- 1Institute for Sustainable Plant Protection, National Research Council of Italy, Legnaro, Padua, Italy
- 2Department of Agronomy, Food, Natural Resources, Animals and Environment, University of Padova, Legnaro, Padua, Italy
- 3Department of Pharmaceutical and Pharmacological Sciences, University of Padova, Padova, Italy
- 4Department of Biomedical, Surgical and Dental Sciences, University of Milan, Milano, Italy
The search for new tools appear to be crucial to ensure sustainable weed management and the study of allelochemicals in plants plays a strategic role in the eco-friendly agrochemical development. This study aims, for the first time, to identify and quantify allelopathic substances in aqueous extracts from leaves (BL) and roots (BR) of the invasive species Baccharis halimifolia L. (Asteraceae) and to assess their phytotoxic activity at different dilutions in both germination tests and greenhouse pot trials for the weed species Abutilon theophrasti Medik., Solanum nigrum L., Lolium rigidum Gaudin and Setaria pumila Roem. & Schult. HPLC-DAD-(ESI)-MS analysis revealed the presence of several saponins and phenolics (e.g. Apigenin and Protocatechuic acid), possibly implicated in the observed phytotoxic action. The most relevant effects were observed in the germination test, where BR was more effective than BL and at 20% v/v dilution caused a 50-75% reduction in root length of all weed species. Maximum reduction (>85%) in root length occurred at 100% v/v dilution of both extracts for all species. Pre- and post-emergence greenhouse trials showed unstable and variable herbicidal activity among the tested species and extract doses. Effects were observed mainly on the grasses S. pumila and L. rigidum, where BR application in post-emergence reduced plant biomass by 42% and 34%. This study suggests that B. halimifolia could be a potential source of natural herbicides; however, it also showed the difficulties in reproducing, under the field-like conditions of pot trials, the phytotoxic effects observed in germination tests due to radical differences in applied doses and exposure conditions. This highlights the importance of studies that consider field application and the need of green extraction methodologies that improve active substances concentration in extract.
1 Introduction
Since weeds are among the main biotic cause of crop yield loss, their Integrated Management is necessary to ensure the productivity and sustainability of agricultural production (Riemens et al., 2022). Herbicides remain the most widely used and effective tools for weed control (Gianessi, 2013; Tudi et al., 2021), but in the last decades, critical issues have arisen on a political, environmental, health, and technical level (Loddo et al., 2021). This adds a criticality to the future of weed control and highlights the need to find new eco-friendly tools for management (Westwood et al., 2018).
The importance of research into new natural herbicides derived from plant extracts and essential oils is also due to the continuing growth of organic farming in Europe (Eurostat, 2024). In this farming system, there is a shortage of technical tools for weed control, which is currently based mainly on cultural, physical and mechanical methods (Merfield, 2023).
A growing number of studies are emphasizing the potential phytotoxic activity of aqueous extracts from which new eco-friendly herbicides can be developed (Chon and Nelson, 2010; Scavo et al., 2018; Hasan et al., 2021; Vitalini et al., 2020, 2021). These studies, however, are mainly based on laboratory tests and rarely include trials under field or field-like conditions even if they are necessary steps in the development of any new agrochemical (Kostina-Bednarz et al., 2023). This is a major limitation given that phytotoxic effects observed during in-vitro experiments do not automatically translate into real herbicidal activity under field conditions. This knowledge gap is currently hindering the development of plant-based herbicides and contributing to the present scarcity of commercially available natural herbicides in comparison with other categories of biopesticides (Marrone, 2024) and the lack of consistent performance in terms of weed control reported for the few existing products (Loddo et al., 2023; Torres-Pagán et al., 2024).
Allelopathy is the natural phenomenon whereby organisms positively or negatively affect other nearby organisms by releasing chemical compounds known as allelochemicals (Kostina-Bednarz et al., 2023). The ability of producing allelochemicals as phenolic compounds and terpenes has been largely described for the Asteraceae botanical family in general (Chon et al., 2003; Chon and Nelson, 2010; La Iacona et al., 2024) and the genus Baccharis in particular (Dias et al., 2017; Ibáñez and Zoppolo, 2011; Miranda-Arámbula et al., 2021). Research on secondary metabolites with allelopathic action is critical to the improvement of sustainable weed management tools, such as the selection and breeding of allelopathic cover crops and the development of natural herbicides (Khamare et al., 2022).
Baccharis halimifolia L. (Asteraceae) is a North American shrub that is invading estuarine communities in Europe and forms extremely dense, monospecific communities (Fried et al., 2016) leading to its inclusion in a list of invasive plants important for the European Union (Commission Implementing Regulation (EU) 2016/1141). Mechanical weeding is currently the most widely used control technique and large quantities of plant residues like leaves, branches, and roots are generated (EPPO, 2016). From a bio-circular economy perspective, the reuse of this plant waste for agrochemical production would be desirable (Mikula et al., 2021).
B. halimifolia can contain several classes of secondary metabolites (Martinez et al., 2005; Fernandes et al., 2022; Desrini et al., 2023). Despite being the most invasive species of its genus, researchers have not yet investigated B. halimifolia allelopathy (Lázaro-Lobo et al., 2022).
In this study, for the first time, the phytotoxic activity of B. halimifolia roots and leaves aqueous extracts was evaluated with three experiments: (i) a germination test to assess effect on weed seed germination, then a (ii) pre- and (iii) post-emergence pot trials in greenhouse simulating field-like conditions. Chemical analyses were carried out to characterize the compounds in the extracts.
2 Materials and methods
2.1 Plant material, weed seeds and aqueous extracts preparation
The species tested in this study were the broadleaf weeds Abutilon theophrasti Medik. (ABUTH hereinafter) and Solanum nigrum L. (SOLNI) and the grasses Lolium rigidum Gaudin (LOLRI) and Setaria pumila Roem. & Schult. (SETPU). These species were chosen because they are all important weeds and have different physiological, such as a C4 photosynthetic pathway for SETPU and a C3 for LOLRI, and morphological characteristics, such as a greater presence of hairs on leaves in SETPU than in LOLRI, different leaf inclination between ABUTH and SOLNI, that could suggest a potential different sensitivity to allelochemicals. Weed seeds were collected at the Experimental Farm of the University of Padova (45°N, 11°E) according to the methodology described by Loddo et al. (2023) and stored in the fridge at 4°C until use.
B. halimifolia leaves and roots were collected from more than thirty plants during the flowering phenological phase in October 2022 on an artificial island located in the Venice Lagoon (45°N, 12°E). Both young and old pathogen-free plants were randomly chosen for sampling. The plants were uprooted entirely by hand, taking care not to break the roots. Roots were then separated from the trunk with a pruning shear. Next, the roots and all branches with leaves were air-dried separately in the dark for three weeks at room temperature ranging from 18 to 25°C. Once dry, the leaves detached from the branches and the roots were powdered in an electric mill (RETSCH Cutting Mill SM 300).
The aqueous extracts were prepared according to the methodology described by Vitalini et al. (2021). Distilled water was added to B. halimifolia powder of leaves and rhizomes in 1:10 v/v. Using an orbital shaker, the mixtures were agitated at room temperature for 24 hours in a flask covered with foil to avoid light contact. After that, it was filtered through double gauzes to remove big solid residues and centrifuged at 4500 rpm for 30 min. Until use, the extracts were stored in the freezer at -20°C. Hereinafter, B. halimifolia extracts obtained from leaves and roots will be named BL and BR, respectively.
2.2 HPLC-DAD-(ESI)-MS analysis
For the analysis, an Agilent 1260 chromatograph equipped with 1260 series diode array and Varian MS 500 mass spectrometer (Varian, Santa Clara, CA, USA) was used. As stationary phase an Agilent C18 SB (4.6 x 250 mm) 5 µm was used, and water 1% Formic acid (A), Acetonitrile (B) and Methanol (C) were used as mobile phase. A gradient program was used as follows: 0 ⟶ 0.5th min: A:B:C (95:5:0) ⟶ A:B:C (95:5:0), 0.5⟶5th min: A:B:C (95:5:0)⟶ A:B:C (85:15:0), 5⟶15th min: A:B:C (85:15:0) ⟶ A:B:C (60:30:10), 15⟶20th min: A:B:C (60:30:10) ⟶ A:B:C (20:70:10), 20⟶25th min: A:B:C (20:70:10) ⟶ A:B:C (0:90:10), 25⟶30th min: A:B:C (0:90:10) ⟶ A:B:C (0:90:10), 30⟶31th min: A:B:C (0:90:10) ⟶ A:B:C (95:5:0), 31 ⟶37th min: A:B:C (95:5:0)⟶ A:B:C (95:5:0). Flow rate was 0.750 ml/min. After the column, a “T” junction split the flow to the DAD and to a Varian MS 500 mass spectrometer equipped with ESI as ion source. MS spectra were acquired using Electrospray (ESI) in negative ion mode acquiring spectra in the range m/z 50–1500. For qualitative purposes, MSn spectra were used to identify the compound, with the use of reference when available. DAD detector was set at 287, 254, 330, and 350 nm. Compounds were identified based on MS data and UV spectra. Liquid extracts were filtrated and the solution was used for LC-MS analysis.
2.3 Germination test
The germination test was conducted in 9 cm diameter Petri dishes covered by 2 filter paper discs Whatman No. 1, (Whatman, Maidstone, UK). BL and BR extracts were diluted with distilled water to obtain solutions diluted to 2.5, 5, 10, 20, 40, and 100% v/v. Twenty seeds of ABUTH, SOLNI, LOLRI, and SETPU were sown in Petri dishes moistened with 4 ml of each solution. Three replicates (Petri dishes) were prepared for each combination of target species (4) * extracts (2) * dilutions (6). Finally, to obtain an untreated reference, 3 Petri dishes with twenty seeds for each target species moistened with distilled water (0% dilution of the plant part extract) were included. The experiment was conducted twice. Thus, a total of 156 Petri dishes were used for each experiment repetition for a total of 312 Petri dishes. Petri dishes were placed in a germination chamber (KW Apparecchi Scientifici) set at an alternate temperature regime of 25 (light) − 15 (dark) °C and a 12 h light photoperiod as already adopted in previous experiments (Loddo et al., 2023).
Seed germination was monitored daily for 7 days for ABUTH and LOLRI and 14 days for SETPU and SOLNI due to the slower germination of these species. The final survey included also the measurement of root length. Germination (Germ%NT hereinafter) and Root Length (RootLenght%NT) of the treated replicates were expressed as percentage of the mean germination or mean root length of the respective untreated for each target species.
2.4 Greenhouse pre-emergence trial
To test the herbicidal effects of BL and BR under field-like conditions of a pre-emergence application, a pot trial was conducted in a greenhouse located at the Experimental Farm of the University of Padova (Italy).
To break dormancy and promote germination of SOLNI, its seeds were sown in plastic boxes containing a mix of 60% silty loam soil, 15% sand, 15% perlite, and 10% peat (potting mix hereinafter). These boxes were kept in a refrigerator at 4°C for 8 days. Subsequently, they were placed for 3 days in a germination chamber at an alternate temperature regime of 25 (light) −15 (dark) °C and a 12 h light photoperiod. Afterward, 25 seeds of SOLNI, LOLRI, SETPU, and ABUTH were sown at 2 cm of depth in plastic pots (15*15*30 cm) containing the potting mix. For the entire duration of the experiment, pots were irrigated to maintain the substrate at field capacity. Irrigation was suspended from 24 h before to 24 h after the application of extracts.
On the third day after sowing, when seedlings emergence had not yet started, BL and BR extracts were applied on the pot surface with a precision bench sprayer equipped with three flat fan hydraulic nozzles (TeeJet TP11001‐VH, Glendale Heights, IL, USA) at a pressure of 215 kPa and speed of 0.6 ms−1.
In order to increase the phytotoxic compounds applied on pot surface, only non-diluted BL and BR extracts were applied and 3 different spray volumes (330, 660 and 990 L ha-1) were tested to identify potential dose-effect of the plant part extracts. Spray volumes were chosen to simulate the volumes normally adopted for field herbicide applications.
Three pots (replicates) were prepared for each combination of target species (4 levels) * type of plant part extracts (2 levels) * spray volume (or doses, 3 levels). In addition, 3 pots for each target species were prepared for the untreated as control for a total of 84 pots. The experimental layout was a completely randomized design. The experiment was conducted twice.
Seedlings emergence and fresh weight of aboveground biomass (fresh biomass hereinafter) were evaluated 2 weeks after treatment. Seedlings emergence of the treated pots was expressed as a percentage of the mean seedlings emergence observed in untreated pots of the same target species (Emerg%NT parameter hereinafter). For each target species, fresh biomass was measured using a precision balance; then, the average individual plant biomass was calculated by dividing total fresh biomass by the number of live plants. For each target species, to assess biomass reduction, the average individual plant biomass of treated replicates was expressed as a percentage of the average individual plant biomass of the untreated (Biomass%NT hereinafter).
2.5 Greenhouse post-emergence trial
Seedlings of ABUTH, LOLRI, SETPU and SOLNI were obtained according to the procedure described by Loddo et al. (2023).
For each target species and replicate, 6 seedlings were transplanted into a plastic pot filled with the same potting mix used in pre-emergence trials. Pots were transferred in the greenhouse with regular irrigation to maintain optimal water availability throughout the experiment. Irrigation was suspended from 24 h before to 24 h after the application of extracts.
BR and BL were sprayed on when plants reached the phenological stage of fourth-sixth leaves (BBCH 14-16).
The application method and the experimental design were the same as in the greenhouse pre-emergence trial (completely randomized experimental design, three spray volumes of the non-diluted BR and BL extracts, three replicates for each treatment, and two experiment repetitions).
Plant survival and fresh biomass of treated plants were evaluated 2 weeks after treatment. Plant survival was expressed as a percentage of the living plants before the treatment. Fresh biomass was measured with the same methodology adopted for the pre-emergence trial and was expressed as a percentual of the untreated (Biomass%NT).
2.6 Statistics analysis
For each of the three experiments (Germination test, Pre- and Post-emergence pot trials in greenhouse) the statistical analysis was conducted separately for each target species. The main aim of this study was indeed to investigate the phytotoxic activity of leaves (BL) and roots (BR) extract of B. halimifolia. Thus, several target species were included to assess whether these extracts had a phytotoxic effect on at least one of them and not to compare their species-specific response. Conversely, including all target species in a single analysis, that is considering Species as an explanatory variable, could have made the result interpretation more challenging due to the potentially different response of the target species and eventually overshadowed the main aim of this study.
To investigate the effect of extracts on weed germination, data normality (Shapiro - Wilk Test, p>0.05) and the homogeneity of the variances (Levene Test, p>0.05) were firstly verified for the dependent variable Germ%NT through R (R Core Team, 2024). The Box-Cox transformation was applied to species that did not follow a normal distribution, in order to determine the best value which maximizes the Likelihood function. A 2-way ANOVA was then performed on a mixed linear model with the dependent variable Germ%NT, the random factor Experiment (2 levels)and the explanatory variables Extracts (2 levels) and Dose (6 levels). The 2-way ANOVA was followed by the post-hoc Tukey test (p>0,05). To include the representation of the untreated in the graphs, a statistical analysis was carried out on its variability (mean, median, standard deviation, confidence interval, standard error).
Given the large variations observed for the response variable RootLenght%NT at the different plant part extracts doses for all target species, a dose-response analysis was performed to assess the extract dilutions (7 levels) on root length using an approach already adopted to evaluate weed sensitivity to herbicides (Loddo et al., 2020; Panozzo et al., 2020). Non-linear regression was conducted to estimate ED50 (Effective Dilution50 i.e. the extract dilution required to reduce root growth by 50% in comparison to the untreated control for each target species) with their Confidence Intervals 95% (CI95 hereinafter). This analysis was done with the Windows Excel macro Bioassay97 (Onofri, 2005) using the following log-logistic model:
Where Y(x) is the root length at the extract dilutions x, C and D are the lower and upper asymptotes, ED50 is the extract dilution required to reduce root length by 50% in comparison with the untreated control, b is the slope. No constraints were imposed on lower or upper asymptotes.
Data of each target species were analyzed with a single curve but including the value of each replicate to estimate a single specific ED50 parameter but taking into account the existing variability between replicates. The two experiments were initially analyzed separately, and then an ad-hoc lack-of-fit test was performed for each target species to evaluate whether data from the two experiments could be pooled. Finally, the ED50 values estimated for target species and plant part extracts were compared using the criteria that two values were considered statistically different only if their CI95 did not overlap.
For the pre- and post-emergence trials in the greenhouse, data analysis was performed with the same methods described for the analysis on germination [check of normal distribution of the data, Box Cox transformation and homoscedasticity, compute 2-way ANOVA followed by a post-hoc Tukey test (p<0.05)] using as a dependent variable the Emerg%NT and the Biomass%NT for the pre-emergence trial and only Biomass%NT for post-emergence trial since no difference in Plant survival was observed across all replicates. For both pre- and post-emergence trials, Experiment (2 levels) was considered as random factor and Extracts (2 levels) and the Dose (3 levels) as fixed factors of the ANOVA.
3 Results
3.1 Chemical characterization
Aqueous plant part extracts were analyzed by LC-DAD-MS and chromatograms at 287 nm presented the most intense signals in BL compared to BR (Figure 1).
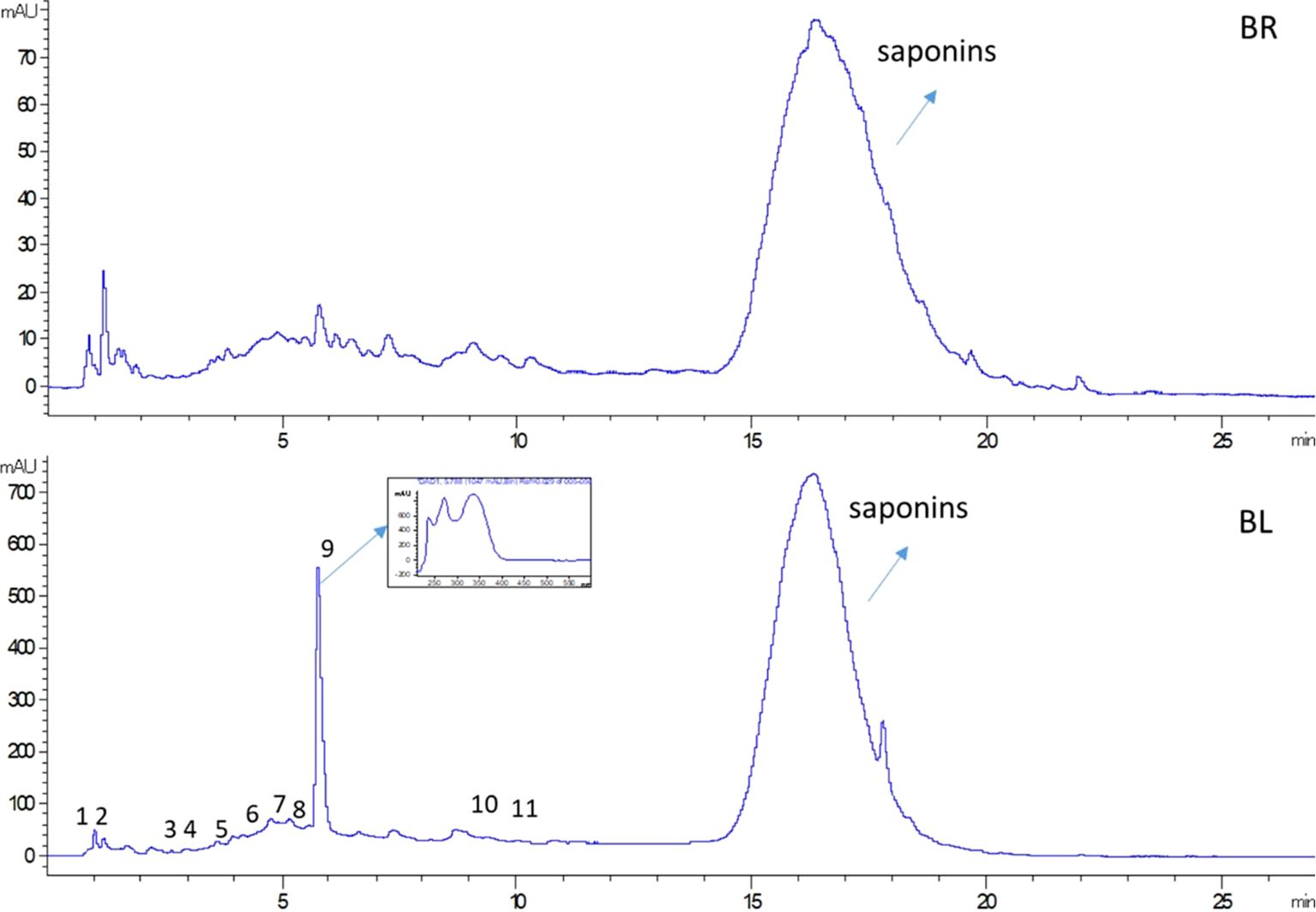
Figure 1. Chromatogram at 287 nm of B. halimifolia leaves extract (BL) and B. halimifolia roots extract (BR); numbers (1-11) indicate the peaks of the identified compounds as reported in Table 2.
The BL chromatogram presents an intense peak at 5.9 min and its UV spectra is related to flavonoid compounds. Other small peaks are present and can be ascribed to general phenolics structure on the basis of UV absorption. In all the chromatograms, a broad peak is present between 15 and 20 minutes, that in mass spectrometry was found to be formed by species that present high molecular showing m/z 827, 811, 829, 825 and 813. The fragmentation of these species is characterized by losses of 86 and 206 Da that can be ascribed to malonyl and sugar moieties as described (Pollier et al., 2011). Considering the high molecular weight and the mass fragmentation pattern, those ion species can be ascribed to different saponin derivatives, as previously reported for Baccharis species (Gené et al., 1995; Martinez et al., 2005).
We estimated the total content of saponin in the two plant part extracts and the results are reported in Table 1. The phytochemical investigation of the obtained plant part extracts revealed the presence of some phenolic compounds, sulphate carbohydrate derivatives and saponins BL and mainly saponins in BR.
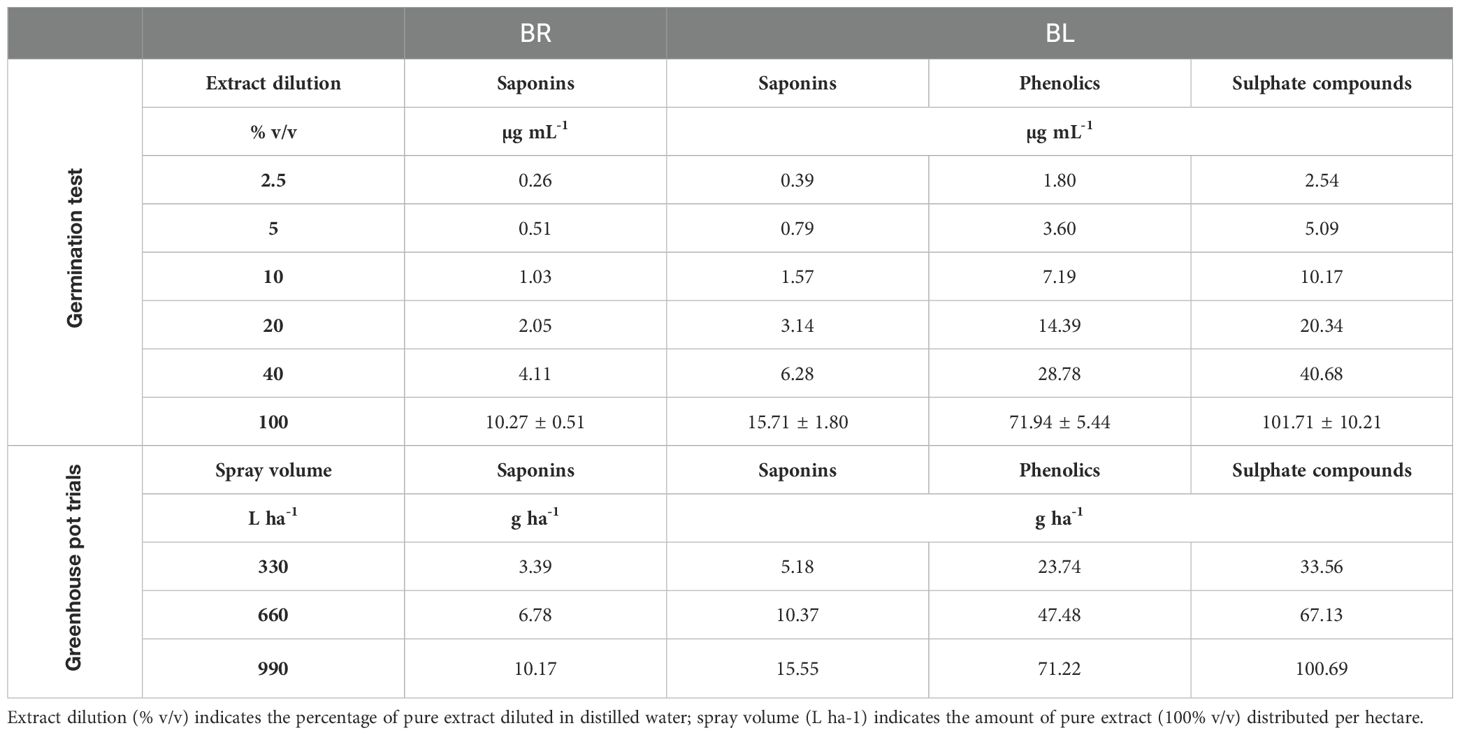
Table 1. Concentrations (means + standard error) and doses of characterized compounds in leaves (BL) and roots (BR) extracts of B. halimifolia used in the germination test (concentration expressed in µg/mL) and in the pre- and post-emergence greenhouse pot trials (doses expressed in g/ha).
Other secondary metabolites were identified in the plant part extracts based on mass fragmentation pathway and comparison with the literature. Apigenin-6,8-di-C-glucoside, protocatechuic acid hexoside sulfate, and several sulphate sugar derivatives are present in BL as reported in Table 2. Different ions are observed with common fragmentation pathways and characteristic ions at 241 m/z and 96 m/z. The signal at m/z 241 is formed through intramolecular rearrangements, in which the sulfate group is transferred to the thioglucose moiety (Bianco et al., 2017; Jara et al., 2022). Different ions are present and are comparable with those reported in Parthenium argentatum A. Gray (Jara et al., 2022). The authors observed the same pattern of fragmentation and indicated such compounds as sulphate compounds.
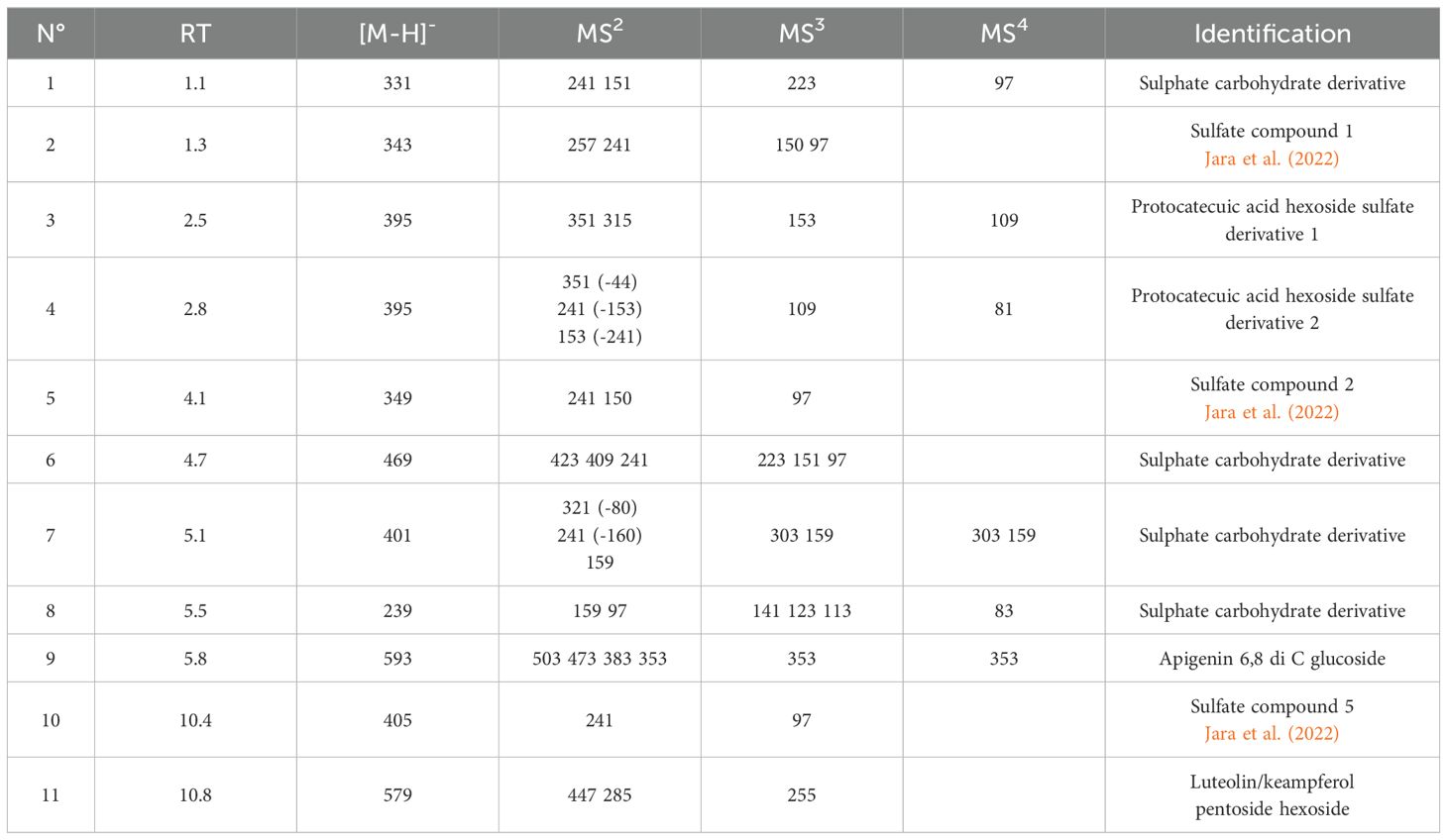
Table 2. Identification of secondary metabolites in B. halimifolia leaves extract (BL); RT, retention time (min); [M-H]-, ion in negative ion mode; MS2 MS3 MS4, mass fragmentation steps.
3.2 Germination test
In the germination test, the ANOVA performed for the dependent variable Germ%NT showed no significant effect for ABUTH and SETPU. Germ%NT remained indeed above 80% across all treatments for ABUTH, while an increase of germination % in comparison with the untreated was even observed for some treatments for SETPU (Figure 2).
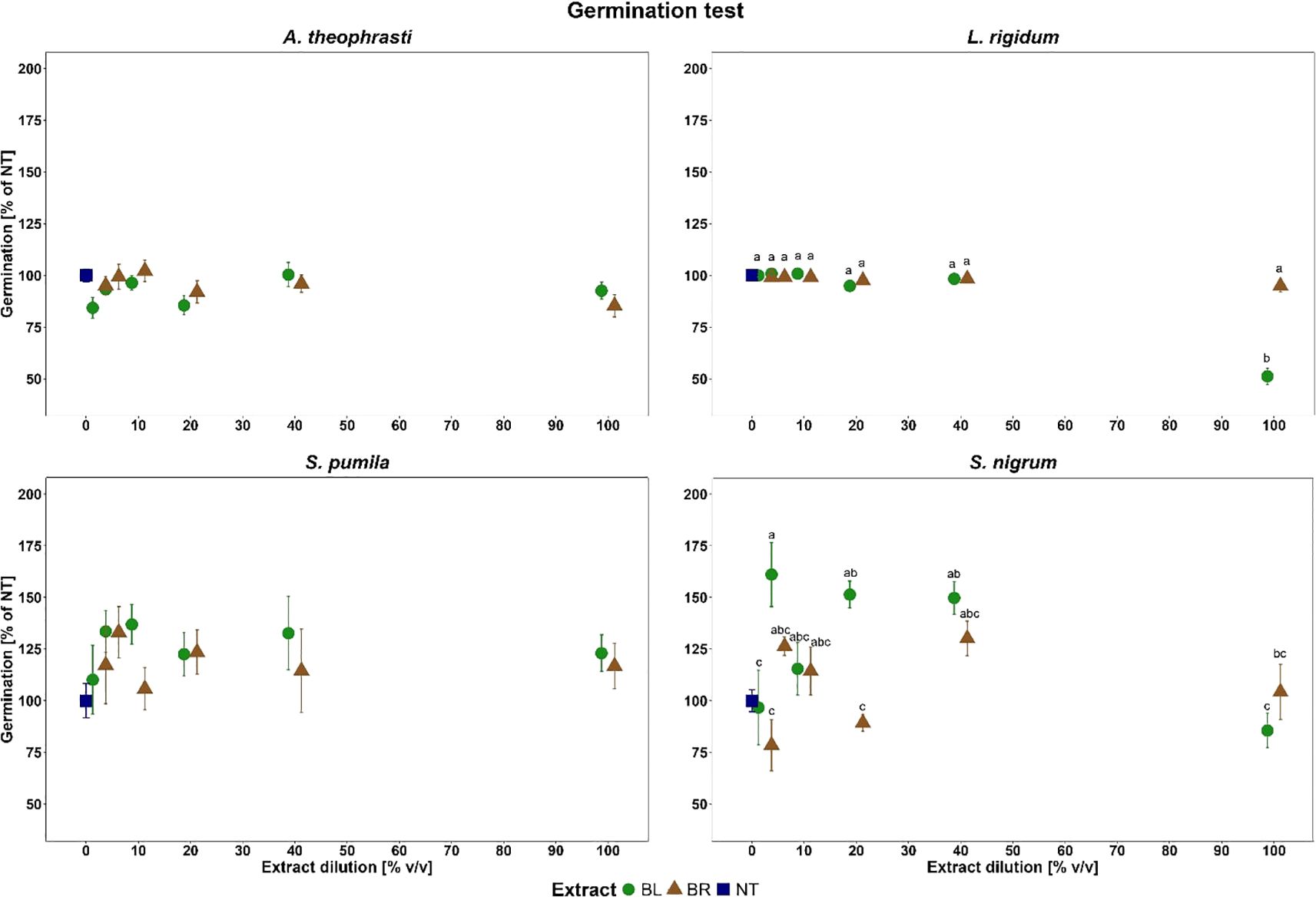
Figure 2. Weeds germination at dilutions of the B. halimifolia extracts (BL leaves extract and BR roots extract). The values, expressed as % of the untreated, are the mean of 6 replicates and the bars are the standard error. Different letters indicate significant differences by Tukey test (p<0.05).
For LOLRI, a significant effect was identified for the factor Dose (F=56.56; p<0.001***), Extract (F=32.32; p<0.001***) and their interaction Extract*Dose (F=37.62; p<0.001***). As evidenced by the Tukey test, BL at the 100% v/v dilution significantly reduced LOLRI germination compared to the other doses of both extracts resulting 48.8% lower than the untreated reference, while Germ%NT was close to 100% for all the other treatments (Figure 2).
For SOLNI, a significant effect of the fixed factors Dose (F=8.75; p<0.001***) and Extract (F=9.61; p<0.01**), and the interaction of Extract*Dose (F=3.23; p<0.05*). On the overall, no clear dose effect on germination was observed and high values of Germ%NT were obtained for most treatments with both plant part extracts (Figure 2). In some cases, as 5 and 40% v/v dilutions for both plant part extracts, a significant increase of Germ%NT was even detected by the Tukey test.
Tables with the results of the ANOVA and Tukey tests for all target species can be found in the appendix.
Dilution-dependent reduction in RootLenght%NT was instead noted for both plant part extracts, with LOLRI and ABUTH being the most sensitive target species showing relevant inhibition already at 20% BL and 10% BR v/v dilutions (Figure 3). Overall, BR was more effective than BL; indeed, BR at 20% v/v dilution provoked a 50-75% RootLenght%NT reduction for all target species. Maximum reduction (>85%) occurred at 100% v/v dilution of both plant part extracts for all target species. Conversely, low dilutions (<5% v/v) of both plant part extracts increased RootLenght%NT of SETPU and SOLNI.
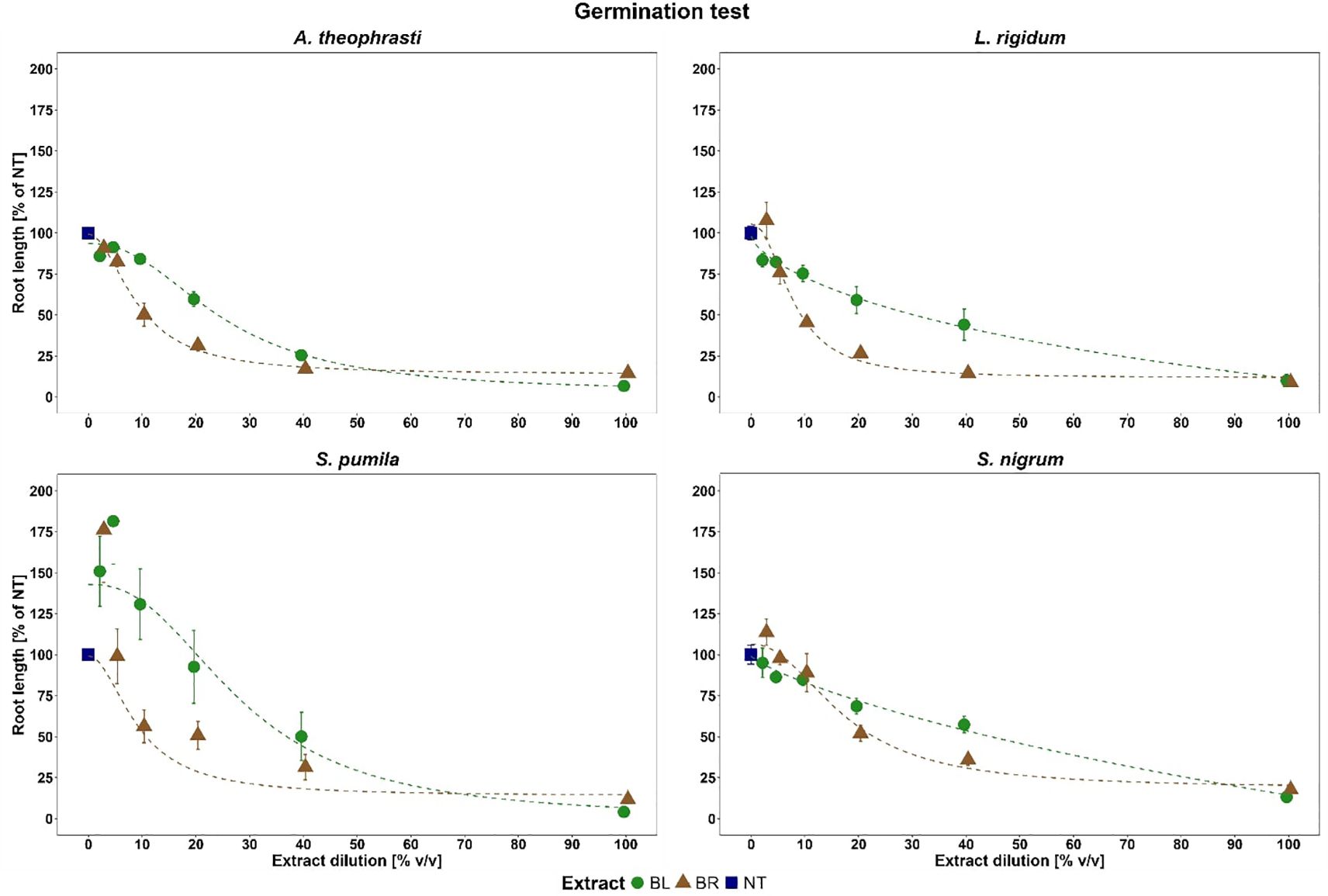
Figure 3. Inhibition effects of B. halimifolia extracts dilutions (BL leaves extract, BR roots extract) on root length for the four weeds. The values expressed as % of the untreated (NT) are the average of 6 replicates and the bars are the standard error. The dotted lines are the log logistic fitted model where b, c, d, e are model parameters.
Since the lack-of-fit F-test identified no significant differences between experiment repetitions, the data were pooled enabling the estimation of a single ED50 value for each target species and plant part extracts. On the overall, significantly higher ED50 values were estimated for BL than for BR for all target species (Figure 4). A proper estimation of an ED50 value was not possible in the case of SOLNI BL due to the lesser inhibition of root length suffered by this species. Mean RootLenght%NT was indeed above 60 and 50% at 20 and 40% v/v of BL, respectively, and a marked decrease was observed only in the undiluted extract (100% v/v). Thus, given that ED50 in the case of SOLNI BL probably lies in the 40-100% v/v interval, we decided to report it simply as ED50 > 40% v/v.
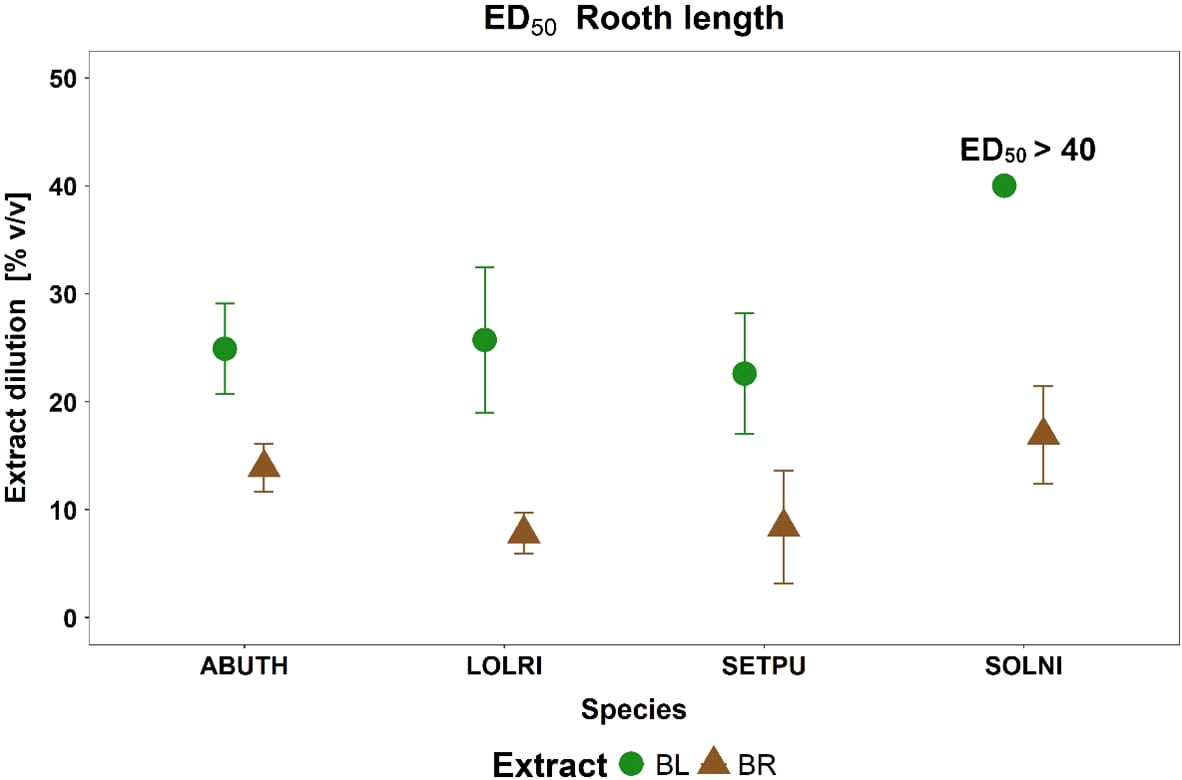
Figure 4. Extract dilution values (% v/v) representing ED50 Root length for the four weeds (ABUTH for A. theophrasti, LOLRI for L. rigidum, SETPU for S. pumila, SOLNI for S. nigrum and the two B. halimifolia extracts (BR, roots extract, brown triangle and BL, leaves extract, green square). Bars are the Confidence Interval 95%.
3.3 Pre-emergence trial in greenhouse
In the greenhouse pre-emergence pot trial, no mortality and no phytotoxicity symptoms were observed. Values of Emerg%NT were close to or even above 100%, so no relevant reduction of seedlings emergence from a reliable crop protection perspective was observed for all treatments (Figure 5). Nonetheless, some statistically significant effects and differences were detected.
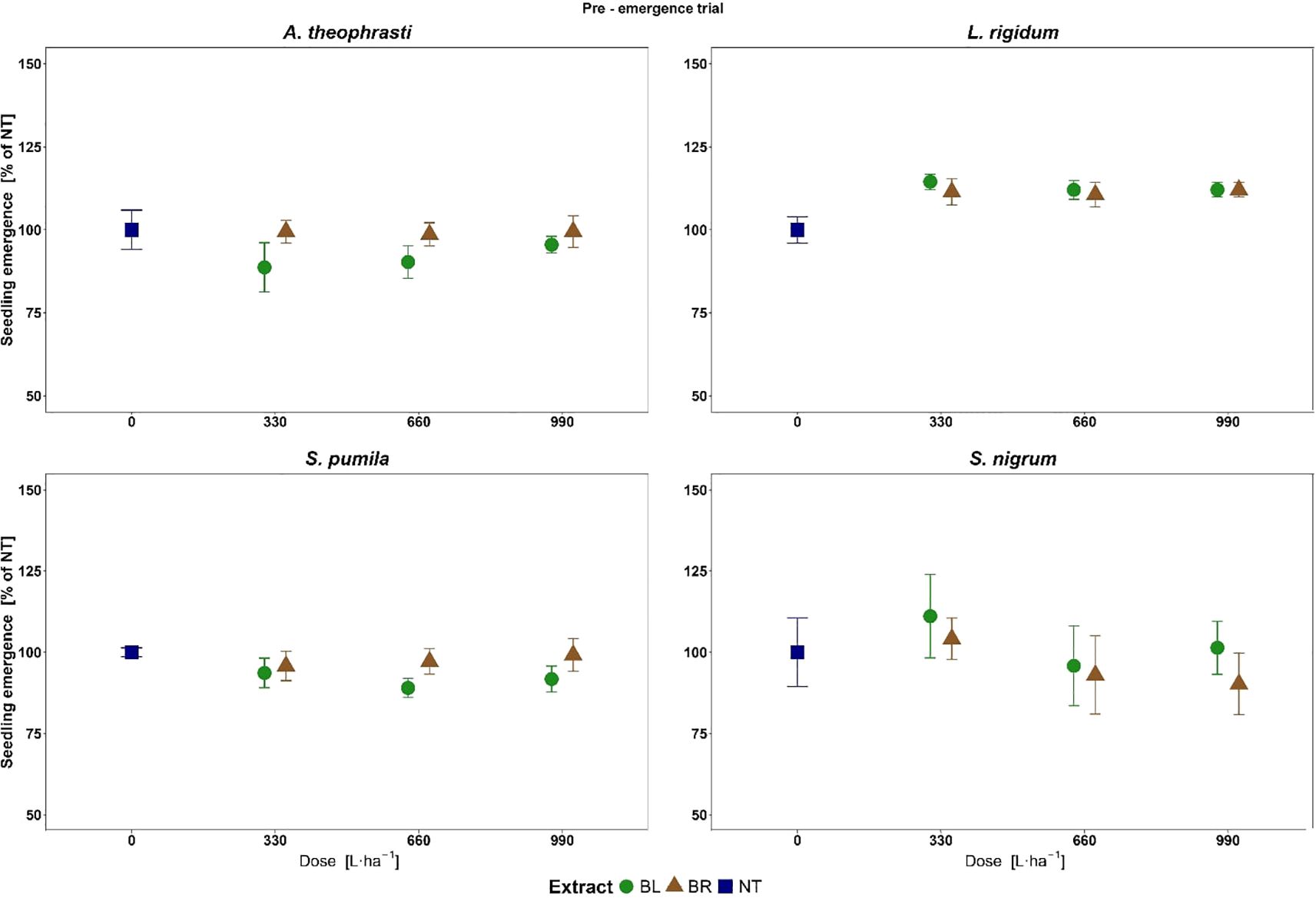
Figure 5. Weeds seedlings emergence at different doses of B. halimifolia leaves (BL) and roots (BR) extracts in the Pre-emergence trial. The values expressed as % of the untreated are the mean of 6 replicates and the bars are the standard error.
The ANOVA showed a significant effect of the fixed factor Extract (F= 6.73, P<0.05*) on Emerg%NT for ABUTH. The BL extract on overall significantly reduced weed emergence compared to BR, with mean Emerg%NT of 91.5 and 99.2%, respectively. For LOLRI, SETPU and SOLNI, the ANOVA did not detect any significant effects on Emerg%NT.
A different response was observed for the target species in terms of Biomass%NT reduction at the different plant part extract doses (Figure 6). No significant decrease in plant biomass was observed across all doses of the two plant part extracts for ABUTH and LOLRI.
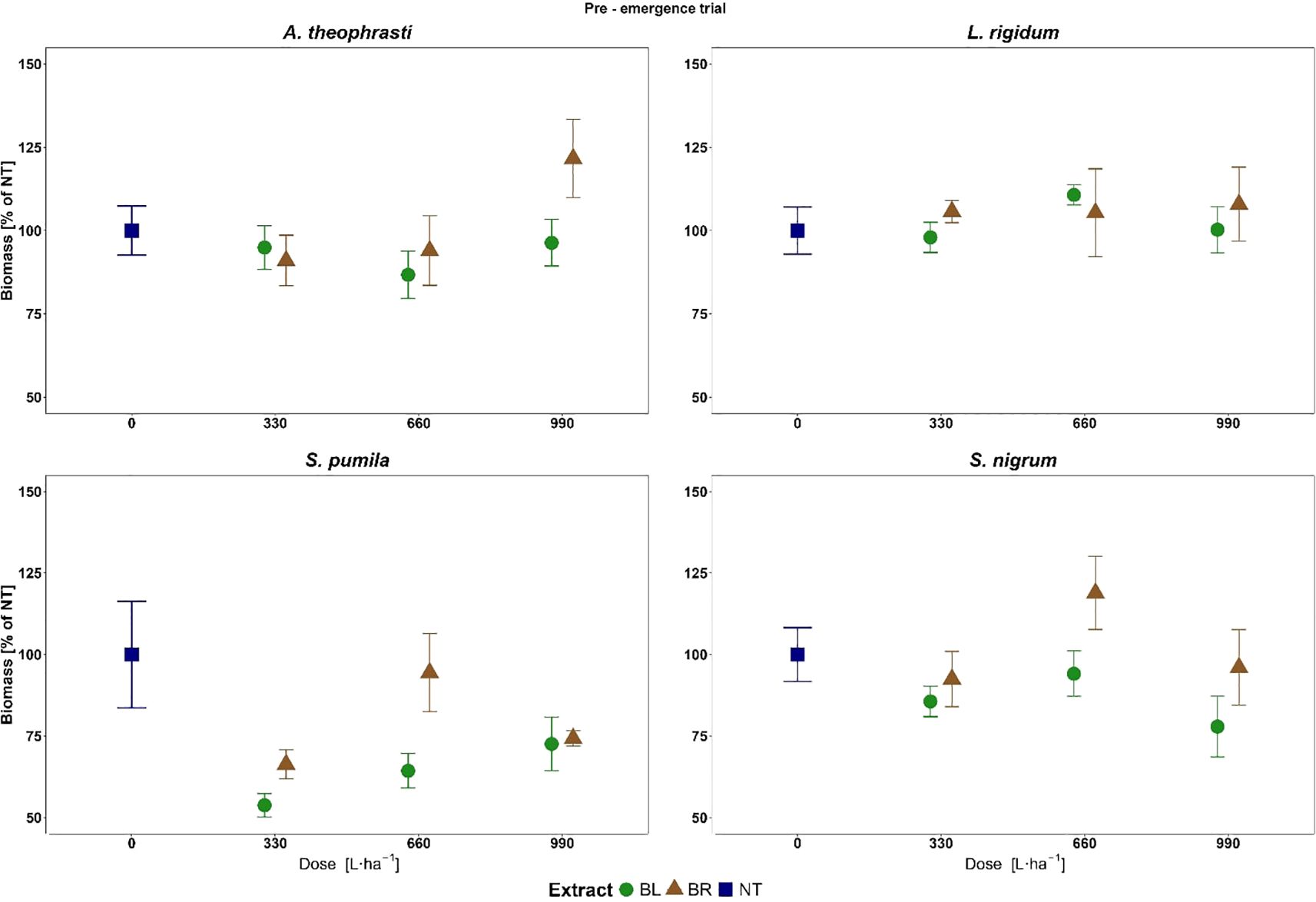
Figure 6. Biomass of weeds treated with the different doses of roots (BR) and leaves BL extracts of B. halimifolia in the Pre-emergence trial. The values expressed as % of the untreated (NT) are the mean of 6 replicates and the bars are the standard error.
SETPU was the species in which the greatest biomass reduction due to the application of BL and BR extracts was observed (Figure 6). The ANOVA performed for SETPU identified the significant effect of factors Dose (F= 4.23; p<0.05*) and Extract (F= 8.39; p<0.01**) on Biomass%NT. On the overall, BL was more effective and provoked a significantly higher Biomass%NT reduction than BR (36.4% and 21.6%, respectively) (Figure 7).
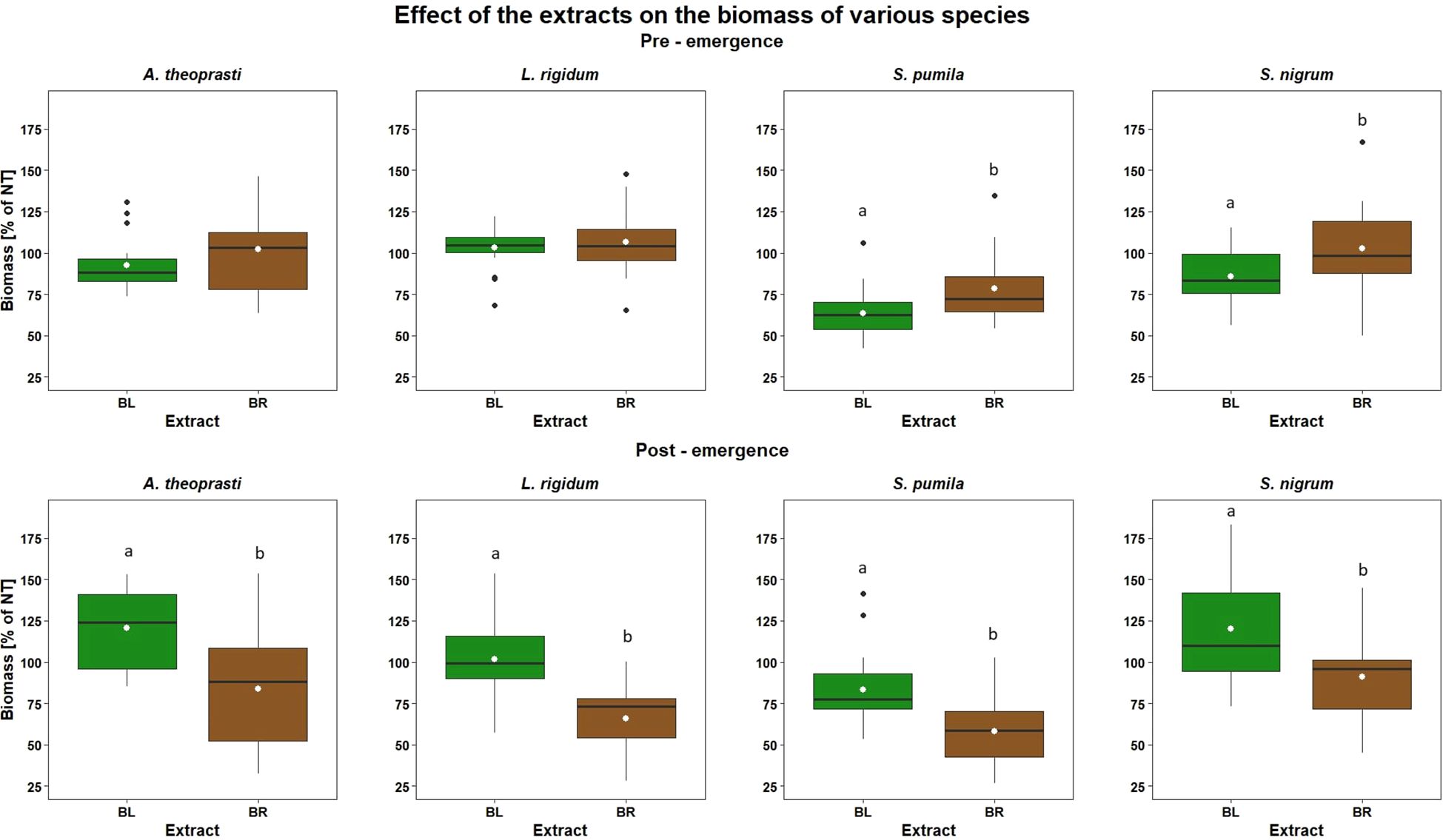
Figure 7. Effect of roots (BR) and leaves (BL) extracts of B. halimifolia on weed biomass expressed as % of the untreated (NT) in the Pre-emergence (top) and Post-emergence (bottom) trials. The box plots show the 25-75% percentiles, the lines indicate the median, the white points is the mean, the whiskers identify the outliner range, and the black points are the outliers for each group. Different letters indicate significant differences by Tukey test (p<0.05).
Furthermore, considering the two plant part extracts together, the 330 L ha-1 dose significantly reduced plant biomass more than the 660 L ha-1 dose (60 and 79.4% Biomass%NT, respectively).
Only a minimal reduction of plant biomass was observed for SOLNI after the BR and BL application, with values of Biomass%NT even exceeding 100% for some treatments (Figure 6). The ANOVA identified the Extract as the only significant explanatory factor (F= 5.08; p<0.05*). As already observed for SETPU, BL provoked on average a significantly higher biomass reduction of the treated plants compared to BR (Figure 7). While biomass of BR-treated plants was similar to the untreated (on average 102.5% Biomass%NT), BL showed a 14.1% average biomass reduction compared to control.
Tables with the results of the ANOVA and Tukey tests for all target species can be found in the appendix.
3.4 Post-emergence trial in greenhouse
No mortality or damage was observed in the plants treated with any of the BR and BL doses. Nevertheless, more relevant effects of plant part extracts application were observed on the weed fresh biomass, with different responses between the studied weeds (Figure 7).
For ABUTH, the ANOVA showed a significant effect for the main factor Extract (F= 18.11; p<0.001***) and almost significant for Dose (F=3.21; p=0.055). ABUTH had indeed a different response to the application of the two plant part extracts (Figure 7). BL on average increased plant biomass by 20.4% (Biomass%NT ranged from 132.8 ± 19.46 at 330 L ha-1 to 106.3 ± 17.84 at 990 L ha-1) while BR reduced biomass by 16.6% compared to untreated control (Biomass%NT ranged from 95.7 ± 34.58 at 330 L ha-1 to 69.4 ± 36.81 at 990 L ha-1). Although BR and BL had overall different effect on ABUTH, the Tukey test showed that a significant reduction of plant biomass was observed for both plant part extracts as application doses increased. The highest dose of 990 L ha-1 indeed significantly reduced plant biomass (-12.2% to untreated) when compared to the lowest dose of 330 L ha-1 (+14.3% to untreated).
The ANOVA for LOLIUM showed a significant effect for the main factor Extract (F= 22.28; p<0.001***). On average, BR extract reduced indeed plant biomass by 34% compared to untreated (Biomass%NT ranged from 62.2 ± 21.75 at 330 L ha-1 to 69.0 ± 30.80 at 990 L ha-1) while plants treated with BL extract achieved similar or even higher biomass than the control (Figure 7).
For SETPU, significant effects for the main factor Extract (F= 15.10; p<0.001***) and the interaction Extract*Dose (F= 3.58; p<0.05*) were observed. SETPU had the highest response among the target species to the post-emergence extract application, with biomass values lower than the untreated for all treatments apart from BL at 660 L ha-1 (Figure 8). On average, a reduction in plant biomass compared to the untreated was noted for both BR (-42%) and BL (-16.6%), showing again the highest post-emergence herbicidal activity of BR (Figure 7).
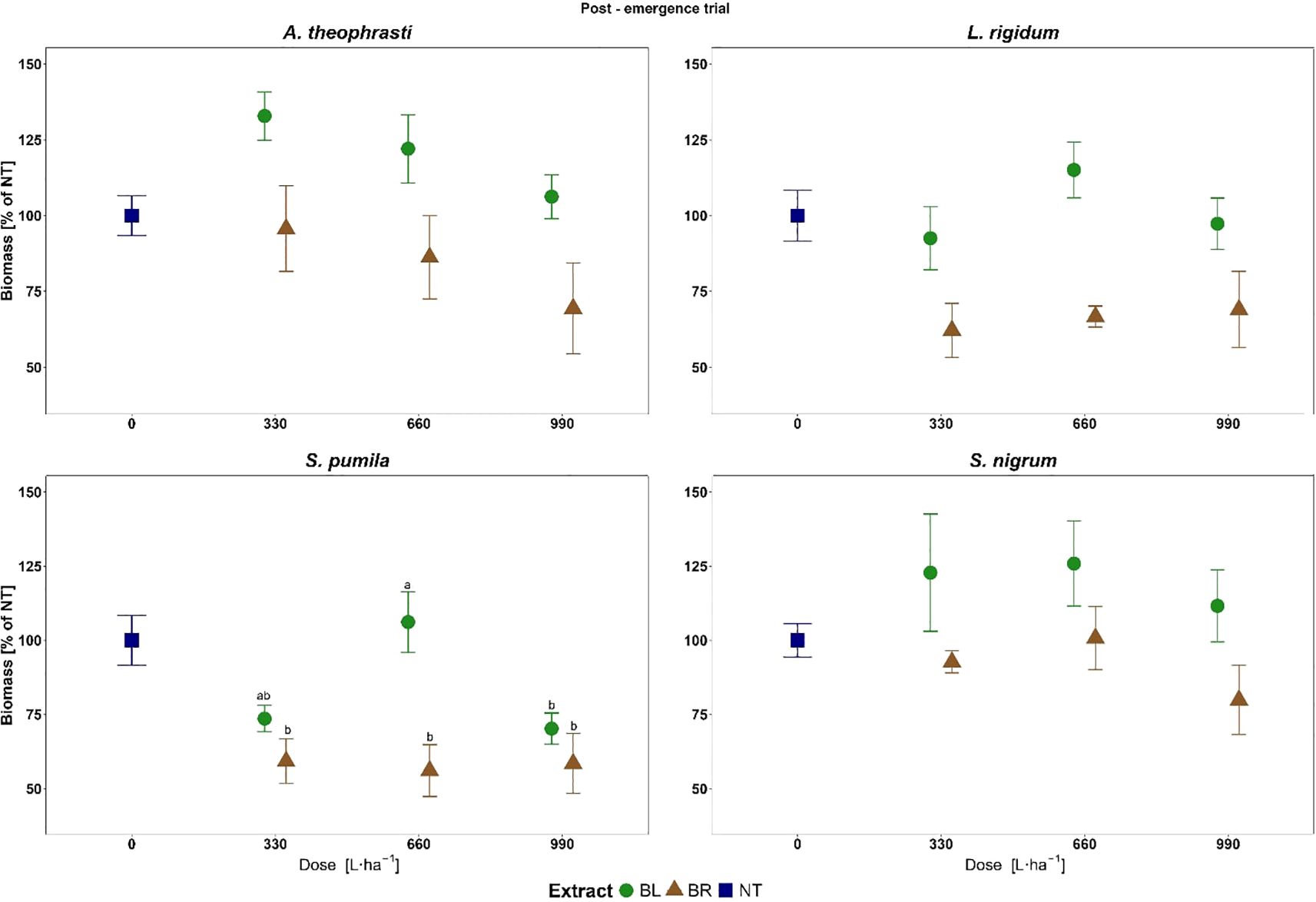
Figure 8. Biomass of weeds treated with the different doses of roots (BR) and leaves (BL) extracts of B. halimifolia in the Post-emergence trial. The values expressed as % of the untreated (NT) are the mean of 6 replicates and the bars are the standard error. Different letters indicate significant differences by Tukey test (p<0.05).
For SOLNI, significant effect for the main factor Extract (F= 7.84; p<0.01**) was only detected. Also for SOLNI BR seemed to have a higher herbicidal activity (average biomass reduction of 8.8% compared to untreated) compared to BL (average biomass increase of 20.1% compared to untreated), as shown in Figure 7.
Tables with the results of the ANOVA and Tukey tests for all target species can be found in the appendix.
4 Discussion
The results confirmed the BR and BL phytotoxic activity, consistent with findings of other Asteraceae and species of the genus Baccharis (Chon and Nelson, 2010; Ibáñez and Zoppolo, 2011; Dias et al., 2017; La Iacona et al., 2024) highlighting the presence of allelopathic compounds that may enhance B. halimifolia competitive ability and invasion capacity (Lázaro-Lobo et al., 2022).
HPLC-DAD-(ESI)-MS analysis revealed the presence of phenolic compounds, sulphate carbohydrate derivatives and saponins in BL and mainly saponins in BR. It is worth noting that this is, to our knowledge, the first report regarding the phytochemical composition of B. halimifolia aqueous plant part extracts as previous studies have only studied essential oils (Desrini et al., 2023). The presence of carbohydrate derivative sulphate compound and saponin mixture seems to be unusual in Baccharis species (Martinez et al., 2005), although saponins have already been identified as the main constituent in Baccharis trimera (Less) DC (Gené et al., 1995). On the contrary, many previous studies reported the presence of phenolics such as flavonoids and coumarins among the main biologically active substances of the genus (Fernandes et al., 2022; Martinez et al., 2005). Among the compounds identified in BL and BR extracts, relevant phytotoxic effects have been usually attributed to saponins and phenolics (Kostina-Bednarz et al., 2023; Li et al., 2010). Sulphate carbohydrate derivatives seem to have less phytotoxic potential. They are mainly known to be involved in the processes of increasing salinity tolerance in plants (Nafees et al., 2014).
The phytotoxic effect of both plant part extracts in the germination test was relevant to root growth, while germination inhibition was minimal for all tested weeds. This contrasting response has been frequently reported across previous studies testing the phytotoxicity of different natural extracts (Chon and Nelson, 2010). Nonetheless, a significant reduction of germination occurred for LOLRI at the BL 100% v/v and this may be due to phenolic compounds. Many studies confirm that these compounds can affect the germination of numerous weed species (Li et al., 2010; Dias et al., 2017; Kostina-Bednarz et al., 2023) and, in agreement with our study, it has been observed that species of the genus Lolium are highly susceptible to extracts containing phenolic compounds like Protocatechuic acid (Vitalini et al., 2020). This compound, which was identified in BL, is reported in previous studies as a main allelochemical with action on germination of Lolium multiflorum, Echinochloa oryzoides, and Lactuca sativa (Li et al., 2010; Vitalini et al., 2020). Apigenin is another compound that may have had a phytotoxic action in BL extract. This substance has already been found in Baccharis species (Del Corral et al., 2012) and its phytotoxic action on germination and root growth has been confirmed (Basile et al., 2000; Cipollini et al., 2008; Vitalini et al., 2020). Nevertheless, the BR and BL effects on germination were smaller than those noted in other studies that have adopted similar methodologies (Dias et al., 2017; Scavo et al., 2018; Miranda-Arámbula et al., 2021; Vitalini et al., 2021) and respect to studies that used organic solvents (e.g. methanol and ethanol) or different extraction methodologies (e.g. essential oil extraction) (Ibáñez and Zoppolo, 2011; Scavo et al., 2019, 2020; Vitalini et al., 2022).
As observed in most studies on the phytotoxicity of aqueous extracts, BL and BR showed their inhibitory activity mainly against root growth. This is because seed imbibition during germination probably facilitated the entry of phytotoxic compounds that then exerted their biological activity in the early stages of growth (De Martino et al., 2012). The reduction in root growth observed is greater or similar to values reported in previous studies on essential oils of Baccharis dracunculifolia DC (Ibáñez and Zoppolo, 2011), aqueous extracts of Baccharis sp (Dias et al., 2017; Miranda-Arámbula et al., 2021) and other phytotoxic species (Vitalini et al., 2020, 2021).
BR provoked a stronger inhibition of root growth than BL in all target species, being the estimated BR ED50 values at least half of the corresponding BL ED50 for each target species. Since chemical analysis showed the presence of only saponins in BR, it would seem that the other compounds detected in BL did not improve the inhibition effect, despite the presence of compounds with phytotoxicity such as phenolics (Li et al., 2010; Kostina-Bednarz et al., 2023). Furthermore, considering that higher saponin concentration was detected in BL than in BR (15.71 and 10.27 µg mL-1, respectively) and different saponins were present in the two plant part extracts as showed by HPLC-DAD-(ESI)-MS analysis, it is likely that the saponins present in BR have greater in vitro phytotoxicity. In contrast, other works have found that the lower content of active substances in roots also corresponded to a lower phytotoxic effect (Scavo et al., 2022).
Saponins are glycosides of triterpene and steroids which in plants have mainly pre-defense functions against disease and herbivory, but it has been shown that they can be the phytochemical compounds with a high phytotoxic activity (Mugford and Osbourn, 2013; Stavropoulou et al., 2017). Some authors argue that their phytotoxicity may be due to their interaction with the cytoplasmatic membrane and its constituents (Faizal and Geelen, 2013; Kostina-Bednarz et al., 2023). According to the available literature, the phytotoxic action of saponins was observed mainly on early root growth rather than germination (Oleszek, 1993; Waller et al., 1995, 1999; Hoagland et al., 1996; Faizal and Geelen, 2013).
Supporting our hypothesis that the phytotoxicity occurred during the germination test was mainly due to saponins, the browning of root apex in treated plants was observed with both plant part extracts (see the Petri dishes germination test photo in the Supplementary Material), a symptom brought back to the action of saponins which can lead to the decay of the root system (Oleszek, 1993; Hoagland et al., 1996).
Interestingly, the increase in root growth observed for SETPU and SOLNI at low dilutions of both plant part extracts agreed with a previous study that reported a root growth stimulation with an aqueous extract of Baccharis uncinella DC. at 2.5% v/v dilution (Dias et al., 2017). This phenomenon, called hormesis, is also described for several herbicides (Duke et al., 2006) and may be caused by saponins and phenolics compounds (e.g. luteolin) at low concentrations as reported by previous studies (Waller et al., 1995; Hoagland et al., 1996; De Martino et al., 2012).
If we assume that the phytotoxic effects observed with BR are caused exclusively by saponins without considering synergistic or addictive effects with other substances, it can be stated that BR, in which saponins are present at 10.27 µg mL-1, provoked stronger inhibition of root growth than those described in other studies conducted with pure saponin molecules such as medicagenic acid glycosides, hederagenic glycosides, zahnic acid glycosides, and soyasaponin I (Oleszek, 1993; Waller et al., 1995; Hoagland et al., 1996). For example, Waller et al. (1995) observed very mild phytotoxicity at concentrations of 10-100 µg mL-1, with significant effects (80-90% reduction of root growth) only at concentrations of 500-1000 µg mL-1.
In the greenhouse pre-emergence trial, no relevant herbicidal effects were observed for both plant part extracts at any doses. Despite this, some phytotoxic effects have been observed. BL reduced seedlings emergence in ABUTH and had greater effects on plant fresh biomass than BR, reducing the average Biomass%NT of SETPU and SOLNI. It has been shown that both saponins and phenolic compounds accumulation in the soil can cause phytotoxicity (Waller et al., 1999; Li et al., 2010) and the allelopathic action of these compounds seems to be carried out mainly through the leaching in the soil from leaf litter (Li et al., 2010). Furthermore, Ibáñez and Zoppolo (2011) have measured a higher amount of phenolics correlated with a noticeable decrease in plant diversity in soils under B. dracunculifolia plantation, so the presence of this allelopathic mechanism in B. halimifolia can be supposed.
In the greenhouse post-emergence trial, no plant mortality and only partial herbicidal activity were observed but, in contrast to what was observed in the pre-emergence trial, BR provoked even larger plant biomass reduction for all target species, while an increase in plant biomass compared to the untreated ones was often noticed after BL application. With an average reduction in plant fresh biomass of up to 34 and 42% compared to the controls, LOLRI and SETPU were the most susceptible species to post-emergence treatment with BR. This result may be explained by the presence of saponins, amphiphilic molecules that may have penetrated the leaf cuticle. A higher sensitivity to phytotoxic effects of saponins has already been observed by Hoagland et al. (1996) and Waller et al. (1995) for the grass species Bromus secalinus L. and Echinochloa crus galli (L.) P. Beauv.
Although some herbicidal activity mainly in terms of plant biomass reduction were detected in pre- and post-emergence trials, no relevant results on seedlings emergence or plant mortality were observed. Moreover, these effects were often variable between replicates or experiment repetitions, indicating that BR and BL herbicidal activity was limited and unstable under the experimental conditions of greenhouse pot trials. This can be related to the low doses of phytotoxic active substances applied in these trials. Even if all phenolics and saponins identified in the two plant part extracts had phytotoxicity, the whole concentration of active substances would be about 85 and 10 µg mL-1 for BL and BR, respectively. Thus, given the highest tested spray volume of 990 L ha-1, the highest dose of active substances applied in pre- and post-emergence pot trials would be about 85 and 10 g ha-1 for BL and BR respectively (Table 1). Such doses are largely lower than label doses of most synthetic chemical herbicides and achieving only limited and unstable herbicidal activity with them is therefore expectable and reasonable.
There are very few studies evaluating the phytotoxicity of aqueous extracts with spray volumes and extract doses comparable to field conditions (Kostina-Bednarz et al., 2023), making difficult to compare the results of the present study with the available literature. Large experimental variability in magnitude of phytotoxicity, e.g. reduction of treated plant biomass, leading to unsatisfactory weed control from a practical perspective, were often observed in studies testing plant-derived natural products as herbicides (Loddo et al., 2023; Torres-Pagán et al., 2024; Khamare et al., 2022).
Finally, substantial differences in the phytotoxicity were observed between the germination test in Petri dishes and the greenhouse pre- and post-emergence pot trials. Several factors probably contributed to this result, but first it is necessary to underline the different conditions of exposure of weeds to the aqueous plant part extracts in the three trials. In the germination test, the phytotoxicity was high because weed were directly and continuously exposed to aqueous extracts during seed imbibition and root growth. Conversely, in pre-emergence pot trials, seeds were in the soil, and plant part extracts were sprayed on the soil surface with water volumes to reproduce field-like conditions of herbicide applications. Exposure to phytotoxic compounds could have occurred if those compounds moved from soil surface and reached the germinating seeds, or when seedlings growing belowground passed through the superficial soil layer where extracts were applied, or later when plant roots started adsorbing water. To have phytotoxicity after soil application, active substances must have relevant soil mobility and persistence. Besides, these substances must be effective at low concentrations, given the dilution effect caused by the soil volume, when few mL of spray solutions diffuse in dozens of Liters of soil, and the buffering effect through the adsorption of allelopathic compounds on organic matter and clay particles. Regarding post-emergence pot trials, plant part extracts were applied as spray droplets on leaves surface, and active substances must penetrate the leaf cuticle and at least spread into the underlying leaf cells to cause relevant damage. This can be difficult for hydrophilic compounds, as those likely contained in an aqueous extract, given the lipophilic nature of the leaf cuticle (Hasan et al., 2021).
5 Conclusions
This study suggests that B. halimifolia could be a potential source of active substances with phytotoxic activity, but both aqueous extracts tested in this study (BR and BL) are not yet directly usable as natural herbicides in field conditions since they did not reach acceptable control efficacy in the greenhouse trials. Given that the main reason for this lack of efficacy was probably the low dose of active substances applicable with spray volumes representative of realistic field conditions, as those adopted in the pre- and post-emergence trials, future efforts should aim at increasing their concentration in the extracts either by changing the extraction method or by improving the formulation. Similarly, it is relevant to identify the compounds of the two extracts with greater phytotoxic activity, by fractioning the extracts and testing the fractions separately.
Data availability statement
The raw data supporting the conclusions of this article will be made available by the authors, without undue reservation.
Author contributions
GT: Conceptualization, Data curation, Formal analysis, Investigation, Methodology, Resources, Visualization, Writing – original draft. VX: Data curation, Formal analysis, Investigation, Visualization, Writing – review & editing. SS: Formal analysis, Methodology, Resources, Validation, Visualization, Writing – review & editing. SO: Investigation, Supervision, Validation, Writing – review & editing. NN: Investigation, Visualization, Writing – review & editing. ES: Investigation, Resources, Writing – review & editing. SV: Methodology, Resources, Writing – review & editing. SD: Resources, Supervision, Writing – review & editing. MI: Supervision, Writing – review & editing. RM: Formal analysis, Writing – review & editing. DL: Conceptualization, Investigation, Methodology, Project administration, Resources, Supervision, Writing – review & editing.
Funding
The author(s) declare that no financial support was received for the research and/or publication of this article.
Conflict of interest
The authors declare that the research was conducted in the absence of any commercial or financial relationships that could be construed as a potential conflict of interest.
Generative AI statement
The author(s) declare that no Generative AI was used in the creation of this manuscript.
Publisher’s note
All claims expressed in this article are solely those of the authors and do not necessarily represent those of their affiliated organizations, or those of the publisher, the editors and the reviewers. Any product that may be evaluated in this article, or claim that may be made by its manufacturer, is not guaranteed or endorsed by the publisher.
Supplementary material
The Supplementary Material for this article can be found online at: https://www.frontiersin.org/articles/10.3389/fagro.2025.1542124/full#supplementary-material
References
Basile A., Sorbo S., Giordano S., Ricciardi L., Ferrara S., Montesano D., et al. (2000). Antibacterial and allelopathic activity of extract from Castanea sativa leaves. Fitoterapia 71, 110–116. doi: 10.1016/s0367-326x(00)00185-4
Bianco G., Pascale R., Lelario F., Bufo S. A., Cataldi T. R. I. (2017). ““Investigation of glucosinolates by mass spectrometry,”,” in Glucosinolates. Eds. Mérillon J. M., K. G. R. (Springer International Publishing, Switzerland), 431–461. doi: 10.1007/978-3-319-25462-3
Chon S. U., Kim Y. M., Lee J. C. (2003). Herbicidal potential and quantification of causative allelochemicals from several Compositae weeds. Weed Res. 43, 444–450. doi: 10.1046/j.0043-1737.2003.00361.x
Chon S. U., Nelson C. J. (2010). Allelopathy in Compositae plants. A review. Agron. Sustain Dev. 30, 349–358. doi: 10.1051/agro/2009027
Cipollini D., Stevenson R., Enright S., Eyles A., Bonello P. (2008). Phenolic metabolites in leaves of the invasive shrub, Lonicera maackii, and their potential phytotoxic and anti-herbivore effects. J. Chem. Ecol. 34, 144–152. doi: 10.1007/s10886-008-9426-2
Commission Implementing Regulation (EU) (2016). 2016/1141 - of 13 July 2016 - adopting a list of invasive alien species of Union concern pursuant to Regulation (EU) No 1143/2014 of the European Parliament and of the Council. Official Journal of the European Union, Brussels.
Del Corral S., Cuffini S. L., Cardoso S. G., Bortoluzzid A. J., Palacios S. M. (2012). Phytotoxic halimanes isolated from Baccharis salicifolia (Ruiz & Pad.) Pers. Phytochem. Lett. 5, 280–283. doi: 10.1016/j.phytol.2012.02.001
De Martino L., Mencherini T., Mancini E., Aquino R. P., De Almeida L. F. R., De Feo V. (2012). In Vitro phytotoxicity and antioxidant activity of selected flavonoids. Int. J. Mol. Sci. 13, 5406–5419. doi: 10.3390/ijms13055406
Desrini S., Ducloux J., Hamion G., Bodet C., Labanowski J., Mustofa M., et al. (2023). Antibiofilm Activity of Invasive Plants against Candida albicans: Focus on Baccharis halimifolia Essential Oil and Its Compounds. Chem. Biodivers 20, e202300130. doi: 10.1002/cbdv.202300130
Dias M. P., Nozari R. M., Santarém E. R. (2017). Herbicidal activity of natural compounds from Baccharis spp. on the germination and seedlings growth of Lactuca sativa and Bidens pilosa. Allelopathy J. 42, 21–35. doi: 10.26651/2017-42-1-1103
Duke S. O., Cedergreen N., Velini E. D., Belz R. G. (2006). Hormesis: is it an important factor in herbicide use and allelopathy? Outlooks Pest Manag. 17, 29–33. doi: 10.1564/16feb10
Faizal A., Geelen D. (2013). Saponins and their role in biological processes in plants. Phytochem. Rev. 12, 877–893. doi: 10.1007/s11101-013-9322-4
Fernandes G. W., Oki Y., Barbosa M. (2022). Baccharis: From evolutionary and ecological aspects to social uses and medicinal applications (Cham, Switzerland: Springer International Publishing). doi: 10.1007/978-3-030-83511-8
Fried G., Caño L., Brunel S., Beteta E., Charpentier A., Herrera M., et al. (2016). Monographs on invasive plants in Europe: Baccharis halimifolia L. Bot. Lett. 163, 127–153. doi: 10.1080/23818107.2016.1168315
Gené R. M., Cartaña C., Adzet T., Marìn E., Parella T., Cañigueral S. (1995). Anti-Inflammatory and Analgesic Activity of Baccharis trimera: Identification of its Active Constituents. Planta Med. 62, 232–235. doi: 10.1055/s-2006-957866
Gianessi L. P. (2013). The increasing importance of herbicides in worldwide crop production. Pest Manag Sci. 69, 1099–1105. doi: 10.1002/ps.3598
Hasan M., Ahmad-Hamdani M. S., Rosli A. M., Hamdan H. (2021). Bioherbicides: An eco-friendly tool for sustainable weed management. Plants 10, 1212. doi: 10.3390/plants10061212
Hoagland R. E., Zablotowicz R. M., Reddy K. N. (1996). Studies of the phytotoxicity of saponins on weed and crop plants. Saponins Used Food Agric. 405, 57–73. doi: 10.1007/978-1-4613-0413-5_6
Ibáñez F., Zoppolo R. (2011). Assessment of allelopathic properties of Baccharis dracunculifolia DC in laboratory and field conditions. Allelopathy J. 28, 77–86.
Jara F. M., Carrión M. E., Angulo J. L., Latorre G., López-Córcoles H., Zalacain A., et al. (2022). Chemical characterization, antioxidant activity and morphological traits in the leaves of guayule (Parthenium argentatum A. Gray) and its hybrids. Ind Crops Prod 182, 1-17. doi: 10.1016/j.indcrop.2022.114927
Khamare Y., Chen J., Marble S. C. (2022). Allelopathy and its application as a weed management tool: A review. Front. Plant Sci. 13. doi: 10.3389/fpls.2022.1034649
Kostina-Bednarz M., Płonka J., Barchanska H. (2023). Allelopathy as a source of bioherbicides: challenges and prospects for sustainable agriculture. Rev. Environ. Sci. Biotechnol. 22, 471–504. doi: 10.1007/s11157-023-09656-1
La Iacona M., Lombardo S., Mauromicale G., Scavo A., Pandino G. (2024). Allelopathic activity of three wild Mediterranean Asteraceae: Silybum marianum, Cynara cardunculus var. sylvestris, Galactites tomentosus. Agronomy 14, 575. doi: 10.3390/agronomy14030575
Lázaro-Lobo A., Ervin G. N., Caño L., Panetta F. D. (2022). ““Biological Invasion by Baccharis,”,” in Baccharis: From Evolutionary and Ecological Aspects to Social Uses and Medicinal Applications (Cham, Switzerland: Springer International Publishing), 185–214. doi: 10.1007/978-3-030-83511-8_8
Li Z. H., Wang Q., Ruan X., De Pan C., Jiang D. A. (2010). Phenolics and plant allelopathy. Molecules 15, 8933–8952. doi: 10.3390/molecules15128933
Loddo D., Imperatore G., Milani A., Panozzo S., Farinati S., Sattin M., et al. (2020). First report of glyphosate-resistant biotype of Eleusine indica (L.) Gaertn. in Europe. Agronomy 10, 1692. doi: 10.3390/agronomy10111692
Loddo D., McElroy J. S., Giannini V. (2021). Problems and perspectives in weed management. Ital. J. Agron. 16, 1854. doi: 10.4081/ija.2021.1854
Loddo D., Jagarapu K. K., Strati E., Trespidi G., Nikolić N., Masin R., et al. (2023). Assessing herbicide efficacy of pelargonic acid on several weed species. Agronomy 13, 1511. doi: 10.3390/agronomy13061511
Marrone P. G. (2024). Status of the biopesticide market and prospects for new bioherbicides. Pest Manag. Sci. 80, 81–86. doi: 10.1002/ps.7403
Martinez M. J. A., Bessa A. L., Benito P. B. (2005). Biologically active substances from (Compositae). Stud. Nat. Prod. Chem. 30, 703–759. doi: 10.1016/S1572-5995(05)80045-6
Merfield C. N. (2023). ““Integrated weed management in organic farming,”,” in Organic Farming: Global Perspectives and Methods, 2nd ed. (Sawston, Cambridge: Elsevier), 31–109. doi: 10.1016/B978-0-323-99145-2.00004-5
Mikula K., Gersz A., Witek-Krowiak A., Skrzypczak D., Izydorczyk G., Chojnacka K. (2021). ““Agrochemicals in view of circular economy,”,” in Smart Agrochemicals for Sustainable Agriculture (Elsevier, Academic Press), 57–80. doi: 10.1016/B978-0-12-817036-6.00004-2
Miranda-Arámbula M., Reyes-Chilpa R., Anaya L. A. L. (2021). Phytotoxic activity of aqueous extracts of ruderal plants and its potential application to tomato crop. Bot. Sci. 1, 487–498. doi: 10.17129/BOTSCI.2727
Mugford S. T., Osbourn A. (2013). ““Saponin synthesis and function,”,” in Isoprenoid Synthesis in Plants and Microorganisms: New Concepts and Experimental Approaches (Springer New York), 405–424. doi: 10.1007/978-1-4614-4063-5_28
Nafees A. K., M Iqbal R. K., Mohd A., Mehar F., Asim M., Shabina S. (2014). Salinity Tolerance in Plants: Revisiting the Role of Sulfur Metabolites. J. Plant Biochem. Physiol. 02, 1063–1074. doi: 10.4172/2329-9029.1000120
Oleszek W. (1993). Allelopathic potentials of alfalfa (Medicago sativa) saponins: their relation to antifungal and hemolytic activities. J. Chem. Ecol. 19, 165. doi: 10.1007/BF00987369
Onofri A. (2005). BIOASSAY97: a new EXCEL VBA macro to perform statistical analyses on herbicide dose-response data. Rivista Italiana di Agrometeorol. 3, 40–45.
Panozzo S., Collavo A., Sattin M. (2020). Sensitivity analysis of italian Lolium spp. to glyphosate in agricultural environments. Plants 9, 165. doi: 10.3390/plants9020165
Pollier J., Morreel K., Geelen D., Goossens A. (2011). Metabolite profiling of triterpene saponins in Medicago truncatula hairy roots by liquid chromatography fourier transform ion cyclotron resonance mass spectrometry. J. Nat. Prod 74, 1462–1476. doi: 10.1021/np200218r
R Core Team (2024).R: a language and environment for statistical computing. In: R Foundation for Statistical Computing (Vienna, Austria) (Accessed September 7, 2024).
Riemens M., Sønderskov M., Moonen A. C., Storkey J., Kudsk P. (2022). An Integrated Weed Management framework: A pan-European perspective. Eur. J. Agron. 133, 126443. doi: 10.1016/j.eja.2021.126443
Scavo A., Pandino G., Restuccia A., Caruso P., Lombardo S., Mauromicale G. (2022). Allelopathy in durum wheat landraces as affected by genotype and plant part. Plants 11, 1021. doi: 10.3390/plants11081021
Scavo A., Pandino G., Restuccia A., Mauromicale G. (2020). Leaf extracts of cultivated cardoon as potential bioherbicide. Sci. Hortic. 261, 109024. doi: 10.1016/j.scienta.2019.109024
Scavo A., Restuccia A., Pandino G., Onofri A., Mauromicale G. (2018). Allelopathic effects of Cynara cardunculus L. leaf aqueous extracts on seed germination of some mediterranean weed species. Ital. J. Agron. 13, 119–125. doi: 10.4081/ija.2018.1021
Scavo A., Rial C., Molinillo J. M. G., Varela R. M., Mauromicale G., Macias F. A. (2019). The extraction procedure improves the allelopathic activity of cardoon (Cynara cardunculus var. altilis) leaf allelochemicals. Ind Crops Prod 128, 479–487. doi: 10.1016/j.indcrop.2018.11.053
Stavropoulou M. I., Angelis A., Aligiannis N., Kalpoutzakis E., Mitakou S., Duke S. O., et al. (2017). Phytotoxic triterpene saponins from Bellis longifolia, an endemic plant of Crete. Phytochemistry 144, 71–77. doi: 10.1016/j.phytochem.2017.08.019
Torres-Pagán N., Muñoz M., Barbero S., Mamone R., Peiró R., Carrubba A., et al. (2024). Herbicidal Potential of the Natural Compounds Carvacrol, Thymol, Eugenol, p-Cymene, Citral and Pelargonic Acid in Field Conditions: Indications for Better Performance. Agronomy 14, 537. doi: 10.3390/agronomy14030537
Tudi M., Ruan H. D., Wang L., Lyu J., Sadler R., Connell D., et al. (2021). Agriculture development, pesticide application and its impact on the environment. Int. J. Environ. Res. Public Health 18, 1–24. doi: 10.3390/ijerph18031112
Vitalini S., Iriti M., Vaglia V., Garzoli S. (2022). Chemical Investigation and Dose-Response Phytotoxic Effect of Essential Oils from Two Gymnosperm Species (Juniperus communis var. saxatilis Pall. and Larix decidua Mill.). Plants 11, 1510. doi: 10.3390/plants11111510
Vitalini S., Orlando F., Palmioli A., Alali S., Airoldi C., De Noni I., et al. (2020). Different phytotoxic effect of Lolium multiflorum Lam. leaves against Echinochloa oryzoides (Ard.) Fritsch and Oriza sativa L. Environ. Sci. Pollut. Res. 27, 33204–33214. doi: 10.1007/s11356-020-09573-8
Vitalini S., Palmioli A., Orlando F., Scarì G., Airoldi C., De Noni I., et al. (2021). Phytotoxicity, nematicidal activity and chemical constituents of Peucedanum ostruthium (L.) W.D.J.Koch (Apiaceae). Ind Crops Prod.Interestingly, 166, 113499. doi: 10.1016/j.indcrop.2021.113499
Waller G. R., Jurzysta M., Thorne R. L. Z. (1995). Root saponins from alfalfa (Medicago sativa L.) and their allelopathic activity on weeds and wheat. Allelopathy J. 2, 21–30.
Waller G. R., Yang C. F., Chen L. F., Su C. H., Liou R. M., Wu S. C., et al. (1999). Saponins produced during the life cycles of mungbeans and their role as allelochemicals. Stud. Plant Sci. 6, 105–130. doi: 10.1016/S0928-3420(99)80015-9
Keywords: phytotoxicity, allelopathy, invasive species, saponins, phenolics, natural herbicides, botanicals
Citation: Trespidi G, Xotta V, Sut S, Otto S, Nikolić N, Strati E, Vitalini S, Dall’Acqua S, Iriti M, Masin R and Loddo D (2025) Phytotoxic activity and chemical characterization of Baccharis halimifolia L. (Asteraceae) aqueous extracts. Front. Agron. 7:1542124. doi: 10.3389/fagro.2025.1542124
Received: 09 December 2024; Accepted: 15 April 2025;
Published: 14 May 2025.
Edited by:
Simerjeet Virk, Auburn University, United StatesReviewed by:
Aurelio Scavo, University of Messina, ItalyArnold Mashingaidze, Chinhoyi University of Technology, Zimbabwe
Copyright © 2025 Trespidi, Xotta, Sut, Otto, Nikolić, Strati, Vitalini, Dall’Acqua, Iriti, Masin and Loddo. This is an open-access article distributed under the terms of the Creative Commons Attribution License (CC BY). The use, distribution or reproduction in other forums is permitted, provided the original author(s) and the copyright owner(s) are credited and that the original publication in this journal is cited, in accordance with accepted academic practice. No use, distribution or reproduction is permitted which does not comply with these terms.
*Correspondence: Giacomo Trespidi, Z2lhY29tby50cmVzcGlkaUBnbWFpbC5jb20=