- 1Marine Bioproducts Research Laboratory, Department of Environmental Sciences, Dalhousie University, Truro, NS, Canada
- 2Acadian Seaplants Limited, Research and Development, Dartmouth, NS, Canada
Plants incessantly encounter abiotic and biotic stresses that limit their growth and productivity. However, conversely, plant growth can also be induced by treatments with various abiotic and biotic elicitors. Carrageenans are sulfated linear polysaccharides that represent major cellular constituents of seaweeds belonging to red algae (Rhodophyta). Recent research has unraveled the biological activity of carrageenans and of their oligomeric forms, the oligo carrageenans (OCs), as promoters of plant growth and as elicitors of defense responses against pests and diseases. In this review, we discuss the molecular mechanisms by which carrageenans and OCs mediate plant growth and plant defense responses. Carrageenans and OCs improve plant growth by regulating various metabolic processes such as photosynthesis and ancillary pathways, cell division, purine and pyrimidine synthetic pathways as well as metabolic pathways involved in nitrogen and sulfur assimilation. Carrageenans and OCs also induce plant defense responses against viroids, viruses, bacteria, fungi and insects by modulating the activity of different defense pathways, including salicylate, jasmonate and ethylene signaling pathways. Further studies will likely substantiate the beneficial effects of carrageenans and of OCs on plant growth and plant defense responses and open new avenues for their use in agriculture and horticultural industry.
Introduction
Marine organisms such as bacteria, microalgae and seaweeds constitute approximately one-half of the global biodiversity and thus, the oceans represent an enormous source of functional compounds produced by these biological systems, including various polysaccharides (Courtois, 2009; de Jesus Raposo et al., 2013, 2015; Ahmadi et al., 2015), polyunsaturated fatty acids (PUFA) (Russell and Nichols, 1999), vitamins, anti-oxidants (Rocha de Souza et al., 2007), enzymes and bioactive peptides (Campo et al., 2009; Shahidi and Ambigaipalan, 2015). Since many of the habitats of marine organisms are harsher, more diverse and extremely competitive as compared to those of terrestrial organisms, they adapted to produce a wide range of unique molecules that are essential for their survival (Vo and Kim, 2010).
Seaweeds are multicellular marine macroalgae found in all coastal ecosystems of the world, with essential roles in maintaining the biodiversity of the in-shore marine environment (Sangha et al., 2014). These algae have long been used in the human nutritional regime as a source of unique carbohydrates. Currently, three carbohydrate polymers from seaweeds are commercially exploited: agar and carrageenans from red seaweeds and alginates from brown seaweeds (Campo et al., 2009). Carrageenans are represented by a number of naturally occurring anionic sulfated linear polysaccharides present in a variety of red algae (Rhodophyta). The term carrageenan is derived from an Irish word, “carrageen” meaning a “little rock” (Campo et al., 2009). Carrageenans found their way in a large number of commercial applications such as food and dairy industry (Prajapati et al., 2014) and drug delivery (Li et al., 2014). In addition to these applications, carrageenans were shown to possess several properties that are of interest for the pharmaceutical industry including antiviral, antitumor, immunomodulatory, antihyperlipidemic and anticoagulant activities (Yuan et al., 2006; Campo et al., 2009; Prajapati et al., 2014; Ahmadi et al., 2015). Carrageenans were also shown to have antioxidant activity by scavenging hydrogen peroxide (Sun et al., 2010). The effects of carrageenans on mammalian systems were examined in several reviews (Campo et al., 2009; Courtois, 2009; Vo and Kim, 2010; de Jesus Raposo et al., 2013, 2015; Prajapati et al., 2014; Ahmadi et al., 2015). More recently, carrageenans were tested for their effects on plant growth and for their roles in enhancing plant immunity. Though the information on the effects of carrageenans on plant systems is still rather limited, in this review we discuss the advancements in this field of research, focusing on the molecular mechanisms through which carrageenans promote plant growth and induce plant defense responses, resulting in increased plant productivity and protection.
Structure and Sources of Carrageenans
Carrageenans are linear, partially hydrophilic sulfated galactans formed by alternate units of D-galactose and 3, 6-anhydro-galactose joined by α-1, 3 and β-1, 4-glycosidic linkage (Prajapati et al., 2014). These polysaccharides are traditionally split into six basic types: iota (ι)-, kappa (κ)-, lambda (λ)-, mu (μ)-, nu (υ)-, and theta (θ)-carrageenans (Campo et al., 2009). κ-carrageenan consists of D-galactose having sulfate at C4 position linked to anhydro-galactose (Vera et al., 2011) and is commercially extracted from the red alga Kappaphycus alvarezii through a hot extraction process. Beta (β)-carrageenan is similar to κ-carrageenan but lacks the sulfate on C4 of the 1, 3-linked units. λ-carrageenan is formed by D-galactose having the sulfate group at C2 position linked to a D-galactose sulfated at C2 and C6 position. λ-carrageenan is more hydrophilic than κ-carrageenan and it is obtained from the red algae of the genera Gigartina and Chondrus by drum dryer or alcohol precipitation process (Vera et al., 2011; Prajapati et al., 2014). ι-carrageenan is obtained mainly from Eucheuma denticulatum by the freeze thaw or gel process and it is composed of D-galactose having the sulfate group at C4 position linked to an anhydrogalactose sulfated at C2 positions (Vera et al., 2011; Prajapati et al., 2014). Sulfate content in carrageenans varies from 20% in κ-carrageenan to 33% in ι-carrageenan and to 41% in λ-carrageenan.
Effect of Carrageenans on Plant Growth and Plant Defense
Plant Growth
Green land plants are autotrophic, immobile and hardy organisms. They evolved various complex metabolic pathways to capture energy and synthesize metabolites required for their growth and development (Yu et al., 2015). Carrageenans and oligo carrageenans (OCs) stimulate the growth of green land plants by modulating various physiological and biochemical processes. OCs are oligomers of sulfated galactose, generally 20 units long, prepared through depolymerization by hot, acid hydrolysis of the commercially available κ-, λ-, and ι-carrageenans. OCs appear to act as signal molecules, enhancing the induction of microspore embryogenesis in Brasssica oleracea var. italica (Lemonnier-Le Penhuizic et al., 2001). The efficiency of OCs in inducing microspore embryogenesis increased with the increase in the number of sulfate substituents (from κ- to λ-carrageenan). In combination with heat stress, λ-carrageenan significantly enhanced the production of B. oleracea var. italica microspore-derived embryos (Lemonnier-Le Penhuizic et al., 2001). OCs were also shown to augment plant height and leaf biomass in tobacco by stimulating photosynthesis and carbon fixation in plants including enhanced ribulose 1, 5 bisphosphate carboxylase/oxygenase (Rubisco) activity Muñoz et al. (2011). Similarly, found that spraying κ-, λ-, and ι-OCs on tobacco leaves increased plant height, leaf biomass, chlorophyll content, and enhanced net photosynthesis through higher PSII activity. Tobacco plants also showed improved carbon fixation and nitrogen assimilation. Rubisco and other enzymes involved in the basal metabolism such as glutamate dehydrogenase, pyruvate dehydrgenase, isocitrate dehydrogenase, inosine monophosphate dehydrogenase and glucose 6-phosphate dehydrogenase displayed higher activity. The activation of NADP(H)-synthesizing enzymes may determine an overall increase in the intracellular content of NADP(H) and a modified redox state. As a result, it has been suggested that the reducing condition probably promoted photosynthesis and ancillary processes (Castro et al., 2012). Saucedo et al. (2015) found that the application of OCs improved the growth of Pinus radiata by inducing the accumulation of C, N, and S. OCs treated plants showed increased activity of several NAD(P)H-synthesizing enzymes involved in the basal metabolism. Similarly, Bi et al. (2011) found that κ-carrageenan initiates early flowering and improves growth in chickpea and maize by increasing plant height, the number of pods in chickpea and leaves in both plants. Spray applications of κ-, λ- and ι-OCs resulted in increased plant growth and cell division in tobacco and these changes were associated with increased cell number but not cell size. The transcript levels of cyclins A and B and of CDKs A and B were found to be increased by the applications of OCs (Castro et al., 2012). Therefore, it has been suggested that the increased biomass is caused by OCs' modulating effects on the expression of cell cycle regulatory proteins (Castro et al., 2012; González et al., 2013a).
Eucalyptus globulus plants treated with carrageenans showed an increase in height and trunk diameter (González et al., 2013b). κ- and ι-carrageenan caused a 58 and 47% increase in plant height, respectively, and a 44 and 40% increase in trunk diameter, respectively. In addition to changes in growth parameters, E. globulus treated with OCs had increased amounts of holocellulose, α-cellulose and essential oils. OCs also altered the levels of polyphenolic compounds such as genistein, rutin, ellagic acid, morin, luteolin, and quercetin that have potential antimicrobial activities in E. globulus (González et al., 2013b). The mechanisms through which κ-carrageenan mediated the growth of E. globulus were also analyzed by González et al. (2014a). It has been suggested that κ-carrageenan improved the growth of E. globulus by regulating a plethora of cellular processes that are subjected to continuous environmental changes; these variations can determine large fluctuations in the redox state. κ-OC improved the redox status of E. globulus by inducing the synthesis of reduced nicotinamide adenine dinucleotide phosphate (NADPH), of ascorbate (ASC) and glutathione (GSH), and of thioredoxin reductase (TRR) activity, and likely modulated the crosstalk between various biological processes, leading to improved plant growth (González et al., 2014a). NAD+ and NADP+ are essential reducing compounds for the plant growth as co-factors of enzymes involved in electron transport and metabolic processes (Hashida et al., 2009). OCs increased the NADPH levels, which are involved in regulating the basal metabolism through TRRs/thioredoxins (TRXs) system. In presence of elevated levels of NADPH the TRR/TRX system reduces the disulfide residues of many key metabolic enzymes modulating, therefore, various pathways (González et al., 2014a). ASC and GSH play important roles in the cell cycle during the transition from G1 to S phase (Liso et al., 1988; Noctor and Foyer, 1998; de Pinto et al., 1999; Vernoux et al., 2000). In addition to cell cycle regulation, GSH may also regulate photosynthesis, growth and carbon assimilation (Jiang et al., 2012). Thus, κ-OC applications appear to affect NADPH, ASC, and GSH synthesis and the activity of TRR/TRX systems, also influencing processes such as photosynthesis, cell division, Krebs cycle, purine and pyrimidine biosynthetic pathways as well as other metabolic pathways involved in C, N, and S assimilation (Gelhaye et al., 2005; Montrichard et al., 2009; González et al., 2014a).
κ-OC was shown to induce the synthesis of plant growth hormones such as indole-3-acetic acid (IAA), gibberellic acid (GA3) and trans-zeatin by activating the TRR/TRX system and altering the redox status of plant cells (González et al., 2014b). Similarly, Saucedo et al. (2015) found that κ-OC increased the levels of IAA and GA3, promoting the growth of Pinus radiata. Though data is still limited these studies indicate that carrageenans can improve plant growth by regulating phytohormone synthesis.
In addition to promoting plant growth, the application of κ-OC also improved the economic value of Eucalyptus by reprogramming its terpenoid metabolism. Treatment of Eucalyptus with κ-OC kappa was found to reduce the synthesis of six terpenoids and to increase the level of 12 other terpenoids (González et al., 2014c). Among the terpenoids shown to be down-regulated were monoterpenoids from the limonene family (eucalyptol, sabinene, and α-terpineol) and pinene family (α-pinene), a sesquiterpenoid of the cadinene family (δ-cadinene) and of the isoledene family (isoledene). The terpenoids whose expression was up-regulated by κ-OC were monoterpenoids of the limonene family (silvestrene, α-phellandrene, γ-terpinene, limonene oxide) and pinene family (β-pinene), a sequiterpenoid of the cadinene family (γ-cadinene), several of the isoledene family (aromadendrene, viriflorene, α-gurjunene, γ-gurjunene, α-guaiene), and a linear monoterpene (myrcene). κ-OC also conferred defense against insects and microbes in Eucalyptus by increasing the synthesis of the monoterpene γ-terpinene (González et al., 2014c). Overall, by changing the redox status and by activating the TRR/TRX system, κ-OC reprogrammed terpenoid metabolism with noticeable effects on the secondary metabolism of Eucalyptus trees (González et al., 2014c). Therefore, in some cases, κ-OC can influence secondary metabolism regulation with beneficial effects on plant growth. The effects of various types of carrageenans on plant growth are summarized in Table 1 and Figure 1.
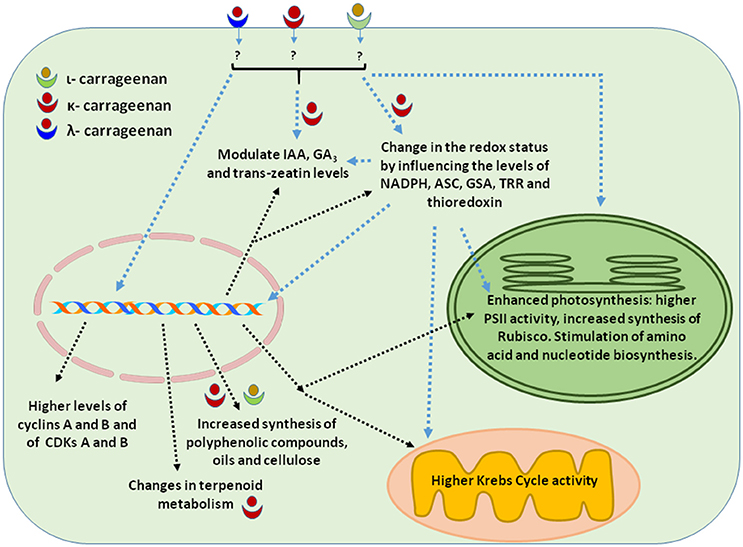
Figure 1. Cellular activities elicited by carrageenans applications that resulted in enhanced plant growth. The drawing summarizes most of the information presented in Section Plant Growth.
Plant Defense
Green land plants are attacked by a multitude of organisms including insects and pathogens such as viruses, bacteria and fungi (Pieterse and Dicke, 2007; Stadnik and De Freitas, 2014). These pathogens impair plant growth and productivity. Plants deploy a wide range of defense mechanisms against pathogens. As plants do not have specialized defense cells and are devoid of a somatic adaptive immune system, plant's immunity relies on the capacity of every cell to detect pathogens and activate defense responses (Jones and Dangl, 2006; Nicaise, 2015). Plants follow either constitutive or inducible defense mechanisms to prevent pathogen ingress (Pieterse and Dicke, 2007). Plants challenged by a pathogen or under insect attack respond through a series of molecular events, including the synthesis of different responsive alarm signals that activate the salicylate (SA), jasmonate (JA) and ethylene (ET) signaling pathways that are part of plant's primary defense response (Koornneef and Pieterse, 2008). Depending on the type of attack, the crosstalk between these signaling pathways determines the plant defense tactics. SA mediated defense responses play a central role in systemic acquired resistance, which occurs mainly against biotrophic pathogens, involving the activation of pathogenesis-related (PR) proteins. JA and ET pathways are required mainly for the induction of nonspecific disease resistance (Dixon et al., 1994; Dong, 1998; Scheel, 1998; Shah, 2003; Maffei et al., 2007; Thaler et al., 2012; Fu and Dong, 2013).
Plant defense responses can be induced by pathogen's cell wall components including chitin, lipo-polysaccharides, proteins such as bacterial flagellin and other chemicals of natural and synthetic origin like chitosan, salicylic acid, jasmonic acid, isonicotinic acid, and benzothiadiazole (Dixon et al., 1994; Mercier et al., 2001; Bektas and Eulgem, 2015; Iriti and Varoni, 2015). Compounds that induce plant defense responses are known as elicitors. These molecules confer protection against pathogens by binding to specific receptors located in the plasma membrane of plants and trigger defense responses by inducing a plethora of genes involved in defense mechanisms (Vera et al., 2011).
Epidemics of infectious disease are rarely observed in the natural seaweed populations, indicating that during the course of evolution these plants have developed efficient defense mechanism against different pathogens (Potin et al., 1999). These seaweeds serve as a source for a large number of compounds such as laminarin, fucans, ulvans, and carrageenans, which all act as elicitor molecules against pathogens (Mercier et al., 2001; Klarzynski et al., 2003; Sangha et al., 2010; Vera et al., 2011).
Carrageenans and the OCs derived from them act as elicitors and activate defense mechanism against plant pathogens (Stadnik and De Freitas, 2014); probably this activity depends on the degree of sulfation (Sangha et al., 2010, 2015). Viroids belonging to Pospiviroidae and Avsunviroidae families are causing more than two dozens of diseases in agricultural, horticultural and ornamental plants (Singh and Teixeria da Silva, 2006). Viroids replicate in the nucleus or chloroplasts from where they can be transferred from cell to cell through plasmodesmata and, for longer distances, through phloem, to eventually establish systemic infections (Ding, 2009). Carrageenans have been shown to elicit resistance against viroids in plants (Sangha et al., 2015). λ-carrageenan treated tomato plants showed higher resistance against Tomato Chlorotic Dwarf Viroid (TCDVd), a devastating pathogen of a large number of plants (Singh et al., 2006; Sangha et al., 2015). TCDVd transcript abundance was relatively higher in the water treated plants as compared to λ-carrageenan treated tomato plants. This result implies that λ-carrageenans exhibit suppressive effects on TCDVd in tomato plants, likely by controlling viroid replication (Sangha et al., 2015). Proteomic analysis of λ-carrageenan treated plants in the presence of TCDVd infection revealed differences in the abundance of 14 proteins with different cellular functions. These results suggest that the reduction of viroid infection was primarily due to biochemical changes that occurred in plants. The up-regulation of lipoxygenase (LOX), allene oxide synthase (AOS) and pathogenesis-related proteins in TCDVd infected tomatoes indicates a possible role of JA response in plant's resistance against viroid infection (Sangha et al., 2015). Carrageenans are selective inhibitors of several enveloped and non-enveloped viruses in plants and animals (González et al., 1987; Vera et al., 2011; Wang et al., 2012; Kalitnik et al., 2013) and act predominantly by inhibiting the binding or internalization of viral particles into the host cells (Ahmadi et al., 2015). Nagorskaya et al. (2008) reported that κ/β-carrageenan from the red marine alga Tichocarpus crinitus reduced the infection of tobacco leaves by the tobacco mosaic virus (TMV). Carrageenans conferred their antiviral activity on plants as well as on the virus itself (Nagorskaya et al., 2008). Also, the treatment with κ/β-carrageenan from T. crinitus stimulated, to some extent, several lytic processes in Datura stramonium, preventing the intracellular accumulation and translocation of Potato Virus X particles (Nagorskaya et al., 2010). Sulfated Polysaccharide 4 (SPS4), containing 98% κ-carrageenan and extracted from the red alga Hypnea musciformis, was found to reduce the TMV infection in tobacco plants (Ghannam et al., 2013). Molecular analysis of SPS4 induced antiviral activity revealed that the expression of SA-dependent defense related genes PR1a (encoding an antimicrobial protein), PR2 (1, 3-glucanase), and PR5 (thaumatin-like antimicrobial protein) was increased. The expression of JA pathway-dependent genes such as PR3 (encoding a basic chitinase) and Def1.2 (encoding a defensin) were also found to be enhanced (Ghannam et al., 2013). Thus, SPS4 modulates defense response in tobacco plants by inducing the crosstalk between the SA and JA-dependent signaling pathways.
ι-, κ-, and λ-oligo carrageenans were found to provide tobacco plants enhanced protection against tobacco mosaic virus (TMV), Pectobacterium carotovorum and Botrytis cinerea likely due to a sustained induction of phenylalanine ammonia lyase activity which determined the accumulation of phenylpropanoid compounds with potential antimicrobial activities (Vera et al., 2012). Patier et al. (1995) showed that κ-OC prepared by the enzymatic hydrolysis of κ-carrageenan elicits laminarinase activity in Rubus fruticosus cells, and this activity is known to be involved in plant defense. Mercier et al. (2001) reported that λ-carrageenan proved to be the most potent elicitor molecule because of its high sulfate content, as compared to that of ι- and κ-carrageenan. λ-carrageenan induced systemic resistance against Phytophthora parasitica var. nicotianae in tobacco cells by increasing the expression of a sesquiterpene cylase involved in the synthesis of the phytoalexin capsidiol, of a basic chitinase (PR3 family), and of a type II proteinase inhibitor. The expression of lipoxygenase (LOX) and of ACC oxidase (ACO), whose gene expression leads to JA and ET biosynthesis, was also found to be induced, and the amount of cellular SA to be increased. In contrast to this report, Sangha et al. (2010), showed λ-carrageenan triggered a differential resistance to Sclerotina sclerotiorum infection in Arabidopsis thaliana, largely by a JA dependent mechanism. Notably, S. sclerotiorum requires the secretion of oxalic acid to infect the plant by suppressing the oxidative burst of the host plant (Cessna et al., 2000). Sangha et al. (2010) showed that λ-carrageenan treatment reduces the oxalic acid concentration in Arabidopsis plants by increasing the in planta oxalate oxidase activity providing, therefore, an explanation for the reduced susceptibility to S. sclerotiorum. The expression of the PR3, PDF1.2 (Plant Defensin 1.2) and AOS genes involved in the defense response were induced in the λ-carrageenan treated plants. Higher levels of AOS correlate with increased biosynthesis of JA (Laudert and Weiler, 1998) and with a strong up-regulation of JA responsive gene PDF1.2, which has antifungal properties (Sangha et al., 2010). λ-carrageenan induced resistance against S. sclerotiorum in salicylic acid deficient mutant of A. thaliana (ics1), but did not rescue the susceptibility jar1 (JA deficient) mutant, suggesting that λ-carrageenan activates only the JA dependent pathway and not the SA dependent pathway. However, these responses against S. sclerotiorum were not observed in Arabidopsis treated with ι-carrageenan (Sangha et al., 2010). Thus, the application of carrageenans elicits JA signaling pathway in most plants and the SA signaling pathway in some plants but the level of activation seems to be influenced by the type of carrageenans used in treatments as well as by the plant model used in experiments.
While the protective effects of carrageenans against viriods, viruses, bacteria, and fungi are rather well documented, not much information is available on the role of carrageenans in defense response against insects. Sangha et al. (2011) reported that carrageenans act as elicitor of defense responses in Arabidopsis against the insect Trichoplusia ni (cabbage looper), which is a polyphagus herbivore that feeds on a number of plant species including Arabidopsis (Jander et al., 2001). T. ni affects various Arabidopsis ecotypes differently and shows specific sensitivity to different plant glucosinolates, defense signaling pathways and proteinase inhibitors (Jander et al., 2001; Zhang et al., 2006). Sangha et al. (2011) showed that foliar application of ι- and κ-carrageenans on Arabidopsis reduced the leaf damage caused by T. ni as compared to those treated with λ-carrageenan and control. On the 10th day of T. ni infestation, inhibition of larval growth was greater in the κ-carrageenan treated than in the ι-carrageenan treated plants while λ-carrageenan had no effect (Sangha et al., 2011). Adaptation of plants in response to insect invasion includes induced biochemical responses which regulate the expression of defense related genes and, hence, the production of secondary metabolites and proteins including toxins, antifeedants and anti-nutrients (Walling, 2000; Zangerl, 2003). Arabidopsis plants treated with ι-carrageenan showed higher expression of JA and SA responsive genes PDF1.2 and PR1, respectively. SA and JA deficient mutant ics1 and jar1 are susceptible to T. ni infestation in the presence of carrageenan, suggesting that both SA and JA dependent pathways are involved in the carrageenan induced Arabidopsis resistance against T. ni (Sangha et al., 2011). Thus, ι-carrageenan mediates the cross-talk between defense signaling pathways and induced plant resistance toward T. ni by regulating different biochemical pathways. Plant defense mechanisms against herbivores comprise the synthesis of various anti-feedant proteinase inhibitors such as trypsin inhibitor protein 1 (TI1) (Ryan, 1990; Broadway, 1995). These proteins inhibit several insect digestive proteases and ultimately determine reduced insect growth rate or, in more severe cases, increased mortality due to reduced nutrient uptake (Ryan, 1990). ι-carrageenan enhanced the expression of TI1, which indeed led to reduced larval growth and development in ι-carrageenan treated plants as compared to healthy control larvae. More feeding damage was observed on λ-carrageenan treated plant which did not show a stimulation of TI1 gene expression while κ-carrageenans had some inhibitory effects on larval growth and development in spite of limited TI1 induction (Sangha et al., 2011). In addition to this, ι-carrageenan was found to induce the expression of genes involved in indole glucosinolate biosynthesis including that of CYP79B2 and CYP83B1 that were associated with a prominent phenotypic effect on T. ni larva (Sangha et al., 2011). Glucosinolates have essential roles in defense responses as they can be easily converted to volatile compounds that have been shown to affect the performance of various herbivores. Up-regulation of glucosinolate biosynthesis genes, in the presence of ι-carrageenan, suggests that these elicitors can regulate the biosynthesis of glucosinolates, conferring carrageenan-induced resistance to T.ni larva but not to adults (Jander et al., 2001; Lambrix et al., 2001; Sangha et al., 2011). The expression of Epithiospecifer Modifier 1 (ESM1), a gene involved in glucosinolate hydrolysis and isothiocyanates production, was also increased in the ι-carrageenan treated plants, causing further damage to T. ni herbivory (Zhang et al., 2006; Sangha et al., 2011). The effects of carrageenans on plant resistance against various pathogens are summarized in Table 2 and Figure 2.
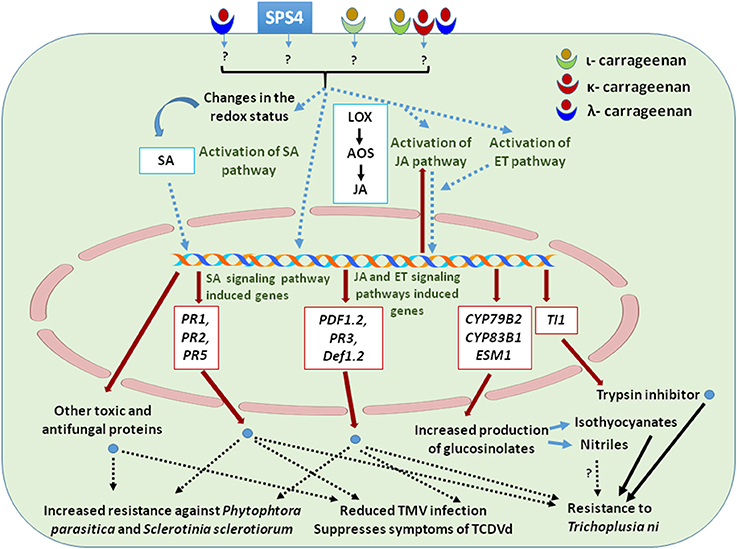
Figure 2. Cellular functions and signaling pathways involved in defense mechanisms elicited by carrageenans applications that resulted in increased resistance to pathogens. The drawing summarizes most of the information presented in Section Plant Defense.
In conclusion, the use of carrageenans in agriculture represents a promising approach for improving plant growth and for inducing plant defense mechanisms. Large scale, field based applications of carrageenans are needed to validate their effects on plant growth and resistance against various pathogens. Additional, more comprehensive and systematic studies of their functions, at molecular level, in model plant systems such as Arabidopsis, are required to further decipher their mode of action. These approaches may lead to the discovery of green plant target molecules that interact with carrageenans and reveal novel regulatory interactions controlling plant growth and defense mechanisms. The detailed study of carrageenans as promoters of plant growth and elicitors of resistance to pathogens may lead to the development of multifunctional crop protectants, conferring resistance against a broad spectrum of plant pathogens as well as better crop yields.
Author Contributions
PS, TB, AC, BP conceived the layout and wrote the review.
Funding
PS is supported by a NSERC CRD grant awarded to BP.
Conflict of Interest Statement
The authors declare that the research was conducted in the absence of any commercial or financial relationships that could be construed as a potential conflict of interest.
The Review Editor BJ declares that, despite having been supervisor of author PS, the review process was handled objectively and no conflict of interest exists.
References
Ahmadi, A., Moghadamtousi, S. Z., Abubakar, S., and Zandi, K. (2015). Antiviral potential of algae polysaccharides isolated from marine sources: a review. Biomed. Res. Int. 41, 42. doi: 10.1155/2015/825203
Bektas, Y., and Eulgem, T. (2015). Synthetic plant defense elicitors. Front. Plant Sci. 5:804. doi: 10.3389/fpls.2014.00804
Bi, F., Iqbal, S., Arman, M., Ali, A., and Hassan, M. U. (2011). Carrageenan as an elicitor of induced secondary metabolites and its effects on various growth characters of chickpea and maize plants. J. Saudi Chem. Soc. 15, 269–273. doi: 10.1016/j.jscs.2010.10.003
Broadway, M. R. (1995). Are insects resistant to plant proteinase inhibitors? Nature 41, 107–116. doi: 10.1016/0022-1910(94)00101-L
Campo, V. L., Kawano, D. F., da Silva Jr, D. B., and Carvalho, I. (2009). Carrageenans: biological properties, chemical modifications and structural analysis – a review. Carbohydr. Polym. 77, 167–180. doi: 10.1016/j.carbpol.2009.01.020
Castro, J., Vera, J., González, A., and Moenne, A. (2012). Oligo-carrageenans stimulate growth by enhancing photosynthesis, basal metabolism, and cell cycle in tobacco plants (var. Burley). J. Plant Growth Regul. 31, 173–185. doi: 10.1007/s00344-011-9229-5
Cessna, S. G., Sears, V. E., Dickman, M. B., and Low, P. S. (2000). Oxalic acid, a pathogenicity factor for Sclerotinia sclerotiorum, suppresses the oxidative burst of the host plant. Plant Cell 12, 2191–2200. doi: 10.1105/tpc.12.11.2191
Courtois, J. (2009). Oligosaccharides from land plants and algae: production and applications in therapeutics and biotechnology. Curr. Opin. Microbiol. 12, 261–273. doi: 10.1016/j.mib.2009.04.007
de Jesus Raposo, M. F., de Morais, A. M. B., and de Morais, R. M. S. C. (2015). Marine polysaccharides from algae with potential biomedical applications. Mar. Drugs 13, 2967–3028. doi: 10.3390/md13052967
de Jesus Raposo, M. F., De Morais, R. M. S. C., and De Morais, A. M. M. B. (2013). Bioactivity and applications of sulphated polysaccharides from marine microalgae. Mar. Drugs 11, 233–252. doi: 10.3390/md11010233
de Pinto, M. C., Francis, D., and De Gara, L. (1999). The redox state of the ascorbate-dehydroascorbate pair as a specific sensor of cell division in tobacco BY-2 cells. Protoplasma 209, 90–97. doi: 10.1007/BF01415704
Ding, B. (2009). The biology of viroid-host interactions. Annu. Rev. Phytopathol. 47, 105–131. doi: 10.1146/annurev-phyto-080508-081927
Dixon, R. A., Harrison, M. J., and Lamb, C. J. (1994). Early events in the activation of plant defense responses. Annu. Rev. Phytopathol. 32, 479–501. doi: 10.1146/annurev.py.32.090194.002403
Dong, X. (1998). SA, JA, ethylene, and disease resistance in plants. Curr. Opin. Plant Biol. 1, 316–323. doi: 10.1016/1369-5266(88)80053-0
Fu, Z. Q., and Dong, X. (2013). Systemic acquired resistance: turning local infection into global defense. Annu. Rev. Plant Biol. 64, 839–863. doi: 10.1146/annurev-arplant-042811-105606
Gelhaye, E., Rouhier, N., Navrot, N., and Jacquot, J. P. (2005). The plant thioredoxin system. Cell. Mol. Life Sci. 62, 24–35. doi: 10.1007/s00018-004-4296-4
Ghannam, A., Abbas, A., Alek, H., Al-Waari, Z., and Al-Ktaifani, M. (2013). Enhancement of local plant immunity against tobacco mosaic virus infection after treatment with sulphated-carrageenan from red alga (Hypnea musciformis). Physiol. Mol. Plant Pathol. 84, 19–27. doi: 10.1016/j.pmpp.2013.07.001
González, A., Castro, J., Vera, J., and Moenne, A. (2013a). Seaweed oligosaccharides stimulate plant growth by enhancing carbon and nitrogen assimilation, basal metabolism, and cell division. J. Plant Growth Regul. 32, 443–448. doi: 10.1007/s00344-012-9309-1
González, A., Contreras, R. A., and Moenne, A. (2013b). Oligo-carrageenans enhance growth and contents of cellulose, essential oils and polyphenolic compounds in Eucalyptus globulus trees. Molecules 18, 8740–8751. doi: 10.3390/molecules18088740
González, A., Contreras, R. A., Zúñiga, G., and Moenne, A. (2014b). Oligo-carrageenan kappa-induced reducing redox status and activation of TRR/TRX system increase the level of indole-3-acetic Acid, gibberellin A3 and trans-zeatin in Eucalyptus globulus trees. Molecules 19, 12690–12698. doi: 10.3390/molecules190812690
González, A., Gutiérrez-Cutiño, M., and Moenne, A (2014c). Oligo-carrageenan kappa-induced reducing redox status and increase in TRR/TRX activities promote activation and reprogramming of terpenoid metabolism in Eucalyptus trees. Molecules 19, 7356–7367. doi: 10.3390/molecules19067356
González, A., Moenne, F., Gómez, M., Sáez, C. A., Contreras, R. A., and Moenne, A. (2014a). Oligo-carrageenan kappa increases NADPH, ascorbate and glutathione syntheses and TRR/TRX activities enhancing photosynthesis, basal metabolism, and growth in Eucalyptus trees. Front. Plant Sci. 5:512. doi: 10.3389/fpls.2014.00512
González, M. E., Alarcón, B., and Carrasco, L. (1987). Polysaccharides as antiviral agents: antiviral activity of carrageenan. Antimicrob. Agents Chemother. 31, 1388–1393. doi: 10.1128/AAC.31.9.1388
Hashida, S. N., Takahashi, H., and Uchimiya, H. (2009). The role of NAD biosynthesis in plant development and stress responses. Ann. Bot. 103, 819–824. doi: 10.1093/aob/mcp019
Iriti, M., and Varoni, E. M. (2015). Chitosan-induced antiviral activity and innate immunity in plants. Environ. Sci. Pollut. Res. 22, 2935–2944. doi: 10.1007/s11356-014-3571-7
Jander, G., Cui, J., Nhan, B., Pierce, N. E., and Ausubel, F. M. (2001). The TASTY locus on chromosome 1 of Arabidopsis affects feeding of the insect herbivore Trichoplusia ni. Plant Physiol. 126, 890–898. doi: 10.1104/pp.126.2.890
Jiang, Y. P., Cheng, F., Zhou, Y. H., Xia, X. J., Mao, W. H., Shi, K., et al. (2012). Cellular glutathione redox homeostasis plays an important role in the brassinosteroid-induced increase in CO2 assimilation in Cucumis sativus. New Phytol. 194, 932–943. doi: 10.1111/j.1469-8137.2012.04111.x
Jones, J. D. G., and Dangl, J. L. (2006). The plant immune system. Nature 444, 323–329. doi: 10.1038/nature05286
Kalitnik, A. A., Byankina Barabanova, A. O., Nagorskaya, V. P., Reunov, A. V., Glazunov, V. P., Solov'eva, T. F., et al. (2013). Low molecular weight derivatives of different carrageenan types and their antiviral activity. J. Appl. Phycol. 25, 65–72. doi: 10.1007/s10811-012-9839-8
Klarzynski, O., Descamps, V., Plesse, B., Yvin, J.-C., Kloareg, B., and Fritig, B. (2003). Sulfated fucan oligosaccharides elicit defense responses in tobacco and local and systemic resistance against tobacco mosaic virus. Mol. Plant. Microbe. Interact. 16, 115–122. doi: 10.1094/MPMI.2003.16.2.115
Koornneef, A., and Pieterse, C. M. J. (2008). Cross talk in defense signaling. Plant Physiol. 146, 839–844. doi: 10.1104/pp.107.112029
Lambrix, V., Reichelt, M., Mitchell-Olds, T., Kliebenstein, D. J., and Gershenzon, J. (2001). The Arabidopsis epithiospecifier protein promotes the hydrolysis of glucosinolates to nitriles and influences Trichoplusia ni herbivory. Plant Cell 13, 2793–2807. doi: 10.1105/tpc.010261
Laudert, D., and Weiler, E. W. (1998). Allene oxide synthase: a major control point in Arabidopsis thaliana octadecanoid signaling. Plant J. 15, 675–684. doi: 10.1046/j.1365-313x.1998.00245.x
Lemonnier-Le Penhuizic, C., Chatelet, C., Kloareg, B., and Potin, P. (2001). Carrageenan oligosaccharides enhance stress-induced microspore embryogenesis in Brassica oleracea var. italica. Plant Sci. 160, 1211–1220. doi: 10.1016/S0168-9452(01)00372-7
Li, L., Ni, R., Shao, Y., and Mao, S. (2014). Carrageenan and its applications in drug delivery. Carbohydr. Polym. 103, 1–11. doi: 10.1016/j.carbpol.2013.12.008
Liso, R., Innocenti, A. M., Bitonti, M. B., and Arrigoni, O. (1988). Ascorbic acid induced progression of quiescent center cells from G1 to S phase. New Phytol. 110, 469–471. doi: 10.1111/j.1469-8137.1988.tb00284.x
Maffei, M. E., Mithöfer, A., and Boland, W. (2007). Before gene expression: early events in plant-insect interaction. Trends Plant Sci. 12, 310–316. doi: 10.1016/j.tplants.2007.06.001
Mercier, L., Lafitte, C., Borderies, G., Briand, X., Esquerré-Tugayé, M. T., and Fournier, J. (2001). The algal polysaccharide carrageenans can act as an elicitor of plant defence. New Phytol. 149, 43–51. doi: 10.1046/j.1469-8137.2001.00011.x
Montrichard, F., Alkhalfioui, F., Yano, H., Vensel, W. H., Hurkman, W. J., and Buchanan, B. B. (2009). Thioredoxin targets in plants: the first 30 years. J. Proteomics 72, 452–474. doi: 10.1016/j.jprot.2008.12.002
Muñoz, A. M., Ponce, J. C., and Araya, J. V. (2011). Method to Stimulate Carbon Fixation in Plants with an Aqueous Solution of Oligo-Carrageenans Selected from Kappa1, Kappa2, Lambda or Iota. U.S. Patent Application 12/911,790.
Nagorskaya, V. P., Reunova, V., Lapshina, L. A., Ermak, I. M., and Barabanova, A O. (2008). Influence of kappa/beta-carrageenan from red alga Tichocarpus crinitus on development of local infection induced by tobacco mosaic virus in Xanthi-nc tobacco leaves. Izv. Akad. Nauk Ser. Biol. 35, 360–364. doi: 10.1134/S1062359008030126
Nagorskaya, V. P., Reunov, A. V., Lapshina, L. A., Ermak, I. M., and Barabanova, A. O. (2010). Inhibitory effect of κ/β carrageenan from red alga Tichocarpus crinitus on the development of a potato virus X infection in leaves of Datura stramonium L. Biol. Bull. 37, 653–658. doi: 10.1134/S1062359010060142
Nicaise, V. (2015). Lost in translation: an antiviral plant defense mechanism revealed. Cell Host Microbe 17, 417–419. doi: 10.1016/j.chom.2015.03.009
Noctor, G., and Foyer, C. H. (1998). Ascorbate amd glutathione:: Keeping active oxygen under control. Annu. Rev. Plant Physiol. Plant Mol. Biol. 49, 249–279. doi: 10.1146/annurev.arplant.49.1.249
Patier, P., Potin, P., Rochas, C., Kloareg, B., Yvin, J., and Licnart, Y. (1995). Free or silica-bound oligokappa-carrageenans elicit laminarinase activity in Rubus cells and protoplasts. Plant Sci. 9452, 27–35. doi: 10.1016/0168-9452(95)04182-T
Pieterse, C. M. J., and Dicke, M. (2007). Plant interactions with microbes and insects: from molecular mechanisms to ecology. Trends Plant Sci. 12, 564–569. doi: 10.1016/j.tplants.2007.09.004
Potin, P., Bouarab, K., Küpper, F., and Kloareg, B. (1999). Oligosaccharide recognition signals and defence reactions in marine plant-microbe interactions. Curr. Opin. Microbiol. 2, 276–283. doi: 10.1016/S1369-5274(99)80048-4
Prajapati, V. D., Maheriya, P. M., Jani, G. K., and Solanki, H. K. (2014). Carrageenan: a natural seaweed polysaccharide and its applications. Carbohydr. Polym. 105, 97–112. doi: 10.1016/j.carbpol.2014.01.067
Rocha de Souza, M. C., Marques, C. T., Guerra Dore, C. M., Ferreira da Silva, F. R., Oliveira Rocha, H. A., and Leite, E. L. (2007). Antioxidant activities of sulfated polysaccharides from brown and red seaweeds. J. Appl. Phycol. 153–160. doi: 10.1007/s10811-006-9121-z
Russell, N. J., and Nichols, D. S. (1999). Polyunsaturated fatty acids in marine bacteria – a dogma rewritten. Microbiology 145, 767–779. doi: 10.1099/13500872-145-4-767
Ryan, C. A. (1990). Protease inhibitors in plants: genes for improving defenses against insects and pathogens. Annu. Rev. Phytopathol. 28, 425–449. doi: 10.1146/annurev.py.28.090190.002233
Sangha, J. S., Kandasamy, S., Khan, W., Bahia, N. S., Singh, R. P., Critchley, A. T., et al. (2015). λ-Carrageenan suppresses Tomato Chlorotic Dwarf Viroid (TCDVd) replication and symptom expression in tomatoes. Mar. Drugs 13, 2875–2889. doi: 10.3390/md13052875
Sangha, J. S., Kelloway, S., Critchley, A. T., and Prithiviraj, B. (2014). Seaweeds (Macroalgae) and their extracts as contributors of plant productivity and quality: the current status of our understanding. Sea Plants 71, 189–219. doi: 10.1016/B978-0-12-408062-1.00007-X
Sangha, J. S., Khan, W., Ji, X., Zhang, J., Mills, A. A. S., Critchley, A. T., et al. (2011). Carrageenans, sulphated polysaccharides of red seaweeds, differentially affect Arabidopsis thaliana resistance to Trichoplusia ni (Cabbage looper). PLoS ONE 6:e26834. doi: 10.1371/journal.pone.0026834
Sangha, J. S., Ravichandran, S., Prithiviraj, K., Critchley, A. T., and Prithiviraj, B. (2010). Sulfated macroalgal polysaccharides λ-carrageenan and ι-carrageenan differentially alter Arabidopsis thaliana resistance to Sclerotinia sclerotiorum. Physiol. Mol. Plant Pathol. 75, 38–45. doi: 10.1016/j.pmpp.2010.08.003
Saucedo, S., Contreras, R. A., and Moenne, A. (2015). Oligo-carrageenan kappa increases C, N and S assimilation, auxin and gibberellin contents, and growth in Pinus radiata trees. J. For. Res. 26, 635–640. doi: 10.1007/s11676-015-0061-9
Scheel, D. (1998). Resistance response physiology and signal transduction. Curr. Opin. Plant Biol. 1, 305–310. doi: 10.1016/1369-5266(88)80051-7
Shah, J. (2003). The salicylic acid loop in plant defense. Curr. Opin. Plant Biol. 6, 365–371. doi: 10.1016/S1369-5266(03)00058-X
Shahidi, F., and Ambigaipalan, P. (2015). Novel functional food ingredients from marine sources. Curr. Opin. Food Sci. 2, 123–129. doi: 10.1016/j.cofs.2014.12.009
Singh, R. P., Dilworth, A. D., Singh, M., and Babcock, K. M. (2006). An alkaline solution simplifies nucleic acid preparation for RT-PCR and infectivity assays of viroids from crude sap and spotted membrane. J. Virol. Methods 132, 204–211. doi: 10.1016/j.jviromet.2005.09.007
Singh, R. P., and Teixeria da Silva, J. A. (2006). “Ornamental plants: silent carrier of evolving viroids,” in Floriculture, Ornamental and Plant Biotechnology, Vol. 3, ed J. A. Teixeria da Silva (London: Global Science Books), 531–539.
Stadnik, M. J., and De Freitas, M. B. (2014). Algal polysaccharides as source of plant resistance inducers. Trop. Plant Pathol. 39, 111–118. doi: 10.1590/S1982-56762014000200001
Sun, T., Tao, H., Xie, J., Zhang, S., and Xu, X. (2010). Degradation and antioxidant activity of κ-carrageenans. J. Appl. Polym. Sci. 117, 194–199. doi: 10.1002/app.31955
Thaler, J. S., Humphrey, P. T., and Whiteman, N. K. (2012). Evolution of jasmonate and salicylate signal crosstalk. Trends Plant Sci. 17, 260–270. doi: 10.1016/j.tplants.2012.02.010
Vera, J., Castro, J., Contreras, R. A., González, A., and Moenne, A. (2012). Oligo-carrageenans induce a long-term and broad-range protection against pathogens in tobacco plants (var. Xanthi). Physiol. Mol. Plant Pathol. 79, 31–39. doi: 10.1016/j.pmpp.2012.03.005
Vera, J., Castro, J., Gonzalez, A., and Moenne, A. (2011). Seaweed polysaccharides and derived oligosaccharides stimulate defense responses and protection against pathogens in plants. Mar. Drugs 9, 2514–2525. doi: 10.3390/md9122514
Vernoux, T., Wilson, R. C., Seeley, K. A, Reichheld, J. P., Muroy, S., Brown, S., et al. (2000). The ROOT MERISTEMLESS1/CADMIUM SENSITIVE2 gene defines a glutathione-dependent pathway involved in initiation and maintenance of cell division during postembryonic root development. Plant Cell 12, 97–110. doi: 10.1016/S1369-5266(00)80035-7
Vo, T. S., and Kim, S. K. (2010). Potential anti-HIV agents from marine resources: an overview. Mar. Drugs 8, 2871–2892. doi: 10.3390/md8122871
Walling, L. L. (2000). The myriad plant responses to herbivores. J. Plant Growth Regul. 19, 195–216. doi: 10.1007/s003440000026
Wang, W., Wang, S. X., and Guan, H. S. (2012). The antiviral activities and mechanisms of marine polysaccharides: an overview. Mar. Drugs 10, 2795–2816. doi: 10.3390/md10122795
Yu, S.-M., Lo, S.-F., and Ho, T.-H. D. (2015). Source–sink communication: regulated by hormone, nutrient, and stress cross-signaling. Trends Plant Sci. 20, 844–857. doi: 10.1016/j.tplants.2015.10.009
Yuan, H., Song, J., Li, X., Li, N., and Dai, J. (2006). Immunomodulation and antitumor activity of kappa-carrageenan oligosaccharides. Cancer Lett. 243, 228–234. doi: 10.1016/j.canlet.2005.11.032
Zangerl, A. R. (2003). Evolution of induced plant responses to herbivores. Basic Appl. Ecol. 4, 91–103. doi: 10.1078/1439-1791-00135
Keywords: carrageenans, plant elicitor, plant growth, plant defense mechanisms, plant signaling pathways, systemic acquired resistance, induced resistance
Citation: Shukla PS, Borza T, Critchley AT and Prithiviraj B (2016) Carrageenans from Red Seaweeds As Promoters of Growth and Elicitors of Defense Response in Plants. Front. Mar. Sci. 3:81. doi: 10.3389/fmars.2016.00081
Received: 07 March 2016; Accepted: 13 May 2016;
Published: 31 May 2016.
Edited by:
Christine Delbarre-Ladrat, Institut Français de Recherche pour l'Exploitation de la Mer, FranceReviewed by:
Bhavanath Jha, CSIR-Central Salt and Marine Chemicals Research Institute, IndiaGiuseppe Colla, University of Tuscia, Italy
Copyright © 2016 Shukla, Borza, Critchley and Prithiviraj. This is an open-access article distributed under the terms of the Creative Commons Attribution License (CC BY). The use, distribution or reproduction in other forums is permitted, provided the original author(s) or licensor are credited and that the original publication in this journal is cited, in accordance with accepted academic practice. No use, distribution or reproduction is permitted which does not comply with these terms.
*Correspondence: Balakrishnan Prithiviraj, YnByaXRoaXZpcmFqQGRhbC5jYQ==