- 1Global Maize Program, International Maize and Wheat Improvement Center (CIMMYT), Nairobi, Kenya
- 2Texas AgriLife Research and Extension Center, Texas A&M University, Beaumont, TX, United States
- 3Biorisk Management Facility (BIMAF), International Institute of Tropical Agriculture (IITA-Benin), Cotonou, Benin
- 4Ecole de Gestion et de Production Végétale et Semencière (EGPVS), Université Nationale d’Agriculture (UNA-Bénin), Kétou, Benin
- 5Crop Health, Kenya Agricultural and Livestock Research Organization (KALRO), Nairobi, Kenya
The fall armyworm (FAW), Spodoptera frugiperda, is a polyphagous pest native to the American continent that was first detected in Africa in 2016, where it has since become a major constraint to agriculture. This species severely damages staple crops like maize, sorghum, and rice, threatening food security and the livelihoods of millions of smallholder farmers. Maize, the most vulnerable crop in sub-Saharan Africa, suffers significant annual losses due to the destructive impact of FAW, which affects agricultural productivity and overall rural economies. The pest displays complex biological and ecological patterns that are highly dependent on environmental factors, host plant availability, and natural enemy diversity, making control efforts challenging. This review explores the traits driving FAW’s invasive success in Africa, summarizing key findings on its biology and ecology while outlining current management strategies. It underscores the importance of Integrated Pest Management (IPM), which includes cultural practices, biological control, mechanical/physical methods, host plant resistance, and judicious application of chemicals. Regular crop monitoring and surveillance principles are also discussed as prevention and early detection measures to mitigate FAW damage. Future directions emphasize the need for collaboration among stakeholders, including international research organizations, to effectively control FAW invasion. Given the economic risks of the FAW outbreak in Africa, adopting IPM solutions is crucial for reducing pesticide reliance and ensuring stable agricultural production. This review offers valuable insights into achieving this goal.
1 Introduction
Fall armyworm (FAW), Spodoptera frugiperda (J. E. Smith, 1797) (Lepidoptera: Noctuidae), originally native to America, has rapidly spread across the African continent as a highly destructive polyphagous pest. Since its first reported occurrence on the continent in early 2016 (Goergen et al., 2016), FAW has severely impacted food security and the livelihoods of millions of smallholder farmers.
Studies have reported that FAW feeds on more than 350 plant species, primarily from the Poaceae, Asteraceae, and Fabaceae families (Wyckhuys and O’Neil, 2006; Badhai et al., 2020; Jing et al., 2021). Affected crops include major staples such as maize, sorghum, and rice, along with other important crops like cotton, sugarcane, cabbage, okra, beet, groundnut, soybean, alfalfa, onion, pearl millet, tomato, and potato, as well as pasture grasses (Day et al., 2017; Montezano et al., 2018; Akutse et al., 2020; Ahissou et al., 2022).
Despite its broad host range, FAW poses the greatest threat to maize in sub-Saharan Africa. This crop provides essential nutrients and supports livestock feed production across the region (Ekpa et al., 2019; Galani et al., 2022; Makuachukwu et al., 2022). With over 27 million hectares cultivated to feed more than 300 million people (Akutse et al., 2020), its reliability is crucial for food security. However, FAW infestations have led to significant yield losses, exacerbating agricultural instability, particularly for vulnerable populations.
In response, many farmers rely heavily on synthetic pesticides, which increase production costs and reduce farm income. The decline in production leads to higher food prices, limiting access to nutritious food. Additionally, FAW outbreaks disrupt agricultural supply chains, creating market uncertainties from farms to consumers in affected regions (Amusan and Olelekan, 2018; Banson et al., 2020).
The persistent and evolving nature of FAW infestations necessitates sustainable and resilient agricultural practices. This review provides a comprehensive analysis of FAW’s biology, ecology, and its impact on agricultural production. It also evaluates current FAW management strategies and highlights integrated pest management (IPM) as a sustainable approach to enhance resilience within African agriculture. Furthermore, the review explores future directions, emphasizing a holistic and adaptive framework to protect crops, sustain livelihoods, and ensure food security across the African continent.
2 Knowledge of FAW biology and ecology
2.1 Adult behavior and dispersal
Spodoptera frugiperda adults are highly active at night, seeking mates and expanding into new territories while staying elusive during the day (Patel Sagarbhai et al., 2021). Research has confirmed that the males of FAW are more attracted to light than females (Vilarinho et al., 2011). Studies have also documented that FAW moths from the same generation can travel more than 500 kilometers before they are ready to lay their eggs (Prasanna et al., 2018; Acharya et al., 2020; Badhai et al., 2020). Male and female S. frugiperda are shown in Figures 1a, b, respectively.
2.2 Reproduction and oviposition
Oviposition typically occurs at night, with females laying inconspicuous egg clusters on the undersides of leaves to shield them from predators (Kasige et al., 2022). In some cases, the eggs are laid in layers and covered with scales for added protection. The upper leaf surface and whorl of host plants may also harbor some egg batches (Sharanabasappa et al., 2018). A single FAW female can lay an average of 1500 eggs, showing the high fecundity of the pest (Prasanna et al., 2018). FAW eggs hatch into tiny neonate larvae within 3 to 4 days at an average temperature of 28°C. Eggs of S. frugiperda are shown in Figure 1c.
2.3 Larval stage, damage and behavior
Soon after hatching, the larvae disperse from the site and infest nearby plants, often aided by the wind (Ortiz-Carreón et al., 2024). The larval stage of FAW consists of six instars and lasts about 14 days in warm conditions and up to 30 days in cooler weather (Prasanna et al., 2018). This stage is the damaging phase of FAW’s life cycle (Sagar et al., 2020). Caterpillars primarily feed at night, but activity may also occur in the late evening or early morning (Schlemmer, 2018; Patel Sagarbhai et al., 2021). They feed on the leaves, the tender stem, the silks, and the ears of host plants. As they grow, their appetite intensifies, causing severe damage to host plants. Day et al. (2017) and Flanders et al. (2017) reported that the largest larvae can cause up to 77% of plant damage.
During the vegetative phase of the host crops, continuous feeding results in skeletonized leaves and heavily windowed whorls filled with larval frass (Goergen et al., 2016).
Cannibalism is an important biological phenomenon within the FAW larval stage. This intraspecific behavior frequently results in a significant reduction in larval numbers, leaving only one larva per plant. The eliminated cohabitant either succumbs to cannibalism or relocates to another plant where competition is less intense. According to Ren et al. (2020) and Ahissou et al. (2022), a high density of larvae on a maize plant suggests that the larvae have not yet reached advanced developmental stages. Chapman et al. (2000) observed that the larvae of FAW exhibit cannibalistic behavior from the third instar (L3) stage onward, allowing them to dominate interspecific competitors and reduce intraspecific competition. Larva of S. frugiperda is shown in Figure 1d.
2.4 Pupation and adult emergence
At the 6th instar, larvae undergo metamorphosis within a woven silky cocoon. Pupation usually occurs in the soil (Figure 1e) at a depth of 2-8 cm (Ojumoola, 2021). During summer, the pupal stage lasts about 8-9 days. Studies conducted by Huang et al. (2021) and Montezano et al. (2018) revealed that the pupal developmental stage was shorter in female FAW than in males. After pupation, adult moths emerge and live for an average of 14 days (Prasanna et al., 2018). The total life cycle of FAW (a generation time) is about 30–40 days at 28°C but extends to approximately 55 days in cooler seasons (Prasanna et al., 2018; Ashok et al., 2020; Lekha et al., 2020). This is supported by Kenis et al. (2022), who found that the FAW life cycle can extend to 90 days at lower temperatures. Tendeng et al. (2019) found that the total cycle is between 22 and 28 days at 25°C with an average of 25 days in laboratory conditions.
2.5 Environmental factors influencing FAW development
Environmental factors such as temperature, relative humidity, soil moisture, and host plant characteristics (quality, diversity, availability and phenology), and the presence of natural enemies influence the development, distribution, abundance and population dynamics of FAW (Kenis et al., 2022).
Several studies have demonstrated the direct impact of temperature and relative humidity on various biological activities, including adult mating, egg production and hatching, larval development, pupal survival, and adult longevity (Table 1). Studies have indicated that FAW egg-to-adult development requires a specific number of degree days, with potential differences in developmental duration between sexes (Schlemmer, 2018; Malekera et al., 2022). Additionally, research has shown that larval and pupal development is optimal at temperatures ranging from 28 to 30°C, while lower temperatures tend to prolong the developmental period compared to warmer temperatures (Du Plessis et al., 2020). Schlemmer (2018) and Kumara et al. (2022) noted that temperatures around 30°C favor the growth and development of FAW, resulting in the fastest larval growth rate and the lowest mortality. Gergs and Baden (2021) found that lower ambient temperatures, combined with poor diet quality, increase the duration of FAW’s life cycle.
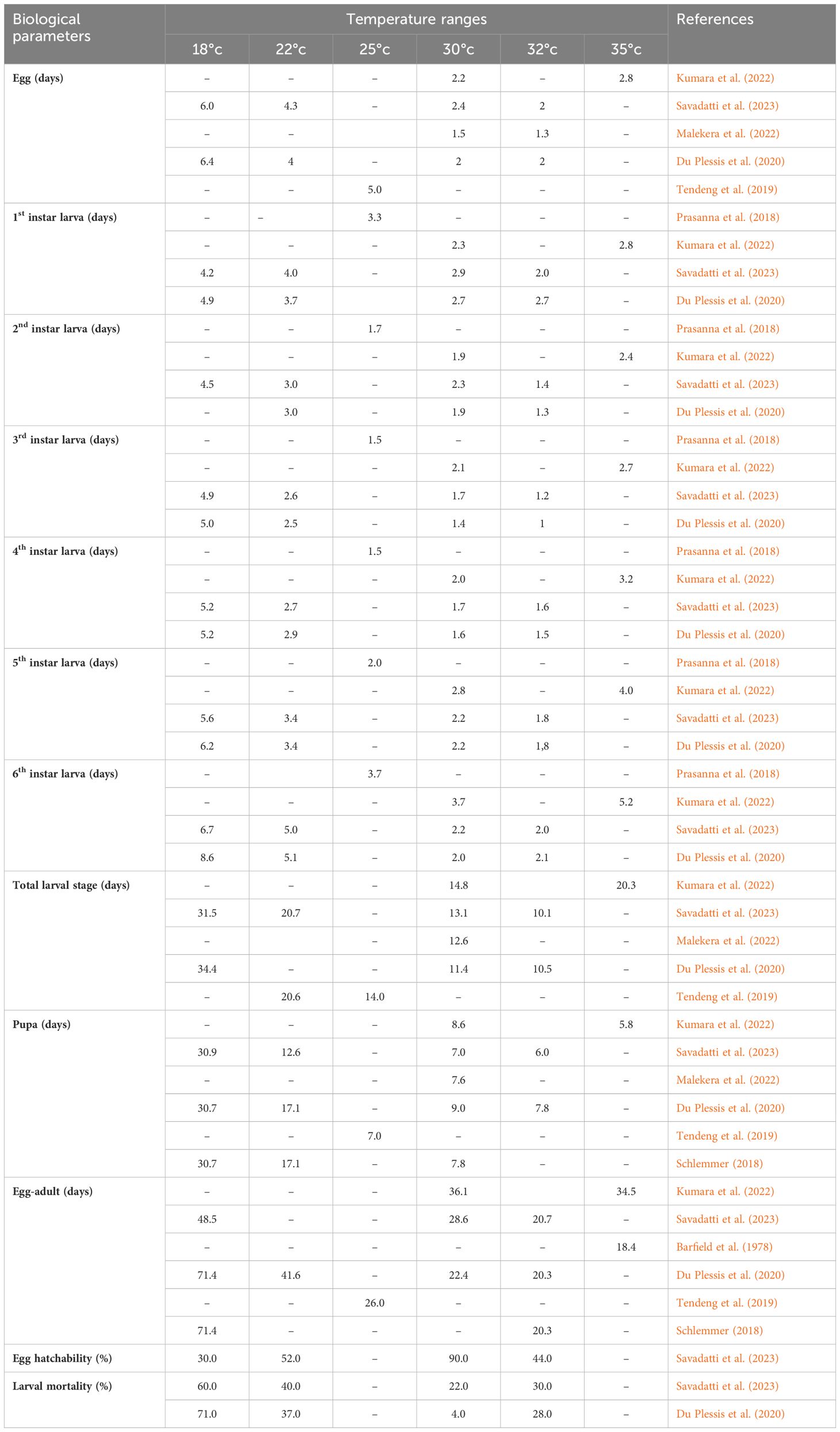
Table 1. Influence of various temperatures on the mean development time (day), egg hatchability and larval mortality of fall armyworm.
Researchers typically observed the effect of temperature on pupal weight, noting the highest weights at 25°C and decreasing weights as temperatures deviate from this optimal point (Huang et al., 2021).
Rising temperatures due to global warming may increase the number of FAW generations, facilitating its spread and establishment in new regions (Garcia et al., 2018; Yan et al., 2022). In African climatic conditions, the pest reproduces continuously, producing up to 15 generations annually (Tendeng et al., 2019; Barkessa et al., 2024).
Studies have shown that relative humidity (RH) also plays an important role in FAW biology. According to He et al. (2021), an RH of 80% was optimal for FAW to achieve its highest intrinsic rate of increase, finite rate of increase, and net reproduction rate. Additionally, the authors noted that soil moisture ranging from 6.80% to 47.59% supports FAW pupation, survival, and eclosion.
Another key factor influencing the ecology and population dynamics of FAW is host plant selection. The preference of FAW for a particular host plant depends on the strain type (Tiwari, 2022). Two distinct strains have been identified within the FAW population: the C-strain and R-strain (Schofl et al., 2009; Groot et al., 2010; Dumas et al., 2015; Jing et al., 2021). Although these strains have morphological similarities, they differ in several key biological aspects including host plant preference, reproductive behaviors, genetic profiles, and pheromone compositions. The C-strain predominantly feeds on maize, sorghum, and cotton, whereas the R-strain demonstrates a greater affinity for rice, sugarcane, and pasture grasses (Dumas et al., 2015; Jing et al., 2021).
Beyond host specificity, these strains also exhibit differences in their dispersal abilities and resistance to environmental stressors. Studies suggest that the C-strain is more adapted to agricultural landscapes with annual crops, while the R-strain thrives in more stable, perennial grassland environments (Nagoshi et al., 2017). Additionally, inter-strain hybridization has been reported, potentially leading to increased genetic diversity and adaptive capabilities in invasive populations (Nagoshi et al., 2019). The presence of both strains in Africa has raised concerns about their combined impact on food security, as they contribute to the rapid expansion and persistence of FAW populations across diverse agroecosystems (Assefa, 2019).
3 The invasive potential of the FAW
The invasive success of the FAW is attributed to its biological, ecological, and behavioral traits. These include its strong migratory capabilities, high reproductive rate, adaptability to diverse habitats and a broad host range (Jing et al., 2021; Barkessa et al., 2024). FAW is a multivoltine pest that lacks diapause, allowing it to maintain continuous infestations in suitable environments (Barkessa et al., 2024).
Africa’s favorable climatic conditions and the abundance of suitable host plants further facilitate FAW’s establishment and spread, increasing the likelihood of it becoming endemic (Chimweta et al., 2020; Kenis et al., 2022). Furthermore, the overlap of maize cropping seasons and relay planting allows FAW to move from rain-fed to flood-recession crops, thereby intensifying its presence and impact (Chimweta et al., 2020). Bioclimatic factors, particularly temperature, rainfall, and land use, significantly influence the pest’s distribution (Fan et al., 2020; Liu et al., 2020; Barkessa et al., 2024; Dessie et al., 2024; Huang et al., 2024). Several studies have confirmed that FAW habitat suitability in Africa is strongly associated with temperature and rainfall patterns (Early et al., 2018; Cokola et al., 2020; Abdel-Rahman et al., 2023).
Other key factors contributing to FAW’s invasive potential in newly invaded regions include the absence of native natural enemies and favorable environmental conditions that support its survival and reproduction, leading to unchecked population growth (Early et al., 2018; Prasanna et al., 2018; Tendeng et al., 2019; Jing et al., 2021). The Enemy Release Hypothesis (Joshi and Vrieling, 2005; Elton, 2020) helps explain why FAW has become a devastating pest in Africa. In its native range in the Americas, FAW populations are regulated by a diverse community of over 150 natural enemies, including various species and families of pathogenic microorganisms, parasitoids, and predators (Wyckhuys and O’Neil, 2006; Meagher et al., 2016; Molina-Ochoa et al., 2003). However, when introduced to new regions, invasive species often escape these natural controls, leading to rapid population expansion and greater ecological and economic impacts (Harrison et al., 2019; Kenis et al., 2019). In Africa, FAW has encountered significantly fewer natural enemies, resulting in severe infestations and crop damage (Early et al., 2018).
Studies across Africa have documented around 20 natural enemy species of FAW, including 11 identified in Zambia alone, but their overall parasitism rate remains low at 10.5% (Sisay et al., 2018; Ahissou et al., 2021; Chipabika et al., 2023). This reduced effectiveness compared to FAW’s native range underscores the lag time before local natural enemies adapt to the pest and highlights the need for biological control interventions (Prasanna et al., 2018; Pal et al., 2024). The introduction of specialized parasitoids from the Americas has been proposed as a potential strategy to enhance FAW management and mitigate its economic impact (Agboyi et al., 2020).
4 Agronomic and economic impact of fall armyworm in Africa
FAW infestations pose significant threats to crop development, agricultural ecosystems, and economic stability across Africa. In maize, damage during early and mid-whorl stages (first to ninth vegetative leaf stages, V1-V9) significantly reduce plant height, stalk thickness, leaf size, and fresh and dry plant biomass (Marenco et al., 1992). Defoliation reduces the photosynthetic area and leads to seedling loss, both of which can lower grain production (Vilarinho et al., 2011; Overton et al., 2021). However, at this stage, maize can compensate and keep defoliation below 50%, unless recovery is hindered by factors such as soil conditions, plant genetics, and poor cultural practices (Kasoma et al., 2020). Infestations during late whorl stage (V9-R1) result in increased ear damage and reduced crop yields (Marenco et al., 1992). FAW feeding during tasseling stage results in decreased pollen production (Kasoma et al., 2020), while silk damage leads to poor fertilization rates and fewer kernels per ear (Kasoma et al., 2020). Infestations at grain filling stage cause direct yield losses as larvae feed on ears, cobs, and seeds (Overton et al., 2021). Additionally, FAW damage facilitates fungal infections, increasing mycotoxin contamination and further reducing both marketability and overall grain quality (Overton et al., 2021). Beyond direct crop damage, FAW infestations threaten biodiversity and disrupt agricultural ecosystems and natural habitats, affecting the balance of local flora and fauna by consuming non-crop plants (FAO, 2018, 2022; Ayra-Pardo et al., 2024). The heavy reliance on chemical pesticides for FAW control harms beneficial insects, soil microorganisms, and aquatic life, leading to ecological imbalance and affecting ecosystem services (FAO, 2022; Mlambo et al., 2024).
Economically, FAW attacks on maize, rice, sorghum, millet, and other important crops result in substantial yield losses, posing a direct threat to food security and livelihoods of the rural population (Kasoma et al., 2020). FAW infestations across sub-Saharan Africa cause estimated annual economic losses of up to US$13 billion in maize, rice, sorghum, and sugarcane (Abrahams et al., 2017; Overton et al., 2021).
FAW’s widespread presence in Africa has severely reduced maize yield, affecting millions of smallholder farmers whose livelihoods depend on this crop (Tambo et al., 2021). The pest has been reported to cause damage ranging from 25% to 50% in Zimbabwe, with farmers noting a 58% yield reduction (Chimweta et al., 2020). In Zambia, estimated yield losses during the 2016/17 cropping season ranged from 38% to 62% (Day et al., 2017). Prasanna et al. (2018) reported that 12 African countries experienced annual maize yield losses ranging from 21% to 53% in 2017. Additionally, FAW-induced yield losses were reported to range from 22% to 67% in West Africa (Day et al., 2017) and 32% in East Africa (Kumela et al., 2019). Day et al. (2017) stated that Sub-Saharan Africa loses approximately 13.5 million tons of maize annually, valued at USD 3.06 billion, due to FAW infestations. Eschen et al. (2021) and Cokola et al. (2023) have noted a total monetary value of approximately USD 9.4 billion due to FAW on maize in Africa. Chimweta et al. (2020) reported that, without effective control measures, FAW could reduce maize yield by 8.3 to 20.6 million tons annually in Africa.
Beyond maize, FAW has also been reported to affect other staple crops. Rice is among the most vulnerable, with infestations significantly reducing both yield and grain quality. Rwomushana et al. (2018) estimated that without effective control measures, annual FAW-induced losses in maize, rice, sorghum, and sugarcane could reach US$13 billion across sub-Saharan Africa. In Ghana, a survey by Koffi et al. (2020) reported FAW impact on rice (13.6% of respondents), millet (5.79%), and sorghum (5.41%), highlighting the pest’s broad impact. FAW infestations in sorghum have also been shown to cause substantial yield reductions (Day et al., 2017), although specific figures on economic losses for sorghum in Africa remain limited. Similarly, household surveys conducted in Ghana and Zambia in 2018, indicated that only 2-4% of farmers reported FAW damage to millet, suggesting a relatively lower economic impact on this crop (Rwomushana et al., 2018). Additionally, FAW outbreaks disrupt agricultural trade at local, regional, and global levels, amplifying economic risks (FAO, 2018). Addressing FAW’s agronomic and economic impacts requires sustainable pest management strategies that balance productivity, ecosystem stability, and farmer resilience.
5 Integrated pest management strategies specific to Spodoptera frugiperda
IPM is a decision-based process that uses a coordinated combination of methods to effectively control complex pests, including insects, pathogens, weeds, and vertebrates, while promoting environmental and economic sustainability (Ehler, 2006). FAW IPM requires a combination of two or more compatible management strategies. It also includes regular field monitoring and surveillance as essential operations for assessing the Economic Injury Level (EIL) and the Economic Threshold (ET). The EIL and ET are used as decision-making tools to determine when to take appropriate control measures to prevent economic losses caused by insect pests (Togola et al., 2023). Additionally, several strategies, including cultural practices, host plant resistance, mechanical control, and biological control, have been employed to manage S. frugiperda (Kenis et al., 2022; Tepa-Yotto et al., 2022a; Mendesil et al., 2023; Obala et al., 2023). To reduce pest-related losses and the risk of hazardous chemicals, several International Research Organizations in Africa have actively conducted research to develop effective IPM strategies for FAW management. They have also made various training materials available, including handouts and guides (FAO, 2018; Prasanna et al., 2018; Tefera et al., 2019; Ahissou et al., 2021; Kasoma et al., 2021). Researchers have implemented a range of community-based educational programs to encourage African farmers to adopt FAW IPM technologies. According to FAO and ASARECA (2018), there is a growing awareness of the limitations and environmental impacts of chemical controls, prompting a gradual shift towards IPM strategies.
5.1 Monitoring and surveillance for early detection
Regular scouting of crops for signs of FAW infestations can help with early detection and prompt action before populations reach the economic threshold levels. According to Prasanna et al. (2018), implementing effective monitoring, surveillance, and scouting systems is a critical step in developing a successful IPM of FAW. Essential tools and practices for field monitoring at the farmer level include regular field scouting, use of pheromones (Tepa-Yotto et al., 2022b), and light traps. Pheromone traps are one of the most prominent and effective scientific tools used in pest management programs worldwide. This device enables early detection of pest infestations, tracks pest population dynamics, and supports pest management decision-making (Chiwamba et al., 2018). For small-scale farmers, several advanced technologies are available for monitoring and surveillance of FAW.
The FAW Monitoring and Early Warning System (FAMEWS) has been widely adopted across the continent, enabling farmers to track pest infestations (FAO, 2020). In six Eastern African countries (Ethiopia, Kenya, Tanzania, Uganda, Rwanda, and Burundi), a community-based initiative involving 650 focal persons has demonstrated its effectiveness (Niassy et al., 2021).
Remote sensing, particularly satellite imagery and NDVI analysis, has proven useful for detecting FAW damage. A Sentinel-2 satellite-based algorithm successfully identified biomass loss in maize fields in Zimbabwe, Kenya, and Tanzania (Buchaillot et al., 2022). In Ghana, integrating field surveys with remote sensing improved FAW distribution modeling (Bilintoh, 2019). Predictive models, such as MaxEnt, have been used to simulate FAW distribution under current and future climate scenarios in Ethiopia (Barkessa et al., 2024).
Machine learning is also being explored, with the University of Zambia developing an automated FAW identification system using Convolutional Neural Networks (CNN) that integrates vision and motion sensors with pheromone traps for real-time pest monitoring, reducing field visits, improving data collection efficiency, and enhancing decision-making (Chiwamba et al., 2018).
5.2 Cultural practices
Studies have found that cultural practices such as crop rotation, regular weeding, and destruction of crop residues help to mitigate FAW infestation (Cokola et al., 2023). Maintaining organic soil fertility was found to prevent high FAW density (Bayissa et al., 2023). Using early-maturing cultivars was reported to be effective against FAW, as these varieties have a shorter growth cycle, reducing their exposure to the pest (Day et al., 2017). Also, adjusting the planting schedule to avoid peak periods of FAW activity can help prevent infestations (Ahissou et al., 2022). Other studies have indicated that destroying the ratoons of sorghum and sugarcane, which serve as reservoirs for FAW, also effectively controls the pest’s population. Intercropping maize or other cereals with non-preferred or repellent crops (e.g., legume crops, desmodium and Dolichos lablab), as well as practicing mixed cropping, has been shown to attract natural enemies that prey on FAW, making it a proven effective pest management strategy (Ratnadass et al., 2006; Chimweta et al., 2020). However, many leguminous crops that are often grown with maize are known to be hosts for FAW and tend to sprout earlier, which could exacerbate the pest infestations in maize (Rwomushana et al., 2018; Ambia, 2023). Other practices like zero-tillage can reduce overwintering larvae and promote higher densities of natural enemies, making the environment less attractive for FAW populations (Baudron et al., 2019). The number of FAW predators can also be maintained through habitat management techniques like building shelterbelts or live fences that increase biodiversity (Hellmich et al., 2008). Additionally, push-pull technology, which combines trap plants to attract FAW and repellent intercrops to deter it, is cited as an ideal approach for mixed farming systems, effectively reducing FAW larval density and damage to maize and other cereals (Joatya et al., 2022; ICIPE, 2024). However, certain cultural practices, like intercropping cereals with pumpkins, should be avoided as they may inadvertently increase FAW damage by providing shelter for moths and facilitating larval migration. Integrating these cultural practices with other FAW control strategies can significantly improve the management of the pest.
5.3 Host plant resistance
Host plant resistance (HPR) is a key component of IPM strategies for controlling FAW. It involves the inherent defense mechanisms of plants, which include both biophysical and biochemical properties (Meihls et al., 2012; Jin, 2017);.
Studies discovered several traits associated with maize resistance against FAW. Callahan et al. (1992) found that a combination of two specific polypeptides (36 and 21 kDa) is predictive of resistance to lepidopteran insects. Furthermore, Smith et al. (2012) revealed that the terpenoid (E)-β-caryophyllene in maize whorl leaves showed that the plant is naturally resistant to FAW. Other compounds associated with resistance include mir1-CP, oxophytodienoic acid (OPDA), a low protein/total nonstructural carbohydrates ratio, thicker leaves, and higher contents of crude fiber, hemicellulose, and thicker cell wall complexes, as well as the constitutive expression of jasmonic acid genes (Singh et al., 2022). Similarly, Darshan et al. (2024) reported significant negative correlations among morphological traits such as plant height, stem girth, and the presence of trichomes related to FAW incidence. Baudron et al. (2019) and Snook et al. (1993) stated that the rapid accumulation of proteins or phytochemicals like maysin in silks can either poison or starve the pest. Chiriboga Morales et al. (2021) and dos Santos et al. (2020) found a negative correlation between maize leaf toughness at the twelfth vegetative leaf stage (V12) and leaf damage from FAW infestation. Jiang et al. (1995) and Pechan et al. (2000) found that higher levels of the 33-kD cysteine proteinase in plant leaves were associated with lower FAW larval weight, suggesting that the enzyme may serve as a novel insect defense mechanism in plants. Baudron et al. (2019) reported that plant characteristics such as the density of leaf hairs or the density of the cuticular wax layer can reduce foliar damage. In response to pest attacks, some plants activate wound-response pathways and release volatile compounds (Smith et al., 2012).
To date, the International Maize and Wheat Improvement Center (CIMMYT) has disseminated several FAW-tolerant maize inbred lines, including CML71, CML124, CML125, CML338, CML333, CML334, CML370, CML372, and CML574, across 34 countries. Also, the center has developed three high yielding maize hybrids (FAWTH2001, FAWTH2002 and FAWTH2003) with native tolerance to FAW, which are being widely released in Africa. CIMMYT undertook these research efforts in collaboration with various National Agricultural Research and Extension Systems (NARES), advanced research institutes (ARIs), and commercial seed companies (Prasanna et al., 2021). Several other studies have identified different maize germplasms with desirable resistance traits or mechanisms to FAW, as illustrated in Table 2.
The identification of resistant donor lines and the introduction of desired traits into elite maize cultivars represent significant advancements in developing FAW-resistant plants. Exploring molecular markers for FAW resistance can accelerate the breeding process (Sharma et al., 2008; Nashath et al., 2023). Two important tools used in modern breeding are marker-assisted selection and quantitative trait loci (QTLs). They facilitate the identification of genetic traits, accelerate breeding cycles, and enhance the development of insect-resistant germplasms (Mihm, 1997; Singh et al., 2022). These practices help incorporate desired traits while avoiding deleterious alleles. According to Abdulmalik et al. (2017), molecular tools make it possible to select for FAW resistance while keeping the beneficial traits of adapted cultivars. Singh et al. (2022) emphasized that rapid screening strategies and genomic selection can enhance the utilization of plant genetic resources. In a GWAS study, Badji et al. (2020) utilized SNP markers to identify 62 quantitative trait nucleotides (QTNs) linked to FAW resistance traits in maize in four SNP regions. Notably, QTLs on chromosomes 4 and 9 correspond to bi-parental QTL mapping, highlighting their importance they in in developing markers for FAW leaf damage resistance (Womack et al., 2018; 2020).
Emerging biotechnological solutions are being explored to combat lepidopteran pests, including stemborers and FAW. In the African context, genetically modified Bt maize, which is resistant to both pests, has been commercialized in South Africa and is expected to expand to other countries where transgenic maize is already approved as an effective management strategy (Prasanna et al., 2022). Furthermore, advanced molecular technologies, including RNA interference (RNAi) (Kebede and Fites, 2022; Kumara et al., 2022; Nwokeoji et al., 2022) and gene editing using CRISPR-Cas9 (Singh et al., 2022), have been investigated to target essential genes in lepidopteran pests, disrupting their development and reducing crop damage. However, these technologies have yet to be implemented for FAW management in Africa.
5.4 Mechanical control
It has been reported that practices like handpicking larvae, crushing egg and neonate masses, and uprooting infested plants increase the resilience to FAW. However, these options are best suited for shorter cultivars, early to mid-whorl stages, and small-sized fields (Chimweta et al., 2020). Traditionally, small-scale farmers in Africa apply soil and ash to leaf whorls to control the pest (Abate et al., 2000; Ambia, 2023). These mechanical methods are still applicable at the farmer level in SSA due to the relatively small areas of production (Hruska, 2019; Cokola et al., 2023). Other mechanical approaches used in SSA to control FAW include the use of pitfall traps, sticky traps, light traps, or pheromone traps (Prasanna et al., 2018; Bhusal and Bhattarai, 2019; Gebreziher and Gebreziher, 2020; Akeme et al., 2021), as well as ash and detergents (Tambo et al., 2020). Also, legumes such as beans (Phaseolus vulgaris), groundnuts (Arachis hypogaea), cowpeas (Vigna unguiculata) and soybean (Glycine max) are intercropped within maize to serve as trap crops for the pest (Akeme et al., 2021).
5.5 Biological control strategies
Biological control is an effective strategy for managing FAW using natural enemies such as predators, parasitoids, entomopathogenic fungi, viruses, nematodes, bacteria, and biopesticides (Assefa and Ayalew, 2019; Abbas et al., 2022). This can be implemented through two primary approaches: augmentative biocontrol, which involves mass rearing and periodic release of beneficial organisms to boost existing populations, and inoculative biocontrol, which entails a single initial release of natural enemies aimed at establishing a self-sustaining population for long-term pest control (Nyamutukwa et al., 2022).
Additionally, proper habitat conservation, maintaining prey availability, and minimizing the use of broad-spectrum insecticides in agricultural and natural ecosystems can support the populations of natural enemies, a practice known as conservation biocontrol. These methods can be effectively integrated as biocontrol-based applications within an IPM framework against FAW (Tefera et al., 2019; Kenis et al., 2022; Ayra-Pardo et al., 2024).
Ideally, biological control should be based on natural enemies already attacking FAW in Africa rather than organisms from other regions, as these may cause unforeseen problems (Chimweta et al., 2020). In Africa, Abbas (2023) reported 48 species of parasitoids, primarily from the Braconidae family (19 species), followed by Ichneumonidae (11 species), Tachinidae (9 species), and Trichogrammatidae (5 species). The remaining species belong to families such as Eulophidae, Heciridae, Pteromalidae, and Scelionidae, with one species each. The egg-larval endoparasitoid Chelonus bifoveolatus (Hymenoptera: Braconidae) commonly targets the eggs of FAW on the African continent, causing the young instars of FAW to hatch alongside the parasitoid’s eggs (Obala et al., 2023; ICIPE, 2024). In Africa, Trichogramma chilonis (Hymenoptera: Trichogrammatidae), Coccygidium luteum, Telenomus remus (Hymenoptera: Scelionidae), and Cotesia icipe are also common FAW parasitoids. Cotesia icipe is known for its strong ability to control FAW in maize crops.
Several studies found that the parasitism rates of those mentioned parasitoids varied across African countries, such as Ghana, Kenya, Niger, Nigeria, Egypt, Uganda, South Africa, Senegal, Tanzania, and Zambia (Agboyi et al., 2020; Abang et al., 2021; Mohamed et al., 2021; Otim et al., 2021; Abbas, 2023; Koffi et al., 2023; ICIPE, 2024).
Trichogramma spp. and Telenomus spp. are widely used parasitoids for FAW control because they are easy to rear in laboratories and effective in managing FAW population (Figueiredo et al., 2002; Gutierrez-Martinez et al., 2012; Tefera et al., 2019).
Abbas (2023) reported a total of 17 predators of FAW in Africa, which include seven coleopterans from the Coccinellidae family (Coleomegilla maculata, Cycloreda sanguinea, Cheilomenes sulphurea, Coccinella transvirsalis, Harmonia octomaculata, Eriopis sp., and Hippodamia sp.), two coleopterans from the Carabidae family (Callida sp. and Calosoma granulatus), one coleopteran from the Lampyridae family (Hematochares obscuripennis), three dermapterans from the Forficulidae family (Diaperasticus crythrocephalus, Forficula senegalensis, and Doru sp.), one hemipteran from the Geocoridae family (Geocoris sp.), one hemipteran from the Pentatomidae family (Podisus sp.), one hymenopteran from the Formicidae family (Pheidole megacephala), and one lepidopteran from the Erebidae family (Perprius modiulipes). Table 3 shows the main families and order/class of FAW’s natural enemies in Africa.
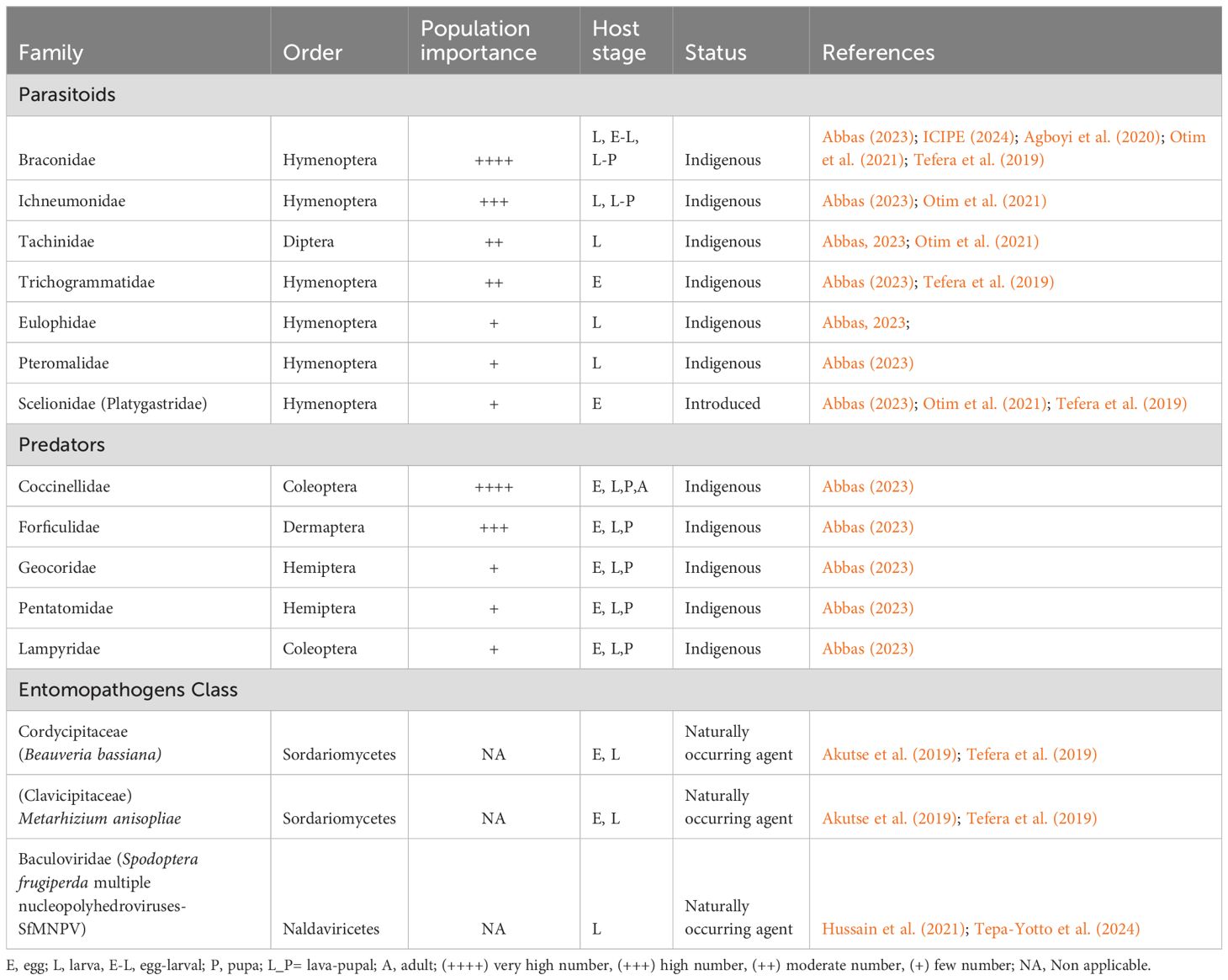
Table 3. Natural enemies (parasitoids, predators and entomopathogenic organisms) of fall armyworm in Africa.
Several bio-rational control agents have shown effectiveness in FAW management. These include the entomopathogenic fungi Metarhizium anisopliae and Beauveria bassiana, as well as the biological pesticide Spodoptera frugiperda nucleo-polyhedrovirus (Fawligen, SfNPV) (Akutse et al., 2019). Nematodes, protozoans, and botanical extracts such as powders, volatile and non-volatile oils, and extracts from Azadirachta indica and Jatropha curcas seed can also be used to control FAW (Adjaoke et al., 2021; Abbas et al., 2022; El-Sappagh et al., 2022; Tepa-Yotto et al., 2022b). Patel Sagarbhai et al. (2021) stated that neem affects insect growth, development, egg laying, and has been shown to have antifeeding and larvicidal activity in FAW. Sisay et al. (2019) noted that extracts from several plants, such as Milletia ferruginea, Phytolacca dodecandra, Schinus molle, Melia abyssinica, Nicotiana tabacum, Lantana camara, Chenopodium ambroides, and Jatropha gossypifolia, induced high mortality of FAW. The deployment of biological control methods against FAW among African farmers has been progressing. However, adoption remains minimal due to the absence of true native natural enemies. In Zambia, CABI launched a village-based biological control initiative to promote the use of natural enemies and biopesticides, aiming to reduce farmers’ reliance on chemical pesticides (CABI, 2023). Additionally, training programs have been conducted to empower young farmers to explore business opportunities in the biocontrol sector, further facilitating the adoption of these methods (CABI, 2024).
5.6 Chemical control options and insect resistance management
At present, most African farmers rely on the application of synthetic insecticides to control the FAW (Assefa and Ayalew, 2019). According to Tambo et al. (2020) and Kumela et al. (2019), the most used control measure in Africa for the management of FAW is the application of synthetic pesticides.
Insecticide applications should be based on FAW infestation thresholds after continuous monitoring. Studies have validated several treatment thresholds that consider not only the pest’s developmental stages but also the phenological stages of the infested crops (FAO, 2017; Prasanna et al., 2018). To be technically and cost-effective, the pesticide choice should respect these action thresholds (Chimweta et al., 2020).
However, chemical insecticides should be considered as the last resort for managing FAW, as they may negatively impact the environment and the non-target organisms, human health, and biodiversity, including beneficial insects (Harrison et al., 2019; Deshmukh et al., 2020; Idrees et al., 2022). Furthermore, their use is associated with high costs, potential environmental contamination, resistance development, and frequent pest resurgences (Gebreziher, 2020). Studies have demonstrated that repeated, indiscriminate use and misuse of chemical insecticides exert strong selection pressure, leading to the evolution of resistance mechanisms (Togola et al., 2018; Zhang et al., 2021). Cases of FAW resistance to multiple classes of insecticides, including pyrethroids, organophosphates, and carbamates, have been widely documented in different regions, posing a significant challenge to pest management (Carvalho et al., 2013; Yu et al., 2023). The increasing resistance not only reduces the efficacy of chemical control but also requires higher application rates and more frequent spraying, further exacerbating environmental and economic burdens (Gutiérrez-Moreno et al., 2019). To prevent insecticide resistance in FAW, it is important to implement integrated pest management practices. These strategies will help maintain resistance at low levels.
Studies have shown that selection pressure from Bacillus thuringiensis (Bt)-resistant maize can lead to FAW resistance. FAW has developed resistance to Bt toxins such as Cry1Ac, Cry1Ab, and Cry1A.105 through mechanisms like metabolic detoxification, target-site insensitivity, and behavioral avoidance (Storer et al., 2010; Huang et al., 2014; Boaventura et al., 2020; Boaventura et al., 2021; Jing et al., 2021; Zhang et al., 2021). This poses significant challenges for FAW- preferred crop growers (Peferoen, 1997; Zhang et al., 2021). Several studies have also shown that FAW has become resistant to different ranges of pesticides, such as carbamates, organophosphates, and pyrethroids (Jing et al., 2021; Zhang et al., 2021).
It is suggested that to break insects from becoming resistant to protein toxins, an insect resistance management (IRM) approach is recommended. One key strategy of IRM is the establishment of refuge areas, either within or adjacent to transgenic fields, to allow non-resistant insect populations to thrive. This promotes random mating between treated and refuge populations, weakening the resistance alleles in the overall population (Vilarinho et al., 2011; Murúa et al., 2019). Several studies have shown that the presence of non-Bt refuges can sustain the long-term efficacy of Bt crops by preventing resistant populations from becoming dominant (Huang et al., 2014; Carrière et al., 2020). Proper implementation of refuge areas requires careful planning, including the selection of appropriate non-Bt host plants, strategic placement, and maintaining a sufficient proportion of the crop area as a refuge. Research suggests that an ideal refuge should be at least 20% of the total crop area (Bourguet et al., 2005; EuropaBio, 2019). Other studies suggest placing refuge areas approximately 800 meters apart to maintain their effectiveness (Vilarinho et al., 2011). Prasanna et al. (2022) suggested that integrating polygenic native resistance with transgenic Bt maize, which typically relies on one gene (monogenic) or a few genes (oligogenic) in conventional cropping systems, could be a key strategy for insect resistance management. This approach would help prevent FAW from overcoming the limited genetic defenses of Bt maize, thereby promoting a more effective and sustainable FAW control strategy.
6 Future directions and recommendations
Emerging technologies such as digital tools (e.g., mobile applications and remote sensing technologies), drones equipped with imaging technology, and computer vision techniques to automatically detect FAW infestation in maize crops can play a significant role in monitoring FAW infestations in real-time, assessing their severity through data analytics and predictive modeling, and allowing timely interventions (Shaurub, 2024; Shinde et al., 2024). Additionally, advanced genetic research, including the development of genetically modified maize as well as native genetic resistance hybrids, offers promising management avenues for FAW (Prasanna et al., 2022; Gouda et al., 2024). Integrated approaches that combine multiple management options are being explored to enhance the efficacy of traditional methods, promoting sustainable agricultural practices against FAW (Anilkumar et al., 2024). These emerging technologies can not only improve management strategies but also help mitigate the environmental impact of pesticide use.
Despite significant progress in FAW management, several research gaps remain that warrant further investigation. For instance, the role of climate change and its impact on the distribution and life cycle and population dynamics of FAW requires deeper exploration, as it could significantly impact the dynamics of the pest and, consequently, agricultural productivity (Ntwari et al., 2024). Also, the socio-economic and environmental aspects of pest management, including farmers’ knowledge, adoption of technologies, and access to resources, need thorough investigation to design comprehensive strategies that enhance resilience (Berg and Plessis, 2024). Furthermore, more research is needed to evaluate the effectiveness of biological control agents and their interaction with native ecosystems.
The battle against FAW in Africa underscores the necessity of collaborative efforts among governments, research institutions, non-governmental organizations, and local farmers. Effective management requires a unified approach, as FAW does not respect borders and can swiftly spread across regions. Collaborative initiatives facilitate the sharing of knowledge, resources, and technology, enabling countries to develop and implement coordinated action plans tailored to local challenges (Karakkottil et al., 2024). For instance, regional networks can enhance surveillance and monitoring efforts, leading to early detection and response to infestations. Moreover, partnerships can support training programs for farmers, empowering them with information on best practices and sustainable pest management techniques.
7 Conclusion
African farmers continue to face regular infestations and outbreaks of FAW, which impact the yields of staple crops, particularly causing severe losses in maize production. Addressing this pest, which hinders crop production across Africa, requires adequate attention. It is important to know about the pest’s biology, ecology, and irreversible impacts, and the range of interventions that can serve as alternative control strategies to minimize pesticide applications.
In this regard, there is an ongoing need for the research community to develop IPM strategies to achieve these goals. The research community has made significant progress in developing, testing, and validating IPM options as holistic management solutions for FAW. CIMMYT and its partners, including National Agricultural Research and Extension Systems (NARES), have developed FAW IPM technologies and made numerous field-guides available for training and capacity building purposes.
Moreover, CIMMYT’s Global Maize Program has developed high-yielding maize hybrids with native genetic tolerance to FAW, which are being widely released in Africa. Several African countries are employing other options, including biological control, cultural practices, mechanical control methods, and the judicious use of chemicals as effective strategies to control the FAW. Researchers have utilized various knowledge-sharing platforms such as pest diagnostic guides, field demonstrations, training sessions, videos, animations, and Information and Communication (ICT) tools to facilitate the adoption of IPM technologies.
Author contributions
AT(1st author): Validation, Writing – original draft, Writing – review & editing. YB: Funding acquisition, Resources, Validation, Writing – review & editing. RB: Validation, Writing – original draft, Writing – review & editing. GT: Validation, Writing – original draft, Writing – review & editing. MG: Validation, Writing – review & editing. AT(6th author): Validation, Writing – original draft, Writing – review & editing. PB: Funding acquisition, Resources, Writing – review & editing.
Funding
The author(s) declare that financial support was received for the research and/or publication of this article. The research was supported by the International Maize and Wheat Improvement Center (CIMMYT).
Acknowledgments
The authors extend their sincere gratitude to the International Maize and Wheat Improvement Center (CIMMYT) for supporting this manuscript, highlighting the valuable efforts of the center to disseminate knowledge and research innovations for combating FAW in maize and enhance global food security.
Conflict of interest
The authors declare that the research was conducted in the absence of any commercial or financial relationships that could be construed as a potential conflict of interest.
Generative AI statement
The author(s) declare that no Generative AI was used in the creation of this manuscript.
Publisher’s note
All claims expressed in this article are solely those of the authors and do not necessarily represent those of their affiliated organizations, or those of the publisher, the editors and the reviewers. Any product that may be evaluated in this article, or claim that may be made by its manufacturer, is not guaranteed or endorsed by the publisher.
References
Abang A. F., Nanga S. N., Fotso Kuate A., Kouebou C., Suh C., Masso C., et al. (2021). Natural enemies of fall armyworm Spodoptera frugiperda (Lepidoptera: Noctuidae) in different agro-ecologies. Insects 12, 509. doi: 10.3390/insects12060509
Abate T., van Huis A., Ampofo J. K. O. (2000). Pest management strategies in traditional agriculture: an african perspective. Annu. Rev. Entomology 45 (1), 631–659.
Abbas M. S. (2023). Entomophagous Insects of The Invasive Fall Armyworm, Spodoptera frugiperda (Nixon) in African and Asian countries. Egyptian Acad. J. Biol. Sci. A Entomol. 16, 51–67. doi: 10.21608/eajbsa.2023.299255
Abbas A., Ullah F., Hafeez M., Han X., Dara M. Z. N., Gul H., et al. (2022). Biological control of fall armyworm, Spodoptera frugiperda. Agronomy 12, 2704. doi: 10.3390/agronomy12112704
Abdel-Rahman E. M., Kimathi E., Mudereri B. T., Tonnang H. E., Mongare R., Niassy S., et al. (2023). Computational biogeographic distribution of the fall armyworm (Spodoptera frugiperda J.E. Smith)moth in eastern Africa. Heliyon 9, e16144. doi: 10.1016/j.heliyon.2023.e16144
Abdulmalik R. O., Menkir A., Meseka S. K., Unachukwu N., Ado S. G., Olarewaju J. D., et al. (2017). Genetic gains in grain yield of a maize population improved through marker assisted recurrent selection under stress and non-stress conditions in West Africa. Front. Plant Sci. 8, 841. doi: 10.3389/fpls.2017.00841
Abrahams P., Bateman M., Beale T., Clottey V., Cock M., Colmenarez Y., et al. (2017). Fall armyworm: Impacts and implications for africa (Wallingford: CABI). https://www.invasive-species.org/wp-content/uploads/sites/2/2019/02/FAW-Evidence-Note-October-2018.pdf?utm_source=chatgpt.com (Access date for the url: 15/02/2025).
Acharya S., Kaphle S., Upadhayay J., Pokhrel A., Paudel S. (2020). Damaging nature of fall armyworm and its management practices in maize: A review. Trop. Agrobiodiversity 1, 82–85. doi: 10.26480/trab.02.2020.82.85
Adjaoke A. M., Adandonon A., Yotto G. T. (2021). Fall armyworm, Spodoptera frugiperda (Lepidoptera, Noctuidae): State of knowledge and control methods. Afr. J. Agric. Res. 17, 1237–1248.
Agboyi L. K., Goergen G., Beseh P., Mensah S. A., Clottey V. A., Glikpo R., et al. (2020). Parasitoid complex of fall armyworm, Spodoptera frugiperda, in Ghana and Benin. Insects 11, 68. doi: 10.3390/insects11020068
Ahissou B. R., Sawadogo W. M., Bonzi S., Baimey H., Somda I., Bokonon-Ganta A., et al. (2021). Natural enemies of the fall armyworm Spodoptera frugiperda (Smith)(Lepidoptera: Noctuidae) in Burkina Faso. Tropicultura 39 (3). doi: 10.25518/2295-8010.1881
Ahissou B. R., Sawadogo W. M., Sankara F., Brostaux Y., Bokonon-Ganta A. H., Somda I., et al. (2022). Annual dynamics of fall armyworm populations in West Africa and biology in different host plants. Sci. Afr. 16, e01227. doi: 10.1016/j.sciaf.2022.e01227
Akeme C. N., Ngosong C., Sumbele S. A., Aslan A., Tening A. S., Krah C. Y., et al. (2021). “Different controlling methods of fall armyworm (Spodoptera frugiperda) in maize farms of small-scale producers in Cameroon,” in IOP Conference Series: Earth and Environmental Science, Vol. 911. 012053 (Bristol, United Kingdom: IOP Publishing).
Akutse K. S., Khamis F. M., Ambele F. C., Kimemia J. W., Ekesi S., Subramanian S. (2020). Combining insect pathogenic fungi and a pheromone trap for sustainable management of the fall armyworm, Spodoptera frugiperda (Lepidoptera: Noctuidae). J. Invertebrate Pathol. 177, 107477. doi: 10.1016/j.jip.2020.107477
Akutse K. S., Kimemia J. W., Ekesi S., Khamis F. M., Ombura O. L., Subramanian S. (2019). Ovicidal effects of entomopathogenic fungal isolates on the invasive fall armyworm Spodoptera frugiperda (Lepidoptera: Noctuidae). J. Appl. Entomol. 143, 626–634. doi: 10.1111/jen.12634
Ambia A. (2023). Efficacy of pheromone-based mass trapping in management of fall armyworm spodoptera frugiperda (Lepidoptera: Noctuidae) in maize farms. (Doctoral dissertation, University of Nairobi).
Anilkumar G., LakshmiSoujanya P., Kumar D. V., Kumar V. M., Yathish K. R., Sekhar J. C., et al. (2024). Integrated approaches for the management of invasive fall armyworm, Spodoptera frugiperda (JE Smith) in maize. J. Plant Dis. Prot. 131, 793–803. doi: 10.1007/s41348-024-00914-0
Ashok K., Kennedy J. S., Geethalakshmi V., Jeyakumar P., Sathiah N., Balasubramani V. (2020). Lifetable study of fall army worm Spodoptera frugiperda (JE Smith) on maize. Indian J. Entomol. 82, 574–579. doi: 10.5958/0974-8172.2020.00143.1
Assefa Y. (2019). Molecular identification of the invasive strain of Spodoptera frugiperda (JE smith) (Lepidoptera: Noctuidae) in Swaziland. Int. J. Trop. Insect Sci. 39, 73–78. doi: 10.1007/s42690-019-00018-5
Assefa F., Ayalew D. (2019). Status and control measures of fall armyworm (Spodoptera frugiperda) infestations in maize fields in Ethiopia: A review. Cogent Food Agric. 5, 1641902. doi: 10.1080/23311932.2019.1641902
Ayra-Pardo C., Huang S., Kan Y., Wright D. J. (2024). Impact of invasive fall armyworm on plant and arthropod communities and implications for crop protection. Int. J. Pest Manage. 70, 180–191. doi: 10.1080/09670874.2021.1968534
Badhai S., Gupta A. K., Koiri B. (2020). Integrated management of fall armyworm (Spodoptera frugiperda) in maize crop. Rev. Food Agric. 1, 27–29. doi: 10.26480/rfna.01.2020.27.29
Badji A., Kwemoi D. B., Machida L., Okii D., Mwila N., Agbahoungba S., et al. (2020). Genetic basis of maize resistance to multiple insect pests: Integrated genome-wide comparative mapping and candidate gene prioritization. Genes 11, 689. doi: 10.3390/genes11060689
Banson K. E., Asare D. K., Dery F. D., Boakye K., Boniface A., ASamoah M., et al. (2020). Impact of fall armyworm on farmer’s maize: systemic approach. Systemic. Pract. Action Res. 33, 237–264. doi: 10.1007/s11213-019-09489-6
Barfield C. S., Mitchell E. R., Poeb S. L. (1978). A temperature-dependent model for fall armyworm development. Ann. Entomol. Soc. America 71, 70–74. doi: 10.1093/aesa/71.1.70
Barkessa M. K., Degaga E. G., Duressa T. F., Muleta M. G., Amenta M. W., Gurmu A. K. (2024). Factors influencing the current and future distribution of the fall armyworm, Spodoptera frugiperda (Lepidoptera: Noctuidae), in Ethiopia. Agric. For. Entomol. 26 (1), 1–11. doi: 10.1111/afe.12671
Baudron F., Zaman-Allah M. A., Chaipa I., Chari N., Chinwada P. (2019). Understanding the factors influencing fall armyworm (Spodoptera frugiperda JE Smith) damage in African smallholder maize fields and quantifying its impact on yield. A case study in Eastern Zimbabwe. Crop Prot. 120, 141–150. doi: 10.1016/j.cropro.2019.01.028
Bayissa W., Abera A., Temesgen J., Abera G., Mendesil E. (2023). Organic soil fertility management practices for the management of fall armyworm, Spodoptera frugiperda (JE Smith), in maize. Front. Insect Sci. 3, 1210719. doi: 10.3389/finsc.2023.1210719
Berg J., Plessis H. (2024). “Managing the invasive fall armyworm in the large-and small-scale maize farming landscapes of East and Southern Africa,” in Arthropod Management and Landscape Considerations in Large-scale Agroecosystems (CABI, GB), 78–103.
Bhusal K., Bhattarai K. (2019). A review on fall armyworm (Spodoptera frugiperda) and its possible management options in Nepal. J. Entomol. Zool. Stud. 7, 1289–1292.
Bilintoh T. (2019). Remote Sensing for Mapping and Modeling Presence of Armyworm Infestation on Maize in Ejura, Ghana (Michigan State University).
Boaventura D., Buer B., Hamaekers N., Maiwald F., Nauen R. (2021). Toxicological and molecular profiling of insecticide resistance in a Brazilian strain of fall armyworm resistant to Bt Cry1 proteins. Pest Manage. Sci. 77, 3713–3726. doi: 10.1002/ps.6061
Boaventura D., Ulrich J., Lueke B., Bolzan A., Okuma D., Gutbrod O., et al. (2020). Molecular characterization of Cry1F resistance in fall armyworm, spodoptera frugiperda from brazil. Insect Biochem. Mol. Biol. 116, 103280.
Bourguet D., Desquilbet M., Lemarié S. (2005). Regulating insect resistance management: the case of non-Bt corn refuges in the US. J. Environ. Manage. 76, 210–220. doi: 10.1016/j.jenvman.2005.01.019
Brooks T. D., Shaun Bushman B., Paul Williams W., McMullen M. D., Buckley P. M. (2007). Genetic basis of resistance to fall armyworm (Lepidoptera: Noctuidae) and southwestern corn borer (Lepidoptera: Crambidae) leaf-feeding damage in maize. J. Econ. Entomol. 100, 1470–1475. doi: 10.1603/0022-0493(2007)100[1470:gbortf]2.0.co;2
Buchaillot M. L., Cairns J., Hamadziripi E., Wilson K., Hughes D., Chelal J., et al. (2022). Regional monitoring of fall armyworm (FAW) using early warning systems. Remote Sens. 14, 5003. doi: 10.3390/rs14195003
CABI (2023). Village-based biological control of fall armyworm in Zambia. Available online at: https://www.cabi.org/projects/village-based-biological-control-of-fall-armyworm-in-Zambia/?utm_source=chatgpt.com (Accessed June 28, 2024).
CABI (2024). CABI empowers youth in Zambia to establish businesses in biocontrol of fall armyworm. Available online at: https://blog.invasive-species.org/2024/08/19/cabi-empowers-youth-in-Zambia-to-establish-businesses-in-biocontrol-of-fall-armyworm/?utm_source=chatgpt.com (Accessed June 28, 2024).
Callahan F. E., Davis F. M., Williams W. P. (1992). Steady-state polypeptide profiles of whorl tissue from lepidoptera-resistant and susceptible corn inbred lines. Crop Sci. 32, 1203–1207. doi: 10.2135/cropsci1992.0011183x003200050029x
Carrière Y., Crickmore N., Tabashnik B. E. (2020). Optimizing pyramided transgenic Bt crops for sustainable pest management. Nat. Biotechnol. 38, 529–540. doi: 10.1038/nbt.3099
Carvalho R. A., Omoto C., Field L. M., Williamson M. S., Bass C. (2013). Investigating the molecular mechanisms of organophosphate and pyrethroid resistance in the fall armyworm Spodoptera frugiperda. PloS One 8, e62268. doi: 10.1371/journal.pone.0062268
Chapman J. W., Williams T., Martínez A. M., Cisneros J., Caballero P., Cave R. D., et al. (2000). Does cannibalism in Spodoptera frugiperda (Lepidoptera: Noctuidae) reduce the risk of predation? Behav. Ecol. Sociobiol. 48, 321–332. doi: 10.1007/s002650000237
Chimweta M., Nyakudya I. W., Jimu L., Bray Mashingaidze A. (2020). Fall armyworm [Spodoptera frugiperda (JE Smith)] damage in maize: management options for flood-recession cropping smallholder farmers. Int. J. Pest Manage. 66, 142–154. doi: 10.1080/09670874.2019.1577514
Chipabika G., Sohati P. H., Khamis F. M., Chikoti P. C., Copeland R., Ombura L., et al. (2023). Abundance, diversity and richness of natural enemies of the fall armyworm, Spodoptera frugiperda (JE Smith)(Lepidoptera: Noctuidae), in Zambia. Front. Insect Sci. 3, 1091084. doi: 10.3389/finsc.2023.1091084
Chiriboga Morales X., Tamiru A., Sobhy I. S., Bruce T. J., Midega C. A., Khan Z. (2021). Evaluation of African maize cultivars for resistance to fall armyworm Spodoptera frugiperda (JE Smith)(Lepidoptera: Noctuidae) larvae. Plants 10, 392. doi: 10.3390/plants10020392
Chiwamba S. H., Phiri J., Nkunika P. O. Y., Nyirenda M., Kabemba M. M. (2018). An application of machine learning algorithms in automated identification and capturing of fall armyworm (FAW) moths in the field (Lusaka, Zambai: ICICT2018).
Cokola M. C., Mugumaarhahama Y. M., Noel G., Bisimwa E. B., Bugeme D. M., Chuma G. B., et al. (2020). Bioclimatic zonation and potential distribution of Spodoptera frugiperda (Lepidoptera: Noctuidae) in south Kivu Province, DR Congo. BMC Ecol. 20, 1–13. doi: 10.1186/s12898-020-00335-1
Cokola M. C., Van Den Bussche R., Noël G., Kouanda N., Sèye F., Yarou B. B., et al. (2023). Managing fall armyworm, Spodoptera frugiperda (Lepidoptera: Noctuidae): Experience from smallholder farmers in central and western Africa. Food Energy Secur. 12, e491. doi: 10.1002/fes3.491
Darshan R., Prasanna P. M., Hegde J. N. (2024). Screening of Popular Maize Hybrids against fall Armyworm, Spodoptera frugiperda. J. Exp. Agric. Int. 46, 306–312. doi: 10.9734/jeai/2024/v46i52379
Day R., Abrahams P., Bateman M., Beale T., Clottey V., Cock M., et al. (2017). Fall armyworm: impacts and implications for Africa. Outlooks Pest Manage. 28, 196–201. doi: 10.1564/v28_oct_02
Deshmukh S., Pavithra H. B., Kalleshwaraswamy C. M., Shivanna B. K., Maruthi M. S., Mota-Sanchez D. (2020). Field efficacy of insecticides for management of invasive fall armyworm, Spodoptera frugiperda (JE Smith)(Lepidoptera: Noctuidae) on maize in India. Florida Entomol. 103, 221–227. doi: 10.1653/024.103.0211
de Souza Crubelati-Mulat N. C., Baleroni A. G., Contreras-Soto R. I., Ferreira C. J. B., Castro C. R., de Albuquerque F. A., et al. (2019). Evaluation of resistance to Spodoptera frugiperda in sweet and field corn genotypes. Maydica 64.
Dessie B., Ferede B., Taye W., Shimelash D. (2024). Field infestation of the invasive fall armyworm, Spodoptera frugiperda (Lepidoptera: Noctuidae) on maize in Southern Ethiopia. Crop Prot. 178, 106587. doi: 10.1016/j.cropro.2024.106587
dos Santos L. F. C., Ruiz-Sánchez E., Andueza-Noh R. H., Garruña-Hernández R., Latournerie-Moreno L., Mijangos-Cortés J. O. (2020). Leaf damage by Spodoptera frugiperda JE Smith (Lepidoptera: Noctuidae) and its relation to leaf morphological traits in maize landraces and commercial cultivars. J. Plant Dis. Prot. 127, 103–109. doi: 10.1007/s41348-019-00276-y
Dumas P., Legeai F., Lemaitre C., Scaon E., Orsucci M., Labadie K., et al. (2015). Spodoptera frugiperda (Lepidoptera: Noctuidae) host-plant variants: two host strains or two distinct species? Genetica 143, 305–316. doi: 10.1007/s10709-015-9829-2
Du Plessis H., Schlemmer M. L., Van den Berg J. (2020). The effect of temperature on the development of Spodoptera frugiperda (Lepidoptera: Noctuidae). Insects 11, 228. doi: 10.3390/insects11040228
Early R., González-Moreno P., Murphy S. T., Day R. (2018). Forecasting the global extent of invasion of the cereal pest Spodoptera frugiperda, the fall armyworm. NeoBiota 40, 25–50. doi: 10.3897/neobiota.40.28165
Ehler L. E. (2006). Integrated pest management (IPM): definition, historical development and implementation, and the other IPM. Pest Manage. Sci. 62, 787–789. doi: 10.1002/ps.1247
Ekpa O., Palacios-Rojas N., Kruseman G., Fogliano V., Linnemann A. R. (2019). Sub-Saharan African maize-based foods-processing practices, challenges and opportunities. Food Rev. Int. 35, 609–639. doi: 10.1080/87559129.2019.1588290
El-Sappagh I. A., Khalil A. E. H., EL-AMIR S. M. (2022). Efficacy of Some Synthetic Insecticides and Botanical oils against Fall Armyworm, Spodoptera frugiperda (Lepidoptera: Noctuidae), on Maize in Egypt. Egyptian Acad. J. Biol. Sci. F. Toxicol. Pest Control 14, 99–107. doi: 10.21608/eajbsf.2022.226726
Elton C. S. (2020). The ecology of invasions by animals and plants (Cham, Switzerland: Springer Nature).
Eschen R., Beale T., Bonnin J. M., Constantine K. L., Duah S., Finch E. A., et al. (2021). Towards estimating the economic cost of invasive alien species to African crop and livestock production. CABI Agric. Biosci. 2, 18. doi: 10.1186/s43170-021-00038-7
EuropaBio E. M. A. (2019). Harmonised insect resistance management (IRM) plan for cultivation of Bt maize (single insecticidal trait) in the EU. Brussels, Belgium: EuropaBio.
Fan J., Wu P., Tian T., Ren Q., Zhang R. (2020). Potential distribution and niche differentiation of Spodoptera frugiperda in Africa. Insects 11, 383. doi: 10.3390/insects11060383
FAO, ASARECA (2018). Eastern Africa Fall Armyworm Management Strategy and Implementation Plan (Rome: FAO and ASARECA), 48 pp. Licence: CC BY-NC-SA 3.0 IGO.
FAO (Food and Agriculture Organization) (2020). Openknowledge guidance note 10. Available online at: https://openknowledge.fao.org/server/api/core/bitstreams/3fd28d9d-ba82-4040-b41f-f3f8188f166a/content?utm_source=chatgpt.com (Accessed February 15, 2025).
FAO (Food and Agriculture Organization) (2022). Technical guidance on fall armyworm – Coordinated surveillance and an early warning system for the sustainable management of transboundary pests, with special reference to fall armyworm (Spodoptera frugiperda [J.E. Smith]) in South and Southeast Asia. Bangkok, Thailand: FAO. doi: 10.4060/cc0227en
FAO (Food and Agriculture Organization of the United Nations) (2017). Training manual on fall armyworm. Available online at: http://www.livestockZimbabwe.com/Publications/Fall%20Army%20Worm%20Training%20Manual.pdf (Accessed September 4, 2024).
FAO (Food and Agriculture Organization of the United Nations) (2018). Integrated Management of the Fall Armyworm on Maize: A Guide for Farmer Field Schools in Africa (Food and Agriculture Organization (FAO) of the United Nations). Available online at: http://www.fao.org/3/I8665EN/i8665en.pdf (Accessed September 4, 2024).
Figueiredo M. L. C., Della Lucia T. M. C., Cruz I. (2002). Effect of Telenomus remus Nixon (Hymenoptera: Scelionidae) density on control of Spodoptera frugiperda (Smith) (Lepidoptera: Noctuidae) egg masses upon release in maize field. Rev. Bras. Milho Sorgo 1, 12–19. doi: 10.18512/1980-6477/rbms.v1n2p12-19
Flanders K. L., Ball D. M., Cobb P. P. (2017). Management of fall armyworm in pastures and hayfields. alabama cooperative extension system (Alabama A&M and Auburn Universities).
Galani Y. J. H., Orfila C., Gong Y. Y. (2022). A review of micronutrient deficiencies and analysis of maize contribution to nutrient requirements of women and children in Eastern and Southern Africa. Crit. Rev. Food Sci. Nutr. 62, 1568–1591. doi: 10.1080/10408398.2020.1844636
Garcia A. G., Godoy W. A. C., Thomas J. M. G., Nagoshi R. N., Meagher R. L. (2018). Delimiting strategic zones for the development of fall armyworm (Lepidoptera: Noctuidae) on corn in the state of Florida. J. Econ. Entomol. 111, 120–126. doi: 10.1093/jee/tox329
Gebreziher H. G. (2020). Review on management methods of fall armyworm (Spodoptera frugiperda JE Smith) in Sub-Saharan Africa. Int. J. Entomol. Res. 5, 09–14.
Gebreziher H. G., Gebreziher F. G. (2020). Effect of integrating night-time light traps and push-pull method on monitoring and deterring adult fall armyworm (Spodoptera frugiperda). Int. J. Entomol. Res. 5, 28–32.
Gergs A., Baden C. U. (2021). A dynamic energy budget approach for the prediction of development times and variability in Spodoptera frugiperda rearing. Insects 12, 300. Recent Advances in the Understanding of Molecular Mechanisms of Resistance in Noctuid Pests, 75. doi: 10.3390/insects12040300
Goergen G., Kumar P. L., Sankung S. B., Togola A., Tamò M. (2016). First report of outbreaks of the fall armyworm Spodoptera frugiperda (JE Smith)(Lepidoptera, Noctuidae), a new alien invasive pest in West and Central Africa. PloS One 11, e0165632. doi: 10.1371/journal.pone.0165632
Gouda M. R., Jeevan H., Shashank H. G. (2024). CRISPR/Cas9: a cutting-edge solution for combatting the fall armyworm, Spodoptera frugiperda. Mol. Biol. Rep. 51, 13. doi: 10.1007/s11033-023-08986-1
Groot A. T., Marr M., Heckel D. G., SchÖfl G. (2010). The roles and interactions of reproductive isolation mechanisms in fall armyworm (Lepidoptera: Noctuidae) host strains. Ecol. Entomol. 35, 105–118. doi: 10.1111/j.1365-2311.2009.01138.x
Gutierrez-Martinez A., Tolon-Becerra A., Lastra-Bravo X. B. (2012). Biological control of Spodoptera frugiperda eggs using Telenomus remus Nixon in maize-bean-squash polyculture. Am. J. Agr. Biol. Sci. 7, 285–292.
Gutiérrez-Moreno R., Mota-Sanchez D., Blanco C. A., Whalon M. E., Terán-Santofimio H., Rodriguez-Maciel J. C., et al. (2019). Field-evolved resistance of the fall armyworm (Lepidoptera: Noctuidae) to synthetic insecticides in Puerto Rico and Mexico. J. Econ. Entomol. 112, 792–802. doi: 10.1093/jee/toy372
Harrison R. D., Thierfelder C., Baudron F., Chinwada P., Midega C., Schaffner U., et al. (2019). Agro-ecological options for fall armyworm (Spodoptera frugiperda JE Smith) management: Providing low-cost, smallholder friendly solutions to an invasive pest. J. Environ. Manage. 243, 318–330. doi: 10.1016/j.jenvman.2019.05.011
He L., Zhao S., Ali A., Ge S., Wu K. (2021). Ambient humidity affects development, survival, and reproduction of the invasive fall armyworm, Spodoptera frugiperda (Lepidoptera: Noctuidae), in China. J. Econ. Entomol. 114, 1145–1158. doi: 10.1093/jee/toab056
Hellmich R. L., Albajes R., Bergvinson D., Prasifka J. R., Wang Z. Y., Weiss M. J. (2008). “The present and future role of insect-resistant genetically modified maize in IPM,” in Integration of insect-resistant genetically modified crops within IPM programs (Dordrecht, Netherlands: Springer), 119–158.
Hruska A. (2019). “Fall armyworm (Spodoptera frugiperda) management by smallholders,” in CAB Reviews: Perspectives in Agriculture, Veterinary Science, Nutrition and Natural Resources, CAB Reviews. United Kingdom: Wallingford, Oxfordshire 14, 1–11. doi: 10.1079/PAVSNNR201914043
Huang Y., Dong Y., Huang W., Guo J., Hao Z., Zhao M., et al. (2024). Predicting the global potential suitable distribution of fall armyworm and its host plants based on machine learning models. Remote Sens. 16, 2060. doi: 10.3390/rs16122060
Huang F., Qureshi J. A., Meagher R. L. Jr., Reisig D. D., Head G. P., Andow D. A., et al. (2014). Cry1F resistance in fall armyworm Spodoptera frugiperda: single gene versus pyramided Bt maize. PloS One 9, e112958. doi: 10.1371/journal.pone.0112958
Huang L. L., Xue F. S., Chen C., Guo X., Tang J. J., Zhong L., et al. (2021). Effects of temperature on life-history traits of the newly invasive fall armyworm, Spodoptera frugiperda in Southeast China. Ecol. Evol. 11, 5255–5264. doi: 10.1002/ece3.7413
Hussain A. G., Wennmann J. T., Goergen G., Bryon A., Ros V. I. D. (2021). Viruses of the fall armyworm Spodoptera frugiperda: a review with prospects for biological control. Viruses 13, 2220. doi: 10.3390/v13112220
ICIPE (International Centre of Insect Physiology and Ecology) (2024). Fall armyworm natural enemies. Available online at: http://www.icipe.org/news/fall-armyworm-natural-enemies (Accessed June 28, 2024).
Idrees A., Qadir Z. A., Afzal A., Ranran Q., Li J. (2022). Laboratory efficacy of selected synthetic insecticides against second instar invasive fall armyworm, Spodoptera frugiperda (Lepidoptera: Noctuidae) larvae. PloS One 17, e0265265. doi: 10.1371/journal.pone.0265265
Jiang B., Siregar U., Willeford K. O., Luthe D. S., Williams W. P. (1995). Association of a 33-kilodalton cysteine proteinase found in corn callus with the inhibition of fall armyworm larval growth. Plant Physiol. 108, 1631–1640. doi: 10.1104/pp.108.4.1631
Jin S. (2017). Maize defense responses to caterpillars at the molecular, phenotypic and ecological scales (Pennsylvania, USA: Pennsylvania State University).
Jing W. A. N., Huang C., LI C. Y., Zhou H. X., Ren Y. L., LI Z. Y., et al. (2021). Biology, invasion and management of the agricultural invader: Fall armyworm, Spodoptera frugiperda (Lepidoptera: Noctuidae). J. Integr. Agric. 20, 646–663.
Joatya J. Y., Rahmana M. M., Amina M. R., Khanb M. A. R. (2021). Fall armyworm outbreaks in Asia: Analyzing the strategies to control. Malays J Sustain Environ. 6, 38–43. doi: 10.26480/mjsa.01.2022.38.43
Joshi J., Vrieling K. (2005). The enemy release and EICA hypothesis revisited: incorporating the fundamental difference between specialist and generalist herbivores. Ecol. Lett. 8, 704–714. doi: 10.1111/j.1461-0248.2005.00769.x
Karakkottil P., Pulamte L., Kumar V. (2024). Strategic analysis of collaborative networks in Spodoptera frugiperda (Lepidoptera: Noctuidae) research for improved pest management strategies. Neotropical Entomol. 53, 1–18. doi: 10.1007/s13744-024-01146-5PubMed+2
Kasige R. H., Dangalle C. D., Pallewatta N., Perera M. T. M. D. R. (2022). Egg cluster characteristics of fall armyworm, Spodoptera frugiperda (Lepidoptera: Noctuidea) in Sri Lanka under laboratory conditions. J. Agric. Sci. (Sri Lanka) 17 (1). doi: 10.4038/jas.v17i1.9620
Kasoma C., Shimelis H., Laing M. D. (2021). Fall armyworm invasion in Africa: implications for maize production and breeding. J. Crop Improv. 35, 111–146. doi: 10.1080/15427528.2020.1802800
Kasoma C., Shimelis H., Laing M., Shayanowako A. I., Mathew I. (2020). Screening of inbred lines of tropical maize for resistance to fall armyworm, and for yield and yield-related traits. Crop Prot. 136, 105218. doi: 10.1016/j.cropro.2020.105218
Kebede M., Fite T. (2022). RNA interference (RNAi) applications to the management of fall armyworm, spodoptera frugiperda (Lepidoptera: Noctuidae): Its current trends and future prospects. Front. Mol. Biosci. 9, 944774.
Kenis M., Benelli G., Biondi A., Calatayud P. A., Day R., Desneux N., et al. (2022). Invasiveness, biology, ecology, and management of the fall armyworm, Spodoptera frugiperda. Entomol. Gen. 43 (2), 187–241. doi: 10.1127/entomologia/2022/1659
Kenis M., Du Plessis H., Van den Berg J., Ba M. N., Goergen G., Kwadjo K. E., et al. (2019). Telenomus remus, a candidate parasitoid for the biological control of Spodoptera frugiperda in Africa, is already present on the continent. Insects 10, 92. doi: 10.3390/insects10040092
Koffi D., Agboka K., Adjevi M. K. A., Adom M., Tounou A. K., Meagher R. L. (2023). The natural control agents of the fall armyworm, Spodoptera frugiperda in Togo: moderating insecticide applications for natural control of the pest? J. Pest Sci. 96, 1405–1416. doi: 10.1007/s10340-023-01662-0
Koffi D., Kyerematen R., Eziah V. Y., Osei-Mensah Y. O., Afreh-Nuamah K., Aboagye E., et al. (2020). Assessment of impacts of fall armyworm, Spodoptera frugiperda (Lepidoptera: Noctuidae) on maize production in Ghana. J. Integr. Pest Manage. 11, 20. doi: 10.1093/jipm/pmaa015
Kumara A. T., Muhandiram A. M. K. G., GKMMK R., Ayeshmanthi M. B., Sarathchandra S. R., Mubarak A. N. M. (2022). Association between temperature and life table development of Fall armyworm Spodoptera frugiperda under control condition. Research Square. doi: 10.21203/rs.3.rs-1295288/v1
Kumela T., Simiyu J., Sisay B., Likhayo P., Mendesil E., Gohole L., et al. (2019). Farmers’ knowledge, perceptions, and management practices of the new invasive pest, fall armyworm (Spodoptera frugiperda) in Ethiopia and Kenya. Int. J. Pest Manage. 65, 1–9. doi: 10.1080/09670874.2017.1423129
Lekha M. K., Swami H., Vyas A. K., Ahir K. C. (2020). Biology of fall armyworm, Spodoptera frugiperda (JE Smith) on different artificial diets. J. Entomol. Zool. Stud. 8, 584–586.
Liu T. M., Wang J. M., Hu X. K., Feng J. M. (2020). Land-use change drives present and future distributions of fall armyworm, Spodoptera frugiperda (J.E. Smith) (Lepidoptera: Noctuidae). Sci. Total Environ. 1, 135872. doi: 10.1016/j.scitotenv.2019.135872
Makuachukwu O. G., Busie M. D., Tahirou A. (2022). Contribution of maize-based products to the livelihood of smallholder processors in rural northern Nigeria. Int. J. Rural Manage. 18, 167–183. doi: 10.1177/0973005221998244
Malekera M. J., Acharya R., Mostafiz M. M., Hwang H. S., Bhusal N., Lee K. Y. (2022). Temperature-dependent development models describing the effects of temperature on the development of the fall armyworm Spodoptera frugiperda (JE Smith)(Lepidoptera: Noctuidae). Insects 13, 1084. doi: 10.3390/insects13121084
Marenco R. J., Foster R. E., Sanchez C. A. (1992). Sweet corn response to fall armyworm (Lepidoptera: Noctuidae) damage during vegetative growth. J. Econ. Entomol. 85, 1285–1292. doi: 10.1093/jee/85.4.1285
Meagher R. L., Nuessly G. S., Nagoshi R. N., Hay-Roe M. N. (2016). Parasitoids attacking fall armyworm (Lepidoptera: Noctuidae) in sweet corn habitats. Biol. Control. 95, 66–72. doi: 10.1016/j.biocontrol.2016.01.006
Meihls L. N., Kaur H., Jander G. (2012). “Natural variation in maize defense against insect herbivores,” in Cold Spring Harbor symposia on quantitative biology. (New York, USA: Cold Spring Harbor), vol. 77, 269–283.
Mendesil E., Tefera T., Blanco C. A., Paula-Moraes S. V., Huang F., Viteri D. M., et al. (2023). The invasive fall armyworm, Spodoptera frugiperda, in Africa and Asia: responding to the food security challenge, with priorities for integrated pest management research. J. Plant Dis. Prot. 130, 1175–1206. doi: 10.1007/s41348-023-00777-x
Mihm J. A. (1997). Insect Resistant Maize: Recent Advances and Utilization: Proceedings of an International Symposium Held at the International Maize and Wheat Improvement Center (CIMMYT), 27 November-3 December 1994. Mexico City, Mexico: CIMMYT.
Miranda-Anaya M., Guevara-Fefer P., García-Rivera B. E. (2002). Circadian locomotor activity in the larva and adult fall armyworm, Spodoptera frugiperda (Noctuidae): Effect of feeding with the resistant variety of maize CML67. Biol. Rhythm. Res. 33, 475–486. doi: 10.1076/brhm.33.5.475.13936
Mlambo S., Mubayiwa M., Tarusikirwa V. L., Machekano H., Mvumi B. M., Nyamukondiwa C. (2024). The fall armyworm and larger grain borer Pest invasions in Africa: drivers, impacts and implications for food systems. Biology 13, 160. doi: 10.3390/biology13030160
Mohamed S. A., Wamalwa M., Obala F., Tonnang H. E., Tefera T., Calatayud P. A., et al. (2021). A deadly encounter: alien invasive Spodoptera frugiperda in Africa and indigenous natural enemy, Cotesia icipe (Hymenoptera, Braconidae). PloS One 16, e0253122. doi: 10.1371/journal.pone.0253122
Molina-Ochoa J., Carpenter J. E., Heinrichs E. A., Foster J. E. (2003). Parasitoids and parasites of Spodoptera frugiperda (Lepidoptera: Noctuidae) in the Americas and Caribbean Basin: An inventory. Fla. Entomol. 86, 254–289. doi: 10.1653/0015-4040(2003)086[0254:paposf]2.0.co;2
Montezano D. G., Sosa-Gómez D. R., Specht A., Roque-Specht V. F., Sousa-Silva J. C., Paula-Moraes S. D., et al. (2018). Host plants of Spodoptera frugiperda (Lepidoptera: Noctuidae) in the Americas. Afr. Entomol. 26, 286–300. doi: 10.4001/003.026.0286
Murúa M. G., Vera M. A., Michel A., Casmuz A. S., Fatoretto J., Gastaminza G. (2019). Performance of field-collected Spodoptera frugiperda (Lepidoptera: Noctuidae) strains exposed to different transgenic and refuge maize hybrids in Argentina. J. Insect Sci. 19, 21. doi: 10.1093/jisesa/iez110
Nagoshi R. N., Fleischer S., Meagher R. L. (2017). Demonstration and quantification of restricted mating between fall armyworm host strains in field collections by SNP comparisons. J. Econ. Entomol. 110, 2568–2575. doi: 10.1093/jee/tox229
Nagoshi R. N., Goergen G., Plessis H. D., van den Berg J., Meagher R. Jr. (2019). Genetic comparisons of fall armyworm populations from 11 countries spanning sub-saharan africa provide insights into strain composition and migratory behaviors. Sci. Rep. 9 (1), 8311.
Nashath M. N. F., Mubarak A. N. M., Kumara A. D. N. T. (2023). Characterization of Sri Lankan maize (zea mays l.) accessions using ssr markers associated with insect-resistant traits. J. Bangladesh Agricultural Univ. 21 (2), 124–131. doi: 10.5455/JBAU.154165
Niassy S., Agbodzavu M. K., Kimathi E., Mutune B., Abdel-Rahman E. F. M., Salifu D., et al. (2021). Bioecology of fall armyworm Spodoptera frugiperda (JE Smith), its management and potential patterns of seasonal spread in Africa. PloS One 16, e0249042. doi: 10.1371/journal.pone.0249042
Nogueira L., Costa E. N., Di Bello M. M., Diniz J. F. S., Ribeiro Z. A., Boiça Júnior A. L. (2019). Oviposition preference and antibiosis to Spodoptera frugiperda (Lepidoptera: Noctuidae) in Brazilian maize landraces. J. Econ. Entomol. 112, 939–947. doi: 10.1093/jee/toy400
Ntwari N., Yocgo R., Ndokoye P., Kagabo D. (2024). Mapping and forecasting of fall armyworm pest distribution in East Africa using climate information. Sustain. Biodivers. Conserv. 3, 90–105.
Nuessly G. S., Scully B. T., Hentz M. G., Beiriger R., Snook M. E., Widstrom N. W. (2007). Resistance to Spodoptera frugiperda (Lepidoptera: Noctuidae) and Euxesta stigmatias (Diptera: Ulidiidae) in sweet corn derived from exogenous and endogenous genetic systems. J. Econ. Entomol. 100, 1887–1895. doi: 10.1093/jee/100.6.1887
Nwokeoji A. O., Nwokeoji E. A., Chou T., Togola A. (2022). A novel sustainable platform for scaled manufacturing of double-stranded RNA biopesticides. Bioresources Bioprocessing 9 (1), 1–16.
Nyamutukwa S., Mvumi B. M., Chinwada P. (2022). Sustainable management of fall armyworm, Spodoptera frugiperda (JE Smith): challenges and proposed solutions from an African perspective. Int. J. Pest Manage. 70, 1–19. doi: 10.1080/09670874.2022.2041192
Obala F., Mohamed S. A., Magomere T. O., Subramanian S. (2023). Old and new association of Cotesia icipe (Hymenoptera: Braconidae) with alien invasive and native Spodoptera species and key stemborer species: implication for their management. Pest Manage. Sci. 79, 5312–5320. doi: 10.1002/ps.7740
Ojumoola O. A. (2021). Bioecology of the Fall armyworm, Spodoptera frugiperda J.E Smith on maize, Zea mays L. in the South-West, Nigeria (Doctoral dissertation). Ibadan, Oyo State, Nigeria: University of Ibadan.
Ortiz-Carreón F. R., Bernal J. S., Rojas J. C., Cruz-López L., Kolomiets M. V., Malo E. A. (2024). Fall armyworm females choose host plants that are detrimental to their offspring’s performance but young larvae amend their mother’s choices. Entomol. Experimentalis Applicata. 173 (1), 21–31. doi: 10.1111/eea.13393
Otim M. H., Adumo Aropet S., Opio M., Kanyesigye D., Nakelet Opolot H., Tek Tay W. (2021). Parasitoid distribution and parasitism of the fall armyworm Spodoptera frugiperda (Lepidoptera: Noctuidae) in different maize producing regions of Uganda. Insects 12, 121. doi: 10.3390/insects12020121
Overton K., Maino J. L., Day R., Umina P. A., Bett B., Carnovale D., et al. (2021). Global crop impacts, yield losses and action thresholds for fall armyworm (Spodoptera frugiperda): A review. Crop Prot. 145, 105641. doi: 10.1016/j.cropro.2021.105641
Pal S., Bhattacharya S., Dhar T., Gupta A., Ghosh A., Debnath S., et al. (2024). Hymenopteran parasitoid complex and fall armyworm: a case study in eastern India. Sci. Rep. 14, 4029. doi: 10.1038/s41598-024-54342-z
Patel Sagarbhai B., Bhande Satish S., Wasu Yogesh H. (2021). A review on management of invasive insect pest fall armyworm, Spodoptera frugiperda (Lepidoptera: Noctuidae). Intern. J. Zool. Invest. 7, 723–732.
Pechan T., Ye L., Chang Y. M., Mitra A., Lin L., Davis F. M., et al. (2000). A unique 33-kD cysteine proteinase accumulates in response to larval feeding in maize genotypes resistant to fall armyworm and other Lepidoptera. Plant Cell 12, 1031–1040. doi: 10.2307/3871253
Peferoen M. (1997). “Insect control with transgenic plants expressing Bacillus thuringiensis crystal pro- teins,” in Advances in Insect Control: The Role of Transgenic Plants. Ed. Carozzi N. B. (Tay- lor & Francis, London, UK), 31–48.
Prasanna B. M., Bruce A. Y., Beyene Y., Makumbi D., Gowda M., Asim M., et al. (2021). Host plant resistance in maize to Fall armyworm. CIMMYT (Mexico: CDMX, CIMMYT), 99–113.
Prasanna B. M., Bruce A., Beyene Y., Makumbi D., Gowda M., Asim M., et al. (2022). Host plant resistance for fall armyworm management in maize: relevance, status and prospects in Africa and Asia. Theor. Appl. Genet. 135, 3897–3916. doi: 10.1007/s00122-022-04073-4
Prasanna B. M., Huesing J. E., Eddy R., Peschke V. M. (2018). Fall armyworm in Africa: a guide for integrated pest management, 1–104 (Mexi: CIMMYT, USAID), 1–102.
Ratnadass A., Michellon R., Randriamanantsoa R., Séguy L. (2006). “Effects of soil and plant management on crop pests and diseases,” in Biological Approaches to Sustainable Soil Systems. (Boca Raton, Florida, USA: CRC Press), vol. 41, 589–602. doi: 10.1201/9781420017113.ch46
Ren Q., Haseeb M., Fan J., Wu P., Tian T., Zhang R. (2020). Functional response and intraspecific competition in the fall armyworm, Spodoptera frugiperda (Lepidoptera: Noctuidae). Insects 11, 806. doi: 10.3390/insects11110806
Rwomushana I., Bateman M., Beale T., Beseh P., Cameron K., Chiluba M., et al. (2018). Fall armyworm: impacts and implications for Africa. Evidence note update (Center for Agriculture and Bioscience International-CABI). Oxfordshire, United Kingdom: CABI, Wallingford. Available at: https://www.invasive-species.org/wp-content/uploads/sites/2/2019/02/FAW-Evidence-Note-October-2018.pdf?utm_source=chatgpt.com (Accessed February 15, 2025).
Sagar G. C., Aastha B., Laxman K. (2020). An introduction of fall armyworm (Spodoptera frugiperda) with management strategies: a review paper. Nippon J. Environ. Sci. 1, 1010. doi: 10.46266/njes.1010
Savadatti E., Ginnu S. A., Mariyanna L., Hosamani A., Desai B. R. K. R., Subbanna A. D., et al. (2023). Temperature effects on the development of life stages of fall armyworm, Spodoptera frugiperda (JE Smith)(Lepidoptera: Noctuidae) on maize. Int. J. Environ. Climate Change 13, 1884–1892. doi: 10.9734/ijecc/2023/v13i92419
Schlemmer M. (2018). Effect of temperature on development and reproduction of Spodoptera frugiperda (Lepidoptera: Noctuidae). Potchefstroom, Northwest Province, South Africa: Doctoral dissertation, North-West University.
Schöfl G., Heckel D. G., Groot A. T. (2009). Time-shifted reproductive behaviours among fall armyworm (Noctuidae: Spodoptera frugiperda) host strains: evidence for differing modes of inheritance. J. Evol. Biol. 22, 1447–1459. doi: 10.1111/j.1420-9101.2009.01759.x
Scott G. E., Davis F. M. (1981). Registration of MpSWCB-4 population of maize (Reg. No. GP 87). Crop Sci. 21, 148–148. doi: 10.2135/cropsci1981.0011183x002100010048x
Sharanabasappa S. D., Kalleshwaraswamy C. M., Maruthi M. S., Pavithra H. B. (2018). Biology of invasive fall army worm Spodoptera frugiperda (JE Smith)(Lepidoptera: Noctuidae) on maize. Indian J. Entomol. 80, 540–543. doi: 10.5958/0974-8172.2018.00238.9
Sharma H., Varshney R., Gaur P., Gowda C. L. (2008). Poten-tial for using morphological, biochemical, and molecular markers forresistance to insect pests in grain legumes. J. Food Legumes 21, 211–217.
Shaurub E. S. H. (2024). Remote sensing and geographic information system applications as early-warning tools in monitoring fall armyworm, Spodoptera frugiperda: a review. Int. J. Trop. Insect Sci. 44, 1–18. doi: 10.1007/s42690-023-00806-3
Shinde M., Suryawanshi K., Kakade K., More V. A. (2024). “Digital maize crop guardian: automated identification of fall armyworm infestation using computer vision,” in International Conference on Agriculture Digitalization and Organic Production (Springer Nature Singapore, Singapore), 147–159.
Singh G. M., Xu J., Schaefer D., Day R., Wang Z., Zhang F. (2022). Maize diversity for fall armyworm resistance in a warming world. Crop Sci. 62, 1–19. doi: 10.1002/csc2.20649
Sisay B., Simiyu J., Malusi P., Likhayo P., Mendesil E., Elibariki N., et al. (2018). First report of the fall armyworm, Spodoptera frugiperda (Lepidoptera: Noctuidae), natural enemies from Africa. J. Appl. Entomol. 142, 800–804. doi: 10.1111/jen.12534
Sisay B., Tefera T., Wakgari M., Ayalew G., Mendesil E. (2019). The efficacy of selected synthetic insecticides and botanicals against fall armyworm, Spodoptera frugiperda, in maize. Insects 10, 45. doi: 10.3390/insects10020045
Smith W. E. C., Shivaji R., Williams W. P., Luthe D. S., Sandoya G. V., Smith C. L., et al. (2012). A maize line resistant to herbivory constitutively releases (E)-β-caryophyllene. J. Econ. Entomol. 105, 120–128. doi: 10.1603/ec11107
Snook M. E., Gueldner R. C., Widstrom N. W., Wiseman B. R., Himmelsbach D. S., Harwood J. S., et al. (1993). Levels of maysin and maysin analogs in silks of maize germplasm. J. Agric. Food Chem. 41, 1481–1485. doi: 10.1021/jf00033a024
Storer N. P., Babcock J. M., Schlenz M., Meade T., Thompson G. D., Bing J. W., et al. (2010). Discovery and characterization of field resistance to Bt maize: Spodoptera frugiperda (Lepidoptera: Noctuidae) in Puerto Rico. J. Econ. Entomol. 103, 1031–1038. doi: 10.1603/EC10040
Tambo J. A., Day R. K., Lamontagne-Godwin J., Silvestri S., Beseh P. K., Oppong-Mensah B., et al. (2020). Tackling fall armyworm (Spodoptera frugiperda) outbreak in Africa: an analysis of farmers’ control actions. Int. J. Pest Manage. 66, 298–310. doi: 10.1080/09670874.2019.1646942
Tambo J. A., Kansiime M. K., Rwomushana I., Mugambi I., Nunda W., Mloza Banda C., et al. (2021). Impact of fall armyworm invasion on household income and food security in Zimbabwe. Food Energy Secur. 10, 299–312. doi: 10.1002/fes3.281
Tefera T., Goftishu M., Ba M. N., Muniappan R. (2019). A guide to biological control of fall armyworm in Africa using egg parasitoids. (Nairobi, Kenya: ICIPE).
Tendeng E., Labou B., Diatte M., Djiba S., Diarra K. (2019). The fall armyworm Spodoptera frugiperda (JE Smith), a new pest of maize in Africa: biology and first native natural enemies detected. Int. J. Biol. Chem. Sci. 13, 1011–1026. doi: 10.4314/ijbcs.v13i2.35
Tepa-Yotto G. T., Chinwada P., Rwomushana I., Goergen G., Subramanian S. (2022a). Integrated management of Spodoptera frugiperda 6 years post detection in Africa: a review. Curr. Opin. Insect Sci. 52, 100928. doi: 10.1016/j.cois.2022.100928
Tepa-Yotto G. T., Douro-Kpindou O. K., Koussihouédé P. S. B., Adjaoké A. M., Winsou J. K., Tognigban G., et al. (2024). Control potential of multiple nucleopolyhedrovirus (SfMNPV) isolated from fall armyworm in Nigeria (West Africa). Insects 15, 225. doi: 10.3390/insects15040225
Tepa-Yotto G. T., Meagher R. L., Winsou J. K., Dahoueto B. T., Tamò M., Sæthre M. G., et al. (2022b). Monitoring Spodoptera frugiperda in Benin: assessing the influence of trap type, pheromone blends, and habitat on pheromone trapping. Florida Entomol. 105, 71–78. doi: 10.1653/024.105.0111
Tiwari S. (2022). Host plant preference by the fall armyworm, Spodoptera frugiperda (JE Smith)(Noctuidae: Lepidoptera) on the range of potential host plant species. J. Agric. Forest. Univ. 6, 25–33.
Togola A., Datinon B., Laouali A., Traore F., Agboton C., Ongom P. O., et al. (2023). Recent advances in cowpea IPM in West Africa. Front. Agron. 5, 1220387. doi: 10.3389/fagro.2023.1220387
Togola A., Meseka S., Menkir A., Badu-Apraku B., Boukar O., Tamò M., et al. (2018). Measurement of pesticide residues from chemical control of the invasive Spodoptera frugiperda (Lepidoptera: Noctuidae) in a maize experimental field in Mokwa, Nigeria. Int. J. Environ. Res. Public Health 15, 849. doi: 10.3390/ijerph15050849
Viana P. A., Guimaraes P. D. O., Mendes S. M. (2022). Maize cultivars with native insect resistance: potential, advances and challenges. Revista Brasileira de Milho e Sorgo, 21. doi: 10.18512/rbms2022vol21e1250
Viana P. A., Potenza M. R. (2000). Non-preference and antibiosis of fall armyworm for selected corn genotypes. Bragantia 59, 27–33. doi: 10.1590/S0006-87052000000100004
Vilarinho E. C., Fernandes O. A., Hunt T. E., Caixeta D. F. (2011). Movement of” Spodoptera frugiperda” adults (Lepidoptera: Noctuidae) in Maize in Brazil. Florida Entomol. 94, 480–488. doi: 10.1653/024.094.0311
Widstrom N. W., Williams W. P., Wiseman B. R., Davis F. M. (1992). Recurrent selection for resistance to leaf feeding by fall armyworm on maize. Crop Sci. 32, 1171–1174. doi: 10.2135/cropsci1992.0011183x003200050022x
Widstrom N. W., Wiseman B. R., Snook M. E., Nuessly G. S., Scully B. T. (2003). Registration of the maize population Zapalote Chico 2451F. Crop Sci. 42, 444–445. doi: 10.2135/cropsci2003.4440
Williams W. P., Davis F. M. (1994). “Mechanisms and bases of resistance in maize to southwestern corn borer and fall armyworm,” in Proc. International Symposium. International Maize and Wheat Improvement Center, vol. 27. (CIMMYT, México), 29–36.
Wiseman B. R., Williams W. P., Davis F. M. (1981). Fall armyworm: Resistance mechanisms in selected corns. J. Econ. Entomol. 74, 622–624.
Womack E. D., Warburton M. L., Williams. W. P. (2018). Mapping of quantitative trait loci for resistance to fall armyworm and southwesterrn corn borer leaf-feeding damage in maize. Crop Sci. 58, 529–539. doi: 10.2135/cropsci2017.03.0155
Womack E. D., Williams W. P., Smith J. S., Warburton M. L., Bhattramakki. D. (2020). Mapping quantitative trait loci for resistance to the fall armyworm (Lepidoptera Noctuidae) leaf-feeding damage in maize inbred line Mp705. J. Econ. Entomol. 2020, 1–8. doi: 10.1093/jee/toz357
Wyckhuys K. A. G., O’Neil R. J. (2006). Population dynamics of Spodoptera frugiperda Smith (Lepidoptera: Noctuidae) and associated arthropod natural enemies in Honduran ubsistence maize. Crop Protect. 25, 1180–1190. doi: 10.1016/j.cropro.2006.03.003
Yan X. R., Wang Z. Y., Feng S. Q., Zhao Z. H., Li Z. H. (2022). Impact of temperature change on the fall armyworm, Spodoptera frugiperda under global climate change. Insects 13, 981. doi: 10.3390/insects13110981
Yu H., Yang X., Dai J., Li Y., Veeran S., Lin J., et al. (2023). Effects of azadirachtin on detoxification-related gene expression in the fat bodies of the fall armyworm, Spodoptera frugiperda. Environ. Sci. Pollut. Res. 30, 42587–42595. doi: 10.1007/s11356-022-19661-6
Keywords: fall armyworm, integrated pest management, food security, crop loss, host plant resistance
Citation: Togola A, Beyene Y, Bocco R, Tepa-Yotto G, Gowda M, Too A and Boddupalli P (2025) Fall armyworm (Spodoptera frugiperda) in Africa: insights into biology, ecology and impact on staple crops, food systems and management approaches. Front. Agron. 7:1538198. doi: 10.3389/fagro.2025.1538198
Received: 02 December 2024; Accepted: 01 April 2025;
Published: 24 April 2025.
Edited by:
Salvatore Arpaia, Energy and Sustainable Economic Development (ENEA), ItalyReviewed by:
Phetole Mangena, Agricultural Research Council of South Africa (ARC-SA), South AfricaLoulou Albittar, Université Catholique de Louvain, Belgium
Erik Smith, Cornell University, United States
Copyright © 2025 Togola, Beyene, Bocco, Tepa-Yotto, Gowda, Too and Boddupalli. This is an open-access article distributed under the terms of the Creative Commons Attribution License (CC BY). The use, distribution or reproduction in other forums is permitted, provided the original author(s) and the copyright owner(s) are credited and that the original publication in this journal is cited, in accordance with accepted academic practice. No use, distribution or reproduction is permitted which does not comply with these terms.
*Correspondence: Abou Togola, YS50b2dvbGFAY2dpYXIub3Jn
†ORCID: Abou Togola, orcid.org/0000-0001-6155-8292
Abel Too, orcid.org/0000-0002-8181-9397