- 1NIAB East Malling, West Malling, United Kingdom
- 2World Vegetable Center Eastern and Southern Africa, Arusha, Tanzania
- 3Tanzania Agricultural Research Institute - Mikocheni (TARI - Mikocheni), Dar es Salaam, Tanzania
- 4NIAB, Park Farm, Cambridge, United Kingdom
The African eggplant (Solanum aethiopicum L., Gilo group) is one of the most common traditional vegetables in Tanzania. The yield of the African eggplant in Tanzania is far lower than the potential yield expected, which is due primarily to biotic and abiotic stresses. We conducted experiments at two locations in Tanzania to study whether or not plant growth and the fruit yield of the Gilo group of African eggplant can be significantly improved through transplanting coupled with the inoculation of arbuscular mycorrhizal fungi (AMF) at sowing. Many plants, particularly for the directly sown treatment, died well before harvesting, and, in addition, many surviving plants showed symptoms of wilting. The transplanting of tray seedlings and using AMF inocula at sowing led to an overall significant increase in fruit yield by 30%–40% and 20%–25%, respectively. Increased fruit yield per experimental plot resulted primarily from a greater number of plants that survived and reached the harvesting stage. The exact effect of planting method (i.e., transplanting or direct sowing) and AMF inoculation, however, varied greatly with plant accessions. Further research is needed to understand the cause(s) for plant mortality in order to develop appropriate plant management practices.
Introduction
The African eggplant (Solanum aethiopicum L.) is a common traditional vegetable in Tanzania and Uganda (Omondi et al., 2016; Ochieng et al., 2018). The Gilo group of S. aethiopicum is grown for its fruit, whereas the Shum group is grown for its leaves. The African eggplant is one of two major strategic African traditional vegetables used for breeding at the World Vegetable Center (Dinssa et al., 2016). The vegetable is grown for both its fruit and leaves. It has the potential for improving livelihoods and production has been shifting from a low-input vegetable for home consumption to a high-input, market-oriented crop (Chadha and Mndiga, 2007). The common Gilo varieties in Tanzania include ‘DB3’ and ‘Tengeru White’, which are grown for their fruits; these are open-pollinated varieties (OPVs). Recently, hybrid varieties have been released by two multinational breeding companies, but their market opportunity over OPVs is yet to be reported.
The African eggplant of the Gilo group is grown by smallholder farmers via transplanting using irrigation or under rainfed conditions with supplemental irrigation. Farmers usually use less fertilizer for traditional vegetables than the amount used in global vegetables, such as tomato, although the situation is changing as the economic benefit of producing African eggplant is increasing. The yield of the African eggplant in Tanzania usually ranges from 5 t ha−1 to 25 t ha−1, which is by far below the average potential yield of about 42 t ha−1 for this crop. This low yield is due primarily to biotic and abiotic stresses (Mwinuka et al., 2021), including low soil fertility, lack of sufficient irrigation, and pests and pathogens, which in turn lead to heterogeneous/variable plant development (including plant death). Poor crop management and the use of low-performing cultivars, partly due to inadequate knowledge among smallholder farmers, have contributed to increased incidences of pests and diseases, and low soil fertility (Lyimo, 2010). The pathogens causing wilt diseases are the most important biotic factor limiting the yield potential of the African eggplants. In addition to the bacterial pathogen Ralstonia solanacearum (Nahar et al., 2019; Phukan et al., 2019), several fungal genera, including Fusarium, Verticillium, and Rhizoctonia, can infect Solanum melongena and cause wilt symptoms (Najar et al., 2011). However, currently there are no published studies on the causal agent(s) of wilt symptoms in field-grown eggplants of S. aethiopicum.
The inoculation of arbuscular mycorrhizal fungi (AMF) in growing media (including soil) has been shown to improve plant resilience to biotic and abiotic stresses and enhance crop productivity (Jeffries et al., 2003; Elahi et al., 2010; Raklami et al., 2019). Compared with the control plants, inoculation of AMF (Funneliformis mosseae and Glomus versiforme) improved the photosynthetic performance, root activity, and growth of S. melongena plants (Ning et al., 2019). Inoculation of several AMF species alleviated cold stress in the eggplant (S. melongena) by improving photochemical reactions, activating antioxidant defense systems, facilitating the accumulation of protecting molecules, and reducing membrane damage (Pasbani et al., 2020). AMF inoculation of S. melongena eggplants enhanced the biomass, metal uptake, and antioxidant activity of the crop (Chaturvedi et al., 2018). AMF inoculation not only reduced arsenic toxicity, but also increased growth and nutrient uptake of S. melongena plants (Elahi et al., 2010). Greenhouse studies suggested that application of AMF biofertilizers could substantially reduce the use of chemical fertilizers without impairing the quality and yield of the eggplants (S. melongena) (Elahi et al., 2010). However, one study showed that inoculation of S. melongena plants with a commercial product containing Glomus spp. had no significant effect on plant growth, physiological responses, and yield attributes in an organic soilless production system (Chaturvedi et al., 2018).
The transplanting operation is one of the most labor intensive in commercial vegetable production, which is still largely done by hand in many developing countries (Kumar and Raheman, 2008); therefore, much effort has been directed into automating this process, thus leading to advances being made in the automation of vegetable transplanting (Khadatkar et al., 2018). Although transplant production requires higher amounts of human labor than direct sowing, there are several advantages to transplanting instead of direct seed sowing in the field. These advantages include (1) the planting of more vigorous and healthier seedlings in the field, (2) ensuring uniform plant growth, maturity, and higher yields, (3) less soil disturbance, and (4) increasing crop competitiveness against weeds. Transplants of tomato grow stronger than field direct-sown plants because of the better-developed root system (Charles, 1998). Consistent improvements in root and shoot traits and yield were observed for transplants of globe artichoke as compared with direct-sown plants (Leskovar and Othman, 2021). Thus, sowing seeds in trays instead of direct sowing in the soil is common practice in the production of the African eggplants in Tanzania.
Using transplants can offer a more efficient and economical way to pre-colonize seedlings with beneficial microbes in trays before sowing. AMF-inoculated tomato seedlings exhibited better transplant performance in terms of higher shoot fresh weight, shoot-to-root ratio, root biomass, growth rate, and leaf area in soilless culture (Elahi et al., 2010). The application of Gigaspora margarita and Glomus intraradices improved the development of S. melongena seedlings, but the exact effects varied greatly with genotype (Keskin et al., 2010).
In this paper, we report two experiments conducted at two sites in Tanzania to assess the effects of transplanting of tray plants coupled with AMF inoculation at sowing on plant growth and fruit yield of the Gilo group of S. aethiopicum.
Materials and methods
The effect of genotype, AMF inoculation at sowing, and planting methods (i.e., transplanting or direct sowing) on the yield and other traits of S. aethiopicum (the Gilo group) was studied in two locations in Tanzania in 2020: one site was at the Chambezi Agricultural Research Sub Station of the Tanzania Agricultural Research Institute (referred to as Chambezi hereafter; latitude 6.2°S, longitude 38.5°E, and altitude 39 m), and the other was in northern Tanzania at the WorldVeg Eastern and Southern Africa Research Station (referred to as WorldVeg-ESA hereafter; latitude 3.38°S, longitude 36.81°E, and altitude 1,235 m). Table 1 gives the soil characteristics of the two sites, whereas Table 2 gives the summary of the temperature and rainfall during the study period at the two sites. The conditions were generally cooler and wetter at the WorldVeg-ESA site than at the Chambezi site.
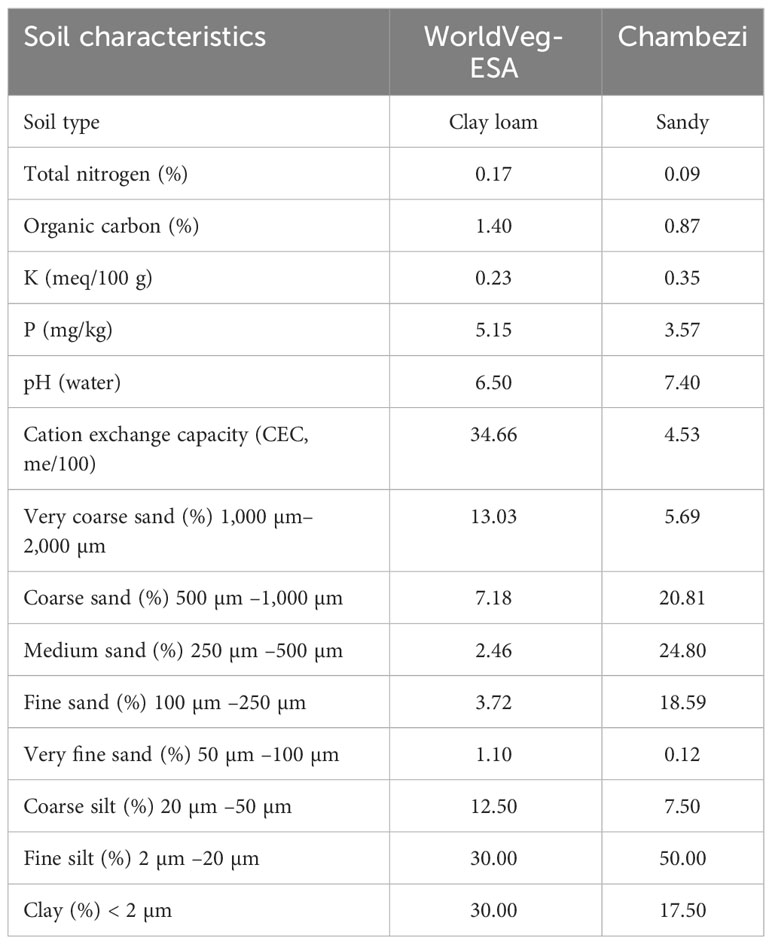
Table 1 Soil chemical and physical characteristics at the two experimental sites: WorldVeg-ESA (World Vegetable Center, Eastern and Southern Africa; altitude 1,235 m, located at 36.8°E and 3.4°S) and Chambezi (Dar es Salaam; altitude 39 m, located at 38.5°E and 6.2°S).
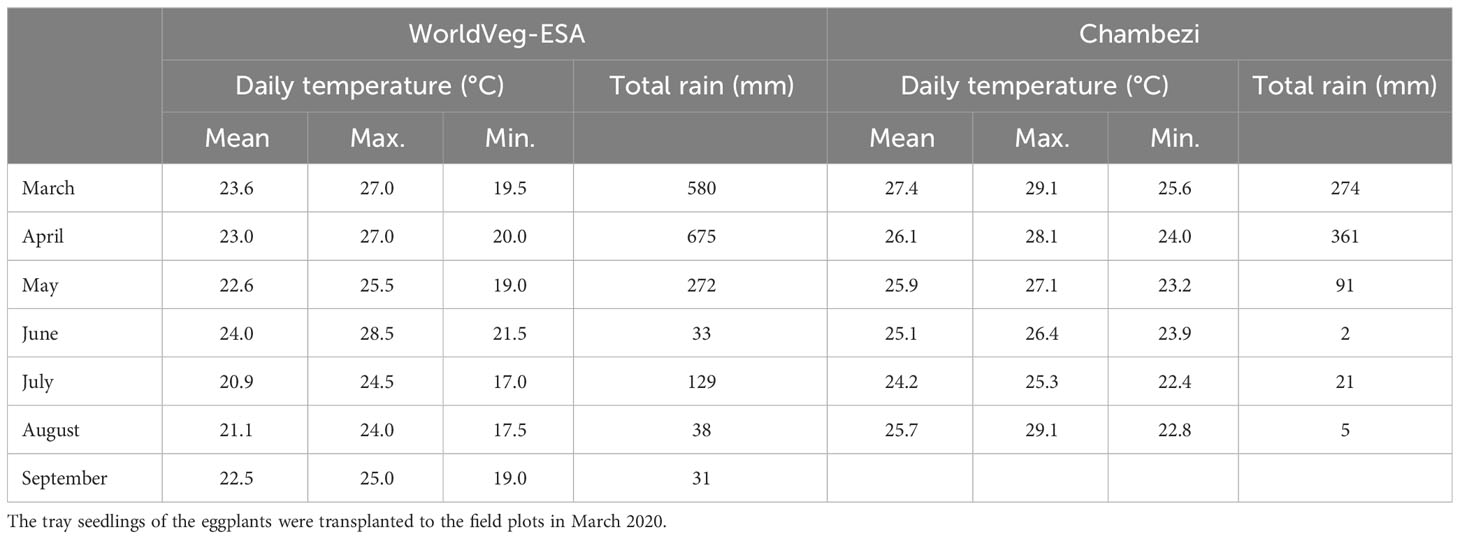
Table 2 Monthly summary of total rainfall and daily temperature during the study period at the two experimental sites: WorldVeg-ESA (World Vegetable Center, Eastern and Southern Africa; altitude 1,235 m, located at 36.8°E and 3.4°S), and Chambezi (Dar es Salaam; altitude 39 m, located at 38.5°E and 6.2°S).
AMF were used in the present research as they can improve the establishment of transplants and subsequent tolerance to abiotic and biotic stresses (e.g., Karagiannidis et al., 2002). A commercial AMF product (RHIZATECH®) was purchased from Dudutech Integrated Pest Management Ltd. (Naivasha, Kenya). All crop management practices of the experimental plots followed the standard practices of the region.
Treatment and planting methods
There were 12 treatments: three African eggplant entities; two planting methods (direct sowing in the field or transplanting of tray plants); with or without AMF inoculation at sowing. Three entities, ‘DB3’ and ‘Tengeru White’ (from the S. aethiopicum Gilo group), and a genebank accession (Solanum anguivi), were grown at two locations—Chambezi and WorldVeg-ESA. At each location, a randomized complete block design was used, with four blocks (replications). Within each replication, there were 12 plots in which the 12 treatments were randomly assigned. A single plot consisted of four rows, each with 12 plants. Both inter-row and within-row inter-plant distances were 60 cm. Thus, the plot size was 17.3 m2, half of which (i.e., the central two rows) was used for plant assessment.
For direct sowing, seeds were individually sown in the appropriate experimental field plots 3 days before sowing in the trays because seeds directly sown under field conditions may take longer to germinate. For the directly sown treatment, seeds were sown at twice the density to guarantee enough plants for assessment. For the direct sowing with AMF treatment, 5 g of AMF per plant (as recommended by the AMF supplier, Dudutech) was placed 1 cm–2 cm directly below each seed and then another soil layer (ca. 1 cm–2 cm) was added on the top of the AMF inocula before sowing the seeds. Similarly, for the AMF application with sowing in trays each cell was first filled with soil to be one-third full before the AMF inocula was added.
Assessment
The number of germinated seeds was counted for seeds sown in the trays before transplanting. Data collection was conducted on the central two rows per plot; the plant in either of the ends of each row was excluded, hence only the central 10 plants were assessed per row.
The experiment at Chambezi
For each plant entity, 12 trays (each with 28 cells) were sown without AMF—a single seed per cell; the other 12 trays were sown with the AMF inocula after each cell was first filled with soil to be one-third full. The seeds were then sown into trays on 10 February 2020, and seedlings of a similar size were transplanted to field plots on 23 March 2020. At the same time of transplanting, field-sown plots were thinned to leave 12 plants per row. Standard crop management was applied to all plots after transplanting.
Plant vigor for each plot was assessed 2 weeks after transplanting on a five-point scale: 0 = very poor vigor (very short plant, very small leaf, and not healthy leaf); 1 = poor vigor (short plant, small leaf, and medium healthy leaf); 2 = good vigor (medium tall plant, medium large leaf, and healthy leaf); 3 = very good vigor (tall plant, large leaf, and healthy leaf); and 4 = excellent vigor (very tall plant, very large leaf, and very healthy leaf). Plant height was measured on 23 June 2020, 6 July 2020, 24 July 2020, 18 August 2020 and 2 September 2020. The number of harvested fruits per plant was counted on 6 July 2020, 24 July 2020, 18 August 2020, and 2 September 2020. The total harvested fruit weight was obtained on five occasions, that is, on 23 June 2020, 6 July 2020, 24 July 2020, 18 August 2020, and 2 September 2020. The fruit length and width, as well as number of locules, were assessed on 18 August 2020. The number of plants showing wilting symptoms was counted on 17 June 2020, 23 June 2020, 6 July 2020, 20 July 2020, 3 August 2020, 18 August 2020, and 2 September 2020. At the final harvest, plants were destructively sampled to estimate root length, root weight, and above-ground plant biomass. However, the number of plants with fruit harvested at each harvest was not recorded; similarly, the total number of surviving plants (or dead plants) was not recorded when wilting symptoms were assessed.
The experiment at WorldVeg-ESA
Direct sowing was conducted on 5 February 2020 and tray sowing on 7 February 2020. The transplanting of the seedlings raised on trays took place on 18 March 2020. For each plant entity, there were 14 trays (each with 66 cells), seven of which were allocated to AMF inoculation.
In addition to seed germination, the wilting of the seedlings in the trays was assessed on 24 February 2020, that is, before transplanting. The field plant assessments included the following traits: (i) the number of branches per plant on 27 April 2020, (ii) plant height (cm) at flowering, (iii) the number of days to flowering, (iv) the number of days to physiological maturity, (v) plant height (cm) at harvest, (vi) the number of plants harvested at each harvest, and (vii) the total fruit yield per plot from five harvests. The wilt symptoms in each plot were assessed on 31 March 2020, 1 April 2020, 8 April 2020, 14 April 2020, 28 April 2020, 5 May 2020, 12 May 2020, 22 May 2020, and 26 May 2020 on a five-point scale: 0 = no wilting; 1 = 1%–20% wilting; 2 = 21%–40% wilting; 3 = 41%–60% wilting; 4 = 61%–80% wilting; and 5 = 81%–100% wilting.
Statistical data analysis
Generalized linear modeling (GLM) was applied to the germination and seedling wilt incidence data in which a single tray was treated as an experimental unit. The plant entity and AMF inoculation were treated as factors in the GLM, with the residuals assumed to follow a binomial distribution. The deviance analysis of nested models was used to assess the significance of the individual treatment factors.
Post-transplanting wilt incidence data were logit-transformed and then subjected to a repeated measurement ANOVA, since wilt was assessed on the same plot over time. For all other post-transplanting variables, only the final assessments and total yield were analyzed with the standard factorial ANOVA with two treatment factors (i.e., planting method and AMF inoculation) for each site separately. For these variables, data were not transformed, as the residuals from the ANOVA were close to being normally distributed.
Finally, plant height and total yield from both the Chambezi and WorldVeg-ESA sites were analyzed together, in which the location was treated as another block factor. All statistical analyses were carried out with R, version 4.1.0 (R Core Development Team, 2019).
Results
The experiment at Chambezi
In total, there were 72 trays used for direct sowing; 12 trays were allocated to a single combination of plant entity and AMF (yes/no) inoculation. The number of germinated seeds per tray (28 seeds in total) ranged from 3 to 26, with an average of 19.5 and median of 21. Figure 1A shows the average germination for each combination of AMF treatment and three plant entities for tray sowing. Applying the AMF inocula led to an increased (p < 0.001) germination rate: 21.4 ± 0.54 (with AMF) vs. 17.6 ± 0.85 (without AMF). The plant entities also differed (p < 0.05): ‘Tengeru White’ (18.5 ± 0.94) had a lower germination than ‘DB3’ (20.1 ± 0.92) and the genebank accession (20.0 ± 0.99). There were no significant interactions between the AMF inoculation and the three plant entities on germination rate.
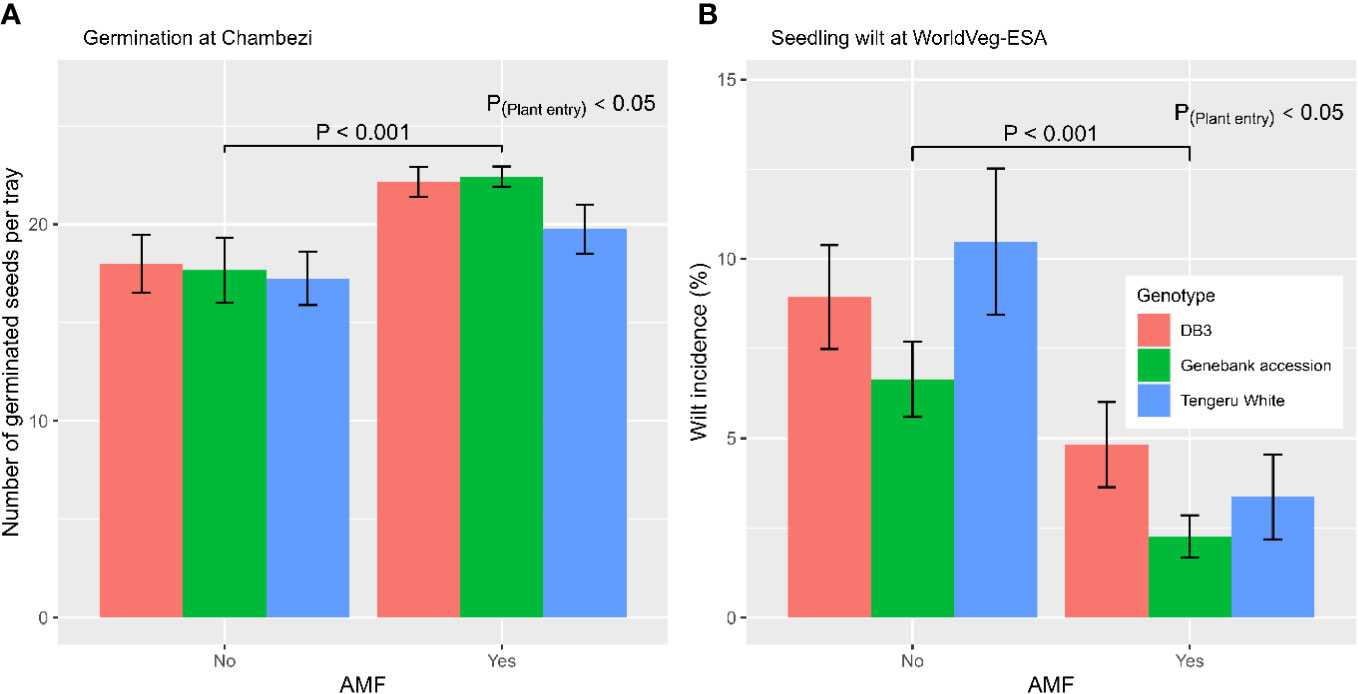
Figure 1 (A) Number of germinated seeds of the three S. aethiopicum entities per tray (28 seeds in each tray) with or without AMF inoculation at sowing at the Chambezi site in Tanzania; and (B) the percentage of seedlings of the three African eggplant entities per tray (66 seeds in each tray) showing wilting in relation to AMF inoculation at sowing for the experiment conducted at the World Vegetable Center Eastern and Southern Africa (WorldVeg-ESA) site in Tanzania.
Four field plots did not establish. Three of the four plots were for ‘DB3’ (block 1—with or without AMF inocula for direct sowing, and block 3 without AMF inocula for direct sowing). The final failed plot was for the direct sowing of the genebank accession without the AMF inocula. These four plots were excluded from all subsequent statistical analysis. Plant vigor was similar among all treatments—all scored three or four.
The temporal wilt dynamics differed (p < 0.001) between the two planting methods (Figure 2A): the wilt incidence increased for the transplanting treatment only. Overall, the three plant entities did not differ significantly in wilting development. In contrast, wilt development was affected by AMF inoculation (p < 0.001), planting method (p < 0.001), and the interaction between AMF and planting method (p < 0.001). Overall, about 3.0 (± 0.33) plants per plot showed wilting symptoms without having been inoculated with AMF, compared with 1.0 (± 0.13) wilting plants in the AMF-inoculated plots. Transplanting had a higher incidence of wilted plants (3.4 ± 0.28 per plot) than direct sowing (0.24 ± 0.04 per plot). The interaction between planting method and AMF inoculation was due primarily to the high incidence of transplanting without AMF inocula (Figure 2B). When GLM was applied to the final wilt assessment, the main results remained the same. The fruit yield of individual plots was positively (p < 0.01) correlated with the wilt incidence (Figure 3).
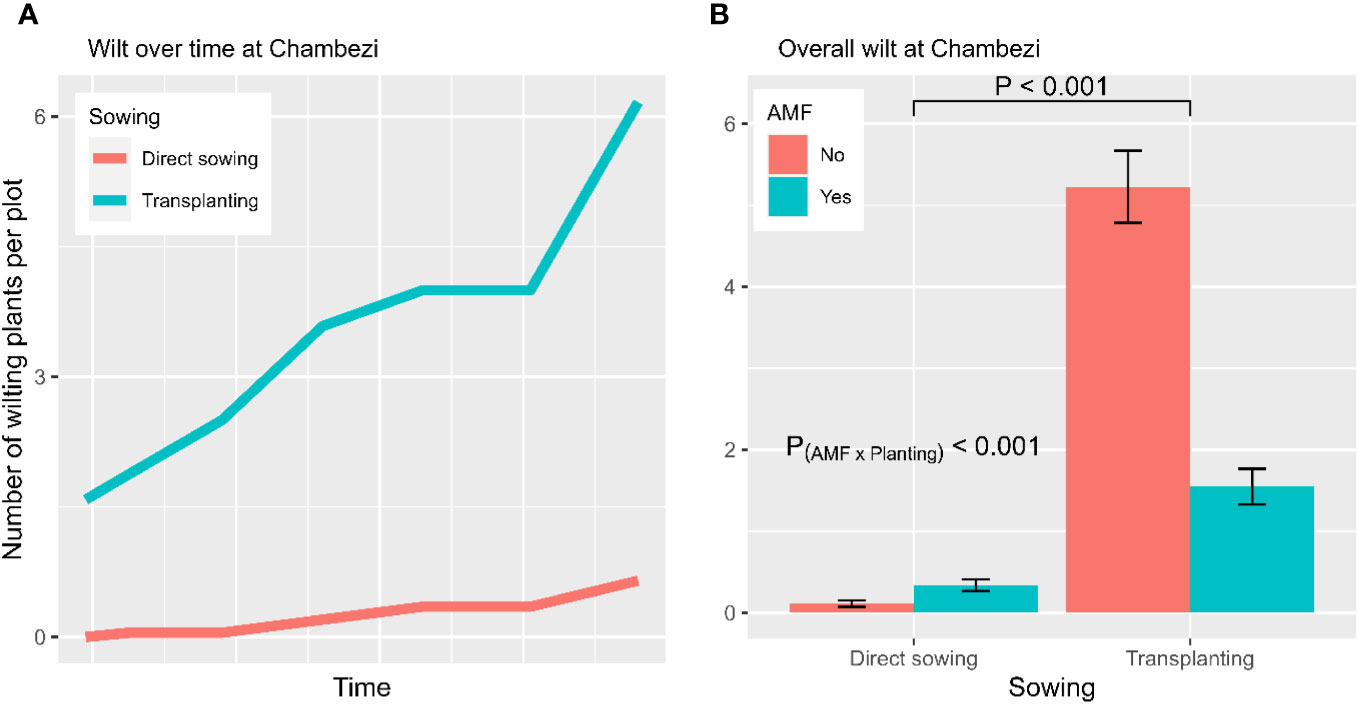
Figure 2 Wilt development from the experiment conducted at Chambezi, Tanzania. (A) The temporal dynamics of wilt development for each combination of planting method and AMF inoculation; and (B) the average number of plants per plot showing wilting symptoms summarized over three African eggplant entities across all assessment times (the line/bar represent one standard error). There were 20 plants assessed per plot.
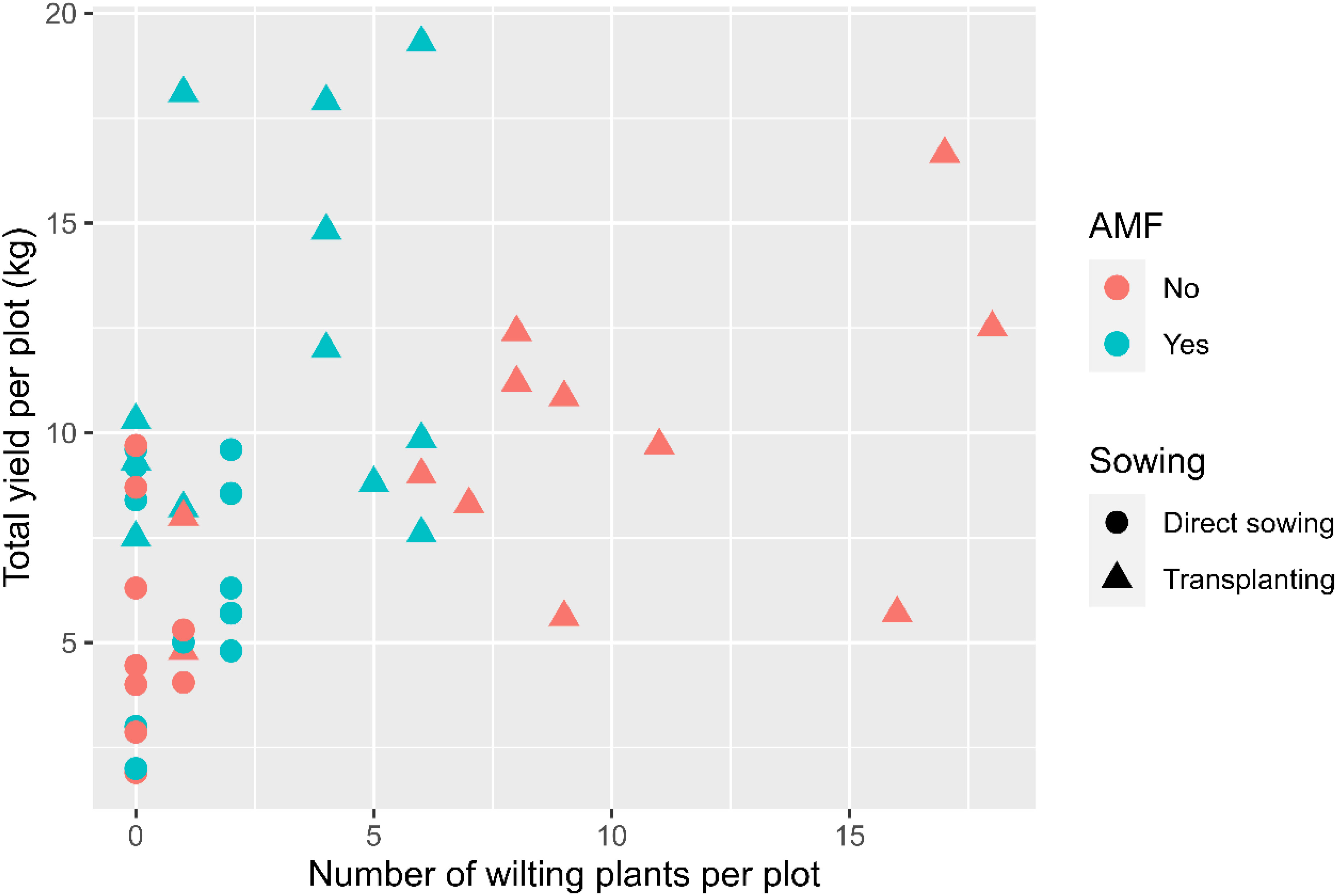
Figure 3 Number of plants with witling symptoms plotted against the total yield in each plot for the experiment at Chambezi, Tanzania in which three African eggplant entities were subjected to each combination of planting method (direct or transplanting) and with/without AMF inoculation at sowing. There were 20 plants assessed per plot.
The ANOVA summary of all other plant traits is given in Table 3. The treatment means for the two planting methods, with or without AMF inoculation, and three plant entities are given in Table 4. The planting method greatly affected (p < 0.001) the number of harvested fruits, total fruit yield, root length and weight, and total biomass, whereas plant entities differed significantly in all traits except root length and weight, and total biomass (Table 3). Although direct sowing had a greater (p < 0.001) number of fruits per harvested plant (ca. 113) than transplanting (ca. 60), its total yield per plot was much lower than that achieved in transplanting: 6.0 kg vs. 10.8 kg. Similarly, the root length was greater (p < 0.001) for direct sowing (57 cm) than for transplanting (32 cm), but the opposite was true for root weight and total biomass (Table 4). Finally, fruit width was less (p < 0.05) for transplanting than for direct sowing. In general, the planting method effect was consistent across the three plant entities, but there were significant interactions between planting method and plant entities for total yield (p < 0.01), root weight (p < 0.01), and total biomass (p < 0.05). The increase in yield, root weight, and total biomass with transplanting was far greater for ‘DB3’ and ‘Tengeru White’ than the genebank accession. For ‘DB3’, the increase was in the range of 90% to 200%, whereas it was about 15%–25% for the other two entities. Figure 4 shows the nature of this interaction between the sowing method and the plant entities for the total yield, root weight, and total biomass. Inoculation with AMF at sowing increased (p < 0.05) the fruit yield by 22%: 7.7 kg (without AMF) vs. 9.4 kg (with AMF). The effect of AMF varied (p < 0.05) with specific plant entities for fruit width: the inoculation with AMF led to a large increase in the fruit width for the genebank accession but reduced fruit width for ‘DB3’ and ‘Tengeru White’.
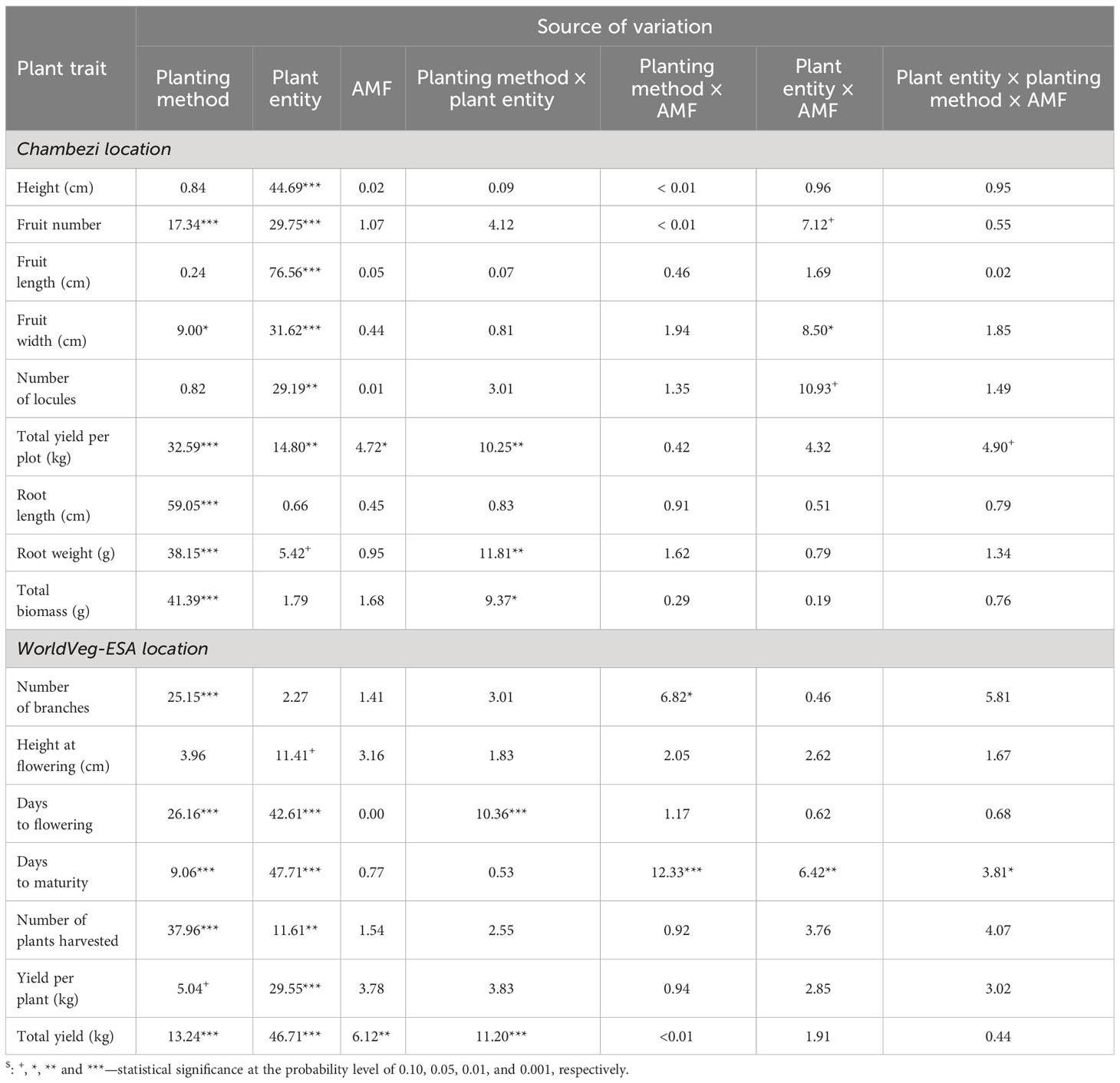
Table 3 Percent sum of squares accounted for by each source of variation in the ANOVA for each assessed trait of the three African eggplant entities subjected to each combination of planting method (direct or transplanting) and AMF inoculation at sowing (with or without) in two experiments (one conducted at Chambezi and the other at the World Vegetable Center Eastern and Southern Africa, Tanzania).
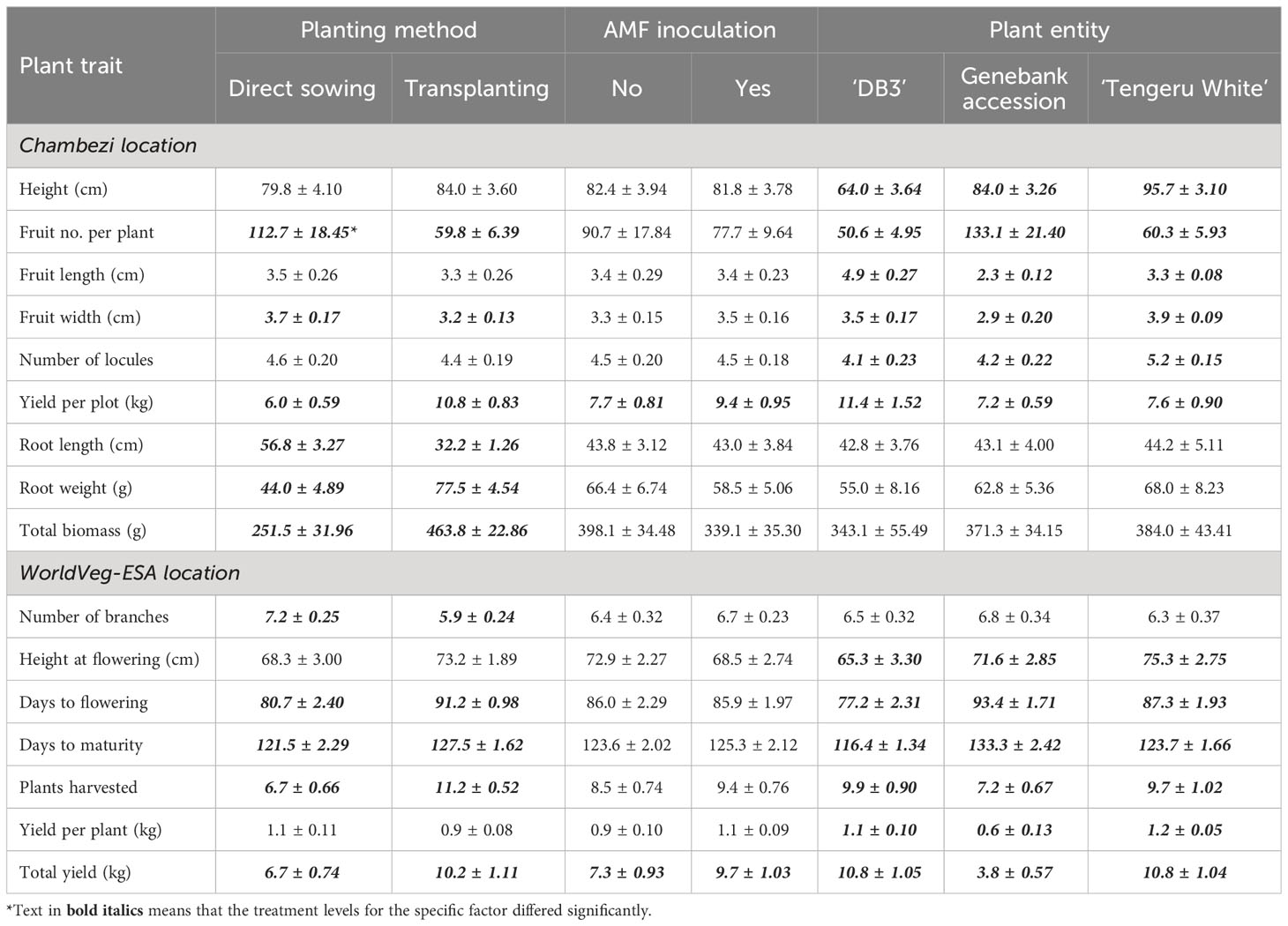
Table 4 Treatment means and standard errors for each trait of the three African eggplant entities in relation to planting method (direct sowing or transplanting) and with/without AMF inoculation at sowing in a randomized complete block design at Chambezi, Tanzania.
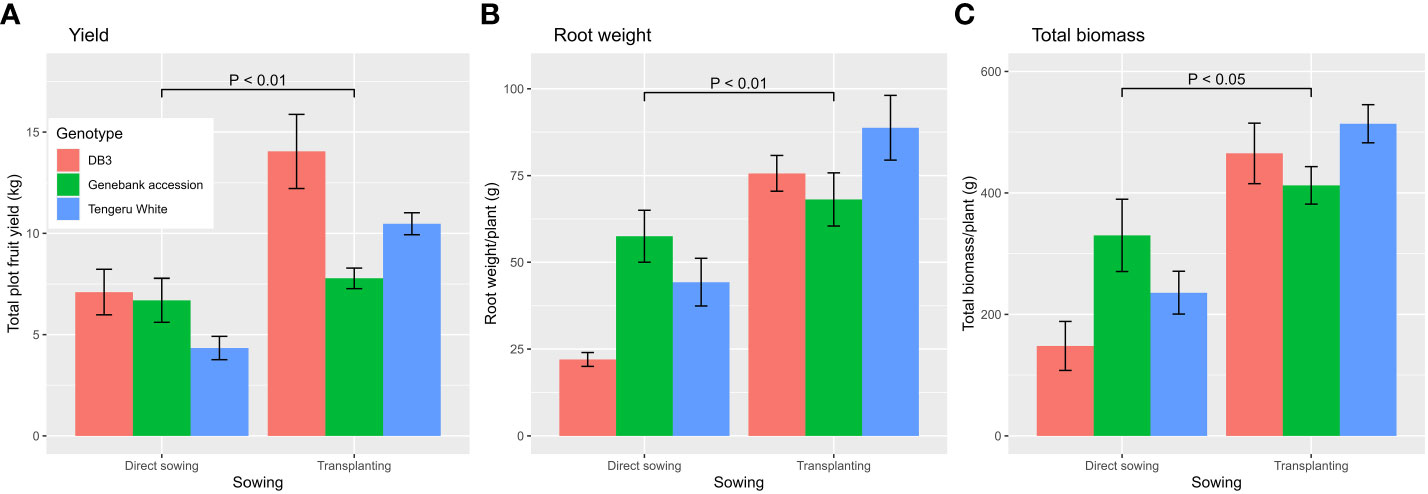
Figure 4 Total fruit yield (A), and root weight (B), and total biomass (C) per plant for three African eggplant entities that were subjected to each combination of planting method (direct or transplanting) and with/without AMF inoculation at sowing at Chambezi, Tanzania. The line/bar represent one standard error. There were 20 plants assessed per plot.
The experiment at WorldVeg-ESA
In total, there were 42 trays used for direct sowing; seven trays were allocated to a single combination of plant entity and AMF (yes/no) inoculation. The number of germinated seeds per tray ranged from 52 to 65, with an average of 60.2 and median of 61. GLM indicated that plant entities differed (p < 0.001) in germination rate: ‘DB3’ (57.2 ± 0.80) had a lower germination rate than ‘Tengeru White’ (60.9 ± 0.91) and the genebank accession (62.6 ± 0.56). Germination was not affected by AMF inoculation at sowing. In contrast, the incidence of wilting in tray seedlings was affected by AMF inoculation (p < 0.001), as well as by plant entity (p < 0.05) (Figure 1B). AMF inoculation led to reduced levels of wilting: 3.5% ± 0.61% (with AMF) vs. 8.7% ± 0.93% (without AMF). The wilting incidence was lower for the genebank accession (4.5% ± 0.84%) than for ‘DB3’ (6.9% ± 1.06%) and ‘Tengeru White’ (6.9% ± 1.50%).
Repeated measurement ANOVA suggested that only entities differed (p < 0.01) in the level of wilt development post planting. When GLM was applied to the final assessment data, wilt incidence was affected (p < 0.001) by planting method, AMF inoculation, entities, and interactions between planting method and AMF, and between planting method and entities. The overall wilt incidence was 32.6% ± 5.09%, 15.4% ± 2.18%, and 15.4% ± 2.18% for ‘DB3’, ‘Tengeru White’ and the Genebank accession, respectively. Direct sowing led to a higher wilt incidence: 25.3% ± 4.00%, compared with 17.0% ± 01.92% for transplanting. Applying AMF inocula led to a higher wilt incidence: 24.4% ± 3.67%, compared with 17.9% ± 2.60% for no amendment. However, the exact effect of planting method depended on the plant entity and AMF inoculation (Figure 5). The reduction in wilt development associated with transplanting was only observed for ‘DB3’ (Figure 5A). The effect of planting method on wilt incidence was greater when there was no AMF inoculation (Figure 5B). There was no correlation between the incidence of wilt at the final assessment and the total yield.
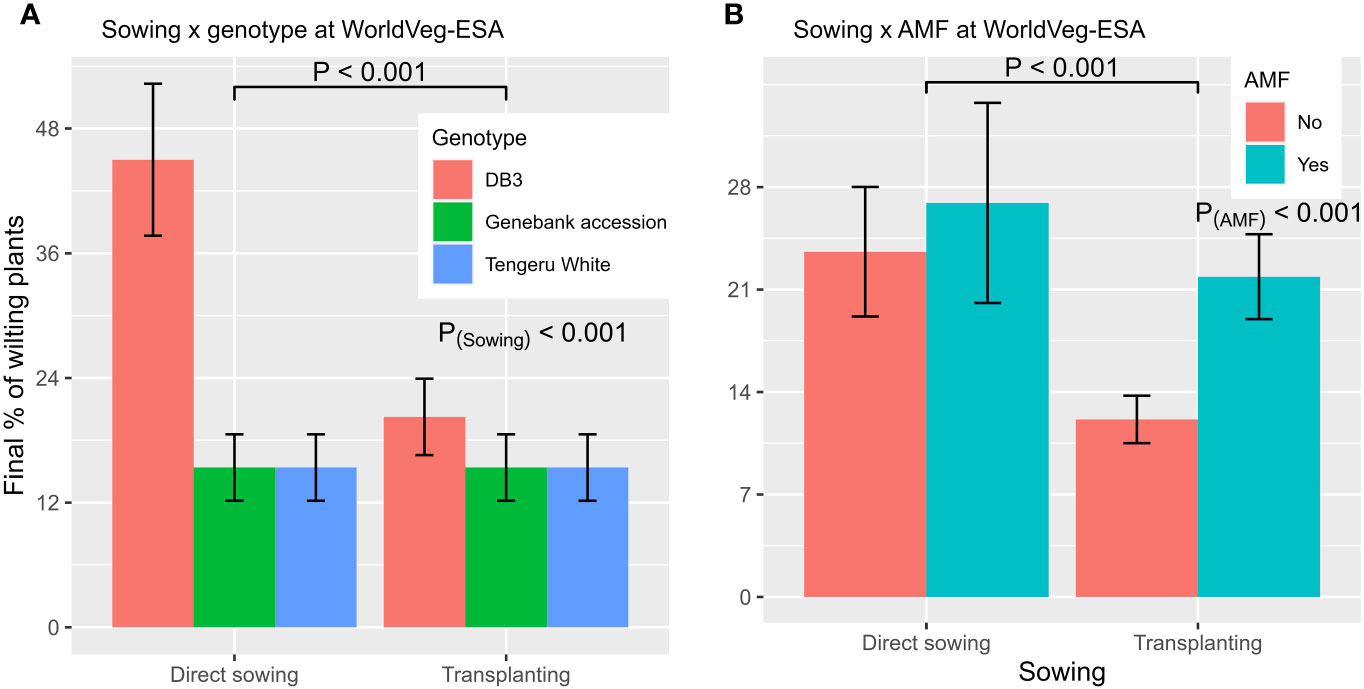
Figure 5 Final incidence of wilted plants from the experiment conducted at the World Vegetable Center Eastern and Southern Africa (WolrdVeg-ESA), Tanzania, demonstrating the nature of interactions of planting method with plant entity (A) and AMF inoculation (B). The line/bar represent one standard error.
The ANOVA summary of all other plant traits is given in Table 3 and the treatment means are presented in Table 4. The planting method greatly affected (p < 0.001) all assessed plant traits, except plant height at flowering and fruit yield per plant. Compared with the direct sowing, transplanting reduced the number of branches from 7.2 to 5.9, delayed flowering and maturity, but increased plant survival from 6.7 plants per plot to 11.2 plants per plot and the total fruit yield from 6.7 kg to 10.2 kg (Table 4). For days to flowering and total yield, the effects of planting methods varied (p < 0.001) with plant entity. The delay to flowering associated with transplanting was much greater in ‘DB3’ (17 days) and ‘Tengeru White’ (13 days) than in the genebank accession (2 days). Similarly, the increase in the total yield associated with transplanting was observed for ‘DB3’ (7.4 kg vs. 14.2 kg; 92%) and ‘Tengeru White’ (8.4 kg vs. 13.2 kg; 56%), whereas for the genebank accession transplanting reduced the total yield (4.2 kg vs. 3.3 kg; 22%).
The inoculation with AMF at sowing only affected (p < 0.01) the total yield per plot, resulting in an increase of 33% in fruit yield (7.3 kg without AMF vs. 9.7 kg with AMF). This AMF effect was consistent over the two planting methods and the three plant entities. There were significant interactions between the planting method and AMF inoculation, and between all three factors for the number of days to maturity. For direct sowing, AMF inoculation delayed maturity for ‘Tengeru White’ and the genebank accession but for transplanting shortened the time for all three plant entities (Figure 6).
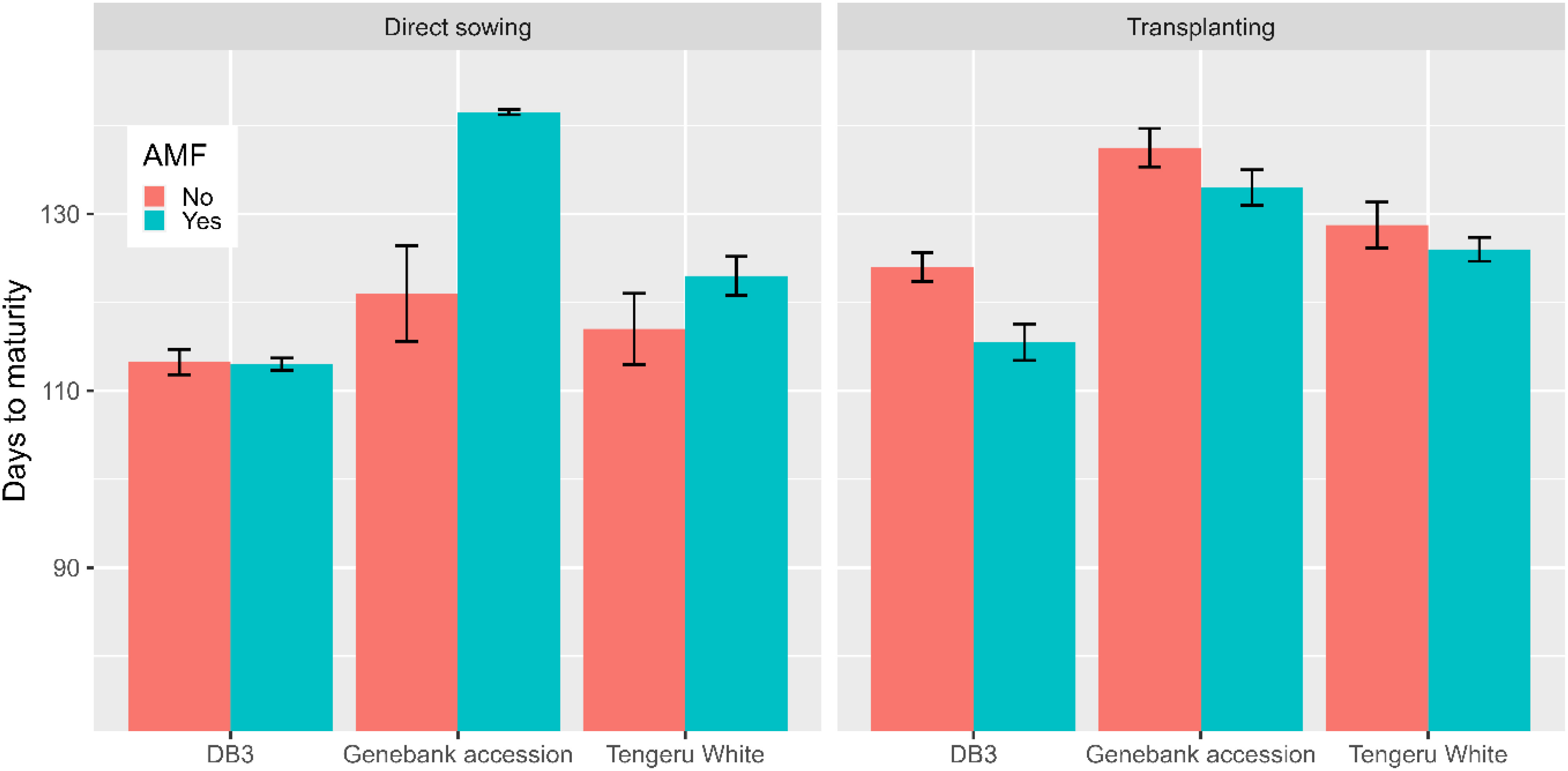
Figure 6 Number of days to maturity for the three African eggplant entities subjected to each combination of planting method and AMF inoculation at the World Vegetable Center Eastern and Southern Africa (WorldVeg-ESA), Tanzania. The line/bar represent one standard error.
Combined analysis of plant height and yield
When plant height and yield were analyzed jointly across the two locations, plant height was primarily affected by plant entity (p < 0.001), accounting for 25.7% of the total variability. The effects of planting method were significant (p < 0.05), but only accounted for 2.5% of the total variability, whereas AMF effects were not significant. For total yield per plot, 18.9% and 5.2% of the observed variability was due to planting method (p < 0.001) and AMF inoculation (p < 0.01), respectively. The three plant entities differed significantly (p < 0.001), accounting for 26.2% of the total variability.
Discussion
We investigated whether adopting the transplanting practice with or without AMF inoculation at sowing could improve plant growth and fruit productivity of the African eggplant (S. aethiopicum, Gilo group and S. anguivi). The present results demonstrated that both transplanting of tray seedlings and the use of AMF inocula at sowing led to an overall significant increase in fruit yield. When interpreting the results from the Chambezi location, we should note that the total number of surviving plants were unfortunately not recorded at each wilting assessment and harvest. It was noted (but not recorded) that many more plants died very early for the directly sown plots than for the transplanted plants; however, these dead plants were not recorded as “wilting” plants. This lack of recording for the total number of surviving plants explains the positive correlation between wilt incidence (namely surviving plants) and yield for the Chambezi location. More transplants survived but showed wilting symptoms, thus resulting in a higher yield than directly sown plots with much fewer surviving plants. Indeed, 4 of the 24 directly sown plots failed to establish completely.
Transplanting significantly increased fruit yield per plot, which is almost all attributable to the increased number of plants that reached the harvest stage for transplanting. At the Chambezi location, direct sowing led to much greater number of fruits per plant than transplanting; at WorldVeg-ESA, direct sowing led to a higher yield per plant than transplanting. However, the increased survival rate associated with transplanting of tray seedlings has more than compensated for the reduced fruit yield per plant, leading to the increased total yield per plot for the transplanting treatment. At the WorldVeg-ESA site, such an increase in the total yield is largely (ca. 75%) attributable to the increased number of plants that produced fruit in the transplanted plots. Fewer plants in the directly sown plots may have experienced less competition for resources, which may partially explain the larger plants and greater yield per plant associated with the direct sowing. Increased survival of transplants may be due to the increased root biomass, supporting water/nutrient uptake and increasing tolerance against water stress and wilt pathogen(s). At the Chambezi location, we underestimated the benefits associated with transplanting as the four directly sown plots that failed to establish in the field were omitted from the statistical analysis (because we could not be certain that the failure of seedling establishment was all due to the direct sowing treatment).
The addition of AMF inocula in trays at sowing led to increased germination rate observed at Chambezi, which cannot be due to the direct effects of AMF establishing in the roots. Similar results were also obtained for several herb species, such as coriander in substrate (Xu, unpublished), when mixed AMF species were applied at sowing. This increased germination might have resulted from nutrients presented in the formulated products and/or volatiles from the applied product. On the other hand, colonization of seedling roots by AMF may account for the observation of the reduction in the wilt incidence of seedlings associated with AMF inoculation at the WorldVeg-ESA site. However, AMF inoculation did not affect post-transplanting wilt incidence at the WorldVeg-ESA site. In contrast, the post-transplanting wilt incidence was lower with AMF inoculation than without AMF at the Chambezi site. The positive effect of using AMF to suppress Verticillium wilt has been demonstrated previously on tomato and eggplant (S. melongena) (Karagiannidis et al., 2002), and cotton (Bu et al., 2009; Kobra et al., 2009). On the other hand, although AMF inoculation improved olive seedling development and establishment in orchards, it failed to reduce Verticillium wilt (Kapulnik et al., 2010). At the Chambezi site, the incidence of post-transplanting wilting was higher for the transplanted plots than for the directly sown plots. As mentioned above, this is likely due to the fact that total number of surviving plants per plot was not recorded. Amending substrate at sowing with AMF led to an overall increase of fruit yield, although to a much lesser extent than transplanting. AMF improved resilience to a limited water supply, and increased yield of solanaceous crops, like the leafy S. aethiopicum, Shum group (Joseph et al., 2022). AMF (Acaulospora laevis) inoculation led to an increased biochemical composition and mineral content of S. melongena eggplants (Sharma et al., 2022). The inoculation of Gigaspora margarita and Glomus intraradices improved development of S. melongena seedlings, but the exact effects varied greatly with genotype (Keskin et al., 2010). The present study suggested that the significant increase in total yield per plot associated with AMF inoculation resulted jointly from an increased plant survival and yield per plant, although the positive effect was not statistically significant for either of the two traits analyzed alone.
Plant wilting in the present study resulted most likely from a pathogen infection; however, water stress cannot be completely excluded. Wilting could have resulted from the legacy of water stress effect associated with the initial establishment of transplants; however, in the present study irrigation was provided when needed. Wilting from water stress is also consistent with the observation that wilting is reduced by AMF inoculation of transplants, as AMF inoculation can improve plants’ tolerance to water stress (Boyer et al., 2015; Oliveira et al., 2022). This may also suggest that, in addition to disease management post transplanting, optimizing irrigation immediately post transplanting could further improve crop establishment and subsequent fruit production. One published study demonstrated that increasing the water supply to transplants led to an increase in yield (Gaveh et al., 2011). It should, however, be noted that even for transplanting there were nearly 45% of plants that failed to reach the harvest stage at the WordVeg-ESA site (data from Chambezi not available). This suggests that there is a greater scope to increase crop production through agronomy management practices to improve plant survival if the precise cause(s) for plant mortality is known. The inoculation of AMF at the time of sowing is expected to increase the probability of early root colonization by AMF, as the AMF are concentrated around the young roots, that is, their presence is much increased, compared with the natural situations where root colonization is by local resident AMF.
In the present study, tray plants were transplanted at a fixed time for all the three plant entities. The exact effect of sowing and AMF inoculation varied greatly with specific plant entities, as indicated by the significant interactions of planting entity with sowing and AMF inoculation. These interactions may have resulted from differential growth dynamics among the three plant entities, indicating the need to optimize transplanting time for specific genotypes/entities. A previous research study showed that transplanting 6-week-old seedlings gave higher-quality leaf production, taller plants, wider crop canopies, broader leaves, and better root development than transplanting 4-week-old seedlings in Solanum macrocarpon plants (Gaveh et al., 2011). Transplanted seedlings performed better than those not transplanted, but the benefit associated with transplanting varied with the three studied genotypes of scarlet eggplants (S. aethiopicum) (Abdulazeez et al., 2020). It should be noted that the observed interactions may have partially resulted from the fact that these plant entities are open-pollinated varieties (OPVs) and hence there are genetic variation within each entity.
In conclusion we showed that using transplanting together with AMF inoculation can significantly increase the fruit yield of the African eggplant (S. aethiopicum, Gilo group). Future research is needed to (1) understand the precise cause(s) of plant mortality in order to develop management practices to minimize the mortality, and (2) optimize seedling physiological age for transplanting to maximize yield gains for individual genotypes.
Data availability statement
The raw data supporting the conclusions of this article will be made available by the authors, without undue reservation.
Author contributions
XX: Conceptualization, Formal Analysis, Funding acquisition, Methodology, Writing – original draft, Writing – review & editing. FD: Data curation, Investigation, Supervision, Writing – review & editing. RM: Data curation, Investigation, Supervision, Writing – review & editing. VM: Data curation, Investigation, Writing – review & editing. OM: Data curation, Investigation, Writing – review & editing. ZM: Data curation, Investigation, Writing – review & editing. ES: Project administration, Writing – review & editing. GB: Funding acquisition, Project administration, Writing – review & editing.
Funding
The author(s) declare financial support was received for the research, authorship, and/or publication of this article. The study was funded by UK Research and Innovation’s Biotechnology and Biological Sciences Research Council (BBSRC) through the GCRF SASSA initiative grant number BB/R020655/1.
Acknowledgments
We acknowledge the World Vegetable Center and its long-term strategic donors for contributing to the implementation of the study. We thank TARI for administrative and technical support.
Conflict of interest
The authors declare that the research was conducted in the absence of any commercial or financial relationships that could be construed as a potential conflict of interest.
The author(s) declared that they were an editorial board member of Frontiers, at the time of submission. This had no impact on the peer review process and the final decision.
Publisher’s note
All claims expressed in this article are solely those of the authors and do not necessarily represent those of their affiliated organizations, or those of the publisher, the editors and the reviewers. Any product that may be evaluated in this article, or claim that may be made by its manufacturer, is not guaranteed or endorsed by the publisher.
References
Abdulazeez O., Daudu Y., Falusi A., Abubakar A., Liman Muhammad M., Anyichie A. R. (2020). Effects of age at transplanting on morphological and yield attributes of three genotypes of Scarlet eggplant. J. Plant Dev. 27, 129–135. doi: 10.33628/jpd.2020.27.1.129
Boyer L. R. L. R., Brain P., Xu X.M.X.-M., Jeffries P. (2015). Inoculation of drought-stressed strawberry with a mixed inoculum of two arbuscular mycorrhizal fungi: effects on population dynamics of fungal species in roots and consequential plant tolerance to water deficiency. Mycorrhiza 25, 215–227. doi: 10.1007/s00572-014-0603-6
Bu J., Cui W., Long X., Bu J., Cui W. D., Lou M., et al. (2009). The effect of inoculation with AMF inoculum on growth and Verticillium wilt of cotton. Xinjiang Agric. Sci. 46, 549–555.
Chadha M. L., Mndiga H. (2007). African eggplant - From underutilized to a commercially profitable venture. Acta Hortic. 752, 521–523. doi: 10.17660/ACTAHORTIC.2007.752.96
Charles M. T. (1998). Physiological basis of UV-C induced disease resistance in post-harvest tomato fruits (PhD) (Canada: Laval University Quebec).
Chaturvedi R., Favas P., Pratas J., Varun M., Paul M. S. (2018). Assessment of edibility and effect of arbuscular mycorrhizal fungi on Solanum melongena L. grown under heavy metal (loid) contaminated soil. Ecotoxicol Environ. Saf. 148, 318–326. doi: 10.1016/J.ECOENV.2017.10.048
Dinssa F. F., Hanson P., Dubois T., Tenkouano A., Stoilova T., Hughes J. A., et al. (2016). AVRDC – The world vegetable center’s women-oriented improvement and development strategy for traditional African vegetables in sub-Saharan Africa. Eur. J. Hortic. Sci. 81, 91–105. doi: 10.17660/EJHS.2016/81.2.3
Elahi F. E., Mridha M. A. U., Aminuzzaman F. M. (2010). Influence of AMF inoculation on growth, nutrient uptake, arsenic toxicity and chlorophyll content of eggplant grown in arsenic amended soil. Adv. Nat. Appl. Sci. 4, 184–192.
Gaveh E. A., Timpo G. M., Agodzo S. K., Shin D. H. (2011). Effect of irrigation, transplant age and season on growth, yield and irrigation water use efficiency of the African eggplant. Hortic. Environ. Biotechnol. 52, 13–28. doi: 10.1007/S13580-011-0054-3/METRICS
Jeffries P., Gianinazzi S., Perotto S., Turnau K., Barea J.-M. (2003). The contribution of arbuscular mycorrhizal fungi in sustainable maintenance of plant health and soil fertility. Biol. Fertil Soils 37, 1–16. doi: 10.1007/s00374-002-0546-5
Joseph M., Geoffrey S., Tom B., Julian N. M., Brian Justus M., Bishop G. J., et al. (2022). Trader acceptability of African eggplant (Solanum aethiopicum Shum) genotypes and effect of bio-control treatments on consumer sensory acceptability. Int. J. Food Sci. Technol. 57, 6165–6180. doi: 10.1111/IJFS.15977
Kapulnik Y., Tsror L., Zipori I., Hazanovsky M., Wininger S., Dag A. (2010). Effect of AMF application on growth, productivity and susceptibility to Verticillium wilt of olives grown under desert conditions. Symbiosis 52, 103–111. doi: 10.1007/s13199-010-0085-z
Karagiannidis N., Bletsos F., Stavropoulos N. (2002). Effect of Verticillium wilt (Verticillium dahliae Kleb.) and mycorrhiza (Glomus mosseae) on root colonization, growth and nutrient uptake in tomato and eggplant seedlings. Sci. Hortic. 94, 145–156. doi: 10.1016/S0304-4238(01)00336-3
Keskin L., Paksoy M., Türkmen Ö. (2010)Effects of arbucular mycorrhizal fungi applications on eggplant seedling development [WWW Document] (Accessed 12.30.22).
Khadatkar A., Mathur S. M., Gaikwad B. B. (2018). Automation in transplanting: A smart way of vegetable cultivation. Curr. Sci. 115, 1884–1892. doi: 10.18520/cs/v115/i10/1884-1892
Kobra N., Jalil K., Youbert G. (2009). Effects of three Glomus species as biocontrol agents against Verticillium-induced wilt in cotton. J. Plant Prot Res. 49, 185–189. doi: 10.2478/v10045-009-0027-z
Kumar G. V. P., Raheman H. (2008). Vegetable transplanters for use in developing countries—A review. Int. J. Vegetable Sci. 14, 232–255. doi: 10.1080/19315260802164921
Leskovar D. I., Othman Y. A. (2021). Direct seeding and transplanting influence root dynamics, morpho-physiology, yield, and head quality of globe artichoke. Plants 10 (5), 899. doi: 10.3390/PLANTS10050899
Lyimo N. E. (2010). Management practices in African egg plant cultivation: A case study of women farmers in mvomero District, Morogoro region Tanzania (MSC). Van Hall Larenstein Univ. Appl. Sci.
Mwinuka P. R., Mbilinyi B. P., Mbungu W. B., Mourice S. K., Mahoo H. F., Schmitter P. (2021). Optimizing water and nitrogen application for neglected horticultural species in tropical sub-humid climate areas: A case of African eggplant (Solanum aethiopicum L.). Sci. Hortic. 276, 109756. doi: 10.1016/J.SCIENTA.2020.109756
Nahar N., Islam M. R., Uddin M. M., de Jong P., Struik P. C., Stomph T. J. (2019). Disease management in eggplant (Solanum melongena L.) nurseries also reduces wilt and fruit rot in subsequent plantings: A participatory testing in Bangladesh. Crop Prot. 120, 113–124. doi: 10.1016/J.CROPRO.2019.02.018
Najar A. G., Anwar A., Masoodi L., Khar M. S. (2011). Evaluation of native biocontrol agents against Fusarium solan f.sp. melongenae causing wilt disease of brinjal in Kashmir. J. Phytology 3, 2075–6240.
Ning C. H., Li W., Zhang C., Liu R. J. (2019). Growth-promotion and disease control effects on chili and eggplant by arbuscular mycorrhizal fungi and plant symbiotic actinomycetes. J. Appl. Ecol. (Chinese) 30, 3195–3202. doi: 10.13287/J.1001-9332.201909.037
Ochieng J., Afari-Sefa V., Karanja D., Kessy R., Rajendran S., Samali S. (2018). How promoting consumption of traditional African vegetables affects household nutrition security in Tanzania. Renewable Agric. Food Syst. 33, 105–115. doi: 10.1017/S1742170516000508
Oliveira T. C., Cabral J. S. R., Santana L. R., Tavares G. G., Santos L. D. S., Paim T. P., et al. (2022). The arbuscular mycorrhizal fungus Rhizophagus clarus improves physiological tolerance to drought stress in soybean plants. Sci. Rep. 12, 1–15. doi: 10.1038/s41598-022-13059-7
Omondi E. O., Debener T., Linde M., Abukutsa-Onyango M., Dinssa F. F., Winkelmann T. (2016). Molecular markers for genetic diversity studies in African leafy vegetables. Adv. Bioscience Biotechnol. 07, 188–197. doi: 10.4236/abb.2016.73017
Pasbani B., Salimi A., Aliasgharzad N., Hajiboland R. (2020). Colonization with arbuscular mycorrhizal fungi mitigates cold stress through improvement of antioxidant defense and accumulation of protecting molecules in eggplants. Sci. Hortic. 272, 109575. doi: 10.1016/J.SCIENTA.2020.109575
Phukan T., Kabyashree K., Singh R., Sharma P. L., Singh N., Barman A., et al. (2019). Ralstonia solanacearum virulence in eggplant seedlings by the leaf-clip inoculation. Phytopathol. Res. 1, 1 1, 1–1 1,11. doi: 10.1186/S42483-019-0030-X
Raklami A., Bechtaoui N., Tahiri A., Anli M., Meddich A., Oufdou K. (2019). Use of rhizobacteria and mycorrhizae consortium in the open field as a strategy for improving crop nutrition, productivity and soil fertility. Front. Microbiol. 10. doi: 10.3389/fmicb.2019.01106
R Core Development Team (2019). R: A language and environment for statistical computing (Vienna, Austria: R Foundation for Statistical Computing).
Keywords: Gilo group eggplant, arbuscular mycorrhizal fungi, transplanting, wilt, yield
Citation: Xu X, Dinssa FF, Minja R, Mwaijande V, Mbwambo O, Mziray Z, Stavridou E and Bishop GJ (2023) Effects of transplanting and AMF inoculation on the fruit yield of African eggplants (Solanum aethiopicum and Solanum anguivi) in Tanzania. Front. Agron. 5:1300553. doi: 10.3389/fagro.2023.1300553
Received: 23 September 2023; Accepted: 23 November 2023;
Published: 18 December 2023.
Edited by:
Ravinder Kumar, Central Potato Research Institute (ICAR), IndiaReviewed by:
Joseph Onyango Gweyi, Kenyatta University, KenyaAurelio Scavo, University of Catania, Italy
Copyright © 2023 Xu, Dinssa, Minja, Mwaijande, Mbwambo, Mziray, Stavridou and Bishop. This is an open-access article distributed under the terms of the Creative Commons Attribution License (CC BY). The use, distribution or reproduction in other forums is permitted, provided the original author(s) and the copyright owner(s) are credited and that the original publication in this journal is cited, in accordance with accepted academic practice. No use, distribution or reproduction is permitted which does not comply with these terms.
*Correspondence: Xiangming Xu, eGlhbmdtaW5nLnh1QG5pYWIuY29t