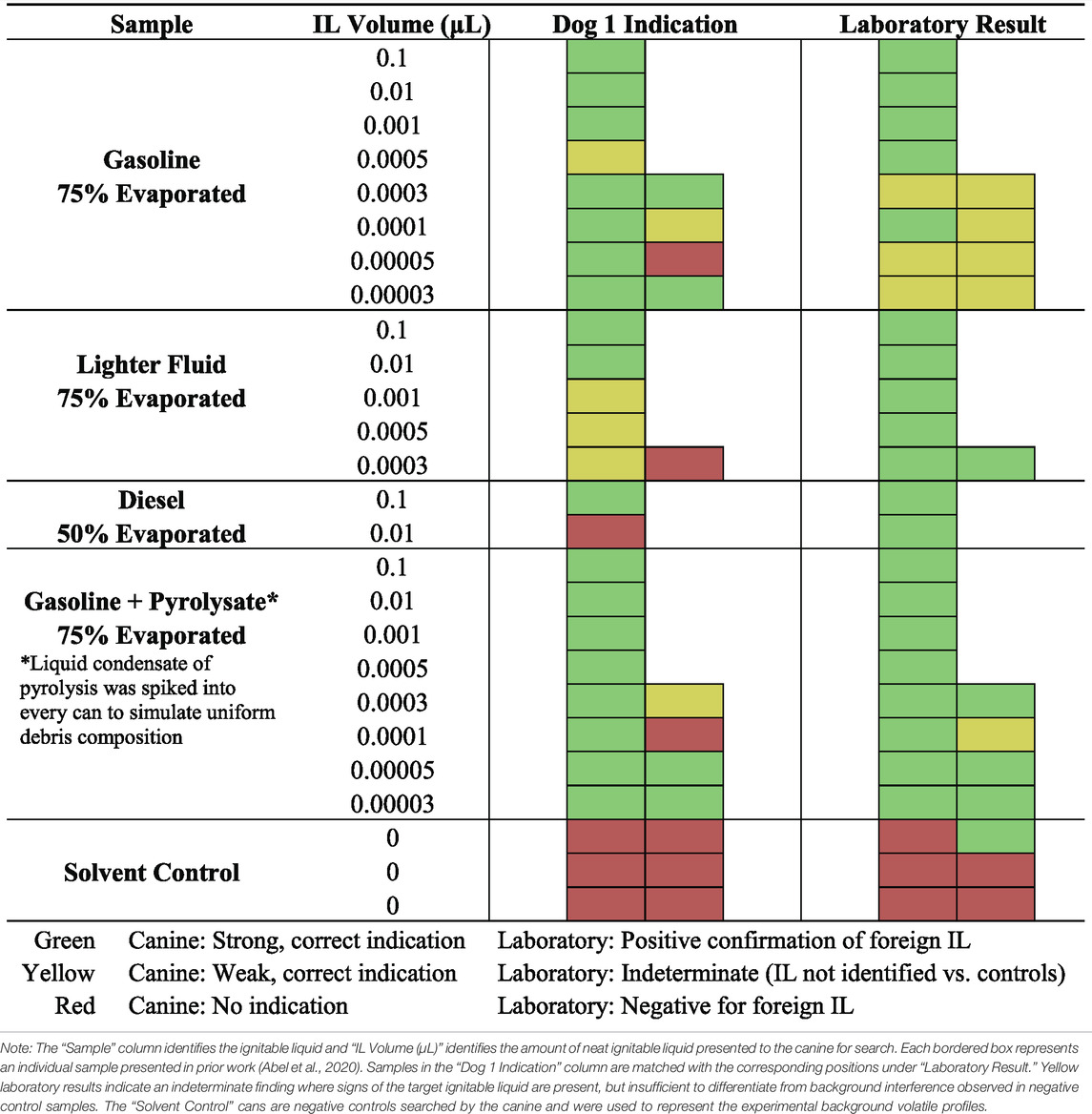
TABLE 1. Indications from Dog 1 for Wheel/Can Search (Abel et al., 2020) vs. Lab Confirmation Result.
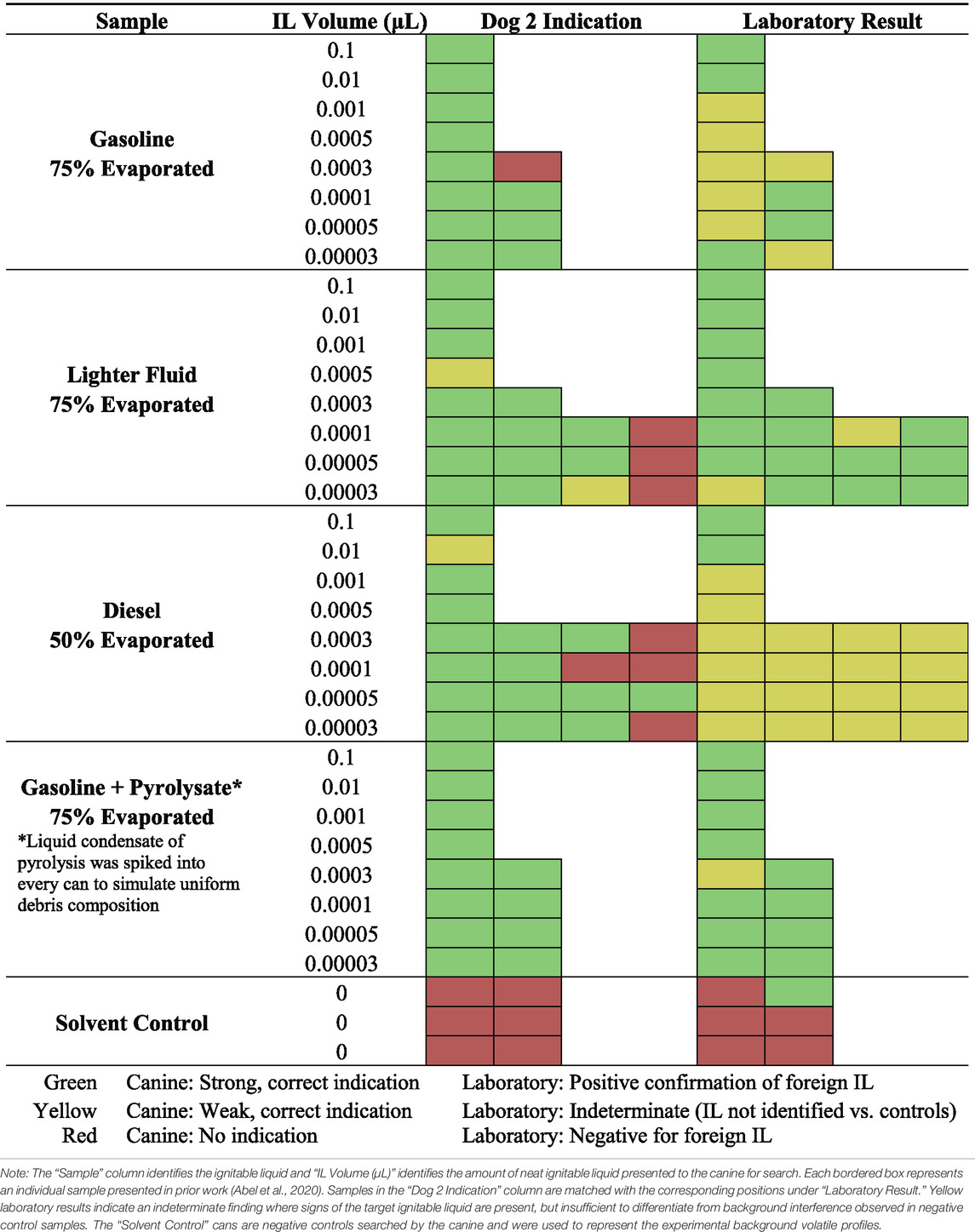
TABLE 2. Indications from Dog 2 for Wheel/Can Search (Abel et al., 2020) vs. Lab Confirmation Result.
Tables 1, 2 present the canine indications for each sample alongside the laboratory result from confirmatory analysis using the SPME Arrow extraction method. In general, the SPME Arrow extraction method allowed confirmation of the canine indications for both “Dog 1” and “Dog 2.” For the purposes of comparison to the canine, indeterminate laboratory findings may be considered as equivalent to negative findings since the lack of an identifiable foreign IL means little-to-no context can be provided for the underlying causes of the canine indication.
Table 1 shows that laboratory confirmation of Dog 1’s indications was generally successful at all IL volumes, apart from the low volume neat gasoline samples which is attributed to background interference (described in the following section). A few differences between the canine and the laboratory are worth noting. Several of the lower abundance gasoline samples resulted in indeterminate findings (i.e. yellow shaded boxes) where the canine indication was a strong positive (i.e. green shaded boxes). An indeterminate lab finding occurred for the 0.00005 μL gasoline can which received no indication from the canine. This may be considered an agreement/correct confirmation, although it may also be a consequence of background interference. A similar result occurred for the 0.0001 μL gasoline + pyrolysate can. However, the laboratory result confirmed the presence of foreign IL in the 0.0003 μL lighter fluid can in spite of the lack of a canine indication. The same also occurred for the 0.01 μL Diesel sample, however as noted in the prior study (Abel et al., 2020), Dog 1 had significant difficulty locating lighter fluid and Diesel at any level below the most abundant samples likely due to having been trained primarily on gasoline. These samples were transferred to the other ILDC team, and Table 2 presents more searches and additional replicates for this reason. One solvent control can was positive for a trace gasoline bearing a less evaporated profile than the interfering highly weathered gasoline. This may have been a consequence of inadvertent vapour transfer from an adjacent high-volume gasoline sample during search, or may have been a cross-transfer between samples caused by the canine’s search itself. Regardless, the majority of positive canine indications were successfully confirmed by the laboratory in spite of the latent interferences from the SPME Arrow fibre and the experimental background interferences further described in the next section.
Table 2 shows that laboratory confirmation of Dog 2’s indications was also generally successful at all IL volumes, with the exception of some samples with low levels of neat gasoline and all samples with low levels of Diesel. However, for the gasoline samples, successful confirmation was achieved for one sample at each of the lower gasoline volumes (0.0001, 0.00005, and 0.00003 μL) demonstrating that the presented method is successful when background interference is minimal or can be well controlled. An indeterminate lab finding occurred for the 0.0003 μL gasoline can which received no indication from the canine. This may be considered an agreement/correct confirmation, or may again be a consequence of background interference. A similar discrepancy occurred for the 0.0003 μL gasoline + pyrolysate can, but confirmatory results were achieved for these samples down to the lowest volume (0.00003 μL). The laboratory result could not confirm Diesel at any of the concentrations at or below 0.001 μL, due to relatively early dropout of the interspersed branched alkane peaks and the confounding influence of a series of n-alkanes arising from the SPME Arrow fibre itself (described in the following section, with chromatographic data shown in the Supplementary Material), representing an inherent deficiency in the method. However, the non-exhaustive nature of the SPME Arrow extraction allows for longer secondary extractions which may serve to correct the issue when a heavy distillate is suspected. A more significant concern was noted for the lighter fluid results where the lab yielded indeterminate findings for two strong canine positives, and provided confirmation for three samples which yielded no indication from the canine. Since light petroleum distillates are generally highly significant due to their low persistence, the two samples which were strongly indicated by the canine but could not be confirmed by the lab (0.0001 and 0.00003 μL) represent a failure of the method. However, numerous other samples at these levels were successfully confirmed possibly indicating that the failed confirmatory analysis may result from volatile losses during search. The ability to confirm the presence of foreign IL in lighter fluid samples not indicated by the canine (i.e. the three red samples at 0.0001, 0.00005, and 0.00003 μL) may indicate either excess laboratory sensitivity or greater discriminating ability than the canine under some circumstances. While any positive result yielded from a putatively negative sample is usually very problematic in forensic examination, in this case the risk is relatively low. This is because any sample not receiving an indication from the canine at the scene would either be used as a negative control or would not be submitted to the laboratory at all. If these positive exhibits were debris selected by other investigative means not involving the canine, the SPME Arrow extraction method would be inappropriately sensitive and should not be used. However, when selected by the canine, the role of the laboratory shifts to scrutinising the reasons for an indication so the analytical sensitivity observed is still acceptable even if it may exceed the canine in some cases. In spite of these considerations, the confirmatory results demonstrate that the SPME Arrow extraction is an appropriately sensitive and effective confirmatory technique for scrutinising canine indications on fire debris.
3.4 Background Interferences Encountered During Confirmatory Analysis
In the confirmatory analysis of the canine search results, the causes for indeterminate findings can be broadly attributed to interference from n-alkanes arising from the background profile of the SPME Arrow fibre (for Diesel samples) and the presence of a weathered gasoline (estimated at ∼90% evaporation) in the experimental background, including the negative ‘Solvent Control’ samples in Tables 1, 2, 3. Example chromatograms of the ambient laboratory air and the blank can controls extracted at 60°C are presented in Figure 4. These data show significant levels of latent interfering ignitable liquid residues in both the laboratory air and in some of the blanks which represent the matrix expected for the target sample cans. A comparison between a positive gasoline sample, a gasoline sample resulting in an indeterminate finding, and a negative control can with a latent interfering gasoline profile are presented in Figure 5, along with a laboratory ambient air blank. In general, the interfering profiles observed in control samples and the exercise of caution during confirmatory interpretation account for the high number of indeterminate results seen in Tables 1, 2. An expanded description of the interpretive approach used to discriminate between foreign ILs and background contamination profiles is provided in the Supplementary Material.
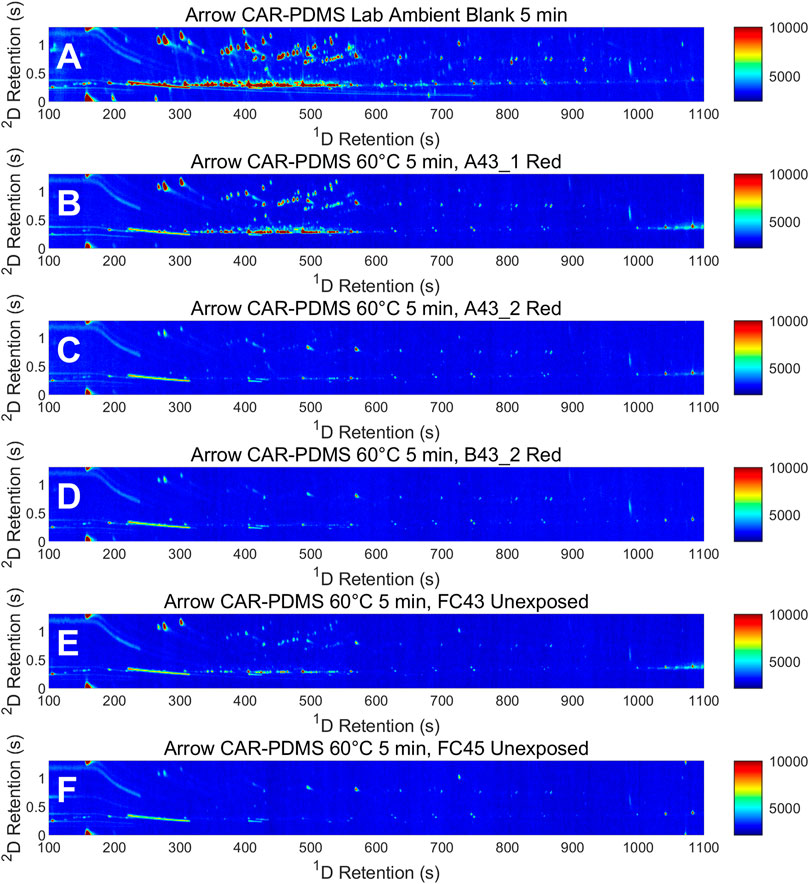
FIGURE 4. Background Profiles of Exposed and Unexposed Can Blanks. The background laboratory air profile (A) contains a highly evaporated gasoline (estimated at 90% evaporation) and indications of a heavy petroleum distillate (likely Diesel). A highly evaporated gasoline similar to (A) is also observed in the blank can (B). Plots (B-D) are three representative blank cans with varied amounts of background interference. Plots (E,F) show the background profile for two unexposed blank cans, with (E) bearing a weak profile similar to (A,B).
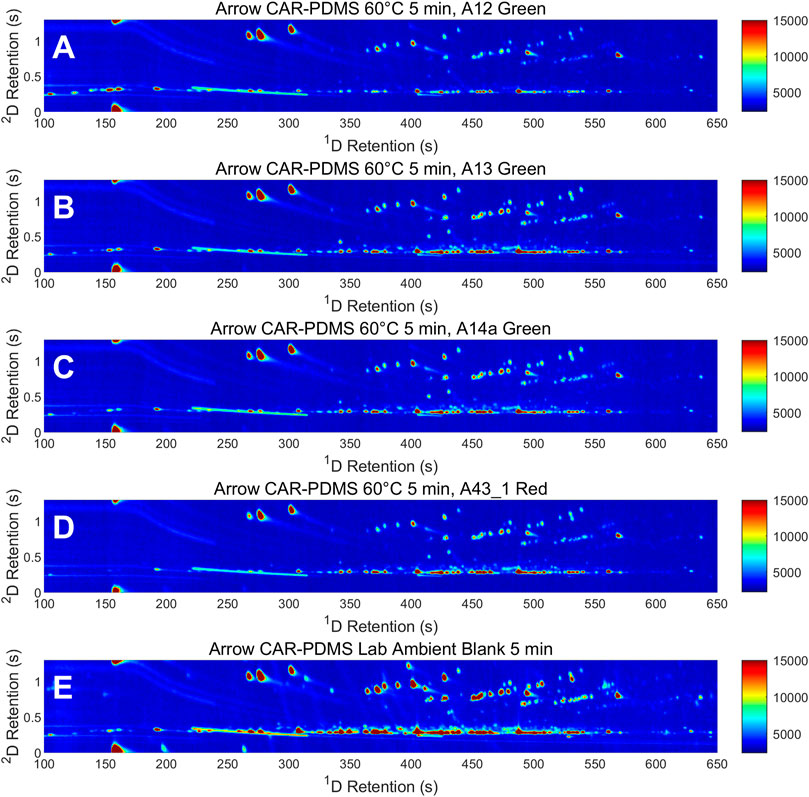
FIGURE 5. Gasoline Detectability vs. Background Interferences. (A) shows the analytical result from a canine hit on 0.001 μL gasoline. The only components differentiating (A) from interferences in laboratory air (E) or the blank control can (D) are alkanes eluting from 1tR 100–250 s. These peaks were used to determine the detectability of gasoline in the canine-searched cans. At lower abundances most gasoline components are either obscured by, or are indistinguishable from, the interferent profile (B,C) show canine hits on 0.0005 and 0.0003 μL gasoline respectively, for which gasoline is confirmed in (B) while (C) is indeterminate.
The samples presented in Table 3 were prepared in the same fashion as the samples in Tables 1, 2, but were not exposed to canine search in order to assess whether volatile losses from the canine-exposed samples could have impacted the confirmatory analysis results. However, Table 3 shows the same indeterminate findings for Diesel as observed for the canine-exposed samples, and shows a positive IL finding for one of the negative control cans. This indicates that the Arrow fibre background (for Diesel) and IL interferences in the experimental background (for gasoline) had a more significant impact on the confirmatory analysis than any losses from the cans. This observation is supported by the relative similarity in profile strength observed for the cans exposed to canine search and the unexposed controls (Figure 6).
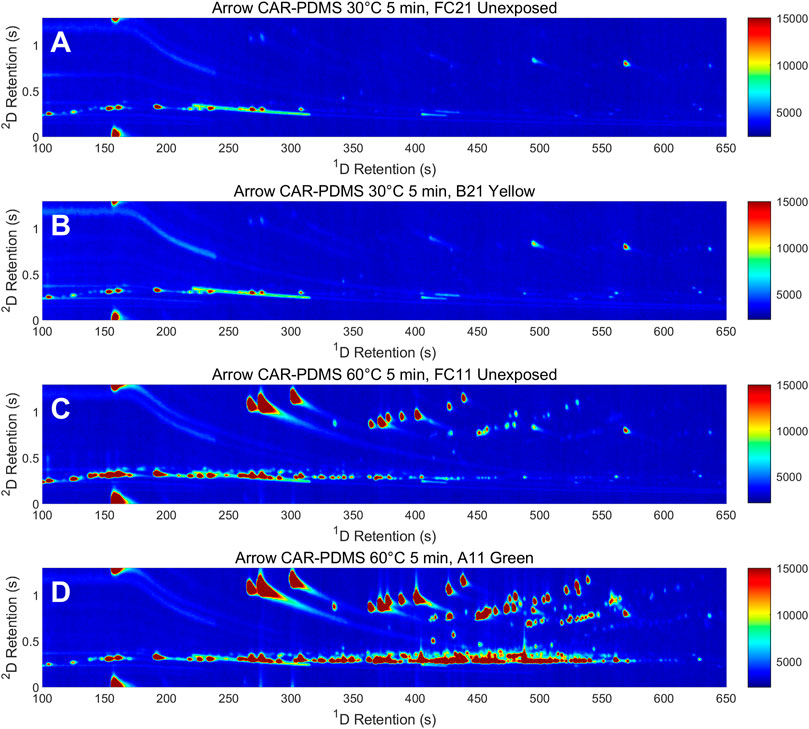
FIGURE 6. Volatile Losses in Exposed vs. Unexposed Cans. The analytical results for lighter fluid are shown in (A) for a sample left sealed and unexposed to canine search, and (B) opened and exposed to search by the canine. The results for gasoline are shown in (C) for the unexposed can, and (D) for the searched can. Notably, the heavier gasoline components are more abundant in the exposed can (D) than the unexposed can (C) showing the impact of high sensitivity and latent petroleum either in the surroundings or in the exhibit packaging.
The interfering gasoline profile showed a higher degree of evaporation than the 75% evaporated gasoline used for the canine search and SPME extraction experiments. Therefore, confirmation of foreign gasoline in the canine-selected cans relied on relative elevation in the early alkane abundances compared to the aromatic peaks observed in the interfering gasoline profile (these abundances decrease with decreasing gasoline concentration as shown in Figures 5A,B,C, as compared to the negative control can in Figure 5D). In the absence of interfering profiles it is reasonable to expect that the rate of laboratory confirmation would improve. However, unless the source of any interfering profile can be identified and controlled, confirmation of foreign ignitable liquid would necessarily depend on a rigorous comparison to appropriate packaging and debris controls in every case.
The source of the interfering gasoline could be passive accumulation from vehicle exhaust during transport, ambient contamination during exposure for canine search, storage with other more abundant samples prior to analysis, or even from diffusion of contaminated laboratory air into the can. These scenarios are all plausible, except for contaminated laboratory air due to the lack of uniformity between samples extracted in an identical fashion.
As for volatile losses during canine search, chromatograms from the exposed cans were of similar abundance to their unexposed equivalents for all ignitable liquids tested (Figure 6). Although some loss of volatiles may occur during exposure of the can contents during the canine search, the presence of interfering profiles is a far stronger effect and a more likely cause of indeterminate findings.
4 Discussion
4.1 Extraction Time
The experiments on extraction time were only conducted with the standard SPME fibres because the selected SPME Arrow and inlet conversion kit had not yet been released commercially when this research was commenced. While some published SPME extractions last hours or even days (de la Mata et al., 2017), our experiments were constrained to ≤25 min to maximise throughput and minimise instrument idle time based on a typical well-optimised separation of fire debris extracts using a standard non-polar GC column (∼30 min). Longer thermodynamically controlled SPME extractions could increase sensitivity and reduce variability (Pawliszyn, 2000), but the chromatographic profile would progressively skew towards heavier components, making interpretation more challenging and increasing the risk of misclassification. When the SPME Arrow configuration became available, it was sourced and tested using the optimised conditions for the standard SPME format. The Arrow fibre outperformed the conventional SPME fibre and provided canine-like sensitivity and IL profiles comparable to the ACS strips. Accordingly, no further optimisation was pursued with the SPME Arrow fibre as there was little practical reason to do so.
4.2 Fibre Selection
While the SPME Arrow fibre provides the required sensitivity and representativeness for confirmatory analysis of canine-selected samples, the new format has other advantages which make it ideal for use with fire debris. The sorptive coating on standard SPME fibres is adhered to a fused silica support, which is attached to the metal plunger rod with adhesive (Figure 2C, Figures 7A,B). This keeps the device compact with an outer diameter small enough to be used with an unmodified GC inlet. However, the delicate bond between the fibre and metal plunger, and the fragile surface coating means the fibres are easily damaged. Chipping or delamination of the fibre coating happened frequently throughout these experiments (Figure 7B). This issue is compounded by the nature of fire debris, which typically contains rigid and irregularly shaped materials such as wood, concrete, and metal, which may sit close to the fibre insertion point even in under-filled containers, resulting in collision and damage to the fibre coating. In contrast, the SPME Arrow uses a metal support which is capped with a conical metal tip (Figures 7C-E). This configuration protects the coating from flexing or contact with debris during exposure. Breaking of the standard SPME fibres requires such little force that it may not be felt by the user, while collision of the SPME Arrow tip during exposure does not cause damage to the coating and the rigid pin translates the force to the user who can adjust the fibre position. The coating may be scraped along the edge of an obstruction, but this can also be felt by the user who can respond accordingly. During this study, such scrapes only resulted in superficial abrasion of the coating surface without meaningful damage or loss of sorbent material. Also, the Arrow tip recesses into the fibre housing which is likely to protect against contamination and losses of volatile analytes relative to the open tip of the conventional SPME format (Figure 7E).
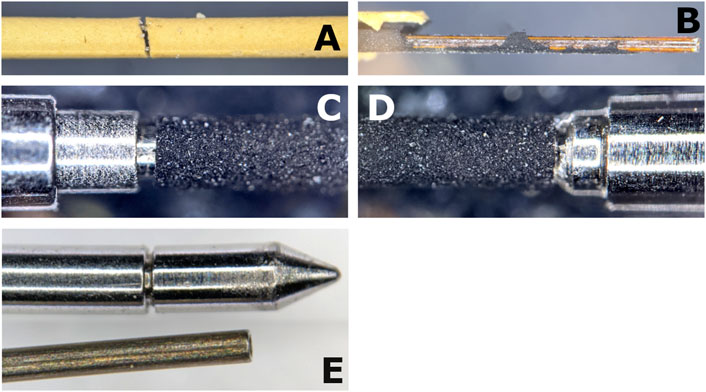
FIGURE 7. Standard SPME and SPME Arrow Fibre Expansions and Sheathed Appearance. The fibre in (A,B) is the standard SPME format. One of numerous cracks along the length of the coating is shown in (A), while chipping/shearing damage is visible in (B). The black material in (B) is the Carboxen phase, and the translucent brown/orange is the linker material connecting the coating to the fused silica support. The fibre in (C,D) is the SPME Arrow, illustrating the rough black coating over a continuous metal support. The retracted fibres are shown in (E) where the SPME Arrow (top) produces a recessed seal of the fibre sheath, while the standard SPME format is simply held a short distance inside the open bore.
4.3 Analytical Observations for Recovered Canine-Searched Cans
The putative identity and amount of ignitable liquid was known for each of the canine-searched cans recovered from the previously published study investigating ILDC sensitivity (Abel et al., 2020). Overall, the SPME Arrow extraction method provided the necessary sensitivity for positive confirmation of the canine’s indications. However, definitive confirmation was not possible for some of the individual canine can samples. The most significant issue with confirmatory analysis by SPME Arrow extraction resulted from interference with the gasoline sample extracts due to the presence of a highly evaporated gasoline residue as latent contaminant most likely arising from the factory-applied oil used to protect and lubricate the steel can surfaces. Interference with the Diesel sample extracts was also caused by a series of n-alkanes arising from the fibre itself. Minor variation in the ignitable liquid profile strength was observed across samples of identical prepared concentration, which generally coincided with differences in the strength of the canine indications. Losses of volatile compounds from cans exposed to canine search was expected, especially for the highest volatility compounds. However, such losses were insignificant based on comparison to unexposed control cans (i.e. cans kept sealed from preparation to analysis) which showed little difference relative to their exposed equivalents (Figure 6). Differences between cans with identical prepared concentrations may be an indicator of differing substrate competition for headspace volatiles. However, any variation in the ignitable liquid profile strength between replicate cans did not affect identification except when background profiles directly interfered.
Confirmation of Diesel was especially problematic since the branched alkanes required to differentiate the profile from the fibre background dropped below the detection threshold at relatively high amounts of ignitable liquid. The overall impact of this shortcoming on confirmatory analysis of canine-selected debris is likely to be minimal, as heavy distillates such as Diesel have limited forensic significance especially in trace amounts. Diesel is combustible but not flammable (National Fire Protection Association, 2017) and heavy distillates are far more persistent than gasoline due to low volatility. Nevertheless, it remains important to confirm the underlying cause of any canine indication even if that cause is of low significance. Sensitivity towards heavy distillates may be improved by longer extraction time, but at the risk of increasing false positives or misclassification of other ignitable liquid profiles. In cases where no ignitable liquid can be identified from an initial SPME Arrow extraction a second, longer SPME Arrow extraction may be advisable. Regardless of the challenges in confirming the presence of Diesel, the overall tabulated results demonstrate that the SPME Arrow provides the high extraction sensitivity required for confirmatory analysis of canine-selected exhibits with more representative extraction than the standard SPME fibres. This closes an important gap in fire investigation and the adjudication of suspected arsons. This method also opens the possibility for more objective assessment of odour detection canines by providing objective data to scrutinise the underlying reasons for false positives or false negatives, for subsequent improvement in training and certification regimens. However, the added sensitivity increases the problem of interference by latent ignitable liquid profiles and more work is required to determine whether these interferences can be resolved or mitigated. The SPME Arrow extraction method is minimally invasive, non-exhaustive, and easily repeatable without degrading subsequent extraction by other techniques. One scenario requiring secondary extraction is overloaded SPME Arrow extracts resulting from samples with relatively strong ignitable liquid, where the overloaded data could be ignored and the sample extracted by PHS via ACS for routine analysis.
5 Conclusion
Highly sensitive and non-exhaustive extraction using the SPME Arrow ‘Carbon Wide Range-PDMS’ fibre was developed and shown to be suitable for confirmatory analysis of fire debris selected by ILDCs. The optimised method was shown to produce ignitable liquid profiles representative of the neat ignitable liquids and ACS extracts which allows comparison to standard reference ignitable liquid and substrate databases, and reduces the risk of misclassification relative to other SPME methods. The optimised method was demonstrated on real fire debris exhibits, further confirming that the extraction generates chromatographic profiles equivalent to ACS. The non-exhaustive nature of the presented method allows for repeated extractions, such as longer extraction in the event of poor recovery of a heavy petroleum distillate, or the use of secondary extraction by more routine methods in the event of an overloaded SPME Arrow extract. While these results resolve a longstanding disparity between the ILDC and the laboratory, forensic laboratories should exercise caution when deciding to apply this technique. The high sensitivity offered by SPME Arrow extraction and the widespread presence of latent petroleum can increase the number of false positives if adequate control exhibits and representative packaging samples are not submitted to the laboratory. The use of rigorous controls is absolutely critical based on the significant impact of latent interfering profiles encountered during this study, which would have produced false positives if adequate controls had not been used. However, when a properly trained and certified ILDC team has identified an exhibit and appropriate control packaging and control debris (i.e. debris not eliciting a canine indication) are provided, this extraction technique is effective for confirmatory testing and should help address the larger problem of unconfirmed canine indications being tendered to the court.
Data Availability Statement
The original contributions presented in the study are included in the article/Supplementary Material, further inquiries can be directed to the corresponding author.
Author Contributions
RA and JH contributed to the conceptualisation and design of the study. RA was responsible for performing experiments, burning a building, collection and analysis of samples, and interpretation of data. RA drafted the manuscript under the guidance of JH. JH was responsible for editing the manuscript and securing funding for the study.
Funding
Funding for this research was provided by the Natural Sciences and Engineering Research Council of Canada (NSERC) grant number RGPIN-2016-05445.
Conflict of Interest
Author RJA was employed by the MBC Group after the completion of this work.
The authors declare that the research was conducted in the absence of any commercial or financial relationships that could be construed as a potential conflict of interest.
Publisher’s Note
All claims expressed in this article are solely those of the authors and do not necessarily represent those of their affiliated organizations or those of the publisher, the editors, and the reviewers. Any product that may be evaluated in this article, or claim that may be made by its manufacturer, is not guaranteed or endorsed by the publisher.
Acknowledgments
The authors would like to extend their sincere gratitude and thanks to the following people/groups. To Restek and Chromatographic Specialties for donating an Arrow fibre for preliminary testing. To Joe Towers and Global Forensics, to Eza and her handler Jeff Lunder, to the unnamed canine team, and to Lawrence Hogg and the Town of Trochu, Alberta for facilitating the canine experiments and our participation in a controlled structure fire which provided critical samples for this paper. Thanks are also given to the machine shop in the Department of Chemistry at the University of Alberta for their excellent work in fabricating the heating sleeves and for the challenging fabrication of the fibre chiller.
Supplementary Material
The Supplementary Material for this article can be found online at: https://www.frontiersin.org/articles/10.3389/frans.2022.857880/full#supplementary-material
References
Abel, R. J., and Harynuk, J. J. Abelrobin/LoadPEG: First Public Release of LoadPEG.M. Edmonton, Canada: University of Alberta (2020). doi:10.5281/zenodo.4035154
Abel, R. J., Lunder, J. L., and Harynuk, J. J. A Novel Protocol for Producing Low-Abundance Targets to Characterize the Sensitivity Limits of Ignitable Liquid Detection Canines. Forensic Chem. (2020) 18:100230. doi:10.1016/j.forc.2020.100230
Abel, R., Zadora, G., Sandercock, P., and Harynuk, J. Modern Instrumental Limits of Identification of Ignitable Liquids in Forensic Fire Debris Analysis. Separations (2018) 5:58. doi:10.3390/separations5040058
Ahmad, U., and Selvaraju, G. Forensic Detection of Fire Accelerants Using a New Solid Phase Microextraction (SPME) Fiber. Internet J. Forensic Sci. (2007) 2:1–28. Available at: https://ispub.com/IJFS/2/1/6781.(Accessed April 20, 2022)
Ahmad, U., and Voon, C. Detection of Accelerants in Fire Debris Using Headspace Solid Phase Microextraction-Capillary Gas Chromatography. Malaysian J. Anal. Sci. (2001) 7:57–63.
Almirall J. R., and Furton K. G., editors. Analysis and Interpretation of Fire Scene Evidence. Boca Raton: CRC Press (2004).
Almirall, J. R., Wang, J., Lothridge, K., and Furton, K. G. The Detection and Analysis of Ignitable Liquid Residues Extracted from Human Skin Using SPME/GC. J. Forensic Sci. (2000) 45:453–461. doi:10.1520/jfs14706j
Almirall, J. R., Bruna, J., and Furton, K. G. The Recovery of Accelerants in Aqueous Samples from Fire Debris Using Solid-phase Microextraction (SPME). Sci. Justice (1996) 36:283–287. doi:10.1016/s1355-0306(96)72615-1
Armstrong, A., Babrauskas, V., Holmes, D. L., Martin, C., Powell, R., Riggs, S., et al. The Evaluation of the Extent of Transporting or “Tracking” an Identifiable Ignitable Liquid (Gasoline) throughout Fire Scenes during the Investigative Process. J. Forensic Sci. (2004) 49:741–748. doi:10.1520/jfs2003155
ASTM International, . ASTM E1386-15 Standard Practice for Separation of Ignitable Liquid Residues from Fire Debris Samples by Solvent Extraction. West Conshohocken, Pennsylvania, United States: ASTM International (2015).
ASTM International, . ASTM E1412-16 Standard Practice for Separation of Ignitable Liquid Residues from Fire Debris Samples by Passive Headspace Concentration with Activated Charcoal. West Conshohocken, Pennsylvania, United States: ASTM International (2016).
ASTM International, . ASTM E1413-13 Standard Practice for Separation of Ignitable Liquid Residues from Fire Debris Samples by Dynamic Headspace Concentration. West Conshohocken, Pennsylvania, United States: ASTM International (2013).
ASTM International, . ASTM E1618-14 Standard Test Method for Ignitable Liquid Residues in Extracts from Fire Debris Samples by Gas Chromatography-Mass Spectrometry. West Conshohocken, Pennsylvania, United States: ASTM International (2014).
ASTM International, . ASTM E2154-15a Standard Practice for Separation and Concentration of Ignitable Liquid Residues from Fire Debris Samples by Passive Headspace Concentration with Solid Phase Microextraction (SPME). West Conshohocken, Pennsylvania, United States: ASTM International (2015).
Bertsch, W., and Ren, Q. Contemporary Sample Preparation Methods for the Detection of Ignitable Liquids in Suspect Arson Cases. Forensic Sci. Rev. (1999) 11:141–156.
Bertsch, W., and Zhang, Q.-W. Sample Preparation for the Chemical Analysis of Debris in Suspect Arson Cases. Analytica Chim. Acta (1990) 236:183–195. doi:10.1016/s0003-2670(00)83312-7
Blumberg, L. M., and Klee, M. S. Optimal Heating Rate in Gas Chromatography. J. Micro. Sep. (2000) 12:508–514. doi:10.1002/1520-667x(2000)12:9<508::aid-mcs5>3.0.co;2-y
Bodle, E. S., and Hardy, J. K. Multivariate Pattern Recognition of Petroleum-Based Accelerants by Solid-phase Microextraction Gas Chromatography with Flame Ionization Detection. Analytica Chim. Acta (2007) 589:247–254. doi:10.1016/j.aca.2007.03.006
Borusiewicz, R. Fire Debris Analysis – a Survey of Techniques Used for Accelerants Isolation and Concentration. Probl. Forensic Sci. (2002) 50:44–63.
Chalmers, D., Yan, S. X., Cassista, A., Hrynchuk, R., and Sandercock, P. M. L. Degradation of Gasoline, Barbecue Starter Fluid, and Diesel Fuel by Microbial Action in Soil. Can. Soc. Forensic Sci. J. (2001) 34:49–62. doi:10.1080/00085030.2001.10757517
Chasteen, C., DeHaan, J., Higgins, M., Armstrong, A., Colver, J., Custer, R., et al. IAAI Forensic Science Committee Position on the Use of Accelerant Detection Canines. J. Forensic Sci. (1995) 40:532–534.
Cornett, C., and Wermeling, J. Detection of Substituted PAH Residues by SPME in Arson Debris Analysis. Wisconsin, USA: US Department of Energy (2005).
de la Mata, A. P., McQueen, R. H., Nam, S. L., and Harynuk, J. J. Comprehensive Two-Dimensional Gas Chromatographic Profiling and Chemometric Interpretation of the Volatile Profiles of Sweat in Knit Fabrics. Anal. Bioanal. Chem. (2017) 409:1905–1913. doi:10.1007/s00216-016-0137-1
DeHaan, J. Canine Accelerant Detection Teams: Validation and Certification. Santa Rosa, CA, USA: The CAC News (1994). p. 17–21. Available at: https://www.firearson.com/Publications-Resources/Fire-Arson-Investigation-Journal/Default.aspx
DeHaan, J. Our Changing World, Part 3: Is More Sensitive Necessarily More Better? and Part 4: A Matter of Time. Fire and Arson Investigator (2002) 52:20–23.
Eckert, K., Carter, D., and Perrault, K. Sampling Dynamics for Volatile Organic Compounds Using Headspace Solid-phase Microextraction Arrow for Microbiological Samples. Separations (2018) 5:45. doi:10.3390/separations5030045
Ferrino-McAllister, J., Carpenter, D., and Roby, R. Comparison of Gasoline Weathering on Carpet Samples Exposed to Various thermal Environments. Cincinnati, Ohio, USA: University of Cincinnati (2006). p. 187–195.
Fettig, I., Krüger, S., Deubel, J. H., Werrel, M., Raspe, T., and Piechotta, C. Evaluation of a Headspace Solid-phase Microextraction Method for the Analysis of Ignitable Liquids in Fire Debris. J. Forensic Sci. (2014) 59:743–749. doi:10.1111/1556-4029.12342
Furton, K. G., Almirall, J. R., and Bruna, J. C. A Novel Method for the Analysis of Gasoline from Fire Debris Using Headspace Solid-phase Microextraction. J. Forensic Sci. (1996) 41:12–22. doi:10.1520/jfs13890j
Furton, K. G., Bruna, J., and Almirall, J. R. A Simple, Inexpensive, Rapid, Sensitive and Solventless Technique for the Analysis of Accelerants in Fire Debris Based on SPME. J. High Resol. Chromatogr. (1995) 18:625–629. doi:10.1002/jhrc.1240181003
Grafit, A., Muller, D., Kimchi, S., and Avissar, Y. Y. Development of a Solid-phase Microextraction (SPME) Fiber Protector and its Application in Flammable Liquid Residues Analysis. Forensic Sci. Int. (2018) 292:138–147. doi:10.1016/j.forsciint.2018.09.004
Haddadi, S. H., and Pawliszyn, J. Cold Fiber Solid-phase Microextraction Device Based on Thermoelectric Cooling of Metal Fiber. J. Chromatogr. A (2009) 1216:2783–2788. doi:10.1016/j.chroma.2008.09.005
Harris, A. C., and Wheeler, J. F. GC-MS of Ignitable Liquids Using Solvent-Desorbed SPME for Automated Analysis. J. Forensic Sci. (2003) 48:1–6. doi:10.1520/jfs2002067
Heitmann, K., Wichmann, H., and Bahadir, M. Chemical Causes of the Typical Burnt Smell after Accidental Fires. Anal. Bioanal. Chem. (2009) 395:1853–1865. doi:10.1007/s00216-009-3071-7
Hetzel, S. S., and Moss, R. D. How Long after Waterproofing a Deck Can You Still Isolate an Ignitable Liquid? J. Forensic Sci. (2005) 50:369–376. doi:10.1520/jfs2004250
Hutches, K. Microbial Degradation of Ignitable Liquids on Building Materials. Forensic Sci. Int. (2013) 232:e38–e41. doi:10.1016/j.forsciint.2013.08.006
Katz, S. R., and Midkiff, C. R. Unconfirmed Canine Accelerant Detection: a Reliability Issue in Court. J. Forensic Sci. (1998) 43:329–333. doi:10.1520/jfs16142j
Kirkbride, K., Yap, S., Andrews, S., Pigou, P., Klass, G., Dinan, A., et al. Microbial Degradation of Petroleum Hydrocarbons: Implications for Arson Residue Analysis. J. Forensic Sci. (1992) 37:1585–1599. doi:10.1520/jfs13349j
Klee, M. S., and Blumberg, L. M. Theoretical and Practical Aspects of Fast Gas Chromatography and Method Translation. J. Chromatogr. Sci. (2002) 40:234–247. doi:10.1093/chromsci/40.5.234
Kremser, A., Jochmann, M. A., and Schmidt, T. C. PAL SPME Arrow-Evaluation of a Novel Solid-phase Microextraction Device for Freely Dissolved PAHs in Water. Anal. Bioanal. Chem. (2016) 408:943–952. doi:10.1007/s00216-015-9187-z
Kremser, A., Jochmann, M. A., and Schmidt, T. C. Systematic Comparison of Static and Dynamic Headspace Sampling Techniques for Gas Chromatography. Anal. Bioanal. Chem. (2016) 408:6567–6579. doi:10.1007/s00216-016-9843-y
Kurz, M. E., Billard, M., Rettig, M., Augustiniak, J., Lange, J., Larsen, M., et al. Evaluation of Canines for Accelerant Detection at Fire Scenes. J. Forensic Sci. (1994) 39:1528–1536. doi:10.1520/jfs13740j
Kurz, M. E., Schultz, S., Griffith, J., Broadus, K., Sparks, J., Dabdoub, G., et al. Effect of Background Interference on Accelerant Detection by Canines. J. Forensic Sci. (1996) 41:868–873. doi:10.1520/jfs14014j
Lashgari, M., Singh, V., and Pawliszyn, J. A Critical Review on Regulatory Sample Preparation Methods: Validating Solid-phase Microextraction Techniques. Trac Trends Anal. Chem. (2019) 119:115618. doi:10.1016/j.trac.2019.07.029
Lentini, J. J., Dolan, J. A., and Cherry, C. The Petroleum-Laced Background. J. Forensic Sci. (2000) 45:968–989. doi:10.1520/jfs14819j
Lentini, J. J. Persistence of Floor Coating Solvents. J. Forensic Sci. (2001) 46:1470–1473. doi:10.1520/jfs15173j
Lloyd, J. A., and Edmiston, P. L. Preferential Extraction of Hydrocarbons from Fire Debris Samples by Solid Phase Microextraction. J. Forensic Sci. (2003) 48:1–5. doi:10.1520/jfs2002223
Mann, D. C., and Gresham, W. R. Microbial Degradation of Gasoline in Soil. J. Forensic Sci. (1990) 35:913–923. doi:10.1520/jfs12904j
Martín-Alberca, C., Ortega-Ojeda, F. E., and García-Ruiz, C. Analytical Tools for the Analysis of Fire Debris. A Review: 2008-2015. A Review: 2016–2015. Analytica Chim. Acta (2016) 928:1–19. doi:10.1016/j.aca.2016.04.056
Menezes, H. C., and de Lourdes Cardeal, Z. Determination of Polycyclic Aromatic Hydrocarbons from Ambient Air Particulate Matter Using a Cold Fiber Solid Phase Microextraction Gas Chromatography-Mass Spectrometry Method. J. Chromatogr. A (2011) 1218:3300–3305. doi:10.1016/j.chroma.2010.10.105
Menezes, H. C., Paiva, M. J. N., Santos, R. R., Sousa, L. P., Resende, S. F., Saturnino, J. A., et al. A Sensitive GC/MS Method Using Cold Fiber SPME to Determine Polycyclic Aromatic Hydrocarbons in spring Water. Microchemical J. (2013) 110:209–214. doi:10.1016/j.microc.2013.03.010
Monfreda, M., and Gregori, A. Differentiation of Unevaporated Gasoline Samples According to Their Brands, by SPME-GC-MS and Multivariate Statistical Analysis. J. Forensic Sci. (2011) 56:372–380. doi:10.1111/j.1556-4029.2010.01644.x
National Fire Protection Association, . Technical Committee on Fire Investigations, NFPA 921: Guide for Fire and Explosion Investigations. Quincy, Massachusetts: National Fire Protection Association (2017).
Pawliszyn, J. Theory of Solid-phase Microextraction. J. Chromatogr. Sci. (2000) 38:270–278. doi:10.1093/chromsci/38.7.270
Ren, Q., and Bertsch, W. A Comprehensive Sample Preparation Scheme for Accelerants in Suspect Arson Cases. J. Forensic Sci. (1999) 44:504–515. doi:10.1520/jfs14501j
Stauffer, É., Dolan, J., and Newman, R. Fire Debris Analysis. 1st ed. Cambridge, Massachusetts, United States: Elsevier/Academic Press (2008).
Steffen, A., and Pawliszyn, J. Determination of Liquid Accelerants in Arson Suspected Fire Debris Using Headspace Solid-phase Microextraction. Anal. Commun. (1996) 33:129–131. doi:10.1039/ac9963300129
Tankiewicz, M., Morrison, C., and Biziuk, M. Application and Optimization of Headspace Solid-phase Microextraction (HS-SPME) Coupled with Gas Chromatography-Flame-Ionization Detector (GC-FID) to Determine Products of the Petroleum Industry in Aqueous Samples. Microchemical J. (2013) 108:117–123. doi:10.1016/j.microc.2012.10.010
Thatcher, P. J. The Scientific Investigation of Fire CausesForensic Sci. Prog. (1986) 1:117–151. doi:10.1007/978-3-642-69400-4_4
Tindall, R., and Lothridge, K. An Evaluation of 42 Accelerant Detection Canine TeamsJ. Forensic Sci. (1995) 40:561–564. doi:10.1520/jfs13825j
Turner, D. A., and Goodpaster, J. V. Comparing the Effects of Weathering and Microbial Degradation on Gasoline Using Principal Components Analysis*. J. Forensic Sci. (2012) 57:64–69. doi:10.1111/j.1556-4029.2011.01989.x
Turner, D. A., Williams, M., Sigman, M. A., and Goodpaster, J. V. A Comprehensive Study of the Alteration of Ignitable Liquids by Weathering and Microbial Degradation,. J. Forensic Sci. (2017) 63:58–65. doi:10.1111/1556-4029.13527
Yoshida, H., Kaneko, T., and Suzuki, S. A Solid-phase Microextraction Method for the Detection of Ignitable Liquids in Fire Debris. J. Forensic Sci. (2008) 53:668–676. doi:10.1111/j.1556-4029.2008.00704.x
Keywords: fire debris analysis (FDA), GC×GC/MS, SPME (solid-phase microextraction), ignitable liquid detection, passive headspace extraction, ignitable liquid detection canine
Citation: Abel RJ and Harynuk JJ (2022) Sensitive and Representative Extraction of Petroleum-Based Ignitable Liquids From Fire Debris For Confirmatory Analysis of Canine-Selected Exhibits. Front. Anal. Sci. 2:857880. doi: 10.3389/frans.2022.857880
Received: 19 January 2022; Accepted: 30 March 2022;
Published: 25 May 2022.
Edited by:
Jorn Chi Chung Yu, Sam Houston State University, United StatesReviewed by:
Rafał Borusiewicz, Institute of Forensic Research (IFR), PolandShokouh Haddadi, Suny Oswego, United States
Copyright © 2022 Abel and Harynuk. This is an open-access article distributed under the terms of the Creative Commons Attribution License (CC BY). The use, distribution or reproduction in other forums is permitted, provided the original author(s) and the copyright owner(s) are credited and that the original publication in this journal is cited, in accordance with accepted academic practice. No use, distribution or reproduction is permitted which does not comply with these terms.
*Correspondence: James J. Harynuk, amFtZXMuaGFyeW51a0B1YWxiZXJ0YS5jYQ==