- 1Institute for Marine and Antarctic Studies, University of Tasmania, Hobart, TAS, Australia
- 2Discipline of Geography and Spatial Sciences, School of Land and Food, University of Tasmania, Hobart, TAS, Australia
- 3Centre for Research in Mathematics, Western Sydney University, Parramatta, NSW, Australia
- 4National Centre for Coasts and Climate, School of Biosciences, University of Melbourne, Melbourne, VIC, Australia
Expanding urbanization in estuaries and the increase in pollutants from anthropogenic point sources can affect nearby benthic assemblages. Using a paired impact-control design, we assessed the effects of pollution from anthropogenic point sources (marinas, storm-water drains, sewage outfalls and fish farms) on algal and sessile invertebrate recruits to pavers placed in an industrialized Tasmanian estuary. Species number and cover of native recruits were lower after 12 months at sites outside marinas relative to paired control sites, whereas non-native and cryptogenic recruits were significantly higher outside marinas and near sewage outfalls. The cover of fast-growing, opportunistic species was significantly higher at sites near fish farms and sewage outfalls, and the cover of native species was also greater at sites near sewage outfalls relative to the paired control sites. Our results suggest an increased management focus on controlling pollution from marinas and sewage outfalls is warranted to limit the spread of non-native and cryptogenic species.
Introduction
Many of the world’s major urban centers are established on the land–sea interface of large estuaries (Waltham and Connolly, 2011). Continued population growth in these areas has resulted in the proliferation of man-made structures including marinas, sewage outfalls, storm water drains, and, in more remote locations, fish farms (Airoldi et al., 2015; Dafforn et al., 2015). Artificial structures can alter flow rates and act as point sources of pollutants, which places pressure on the adjacent near-shore rocky reef biota (Vitousek et al., 2007; Airoldi and Bulleri, 2011; Strain et al., 2015). Their impacts can vary temporally and spatially, and are often amplified in estuaries which are subject to a variety of other environmental stressors (Edgar et al., 2000; Roy et al., 2001).
The successful establishment of sessile species onto rocky reefs is a key process in temperate marine systems (Airoldi and Beck, 2007; Mangialajo et al., 2008; Gorman and Connell, 2009; Gorman et al., 2009). Pollution from anthropogenic point sources can reduce the survival of new recruits (Vadas et al., 1992) and are an important force in structuring the existing species assemblage (Johnston et al., 2002). Nutrient enrichment from sewage outfalls and fish farms can negatively affect the fertilization of canopy-forming algae and increase the mortality of juveniles (Coelho et al., 2000; Kevekordes and Clayton, 2000). High sediment from sewage outfalls and storm water drains can smother canopy algae recruits (Strain et al., 2015) and promote the growth of more tolerant opportunistic species (Eriksson et al., 2002). Ports and marinas are rich in heavy metals and biocides, and can cause selective mortality of native algae (Coelho et al., 2000) and sessile invertebrates (Schiff et al., 2004; Medina et al., 2005; Johnston and Roberts, 2009). Despite widespread concern, single impacts are often studies in isolation (e.g., Crooks et al., 2011; Megina et al., 2016) and studies comparing the relative impacts of different sources of urban pollution on the recruitment of sessile species are rare.
The loss of native sessile algae and invertebrate species in estuaries (Johnston et al., 2011; Dafforn et al., 2012) represents a form of disturbance. Recent succession theory suggests that invasive and rapidly growing opportunistic species may capitalize on these empty niches (Levine, 2000; Ruiz et al., 2000; Piola and Johnston, 2008b; Johnston et al., 2011). In addition, certain types of anthropogenic point sources (such as marinas, and jetties) provide bare substrate and altered environmental conditions (i.e., reduced light and wave action or increased turbidity and sedimentation) which result in increased colonization of non-native species (Dafforn et al., 2009) and may act as sources and “corridors” for the spread of invasive species into natural habitats (Airoldi et al., 2015). Yet relatively little is known about the effects of different anthropogenic point sources in promoting the successful establishment of invasive species on nearby rocky reefs (but see Dafforn et al., 2008; Sheehy and Vik, 2010).
In the present paper, we used a paired control-impact design to assess the effects of pollution from four different anthropogenic point sources on the species richness and cover of recruited algae and sessile invertebrates in the Derwent Estuary, Australia. This design allowed us to distinguish the effects of these anthropogenic point sources from the site variation in other environmental stressors. We hypothesized that sites outside marinas (50 m) and near storm water drains (20 m), where inorganic and toxic pollutants or sediment (storm water drains only) enter the system, would have an early successional sessile assemblage comprising reduced native species richness and cover relative to nearby control sites ( > 1 km away) (Whitehead et al., 2010; Coughanowr et al., 2015). In contrast, sites near fish farms (100 m) and sewage drains (20 m), which release organic pollution or sediment, would have higher native species richness and cover of opportunistic species, and lower cover of non-opportunistic species, compared with paired control sites ( > 1 km away), (Coughanowr et al., 2015; Oh et al., 2015). We also hypothesized that species richness and cover of non-indigenous species would be higher at sites outside marinas because of higher pollution loads, proximity to donor populations (e.g., boats) and bare space relative to the other anthropogenic point sources.
Materials and Methods
Study Sites
Effect of Pollution From Anthropogenic Point Sources on Algae and Sessile Invertebrate Recruits
A total of one hundred and twenty eight 0.3 × 0.3 m concrete pavers were deployed for 12 months (March 2011–2012) at 27 sites along two connected marine embayments on the south-eastern coast of Tasmania, Australia (Figure 1): the Derwent Estuary, located adjacent to the state capital city of Hobart, and the D’Entrecasteaux Channel, which lies between Tasmania and Bruny Island (Fowles, 2017). The two estuaries are micro-tidal (0.8 m), with exposure to moderated oceanic swells, and wide entrances that promote efficient marine flushing (Whitehead et al., 2010).
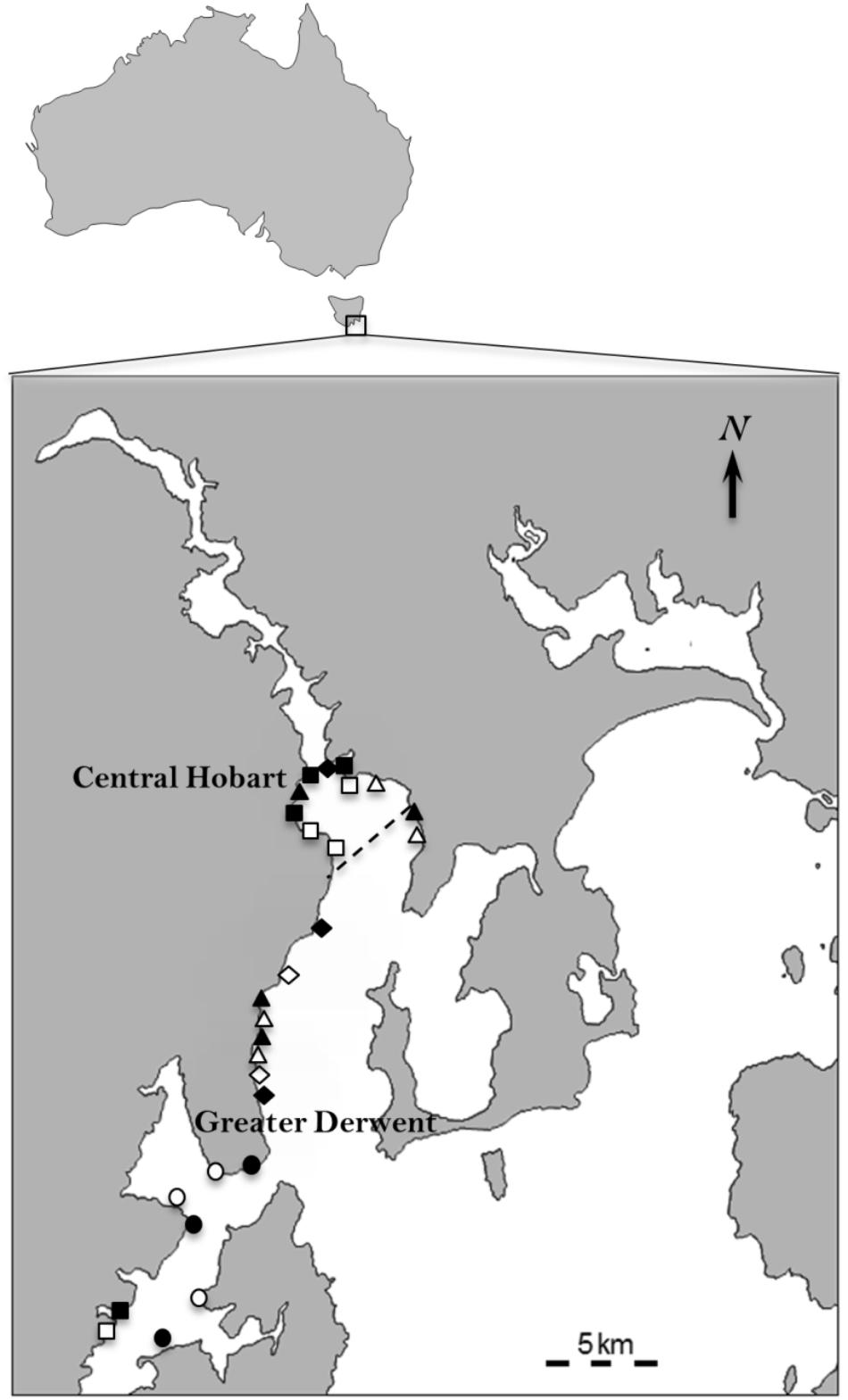
FIGURE 1. Location of the 27 sites used to test the effects of pollution from anthropogenic point sources on algal and sessile invertebrate recruits in the Derwent and D’Entrecasteaux Channel, Tasmania (43.03° S 147.34° E). The dashed line indicates 5 km from Hobart CBD that separates sites in the central Hobart region from the greater Derwent region. Solid symbols are impact sites, open symbols control sites. Diamonds = sewage outfalls, squares = marinas, circles = fish farms, triangles = storm water drains. The study area has a long history of extensive foreshore development, including establishment of commercial and recreational boating marinas, storm water drains and sewage outfalls starting in the early 1900’s, and more recently the introduction of salmon farming in the D’Entrecasteaux Channel in the 1980s.
In the study region, historical contamination from industry has resulted in sediment heavy metal contamination levels that are among the highest in the world (Whitehead et al., 2010). More recent point sources of metal contamination include storm water run-off, antifouling paint fragments, and wastes associated with marinas (Whitehead et al., 2010; Coughanowr et al., 2015). The other major point sources of pollution are sediment from storm water drains, nutrients from sewage outfalls, and fish waste associated with salmon aquaculture farms (Whitehead et al., 2010; Coughanowr et al., 2015; Oh et al., 2015). These elevated nutrient concentrations are largely localized and retained within the estuarine systems (Coughanowr et al., 2015).
Concrete pavers presented a rough surface to facilitate recruitment by algal spores and sessile invertebrates (Park et al., 2014) and support growth of dense native algal communities similar to that found on natural rocky shores (Fowles, 2017). Four pavers were placed at each of the four sites, each in close proximity to four different anthropogenic point sources (fish farm, marinas, sewage outfalls and storm water drains). Four pavers were also placed in nearby control rocky reefs located ∼1 km (upstream in as many cases as possible) from the treatment sites as well as 1 km from any other known point source of pollution (Figure 1). This 1 km distance was chosen based on previous research in this region which has shown that the outputs of storm water drains, and enrichment from fish farms and sewage outfalls attenuate rapidly from point sources (Roberts et al., 2007; Whitehead et al., 2010; Wild-Allen et al., 2013; Coughanowr et al., 2015; Oh et al., 2015; Condie et al., 2017). Pavers were deployed on rocky reef along the 2–4 m depth contour, approximately 0.5 to 1.0 m apart and at least 3 m from the reef-sand interface. Marina sites were located 50 m from permanent boat moorings that harbored between 200 and 400 recreational and commercial vessels. Fish farm sites were all located in the D’Entrecasteaux Channel 100 m from stocked cages. Storm water pipe sites were located 20 m from the pipe outlet, which consisted of a large concrete pipe (30–50 cm in diameter) that extended into the estuary perpendicular to the shoreline. Sewage outfall sites were located 20 m from the pipe outlet. The impacted sites had high pollution loads (Whitehead et al., 2010; Coughanowr et al., 2015; Oh et al., 2015) and were based on distances to the nearest rocky reef.
At the conclusion of the experiment (March 2012), a 0.3 m × 0.3 m quadrat was placed on each paver. The percentage cover of all sessile taxa was estimated by counting the number of times each taxon occurred directly under 18 string intersection points, dividing by the number of points counted, and multiplying by 100. Taxa were identified to the highest taxonomic resolution practical. Abiotic variables (sediment and bare paver surface) were considered separately in the analyses as a measure of potential recruitment space. During the experiment, 30 pavers were lost or turned over by storms. The missing pavers were not spread evenly across all sites and resulted in 5 sites (1 fish farm impact and 1 fish farm control site; 1 sewage outfall and 2 sewage control sites) being excluded from analyses due to lack of replication. Pavers were collected after visual assessment of percent cover, and the species identity checked where necessary using microscopes in the laboratory.
Statistical Analyses
The effect of the different anthropogenic point sources on the benthic community assemblage was assessed using canonical analysis of principal coordinates (CAP) (Anderson, 2003). CAP was “constrained” by the point source type to maximize the separation between the impact and control sites. To reduce variability in outputs, data were averaged at the site level. The resultant “site × species” matrix was square root transformed and converted to a Bray Curtis similarity matrix. We used a Spearman correlation (r) between the canonical axes and density of taxa to measure the contribution of individual taxa to the differences between the impact and paired control sites. These correlations are shown visually as a vector plot for taxa with r > 0.5. All multivariate analyses and plots were conducted with the package PRIMER 6.1.13 (Clarke, 1993; Anderson et al., 2008).
The taxa identified during this study were classified into broad functional groups: non-indigenous/cryptogenic or native; and opportunistic or non-opportunistic species based on the literature in Table 1. Opportunistic species exhibited at least two of the following traits; fast growing, ephemeral, and with continuous periods of reproduction (Lobban and Harrison, 1995). Non-opportunistic species exhibited at least two of the following traits; slower growing, relatively long life span, perennial, and shorter periods of reproduction (Littler and Littler, 1980).
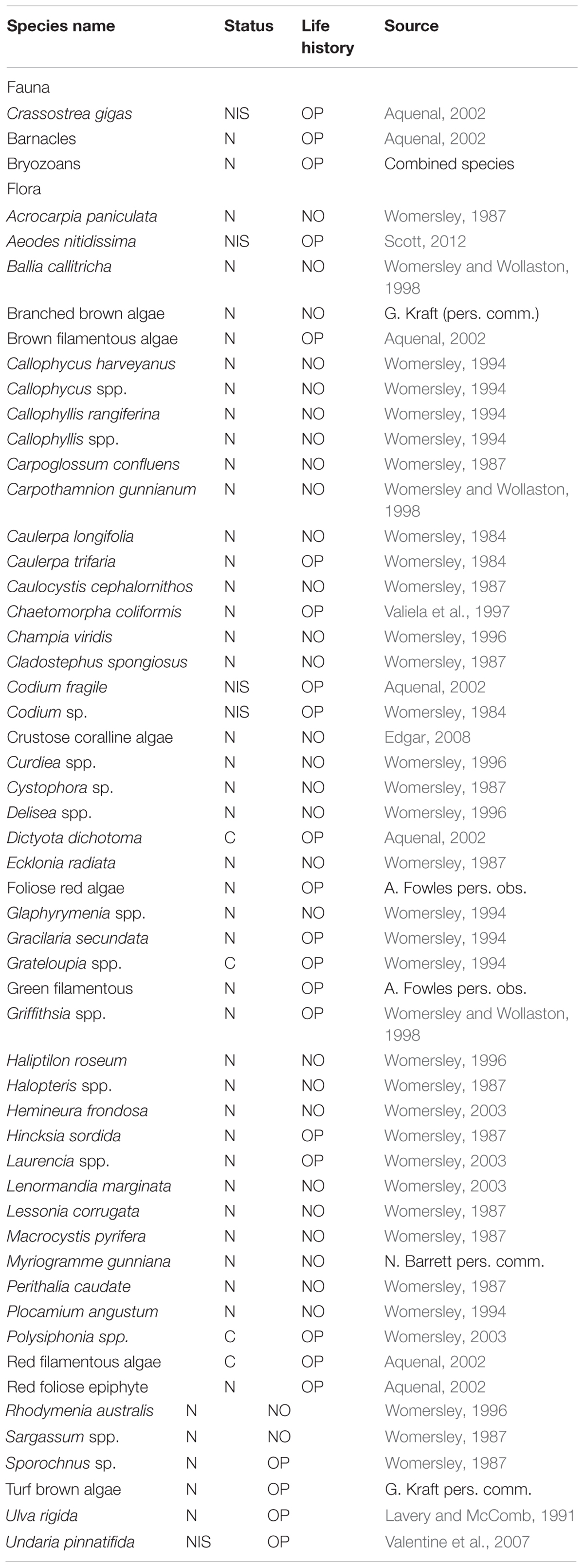
TABLE 1. Summary of taxa found during the experiment and the information source used to classify them as native (N) or non-indigenous (NIS), cryptogenic (C), opportunistic (OP) or non-opportunistic (NO) based on the criteria outlined in the methods.
The effects of the pollution from anthropogenic point sources on the number of species and the cover of the different functional groups were tested using generalized linear mixed models. A quasi-poisson distribution was used for the number of species and a quasi-binomial distribution was used for the cover data. The models included the fixed effects of anthropogenic point source (fish farms, marinas, sewage outfalls, and storm water drains) relative to the paired controls, region, wave exposure, and the random effect of site. The random effect of site was included in the model to adjust for the natural variability between sites. Region was a categorical variable with two levels that distinguished sites within 5 km of the Hobart CBD (called central Hobart region), from sites greater than 5 km from Hobart CBD (called greater Derwent region; See Figure 1). Sites near the capital were assumed to be more heavily impacted by historical contamination and cumulative pressures (Coughanowr et al., 2015). Exposure was measured using a fetch model, which used the distance of 14 vectors from a site to any mass of land in multiple directions as a proxy for potential wind-generated waves (Hill et al., 2010).
All univariate analyses were carried out with the Rgui version 3.1.0 (R Core Team, 2013). Confidence intervals (95%; CIs) and p-values were obtained for the model estimates (or log response ratios), assuming that they are t-distributed with the appropriate degrees of freedom. The log response ratios for the anthropogenic point sources (and associated CIs) were further converted to percentage change (or n-fold increase if>100%) relative to the paired control sites. The percentage change was calculated as 100∗[exp(estimate)-1]. If the percentage change was greater than 100%, the n-fold increase was reported for ease of interpretation [n-fold increase as exp(estimate)]. CIs and p-values were used to assess significance of differences in the number of species or percentage cover of the different functional groups at impacted sites relative to paired controls.
Results
Effect of Pollution From Anthropogenic Point Sources on Species Assemblage
During the experiment, fifty-one species of algae (94%) and three species of (6%) sessile invertebrates recruited to the pavers (Table 1). In general, cover was low on the pavers. Sessile invertebrates were not prevalent or abundant enough to analyse separately and so were analyzed in combination with algae in each of the broad functional groups. The most abundant species were Ulva rigida, Polysiphonia spp., brown algal turf, Aeodes nitidissima and Codium fragile.
The anthropogenic point sources (fish farms, marinas, sewage outfalls and storm water drains) and their paired control sites were generally separated along the first two axes of canonical space (Figure 2). The greatest difference in community assemblage were observed between the marina impact and paired control sites (Codium spp. r = 0.52; Grateloupia sp. r = -0.60; Figure 2). There were also significant differences between the fish farm and sewage outfall paired impact sites and control sites (Chaetomorpha coliformis r = 0.59, Polysiphonia sp. r = -0.51; Figure 2). In contrast, no significant differences were detected between the paired storm water drain impact and control sites (Figure 2). Supplementary Table S1 shows absolute values for each functional group in each treatment and control.
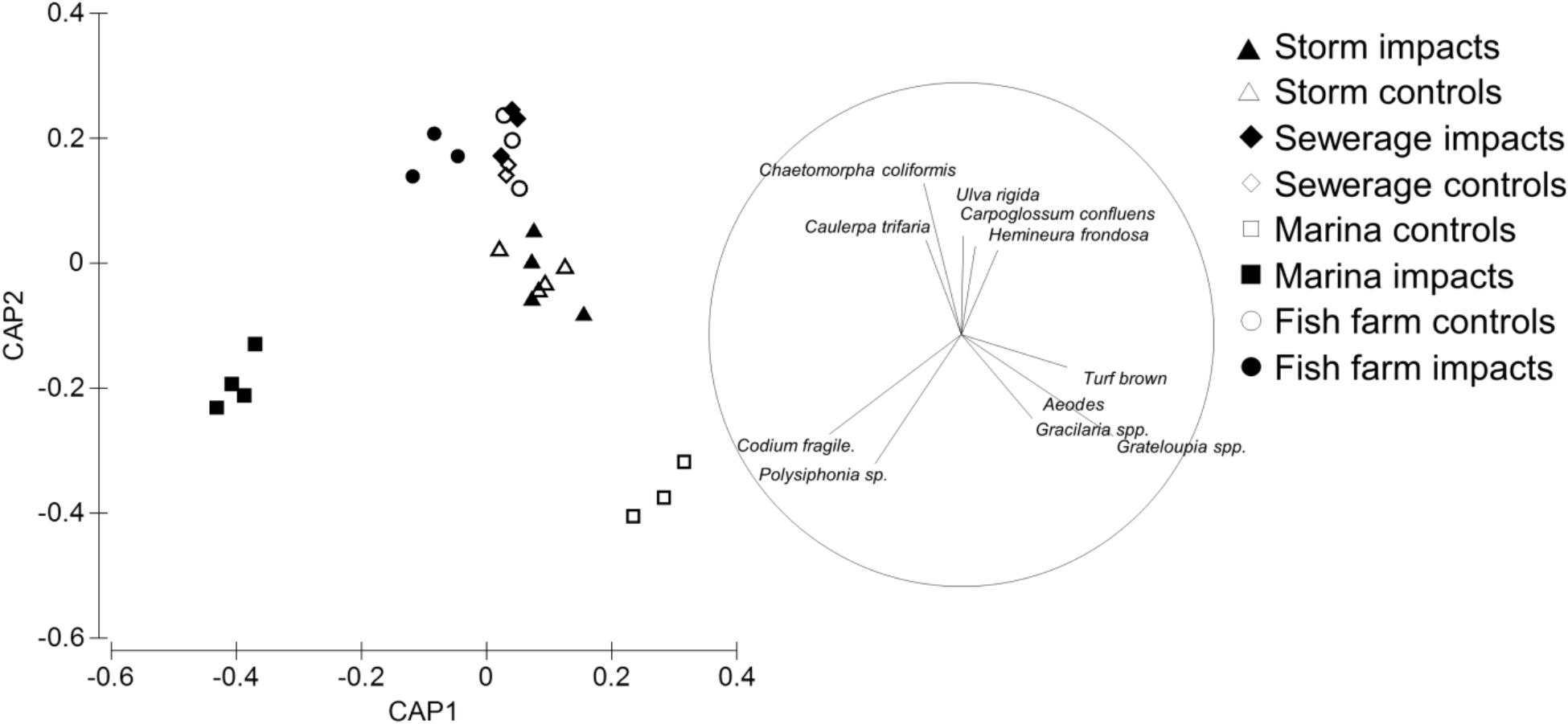
FIGURE 2. Canonical analysis of principal coordinates (CAP) ordination showing differences in the recruited sessile assemblage on pavers near anthropogenic point sources (solid symbols) relative to their paired control sites (open symbols). Taxa that contributed to the greatest dissimilarity between the impact and paired control sites are shown by vector plot, with circle diameter showing correlation of 1. Anthropogenic point sources are: sew = sewerage outfall, storm = storm water drains, fish farm = fish farms, marina = marinas.
Effect of Pollution From Anthropogenic Point Sources on the Species Richness of Different Functional Groups
As expected, the number of native species at sites outside marinas was significantly lower than at paired control sites (-96% change, t = -3.45, df = 80, p < 0.001, Figure 3). There was also a significantly greater number of cryptogenic and non-indigenous species (e.g., Crassostrea gigas, Polysiphonia spp. and Codium fragile) at the sites outside marinas (2.7- fold increase, t = 2.9, df = 79, p < 0.01) and sewage outfalls (6.1- fold increase, t = 2.11, df = 79, p = 0.04) than at paired control sites, although there was high variation between pairs of sites (Figure 3). In contrast, no difference was evident in the number of native species at sites near fish farms (16% change, t = 0.51, df = 79, p > 0.05), storm water drains (-34% change, t = -1.63, df = 79, p > 0.05), or sewage outfall (8% change, t = 0.36, df = 79, p > 0.05) when related to paired control sites (Figure 3). There were also no detectable differences in the number of non-indigenous species between sites near storm water drains (78% increase, t = 1.61, df = 79, p > 0.05) and fish farms (25% increase, t = 0.26, df = 79, p > 0.05), and their paired control sites (Figure 3).
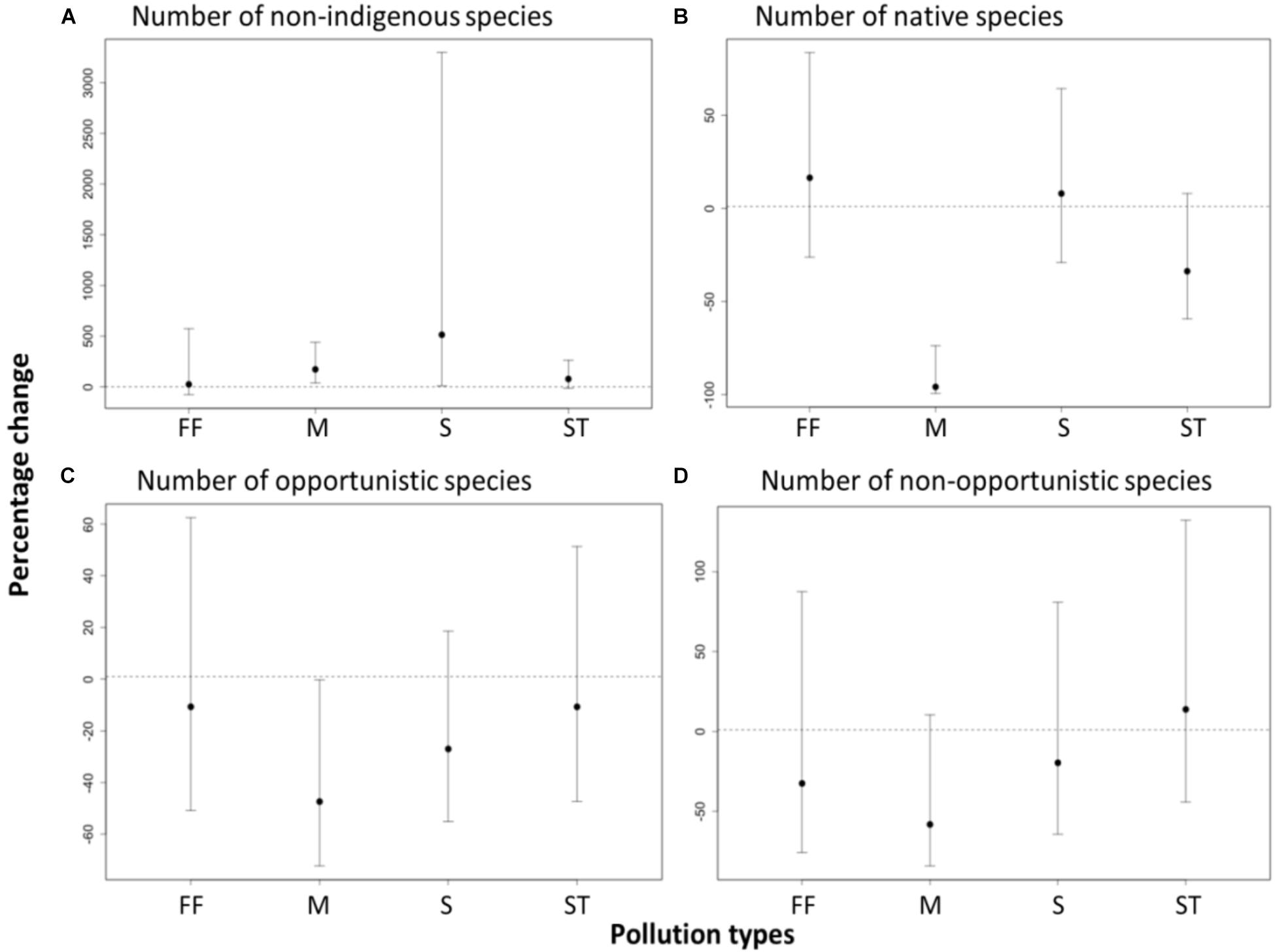
FIGURE 3. Effects of pollution from anthropogenic point sources [fish farms (FF), marinas (M), sewage outfalls (S), and stormwater drains (ST)] on the percentage change in the number of recruited (A) non-indigenous, (B) native, (C) opportunistic and (D) non-opportunistic species. Effects are significant if confidence intervals do not overlap zero. Percentage change was calculated from GLMM estimates based on a quasi-poisson, mixed effect model with a log link.
Contrary to our hypotheses, the recruitment of opportunistic species but not the non-opportunistic species differed between the anthropogenic point sources (Figure 3). There were significantly fewer opportunistic species at marina sites than at the paired control sites (47% change, t = -2.0, df = 80, p > 0.05). In contrast, there were no detectable differences in the number of opportunistic species in the sites near fish farms (-11% change, t = -0.77, df = 80, p > 0.05), storm water drains (-11% change, t = -0.43, df = 80, p > 0.05), or sewage outfall (-27% change, t = -1.29, df = 80, p > 0.05) relative to their paired control sites (Figure 3).
Supplementary Table S1 shows absolute values for each functional group in each treatment and control and shows that in absolute terms marina impacts sites are devoid of native species cover, high in opportunistic species cover and high in non-indigenous species richness compared to both marina control sites and all other control and impact sites.
Effect of Pollution From Anthropogenic Point Sources on the Cover of Different Functional Groups
As expected, the biotic (i.e., visible algae and sessile invertebrates) cover was significantly higher at sites near sewage outfall (72% increase, t = 2.37, df = 79, p < 0.02) relative to their paired control sites (Figure 4). The sites near fish farms had slightly higher, but not significant, biotic cover (46% increase, t = 1.61, df = 79, p > 0.05) than their paired control sites (Figure 4). In contrast, the biotic cover was slightly lower, but not significant, at sites outside marinas and near storm water drains relative to the paired control sites (Figure 4).
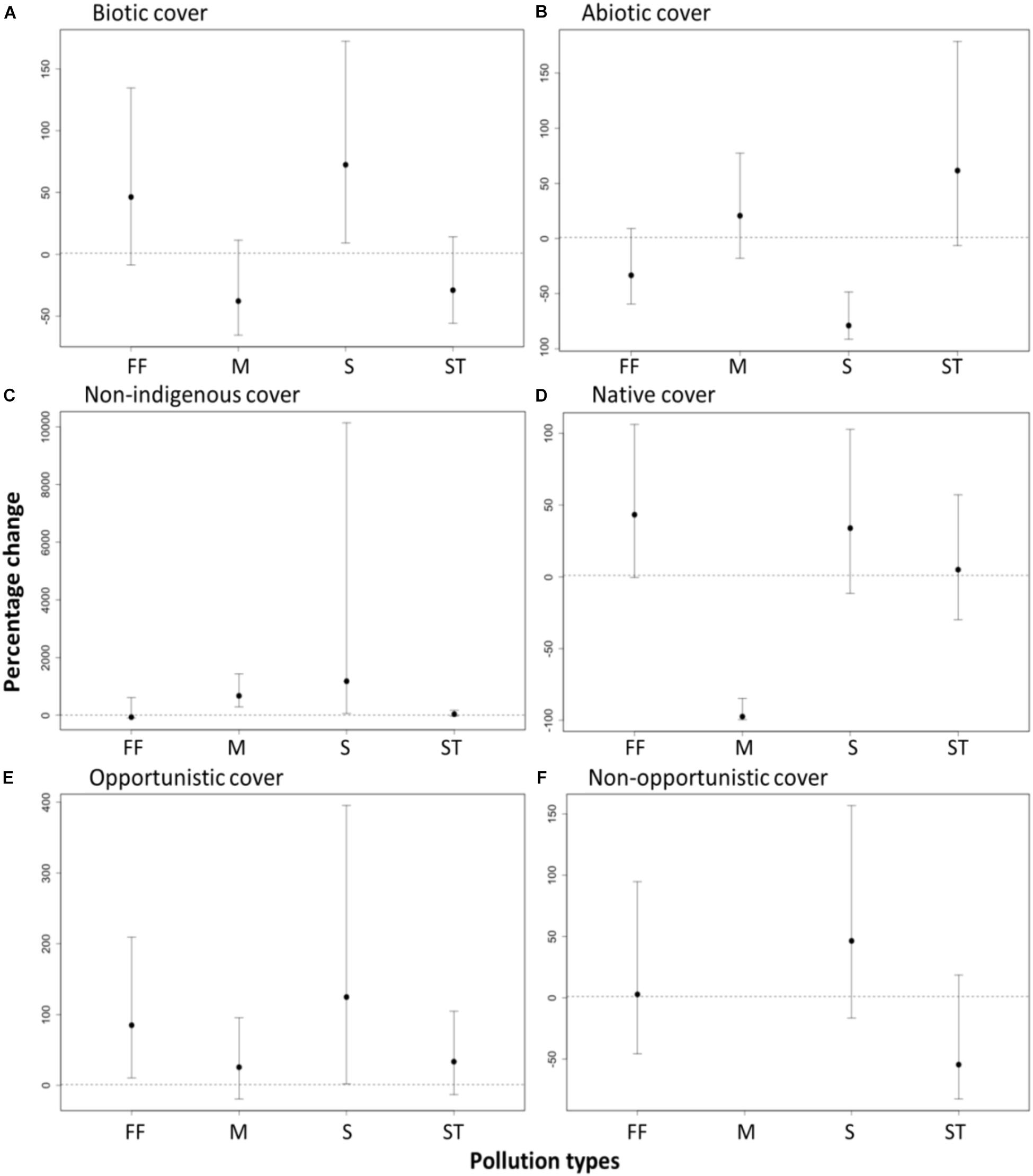
FIGURE 4. Effects of pollution from anthropogenic point sources [fish farms (FF), marinas (M), sewage outfalls (S), and storm water drains (ST)] on the change in percentage cover of recruited (A) biotic species (i.e., visible algae and sessile invertebrates), (B) abiotic (bare space and sediment), (C) non-indigenous species (D) native species, (E) opportunistic species and (F) non-opportunistic species. Effects are significant if confidence intervals do not overlap zero. Percentage change was calculated from the GLMM estimates based on a quasi-binomial, mixed effect model with a log link.
Consistent with our expectations, significantly lower cover of native species (97% decrease, t = -4.1, df = 79, p < 0.01), and higher cover of non-indigenous species (7.7- fold increase t = 2.04, df = 79, p > 0.001), were found at sites outside marinas than at paired control sites (Figure 4). Sites near sewage outfalls also had a higher cover of non-indigenous species (12.8-fold increase, t = 2.44, df = 79, p = 0.02), but no significant difference in cover of native species (33% increase, t = 1.4, df = 79, p > 0.05), compared with their paired control sites (Figure 4). In contrast, no significant differences in the cover of native species (5% increase, t = 0.24, df = 79, p > 0.05) or the cover of non-indigenous species (37% increase, t = 0.9, df = 79, p > 0.05) were found at sites near storm water drains relative to paired control sites (Figure 4). Sites near fish farm sites also had significantly higher cover of native species (43% increase, t = 1.97, df = 79, p = 0.05) than at paired control sites but no detectable differences in the cover of non-indigenous species (66% decrease, t = -0.71, df = 79, p > 0.05), (Figure 4).
Sites near fish farms had significantly higher cover of opportunistic species relative to their paired control sites (85%, t = 2.38, df = 79, p < 0.05), as did sewage outfalls (2.3-fold increase, t = 2.04, df = 79, p = 0.05, Figure 4). Overall, there were no detectable differences in the cover of non-opportunistic species between the impact sites relative to the paired control sites (Figure 4).
Discussion
The paired study design applied here allowed us to distinguish the effects of pollution from anthropogenic point sources (marinas, storm-water drains, sewage outfalls and fish farms) from the natural site variation in environmental conditions (i.e., > 5 km of the capital city, wave exposure), extending prior studies of estuaries that have focused on single impacts (e.g., Johnston et al., 2011; Hedge and Johnston, 2012). Whilst a paired design was optimal, the choice of control sites is important but difficult in estuaries that are subject to multiple current and historical sources of pollution. Including a factor that accounted for expected differences in historical contamination assisted analyses and our results support the growing body of literature that indicates marinas, storm water drains, sewage outfalls and fish farms have different influences on the nearby rocky reef biota (Airoldi and Beck, 2007; Mangialajo et al., 2008; Gorman and Connell, 2009; Gorman et al., 2009).
Marinas had the greatest impacts on recruitment of the algal-dominated species assemblage. Pavers located in sites outside marinas were largely devoid of biotic cover (i.e., visible algae and sessile invertebrates), with half the species richness and cover of native species relative to associated control sites and lower absolute coverage and richness compared to all other treatments (Supplementary Table S1). The impacts of marinas probably stem from the high levels of heavy metals and biocides released into the surrounding waterways at these location (Whitehead et al., 2010; Johnston et al., 2011). Previous studies have consistently demonstrated that the heavy metal and biocides used in anti-fouling paints can dramatically reduce the recruitment success of native algae and invertebrates (Piola and Johnston, 2008a,b; Piola et al., 2009).
Similar to other studies, we found higher cover but not species richness of opportunistic species such as Ulva rigida at sites located near sewage outfalls and fish farms relative to nearby control sites (Lavery and McComb, 1991; Munda and Veber, 2004; Oh et al., 2015). Both of these anthropogenic point sources contribute to high nutrient levels in the study region (Whitehead et al., 2010; Coughanowr et al., 2015; Oh et al., 2015). Ulva rigida is ephemeral, with the ability to assimilate high levels of nutrients, reproduce year-round, and tolerate substantial sedimentation (Littler and Murray, 1975; Correa et al., 1999). Contrary to our expectations, a higher cover of native algae recruits (e.g., Carpoglossum confluens, Ulva rigida, and Acrocarpia paniculata) was also found at sites near sewage outfalls relative to paired control sites. Previous research suggests the elevated nutrient inputs from sewage outfalls and fish farms may allow some native algal taxa to colonize and grow on nearshore rocky reefs (Campbell, 2001).
Studies have demonstrated that anthropogenic point sources can support high densities of non-native species (Carlton, 1987; Dafforn et al., 2009; Ling et al., 2012). Our results demonstrated a much higher number and cover of non-indigenous and cryptic species at sites outside marinas and near sewage outfalls relative to their paired control sites. A dominant non-indigenous species observed was Codium fragile subsp. fragile (Suringar) Hariot, 1889 [formerly C. fragile subsp. tomentosoides (van Goor) Silva, 1955], an alga regarded as native to Japan and Korea (Lyons and Scheibling, 2009), and that has only recently been identified in South Australian and Tasmanian waters (McDonald et al., 2015). Previous research has demonstrated this species has high tolerance for heavy metals and elevated nutrients, and the ability to withstand sedimentation (Gorgula and Connell, 2004). It can also spread, regenerate and grow quicker than many native algal species (Bégin and Scheibling, 2003). The continuing spread and establishment of this alga in sites adjacent to marinas and sewage outfalls is concerning given the additional stress from competition on native species in addition to stresses from these sources of contamination (Vye et al., 2015).
Polysiphonia spp. also recruited disproportionately to locations outside marinas and near sewage outfalls. The introduced red filamentous alga Polysiphonia senticulosa occurs in moderate water movement, an important trait for establishing permanent populations in newly invaded habitats (Thomsen et al., 2007). Polysiphonia spp. have frequently been recorded on artificial structures in marinas and ports (Womersley, 2003), suggesting that they may quickly establish on newly available substrata, but are perhaps less able to compete with native communities when present. In this study, Polysiphonia spp. were able to colonize free space more efficiently than any native species so appears to be relatively tolerant of contamination by heavy metals and other pollutants found at sites located outside marinas in the Derwent Estuary (Whitehead et al., 2010; Coughanowr et al., 2015).
Estuaries are rapidly undergoing transformation by anthropogenic point sources, which can adversely affect nearby intertidal and subtidal marine habitats, both rocky reef and soft sediment (Schiff et al., 2004; Dafforn et al., 2009). Our study indicates the pollution from these anthropogenic point sources can cause shifts in algal- dominated rocky coastal habitats from native to more opportunistic/non-indigenous species. These opportunistic and non-indigenous species could potentially benefit from reduced competition with native species, increased tolerance of heavy metals and biocides and the presences of empty niches in locations where their propagule pressure is greatest.
Our results suggest that management needs to focus on reducing the pollution loads from these anthropogenic point sources, in particular marinas and sewage outfalls, to minimize declines in populations of native species and to prevent the spread of non-native species in nearby rocky reefs. Further study is, however, required to understand what contaminants (i.e., heavy metals, biocides, or high sediment loads) from marinas and sewage outfalls have the greatest impacts on algal and sessile invertebrate recruits. New research has identified a range of potential solutions including, non-toxic antifouling paints (Maréchal and Hellio, 2009), and filtration systems (bioretention systems, rain gardens, vegetated filter strips, permeable asphalt or concrete pavement and drainage wells) which could be used to treat the runoff from urban infrastructure (McNabb, 2017). Future research should assess the effectiveness of these solutions in providing positive benefits in marine estuarine environments.
Author Contributions
AF, GE, JK, and RS-S conceived the study. AF led fieldwork and data analysis with assistance from RT, NH, and ES. AF drafted the paper with contributions from all other authors.
Funding
Scholarship funding was provided by the Marine Biodiversity Hub, a collaborative partnership supported through the Australian Government’s National Environmental Research Program, administered by the Department of the Environment. Marine Biodiversity Hub partners include the Institute for Marine and Antarctic Studies, University of Tasmania; CSIRO, Geoscience Australia, Australian Institute of Marine Science, Museum Victoria, Charles Darwin University and the University of Western Australia. Additional costs were supported by the Australian Research Council.
Conflict of Interest Statement
The authors declare that the research was conducted in the absence of any commercial or financial relationships that could be construed as a potential conflict of interest.
Acknowledgments
The authors thank the University of Tasmania divers for data collection and logistics support, particularly Tania Mendo, Luis Henriquez, Lara Marcus, Justin Hulls and Tim Alexander. Gerald Kraft and Fiona Scott from the Tasmanian Herbarium provided advice on macro-algal species identification.
Supplementary Material
The Supplementary Material for this article can be found online at: https://www.frontiersin.org/articles/10.3389/fmars.2018.00417/full#supplementary-material
References
Airoldi, L., and Beck, M. W. (2007). Loss, status and trends for coastal marine habitats of Europe. Oceanogr. Mar. Biol. 45, 345–405.
Airoldi, L., and Bulleri, F. (2011). Anthropogenic disturbance can determine the magnitude of opportunistic species responses on marine urban infrastructures. PLoS One 6:e22985. doi: 10.1371/journal.pone.0022985
Airoldi, L., Turon, X., Perkol-Finkel, S., and Rius, M. (2015). Corridors for aliens but not for natives: effects of marine urban sprawl at a regional scale. Divers. Distrib. 21, 755–768. doi: 10.1111/ddi.12301
Anderson, M. J. (2003). CAP: a FORTRAN Computer Program for Canonical Analysis of Principal Coordinates. Auckland: University of Auckland.
Anderson, M. J., Gorley, R. N., and Clarke, K. R. (2008). PERMANOVA + for PRIMER: Guide to Software and Statistical Methods. Plymouth: PRIMER-E.
Aquenal. (2002). Exotic Marine Pests Survey. Port of Hobart, Tasmania. Final report Prepared for Hobart Ports Corporation Pty Ltd. Hobart, TAS: Aquenal.
Bégin, C., and Scheibling, R. E. (2003). Growth and survival of the invasive green alga Codium fragile ssp. tomentosoides in tide pools on a rocky shore in Nova Scotia. Bot. Mar. 46, 404–412. doi: 10.1515/BOT.2003.040
Campbell, S. (2001). Ammonium requirements of fast-growing ephemeral macroalgae in a nutrient-enriched marine embayment (Port Phillip Bay. Australia). Mar. Ecol. Prog. Ser. 209, 99–107. doi: 10.3354/meps209099
Carlton, J. T. (1987). Patterns of transoceanic marine biological invasions in the Pacific Ocean. Bull. Mar. Sci. 31, 452–465.
Clarke, K. R. (1993). Non-parametric multivariate analyses of changes in community structure. Aust. J. Ecol. 18, 117–143. doi: 10.1111/j.1442-9993.1993.tb00438.x
Coelho, S. M., Rijstenbil, J. W., and Brown, M. T. (2000). Impacts of anthropogenic stresses on the early development stages of seaweeds. J. Aquat. Ecosyst. Stress Recov. 7, 317–333. doi: 10.1023/A:1009916129009
Condie, S., Gorton, R., Hadley, S. A., Little, R., MacLeod, C., Ogier, E. M. et al. (2017). INFORMD2: Risk-Based Tools Supporting Consultation, Planning and Adaptive Management for Aquaculture and Other Multiple-Uses of the Coastal Waters of Southern Tasmania. Hobart, TAS: Fisheries Research and Development Corporation.
Correa, J. A., Castilla, J. C., Ramírez, M., Varas, M., Lagos, N., Vergara, S., et al. (1999). Copper, copper mine tailings and their effect on marine algae in Northern Chile. J. Appl. Phycol. 11, 57–67. doi: 10.1023/A:1008027610826
Crooks, J. A., Chang, A. L., and Ruiz, G. M. (2011). Aquatic pollution increases the relative success of invasive species. Biol. Invasions 13, 165–176.
Coughanowr, C., Whitehead, S., Whitehead, J., Einoder, L., Taylor, U., and Weeding, B. (2015). State of the Derwent Estuary: A Review of Environmental Data from 2009 to 2014. Hobart, TAS: Derwent Estuary Program.
Dafforn, K. A., Glasby, T. M., Airoldi, L., Rivero, N. K., Mayer-Pinto, M., and Johnston, E. L. (2015). Marine urbanization: An ecological framework for designing multifunctional artificial structures. Front. Ecol. Environ. 13, 82–90. doi: 10.1890/140050
Dafforn, K. A., Glasby, T. M., and Johnston, E. L. (2008). Differential effects of tributyltin and copper antifoulants on recruitment of non-indigenous species. Biofouling 24, 23–33. doi: 10.1080/08927010701730329
Dafforn, K. A., Glasby, T. M., and Johnston, E. L. (2009). Links between estuarine condition and spatial distributions of marine invaders. Divers. Distrib. 15, 807–821. doi: 10.1111/j.1472-4642.2009.00587.x
Dafforn, K. A., Glasby, T. M., and Johnston, E. L. (2012). Comparing the invasibility of experimental “reefs” with field observations of natural reefs and artificial structures. PLoS One 7:e38124. doi: 10.1371/journal.pone.0038124
Edgar, G. J. (2008). Australian Marine Life, Revised Edition. Melbourne, VIC: New Holland Publishers.
Edgar, G. J., Barrett, N. S., Graddon, D. J., and Last, P. R. (2000). The conservation significance of estuaries: a classification of Tasmanian estuaries using ecological, physical and demographic attributes as a case study. Biol. Conserv. 92, 383–397. doi: 10.1016/S0006-3207(99)00111-1
Eriksson, B. K., Johansson, G., and Snoeijs, P. (2002). Long-term changes in the macroalgal vegetation of the inner Gullmar Fjord, Swedish Skagerrak coast. J. Phycol. 38, 284–296. doi: 10.1046/j.1529-8817.2002.00170.x
Fowles, A. E. (2017). Assessing Anthropogenic Impacts on Reef Communities: Patterns, Indicators and Processes. Hobart, TAS: University of Tasmania.
Gorgula, S. K., and Connell, S. D. (2004). Expansive covers of turf-forming algae on human-dominated coast: the relative effects of increasing nutrient and sediment loads. Mar. Biol. 145, 613–619. doi: 10.1007/s00227-004-1335-5
Gorman, D., and Connell, S. D. (2009). Recovering subtidal forests in human-dominated landscapes. J. Appl. Ecol. 46, 1258–1265. doi: 10.1111/j.1365-2664.2009.01711.x
Gorman, D., Russell, B. D., and Connell, S. D. (2009). Land-to-sea connectivity: linking human-derived terrestrial subsidies to subtidal habitat change on open rocky coasts. Ecol. Appl. 19, 1114–1126. doi: 10.1890/08-0831.1
Hedge, L. H., and Johnston, E. L. (2012). Propagule pressure determines recruitment from a commercial shipping pier. Biofouling 28, 73–85. doi: 10.1080/08927014.2011.652622
Hill, N. A., Pepper, A. R., Puotinen, M. L., Hughes, M. G., Edgar, G. J., Barrett, N. S., et al. (2010). Quantifying wave exposure in shallow temperate reef systems: applicability of fetch models for predicting algal biodiversity. Mar. Ecol. Prog. Ser. 417, 83–95. doi: 10.3354/meps08815
Johnston, E. L., Keough, M. J., and Qian, P. Y. (2002). Maintenance of species dominance through pulse disturbances to a sessile marine invertebrate assemblage in Port Shelter. Hong Kong. Mar. Ecol. Prog. Ser. 226, 103–114. doi: 10.3354/meps226103
Johnston, E. L., Marzinelli, E. M., Wood, C. A., Speranza, D., and Bishop, J. D. D. (2011). Bearing the burden of boat harbours: heavy contaminant and fouling loads in a native habitat-forming alga. Mar. Pollut. Bull. 62, 2137–2144. doi: 10.1016/j.marpolbul.2011.07.009
Johnston, E. L., and Roberts, D. A. (2009). Contaminants reduce the richness and evenness of marine communities: a review and meta-analysis. Environ. Pollut. 157, 1745–1752. doi: 10.1016/j.envpol.2009.02.017
Kevekordes, K., and Clayton, M. N. (2000). Development of hormosira banksii (PHAEOPHYCEAE) embryos in selected components of secondarily-treated sewage effluent. J. Phycol. 36, 25–32. doi: 10.1046/j.1529-8817.2000.99059.x
Lavery, P. S., and McComb, A. J. (1991). Macroalgal-sediment nutrient interactions and their importance to macroalgal nutrition in a eutrophic estuary. Estuar. Coast. Shelf Sci. 32, 281–295. doi: 10.1016/0272-7714(91)90021-3
Levine, J. M. (2000). Species diversity and biological invasions: relating local process to community pattern. Science 288, 852–854. doi: 10.1126/science.288.5467.852
Ling, S. D., Johnson, C. R., Mundy, C. N., Morris, A., and Ross, D. J. (2012). Hotspots of exotic free-spawning sex: man-made environment facilitates success of an invasive seastar. J. Appl. Ecol. 49, 733–741. doi: 10.1111/j.1365-2664.2012.02133.x
Littler, M. M., and Littler, D. S. (1980). The evolution of thallus form and survival strategies in benthic marine macroalgae: filed and laboratory tests of a functional form model. Am. Nat. 116, 25–44. doi: 10.1086/283610
Littler, M. M., and Murray, S. N. (1975). Impact of sewage on the distribution, abundance and community structure of rocky intertidal macro organisms. Mar. Biol. 30, 277–291. doi: 10.1007/BF00390633
Lyons, D. A., and Scheibling, R. E. (2009). Range expansion by invasive marine algae: rates and patterns of spread at a regional scale. Divers. Distrib. 15, 762–775. doi: 10.1111/j.1472-4642.2009.00580.x
Mangialajo, L., Chiantore, M., and Cattaneo-Vietti, R. (2008). Loss of fucoid algae along a gradient of urbanisation, and structure of benthic assemblages. Mar. Ecol. Prog. Ser. 358, 63–74. doi: 10.3354/meps07400
Maréchal, J.-P., and Hellio, C. (2009). Challenges for the development of new non-toxic antifouling solutions. Int. J. Mol. Sci. 10, 4623–4637. doi: 10.3390/ijms10114623
McDonald, J., Huisman, J., Hart, F., Dixon, R., and Lewis, J. (2015). The first detection of the invasive macroalga Codium fragile subsp. fragile (Suringar) Hariot in Western Australia. Bioinvasions Rec. 4, 75–80. doi: 10.3391/bir.2015.4.2.01
McNabb, D. E. (2017). Managing Storm, Flood, and Runoff Water: Water Resource Management. Cham: Palgrave Macmillan, 263–281 doi: 10.1007/978-3-319-54816-6_11
Medina, M., Andrade, S., Faugeron, S., Lagos, N., Mella, D., and Correa, J. A. (2005). Biodiversity of rocky intertidal benthic communities associated with copper mine tailing discharges in northern Chile. Mar. Pollut. Bull. 50, 396–409. doi: 10.1016/j.marpolbul.2004.11.022
Megina, C., González-Duarte, M. M., and López-González, P. J. (2016). Benthic assemblages, biodiversity and invasiveness in marinas and commercial harbours: an investigation using a bioindicator group. Biofouling 32, 465–475. doi: 10.1080/08927014.2016.1151500
Munda, I. M., and Veber, M. (2004). Interactive effects of macronutrients and metals (Mn, Co, Zn) on the ephemeral green alga Ulva rigida C. Agardh (Chlorophyta, Ulvophyceae, Ulvales). Nova Hedwigia 79, 353–375. doi: 10.1127/0029-5035/2004/0079-0353
Oh, E. S., Edgar, G. J., Kirkpatrick, J. B., Stuart-Smith, R. D., and Barrett, N. S. (2015). Broad-scale impacts of salmon farms on temperate macroalgal assemblages on rocky reefs. Mar. Pollut. Bull. 98, 201–209. doi: 10.1016/j.marpolbul.2015.06.049
Park, S. R., Kang, Y. H., Lee, H. J., Ko, Y. W., and Kim, J. H. (2014). The importance of substratum and elevation in recruitment and persistence of ulvoid algal blooms on rocky intertidal shores of the southern Korean coast. Bot. Mar. 57, 55–66. doi: 10.1515/bot-2013-0024
Piola, R. F., Dafforn, K. A., and Johnston, E. L. (2009). The influence of antifouling practices on marine invasions. Biofouling 25, 633–644. doi: 10.1080/08927010903063065
Piola, R. F., and Johnston, E. L. (2008a). Pollution reduces native diversity and increases invader dominance in marine hard-substrate communities. Divers. Distrib. 14, 329–342. doi: 10.1111/j.1472-4642.2007.00430.x
Piola, R. F., and Johnston, E. L. (2008b). The potential for translocation of marine species via small-scale disruptions to antifouling surfaces. Biofouling 24, 145–155. doi: 10.1080/08927010801930480
R Core Team (2013). R: A Language and Environment for Statistical Computing. Vienna: R Foundation for Statistical Computing.
Roberts, D. A., Poore, A. G. B., and Johnston, E. L. (2007). MBACI sampling of an episodic disturbance: Stormwater effects on algal epifauna. Mar. Environ. Res. 64, 514–523. doi: 10.1016/j.marenvres.2007.04.005
Roy, P. S., Williams, R. J., Jones, A. R., Yassini, I., Gibbs, P. J., Coates, B., et al. (2001). Structure and function of south-east Australian estuaries. Estuar. Coast. Shelf Sci. 53, 351–384. doi: 10.1006/ecss.2001.0796
Ruiz, G. M., Fofonoff, P. W., Carlton, J. T., Wonham, M. J., and Hines, A. H. (2000). Invasion of coastal marine communities in North America: apparent patterns, processes, and biases. Annu. Rev. Ecol. Syst. 31, 481–531. doi: 10.1146/annurev.ecolsys.31.1.481
Schiff, K., Diehl, D., and Valkirs, A. (2004). Copper emissions from antifouling paint on recreational vessels. Mar. Pollut. Bull. 48, 371–377. doi: 10.1016/j.marpolbul.2003.08.016
Sheehy, D. J., and Vik, S. F. (2010). The role of constructed reefs in non-indigenous species introductions and range expansions. Ecol. Eng. 36, 1–11. doi: 10.1016/j.ecoleng.2009.09.012
Strain, E. M. A., van Belzen, J., van Dalen, J., Bouma, T. J., and Airoldi, L. (2015). Management of local stressors can improve the resilience of marine canopy algae to global stressors. PLoS One 10:e0120837. doi: 10.1371/journal.pone.0120837
Thomsen, M. S., Silliman, B. R., and McGlathery, K. J. (2007). Spatial variation in recruitment of native and invasive sessile species onto oyster reefs in a temperate soft-bottom lagoon. Estuar. Coast. Shelf Sci. 72, 89–101. doi: 10.1016/j.ecss.2006.10.004
Vadas, R. L., Johnson, S., and Norton, T. A. (1992). Recruitment and mortality of early post-settlement stages of benthic algae. Br. Phycol. J. 27, 331–351. doi: 10.1080/00071619200650291
Valentine, J. P., Magierowski, R. H., and Johnson, C. R. (2007). Mechanisms of invasion: establishment, spread and persistence of introduced seaweed populations. Bot. Mar. 50, 351–360. doi: 10.1007/s00442-012-2510-x
Valiela, I., Collins, G., Kremer, J., Lajtha, K., Geist, M., Seely, B., et al. (1997). Nitrogen loading from coastal watersheds to receiving estuaries: new method and application. Ecol. Appl. 7, 358–380. doi: 10.1890/1051-0761(1997)007[0358:NLFCWT]2.0.CO;2
Vitousek, P., Aber, J., Howarth, R., Likens, G., and Wallentinus, P. (2007). Human alterations of the global nitrogen cycle: sources and consequences. Ecol. Appl. 7, 737–750.
Vye, S. R., Emmerson, M. C., Arenas, F., Dick, J. T. A., and O’Connor, N. E. (2015). Stressor intensity determines antagonistic interactions between species invasion and multiple stressor effects on ecosystem functioning. Oikos 124, 1005–1012. doi: 10.1111/oik.01583
Waltham, N. J., and Connolly, R. M. (2011). Global extent and distribution of artificial, residential waterways in estuaries. Estuar. Coast. Shelf Sci. 94, 192–197. doi: 10.1016/j.ecss.2011.06.003
Wild-Allen, K., Skerratt, J., Whitehead, J., Rizwi, F., and Parslow, J. (2013). Mechanisms driving estuarine water quality: a 3D biogeochemical model for informed management. Estuar. Coast. Shelf Sci. 135, 33–45. doi: 10.1016/j.ecss.2013.04.009
Whitehead, J., Coughanowr, C., Agius, J., Chrispijn, J., Taylor, U., and Wells, F. (2010). State of the Derwent Estuary 2009: a Review of Pollution Sources, Loads and Environmental Quality Data from 2003 – 2009. Tasmania: Derwent Estuary Program, DPIPWE.
Womersley, H. B. S. (1984). The Marine Benthic Flora of Southern Australia, Part I. Adelaide, SA: Government Printer.
Womersley, H. B. S. (1987). The Marine Benthic Flora of Southern Australia, Part II. Adelaide, SA: Government Printer.
Womersley, H. B. S. (1994). The Marine Benthic Flora of Southern Australia, Part IIIA. Canberra, ACT: ABRS.
Womersley, H. B. S. (1996). The Marine Benthic Flora of Southern Australia, Part IIIB. Canberra, ACT: Australian Biodiversity Resources Survey.
Womersley, H. B. S. (2003). The Marine Benthic Flora of Southern Australia, Part IIID. Canberra, ACT: Australian Biological Resources Study and the State Herbarium of South Australia.
Keywords: introduced species, macroalgae, sewage outfall, fish farm, marina, stormwater drainage
Citation: Fowles AE, Edgar GJ, Stuart-Smith RD, Kirkpatrick JB, Hill N, Thomson RJ and Strain EMA (2018) Effects of Pollution From Anthropogenic Point Sources on the Recruitment of Sessile Estuarine Reef Biota. Front. Mar. Sci. 5:417. doi: 10.3389/fmars.2018.00417
Received: 10 April 2018; Accepted: 18 October 2018;
Published: 09 November 2018.
Edited by:
Rodrigo Riera, Catholic University of the Most Holy Conception, ChileReviewed by:
Angel Pérez-Ruzafa, Universidad de Murcia, SpainChristian Joshua Sanders, Southern Cross University, Australia
Fernando Tuya, Universidad de Las Palmas de Gran Canaria, Spain
Copyright © 2018 Fowles, Edgar, Stuart-Smith, Kirkpatrick, Hill, Thomson and Strain. This is an open-access article distributed under the terms of the Creative Commons Attribution License (CC BY). The use, distribution or reproduction in other forums is permitted, provided the original author(s) and the copyright owner(s) are credited and that the original publication in this journal is cited, in accordance with accepted academic practice. No use, distribution or reproduction is permitted which does not comply with these terms.
*Correspondence: Amelia E. Fowles, YWZvd2xlc0Bucm1zb3V0aC5vcmcuYXU= Rick D. Stuart-Smith, cnN0dWFydHNAdXRhcy5lZHUuYXU=