- 1Centre for Integrative Ecology, School of Life and Environmental Sciences, Deakin University, Warrnambool, VIC, Australia
- 2Centre d'Estudis Avançats de Blanes, CSIC, Blanes, Spain
- 3Oceans and Coasts Program, Nature Conservation Foundation, Mysore, India
- 4Institute for Wetland and Water Research, Radboud University Nijmegen, Nijmegen, Netherlands
- 5Red Sea Research Center, King Abdullah University of Science and Technology, Thuwal, Saudi Arabia
- 6College of Science and Engineering, James Cook University, Townsville, QLD, Australia
- 7Centre for Tropical Water and Aquatic Ecosystem Research (TropWATER), James Cook University, Cairns, QLD, Australia
- 8Australian Institute of Marine Science, Indian Ocean Marine Research Centre, University of Western Australia, Crawley, WA, Australia
- 9Department of Biosciences, Swansea University, Swansea, United Kingdom
- 10Project Seagrass, Cardiff, United Kingdom
Seagrasses are hugely valuable to human life, but the global extent of seagrass meadows remains unclear. As evidence of their value, a United Nations program exists (http://data.unep-wcmc.org/datasets/7) to try and assess their distribution and there has been a call from 122 scientists across 28 countries for more work to manage, protect and monitor seagrass meadows (http://www.bbc.com/news/science-environment-37606827). Emerging from the 12th International Seagrass Biology Workshop, held in October 2016, has been the view that grazing marine megafauna may play a useful role in helping to identify previously unknown seagrass habitats. Here we describe this concept, showing how detailed information on the distribution of both dugongs (Dugong dugon) and green sea turtles (Chelonia mydas) obtained, for example, by aerial surveys and satellite tracking, can reveal new information on the location of seagrass meadows. We show examples of how marine megaherbivores have been effective habitat indicators, revealing major, new, deep-water seagrass meadows and offering the potential for more informed estimates of seagrass extent in tropical and sub-tropical regions where current information is often lacking.
Background
Seagrasses are among the most valuable ecosystems on earth, are of fundamental importance to human life and yet the lack of data on their distribution for much of the globe has limited the ability of scientists to truly quantify and understand their roles on global scales (Duarte, 2017). Each year seagrasses provide ecosystem services worth many trillions of dollars (Costanza et al., 2014). For example, seagrass meadows provide habitat for fish that are the primary source of protein for millions of people from developing nations (Orth et al., 2006; Unsworth and Cullen, 2010; Nordlund et al., 2017); play an important role in mitigating climate change (by storing carbon in sediment 40 times faster than a typical terrestrial forest) (Duarte et al., 2013); protect coasts by raising the seafloor, dissipating waves, stabilizing sediment and preventing erosion (Duarte et al., 2013); can reduce the abundance of bacterial pathogens capable of causing disease in humans and marine organisms and can help improve the health of adjacent corals (Lamb et al., 2017).
One of the greatest issues facing the effective management and assessment of the global role of seagrass ecosystems is the difficulty in mapping their distribution. At present there is high uncertainty around how much seagrass exists globally, especially in sub-tidal environments and particularly within the tropics. Current estimates of global seagrass area range from 150,000 to 600,000 km2, with no progress over the past two decades in narrowing this uncertainty band (Duarte, 2017). However, the potential global seagrass area determined by light regime, bathymetry, and seagrass light requirements is estimated to be 4,320,000 km2 (Gattuso et al., 2006), potentially yielding a 35-fold increase of recent estimates of seagrass ecosystem services value. The need to understand and effectively model our global carbon cycle together with a growing desire to incorporate seagrass “blue carbon” into climate mitigation strategies necessitates that we improve our understanding of global seagrass extent.
The spatial extent of seagrass remains difficult to assess using conventional remote sensing tools, particularly in either turbid, deep environments or shallow waters where density can be low. Historically poor seagrass research and conservation efforts, together with the charisma problem that seagrass has been faced with (Duarte et al., 2008), means that there are key global gaps in mapping efforts. One of the reasons that the global extent of seagrass meadows is so uncertain is because, unlike terrestrial habitats, benthic habitats are generally not clearly visible from the surface and are therefore more difficult, or in many cases impossible, to map and monitor with conventional remote sensing techniques. Nowhere is this truer than in tropical and subtropical areas like SE Asia; which likely represent a substantial component of the world's seagrass resources but remain largely unmapped (UNEP-WCMC and Short, 2016).
Given this long-standing uncertainty with global estimates of seagrass abundance, new methods to tackle this problem may be needed. Scientists from diverse disciplines attending the 12th International Seagrass Biology Workshop, held in October 2016 (Hind-Ozan and Jones, 2017), came to the realization that grazing marine megafauna may be used to help identify previously unknown seagrass habitats. Here we describe this idea that seagrass grazers, whose distribution can be remotely assessed, may be used as a new tool to help identify the location of seagrass meadows and to help to improve estimates of the global distribution of seagrasses. So we build on the long tradition of using indicator species to indicate habitat types.
Two widely distributed, largely tropical and subtropical, marine megavertebrates feed on seagrass: the green sea turtle (Chelonia mydas) feeds primarily on seagrass in many regions while the dugong (Dugong dugon) is a seagrass community specialist (Figures 1A,B,E,F). So by assessing the distribution of green turtles and dugongs, using existing data from surveys and satellite tracking, we can potentially gain a new level of understanding on the global extent of seagrass meadows. Knowing the distribution of these seagrass grazers will not, in itself, allow remote quantitative estimates of seagrass abundance. Rather, knowing the location of these grazers will allow directed in-water sampling of seagrass in areas hitherto ignored. So it is this synergistic use of recording animal locations with in-water surveys that may allow new knowledge to be gained about seagrass distribution. We use two case studies to illustrate the new insights that can be obtained in this way. The same methodology could also apply to two other sirenian species in the Atlantic, the West Indian and African manatees (Trichechus spp.) that also feed on seagrass in some parts of their ranges (Lefebvre et al., 1999), although their diet is much less specialized than that of the dugong (Marsh et al., 2011).
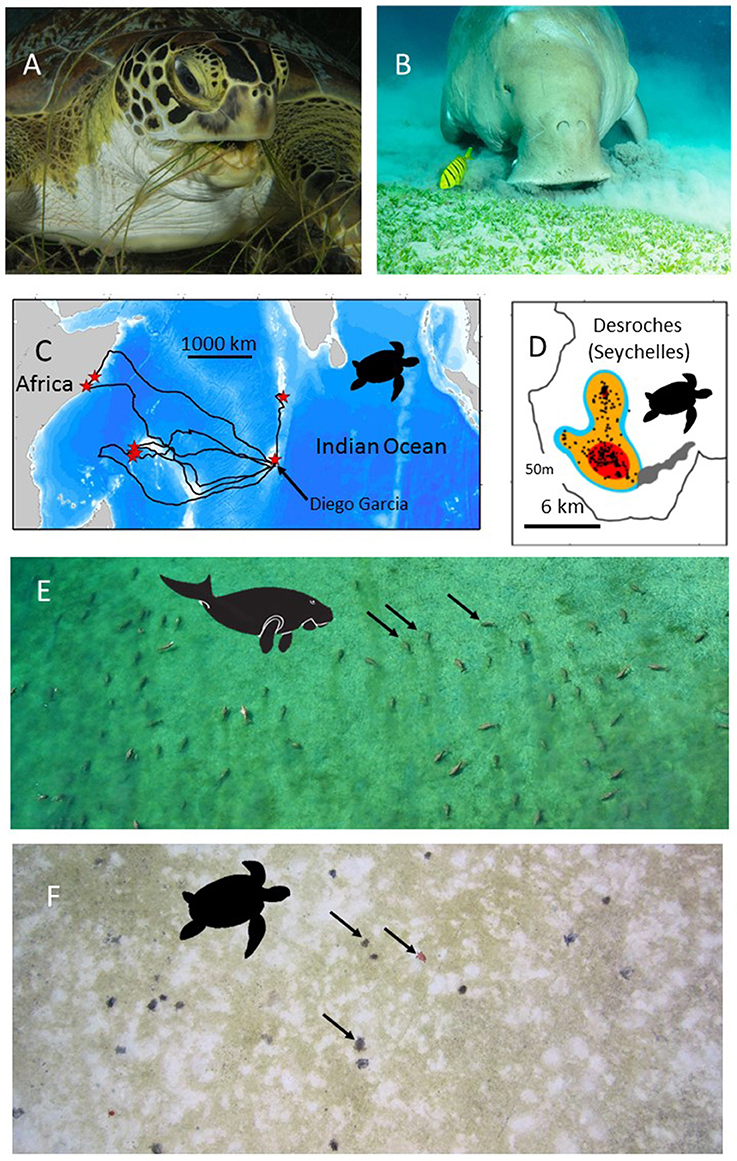
Figure 1. The use of green turtles and dugongs as habitat indicators. (A) A green turtle (Chelonia mydas) (B) A dugong (Dugong dugon). Both species feed on seagrasses and so the location of their foraging sites may indicate seagrass habitat. (C) Green turtles can be satellite tracked from their nesting beaches to their, sometimes, very discrete foraging grounds. Here the tracks of 8 green turtles that had nested on Diego Garcia, in the Chagos Archipelago, are shown. Red stars show the end point of each track, i.e., the location of each turtle's foraging grounds. (D) Modern satellite tracking can provide very high accuracy locations (e.g., Fastloc-GPS) (black dots) allowing space use on foraging grounds to be accurately assessed. In this case the foraging home-range is shown for one of the turtles tracked in (C). Red, orange, blue colors show the 50, 90, and 95% kernel home-range estimates. On-site validation of the foraging habitats used by (E) dugongs and (F) green turtles in the Indo-Pacific has confirmed the hitherto unknown location of extensive seagrass beds. (E) An aerial photo of 50+ foraging dugongs. Black arrows indicate three of the dugongs. (F) An aerial photo (from a drone survey) of 20+ foraging green turtles. Black arrows indicate three of the green turtles. Note the grazed area (white) around each green turtle. Photo in (A) courtesy of RD and BS Kirkby in (B) courtesy of EH Meesters, (C,D) from Hays et al. (2014), (E) courtesy of Susan Sobtzick and (F) courtesy of MC.
Case Study: Green Sea Turtles and the Great Chagos Bank
Adult female green turtles (adults can be up to 1.5 m long, weigh >150 kg, and eat up to 2 kg of seagrass day−1) come ashore to nest on sandy beaches, but then at the end of the nesting season they may travel 100s or 1,000s of km to foraging grounds, with each individual often having fidelity to a specific foraging location (Hays et al., 2014). In many parts of their range, seagrass is thought to form a major component of the diet of green turtles. For example, in the Caribbean green turtles often cultivate mono-specific meadows of the seagrass Thalassia testudinum, feeding on the more easily digestible new growth that develops after cropping (Bjorndal, 1980). Similarly in Mayotte in the western Indian Ocean, several seagrass species are thought to form the mainstay of the diet of green turtles (Ballorain et al., 2010). Where the density of green turtles is very high, green turtles may even overgraze seagrass meadows causing habitat degradation (Christianen et al., 2014). However, at some coastal foraging sites green turtles may also consume macroalgae such as in parts of the Torres Strait, Australia (André et al., 2005). Macroalgae may even dominate their diet, for example in some areas of the eastern Pacific (Seminoff et al., 2002). On occasion, green turtles may even consume invertebrates and be considered omnivorous (Cardona et al., 2009; Burkholder et al., 2011). However, at some sites where turtles are omnivorous, age/size based shifts have been reported with larger turtles being more likely to forage on seagrass (Cardona et al., 2009). In short green turtles do not exclusively consume seagrass in all areas. So tracking green turtles to their foraging grounds will not definitively allow seagrass beds to be identified, but will certainly give a good indication of where in-situ surveys can be targeted to potentially document areas of seagrass that were previously unknown. For green turtles it is known that they migrate between disparate nesting areas and foraging areas. So, for example, female turtles can be equipped with satellite tags while nesting and then tracked back to their foraging grounds. On the foraging grounds individuals typically show a movement between night-time resting areas and day-time feeding areas (Christiansen et al., 2017). So the day-time foraging locations inform on where in situ surveys for seagrasses should be targeted.
The range of green sea turtles extends throughout tropical and subtropical oceans worldwide. From some nesting areas, green turtles have been tracked by satellite and their post-nesting migrations to their foraging grounds have been astounding and unexpected (Figures 1C,D). For example, green turtles equipped with satellite transmitters on nesting beaches on Diego Garcia, in the Chagos Archipelago, have traveled to deep water sites (30 m) on the Great Chagos Bank (Hays et al., 2014) and have remained there with a restricted home range of 36 km2 for over 19 months until satellite transmissions ceased (Christiansen et al., 2017). The first tagged turtle recorded traveling to the Great Chagos Bank was one of eight nesting females we equipped in 2012. We have subsequently (as on 1 December 2017) tracked a total of 5 green turtles to the Great Chagos Bank of 23 nesting turtles equipped (Hays, Esteban, unpublished). The Great Chagos Bank extends over approximately 13,000 km2 and was not known to contain seagrass meadows. In 2016 the first opportunity arose to visit some of the areas that turtle tracking had identified as likely foraging grounds (evidenced by high residency in discrete areas) and use SCUBA and remote cameras to assess the benthic habitat. Two of the green turtle foraging sites were visited with three submarine surveys conducted at each site. Extensive Thalassodendron ciliatum seagrass meadows occurred on all six of these submarine surveys (depth range 12–29 m). So where seagrass meadows were previously unknown they may, in fact, be extensive.
There are now many 100s of adult green turtles that have been satellite tracked (see seaturtle.org), offering huge potential to use these tracking data to identify and map the likely extent of seagrass habitat. Importantly, there have been around 400 adult green turtles tracked in the Indian Ocean and SE Asia region and thus knowledge gained from those tracks could add substantially to our understanding of the distribution of seagrass meadows in this important, but unexplored region. Furthermore, advances in tracking technology mean that very accurate locations (i.e., within a few 10s of meters) can now be obtained via satellite tags (e.g., Dujon et al., 2014), meaning that patterns of space use can be assessed in great detail (Hays et al., 2014). This high resolution tracking data could be used to direct in situ surveys and hence help validate models of seagrass distribution based on physical properties alone. For example, we have used green turtle tracking data in this way to show the existence of extensive seagrass meadows at much deeper sites than expected. Some satellite tags also collect data on water temperature, which may allow information on the temperature regime required by seagrasses to be refined.
Case Study: Dugongs in the Torres Strait
Dugongs are large air-breathing mammals (adults up to ~3 m long, weigh up to 500 kg and consume up to 35 kg of seagrass day−1; Marsh et al., 2011). Dugongs are visible during aerial surveys, when they are at the surface or in a sub-surface detection zone, the depth of which varies according to water clarity and location (Figure 1E; Pollock et al., 2006; Hagihara et al., 2014, 2018). Depending on the nature of the sediment and the species of seagrass, dugongs use two modes of feeding: excavating whole plants or cropping leaves (Marsh et al., 2011). When feeding in excavating mode, dugongs leave feeding trails in seagrass meadows that are visible from the air in exposed intertidal meadows or in shallow clear water. Some animals and feeding trails might even be visible from very high resolution satellite imagery such as WV3 with 30 cm on ground resolution or from orthorectified images collected from unmanned aerial surveys (Hodgson et al., 2013). Automated systems that use machine learning to detect dugong from the large number of images captured in these aerial surveys are already under development (Maire et al., 2015).
Areas occupied by dugongs can guide ground surveys to establish the extent of seagrass meadows. For example, information on the distribution of dugongs in Torres Strait between northern Australia and Papua New Guinea (Marsh et al., 1997) was used to direct ground surveys that revealed a total seagrass area in excess of 30,000 km2, including the discovery of the largest continuous seagrass meadow mapped in Australia, totaling over 8,750 km2 of mostly deep-water (>15 m) seagrasses growing to around 30 m (Taylor and Rasheed, 2010). Similarly, the location of large numbers of dugong obtained both by aerial surveys and through local knowledge of hunters was used to guide seagrass surveys in the Northern Torres Strait, leading to extensive intertidal and subtidal seagrasses being mapped in the vicinity of Orman Reefs (Rasheed et al., 2008). This significantly expanded the known mapped area of seagrass in the region and led to an understanding of the large amount of carbon incorporated by Torres Strait seagrass productivity (Rasheed et al., 2008).
Satellite tracking of dugong is less prevalent than for turtles. In the tracked individuals, we observed that dugong movements tend to be irregular and localized although some individuals undertake highly directional movements exceeding 500 km, sometimes including return to the start point (Sheppard et al., 2006; Gredzens et al., 2014). As dugong are seagrass community specialists, their use of space should provide greater certainty of seagrass presence than that of green turtles due to their closer association with this habitat (Sheppard et al., 2007; Marsh et al., 2011, 2018). In fact, the fossil record of dugong relatives (Sirenia) has already been used to provide a paleobiogeographic overview of seagrass distribution through time (Vélez-Juarbe, 2014), reiterating how present-day work may couple information on dugong occurrence with seagrass assessment studies.
Conclusions
These case studies highlight the huge potential for productive collaborations between scientists studying the distribution and movement of green turtles and dugong (and manatees) and those attempting to assess the global roles of seagrass ecosystems, but we also note the caveats of this approach. Information on the location of dugongs and green turtles can be used to direct in-water surveys of hitherto unknown seagrass meadows. Furthermore, potentially knowing the location of these grazers may help to inform models that are used to assess seagrass extent, such as those based on light and ocean bathymetry (Gattuso et al., 2006). Changes in the movement of grazers over time may potentially also inform on the dynamics of seagrass meadows. Our proof-of-concept discovery of seagrass meadows on the Great Chagos Bank emphasizes how little is known of deeper-water seagrasses and points to the likelihood of extensive undiscovered deeper-water seagrass meadows elsewhere. We hope that this article stimulates discussion and new approaches to narrow down uncertainties in estimates of the global extent of seagrass.
Author Contributions
GH and NE conceived this project. NE, MC, and CD hosted a workshop at the 12th International Seagrass Biology Workshop to bring the group together. All authors contributed intellectually and commented on the drafts and approved the manuscript for publication.
Funding
We would like to thank Ernesto and Kirsty Bertarelli, and the Bertarelli Foundation, for their support of research in the Chagos Archipelago. MR, HM, MH, PY, and PM were supported through an Australian Research Council grant LP160100492.
Conflict of Interest Statement
The authors declare that the research was conducted in the absence of any commercial or financial relationships that could be construed as a potential conflict of interest.
The handling Editor declared a past co-authorship with some of the authors MT, CD, and GH.
Acknowledgments
We acknowledge and thank the British Indian Ocean Territory (BIOT) Administration for assistance and permits to carry out research within the Chagos Archipelago. We would like to thank the Torres Strait Regional Authority Land and Sea Management Unit for the support of seagrass and dugong assessments. Thanks to E. Meesters, S. Sobtzick, R. & B. Kirkby, and MC for use of photographic material.
References
André, J., Gyuris, E., and Lawler, I. R. (2005). Comparison of the diets of sympatric dugongs and green turtles on the Orman Reefs, Torres Strait, Australia. Wildlife Res. 32, 53–62. doi: 10.1071/WR04015
Ballorain, K., Ciccione, S., Bourjea, J., Grizel, H., Enstipp, M., and Georges, J.-Y. (2010). Habitat use of a multispecific seagrass meadow by green turtles Chelonia mydas at Mayotte Island. Mar. Biol. 157, 2581–2590. doi: 10.1007/s00227-010-1520-7
Bjorndal, K. A. (1980). Nutrition and grazing behavior of the green turtle Chelonia mydas. Mar. Biol. 56, 147–154. doi: 10.1007/BF00397131
Burkholder, D. A., Heithaus, M. R., Thomson, J. A., and Fourqurean, J. W. (2011). Diversity in trophic interactions of green sea turtles Chelonia mydas on a relatively pristine coastal foraging ground. Mar. Ecol. Prog. Ser. 439, 277–293. doi: 10.3354/meps09313
Cardona, L., Aguilar, A., and Pazos, L. (2009). Delayed ontogenic dietary shift and high levels of omnivory in green turtles (Chelonia mydas) from the NW coast of Africa. Mar. Biol. 156, 1487–1495. doi: 10.1007/s00227-009-1188-z
Christianen, M. J., Herman, P. M. J., Bouma, T. J., Lamers, L. P. M., van Katwijk, M. M., van der Heide, T., et al. (2014). Habitat collapse due to overgrazing threatens turtle conservation in marine protected areas. Proc. R. Soc. Lond. B 281:20132890. doi: 10.1098/rspb.2013.2890
Christiansen, F., Esteban, N., Mortimer, J. A., Dujon, A. M., and Hays, G. C. (2017). Diel and seasonal patterns in activity and home range size of green turtles on their foraging grounds revealed by extended Fastloc-GPS tracking. Mar. Biol. 164, 10. doi: 10.1007/s00227-016-3048-y
Costanza, R., de Groot, R., Sutton, P., van der Ploeg, S., Anderson, S. J., Kubiszewski, I., et al. (2014). Changes in the global value of ecosystem services. Glob. Environ. Change 26, 152–158. doi: 10.1016/j.gloenvcha.2014.04.002
Duarte, C. M. (2017). Reviews and syntheses: hidden forests, the role of vegetated coastal habitats in the ocean carbon budget. Biogeosciences 14, 301–310. doi: 10.5194/bg-14-301-2017
Duarte, C. M., Dennison, W. C., Orth, R. J., and Carruthers, T. J. (2008). The charisma of coastal ecosystems: addressing the imbalance. Estuar. Coasts 31, 233–238. doi: 10.1007/s12237-008-9038-7
Duarte, C. M., Losada, I. J., Hendriks, I. E., Mazarrasa, I., and Marbà, N. (2013). The role of coastal plant communities for climate change mitigation and adaptation. Nat. Clim. Change 3, 961–968. doi: 10.1038/nclimate1970
Dujon, A. M., Lindstrom, R. T., and Hays, G. C. (2014). The accuracy of Fastloc-GPS locations and implications for animal tracking. Methods Ecol. Evol. 5, 1162–1169. doi: 10.1111/2041-210X.12286
Gattuso, J. P., Gentili, B., Duarte, C. M., Kleypas, J. A., Middelburg, J. J., and Antoine, D. (2006). Light availability in the coastal ocean: impact on the distribution of benthic photosynthetic organisms and their contribution to primary production. Biogeosciences 3, 489–513. doi: 10.5194/bg-3-489-2006
Gredzens, C., Marsh, H., Fuentes, M. M. P. B., Limpus, C. J., Shimada, T., and Hamann, M. (2014). Satellite tracking of sympatric marine megafauna can inform the biological basis for species co-management. PLoS ONE 9:e98944. doi: 10.1371/journal.pone.0098944
Hagihara, R., Jones, R. E., Grech, A., Lanyon, J. M., Sheppard, J. K., and Marsh, H. (2014). Improving population estimates by quantifying diving and surfacing patterns: a dugong example. Mar. Mammal Sci. 30, 348–366. doi: 10.1111/mms.12041
Hagihara, R., Jones, R. E., Sobtzick, S., Cleguer, C., Garrigue, C., and Marsh, H. (2018). Compensating for geographic variation in detection probability with water depth improves abundance estimates of coastal marine megafauna. PLoS ONE 13:e0191476. doi: 10.1371/journal.pone.0191476
Hays, G. C., Mortimer, J. A., Ierodiaconou, D., and Esteban, N. (2014). Use of long-distance migration patterns of an endangered species to inform conservation planning for the world's largest marine protected area. Cons. Biol. 28, 1636–1644. doi: 10.1111/cobi.12325
Hind-Ozan, E. J., and Jones, B. L. (2017). Seagrass science is growing: a report on the 12th International Seagrass Biology Workshop. Mar. Pollut. Bull. doi: 10.1016/j.marpolbul.2017.08.017. [Epub ahead of print].
Hodgson, A., Kelly, N., and Peel, D. (2013). Unmanned aerial vehicles (UAVs) for surveying marine fauna: a dugong case study. PLoS ONE 8:e79556. doi: 10.1371/journal.pone.0079556
Lamb, J. B., van de Water, J. A. J. M., Bourne, D. G., Altier, C., Hein, M. Y., Fiorenza, E. A., et al. (2017). Seagrass ecosystems reduce exposure to bacterial pathogens of humans, fishes, and invertebrates. Science 355, 731–733. doi: 10.1126/science.aal1956
Lefebvre, L. W., Reid, J. P., Kenworthy, W. J., and Powell, J. A. (1999). Characterizing manatee habitat use and seagrass grazing in Florida and Puerto Rico: implications for conservation and management. Pacific Conserv. Biol. 5, 289–298. doi: 10.1071/PC000289
Maire, F., Alvarez, L. M., and Hodgson, A. (2015). “Automating marine mammal detection in aerial images captured during wildlife surveys: a deep learning approach,” in AI 2015: Advances in Artificial Intelligence. Lecture Notes in Computer Science, Vol. 9457, eds B. Pfahringer and J. Renz (Cham: Springer), 379–385.
Marsh, H., Grech, H., and McMahon, K. (2018). “Dugongs; seagrass community specialists,” in Seagrasses of Australia, eds A. W. D. Larkum, P. Ralph, and G. Kendrick (Springer).
Marsh, H., Harris, A. N. M., and Lawler, I. R. (1997). The sustainability of the Indigenous dugong fishery in Torres Strait, Australia/Papua New Guinea. Conserv. Biol. 11, 1375–1386. doi: 10.1046/j.1523-1739.1997.95309.x
Marsh, H., O'Shea, T. J., and Reynolds, J. E. I. I. I. (2011). The Ecology and Conservation of Sirenia: Dugongs and Manatees. Cambridge: Cambridge University Press.
Nordlund, L. M., Unsworth, R. K. F., Gullström, M., and Cullen-Unsworth, L. C. (2017). Global significance of seagrass fishery activity. Fish Fish. doi: 10.1111/faf.12259. [Epub ahead of print].
Orth, R. J., Carruthers, T. J., Dennison, W. C., Duarte, C. M., Fourqurean, J. W., Heck, K. L., et al. (2006). A global crisis for seagrass ecosystems. AIBS Bull. 56, 987–996. doi: 10.1641/0006-3568(2006)56[987:AGCFSE2.0.CO2]
Pollock, K., Marsh, H., Lawler, I., and Alldredge, M. (2006). Modelling availability and perception processes for strip and line transects: an application to dugong aerial surveys. J. Wildl. Manag. 70, 255–262. doi: 10.2193/0022-541X(2006)70[255:EAAIHE]2.0.CO;2
Rasheed, M. A., Dew, K. R., McKenzie, L. J., Coles, R. G., Kerville, S., and Campbell, S. J. (2008). Productivity, carbon assimilation and intraannual change in tropical reef platform seagrass communities of the Torres Strait, north-eastern Australia. Cont. Shelf Res. 28, 2292–2303. doi: 10.1016/j.csr.2008.03.026
Seminoff, J. A., Resendiz, A., and Nichols, W. J. (2002). Diet of East Pacific green turtles (Chelonia mydas) in the central Gulf of California, Mexico. J. Herpetol. 36, 447–453. doi: 10.1670/0022-1511(2002)036[0447:DOEPGT]2.0.CO;2
Sheppard, J. K., Lawler, I. R., and Marsh, H. (2007). Seagrass as pasture for seacows: landscape-level dugong habitat evaluation. Estuar. Coast. Shelf Sci. 71, 117–132. doi: 10.1016/j.ecss.2006.07.006
Sheppard, J. K., Preen, A. R., Marsh, H., Lawler, I. R., Whiting, S. D., and Jones, R. E. (2006). Movement heterogeneity of dugongs, Dugong dugon (Müller), over large spatial scales. J. Exp. Mar. Biol. Ecol. 334, 64–83. doi: 10.1016/j.jembe.2006.01.011
Taylor, H. A., and Rasheed, M. A. (2010). Torres Straits Dugong Sanctuary Seagrass Baseline Survey, March 2010. Cairns, QLD: DEEDI Publication (Fisheries Queensland, Northern Fisheries Centre).
UNEP-WCMC and Short, F. T. (2016). Global Distribution of Seagrasses (Version 4.0). Fourth Update to the Data Layer Used in Green and Short (2003). Cambridge: UNEP World Conservation Monitoring Centre. Available online at: http://data.unep-wcmc.org/datasets/7
Unsworth, R. K., and Cullen, L. C. (2010). Recognising the necessity for Indo-Pacific seagrass conservation. Conserv. Lett. 3, 63–73. doi: 10.1111/j.1755-263X.2010.00101.x
Keywords: blue carbon, ecosystem services, climate change mitigation, drone surveys, satellite tracking, animal movement, benthic habitat mapping
Citation: Hays GC, Alcoverro T, Christianen MJA, Duarte CM, Hamann M, Macreadie PI, Marsh HD, Rasheed MA, Thums M, Unsworth RKF, York PH and Esteban N (2018) New Tools to Identify the Location of Seagrass Meadows: Marine Grazers as Habitat Indicators. Front. Mar. Sci. 5:9. doi: 10.3389/fmars.2018.00009
Received: 07 September 2017; Accepted: 11 January 2018;
Published: 21 February 2018.
Edited by:
Alastair Martin Mitri Baylis, South Atlantic Environmental Research Institute, Falkland IslandsReviewed by:
Luke Einoder, Northern Territory Government, AustraliaDavid Ainley, H. T. Harvey & Associates, United States
Copyright © 2018 Hays, Alcoverro, Christianen, Duarte, Hamann, Macreadie, Marsh, Rasheed, Thums, Unsworth, York and Esteban. This is an open-access article distributed under the terms of the Creative Commons Attribution License (CC BY). The use, distribution or reproduction in other forums is permitted, provided the original author(s) and the copyright owner are credited and that the original publication in this journal is cited, in accordance with accepted academic practice. No use, distribution or reproduction is permitted which does not comply with these terms.
*Correspondence: Graeme C. Hays, Zy5oYXlzQGRlYWtpbi5lZHUuYXU=