- 1Department of Anatomy, Division of Histology and Cell Biology, School of Medicine, Jichi Medical University, Shimotsuke, Japan
- 2Division of Ultrastructural Research, National Institute for Physiological Sciences, Okazaki, Japan
White matter in the central nervous system comprises bundled nerve fibers myelinated by oligodendrocytes. White matter injury, characterized by the loss of oligodendrocytes and myelin, is common after ischemic brain injury, inflammatory demyelinating diseases including multiple sclerosis, and traumatic damage such as spinal cord injury. Currently, no therapies have been confirmed to promote remyelination in these diseases. Over the past decade, various reports have suggested that the anti-muscarinic drug clemastine can stimulate remyelination by oligodendrocytes. Consequently, the repurposing of clemastine as a potential treatment for a variety of neurological disorders has gained significant attention. The therapeutic effects of clemastine have been demonstrated in various animal models, and its mechanisms of action in various neurological disorders are currently being investigated. In this review, we summarize reports relating to clemastine administration for white matter injury and neurological disease and discuss the therapeutic potential of remyelination promotion.
1 Introduction
Oligodendrocytes are a form of glial cell in the central nervous system (CNS) (Bercury and Macklin, 2015), which extend processes around nerve cell axons and generate myelin to enhance conduction velocity by saltatory conduction (Nave and Werner, 2014; Osanai et al., 2022). To ensure the rapid transmission of information required to maintain brain function, white matter tracts of the CNS consist predominantly of myelinated fibers (Fields, 2010), critical for facilitating communication between different brain regions (Filley and Fields, 2016; Ribeiro et al., 2024). White matter injury is brain damage characterized by the loss or demyelination of these fibers (Ohno and Ikenaka, 2019). Reduced blood flow to brain tissue also often results in white matter damage and the loss of oligodendrocytes (Wang et al., 2016; Youssef et al., 2021). Ischemic stroke is therefore a common cause, alongside demyelinating diseases such as multiple sclerosis (MS) (Compston and Coles, 2008; Wang et al., 2016; Reich et al., 2018). To study white matter injury, focal lesions have been generated in animal models, targeting tracts of the corpus callosum, internal capsule, and spinal cord (Blakemore and Franklin, 2008; Huang et al., 2011; Keough et al., 2015; Yamazaki et al., 2021). Therapies for diseases associated with white matter injury have focused on the induction of remyelination by oligodendrocytes (Ohtomo and Arai, 2020; Youssef et al., 2021; Huang et al., 2023). Pharmacological options that promote remyelination are important for functional recovery after white matter injury.
High-throughput screening platforms using micropillar arrays have been used to identify candidate medications for demyelinating diseases that promote remyelination by oligodendrocytes; clemastine was first identified as a potential remyelinating agent in this way by Mei et al. (2014). Clemastine and its salt form, clemastine fumarate, are first-generation antihistamines used to treat allergy symptoms and relieve itching, with sedative and anticholinergic effects (Simons, 2004). Clemastine fumarate has enhanced solubility and bioavailability, and so is the commonly used form for pharmaceutical purposes, basic research, and clinical settings. Because it is already widely used in the clinic, the potential repurposing of the drug for the treatment of white matter injury and other neurological diseases has recently gained attention (Jiang et al., 2023; Zhu et al., 2023).
In this review, we summarize the therapeutic potential of clemastine in white matter injury and neurological diseases, including the latest findings and insights.
2 Therapeutic effects of clemastine in animal models and potential molecular mechanisms
Since being identified for potential repurposing, clemastine has been administered in various animal models associated with demyelination (Figure 1A).
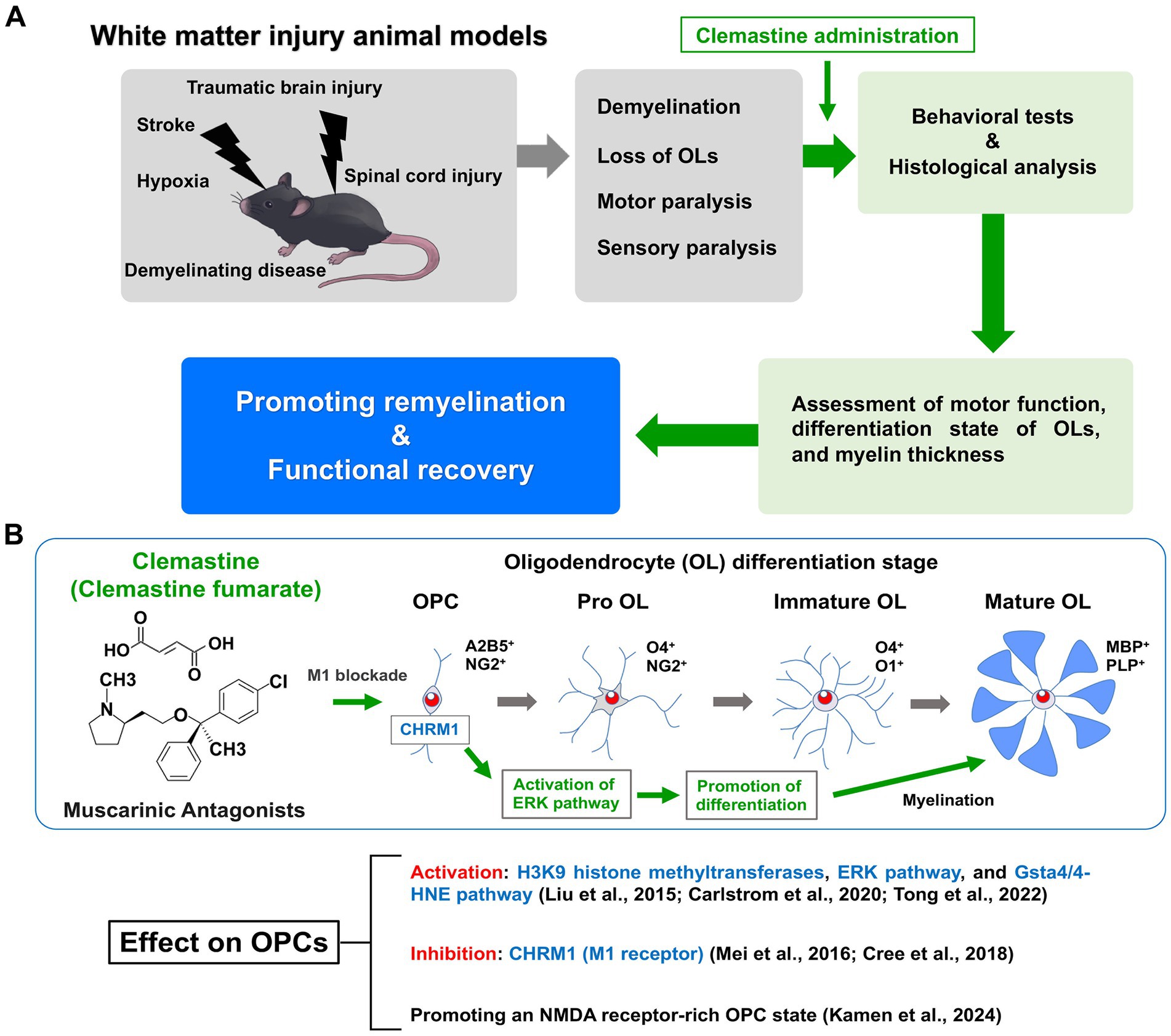
Figure 1. Therapeutic effects of clemastine in a mouse model of white matter injury and molecular mechanisms of action in oligodendrocyte precursor cells. (A) The animal models of white matter injury are induced demyelination, oligodendrocyte loss, motor paralysis and sensory paralysis. Motor function and remyelination state of oligodendrocytes (OLs) are assessed by behavioral tests and histological analysis. Clemastine administration in these animal models promotes remyelination and functional recovery. (B) Clemastine administration activates the extracellular signal-regulated kinase (ERK) pathway to promote differentiation via inhibition of the M1 muscarinic acetylcholine receptor (CHRM1) in oligodendrocyte precursor cells (OPCs). Clemastine also induces the activation of H3K9 histone methyltransferases and the glutathione S-transferase 4α (Gsta4)/4-hydroxynonenal (4-HNE) pathway in OPCs. In addition, clemastine promotes the N-methyl-D-aspartate (NMDA) receptor-rich state in OPCs. Shown are the schematic diagram of oligodendrocyte (OL) differentiation and stage-specific markers.
2.1 Summary of the effects of clemastine on remyelination in different animal models
Lysophosphatidylcholine (LPC)-induced demyelination is a representative animal model in which demyelination lesions are generated by focal injection; the model is highly informative for the assessment of remyelination (Blakemore and Franklin, 2008; Keough et al., 2015). Clemastine was shown to promote remyelination in an LPC-induced mouse model of spinal cord demyelination (Mei et al., 2014; Jensen et al., 2018). Recently, we have developed a mouse model of internal capsule demyelination that allows the evaluation of remyelination-induced functional recovery (Yamazaki et al., 2021; Yamazaki and Ohno, 2024). In this model, an asymmetric motor deficit is induced by internal capsule demyelination; recovery is associated with subsequent remyelination (Yamazaki et al., 2021; Yamazaki and Ohno, 2024). Using this model, we have also shown that clemastine administration promotes remyelination and related functional recovery (Yamazaki et al., 2023). A mouse model of demyelination induced by cuprizone (CPZ) diet intoxication has also been widely used to evaluate the efficacy of remyelination-promoting treatments (Matsushima and Morell, 2001; Yamazaki et al., 2018). In the CPZ-induced model, clemastine enhanced remyelination in the corpus callosum, cerebral cortex, and hippocampus (Li et al., 2015). Overall, clemastine has been reported to have therapeutic effects on multiple white matter regions, including the corpus callosum, spinal cord, and internal capsule. Another relevant mouse model is the experimental autoimmune encephalomyelitis (EAE) model, in which demyelination is mediated by the immune system (Constantinescu et al., 2011; Robinson et al., 2014). Clemastine administration in the EAE model improved clinical scores and enhanced remyelination (Motawi et al., 2023; Ibrahim et al., 2024).
Different types of injury models have also been used to evaluate the therapeutic potential of clemastine. Spinal cord injury is a neurological disorder associated with traumatic damage to white matter tracts. In a rat model of spinal cord injury, clemastine was shown to improve functional recovery (Du et al., 2022; Tong et al., 2022). Traumatic brain injury is also known to affect white matter and cognitive function (Huntemer-Silveira et al., 2020; Strogulski et al., 2023). Clemastine was shown to enhance myelination of the cortex and hippocampus and improve cognitive function in a rat model of mild traumatic brain injury (Huang et al., 2024).
Stroke is a common cause of hypoxic brain injury (Wang et al., 2016; Youssef et al., 2021). Hypoxic conditions are known to induce oligodendrocyte loss in white matter regions (Dewar et al., 2003; Huang et al., 2023); promoting remyelination is therefore a potential therapeutic approach for resolving white matter damage associated with cerebral ischemia (Wang et al., 2016; Youssef et al., 2021; Huang et al., 2023; Fernandes et al., 2025). The middle cerebral artery occlusion (MCAO) model is one of the most widely used in stroke research (Ma et al., 2020; Li et al., 2023). Recently, it was reported that clemastine treatment preserved white matter integrity, promoted neuronal survival, and accelerated functional recovery after transient MCAO (Cheng et al., 2024). In models of neonatal and adult hypoxic brain injury, enhancing myelination with clemastine treatment led to functional recovery and improved motor coordination (Cree et al., 2018; Wang et al., 2018; Chen et al., 2021).
White matter changes have also been reported in patients with depressive disorders, bringing attention to the potential role of oligodendrocytes in depression and the stress response (Wang et al., 2014; van Velzen et al., 2020; He et al., 2022). Social isolation of adult mice impaired myelination in the prefrontal cortex (PFC) (Liu et al., 2012), while clemastine rescued the behavioral changes (Liu et al., 2016). The depressive-like behavior induced by social defeat stress in adolescent mice was also ameliorated by clemastine treatment (Shimizu et al., 2020). Chemotherapy is also reported to induce cognitive impairments, associated with the alteration of white matter integrity; clemastine was able to rescue such chemotherapy-induced abnormalities (Chen et al., 2022). Interestingly, clemastine administration in a mouse model of glaucoma was reported to attenuate optic nerve and retinal neuropathy by promoting remyelination by enhancing the differentiation of oligodendrocyte precursor cells (OPCs) (Liu et al., 2024). This suggests that promoting remyelination also has therapeutic potential for glaucoma. Finally, clemastine was reported to improve electrophysiological changes and promote peripheral myelin repair in a murine model of compression neuropathy (Lee et al., 2021). Overall, therapeutic effects of clemastine have been reported in various pathological animal models in preclinical studies (Table 1).
2.2 The potential molecular mechanisms underlying the therapeutic effects of clemastine
The M1 muscarinic acetylcholine receptor (CHRM1) was identified as the major target of clemastine by OPC culture studies; CHRM1 knockout mice exhibited accelerated remyelination and reduced axonal loss after EAE induction (Mei et al., 2016). In neonatal and adult hypoxic brain injury models, enhancing myelination with clemastine treatment led to functional recovery via CHRM1-mediated effects on OPCs (Cree et al., 2018; Wang et al., 2018). Therefore, CHRM1 is an important target receptor for clemastine administration. Recent reports have shown that clemastine administration in EAE mice activates F3/Contactin-1 through non-canonical Notch-1 signaling, while inhibiting p38 mitogen-activated protein kinase (MAPK)/NOD-like receptor protein-3 (NLRP3) signaling (Motawi et al., 2023; Ibrahim et al., 2024). Evidence has also shown that clemastine-induced activation of the glutathione S-transferase 4α (Gsta4)/4-hydroxynonenal (4-HNE) pathway promotes remyelination by oligodendrocytes (Carlström et al., 2020). Overexpression of Gsta4 has also been reported to contribute to the amelioration of the EAE phenotype (Carlström et al., 2020). Clemastine was shown to promote the differentiation of OPCs by activating extracellular signal-regulated kinase (ERK) signaling through the muscarinic receptor in the spinal cord injury model (Du et al., 2022; Tong et al., 2022). It has also been reported to reduce inflammation and induce the downregulation of NLRP3 and IL-1β, through the inhibition of the P38 signaling pathway in microglia (Xie et al., 2020). Clemastine enhanced the activity of H3K9 histone methyltransferases in PFC oligodendrocytes in a social isolation mouse model (Liu et al., 2016). Recently, Kamen et al. (2024) performed whole-cell patch-clamping and reported that clemastine induces an N-methyl-D-aspartate (NMDA) receptor-rich state in OPCs by altering membrane properties. Taken together, the molecular mechanisms of clemastine action in OPCs are currently being elucidated (Figure 1B).
3 Clinical studies, remaining questions, and future directions
Clinical trials of clemastine began in multiple sclerosis patients in 2014 (Green et al., 2017). In a double-blind, randomized, placebo-controlled, crossover trial (ReBUILD) in patients with relapsing multiple sclerosis, clemastine delayed the decline in visual-evoked potentials (Green et al., 2017). MRI analysis from the ReBUILD trial showed that normal-appearing regions of corpus callosum white matter exhibited increased derived myelin water fraction values after clemastine administration (Caverzasi et al., 2023). Because clemastine is safe to use, with a relatively low incidence of side effects, the results suggest promising benefits for the treatment of multiple sclerosis patients.
However, several questions remain in terms of human application. Clemastine treatment impaired myelination in the developmental stages in mice, despite an increase in the number of oligodendrocytes (Palma et al., 2022). A recent preprint clinical report also suggests that clemastine enhances pyroptosis and accelerates the advance of disability in progressive MS (Kocot et al., 2024). In addition, in a rabbit model of LPC-induced demyelination, short-term administration of clemastine reduced the number of OPCs, while delayed administration resulted in the accumulation of OPCs expressing markers of senescence (Cooper et al., 2024). However, long-term treatment increased the density of oligodendrocytes at the lesion site (Cooper et al., 2024). These results may reflect differences in oligodendrocyte numbers and the CNS environment between biological species. Optimization of the timing and duration of clemastine administration is therefore critical for achieving maximal therapeutic effects for different diseases. In addition, while clemastine may be more clinically effective when used in combination with existing treatments, such benefits are still unconfirmed. However, other muscarinic antagonists such as quetiapine and benztropine have also been identified as potential treatments in high-throughput screens (Deshmukh et al., 2013; Mei et al., 2014). Benztropine is currently used for Parkinson’s disease, while quetiapine is used as an atypical antipsychotic; both have been reported to promote OPC differentiation and myelin repair (Xiao et al., 2008; Zhang et al., 2012; Deshmukh et al., 2013; Wang et al., 2021). Clinical trials in multiple sclerosis patients have not yet been initiated for benztropine, but results from those of quetiapine have been reported. A phase I/II dose-finding study (NCT02087631) in patients with relapsing–remitting and progressive multiple sclerosis reported adverse effects including sedation and paraparesis (Metz et al., 2020). These results highlight the need to demonstrate the efficacy of low-dose quetiapine in preclinical studies. Recently, the selective muscarinic M1 receptor antagonists PIPE-359 and PIPE-307 were reported to improve clinical scores in an EAE model (Schrader et al., 2021; Poon et al., 2024; Chen et al., 2025). These novel drug candidates may attenuate side effects through the selective blockade of the M1 receptor. PIPE-307 has completed Phase I trials in healthy volunteers (NCT04725175 and NCT04941781) and is currently in Phase II in MS patients (NCT06083753) (Poon et al., 2024; Chen et al., 2025).
Recently, ApTOLL—a single-stranded DNA aptamer—and alpha-keto acids generated by the amino acid oxidase interleukin-4 induced 1 (IL4I1) have been reported as new drug candidates for MS (Fernández-Gómez et al., 2024; Hu et al., 2024). Previously, creatine, a nitrogenous organic acid, has also been reported to promote remyelination (Chamberlain et al., 2017; Rosko et al., 2023). These drug candidates are being evaluated in several types of demyelinating mouse models and may have different potential molecular mechanisms. However, these drugs have not been directly compared in their efficacy in promoting remyelination. Therefore, it may be important to compare their relative strengths against the expected effects of clemastine.
Meanwhile, in hypoxic–ischemic rats, clemastine was shown to promote exercise-induced motor improvement (Goto et al., 2025). Interestingly, synthetic MRI measurements of acute stroke patients suggested that higher myelin content in the brain leads to better prognosis (Toko et al., 2025). Therefore, the combination of clemastine administration and exercise may have a synergistic effect on rehabilitation. In the near future, clinical trials may be conducted combining rehabilitation and clemastine administration in stroke patients.
4 Conclusion
Over the past decade, the efficacy of clemastine for treating neurological disorders has been reported in various preclinical models, and the possibility of repurposing clemastine has been discussed. In this review, we have summarized previous reports on clemastine administration in models of white matter injury and neurological disease, and discussed the therapeutic effects and potential mechanisms of action. Taken together, clemastine has the potential to be repurposed for various neurological disorders. However, further investigation is needed to overcome outstanding questions, such as the optimization of the timing and duration of administration.
Author contributions
RY: Writing – original draft, Writing – review & editing. NO: Writing – review & editing.
Funding
The author(s) declare that financial support was received for the research and/or publication of this article. This work was supported by Takeda Science Foundation, Kobayashi Foundation, and Japan Society for the Promotion of Science (JSPS) KAKENHI grants #23K14432 and #25K02967 to RY and #24H00583 to NO.
Acknowledgments
We thank members of the Ohno lab, especially Yasuyuki Osanai for valuable discussion. We thank Wilf Gardner, from Edanz (https://jp.edanz.com/ac) for editing a draft of this manuscript.
Conflict of interest
The authors declare that the research was conducted in the absence of any commercial or financial relationships that could be construed as a potential conflict of interest.
Generative AI statement
The authors declare that no Gen AI was used in the creation of this manuscript.
Publisher’s note
All claims expressed in this article are solely those of the authors and do not necessarily represent those of their affiliated organizations, or those of the publisher, the editors and the reviewers. Any product that may be evaluated in this article, or claim that may be made by its manufacturer, is not guaranteed or endorsed by the publisher.
References
Bercury, K. K., and Macklin, W. B. (2015). Dynamics and mechanisms of CNS myelination. Dev. Cell 32, 447–458. doi: 10.1016/j.devcel.2015.01.016
Blakemore, W. F., and Franklin, R. J. (2008). Remyelination in experimental models of toxin-induced demyelination. Curr. Top. Microbiol. Immunol. 318, 193–212. doi: 10.1007/978-3-540-73677-6_8
Carlström, K. E., Zhu, K., Ewing, E., Krabbendam, I. E., Harris, R. A., Falcão, A. M., et al. (2020). Gsta4 controls apoptosis of differentiating adult oligodendrocytes during homeostasis and remyelination via the mitochondria-associated Fas-Casp8-bid-axis. Nat. Commun. 11:4071. doi: 10.1038/s41467-020-17871-5
Caverzasi, E., Papinutto, N., Cordano, C., Kirkish, G., Gundel, T. J., Zhu, A., et al. (2023). MWF of the corpus callosum is a robust measure of remyelination: results from the ReBUILD trial. Proc. Natl. Acad. Sci. USA 120:e2217635120. doi: 10.1073/pnas.2217635120
Chamberlain, K. A., Chapey, K. S., Nanescu, S. E., and Huang, J. K. (2017). Creatine enhances mitochondrial-mediated oligodendrocyte survival after demyelinating injury. J. Neurosci. 37, 1479–1492. doi: 10.1523/JNEUROSCI.1941-16.2016
Chen, K., Park, E., and Abd-Elrahman, K. S. (2025). Enhancing Remyelination in multiple sclerosis via muscarinic acetylcholine receptor M1. Mol. Pharmacol. 107:100027. doi: 10.1016/j.molpha.2025.100027
Chen, L., Ren, S. Y., Li, R. X., Liu, K., Chen, J. F., Yang, Y. J., et al. (2021). Chronic exposure to hypoxia inhibits myelinogenesis and causes motor coordination deficits in adult mice. Neurosci. Bull. 37, 1397–1411. doi: 10.1007/s12264-021-00745-1
Chen, Y., Sheng, J., Tang, X., Zhao, Y., Zhu, S., and Liu, Q. (2022). Clemastine rescues chemotherapy-induced cognitive impairment by improving white matter integrity. Neuroscience 484, 66–79. doi: 10.1016/j.neuroscience.2022.01.001
Cheng, Y. J., Wang, F., Feng, J., Yu, B., Wang, B., Gao, Q., et al. (2024). Prolonged myelin deficits contribute to neuron loss and functional impairments after ischaemic stroke. Brain 147, 1294–1311. doi: 10.1093/brain/awae029
Compston, A., and Coles, A. (2008). Multiple sclerosis. Lancet 372, 1502–1517. doi: 10.1016/S0140-6736(08)61620-7
Constantinescu, C. S., Farooqi, N., O'brien, K., and Gran, B. (2011). Experimental autoimmune encephalomyelitis (EAE) as a model for multiple sclerosis (MS). Br. J. Pharmacol. 164, 1079–1106. doi: 10.1111/j.1476-5381.2011.01302.x
Cooper, J. J. M., Muthaiah, R., Frost, J. R., Buck, G. T., Ravichandar, R., Gadelkarim, F., et al. (2024). Clemastine induces oligodendrocyte progenitor Pool exhaustion and senescence in the context of chronic demyelination in a rabbit model. Ann. Neurol. 97, 116–133. doi: 10.1002/ana.27098
Cree, B. A. C., Niu, J., Hoi, K. K., Zhao, C., Caganap, S. D., Henry, R. G., et al. (2018). Clemastine rescues myelination defects and promotes functional recovery in hypoxic brain injury. Brain 141, 85–98. doi: 10.1093/brain/awx312
Deshmukh, V. A., Tardif, V., Lyssiotis, C. A., Green, C. C., Kerman, B., Kim, H. J., et al. (2013). A regenerative approach to the treatment of multiple sclerosis. Nature 502, 327–332. doi: 10.1038/nature12647
Dewar, D., Underhill, S. M., and Goldberg, M. P. (2003). Oligodendrocytes and ischemic brain injury. J. Cereb. Blood Flow Metab. 23, 263–274. doi: 10.1097/01.WCB.0000053472.41007.F9
Du, W., Deng, Y., Jiang, R., Tong, L., Li, R., and Jiang, X. (2022). Clemastine enhances myelination, delays axonal loss and promotes functional recovery in spinal cord injury. Neurochem. Res. 47, 503–515. doi: 10.1007/s11064-021-03465-0
Fernandes, M. G. F., Pernin, F., Antel, J. P., and Kennedy, T. E. (2025). From BBB to PPP: bioenergetic requirements and challenges for oligodendrocytes in health and disease. J. Neurochem. 169:e16219. doi: 10.1111/jnc.16219
Fernández-Gómez, B., Marchena, M. A., Piñeiro, D., Gómez-Martín, P., Sánchez, E., Laó, Y., et al. (2024). ApTOLL: A new therapeutic aptamer for cytoprotection and (re)myelination after multiple sclerosis. Br. J. Pharmacol. 181, 3263–3281. doi: 10.1111/bph.16399
Fields, R. D. (2010). Neuroscience. Change in the brain's white matter. Science 330, 768–769. doi: 10.1126/science.1199139
Filley, C. M., and Fields, R. D. (2016). White matter and cognition: making the connection. J. Neurophysiol. 116, 2093–2104. doi: 10.1152/jn.00221.2016
Goto, T., Tsurugizawa, T., Komaki, Y., Takashima, I., Iwaki, S., and Kunori, N. (2025). Clemastine enhances exercise-induced motor improvement in hypoxic ischemic rats. Brain Res. 1846:149257. doi: 10.1016/j.brainres.2024.149257
Green, A. J., Gelfand, J. M., Cree, B. A., Bevan, C., Boscardin, W. J., Mei, F., et al. (2017). Clemastine fumarate as a remyelinating therapy for multiple sclerosis (ReBUILD): a randomised, controlled, double-blind, crossover trial. Lancet 390, 2481–2489. doi: 10.1016/S0140-6736(17)32346-2
He, E., Liu, M., Gong, S., Fu, X., Han, Y., and Deng, F. (2022). White matter alterations in depressive disorder. Front. Immunol. 13:826812. doi: 10.3389/fimmu.2022.826812
Hu, J., Melchor, G. S., Ladakis, D., Reger, J., Kim, H. W., Chamberlain, K. A., et al. (2024). Myeloid cell-associated aromatic amino acid metabolism facilitates CNS myelin regeneration. NPJ Regen Med 9:1. doi: 10.1038/s41536-023-00345-9
Huang, Z., Feng, Y., Zhang, Y., Ma, X., Zong, X., Jordan, J. D., et al. (2024). Enhancing axonal myelination: Clemastine attenuates cognitive impairment in a rat model of diffuse traumatic brain injury. Transl. Res. 268, 40–50. doi: 10.1016/j.trsl.2024.01.008
Huang, J. K., Jarjour, A. A., Nait Oumesmar, B., Kerninon, C., Williams, A., Krezel, W., et al. (2011). Retinoid X receptor gamma signaling accelerates CNS remyelination. Nat. Neurosci. 14, 45–53. doi: 10.1038/nn.2702
Huang, S., Ren, C., Luo, Y., Ding, Y., Ji, X., and Li, S. (2023). New insights into the roles of oligodendrocytes regulation in ischemic stroke recovery. Neurobiol. Dis. 184:106200. doi: 10.1016/j.nbd.2023.106200
Huntemer-Silveira, A., Patil, N., Brickner, M. A., and Parr, A. M. (2020). Strategies for oligodendrocyte and myelin repair in traumatic CNS injury. Front. Cell. Neurosci. 14:619707. doi: 10.3389/fncel.2020.619707
Ibrahim, S. M., Kamel, A. S., Ahmed, K. A., Mohammed, R. A., and Essam, R. M. (2024). The preferential effect of Clemastine on F3/Contactin-1/Notch-1 compared to Jagged-1/Notch-1 justifies its remyelinating effect in an experimental model of multiple sclerosis in rats. Int. Immunopharmacol. 128:111481. doi: 10.1016/j.intimp.2023.111481
Jensen, S. K., Michaels, N. J., Ilyntskyy, S., Keough, M. B., Kovalchuk, O., and Yong, V. W. (2018). Multimodal enhancement of Remyelination by exercise with a pivotal role for oligodendroglial PGC1α. Cell Rep. 24, 3167–3179. doi: 10.1016/j.celrep.2018.08.060
Jiang, S., Wang, X., Cao, T., Kang, R., and Huang, L. (2023). Insights on therapeutic potential of clemastine in neurological disorders. Front. Mol. Neurosci. 16:1279985. doi: 10.3389/fnmol.2023.1279985
Kamen, Y., Evans, K. A., Sitnikov, S., Spitzer, S. O., De Faria, O., Yucel, M., et al. (2024). Clemastine and metformin extend the window of NMDA receptor surface expression in ageing oligodendrocyte precursor cells. Sci. Rep. 14:4091. doi: 10.1038/s41598-024-53615-x
Keough, M. B., Jensen, S. K., and Yong, V. W. (2015). Experimental demyelination and remyelination of murine spinal cord by focal injection of lysolecithin. J. Vis. Exp. e52679. doi: 10.3791/52679-v
Kocot, J., Kosa, P., Ashida, S., Pirjanian, N., Goldbach-Mansky, R., Peterson, K., et al. (2024). Clemastine fumarate accelerates accumulation of disability in progressive multiple sclerosis by enhancing pyroptosis. [Epubh ahead of preprint]. doi: 10.1101/2024.04.09.24305506
Lee, J. I., Park, J. W., Lee, K. J., and Lee, D. H. (2021). Clemastine improves electrophysiologic and histomorphometric changes through promoting myelin repair in a murine model of compression neuropathy. Sci. Rep. 11:20886. doi: 10.1038/s41598-021-00389-1
Li, Z., He, Y., Fan, S., and Sun, B. (2015). Clemastine rescues behavioral changes and enhances remyelination in the cuprizone mouse model of demyelination. Neurosci. Bull. 31, 617–625. doi: 10.1007/s12264-015-1555-3
Li, Y., Tan, L., Yang, C., He, L., Liu, L., Deng, B., et al. (2023). Distinctions between the Koizumi and Zea Longa methods for middle cerebral artery occlusion (MCAO) model: a systematic review and meta-analysis of rodent data. Sci. Rep. 13:10247. doi: 10.1038/s41598-023-37187-w
Liu, J., Dietz, K., Deloyht, J. M., Pedre, X., Kelkar, D., Kaur, J., et al. (2012). Impaired adult myelination in the prefrontal cortex of socially isolated mice. Nat. Neurosci. 15, 1621–1623. doi: 10.1038/nn.3263
Liu, J., Dupree, J. L., Gacias, M., Frawley, R., Sikder, T., Naik, P., et al. (2016). Clemastine enhances myelination in the prefrontal cortex and rescues behavioral changes in socially isolated mice. J. Neurosci. 36, 957–962. doi: 10.1523/JNEUROSCI.3608-15.2016
Liu, K., Yang, Y., Wu, Z., Sun, C., Su, Y., Huang, N., et al. (2024). Remyelination-oriented clemastine treatment attenuates neuropathies of optic nerve and retina in glaucoma. Glia 72, 1555–1571. doi: 10.1002/glia.24543
Ma, R., Xie, Q., Li, Y., Chen, Z., Ren, M., Chen, H., et al. (2020). Animal models of cerebral ischemia: A review. Biomed. Pharmacother. 131:110686. doi: 10.1016/j.biopha.2020.110686
Matsushima, G. K., and Morell, P. (2001). The neurotoxicant, cuprizone, as a model to study demyelination and remyelination in the central nervous system. Brain Pathol. 11, 107–116. doi: 10.1111/j.1750-3639.2001.tb00385.x
Mei, F., Fancy, S. P. J., Shen, Y. A., Niu, J., Zhao, C., Presley, B., et al. (2014). Micropillar arrays as a high-throughput screening platform for therapeutics in multiple sclerosis. Nat. Med. 20, 954–960. doi: 10.1038/nm.3618
Mei, F., Lehmann-Horn, K., Shen, Y. A., Rankin, K. A., Stebbins, K. J., Lorrain, D. S., et al. (2016). Accelerated remyelination during inflammatory demyelination prevents axonal loss and improves functional recovery. eLife 5:8246. doi: 10.7554/eLife.18246
Metz, L. M., Al Malik, Y., Makkawi, S., Zedde, A., and Cerchiaro, G. (2020). Quetiapine is not tolerable to people with MS in doses potentially required to enhance myelin repair (2868). Neurology 94:2868. doi: 10.1212/WNL.94.15_supplement.2868
Motawi, T. K., El-Maraghy, S. A., Kamel, A. S., Said, S. E., and Kortam, M. A. (2023). Modulation of p38 MAPK and Nrf2/HO-1/NLRP3 inflammasome signaling and pyroptosis outline the anti-neuroinflammatory and remyelinating characters of Clemastine in EAE rat model. Biochem. Pharmacol. 209:115435. doi: 10.1016/j.bcp.2023.115435
Nave, K. A., and Werner, H. B. (2014). Myelination of the nervous system: mechanisms and functions. Annu. Rev. Cell Dev. Biol. 30, 503–533. doi: 10.1146/annurev-cellbio-100913-013101
Ohno, N., and Ikenaka, K. (2019). Axonal and neuronal degeneration in myelin diseases. Neurosci. Res. 139, 48–57. doi: 10.1016/j.neures.2018.08.013
Ohtomo, R., and Arai, K. (2020). Recent updates on mechanisms of cell-cell interaction in oligodendrocyte regeneration after white matter injury. Neurosci. Lett. 715:134650. doi: 10.1016/j.neulet.2019.134650
Osanai, Y., Yamazaki, R., Shinohara, Y., and Ohno, N. (2022). Heterogeneity and regulation of oligodendrocyte morphology. Front. Cell Dev. Biol. 10:1030486. doi: 10.3389/fcell.2022.1030486
Palma, A., Chara, J. C., Montilla, A., Otxoa-De-Amezaga, A., Ruíz-Jaén, F., Planas, A. M., et al. (2022). Clemastine induces an impairment in developmental myelination. Front. Cell Dev. Biol. 10:841548. doi: 10.3389/fcell.2022.841548
Poon, M. M., Lorrain, K. I., Stebbins, K. J., Edu, G. C., Broadhead, A. R., Lorenzana, A. J., et al. (2024). Targeting the muscarinic M1 receptor with a selective, brain-penetrant antagonist to promote remyelination in multiple sclerosis. Proc. Natl. Acad. Sci. USA 121:e2407974121. doi: 10.1073/pnas.2407974121
Reich, D. S., Lucchinetti, C. F., and Calabresi, P. A. (2018). Multiple Sclerosis. N. Engl. J. Med. 378, 169–180. doi: 10.1056/NEJMra1401483
Ribeiro, M., Yordanova, Y. N., Noblet, V., Herbet, G., and Ricard, D. (2024). White matter tracts and executive functions: a review of causal and correlation evidence. Brain 147, 352–371. doi: 10.1093/brain/awad308
Robinson, A. P., Harp, C. T., Noronha, A., and Miller, S. D. (2014). The experimental autoimmune encephalomyelitis (EAE) model of MS: utility for understanding disease pathophysiology and treatment. Handb. Clin. Neurol. 122, 173–189. doi: 10.1016/B978-0-444-52001-2.00008-X
Rosko, L. M., Gentile, T., Smith, V. N., Manavi, Z., Melchor, G. S., Hu, J., et al. (2023). Cerebral Creatine deficiency affects the timing of oligodendrocyte myelination. J. Neurosci. 43, 1143–1153. doi: 10.1523/JNEUROSCI.2120-21.2022
Schrader, T. O., Xiong, Y., Lorenzana, A. O., Broadhead, A., Stebbins, K. J., Poon, M. M., et al. (2021). Discovery of PIPE-359, a brain-penetrant, selective M(1) receptor antagonist with robust efficacy in murine MOG-EAE. ACS Med. Chem. Lett. 12, 155–161. doi: 10.1021/acsmedchemlett.0c00626
Shimizu, T., Ishida, A., Hagiwara, M., Ueda, Y., Hattori, A., Tajiri, N., et al. (2020). Social defeat stress in adolescent mice induces depressive-like behaviors with reduced Oligodendrogenesis. Neuroscience 443, 218–232. doi: 10.1016/j.neuroscience.2020.07.002
Simons, F. E. (2004). Advances in H1-antihistamines. N. Engl. J. Med. 351, 2203–2217. doi: 10.1056/NEJMra033121
Strogulski, N. R., Portela, L. V., Polster, B. M., and Loane, D. J. (2023). Fundamental neurochemistry review: microglial immunometabolism in traumatic brain injury. J. Neurochem. 167, 129–153. doi: 10.1111/jnc.15959
Toko, M., Nezu, T., Eto, F., Aoki, S., Ohshita, T., Ueno, H., et al. (2025). Usefulness of myelin quantification using synthetic magnetic resonance imaging for predicting outcomes in patients with acute ischemic stroke. Stroke 56, 649–656. doi: 10.1161/STROKEAHA.124.049851
Tong, L. Y., Deng, Y. B., Du, W. H., Zhou, W. Z., Liao, X. Y., and Jiang, X. (2022). Clemastine promotes differentiation of oligodendrocyte progenitor cells through the activation of ERK1/2 via muscarinic receptors after spinal cord injury. Front. Pharmacol. 13:914153. doi: 10.3389/fphar.2022.914153
Van Velzen, L. S., Kelly, S., Isaev, D., Aleman, A., Aftanas, L. I., Bauer, J., et al. (2020). White matter disturbances in major depressive disorder: a coordinated analysis across 20 international cohorts in the ENIGMA MDD working group. Mol. Psychiatry 25, 1511–1525. doi: 10.1038/s41380-019-0477-2
Wang, L., Leonards, C. O., Sterzer, P., and Ebinger, M. (2014). White matter lesions and depression: a systematic review and meta-analysis. J. Psychiatr. Res. 56, 56–64. doi: 10.1016/j.jpsychires.2014.05.005
Wang, Y., Liu, G., Hong, D., Chen, F., Ji, X., and Cao, G. (2016). White matter injury in ischemic stroke. Prog. Neurobiol. 141, 45–60. doi: 10.1016/j.pneurobio.2016.04.005
Wang, X., Su, Y., Li, T., Yu, G., Wang, Y., Chen, X., et al. (2021). Quetiapine promotes oligodendroglial process outgrowth and membrane expansion by orchestrating the effects of Olig1. Glia 69, 1709–1722. doi: 10.1002/glia.23986
Wang, F., Yang, Y. J., Yang, N., Chen, X. J., Huang, N. X., Zhang, J., et al. (2018). Enhancing oligodendrocyte myelination rescues synaptic loss and improves functional recovery after chronic hypoxia. Neuron 99, 689–701.e5. doi: 10.1016/j.neuron.2018.07.017
Xiao, L., Xu, H., Zhang, Y., Wei, Z., He, J., Jiang, W., et al. (2008). Quetiapine facilitates oligodendrocyte development and prevents mice from myelin breakdown and behavioral changes. Mol. Psychiatry 13, 697–708. doi: 10.1038/sj.mp.4002064
Xie, D., Ge, X., Ma, Y., Tang, J., Wang, Y., Zhu, Y., et al. (2020). Clemastine improves hypomyelination in rats with hypoxic-ischemic brain injury by reducing microglia-derived IL-1β via P38 signaling pathway. J. Neuroinflammation 17:57. doi: 10.1186/s12974-019-1662-6
Yamazaki, R., Baba, H., and Yamaguchi, Y. (2018). Unconventional myosin ID is involved in Remyelination after Cuprizone-induced demyelination. Neurochem. Res. 43, 195–204. doi: 10.1007/s11064-017-2413-7
Yamazaki, R., and Ohno, N. (2024). The mouse model of internal capsule demyelination: a novel tool for investigating motor functional changes caused by demyelination and for evaluating drugs that promote Remyelination. Acta Histochem. Cytochem. 57, 1–5. doi: 10.1267/ahc.24-00005
Yamazaki, R., Ohno, N., and Huang, J. K. (2021). Acute motor deficit and subsequent remyelination-associated recovery following internal capsule demyelination in mice. J. Neurochem. 156, 917–928. doi: 10.1111/jnc.15142
Yamazaki, R., Osanai, Y., Kouki, T., Huang, J. K., and Ohno, N. (2023). Pharmacological treatment promoting remyelination enhances motor function after internal capsule demyelination in mice. Neurochem. Int. 164:105505. doi: 10.1016/j.neuint.2023.105505
Youssef, M. I., Ma, J., Chen, Z., and Hu, W. W. (2021). Potential therapeutic agents for ischemic white matter damage. Neurochem. Int. 149:105116. doi: 10.1016/j.neuint.2021.105116
Zhang, Y., Zhang, H., Wang, L., Jiang, W., Xu, H., Xiao, L., et al. (2012). Quetiapine enhances oligodendrocyte regeneration and myelin repair after cuprizone-induced demyelination. Schizophr. Res. 138, 8–17. doi: 10.1016/j.schres.2012.04.006
Keywords: remyelination, oligodendrocyte, clemastine, white matter injury, drug repositioning
Citation: Yamazaki R and Ohno N (2025) The potential of repurposing clemastine to promote remyelination. Front. Cell. Neurosci. 19:1582902. doi: 10.3389/fncel.2025.1582902
Edited by:
Kyoji Ohyama, Tokyo Medical University, JapanReviewed by:
Fernando de Castro, Spanish National Research Council (CSIC), SpainIva D. Tzvetanova, European University Cyprus, Cyprus
Junji Yamauchi, Tokyo University of Pharmacy and Life Sciences, Japan
Rongchen Huang, University of Colorado, United States
Copyright © 2025 Yamazaki and Ohno. This is an open-access article distributed under the terms of the Creative Commons Attribution License (CC BY). The use, distribution or reproduction in other forums is permitted, provided the original author(s) and the copyright owner(s) are credited and that the original publication in this journal is cited, in accordance with accepted academic practice. No use, distribution or reproduction is permitted which does not comply with these terms.
*Correspondence: Reiji Yamazaki, cnlhbWF6YWtpQGppY2hpLmFjLmpw
†ORCID: Reiji Yamazaki, https://orcid.org/0000-0001-8052-6624
Nobuhiko Ohno, https://orcid.org/0000-0002-6536-2753