- 1NSW Department of Primary Industries, Narrandera Fisheries Centre, Narrandera, NSW, Australia
- 2Gubali Institute for Agriculture, Water and Environment, Charles Sturt University, Albury, NSW, Australia
- 3Fishway Consulting Services, St Ives Chase, NSW, Australia
- 4Fisheries Conservation Technology Unit, NSW Department of Primary Industries, National Marine Science Centre, Southern Cross University, Coffs Harbour, NSW, Australia
- 5School of the Environment, The University of Queensland, Brisbane, QLD, Australia
River development affects fish connectivity, with intra-river issues exacerbated via sequential barriers. Remediation typically involves installing ‘fishways' to facilitate upstream movements. Here we evaluated species-specific upstream fish-passage efficiencies through three sequential vertical-slot fishways along the Nepean River in Australia via paired entry and exit trapping. Species-specific water velocity preferences associated with fishway entrance were informed by restricting head loss at the entry traps, but not at the exit traps. During 78 paired trap deployments 26,139 fish were caught, comprising 19 species; most of which successfully negotiated the fishways—albeit with considerable inter- and intra-specific variability among fishways. Catches of the most abundant species (38% of total), the amphidromous Gobiomorphus coxii (20–160 mm total length; TL), in the entry and exit traps were negatively and positively affected by water velocity, respectively at the second and third fishways, but not at the first. Catches of other species were also directly or indirectly affected by water velocity, with fewer catadromous Trachystoma petardi (145–460 mm fork length; FL) and Mugil cephalus (35–410 mm FL) recorded in entry than exit traps, implying (1) insufficient water velocity to permit entry and/or (2) confounding effects of the entry-trap design on capture. Conversely, two gudgeons [the potamodromous Philypnodon grandiceps (29–77 mm TL) and Hypseleotris galii (31–49 mm TL)] were caught in significantly greater abundances in the entry than exit traps implying some restriction to their passage and possibly due to deficits in fishway hydraulics and/or a lack of motivation to migrate in these species. The study highlights the value of location-specific monitoring for identifying key factors affecting fishway performance.
1 Introduction
Growing demand for reliable water supplies to support agriculture, hydropower and human consumption is increasing pressure on global water resources and their associated biota, especially fish (1). Currently, only ~23% of rivers worldwide flow unimpeded to oceans (2), with the rest subjected to instream barriers such as dams and weirs that modify hydrological regimes, often reducing water quality and causing sedimentation that affects feeding or spawning grounds (3, 4). These cumulative impacts restrict connectivity, creating fragmentated fish populations with increased risks of local extinctions (5, 6). Addressing the loss of riverine connectivity involves either removing problematic barriers or, more commonly, retroactively installing so-called ‘fishways' that facilitate upstream fish movements around or through obstructions (7). Restoring connectivity via the latter approach necessitates detailed location considerations, understanding of the life-history requirements of the target species, and assessing the suitability of any reconnected habitats (7).
A successful fishway design requires satisfying three processes for a particular species: approach, entry and passage (7). There are considerations for each process that are affected by the barrier and site-level hydraulics (and hydrology), as well as the biological characteristics of the fish (8–11). For example, fishways constructed throughout New Zealand and especially southern Australia before ~1985 were generally based on designs for salmonids, and inefficient at facilitating the passage of comparatively poorer swimming native species (12–15). Such deficits in design precipitated critical thinking concerning the factors required to suit the swimming abilities of native fish communities (16–18). Fundamental to this change was the collection of empirical evidence to inform effectiveness of the structures and future management options.
While an empirically informed fishway can facilitate multiple upstream species movements past a barrier, in some cases efficiencies can still reduce with sequential use within a single watershed (19, 20). For example, Gowans et al. (19) demonstrated cumulative losses of Atlantic salmon (Salmo salar) migrating upstream to reach spawning grounds, while Caudill et al. (20) identified unsuccessful migrations of adult Chinook salmon (Oncorhynchus tshawytscha) and steelhead (O. mykiss) were associated with delayed passage through a multiple-dam reach. Similarly, Castro-Santos et al. (21) noted delays at fishways limited the migratory range of the sea lamprey (Petromyzon marinus), and the effects were cumulative across four fishways.
Following refinement and re-evaluation, appropriate fishways at sequential barriers can improve connectivity within a system (22, 23). One example is the Hawkesbury–Nepean River system (hereafter the Nepean River) in south-eastern Australia, where many fish species require access to freshwater and marine environments to complete their lifecycles, but there are at least 278 structures (construction dating back to 1888) affecting passage (24) (NSW Passage Database, NSW DPI 2023). Between 1913 and 1985, some 44 fishways were built in New South Wales (NSW); albeit with poor performances for native species (13, 16, 25, 26). During 2009/10, ten low-gradient, vertical-slot fishways [following the designs described by Mallen-Cooper (27)] were installed at consecutive weirs in the Nepean River to reconnect ~250 km of riverine habitat to the estuary.
Rourke et al. (22) subsequently detected improvements in species richness between weirs in the Nepean River, presumably owing to improved fish passage, but there was species-specific variability, and in some cases individual species performances remain unknown. Generally, there was an overall upstream improvement among the distributions of native species, including freshwater herring (Potamalosa richmondia), sea mullet (Mugil cephalus), and freshwater mullet (Trachystoma petardi). However, several other species including bullrout (Notesthes robusta), empire gudgeon (Hypseleotris compressa) and striped gudgeon (Gobiomorphus australis) showed no changes in distributions. Data are required to determine if these latter species were unsuccessfully attempting to pass through the fishways or were simply remaining in situ. Such information will facilitate progressing future fishway design refinements and operation, and inform the mechanisms contributing toward population recoveries, or lack thereof, within this river system (22).
Considering the deficit described above, the aim of this study was to assess the fish-passage efficiencies of three Nepean River fishways to determine whether their design was limiting the upstream movement of abundant species. The specific objectives were to determine relative species and size compositions at the entrance and exit of the fishways. Satisfying these objectives will not only aid in the future refinement of fishways for fish communities in south-eastern Australia, but also their application and design more broadly.
2 Methods
2.1 Study site and fishways
The Hawkesbury–Nepean catchment encompasses 21,400 km2 and it supplies most of metropolitan Sydney's water (28). Land use along the Nepean River includes agriculture, urban development and native vegetation, which varies in condition. The river's morphology varies along its course, with steep and rocky upper reaches transitioning into wider, slower-flowing sections in mid and lower reaches. The Nepean River supports diverse fish communities including many diadromous species (57). Maximum total lengths (TL) range from ~7 cm (e.g., Australian smelt, Retropinna semoni, and gudgeons) to >1 m (long-finned eel, Anguilla reinhardtii). The Nepean River has also been highly modified, with five dams and twelve weirs starting downstream at Penrith and to Pheasants Nest (over ~250 km; Figure 1).
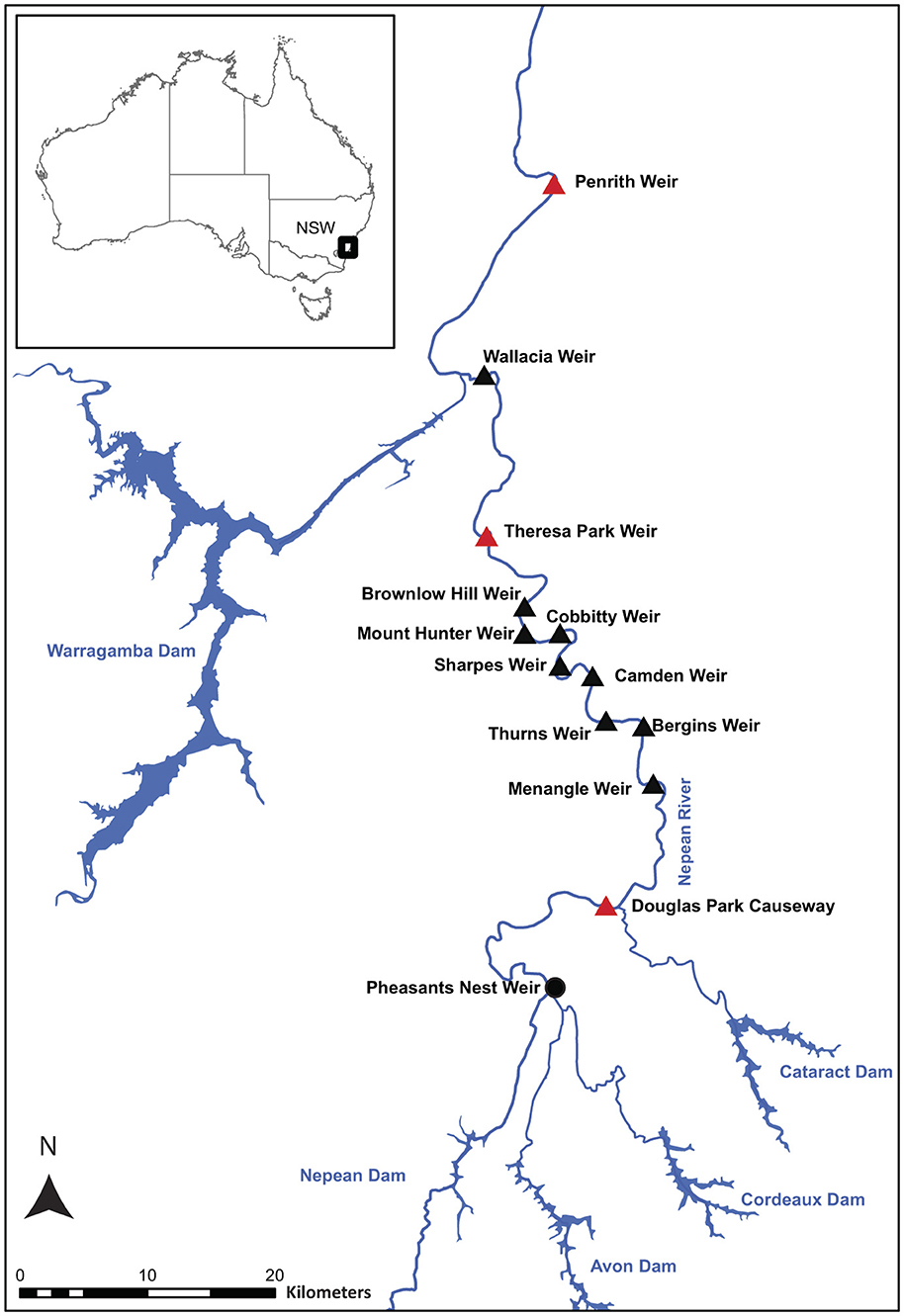
Figure 1. Map showing the Nepean River in New South Wales (NSW) with all weirs that have been retroactively fitted with vertical-slot fishways shown as black triangles, and those fishways that were trapped are shown as red triangles.
Historically, some weirs were equipped with a fishway, but these were not operating effectively, and many diadromous species including freshwater herring, and sea mullet, were absent at upstream locations (22, 24, 29–32). Consequently, between January 2009 and December 2010, ten vertical-slot fishways were installed with the same design criteria: low-gradient (1:21.4), low head loss between pools of 100 mm (producing a maximum water velocity of 1.4 ms−1), pool size (2.0 m long by 1.5 m wide), and a variable baffle shape that produced low turbulence (34 W/m−3) at low water levels and a higher turbulence (52 W/m−3) and attraction flow at higher water levels [see (32) for details and Supplementary Figure 1 and Supplementary Table 1]. All fishways varied in length, bends and layout (Supplementary Figures 2–4). The entrance design of each fishway followed the Mekong River Commission (33) guidelines on fishway design, ensuring the entrance: (i) was at the upstream limit of migration, (ii) the entrance flow was not masked by flow passing over the spillway, and (iii) that additional attraction flow was provided by a lower section of weir crest near the fishway (see Supplementary Figures 2–4).
Three of the ten vertical-slot fishways were sampled; the most downstream (Penrith Weir), central (Theresa Park Weir) and upstream barriers (i.e., Douglas Park Causeway) in the system (Figure 1). The Penrith Weir fishway was the shortest (~40 m) with 16 baffles in a relatively straight channel with two minor bends (Supplementary Figure 2), while the Douglas Park Causeway fishway was also 40 m long, but with four bends and 11 baffles, and it passed under a road resulting in lower diurnal light levels (Supplementary Figure 4). The Theresa Park fishway was the longest at 90 m, with five bends and 36 baffles (Supplementary Figure 3).
2.2 Fish traps and experimental design
One fish trap was constructed for each fishway and was designed to be compatible with both the entrance and exit slots (Supplementary Figure 5). Each trap comprised an aluminum frame and measured 1,600 mm long, 1,300 mm wide, and 1,400 mm high (except for the Douglas Park Causeway fish trap which was 1,100 mm high) (Supplementary Figure 5). The funnels on all traps were 350 mm wide at the entrance and extended back into the trap 600 mm on one side and 350 mm on the other. The exit of the funnel (where fish entered the main body of the fish trap) was 200 mm wide and 550 mm high (Supplementary Figure 5). All traps were covered in 4-mm square aluminum mesh to ensure small fish were retained, and the funnel was fitted with 80-mm long polyamide brushes to prevent any egress/ingress over the entire vertical slot. The traps were deployed and retrieved from the fishway using a gantry crane (Graham Handling Equipment) and Hitachi electric hoist (Model 1S1, capacity 1,000 kg) (Supplementary Figure 6).
Trapping was done during spring and summer (during the peak austral migration period for diadromous fish) from 2010 to 2013. Monitoring involved determining the numbers of fish (1) attempting to ascend the fishway (‘entry trapping'; i.e., attempting to migrate upstream), and (2) successfully ascending the fishway (‘exit trapping'; i.e., successfully migrating upstream). Trapping was stratified so that a paired entry and exit sample were performed over a ~48-h period, with traps set for ~24 h at each location. The order of entry and exit trapping samples was randomized during each sampling week to avoid biases and any fish escape from the entrance and exit was assumed to be the same.
The entry trapping sample was used to infer that fish could locate the fishway entrance and there were no behavioral inhibitions. Because the swimming capacity of fish is positively influenced by their size (34, 56) high water velocity could preclude some smaller individuals from entering fishways and so we reduced the head loss at the entrance baffle from 100 mm to ~50 mm (~1.0 ms−1) by inserting stop logs in the exit baffle to restrict the discharge of water through the fishway (15, 27). Head loss was used as a proxy for water velocities through the fishway pools: higher head losses increased velocities, while lower head losses reduced velocities. The entrance head loss was measured at the start and end of trapping to provide an average for each event, thus accounting for natural variations in river flow that could affect head loss height. Exit trapping was conducted with the fishway under normal operation and without flow-control stop logs, with head loss similarly measured. We considered the fishway to be operating successfully if fish abundances and biomasses at the fishway exit were similar to those at the entrance.
2.3 Data collected and analyses
The deployment and retrieval times of all traps were recorded. After each 24-h trapping event, all fish were transferred to an aerated 60- or 200-l container of river water, identified, counted and up to 50 of each species were measured for fork length (FL – fork-tailed species) or TL (all other species) to the nearest 1 mm. Known length-weight relationships for key species were used to derive their catch weights. All fish were released alive. We classified species by migratory strategy: catadromous, anadromous, amphidromous or potamodromous. We classified fish as potamodromous (defined here as freshwater species that migrate between two or more habitats, which is essential for their life history) either from the literature or if they were abundant in a fishway exit. The latter showed that there was motivation and bioenergetic cost used in ascending the fishway, which indicated migration—although it is unknown if this was migration between two habitats, countering displacement as larvae or juveniles, or intergenerational dispersal.
The numbers and weights of individual species trapped in sufficient quantities were standardized to 24 h−1 and log-transformed to act multiplicatively before being separately analyzed in linear mixed models (LMMs). In these analyses, ‘locations' (up to three), ‘traps' (entry vs. exit) and ‘head loss' (as a default for water velocity) were fixed factors, with all second- and third-order interactions included. Because water velocity was always greater at the exit than entrance, head loss was normalized to ensure its variation was included yet remained independent of trap location. Reduced LMMs were used for those species only occurring at one or two locations. Random blocking effects in all LMMs included ‘pairs' of trapping events, ‘months' (within years) and ‘years'. The distributional assumptions and model fits were assessed via QQ tests and plots of residuals, respectively before the significance of fixed effects (5% level) were determined using exact Wald-F tests. Models were fitted using the ASReml function in R (35, 36). Raw means of interest were plotted.
Relative selectivity curves were fitted to the length frequencies of abundant trapped species at each location using generalized additive modeling (GAM). Data were first scaled up by subsampling fractions to estimate total frequencies trap deployment−1. Relative selectivity was assessed whereby and denote the number of length l fish caught in those traps. Then
is the proportion caught in the entry trap. The expected value of pl was modeled on the logit scale using cubic regression splines of dimension three, denoted s(l), whereby
The error distribution of pl was specified to be quasi-binomial and so incorporate overdispersion. The GAMs were fitted using the gam function within the mgcv package in R (37), while spline confidence intervals were obtained using a 1,000 iteration double bootstrap (38, 39). This catch-comparison analysis was implemented using the SELECT R package (40, 41). A permutation test was used (1,000 resamples) to test for no FL/TL effects due to trap location (exit or entry), whereby the relative selectivities between the entry and exit traps were the same for all FL/TLs (42).
3 Results
3.1 Head loss/water differential among locations
The mean (±SE) head losses (mm) at the fishway entrances at Penrith Weir, Theresa Park Weir and Douglas Park Causeway were 55.7 (2.2), 58.5 (3.3) and 67.8 (2.7) mm, while exit head losses were 108.3 (2.3), 121.4 (3.6) and 98.9 (2.3) mm, respectively (Supplementary Table 2). Two head-loss replicates were missing for the exit traps at Theresa Park Weir fishway and so the associated catch data were not included in analyses (although length data were).
3.2 Species composition
A total of 26,139 fish representing 19 species were trapped during 27 paired entry and exit samples at Penrith and Theresa Park Weir fishways and 24 paired samples at Douglas Park Causeway (Table 1). Most fish were trapped at Penrith Weir (59%) and Douglas Park Causeway (36%). Empire gudgeon was the most common species at Penrith Weir (43% of catch) but was not caught at the other locations, while Cox's gudgeon (Gobiomorphus coxii) dominated catches at Theresa Park Weir (35%) and Douglas Park Causeway (80%) and was also frequently caught at Penrith weir (Table 1). Australian bass (Percalates novemaculeata), Australian smelt and flat-headed gudgeon (Philypnodon grandiceps) were also collected from all three fishways. These species, along with freshwater herring (nearly all at Penrith Weir and in one trapping event), striped gudgeon, freshwater mullet, sea mullet, firetail (Hypseleotris galii) and bullrout accounted for 99.6% of the total number and so, excluding freshwater herring, formed the basis of the catch analyses. Very few introduced species [common carp (Cyprinus carpio), goldfish (Carassius auratus) and eastern gambusia (Gambusia holbrooki)] were trapped at any of the fishways (Table 1).
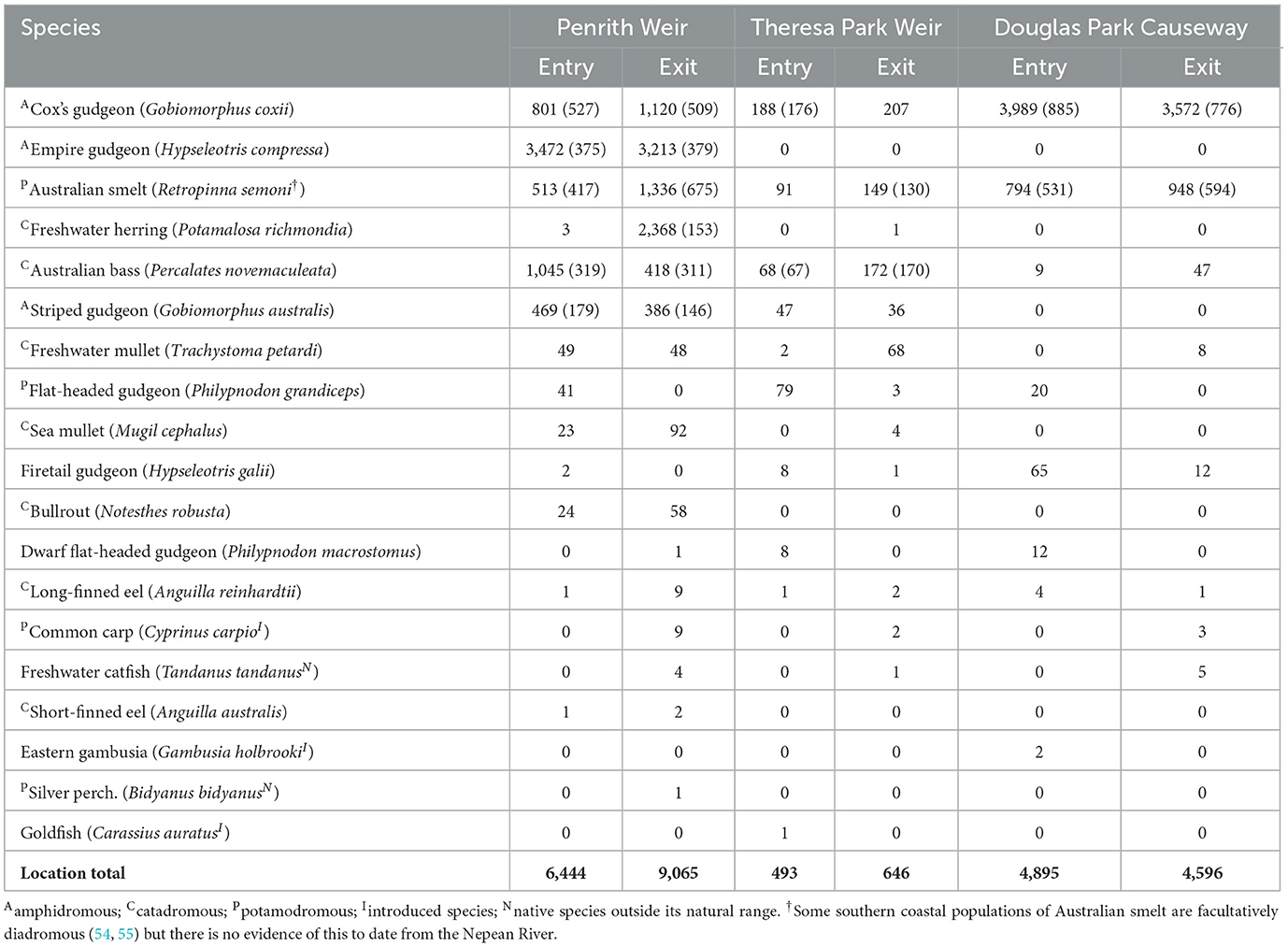
Table 1. The total numbers (in descending order) of trapped species and their measured numbers in parentheses (if not all done) at the entry and exits of verticle-slot fishways at Penrith and Theresa Park weirs and Douglas Park Causeway during 27, 27, and 24 respective paired sample days.
3.3 Analyses of catches
Four groups of LMMs were done. The first group of models were used for Cox's gudgeon, Australian smelt, Australian bass and flat-headed gudgeon at all three locations (Table 2). These models returned a significant third-order interaction for the number and weight of Cox's gudgeon, second-order interactions between location and head loss for the numbers and weights of Australian smelt and Australian bass and between location and traps for the catches of Australian bass, and a significant main effect of traps on the number of flat-headed gudgeon 24-h trapping event−1 (LMM, p < 0.05; Table 2, Supplementary Table 3, Figures 2A–C, 3A–C).
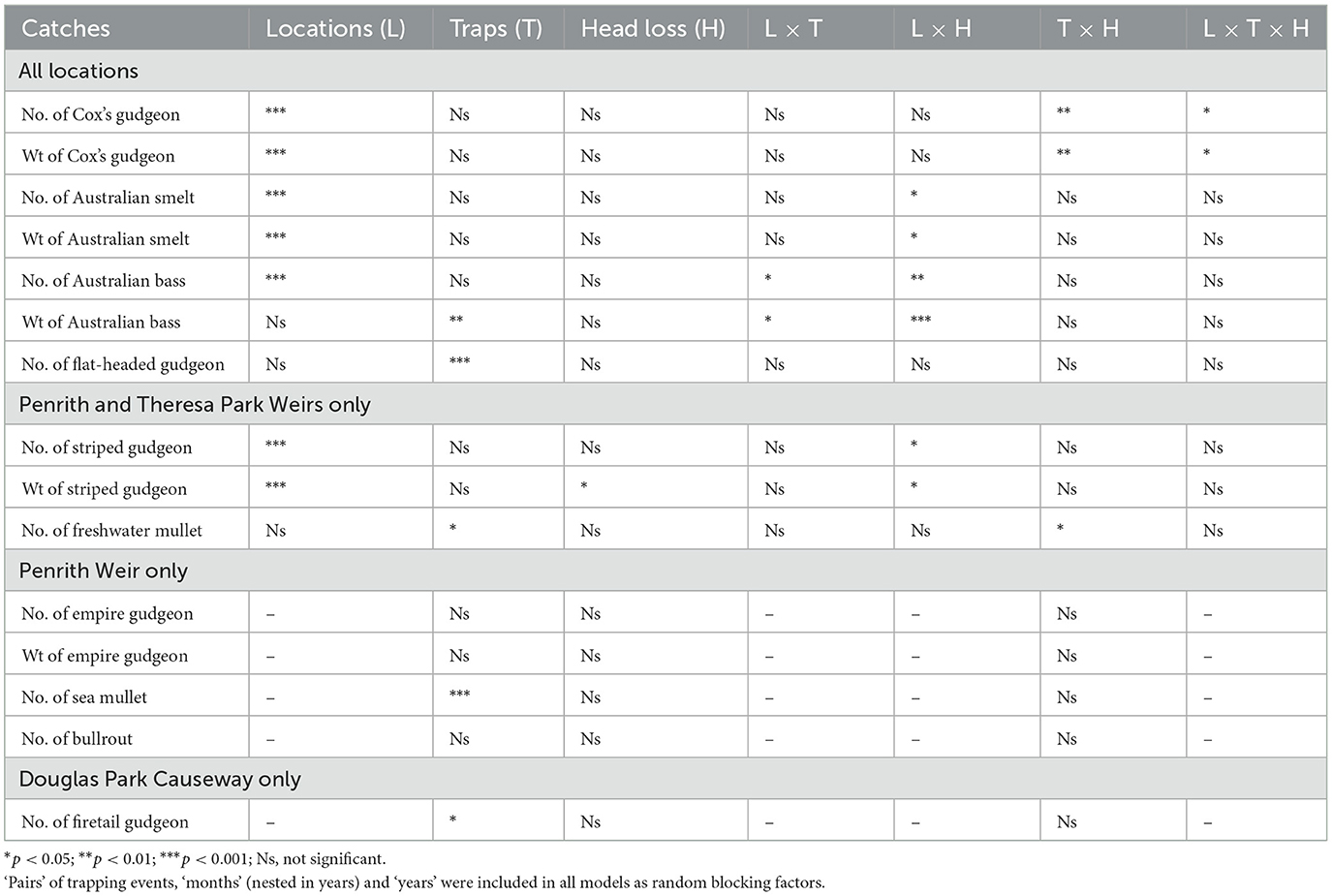
Table 2. Summaries of Wald F-value significance (or otherwise) from linear mixed models assessing the importance of ‘locations' (up to three), ‘traps' (entry vs. exit), normalized ‘head loss' (continuous) and all interactions on the numbers (no.) and weights (wt) of key species caught.
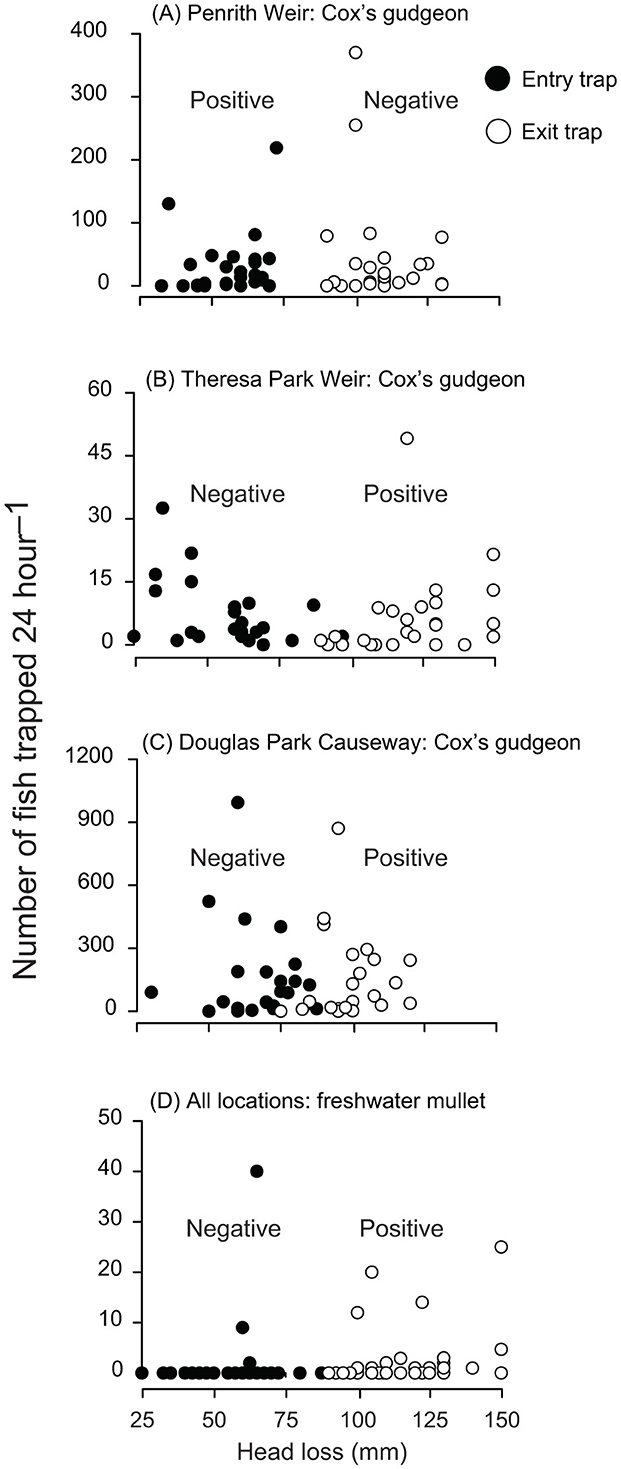
Figure 2. Scatter plots of the relationships between head loss and traps in fishways for the numbers caught 24 h−1 of Cox's gudgeon, (Gobiomorphus coxii) at (A) Penrith Weir, (B) Theresa Park Weir and (C) Douglas Park Causeway and of (D) freshwater mullet (Trachystoma petardi) across Penrith and Theresa Park weirs. Untransformed data are shown for ease of interpretation, but analyses included log-transformed abundances and normalized head loss (a proxy for water velocity), with the directions of regression coefficients (negative or positive) indicated (see Supplementary Tables 3, 4).
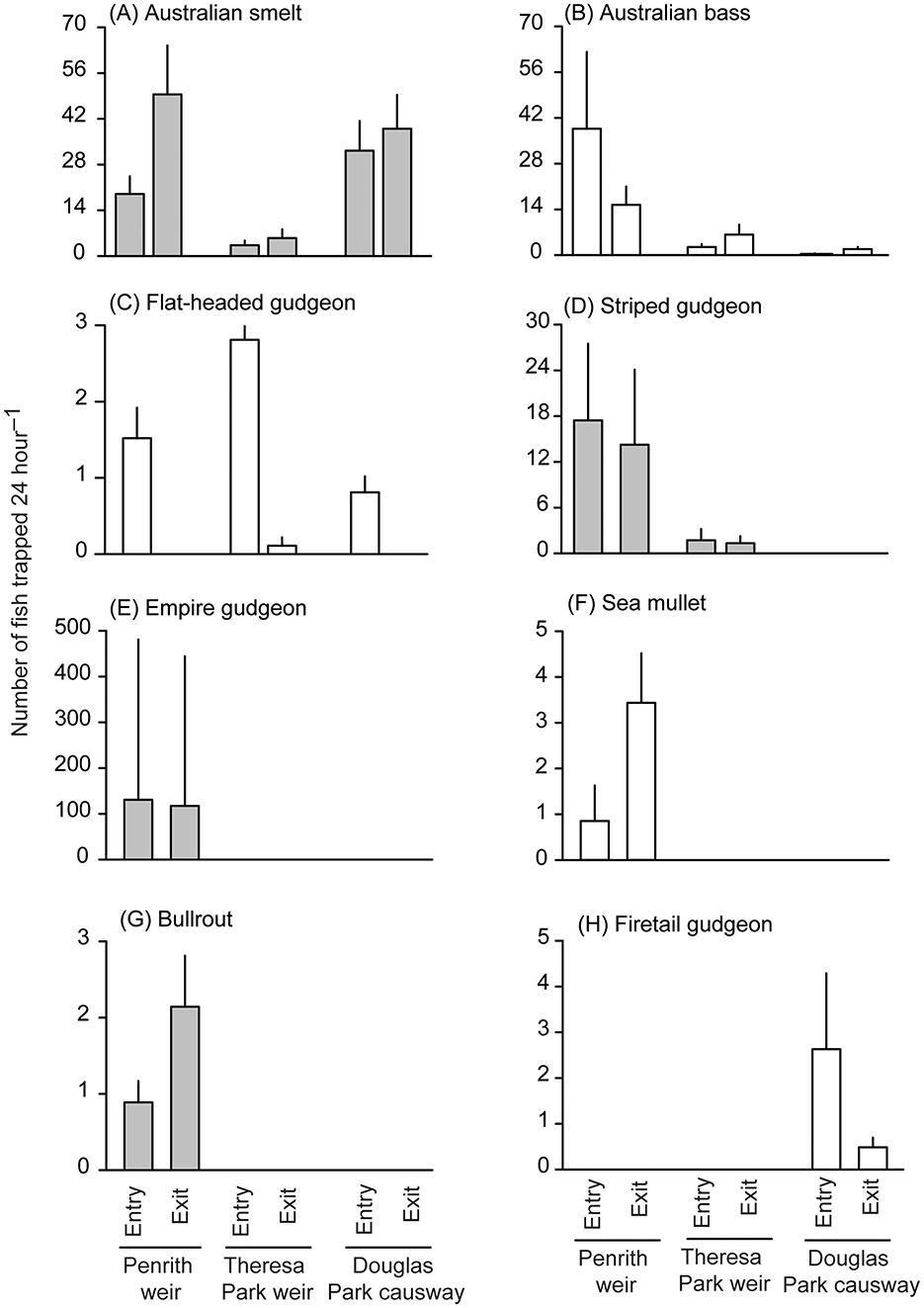
Figure 3. Differences in mean (+SE) numbers caught 24 h−1 in entry and exit traps installed in fishways at relevant locations (Penrith and Theresa Park weirs and/or Douglas Park Causeway) for (A) Australian smelt (Retropinna semoni), (B) Australian bass (Percalates novemaculeata), (C) flat-headed gudgeon (Philypnodon grandiceps), (D) striped gudgeon (Gobiomorphus australis), (E) empire gudgeon (Hypseleotris compressa), (F) sea mullet (Mugil cephalus), (G) bullrout (Notesthes robusta), and (H) firetail gudgeon (Hypseleotris galii). White and gray histograms indicate significant (p < 0.05) and non-significant (p > 0.05) effects, respectively involving traps that were detected in linear mixed models.
Owing to similarity in significance for weights and numbers, only data for the latter were plotted in Figures 2, 3. For Cox's gudgeon, head loss was positively and negatively associated with entry and exit trapping, respectively at Penrith Weir, but the opposite trend occurred at Theresa Park Weir and Douglas Park Causeway (Supplementary Table 3, Figures 2A–C). Among locations (and regardless of traps) the catches of trapped Australian smelt and Australian bass were both positively and negatively associated with head loss at Penrith and Douglas Park, respectively, while at Theresa Park head loss had a negative effect on the catches of Australian smelt and a positive effect on Australian bass (Supplementary Table 3). In terms of trap effects, there were no significant differences in catches 24 h−1 between entry and exit traps for Australian smelt (LMM, p > 0.05; although the means were greater in exit traps at all locations), but there were divergent trends for Australian bass, with more caught in entry than exit traps at Penrith, and the opposite occurring at Theresa and Douglas parks (LMM, p < 0.05; Table 2, Supplementary Table 3, Figures 3A, B). Regardless of location, ~98% of flat-headed gudgeon were caught in the entry traps (LMM, p < 0.001; Table 2, Supplementary Table 3, Figure 3C).
The second group of models was done for the number and weight of striped gudgeon and the numbers of freshwater mullet at Penrith Weir and Theresa Weir fishways (Table 2, Supplementary Table 4). There was a significant interaction between locations and head loss for striped gudgeon (LMM, p < 0.05), with negative and positive coefficients at Penrith and Theresa Park, but no significant effects involving traps (LMM, p > 0.05; Table 2, Supplementary Table 4, Figure 3D). There was a significant interaction between traps and head loss for the number of freshwater mullet: producing negative and positive coefficients for entry and exit traps, respectively regardless of locations (LMM, p < 0.05; Table 2, Supplementary Table 4, Figure 2D).
The third and fourth groups of models were restricted to the number and weight of empire gudgeon and numbers of sea mullet and bullrout at Penrith Weir and the number of firetail gudgeon at Douglas Park (Table 2, Supplementary Tables 5, 6). The only significant effects involved traps on the numbers of sea mullet and firetail gudgeon, with more of the former in the exit trap at Penrith Weir fishway and more of the latter in the entry trap at Douglas Park Causeway (LMM, p < 0.05; Table 2, Supplementary Tables 5, 6, Figures 3F, H).
3.4 Analyses of sizes
Sufficient data were collected for most species to permit relative size-selectivity analyses, which were separated by location (Figures 4, 5). For nearly all species at all locations, the p-values for the hypothesis of no FL/TL effects on the proportions retained in the exit traps exceeded 0.1 (permutation test, Figures 4, 5). The exceptions were Cox's gudgeon at Douglas Park (p = 0.08) and Australian smelt at Theresa Park (p = 0.06), with a bias toward smaller Cox's gudgeon (< 70 mm TL) and larger Australian smelt (>~45 mm FL) being retained at greater proportions in the exit traps (Figures 4C, E). There were also trends of proportionally more large Australian bass retained in the exit traps at Penrith and Theresa Park fishways and freshwater mullet at Penrith (Figures 4G, H, 5D).
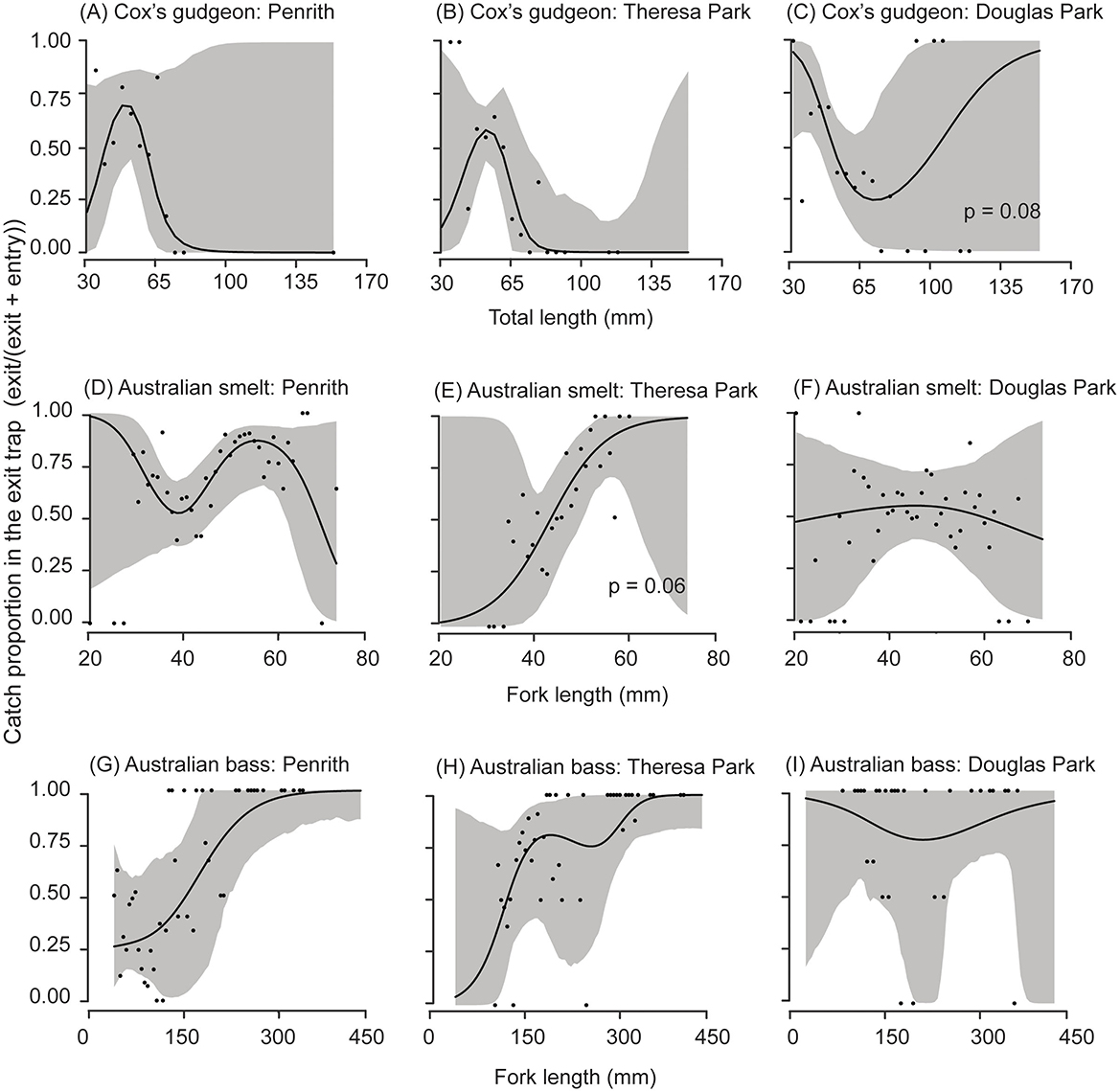
Figure 4. Cubic regression splines (solid lines) with 95% pointwise confidence intervals (gray bands) fitted to the proportions (black circles) of the combined catches (entry + exit traps) of each total or fork length retained in the exit trap at three fishways (Penrith Weir, Theresa Park Weir and Douglas Park Causeway) for (A–C) Cox's gudgeon (Gobiomorphus coxii), (D–F) Australian smelt (Retropinna semoni), and (G–I) Australian bass (Percalates novemaculeata). Unless stated, p-values from permutation tests for an effect of length were > 0.1.
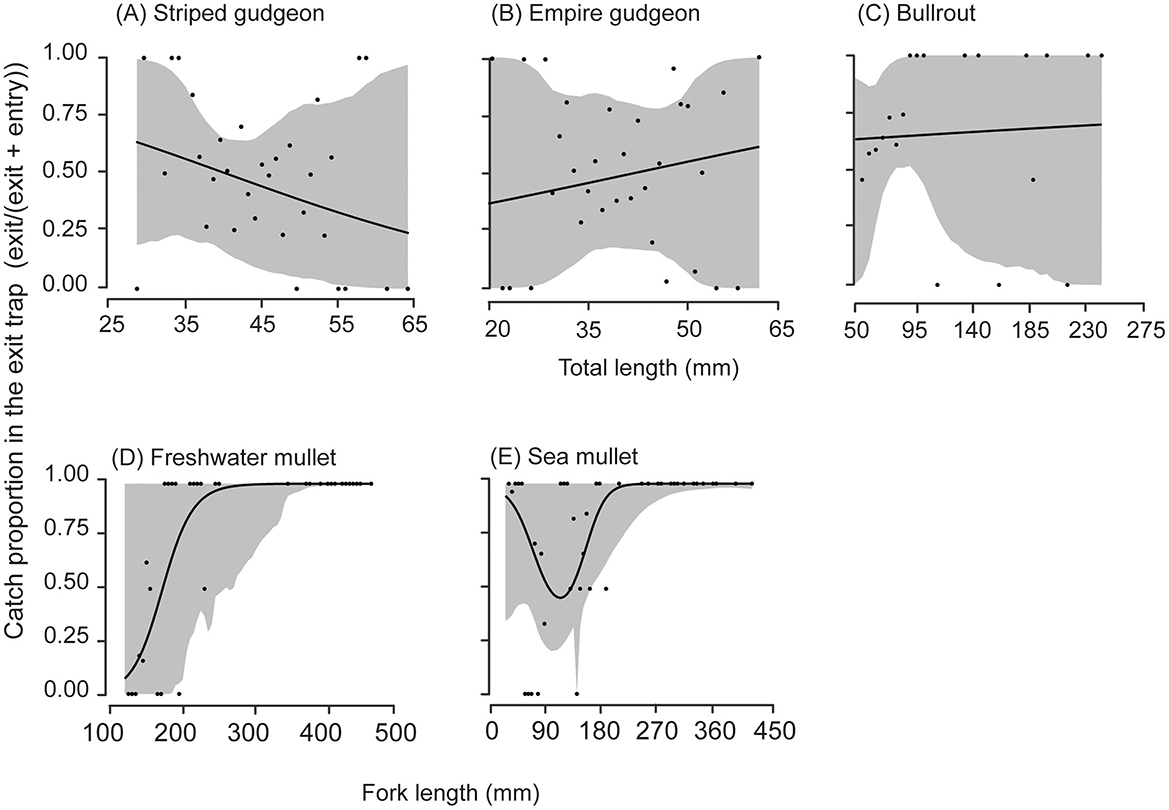
Figure 5. Cubic regression splines (solid lines) with 95% pointwise confidence intervals (gray bands) fitted to the proportions (black circles) of the combined catches of each total or fork length retained in the exit trap of the fishway at Penrith Weir for (A) striped gudegon (Gobiomorphus australis), (B) empire gudgeon (Hypseleotris compressa), (C) bullrout (Notesthes robusta), (D) freshwater mullet (Trachystoma petardi), and (E) sea mullet (Mugil cephalus). All p-values from permutation tests for an effect of length were > 0.1.
4 Discussion
This study reiterates the utility of a vertical-slot fishway system for maintaining the upstream migration of most fish and size classes in the Nepean River (22, 32) and contributes toward the broader literature supporting retroactively fitted barrier modifications globally (11, 19, 43, 44). Nevertheless, by incorporating a hierarchical analysis of trapped catches at the entrances (water velocity controlled) and exits of sequential fishways, we have identified considerable inter- and intra-specific variability in fish passage. Because we accepted the hypothesis of no size effects for all species, the observed trapped catch variabilities might be attributed to broader species-specific behavioral/physiological responses, and these are ultimately discussed to propose guidance for fishway management into the future.
Most analyzed species, and certainly all those that were amphidromous or catadromous, moved through the fishways—evidenced by similar or greater catches in at least some exit than entry traps. However, several species' passages were either directly or indirectly affected by water velocity and with variable spatial influence. Specifically, catches of the most abundant species (38% of total), the amphidromous Cox's gudgeon (20–160 mm TL) in the entry and exit traps were negatively and positively affected by water velocity, respectively at the Theresa Park and Douglas Park fishways, but not at Penrith (i.e., the first of the sequential fishways). Like all gudgeons, this species has a round tail (hence the TL measure); a characteristic, along with their general body form, implying they would be less proficient at swimming than similar-sized fish with forked or lunate tails presenting greater caudal fin ratios (i.e., those species measured for FL) (45). Nevertheless, in 50% of the traps at the three fishways (entry at Penrith and exits at Theresa Park and Douglas Park), catches of Cox's gudgeon were positively affected by water velocity (indicating no issues with swimming within the range of flows experienced), while in the other 50% of the traps, the opposite occurred.
Although speculative, such differences in the effects of water velocity on the capture of Cox's gudgeon could simply reflect localized behavioral responses to the fishways and/or site-specific confounding effects of the trap design when used at the entry. For example, at least some Cox's gudgeon may have simply sought shelter at the entrance chamber and were not migrating, whereby lower water velocity would be expected to facilitate trapped catches, which is what occurred at the upstream Theresa Park and Douglas Park fishways (and consistent across two slightly different-sized traps). Alternatively, because the types of traps used here are typically more efficient with greater water velocity (i.e., after entry, with faster flows fish might find it more difficult to relocate the brushed funnel and escape) (46), there may have been some confounding effects of the design on capture at these fishway entrances. The latter hypothesis might also explain the negative relationship between water velocity and trapped catches of freshwater mullet at the Penrith and Theresa Park fishways—although this species has a lunate tail and would be a more proficient swimmer than any of the gudgeons. Possibly there was insufficient water velocity to facilitate entry and/or preclude any escape back though the brushed entry funnel of the trap.
While there were no other direct effects of water velocity on trapped catches, significantly fewer sea mullet (35–410 mm FL and catadromous) were trapped at the slower-velocity entry than faster velocity exit of the Penrith fishway, implying indirect effects. Also, the potamodromous Australian smelt showed a similar non-significant trend as both mullets with lower mean catches at all entry traps and almost returned a significant size effect, manifesting as proportionally larger (i.e., faster swimming) fish at the exit of the Theresa Park fishway (characterized by the greatest mean velocity of all fishways). A similar non-significant trend in sizes was observed for freshwater mullet at the Penrith fishway.
Regardless of the mechanisms contributing toward the lower catches of some species in the entry than exit traps, these did not extend to two of the lesser abundant species of potamodromous gudgeons (flat-headed gudgeon [31–49 mm TL] and firetail gudgeon [29–77 mm TL]). Rather, these species were almost entirely caught in the entry traps; a result that implies limitations to their passage up the fishways. The life-history movements of these species are not well understood, though there is some evidence that flat-headed gudgeon undertake both downstream and upstream migrations [(47) and references therein (48–50)]. Similarly, firetail gudgeon also undertake upstream migrations following increases in discharge (47).
Assuming flat-headed and firetail gudgeons would normally seek to migrate upstream, their small sizes recorded here may have affected their ability to ascend the fishways, although the same-sized Cox's gudgeon were not similarly affected and other studies have detected the passage of flat-headed gudgeon through faster-flowing fishways in the Murray River (50). Also, during laboratory studies, Kilsby and Walker (51) noted that flat-headed gudgeon were capable of high burst swimming speeds. Possibly, despite capacity to move up the fishway, and as discussed above, flat-headed and firetail gudgeons were simply using the entrance for shelter and were not migrating upstream. Because these species are capable of completing their lifecycle solely in freshwater, any affected small-scale migrations might be less important than for amphidromous (e.g., Cox's gudgeon) or catadromous (e.g., Australian bass and sea mullet) species. Certainly, the similar-sized amphidromous empire gudgeons, which need to migrate upstream to maintain populations in freshwater habitats, were caught in the same (albeit variable) amounts in entry and exit traps. Ongoing research is warranted to investigate the observed inter-specific differences in gudgeon movements.
The small sizes of species like flat-headed and firetail gudgeons (and others) in the Nepean River precludes tagging with passive transponders to better understand their movements. Coded wire tags and visual implant elastomers might be applicable for some larger individuals, but these require recaptures (lethal in some cases) which can be labor intensive for routine application. Other methods such as eDNA are likely to have practical future applications (58). Nevertheless, as necessary and existing structures, fishway monitoring via non-lethal trapping will likely remain a useful tool for understanding fish movements in the Nepean River and so, considering the data here, future research efforts warrant assessing for, and eliminating, any confounding effects when positioning traps at fishway entrances.
In the interim, reducing velocity at the entrance of fishways doesn't seem to be an essential requirement for most fish and in fact seems to hinder some species, including freshwater mullet. This result implies fishways should be regularly maintained to ensure that natural debris doesn't block compartments and reduce head loss at the entry. Another design feature that warrants attention are the effects of light and/or visibility on fishway passage (52). Neither parameter was investigated here, although the Douglas Park Causeway fishway passes under a road and with lower ambient diurnal light. While confounded by a plethora of other technical parameters (head loss, length of fishway and bends), the species-specific trends in catches at this fishway were maintained with either Penrith or Theresa Park, which may suggest few light-related impacts and/or possibly most fish were nocturnally moving.
Notwithstanding the observed intra- and inter-specific variability in passage, the catches in the exit traps [and other methods involving electrofishing by Rourke et al. (22)] indicate fishways offer a solution for facilitating the upstream passage of most amphidromous and catadromous fish around barriers and across the range of coherent water velocities within the Nepean River. These data support similar conclusions for other waterways and warrant the widespread use of fishways at anthropogenic barriers, albeit with ongoing monitoring to assess and refine operations (53).
Data availability statement
The raw data supporting the conclusions of this article will be made available by the authors, without undue reservation.
Ethics statement
This work was conducted under the NSW DPI Fisheries Animal Care and Ethics permit 09/02. The study was conducted in accordance with the local legislation and institutional requirements.
Author contributions
MR: Conceptualization, Data curation, Formal analysis, Funding acquisition, Investigation, Methodology, Project administration, Supervision, Writing – original draft, Writing – review & editing. WR: Data curation, Formal analysis, Writing – original draft, Writing – review & editing. LB: Conceptualization, Funding acquisition, Methodology, Supervision, Writing – original draft, Writing – review & editing. JD: Data curation, Investigation, Writing – original draft, Writing – review & editing. MM-C: Formal analysis, Writing – original draft, Writing – review & editing. JT: Formal analysis, Writing – original draft, Writing – review & editing. MB: Data curation, Formal analysis, Writing – original draft, Writing – review & editing.
Funding
The author(s) declare that financial support was received for the research and/or publication of this article. This study was funded by the Sydney Catchment Authority (now WaterNSW) and the New South Wales Department of Primary Industries and Regional Development.
Acknowledgments
We are grateful for discussions with Tony Paul and for technical assistance and or/reviews of earlier versions of the manuscript from Joe Pera, Dylan Van Der Meulen, Jamie Hutchison, John Stuart, Frances Corey, Martin Hill, Andrew Bruce, Dylan Galt, Jack Simpson, Michael Rodgers, Chris Smith, Duncan McLay, Tim McGarry, Jarrod McPherson, Rohan Rehwinkel, Julie Rehwinkel, Chris Walsh, Ivor Growns, Dean Gilligan and Md Golam Sarwer.
Conflict of interest
MM-C was employed by Fishway Consulting Services.
The remaining authors declare that the research was conducted in the absence of any commercial or financial relationships that could be construed as a potential conflict of interest.
Generative AI statement
The author(s) declare that no Gen AI was used in the creation of this manuscript.
Publisher's note
All claims expressed in this article are solely those of the authors and do not necessarily represent those of their affiliated organizations, or those of the publisher, the editors and the reviewers. Any product that may be evaluated in this article, or claim that may be made by its manufacturer, is not guaranteed or endorsed by the publisher.
Supplementary material
The Supplementary Material for this article can be found online at: https://www.frontiersin.org/articles/10.3389/frish.2025.1571172/full#supplementary-material
References
1. Boretti A, Rosa L. Reassessing the projections of the world water development report. npj Clean Water. (2019) 2:15. doi: 10.1038/s41545-019-0039-9
2. Grill G, Lehner B, Thieme M, Geenen B, Tickner D, Antonelli F, et al. Mapping the world's free-flowing rivers. Nature. (2019) 569:215–21. doi: 10.1038/s41586-019-1111-9
3. Brink K, Gough P, Royte J, Schollema PP, Wanningen H. From Sea to Source 2.0. Protection and Restoration of Fish Migration in Rivers Worldwide. The Netherlands: World Fish Migration Foundation (2018).
4. Barbarossa V, Schmitt RJP, Huijbregts MAJ, Zarfl C, King H, Schipper AM. Impacts of current and future large dams on the geographic range connectivity of freshwater fish worldwide. Proc Natl Acad Sci. (2020) 117:3648–55. doi: 10.1073/pnas.1912776117
5. Gehrke PC, Gilligan DM, Barwick M. Changes in fish communities of the Shoalhaven River 20 years after construction of Tallowa Dam, Australia. River Res Appl. (2002) 18:265–86. doi: 10.1002/rra.669
6. Morita K, Yamamoto S. Effects of habitat fragmentation by damming on the persistence of stream-dwelling charr populations. Conserv Biol. (2002) 16:1318–23. doi: 10.1046/j.1523-1739.2002.01476.x
7. Silva AT, Lucas MC, Castro-Santos T, Katopodis C, Baumgartner LJ, Thiem JD, et al. The future of fish passage science, engineering, and practice. Fish Fish. (2018) 19:340–62. doi: 10.1111/faf.12258
8. Clay CH. Design of Fishways and other Fish Facilities, 2nd Edn. Boca Raton: Lewis Publishers. (1995).
9. Larinier M. Biological factors to be taken into account in the design of fishways, the concept of obstruction to upstream migration. Bull Fr Pêche Piscic. (2002) 364:28–38. doi: 10.1051/kmae/2002105
10. Bunt CM, Castro-Santos T, Haro A. Performance of fish passage structures at upstream barriers to migration. River Res Appl. (2012) 28:457–78. doi: 10.1002/rra.1565
11. Noonan MJ, Grant JWA, Jackson CD. A quantitative assessment of fish passage efficiency. Fish and Fish. (2012) 13:450–64. doi: 10.1111/j.1467-2979.2011.00445.x
12. Kowarsky J, Ross AH. Fish movement upstream through a central Queensland (Fitzroy River) coastal fishway. Mar Freshwater Res. (1981) 32:93–109. doi: 10.1071/MF9810093
13. Harris JH. A survey of fishways in streams of coastal South-eastern Australia. Aust J Zool. (1984) 21:219–33.
14. Russell DJ. Fish movements through a fishway on a tidal barrage in subtropical Queensland. Proc R Soc Queensland. (1991) 101:109–18.
15. Mallen-Cooper M, Brand DA. Non-salmonids in a salmonid fishway: what do 50 years of data tell us about past and future fish passage? Fish Manage Ecol. (2007) 14:319–32. doi: 10.1111/j.1365-2400.2007.00557.x
16. Mallen-Cooper M. Swimming ability of juvenile Australian bass, Macquaria novemaculeata (Steindachner), and juvenile barramundi, Lates calcarifer (Bloch), in an experimental vertical-slot fishway. Mar Freshwater Res. (1992) 43:823–33. doi: 10.1071/MF9920823
17. Mallen-Cooper M. Swimming ability of adult golden perch, Macquaria ambigua (Percicthyidae), and adult silver perch, Bidyanus bidyanus (Teraponidae), in an experimental vertical-slot fishway. Aust J Mar Freshwater Res. (1994) 45:191–8. doi: 10.1071/MF9940191
18. Cooke SJ, Hinch SG. Improving the reliability of fishway attraction and passage efficiency estimates to inform fishway engineering, science, and practice. Ecol Eng. (2013) 58:123–32. doi: 10.1016/j.ecoleng.2013.06.005
19. Gowans ARD, Armstrong JD, Priede IG, McKelvey S. Movements of Atlantic salmon migrating upstream through a fish-pass complex in Scotland. Ecol Freshwater Fish. (2003) 12:177–89. doi: 10.1034/j.1600-0633.2003.00018.x
20. Caudill CC, Daigle WR, Keefer ML, Boggs CT, Jepson MA, Burke BJ, et al. Slow dam passage in adult Columbia river salmonids associated with unsuccessful migration: delayed negative effects of passage obstacles or condition-dependent mortality? Can. J Fish Aquat Sci. (2007) 64:979–95. doi: 10.1139/f07-065
21. Castro-Santos T, Shi X, Haro A. Migratory behavior of adult sea lamprey and cumulative passage performance through four fishways. Can J Fish Aquat Sci. (2016) 74:790–800. doi: 10.1139/cjfas-2016-0089
22. Rourke ML, Robinson W, Baumgartner LJ, Doyle J, Growns I, Thiem JD, et al. Sequential fishways reconnect a coastal river reflecting restored migratory pathways for an entire fish community. Restor Ecol. (2019) 27:399–407. doi: 10.1111/rec.12886
23. Ovidio M, Sonny D, Watthez Q, Goffaux D, Detrait O, Orban P, et al. Evaluation of the performance of successive multispecies improved fishways to reconnect a rehabilitated river. Wetlands Ecol Manage. (2020) 28:641–54. doi: 10.1007/s11273-020-09737-w
24. Gehrke PC, Astles KL, Harris JH. Within-catchment effects of flow alteration on fish assemblages in the Hawkesbury-Nepean River system, Australia. Regul Rivers: Res Manag. (1999) 15:181–98. doi: 10.1002/(SICI)1099-1646(199901/06)15:1/3<181::AID-RRR528>3.0.CO;2-5
25. Mallen-Cooper M, Harris J. Fishways in mainland south-eastern Australia. In:Korura S, , editor. Proceedings of the International Symposium on Fishways '90 in Gifu Japan, October 8-10, 1990, Gifu, Japan. (1990). p. 221−9.
26. Thorncraft G, Harris JH. Fish Passage and Fishways in New South Wales: A Status Report. Cooperative Research Centre for Freshwater Ecology Technical Report 1/2000. Sydney: Office of Conservation, NSW Fisheries (2000).
27. Mallen-Cooper M. Developing fishways for nonsalmonid fishes: a case study from the murray river in Australia. In:Odeh M, , editor. Innovations in Fish Passage Technology. Bethseda, MD: American Fisheries Society (1999). p.173–95
28. Anon. Riverine Ecosystems, Hawkesbury-Nepean region (State of the catchments 2010). NSW, Sydney South: Department of Environment, Climate Change and Water (2010).
29. Gehrke PC, Astles KL, Harris JH. Comparison of Present Day and Historical Fish Communities in the Hawkesbury-Nepean River. Cronulla: NSW Fisheries Research Institute and Cooperative Research Centre for Freshwater Ecology (1996).
30. Harris JH, Silveira R, Krogh M, Gehrke PC. Historical (1985–86) composition of fish communities in the lowland fluvial reaches of the Nepean River and the upper Hawkesbury estuary. In:Gehrke PC, Harris JH, , editors. Fish and Fisheries of the Hawkesbury-Nepean River System. Final Report to the Sydney Water Corporation. NSW Fisheries Research Institute and Cooperative Research Centre for Freshwater Ecology, Cronulla (1996).
31. Baumgartner L, Reynoldson N. Fish Communities of the Nepean River in the Vicinity of Pheasants Nest Weir. Fisheries Final Report Series: No. 15. Cronulla: NSW Department of Primary Industries (2007).
32. Mallen-Cooper M. Environmental Flows for the Upper-Hawkesbury-Nepean River: Nepean River Fish Passage Design Report. St Ives, NSW: Fishway Consulting Services (2009).
33. Mekong River Commission. Fish-Friendly Irrigation: Guidelines on Fishway Design, Construction, Operation, Maintenance and Adjustment. Vientiane: MRC Secretariat (2023).
34. Beamish FWH. Swimming capacity. In:Hoar WS, Randall DJ, , editors. Fish Physiology, Vol. VII, Locomotion. New York: Academic Press (1978). p. 101–87. doi: 10.1016/S1546-5098(08)60164-8
35. Butler DG, Cullis BR, Gilmour AR, Gogel BG, Thompson R. ASReml-R Reference Manual Version 4.2. Hemel Hempstead: VSN International Ltd (2023).
36. R Core Team. R: A Language and Environment for Statistical Computing. Vienna: R Foundation for Statistical Computing. (2021).
37. Wood SN. Generalized Additive Models: An Introduction with R, 2nd Edn. New York, NY: Chapman and Hall/CRC Press (2017). doi: 10.1201/9781315370279
38. Millar RB. Incorporation of between-haul variation using bootstrapping and nonparametric estimation of selection curves. Fish Bull. (1993) 91:564–72.
39. Xu X, Millar RB. Estimation of trap selectivity for male snow crab (Chionoecetes opilio) using the SELECT modeling approach with unequal sampling effort. Can J Fish Aquat Sci. (1993) 50:2485–90. doi: 10.1139/f93-273
40. Millar RB, Broadhurst MK, MacBeth WG. Modelling between-haul variability in the size selectivity of trawls. Fish Res. (2004) 67:171–81. doi: 10.1016/j.fishres.09, 040.
41. Millar RB. R package SELECT for estimation of the size selectivity of fishing gears. Version 1.0.0.0. (2021). Available online at: https://github.com/rbmillar/SELECT (accessed July 2024).
42. Millar RB, Broadhurst MK. Incorrect inference from size-selectivity studies due to widespread misuse of bootstrap intervals. Fish Res. (2025) 281:107225. doi: 10.1016/j.fishres.2024.107225
43. Hodge BW, Fetherman ER, Rogers KB, Henderson R. Effectiveness of a fishway for restoring passage of colorado river cutthroat trout. N Am J Fish Manage. (2017) 37:1332–40. doi: 10.1080/02755947.2017.1386144
44. O'Connor J, Pickworth A, Fanson B, Lovric D. Assessment of a vertical slot fishway in south-eastern Australia designed to pass numerous species and size classes of fish. Ecol Manage Restor. (2019) 20:151–5. doi: 10.1111/emr.12367
46. Gabriel O, Lange K, Dahm E, Wendt T. Von Brandt's Fish Catching Methods of the World, 4th Edn. Oxford: Blackwell Publishing. (2005) 523. doi: 10.1002/9780470995648
47. Pusey B, Kennard M, Arthington A. Freshwater Fishes of North-Eastern Australia. Collingwood, VIC: CSIRO Publishing. (2004). doi: 10.1071/9780643092082
48. Jennings PR, Zampatti BP, Stuart IG, Baumgartner LJ. Fish Passage at the Murray River Barrages. The Sea to Hume Dam: Restoring Fish Passage in the Murray River. Canberra: Murray-Darling Basin Commission (2008).
49. Stuart IG, Zampatti BP, Baumgartner LJ. Can a low-gradient vertical-slot fishway provide passage for a lowland river fish community? Mar. Freshwater Res. (2008) 59:332–46. doi: 10.1071/MF07141
50. Bice CM, Zampatti BP, Mallen-Cooper M. Paired hydraulically distinct vertical-slot fishways provide complementary fish passage at an estuarine barrier. Ecol Eng. (2017) 98:246–56. doi: 10.1016/j.ecoleng.11.001
51. Kilsby NN, Walker KF. Linking the swimming ability of small freshwater fish to body form and ecological habit. Trans R Soc South Aust. (2010) 134:89–96. doi: 10.1080/3721426.2010.10887132
52. Jones MJ, Baumgartner LJ, Zampatti BP, Beyer K. Low light inhibits native fish movement through a vertical-slot fishway: implications for engineering design. Fish Manage Ecol. (2017) 24:177–85. doi: 10.1111/fme.12205
53. Baumgartner LJ, Boys CA, Stuart IG, Zampatti BP. Evaluating migratory fish behaviour and fishway performance: testing a combined assessment methodology. Aust J Zool. (2010) 58:154–64. doi: 10.1071/ZO10035
54. Hughes JM, Schmidt DJ, Macdonald JI, Huey JA, Crook DA. Low interbasin connectivity in a facultatively diadromous fish: evidence from genetics and otolith chemistry. Mol Ecol. (2014) 23:1000–13. doi: 10.1111/mec.12661
55. Crook DA, Macdonald JI, Raadik TA. Evidence of diadromous movements in a coastal population of southern smelts (Retropinninae: Retropinna) from Victoria, Australia. Mar Freshwater Res. (2008) 59:638–46. doi: 10.1071/MF07238
56. Bainbridge R. The speed of swimming of fish as related to size and to the frequency and amplitude of the tail beat. J Exp Biol. (1958) 35:109–33.
57. Gehrke PC. Native, introduced and alien species. In: Gehrke PC, Harris JH, , editors. Fish and Fisheries of the Hawkesbury-Nepean River System. Final Report to the Sydney Water Corporation. Cronulla: NSW Fisheries Research Institute and Cooperative Research Centre for Freshwater Ecology (1996).
Keywords: barriers, vertical-slot fishway, migration, diadromy, fishway trapping
Citation: Rourke ML, Robinson W, Baumgartner LJ, Doyle J, Mallen-Cooper M, Thiem JD and Broadhurst MK (2025) Multiple fishways mostly maintain upstream teleost movement in a south-eastern Australian river. Front. Fish Sci. 3:1571172. doi: 10.3389/frish.2025.1571172
Received: 05 February 2025; Accepted: 21 March 2025;
Published: 30 April 2025.
Edited by:
Géraldine Lassalle, l'alimentation et l'environnement (INRAE), FranceReviewed by:
Marc Ordeix Rigo, Central Unviersity of Catalonia, SpainSerhat Kucukali, Hacettepe University, Türkiye
Copyright © 2025 Rourke, Robinson, Baumgartner, Doyle, Mallen-Cooper, Thiem and Broadhurst. This is an open-access article distributed under the terms of the Creative Commons Attribution License (CC BY). The use, distribution or reproduction in other forums is permitted, provided the original author(s) and the copyright owner(s) are credited and that the original publication in this journal is cited, in accordance with accepted academic practice. No use, distribution or reproduction is permitted which does not comply with these terms.
*Correspondence: Meaghan L. Rourke, bWVhZ2hhbi5kdW5jYW5AZHBpLm5zdy5nb3YuYXU=