- 1Neurology Section of Department of Neurological and Movement Sciences, University of Verona, Verona, Italy
- 2Division of Brain Sciences, Department of Medicine, Imperial College London, London, United Kingdom
- 3Department of Oncology and Molecular Medicine, National Institute of Health, Rome, Italy
Background: Intrathecal immunoglobulin M (IgM) synthesis has been demonstrated in the early disease stages of multiple sclerosis (MS) as a predictor factor of a worsening disease course. Similarly, increased cerebrospinal fluid (CSF) molecules related to B-cell intrathecal activity have been associated with a more severe MS progression. However, whether CSF levels of IgM are linked to specific inflammatory and clinical profile in MS patients at the time of diagnosis remains to be elucidated.
Methods: Using customized Bio-Plex assay, the protein levels of IgG, IgA, IgM, and of 34 other inflammatory molecules, related to B-cell, T-cell, and monocyte/macrophage activity, were analyzed in the CSF of 103 newly diagnosed relapsing–remitting MS patients and 36 patients with other neurological disorders. CSF IgM levels were also correlated with clinical and neuroradiological measures [advanced 3-T magnetic resonance imaging (MRI) parameters], at diagnosis and after 2 years of follow-up.
Results: A 45.6% increase in CSF IgM levels was found in MS patients compared to controls (p = 0.013). CSF IgM levels correlated with higher CSF levels of CXCL13 (p = 0.039), CCL21 (p = 0.023), interleukin 10 (IL-10) (p = 0.025), IL-12p70 (p = 0.020), CX3CL1 (p = 0.036), and CHI3L1 (p = 0.048) and were associated with earlier age of patients at diagnosis (p = 0.008), white matter lesion (WML) number (p = 0.039) and disease activity (p = 0.033) after 2 years of follow-up.
Conclusion: IgMs are the immunoglobulins mostly expressed in the CSF of naive MS patients compared to other neurological conditions at the time of diagnosis. The association between increased CSF IgM levels and molecules related to both B-cell immunity (IL-10) and recruitment (CXCL13 and CCL21) and to macrophage/microglia activity (IL-12p70, CX3CL1, and CHI3L1) suggests possible correlation between humoral and innate intrathecal immunity in early disease stage. Furthermore, the association of IgM levels with WMLs and MS clinical and MRI activity after 2 years supports the idea of key role of IgM in the disease course.
Introduction
Multiple sclerosis (MS) is a chronic inflammatory demyelinating and neurodegenerative disease that affects the central nervous system (CNS). The presence of oligoclonal bands (OCBs) in the cerebrospinal fluid (CSF), but not within the serum, is a strong indicator of intrathecal antibody synthesis (Ziemssen et al., 2019) and has been considered a hallmark of MS that contributes to the diagnosis (Thompson et al., 2018). Immunoglobulin G (IgG) and IgM antibodies are major contributors to the OCBs formation in the CSF (Ziemssen and Ziemssen, 2005; Ziemssen et al., 2019). Elevated intrathecal IgM synthesis, but not other immunoglobulins, has been suggested to predict a worse disease evolution since the early MS stages, being associated in patients with a more disability progression and with a more aggressive form of the disease (Walsh and Tourtellotte, 1986; Villar et al., 2002, 2003; Cepok et al., 2006; Durante et al., 2012; Beltrán et al., 2014; Frau et al., 2018). In patients with clinically isolated syndrome (CIS), IgM OCB detection was associated with higher risks of conversion to clinically definite MS and in relapsing–remitting (RRMS) patients predicted a higher probability of converting to secondary progressive MS (Villar et al., 2002). In addition, the presence of colocalizing immunoglobulins and complement depositions in ongoing MS lesions (Genain et al., 1999) and studies demonstrating that antibodies isolated from the CSF of MS patients induce axonal damage and complement-mediated demyelination when applied to human CNS tissue ex vivo or in vitro (Elliott et al., 2012; Blauth et al., 2015), strongly support a key role of plasma cells and immunoglobulins, in MS pathology. It is known that B cells play a pathogenic role in MS both through the production of antibodies in the CNS and the release of proinflammatory factors in the CSF (Li et al., 2018; Sabatino et al., 2019), such as cytokines [tumor necrosis factor (TNF), interferon IFN (IFNγ), interleukin 6 (IL-6), IL-10, IL-34, IL-35, and granulocyte–macrophage colony-stimulating factor (GM-CSF)] and lymphoid chemokines (CXCL10, CXCL12, CXCL13) (Gardner et al., 2013; Li et al., 2015; Magliozzi et al., 2018). The release of B cells–related factors in the CSF has been suggested to have a key role in intrathecal inflammation, which could be linked to neuronal loss and microglia/macrophage activation and a worse MS course (Magliozzi et al., 2007, 2010; Haider et al., 2016; Lisak et al., 2017; Touil et al., 2017). More recently, increased CSF expression of similar inflammatory pattern related to B-cell immunity and lymphoid neogenesis was found associated with increased cortical lesion (CL) load, as revealed by advanced 3-T double inversion recovery magnetic resonance imaging (MRI) analysis, also in MS patients at time of diagnosis (Magliozzi et al., 2018). These evidences support the hypothesis of complex intrathecal immune interactions and potential correlations between intrathecal antibody syntheses by plasma cells, B cells–related factors release innate immune activities, which occur since early disease stages (Magliozzi et al., 2019). However, the definite inflammatory CSF milieu associated with increased CSF IgM levels remains to be better clarified.
The objective of this study was to evaluate the presence of IgM, and others immunoglobulins (IgG and IgA) in the CSF of both MS patients and controls with other neurological disorders. Furthermore, we investigated the existence of specific correlations between CSF IgM levels and the CSF inflammatory profiling at the time of diagnosis and the clinical and MRI activity in addition to baseline, even after 2 years of follow-up in a cohort of MS patients.
Materials and Methods
Patients Cohort
We recruited 103 treatment-naive RRMS patients (26 males and 77 females), followed at the MS Centre at Verona University Hospital (Italy), who received a diagnosis of MS from January 2012 to December 2019. All MS patients underwent at the time of diagnosis (T0) a detailed neurological evaluation including the Expanded Disability Status Scale (EDSS) assessment (Kurtzke, 1983) and the 3-T MRI and the CSF examination. Seventy patients who did not undergo second-line therapies, in particular anti–B-cell drug treatments, were also monitored from a clinical and radiological point of view for 2 years [24 months (T24)]. The evidence (EDA) and no evidence (NEDA) of disease activity, based on the presence of relapses and/or disability progression and/or any MRI activity (Giovannoni et al., 2015), were evaluated.
Demographic, clinical, and MRI data of MS patients are reported in Table 1.
Thirty-six age- and sex-matched available patients affected by other neurological diseases, who underwent neurological evaluation and CSF examination at the time of the diagnosis, were included in the study. This group included 21 individuals with non-inflammatory neurological diseases, NIND (one idiopathic tremor, two migraine, two amyloid angiopathy, two fibromyalgia, four ischemic stroke, one spondylotic myelopathy, two amyotrophic lateral sclerosis, one olivopontocerebellar atrophy, one idiopathic spastic paraparesis, one idiopathic ataxia, one myopathy, one endocranial hypertension, two peripheral neuropathy), and 15 subjects with other inflammatory neurological diseases, OIND (one infective myelopathy, two CNS lymphoma, two intracranial abscess, one peripheral neuropathy, two Behçet disease, three neuromyelitis optica spectrum disorder, three autoimmune encephalitis, one aseptic meningitis).
The Ethics Committee of the University of Verona approved the study, and informed consent was obtained from all participants.
CSF Collection and Analysis
CSF samples were obtained at least 1 month after the last relapse and within 2 months from the MRI acquisition, according to the Consensus Guidelines for CSF and Blood Biobanking (Teunissen et al., 2009). Once collected, the CSF was centrifuged, and the supernatant was divided from the cell pellet and stored at −80°C until use (Ethics Committee Protocol n° 66418). OCB presence was evaluated by isoelectric focusing on agarose gel for each MS patient group, as reported in Table 1. Protein presence and levels in the CSF were assessed by multiplex technology using a Bioplex assay (Bio-Plex X200 System equipped with a magnetic workstation, BioRad, Hercules, CA, United States) as previously described (Magliozzi et al., 2018). CSF samples were diluted 1:2 in phosphate-buffered saline to reach the optimal concentration. The immunoglobulin protein analysis (IgG1, IgA, IgM) was performed by using a custom Bio-Plex Pro-Human Isotyping Panel (3-plex). In addition, levels of specific inflammatory chemokines and cytokines associated with several pathways were evaluated including B-cell pathway (APRIL, LIGHT, TWEAK, BAFF, CXCL12, CXCL13, CCL21, IL-10, IL-34, IL-35, and GM-CSF), T-cell pathway (IFNγ, IFNα2, IL-4, IL-8, IL-22, CCL19, CCL20, and CCL25), monocyte/macrophage pathway (IL-1β, IL-6, CCL2, CCL8, CX3CL1, CXCL10, CXCL11, CHI3L1, sCD163, MMP1, and MMP2), and TNF pathway (TNFα, sTNFR1, and sTNFR2), as previously optimized (Magliozzi et al., 2018). These analytes were assayed by using customized kits Bio-Plex Pro Human Chemokine Panel-40-Plex and Bio-Plex Pro Human Inflammation Panel 1-37-Plex as previously described (Magliozzi et al., 2018) (see Supplementary Material). All samples were run in duplicate in the same experiment and in two consecutive experiments in order to verify the reproducibility and consistency of the results. The CSF analysis was performed by two independent investigators (RM and SR), blinded with respect to the clinical/radiological characteristics of each patient. Index of blood–brain barrier damage was calculated considering the CSF/serum albumin ratio and used to verify the potential correlation between IgM intrathecal synthesis and peripheral one.
MRI Acquisition Protocol and Analysis
At the time of diagnosis, 3-T MRI was performed in all MS patients, and this was repeated 2 years after diagnosis on 70 of these patients. MRI sequences were acquired at the Radiology Unit of the University Hospital of Borgo Trento (Verona, Italy) using a Philips Achieva 3T MR Scanner (Philips Medical Systems, Best, Netherlands) as previously described (Magliozzi et al., 2018). The following image sets were acquired:
• 3D T1-weighted turbo field echo [repetition time (TR)/echo time (TE) = 8.4/3.7 ms, voxel size of 1 mm × 1 mm × 1 mm), total acquisition time of 5:51 min;
• 3D double inversion recovery (DIR) (TR/TE = 5,500/292 ms, inversion times (TI) TI1/TI2 = 525/2,530 ms voxel size of 1 mm × 1 mm × 1 × mm), turbo spin echo (TSE) readout with an optimal variable flip angle scheme and number of excitations (3, with total acquisition time of 10:49 min;
• 3D fluid-attenuated inversion recovery (FLAIR) (TR/TE = 5,500/292 ms, TI = 1,650 ms voxel size of 1 mm × 1 mm × 1 mm), same TSE readout as the DIR sequence, number of excitations 1, with total acquisition time 5:44 min.
The number of T2 hyperintense white matter lesions (WMLs) and CLs were identified on FLAIR and DIR images by an observer with a large experience on MS. The number of CLs was assessed following the recommendations for CLs scoring in patients with MS (Geurts et al., 2011). Owing to the suboptimal performance of the image-acquisition sequences on MRI in visualizing subpial lesions, the present analysis has taken into account mainly the intracortical and leukocortical lesions. MRI data are reported in Table 1.
Statistical Analysis
Mann–Whitney U test was used to test differences between MS patients and control group, as well as differences between MS patients stratified by CL number at diagnosis (</>4, where 4 was the mean of the CL number in all the examined patients) and by the presence or not of the EDA after 2 years of follow-up. Analysis of variance (ANOVA) followed by post hoc pairwise comparison using the Tukey test was used to evaluate difference among immunoglobulin CSF levels in MS patients.
Pairwise univariate Spearman rank index was used to evaluate the correlation between CSF IgM levels and demographic and clinical MRI parameters (both at diagnosis and after 2 years of follow-up) and several inflammatory/immune-mediated pathways. Differences between males and females and between those patients with or without OCBs were tested by Mann–Whitney U test. p < 0.05 was considered statistically significant. GraphPad (version 5.0) and R software (version 3.5.3) were used to perform the analysis.
Results
Immunoglobulin CSF Expression in MS Patients and Controls
Intrathecal levels of IgG, IgA, and IgM were investigated in the CSF of 103 RRMS patients and 36 controls with other neurological disorders (Table 2).
CSF IgG levels in MS patients were significantly higher compared to IgA (fold change = 2.65, p < 0.001; Figure 1A) and IgM (fold change = 2.15, p < 0.001; Figure 1A). However, only the CSF IgM levels reached statistical significance in MS patients when compared to controls (fold change = 1.46, p = 0.013; Figure 1B), while there was no difference in IgG (fold change = 0.96, p = 0.360; Figure 1C) and IgA (fold change = 0.27, p = 0.700; Figure 1D) levels between the two groups. In order to understand whether CSF IgM levels were related to peripheral ones and inflammation, we have further correlated CSF IgM concentration with measurement of blood–brain barrier damage (calculated considering the CSF–to–serum albumin ratio). However, we found no correlation (R = −0.12, p = 0.270) between CSF IgM levels and blood–brain barrier alteration, suggesting that IgM intrathecal levels were not related to peripheral ones.
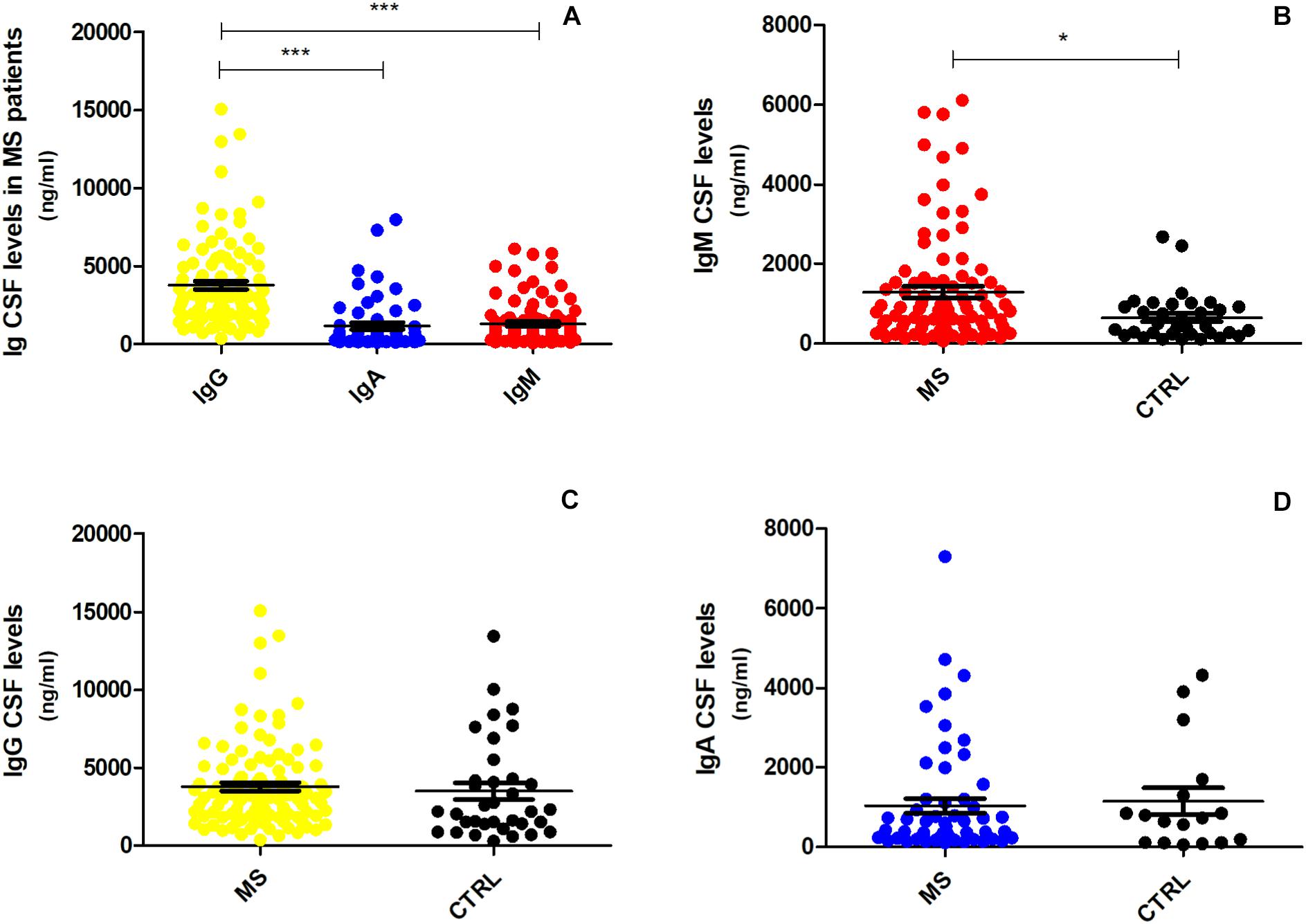
Figure 1. CSF expression levels of IgG, IgA, and IgM in MS and controls patients. (A) IgG levels were significantly higher in MS patients (n = 103) compared to IgA and IgM levels (one-way ANOVA, p < 0.001); (B) IgM was the only immunoglobulin found to be higher in MS patients (n = 103) compared to controls (n = 36) (Mann–Whitney U test, p = 0.013). The difference between (C) IgG and (D) IgA expression levels was not significant (respectively, p = 0.360, p = 0.700) between MS patients and controls. *p < 0.05, ***p < 0.001.
Correlation Between CSF IgM Levels With Demographic, Clinical, and MRI Data
CSF IgM levels of MS patients were correlated with demographic, clinical, and MRI data, at the time of diagnosis and after 2 years of follow-up. The results of these correlations were reported in Table 3.
At T0, CSF IgM levels were correlated negatively with the age of MS patients (R = −0.26, p = 0.008; Figure 2A); on the contrary, no correlation was found between CSF IgM levels and EDSS and MRI data. Although there were no significant correlations between the CSF IgM levels and WMLs and CL number at diagnosis, when MS patients were stratified according to the CL load, we found a trend to increase of CSF IgM levels that were almost twice higher in MS patients with CLs ≥ 4 compared to MS patients with CLs < 4 (fold change = 1.74, p = 0.072; Figure 2B).
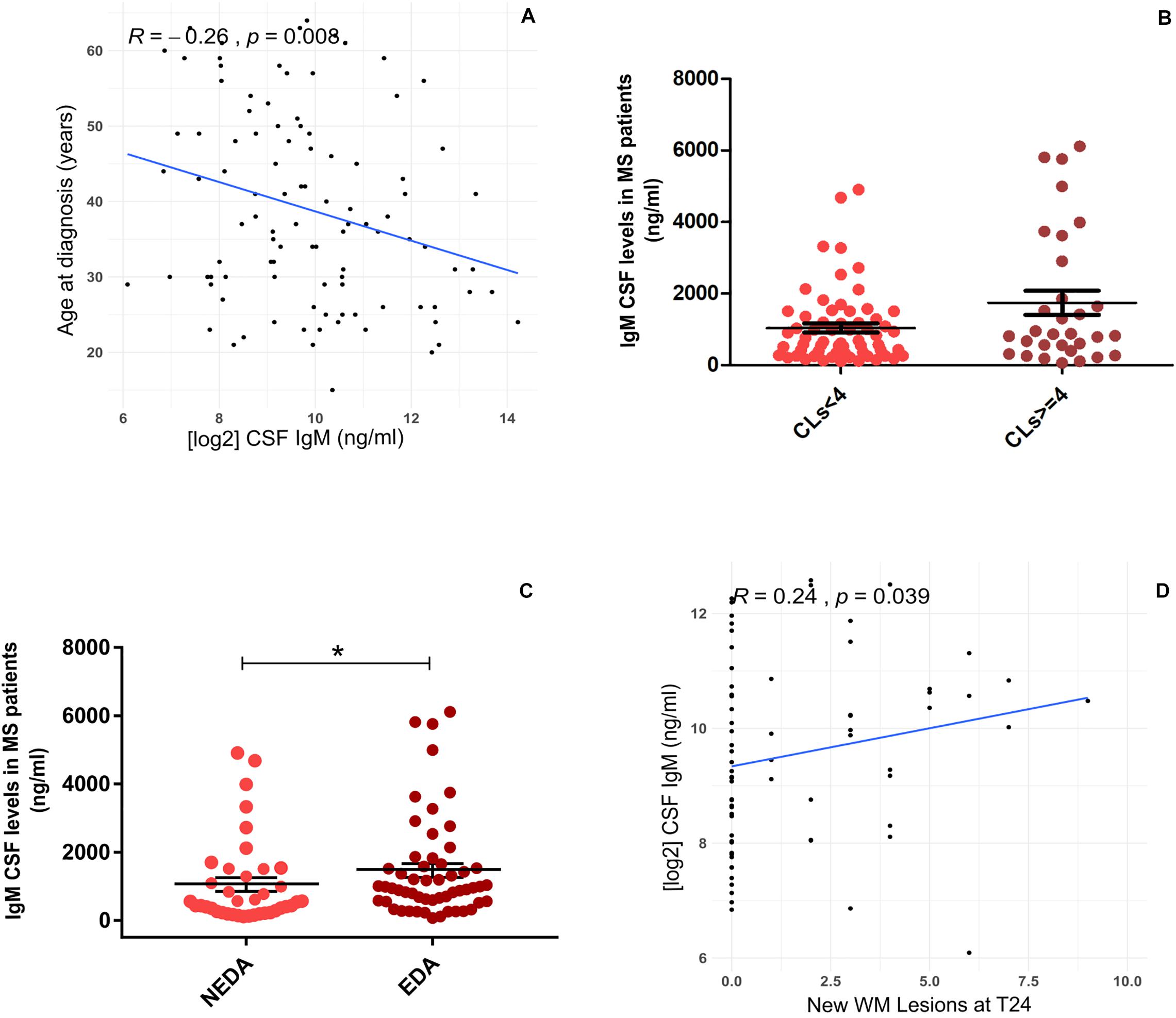
Figure 2. Association between CSF IgM levels in MS patients with demographic, clinical, and MRI data: (A) CSF IgM levels correlates negatively with the age at diagnosis (R = −0.26, p = 0.008); (B) CSF IgM levels were higher in MS patients with CLs ≥ 4 compared to MS patients with CLs < 4, at the time of diagnosis (Mann–Whitney U test, p = 0.072); (C) CSF IgM levels were higher in EDA patients compared to NEDA after 2 years of follow-up (p = 0.033); (D) CSF IgM levels correlate with the presence of new WMLs after 2 years of follow-up (R = 0.24, p = 0.039). *p < 0.05.
Considering the second year of follow-up, CSF IgM levels (measured at the time of diagnosis) were significantly higher in patients with EDA compared to NEDA (fold change = 1.61, p = 0.033; Figure 2C), and a mild correlation was found between CSF IgM levels and the presence of new WMLs (R = 0.24, p = 0.039; Figure 2D). By further analyzing the potential correlation between both IgG and IgA with all the tested clinical and radiological parameters, either at T0 and T24, we did not find any significant correlation.
Correlation Between CSF IgM Levels and Inflammatory Profiles
CSF IgM levels of MS patients were correlated with specific inflammatory/immune-mediated molecules, which were categorized in several pathways (Table 4). Among all the B cell–related molecules, we found that CSF IgM levels mildly correlated with CSF IL-10 (R = 0.22, p = 0.025; Figure 3A), CCL21 (R = 0.23, p = 0.023; Figure 3B), and CXCL13 (R = 0.20, p = 0.039; Figure 3C). Furthermore, the CSF IgM levels also weakly correlated with macrophage and microglia-related biomarkers such as CHI3L1 (R = 0.19, p = 0.048; Figure 3D), CX3CL1 (R = 0.21, p = 0.036; Figure 3E) and IL-12p70 (R = 0.25, p = 0.020; Figure 3F).
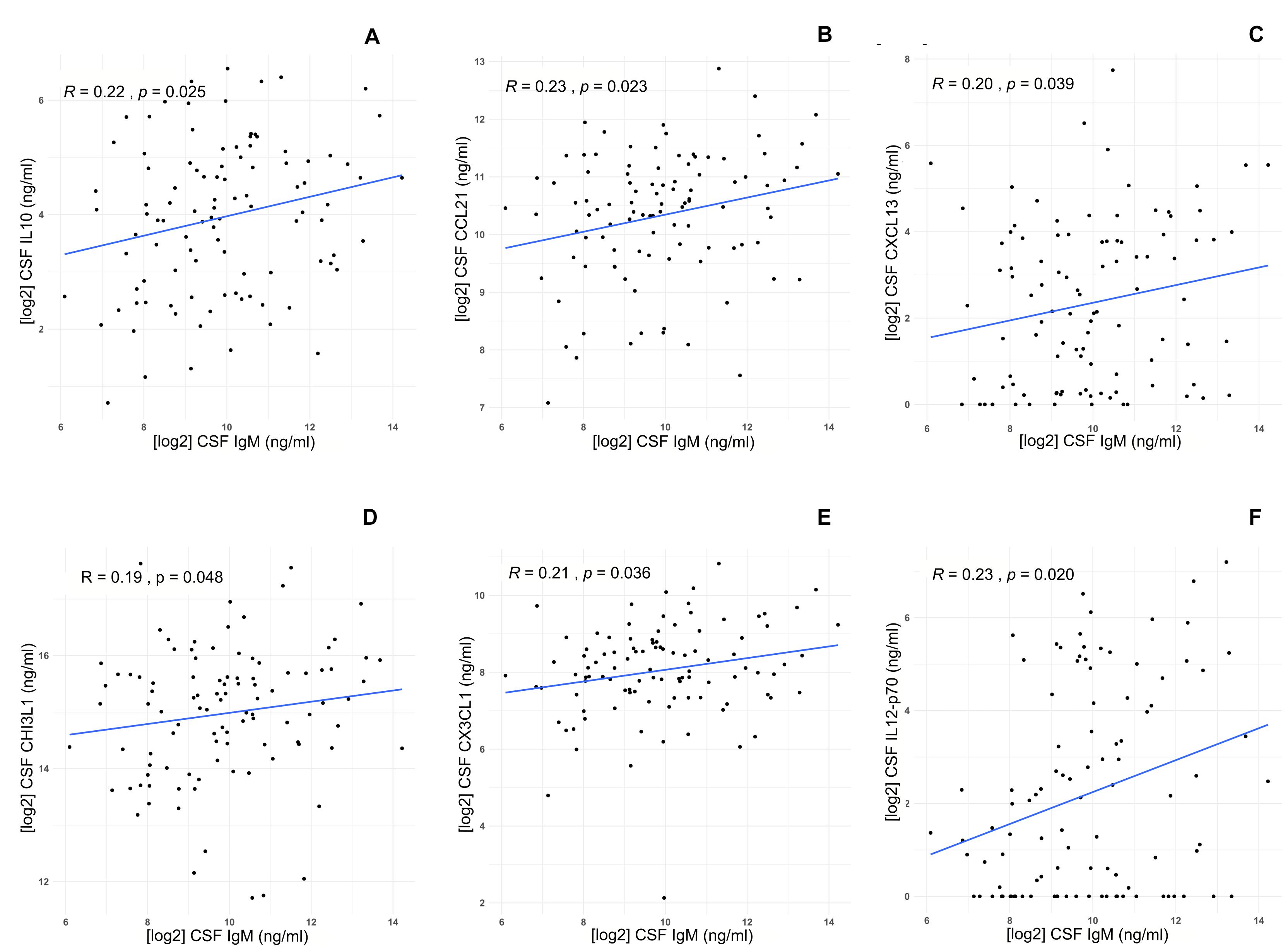
Figure 3. Correlations between IgM CSF levels and specific biomarkers in MS patients (n = 103). IgM CSF levels correlate with high CSF levels of (A) IL-10 (R = 0.22, p = 0.025), (B) CCL21 (R = 0.22, p = 0.023), (C) CXCL13 (R = 0.20, p = 0.039), (D) CHI3L1 (R = 0.19, p = 0.048), (E) CX3CL1 (R = 0.21, p = 0.036), (F) IL-12p70 (p = 0.020).
Discussion
In the last decade, several studies have demonstrated a relevant association between intrathecally produced IgM and a more severe MS course (Villar et al., 2005, 2008; Calabrese et al., 2012). However, other studies did not find an association between IgM and a more severe MS course (Schneider et al., 2007; Stauch et al., 2011). In our study, we aimed to define quantitatively the intrathecal IgM levels and its possible association with combined specific inflammatory profile in the CSF of MS patients.
We found that higher levels of IgM, but not IgG and IgA, were present already at diagnosis in the CSF of MS patients when compared with patients with other neurological diseases. This result corroborates the hypothesis that IgM production occurs from the early stages of MS. As known in MS, B cells migrate from the periphery into the meninges, CSF, and the CNS parenchyma (Levinson et al., 1983; Sandberg et al., 1986), where these cells showed a local activation and clonal expansion (Weber et al., 2010). Plasma blasts and plasma cells, maturing from these B cells, were capable to secrete oligoclonal antibodies in the CSF of MS patients (Weber et al., 2010). MS patients showed an intrathecal production of IgG, IgM, and IgA (Lolli et al., 1989); about 95% of the MS patients displayed IgG OCBs (Link, 1978; Villar et al., 2005), and around 40% also showed intrathecal IgM production (Villar et al., 2005), while CSF IgA synthesis was only occasionally observed (in 13% of cases) (Link and Müller, 1971; Leary et al., 2000). Therefore, IgG (predominantly IgG1), and IgM are considered the major contributors to the OCB formation in the CSF (Ziemssen and Ziemssen, 2005; Ziemssen et al., 2019).
Intrathecal IgM synthesis is involved in demyelination and axonal injury (Piddlesden et al., 1993; Villar et al., 2005), the main source of disability in MS patients (Mead et al., 2002). In particular, IgMs are the only immunoglobulins capable of recognizing myelin lipids, such as myelin oligodendrocyte glycoprotein, proteolipid protein, and myelin basic protein, in most of MS patients (Villar et al., 2005, 2008; Owens et al., 2009). In relation with these studies, we decided to analyze the association between the CSF IgM levels, detected at diagnosis (T0), and the clinical and MRI parameters after 2 years of follow-up [24 months (T24)]. We found that while CSF IgM levels did not correlate with any clinical/MRI parameters at time of diagnosis, they were correlated, even if moderately, with the presence of new WMLs at T24 and were significantly higher in EDA patients compared to NEDA, thus suggesting a possible prognostic role of IgM levels in terms of disease activity. These results are in line with previously mentioned studies (Villar et al., 2005, 2008; Owens et al., 2009) and with studies showing an association between IgM with a severe MS course, according to both clinical and MRI outcomes (Villar et al., 2003, 2005;Ozakbas et al., 2017).
Analyzing the correlations between CSF IgM levels and the clinical and MRI parameters even at the time of diagnosis, we detected only a negative and low correlation between CSF IgM levels with and the age of patients at diagnosis, although previous studies showed that IgM levels were strongly associated with a younger age at first clinical symptoms (Tintore et al., 2008; Huss et al., 2018; Pfuhl et al., 2019). Moreover, despite that CSF IgM levels were higher in MS patients with high (CLs ≥ 4) compared to MS patients with low (CLs < 4) CL load, such a difference did not reach the statistical significance. On the contrary, we detected low negative correlation with age of the patients. These results might be explained by the small number of examined patients and by the possibility that other underlying immunological mechanisms are involved in brain damage besides the immunoglobulin production (Lassmann, 2008).
Recent studies have shown that intrathecal IgM synthesis, mainly mediated by CD5+ B cells, contributes to B-cell activation and differentiation within the CNS (Villar et al., 2010). In particular, positive correlations between CSF inflammatory biomarkers, especially of humoral immunity, with MS severity support a pathogenic role of intrathecal inflammation, particularly linked to B-cell immunity, in CNS tissue destruction in MS patients (Milstein et al., 2019), causing a more severe and rapid disease course (Magliozzi et al., 2007; Howell et al., 2011). For all these reasons, we investigated whether IgM overexpression in the CSF of MS patients at diagnosis might be associated with a specific inflammatory intrathecal milieu, by analyzing other cytokine/chemokine CSF molecules related to either B cells or other immune cell pathways. First, we observed mild correlation between CSF IgM levels with some B cell–related factors, such as CXCL13, CCL21, and IL-10. The chemokines CXCL13 and CCL21 are particularly known to regulate B-cell migration into the CNS and to favor the intrathecal accumulation of B cells (Kowarik et al., 2012). In particular, CXCL13 has recently been suggested as a prognostic marker for CIS and MS (Brettschneider et al., 2010; Ferraro et al., 2015; Magliozzi et al., 2020) and seems to play a role in the formation of ectopic lymphoid tissues within the CNS in MS (Magliozzi et al., 2007). In addition, CSF CXCL13 levels were found incremented in MS patients (Khademi et al., 2011), in which they are correlated with a high number of CSF CD5+ B cells and with intrathecal IgM production (Krumbholz et al., 2006; Villar et al., 2010; Ferraro et al., 2015). Moreover, the correlation between CSF IgM levels and IL-10 supports the hypothesis that the B-cell activity could regulate activation of further immune reactions, even independently by immunoglobulin/complement–mediated response, since the earliest phase of the disease (Li et al., 2018). IL-10 is a potent immunomodulatory cytokine that can promote humoral immune responses by enhancing class II expression on B cells and inducing immunoglobulin production (Saxena et al., 2015). However, IL-10 could be also released, together with IL-1, IL-6, IL-15, and TNF, by activated macrophages, which can have a key role in B-cell activation. We also found that the CSF IgM levels in MS patients weakly correlated also with molecules related to monocyte/macrophage activity and response, such as CHI3L1, IL-12p70, and CX3CL1, suggesting a mild, but significant, association between humoral and innate immune inflammatory processes. CHI3L1, also named YKL-40, is a molecule released by activated macrophages and astrocytes, and it was proposed as a putative CSF biomarker of disease activity, indicating higher risk of conversion from CIS to MS (Comabella et al., 2010). IL-12 protein is composed by p35 and p40 subunits; when combined, these subunits form the bioactive IL-12p70, which is mainly produced by dendritic cells, macrophages, neutrophils, and probably by naive B cells. IL-12p70 is involved in the response to the antigen presentation, possibly with anti-inflammatory function (Gee et al., 2009). CX3CL1, also named fractalkine, is a chemokine mainly produced by neurons and can be soluble, as well as membrane-bound capable of attracting T cells, NK cells, and myeloid cells, including microglia (Hatori et al., 2002; Savarin-Vuaillat and Ransohoff, 2007). It has a key role in neuron–microglia cross-talk in physiology and aging, but the exact role of this chemokine in MS pathology still remains unclear, because CX3CL1 appeared to interfere with proinflammatory microglia activity, therefore with neuroprotective effect (Ransohoff and El Khoury, 2015). We previously demonstrated that the CSF levels of other specific markers of activated macrophages, such as soluble CD163, positively correlated with CSF levels of neurofilament, fibrinogen, and B cell–related molecules, such as CXCL13, CXCL12, IL-10, and BAFF (Magliozzi et al., 2019). Therefore, although the correlations that we identified in the CSF at time of diagnosis between all these mediators and IgM were modest and need to be validated in a larger and independent MS population, it might be hypothesized that IgM could possibly reflect the interactions between innate and adaptive humoral immune responses, as previously suggested (Boes, 2000; Villar et al., 2010).
This analysis was performed using a multiple, advanced immunoassay methodology (Bio-Plex), to obtain a simultaneous, sensitive, and reproducible evaluation of immunoglobulins and several other inflammatory mediators in the CSF of MS patients. Despite further studies are needed to confirm these preliminary results, the sensitivity and reproducibility of this easily performed procedure could allow extending such a detailed CSF proteomic analysis to the clinical practice.
This study is not without limitations. The low number of patients recruited, compared to the number of parameters analyzed, and the limited MS phenotypes suggest that these data need to be further validated, considering an independent validation cohort, and confirmed by other studies. Moreover, only 2 years of follow-up have been analyzed at the moment in order to select only MS patients treated by first-line therapies, in order to avoid further confounding factor of the correlation analysis. All together, these limitations suggest that this study needs to be confirmed by further analysis of a larger sample size and longer follow-up and paired serum samples.
Conclusion
In this study, we used a comprehensive, advanced proteomic approach for quantitative and qualitative CSF protein analyses in combination with clinical and radiological assessment of MS patients, demonstrating that intrathecal IgM levels are increased in the CSF of treatment-naive MS patients compared to controls and that there is significant correlation between CSF IgM levels and further CSF molecules related to B-cell, macrophage, and microglia activity. Moreover, we found an association between CSF IgM production and the number of new WMLs and MRI activity after 2 years of follow-up, suggesting that CSF IgM levels might reflect the relationship between humoral and innate intrathecal immune response in MS and might represent an early biomarker of underling disease activity.
Data Availability Statement
The raw data supporting the conclusions of this article will be made available by the authors, without undue reservation.
Ethics Statement
The Ethics Committee of the University of Verona approved the study. The patients/participants provided their written informed consent to participate in this study. Biological material and associated data were obtained from MSBioB Biological bank – A.O.U.I., Verona (Protocol number 66418, 25/11/2019). Biological material was obtained from voluntary donors in compliance with the Legislative Decree 196/2003 “Personal Data Protection Code.”
Author Contributions
RM and MC contributed to the conception and design of the work. RM, VM, LM, AP, DM, AT, and SR contributed to the acquisition, analysis, and interpretation of data for the work. RM, VM, LM, AP, DM, and FC contributed to the data interpretation. RM, VM, LM, AP, DM, AT, and MC contributed to writing the manuscript. RM, VM, DM, FC, AP, AT, and MC contributed to revise the manuscript. All authors provided approval for publication of the final content.
Funding
RM and AT were supported by grant from the Italian MS Foundation (FISM 16/17/F14). MC and SR were supported by the GR-2013-02-355322 grant from Italian Ministry of Health.
Conflict of Interest
The authors declare that the research was conducted in the absence of any commercial or financial relationships that could be construed as a potential conflict of interest.
Acknowledgments
We would like to thank all the patients and their families from the Multiple Sclerosis Center of University Hospital of Verona.
Supplementary Material
The Supplementary Material for this article can be found online at: https://www.frontiersin.org/articles/10.3389/fncel.2020.569827/full#supplementary-material
References
Beltrán, E., Obermeier, B., Moser, M., Coret, F., Simó-Castelló, M., Boscá, I., et al. (2014). Intrathecal somatic hypermutation of IgM in multiple sclerosis and neuroinflammation. Brain 137, 2703–2714. doi: 10.1093/brain/awu205
Blauth, K., Soltys, J., Matschulat, A., Reiter, C. R., Ritchie, A., Baird, N. L., et al. (2015). Antibodies produced by clonally expanded plasma cells in multiple sclerosis cerebrospinal fluid cause demyelination of spinal cord explants. Acta Neuropathol. 130, 765–781. doi: 10.1007/s00401-015-1500-6
Boes, M. (2000). Role of natural and immune IgM antibodies in immune responses. Mol. Immunol. 37, 1141–1149. doi: 10.1016/s0161-5890(01)00025-6
Brettschneider, J., Czerwoniak, A., Senel, M., Fang, L., Kassubek, J., Pinkhardt, E., et al. (2010). The chemokine CXCL13 is a prognostic marker in clinically isolated syndrome (CIS). PLoS One 5:e11986. doi: 10.1371/journal.pone.0011986
Calabrese, M., Federle, L., Bernardi, V., Rinaldi, F., Favaretto, A., Varagnolo, M. C., et al. (2012). The association of intrathecal immunoglobulin synthesis and cortical lesions predicts disease activity in clinically isolated syndrome and early relapsing-remitting multiple sclerosis. Mult. Scler. 18, 174–180. doi: 10.1177/1352458511418550
Cepok, S., von Geldern, G., Grummel, V., Hochgesand, S., Celik, H., Hartung, H., et al. (2006). Accumulation of class switched IgD-IgM- memory B cells in the cerebrospinal fluid during neuroinflammation. J. Neuroimmunol. 180, 33–39. doi: 10.1016/j.jneuroim.2006.06.031
Comabella, M., Fernández, M., Martin, R., Rivera-Vallvé, S., Borrás, E., Chiva, C., et al. (2010). Cerebrospinal fluid chitinase 3-like 1 levels are associated with conversion to multiple sclerosis. Brain 133, 1082–1093. doi: 10.1093/brain/awq035
Durante, L., Zaaraoui, W., Rico, A., Crespy, L., Wybrecht, D., and Faivre, A. (2012). Intrathecal synthesis of IgM measured after a first demyelinating event suggestive of multiple sclerosis is associated with subsequent MRI brain lesion accrual. Mul. Scler. 18, 587–591. doi: 10.1177/1352458511424589
Elliott, C., Lindner, M., Arthur, A., Brennan, K., Jarius, S., Hussey, J., et al. (2012). Functional identification of pathogenic autoantibody responses in patients with multiple sclerosis. Brain 135, 1819–1833. doi: 10.1093/brain/aws105
Ferraro, D., Galli, V., Vitetta, F., Simone, A. M., Bedin, R., Del Giovane, C., et al. (2015). Cerebrospinal fluid CXCL13 in clinically isolated syndrome patients: association with oligoclonal IgM bands and prediction of Multiple Sclerosis diagnosis. J. Neuroimmunol. 283, 64–69. doi: 10.1016/j.jneuroim.2015.04.011
Frau, J., Villar, L. M., Sardu, C., Secci, M. A., Schirru, L., Ferraro, D., et al. (2018). Intrathecal oligoclonal bands synthesis in multiple sclerosis: is it always a prognostic factor? J. Neurol. 265, 424–430. doi: 10.1007/s00415-017-8716-4
Gardner, C., Magliozzi, R., Durrenberger, P. F., Howell, O. W., Rundle, J., and Reynolds, R. (2013). Cortical grey matter demyelination can be induced by elevated pro-inflammatory cytokines in the subarachnoid space of MOG-immunized rats. Brain 136, 3596–3608. doi: 10.1093/brain/awt279
Gee, K., Guzzo, C., Che Mat, N. F., Ma, W., and Kumar, A. (2009). The IL-12 family of cytokines in infection, inflammation and autoimmune disorders. Inflamm. Allergy Drug Targets 8, 40–52. doi: 10.2174/187152809787582507
Genain, C. P., Cannella, B., Hauser, S. L., and Raine, C. S. (1999). Identification of autoantibodies associated with myelin damage in multiple sclerosis. Nat. Med. 5, 170–175. doi: 10.1038/5532
Geurts, J. J., Roosendaal, S. D., Calabrese, M., Ciccarelli, O., Agosta, F., Chard, D. T., et al. (2011). Consensus recommendations for MS cortical lesion scoring using double inversion recovery MRI. Neurology 76, 418–424. doi: 10.1212/WNL.0b013e31820a0cc4
Giovannoni, G., Turner, B., Gnanapavan, S., Offiah, C., Schmierer, K., and Marta, M. (2015). Is it time to target no evident disease activity (n.d.) in multiple sclerosis? Mult. Scler. Relat. Disord. 4, 329–333. doi: 10.1016/j.msard.2015.04.006
Haider, L., Zrzavy, T., Hametner, S., Höftberger, R., Bagnato, F., Grabner, G., et al. (2016). The topograpy of demyelination and neurodegeneration in the multiple sclerosis brain. Brain 139, 807–815. doi: 10.1093/brain/awv398
Hatori, K., Nagai, A., Heisel, R., Ryu, J. K., and Kim, S. U. (2002). Fractalkine and fractalkine receptors in human neurons and glial cells. J. Neurosci. Res. 69, 418–426. doi: 10.1002/jnr.10304
Howell, O. W., Reeves, C. A., Nicholas, R., Carassiti, D., Radotra, B., Gentleman, S. M., et al. (2011). Meningeal inflammation is widespread and linked to cortical pathology in multiple sclerosis. Brain 134, 2755–2771. doi: 10.1093/brain/awr182
Huss, A., Abdelhak, A., Halbgebauer, S., Mayer, B., Senel, M., Otto, M., et al. (2018). Intrathecal immunoglobulin M production: a promising high-risk marker in clinically isolated syndrome patients. Ann. Neurol. 83, 1032–1036. doi: 10.1002/ana.25237
Khademi, M., Kockum, I., Andersson, M. L., Iacobaeus, E., Brundin, L., Sellebjerg, F., et al. (2011). Cerebrospinal fluid CXCL13 in multiple sclerosis: a suggestive prognostic marker for the disease course. Mult. Scler. 17, 335–343. doi: 10.1177/1352458510389102
Kowarik, M. C., Cepok, S., Sellner, J., Grummel, V., Weber, M. S., Korn, T., et al. (2012). CXCL13 is the major determinant for B cell recruitment to the CSF during neuroinflammation. J. Neuroinflam. 16:93. doi: 10.1186/1742-2094-9-93
Krumbholz, M., Theil, D., Cepok, S., Hemmer, B., Kivisäkk, P., Ransohoff, R. M., et al. (2006). Chemokines in multiple sclerosis: CXCL12 and CXCL13 up-regulation is differentially linked to CNS immune cell recruitment. Brain 129, 200–211. doi: 10.1093/brain/awh680
Kurtzke, J. F. (1983). Rating neurological impairment in multiple sclerosis: an expanded disability status scale (EDSS). Neurology 33, 1444–1452. doi: 10.1212/wnl.33.11.1444
Lassmann, H. (2008). Mechanisms of inflammation induced tissue injury in multiple sclerosis. J. Neurol. Sci. 274, 45–47. doi: 10.1016/j.jns.2008.04.003
Leary, S. M., McLean, B. N., and Thompson, E. J. (2000). Local synthesis of IgA in the cerebrospinal fluid of patients with neurological diseases. J. Neurol. 247, 609–615. doi: 10.1007/s004150070129
Levinson, A. I., Sandberg-Wollheim, M., Lisak, R. P., Zweiman, B., Sjogren, K., Laramore, C., et al. (1983). Analysis of B-cell activation of cerebrospinal fluid lymphocytes in multiple sclerosis. Neurology 33, 1305–1310. doi: 10.1212/wnl.33.10.1305
Li, R., Patterson, K. R., and Bar-Or, A. (2018). Reassessing B cell contributions in multiple sclerosis. Nat. Immunol. 19, 696–707. doi: 10.1038/s41590-018-0135-x
Li, R., Rezk, A., Miyazaki, Y., Hilgenberg, E., Touil, H., Shen, P., et al. (2015). Proinflammatory GM-CSF-producing B cells in multiple sclerosis and B cell depletion therapy. Sci. Transl. Med. 7:310ra166. doi: 10.1126/scitranslmed.aab4176
Link, H. (1978). The immune response in human demyelinating diseases. Adv. Exp. Med. Biol. 100, 529–543. doi: 10.1007/978-1-4684-2514-7_39
Link, H., and Müller, R. (1971). Immunoglobulins in multiple sclerosis and infections of the nervous system. Arch. Neurol. 25, 326–344. doi: 10.1001/archneur.1971.00490040052007
Lisak, R. P., Nedelkoska, L., Benjamins, J. A., Schalk, D., Bealmear, B., Touil, H., et al. (2017). B cells from patients with multiple sclerosis induce cell death via apoptosis in neurons in vitro. J. Neuroimmunol. 309, 88–99. doi: 10.1016/j.jneuroim.2017.05.004
Lolli, F., Halawa, I., and Link, H. (1989). Intrathecal synthesis of IgG, IgA, IgM and IgD in untreated multiple sclerosis and controls. Acta Neurol. Scand. 80, 238–247. doi: 10.1111/j.1600-0404.1989.tb03869.x
Magliozzi, R., Hametner, S., Facchiano, F., Marastoni, D., Rossi, S., Castellaro, M., et al. (2019). Iron homeostasis, complement, and coagulation cascade as CSF signature of cortical lesions in early multiple sclerosis. Ann. Clin. Transl. Neurol. 6, 2150–2163. doi: 10.1002/acn3.50893
Magliozzi, R., Howell, O., Vora, A., Serafini, B., Nicholas, R., Puopolo, M., et al. (2007). Meningeal B-cell follicles in secondary progressive multiple sclerosis associate with early onset of disease and severe cortical pathology. Brain 130, 1089–1104. doi: 10.1093/brain/awm038
Magliozzi, R., Howell, O. W., Nicholas, R., Cruciani, C., Castellaro, M., Romualdi, C., et al. (2018). Inflammatory intrathecal profiles and cortical damage in multiple sclerosis. Ann. Neurol. 83, 739–755. doi: 10.1002/ana.25197
Magliozzi, R., Howell, O. W., Reeves, C., Roncaroli, F., Nicholas, R., Serafini, B., et al. (2010). A gradient of neuronal loss and meningeal inflammation in multiple sclerosis. Ann. Neurol. 68, 477–493. doi: 10.1002/ana.22230
Magliozzi, R., Scalfari, A., Pisani, A. I., Ziccardi, S., Marastoni, D., Pizzini, F. B., et al. (2020). The CSF profile linked to cortical damage predicts Multiple Sclerosis activity. Ann. Neurol. doi: 10.1002/ana.25786 [Online ahead of print]
Mead, R. J., Singhrao, S. K., Neal, J. W., Lassmann, H., and Morgan, B. P. (2002). The membrane attack complex of complement causes severe demyelination associated with acute axonal injury. J. Immunol. 168, 458–465. doi: 10.4049/jimmunol.168.1.458
Milstein, J. L., Barbour, C. R., Jackson, K., Kosa, P., and Bielekova, B. (2019). Intrathecal, not systemic inflammation is correlated with multiple sclerosis severity, especially in progressive multiple sclerosis. Front. Neurol. 10:1232. doi: 10.3389/fneur.2019.01232
Owens, G. P., Bennett, J. L., Lassmann, H., O’Connor, K. C., Ritchie, A. M., Shearer, A., et al. (2009). Antibodies produced by clonally expanded plasma cells in multiple sclerosis cerebrospinal fluid. Ann. Neurol. 65, 639–649. doi: 10.1002/ana.21641
Ozakbas, S., Cinar, B. P., Özcelik, P., Baser, H., and Kosehasanoğullari, G. (2017). Intrathecal IgM index correlates with a severe disease course in multiplesclerosis: clinical and MRI results. Clin. Neurol. Neurosurg. 160, 27–29. doi: 10.1016/j.clineuro.2017.05.026
Pfuhl, C., Grittner, U., Gieß, R. M., Scheel, M., Behrens, J. R., Rasche, L., et al. (2019). Intrathecal IgM production is a strong risk factor for early conversion to multiple sclerosis. Neurology 93, e1439–e1451. doi: 10.1212/WNL.0000000000008237
Piddlesden, S. J., Lassmann, H., Zimprich, F., Morgan, B. P., and Linington, C. (1993). The demyelinating potential of antibodies to myelin oligodendrocyte glycoprotein is related to their ability to fix complement. Am. J. Pathol. 143, 555–564.
Ransohoff, R. M., and El Khoury, J. (2015). Microglia in health and disease. Cold Spring Harb. Perspect. Biol. 8:a020560. doi: 10.1101/cshperspect.a020560
Sabatino, J. J. Jr., Pröbstel, A. K., and Zamvil, S. S. (2019). B cells in autoimmune and neurodegenerative central nervous system diseases. Nat. Rev. Neurosci. 20, 728–745. doi: 10.1038/s41583-019-0233-2
Sandberg, M., Levinson, A. I., Zweiman, B., and Lisak, R. P. (1986). B cell activation in multiple sclerosis. Acta Neurol. Scand. 74, 417–424. doi: 10.1111/j.1600-0404.1986.tb07866.x
Savarin-Vuaillat, C., and Ransohoff, R. M. (2007). Chemokines and chemokine receptors in neurological disease: raise, retain, or reduce? Neurotherapeutics 4, 590–601. doi: 10.1016/j.nurt.2007.07.004
Saxena, A., Khosraviani, S., Noel, S., Mohan, D., Donner, T., and Hamad, A. R. (2015). Interleukin-10 paradox: a potent immunoregulatory cytokine that has been difficult to harness for immunotherapy. Cytokine 74, 27–34. doi: 10.1016/j.cyto.2014.10.031
Schneider, R., Euler, B., and Rauer, S. (2007). Intrathecal IgM-synthesis does not correlate with the risk of relapse in patients with a primary demyelinating event. Eur. J. Neurol. 14, 907–911. doi: 10.1111/j.1468-1331.2007.01871.x
Stauch, C., Reiber, H., Rauchenzauner, M., Strasak, A., Pohl, D., Hanefeld, F., et al. (2011). Intrathecal IgM synthesis in pediatric MS is not a negative prognostic marker of disease progression: quantitative versus qualitative IgM analysis. Mult. Scler. 17, 327–334. doi: 10.1177/1352458510388543
Teunissen, C. E., Petzold, A., Bennett, J. L., Berven, F. S., Brundin, L., Comabella, M., et al. (2009). A consensus protocol for the standardization of cerebrospinal fluid collection and biobanking. Neurology 73, 1914–1922. doi: 10.1212/WNL.0b013e3181c47cc2
Thompson, A. J., Banwell, B. L., Barkhof, F., Carroll, W. M., Coetzee, T., Comi, G., et al. (2018). Diagnosis of multiple sclerosis: 2017 revisions of the McDonald criteria. Lancet Neurol. 17, 162–173. doi: 10.1016/S1474-4422(17)30470-2
Tintore, M., Rovira, A., Rio, J., Tintoré, M., Rovira, A., Río, J., et al. (2008). Do oligoclonal bands add information to MRI in first attacks of multiple sclerosis? Neurology 70, 1079–1083. doi: 10.1212/01.wnl.0000280576.73609.c6
Touil, H., Li, R., Moore, C., Antel, J. P., and Bar-Or, A. (2017). Cross-talk between human glial cells and B cells help propagation of CNS-compartmentalized in progressive MS. J. Immunol. 198:132.5.
Villar, L., García-Barragán, N., Espiño, M., Roldán, E., Sádaba, M., Gómez-Rial, J., et al. (2008). Influence of oligoclonal IgM specificity in multiple sclerosis disease course. Mult. Scler. 14, 183–187. doi: 10.1177/1352458507082046
Villar, L. M., Espiño, M., Cavanillas, M. L., Roldán, E., Urcelay, E., de la Concha, E. G., et al. (2010). Immunological mechanisms that associate with oligoclonal IgM band synthesis in multiple sclerosis. Clin. Immunol. 137, 51–59. doi: 10.1016/j.clim.2010.06.007
Villar, L. M., Masjuan, J., González-Porqué, P., Plaza, J., Sádaba, M. C., Roldán, E., et al. (2002). Intrathecal IgM synthesis in neurological diseases. Relationship with disability in MS. Neurology 58, 824–826. doi: 10.1212/WNL.58.5.824
Villar, L. M., Masjuan, J., González-Porqué, P., Plaza, J., Sádaba, M. C., Roldán, E., et al. (2003). Intrathecal IgM synthesis is a prognostic factor in multiple sclerosis. Ann. Neurol. 53, 222–226. doi: 10.1002/ana.10441
Villar, L. M., Sádaba, M. C., Roldán, E., Masjuan, J., González-Porqué, P., Villarrubia, N., et al. (2005). Intrathecal synthesis of oligoclonal IgM against myelin lipids predicts an aggressive disease course in MS. J. Clin. Invest. 115, 187–194. doi: 10.1172/JCI22833
Walsh, M. J., and Tourtellotte, W. W. (1986). Temporal invariance and clonal uniformity of brain and cerebrospinal IgG, IgA, and IgM in multiple sclerosis. J. Exp. Med. 163, 41–53. doi: 10.1084/jem.163.1.41
Weber, M. S., Prod’homme, T., Patarroyo, J. C., Molnarfi, N., Karnezis, T., Lehmann-Horn, K., et al. (2010). B-cell activation influences T-cell polarization and outcome of anti-CD20 B-cell depletion in central nervous system autoimmunity. Ann. Neurol. 68, 369–383. doi: 10.1002/ana.22081
Ziemssen, T., Akgün, K., and Brück, W. (2019). Molecular biomarkers in multiple sclerosis. J Neuroinflam. 16:272. doi: 10.1186/s12974-019-1674-2
Keywords: CSF, multiple sclerosis, B cell, lesion activity, immunoglobulin M
Citation: Magliozzi R, Mazziotti V, Montibeller L, Pisani AI, Marastoni D, Tamanti A, Rossi S, Crescenzo F and Calabrese M (2020) Cerebrospinal Fluid IgM Levels in Association With Inflammatory Pathways in Multiple Sclerosis Patients. Front. Cell. Neurosci. 14:569827. doi: 10.3389/fncel.2020.569827
Received: 05 June 2020; Accepted: 22 September 2020;
Published: 16 October 2020.
Edited by:
João J. Cerqueira, University of Minho, PortugalReviewed by:
Catharina C. Gross, University of Münster, GermanyLuisa María Villar, Ramón y Cajal University Hospital, Spain
Copyright © 2020 Magliozzi, Mazziotti, Montibeller, Pisani, Marastoni, Tamanti, Rossi, Crescenzo and Calabrese. This is an open-access article distributed under the terms of the Creative Commons Attribution License (CC BY). The use, distribution or reproduction in other forums is permitted, provided the original author(s) and the copyright owner(s) are credited and that the original publication in this journal is cited, in accordance with accepted academic practice. No use, distribution or reproduction is permitted which does not comply with these terms.
*Correspondence: Roberta Magliozzi, cm9iZXJ0YS5tYWdsaW96emlAdW52ci5pdA==; cm1hZ2xpb3p6aTczQGdtYWlsLmNvbQ==
†These authors have contributed equally to this work