- 1International Center for Maize and Wheat Improvement (CIMMYT), Southern Africa Regional Office, Harare, Zimbabwe
- 2University of KwaZulu Natal, School of Agricultural, Earth and Environmental Sciences, Pietermaritzburg, South Africa
- 3Department of Plant Production Sciences and Technologies, University of Zimbabwe, Harare, Zimbabwe
- 4Consultative Group on International Agricultural Research (CGIAR), c/o International Institute of Tropical Agriculture (IITA) Headquarters & West Africa Hub, Ibadan, Oyo State, Nigeria
Introduction: Fertilizer use in sub-Saharan Africa (SSA) is the lowest in theworld and has stagnated. Consequently low nitrogen (N) stress is one of the principal constraints to maize yields in this region. Therefore improving nitrogen use efficiency of maize varieties will result in higher nitrogen recovery rates, leading to less leaching of nitrogen as well as loss through nitrification and ammonification. This study aimed to: 1) Investigate the relationship between grain yield under low N and optimal conditions; and 2) Establish the level of variability in low N tolerance among elite Eastern and Southern African (ESA) maize varieties.
Methods: Fifty-eight paired trials, each consisting of 40 to 65 maize hybrids, were conducted under low N and optimal (i.e.,high N) conditions in five countries, in Eastern and Southern Africa during 2013-2015.
Results and discussion: The level of yield reduction as a result of low N stress ranged from 8% to 91% across the 58 paired trails. Grain yield of hybrids ranged from1.69Mg ha-1 to 3.44 Mg ha-1 in the early maturity group and 1.71 Mg ha-1 to 3.35 Mg ha-1 in the intermediate to late maturity group, with heritability ranging from 0.25 to 0.53 and 0.29 to 0.76, in the respective two maturity groups. Under the low N stress. Pre-commercial hybrids that were bred for low N tolerance performed better than the old commercial hybrids and open pollinated varieties (OPVs). These results suggest that if more effort is devoted to selecting maize under low N conditions, significant yield gains can be realized with profound impact on maize productivity in SSA.
Introduction
Intensification of maize production in sub-Saharan Africa (SSA) requires sufficient and efficient use of inorganic fertilizers (Van Ittersum et al., 2019). Maize production is severely impacted by the increasing frequency, intensity and severity of drought combined with heat stress (Wahid et al., 2007; Cairns et al., 2013; Serdeczny et al., 2017; Niu et al., 2021) as well as low soil nitrogen (N) stress (Holden, 2018; Dimkpa et al., 2023). Poor soil management and inadequate addition of both organic and inorganic fertilizer has resulted in severe nutrient mining in SSA, as a result, most farmers in SSA end up producing maize in fields that are deficient in most soil nutrients especially nitrogen. Average fertilizer application rates in the region have stagnated between 13 and 20kg of nutrient per hectare (Sheahan and Barrett, 2017; Jayne and Sanchez, 2021), as opposed to 120kg and 73 kgha-1 in South Asia and Latin America, respectively (Crawford et al., 2005; Begho et al., 2022). In sharp contrast to other regions of the world, fertilizer use in SSA has decreased over the past decade (Kostandini et al., 2015). Coupled with the low fertilizer application rates in SSA, is the low agronomic efficiency of nitrogen, making the application of inorganic fertilizers unprofitable. The agronomic efficiency of N in SSA is about a third of the world average (Jayne and Sanchez, 2021), thereby making fertilizer use unattractive to resource constrained farmers. However, fertilizer use in SSA must increase enormously to meet the required yield increases capable of meeting the demand for food from the rapidly increasing population (Van Ittersum et al., 2019; Cassman and Dobermann, 2022). Low fertilizer use is one of the major causes of the huge yield gaps experienced in SSA despite the genetic gains in maize breeding.
There is need to change from extensification to intensification of smallholder agriculture in order to reduce the conversion of marginal lands into agricultural lands. Extensification has been the major driver of maize production increase in SSA (Giller et al., 2021; Jayne and Sanchez, 2021). Intensification with sufficient and efficient use of both organic and inorganic fertilizers and adapted cultivars as well as best management practices will reduce the environmental impacts (Van Ittersum et al., 2019) while maintaining biodiversity. Improvement in agricultural productivity has many synergistic effects on the environment as well as the economy due to the downstream industries involved. Transitioning to intensification will require a holistic approach to crop and soil management.
The SSA region is extremely heterogenous ecologically, economically as well as in-terms of farmer’s capacity to adapt to yield-constraining shocks. As a result, technical advancement and innovation are not readily adopted thus despite several interventions to increase crop productivity, only Ethiopia has had a major increase in crop productivity (Abate et al., 2015; Jayne and Sanchez, 2021). Unlike climate-related stresses, such as drought and heat, which vary in occurrence, duration, and severity each season, low N stress is a consistent problem for most smallholder farmers. This is particularly true for many female farmers in SSA, who tend to have less access to inputs, including fertilizers (Kilic et al., 2015; Sheahan and Barrett, 2017), creating a large gender gap in agricultural productivity. In SSA, women farmers produce 30% less than men farmers per hectare and one of the main reasons for the low productivity is the 21% gender gap in the use of inorganic fertilizers in maize production (Peterman et al., 2011; Abdisa and Mehare, 2024) The agricultural productivity gender gap is one of the significant and costly inefficiencies derailing the SSA region progress (Buehren, 2023). Increasing agricultural productivity is an important step in poverty reduction as well as ensuring food and nutrition security.
Improved maize varieties with a yield advantage at below recommended fertilization rates would provide an immediate benefit to smallholder farmers. An ex-ante impact assessment of low N stress tolerant maize previously projected new varieties with a yield gain of 16% under very low fertilization rates (0-29 kg N ha-1) and low fertilization rates (30-59 kg N ha-1) would have the potential to deliver a total of US$586 million in gross benefits across SSA, with US$136 million and US$100 million of benefits to maize producers in Kenya and South Africa over nine years (Kostandini et al., 2015). The simultaneous improvement of maize grain yield and the Nitrogen use efficiency (NUE) is an important breeding objective which will reduce environmental pollution as well as reduce fertilizer cost for the resource constrained smallholder farmers. Rongfa et al. (2022) noted an increase in the nitrogen use efficiency of newer hybrids produced between 1990 and 2000 in China compared to the older hybrids. The improved nitrogen use efficiency emanated from the improved remobilization of nitrogen from the pre-anthesis structures.
Bänziger et al. (1997) first investigated the efficiency of direct selection for grain yield under low N stress conditions relative to indirect selection under optimal N in 14 trials in Mexico. The genetic correlation between grain yield under low N and optimal N decreased as yield under low N decreased. The authors concluded that direct selection for low N tolerance was more efficient under low N conditions where yields were at least 40% less than those under optimal conditions. Presterl et al. (2003) found a similar relationship in temperate maize using 21 experiments under low N and optimal conditions in eight locations across France and Germany where N stress reduced grain yields by 14% to 55%. In the current study, we aim to: 1) Investigate the relationship between grain yield under low N and optimal conditions; and 2) Establish the level of variability in low N tolerance among elite Eastern and Southern African (ESA) maize varieties. We hypothesize that a better understanding of the variability that exists in maize for yield performance under low N conditions could guide objective setting of maize breeding programs which will lead to rapid genetic gains under low nitrogen stress conditions in the region.
Materials and methods
Study designs, experiment management and data collection
The study was separated into two parts in order to answer two major objectives: 1) Investigating the relationship between grain yield under low N and optimal conditions; and 2) Establishing the level of variability in low N tolerance among elite Eastern and Southern African (ESA) maize varieties.
Part 1: investigating the relationship between grain yield under low N and optimal conditions
Germplasm
The germplasm used for this study was from the International Maize and Wheat Improvement Centre (CIMMYT) regional (stage 4) trials that are comprised of advanced elite and pre-commercial hybrids which are tested throughout ESA (Masuka et al., 2017a; Setimela et al., 2017a). Regional trials were separated into two maturity groups: early (less than 68 days to anthesis) and intermediate to late (more than 68 days to anthesis). In the early maturity group (EMG), a total of 14 varieties were used, comprising of ten hybrids and four OPVs (see Table 1). In the intermediate to late maturity group (ILMG), a total of 35 varieties (comprised of 28 hybrids and 7 OPVs) were used (see Table 2).
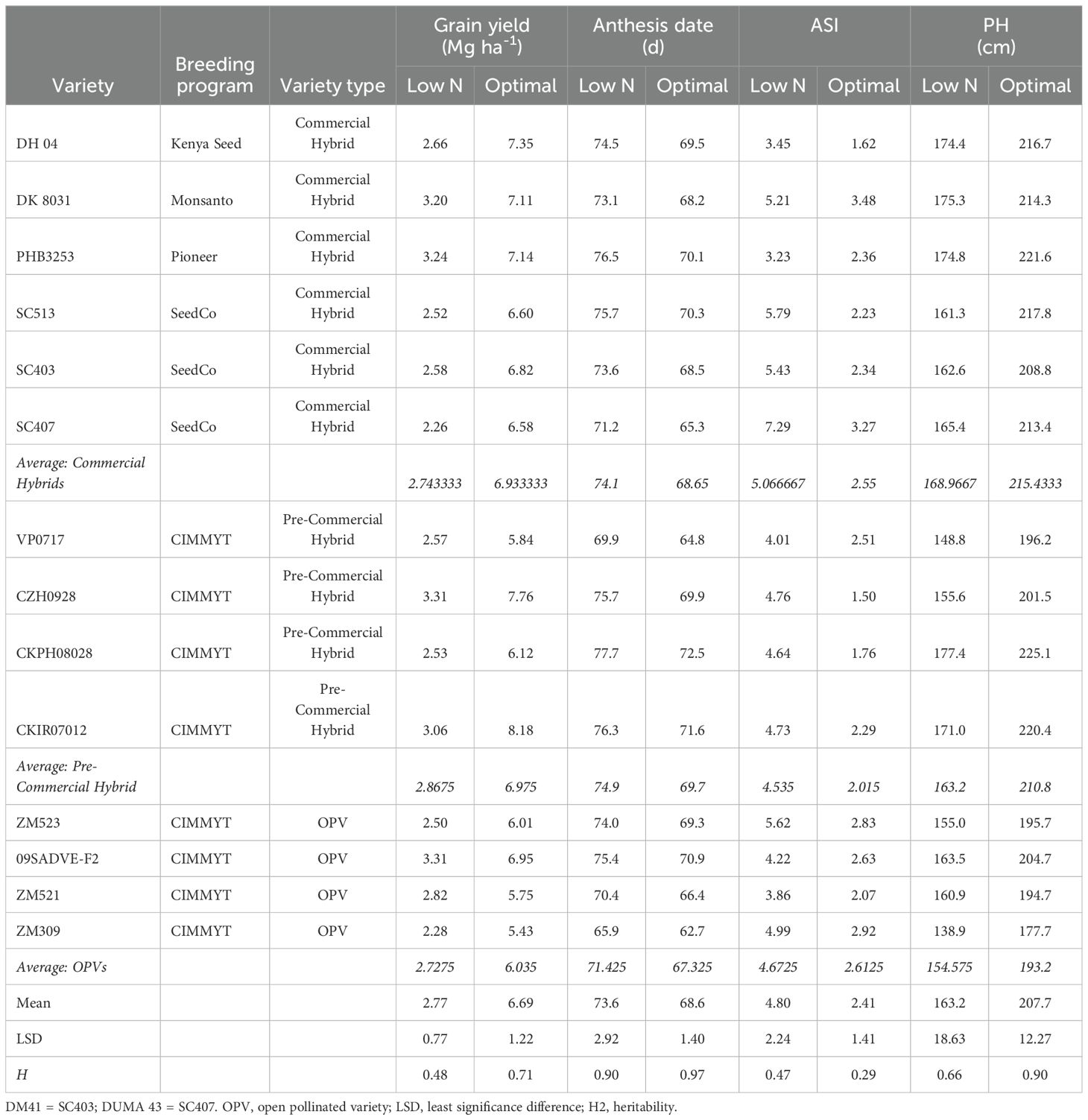
Table 1. Best linear unbiased predictions (BLUPS) for grain yield and best linear unbiased estimates (BLUES) anthesis date, anthesis-silking interval (ASI) and plant height (PH) of under low nitrogen and optimal conditions for early maturity varieties.
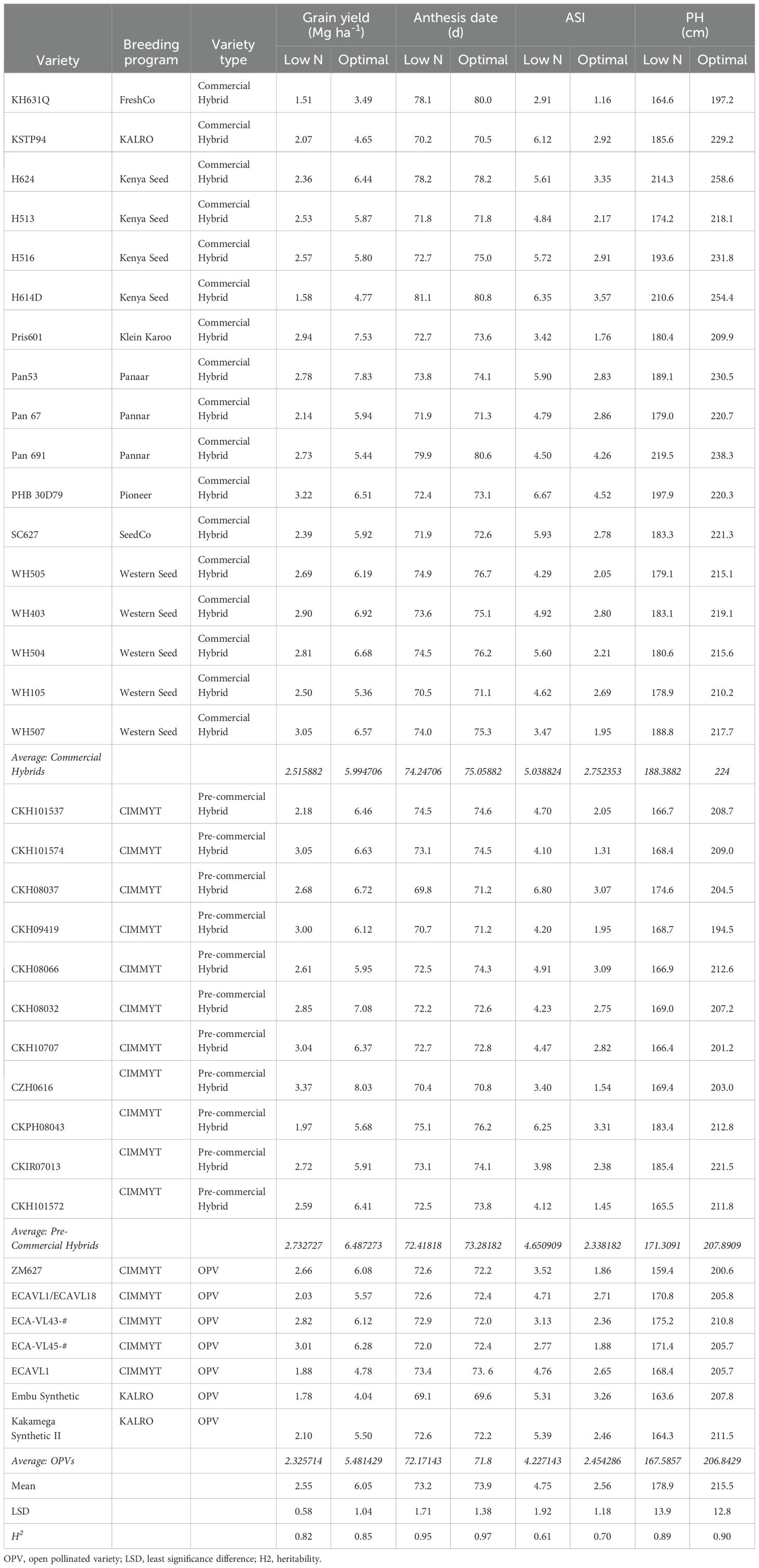
Table 2. Best linear unbiased predictors (BLUPS) for grain yield, anthesis date, anthesis-silking interval (ASI) and plant height (PH) under low nitrogen (N) and optimal conditions for intermediate-late maturity varieties.
Field trials
Experiments were conducted at 13 locations across five countries in ESA between 2013 and 2015 (Table 3). An alpha-lattice design replicated twice was used. At all locations, two seeds per hill were sown, then thinned to one seed per hill three weeks after emergence. Trials were planted at the same time. Experiments were planted in two-row plots, with a final plant density of 5.33 plants m-2. In East Africa optimal trials, Calcium ammonium nitrate (CAN) fertilizer was used at the rate of 54 kg N ha-1, followed by top dressing of urea fertilizer at the rate of 138 kg N ha-1 three weeks after planting. As for southern Africa optimal trials, all plots received 400 kg ha-1 as compound D (7% N, 14% P2O5 and 7% K2O) at sowing. A second application of N (66 kg N ha-1) was applied as urea at the V6 stage. In East Africa managed low N trials, triple super phosphate (46% P2O5) was applied at planting at the rate of 50 kg P2O5 ha-1, with no further top dressing. In southern Africa low N trials, all plots received basal application of P as triple super phosphate at 200 kgha-1 and K as muriate of potash (200 kgha-1). The number of years of depletion of residual N at each location varied from two to six years. The field site in Harare consisted of five separate blocks that had been N-depleted for different lengths of time. A total of 116 experiments were conducted under optimal and low N conditions giving 58 paired sites (low N and well fertilized). At all sites, the blocks were ploughed using a tractor drawn disc plough and harrowed using a disc harrow to obtain a fine tilth to ensure good crop establishment. All recommended plant, weed, and insect control measures were followed.
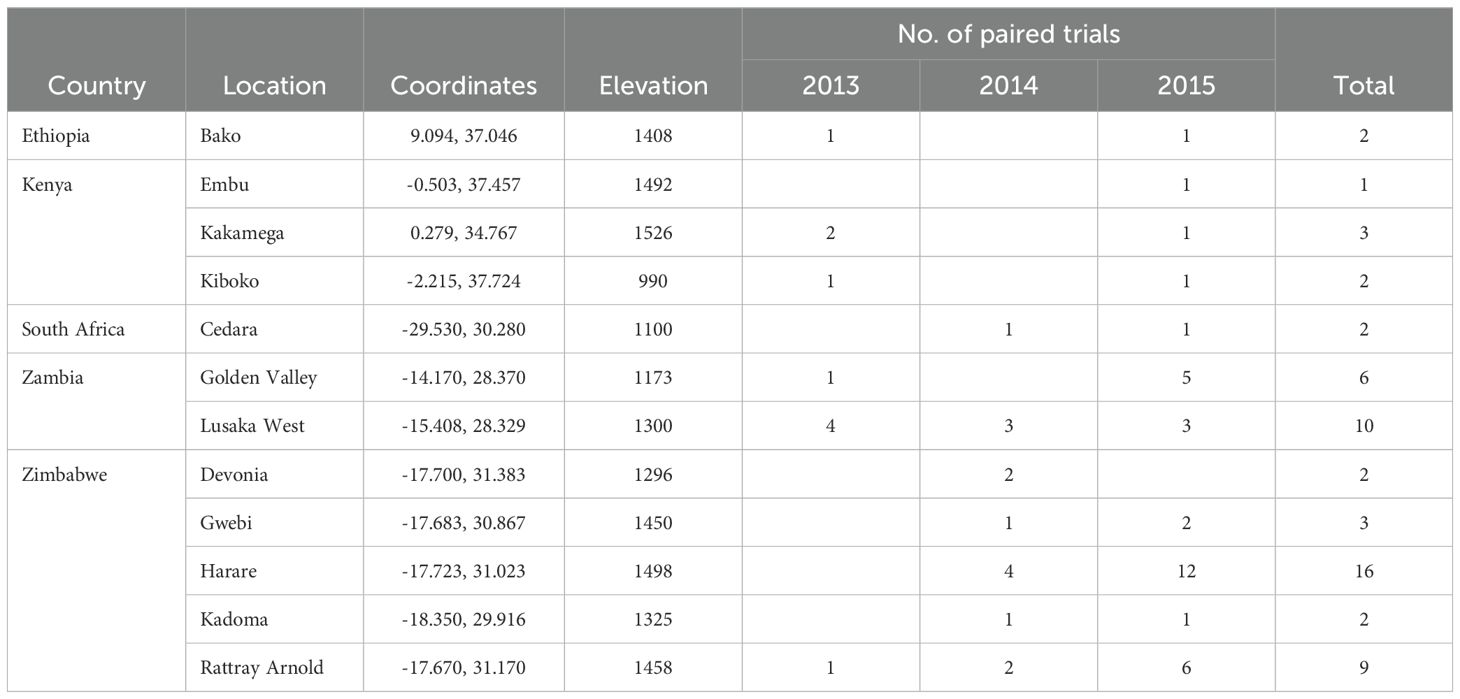
Table 3. Summary of trial locations and the number of trials per site used to determine the relationship between grain yield under low nitrogen and optimal conditions.
Measurements
At physiological maturity, all plants were hand harvested and shelled. The shelled grain was weighed using a scale. Grain yield was estimated as ‘sheled grain weight’ per plot adjusted to 12.5% grain moisture content and expressed as Mgha-1.
Part 2: Establishing the level of genetic variability among elite ESA maize varieties in low N tolerance
Germplasm
Germplasm was selected to represent current commercial varieties available in ESA and experimental varieties. Commercial varieties were not selected under low N stress, with yield potential under optimal conditions being the primary trait of interest. CIMMYT experimental hybrids were primarily selected for drought and low N stress tolerance, and yield potential under optimal conditions. Germplasm was separated into two maturity groups: early and intermediate to late. In the EMG, a total of 14 varieties were used, comprising of ten hybrids and four OPVs. In the ILMG, a total of 35 varieties (comprised of 28 hybrids and 7 OPVs) were used.
Field trials
Experiments were conducted at five locations in Kenya (Embu, Kakamega and Kiboko), Zambia (GART) and Zimbabwe (Harare) in 2011 (Table 4). Details of site coordinates and elevation are presented in Table 3. Two treatments were used: low N stress and optimal. Experiments were planted and managed as described above under Part 1.
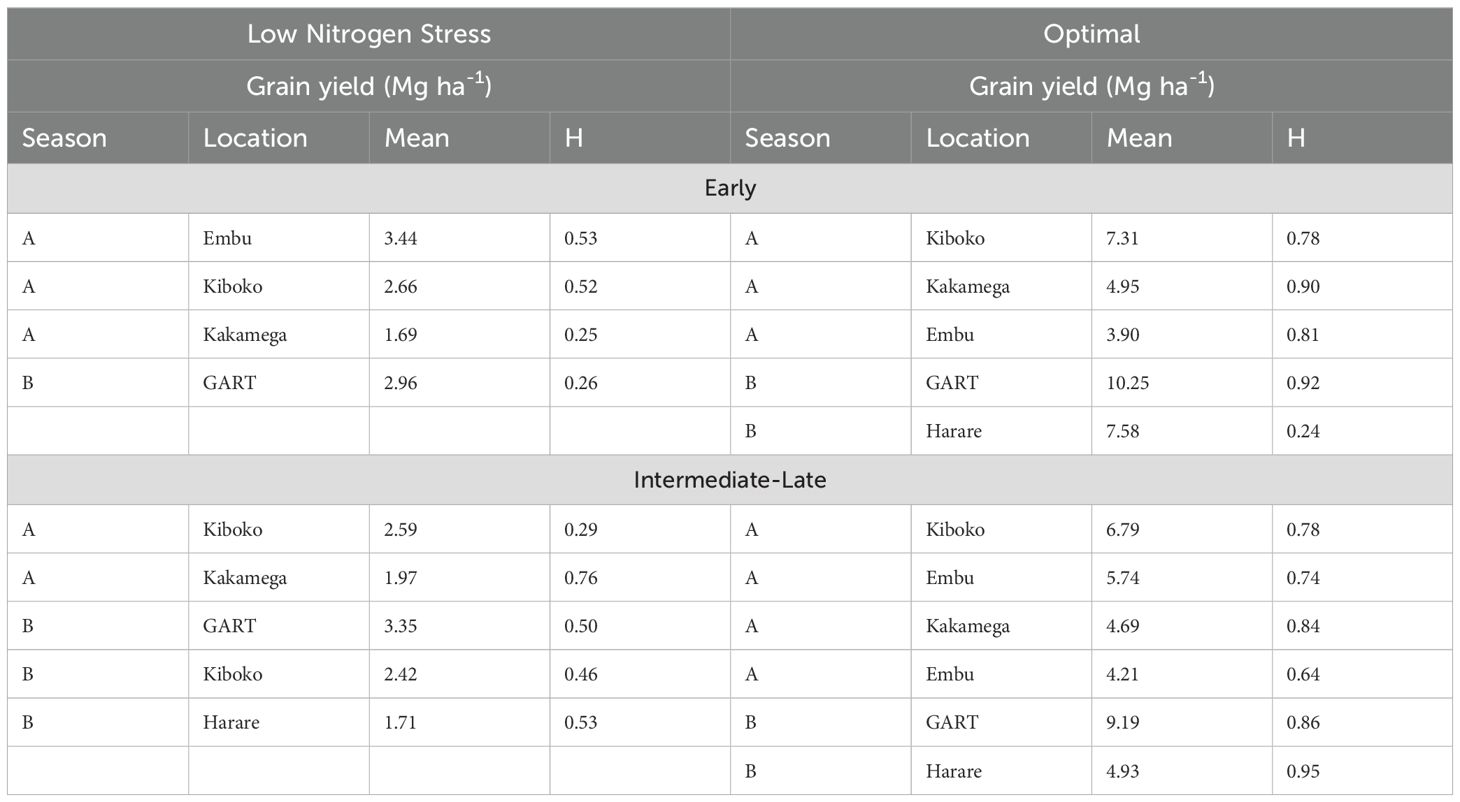
Table 4. Summary of locations and grain yield and repeatability (H) of trials under low nitrogen stress and optimal conditions of early and intermediate-late commercial hybrids and OPVs.
Measurements
Days to anthesis and silking were recorded when 50% of the plants had shed pollen and 50% of the plants had silks, respectively. The anthesis-silking interval (ASI) was calculated as days to silking - days to anthesis. At physiological maturity, plant height was measured on five representative plants per plot, then all plants were hand harvested, and grain yield measured.
Statistical analysis
Data from the two clusters of experiments were analyzed in Meta-R version 3.3 (Alvarado et al., 2020). The linear models are implemented in lmer from package lme4 of R using the Residual Maximum Likelihood (REML) to calculate BLUEs and BLUPs and estimate the variance components. REML uses a linear transformation to remove fixed effects from the model it is often preferred over maximum likelihood e stimation because it produces unbiased estimates of variance parameters and accounts for the loss of degrees of freedom. For analysis of individual environments, the following model was used:
Where; Yijk was the trait of interest, µ was the mean effect, Repi is the effect of the ith replicate, Blockj(Repi) is the effect of the jth incomplete block within the ith replicate, Genk is the effect of the kth genotype, Cov is the effect of the covariate (plant height), εijk is the error associated with the ith replication, jth incomplete block and the kth genotype. Both genotype and covariate were considered as fixed effects.
For combined analysis, the following model was used:
Where, Envi and Envi x Genl are the effects of the ith environment and environment x genotype interaction.
Broad-sense heritability (H2) of a given trait at an individual environment was calculated as:
Where, σ2g and σ2e are the genotype and error variance components respectively, and nreps is the number of replicates.
For the combined analysis, H2 was calculated as:
Where; σ2ge is the genotype x environment interaction variance component and nEnvs is the number of environments in the analysis.
Regression analysis to study the relationship between grain yield under low N and optimal conditions was performed using the ‘lm’ function in the R statistical package and these relationships were visualized on a regression plots using the ‘plot’ function in the R package (R Core Team, 2013).
Results
Relationship between grain yield under low N and optimal conditions
The level of yield reduction as a result of nitrogen stress ranged from 8% to 91% across 58 paired experiments. Over two-thirds of low N trials had a yield reduction of over 50% relative to the optimal trials, while one-fifth of low N trials had a yield reduction of over 75%. Broad sense heritability (H2) ranged from 0.05 to 0.85 under low N stress and 0.28 to 0.95 under optimal conditions (Table 4). For optimal trials, 79% had an H2 greater than 0.5. Four trials under low N had an H2 of less than 0.20. In almost two-thirds of paired trials, H2 was higher under optimal conditions than low N stress. Within these paired trials, H2 was higher under optimal conditions than under low N stress by an average of 0.38 (Table 5).
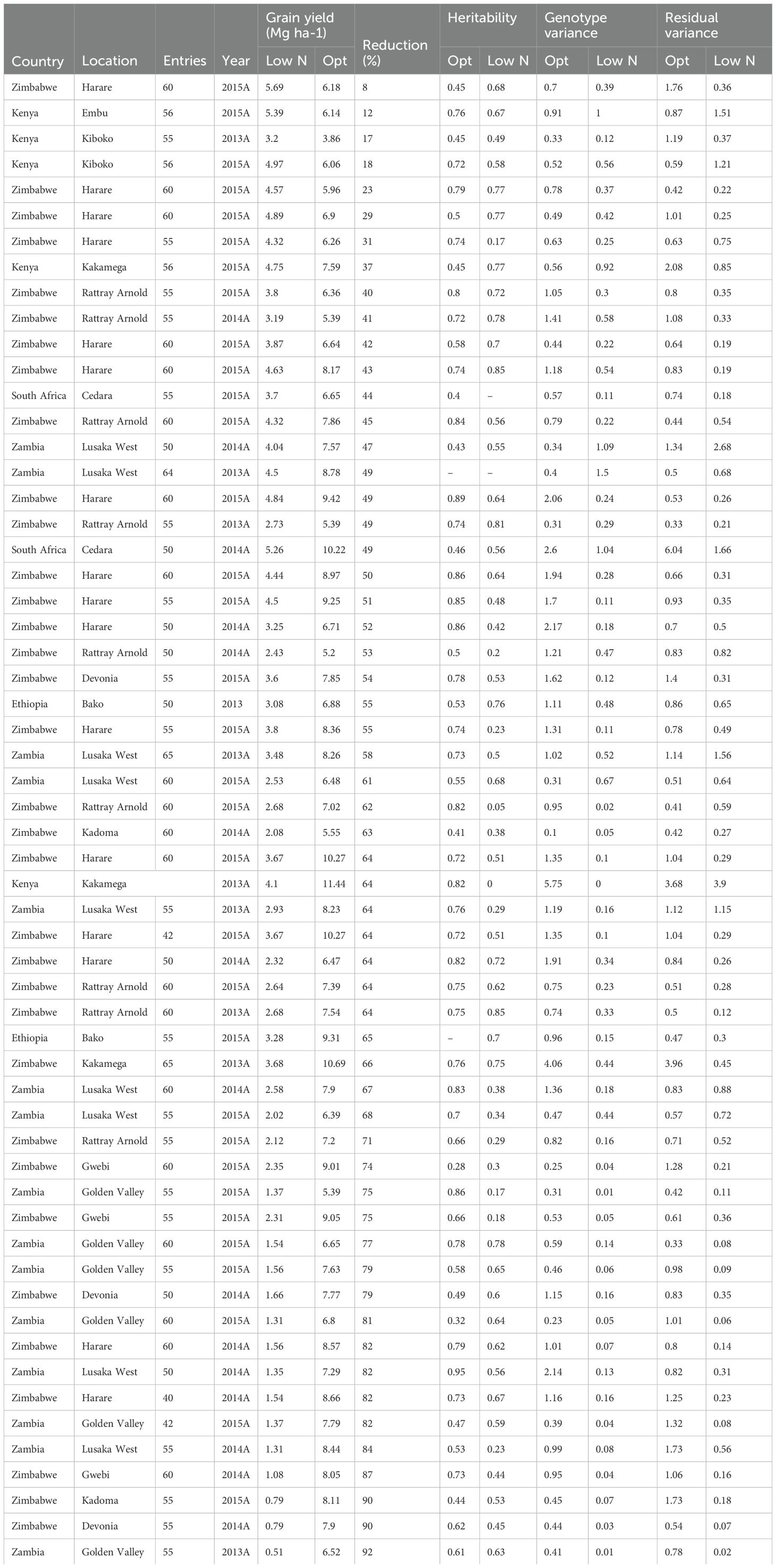
Table 5. Means for grain yield under low nitrogen and optimal conditions and the yield reduction under low nitrogen stress relative to optimal conditions, repeatability and variance components under low nitrogen and optimal conditions.
Although H2 decreased as the level of yield reduction under low N stress increased (Figure 1), over two-thirds of low N stress trials had an H2 greater than 0.5 (see Table 5). Regression analysis also showed a positive association between ‘genetic variance components under low nitrogen stress’ and ‘yield reduction under low nitrogen stress’ (Figure 1). But a negative association was observed between ‘genetic correlation between grain yield under low nitrogen stress and optimal conditions’ against ‘yield reduction under low nitrogen stress’ (Figure 2).
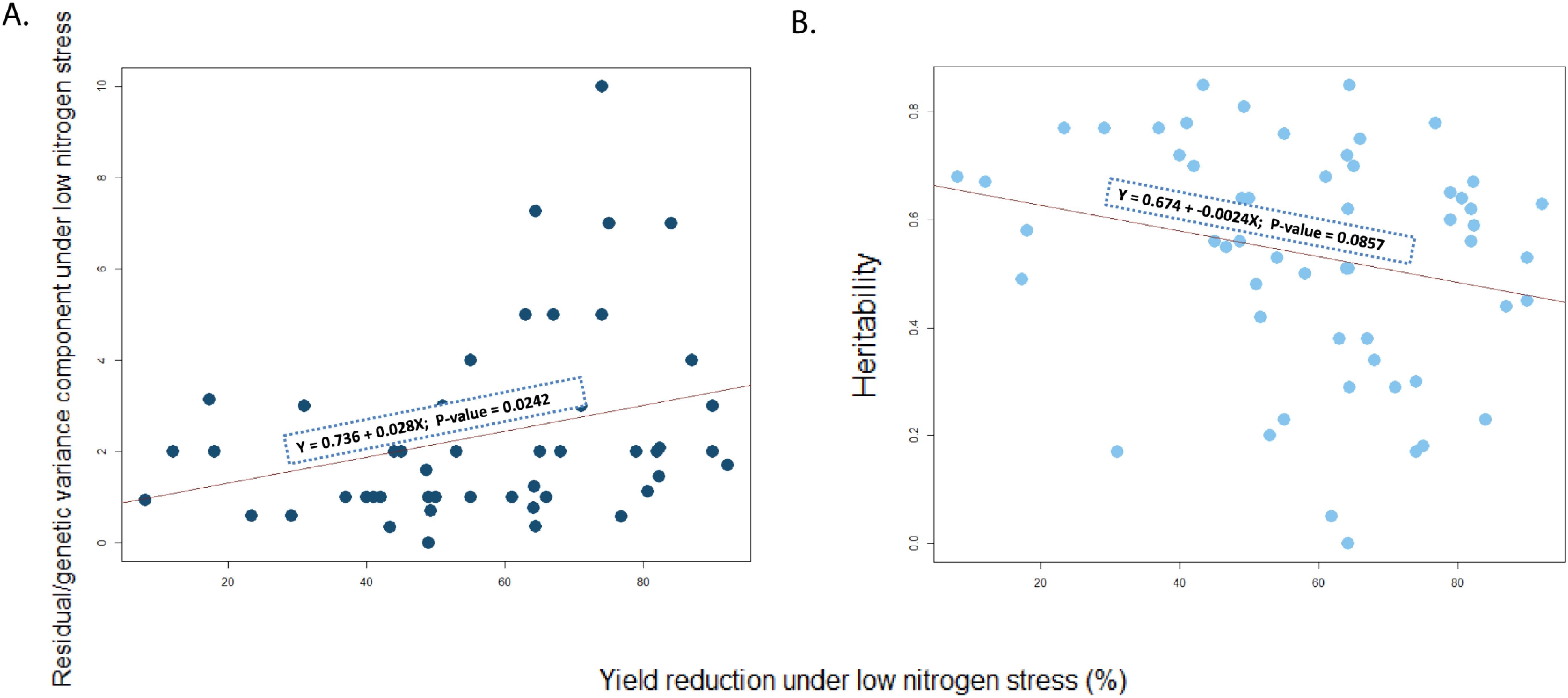
Figure 1. Regression between % yield reduction under low N and (A) Residual/genetic variance component under low N stress and (B) Heritability.
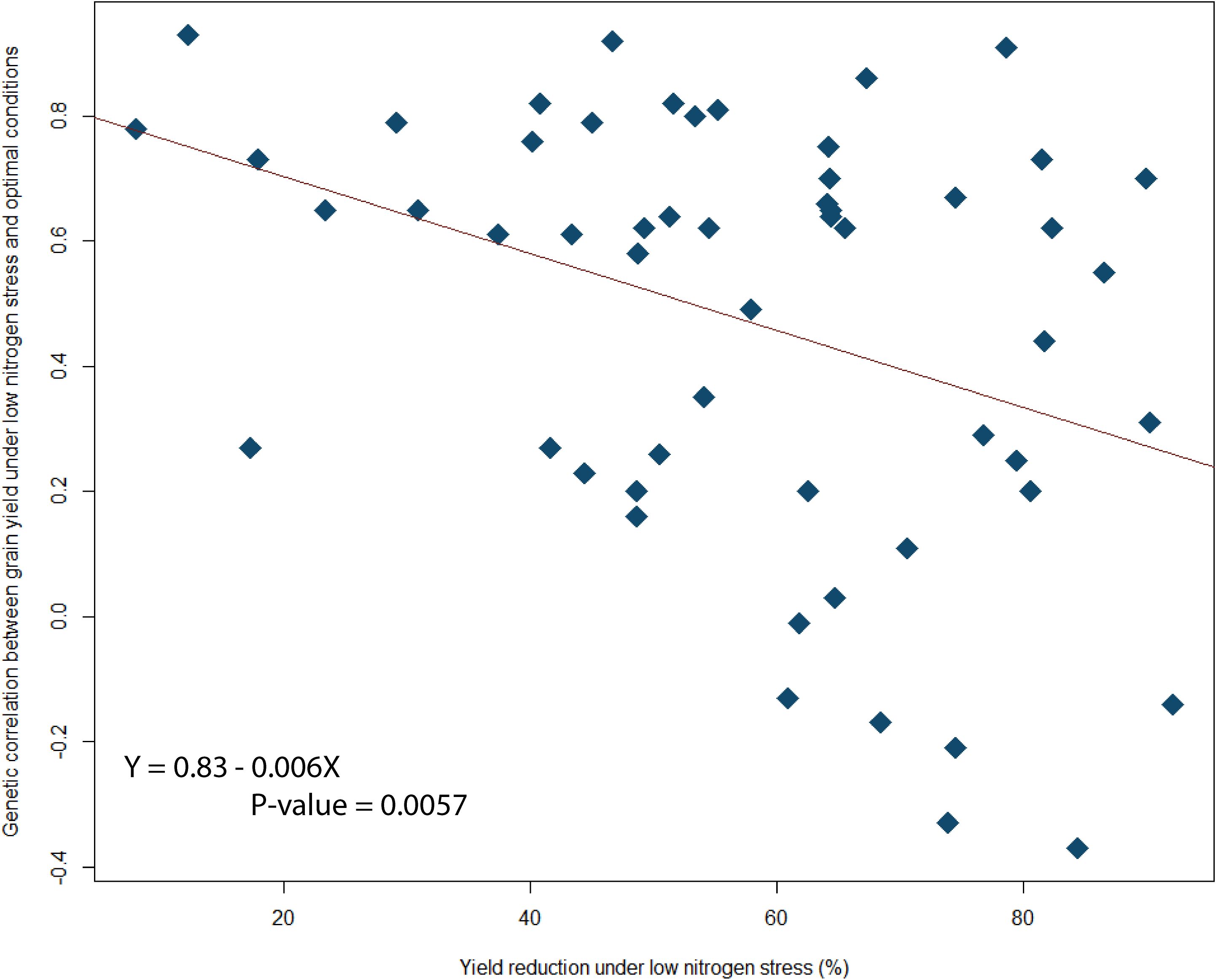
Figure 2. Regression between % yield reduction genetic correlation between grain yield under low nitrogen stress and optimal conditions.
Variability in low N tolerance among elite Eastern and Southern African maize varieties
Under low N stress, grain yield ranged from 1.69 Mg ha-1 to 3.44 Mg ha-1 in the EMG and 1.71 Mg ha-1 to 3.35 Mg ha-1 in the ILMG. Grain yield under optimal conditions ranged from 3.90 Mg ha-1 to 10.25 Mg ha-1 in the EMG and 4.21 Mg ha-1 to 9.19 Mg ha-1 in the ILMG (Table 4). Under optimal conditions, H2 ranged from 0.24 to 0.90 and 0.64 to 0.95 in the EMG and ILMG, respectively. Under low N stress, H2 ranged from 0.25 to 0.53 and 0.29 to 0.76 in the EMG and ILMG, respectively.
There was large genetic variability for grain yield under low N stress (Tables 1, 2). In the EMG (see Table 1), there was over a 1 Mg ha-1 difference in yield in both the hybrids and OPVs, with hybrid CZH0928 and OPV 09ADVE-F2 both yielding 3.31 Mg ha-1. Two commercial hybrids (PHB3253 and DK8031) yielded more than 3 Mg ha-1 under low N stress. OPV 09ADVE-F2 yielded significantly more than three hybrids under low N stress (CKPH08028, SC513 and SC403), and no hybrid out-yielded this OPV under low N stress.
In the ILMG (see Table 2), there was over a one-fold difference in yields under low N stress. Yields ranged from 1.51 Mg ha-1 and 1 Mg ha-1 to 3.37Mg ha-1 and 3.01 Mg ha-1 for hybrids and OPVs, respectively. Pre-commercial hybrids, both within the EMG (LSD = 0.77 Mg ha-1) and ILMG (LSD = 0.5 Mg ha-1), averagely yielded more than the commercial hybrids and the OPVs under low N conditions, although the differences were not significant (Tables 1, 2). Low N stress significantly reduced plant height, while significantly increasing days to anthesis and anthesis to silking interval (ASI) in both maturity groups (Tables 1, 2).
Discussion
In this study, as the level of N stress intensity increased the genetic correlation between yields under low N and optimal conditions decreased, indicating that the efficiency of indirect selection under optimal conditions for grain yield under low N stress also decreased, concurring with the findings of Bänziger et al. (1997) and Presterl et al. (2003). These results confirm increased yields under low fertility conditions will be achieved much more slowly by selecting under optimal fertilizer rates than selecting for low N directly. Our results suggest low N stress tolerance should target a yield reduction of approximately 60% as previously low N trials in ESA have been classified quantitatively on yield levels, of 3 Mg ha-1 for combined analyses (Weber et al., 2012). Our results suggest that at sites of higher yield potential (e.g. Kakamega and Harare), trials between 3-4 Mg ha-1 can still have a yield reduction of greater than 60% and can be used as low N stress sites as opposed to the established classification of 3 Mg ha-1.
The commercial varieties used in this study were selected to represent genetic diversity within commercial varieties in ESA at the time and provide a baseline for low N stress breeding in the region. Varietal replacement in ESA is sub-optimal but is increasing (Abate et al., 2017; Atlin et al., 2017; Chivasa et al., 2022) and these varieties are still widely grown in this region and represent the current genetic diversity within the region to a certain extent. Several varieties had very low yields under low N stress, particularly within the ILMG (CKPH08043, ECAVL1, Embu Synthetic, H614D and KH631Q all yielded less than 2 Mg ha-1). However, despite initially limited screening capacity for low N stress tolerance in the public and private sector in ESA, large variability for grain yield under low N stress was observed. This would suggest that there were spill-over effects resulting in indirect selection for low N tolerance. Several early and intermediate to late hybrids and OPV varieties yielded over 3 Mg ha-1 under severe N stress. In the EMG, several hybrids (CZH0928 and PHB3253) and OPVs (O9ADVE-F2) yielded over 1 Mg ha-1 more under severe N stress than the widely grown hybrid SC407 (sold in Kenya as Duma 43), while there were significant differences in yield under optimal conditions. In the ILMG, CZH0616, PHB30D79, WH507 and ECA-VL45-# yielded more than 3 Mg ha-1 under severe N stress. These results are likely to reflect breeding for drought tolerance. The highest yielding intermediate to late maturity variety, CZH0616, was selected for drought tolerance (Setimela et al., 2017a, b). Similarly, CZH0928 (early maturity variety) and Pris061 (intermediate to late maturity variety) were also developed primarily for drought tolerance. Tolerance to mid-season drought stress is closely related to tolerance to low N stress (Bänziger et al., 1999). Selection for mid-season drought tolerance was associated with changes in the establishment of reproductive structures related to low N stress tolerance (Bänziger et al., 2002). Consequently, selection for drought tolerance tends to result in improved tolerance to low N stress. However genetic gain for low N stress is lower when selection is indirect (Masuka et al., 2017a).
This study suggests that in very low yield environments, OPVs could potentially yield more than commercial hybrids. For example, within the EMG, O9ADVE-F2 yielded significantly more than two commercial hybrids (SC407 and SC513) and was not out-yielded by any hybrid under low N. The OPV O9ADVE-F2 had previously been identified as the highest yielding in regional (stage 4) trials within the medium maturity group for maize mega-environments A and C. In subsequent on-farm trials, under farmer management, O9ADVE-F2 yielded more than the commercial hybrid SC513. Intermediate to late maturity (ILMG) OPVs, i.e., ECA-VL45-# and ECA-VL43-#, yielded significantly more than four commercial hybrids (Pan67, KSTP64, H614D and KH631Q). This is in direct contrast to studies of experimental hybrids and OPVs which showed that hybrids yield significantly more than OPVs under low N stress (Masuka et al., 2017a, b) and an earlier study by Chiduza et al. (1994). However, the commercial hybrids in this study were released up to 30 years ago (H614D was released in 1986 and SC513 in 1999). Thus, in regions of ESA where only obsolete hybrid varieties are available to smallholder farmers, OPVs may offer an important option for increased food security (Masuka et al., 2017b). Because no hybrid from the private sector out-yielded O9ADVE-F2 under low-N, it is likely to be a cost-effective varietal choice for farmers in ESA managing under low-N conditions (Pixley and Bänziger, 2004).
Pre-commercial hybrids, both within the EMG (LSD = 0.77 Mg ha-1) and ILMG (LSD = 0.5 Mg ha-1), averagely yielded more than the commercial hybrids and the OPVs under low N conditions, although the differences were not significant (Tables 1, 2). These results suggest that if more effort is devoted to selecting maize under low N, yield gains can be realized, resulting in increased maize productivity in SSA. Kostandini et al. (2015) estimated that an increase in yield of 16% under very low N fertilizer application rates (0-29 kg N ha-1) has the potential to deliver a total of US$586 million in gross benefits with US$136 million and US$100 million of benefits to maize producers in Kenya and South Africa respectively within nine years. These results suggest that breeding for low N stress tolerant maize could have substantial impact. For example, SC513 (sold as SC Punda Mulia 55 in Kenya) is one of the most widely grown varieties by smallholder farmers in ESA. Under optimal conditions, there was no significant difference in yield between SC513 and all other hybrids and OPVs, with the exception of CKIR07012 (a hybrid which yielded significantly more than SC513). However, under low N stress conditions faced by most smallholders in SSA, SC513 yielded nearly 0.8 Mg ha-1 less than the highest-yielding OPV and hybrid. Replacing this obsolete variety with the OPV 09SADVE-F2 or the hybrid CZ0928 could increase yields for smallholders as much as 30% under low-N conditions.
Conclusions
Low N stress as a result of low fertilizer use, organic matter removal, and highly depleted soils will likely persist for many years to come in most maize systems in SSA. There is an urgent need to increase genetic gain for grain yield under environmental conditions experienced in farmers’ fields (Atlin et al., 2017). Our results concur with previous studies showing that yield under low N stress is not closely related to yield under optimal conditions (Bänziger et al., 1997; Presterl et al., 2003) and direct selection for yield under low N stress is required to increase yields in conditions similar to those of smallholder farmers. It is essential that low N stress screening is included in all maize breeding programs and there is need to increase the area available for Low N screening in SSA (see Table 6). This will enable the development of varieties that are suited to the smallholder farmers’ conditions and help mitigate climate change as well as reduce input costs. Although experiments were conducted six years ago, varietal replacement in this region is very slow (>15 years) (Abate et al., 2017; Atlin et al., 2017) and these varieties still represent current genetic variability within ESA.
Data availability statement
The original contributions presented in the study are included in the article/supplementary material. Further inquiries can be directed to the corresponding author.
Author contributions
SG: Formal Analysis, Investigation, Writing – original draft. CK: Formal Analysis, Methodology, Supervision, Writing – review & editing. MS: Project administration, Supervision, Writing – review & editing. JD: Project administration, Supervision, Writing – review & editing.
Funding
The author(s) declare that financial support was received for the research and/or publication of this article. This work was supported by the Improved Maize for African Soils (IMAS), Stress Tolerant Maize for Africa (STMA) and Drought Tolerant Maize for Africa (DTMA) projects funded by the Bill & Melinda Gates Foundation and USAID, and the MAIZE CGIAR research programme and CGIAR Excellence in Breeding Platform.
Acknowledgments
We thank the CIMMYT-Zimbabwe and CIMMYT-Kenya Maize Breeders and their Technical Assistants at each site for conducting the trials.
Conflict of interest
The authors declare that the research was conducted in the absence of any commercial or financial relationships that could be construed as a potential conflict of interest.
Publisher’s note
All claims expressed in this article are solely those of the authors and do not necessarily represent those of their affiliated organizations, or those of the publisher, the editors and the reviewers. Any product that may be evaluated in this article, or claim that may be made by its manufacturer, is not guaranteed or endorsed by the publisher.
Abbreviations
ASI, anthesis-silking interval; BLUES, best linear unbiased estimates; BLUPS, best linear unbiased predictions; CIMMYT, International Maize and Wheat Improvement Centre; ESA, eastern and southern Africa; N, nitrogen; PH, plant height; SSA, sub-Saharan Africa.
References
Abate T., Fisher M., Abdoulaye T., Kassie G. T., Lunduka R. (2017). Characteristics of maize cultivars in Africa : How modern are they and how many do smallholder farmers grow? Agric. Food Secur. doi: 10.1186/s40066-017-0108-6
Abate T., Shiferaw B., Menkir A., Wegary D., Kebede Y., Tesfaye K., et al. (2015). Factors that transformed maize productivity in Ethiopia. Food Secur. 7. doi: 10.1007/s12571-015-0488-z
Abdisa T., Mehare A. (2024). Analysing gender gap in Agricultural productivity: Evidence from Ethiopia. J. Agric. Food Res. 15, article 100960. doi: 10.1016/j.jafr.2023.100960
Alvarado G., Rodríguez F. M., Pacheco A., Burgueño J., Crossa J., Vargas M., et al. (2020). META-R: A software to analyze data from multi-environment plant breeding trials. Crop J. 8, 745–756. doi: 10.1016/j.cj.2020.03.010
Atlin G. N., Cairns J. E., Das B. (2017). Rapid breeding and varietal replacement are critical to adaptation of cropping systems in the developing world to climate change. Global Food Secur. 12, 31–37. doi: 10.1016/j.gfs.2017.01.008
Bänziger M., Betrán F. J., Lafitte H. R. (1997). Efficiency of high-nitrogen selection environments for improving maize for low-nitrogen target environments. Crop Sci. 37 (4), 1103–1109.
Bänziger M., Edmeades G. O., Lafitte H. R. (1999). Selection for drought tolerance increases maize yields across a range of nitrogen levels. Crop Sci. 39 (4), 1035–1040.
Bänziger M., Edmeades G. O., Lafitte H. R. (2002). Physiological mechanisms contributing to the increased n stress tolerance of tropical maize selected for drought tolerance. Field Crops Res. 75 (2-3), 223–233.
Begho T., Eory V., Glenk K. (2022). Demystifying risk attitudes and fertilizer use: A review focusing on the behavioral factors associated with agricultural nitrogen emissions in South Asia. Front. Sustain. Food Syst. 6. doi: 10.3389/fsufs.2022.991185
Buehren N. (2023). Gender and Agriculture in Sub Saharan Africa: Review of constraints and effective interventions (Washington DC: Gender Innovation Lab. The World Bank). Available at: http://hdl.handle.net/10986/39994 (Accessed August, 24, 2024).
Cairns J. E., Crossa J., Zaidi P. H., Grudloyma P., Sanchez C., Araus J. L., et al. (2013). Identification of drought, heat, and combined drought and heat tolerant donors in maize. Crop Sci. doi: 10.2135/cropsci2012.09.0545
Cassman K. G., Dobermann A. (2022). Nitrogen and the future of agriculture : 20 years on. Ambio 51, 17–24. doi: 10.1007/s13280-021-01526-w
Chiduza C., Waddington S. R., Mariga I. K. (1994). Grain yield and economic performance of experimental open-pollinated varieties and released hybrids of maize in a remote semi-arid area of Zimbabwe. Zimbabwe J. Agric. Res. 32, 33–43.
Chivasa W., Worku M., Teklewold A., Setimela P., Gethi J., Magorokosho C., et al. (2022). Maize varietal replacement in Eastern and Southern Africa: Bottlenecks, drivers and strategies for improvement. Global Food Secur. 32, 100589. doi: 10.1016/j.gfs.2021.100589
Crawford W. E., Jayne T. S., Kelly V. A. (2005). Alternative approaches for promoting fertiliser use in Africa, with particular reference to the role of fertiliser subsidies. Staff paper No. 15-19 (East Lansing, USA: Michigan State University).
Dimkpa C., Adzawla W., Pandey R., Atakora W. K., Kouame A. K., Jemo M., et al. (2023). Fertilizers for food and nutrition security in sub-Saharan Africa: An overview of soil health implications. Front. Soil Sci. 3. doi: 10.3389/fsoil.2023.1123931
Giller K. E., Delaune T., Silva J. V., Descheemaeker K., van de Ven G., Schut A. G. T., et al. (2021). The future of farming: Who will produce our food? Food Secur. 13, 1073–1099. doi: 10.1007/s12571-021-01184-6
Holden S. T. (2018). Fertilizer and sustainable intensification in Sub-Saharan Africa. Global Food Secur. 18, 20–26. doi: 10.1016/j.gfs.2018.07.001
Jayne T. S., Sanchez P. A. (2021). Agricultural productivity must improve in sub-Saharan Africa. Sci 372, 1045–1047. doi: 10.1126/science.abf5413
Kilic T., Winters P., Carletto C. (2015). Gender and Agriculture in sub Saharan Africa: Introduction to the special Issue. Agric. Economics Int. Assoc. Agric. economics 46, 281–284.
Kostandini G., La Rovere R., Guo Z. (2015). Ex Ante Welfare Analysis of Technological Change: The case of nitrogen efficient maize for African soils. Can. J. Agric. Economics/Revue Can. D’agroeconomie 64, 147–168. doi: 10.1111/cjag.12067
Masuka B., Atlin G. N., Olsen M., Magorokosho C., Labuschagne M., Crossa J., et al. (2017b). Gains in maize genetic improvement in Eastern and Southern Africa: I. CIMMYT hybrid breeding pipeline. Crop Sci. 57 (1), 168–179.
Masuka B. P., van Biljon A., Cairns J. E., Das B., Labuschagne M., MacRobert J., et al. (2017a). Genetic diversity among selected elite CIMMYT maize hybrids in East and Southern Africa. Crop Sci. 57 (5), 2395–2404.
Niu S., Du X., Wei D., Liu S., Tang Q., Bian D., et al. (2021). Heat stress after pollination reduces kernel number in maize by insufficient assimilates. Front. Genet. 12. doi: 10.3389/fgene.2021.728166
Peterman A., Quisumbine A., Behman J., Nkonya E. (2011). Understanding the complexities sdurrounding gender differences in Agricultural productivity in Nigeria and Uganda. J. Dev. Stud. 47, 1482–1509. doi: 10.1080/00220388.2020.536222
Pixley K., Banziger M. (2004). “Open-pollinated maize varieties: A backward step or valuable option for farmers,” in Seventh eastern and southern africa regional maize conference (Mexico City, Mexico: CIMMYT), 22–28.
Presterl T., Seitz G., Landbeck M., Thiemt E. M., Schmidt W., Geiger H. H. (2003). Improving nitrogen-use efficiency in european maize: Estimation of quantitative genetic parameters. Crop Sci. 43 (4), 1259–1265.
Rongfa L., Gao J., Li Y., Yu S., Wang Z. (2022). Heterosis for nitrogen use efficiecny of maize hybrids enhanced over decades in China. Agriculture. doi: 10.3390/Agriculture12060764
Serdeczny O., Adams S., Baarsch F., Coumou D., Robinson A., Hare W., et al. (2017). Climate change impacts in Sub-Saharan Africa: from physical changes to their social repercussions. Regional Environ. Change 17, 1585–1600. doi: 10.1007/s10113-015-0910-2
Setimela P. S., Gasura E., Tarekegne A. T. (2017b). Evaluation of grain yield and related agronomic traits of quality protein maize hybrids in Southern Africa. Euphytica 213 (12), 289.
Setimela P. S., Magorokosho C., Lunduka R., Gasura E., Makumbi D., Tarekegne A., et al. (2017a). On-farm yield gains with stress-tolerant maize in eastern and southern africa. Agron. J. 109 (2), 406–417.
Sheahan M., Barrett C. B. (2017). Review : Food loss and waste in Sub-Saharan Africa. Food Policy 70, 1–12. doi: 10.1016/j.foodpol.2017.03.012
Van Ittersum M. K., Hijbeek R., Ten Berge H., Van Loon M., Boogaard H., Tesfaye K. (2019). Minimum emission pathways to triple Africa’s cereal production by 2050 Key messages. November, 1–5. Available online at: www.yieldgap.org (Accessed November, 09, 2024).
Wahid A., Gelani S., Ashraf M., Foolad M. R. (2007). Heat tolerance in plants: An overview. Environ. Exp. Bot. 61, 199–223. doi: 10.1016/j.envexpbot.2007.05.011
Keywords: low N, grain yield, maize, SSA, hybrids
Citation: Gokoma S, Kamutando CN, Siwela M and Derera J (2025) A retrospective analysis of maize performance under low nitrogen stress conditions in sub-Saharan Africa. Front. Agron. 7:1490815. doi: 10.3389/fagro.2025.1490815
Received: 03 September 2024; Accepted: 31 March 2025;
Published: 25 April 2025.
Edited by:
Hang Zhao, Qufu Normal University, ChinaReviewed by:
Dan Makumbi, International Maize and Wheat Improvement Center, MexicoHewan Degu, Hawassa University, Ethiopia
Copyright © 2025 Gokoma, Kamutando, Siwela and Derera. This is an open-access article distributed under the terms of the Creative Commons Attribution License (CC BY). The use, distribution or reproduction in other forums is permitted, provided the original author(s) and the copyright owner(s) are credited and that the original publication in this journal is cited, in accordance with accepted academic practice. No use, distribution or reproduction is permitted which does not comply with these terms.
*Correspondence: Casper N. Kamutando, a2FtdXRhbmRvY25AZ21haWwuY29t