- 1Department of Rehabilitation Medicine, The First Affiliated Hospital, Sun Yat-sen University, Guangzhou, China
- 2Guangdong Provincial Clinical Research Center for Rehabilitation Medicine, Sun Yat-sen University, Guangzhou, China
Background: Repetitive transcranial magnetic stimulation (rTMS) is emerging as a promising non-invasive intervention for Alzheimer’s disease (AD), yet therapeutic outcomes remain inconsistent across studies. This meta-analysis aimed to evaluate the cognitive benefits of rTMS in AD patients, with a specific focus on stimulation targets and protocols variations.
Methods: A systematic literature search was conducted in PubMed, Web of Science, Embase, and Cochrane Library for relevant English-language studies published up to 31 May 2024. Cognitive outcomes were assessed using the Mini-Mental State Examination (MMSE) and Alzheimer’s Disease Assessment Scale-Cognitive Section (ADAS-Cog). Data were pooled using a random-effects model, with standardized mean difference (SMD) or mean differences (MD) and 95% confidence intervals (CI) calculated. Subgroup analyses were performed to examine the effects of stimulation targets, protocol variations and population demographics on rTMS efficacy.
Results: Twenty-two studies involving 874 participants were included in this meta-analysis. Overall, rTMS significantly improved cognitive function (SMD = 0.27; 95% CI = 0.14–0.41; p < 0.0001), showing that the efficacy of rTMS varied by stimulation target and protocol. Stimulation of the dorsolateral prefrontal cortex (DLPFC) led to significant cognitive improvement (SMD = 0.49, 95% CI = −0.26 to 0.73; p < 0.0001), whereas bilateral DLPFC stimulation showed no significant improvement (SMD = 0.13; 95% CI = −0.40 to 0.66; p = 0.62). Stimulating the parietal lobe or associated regions produced moderate cognitive benefits (SMD = 0.29; 95% CI = 0.03–0.55; p = 0.03). Notably, multi-target stimulation over the bilateral DLPFC, parietal lobes, Wernicke’s area, and Broca’s area also showed substantial cognitive improvement (MD = 2.85; 95% CI = 1.69–4.00; p < 0.00001). Additionally, subgroup analysis based on geographical background revealed greater effects in studies conducted in Asia (SMD = 0.40, 95% CI = 0.14–0.65; p < 0.003).
Conclusion: rTMS is an effective intervention for cognitive enhancement in AD, with its efficacy significantly influenced by stimulation target and protocol. Notably, the greater cognitive benefits observed in Asian populations suggest a potential role of genetic and demographic factors that warrant further investigation. These findings contribute to the development of optimized, personalized rTMS protocols for AD treatment.
Systematic review registration: https://www.crd.york.ac.uk/PROSPERO/recorddashboard, CRD42023434084.
Introduction
Alzheimer’s disease (AD) is the most common cause of dementia (Plassman et al., 2007), emerging as one of the most pressing global health challenges of the 21st century. Currently, approximately 50 million people worldwide are affected by AD, with projections indicting a rise to 150 million by 2050 (Lane et al., 2018). AD is characterized by a progressive decline of cognitive functions (e.g., memory, language, executive function, and visuospatial skill) that are accompanied by widespread disruptions in functional connectivity within crucial neural networks (Chou et al., 2022). Despite decades of research, pharmacological interventions remain largely ineffective in mitigating disease progression, offering only symptomatic relief with minimal impact on underlying neurodegenerative mechanisms (Canter et al., 2016; Livingston et al., 2017). This therapeutic gap highlights the urgent need to explore and develop alternative, more effective treatment strategies.
A growing body of research suggests that AD is not only a disorder of neuronal loss but also a disease of large-scale network dysfunction, affecting both structural and functional connectivity (Dennis and Thompson, 2014). Specifically, impaired connectivity within major neural networks, including the default mode network (DMN), dorsal attention network (DAN), salience network (SAL), executive control network (ECN), and sensory-motor network (SMN), has been strongly linked to cognitive deterioration in AD (Brem et al., 2020). The DMN, which includes the posterior cingulate cortex, precuneus, medial prefrontal cortex, inferior parietal lobule, and bilateral temporal cortex, has received particular attention due to its important role in memory consolidation, self-referential thinking, and cognitive processing (Horn et al., 2014; Whitfield-Gabrieli and Ford, 2012). Studies have revealed a close relationship between functional connectivity abnormalities in the DMN and cognitive impairments in AD. For example, Talwar et al. (2021) found that AD patients exhibited widespread impairment of functional connectivity, with the precuneus and posterior cingulate cortex being severely affected. Similarly, Zheng et al. (2019) reported disruptions in the posterior parts of the DMN in AD patients. In addition, Sorg et al. (2007) found that patients with mild cognitive impairment (MCI) exhibited impaired connectivity in the left posterior cingulate cortex and the right medial prefrontal cortex of the DMN as well as the bilateral superior parietal lobules and inferior frontal gyri of the ECN. Interestingly, compensatory increase in ECN connectivity was observed in AD patients (Agosta et al., 2012). These studies highlight the complex interplay between cognitive impairments in AD and disruptions in network connectivity. Therefore, modulating these disrupted neural networks holds potential for improving cognitive function in AD patients (Pennisi et al., 2011).
Non-invasive brain stimulation (NIBS) techniques have emerged as promising tools for modulating cortical excitability (Di Pino et al., 2014) and inducing neuroplasticity (Cirillo et al., 2017). Among these techniques, repetitive transcranial magnetic stimulation (rTMS) has received significant attention for its ability to modulate neural activity and functional connectivity (Brignani et al., 2008; Maeda et al., 2000), offering a therapeutic potential for neurological and psychiatric disorders (Esposito et al., 2022). The neurophysiological effects of rTMS are highly dependent on stimulation parameters, including frequency, intensity, target, and duration. High-frequency rTMS (HF-rTMS, ≥ 10 Hz) and intermittent theta-burst stimulation (iTBS) are known to induce long-term potentiation (LTP)-like plasticity, enhancing synaptic strength. In contrast, low-frequency rTMS (LF-rTMS, ≤ 1 Hz) and continuous theta-burst stimulation (cTBS) typically induce long-term depression (LTD)-like plasticity, reducing cortical excitability (Fox et al., 1997). Note that conventional rTMS faces limitations in spatial focality, as the widely used international 10–20 EEG-based targeting approach contributes to variability in treatment outcomes. Neuronavigation-guided rTMS is thus increasingly employed, integrating individualized neuroimaging data to optimize stimulation precision and maximize therapeutic outcomes (Lefaucheur et al., 2014; Lefaucheur et al., 2020; Terao and Ugawa, 2002).
A growing body of evidence supports rTMS as a promising intervention for neurodegenerative disorders including AD (Anderkova and Rektorova, 2014; Hsu et al., 2015; Luber and Lisanby, 2014; Zhang W. et al., 2022), showing sustained cognitive improvements in memory, attention, and executive function that can persist beyond the treatment phase (Sabbagh et al., 2020). To date, six meta-analyses have examined the cognitive effects of rTMS in AD patients (Dong et al., 2018; Liao et al., 2015; Lin et al., 2019; Wang et al., 2020; Wei Z. et al., 2022; Zhang T. et al., 2022). While these studies generally support the therapeutic effects of HF-rTMS in enhancing cognitive function, their findings exhibited considerable variability due to heterogeneity in stimulation parameters such as frequency, intensity, target, and duration. Notably, much attention has been given to optimizing rTMS frequencies, but fewer studies have systematically examined the role of stimulation target. Single-site rTMS over the DLPFC has shown efficacy in improving memory and executive functions in AD patients (Wang et al., 2014). However, emerging evidence suggests that alternative targets, such as the parietal lobe, may also play a crucial role in modulating cortical-hippocampal networks essential for memory function (Wang et al., 2014). Despite these promising findings, there remains a lack of a systematic comparisons evaluating the cognitive benefits of rTMS across different cortical regions in AD. To address this gap, we conducted a comprehensive systematic meta-analysis to assess the cognitive effects of rTMS over different cortical targets in AD patients. By examining how different stimulation targets influence cognitive improvements, this study provides critical insights for refining rTMS protocols, thereby guiding the development of optimized, personalized neuromodulation strategies for effective treatment of cognitive impairment in AD.
Materials and methods
Search strategy
This study protocol was registered in the PROSPERO database (CRD42023434084) and conducted in accordance with the Preferred Reporting Items for Systematic Reviews and Meta-Analyses (PRISMA) guidelines, specifically following the Cochrane extension statement. A systematic literature search was performed across PubMed, Web of Science, and EMBASE databases to identify relevant studies published between 1 January 2010 and 31 May 2024. The search strategy incorporated controlled vocabulary and free-text keywords using the following query structure: (Alzheimer’s disease OR Alzheimer Dementia OR related MeSH entry terms) AND (Transcranial Magnetic Stimulation OR Magnetic Stimulation, Transcranial OR related MeSH entry terms) AND (filters for maximum sensitivity in identifying controlled trials).
Inclusion and exclusion criteria
Studies were included in this meta-analysis if they met the following criteria: (1) participants were diagnosed with AD based on clinical diagnostic criteria; (2) rTMS was the sole intervention; (3) cognitive function was assessed as the primary outcome measure; (4) study design included either a parallel-group or crossover sham-controlled group; (5) published in English in a peer-reviewed journal; (6) patients were allowed to continue their standard medication regimens, provided these remained unchanged throughout the rTMS treatment. Initial screening was conducted based on titles and abstracts, and full-text review was performed for studies with unclear eligibility. Studies were excluded if they were irrelevant, lacked a sham-control condition, or did not report cognitive outcomes. If relevance or eligibility remained uncertain, the full text of the paper was reviewed. Additionally, conference abstracts, case reports, and non-peer-reviewed publications were systematically excluded.
Primary outcomes
The primary outcome measure was cognitive function, assessed using the Mini-Mental State Examination (MMSE) and the Alzheimer’s Disease Assessment Scale-Cognitive Subscale (ADAS-Cog). The analysis focused on evaluating the effect of rTMS on cognitive function across different stimulation targets. For continuous outcome data, results were synthesized using the mean and standard deviation (Mean ± SD) of changes in cognitive measurements following rTMS administration.
Evaluation of risk of bias
The risk of bias in the included studies was assessed using a modified Cochrane Risk of Bias tool. The assessment focused on the following factors: (1) adherence to standardized diagnostic criteria for AD; (2) random sequence generation and allocation concealment; (3) blinding of participants and study personnel; (4) blinding of outcome assessments; (5) baseline comparability between rTMS and sham groups; and (6) completeness of data reporting, including dropout rates and handling of missing data. Each study was assigned a qualitative risk rating for each domain: Low risk (1), High risk (0), or Unclear (unreported information). Studies with higher cumulative scores were considered to have a lower overall risk of bias. In cases of discrepancies in risk assessment, a third independent reviewer (YJJ) was consulted to reach a consensus.
Data analysis
All statistical analyses were performed using RevMan 5.3 software (Review Manager of Cochrane Collaboration) and R language. For continuous outcomes, effect sizes were expressed as either the standardized mean difference (SMD) or mean difference (MD) with 95% confidence intervals (CIs). Heterogeneity among studies was assessed using the I2 statistic, where I2 < 50% indicated low heterogeneity and a fixed-effects model was applied. I2 > 50% indicated substantial heterogeneity, resulting in the use of a random-effects model unless heterogeneity could be reduced through subgroup or sensitivity analyses. If heterogeneity persisted despite these adjustments, results were reported descriptively. Forest plots were used to visually represent the findings, with each study displayed as a colored circle (red, pink or blue) indicating SMD, MD, or risk ratio, while the overall pooled effect size was shown as a hollow orange diamond.
Results
Search and selection of studies
A comprehensive literature research across PubMed, Web of Science, EMBASE, and the Cochrane Library yielded 196 studies. After removing duplicates and screening titles and abstracts for relevance, 38 articles were selected for full-text review. Following rigorous eligibility assessment, 22 studies (Ahmed et al., 2012; Bagattini et al., 2020; Brem et al., 2020; Chen et al., 2023; Cotelli et al., 2011; Hu et al., 2022; Jia et al., 2021; Koch et al., 2018; Lee et al., 2016; Leocani et al., 2020; Li et al., 2021; Padala et al., 2020; Rabey et al., 2013; Saitoh et al., 2022; Vecchio et al., 2022; Wei L. et al., 2022; Wu et al., 2015; Wu et al., 2022; Yao et al., 2022; Zhang et al., 2019; Zhao et al., 2017; Zhou et al., 2022) met the inclusion criteria and were included in the meta-analysis. The study selection process is detailed in Figure 1, and the primary clinical and demographic characteristics of the included studies are summarized in Table 1.
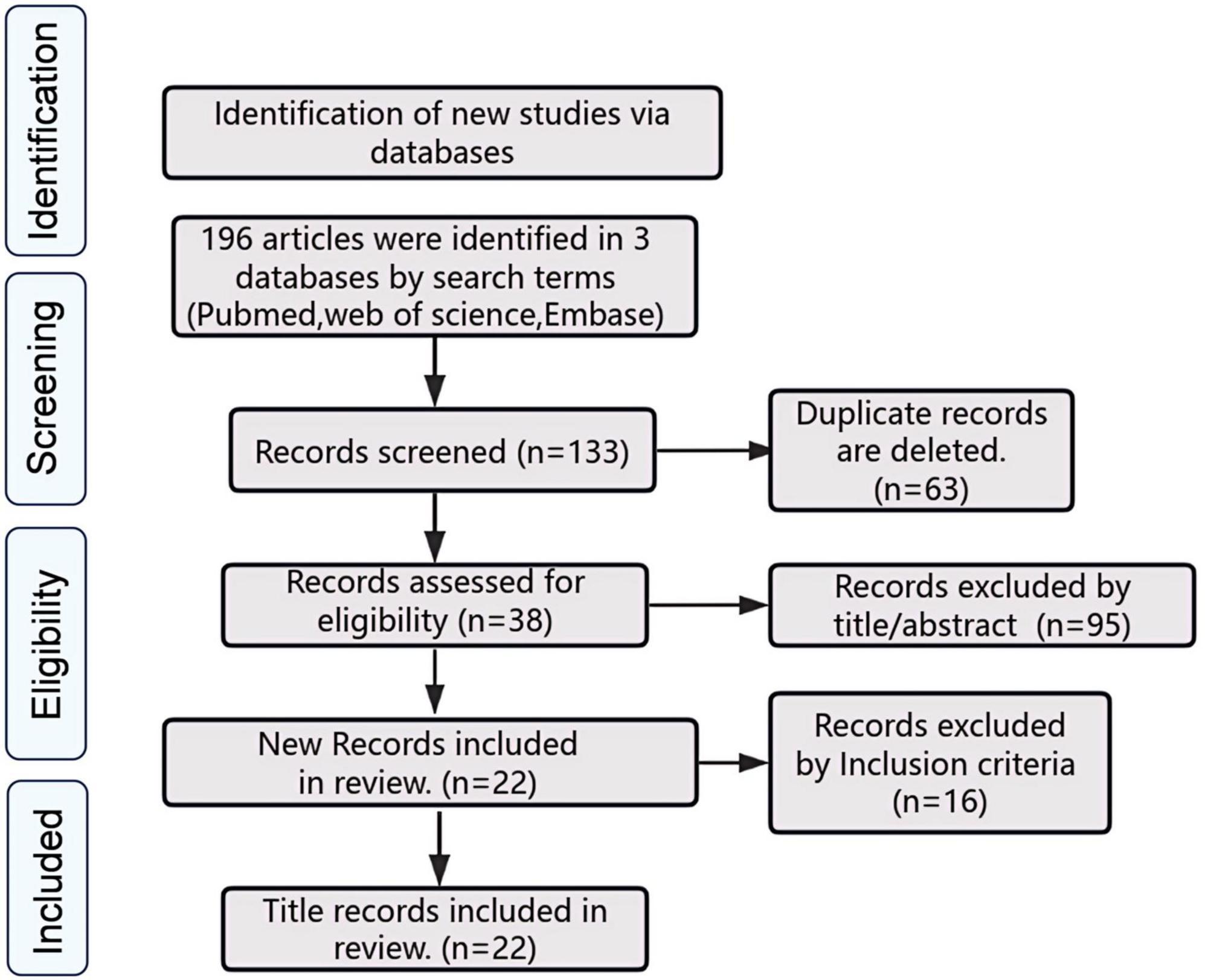
Figure 1. Flow diagram showing the search and selection procedure that was used for this meta-analysis.
Global cognitive function (immediately after the intervention)
A total of 22 studies encompassing 874 participants with AD evaluated the effects of rTMS on global cognitive function. If both MMSE and ADAS-Cog were reported, MMSE was prioritized as the primary outcome measure. The results showed that rTMS significantly improved cognitive function score in AD patients, with an SMD of 0.27 (95% CI = 0.14–0.41; z = 3.95; p < 0.0001) and moderate heterogeneity (I2 = 38%; p < 0.03) (see Figure 2). To further explore the potential sources of heterogeneity, additional subgroup analyses were conducted considering stimulation targets, rTMS protocols, and genetic backgrounds.
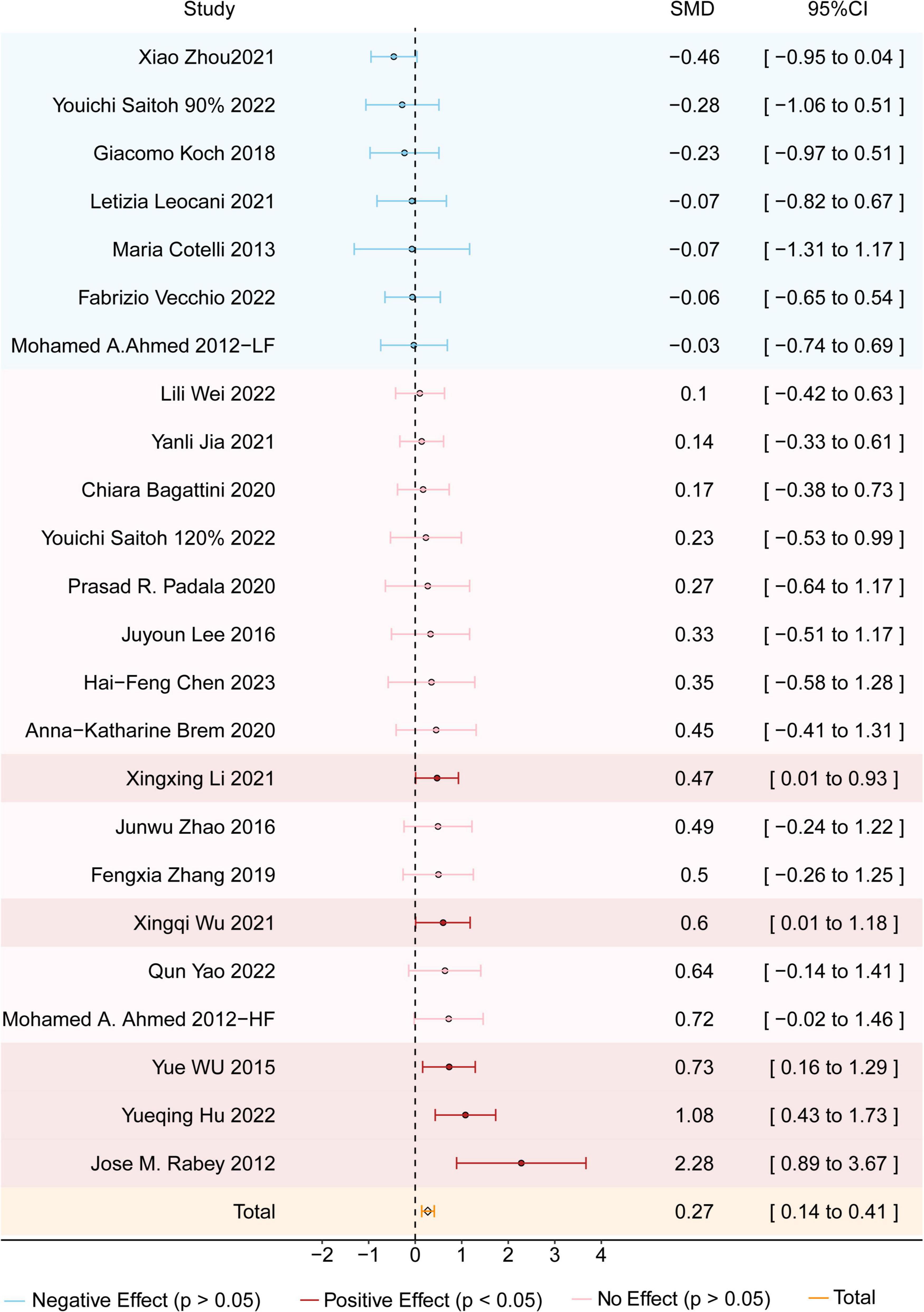
Figure 2. Forest plot shows the SMD (Standardized Mean Difference) and 95% CI (Confidence Interval) for all included studies compared to the sham group. Different effects are color-coded for clarity: red indicates a positive effect, blue signifies a negative effect, and pink denotes no effect. The orange diamond symbolizes the overall effect. If the diamond does not intersect the line of no effect, the overall effect is statistically significant; otherwise, it is not statistically significant.
Subgroup analysis of global cognitive function (rTMS on different targets)
Subgroup analyses were conducted to assess the effects of rTMS over different stimulation targets. Across all three subgroups, the experimental group showed significantly greater improvement in global cognitive function than the sham group (p < 0.05) (see Figure 3). DLPFC stimulation resulted in an SMD of 0.25 (95% CI = 0.07–0.44; z = 2.67; p = 0.008) with moderate heterogeneity (I2 = 37%; p = 0.09). Stimulation over the parietal lobe or its associated areas showed a slightly greater effect, with an SMD of 0.29 (95% CI = 0.03–0.55; z = 2.22; p = 0.03) and moderate heterogeneity (I2 = 42%; p = 0.12). Additionally, multi-target stimulation over the bilateral DLPFC, Broca’s area, Wernicke’s area, and bilateral parietal lobes yielded the greatest cognitive improvement, with an MD of 2.85 (95% CI = 1.69–4.02; z = 4.78; p < 0.00001) and low heterogeneity (I2 = 0%; p = 0.79).
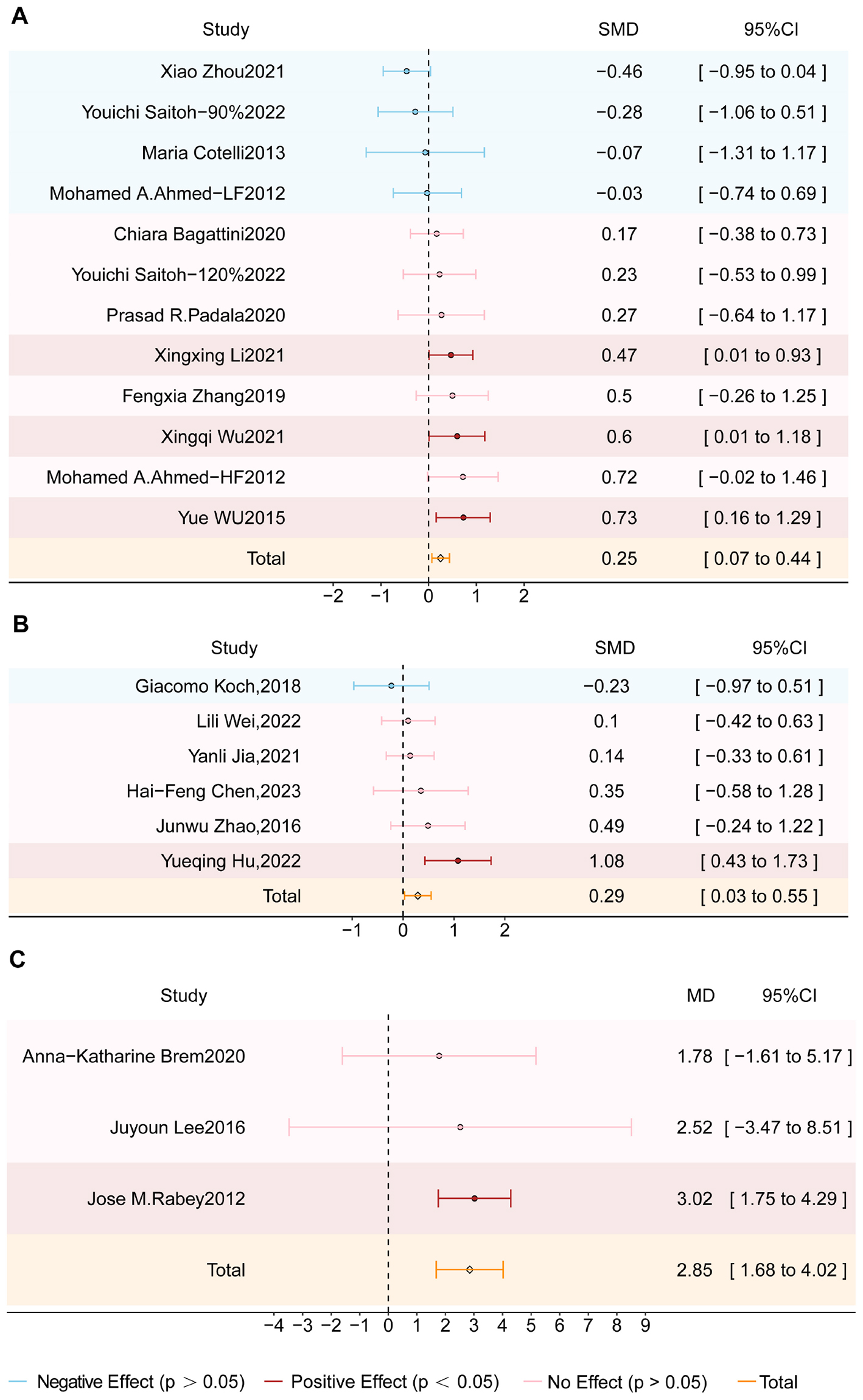
Figure 3. Forest plot shows the SMD (Standardized Mean Difference) or the MD (Mean Difference) and 95% CI (Confidence Interval) for three subgroups: (A) Dorsolateral prefrontal cortex (DLPFC), (B) Parietal lobe or associated regions, and (C) Multi-target, each compared to the sham group. Different effects are color-coded for clarity: red indicates a positive effect, blue signifies a negative effect, and pink denotes no effect. The orange diamond symbolizes the overall effect. If the diamond does not intersect the line of no effect, the overall effect is statistically significant; otherwise, it is not statistically significant.
Subgroup analysis of global cognitive function (rTMS on different protocols)
The effects of different stimulation protocols were further analyzed (see Figure 4). Excitatory rTMS significantly improved cognitive function, with an SMD of 0.27 (95% CI = 0.08–0.47; z = 0.78; p = 0.005) and moderate heterogeneity (I2 = 41%; p = 0.08). Bilateral DLPFC stimulation did not show improvement compared to the sham group, with an SMD of 0.13 (95% CI = −0.40–0.66; z = 0.49; p = 0.62) and high heterogeneity (I2 = 70%; p = 0.01). In contrast, left DLPFC stimulation yielded significant cognitive improvement, with an SMD of 0.49 (95% CI = 0.26–0.73; z = 4.11; p < 0.0001) and low heterogeneity (I2 = 0%; p = 0.78).
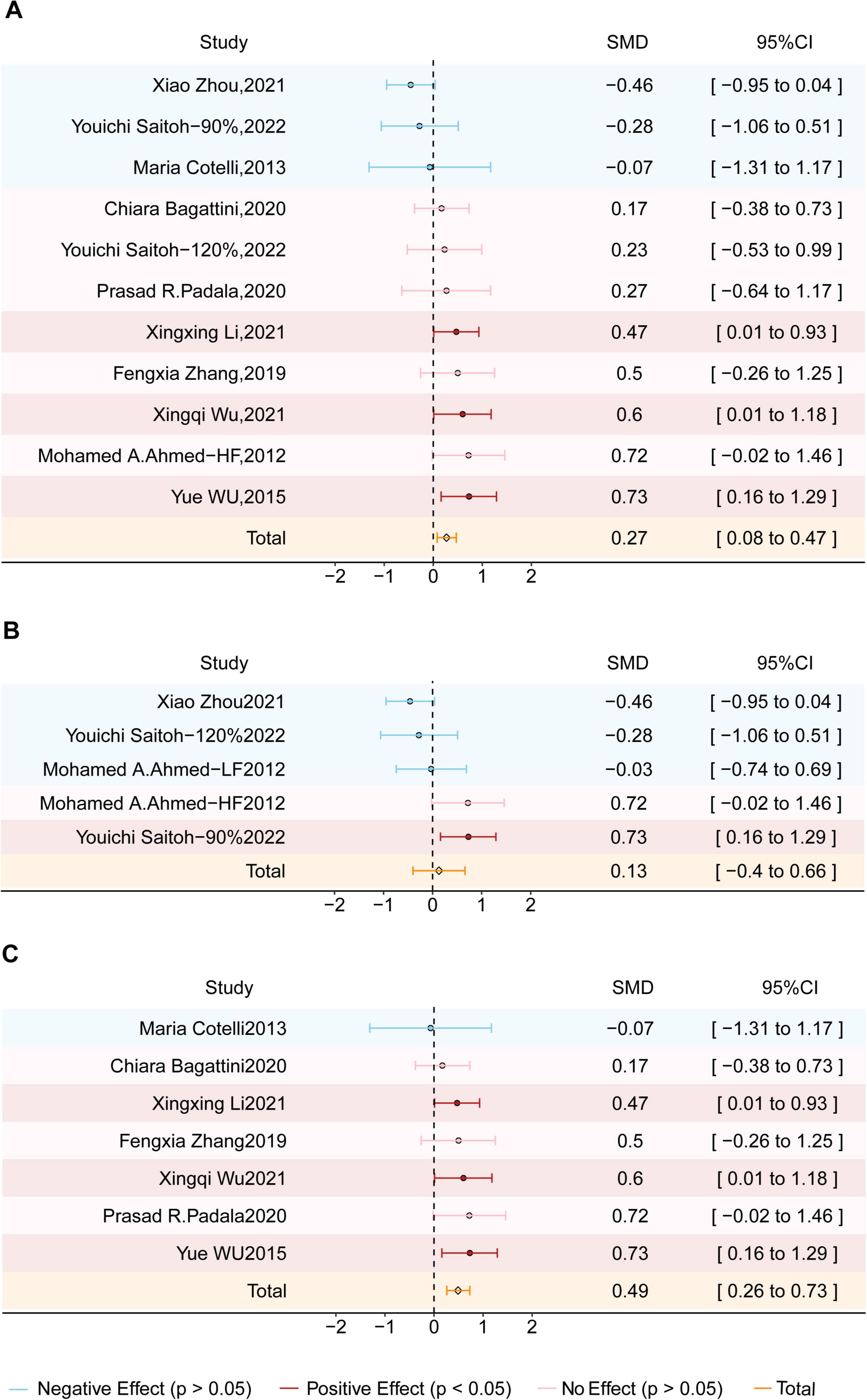
Figure 4. Forest plot shows the SMD (Standardized Mean Difference) and 95% CI (Confidence Interval) for three subgroups: (A) Excitatory stimulation, (B) Bilateral dorsolateral prefrontal cortex (DLPFC) stimulation, and (C) Left DLPFC stimulation, each compared to the sham group. Different effects are color-coded for clarity: red indicates a positive effect, blue signifies a negative effect, and pink denotes no effect. The orange diamond symbolizes the overall effect. If the diamond does not intersect the line of no effect, the overall effect is statistically significant; otherwise, it is not statistically significant.
Subgroup analysis of global cognitive function (rTMS on different genetic backgrounds)
To evaluate the impact of genetic background, subgroup analyses were conducted based on geographic origin (see Figure 5). Among the included studies, 14 were conducted in Asia, one in Africa, six in Europe, and one in North America. The Asian subgroup demonstrated a significant cognitive benefit, with an SMD of 0.42 (95% CI = 0.16–0.67; z = 3.21; p = 0.001) and moderate heterogeneity (I2 = 56%; P = 0.004). In contrast, studies conducted in Europe, Africa, and North America did not yield significant effects, which may be due to variability in sample size, treatment protocols, and genetic factors.
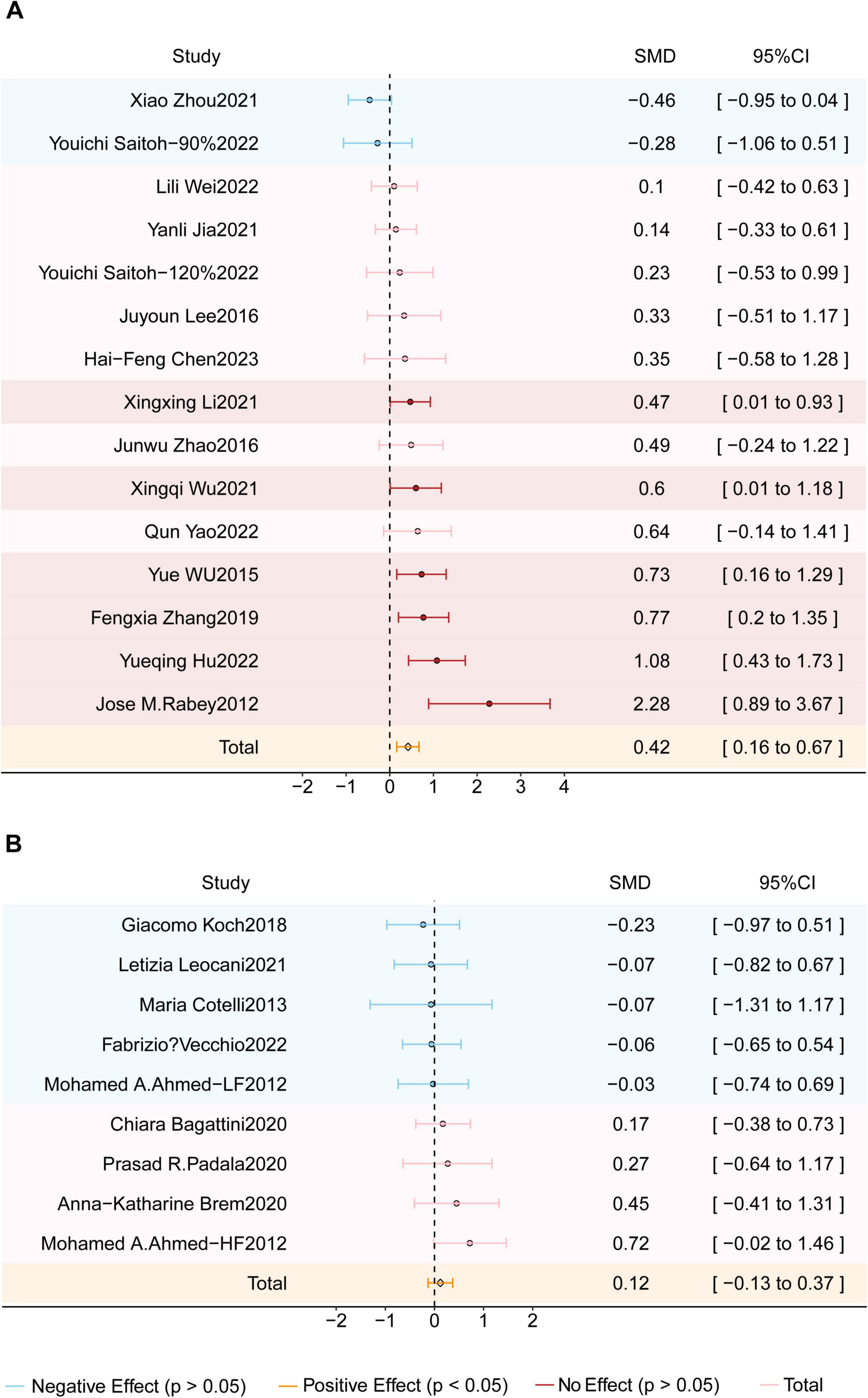
Figure 5. Forest plot shows the SMD (Standardized Mean Difference) and 95% CI (Confidence Interval) for two subgroups: (A) the Asian studies group and (B) the Europe-America-Africa studies group, each compared to the sham group. Different effects are color-coded for clarity: red indicates a positive effect, blue signifies a negative effect, and pink denotes no effect. The orange diamond symbolizes the overall effect. If the diamond does not intersect the line of no effect, the overall effect is statistically significant; otherwise, it is not statistically significant.
Risk of bias assessment
The risk of bias assessment was conducted independently by two reviewers, following the Cochrane Intervention Systematic Review Manual 5.1.0. Any discrepancies were resolved through consultation with a third reviewer. Overall, the included studies were rated as having low to moderate risk of bias, as summarized in Figure 6.
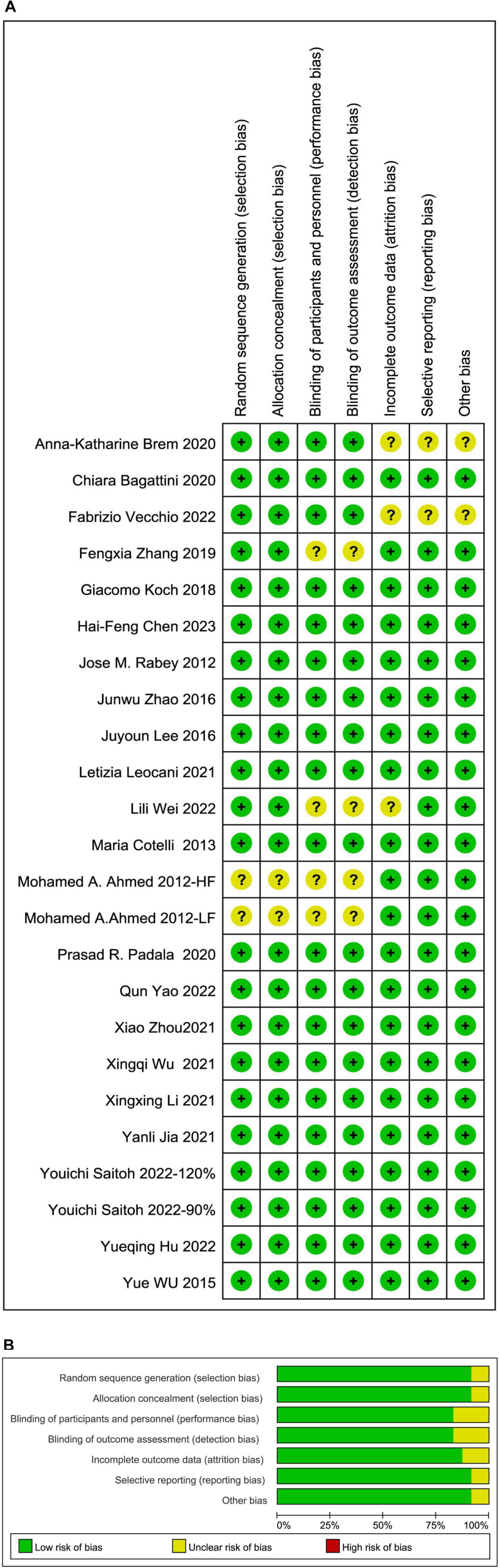
Figure 6. (A) Risk of bias summary and (B) Risk of bias graph present the quality of included studies.
Discussion
This systematic meta-analysis, synthesizing data from 22 randomized, sham-controlled trials involving 874 participants, provided a comprehensive evaluation of the efficacy of rTMS on cognitive function in AD. Our findings confirmed that rTMS significantly enhanced global cognitive performance, with therapeutic effects varying based on stimulation targets. Notably, unilateral DLPFC and parietal lobe stimulation produced moderate cognitive improvements, whereas bilateral DLPFC stimulation did not yield significant effects. In contrast, multi-target rTMS over the bilateral DLPFC, parietal lobes, Wernicke’s area, and Broca’s area produced the most pronounced cognitive benefits. These findings demonstrate the potential of rTMS as a therapeutic intervention for AD, with multi-site stimulation strategies offering greater efficacy than single-site approaches by enhancing network-level plasticity and promoting functional connectivity across cognitive domains.
AD is characterized by profound cognitive impairments, accompanied by widespread structural and functional disruptions in brain networks. Alterations in functional connectivity between the parietal and frontal cortices have been closely linked to cognitive decline in AD (Jia et al., 2021; Velioglu et al., 2021). The parietal lobe, particularly the precuneus, plays an important role in the cortical-hippocampal network that supports memory processing and higher-order cognitive functions (Cavanna and Trimble, 2006). Likewise, the DLPFC is a key hub in the DMN and the ECN essential for working memory, executive function, and cognitive control (Andrews-Hanna et al., 2010; Cai et al., 2017; Cavanna and Trimble, 2006; Crippa et al., 2011). Given the progressive dysfunctions of these networks observed in AD, rTMS over these key regions offers a promising approach to enhancing functional connectivity and attenuating cognitive decline.
Our meta-analysis showed that HF-rTMS over the left DLPFC was effective in improving cognitive function in AD patients. However, several studies (Ahmed et al., 2012; Saitoh et al., 2022; Zhou et al., 2022) employing bilateral DLPFC stimulation yielded inconsistent results, potentially due to differences in stimulation parameters. For example, Ahmed et al. (2012) examined the effects of 20, 1 Hz, or sham rTMS over the bilateral DLPFC, showing that HF-rTMS significantly improved cognitive function whereas LF-rTMS did not elicit cognitive enhancement. These findings suggest that excitatory rTMS is more effective in AD, possibly due to its role in promoting LTP-like plasticity and enhancing synaptic efficiency. Similarly, Saitoh et al. (2022) reported that HF-rTMS over the bilateral DLPFC resulted in cognitive improvements, while another study (Cotelli et al., 2006) found enhanced action naming abilities following bilateral DLPFC stimulation. The neural basis for these effects may involve rTMS-induced modulation of functional connectivity across the DMN, ECN, and FPN, thereby strengthening network integration and cognitive performance (Yuan et al., 2021).
Notably, differential effects of unilateral DLPFC stimulation have also been reported. Zhou et al. (2022) found that 20 Hz rTMS over the left DLPFC combined with 1 Hz rTMS over the right DLPFC led to significant cognitive improvements. This finding aligns with research suggesting that inhibitory rTMS over the right DLPFC may counteract dysfunctional network activity, thereby restoring functional balance in patients with MCI (Fregni and Pascual-Leone, 2007). Given that the right DLPFC is involved in cognitive inhibition (Anderson et al., 2004), LF-rTMS over this region has been shown to reduce hyperactive frontoparietal activity, suppress DMN overactivation (Cui et al., 2019; Wang et al., 2012), and ultimately enhance episodic memory (Turriziani et al., 2019) and recognition performance (Turriziani et al., 2012).
The present study incorporated six studies (Chen et al., 2023; Hu et al., 2022; Jia et al., 2021; Koch et al., 2018; Wei L. et al., 2022; Zhao et al., 2017) that examined the effects of rTMS over the parietal regions such as the left parietal cortex, bilateral parietal lobes, bilateral angular gyrus (AG), and precuneus. Neuroimaging evidence highlights the critical role of the parietal cortex in cognitive processing, particularly in memory consolidation, language processing, and large-scale network integration. Notably, HF-rTMS over the AG has been shown to significantly enhance functional connectivity between the AG and language-related regions such as the left inferior frontal gyrus (IFG) and anterior middle temporal gyrus (aMTG) (Garcia et al., 2022). This network-level modulation may underlie the rTMS-induced improvements in verbal fluency and semantic processing observed in AD patients. Additionally, HF-rTMS over the left AG has been found to increase connectivity with the dorsal medial prefrontal cortex (dMPFC), which plays a fundamental role in memory retrieval and executive function (Chen et al., 2023). There is evidence showing that parietal lobe stimulation induces multiple neurophysiological changes, including increased regional cerebral blood flow, enhanced cortical-subcortical connectivity, and activation of residual hippocampal neurons, all of which contribute to improved synaptic plasticity (Wang et al., 2014). These effects further support the therapeutic potential of this novel stimulation target (Jia et al., 2021). Beyond the AG, the precuneus has emerged as another promising stimulation site due to its central role in episodic memory retrieval (Nellessen et al., 2015). Structural and functional neuroimaging studies have consistently shown that AD patients exhibit reduced precuneus thickness, abnormal task-related activation, and impaired functional connectivity within this region (Chen et al., 2017). Notably, HF-rTMS over the precuneus has been shown to restore DMN connectivity (Wei L. et al., 2022) and modulate long-term memory function (Bonnì et al., 2015; Koch et al., 2018), providing a potential basis for cognitive improvement in AD patients.
Our findings support and extend previous meta-analytic evidence demonstrating the beneficial effects of rTMS on cognitive functions in AD. A large-scale meta-analysis incorporating nine clinical trials with 361 participants demonstrated that rTMS over the DLPFC significantly improved cognitive function in AD patients (Zhang T. et al., 2022). Two additional studies provide compelling evidence that rTMS over the left lateral parietal cortex enhanced functional connectivity within neural networks and improved memory-related cognitive outcomes (Jia et al., 2021; Velioglu et al., 2021). These converging findings underscore the therapeutic potential of combining DLPFC and parietal cortex stimulation, suggesting that multi-target rTMS protocols may be more effective than single-site stimulation for cognitive restoration in AD.
Notably, one study (Yao et al., 2022) included in our meta-analysis explored the effects of HF-rTMS over the bilateral cerebellum, showing that rTMS-induced neuroplastic changes in cerebellar nodes selectively enhanced connectivity with key cortical regions, particularly the DLPFC, cingulate cortex, and medial frontal cortex. These network-level enhancements were associated with significant cognitive improvements, particularly in memory consolidation and language processing. These findings highlight the role of the cerebellum role in higher-order cognitive functions, suggesting that its extensive connectivity with cerebral cortical networks may contribute to rTMS-induced cognitive benefits in AD.
Cognitive impairment in AD is predominantly driven by deficits in synaptic plasticity and neural network dysfunction within the DMN (Brier et al., 2012; Grieder et al., 2018; Menon, 2023). This network encompasses key regions such as the frontal lobes (affected by cholinergic neuron degeneration), the cingulate cortex, posterior parietal areas (including the precuneus), and temporal regions (Boublay et al., 2016; Mohan et al., 2016). Applying rTMS to these regions may enhance network connectivity and functional integration, thereby supporting cognitive improvements in AD patients. Neuroimaging studies provide further insights into the network-level alterations observed in AD. For example, AD-related network disruptions primarily involve bilateral prefrontal-parietal disconnections, as well as disrupted dominant-hemisphere connectivity between posteroinferior frontal and superior temporal regions (Alcalá-Lozano and Garza-Villarreal, 2018; Nguyen et al., 2018).
Our meta-analysis provides one of the first comprehensive evaluations of multi-target rTMS protocols in AD treatment. The subgroup analysis included three studies employing multi-target stimulation, specifically targeting the bilateral DLPFC, Broca’s and Wernicke’s areas, and bilateral parietal somatosensory association cortex (pSAC). These studies followed a structured sequential stimulation approach, selecting three distinct targets daily with no repetition of stimulation sites across consecutive days. Participants underwent 30 treatment sessions over 6 weeks (five sessions/week). While Brem et al. (2020), applied suprathreshold stimulation to all six targets, other studies (Lee et al., 2016; Rabey et al., 2013) employed subthreshold stimulation for Broca’s area and bilateral DLPFC, while using suprathreshold stimulation for other targets. These findings demonstrate significant cognitive improvements across multiple domains, including syntax processing, grammatical comprehension, and spatial memory. The neurobiological mechanisms underlying multi-target rTMS-induced cognitive improvements may involve several neural pathways. The frontal lobe is integral to episodic memory processing (Budson and Price, 2005), while the medial temporal lobe regulates recent memory formation. Additionally, the left prefrontal cortex is critical for verbal working memory tasks (Barbey et al., 2013). Accumulating evidence suggests that rTMS can enhance synaptic plasticity through repeated stimulation of specific neural circuits, leading to cognitive improvements (Brem et al., 2020; Casarotto et al., 2023; Luber and Lisanby, 2014) and strengthening functional connectivity (Johansen et al., 2014). Further, enhanced functional connectivity between the orbitofrontal cortex, DLPFC, and parietal regions facilitates the integration of rewards processing, executive control, and spatial attention during reinforcement learning (Jarbo and Verstynen, 2015).
A large-scale meta-analysis of 831 fMRI studies focused on DLPFC activation patterns demonstrated significant co-activation between the left DLPFC and several key regions, including the right DLPFC, bilateral pSAC, and the anterior cingulate cortex (Alcalá-Lozano and Garza-Villarreal, 2018). These findings highlight the potential of rTMS applied to specific cortical sites to modulate functionally connected neural networks, thereby enhancing cognitive and linguistic capabilities. This effect is thought to be mediated by mechanisms of LTP and LTD, both of which are fundamental to synaptic plasticity and network reorganization (Andersen et al., 2017). HF-rTMS applied in a multi-target sequential stimulation paradigm has been shown to increase synaptic efficacy, elicit LTP-like effects, and strengthen interregional connectivity (Buch et al., 2011; Koch et al., 2013). These mechanisms suggest that network-based rTMS approaches may drive lasting cognitive improvements in AD by enhancing synaptic plasticity and restoring disrupted functional connectivity.
Limitations
This study has several limitations that should be acknowledged. First, the limited number of included studies and small sample sizes constrained the scope of our subgroup analyses, particularly regarding the differential effects of rTMS on distinct cognitive domains. Second, we did not distinguish between left- and right-hemispheric stimulation or different frequency protocols (e.g., HF- vs. LF-rTMS), factors that may contribute to variability in treatment efficacy. Third, cognitive outcomes were predominantly assessed using the MMSE and ADAS-Cog. These measures exhibit variations in sensitivity and reliability across different stages of AD, which may lead to an overestimation or underestimation of the therapeutic effects of rTMS. Future studies incorporating multimodal cognitive assessments, functional neuroimaging markers, and standardized rTMS protocols are essential for refining our understanding of optimal stimulation targets and individual treatment responses in AD.
Conclusion
This meta-analysis provides a comprehensive synthesis of existing evidence on the effects of rTMS on cognitive function in AD. Despite variability across studies, our findings demonstrate that rTMS targeting the DLPFC and parietal lobe leads to significant cognitive improvements. Notably, multi-target rTMS stimulation engaging both prefrontal and parietal regions enhances cognitive outcomes more effectively than single-site stimulation. These results underscore the therapeutic promise of targeted rTMS interventions in mitigating AD-related cognitive decline. Future research should focus on optimizing stimulation protocols, integrating neuroimaging-guided targeting, and exploring individualized approaches to maximize the clinical efficacy of rTMS in the treatment of AD.
Data availability statement
The raw data supporting the conclusions of this article will be made available by the authors, without undue reservation.
Author contributions
YuZ: Formal Analysis, Investigation, Writing – original draft, Writing – review and editing. KD: Software, Writing – review and editing. JY: Data curation, Methodology, Writing – review and editing. QG: Methodology, Writing – review and editing. YaZ: Software, Writing – review and editing. XZ: Funding acquisition, Supervision, Writing – original draft. DL: Funding acquisition, Project administration, Resources, Writing – review and editing. PL: Funding acquisition, Writing – review and editing.
Funding
The author(s) declare that financial support was received for the research and/or publication of this article. The study was supported by grants from the National Natural Science Foundation of China (Nos. 82372558, 82302848, 82402971), Guangdong Basic and Applied Basic Research Foundation (No. 2023A1515011758), and the program of Guangdong Provincial Clinical Research Center for Rehabilitation Medicine (2023B110003).
Conflict of interest
The authors declare that the research was conducted in the absence of any commercial or financial relationships that could be construed as a potential conflict of interest.
Generative AI statement
The authors declare that no Generative AI was used in the creation of this manuscript.
Publisher’s note
All claims expressed in this article are solely those of the authors and do not necessarily represent those of their affiliated organizations, or those of the publisher, the editors and the reviewers. Any product that may be evaluated in this article, or claim that may be made by its manufacturer, is not guaranteed or endorsed by the publisher.
References
Agosta, F., Pievani, M., Geroldi, C., Copetti, M., Frisoni, G., and Filippi, M. (2012). Resting state fMRI in Alzheimer’s disease: Beyond the default mode network. Neurobiol. Aging 33, 1564–1578. doi: 10.1016/j.neurobiolaging.2011.06.007
Ahmed, M., Darwish, E., Khedr, E., El Serogy, Y., and Ali, A. (2012). Effects of low versus high frequencies of repetitive transcranial magnetic stimulation on cognitive function and cortical excitability in Alzheimer’s dementia. J. Neurol. 259, 83–92. doi: 10.1007/s00415-011-6128-4
Alcalá-Lozano, R., and Garza-Villarreal, E. (2018). Overlap of large-scale brain networks may explain the similar cognitive improvement of single-site vs multi-site rTMS in Alzheimer’s disease. Brain Stimul. 11, 942–944. doi: 10.1016/j.brs.2018.03.016
Anderkova, L., and Rektorova, I. (2014). Cognitive effects of repetitive transcranial magnetic stimulation in patients with neurodegenerative diseases - Clinician’s perspective. J. Neurol. Sci. 339, 15–25. doi: 10.1016/j.jns.2014.01.037
Andersen, N., Krauth, N., and Nabavi, S. (2017). Hebbian plasticity in vivo: Relevance and induction. Curr. Opin. Neurobiol. 45, 188–192. doi: 10.1016/j.conb.2017.06.001
Anderson, M., Ochsner, K., Kuhl, B., Cooper, J., Robertson, E., Gabrieli, S., et al. (2004). Neural systems underlying the suppression of unwanted memories. Science 303, 232–235. doi: 10.1126/science.1089504
Andrews-Hanna, J., Reidler, J., Sepulcre, J., Poulin, R., and Buckner, R. (2010). Functional-anatomic fractionation of the brain’s default network. Neuron 65, 550–562. doi: 10.1016/j.neuron.2010.02.005
Bagattini, C., Zanni, M., Barocco, F., Caffarra, P., Brignani, D., Miniussi, C., et al. (2020). Enhancing cognitive training effects in Alzheimer’s disease: Rtms as an add-on treatment. Brain Stimul. 13, 1655–1664. doi: 10.1016/j.brs.2020.09.010
Barbey, A., Koenigs, M., and Grafman, J. (2013). Dorsolateral prefrontal contributions to human working memory. Cortex 49, 1195–1205. doi: 10.1016/j.cortex.2012.05.022
Bonnì, S., Veniero, D., Mastropasqua, C., Ponzo, V., Caltagirone, C., Bozzali, M., et al. (2015). TMS evidence for a selective role of the precuneus in source memory retrieval. Behav. Brain Res. 282, 70–75. doi: 10.1016/j.bbr.2014.12.032
Boublay, N., Schott, A., and Krolak-Salmon, P. (2016). Neuroimaging correlates of neuropsychiatric symptoms in Alzheimer’s disease: A review of 20 years of research. Eur. J. Neurol. 23, 1500–1509. doi: 10.1111/ene.13076
Brem, A., Di Iorio, R., Fried, P., Oliveira-Maia, A., Marra, C., Profice, P., et al. (2020). Corticomotor plasticity predicts clinical efficacy of combined neuromodulation and cognitive training in Alzheimer’s disease. Front. Aging Neurosci. 12:200. doi: 10.3389/fnagi.2020.00200
Brier, M., Thomas, J., Snyder, A., Benzinger, T., Zhang, D., Raichle, M., et al. (2012). Loss of intranetwork and internetwork resting state functional connections with Alzheimer’s disease progression. J. Neurosci. 32, 8890–8899. doi: 10.1523/JNEUROSCI.5698-11.2012
Brignani, D., Manganotti, P., Rossini, P., and Miniussi, C. (2008). Modulation of cortical oscillatory activity during transcranial magnetic stimulation. Hum. Brain Mapp. 29, 603–612. doi: 10.1002/hbm.20423
Buch, E., Johnen, V., Nelissen, N., O’Shea, J., and Rushworth, M. (2011). Noninvasive associative plasticity induction in a corticocortical pathway of the human brain. J. Neurosci. 31, 17669–17679. doi: 10.1523/JNEUROSCI.1513-11.2011
Budson, A., and Price, B. (2005). Memory dysfunction. N. Engl. J. Med. 352, 692–699. doi: 10.1056/NEJMra041071
Cai, S., Peng, Y., Chong, T., Zhang, Y., von Deneen, K., Huang, L., et al. (2017). Differentiated effective connectivity patterns of the executive control network in progressive MCI: A potential biomarker for predicting AD. Curr. Alzheimer Res. 14, 937–950. doi: 10.2174/1567205014666170309120200
Canter, R., Penney, J., and Tsai, L. (2016). The road to restoring neural circuits for the treatment of Alzheimer’s disease. Nature 539, 187–196. doi: 10.1038/nature20412
Casarotto, A., Dolfini, E., Cardellicchio, P., Fadiga, L., D’Ausilio, A., and Koch, G. (2023). Mechanisms of Hebbian-like plasticity in the ventral premotor - primary motor network. J. Physiol. 601, 211–226. doi: 10.1113/JP283560
Cavanna, A., and Trimble, M. (2006). The precuneus: A review of its functional anatomy and behavioural correlates. Brain 129, 564–583. doi: 10.1093/brain/awl004
Chen, H., Sheng, X., Yang, Z., Shao, P., Xu, H., Qin, R., et al. (2023). Multi-networks connectivity at baseline predicts the clinical efficacy of left angular gyrus-navigated rTMS in the spectrum of Alzheimer’s disease: A sham-controlled study. CNS Neurosci. Ther. 29, 2267–2280. doi: 10.1111/cns.14177
Chen, Y., Liu, Z., Zhang, J., Chen, K., Yao, L., Li, X., et al. (2017). Precuneus degeneration in nondemented elderly individuals with APOE ε4: Evidence from structural and functional MRI analyses. Hum. Brain Mapp. 38, 271–282. doi: 10.1002/hbm.23359
Chou, Y., Sundman, M., Ton That, V., Green, J., and Trapani, C. (2022). Cortical excitability and plasticity in Alzheimer’s disease and mild cognitive impairment: A systematic review and meta-analysis of transcranial magnetic stimulation studies. Ageing Res. Rev. 79:101660. doi: 10.1016/j.arr.2022.101660
Cirillo, G., Di Pino, G., Capone, F., Ranieri, F., Florio, L., Todisco, V., et al. (2017). Neurobiological after-effects of non-invasive brain stimulation. Brain Stimul. 10, 1–18. doi: 10.1016/j.brs.2016.11.009
Cotelli, M., Calabria, M., Manenti, R., Rosini, S., Zanetti, O., Cappa, S., et al. (2011). Improved language performance in Alzheimer disease following brain stimulation. J. Neurol. Neurosurg. Psychiatry 82, 794–797. doi: 10.1136/jnnp.2009.197848
Cotelli, M., Manenti, R., Cappa, S., Geroldi, C., Zanetti, O., Rossini, P., et al. (2006). Effect of transcranial magnetic stimulation on action naming in patients with Alzheimer disease. Arch. Neurol. 63, 1602–1604. doi: 10.1001/archneur.63.11.1602
Crippa, A., Cerliani, L., Nanetti, L., and Roerdink, J. (2011). Heuristics for connectivity-based brain parcellation of SMA/pre-SMA through force-directed graph layout. Neuroimage 54, 2176–2184. doi: 10.1016/j.neuroimage.2010.09.075
Cui, H., Ren, R., Lin, G., Zou, Y., Jiang, L., Wei, Z., et al. (2019). Repetitive transcranial magnetic stimulation induced hypoconnectivity within the default mode network yields cognitive improvements in amnestic mild cognitive impairment: A randomized controlled study. J. Alzheimers Dis. 69, 1137–1151. doi: 10.3233/JAD-181296
Dennis, E., and Thompson, P. (2014). Functional brain connectivity using fMRI in aging and Alzheimer’s disease. Neuropsychol. Rev. 24, 49–62. doi: 10.1007/s11065-014-9249-6
Di Pino, G., Pellegrino, G., Assenza, G., Capone, F., Ferreri, F., Formica, D., et al. (2014). Modulation of brain plasticity in stroke: A novel model for neurorehabilitation. Nat. Rev. Neurol. 10, 597–608. doi: 10.1038/nrneurol.2014.162
Dong, X., Yan, L., Huang, L., Guan, X., Dong, C., Tao, H., et al. (2018). Repetitive transcranial magnetic stimulation for the treatment of Alzheimer’s disease: A systematic review and meta-analysis of randomized controlled trials. PLoS One 13:e0205704. doi: 10.1371/journal.pone.0205704
Esposito, S., Trojsi, F., Cirillo, G., de Stefano, M., Di Nardo, F., Siciliano, M., et al. (2022). Repetitive transcranial magnetic stimulation (rTMS) of dorsolateral prefrontal cortex may influence semantic fluency and functional connectivity in fronto-parietal network in Mild cognitive impairment (MCI). Biomedicines 10:994. doi: 10.3390/biomedicines10050994
Fox, P., Ingham, R., George, M., Mayberg, H., Ingham, J., Roby, J., et al. (1997). Imaging human intra-cerebral connectivity by PET during TMS. Neuroreport 8, 2787–2791. doi: 10.1097/00001756-199708180-00027
Fregni, F., and Pascual-Leone, A. (2007). Technology insight: Noninvasive brain stimulation in neurology-perspectives on the therapeutic potential of rTMS and tDCS. Nat. Clin. Pract. Neurol. 3, 383–393. doi: 10.1038/ncpneuro0530
Garcia, A., Cohen, R., Porges, E., Williamson, J., and Woods, A. (2022). Functional connectivity of brain networks during semantic processing in older adults. Front. Aging Neurosci. 14:814882. doi: 10.3389/fnagi.2022.814882
Grieder, M., Wang, D., Dierks, T., Wahlund, L., and Jann, K. (2018). Default mode network complexity and cognitive decline in mild Alzheimer’s disease. Front. Neurosci. 12:770. doi: 10.3389/fnins.2018.00770
Horn, A., Ostwald, D., Reisert, M., and Blankenburg, F. (2014). The structural-functional connectome and the default mode network of the human brain. Neuroimage 102, 142–151. doi: 10.1016/j.neuroimage.2013.09.069
Hsu, W., Ku, Y., Zanto, T., and Gazzaley, A. (2015). Effects of noninvasive brain stimulation on cognitive function in healthy aging and Alzheimer’s disease: A systematic review and meta-analysis. Neurobiol. Aging 36, 2348–2359. doi: 10.1016/j.neurobiolaging.2015.04.016
Hu, Y., Jia, Y., Sun, Y., Ding, Y., Huang, Z., Liu, C., et al. (2022). Efficacy and safety of simultaneous rTMS-tDCS over bilateral angular gyrus on neuropsychiatric symptoms in patients with moderate Alzheimer’s disease: A prospective, randomized, sham-controlled pilot study. Brain Stimul. 15, 1530–1537. doi: 10.1016/j.brs.2022.11.009
Jarbo, K., and Verstynen, T. (2015). Converging structural and functional connectivity of orbitofrontal, dorsolateral prefrontal, and posterior parietal cortex in the human striatum. J. Neurosci. 35, 3865–3878. doi: 10.1523/JNEUROSCI.2636-14.2015
Jia, Y., Xu, L., Yang, K., Zhang, Y., Lv, X., Zhu, Z., et al. (2021). Precision repetitive transcranial magnetic stimulation over the left parietal cortex improves memory in Alzheimer’s disease: A randomized, double-blind, sham-controlled study. Front. Aging Neurosci. 13:693611. doi: 10.3389/fnagi.2021.693611
Johansen, J., Diaz-Mataix, L., Hamanaka, H., Ozawa, T., Ycu, E., Koivumaa, J., et al. (2014). Hebbian and neuromodulatory mechanisms interact to trigger associative memory formation. Proc. Natl. Acad. Sci. U S A. 111, E5584–E5592. doi: 10.1073/pnas.1421304111
Koch, G., Bonnì, S., Pellicciari, M., Casula, E., Mancini, M., Esposito, R., et al. (2018). Transcranial magnetic stimulation of the precuneus enhances memory and neural activity in prodromal Alzheimer’s disease. Neuroimage 169, 302–311. doi: 10.1016/j.neuroimage.2017.12.048
Koch, G., Ponzo, V., Di Lorenzo, F., Caltagirone, C., and Veniero, D. (2013). Hebbian and anti-Hebbian spike-timing-dependent plasticity of human cortico-cortical connections. J. Neurosci. 33, 9725–9733. doi: 10.1523/JNEUROSCI.4988-12.2013
Lane, C., Hardy, J., and Schott, J. (2018). Alzheimer’s disease. Eur. J. Neurol. 25, 59–70. doi: 10.1111/ene.13439
Lee, J., Choi, B., Oh, E., Sohn, E., and Lee, A. (2016). Treatment of Alzheimer’s disease with repetitive transcranial magnetic stimulation combined with cognitive training: A prospective, randomized, double-blind, placebo-controlled study. J. Clin. Neurol. 12, 57–64. doi: 10.3988/jcn.2016.12.1.57
Lefaucheur, J., Aleman, A., Baeken, C., Benninger, D., Brunelin, J., Di Lazzaro, V., et al. (2020). Evidence-based guidelines on the therapeutic use of repetitive transcranial magnetic stimulation (rTMS): An update (2014-2018). Clin. Neurophysiol. 131, 474–528. doi: 10.1016/j.clinph.2019.11.002
Lefaucheur, J., André-Obadia, N., Antal, A., Ayache, S., Baeken, C., Benninger, D., et al. (2014). Evidence-based guidelines on the therapeutic use of repetitive transcranial magnetic stimulation (rTMS). Clin. Neurophysiol. 125, 2150–2206. doi: 10.1016/j.clinph.2014.05.021
Leocani, L., Dalla Costa, G., Coppi, E., Santangelo, R., Pisa, M., Ferrari, L., et al. (2020). Repetitive transcranial magnetic stimulation with H-Coil in Alzheimer’s disease: A double-blind, placebo-controlled pilot study. Front. Neurol. 11:614351. doi: 10.3389/fneur.2020.614351
Li, X., Qi, G., Yu, C., Lian, G., Zheng, H., Wu, S., et al. (2021). Cortical plasticity is correlated with cognitive improvement in Alzheimer’s disease patients after rTMS treatment. Brain Stimul. 14, 503–510. doi: 10.1016/j.brs.2021.01.012
Liao, X., Li, G., Wang, A., Liu, T., Feng, S., Guo, Z., et al. (2015). Repetitive transcranial magnetic stimulation as an alternative therapy for cognitive impairment in Alzheimer’s disease: A meta-analysis. J. Alzheimers Dis. 48, 463–472. doi: 10.3233/JAD-150346
Lin, Y., Jiang, W., Shan, P., Lu, M., Wang, T., Li, R., et al. (2019). The role of repetitive transcranial magnetic stimulation (rTMS) in the treatment of cognitive impairment in patients with Alzheimer’s disease: A systematic review and meta-analysis. J. Neurol. Sci. 398, 184–191. doi: 10.1016/j.jns.2019.01.038
Livingston, G., Sommerlad, A., Orgeta, V., Costafreda, S., Huntley, J., Ames, D., et al. (2017). Dementia prevention, intervention, and care. Lancet 390, 2673–2734. doi: 10.1016/S0140-6736(17)31363-6
Luber, B., and Lisanby, S. (2014). Enhancement of human cognitive performance using transcranial magnetic stimulation (TMS). Neuroimage 85, 961–970. doi: 10.1016/j.neuroimage.2013.06.007
Maeda, F., Keenan, J., Tormos, J., Topka, H., and Pascual-Leone, A. (2000). Interindividual variability of the modulatory effects of repetitive transcranial magnetic stimulation on cortical excitability. Exp. Brain Res. 133, 425–430. doi: 10.1007/s002210000432
Menon, V. (2023). 20 years of the default mode network: A review and synthesis. Neuron 111, 2469–2487. doi: 10.1016/j.neuron.2023.04.023
Mohan, A., Roberto, A., Mohan, A., Lorenzo, A., Jones, K., Carney, M., et al. (2016). The significance of the default mode network (DMN) in neurological and neuropsychiatric disorders: A review. Yale J. Biol. Med. 89, 49–57.
Nellessen, N., Rottschy, C., Eickhoff, S., Ketteler, S., Kuhn, H., Shah, N., et al. (2015). Specific and disease stage-dependent episodic memory-related brain activation patterns in Alzheimer’s disease: A coordinate-based meta-analysis. Brain Struct. Funct. 220, 1555–1571. doi: 10.1007/s00429-014-0744-6
Nguyen, J., Suarez, A., Le Saout, E., Meignier, M., Nizard, J., and Lefaucheur, J. (2018). Combining cognitive training and multi-site rTMS to improve cognitive functions in Alzheimer’s disease. Brain Stimul. 11, 651–652. doi: 10.1016/j.brs.2018.02.013
Padala, P., Boozer, E., Lensing, S., Parkes, C., Hunter, C., Dennis, R., et al. (2020). Neuromodulation for apathy in Alzheimer’s disease: A double-blind, randomized, sham-controlled pilot study. J. Alzheimers Dis. 77, 1483–1493. doi: 10.3233/JAD-200640
Pennisi, G., Ferri, R., Lanza, G., Cantone, M., Pennisi, M., Puglisi, V., et al. (2011). Transcranial magnetic stimulation in Alzheimer’s disease: A neurophysiological marker of cortical hyperexcitability. J. Neural Transm. 118, 587–598. doi: 10.1007/s00702-010-0554-9
Plassman, B., Langa, K., Fisher, G., Heeringa, S., Weir, D., Ofstedal, M., et al. (2007). Prevalence of dementia in the United States: The aging, demographics, and memory study. Neuroepidemiology 29, 125–132. doi: 10.1159/000109998
Rabey, J., Dobronevsky, E., Aichenbaum, S., Gonen, O., Marton, R., and Khaigrekht, M. (2013). Repetitive transcranial magnetic stimulation combined with cognitive training is a safe and effective modality for the treatment of Alzheimer’s disease: A randomized, double-blind study. J. Neural Transm. 120, 813–819. doi: 10.1007/s00702-012-0902-z
Sabbagh, M., Sadowsky, C., Tousi, B., Agronin, M., Alva, G., Armon, C., et al. (2020). Effects of a combined transcranial magnetic stimulation (TMS) and cognitive training intervention in patients with Alzheimer’s disease. Alzheimers Dement. 16, 641–650. doi: 10.1016/j.jalz.2019.08.197
Saitoh, Y., Hosomi, K., Mano, T., Takeya, Y., Tagami, S., Mori, N., et al. (2022). Randomized, sham-controlled, clinical trial of repetitive transcranial magnetic stimulation for patients with Alzheimer’s dementia in Japan. Front. Aging Neurosci. 14:993306. doi: 10.3389/fnagi.2022.993306
Sorg, C., Riedl, V., Mühlau, M., Calhoun, V., Eichele, T., Läer, L., et al. (2007). Selective changes of resting-state networks in individuals at risk for Alzheimer’s disease. Proc. Natl. Acad. Sci. U S A. 104, 18760–18765. doi: 10.1073/pnas.0708803104
Talwar, P., Kushwaha, S., Chaturvedi, M., and Mahajan, V. (2021). Systematic review of different neuroimaging correlates in mild cognitive impairment and Alzheimer’s disease. Clin. Neuroradiol. 31, 953–967. doi: 10.1007/s00062-021-01057-7
Terao, Y., and Ugawa, Y. (2002). Basic mechanisms of TMS. J. Clin. Neurophysiol. 19, 322–343. doi: 10.1097/00004691-200208000-00006
Turriziani, P., Smirni, D., Mangano, G., Zappalà, G., Giustiniani, A., Cipolotti, L., et al. (2019). Low-frequency repetitive transcranial magnetic stimulation of the right dorsolateral prefrontal cortex enhances recognition memory in Alzheimer’s disease. J. Alzheimers Dis. 72, 613–622. doi: 10.3233/JAD-190888
Turriziani, P., Smirni, D., Zappalà, G., Mangano, G., Oliveri, M., and Cipolotti, L. (2012). Enhancing memory performance with rTMS in healthy subjects and individuals with Mild cognitive impairment: The role of the right dorsolateral prefrontal cortex. Front. Hum. Neurosci. 6:62. doi: 10.3389/fnhum.2012.00062
Vecchio, F., Quaranta, D., Miraglia, F., Pappalettera, C., Di Iorio, R., L’Abbate, F., et al. (2022). Neuronavigated magnetic stimulation combined with cognitive training for Alzheimer’s patients: An EEG graph study. Geroscience 44, 159–172. doi: 10.1007/s11357-021-00508-w
Velioglu, H., Hanoglu, L., Bayraktaroglu, Z., Toprak, G., Guler, E., Bektay, M., et al. (2021). Left lateral parietal rTMS improves cognition and modulates resting brain connectivity in patients with Alzheimer’s disease: Possible role of BDNF and oxidative stress. Neurobiol. Learn. Mem. 180:107410. doi: 10.1016/j.nlm.2021.107410
Wang, J., Rogers, L., Gross, E., Ryals, A., Dokucu, M., Brandstatt, K., et al. (2014). Targeted enhancement of cortical-hippocampal brain networks and associative memory. Science 345, 1054–1057. doi: 10.1126/science.1252900
Wang, X., Mao, Z., Ling, Z., and Yu, X. (2020). Repetitive transcranial magnetic stimulation for cognitive impairment in Alzheimer’s disease: A meta-analysis of randomized controlled trials. J. Neurol. 267, 791–801. doi: 10.1007/s00415-019-09644-y
Wang, Z., Liang, P., Jia, X., Jin, G., Song, H., Han, Y., et al. (2012). The baseline and longitudinal changes of PCC connectivity in mild cognitive impairment: A combined structure and resting-state fMRI study. PLoS One 7:e36838. doi: 10.1371/journal.pone.0036838
Wei, L., Zhang, Y., Wang, J., Xu, L., Yang, K., Lv, X., et al. (2022). Parietal-hippocampal rTMS improves cognitive function in Alzheimer’s disease and increases dynamic functional connectivity of default mode network. Psychiatry Res. 315:114721. doi: 10.1016/j.psychres.2022.114721
Wei, Z., Fu, J., Liang, H., Liu, M., Ye, X., Zhong, P., et al. (2022). The therapeutic efficacy of transcranial magnetic stimulation in managing Alzheimer’s disease: A systemic review and meta-analysis. Front. Aging Neurosci. 14:980998. doi: 10.3389/fnagi.2022.980998
Whitfield-Gabrieli, S., and Ford, J. (2012). Default mode network activity and connectivity in psychopathology. Annu. Rev. Clin. Psychol. 8, 49–76. doi: 10.1146/annurev-clinpsy-032511-143049
Wu, X., Ji, G., Geng, Z., Wang, L., Yan, Y., Wu, Y., et al. (2022). Accelerated intermittent theta-burst stimulation broadly ameliorates symptoms and cognition in Alzheimer’s disease: A randomized controlled trial. Brain Stimul. 15, 35–45. doi: 10.1016/j.brs.2021.11.007
Wu, Y., Xu, W., Liu, X., Xu, Q., Tang, L., and Wu, S. (2015). Adjunctive treatment with high frequency repetitive transcranial magnetic stimulation for the behavioral and psychological symptoms of patients with Alzheimer’s disease: A randomized, double-blind, sham-controlled study. Shanghai Arch. Psychiatry 27, 280–288. doi: 10.11919/j.issn.1002-0829.215107
Yao, Q., Tang, F., Wang, Y., Yan, Y., Dong, L., Wang, T., et al. (2022). Effect of cerebellum stimulation on cognitive recovery in patients with Alzheimer disease: A randomized clinical trial. Brain Stimul. 15, 910–920. doi: 10.1016/j.brs.2022.06.004
Yuan, L., Zeng, Q., Wang, D., Wen, X., Shi, Y., Zhu, F., et al. (2021). Neuroimaging mechanisms of high-frequency repetitive transcranial magnetic stimulation for treatment of amnestic mild cognitive impairment: A double-blind randomized sham-controlled trial. Neural Regen. Res. 16, 707–713. doi: 10.4103/1673-5374.295345
Zhang, F., Qin, Y., Xie, L., Zheng, C., Huang, X., and Zhang, M. (2019). High-frequency repetitive transcranial magnetic stimulation combined with cognitive training improves cognitive function and cortical metabolic ratios in Alzheimer’s disease. J. Neural Transm. 126, 1081–1094. doi: 10.1007/s00702-019-02022-y
Zhang, T., Sui, Y., Lu, Q., Xu, X., Zhu, Y., Dai, W., et al. (2022). Effects of rTMS treatment on global cognitive function in Alzheimer’s disease: A systematic review and meta-analysis. Front. Aging Neurosci. 14:984708. doi: 10.3389/fnagi.2022.984708
Zhang, W., Deng, B., Xie, F., Zhou, H., Guo, J., Jiang, H., et al. (2022). Efficacy of repetitive transcranial magnetic stimulation in Parkinson’s disease: A systematic review and meta-analysis of randomised controlled trials. EClinicalMedicine 52:101589. doi: 10.1016/j.eclinm.2022.101589
Zhao, J., Li, Z., Cong, Y., Zhang, J., Tan, M., Zhang, H., et al. (2017). Repetitive transcranial magnetic stimulation improves cognitive function of Alzheimer’s disease patients. Oncotarget 8, 33864–33871. doi: 10.18632/oncotarget.13060
Zheng, W., Cui, B., Han, Y., Song, H., Li, K., He, Y., et al. (2019). Disrupted regional cerebral blood flow, functional activity and connectivity in Alzheimer’s disease: A combined ASL perfusion and resting state fMRI study. Front. Neurosci. 13:738. doi: 10.3389/fnins.2019.00738
Keywords: repetitive transcranial magnetic stimulation, Alzheimer’s disease, cognitive function, stimulation targets, meta-analysis
Citation: Zhang Y, Dong K, Yang J, Guo Q, Zhao Y, Zhu X, Liu D and Liu P (2025) Comparative efficacy of rTMS on different targets in Alzheimer’s disease: a systematic review and meta-analysis. Front. Aging Neurosci. 17:1536573. doi: 10.3389/fnagi.2025.1536573
Received: 29 November 2024; Accepted: 31 March 2025;
Published: 22 April 2025.
Edited by:
Valia Rodríguez-Rodríguez, Aston University, United KingdomReviewed by:
Anita Monteverdi, Neurological Institute Foundation Casimiro Mondino (IRCCS), ItalyAvishek Roy, Karolinska Institutet (KI), Sweden
Copyright © 2025 Zhang, Dong, Yang, Guo, Zhao, Zhu, Liu and Liu. This is an open-access article distributed under the terms of the Creative Commons Attribution License (CC BY). The use, distribution or reproduction in other forums is permitted, provided the original author(s) and the copyright owner(s) are credited and that the original publication in this journal is cited, in accordance with accepted academic practice. No use, distribution or reproduction is permitted which does not comply with these terms.
*Correspondence: Peng Liu, bGl1cGVuZzJAbWFpbC5zeXN1LmVkdS5jbg==; Dongxu Liu, bGl1ZHgyNkBtYWlsLnN5c3UuZWR1LmNu
†These authors have contributed equally to this work