- 1Centre for Ecology and Conservation, College of Life and Environmental Sciences, University of Exeter, Penryn Campus, Penryn, Cornwall, United Kingdom
- 2Fishtek Marine, Dartington, Devon, United Kingdom
- 3Whale and Dolphin Conservation, Chippenham, United Kingdom
- 4Independent Statistical Consultant, Forston, Dorchester, United Kingdom
- 5Chelonia Limited, Mousehole, Cornwall, United Kingdom
- 6Cornwall Wildlife Trust, Truro, Cornwall, United Kingdom
Bycatch is a significant cause of population declines of marine megafauna globally. While numerous bycatch mitigation strategies exist, acoustic alarms, or pingers, are the most widely adopted strategy for small cetaceans. Although pingers have been shown to be an effective measure for numerous species, there are some concerns about their long-term use. Bycatch is recognized as a persistent problem in waters around Cornwall, United Kingdom, where several cetacean species are resident, with harbour porpoises (Phocoena phocoena) being the most-commonly sighted. In this study, we assessed the effects of a Banana Pinger (Fishtek Marine Limited) on harbour porpoises in Cornwall between August 2012 and March 2013. Two passive acoustic loggers (C-PODs; Chelonia Limited) were deployed 100 m apart to record cetacean activity during cycles of active and inactive pinger periods. Harbour porpoises were 37% less likely to be detected at the C-POD near the pinger when the pinger was active, while they were only 9% less likely to be detected 100 m further away. The effect of the pinger was constant over the study period at both C-PODs despite the temporal variation in harbour porpoise detections. In addition, we found no evidence of reduced pinger effect with changing environmental conditions. Furthermore, harbour porpoise detections at the C-POD near the pinger did not depend on the time elapsed since the pinger turned off, with harbour porpoises returning to the ensonified area with no delay. Together these results suggest that (1) harbour porpoises did not habituate to the pinger over an 8-month period, (2) the pinger effect is very localized, and (3) pinger use did not lead to harbour porpoise displacement over the study period, suggesting an absence of long-term behavioral effects. We suggest that the deployment of pingers on fishing nets would likely reduce net-porpoise interactions, thereby mitigating bycatch of harbour porpoises and potentially other cetacean species. As the small-scale fishery dominates in United Kingdom waters, there is an acute need for cost-effective mitigation strategies with concurrent monitoring to be implemented rapidly in order to address the problem of harbour porpoise, and more generally, cetacean bycatch.
Introduction
Bycatch is defined as the incidental capture of unwanted, unmanaged or discarded catch (Davies et al., 2009). It is believed to be the greatest threat to marine megafauna globally (Lewison et al., 2014), and one of the primary causes of population declines of seabirds (e.g., Clay et al., 2019b), marine mammals (e.g., Read et al., 2006), sharks (e.g., Molina and Cooke, 2012) and sea turtles (e.g., Lewison et al., 2004). Life-history traits of these marine vertebrates, especially delayed sexual maturity and low reproductive rates, make them particularly vulnerable to bycatch mortality, which can lead to relatively rapid population declines when bycatch rates exceed intrinsic population growth (Baum et al., 2003; Mei et al., 2012; Jaramillo-Legorreta et al., 2017). Several management strategies are available to reduce the frequency of interactions between fisheries and marine megafauna, including fishing gear modifications (e.g., Lewison et al., 2003; Sullivan et al., 2018), time-area closures (e.g., O’Keefe et al., 2014), and acoustic alarms or deterrents (e.g., Amano et al., 2017; Clay et al., 2019a). However, the effectiveness of each management strategy is often both species- and fishery-specific (Dawson et al., 2013; Wakefield et al., 2017), and management is most effective when multiple strategies are used simultaneously (Read, 2013; Van Beest et al., 2017).
Acoustic alarms, also known as pingers, exploit the use of hearing by cetaceans (Kraus et al., 1997). They are the most widely adopted bycatch-mitigation strategy for this taxon, due to relatively low cost compared to alternative strategies, and requiring little to no change in fishing gear or practices (Dawson et al., 2013). Pingers emit low-intensity intermittent sounds, usually ranging between 10 and 140 kHz in frequency. They can be deployed with fishing nets to reduce the likelihood of entanglement (reviewed in Dawson et al., 2013). Pingers have been shown to effectively reduce incidental capture of several species of dolphins (e.g., Carretta and Barlow, 2011; Mangel et al., 2013), whales (e.g., Carretta and Barlow, 2011; Mangel et al., 2013), and porpoises (e.g., Mangel et al., 2013).
While pingers are mandatory in some fisheries around the world (e.g., Barlow and Cameron, 2003; Read, 2013; Sørensen and Kindt-Larsen, 2016), there are concerns that their effectiveness might decline over time. Indeed, some studies have found evidence of pinger habituation after only 4 days (Cox et al., 2001; Carlström et al., 2009; Bowles and Anderson, 2012; Kyhn et al., 2015; Amano et al., 2017), while others suggest no such effect after more than a decade of use in active fisheries (Carretta and Barlow, 2011; Read, 2013; Sørensen and Kindt-Larsen, 2016). In addition, pingers have been suggested to be particularly effective for harbour porpoises as they result in their displacement from the area ensonified (filled with sound) by the pinger (Dawson et al., 2013). The evidence of aversion has led some authors to suggest that the long-term use of pingers could result in habitat exclusion, and thus, long-term population displacement, particularly for in-shore populations (Dawson et al., 2013; Kyhn et al., 2015).
Several cetacean species occupy waters around Cornwall, United Kingdom (UK). Harbour porpoises (Phocoena phocoena) and short-beaked common dolphins (Delphinus delphis) are the most commonly sighted and stranded species in the region, while the South-West population of common bottlenose dolphin (Tursiops truncatus) is very small (Pikesley et al., 2011; Leeney et al., 2012; Clear et al., 2018). Harbour porpoises are classified as a priority species under the United Kingdom Post-2010 Biodiversity Framework and are protected under the European Union (EU) Habitats Directive and Habitats Regulations as transposed into United Kingdom law, under which there are explicit bycatch requirements. To comply with the Habitats Directive, the United Kingdom has recently designated five Special Areas of Conservation for harbour porpoises, however, there is currently no management in place for these.
The seas of the South-West of England are an important porpoise habitat, but also represent an area of concern. This region has the highest level of gillnet and ring net effort in the United Kingdom, which largely overlaps with the distribution of harbour porpoises (Calderan and Leaper, 2019). While cetacean bycatch is recognized as a long-term problem around Cornwall (Tregenza, 1992), the occurrence of stranded cetaceans has increased over the last two decades. In 2018, a third of recorded stranded cetaceans exhibited features consistent with bycatch or entanglement in fishing gear (Clear et al., 2018). Although the use of pingers is mandatory for fishing vessels over 12 m in the EU, such vessels only represented 2% by number of those deploying static nets in 2017 in the United Kingdom (Northridge et al., 2018). This is despite vessels both under and above 12 m fishing where harbour porpoises are known to be present (Leeney et al., 2012).
In this study in Cornwall, we assessed the effects of Banana Pingers (Fishtek Marine Limited) on harbour porpoises between August 2012 and March 2013. We sought to answer four questions: (1) are harbour porpoises showing signs of pinger habituation over the study period; (2) are the pinger effects similar at the two C-PODs deployed 100 m apart; (3) does the pinger result in long-term displacement of harbour porpoises; and (4) are pingers likely to be effective deterrents and bycatch mitigation devices in the region?
Materials and Methods
Study Design and Data Collection
The study was conducted in waters off the coast of Mousehole in the South-West of England, United Kingdom between July 2012 and April 2013 (Figure 1A), as part of the Cornwall Wildlife Trust led pinger trial project 2012–2013. A specialist acoustic deterrent (Banana Pinger; frequency range: 50–120 kHz (randomized), SPL: 145 dB re 1 μPa at 1 m, ping duration: 300 ms with randomized ping intervals between 4 and 12 s) was programmed to cycle on and off underwater. The pinger alternated active periods of 24 h (on) with inactive periods of 21 h (off) to investigate cetacean response while ensuring that the pinger activity pattern did not stay in phase with tide or day. The pinger and one passive acoustic logger (C-POD; Chelonia Limited, chelonia.co.uk) were deployed together on July 29th 2012. This C-POD, hereafter referred to as the “near C-POD,” recorded cetacean activity (clicks) continuously throughout the study to measure the effects of the pinger (Tregenza et al., 2016). The near C-POD ceased recording on March 17th 2013 at 02:00 (229 days of records) and was subsequently retrieved. A second C-POD was deployed on November 8th 2012 at 17:00, 100 m away from the pinger, which represents the mid-distance between two pingers based on EU Regulation No. 812/2004 (200 m between pingers at 145 dB devices). This C-POD, hereafter referred to as the “remote C-POD,” recorded cetacean activity away from the area ensonified by the pinger, to assess harbour porpoise displacement. The remote C-POD ceased recording on April 27th 2013 at 21:00 and was subsequently retrieved (170 days of records; see Figure 1B for study design). All applicable international, national and/or institutional guidelines concerning the care and use of animals were followed. Fieldwork was approved by the Cornwall Wildlife Trust and the University of Exeter Ethics Committee.
Data Processing
C-POD files from both locations were downloaded and processed using the proprietary C-POD software (C-POD.exe). Hours at the end of the logger data with less than 60 min of recording were removed to maintain uniform sampling intervals. To identify porpoise detections, all click trains identified by the software that had porpoise-like features (specifically a modal frequency in the range of 125–145 kHz and a click rate in the range of 15–100 per second) were used to determine the number of porpoise-like clicks per hour. This classification is expected to have a substantive false positive rate which is acceptable because the potential sources of false positives (the pinger itself and sea surface and sediment transport noise) are independent of the pinger state. Consequently, the use of a weak classifier here creates a bias against finding that the pinger reduces detections but does not reduce the validity of any positive effect found. Pinger activity was confirmed based on the number of clicks having a frequency of 62–64 kHz and a duration of over 20 cycles, being over 100 in each hour at the near C-POD. This allowed on and off pinger hours to be defined with no outliers or gaps, with “on” hours at the ends of active periods generally having some “off” time before the pinger starts or stops.
Harbour porpoises were categorized as either (1) absent if no clicks were recorded or (2) present if one or more clicks were recorded in an hour for the analysis. We used presence/absence data to account for within- and between-individual variation in click trains. Data were analysed on a monthly basis and ranged from August 1st, 2012, 00:00 until March 17th, 2013, 02:00. Weather data were included to account for varying sea conditions in detections and propagation of sound. Weather data were obtained from the Culdrose weather station, which is the nearest provider to the study site (Figure 1A). Wind speed and wind bearing were each provided as hourly averages. Wind speed was averaged to the nearest knot and wind bearing to the closest multiple of 10°. Wind bearing was classified into eight categories for the analysis: North-East (22.5–67.5°), East (67.5–112.5°), South-East (112.5–157.5°), South (157.5–202.5°), South-West (202.5–247.5°), West (247.5–292.5°), North-West (292.5–337.5°), and North (337.5–360° and 0–22.5°).
Statistical Analysis
We considered three models: (1) habituation: to determine whether the pinger effect varied over the study period as a result of the repeated stimulus; (2) ensonification: to investigate the pinger effect 100 m away from the pinger; and (3) displacement: to explore any long-term behavioral effects of the pinger. For all models, the response variable was the probability of detecting harbour porpoises. The habituation and ensonification models comprised seven fixed effects: pinger activity (on/off); month and its interaction with pinger activity to investigate any habituation effect; mean hourly wind direction and its interaction with month (Table 1 and Supplementary Figure S1); and mean hourly wind speed and its interaction with month (Table 1 and Supplementary Figure S2). To investigate whether the pinger activity resulted in long-term displacement, we looked at the probability of detecting harbour porpoises at the near C-POD within 10 h of the pinger turning off, i.e., a time period longer than half a tidal cycle which would represent significant long-term displacement. This model comprised the following fixed effects: the number of hours since the pinger turned off; month; whether harbour porpoises were detected as present the hour before the pinger turned on and its interaction with month; mean hourly wind direction and its interaction with month; and mean hourly wind speed and its interaction with month. For all models, wind speed and direction were included to investigate whether the probability of detecting harbour porpoises varied with environmental conditions.
All analyses were tested using binomial generalised linear models (GLMs) in the MASS package (Venables and Ripley, 2002) in R v3.5.1 (R Core Team, 2018). A logit link function was used for the habituation model, whereas a cloglog link function was used for the ensonification and displacement models to allow for more asymmetry in the distribution. All combinations of terms were examined and ranked by Akaike’s Information Criteria (AIC) using subset selection of the maximal model using the MuMIn package v1.42.1 (Barton, 2015). Top ranked models were defined as models ΔAIC ≤ 2 units of the best supported model, after excluding further models where a simpler model attained stronger weighting (“nesting rule”; Richards et al., 2011). Where more than one model fitted these criteria, the best supported model was used (Burnham and Anderson, 2002).
Results
Habituation
A total of 5452 complete hours were recorded at the near C-POD between August 2012 and March 2013. Twenty-two hours of recordings from the near C-POD were removed due to being incomplete (<1% of data). Harbour porpoises were present 54% of the time at the near C-POD (n = 2942 h). The modal prevailing wind directions over the study period were West (n = 1356) and South-West (n = 1002), followed by East (n = 722) and North-West (n = 592; Supplementary Figure S3). Gentle to fresh breezes (Beaufort scale 2–3: 7–21 knots) dominated over the whole period (n = 3154), with extreme hourly averages ranging from 0 (Beaufort scale 1: calm) to 39 knots (Beaufort scale 8: gale/fresh gale; mean ± SD = 13.5 ± 6.5).
Harbour porpoises were 37% less likely to be detected at the near C-POD when the pinger was on (Figure 2A) and this effect was constant over the study period (Figure 3A). The probability of detecting harbour porpoises varied across months, decreasing either side of December 2012 (Figure 3A). It was also influenced by mean hourly wind speed and direction, but both also varied with month (Supplementary Figures S4, S5). Overall, detection probability increased with increasing wind speeds over the study period at the near C-POD (Supplementary Figure S6) in all months except January and March 2013 (Supplementary Figure S4). While the probability of detections varied temporally, a similar pattern was seen across wind directions, decreasing either side of December 2012 (Supplementary Figure S5).
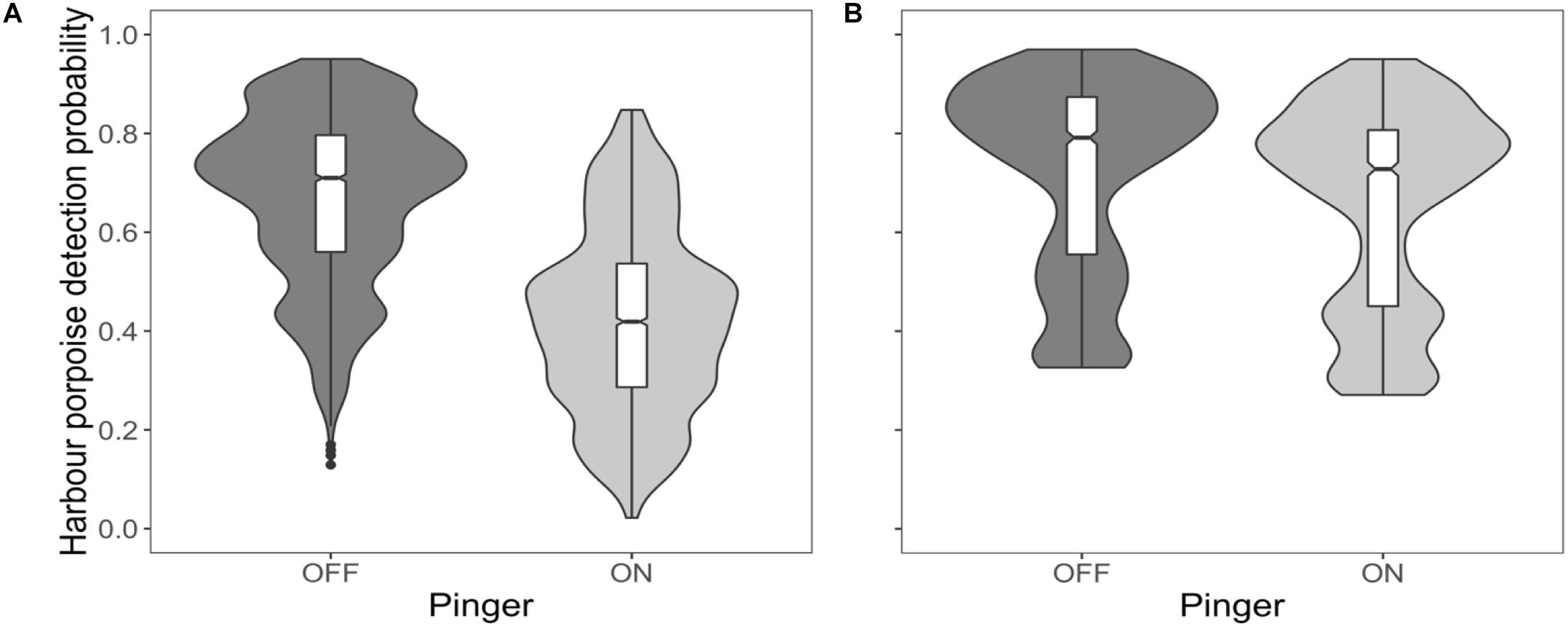
Figure 2. Effect of the pinger. Probability of detecting harbour porpoises based on pinger activity at the near C-POD (A) and at the remote C-POD (B) presented as boxplots, indicating the median and interquartile range, using predictions from top ranked models presented in Table 1. Whiskers indicate minimum and maximum values excluding outliers which are shown as dots. Violin outlines around each boxplot show kernel density of data at different values.
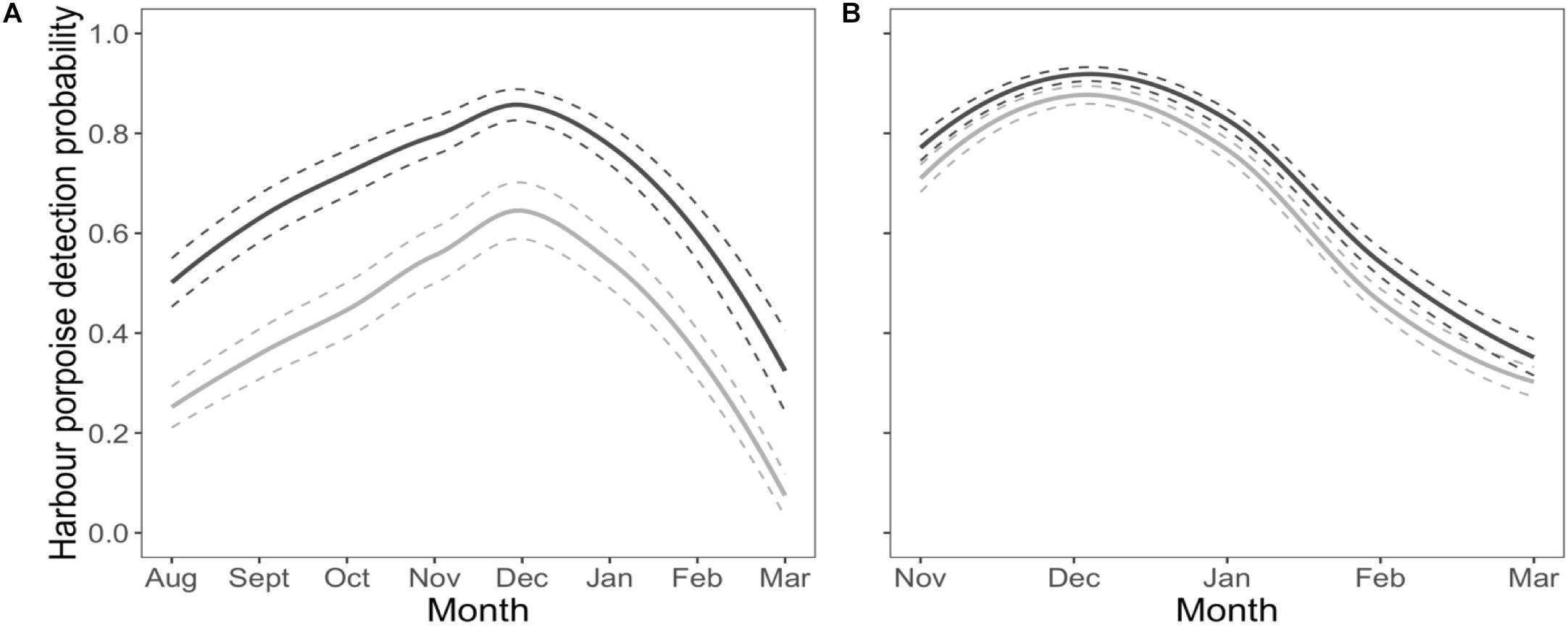
Figure 3. Habituation. Temporal variation of the probability of detecting harbour porpoises as a function of pinger activity (dark gray line: pinger off; light gray line: pinger on) at the near C-POD (A) and at the remote C-POD (B). Solid lines denote predictions from top ranked models presented in Table 1. Standard errors are shown by the colored dashed lines.
Ensonification
A total of 3078 complete hours were recorded at the remote C-POD between November 2012 and March 2013. A further 75 h (2% of deployment period used for the analysis) were recorded but were removed as they were incomplete. Harbour porpoises were present 69% of the time at the remote C-POD between November 2012 and March 2013 (n = 2111).
The probability of detections 100 m away from the pinger was influenced by pinger activity, but to a lesser extent than at the near C-POD. The probability of detections decreased by 9% at the remote C-POD when the pinger was on (Figure 2B), and this effect was constant over the study period (Figure 3B). The probability of detections at the remote C-POD was also influenced by wind speed but not by wind direction, however, the effect of wind speed also varied with month (Supplementary Figure S4). Overall, detection probability increased with higher wind speeds (Supplementary Figure S6).
Displacement
The probability of detections at the near C-POD was not dependent on the number of hours since the pinger turned off. Detections in the hour immediately prior to the pinger turning on positively influenced detection probability. However, this effect was not consistent across months (Supplementary Figure S7). Overall, detections were 24% more likely after the pinger turned off when they were also present in the hour before the pinger turning on (Figure 4). The probability of detections was also influenced by wind speed, increasing with higher wind speeds in all months except January and March 2013 (Supplementary Figure S8). Overall, detections were more likely with higher wind speeds at the near C-POD when the pinger was off.
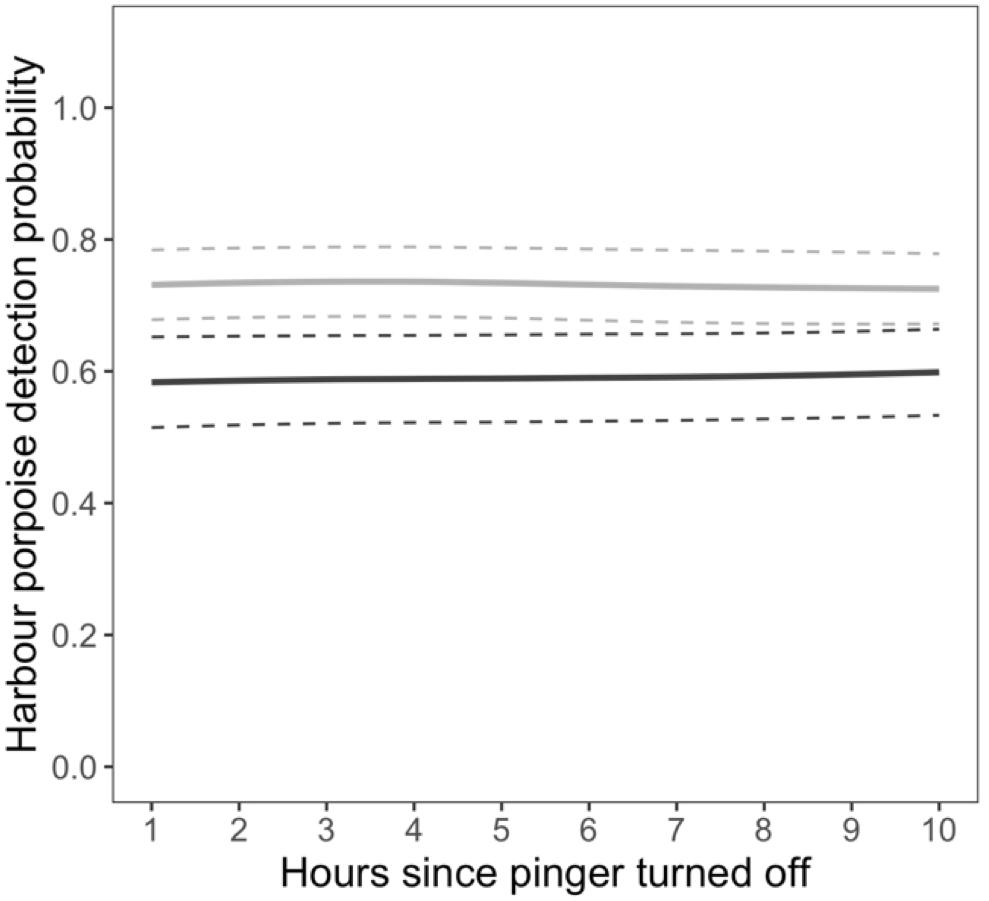
Figure 4. Displacement. Relationship between the probability of detecting harbour porpoises and the number of hours since the pinger turned off as a function harbour porpoise presence 1 h before the pinger turned on (absent: dark gray, present: light gray) at the C-POD near the pinger. Lines denote predictions from the top ranked model presented in Table 1. Standard errors are shown by the colored dashed lines.
Discussion
Here, we address the effectiveness of Banana Pingers as a potential cetacean bycatch mitigation device in waters off the coast of Cornwall, United Kingdom. We highlight the absence of long-term behavioral effects over an 8-month period, suggesting that pingers would likely be effective bycatch mitigation devices in the region.
Pinger Effectiveness
Pingers have been shown to decrease the probability of individual presence for a number of species (e.g., common dolphins and dusky dolphins (Lagenorhynchus obscurus) Mangel et al., 2013, narrow-ridged finless porpoises (Neophocaena asiaeorientalis) Amano et al., 2017, Burmeister’s porpoise (Phocoena spinipinnis) Clay et al., 2019a), including harbour porpoises (e.g., Palka et al., 2008; Carretta and Barlow, 2011; Kyhn et al., 2015), as found in this study. While we did not visually confirm the occurrences of harbour porpoises during the study period, it is unlikely that the decrease in harbour porpoise detection probability when the pinger was on was due to changes in vocalization rates. Indeed, this would imply that porpoises closer to the pinger were less likely to be using echolocation. As pingers are known to reduce bycatch strongly in studies directly measuring bycatch rates, there would be a further, and implausible, implication that a porpoise was more at risk when using its sonar than when silent. Furthermore, observational studies on harbour porpoise behavioral responses to Banana Pingers, such as cliff top theodolite tracking (ICES, 2017) and more recently drone tracking (pers. comm. L. Kindt-Larsen, 2019), all confirm that the reduced detection of harbour porpoise vocalizations when acoustic deterrents are active is due to individuals being deterred from the sound source, and thus, detectability by passive acoustic monitoring. In addition, harbour porpoise are a highly vocal species, relying on echolocation to forage and communicate (Sørensen et al., 2018), and are required to feed nearly continuously to support high metabolic demands (Kastelein et al., 1997; Lockyer et al., 2003; Wisniewska et al., 2016). Therefore, it is unlikely that individuals would remain silent for such extended periods.
It has been suggested that pingers may not be successful bycatch mitigation devices for all cetacean and pinniped species (Dawson et al., 2013; Hamilton and Baker, 2019). Indeed, they have been described as acting as “dinner bells” for California sea lions (Zalophus californianus), South American sea lions (Otaria flavescens), and common bottlenose dolphins (Tursiops truncatus) for example, with individuals of these species having been observed depredating nets equipped with pingers (e.g., Bordino et al., 2002; Read et al., 2003; Carretta and Barlow, 2011; Snape et al., 2018). While early studies deployed low frequency (10 kHz) pingers (e.g., Bordino et al., 2002), such a “dinner bell” effect was eliminated in more recent trials using higher frequency pingers (50–120 kHz; e.g., Øien and Haug, 2017), deemed “seal safe,” as they were tailored to transmit outside of the optimal hearing thresholds of pinnipeds (Mulsow et al., 2012; Erbe et al., 2016). In instances where cetaceans actively depredate fisheries, the evidence underpinning the efficacy of pingers at reducing fisher-cetacean interactions is unclear (Dawson et al., 2013). For example, Snape et al. (2018) highlighted that for common bottlenose dolphins in North Cyprus, the reduction or absence of a pinger effect for some species might ultimately be the result of increased competition for shared but depleting resources (Read, 2008; Wild et al., 2017). If individuals are biologically and/or physically constrained to the area in which the pingers are deployed, they might have no other alternative than to remain despite the aversive sounds (Forney et al., 2017).
In addition to inter-specific differences in pinger effects, changes in environmental conditions and associated background noise levels could mask pings, resulting in a decreased pinger response rate with worsening environmental conditions (Hardy et al., 2012). In this study, we found that the detection rate increased with higher mean hourly wind speeds at both C-PODs, but we cannot substantiate this as a real effect on porpoises. The occurrence of porpoise-like clicks from non-porpoise sources in ambient noise in this study may have occurred, which would cause an underestimation of the effect of the pinger on porpoises and might cause an increase in detections in conditions such a high sea states.
Overall, our findings are consistent with previous literature, highlighting the success of pingers in reducing the detection probability of individuals, and therefore, decreasing the likelihood of localized net-individual interactions across a wide range of species, especially for neophobic species with large home ranges (Dawson et al., 2013; Hamilton and Baker, 2019). Thus, if pingers were to be implemented on vessels under 12 m in Cornwall, we would expect reduced bycatch rates of harbour porpoises and possibly of other resident cetacean species, such as bottlenose dolphins, whose small resident population would greatly benefit from management to prevent further decline in the region (Tregenza, 1992).
Pinger Habituation
Pingers are mandatory in many fisheries around the world, where they are used continuously (e.g., Barlow and Cameron, 2003; Carretta and Barlow, 2011; Geijer and Read, 2013; Read, 2013; Sørensen and Kindt-Larsen, 2016). Carretta and Barlow (2011), for example, showed that cetacean bycatch rates were nearly 50% lower in nets with pingers compared to those without, even after over a decade of deployment. In our study, we found no evidence of harbour porpoise pinger habituation at either C-PODs, which is consistent with a number of studies for the species (e.g., Palka et al., 2008; Carretta and Barlow, 2011; Hardy et al., 2012; Geijer and Read, 2013). Although the concern persists in the literature (Dawson et al., 2013; Hamilton and Baker, 2019), our and such case studies highlight an absence of long-term pinger habituation in operating fisheries. Furthermore, it should be emphasized that, without assessing differences in bycatch levels between nets with or without pingers, it cannot be concluded that a decreased pinger effect would ultimately translate into an increase in cetacean bycatch over time (Dawson et al., 2013). Indeed, pinger habituation that did not result in increased net-individual interactions would beneficially demonstrate a reduction in habitat exclusion resulting from anthropogenic activities (Graham et al., 2019).
Displacement and Ensonification
Displacement appears to be the mechanism through which pingers confer their benefit, although true alerting may be part of the process in some cases. Quantifying displacement is important in order to assess individual- and population-level impacts, and can help to understand whether individuals are at risk of being displaced from critical habitats. Although some studies have reported a decrease in harbour porpoise echolocation activity several kilometers away from the ensonified area (Johnston, 2002; Olesiuk et al., 2002; Kyhn et al., 2015), we found that the pinger effect on detection rates was substantially reduced 100 m away. In addition, we found no evidence of long-term habitat displacement during the study period, with harbour porpoises returning to the ensonified area with no delay, as opposed to studies which investigated displacement during offshore wind farm constructions which involved vastly louder sources, for example (Brandt et al., 2018; Nabe-Nielsen et al., 2018). We note that the one-hour samples used here have the effect that return delays shorter than 1 h are more likely to be undetected.
The Wildlife and Countryside Act of 1981 in the United Kingdom prevents the disturbance of wildlife and has been invoked to prevent the implementation of pingers on vessels under 12 m in Cornwall. This study, however, suggests that the pinger effect is very localized, short-lived and has no long-term behavioral effects. Thus, pingers should be considered as likely to cause less disturbance than other much louder anthropogenic activities, such as boat sonars and naval exercises. The latter are thought to lead to cetacean mass stranding events in this region (Jepson et al., 2013) and elsewhere (Parsons, 2017).
Challenges
The deployment and implementation of pingers as bycatch mitigation strategies for vessels under 12 m in the United Kingdom has been hampered, in part, by uncertainty surrounding the success of their use in other waters. In many cases, however, factors including poor pinger uptake, misuse and lack of compliance can explain the more negative outcomes. A study by Palka et al. (2008), for example, influenced pinger use worldwide, as it presented evidence that pinger misuse increased cetacean bycatch compared to when no pingers were used. This has been incorrectly interpreted as requiring a precautionary approach, i.e., not implementing these measures. However, the study lacked some key information. Most significantly, it did not sufficiently address pinger functionality although in some years, 64% of pingers were inactive, with nets classified as having a “complete set of pingers” or “some pingers,” which may have resulted in effectively no pingers. Furthermore, while it is often concluded from that study that pinger misuse could be creating acoustic holes increasing bycatch, Larsen et al. (2013) found that bycatch was reduced by 78% when pingers were placed almost 600 m apart, i.e., almost six times the minimum required distance stated in the EU Council Regulation No. 812/2004 and that of the United States requirements (NOAA, 1999). In addition, with the level of anthropogenic noise introduced in the marine environment on the rise (Hildebrand, 2009; Findlay et al., 2018), some have questioned the use of pingers to reduce bycatch, considering that anthropogenic noise has been found to disrupt natural behaviors, such as foraging in harbour porpoises (Wisniewska et al., 2018) and communication in bottlenose dolphins (Fouda et al., 2018). However, findings from Larsen et al. (2013) also suggest that the amount of marine anthropogenic noise introduced due to pingers could be reduced if the latter are placed further apart than currently stated in the EU’s Council Regulation.
Pinger effectiveness is influenced by uptake and compliance, with the former generally influencing the latter. Geijer and Read (2013) showed that cetacean bycatch mitigation efforts were more successful in the United States Fisheries in the Pacific Ocean as a result of higher levels of compliance. In contrast, in the Gulf of Maine, where fishers have resisted the implementation of pingers due to their purchasing and maintenance costs, over three quarters of observed hauls (78%) were not compliant with pinger regulations. It is evident that a bottom-up approach to the implementation of pingers would be more successful to reduce bycatch than top-down management measures, such as reduced fishing effort, gear switching, and spatial and temporal closures, which are slow to implement and often met with resistance and non-compliance in the absence of monitoring or enforcement programmes. For example, due to environmental conditions, some fishers in Cornwall change their fishing practices during the winter months. Based on strandings data and fisher knowledge, it is estimated that approximately 1000–2000 harbour porpoises are killed every winter in inshore gillnets in Cornwall alone. The small fishing fleet (12 vessels) in Mevagissey, Cornwall bycaught two to three individuals per vessel per week before fishers willingly started using pingers 2 years ago. They have not caught a single harbour porpoise or common dolphin since (pers. comm. B. Preston), thus highlighting how implementing pingers in a very temporal and short-lived fishery can be very effective at reducing high bycatch rates rapidly and with likely no long-term impacts.
Conclusion
This study reiterates the effectiveness of pingers in reducing the probability of detecting harbour porpoises in Cornwall, United Kingdom. We highlight that the pinger effect is very localized, short-lived and has no long-term behavioral effects over the study period, which is representative of the local fishing season. Thus, if pingers were to be deployed with static fishing nets, we would expect reduced net-porpoise interactions, thereby mitigating bycatch of harbour porpoises and, possibly, other cetacean species in the region. As small-scale fisheries dominate in United Kingdom waters, there is an acute need for cost-effective mitigation strategies to be implemented rapidly, and with concurrent monitoring, in order to address the long-term problem of harbour porpoise, and more generally, cetacean bycatch in Cornwall.
Data Availability Statement
The raw data supporting the conclusions of this article will be made available by the authors, without undue reservation, to any qualified researcher.
Ethics Statement
The animal study was reviewed and approved by the Cornwall Wildlife Trust and by the University of Exeter Ethics Committee.
Author Contributions
NT conceived the study, carried out the fieldwork and processed the data, as part of the Cornwall Wildlife Trust led pinger trial project 2012–2013. AR and LO independently analyzed the data, with input from PD. LO led the writing of the manuscript with contributions from all authors. All authors read and approved the submitted version of the manuscript.
Funding
LO was supported by the Whale and Dolphin Conservation and by Fishtek Marine Limited.
Conflict of Interest
NT and RE have an interest in the Banana Pinger. NT owns Chelonia Limited which manufactures the C-POD loggers and RE is employed by Fishtek Marine which manufactures the Banana Pingers.
The remaining authors declare that the research was conducted in the absence of any commercial or financial relationships that could be construed as a potential conflict of interest.
Acknowledgments
We thank the reviewers and the editor, whose input improved the manuscript. We also thank Julia Haywood for the map presented in Figure 1.
Supplementary Material
The Supplementary Material for this article can be found online at: https://www.frontiersin.org/articles/10.3389/fmars.2020.00285/full#supplementary-material
References
Amano, M., Kusumoto, M., Abe, M., and Akamatsu, T. (2017). Long-term effectiveness of pingers on a small population of finless porpoises in Japan. Endanger. Species Res. 32, 35–40.
Barlow, J., and Cameron, G. A. (2003). Field experiments show that acoustic pingers reduce marine mammal bycatch in the California drift gill net fishery. Mar. Mammal Sci. 19, 265–283.
Barton, K. (2015). MuMIn: Multi-model inference. Available online at: https://CRAN.R-project.org/package=MuMIn.
Baum, J. K., Myers, R. A., Kehler, D. G., Worm, B., Harley, S. J., and Doherty, P. A. (2003). Collapse and conservation of shark populations in the Northwest Atlantic. Science (80-) 299, 389–392. doi: 10.1126/science.1079777
Bordino, P., Kraus, S., Albareda, D., Fazio, A., Palmerio, A., Mendez, M., et al. (2002). Reducing incidental mortality of Franciscana dolphin Pontoporia blainvillei with acoustic warning devices attached to fishing nets. Mar. Mammal Sci. 18, 833–842.
Bowles, A. E., and Anderson, R. C. (2012). Behavioral responses and habituation of pinnipeds and small cetaceans to novel objects and simulated fishing gear with and without a pinger. Aquat. Mamm. 38, 161–188.
Brandt, M. J., Dragon, A. C., Diederichs, A., Bellmann, M. A., Wahl, V., Piper, W., et al. (2018). Disturbance of harbour porpoises during construction of the first seven offshore wind farms in Germany. Mar. Ecol. Prog. Ser. 596, 213–232.
Burnham, K., and Anderson, D. (2002). Model Selection and Multimodel Inference: A Practical Information-Theoretic Approach, 2 Edn. New York: Springer.
Calderan, S., and Leaper, R. (2019). Review of harbour porpoise Bycatch in UK Waters and Recommendations for Management. Nairobi: United Nations Environment Programme.
Carlström, J., Berggren, P., and Tregenza, N. J. C. (2009). Spatial and temporal impact of pingers on porpoises. Can. J. Fish. Aquat. Sci. 66, 72–82.
Carretta, J. V., and Barlow, J. (2011). Long-term effectiveness, failure rates, and “dinner bell” properties of acoustic pingers in a gillnet fishery. Mar. Technol. Soc. J. 13, 7–19.
Clay, T. A., Alfaro-Shigueto, J., Godley, B., Tregenza, N., and Mangel, J. (2019a). Pingers reduce the activity of Burmeister’s porpoise around small-scale gillnet vessels. Mar. Ecol. Prog. Ser. 626, 197–208.
Clay, T. A., Small, C., Tuck, G. N., Pardo, D., Carneiro, A. P. B., Wood, A. G., et al. (2019b). A comprehensive large-scale assessment of fisheries bycatch risk to threatened seabird populations. J. Appl. Ecol. 56, 1–12.
Clear, N., Hawtrey-Collier, A., and Williams, R. (2018). Marine Strandings in Cornwall and the Isles of Scilly Annual Report. 1–22.
Cox, T. M., Read, A. J., Solow, A., and Tregenza, N. (2001). Will harbour porpoises (Phocoena phocoena) habituate to pingers? J. Cetacean Res. Manage. 3, 81–86.
Davies, R. W. D., Cripps, S. J., Nickson, A., and Porter, G. (2009). Defining and estimating global marine fisheries bycatch. Mar. Policy 33, 661–672.
Dawson, S. M., Northridge, S., Waples, D., and Read, A. J. (2013). To ping or not to ping: the use of active acoustic devices in mitigating interactions between small cetaceans and gillnet fisheries. Endanger. Species Res. 19, 201–221.
Erbe, C., Reichmuth, C., Cunningham, K., Lucke, K., and Dooling, R. (2016). Communication masking in marine mammals: a review and research strategy. Mar. Pollut. Bull. 103, 15–38. doi: 10.1016/j.marpolbul.2015.12.007
Findlay, C. R., Ripple, H. D., Coomber, F., Froud, K., Harries, O., Geel, N. C. F., et al. (2018). Mapping widespread and increasing underwater noise pollution from acoustic deterrent devices. Mar. Pollut. Bull. 135, 1042–1050. doi: 10.1016/j.marpolbul.2018.08.042
Forney, K. A., Southall, B. L., Slooten, E., Dawson, S., Read, A. J., Baird, R. W., et al. (2017). Nowhere to go: noise impact assessments for marine mammal populations with high site fidelity. Endanger. Species Res. 32, 391–413.
Fouda, L., Wingfield, J. E., Fandel, A. D., Garrod, A., Hodge, K. B., Rice, A. N., et al. (2018). Dolphins simplify their vocal calls in response to increased ambient noise. Biol. Lett. 14:20180484. doi: 10.1098/rsbl.2018.0484
Geijer, C. K. A., and Read, A. J. (2013). Mitigation of marine mammal bycatch in U.S. fisheries since 1994. Biol. Conserv. 159, 54–60.
Graham, I. M., Merchant, N. D., Farcas, A., Barton, T. R., Cheney, B., Bono, S., et al. (2019). Harbour porpoise responses to pile-driving diminish over time. R Soc Open Sci 6:190335. doi: 10.1098/rsos.190335
Hamilton, S., and Baker, G. B. (2019). Technical mitigation to reduce marine mammal bycatch and entanglement in commercial fishing gear: lessons learnt and future directions. Rev. Fish. Biol. Fish. 29, 223–247.
Hardy, T., Williams, R., Caslake, R., and Tregenza, N. (2012). An investigation of acoustic deterrent devices to reduce cetacean bycatch in an inshore set net fishery. J. Cetacean Res. Manage. 12, 85–90.
Hildebrand, J. A. (2009). Anthropogenic and natural sources of ambient noise in the ocean. Mar. Ecol. Prog. Ser. 395, 5–20. doi: 10.1146/annurev-marine-121211-172423
ICES (2017). Report of the Working Group on Bycatch of Protected Species (WGBYC). Woods Hole, MA: ICES.
Jaramillo-Legorreta, A., Cardenas-Hinojosa, G., Nieto-Garcia, E., Rojas-Bracho, L., Hoef, J., Ver Moore, J., et al. (2017). Passive acoustic monitoring of the decline of Mexico’s critically endangered vaquita. Conserv. Biol. 31, 183–191. doi: 10.1111/cobi.12789
Jepson, P. D., Deaville, R., Acevedo-Whitehouse, K., Barnett, J., Brownlow, A., Brownell, R. L., et al. (2013). What caused the UK’s largest common dolphin (Delphinus delphis) mass stranding event? PLoS ONE 8:e60953. doi: 10.1371/journal.pone.0060953
Johnston, D. W. (2002). The effect of acoustic harassment devices on harbour porpoises (Phocoena phocoena) in the Bay of Fundy. Canada. Biol Conserv. 108, 113–118.
Kastelein, R. A., Hardeman, J., and Boer, H. (1997). Food Consumption and Body Weight of Harbour Porpoises (Phocoena phocoena). Biol Harb Porpoise A J Read, P R Wiepkema P E Nachtigall (eds). Netherlands: Spil Publ Woerden, 217–233.
Kraus, S. D., Read, A. J., Solow, A., Baldwin, K., Spradlin, T., Anderson, E., et al. (1997). Acoustic alarms reduce porpoise mortality [4]. Nature 388:525.
Kyhn, L. A., Jørgensen, P. B., Carstensen, J., Bech, N. I., Tougaard, J., Dabelsteen, T., et al. (2015). Pingers cause temporary habitat displacement in the harbour porpoise Phocoena phocoena. Mar. Ecol. Prog. Ser. 526, 253–265.
Larsen, F., Krog, C., and Eigaard, O. R. (2013). Determining optimal pinger spacing for harbour porpoise bycatch mitigation. Endanger. Species Res. 20, 147–152.
Leeney, R. H., Witt, M. J., Broderick, A. C., Buchanan, J., Jarvis, D. S., Richardson, P. B., et al. (2012). Marine megavertebrates of Cornwall and the Isles of Scilly: relative abundance and distribution. J. Mar. Biol. Assoc. U.K. 92, 1823–1833.
Lewison, R. L., Crowder, L. B., and Shaver, D. J. (2003). The impact of turtle excluder devices and fisheries closures on loggerhead and Kemp’s ridley strandings in the western Gulf of Mexico. Conserv. Biol. 17, 1089–1097.
Lewison, R. L., Crowder, L. B., Wallace, B. P., Moore, J. E., Cox, T., Zydelis, R., et al. (2014). Global patterns of marine mammal, seabird, and sea turtle bycatch reveal taxa-specific and cumulative megafauna hotspots. Proc. Natl. Acad. Sci. U.S.A. 111, 5271–5276. doi: 10.1073/pnas.1318960111
Lewison, R. L., Freeman, S. A., and Crowder, L. B. (2004). Quantifying the effects of fisheries on threatened species: the impact of pelagic longlines on loggerhead and leatherback sea turtles. Ecol. Lett. 7, 221–231.
Lockyer, C., Desportes, G., Hansen, K., Labberté, S., and Siebert, U. (2003). Monitoring growth and energy utilisation of the harbour porpoise (Phocoena phocoena) in human care. NAMMCO Sci. Publ. 5, 107–120.
Mangel, J. C., Alfaro-Shigueto, J., Witt, M. J., Hodgson, D. J., and Godley, B. J. (2013). Using pingers to reduce bycatch of small cetaceans in Peru’s small-scale driftnet fishery. Oryx 47, 595–606.
Mei, Z., Huang, S. L., Hao, Y., Turvey, S. T., Gong, W., and Wang, D. (2012). Accelerating population decline of Yangtze finless porpoise (Neophocaena asiaeorientalis asiaeorientalis). Biol. Conserv. 153, 192–200.
Molina, J. M., and Cooke, S. J. (2012). Trends in shark bycatch research: current status and research needs. Rev. Fish. Biol. Fish. 22, 719–737.
Mulsow, J., Houser, D. S., and Finneran, J. J. (2012). Underwater psychophysical audiogram of a young male California sea lion (Zalophus californianus). J. Acoust. Soc. Am. 131, 4182–4187. doi: 10.1121/1.3699195
Nabe-Nielsen, J., Beest, F. M., van, Grimm, V., Sibly, R. M., Teilmann, J., et al. (2018). Predicting the impacts of anthropogenic disturbances on marine populations. Conserv. Lett. 11:e12563.
NOAA (1999). “Taking of marine mammals incidental to commercial fishing operations,” in Pacific Offshore Cetacean Take Reduction Plan Regulations, ed A. A. Rosenberg (Silver Spring, MD: NOAA).
Northridge, S., Kingston, A., and Thomas, L. (2018). Annual Report on the Implementation of Council Regulation (EC) No 812/2004 During 2017. St Andrews: University of St Andrews.
Øien, N., and Haug, T. (2017). Norway – Progress report on Marine Mammals. Nuuk: North Atlantic Marine Mammal Commission.
O’Keefe, C. E., Cadrin, S. X., and Stokesbury, K. D. E. (2014). Evaluating effectiveness of time/area closures, quotas/caps, and fleet communications to reduce fisheries bycatch. ICES J. Mar. Sci. 71, 1286–1297.
Olesiuk, P. F., Nichol, L. M., Sowden, M. J., and Ford, J. K. B. (2002). Effect of the sound generated by an acoustic harassment device on the relative abundance and distribution of harbor porpoises (Phocoena phocoena) in Retreat Passage, British Columbia. Mar. Mammal Sci. 18, 843–862.
Palka, D. L., Rossman, M. C., VanAtten, A. S., and Orphanides, C. D. (2008). Effect of pingers on harbour porpoise (Phocoena phocoena) bycatch in the US Northeast gillnet fishery. J. Cetacean Res. Manage. 10, 217–226.
Parsons, E. C. M. (2017). Impacts of Navy sonar on whales and dolphins: now beyond a smoking gun? Front. Mar. Sci. 4:295. doi: 10.3389/fmars.2017.00295
Pikesley, S. K., Witt, M. J., Hardy, T., Loveridge, J., Williams, R., and Godley, B. (2011). Cetacean sightings and strandings: evidence for spatial and temporal trends? J. Mar. Biol. Assoc. U. K. 92, 1809–1820.
R Core Team (2018). R: A Language and Environment for Statistical Computing. Vienna: R Found Stat Comput.
Read, A. J. (2008). The looming crisis: interactions between marine mammals and fisheries. J Mammal 89, 541–548.
Read, A. J. (2013). Development of conservation strategies to mitigate the bycatch of harbor porpoises in the Gulf of Maine. Endanger. Species Res. 20, 235–250.
Read, A. J., Drinker, P., and Northridge, S. (2006). Bycatch of marine mammals in U.S. and global fisheries. Conserv. Biol. 20, 163–169. doi: 10.1111/j.1523-1739.2006.00338.x
Read, A. J., Waples, D. M., Urian, K. W., and Swanner, D. (2003). Fine-scale behaviour of bottlenose dolphins around gillnets. Proc. R. Soc. B Biol. Sci. 270, 90–92. doi: 10.1098/rsbl.2003.0021
Richards, S. A., Whittingham, M. J., and Stephens, P. A. (2011). Model selection and model averaging in behavioural ecology: the utility of the IT-AIC framework. Behav. Ecol. Sociobiol. 65, 77–89.
Snape, R. T. E., Broderick, A. C., Çiçek, B. A., Fuller, W. J., Tregenza, N., Witt, M. J., et al. (2018). Conflict between dolphins and a data-scarce fishery of the European Union. Hum. Ecol. 46, 423–433. doi: 10.1007/s10745-018-9989-7
Sørensen, P. M., Wisniewska, D. M., Jensen, F. H., Johnson, M., Teilmann, J., and Madsen, P. T. (2018). Click communication in wild harbour porpoises (Phocoena phocoena). Sci. Rep. 8:9702. doi: 10.1038/s41598-018-28022-8
Sørensen, T. K., and Kindt-Larsen, L. (2016). Uncovering governance mechanisms surrounding harbour porpoise conservation in the Danish Skagerrak Sea. Mar. Policy 71, 318–324.
Sullivan, B. J., Kibel, B., Kibel, P., Yates, O., Potts, J. M., Ingham, B., et al. (2018). At-sea trialling of the Hookpod: a ‘one-stop’ mitigation solution for seabird bycatch in pelagic longline fisheries. Anim. Conserv. 21, 159–167.
Tregenza, N., Dawson, S., Rayment, W., and Verfuss, U. (2016). “Listening to echolocation clicks with PODs,” in Listening in the Ocean, eds W. W. Au and M. Lammers (New York, NY: Springer-Verlag), 163–306.
Tregenza, N. J. C. (1992). Fifty years of cetacean sightings from the Cornish coast, SW England. Biol. Conserv. 59, 65–70.
Van Beest, F. M., Van, Kindt-Larsen, L., Bastardie, F., Bartolino, V., and Nabe-Nielsen, J. (2017). Predicting the population-level impact of mitigating harbor porpoise bycatch with pingers and time-area fishing closures. Ecosphere 8:e01785.
Venables, W. N., and Ripley, B. D. (2002). Modern Applied Statistics with S, 4th Edn. New York, NY: Springer.
Wakefield, C. B., Santana-Garcon, J., Dorman, S. R., Blight, S., Denham, A., Wakeford, J., et al. (2017). Performance of bycatch reduction devices varies for chondrichthyan, reptile, and cetacean mitigation in demersal fish trawls: assimilating subsurface interactions and unaccounted mortality. ICES J. Mar. Sci. 74, 343–358.
Wild, L., Thode, A., Straley, J., Rhoads, S., Falvey, D., and Liddle, J. (2017). Field trials of an acoustic decoy to attract sperm whales away from commercial longline fishing vessels in western Gulf of Alaska. Fish. Res. 196, 141–150.
Wisniewska, D. M., Johnson, M., Teilmann, J., Siebert, U., Galatius, A., Dietz, R., et al. (2018). High rates of vessel noise disrupt foraging in wild harbour porpoises (Phocoena phocoena). Proc. R. Soc. B Biol. Sci. 285:20172314. doi: 10.1098/rspb.2017.2314
Keywords: acoustic deterrent, C-POD, cetacean, marine mammal, passive acoustic monitoring
Citation: Omeyer LCM, Doherty PD, Dolman S, Enever R, Reese A, Tregenza N, Williams R and Godley BJ (2020) Assessing the Effects of Banana Pingers as a Bycatch Mitigation Device for Harbour Porpoises (Phocoena phocoena). Front. Mar. Sci. 7:285. doi: 10.3389/fmars.2020.00285
Received: 21 February 2020; Accepted: 07 April 2020;
Published: 13 May 2020.
Edited by:
Mark Meekan, Australian Institute of Marine Science, AustraliaReviewed by:
Vinay Udyawer, Australian Institute of Marine Science, AustraliaDavid Ainley, H. T. Harvey & Associates, United States
Copyright © 2020 Omeyer, Doherty, Dolman, Enever, Reese, Tregenza, Williams and Godley. This is an open-access article distributed under the terms of the Creative Commons Attribution License (CC BY). The use, distribution or reproduction in other forums is permitted, provided the original author(s) and the copyright owner(s) are credited and that the original publication in this journal is cited, in accordance with accepted academic practice. No use, distribution or reproduction is permitted which does not comply with these terms.
*Correspondence: Lucy C. M. Omeyer, TC5PbWV5ZXJAZXhldGVyLmFjLnVr; Philip D. Doherty, UC5Eb2hlcnR5QGV4ZXRlci5hYy51aw==
†These authors share first authorship