- BIOTARGEN, UNICAEN, Normandie University, Caen, France
Osteoarthritis (OA) is a degenerative disease that eventually leads to the complete degradation of articular cartilage. Articular cartilage has limited intrinsic capacity for self-repair and, to date, there is no curative treatment for OA. Humans and horses have a similar articular cartilage and OA etiology. Thus, in the context of a One Health approach, progress in the treatment of equine OA can help improve horse health and can also constitute preclinical studies for human medicine. Furthermore, equine OA affects horse welfare and leads to significant financial losses in the equine industry. In the last few years, the immunomodulatory and cartilage regenerative potentials of mesenchymal stromal cells (MSCs) have been demonstrated, but have also raised several concerns. However, most of MSC therapeutic properties are contained in their secretome, particularly in their extracellular vesicles (EVs), a promising avenue for acellular therapy. From tissue origin to in vitro culture methods, various aspects must be taken into consideration to optimize MSC secretome potential for OA treatment. Immunomodulatory and regenerative properties of MSCs can also be enhanced by recreating a pro-inflammatory environment to mimic an in vivo pathological setting, but more unusual methods also deserve to be investigated. Altogether, these strategies hold substantial potential for the development of MSC secretome-based therapies suitable for OA management. The aim of this mini review is to survey the most recent advances on MSC secretome research with regard to equine OA.
1. Introduction
Articular cartilage is the connective tissue that covers the extremities of bones in diarthrodial joints. Its viscoelasticity allows for shock absorption and joint mobility without friction (1). Articular cartilage is composed of specialized cells called chondrocytes, and an abundant extracellular matrix mainly enriched in type II collagen (Col II) and aggrecan. Osteoarthritis (OA) is a degenerative joint disease that, in its later stages, affects the whole joint and leads to decreased joint mobility, pain and impaired quality of life. During OA, articular cartilage homeostasis is disrupted and the overproduction of catabolic enzymes, such as matrix metalloproteinases (MMP) and aggrecanases, leads to cartilage degradation, articular inflammation and, eventually, subchondral bone exposure (1). OA management is challenging because cartilage has a limited capacity for self-repair. To date, there are no curative OA treatments.
As in humans, horses can develop OA due to aging or intense physical activity, directly affecting horse health and welfare, and diminishing performance in sport and race horses (2). OA can thus put an early end to a horse career, leading to economic losses (3). The horse is also an excellent preclinical model for OA, because human and equine articular cartilage share many similarities in terms of anatomy, mechanical functioning, and cellular and molecular composition (2, 4).
Current clinical treatments of equine OA such as anti-inflammatory drugs, dietary supplements or viscosupplementation are only symptomatic and do not prevent the degenerative process of the disease (2). However, among the various emerging regenerative therapies, strategies based on mesenchymal stromal cells (MSCs) appear to hold promise. MSCs possess immunomodulatory and anti-inflammatory effects and regenerative properties that have direct effects or act indirectly through the release of bioactive molecules free or enclosed in extracellular vesicles (EVs) such as exosomes or ectosomes (5).
Here, we explore the relevance and future challenges of MSC-derived EVs (MSC-EVs) as a new orthobiologic approach to manage equine OA.
2. Mesenchymal stromal cell-based therapies
MSCs are defined as multipotent cells able to self-replicate and differentiate into distinct cell types, such as adipocytes, osteoblasts or chondrocytes. Bone marrow (BM) is the most common source of MSCs, even though these cells can be found in several other niches in an organism [adipose tissue (AT), umbilical cord (UC), dental pulp, synovium, etc.] (5). Research over the last few years has suggested that MSCs hold great potential for diverse therapeutic applications through putative immunomodulatory, anti-inflammatory effects or by stimulating tissue regeneration (6–9).
Regarding articular diseases, MSCs have shown the potency to reduce OA-related pain and increase cartilage repair (6, 9). Additionally, OA-afflicted horses treated with intra-articular injections of MSCs show improvement in clinical signs, cartilage appearance and athletic performance (10–12). In the context of autologous chondrocyte transplantation (ACT), equine MSC (eMSC)-derived cartilage organoids overcome the limitations inherent to the use of dedifferentiated chondrocytes and may provide an accurate and reliable drug screening model for OA (13–17).
Although the direct use of MSCs remains promising for equine OA treatments, several challenges have been identified, including their in vivo distribution, a low engraftment rate, their immunogenicity and their possible tumorigenicity risk (18–24) (Figure 1).
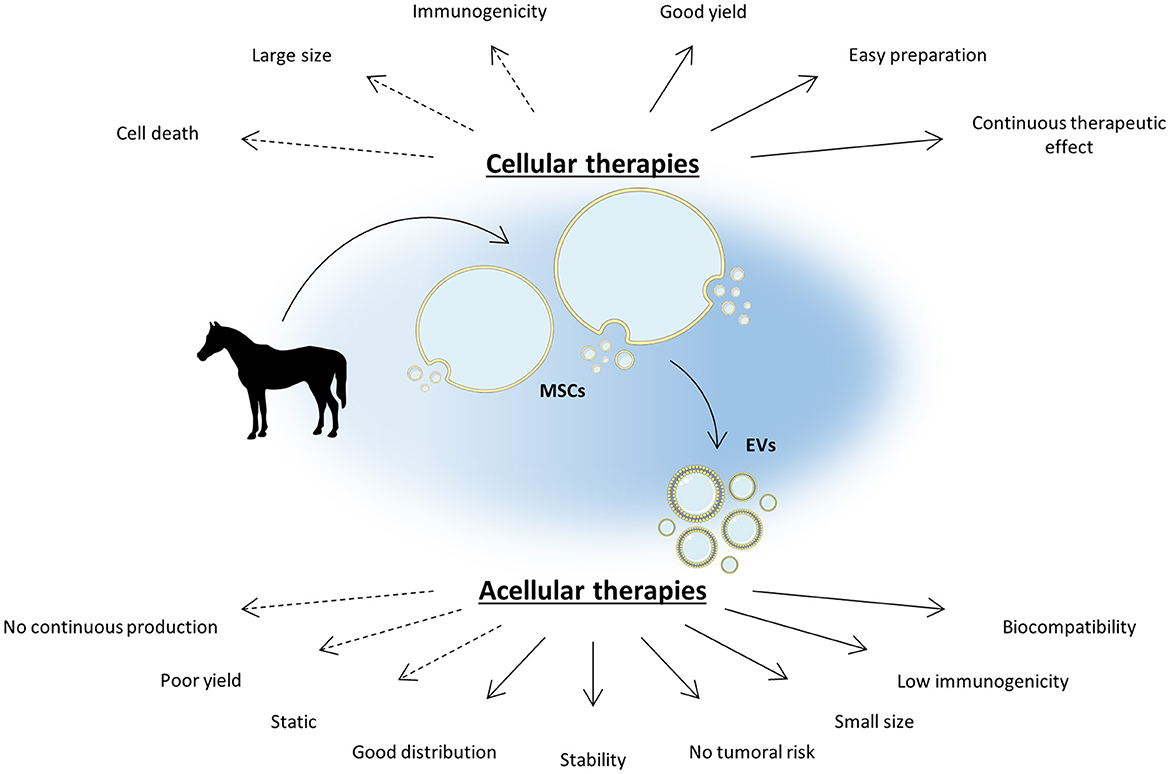
Figure 1. MSC-based acellular therapies are an appealing strategy to bypass cellular therapy limitations. Cellular therapies based on mesenchymal stromal cells (MSCs) demonstrate a promising potential in the management of equine osteoarthritis (OA) by reducing joint inflammation and enhancing cartilage regeneration. However, MSC intra-articular injection can be immunogenic and brings several concerns inherent to their nature. As the main part of MSC therapeutic potential lies in their secretome, notably in extracellular vesicles (EVs), acellular therapies appear to be a promising alternative to increase the safety of MSC-based therapy while improving its efficacy. EVs are highly biocompatible and can easily diffuse into tissues thanks to their small size. To date, acellular therapies still exhibit a few limitations but several strategies are in progress to overcome them. The Figure was partly generated using Servier Medical Art, provided by Servier, licensed under a Creative Commons Attribution 3.0 unported license.
3. Mesenchymal stromal cell-derived extracellular vesicles as a new orthobiologic therapy
The MSC secretome contains a broad spectrum of compounds including nucleic acids, proteins such as cytokines, growth factors or even lipids. Some of these compounds can be encapsulated in vesicles, called EVs. EVs include apoptotic bodies (>1 μm diameter), ectosomes or microvesicles (100–1,000 nm) and exosomes (30–200 nm). Among the MSC secretome, exosomes contain numerous molecules with proven pro-regenerative and anti-inflammatory properties as reviewed in Hade et al. (25). In addition, numerous studies have demonstrated the cartilage regeneration potential of EVs (26).
Exosomes originate from the endocytic pathway, develop within multivesicular bodies (MVBs) and are delivered to the extracellular environment when MVBs fuse with the plasma membrane (27). Exosomes enter the cells through membrane fusion, endocytosis or interaction with a receptor that is subsequently internalized.
Given the disadvantages attributed to MSC-based cellular therapy, secretome-, EV- and exosome-based strategies are an appealing alternative to explore the therapeutic potential of eMSCs in equine OA management. To date, only a few studies have demonstrated the therapeutic potential of eMSC-EVs in the context of horse OA. Using an in vitro cartilage organoid model, our research group has already demonstrated the pro-anabolic potential of eMSC-conditioned media (CM) and the presence of exosomes in eMSC-CM (28). The CM corresponds to the medium in which cell were cultured, hence it contains components that had been secreted by the cells. Noteworthy, because cells cultured in vitro do not have exactly the same features than their in vivo counterparts, the CM and the secretome of MSC in vivo might differ. MSC-EVs can decrease the transcript levels of MMP and pro-inflammatory molecules (29, 30). Furthermore, EVs can be used as biomarkers to evaluate the progression of OA (31). However, to date, in vivo cartilage regeneration using EVs in the equine model remains to be demonstrated.
Nevertheless, environment deeply influences MSC secretion and constitutes a variable worth of consideration to improve the capacities attributed to their therapeutic effect (32–34). The MSC therapeutic potential and secretome differ according to the tissue they derive from and can be modulated by several factors as discussed below.
4. Therapeutic potential of mesenchymal stromal cells and their derivatives depends on the source and the culture procedure
MSCs from all sources share similarities, regarding in particular their self-renewal, multipotency and immunomodulation capacities. Nevertheless, the individual, the age, the tissue and the niche MSCs are isolated from lead to slight variations of their properties (35) including their secretory production. For example, AT-eMSCs, peripheral blood (PB)-eMSCs, BM-eMSCs and UC-eMSCs display significant variation in inflammation-related gene expression, although interferon-γ (IFN-γ) stimulation homogenizes the gene expression profile between the studied MSC sources (36). Furthermore, their immunomodulatory properties can be induced through different mechanisms. For example, AT-eMSCs and UC-eMSCs can induce lymphocyte apoptosis, whereas BM-eMSCs, PB-eMSCs and cord blood (CB)-eMSCs induce lymphocyte cell cycle arrest (37). Our research group has demonstrated differences in proliferation and differentiation capacity between BM-eMSCs, CB-eMSCs and UC-eMSCs (13–17). BM-eMSCs are more prone to produce hyaline-like cartilage extracellular matrix (ECM) with low amounts of atypical molecules than are CB-eMSCs and UC-eMSCs. The impact of eMSC origin on antibacterial activity has also been demonstrated (38) and the eMSC secretome also depends on the tissue source they derive from. Indeed, Navarette et al. have reported that the miRNA content of EVs differs between AT- and endometrial eMSCs from the same animal (39). In addition to the inter-tissue origin heterogeneity, eMSCs derived from the same tissue source can show differences in gene expression and functional heterogeneity (40). Ultimately, the differentiation status of MSCs modulates their properties. As an example, BM-eMSCs engaged in a chondrogenic differentiation process exert a weaker inflammatory response to IL-1β than naive BM-eMSCs (41). eMSC heterogeneity suggests that these cells are highly influenced by their environment. Considering the impact of MSC origin on their properties, tissue source should be wisely selected before exploiting their secretome. Hence, in the study of the therapeutic potential of eMSCs, medium composition and culture conditions must be carefully selected.
Culture medium supplementation with fetal bovine serum (FBS) is widely used to support in vitro MSC proliferation. One of the challenges in the use of FBS resides in its non-standardized and variable composition between batches (42). This xenogeneic supplement can interfere with MSC metabolism, phenotype and, by consequence, the properties of their secretome. Additionally, FBS is a limiting factor in in vivo applications because it can trigger an immune response. For instance, eMSCs cultured with FBS have exacerbated immunogenicity compared with eMSCs cultured with allogenic or autogenic equine BM supernatant-supplemented culture medium (43). The use of autologous equine serum can be considered for the culturing eMSCs because horses can tolerate the removal of 25% of their blood volume (44). Replacing FBS with equine platelet lysate as a medium supplement has also been tested, and resulted in similar growth and phenotypical BM-MSC characteristics (45) as well in moderately increased immunomodulatory marker expression (46). In contrast, Pezzanite et al. demonstrated the superiority of FBS over equine serum supplementation to generate functional eMSCs (47). Serum-free medium is another alternative to FBS supplementation during MSC expansion. This option is being investigated, especially during CM and EV harvest, to avoid the co-isolation of xenogeneic contaminants that can reduce the therapeutic efficacy of EVs. Serum-free-cultured eMSCs decrease the pro-inflammatory mediator secretion of activated T-cells, but to a lesser extent than eMSCs cultured with FBS (48). In the last few decades, efforts have been made to culture the cells in vitro in contexts similar to those in vivo, particularly using three-dimensional (3D) cultures instead of monolayers (2D). MSCs cultured in 3D undergo morphological and metabolic changes, and their proliferation and survival rate are increased (49). Compared with a monolayer culture, CM from MSCs cultured in spheroids suppress macrophage pro-inflammatory cytokine secretion and enhance the production of the anti-inflammatory cytokine interleukin (IL)-10 (50). Additionally, MSCs grown in dynamic 3D cultures—spinner flasks and a rotating bioreactor—show enhanced therapeutic properties, but mRNA profiles differ according to the method used (51). To date, these culture methods have not yet been tested in the equine model.
Long-term in vitro expansion affects MSCs (52). A large proportion of MSCs become senescent and display altered differentiation and immunosuppressive potential (53, 54). Therefore, early passages should be preferred to harness eMSC therapeutic properties. Noteworthily, cryopreservation does not appear to interfere with eMSC differentiation potential and therapeutic potential (55, 56), but the isolation protocol can affect the characteristics of these cells (57). Nevertheless, the MSC secretome, particularly EVs, can exhibit diminished immunosuppressive properties after freeze-thawing (58).
The surrounding environment inevitably affects eMSCs. Controlling it remains a real challenge that must be addressed to increase the reproducibility of the therapeutic effects of MSCs or MSC-derived products such as EVs. On the other hand, the ability of MSCs to adapt to their environment also represents a tremendous opportunity to improve their therapeutic potential (Figure 2).
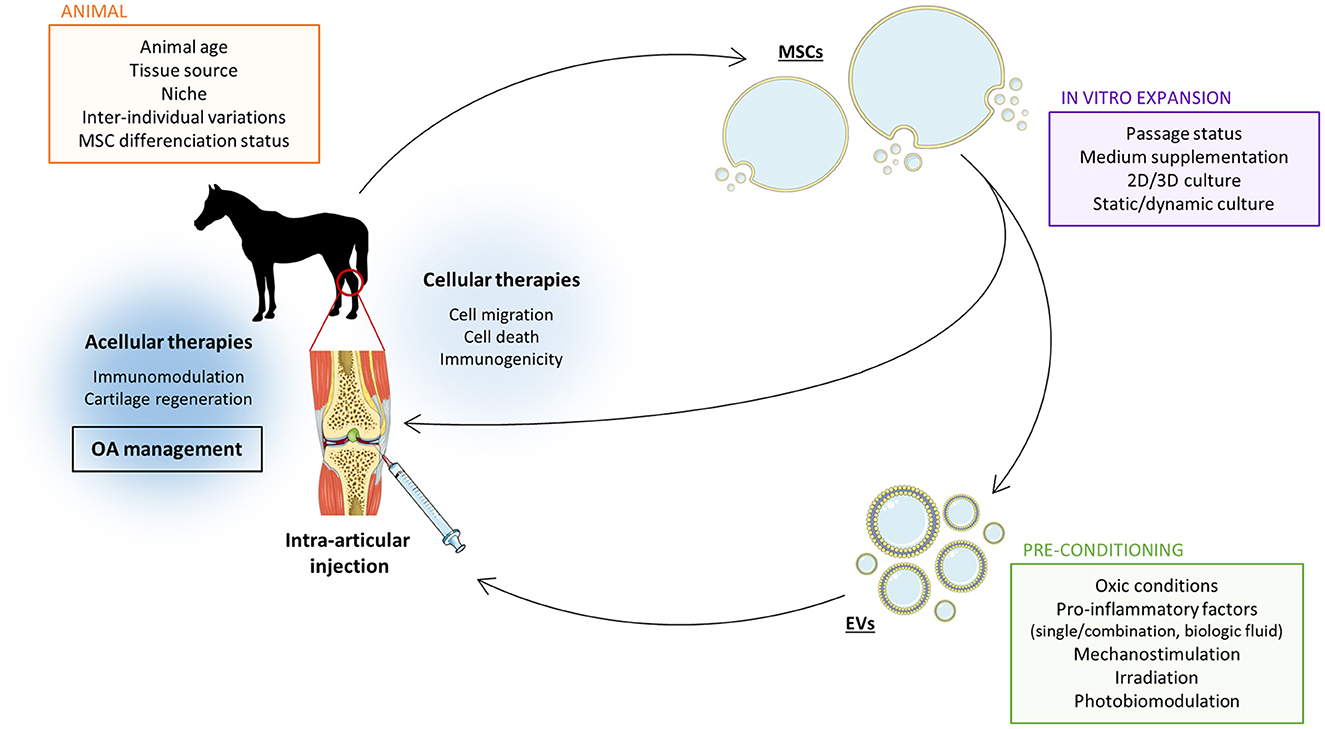
Figure 2. Several parameters must be considered to improve the therapeutic potential of MSC-derived EVs in the treatment of equine OA. Mesenchymal stromal cells (MSC)-derived extracellular vesicles (EVs) have the capacity to improve equine osteoarthritis (OA) management via immunomodulation and stimulation of cartilage matrix synthesis. However, this therapeutic potential can be enhanced by adjusting the steps in the EV production protocol. The source of MSCs affects EV properties and their therapeutic capacities. The MSC culture methods can be used as a tool to optimize the therapeutic effect of MSC-derived EVs. In the last years, it has been demonstrated that EV properties can be refined by adjusting oxic conditions, adding pro-inflammatory cytokines or other physical parameters such as mechanical stress or light exposition. Setting the balance between all these factors is crucial to achieve the most effective therapeutic effect of MCS-derived EVs for equine OA. Moreover, acellular therapy has the advantage to avoid most of direct MSC intra-articular injections concerns such as immunogenicity. The Figure was partly generated using Servier Medical Art, provided by Servier, licensed under a Creative Commons Attribution 3.0 unported license.
5. Future directions to enhance the therapeutic potential of MSC and their derivatives
It has already been proven that the unstimulated eMSC secretome can enhance the equine articular chondrocyte phenotype and increase their migratory capacity (28, 30). Nevertheless, therapeutic capacities of naive MSCs developing in a healthy environment have not been optimized. To maximize the MSC therapeutic properties, stimulation by extrinsic factors can mimic a pathological situation, leading to a boost in the MSC immunomodulatory and therapeutic capacities (8). In OA, this is illustrated in vivo by a pro-inflammatory environment induced by a cartilage lesion, triggering an MSC reaction to external aggression. These diverse procedures are collectively called preconditioning, licensing or priming and are probably the key to improvements in the regenerative potential of the MSC secretome (Figure 2).
Given that MSCs evolve in vivo in hypoxic conditions (2%−9% oxygen) (59), growing them under a 21% oxygen atmosphere can alter their phenotype and their secretome. Low oxygen tension regulates hypoxia-inducible factor (HIF-1α) activity that triggers the transcription of diverse genes involved in eMSC stemness-associated features, differentiation and self-renewal (60). Because EV cargo reflects the nature and composition of their cell source, these factors are likely to be found in the eMSC secretome and modify their properties (61). Recently, Zhang et al. (62) showed that the secretome of hypoxia-preconditioned MSCs enhanced rat chondrocyte proliferation and migration and inhibited apoptosis compared with rat chondrocytes cultured with the secretome from MSCs grown in normoxia. To our knowledge, none of the hypoxia preconditioning advantages described previously have yet been demonstrated in equines.
When tissue is damaged, inflammatory factors and chemokines are released by immune cells recruited to the inflammation site, triggering eMSC activation. Reproducing this process in vitro is one way to enhance eMSC-EV therapeutic capacities. Interferon γ (IFN-γ) is known as the gold standard cytokine priming for MSCs. Many studies confirm its abilities to enhance eMSC secretome-mediated chondroprotection and downregulate inflammatory genes in equine chondrocytes (63, 64). IFN-γ can also increase the immunosuppressive properties of murine BM-MSCs, but priming does not enhance the capacities of EVs (58). Depending on their source, eMSCs vary in their response to IFN-γ, but this cytokine lowers inter-tissue differences in unstimulated eMSC immunomodulatory gene expression (36). Therefore, tissue of origin may not be a crucial parameter when IFN-γ is used to license eMSCs. Moreover, eMSC surface expression of major histocompatibility complex (MHC)-II in horses is increased by IFN-γ and decreased by IL-1β. However, tumor necrosis factor-α (TNF-α) has no impact on the expression of MHC-II, demonstrating the importance of the nature of the cytokine used for eMSC stimulation on their antigenicity and immunomodulation (65).
However, a single molecule is not an accurate replication of the whole inflammatory environment. Pro-inflammatory cytokines can exert distinct actions. For example, preconditioning human MSCs with TNF-α enhances the chondrogenic differentiation potential of the cells, whereas IL-1β does not enhance the chondrogenic potential of MSCs (66). Thus, a combination of several of these factors may be more accurate. Stimulation of eMSCs with IFN-γ and TNF-α resulted in the overexpression of immunomodulation-related genes (67). Alone or in combination, these cytokines also significantly increased the expression of prostaglandin-endoperoxide synthase 2 (PGE2) and indoleamine 2,3-dioxygenase (IDO) in eMSCs (68). PGE2 (37) and IDO both mediate most of the inhibition of equine lymphocyte proliferation, although the involvement of IDO in the horse model is a subject of debate (69). Recently, injection of the secretome from TNFα and IFNγ-stimulated eMSCs in LPS-induced osteoarthritic equine joints (70) led to reduced inflammatory symptoms and higher ECM marker expression in joints treated with concentrated CM. Nonetheless, no differences were noted between MSC-secretome and MSC groups. To our knowledge, this is the only MSC-CM in vivo experiment that has been carried out in the equine model.
However, combining cytokines only considers a small part of in vivo molecular content and interactions. Cytokine priming can have a deleterious effect on eMSC viability and trilineage differentiation, which is not observed when they are primed with inflammatory synovial fluid (SF) (71). Because immunomodulatory cytokines are also released by activated T-cells, CM from PB mononuclear cell-activated eMSCs can diminish T-cell proliferation in a significative manner compared with naive eMSCs (69). Platelet-rich plasma (PRP) and bone marrow concentrate (BMC) can stimulate the migration of eMSCs (72). These biological fluids contain various healing-related factors and, because migration is linked to immunomodulation (73), they may represent a strategy for eMSC preconditioning.
Hypoxia and cytokine priming are the most investigated strategies for improving eMSC therapeutic potential. Nevertheless, some less well-known methods may be promising. eMSCs are naturally exposed to mechanical forces such as fluid shear stress, hydrostatic compression or mechanical loading that affect MSC proliferation, differentiation and migration (74). Moreover, the human MSC secretome's ability to modulate angiogenesis is influenced by the mechanical environment of MSCs in both 2D and 3D culture systems (75). To date, mechanostimulation efficiency has not been demonstrated in the equine model. Extracorporeal shock wave therapy (ESWT) is a type of mechanical sensing using acoustic waves already employed in the therapies for tendon and ligament affections, but only as an auxiliary treatment in equine OA management. ESWT can increase metabolic activity and differentiation of eMSCs, but no effects on immunomodulatory potential have been observed (76). Furthermore, CM from human MSCs exposed to pulsed electromagnetic fields can also enhance articular chondrocyte migration and reduce the inflammatory state and apoptosis of these cells (77), but no proof is available regarding equine cells. Lastly, 1064 nm irradiation enhances IL-10 and VEGF expression in naive eMSCs (78). Photobiomodulation may be another way of maximizing stimulation and therapeutic potential of eMSCs in the treatment of equine OA.
6. Conclusion
The relevance of the equine model in OA therapy research contributes to the emergence of new studies and better understanding of the therapeutic potential of eMSCs. Despite recent advances in MSC-based therapies, several hurdles still need be overcome to propose a MSC therapy to treat equine OA. Notwithstanding the difficulties of quantification and large-scale production due to the novelty of the approach, eMSC-EVs may be an appropriate adjunct to improve MSC-based equine OA management. This strategy can benefit from the immunomodulatory, anti-inflammatory and regenerative properties of MSCs without inducing side effects such as immunogenicity or tumoral transformation (Figure 1).
Nonetheless, there still are numerous questions before considering therapies based on MSC-EVs for equine joint disorders. Issues involving eMSC origin, culture and preconditioning conditions, method of EV isolation, enrichment, storage and dosing need to be addressed, as well as the safety of allogenic or autologous EVs (Figure 2). Another critical issue that needs to be examined is the in vivo targeting of cartilage. Currently, a promising strategy is the use of a cationic molecule that can coat EVs and reverse their negative surface charge to infiltrate the negatively charged cartilage more easily (79).
To address all these considerations, in vitro organoid models of equine chondrocytes or eMSCs can be useful to optimize EV preparation and to identify the ideal treatment for use in controlled clinical trials on horses affected by OA.
Finally, progress in equine OA treatment using the therapeutic potential of MSC-EVs is critical for horse welfare and the equine industry, and may even eventually be transposable to humans as part of a One Health approach.
Author contributions
Conceptualization, literature search, and writing: MJ, RC, and FC. Review and editing: MJ, RC, FC, and PG. Supervision and approval: PG. All authors contributed to this review and approved the submitted version.
Funding
The publication of this review was supported by the European project OA-ACTIVE funded by the European Union within the frame-work of the Operational Programme ERDF (European Regional Development Funds)/ESF 2014−20. This work was also funded by an EQUISTEM ERDF grant, by Fonds Eperon (EQUISTEM), by the GIS CENTAURE-equine research (EQUISTEM-G), by ERDF and Regional Council of Basse-Normandie grant in the CPER CENTAURE program (2014–2020, CENTAURE Biotechnologies 2015 and CENTAURE PREACH 2016–17). MJ was supported by a Ph.D. scholarship from the Normandy Region Council (France) and the GIS CENTAURE-equine research (Nanovexstem project). RC was supported by a PhD fellowship co-financed by the Fonds Eperon and the Regional Council of Basse-Normandie. All the funding sources had absolutely no involvement in the study design, collection, analysis, and interpretation of data; in the writing of the manuscript; or in the decision to submit the manuscript for publication.
Conflict of interest
The authors declare that the research was conducted in the absence of any commercial or financial relationships that could be construed as a potential conflict of interest.
Publisher's note
All claims expressed in this article are solely those of the authors and do not necessarily represent those of their affiliated organizations, or those of the publisher, the editors and the reviewers. Any product that may be evaluated in this article, or claim that may be made by its manufacturer, is not guaranteed or endorsed by the publisher.
References
1. Armiento AR, Alini M, Stoddart MJ. Articular fibrocartilage - why does hyaline cartilage fail to repair? Adv Drug Deliv Rev. (2019) 146:289–305. doi: 10.1016/j.addr.2018.12.015
2. McIlwraith CW, Frisbie DD, Kawcak CE. The horse as a model of naturally occurring osteoarthritis. Bone Joint Res. (2012) 1:297–309. doi: 10.1302/2046-3758.111.2000132
3. Preston SA, Trumble TN, Zimmel DN, Chmielewski TL, Brown MP, Hernandez JA. Lameness, athletic performance, and financial returns in yearling thoroughbreds bought for the purpose of resale for profit. J Am Vet Med Assoc. (2008) 232:85–90. doi: 10.2460/javma.232.1.85
4. McCoy AM. Animal models of osteoarthritis: comparisons and key considerations. Vet Pathol. (2015) 52:803–18. doi: 10.1177/0300985815588611
5. Merimi M, El-Majzoub R, Lagneaux L, Moussa Agha D, Bouhtit F, Meuleman N, et al. The therapeutic potential of mesenchymal stromal cells for regenerative medicine: current knowledge and future understandings. Front Cell Dev Biol. (2021) 9:661532. doi: 10.3389/fcell.2021.661532
6. Chahal J, Gómez-Aristizábal A, Shestopaloff K, Bhatt S, Chaboureau A, Fazio A, et al. Bone marrow mesenchymal stromal cell treatment in patients with osteoarthritis results in overall improvement in pain and symptoms and reduces synovial inflammation. Stem Cells Transl Med. (2019) 8:746–57. doi: 10.1002/sctm.18-0183
7. Harrell CR, Jovicic N, Djonov V, Arsenijevic N, Volarevic V. Mesenchymal stem cell-derived exosomes and other extracellular vesicles as new remedies in the therapy of inflammatory diseases. Cells. (2019) 8:E1605. doi: 10.3390/cells8121605
8. Theeuwes WF, van den Bosch MHJ, Thurlings RM, Blom AB, van Lent PLEM. The role of inflammation in mesenchymal stromal cell therapy in osteoarthritis, perspectives for post-traumatic osteoarthritis: a review. Rheumatology. (2021) 60:1042–53. doi: 10.1093/rheumatology/keaa910
9. Song Y, Jorgensen C. Mesenchymal stromal cells in osteoarthritis: evidence for structural benefit and cartilage repair. Biomedicines. (2022) 10:1278. doi: 10.3390/biomedicines10061278
10. Fortier LA, Potter HG, Rickey EJ, Schnabel LV, Foo LF, Chong LR, et al. Concentrated bone marrow aspirate improves full-thickness cartilage repair compared with microfracture in the equine model. JBJS. (2010) 92:1927–37. doi: 10.2106/JBJS.I.01284
11. McIlwraith CW, Frisbie DD, Rodkey WG, Kisiday JD, Werpy NM, Kawcak CE, et al. Evaluation of intra-articular mesenchymal stem cells to augment healing of microfractured chondral defects. Arthroscopy. (2011) 27:1552–61. doi: 10.1016/j.arthro.2011.06.002
12. Ferris DJ, Frisbie DD, Kisiday JD, McIlwraith CW, Hague BA, Major MD, et al. Clinical outcome after intra-articular administration of bone marrow derived mesenchymal stem cells in 33 horses with stifle injury. Vet Surg. (2014) 43:255–65. doi: 10.1111/j.1532-950X.2014.12100.x
13. Branly T, Bertoni L, Contentin R, Rakic R, Gomez-Leduc T, Desancé M, et al. Characterization and use of equine bone marrow mesenchymal stem cells in equine cartilage engineering. study of their hyaline cartilage forming potential when cultured under hypoxia within a biomaterial in the presence of BMP-2 and TGF-ß1. Stem Cell Rev Rep. (2017) 13:611–30. doi: 10.1007/s12015-017-9748-y
14. Branly T, Contentin R, Desancé M, Jacquel T, Bertoni L, Jacquet S, et al. Improvement of the chondrocyte-specific phenotype upon equine bone marrow mesenchymal stem cell differentiation: influence of culture time, transforming growth factors and type I collagen siRNAs on the differentiation Index. IJMS. (2018) 19:435. doi: 10.3390/ijms19020435
15. Desancé M, Contentin R, Bertoni L, Gomez-Leduc T, Branly T, Jacquet S, et al. Chondrogenic differentiation of defined equine mesenchymal stem cells derived from umbilical cord blood for use in cartilage repair therapy. Int J Mol Sci. (2018) 19:E537. doi: 10.3390/ijms19020537
16. Rakic R Bourdon B Demoor M Maddens S Saulnier N Galymal Galymal stem cells derived from umbilical cord bloo potential of equine umbilical cord matrix and cord blood mesenchymal stromal/stem cells for cartilage regeneration. Sci Rep. (2018) 8:13799. doi: 10.1038/s41598-018-28164-9
17. Contentin R, Demoor M, Concari M, Desancé M, Audigié F, Branly T, et al. Comparison of the chondrogenic potential of mesenchymal stem cells derived from bone marrow and umbilical cord blood intended for cartilage tissue engineering. Stem Cell Rev and Rep. (2020) 16:126–43. doi: 10.1007/s12015-019-09914-2
18. Herberts CA, Kwa MS, Hermsen HP. Risk factors in the development of stem cell therapy. J Transl Med. (2011) 9:29. doi: 10.1186/1479-5876-9-29
19. Pigott JH, Ishihara A, Wellman ML, Russell DS, Bertone AL. Investigation of the immune response to autologous, allogeneic, and xenogeneic mesenchymal stem cells after intra-articular injection in horses. Vet Immunol Immunopathol. (2013) 156:99–106. doi: 10.1016/j.vetimm.2013.09.003
20. Paterson YZ, Rash N, Garvican ER, Paillot R, Guest DJ. Equine mesenchymal stromal cells and embryo-derived stem cells are immune privileged in vitro. Stem Cell Res Ther. (2014) 5:90. doi: 10.1186/scrt479
21. Schnabel LV, Pezzanite LM, Antczak DF, Felippe MJB, Fortier LA. Equine bone marrow-derived mesenchymal stromal cells are heterogeneous in MHC class II expression and capable of inciting an immune response in vitro. Stem Cell Res Ther. (2014) 5:13. doi: 10.1186/scrt402
22. Bertoni L, Branly T, Jacquet S, Desanc Felippe MJB, Fortier LA. E, et al. Intra-articular injection of 2 different dosages of autologous and allogeneic bone marrow- and umbilical cord-derived mesenchymal stem cells triggers a variable inflammatory response of the fetlock joint on 12 sound experimental horses. Stem Cells Int. (2018) 2019:9431894. doi: 10.1155/2019/9431894
23. Wang Y, Huso DL, Harrington J, Kellner J, Jeong DK, Turney J, et al. Outgrowth of a transformed cell population derived from normal human BM mesenchymal stem cell culture. Cytotherapy. (2005) 7:509–19. doi: 10.1080/14653240500363216
24. Pan Q, Fouraschen SMG, de Ruiter PE, Dinjens WNM, Kwekkeboom J, Tilanus HW, et al. Detection of spontaneous tumorigenic transformation during culture expansion of human mesenchymal stromal cells. Exp Biol Med. (2014) 239:105–15. doi: 10.1177/1535370213506802
25. Hade MD, Suire CN, Suo Z. Mesenchymal stem cell-derived exosomes: applications in regenerative medicine. Cells. (2021) 10:1959. doi: 10.3390/cells10081959
26. Velot 1, Madry H, Venkatesan JK, Bianchi A, Cucchiarini M. Is extracellular vesicle-based therapy the next answer for cartilage regeneration? Front Bioeng Biotechnol. (2021) 9:645039. doi: 10.3389/fbioe.2021.645039
27. Liang Y, Duan L, Lu J, Xia J. Engineering exosomes for targeted drug delivery. Theranostics. (2021) 11:3183–95. doi: 10.7150/thno.52570
28. Contentin R, Jammes M, Bourdon B, Cassé F, Bianchi A, Audigié F, et al. Bone marrow MSC secretome increases equine articular chondrocyte collagen accumulation and their migratory capacities. Int J Mol Sci. (2022) 23:5795. doi: 10.3390/ijms23105795
29. Arévalo-Turrubiarte M, Baratta M, Ponti G, Chiaradia E, Martignani E. Extracellular vesicles from equine mesenchymal stem cells decrease inflammation markers in chondrocytes in vitro. Equine Vet J. (2021) 54:1133. do doi: 10.1111/evj.13537
30. Hotham WE, Thompson C, Szu-Ting L, Henson FMD. The anti-inflammatory effects of equine bone marrow stem cell-derived extracellular vesicles on autologous chondrocytes. Vet Rec Open. (2021) 8:e22. doi: 10.1002/vro2.22
31. Anderson JR, Jacobsen S, Walters M, Bundgaard L, Diendorfer A, Hackl M, et al. Small non-coding RNA landscape of extracellular vesicles from a post-traumatic model of equine osteoarthritis. Front Vet Sci. (2022) 9:901269. doi: 10.3389/fvets.2022.901269
32. Madrigal M, Rao KS, Riordan NH. A review of therapeutic effects of mesenchymal stem cell secretions and induction of secretory modification by different culture methods. J Transl Med. (2014) 12:260. doi: 10.1186/s12967-014-0260-8
33. Hu C, Li L. Preconditioning influences mesenchymal stem cell properties in vitro and in vivo. J Cell Mol Med. (2018) 22:1428–42. doi: 10.1111/jcmm.13492
34. Noronha NC, Mizukami A, Cali11/jcmm.13492senchymal stem cell properties chy, et al. Priming approaches to improve the efficacy of mesenchymal stromal cell-based therapies. Stem Cell Res Ther. (2019) 10:131. doi: 10.1186/s13287-019-1224-y
35. Costa LA, Eiro N, Fraile M, Gonzalez LO, Saá J, Garcia-Portabella P, et al. Functional heterogeneity of mesenchymal stem cells from natural niches to culture conditions: implications for further clinical uses. Cell Mol Life Sci. (2021) 78:447–67. doi: 10.1007/s00018-020-03600-0
36. Cassano JM, Fortier LA, Hicks RB, Harman RM, Van de Walle GR. Equine mesenchymal stromal cells from different tissue sources display comparable immune-related gene expression profiles in response to interferon gamma (IFN)-γ. Vet Immunol Immunopathol. (2018) 202:25–30. doi: 10.1016/j.vetimm.2018.06.008
37. Carrade Holt DD, Wood JA, Granick JL, Walker NJ, Clark KC, Borjesson DL. Equine mesenchymal stem cells inhibit T cell proliferation through different mechanisms depending on tissue source. Stem Cells Dev. (2014) 23:1258–65. doi: 10.1089/scd.2013.0537
38. Cortés-Araya Y, Amilon K, Rink BE, Black G, Lisowski Z, Donadeu FX, et al. Comparison of antibacterial and immunological properties of mesenchymal stem/stromal cells from equine bone marrow, endometrium, and adipose tissue. Stem Cells Dev. (2018) 27:1518–25. doi: 10.1089/scd.2017.0241
39. Navarrete F, Wong YS, Cabezas J, Riadi G, Manríquez J, Rojas D, et al. Distinctive cellular transcriptomic signature and microRNA cargo of extracellular vesicles of horse adipose and endometrial mesenchymal stem cells from the same donors. Cell Reprogram. (2020) 22:311–27. doi: 10.1089/cell.2020.0026
40. Harman RM, Patel RS, Fan JC, Park JE, Rosenberg BR, Van de Walle GR. Single-cell RNA sequencing of equine mesenchymal stromal cells from primary donor-matched tissue sources reveals functional heterogeneity in immune modulation and cell motility. Stem Cell Res Ther. (2020) 11:524. doi: 10.1186/s13287-020-02043-5
41. Bundgaard L, Stensballe A, Elbæk KJ, Berg LC. Mass spectrometric analysis of the in vitro secretome from equine bone marrow-derived mesenchymal stromal cells to assess the effect of chondrogenic differentiation on response to interleukin-1β treatment. Stem Cell Res Ther. (2020) 11:187. doi: 10.1186/s13287-020-01706-7
42. Pilgrim CR, McCahill KA, Rops JG, Dufour JM, Russell KA, Koch TG, et al. Review of fetal bovine serum in the culture of mesenchymal stromal cells and potential alternatives for veterinary medicine. Front Vet Sci. (2022) 9:859025. doi: 10.3389/fvets.2022.859025
43. Rowland AL, Burns ME, Levine GJ, Watts AE. Preparation technique affects recipient immune targeting of autologous mesenchymal stem cells. Front Vet Sci. (2021) 8:724041. doi: 10.3389/fvets.2021.724041
44. Malikides N, Hodgson JL, Rose RJ, Hodgson DR. Cardiovascular, haematological and biochemical responses after large volume blood collection in horses. Vet J. (2001) 162:44–55. doi: 10.1053/tvjl.2001.0583
45. Naskou MC, Sumner SM, Chocallo A, Kemelmakher H, Thoresen M, Copland I, et al. Platelet lysate as a novel serum-free media supplement for the culture of equine bone marrow-derived mesenchymal stem cells. Stem Cell Res Ther. (2018) 9:75. doi: 10.1186/s13287-018-0823-3
46. Yaneselli K, Barrachina L, Remacha AR, Algorta A, Vitoria A, Cequier A, et al. Effect of allogeneic platelet lysate on equine bone marrow derived mesenchymal stem cell characteristics, including immunogenic and immunomodulatory gene expression profile. Vet Immunol Immunopathol. (2019) 217:109944. doi: 10.1016/j.vetimm.2019.109944
47. Pezzanite L, Chow L, Griffenhagen G, Dow S, Goodrich L. Impact of three different serum sources on functional properties of equine mesenchymal stromal cells. Front Vet Sci. (2021) 8:634064. doi: 10.3389/fvets.2021.634064
48. Clark KC, Kol A, Shahbenderian S, Granick JL, Walker NJ, Borjesson DL. Canine and equine mesenchymal stem cells grown in serum free media have altered immunophenotype. Stem Cell Rev Rep. (2016) 12:245–56. doi: 10.1007/s12015-015-9638-0
49. Jauković A, Abadjieva D, Trivanović D, Stoyanova E, Kostadinova M, Pashova S, et al. Specificity of 3D MSC spheroids microenvironment: impact on msc behavior and properties. Stem Cell Rev Rep. (2020) 16:853–75. doi: 10.1007/s12015-020-10006-9
50. Ylostalo JH, Bazhanov N, Mohammadipoor A, Bartosh TJ. Production and administration of therapeutic mesenchymal stem/stromal cell (MSC) spheroids primed in 3-D cultures under xeno-free conditions. J Vis Exp. (2017) 121:55126. doi: 10.3791/55126
51. Frith JE, Thomson B, Genever PG. Dynamic three-dimensional culture methods enhance mesenchymal stem cell properties and increase therapeutic potential. Tissue Eng Part C Methods. (2010) 16:735–49. doi: 10.1089/ten.tec.2009.0432
52. Siennicka K, Zołocińska A, Debski T, Pojda Z. Comparison of the donor age-dependent and In Vitro culture-dependent Mesenchymal stem cell aging in rat model. Stem Cells Int. (2021) 2021:6665358. doi: 10.1155/2021/6665358
53. Sepúlveda JC, Tomé M, Fernández ME, Delgado M, Campisi J, Bernad A, et al. Cell senescence abrogates the therapeutic potential of human mesenchymal stem cells in the lethal endotoxemia model. Stem Cells. (2014) 32:1865–77. doi: 10.1002/stem.1654
54. Zhang Y, Ravikumar M, Ling L, Nurcombe V, Cool SM. Age-related changes in the inflammatory status of human mesenchymal stem cells: implications for cell therapy. Stem Cell Rep. (2021) 16:694–707 doi: 10.1016/j.stemcr.2021.01.021
55. Martinello T, Bronzini I, Maccatrozzo L, Iacopetti I, Sampaolesi M, Mascarello F, et al. Cryopreservation does not affect the stem characteristics of multipotent cells isolated from equine peripheral blood. Tissue Eng Part C Methods. (2010) 16:771–81. doi: 10.1089/ten.tec.2009.0512
56. Williams LB, Tessier L, Koenig JB, Koch TG. Post-thaw non-cultured and post-thaw cultured equine cord blood mesenchymal stromal cells equally suppress lymphocyte proliferation in vitro. PLoS ONE. (2014) 9:e113615. doi: 10.1371/journal.pone.0113615
57. Bourzac C, Smith LC, Vincent P, Beauchamp G, Lavoie JP, Laverty S. Isolation of equine bone marrow-derived mesenchymal stem cells: a comparison between three protocols. Equine Vet J. (2010) 42:519–27. doi: 10.1111/j.2042-3306.2010.00098.x
58. Cosenza S, Toupet K, Maumus M, Luz-Crawford P, Blanc-Brude O, Jorgensen C, et al. Mesenchymal stem cells-derived exosomes are more immunosuppressive than microparticles in inflammatory arthritis. Theranostics. (2018) 8:1399–410. doi: 10.7150/thno.21072
59. Jagannathan L, Cuddapah S, Costa M. Oxidative stress under ambient and physiological oxygen tension in tissue culture. Curr Pharmacol Rep. (2016) 2:64–72. doi: 10.1007/s40495-016-0050-5
60. Chen S, Sun F, Qian H, Xu W, Jiang J. Preconditioning and engineering strategies for improving the efficacy of mesenchymal stem cell-derived exosomes in cell-free therapy. Stem Cells Int. (2022) 2022:1779346. doi: 10.1155/2022/1779346
61. Jiang H, Zhao H, Zhang M, He Y, Li X, Xu Y, et al. Hypoxia Induced changes of exosome cargo and subsequent biological effects. Front Immunol. (2022) 13:824188. doi: 10.3389/fimmu.2022.824188
62. Zhang B, Tian X, Qu Z, Hao J, Zhang W. Hypoxia-preconditioned extracellular vesicles from mesenchymal stem cells improve cartilage repair in osteoarthritis. Membranes. (2022) 12:225. doi: 10.3390/membranes12020225
63. Maumus M, Roussignol G, Toupet K, Penarier G, Bentz I, Teixeira S, et al. Utility of a Mouse model of osteoarthritis to demonstrate cartilage protection by IFNom mesenchymal stem cells improve cartilFront Immunol. (2016) 7:392. doi: 10.3389/fimmu.2016.00392
64. Cassano JM, Schnabel LV, Goodale MB, Fortier LA. Inflammatory licensed equine MSCs are chondroprotective and exhibit enhanced immunomodulation in an inflammatory environment. Stem Cell Res Ther. (2018) 9:82. doi: 10.1186/s13287-018-0840-2
65. Hill JA, Cassano JM, Goodale MB, Fortier LA. Antigenicity of mesenchymal stem cells in an inflamed joint environment. Am J Vet Res. (2017) 78:867–75. doi: 10.2460/ajvr.78.7.867
66. Voskamp C, Koevoet WJLM, Somoza RA, Caplan AI, Lefebvre V, van Osch GJVM, et al. Enhanced chondrogenic capacity of mesenchymal stem cells after TNFα pre-treatment. Front Bioeng Biotechnol. (2020) 8:658. doi: 10.3389/fbioe.2020.00658
67. Barrachina L, Remacha AR, Romero A, Vázquez FJ, Albareda J, Prades M, et al. Priming equine bone marrow-derived mesenchymal stem cells with proinflammatory cytokines: implications in immunomodulation-immunogenicity balance, cell viability, and differentiation potential. Stem Cells Dev. (2017) 26:15–24. doi: 10.1089/scd.2016.0209
68. Caffi V, Espinosa G, Gajardo G, Morales N, Dural stem cells w, et al. Pre-conditioning of equine bone marrow-derived mesenchymal stromal cells increases their immunomodulatory capacity. Front Vet Sci. (2020) 7:318. doi: 10.3389/fvets.2020.00318
69. Carrade DD, Lame MW, Kent MS, Clark KC, Walker NJ, Borjesson DL. Comparative analysis of the immunomodulatory properties of equine adult-derived mesenchymal stem cells. Cell Med. (2012) 4:1–11. doi: 10.3727/215517912X647217
70. Kearney CM, Khatab S, van Buul GM, Plomp SGM, Korthagen NM, Labberté MC, et al. Treatment effects of intra-articular allogenic mesenchymal stem cell secretome in an equine model of joint inflammation. Front Vet Sci. (2022) 9:907616. doi: 10.3389/fvets.2022.907616
71. Barrachina L, Remacha AR, Romero A, Vázquez FJ, Albareda J, Prades M, et al. Inflammation affects the viability and plasticity of equine mesenchymal stem cells: possible implications in intra-articular treatments. J Vet Sci. (2017) 18:39–49. doi: 10.4142/jvs.2017.18.1.39
72. Holmes HL, Wilson B, Goerger JP, Silverberg JL, Cohen I, Zipfel WR, et al. Facilitated recruitment of mesenchymal stromal cells by bone marrow concentrate and platelet rich plasma. PLoS ONE. (2018) 13:e0194567. doi: 10.1371/journal.pone.0194567
73. Volarevic V, Gazdic M, Simovic Markovic B, Jovicic N, Djonov V, Arsenijevic N. Mesenchymal stem cell-derived factors: immuno-modulatory effects and therapeutic potential. Biofactors. (2017) 43:633–44. doi: 10.1002/biof.1374
74. Hao J, Zhang Y, Jing D, Shen Y, Tang G, Huang S, et al. Mechanobiology of mesenchymal stem cells: perspective into mechanical induction of MSC fate. Acta Biomater. (2015) 20:1–9. doi: 10.1016/j.actbio.2015.04.008
75. Kasper G, Dankert N, Tuischer J, Hoeft M, Gaber T, Glaeser JD, et al. Mesenchymal stem cells regulate angiogenesis according to their mechanical environment. Stem Cells. (2007) 25:903–10. doi: 10.1634/stemcells.2006-0432
76. Salcedo-Jiménez R, Koenig JB, Lee OJ, Gibson TWG, Madan P, Koch TG. Extracorporeal shock wave thrapy enhances the in vitro metabolic activity and differentiation of equine umbilical cord blood mesenchymal stromal cells. Front Vet Sci. (2020) 7:554306. doi: 10.3389/fvets.2020.554306
77. Parate D, Kadir ND, Celik C, Lee EH, Hui JHP, Franco-Obregón A, et al. Pulsed electromagnetic fields potentiate the paracrine function of mesenchymal stem cells for cartilage regeneration. Stem Cell Res Ther. (2020) 11:46. doi: 10.1186/s13287-020-1566-5
78. Peat FJ, Colbath AC, Bentsen LM, Goodrich LR, King MR. In vitro effects of high-intensity laser photobiomodulation on equine bone marrow-derived mesenchymal stem cell viability and cytokine expression. Photomed Laser Surg. (2018) 36:83–91. doi: 10.1089/pho.2017.4344
Keywords: osteoarthritis, mesenchymal stromal cells, extracellular vesicles, acellular therapy, horse
Citation: Jammes M, Contentin R, Cassé F and Galéra P (2023) Equine osteoarthritis: Strategies to enhance mesenchymal stromal cell-based acellular therapies. Front. Vet. Sci. 10:1115774. doi: 10.3389/fvets.2023.1115774
Received: 04 December 2022; Accepted: 26 January 2023;
Published: 10 February 2023.
Edited by:
Janina Burk, Justus-Liebig-University Giessen, GermanyReviewed by:
Luisa Pascucci, University of Perugia, ItalyCopyright © 2023 Jammes, Contentin, Cassé and Galéra. This is an open-access article distributed under the terms of the Creative Commons Attribution License (CC BY). The use, distribution or reproduction in other forums is permitted, provided the original author(s) and the copyright owner(s) are credited and that the original publication in this journal is cited, in accordance with accepted academic practice. No use, distribution or reproduction is permitted which does not comply with these terms.
*Correspondence: Philippe Galéra, philippe.galera@unicaen.fr
†These authors have contributed equally to this work