- 1Eawag, Swiss Federal Institute of Aquatic Science and Technology, Dübendorf, Switzerland
- 2International Institute for Water and Environmental Engineering (2iE), Ouagadougou, Burkina Faso
As compared to the Asian lowlands, environmental exposure to arsenic (As) in West Africa has received little attention. Recent studies have found geogenic As contamination of groundwater in many regions in Burkina Faso. As-contaminated groundwater is used for drinking and increasingly also for the irrigation of staple foods. This study assesses the extent to which irrigation and cooking of staple foods in Burkina Faso influence plant uptake and dietary consumption of As, respectively. Using a greenhouse experimental setup, we evaluated the transfer of As from irrigation water spiked with 0, 100, 500, and 1,000 μg/L As(V) to the organs and edible parts of seven commonly consumed vegetables (amaranth, carrot, green bean, lettuce, okra, spinach, and tomato). Next, we cooked the greenhouse-cultivated vegetables and externally purchased foods with As-free and As-spiked waters. The As content in all plant organs increased with increasing As in the irrigation water. With 500 μg/L, the concentrations of As in the edible parts (ordered from highest to lowest) were as follows: spinach (6.6 ± 0.5 μg/g); lettuce (3.9 ± 0.1 μg/g); carrot (3.5 ± <0.1 μg/g); amaranth (2.2 ± <0.1 μg/g); okra (0.9 ± <0.1 μg/g); green bean (0.8 ± <0.1 μg/g); and tomato (0.2 ± <0.1 μg/g). The edible parts of leafy vegetables irrigated with As-spiked water had a higher average As content (4.9 ± 4.5 μg/g) than root (2.9 ± 2.0 μg/g) and fruit/pod vegetables (0.8 ± 1.1 μg/g). Cooking with an excess volume of As-free water reduced the As content in the cooked vegetables by 39% on average, while cooking with As-contaminated water transferred As to the cooked food. The As content in steamed foods was 8 to 18 times lower than in boiled foods. Based on human health risk estimates, we generally recommend to avoid planting leafy and root vegetables in areas with As concentrations above 100 μg/L in irrigation water. In areas with elevated As contamination, mitigation strategies include the cultivation of fruit/pods vegetables such as tomato and okra and steaming the food instead of boiling.
Introduction
The contamination of groundwater by naturally occurring arsenic (As) in West Africa has received little attention compared to the widespread As-endemic areas in Asian lowlands (e.g., Pakistan, Vietnam, Cambodia, and Bangladesh) (Podgorski et al., 2017; Stopelli et al., 2020). However, groundwater of some areas in Burkina Faso shows high concentrations of this toxic metalloid (Smedley et al., 2007; Some et al., 2012; Ahoule et al., 2015; Sako et al., 2016; Bretzler et al., 2017). The contamination stems from As-bearing sulfide minerals, commonly occurring in mineralized zones and associated with gold ores. In Burkina Faso groundwater, As is predominantly present as arsenate [As(V)], and concentrations can exceed 1,000 μg/L.
Prolonged exposure to As increases the risk of developing a number of serious health effects, such as skin lesions and cancer of the liver, lung, bladder, kidney, and skin, and neurological, respiratory, cardiovascular, and developmental effects (Smith et al., 1992; Naujokas et al., 2013; Schmidt, 2014). It is estimated that about 560,000 people in Burkina Faso (~3% of the population) are potentially exposed to As concentrations exceeding the national guideline value of 10 μg/L for drinking water (Bretzler et al., 2017). As in food may add to the overall consumption-related As exposure of the population.
Arsenic is known for its toxicity not only to humans, who are exposed through drinking water, cooking water, and food products (Podgorski and Berg, 2020), but also to plants that are exposed via irrigation water or soil. Contamination of rice with As has been especially well-studied. In Bangladesh, As-rich groundwater used for cultivation leads to long-term As accumulation in soils, and this results in increasing levels of As in rice and, eventually, in decreasing rice yields due to As phytotoxicity (Dittmar et al., 2010). Subsequently, the guideline value for As in irrigation water has been set as 100 μg/L by the Food and Agriculture Organization (FAO) [FAO, accessed on 18/02/2020]. The potential uptake of As by vegetable crops has been studied using soil or hydroponic systems irrigated with various concentrations of As in water. These studies indicate that uptake of As depends on plant species and other parameters, such as soil characteristics or cultivation methods (Miteva, 2002; Gousul Azam et al., 2017). Furthermore, the consumption of contaminated vegetables could be associated with human health risks (Gupta et al., 2019).
However, little is known about the contamination of staple foods typical to West Africa with toxic trace elements. Specifically, the extent to which As is taken up by irrigated vegetables in this region remains unclear (Chen et al., 2015). For example, in a small number of randomly collected samples from tomatoes, cabbages, and potatoes produced in villages in the province of Yatenga (in northern Burkina Faso) that were irrigated with water low in As from rivers or dug wells, As was below the detection limit (not specified in this study) (Some et al., 2012). Associated health risks have been explored in some West African countries, including Nigeria (Onakpa et al., 2018) and, to a lesser extent, Benin (Fangnon et al., 2012; Koumolou et al., 2013), Ghana (Odai et al., 2008; Adomako et al., 2011), and Cote d'Ivoire (Kinimo et al., 2018a,b). However, to the knowledge of the authors, there has been no such study in Burkina Faso with any of the commonly cultivated vegetables of this country. Hence, there is a need to characterize the As uptake of vegetable crops locally grown in Burkina Faso under varying concentrations of As groundwater contamination. Furthermore, there is little information on whether certain organs of these vegetables accumulate As more than the others. Such investigations, however, are necessary in order to determine whether typical consumption patterns of this region could present a risk to human health.
In Burkina Faso, 85–95% of the population makes a living on agriculture (Ouédraogo et al., 2011). The majority of the population relies on cereals as their principal diet. One of the most commonly consumed dishes is tô, a traditional porridge cooked with flour (maize, millet, or sorghum) and water. Vegetables are also part of the daily diets and are especially found in soups and sauces accompanied by carbohydrate staples (Smith and Eyzaguirre, 2007). Market gardening represents 15% of the agricultural production of Burkina Faso: Cereals, vegetables, and leafy vegetables are the most cultivated products (Konkobo et al., 2002). The cultivation of cereals is traditionally carried out during the rainy season (June to September). However, since the 1990s, irrigated vegetable production has significantly expanded following the widespread construction of small dams, and small-scale irrigation is one of the main topics of the current agricultural policy drafted in 2017 (Gross and Jaubert, 2019). Consequently, there is an increasing use of groundwater for irrigation purposes: Boreholes and shallow wells are widely used as a supplement to surface water during the long dry season (October to June) (Gross and Jaubert, 2019). As a result, there is a growing practice of using potentially As-contaminated groundwater for irrigation purposes despite little information being available regarding the potential for As accumulation in locally grown staple crops.
In addition to the uptake of As by plants, it has been shown that cooking can either increase or decrease the As content in foods, depending on the relative concentrations of As in the food and the cooking water. For instance, contaminated rice prewashed and cooked in a 6:1 ratio of water to rice removed up to 57% of As (Sengupta et al., 2006). On the other hand, several studies have explored the transfer of As from cooking water to rice, highlighting the increased health risks of rice cooked in As-contaminated water (Bae et al., 2002). However, no such studies have been undertaken with African dishes such as tô (maize flour), bean/niébé (black-eyed peas), or gari (cassava).
Based on these knowledge gaps, the motivation of the present study is 2-fold: first, to characterize the uptake of As by staple vegetables in Burkina Faso when irrigated with As-contaminated groundwater and, second, to assess the extent to which the cooking practices for these traditional foods determine the As content of the consumed cooked foods. Taking into consideration the prevalence of elevated groundwater concentrations of As in Burkina Faso, we hypothesize that there is a high likelihood of transfer of As to irrigated plants and cooked food. Based on the findings of this study, we discuss the potential risks to human health due to their ingestion.
Materials and Methods
Irrigation of Vegetables With Various Concentration of Arsenic in Water: Greenhouse Experiment Characterization
Seven commonly produced and consumed vegetables in Burkina Faso [amaranth (Amaranthus), carrot (Daucus carota), green bean (Phaseolus vulgaris), lettuce (Lactuca sativa), okra (Abelmoschus esculentus), spinach (Spinacia oleracea), and tomato (Solanum lycopersicum)] were grown in greenhouses with irrigation water spiked with four concentrations of As (0, 100, 500, and 1,000 μg/L). According to the classification based on edible parts, the plants were classified as leafy vegetables (amaranth, lettuce, and spinach), fruit/pod vegetables (tomato, okra, and green bean), and root vegetables (carrot). The experimental setup was similar as in previous studies (Carbonell-Barrachina et al., 1997; Dittmar et al., 2010; Bhatti et al., 2013). A total of 168 plastics pots (7 species * 4 As concentrations * 6 replicates) were installed on wooden planks 20 cm above the soil in three separate greenhouses built with plastic roofs and metallic mosquito net mesh walls to prevent intrusion from rain, insects, or other small animals. The experiment was carried out during the dry season between November 2017 and February 2018 at the International Institute for Water and Environmental Engineering (2iE). The greenhouses were located in the compound of 2iE in Kamboinsin (15 km north of Ouagadougou), while the laboratory was located on the main campus of 2iE Institute in Ouagadougou.
Sandy loam soil (according to the texture classifications defined by the United States Department of Agriculture) was collected from the subsurface layer (2–25 cm) in the compound of 2iE-Kamboinsin, dried in the sun, crushed, and passed through a 1 mm sieve. Approximately 4.5 L of homogenized soil was distributed into every pot (diameter 16 cm, height 26 cm, and volume 5.2 L) that was previously cleaned and drilled at the bottom (five holes per pot). The soil used as a substrate was characterized at the beginning of the trial. A composite sample was created out of six soil subsamples and sent for analysis to the National Laboratory of Soil (BUNASOLS, Ouagadougou) for the determination of granulometry, pH, available phosphorous, organic carbon, total nitrogen, and cation-exchange capacity. The samples were weighed by the independent laboratory prior to analysis, and the initial concentration of As in the composite soil sample was determined by HNO3/H2O2 digestion and inductively coupled plasma (ICP) determination methods as described below. Irrigation water was artificially spiked with As(V) in a concentration range that is found in groundwater in Burkina Faso. Seven plant species were watered with four different As(V) concentrations: an As-uncontaminated control (water not spiked with As) and three As(V) concentrations (100, 500, and 1,000 μg/L). Irrigation waters were prepared using a 1 g/L stock solution of sodium arsenate dibasic heptahydrate (Na2HAsO4, 7H2O; Sigma-Aldrich ACS reagent, assay ≥ 98%) and As-free water from a nearby borehole.
Seeds were purchased from the company Nankosem (6502 Ouagadougou 01) in September 2017. The seeds were germinated directly in the pots, and the most vigorous plants were selected so that one healthy plant remained per pot. Irrigation with the As-spiked waters started with the germination step and continued until the end of the experiment.
Irrigation water was frequently added with a watering can to maintain the soil moisture equally in all pots, at an estimated volume of 0.2 to 0.5 L/pot/day. Four watering cans were used independently for each of the four concentrations of As. Interviews conducted with professionals from the agricultural network (market gardeners, market sellers, and scientists) confirmed that external fertilizer input is commonly used by farmers in Burkina Faso. In order to fertilize the nutrient-poor experimental soil and to reproduce local conventions, plants were fertilized with nitrogen, phosphorus, and potassium (NPK) (14% total N, 23% P2O5, and 14% K2O, obtained from Yara Company, Abidjan, Côte d'Ivoire). The equivalent of a handful of NPK was applied to each of the plants two times during the study. Every week, plant growth was monitored by four measurements (height and diameter of the main stem, number of branches, and diameter of leaves) on amaranth, okra, spinach, and tomato. One-sided ANOVA tests were used to examine whether different concentrations of As in irrigation water were associated with differences in plant growth by harvest time.
After a culture period of 65 to 130 days, plants were harvested at maturity (defined as the point of readiness for human consumption) with nitrile hand gloves and divided into different organs (roots, stem, leaves, and fruits). Visible physical characteristics such as root length and fresh mass (FM) of the plants were measured. Samples were transported to the laboratory in polyester tubes, rinsed with distilled water with special attention to roots as mentioned by Queirolo et al. (2000), and cut into small pieces (1–2 cm in size). Composite samples of the six replicates were mixed together in polyester tubes.
Cooking of Contaminated Greenhouse Vegetables With As-Free Water
Vegetables grown in our greenhouse experiment with high concentrations of As were expected to have higher As contents than the food items that can be bought in the market. In order to characterize the transfer of As of our As-contaminated vegetables to the cooking water, the remaining vegetables from the greenhouse experiment were cooked with As-free water. The edible parts of amaranth, okra, spinach, and tomato were boiled in excess distilled water in a stainless steel saucepan. For each type of vegetable, the same mass of vegetables was cooked in the same volume of distilled water. Samples were collected with a spoon, transferred in polyester tubes, weighed, and stored at −18°C before being freeze-dried.
Cooking of Externally Purchased Food Items With As-Contaminated Water
Food items were bought in Zogona market (Ouagadougou) in February 2018 and transported to the laboratory in plastic bags. Local rice came from Boulkiemdé (central-west region), spinach leaves from market gardens of Ouagadougou, and corn flour and bean/niébé from the Kossodo neighborhood (Ouagadougou). Food items were then prepared and cooked in the laboratory at 2iE main campus according to local customs and the traditional recipes typically used in the country (see Supplementary Table 1). The traditional cooking methods were provided by the Burkinabe research team members (N. D. N and S. F. K. T.) and other colleagues of 2iE based on their personal experience in cooking the same dishes on a regular basis (see Bae et al., 2002; Rahman et al., 2006) for justification of local recipes and cookware in As uptake experiments. Spinach leaves, bean/niébé, and yam were rinsed with distilled water several times, whereas rice, maize flour, and manioc flour were not processed after being purchased. Vegetables and foods were prepared in water with the same four As(V) concentrations (0, 100, 500, and 1,000 μg/L) as used for the irrigation. To minimize cross-contamination, a set of four cooking accessories (stainless steel saucepan, covers, and metallic cutlery) was dedicated to each of the four treatments. In order to characterize the influence of the cooking method, separate preparations by boiling, steaming, and cold water were compared. Rice, tô, yam, bean/niébé, and spinach were boiled using a heating plate; rice, yam, and spinach were steamed; and gari was prepared with cold water according to the recipes in Supplementary Table 1. Samples of prepared foods were collected with a spoon and transferred in a ceramic crucible before being dried.
Analytical Methods: Digestion
Vegetable samples harvested from the greenhouse irrigation experiment (seven raws and four cooked with As-free water) were stored in a freezer at −18°C before being freeze-dried (model Alpha 2–4 LDplus, Martin Christ GmbH, Germany). Externally purchased market food samples were dried in the oven at 80°C for 48 h and then allowed to cool in a desiccator.
Digestion With HNO3 at 80°C in Ouagadougou
The lyophilized vegetables and oven-dried market foods were ground into powder of 0.25 mm with a laboratory grinder (IKA® MF 10 grinder, IKA, 79219 Staufen, Germany) and stored at ambient temperature before being digested. The main digestion method used in this study to extract As from the vegetables or dish samples in the laboratory at 2iE main campus was adapted from a protocol developed by Bhatti et al. (2013). Briefly, 0.5 g of powder sample was introduced into clean and dry quartz tubes and 5 ml of HNO3 (Fluka TraceSELECT, purity > 69.0%) was added. Samples were predigested at ambient temperature overnight under a fume hood. The subsequent day, samples were placed in a heating water bath at 80°C for 2–3 h. After this time, no fumes were visible and the volume had reduced to 1–2 ml. The samples were subsequently cooled down to ambient temperature, diluted with 10 ml of distilled water directly added to the quartz tubes, filtered through a Whatman filter paper No. 42, and further diluted with distilled water to a final volume of 25 ml.
Digestion With HNO3/H2O2 at 240°C in Switzerland
For comparison, a second digestion method was carried out in the laboratories of Eawag for both the dried vegetable powder samples (as quality control) and potted soil samples.
In this method, each digestion tube contained 50 mg of sample, 6 ml of concentrated nitric acid (65% Suprapur, Merck AG, Switzerland), and 1 ml of hydrogen peroxide (30%, Merck AG, Switzerland). The tubes were placed in a pressurized ultraCLAVE microwave oven (MLS GmbH, Germany) and subjected to 1,000 Watts of energy for 15 min at 240°C. After cooling, the samples were removed from the microwave and diluted in 50 ml of ultrapure water (NanoPure, Barnstead, ThermoFisher Scientific Inc.).
The two digestion methods were compared for the digestion of spinach leaves and okra pods (N = 46 samples in total, including replicates, see Supplementary Figure 1 and Supplementary Table 2). For the important concentration range from 0.5 to 20 μg/g, the two digestion methods agreed sufficiently well for the determination of concentrations of As in the food samples with either of the two digestion methods. Standard reference materials (rice flour, NIST 1568b, and tomato leaves, NIST 1573a) obtained from the National Institute of Standard and Technologies (Gaithersburg, USA) were used to calibrate the digestion methods of As in our food samples. Good agreement with the mean certified values was observed for NIST 1568b (118% mean recovery) and for NIST 1573a (125% mean recovery) (Table 1).
Analytical Methods: Determination
Analysis of trace elements (As, Cu, and Pb) from digested soil, vegetables, and food samples was performed in the Eawag laboratories using ICP-MS (Agilent 7500cx, Agilent Technologies, Inc., USA). All glassware were washed with 2% HNO3, rinsed with distilled water, and dried in a laminar flow clean bench with particle-filtered air (SKAN AG, Pure Solutions, Allschwil, Switzerland). About 1,000 mg/L stock solutions of As(V) were prepared with sodium arsenate dibasic heptahydrate (Na2HAsO4· 7H2O; Sigma-Aldrich ACS reagent, assay ≥98%) and stored in borosilicate glass vials in the dark. Distilled water was produced by a Milli-Q Integral 3 water system (Millipore, USA). Digests from Burkina Faso (20.0 g/L) were diluted 5- to 20-fold into 1% HNO3. Digests from Eawag (1.00 g/L) were measured undiluted. The detection limits in the liquids were 0.05 μg/L, corresponding to the detection limits in food samples of 0.05 μg/g. Of the edible parts of the plants, all three triplicate digestions were analyzed by ICP-MS; of the non-edible parts, only one of three triplicate digestions was analyzed.
Translocation Factor
The translocation factor (TF) is calculated to determine the relative translocation of metals from the roots to the different parts (stalk, leaf, grain, husk, etc.) of the plant species (Rauf et al., 2011). It is calculated according to Equation 1 (Rezvani and Zaefarian, 2011):
where [As(shoot,DM)] is the total As concentration in the parts of shoot plants (stem and leaves) and [As(root,DM)] is the total As concentration in the roots. Here and throughout the study, DM stands for “Dry Mass” and FM stands for “Fresh Mass.”
Human Health Risk Characterization
Estimated Daily Intake
The estimated daily intake (EDI) of As was calculated using Equation 2:
where EDI is the estimated daily intake of As (μg/kg body mass/day), Asediblepart, FM is the concentration of As in fresh edible part of the vegetable (μg/g, FM), CRvegetable is the daily vegetable consumption rate (g FM/person/day), and BMaverage is the average body mass of 60 kg (Walpole et al., 2012). The concentration of As in the fresh edible part of the vegetable was calculated as a product of the concentration of As in the dry edible part of the vegetable (μg/g, DM) and the mean water content of edible part of each vegetable species according to Equation 3:
Calculation of the daily intake requires the quantification of the average daily food consumption rate. However, estimates of daily intake vary among countries and data were unavailable at the time of publication for Burkina Faso. The weighted average portion estimates of okra and tomato (respectively, 10 and 70 g FM/person/day) were taken from the study of Lehmann et al. (2017). For the other vegetables, the daily consumption rate was assumed to be 10 g/person/day for amaranth and spinach, 30 g/person/day for lettuce, and 100 g/person/day for carrot and green bean. Those assumptions (see Supplementary Table 7) were compared with the Global Nutrition and Policy Consortium (Friedman and Friedman, 2019) that indicates consumption of total vegetables of 120.5 g/person/day for Burkina Faso in 2010. This is in the same range of what is reported by Ganry and Le Guilloux (2007) for Ivory Coast and Benin, two neighboring countries, indicating an average daily vegetable consumption of 40 and 44 kg/person/year, which represents 110 and 121 g/person/day, respectively. For bean/niébé, gari, tô, rice, and yam, the daily consumption rate was assumed to be 150 g of cooked food/person/day (FAO, accessed on 13 April 2021).
The cadmium (Cd) monthly intake was calculated using Equation (4)
where EMICd is the estimated monthly intake of Cd (μg/kg body mass/month), Cdfood, FM is the concentration of Cd in cooked food (μg/g, FM), CRfood is the daily food consumption rate (g FM/person/day), EFr is the exposure frequency (365 days/year), BMaverage is the average body mass of 60 kg, and month is the number of months (12 months/year).
Calculation of Hazard Quotient (HQ) and Cancer Risk (CR)
As reported by Antoine et al. (2017), there are strict regulations of intakes of many trace elements by various international, national, and regional bodies. However, in 2011, the Joint FAO/ WHO Expert Committee on Food Additives (JECFA) withdrew the provisional tolerable weekly intake (PTWI) for inorganic As because it was no longer appropriate (WHO, 2011). The risks associated with consumption of foodstuff with significant levels of As were characterized following the definitions of the United States Environmental Protection Agency (US EPA). The non-carcinogenic and carcinogenic risks were characterized by the HQ and CR. A HQ of 1 or lower means adverse non-cancer effects are unlikely. The potential for adverse non-carcinogenic health effects increases for HQ > 1. The CR is “estimated as the incremental probability of an individual developing cancer over a lifetime” (Uddh-Söderberg et al., 2015). HQ and CR were calculated according to Equations 5 and 6, respectively, as defined by US EPA (IRIS, 2019),
where EDI is the estimated daily intake (mg/kg BM/day, see Equation 2), EFr is the exposure frequency (365 days/year), ED is the exposure duration (70 years), AT is the period over which exposure is averaged in days for non-carcinogens (365 days/year * number of exposure years), and RfD is the oral reference dose (0.0003 mg/kg/day). According to USEPA, RfD is “an estimate of a daily oral exposure for an acute duration to the human population (including sensitive subgroups) that is likely to be without an appreciable risk of deleterious effects during a lifetime” (US EPA glossary, accessed on 09/06/2020). OSF is the cancer oral slope factor for inorganic As (1.5 mg/kg/day) (IRIS, 2019).
Results
Soil Properties
The sandy loam soil used in the experiment is representative of the majority of soils found in Burkina Faso (Pallo and Thiombiano, 1989 citing FAO). It was characterized by low concentrations of clay, a low pH, low nitrogen content, and a cation-exchange capacity of 2.22 mol/kg. The soil contained As (0.684 μg/g), Cd (0.029 μg/g), and lead (6.620 μg/g) (Supplementary Table 3). The concentration of As was lower than the maximum acceptable limit for agricultural soil (20 μg/g) recommended by the European Union (Rahaman et al., 2013).
Arsenic Concentration in Vegetables Irrigated With As-Contaminated Water
For all plants, the As content (whether in an edible part or not) increased with increasing As in the irrigation water. The trend of As bioaccumulation was leaf > stem (spinach), root > leaf (lettuce), root > leaf > stem (amaranth), leaf > stem > fruit (green bean), root > leaf > stem > fruit (tomato), and leaf > root (carrot). One-way ANOVA tests did not reveal a statistically significant difference in plant growth when different concentrations of As were applied. In the following, the observations for each of the vegetables irrigated with As-spiked water are briefly discussed.
Translocation of As to Different Parts of Plants
In spinach, concentrations in the leaves rose sharply from <1.5 to 15.2 μg/g with increasing concentrations of As from 0 to 1,000 μg/L in the irrigation water. The mean concentrations of As in the leaves were 2.5–6.6 times higher than in the stems (Figure 1). In contrast to the expectations, the control plants irrigated with As-free water had measurable concentrations of As in their leaves (1.0 μg/g ± 0.3). Growth curves show no difference in plant heights after 52 days within the four As treatments (Supplementary Figure 5).
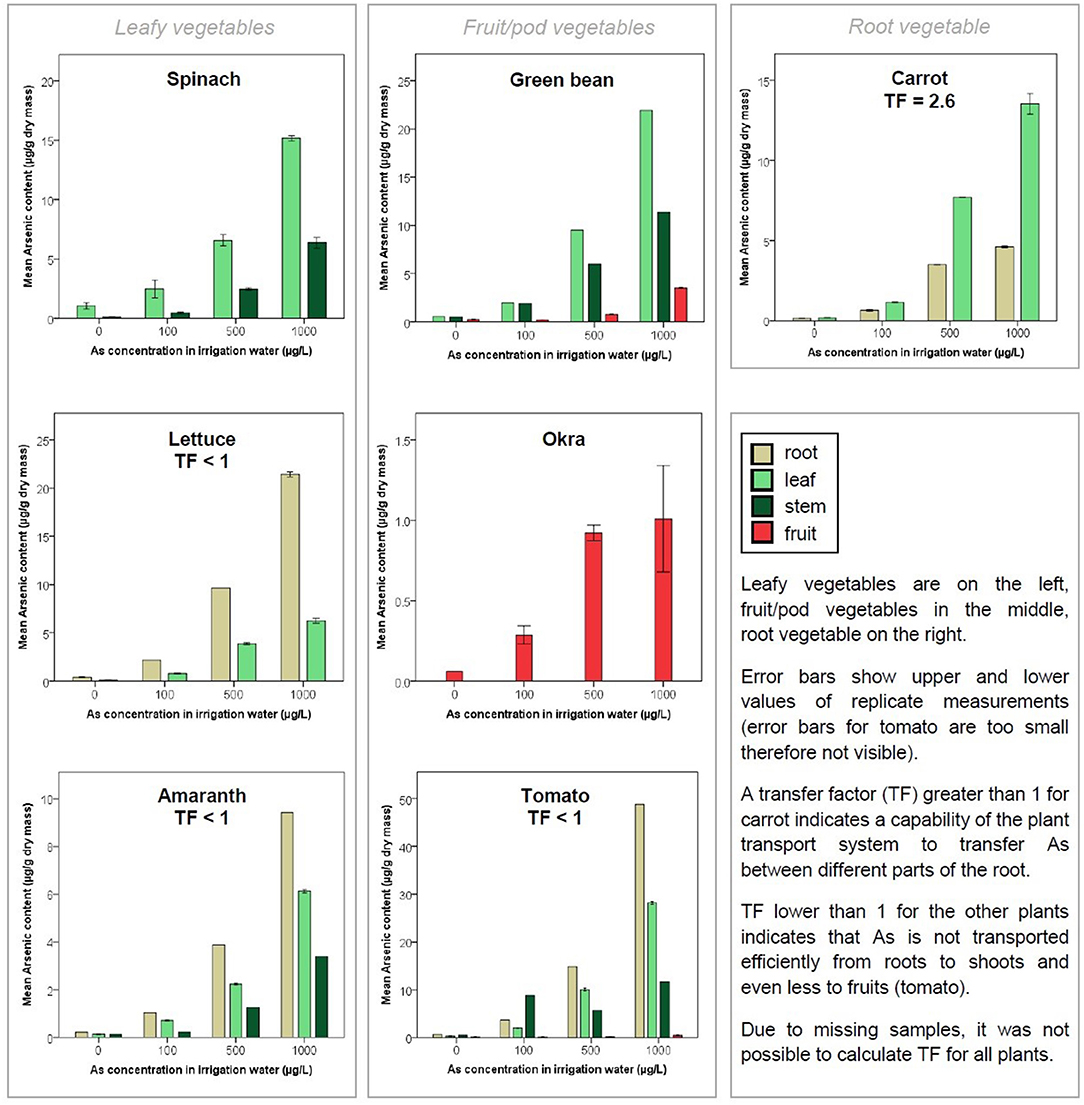
Figure 1. Mean Arsenic (As) content [μg/g, dry mass (DM)] in different organs of cultivated plants as a function of As in the irrigation water (μg/L).
In lettuce, concentrations in the leaves rose from <0.5 to 6.3 μg/g with increasing As in the irrigation water. As accumulated more in the roots than in the leaves (around three times more on average). The TF (concentration of As in the shoot parts relative to the concentration in the roots) was lower than 1 (Figure 1). With increasing concentration of As in the irrigation water, leaves were drier, i.e., water content decreased.
In amaranth, concentrations in the leaves rose from <1 to 6.1 μg/g with increasing As in the irrigation water (Figure 1). Plants showed a higher concentration in their roots than in leaves (1.6 times higher on average) and stems (3 times higher). TF was lower than 1. Similarly to spinach, green bean, and tomato, concentration of As was higher in the leaf than in the stem. With increasing concentration of As in irrigation water, the water content in roots and stem decreased while it remained stable in leaves (Supplementary Figure 4).
In green bean, concentrations in the fruits rose from <1 to 3.5 μg/g with increasing As in the irrigation water. As mainly translocated to leaves, stems, and then fruits (Figure 1). For plants irrigated with As-spiked water, the concentration in fruits was on average 9.4 times lower than that in leaves and 5.4 times lower than that in the stems. The TF could not be calculated (due to a breakdown of the freeze dryer, roots could not be dried and therefore not analyzed).
In okra, concentrations in the fruits rose from <0.1 to 1.0 μg/g with increasing As in the irrigation water. Due to the same laboratory problems mentioned earlier, analysis of As was only possible in the fruits of okra. As content in fruits increased with increasing concentration of As in the irrigation water (Figure 1). The evolution of the height of the stem (measured 52 days after germination) showed that treatment with 100 and 500 μg/L seemed beneficial for plant growth, with greater heights than plants irrigated at 1,000 and 0 μg/L (Supplementary Figure 5). However, one-way ANOVA tests did not reveal that the differences in stem height were statistically significant at the p <0.05 level.
In tomato, concentrations in the fruits rose from 0.1 to 0.4 μg/g with increasing As in the irrigation water. With the exception of treatment at 100 μg/L, As mainly translocated to roots, leaves, stems, and then fruits (Figure 1). For plants irrigated with As-spiked water, the concentration of As in fruits (0.2 μg/g ± 0.1) was on average 90 times lower than in roots (22.5 μg/g ± 23.5) and 54 times lower than in leaves (13.4 μg/g ± 12.0) (Supplementary Table 5). Among all plants, the tomato had the lowest accumulation of As in its edible part with an average TF of 0.6. Tomato growth curves (Supplementary Figure 5) show that plants irrigated with As treatment at 1,000 and 500 μg/L had greater heights and greater leaf diameters compared to plants irrigated at 100 and 0 μg/L.
In carrot, concentrations in the roots rose from <1 to 4.6 μg/g with increasing As in the irrigation water. For plants irrigated with As-spiked water, the concentration of As in leaves (7.5 ± 5.5 μg/g) was on average 2.6 times higher than in roots (2.9 ± 1.8 μg/g) (Figure 1) and TF was >1.
Accumulation of As in the Edible Parts
The As content in the dried edible parts of each plant increased approximately linearly with the concentration of As in irrigation water (Figure 2; see also Supplementary Figure 2 for concentrations of As based on the FM). When examining all levels of As-spiked irrigation water used, the greatest average uptake of As based on DM was observed in spinach (8.1 ± 5.6 μg/g), lettuce (3.6 ± 2.5 μg/g), and amaranth (3.0 ± 2.5 μg/g), whereas the lowest concentrations were observed in green bean (1.5 ± 1.5 μg/g), okra (0.7 ± 0.4 μg/g), and tomato (0.3 ± 0.2 μg/g) (Supplementary Table 5). On the whole, the edible parts of leafy vegetables irrigated with As-spiked water had a higher average As content (4.9 ± 4.5 μg/g) than root vegetable (2.9 ± 2.0 μg/g) and fruit/pod vegetables (0.8 ± 1.1 μg/g). At the mid-point level of spiked irrigation water (500 μg/L), the concentrations of As in the edible parts (from highest to lowest) were as follows: spinach (6.6 ± 0.5 μg/g); lettuce (3.9 ± 0.1 μg/g); carrot (3.5 ± <0.1 μg/g); amaranth (2.2 ± <0.1 μg/g); okra (0.9 ± <0.1 μg/g); green bean (0.8 ± <0.1 μg/g); and tomato (0.2 ± <0.1 μg/g) (Supplementary Table 4).
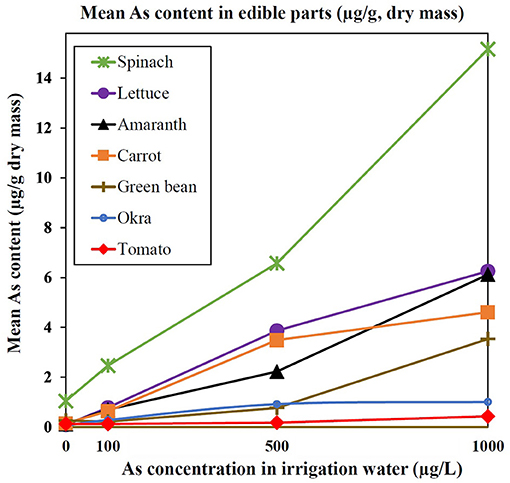
Figure 2. Mean As content (μg/g, DM) in edible parts of cultivated plants as a function of As in the irrigation water (μg/L). Error bars are given in Supplementary Table 4.
Cooking of As-Contaminated Vegetables and Market Food Products
We first analyzed the residual As concentrations of our contaminated vegetables after being cooked with As-free water. The extraction of As was highest for amaranth (61% on average), reaching up to 69% (Table 2). On average, about a third (3–49%) of the As was extracted from spinach and okra. The As content in tomato was too low for reliable measurement. Cooking with As-free water reduced the As content in amaranth, spinach, and okra by 39% on average.
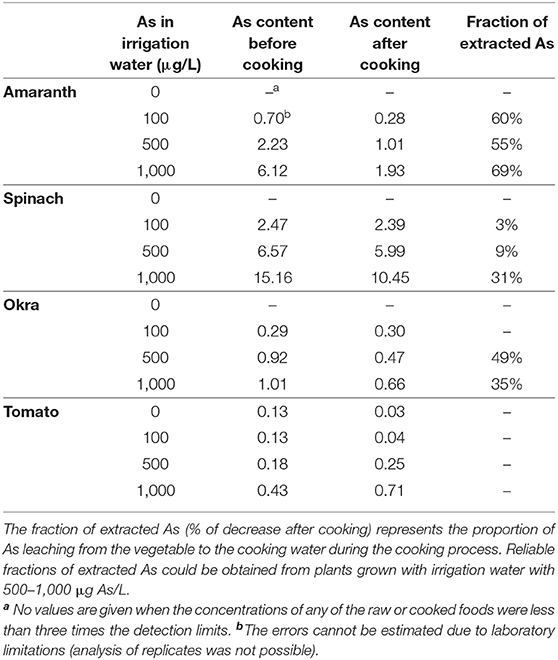
Table 2. Mean As content (μg/g DM) in the edible part of contaminated vegetables before and after cooking with As-free water and fractions (%) of extracted As (DM = dry mass).
We then analyzed the initial concentrations of As of food products purchased in the local market and the final concentrations of As after the products were cooked with As-contaminated water. Local rice originating from Burkina Faso had an initial As content of 0.127 μg/g, local maize flour (used for the preparation of tô) of 0.06 μg/g, and local black-eyed peas (used for the preparation of bean/niébé) of 0.04 μg/g. The As content was not measured in yam, spinach, and cassava (used for the preparation of gari) and was assumed to be zero in the next calculations.
The As content in the cooked purchased foods increased with increasing concentration of As in cooking water (Figure 3). The average transfer of As from cooking water to the prepared food (% of increase after cooking) was 96% for gari, 117% for bean/niébé, 133% for spinach, and 137% for tô. The As content in steamed yam was 7.9 times less than in boiled yam (Figure 4). Similarly, the As content in steamed rice was 17.9 times less than in rice that was boiled with all the water being absorbed. The cooking time influenced the As content of the food products (Figure 5). In spinach, As content increased with increasing cooking time. In boiled rice and tô, the cooking time did not affect the final As content.
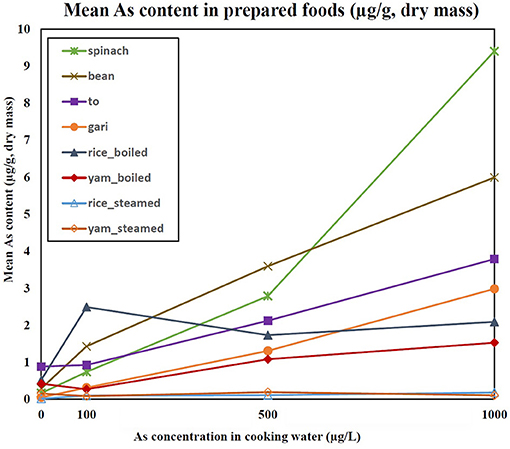
Figure 3. Mean As content in prepared foods (μg/g, DM) as a function of As concentration in cooking water (μg/L). Errors bars are given in Supplementary Table 6.
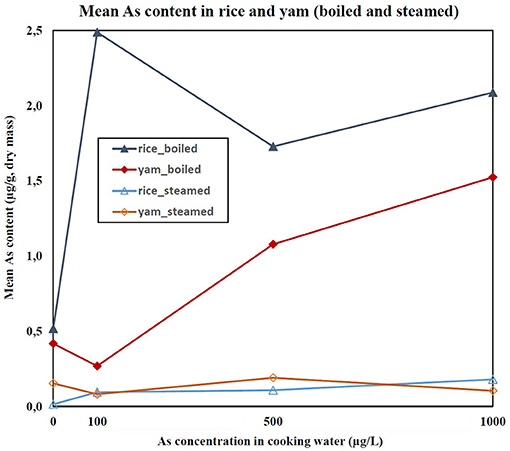
Figure 4. Mean As content in boiled and steamed rice and yam (μg/g, DM) as a function of As concentration in cooking water (μg/L). Errors bars are given in Supplementary Table 6.
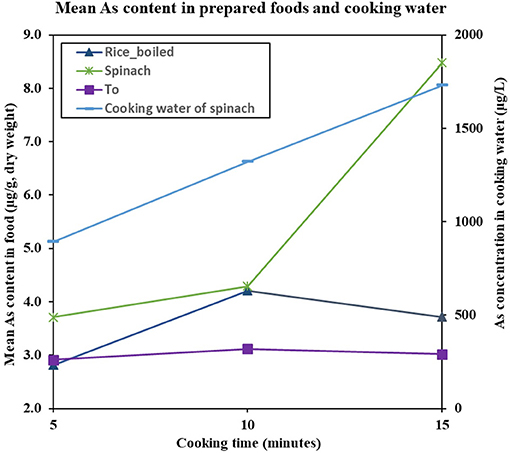
Figure 5. Mean As concentration in prepared foods (μg/g, DM) and cooking water (μg/L) as a function of cooking time. Errors bars are given in Supplementary Table 6.
Human Health Risk Characterization
For our contaminated vegetables, the HQs were >1 for green bean and lettuce irrigated at 1,000 μg/L, as well as carrot irrigated at 500 and 1,000 μg/L (Table 3). Therefore, for those species, there is an increased potential for adverse non-carcinogenic effects compared to tomato, okra, amaranth, and spinach. The CR exceeded 1 in 10,000 for green bean, lettuce, and spinach cultivated under 500 and 1,000 μg/L, as well as for amaranth cultivated under 1,000 μg/L and carrot cultivated under all treatment concentrations. Only the resistant varieties of tomato and okra had a CR lower than 1 in 10,000. The HQ and the CR were lower if the contaminated vegetables were cooked in As-free water (Table 3). For instance, the CR was 2.6E-04 for amaranth cultivated at 1,000 μg/L. After amaranth was cooked with As-free water, the CR was reduced to 8.2E-05 and, therefore, likely to be without an appreciable risk of deleterious effects.
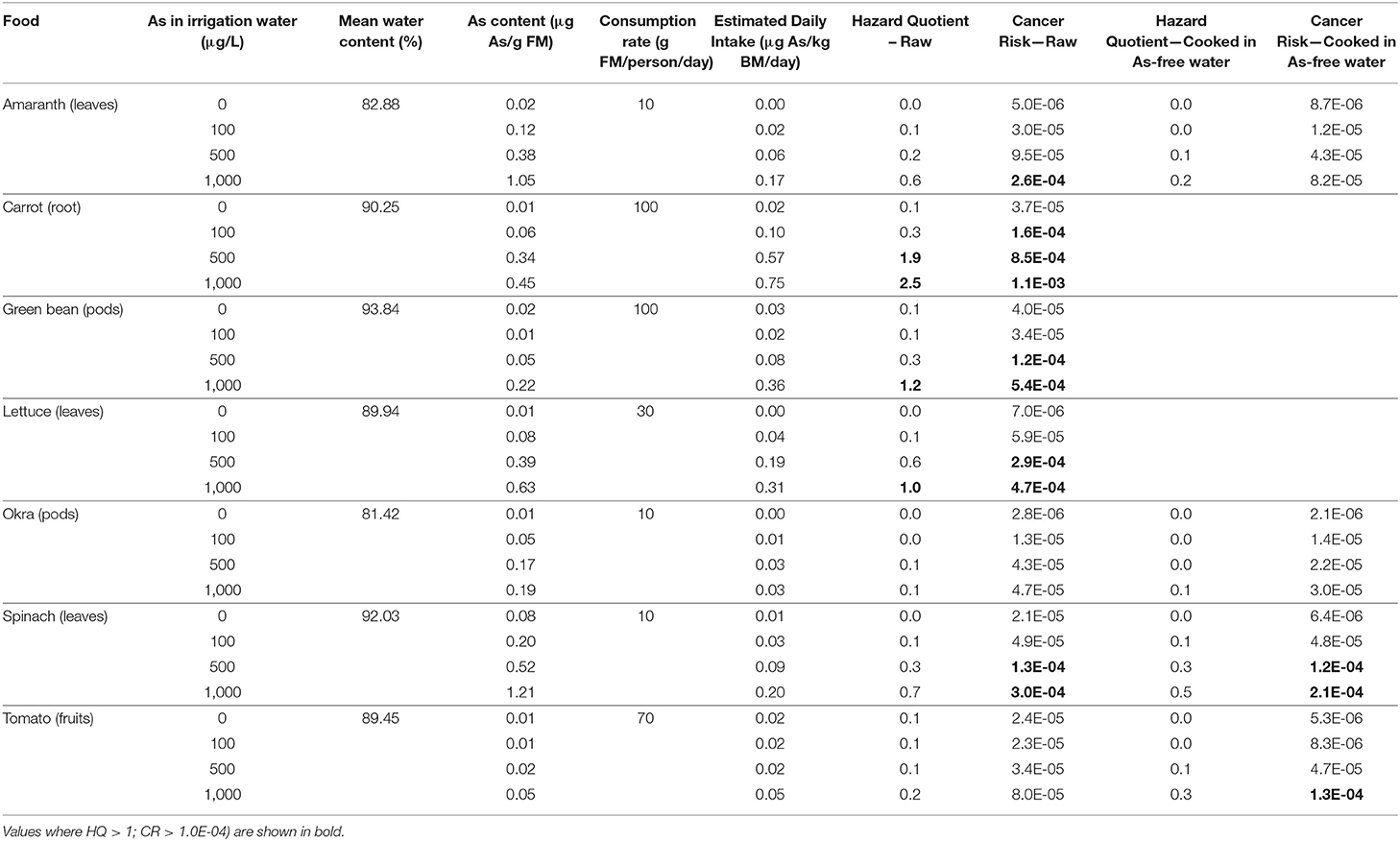
Table 3. Hazard quotient (HQ) and cancer risk (CR) for the ingestion of the edible part of contaminated vegetables (raw and cooked) as a function of As in irrigation water (FM = fresh mass and BM = body mass).
For food products purchased in the local market, the HQs were lower than 1 for boiled spinach, steamed rice, and steamed yam for all concentrations of As in cooking waters. The HQs were >1 for bean/niébé, gari, and tô cooked with waters at 500 and 1,000 μg/L, as well as yam boiled with water at 1,000 μg/L and rice boiled with waters at 100, 500, and 1,000 μg/L (Table 4). The CR exceeded 1 in 10,000 for bean/niébé and gari cooked with waters at 100, 500, and 1,000 μg/L, for boiled rice and tô, whatever the concentration of As in cooking waters. The CR exceeded 1 in 10,000 for spinach only when it was cooked at the highest concentration of As (1,000 μg/L). Surprisingly, the CR exceeded 1 in 10,000 for boiled rice and boiled yam prepared with As-free water. The HQ and CR were lower when food (rice or yam) was steamed rather than boiled.
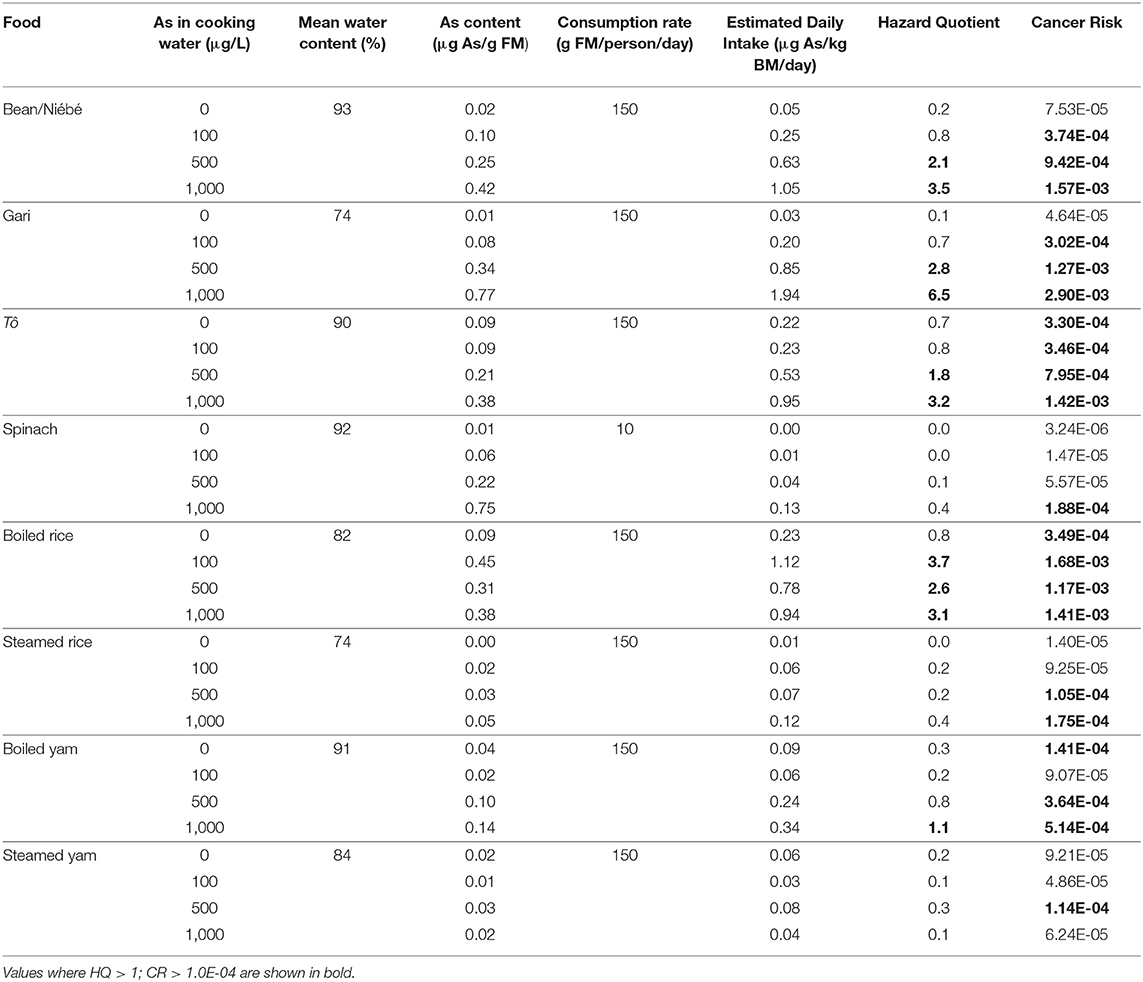
Table 4. Hazard quotient (HQ) and cancer risk (CR) for the ingestion of prepared food as a function of As in cooking water (FM = fresh mass and BM = body mass).
Discussion
Arsenic Concentration in Vegetables Irrigated With As-Contaminated Water
Translocation of As to Different Plant Organs
Amaranth, green bean, lettuce, spinach, and tomato verified the accumulation trend orders of organs (root > leaf > stem > fruit) described in previous studies (Arslan et al., 2016). Carrot also verified the trend order leaf > root as described by Mayorga et al. (2013).
Root Vegetable (Carrot)
Our results showed that carrot had the capability to translocate As from roots to leaves, which minimized the contamination of As in the edible part. Among the four species where the TF could be calculated, only carrots had a TF > 1. Liu et al. (2006) and Mayorga et al. (2013) also reported higher concentrations of As in carrot leaves that are described as efficient bio-accumulators.
Leafy Vegetables
Amaranth and lettuce had TF lower than 1, which indicates that these two plants prevent efficient translocation of As from root to shoots, resulting in lower concentrations of As in edible parts than in the roots. Previous studies (Bhatti et al., 2013) demonstrated that the concentration of As in spinach roots was higher than in its leaves, confirming that spinach is not an accumulator of As.
With increasing concentrations of As in the irrigation water, lettuce leaves had a lower water content, i.e., they became drier (Supplementary Figure 3). This is consistent with a previous study (Yanez et al., 2019) that observed that lettuce plant growth was significantly affected by irrigation with As-contaminated water (in terms of biomass reduction). However, there was no difference in plant mass between treatments, as also observed by Caporale et al. (2014).
Fruit and Pod Vegetables
Tomato had a TF much lower than 1, which is consistent with the literature study (Stazi et al., 2016b). Previous research studies (Meharg and Macnair, 1992; Burlo et al., 1999) reported that this could be due to a strategy adopted by tomato plants to tolerate As by avoiding and/or limiting transportation of As from roots to other parts of the plants, particularly to the fruit.
Translocation factors <1 were observed in two other studies for okra and green bean (Chandra et al., 2016, 2017). Chandra et al. (2016) also observed that okra is more efficient at concentrating As(V) in the shoots than As(III). Thus, the two plants are not As accumulators and have the capacity to tolerate As stress, extract As from soil, and concentrate it into their roots. They can be considered as resistant varieties.
We observed increased growth of tomato plants receiving higher concentrations of As in irrigation water (Supplementary Figure 5). From a study in Bulgaria, Miteva (2002) reported an increase in tomato stem height at lower concentrations of As in soil (15 and 25 mg/kg) and a decrease in tomato stem height at higher concentrations (50 and 100 mg/kg). Barrachina et al. (1995) also reported lower height in tomato plants when concentrations of As in the nutrient solution increased to 2,000, 5,000, and 10,000 μg/L. By contrast, we observed increased growth in okra for the mid-range treatments of 100 and 500 μg/L and lower growth at 0 and 1,000 μg/L. However, these and other observed variations in plant height under different irrigation conditions were not statistically significant; therefore, further research studies regarding the influence of the concentration of As on the growth of plants consumed in Burkina Faso are needed to address this question.
Accumulation of As in the Edible Parts
The As content in all edible parts of the plants increased as the concentration of As increased in irrigation water. Similar observations are well-documented in the literature studies (Table 5). The As content in edible parts of okra and tomato was in the range of what was previously reported in the literature studies. The As content in green bean was also in the range of previous studies. However, in one study carried out in India (Chandra et al., 2017), using contaminated soil at 20 mg/kg, the As content was 2.6 times higher than in the plants irrigated with 1,000 μg/L in our study. For carrot roots, amaranth leaves, and spinach leaves, the As contents in our study were 1, 8, 2.2, and 10.9 times higher than the highest values reported in the literature studies (Table 5). For lettuce leaves, the As content was higher than what was previously reported in the literature studies. However, the As content in the leaves of lettuce irrigated with 1,000 μg/L in this study was 0.7 times lower than one study carried out in Argentina (Yanez et al., 2019) using soil at 8 mg As/kg and As-contaminated water at 1,440 μg/L.
There are several possible reasons explaining the higher levels of As observed in carrot and leafy vegetables: First, leafy vegetables have a greater capability to accumulate high levels of trace metals and minerals from soil than other vegetables (Jamali et al., 2008; Baig and Kazi, 2012). The climate of Burkina Faso, characterized by low relative humidity and high ambient temperatures, could have increased the transpiration rate and caused the higher As uptake of leafy vegetables compared to non-leafy vegetables (Tani and Barrington, 2005; Luo et al., 2011; Hao et al., 2012).
Second, the sandy loam texture of the soil used in our experiment may explain the overall higher As contents observed among the plants in this study. The high soil density sometimes resulted in a flooded pot (after irrigation, water remained above the soil surface), which could have created anaerobic conditions. According to Bhatti et al. (2013), excessive use of irrigation water will develop an anaerobic condition in soil, which will result in increased solubility and release of As from As-binding minerals.
Third, experimental features of this study could explain the differences observed in the uptake of As as compared to previously reported values. These features include the relatively low volume of pots, the use of As-contaminated water directly for germination, and the irrigation water dispersion on the leaves.
Finally, it is possible that the rinsing of vegetables (without hydrochloric acid) was not sufficiently thorough to remove all the As present on the leaves. However, this simple rinsing with water reflects a more realistic scenario after the plants are harvested. Further details on the reasons explaining higher As content in carrot and leafy vegetables can be found in the Supplementary Material.
Management Strategies for Reducing the Risks of As Exposure
Irrigation With As-Contaminated Water
To alleviate As pollution in local vegetables, it is suggested to avoid planting leafy vegetables in areas with As concentrations in irrigation water. It has been shown that rinsing the leafy vegetables does not seem to be an effective measure to remove contaminants like As (HAS, 2020). For root vegetable, such as carrot, one measure is to peel the skin as it accumulates a higher As concentration than edible root (Bhatti et al., 2013). Therefore, fruit vegetables and pod vegetables could be considered a safer option in As-contaminated areas, particularly tomato or okra.
Reducing As in Cooked Food Products
Cooking As-contaminated vegetables with As-free water reduces the As content in the cooked vegetables and, therefore, decreases the HQ and CR associated with their consumption. As concentrations in edible parts decreased after cooking amaranth, okra, and spinach irrigated with 500 and 1,000 μg/L. Similar results were found by Devesa et al. (2008), reporting that vegetables cooked with As-free water had lower inorganic As contents than those detected in the product prior to cooking. Surprisingly, amaranth released more As to cooking water than spinach. This might be explained by the higher surface to volume ratio of amaranth.
Cooking with As-contaminated water transfers As to the prepared foods. Adapting the cooking method could be a solution; for instance, the transfer of As, CR, and HQ are lower if yams or rice are cooked with steam rather than in As-contaminated boiling water.
We observed unexpected Cd levels in cooked foods (Supplementary Figure 8). With the highest observed Cd concentration in samples of tô (17.4 μg/g), the monthly intake of Cd associated with the consumption of 150 g of tô per day would be 132 μg/kg/month. This is 5.3 times higher than the oral provisional tolerable monthly intake (PTMI) defined by WHO/JECFA (25 μg/kg/month). The non-carcinogenic and carcinogenic risks associated with the consumption of Cd are high. It is possible that high concentrations of Cd in cooked foods could originate from the cooking materials (e.g., metal pot and cooking spoons). Cheap and low-quality materials are abundant in low-income countries such as Burkina Faso. However, the source of the contamination with Cd has to be investigated in future studies, as contamination during sample collection or sample processing (especially grinding tô a powder and digestion) in the laboratories in Burkina Faso cannot be excluded. Furthermore, Hashempour-Baltork et al. (2019) reported high levels of mercury in imported rice. This might be a concern in Burkina Faso, where large quantities of rice are imported.
Reducing Human Health Risks
To limit CR, irrigation water containing more than 100 μg/L As should be avoided for the cultivation of carrot. For the cultivation of green bean, spinach, lettuce and amaranth, irrigation water should not contain more than 500 μg/L. In areas where those As concentration thresholds in irrigation water cannot be ensured, or information regarding the concentration of As in groundwater is unavailable, it is recommended to cultivate resistant varieties such as tomato or okra and to avoid planting leafy vegetables.
To limit CR, cooking water containing more than 100 μg/L As should be avoided for boiling food. In this case, our results show that the CR is lower when the food is steamed. However, cooking water containing more than 500 μg/L As should be avoided for any cooking method. Spinach had lower CRs compared to other foods (the CR exceeded 1 in 10,000 for spinach only when it was cooked at the highest As concentration of 1,000 μg/L). This is due to the estimates of small spinach portion (consumption rate of 10 g FM/person/day compared to 150 for the other prepared foods, see assumptions in Supplementary Table 7).
According to As measurements in 1,498 rural drinking water boreholes in Burkina Faso collected by Bretzler et al. (2017), 2.3% of wells were affected by As > 50 μg/L and only 1% affected by As > 100 μg/L, which is within the permissible limit of FAO for irrigation water. Groundwater irrigation for smallholder farmers is growing in extent and importance (Villholth, 2013), and a study in Kenya underlined that drip irrigation could reduce the drought vulnerability of households (Wens et al., 2020). Shallow groundwater represents a neglected opportunity for small-scale irrigated agriculture in sub-Saharan Africa (Gowing et al., 2020). In northern regions of Burkina Faso concerned with high concentrations of As in groundwater, access to a sufficient quantity of surface water throughout the year and especially during the dry season could be problematic. Thus, these regions may be more likely to depend on groundwater than southern and eastern regions and, therefore, at risk for exposure to elevated As. Additional at-risk populations in Burkina Faso include those living next to mining sites, where high concentrations of heavy metals can be released locally into the environment without proper treatment, leading to contamination of soil and water. Above all, the risks from vegetable consumption may be higher in areas where As-contaminated water is used for both drinking and cooking.
Limitations of This Study
First, the current study did not evaluate As speciation. However, in food products of vegetable origin, inorganic As species predominate over organic species (Yost et al., 1998; Koch et al., 2000) and the measured total concentrations of As are thus an appropriate measure of the inorganic As contents. In our human health risk assessment, As was assumed to be inorganic, which is a good approximation as explained above. Furthermore, this study did not evaluate the bioavailability/bioaccessibility of As in the intestinal track. A complete food safety assessment should also evaluate the intake of As from food on the basis of the fraction of the substance that is available to promote its action in the organism (Reeder et al., 2006; Llorente-Mirandes et al., 2016). The measured concentrations of As in control boiled foods (0.01 and 0.15 μg/g in steamed rice and yam, respectively) were surprisingly high. It is possible that the washing of the cooking pans used for boiling was not thorough enough, and/or some As remained at the beginning of the experiment.
Conclusions
As our investigations demonstrate, groundwater used for drinking is not the only source of As exposure in Burkina Faso. This study presents evidence-based estimations of risks of As exposure posed by various foods grown and consumed in Burkina Faso. Foods grown by irrigation with As-contaminated groundwater can be a significant additional source of As intake contributing to total As exposure and can become the dominant source if drinking water has no or low concentrations of As. The concentration of As in all vegetables increased as a function of the As concentration in irrigation water. The effect of irrigation with 1,000 μg/L was significant in all vegetable species relative to the other treatments and increased the concentration of As of each plant. When examining all irrigation treatments, the average concentrations of As in the edible parts of vegetables (from highest to lowest) were as follows: spinach > lettuce > amaranth > carrot > green bean > okra > and tomato. Interestingly, most of the plants stored the As in the parts that humans do not consume. However, the daily consumption of edible parts still poses risks of carcinogenic and non-carcinogenic effects to the population. Those risks can be reduced by cooking the vegetables in an excess amount of As-free water, which serves as a reservoir into which a portion of the As present in the food migrates. However, when As-contaminated water is used for cooking, the As is transferred to the cooked food, and this increases the risks for both carcinogenic and non-carcinogenic effects. These findings indicate that food should be steamed instead of boiled if As-free water is not available. In areas with As-contaminated irrigation water, priority should be given to the cultivation of fruit/pod vegetables (such as tomato or okra). Based on the health risk assessment, an upper As concentration limit of 100–500 μg/L is recommended for most vegetables. Those recommendations are valid in As-contaminated areas of Burkina Faso and other countries of West Africa with similar cooking practices, soil characteristics, groundwater contamination profiles, and staple food crops.
Data Availability Statement
The datasets analyzed for this study can be found in the Eawag Research Data Institutional Collection: https://doi.org/10.25678/00046Q.
Author Contributions
GC-C, FL, and SM conceived and designed the research. GC-C, NN, and ST conducted the field research. GC-C, NN, ST, FL, SM, and SH analyzed the samples/results. GC-C, SM, SH, and AB wrote the first draft of the manuscript. GC-C, SM, SH, AB, and FL contributed to the writing of the article. All authors contributed to the article and approved the submitted version.
Funding
We gratefully acknowledge the Swiss Agency for Development and Cooperation (SDC) for financing the present research as part of the 3E program (contract no. 81016359).
Conflict of Interest
The authors declare that the research was conducted in the absence of any commercial or financial relationships that could be construed as a potential conflict of interest.
Publisher's Note
All claims expressed in this article are solely those of the authors and do not necessarily represent those of their affiliated organizations, or those of the publisher, the editors and the reviewers. Any product that may be evaluated in this article, or claim that may be made by its manufacturer, is not guaranteed or endorsed by the publisher.
Acknowledgments
We thank Thomas Rüttimann (Eawag) for the ICP-MS analysis of food and water samples and Brian Sinnet (Eawag) for the microwave digestions of solid samples. We greatly thank Mr. Rodrigue Nougbodohoue, Mr. Moustapha Ouedraogo, Mr. Boukary Sawadogo, Mr. Noël Tindoure, Mr. Londja Yonli, Miranise Ouedraogo and Mrs. Linda Zougmoure for their logistic support in Burkina Faso and their assistance in the laboratory. We also particularly acknowledge Dr. Cécile Caliot, Dr. Claire Jacquet, Dr. Ralf Kägi, Mr. Yao Désiré Kouakou, Dr. Seyram Sossou, Mr. Ibrahima Zerbo and Dr. Christian Zurbrügg for their contributions and helpful discussions.
Supplementary Material
The Supplementary Material for this article can be found online at: https://www.frontiersin.org/articles/10.3389/frwa.2021.667308/full#supplementary-material
References
Adomako, E. E., Williams, P. N., Deacon, C., and Meharg, A. A. (2011). Inorganic arsenic and trace elements in Ghanaian grain staples. Environ. Pollut. 159, 2435–2442. doi: 10.1016/j.envpol.2011.06.031
Ahoule, D. G., Lalanne, F., Mendret, J., Brosillon, S., and Maiga, A. H. (2015). Arsenic in African waters: a review. Water Air Soil Pollut. 226:4400874. doi: 10.1007/s11270-015-2558-4
Antoine, J. M. R., Fung, L. A. H., and Grant, C. N. (2017). Assessment of the potential health risks associated with the aluminium, arsenic, cadmium and lead content in selected fruits and vegetables grown in Jamaica. Toxicol. Rep. 4, 181–187. doi: 10.1016/j.toxrep.2017.03.006
Arain, M. B., Kazi, T. G., Baig, J. A., Jamali, M. K., Afridi, H. I., Shah, A. Q., et al. (2009). Determination of arsenic levels in lake water, sediment, and foodstuff from selected area of Sindh, Pakistan: estimation of daily dietary intake. Food Chem. Toxicol. 47, 242–248. doi: 10.1016/j.fct.2008.11.009
Arslan, B., Djamgoz, M. B. A., and Akun, E. (2016). ARSENIC: a review on exposure pathways, accumulation, mobility and transmission into the human food chain. Rev. Environ. Contam. Toxicol. 243, 27–51. doi: 10.1007/398_2016_18
Bae, M., Watanabe, C., Inaoka, T., Sekiyama, M., Sudo, N., Bokul, M. H., et al. (2002). Arsenic in cooked rice in Bangladesh. Lancet 360, 1839–1840. doi: 10.1016/S0140-6736(02)11738-7
Baig, J. A., and Kazi, T. G. (2012). Translocation of arsenic contents in vegetables from growing media of contaminated areas. Ecotoxicol. Environ. Saf. 75, 27–32. doi: 10.1016/j.ecoenv.2011.09.006
Barrachina, A. C., Carbonell, F. B., and Beneyto, J. M. (1995). Arsenic uptake, distribution, and accumulation in tomato plants: effect of arsenite on plant growth and yield. J. Plant Nutr. 18, 1237–1250. doi: 10.1080/01904169509364975
Bhattacharya, P., Samal, A. C., Majumdar, J., and Santra, S. C. (2010). Arsenic contamination in rice, wheat, pulses, and vegetables: a study in an arsenic affected area of West Bengal, India. Water Air Soil Pollut. 213, 3–13. doi: 10.1007/s11270-010-0361-9
Bhatti, S. M., Anderson, C. W. N., Stewart, R. B., and Robinson, B. H. (2013). Risk assessment of vegetables irrigated with arsenic-contaminated water. Environ. Sci. Proc. Impacts 15, 1866–1875. doi: 10.1039/c3em00218g
Biswas, A., Biswas, S., and Santra, S. C. (2012). Risk from winter vegetables and pulses produced in arsenic endemic areas of Nadia district: field study comparison with market basket survey. Bull. Environ. Contam. Toxicol. 88, 909–914. doi: 10.1007/s00128-012-0569-z
Bretzler, A., Lalanne, F., Nikiema, J., Podgorski, J., Pfenninger, N., Berg, M., et al. (2017). Groundwater arsenic contamination in Burkina Faso, West Africa: predicting and verifying regions at risk. Sci. Total Environ. 584–585, 958–970. doi: 10.1016/j.scitotenv.2017.01.147
Burlo, F., Guijarro, I., Carbonell-Barrachina, A. A., Valero, D., and Martinez-Sanchez, F. (1999). Arsenic species: effects on and accumulation by tomato plants. J. Agric. Food Chem. 47, 1247–1253. doi: 10.1021/jf9806560
Caporale, A. G., Pigna, M., Sommella, A., Dynes, J. J., Cozzolino, V., and Violante, A. (2013). Influence of compost on the mobility of arsenic in soil and its uptake by bean plants (Phaseolus vulgaris L.) irrigated with arsenite-contaminated water. J. Environ. Manage. 128, 837–843. doi: 10.1016/j.jenvman.2013.06.041
Caporale, A. G., Sommella, A., Lorito, M., Lombardi, N., Azam, S. M. G. G., Pigna, M., et al. (2014). Trichoderma spp. alleviate phytotoxicity in lettuce plants (Lactuca sativa L.) irrigated with arsenic-contaminated water. J. Plant Physiol. 171, 1378–1384. doi: 10.1016/j.jplph.2014.05.011
Carbonell-Barrachina, A. A., Burlo, F., Burgos-Hernandez, A., Lopez, E., and Mataix, J. (1997). The influence of arsenite concentration on arsenic accumulation in tomato and bean plants. Sci. Hortic. 71, 167–176. doi: 10.1016/S0304-4238(97)00114-3
Chandra, S., Saha, R., and Pal, P. (2016). Arsenic uptake and accumulation in okra (Abelmoschus esculentus) as affected by different arsenical speciation. Bull. Environ. Contam. Toxicol. 96, 395–400. doi: 10.1007/s00128-015-1712-4
Chandra, S., Saha, R., and Pal, P. (2017). Assessment of arsenic toxicity and tolerance characteristics of bean plants (Phaseolus vulgaris) exposed to different species of arsenic. J. Plant Nutr. 41, 340–347. doi: 10.1080/01904167.2017.1385801
Chen, T. W., Huang, L. H., Lai, G. X., and Chen, G. Y. (2015). Inorganic arsenic in starchy roots, tubers, and plantain and assessment of cancer risk of Sub-Saharan African populations. Food Control 53, 104–108. doi: 10.1016/j.foodcont.2015.01.016
Devesa, V., Vélez, D., and Montoro, R. (2008). Effect of thermal treatments on arsenic species contents in food. Food Chem. Toxicol. 46, 1–8. doi: 10.1016/j.fct.2007.08.021
Díaz, O. P., Leyton, I., Muñoz, O., Núñez, N., Devesa, V., Súñer, M. A., et al. (2004). Contribution of water, bread, and vegetables (raw and cooked) to dietary intake of inorganic arsenic in a rural village of Northern Chile. J. Agric. Food Chem. 52, 1773–1779. doi: 10.1021/jf035168t
Dittmar, J., Voegelin, A., Maurer, F., Roberts, L. C., Hug, S. J., Saha, G. C., et al. (2010). Arsenic in soil and irrigation water affects arsenic uptake by rice: complementary insights from field and pot studies. Environ. Sci. Technol. 44, 8842–8848. doi: 10.1021/es101962d
Fangnon, B., Tohozin, A. Y., Guedenon, P., and Edorh, A. P. (2012). Conservation des produits agricoles et accumulation des métaux lourds dans les produits vivriers dans le département du Couffo (Benin). J. Appl. Biosci. 57, 4168–4176.
Farid, A., Roy, K., and Sen, R. (2003). A study of arsenic contaminated irrigation water and its carried over effect on vegetable. Fate Arsenic Environ, 113–121.
Friedman, G. J., and Friedman, D. R. (2019). Global Dietary Database. Available online at: https://www.globaldietarydatabase.org (accessed August 20, 2019).
Ganry, J., and Le Guilloux, M. (2007). Synthése des réponses au questionnaire d'enquête sur la situation dans les pays d'Afrique francophone. Report for La Promotion des Fruits et L?gumes (PROFEL) dans les pays d'Afrique francophone. Yaoundé: WHO/FAO.
Gousul Azam, S. M. G., Afrin, S., and Naz, S. (2017). Arsenic in cereals, their relation with human health risk, and possible mitigation strategies. Food Rev. Int. 33, 620–643. doi: 10.1080/87559129.2016.1210633
Gowing, J., Walker, D., Parkin, G., Forsythe, N., Haile, A. T., and Ayenew, D. A. (2020). Can shallow groundwater sustain small-scale irrigated agriculture in sub-Saharan Africa? Evidence from N-W Ethiopia. Groundw. Sustain. Dev. 10:100290. doi: 10.1016/j.gsd.2019.100290
Gross, B., and Jaubert, R. (2019). Vegetable gardening in Burkina Faso: drip irrigation, agroecological farming and the diversity of smallholders. Water Altern. 12, 46–67.
Gupta, N., Yadav, K. K., Kumar, V., Kumar, S., Chadd, R. P., and Kumar, A. (2019). Trace elements in soil-vegetables interface: translocation, bioaccumulation, toxicity and amelioration - a review. Sci. Total Environ. 651, 2927–2942. doi: 10.1016/j.scitotenv.2018.10.047
Hao, H. Z., Zhong, R. G., Xiao, R., Liu, C. W., and Zhong, X. B. (2012). The effect of transpiration for heavy metal uptake of hyperaccumulators. Appl. Mech. Mater. 178–181, 901–904. doi: 10.4028/www.scientific.net/AMM.178-181.901
HAS (2020). Dépistage, prise en charge et suivi des personnes potentiellement surexposées à l'arsenic inorganique du fait de leur lieu de résidence. Recommendations for Best Practice. Saint-Denis: Haute Autorité de Santé. doi: 10.1016/j.toxac.2020.10.052
Hashempour-Baltork, F., Hosseini, H., Houshiarrad, A., and Esmaeili, M. (2019). Contamination of foods with arsenic and mercury in Iran: a comprehensive review. Environ. Sci. Pollut. Res. 26, 25399–25413. doi: 10.1007/s11356-019-05863-y
IRIS (2019). Arsenic, Inorganic (CASRN 7440-38-2). Integrated Risk Information System (IRIS). U.S. Environmental Protection Agency.
Jamali, M. K., Kazi, T. G., Arain, M. B., Afridi, H. I., Memon, A. R., Jalbani, N., et al. (2008). Use of sewage sludge after liming as fertilizer for maize growth. Pedosphere 18, 203–213. doi: 10.1016/S1002-0160(08)60008-9
Kinimo, K. C., Yao, K. M., Marcotte, S., Kouassi, N. G. L. B., and Trokourey, A. (2018a). Distribution trends and ecological risks of arsenic and trace metals in wetland sediments around gold mining activities in central-southern and southeastern Côte d'Ivoire. J. Geochem. Explor. 190, 265–280. doi: 10.1016/j.gexplo.2018.03.013
Kinimo, K. C., Yao, K. M., Marcotte, S., Kouassi, N. G. L. B., and Trokourey, A. (2018b). Preliminary data on arsenic and trace metals concentrations in wetlands around artisanal and industrial mining areas (Cote d'Ivoire, West Africa). Data Brief 18, 1987–1994. doi: 10.1016/j.dib.2018.04.105
Koch, I., Wang, L., Ollson, C. A., Cullen, W. R., and Reimer, K. J. (2000). The predominance of inorganic arsenic species in plants from Yellowknife, Northwest Territories, Canada. Environ. Sci. Technol. 34, 22–26. doi: 10.1021/es9906756
Konkobo, Y. C., Karimou, A. R., Kabore, S., and Diasso, K. (2002). Les Pratiques Alimentaires à Ouagadougou, Burkina Faso. Montpellier: CIRAD.
Koumolou, L., Edorh, P., Montcho, S., Aklikokou, K., Loko, F., Boko, M., et al. (2013). Health-risk market garden production linked to heavy metals in irrigation water in Benin. C. R. Biol. 336, 278–283. doi: 10.1016/j.crvi.2013.04.002
Lehmann, E., Turrero, N., Kolia, M., Konate, Y., and de Alencastro, L. F. (2017). Dietary risk assessment of pesticides from vegetables and drinking water in gardening areas in Burkina Faso. Sci. Total Environ. 601–602, 1208–1216. doi: 10.1016/j.scitotenv.2017.05.285
Liu, W. X., Li, H. H., Li, S. R., and Wang, Y. W. (2006). Heavy metal accumulation of edible vegetables cultivated in agricultural soil in the suburb of Zhengzhou city, People's Republic of China. Bull. Environ. Contam. Toxicol. 76, 163–170. doi: 10.1007/s00128-005-0903-9
Llorente-Mirandes, T., Llorens-Muñoz, M., Funes-Collado, V., Sahuquillo, À., and López-Sánchez, J. F. (2016). Assessment of arsenic bioaccessibility in raw and cooked edible mushrooms by a PBET method. Food Chem. 194, 849–856. doi: 10.1016/j.foodchem.2015.08.047
Luo, C., Liu, C., Wang, Y., Liu, X., Li, F., Zhang, G., et al. (2011). Heavy metal contamination in soils and vegetables near an e-waste processing site, South China. J. Hazard. Mater. 186, 481–490. doi: 10.1016/j.jhazmat.2010.11.024
Mayorga, P., Moyano, A., Anawar, H. M., and Garcia-Sanchez, A. (2013). Uptake and accumulation of arsenic in different organs of carrot irrigated with As-rich water. CLEAN Soil Air Water 41, 587–592. doi: 10.1002/clen.201100697
Meharg, A. A., and Macnair, M. R. (1992). Suppression of the high affinity phosphate uptake system: a mechanism of arsenate tolerance in Holcus lanatus L. J. Exp. Bot. 43, 519–524. doi: 10.1093/jxb/43.4.519
Miteva, E. (2002). Accumulation and effect of arsenic on tomatoes. Commun. Soil Sci. Plant Anal. 33, 1917–1926. doi: 10.1081/CSS-120004832
Muñoz, O., Diaz, O. P., Leyton, I., Nuñez, N., Devesa, V., Súñer, M. A., et al. (2002). Vegetables collected in the cultivated andean area of Northern Chile: total and inorganic arsenic contents in raw vegetables. J. Agric. Food Chem. 50, 642–647. doi: 10.1021/jf011027k
Naujokas, M. F., Anderson, B., Ahsan, H., Aposhian, H. V., Graziano, J. H., Thompson, C., et al. (2013). The broad scope of health effects from chronic arsenic exposure: update on a worldwide public health problem. Environ. Health Perspect. 121, 295–302. doi: 10.1289/ehp.1205875
Odai, S., Mensah, E., Sipitey, D., Shoji, R., and Awuah, E. (2008). Heavy metals uptake by vegetables cultivated on urban waste dumpsites: case study of Kumasi, Ghana. Res. J. Environ. Toxicol. 2, 92–99. doi: 10.3923/rjet.2008.92.99
Onakpa, M. M., Njan, A. A., and Kalu, O. C. (2018). A review of heavy metal contamination of food crops in Nigeria. Annal. Glob. Health 84, 488–494. doi: 10.29024/aogh.2314
Ouédraogo, M., Toé, A. M., Ouédraogo, T. Z., and Guissou, P. I. (2011). “Pesticides in Burkina Faso: overview of the situation in a Sahelian African country,” in Pesticides in the Modern World-Pesticides Use and Management (IntechOpen), 35–48. doi: 10.5772/16507
Pallo, F. J. P., and Thiombiano, L. (1989). Les Sols Ferrugineux Tropicaux Lessives à Concrétions du Burkina Faso: Caractéristiques et Contraintes pour L'utilisation Agricole. Bunasol: ORSTOM.
Pigna, M., Caporale, A. G., Cozzolino, V., Lopez, C. F., Mora, M. L., Sommella, A., et al. (2012). Influence of phosphorus on the arsenic uptake by tomato (Solanum lycopersicum L) irrigated with arsenic solutions at four different concentrations. J. Soil Sci. Plant Nutr. 12, 775–784. doi: 10.4067/S0718-95162012005000031
Podgorski, J., and Berg, M. (2020). Global threat of arsenic in groundwater. Science 368, 845–850. doi: 10.1126/science.aba1510
Podgorski, J. E., Eqani, S. A. M. A.S., Khanam, T., Ullah, R., Shen, H., and Berg, M. (2017). Extensive arsenic contamination in high-pH unconfined aquifers in the Indus valley. Sci. Adv. 3:e1700935. doi: 10.1126/sciadv.1700935
Queirolo, F., Stegen, S., Restovic, M., Paz, M., Ostapczuk, P., Schwuger, M. J., et al. (2000). Total arsenic, lead, and cadmium levels in vegetables cultivated at the Andean villages of northern Chile. Sci. Total Environ. 255, 75–84. doi: 10.1016/S0048-9697(00)00450-2
Rahaman, S., Sinha, A. C., Pati, R., and Mukhopadhyay, D. (2013). Arsenic contamination: a potential hazard to the affected areas of West Bengal, India. Environ. Geochem. Health 35, 119–132. doi: 10.1007/s10653-012-9460-4
Rahman, M. A., Hasegawa, H., Rahman, M. A., Rahman, M. M., and Miah, M. A. M. (2006). Influence of cooking method on arsenic retention in cooked rice related to dietary exposure. Sci. Total Environ. 370, 51–60. doi: 10.1016/j.scitotenv.2006.05.018
Rauf, M. A., Hakim, M. A., Hanafi, M. M., Islam, M. M., Rahman, G. K. M. M., and Panaullah, G. M. (2011). Bioaccumulation of arsenic (As) and phosphorous by transplanting Aman rice in arsenic-contaminated clay soils. Aust. J. Crop Sci. 5, 1678–1684.
Reeder, R. J., Schoonen, M. A. A., and Lanzirotti, A. (2006). Metal speciation and its role in bioaccessibility and bioavailability. Rev. Mineral. Geochem. 64, 59–113. doi: 10.2138/rmg.2006.64.3
Rezvani, M., and Zaefarian, F. (2011). Bioaccumulation and translocation factors of cadmium and lead in Aeluropus littoralis. Aust. J. Agric. Eng. 2, 114–119.
Sako, A., Bamba, O., and Gordio, A. (2016). Hydrogeochemical processes controlling groundwater quality around Bombore gold mineralized zone, Central Burkina Faso. J. Geochem. Explor. 170, 58–71. doi: 10.1016/j.gexplo.2016.08.009
Schmidt, C. W. (2014). Low-dose arsenic: in search of a risk threshold. Environ. Health Perspect. 122, A130–A134. doi: 10.1289/ehp.122-A130
Sengupta, M. K., Hossain, M. A., Mukherjee, A., Ahamed, S., Das, B., Nayak, B., et al. (2006). Arsenic burden of cooked rice: traditional and modern methods. Food Chem. Toxicol. 44, 1823–1829. doi: 10.1016/j.fct.2006.06.003
Smedley, P. L., Knudsen, J., and Maiga, D. (2007). Arsenic in groundwater from mineralised Proterozoic basement rocks of Burkina Faso. Appl. Geochem. 22, 1074–1092. doi: 10.1016/j.apgeochem.2007.01.001
Smith, A. H., Hopenhayn-Rich, C., Bates, M. N., Goeden, H. M., Hertz-Picciotto, I., Duggan, H. M., et al. (1992). Cancer risks from arsenic in drinking water. Environ. Health Perspect. 97, 259–267. doi: 10.1289/ehp.9297259
Smith, E., Juhasz, A. L., and Weber, J. (2009). Arsenic uptake and speciation in vegetables grown under greenhouse conditions. Environ. Geochem. Health 31, 125–132. doi: 10.1007/s10653-008-9242-1
Smith, F. I., and Eyzaguirre, P. (2007). African leafy vegetables: their role in the World Health Organization's global fruit and vegetables initiative. Afr. J. Food Agric. Nutr. Dev. 7, 1–17.
Some, I. T., Sakira, A. K., Ouedraogo, M., Ouedraogo, T. Z., Traore, A., Sondo, B., et al. (2012). Arsenic levels in tube-wells water, food, residents' urine and the prevalence of skin lesions in Yatenga province, Burkina Faso. Interdiscipl. Toxicol. 5, 38–41. doi: 10.2478/v10102-012-0007-4
Stazi, S. R., Cassaniti, C., Marabottini, R., Giuffrida, F., and Leonardi, C. (2016a). Arsenic uptake and partitioning in grafted tomato plants. Hortic. Environ. Biotechnol. 57, 241–247. doi: 10.1007/s13580-016-0036-6
Stazi, S. R., Mancinelli, R., Allevato, E., Marabottini, R., Campiglia, E., and Marinari, S. (2016b). Phytoavailability of geogenic arsenic and its partitioning in soil: a case of study in a thermal area of Central Italy. Eqa Int. J. Environ. Qual. 20, 27–34. doi: 10.6092/issn.2281-4485/6305
Stopelli, E., Duyen, V. T., Mai, T. T., Trang, P. T. K., Viet, P. H., Lightfoot, A., et al. (2020). Spatial and temporal evolution of groundwater arsenic contamination in the Red River delta, Vietnam: interplay of mobilisation and retardation processes. Sci. Total Environ. 717:137143. doi: 10.1016/j.scitotenv.2020.137143
Tani, F. H., and Barrington, S. (2005). Zinc and copper uptake by plants under two transpiration rates. Part II. Buckwheat (Fagopyrum esculentum L.). Environ. Pollut. 138, 548–558. doi: 10.1016/j.envpol.2004.06.004
Uddh-Söderberg, T. E., Gunnarsson, S. J., Hogmalm, K. J., Lindegård, M. I. B. G., and Augustsson, A. L. M. (2015). An assessment of health risks associated with arsenic exposure via consumption of homegrown vegetables near contaminated glassworks sites. Sci. Total Environ. 536, 189–197. doi: 10.1016/j.scitotenv.2015.07.018
Villholth, K. G. (2013). Groundwater irrigation for smallholders in Sub-Saharan Africa – a synthesis of current knowledge to guide sustainable outcomes. Water Int. 38, 369–391. doi: 10.1080/02508060.2013.821644
Walpole, S. C., Prieto-Merino, D., Edwards, P., Cleland, J., Stevens, G., and Roberts, I. (2012). The weight of nations: an estimation of adult human biomass. BMC Public Health 12:439. doi: 10.1186/1471-2458-12-439
Wens, M., Veldkamp, T. I. E., Mwangi, M., Johnson, J. M., Lasage, R., Haer, T., et al. (2020). Simulating small-scale agricultural adaptation decisions in response to drought risk: an empirical agent-based model for semi-Arid Kenya. Front. Water 2:15. doi: 10.3389/frwa.2020.00015
WHO (2011). Evaluation of Certain Contaminants in Food: Seventy-Second Report of the Joint FAO/WHO Expert Committee on Food Additives (WHO Technical Report Series). Joint FAO/WHO Expert Committee on Food Additives (JECFA).
Yanez, L. M., Alfaro, J. A., Avila Carreras, N. M. E., and Bovi Mitre, G. (2019). Arsenic accumulation in lettuce (Lactuca sativa L.) and broad bean (Vicia faba L.) crops and its potential risk for human consumption. Heliyon 5:e01152. doi: 10.1016/j.heliyon.2019.e01152
Keywords: arsenic, irrigation water, accumulation, vegetable, uptake, cooking, risk assessment, Burkina Faso
Citation: Clair-Caliot G, Marks SJ, Hug SJ, Bretzler A, N'guessan ND, Tihe SFK and Lalanne F (2021) Uptake of Arsenic by Irrigated Vegetables and Cooked Food Products in Burkina Faso. Front. Water 3:667308. doi: 10.3389/frwa.2021.667308
Received: 12 February 2021; Accepted: 03 May 2021;
Published: 26 July 2021.
Edited by:
Katherine A. James, University of Colorado Anschutz Medical Campus, United StatesReviewed by:
M. Safiur Rahman, Bangladesh Atomic Energy Commission, BangladeshAdewale Matthew Taiwo, Federal University of Agriculture, Abeokuta, Nigeria
Copyright © 2021 Clair-Caliot, Marks, Hug, Bretzler, N'guessan, Tihe and Lalanne. This is an open-access article distributed under the terms of the Creative Commons Attribution License (CC BY). The use, distribution or reproduction in other forums is permitted, provided the original author(s) and the copyright owner(s) are credited and that the original publication in this journal is cited, in accordance with accepted academic practice. No use, distribution or reproduction is permitted which does not comply with these terms.
*Correspondence: Guillaume Clair-Caliot, Z3VpbGxhdW1lLmNsYWlyQGVjb21haWwucHJv
†Present address: Anja Bretzler, Swiss Academy of Sciences (SCNAT), Bern, Switzerland