- 1Department of Ecology, Evolution and Natural Resources, Rutgers University, New Brunswick, NJ, United States
- 2Australian Institute of Marine Science, Crawley, WA, Australia
- 3Center for Environment and Water, King Fahd University of Petroleum and Minerals, Dhahran, Saudi Arabia
- 4Marine Fisheries Department, Food and Agriculture Organization of the United Nations, Karachi, Pakistan
- 5Life and Environmental Sciences, University of Iceland, Reykjavik, Iceland
Conservation and management strategies for endangered and threatened species require accurate estimates of demographic parameters such as age and growth. The whale shark, Rhincodon typus, is the largest fish in the world and is highly valued in the eco-tourism sector. Despite conservation concerns and advances in our understanding of their life history, basic demographic parameters for growth, longevity and mortality are of questionable accuracy; previous growth studies could not agree whether the vertebral growth bands were formed annually or biannually. Here, we provide the first validation of the annual formation of growth bands within the vertebrae of the whale shark using bomb radiocarbon assays. Ages of up to 50 years were estimated from sectioned vertebrae of sharks collected in Taiwan and Pakistan. There was no cessation of the formation of growth bands in the vertebrae of older sharks and our study provides the oldest observed longevity for this species. Initial estimates of growth (k = 0.01–0.12) and natural mortality rates (M = 0.09–0.14) are consistent with those expected of long-lived sharks, which highlights their sensitivity to fishing pressure and conservation concerns.
Introduction
Accurate and reliable estimates of the age and growth of individuals in a population are central to effective strategies for the management and conservation of any species. For teleost marine fishes, estimates of age are usually obtained from counts of the annual growth bands formed within otoliths, which are calcified structures within the skull case (Campana, 2001). For elasmobranchs such as sharks, skates and rays, which lack otoliths, age estimates have been calculated from growth bands formed in the vertebrae (Cailliet, 1990).
It is critical that age estimates provided by otoliths and vertebrae are accurate, since uncertainty or underestimates surrounding these ages can lead to stock collapses of exploited species (e.g., orange roughy Hoplostethus atlanticus; Smith et al., 1995), or compromise the effectiveness of recovery programs for species that are threatened or endangered. For this reason, many studies have sought to validate the timing of the production of growth bands (Campana, 2001). A common approach is to tag individuals with a chemical marker such as oxytetracycline (OTC) that is laid down within an otolith or vertebrae. Individuals are released and when recaptured at some time in the future, the tag acts as a time stamp that allows the rate of deposition of subsequent growth bands to be determined. For large fishes and sharks that are relatively long-lived and difficult to tag and recapture, validation of annual banding patterns can also be obtained through an analysis of bomb radiocarbon in vertebrae. Above-ground testing of thermonuclear weapons in the 1950s and 60s increased the ratio of carbon 14 isotopes in the atmosphere that were then mixed into the ocean, passed up food webs and incorporated into marine organisms. As a result, the timing of the deposition of bands can be validated by comparing carbon isotope values within vertebrae, with an isotope baseline chronology of known age (Campana, 2001; Campana et al., 2002; Goldman et al., 2012).
The whale shark, Rhincodon typus, is a huge (up to 18 m length; Mcclain et al., 2015), highly migratory, filter-feeding shark found in all tropical and warm temperate seas (Compagno, 2001; Chen et al., 2002; Stevens, 2007). It forms aggregations in productive coastal areas and is a highly valued target for marine eco-tourism (e.g., Huveneers et al., 2017). However, the whale shark has recently been classified as Endangered (IUCN Red List; Pierce and Norman, 2016) and there is now an urgent need for reliable and accurate information on age and growth of the species in order to develop effective conservation and management strategies. At present, there is relatively little demographic data available, especially for large or mature individuals. Using X-radiography, Wintner (2000) analyzed the growth bands in whole vertebrae of juveniles that had stranded on the coast of South Africa to develop an initial growth curve for the species. More recently, Hsu et al. (2014) provided growth and age estimates for individuals collected from a fishery in Taiwan and used marginal increment ratios and centrum edge analysis to conclude that growth bands were deposited biannually in both whole and sectioned vertebrae. The reliability of the age estimates of these studies remains unknown and is of concern, because other studies show that whole vertebrae are known to provide underestimates of age and longevity, and thus overestimates of growth rate in slow-growing sharks (Cailliet and Goldman, 2004; Harry, 2018; Natanson et al., 2018). To our knowledge, only one study has attempted to validate an aging method for whale sharks, which involved a captive immature shark reared in an aquarium after being fed OTC. When the animal died 2 years later, two growth bands were observed following the OTC mark (Wintner, 2000).
Here, we provide the first age validation of whale sharks using bomb radiocarbon assays. We then used sectioned vertebrae from a small sample of sharks to provide initial estimates of growth, longevity and mortality data that can be used in support of current conservation and management efforts.
Methods
Sample Collection
A subset of vertebral samples were taken from 92 vertebral samples that were previously published in Hsu et al. (2014). These were dead individuals that had been landed by the Taiwanese fishery, before the whale shark fishery was closed in November 2007 (Hsu et al., 2012). The vertebral sample from Pakistan was obtained from a dead stranded whale shark.
Sample Preparation and Age Interpretation
The vertebral samples from Hsu et al. (2014) were sectioned with a single cut using paired blades separated by a spacer on an Isomet low-speed diamond-bladed saw. Sections were digitally photographed at 2048 × 1536 resolution using a digital color Leica camera DFC295 mounted on a stereo microscope Leica M205C (Leica Microsystems, Germany), while immersed in ethanol. Age interpretation was based on images enhanced for contrast using Adobe Photoshop CS6, following the interpretation criteria of Natanson et al. (2002). The precision of the age determinations was quantified with both average percent error (APE) and coefficient of variation (CV) (Campana, 2001).
Bomb Radiocarbon Analyses
Vertebrae used for bomb radiocarbon age validation were taken from two specimens that had died after becoming entangled in fishing gear. A 10 m total length (TL) female with an estimated weight of 7000 kg was landed in Karachi, Pakistan in February 2012. One of the cervical vertebrae was cleaned of tissue and then stored frozen. A second individual, a mature male weighing 8500 kg with TL of 9.9 m was landed in Taiwan in April 2005. A cervical vertebra over the gills was extracted and stored in ethanol until assay. Vertebral growth bands from both sharks were isolated from 1 mm thick longitudinal sections of the vertebrae. All sections were prepared using the same procedure outlined above. Sections were digitally photographed at 2048 × 2048 resolution under a binocular microscope at 16–40X magnification using reflected light while immersed in ethanol.
Multiple samples from each of the vertebral sections (N = 11 samples; 5–13 mg each) were extracted from growth bands visible in the corpus calcareum region while working at 16X magnification under a binocular microscope. For the shark landed in Taiwan, the first three growth bands were extracted as a single sample from the vertebral section. For the shark landed in Pakistan, the first-formed growth band (distal to the birth band) was extracted, as were individual growth bands corresponding to later growth. Extracted samples were isolated as solid pieces using a Gesswein high-speed hand tool fitted with steel bits < 1 mm in diameter. The presumed date of sample formation was calculated as the year of shark collection minus the annulus count from the birth band to the mid-point of the sample. After sonification in Super Q water and drying, the sample was weighed to the nearest 0.1 mg in preparation for 14C assay with accelerator mass spectrometry (AMS). AMS assays also provided δ13C (‰) values, which were used to correct for isotopic fractionation effects. Radiocarbon values were subsequently reported as Δ14C, which is the per mil (‰) deviation of the sample from the radiocarbon concentration of 19th-century wood, corrected for sample decay prior to 1950 according to methods outlined by Stuiver and Polach (1977). The mean standard deviation of the individual radiocarbon assays was about 4‰.
To assign dates of formation to an unknown sample, it is necessary that the Δ14C of the unknown sample be compared with a Δ14C chronology based on known-age material (a reference chronology). Since whale sharks are surface planktivores, we assumed that a reference chronology for dissolved inorganic carbon (DIC) in surface waters was most appropriate for our analysis. Therefore, we used a reference chronology developed from young known-age otoliths (calcium carbonate) in the northwest Atlantic (Campana et al., 2008), which has a period of increasing bomb radiocarbon values nearly identical to that of surface waters off of both Pakistan and Taiwan (Andrews et al., 2011a). We also included another reference chronology based on corals from the Mentawai Islands in Sumatra, Indonesia (Grumet et al., 2004).
Growth Models
Preliminary growth estimates were obtained from length-at-age data using two types of growth model. The first was a conventional 3-parameter von Bertanlanffy growth function (Von Bertalanffy, 1938) and the second was a logistic growth function (Smart et al., 2013) with length-at-birth fixed at 60 cm (Chang et al., 1997).
3-parameter von Bertanlanffy growth function:
Logistic growth function with fixed length-at-birth:
where Lt is length-at-age t, L0 is length-at-age 0, L∞ is asymptotic length and k is the growth coefficient.
Longevity estimates generally require either a precisely defined growth model or an estimate of mortality rate, neither of which were available here. Therefore, only the observed maximum age is reported here. Natural mortality was estimated from two equations. The first was based on the linear regression equation of observed maximum age (Hoenig, 1983):
The second natural mortality estimate was based on the non-linear least squares equation of observed maximum age, with a prediction error of 0.32 (Then et al., 2015):
where M is the estimated instantaneous rate of natural mortality and tmax is the observed maximum age.
Results
Counts of Growth Bands in Vertebrae Samples
All vertebrae exhibited distinct growth band (annulus) patterns (Figure 1). The birth mark was identified as the most pronounced first band. Subsequent annuli consisted of a pair of alternating opaque and translucent bands that crossed the entire centrum, except in the oldest sharks. Band width decreased with age, narrowing substantially in the oldest individuals (Figure 1). Counts of growth bands in 20 sharks ranged from 15 to 50 (Table 1). Aging precision was acceptable across both readers, with an APE of 5.5% and CV of 8.2%.
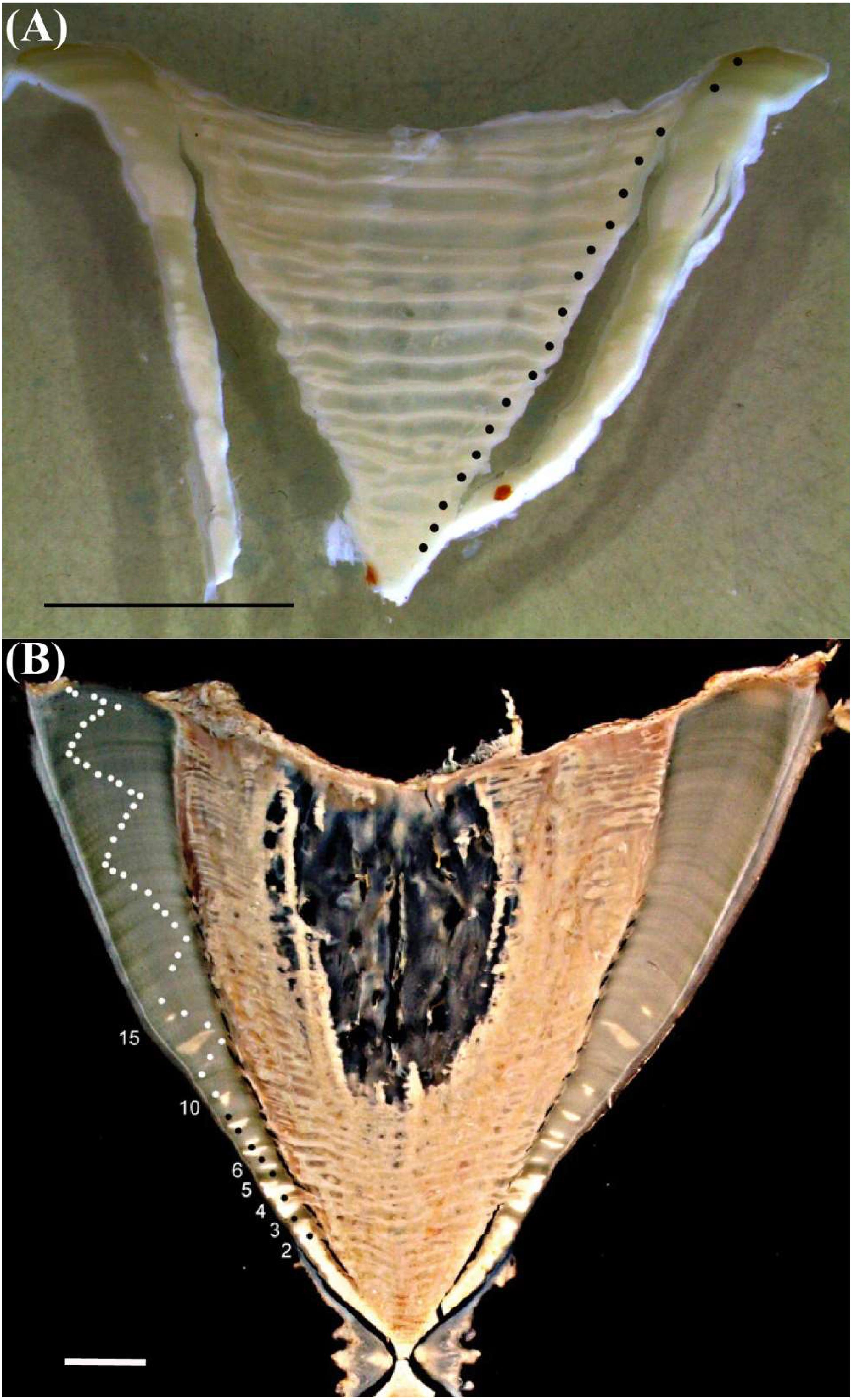
Figure 1. Images of sectioned whale shark vertebrae with annotations of growth bands. (A) Vertebra from Taiwan (RT04) showing 18 growth bands. (B) Vertebra from Pakistan (RT20) used for bomb radiocarbon assay showing 50 growth bands. Scale bars – 1 cm.
Bomb Radiocarbon Assays and Age Validation
The date of formation of the vertebral samples was estimated in two ways: (1) through age determination of the shark based on growth band (annulus) counts; and (2) through comparison of annulus Δ14C values with the values known to be present in surface marine waters at the time (the NWA reference chronology). Agreement between the annulus- and Δ14C -based dates would confirm that the annuli were interpreted correctly for age estimation, at least on average. Under- or over-aging of annuli would be apparent as a left or right phase-shifting of the reference curve relative to the assay values.
Eleven samples from two whale sharks, aged 35 and 50 years based on growth band counts, were analyzed for Δ14C (Table 2). Assay values ranged between 15.1 and 70.0. Two of the samples, including one with the earliest date of formation (1962.5), were too depleted in Δ14C (15.1 and 20.6) to have formed post-bomb (Figure 2), but no pre-bomb samples were identified. The remaining Δ14C values all ranged between 40 and 70, which is consistent with a post-bomb year of formation. All samples were characterized by δ13C values consistent with typical shark vertebrae of metabolic origin (mean = −13.6; SE = 0.4; Table 2).
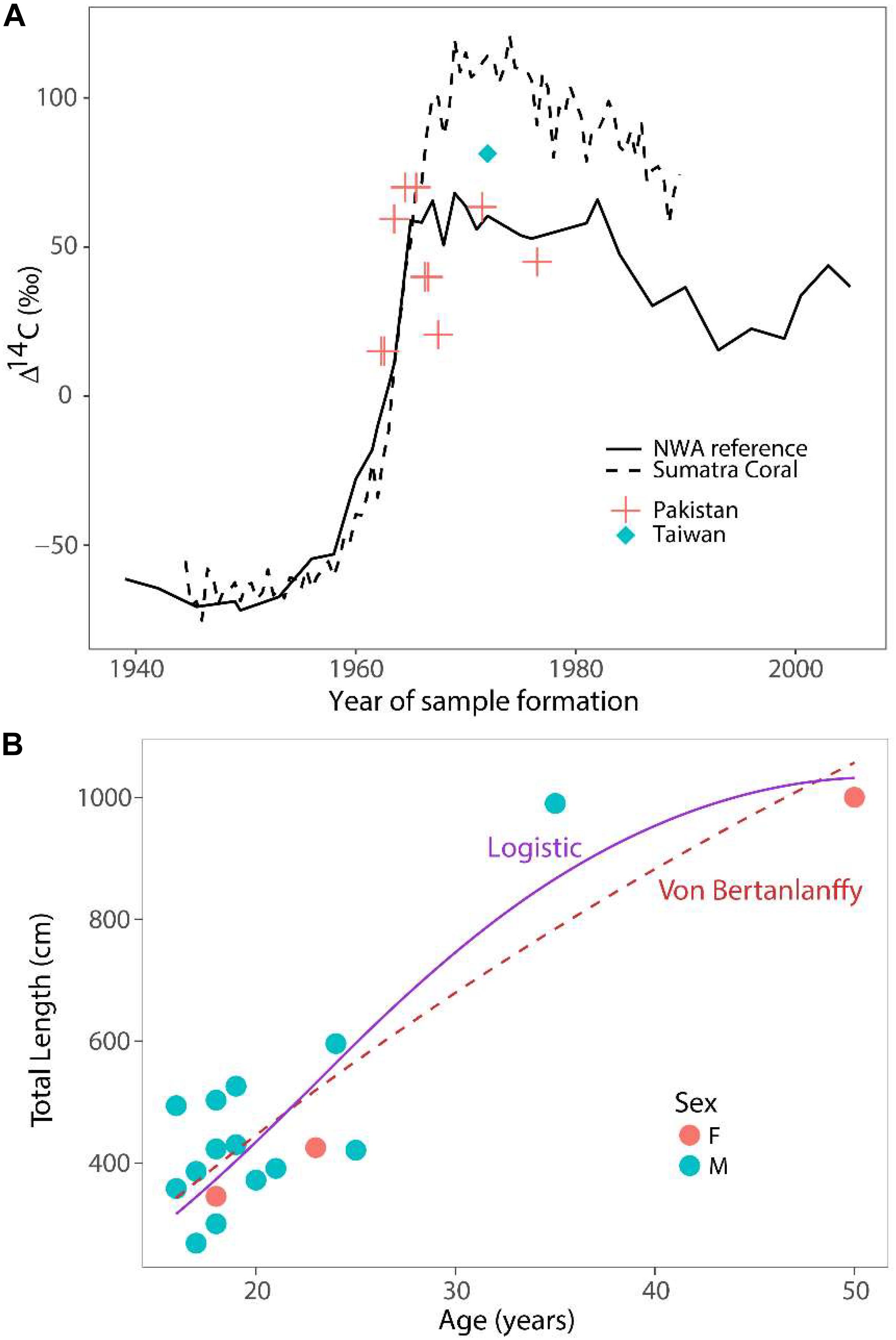
Figure 2. Results of bomb radiocarbon assays and growth models. (A) Bomb radiocarbon (Δ14C) results of whale shark vertebrae from two locations (Pakistan – red crosses; Taiwan – blue diamond) compared to two reference chronologies (carbonate surface water reference from north west Atlantic – solid line; coral reference from Sumatra – dashed line). (B) Length-at-age data fitted with logistic (solid purple) and von Bertanlanffy (dashed red) growth models. Data pooled between sexes for both models.
All of the assay values aligned well with the reference chronologies (Figure 2A), with no obvious bias to one side or the other. Since errors in growth band counts would result in misalignment of the reference and assay values, the assay results indicate that the two sharks must have been aged correctly, at least on average. The 35-year old shark from Taiwan was least informative in this respect, since its post-bomb assay value indicated only that the shark could not have been over-aged by more than 10 years. Conversely, aging error of more than about 5 years would have been apparent as an obvious misalignment in the 50-year old Pakistan shark.
Preliminary Growth, Longevity and Mortality Estimates
Both sexes were combined for estimation of growth (Figure 2B). The von Bertanlanffy growth function produced an asymptotic length L∞ = 2189 cm and a growth coefficient k = 0.014 year–1. The logistic growth function with a fixed length-at-birth L0 = 60 cm produced estimates of L∞ = 1071 cm and k = 0.122 year–1. We caution, however, that the estimates of asymptotic length and growth coefficients are uncertain because of low sample size. The maximum observed age was 50 years based on vertebral aging and bomb radiocarbon assays. The Hoenig (1983) estimated rate of instantaneous natural mortality was 0.09 year–1, while the estimate from Then et al. (2015) was 0.14 year–1.
Discussion
Our study used bomb radiocarbon assays to provide the first validated age estimates for whale sharks. We showed that growth bands in sectioned vertebrae can provide accurate estimates of sharks aged up to 50 years old. These results confirm the use of sectioned vertebrae as age indicators for these sharks, as is also the case for other large species, such as white (Hamady et al., 2014), shortfin mako (Natanson et al., 2006), sandbar (Andrews et al., 2011b), and porbeagle (Natanson et al., 2002) sharks. We found no evidence that vertebral counts underestimated the age of older individuals, as can be the case for porbeagle and white sharks (Francis et al., 2007; Hamady et al., 2014), presumably because the much larger asymptotic body size means that there is no cessation of vertebral growth in the older sharks we sampled in our study.
Although our understanding of the movements, behavior, connectivity and distribution of whale sharks have improved dramatically over the last 10 years (Schmidt et al., 2009; Sequeira et al., 2012, 2013), basic life history traits such as age, longevity and mortality remain unknown and are frequently inferred (e.g., Bradshaw et al., 2007). This lack of basic demographic information has been consistently highlighted in multiple reviews of the biology and ecology of whale sharks (Colman, 1997; Stevens, 2007; Rowat and Brooks, 2012). Few studies have directly estimated age, growth and longevity from vertebral samples of wild populations, due to the lack of samples. To our knowledge, only two studies (Wintner, 2000; Hsu et al., 2014) have analyzed vertebral growth bands to provide age estimates. In the first of these, Wintner (2000) used x-radiography to count bands within the vertebral centra of 15 whale sharks from South Africa and assumed that these were formed annually. The oldest specimen was a male with 31 growth bands (770 cm precaudal length), but a von Bertanlanffy growth model could not be fitted to the data. A second, more recent study by Hsu et al. (2014) analyzed the vertebrae of 92 whale sharks collected by a fishery off the coast of Taiwan. Age validation was based on two forms of marginal increment analysis, which gave inconsistent results. This approach has been criticized as a problematic form of age validation, but is often the only technique available to researchers when mark-recapture studies are not feasible (Campana, 2001; Cailliet and Goldman, 2004). Based on this validation, Hsu et al. (2014) assumed that two growth bands were formed each year. In their study, the oldest specimen (a male, 988 cm total length) had 42 growth bands and was thus assumed to be 21 years old. Perhaps more importantly, counts of growth bands by Hsu et al. (2014) were made in the intermedialia region, which contrasts to most other studies that use the corpus calcareum region of shark vertebrae for age interpretation (e.g., Campana et al., 2002; Christiansen et al., 2016). Our results, based on growth bands visible in the corpus calcareum region of sectioned vertebrae and validated with bomb radiocarbon assays, confirmed that growth bands must have formed annually, suggesting that the study of Hsu et al. (2014) overestimated growth rates of the species.
Our estimate of k = 0.014 year–1 for whale sharks from the von Bertanlanffy growth model was lower than the estimates provided by both Wintner (2000) and Hsu et al. (2014). The study by Wintner (2000) reported linear growth for 15 individuals, all of which were less than 8 m in length and under 30 years old. To constrain the growth curve, Wintner (2000) added two theoretical data points (60 and 100 years with 14 m TL) to obtain k = 0.032 or 0.021 year–1, respectively, with L∞ of 13.7 m TL. The more recent study by Hsu et al. (2014) included the lengths of 3 full-term embryos and used a modified 2-parameter von Bertanlanffy growth model to obtain two growth curves that were based on either biannual or annual deposition of growth bands. For annual growth bands, they reported estimates of k = 0.021 year–1 with L∞ of 15.3 m TL. In our study, the predicted L∞ (21.9 m TL) was close to the largest maximum length ever recorded in the wild, estimated at 20 m from Taiwan in March 1987 (Chen et al., 2002) and close to maximum sizes recorded in other locations (Mcclain et al., 2015). This suggests that the growth models of both Wintner (2000) and Hsu et al., 2014 underestimated maximum sizes of whale sharks. We did, however, find a large difference between the growth coefficients of the von Bertanlanffy and the logistic growth models, with the latter having higher growth coefficients but seeming to underestimate L∞. In this context, it is important to note that our dataset represents a small sample of individuals and only included two mature individuals, hence it is likely that the asymptotic length estimated in the von Bertanlanffy growth model was poorly constrained and thus unrealistic. Actual growth parameters are probably bracketed by the results of the two growth models. Given the closure of the fishery in Taiwan and the protection of whale sharks in the waters of many of the countries where they occur (Rowat and Brooks, 2012), increased sample sizes are likely to rely on unfortunate but opportunistic events such as stranding (Wintner, 2000; Speed et al., 2009) to provide new vertebrae for analysis. Alternatively, photo-identification and imagery techniques may now offer a means to estimate in-situ growth rates for whale sharks, for at least the individuals and size classes participating in nearshore aggregations (e.g., Perry et al., 2018).
Our estimates of natural mortality for whale sharks, ranging from 0.09 to 0.14 year–1 was close to those of other large species of sharks, such as the filter-feeding basking shark (0.07 year–1; Pauly, 2002; Campana et al., 2008), white (0.08 year–1; Mollet and Cailliet, 2002), and scalloped hammerhead (0.10 year–1; Cortés and Brooks, 2018) sharks. These estimates are generally considered low, however, for smaller whale sharks (< 3 m TL), mortality rates may be higher, since the early juvenile stage is likely to be the most vulnerable to predators (Rowat and Brooks, 2012). Information on this life history stage is difficult to gather, because neonatal and very young whale sharks are only rarely encountered and are assumed to reside in the open ocean away from coasts (Rowat et al., 2008).
Our estimates of slower growth and greater observed longevity have important implications for conservation of whale sharks. Underestimation of longevity and overestimation of growth is a serious concern for management strategies for fisheries, because it has led to population crashes due to overharvesting (e.g., orange roughy; Smith et al., 1995). The case for whale sharks is somewhat different from other species that are targeted in fisheries, in part because they are protected across most of their distribution (Bradshaw et al., 2008; Hsu et al., 2012). This status reflects the continuing rise and value of eco-tourism in sites where they aggregate, such as Ningaloo Reef in Western Australia (Meekan et al., 2006). Although the harvesting of whale sharks has been reduced for over a decade, the sizes and abundances of populations have declined in multiple regions (Theberge and Dearden, 2006; Bradshaw et al., 2008), which is reflected in the recent upgrade of the species from Threatened to Endangered by the IUCN Red List (Pierce and Norman, 2016). Given the slow growth rates, extended longevity, late maturity and global connectivity of this species (Bradshaw et al., 2007; Graham and Roberts, 2007; Sequeira et al., 2013), this species is likely to be highly susceptible to sources of anthropogenic mortality such as ship-strike (Bradshaw et al., 2007; Speed et al., 2008). We are hopeful that the demographic data we have provided in this study will help to improve the accuracy of population models (e.g., persistence, survival) and hence, better inform management and conservation efforts for this iconic species.
Data Availability Statement
All datasets generated for this study are included in the article/supplementary material.
Ethics Statement
This study was carried out in accordance with the guidelines of use of animal tissue/cadaver and the protocol was approved by the University of Western Australia Institutional Biosafety Committee.
Author Contributions
MM and SC conceived of the idea, HH and LF provided the vertebral samples. JO did the sample preparation and analyses for vertebrae aging. SC contributed to bomb radiocarbon results and verified aging results. JO wrote the manuscript, with critical feedback and help from SC, MM, LF, and HH.
Funding
This work was supported by the Fisheries and Oceans Canada, US National Science Foundation Grant OCE-9985884, and the University of Iceland. Travel funding was provided by the Australian Institute of Marine Science.
Conflict of Interest
The authors declare that the research was conducted in the absence of any commercial or financial relationships that could be construed as a potential conflict of interest.
Acknowledgments
We are grateful to Warren Joyce for his help in the sectioning of the vertebral samples, and Prof. Shoou Jeng Joung at National Taiwan Ocean University for the use of his laboratory space to section and image the vertebral samples. We thank Brett Taylor for helping to improve the manuscript.
References
Andrews, A. H., Kalish, J. M., Newman, S. J., and Johnston, J. M. (2011a). Bomb radiocarbon dating of three important reef-fish species using Indo-Pacific 14C chronologies. Mar. Freshw. Res. 62, 1259–1269.
Andrews, A. H., Natanson, L. J., Kerr, L. A., Burgess, G. H., and Cailliet, G. M. (2011b). Bomb radiocarbon and tag-recapture dating of sandbar shark (Carcharhinus plumbeus). Fish. Bull. 109, 454–465.
Bradshaw, C. J. A., Fitzpatrick, B. M., Steinberg, C. C., Brook, B. W., and Meekan, M. G. (2008). Decline in whale shark size and abundance at ningaloo reef over the past decade: the world’s largest fish is getting smaller. Biol. Conserv. 141, 1894–1905. doi: 10.1016/j.biocon.2008.05.007
Bradshaw, C. J. A., Mollet, H. F., and Meekan, M. G. (2007). Inferring population trends for the world’s largest fish from mark-recapture estimates of survival. J. Anim. Ecol. 76, 480–489. doi: 10.1111/j.1365-2656.2006.01201.x
Cailliet, G. M. (1990). Elasmobranch Age Determination and Verification: an Updated Review. Silver Spring, MA: NOAA.
Cailliet, G. M., and Goldman, K. J. (2004). “Age determination and validation in chondrichthyan fishes,” in Biology of Sharks and Their Relatives, eds J. C. Carrier, J. A. Musick, and M. R. Heithaus, (Boca Raton, FL: CRC Press), 404–453.
Campana, S. E. (2001). Accuracy, precision and quality control in age determination, including a review of the use and abuse of age validation methods. J. Fish Biol. 59, 197–242. doi: 10.1111/j.1095-8649.2001.tb00127.x
Campana, S. E., Natanson, L. J., and Myklevoll, S. (2002). Bomb dating and age determination of large pelagic sharks. Can. J. Fish. Aquat. Sci. 59, 450–455. doi: 10.1139/f02-027
Campana, S. E., Shelton, P., Simpson, M., and Lawson, J. (2008). “Status of basking sharks in Atlantic Canada,” in Canadian Science Advisory Secretariat, (Ottawa ON: Fisheries and Oceans Canada), 2008/004.
Chang, W.-B., Leu, M.-Y., and Fang, L.-S. (1997). Embryos of the Whale Shark, Rhincodon typus: early growth and size distribution. Copeia 1997, 444–446.
Chen, C. T., Liu, K. M., and Joung, S. J. (2002). “Preliminary report on Taiwan’s whale shark fishery,” in Elasmobranch biodiversity, Conservation and Management: Proceedings of the International Seminar and Workshop, Sabah, Malaysia, July 1997, eds S. L. Fowler, T. M. Reed, and F. A. Dipper, (Gland: IUCN SSC Shark Specialist Group), 162–167.
Christiansen, H. M., Campana, S. E., Fisk, A. T., Cliff, G., Wintner, S. P., Dudley, S. F. J., et al. (2016). Using bomb radiocarbon to estimate age and growth of the white shark, Carcharodon carcharias, from the southwestern Indian Ocean. Mar. Biol. 163:144.
Colman, J. G. (1997). A review of the biology and ecology of the whale shark. J. Fish Biol. 51, 1219–1234. doi: 10.1111/j.1095-8649.1997.tb01138.x
Compagno, L. J. V. (2001). “Sharks of the world: an annotated and illustrated catalogue of shark species known to date, vol2: Bullhead, mackerel and carpet sharks (Heterodontiformes, Lamniformes and Orectolobiformes),” in FAO Species Catalogue for Fishery Purposes, (Rome: FAO), 269.
Cortés, E., and Brooks, E. N. (2018). Stock status and reference points for sharks using data-limited methods and life history. Fish Fish. 19, 1110–1129. doi: 10.1111/faf.12315
Francis, M. P., Campana, S. E., and Jones, C. M. (2007). Age under-estimation in New Zealand porbeagle sharks (Lamna nasus): is there an upper limit to ages that can be determined from shark vertebrae? Mar. Freshw. Res. 58, 10–23.
Goldman, K. J., Cailliet, G. M., Andrews, A. H., and Natanson, L. J. (2012). “Assessing the age and growth of chondrichthyan fishes,” in Biology of Sharks and their Relatives, eds J. C. Carrier, J. A. Musick, and M. R. Heithaus, (Boca Raton, FL: CRC Press), 423–452.
Graham, R. T., and Roberts, C. M. (2007). Assessing the size, growth rate and structure of a seasonal population of whale sharks (Rhincodon typus Smith 1828) using conventional tagging and photo identification. Fish. Rese.84, 71–80. doi: 10.1016/j.fishres.2006.11.026
Grumet, N. S., Abram, N. J., Beck, J. W., Dunbar, R. B., Gagan, M. K., Guilderson, T. P., et al. (2004). Coral radiocarbon records of Indian Ocean water mass mixing and wind-induced upwelling along the coast of Sumatra, Indonesia. J. Geophys. Res. 109:C05003.
Hamady, L. L., Natanson, L. J., Skomal, G. B., and Thorrold, S. R. (2014). Vertebral bomb radiocarbon suggests extreme longevity in white sharks. PLoS One 9:e84006. doi: 10.1371/journal.pone.0084006
Harry, A. V. (2018). Evidence for systemic age underestimation in shark and ray ageing studies. Fish Fish. 19, 185–200. doi: 10.1111/faf.12243
Hoenig, J. M. (1983). Empirical use of longevity data to estimate mortality rates. Fish. Bull. 82, 898–903.
Hsu, H. H., Joung, S. J., Hueter, R. E., and Liu, K. M. (2014). Age and growth of the whale shark (Rhincodon typus) in the north-western Pacific. Mar. Freshw. Res. 65, 1145–1154.
Hsu, H. H., Joung, S. J., and Liu, K. M. (2012). Fisheries, management and conservation of the whale shark Rhincodon typus in Taiwan. J. Fish Biol. 80, 1595–1607. doi: 10.1111/j.1095-8649.2012.03234.x
Huveneers, C., Meekan, M. G., Apps, K., Ferreira, L. C., Pannell, D., and Vianna, G. M. S. (2017). The economic value of shark-diving tourism in Australia. Rev. Fish Biol. Fish. 27, 665–680. doi: 10.1007/s11160-017-9486-x
Mcclain, C. R., Balk, M. A., Benfield, M. C., Branch, T. A., Chen, C., Cosgrove, J., et al. (2015). Sizing ocean giants: patterns of intraspecific size variation in marine megafauna. PeerJ 3, e715. doi: 10.7717/peerj.715
Meekan, M. G., Bradshaw, C. J. A., Press, M., Mclean, C., Richards, A., Quasnichka, S., et al. (2006). Population size and structure of whale sharks Rhincodon typus at Ningaloo Reef. Western Australia. Mar. Ecol. Prog. Ser. 319, 275–285. doi: 10.3354/meps319275
Mollet, H. F., and Cailliet, G. M. (2002). Comparative population demography of elasmobranchs using life history tables, Leslie matrices and stage-based matrix models. Mar. Freshw. Res. 53, 503–515.
Natanson, L. J., Kohler, N. E., Ardizzone, D., Cailliet, G. M., Wintner, S. P., and Mollet, H. F. (2006). ““Validated age and growth estimates for the shortfin mako, Isurus oxyrinchus,” in the North Atlantic Ocean,” in Special Issue: Age and Growth of Chondrichthyan Fishes: New Methods, Techniques and Analysis, eds J. K. Carlson, and K. J. Goldman, (Dordrecht: Springer Netherlands), 367–383. doi: 10.1007/978-1-4020-5570-6_16
Natanson, L. J., Mello, J. J., and Campana, S. E. (2002). Validated age and growth of the porbeagle shark (Lamna nasus) in the western North Atlantic Ocean. Fish. Bull. 100, 266–278.
Natanson, L. J., Skomal, G. B., Hoffmann, S. L., Porter, M. E., Goldman, K. J., and Serra, D. (2018). Age and growth of sharks: do vertebral band pairs record age? Mar. Freshw. Res. 69, 1440–1452.
Pauly, D. (2002). “Growth and mortality of the Basking Shark Cetorhinus maximus and their implications for management of Whale Sharks Rhincodon typus,” in Elasmobranch Biodiversity, Conservation and Management: Proceedings of the International Seminar and Workshop, eds S. L. Fowler, T. M. Reed, and F. A. Dipper, (Gland: IUCN SSC Shark Specialist Group), 199–206.
Perry, C. T., Figueiredo, J., Vaudo, J. J., Hancock, J., Rees, R., and Shivji, M. (2018). Comparing length-measurement methods and estimating growth parameters of free-swimming whale sharks (Rhincodon typus) near the South Ari Atoll, Maldives. Mar. Freshw. Res. 69, 1487–1495.
Pierce, S. J., and Norman, B. (2016). “Rhincodon typus,” in The IUCN Red List of Threatened Species 2016, e.T19488A2365291 (Gland: International Union for Conservation of Nature and Natural Resources).
Rowat, D., and Brooks, K. S. (2012). A review of the biology, fisheries and conservation of the whale shark Rhincodon typus. J. Fish Biol. 80, 1019–1056. doi: 10.1111/j.1095-8649.2012.03252.x
Rowat, D., Gore, M. A., Baloch, B. B., Islam, Z., Ahmad, E., Ali, Q. M., et al. (2008). New records of neonatal and juvenile whale sharks (Rhincodon typus) from the Indian Ocean. Environ. Biol. Fishes 82, 215–219. doi: 10.1007/s10641-007-9280-z
Schmidt, J. V., Schmidt, C. L., Ozer, F., Ernst, R. E., Feldheim, K. A., Ashley, M. V., et al. (2009). Low genetic differentiation across three major ocean populations of the whale shark. Rhincodon typus. PLoS One 4:e4988. doi: 10.1371/journal.pone.0004988
Sequeira, A., Mellin, C., Rowat, D., Meekan, M. G., and Bradshaw, C. J. A. (2012). Ocean-scale prediction of whale shark distribution. Divers. Distrib. 18, 504–518. doi: 10.1111/j.1472-4642.2011.00853.x
Sequeira, A. M. M., Mellin, C., Meekan, M. G., Sims, D. W., and Bradshaw, C. J. A. (2013). Inferred global connectivity of whale shark Rhincodon typus populations. J. Fish Biol. 82, 367–389. doi: 10.1111/jfb.12017
Smart, J. J., Harry, A. V., Tobin, A. J., and Simpfendorfer, C. A. (2013). Overcoming the constraints of low sample sizes to produce age and growth data for rare or threatened sharks. Aquat. Conserv.: Mar. Freshwat. Ecosyst. 23, 124–134. doi: 10.1002/aqc.2274
Smith, D. C., Robertson, S. G., Fenton, G. E., and Short, S. A. (1995). Age determination and growth of orange roughy (Hoplostethus atlanticus): a comparison of annulus counts with radiometric ageing. Can. J. Fish. Aquat. Sci. 52, 391–401. doi: 10.1139/f95-041
Speed, C. W., Meekan, M. G., Rowat, D., Pierce, S. J., Marshall, A. D., and Bradshaw, C. J. A. (2008). Scarring patterns and relative mortality rates of Indian Ocean whale sharks. J. Fish Biol. 72, 1488–1503. doi: 10.1111/j.1095-8649.2008.01810.x
Speed, C. W., Meekan, M. G., Russell, B. C., and Bradshaw, C. J. A. (2009). Recent whale shark (Rhincodon typus) beach strandings in Australia. Mar. Biodiv. Records 2:E15.
Stevens, J. D. (2007). Whale shark (Rhincodon typus) biology and ecology: a review of the primary literature. Fish. Res. 84, 4–9. doi: 10.1016/j.fishres.2006.11.008
Stuiver, M., and Polach, H. A. (1977). Discussion reporting of 14C data. Radiocarbon 19, 355–363. doi: 10.1017/s0033822200003672
Theberge, M. M., and Dearden, P. (2006). Detecting a decline in whale shark Rhincodon typus sightings in the Andaman Sea, Thailand, using ecotourist operator-collected data. Oryx 40, 337–342. doi: 10.1017/s0030605306000998
Then, A. Y., Hoenig, J. M., Hall, N. G., and Hewitt, D. A. (2015). Evaluating the predictive performance of empirical estimators of natural mortality rate using information on over 200 fish species. ICES J. Mar. Sci. 72, 82–92. doi: 10.1093/icesjms/fsu136
Von Bertalanffy, L. (1938). A quantitative theory of organic growth (inquiries on growth laws. II). Hum Biol 10, 181–213.
Keywords: whale shark, vertebrae, age determination, radiocarbon, longevity, growth bands
Citation: Ong JJL, Meekan MG, Hsu HH, Fanning LP and Campana SE (2020) Annual Bands in Vertebrae Validated by Bomb Radiocarbon Assays Provide Estimates of Age and Growth of Whale Sharks. Front. Mar. Sci. 7:188. doi: 10.3389/fmars.2020.00188
Received: 12 December 2019; Accepted: 10 March 2020;
Published: 06 April 2020.
Edited by:
Benjamin D. Walther, Texas A&M University Corpus Christi, United StatesReviewed by:
John R. Wallace, Northwest Fisheries Science Center (NOAA), United StatesJohn Austin Mohan, Texas A&M University, United States
Copyright © 2020 Ong, Meekan, Hsu, Fanning and Campana. This is an open-access article distributed under the terms of the Creative Commons Attribution License (CC BY). The use, distribution or reproduction in other forums is permitted, provided the original author(s) and the copyright owner(s) are credited and that the original publication in this journal is cited, in accordance with accepted academic practice. No use, distribution or reproduction is permitted which does not comply with these terms.
*Correspondence: Joyce J. L. Ong, am95Y2Uub25nLmpsQGdtYWlsLmNvbQ==