- 1Department of Veterinary Sciences, University of Messina, Messina, Italy
- 2Soil and Crop Science Department, Texas A&M AgriLife Extension, Lubbock, TX, United States
- 3Legume and Rhizobium Sciences, Murdoch University, Murdoch, WA, Australia
- 4Consorzio di Ricerca Filiera Carni, University of Messina, Messina, Italy
Forage legumes play a fundamental role in the sustainability of cropping systems, as rotating species with grain crops, intercrops, or winter cover crops. However, their compatibility with rhizobial inoculants needs context-specific studies. The objectives were to evaluate the effectiveness of three species-specific inoculants [Australian granular (AUG), Australian peat (AUP), and American peat (USP)], compared with a non-inoculated control (CNT). These were applied at the recommended and double dose on five Mediterranean forage legumes (Vicia sativa, Medicago polymorpha, Trifolium michelianum, T. subterraneum, and T. pratense). Plant growth, nodulation, and relative N2 fixation were measured. Species-specific variations were observed for each inoculant. Across the average of legume species, AUG demonstrated the highest growth- and nodulation-promoting effects at both standard and double inoculum doses. The USP was the worst inoculant at the standard dose but induced positive effects at double dose. The relative N2 fixation was only improved at double dose, especially by USP and AUG, whereas only AUP provided significant N2 fixation enhancements at standard dose. Overall, the double dose was the best strategy for all tested forage legumes. These findings suggest that inoculating Mediterranean forage legumes with selected inoculants, especially at double dose, may be an effective solution to increase their N2 fixation ability, reduce the use of mineral N fertilizers, and identify the optimal forage legume × inoculant combinations for intercropping systems with cereals.
1 Introduction
Agroecosystems are facing a significant decline in soil fertility due to the intensification of agricultural practices and climate change. This is especially true in semi-arid regions where erratic rainfall, frequent soil erosion events, increased salinity, and severe weed pressure are characteristic features (Abdelhak, 2022). Under these conditions of low soil organic matter, nitrogen (N) is generally the most limiting soil macronutrient. It is estimated applied N for plant growth is effectively incorporated into agricultural products at a rate of 40%–50%. While the remaining is subjected to losses by nitrate leaching (NO3), ammonia volatilization (NH3), and nitrous oxide (N2O) emissions, causing environmental burdens (Mahmud et al., 2021). The environmental impact, coupled with increasing costs of mineral N fertilizers, demands the scouting for alternative sources of N (Allito et al., 2020).
The symbiotic biological N fixation (BNF) by N-fixing bacteria, especially those of the family Rhizobiaceae, is a sustainable and alternative source of available N. The bacteria allow reduced N mineral fertilizer application while maintaining high crop yields, in agreement with the European Green Deal and the United Nations (UN) Sustainable Development Goals (United Nation (UN), 2015; European Commission (EC), 2019). Effective root nodule symbiosis not only allows legumes to grow in N-poor soils but also increases the soil N levels for the subsequent rotational cash crops or for companion crops in polycultural systems (Drevon et al., 2015; Schwember et al., 2019; Scordia et al., 2024). However, BNF is species specific, and its efficiency depends on legume genotype and bacterial strain (Allito et al., 2021; Kohlmeier et al., 2023). Soil bacteria associated with legumes for BNF are commonly termed rhizobia. The family Rhizobiaceae includes at least 168 species in 17 genera, of which those with the highest number of described species are Rhizobium, Bradyrhizobium, Mesorhizobium, and Sinorhizobium (Kuzmanović et al., 2022). Rhizobial strains are supplied through legume inoculants, crop species-specific products containing isolates of live rhizobia protected by organic carrier material (Lupwayi et al., 2000). Although large-scale production of legume inoculants is complex, the legume inoculant industry is now well established (O'Callaghan et al., 2022), with several different commercial inoculant formulations such as peat, granular, liquid, and freeze-dried powders (Howieson and Dilworth, 2016). Inoculant quality depends on the cell numbers of a selected rhizobial strain, an easy-to-apply and effective formulation, an adequate shelf life, and the dose of application (Lupwayi et al., 2000). Nonetheless, it has been demonstrated that applying inoculants at higher than recommended rates could be beneficial, especially where forage legumes are grown in adverse soil conditions such as low pH (Farquharson et al., 2022; Frame and Laidlaw, 2005).
Forage legumes are a key component for the sustainability of pastures and are key to livestock production, BNF, soil organic matter levels, and soil erosion mitigation (Sheaffer and Seguin, 2003). They are commonly cultivated in rotation with grain crops, but in recent years, they also emerged as intercrops or winter cover crops (Holman et al., 2018; Vujić et al., 2021; Scordia et al., 2024). Carlsson and Huss-Danell (2003), who investigated the N2 fixation in three perennial forage legumes primarily relating to ungrazed northern temperate/boreal areas, reported BNF rates up to 545 kg N ha−1 year−1 in white clover (Trifolium repens), 350 kg N ha−1 year−1 in alfalfa (Medicago sativa L.), and 373 kg N ha−1 year−1 in red clover (T. pratense). In Australia, it is estimated forage legumes fix 3.5 million tonnes of N annually on about 45 million hectares, equivalent to a national value of up to Aus$ 3.5 billion annually (Farquharson et al., 2022). Forage legumes are a traditional component of Mediterranean grassland communities such as Syria, Greece, Sardinia, Sicily, Morocco, and Tunisia, coevolving over the last 1,000 years with native rhizobial populations (Howieson et al., 2000). Unfortunately, the high soil temperatures of arid and semi-arid Mediterranean regions, coupled with severe salinity levels and desertification processes that are increasing under the climate change effects, may affect negatively forage legume-rhizobia associations (Rejili et al., 2012). Furthermore, most farmers of legumes in the Mediterranean region and elsewhere assume that their fields nodulate and fix nitrogen as they have not been adequately trained; thus, they have never examined the roots for active nodulation. This, too, presents a risk to productive farming if legumes are undernodulated. Hence, there is a need for legume inoculation with elite rhizobia to provide and to ensure optimal BNF, which in turn is required to offset increasing agronomic challenges. These include farming practices such as monoculture plantings, low-frequency (over 5 years) legume rotational break crops, soil acidification, detrimental residual soil herbicides, lack of certainty in field nodulation, and uncertain climatic conditions (Yates et al., 2024). However, the compatibility of new forage legume species or cultivars to agriculture with commercial rhizobial inoculants requires ongoing research support to optimise the symbiosis (Rigg et al., 2021; Shi et al., 2023).
In this preliminary work, considering the increasing diffusion of cereal-legume double cropping, five forage legumes common to Mediterranean regions (Vicia sativa L., M. polymorpha, Trifolium michelianum Savi, T. subterraneum, and T. pratense) were screened in pot bioassays under edaphic and climatic uncontrolled conditions to select the optimal inoculant formulation and dose of application for each legume-rhizobial strain combination for testing in future intercropping systems. These forage legumes exhibit different rhizobial associations and are highly specific to the micro-symbiont they nodulate to achieve an effective symbiosis (Kohlmeier et al., 2025). For instance, V. sativa forms a symbiosis with the micro-symbiont Rhizobium leguminosarum bv. viciae, although differences in host plant preference for specific rhizobial genotypes within natural populations have been observed (Laguerre et al., 2003). Clovers and medics are nodulated and can form an effective symbiosis with R. leguminosarum biovar trifolii and Sinorhizobium spp., respectively (Charman and Ballard, 2004; Farquharson et al., 2022). Our goals were (1) to evaluate the effect of inoculant formulations and rhizobial strains on plant growth, nodulation, and N2 fixation of selected forage legumes, (2) to assess whether increasing the inoculant rate could further improve the dependent variables, and (3) whether the phenological growth stage could affect the inoculation efficiency.
2 Materials and methods
2.1 Experimental design
Two different pot trials under natural conditions, hereafter referred to as Experiments A and B, were set up in 2022/2023 in a private farm located in Milazzo (Messina, 38°11’25’’ N, 15°14’28’’ E) according to a complete randomized block design with three replications. In Experiment A, four forage legumes were inoculated with three species-specific different inoculants (an Australian granular, AUG; an Australian peat, AUP; an American peat from Visjon Exceed®, USP), versus a non-inoculated control (CNT). Inoculants were seed applied at the recommended application method and dose at sowing, and crops were harvested at the vegetative growth stage (just prior to reproductive growth). In Experiment B, five forage legumes were inoculated with the same Experiment A inoculants but at double dose, and crops were harvested in the reproductive growth stage to respectively assess the second and third research objectives.
2.2 Plant material
The forage legumes employed in Experiment A were Vicia sativa L. var. Buza, M. polymorpha var. Scimitar, Trifolium michelianum Savi (local ecotype), and T. subterraneum var. Urana. In Experiment B, T. pratense var. Rozeta was added. M. polymorpha, T. subterraneum, and T. michelianum, annual self-seeding species with autumn-winter-spring cycle (Charman and Ballard, 2004; Scavo et al., 2021), were purchased from Padana sementi (Tombolo, Padua, Italy). V. sativa and T. pratense were two local ecotypes. Although the legume species investigated in the present study differ in growth/biomass production potentials, growth habits, and life cycle length due to inherent genetic differences, they are adapted to semiarid environments and were selected based on predominant winter growth in Mediterranean climates (Blackwell et al., 2018; Enriquez-Hidalgo et al., 2020; Scavo et al., 2021).
2.3 Pot trials
Experiments were conducted in dark plastic pots (diameter 20 cm; height 20 cm; volume 5 L) filled with a substrate composed of 3 cm of stones at the base to prevent soil saturation, soil from the transition zone between natural pastures and forest in Messina mountains, and peat (Pindstrup Mosebrug A/S, Denmark). The substrate components are described in Table 1. Ten seeds pot−1 for each species were sown at 1 cm depth and then thinned to ensure a homogeneous population density of three plants pot−1. Detailed information on sowing, thinning, and harvest dates is shown in Table 2. Starting from sowing, pots were drip irrigated every 2 days for a total of 12,600 ml pot−1 in Experiment A and 9,400 ml pot−1 in Experiment B. No fertilization or pest control treatments were applied.
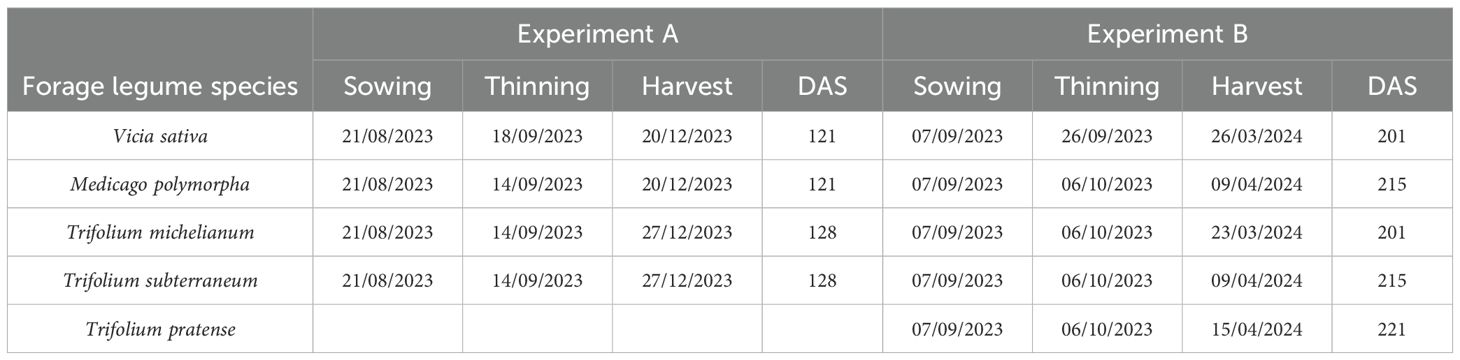
Table 2. Sowing, thinning and harvest dates, and length of the biological cycle expressed as days after sowing (DAS) for each legume species in Experiments A and B.
Inoculation was carried out at sowing on 21 August 2023 for Experiment A and 7 September 2023 for Experiment B following the recommendations reported in the product labels. In detail, AUG and AUP inoculants were kindly provided by Murdoch University (Australia). AUG and AUP specific strains were Rhizobium leguminosarum bv. viciae (Australian Group E – WSM4643) for common vetch, Sinorhizobium spp. (Australian Group AM – WSM1115) for burr medic and Rhizobium leguminosarum bv. trifolii (Australian Group C – WSM1325) for clovers. USP consisted of three species-specific inoculants containing either Rhizobium leguminosarum bv. viciae or bv. trifolii depending on the legume species. Australian inoculants at standard doses (Experiment A) were inoculated as slurry to coat the seed by mixing 1.5 g (for AUP) or 2.5 g (for AUG) of each inoculant strain with 30 ml of water and injecting it into a moist seedbed (25 g of peat substrate with 500 ml of water) at sowing. For double doses (Experiment B), double inoculant rates were applied in the same amount of water as the standard rate. The USP was seed applied by mixing 0.75 g of inoculum on 100 g of seeds for each forage legume previously soaked with 1.1 ml of water. Detailed information about inoculants is summarized in Table 3.
Weather conditions were obtained from a weather station of the SIAS (Servizio Informativo Agrometeorologico Siciliano, www.sias.regione.sicilia.it), close to the experimental site. Total rainfall and reference evapotranspiration (ET0) during the experimental period (August–April) were 469 and 780.2 mm, respectively (Table 4). Except for the November–February period, the remaining months experienced water deficits, especially August (−149 mm) and October (−97 mm). In general, weather conditions were optimal for the growth of forage legumes and supplemented with drip irrigation when required.
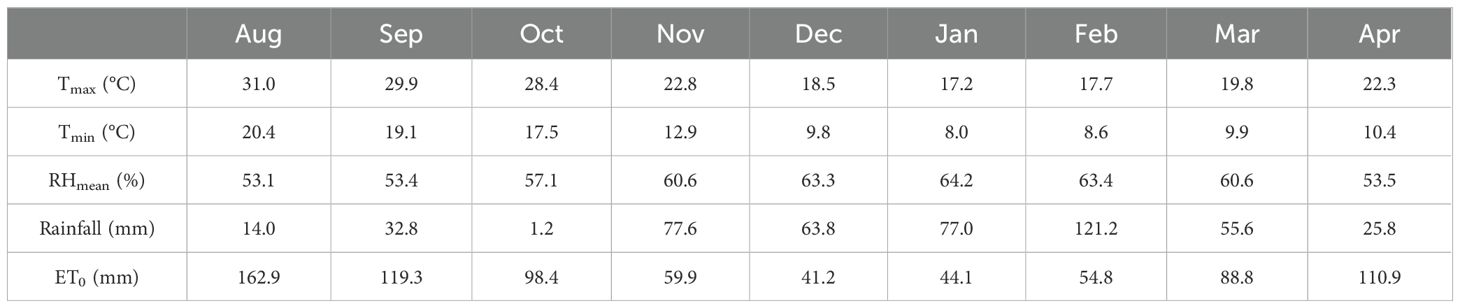
Table 4. Weather conditions [maximum and minimum temperatures, mean relative humidity (RH), rainfall, and reference evapotranspiration (ET0)] at the experimental site (Milazzo, 38°11’25’’ N, 15°14’28’’ E) during the 2023/2024 growing seasons of Experiments A and B.
2.4 Measurements
After harvest (Table 1), soil substrates were removed from pots, the roots were gently washed with tap water, and the aboveground and belowground plant parts were carefully separated. Aboveground and belowground fresh weight, root nodulation (nodule counts), and total nodule fresh weight were measured on the three plants pot–1. Dry matter was determined by oven-drying biomass samples at 65°C up to constant weight.
Aboveground and belowground samples were ground to pass a 1-mm sieve (Cyclotec™ 1093 Sample Mill, Foss, Denmark), and the total nitrogen was determined according to the Kjeldahl method (UDK 169, Velp Scientifica, Italy).
2.5 Estimation of relative N2 fixation
N2 fixation was estimated by the N-difference method, in which the N yields of fixing plants are compared with an uninoculated legume of the same species (control). This is a simple and low-cost method based on the arbitrary assumption that plants absorb the same amount of N from the soil and translocate equal amounts of soil-derived N (Hardarson and Danso, 1993). Here, we adapted the method by considering the uninoculated legumes of the same species as control plants, aware that uninoculated control plants can nodulate due to the presence of native rhizobial communities in the experimental soils. We did not use sterile soil in control pots because it would have changed the soil microbiome and thus the inoculation efficiency, given that they act in synergism with plant-growth-promoting rhizobacteria (Tilak et al., 2006). N yields of aboveground (ANY) and belowground (BNY) plant parts were calculated as:
where %Nshoots and %Nroots are the total nitrogen in above and belowground of legume species, respectively. The amount of relative N2 fixation was quantified in accordance with the equation proposed by Howieson and Dilworth (2016):
where total N yield is the sum of aboveground and belowground N yields. Given that inoculated and non-inoculated plants grew with the same pedo-climatic conditions and management, we assume that the difference in N fixed could be attributable to the inoculation effect.
2.6 Statistical analysis
Data for each experiment were analyzed separately by two-way analysis of variance (ANOVA), according to the complete randomized design. The legume species and the inoculant type (rhizobial strain × inoculant formulation combination) were the fixed effects. Before the ANOVA, the homogeneity of variance was assessed with the Bartlett’s test and normality by a graphical inspection of the residuals. Percentage data were arcsine √% transformed before the analysis. The significance of differences among groups was tested using the Tukey’s HSD test at p ≤ 0.05. All analyses were conducted using CoStat® software version 6.003 (Cohort Software, Monterey, CA, USA).
3 Results
3.1 Experiment A
3.1.1 Plant growth and nodulation
The ANOVA showed that legume species contributed the most to the overall variance for all the investigated parameters (Table 5). The effect of inoculant formulation was significant for root nodulation and total nodule weight, whereas aboveground and belowground biomass were significantly affected only by legume species. Moreover, a significant 'species × inoculant’ effect was found for belowground biomass and root nodulation.

Table 5. Analysis of variance (ANOVA) according to the complete randomized design for main factors and their interactions in Experiment A for aboveground dry weight (ADW), belowground dry weight (BDW), root nodulation (RN), total nodule weight (NW), aboveground nitrogen yield (ANY), belowground nitrogen yield (BNY) and relative N2 fixation.
The effects on aboveground and belowground biomass, which were the highest in T. subterraneum (17.2 and 16.6 g pot−1, respectively) and T. michelianum (13.0 and 15.4 g pot−1), were species specific (Figure 1). In fact, all inoculants investigated decreased belowground biomass of T. subterraneum and T. michelianum compared with the control (CNT), while an opposite trend was observed for V. sativa. In M. polymorpha, except for USP that reduced belowground biomass, AUG and AUP showed not significant differences compared to CNT.
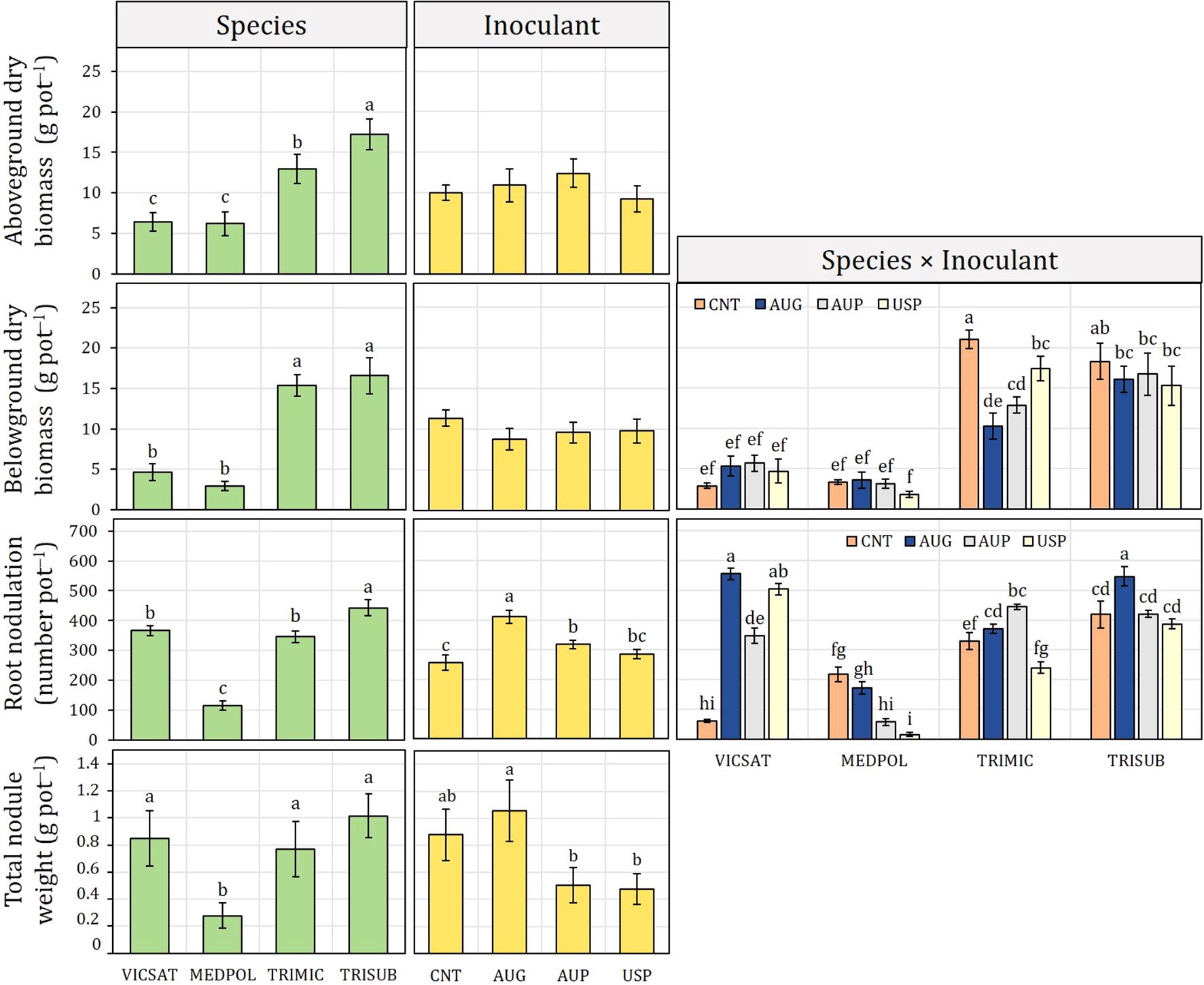
Figure 1. Inoculant formulation effect (non-inoculated control, CNT; Australian granular, AUG; Australian peat, AUP; American peat from Visjon Exceed®, USP) on aboveground and belowground dry biomass, root nodulation, and total nodule fresh weight of Vicia sativa (VICSAT), Medicago polymorpha (MEDPOL), Trifolium michelianum (TRIMIC), and Trifolium subterraneum (TRISUB) from Experiment A. Bars are standard error. Different letters indicate statistical significance at p ≤ 0.05 (Tukey’s HSD test).
Root nodulation was significantly improved by AUG (+60% than control), followed by AUP (+23.6%) and USP (+11.5%) (Figure 1). The greatest root nodulation was found in V. sativa and T. subterraneum inoculated with AUG (555 and 547 number pot−1, respectively). AUP induced the highest root nodulation in T. michelianum (445 number pot−1), while no positive effect was observed in M. polymorpha.
Total nodule fresh weight showed a similar trend to root nodulation (Figure 1). AUG determined the highest total nodule weight (1.1 g pot−1), while both AUP and USP caused lower values than CNT. Across the average of inoculants, no significant differences were observed between V. sativa, T. michelianum, and T. subterraneum, while M. polymorpha had the lowest total nodule weight (0.3 g pot−1).
3.1.2 Relative N2 fixation
From the ANOVA emerged that the “species × inoculant” interaction was significant on BNY and N2 fixation (Table 5). ANY, BNY, and relative N2 fixation were mostly affected by legume species, and the inoculant effect was not significant for ANY and BNY.
Across the average of inoculants, T. subterraneum and T. michelianum showed the highest ANY (43.5 and 33.0 mg N pot−1, respectively) and BNY (24.9 and 18.2 mg N pot−1), while no significant effects were observed between the inoculants (Figure 2). Concerning BNY, the two-way interaction showed that it was increased by all inoculants in V. sativa and decreased in T. michelianum, although without significant differences. No significant differences among the inoculants were also detected in T. subterraneum. USP was the worst inoculant for all forage legumes.
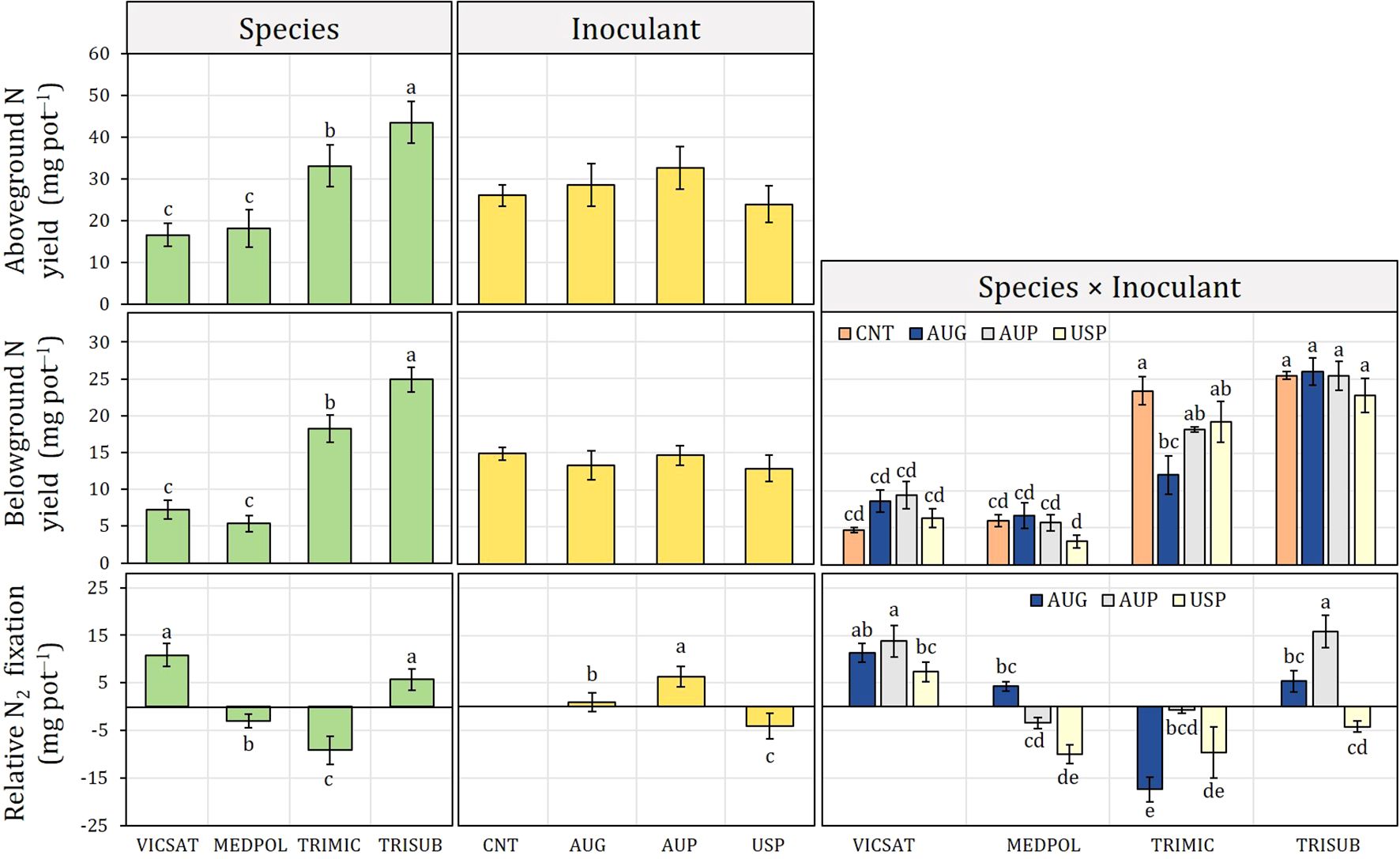
Figure 2. Inoculant formulation effect (non-inoculated control: CNT; Australian granular: AUG; Australian peat, AUP; American peat from Visjon Exceed®, USP) on aboveground N yield, belowground N yield and relative N2 fixation of Vicia sativa (VICSAT), Medicago polymorpha (MEDPOL), Trifolium michelianum (TRIMIC), and Trifolium subterraneum (TRISUB) from Experiment A. Bars are standard error. Different letters indicate statistical significance at p ≤ 0.05 (Tukey’s HSD test).
Across the average of species, AUP induced the greatest relative N2 fixation (6.4 mg N pot−1), followed by AUG (0.8 mg N pot−1), whereas USP fixed less than CNT (Figure 2). V. sativa was the best fixing species among the forage legumes investigated (10.8 mg N pot−1), followed by T. subterraneum (5.7 mg N pot−1), while M. polymorpha and T. michelianum fixed less than CNT with the investigated inoculants at the standard application rate. In detail, the inoculants improved the relative N2 fixation in V. sativa and T. subterraneum, excluding USP for the latter species, with AUP that determined the highest values for both species (13.7 and 15.7 mg N pot−1, respectively). Only AUG induced relative N2 fixation in M. polymorpha (4.3 mg N pot−1), while none of the inoculants was effective in T. michelianum.
3.2 Experiment B
3.2.1 Plant growth and nodulation
Except for the aboveground biomass, the ANOVA highlighted a significant “species × inoculant” effect for all the investigated parameters (Table 6). Legume species were confirmed as the major factor affecting variance.
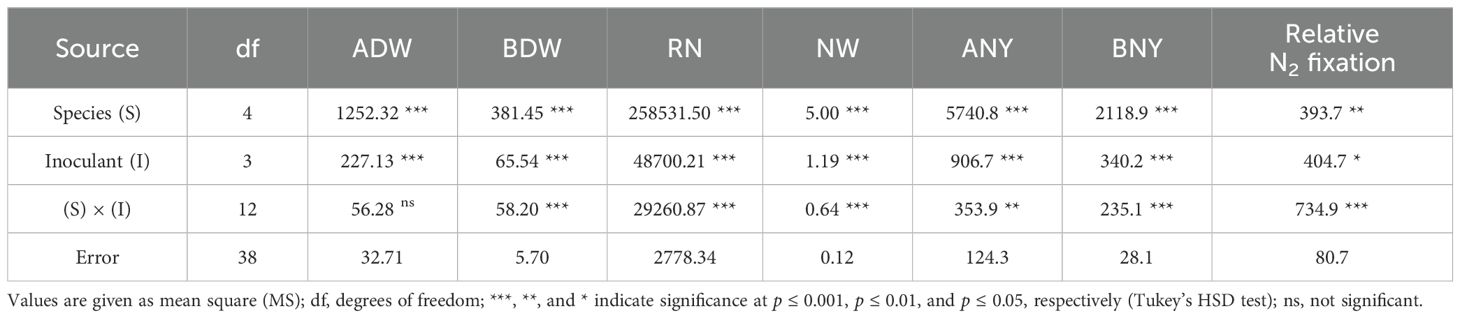
Table 6. Analysis of variance (ANOVA) according to the complete randomized design for main factors and their interactions in Experiment B for aboveground dry weight (ADW), belowground dry weight (BDW), root nodulation (RN), total nodule weight (NW), aboveground nitrogen yield (ANY), belowground nitrogen yield (BNY), and relative N2 fixation.
Across the average of species, USP had the highest stimulating effect on aboveground biomass (23.3 g pot−1, +63.8% than CNT), followed by AUG (19.5 g pot−1) (Figure 3). Concerning belowground biomass, no significant differences were detected between inoculants and the control for V. sativa. The highest belowground biomass for M. polymorpha was induced by USP (14.5 g pot−1), for T. michelianum and T. pratense by AUG (18.2 and 20.6 g pot−1, respectively), and for T. subterraneum by AUP (30.1 g pot−1). All inoculants increased the belowground biomass versus the control, with no significant differences among inoculant types. Across the average of inoculants, T. subterraneum had the highest belowground biomass (20 g pot−1), followed by T. pratense (15.9 g pot−1), and V. sativa the lowest (5.1 g pot−1).
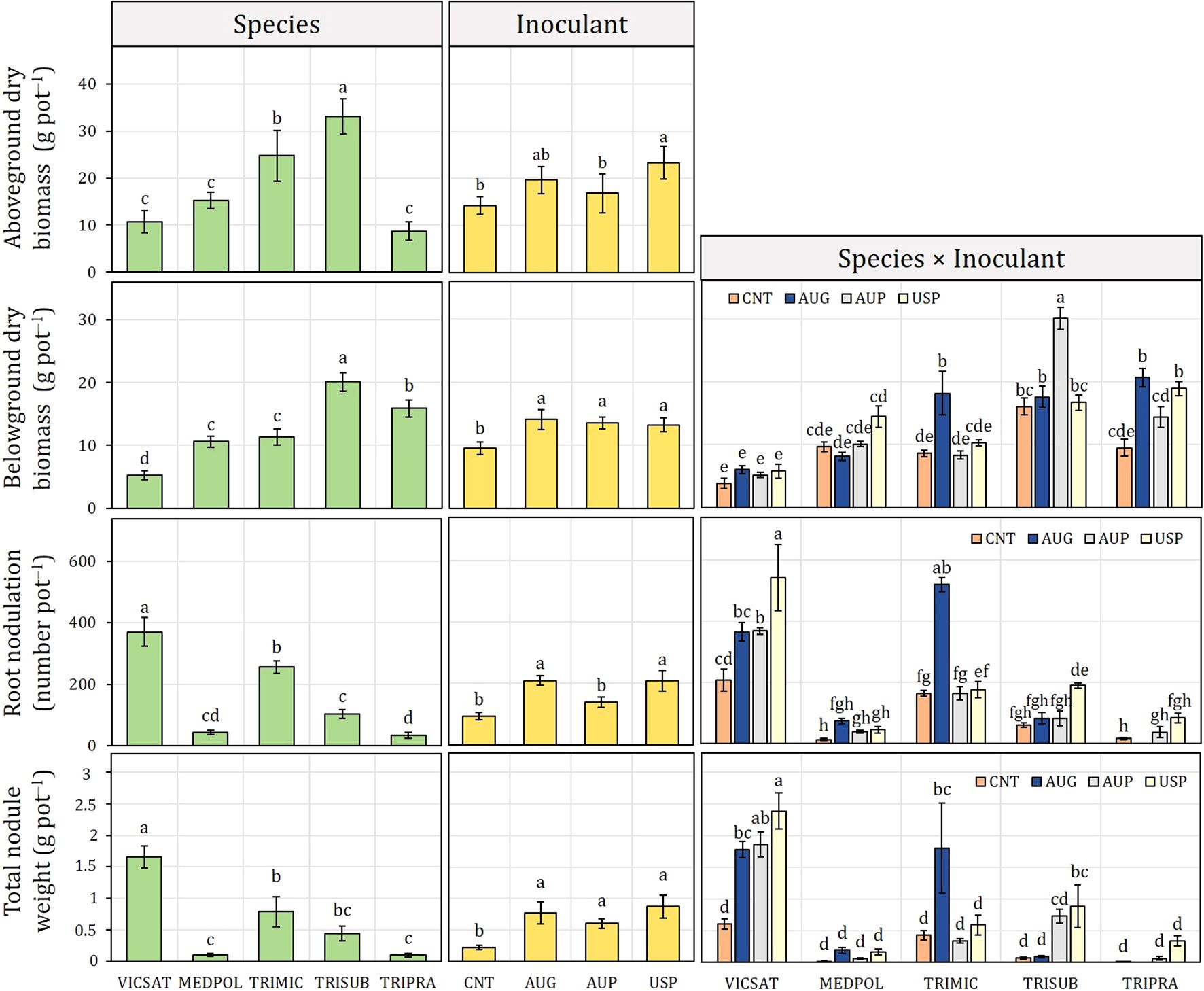
Figure 3. Inoculant formulation effect (non-inoculated control, CNT; Australian granular, AUG; Australian peat, AUP; American peat from Visjon Exceed®, USP) on aboveground and belowground dry biomass, root nodulation, and total nodule fresh weight of Vicia sativa (VICSAT), Medicago polymorpha (MEDPOL), Trifolium michelianum (TRIMIC), Trifolium subterraneum (TRISUB) and Trifolium pratense (TRIPRA) from Experiment B. Bars are standard error. Different letters indicate statistical significance at p ≤ 0.05 (Tukey’s HSD test).
Both root nodulation and total nodule weight were highly affected by the “species × inoculant” interaction (Table 6). USP induced the highest values in V. sativa (543 number pot−1 and 2.4 g pot−1), T. subterraneum (190 number pot−1 and 0.3 g pot−1) and T. pratense (84 number pot−1 and 0.1 g pot−1), while AUG in M. polymorpha (75 number pot−1 and 0.2 g pot−1) and T. michelianum (520 number pot−1 and 1.8 g pot−1) (Figure 3). Interestingly, AUG inhibited root nodulation on T. pratense. V. sativa had the highest root nodulation and total nodule weight, followed by T. michelianum. Regardless of legume species, all inoculant formulations increased both parameters versus the control.
3.2.2 Relative N2 fixation
The “species × inoculant” interaction was significant for ANY (p ≤ 0.01), BNY (p ≤ 0.001), and relative N2 fixation (p ≤ 0.001) (Table 6). Legume species had the greatest influence on variance for ANY and BNY, while N2 fixation was more affected by inoculant formulation.
ANY and BNY showed the same trend, respectively, observed for aboveground and belowground biomass (Figure 4). Concerning ANY, the highest value was found in T. subterraneum (73.9 mg N pot−1) and T. michelianum (47.9 mg N pot−1) for legume species, and in USP (48.3 mg N pot−1, +58.7% than CT) and AUG (43.3 mg N pot−1, +42.4% than CT) for inoculant formulations. In particular, USP induced the highest ANY for T. subterraneum (96.8 mg N pot−1), M. polymorpha (56.6 mg N pot−1), and T. pratense (23.9 mg N pot−1), whereas AUG was the best inoculant for V. sativa (30.7 mg N pot−1) and T. michelianum (59.2 mg N pot−1). Concerning BNY, T. subterraneum and T. pratense showed the greatest values (44.2 and 38.2 mg N pot−1, respectively), while V. sativa confirmed as the poorest legume species (11.1 mg N pot−1). Across the average of species, no significant differences were found between inoculant formulations, as observed for belowground biomass, all of which have increased BNY compared to CNT. In accordance with the belowground biomass trend, AUG determined the highest BNY in T. michelianum (38.4 mg N pot−1) and T. pratense (50.3 mg N pot−1), USP in M. polymorpha (31.4 mg N pot−1), and AUP in T. subterraneum (62.4 mg N pot−1), whereas no significant differences were observed in V. sativa.
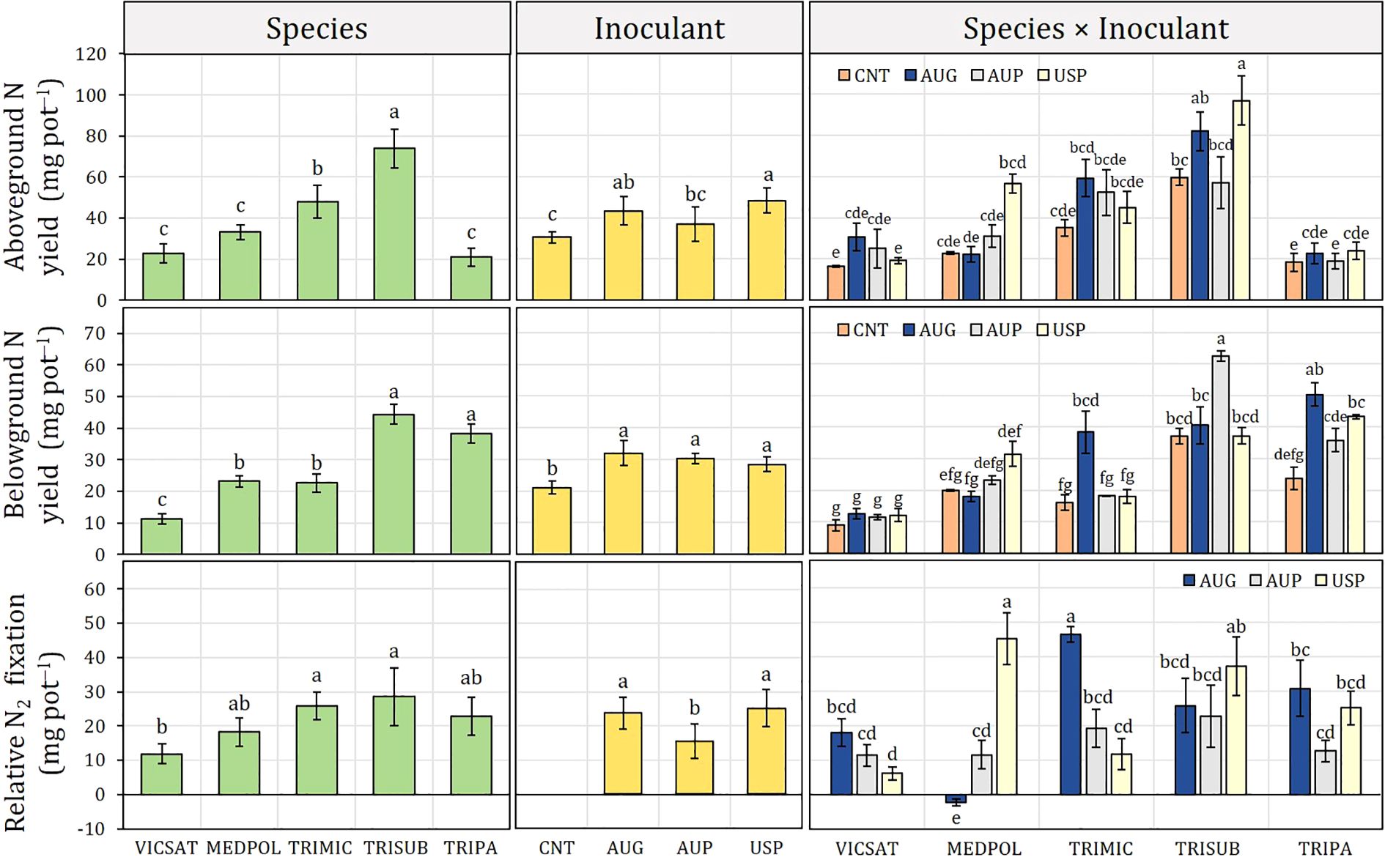
Figure 4. Inoculant formulation effect (non-inoculated control, CNT; Australian granular, AUG; Australian peat, AUP; American peat from Visjon Exceed®, USP) on aboveground N yield, belowground N yield and N2 fixation of Vicia sativa (VICSAT), Medicago polymorpha (MEDPOL), Trifolium michelianum (TRIMIC), Trifolium subterraneum (TRISUB), and Trifolium pratense (TRIPRA) from Experiment B. Bars are standard error. Different letters indicate statistical significance at p ≤ 0.05 (Tukey’s HSD test).
Across the average of inoculant formulations, T. subterraneum and T. michelianum showed the highest relative N2 fixation rates (28.6 and 25.7 mg N pot−1, respectively) and V. sativa the lowest one (11.8 mg N pot−1) (Figure 4). On the average of legume species, the relative N2 fixation of AUG and USP inoculants was similar and higher than AUP. From the “species × inoculant” interaction emerged that AUG in V. sativa, T. michelianum, and T. pratense fixed N2 at levels greater than the other inoculants (18.0, 46.5, and 30.7 mg N pot−1), but performed worse than CNT in M. polymorpha (−2.3 mg N pot−1). At the same time, M. polymorpha and T. subterraneum inoculated with USP had significantly higher relative fixed N2 than the other inoculants, with fixation rates of 45.4 and 37.2 mg N pot−1, respectively.
4 Discussion
Positive effects of rhizobia inoculants on forage legume growth and nodulation have been previously reported (Carlsson and Huss-Danell, 2003; Shockley et al., 2004), including common vetch (Albayrak et al., 2006) and annual medics (Materon, 1988). The enhancement of plant growth induced by rhizobial inoculation could be attributed to the direct fixed N provided by rhizobia strains, the increase in plant nutrient uptake, and the production of plant growth-promoting hormones such as indole-3-acetic acid (Yadav and Verma, 2014; Allito et al., 2021). Although background rhizobial populations are common in Mediterranean regions due to the long history of forage legume cultivation, their N-fixing effectiveness varies widely (Rejili et al., 2012). Two main strategies can be pursued to enhance forage legumes N-fixation: inoculating with commercial elite strains or inoculating with selected native rhizobia. This research focused on the former strategy, given that most of these strains, such as the Australians, were selected for high effectiveness, desiccation tolerance, and persistence in harsh conditions, which are in part exacerbated by climate change and can adversely impact legume-rhizobia associations.
In the present study, inoculation with elite species-specific rhizobia strains and different inoculant formulations significantly improved plant growth and nodulation of the forage legumes investigated only in Experiment B.
The native rhizobial populations likely present in the substrate may have been sufficient in Experiment A, as evidenced by nodulation in the control plants. However, assuming that native rhizobia are always effective is misguided. Some fields lack a history of specific legumes, and certain rhizobial strains can form nodules without efficiently fixing N, as indicated by nodules that lack pink or red coloration, signifying low leghemoglobin content. From a practical perspective, farmers often assume adequate nodulation without examining root nodules, risking undernodulation and reduced N-fixation. Insufficient inoculation, combined with challenges such as monoculture, limited crop rotation, soil acidification, residual herbicides, and unpredictable climates, can significantly reduce legume productivity and N-fixation (Yates et al., 2024).
Only root nodulation and total nodule weight were affected in Experiment A, in which the AUG and the American peat inoculant (USP) showed, respectively, the best and the worst promoting effect among the inoculants. The optimal performance of AUG may have a dual explanation. First, higher efficacy of granular inoculants compared to liquid or peat-based powder inoculation treatments has been demonstrated by Kyei-Boahen et al. (2002) for chickpea, Rice and Olsen (1992) for alfalfa, and Ocumpaugh (1991) for arrowleaf clover, especially under unfavourable conditions for rhizobia survival. Furthermore, the long transport distance from Australia and the United States could have compromised the quality of peat inoculants, whereas granules could have maintained stable cell numbers (1 × 109 CFU g−1). Second, native rhizobia strains may exert better performances than non-native commercial inoculants. Batista et al. (2015), for example, indicated that red clovers inoculated with the native isolate of R. leguminosarum bv. trifolii (strain 317) in Uruguay grasslands produced more biomass than those inoculated with the commercial strain U204 thanks to the increased nodulation competitiveness of indigenous isolate 317 than U204. A similar finding was reported by Roughley et al. (1976) for subterranean clover across five sites in New South Wales (Australia). However, the symbiotic performance of naturalised soil rhizobia is not always constant and can be compromised if diverse rhizobia populations have naturalised in soils, as demonstrated by Drew et al. (2011) for several annual clover species of Mediterranean origin.
Rhizobial inoculation was ineffective in its nodulating and growth promotion effects of M. polymorpha, in agreement with Charman and Ballard (2004), who concluded that none of the 222 screened lines of burr medic formed effective symbioses with a wide range of soil rhizobia. The reasons for poor nodulation are numerous and can be attributed to the high specificity of burr medic rhizobial strains, the inoculation of inappropriate rhizobia strains, the presence of competing indigenous rhizobia in the commercial soil substrate, or the decline of viable rhizobia contained in the inoculant. About the former aspect, it is known that rhizobia strains differ in nodulation effectiveness. Hence, rhizobiologists are continuing to search rhizobia strains for improved N fixation and subsequent forage quality (Yates et al., 2021). More specifically, for an efficient translation to the field of the inoculant-strain selection, it should be considered the overall symbiont genotype × host genotype × environment interaction (Bellabarba et al., 2023). In this regard, selecting legumes with inherent diversity in growth potential, biomass production, growth habits, and life cycle length offers a range of options to fit specific management goals, such as forage production, soil cover, or improved BNF.
In Experiment B, a greater influence of the “species × inoculant” interaction than Experiment A was observed, denoting a higher species- and inoculant-dependent effect. Overall, the trend was similar to Experiment A, but the effects were more pronounced, probably by virtue of the double dosage of inoculant application on the one hand, and the different phenological growth stage on the other hand. Doubling the rate of application of inoculants is often reported as a good strategy to improve the nodulation effectiveness (Jakhar et al., 2018; Jesus et al., 2018). This improvement is generally suggested under poor soils with no legume history and soil pH ≥ 5.5 or where host plants are stressed (Farquharson et al., 2022). Increasing the application rates of peat inoculants on seeds enhances, in turn, the likelihood of sufficient rhizobia survival until plant germination. Moreover, it is known that the effects of rhizobial inoculation are exacerbated at flowering. Our results are consistent with Lamptey et al. (2014), who found that inoculated soybean plants harvested at flowering recorded higher fresh and dry shoot matter versus plants harvested at the vegetative stage. Hossain and Solaiman (2004) reported a similar finding for mungbean varieties.
Overall, AUG and USP showed the best growth-promoting effects and N fixation rates in Experiment B, with species-specific results. According to Zdor and Pueppke (1990), peat formulation may help protect rhizobial strains from adverse environmental effects versus liquid carriers. Moreover, granular inoculants, which contain less moisture, could offer even greater protection. Therefore, although all inoculants under investigation were applied directly to the seeds, granular and peat inoculants could have increased the strain survival. The worse results provided by AUP compared to USP may be related to the use of native rhizobia strains.
Interestingly, AUG inhibited T. pratense root nodulation. We suppose this negative effect may be attributed to the not-appropriate choice of rhizobial strain. In this regard, Valverde et al. (2005) isolated and described from T. pratense a novel Trifolium-nodulating species (Phyllobacterium trifolii sp. nov.) that produces nodules on Trifolium spp. and Lupinus spp. Rodríguez-Navarro et al. (2022) selected and characterized Trifolium-nodulating rhizobia for pasture inoculation in Spain, indicating that several Rhizobium species can nodulate Trifolium spp. better than R. leguminosarum bv. trifolii and that some Trifolium species growth could be improved through appropriate rhizobial selection.
In both experiments, aboveground and belowground N yields were respectively consistent with aboveground and belowground dry weights, with significant differences between forage legume species. T. subterraneum showed the highest aboveground and belowground N yields among the investigated forage legumes. Similar N shoot concentrations were reported by Ovalle et al. (2006). To the best of our knowledge, root N content of forage legumes has been poorly investigated. It was estimated to be 15–111 kg N ha−1 for temperate species. Nnadi and Haque (1988) reported root N contents of 2.43% for V. benghalensis, 2.25% for V. dasycarpa, 2.08% for M. truncatula, 2.01% for T. steudneri cv. Shola, 1.23% for M. scutellata, 1.10% for Lablab purpureus cv. Rongai, and 0.87% for L. purpureus cv. Highworth, with positive but not significant interactions between N concentrations in the roots and aerial parts. Most studies estimate N2 fixation only from aboveground plant parts, but according to Danso et al. (1988) up to 60% of the total fixed N of forage legumes may derive from roots. Indeed, the variation in N2 fixation may vary based not only on the method adopted but also on the plant parts analyzed, dry matter yields, and C/N ratio (Carlsson and Huss-Danell, 2003). In general, N2 fixation is positively correlated to dry weights and high C/N ratios (Carlsson and Huss-Danell, 2003; Zhang et al., 2022), which explains why aboveground and belowground N yields did not reflect the N2 fixation in the present work. In addition, considering the significant contribution of belowground N, as demonstrated by the high root:shoot ratios here obtained (data not shown), we consider our estimation of the total N2 fixation more realistic than the sole shoots N2 fixation. Although we did not use sterile soil in the present experiments for BNF estimation, a natural soil with its native microbiota allows for evaluating the effectiveness of the additional inoculum in a more realistic context. Indeed, a sterile soil not only eliminates natural rhizobia but also other beneficial microorganisms, altering microbial interactions and making it difficult interpreting data in relation to real agricultural soil conditions.
5 Conclusions
From this preliminary study, we concluded that inoculation of selected forage legumes (V. sativa, M. polymorpha, T. michelianum, T. subterraneum, and T. pratense) with specific inoculants at double the recommended dose may be an efficient approach to enhance plant growth, nodulation, and N2 fixation. Unfortunately, there is still a low availability of elite commercial strains across the Mediterranean area due to the lack of rhizobia inoculant companies in Europe. The implementation in the Mediterranean agriculture of elite rhizobia strains supported by high-quality research, such as from Australia and the United States, could be a partial solution, avoiding long transport distances that could compromise the quality of inoculants. The cost of increased inoculant use is minimal compared to the potential risk in yield and profit due to unknown nodulation (lack of field knowledge by the farmer), poor nodulation, and N deficiency. Given the ongoing decline in the fertility of Mediterranean soils, this strategy could enhance legume production under restrictive conditions while reducing the need for mineral N fertilizers for subsequent crops. Future research steps will focus on field trials to validate the optimal forage legume × inoculant combinations identified here, particularly in intercropping with cereals, as it represents a promising agroecological practice for Mediterranean cropping systems.
Data availability statement
The raw data supporting the conclusions of this article will be made available by the authors, without undue reservation.
Author contributions
AS: Data curation, Formal analysis, Investigation, Software, Validation, Visualization, Writing – original draft, Writing – review & editing. AM: Data curation, Investigation, Methodology, Writing – review & editing. FC: Data curation, Investigation, Methodology, Writing – review & editing. TM: Data curation, Investigation, Methodology, Writing – review & editing. CT: Conceptualization, Investigation, Methodology, Writing – review & editing. RY: Conceptualization, Investigation, Methodology, Writing – review & editing. ST: Formal analysis, Investigation, Writing – review & editing. CC: Data curation, Investigation, Writing – review & editing. MO: Conceptualization, Funding acquisition, Investigation, Project administration, Resources, Writing – review & editing. MF: Writing – review & editing, Data curation, Formal analysis. AV: Data curation, Writing – review & editing. DS: Methodology, Project administration, Resources, Validation, Writing – review & editing, Conceptualization, Data curation, Formal analysis, Funding acquisition, Investigation. FG: Conceptualization, Data curation, Formal analysis, Funding acquisition, Investigation, Methodology, Project administration, Resources, Supervision, Validation, Writing – review & editing.
Funding
The author(s) declare that financial support was received for the research and/or publication of this article. This work was supported in part by the Italian Ministry of Foreign Affairs and International Cooperation, grant number US23GR18 (RE-FARM project, CUP: J43C23000110001).
Conflict of interest
The authors declare that the research was conducted in the absence of any commercial or financial relationships that could be construed as a potential conflict of interest.
The author(s) declared that they were an editorial board member of Frontiers, at the time of submission. This had no impact on the peer review process and the final decision.
Generative AI statement
The author(s) declare that no Generative AI was used in the creation of this manuscript.
Publisher’s note
All claims expressed in this article are solely those of the authors and do not necessarily represent those of their affiliated organizations, or those of the publisher, the editors and the reviewers. Any product that may be evaluated in this article, or claim that may be made by its manufacturer, is not guaranteed or endorsed by the publisher.
References
Abdelhak M. (2022). “Soil improvement in arid and semiarid regions for sustainable development,” in Natural Resources Conservation and Advances for Sustainability. Eds. Jhariya M. K., Banerjee A. (Elsevier, Amsterdam, The Netherlands), 73–90.
Albayrak S., Sevimay C. S., Çöçü S. (2006). Effect of Rhizobium inoculation on forage and seed yield and yield components of common vetch (Vicia sativa L.) under rainfed conditions. Acta Agric. Scand. - B Soil Plant Sci. 56, 235–240. doi: 10.1080/0906471051003140
Allito B. B., Ewusi-Mensah N., Logah V. (2020). Legume-rhizobium strain specificity enhances nutrition and nitrogen fixation in faba bean (Vicia faba L.). Agronomy 10, 826. doi: 10.3390/agronomy10060826
Allito B. B., Ewusi-Mensah N., Logah V., Hunegnaw D. K. (2021). Legume-rhizobium specificity effect on nodulation, biomass production and partitioning of faba bean (Vicia faba L.). Sci. Rep. 11, 3678. doi: 10.1038/s41598-021-83235-8
Batista L., Irisarri P., Rebuffo M., Cuitiño M. J., Sanjuán J., Monza J. (2015). Nodulation competitiveness as a requisite for improved rhizobial inoculants of Trifolium pratense. Biol. Fertil. Soils 51, 11–20. doi: 10.1007/s00374-014-0946-3
Bellabarba A., Decorosi F., Fagorzi C., El Hadj Mimoune A., Buccioni A., Santoni M., et al. (2023). Salt stress highlights the relevance of genotype × Genotype interaction in the nitrogen-fixing symbiosis between sinorhizobium meliloti and alfalfa. Soil Syst. 7, 112. doi: 10.3390/soilsystems7040112
Blackwell M. S. A., Jarvis S. C., Wilkins R. J. (2018). The importance of sustained grassland and environmental research: A case study from North Wyke Research station, UK 1982–2017. Adv. Agron. 149, 161–235. doi: 10.1016/bs.agron.2018.01.004
Carlsson G., Huss-Danell K. (2003). Nitrogen fixation in perennial forage legumes in the field. Plant Soil 253, 353–372. doi: 10.1023/A:1024847017371
Charman N., Ballard R. A. (2004). Burr medic (Medicago polymorpha L.) selections for improved N2 fixation with naturalised soil rhizobia. Soil Biol. Biochem. 36, 1331–1337. doi: 10.1016/j.soilbio.2004.04.014
Danso S. K. A., Hardarson G., Zapata F. (1988). Dinitrogen fixation estimates in alfalfa-ryegrass swards using different nitrogen-15 labeling methods. Crop Sci. 28, 106–110. doi: 10.2135/cropsci1988.0011183X002800010023x
Drevon J. J., Alkama N., Bargaz A., Rodiño A. P., Sungthongwises K., Zaman-Allah M. (2015). “The Legume–Rhizobia Symbiosis,” in Grain Legumes. Ed. De Ron A. M. (Springer Science + Business Media, New York, USA). doi: 10.1007/978-1-4939-2797-5_9
Drew E. A., Charman N., Dingemanse R., Hall E., Ballard R. A. (2011). Symbiotic performance of Mediterranean Trifolium spp. with naturalised soil rhizobia. Crop Pasture Sci. 62, 903–913. doi: 10.1071/CP11047
Enriquez-Hidalgo D., Cruz T., Teixeira D. L., Steinfort U. (2020). Phenological stages of Mediterranean forage legumes, based on the BBCH scale. Ann. Appl. Biol. 176, 357–368. doi: 10.1111/aab.12578
European Commission (EC) (2019). Communication from the Commission. The European Green Deal. Available online at: https://ec.europa.eu/info/strategy/priorities-2019-2024/european-green-deal_en (Accessed September 5, 2024).
Farquharson E. A., Ballard R. A., Herridge D. F., Ryder M. H., Denton M. D., Webster A., et al. (2022). Inoculating Legumes: Practice and Science (Australia: Grains Research and Development Corporation).
Frame J., Laidlaw A. J. (2005). “Prospects for temperate forage legumes,” in Grasslands: Developments, Opportunities, Perspectives. Eds. Reynolds S. G., Frame J. (Science Publishers, Inc, Enfield, USA), 1–28.
Hardarson G., Danso S. K. A. (1993). Methods for measuring biological nitrogen fixation in grain legumes. Plant Soil 152, 19–23. doi: 10.1007/BF00016330
Holman J. D., Arnet K., Dille J., Maxwell S., Obour A., Roberts T., et al. (2018). Can cover or forage crops replace fallow in the semiarid central Great Plains? Crop Sci. 58, 932–944. doi: 10.2135/cropsci2017.05.0324
Hossain D., Solaiman A. R. M. (2004). Performance of mungbean varieties as affected by Rhizobium inoculants. Bull. Inst. Trop. Agric. 27, 35–43. doi: 10.11189/bita.27.35
Howieson J. G., Dilworth M. J. (2016). Working with Rhizobia (Canberra: Australian Centre for International Agricultural Research).
Howieson J. G., O’Hara G. W., Carr S. J. (2000). Changing roles for legumes in Mediterranean agriculture: developments from an Australian perspective. Field Crops Res. 65, 107–122. doi: 10.1016/S0378-4290(99)00081-7
Jakhar S. R., Kumar V., Mitra N. G. (2018). Effect of seed inoculation with liquid and carrier based Rhizobium cultures and phosphorus levels on rhizobia population and yield of soybean (Glycine max). Ann. Plant Sci. Soil Res. 20, 197–202.
Jesus E. D. C., Leite R. D. A., Bastos R. D. A., Aragão O. O. D. S., Araújo A. P. (2018). Co-inoculation of Bradyrhizobium stimulates the symbiosis efficiency of Rhizobium with common bean. Plant Soil 425, 201–215. doi: 10.1007/s11104-017-3541-1
Kohlmeier M. G., Farquharson E. A., Ballard R. A., O’Hara G. W., Terpolilli J. J. (2023). Complete genome sequence of Rhizobium leguminosarum bv. viciae SRDI969, an acid-tolerant, efficient N2-fixing microsymbiont of Vicia faba. Microbiol. Resour. Announc. 12, e00489–e00423. doi: 10.1128/MRA.00489-23
Kohlmeier M. G., O'Hara G. W., Ramsay J. P., Terpolilli J. J. (2025). Closed genomes of commercial inoculant rhizobia provide a blueprint for management of legume inoculation. Appl. Environ. Microbiol. 91, e02213–e02224. doi: 10.1128/aem.02213-24
Kuzmanović N., Fagorzi C., Mengoni A., Lassalle F., DiCenzo G. C. (2022). Taxonomy of Rhizobiaceae revisited: proposal of a new framework for genus delimitation. Int. J. Syst. Evol. Microbiol. 72, 5243. doi: 10.1099/ijsem.0.005243
Kyei-Boahen S., Slinkard A. E., Walley F. L. (2002). Evaluation of rhizobial inoculation methods for chickpea. Agron. J. 94, 851–859. doi: 10.2134/agronj2002.8510
Laguerre G., Louvrier P., Allard M., Amarger N. (2003). Compatibility of rhizobial genotypes within natural populations of Rhizobium leguminosarum biovar viciae for nodulation of host legumes. Appl. Environ. Microbiol 69, 4. doi: 10.1128/AEM.69.4.2276-2283.2003
Lamptey S., Ahiabor B. D. K., Yeboah S., Osei D. (2014). Effect of Rhizobium inoculants and reproductive growth stages on shoot biomass and yield of soybean (Glycine max (L.) Merril). J. Agric. Sci. 6, 44–54. doi: 10.5539/jas.v6n5p44
Lupwayi N. Z., Olsen P. E., Sande E. S., Keyser H. H., Collins M. M., Singleton P. W., et al. (2000). Inoculant quality and its evaluation. Field Crops Res. 65, 259–270. doi: 10.1016/S0378-4290(99)00091-X
Mahmud K., Panday D., Mergoum A., Missaoui A. (2021). Nitrogen losses and potential mitigation strategies for a sustainable agroecosystem. Sustainability 13, 2400. doi: 10.3390/su13042400
Materon L. A. (1988). “Maximizing Biological Nitrogen Fixation by Forage and Pasture Legumes in Semi-Arid Areas,” in Nitrogen Fixation by Legumes in Mediterranean Agriculture. Developments in Plant and Soil Sciences, vol. 32 . Eds. Beck D. P., Materon L. A. (Springer, Dordrecht), 33–40. doi: 10.1007/978-94-009-1387-5_4
Nnadi L. A., Haque I. (1988). Root nitrogen transformation and mineral composition in selected forage legumes. J. Agric. Sci. 111, 513–518. doi: 10.1017/S0021859600083714
O'Callaghan M., Ballard R. A., Wright D. (2022). Soil microbial inoculants for sustainable agriculture: Limitations and opportunities. Soil Use Manage. 38, 1340–1369. doi: 10.1111/sum.12811
Ocumpaugh W. R. (1991). Granular inoculum enhances establishment and forage production of arrowleaf clover. J. Prod. Agric. 4, 219–224. doi: 10.2134/jpa1991.0219
Ovalle C., Urquiaga S., Pozo A. D., Zagal E., Arredondo S. (2006). Nitrogen fixation in six forage legumes in Mediterranean central Chile. Acta Agric. Scand. 56, 277–293. doi: 10.1080/09064710500310246
Rejili M., Mahdhi M., Fterich A., Dhaoui S., Guefrachi I., Abdeddayem R., et al. (2012). Symbiotic nitrogen fixation of wild legumes in Tunisia: Soil fertility dynamics, field nodulation and nodules effectiveness. Agric. Ecosyst. Environ. 157, 60–69. doi: 10.1016/j.agee.2012.01.015
Rice W. A., Olsen P. E. (1992). Effects of inoculation method and size of Rhizobium meliloti population in the soil on nodulation of alfalfa. Can. J. Soil Sci. 72, 57–67. doi: 10.4141/cjss92-006
Rigg J. L., Webster A. T., Harvey D. M., Orgill S. E., Galea F., Dando A. G., et al. (2021). Cross-host compatibility of commercial rhizobial strains for new and existing pasture legume cultivars in south-eastern Australia. Crop Pasture Sci. 72, 652–665. doi: 10.1071/CP20234
Rodríguez-Navarro D. N., Lorite M. J., Vera F. J. T., Camacho M. (2022). Selection and characterization of Spanish Trifolium-nodulating rhizobia for pasture inoculation. Syst. Appl. Microbiol. 45, 126290. doi: 10.1016/j.syapm.2021.126290
Roughley R. J., Blowes W. M., Hurridge D. F. (1976). Nodulation of Trifolium subterraneum by introduced rhizobia in competition with naturalized strains. Soil Biol. Biochem. 8, 403–407. doi: 10.1016/0038-0717(76)90041-9
Scavo A., Restuccia A., Abbate C., Lombardo S., Fontanazza S., Pandino G., et al. (2021). Trifolium subterraneum cover cropping enhances soil fertility and weed seedbank dynamics in a Mediterranean apricot orchard. Agron. Sustain. Dev. 41, 70. doi: 10.1007/s13593-021-00721-z
Schwember A. R., Schulze J., del Pozo A., Cabeza R. A. (2019). Regulation of symbiotic nitrogen fixation in legume root nodules. Plants 8, 333. doi: 10.3390/plants8090333
Scordia D., Guarnaccia P., Calderone F., Maio A., La Malfa T., Scavo A., et al. (2024). Adoption of cereal–legume double cropping toward more sustainable organic systems in the mediterranean area. Agronomy 14, 772. doi: 10.3390/agronomy14040772
Sheaffer C. C., Seguin P. (2003). Forage legumes for sustainable cropping systems. J. Crop Prod. 8, 187–216. doi: 10.1300/J144v08n01_08
Shi S., Wakelin S., Gerard E., Young S., van Koten C., Caradus J., et al. (2023). Screening and field evaluation of white clover rhizobia for New Zealand pastures. Crop Pasture Sci. 74, 1258–1271. doi: 10.1071/CP22405
Shockley F., McGraw R., Garrett H. (2004). Growth and nutrient concentration of two native forage legumes inoculated with Rhizobium and Mycorrhiza in Missouri, USA. Agrofor. Syst. 60, 137–142. doi: 10.1023/B:AGFO.0000013269.19284.53
Tilak K. V. B. R., Ranganayaki N., Manoharachari C. (2006). Synergistic effects of plant-growth promoting rhizobacteria and Rhizobium on nodulation and nitrogen fixation by pigeonpea (Cajanus cajan). Eur. J. Soil Sci. 57, 67–71. doi: 10.1111/j.1365-2389.2006.00771.x
United Nation (UN) (2015). Transforming our world: the 2030 Agenda for Sustainable Development (New York: United Nations). Available online at: https://www.un.org/sustainabledevelopment/development-agenda/ (Accessed September 5, 2024).
Valverde A., Velázquez E., Fernández-Santos F., Vizcaíno N., Rivas R., Mateos P. F., et al. (2005). Phyllobacterium trifolii sp. nov., nodulating Trifolium and Lupinus in Spanish soils. Int. J. Syst. Evol. Microbiol 55, 1985–1989. doi: 10.1099/ijs.0.63551-0
Vujić S., Krstić D., Mačkić K., Čabilovski R., Radanović Z., Zhan A., et al. (2021). Effect of winter cover crops on water soil storage, total forage production, and quality of silage corn. Eur. J. Agron. 130, 126366. doi: 10.1016/j.eja.2021.126366
Yadav J., Verma J. P. (2014). Effect of seed inoculation with indigenous Rhizobium and plant growth promoting rhizobacteria on nutrients uptake and yields of chickpea (Cicer arietinum L.). Eur. J. Soil Biol. 63, 70–77. doi: 10.1016/j.ejsobi.2014.05.001
Yates R. J., Harrison R. J., Loi A., Steel E. J., Edwards T. J., Nutt B. J., et al. (2021). Sourcing Rhizobium leguminosarum biovar viciae strains from Mediterranean centres of origin to optimize nitrogen fixation in forage legumes grown on acid soils. Grass Forage Sci. 76, 33–43. doi: 10.1111/gfs.12524
Yates R. J., Steel E. J., Edwards T. J., Harrison R. J., Hackney B. F., Howieson J. G. (2024). Adverse consequences of herbicide residues on legumes in dryland agriculture. Field Crops Res. 308, 109271. doi: 10.1016/j.fcr.2024.109271
Zdor R. E., Pueppke S. G. (1990). Competition for nodulation of soybean by Bradyrhizobium japonicum 123 and 138 in soil containing indigenous rhizobia. Soil Biol. Biochem. 22, 607–613. doi: 10.1016/0038–0717(90)90005-K
Keywords: agroecology, Rhizobium, biological nitrogen fixation, plant growth, nodulation, sustainability
Citation: Scavo A, Maio A, Calderone F, La Malfa T, Trostle C, Yates RJ, Toscano S, Cavallo C, Oteri M, Furfaro ME, Virga AN, Scordia D and Gresta F (2025) Enhancing Mediterranean forage legume production through inoculation with elite rhizobia. Front. Agron. 7:1551176. doi: 10.3389/fagro.2025.1551176
Received: 24 December 2024; Accepted: 09 April 2025;
Published: 08 May 2025.
Edited by:
Desouza Blaise, Central Institute for Cotton Research (ICAR), IndiaReviewed by:
Esther Menendez, University of Salamanca, SpainEndalkachew Woldemeskel, World Agroforestry, Ethiopia
Copyright © 2025 Scavo, Maio, Calderone, La Malfa, Trostle, Yates, Toscano, Cavallo, Oteri, Furfaro, Virga, Scordia and Gresta. This is an open-access article distributed under the terms of the Creative Commons Attribution License (CC BY). The use, distribution or reproduction in other forums is permitted, provided the original author(s) and the copyright owner(s) are credited and that the original publication in this journal is cited, in accordance with accepted academic practice. No use, distribution or reproduction is permitted which does not comply with these terms.
*Correspondence: Danilo Scordia, ZGFuaWxvLnNjb3JkaWFAdW5pbWUuaXQ=