- 1Department of Civil Engineering, Indian Institute of Technology Palakkad, Palakkad, India
- 2Environmental Sciences and Sustainable Engineering Centre, Indian Institute of Technology Palakkad, Palakkad, India
- 3Global Sanitation Centre of Excellence, Indian Institute of Technology Palakkad, Palakkad, India
Source separated urine comprises nitrogen, phosphorus, and potassium which are essential nutrients for plant growth. Although numerous methods and techniques exists to recover these nutrients from urine, factors such as chemical addition, high cost, operational complexities, and energy consumption limit their widespread adoption. Of late, magnesium air fuel cell (MAFC) has emerged as a promising candidate for concurrent nutrient recovery and energy production from source separated urine, owing to its electrochemical spontaneity. In our previous study, we investigated the concurrent resource (nutrient and energy) recovery from real source separated urine, where the preferential formation of magnesium carbonates (nesquehonite, MgCO3.3H2O) was observed. It was hypothesised that, bicarbonate (HCO3−) ions resulting from urea hydrolysis compete with phosphate (PO43−) ions to react with magnesium (Mg2+) ions. The objectives of this study were formulated to investigate the preferential formation of magnesium carbonates. To explore the influence of HCO3− ions on struvite formation, experiments were conducted with molar ratio from Mg2+: NH4+: PO43−: HCO3− = 1:1:1:1 to the real urine condition (Mg2+: NH4+: PO43−: HCO3− = 1:90:1:120). The experiments were conducted using both MAFC and sacrificial chemical precipitation (sacrificial chemical addition) methods. The recovered precipitates were characterised using X-Ray diffraction. The investigation revealed that the presence of HCO3− ions at a molar ratio > 1.5 hinders the struvite formation and imparts an amorphous nature to the precipitates. Additionally, a techno-economic assessment of MAFCs as a resource recovery system revealed that, the magnesium anode cost accounted for 47% of the capital expenditure (CAPEX). Furthermore, the revenue generation contributed to 0.85% of the total expenditure, emphasizing the need to increase the value proposition of source separated urine through recovery of additional nutrients and water.
1 Introduction
The anticipated global population of 9.8 billion by 2050 presents a significant challenge to food security and fertiliser consumption (Penuelas et al., 2023). Global nitrogen (N) and phosphorus (P) demand is expected to increase by 50%–100%, while the phosphate rock reserves may approach near depletion (Cordell et al., 2011). The linear flow of P from the cradle to grave contributes to eutrophication in aquatic ecosystems, involving a significant P loss from the value chain (around 30% of rock reserves mined) (Liu et al., 2021). A radically new and sustainable method is essential to harness P from alternate sources. One such source is the domestic wastewater, in which, urine is the major contributor of P (50%), N (70%–90%), and Potassium (K) (75%), yet constitute only <1% by volume (Taylor et al., 2014). Therefore, source separation of urine is a deep-seated approach to close the P loop in the environment. Globally, the P recovered from human urine is estimated to reach 2.16 × 106 metric tons by 2050 (Cid et al., 2018).
Urine has been explored as a sustainable fertilizer alternative, with reduced dependence on phosphate rock-derived fertilizers. Struvite (MgNH4PO4.6H2O) or magnesium ammonium phosphate is the most common method of nutrient recovery from urine due to its agronomic advantages (Dong et al., 2024; Hall et al., 2020). The techniques to harness N and P from urine include physical separation (stripping, ion exchange, adsorption) (Wu and Vaneeckhaute, 2022), chemical precipitation (Mg salts addition) (Sangeetha et al., 2022), electrochemical techniques (electrocoagulation, electrodialysis) (Inan and Alaydin, 2014; Zaffar et al., 2022), and bioelectrochemical methods (microbial fuel cell, microbial electrolysis cell) (Gangadharan et al., 2022; 2021; Sabin et al., 2022). Physical separation techniques are energy intensive and rely on expensive membranes for ion separation; chemical precipitation requires chemical inputs; and electrochemical techniques are non-spontaneous (requires external energy). Bioelectrochemical approaches are sustainable, however, they are time intensive and electricity production is minimal.
Recently, Magnesium air fuel cell (MAFC) technology has emerged as a promising candidate for struvite precipitation coupled with energy production (Mahmood et al., 2023). The redox reactions in MAFC are spontaneous and do not require external power supply. The MAFC uses magnesium (pure metal or alloy) as anode and air cathode (gas diffusion electrode) as the cathode. At the anode, magnesium oxidises to release Mg2+ ions and two electrons (Equation 1), while oxygen reduction occurs at the cathode to produce OH− ions by accepting the electrons (four electron transfer pathway) (Equation 2). The Mg2+ ions react with PO43- and NH4+ ions in the urine to precipitate as struvite under supersaturation and optimal pH conditions (8.5–9) (Equation 3).
The MAFCs have advantages over conventional systems, including reduced sludge production, no external addition of chemical and energy, and no stirring requirements. However, excess magnesium dissolution and accelerated oxygen reduction at the cathode can reduce system efficiency, limiting field scale applications (Mahmood et al., 2023). The first MAFC study on simultaneous nutrient recovery and energy production was reported by (Kim et al., 2018) achieving 99% struvite purity from aqueous solution of NH4Cl and KH2PO4. Subsequent studies explored synthetic hydrolysed urine (Liao et al., 2020) and solution comprising Na3PO4 and NH4Cl (Wu et al., 2022), reporting >99% PO43- removal efficiency and struvite purity >98%. However (Sangeetha et al., 2024), evaluated MAFC with real source separated urine and highlighted the challenge with the preferential formation of nesquehonite (MgCO3.3H2O), a meta stable precursor of magnesite (MgCO3). Magnesium carbonate precipitation, including nesquehonite, brucite (Mg(OH)2), magnesite (MgCO3), barringtonite (MgCO3. 2H2O), dypingite (Mg5(CO3)4(OH)2.5H2O), hydro magnesite (Mg5(CO3)4(OH)2.5H2O), pokrovskite (Mg2(CO3) (OH)2.0.5H2O) occurs under ambient temperature (25°C) (Pokharel et al., 2024; Glasser et al., 2016). However, these precipitates impair the struvite purity, reducing its economic value in the fertiliser market (Dong et al., 2024). The impact of HCO3− ions resulting from urea hydrolysis, on struvite precipitation, the competition between HCO3− and PO43− ions during precipitation and the self-buffering capacity of urine remain unexplored.
In this study, the authors evaluate the influence of HCO3− ion concentration on the preferential formation of magnesium carbonates over struvite in MAFCs. The specific objectives include: (i) determination of Mg2+ ion dissolution rate; (ii) investigation of the impact of HCO3− ions on struvite precipitation; (iii) assessment of the role of electrochemical reactions on magnesium carbonate formation using the sacrificial chemical precipitation (SCP) method; and (iv) evaluation of the techno-economic feasibility of a 100 L.d−1 MAFC system installed downstream of urinals. The findings aim to address the challenges associated with nutrient recovery as struvite from source separated urine and provide insights for optimising the operational parameters of an MAFC.
2 Materials and methods
2.1 Materials
Magnesium alloy AZ31 with a thickness of 4 mm was procured from NextGen steels and alloys, Maharashtra, India. The alloy is composed of 95.68% Mg, 2.85% Al, 0.91% Zn, and 0.31% Mn. A 60 Wt.% solution of Polytetrafluoroethylene (PTFE) and vulcan carbon black powder were purchased from Sainergy Fuel Cells, Chennai, India. Stainless steel wire mesh with 1 mm aperture was procured from Banaraswala wire mesh, Coimbatore, India. All chemicals and solvents used for physio-chemical analysis were of analytical reagent grade with a purity >99%, obtained from Merck, India, and used without further purification. Deionised water (<2 μS.cm−1) was used throughout the study.
2.2 Construction of MAFC
The MAFCs, with an effective volume of 500 mL, were constructed using transparent plexiglass with a thickness of 5 mm as shown in Supplementary Figures S1A–C. Magnesium alloy AZ31 (dimensions: 7 × 7 cm) and an air cathode (dimensions: 10 × 10 cm) were used as the anode and cathode, respectively. The air cathode constitutes three layers: (i) a hydrophilic catalyst layer facing the electrolyte; (ii) a stainless-steel wire mesh acting as current collector; and (iii) a hydrophobic gas diffusion layer exposed to the atmosphere (Supplementary Figure S1D). The air cathode (600 µm thickness) was prepared as described in our previous study (Sangeetha et al., 2024). The interelectrode distance was maintained as 2.1 cm and an external resistance of 22 Ω was applied between the anode and cathode.
2.3 Experimental design
2.3.1 Magnesium dissolution rate
In an MAFC, Mg2+ ions are released into the electrolyte through spontaneous oxidation of the anode. Determining the Mg2+ dissolution rate is crucial to estimate the quantity of Mg2+ required when employing the SCP method. To compute the Mg2+ dissolution rate, synthetic urine was used as an electrolyte and Mg2+ ion concentration was measured at hourly intervals. The composition of synthetic urine is presented in Supplementary Table S1. The Mg2+ dissolution rate was determined to be 10 mg. L−1 h−1, and followed the zero-order kinetics (Supplementary Figure S2).
2.3.2 Nutrient recovery experiments
Nutrient recovery experiments were conducted in both MAFC and SCP methods under the conditions outlined in Table 1. The experimental condition EX1 represents 1:1:1:0 M ratio of Mg2+: NH4+: PO43−: HCO3−, where X denotes “MAFC” for experiments conducted in the MAFC system and “SCP” for those conducted using SCP method. The preparation of aqueous solutions for the experimental conditions are provided in the Supplementary Table S2. In the MAFC experiments, the system was operated in closed circuit mode with a resistance of 22 Ω, connected across the anode and cathode. For the SCP method, MgCl2 was added at a rate mimicking the Mg2+ dissolution rate in an MAFC. Specifically, 1 mL of MgCl2 solution (1 M concentration) was added drop wise to maintain a consistent Mg2+ ion introduction rate of 10 mg. L−1h−1. All experiments were performed in duplicates to minimise the errors, and the results are presented as average values ±standard deviation.
2.4 Analytical methods
Samples were collected from the experimental setups at intervals of t = 4 h and filtered through 0.2 μm syringe filters (Omicron, diameter: 25 mm, pore size: 0.2 μm). The filtrate was analyzed for various physicochemical parameters, as outlined Table 2. The removal efficiency was calculated using Equation 4.
where, Ci and Cf are the initial and final concentrations, respectively, in mg. L−1. The open-circuit voltage (OCV) of MAFCs was recorded using a data acquisition system (Keithley DAQ6510). Polarization studies were conducted by varying the external resistors (100 KΩ - 10 Ω), and power density (W. m−2) and current density (A. m−2) were calculated based on the anode surface area under all experimental conditions.
2.5 Analysis of precipitates
The precipitates were separated from the supersaturated aqueous solution after achieving the PO43− removal efficiency >95%. The supersaturated solution was centrifuged at 7,000 rpm for 30 min. The precipitates were repeatedly washed and centrifuged with deionized water to remove the impurities, followed by oven drying at 60°C for 12 h (Thermoscientific, Heratherm, Massachusetts, United States). The dried precipitates were homogenized and characterized for their X-ray diffraction (XRD) pattern. The analysis was conducted over 2θ range from 10° to 80° using an X-ray diffractometer (Rigaku, XRD Smart Lab, Japan) equipped with Cu Kα radiation with a wavelength (λ) of 1.5406 Å. Also, the chemical bonds and functional groups in the dried precipitates were identified using Fourier transform infrared spectroscopy (FTIR) (Shimadzu Scientific Instruments, IR Tracer 100, Japan) in the range of 400–4,000 cm−1 (transmission mode) using KBr pellet method.
3 Results and discussion
3.1 Phosphate removal efficiency
The PO43− removal efficiencies for EMAFC1, EMAFC2, EMAFC3, EMAFC4, EMAFC5, and EMAFC6 at t = 24 h were 82.19 ± 3.33, 75.72 ± 0.5, 47.04 ± 1.82, 31.41 ± 3.65, 31.89 ± 4.54, and 46.42% ± 0.975%, respectively (Figure 1a). Similarly, PO43− removal efficiencies for ESCP1, ESCP2, ESCP3, ESCP4, ESCP5, and ESCP6 at t = 24 h were 45.2 ± 2.5, 48.5 ± 4.4, 28.42 ± 0.42, 24.76 ± 1.04, 25.74 ± 2.295, and 21.3% ± 2.66%, respectively. It was observed that during the first 24 h, the PO43− removal efficiency in the MAFC systems was higher than in the SCP system. This can be ascribed to the alkaline condition in the MAFC, resulting from the generation of OH− ions, as a product of oxygen reduction reaction (ORR) at the electrolyte-cathode interface. The ORR increases the pH, creating a super saturated urine environment conducive for struvite precipitation when Mg2+ ions are introduced into the solution. Conversely, in the SCP method, the PO43- removal exhibited a slower trend, likely due to the absence of ORR, which delayed the attainment of supersaturation.
At t = 48 h, the PO43− removal efficiencies for EMAFC1, EMAFC2, EMAFC3, EMAFC4, EMAFC5, and EMAFC6 increased to 95.33 ± 0.37, 86.02 ± 0.7, 71.62 ± 3.53, 59.65 ± 4.5, 54.48 ± 9.86, and 73.64% ± 0.45%, respectively (Figure 1b). Similarly, the efficiencies for ESCP1, ESCP2, ESCP3, ESCP4, ESCP5, and ESCP6 were determined to be 96.9 ± 0.5, 94.1 ± 0.3, 86.98 ± 2.24, 84.82 ± 6.71, 82.93 ± 1.715, and 92.7% ± 0.37%, respectively. It is evident that, after 24 h, the PO43− removal efficiency in MAFC systems decreased, which can be attributed to the passivation phenomenon hindering Mg dissolution and, consequently, PO43− removal. In contrast, the SCP systems exhibited near complete PO43− removal due to the gradual attainment of supersaturation conditions, as evidenced by increasing electrical conductivity (Supplementary Figures S4C, D). This facilitated effective PO43− removal in all SCP cases.
3.2 Influence of bicarbonate ions
At t = 24 h, approximately 40% of the HCO3− ions were precipitated in both MAFC and SCP systems in all conditions, indicating co-precipitation with PO43− ions (Figures 2a–d). In the MAFC system, the HCO3− ions were removed either through precipitation into the solution or via anode passivation. A decline in PO43− removal efficiency was observed in both MAFC and SCP system as the relative molar ratio of HCO3− ions increased (Figures 1a,b). In the MAFC system, this decline could be attributed to anode passivation caused by magnesium carbonates. Conversely, in the SCP systems, the reduction in PO43− removal efficiency was likely due to failure to attain super saturation condition. However, in EMAFC6 and ESCP6, the electrolyte reached supersaturation with electrical conductivity values of 90–96 mS.cm−1 (Supplementary Figures S4C, D). This supersaturation would have facilitated co-precipitation of HCO3− and PO43− ions, improving the removal efficiency under these conditions.
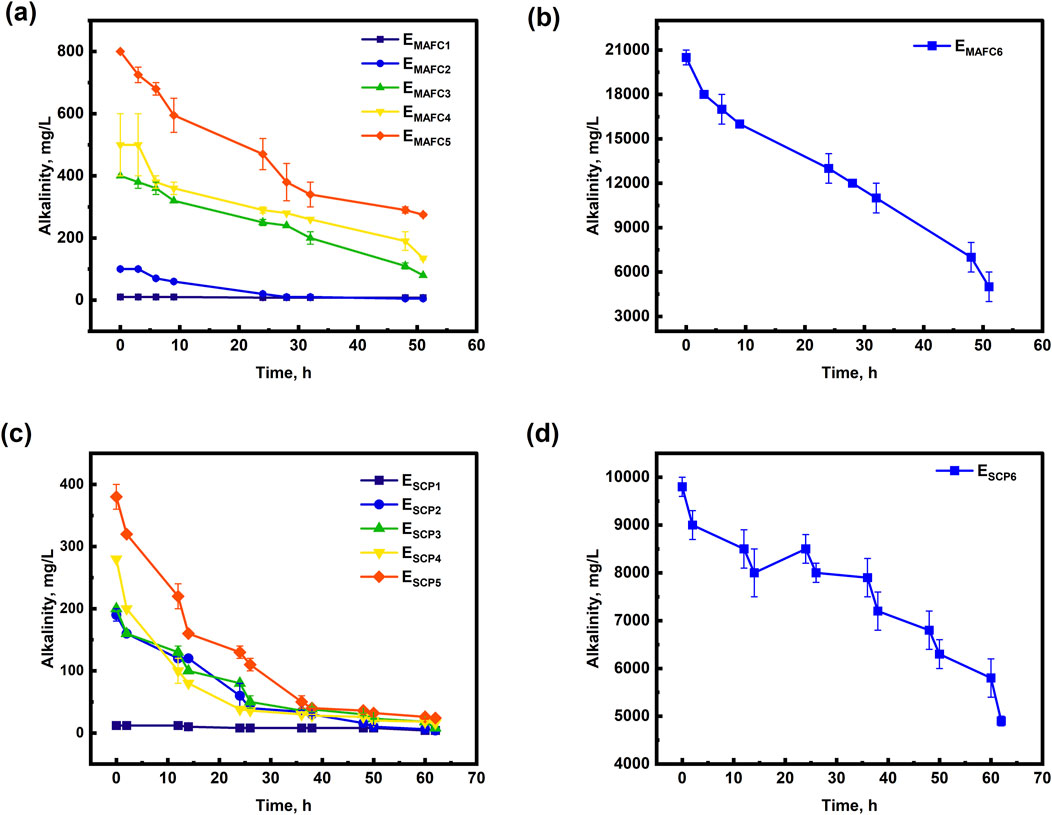
Figure 2. Temporal variation of alkalinity in experimental conditions (a) EMAFC1–5, (b) EMAFC6 and (c) ESCP1-5 (d) ESCP6.
3.3 Characterisation of recovered precipitate
The X-ray diffractograms of the precipitates EX1, EX2, and EX4, correlated well with standard struvite (JCPDS card no: 00-015-0762) (Figures 3a,b,d) in both MAFC and SCP systems. In these cases, only PO43− and NH4+ were present, with Mg2+ as the limiting factor. Since no interfering ions were present, struvite readily precipitated upon the addition of Mg2+ ions. Whereas, the precipitate from EX3, was identified as K-Struvite (MgKPO4. 6H2O) (card no: 01-075-1076) under both MAFC and SCP conditions (Figure 3c). K-Struvite forms under similar conditions to those for struvite precipitation, but requires K+ ions instead of NH4+ ions. Although, an equimolar ratio of PO43− and NH4+ was maintained in EX3, NH4+ did not participate in struvite formation. Instead, K+ from KH2PO4 reacted with PO43− and Mg2+, resulting in K-Struvite precipitation. In EX4, the relatively high pH (ranging from 9 to 9.5) (Supplementary Figures S3A, B) likely caused NH4+ to deprotonate into NH3 gas, which facilitated K-struvite precipitation. However, NH4+ removal was also observed, suggesting that the deprotonated NH4+ was removed from the system as NH3 (Supplementary Figure S6A). The precipitate from EX5 was identified as hazenite (KNaMg2 (PO4)2·14H2O) (Figure 3e), which is attributed to the high alkalinity in the system due to HCO3− ions resulting from urea hydrolysis (Figures 2a,c). Hazenite is also a potential fertiliser that releases P at a faster rate than struvite and typically precipitates under strongly alkaline conditions (Lapinkangas et al., 2022). In EX6, which mimicked the molar ratio of real hydrolysed urine, the presence of pokrovskite (Mg2(CO3) (OH)2·0.5(H2O) (Figure 3f) was identified in MAFC and SCP systems. This highlights that excess of alkalinity (HCO3− ions) (Figures 2b,d) impairs PO43− removal and reduces struvite purity.
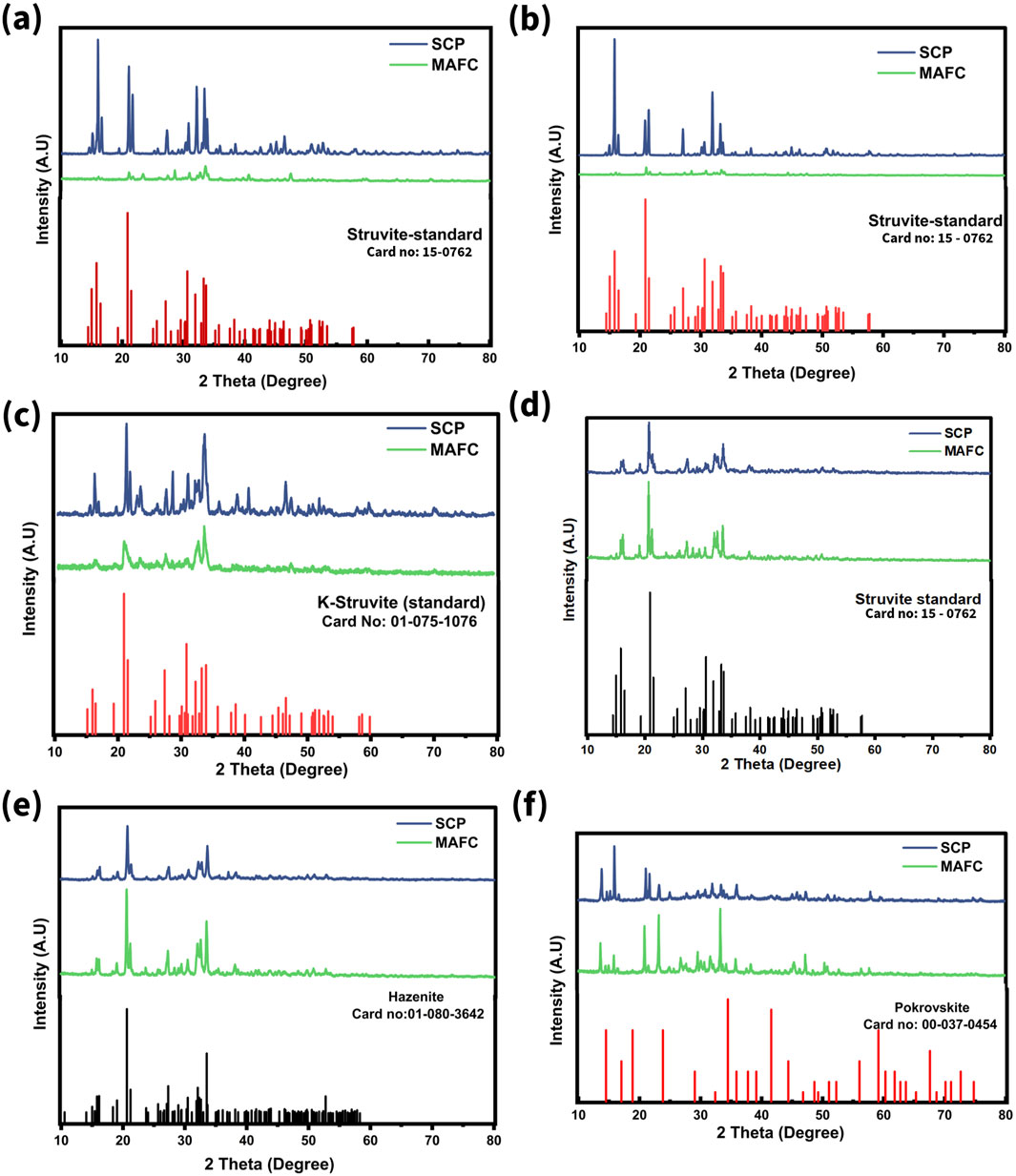
Figure 3. X-ray diffractogram of precipitates recovered in magnesium air fuel cell and by sacrificial chemical precipitation method (a) EX1, (b) EX2, (c) EX3, (d) EX4, (e) EX5, and (f) EX6.
Moreover, the XRD patterns of ESCP1 and ESCP2 displayed sharp peaks, indicating high crystallinity. In the SCP systems, continuous stirring promoted nucleation and adequate crystal growth. Conversely, in the MAFC systems, despite continuous stirring, factors such as the presence of electrodes, reactor dimensions, and electrochemical reactions may have hindered nucleation and crystal growth. As the HCO3− ion concentration increased, peak broadening effect (evidenced by high full width half maxima), were observed, indicating the formation of an amorphous precipitate. This phenomenon negatively impacted struvite purity in the MAFC systems. Furthermore, the FT-IR spectra of the precipitates are presented in Supplementary Figures S7A–F. The bands from 560 to 750 cm−1 represent the stretching vibrations of Mg-O bond. The characteristic peaks around 854–892 cm−1 denote the asymmetric stretching vibration of PO4. The bands at 1,023 cm−1 are due to the ν1 asymmetric stretching of PO4. The broad bands at 3,284, 3,340 cm−1 and peaks between 1,420–1,645 cm−1 correspond to the N-H stretching vibrations. The H-O-H stretching of water of crystallisation is observed in the range of 2,318–2,478 cm‒1. Moreover, the asymmetric stretching and bending vibration bands of CO32− were observed at 1,420 cm−1 and in the range of 700–880 cm−1, respectively (Wang et al., 2023).
3.4 Electrochemical performance of the MAFC
The OCV of the MAFCs under various experimental conditions remained consistent ranging from 1.4 to 1.6 V. However, the power densities increased as the concentration of HCO3− ions increased. The maximum power densities observed for EMAFC1, EMAFC2, EMAFC3, EMAFC4, EMAFC5, and EMAFC6 were 0.173, 0.137, 0.20, 0.238, 0.260, and 2.06 W.m−2, respectively (Figures 4a–f). This increase in power densities correlates with the electrical conductivities of the aqueous solutions (Supplementary Figures S4A–D). At t = 3 h, Mg2+ dissolution and subsequent struvite precipitation would have led to a CE < 100% (Figure 5). By t = 24 h, the CE exceeded 100%, suggesting that Mg2+ ions are no longer participating in PO43− removal. Instead, they either remain in the aqueous solution or precipitate as magnesium carbonates. At this stage, struvite precipitation becomes sluggish as evidenced from the PO43− removal efficiencies for experimental cases from EMAFC2 to EMAFC5, likely due to the non-participation of Mg2+ ions, which is also reflected in the high Mg2+ ion concentration (Supplementary Figure S5A). However, at t = 48 h, the CE remained below 100%, indicating that Mg2+ ions are actively facilitating PO43− removal.
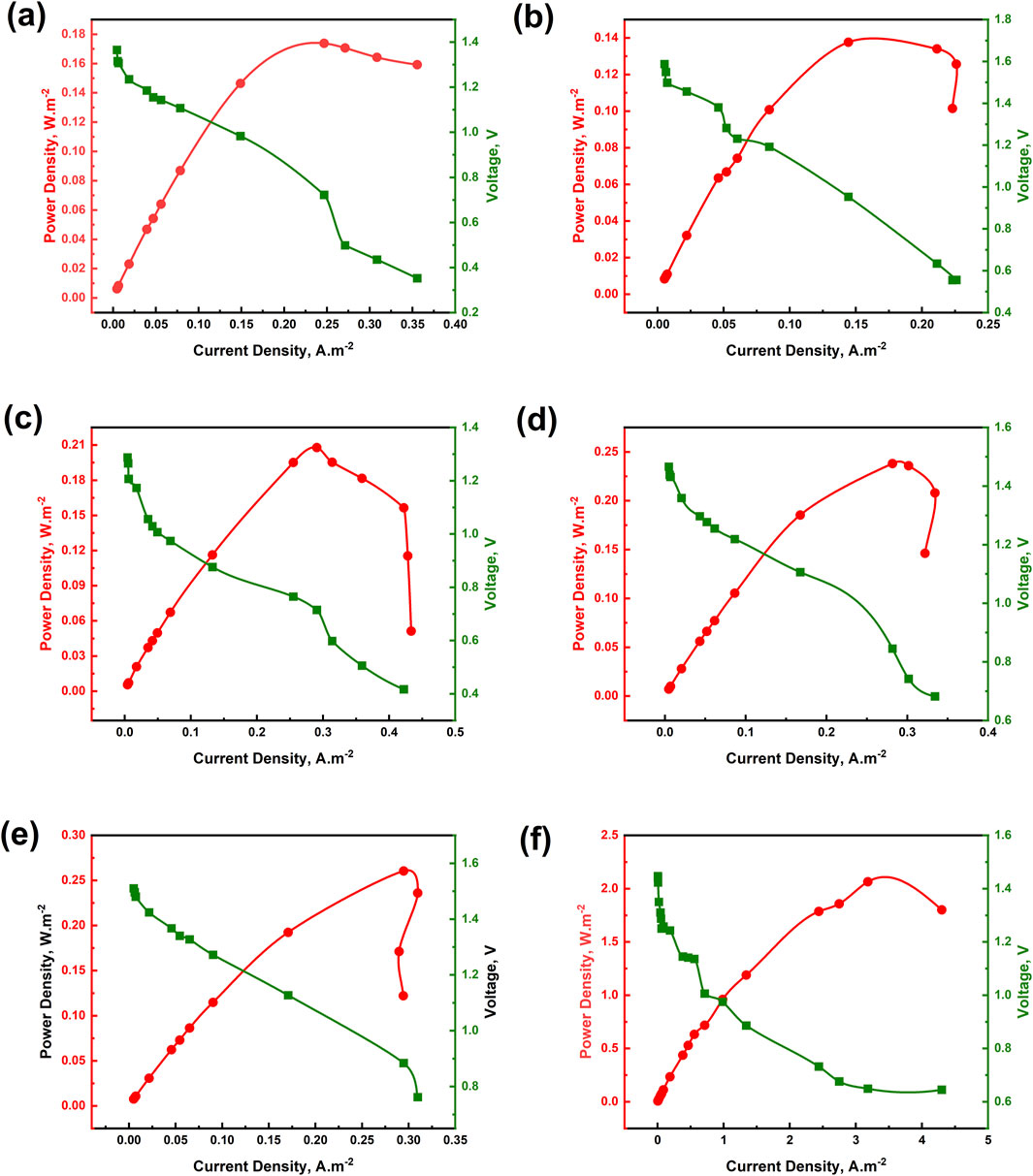
Figure 4. Polarisation curve of MAFC at different experimental conditions (a) EMAFC1, (b) EMAFC1, (c) EMAFC1, (d) EMAFC1, (e) EMAFC1, and (f) EMAFC1.
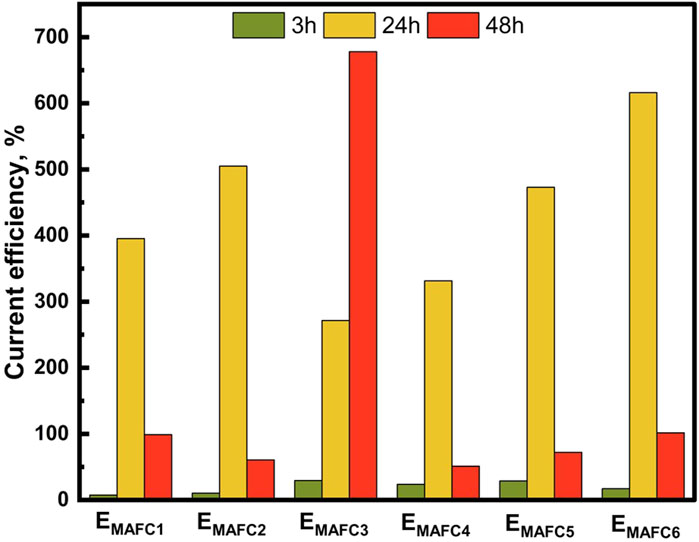
Figure 5. Current efficiency at t = 3, 24, and 48 h in MAFCs under different experimental conditions.
4 Elucidation of preferential formation of carbonates of magnesium over struvite
In an MAFC, magnesium is spontaneously oxidised at the anode to release Mg2+ ions, which react with PO43− and NH4+ ions to form struvite under favourable conditions. Simultaneously, oxygen diffuses across the GDE and is reduced to OH− ions at the CL-electrolyte interface by accepting electrons from the anode through the current collector. This ORR reaction increases the pH, making the environment alkaline, and facilitates struvite recovery. The aqueous solutions in this study contained cations such as K+, Na+, NH4+, as well as anions like Cl−, H2PO4−, HCO3−.
In the case of EX1, only PO43− and NH4+ ions were present. Upon addition of Mg2+, high quality struvite is formed. However, for EX2 to EX5, HCO3− ion were present along with other anions (Cl−, H2PO4−). Based on the X-ray diffractogram, struvite or K-struvite was precipitated in all cases except EX5 and EX6. It is hypothesised that during early stages (phase I), the H2PO4− buffer system is activated by the addition of OH− ions through the ORR, owing to its low pKa value of 7.2 (Equation 5). The resulting PO43- ions participate in struvite precipitation, gradually decreasing the PO43- concentration in the system. In phase II, the HCO3− ions buffer the incoming OH− ions, and the forward reaction is favoured, producing CO32− ions (Equation 6). The buffering action of HCO3− ions follows H2PO4− ion due to the former’s higher pKa value (10.3) compared to pKa value of H2PO4− (7.3) (Figure 6). The produced CO32− ions react with Mg2+ ions to precipitate as pokrovskite (Mg2(CO3) -(OH)2·0.5(H2O) or any other magnesium carbonates. Moreover, the pH stabilisation at 9–9.5 (Supplementary Figure S3) under high bicarbonate molar ratio, supports the hypothesis that HCO3− buffers the incoming OH−, shifting the equilibrium towards CO32− formation (Equation 6). The formation of CO32− ions at these pH levels leads to the precipitation of magnesium carbonates rather than struvite. In EX2 to EX5, the precipitation of magnesium carbonates was limited since the reaction was stopped as the PO43− removal efficiency reached 90%.
For EX6, the molar ratio of ions mimics that of real hydrolysed source separated urine (Mg2+: NH4+:PO43−: HCO3− = 1:90:1:120). While PO43− ions are present, the molar ratio of HCO3− ion was 120 times greater than PO43− ions, causing HCO3− ions to predominate and precipitate as magnesium carbonates. This highlights the importance of removing alkalinity or HCO3− ions from source separated urine to recover high quality struvite. However, there is a possibility of co-precipitation of PO43− along with HCO3− during the removal of alkalinity. The HCO3− ions or alkalinity in source separated urine are introduced through urea hydrolysis (Equation 7).
Studies have reported that urea hydrolysis can be inhibited by the addition of acids (e.g., acetic acid, citric acid, vinegar) (Ray et al., 2018) or alkalis (MgO, Mg (OH)2, and their mixtures) (Vasiljev et al., 2022).
5 Techno-economic assessment
The techno economic assessment was conducted based on the following assumptions: (i) The MAFC nutrient recovery system is to be installed downstream of a urinal complex (Figure 7). The quantity of urine generated is assumed to be 100 L.d−1. Additionally, the collected urine is source separated, undiluted, and stored in a collection tank, which also serves as an equalisation tank; (ii) The working volume of a single MAFC reactor is 500 mL. It is assumed that, approximately 100 MAFCs will be required to recover nutrients (1 Kg from 1,000 L) from the urine, with an operating hydraulic retention time of 12 h; (iii) The total cost of constructing the nutrient recovery system was calculated based on the Indian market rates, but it is presented in US dollars to provide a global perspective; (iv) The quantity of struvite recovered was 1 g.L−1 as referenced from literature (Krishnamoorthy et al., 2021). The energy generated by the MAFC system was calculated based on our observations, where one MAFC generates 6.4 W of power from 500 mL of urine; (v) To pump the collected urine from the equalisation tank, a pump requiring 23 W for operation is used; and (vi) The revenue generated from sale of struvite was calculated based on its agronomic performance, which is similar to triple super phosphate, a commercial fertiliser (Hall et al., 2020). In India, the market rate of TSP is INR 483 per 50 Kg bag (USD 5.78 approximately) (Source: https://agritech.tnau.ac.in/agriculture/agri_nutrientmgt_priceoffertilizers.html). Additonally, the cost of 1 unit electricity (1 KWh) is INR 6.9 (USD 0.083 approximately). The cost breakdown for capital expenditure (CAPEX), operating expenditure (OPEX), revenue generated, and maintenance costs are outlined in Table 3.
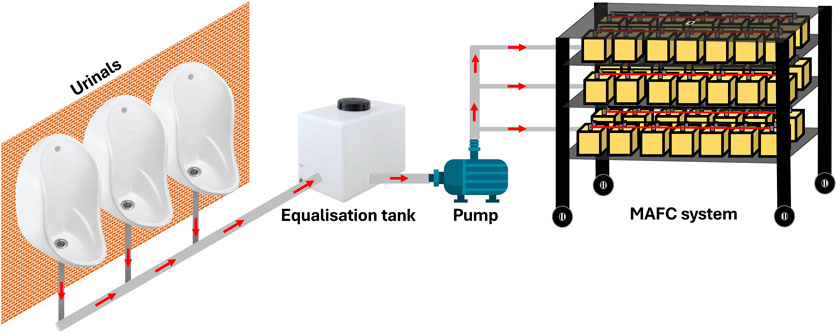
Figure 7. Illustration of application of MAFC system for resource (nutrient and energy) from the urinals.
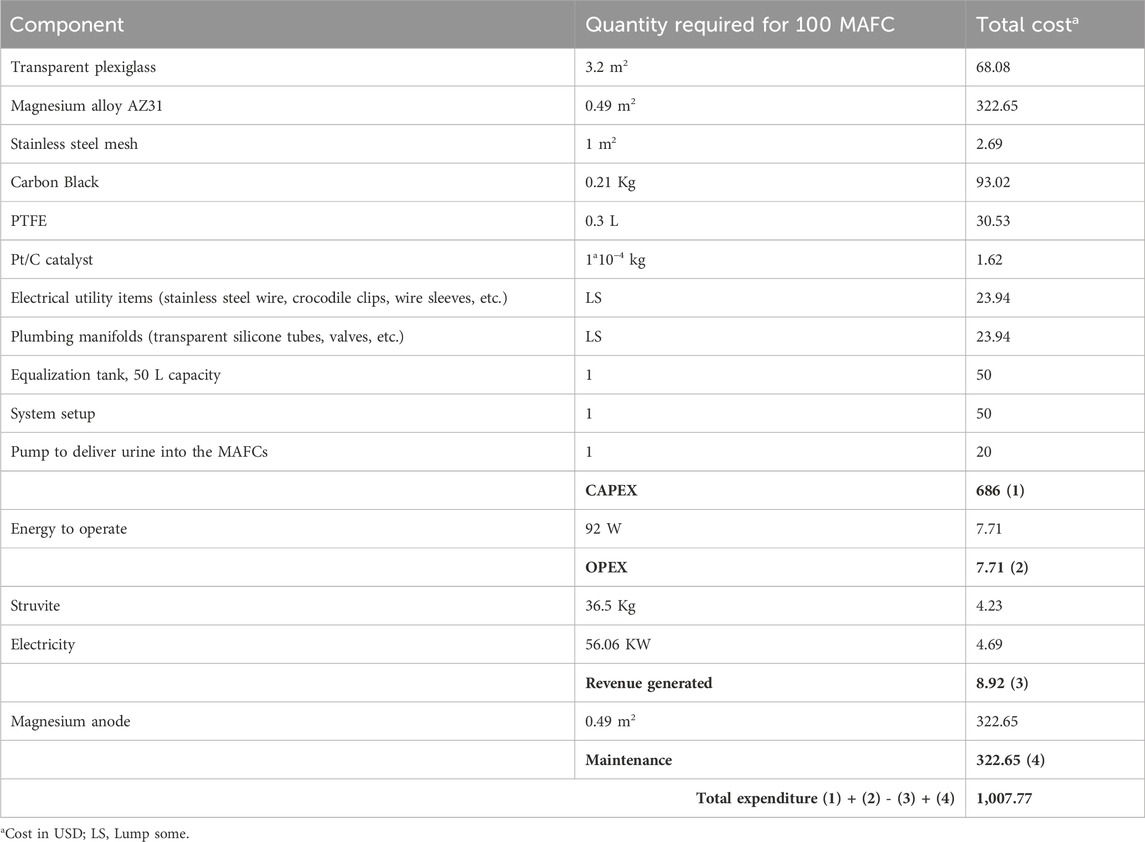
Table 3. Breakdown of cost for MAFC as resource (nutrient and energy) recovery system in the downstream of urinals.
As shown in Figure 8, the cost of magnesium alone contributes to 47% of the CAPEX. Magnesium is essential as the anode material in the MAFC, facilitating both struvite precipitation and energy production from source separated urine. However, the longevity of the magnesium anode can be improved by: (i) modifying its microstructure; (ii) alloying with aluminium (Al), tin (Sn), zinc (Zn), lithium (Li), manganese (Mn), lead (Pb), indium (In), and numerous rare earth metals; and (iii) applying surface modifications with metal oxide or metal hydroxide coatings (Shahzira and Sahriah, 2021). By improving the longevity of magnesium anode, the maintenance cost, which is currently 47% of the CAPEX could be delayed or reduced. The components of the air cathode represent 18.6% of the CAPEX, which is about 39.4% of the anode cost, even though a Pt/C catalyst is used. Other major contributors to the CAPEX include plexiglass (9.9%), the equalisation tank (7.3%), and system setup (7.3%), which are integral parts of the resource recovery system. However, replacing plexiglass with low-cost polymer or refurbished materials could significantly reduce the CAPEX. The only operating expenditure (OPEX) is the power for equalising the urine flow into the MAFC system, which forms only 0.76% of the total expenditure. The revenue generation from the sale of struvite is USD 8.92, which is 0.85% of the total expenditure. This necessitates increasing the value proposition by recovering ammonia and water from source separated urine. It is very certain that MAFCs are an ideal choice for concurrent resource (nutrient and energy) recovery with a theoretical energy density and voltage of 2,843 Wh. Kg−1 and 3.09 V, respectively (Shahzira and Sahriah, 2021).
6 Conclusion
This study aimed to investigate the influence of the relative molar concentration of HCO3− ions on struvite precipitation. A 90% phosphate removal efficiency was achieved within 48 h using both MAFC and sacrificial chemical precipitation methods. Additionally, XRD analysis revealed a transition of the recovered precipitate from a crystalline to an amorphous form, ascribed to the presence of HCO3− ions. It is evident that the Mg2+: NH4+:PO43−: HCO3− molar ratios play a significant role in struvite precipitation, when real source separated urine is used. The study also provided insights to optimize the system for a hydraulic retention time well below the time required for bicarbonate buffer system to act. Moreover, the techno-economic assessment revealed that 47% of the CAPEX is attributed to the cost of the magnesium anode. However, revenue generation is only 8.92 USD, representing just 0.85% of the total expenditure, highlighting the need to enhance the value proposition by recovering ammonia and water from source separated urine.
Data availability statement
The original contributions presented in the study are included in the article/Supplementary Material, further inquiries can be directed to the corresponding author.
Author contributions
VS: Conceptualization, Data curation, Formal Analysis, Investigation, Methodology, Writing – original draft. RK: Writing – review and editing, Formal Analysis, Investigation. GK: Formal Analysis, Investigation, Writing – review and editing, Methodology. PG: Methodology, Writing – review and editing, Conceptualization, Funding acquisition, Project administration, Resources, Supervision, Visualization.
Funding
The author(s) declare that financial support was received for the research and/or publication of this article. The authors wish to thank the Science for Equity Empowerment and Development (SEED) division of Department of Science and Technology (DST), Government of India, for providing the financial support in the execution of the project (Project grant No. SEED/SCSP/2021/166/G1).
Acknowledgments
The authors are also thankful for facilitating material characterization by the central instrumentation facility (CIF) of Indian Institute of Technology Palakkad.
Conflict of interest
The authors declare that the research was conducted in the absence of any commercial or financial relationships that could be construed as a potential conflict of interest.
Generative AI statement
The author(s) declare that no Generative AI was used in the creation of this manuscript.
Publisher’s note
All claims expressed in this article are solely those of the authors and do not necessarily represent those of their affiliated organizations, or those of the publisher, the editors and the reviewers. Any product that may be evaluated in this article, or claim that may be made by its manufacturer, is not guaranteed or endorsed by the publisher.
Supplementary material
The Supplementary Material for this article can be found online at: https://www.frontiersin.org/articles/10.3389/fenvc.2025.1538100/full#supplementary-material
References
Cordell, D., Rosemarin, A., Schröder, J. J., and Smit, A. L. (2011). Towards global phosphorus security: A systems framework for phosphorus recovery and reuse options. Chemosphere 84 (6), 747–758. doi:10.1016/j.chemosphere.2011.02.032
Cid, C. A., Jasper, J. T., and Hoffmann, M. R. (2018). Phosphate recovery from human waste via the formation of hydroxyapatite during electrochemical wastewater treatment. ACS Sustain Chem Eng. 6 (3), 3135–3142. doi:10.1021/acssuschemeng.7b03155
Dong, T., Li, X., Zhao, Z., and Wang, R. (2024). Comprehensive impacts of the presence of proton exchange membrane on the efficiency of Mg-air fuel cell to remove nutrients and recover struvite. J. Water Process Eng. 62, 105340. doi:10.1016/j.jwpe.2024.105340
Gangadharan, P., Rajumon, R., Sibi, R., and Peter, A. E. (2022). Osmotic urine fuel cell to recover water, energy, and nutrients along with salinity reduction. J. Appl. Electrochem 52, 1597–1606. doi:10.1007/s10800-022-01738-2
Gangadharan, P., Vadekeetil, A., Sibi, R., and Sheelam, A. (2021). Concentrating nutrients and recovering water and energy from source separated urine using osmotic microbial fuel cell. Chemosphere 285, 131548. doi:10.1016/j.chemosphere.2021.131548
Glasser, F. P., Jauffret, G., Morrison, J., Galvez-Martos, J. L., Patterson, N., and Imbabi, M. S. E. (2016). Sequestering CO 2 by mineralization into useful nesquehonite-based products. Front. Energy Res. 4. doi:10.3389/fenrg.2016.00003
Hall, R. L., Boisen Staal, L., Macintosh, K. A., McGrath, J. W., Bailey, J., Black, L., et al. (2020). Phosphorus speciation and fertiliser performance characteristics: a comparison of waste recovered struvites from global sources. Geoderma 362, 114096. doi:10.1016/j.geoderma.2019.114096
Inan, H., and Alaydin, E. (2014). Phosphate and nitrogen removal by iron produced in electrocoagulation reactor. Desalination Water Treat. 52, 1396–1403. doi:10.1080/19443994.2013.787950
Kim, J. H., An, B. min, Lim, D. H., and Park, J. Y. (2018). Electricity production and phosphorous recovery as struvite from synthetic wastewater using magnesium-air fuel cell electrocoagulation. Water Res. 132, 200–210. doi:10.1016/j.watres.2018.01.003
Krishnamoorthy, N., Arunachalam, T., and Paramasivan, B. (2021). A comparative study of phosphorus recovery as struvite from cow and human urine. Mater Today Proc. 47, 391–395. doi:10.1016/j.matpr.2021.04.587
Lapinkangas, S., Rautio, L., Kauppinen, T., Hu, T., Pesonen, J., and Lassi, U. (2022). Precipitation of potassium as hazenite from washing water of spent alkaline batteries. Chem. Eng. J. Adv. 12, 100426. doi:10.1016/j.ceja.2022.100426
Liao, M., Liu, Y., Tian, E., Ma, W., and Liu, H. (2020). Phosphorous removal and high-purity struvite recovery from hydrolyzed urine with spontaneous electricity production in Mg-air fuel cell. Chem. Eng. J. 391, 123517. doi:10.1016/j.cej.2019.123517
Liu, Y., Deng, Y. Y., Zhang, Q., and Liu, H. (2021). Overview of recent developments of resource recovery from wastewater via electrochemistry-based technologies. Sci. Total Environ. 757, 143901. doi:10.1016/j.scitotenv.2020.143901
Mahmood, A., Kim, J. H., and Park, J. W. (2023). Development of an effective operation system in a magnesium-air desalination cell for electricity production with nitrogen and phosphorus removal. Desalination 545, 116164. doi:10.1016/j.desal.2022.116164
Penuelas, J., Coello, F., and Sardans, J. (2023). A better use of fertilizers is needed for global food security and environmental sustainability. Agricul. Food. Secur. 12:5, 1–9. doi:10.1186/s40066-023-00409-5
Pokharel, R., Popa, I. C., de Kok, Y., and King, H. E. (2024). Enhanced nesquehonite formation and stability in the presence of dissolved silica. Environ. Sci. Technol. 58, 362–370. doi:10.1021/acs.est.3c06939
Ray, H., Saetta, D., and Boyer, T. H. (2018). Characterization of urea hydrolysis in fresh human urine and inhibition by chemical addition. Environ. Sci. (Camb) 4, 87–98. doi:10.1039/c7ew00271h
Sabin, J. M., Leverenz, H., and Bischel, H. N. (2022). Microbial fuel cell treatment energy-offset for fertilizer production from human urine. Chemosphere 294, 133594. doi:10.1016/j.chemosphere.2022.133594
Sangeetha, V., Devasena, M., Nambi, I. M., and Dwarakanathan, S. (2022). Crystallization of struvite family crystals from cow urine: analysis, characterization, and effects of crystallization method, retention time, rate of mixing, and competing ions. Biomass Convers. Biorefin 14, 2357–2368. doi:10.1007/s13399-022-02452-x
Sangeetha, V., Kuppurangan, G., and Gangadharan, P. (2024). Stale urine catalysed resource recovery from source separated urine using magnesium air fuel cell: insights into the mechanism, its implications and challenges. Sep. Purif. Technol. 330, 125295. doi:10.1016/j.seppur.2023
Shahzira, N., and Sahriah, H. (2021). Critical review on development of magnesium alloy as anode in Mg-Air fuel cell and additives in electrolyte. Int. J. Energy Res 45, 15739–15759. doi:10.1002/er.6881
Taylor, P., Liu, X., Hu, Z., Mu, J., Zang, H., and Liu, L. (2014). Phosphorus recovery from urine with different magnesium resources in an air-agitated reactor. Environ. Technol. 37–41. doi:10.1080/09593330.2014.921732
Vasiljev, A., Simha, P., Demisse, N., Karlsson, C., Randall, D. G., and Vinnerås, B. (2022). Drying fresh human urine in magnesium-doped alkaline substrates: capture of free ammonia, inhibition of enzymatic urea hydrolysis and minimisation of chemical urea hydrolysis. Chem. Eng. J. 428, 131026. doi:10.1016/j.cej.2021
Wang, W., Li, W., Huang, F., Zheng, C., Liu, T., and Liu, C. (2023). Study on the application of two magnesium salts in low temperature adsorption refining of fragrant rapeseed oil. LWT - Food Sci. Technol. 188, 115399. doi:10.1016/j.lwt.2023.115399
Willis, R. B., Montgomery, M. E., and Allen, P. R. (1996). Improved method for manual, colorimetric determination of total kjeldahl nitrogen using salicylate. J. Agric. Food Chem. 44, 1804–1807. doi:10.1021/jf950522b
Wu, H., and Vaneeckhaute, C. (2022). Nutrient recovery from wastewater: a review on the integrated Physicochemical technologies of ammonia stripping, adsorption and struvite precipitation. Chem. Eng. J. 433, 133664. doi:10.1016/j.cej.2021.133664
Wu, X., Xie, R., Ding, J., Dai, L., Ke, X., Liu, Y., et al. (2022). Recovery of phosphate and ammonium nitrogen as struvite from aqueous solutions using a magnesium – air cell system. Sci. Total Environ. 819, 152006. doi:10.1016/j.scitotenv.2021.152006
Keywords: magnesium air fuel cell, struvite, magnesium carbonates, sacrificial chemical precipitation, hazenite, pokrovskite
Citation: Sangeetha V, Koshy RA, Kuppurangan G and Gangadharan P (2025) Comprehensive insights into the preferential precipitation of carbonates of magnesium in magnesium air fuel cell system. Front. Environ. Chem. 6:1538100. doi: 10.3389/fenvc.2025.1538100
Received: 02 December 2024; Accepted: 28 April 2025;
Published: 12 May 2025.
Edited by:
Muhammad Usman Khan, Washington State University, United StatesReviewed by:
Yu Rong tai, Jingdezhen Ceramic University, ChinaHector Alfredo Calderon, National Polytechnic Institute (IPN), Mexico
Copyright © 2025 Sangeetha, Koshy, Kuppurangan and Gangadharan. This is an open-access article distributed under the terms of the Creative Commons Attribution License (CC BY). The use, distribution or reproduction in other forums is permitted, provided the original author(s) and the copyright owner(s) are credited and that the original publication in this journal is cited, in accordance with accepted academic practice. No use, distribution or reproduction is permitted which does not comply with these terms.
*Correspondence: Praveena Gangadharan, cHJhdmVlbmFnQGlpdHBrZC5hYy5pbg==