- 1Mazingira Centre, International Livestock Research Institute (ILRI), Nairobi, Kenya
- 2Faculty of Agriculture and Veterinary Sciences, University of Melbourne, Melbourne, VIC, Australia
- 3Integrative Agroecology Group, Research Division Agroecology and Environment, Agroscope, Zurich, Switzerland
Ruminant production systems in the arid and semi-arid regions of Sub-Saharan Africa confront severe challenges due to recurring droughts and the intensifying effects of climate change (CC). These systems grapple with numerous stress factors, including poor animal nutrition, water scarcity, gastrointestinal (GIT) parasite burdens, and heat stress, which contribute to below optimal animal productivity and a high environmental footprint. Addressing these issues urgently, by creating livestock systems resilient to CC that also promote better animal health, enhanced productivity, and reduced environmental impact, is paramount to safeguarding the livelihoods of the rural population. This 50-day study aimed to evaluate the effects of improved feeding and nutritional management in sheep, focusing on GIT parasite infections, feed intake and digestibility, liveweight (LW) gain, and enteric methane (CH4) emissions. We investigated the legume forage tree, Calliandra (Calliandra calothyrsus), as a high-quality feed source because of its rich crude protein content and its potential as a remedy for gastrointestinal tract (GIT) parasite infections, attributed to its high condensed tannin (CT) content. Twenty-eight Dorper lambs underwent random allocation across four treatments, each consisting of seven lambs. These treatments combined either a trickle infection or no infection with Haemonchus contortus with a supplementation (40% of diet on a dry matter basis or lack thereof) of the basal diet (Rhodes grass hay) with dried Calliandra leaflets. The treatments were: UnHay (uninfected, fed on hay-only), InHay (infected, fed on hay-only), InHay+Cal (infected, fed on hay plus Calliandra), and InHay+Cal+PEG [infected, fed on hay, Calliandra, and polyethylene glycol (PEG)]. The latter was to evaluate the potential effects of Calliandra’s condensed tannins. The results show that lambs in the InHay+Cal treatment exhibited a higher packed cell volume (PCV) and lower faecal egg counts (FEC) compared to the InHay group. No effects of infection on the other measured variables were observed in unsupplemented lambs. Calliandra supplementation increased total feed dry matter intake (DMI) by 20% (61.8 vs. 51.7 g DM/kg LW0.75) (InHay+Cal vs. InHay) and enhanced LW gain (7.2 g/d) in the InHay+Cal group, whereas the unsupplemented infected group (InHay) experienced LW loss (-26.6 g/d). Calliandra supplementation to infected lambs reduced daily CH4 emission by 15% (13.9 vs. 16.2 g/d) and CH4 yield (g/kg DMI) by 30% (18.7 vs. 26.5 g/kg DMI), compared to emissions from unsupplemented infected lambs. Nonetheless, Calliandra supplementation decreased the digestibility of crude protein and fibre and raised the faecal nitrogen (N) output to N intake (FN/NI) ratio. The effects of PEG supplementation on CT activity remained inconclusive. The study concludes that a 40% replacement of a protein-deficient basal diet with Calliandra may be excessive. However, the findings underscore the considerable advantages of integrating Calliandra into farming systems. Such nature-based solution control GIT parasite infections and their lifecycle, bolster the nutritional value of a deficient basal diet, improve animal productivity cost-effectively, and mitigate enteric methane emissions both in absolute terms and intensity.
1 Introduction
According to the FAO database (FAO, 2018), the global population of sheep and goats is 1.18 billion and one billion, respectively. Africa has the second-highest population of small ruminants in the world at 352 million and 388 million heads of sheep and goats, respectively. These small ruminants play an important socioeconomic role as a source of nutrition, income, and other intangible benefits, such as savings, insurance against emergencies, and cultural and ceremonial purposes (Kosgey et al., 2008). They also play a complementary role to other livestock by utilizing feed resources in natural grasslands (Baker & Rege, 1994). Globally, the livestock sector greenhouse gas (GHG) emissions are equivalent to 7.1 Gt CO2-eq/year (14.5% of anthropogenic GHG emissions), of which the share of small ruminants is 6.5% (Gerber et al., 2013). Over 55% of emissions from small ruminants are attributed to enteric methane (CH4) (Gerber et al., 2013).
The most significant production constraints facing small ruminant production systems in the tropics are gastrointestinal tract (GIT) parasite infection, shortage of feed and grazing land, and water scarcity due to recurrent droughts and climate change (CC) (Armson et al., 2021; Rahimi et al., 2021). Finding cost-effective solutions to these challenges is crucial for improving animal productivity, health, and welfare, and safeguarding the livelihoods of smallholder livestock keepers.
Feeding ruminants during dry seasons in the tropics is characterized by the provision of forages of poor feeding value (poor digestibility, low concentrations of crude protein (CP), and high concentrations of fibre and lignin). This is often below maintenance energy requirements of the animals and consequently leads to low productivity, loss of liveweight (LW), high enteric CH4 emissions and results to high emission intensity (emissions per unit of animal product) (Kurihara et al., 1999; Chaokaur et al., 2015; Goopy et al., 2020). In the tropics, there is a diversity of forage plants, especially legumes, which contain secondary compounds such as condensed tannins (CT) (Bhatta et al., 2013). Condensed tannins can directly affect rumen microorganisms, thereby decreasing CH4 production (Tavendale et al., 2005; Berça et al., 2023). Furthermore, consumption of CT-containing forages can lead to reduced shedding of GIT nematode (GIN) eggs, hence reducing the contamination of pastures (Min et al., 2005) as well as lower host infection through reduced parasite numbers in the GIT (Brunet et al., 2008; Oliveira et al., 2013). There is currently a challenge with GIN resistance to most commonly used deworming drugs. This widespread increase in anthelmintic resistance (Greiffer et al., 2022) has prompted the search for alternative GIN control solutions, and efforts are also supported by increased consumer demand for more sustainable livestock production with reduced use of chemicals (Waller, 1999).
Supplementing grazing ruminants with legume forage trees, such as Calliandra (Calliandra calothyrsus), which is high in CP and CT, during the dry seasons may provide various benefits to livestock production systems, including reducing LW loss, providing nutrients to enhance animal resilience to GIN, and reducing CH4 emissions (Kaitho et al., 1996; Min et al., 2005; Piñeiro-Vázquez et al., 2018). Calliandra is a multipurpose tree legume native to humid and sub-humid regions of Central America and Mexico. It was introduced to the Central Highlands of Kenya in the 1980s and has since been widely promoted and adopted as a supplement for ruminants on low-quality forages (Wambugu et al., 2001). Various studies conducted in Sub-Saharan Africa (SSA) have shown that supplementation with tropical tannin-rich forage legumes increases the productivity of animals fed on poor-quality diets (Kinuthia et al., 2007; Yisehak et al., 2014). Forage trees containing CT have also demonstrated anti-methanogenic activity when fed to ruminants (Tiemann et al., 2008; Piñeiro-Vázquez et al., 2018). However, to our knowledge, no research in SSA has evaluated the effects of these forages on the health of animals in combination with enteric methane emissions. In this context, our study assesses the potential of Calliandra as a supplement to sheep consuming poor-quality Rhodes grass (Chloris gayana) hay evaluating its effects on voluntary feed intake, LW gain (LWG), nitrogen and energy partitioning, GIN infection (Haemonchus contortus) response, and enteric CH4 emissions. We hypothesized that supplementation of poor-quality Rhodes grass hay with Calliandra would enhance sheep productivity (LWG) and health while reducing enteric CH4 emissions.
2 Materials and methods
This study was conducted at the Mazingira Centre, International Livestock Research Institute (ILRI), Nairobi, Kenya. The experimental protocol was reviewed and approved by the ILRI Institutional Animal Care and Use Committee (IACUC; Reference number 2022-03).
2.1 Animal management and experimental design
Dorper lambs aged 10−11 months old, mixed sex, and 28.1 ± 2.7 kg liveweight (LW) were selected from a cohort of lambs born at the ILRI Kapiti Research Station & Wildlife Conservancy, located 60 km South-East of Nairobi. In this conservancy, sheep flocks are kept on a grassland interspersed with Acacia trees and shrubs. These lambs were then transported to the ILRI farm in Nairobi where they underwent a 3-week quarantine period. During this period, they were kept as a single group and fed Rhodes grass (Chloris gayana) hay before starting the experiment. At the beginning of the quarantine period, each lamb was given anthelmintic treatment using 10% albendazole (albafas) at a dose of 8 mg/kg LW. Subsequently, faecal examinations were conducted regularly to ensure that they attained a worm-free status and remained worm-free.
At the end of the quarantine period, the animals were acclimatized to their experimental diets for a period of seven days. Thereafter, the experiment was started lasting 50 days (Days 1–50). For the initial 40 days (Days 140), the lambs were housed in individual pens within an animal shed each equipped with feed and water bins. On Day 41, lambs were moved to individual metabolic crates and kept for seven days during which total faeces and urine were collected over the last five days (Days 43−47). Afterwards, the lambs were transferred to respiration chambers to measure CH4 emission over the last three days of the experiment (Days 48−50).
The experiment used a completely randomized design. Twenty-eight Dorper lambs were subdivided in a balanced manner (LW and sex) into four experimental groups (seven animals each; three castrated males and four females). The lambs were then randomly allocated to four experimental treatments that combined infection status (infected or uninfected with Haemonchus contortus) and Calliandra supplementation (supplemented or unsupplemented), and fed a basal diet of poor-quality Rhodes grass hay. The experimental treatments were uninfected fed on hay only (UnHay), infected fed on hay only (InHay), infected fed on hay plus Calliandra supplement (InHay+Cal), and infected fed on hay plus Calliandra supplement plus polyethylene glycol (PEG) supplement (InHay+Cal+PEG). InHay+Cal+PEG treatment was used to evaluate the anticipated role of CT in Calliandra on feed intake, digestibility, N metabolism, and CH4 emissions, as PEG binds to tannins to form stable complexes (Makkar et al., 1995). The level of Calliandra supplementation was set to replace 40% of the voluntary feed intake (dry matter basis) of the Rhodes grass hay, whereas the level of PEG supplementation was set to neutralize the total concentration of CT in the diet (PEG : CT ratio of 1:1), based on results of preliminary laboratory analyses.
Given the availability of only three animal respiration chambers, the experimental animals were subdivided into ten batches (Eight batches of three animals each and two batches of two animals each). The batches entered the experiments in a staggered manner, every three days. Batches were balanced as much as possible for sex and experimental treatment, each batch included only one lamb from either of the four treatment groups.
2.2 Feeds and feeding
The Rhodes grass hay had 92.2% dry matter (DM) and on DM basis it contained 90.36% organic matter (OM), 73.0% neutral detergent fibre (NDF), and 5.6% crude protein (CP) (Table 1). Calliandra leaflets had a DM content of 91.8%, 94.1% OM, 17.4% NDF, 21.1% CP, and 9.2% total condensed tannins (CT) (Table 1). Calliandra leaves were harvested from a single farm in Kakamega County (northwest of Nairobi). Branches were cut and allowed to dry in the field over PVC sheets until some primary leaflets started self-detaching from the leaf rachis. Primary leaflets (pinnae) were then manually harvested. The harvested material was transported in jute sacks and stored under protection from rain and sun until the start of the experiment. Thus, the Calliandra material used in this study was composed of secondary leaflets in the pinnae.
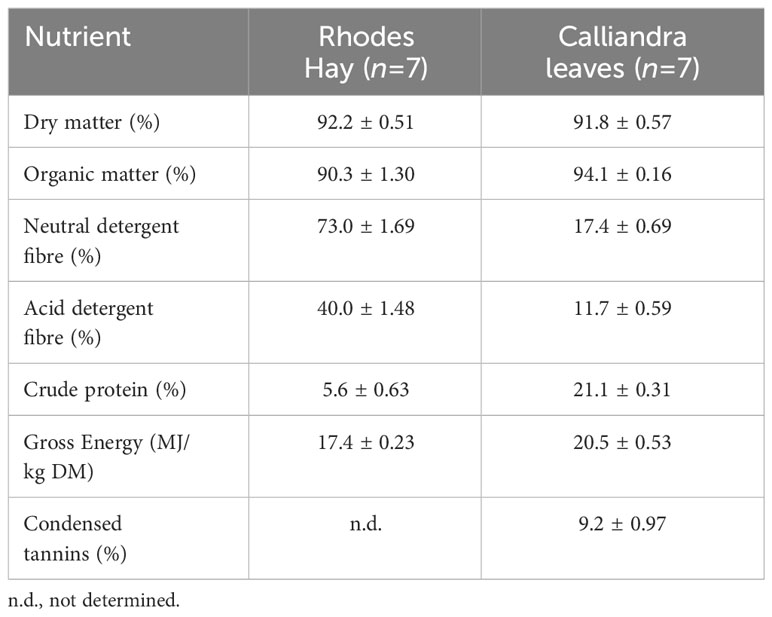
Table 1 Mean ( ± standard deviation) chemical composition (dry matter (DM) basis) of Rhodes grass (Chloris gayana) hay and air-dried Calliandra (Calliandra calothyrsus) leaves.
During the seven-day acclimatization period, all animals were fed on chaffed Rhodes grass hay ad libitum. During the first five days of acclimatization, lambs in the supplemented experimental treatments (InHay+Cal and InHay+Cal+PEG) were gradually offered Calliandra. By the end of the acclimatization period, the supplemented animals had adjusted to their full allowance of Calliandra, with no refusals. Voluntary feed intake (VFI) of Rhodes grass was determined during the acclimatization period. The average VFI of the hay was determined on a metabolic LW basis (LW0.75). The unsupplemented animals were fed hay at 1.1 VFI. Calliandra supplementation was set to replace 40% of the VFI of hay (DM basis); hence, the hay offered to Calliandra-supplemented animals was set at 0.7 VFI of hay. This feeding level remained consistent throughout the study.
Lambs in the InHay+Cal+PEG treatment were offered the Calliandra supplement sprayed with PEG. The amount of PEG used was based on preliminary sampling and analysis of Calliandra samples that had a CT concentration of 28.2 g CT/kg DM. The preliminary sampling was done 12 weeks prior to collection of the Calliandra forage used in the experiment (Both the preliminary and experimental samples were collected from the same farms). A solution of PEG was prepared daily using 250 g of PEG 6000 dissolved in 1250 ml distilled water and depending on the intake of Calliandra in the ration, a fixed volume of the solution was sprayed onto the Calliandra forage twice a day. The average daily dose of PEG solution was 50 ml (i.e., 10 g PEG/animal), providing a PEG : CT ratio of 1:1, a level slightly higher than 1:1 required to neutralize CT activity. Further analysis of CT in the Calliandra fed during the experiment (using specific standards) was carried out at the end of the study, which showed a higher concentration of total CT (92.1 g/kg DM), resulting in a PEG : CT ratio of 1:2.8.
The daily rations of hay and Calliandra were divided into two equal portions and fed at 0830 and 1630 h. The lambs were offered water ad libitum using a plastic bucket, and a mineral block was provided free of choice.
2.3 Experimental larvae preparation and infection
Larvae were prepared by infecting three adult donor sheep of the Dorper breed with a single dose of 5,000 H. contortus L3 larvae suspended in distilled water, prior to deworming them by oral dosing with 10% albendazole (albafas) at a dosage of 8 mg/kg LW. After a 21-day prepatent period, following L3 larvae dosing, the total faecal output was collected from each donor sheep. Larval production was performed according to the process described by Mwangi et al. (2023). In brief, the jars containing the faeces-vermiculite mix were incubated at 27°C (Lovibond, Germany). Hatched larvae were washed off the sides of the jars into cell culture flasks, and each flask was filled up to 60 ml (distilled water containing larvae) and stored at 4°C until they were administered to the lambs in the infection experimental treatments. The viability of the larvae was confirmed under a dissection microscope (Zeiss, Model Axiocam ERc 5s, Germany).
The larvae doses used for the experiment were calculated as described by Mwangi et al. (2023). Starting from Day 1 of the study, lambs in the infection experimental treatments (InHay, InHay+Cal and InHay+Cal+PEG) were dosed with 1000 H. contortus L3 stage larvae over five consecutive days, leading to a total dose of 5,000 larvae per animal. The uninfected lambs were sham-infected with clean water as a control. The donor lambs were not included in the experiment.
2.4 Measurements and sample collection
2.4.1 Parasitological examination and haematology
A faecal sample for parasitological examination was collected per animal on Day 1 of the experiment, and weekly thereafter. The sample was collected directly from the rectum and placed in a plastic sampling bottle. Faecal egg counting was conducted using a modified McMaster technique (Whitlock, 1948).
At the times of faecal sampling, blood samples were collected via jugular venipuncture into EDTA sampling bottles for hematological examination. Packed cell volume (PCV, %) was determined using a hematology analyzer (Nihon Kodehn, MEK 6550K, Japan).
2.4.2 Feed intake
The lambs were fed individually throughout the study. A sample of the feeds on offer (hay and Calliandra) were collected daily during meal weighing. These samples were stored at -20°C, weekly, and subsequently oven-dried for DM determination. Feed refusals were collected daily from each animal before the morning feeding and treated similarly to the feeds on offer, pooled weekly per animal and then oven-dried.
Feed intake by individual animals (feed on offer minus refusals, for each feed item) was calculated on DM basis for the pen period (Days 1−40), during their time in metabolic crates (Days 43−47) and respiration chambers (Days 48−50). Lambs supplemented with Calliandra showed no refusal of Calliandra forage.
2.4.3 Liveweight and liveweight gain
Liveweight (LW) measurement of each lamb was recorded before morning feeding on Day 1, and weekly thereafter until the end of the experimental period (Day 50) using an outdoor automatic digital weighing scale (Gallagher, Model 3B4092, Hamilton, New Zealand).
2.4.4 Total faeces and urine outputs (balance period)
A total collection of daily outputs of faeces and urine was conducted over Days 43−47, while the lambs were kept in individual metabolic crates. Collections were not carried out over the first two days in metabolic crates (Days 41 and 42 due to disruption in feed intake caused by animal movement from pens to crates). The metabolic crates allowed for separate collection of faeces and urine for each animal. Faeces passed through the crate floor to a mesh below, while urine passed through this mesh and flowed into a Perspex tray, eventually being collected in a bottle containing 70 ml of 10% HCl. This setup ensured the urine pH remained below 3 at the conclusion of the 24-hour collection period and prevented ammonia volatilization. The urine tray was sprayed with fresh water five times daily during daylight to prevent nitrogen loss from urine droplets. Roughly 0.5 L of water was used for this, and its addition did not influence urine nitrogen and energy calculations.
Every morning before feeding, the daily faecal and urine outputs were collected. Faecal outputs from each lamb were weighed and recorded, a 10% sub-sample was stored at -20°C in a zip lock bag. Simultaneously, the day’s total urine (plus water) output was sieved and its volume recorded, and a 10% sub-sample was stored at -20°C in an airtight bottle. The daily faecal and urine samples were then pooled by individual animals for future processing for determination of DM (faeces) and subsequent chemical analyses.
2.4.5 Methane emissions from enteric fermentation
Enteric CH4 emission from each lamb was measured over three consecutive days (Days 48 to 50) using three open-circuit respiration chambers (No Pollution Industrial Systems Limited, UK) as described by Mwangi et al. (2023). During this measurement period, each lamb spent one day in each of the three chambers. On each day, the CH4 measurement was conducted over 23.5 hours (starting at 0830 h and finishing to 0800 h following day), with chambers opened twice a day for 15 min each for feeding and watering (morning and afternoon) and cleaning (before morning feeding). Emissions calculations were extrapolated to 24-h periods.
During measurement, the internal temperature of the chambers was maintained at 22°C with a relative humidity of 47%. The ventilation rate of the chambers was set to 8 l/s. A cavity ringdown laser absorption spectrometer (Picarro G2508 analyzer, Santa Clara, USA) was used to measure the CH4 concentrations (in ppm) at intervals of approximately five minutes (including the incoming background gas sample). CH4 emissions were calculated using the difference between inlet and outlet gas concentrations multiplied by volumetric air flow and corrected to standard temperature and pressure (25°C and 101,300 Pa).
A whole-system recovery of known volumes of pure CH4 into the respiration chamber system was carried out before the start of the methane measuring period and at the end of the measurement period using a gas phase titration unit (Environics 4020, Environics Inc., Tolland, USA). The CH4 recovery rate was 98−106%.
2.4.6 Rumen sampling and pH measurement
Once the lambs exited from the respiration chambers (0900 h, before feeding), rumen samples were collected from each sheep using an orogastric tube. With the animal properly restrained and the neck extended forward, a PVC tube speculum was placed in the mouth. A flexible orogastric tube was gently passed through the speculum to the base of the tongue. While the animals were allowed to swallow, the tube was slowly pushed through the oesophagus and into the rumen. Sample extraction was performed once the tube was in the rumen using a pump connected to the tube. The first 10–20 ml of rumen sample collected was discarded, and a second sample (~40 ml) was collected, and pH was measured immediately using a pH and conductivity meter (Jenway 3540 model, UK).
2.5 Laboratory analyses
Samples of feeds on offer (pooled on a weekly basis) and within-animal pooled samples of feed refusals and faeces were oven-dried (Genlab oven, Model SDO/425/DIG, UK) at 50°C for 72 h. The dried samples were ground to pass a 5 mm sieve using a Wiley mill (Model MF 10B, IKA Werke, Willmington, N.C., USA) and then passed a 1 mm sieve using a hammer mill (MF 10 basic, IKA, Werke GmbH & CO. KG, Staufen, Germany). The total DM was determined by drying the samples at 105°C in an oven (Genlab oven, model SDO/425/TDIG, UK) for 24 h while ash was determined by combustion in a muffle furnace (Nabertherm GmbH, Germany) at 550°C for six hours according to the methods of the Association of Official Analytical Chemists (AOAC, 1990 methods no. 924.05). Feed, refusals, and faecal samples were analysed for NDF and ADF using the methods of Van Soest et al. (1991) with an Ankom 200 fibre analyzer (model A2001, USA). The total nitrogen (N) content in urine samples was determined using the micro-Kjeldahl procedure (AOAC, 1990, method no. 988.05). The total N content in feed, refusals, and faecal samples was determined using an organic elemental analyzer (Vario Max, model vario Solid Sampler, Germany). Total condensed tannins (CT) in Calliandra were preliminarily analysed in the Competence Division Method Development and Analytics Laboratory of Agroscope (Posieux, Switzerland) using the modified butanol-HCI procedure described by Terrill et al. (1992), using cyanidin to set the standard calibration curve. Subsequent analyses of CT in Calliandra, conducted at the end of the study, were performed at the Texas A&M Agrilife Center (Stephenville, TX) using the simplified butanol-HCl method and specific plant standards, as described by Wolfe et al. (2008).
Gross energy (GE) content of feed on offer, feed refusal, and faeces was determined according to the methods of Harris (1970) using a bomb calorimeter (Parr 6200, model A1290DDEE, UK). The gross energy contents of the urine samples were determined by a method described by Zhao et al. (2016), where the urine was soaked in a filter paper with a predetermined GE, the soaked filter paper was dried (50° C, 2 h), weighed, and its GE determined in a bomb calorimeter. To calculate the GE of urine, the GE value of the filter paper was subtracted from the GE value of the urine-soaked filter paper.
2.6 Calculations
Daily feed intake of DM and its constituents (OM, N, NDF, ADF, GE on a DM basis) by individual animals was calculated from the amounts on offer and refusals. Feed intake was calculated for the periods when the animals were in the individual pens, metabolic crates, and respiration chambers. Daily feed intake was expressed both daily (g/d) and as g/kg metabolic LW (g/kg LW0.75).
The average daily liveweight gain (LWG, g/d) was calculated both for the pen period (Days 1−40) and entire experimental period (Days 1−50).
Apparent total tract digestibility (%) of feed DM and its constituents (OM, N, NDF, ADF, GE; DM basis) was calculated from the ingested and excreted amounts in faeces of each component during the balance period (Days 43–47). For the same period, nitrogen partitioning into faeces, urine, and retained was calculated. Partitioning was expressed as % of N intake.
Daily gross energy intake (GEI) during the balance period was calculated using the DMI and GE concentrations. The partitioning of GEI into faeces, urine, and CH4 was calculated, as well as digestible energy (DE) and metabolisable energy (ME). Digestible energy (DE) was calculated by subtracting faecal energy (FE) from GEI (DE=(GEI-FE)), while ME was calculated by subtracting FE, urine energy (UE) and energy lost in CH4 from the GEI (ME=GEI-(FE+UE+CH4E). The methane energy for the balance period was calculated using the CH4 energy conversion factor (Ym, MJ CH4/MJ GEI) determined during the chamber period.
Given that each animal rotated throughout the three respiration chambers (one day per chamber), the average daily CH4 emissions from each animal were calculated by averaging the emissions over the three days. Calculations of the daily emissions in each chamber accounted for the ventilation rate and net concentrations at each data point, gas conditions, and CH4 recovery rate. Daily CH4 emissions were expressed on an absolute basis (g/d), CH4 yield [g/kg DMI, g/kg organic matter (OM) intake, OMI; g/kg digestible DMI (DDMI) and g/kg digestible OMI (DOMI)], and Ym (CH4 energy/GEI).
2.7 Data analysis
Data were analysed using a linear mixed-effects model in R statistical software (version 4.1.2; R Development Core Team, USA). The data sets were first tested for normality by producing residual vs fitted plots, normal quantile-quantile plots, and density plots of the residuals in R., Visual inspection of the plots was done to confirm the normal distribution of data. The model for each variable was fitted using the “lmerTest” package in R. The treatment was set as the fixed effect, while the sex of the lambs and batch number were set as random effects. ANOVA type 3 was used to test the significance of the fixed factors. The least square means were calculated using the “lsmeans” package and Tukey’s built-in “Multicompview” package in R was used to separate the means per treatment. The significance level was set at P<0.05.
3 Results
3.1 Packed cell volume and faecal egg count
The packed cell volumes (PCV) on Day 1 were comparable across treatments (P>0.05), a trend that persisted until Day 21 post-infection (Table 2). However, by Day 50, the PCV of infected, unsupplemented lambs (InHay) was significantly lower than that of lambs in other treatments (P<0.05). At this stage, there was no difference in PCV between Calliandra supplemented lambs, regardless of PEG supplementation (InHay+Cal and InHay+Cal+PEG) (Table 2). The faecal egg counts (FEC) on Day 1 confirmed that all lambs were worm-free (Table 2). By Day 21 post-infection, there was no significant difference in FEC among the infected lambs (P>0.05). Yet, on Day 50, FEC in InHay lambs was significantly higher than in Calliandra-supplemented lambs (P<0.05) (Table 2). Throughout the study, uninfected lambs remained worm-free.
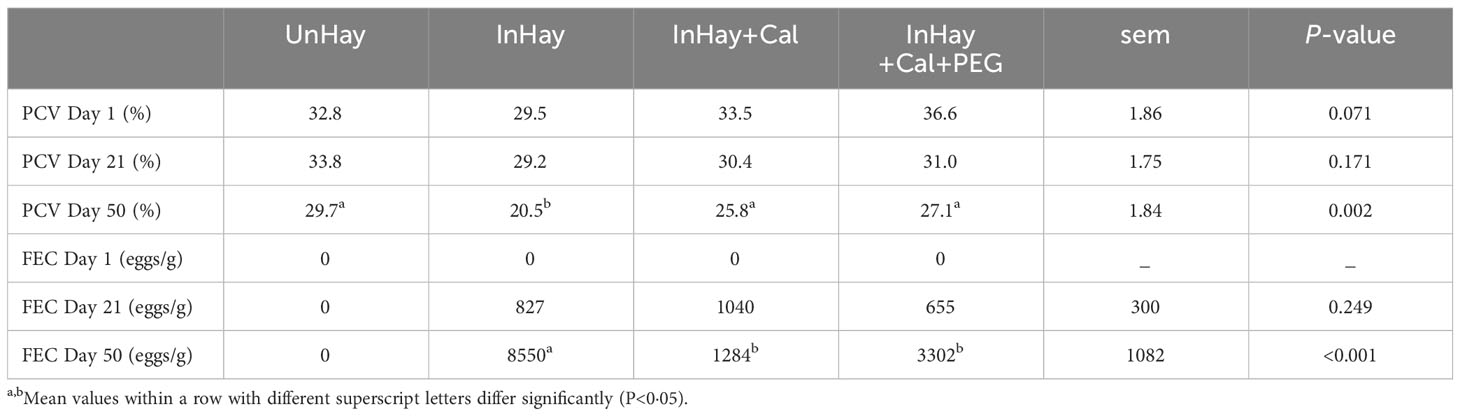
Table 2 Mean ( ± standard error of means, sem) packed cell volume (PCV, %) and faecal egg counts (FEC, eggs/g) on Days 1, 21 and 50 of the study for lambs fed on Rhodes grass hay (control diet, Hay) and either uninfected with H. contortus (UnHay), infected with H.contortus (InHay), infected and supplemented with Calliandra forage (InHay+Cal) or infected and supplemented with Calliandra forage and polyethylene glycol (InHay+Cal+PEG).
3.2 Feed intake, liveweight and liveweight gain
Experimental treatments significantly influenced feed DMI (g/d and g/kg LW0.75) (Table 3). Calliandra-supplemented lambs had a significantly higher DMI than unsupplemented lambs (P<0.05), but PEG supplementation did not alter DMI (P>0.05). Moreover, infection status did not influence feed intake in unsupplemented lambs (Table 3). There was no significant difference in lamb LW across treatments on days 1, 40, and 50 (P>0.05) (Table 3). However, treatments significantly affected LWG during both the pen period (Days 1−40) and the whole experimental period (days 1−50) (Table 3). Notably, Calliandra-supplemented lambs exhibited positive LWG, while unsupplemented lambs experienced weight loss. Supplementation with PEG did not influence the LWG of Calliandra-supplemented lambs. PEG supplementation had no effect on LWG in Calliandra-supplemented lambs (InHay+Cal+PEG vs. InHay+Cal) (P>0.05). Furthermore, infection status did not affect LWG in unsupplemented lambs (P>0.05) (Table 3).
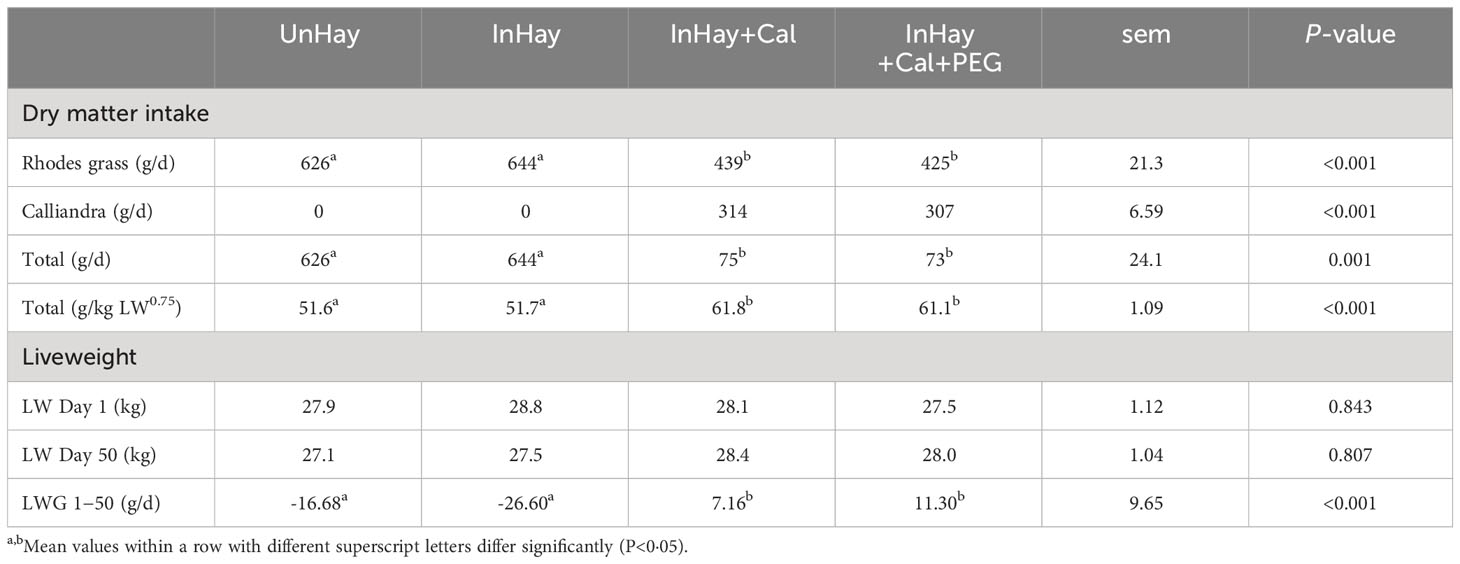
Table 3 Mean ( ± standard error of means, sem) intakes of dry matter (DM) in Rhodes grass hay, Calliandra and total intake (g/d and per unit of metabolic weight, LW0.75) over the entire experiment (50 days) by lambs fed on Rhodes grass hay (control diet, Hay) and either uninfected with H. contortus (UnHay), infected with H.contortus (InHay), infected and supplemented with Calliandra forage (InHay+Cal) or infected and supplemented with Calliandra forage and polyethylene glycol (InHay+Cal+PEG).
3.3 Nutrient intakes, apparent feed digestibility, nitrogen and energy partitioning (balance period)
Calliandra-supplemented lambs (InHay+Cal and InHay+Cal+PEG) had higher (P<0.05) intakes of DM, OM, and CP than the unsupplemented lambs (UnHay and InHay) (Table 4). Unsupplemented lambs had higher (P<0.05) NDF intake than Calliandra-supplemented lambs, while there was no significant treatment effect on ADF intake (Table 4). Similarly, PEG supplementation had no effect on DMI or its constituents in Calliandra-supplemented lambs. Infection had no effect on the feed intake of Calliandra-unsupplemented lambs (UnHay and InHay) (Table 4).
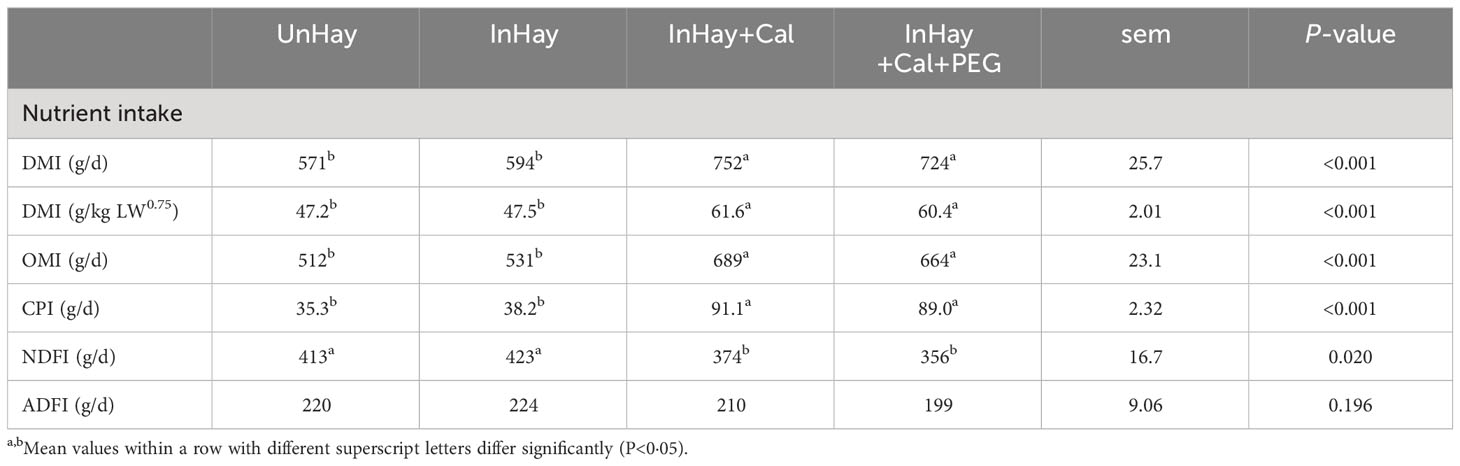
Table 4 Mean ( ± standard error of means, sem) dry matter intake (DMI), organic matter (OMI), crude protein (CPI), neutral detergent fibre (NDFI) and acid detergent fibre (ADFI) by lambs over the balance period fed on Rhodes grass hay (control diet, Hay) and either uninfected with H. contortus (UnHay), infected with H.contortus (InHay), infected and supplemented with Calliandra forage (InHay+Cal) or infected and supplemented with Calliandra forage and polyethylene glycol (InHay+Cal+PEG).
The apparent total tract digestibility of DM, OM, CP, NDF, and ADF differed significantly (P<0.05) among the experimental treatments (Table 5). Calliandra-supplemented lambs (InHay+Cal and InHay+Cal+PEG) had similar digestibility. However, lambs not receiving PEG (InHay+Cal) had significantly lower crude protein (CP) digestibility (P<0.05) than those that did receive PEG (InHay+Cal+PEG). While the digestibility of DM, OM, NDF, and ADF was numerically and slightly higher in PEG-supplemented lambs, it wasn’t statistically significant (P>0.05) compared to lambs without PEG. Calliandra-supplemented lambs (InHay+Cal and InHay+Cal+PEG) had significantly lower (P<0.05) feed digestibility than the unsupplemented ones (UnHay and InHay). However, there were no discernable differences (P>0.05) in feed digestibility between the uninfected and infected lambs that were solely on a hay diet (UnHay vs. InHay) (Table 5).
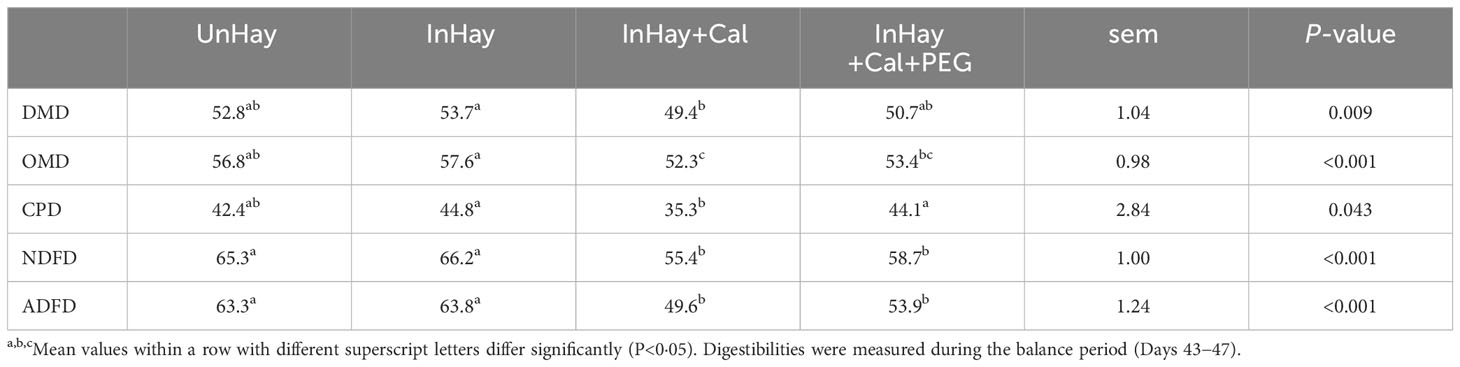
Table 5 Mean ( ± standard error of means, sem) digestibilities (%) of dry matter (DMD), organic matter (OMD), crude protein (CPD), neutral detergent fibre (NDFD), acid detergent fibre (ADFD) and gross energy (GED) by lambs fed on Rhodes grass hay (control diet, Hay) and either uninfected with H. contortus (UnHay), infected with H.contortus (InHay), infected and supplemented with Calliandra forage (InHay+Cal) or infected and supplemented with Calliandra forage and polyethylene glycol (InHay+Cal+PEG).
The experimental treatments exhibited significant differences (P<0.05) in nitrogen (N) intake and its partitioning (g/d) into faeces (FN), urine (UN), and retained (RN). Calliandra-supplemented lambs (InHay+Cal and InHay+Cal+PEG) had similar (P>0.05) daily intake and partitioning of N. However, lambs not given PEG had higher (P<0.05) faecal partitioning than those given PEG (InHay+Cal vs. InHay+Cal+PEG) (Table 6). As expected, lambs without Calliandra supplementation (UnHay and InHay) had lower (P<0.05) daily N intake, faecal partitioning, and retained N compared to Calliandra-supplemented lambs (InHay+Cal vs. InHay+Cal+PEG) (Table 6). There was no significant difference (P>0.05) in daily N partitioning into urine between the unsupplemented lambs (UnHay and InHay) and the Calliandra-supplemented lambs without PEG (InHay+Cal). However, lambs given both Calliandra and PEG (InHay+Cal+PEG) had the most pronounced (P<0.05) daily partitioning of N into urine. The infection status did not influence (P>0.05) the daily N intake or partitioning in unsupplemented lambs (UnHay vs. InHay) (Table 6).
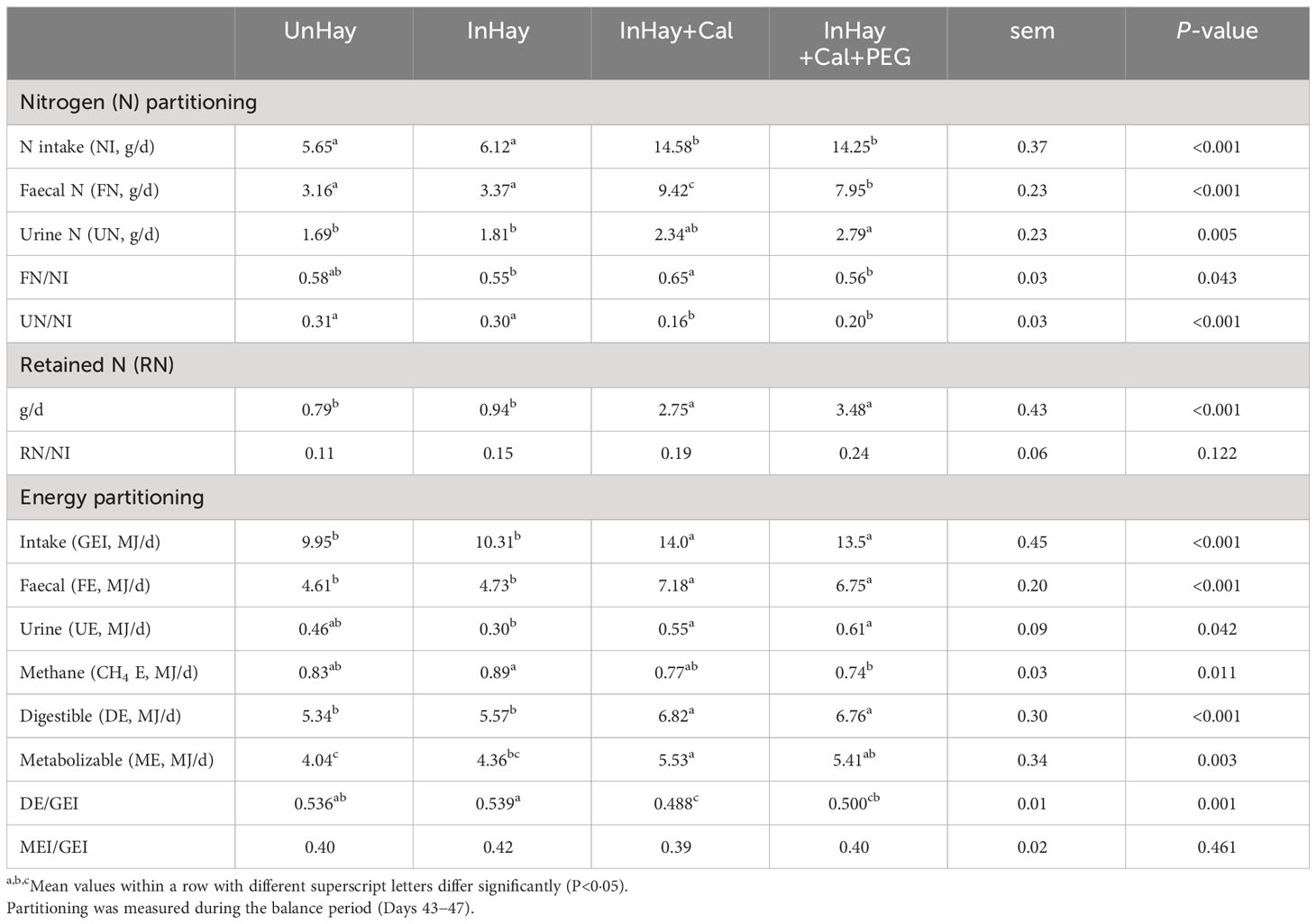
Table 6 Mean ( ± standard error of means, sem) intakes of nitrogen (N) and gross energy (GEI) and their partitioning by lambs fed on Rhodes grass hay (control diet, Hay) and either uninfected with H. contortus (UnHay), infected with H.contortus (InHay), infected and supplemented with Calliandra forage (InHay+Cal) or infected and supplemented with Calliandra forage and polyethylene glycol (InHay+Cal+PEG).
The ratio of faecal N to N intake (FN/NI) was the highest (P<0.05) in lambs that received Calliandra supplementation without PEG (InHay+Cal), with no differences (P>0.05) between the other treatments (UnHay vs. InHay vs. InHay+Cal+PEG) (Table 6). In contrast, the ratio of urinary N to N intake (UN/NI) did not differ (P>0.05) between the Calliandra-supplemented lambs (InHay+Cal and InHay+Cal+PEG). However, these lambs had lower (P<0.05) UN/NI ratios than the unsupplemented lambs (UnHay and InHay). Infection status did not impact the FN/NI or UN/NI ratios of unsupplemented lambs (P > 0.05) (Table 6). The retained N to N intake ratio (RN/NI) did not significantly vary (P>0.05) across experimental treatments. However, lambs in the combined Calliandra and PEG treatment (InHay+Cal+PEG) showed a higher RN/NI, particularly when compared to unsupplemented lambs (P=0.12) (Table 6).
Experimental treatments differed in the daily intake of GE (GEI) and its daily partitioning (megajoules/d, MJ/d) into faecal energy (FE), urinary energy (UE), CH4 energy (CH4 E), digestible energy (DE), and metabolisable energy (ME). Overall, both GEI and its partitioning were higher (P<0.05) in Calliandra-supplemented lambs (InHay+Cal and InHay+Cal+PEG) than in those without supplementation (UnHay and InHay) (Table 6). PEG supplementation did not affect (P>0.05) the daily GEI or its partitioning in Calliandra-supplemented lambs (InHay+Cal+PEG vs. InHay+Cal). Similarly, the infection status did not affect (P>0.05) the daily GEI and its partitioning in unsupplemented lambs (UnHay and InHay) (Table 6). The ratio of digestible energy (DE) to GE (DE/GEI) differed among the experimental treatments. However, the ME/GEI ratio did not differ (P>0.05) between the experimental treatments (Table 6).
3.4 Enteric methane emission
Daily CH4 emissions CH4 yield, and CH4 conversion factor (Ym) differed significantly among treatments (Table 7). Calliandra-supplemented lambs emitted less CH4 compared to their unsupplemented counterparts (Table 7). PEG supplementation had no discernible effect on these measures. Infection had no effect (P>0.05) on CH4 emissions in unsupplemented lambs (Table 7).
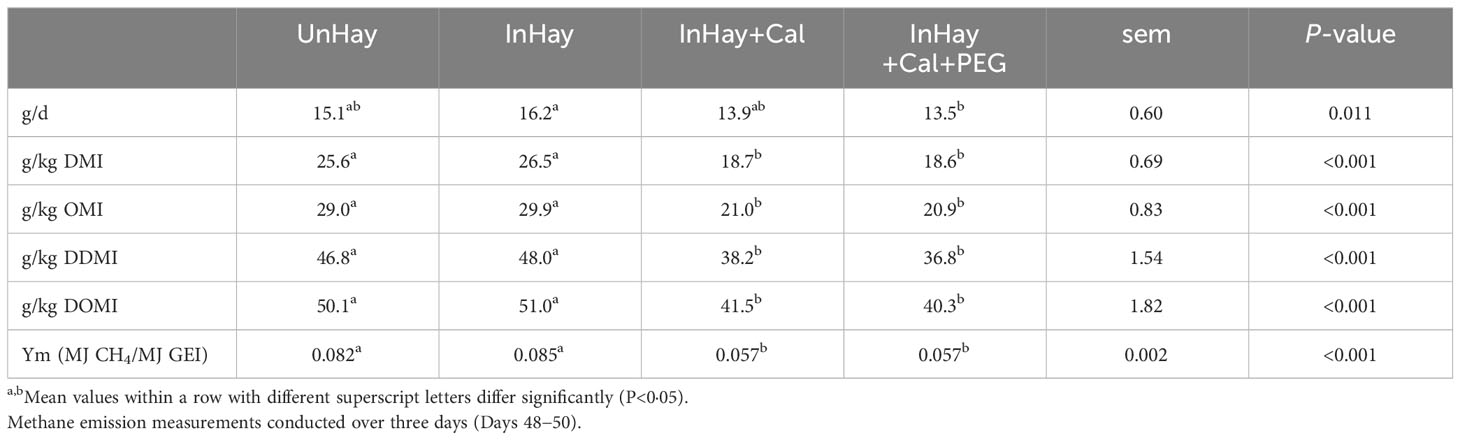
Table 7 Mean ( ± standard error of means, sem) daily methane emission (g/d), methane yield [expressed per kg of intake of dry matter (DMI), organic matter (OMI), digestible DMI (DDMI), digestible organic matter intake (DOMI)] and methane conversion factor (Ym, MJ CH4/MJ GEI) by lambs fed on Rhodes grass hay (control diet, Hay) and either uninfected with H. contortus (UnHay), infected with H.contortus (InHay), infected and supplemented with Calliandra forage (InHay+Cal) or infected and supplemented with Calliandra forage and polyethylene glycol (InHay+Cal+PEG).
3.5 Rumen pH
Rumen pH did not differ significantly (P>0.05) across treatments. The average pH for the respective treatments were: UnHay (7.06), InHay (7.06), InHay+Cal (7.15), and InHay+Cal+PEG (7.12).
4 Discussion
4.1 Effects of Haemonchus contortus infection on animal health, feed intake and digestibility, nutrient partitioning, liveweight gain and CH4 emission
This study found reduced Haemonchus contortus infection with Calliandra supplementation. However, there were no effects of infection on feed intake and digestibility, nutrient partitioning, liveweight gain, or CH4 emission. These findings confirmed previous observations in lambs infected with H. contortus (Mwangi et al., 2023). However, Mwangi et al. (2023) found that LW loss by infected lambs was significantly higher and twice as high than the LW loss in uninfected lambs, similarly in the current study, the LW loss in the infected lambs fed solely on hay was 37% higher than that of their uninfected counterparts, however this difference was not statistically significant.
A decrease in PCV and increase in FEC are reliable indicators of GIN infection (Xiang et al., 2021). In this study, parasite infection in the infected treatment groups was successful. Our results also indicate the effects of infection on PCV and FEC were evident from 3-week post-infection.
Haemonchus contortus infection results in the destruction of the abomasal mucosa and increased mucus production (Ramos-Bruno et al., 2021), consequently compromising feed intake and digestibility, nutrient absorption, and feed conversion efficiency (Kyriazakis, 2014; Hoste et al., 2016; Sahoo and Khan, 2016). A recent study conducted by Xiang et al. (2021) concluded that lambs infected with H. contortus (10,000 L3 larvae) had decreased feed intake and nutrient digestibility (especially of protein and lipids), reduced LWG, disturbed protein absorption, altered plasma amino acid profiles, and changes in gastrointestinal microbial community composition. In contrast, a meta-analysis conducted by Méndez-Ortíz et al. (2019) on the effects of gastrointestinal nematodes on feed intake and LWG by lambs revealed that 41% and 27% of the studies did not observe a negative effect of GIN on feed intake and LWG, respectively. In the present study, however, there was no effect of H. contortus infection on feed intake, feed digestibility, nitrogen retention, or diet metabolisability and the rumen pH was not altered. In line with the above, various authors (Sykes and Coop, 1977; Kyriazakis et al., 1996; Hoste et al., 2016) have suggested that the effects of H. contortus on feed intake and animal performance are much less pronounced and transient than those of infection by other gastrointestinal nematodes.
It is plausible to assess the nutritional cost of infection in terms of nitrogen and energy metabolism (Retama-Flores et al., 2012; Méndez-Ortíz et al., 2019; Ramos-Bruno et al., 2021). For example, Ramos-Bruno et al. (2021) suggested that the nutritional cost of infection depends on the animal’s ability to withstand the parasitic impact (resilience) or the ability to mount an immune response (resistance). In line with the latter, Blackburn et al. (2013) suggested that the host immune response rather than direct parasite damage is the major cause of loss in productivity. In the present study, infection did not affect feed digestibility, N retention, or diet metabolisability. Therefore, there was no significant impact of infection on animal performance. The LW loss by infected and uninfected lambs could be explained by the poor quality of the Rhodes grass hay.
GIN infection in small ruminants substantially alters the anatomy, function, microbial structure, and composition of the rumen and abomasum (Li et al., 2016; El-Ashram et al., 2017; Corrêa et al., 2020, 2021; Xiang et al., 2021), resulting in decreased daily emissions of CH4 (arising from reduced feed intake) but increased CH4 yields (emissions per gram of intake). In fact, studies involving parasite-naïve sheep or mixed GIN infection (Fox et al., 2018; Lima et al., 2019; Corrêa et al., 2021; Fernandes et al., 2022) showed depressed feed intake and high CH4 yields associated with parasite infection. The lambs in this study, sourced from a flock kept on rangelands, had previous exposure to H. contortus and other GIN. Thus, the lack of GIN infection effect on feed intake, digestibility, and CH4 emissions in the current study supports the hypothesis of Mwangi et al. (2023) that prior exposure of lambs to GIN confers them with a degree of resilience to the negative effects of infection on feed intake and digestibility (and consequently CH4 emission) and animal performance.
4.1.1 Effects of Calliandra supplementation in alleviating H. contortus infection
Consumption of tannin-rich forages has been associated with the effects of these compounds on different stages of the GIN life cycle. In vitro studies involving condensed tannin (CT) extracts (including those of Calliandra) have reported that CT is effective in inhibiting the egg, larvae, and adult stages of H. contortus and disrupts its life cycle (Wabo et al., 2011; Hoste et al., 2012; Mustabi et al., 2019). Similarly, in vivo studies (Cresswell, 2007; Manolaraki et al., 2010) have reported decreased egg production when CT-containing forages were fed. For example, Cresswell (2007) fed fresh Calliandra forage (114 g total CT/kg DM) to lambs infected with 3,200 H. contortus L3 larvae, which showed 24−68% lower egg production, compared with control animals. Reduced egg production has been attributed to the reduced fertility of female worms (Manolaraki et al., 2010; Martínez-Ortíz-de-Montellano et al., 2010). Reduced establishment rates of the infective stage of larvae have also been reported when CT-containing diets are fed to goats (Paolini et al., 2003; Brunet et al., 2008). Furthermore, tannin-rich fodder may also disrupt GIN egg development becoming infective L3 larvae, thereby disrupting the life cycle of nematodes and reducing pasture contamination (Molan et al., 2000; Min et al., 2005). In the present study, the FEC in Calliandra-supplemented lambs was 46−63% lower than that in unsupplemented lambs, confirming the anthelmintic effect of CT in Calliandra.
In the present study, H. contortus infected lambs fed only hay had significantly lower PCV than their counterparts supplemented with Calliandra (InHay vs. InHay+Cal). This can be partially attributed to the improved N nutrition provided by Calliandra. Studies involving sheep infected with H. contortus and supplementation of a basal diet (low in CP) with higher protein sources (Abbott et al., 1988; Wallace et al., 1996; Bricarello et al., 2005) have reported improved animal health (e.g., higher PCV and total plasma proteins). However, in this study, Calliandra supplementation, which contained a high concentration of CT, likely turned the CP at least partially unavailable for the host. Although the amount of retained N (RN) was higher in supplemented lambs, RN/NI did not differ between the supplemented and unsupplemented lambs (InHay+Cal vs InHay).
4.1.2 Effects of Calliandra supplementation on feed intake and liveweight gain
The total DMI of lambs supplemented with Calliandra was significantly higher (by 17−20%) than that of their unsupplemented counterparts (InHay+Cal vs. InHay or UnHay). However, care must be exercised when interpreting this difference. Unsupplemented lambs ate more of the basal diet (hay) than their Calliandra-supplemented counterparts, that is, the intake of Calliandra effectively substituted the intake of hay. The astringent properties of CT reduce feed intake and animal performance when the CT content in the diet is >50 g/kg DM (Barry and McNabb, 1999; Naumann et al., 2017). In the present study, the concentration of total CT in the InHay+Cal diet was approximately 39 g/kg DMI, with no apparent impact on feed intake. However, literature shows both negative effects of CT on feed intake (and digestibility) at concentrations <50 g CT/kg DM, and no impact at >50 g CT/kg DM. Thus, it is likely (Méndez-Ortiz et al., 2018) that the impact of CT on feed intake depends more on the structure of CT rather than its concentration. The astringency arising from CT-protein complexes is responsible for low palatability; the greater the protein bound by CT, the greater the astringency and the lower the palatability (Naumann et al., 2017). The level of astringency depends on the ability of CT to bind to proteins (McMahon et al., 2000; Waghorn, 2008; Naumann et al., 2017). A reduced rate of fibre digestion associated with CT activity slows the clearance of feed residues from the rumen, thereby reducing voluntary feed intake (Waghorn, 2008).
The improved total feed intake found in this study confirms reports from other studies where low-quality forages were supplemented with tannin-rich forages (Yisehak et al., 2014; Korir et al., 2016; Gaviria-Uribe et al., 2020). For example, Yisehak et al. (2014) reported improved feed intake by sheep when Albizia gummifera, a leguminous fodder tree, was supplemented at 30% of the basal diet consisting of forage from natural mixed species. Korir et al. (2016), in their study with cattle fed on wheat straw, reported an improved feed intake when a straw basal diet in cattle was supplemented with Calliandra dry leaves (20 g/kg LW). The increase in feed intake observed as a result of legume forage supplementation is also in agreement with other studies where ruminants fed on a low-protein diet were supplemented with non-forage protein sources (for example, McGuire et al., 2013). The increase in feed intake when sources of protein are supplemented is attributed to increased ruminal microbial activity and increased rumen turnover (Köster et al., 1996). The higher fibre content of poor-quality diets reduces voluntary feed intake (Minson, 1990; Bosa et al., 2012). Hence, it is likely that the increased intake observed when higher-quality forages are supplemented results from an increased rate of passage (Barahona et al., 1997). In this study, the basal diet contained 73.0 and 5.6% NDF and CP, respectively, whereas the corresponding contents of Calliandra were 17.4 and 21.1%, respectively.
In the present study, Calliandra-supplemented lambs had a higher LWG than their unsupplemented counterparts (InHay+Cal vs. InHay or UnHay), which is consistent with the findings of other studies. For example, Yisehak et al. (2014) reported a LWG of 14 g/d when Bonga lambs were supplemented with Albizia gummifera, while their unsupplemented counterparts fed on hay alone gained 3 g/d. In another study, Nherera et al. (1998) reported 44 g/d LWG in goats fed on maize stover and supplemented with Calliandra which was higher than goats supplemented with Leucaena. Similarly, Palmer and Ibrahim (1996) reported that sheep supplemented with Calliandra at inclusion of 27% on DM basis had higher LWG than their counterparts fed on a tropical grass alone (25 vs. -27 g/d). In Kenya, Kinuthia et al. (2007) observed that weaner goats supplemented with 200 g air-dried Calliandra leaves over seven months had higher LWG than those fed Rhodes hay alone (57 vs. 17 g/d). The superior LWG of Calliandra-supplemented lambs observed in the present study could be attributed to improved feed intake and nutrition (increased N retention and metabolisable energy).
4.1.3 Effect of Calliandra supplementation on nutrient intakes, apparent feed digestibility, and nitrogen and energy partitioning
During the balance period, Calliandra-supplemented lambs had significantly higher daily intakes of DM, OM, and CP than their unsupplemented counterparts. The reasons for these higher intakes are discussed in the preceding subsection. The unchanged intake of NDF and ADF with supplementation can be attributed to the low level of fiber in the Calliandra. In this study, the Calliandra consisted not of whole leaves, but of their primary leaflets, which had low NDF content (Table 1).
In this study, lambs supplemented with Calliandra had 9% lower OM digestibility than their unsupplemented counterparts (InHay+Cal vs. InHay). However, the decrease in CP and ADF digestibilities were much higher (22%). The latter is in close agreement with the findings of Tiemann et al. (2008), in which lambs fed on a tropical grass and supplemented with Calliandra (CT=175 g/kg DM) at 30% of the daily diet (DM basis) had depression in CP and ADF digestibilities of the order of 38%, compared to those receiving no supplement. Similarly, Fassler and Lascano Aguilar (1995) and Hove et al. (2001) reported a much greater effect on ADF digestion than NDF digestion when CT-rich tropical legumes were supplemented to small ruminants.
In the current study, the only measured aspect of CT was its concentration, and the estimated total concentration of CT in the diet of supplemented lambs (InHay+Cal) was ~39 g/kg DM, which is far below the suggested limit of tolerance of 50 g/kg DM) (Barry & McNabb, 1999). However, as stated earlier, the anti-nutritional effects of CT cannot be ascribed to its concentration alone (Waghorn, 2008; Naumann et al., 2017; Besharati et al., 2022). Waghorn (2008) concluded that the effects of CT on ruminal proteolysis, crude protein digestibility, OM digestibility, and N balance are well understood, and that the degree to which CT interferes with these processes is a function of the astringency, concentration, and chemical and physical properties of CT. The negative effects of CT on CP digestibility and, to a minor degree, on fibre digestibility are common features reported in the literature (e.g., Jayanegara et al., 2012; Cabral Filho et al., 2013; Méndez-Ortiz et al., 2021). Besharati et al. (2022) concluded that CT mainly affects CP digestibility by creating hydrogen bonds that are stable in the pH range of 3.5−8.0, whereas Naumann et al. (2017) concluded that at a high tannin:protein ratio, the protein becomes coated with CT, leading to insoluble precipitates, which is especially true in CT-rich Calliandra (Rira et al., 2022). High concentrations of dietary CT increase dietary faecal nitrogen excretion; however, tannin consumption may also increase endogenous N loss (Besharati et al., 2022). Rira et al. (2022) reported that tannins interfere with the microbial colonisation of plant material, especially by fibrolytic bacteria, thereby affecting fibre digestion. McMahon et al. (2000) from a review of in vitro studies concluded that CT could inhibit cellulose digesting ruminal bacteria and fungi. Furthermore, the strong chelating properties of CT may reduce the availability of metal ions necessary for microbial metabolism (McMahon et al., 2000).
Compared to unsupplemented lambs (InHay+Cal vs. InHay), Calliandra supplementation significantly increased faecal N partitioning (FN/NI) and reduced urinary N (UN/NI), whereas retained N (RN/NI) remained unaltered. This is in close agreement with previous reports (McSweeney et al., 2001; Tiemann et al., 2008; Besharati et al., 2022). For example, Tiemann et al. (2008) with sheep supplemented with Calliandra, found increased FN/NI and lowered UN/NI, whereas RN/NI was negative. Similar findings were also observed when Gymnopodium floribundum, a Polygonaceae plant species, was supplemented to sheep in a diet providing 86 g CT/kg DM (Méndez-Ortiz et al., 2021). The increased faecal N partitioning is in line with the depressed digestibility of CP, whereas decreased urinary N excretion likely reflects the effect of CT on ruminal proteolysis by complexing proteins, making them less available to proteolytic microorganisms (Mueller-Harvey, 2006). The latter suggests that CT may have an additional role in reducing urinary sources of NH3 and N2O (Śliwiński et al., 2004; Waghorn, 2008). In the present study, despite the higher N loss in faeces, the retained N (g/d) of Calliandra-supplemented lambs was significantly higher than that of the unsupplemented lambs.
In this study, Calliandra-supplemented lambs had higher daily energy intake (GEI), daily faecal energy (FE), and urinary energy (UE) outputs than their unsupplemented counterparts (InHay+Cal vs. InHay). The DE/GEI ratio was lower for the supplemented lambs, whereas the ME/GEI ratio did not differ between these groups of lambs. The former indicates the effects of compromised feed digestibility discussed earlier. Our findings are in partial agreement with those of Tiemann et al. (2008), who observed a 12% decrease in DE/GE and ME/GE ratios in Calliandra-supplemented lambs compared with the control (grass only). Similarly, Méndez-Ortiz et al. (2021) reported depressed DE/GE (by 26%) and ME/GE (by 29%) ratios in lambs supplemented with Gymnopodium floribundum compared to unsupplemented lambs. The differences between these studies may be due to the nature and concentration of CT (Waghorn, 2008). In our study, the digestible (DE) and metabolisable (ME) energies available for supplemented lambs were higher than those for their unsupplemented counterparts.
4.1.4 Effect of Calliandra supplementation on enteric methane emission
In this study, daily CH4 emissions (g/d), CH4 yield (per unit of intake), and Ym were decreased by Calliandra supplementation (InHay+Cal vs. InHay). Our findings confirm those from other in vitro and in vivo studies that reported significant reductions in CH4 emissions when CT-containing forages were fed (Pinares-Patiño et al., 2003; Puchala et al., 2005; Tiemann et al., 2008; Jayanegara et al., 2012; Rira et al., 2022). Forages rich in CT can reduce daily CH4 emissions. For example, Pinares-Patiño et al. (2003) and Piñeiro-Vázquez et al. (2018), working with sheep and heifers, respectively, reported 62−65% reduction in CH4 yield (emissions per unit of feed intake) with diets comprising >80% CT-rich legumes. Tiemann et al. (2008) reported a 17% decrease when lambs were supplemented with Calliandra at a rate of 30% daily DMI. The negative effects of CT on CH4 emissions can be associated with the reduced digestion of CP and fibre, hence reducing the availability of reducing equivalents for methanogenesis, as well as direct anti-methanogenic action (McMahon et al., 2000; Tavendale et al., 2005; Rira et al., 2022). In fact, Rira et al. (2022), from in situ studies, reported that archaea diversity was reduced in high CT-containing Calliandra calothyrsus, and concurrently, in vitro studies revealed that CH4 production was low. Furthermore, Kelln et al. (2020) indicated that CT (Catechins) act as H2 sinks, reducing the availability of H2 to archaea.
4.3 Effect of PEG treatment in reducing condensed tannins activity in Calliandra supplemented lambs
Polyethylene glycol (PEG) is a non-ionic detergent with high affinity for CT (Bento et al., 2005). Because of PEG’s ability to bind CT, the addition of PEG to diets can reduce the effect of CT and has led to its use in testing the effects of CT on feed intake, rumen function, and metabolism of N and energy (de Frutos Fernández et al., 2004), and methane emissions (Wang et al., 2018). The ability of PEG to bind CT has been suggested to improve the feeding value of CT-rich plant species, especially in the tropics (Waghorn, 2008). In the current study, the PEG dose was calculated based on a preliminary analysis of the CT concentration in the Calliandra forage material collected prior to collection of the actual experimental Calliandra forage, which showed a total CT of 28.2 g/kg DM. However, analysis of samples of the Calliandra forage used in the experiment (carried out at the end of the study) in a different laboratory yielded a higher concentration of total CT (92.1 g/kg DM). The reason for this large difference in the analytical results may be due to differences in CT concentration between the samples or due to the different analytical procedures used. Studies in Sub-Saharan Africa have reported total CT concentrations in Calliandra in the range of 10−200 g/kg DM (Dzowela et al., 1995; Hove et al., 2001). The large range can be attributed to various factors, including the environment where the sample was collected, sample drying, and method of analysis (Wolfe et al., 2008). Based on the lack of differences in the effects of InHay+Cal and InHay+Cal+PEG treatments on infection response, DMI, LWG, feed digestibility, N and gross energy partitioning, and CH4 emission, we are confident that the initial analyses of CT concentrations underestimated the real concentrations, resulting in undercalculation of PEG dosage required to neutralize CT activities. Other studies consider Calliandra to be a high CT-rich legume (Perez-Maldonado and Norton, 1996; Rakhmani et al., 2005; Cresswell, 2007; Tiemann et al., 2008; Rira et al., 2022).
In this study, underestimating CT concentration in Calliandra led to a lower PEG dosage in the InHay+Cal+PEG treatment. The intended ratio of PEG: CT was 1.1, while an actual PEG : CT ratio of 1:2.8 was achieved. Consequently, the PEG level used was insufficient to fully counteract the CT properties. In effect, the mean values of PCV and FEC, feed intake, LWG, nutrient intake in feed, feed digestibility, N partitioning to urine and retained N, energy partitioning, and CH4 emission did not differ between Calliandra-supplemented treatments with and without PEG supplementation (InHay+Cal vs. InHay+Cal+PEG). On the other hand, CP digestibility and partitioning of N intake to faecal N were higher and lower, respectively, in PEG-supplemented lambs than in lambs receiving no PEG supplement (InHay+Cal+PEG vs. InHay+Cal). However, the extent to which PEG supplementation reduced the protein-binding property of CT remains uncertain.
5 Conclusion and recommendations
In the present study, supplementation of a poor-quality basal diet with Calliandra resulted in reduced GIN infection, improved feed intake, improved liveweight gain and reduced CH4 emission from sheep. However, these effects were partially offset by reduced crude protein and fibre digestibility. Small-holder livestock keepers in SSA may not be able to achieve high (40%) supplementation levels of forage from fodder trees because of competing interests from other food crops. Hence, lower supplementation levels may be optimal and more achievable in local farming situations. Calliandra crops, when well-managed, could potentially provide year-round green forage that helps control GIT parasite infection, improves the feeding value of the basal diet, hence lowering the cost of animal production. Furthermore, incorporating Calliandra can lead to a notable reduction in enteric CH4 emissions, both in absolute (g/animal/day) and yield (g/kg DMI) basis.
Data availability statement
The raw data supporting the conclusions of this article will be made available by the authors, without undue reservation.
Ethics statement
The animal study was approved by International Livestock Research Institute’s Institutional Animal Care and Use Committee (IACUC, Reference number 2022-03). The study was conducted in accordance with the local legislation and institutional requirements.
Author contributions
PM: Conceptualization, Data curation, Formal analysis, Investigation, Methodology, Writing – original draft. RE: Conceptualization, Methodology, Supervision, Validation, Writing – review & editing. IG: Conceptualization, Methodology, Supervision, Writing – review & editing. LM: Funding acquisition, Writing – review & editing. DM: Investigation, Writing – review & editing. JG: Investigation, Writing – review & editing, Data curation. SM: Conceptualization, Methodology, Supervision, Writing – review & editing. CP: Conceptualization, Investigation, Methodology, Project administration, Resources, Supervision, Validation, Writing – review & editing.
Funding
The author(s) declare financial support was received for the research, authorship, and/or publication of this article. The study was funded by the International Development Research Centre (IDRC) -Canada, Project 109211 -Livestock Keeping in a Changing Climate. Additional financial support for CGIAR authors came through the CGIAR Research Initiatives, ‘Livestock and Climate’ and ‘Mitigate+: Low-Emission Food Systems’ which are supported by contributions to the CGIAR Trust Fund (https://www.cgiar.org/funders/). Lutz Merbold acknowledges funding received from the European Union’s Horizon Europe Programme (grant agreement number 101058525) for the project “Knowledge and climate services from an African observation and Data Research Infrastructure (KADI).”
Acknowledgments
The authors acknowledge the Tick Unit, Animal and Human health, ILRI for facilitating faecal egg counts and packed cell volume examination in their facility. We also thank CLINGLOBAL South Africa for providing the H. contortus L3 used for infection of experimental animals. We appreciate Erick Kiprotich and Nelson Saya for assisting with animal handling and care, sample collection and preparation, and respiration chamber management. We also appreciate Dr. Alice Onyango, Rodgers Rogito, Francis Njenga, and Collins Oduor for carrying out chemical analysis of the samples. The authors are thankful to Jane Poole and Nicholas Ndiwa for their guidance on experimental design and statistical analyses. We also acknowledge Professor Graham Hepworth (The University of Melbourne) for his guidance on the experimental design and statistical analysis. The authors also acknowledge Silvia Ampuero (Agroscope, Zurich, Switzerland) for the preliminary analyses of condensed tannins in Calliandra and Nichole Cherry, James P. Muir and Luis O. Tedeshi (Texas A&M AgriLife Research, TX) for the final analyses of condensed tannins in Calliandra. We sincerely appreciate Daniel Korir for offering data management and analysis support to the experimental team.
Conflict of interest
The authors declare that the research was conducted in the absence of any commercial or financial relationships that could be construed as a potential conflict of interest.
Publisher’s note
All claims expressed in this article are solely those of the authors and do not necessarily represent those of their affiliated organizations, or those of the publisher, the editors and the reviewers. Any product that may be evaluated in this article, or claim that may be made by its manufacturer, is not guaranteed or endorsed by the publisher.
References
Śliwiński B. J., Kreuzer M., Sutter F., Machmüller A., Wettstein H. R. (2004). Performance, body nitrogen conversion and nitrogen emission from manure of dairy cows fed diets supplemented with different plant extracts. J. Anim. Feed Sci. 13, 73–91. doi: 10.22358/jafs/67390/2004
Abbott E. M., Parkins J. J., Holmes P. H. (1988). Influence of dietary protein on the pathophysiology of haemonchosis in lambs given continuous infections. Res. Veterinary Sci. 45, 41–49. doi: 10.1016/S0034-5288(18)30892-0
AOAC (1990). Official methods of analysis of the Association of Official Analytical Chemists (Issue BOOK) (Washington DC: Association of official analytical chemists).
Armson B., Ekiri A. B., Alafiatayo R., Cook A. J. (2021). Small ruminant production in Tanzania, Uganda, and Ethiopia: A systematic review of constraints and potential solutions. Veterinary Sci. 8, 1–13. doi: 10.3390/vetsci8010005
Baker R. L., Rege J. E. O. (1994). “Genetic resistance to diseases and other stresses in improvement of ruminant livestock in the tropics,” in 7th world congress on genetics applied to livestock production (Guelph Canada: International Committee for World Congresses on Genetics Applied to Livestock Production).
Barahona R., Lascano C. E., Cochran R., Morrill J., Titgemeyer E. C. (1997). Intake, digestion, and nitrogen utilization by sheep fed tropical legumes with contrasting tannin concentration and astringency. J. Anim. Sci. 75, 1633–1640. doi: 10.2527/1997.7561633x
Barry T. N., McNabb W. C. (1999). The implications of condensed tannins on the nutritive value of temperate forages fed to ruminants. Br. J. Nutr. 81, 263–272. doi: 10.1017/S0007114599000501
Bento M. H. L., Acamovic T., Makkar H. P. S. (2005). The influence of tannin, pectin and polyethylene glycol on attachment of 15N-labelled rumen microorganisms to cellulose. Anim. Feed Sci. Technol. 122, 41–57. doi: 10.1016/j.anifeedsci.2005.04.010
Berça A. S., Tedeschi L. O., da Silva Cardoso A., Reis R. A. (2023). Meta-analysis of the relationship between dietary condensed tannins and methane emissions by cattle. Anim. Feed Sci. Technol. 298, 115564. doi: 10.1016/j.anifeedsci.2022.115564
Besharati M., Maggiolino A., Palangi V., Kaya A., Jabbar M., Eseceli H., et al. (2022). Tannin in ruminant nutrition. Molecules 27, 8273. doi: 10.3390/molecules27238273
Bhatta R., Enishi O., Yabumoto Y., Nonaka I., Takusari N., Higuchi K., et al. (2013). Methane reduction and energy partitioning in goats fed two concentrations of tannin from Mimosa spp. J. Agric. Sci. 151, 119–128. doi: 10.1017/S0021859612000299
Blackburn P. J., Carmichael I. H., Walkden-Brown S. W., Greenslade S. (2013). “Cost of immune response to Trichostrongylus vitrinus infection in meat sheep,” in Proceedings of the Australian Sheep Veterinarians 2013 Conference, Albany (Sydney, Australia: Sheep Veterinary Association). 41–46.
Bosa R., Faturi C., Vasconcelos H. G. R., Cardoso A. M., Ramos A. F. O., de Azevedo J. C. (2012). Intake and apparent digestibility with different inclusion levels of coconut meal for sheep feeding/Consumo e digestibilidade aparente de dietas com diferentes niveis de inclusao de torta de coco para alimentacao de ovinos. Acta Scientiarum. Anim. Sci. 34, 57–63. doi: 10.4025/actascianimsci.v34i1.11936
Bricarello P. A., Amarante A. F. T., Rocha R. A., Cabral Filho S. L., Huntley J. F., Houdijk J. G. M., et al. (2005). Influence of dietary protein supply on resistance to experimental infections with Haemonchus contortus in Ile de France and Santa Ines lambs. Veterinary Parasitol. 134, 99–109. doi: 10.1016/j.vetpar.2005.05.068
Brunet S., de Montellano C., Torres-Acosta J. F. J., Sandoval-Castro C. A., Aguilar-Caballero A. J., Capetillo-Leal C., et al. (2008). Effect of the consumption of Lysiloma latisiliquum on the larval establishment of gastrointestinal nematodes in goats. VETERINARY Parasitol. 157, 81–88. doi: 10.1016/j.vetpar.2008.07.013
Cabral Filho S. L. S., Abdalla A. L., Bueno I. C., da S., Gobbo S. P., Oliveira A. A. M. (2013). Effect of sorghum tannins in sheep fed with high-concentrate diets. Arquivo Brasileiro Medicina Veterinária e Zootecnia 65, 1759–1766. doi: 10.1590/S0102-09352013000600025
Chaokaur A., Nishida T., Phaowphaisal I., Sommart K. (2015). Effects of feeding level on methane emissions and energy utilization of Brahman cattle in the tropics. Agricul. Ecosyst. Environ. 199, 225–230. doi: 10.1016/j.agee.2014.09.014
Corrêa P. S., Mendes L. W., Lemos L. N., Crouzoulon P., Niderkorn V., Hoste H., et al. (2020). Tannin supplementation modulates the composition and function of ruminal microbiome in lambs infected with gastrointestinal nematodes. FEMS Microbiol. Ecol. 96, fiaa024. doi: 10.1093/femsec/fiaa024
Corrêa P. S., Mendes L. W., Lemos L. N., Sampaio A. C. K., Issakowicz J., McManus C. M., et al. (2021). The effect of Haemonchus contortus and Trichostrongylus colubriforms infection on the ruminal microbiome of lambs. Exp. Parasitol. 231, 108175. doi: 10.1016/j.exppara.2021.108175
Cresswell K. J. (2007). Anthelmintic effects of tropical shrub legumes in ruminant animals (Australia: James Cook University). Available at: https://doi.org/http://eprints.jcu.edu.au/2086.
de Frutos Fernández P., Mantecón Á.R., Angulo G. H., García F. J. G. (2004). Tannins and ruminant nutrition. Spanish J. Agric. Res. 2, 191–202. doi: 10.5424/sjar/2004022-73
Dzowela B. H., Hove L., Topps J. H., Mafongoya P. L. (1995). Nutritional and anti-nutritional characters and rumen degradability of dry matter and nitrogen for some multipurpose tree species with potential for agroforestry in Zimbabwe. Anim. Feed Sci. Technol. 55, 207–214. doi: 10.1016/0377-8401(95)00803-U
El-Ashram S., Al Nasr I., Abouhajer F., El-Kemary M., Huang G., Dinçel G., et al. (2017). Microbial community and ovine host response varies with early and late stages of Haemonchus contortus infection. Veterinary Res. Commun. 41, 263–277. doi: 10.1007/s11259-017-9698-5
FAO (2018). “Food and agriculture organisation of the united nations, faostat. Stat,” in Database, vol. 16. (Rome, Italy: FAO). Available at: http://www.fao.org/faostat/en/#data/QC.
Fassler O. M., Lascano Aguilar C. E. (1995). The effect of mixtures of sun-dried tropical shrub legumes on intake and nitrogen balance by sheep. Trop. Grasslands. 29, 92–96. Available at: https://hdl.handle.net/10568/44164.
Fernandes M. A., Lima P., de M. T., do Amarante A. F. T., Abdalla A. L., Louvandini H. (2022). Hematological, biochemical alterations and methane production in sheep submitted to mixed infection of Haemonchus contortus and Trichostrongylus colubriformis. Small Ruminant Res. 216, 106798. doi: 10.1016/j.smallrumres.2022.106798
Fox N. J., Smith L. A., Houdijk J. G. M., Athanasiadou S., Hutchings M. R. (2018). Ubiquitous parasites drive a 33% increase in methane yield from livestock. Int. J. Parasitol. 48, 1017–1021. doi: 10.1016/j.ijpara.2018.06.001
Gaviria-Uribe X., Bolivar D. M., Rosenstock T. S., Molina-Botero I. C., Chirinda N., Barahona R., et al. (2020). Nutritional quality, voluntary intake and enteric methane emissions of diets based on novel cayman grass and its associations with two leucaena shrub legumes. Front. Veterinary Sci. 7 (October), 1–12. doi: 10.3389/fvets.2020.579189
Gerber P. J., Steinfeld H., Henderson B., Mottet A., Opio C., Dijkman J., et al. (2013). Tackling climate change through livestock: a global assessment of emissions and mitigation opportunities (Rome: Food and Agriculture Organization of the United Nations (FAO).
Goopy J. P., Korir D., Pelster D., Ali A. I. M., Wassie S. E., Schlecht E., et al. (2020). Severe below-maintenance feed intake increases methane yield from enteric fermentation in cattle. Br. J. Nutr. 123, 1239–1246. doi: 10.1017/S0007114519003350
Greiffer L., Liebau E., Herrmann F. C., Spiegler V. (2022). Condensed tannins act as anthelmintics by increasing the rigidity of the nematode cuticle. Sci. Rep. 12, 18850. doi: 10.1038/s41598-022-23566-2
Hoste H., Martinez-Ortiz-De-Montellano C., Manolaraki F., Brunet S., Ojeda-Robertos N., Fourquaux I., et al. (2012). Direct and indirect effects of bioactive tannin-rich tropical and temperate legumes against nematode infections. Veterinary Parasitol. 186, 18–27. doi: 10.1016/j.vetpar.2011.11.042
Hoste H., Torres-Acosta J. F. J., Quijada J., Chan-Perez I., Dakheel M. M., Kommuru D. S., et al. (2016). Interactions between nutrition and infections with Haemonchus contortus and related gastrointestinal nematodes in small ruminants. Adv. Parasitol. 93, 239–351. doi: 10.1016/bs.apar.2016.02.025
Hove L., Topps J. H., Sibanda S., Ndlovu L. R. (2001). Nutrient intake and utilisation by goats fed dried leaves of the shrub legumes Acacia angustissima, Calliandra calothyrsus and Leucaena leucocephala as supplements to native pasture hay. Anim. Feed Sci. Technol. 91, 95–106. doi: 10.1016/S0377-8401(01)00233-4
Jayanegara A., Leiber F., Kreuzer M. (2012). Meta-analysis of the relationship between dietary tannin level and methane formation in ruminants from in vivo and in vitro experiments. J. Anim. Physiol. Anim. Nutr. 96, 365–375. doi: 10.1111/j.1439-0396.2011.01172.x
Kaitho R. J., Umunna N. N., Nsahlai I. V., Tamminga S., Van Bruchem J., Hanson J., et al. (1996). Palatability of multipurpose tree species: effect of species and length of study on intake and relative palatability by sheep. Agroforestry Systems. 33, 249–261. doi: 10.1007/BF00055426
Kelln B. M., Penner G. B., Acharya S. N., McAllister T. A., Lardner H. A. (2020). Impact of condensed tannin-containing legumes on ruminal fermentation, nutrition, and performance in ruminants: a review. Can. J. Anim. Sci. 101, 210–223. doi: 10.1139/cjas-2020-0096
Kinuthia M. N., Wanyoike M. M., Gachuiri C. K., Wakhungu J. W. (2007). Effect of supplementing weaner goats with graded levels of Calliandra calothyrsus and Lucerne (Medicago sativa) on feed intake and weight gain. Livestock Res. Rural Dev. 19, 1–9.
Korir D., Goopy J. P., Gachuiri C., Butterbach-Bahl K. (2016). Supplementation with Calliandra calothyrsus improves nitrogen retention in cattle fed low-protein diets. Anim. Production Sci. 56, 619–626. doi: 10.1071/AN15569
Kosgey I. S., Rowlands G. J., van Arendonk J. A. M., Baker R. L. (2008). Small ruminant production in smallholder and pastoral/extensive farming systems in Kenya. Small Ruminant Res. 77, 11–24. doi: 10.1016/j.smallrumres.2008.02.005
Köster H. H., Cochran R. C., Titgemeyer E. C., Vanzant E. S., Abdelgadir I., St-Jean G. (1996). Effect of increasing degradable intake protein on intake and digestion of low-quality, tallgrass-prairie forage by beef cows. J. Anim. Sci. 74, 2473–2481. doi: 10.2527/1996.74102473x
Kurihara M., Magner T., Hunter R. A., McCrabb G. J. (1999). Methane production and energy partition of cattle in the tropics. Br. J. Nutr. 81, 227–234. doi: 10.1017/S0007114599000422
Kyriazakis I. (2014). Pathogen-induced anorexia: a herbivore strategy or an unavoidable consequence of infection? Anim. Production Sci. 54, 1190–1197. doi: 10.1071/AN14431
Kyriazakis I., Anderson D. H., Oldham J. D., Coop R. L., Jackson F. (1996). Long-term subclinical infection with Trichostrongylus colubriformis: effects on food intake, diet selection and performance of growing lambs. Veterinary Parasitol. 61, 297–313. doi: 10.1016/0304-4017(95)00824-1
Li R. W., Li W., Sun J., Yu P., Baldwin R. L., Urban J. F. (2016). The effect of helminth infection on the microbial composition and structure of the caprine abomasal microbiome. Sci. Rep. 6, 1–10. doi: 10.1038/srep20606
Lima P., de M. T., Crouzoulon P., Sanches T. P., Zabré G., Kabore A., et al. (2019). Effects of Acacia mearnsii supplementation on nutrition, parasitological, blood parameters and methane emissions in Santa Inês sheep infected with Trichostrongylus colubriformis and Haemonchus contortus. Exp. Parasitol. 207, 107777. doi: 10.1016/j.exppara.2019.107777
Manolaraki F., Sotiraki S., Stefanakis A., Skampardonis V., Volanis M., Hoste H. (2010). Anthelmintic activity of some Mediterranean browse plants against parasitic nematodes. Parasitology 137, 685–696. doi: 10.1017/S0031182009991399
Martínez-Ortíz-de-Montellano C., Vargas-Magaña J. J., Canul-Ku H. L., Miranda-Soberanis R., Capetillo-Leal C., Sandoval-Castro C. A., et al. (2010). Effect of a tropical tannin-rich plant Lysiloma latisiliquum on adult populations of Haemonchus contortus in sheep. Veterinary Parasitol. 172, 283–290. doi: 10.1016/j.vetpar.2010.04.040
McGuire D. L., Bohnert D. W., Schauer C. S., Falck S. J., Cooke R. F. (2013). Daily and alternate day supplementation of urea or soybean meal to ruminants consuming low-quality cool-season forage: I—Effects on efficiency of nitrogen use and nutrient digestion. Livestock Sci. 155, 205–213. doi: 10.1016/j.livsci.2013.05.015
McMahon L. R., McAllister T. A., Berg B. P., Majak W., Acharya S. N., Popp J. D., et al. (2000). A review of the effects of forage condensed tannins on ruminal fermentation and bloat in grazing cattle. Can. J. Plant Sci. 80, 469–485. doi: 10.4141/P99-050
McSweeney C. S., Palmer B., McNeill D. M., Krause D. O. (2001). Microbial interactions with tannins: nutritional consequences for ruminants. Anim. Feed Sci. Technol. 91, 83–93. doi: 10.1016/S0377-8401(01)00232-2
Méndez-Ortiz F. A., Sandoval-Castro C. A., Sarmiento-Franco L. A., Ventura-Cordero J., González-Pech P. G., Vargas-Magaña J. J., et al. (2021). Impact of dietary condensed tannins and haemonchus contortus infection in growing sheep: effects on nutrient intake, digestibility, and the retention of energy and nitrogen. Acta Parasitologica. 67 (1), 196–206. doi: 10.1007/s11686-021-00441-0
Méndez-Ortíz F. A., Sandoval-Castro C. A., Vargas-Magaña J. J., Sarmiento-Franco L., Torres-Acosta J. F. J., Ventura-Cordero J. (2019). Impact of gastrointestinal parasitism on dry matter intake and live weight gain of lambs: A meta-analysis to estimate the metabolic cost of gastrointestinal nematodes. Veterinary Parasitol. 265, 1–6. doi: 10.1016/j.vetpar.2018.11.008
Méndez-Ortiz F. A., Sandoval-Castro C. A., Ventura-Cordero J., Sarmiento-Franco L. A., Torres-Acosta J. F. J. (2018). Condensed tannin intake and sheep performance: A meta-analysis on voluntary intake and live weight change. Anim. Feed Sci. Technol. 245, 67–76. doi: 10.1016/j.anifeedsci.2018.09.001
Min B. R., Hart S. P., Miller D., Tomita G. M., Loetz E., Sahlu T. (2005). The effect of grazing forage containing condensed tannins on gastro-intestinal parasite infection and milk composition in Angora does. VETERINARY Parasitol. 130, 105–113. doi: 10.1016/j.vetpar.2005.03.011
Minson D. J. (1990). Intake of grazed forage In ‘Forage in ruminant nutrition’. (Ed. Minson D. J.) pp. 60–84. London, Uk: Academic Press Inc.–Harcourt Brace Jovanovich Publishers.
Molan A. L., Waghorn G. C., Min B. R., McNabb W. C. (2000). The effect of condensed tannins from seven herbages on Trichostrongylus colubriformis larval migration in vitro. Folia Parasitologica 47, 39–44. doi: 10.14411/fp.2000.007
Mueller-Harvey I. (2006). Unravelling the conundrum of tannins in animal nutrition and health. J. Sci. Food Agriculture. 86 (13), 2010–2037. doi: 10.1002/jsfa.2577
Mustabi J., Prahesti K. I., Nurpaidah. (2019). Efficacy of calliandra (Calliandra calothyrsus) leaf extract on Haemonchus contortus mortality in vitro. IOP Conference Series: Earth and Environmental Science. 343 (1). doi: 10.1088/1755-1315/343/1/012032
Mwangi P. M., Eckard R., Gluecks I., Merbold L., Mulat D. G., Gakige J., et al. (2023). “Impact of Haemonchus contortus infection on feed intake, digestion, liveweight gain, and enteric methane emission from Red Maasai and Dorper sheep,” in Frontiers in animal science, vol. Vol. 4. . doi: 10.3389/fanim.2023.1212194
Naumann H. D., Tedeschi L. O., Zeller W. E., Huntley N. F. (2017). The role of condensed tannins in ruminant animal production: advances, limitations and future directions. Rev. Bras. Zootecnia 46, 929–949. doi: 10.1590/s1806-92902017001200009
Nherera F. V., Ndlovu L. R., Dzowela B. H. (1998). Utilisation of Leucaena diversifolia, Leucaena esculenta, Leucaena pallida and Calliandra calothyrsus as nitrogen supplements for growing goats fed maize stover. Anim. Feed Sci. Technol. 74, 15–28. doi: 10.1016/S0377-8401(98)00164-3
Oliveira L. M. B., Macedo I. T. F., Vieira L. S., Camurça-Vasconcelos A. L. F., Tomé A. R., Sampaio R. A., et al. (2013). Effects of Mimosa tenuiflora on larval establishment of Haemonchus contortus in sheep. Veterinary Parasitol. 196, 341–346. doi: 10.1016/j.vetpar.2013.04.014
Palmer B., Ibrahim T. M. (1996). “Callandra calothyrsus forage for the tropics-a current assessment,” in International workshop on the genus calliandra (Bogor, Indonesia: Winrock International). Available at: http://hdl.handle.net/102.100.100/227088?index=1.
Paolini V., Bergeaud J.-P., Grisez C., Prevot F., Dorchies P., Hoste H. (2003). Effects of condensed tannins on goats experimentally infected with Haemonchus contortus. Veterinary Parasitol. 113, 253–261. doi: 10.1016/S0304-4017(03)00064-5
Perez-Maldonado R. A., Norton B. W. (1996). The effects of condensed tannins from Desmodium intortum and Calliandra calothyrsus on protein and carbohydrate digestion in sheep and goats. Br. J. Nutr. 76, 515–533. doi: 10.1079/BJN19960060
Pinares-Patiño C. S., Ulyatt M. J., Waghorn G. C., Lassey K. R., Barry T. N., Holmes C. W., et al. (2003). Methane emission by alpaca and sheep fed on lucerne hay or grazed on pastures of perennial ryegrass/white clover or birdsfoot trefoil. J. Agric. Sci. 140, 215–226. doi: 10.1017/S002185960300306X
Piñeiro-Vázquez A. T., Canul-Solis J. R., Jiménez-Ferrer G. O., Alayón-Gamboa J. A., Chay-Canul A. J., Ayala-Burgos A. J., et al. (2018). Effect of condensed tannins from Leucaena leucocephala on rumen fermentation, methane production and population of rumen protozoa in heifers fed low-quality forage. Asian-Australasian J. Anim. Sci. 31, 1738. doi: 10.5713/ajas.17.0192
Puchala R., Min B. R., Goetsch A. L., Sahlu T. (2005). The effect of a condensed tannin-containing forage on methane emission by goats. J. Anim. Sci. 83, 182–186. doi: 10.2527/2005.831182x
Rahimi J., Mutua J. Y., Notenbaert A. M. O., Marshall K., Butterbach-Bahl K. (2021). Heat stress will detrimentally impact future livestock production in East Africa. Nat. Food 2, 88–96. doi: 10.1038/s43016-021-00226-8
Rakhmani S., Brooker J. D., Jones G. P., Palmer B. (2005). Composition of condensed tannins from Calliandra calothyrsus and correlation with in sacco digestibility. Anim. Feed Sci. Technol. 121, 109–124. doi: 10.1016/j.anifeedsci.2005.02.010
Ramos-Bruno E., Sandoval-Castro C. A., Torres-Acosta J. F. J., Sarmiento-Franco L. A., Torres-Fajardo R., Chan-Pérez J. I., et al. (2021). Nitrogen retention in hair sheep lambs with a gradient of Haemonchus contortus infection. Veterinary Parasitol. 296, 109488. doi: 10.1016/j.vetpar.2021.109488
Retama-Flores C., Torres-Acosta J. F. J., Sandoval-Castro C. A., Aguilar-Caballero A. J., Cámara-Sarmiento R., Canul-Ku H. L. (2012). Maize supplementation of Pelibuey sheep in a silvopastoral system: fodder selection, nutrient intake and resilience against gastrointestinal nematodes. Animal 6, 145–153. doi: 10.1017/S1751731111001339
Rira M., Morgavi D. P., Popova M., Maxin G., Doreau M. (2022). Microbial colonisation of tannin-rich tropical plants: Interplay between degradability, methane production and tannin disappearance in the rumen. Animal 16, 100589. doi: 10.1016/j.animal.2022.100589
Sahoo A., Khan F. (2016). Nutritional and biological control synergism against gastrointestinal nematodes in small ruminants. J. Vet. Sci. Anim. Husb 4, 104. doi: 10.15744/2348-9790.4.104
Sykes A. R., Coop R. L. (1977). Intake and utilization of food by growing sheep with abomasal damage caused by daily dosing with Ostertagia circumcincta larvae. J. Agric. Sci. 88, 671–677. doi: 10.1017/S0021859600037369
Tavendale M. H., Meagher L. P., Pacheco D., Walker N., Attwood G. T., Sivakumaran S. (2005). Methane production from in vitro rumen incubations with Lotus pedunculatus and Medicago sativa, and effects of extractable condensed tannin fractions on methanogenesis. Anim. Feed Sci. Technol. 123, 403–419. doi: 10.1016/j.anifeedsci.2005.04.037
Terrill T. H., Rowan A. M., Douglas G. B., Barry T. N. (1992). Determination of extractable and bound condensed tannin concentrations in forage plants, protein concentrate meals and cereal grains. J. Sci. Food Agric. 58, 321–329. doi: 10.1002/jsfa.2740580306
Tiemann T. T., Lascano C. E., Wettstein H.-R., Mayer A. C., Kreuzer M., Hess H. D. (2008). Effect of the tropical tannin-rich shrub legumes Calliandra calothyrsus and Flemingia macrophylla on methane emission and nitrogen and energy balance in growing lambs. Animal 2, 790–799. doi: 10.1017/S1751731108001791
Wabo P. J., Yondo J., Fossi T. O., Marie C. K., Bilong B. C. F., Mpoame M. (2011). The in vitro effects of Chenopodium ambrosioides (Chenopodiaceae) extracts on the parasitic nematode Heligmosomoides bakeri (Nematoda, Heligmosomatidae). J. Pharmacog. Phytother. 3, 56–62.
Waghorn G. (2008). Beneficial and detrimental effects of dietary condensed tannins for sustainable sheep and goat production—Progress and challenges. Anim. Feed Sci. Technol. 147, 116–139. doi: 10.1016/j.anifeedsci.2007.09.013
Wallace D. S., Bairden K., Duncan J. L., Fishwick G., Holmes P. H., McKellar Q. A., et al. (1996). Influence of soyabean meal supplementation on the resistance of Scottish blackface lambs to haemonchosis. Res. IN VETERINARY Sci. 60, 138–143. doi: 10.1016/S0034-5288(96)90008-9
Waller P. J. (1999). International approaches to the concept of integrated control of nematode parasites of livestock. Int. J. Parasitol. 29, 155–164. doi: 10.1016/S0020-7519(98)00178-7
Wambugu C., Franzel S., Tuwei P., Karanja G. (2001). Scaling up the use of fodder shrubs in central Kenya. Dev. Pract. 11, 487–494. doi: 10.1080/09614520120066765
Wang S., Terranova M., Kreuzer M., Marquardt S., Eggerschwiler L., Schwarm A. (2018). Supplementation of pelleted hazel (Corylus avellana) leaves decreases methane and urinary nitrogen emissions by sheep at unchanged forage intake. Scientific Reports. 8 (1), 1–10. doi: 10.1038/s41598-018-23572-3
Wolfe R. M., Terrill T. H., Muir J. P. (2008). Drying method and origin of standard affect condensed tannin (CT) concentrations in perennial herbaceous legumes using simplified butanol-HCl CT analysis. J. Sci. Food Agric. 88, 1060–1067. doi: 10.1002/jsfa.3188
Xiang H., Fang Y., Tan Z., Zhong R. (2021). Haemonchus contortus infection alters gastrointestinal microbial community composition, protein digestion and amino acid allocations in lambs. Front. Microbiol. 12. doi: 10.3389/fmicb.2021.797746
Yisehak K., Biruk K., Abegaze B., Janssens G. P. J. (2014). Growth of sheep fed tannin-rich Albizia gummifera with or without polyethylene glycol. Trop. Anim. Health Production 46, 1113–1118. doi: 10.1007/s11250-014-0605-6
Keywords: Rhodes grass, fodder tree, gastrointestinal parasite, greenhouse gas, ruminants, condensed tannins
Citation: Mwangi PM, Eckard R, Gluecks I, Merbold L, Mulat DG, Gakige J, Marquardt S and Pinares-Patino CS (2024) Supplementation of a tropical low-quality forage with Calliandra calothyrsus improves sheep health and performance, and reduces methane emission. Front. Anim. Sci. 5:1296203. doi: 10.3389/fanim.2024.1296203
Received: 18 September 2023; Accepted: 05 April 2024;
Published: 19 April 2024.
Edited by:
Marcos Inacio Marcondes, Washington State University, United StatesReviewed by:
Vincenzo Lopreiato, University of Messina, ItalyZhongyue Yang, Stanford University, United States
Juana Catarina Cariri Chagas, Swedish University of Agricultural Sciences, Sweden
Copyright © 2024 Mwangi, Eckard, Gluecks, Merbold, Mulat, Gakige, Marquardt and Pinares-Patino. This is an open-access article distributed under the terms of the Creative Commons Attribution License (CC BY). The use, distribution or reproduction in other forums is permitted, provided the original author(s) and the copyright owner(s) are credited and that the original publication in this journal is cited, in accordance with accepted academic practice. No use, distribution or reproduction is permitted which does not comply with these terms.
*Correspondence: Cesar S. Pinares-Patino, dHJvdXRhcmNvaXJpc0BnbWFpbC5jb20=