- 1Laboratory of Genetic Animal and Feed Resources, University of Carthage, National Agronomic Institute of Tunisia, Tunis, Tunisia
- 2Laboratory of Materials, Molecules, and Application, Preparatory Institute for Scientific and Technical Studies, University of Carthage, Tunis, Tunisia
- 3Institute of Veterinary Research of Tunisia, University of Tunis El Manar, Tunis, Tunisia
- 4Technopark of Sidi Thabet, National Institute of Research and Physico-Chemical Analysis (INRAP) Laboratory of Useful Materials, University of Carthage, Ariana, Tunisia
- 5Department of Chemistry, Faculty of Sciences of Bizerte, University of Carthage, Bizerte, Tunisia
- 6Animal and Food Resources Laboratory (LRAA), University of Carthage, National Agronomic Institute of Tunisia, Tunis, Tunisia
- 7Unité Transformations and Agroressources, ULR7519, Université d’Artois-UniLaSalle, Bethune, France
Introduction: It has been suggested that reducing the bioenergetic costs of gut inflammation and stimulating the immune system of birds lead to improved growth and feed efficiency in poultry. Essential oils and their chemotypes have been recognized for their antimicrobial properties and emerged as promising molecules that may be used for sustainable poultry production and respond to the challenges posed by the One Health concept.
Methods: This trial was conducted to evaluate the effects of carvacrol at two levels (0 and 20 mg/Kg of diet) on growth performances, carcass traits, serum biochemicals, and intestinal gut microflora counts in broiler chickens. One thousand six hundred 1-day-old broiler chicks were divided equally into 2 groups: CON, the control group, and CARV, the experimental one.
Results: Each group consisted of 08 replicates of 100 chicks each, from 1 to 35 days. Carvacrol supplementation linearly reduced feed intake (P<0.05) and improved the feed conversion ratio (P<0.05). The highest body weight gain (P<0.0001) and daily weight gain (P<0.0001) were recorded in the CARV group. However, the weights of hot and cold carcasses, as well as lymphoid organs were not affected. Similarly, blood biochemistry parameters, except for glucose, and protein electrophoresis fractions were not changed. Carvacrol supplementation modified the immune response by reducing the Albumine/Globuline ratio (P<0.05) and lowering lactic bacteria (P<0.05), Enterobacteriaceae (P<0.0001), and Salmonella (P<0.001) counts in the intestinal gut. Clostridium count wasn’t affected.
Discussion: It can be concluded that carvacrol supplementation enhanced growth performances, decreased intestinal bacterial pathogens, and improved the immune status of broiler chickens. Carvacrol can be consequently recommended as an alternative to antibiotic growth promoters for broilers’ production.
1 Introduction
The escalating global demand for broiler meat to fulfill the surged need for animal proteins (Devi et al., 2018) has urged poultry producers to improve chicks’ health, growth rates, and feed efficiency. Broilers were then treated with antibiotics growth promoters (AGPs) to boost growth, control infections, maintain gut health, and obtain heavy carcasses at slaughter (Lin et al., 2013). Regrettably, the anarchic use of AGPs has led to antibiotic resistance, which has resulted in serious public health risks (Lekshmi et al., 2017; Jeżak and Kozajda, 2022). Increased antibiotic resistance has led to the complete ban of APGs in animal feed by the European Union in 2006 (E.U. regulation 1831/2003/EC, 2003) and the further FDA request to remove APGs from animal feed in the United States (Thanner et al., 2016).
The ban on the use of such AGPs in many countries as a means to tackle antibacterial resistance accelerated the shift toward herbal medicine and phytobiotics. Essential oils (EO) have gained attention because of their antimicrobial properties that allow achieving high broiler production (Amiri et al., 2020). Nevertheless, the use of these phyto-bioactive molecules is hindered by their high volatility, low water solubility, poor intestinal absorption, and environmental instability. Synthetized phytobiotics demonstrated enhanced performances, greater feed utilization, boosted immune function, and improved meat and egg quality in broilers and layers (Gholami-Ahangaran et al., 2022; Mountzouris and Brouklogiannis, 2024).
Carvacrol (C10H14O), or 5-isopropyl-2-methylphenol (Figure 1), an oxygenated monoterpenoid phenol, is found in the essential oil fraction of some medicinal and aromatic plants such as Origanum vulgare, Origanum dictamnus, Origanum microphyllum, Origanum onites, Origanum scabrum, Thymus vulgaris, Thymus capitatus, Corido thymus, Thymus glandulosus, Nigella sativa and Lepidium flavum (Marinelli et al., 2018; Gholami-Ahangaran et al., 2022). Carvacrol is insoluble in water but very soluble in ethanol, acetone, and diethyl ether. It has a boiling point of 236–237°C and a density of 0.976 g/mL at 20°C (Yadav and Kamble, 2009).
In plants, the biosynthesis pathway of carvacrol relies on γ-terpinene, which is oxidized by cytochrome P450 monooxygenases to an unstable cyclohexadienol intermediate. The latter is dehydrogenated by a short-chain dehydrogenase/reductase to yield its corresponding ketone, which is converted to carvacrol (Hao et al., 2022). Indeed, carvacrol can be obtained by organic synthesis via Friedel-Crafts alkylation of o-cresol (Yadav and Kamble, 2009), sulfonation of p-cymene (Phillips, 1924), chlorination of α-pinene (Ritter and Ginsburg, 1950), or aromatization of carvone (Vrbková et al., 2021).
Carvacrol has been approved by the FDA for food use, recognized by the Council of Europe as a chemical flavoring category B (De Vincenzi et al., 2004), and allowed by the EFSA (European Food Safety Authority) Panel on Additives and Products or Substances used in Animal Feed to be supplemented for all poultry species as a safe feed additive (EFSA et al., 2022). In addition to its antioxidant potential (Kohen and Nyska, 2002; Hariri et al., 2010), carvacrol elicits a wide range of biological activities such as antibacterial (Lee, 2002; Imran et al., 2022), antifungal (Nazzaro et al., 2017), antiviral (Alagawany et al., 2015; Javed et al., 2021), anti-inflammatory (Imran et al., 2022; Mączka et al., 2023), anti-carcinogenic (Imran et al., 2022; Elbe et al., 2020) and immune-modulatory (Imran et al., 2022).
Researchers have focused on carvacrol as an alternative to APGs in the poultry industry, and efforts to elucidate its mechanistic aspects are ongoing. The present study aimed to investigate the effects of carvacrol on the growth performances, carcass quality, serum biochemical, and gut health of broiler chickens.
2 Materials and methods
The Official Animal Care and Use Committee of Tunisia’s National Institute of Agronomy approved all procedures used in the study.
2.1 Birds, experimental design, diets, and management
A total of 1600 one-day-old healthy unsexed Arbor Acres broiler chicks with an average weight of 42 ± 0.77 g were obtained from a commercial hatchery (SOTAVI, Ben Arous, Tunisia). The experiment was conducted in a completely randomized design. Chicks were randomly allocated to 2 groups: CON, the control group, and CARV, the experimental one. Each group consisted of 08 replicates of 100 chicks each. Birds in the two groups were fed a basal diet, and the CARV group was supplemented with Aviance (0.05%). This dose was recommended by the product’s manufacturer. Aviance is a carvacrol feed additive, which was developed and provided by Techna Group, Tunisia. An inclusion level of 0.05% of Aviance corresponded to 20 mg/Kg of carvacrol in the avian feed. The form of presentation, composition, and nutrient levels of the control and the experimental diets are shown in Table 1. The experiment was held in Zitouna Nutrition (Sousse, Tunisia), the experimental site of Techna, Tunisia. All broilers were housed in floor pens (length 3.7 × width 2.05 × height 1.5 m). They had free access to water and feed. The temperature was set to 32°C for the first 3 days and then gradually decreased until it reached 20°C. The relative humidity was between 75–79% during the first week of age. The light was provided 24 hours per day for the first two days and then reduced to 18 hours of light per day.
2.2 Data and sample collection
At 10, 21, and 35 days of age, feed refusal and birds’ weight were recorded. Body weight (BW), daily WEIGHT gain (DWG), feed intake (FI), feed conversion ratio (FCR), and mortality rate were calculated for the periods of 0–10, 11–21, and 21–35 d of age. At 35 d old, one bird from each replicate was randomly selected for carcass traits, organ weights, and blood collection (n = 16). Chickens were euthanized following the decapitation method, and hot and cold carcass weights were recorded. The liver, spleen, heart, gizzard with the intact yellow lining membrane, and intestines were removed by a trained veterinarian and weighed. Relative organ weight (% of live body weight) was calculated to indicate gut development (gizzard and intestines) and as associated with the immune system (spleen).
2.3 Blood variables
At slaughter, 5 mL of blood were collected and placed into heparinized tubes. For glucose determination, an additional 3 mL of blood was collected into sodium fluoride tripotassium Ethylene Diamine ethylenediaminetetraacetic acid (K3 EDTA) tubes. Sodium fluoride is a glycolysis inhibitor, whereas K3 EDTA prevents blood from clotting. For protein electrophoresis, non-heparinized tubes were used for blood collection. Glucose, creatinine, and total cholesterol were analyzed by an auto-analyzer (ROCHE cobas integra 400 plus Mannheim, Germany). Serum total protein (TP), albumin, α-globulins, β-globulins, and γ-globulins were analyzed using capillary protein electrophoresis equipment (SEBIA minicap flex piercing Lisses, France).
2.4 Intestinal bacterial count
This analysis aimed to evaluate the effect of the additive on the microflora of the digestive tract of broiler chickens by counting lactic acid bacteria (LAB), enterobacteria, Salmonella spp., and anaerobic bacteria. On the thirty-second day of age (d32), 16 broilers (08 controls and 08 experimental) were slaughtered, at a rate of one bird from each pen. The small intestine tract of each slaughtered subject was collected in sterile spittoons and transferred at 4°C to the bacteriology laboratory for bacteriological analyses on the same day. The digesta contents of the duodenum, jejunum, and ileum were collected and pooled. One gram of each sample was diluted in 9 ml of sterile peptone water and homogenized. Then, 1 ml of each solution was used to make a series of dilutions of 10−1 up to the 10−10 dilution. Then 100 µl of each dilution was used to inoculate the different selective solid media, namely, Slanetz-Bartley (SB) agar (BioKar diagnostics, France) for the enumeration of Enterococcus spp. (belonging to LAB); Violet Red Bile Glucose (VRBG) agar (BioMérieux, France) intended for the enumeration of enterobacteria (Escherichia coli and others); Salmonella-Shigellla (SS) agar (Merck, Darmstadt, Germany) for the enumeration of Shigella spp. and Salmonella spp. All those plates were incubated at 37°C for 18–24 h. Tryptone sulfite neomycin (TSN) agar (Merck, Darmstadt, Germany) was used for the detection and enumeration of strictly anaerobic Clostridium spp. TSN plates were incubated at 37°C for 24 h under anaerobic conditions in a gas pack system (Gas Pak, BBL, Merck, Darmstadt, Germany) (Baurhoo et al., 2007).
2.5 Statistical analysis
The results were subjected to the Shapiro-Wilk normality test. When normal, the one-way ANOVA was used. All analyses were performed at a 5% significance level using the R statistical program. The statistical model was as follows (Equation 1):
Where Yij = dependent variable, μ = variable mean, βi = effect of carvacrol, and ϵij = experimental error associated with observation Yij.
3 Results
3.1 Growth performances
Table 2 shows the effect of carvacrol-containing diets on BWG, DWG, FI, FCR, and mortality rate of broiler chicks during the starter, grower, and finisher phases. Significant effects of carvacrol supplementation on BWG and DWG were noted. Final BW and total WG were higher (P<0.0001) in CARV chicks as compared to CON pullets at 35 days old. BW increased from 41.88 ± 74.42 g to 1877 ± 34.86 g and from 42.54 ± 0.68 g to 1937.44 ± 19.05 g in CON and CARV broiler chicks, respectively. The total weight gain was higher in the CARV group than in the CON group by an average of 103.23%. The highest values of DWG were recorded in CARV chicks during the trial. Interestingly, statistically significant differences in BWG between CON and CARV groups were observed during the starter (P<0.0001) and the grower (P=0.005) phases of the trial. The DWG of CON and CARV broilers during the 35 days of the trial were 52.44 ± 0.98 g/d and 54.13 ± 0.55 g/d, respectively (P<0.0001). The lowest FI values were recorded for CARV broiler chicks during all phases of the growing period. FI varied significantly (P=0.02) between CON and CARV chicks, especially during the finisher phase, where averages of 136.51 ± 8.19 g/d and 127.83 ± 5.43 g/d were observed respectively for CON and CARV groups. The difference in total FI between the treatments was also significant (P=0.02). Likewise, the lowest values of FCR were noted in the CARV group. Significant differences in FCR between CON and CARV chicks were calculated, especially during the grower (P=0.01) and the total trial (P=0.03) periods. Similarly, the mortality rate was lower in CARV than in the CON group during the starter, the finisher, and the total trial. However, this difference was not significant (P>0.05).
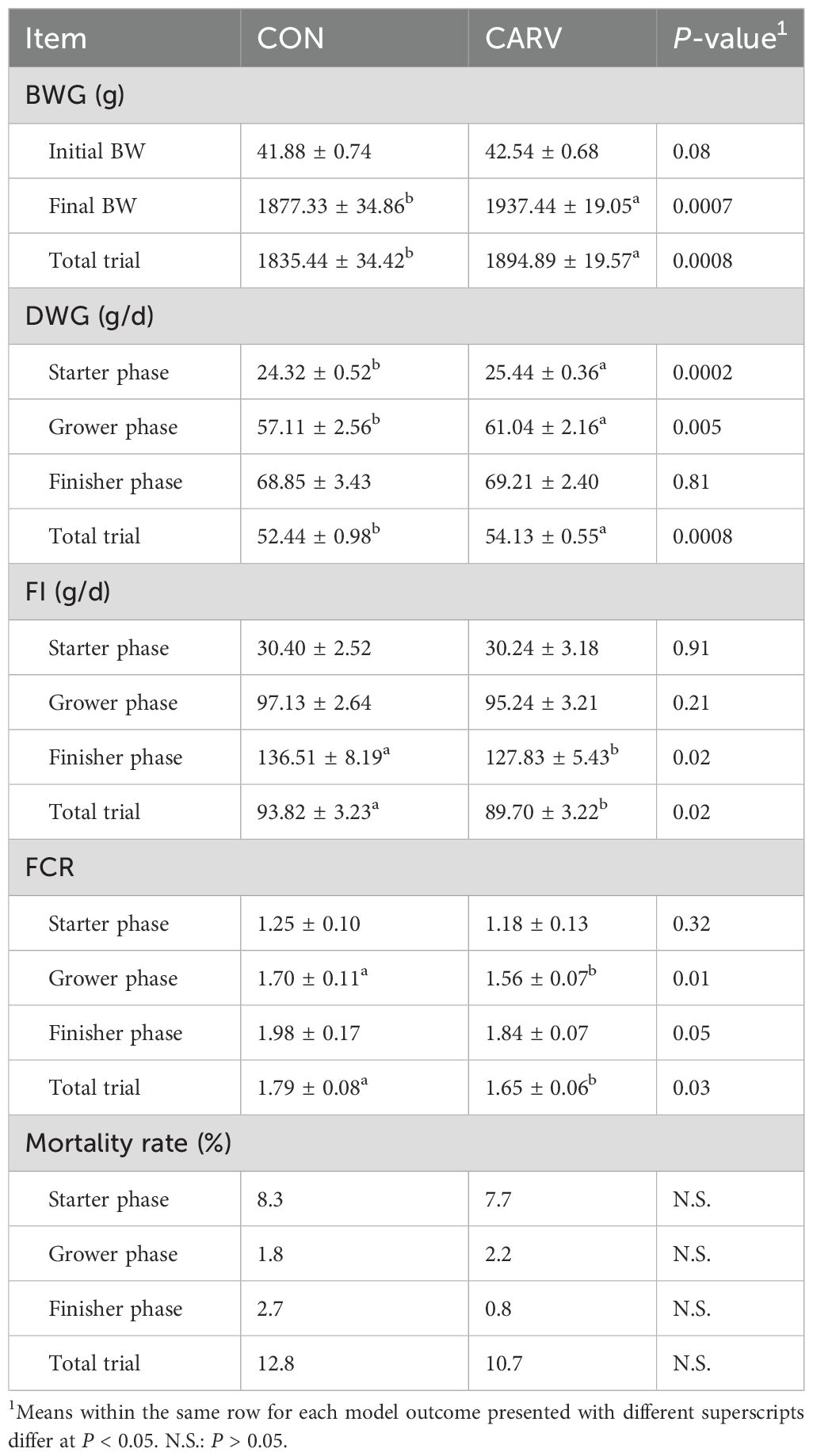
Table 2. Average Body Weight Gain (BWG), Daily Weight Gain (DWG), Feed Intake (FI), Feed Conversion Ratio (FCR), and mortality rate during the starter phase (days 0–10), grower phase (days 10–21), finisher phase (days 21–35) and total trial (days 0–35) of control (CON) and experimental (CARV) broilers.
3.2 Carcass yield and organ weights
Most read-outs on carcass yield and organ weights were not affected by the experimental treatment (Table 3), which averaged in the treated chicks 74.85% for hot carcass yield, 72.76% for cold carcass yield, 161.18 ± 22.28 g for intestines, 57.87 ± 4.95 g for liver, 2.44 ± 0.54 g for spleen and 35.42 ± 4.57 g for gizzard. Nevertheless, carvacrol treatment reduced significantly (P=0.04) heart weight, which averaged 13.9 ± 2.97 g and 10.84 ± 1.89 g respectively in CON and CARV chicks, and tended (P=0.08) to decrease the spleen/BW ratio in the CARV group.
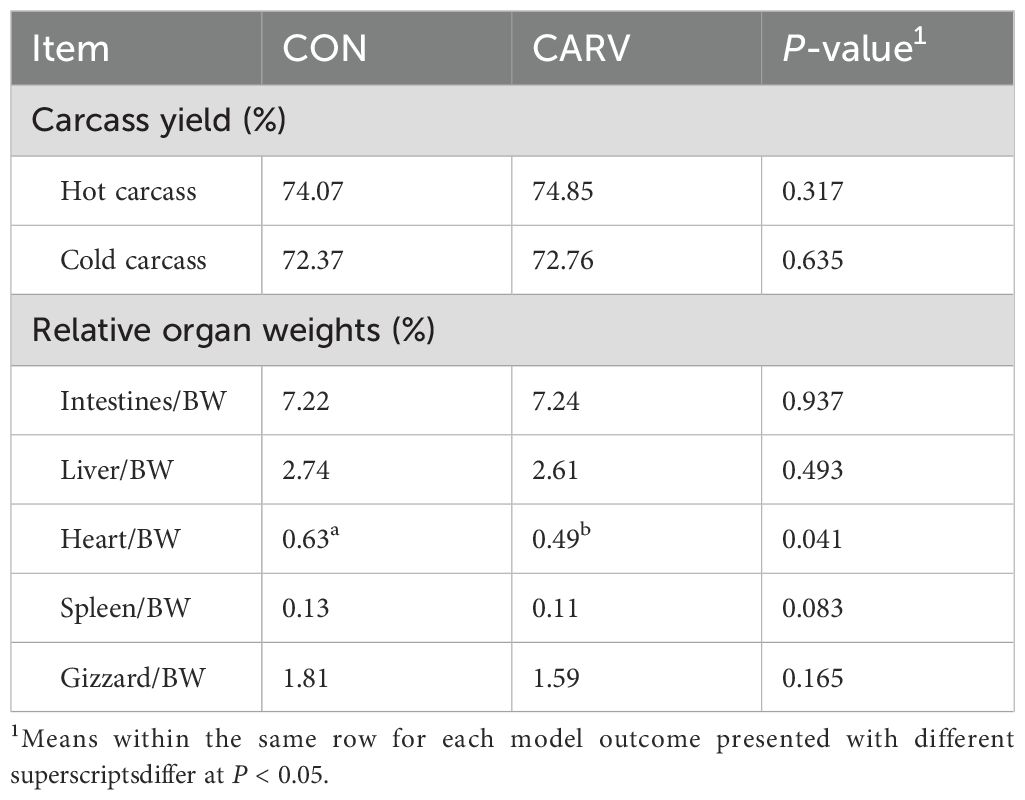
Table 3. Average carcass yield and relative organ weights in control and carvacrol-treated broiler chicks.
3.3 Blood biochemicals
Carvacrol supplementation tended to increase blood glucose (P=0.07) but didn’t have any effect (P>0.05) on total proteins, total cholesterol, and creatinine (Table 4). For all the flock of chicks, the electrophoretic pattern of serum proteins of broilers at 32 days old assessed by capillary zone electrophoresis showed 08 fractions: prealbumin, albumin, α1, α2, α3, β1, β2 and γ (Figure 2). No significant (P>0.05) differences in these protein electrophoresis fractions were noted between control and experimental groups, except the A/G ratio (P<0.05), which averaged (+SD) 0.79 ± 0.07 and 0.72 ± 0.07, respectively for control and carvacrol supplemented broilers.
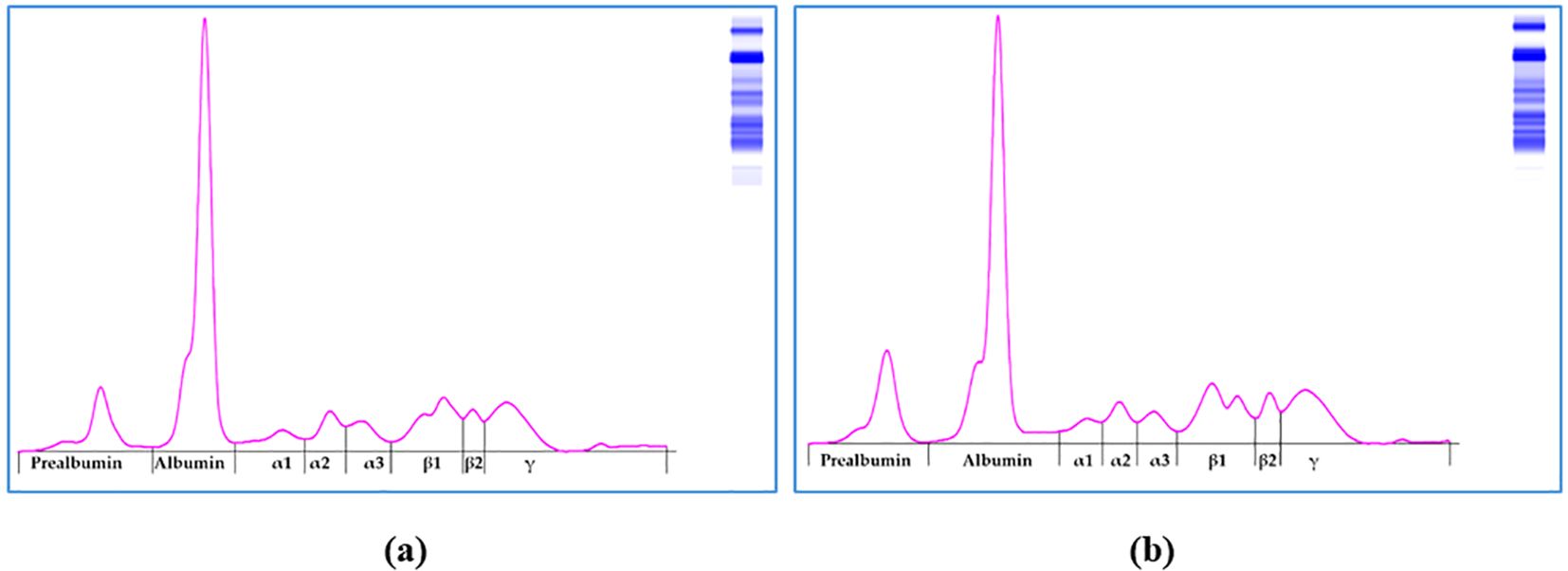
Figure 2. Capillary zone electrophoresis of (a) Control and (b) Carvacrol-treated broilers at 32 days old.
3.4 Intestinal bacterial count
The effect of carvacrol supplementation on the intestinal microbial population of broilers at 32 days old is shown in Table 5. Lactic acid bacteria, Enterobacteriaceae, and Salmonella spp. counts dropped in CARV group (P<0.05; P<0.0001 and P<0.001, respectively). Clostridium counts were not affected by the treatment.
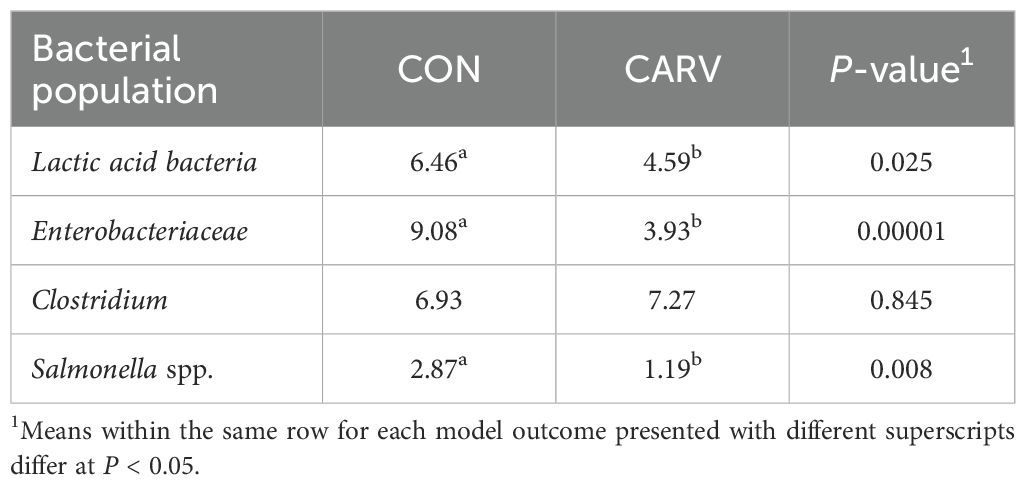
Table 5. Effect of carvacrol supplementation on intestinal bacterial population (log CFU/g of digesta) of broilers at 32 days of age.
4 Discussion
In recent decades, the shift towards phytobiotics away from chemical drugs has been increasing due to their safety, availability, and the multiple biological effects they exert. Here, we hypothesized that growth performances, carcass yield, organ weights, blood biochemistry, and intestinal microflora of broiler chickens could be improved by carvacrol supplementation. In our study, we assessed Aviance as a carvacrol feed additive. The tested level (0.05%) represents the level recommended by the product’s manufacturer. It corresponds to 20 mg of carvacrol per Kg of starter and grower feed and 15 mg of carvacrol per Kg of finisher diet. Our findings displayed beneficial effects on chicks’ growth performances. An improvement in BWG, ADG, and FCR and decreased FI were noted. Peng et al. (2016) and Zhang et al. (2021) described similar results in broiler chickens from 1 to 21 days old in response to 200, 300, and 600 mg/Kg of oregano essential oil (EO). Likewise, Lillehoj et al. (2011) reported improved BWG in response to 5 ppm carvacrol supplementation from 1-day-old for 7 days. Several studies (Jamroz et al., 2005; Feng et al., 2021; Flees et al., 2021; Mountzouris and Brouklogiannis, 2024) suggested that EOs and derived molecules such as carvacrol improve growth performances by improving gut transit rate and stimulating the secretion of digestive enzymes, which enhances the digestion, absorption, and utilization of nutrients. In this context, it was stated that EOs exert a positive effect on amylase and trypsin’s activities once incorporated in poultry feed (Jamroz et al., 2005). In addition, the increase of BWG could be attributed to the antimicrobial activity of carvacrol, allowing it to reduce intestinal diseases and promote gut health (Bento et al., 2013). The FCR indicates how efficiently feed is converted to body mass over a fixed time. The observed effects of carvacrol in lowering FI and enhancing FCR could be attributed to the subsequent increase of the absorption of the diet as well as the reduction of the bioenergetic costs of gut inflammation. The villus height directly affects the absorption capacity of the intestine mucosa membrane and Goblet cells secrete a sticky protein that exerts a protective effect on intestinal epithelium (Yamauchi et al., 2006). Liu et al. (2019) reported that 200 µl of carvacrol allowed a significant increase in the villus height and the Goblet cell count of the small intestine in broilers. Similar findings were described by Reisinger et al. (2011) in response to an administration of a blend of EOs containing 15 mg kg−1 of carvacrol and by Du et al. (2016) as a result of a supplementation of 25% thyme + 25% carvacrol in the broiler diet. In another hand, there is growing evidence of the connection between the metabolizable energy of the diet and the microbiota composition of the hindgut. Assuming that only the antimicrobial activity of carvacrol is responsible for decreasing harmful bacteria, thus enhancing intestinal integrity, increasing the metabolizable energy available for growth, and improving animal performance. In this trend, Kirkpinar et al. (2011) described a significant decrease of total Clostridium spp. counts in birds supplemented with oregano EO. Furthermore, Giannenas et al. (2004) reported that Origanum vulgare was described for its efficiency against intestinal parasites, including the Eimeria species.
Notwithstanding our findings regarding the increase of BWG and the decrease of FI, Saadat Shad et al. (2016) didn’t point to any improvement of BWG and FI in broiler chickens receiving carvacrol at 300 and 500 mg/Kg of diet during 42 days. In the same way, Botsoglou et al. (2002) didn’t state any beneficial effect on growth performances with various doses of oregano EO (from 50 to 1000 mg/Kg). While Jaafari et al. (2012) reported reduced FI and BWG at 200 ppm carvacrol supplementation for 4 weeks from 1 day old, Bosetti et al. (2020) described increased FI in response to a microencapsulated EO containing g 60% cinnamaldehyde and 30% carvacrol, supplemented at 100, 200 and 400 mg/kg. Such inconsistencies could be attributed to the odor and the flavor of the supplemented feed, which may stimulate or suppress the bird’s appetite. It could also vary with the supplied types and doses (Russo et al., 1998), the phytobiotics’ combination (Skoufos et al., 2016), the bird type, and the rearing conditions (Pirgozliev, 2014). Improvement in chicken meat production suggests enhanced growth, increased carcass yield and muscle proportion, as well as reduced abdominal fat. In our study, although birds receiving a carvacrol had heavier hot and cold carcass yields, the difference between the control and experimental groups wasn’t statistically significant. A non-significant impact of carvacrol on internal organ weights was noted as well. The current findings comply with the results of Alp et al. (2012), indicating no additional beneficial effect of dietary supplementation of oregano EO at 300 mg/Kg on broilers’ carcass characteristics. Similarly, Alcicek et al. (2003) showed that supplementation of a dose above 48 mg/Kg of a selection of EOs (including Oregano EO) had any positive effect on broilers’ carcass yield. Nonetheless, Khattak et al. (2014) described an increase in carcass and breast weights in response to a feed additive containing a blend of EOs, including Oregano and thyme EOs. This was also the case with Alcicek et al. (2003), who concluded that their selection of EOs was positively effective on broilers’ carcass yield at a dose lower than 48 mg/Kg.
Reports on the effect of carvacrol on internal organ growth are very scarce. Our results corroborate those of Rahimi et al. (2011) and Hashemipour et al. (2013), who reported that the relative weights of the spleen and other lymphoid organs were not affected by thymol and carvacrol supplementation. An elevated weight of these lymphoid organs may be a sign of infections and increased mortality in birds. A balanced metabolism suggests an equilibrium between the rate of catabolic and anabolic reactions. Blood biochemistry is an indicator of the bird’s metabolism and is affected by the feed composition, as dietary nutrients can influence cell homeostasis. In the present study, carvacrol tended to increase blood glucose levels, which may be an indicator of a stimulation of glucose metabolism and a better availability of this carbohydrate for cells. The other blood biochemical parameters (total proteins, cholesterol, creatinine, albumin, globulins) were not affected by carvacrol, except the A/G ratio. Our values were in harmony with the normal range for broilers. This result was in agreement with those of Li et al. (2023), who showed that thymol + carvacrol supplementation did not have a significant effect on serum biochemical indexes (total proteins, albumin, globulin, uric acid, IgE, IgG, and IgM) of broilers at 21 and 42 days of age. Our results were also in line with Lee et al. (2003), who indicated no hypocholesterolaemic effect of dietary carvacrol in female broilers at 28 d of age. However, Cho et al. (2014) described reduced blood total cholesterol in broilers fed a mixture of EOs with thyme and star anise as active components (17.0% and 17.0%). These inconsistencies could be assigned to the applied dose, the composition of the EO mix, the stability of the product, or the rearing conditions of the birds.
In healthy animals, albumin corresponds to the largest peak in the protein electrophoretogram. The liver synthesizes albumin, like the other plasma proteins. It is involved in the regulation of colloid oncotic pressure in both intravascular and extravascular spaces, as well as the transport of a large number of compounds (Kaneko et al., 1997). Globulins are implicated in various processes, including the transport of ions, hormones, and lipids, acute phase response, and immune response (Kaneko et al., 1997). The α fraction is the fastest migrating globulin fraction. It includes α-lipoproteins, α1 acid glycoproteins, α1 antitrypsin, α2 macroglobulin, haptoglobin, etc. The β fraction consists of heterogeneous proteins such as β-lipoprotein LDL, transferrin, ferritin, complement (C3, C4), C-Reactive protein (CRP), etc (Kaneko et al., 1997). The γ fraction comprises immunoglobulins synthesized by β cells, such as IgM, IgA, IgE, and IgY (Kaneko et al., 1997). In birds, the A/G ratio is calculated by dividing the albumin fraction by the sum of globulins. It is used to assess the global status of birds’ health since it reflects the global evolution of all globulin fractions. Although carvacrol didn’t significantly affect albumin and globulin levels, the A/G ratio was significantly decreased in the experimental birds. This effect can be explained by the enhancement of the immune response, taking into consideration the observed decrease of mortality rate, as well as Enterobacteriaceae and Salmonella spp. counts in the experimental group. This effect may be owed to the anti-inflammatory and antimicrobial activities of carvacrol, which was reported to lower the expression of COX2 mRNA and proteins triggered by lipopolysaccharides present in the outer leaflet of the outer membrane of Gram-negative bacteria and recognized as endotoxins. It was also reported as an inhibitor of PPAR-α and a γ-regulator (Hotta et al., 2010). These effects are at the origin of its biological activities, including its anti-inflammatory response (Hotta et al., 2010).
Our findings confirmed the antibacterial activity of carvacrol. We experienced a significant decrease in lactic bacteria, Enterobacteriaceae, and Salmonella counts in the intestinal gut of experimental broilers. Dahiya et al. (2006) described an antimicrobial activity of carvacrol against S. aureus, Clostridia spp., E. coli, and S. pullorum. Broiler chicken productivity is optimal with a healthy gastrointestinal tract (GIT) (Sugiharto, 2016). A balanced population of pathogenic and beneficial microflora in the GIT plays a crucial role in the digestion and absorption of nutrients (Patterson and Burkholder, 2003; Kročko et al., 2012). Escherichia coli, Salmonella typhimurium, and Clostridium perfringens are the 3 major pathogens found in the poultry gut (La Ragione et al., 2004). Therefore, antibiotics are usually used to reduce the risk of bacterial diseases. However, the selection and spread of antibiotic-resistant bacteria caused by heavy use of antibiotics, especially as growth promoters, is a cause for concern. Therefore, exploring biological alternatives such as food additives to reduce antibiotics as growth promoters has been reported (Hajati and Rezaei, 2010). Indeed, essential oils and plant extracts (e.g. thyme, garlic, cinnamaldehyde, oleoresin, pennywort, and lavender) have been reported to increase the number of beneficial bacteria by altering the intestinal microflora (mainly Gram-negative bacteria) due to their antimicrobial properties (Sariçoban and Yilmaz, 2014). In addition, they have been reported to suppress pathogenic microorganisms and reduce their numbers in all intestinal segments of broiler chickens and pigs (Adaszýnska-Skwirzýnska and Szczerbínska, 2019; Zhu et al., 2019).
The bactericidal activity of carvacrol is obviously explained by its ability to change the bacterial cell membrane structure and conformation. It induces depolarization, increased permeability, and leakage of the cytoplasmic contents, causing bacterial cell death. Carvacrol is an oxygenated lipophilic monoterpenoid phenol that holds a hydroxyl group on its aromatic ring. This hydroxyl group is at the origin of the bactericidal activity of carvacrol since it acts as a proton exchanger and drops the pH gradient across the bacterial membrane. The subsequent collapse of the proton motive force and depletion of the ATP pool lead to cell death (Ultee et al., 2002). Moreover, carvacrol preferentially binds to phosphatidylglycerol and phosphatidylethanolamine and partially to cardiolipins in the bacterial membrane (Wijesundara et al., 2022). By integrating the phospholipid monolayer, carvacrol forms aggregates of antibacterial-lipid complexes. The packing effectiveness of the acyl chains (tails) of these phospholipids is then lowered. As a result, the bacterial cell membrane fluidity increases, and its related total dipole moment is altered (Nowotarska et al., 2014). Fluidization of the membrane lipids is a consequence of the disturbance of the non-covalent van der Waals bonds that connect the phospholipid acyl chains in the membrane lipids (Sohlenkamp and Geiger, 2015). Such a fluidization mechanism was reported for thymol, which is an isomer to carvacrol. Based on these reports, we suggest that carvacrol exerts a similar fluidization effect on the bacterial lipid membrane.
In our study, treatment of chickens with carvacrol as a dietary supplement significantly reduced the growth of enterobacteria, including Salmonella spp. and lactic acid bacteria; however, a non-significant increase in anaerobic bacteria was observed. Inhibition of enterobacteria in general minimizes the risk of avian pathologies caused by this bacterial family, mainly colibacillosis caused by Escherichia coli. This result (decrease in coliforms and Salmonella) has been often reported by other studies (Alagawany et al., 2021; Yilmaz and Gul, 2023).
However, the decrease in the total count of lactic acid bacteria (on SB medium) is in contradiction with other studies where the number of lactic acid bacteria is significantly improved by the treatment of essential oils (Chen et al., 2020). Lactic acid bacteria, such as Lactobacillus, are largely used as probiotics in poultry production because they are able to thrive under harsh gastrointestinal conditions, confer beneficial effects on growth performance, and act as antagonism against enteric pathogens (El-Sawah et al., 2020). The decrease in lactic acid bacteria in our study could be linked to an anti-LAB effect exerted by carvacrol. Despite all these desired beneficial effects of lactic bacteria, it seems that the growth promoter and antimicrobial properties have made it possible to remedy these intended effects.
Carvacrol promoted the growth performance of broiler chickens. It allowed for the reduction of FI and improved ADG and FCR, leading to an enhanced BWG. Moreover, we found that carvacrol tended to increase blood glucose levels without affecting total proteins and cholesterol. Besides, carvacrol improved gut health by decreasing the Enterobacteriaceae and Salmonella spp. counts, which resulted in a reduced mortality rate and A/G ratio. In addition, this bactericidal activity of carvacrol targeted lactic acid bacteria but had no drastic effect on the global health status and the growth performance of the birds. Therefore, as an antibacterial and growth promoter agent, having a plant origin, and being regarded as safe, carvacrol is a potent natural molecule that can be used as a substitute for AGPs.
The findings of this study support the need for more investigation in this field. It is crucial to recognize that this study only employed a small number of duplicates, which might have an impact on the findings’ statistical power and generalizability. The results should thus be regarded as preliminary evidence and interpreted cautiously. Housing circumstances, the nutritional and health state of the birds, and the product’s stability are some of the intrinsic and extrinsic elements that affect the effectiveness of phytobiotics like carvacrol.
Consequently, more research with more duplicates and more expansive experimental setups is required to confirm these results. The effects of carvacrol, in particular, on broilers raised in less-than-ideal housing circumstances need careful examination, and figuring out the ideal lowest active dosage is still a crucial topic for further study.
5 Conclusion
Overall, this study highlights the potential of carvacrol as a promising growth promotor and antibacterial agent for the poultry industry. Carvacrol enhanced body weight gain and feed conversion in broiler chickens while reducing feed intake. Furthermore, carvacrol promoted gut health by reducing Enterobacteriaceae and Salmonella spp. counts, which resulted in an enhanced global health status despite the decrease of lactic acid bacteria population. These findings suggest that carvacrol is a possible candidate for substituting AGPs. Further studies are warranted to determine its full potential as a prebiotic for enhancing poultry health, improving growth performance, and reducing breeding costs.
Data availability statement
The raw data supporting the conclusions of this article will be made available by the authors, without undue reservation.
Ethics statement
The animal study was approved by the Official Animal Care and Use Committee of the National Institute of Agronomy of Tunisia (Protocol No. 05/15). The study was conducted in accordance with the local legislation and institutional requirements.
Author contributions
IB: Conceptualization, Formal Analysis, Methodology, Software, Supervision, Validation, Writing – original draft, Writing – review & editing. MV: Conceptualization, Methodology, Software, Writing – original draft. SL: Conceptualization, Methodology, Validation, Writing – original draft. BB: Formal Analysis, Writing – original draft. HS: Methodology, Validation, Writing – original draft. MA: Resources, Validation, Writing – original draft. NM: Validation, Writing – review & editing. LC: Methodology, Writing – review & editing. PM: Conceptualization, Resources, Validation, Writing – review & editing. MA: Conceptualization, Resources, Validation, Writing – review & editing. TN: Conceptualization, Funding acquisition, Resources, Validation, Writing – review & editing.
Funding
The author(s) declare that financial support was received for the research and/or publication of this article. Techna group, Tunisia funded this study.
Acknowledgments
The authors are grateful to Techna Group, Tunisia for providing the birds and experimental facilities.
Conflict of interest
The authors declare that the research was conducted in the absence of any commercial or financial relationships that could be construed as a potential conflict of interest.
Generative AI statement
The author(s) declare that no Generative AI was used in the creation of this manuscript.
Publisher’s note
All claims expressed in this article are solely those of the authors and do not necessarily represent those of their affiliated organizations, or those of the publisher, the editors and the reviewers. Any product that may be evaluated in this article, or claim that may be made by its manufacturer, is not guaranteed or endorsed by the publisher.
References
Adaszýnska-Skwirzýnska M. and Szczerbínska D. (2019). The effect of lavender (Lavandula angustifolia) essential oil as a drinking water supplement on the production performance, blood biochemical parameters, and ileal microflora in broiler chickens. Poult. Sci. 98, 358–365. doi: 10.3382/ps/pey385
Alagawany M., El-Hack M. E. A., Farag M. R., Tiwari R., and Dhama K. (2015). Biological effects and modes of action of carvacrol in animal and poultry production and health - A review. Adv. Anim. Vet. Sci. 3, 73–84. doi: 10.14737/journal.aavs/2015/3.2s.73.84
Alagawany M., El-Saadony M. T., Elnesr S. S., Farahat M., Attia G., Madkour M., et al. (2021). Use of lemongrass essential oil as a feed additive in quail’s nutrition: its effect on growth, carcass, blood biochemistry, antioxidant and immunological indices, digestive enzymes, and intestinal microbiota. Poult. Sci. 100, 101172. doi: 10.1016/j.psj.2021.101172
Alcicek A., Bozkurt M., and Çabuk. M. (2003). The effect of an essential oil combination derived from selected herbs growing wild in Turkey on broiler performance. S. Afr. J. Anim. Sci. 33, 89–94. doi: 10.4314/sajas.v33i2.3761
Alp M., Midilli M., Kocabagli N., Yilmaz H., Turan N., Gargili A., et al. (2012). The effects of dietary oregano essential oil on live performance, carcass yield, serum immunoglobulin G level, and oocyst count in broilers. J. App. Poult. Res. 21, 630–636. doi: 10.3382/japr.2012-00551
Amiri N., Afsharmanesh M., Salarmoini M., Meimandipour A., Hosseini S. A., and Ebrahimnejad H. (2020). Effects of nanoencapsulated cumin essential oil as an alternative to the antibiotic growth promoter in broiler diets. J.f App. Poult. Res. 29, 875–885. doi: 10.1016/j.japr.2020.08.004
Baurhoo B., Letellier A., Zhao X., and Ruiz-Feria C. A. (2007). Caecal populations of Lactobacilli and Bifidobacteria and Escherichia coli after in vivo Escherichia coli challenge in birds fed diets with purified lignin or mannan oligosaccharides. J. Poult. Sci. 86, 2509–2516. doi: 10.3382/ps.2007-00136
Bento M. H. L., Ouwehand A. C., Tiihonen K., Lahtinen S., Nurminen P., Saarinen M. T., et al. (2013). Essential oils and their use in animal feeds for monogastric animals – effects on feed quality, gut microbiota, growth performance and food safety: a review. Vet. Med. 58, 449–458. doi: 10.17221/7029-VETMED
Bosetti G. E., Griebler L., Aniecevski E., Facchi C. S., Baggio C., Rossatto G., et al. (2020). Microencapsulated carvacrol and cinnamaldehyde replace growth-promoting antibiotics: Effect on performance and meat quality in broiler chickens. Anais Acad. Bras. Ciên. 92, 109–111. doi: 10.1590/0001-3765202020200343
Botsoglou N. A., Florou-Paner P., Christaki E., Fletouris D. J., and Spais A. B. (2002). Effect of dietary oregano essential oil on performance of chickens and on iron-induced lipid oxidation of breast, thigh and abdominal fat tissues. Br. Poult. Sci. 43, 223–230. doi: 10.1080/00071660120121436
Chen Y., Wang J., Yu L., Xu T., and Zhu N. (2020). Microbiota and metabolome responses in the cecum and serum of broiler chickens fed with plant essential oils or virginiamycin. Sci. Rep. 10, 5382. doi: 10.1038/s41598-020-60135-x
Cho J. H., Kim H. J., and Kim I. H. (2014). Effects of phytogenic feed additive on growth performance, digestibility, blood metabolites, intestinal microbiota, meat color, and relative organ weight after oral challenge with Clostridium perfringens in broilers. Livest. Sci. 160, 82–88. doi: 10.1016/j.livsci.2013.11.006
Dahiya J. P., Wilkie D. C., Van Kessel A. G., and Drew M. D. (2006). Potential strategies for controlling necrotic enteritis in broiler chickens in a post-antibiotic era. Anim. Feed Sci. Technol. 129, 60–88. doi: 10.1016/j.anifeedsci.2005.12.003
Devi P. C., Samanta A. K., Das B., Kalita G., Behera P. S., and Barman S. (2018). Effect of plant extracts and essential oil blend as alternatives to antibiotic growth promoters on growth performance, nutrient utilization and carcass characteristics of broiler chicken. Ind. J. Anim. Nutr. 35, 421–427. doi: 10.5958/2231-6744.2018.00064.6
De Vincenzi M., Stammati A., De Vincenzi A., and Silano M. (2004). Constituents of aromatic plants: carvacrol. Fitoter. 75, 801–804. doi: 10.1016/j.fitote.2004.05.002
Du E., Wang W., Gan L., Li Z., Guo S., and Guo Y. (2016). Effects of thymol and carvacrol supplementation on intestinal integrity and immune responses of broiler chickens challenged with Clostridium perfringens. J. Anim. Sci. Biotechnol. 7, 19–29. doi: 10.1186/s40104-016-0079-7
E.U. regulation 1831/2003/EC. (2003). European Commission Regulation (EC) No 1831/2003 of the European Parliament and of the Council of 22 September 2003 on additives for use in animal nutrition. Off. J. Eur. Union 268, 29–43.
EFSA, Bampidis V., Azimonti G., Bastos M. L., Christensen H., Dusemund B., et al. (2022). Safety and efficacy of a feed additive consisting of apreparation of carvacrol, thymol, D-carvone, methylsalicylate and L-menthol (Biomin® DC-P) for all poultryspecies (Biomin GmbH). EFSA J. 20, 7429. doi: 10.2903/j.efsa.2022.7429
El-Sawah A. A., Aboelhadid S. M., El-Nahass E. N., Helal H. O., Korany A.M., and El-Ashram S. (2020). Efficacy of probioticEnterococcus faecium in combination with diclazuril against coccidiosisin experimentally infected broilers. J. App. Microbiol. 129, 1020–1028. doi: 10.1111/jam.14691
Elbe H., Yigitturk G., Cavusoglu T., Baygar T., Ozgul Onal M., and Ozturk F. (2020). Comparison of ultrastructural changes and the anticarcinogenic effects of thymol and carvacrol on ovarian cancer cells: which is more effective? Ultrastruct. Pathol. 44, 193–202. doi: 10.1080/01913123.2020.1740366
Feng J., Lu M., Wang J., Zhang H., Qiu K., Qi G., et al. (2021). Dietary oregano essential oil supplementation improves intestinal functions and alters gut microbiota in late-phase laying hens. J. Anim. Sci. Biotechnol. 12, 1–15. doi: 10.1186/s40104-021-00600-3
Flees J. J., Ganguly B., and Dridi S. (2021). Phytogenic feed additives improve broiler feed efficiency via modulation of intermediary lipid and protein metabolism–related signaling pathways. Poult. Sci. 100, 100963. doi: 10.1016/j.psj.2020.12.060
Gholami-Ahangaran M., Ahmadi-Dastgerdi A., Azizi S., Basiratpour A., Zokaei M., and Derakhshan M. (2022). Thymol and carvacrol supplementation in poultry health and performance. Vet. Med. Sci. 8, 267–288. doi: 10.1002/vms3.v8.1
Giannenas I. A., Florou-Paneri P., Papazahariadou M., Botsoglou N. A., Christaki E., and Spais. A. (2004). Effect of diet supplementation with ground oregano on performance of broiler chickens challenged with Eimeria tenella. Archiv. Fur. Geflugelkunde 68, 247–252. doi: 10.1016/S0003-9098(25)00054-2
Hajati H. and Rezaei M. (2010). The application of prebiotics in poultry production. Int. J. Poult. Sci. 9, 298–304. doi: 10.3923/ijps.2010.298.304
Hao Y., Guo X., Yang R., Yan Y., Sun M., Li H., et al. (2022). Unraveling the biosynthesis of carvacrol in different tissues of Origanum vulgare. Int. J. Mol. Sci. 23, 13231. doi: 10.3390/ijms232113231
Hariri A. T., Moallem S. A., Mahmoudi M., Memar B., and Hosseinzadeh H. (2010). Sub-acute effects of diazinon on biochemical indices and specific biomarkers in rats: Protective effects of crocin and safranal. Food Chem. Toxicol. 48, 2803–2808. doi: 10.1016/j.fct.2010.07.010
Hashemipour H., Kermanshahi H., Golian A., and Veldkamp T. (2013). Effect of thymol and carvacrol feed supplementation on performance, antioxidant enzyme activities, fatty acid composition, digestive enzyme activities, and immune response in broiler chickens. Poult. Sci. 92, 2059–2069. doi: 10.3382/ps.2012-02685
Hotta M., Nakata R., Katsukawa M., Hori K., Takahashi S., and Inoue H. (2010). Carvacrol, a component of thyme oil, activates PPARand suppresses COX-2 expression. J. Lipid Res. 51, 132–139. doi: 10.1194/jlr.M900255-JLR200
Imran M., Aslam M., Alsagaby S. A., Saeed F., Ahmad I., Afzaal M., et al. (2022). Therapeutic application of carvacrol: A comprehensive review. Food Sci. Nutr. 10, 3544–3561. doi: 10.1002/fsn3.v10.11
Jaafari A., Tilaou M., Mouse H. A., M’bark L. A., Aboufatima R., Chait A., et al. (2012). Comparative study of the antitumor effect of natural monoterpenes: Relationship to cell cycle analysis. Braz. J. Pharmacog. 22, 534–540. doi: 10.1590/S0102-695X2012005000021
Jamroz D., Wiliczkiewicz A., Wertelecki T., Orda J., and Skorupińska J. (2005). Use of active substances of plant origin in chicken diets based on maize and locally grown cereals. Br. Poult. Sci. 46, 485–493. doi: 10.1080/00071660500191056
Javed H., Meeran M. F. N., Jha N. K., and Ojha S. (2021). Carvacrol, a plant metabolite targeting viral protease (Mpro) and ACE2 in host cells can be a possible candidate for COVID-19. Front. Plant Sci. 11, 1–10. doi: 10.3389/fpls.2020.601335
Jeżak K. and Kozajda A. (2022). Occurrence and spread of antibiotic-resistant bacteria on animal farms and in their vicinity in Poland and Ukraine. Env. Sci. Pol. Res. 29, 9533–9559.
Kaneko J. J., Harvey J. W., and Bruss M. L. (1997). “Serum proteins and the disproteinemias,” in Clinical biochemistry of domestic animals, 5th Edn. Ed. Kaneko J. J. (Academic Press, Sn Diego), 117–138.
Khattak F., Ronchi A., Castelli P., and Sparks N. (2014). Effects of natural blend of essential oil on growth performance, blood biochemistry, cecal morphology, and carcass quality of broiler chickens. Poult. Sci. 93, 132–137. doi: 10.3382/ps.2013-03387
Kirkpinar F., Bora Ünlü H., and Özdemir G. (2011). Effects of oregano and garlic essential oils on performance, carcass, organ and blood characteristics and intestinal microflora of broilers. Livest. Sci. 137, 219–225. doi: 10.1016/j.livsci.2010.11.010
Kohen R. and Nyska A. (2002). Oxidation of biological systems: Oxidative stress phenomena, antioxidants, redox reactions, and methods for their quantification. Toxic. Pathol. 30, 620–650. doi: 10.1080/01926230290166724
Kročko M., Čanigová M., Bezeková J., Lavová M., Haščík P., and Ducková V. (2012). Effect of nutrition with propolis and bee pollen supplements on bacteria colonization pattern in gastrointestinal tract of broiler chickens. J. Anim. Sci. Biotechnol. 45, 63–67.
La Ragione R. M., Narbad A., Gasson M. J., and Woodward M. J. (2004). In vivo characterization of Lactobacillus johnsonii F19785 for use as a defined competitive exclusion agent against bacterial pathogens in poultry. Lett. App. Microbiol. 38, 197–205. doi: 10.1111/j.1472-765X.2004.01474.x
Lee K. W. (2002). Essential oils in broiler nutrition. Doctoral dissertation. Utrecht University, Utrecht, Netherlands.
Lee K. W., Everts H., Kappert H. J., Yeom K.-H., and Beynen A. C. (2003). Dietary carvacrol lowers body weight gain but improves feed conversion in female broiler chickens. J. App. Poult. Res. 12, 394–399. doi: 10.1093/japr/12.4.394
Lekshmi M., Ammini P., Kumar S., and Varela M. F. (2017). The food production environment and the development of antimicrobial resistance in human pathogens of animal origin. Microorganisms 5, 11. doi: 10.3390/microorganisms5010011
Li L., Chen X., Zhang K., Tian G., Ding X., Bai S., et al. (2023). Effects of thymol and carvacrol eutectic on growth performance, serum biochemical parameters, and intestinal health in broiler chickens. Animals 13, 2242. doi: 10.3390/ani13132242
Lillehoj H. S., Kim D. K., Bravo D. M., and Lee S. H. (2011). Effects of dietary plant-derived phytonutrients on the genome-wide profiles and coccidiosis resistance in the broiler chickens. BMC Proceed. 5, S34. doi: 10.1186/1753-6561-5-S4-S34
Lin J., Hunkapiller A. A., Layton A. C., Chang Y. J., and Robbins K. R. (2013). Response of intestinal microbiota to antibiotic growth promoters in chickens. Foodborne Pathog. Dis. 10, 331–337. doi: 10.1089/fpd.2012.1348
Liu S. D., Song M. H., Yun W., Lee J. H., Kim H. B., and Cho J. H. (2019). Effects of oral administration of essential oils on anti-immune stress, antimicrobial properties, and repairing the intestinal damage in broilers challenged by lipopolysaccharide. Can. J. Anim. Sci. 99, 377–383. doi: 10.1139/cjas-2018-0055
Mączka W., Twardawska M., Grabarczyk M., and Wińska K. (2023). Carvacrol—A natural phenolic compound with antimicrobial properties. Antibiotics 12, 824. doi: 10.3390/antibiotics12050824
Marinelli L., di Stefano A., and Cacciatore I. (2018). Carvacrol and its derivatives as antibacterial agents. Phytochem. Rev. 17, 903–921. doi: 10.1007/s11101-018-9569-x
Mountzouris K. C. and Brouklogiannis I. (2024). Phytogenics as natural gut health management tools for sustainable poultry production. Livest. Sci. 286, 105525. doi: 10.1016/j.livsci.2024.105525
Nazzaro F., Fratianni F., Coppola R., and Feo V. D. (2017). Essential oils and antifungal activity. Pharmaceuticals 10, 86. doi: 10.3390/ph10040086
Nowotarska S. W., Nowotarski K. J., Friedman M., and Situ C. (2014). Effect of structure on the interactions between five natural antimicrobial compounds and phospholipids of bacterial cell membrane on model monolayers. Molecules 19, 7497–7515. doi: 10.3390/molecules19067497
Patterson J. A. and Burkholder K. M. (2003). Application of prebiotics and probiotics in poultry production. Poult. Sci. 82, 627–631. doi: 10.1093/ps/82.4.627
Peng Q. Y., Li J. D., Li Z., Duan Z. Y., and Wu Y. P. (2016). Effects of dietary supplementation with oregano essential oil on growth performance, carcass traits and jejunal morphology in broiler chickens. Anim. Feed Sci. Technol. 214, 148–153. doi: 10.1016/j.anifeedsci.2016.02.010
Phillips M. (1924). The sulfonation of para-cymene. J. Am. Chem. Soc 46, 686–694. doi: 10.1021/ja01668a019
Pirgozliev V. (2014). Rearing conditions influence nutrient availability of plant extracts supplemented diets when fed to broiler chickens. J. Anim. Physiol. Anim. Nutr. 98, 667–671. doi: 10.1111/jpn.2014.98.issue-4
Rahimi S., Teymouri Zadeh Z., Karimi Torshizi M. A., Omidbaigi R., and Rokni H. (2011). Effect of the three herbal extracts on growth performance, immune system, blood factors and intestinal selected bacterial population in broiler chickens. J. Agric. Sci. Technol. 13, 527–539.
Reisinger N., Steiner T., Nitsch S., Schatzmayr G., and Applegate T. J. (2011). Effects of a blend of essential oils on broiler performance and intestinal morphology during coccidial vaccine exposure. J. Appl. Poult. Res. 20, 272–283. doi: 10.3382/japr.2010-00226
Ritter J. J. and Ginsburg D. (1950). Preparation of chlorination of alpha-pinene with tert-butyl hypochlorite. J. Am. Chem. Soc 72, 2381–2384. doi: 10.1021/ja01162a009
Russo M., Galletti G. C., Bocchini P., and Carnacini. A. (1998). Essential oil chemical composition of wild populations of Italian Oregano spice (Origanum vulgare ssp. hirtum (link) lestwaart). J. Agric. Food Chem. 46, 3741–3746. doi: 10.1021/jf980087w
Saadat Shad H., Mazhari M., Esmaeilipour O., and Khosravinia H. (2016). Effects of thymol and carvacrol on productive performance, antioxidant enzyme activity and certain blood metabolites in heat-stressed broilers. Ir. J. App. Anim. Sci. 6, 195–202.
Sariçoban C. and Yilmaz M. T. (2014). Effect of thyme/cumin essential oils and butylated hydroxyl anisole/butylated hydroxyl toluene on physicochemical properties and oxidative/microbial stability of chicken patties. Poult. Sci. 93, 456–463. doi: 10.3382/ps.2013-03477
Skoufos I., Giannenas I., Tontis D., Bartzanas T., Kittas C., Panagakis P., et al. (2016). Effects of oregano essential oil and attapulgite on growth performance, intestinal microbiota and morphometry in broilers. S. Afr. J. Anim. Sci. 46, 77–88. doi: 10.4314/sajas.v46i1.10
Sohlenkamp C. and Geiger O. (2015). Bacterial membrane lipids: Diversity in structures and pathways. FEMS Microbiol. Rev. 40, 133–159. doi: 10.1093/femsre/fuv008
Sugiharto S. (2016). Role of nutraceuticals in gut health and growth performance of poultry. J. Saudi Soc Agric. Sci. 15, 99–111. doi: 10.1016/j.jssas.2014.06.001
Thanner S., Drissner D., and Walsh F. (2016). Antimicrobial resistance in agriculture. mBio 7, e02227–e02215. doi: 10.1128/mBio.02227-15
Ultee A., Bennik M. H. J., and Moezelaar R. (2002). The phenolic hydroxyl group of carvacrol is essential for action against the food-borne pathogen Bacillus cereus. Appl. Environ. Microbiol. 68, 1561–1568. doi: 10.1128/AEM.68.4.1561-1568.2002
Vrbková E., Šímová A., Vyskocilová E., Lhotka M., and Cervený L. (2021). Acid treated montmorillonite—Eco-Friendly clay as catalyst in carvone isomerization to carvacrol. Reactions 2, 486–498. doi: 10.3390/reactions2040031
Wijesundara N. M., Lee S. F., Cheng Z., Davidson R., Langelaan D. N., and Rupasinghe H. P. V. (2022). Bactericidal Activity of Carvacrol against Streptococcus pyogenes Involves Alteration of Membrane Fluidity and Integrity through Interaction with Membrane Phospholipids. Pharmaceutics 14, 1992. doi: 10.3390/pharmaceutics14101992
Yadav G. and Kamble S. B. (2009). Synthesis of carvacrol by Friedel–Crafts alkylation of o-cresol with isopropanol using superacidic catalyst UDCaT-5. J. Chem. Technol. Biotechnol. 84, 1499–1508. doi: 10.1002/jctb.v84:10
Yamauchi K., Buwjoom T., Koge K., and Ebashi T. (2006). Histological alterations of the intestinal villi and epithelial cells in chickens fed dietary sugar cane extract. Br. Poult. Sci. 47, 544–553. doi: 10.1080/00071660600963149
Yilmaz E. and Gul M. (2023). Effects of cumin (Cuminum cyminum L.) essential oil and chronic heat stress on growth performance, carcass characteristics, serum biochemistry, antioxidant enzyme activity, and intestinal microbiology in broiler chickens. Vet. Res. Com. 47, 861–875. doi: 10.1007/s11259-022-10048-z
Zhang L. Y., Peng Q. Y., Liu Y. R., Ma Q. G., Zhang J. Y., Guo Y. P., et al. (2021). Effects of oregano essential oil as an antibiotic growth promoter alternative on growth performance, antioxidant status and intestinal health of broilers. Poult. Sci. 100, 101163. doi: 10.1016/j.psj.2021.101163
Keywords: carvacrol, broilers, growth performance, intestinal microflora, protein electrophoresis, immunity, AGPs
Citation: Belhadj Slimen I, Val Boa M, Lengliz S, Bejaoui B, Samti H, Abbassi MS, M’Hamdi N, Chaveriat L, Martin P, Abderrabba M and Najar T (2025) Effects of carvacrol on growth performance, carcass traits, serum biochemistry, and intestinal gut microflora of broiler chickens. Front. Anim. Sci. 6:1563915. doi: 10.3389/fanim.2025.1563915
Received: 20 January 2025; Accepted: 24 April 2025;
Published: 14 May 2025.
Edited by:
Bianca Castiglioni, National Research Council (CNR), ItalyReviewed by:
Othusitse Ricky Madibela, Botswana University of Agriculture and Natural Resources, BotswanaVishal Manjunatha, Clemson University, United States
Copyright © 2025 Belhadj Slimen, Val Boa, Lengliz, Bejaoui, Samti, Abbassi, M’Hamdi, Chaveriat, Martin, Abderrabba and Najar. This is an open-access article distributed under the terms of the Creative Commons Attribution License (CC BY). The use, distribution or reproduction in other forums is permitted, provided the original author(s) and the copyright owner(s) are credited and that the original publication in this journal is cited, in accordance with accepted academic practice. No use, distribution or reproduction is permitted which does not comply with these terms.
*Correspondence: Imen Belhadj Slimen, aW1lbi5iZWxoYWRqc2xpbWVuQGluYXQudWNhci50bg==