- 1School of Pharmaceutical Science & Yunnan Provincial Key Laboratory of Pharmacology for Natural Products, Kunming Medical University, Kunming, China
- 2College of Modern Biomedical Industry, Kunming Medical University, Kunming, China
Major depressive disorder (MDD) is one of the most common mental health conditions, characterized by pervasive and persistent low mood, low self-esteem, and a loss of interest or pleasure in activities that are typically enjoyable. Despite decades of research into the etiology and pathophysiological mechanisms of depression, the therapeutic outcomes for many individuals remain less than expected. A promising new area of research focuses on stress-induced neuroinflammatory processes, such as the excessive activation and crosstalk of microglia and astrocytes in the central nervous system under stress, as well as elevated levels of pro-inflammatory cytokines, which are closely linked to the onset and progression of depression. This review summarizes the mechanisms through which neuroinflammation induces or promotes the development of depression, and also highlights the effective roles of small molecules with anti-inflammatory activity in the treatment of MDD. Understanding the specific mechanisms through which stress-induced neuroinflammation further impacts depression, and using technologies such as single-cell RNA sequencing to elucidate the specific subtypes and interactions of microglia and astrocytes in depression, is of great importance for developing more effective therapeutic strategies for MDD.
1 Introduction
Depression is the most common neuropsychiatric disorder and a leading cause of disability (Clayton et al., 2024; Zeng et al., 2024). According to the World Health Organization, about 350 million people worldwide suffer from depression, and among them about 1 million people commit suicide each year (Ara, 2022). The major clinical symptoms of depression include persistent feelings of sadness, anhedonia, worthlessness, hopelessness or guilt, difficulty with thinking and decision-making, suicidal ideation, and changes in weight, appetite, and sleep (Otte et al., 2016; Nestler et al., 2002). At present, the pathogenic factors of depression include environmental factors, biological factors, psychological factors, genetic factors, etc., (Cui et al., 2024).
Currently, there are several main treatments for depression, such as (1) antidepressants, (2) evidence-based psychotherapy, (3) somatic non-drug therapies (Marwaha et al., 2023). Antidepressants are mainly classified according to their mechanism of action, and the more common types are listed below: (1) Tricyclic drugs (TCAs): Imipramine, Amitriptyline, Clomipramine, etc., (2) Monoamine oxidase inhibitors (MAOIs): Tranylcypromine, Phenelzine, Selegiline, Rasagiline, etc., (3) Serotonin reuptake inhibitors (SSRIs): Fluoxetine, paroxetine, Escitalopram, etc., (4) Norepinephrine reuptake inhibitors (NERIs): Bupropion, Reboxetine, Atomoxetine, etc., (5) Serotonin-norepinephrine reuptake inhibitors (SNRIs): Venlafaxine, Desvenlafaxine, Duloxetine, etc., (6) Norepinephrine and specific serotonergic antidepressants (NaSSAs): Mirtazapine, etc., (Ménard et al., 2016; Li and Zhang, 2020). Most treatment for depression have not achieved satisfactory clinical results, in approximately 50% of previously untreated depression patients, monotherapy with antidepressants or evidence-based psychotherapy provides some relief, but does not reverse depressive symptoms and return patients to their pre-illness state (Hengartner, 2020; Malhi and Mann, 2018). Clinical studies suggest that the poor efficacy of clinical antidepressants may be related to the complex pathogenesis of depression (Ye et al., 2023). At present, the known pathophysiological mechanisms of depression include the monoamine hypothesis, receptor hypothesis, neuroendocrine hypothesis, neuroplasticity hypothesis, inflammation hypothesis, excitatory amino acid hypothesis, and intestinal flora imbalance hypothesis (Jesulola et al., 2018; Stetler and Miller, 2011). Among these hypotheses, the neuroinflammatory hypothesis has attracted increasing attention in recent years.
Immune activation and inflammatory responses are believed to be important causes of many brain diseases, such as Parkinson’s disease, Alzheimer’s disease, and Huntington’s disease (Hurley and Tizabi, 2013; Wu et al., 2021). Ongoing studies in neurophysiology and neuropsychiatry are increasingly focusing on the relationship between neuroinflammation and depression, suggesting that the immune system is involved in the pathophysiology of depression (Troubat et al., 2021). Microglia and astrocytes are important participants in the neuroimmune response. Microglia play a crucial role in brain development by regulating neurogenesis, synapse formation and elimination, and the assembly of neuronal circuits (Kreisel et al., 2014). Astrocytes, the most abundant glial cells in the central nervous system, are fundamental in regulating normal brain function and are involved in the pathologies of psychiatric and neurodegenerative diseases. Reactive astrocytes are highly heterogeneous and play an important role in restoring homeostasis and limiting tissue damage in the central nervous system (Leng et al., 2018). However, in the presence of stress or endotoxin stimulation, overactivated microglia and astrocytes can release an excessive amount of inflammatory factors. These overproduced inflammatory factors can lead to neuronal damage and are considered to induce depression (Nettis and Pariante, 2020).
This paper summarizes the roles of different polarization phenotypes of microglia and astrocytes in stress-induced neuroinflammation and their potential mechanisms in depression. Additionally, it reviews recent research on the therapeutic potential of natural compounds with anti-inflammatory properties for treating depression. The importance of identifying specific subtypes of microglia and astrocytes, as well as effective genetic targets, for depression therapy is discussed. Furthermore, the paper explores the therapeutic potential of natural compounds in modulating these distinct phenotypes and genes in the treatment of depression.
2 Manuscript formatting
2.1 Neuroinflammation
The human immune system can be viewed as a multi-layered defense network that comprehensively protects the body from external threats and internal damage. It crucially prevents the invasion of foreign microorganisms, mitigates the pathogenicity of microorganisms within the body, inhibits the proliferation of cancer cells, and promotes the rejection of transplanted tissues (Kölliker-Frers et al., 2021; Berk et al., 2013). Immune defense includes physical barriers such as the skin, various epithelia, and blood-brain barrier. The innate immune system, which relies on leukocytes, responds to infections or tissue damage through early inflammatory reactions. The adaptive immune system, which is composed of T lymphocytes and B lymphocytes, interacts with specific antigens and forms immunological memory (Zhou et al., 2024).
Inflammatory responses play a protective role in the body. Transient inflammation in the nervous system typically occurs in response to central nervous system (CNS) injury, infection, toxin exposure, or autoimmune reactions (Sarno et al., 2021; Table 1). This response is beneficial during tissue repair and development (Heneka et al., 2018). Neuroinflammation activates innate immune molecules and cellular pathways (Parsi et al., 2024). In particular, peripheral immune cells, including monocytes, granulocytes, and dendritic cells, migrate to the brain through the blood and lymphatic systems to survey for pathogens or damage and support neurological function. Animal studies have shown that endotoxin administration triggers perivascular macrophage-derived monocytes to initiate an adaptive neuroinflammatory response, involving prostaglandins and anti-inflammatory feedback mechanisms (Serna-Rodríguez et al., 2022; Balistreri and Monastero, 2023). Furthermore, exogenous immune cells, such as lymphocytes, play a critical role in limiting damage spread, providing neuroprotection, and influencing cognitive function after brain injury (Wohleb et al., 2016).
Chronic inflammatory responses may lead to excessive production of inflammatory factors and abnormal activation of immune cells, ultimately resulting in tissue damage. These inflammatory processes are mediated by pro-inflammatory cytokines [e.g., interleukin-1β (IL-1β), interleukin-6 (IL-6), tumor necrosis factor-α (TNF-α)], chemokines [e.g., C-C motif chemokine ligand 2 (CCL2), C-C motif chemokine ligand 5 (CCL5), C-X-C motif chemokine ligand 1 (CXCL1)], secondary messengers [e.g., nitric oxide (NO), prostaglandins], and reactive oxygen species (ROS) (Baumeister et al., 2014). Studies have shown that under pathological conditions, the permeability of the blood-brain barrier (BBB) increases, allowing peripheral cytokines to stimulate the activation of microglia and astrocytes, thereby exacerbating the inflammatory response (Abbott et al., 2010). These pro-inflammatory factors, by reducing the activity of glutamine synthetase, lead to the accumulation of glutamate, which enhances the activation of excitatory neurons, thereby triggering excitotoxicity and cell apoptosis (Alzarea et al., 2024). Meanwhile, inflammatory factors can also lead to mitochondrial dysfunction, cytochrome C release, adenosine triphosphate (ATP) depletion, free radical generation, and oxidative damage (Bhatt et al., 2023; Culmsee et al., 2018). Some therapeutic agents, such as interferon-α (IFN-α), are effective in alleviating somatic diseases; however, due to their pro-inflammatory effects, they often induce mild to moderate depressive symptoms by impairing the function of brain regions involved in emotional regulation, such as the prefrontal cortex (PFC) and the amygdala (Vignau et al., 2005; Pinto and Andrade, 2016). Important emotional regulation areas in the brain, such as the PFC and amygdala, are directly affected by the overactivation of cytokine networks. Pro-inflammatory cytokines have been reported to reduce neurotrophic supply and down-regulate neurogenesis via the brain-derived neurotrophic factor (BDNF) signaling pathway, and to debilitate hippocampal cell proliferation via the nuclear factor kappa B (NF-κB) signaling pathway. Moreover, they lead to damage to the body by increasing glutamate levels through N-methyl-D-aspartic acid receptor (NMDA) receptor activation, leading to excitotoxicity and reduced neurogenesis (Kiecolt-Glaser et al., 2015).
In recent years, the role of inflammation in neurological disorders has attracted significant attention. Research has shown that the excessive activation of pro-inflammatory cytokines, such as IL-1β, TNF-α, and IL-6, is closely associated with the pathogenesis of many central nervous system disorders, including depression (Dantzer et al., 2008; Na et al., 2014). These inflammatory mediators contribute to the development of depressive symptoms by affecting brain tissue, modulating the monoaminergic system, and triggering neurotoxic processes (Arioz et al., 2019; Shi et al., 2023; Zhou et al., 2024). Animal models established through the in vivo injection of lipopolysaccharide (LPS) or inflammatory factors also exhibit typical depressive symptoms, such as a decrease in aggression and curiosity (Zhang et al., 2023; Zhou et al., 2024). Inhibition of the production of inflammatory cytokines, such as IL-1β, IL-6, and TNF-α, exerts antidepressant effects (Şahin et al., 2015; Zhang et al., 2017; Cheng et al., 2016). These findings suggest that the activation of microglia is closely associated with depression, and inhibiting neuroinflammation may provide a novel therapeutic target for the treatment of depression.
2.1.1 Microglia
Microglia are the primary immune cells in the brain and serve as the first line of defense. In recent years, they have garnered significant attention due to their roles in immune responses and neuroinflammation. They play a crucial role in brain development by regulating neurogenesis, synaptogenesis, synapse elimination, and the formation of neuronal circuits. Furthermore, microglia possess the ability to recognize pathogens, perform phagocytosis, present antigens, and remodel synapses (DiSabato et al., 2016).
Under normal conditions, resting microglia continuously monitor the surrounding environment. Upon injury or changes in the external environment, they become activated and undergo morphological changes. Microglial cells transform from a branched form to an amoeboid shape, with cell body swelling, shortened processes, increased phagocytic activity, and elevated cytokine production. This process is referred to as microglial activation (Boche et al., 2013). Microglial activation is triggered by the recognition of pattern recognition receptors (PRRs), pathogen-associated molecular patterns (PAMPs), and damage-associated molecular patterns (DAMPs). PAMPs and DAMPs interact with microglial receptors such as Toll-like receptors (TLRs) and the receptor for advanced glycation end products (RAGE), thereby initiating the synthesis and release of inflammatory mediators and promoting the transmission of inflammatory signals (Liu et al., 2023). Microglial activation can occur through two main pathways: the classical M1 activation and the selective M2 activation (Jia et al., 2021). Various factors, such as cellular aging, endotoxins, inflammatory cytokines, and ROS, can drive microglia to polarize toward the M1 phenotype. M1 microglia produce pro-inflammatory factors, including IL-1β, TNF-α, IL-6, and superoxide radicals, which help clear infections and repair tissues (Takahashi et al., 2024). In contrast, M2 activation is induced by cytokines such as interleukin-4 (IL-4) and interleukin-13 (IL-13), accompanied by the release of anti-inflammatory factors like Interleukin-10 (IL-10), insulin-like growth factor-1 (IGF 1), and transforming growth factor-β (TGF-β), which promote tissue healing, regeneration, and angiogenesis, and also repair neuronal damage (Butovsky et al., 2014; Parkhurst et al., 2013; Yi et al., 2020). It promotes healing, tissue regeneration, and angiogenesis, and can inhibit or promote the repair of neuronal injury (Wang et al., 2022; Figure 1).
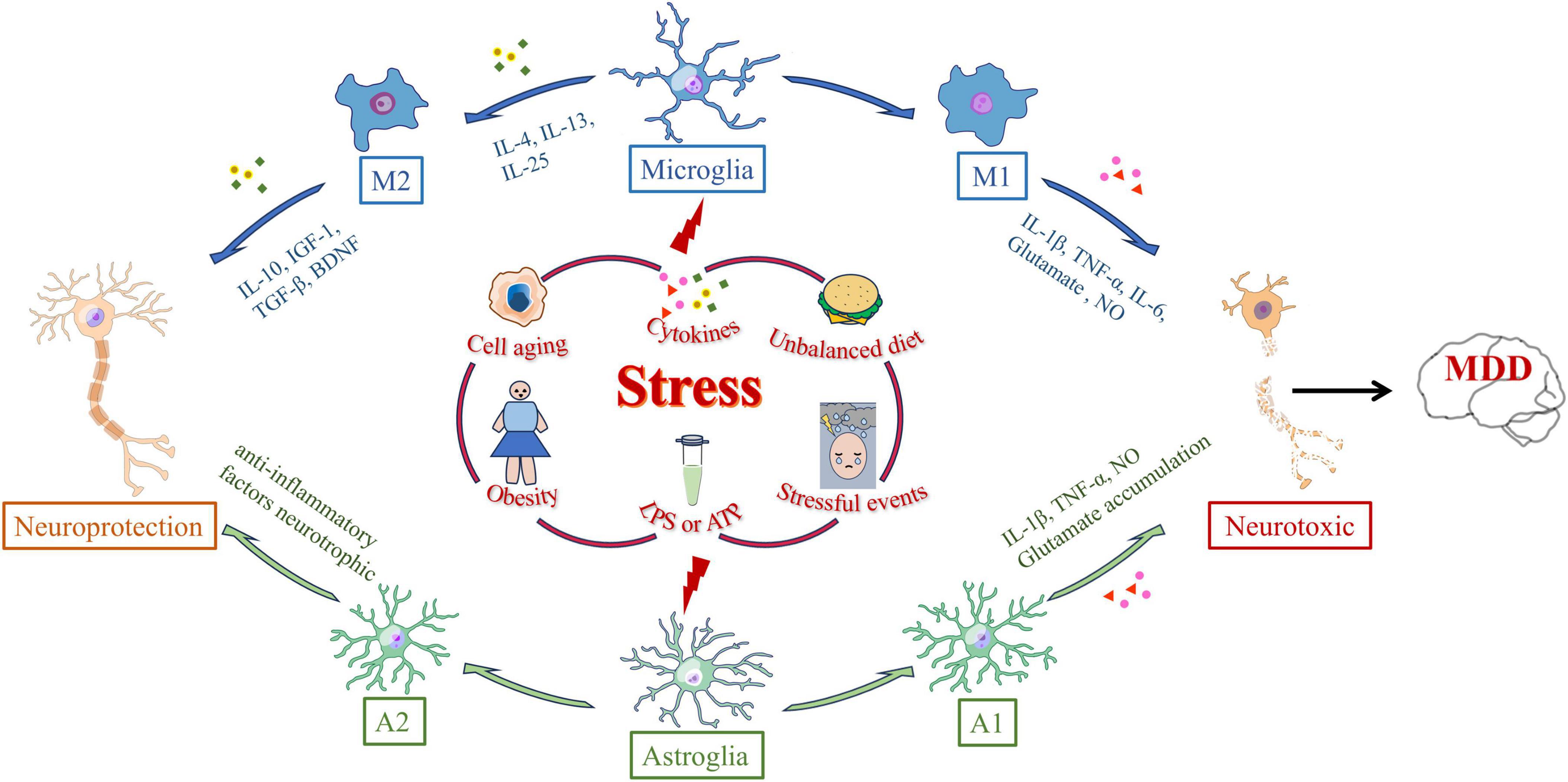
Figure 1. Polarization-inducing factors and dynamic properties of microglia and astrocyte. Microglia and astrocytes are polarized by many external stressors, such as prolonged stressful events, cell aging, obesity, cytokine, lipopolysaccharide (LPS) or adenosine triphosphate (ATP) stimulation, and dietary imbalance. External stress activates microglia through microglia receptors. Microglia polarized to the M1 phenotype synthesize interleukin-1β (IL-1β), tumor necrosis factor-α (TNF-α), interleukin-6 (IL-6), superoxide anion radicals, glutamate, and NO, ultimately clearing infection and repairing tissue. Cytokines such as IL-4, IL-13, or IL-25 trigger M2 activation and promote M2 microglia to release anti-inflammatory cytokines such as IL-10, insulin-like growth factor-1 (IGF-1), transforming growth factor-β (TGF-β), and brain-derived neurotrophic factor (BDNF). Astrocytes can differentiate into A1 reactive astrocytes or A2 astrocytes in response to central nervous system injury, such as injury, neurodegeneration, or infection. The expressions of complement cascade gene, IL-1β, TNF-α and NO in A1 astrocytes were significantly up-regulated. A2 astrocytes can up-regulate neurotrophic or anti-inflammatory genes, promoting the survival and growth of neurons.
However, recent research has revealed that categorizing microglia solely into M1 and M2 phenotypes is an oversimplification. Through high-throughput single-cell RNA sequencing, researchers have analyzed the RNA expression patterns of over 76,000 microglial cells from mice at different developmental stages, during aging, and in response to brain injury. The study identified at least nine distinct microglial states, which vary according to development, aging, and injury (Hammond et al., 2019). Refining the classification of microglia will help to better understand the functions, signaling mechanisms, and interactions of these subtypes with other brain cells. This, in turn, could facilitate the identification of specific microglial biomarkers for assessing human health and disease states.
Activated microglia show different responses to external stimuli, which is a double-edged sword: Acutely activated microglia usually promote tissue repair by removing invading pathogens and cell debris; Sustained microglial activation causes chronic neuroinflammation, which worsens the damage and promotes disease progression. With the deepening of the research on the pathogenesis of depression, the role of microglia in the pathogenesis of depression has also been proved in large numbers, so depression is also considered to be a microglia-related disease (microgliosis) (Yirmiya et al., 2015; Wang et al., 2022; Deng et al., 2020). Here, we summarize recent research over the past 3 years on the mechanisms through which the modulation of microglial phenotype exerts anti-inflammatory and antidepressant effects. Understanding these mechanisms is crucial for identifying new directions in the treatment and drug development for depression (Table 2).
2.1.2 Astrocytes
Traditionally, astrocytes have been regarded as supportive cells for neurons, playing a critical role in maintaining brain homeostasis and the normal function of neurons. As the largest cell type in the CNS, astrocytes provide energy, recycle neurotransmitters, supply neurotrophic factors, and regulate synaptic formation and elimination. They also maintain the BBB and participate in immune signaling (Colombo and Farina, 2016). When the CNS undergoes damage, such as trauma, neurodegenerative diseases, or infections, astrocytes exhibit rapid changes in gene expression, morphology, and function, a response known as astrocyte reactivity (Stoklund Dittlau and Freude, 2024). Research indicates that reactive astrocytes may have detrimental effects, such as exacerbating neuroinflammation, inhibiting synaptic sprouting, or axonal growth. However, some studies suggest that A1 and A2 reactive astrocytes have beneficial roles, including anti-inflammatory effects, neuroprotection, and BBB repair (Rupareliya et al., 2023). Compared to normal astrocytes, A1 astrocytes lose many critical functions, particularly the maintenance of synaptic activity. Furthermore, A1 astrocytes significantly upregulate substances that are harmful to synapses, such as complement cascade factors, IL-1β, TNF-α, and NO (Cong et al., 2023). In contrast, A2 astrocytes can upregulate neurotrophic factors or anti-inflammatory genes, promoting neuronal survival and growth, and playing an active role in neurorepair. Astrocytes are also responsible for the uptake and metabolism of over 90% of glutamate in the brain (Mahmoud et al., 2019). When astrocytes are deficient, excessive accumulation of glutamate in the synaptic cleft may lead to excitotoxicity and an imbalance in neuronal activity (Wang et al., 2017; Figure 1).
Recent studies, however, have shown that A1 and A2 types only represent two of the potential astrocyte transcriptomes when classifying astrocytes using multi-dimensional data and co-clustering methods. Moreover, research has found that astrocytes in a healthy brain are highly diverse and perform specific roles in different CNS circuits. Reactive astrocytes are equally heterogeneous, with RNA sequencing and microarray analysis data indicating that reactive astrocytes in various disease models exhibit distinct molecular characteristics (Henrik Heiland et al., 2019).
Current research has confirmed that astrocytes are closely involved in the pathophysiology of depression. In rodent models, chronic mild stress induces overexpression of glial fibrillary acidic protein (GFAP). Increased numbers of astrocytes have also been found in the hippocampus and medial prefrontal cortex (mPFC) of patients with major depressive disorder (Wen et al., 2024; Yuan et al., 2024). Additionally, elevated levels of glutamate have been observed in the brains and cerebrospinal fluid of depression patients, and chronic stress appears to induce brain structural atrophy by disrupting the GFAP astrocytic network (Rajkowska and Stockmeier, 2013). We summarize researches conducted over the past 3 years on the mechanisms through which astrocytes mediate antidepressant effects (Table 3). These mechanistic insights may serve as potential targets for the prevention and treatment of depression (Wang J. Y. et al., 2024).
2.1.3 Crosstalk between microglia and astrocytes in neuroinflammation
Microglia and astrocytes play dual roles in brain diseases. They not only enhance immune responses and promote neurodegeneration, but also modulate the inflammatory responses in the central nervous system (Goshi et al., 2020). Furthermore, the interaction between astrocytes and microglia plays a critical role in neuroinflammatory responses (Olude et al., 2022).
These two types of glial cells regulate inflammation in the central nervous system through the secretion of cytokines and inflammatory mediators (Kim and Son, 2021). For example, LPS-activated microglia can induce a neurotoxic phenotype in astrocytes by secreting Interleukin-1α (IL-1α), TNF-α, and complement component C1q, triggering transcriptional responses in astrocytes that lead to the production of neurotoxic factors while inhibiting phagocytic function and the expression of neurotrophic factors (Li S. et al., 2022). Moreover, aryl hydrocarbon receptor (AHR) in microglia regulates the expression of vascular endothelial growth factor B (VEGF-B) and Transforming growth factor-α (TGF-α), further promoting the expression of pro-inflammatory genes in astrocytes, such as CCL2, IL-1β, and nitric oxide synthase 2 (NOS2) (Ge et al., 2021). The production of TNF-α enhances the release of glutamate from astrocytes, thereby increasing neuronal excitotoxicity. Studies have also shown that NF-κB signaling in microglia activates the NOD-like receptor thermal protein domain associated protein 3 (NLRP3) inflammasome, which in turn triggers A1-type astrocytes through caspase-1 activation. A1 astrocytes secrete factors such as CCL2, C-X3-C motif chemokine ligand 1 (CX3CL1), C-X-C motif chemokine ligand 10 (CXCL10), granulocyte-macrophage colony-stimulating factor (GM-CSF), and interleukin-1 (IL-1), which in turn activate pro-inflammatory microglia (Linnerbauer et al., 2020; Jha et al., 2019). Furthermore, the deficiency of sigma-1 receptors in astrocytes leads to the activation of the NF-κB pathway, thereby amplifying the interaction between reactive astrocytes and activated microglia, exacerbating neuroinflammation and triggering stress-induced neuronal apoptosis, ultimately resulting in depressive-like behavior in mice (Figure 2).
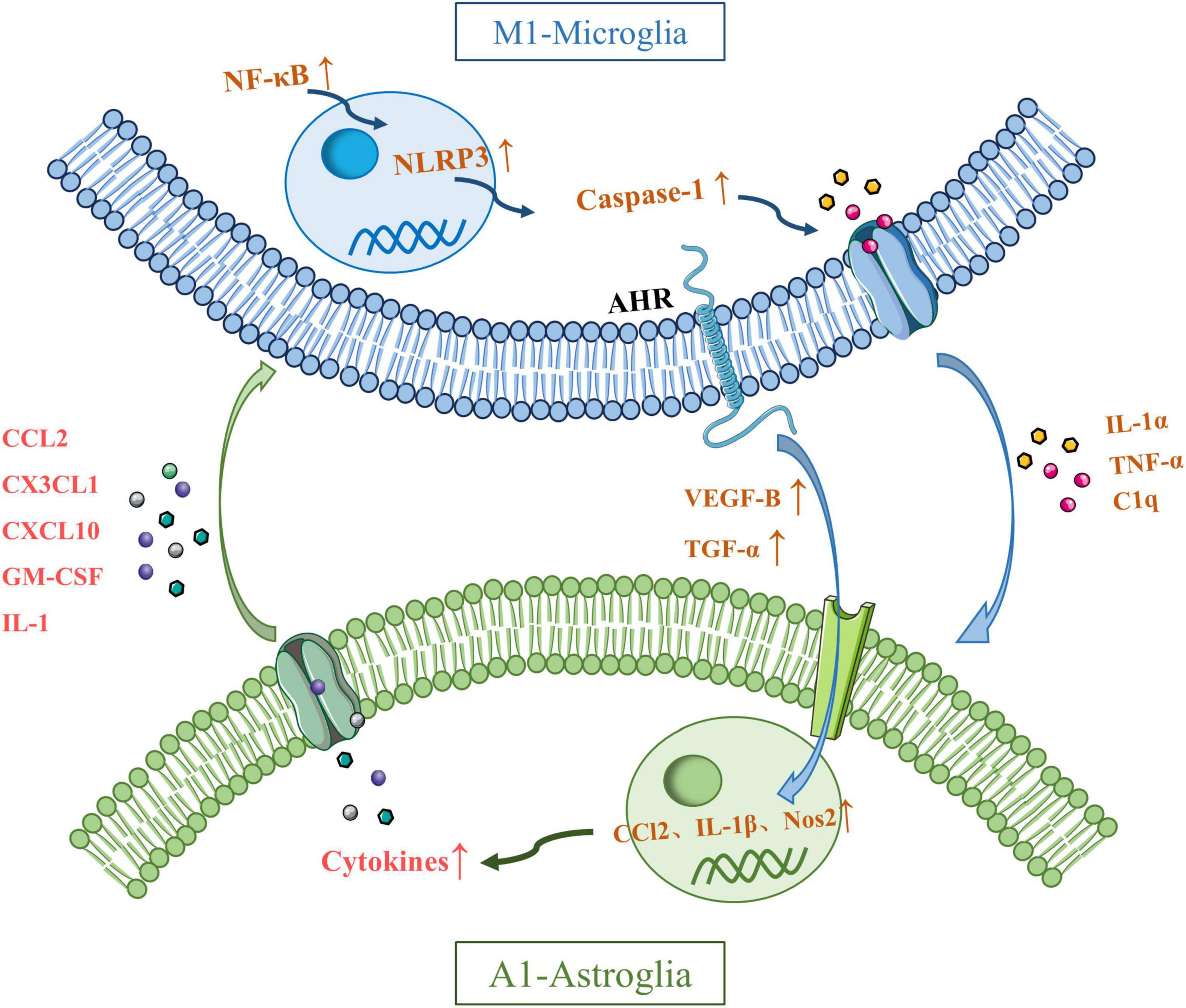
Figure 2. Possible mechanisms underlying crosstalk between microglia and astroglia. Interleukin-1α (IL-1α), tumor necrosis factor-α (TNF-α), and C1q secreted by activated microglia induce transcription of astrocytes to produce neurotoxic factors. Microglia can regulate the expression of CCl2, IL-1β and nitric oxide synthase 2 (NOS2) by regulating the expression of vascular endothelial growth factor B (VEGF-B) and transforming growth factor-β (TGF-α) through aryl hydrocarbon receptor (AHR). Microglial TNF-α promotes the release of glutamate from astrocytes and produces neuronal excitotoxicity. The NF-kB pathway in microglia activates the NLRP3 inflammasome, which in turn activates caspase-1 and induces the activation of A1 astrocytes. A1 astrocytes secrete CCL2, CX3CL1, CXCL10, GM-CSF, and IL-1, which in turn activate proinflammatory microglia. The deficiency of sigma-1 receptors in astrocytes leads to the activation of the NF-κB pathway, thereby amplifying the interaction between reactive astrocytes and activated microglia.
2.2 Stress induces neuroinflammation in depression
Stress is an external stimulus that affects both the body and mind, often manifesting as emotional responses. Research suggests that the onset of depression may be related to an individual’s ability to cope with stress (Yaribeygi et al., 2017). Studies have shown that individuals who experience significant stressful events (such as the loss of a loved one, divorce, relocation, or social failure) are at a 5–6-fold increased risk of depression within 6 months (Kessler, 1997). Extensive research has demonstrated a significant causal relationship between stressful life events and the occurrence of major depressive episodes (Du Preez et al., 2021). Acute stressors are a natural physiological response to sudden events, whereas prolonged exposure to stress may lead to neuroendocrine dysfunction and emotional blunting, which can trigger mental health issues such as anxiety and depression (Lee et al., 2021).
Stress exposure experiments in rodents have shown that stress can induce the excessive secretion of cytokines (Munhoz et al., 2008). Studies have found that stress leads to elevated levels of the cytokine IL-6 in the plasma of rodents (Xu et al., 2020; Xu K. et al., 2024). Furthermore, acute restraint stress has been shown to increase the expression of IL-1β mRNA in the hypothalamus of rats (Liu H. et al., 2022; Liu et al., 2021). These findings suggest that psychosocial stressors may play an important role in the pathophysiology of stress-related disorders, such as depression, by regulating the production of pro-inflammatory and anti-inflammatory cytokines. In addition, research has revealed that stress mediators can cross the BBB and influence the immune system. Microglial cells are considered the primary source of these cytokines, and chronic stress can alter their morphology (Yao et al., 2022). In summary, the close relationship between microglial activation and neuroinflammation has been well-established. Therefore, psychological stress may induce neuroinflammation, ultimately leading to the development of depressive-like behavior.
2.3 Anti-inflammatory treatment can alleviate depression
Based on the impact of various neuroinflammatory lesions on the pathogenesis of depression, exploring the mechanisms and treatment methods of depression from an anti-inflammatory perspective has become a research focus in recent years (Patil et al., 2023). It is noteworthy that some of the aforementioned marketed antidepressants may alleviate depression to some extent through anti-inflammatory effects (Eyre and Baune, 2012). The serotonin reuptake inhibitor vortioxetine can inhibit the NLRP3 inflammasome pathway through its immunomodulatory effects, thus exerting antidepressant and cognitive improvement effects (Ciani et al., 2025). Additionally, a study showed that administering 10 mg/kg of ketamine to depressed model animals significantly reduced the IL-1β levels in their hippocampus (Wang et al., 2015). This suggests that anti-inflammatory treatment for depression may be an effective strategy. Common anti-inflammatory medications, such as non-steroidal anti-inflammatory drugs (NSAIDs), have shown in a meta-analysis that these drugs can effectively treat depression in animal models when used alone or in combination with antidepressants (Bai et al., 2020). However, some studies indicate that these medications may affect the efficacy of antidepressants. These mixed results may be attributed to various experimental design factors. For instance, some studies involve middle-aged patients, while others primarily focus on younger individuals. The use of selective COX-1 and COX-2 inhibitors NSAIDs has also demonstrated varying antidepressant efficacy. Furthermore, the stage of depression in patients across different studies may contribute to the observed differences in the efficacy of NSAIDs (Eyre et al., 2015; Baune, 2017).
2.3.1 Natural compounds with anti-inflammatory properties have antidepressant effects
Increasingly, studies are concentrating on the mechanisms and effects of natural products with anti-inflammatory activities in improving depressive-like behaviors. The structural diversity and broad pharmacological effects of natural products are notable characteristics that are not commonly found in synthetic antidepressants (Dai et al., 2022). Natural products can modulate neural function through various mechanisms, such as affecting receptors or regulating immune processes, thereby achieving anti-inflammatory and antidepressant effects (Noori et al., 2022).
Compound 3C is a derivative of (+)-balasubramide, an 8-metalactam compound extracted from the yellow peel leaf of the Sri Lankan plant, which has been shown to have significant anti-inflammatory effects in microglia. Further investigation of the pharmacological activity of compound 3C showed that compound 3C could improve the depressive behavior of mice with endotoxins induced neuroinflammation by promoting the anti-inflammatory activity of microglia through adenosine 5’-monophosphate-activated protein kinase (AMPK)/peroxisome proliferator-activated receptor gamma coactivator 1α (PGC-1α) signaling pathway, enhancing the expression of a variety of anti-inflammatory mediators, and inhibiting the pro-inflammatory activity of microglia (Wang et al., 2018). Astragaloside IV (AS-IV) has antioxidant, anti-inflammatory, anti-hypertensive and neuroprotective effects (Abd Elkader et al., 2021). It has been reported that AS-IV may alleviate peroxisome proliferator-activated receptor γ (PPARγ)/ axis-mediated neuroinflammation and relieve depression-like behaviors in chronic restraint stress-induced and LPS-induced mice by up-regulating PPARγ expression. Baicalin, a widely used drug, has strong anti-inflammatory, anti-oxidation and anti-apoptosis activities (Song et al., 2018). Recent studies using chronic unpredictable mild stress (CUMS)-induced and endotoxin-induced depression mice have demonstrated that baicalin can improve depressive-like behavior and neuroinflammation by inhibiting the harmful overexpression of Toll-like receptor 4 (TLR4) by inhibiting the phosphatidylinositol-3-kinase (PI3K)/protein kinase B (AKT)/forkhead Box O1 (FOXO1) pathway (Guo et al., 2019). Ginsenoside Rg1 is widely reported to have a strong neuroprotective effect (Wang et al., 2023). Further evidence suggests that Rg1 may inhibit the transcriptional activity of NF-κB by increasing anti-inflammatory and inhibiting pro-inflammatory cytokines, neurotoxic mediators, pro-apoptotic proteins and microglia activation, as well as regulating mitogen activation and sirtuin 1 (SIRT1) signaling pathways. Thus, it can reduce chronic social defeat stress (CSDS) -induced hippocampal neuroinflammation and improve adult hippocampal neurogenesis, and play an antidepressant role (Jiang et al., 2020). Many previous studies have found that pinocembrin exhibit antioxidant, anti-inflammatory and neuroprotective effects both in vitro and in vivo (Li J. M. et al., 2022). Current studies have shown that Pinocembrin can reverse CUMS-induced depression-like behaviors by acting against neuroinflammation and apoptosis through nuclear factor erythroid 2-related factor 2 (Nrf2)/heme oxygenase 1 (HO-1) and NF-κB signaling pathways (Wang et al., 2020). In addition, catalpol has been shown to have anti-inflammatory, anti-tumor and anti-oxidative effects (Liang et al., 2023). Recently, catalpol has been shown to improve depression-like behaviors in CUMS mice by alleviating oxidative stress-mediated NLRP3 inflammasome activation and neuroinflammation (Wang et al., 2021). Previous studies have shown that cinnamic acid can attenuate LPS-induced depression-like behaviors by reducing LPS-induced inflammation and oxidative stress, and ameliorating LPS-induced BDNF damage (Zhuo et al., 2022). In addition to the above related findings, recent studies have also found that Asperosaponin VI exerts antidepressant effects by inhibiting TLR4/NF-κB signaling pathway, inhibiting microglia-mediated neuroinflammation, down-regulating the expression of indoleamine 2, 3-dioxygenase (IDO) and normalizing abnormal glutamate transmission (Zhang et al., 2020).
By exploring the specific mechanisms and effects of these compounds in anti-inflammatory and anti-depressive effects in vivo and in vitro depression models, it lays a foundation for the pathogenesis and treatment of depression (Figure 3 and Table 4).
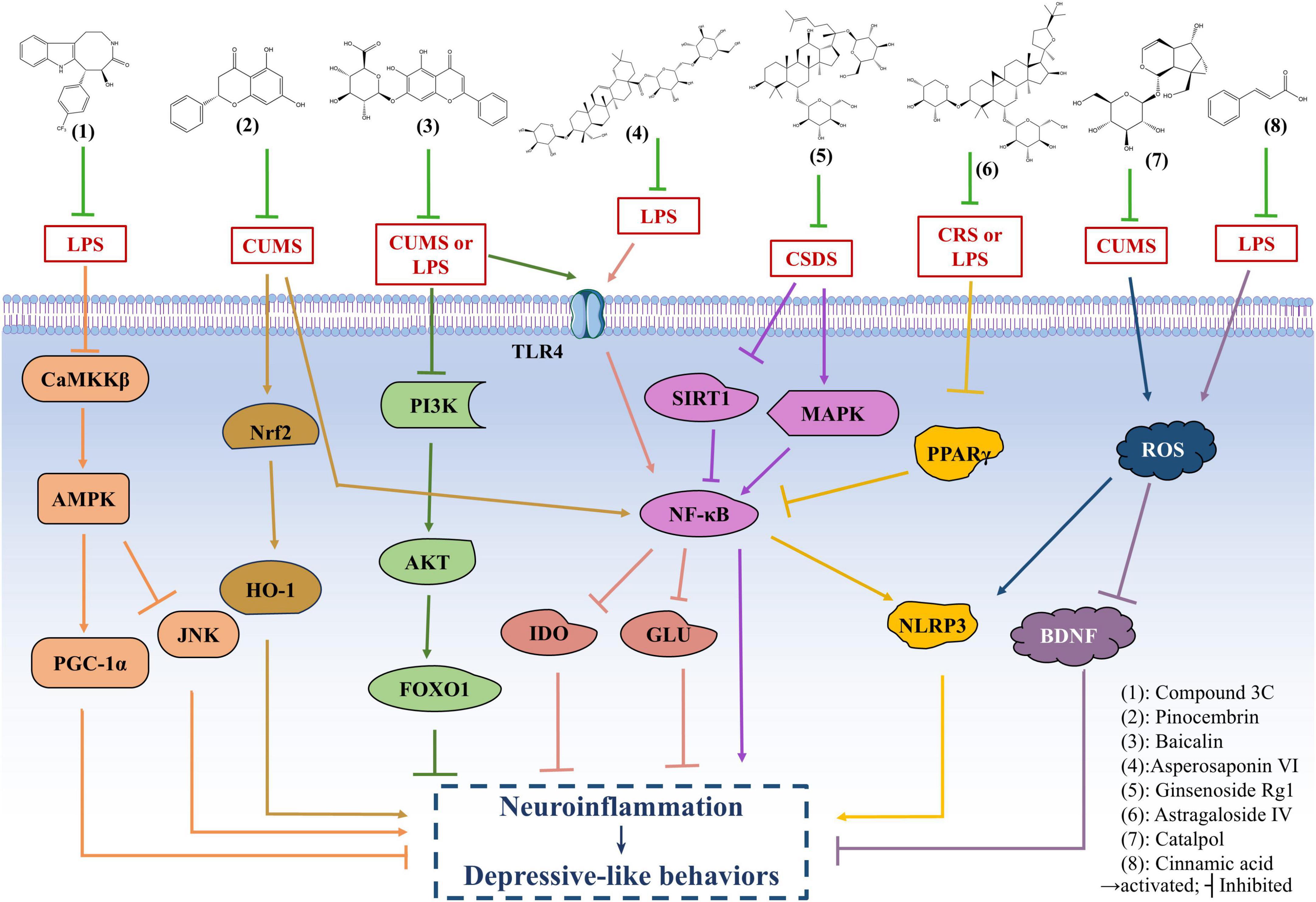
Figure 3. The antidepressant mechanism of natural anti-inflammatory products. Compound 3C improves the depressive behavior of mice with endotoxins induced neuroinflammation by promoting the anti-inflammatory activity of microglia through adenosine 5’-monophosphate-activated protein kinase (AMPK)/ peroxisome proliferator-activated receptor gamma coactivator 1α (PGC-1α) signaling pathway. Astragaloside IV (AS-IV) alleviates peroxisome proliferator-activated receptor γ (PPARγ)/NOD-like receptor thermal protein domain associated protein 3 (NLRP3) axis-mediated neuroinflammation and relieve depression-like behaviors in chronic unpredictable mild stress (CUMS) and lipopolysaccharide (LPS)-induced mice by up-regulating PPARγ expression. Baicalin can improve depressive-like behavior and neuroinflammation in CUMS and LPS-induced mice by inhibiting the Toll-like receptor 4 (TLR4) and phosphatidylinositol-3-kinase (PI3K)/protein kinase B (AKT)/forkhead Box O1 (FOXO1) pathway. Ginsenoside Rg1 may reduce chronic social defeat stress (CSDS)-induced depressive-like behavior in mice by inhibiting the transcriptional activity of NF-κB and regulating mitogen activation and SIRT1 signaling pathways. Pinocembrin can reverse CUMS-induced depression-like behaviors by regulating nuclear factor erythroid 2-related factor 2 (Nrf2)/heme oxygenase 1 (HO-1) and NF-kB signaling pathways. Catalpol improves depression-like behaviors in CUMS mice by alleviating oxidative stress-mediated NLRP3 inflammasome activation and neuroinflammation. Cinnamic acid can attenuate LPS-induced depression-like behaviors by reducing oxidative stress, and ameliorating LPS-induced BDNF damage. Asperosaponin VI exerts antidepressant effects by inhibiting TLR4/NF-κB signaling pathway, down-regulating the expression of indoleamine 2, 3-dioxygenase (IDO) and normalizing abnormal glutamate transmission.
2.4 Depression increases neuroinflammation
In summary, studies have found that depression and inflammation are mutually reinforcing. As mentioned above, inflammation plays a key role in the pathogenesis of depression (Slavich and Irwin, 2014). It has been mentioned in many studies related to the pathogenesis of depression that the activation of microglial in the prefrontal cortex or hippocampus and the release of pro-inflammatory factors such as IL-1β, TNF-α and IL-6 in depressed animals with stress models (Su et al., 2017; McWhirt et al., 2019). In addition, the presence of depression also triggers more cytokines in the face of stressors and pathogens (Glaser et al., 2003). Consistent with the animal literature, human studies have shown that depression triggers an inflammatory response that promotes an increase in cytokines that respond to stressors and pathogens (Rohleder and Miller, 2008; Fagundes et al., 2013). For example, in women who had just given birth, those with a lifetime history of MDD had greater increases in serum levels of IL-6 and soluble IL-6 receptors than those without a history of depression (Maes et al., 2001). Similarly, MDD patients had greater increases in inflammatory markers after stressor stimulation than non-depressed controls. A similar conclusion was reached in another study that individuals with more severe depressive symptoms were more likely to induce an increase in IL-6 in laboratory stressors. As a result, patients who are in the midst of depression are exposed to stress again, and they may continue to experience severe and recurring inflammatory responses (Pace et al., 2006; Figure 4). This suggests that depression may enhance stress response systems by promoting hyperinflammation. These findings lead to a new understanding of the complex interplay between stress, depression, and immune disorders, and the possibility that combined treatment may promote recovery and reduce relapse risk when inflammation and depression occur simultaneously. Effective depression treatment can have profound effects on mood, inflammation, and health (Kaye et al., 2000).
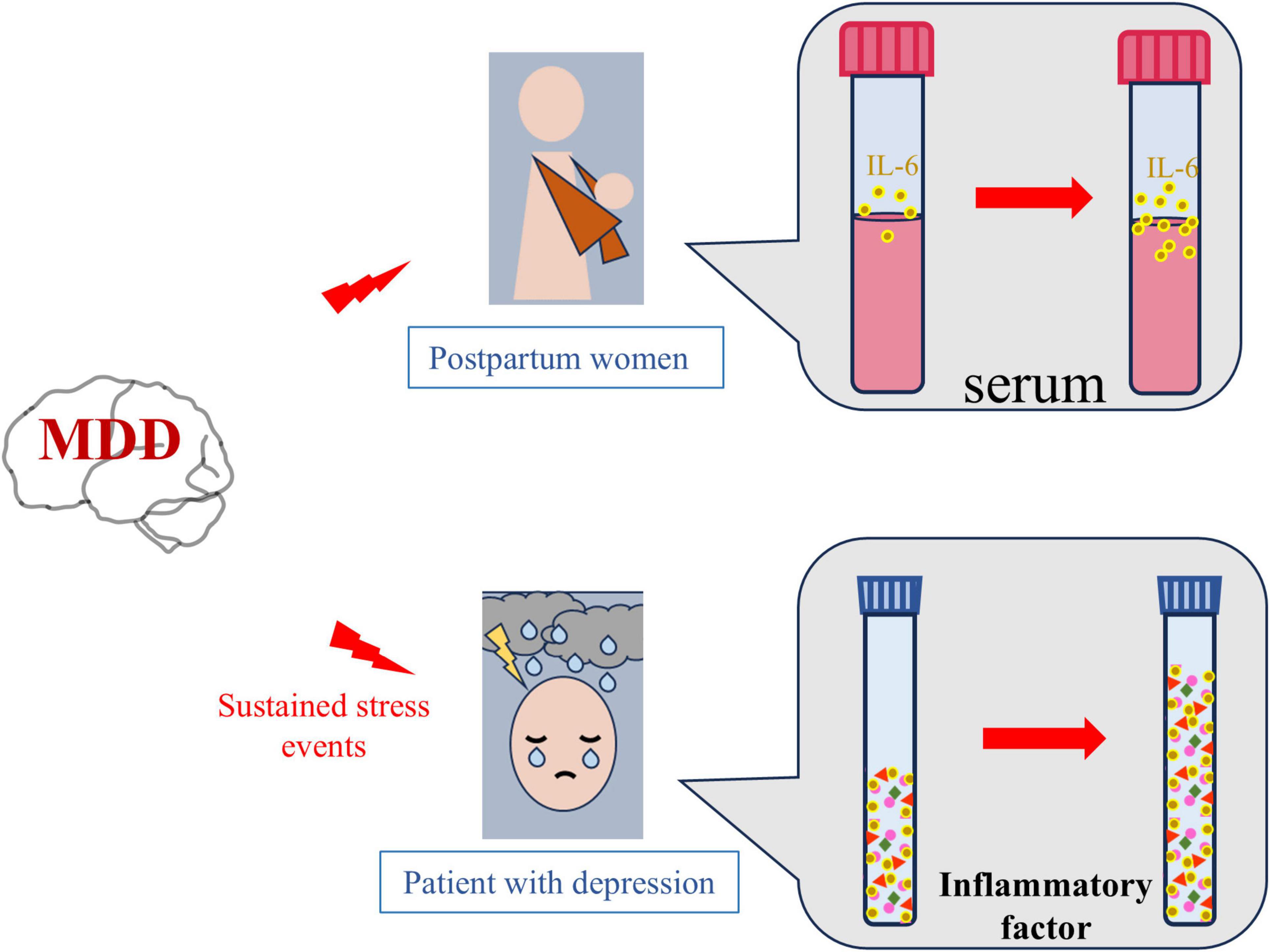
Figure 4. Depression increases neuroinflammation. Compared with healthy women, pregnant women with depression have higher serum levels of the inflammatory factor interleukin-6 (IL-6) after delivery. Similarly, continuous stress stimulation is more likely to lead to increased levels of inflammatory factors in patients with depression than in healthy people.
2.5 Conclusion and future perspectives
This review summarizes the relationship between long-term stress-induced neuroinflammation and the increased incidence of depression. Stress stimuli can activate the central immune system, triggering neuroinflammation, which ultimately leads to the emergence of depressive symptoms. Chronic neuroinflammation promotes the polarization of stress-induced microglia and astrocytes, stimulating the release of neurotoxic inflammatory mediators, which in turn induce symptoms such as anhedonia, memory loss, and insomnia. Furthermore, the occurrence of depression exacerbates neuroinflammation, leading to the production and release of more pro-inflammatory mediators. This review also discusses the specific mechanisms by which microglia and astrocytes modulate depression. Modulating the phenotype and function of these glial cells may provide effective strategies for the prevention and treatment of depression. Additionally, we highlight the potential of natural products with anti-inflammatory properties in improving depressive symptoms, underscoring their significant potential in the development of depression therapies. In the future, technologies such as single-cell RNA sequencing, PET, MRI, and CRISPR-Cas9 can be employed to explore the specific activation phenotypes and gene expression targets of microglia and astrocytes in depression, enabling real-time monitoring of the activation of these two glial cells. Furthermore, by targeting the specific polarization phenotypes and gene targets of microglia and astrocytes in depression, natural products that can modulate these phenotypes and act on multiple targets in combination may become a promising strategy for the prevention and treatment of depression.
Author contributions
KZ: Writing – original draft. YZ: Writing – original draft. SY: Visualization, Writing – original draft. LX: Visualization, Writing – original draft. SW: Visualization, Writing – original draft. JD: Visualization, Writing – original draft. HL: Visualization, Writing – original draft. HY: Writing – review and editing. WH: Writing – review and editing.
Funding
The author(s) declare that financial support was received for the research and/or publication of this article. This work was funded by First-Class Discipline Team of Kunming Medical University (2024XKTDPY11), and Yunnan Province Young Academic and Technical Leaders Project (202105AC160078, WH).
Acknowledgments
We would like to thank Yunnan Key Laboratory of Pharmacology for Natural Products, Kunming Medical University for the support.
Conflict of interest
The authors declare that the research was conducted in the absence of any commercial or financial relationships that could be construed as a potential conflict of interest.
Publisher’s note
All claims expressed in this article are solely those of the authors and do not necessarily represent those of their affiliated organizations, or those of the publisher, the editors and the reviewers. Any product that may be evaluated in this article, or claim that may be made by its manufacturer, is not guaranteed or endorsed by the publisher.
References
Abbott, N. J., Patabendige, A. A., Dolman, D. E., Yusof, S. R., and Begley, D. J. (2010). Structure and function of the blood-brain barrier. Neurobiol. Dis. 37, 13–25. doi: 10.1016/j.nbd.2009.07.030
Abd Elkader, H. A. E., Abdou, H. M., Khamiss, O. A., and Essawy, A. E. (2021). Anti-anxiety and antidepressant-like effects of astragaloside IV and saponins extracted from Astragalus spinosus against the bisphenol A-induced motor and cognitive impairments in a postnatal rat model of schizophrenia. Environ. Sci. Pollut. Res. Int. 28, 35171–35187. doi: 10.1007/s11356-021-12927-5
Alzarea, S., Khan, A., Ronan, P. J., Lutfy, K., and Rahman, S. (2024). The α-7 nicotinic receptor positive allosteric modulator alleviates lipopolysaccharide induced depressive-like behavior by regulating microglial function, trophic factor, and chloride transporters in mice. Brain Sci. 14:290. doi: 10.3390/brainsci14030290
Ara, S. K. (2022). Analysis of the prescribing pattern of antidepressants and the side effects in depression patients. J. Family Med. Prim. Care 11, 6640–6645. doi: 10.4103/jfmpc.jfmpc_1288_21
Arioz, B. I., Tastan, B., Tarakcioglu, E., Tufekci, K. U., Olcum, M., Ersoy, N., et al. (2019). Melatonin attenuates LPS-Induced acute depressive-like behaviors and microglial NLRP3 inflammasome activation through the SIRT1/Nrf2 pathway. Front. Immunol. 10:1511. doi: 10.3389/fimmu.2019.01511
Bai, S., Guo, W., Feng, Y., Deng, H., Li, G., Nie, H., et al. (2020). Efficacy and safety of anti-inflammatory agents for the treatment of major depressive disorder: A systematic review and meta-analysis of randomised controlled trials. J. Neurol. Neurosurg. Psychiatry 91, 21–32. doi: 10.1136/jnnp-2019-320912
Balistreri, C. R., and Monastero, R. (2023). Neuroinflammation and neurodegenerative diseases: How MUCH DO WE STILL NOT KNOW? Brain Sci. 14:19. doi: 10.3390/brainsci14010019
Baumeister, D., Russell, A., Pariante, C. M., and Mondelli, V. (2014). Inflammatory biomarker profiles of mental disorders and their relation to clinical, social and lifestyle factors. Soc. Psychiatry Psychiatr. Epidemiol. 49, 841–849. doi: 10.1007/s00127-014-0887-z
Baune, B. T. (2017). Are non-steroidal anti-inflammatory drugs clinically suitable for the treatment of symptoms in depression-associated inflammation? Curr. Top. Behav. Neurosci. 31, 303–319. doi: 10.1007/7854_2016_19
Berk, M., Williams, L. J., Jacka, F. N., O’Neil, A., Pasco, J. A., Moylan, S., et al. (2013). So depression is an inflammatory disease, but where does the inflammation come from? BMC Med. 11:200. doi: 10.1186/1741-7015-11-200
Bhatt, S., Dhar, A. K., Samanta, M. K., and Suttee, A. (2023). Effects of current psychotropic drugs on inflammation and immune system. Adv. Exp. Med. Biol. 1411, 407–434. doi: 10.1007/978-981-19-7376-5_18
Boche, D., Perry, V. H., and Nicoll, J. A. (2013). Review: Activation patterns of microglia and their identification in the human brain. Neuropathol. Appl. Neurobiol. 39, 3–18. doi: 10.1111/nan.12011
Butovsky, O., Jedrychowski, M. P., Moore, C. S., Cialic, R., Lanser, A. J., Gabriely, G., et al. (2014). Identification of a unique TGF-β-dependent molecular and functional signature in microglia. Nat. Neurosci. 17, 131–143. doi: 10.1038/nn.3599
Cheng, Y., Pardo, M., Armini, R. S., Martinez, A., Mouhsine, H., Zagury, J. F., et al. (2016). Stress-induced neuroinflammation is mediated by GSK3-dependent TLR4 signaling that promotes susceptibility to depression-like behavior. Brain Behav. Immun. 53, 207–222. doi: 10.1016/j.bbi.2015.12.012
Ciani, M., Rigillo, G., Benatti, C., Pani, L., Blom, J. M. C., Brunello, N., et al. (2025). Time- and region-specific effect of vortioxetine on central LPS-induced transcriptional regulation of NLRP3 inflammasome. Curr. Neuropharmacol. 23, 196–208. doi: 10.2174/1570159x22666240705143649
Clayton, A. H., Suthoff, E., Jain, R., Kosinski, M., Fridman, M., Deligiannidis, K. M., et al. (2024). The magnitude and sustainability of treatment benefit of zuranolone on function and well-being as assessed by the SF-36 in adult patients with MDD and PPD: An integrated analysis of 4 randomized clinical trials. J. Affect. Disord. 351, 904–914. doi: 10.1016/j.jad.2024.01.268
Colombo, E., and Farina, C. (2016). Astrocytes: Key regulators of neuroinflammation. Trends Immunol. 37, 608–620. doi: 10.1016/j.it.2016.06.006
Cong, T., Sun, Y., Zhou, Y., Wu, H., Li, L., Chu, Z., et al. (2023). Blocking two-pore domain potassium channel TREK-1 inhibits the activation of A1-like reactive astrocyte through the NF-κB Signaling pathway in a rat model of major depressive disorder. Neurochem. Res. 48, 1737–1754. doi: 10.1007/s11064-023-03857-4
Cui, L., Li, S., Wang, S., Wu, X., Liu, Y., Yu, W., et al. (2024). Major depressive disorder: Hypothesis, mechanism, prevention and treatment. Signal. Transduct. Target Ther. 9:30. doi: 10.1038/s41392-024-01738-y
Culmsee, C., Michels, S., Scheu, S., Arolt, V., Dannlowski, U., and Alferink, J. (2018). Mitochondria, microglia, and the immune system-how are they linked in affective disorders? Front. Psychiatry 9:739. doi: 10.3389/fpsyt.2018.00739
Dai, W., Feng, K., Sun, X., Xu, L., Wu, S., Rahmand, K., et al. (2022). Natural products for the treatment of stress-induced depression: Pharmacology, mechanism and traditional use. J. Ethnopharmacol. 285:114692. doi: 10.1016/j.jep.2021.114692
Dantzer, R., O’Connor, J. C., Freund, G. G., Johnson, R. W., and Kelley, K. W. (2008). From inflammation to sickness and depression: When the immune system subjugates the brain. Nat. Rev. Neurosci. 9, 46–56. doi: 10.1038/nrn2297
Deng, S. L., Chen, J. G., and Wang, F. (2020). Microglia: A central player in depression. Curr. Med. Sci. 40, 391–400. doi: 10.1007/s11596-020-2193-1
DiSabato, D. J., Quan, N., and Godbout, J. P. (2016). Neuroinflammation: The devil is in the details. J. Neurochem. 139, 136–153. doi: 10.1111/jnc.13607
Du Preez, A., Onorato, D., Eiben, I., Musaelyan, K., Egeland, M., Zunszain, P. A., et al. (2021). Chronic stress followed by social isolation promotes depressive-like behaviour, alters microglial and astrocyte biology and reduces hippocampal neurogenesis in male mice. Brain Behav. Immun. 91, 24–47. doi: 10.1016/j.bbi.2020.07.015
Duan, N., Zhang, Y., Tan, S., Sun, J., Ye, M., Gao, H., et al. (2022). Therapeutic targeting of STING-TBK1-IRF3 signalling ameliorates chronic stress induced depression-like behaviours by modulating neuroinflammation and microglia phagocytosis. Neurobiol. Dis. 169:105739. doi: 10.1016/j.nbd.2022.105739
Eyre, H. A., Air, T., Proctor, S., Rositano, S., and Baune, B. T. (2015). A critical review of the efficacy of non-steroidal anti-inflammatory drugs in depression. Prog Neuropsychopharmacol Biol. Psychiatry 57, 11–16. doi: 10.1016/j.pnpbp.2014.10.003
Eyre, H., and Baune, B. T. (2012). Neuroplastic changes in depression: A role for the immune system. Psychoneuroendocrinology 37, 1397–1416. doi: 10.1016/j.psyneuen.2012.03.019
Fagundes, C. P., Glaser, R., Hwang, B. S., Malarkey, W. B., and Kiecolt-Glaser, J. K. (2013). Depressive symptoms enhance stress-induced inflammatory responses. Brain Behav. Immun. 31, 172–176. doi: 10.1016/j.bbi.2012.05.006
Fan, J., Guo, F., Mo, R., Chen, L. Y., Mo, J. W., Lu, C. L., et al. (2023). O-GlcNAc transferase in astrocytes modulates depression-related stress susceptibility through glutamatergic synaptic transmission. J. Clin. Invest. 133:e160016. doi: 10.1172/jci160016
Fu, A., Qiao, F., Feng, H., and Luo, Q. (2023). Inhibition of TREM-1 ameliorates Lipopolysaccharide-induced depressive-like behaviors by alleviating neuroinflammation in the PFC via PI3K/Akt signaling pathway. Behav. Brain Res. 449:114464. doi: 10.1016/j.bbr.2023.114464
Gao, R., Ali, T., Liu, Z., Li, A., He, K., Yang, C., et al. (2024). NMDAR (2C) deletion in astrocytes relieved LPS-induced neuroinflammation and depression. Int. Immunopharmacol. 132:111964. doi: 10.1016/j.intimp.2024.111964
Ge, T., Yao, X., Zhao, H., Yang, W., Zou, X., Peng, F., et al. (2021). Gut microbiota and neuropsychiatric disorders: Implications for neuroendocrine-immune regulation. Pharmacol. Res. 173:105909. doi: 10.1016/j.phrs.2021.105909
Geng, M., Shao, Q., Fu, J., Gu, J., Feng, L., Zhao, L., et al. (2024). Down-regulation of MKP-1 in hippocampus protects against stress-induced depression-like behaviors and neuroinflammation. Transl. Psychiatry 14:130. doi: 10.1038/s41398-024-02846-7
Glaser, R., Robles, T. F., Sheridan, J., Malarkey, W. B., and Kiecolt-Glaser, J. K. (2003). Mild depressive symptoms are associated with amplified and prolonged inflammatory responses after influenza virus vaccination in older adults. Arch. Gen. Psychiatry 60, 1009–1014. doi: 10.1001/archpsyc.60.10.1009
Goshi, N., Morgan, R. K., Lein, P. J., and Seker, E. (2020). A primary neural cell culture model to study neuron, astrocyte, and microglia interactions in neuroinflammation. J. Neuroinflammation 17:155. doi: 10.1186/s12974-020-01819-z
Guo, F., Fan, J., Liu, J. M., Kong, P. L., Ren, J., Mo, J. W., et al. (2024). Astrocytic ALKBH5 in stress response contributes to depressive-like behaviors in mice. Nat. Commun. 15:4347. doi: 10.1038/s41467-024-48730-2
Guo, L. T., Wang, S. Q., Su, J., Xu, L. X., Ji, Z. Y., Zhang, R. Y., et al. (2019). Baicalin ameliorates neuroinflammation-induced depressive-like behavior through inhibition of toll-like receptor 4 expression via the PI3K/AKT/FoxO1 pathway. J. Neuroinflammation 16:95. doi: 10.1186/s12974-019-1474-8
Hammond, T. R., Dufort, C., Dissing-Olesen, L., Giera, S., Young, A., Wysoker, A., et al. (2019). Single-Cell RNA sequencing of microglia throughout the mouse lifespan and in the injured brain reveals complex cell-state changes. Immunity 50, 253–271.e6. doi: 10.1016/j.immuni.2018.11.004
Han, C., Pei, H., Sheng, Y., Wang, J., Zhou, X., Li, W., et al. (2024). HIPK2 mediates M1 polarization of microglial cells via STAT3: A new mechanism of depression-related neuroinflammation. J. Cell. Physiol. 239:e30994. doi: 10.1002/jcp.30994
He, L., Zheng, Y., Huang, L., Ye, J., Ye, Y., Luo, H., et al. (2022). Nrf2 regulates the arginase 1(+) microglia phenotype through the initiation of TREM2 transcription, ameliorating depression-like behavior in mice. Transl. Psychiatry 12:459. doi: 10.1038/s41398-022-02227-y
He, Y., Wang, Y., Yu, H., Tian, Y., Chen, X., Chen, C., et al. (2023). Protective effect of Nr4a2 (Nurr1) against LPS-induced depressive-like behaviors via regulating activity of microglia and CamkII neurons in anterior cingulate cortex. Pharmacol. Res. 191:106717. doi: 10.1016/j.phrs.2023.106717
Heneka, M. T., McManus, R. M., and Latz, E. (2018). Inflammasome signalling in brain function and neurodegenerative disease. Nat. Rev. Neurosci. 19, 610–621. doi: 10.1038/s41583-018-0055-7
Hengartner, M. P. (2020). How effective are antidepressants for depression over the long term? A critical review of relapse prevention trials and the issue of withdrawal confounding. Ther. Adv. Psychopharmacol. 10:2045125320921694. doi: 10.1177/2045125320921694
Henrik Heiland, D., Ravi, V. M., Behringer, S. P., Frenking, J. H., Wurm, J., Joseph, K., et al. (2019). Tumor-associated reactive astrocytes aid the evolution of immunosuppressive environment in glioblastoma. Nat. Commun. 10:2541. doi: 10.1038/s41467-019-10493-6
Hu, K., Chen, H., Gao, Y., Hua, R., Shi, X., Li, L., et al. (2023). Astrocytic SIRT6 is a potential anti-depression and anti-anxiety target. Prog. Neuropsychopharmacol. Biol. Psychiatry 123:110702. doi: 10.1016/j.pnpbp.2022.110702
Hurley, L. L., and Tizabi, Y. (2013). Neuroinflammation, neurodegeneration, and depression. Neurotox Res. 23, 131–144. doi: 10.1007/s12640-012-9348-1
Jesulola, E., Micalos, P., and Baguley, I. J. (2018). Understanding the pathophysiology of depression: From monoamines to the neurogenesis hypothesis model - are we there yet? Behav. Brain Res. 341, 79–90. doi: 10.1016/j.bbr.2017.12.025
Jha, M. K., Jo, M., Kim, J. H., and Suk, K. (2019). Microglia-astrocyte crosstalk: An intimate molecular conversation. Neuroscientist 25, 227–240. doi: 10.1177/1073858418783959
Jia, X., Gao, Z., and Hu, H. (2021). Microglia in depression: Current perspectives. Sci. China Life Sci. 64, 911–925. doi: 10.1007/s11427-020-1815-6
Jiang, N., Lv, J., Wang, H., Huang, H., Wang, Q., Lu, C., et al. (2020). Ginsenoside Rg1 ameliorates chronic social defeat stress-induced depressive-like behaviors and hippocampal neuroinflammation. Life Sci. 252:117669. doi: 10.1016/j.lfs.2020.117669
Kaye, J., Morton, J., Bowcutt, M., and Maupin, D. (2000). Stress, depression, and psychoneuroimmunology. J. Neurosci. Nurs. 32, 93–100. doi: 10.1097/01376517-200004000-00005
Kessler, R. C. (1997). The effects of stressful life events on depression. Annu. Rev. Psychol. 48, 191–214. doi: 10.1146/annurev.psych.48.1.191
Kiecolt-Glaser, J. K., Derry, H. M., and Fagundes, C. P. (2015). Inflammation: Depression fans the flames and feasts on the heat. Am. J. Psychiatry 172, 1075–1091. doi: 10.1176/appi.ajp.2015.15020152
Kim, S., and Son, Y. (2021). Astrocytes stimulate microglial proliferation and M2 polarization in vitro through crosstalk between astrocytes and microglia. Int. J. Mol. Sci. 22:8800. doi: 10.3390/ijms22168800
Kölliker-Frers, R., Udovin, L., Otero-Losada, M., Kobiec, T., Herrera, M. I., Palacios, J., et al. (2021). Neuroinflammation: An integrating overview of reactive-neuroimmune cell interactions in health and disease. Mediators Inflamm. 2021:9999146. doi: 10.1155/2021/9999146
Kreisel, T., Frank, M. G., Licht, T., Reshef, R., Ben-Menachem-Zidon, O., Baratta, M. V., et al. (2014). Dynamic microglial alterations underlie stress-induced depressive-like behavior and suppressed neurogenesis. Mol. Psychiatry 19, 699–709. doi: 10.1038/mp.2013.155
Lee, E. H., Park, J. Y., Kwon, H. J., and Han, P. L. (2021). Repeated exposure with short-term behavioral stress resolves pre-existing stress-induced depressive-like behavior in mice. Nat. Commun. 12:6682. doi: 10.1038/s41467-021-26968-4
Leng, L., Zhuang, K., Liu, Z., Huang, C., Gao, Y., Chen, G., et al. (2018). Menin deficiency leads to depressive-like behaviors in mice by modulating astrocyte-mediated neuroinflammation. Neuron 100, 551–563.e7. doi: 10.1016/j.neuron.2018.08.031
Li, F., Jiang, S. Y., Tian, T., Li, W. J., Xue, Y., Du, R. H., et al. (2022). Kir6.1/K-ATP channel in astrocytes is an essential negative modulator of astrocytic pyroptosis in mouse model of depression. Theranostics 12, 6611–6625. doi: 10.7150/thno.77455
Li, J. M., Hu, T., Jiang, C. L., and Wang, W. (2022). Pinocembrin ameliorates depressive-like behaviors by regulating P2X7/TRL4 receptors expression in mouse hippocampus. Behav. Pharmacol. 33, 301–308. doi: 10.1097/fbp.0000000000000677
Li, S., Fang, Y., Zhang, Y., Song, M., Zhang, X., Ding, X., et al. (2022). Microglial NLRP3 inflammasome activates neurotoxic astrocytes in depression-like mice. Cell Rep. 41:111532. doi: 10.1016/j.celrep.2022.111532
Li, W., Ali, T., Zheng, C., He, K., Liu, Z., Shah, F. A., et al. (2022). Anti-depressive-like behaviors of APN KO mice involve Trkb/BDNF signaling related neuroinflammatory changes. Mol. Psychiatry 27, 1047–1058. doi: 10.1038/s41380-021-01327-3
Li, X., and Zhang, C. (2020). Comparative efficacy of nine antidepressants in treating Chinese patients with post-stroke depression: A network meta-analysis. J. Affect. Disord. 266, 540–548. doi: 10.1016/j.jad.2020.02.005
Li, Y., Zhan, B., Zhuang, X., Zhao, M., Chen, X., Wang, Q., et al. (2024). Microglial Pdcd4 deficiency mitigates neuroinflammation-associated depression via facilitating Daxx mediated PPARγ/IL-10 signaling. J. Neuroinflammation 21:143. doi: 10.1186/s12974-024-03142-3
Liang, X., Zhao, Y., Xu, T., Wang, W., Sun, W., and Wang, R. (2023). Catalpol alleviates depression by inhibiting NLRP3 inflammasome via TLR4/MAPK/NF-Kb Pathway. Iran J. Public Health 52, 722–731. doi: 10.18502/ijph.v52i4.12440
Linnerbauer, M., Wheeler, M. A., and Quintana, F. J. (2020). Astrocyte crosstalk in CNS inflammation. Neuron 108, 608–622. doi: 10.1016/j.neuron.2020.08.012
Liu, B., Zhang, Y., Yang, Z., Liu, M., Zhang, C., Zhao, Y., et al. (2021). ω-3 DPA protected neurons from neuroinflammation by balancing microglia M1/M2 polarizations through inhibiting NF-κB/MAPK p38 signaling and activating neuron-BDNF-PI3K/AKT pathways. Mar. Drugs 19:587. doi: 10.3390/md19110587
Liu, H., Liu, L. L., Chen, J., Chen, Y. W., Chai, Y., Liu, Q. S., et al. (2022). Muscone with attenuation of neuroinflammation and oxidative stress exerts antidepressant-like effect in mouse model of chronic restraint stress. Oxid. Med. Cell. Longev. 2022:3322535. doi: 10.1155/2022/3322535
Liu, X., Jiang, N., and Zhou, W. (2023). Various energetic metabolism of microglia in response to different stimulations. Molecules 28:4501. doi: 10.3390/molecules28114501
Liu, X., Tang, S. S., Liu, S. M., Zeng, J., Chen, Z. G., Liu, C. H., et al. (2022). Deficiency of astrocyte CysLT(1)R ameliorates depression-like behaviors in mice by modulating glutamate synaptic transmission. Neurobiol. Dis. 175:105922. doi: 10.1016/j.nbd.2022.105922
Maes, M., Ombelet, W., De Jongh, R., Kenis, G., and Bosmans, E. (2001). The inflammatory response following delivery is amplified in women who previously suffered from major depression, suggesting that major depression is accompanied by a sensitization of the inflammatory response system. J. Affect. Disord. 63, 85–92. doi: 10.1016/s0165-0327(00)00156-7
Mahmoud, S., Gharagozloo, M., Simard, C., and Gris, D. (2019). Astrocytes maintain glutamate homeostasis in the CNS by controlling the balance between glutamate uptake and release. Cells 8:184. doi: 10.3390/cells8020184
Malhi, G. S., and Mann, J. J. (2018). Depression. Lancet 392, 2299–2312. doi: 10.1016/s0140-6736(18)31948-2
Mao, L., You, J., Xie, M., Hu, Y., and Zhou, Q. (2024). Arginine methylation of β-Catenin induced by PRMT2 aggravates LPS-induced cognitive dysfunction and depression-like behaviors by promoting ferroptosis. Mol. Neurobiol. 61, 7796–7813. doi: 10.1007/s12035-024-04019-5
Marwaha, S., Palmer, E., Suppes, T., Cons, E., Young, A. H., and Upthegrove, R. (2023). Novel and emerging treatments for major depression. Lancet 401, 141–153. doi: 10.1016/s0140-6736(22)02080-3
McWhirt, J., Sathyanesan, M., Sampath, D., and Newton, S. S. (2019). Effects of restraint stress on the regulation of hippocampal glutamate receptor and inflammation genes in female C57BL/6 and BALB/c mice. Neurobiol. Stress 10:100169. doi: 10.1016/j.ynstr.2019.100169
Ménard, C., Hodes, G. E., and Russo, S. J. (2016). Pathogenesis of depression: Insights from human and rodent studies. Neuroscience 321, 138–162. doi: 10.1016/j.neuroscience.2015.05.053
Meng, Z., Guo, S., Dong, X., Wang, Q., Hu, D., Liu, X., et al. (2023). Astrocyte-ablation of Mtnr1b increases anxiety-like behavior in adult male mice. J. Integr. Neurosci. 22:154. doi: 10.31083/j.jin2206154
Mo, J. W., Kong, P. L., Ding, L., Fan, J., Ren, J., Lu, C. L., et al. (2024). Lysosomal TFEB-TRPML1 Axis in astrocytes modulates depressive-like behaviors. Adv. Sci. 11:e2403389. doi: 10.1002/advs.202403389
Munhoz, C. D., García-Bueno, B., Madrigal, J. L., Lepsch, L. B., Scavone, C., and Leza, J. C. (2008). Stress-induced neuroinflammation: Mechanisms and new pharmacological targets. Braz. J. Med. Biol. Res. 41, 1037–1046. doi: 10.1590/s0100-879x2008001200001
Na, K. S., Jung, H. Y., and Kim, Y. K. (2014). The role of pro-inflammatory cytokines in the neuroinflammation and neurogenesis of schizophrenia. Prog Neuropsychopharmacol Biol. Psychiatry 48, 277–286. doi: 10.1016/j.pnpbp.2012.10.022
Nestler, E. J., Barrot, M., DiLeone, R. J., Eisch, A. J., Gold, S. J., and Monteggia, L. M. (2002). Neurobiology of depression. Neuron 34, 13–25. doi: 10.1016/s0896-6273(02)00653-0
Nettis, M. A., and Pariante, C. M. (2020). Is there neuroinflammation in depression? Understanding the link between the brain and the peripheral immune system in depression. Int. Rev. Neurobiol. 152, 23–40. doi: 10.1016/bs.irn.2019.12.004
Noori, T., Sureda, A., Sobarzo-Sánchez, E., and Shirooie, S. (2022). The role of natural products in treatment of depressive disorder. Curr. Neuropharmacol. 20, 929–949. doi: 10.2174/1570159x20666220103140834
Novakovic, M. M., Korshunov, K. S., Grant, R. A., Martin, M. E., Valencia, H. A., Budinger, G. R. S., et al. (2023). Astrocyte reactivity and inflammation-induced depression-like behaviors are regulated by Orai1 calcium channels. Nat. Commun. 14:5500. doi: 10.1038/s41467-023-40968-6
Olude, M. A., Mouihate, A., Mustapha, O. A., Farina, C., Quintana, F. J., and Olopade, J. O. (2022). Astrocytes and Microglia in Stress-Induced Neuroinflammation: The African Perspective. Front. Immunol. 13:795089. doi: 10.3389/fimmu.2022.795089
Otte, C., Gold, S. M., Penninx, B. W., Pariante, C. M., Etkin, A., Fava, M., et al. (2016). Major depressive disorder. Nat. Rev. Dis. Primers 2:16065. doi: 10.1038/nrdp.2016.65
Pace, T. W., Mletzko, T. C., Alagbe, O., Musselman, D. L., Nemeroff, C. B., Miller, A. H., et al. (2006). Increased stress-induced inflammatory responses in male patients with major depression and increased early life stress. Am. J. Psychiatry 163, 1630–1633. doi: 10.1176/ajp.2006.163.9.1630
Parkhurst, C. N., Yang, G., Ninan, I., Savas, J. N., Yates, J. R., Lafaille, J. J., et al. (2013). Microglia promote learning-dependent synapse formation through brain-derived neurotrophic factor. Cell 155, 1596–1609. doi: 10.1016/j.cell.2013.11.030
Parsi, S., Zhu, C., Motlagh, N. J., Kim, D., Küllenberg, E. G., Kim, H. H., et al. (2024). Basic science of neuroinflammation and involvement of the inflammatory response in disorders of the nervous system. Magn. Reson. Imaging Clin. N. A. 32, 375–384. doi: 10.1016/j.mric.2024.01.003
Patil, C. R., Suryakant Gawli, C., and Bhatt, S. (2023). Targeting inflammatory pathways for treatment of the major depressive disorder. Drug Discov. Today 28:103697. doi: 10.1016/j.drudis.2023.103697
Pinto, E. F., and Andrade, C. (2016). Interferon-related depression: A primer on mechanisms, treatment, and prevention of a common clinical problem. Curr. Neuropharmacol. 14, 743–748. doi: 10.2174/1570159x14666160106155129
Rajkowska, G., and Stockmeier, C. A. (2013). Astrocyte pathology in major depressive disorder: Insights from human postmortem brain tissue. Curr. Drug Targets 14, 1225–1236. doi: 10.2174/13894501113149990156
Rohleder, N., and Miller, G. E. (2008). Acute deviations from long-term trait depressive symptoms predict systemic inflammatory activity. Brain Behav. Immun. 22, 709–716. doi: 10.1016/j.bbi.2007.10.012
Rupareliya, V. P., Singh, A. A., Butt, A. M., and Kumar, H. (2023). The “molecular soldiers” of the CNS: Astrocytes, a comprehensive review on their roles and molecular signatures. Eur. J. Pharmacol. 959:176048. doi: 10.1016/j.ejphar.2023.176048
Şahin, T. D., Karson, A., Balcı, F., Yazır, Y., Bayramgürler, D., and Utkan, T. (2015). TNF-alpha inhibition prevents cognitive decline and maintains hippocampal BDNF levels in the unpredictable chronic mild stress rat model of depression. Behav. Brain Res. 292, 233–240. doi: 10.1016/j.bbr.2015.05.062
Sarno, E., Moeser, A. J., and Robison, A. J. (2021). Neuroimmunology of depression. Adv. Pharmacol. 91, 259–292. doi: 10.1016/bs.apha.2021.03.004
Serna-Rodríguez, M. F., Bernal-Vega, S., de la Barquera, J. A. O., Camacho-Morales, A., and Pérez-Maya, A. A. (2022). The role of damage associated molecular pattern molecules (DAMPs) and permeability of the blood-brain barrier in depression and neuroinflammation. J. Neuroimmunol. 371:577951. doi: 10.1016/j.jneuroim.2022.577951
Shi, L., Xia, Z., Guo, J., Wang, L., Peng, Z., Qiu, D., et al. (2023). Maresin-1 improves LPS-induced depressive-like behavior by inhibiting hippocampal microglial activation. J. Affect. Disord. 328, 261–272. doi: 10.1016/j.jad.2023.02.016
Slavich, G. M., and Irwin, M. R. (2014). From stress to inflammation and major depressive disorder: A social signal transduction theory of depression. Psychol. Bull. 140, 774–815. doi: 10.1037/a0035302
Song, M. T., Ruan, J., Zhang, R. Y., Deng, J., Ma, Z. Q., and Ma, S. P. (2018). Astragaloside IV ameliorates neuroinflammation-induced depressive-like behaviors in mice via the PPARγ/NF-κB/NLRP3 inflammasome axis. Acta Pharmacol. Sin. 39, 1559–1570. doi: 10.1038/aps.2017.208
Stetler, C., and Miller, G. E. (2011). Depression and hypothalamic-pituitary-adrenal activation: A quantitative summary of four decades of research. Psychosom. Med. 73, 114–126. doi: 10.1097/PSY.0b013e31820ad12b
Stoklund Dittlau, K., and Freude, K. (2024). Astrocytes: The stars in neurodegeneration? Biomolecules 14:289. doi: 10.3390/biom14030289
Su, W. J., Zhang, Y., Chen, Y., Gong, H., Lian, Y. J., Peng, W., et al. (2017). NLRP3 gene knockout blocks NF-κB and MAPK signaling pathway in CUMS-induced depression mouse model. Behav. Brain Res. 322, 1–8. doi: 10.1016/j.bbr.2017.01.018
Sun, C., Shen, Y., Liu, P., Shen, Y., Hu, Y., Li, P., et al. (2023). NLRC5 deficiency reduces LPS-induced microglial activation via inhibition of NF-κB signaling and ameliorates Mice’s depressive-like behavior. Int. J. Mol. Sci. 24:13265. doi: 10.3390/ijms241713265
Takahashi, K., Tsuji, M., Nakagawasai, O., Katsuyama, S., Miyagawa, K., Kurokawa, K., et al. (2024). Polarization to M1-type microglia in the hippocampus is involved in depression-like behavior in a mouse model of olfactory dysfunction. Neurochem. Int. 175:105723. doi: 10.1016/j.neuint.2024.105723
Troubat, R., Barone, P., Leman, S., Desmidt, T., Cressant, A., Atanasova, B., et al. (2021). Neuroinflammation and depression: A review. Eur. J. Neurosci. 53, 151–171. doi: 10.1111/ejn.14720
Vignau, J., Karila, L., Costisella, O., and Canva, V. (2005). [Hepatitis C, interferon a and depression: Main physiopathologic hypothesis]. Encephale 31, 349–357. doi: 10.1016/s0013-7006(05)82400-5
Wang, H., He, Y., Sun, Z., Ren, S., Liu, M., Wang, G., et al. (2022). Microglia in depression: An overview of microglia in the pathogenesis and treatment of depression. J. Neuroinflammation 19:132. doi: 10.1186/s12974-022-02492-0
Wang, J. Y., Ren, P., Cui, L. Y., Duan, J. Y., Chen, H. L., Zeng, Z. R., et al. (2024). Astrocyte-specific activation of sigma-1 receptors in mPFC mediates the faster onset antidepressant effect by inhibiting NF-κB-induced neuroinflammation. Brain Behav. Immun. 120, 256–274. doi: 10.1016/j.bbi.2024.06.008
Wang, N., Yu, H. Y., Shen, X. F., Gao, Z. Q., Yang, C., Yang, J. J., et al. (2015). The rapid antidepressant effect of ketamine in rats is associated with down-regulation of pro-inflammatory cytokines in the hippocampus. Ups J. Med. Sci. 120, 241–248. doi: 10.3109/03009734.2015.1060281
Wang, Q., Jie, W., Liu, J. H., Yang, J. M., and Gao, T. M. (2017). An astroglial basis of major depressive disorder? An overview. Glia 65, 1227–1250. doi: 10.1002/glia.23143
Wang, R., Ji, L., Yuan, S., Liu, X., Liang, Z., Chen, W., et al. (2024). Microglial forkhead box O3a deficiency attenuates LPS-induced neuro-inflammation and depressive-like behaviour through regulating the expression of peroxisome proliferator-activated receptor-γ. Br. J. Pharmacol. 181, 3908–3925. doi: 10.1111/bph.16474
Wang, W., Wang, L., Wang, L., Li, Y., Lan, T., Wang, C., et al. (2023). Ginsenoside-Rg1 synergized with voluntary running exercise protects against glial activation and dysregulation of neuronal plasticity in depression. Food Funct. 14, 7222–7239. doi: 10.1039/d3fo00496a
Wang, W., Zheng, L., Xu, L., Tu, J., and Gu, X. (2020). Pinocembrin mitigates depressive-like behaviors induced by chronic unpredictable mild stress through ameliorating neuroinflammation and apoptosis. Mol. Med. 26:53. doi: 10.1186/s10020-020-00179-x
Wang, Y. L., Wu, H. R., Zhang, S. S., Xiao, H. L., Yu, J., Ma, Y. Y., et al. (2021). Catalpol ameliorates depressive-like behaviors in CUMS mice via oxidative stress-mediated NLRP3 inflammasome and neuroinflammation. Transl. Psychiatry 11:353. doi: 10.1038/s41398-021-01468-7
Wang, Y., Ruan, W., Mi, J., Xu, J., Wang, H., Cao, Z., et al. (2018). Balasubramide derivative 3C modulates microglia activation via CaMKKβ-dependent AMPK/PGC-1α pathway in neuroinflammatory conditions. Brain Behav. Immun. 67, 101–117. doi: 10.1016/j.bbi.2017.08.006
Wen, G., Zhan, X., Xu, X., Xia, X., Jiang, S., Ren, X., et al. (2024). Ketamine improves the Glymphatic pathway by reducing the pyroptosis of hippocampal astrocytes in the chronic unpredictable mild stress model. Mol. Neurobiol. 61, 2049–2062. doi: 10.1007/s12035-023-03669-1
Wohleb, E. S., Franklin, T., Iwata, M., and Duman, R. S. (2016). Integrating neuroimmune systems in the neurobiology of depression. Nat. Rev. Neurosci. 17, 497–511. doi: 10.1038/nrn.2016.69
Wu, A. G., Zhou, X. G., Qiao, G., Yu, L., Tang, Y., Yan, L., et al. (2021). Targeting microglial autophagic degradation in NLRP3 inflammasome-mediated neurodegenerative diseases. Ageing Res. Rev. 65:101202. doi: 10.1016/j.arr.2020.101202
Wu, H., Bao, H., Liu, C., Zhang, Q., Huang, A., Quan, M., et al. (2022). Extracellular nucleosomes accelerate microglial inflammation via C-Type lectin receptor 2D and Toll-Like Receptor 9 in mPFC of mice with chronic stress. Front. Immunol. 13:854202. doi: 10.3389/fimmu.2022.854202
Wu, X., Li, L., Zhou, B., Wang, J., and Shao, W. (2023). Connexin 43 regulates astrocyte dysfunction and cognitive deficits in early life stress-treated mice. Exp. Brain Res. 241, 1207–1214. doi: 10.1007/s00221-023-06587-9
Xiao, X., Zhang, H., Ning, W., Yang, Z., Wang, Y., and Zhang, T. (2022). Knockdown of FSTL1 inhibits microglia activation and alleviates depressive-like symptoms through modulating TLR4/MyD88/NF-κB pathway in CUMS mice. Exp. Neurol. 353:114060. doi: 10.1016/j.expneurol.2022.114060
Xu, D. D., Hou, Z. Q., Xu, Y. Y., Liang, J., Gao, Y. J., Zhang, C., et al. (2024). Potential role of Bmal1 in Lipopolysaccharide-Induced Depression-Like Behavior And Its Associated “Inflammatory Storm”. J. Neuroimmune Pharmacol. 19:4. doi: 10.1007/s11481-024-10103-3
Xu, K., Wang, M., Wang, H., Zhao, S., Tu, D., Gong, X., et al. (2024). HMGB1/STAT3/p65 axis drives microglial activation and autophagy exert a crucial role in chronic Stress-Induced major depressive disorder. J. Adv. Res. 59, 79–96. doi: 10.1016/j.jare.2023.06.003
Xu, X., Piao, H. N., Aosai, F., Zeng, X. Y., Cheng, J. H., Cui, Y. X., et al. (2020). Arctigenin protects against depression by inhibiting microglial activation and neuroinflammation via HMGB1/TLR4/NF-κB and TNF-α/TNFR1/NF-κB pathways. Br. J. Pharmacol. 177, 5224–5245. doi: 10.1111/bph.15261
Yao, W., Cao, Q., Luo, S., He, L., Yang, C., Chen, J., et al. (2022). Microglial ERK-NRBP1-CREB-BDNF signaling in sustained antidepressant actions of (R)-ketamine. Mol. Psychiatry 27, 1618–1629. doi: 10.1038/s41380-021-01377-7
Yaribeygi, H., Panahi, Y., Sahraei, H., Johnston, T. P., and Sahebkar, A. (2017). The impact of stress on body function: A review. Excli J. 16, 1057–1072. doi: 10.17179/excli2017-480
Ye, Q., Lin, S. S., Ulrich, H., and Tang, Y. (2023). Decoupling SERT-nNOS interaction to generate fast-onset antidepressants. Neurosci. Bull. 39, 1327–1329. doi: 10.1007/s12264-023-01049-2
Ye, Y., Liang, J., Xu, C., Liu, Y., Chen, J., and Zhu, Y. (2024). Inhibition of HMOX1 by MAFG potentiates the development of depression-like behavior in mice associated with astrocyte-mediated neuroinflammation. Brain Res. 1843:149115. doi: 10.1016/j.brainres.2024.149115
Yi, S., Jiang, X., Tang, X., Li, Y., Xiao, C., Zhang, J., et al. (2020). IL-4 and IL-10 promotes phagocytic activity of microglia by up-regulation of TREM2. Cytotechnology 72, 589–602. doi: 10.1007/s10616-020-00409-4
Yirmiya, R., Rimmerman, N., and Reshef, R. (2015). Depression as a microglial disease. Trends Neurosci. 38, 637–658. doi: 10.1016/j.tins.2015.08.001
Yuan, Q., Lei, Y., Yu, K., Wu, J., Xu, Z., Wen, C., et al. (2024). Repetitive transcranial magnetic stimulation and fluoxetine attenuate astroglial activation and benefit behaviours in a chronic unpredictable mild stress mouse model of depression. World J. Biol. Psychiatry 25, 82–94. doi: 10.1080/15622975.2023.2279958
Zeng, Y., Li, W., Chen, X., You, Z., Mai, S., Lan, X., et al. (2024). Mediating effect of inflammation on the relationship between sleep disruption and suicidal ideation in major depressive disorder. J. Affect. Disord. 352, 371–378. doi: 10.1016/j.jad.2024.02.078
Zhang, J. C., Yao, W., Dong, C., Yang, C., Ren, Q., Ma, M., et al. (2017). Blockade of interleukin-6 receptor in the periphery promotes rapid and sustained antidepressant actions: A possible role of gut-microbiota-brain axis. Transl. Psychiatry 7:e1138. doi: 10.1038/tp.2017.112
Zhang, J., Li, L., Liu, Q., Zhao, Z., Su, D., Xiao, C., et al. (2023). Gastrodin programs an Arg-1(+) microglial phenotype in hippocampus to ameliorate depression- and anxiety-like behaviors via the Nrf2 pathway in mice. Phytomedicine 113:154725. doi: 10.1016/j.phymed.2023.154725
Zhang, J., Yi, S., Li, Y., Xiao, C., Liu, C., Jiang, W., et al. (2020). The antidepressant effects of asperosaponin VI are mediated by the suppression of microglial activation and reduction of TLR4/NF-κB-induced IDO expression. Psychopharmacology 237, 2531–2545. doi: 10.1007/s00213-020-05553-5
Zhou, Y., Huang, Y., Ye, W., Chen, Z., and Yuan, Z. (2024). Cynaroside improved depressive-like behavior in CUMS mice by suppressing microglial inflammation and ferroptosis. Biomed. Pharmacother. 173:116425. doi: 10.1016/j.biopha.2024.116425
Keywords: depression, neuroinflammation, microglia, astrocytes, anti-inflammatory
Citation: Zhao K, Zhang Y, Yang S, Xiang L, Wu S, Dong J, Li H, Yu H and Hu W (2025) Neuroinflammation and stress-induced pathophysiology in major depressive disorder: mechanisms and therapeutic implications. Front. Cell. Neurosci. 19:1538026. doi: 10.3389/fncel.2025.1538026
Received: 02 January 2025; Accepted: 03 April 2025;
Published: 23 April 2025.
Edited by:
Yutaka Koyama, Kobe Pharmaceutical University, JapanReviewed by:
Yasuhiko Izumi, Kobe Pharmaceutical University, JapanCharles Elias Assmann, Federal University of Santa Maria, Brazil
Copyright © 2025 Zhao, Zhang, Yang, Xiang, Wu, Dong, Li, Yu and Hu. This is an open-access article distributed under the terms of the Creative Commons Attribution License (CC BY). The use, distribution or reproduction in other forums is permitted, provided the original author(s) and the copyright owner(s) are credited and that the original publication in this journal is cited, in accordance with accepted academic practice. No use, distribution or reproduction is permitted which does not comply with these terms.
*Correspondence: Weiyan Hu, aHV3ZWl5YW4yMDA0QDE2My5jb20=; Haofei Yu, eXVoYW9mZWlAa21tdS5lZHUuY24=
†These authors have contributed equally to this work and share first authorship