- 1Livestock Issues Research Unit, Agricultural Research Service, United States Department of Agriculture, Lubbock, TX, United States
- 2Department of Veterinary Sciences, Texas Tech University, Lubbock, TX, United States
- 3Department of Animal and Food Sciences, Texas Tech University, Lubbock, TX, United States
- 4Division of Animal Sciences, University of Missouri, Columbia, MO, United States
Ghrelin is a hormone that is mainly produced in the stomach and is known to stimulate feed intake. Recent evidence suggests that ghrelin may affect immunity. This study evaluated whether repeated administrations of ghrelin prior to and following an inflammatory challenge (lipopolysaccharide, LPS) would alter the innate immune response. Weaned pigs (n = 36; age, 21 days) were housed in individual pens and were fitted with temperature loggers on day −7 and jugular vein catheters for serial blood collection on day −3. Based on body weight (BW), the pigs were separated into two treatments: 1) Ghrelin—administered human ghrelin (5 µg/kg BW, i.v.) every 12 h from −48 to 36 h relative to the administration of LPS at 0 h; and 2) Control—administered a similar volume of saline and LPS. Blood samples were collected at various time points relative to the administration of LPS. At 48 h, the pigs were humanely euthanized, and samples of the jejunum and ileum were collected for histology. Ghrelin pigs gained more weight (p = 0.04) and had greater average daily gain (ADG) (p = 0.02) compared with the Control pigs. There was a treatment × time interaction (p = 0.03) for flank temperature, in which Ghrelin pigs had greater body temperature at 0, 2, 4, and 48 h post-LPS administration. The neutrophil concentrations and the neutrophil:lymphocyte ratio were reduced (p ≤ 0.04) in Ghrelin compared with Control pigs. There was a treatment × time interaction (p = 0.03) for eosinophil concentrations, in which Ghrelin pigs had reduced eosinophils compared with Control pigs at 0 and 36 h post-LPS administration. There was a treatment × time interaction for serum concentrations of tumor necrosis factor alpha (TNF-α), interferon gamma (IFN-γ), granulocyte–macrophage colony-stimulating factor (GM-CSF), and interleukin 4 (IL-4) (p ≤ 0.04), with greater concentrations observed in Ghrelin compared with Control pigs. In the ileum, Ghrelin pigs had greater villus length and reduced villus blunting scores, lacteal dilation scores, and lamina propria eosinophil counts compared with Control pigs (p ≤ 0.04). The data from this study suggest that ghrelin may provide some protection against endotoxin-induced inflammation in the ileum while increasing the basal concentrations of cytokines. Further research is necessary to fully understand the impact of ghrelin on the inflammatory response in weaned pigs.
1 Introduction
Ghrelin is a 28-amino acid peptide hormone that is mainly produced in the stomach and is known to stimulate feed intake, among other functions in the body (Muller et al., 2015). It binds to the growth hormone secretagogue receptor (GHSR1a) in the hypothalamus, resulting in an increase in growth hormone (GH) concentrations. However, both ghrelin and GHSR1a are found in other tissues of the body, including within the gastrointestinal tract (GIT), lung, heart, kidneys, pancreas, and leukocytes (Muller et al., 2015), suggesting a wider physiological impact.
Evidence in humans and rodents suggests that ghrelin also has a role in modulating inflammation (Baatar et al., 2011; Mathur et al., 2020). Specifically, a study in mice found that ghrelin inhibited the secretion of the pro-inflammatory cytokines tumor necrosis factor-α (TNF-α) and interleukin-1β (IL-1β) from isolated macrophages; however, ghrelin increased the concentrations of the anti-inflammatory cytokine IL-12 (Correa da Silva et al., 2019). In addition, macrophage phagocytosis, but not microbicidal activity, was decreased, and treatment with ghrelin also prevented mitochondrial damage induced by lipopolysaccharide (LPS). Other research has observed that administration of LPS and inflammatory cytokines, such as TNF-α, decreased feed intake and serum ghrelin concentrations (Wang et al., 2006; Endo et al., 2007), while the administration of ghrelin has been demonstrated in humans to decrease pro-inflammatory cytokine concentrations (Dixit et al., 2004).
Much of the research on ghrelin has been conducted using human and rodent models, with limited studies in pigs. Previous work reported that infusion of weaned pigs with ghrelin [2 µg/kg body weight (BW)] three times per day for 5 days increased weight gain and serum concentrations of ghrelin, GH, insulin, and cortisol (Salfen et al., 2004). With evidence that ghrelin may modulate inflammation in rodents and humans, it was hypothesized that ghrelin would decrease inflammatory markers in weaned pigs. Therefore, this study was designed to determine the effect of repeated ghrelin administrations on the acute phase response of weaned pigs to LPS administration.
2 Materials and methods
All experimental procedures were in compliance with the Guide for the Care and Use of Agricultural Animals in Research and Teaching and approved by the Institutional Animal Care and Use Committee at the USDA-ARS Livestock Issues Research Unit (protocol no. 2022F).
2.1 Experimental design
Newly weaned gilts (n = 36; 4.6 ± 0.2 kg BW; PIC 800 × Camborough, PIC, Inc., Hendersonville, TN, USA) were acquired and transported approximately 10 km to the Swine Facility of the USDA-ARS Livestock Issues Research Unit. Upon arrival, pigs were placed in individual stainless-steel pens (0.6 × 1.2 m) with ad libitum access to water via nipple waterers and a standard nursery ration. Pigs received a diet formulated to meet or exceed NRC (2012) with an energy density of 2.4 net energy (NE) Mcal/kg and a standardized ileal digestible (SID) lysine level of 1.46%. The animal housing room in the Swine Facility is temperature-controlled, and the temperature was adjusted daily to meet the needs of the pigs (starting temperature of approximately 31°C and ending temperature of approximately 25°C). Pig body weights and feeder weights were recorded on days −10, −7, −3, 0 (feeders only), and 2 relative to the administration of LPS on day 0.
After a 3-day acclimation period, pigs were individually removed from their pens and carried to the surgery room, where they were anesthetized for placement of a temperature logger (DST nano-T; Star Oddi, Garðabær, Iceland) in the intraperitoneal (IP) cavity and subcutaneously in the skin fold between the abdomen and the right hind leg (flank) as previously described (Burdick Sanchez et al., 2023). Following this procedure, pigs were weighed and returned to their pens to rest for 4 days. After the rest period, pigs were individually carried to the surgery room for non-surgical placement of a catheter in the jugular vein under anesthesia (Carroll et al., 1999). Following placement of the jugular catheter, pigs were weighed and returned to their pens. Based on the pig BW recorded following jugular catheterization, pigs were separated into two treatment groups of similar BW: 1) Ghrelin and 2) Control. Pigs in the Ghrelin treatment received human acyl-ghrelin (Enzo Life Sciences, Inc., Farmingdale, NY, USA) at 5 µg/kg of BW (i.v.) every 12 h from −48 to 36 h relative to i.v. administration of LPS (LPS from Escherichia coli O111:B4, 15 µg/kg of BW; Sigma Aldrich, St. Louis, MO, USA) at 0 h. Control pigs were administered a similar volume of saline and LPS at the previously mentioned time points. The ghrelin dose was selected based on previous work (Salfen et al., 2004). A lower dose of ghrelin was used by Salfen et al. (2004) (2 µg/kg BW), but was administered three times a day. This study used a greater dose of ghrelin administered twice a day in order to achieve similar results. The dose and serotype of LPS used were similar to those in previous studies conducted by the laboratory in pigs (Burdick Sanchez et al., 2019, 2024) and is known to cause a mild to moderate inflammatory response without causing mortality. Blood samples (4 mL) for the isolation of serum were collected via jugular catheters into vacutainers containing no additive at −48, −36, −24, −12, −2, −1, 0, 1, 2, 3, 4, 5, 6, 12, 24, 36, and 48 h relative to the administration of LPS at 0 h. Two additional 4-mL samples were collected in one vacutainer containing EDTA for hematology measurements and one vacutainer containing Li heparin for plasma isolation at −48, −24, −2, 0, 2, 4, 6, 12, 24, 36, and 48 h relative to LPS administration. In addition, sickness behavior score, measured on a scale from 1 (displaying normal maintenance behaviors) to 4 (lying on side with labored breathing), and skin temperature (measured along the midsection of the back using an infrared thermometer) were recorded prior to the collection of each blood sample. After collection of the 48-h blood sample, pigs were humanely euthanized using an AVMA-approved method (AVMA, 2020), and sections of the duodenum and ileum were collected for histological analysis.
2.2 Serum analysis
Serum concentrations of IL-1β, IL-4, IL-6, IL-8, IL-10, IL-12p40p70, granulocyte–macrophage colony-stimulating factor (GM-CSF), interferon-γ (IFN-γ), transforming growth factor-β1 (TGF-β1), and TNF-α were measured by RayBiotech using a Quantibody Multiplex ELISA (Porcine Cytokine Array Q1, RayBiotech, Peachtree Corners, GA, USA) on samples collected at −2, 0, 1, 2, 3, 4, 5, 6, 12, and 24 h relative to LPS administration. Serum cortisol concentrations were determined using an enzyme immunoassay according to the manufacturer’s instructions (RayBiotech). Concentrations were determined based on a standard curve of known cortisol concentrations. The intra- and inter-assay coefficients of variation were 4.5% and 13.3%, respectively. Serum glucose concentrations were determined by modification of the enzymatic Autokit Glucose (Wako Diagnostics, Richmond, VA, USA) to fit a 96-well format as previously described (Burdick Sanchez et al., 2016). The intra- and inter-assay coefficients of variation were 9.9% and 17.8%, respectively. Serum ghrelin concentrations were determined using an enzyme immunoassay according to the manufacturer’s instructions (RayBiotech). Concentrations were determined based on a standard curve of known ghrelin concentrations. The intra- and inter-assay coefficients of variation were 14.9% and 16.1%, respectively.
2.3 Histopathology
Ileal and duodenal samples were collected at necropsy. The samples were washed with sterile saline and immediately placed in 10% buffered formalin. Slides were created at the Anatomical Pathology Laboratory at Texas Tech University Health Science Center. The slides were analyzed with an anatomical histopathologist at the Texas Tech University School of Veterinary Medicine and scanned using an Olympus slide scanner for imaging and counting (Olympus Life Sciences, Center Valley, PA, USA). For each sample, five villus height and five crypt depth measurements were recorded per slide. The slides were scored according to the method reported by Davis et al. (2022).
2.4 Statistical analysis
Prior to analysis, body temperature measurements (IP and subcutaneous measures) were averaged into 1-h intervals. All performance, temperature, sickness behavior, hematology, and serum analysis data were analyzed using the MIXED procedure of SAS (SAS Institute Inc., Cary, NC, USA) specific for repeated measures, with fixed effects of treatment, time, and their interaction. Pig within treatment was the experimental unit. When significant, treatment means were separated using the PDIFF option in SAS. Histopathology data were analyzed using the MIXED procedure of SAS with the fixed effect of treatment and the Kenward–Roger adjustment. Means were separated using the PDIFF option in SAS. All data are reported as the LSM ± SEM, with p ≤ 0.05 considered significant and 0.05 > p ≤ 0.10 considered a tendency.
3 Results
3.1 Performance
There was a treatment × time interaction (p = 0.03) for BW; however, there were no specific time point differences between treatments (Table 1). The change in BW from day −3 to day 2 was also analyzed, where Ghrelin pigs gained more weight (1.8 ± 0.1 kg) compared with Control pigs (1.4 ± 0.01 kg; treatment: p = 0.04). There was a treatment and time effect (p ≤ 0.02) for average daily gain (ADG), but no treatment × time interaction (p = 0.16). Specifically, Ghrelin pigs had a greater ADG than Control pigs (0.19 vs. 0.12 ± 0.02 kg/day for Ghrelin and Control pigs, respectively). There was no treatment effect or treatment × time interaction for average daily feed intake (ADFI) (p ≥ 0.13), but intake increased over time (p < 0.01).
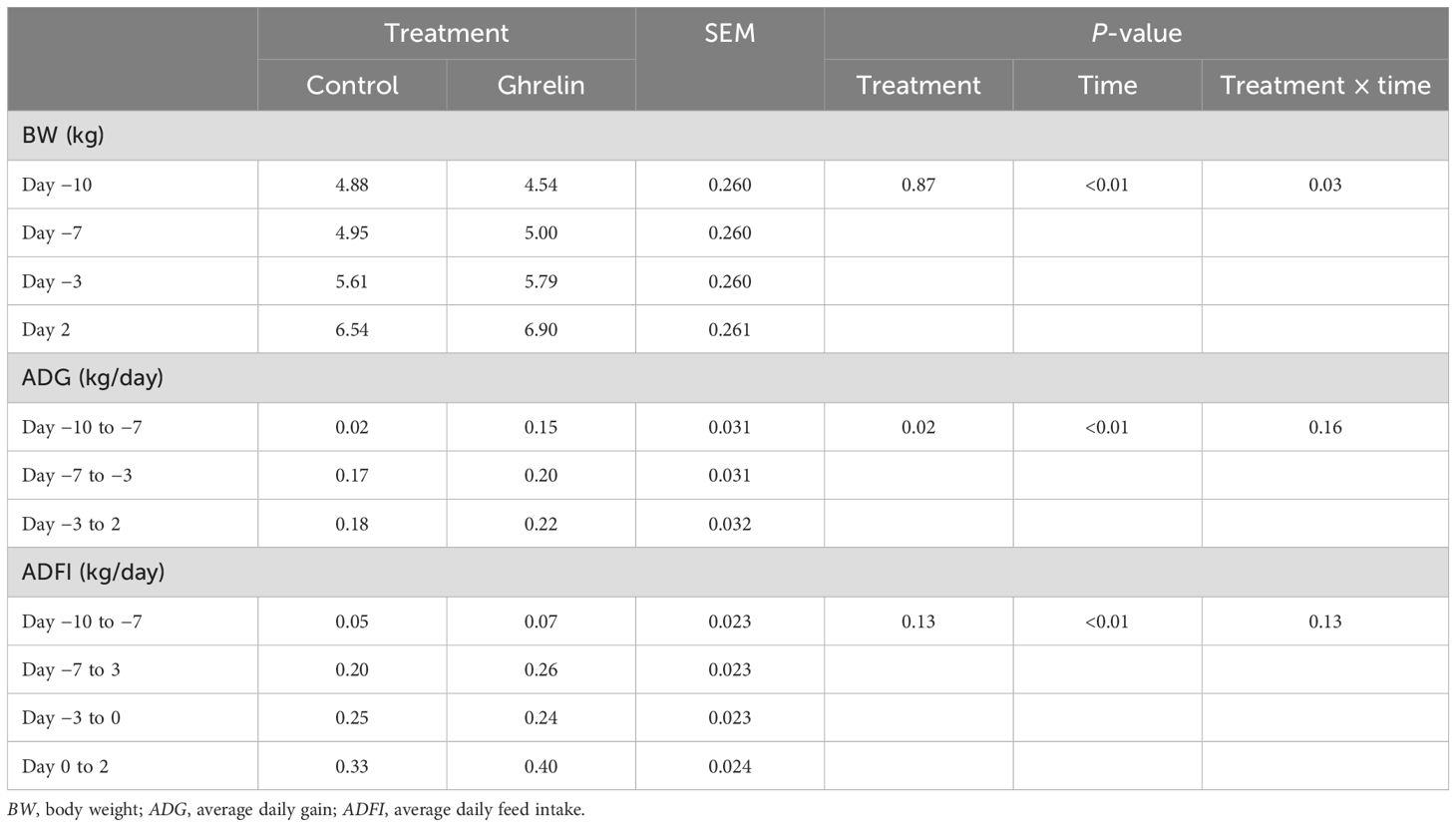
Table 1. Influence of ghrelin administration on performance variables relative to the administration of lipopolysaccharide on day 0.
3.2 Body temperature and sickness behavior
For body temperature measured within the IP cavity, there was a tendency (p = 0.06) for a treatment × time interaction (Figure 1A). Specifically, IP temperature was greater in Ghrelin pigs at 2 h post-LPS compared with Control pigs. Similarly, there was a treatment × time interaction (p = 0.03) for temperature measured subcutaneously in the flank (Figure 1B). Subcutaneous temperature was greater in Ghrelin pigs at 0, 2, and 4 h compared with Control pigs (p ≤ 0.05). In a similar manner, the skin temperature, measured using an infrared thermometer along the midsection of the back, was greater (p = 0.04) in Ghrelin pigs compared with Control pigs (35.8°C vs. 35.6 ± 0.1°C, respectively). Sickness behavior scores tended (p = 0.06) to be greater in Ghrelin pigs compared with Control pigs (1.35 vs. 1.40 ± 0.02, respectively).
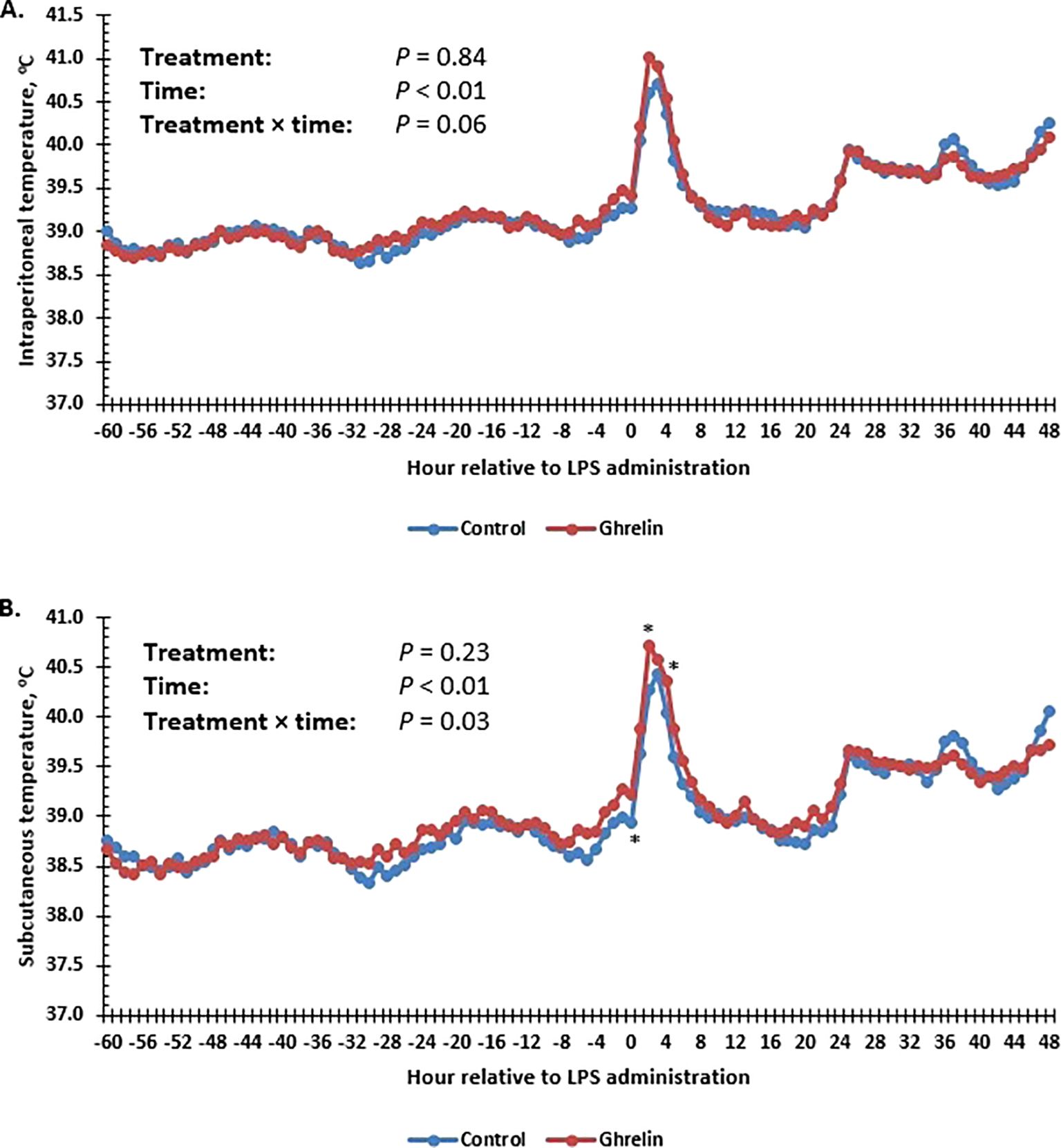
Figure 1. Influence of ghrelin administration (0.5 µg/kg body weight; n = 18) or saline (control; n = 18) administered every 12 h from day −2 to day 2 on the intraperitoneal (A) and subcutaneous flank (B) temperature of pigs in response to lipopolysaccharide administration (0.15 µg/kg body weight). Pooled SEM ± 0.113°C for the intraperitoneal and ±0.095°C for the subcutaneous flank temperature. Data are presented as the LSM ± SEM. *Treatments differ (p ≤ 0.05).
3.3 Hematology
The hematology variables are summarized in Table 2. All of the hematology variables changed over time (p < 0.01). There was no treatment effect or a treatment × time interaction (p ≥ 0.32) for red blood cells, hemoglobin, or hematocrit. Total white blood cell concentrations tended (p = 0.08) to be less in Ghrelin pigs compared with Control pigs (Figure 2A). Neutrophil concentrations were less in Ghrelin compared with Control pigs (p = 0.01); however, there was no treatment × time interaction (p = 0.11) (Figure 2B). In contrast, there was no treatment effect or treatment × time interaction (p ≥ 0.50) for lymphocyte or monocyte concentrations (Figures 2C, E). The neutrophil:lymphocyte ratio was less in Ghrelin pigs compared with Control pigs (p = 0.01), but there was no treatment × time interaction (p = 0.14) (Figure 2D). There was a treatment × time interaction (p = 0.03) for eosinophils, such that Ghrelin pigs had less eosinophils at 0 and 36 h compared with Control pigs (p ≤ 0.01) (Figure 2F).
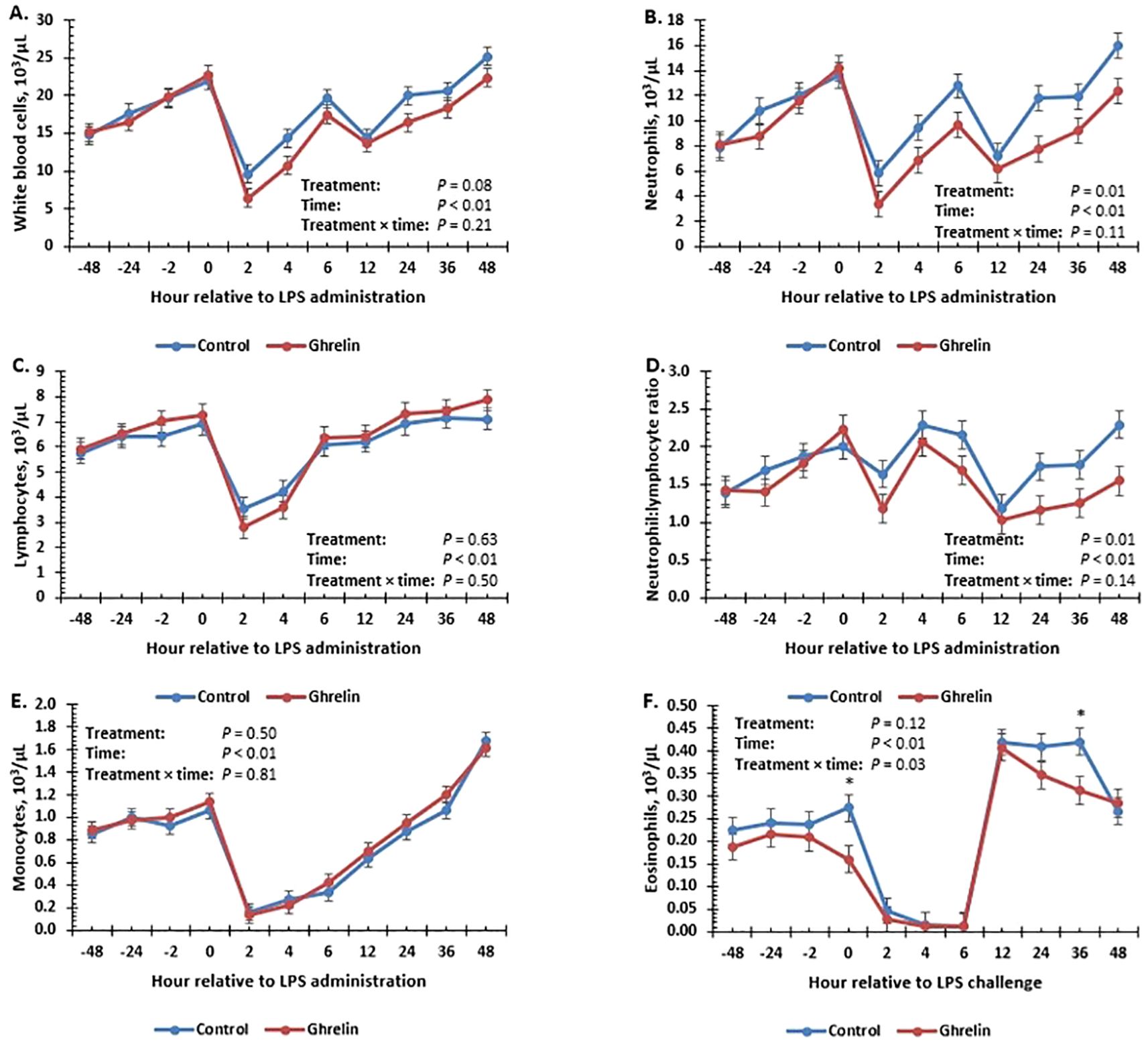
Figure 2. Influence of ghrelin administration (0.5 µg/kg body weight; n = 18) or saline (control; n = 18) administered every 12 h from day −2 to day 2 on concentrations of total white blood cells (A), neutrophils (B), lymphocytes (C), neutrophil:lymphocyte ratio (D), monocytes (E), and eosinophils (F) of pigs in response to lipopolysaccharide administration (0.15 µg/kg body weight). Data are presented as the LSM ± SEM. *Treatments differ (p ≤ 0.03).
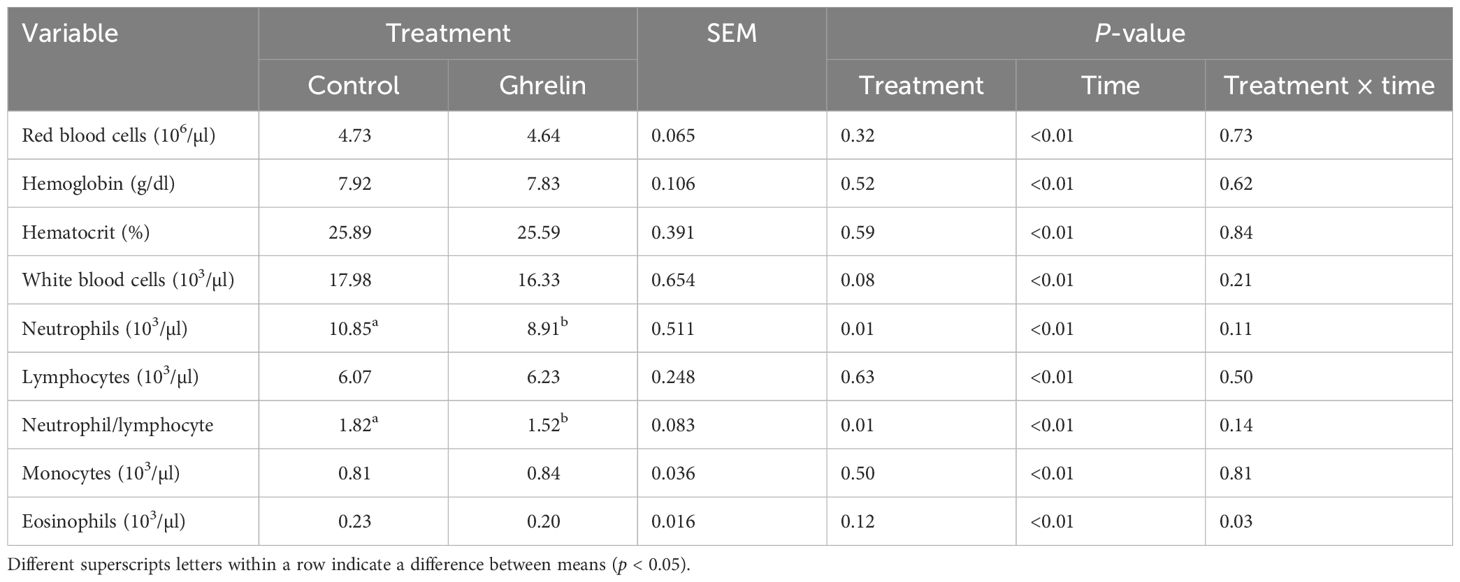
Table 2. Influence of ghrelin administration on hematology variables relative to the administration of lipopolysaccharide.
3.4 Serum analysis
There was no treatment or treatment × time interaction (p ≥ 0.31) for serum concentrations of IL-1β, IL-8, and IL-12p40p70, but values changed over time (p ≤ 0.01) (Table 3). There was a treatment × time interaction (p = 0.02) (Figure 3A) for serum IL-4 concentrations, where concentrations tended (p = 0.06) to be greater in Ghrelin than in Control pigs at −2 h relative to LPS administration. There was a tendency (p = 0.10) for a treatment × time interaction for IL-6 concentrations; however, there were no significant time point differences between treatments. There was a tendency (p = 0.08) for Ghrelin pigs to have greater concentrations of IL-10 compared with Control pigs. There was a treatment × time interaction (p = 0.04) (Figure 3B) for serum GM-CSF concentrations, where pigs administered Ghrelin had greater concentrations at 0 and 12 h (p ≤ 0.05) and tended (p = 0.06) to have greater concentrations at −2 h compared with Control pigs. Similar to GM-CSF, there was a treatment × time interaction (p < 0.01) (Figure 3C) for serum IFN-γ concentrations, where concentrations were greater in Ghrelin pigs at −2, 0, 6, and 12 h (p ≤ 0.04) and tended to be greater at 3 h compared with Control pigs. Serum TGF-β1 concentrations tended (p = 0.07) to be greater in Ghrelin compared with Control pigs. There was a treatment × time interaction (p < 0.01) (Figure 3D) for serum concentrations of TNF-α, such that peak concentrations at 1 h were greater in Ghrelin compared with Control pigs.
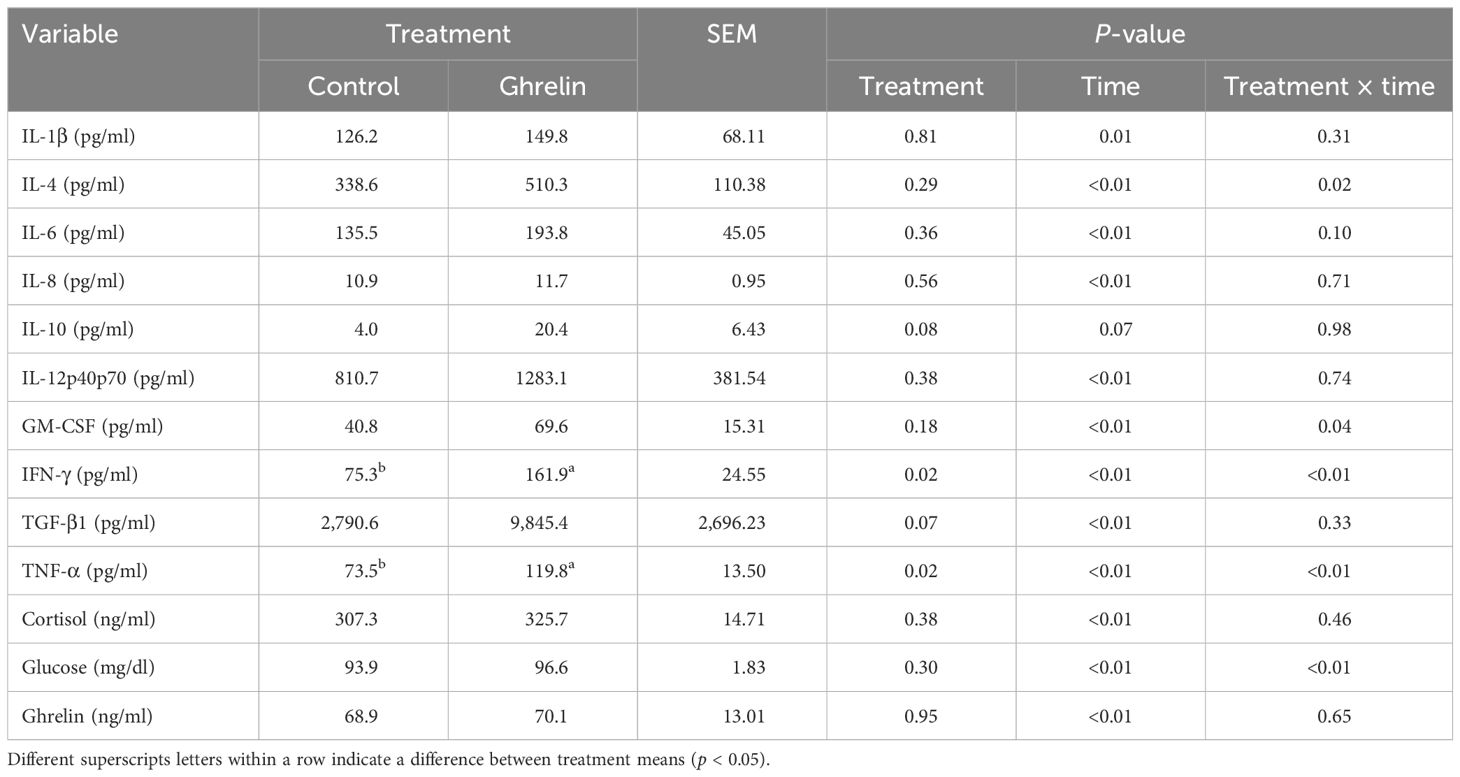
Table 3. Influence of ghrelin administration on the serum cytokine, cortisol, glucose, and ghrelin concentrations relative to the administration of lipopolysaccharide.
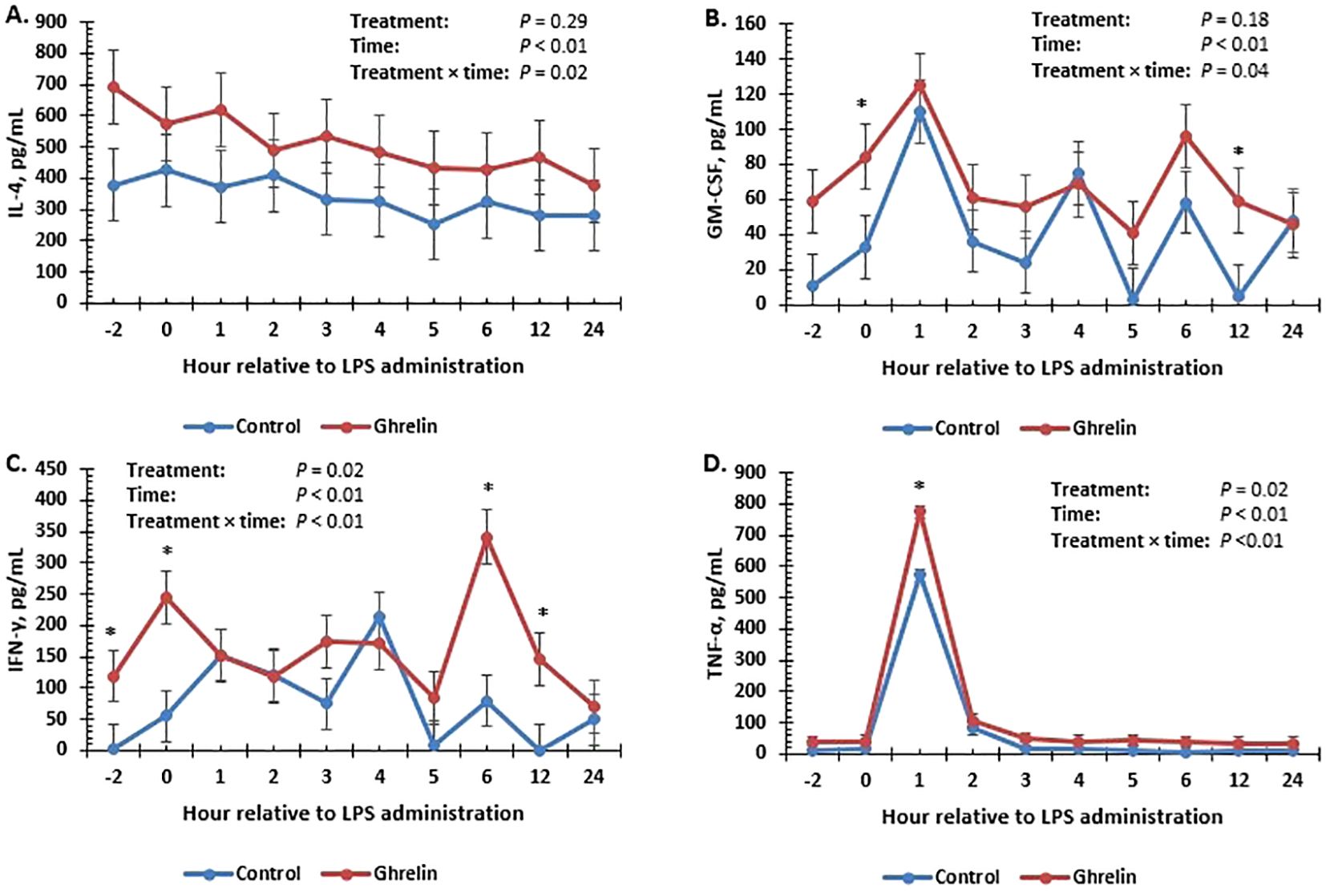
Figure 3. Influence of ghrelin administration (0.5 µg/kg body weight; n = 18) or saline (control; n = 18) administered every 12 h from day −2 to day 2 on concentrations of IL-4 (A), GM-CSF (B), IFN-γ (C), and TNF-α (D) in pigs in response to lipopolysaccharide administration (0.15 µg/kg body weight). Data are presented as the LSM ± SEM. *Treatments differ (p ≤ 0.05).
There was no treatment effect or a treatment × time interaction for the serum cortisol or Ghrelin concentrations (p ≥ 0.38), although values changed over time (p < 0.01) (Table 3). Specifically, Ghrelin concentrations fluctuated over time, while cortisol concentrations increased beginning at 1 h, peaked at 2 h, and returned to baseline values within 5 h. However, there was a treatment × time interaction (p < 0.01) for serum glucose concentrations, where Ghrelin pigs had less glucose concentrations than Control pigs at −36 and 48 h (p ≤ 0.01); however, Ghrelin pigs had greater glucose concentrations than Control pigs at −48 h (p = 0.01).
3.5 Histopathology
There was no effect of Ghrelin on the histopathological measurements in the duodenum (p ≥ 0.25; data not shown), with the exception of the lacteal dilation score, which was reduced in the ghrelin-supplemented pigs compared with the Control pigs (0.22 vs. 0.89 ± 0.16 for Ghrelin vs. Control, p < 0.01). In the ileum, pigs supplemented with Ghrelin had greater villus length (p = 0.01) and tended (p = 0.09) to have a greater villi length:crypt depth ratio (Table 4). In addition, the villus blunting and lacteal dilation scores were less (p < 0.01) in Ghrelin-supplemented pigs compared with Control pigs. Lamina propria eosinophil counts were also less (p = 0.04) in Ghrelin compared with Control pigs.
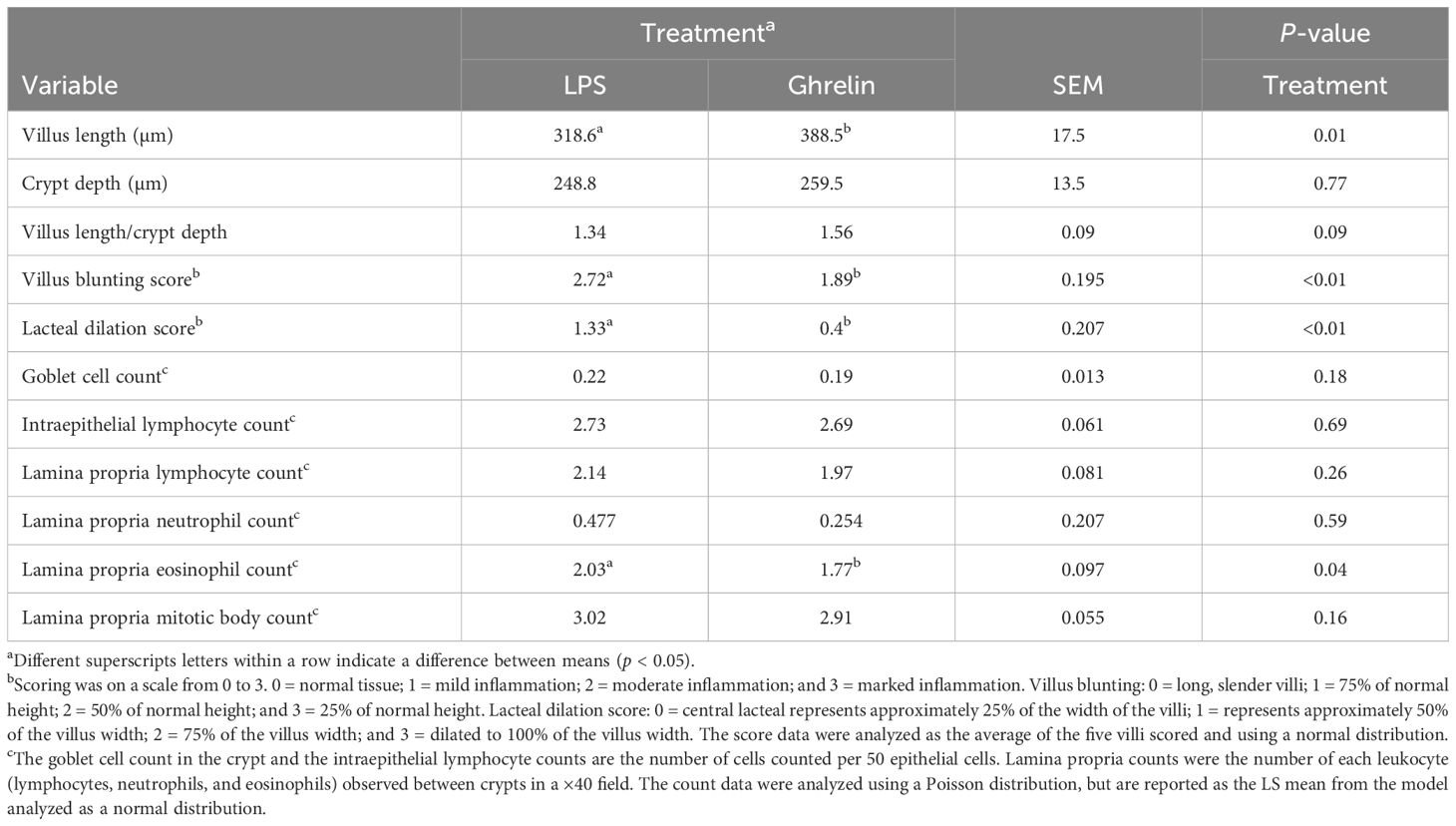
Table 4. Influence of ghrelin administration on histology samples collected 48 h following the administration of lipopolysaccharide on day 0.
4 Discussion
There are limited studies where ghrelin has been administered to pigs in an experimental setting. Many studies analyzing the effects of ghrelin administration on immunity have utilized rodent models or humans. Thus, this is one of the first studies to describe the effects of ghrelin on the innate immune response in pigs.
The work by Salfen et al. (2004), where ghrelin was administered to pigs three times a day for 5 days, supports the BW gain and ADG data from the current study, as both observed an increase in weight gain in pigs in response to ghrelin administration, but no effect on the feed intake. These data suggest that the ghrelin dosing regimen in the current study was sufficient to modulate BW. Vizcarra et al. (2007) reported that immunization against ghrelin decreased feed intake and weight gain in pigs, further supporting the role of ghrelin in growth performance in pigs. Nonetheless, Wu et al. (2008) reported no effect of ghrelin infusion (1 µg/day) on ADG or ADFI, which may be because of the lower dose administered. Administration of LPS is well known to cause a decrease in feed intake (Langhans, 2007). Although no treatment differences in feed intake were observed in the current study, this might have been because of the short duration of the study following LPS administration.
Several studies have demonstrated that administration of ghrelin following immune stimulation improved the overall mortality rate in rodent models. For example, administration of ghrelin either early (2 h post-LPS) or late (12 h post-LPS) reduced LPS-induced mortality in rats (Chang et al., 2003). This effect may be facilitated through decreases in high mobility group box 1 (HMGB1), which is decreased by ghrelin (Chorny et al., 2008). Data suggest that HMGB1 has later effects related to increasing or sustaining inflammation and may explain the positive effects observed when ghrelin was administered hours following LPS (Wang et al., 1999; Chorny et al., 2008). In the current study, ghrelin was administered periodically both before and after administration of LPS. This was modeled after Salfen et al. (2004), where pigs were administered ghrelin three times daily for 5 days while changes in the growth performance were measured. While the dose of LPS used in this study was selected in order to induce mild morbidity and no mortality, the tendency for an increase in sickness behavior in ghrelin pigs suggests that the timing of the administration of ghrelin relative to the immune challenge in pigs requires further study. It is possible that, with regard to the anti-inflammatory activity of ghrelin, repeated administrations are not beneficial. Rather, a single dose prior to or following LPS exposure would have more pronounced anti-inflammatory effects, as seen in other models. However, there are limited data associated with the anti-inflammatory effects of ghrelin in pigs; thus, further research is necessary before conclusions can be made.
Pigs administered ghrelin had a greater peak in temperature response compared with Control pigs. An increase in body temperature is an important component of an immune response as it promotes the release of immune mediators, such as cytokines (Kluger et al., 1995). In addition, the increase in body temperature is a method the body uses to kill invading bacteria, as bacteria can be sensitive to elevated temperatures (Roth and Blatteis, 2014). In support of this, ghrelin has a role in reducing the bacterial load during times of sepsis (Chorny et al., 2008; Mathur et al., 2020). In contrast, work in mice found that ghrelin decreased bacteremia early, but increased it later in response to LPS (Chowen et al., 2015). Alternatively, it is possible that the increase in peak body temperature in ghrelin pigs is associated with the greater weight gain by the pigs or is because of effects of ghrelin on metabolism and thus producing greater heat (Morales et al., 2018; Cook et al., 2020).
Both ghrelin and its receptor have been found in immune cells, including neutrophils, lymphocytes, and monocytes, indicating that these cells are receptive to ghrelin signaling (Mathur et al., 2020). Work by Chorny et al. (2008) indicated that the main cellular target for ghrelin in the immune system is macrophages for some of its anti-inflammatory effects. An increase in peripheral white blood cells was observed in young mice treated with ghrelin (Koo et al., 2001). The increase in circulating leukocytes could be associated with the direct effects of ghrelin on the thymus (Koo et al., 2001). This is in contrast to the current data, which found a decrease in circulating neutrophils and a tendency for a decrease in total white blood cells. Alternatively, reports have indicated a reduced recruitment of leukocytes in mice following LPS administration (Pereira et al., 2017). Another possibility is an effect of ghrelin on diapedesis of leukocytes in an effort to locate the assumed infection. Thus, it appears that further research is necessary to more fully understand the effects of ghrelin administration on the circulating leukocyte concentrations and changes in leukocyte concentrations in response to immune stimulation.
Studies have reported an improved survival rate in rodent models of sepsis where ghrelin was administered, with ghrelin decreasing concentrations of cytokines (Wu et al., 2007; Baatar et al., 2011). Ghrelin has been demonstrated to reduce cytokine expression and serum concentrations in both human and rodent models of sepsis following LPS administration (Dixit et al., 2004; Chowen et al., 2015). These effects on cytokines appear to be a direct effect of ghrelin on transcription factors, such as NF-κB, which are key regulators of cytokine gene expression (Dixit et al., 2004). It is possible that the dose administered to the pigs in the current study was not great enough to reduce cytokine concentrations as observed in other models. In contrast, this could have been because of differences in the response to ghrelin and LPS in rodent models compared with pigs. Another possibility for the observed differences is the timing of ghrelin administration relative to immune stimulation, as discussed previously. The increase in cytokine concentrations observed in the current study included both pro- and anti-inflammatory cytokines and thus appeared to be a broad response. This is interesting considering the reduction in circulating leukocytes. Therefore, the differences in the cytokine response to immune stimulation in rodents and pigs remain to be elucidated.
There was no effect of ghrelin administration on serum cortisol or ghrelin concentrations. This was surprising as studies have indicated a role of ghrelin in increasing cortisol through stimulating the production of corticotropins (Arvat et al., 2001). However, this may have been because of the sampling frequency used in the current study, and thus, any increases may have been missed. Previous work in pigs observed an increase in ghrelin and cortisol concentrations following ghrelin administration (Salfen et al., 2004), but the increase in ghrelin was observed within 15 min and cortisol within 30 min of administration of ghrelin, further supporting an acute response. In a study where humans were administered LPS, ghrelin concentrations peaked 2 h post-LPS administration (Vila et al., 2007). However, there have been mixed reports of LPS both increasing and decreasing concentrations of ghrelin (Baatar et al., 2011). Changes in cortisol may be one way ghrelin elicits anti-inflammatory effects; however, this was not observed in the current study. Ghrelin concentrations in rats were observed to decrease by 51% within 3 h after administration of LPS, which is in contrast to the current study (Basa et al., 2003). However, this difference may be because of differences in the dose, serotype, or administration route of ghrelin and LPS between studies (Basa et al., 2003). According to Mathur et al. (2020), the benefits of ghrelin administration during sepsis may be mediated by its actions in helping to maintain glucose homeostasis. Studies in rodents have observed increases in glucose concentrations following ghrelin administration (Broglio et al., 2003; Chang et al., 2003; Muller et al., 2015). Specifically, ghrelin has a negative effect on insulin sensitivity while increasing glucose through stimulation of gluconeogenesis (Baatar et al., 2011). Nonetheless, the differences in glucose between treatments only occurred at three time points during the study and thus may not be of biological significance.
Administration of LPS can cause gut barrier dysfunction (Parra et al., 2011). Ghrelin has known effects on gut motility, an activity that is closely related to that of motilin (Chen et al., 2010). Work in neonatal pigs reported that ghrelin had an impact on mucosa remodeling when given enterally in milk formula (Słupecka and Woliński, 2007). In addition, because of reductions in cytokine concentrations, it has been suggested that ghrelin can reduce inflammation within the lower GIT (Gonzalez-Rey et al., 2006). Ghrelin significantly ameliorated the negative histological effects associated with a model of colitis in mice (Gonzalez-Rey et al., 2006). Further supporting this, rat mucosa exposed to LPS released ghrelin, suggesting a role for ghrelin in protecting the mucosa during endotoxemia (Chang et al., 2003). This is in agreement with the histopathological results observed in the current study, where the villi length, villi length:crypt depth (tendency), and the villus blunting and lacteal dilation scores were increased and the lamina propria eosinophil counts were decreased with ghrelin supplementation. Słupecka and Woliński (2007) observed an increase in crypt depth and either no change or a decrease in villi length in neonatal pigs supplemented enterally with ghrelin. The lack of an effect of ghrelin administration in the duodenum in the current study is interesting, as cells that secrete ghrelin have been found in high density in the duodenum and jejunum in humans (Wierup et al., 2007).
Ghrelin is a potent stimulator of GH release, and it can be questioned whether some of the observed effects of ghrelin are secondary relative to the effects of GH or perhaps insulin-like growth factor-1 (IGF-1). Both GH and IGF-1 have been found to positively affect the immune system (Clark, 1997). Nevertheless, LPS decreases the sensitivity of the body to GH (Denson et al., 2001; Hattori, 2009). In addition, LPS causes an uncoupling of the GH/IGF-1 axis such that the GH concentrations increase while IGF-1 concentrations decrease in response to LPS (Carroll and Allee, 2009). Thus, it is more likely that the observed effects are a result of direct effects of ghrelin and not secondary effects of GH or downstream mediators.
5 Conclusions
Infusion of ghrelin in weaned pigs increased body temperature, reduced circulating leukocytes, increased basal cytokine concentrations, and reduced endotoxin-induced damage in the ileum. The data from this study suggest that ghrelin may provide some protection against endotoxin-induced inflammation in the ileum while increasing basal cytokine concentrations. Nonetheless, further research is necessary to understand the role of ghrelin in modulating inflammatory responses in weaned pigs. In particular, future research should study the timing of ghrelin administration relative to immune challenge/activation to maximize anti-inflammatory effects.
Data availability statement
The raw data supporting the conclusions of this article will be made available by the authors, without undue reservation.
Ethics statement
The animal study was approved by the USDA-ARS Livestock Issues Research Unit Institutional Animal Care and Use Committee. The study was conducted in accordance with the local legislation and institutional requirements.
Author contributions
NB: Conceptualization, Formal analysis, Investigation, Methodology, Project administration, Writing – original draft. JD: Investigation, Methodology, Writing – review & editing. PB: Investigation, Writing – review & editing. ED: Formal analysis, Investigation, Writing – review & editing. BB: Investigation, Writing – review & editing. AP: Investigation, Resources, Writing – review & editing. MB: Formal analysis, Investigation, Resources, Writing – review & editing. KH: Investigation, Resources, Writing – review & editing. JC: Conceptualization, Investigation, Methodology, Resources, Supervision, Writing – review & editing.
Funding
The author(s) declare that no financial support was received for the research and/or publication of this article.
Acknowledgments
The data presented is an expansion of data previously presented in abstract form (Burdick Sanchez NC, Dailey JW, Broadway PR, Davis EM, Bowen BM, Petry AL, Ballou MA, Hales KE and Carroll J. Anim Sci. (2023) 101(Suppl. 1):70-71 (Burdick Sanchez et al., 2023). The authors would like to thank J. R. Carroll (USDA-ARS) for her excellent technical support.
Conflict of interest
The authors declare that the research was conducted in the absence of any commercial or financial relationships that could be construed as a potential conflict of interest.
Generative AI statement
The author(s) declare that no Generative AI was used in the creation of this manuscript.
Publisher’s note
All claims expressed in this article are solely those of the authors and do not necessarily represent those of their affiliated organizations, or those of the publisher, the editors and the reviewers. Any product that may be evaluated in this article, or claim that may be made by its manufacturer, is not guaranteed or endorsed by the publisher.
Author disclaimer
Mention of trade names or commercial products in this article is solely for the purpose of providing specific information and does not imply recommendation or endorsement by the U.S. Department of Agriculture. USDA is an equal opportunity provider and employer.
References
Arvat E., Maccario M., Di Vito L., Broglio F., Benso A., Gottero C., et al. (2001). Endocrine activities of ghrelin, a natural growth hormone secretagogue (GHS), in humans: comparison and interactions with hexarelin, a nonnatural peptidyl GHS, and GH-releasing hormone. J. Clin. Endocrinol. Metab. 86, 1169–1174. doi: 10.1210/jcem.86.3.7314
Baatar D., Patel K., Taub D. D. (2011). The effects of ghrelin on inflammation and the immune system. Mol. Cell Endocrinol. 340, 44–58. doi: 10.1016/j.mce.2011.04.019
Basa N. R., Wang L., Arteaga J. R., Heber D., Livingston E. H., Taché Y. (2003). Bacterial lipopolysaccharide shifts fasted plasma ghrelin to postprandial levels in rats. Neurosci. Lett. 343, 25–28. doi: 10.1016/s0304-3940(03)00312-4
Broglio F., Gottero C., Benso A., Prodam F., Destefanis S., Gauna C., et al. (2003). Effects of ghrelin on the insulin and glycemic responses to glucose, arginine, or free fatty acids load in humans. J. Clin. Endocrinol. Metab. 88, 4268–4272. doi: 10.1210/jc.2002-021940
Burdick Sanchez N. C., Carroll J. A., Broadway P. R., Bass B. E., Frank J. W. (2019). Supplementation of a Lactobacillus acidophilus fermentation product can attenuate the acute phase response following a lipopolysaccharide challenge in weaned pigs. Animal 13, 144–152. doi: 10.1017/S1751731118001222
Burdick Sanchez N. C., Carroll J. A., Broadway P. R., Hughes H. D., Roberts S. L., Richeson J. T., et al. (2016). Cattle temperament influences metabolism: metabolic response to glucose tolerance and insulin sensitivity tests in beef steers. Domest. Anim. Endocrinol. 56, 85–95. doi: 10.1016/j.domaniend.2016.02.009
Burdick Sanchez N. C., Dailey J. W., Broadway P. R., Davis E. M., Bowen B. M., Petry A. L., et al. (2023). A viable less-invasive alternative for continuous temperature measurement in weaned pigs. Livest Sci. 267, 105126. doi: 10.1016/j.livsci.2022.105126
Burdick Sanchez N. C., Mitchell T., Broadway P. R., Bowen B. M., Davis E. M., Dobbins T., et al. (2024). Evaluation of the use of prenatal immune stimulation to alter postnatal immune function in weaned pigs. Front. Anim. Sci. 5. doi: 10.3389/fanim.2024.1418557
Carroll J. A., Allee G. L. (2009). Hormonal control of feed intake in swine, Voluntary Feed Intake in Pigs. 155–187. (Wageningen, Netherlands: Wageningen Academic Publishers)
Carroll J. A., Daniel J. A., Keisler D. H., Matteri R. L. (1999). Non-surgical catheterization of the jugular vein in young pigs. Lab. Anim. 33, 129–134. doi: 10.1258/002367799780578345
Chang L., Zhao J., Yang J., Zhang Z., Du J., Tang C. (2003). Therapeutic effects of ghrelin on endotoxic shock in rats. Eur. J. Pharmacol. 473, 171–176. doi: 10.1016/s0014-2999(03)01972-1
Chen Y. T., Tsai S. H., Sheu S. Y., Tsai L. H. (2010). Ghrelin improves LPS-induced gastrointestinal motility disturbances: roles of NO and prostaglandin E2. Shock 33, 205–212. doi: 10.1097/SHK.0b013e3181ae841b
Chorny A., Anderson P., Gonzalez-Rey E., Delgado M. (2008). Ghrelin protects against experimental sepsis by inhibiting high-mobility group box 1 release and by killing bacteria. J. Immunol. 180, 8369–8377. doi: 10.4049/jimmunol.180.12.8369
Chowen J. A., Siegl D., Midura E. F., Annecke T., Conzen P., Caldwell C. C., et al. (2015). The Effect of Ghrelin upon the Early Immune Response in Lean and Obese Mice during Sepsis. PloS One 10, e0122211. doi: 10.1371/journal.pone.0122211
Clark R. (1997). The somatogenic hormones and insulin-like growth factor-1: stimulators of lymphopoiesis and immune function. Endocr. Rev. 18, 157–179. doi: 10.1210/edrv.18.2.0296
Cook N., Chabot B., Liu T., Froehlich D., Basarab J., Juarez M. (2020). Radiated temperature from thermal imaging is related to feed consumption, growth rate and feed efficiency in grower pigs. J. Therm. Biol. 94, 102747. doi: 10.1016/j.jtherbio.2020.102747
Correa da Silva F., Aguiar C., Pereira J. A. S., de Brito Monteiro L., Davanzo G. G., Codo A. C., et al. (2019). Ghrelin effects on mitochondrial fitness modulates macrophage function. Free Radic. Biol. Med. 145, 61–66. doi: 10.1016/j.freeradbiomed.2019.09.012
Davis E. M., Wallace K. P., Cruz Penn M. J., Petry A. L., Broadway R., Burdick Sanchez N. C., et al. (2022). A dose-response investigation of a micronized porous ceramic particle to improve the health and performance of post-weaned pigs infected with Salmonella enterica serotype typhimurium. Front. Anim. Sci. 3. doi: 10.3389/fanim.2022.872776
Denson L. A., Menon R. K., Shaufl A., Bajwa H. S., Williams C. R., Karpen S. J. (2001). TNF-alpha downregulates murine hepatic growth hormone receptor expression by inhibiting Sp1 and Sp3 binding. J. Clin. Invest. 107, 1451–1458. doi: 10.1172/JCI10994
Dixit V. D., Schaffer E. M., Pyle R. S., Collins G. D., Sakthivel S. K., Palaniappan R., et al. (2004). Ghrelin inhibits leptin- and activation-induced proinflammatory cytokine expression by human monocytes and T cells. J. Clin. Invest. 114, 57–66. doi: 10.1172/JCI21134
Endo M., Masaki T., Seike M., Yoshimatsu H. (2007). Involvement of stomach ghrelin and hypothalamic neuropeptides in tumor necrosis factor-alpha-induced hypophagia in mice. Regul. Pept. 140, 94–100. doi: 10.1016/j.regpep.2006.11.022
Gonzalez-Rey E., Chorny A., Delgado M. (2006). Therapeutic action of ghrelin in a mouse model of colitis. Gastroenterology 130, 1707–1720. doi: 10.1053/j.gastro.2006.01.041
Hattori N. (2009). Expression, regulation and biological actions of growth hormone (GH) and ghrelin in the immune system. Growth Horm. IGF Res. 19, 187–197. doi: 10.1016/j.ghir.2008.12.001
Kluger M. J., Kozak W., Leon L. R., Soszynski D., Conn C. A. (1995). Cytokines and fever. Neuroimmunomodulation 2, 216–223. doi: 10.1159/000097199
Koo G. C., Huang C., Camacho R., Trainor C., Blake J. T., Sirotina-Meisher A., et al. (2001). Immune enhancing effect of a growth hormone secretagogue. J. Immunol. 166, 4195–4201. doi: 10.4049/jimmunol.166.6.4195
Langhans W. (2007). Signals generating anorexia during acute illness. P Nutr. Soc. 66, 321–330. doi: 10.1017/S0029665107005587
Mathur N., Mehdi S. F., Anipindi M., Aziz M., Khan S. A., Kondakindi H., et al. (2020). Ghrelin as an anti-sepsis peptide: review. Front. Immunol. 11. doi: 10.3389/fimmu.2020.610363
Morales A., Ibarra N., Chavez M., Gomez T., Suarez A., Valle J. A., et al. (2018). Effect of feed intake level and dietary protein content on the body temperature of pigs housed under thermo neutral conditions. J. Anim. Physiol. Anim. Nutr. 102, e718–e725. doi: 10.1111/jpn.12824
Muller T. D., Nogueiras R., Andermann M. L., Andrews Z. B., Anker S. D., Argente J., et al. (2015). Ghrelin. Mol. Metab. 4, 437–460. doi: 10.1016/j.molmet.2015.03.005
Parra J., Agudelo J., Ortiz L., Ramirez M. C., Rodriguez B., Herrera A. L. (2011). Lipopolysaccharide (LPS) from E. coli has detrimental effects on the intestinal morphology of weaned pigs. Rev. Colomb. Cienc. Pec. 24, 598–608. doi: 10.17533/udea.rccp.324719
Pereira J., da Silva F. C., de-Moraes-Vieira P. M. M. (2017). The impact of ghrelin in metabolic diseases: an immune perspective. J. Diabetes Res. 2017, 4527980. doi: 10.1155/2017/4527980
Roth J., Blatteis C. M. (2014). Mechanisms of fever production and lysis: lessons from experimental LPS fever. Compr. Physiol. 4, 1563–1604. doi: 10.1002/cphy.c130033
Salfen B. E., Carroll J. A., Keisler D. H., Strauch T. A. (2004). Effects of exogenous ghrelin on feed intake, weight gain, behavior, and endocrine responses in weanling pigs. J. Anim. Sci. 82, 1957–1966. doi: 10.2527/2004.8271957x
Słupecka M., Woliński J. (2007). Preliminary results on exogenous ghrelin administration via stomach tube influence on the crypt cell proliferation in the small intestine mucosa of neonatal piglets. J. Anim. Feed Sci. 16, 445–451. doi: 10.22358/jafs/66800/2007
Vila G., Maier C., Riedl M., Nowotny P., Ludvik B., Luger A., et al. (2007). Bacterial endotoxin induces biphasic changes in plasma ghrelin in healthy humans. J. Clin. Endocrinol. Metab. 92, 3930–3934. doi: 10.1210/jc.2007-1194
Vizcarra J. A., Kirby J. D., Kim S. K., Galyean M. L. (2007). Active immunization against ghrelin decreases weight gain and alters plasma concentrations of growth hormone in growing pigs. Domest. Anim. Endocrinol. 33, 176–189. doi: 10.1016/j.domaniend.2006.05.005
Wang L., Basa N. R., Shaikh A., Luckey A., Heber D., St-Pierre D. H., et al. (2006). LPS inhibits fasted plasma ghrelin levels in rats: role of IL-1 and PGs and functional implications. Am. J. Physiol. Gastrointest. Liver Physiol. 291, G611–G620. doi: 10.1152/ajpgi.00533.2005
Wang H. C., Bloom O., Zhang M. H., Vishnubhakat J. M., Ombrellino M., Che J. T., et al. (1999). HMG-1 as a late mediator of endotoxin lethality in mice. Science 285, 248–251. doi: 10.1126/science.285.5425.248
Wierup N., Bjorkqvist M., Westrom B., Pierzynowski S., Sundler F., Sjolund K. (2007). Ghrelin and motilin are cosecreted from a prominent endocrine cell population in the small intestine. J. Clin. Endocrinol. Metab. 92, 3573–3581. doi: 10.1210/jc.2006-2756
Wu R., Dong W., Zhou M., Zhang F., Marini C. P., Ravikumar T. S., et al. (2007). Ghrelin attenuates sepsis-induced acute lung injury and mortality in rats. Am. J. Respir. Crit. Care Med. 176, 805–813. doi: 10.1164/rccm.200604-511OC
Keywords: acute phase response, cytokines, ghrelin, hematology, histology, inflammatory response, pigs
Citation: Burdick Sanchez NC, Dailey JW, Broadway PR, Davis EM, Bowen BM, Petry AL, Ballou MA, Hales KE and Carroll JA (2025) Ghrelin modulates innate immune and ileal responses to lipopolysaccharide administration in weaned pigs. Front. Anim. Sci. 6:1572264. doi: 10.3389/fanim.2025.1572264
Received: 06 February 2025; Accepted: 10 March 2025;
Published: 16 April 2025.
Edited by:
Vishwajit S. Chowdhury, Kyushu University, JapanReviewed by:
Guofeng Han, Jiangsu Academy of Agricultural Sciences (JAAS), ChinaMayra A. D. Saleh, University of the Azores, Portugal
At least a portion of this work is authored by Nicole C. Burdick Sanchez, Jeffery W. Dailey, Paul R. Broadway, and Jeffery A. Carroll on behalf of the U.S. Government and as regards Dr. Burdick Sanchez, Dr. Dailey, Dr. Broadway, and Dr. Carroll and the U.S. Government, is not subject to copyright protection in the United States. Foreign and other copyrights may apply. This is an open-access article distributed under the terms of the Creative Commons Attribution License (CC BY). The use, distribution or reproduction in other forums is permitted, provided the original author(s) and the copyright owner(s) are credited and that the original publication in this journal is cited, in accordance with accepted academic practice. No use, distribution or reproduction is permitted which does not comply with these terms.
*Correspondence: Nicole C. Burdick Sanchez, bmljb2xlLnNhbmNoZXpAdXNkYS5nb3Y=