- 1US Integrated Ocean Observing System Office, National Ocean Service, National Oceanic and Atmospheric Administration, Silver Spring, MD, United States
- 2Australian Institute of Marine Science, Townsville, QLD, Australia
- 3Commonwealth Scientific and Industrial Research Organisation Oceans and Atmosphere, Hobart, TAS, Australia
- 4Australian Ocean Data Network, Integrated Marine Observing System, University of Tasmania, Hobart, TAS, Australia
- 5Department of Oceanography, University of Hawai‘i at Mânoa, Honolulu, HI, United States
- 6Office of Observations, National Weather Service, National Oceanic and Atmospheric Administration, Silver Spring, MD, United States
- 7Applied Physics Laboratory, University of Washington, Seattle, WA, United States
- 8Coastal and Regional Oceanography Laboratory, School of Mathematics and Statistics, University of New South Wales, Sydney, NSW, Australia
Instrumented moorings (hereafter referred to as moorings), which are anchored buoys or an anchored configuration of instruments suspended in the water column, are highly valued for their ability to host a variety of interchangeable oceanographic and meteorological sensors. This flexibility makes them a useful technology for meeting end user and science-driven requirements. Overall, societal needs related to human health, safety, national security, and economic prosperity in coastal areas are met through the availability of continuous data from coastal moorings and other complementary observing platforms within the Earth-observing system. These data streams strengthen the quality and accuracy of data products that inform the marine transportation industry, the tourism industry, fisheries, the military, public health officials, coastal and emergency managers, educators, and research scientists, among many others. Therefore, it is critical to sustain existing observing system networks, especially during this time of extreme environmental variability and change. Existing fiscal and operational challenges affecting the sustainability of observing networks will likely continue into the next decade, threatening the quality of downstream data and information products – especially those used for long-term monitoring, planning, and decision-making. This paper describes the utility of coastal moorings as part of an integrated coastal observing system, with an emphasis on stakeholder engagement to inform observing requirements and to ensure data products are tailored to user needs. We provide 10 recommendations for optimizing moorings networks, and thus downstream data products, to guide regional planners, and network operators:
1. Develop strategies to increase investment in coastal mooring networks
2. Collect stakeholder priorities through targeted and continuous stakeholder engagements
3. Include complementary systems and emerging technologies in implementation planning activities
4. Expand and sustain water column ecosystem moorings in coastal locations
5. Coordinate with operators and data managers across geographic scales
6. Standardize and integrate data management best practices
7. Provide open access to data
8. Promote environmental health and operational safety stewardship and regulatory compliance
9. Develop coastal mooring observing network performance metrics
10. Routinely monitor and assess the design of coastal mooring networks
Introduction
Human health, safety, and economic prosperity are tightly connected to the health and state of the ocean, particularly in coastal areas and major lakes. Coastal observations of physical, chemical, and biological variables provide the backbone of coastal intelligence, and contribute to evidence-based decisions in response to societal challenges such as food and water availability, energy security, and the development of sustainable economies. The societal benefits of ocean observations are interconnected at local, regional, national, and global scales, and these observations are indispensable tools for addressing and mitigating risks and producing skillful predictions, especially in coastal areas (Malone et al., 2014).
Observations from instrumented coastal moorings (hereafter referred to as “coastal moorings”) are critical components of the Global Ocean Observing System (GOOS). Coastal moorings are valued for their ability to measure temporal variability through the collection of continuous oceanographic and meteorological data sets on appropriate time-space scales. These observations enable the assessment of environmental or ecosystem conditions and variability and the impact of events to coastal areas, which will then allow us to forecast, adapt to, and mitigate changes. Federal, tribal, state, academic, industry, and public stakeholders rely on this information for a wide range of applications, including shipping, fishing, tourism, energy generation, and scientific research.
Coastal moorings are anchored buoys or an anchored configuration of instruments suspended in the water column collecting near real-time or delayed mode atmospheric and/or oceanographic observations at one or more depths. The coastal area is from the head of tide out to the edge of the continental shelf. The ability to monitor in near real-time is essential for ocean state forecasts, as well as for the long-term maintenance of the monitoring systems. The moorings may have a surface expression such as an instrumented buoy (enabling near real-time transmission of data) or may be configured in buoyant suspension below the surface of the ocean (Figure 1). Some vertically profiling moorings have an instrument package that “crawls” up and down along the mooring line, which allows a single set of sensors to take measurements at multiple depths (McArthur et al., 2017). Only moorings intended for long-term monitoring are considered in scope, which includes seasonal and long-term research moorings as opposed to experimental or short-term project moorings.
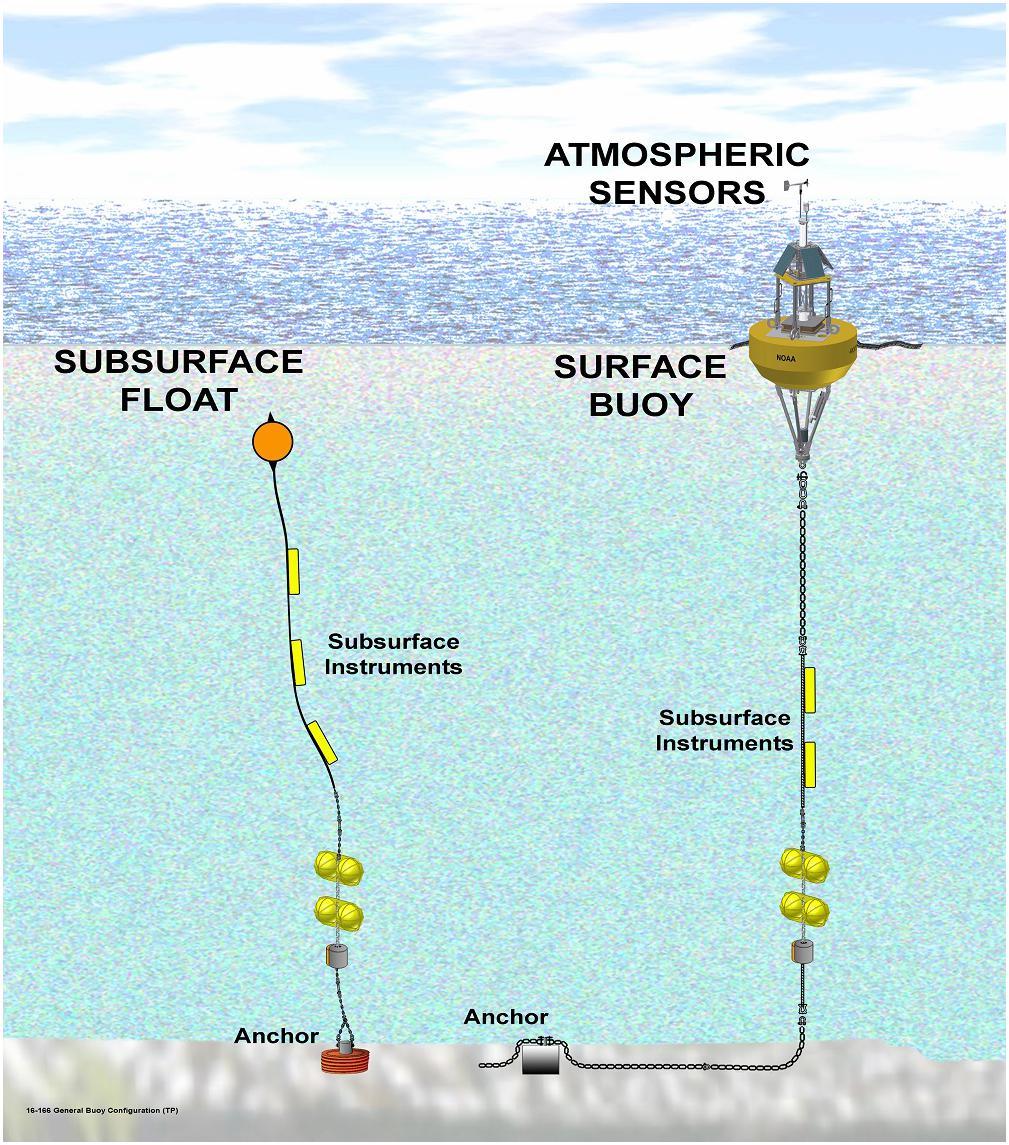
Figure 1. Examples of coastal moorings. Coastal moorings may have a surface expression such as an instrumented data buoy or may be configured as an instrumented mooring in buoyant suspension below the surface of the ocean. Source: NOAA.
This paper describes the utility of coastal moorings as part of an integrated coastal observing system (observations – data management – products), with an emphasis on stakeholder engagement to inform observing requirements and to ensure data products are tailored to user needs. Existing observing capabilities, plus recommendations for improved ecosystem monitoring from moorings are discussed, as well as network design considerations. The importance of data management standards, especially quality control, is emphasized as vital to the integration of moorings data with other observations. Finally, a sample of products are provided to demonstrate moorings data integrated with other observing system data to successfully deliver real-time or forecast information for public consumption. We conclude with recommendations toward sustained moorings networks that deliver continuous, high quality data in order to optimize downstream data products to meet stakeholder and science-driven needs.
Observations Collected by Moorings
Coastal moorings can provide systematic and simultaneous observations in the air, at the sea surface, and throughout the water column all the way to the seafloor. They can support complex payloads, allowing co-located measurements of many of the GOOS Essential Ocean Variables1, and are relatively easy to upgrade and equip with additional sensors, especially as the adaptive management needs of mission requirements evolve (McArthur et al., 2017). Rather than list all of the variables an instrumented mooring is capable of measuring, it is simpler to categorize the moorings into common combinations of the ocean, meteorological, and biological variables selected to meet a primary mission requirement.
• Physical Oceanographic moorings: These moorings are used to monitor the physical environment of the water column, and the main measurements along an in-line mooring consist of temperature, salinity and currents. Since the 1990s, mechanical single point rotary current meters have been replaced by acoustic Doppler current meters that can be point or profiling. A major use is to help calibrate and validate numerical hydrodynamic models.
• Meteorological moorings: These typically include measurements of the WMO essential variables for weather (wind speed and direction, air temperature, atmospheric pressure, and relative humidity) and GOOS physical EOVs at the surface (sea surface temperature, sea state). These measurements are critical for marine weather forecasting and navigation (WMO, 2015).
• Wave moorings: These moorings are primarily intended for monitoring surface GOOS physical EOVs (sea state, sea surface temperature, and/or surface currents). Thus, they are a type of physical oceanographic mooring that requires specialized, single-purposed platforms (e.g., Datawell buoys) to monitor wave height, period, and direction, and do not include instrumentation attached to the mooring line. Wave moorings are used for swell modeling, forecasting, and analysis of coastal environment data for use by coastal engineers, planners, managers, scientists, and mariners.
• Ecosystem moorings: These moorings measure a blend of GOOS EOVs across the physics, biogeochemistry, and biology and ecosystems categories, depending on their application, but always include at least one biological EOV. Surface observations are used for monitoring community structure and changes, detecting harmful algal blooms (HABs), and observing water quality. Subsurface observations are used to examine the water column structure to assess ecosystem characteristics. Physical, chemical and biological variables are often monitored simultaneously in time and space on these moorings.
Existing Networks of Coastal Moorings
Coastal moorings networks are operated and maintained by several countries around the world to meet local, regional, or national stakeholder observing requirements and scientific research needs. The Joint Technical Commission for Oceanography and Marine Meteorology (JCOMM) Data Buoy Cooperation Panel (DBCP), coordinates the use of over 400 of these moorings, and ensures the meteorological and oceanographic data are available in real-time (Figure 2) to support global forecasts of weather and ocean conditions. The DBCP inventory relies on meteorological and ocean observations delivered to and accepted by the World Meteorological Organization (WMO) Global Telecommunication System (GTS), therefore does not provide an exhaustive inventory of global coastal moorings (especially ecosystem moorings); however, it does illustrate the breadth of meteorological and ocean observing from coastal moorings.
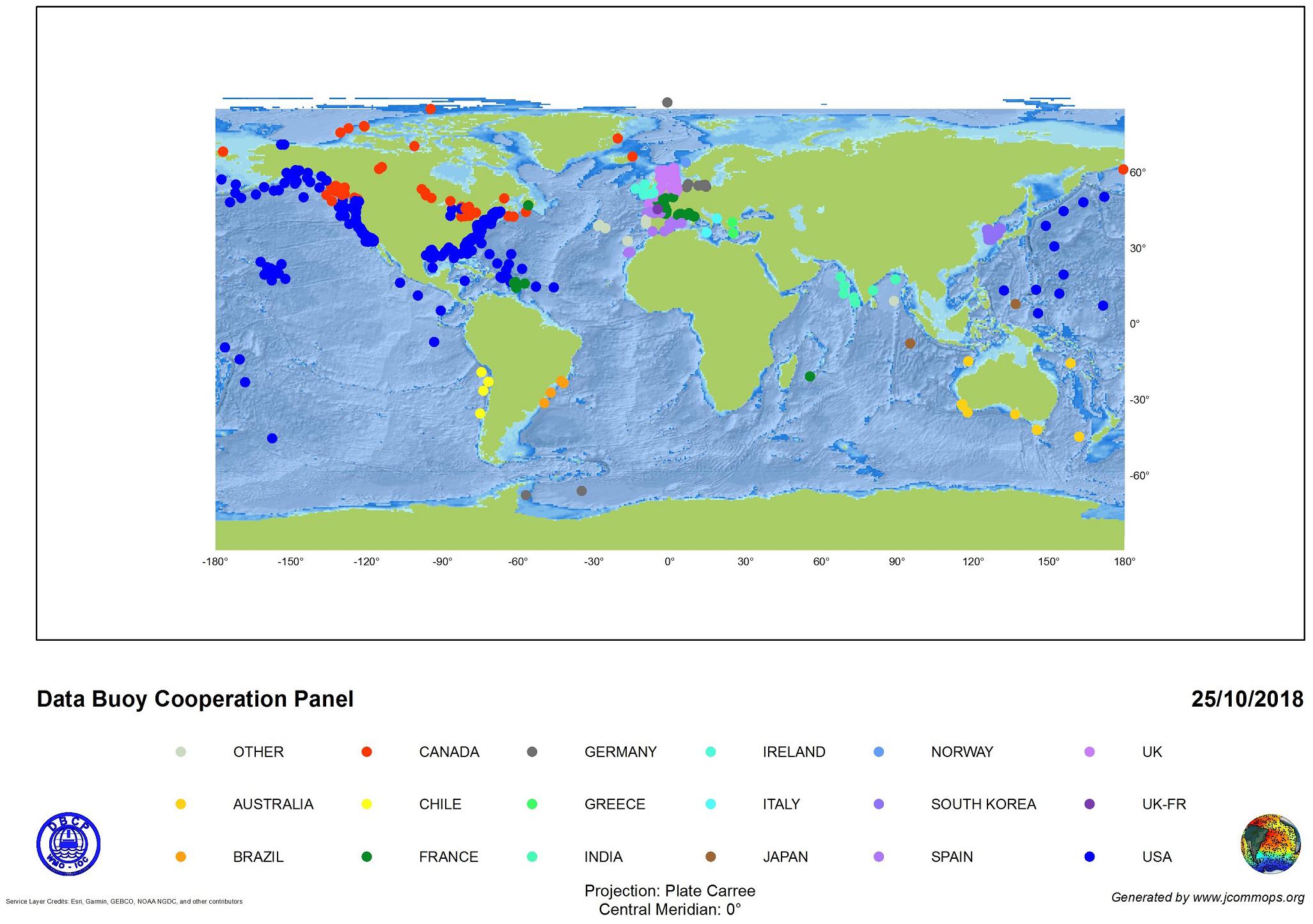
Figure 2. Operational coastal moorings that are coordinated by the Data Buoy Cooperation Panel, as of October 2018. Source: JCOMMOPS.
A small selection of the many countries operating coastal moorings networks, who also contribute to the DBCP are:
The United States (US): About 370 moorings intended for long-term operations are deployed around the US coasts (including Pacific and Caribbean islands) and in the Great Lakes. Most notably, the National Oceanic and Atmospheric Administration (NOAA) National Data Buoy Center (NDBC)2 operates 106 meteorological moorings for operational forecasting, warnings and atmospheric models, scientific and research programs, and emergency response to chemical spills. The US Army Corps of Engineers (USACE) Coastal Data Information Program (CDIP) network3 of about 70 wave moorings are used by coastal engineers and planners, scientists, mariners, and recreational users. Almost half (145) of the US coastal moorings are operated by nonfederal groups that are part of (or partner with) the 11 US Integrated Ocean Observing System (IOOS) Regional Associations (RAs)4. The RAs are the regional component of the US IOOS5, and provide integrated observations and data products in support of local stakeholders and scientific needs.
Canada: Canada’s Weather Buoy Network6 consists of 40 meteorological moorings deployed in coastal waters on each coast, as well as the Great Lakes and other major Canadian waterways. Real-time meteorological and oceanographic observations are delivered to and are managed by Environment Canada. The network provides mariners early detection and warning of incoming severe storms. At inshore locations, 20 of the 40 moorings are deployed seasonally during ice-free periods.
Australia: The Integrated Marine Observing System (IMOS)7 operates a network of 7 National Reference Stations, which include vessel-based biogeochemical water column sampling (Lynch et al., 2014), as well as ocean acidification moorings, acoustic observatories, and regional arrays of over 30 moorings across the continental shelf. IMOS observations are guided by science planning undertaken collaboratively across the regional nodes of the Australian marine and climate science community with input from government, industry and other stakeholders. Observations are primarily used for studying ocean acidification and climate impacts to marine ecosystems. Outside of IMOS, Australian state governments operate coastal wave buoy networks in support of wave forecasts and maritime safety.
The United Kingdom (UK): The UK Met Office operates three coastal meteorological moorings that are part of the Marine Automatic Weather Stations (MAWS) network8 of moorings and shore stations. Meteorological and wave observations are used for weather forecasting and real-time monitoring, climate studies, and ground-truth for satellite calibrations.
India: The Earth System Science and National Institute of Ocean Technology operates physical oceanographic and meteorological moorings for weather forecasting and climate research, among other applications, to understand and predict the Indian monsoon, tropical cyclone impacts, and air-sea interactions. Venkatesan et al. (2016) provides a detailed overview.
Ireland: The Marine Institute in collaboration with the Met Éireann and the UK Met Office operates the Irish Weather Buoy Network9, five meteorological buoys around the Ireland coast that collect real-time meteorological and wave observations. The network is used to support maritime safety, as well as improvements in weather forecasts. Data are used for gale and swell warnings, search and rescue, validation of operational models, and research. The Marine Institute also operates four real-time wave buoys at two offshore test sites in support of marine renewable energy needs and climate research.
Spain: The Puertos del Estado operates about 15 coastal moorings as part of their coastal network10. These are mainly deployed in or near ports, primarily to provide real-time meteorological and wave data to support safe and efficient navigation, and for validation of operational wave models. Spain’s port systems are closely tied to their economy, and the real-time weather and ocean information is vital to port safety and operations (Puertos del Estado and Ministerio de Fomento, 2015).
The Republic of Korea: The Korea Meteorological Administration operates a network of eight meteorological moorings located around the Korean peninsula11. These moorings measure wave observations for maritime safety and weather forecasting.
The abundance of global ecosystem moorings networks are not well-captured, and ecosystem moorings are likely scarcer than meteorological or wave moorings, given higher costs and/or feasibility of deploying newer sensor technologies on moorings. In the US, only about 10% of the 370 federal and nonfederal coastal moorings measure a combination of variables necessary for basic ecosystem monitoring. Specifically, physical variables like temperature and salinity measured at multiple depths and biogeochemical variables like oxygen and chlorophyll measured at least at one depth within the water column (McArthur et al., 2017). This subsurface coverage is critical for monitoring chemical and biological conditions and processes in the coastal ocean. Some of these moorings are part of the US Ocean Observatories Initiative12 (OOI), which is an integrated infrastructure program for long-term physical, biogeochemical and ecological monitoring to inform research on climate change, ecosystem variability, ocean acidification, and carbon cycling. Similarly, long-term ecosystem moorings are included in the European Multidisciplinary Seafloor and water column Observatory (EMSO)13, which is a research infrastructure consortium of regional mooring facilities in France, Greece, Ireland, Italy, Portugal, Romania, Spain, and United Kingdom intended for sustained, real-time monitoring. Beyond ocean observatories, sustained coastal ecosystem moorings are rare in observing networks.
Coastal Moorings Data Collection
The Framework for Ocean Observing discusses a systems engineering approach toward a global sustained ocean observations network that integrates new biogeochemical, ecosystem, and physical observations while sustaining present observations without necessarily deploying new platforms (Lindstrom et al., 2012). The structure of the Framework for Ocean Observing consists of a feedback loop between science-driven requirements and observing system outputs (Figure 3). The process of determining what EOVs to measure and how begins and ends with stakeholder input. Once those requirements are gathered and EOVs are identified, a technology is then selected to measure those variables. Data are assembled, products are developed and distributed, and continued stakeholder engagement determines how the data collection process and/or products might need to change or evolve. Coastal mooring data collection requirements, therefore, hinge on stakeholder needs (both end users and intermediaries who develop value-added products) and engagement strategies to inform observing requirements. They also hinge on the state of sensor technologies for newer EOVs that are being implemented, such as biology and ecosystem variables.
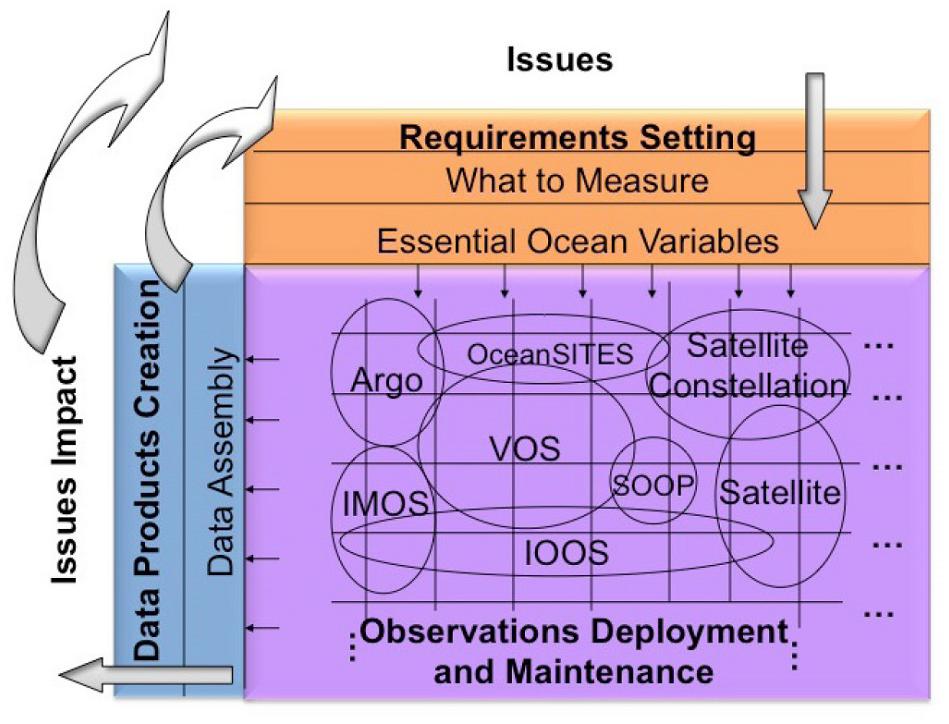
Figure 3. The Structure of the Framework for Ocean Observing. Source: Lindstrom et al. (2012).
Stakeholder Engagement
There are numerous and diverse stakeholders of coastal mooring data representing federal, state, industry, academic, tribal, and other public groups. Stakeholder engagement across these groups is critical for successful partnering, stakeholder identification, and network change management solutions. For operators and data providers to fully capture and understand user requirements, stakeholders must be engaged throughout the lifecycle of the deployed technology and the downstream data produced. The US IOOS RAs excel at this continuous engagement, through annual meetings, workshops, and through their organizational structures, which lend to close connectivity with researchers and coastal communities. Their process (Figure 4) mirrors the approach recommended by the Framework for Ocean Observing, and has been successful for connecting ocean observations and information products to societal needs. The US Pacific Islands Ocean Observing System (PacIOOS) stakeholder-driven approach toward prioritization and strategic planning serves as a model for ocean observing network operators – especially those operating with limited resources in a vast and diverse region. PacIOOS stakeholders have varying degrees of access to and/or understanding of ocean data. Therefore, PacIOOS categorizes stakeholders based on how much data they consume and their knowledge or understanding of ocean data, and this drives their level of engagement and outreach efforts, which then enables them to effectively identify and prioritize user needs (Iwamoto et al., 2016). Operators around the world should establish this type of strategic approach to effectively maximize stakeholder engagements.
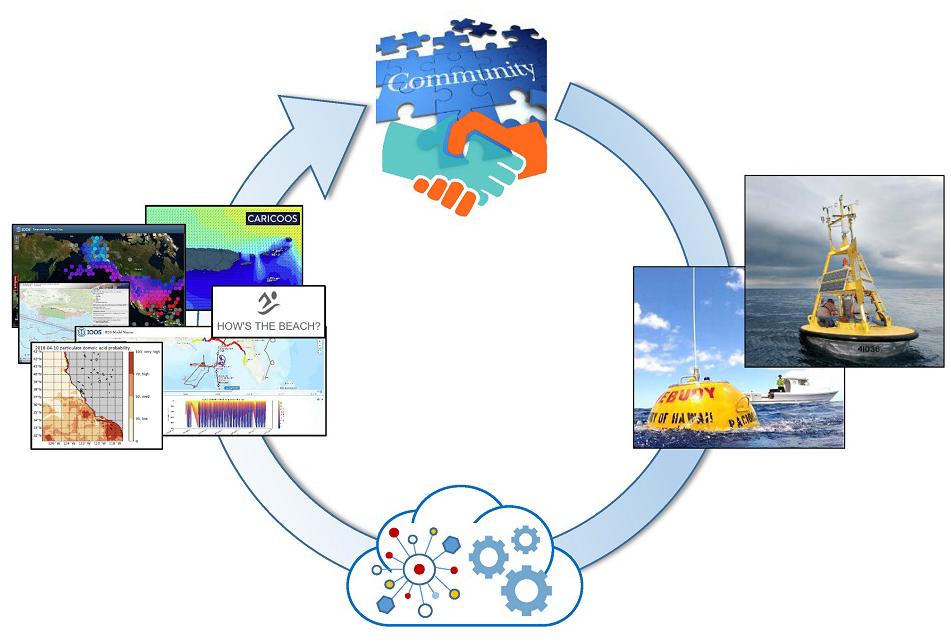
Figure 4. The process by which IOOS RAs produce quality information products based on ocean observations to meet stakeholder needs. The process begins with stakeholder outreach discussions to determine what variables and measuring technologies are needed. Data are ingested into RA systems where they are quality controlled and distributed through regional portals and data access services. Data products developed by the RAs are tuned to stakeholder needs, and the RAs receive feedback through continued stakeholder engagements. Wave buoy source: Pacific Islands Ocean Observing System (PacIOOS); Meteorological buoy source: NOAA.
Operational Observing Capabilities
Once stakeholder requirements are gathered, variables must be selected, though are constrained by the availability of measurement technologies. The GOOS categorizes ocean and atmospheric observations in terms of their readiness for operational capabilities (Nowlin et al., 2001; Nowlin and Malone, 2003). A summary of these are:
1. Research: Development of an observational/analysis technique within the ocean and meteorological community
2. Pilot Project: Community acceptance of the methodology gained
3. Pre-operational Projects: Use of the methods and data
4. Observing System: Incorporation of the methods and data into an operational framework with sustained support and sustained use to meet societal objectives (Nowlin et al., 2001; Nowlin and Malone, 2003).
Physical EOVs are routinely measured at physical oceanographic, meteorological and wave moorings in ocean observing systems like the US NDBC and CDIP networks, and the IMOS network. These measured parameters and methods have been incorporated into an operational framework with sustained support and sustained use to meet societal objectives; however, biogeochemical measurements are not as mature. Biogeochemical instrumentation ranges in readiness for operational capabilities from category one (Research) to category three (Pre-operational Projects). With the exception of chlorophyll, very few biological variables are operational. Activities toward implementing biological essential ocean variables are ongoing, and are coordinated by the GOOS Biology and Ecosystem Panel (GOOS BioEco).
In response to a need for defined ecosystem observing capabilities on moorings, the US Alliance for Coastal Technologies14 (ACT) coordinated a National Coastal Ecosystem Moorings Workshop to identify a combination of required variables for robust ecosystem monitoring, depending on the environmental process being measured. Participants focused on identification of stakeholders and use cases of coastal marine ecosystem mooring data in the US, then discussed challenges and technical requirements, and concluded with recommended configurations of an ecosystem mooring:
1. A backbone of core biogeochemical and physical measurements, which are ancillary to ecosystem observations and which all sites should collect and have in common: temperature and salinity at the surface and subsurface to resolve relevant stratification; dissolved oxygen (at least subsurface); pressure or depth where the sensors are; and chlorophyll/backscatter in the surface layer. This backbone of core biogeochemical and physical measurements informs issues like hypoxia as well as bloom dynamics. It also gives insights into upwelling and thus is important to inform ecosystem processes.
2. A recommended suite of measurements includes the backbone measurements described above in addition to all or a subset of the following, based on the regional needs and applications: pH/pCO2 (at surface both are recommended, but subsurface pH only is acceptable); color dissolved organic matter (CDOM), nitrate, current velocity, meteorological variables, passive bioacoustics (including fish tag receivers), active bioacoustics, and photosynthetically active radiation (PAR). This suite of measurements will help uncover processes driving ecosystem variation, advance direct measurement of biology, help validate ecosystem models for target species and protected species, and may also lead to understanding and predictability of events.
3. A high-capability suite consists of variables measured by technologies that are available but costly, and therefore recommended at a subset of sentinel or demonstration sites. This includes the backbone measurements plus a subset of the following, based on regional needs and applications: carbon system variables (beyond just pH and CO2), CDOM, nutrients, current velocity, meteorological variables, passive bioacoustics, active bioacoustics, and PAR, with spectral, genomic sensors and imaging sensors. This high-capability suite enables real-time detection of toxins, food-web members (plankton and fish) and productivity for informing fisheries management (McManus et al., 2018).
The recommended suite in particular should be used to guide regional planning and should be adopted by operators across the GOOS.
Supplemental Sampling
A regime for vessel-based supplementary biogeochemical sampling and laboratory analysis is recommended to add value to ecosystem moorings measurements. Supplemental measurements also improve the quality of moored observations. In particular, due to the significant drift and bio-fouling of optical sensors, additional conductivity, temperature depth (CTD) casts or water samples should be taken regularly (e.g., at each mooring service). The data can be used to calibrate instrument data throughout its deployment. This requires a systematic comparison and correction of the datasets.
The CTD or water samples can be taken throughout the whole water column to give more detail on stratification and mixing depths and conditions. For example, physical collection and analysis of nutrients, pigments, plankton and microbial sampling can describe the biological community structure at a higher taxonomic resolution for ecosystem assessment, and can be used as inputs to nutrient-phytoplankton-zooplankton (NPZ) models, and describe seasonality and interannual biological community responses to physical drivers. This collection and analysis can also lead to better understanding of biological responses and higher-resolution data, which is not yet available through sensor deployment. Species-level biological data is a future capability for in situ equipment on moorings, and microscopic analysis of physical samples remain the best method to get detailed information on community structures. Thompson et al. (2009) demonstrates that long-term monthly sampling at three moorings in Australia is an adequate sampling frequency to detect trends in parameters, such as water temperature, affecting nutrients and phytoplankton community structures. However, the temporal resolution required to resolve trends in plankton dynamics varies depending on the ecosystem and on the types of functional plankton groups present. Many studies have shown the inadequacy of monthly sampling when it comes to plankton dynamics. Thus, there is a critical need for sustained, continuous, high resolution observations of the plankton.
Other types of recommended supplemental sampling include:
• CTD and Secchi disc – to ground truth sensors, provide a complete water column profile, and clarify the degree of mixing or stratification.
• Variables for carbon monitoring, total organic carbon, total alkalinity, salinity, measurements important to ocean acidification.
• Suspended particulates, which are useful for validation of satellite retrieved estimates of total suspended matter and chlorophyll a concentrations.
Coastal Mooring Network Design and Configuration
The location of mooring arrays have traditionally used advice from experts or those that are aware of certain phenomena that need to be better understood. With long term observations it is important to also ensure that the mooring observations are of value over as wide area as possible so they are useful to as many people as possible.
In order to palliate the spatial limitations of moored observations, clusters and arrays are necessary, and complementary platforms are recommended. For example, while a single temperature mooring provides climate change baselines, a single velocity mooring is of less value; however, an array of just three velocity and or temperature moorings (e.g., across the shelf) provides significantly more detail. We recommend an initial study prior to the design of any observational system to provide the spatial context to identify the optimal locations and recommended variables to measure (Roughan et al., 2013). This is best conducted from observational and modeling studies. Where a surface expression is possible, we also recommend extending the mooring with near surface observations, and a meteorological station, including critical measurements for satellite validation, and meteorological information to enable a better understanding of the local dynamical drivers.
To ensure the most economic spread of observations, International observational programs have been assessed using Observation System Simulation Experiments (OSSE) in order to optimize temporal and spatial coverage when designing the array (Oke et al., 2015). Observation System Simulation Experiments use a model simulation to measure the effectiveness of observation techniques and observation locations needed to examine the state or phenomena of interest. Oke and Sakov (2012) used a model hindcast to determine how well the IMOS National Reference Stations monitor the shelf circulation, which identified several gaps around Australia. OSSEs can also use other spatial observations such as satellite derived chlorophyll-a to determine the footprint of existing observational arrays. This technique was applied to assess the value of the IMOS National Reference Stations and resulted in a re-design of the network (Jones et al., 2015). As another example, a wave model hindcast can be used to look at spatial coherence of the wave field around Australia to help identify gaps in the wave buoy data network. This has led to the prioritization of those locations for any expansion (Greenslade et al., 2018).
An Integrated System
In the coastal ocean, physical and biogeochemical processes occur continuously over time and vary on smaller time-space scales than in the open ocean. Moored observations allow a good representation of temporal variability through the water column, with in situ measurements often every few minutes, at fine (cm–m) vertical resolution. Provided we succeed at sustaining coastal mooring sites over decades (e.g., off Sydney and Tasmania, Roughan et al., 2013), these observations allow us to investigate a range of temporal scales from turbulent mixing to inter-annual climate variability (Ruhl et al., 2011) at the mooring site.
Spatial representation is however an issue with fixed-point systems. Integration with data from other platforms is critical to complement moored observations in space over larger areas. For instance, satellite temperature, ocean color or altimetry observations, as well as coastal High Frequency Radars (Archer et al., 2017) can be used to provide a horizontal spatial context (at the surface) and identify spatial scales of variability, or how far from the mooring location the ocean variables are correlated. Moreover, moored data can be used to provide information on de-correlation timescales for satellite data, which is useful for gap filling (Lee et al., 2018) and validate radar observations (Mantovanelli et al., 2017; Wyatt et al., 2017).
Autonomous ocean gliders provide observations with high vertical resolution, and reasonable spatial coverage. Sensors for parameters typically include; temperature, salinity, and other biogeochemical variables such as chlorophyll fluorescence, light and dissolved oxygen. With sufficient data de-correlation length scales can be estimated throughout the water column (Todd et al., 2013; Schaeffer et al., 2016). When combined with HF radar data and moored observations, a comprehensive three-dimensional picture emerges of the coastal ocean, e.g., off the coast of SE Australia (Roughan et al., 2015; Schaeffer et al., 2017).
Finally, moorings data can complement vessel-based hydrographic sampling, giving temporal context to vertical profile data. For example, off the coast of Sydney, Australia, a dataset of more than 65 years of hydrographic sampling was augmented with a thermistor string approximately 10 years ago, thereby adding significant value to both datasets over many timescales (Schaeffer and Roughan, 2017). Richardson et al. (2015) used the IMOS mooring arrays with the complementary vessel-based sampling information to complete an assessment of Australia’s oceans using plankton data as indicators of change to link science and policy in one document. Again, this shows a significant added value to both datasets when used in conjunction with each other.
By-products from complementary platforms can also help place the moored observations in context. Local upwelling indices (e.g., Alvarez et al., 2008 based on nearby meteorological station, satellite scatterometers or atmospheric models), and distance from the boundary current (when applicable, potentially calculated from satellite altimetry) provide valuable information on the main drivers of the local dynamics.
Data Management
The quality and availability of observations from moorings are increasingly critical for developing data products that adequately meet user needs. Thus, continued emphasis is placed on adoption of and adherence to data management standards and provision of open access to observations. This includes robust metadata, implementation of quality control procedures (e.g., QARTOD – the Quality Assurance and quality control for Real-Time Oceanographic Data), and widespread use of data access services that enable automated discovery and access to global data sets. Adoption of these standards will also facilitate integration of moorings data with other complementary observing systems, increasing the production of multi-use data and information products that target a wide audience.
These standards will also facilitate use by the modeling community. Coastal moorings information is used to ensure the quality and accuracy of ocean models through cal/val and assimilation. Increasingly, operational models are becoming more commonplace and so near real-time data streams that undergo near real-time quality control are highly sought after to better assess model performance. This also requires uncertainty estimates of the data supplied. Therefore, these “model-ready” data, in easily accessed and ingested data formats, are increasingly vital.
Production
Along with the data itself, including detailed and consistent metadata and quality control information is crucial when generating datasets. A data producer should have all this information at hand, yet it is not always homogenous and available in a single place. For example, the Australian Ocean Data Network (AODN) and the Australian National Mooring Network (ANMN) have developed an imos-toolbox15 to process data manually retrieved from long-term mooring sites. As shown in Figure 5, this toolbox can read the data from instrument files and the metadata from a deployment database in order to produce NetCDF files that include both data and metadata. In addition, the toolbox can perform pre-processing and quality control operations, and add the results to the NetCDF files. These files are compliant with the Climate and Forecast (CF) 1.616 and IMOS 1.417 conventions. The toolbox is operated by data producers since they have expertise over the data, metadata and quality control of this data. It produces consistent NetCDF files ready for ingestion by the AODN. This open source software can be adopted by any institution to process their moorings data.
Ingestion
With improved instrumentation and advancing technologies, the volume, variety and complexity of data from moorings (and other observing systems) is increasing. Data providers are also numerous and diverse. Data management systems need to be able to ingest data from all sources in a reliable and consistent way to ensure the safe archival and integrity of the data. They need to maintain accurate catalogs of metadata to enable data discovery and access services, reporting of data holdings, and preservation of data lineage, including metadata lineage. Systems also need to be able to handle real-time ingestion for real-time data access.
Some recommended solutions to these challenges for moorings operators to consider are:
• Automated systems, with human intervention limited to high-level monitoring and dealing with unexpected cases;
• Generic and extendable software infrastructure to accommodate a variety of data types/sources;
• Data providers follow standards and conventions (e.g., CF). Compliance is verified early in the ingestion workflow, and subsequently assumed;
• All operations are recorded and easily retrieved for data lineage reporting;
• “Serverless” architecture (e.g., AWS Batch) for modularity, scalability, and isolation of individual data streams.
As an example, some of these aspects have been incorporated into the data ingestion system being developed at the AODN (Hidas et al., 2016), which handles a broad range of ocean data from numerous providers around Australia. While the detailed ingestion workflow varies from one data source to the next, each can be broken down into a set of standard tasks, such as validation, processing, and publication (Figure 6). The behavior of each standard task can be customized, and additional steps can be included to extend their functionality. The system is implemented as an open-source Python package18. Although the package is designed for the AODN infrastructure, elements of it may be adapted, or used as a guide, by other operators to develop their ingestion systems.
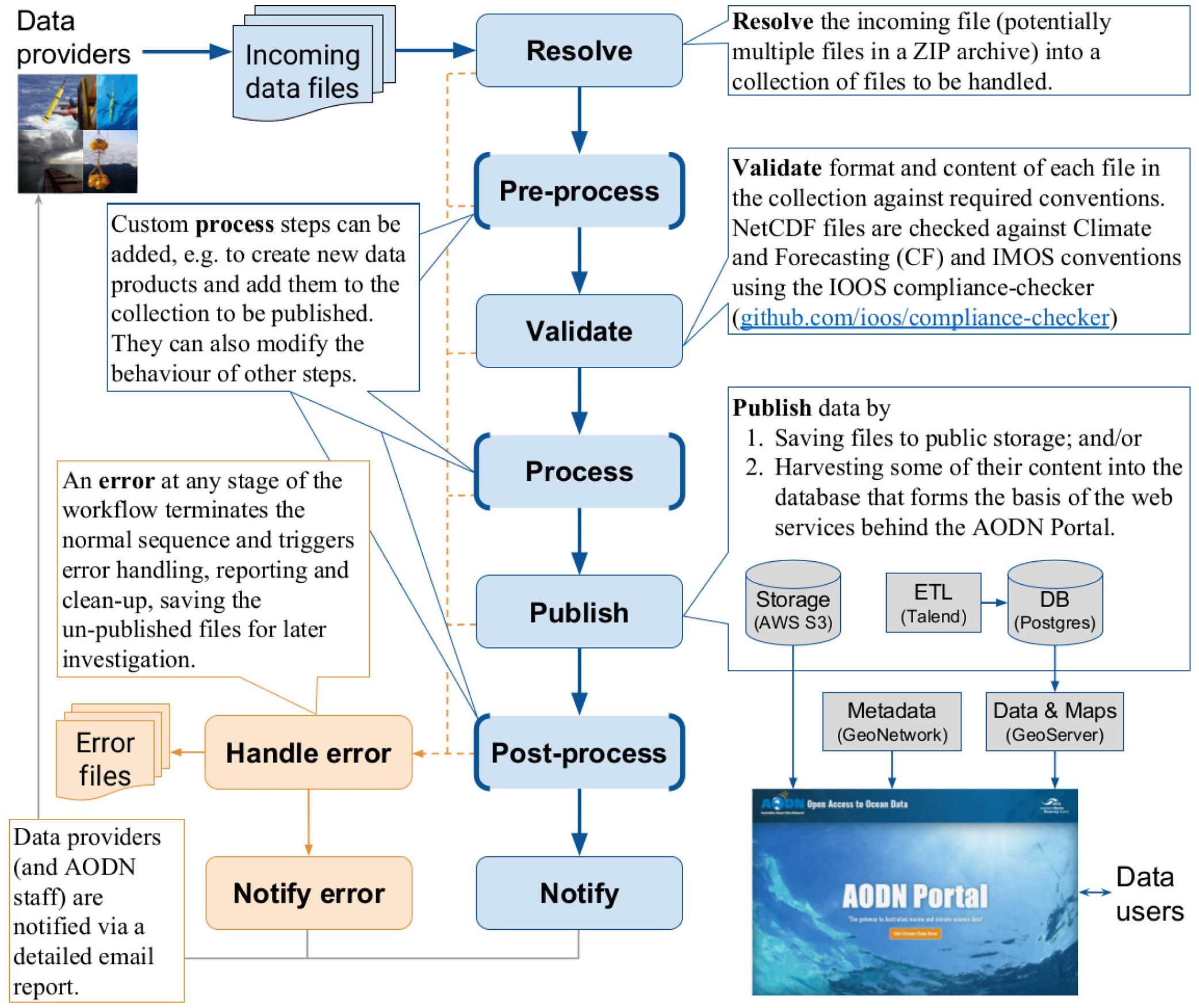
Figure 6. Generic, customizable workflow of the AODN data ingestion system (Hidas et al., 2018).
Quality Control (QC)
Quality control processes and standards are critical for precise and accurate data, which in turn is critical for producing quality data products that serve stakeholder and societal needs. Data quality is the cornerstone of the US IOOS QARTOD project, which establishes data quality standards for IOOS core variables. Manuals that are relevant to coastal moorings operators are: passive acoustics, phytoplankton, dissolved nutrients, winds, in situ surface wave, ocean optics, in situ temperature and salinity, dissolved oxygen, and in situ currents data. A manual on pH is currently in development. These manuals detail QA/QC tests as well as information about the sensors and procedures used to measure the variables.
Successful QC requires convergence in standards and best practices in flag schemes, provenance, and algorithms and thresholds. Some challenges and/or considerations for the coming decade are:
• QC flag schemes: The QARTOD flag scheme (US IOOS, 2017) is based on the Intergovernmental Oceanographic Commission (IOC) 54:V3 primary level flag scheme (Intergovernmental Oceanographic Commission [IOC], 2013) while IMOS and many other institutions are still using an older IOC version used by ARGO.
• QC flags for provenance: users interested in QC are increasingly asking for information about which tests have passed/failed for a particular data sample, and not only the global result of all tests. CF allows for the description of such flags using bit field notation in flag_masks and flag_meanings attributes19.
• QC tests algorithms and parameters: QARTOD defines automated and semi-automated QC tests for real-time observing. Many operators are struggling with assigning QC thresholds for parameters and tests. For example, the IMOS ANMN facility collects a lot of data (57 different instruments collecting more than 10 types of parameters at 137 sites), which makes QC operations a challenging activity in terms of human resources, so an automated approach20 has been adopted. Any semi-automated QC test requiring defining thresholds/parameters that may be site and/or dataset dependent has been left optional.
While some QC tests can be automated, there is still a need for visual validation and manual QC to determine the validity of a flag, catch erroneous data that automated tests missed, and to ensure the automated tests are functioning properly. Dashboards and auto-generated reports can quickly identify sensor problems or determine the validity of a flag (Figure 7). To effectively use this type of dashboard, operators should document QC protocols describing which QC tests to use, how to parameterize them, and what features to examine. For example, IMOS is in the process of documenting a QC protocol for temperature, salinity and pressure collected on moorings and using the imos-toolbox in order to achieve consistent QC across the national facility. This documentation is vital, especially if there is any divergence in QC practices. Standards and practices need to be described in order to potentially implement mapping between the different standards.
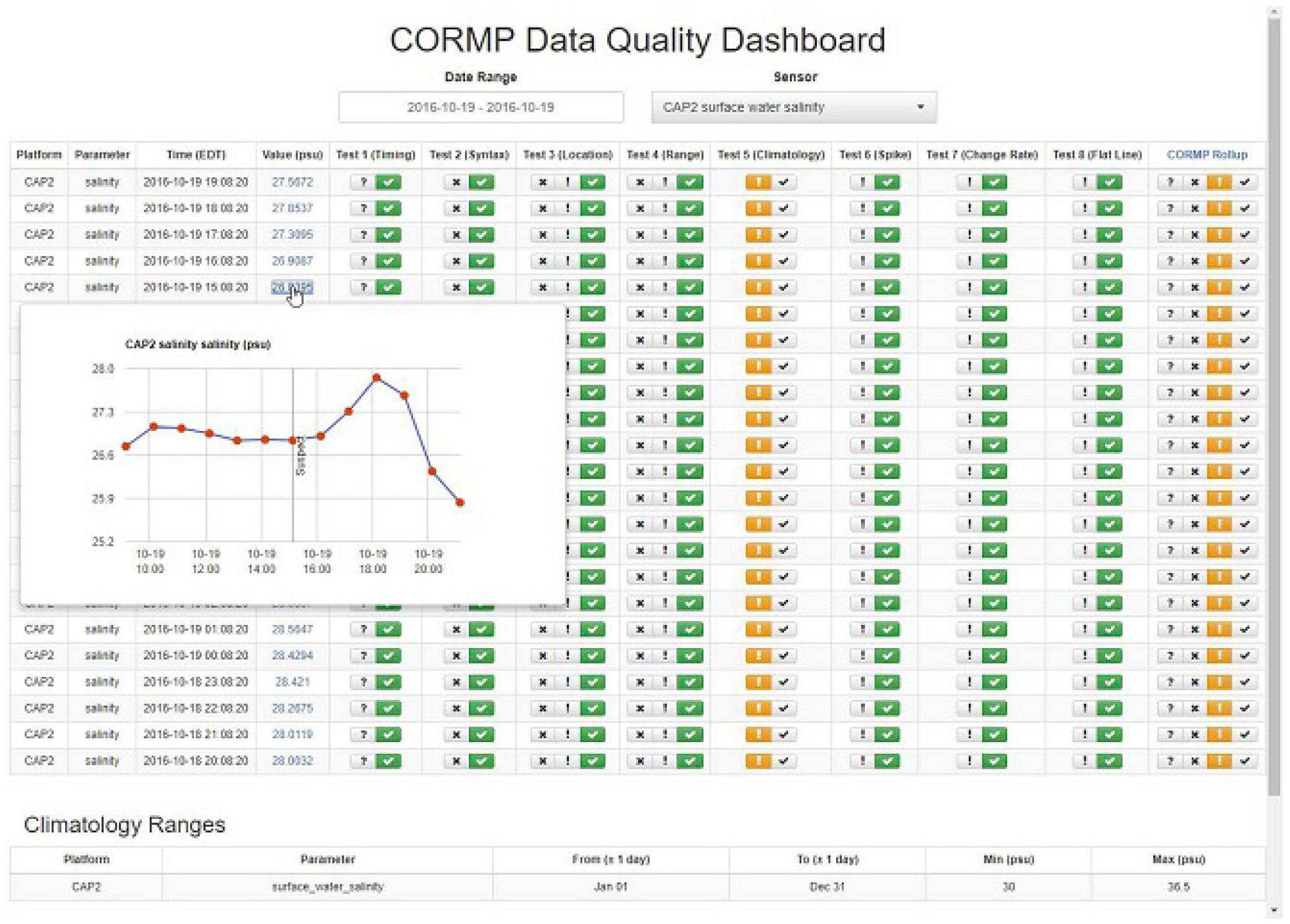
Figure 7. The University of North Carolina-Wilmington Coastal Ocean Research and Monitoring Program (CORMP) recently implemented the Data Quality Dashboard and an auto-generated daily email with flags, which allowed operators to manually review flags to assess their validity, identify any trends, and accept or override flags. This example shows a flag triggered for coastal mooring salinity data after major rain event). Source: Jennifer Dorton, Southeast Coastal Ocean Observing Regional Association (SECOORA).
The QARTOD tests have been implemented by numerous operators around the world, and will continue to evolve over the next decade as technological capabilities evolve, particularly for biological variables. Furthermore, the GOOS EOVs will be reviewed to determine if any other variables are viable for a new QARTOD manual. It is highly recommended these tests continue to be adopted by coastal moorings operators for real-time observations. Other areas of QC within focus for the next 10 years that are applicable to moorings operators include:
• Automated cross-validation QC: QARTOD is already suggesting some tests in this field [e.g., the suggested Neighbor Test for real-time QC of in situ temperature and salinity data (US IOOS, 2016a)]. This effort should expand within QARTOD and these types of tests become elevated to “Required/Recommended.”
• Use of artificial intelligence technologies (e.g., machine learning).
• Online collaborative (web 2.0) QC.
In addition to these focus areas, the International Quality controlled Ocean Database (IQuOD) group21 is exploring both machine learning22 and online collaborative QC approaches for profile data.
Data Curation
The sentiments expressed by the FAIR data principles (Findable, Accessible, Interoperable, and Reusable)23 are readily applied to ocean observations collected on moorings. Data that have undergone quality control to published standards, with complete metadata records, must be preserved and archived with a data center for discovery and access. Properly curated datasets are vital for ongoing research that depends on high-quality ocean information. The ocean observing community need to not only ensure current and future efforts conform to the above standards, but identify and correct historical time-series data out of compliance with these standards.
As observing networks expand, and as programs also improve modeling capabilities, cyberinfrastructure components must evolve to handle space requirements. Cloud services should be explored as a cyberinfrastructure solution to meet rising storage needs. For example, the AODN has adopted Amazon Web Services (AWS) for cloud storage and is also building its data delivery services on AWS. This allows them to meet rising storage requirements, plus AWS provides incremental backups and near 100% uptime for services.
Proper documentation of data management practices is essential, and these practices should be published. In the US, this is a NOAA requirement24 for any funding recipients, and data management templates25 are provided to guide operators, requiring descriptions of ingestion, QC, and archival of datasets. IODE Ocean Best Practices templates26 are also publicly available, which some countries (e.g., Australia’s IMOS) are in the process of implementing.
Data Products
Open data access is a guiding principle toward a successful integrated GOOS. The research community relies on data from all available sources to advance coastal modeling capabilities and studies focused on nearshore processes. Coastal moorings information is vital for these efforts, especially for real-time data assimilation systems that rely on the observations. Furthermore, openly accessible data bolsters the blue economy, which facilitates the development of value-added products across the Ocean Enterprise (profit and nonprofit groups in the private sector that engage in ocean observing and product development). In the US, more than 400 businesses across the Ocean Enterprise generate over $7 billion USD in revenue annually from ocean data and products (US IOOS, 2016b). Intermediaries tap into the enormous pool of ocean data to create value-added products that can be easily digested by a diverse audience. One area of focus is the development of mobile apps that turn open data into services. A recent “Big Button Ocean Data Challenge”27 was sponsored by the XPrize Ocean Initiative on HeroX to motivate developers to harness ocean data to create mobile apps tailored to meet user needs (e.g., fishing and surfing conditions, marine navigation, educational tools, water quality status, etc.), targeting specific societal benefit areas. This challenge builds upon and advances the vision behind the New Blue Economy (Spinrad, 2016) – leveraging ocean data to further economic development – and also encourages coordination and collaboration between private industry developers and scientists. Mobile apps are an area of the new blue economy that is exploding, and over the next 10 years will likely become a primary data product type for users across the world, which makes open data access all the more critical.
One way of promoting open access (beyond public data portals and data services) is ensuring observations are delivered to the GTS for operational ocean and climate forecast and analysis centers. The GTS is designed for international data exchange that enables development of early warnings and forecasts for hazardous events, as well as weather, water, and climate analyses and forecasts. Data are required in specific formats (e.g., BUFR), delivered through regional nodes. Unfortunately, the GTS was primarily designed with physical datasets in mind, whereas the ocean observing community (particularly modelers) have a growing need for biological datasets. BUFR templates are needed for this global distribution and are recommended as a focus for the coming decade.
Data Discovery and Access Requirements and Tools
Observing system programs require data systems that promote interoperability in order to enable user discovery and access of relevant data products. Different users have different needs and ways of interacting with data to advance research and product development. Uses range from simple summary plots to interactive plotting tools, from data download as simple CSV files to more complex formats such as netCDF and other machine-readable formats. Data required by a user is usually a subset and/or aggregation of available data collections, possibly from different sources and in different formats. Raw observation data required by a user may be large in volume but will often be processed into a much smaller final product for analysis. Furthermore, many “users” of observation data are actually software systems (e.g., models) that routinely and autonomously ingest large volumes of data. Users also require access to detailed data lineage information for transparency and repeatability.
Recommendations to improve delivery of moorings data are:
Standards and conventions for data discovery (e.g., ISO19115 metadata records), formats (e.g., CF), controlled vocabularies (e.g., the BODC Parameter Dictionary P0128), and access methods (e.g., Open Geospatial Consortium web services, see below) need to be more widely adopted and strictly followed. This will enable interoperability between distinct data access portals, and allow generic discovery, analysis and visualization tools to operate on all available data products. Coastal moorings datasets should be registered in data catalogs to promote discovery and access, following international standards and conventions.
Controlled vocabularies can be applied to a variety of key vocabularies like measured parameters, platforms and organizations, which can be defined with various levels of detail. These controlled vocabularies can drive faceted search so that portal users can discover data collections that are relevant to their needs without a prior knowledge of what is collected by an observing system.
Web services allow users (or software) to query, filter, transform, download or visualize data via web-based Application Programming Interfaces (API), without first downloading the entire data set. They also allow data access to be standardized, while allowing some flexibility in the format and storage method used for the underlying data. In particular, the Open Geospatial Consortium (OGC) defines the following standard services:
• Web Feature Service (WFS), for access to tabular data;
• Web Coverage Service (WCS), for access to multi-dimensional data;
• Web Map Service (WMS), visualizing spatial data as maps;
• Web Processing Service (WPS), allowing pre-defined operations to be applied to data by the server before downloading the result.
Metadata records are used to describe data collections in detail. They should also include information about relevant controlled vocabularies that are used, and online resources like access to files on servers and/or web services mentioned above.
Networks of data services Individual services can present data focused on specific regions or themes. If they are interoperable (based on the same standards), then broader services can easily integrate these to provide a more global view.
As an example, the infrastructure developed by the AODN (Hidas et al., 2016) uses WMS to display the spatial extent of data collections on a map, and WFS to provide access to one-dimensional data, or summary information on files containing multi-dimensional data. The open-source Open Geospatial Portal29 provides a higher level, more user-friendly web interface to these base services, allowing users to discover, preview, subset, and download data collections. Collections from other organizations that have adopted the same infrastructure for their own data portals (such as the Australian Institute for Marine and Antarctic Studies30) are interoperable with, and easily imported into, the main AODN Portal (Figure 8). A key lesson learned from delivering moorings data via the AODN Portal is that, while it is able to display mooring sites as “dots on a map,” this is not sufficient for complete discovery and visualization. The time and depth dimensions also need to be represented.
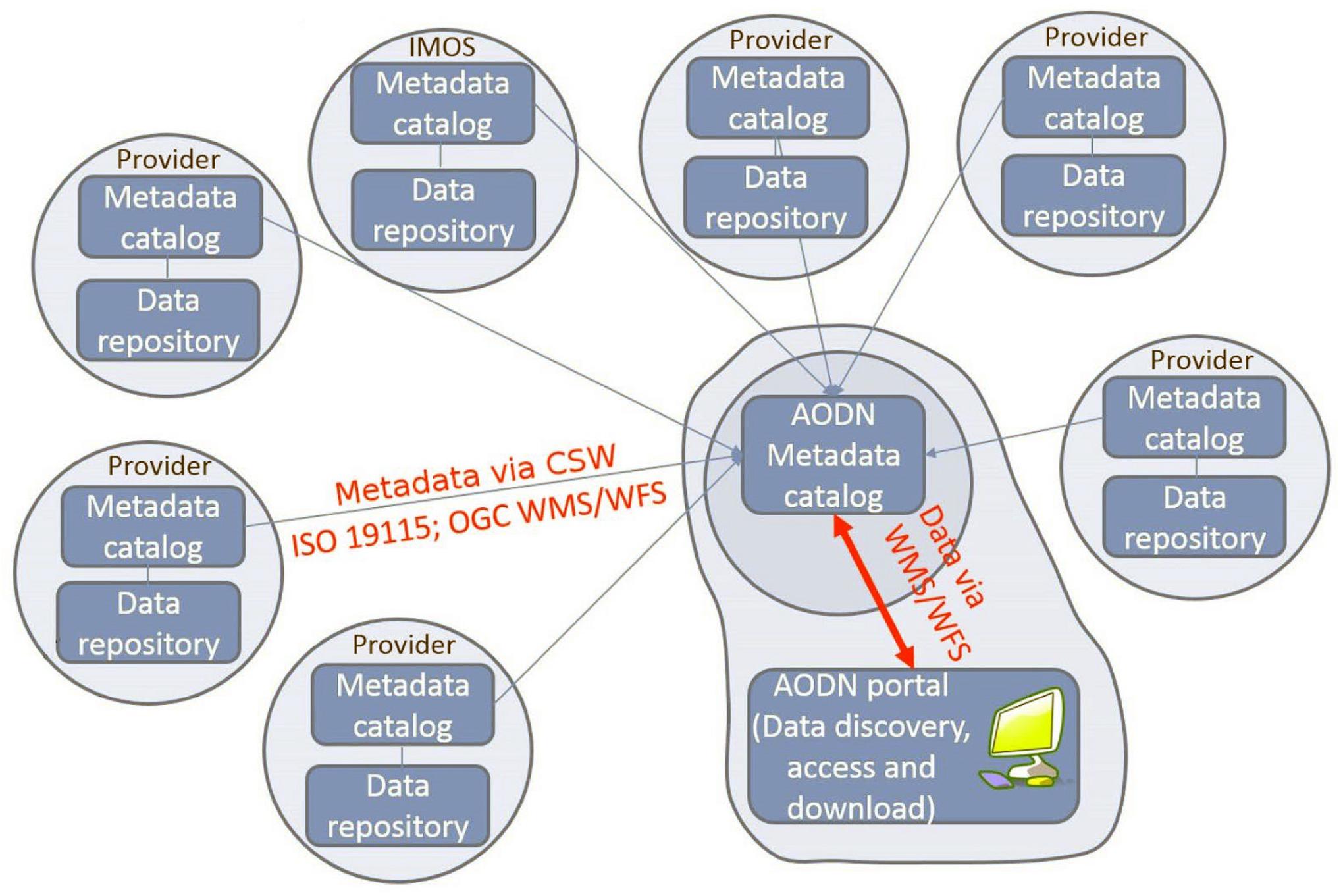
Figure 8. The AODN portal as an example of a network of interoperable data services. Source: Hidas et al. (2016). Used under CC BY (http://creativecommons.org/licenses/by/4.0)/updated to remove the IMOS portal, which no longer exists.
Our observation systems should include easy data visualization tools for end-users without the need for advanced programming skills. Interactive portals with a good graphical user interface are needed. If data can be provided in API format (application programming interface) then data can be readily ingested into a range of existing visualization platforms. ERDDAP is one solution toward this.
ERDDAP is a data server that was developed within NOAA to provide a consistent way for users (and machines) to access subsets of data in common formats. It is relatively low-effort to install and use, and it provides numerous benefits. ERDDAP can be installed alongside existing services and makes them interoperable, and can use those services as a data source, or it can directly access the data from source files. Ultimately, it serves as a broker between observing platforms and data users. Data that are produced in disparate formats are served in several different types of common formats, like.csv.html, ESRI.asc, Google Earth.kml, and netCDF; therefore, users do not need to convert datasets, they simply request the output format desired. A simple data access form within a web browser interface allows users to search, visualize, and download data, and it offers RESTful API services for automated machine-to-machine access.
ERDDAP has been gaining momentum among international operators and is being adopted to support data products. Over 70 institutions worldwide have installed ERDDAP, including Canada, Australia, France, Spain, and South Africa31. The US IOOS is also moving toward using ERDDAP as the standard source for the IOOS Data Catalog and other data products, and IOOS RAs will be adopting this service as a data broker for regional partner data. ERDDAP is highly recommended as a standardized data service that should be adopted by international data providers.
Societal Benefits and Stakeholders Who Rely on Data Products
The open availability of coastal mooring data has enabled scientific research, supported economic and social activities and critical government functions such the protection of life and property. Coastal mooring networks are also important economic elements of the Ocean Enterprise, as the businesses therein deliver products and services that enhance the Coastal Economy (US IOOS, 2016b). Coastal moorings and their data products are used for and/or support:
• Discovery: As newer technologies are developed and added to moorings (e.g., Environmental Sample Processors), discoveries are made particularly in the area of biology, and biodiversity.
• Ecosystem Health and Biodiversity: Information from coastal moorings enhances our understanding of processes related to ecosystem health, and changes in biodiversity. This helps to protect coastal populations and resources, including fisheries, aquaculture, and marine ecosystems.
• Climate Variability and Change: Climate-quality observations from coastal moorings enhances our ability to understand, assess, predict, mitigate, and adapt to climate variability and change. For example, observations that enable carbon cycle research, or examination of sea temperature variations to assess impacts on local fishing in a warming climate.
• Water, Food, and Energy Security: Coastal observations uncover impacts of ocean acidification, hypoxia, or invasive species on aquaculture, fishing, tribal sustenance, and local economies.
• Pollution and Human Health: Coastal moorings data are used to monitor and predict environmental factors and hazards affecting human health and well-being, such as HABs and toxins.
• Hazards and Maritime Safety: Real-time data from moorings leads to reduced loss of life, property, and ecosystem damage from natural and human-induced disasters, through improved marine forecasts and warnings, by improving the safety and efficiency of all forms of marine transportation.
• Blue Economy: Open access to moorings data allows intermediaries to make use of the data as an input to value-added information products (US IOOS, 2016b). Real-time observations and data products support Blue Economy sectors such as tourism, ocean renewable energy, biotechnology, shipping, offshore oil and gas, and fishing and aquaculture.
Data Products and User Needs
Over the next decade, end users increasingly require global access to data products and visualizations that are accurate, high quality, easily understandable, and continuously updated. When the data from coastal moorings are integrated, they contribute to information products that become powerful tools for monitoring and prediction. Furthermore, since mobile devices have become more prevalent, data product owners must consider these types of technologies as products are developed and tuned to user needs. Some network owners have created mobile-friendly versions of web-based products for visualization on a mobile device. For example, NDBC provides a separate “mobile access” webpage showing a text-only version of the data for display on a mobile device.
Coastal Mooring Data Products
Coastal mooring data are accessed daily by millions of national and international stakeholders, and are integrated into a variety of products and services. These stakeholders include a broad spectrum of federal, tribal, state, and local agencies, private industry, nonprofit organizations, and the public. One of the keys to sustaining quality ocean observations is to develop both products and tools that address the needs of end users and that become indispensable to users. Furthermore, targeted products should be developed to help end users understand the context of the observations. The following examples illustrate the types of products that rely on or use coastal moorings data to present information that meets science-driven or stakeholder needs. These examples are provided to motivate regional programs looking to hone or develop new products.
Most common are products that display real-time information of weather and ocean conditions, which is particularly critical to port and harbor authorities for safe marine navigation. For example, Figure 9A shows real-time data from coastal moorings that are used by port operators to assess current conditions experienced at the harbor mouth. In this case, the mooring instruments captured extreme winds during a storm, and Figure 9B shows the program leveraging social media to report those extreme measurements. Real-time data in ports and harbors are also used as boundary conditions for operational models. Together, the information from the models and the real-time data improve port and harbor authorities and commercial and recreational mariners’ ability to navigate safely.
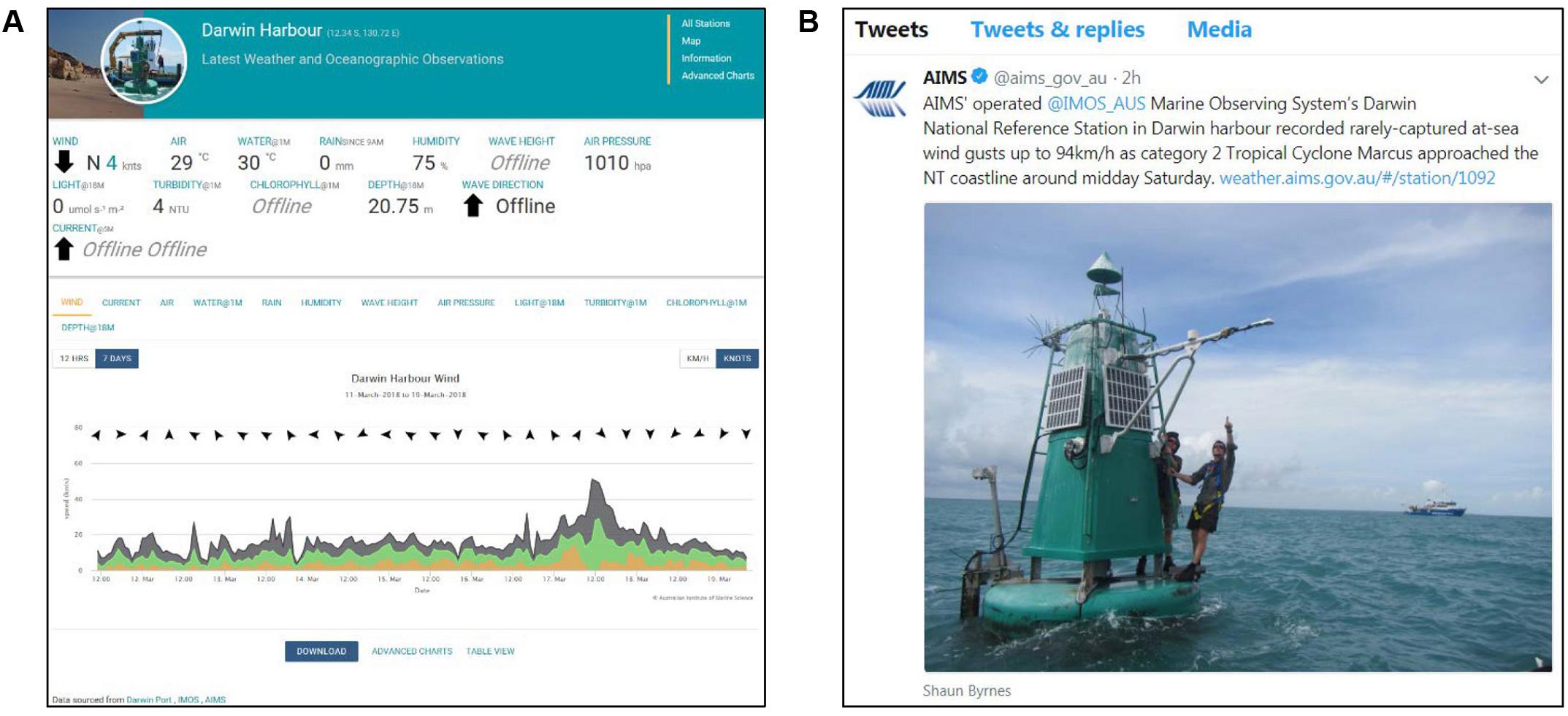
Figure 9. Screenshot of the winds observed at the IMOS Darwin National Reference Station in Darwin Harbor, Northern Australia, during the passage of Tropical Cyclone Marcus in March 2018 (A) and a tweet generated to publicize the recorded event (B). These data have improved the utility of a new East Arm wharf development where safe shipping movements have increased from 15 to 60% of the time. Source: IMOS.
Other products integrate model output and observations in a map-based visualization to allow users to make decisions using complementary information. For example, the US Northwest Association of Networked Ocean Observing Systems (NANOOS) Visualization System (Figure 10) allows users to examine coastal mooring data alongside Regional Ocean Modeling System (ROMS) model output for sea surface temperature and currents. In Figure 10, the ROMS output is color-coded to temperatures where tuna are most likely to be found (red) versus not (blue), to help commercial and recreational fishers plan efficient outings.
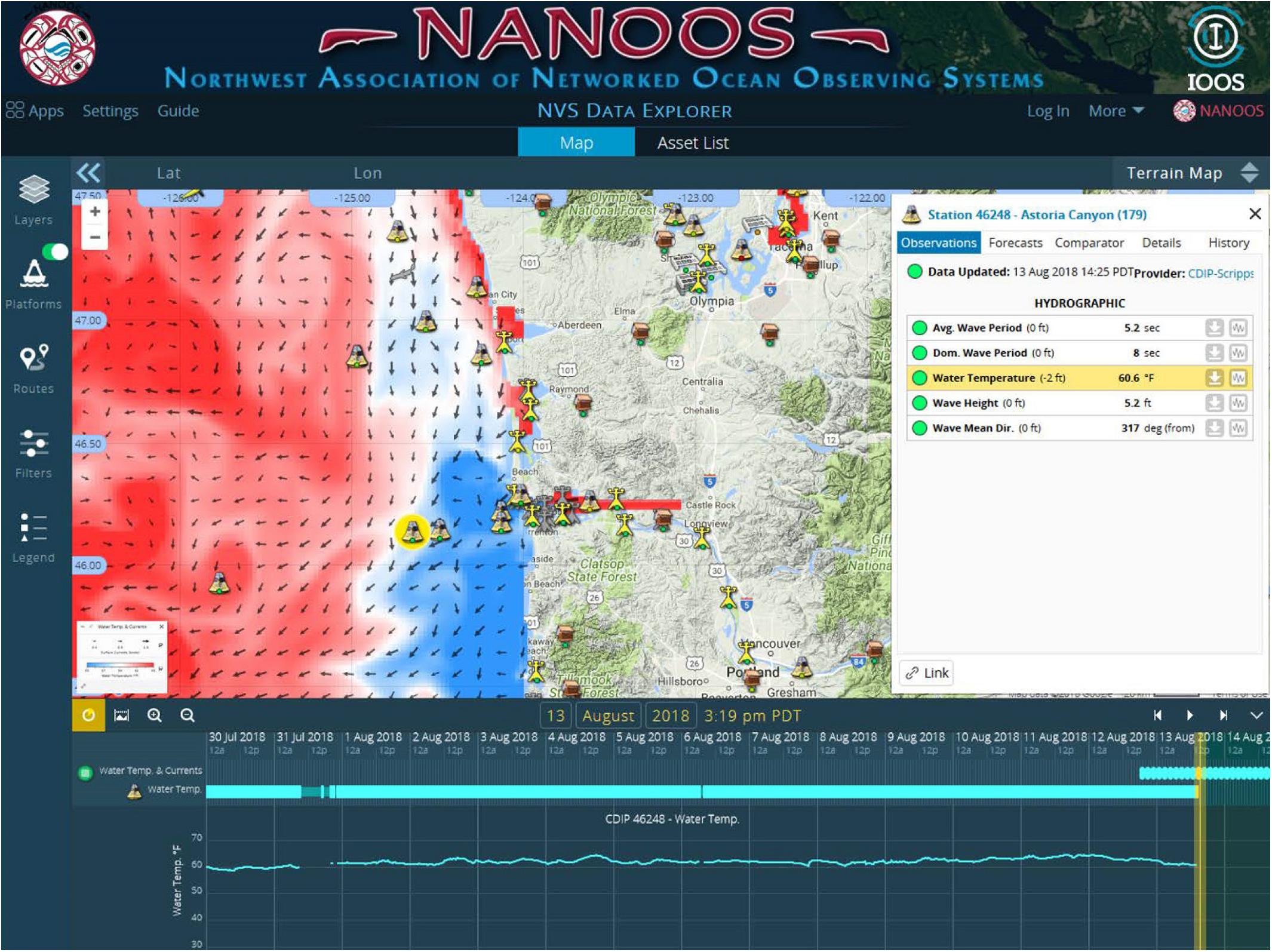
Figure 10. The (NANOOS) Visualization System data portal for the US Pacific Northwest. Shown is Oregon State University Regional Ocean Modeling System (ROMS) model output for surface water temperature and currents, along with near real-time buoy data readings for several variables, including surface water temperature. Source: NANOOS (http://nvs.nanoos.org/Explorer).
The US Mid-Atlantic Regional Association Coastal Ocean Observing System (MARACOOS) OceansMap portal (Figure 11) provides another type of map-based visualization that also enables interactive time-series comparisons between observations and model output, at the mooring location for a user-specified timeframe. This feature allows research scientists to assess model accuracy and perform model validation. Furthermore, in this region the product has been valuable to coastal engineers who require all available observations (gridded surface currents, in situ observations, remote sensing data) in a particular area to inform design criteria for offshore wind farms.
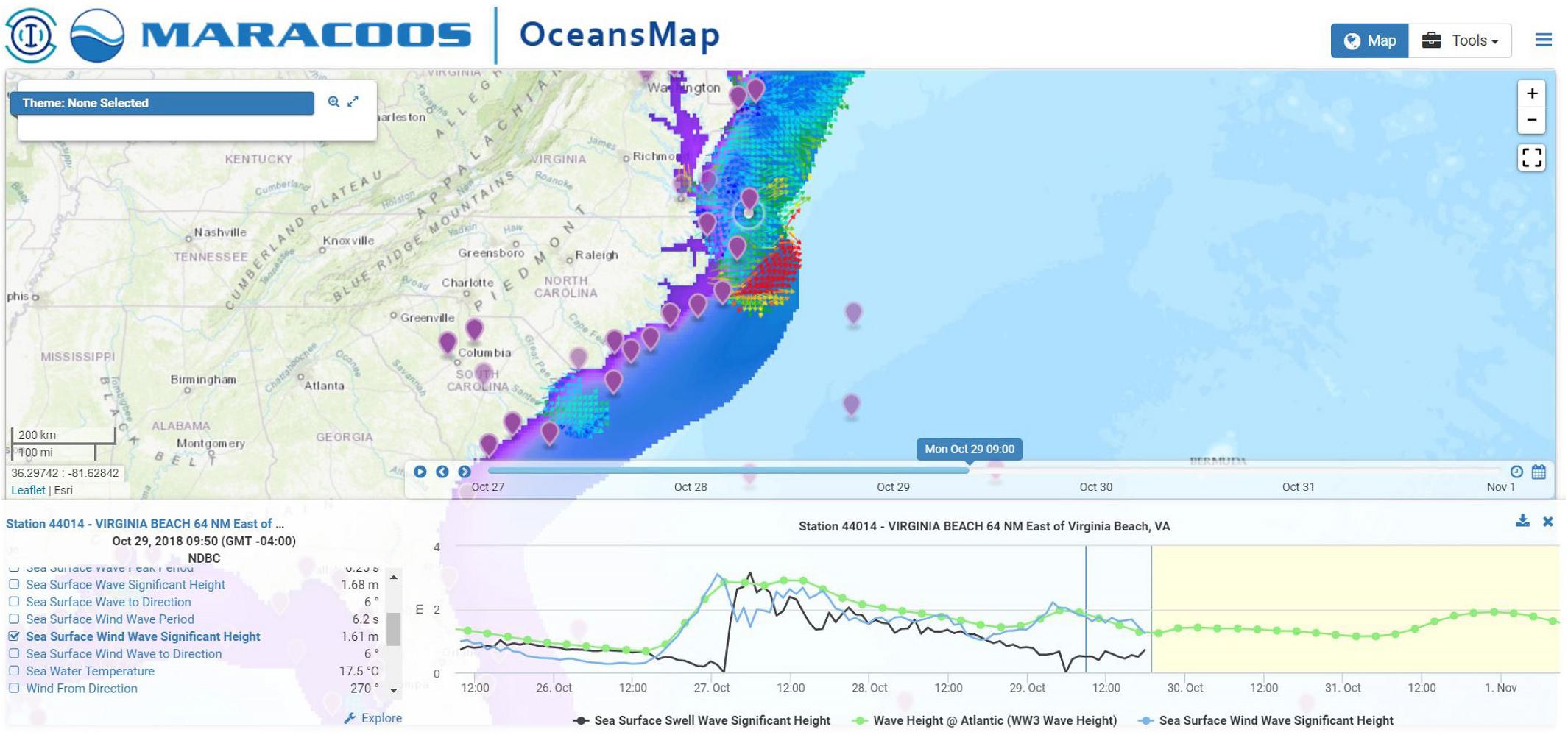
Figure 11. NOAA Wave Watch 3 wave height (modeled), and High Frequency Radar surface currents data (observed) produced by MARACOOS, alongside real-time NDBC data (observed) from coastal moorings (purple markers). This product enables time-series comparisons at the coastal mooring location between modeled and observed variables. Source: MARACOOS (http://oceansmap.maracoos.org).
The IMOS OceanCurrent product (Figure 12) focuses on observations, and combines all available sources in a map-based visualization (satellite sea surface temperature, altimeter-derived currents, Argo profilers, Surface Velocity Program drifters, ocean surface radar, and mooring currents through the water column). This provides a variety of users (emergency managers, public health officials, forecast offices, oil spill responders, mariners, and educators) with an understanding of current oceanographic conditions, and an archive also allows users to go back and look at an event to help understand what oceanographic conditions were at the time.
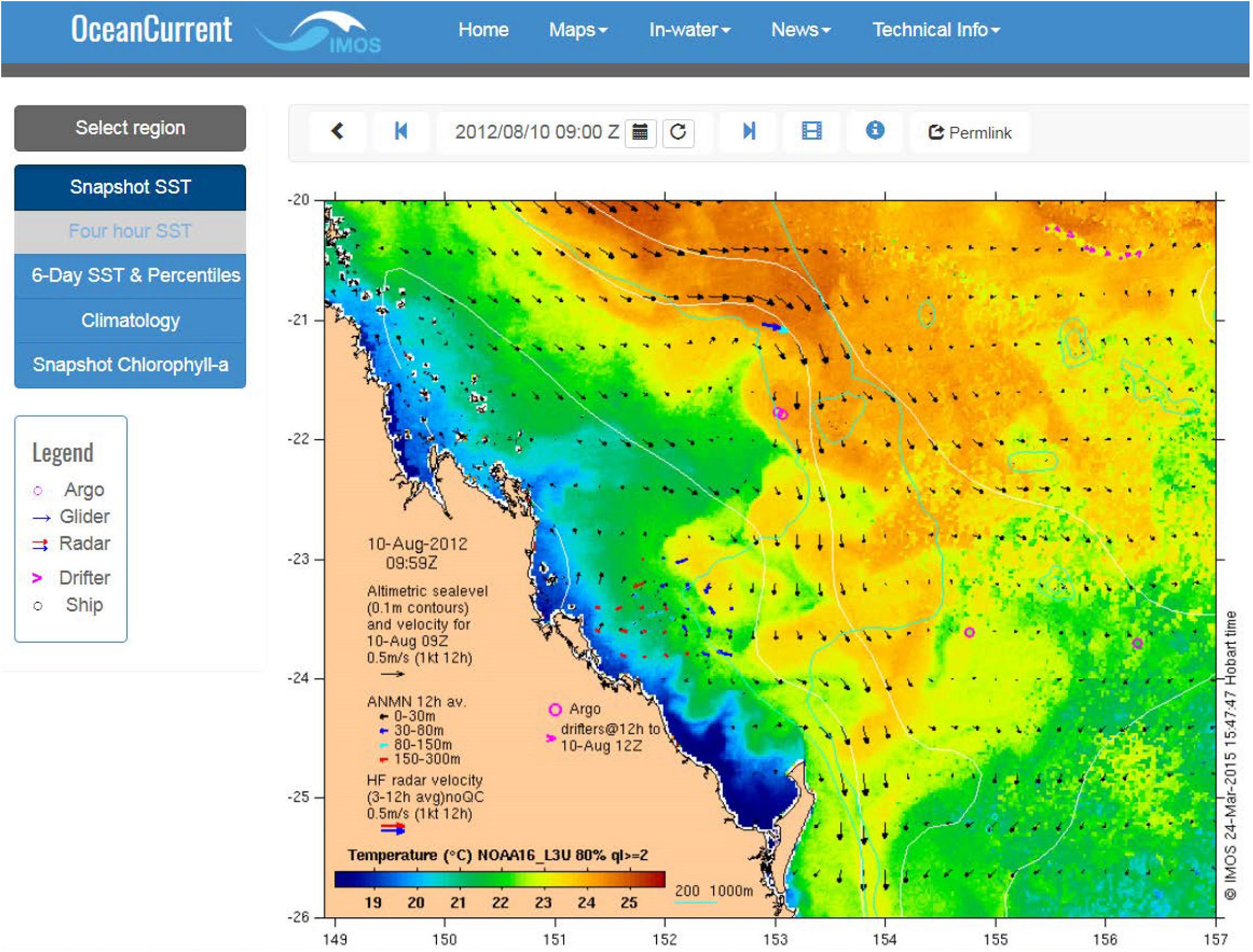
Figure 12. Screen capture of the IMOS OceanCurrent map of the Southern Great Barrier Reef August 10, 2012. Source: IMOS (http://oceancurrent.imos.org.au/).
Other products target environmental events, such as HABs or hypoxia events. A NANOOS “Real-time HABs” app (Figure 13) allows health officials, coastal and environmental managers, water treatment facility operators and the marine industries (among others) to track the movement of toxic algae and monitor conditions that may influence toxic blooms. The Real-Time HABs webpage where the app is hosted was created with manager input. In this product, the observations are measured by a new Environmental Sample Processor (ESP) attached to a mooring, which identifies the presence of organisms and/or biological toxins. These data inform a NOAA HAB Bulletin that is delivered to state and tribal resource managers. Resource managers rely on the ESP “…as a tool [that] should really help us understand what is going on in the off-shore and how it relates to what we are seeing near-shore…” (Dan Ayres, Washington Department of Fish and Wildlife).
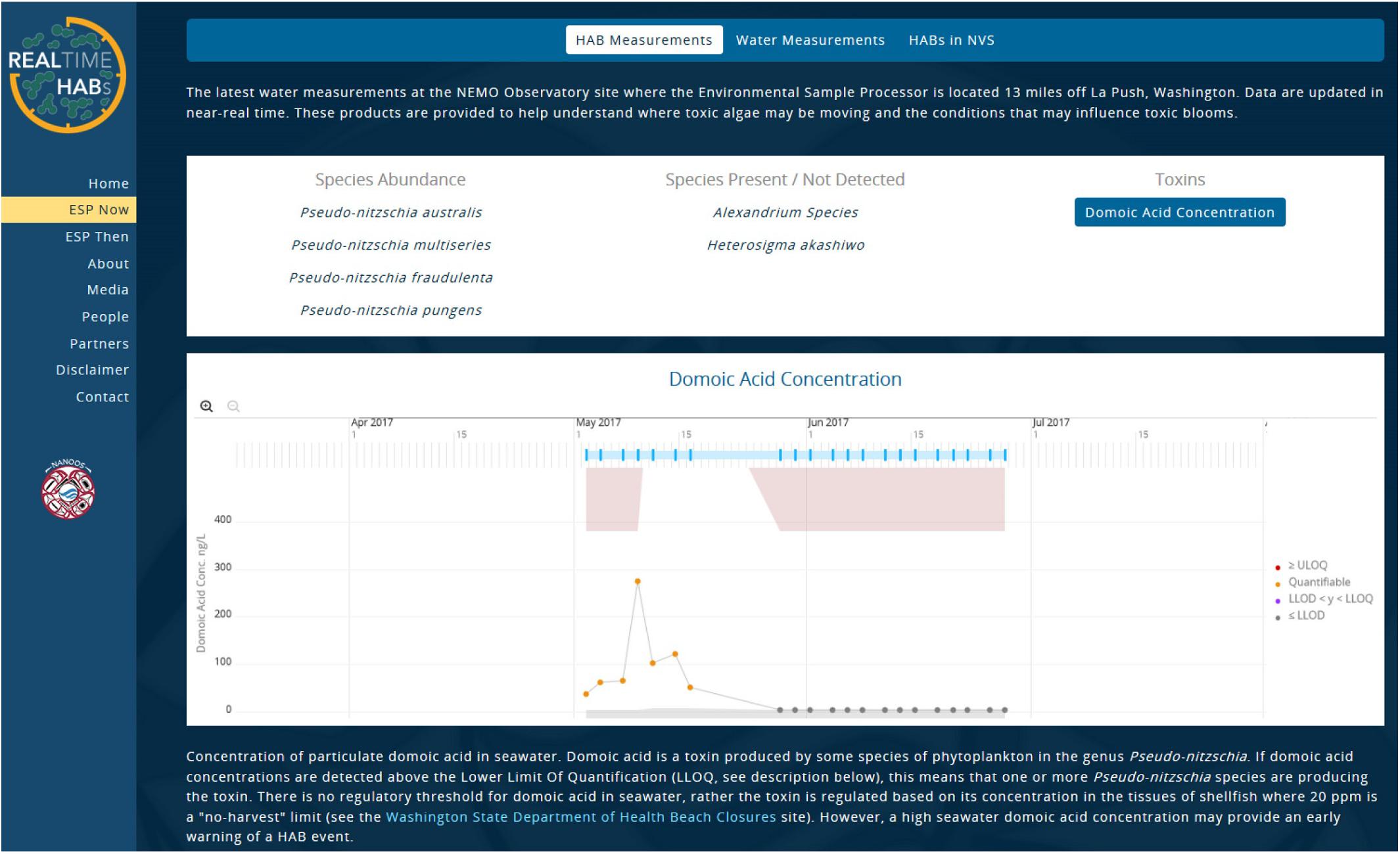
Figure 13. Domoic Acid concentration from an ESP, deployed on a mooring near La Push, Washington. The ESP was deployed by University of Washington and NOAA Northwest Fisheries Science Center through US IOOS funding to NANOOS. Source: NANOOS (http://www.nanoos.org/products/habs/real-time/esp_now/hab_measurements.php).
Another example of a targeted product is the PacIOOS turbidity plume forecast (Figure 14), which is helps recreational users and water quality managers assess the presence of pollutants and contaminants in coastal waters. The turbidity data collected by coastal moorings in the vicinity feed a ROMS numerical model that calculates the advection and dispersion of the turbidity plume over time. A near real-time map of freshwater movements during large storms identifies the location and movement of plumes of “brown-water.”
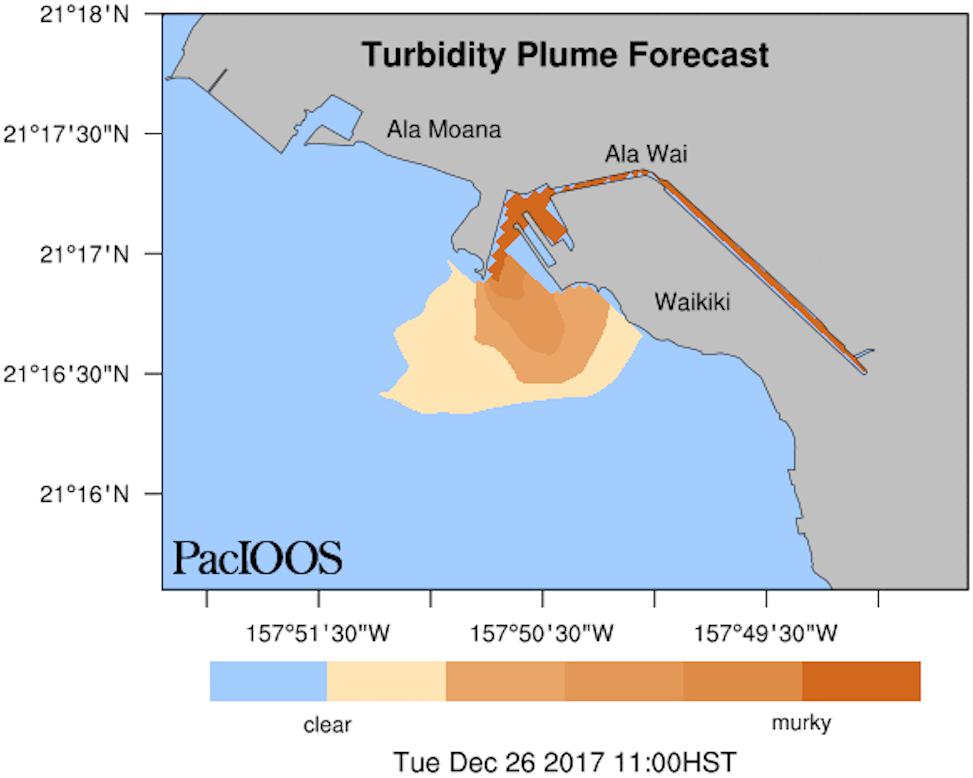
Figure 14. An extreme high rainfall event in December 2017 caused a large turbidity plume off the coast of Waikîkî, Oahu, Hawai‘i. This is one image from a longer animation of the time period produced by PacIOOS. The movement and lifespan of the turbidity plume through time depends on oceanic and atmospheric conditions. Source: PacIOOS (https://www.pacioos.hawaii.edu/water/model-plume-alawai).
Models are a vital tool for assessing and understanding coastal ecosystem changes, and are (as demonstrated) central to many data products. Modern ocean models require as much high-resolution data driven by long time-series measurements as possible to improve their accuracy. The fine scale (2 km) sea surface temperature atlas of the Australian regional seas (SSTARS) is a product that relied on moored sea surface temperature observations for validating the remote-sensing SST data. The atlas covers the 25 years satellite record and is of significant duration to identify warming trends and spatial anomalies. This product is an excellent resource to regional modelers and will also inform research on ecosystem processes (Wijffels et al., 2018).
Many of the above examples are map-based visualizations; however, other value-added products derived from moorings observations are easy to construct and should be made available to facilitate real-time data uptake and to provide operational guidance in a decision-making timescale. For example, mean climatologies at different timescales (daily, monthly, seasonally, and yearly) can uncover anomalous ocean conditions in ocean properties. And historic data can be useful for extracting statistics for a range of purposes including design criteria for structures (waves and currents), workability reports (wind, waves, currents) etc. thermal thresholds, for installation of new aquaculture facilities, etc. Percentiles of anomalies (Figure 15) can be used to identify extreme events such as marine heatwaves easily and routinely (Schaeffer and Roughan, 2017). Historical information is essential to construct the climatology, but real-time data is necessary if operational needs are to be met. For example, marine heatwaves (temperature extremes) are getting more frequent and intense (Oliver et al., 2018), but to date the majority of studies have focused on satellite data which only reveals the surface signature. However subsurface information is also important for marine industries such as the seafood sector (both wild caught and aquaculture) where animals live beneath the surface. Presently real-time monitoring of marine heatwaves is generally only possible at the surface due to a lack of long term in situ observations and climatologies of extremes, and real-time data streams.
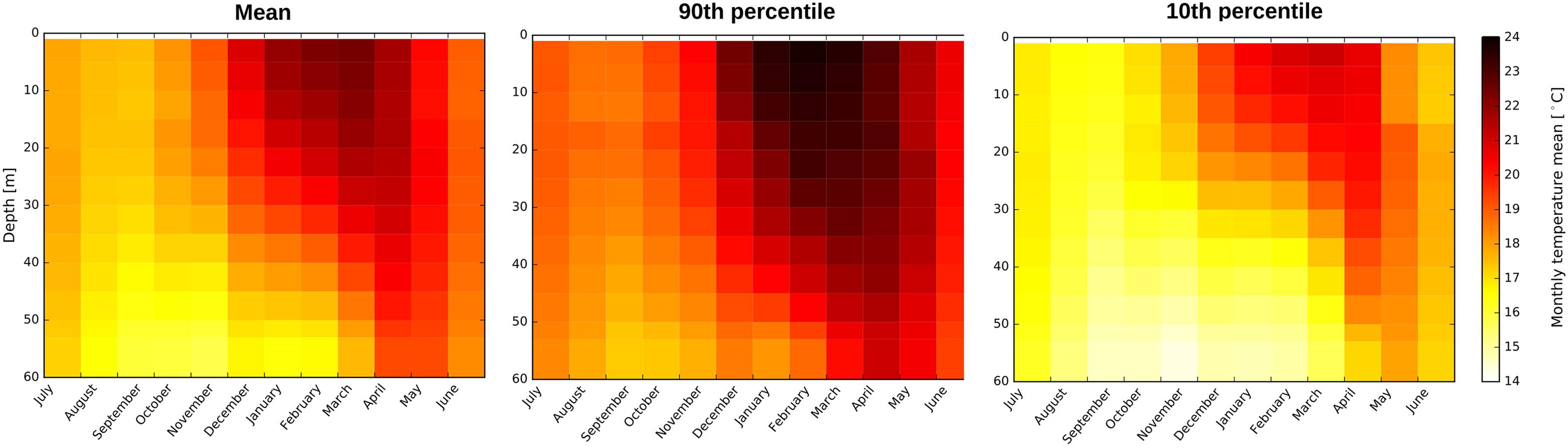
Figure 15. Gridded monthly climatology for the mean, 90th and 10th percentiles of water temperature. Data are from a coastal mooring off Sydney Australia (ORS065) based on hourly observations from 1991 to 2016.
Through the water column, gridded products for moored lines with different instruments should be provided to expand subsurface information, also specifying the criteria for vertical interpolation. Extra subsurface diagnostics could also be included in mooring products, such as the mixed layer depth, thermocline depth and Brunt–Väisälä frequency. Time-series of low-pass filtered and tidal-filtered dataset are also useful.
Operational Challenges
Coastal moorings operators may be distributed across the world, but experience many of the same challenges. The biggest shared challenge is sustaining the networks, particularly in the face of increasingly harsher climates/environments, rising operations and maintenance costs, vandalism, and/or reduced budgets. The National Coastal Ecosystem Moorings Workshop also identified some additional operational challenges that affect data quality and availability:
• Sensor calibration and accuracy estimates, which depend on the local laboratory facilities and the type of ship used for the mooring deployment.
• Biofouling, leading to higher maintenance costs. Sensors require more servicing with extended calibration intervals. For sustained measurements, the community needs field-proven methods to reduce biofouling.
• Ice cover, preventing year-round, real-time observations.
• Lack of new technologies on existing moorings. In some cases, there are no suitable sensors to measure the parameters required or these sensors are too expensive. Although, as the technology continues to evolve it is possible that these sensors may become more accessible due to reduced costs (McManus et al., 2018).
Over the past 20 years, instrumentation to measure biological and chemical properties in the ocean has seen rapid development, but still needs improvement. These sensors remain expensive and often require significant effort to maintain calibration, can be affected by biofouling and are often proxies for the desired observation, such as fluorometry in lieu of chlorophyll. These difficulties mean that such observations remain sparse and are often at risk of discontinuation in lean funding periods. This is a critical time for funders to invest in the development of these sensors in order to bring down the costs and make these sensors more affordable.
Resolving gaps in data products resulting from gaps in coastal measurements should be a focus for the coming decade. This is an issue primarily related to funding and technology limitations. For example, the following needs were identified in the US coastal regions:
• There are insufficient observations for HAB species and the detection of their toxins. Real-time, in situ data on HAB toxins are needed to validate and initialize toxicity forecast models. Seasonal but sustained observations of HAB species and toxins can provide valuable early warnings for potential coastal shellfish toxicity.
• Observations are needed to validate and initialize hypoxia forecast models.
• The local shellfish (e.g., lobster) harvest is impacted by low levels of dissolved oxygen and decreases in the benthic water temperature. More data are required to refine the models for improving commercial and recreational catch limits.
• Fisheries management would be improved by the wider use of acoustics for aggregation, fish spawning, and tracking. Acoustic monitoring baselines are necessary to understand biological impacts for fisheries management.
• An ice breaking buoy system should be developed to enable wider measurements in colder climates (McManus et al., 2018).
Coordinated Groups and Activities to Address Challenges
There are numerous coordinating groups and activities across the world at the regional, national, and international level. Here we provide a few examples of existing international and national coordination efforts underway to address technological challenges.
The DBCP develops best practices and other guidance to help mitigate some of these challenges, and coordinates activities through task teams. The DBCP Task Team on Moored Buoys coordinates across other JCOMM groups and national moorings operators to identify networks and underlying requirements, promote the development of multi-disciplinary mooring systems, leverage advances in operational and technological capabilities, and to monitor best practices documentation. Recently, the DBCP published an outreach strategy to address challenges related to vandalism. It describes a framework to promote public awareness of the value of data and services that instrumented buoys provide, as well as improved collaboration among operators to devise solutions for preventing vandalism (Joint Technical Commission for Oceanography Marine Meteorology [JCOMM] and Data Buoy Cooperation Panel [DBCP], 2017).
The European National Meteorological Services Network’s (EuMetNet) E-SURFMAR program is another international coordination group and integrates surface marine observations and observing activities across Europe for operational weather and climate applications. Actions include coordinating deployments and maintenance activities through voluntary ships, shared equipment procurements, exchange of best practices among members and with other international groups, and data processing and delivery through portals and to the GTS.
At a national level, technological challenges are addressed through engagement and collaboration across societal sectors and across shared borders. For example the US ACT, which is funded through the US IOOS Office, is a partnership of research institutions, resource managers, and private sector companies, and transitions emerging technologies to operational use, engages technology users, developers and providers, identifies technology needs, and documents technology performance and potential. Recently, the ACT coordinated the National Ecosystem Moorings Workshop, which produced a recommendation on ecosystem mooring measurements from experts across public and private sectors. Another US coordination activity is the joint NOAA-Environment and Climate Change Canada (ECCC) pilot project that will focus on solutions for operating moorings in harsh climates. The project covers the development and testing of high-latitude batteries where limited sunlight in the winter months hampers the effectiveness of solar panels. The NDBC will install equipment at ECCC facilities in Ontario and Newfoundland, Canada and will monitor the performance of these two observing systems. Results will be shared at the conclusion of this project in 2020. Additionally, NOAA hosts an annual Emerging Technologies for Observations Workshop (ETW), aimed at identifying observing system technology to either replace existing capabilities at lower cost or fill current geographical gaps. The workshop sessions have focused on technologies the agency could incorporate within 3–5 years, and promoted collaboration between NOAA and private industry, academia, and other federal agencies (Goldstein et al., 2018).
In Australia, IMOS is responding to technological challenges by including assessment of new technology and developments into its decision-making process, following the GOOS Framework for Ocean Observing (Sloyan et al., 2018). When IMOS was established the emphasis was to deploy proven, reliable and robust observing platforms. Ten years later, it is clear that newer technologies need to be considered and integrated into the observing network without diminishing the continuity or quality of data streams. A new advisory mechanism to the IMOS Board is the Science and Technology Advisory Committee (STAC). This broadens the advice from regional science community nodes to ensure uptake of new technology is assessed and introduced in an appropriate manner.
It is clear that technological challenges are addressed at national and even regional levels, but it is unclear if solutions, methodologies, best practices, or lessons learned by those groups are effectively shared and distributed, for the benefit of other network operators. Therefore, we encourage continued involvement in and contribution to international groups like the DBCP, the E-SURFMAR, and the IODE Ocean Best Practices working group to ensure efficient distribution of project results and expertise, through this bottom-up approach.
Vision and Recommendations
In the US, the loss of several critical moorings as a result of funding problems motivated the development of the National Strategy for a Sustained Network of Coastal Moorings. The vision described in the Strategy is applicable to coastal moorings networks around the world: “A sustainable national network of coastal moorings integrated with other environmental observing systems to improve management of resources, safety of life, protection of property, enhancement of the economy, protection of the environment, and science and information about the coastal system” (McArthur et al., 2017). The Strategy concludes with recommendations toward achieving this vision. We use those as a foundation to build upon, and pose the following recommendations for coastal moorings operators and institutions to consider for the following decade:
1. Develop strategies to increase investment in coastal mooring networks. Sustainable funding mechanisms must be identified to ensure and preserve the continuity and accuracy of the coastal environmental record by supporting capital purchases and through-life costs for a network of moorings. To do so, specific gaps must be targeted and presented to potential investors with a clear and compelling tie to stakeholder needs, particularly high-profile user needs, as well as a tie to data products that deteriorate as a result of observing gaps. Data denial exercises like Observing System Experiments could be used as a way to demonstrate the negative impacts of observing gaps on models, and thus products that rely on the observations and predictions.
2. Collect stakeholder priorities through targeted and continuous stakeholder engagements. A stakeholder engagement approach is essential to integrate the collective input of national and regional marine operators, federal and nonfederal moorings operators, and scientists. Stakeholder and scientific needs are the foundation for ocean observing activities, and drive technology and product requirements.
3. 2.1. Establish well-defined use cases to demonstrate and emphasize the value of data products that rely on coastal moorings. Connectivity to coastal communities and stakeholders enforces the value of observing systems by increasing dependency on data products. Improved data products and well-defined use cases will ensure greater understanding and engagement by stakeholders (McManus et al., 2018).
4. Include complementary systems and emerging technologies in coastal moorings implementation planning activities. When observations from the global earth-observing system are fully integrated, they provide a comprehensive picture of the environment, and greatly improve our ability to monitor and predict changes in weather, climate, ecosystems, natural resources and extreme events.
5. 3.1 Use OSSEs to inform optimal siting locations prior to designing a network as well as the initial study recommended in Roughan et al. (2013). Moorings site selection considerations must include proximity to ships, ports, and mooring groups for deployment and servicing, cross-calibration, and for providing complementary measurements (McManus et al., 2018). Complementary platforms (clusters and arrays) are recommended. Where possible, extend the mooring with near surface observations and a meteorological station for satellite validation and to uncover local dynamic drivers.
6. 3.2 Invest in improvements to biological instrumentation to help reduce costs and complexity of operations so that they can be made suitable for deployment on coastal moorings (McManus et al., 2018).
7. Expand and sustain water column ecosystem moorings in coastal locations.
8. 4.1 Expand existing moorings with surface and subsurface measurements of physics, biogeochemistry, and biology and ecosystems variables, following the recommended configuration for coastal ecosystem moorings described in McManus et al. (2018).
9. 4.2 Conduct vessel-based, supplementary biogeochemical sampling and laboratory analysis to add value to these measurements.
10. Coordinate with operators and data managers across geographic scales. Coordinated engagements promote sharing of experiences, best practices, and advances in observations related to platforms, instrumentation, and data management and research applications, and lead to more efficient operations.
11. 5.1 Actively engage in coordination activities across local, regional, national, and international domains (e.g. E-SURFMAR, the Biogeochemical Argo Initiative, the IOOS QARTOD project, the DBCP Task Team on Data Management, and the IODE Ocean Best Practices working group).
12. 5.2 Coordinate maintenance activities with local ship operators (federal, state, university, private).
13. 5.3 Use collaborative platforms like Github to promote open communications among moorings operators, to assist with troubleshooting and other information exchange.
14. Standardize and integrate data management best practices. Compliance with data standards and best practices (e.g., data sharing policies, metadata standards, and QARTOD) promotes interoperability, and common web services and data formats.
6.1 Document and publish data management practices to provide transparency on protocols applied to datasets. The IODE Ocean Best Practices templates are recommended.
6.2 Implement ERDDAP and OGC standard web services (as applicable).
6.3 Produce standardized calibration procedures utilizing national or regional calibration facilities, where possible. Produce and publish uncertainty estimates.
15. Provide open access to data. Publish data through products and services and deliver real-time data to the GTS for applicable variables. Contribute to other coordinated networks and systems that facilitate open data access for advancing studies on global issues (e.g., NOAA’s open ocean moorings32 contribute to the Global Ocean Acidification Observing Network33).
16. 7.1 Develop BUFR templates for biological variables to enable global exchange of real-time biological variables via the GTS.
7.2 Expand the Ocean Data Interoperability Platform (ODIP)34, the international (EU-USA-Australia) project, which contributes to removing barriers hindering effective data sharing across scientific domains and international boundaries.
17. Promote environmental health and operational safety stewardship and regulatory compliance. Check safety rules of the funding agencies, the universities, and any certifications or insurance of the vessels to be used. Written and regularly reviewed standard operating procedures and pre-deployment task risk analyses are also recommended (McManus et al., 2018).
18. Develop coastal mooring network performance metrics. Performance metrics should align with key stakeholder needs and promote key performance indicators (KPI) to be used by all network operators. KPIs should span areas of funding assessment and sustainability, data quality and availability, environmental compliance, operational safety, and adherence to standards and best practices (McArthur et al., 2017).
19. Routinely monitor and assess the design of coastal mooring networks. Routine assessment and coordinated stakeholder planning will promote adaptive management of the network. This review should include a measure of sustainability (continuity of funding) for each coastal mooring in the network (McArthur et al., 2017).
We recommend focused workshops involving coastal moorings operators and institutions to identify and coordinate next steps toward achieving this vision of sustained, robust, integrated networks that meet societal needs. Recommendations for coastal zones from the Implementation of Multi-Disciplinary Sustained Ocean Observations Workshop (Palacz et al., 2017) should also be included as part of these next steps.
Author Contributions
KB and CS led the development of the manuscript. All authors contributed to text, figures, and edits.
Conflict of Interest Statement
The authors declare that the research was conducted in the absence of any commercial or financial relationships that could be construed as a potential conflict of interest.
Acknowledgments
Thanks to all the ship masters and crew, technicians, engineers, and scientists that allow the coastal ocean to be effectively observed around the world. IMOS is a National Collaborative Research Infrastructure Strategy (NCRIS) supported by Australian Government. We also thank the reviewers for their improvements to this paper, especially Shannon McArthur (NOAA NDBC) and Tiffany Vance (US IOOS). Thanks to the Alliance for Coastal Technologies.
Footnotes
- ^www.goosocean.org/eov
- ^https://www.ndbc.noaa.gov/
- ^http://cdip.ucsd.edu/
- ^http://www.ioosassociation.org/
- ^https://ioos.noaa.gov/
- ^https://www.canada.ca/en/environment-climate-change/services/general-marine-weather-information/observations/buoy.html
- ^http://imos.org.au/facilities/nationalmooringnetwork/
- ^https://www.canada.ca/en/environment-climate-change/services/general-marine-weather-information/observations/buoy.html
- ^http://www.marine.ie/Home/site-area/data-services/real-time-observations/real-time-observations
- ^http://www.puertos.es/en-us/oceanografia/Pages/portus.aspx
- ^https://web.kma.go.kr/eng/biz/observation_07.jsp
- ^https://oceanobservatories.org/
- ^http://emso.eu/
- ^http://www.act-us.info
- ^https://github.com/aodn/imos-toolbox
- ^http://cfconventions.org/cf-conventions/v1.6.0/cf-conventions.html
- ^https://s3-ap-southeast-2.amazonaws.com/content.aodn.org.au/Documents/IMOS/Conventions/IMOS_NetCDF_Conventions.pdf
- ^https://github.com/aodn/python-aodncore
- ^http://cfconventions.org/Data/cf-conventions/cf-conventions-1.7/cf-conventions.html#flags
- ^https://github.com/aodn/imos-toolbox/wiki/QCProcedures#moored-time-series
- ^http://www.iquod.org/
- ^https://github.com/IQuOD/machinelearn
- ^https://www.force11.org/group/fairgroup/fairprinciples
- ^http://www.corporateservices.noaa.gov/ames/administrative_orders/chapter_212/212-15.html
- ^https://nosc.noaa.gov/EDMC/documents/EDMC-PD-DMP-2.0.1.pdf
- ^https://www.oceanbestpractices.net/handle/11329/400
- ^https://www.herox.com/bigoceanbutton
- ^https://www.bodc.ac.uk/resources/vocabularies/parameter_codes/
- ^https://github.com/aodn/aodn-portal
- ^https://data.imas.utas.edu.au/static/landing.html
- ^https://coastwatch.pfeg.noaa.gov/erddap/index.html
- ^https://www.pmel.noaa.gov/co2/story/Open+Ocean+Moorings
- ^http://goa-on.org/
- ^http://odip.org/
References
Alvarez, I., Gomez-Gesteira, M., de Castro, M., and Dias, J. M. (2008). Spatio-temporal evolution of upwelling regime along the western coast of the Iberian Peninsula. J. Geophys. Res. 113:C07020. doi: 10.1029/2008JC004744
Archer, M., Roughan, M., Keating, S., and Schaeffer, A. (2017). On the variability of the East Australian current: jet structure, meandering, and influence on shelf circulation. J. Geophys. Res. 122, 8464–8481. doi: 10.1002/2017JC013097
Goldstein, J. C., Kennerley, A. J., and Steckel, A. (2018). “NOAA seeks emerging technologies to further its mission,” in Proceedings of the National Oceanic and Atmospheric Administration Emerging Technologies for Observations Workshop, (College Park, MD).
Greenslade, D. J. M., Zanca, A., Zieger, S., and Hemer, M. A. (2018). “Optimising the Australian wave observation network,” in proceedings of the 1st International Workshop on Waves, Storm Surges and Coastal Hazards, (Liverpool: Liverpool University Press).
Hidas, M., Proctor, R., Mancini, S., Gordon, L., Scheibner, A., Besnard, L., et al. (2018). “Automated data ingestion for the Australia Observing Data Network,” in Poster at EGU. European Geosciences Union, (Vienna).
Hidas, M. G., Proctor, R., Atkins, N., Atkinson, J., Besnard, L., Blain, P., et al. (2016). Information infrastructure for Australia’s integrated marine observing system. Earth Sci. Inform. 9, 525–534. doi: 10.1007/s12145-016-0266-2
Intergovernmental Oceanographic Commission [IOC] (2013). Ocean Data Standards Volume 3. Recommendation for a Quality Flag Scheme for the Exchange of Oceanographic and Marine Meteorological Data.Paris: UNESCO-IOC.
Iwamoto, M. M., Langenberger, F., and Ostrander, C. (2016). Ocean observing: serving stakeholders in the pacific islands. Mar. Technol. Soc. J. 50, 47–54. doi: 10.4031/MTSJ.50.3.2
Joint Technical Commission for Oceanography and Marine Meteorology [JCOMM], and Data Buoy Cooperation Panel [DBCP] (2017). Outreach Strategy to Reduce Damage to Ocean Data Buoys from Vandalism. Available at: http://www.jcommops.org/dbcp/doc/2017-MISC-OBS-Data-Buoys-Vandalism-Strategy-171246_en.pdf (accessed March 26, 2019).
Jones, E., Doblin, M., Matear, R., and King, E. (2015). Assessing and evaluating the ocean-colour footprint of a regional observing system. J. Mar. Syst. 143, 49–61. doi: 10.1016/j.jmarsys.2014.10.012
Lee, K. A., Roughan, M., Malcolm, H. A., and Otway, N. M. (2018). Assessing the use of area- and time-averaging based on known de-correlation scales to provide satellite derived sea surface temperatures in coastal areas. Front. Mar. Sci. 5:261. doi: 10.3389/fmars.2018.00261
Lindstrom, E., Gunn, J., Fischer, A., McCurdy, A., and Glover, L. K. (2012). A Framework for Ocean Observing. By the Task Team for an Integrated Framework for Sustained Ocean Observing. Paris: UNESCO.
Lynch, T. P., Morello, E. B., Evans, K. E., Richardson, A. J., Rochester, W., Steinberg, C. R., et al. (2014). IMOS national reference stations: a continental -wide physical, chemical and biological observing system. PLoS One 9:e113652. doi: 10.1371/journal.pone.0113652
Malone, T. C., DiGiacomo, P. M., Gonçalves, E., Knap, A. H., Talaue–McManus, L., and de Mora, S. (2014). A global ocean observing system framework for sustainable development. Mar. Policy 43, 262–272. doi: 10.1016/j.marpol.2013.06.008
Mantovanelli, A., Keating, S. R., Wyatt, L. R., Roughan, M., and Schaeffer, A. (2017). Eulerian and lagrangian characterization of two counter-rotating sub mesoscale eddies in a western boundary current. J. Geophys. Res. Oceans 122, 4902–4921. doi: 10.1002/2016JC011968
McArthur, S., Bailey, K., Janzen, C., Morrison, R., Murphy, T., Newton, J., et al. (2017). A National Strategy for a Sustained Network of Coastal Moorings. Available at: https://cdn.ioos.noaa.gov/media/2017/12/NationalStrategyforSustainedNetworkofCoastalMoorings_FINAL.pdf.
McManus, M. A., Bailey, K., Send, U., Burt, R., Newton, J. A., Schar, D., et al. (2018). “Alliance for Coastal Technologies,” in Proceedings of the National Coastal Ecosystem Moorings Workshop, (Seattle, WA).
Nowlin, W. D., Briscoe, M., Smith, N., McPhaden, M. J., Roemmich, D., Chapman, P., et al. (2001). Evolution of a sustained ocean observing system. Bull. Am. Meteorol. Soc. 82, 1369–1376. doi: 10.1175/1520-0477(2001)082<1369:EOASOO>2.3.CO;2
Nowlin, W. D., and Malone, T. (2003). Research and GOOS. Mar. Technol. Soc. J. 37, 42–46. doi: 10.4031/002533203787537168
Oke, P. R., Larnicol, G., Jones, E. M., Kourafalou, V., Sperrevik, A. K., Carse, F., et al. (2015). Assessing the impact of observations on ocean forecasts and reanalyses: part 2, regional applications. J. Oper. Oceanogr. 8, s49–s62. doi: 10.1080/1755876X.2015.1022080
Oke, P. R., and Sakov, P. (2012). Assessing the footprint of a regional ocean observing system. J. Mar. Syst. 105, 30–51. doi: 10.1016/j.jmarsys.2012.05.009
Oliver, E. C., Donat, M. G., Burrows, M. T., Moore, P. J., Smale, D. A., Alexander, L. V., et al. (2018). Longer and more frequent marine heatwaves over the past century. Nat. Comm. 9:1324. doi: 10.1038/s41467-018-03732-9
Palacz, A. P., Pearlman, J., Simmons, S., Hill, K., Miloslavich, P., Telszewski, M., et al. (2017). Report of the workshop on the Implementation of Multi-disciplinary Sustained Ocean Observations (IMSOO). Global Ocean Observing System (GOOS) Report No. 223. North Miami, FL.
Puertos del Estado and Ministerio de Fomento. (2015). Conjunto de Datos: REDCOS. Available at: http://calipso.puertos.es//BD/informes/INT_1.pdf (accessed March 26, 2019).
Richardson, A. J., Eriksen, R. S., and Rochester, W. (2015). Plankton 2015: State of Australia’s Oceans. Linking science and policy: an assessment of our oceans using plankton indicators of ecological change. CSIRO report. ISBN 978-1-4863-0565-0 (PRINT) 978-1-4863-0566-7 (EPDF). Canberra.
Roughan, M., Schaeffer, A., and Kioroglou, S. (2013). Assessing the design of the NSW-IMOS moored observation array from 2008–2013: Recommendations for the future. San Diego, CA: IEEE.
Roughan, M., Schaeffer, A., and Suthers, I. M. (2015). “Sustained ocean observing along the coast of Southeastern Australia: NSW-IMOS 2007-2014,” in Coastal Ocean Observing Systems, eds Y. Liu, H. Kerkering, and R. H. Weisberg (Amsterdam: Elsevier). doi: 10.1016/B978-0-12-802022-7.00006-7
Ruhl, H. A., André, M., Beranzoli, L., Çaðatay, M. N., Colaço, A., Cannat, M., et al. (2011). Societal need for improved understanding of climate change, anthropogenic impacts, and geo-hazard warning drive development of ocean observatories in European Seas. Progr. Oceanogr. 91, 1–33. doi: 10.1016/j.pocean.2011.05.001
Schaeffer, A.,Roughan, M., and Mantovanelli, A. (2017). Characterizing frontal eddies along the East Australian current from HF radar observations. J. Geophys. Res. Oceans 122, 3964–3980. doi: 10.1002/2016JC012171
Schaeffer, A., and Roughan, M. (2017). Sub-surface intensification of marine heatwaves off southeastern Australia: the role of stratification and local winds. Geophys. Res. Lett. 44, 5025–5033. doi: 10.1002/2017GL073714
Schaeffer, A., Roughan, M., Jones, E., and White, D. (2016). Physical and biogeochemical spatial scales of variability in the East Australian Current separation zone from shelf glider measurements. Biogeosciences 12, 20101–20121. doi: 10.5194/bg-13-1967-2016
Sloyan, B. M., Roughan, M., and Hill, K. (2018). “The global ocean observing system,” in New Frontiers in Operational Oceanography, eds E. Chassignet, A. Pascual, J. Tintoré, J. Verron, and OceanView (Scotts Valley, CA: CreateSpace). doi: 10.17125/gov2018.ch03
Spinrad, R. W. (2016). The new blue economy: a vast oceanic frontier. Eos 97, 1–6. doi: 10.1029/2016EO053793
Thompson, P., Ingleton, T., Baird, M., and Doblin, M. (2009). Long-term changes in temperate Australian coastal waters: implications for phytoplankton. MEPS 394, 1–19. doi: 10.3354/meps08297l
Todd, R. E., Gawarkiewicz, G. G., and Owens, W. B. (2013). Horizontal scales of variability over the Middle Atlantic Bight shelf break and continental rise from finescale observations. J. Phys. Oceanog. 43, 222–230. doi: 10.1175/JPO-D-12-099.1
US IOOS (2016a). Manual for Real-Time Quality Control of In Situ Temperature and Salinity Data Version 2.0: A Guide to Quality Control and Quality Assurance of In Situ Temperature and Salinity Observations. Silver Spring, MD: U.S. Integrated Ocean Observing System Program.
US IOOS (2016b). The Ocean Enterprise: A Study of US Business Activity in Ocean Measurement, Observation and Forecasting. Silver Spring, MD: U.S. Integrated Ocean Observing System Program.
US IOOS (2017). Manual for Real-Time Oceanographic Data Quality Control Flags Version 1.1. Silver Spring, MA: U.S. Integrated Ocean Observing System Program.
Venkatesan, R., Lix, J. K., Phanindra Reddy, A., Arul Muthiah, M., and Atmanand, M. A. (2016). Two decades of operating the Indian moored buoy network: significance and impact. J. Oper. Oceanogr. 9, 45–54. doi: 10.1080/1755876X.2016.1182792
Wijffels, S. E., Beggs, H., Griffin, C., Middleton, J. F., Cahill, M., King, E., et al. (2018). A fine spatial-scale sea surface temperature atlas of the Australian regional seas (SSTAARS): Seasonal variability and trends around Australasia and New Zealand revisited. J. Mar. Sys. 187, 156–196. doi: 10.1016/j.jmarsys.2018.07.005
WMO. (2015). Manual on the Global Observing System Volume 1 (Global Aspects). Geneva: World Meteorological Organization.
Keywords: coastal, mooring, buoy, ocean, data product, observing systems, user needs
Citation: Bailey K, Steinberg C, Davies C, Galibert G, Hidas M, McManus MA, Murphy T, Newton J, Roughan M and Schaeffer A (2019) Coastal Mooring Observing Networks and Their Data Products: Recommendations for the Next Decade. Front. Mar. Sci. 6:180. doi: 10.3389/fmars.2019.00180
Received: 02 November 2018; Accepted: 21 March 2019;
Published: 05 April 2019.
Edited by:
Maria Snoussi, Mohammed V. University, MoroccoReviewed by:
Naomi Greenwood, Centre for Environment, Fisheries and Aquaculture Science (CEFAS), United KingdomThomas Malone, University of Maryland Center for Environmental Science (UMCES), United States
Jose Henrique Muelbert, Federal University of Rio Grande, Brazil
Copyright © 2019 Bailey, Steinberg, Davies, Galibert, Hidas, McManus, Murphy, Newton, Roughan and Schaeffer. This is an open-access article distributed under the terms of the Creative Commons Attribution License (CC BY). The use, distribution or reproduction in other forums is permitted, provided the original author(s) and the copyright owner(s) are credited and that the original publication in this journal is cited, in accordance with accepted academic practice. No use, distribution or reproduction is permitted which does not comply with these terms.
*Correspondence: Kathleen Bailey, S2F0aGxlZW4uQmFpbGV5QG5vYWEuZ292