- 1Terra Gaia Ecological Services, Berlin, Germany
- 2Cooperative Research Centre for Water Sensitive Cities (CRCWSC), Clayton, VIC, Australia
- 3School of Engineering, Civil, Environmental and Mining Engineering, The University of Western Australia (M051), Perth, WA, Australia
- 4School of Biological Sciences, The University of Western Australia (M004), Perth, WA, Australia
- 5Department of Environmental Engineering, Technical University of Denmark, Kongens Lyngby, Denmark
- 6IHE Delft Institute for Water Education (UNESCO), Delft, Netherlands
- 7ResilienServices, Delft, Netherlands
- 8School of Agriculture and Environment, The University of Western Australia (M004), Perth, WA, Australia
An estimated 90% of the Swan Coastal Plain (SCP) wetlands, located in southwestern Australia, have been lost because of infilling or drainage. This loss continues despite the well-known causes, which include nutrient enrichment; the invasion of exotic flora and fauna; loss of fringing vegetation; and altered hydrological regimes caused by groundwater abstraction; urbanization; and a drying climate. Further loss is expected with climate change exacerbating the undesirable ecosystem changes of remaining wetlands. In this study, we consider these wetlands as examples of social-ecological systems (SES) which are characterized by a close interaction of the ecosystem with the social system. We take the theory of resilient SES as a starting point to identify the adaptive capacity and resilience of the wetlands. We argue that resilience provides a useful framework to analyze adaptation processes and to identify appropriate policy responses. We explore incremental adjustments and transformative action and demonstrate that policy responses arise across multiple scales and levels of jurisdiction and institution. By applying the theoretical framework of resilience to the SCP wetlands, we identified (un)desired ecosystem states of wetlands (hydrology and ecology) through different set of policy actions. Our results show that current wetland management is inadequate to maintain the ecosystem's functioning. We recommend cross-jurisdictional collaboration and the use of conceptual eco-hydrological models to depict gradual ecological change and types of regime shifts (thresholds, hysteresis, and irreversible changes). The different adaption options inform decision-makers to adequately adapt wetland management practices when uncertainty in ecosystem responses exist. Empirical data on how multiple jurisdictions operate and decide could help to further support decision-making. With this research we aim to narrow the science-policy interface which depends on corresponding cross-jurisdictional and institutional responses to coordinate wetland management policies and actions.
Introduction
The aim of environmental management (or ecosystem management) is to create and maintain ecological systems so that they provide benefits to humans. Ecological systems are closely linked to social, economic, and environmental considerations. In social-ecological systems (SES) the social and economic considerations can change processes, structures, and feedbacks that may be unwanted from an environmental management perspective (Hughes et al., 2014a; Poff et al., 2015). The resilience of social-ecological systems is related to (i) the ability of the ecosystem to absorb disturbances and still persist or remain in the same state defined by its processes, structures and feedbacks and (ii) the degree to which the system can build capacity for learning and adaptation (Holling, 1973; Folke et al., 2002; Liao, 2012). Two major resilience interpretations exist: (i) system resilience which refers to the amount of change a system can undergo and still retain the same function and structure while maintaining options to adapt (prescriptive, normative, and actor based) (Gersonius et al., 2012), and (ii) the ability of a system to remain stable, unchanged, or to have minimum fluctuations (modeling and a mathematical foundation) (Holling, 1973, 1996; Folke et al., 2004; Carpenter et al., 2015; Scheffer et al., 2015). As ecosystems rarely operate near equilibrium and the magnitude of change is not predictable, system resilience aims to manage systems with flexibility rather than maintaining stability (Liao, 2012).
Resilience provides a useful framework to study adaptation (management) processes and to identify appropriate policy responses. To maintain the capacity to deal with current or future predicted change, a decision-making process and set of actions are undertaken (adaptation). Most research on adaptation primarily considers an actor-centered view to respond to specific perturbations to reduce vulnerabilities (Nelson et al., 2007). The resilience approach is system orientated and enables adaptation, including social and physical elements, and the ability to mobilize these elements [adaptive capacity (Engle, 2011)]. To analyze adaptation processes and to identify appropriate policy responses it is useful to converge the two approaches. This allows to distinguish between a fundamental alteration of the nature of a system [transformative (Chaffin et al., 2016)] and incremental adjustments once the current ecological or social conditions become undesirable.
While several studies have illustrated ecosystems can be transformed by human action (Scheffer et al., 2001; Folke et al., 2004); these transformations are challenging as undesirable stable ecosystem states may not shift to an alternate state with the desired ecosystems functions (Werner and McNamara, 2007; Hastings and Wysham, 2010; Pace et al., 2015; Reyers et al., 2018).
Ecosystem management (biodiversity and alternate regimes) needs to address how external drivers interact with internal dynamics according to four attributes (Walker et al., 2004, 2012): (10 stability, the maximum amount the system can be changed while remaining in the same state; (2) resistance, the ease or difficulty of changing the system; (3) precariousness, how close the current trajectory of the system is to crossing a threshold and change into an undesirable stable state; (4) cross-scale relations, how stability, resistance, and precariousness are influenced by the states and cross-scale dynamics of the (sub)systems. Understanding cross-scale interactions are at the heart of adoption of a system-based approach (Zevenbergen et al., 2008). To adapt current strategies for ecosystem resilience; integration of adaptation measures for ecology need to include multiple spatial and temporal scales (robustness and flexibility to future change), ecosystem scales, and institutional and jurisdictional boundaries (Salinas Rodriguez et al., 2014). Cross-scale interactions in SES means that multiple institutions and jurisdictions (e.g., management authorities or community groups) are involved in an adaptation strategy. Adaptation requires active involvement of multiple actors to provide transformative changes rather than only incremental adjustments (Holling, 2001; Folke et al., 2002; Walker et al., 2004).
The resilience framework broadens the expanse of adaptation while also providing cross-jurisdictional connections through negotiation, decision-making and actions within actor-based analyses for adaption (Nelson et al., 2007). Adaptation is closely linked to the dynamics of decisions of different actors with different stakes or aims for ecosystem management (Folke et al., 2005; Nelson et al., 2007; Ostrom, 2009). In other words, decision-making needs to be informed by various tools, methods and approaches that can interrelate external drivers, social factors, and ecological factors (Hughes et al., 2014a,b). As different scenarios of ecosystem changes and adaptation measures target different spatial scales (e.g., local, catchment, regional scale); decision-making often occurs across multiple stakeholders with different jurisdictions (Brondizio et al., 2009).
A lack of quantitative and qualitative data makes adaptation and decision-making under high uncertainty challenging to prevent ecosystems to alternate between stable states (Scheffer et al., 2001, 2015; Schultz et al., 2015). For example, the projection of ecosystem responses requires sufficient ecological data which is not always available (Fidelman et al., 2013; Hughes et al., 2017). Climate change scenarios rely on projections and data which are often not representative on the local scale (Lawrence and Haasnoot, 2017; Radhakrishnan et al., 2017; Romm et al., 2018). Understanding uncertainty and reducing vulnerability supports knowledge for learning and creates opportunities for self-organization (Berkes, 2007; Miller et al., 2010).
The second major complexity of decision-making is scale; that is understanding the scale at which these decisions are made and the scale of problems that occur (Folke et al., 2007). Ideally, adaptation measures match the scale of the problem and the jurisdiction of the actor that is responsible for ecosystem management (Young, 2002, 2011). A scale mismatch between ecosystem and jurisdiction could result in uncertainty about the appropriate jurisdictional scale an adaptation measures needs to be taken (Borgström et al., 2006; Cumming et al., 2006). Large ecosystems risk being managed by several jurisdictions that could cover one or multiple spatial scales (Cash et al., 2006). An example is the understanding of how land-use changes impact aquatic ecosystems, which include local and catchment scale effects (Allan, 2004; Hughes et al., 2014b).
At last an adaptation approach depends on views and preferences of stakeholders and data input that support the decision-making process (Brussard et al., 1998; Lebel et al., 2013; Dewulf et al., 2015).
Linking qualitative and quantitative assessments with actors and experts is crucial for advancement of complex social-ecological interactions (Nelson et al., 2007). There is a significant body of literature that discusses the potential problems associated with expert elicitation and methods for avoiding those issues. One issue is uncertainty of results from quantitative assessments. This problem can be overcome by choosing several modeling frames that cover a range of spatial scales and by setting quantifiable objectives (Burgman et al., 2005; Martin et al., 2012). However, the use of modeling frames may be compromised due to a lack of data and the difficulty to interpret data.
In general, an expert-elicitation approach could help to define how information will be used, what to elicit, how the elicitation is performed, and how these are translated into quantitative statements that can be used either in a model or directly for decision-making (Martin et al., 2012). The last step in an elicitation a process may be affected by a lack of experts. A small sample of experts makes it difficult to quantitatively analyze experts' information. Research shows that choosing a participatory and multi-level approach with experts provides an adequate method to address a mix of problem scales [environmental and governance (Chaffin et al., 2016)], participation scales [vertical interplay (Young, 2006)], and authority [decision-making actors (Newig et al., 2005; Brondizio et al., 2009)].
We propose a modified analytical framework, which combines principles of resilience theory related to SES management with policy needs for identifying adaptation actions, to evaluate how a broader set of policy actions can align drivers of long-term ecosystem resilience through human management actions when data is lacking. We use a case study to demonstrate the implementation of this proposed modified framework and describe results through qualitative and quantitative analyses.
Materials and Methods
Adaptation Tipping Points Framework
Two useful tools for resilience-building in social-ecological systems, are structured scenarios and active adaptive management (Olsson et al., 2006). These consider the alteration of the magnitude, frequency, and duration of disturbance regimes to which the system is adapted (Radhakrishnan et al., 2017). Due to a lack of data on the local scale we include a model framework that relies on regional projections. One of the resilience frameworks originates from risk and uncertainty assessment under climate change.
This method enhances reproducibility and is applicable to water, land, and ecosystem management adaptation (Werners et al., 2015). With the Adaptation Tipping Points (ATP) framework policy and management actions are determined when “the magnitude of change (e.g., climate change) is such that the current management strategies are deemed to be inadequate to meet the social-ecological objectives”(Kwadijk et al., 2010).
The application of this framework requires information about water management and land-use planning strategies (Rijke et al., 2013; Werners et al., 2013a). The advantages of applying this method, include stakeholder engagement and the definition of objectives and aims in management of the SES. Challenges remain to overcome when applying the ATP method to ecosystems. These include a lack of data availability and unclear or undefined ecological thresholds (Bölscher et al., 2013; Werners et al., 2013a,b; Nanda et al., 2018a). Overcoming these challenges will help to shape adaptation strategies that are essential to transit to resilience climate change strategies for ecosystems (Haasnoot et al., 2012; Ahmed et al., 2018). The five-step ATP methodology includes: (i) the determination of climate change effects on the system; followed by (ii) identifying key objectives and thresholds; (iii) the determination when standards were compromised in the past; (iv) analyzing when standards will be compromised in the future; and (v) to repeat step 1–4 for alternative strategies (Kwadijk et al., 2010).
The original methodology was modified in step 3. We identified eight ATPs for different social-ecological objectives and thresholds with the assessment of historical hydrological time series in a previous study (Nanda et al., 2018a). Step 3 was extended to interpret ATPs in conjunction with the hydrological response and variation; temporal scale ecosystem responses; and recovery rate and alternative stable state of ecological processes (Figure 1). Steps 4A till 4C were also conducted in a previous study (Nanda et al., 2018b) and focused on governance of the SES which described the mismatches between the ecosystem organization, jurisdiction, and institutions. Based on the previous results we focus here on a repeat of steps 4A and 4B in which we focus on adaptation measures that go beyond the eight ecological objectives (Supplementary Tables 1–4).
Interviews from previous studies (Nanda et al., 2018a,b) were used to add ecosystem processes/patterns caused by alterations of the hydrological system, mainly due to decreased rainfall. From observed seasonal surface- and groundwater levels of Lake Forestdale we created a conceptual eco-hydrological model that highlights the seasonal processes of the wetland. To illustrate the ecological implications of lower surface water levels we collected information about management implications (from stakeholders) of the deep water and shallow water zones and the wetland buffer zone.
As wetlands of the SCP are often steppingstones in linear corridors in the landscape, adaptive management focuses on the integration of water resources and ecosystem management. Therefore, the wetland buffer zone guidelines and ecological water requirements are explained with the available literature that draws on the theories of resilience and ecology. The objective to maintain a wetland buffer zone defined in scientific literature, depends on radial areas measured from the furthermost extent of wetland vegetation and depending on the width of the zone to protect the integrity of the wetland (Castelle et al., 1994). We rated the buffer zone according to main landscape components (Ignatieva et al., 2010): (1) patch quality (habitat value for species), (2) boundary effects [the surroundings of a patch that influence movement of species and protects from disturbance (Weston et al., 2009)], (3) patch context (the spatial location of a patch), and (4) connectivity (movements of species between patches).
Step 4C incorporated the use of eco-hydrological conceptual models of the case study area in interview questions. These were aimed to complement the eco-hydrological conceptual model and inform whether adaptation measures were beneficial for one SES objective and simultaneously negative for another (maladaptation).
Case Study Description
The SCP wetlands in southwest Western Australia provide habitat for endemic flora and fauna and they are located within one of the international biodiversity hotspots (Myers et al., 2000). Forrestdale Lake is a Ramsar listed wetland with extensive fringing sedgeland which is typical of the Swan Coastal Plain (Figure 2). In a regional context, the lake is a major breeding site, migration stopover and semi-permanent drought refuge area for waterbirds and migratory birds. The lake contains open water and is fringed by rushes and a continuous belt of bulrushes of rare native Typha domingensis and introduced/invasive Typha orientalis, behind which are belts of trees tolerant of water-logging (CCWA, 2005). The higher ground around the lake supports open woodland while the aquatic zone consists of macrophytes. The margins of the lake support many terrestrial birds and other vertebrate species, such as the quenda (Isoodon obesulus fusciventer) and invasive European red fox foxes (Vulpes vulpes).
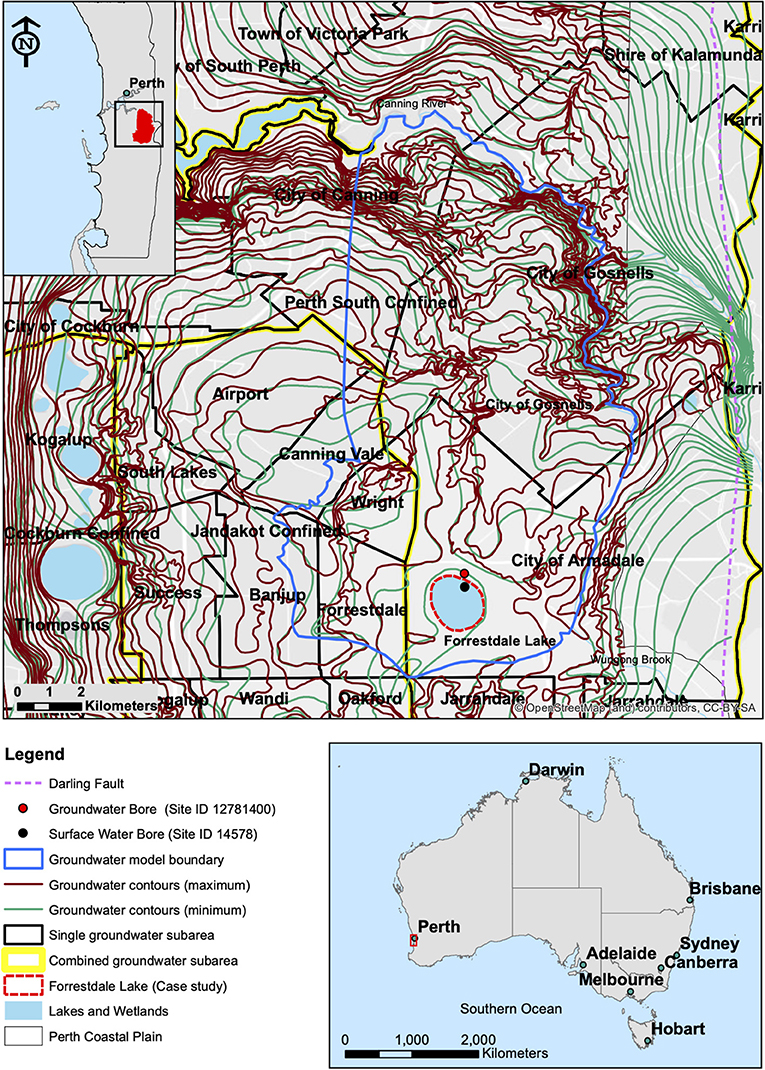
Figure 2. Location of Forrestdale Lake (32° 09′ 30″ S, 115° 56′ 16″ E) with the groundwater model boundary of the superficial aquifer, the minimum and maximum groundwater contours of the superficial aquifer, groundwater management areas in the catchment (subareas), and the various local governments (Map projection: GDA94).
The sediments of the SCP are made of wind and riverine depositions. These sediments form four main geomorphic units with: distinctive geology, topography, drainage patterns, soil characteristics, and vegetation (Semeniuk, 1987). Wetlands in the SCP lie in depressions between dunes which are located where a shallow water table permanently or seasonally intersects the land surface. The depressions appear to act as flow-through lakes, which capture groundwater on the eastern side (Darling Scarp) and discharge water westwards toward the Indian Ocean (Townley et al., 1993).
The geomorphic elements of the Southern River Catchment are typical of the SCP located in the Perth Basin. On the eastern edge of the Perth Basin are the Bassendean Dunes which comprise sandy soils. Lakebed sediments comprise sand to sandy organic mud overlying soft marly limestone and clayey sand (McArthur et al., 1980; Davidson, 1995). The Jandakot Groundwater Mound is situated in the managed Perth Groundwater Area, which is a region of elevated groundwater table beneath the SCP. Groundwater discharges from the mound into low lying depressions that support groundwater dependent vegetation and extensive wetland systems. Wetlands are surface expressions of groundwater where a shallow water table intersects with the ground surface (Semeniuk, 1987; Hill, 1996).
Wetlands of the SCP are classified by water periodicity (permanently or seasonally inundated/waterlogged) and by landform (basin, flat, channel, slope, or highland) with damplands, sumplands, and palusplains the most frequent occurring type of wetlands (Semeniuk, 1987; Semeniuk and Semeniuk, 1995; Hill et al., 1996). Seasonally inundated refers to wetlands depending on rainwater (surface water), while seasonally waterlogged wetlands depend on groundwater which have soils that are saturated throughout summer). Further differentiation between wetlands of the same classification is possible using wetland descriptors such as water quality, size, shape, and vegetation. The wetland types found in the SCP vary in size, shape, hydrology, stratigraphy, and vegetation. The most frequently used classification is shown in Table 1. Wetlands can move through different hydroperiod states as a result from declining rainfall and increases or decrease of the groundwater table. Forrestdale Lake is a groundwater lake and surface run-off had little effect on its depth when it was in an undisturbed condition. Alternative system states have not been extensively studied and are often limited to the hydroperiod of a wetland (Davis and Brock, 2008; Davis et al., 2015).
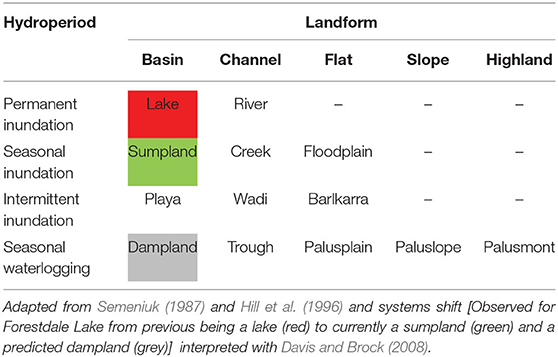
Table 1. Classification of Swan Coastal Plain based on their morphology, hydrology, stratigraphy, and geomorphic units.
The wetlands of the SCP are likely to experience increasing stress, as the population is expected to double by 2050 to 4 million inhabitants (Australian Bureau of Statistics, 2013a,b). With a growing population demand will increase for (ground)water and land for development, posing risks to hydrological alteration that support the critical ecological processes. Climate change studies predict decreasing rainfall for southwest Western Australia adding to an increase of hydrological stress (Smith and Power, 2014). Wetland management needs to respond to a drying climate so that the integrity of the wetland's ecosystem functions is maintained. The responsibility for managing the wetland is shared among different actors (e.g., governments or communities).
Results
The lake can be represented in three spatial zones (Figure 3): (1) A dry buffer zone with vegetation that extend their roots to the deeper groundwater aquifer and does not depend on the availability of surface water or high groundwater table; (2) A shallow water zone (<0.5 m) that depends on surface- and groundwater availability and seasonally dries; and (3) a deep-water zone (1.5–2.0 m) with permanent water year-round that depends on surface water and high groundwater table. The shallow and deep surface water zone provide habitat for migratory bird species, wading birds, aquatic invertebrates, macrophytes, and tortoises.
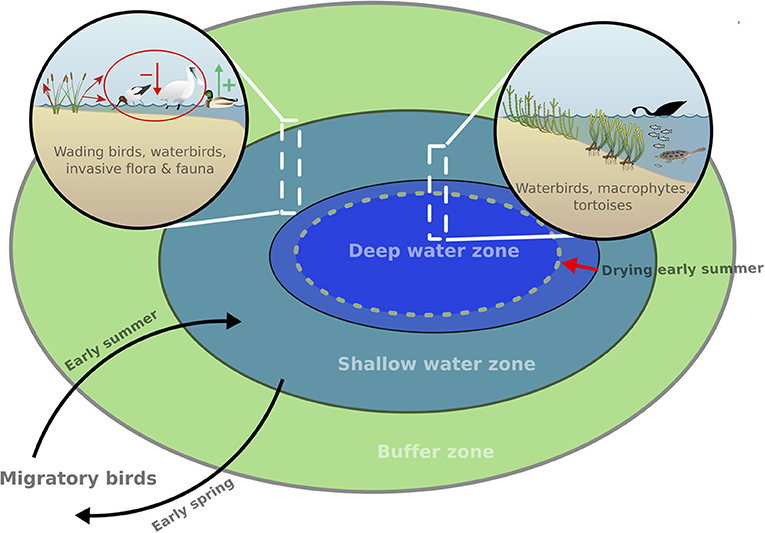
Figure 3. Seasonal representation of the shallow (<0.5 m) and deep surface water zones (<1.5 m) of the lake. Prolonged droughts impact on wading birds due to an increase of invasive flora in the shallow water zone. Reduced habitat for waterbirds occurs when the surface area of the deep-water zone decreases and becomes shallower after prolonged dry periods. Wading birds and migratory birds decline due to the loss of muddy shorelines and increase of invasive flora species in the shallow water zone. Integration and Application Network [ian.umces.edu/media-library (Integration Application Network, 2007)].
Waterbird species decrease when permanent water is unavailable while migratory and wading birds benefit from muddy shorelines in the intermediate zone. Combined with lower water table in spring and summer over consecutive years groundwater drops below the stratigraphic layer. There are locations of downward groundwater flux and locations with upward fluxes due to local head gradients which provide muddy areas. A dry lakebed increases the likelihood of invasive flora that limits feeding grounds for wading birds. In contrary to wading birds, waterbirds benefit from these conditions as fringing vegetation provides resting and nesting habitat. Early summer drying results in a smaller deep-water zone (habitat for waterbirds, tortoises; aquatic vertebrates) and larger intermediate zone increasingly invaded by invasive bulrush Typha orientalis.
The conceptual eco-hydrological model of Forrestdale Lake shows the seasonal deep-water and shallow water phases and inter-annual variability of surface water (Figure 4). Ecological character identifiers are included that serve as an early warning sign of potential negative ecological effects and are explained for each hydrological phase. Groundwater levels remain too low to provide a saturated lakebed for aestivating tortoises and to limit the spread of weeds in the deep-water zone. The lakebed has become more densely vegetated due to an increase of Typha orientalis on the fringes of the shallow water zone because as less surface water availability allows its growth.
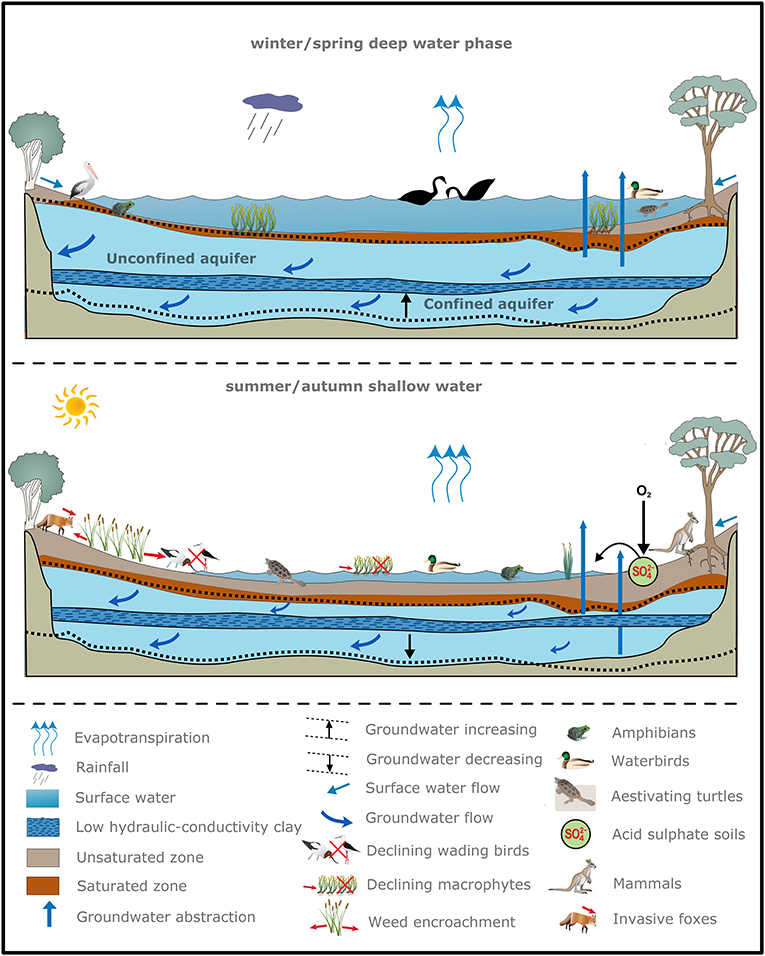
Figure 4. A conceptual, seasonal representation of the hydrology of Forrestdale Lake in a cross-sectional form. Representing the seasonal deep-water and shallow water phases and inter-annual variability. Ecological character identifiers that serve as an early warning sign of potential negative ecological effects are explained for each phase. The superficial groundwater head level provides limited connectivity to surface water level due to a clay layer of the lakebed. This limits groundwater fed surface water in summer but provides a saturated lakebed during summer to support critical ecological functions [ian.umces.edu/media-library (Integration Application Network, 2007)]. Model not-to-scale. Wetland and aquifer system of the Swan Coastal Plain is continuous.
The duration, timing, and rate of seasonal droughts are important to the ecology of the wetland. When there are consecutive years of low rainfall this leads to a lower groundwater table. The lakebed becomes unsaturated and negatively impacts macrophytes and the tortoises which depend on muddy soils to aestivate during summertime.
Stakeholders used the information about maladaptation, to reconsider the social-ecological objectives. The conceptual eco-hydrological model was used as a starting point to explore ecosystem responses when new adaptation measures were considered. The conceptual representation aided the ongoing discussions to set priorities for species groups to be protected. Also, future land-use scenarios were discussed among stakeholders. The 15 proposed adaptation measures were categorized as ecosystem-based, structural, or as combined action (Supplementary Tables 5–7). Stakeholders preferred a decision-process to implement each adaptation measure, since multiple stakeholders would be involved for adequate implementation.
The conceptual eco-hydrological representation indicates that lowering of the groundwater table, and drainage infrastructure negatively impact the hydrology. Removal of invasive weeds and native vegetation increases the risk of acid sulfate soils and reduced sediment processes due to soil disturbance. Removing the weeds may impact waterbird roosting sites and breeding habitat. The optimum time for spraying is usually the end of December through to February but becomes increasingly difficult when water levels are more frequently below 0.1 m during this period of water availability (minimum water depth required according to the Lake Management Plan). This provides a major challenge, as weeds are a major problem and considered as a priority within current and future management of the wetland.
Adaptation measures, such as removing weeds or water suppletion, lead to positive and negative ecological effects (maladaptation). Due to these conflicting ecological impacts; stakeholders could not agree on which species groups or socio-ecological objectives needed to be prioritized (Supplementary Tables 3, 5). Initially there was a strong focus on technical solutions in the discussions, but ecosystem-based solutions were considered as trade-offs for each measure were visualized in the conceptual eco-hydrological overview (Figure 4).
Prior to land-use changes, water levels were more reflective of rainfall patterns. Due to recent urbanization many wetlands are now intensively managed. Management of wetlands is organized under several levels within the institution (policies or legislation) with multiple jurisdictions (government, private landowners, community) mandated to manage wetlands (Figure 5). There is a lack of cross-jurisdictional cooperation; integration of land-use planning, water resources planning, and ecosystem management. Due to this fragmentation in both, the institutional and jurisdictional frameworks, management currently focuses on incremental adaptation which can be defined as:
• Ecological objectives: the protection of local and regional bushland, maintaining buffer zones (Figure 6), species protection, environmental water provisions (Table 2)
• Social-political objectives: mosquito and midge prevention, flood protection, groundwater abstraction
• Institutional objectives: providing guidance to which jurisdiction coordinates wetland management
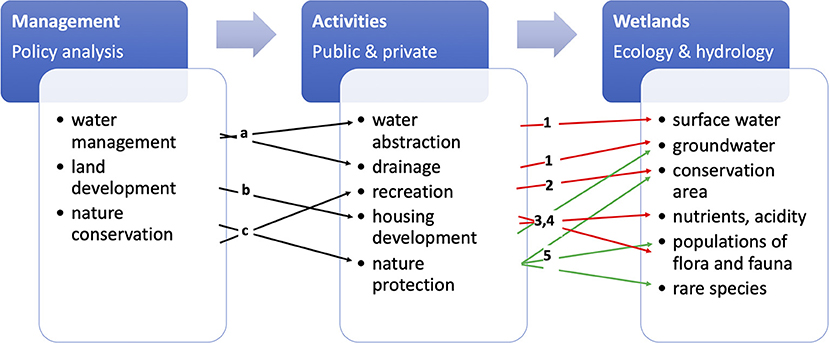
Figure 5. Conceptual overview of the regulation and impacts of the wetland system. Regulation of activities: (a) water authority, (b) planning council, (c) municipal, state, and national department of conservation. Impact on physical wetland system (red: negative impact; green: positive impact): (1) dehydration, (2) disturbance, (3) fragmentation, (4) eutrophication, (5) nature restoration.
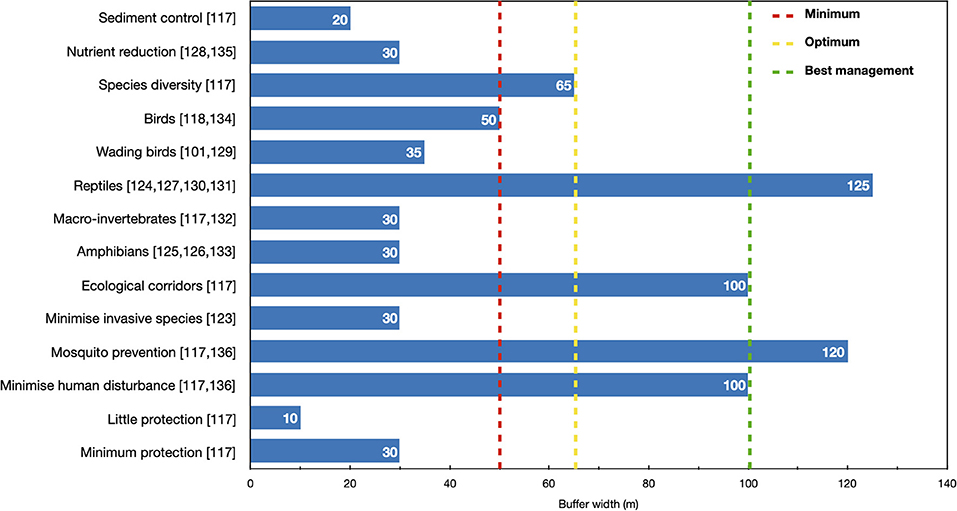
Figure 6. Range of buffer widths for providing specific buffer function from literature and minimum (red, 50 m), optimum (yellow, 65 m), and best management (green, 100 m) from wetland buffer zone policy (Western Australian Planning Commission, 2005). Adapted from Castelle et al. (1994).
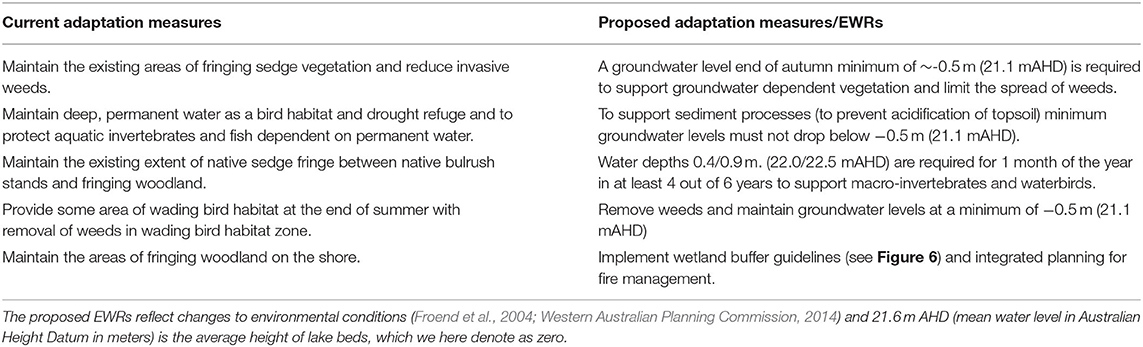
Table 2. Ecological water requirements (EWRs) are the water depths and hydrological regimes necessary to maintain a low level of risk to the ecological values which is based on the state water requirements policy (EPA, 1992).
The current buffer zone of Forrestdale Lake provides for habitat for endemic species, corridors, and reduces disturbance. With other patches of wetlands on a landscape scale they form a mosaic that vary individually on local scale in quality. Currently species can move between different wetlands via existing wildlife corridors (riparian zones and urban water streams).
Discussions with stakeholders included local processes that are observable only on larger scales due to cumulative effects, such as small patch clear cuts of wetland buffers and conversion or fragmentation of land between the remaining wetlands. Local scale improvement of a wetland that supports rich biodiversity, was indicated as inadequate, since populations of threatened species in fragmented habitats exist within the same watershed. There are conflicting management strategies on a local and regional level due to the different objectives of jurisdictions of stakeholders. A major challenge, are future plans to urbanize the area around Forrestdale Lake which need careful planning for maintaining riparian zones and wildlife corridors.
Discussion
System Changes and Constraints for Adaptive Management
The ideal situation for adaptive ecosystem management, is to take timely decisions that maintain systems in desirable stable states that provide the necessary ecosystem services such as biodiversity and to keep this system for the future (Folke et al., 2005; Schultz et al., 2015). This is consistent to systems behavior, which after perturbation, can shift systems in alternative and often undesirable states with few ecosystem services (Scheffer et al., 2001; Folke et al., 2004; Carpenter et al., 2015). The uncertainty about systems behavior and perturbations are major challenges for ecosystem management (Berkes, 2007). Different system states are difficult to determine and often are evaluated from limited data and qualitative assessments to distinguish important ecosystem processes. Once ecosystems have changed; it is often challenging to revert, restore, or remediate them (Folke et al., 2004).
The behavior of an ecosystem depends on many feedback processes that interact with the social system (Holling, 2001). As studies often focus on single drivers of system change, such as climate change, other drivers may be overlooked (Lavery and Donovan, 2005; Kwadijk et al., 2010; Bölscher et al., 2013). Ecosystem resilience is addressed by known system characteristics but becomes very complex when more drivers for change are identified. The interpretation of results requires careful consideration of other external drivers that are responsible for ecosystem performance and possible positive or negative responses (trade-off) within a SES (Cohen-Shacham et al., 2011; van Oudenhoven, 2011; van Slobbe et al., 2016).
Internal drivers could also be important to biophysical processes, such as diseases known to decrease plant resilience (internal) and future land-use change (external). Despite previous research which has pointed to climate change as the current main external driver for change, land use changes need to be considered in the context of adaptation strategies (Seitz et al., 2011; Westbrook and Noble, 2013). Land-use changes (e.g., urbanization and stormwater collection) could be a source of water and thus could support the ecosystem processes, although, under the event of extreme drying these adaptation measures may not be sufficient (Barron and Barr, 2009; Barron et al., 2013).
Involvement of all stakeholders of the water management system is crucial to deliver adaptive strategies and to overcome barriers by science informed planning (MacKay, 2006). To support ecosystem functions patches or habitats must be large enough to support viable populations of endangered species and to support complicated food webs. Planning (spatial and temporal) of these wetland ecosystem services must be coordinated by different jurisdictions under different institutions (Noble et al., 2011; Schleupner and Schneider, 2013).
Resilience of ecosystems is problematic to assess when past or future conditions cannot be compared to baseline ecosystem states (Bloemen et al., 2017). This follows from the definition of ecological resilience, which states that ecosystems are not in an equilibrium, but rather move from one to another stable state (Folke et al., 2004). This is contrary to management, that follows a one-direction and incremental approach (Olsson et al., 2006). However, this does not take into account that individual processes within a system can increase resilience beyond that single ecosystem (Reyers et al., 2018). For example, migratory bird species may find suitable habitat in other wetlands if the original wetland lost the ability to retain them. From a management perspective, this does not immediately lead to a response for restoring this ecosystem service, since sociological or economic considerations are also involved in the process of decision-making (Schultz et al., 2015). Individual processes need to be assessed regarding the likelihood that they can be sustained under extreme conditions (Hughes et al., 2017), so that management authorities can better prioritize their resources (Ostrom, 2009) and select ecological objectives that they are willing to support.
The principle of management for resilience depends on spatial and temporal scales, both for institutional and ecosystem processes (Young, 2002; Huitema et al., 2009). Especially, when these two processes are not similarly organized, assessments for ecosystem resilience must consider multiple spatial scales (Dewulf et al., 2015). Since the adaptive capacity of an ecosystem also depends on the decision-making process; institutional organization over spatial scales; and levels of governance must also be analyzed (Brooks et al., 2005; Pahl-Wostl, 2009). Quick wins and long-term solutions emerge from multi-scale analyses while implementation of adaptation measures on local level without the need for higher level interventions requires consensus (Fünfgeld, 2015; Moloney and Fünfgeld, 2015). Higher level legislative changes require more negotiation at institutional levels and may take longer for implementation. Future research needs to consider the decision pathways for different organizational structures. These may be different and require other institutions or jurisdictions to implement strategic decisions to create a decision space (Carpenter et al., 2015; Hermans et al., 2017).
Informed Decision-Making Through Resilience and Adaptive Capacity of SES
Resilience and adaptive capacity provide information about the vulnerability of SES and closely link social and ecological interactions (Smit and Wandel, 2006; Berkes, 2007); even if clear feedbacks are not known. Feedbacks with actors provide implementation of adaptation measures on the ground which also need to distinguish slow and fast responses to changing systems when thresholds are exceeded (Walker et al., 2012). Even though thresholds are not “fixed” for biophysical processes; exceedance of thresholds can be interpreted with the available ecological data and ATPs to assess individual management objectives [Examples for salmon re-introduction (Hanna, 2008; Bölscher et al., 2013; van Slobbe et al., 2016)]. Future scenarios could inform management timely to prevent exceedance of individual objectives while collecting data and building knowledge with stakeholders is critical to identify information gaps of ecosystem functions and feedbacks.
Current thresholds and objectives give an indication of the ecological success of the ecosystem; however, the advanced conceptual understanding of the ecosystem was more effective to guide the process on effective (structural and non-structural) adaptation measures. Adaptation is managed through cross-scale interactions to avoid a decrease of resilience (Holling, 1996, 2001). In addition, actors in our case study could (i) move thresholds closer to the current state of the system by altering latitude (water suppletion) and (ii) make the threshold easier to reach by lowering these (Walker et al., 2004). Increased system understanding could be helpful to identify the impact of climate change on eco-hydrological processes, but there is still a challenge to identify when other parameters (e.g., water quality) become more relevant when a system may shift to alternate states. Social-economic concerns were not considered in our overview of responses to change, but research shows this is an important link (Biggs et al., 2012; Reyers et al., 2018). Also, we did not consider environmental and citizen groups that may have limited influence on the socio-economic considerations of government actors. Citizen groups often depend on the funding from government for ecosystem-based measures (e.g., removing weeds, revegetation).
Stakeholders represent multiple jurisdictions in the case study area and therefore are not limited to their own jurisdictions. Cross-scale interactions could enhance the connectivity of multi-level SESs (Brondizio et al., 2009; Biggs et al., 2012; Reyers et al., 2018). Cross-jurisdictional collaboration often lead to ecosystem-based considerations aimed to match with ecosystem organization (Young, 2006). Workshops can encourage discussions between government representatives, researchers, and environmental and citizen groups about (un)acceptable change and definition of critical indicator values (Ferguson et al., 2013; Chaffin et al., 2016). Projections in different time periods in the future, enables to provide the level of uncertainty for existing policy and to support new adaptation responses.
Without an integrated and coupled SES model, future decisions and ecosystem responses cannot be easily predicted. Often, much data is required, whereas a coupling between the social and ecological domains can be realized by engaging stakeholders. Resilience approaches deal with uncertainty and data gaps due to simplification of understanding of SES functions to build knowledge for conceptual and hydrological models (Sivapalan et al., 2012). The intensification of policy oriented and bottom-up processes could further increase cross-jurisdictional collaboration and further support the science-policy interface for adaptive ecosystem management.
Natural climate variability, patterns of land-use change, patterns of water regulation, structural design of infrastructure, and distal societal drivers, are just some of many elaborated processes that influence SESs. With drivers (e.g., climate) increasingly leading to changes of complex ecosystem processes, there is a risk that ecosystem management becomes increasingly misaligned with legal, social, ecological, and economic considerations. In the past-anthropogenic factors have already caused the complete collapse of ecosystems (Jackson et al., 2001). This makes the political and public support for ecosystem protection important, to prevent them from becoming lost entirely.
Conclusion
The main aim of this study was to use a modified analytical framework that combined resilience theories related to SES with policy adaptation needs. In a case study we demonstrated the implementation of this framework through a qualitative and quantitative analyses. The modified framework is a valuable addition to inform complex SES when data lacks. We provided policy based management responses through ATP analysis in a case when (1) quantitative boundaries and clear risk thresholds are lacking and are discussed with stakeholders; (2) flexible decision support is required by different institutions that operate on multiple scales; (3) communication to stakeholders is provided when acceptable ecological change is uncertain or there are (in)direct links to climate change; and (4) formulating ecosystem based and structural adaption measures that consider different jurisdictions. Information to stakeholders was provided with eco-hydrological conceptual representations of the ecosystem. These representations supported decision-making and adaptive management as information was provided on cross-jurisdictional collaboration, ecological change, types of regime shifts (thresholds, hysteresis, and irreversible changes), and current and future management trade-offs. Exceedance of thresholds under climate change compromises short-term and long-term ecological processes. We suggest a system-based approach to determine the adaptive capacity of ecosystems which includes data collection from interactions with scientists and management authorities. To enhance local scale adaptation, we suggest investigating hydrological improvements of the wetland system. Urbanization scenarios and interventions such as water suppletion should be considered across multiple spatial scales and sectoral management authorities.
Author Contributions
AN wrote the manuscript under the supervision of BG, MH, and AG. LB reviewed and provided feedback on the structure of the manuscript. LL assisted with the hydrological concepts. All authors contributed to the article and approved the submitted version.
Funding
This research was funded within program B4.2 of the Cooperative Research Centre of Water Sensitive Cities. AN was supported by a Scholarship for International Research Fees (SIRF) funded by the University of Western Australia.
Conflict of Interest
The authors declare that the research was conducted in the absence of any commercial or financial relationships that could be construed as a potential conflict of interest.
Publisher's Note
All claims expressed in this article are solely those of the authors and do not necessarily represent those of their affiliated organizations, or those of the publisher, the editors and the reviewers. Any product that may be evaluated in this article, or claim that may be made by its manufacturer, is not guaranteed or endorsed by the publisher.
Acknowledgments
We thank the stakeholder representatives from each of the government departments that provided case study specific data. The author would like to thank Marjolein Bakx and Dr. Laura Bertola for constructive criticism during the writing of the manuscript.
Supplementary Material
The Supplementary Material for this article can be found online at: https://www.frontiersin.org/articles/10.3389/frwa.2021.754564/full#supplementary-material
References
Ahmed, F., Moors, E., Khan, M. S. A., Warner, J., and Van Scheltinga, C. T. (2018). Tipping points in adaptation to urban flooding under climate change and urban growth: the case of the Dhaka megacity. Land Use Policy.79, 496–506. doi: 10.1016/j.landusepol.2018.05.051
Allan, J. D. (2004). Landscapes and riverscapes: the influence of land use on stream ecosystems. Ann. Rev. Ecol. Evol. Syst. 35, 257–284. doi: 10.1146/annurev.ecolsys.35.120202.110122
Australian Bureau of Statistics (2013a). 3101.0- Australian Demographic Statistics. Canberra, ACT: Australian Bureau of Statistics (ABS). Available online at: http://abs.gov.au/ausstats (accessed November 2, 2017).
Australian Bureau of Statistics (2013b). 3222.0- Population Projections, Australia, 2012 (base) to 2101. Canberra, ACT: Australian Bureau of Statistics (ABS). Available online at: http://abs.gov.au/ausstats (accessed November 2, 2017).
Barron, O., and Barr, A. (2009). Effect of Urban Development on Water Balance in the Southern River Catchment. Canberra, ACT: CSIRO Water for a Healthy Country National Research Flagship, 259.
Barron, O. V., Donn, M. J., and Barr, A. D. (2013). Urbanisation and shallow groundwater: predicting changes in catchment hydrological responses. Water Resour. Manag. 27, 95–115. doi: 10.1007/s11269-012-0168-0
Berkes, F. (2007). Understanding uncertainty and reducing vulnerability: lessons from resilience thinking. Natural Hazards 41, 283–295. doi: 10.1007/s11069-006-9036-7
Biggs, R., Schlüter, M., Biggs, D., Bohensky, E. L., BurnSilver, S., Cundill, G., et al. (2012). Toward principles for enhancing the resilience of ecosystem services. Ann. Rev. Environ. Resour.37, 421–448. doi: 10.1146/annurev-environ-051211-123836
Bloemen, P., Reeder, T., Zevenbergen, C., Rijke, J., and Kingsborough, A. (2017). Lessons learned from applying adaptation pathways in flood risk management and challenges for the further development of this approach. Mitig. Adapt. Strateg. Glob. Change 23, 1083–1108. doi: 10.1007/s11027-017-9773-9
Bölscher, T., van Slobbe, E., van Vliet, M. T., and Werners, S. E. (2013). Adaptation turning points in river restoration? The Rhine salmon case. Sustainability 5, 2288–2304. doi: 10.3390/su5062288
Borgström, S., Elmqvist, T., Angelstam, P., and Alfsen-Norodom, C. (2006). Scale mismatches in management of urban landscapes. Ecol. Society 11:16. doi: 10.5751/ES-01819-110216
Brondizio, E. S., Ostrom, E., and Young, O. R. (2009). Connectivity and the governance of multilevel social-ecological systems: the role of social capital. Ann. Rev. Environ. Resour. 34, 253–278. doi: 10.1146/annurev.environ.020708.100707
Brooks, N., Adger, W. N., and Kelly, P. M. (2005). The determinants of vulnerability and adaptive capacity at the national level and the implications for adaptation. Glob. Environ. Change 15, 151–163. doi: 10.1016/j.gloenvcha.2004.12.006
Brussard, P. F., Reed, J. M., and Tracy, C. R. (1998). Ecosystem management: what is it really? Landsc. Urban Plan. 40, 9–20. doi: 10.1016/S0169-2046(97)00094-7
Burgman, M. A., Lindenmayer, D. B., and Elith, J. (2005). Managing landscapes for conservation under uncertainty. Ecology 86, 2007–2017. doi: 10.1890/04-0906
Carpenter, S. R., Brock, W. A., Folke, C., van Nes, E. H., and Scheffer, M. (2015). Allowing variance may enlarge the safe operating space for exploited ecosystems. Proc. Natl. Acad. Sci. U.S.A.112, 14384–14389. doi: 10.1073/pnas.1511804112
Cash, D., Adger, W. N., Berkes, F., Garden, P., Lebel, L., Olsson, P., et al. (2006). Scale and cross-scale dynamics: governance and information in a multilevel world. Ecol. Society 11:8. doi: 10.5751/ES-01759-110208
Castelle, A. J., Johnson, A., and Conolly, C. (1994). Wetland and stream buffer size requirements—a review. J. Environ. Qual. 23, 878–882. doi: 10.2134/jeq1994.00472425002300050004x
CCWA (2005). Forrestdale Lake Nature Reserve Management Plan 2005. Perth, WA: Management Plan No. 53.
Chaffin, B. C., Garmestani, A. S., Gunderson, L. H., Benson, M. H., Angeler, D. G., Arnold, C. A., et al. (2016). Transformative environmental governance. Ann. Rev. Environ. Resour. 41, 399–423. doi: 10.1146/annurev-environ-110615-085817
Cohen-Shacham, E., Dayan, T., Feitelson, E., and de Groot, R. S. (2011). Ecosystem service trade-offs in wetland management: drainage and rehabilitation of the Hula, Israel. Hydrol. Sci. J. 56, 1582–1601. doi: 10.1080/02626667.2011.631013
Cumming, G., Cumming, D. H., and Redman, C. (2006). Scale mismatches in social-ecological systems: causes, consequences, and solutions. Ecol. Society 11:14. doi: 10.5751/ES-01569-110114
Davidson, W. A. (1995). Hydrogeology and Groundwater Resources of the Perth Region, Western Australia. Perth, WA: Geological Survey of WA.
Davis, J., and Brock, M. (2008). Detecting unacceptable change in the ecological character of Ramsar wetlands. Ecol. Manag. Restor.9, 26–32. doi: 10.1111/j.1442-8903.2008.00384.x
Davis, J., O'Grady, A. P., Dale, A., Arthington, A. H., Gell, P. A., Driver, P. D., et al. (2015). When trends intersect: The challenge of protecting freshwater ecosystems under multiple land use and hydrological intensification scenarios. Sci. Total Environ. 534, 65–78. doi: 10.1016/j.scitotenv.2015.03.127
Dewulf, A., Meijerink, S., and Runhaar, H. (2015). Editorial: The governance of adaptation to climate change as a multi-level, multi-sector and multi-actor challenge: a European comparative perspective. J. Water Climate Change 6, 1–8. doi: 10.2166/wcc.2014.000
Engle, N. L. (2011). Adaptive capacity and its assessment. Glob. Environ. Change 21, 647–656. doi: 10.1016/j.gloenvcha.2011.01.019
EPA (1992). Jandakot Mound Groundwater Resources, Bulletin 1155. Perth, WA: Environmental Protection Authority.
Ferguson, B. C., Brown, R. R., and Deletic, A. (2013). A diagnostic procedure for transformative change based on transitions, resilience, and institutional thinking. Ecol. Society 18:57. doi: 10.5751/ES-05901-180457
Fidelman, P. I. J., Leitch, A. M., and Nelson, D. R. (2013). Unpacking multilevel adaptation to climate change in the Great Barrier Reef, Australia. Glob. Environ. Change 23, 800–812. doi: 10.1016/j.gloenvcha.2013.02.016
Folke, C., Carpenter, S., Elmqvist, T., Gunderson, L., Holling, C. S., and Walker, B. (2002). Resilience and sustainable development: building adaptive capacity in a world of transformations. J Human Environ. 31, 437–440. doi: 10.1579/0044-7447-31.5.437
Folke, C., Carpenter, S., Walker, B., Scheffer, M., Elmqvist, T., Gunderson, L., et al. (2004). Regime shifts, resilience, and biodiversity in ecosystem management. Ann. Rev. Ecol. Evol. Syst. 35, 557–581. doi: 10.1146/annurev.ecolsys.35.021103.105711
Folke, C., Hahn, T., Olsson, P., and Norberg, J. (2005). Adaptive governance of social-ecological systems. Ann. Rev. Environ. Resour. 30, 441–473. doi: 10.1146/annurev.energy.30.050504.144511
Folke, C., Pritchard, L. Jr, Berkes, F., Colding, J., and Svedin, U. (2007). The problem of fit between ecosystems and institutions: ten years later. Ecol. Society 12:30. doi: 10.5751/ES-02064-120130
Froend, R., Loomes, R., Horwitz, P., Bertuch, M., Storey, M., and Bamford, M. (2004). Study of Ecological Water Requirements on the Gnangara and Jandakot Mounds under Section 46 of the Environmental Protection Act, Task 2: Determination of Ecological Water Requirements. Perth, WA: Water and Rivers Commission.
Fünfgeld, H. (2015). Facilitating local climate change adaptation through transnational municipal networks. Curr. Opin. Environ. Sustain. 12, 67–73. doi: 10.1016/j.cosust.2014.10.011
Gersonius, B., Ashley, R., Pathirana, A., and Zevenbergen, C. (2012). Climate change uncertainty: building flexibility into water and flood risk infrastructure. Climatic Change 116, 411–423. doi: 10.1007/s10584-012-0494-5
Haasnoot, M., Middelkoop, H., Offermans, A., Beek, E. V., and Deursen, W. P. A. (2012). Exploring pathways for sustainable water management in river deltas in a changing environment. Climatic Change. 115, 795–819. doi: 10.1007/s10584-012-0444-2
Hanna, S. (2008). Institutions for managing resilient salmon (Oncorhynchus spp.) ecosystems: the role of incentives and transaction costs. Ecol. Society 13:35. doi: 10.5751/ES-02606-130235
Hastings, A., and Wysham, D. B. (2010). Regime shifts in ecological systems can occur with no warning. Ecol. Lett. 13, 464–472. doi: 10.1111/j.1461-0248.2010.01439.x
Hermans, L. M., Haasnoot, M., ter Maat, J., and Kwakkel, J. H. (2017). Designing monitoring arrangements for collaborative learning about adaptation pathways. Environ. Sci. Policy 69, 29–38. doi: 10.1016/j.envsci.2016.12.005
Hill, A. L. (1996). Wetlands of the Swan Coastal Plain: Wetland Mapping, Classification, and Evaluation, Wetland Atlas, Vol. 2b. Perth, WA: Water and Rivers Commission; Department of Environmental Protection.
Hill, A. L., Australia, W., Australia, W., and Water Commission R. (1996). Wetlands of the Swan Coastal Plain: Wetland Mapping, Classification and Evaluation, Main Report, Vol. 2a. Perth, WA: Water and Rivers Commission and Department of Environmental Protection.
Holling, C. S. (1973). Resilience and Stability of Ecological Systems. Ann. Rev. Ecol. Syst. 4, 1–23. doi: 10.1146/annurev.es.04.110173.000245
Holling, C. S. (1996). “Engineering resilience versus ecological resilience,” in Engineering Within Ecological Constraints, Washington: The National Academies Press. 31–44.
Holling, C. S. (2001). Understanding the complexity of economic, ecological, and social systems. Ecosystems 4, 390–405. doi: 10.1007/s10021-001-0101-5
Hughes, R. M., Dunham, S., and Maas-Hebner, K. G. (2014a). A review of urban water body challenges and approaches: (1) rehabilitation and remediation. Fisheries 39, 18–29. doi: 10.1080/03632415.2013.836500
Hughes, R. M., Dunham, S., Maas-Hebner, K. G., Yeakley, J. A., Harte, M., Molina, N., et al. (2014b). A review of urban water body challenges and approaches: (2) mitigating effects of future urbanization. Fisheries 39, 30–40. doi: 10.1080/03632415.2014.866507
Hughes, T. P., Barnes, M. L., Bellwood, D. R., Cinner, J. E., Cumming, G. S., Jackson, J. B. C., et al. (2017). Coral reefs in the anthropocene. Nature 546, 82–90. doi: 10.1038/nature22901
Huitema, D., Mostert, E., Egas, W., Moellenkamp, S., Pahl-Wostl, C., and Yalcin, R. (2009). Adaptive water governance: assessing the institutional prescriptions of adaptive (co-) management from a governance perspective and defining a research agenda. Ecol. Society 14:26. doi: 10.5751/ES-02827-140126
Ignatieva, M., Stewart, G. H., and Meurk, C. (2010). Planning and design of ecological networks in urban areas. Landsc. Ecol. Eng. 7, 17–25. doi: 10.1007/s11355-010-0143-y
Integration and Application Network (2007). Conceptual Diagrams - Tools for Science. Cambridge: University of Maryland, Center for Environmental Science.
Jackson, J. B. C., Kirby, M. X., Berger, W. H., Bjorndal, K. A., Botsford, L. W., Bourque, B. J., et al. (2001). Historical overfishing and the recent collapse of coastal ecosystems. Science 293, 629–637. doi: 10.1126/science.1059199
Kwadijk, J., Haasnoot, M., Mulder, J., Hoogvliet, M., Jeuken, A., van der Krogt, R., et al. (2010). Using adaptation tipping points to prepare for climate change and sea level rise: a case study in the Netherlands. Climate Change 1, 729–740. doi: 10.1002/wcc.64
Lavery, S., and Donovan, B. (2005). Flood risk management in the Thames Estuary looking ahead 100 years. Philos. Trans. R. Soc. London A 363, 1455–1474. doi: 10.1098/rsta.2005.1579
Lawrence, J., and Haasnoot, M. (2017). What it took to catalyse uptake of dynamic adaptive pathways planning to address climate change uncertainty. Environ. Sci. Policy 68, 47–57. doi: 10.1016/j.envsci.2016.12.003
Lebel, L., Nikitina, E., Pahl-Wostl, C., and Knieper, C. (2013). Institutional fit and river basin governance: a new approach using multiple composite measures. Ecol. Society 18:1. doi: 10.5751/ES-05097-180101
Liao, K. H. (2012). A theory on urban resilience to floodsóa basis for alternative planning practices. Ecol. Society 17:48. doi: 10.5751/ES-05231-170448
MacKay, H. (2006). Protection and management of groundwater-dependent ecosystems: emerging challenges and potential approaches for policy and management. Austr. J. Botany 54, 231–237. doi: 10.1071/BT05047
Martin, T. G., Burgman, M. A., Fidler, F., Kuhnert, P. M., Low-Choy, S., Mcbride, M., et al. (2012). Eliciting expert knowledge in conservation science. Conserv. Biol.26, 29–38. doi: 10.1111/j.1523-1739.2011.01806.x
McArthur, W. M., Bartle, G. A., and Scientific, C. (1980). Landforms and Soils as an Aid to Urban Planning in the Perth Metropolitan Northwest Corridor, Western Australia. Perth, WA: Commonwealth Scientific and Industrial Research Organization.
Miller, F., Osbahr, H., Boyd, E., Thomalla, F., Bharwani, S., Ziervogel, G., et al. (2010). Resilience and vulnerability: complementary or conflicting concepts? Ecol. Society 15:11. doi: 10.5751/ES-03378-150311
Moloney, S., and Fünfgeld, H. (2015). Emergent processes of adaptive capacity building: local government climate change alliances and networks in Melbourne. Urban Climate 14, 30–40. doi: 10.1016/j.uclim.2015.06.009
Myers, N., Mittermeier, R. A., Mittermeier, C. G., Da Fonseca, G. A., and Kent, J. (2000). Biodiversity hotspots for conservation priorities. Nature.403, 853–858. doi: 10.1038/35002501
Nanda, A. V. V., Beesley, L., Locatelli, L., Gersonius, B., Hipsey, M. R., and Ghadouani, A. (2018a). Adaptation tipping points of a wetland under a drying climate. Water 10:234. doi: 10.3390/w10020234
Nanda, A. V. V., Rijke, J., Beesley, L., Gersonius, B., Hipsey, M., and Ghadouani, A. (2018b). Matching ecosystem functions with adaptive ecosystem management: decision pathways to overcome institutional barriers. Water 10:672. doi: 10.3390/w10060672
Nelson, D. R., Adger, W. N., and Brown, K. (2007). Adaptation to environmental change: contributions of a resilience framework. Ann. Rev. Environ. Resour. 32, 395–419. doi: 10.1146/annurev.energy.32.051807.090348
Newig, J., Pahl-Wostl, C., and Sigel, K. (2005). The role of public participation in managing uncertainty in the implementation of the Water Framework Directive. Euro. Environ.15, 333–343. doi: 10.1002/eet.398
Noble, B., Hill, M., and Nielsen, J. (2011). Environmental assessment framework for identifying and mitigating the effects of linear development to wetlands. Landsc. Urban Plan. 99, 133–140. doi: 10.1016/j.landurbplan.2010.09.004
Olsson, P., Gunderson, L., Carpenter, S., Ryan, P., Lebel, L., Folke, C., et al. (2006). Shooting the rapids: navigating transitions to adaptive governance of social-ecological systems. Ecol. Society 11:18. doi: 10.5751/ES-01595-110118
Ostrom, E. (2009). A general framework for analyzing sustainability of social-ecological systems. Science 325, 419–422. doi: 10.1126/science.1172133
Pace, M. L., Carpenter, S. R., and Cole, J. J. (2015). With and without warning: managing ecosystems in a changing world. Front. Ecol. Enviro. 13, 460–467. doi: 10.1890/150003
Pahl-Wostl, C. (2009). A conceptual framework for analysing adaptive capacity and multi-level learning processes in resource governance regimes. Glob. Environ. Change 19, 354–365. doi: 10.1016/j.gloenvcha.2009.06.001
Poff, N. L., Brown, C. M., Grantham, T. E., Matthews, J. H., Palmer, M. A., Spence, C. M., et al. (2015). Sustainable water management under future uncertainty with eco-engineering decision scaling. Nature Clim Change 6, 25–34. doi: 10.1038/nclimate2765
Radhakrishnan, M., Pathirana, A., Ashley, R., and Zevenbergen, C. (2017). Structuring climate adaptation through multiple perspectives: framework and case study on flood risk management. Water 9:129. doi: 10.3390/w9020129
Reyers, B., Folke, C., Moore, M.-L., Biggs, R., and Galaz, V. (2018). Social-ecological systems insights for navigating the dynamics of the anthropocene. Ann. Rev. Environ. Resour.43, 11–23. doi: 10.1146/annurev-environ-110615-085349
Rijke, J., Farrelly, M., Brown, R., and Zevenbergen, C. (2013). Configuring transformative governance to enhance resilient urban water systems. Environ. Sci. Policy 25, 62–72. doi: 10.1016/j.envsci.2012.09.012
Romm, J., Conrad, E., and Måren, I. (2018). Resilient governance of water regimes in variable climates: lessons from California's hydro-ecological zones. Water 10:196. doi: 10.3390/w10020196
Salinas Rodriguez, C. N.a, Ashley, R., Gersonius, B., Rijke, J., Pathirana, A., and Zevenbergen, C. (2014). Incorporation and application of resilience in the context of water-sensitive urban design: linking European and Australian perspectives. Water 1, 173–186. doi: 10.1002/wat2.1017
Scheffer, M., Barrett, S., Carpenter, S., Folke, C., Green, A. J., Holmgren, M., et al. (2015). Creating a safe operating space for iconic ecosystems. Science 347, 1317–1319. doi: 10.1126/science.aaa3769
Scheffer, M., Carpenter, S., Foley, J. A., Folke, C., and Walker, B. (2001). Catastrophic shifts in ecosystems. Nature 413, 591–596. doi: 10.1038/35098000
Schleupner, C., and Schneider, U. A. (2013). Allocation of European wetland restoration options for systematic conservation planning. Land Use Policy 30, 604–614. doi: 10.1016/j.landusepol.2012.05.008
Schultz, L., Folke, C., Osterblom, H., and Olsson, P. (2015). Adaptive governance, ecosystem management, and natural capital. Proc. Natl. Acad. Sci. U.S.A. 112, 7369–7374. doi: 10.1073/pnas.1406493112
Seitz, N. E., Westbrook, C. J., and Noble, B. F. (2011). Bringing science into river systems cumulative effects assessment practice. Environ. Impact Assess. Rev. 31, 172–179. doi: 10.1016/j.eiar.2010.08.001
Semeniuk, C. A. (1987). Wetlands of the Darling System- a geomorphic approach to habitat classification. J. R. Soc. West Austr. 69, 95–112.
Semeniuk, C. A., and Semeniuk, V. (1995). A geomorphic approach to global classification for inland wetlands. Vegetatio 118, 103–124. doi: 10.1007/BF00045193
Sivapalan, M., Savenije, H. H. G., and Blöschl, G. (2012). Socio-hydrology: a new science of people and water. Hydrol. Proc. 26, 1270–1276. doi: 10.1002/hyp.8426
Smit, B., and Wandel, J. (2006). Adaptation, adaptive capacity and vulnerability. Glob. Environ. Change 16, 282–292. doi: 10.1016/j.gloenvcha.2006.03.008
Smith, I., and Power, S. (2014). Past and future changes to inflows into Perth (Western Australia) dams. J. Hydrol. 2, 84–96. doi: 10.1016/j.ejrh.2014.08.005
Townley, L., Turner, J., Barr, A. D., and Trefry, M. (1993). Wetlands of the Swan Coastal Plain Volume 3: Interaction Between Lakes, Wetlands and Unconfined Aquifers. Perth, WA: Education Department ofWestern Australia.
van Oudenhoven, A. P. E. (2011). Managing ecosystem services and natural capital – trade-offs, synergies and challenges. Int. J. Biodiv. Sci. Ecosyst. Serv. Manag. 7, 141–142. doi: 10.1080/21513732.2011.655100
van Slobbe, E., Werners, S. E., Riquelme-Solar, M., Bölscher, T., and van Vliet, M. T. H. (2016). The future of the Rhine: stranded ships and no more salmon? Reg. Environ. Change 16, 31–41. doi: 10.1007/s10113-014-0683-z
Walker, B., Holling, C. S., Carpenter, S., and Kinzig, A. (2004). Resilience, adaptability and transformability in social–ecological systems. Ecol. Society 9:5. doi: 10.5751/ES-00650-090205
Walker, B. H., Carpenter, S. R., Rockstrom, J., Crépin, A.-S., and Peterson, G. D. (2012). Drivers, “slow” variables, “fast” variables, shocks, and resilience. Ecol. Soc. 17:30. doi: 10.5751/ES-05063-170330
Werner, B., and McNamara, D. (2007). Dynamics of coupled human-landscape systems. Geomorphology.91, 393–407. doi: 10.1016/j.geomorph.2007.04.020
Werners, S., Pfenninger, S., van Slobbe, E., Haasnoot, M., Kwakkel, J., and Swart, R. (2013b). Thresholds, tipping and turning points for sustainability under climate change. Curr. Opin. Environ. Sustain. 5, 334–340. doi: 10.1016/j.cosust.2013.06.005
Werners, S., Swart, R., van Slobbe, E., and Bölscher, T. (2013a). Turning points in climate change adaptation. Glob. Environ. Change 16, 253–267.
Werners, S. E., van Loon-Steensma, J. M., and Oost, A. P. (2015). Method selection in adaptation research: the case of the Delta Programme for the Dutch Wadden region. Reg. Environ. Change 16, 111–122. doi: 10.1007/s10113-015-0799-9
Westbrook, C. J., and Noble, B. F. (2013). Science requisites for cumulative effects assessment for wetlands. Impact Assess. Project Appraisal 31, 318–323. doi: 10.1080/14615517.2013.833408
Western Australian Planning Commission (2005). Guideline for the Determination of Wetland Buffer Requirements. Perth, WA: Western Australian Planning Commission.
Western Australian Planning Commission (2014). Draft State Planning Policy 2.3, Jandakot Groundwater Protection Policy. Perth, WA: Western Australian Planning Commission.
Weston, M. A., Antos, M. J., and Glover, H. K. (2009). Birds, buffers and bicycles: a review and case study of wetland buffers. Victor. Natural.126, 79–86. doi: 10.3316/informit.669265729456182
Young, O. (2006). Vertical interplay among scale-dependent environmental and resource regimes. Ecol. Society 11:27. doi: 10.5751/ES-01519-110127
Young, O. R. (2002). The Institutional Dimensions of Environmental Change: Fit, Interplay, and Scale. Cambridge: MIT Press. doi: 10.7551/mitpress/3807.001.0001
Young, O. R. (2011). Effectiveness of international environmental regimes: existing knowledge, cutting-edge themes, and research strategies. Proc. Natl. Acad. Sci. U.S.A. 108, 19853–19860. doi: 10.1073/pnas.1111690108
Keywords: ecosystem resilience, social-ecological systems, wetlands, decision-making, cross-scale dynamics
Citation: Nanda AVV, Beesley L, Locatelli L, Gersonius B, Hipsey MR and Ghadouani A (2021) Resilience and Adaptive Capacity of the Swan Coastal Plain Wetlands. Front. Water 3:754564. doi: 10.3389/frwa.2021.754564
Received: 06 August 2021; Accepted: 07 October 2021;
Published: 01 November 2021.
Edited by:
Chingwen Cheng, Arizona State University, United StatesReviewed by:
Daniel L. Childers, Arizona State University, United StatesErik Porse, California State University, Sacramento, United States
Copyright © 2021 Nanda, Beesley, Locatelli, Gersonius, Hipsey and Ghadouani. This is an open-access article distributed under the terms of the Creative Commons Attribution License (CC BY). The use, distribution or reproduction in other forums is permitted, provided the original author(s) and the copyright owner(s) are credited and that the original publication in this journal is cited, in accordance with accepted academic practice. No use, distribution or reproduction is permitted which does not comply with these terms.
*Correspondence: Anas Ghadouani, YW5hcy5naGFkb3VhbmlAdXdhLmVkdS5hdQ==