- Department of Agriculture, Food and Environment, University of Pisa, Pisa, Italy
The implementation of agroecological practices aims at promoting productivity and reducing environmental impacts due to the excessive use of mineral fertilizers and pesticides. It relies on soil microbiota beneficial activities, such as the efficient use of water and natural soil resources and the provision of important ecosystem services. This review will focus on arbuscular mycorrhizal fungi (AMF) and their role in weed management. AMF are soil beneficial microorganisms establishing mutualistic symbiotic associations with the roots of most food crops and playing key roles in plant growth, nutrition and health. Several plant species are unable to form functional mycorrhizal symbioses (nonhost plants), lacking “symbiotic-specific” genes, as shown by genomic, transcriptomic and phylogenomic analyses. The majority of nonhost plants belong to families encompassing some of the world’s worst agricultural weed species, such as Chenopodium album, Raphanus raphanistrum, Rapistrum rugosum, Capsella bursa-pastoris and Sinapis arvensis. The nonhost mycorrhizal status entails adverse effects on nonhost weeds due to attempted fungal colonisation, leading to reduced plant survival, growth and nutrient acquisition, particularly when grown in the presence of active AMF extraradical hyphae originating from host plants. These effects have been attributed to the activation of plant root defenses diverting resources from plant growth. This review provides qualitative and quantitative data on the interactions between AMF and nonhost weeds and on the mechanisms underlying weed fitness reduction. The lack of extensive field studies highlights the need for experimental works under real crop conditions to determine whether the combination of AMF with cover crops – a weed management practice adopted in agroecology – could serve as a valuable strategy for weed control, promoting the agroecological transition towards low-input, safe, and resilient agroecosystems.
1 Introduction
The main challenge for global agriculture in the years to come is represented by the safe production of high-quality food for a growing world population, while conserving natural resources for future generations, reducing the use of pesticides and chemical fertilizers and protecting biological soil fertility. Even more so, when considering climate change and global warming, which have a negative impact on food security, mainly due to increasing heat and drought (IPCC, 2023).
The implementation of novel agroecological practices may enhance soil structure and biodiversity, improve ecosystem services and nutrient cycling, while promoting productivity and reducing environmental impacts caused by the excessive use of mineral fertilizers and pesticides. Such practices rely on the beneficial activities of the soil microbiota, which is related to the efficient use of water and natural soil resources, the modulation of soil biochemical, biological and nutritional processes, and the provision of ecosystem services that are of great economic importance for agriculture, forestry and society (Philippot et al., 2013; Azcón-Aguilar and Barea, 2015; Wang and Qiu, 2006).
Among soil beneficial microorganisms, the most significant groups are represented by arbuscular mycorrhizal (AM) fungi (AMF, Glomeromycota), that establish mutualistic symbiotic associations with the roots of about 71–80% of land plants, including most staple food crops, i.e. wheat, rice, maize, sorghum, potatoes, soybeans, cassava, the majority of horticultural plants and fruit trees, and many economically important industrial crops, such as sunflower, cotton, flax, tobacco, sugarcane (Wang and Qiu, 2006; Smith and Read, 2010; Brundrett, 2017). AMF play key roles in plant growth and nutrition, facilitating the uptake and transfer of mineral nutrients from the soil to the host plants, by means of an extensive network of extraradical hyphae. In exchange, they receive plant organic compounds, mainly sugars and lipids, on which they depend as chemoheterotrophic organisms (Smith and Read, 2010; Helber et al., 2011; Luginbuehl et al., 2017). Moreover, AMF are fundamental factors in soil nutrient flows and biogeochemical cycles, enhancing also carbon sequestration and water supply, and plant tolerance to biotic and abiotic stresses (Gianinazzi et al., 2010). Given their multifunctional roles in soil fertility and health, AMF have recently been included in the specific agricultural products category of biostimulants by the EU Regulation 2019/1009 of the European Parliament and of the Council (European Union, 2019).
Many works reported that AMF may act as determinants of weed community structure and composition, reducing the development and fitness of several agricultural weed species, mainly those unable to establish a functional mycorrhizal symbiosis (nonhost species) (Li et al., 2016). Although weed communities can sometimes contribute to agroecosystem services and provide diverse benefits (Gazoulis et al., 2024), their excessive growth represents a major problem in agriculture, responsible for 31–34% of global crop yield loss (Oerke, 2006; Kubiak et al., 2022), despite the growing use of herbicides, whose global application increased by 121 percent since the 1990s (FAO, 2024). Such a large utilisation of herbicides and the resulting selective pressure on weeds, which led to the development of herbicide resistance in many aggressive species, have boosted the search for innovative non-chemical weed management practices, including crop competition, the adoption of cover crops (Peterson et al., 2018; Little et al., 2021) and AMF, which negatively affect the survival and growth of several pernicious and widespread weeds in agriculture (Li et al., 2016). Thus, it is fundamental to gather existing knowledge on AMF-weeds interactions that could lead to weed growth control, with the aim of advancing their implementation as biocontrol agents, in order to reduce herbicide utilization in agroecology. Weed responsiveness to AMF is related to two main factors: weed symbiotic competence, producing negative growth responses in nonhost species, and the performance of the diverse AMF taxa, which differentially affect plant growth and nutrition (Rinaudo et al., 2010; Veiga et al., 2012; Säle et al., 2022).
In this review AMF-weeds interactions are discussed, in relation to i) the main developmental steps leading to the establishment of the mycorrhizal symbiosis, ii) the nonhost mycorrhizal status of weeds, iii) the growth responses of nonhost weeds and the direct and indirect mechanisms underlying such responses. The main and final objective of the review is to provide insights into the research directions aimed at implementing innovative strategies for weed management in agroecology.
2 AMF life cycle and symbiosis establishment
Knowledge of the complex life cycle of AMF is fundamental in order to understand some of the mechanisms possibly involved in their interactions with weeds. Indeed, the development of the different fungal structures essential for root colonization may be hindered by nonhost weeds, which may trigger diverse defence mechanisms to control, avoid or restrict fungal root penetration, with implications for plant growth and functioning (Delaux et al., 2014; Fernández et al., 2019).
AMF life cycle can be completed only after the establishment of the mycorrhizal symbiosis (Figure 1). AMF show an apparently inconsistent behaviour as obligate symbionts, since their spores lack of host-regulated germination, being able to germinate in the soil even in the absence of a host plant. Germlings are not capable of extensive independent development and cease growth within 8–20 days (Giovannetti et al., 2010). Prior to physical contact with plant roots, a complex chemical and molecular dialogue takes place, as the fungal symbiont release signals – a mixture of sulphated and non-sulphated simple lipochitooligosaccharides (LCOs, called Myc factors) – which stimulate the expression of genes allowing mycorrhizal establishment (Maillet et al., 2011; MacLean et al., 2017). On the other side, plant roots secrete particular signalling molecules, represented by carotenoid-based phytohormones called strigolactones, able to reorient the direction of hyphal elongation to their source – the roots – and to trigger a differential hyphal morphogenesis and branching, facilitating the location of plant roots and successive adhesion and colonization (Giovannetti et al., 1993, 1994; Akiyama et al., 2005; Besserer et al., 2006). At the same time, plant “symbiosis-specific” genes produce the cascade of events leading to the development of the mycorrhizal symbiosis (Delaux et al., 2014; MacLean et al., 2017).
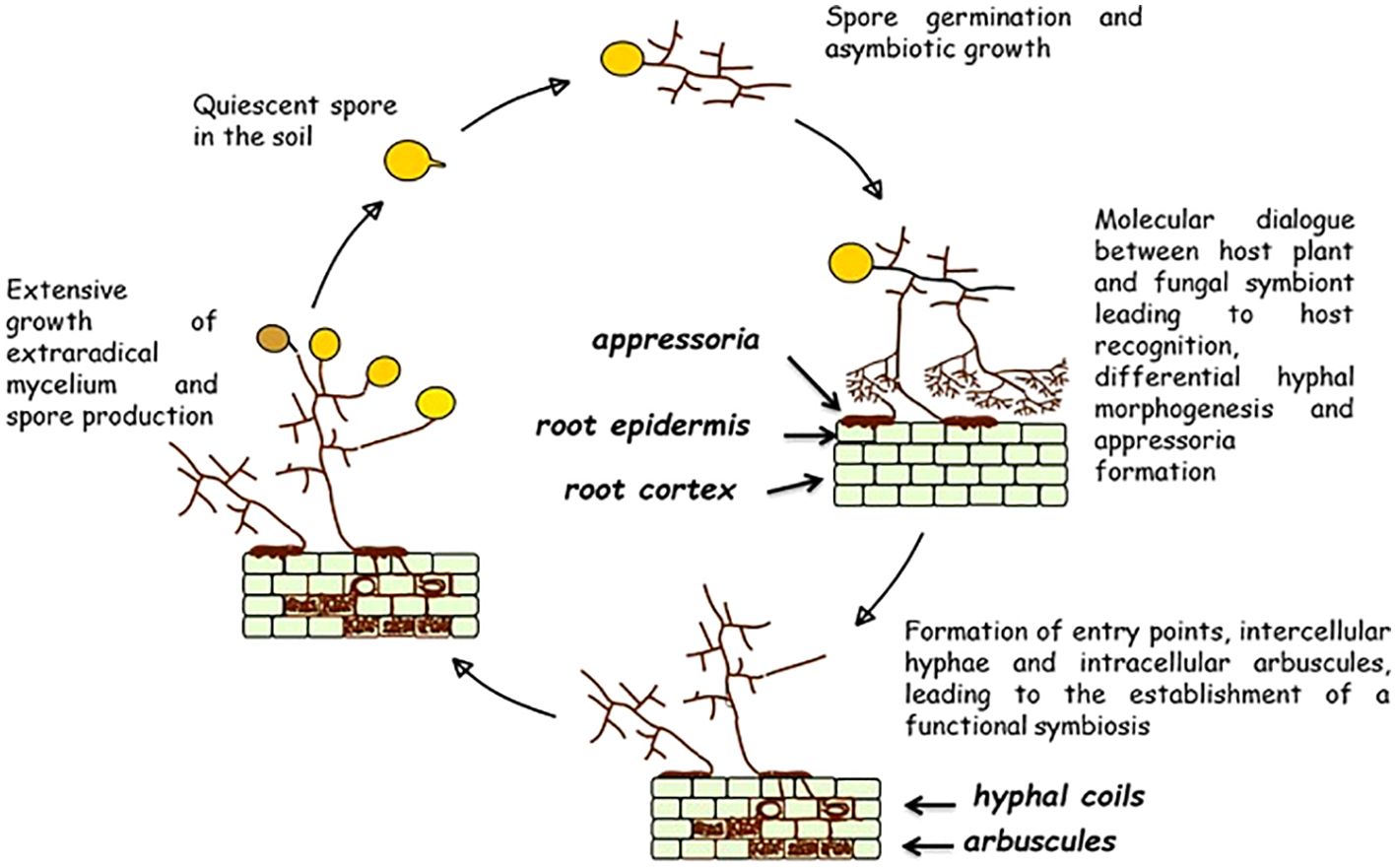
Figure 1. Flow diagram representing the life cycle of obligate biotrophic arbuscular mycorrhizal fungi and the key developmental steps leading to the establishment of the mycorrhizal symbiosis. Source: Modified from Giovannetti (2000), courtesy of Kluwer Academic Press.
The large adhesion structures, appressoria (called also hyphopodia), are formed as early as 36 h after the first contact (Giovannetti and Citernesi, 1993). The role of appressoria is essential not only for the establishment of the symbiosis, but, more importantly, for plant nutrition, as they are the only fungal structures connecting soil-based to root-based hyphae, through which the transfer of P, N and other mineral nutrients from extraradical hyphae to the host plant can be accomplished (Pepe et al., 2020). Appressoria germinate producing entry points (called also infection pegs) and intraradical hyphae, which colonise the roots by forming intracellular hyphal coils and growing intercellularly, before producing, in the inner cortex, intracellular highly branched tree-like structures, similar to haustoria, named arbuscules. Arbuscules are the structures where nutrient exchanges between host plants and fungal symbionts are realized, as at their level plant carbon and lipids are released to the symbionts, while mineral nutrients imported by the extraradical hyphae are released to the hosts (Kiers et al., 2011; Wyatt et al., 2014). Moreover, several AMF taxa form intraradical spore-like lipid-rich vesicles, considered as storage organs.
Once established in their distinctive ecological niche and after obtaining host carbon, AMF grow from the host roots into the surrounding soil, developing wide networks of interconnected extraradical mycelium (ERM), which are real extensions of the absorbing root system, capable of long-term survival in the soil, indipendent of the host plant’s lifespan (Pepe et al., 2018). Besides increasing the uptake of soil mineral nutrients and transferring them to the host plant, ERM can establish belowground interconnections among plants by means of hyphal fusion (anastomosis) (Giovannetti et al., 2004) and translocate soil nutrients from one plant to another (Mikkelsen et al., 2008; Weremijewicz et al., 2016; Řezačová et al., 2025). Eventually, AMF life cycle is completed by the formation of asexual spores by extraradical hyphae, that germinate and give rise to a new cycle (Figure 1).
3 The nonhost mycorrhizal status of weeds
Many plant species are unable to establish functional AM symbioses and are considered nonhost or non-mycorrhizal plants. They represent an estimated 18% of all vascular plants and mainly belong to the families Amaranthaceae, Brassicaceae, Caryophyllaceae, Chenopodiaceae, Cyperaceae, Polygonaceae and Proteaceae (Harley and Harley, 1987; Tester et al., 1987; Brundrett, 2002, 2009; Wang and Qiu, 2006). Nonhost plants encompass some of the world’s worst agricultural weed species, such as Chenopodium album, reported amongst the ten most problematic weeds having developed a wide-scale herbicide resistance, Raphanus raphanistrum, which developed multiple herbicide-resistant populations, Rapistrum rugosum, a highly competitive weed rapidly increasing worldwide, the cosmopolitan Capsella bursa-pastoris and Sinapis arvensis widely distributed around the world with a persistent seedbank (Baskin et al., 2004; Walsh et al., 2004; Manalil et al., 2018; Singh et al., 2022).
The nonhost mycorrhizal status entails the absence of arbuscules, that are the defining feature of the physiological functionality of AM symbioses (Koide and Schreiner, 1992; Brundrett, 2009). Thus, plants are considered nonhosts even in cases where low levels of hyphal root penetration and/or vesicles formation occur (Hirrel et al., 1978; Harley and Harley, 1987; Tester et al., 1987; Wang and Qiu, 2006; Brundrett, 2002, 2009, 2017). In addition, it has long been known that nonhost plants do not elicit the differential hyphal morphogenesis that is the first sign of fungal recognition of a host plants (Giovannetti et al., 1994; Akiyama et al., 2005; Akiyama and Hayashi, 2006; Besserer et al., 2006).
The absence of mycorrhizal colonization and the lack of arbuscules and successful entry points has been reported in several weeds, such as Arabidopsis thaliana, Amaranthus retroflexus (Amaranthaceae), Brassica campestris, B. napus, B. nigra, C. bursa-pastoris, Sinapis arvensis, Sisymbrium altissimum (Brassicaceae), Stellaria media (Caryophyllaceae), Chenopodium album (Chenopodiaceae), Urtica dioica (Urticaceae) inoculated with either of the species Rhizophagus fasciculatus, Rhizophagus intraradices, Funneliformis mosseae, Funneliformis coronatum, Gigaspora margarita and Gigaspora gigantea (Glenn et al., 1985; Fontenla et al., 1999; Rinaudo et al., 2010). Moreover, a large experimental survey of 646 taxa within the Brassicaceae family inoculated with R. intraradices reported that only 122 (19%) showed root penetration, represented by intraradical hyphae and vesicles, while no arbuscules were observed across the 3,230 root sections examined (Demars and Boerner, 1996). Accordingly, no arbuscules were detected in any of the 7,200 root segments examined of C. bursa-pastoris and among 8 nonhost weeds from two field sites (Demars and Boerner, 1994; Wang et al., 2021).
Investigations on a number of Brassicaceae and Chenopodiaceae species showed that no root colonization occurred after AMF inoculation when grown alone, while when a mycorrhizal companion host plant was present, the fungus was able to penetrate the roots, in some cases dead or senescing ones, producing only intercellular hyphae and vesicles, but no functionally active arbuscules (Hirrel et al., 1978; Ocampo et al., 1980, 1986; Demars and Boerner, 1995; Veiga et al., 2013; Fernández et al., 2019). Such a phenomenon was explained by the vigorous activity of extraradical hyphae spreading from mycotrophic plant roots growing nearby (Koide and Schreiner, 1992; Francis and Read, 1995; Lambers and Teste, 2013). This type of endophytic colonization in nonhosts by AMF has been classified as Glomalean Fungus Colonisation (GFC), or rudimentary arbuscular mycorrhizal (RAM) phenotype, in order to discriminate such fungal penetration from well-established functional symbioses in host plants (Brundrett, 2009, 2017; Cosme et al., 2018) (Table 1).

Table 1. Type of AMF structures in the roots and growth responses of nonhost weeds, as assessed in microcosms inoculated with selected AMF or in the field (dash indicates no information on AMF occurrence in the roots or on growth responses).
Alas, many of the quoted works did not follow the dynamics of the colonization process, which was reported in a detailed early investigation on the development of root colonization in the host Trifolium subterraneum and the nonhost Brassica napus (Tommerup, 1984). After 4 weeks’growth no arbuscules were formed in the nonhost, compared with the 86 of the host, while the hyphal swellings developed on the root surface of the nonhost produced few penetration pegs, some of which ceased growth, retracted the cytoplasm and formed septa separating living from dead hyphal parts (abortive entry points). This latter cellular event was detected in other nonhost weeds, such as Sinapis arvensis and Chenopodium album, the latter showing also the formation of rare abortive arbuscules (Rinaudo et al., 2010; Daisog et al., 2012) (Figure 2).
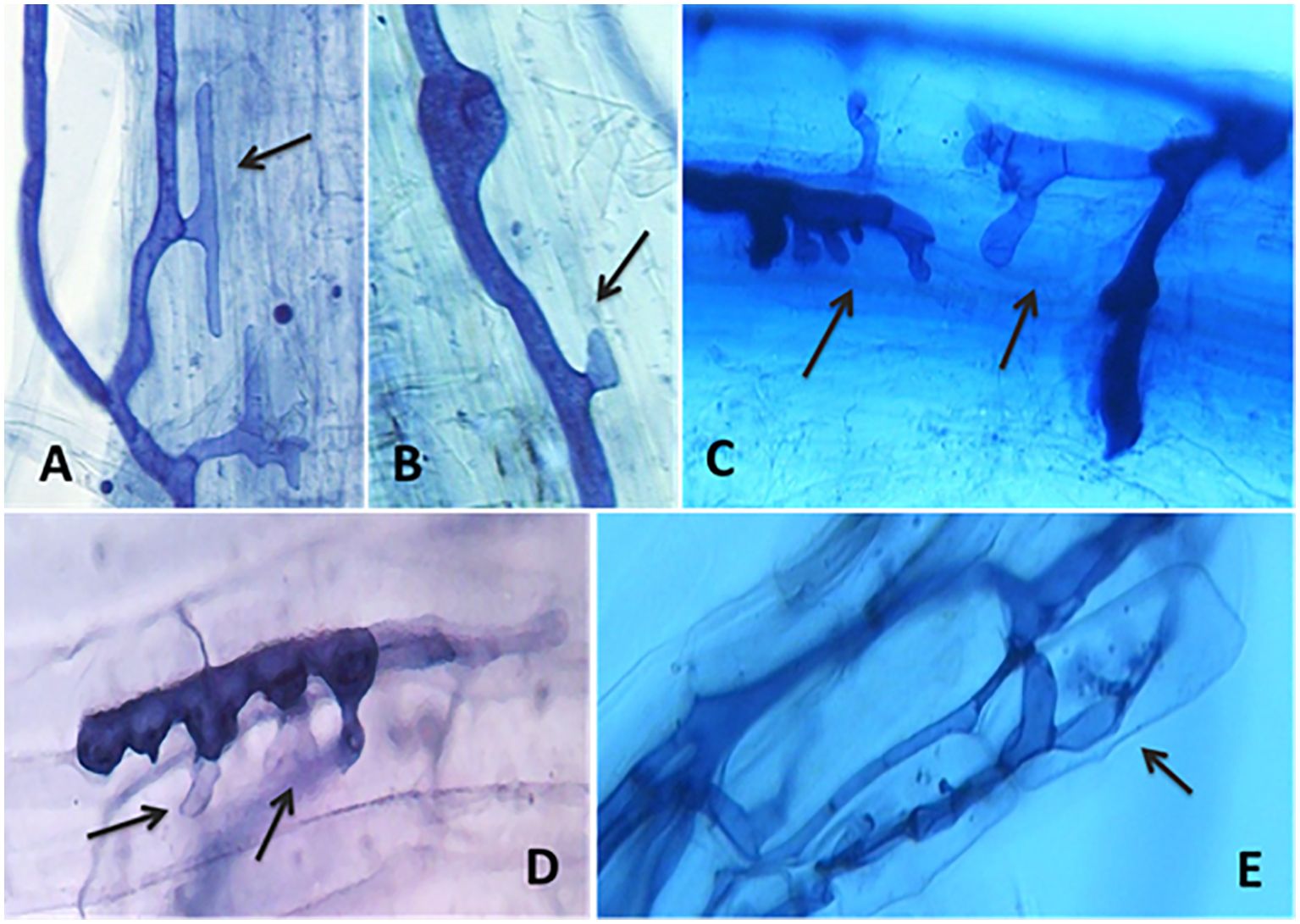
Figure 2. Light microscope pictures of the interactions between the arbuscular mycorrhizal fungus Funneliformis mosseae and roots of the nonhost weeds Sinapis arvensis and Chenopodium album, as shown by Trypan blue differential staining. (A) Hyphal swelling on the root surface of S. arvensis, ceasing growth and lacking cytoplasm (black arrow); (B–D) hyphal swellings and abortive entry points on the root surface of C. album, ceasing growth and lacking cytoplasm (black arrows); (E) a rare abortive arbuscule formed in the root of C. album (black arrow). Source: Courtesy of Dr. Valeria Rinaudo and Dr. Chandra Ramasamy Kamatchi
Such findings, reported also in successive studies with diverse nonhost plants, suggested that plant resistance to fungal colonization is expressed after adhesion, resembling defence responses, such as the production of thick cell wall appositions and intense yellow root autofluorescence indicative of phenolic depositions in incompatible interactions, causing root browning and in some cases root death (Tommerup, 1984; Glenn et al., 1985; Allen et al., 1989; Gollotte et al., 1993; Giovannetti et al., 1994; Veiga et al., 2013) (Figure 3).
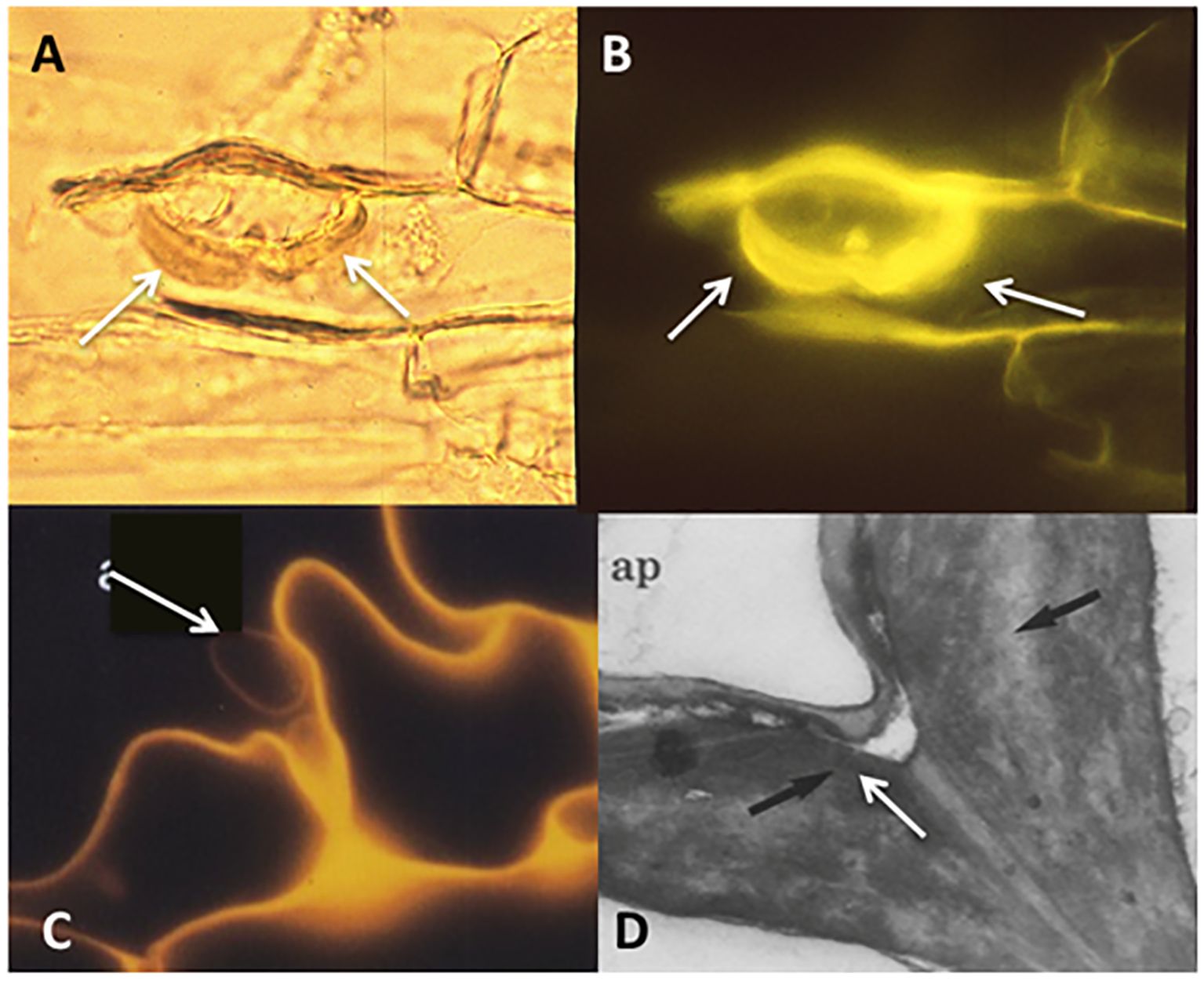
Figure 3. Micrographs of the interactions between Funneliformis mosseae hyphae in contact with the root surface of the nonhost Pisum sativum P2 mutant. (A–C) Wall thickenings below appressoria (A), showing strongly autofluorescence under epifluorescent microscope (B, C); (D) thickening of the plant cell wall below the appressorium (ap), visualised under transmission electron microscope. Source: (A, C, D) after Gollotte et al., 1993, courtesy of Springer-Verlag.
4 Direct and indirect mechanisms underlying the nonhost mycorrhizal status
The phenomenon of nonhost plants sparked interest into the mechanisms underlying the hindering of mycorrhizal development (Tester et al., 1987). One prevalent hypothesis of a role played by inhibitory compounds, such as root glucosinolates, was ruled out by investigations on different cultivars of Brassica spp. containing genetically determined levels of such compounds, whose different concentrations were not correlated with AMF ability to colonise the roots (Glenn et al., 1988). More recent data showed that AMF colonization of transgenic Arabidopsis lines differing in indolic glucosinolates levels was limited to vesicle formation, few intraradical hyphae and no arbuscules (Anthony et al., 2020).
Successive genomic, transcriptomic and comparative phylogenomic analyses revealed that the inability to establish the mycorrhizal symbiosis resulted from the multiple loss of “symbiotic-specific” genes (NFP, DMI2, CASTOR, DMI3, IPD3, RAM1, RAM2, VAPYRIN, STR, STR2, PT4), operating as regulators of the key steps of plant/AMF interactions, such as presymbiotic dialogue, fungal entry into the root, intraradical hyphal proliferation and arbuscule development (Delaux et al., 2014). Such loss was reported to have occurred independently in different plant lineages (Delaux et al., 2014; Bravo et al., 2016; MacLean et al., 2017). It is important to note that the nonhost plants lacking the “symbiotic toolkit” included the weeds Arabidopsis thaliana, Brassica rapa, Camelina sativa, Reseda odorata, Thlaspi arvense (Brassicaceae), Cleome spinosa (Cleomaceae), Utricularia gibba (Lentibulariaceae), Cuscuta pentagona (Cuscutaceae) and also species of the genus Lupinus, the only non-mycorrhizal legume (Delaux et al., 2014) (Figure 4). Actually, recent RNA-seq transcriptome analyses revealed that in the nonhost weed A. thaliana symbiosis-related genes were not expressed and diverse defence-related genes were upregulated (Fernández et al., 2019). These data clearly showed that the fungus was recognized as a potential enemy, whose root colonisation triggered the activation of plant defence responses, possibly leading to negative effects on plant growth (see next section).
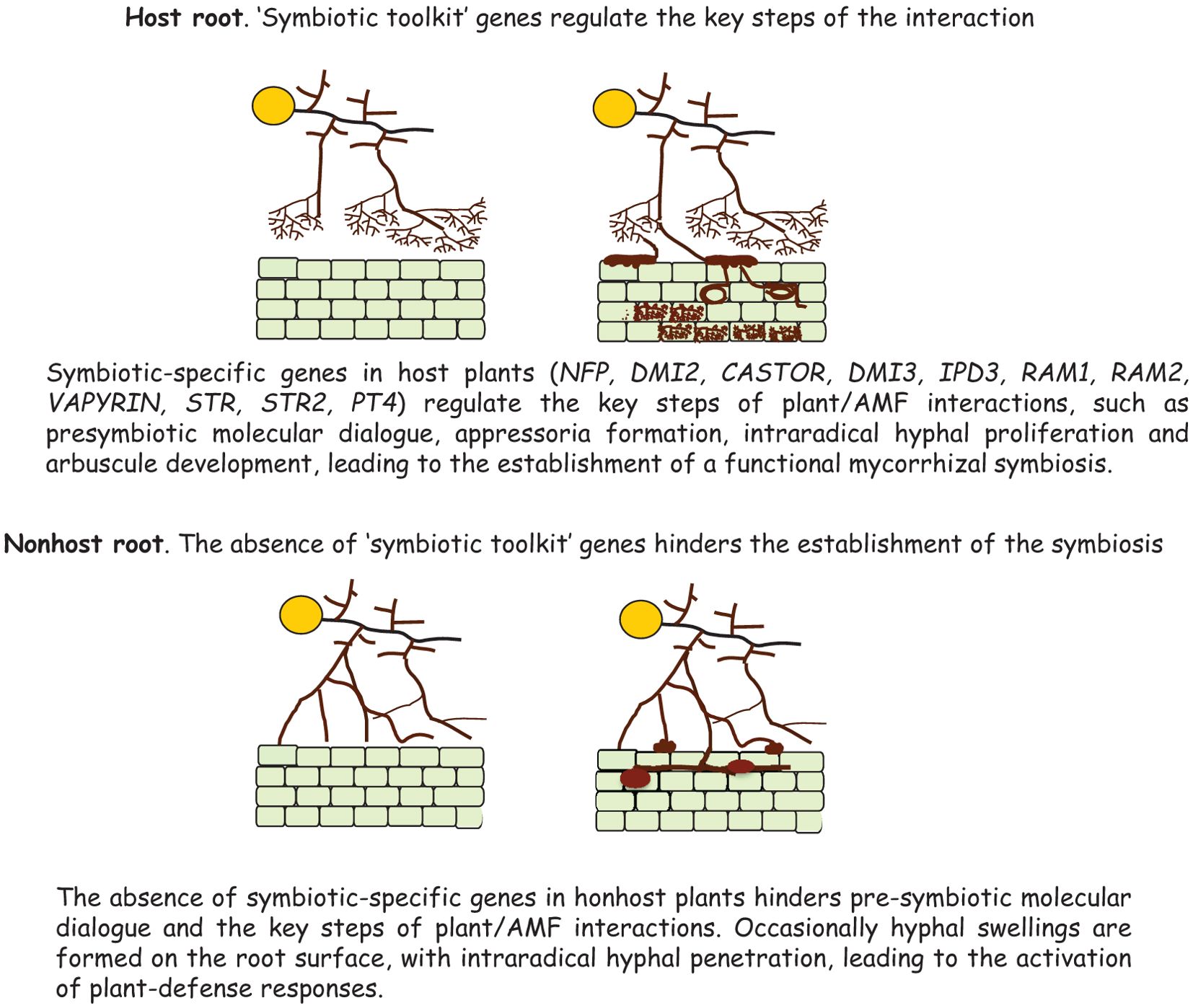
Figure 4. Schematic representation of the key steps of plant/AMF interactions regulated by symbiotic-specific genes, leading to the success or failure of mycorrhizal symbiosis establishment in host or nonhost plants.
5 Growth responses of nonhost weeds to AMF
Since the 1980s, scientists reported AMF adverse effects on nonhost plants by attempted colonisation, such as root damages and reduced plant survival, growth and nutrient acquisition, especially when grown in the presence of active AMF extraradical hyphae originated from a host companion plant. For example, the nonhost Brassica oleracea showed decreases in dry weight and P content of 71 and 72%, respectively, when challenged by F. mosseae extraradical hyphae originated from mycorrhizal roots of the host Sorghum vulgare growing nearby (Ocampo, 1986). In experimental field plots where the mycotrophic weeds Abutilon theophrasti and Setaria lutescens were grown together with the nonhost weed Amaranthus retroflexus, AMF – both native and R. intraradices – reduced the growth and P content of the latter (Sanders and Koide, 1994), whose biomass was reduced also when challenged with multispecies AMF inoculum (Jordan et al., 2000). On the other hand, no growth responses were found when A. retroflexus was inoculated with other AMF species, i.e. native AMF and F. coronatum, F. mosseae or R. irregularis (Vatovec et al., 2005; Rinaudo et al., 2010). Similar results were obtained with Chenopodium album, one of the most aggressive weeds, whose biomass was reduced by F. mosseae, F. coronatum or R. irregularis mycelium (Rinaudo et al., 2010) or by UK native soil AMF (Francis and Read, 1995), while no growth responses were shown when the weed was inoculated with native AMF inoculum of different geographic origin (Vatovec et al., 2005) (Table 1). Such inconsistent effects may be explained by the utilisation of diverse AMF taxa, which may show large differences among genera, species and also isolates, as evidenced by genome sequencing, transcriptomic data (Chen et al., 2018; Morin et al., 2019) and symbiotic performance studies (Lewandowski et al., 2013; Giovannini et al., 2020). Since only a small proportion of AMF have been investigated so far, further in-depth studies should explore the full potential of their wide interspecific and intraspecific physiological and functional diversity, particularly in relation to weeds growth, nutrition and health.
The major role played by AMF in growth control of nonhost weeds was confirmed using one of the worst perennial weeds of agricultural crops worldwide, Cyperus rotundus (Muthukumar et al., 1997). In experimental microcosms the native AMF Rhizophagus aggregatus, Funneliformis geosporus and Sclerocystis sinuosa significantly decreased shoot dry weight by 67% when C. rotundus was grown together with the mycotrophic plant onion, compared with reductions of 36% when grown alone. Interestingly, dry weights of the tubers, the organs perpetuating such weed species belowground, decreased by 94% when grown together with onions (Muthukumar et al., 1997) (Table 1). The importance of mycorrhizal mycelium of companion or “nurse” plants was revealed using experimental microcosms, where nonhost plants were challenged by AMF extraradical hyphae spreading from host plants growing nearby, but separated by a cylinder of nylon mesh with a pore size enabling only the passage of fungal hyphae. In such microcosms, the nonhost weeds Arenaria serpyllifolia, Chenopodium album, Echium vulgare, Rumex acetosella, Spergula arvensis, Verbascum thapsus were strongly inhibited in their growth by the presence of native AMF mycelium and showed lower survivorship, with A. serpyllifolia suffering severe mortality (Francis and Read, 1995) (Table 1). Other works utilized the same device, showing that the nonhost weed Stellaria media had an 8-fold biomass reduction when challenged with the active mycelium of R. irregularis, growing from neighboring wheat plants, which produced some root colonization, only represented by hyphae and vesicles, but no arbuscules (Veiga et al., 2012). The study excluded allelopathy as a mechanism responsible for such growth suppression, but could not assess other possible operative mechanisms (Lambers and Teste, 2013). A successive work confirmed the key role played by active extraradical mycorrhizal hyphae in growth control of the nonhost A. thaliana, whose biomass was reduced by more than 50% when grown together with both T. pratense and Lolium multiflorum inoculated with R. irregularis. Investigations on the cellular interactions between nonhost roots and the AMF fungus by confocal and transmission electron microscopy confirmed early findings by Allen et al. (1989), showing that root tissues colonised by AMF appeared senescent, with dead and partially collapsed cell walls, that appeared degraded at the point of contact with the fungal hyphae (Veiga et al., 2013) (Table 1). Utilizing a similar microcosm setup with Medicago truncatula as source of the active mycorrhizal network, Fernández et al. (2019) showed that the low (5%) hyphal colonization of A. thaliana roots by R. irregularis caused a 50% biomass reduction and was not associated with the expression of the symbiosis-related genes GintPT, GintAMT2, GintMST2 and GintMST4, that are considered markers for a functional AM symbiosis. At the same time, the plant was able to recognise the fungus as an “unwanted invader” which activated root defences, possibly responsible of the growth decrease (Fernández et al., 2019). However, the specific mechanisms at the base of plant defence activation remain poorly understood, as well as the roles of certain fungal species in growth inhibition of diverse plant species. Further comprehensive studies are essential, including various crops, weeds and AMF species other than the model organisms A. thaliana and R. irregularis, as the magnitude of responses may depend on the different plant/fungus combinations and also by the functional traits of each fungal species, in particular colonization ability and mutualistic performance (Giovannetti and Citernesi, 1993; Klironomos, 2003; Giovannini et al., 2020). Moreover, considering that most of the data collected so far have been carried out in microcosms/greenhouses (Table 1), field studies are needed under real crop conditions, as pH, organic matter, nutrient availability, edaphic factors and agricultural management can modulate the effectiveness of AMF in controlling nonhost weeds.
6 Research directions in agroecology
Altogether, all the experimental data obtained so far show that an active extraradical mycelium spreading from colonised host plants represents the main fungal factor responsible for negative impacts on nonhost weeds, ranging from biomass reduction to decreased survivorship. This is a very important, additional function of the soil mycorrhizal fungal networks, which are able to interconnect the roots of host plants belonging to different families, genera and species and transfer nutrients among them (Giovannetti et al., 2004; Mikkelsen et al., 2008; Weremijewicz et al., 2016; Wang et al., 2022; Řezačová et al., 2025). In agricultural fields AMF may produce differential effects on co-occurring species and alter the structure and functioning of plant communities, i.e. by favouring the growth and performance of host species, while simultaneously reducing the fitness and abundance of nonhosts. As to the biocontrol of nonhost weeds, it can be effective whenever crop plants are host species – such as most agricultural crops – able to produce extensive soil hyphal networks that may encounter the roots of nonhost weeds and lower their fitness by at least two diverse mechanisms. Firstly, hyphal root penetration in nonhost weeds without the formation of arbuscules – the site of nutrient transfer from the fungus to the host plant – does not provide any nutritional plant benefit, while the absence of “symbiotic-specific” genes can activate costly plant defence responses, diverting resources from plant growth (Delaux et al., 2014; Fernández et al., 2019). Secondly, the extraradical hyphal network may very efficiently uptake nutrients from the soil and transfer them to the host crops, promoting their growth, while reducing nutrient access by nonhost weeds. In this regard, the selection of crop genotypes with high AMF compatibility and able to produce extensive mycorrhizal networks may represent a good strategy in order to enhance weed control (Sawers et al., 2017; Giovannini et al., 2022).
The implementation of agroecological practices and the sustainable production of food crops, aimed at reducing chemical inputs into the soil, entail a careful and rational use of synthetic herbicides for weeds control. In this regard cover crops are increasingly being used as they provide many documented environmental benefits, including improved soil protection and health, enhancement of biodiversity and biological soil fertility, and the suppression of aggressive agricultural weeds (Adetunji et al., 2020; Haring and Hanson, 2022; Kumar et al., 2020; Tataridas et al., 2022). Mycorrhizal symbionts may boost cover crops performance, through the two main mechanisms already described. The first one concerns host cover crop species, which, when colonized by AMF, may benefit from the enhanced availability of soil nutrients provided by the extraradical hyphae and become more competitive against weeds. The second one involves nonhost weed species, whose growth may be negatively affected by AMF, due to the limited access to nutrients and to negative interactions involving plant defence responses activated in the absence of “symbiotic-specific” genes. Moreover, the AMF networks produced by mycorrhizal cover crops may represent a rich source of inoculum for the successive crops, contributing to the maintenance of a high soil mycorrhizal potential, given the long-term survival of the extraradical mycelium (Pepe et al., 2018).
The detection of the best device to deliver AMF inoculum to crops and cover crops represents a tough challenge. One of the viable strategies to be adopted in agroecology could be represented by experimental seed inoculation with very infective and efficient AMF isolates, able to establish the mycorrhizal symbiosis immediately after seed germination, thus enhancing their competitive ability, in particular against host weeds. Seed inoculation could represent a strategy for broader use across agricultural systems, where viability and economic efficiency are of primary importance.
In order to understand whether the combination of cover crops with the use of beneficial AMF seed inoculation may represent effective agroecological tools for weed management, further work is underway within the framework of the research project Horizon Europe GOOD (AGrOecOlogy for weeDs, 101083589). In particular, we will study the possibility of enrichment cultures of autochthonous AMF to be reused for seed inoculation of a number of cover crops grown in rotation or as companion crops in the fields of annual main crops, such as wheat, rice, triticale, maize, soybean, cowpea, onion and perennial crops including pome and stone fruits, citrus, grapevine and olive. Such work is an example of the experimental studies to be performed under field conditions, needed in order to investigate AMF-cover crops-weeds interactions, implement innovative, systemic and sustainable strategies for weed management, and promote agroecological transition towards low-input, safe and resilient agroecosystems.
Author contributions
IP: Investigation, Writing – review & editing, Conceptualization. AG: Writing – review & editing, Investigation. MA: Data curation, Writing – review & editing. AT: Funding acquisition, Writing – review & editing. LA: Writing – review & editing, Writing – original draft, Data curation. MG: Writing – original draft, Writing – review & editing, Investigation, Conceptualization.
Funding
The author(s) declare that financial support was received for the research and/or publication of this article. This study was supported by the European Union under Grant Agreement No. 101083589 (Agroecology is GOOD project). Views and opinions expressed are however those of the author(s) only and do not necessarily reflect those of the European Union or REA. Neither the European Union nor the granting authority can be held responsible for them.
Conflict of interest
The authors declare that the research was conducted in the absence of any commercial or financial relationships that could be construed as a potential conflict of interest.
Generative AI statement
The author(s) declare that no Generative AI was used in the creation of this manuscript.
Publisher’s note
All claims expressed in this article are solely those of the authors and do not necessarily represent those of their affiliated organizations, or those of the publisher, the editors and the reviewers. Any product that may be evaluated in this article, or claim that may be made by its manufacturer, is not guaranteed or endorsed by the publisher.
References
Adetunji A. T., Ncube B., Mulidzi R., and Lewu F. B. (2020). Management impact and benefit of cover crops on soil quality: A review. Soil Tillage Res. 204, 104717. doi: 10.1016/j.still.2020.104717
Akiyama K. and Hayashi H. (2006). Strigolactones: chemical signals for fungal symbionts and parasitic weeds in plant roots. Ann. Bot. 97, 925–931. doi: 10.1093/aob/mcl063
Akiyama K., Matsuzaki K. I., and Hayashi H. (2005). Plant sesquiterpenes induce hyphal branching in arbuscular mycorrhizal fungi. Nature 435, 824–827. doi: 10.1038/nature03608
Allen M. F., Edith B., and Friese C. F. (1989). Responses of the non-mycotrophic plant Salsola kali to invasion by vesicular–arbuscular mycorrhizal fungi. New Phytol. 111, 45–49. doi: 10.1111/j.1469-8137.1989.tb04216.x
Anthony M. A., Celenza J. L., Armstrong A., and Frey S. D. (2020). Indolic glucosinolate pathway provides resistance to mycorrhizal fungal colonization in a non-host Brassicaceae. Ecosphere 11, e03100. doi: 10.1002/ecs2.3100
Azcón-Aguilar C. and Barea J. M. (2015). Nutrient cycling in the mycorrhizosphere. J. Soil Sci. Plant Nutr. 15, 372–396. doi: 10.4067/S0718-95162015005000035
Baskin C. C., Milberg P., Andersson L., and Baskin J. M. (2004). Germination ecology of seeds of the annual weeds Capsella bursa-pastoris and Descurainia sophia originating from high northern latitudes. Weed Res. 44, 60–68. doi: 10.1046/j.1365-3180.2003.00373.x
Besserer A., Puech-Pagès V., Kiefer P., Gomez-Roldan V., Jauneau A., Roy S., et al. (2006). Strigolactones stimulate arbuscular mycorrhizal fungi by activating mitochondria. PloS Biol. 4, e226. doi: 10.1371/journal.pbio.0040226
Bravo A., York T., Pumplin N., Mueller L. A., and Harrison M. J. (2016). Genes conserved for arbuscular mycorrhizal symbiosis identified through phylogenomics. Nat. Plants 2, 15208. doi: 10.1038/nplants.2015.208
Brundrett M. C. (2002). Coevolution of roots and mycorrhizas of land plants. New Phytol. 154, 275–304. doi: 10.1046/j.1469-8137.2002.00397.x
Brundrett M. C. (2009). Mycorrhizal associations and other means of nutrition of vascular plants: understanding the global diversity of host plants by resolving conflicting information and developing reliable means of diagnosis. Plant Soil 320, 37–77. doi: 10.1007/s11104-008-9877-9
Brundrett M. C. (2017). “Global diversity and importance of mycorrhizal and nonmycorrhizal plants,” in Biogeography of Mycorrhizal Symbiosis. Ed. Tedersoo L. (Springer International Publishing, Cham), 533–556. doi: 10.1007/978-3-319-56363-3_21
Chen E. C. H., Morin E., Beaudet D., Noel J., Yildirir G., Ndikumana S., et al. (2018). High intraspecific genome diversity in the model arbuscular mycorrhizal symbiont Rhizophagus irregularis. New Phytol. 220, 1161–1171. doi: 10.1111/nph.14989
Cosme M., Fernández I., van der Heijden M. G., and Pieterse C. M. (2018). Non-mycorrhizal plants: the exceptions that prove the rule. Trends Plant Sci. 23, 577–587. doi: 10.1016/j.tplants.2018.04.004
Daisog H., Sbrana C., Cristani C., Moonen A. C., Giovannetti M., and Bàrberi P. (2012). Arbuscular mycorrhizal fungi shift competitive relationships among crop and weed species. Plant Soil 353, 395–408. doi: 10.1007/s11104-011-1040-3
Delaux P. M., Varala K., Edger P. P., Coruzzi G. M., Pires J. C., and Ané J. M. (2014). Comparative phylogenomics uncovers the impact of symbiotic associations on host genome evolution. PloS Genet. 10, e1004487. doi: 10.1371/journal.pgen.1004487
Demars B. G. and Boerner R. E. J. (1994). Vesicular-arbuscular mycorrhizal fungi colonization in Capsella bursa-pastoris (Brassicaceae). Am. Midl. Nat. 132, 377–380. doi: 10.2307/2426593
Demars B. G. and Boerner R. E. J. (1995). Arbuscular mycorrhizal development in three crucifers. Mycorrhiza 5, 405–408. doi: 10.1007/BF00213440
Demars B. G. and Boerner R. E. J. (1996). Vesicular arbuscular mycorrhizal development in the Brassicaceae in relation to plant life span. Flora 191, 179–189. doi: 10.1016/S0367-2530(17)30711-9
Eriksen M., Bjureke K. E., and Dhillion S. S. (2002). Mycorrhizal plants of traditionally managed boreal grasslands in Norway. Mycorrhiza 12, 117–123. doi: 10.1007/s00572-002-0165-x
Ernst W. H. O., Van Duin W. E., and Oolbekking G. T. (1984). Vesicular-arbuscular mycorrhiza in dune vegetation. Acta Bot. Neerl. 33, 151–160. doi: 10.1111/j.1438-8677.1984.tb01794.x
European Union (2019).Regulation (EU) 2019/1009 of the European parliament and of the council of 5 June 2019. Available online at: https://eur-lex.europa.eu/eli/reg/2019/1009/oj/eng (Accessed February 18, 2025).
FAO (2024). Pesticides use and trade – 1990–2022 (Rome: FAOSTAT Analytical Briefs, No. 89). Accessed February 18, 2025. doi: 10.4060/cd1486en
Fernández I., Cosme M., Stringlis I. A., Yu K., de Jonge R., van Wees S. C. M., et al. (2019). Molecular dialogue between arbuscular mycorrhizal fungi and the nonhost plant Arabidopsis thaliana switches from initial detection to antagonism. New Phytol. 223, 867–881. doi: 10.1111/nph.15798
Fitter A. H. and Nichols R. (1988). The use of benomyl to control infection by vesicular–arbuscular mycorrhizal fungi. New Phytol. 110, 201–206. doi: 10.1111/j.1469-8137.1988.tb00253.x
Fontenla S., García-Romera I., and Ocampo J. A. (1999). Negative influence of non-host plants on the colonization of Pisum sativum by the arbuscular mycorrhizal fungus Glomus mosseae. Soil Biol. Biochem. 31, 1591–1597. doi: 10.1016/S0038-0717(99)00087-5
Francis R. M. and Read D. J. (1995). Mutualism and antagonism in the mycorrhizal symbiosis, with special reference to impacts on plant community structure. Can. J. Bot. 73, 1301–1309. doi: 10.1139/b95-391
Gazoulis I., Kanatas P., Zannopoulos S., Kokkini M., Kontogeorgou V., Antonopoulos N., et al. (2024). Agroecology and beyond: enhancing ecosystem services provided by natural vegetation and inventing “service weeds. Front. Plant Sci. 15. doi: 10.3389/fpls.2024.1436310
Gianinazzi S., Gollotte A., Binet M. N., van Tuinen D., Redecker D., and Wipf D. (2010). Agroecology: the key role of arbuscular mycorrhizas in ecosystem services. Mycorrhiza 20, 519–530. doi: 10.1007/s00572-010-0333-3
Giovannetti M. (2000). Spore germination and pre-symbiotic mycelial growth. In: Giovannetti, M. (ed.) Arbuscular mycorrhizae: molecular biology and physiology. Kluwer Academic Press: Dordrecht, The Netherlands.
Giovannetti M., Avio L., and Sbrana C. (2010). “Fungal spore germination and pre-symbiotic mycelial growth – physiological and genetic aspects,” in Arbuscular Mycorrhizas: Physiology and Function. Eds. Varma A. and Gaur R. K. (Springer, Dordrecht), 3–32. doi: 10.1007/978-90-481-9489-6_1
Giovannetti M. and Citernesi A. S. (1993). Time-course of appressorium formation on host plants by arbuscular mycorrhizal fungi. Mycol. Res. 97, 1140–1142. doi: 10.1016/S0953-7562(09)80517-0
Giovannetti M. and Nicolson T. H. (1983). Vesicular-arbuscular mycorrhizas in Italian sand dunes. Trans. Br. Mycol. Soc 80, 552–557. doi: 10.1016/S0007-1536(83)80058-8
Giovannetti M., Sbrana C., Avio L., Citernesi A. S., and Logi C. (1993). Differential hyphal morphogenesis in arbuscular mycorrhizal fungi during pre-infection stages. New Phytol. 125, 587–593. doi: 10.1111/j.1469-8137.1993.tb03907.x
Giovannetti M., Sbrana C., Avio L., and Strani P. (2004). Patterns of below-ground plant interconnections established by means of arbuscular mycorrhizal networks. New Phytol. 175, 175–181. doi: 10.1111/j.1469-8137.2004.01145.x
Giovannetti M., Sbrana C., and Logi C. (1994). Early processes involved in host recognition by arbuscular mycorrhizal fungi. New Phytol. 127, 703–709. doi: 10.1111/j.1469-8137.1994.tb02973.x
Giovannini L., Palla M., Agnolucci M., Avio L., Sbrana C., Turrini A., et al. (2020). Arbuscular mycorrhizal fungi and associated microbiota as plant biostimulants: research strategies for the selection of the best performing inocula. Agronomy 10, 106. doi: 10.3390/agronomy10010106
Giovannini L., Sbrana C., Giovannetti M., Avio L., Lanubile A., Marocco A., et al. (2022). Diverse mycorrhizal maize inbred lines differentially modulate mycelial traits and the expression of plant and fungal phosphate transporters. Sci. Rep. 12, 21279. doi: 10.1038/s41598-022-25834-7
Glenn M. G., Chew F. S., and Williams P. H. (1985). Hyphal penetration of Brassica (Cruciferae) roots by a vesicular–arbuscular mycorrhizal fungus. New Phytol. 99, 463–472. doi: 10.1111/j.1469-8137.1985.tb03673.x
Glenn M. G., Chew F. S., and Williams P. H. (1988). Influence of glucosinolate content of Brassica (Cruciferae) roots on growth of vesicular–arbuscular mycorrhizal fungi. New Phytol. 110, 217–225. doi: 10.1111/j.1469-8137.1988.tb00255.x
Gollotte A., Gianinazzi-Pearson V., Giovannetti M., Sbrana C., Avio L., and Gianinazzi S. (1993). Cellular localization and cyto-chemical probing of resistance reactions to arbuscular mycorrhizal fungi in a “locus a’ myc” mutant of Pisum sativum L. Planta 191, 112–122. doi: 10.1007/BF00240902
Grime J. P., Mackey J. M. L., Hillier S. H., and Read D. J. (1987). Floristic diversity in a model system using experimental microcosms. Nature 328, 420–422. doi: 10.1038/328420a0
Haring S. C. and Hanson B. D. (2022). Agronomic cover crop management supports weed suppression and competition in California orchards. Weed Sci. 70, 595–602. doi: 10.1017/wsc.2022.48
Harley J. L. and Harley E. L. (1987). A check-list of mycorrhiza in the British flora. New Phytol. 107, 741–749. doi: 10.1111/j.1469-8137.1987.tb00674.x
Helber N., Wippel K., Sauer N., Schaarschmidt S., Hause B., and Requena N. (2011). A versatile monosaccharide transporter that operates in the arbuscular mycorrhizal fungus Glomus sp is crucial for the symbiotic relationship with plants. Plant Cell 23, 3812–3823. doi: 10.1105/tpc.111.089813
Hirrel M. C., Mehravaran H., and Gerdemann J. W. (1978). Vesicular-arbuscular mycorrhizae in the Chenopodiaceae and Cruciferae: do they occur? Can. J. Bot. 56, 2813–2817. doi: 10.1139/b78-336
IPCC. (2023). Summary for policymakers. In: Climate change 2023: synthesis report Intergovernmental Panel on Climate Change: Geneva, Switzerland. Available online at: https://www.ipcc.ch/report/ar6/syr/downloads/report/IPCC_AR6_SYR_SPM.pdf (accessed February 18, 2025).
Johnson N. C. (1998). Responses of Salsola kali and Panicum virgatum to mycorrhizal fungi, phosphorus and soil organic matter: implications for reclamation. J. Appl. Ecol. 35, 86–94. doi: 10.1046/j.1365-2664.1998.00277.x
Jordan N., Zhang L., and Huerd S. (2000). Arbuscular-mycorrhizal fungi: Potential roles in weed management. Weed Res. 40, 397–410. doi: 10.1046/j.1365-3180.2000.00207.x
Kasowska D. (2002). Mycorrhizal status of plants in two successional stages on spoil heaps from fireloam mining in Lower Silesia (SW Poland). Acta Soc Bot. Pol. 71, 155–161. doi: 10.5586/asbp.2002.018
Kiers E. T., Duhamel M., Beesetty Y., Mensah J. A., Franken O., Verbruggen E., et al. (2011). Reciprocal rewards stabilize cooperation in the mycorrhizal symbiosis. Science 333, 880–882. doi: 10.1126/science.1208473
Klironomos J. N. (2003). Variation in plant response to native and exotic arbuscular mycorrhizal fungi. Ecology 84, 2292–2301. doi: 10.1890/02-0413
Koide R. T. and Schreiner R. P. (1992). Regulation of the vesicular-arbuscular mycorrhizal symbiosis. Annu. Rev. Plant Biol. 43, 557–581. doi: 10.1146/annurev.pp.43.060192.003013
Kubiak A., Wolna-Maruwka A., Niewiadomska A., and Pilarska A. A. (2022). The problem of weed infestation of agricultural plantations vs. the assumptions of the European biodiversity strategy. Agronomy 12, 1808. doi: 10.3390/agronomy12081808
Kumar V., Obour A., Jha P., Liu R., Manuchehri M. R., Dille J. A., et al. (2020). Integrating cover crops for weed management in the semiarid US Great Plains: Opportunities and challenges. Weed Sci. 68, 311–323. doi: 10.1017/wsc.2020.29
Lambers H. and Teste F. P. (2013). Interactions between arbuscular mycorrhizal and non-mycorrhizal plants: do non-mycorrhizal species at both extremes of nutrient availability play the same game? Plant Cell Environ. 36, 1911–1915. doi: 10.1111/pce.12
Laursen G. A., Treu R., Seppelt R. D., and Stephenson S. L. (1997). Mycorrhizal assessment of vascular plants from subantarctic Macquarie Island. Arctic Alpine Res. 29, 483–491. doi: 10.1080/00040851.1997.12003269
Lewandowski T. J., Dunfield K. E., and Antunes P. M. (2013). Isolate identity determines plant tolerance to pathogen attack in assembled mycorrhizal communities. PloS One 8, e61329. doi: 10.1371/journal.pone.0061329
Li M., Jordan N. R., Koide R. T., Yannarell A. C., and Davis A. S. (2016). Meta-analysis of crop and weed growth responses to arbuscular mycorrhizal fungi: implications for integrated weed management. Weed Sci. 64, 642–652. doi: 10.1614/WS-D-16-00032.1
Little N. G., DiTommaso A., Westbrook A. S., Ketterings Q. M., and Mohler C. L. (2021). Effects of fertility amendments on weed growth and weed–crop competition: a review. Weed Sci. 69, 132–146. doi: 10.1017/wsc.2021.1
Luginbuehl L. H., Menard G. N., Kurup S., Van Erp H., Radhakrishnan G. V., Breakspear A., et al. (2017). Fatty acids in arbuscular mycorrhizal fungi are synthesized by the host plant. Science 356, 1175–1178. doi: 10.1126/science.aan0081
MacLean A. M., Bravo A., and Harrison M. J. (2017). Plant signaling and metabolic pathways enabling arbuscular mycorrhizal symbiosis. Plant Cell 29, 2319–2335. doi: 10.1105/tpc.17.00555
Maillet F., Poinsot V., André O., Puech-Pagès V., Haouy A., Gueunier M., et al. (2011). Fungal lipochitooligosaccharide symbiotic signals in arbuscular mycorrhiza. Nature 469, 58–63. doi: 10.1038/nature09622
Manalil S., Ali H. H., and Chauhan B. S. (2018). Germination ecology of turnip weed (Rapistrum rugosum (L.) All.) in the northern regions of Australia. PloS One 13, e0201023. doi: 10.1371/journal.pone.0201023
Mikkelsen B. L., Rosendahl S., and Jakobsen I. (2008). Underground resource allocation between individual networks of mycorrhizal fungi. New Phytol. 180, 890–898. doi: 10.1111/j.1469-8137.2008.02623.x
Morin E., Miyauchi S., San Clemente H., Chen E. C. H., Pelin A., de la Providencia I., et al. (2019). Comparative genomics of Rhizophagus irregularis, R. cerebriforme, R. diaphanus, and Gigaspora rosea highlights specific genetic features in Glomeromycotina. New Phytol. 222, 1584–1598. doi: 10.1111/nph.15687
Muthukumar T., Udaiyan K., Karthikeyan A., and Manian S. (1997). Influence of native endomycorrhiza, soil flooding, and nurse plant on mycorrhizal status and growth of purple nutsedge (Cyperus rotundus L.). Agric. Ecosyst. Environ. 61, 51–58. doi: 10.1016/S0167-8809(96)01073-0
Neeraj, Shanker A., Mathew J., and Varma A. (1991). Occurrence of vesicular-arbuscular mycorrhizae with Amaranthaceae in soils of the Indian semi-arid region. Biol. Fertil. Soils 11, 140–144. doi: 10.1007/BF00336379
Ocampo J. A. (1986). Vesicular–arbuscular mycorrhizal infection of “host” and “non-host” plants: effect on the growth responses of the plants and competition between them. Soil Biol. Biochem. 18, 607–610. doi: 10.1016/0038-0717(86)90083-0
Ocampo J. A., Martin J., and Hayman D. S. (1980). Influence of plant interactions on vesicular–arbuscular mycorrhizal infection. I. Host and non-host plants grown together. New Phytol. 84, 27–35. doi: 10.1111/j.1469-8137.1980.tb00746.x
Parra-Garcia M. D., Lo Giudice V., and Ocampo J. A. (1992). Absence of VA colonization in Oxalis pes-caprae inoculated with Glomus mosseae. Plant Soil 145, 298–300. doi: 10.1007/BF00010359
Pepe A., Giovannetti M., and Sbrana C. (2018). Lifespan and functionality of mycorrhizal fungal mycelium are uncoupled from host plant lifespan. Sci. Rep. 8, 10235. doi: 10.1038/s41598-018-28354-5
Pepe A., Giovannetti M., and Sbrana C. (2020). Appressoria and phosphorus fluxes in mycorrhizal plants: connections between soil- and plant-based hyphae. Mycorrhiza 30, 589–600. doi: 10.1007/s00572-020-00972-w
Peterson M. A., Collavo A., Ovejero R., Shivrain V., and Walsh M. J. (2018). The challenge of herbicide resistance around the world: a current summary. Pest Manage. Sci. 74, 2246–2259. doi: 10.1002/ps.4821
Philippot L., Raaijmakers J. M., Lemanceau P., and van der Putten W. H. (2013). Going back to the roots: the microbial ecology of the rhizosphere. Nat. Rev. Microbiol. 11, 789–799. doi: 10.1038/nrmicro3109
Regvar M., Vogel K., Irgel N., Wraber T., Hildebrandt U., Wilde P., et al. (2003). Colonization of pennycresses (Thlaspi spp.) of the Brassicaceae by arbuscular mycorrhizal fungi. J. Plant Physiol. 160, 615–626. doi: 10.1078/0176-1617-00988
Řezačová V., Weremijewicz J., and Michalová T. (2025). Nutrient exchange within common mycorrhizal networks is altered in a multispecies environment. Funct. Ecol. 39, 418–431. doi: 10.1111/1365-2435.14723
Rinaudo V., Bàrberi P., Giovannetti M., and van der Heijden M. G. (2010). Mycorrhizal fungi suppress aggressive agricultural weeds. Plant Soil 333, 7–20. doi: 10.1007/s11104-009-0202-z
Safari Sinegani A. A. and Elyasi Yeganeh M. (2017). The occurrence of arbuscular mycorrhizal fungi in soil and root of medicinal plants in Bu-Ali Sina garden in Hamadan, Iran. J. Microbial Biol. 5, 43–59. doi: 10.22108/bjm.2017.21145
Säle V., Sieverding E., and Oehl F. (2022). Growth responses of three European weeds on different AMF species during early development. Plants 11, 2020. doi: 10.3390/plants11152020
Sanders I. R. and Koide R. T. (1994). Nutrient acquisition and community structure in co-occurring mycotrophic and non-mycotrophic oldfield annuals. Funct. Ecol. 8, 77–84. doi: 10.2307/2390114
Sawers R. J., Svane S. F., Quan C., Grønlund M., Wozniak B., Gebreselassie M. N., et al. (2017). Phosphorus acquisition efficiency in arbuscular mycorrhizal maize is correlated with the abundance of root-external hyphae and the accumulation of transcripts encoding PHT1 phosphate transporters. New Phytol. 214, 632–643. doi: 10.1111/nph.14403
Singh A., Mahajan G., and Chauhan B. S. (2022). Germination ecology of wild mustard (Sinapis arvensis) and its implications for weed management. Weed Sci. 70, 103–111. doi: 10.1017/wsc.2021.66
Smith S. E. and Read D. J. (2010). Mycorrhizal Symbiosis (London: Academic Press). doi: 10.1016/B978-0-12-370526-6.X5001-6
Tataridas A., Kanatas P., Chatzigeorgiou A., Zannopoulos S., and Travlos I. (2022). Sustainable crop and weed management in the era of the EU Green Deal: A survival guide. Agronomy 12, 589. doi: 10.3390/agronomy12030589
Tester M., Smith S. E., and Smith F. A. (1987). The phenomenon of “nonmycorrhizal” plants. Can. J. Bot. 65, 419–431. doi: 10.1139/b87-051
Tommerup I. C. (1984). Development of infection by a vesicular–arbuscular mycorrhizal fungus in Brassica napus L. and Trifolium subterraneum L. New Phytol. 98, 487–495. doi: 10.1111/j.1469-8137.1984.tb04142.x
van der Heijden M. G. A., Klironomos J. N., Ursic M., Moutoglis P., Streitwolf-Engel R., Boller T., et al. (1998). Mycorrhizal fungal diversity determines plant biodiversity, ecosystem variability and productivity. Nature 396, 69–72. doi: 10.1038/23932
Vatovec C., Jordan N., and Huerd S. (2005). Responsiveness of certain agronomic weed species to arbuscular mycorrhizal fungi. Renew. Agric. Food Syst. 20, 181–189. doi: 10.1079/RAF2005115
Veiga R. S., Howard K., and van der Heijden M. G. (2012). No evidence for allelopathic effects of arbuscular mycorrhizal fungi on the non-host plant Stellaria media. Plant Soil 360, 319–331. doi: 10.1007/s11104-012-1256-x
Veiga R. S., Faccio A., Genre A., Pieterse C. M., Bonfante P., and Heijden M. G. (2013). Arbuscular mycorrhizal fungi reduce growth and infect roots of the non-host plant Arabidopsis thaliana. Plant Cell Environ. 36, 1926–1937. doi: 10.1111/pce.12102
Walsh M. J., Powles S. B., Beard B. R., Parkin B. T., and Porter S. A. (2004). Multiple-herbicide resistance across four modes of action in wild radish (Raphanus raphanistrum). Weed Sci. 52, 8–13. doi: 10.1614/WS-03-016R
Wang Y., He X., and Yu F. (2022). Non-host plants: Are they mycorrhizal networks players? Plant Diversity 44, 127–134. doi: 10.1016/j.pld.2021.06.005
Wang Y., Li Y., Li S., and Rosendahl S. (2021). Ignored diversity of arbuscular mycorrhizal fungi in co-occurring mycotrophic and non-mycotrophic plants. Mycorrhiza 31, 93–102. doi: 10.1007/s00572-020-00997-1
Wang B. and Qiu Y. L. (2006). Phylogenetic distribution and evolution of mycorrhizas in land plants. Mycorrhiza 16, 299–363. doi: 10.1007/s00572-005-0033-6
Weremijewicz J., Sternberg L., and Janos D. P. (2016). Common mycorrhizal networks amplify competition by preferential mineral nutrient allocation to large host plants. New Phytol. 212, 461–471. doi: 10.1111/nph.14041
Wyatt G. A. K., Kiers E. T., Gardner A., and West S. A. (2014). A biological market analysis of the plant-mycorrhizal symbiosis. Evolution 68, 2603–2618. doi: 10.1111/evo.12466
Yaseen T., Ahmad S., Rehman K. U., Asad F., Waheed A., Gul R., et al. (2020a). Arbuscular mycorrhizal fungal spore density and root colonization in weeds of carrot field at Charsadda, Pakistan. Pakistan. J. Agric. Res. 33, 341. doi: 10.17582/journal.pjar/2020/33.4.841.848
Yaseen T., Shah M., and Nawaz G. (2020b). Arbuscular mycorrhizal fungal investigation in the weeds of the wheat field of Tehsil Tangi, district Charsadda, Khyber Pakhtunkhwa, Pakistan. J. Agric. Res. 26, 299–311. doi: 10.28941/pjwsr.v26i3.846
Keywords: nonhost weeds, arbuscular mycorrhizal fungi, plant root defenses, mycorrhizal networks, agroecological practices
Citation: Pagliarani I, Grassi A, Agnolucci M, Turrini A, Avio L and Giovannetti M (2025) The nonhost mycorrhizal status of weeds and its relevance to weed management in agroecology. Front. Agron. 7:1601329. doi: 10.3389/fagro.2025.1601329
Received: 27 March 2025; Accepted: 27 April 2025;
Published: 22 May 2025.
Edited by:
Aurelio Scavo, University of Messina, ItalyReviewed by:
Sheikh Muhammad Masum, Sher-e-bangla Agricultural University, BangladeshJadson Belem De Moura, Evangelical School of Goianésia, Brazil
Ioannis Gazoulis, Agricultural University of Athens, Greece
Copyright © 2025 Pagliarani, Grassi, Agnolucci, Turrini, Avio and Giovannetti. This is an open-access article distributed under the terms of the Creative Commons Attribution License (CC BY). The use, distribution or reproduction in other forums is permitted, provided the original author(s) and the copyright owner(s) are credited and that the original publication in this journal is cited, in accordance with accepted academic practice. No use, distribution or reproduction is permitted which does not comply with these terms.
*Correspondence: Irene Pagliarani, aXJlbmUucGFnbGlhcmFuaUBwaGQudW5pcGkuaXQ=
†These authors share first authorship