- Geographic Information Systems (GIS) and Spatial Ecology Lab, Halmos College of Arts and Sciences, Nova Southeastern University, Dania Beach, FL, United States
Stony coral tissue loss disease (SCTLD) has persisted since 2014 in the Southeast Florida Coral Reef Ecosystem Conservation Area (Coral ECA) where it was first discovered. Most of the highly susceptible corals have perished, leaving Montastraea cavernosa as the most abundant reef-building species with high SCTLD prevalence. Disease interventions (DI) have been conducted throughout Florida’s Coral Reef to save the remaining corals and reduce the disease prevalence with varying degrees of success. The two main treatments were chlorinated (Chl) epoxy and an antibiotic paste. The antibiotic paste was highly effective in the Florida Keys, but its effectiveness in the Coral ECA was questionable. Therefore, we compared the effectiveness of the antibiotic paste and Chl epoxy treatments on M. cavernosa to optimize DI efforts on this species in the Coral ECA. Significant differences were found between the treatment materials and applications related to the proportion of quiesced lesions and corals where antibiotic paste (91.2% success) outperformed Chl epoxy (20% success). By day 351, 50.6% of the antibiotic paste disease-break tissue was fully healed compared to 2.2% of the total Chl epoxy-filled disease-break area. During the study, new lesions occurred on previously treated colonies, as well as colonies not previously treated and new lesion rates varied through time, indicating revisitation is necessary to eliminate disease. Most margin treatments failed within the first 9 days, however, most disease-breaks failed before 44 days. Considering the high treatment success of the antibiotic paste and the conditional variation of new lesion rates, about 1 month is a good practical re-visitation time for retreating failures and any new lesions. DI using antibiotic paste is currently the most effective way to intervene the SCTLD epidemic, but this is only effective as a stopgap measure while the larger causative agents are identified and remediated. Conducting DI at a reef-scape scale is time consuming and requires extensive person-power and resources, making it very expensive. But this expense pales in comparison to the current cost to restore the diversity and live tissue saved with DI. This method also comes with the risk of introducing antibiotics into coral reef environments, which may have unintended outcomes.
Introduction
Considering the ongoing global COVID-19 pandemic, the extreme effect of a pathogen without a treatment is more apparent than ever. While the global human population is reeling from the COVID-19 virus, the reefs of South Florida continue battling their own epizootic event. First discovered on Miami-Dade reefs in 2014, the novel disease, stony coral tissue loss disease (SCTLD), rapidly spread across Florida’s Coral Reef (FCR) and has been found at many locations throughout the Tropical Western Atlantic (Precht et al., 2016; Alvarez-Filip et al., 2019; Meiling et al., 2020; Heres et al., 2021; Thome et al., 2021). In Florida, SCTLD has occurred year-round since 2014 and infected up to 22 of the 45 species of scleractinian corals found on FCR (Aeby et al., 2019; Meyer et al., 2019) including several important reef-building species and several classified as endangered on the International Union for Conservation of Nature (IUCN)’s Red List.
The Tropical Western Atlantic is a known disease “hotspot” having 66% of recorded disease events despite hosting only 8% of the world’s coral reefs (Green and Bruckner, 2000), where some coral diseases, including tissue loss diseases, persist year round and affect numerous coral species (Harvell et al., 2009; Weil et al., 2009; Weil and Rogers, 2011; Muller and van Woesik, 2014; Bruckner, 2015; Weil, 2019). The cause and pathogen(s) responsible for SCTLD are currently unknown and its distinction from other tissue loss diseases is challenging, however, a plausible cause is toxicosis related to a breakdown of the host-symbiont relationship in the gastrodermis resulting in necrosis and a bacterial infection (Landsberg et al., 2020; Thome et al., 2021). SCTLD is highly virulent and able to spread both through direct contact and through the water column (Aeby et al., 2019; Landsberg et al., 2020; Muller et al., 2020; Thome et al., 2021). Spatial epidemiological models have estimated a linear epizootic spread in Florida of about 100 m d–1 over 3 years (Muller et al., 2020). Disease presentation and lethality vary greatly between and among species and regions (Aeby et al., 2019; Voss et al., 2019). Meandroid colonies (Dendrogyra cylindrus, Dichocoenia stokesii, Eusmilia fastigiata, and other Meandroid meandrites) are the most susceptible followed by star corals (e.g., Orbicella spp., Montastraea cavernosa, Siderastrea siderea) (Aeby et al., 2019; Sharp et al., 2020; Heres et al., 2021). Therefore, when SCTLD reaches a new location, it rapidly infects the highly susceptible species first, but can then remain prevalent a long time by infecting the less susceptible ones (Sharp et al., 2020; Thome et al., 2021). Locations where the disease has persisted for several years resulting in widespread regional declines in both colony density and live tissue cover (Precht et al., 2016; Walton et al., 2018; Sharp et al., 2020), like southeast Florida, are considered endemic.
In 2018, disease interventions (DI) were needed as part of the response to Florida’s SCTLD outbreak to simultaneously reduce the pathogen load in the environment and attempt to save the remaining diseased corals. Aeby et al. (2015) previously reported successful disease intervention using a mixture of marine epoxy and chlorine powder (Chl epoxy) to cease black band disease in Hawaii. Considering the immediate need for disease intervention in the Southeast Florida Coral Reef Ecosystem Conservation Area (Coral ECA), similar techniques and the same materials as Aeby et al. (2015) were employed while laboratory trials on many other materials occurred. Chl epoxy was ideal to get started because of easy permitting and material availability. In the Coral ECA, these treatments had moderate success on Orbicella spp. (77%) but not for M. cavernosa (<40%) (section 4.2 in Walker et al., 2020).
Concomitantly, an antibiotic paste was developed by Ocean Alchemists (CoreRx Base 2b with amoxicillin) for experimental use to increase DI success because amoxicillin halted SCTLD progression on D. cylindrus fragments (O’Neil et al., 2018) and amoxicillin and kanamycin halted disease progression on M. cavernosa and Meandrina meandrites in aquaria (Aeby et al., 2019). Once permitted for experimental use, the antibiotic paste was effective on many species in the lower Keys including 90% success on M. cavernosa (Neely et al., 2020), but its effectiveness in the Coral ECA was questionable because antibiotic resistant genes were recently found in the ECA associated with sewage outfalls (Griffin et al., 2020). Therefore, we tested the efficacy (effectiveness) of the antibiotic paste and Chl epoxy treatments on M. cavernosa to optimize DI efforts on this species in the Coral ECA where SCTLD was endemic for 5 years. Our results provide information on treatment success, timing of lesion treatment failures, new infections, and wound healing, which are used to provide recommendations to assist others planning future disease interventions.
Materials and Methods
Site Establishment
Three experimental treatment sites were established in northern Miami-Dade County in the southern part of the Coral ECA (Figure 1). Each site was located 1–1.5 km from shore, on the Broward-Miami Ecoregion Ridge-Shallow habitat (Walker et al., 2008; Walker, 2012) in about 7 m depth. At these locations, all visibly infected M. cavernosa colonies were tagged, measured, photographed, and mapped from a central GPS location by distance and heading. A floating GPS was used to obtain coordinates for all treated colonies.
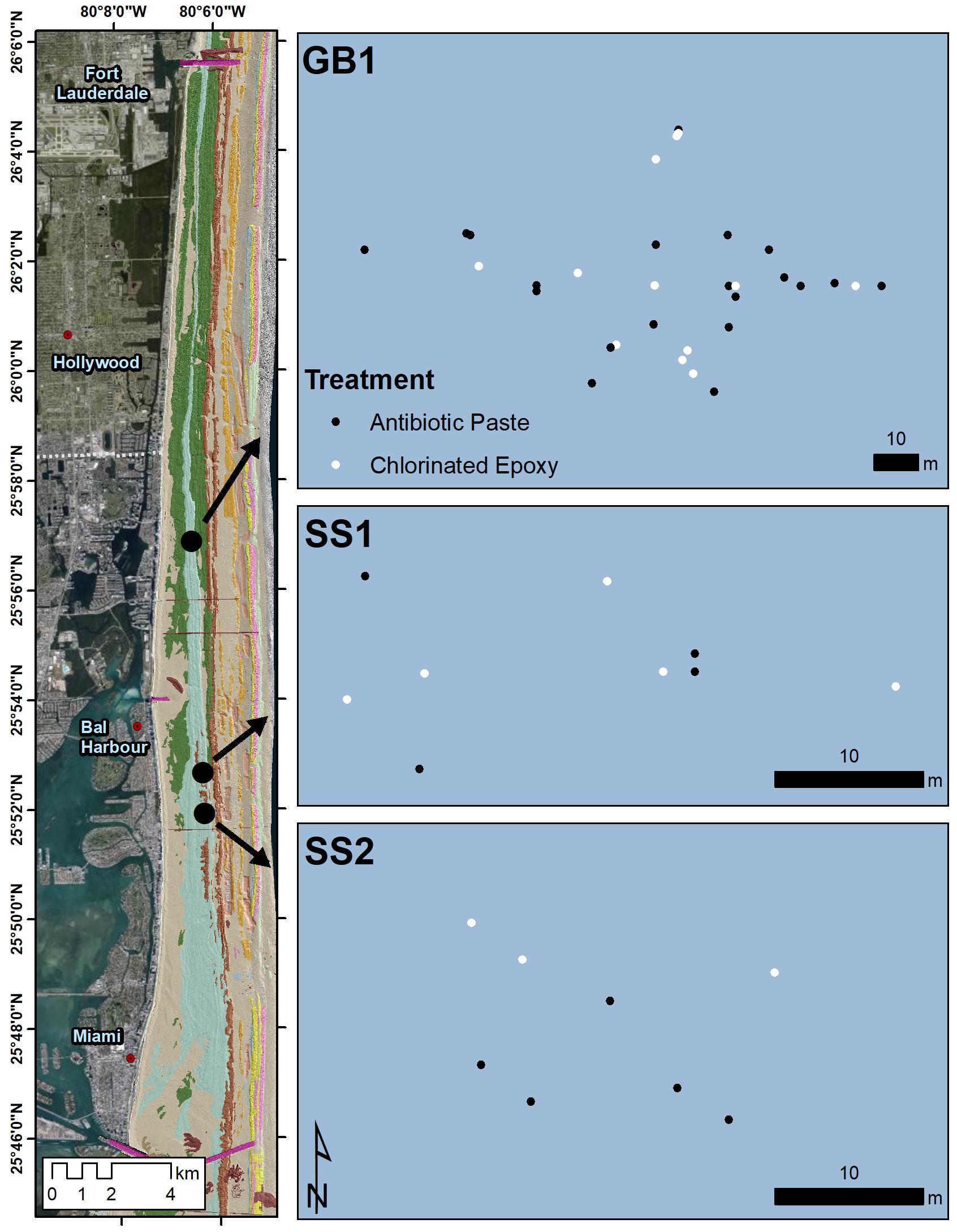
Figure 1. Map of the M. cavernosa corals at the three northern Miami-Dade County treatment sites. Black dots and gray dots represent corals treated with antibiotic paste or chlorinated epoxy respectively.
Treatments for each coral were determined by evaluating the percentage of diseased vs. healthy tissue, number of lesions, colony location, and size to maintain as much consistency between treatment types as possible, and to avoid any biases in the analyses from these factors. Initially, 18 colonies were treated with Chl epoxy and 22 colonies with antibiotic paste. On May 6, 2019 at GB1, 23 lesions were treated on 10 colonies with Chl epoxy and 36 lesions on 14 colonies with antibiotic paste. At SS1, eight lesions were treated on five colonies with Chl epoxy and five lesions on four colonies with antibiotic paste. On May 8, 2019 at SS2, three lesions were treated on three colonies with Chl epoxy and 11 lesions on four colonies with antibiotic paste. Upon subsequent visits, newly diseased corals and treatments were added as they were found, but were kept independent from statistical analysis regarding success of the two treatment materials used.
Treatment Materials
Two disease intervention treatments were tested, an antibiotic paste and Chl epoxy. The antibiotic paste was a mixture of amoxicillin trihydrate from PhytoTechnology Laboratories and a silicone-based paste labeled Base 2b designed to leach antibiotics slowly over 36 h (originally from CoreRX Pharma, now Ocean Alchemists). The Base 2b and amoxicillin were mixed by a weight ratio of 8:1 (Base 2b: amoxicillin). The resulting antibiotic paste was thoroughly mixed then spread in an approximate two-centimeter-thick layer for at least 15 min to allow any of the ethanol-based preservative in the Base 2b to evaporate. The antibiotic paste was then transferred to 60 ml catheter-tip syringes, with the tips cut to facilitate application, and kept on ice until use.
The Chl epoxy treatment utilized ZSPAR A-788 Splash ZoneTM two-part marine epoxy. Part A was premixed with chlorine powder (PoolifeTM Turboshock®) at a ratio of 15 g of chlorine to approximately 50 mL of Part A epoxy. Equal epoxy parts (Part A with Chl and Part B) were kept separate and then mixed underwater before application.
Treatment Application
Treatment application consisted of methods outlined in Aeby et al. (2015). A disease-break [analogous to the “firebreak” in Aeby et al. (2015)] about 5 cm from the visibly diseased marginand ∼1 cm deep was created using a hammer and chisel and a Nemo underwater angle grinder (AG-22-5Li-50) with a 4.5-inch masonry grinding disk to isolate the visually diseased portion from the rest of the colony (white arrows in Figure 2). Then the disease margin was smothered with treatment material covering all visibly infected polyps and the “disease-break” was filled completely (black arrows in Figure 2). After the initial ineffectiveness of Chl epoxy was evident, the remaining Chl epoxy treatments with active disease were treated with antibiotic paste, hereafter referred to as mixed treatments. This was done to test the antibiotic paste treatment on corals with lesions where Chl epoxy failed. No colonies had mixed treatments at the onset of the study. Antibiotic paste success on lesions where Chl epoxy failed would eliminate the possibility of the Chl epoxy lesions being unique. This resulted in a much greater overall number of antibiotic paste treatments by the end of the study.
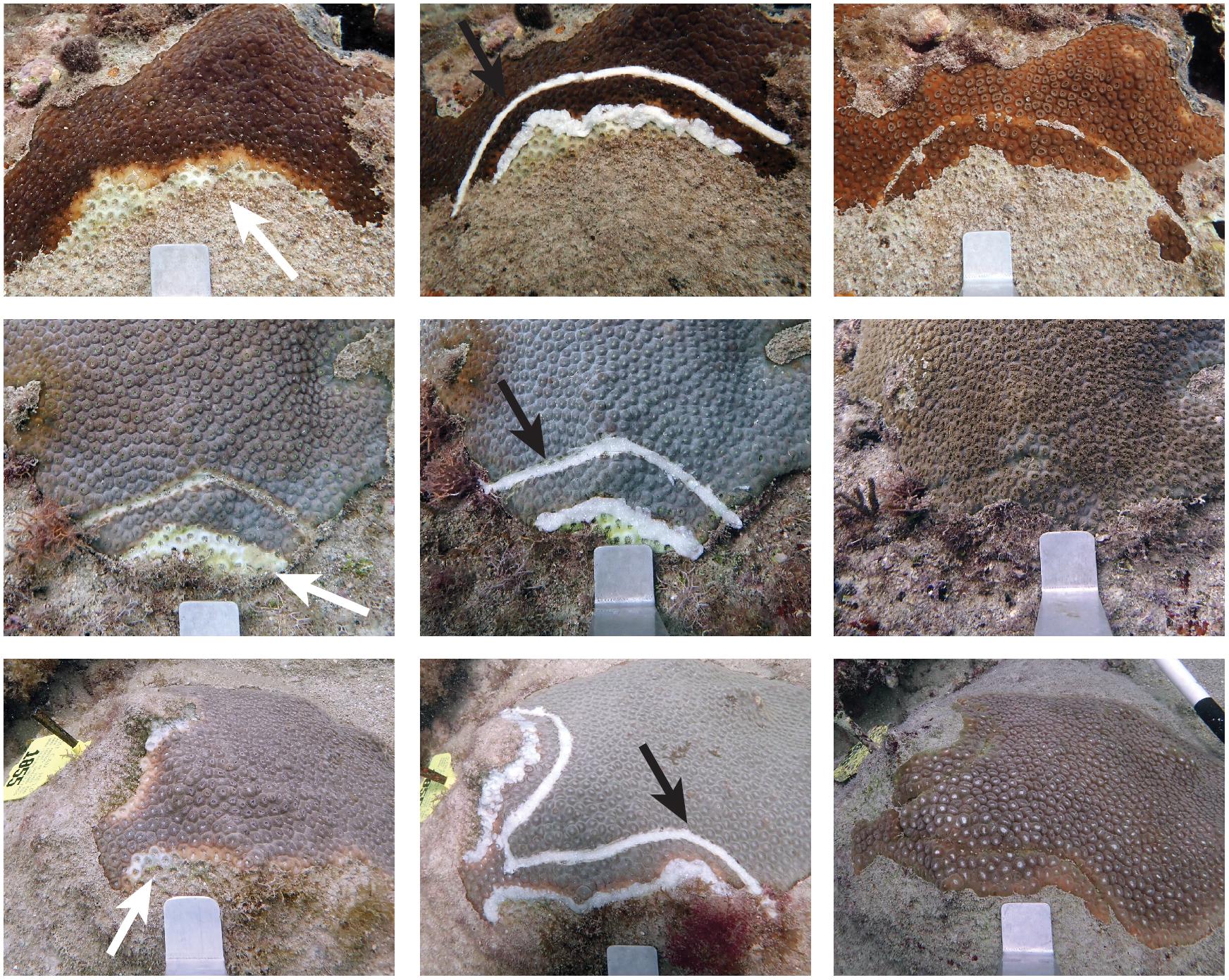
Figure 2. Examples of disease-break application and healing. Disease lesions before treatment are indicated by white arrows and black arrows depict the disease-breaks. The rows are the same lesions over time showing the lesion before treatment (left), upon treatment (Middle), and sometime after treatment (right). The top row lesion was treated on May 6, 2019 and showed disease cessation and healing by June 5, 2019 (1 month). Note that the disease-break contained the spread of the disease where the margin treatment was not effective (right side). The middle row coral was treated on May 15, 2019 and was fully healed by January 31, 2020 (eight and a half months). The bottom row colony was treated on May 19, 2019 and was fully healed on Oct 9, 2019 (5 months).
Monitoring
Corals were initially treated on May 6 and 8, 2019 and revisited weekly until June 5 [May 15 (day 9), May 21 (day 15), May 29 (day 23), June 5 (day 30)], after which they were revisited on June 19 (day 44), June 27 (day 52), July 10 (day 65), July 30 (day 85), August 13 (day 99), and October 10, 2019 (day 157), and January 31 (day 270) and April 21, 2020 (day 351). During treatment and monitoring, high resolution photographs and videos were taken to record the total coral condition, each treatment, and new infections. These consisted of whole-colony nadir photographs and individual treatments taken perpendicularly to the colony center at a fixed distance away with a standard measuring scale in the image. We used these photographs to visually assess colony health, disease progression, treatment effectiveness, and healing. Lesion treatments were considered successful until disease was observed progressing past the treatment on subsequent visits. These cases were recorded as treatment failures. Disease-breaks were considered successful until disease reached and crossed the disease-break. Percentage success was calculated as 100—(number of lesion failures/total number of treatments ∗ 100). Colony treatments were considered successful until one of the lesion treatments failed. Healing was assessed during the final two monitoring visits (January 31 and April 21, 2020) by measuring the length of fully healed tissue along the disease-breaks. To be considered “fully healed,” the live tissue had to grow over and make contact across the disease-break. Because all lesions were treated at every visit, new treatments unrelated to previously treated lesions were used as a surrogate for new lesions. A daily infection rate was calculated by dividing the number of new lesions and corals with new lesions by the number of days since the last visit.
Statistical Analyses
All statistical analyses were completed using R statistical software, version 4.0.4 (R Core Team, 2021) and the rstatix package (Kassambara, 2021). Datasets were not normally distributed (Shapiro-Wilk p < 0.05) and required non-parametric methods for determining whether there were differences between groups (site or treatment application method). Kruskal-Wallis one-way analysis of variance tests were used to determine if live colony surface area, number of initial lesions treated, length of margin or disease-break treatment, and the percent old and new mortality on each coral was different between sites or initial treatment application method. Results of these analyses promoted the grouping of colonies from all sites by treatment application to evaluate the differences in success at halting disease by lesions and pooled by colony.
Fisher’s exact tests and pairwise Fisher’s tests were used to detect significant differences between the success of both treatment materials (antibiotic paste and Chl epoxy). For the purpose of analysis, treatments were considered successful if the intervention halted disease progression and did not fail by the end of the monitoring period. Initial lesion treatments consisted of a margin and a disease-break in combination; the success of both were assessed independently, and in combination, to determine if any significant differences in application method were sequestered to one portion of the treatment. Additionally, both the lesion and colony treatment successes were assessed independently in all statistical tests to determine if disease intervention effectiveness was significantly different between treatments.
The amount of tissue fully healed along the disease-breaks was used to assess differences in healing between treatment materials and application methods. For both treatment types, the proportion of disease-breaks healed for each coral were not normal (Shapiro-Wilk p = 1.58–10) thus non-parametric Kruskal-Wallis one-way analysis of variance were used to determine if differences were significant. A categorical treatment-month variable was used to simultaneously assess the change in healing over time of each treatment, but also the difference in healing between each treatment material at each time. Pairwise multiple comparisons were performed on the treatment-month variable to indicate the level of difference between each treatment material, at each time.
Results
Statistical assessment indicated that there were very few significant differences between sites, and any of the tested coral metrics (Table 1). When pooled by coral, the colony live surface area, number of lesions treated, margin treatment length, disease-break length, the percentage of disease, the percent of old mortality, or the percent of new mortality were not significantly different between the three sites investigated, or between the treatment materials (p > 0.05). When analyzed by lesion, there was a significant difference in disease-break length between sites (p = 0.01913), but pairwise comparisons failed to resolve those differences. Additionally, antibiotic paste disease-breaks were significantly longer than Chl epoxy disease-breaks (p = 0.02485). However, when pooled by coral, disease-break length was not significantly different between sites or treatment materials (p > > 0.05).
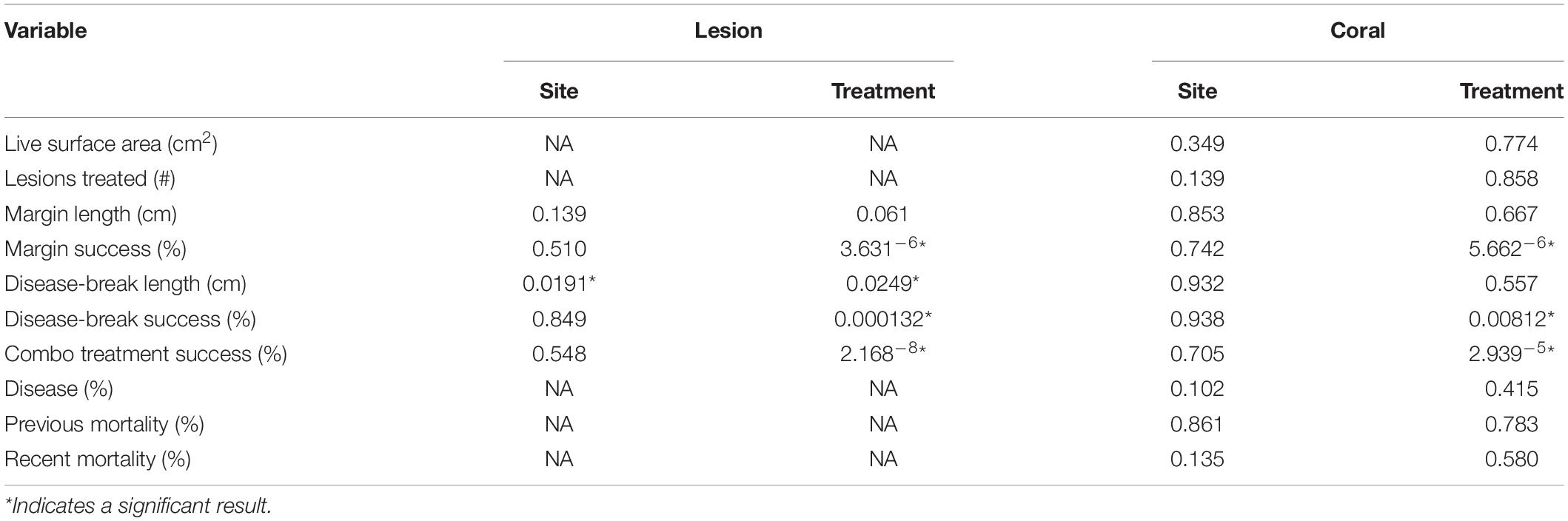
Table 1. Results of the statistical assessment (Kruskal-Wallis p-values) comparing the variable between sites or treatment materials.
Treatment Success
Of the original 41 colonies treated, 34 lesions were treated on 21 colonies with antibiotic paste and 34 lesions on 20 colonies with Chl epoxy (Table 2). Twenty of the antibiotic paste margin treatments stopped the disease and 14 failed (58.8% success), quiescing the disease on 10 colonies (47.6%). All margin failures led to the disease reaching the disease-break, of which 11 quiesced the disease and 3 failed (78% success), quiescing the disease on 8 colonies (38.1%). Thus, the antibiotic paste margin and disease-break treatment combination succeeded 31 out of 34 times (91.2% success), quiescing the disease on 18 out of 21 colonies (85.7%). Conversely, two of the Chl epoxy margin treatments stopped the disease and 32 failed (5.9% success), quiescing the disease on 1 colony (5%). All Chl epoxy margin failures led to the disease reaching the disease-break, of which 6 stopped the disease and 26 failed (19% success), quiescing the disease on 3 colonies (15%). Therefore, the Chl epoxy margin and disease-break treatment combination succeeded 8 out of 34 times (23.5% success), quiescing the disease on 4 out of 20 colonies (20%).
Significant differences were found between the treatment materials and applications related to the proportion of quiesced lesions and corals. When analyzed by lesions, antibiotic paste treatments had a significantly higher proportion of succeeded margins (Fisher’s p = 0.00083), disease-breaks (Fisher’s p = 0.0203), and combination treatments (Fisher’s p = 0.00372) than the Chl epoxy treatments. When pooled by corals, antibiotic paste treatments had a significantly higher proportion of succeeded margins (Fisher’s p = 0.0344) and combination treatments (Fisher’s p = 0.0284) than Chl epoxy, but the difference in disease-break success was not significant (Fisher’s p = 0.308).
The original treatment failure timing varied between materials and application (Figure 3). Most of the margin failures (91.3%) occurred within 9 days of initial treatment, regardless of treatment material (Figures 3A,C), although the proportion of antibiotic paste failures was significantly lower than Chl epoxy failures (Fisher’s p = 0.0169). Very few margin failures occurred after 9 days (8.7%), and all margins that lasted longer than 44 days did not fail (32.3%). Disease-break failures varied in timing and occurred over a longer time period (Figures 3B,D). Chl epoxy disease-breaks failed between 9 and 52 days, with the majority failing between 23 and 30 days post treatment. At 44 days, 61.8% (21/34) of the chlorinated disease-breaks had failed. Conversely at 99 days, only 8.8% (3/34) of the antibiotic disease-breaks had failed.
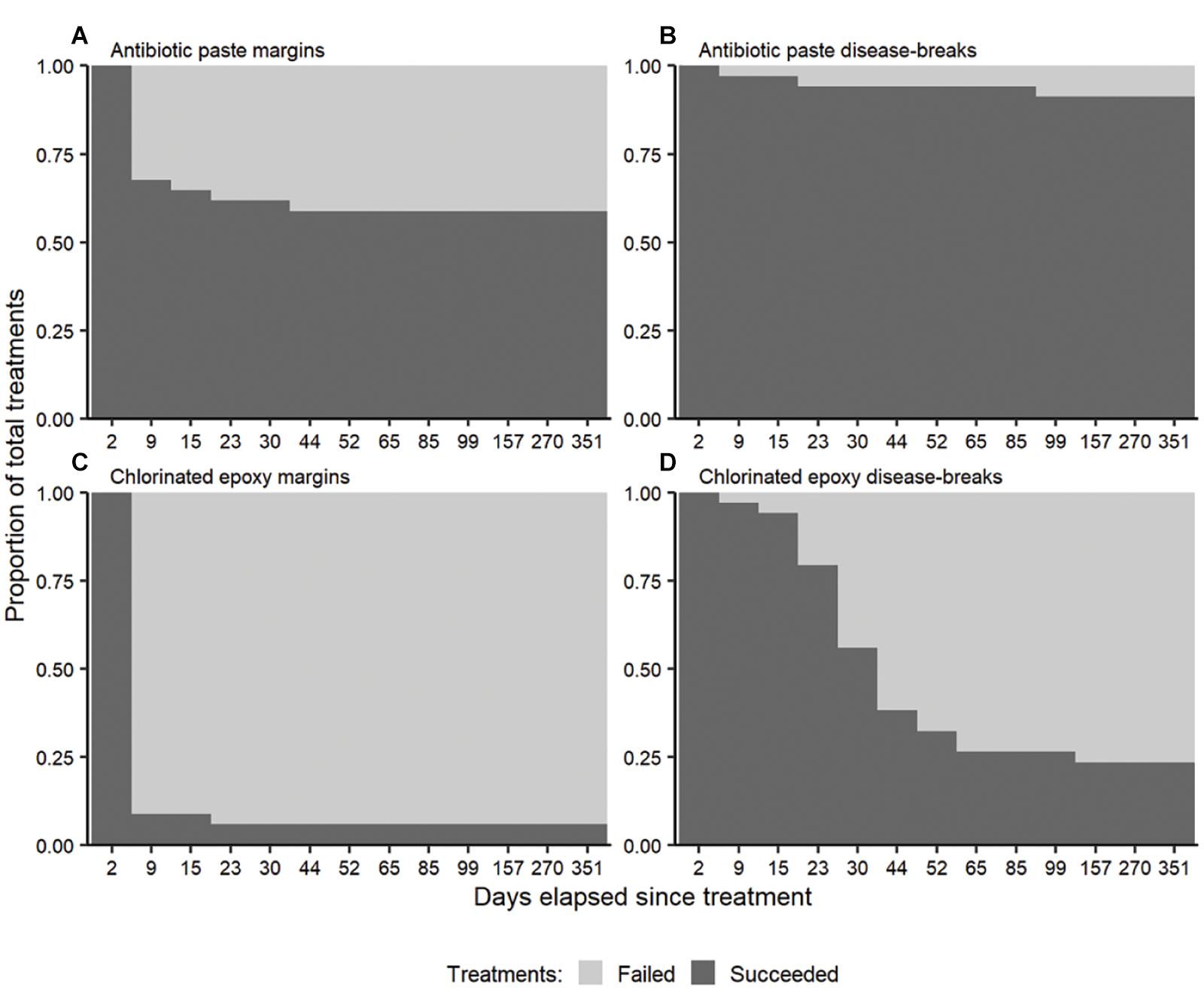
Figure 3. The proportion of treatments (n = 34 per panel) succeeding at halting the disease following antibiotic (A) disease margin and (B) disease-break treatments, and chlorinated epoxy (C) disease margin and (D) disease-break treatments over time. The high initial disease-break successes were from the disease lesions not yet reaching the treatment.
After the Chl epoxy disease-break failures, those lesion margins were retreated with antibiotic paste (no disease-breaks) to test any differences in antibiotic paste treatments between the corals originally treated with different treatment material. On day 23, seven Chl epoxy disease-break failures were retreated on six corals (Table 3). On day 30, seven failures were retreated on seven corals. On day 44, five failures were retreated on four corals. On day 65, five failures were retreated on five corals. And on days 85 and 157, one failure was retreated on one coral. In this mixed-treatment group, the antibiotic paste successfully halted disease progression in 21 of 26 lesions (80.8%) on 11 of the 16 colonies (68.8%). The mixed treatment success was significantly higher than the original Chl epoxy margin treatments (Fisher’s p = 0.0003) and not different from antibiotic paste margin treatment success (Fisher’s p > 0.05). The proportion of mixed treatment corals quiesced by antibiotic paste was not significantly different to the original antibiotic paste treatments (Fisher’s p = 1), nor the Chl epoxy treatments (Fisher’s p = 0.44).
None of the antibiotic paste margin treatment failures quiesced before reaching the disease-break, indicating that the antibiotics in the disease-breaks offered little, if any, effect to the success of the margin treatment. Although, the success of antibiotic paste margin-only treatments on previously untreated colonies (82.8%) was higher than the antibiotic paste margin treatment success of the combination treatments (58.8%), this difference was not significant (Fisher’s p = 0.454) indicating no effect of the disease-break on margin treatment success.
New Lesions
During the study, new lesions occurred on previously treated colonies, as well as colonies not previously treated (Table 3). In total, eight additional colonies were treated after site establishment: three were added on day 15; two on day 23; and one each on days 44, 85, and 157. Six of the eight corals were added during May and early June 2019, which corresponded closely to the peak of new lesions at these sites during the monitoring.
The number of new lesions also varied through time. No new lesions were treated for 2 weeks immediately following initial treatment, but after 44 days, 33 new lesions had been treated, with an additional 18 lesions treated in July (Table 3). Whereas, between August 2019 and January 2020, there were just 12 new lesions treated. The daily infection rate was highest for corals on day 23 with 1 newly infected coral per day since last visit, and for lesions on day 30 with 1.6 new lesions per day since last visit (Figure 4). On day 44 these rates dropped to 0.3 corals with new infections per day and 0.5 new lesions per day since last visit, and stayed relatively stable until dropping noticeably on Day 99 and staying low the remainder of the study.
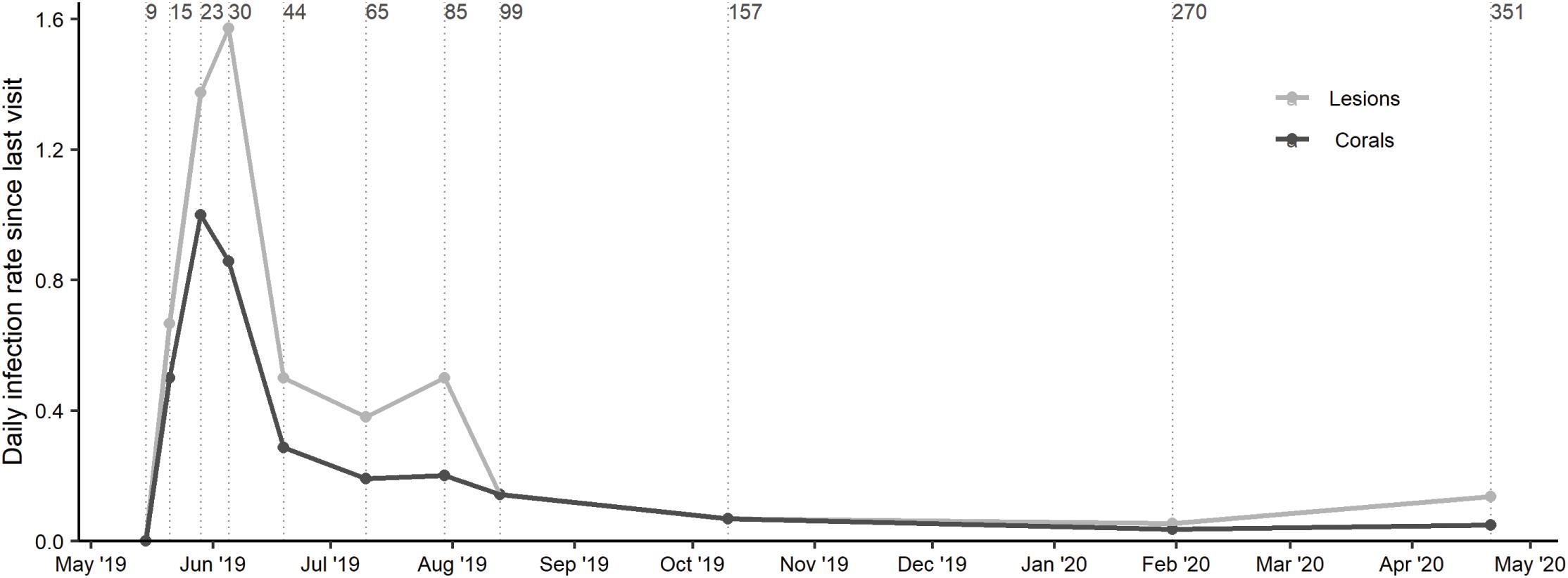
Figure 4. Rates are calculated as the number of new lesions (or new corals)/number of days since last assessed. The rate since last visit of new disease lesions and newly diseased corals. Vertical lines indicate the cumulative number of days elapsed since initial treatments.
There were no statistical differences in new lesions on corals receiving different original treatment materials, indicating no bias between groups of corals. After the original treatments, colonies in both treatment groups had a similar proportion of new lesions: 61.9% (13/21) of the antibiotic paste treatment corals and 55% (11/20) of the mixed treatment corals (Fisher’s p = 1). However, it is unknown if the Chl epoxy treatment corals would have had a different proportion of new lesions if they had not received subsequent antibiotic paste treatments.
The success of new lesion margin treatments on previously treated corals was very high when analyzed by lesion (96.4%; 54/56) and pooled by coral (91.7%; 22/24) (Table 2). However, these differences were not significantly higher than the original margin treatment success analyzed by lesion (Fisher’s p = 0.182) and pooled by coral (Fisher’s p = 0.239).
Disease-Break Healing
Tissue healing over disease-breaks treated with antibiotic paste was more frequent than to those treated with Chl epoxy. Of the original treatments, 90.5% of antibiotic paste-filled disease-breaks exhibited some tissue regrowth compared to just 15% of the disease-breaks filled with Chl epoxy. There was a significantly higher mean proportion of fully healed disease-break area following treatment with amoxicillin, when compared to Chl epoxy in both January (Kruskal-Wallis p = 3.6–5) and April (Kruskal-Wallis p = 1.7–5) (Figure 5). By day 351, the mean disease-break area fully healed for antibiotic paste disease-break treatments was 50.6% (± 7.8%), compared to 2.2% (± 1.9%) for Chl epoxy-filled disease-breaks.
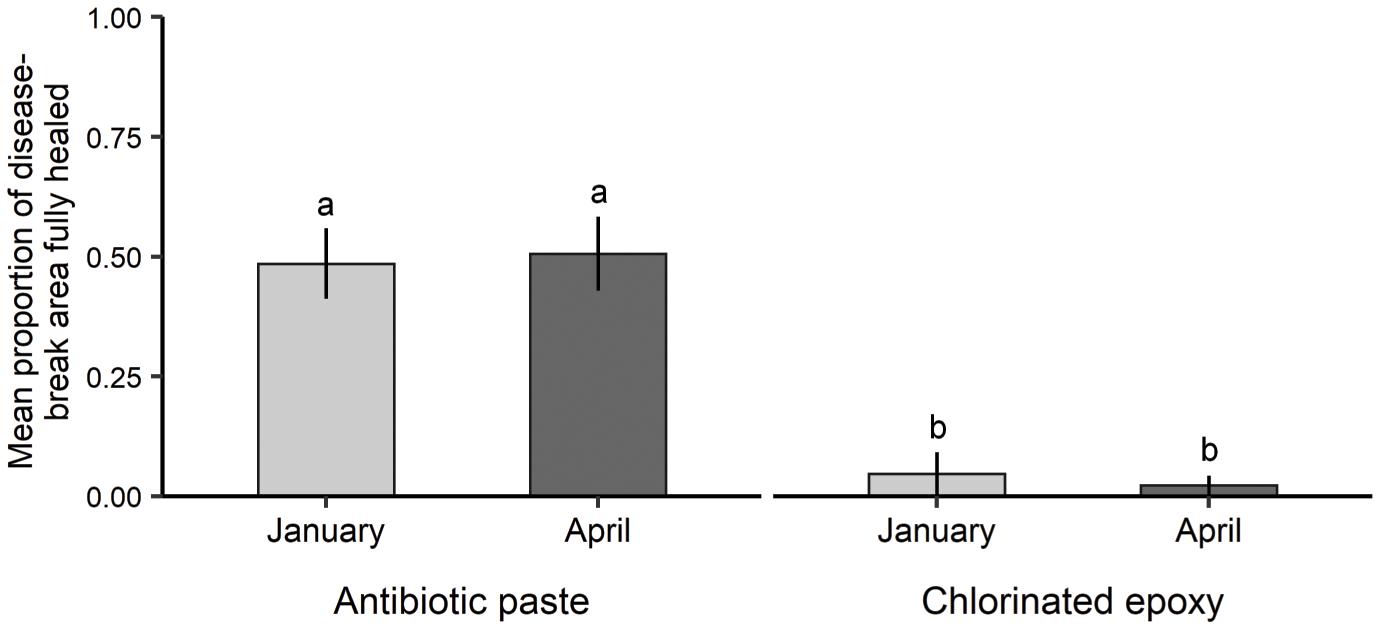
Figure 5. The mean proportion of disease-break area that was fully healed by antibiotic paste and chlorinated epoxy treatments in both months visited. The letters above the bars indicate statistical differences between treatment materials during both months visited. Error bars = standard error.
Discussion
The antibiotic paste used in this study is a highly effective treatment for SCTLD on M. cavernosa when applied both to the disease margin and to a disease-break cut into healthy tissue. Our success rates were consistent with other recent SCTLD interventions using the same materials. For example, Neely et al. (2020) found that Base 2b and amoxicillin margin treatments were 80% effective vs. our result of 82.8% (29/35) effectiveness of margin only treatments. Shilling et al. (2021) found 95% quiescence using margin and disease-break combination treatments vs. 91.2% in this study.
Chlorinated (Chl) epoxy proved far less effective at halting M. cavernosa SCTLD progression (28.6%). This compared closely to the 26% of colonies quiesced in Shilling et al. (2021) with Chl epoxy treatments which were not significantly different than the untreated control colonies. Our study purposefully did not include untreated controls for several reasons. First, two other nearby studies, Aeby et al. (2019) and Shilling et al. (2021), were monitoring control corals that we could reference. Second, it was anecdotally recognized that untreated corals were dying at alarming rates, therefore we chose to include more corals per treatment than to sacrifice some as controls. Thirdly, SCTLD was thought to be a waterborne pathogen and leaving diseased corals at the site might affect the treatment results by providing a nearby source of disease. We do not recommend using Chl epoxy on M. cavernosa, as it may not be different from taking no action.
There are numerous possible reasons for disease intervention (DI) treatment failures and the discrepancy of success between the antibiotic paste and Chl epoxy. First, inconsistent treatment applications are a recognized problem. The objective is to cover all bleached and diseased-looking tissue with treatment material. Neely et al. (2020) found reduced success when paste was not fully contacted with tissue. Treating M. cavernosa is especially challenging because of the species’ polyp size and morphology, tissue thickness, and mucous production. Both treatment materials adhered well to the M. cavernosa rugose dead skeleton, but not to the live tissues. This is problematic because the live tissue at the disease margin is the target of the treatment. Sometimes the disease margin is too wide for the application to adhere solely to the dead skeleton at the margin. The lack of adhesion can lead to an early treatment sloughing, rendering it ineffective.
Another reason for failures and success discrepancy is treatment dosing time. The antibiotic paste was formulated to deliver a high dose of amoxicillin over a 36 h period (Neely et al., 2020), whereas the Chl epoxy has a short hardening time (∼2 h) that bounds the chlorine inside rendering it less effective. A longer exposure time of chlorine may prove more effective, however there are currently no proven treatment methods demonstrating this. Chlorine is difficult to administer in a time release medium as it reacts or dilutes away quickly when exposed to seawater. Chl epoxy has been found more successful on species with smaller polyps and thinner tissues where it can smother live tissue and bind with the skeleton more easily like Orbicella spp. and Montipora spp. (Aeby et al., 2015; Walker et al., 2020), but the antibiotic paste is still more effective.
Finally, it is important to ensure a consistent treatment concentration throughout the treatment material. A thorough mixing of the active agents into the application material is critical to ensuring consistent treatment along the disease margin. Uneven mixing will create portions of application material with little active ingredients which may allow the disease to progress after treatment.
Recent studies indicate that some level of natural disease quiescence can be expected on untreated colonies, but there is high variation depending on the monitoring time of the study, location, environmental conditions, and perhaps time of year. In southeast Florida, Shilling et al. (2021) found that about 40% of untreated colonies quiesced after 46 weeks, whereas Aeby et al. (2019) found 60% (12/20) quiesced after 52 weeks. Neely et al. (2020) found no (0/4) colonies quiesced after 8 weeks in the Florida Keys, while Meiling et al. (2020) found 20% (1/5) of colonies quiesced after 22 weeks in St, Thomas, V.I. Meiling et al. (2020) also reported significant drops in tissue loss rates during high temperature stress in St. Thomas during the late summer and fall. In southeast Florida, we found a drop in new lesion rates without temperature stress during the same time. Additionally, Shilling et al. (2021) first treated colonies in April 2019 and reported quiescence in untreated colonies at 14, 23, and 46 weeks (July, September, and February). Meiling et al. (2020) attributed the slowing of tissue loss to increased temperature stress, however, the coincidence of the slowing of disease in southeast Florida during the same time bears additional investigation. In southeast Florida after monitoring and treating the same colonies monthly for 1.5 years, Walker et al. (2020) found that the number of new infections on Orbicella faveolata colonies varied seasonally with most occurring between June and September, thus it is possible that disease quiescence also has temporal patterns. Conducting intervention studies during different seasons and monitoring for a longer period would help determine seasonal effects on disease quiescence and new lesion occurrence.
Frequent monitoring provided information on optimizing treatment success by capturing the timing of treatment failures and informing when to retreat colonies. The antibiotic paste treatment did not prevent subsequent new lesions and the new lesion rates were similar in each treatment group, indicating that revisitation is necessary to keep the corals alive using the antibiotic paste. The timing and frequency of revisiting likely depends on the treatment method, treatment material, rate of tissue loss, frequency of new lesions, environmental factors, season, and perhaps species. Shilling et al. (2021) recommended revisiting between 2 and 3 months to treat new infections, however most of our failed margin treatments occurred within the first 9 days (Figures 3A,C) indicating the optimum revisiting time was around 10–14 days posttreatment for antibiotic paste margin treatments. Most of our disease-breaks failed before 44 days, extending the necessary return time to 1.5 months. Yet, the new lesion rates were highest in June indicating a new lesion on a coral every day whereas October through May, these rates were extremely low. Considering the high treatment success of the antibiotic paste and the conditional variation of new lesion rates, about 1 month is a good practical re-visitation time for retreating failures and any new lesions.
When deciding on the use of disease-breaks, the increased success must be weighed against the increased treatment application time, materials, coral stress, and healing rates. Disease-breaks kill the live tissue upon contact, carve a trench into the coral skeleton, and isolate the tissues nearest to the disease margin. Aside from local mucus production at the site during creation, there were no other visible signs of coral stress from the trenching. However, physically isolating the disease margin may reduce the colony’s ability to fight the disease and isolating the margin treatment may inhibit any possible benefits of the margin treatment antibiotics from reaching other parts of the colony. This could help explain the lower success of margin treatments when accompanied with a disease-break (58.8%) vs. solo margin treatments (82.8%). Although these successes were not significantly different, the percentage difference is high and bears consideration.
Regardless of the lower margin success, the combined margin and disease-break antibiotic paste treatment was the most effective (91.2%). The high success of the antibiotic paste margin and disease-break combination makes it a good option, especially where re-visitation is not planned, the colonies are of particularly high value, treatment material is in abundant supply, and/or disease prevalence is relatively low. However, creating and treating disease-breaks takes time that may be better spent treating the margins of other colonies if disease prevalence is high.
Antibiotic paste disease-breaks showed extensive healing where the intervention was virtually unnoticeable after 1 year on many corals (Figure 2). Since 50.6% of the disease-break area treated with amoxicillin reconnected and fully healed during our study, it appears tissue isolation was temporary in many cases. Disease-breaks filled with Chl epoxy were far less likely to yield tissue regrowth (<5%). It appeared that the permanent presence of the epoxy and subsequent colonizers hindered the natural coral wound healing process.
There are environmental concerns that releasing antibiotics into the ocean may have unintended negative environmental impacts, like promoting antibiotic resistant bacteria or slowing coral growth (Sweet et al., 2011; Zhang et al., 2018). Unfortunately, antibiotics and other pollutants have been released into the coastal system for many years in southeast Florida through multiple wastewater ocean outfalls that expel over 510 million gallons per day of treated effluent (Koopman et al., 2006). The Miami-Dade Central Wastewater Treatment Plant located on Virginia Key, releases ∼143 million gallons of wastewater daily (Koopman et al., 2006). This effluent is partially treated but still contains nutrients and chemicals such as hormones and antibiotics (Englehardt et al., 2001). Recently, multiple antibiotic resistance genes, including ampC (which bestows amoxicillin resistance), were found year-round in samples taken from the water column and in the sediments around outfall pipes (Griffin et al., 2020). There were no observed impacts of the antibiotic treatments on the treated corals or surrounding organisms other than stopping the disease lesion progression (e.g., no fish or invertebrate grazing on material, no obvious new maladies in surrounding organisms, no new blooms or mortality). To minimize the risk of developing antibiotic resistance, we used a highly concentrated dose which killed both the pathogen and the underlying coral tissue. This dose is less likely to foster/generate antibiotic resistance than multiple lower doses designed to boost the coral’s immune response (Roberts et al., 2008). Although the scale of what is released into the ocean by the outfalls dwarfs the amounts released in SCTLD interventions, the negative ecological impacts of antibiotics to the reef system remains unknown and should be considered in DI efforts, especially in more pristine locales.
The bottom line is that coral disease interventions can save corals. These activities are currently the most effective way to intervene the SCTLD epidemic, but they are only effective as a stopgap measure while the larger causative agents are identified and remediated. Conducting DI at a reef-scape scale (1–10 km2) is time consuming and requires extensive person-power and resources, making it very expensive. But this expense pales in comparison to the current cost to restore the diversity and live tissue saved with DI. DI also comes with the risk of introducing antibiotics into coral reef environments, which may have unintended outcomes (Zhang et al., 2018). Since interventions are not yet practical at a seascape scale, strategies should be employed to target specific disease intervention goals such as prioritizing specific locations, species, and/or individuals to save. We encourage others to continue testing new treatments and addressing other factors affecting coral health. Ideally these are fast one-time highly effective treatments with materials that have a low risk of adverse impacts to the corals or other reef organisms and would heal quickly.
Data Availability Statement
The datasets presented in this study can be found in online repositories. The names of the repository/repositories and accession number(s) can be found below. Please visit our Story map to stay up to date on the latest activities: https://novasoutheastern.maps.arcgis.com/apps/Cascade/index.html?appid=cf2bf16f698d40968d437d402199e027. The data for this study can be found in the Florida Fish and Wildlife Conservation Commission Coral Disease Intervention Dashboard: https://www.arcgis.com/apps/opsdashboard/index.html#/55a759f02f3c486eb1d29a95f80fba0a.
Author Contributions
BW conceived the research idea and design, funded the project, helped collect data, analyzed the data, and wrote most of the manuscript. NT setup the database in R, ran the statistics, wrote some methods and results, and produced the figures. HN collected, analyzed the data, and assisted with writing. SB and KP helped with initial setup and field work, collected, entered, managed data, and helped with stats. All authors contributed to the article and approved the submitted version.
Funding
Funding was provided by the Florida Department of Environmental Protection Award No. B558F2. The views, statements, findings, conclusions, and recommendations expressed herein are those of the authors and do not necessarily reflect the views of the State of Florida or any of its sub-agencies.
Conflict of Interest
The authors declare that the research was conducted in the absence of any commercial or financial relationships that could be construed as a potential conflict of interest.
Acknowledgments
We thank the Florida Department of Environmental Protection’s Office of Resilience and Coastal Protection (DEP RCP) for funding support. We thank the Florida Coral Disease Advisory Committee for the large number of volunteers assisting in the meeting and planning of coral disease efforts. This work was conducted under the State of Florida Special Activity License Permit SAL-19-2022A-SRP. We thank the Florida Fish and Wildlife Conservation Commission and Lisa Gregg for assisting with permitting. We thank the DEP RCP staff including Kristi Kerrigan for contract and project coordination. We thank to Elizabeth Fromuth and Kristin Anderson at the NSU GIS and Spatial Ecology lab.
References
Aeby, G. S., Ushijima, B., Campbell, J. E., Jones, S., Williams, G. J., Meyer, J. L., et al. (2019). Pathogenesis of a tissue loss disease affecting multiple species of corals along the Florida reef tract. Front. Mar. Sci. 6:678. doi: 10.3389/fmars.2019.00678
Aeby, G. S., Work, T. M., Runyon, C. M., Shore-Maggio, A., Ushijima, B., Videau, P., et al. (2015). First record of black band disease in the Hawaiian Archipelago: response, outbreak status, virulence, and a method of treatment. PLoS One 10:e0120853. doi: 10.1371/journal.pone.0120853
Alvarez-Filip, L., Estrada-Saldívar, N., Pérez-Cervantes, E., Molina-Hernández, A., and González-Barrios, F. J. (2019). A rapid spread of the stony coral tissue loss disease outbreak in the Mexican Caribbean. PeerJ 7:e8069. doi: 10.7717/peerj.8069
Bruckner, A. W. (2015). “White syndromes of western atlantic reef-building corals,” in Diseases of Coral, eds C. M. Woodley, C. A. Downs, A. W. Bruckner, J. W. Porter, and S. B. Galloway (Hoboken, NJ: Wiley), 316–332. doi: 10.1002/9781118828502.ch22
Englehardt, J., Amy, V., Bloetscher, F., Chin, D., Fleming, L., Gokgoz, S., et al. (2001). Comparative Assessment of Human and Ecological Impacts from Municipal Wastewater Disposal Methods in Southeast Florida. Coral Gables, FL: University of Miami.
Green, E. P., and Bruckner, A. W. (2000). The significance of coral disease epizootiology for coral reef conservation. Biol. Conserv. 96, 347–361. doi: 10.1016/S0006-3207(00)00073-2
Griffin, D. W., Banks, K., Gregg, K., Shedler, S., and Walker, B. K. (2020). Antibiotic resistance in marine microbial communities proximal to a Florida sewage outfall system. Antibiotics 9:118. doi: 10.3390/antibiotics9030118
Harvell, D., Altizer, S., Cattadori, I. M., Harrington, L., and Weil, E. (2009). Climate change and wildlife diseases: when does the host matter the most? Ecology 90, 912–920. doi: 10.1890/08-0616.1
Heres, M. M., Farmer, B. H., Elmer, F., and Hertler, H. (2021). Ecological consequences of stony coral tissue loss disease in the Turks and Caicos Islands. Coral Reefs 40, 609–624. doi: 10.1007/s00338-021-02071-4
Kassambara, A. (2021). rstatix: Pipe-Friendly Framework for Basic Statistical Tests. Available online at: https://CRAN.R-project.org/package=rstatix (accessed February 2021).
Koopman, B., Heaney, J., Cakir, F., Rembold, M., Indeglia, P., and Kini, G. (2006). Ocean Outfall Study: Final Report. Tallahassee, FL: Prepared for the Florida Department of Environmental Protection.
Landsberg, J. H., Kiryu, Y., Peters, E. C., Wilson, P. W., Perry, N., Waters, Y., et al. (2020). Stony coral tissue loss disease in Florida is associated with disruption of host–zooxanthellae physiology. Front. Mar. Sci. 7:576013. doi: 10.3389/fmars.2020.576013
Meiling, S., Muller, E. M., Smith, T. B., and Brandt, M. E. (2020). 3D photogrammetry reveals dynamics of stony coral tissue loss disease (SCTLD) lesion progression across a thermal stress event. Front. Mar. Sci. 7:597643. doi: 10.3389/fmars.2020.597643
Meyer, J. L., Castellanos-Gell, J., Aeby, G. S., Häse, C. C., Ushijima, B., and Paul, V. J. (2019). Microbial community shifts associated with the ongoing stony coral tissue loss disease outbreak on the Florida reef tract. Front. Microbiol. 10:2244. doi: 10.3389/fmicb.2019.02244
Muller, E. M., and van Woesik, R. (2014). Genetic susceptibility, colony size, and water temperature drive white-pox disease on the coral Acropora palmata. PLoS One 9:e110759. doi: 10.1371/journal.pone.0110759
Muller, E. M., Sartor, C., Alcaraz, N. I., and van Woesik, R. (2020). Spatial epidemiology of the stony-coral-tissue-loss disease in Florida. Front. Mar. Sci. 7:163. doi: 10.3389/fmars.2020.00163
Neely, K. L., Macaulay, K. A., Hower, E. K., and Dobler, M. A. (2020). Effectiveness of topical antibiotics in treating corals affected by stony coral tissue loss disease. PeerJ 8:e9289. doi: 10.7717/peerj.9289
O’Neil, K., Neely, K., and Patterson, J. (2018). Nursery management and treatment of disease ravaged pillar coral (Dendrogyra cylindrus) on the Florida Reef Tract. Rep. Fl. DEP. 1–13.
Precht, W. F., Gintert, B. E., Robbart, M. L., Fura, R., and van Woesik, R. (2016). Unprecedented disease-related coral mortality in Southeastern Florida. Sci. Rep. 6:31374. doi: 10.1038/srep31374
R Core Team (2021). R: A Language and Environment for Statistical Computing. Vienna: R Foundation for Statistical Computing.
Roberts, J. A., Kruger, P., Paterson, D. L., and Lipman, J. (2008). Antibiotic resistance—what’s dosing got to do with it? Crit. Care Med. 36, 2433–2440. doi: 10.1097/CCM.0b013e318180fe62
Sharp, W. C., Shea, C. P., Maxwell, K. E., Muller, E. M., and Hunt, J. H. (2020). Evaluating the small-scale epidemiology of the stony-coral -tissue-loss-disease in the middle Florida Keys. PLoS One 15:e0241871. doi: 10.1371/journal.pone.0241871
Shilling, E. N., Combs, I. R., and Voss, J. D. (2021). Assessing the effectiveness of two intervention methods for stony coral tissue loss disease on Montastraea cavernosa. Sci. Rep. 11:8566. doi: 10.1038/s41598-021-86926-4
Sweet, M. J., Croquer, A., and Bythell, J. C. (2011). Dynamics of bacterial community development in the reef coral Acropora muricata following experimental antibiotic treatment. Coral Reefs 30:1121. doi: 10.1007/s00338-011-0800-0
Thome, P. E., Rivera-Ortega, J., Rodríguez-Villalobos, J. C., Cerqueda-García, D., Guzmán-Urieta, E. O., García-Maldonado, J. Q., et al. (2021). Local dynamics of a white syndrome outbreak and changes in the microbial community associated with colonies of the scleractinian brain coral Pseudodiploria strigosa. PeerJ 9:e10695. doi: 10.7717/peerj.10695
Voss, J., Shilling, E., and Combs, I. (2019). Intervention and Fate Tracking for Corals Affected by Stony Coral Tissue Loss Disease in the Northern Florida Reef Tract. Miami, FL: Florida DEP.
Walker, B. K. (2012). Spatial analyses of benthic habitats to define coral reef ecosystem regions and potential biogeographic boundaries along a latitudinal gradient. PLoS One 7:e30466. doi: 10.1371/journal.pone.0030466
Walker, B. K., Riegl, B., and Dodge, R. E. (2008). Mapping coral reef habitats in southeast Florida using a combined technique approach. J. Coast. Res. 24, 1138–1150. doi: 10.2112/06-0809.1
Walker, B., Noren, H., Brunelle, A., and Buckley, S. (2020). SE FL ECA Reef-Building-Coral Disease Intervention and Preparation for Restoration: Final Report. Miami, FL: Florida DEP.
Walton, C. J., Hayes, N. K., and Gilliam, D. S. (2018). Impacts of a regional, multi-year, multi-species coral disease outbreak in Southeast Florida. Front. Mar. Sci. 5:323. doi: 10.3389/fmars.2018.00323
Weil, E. (2019). “Disease problems,” in Mesophotic Coral Ecosystems, eds Y. Loya, K. A. Puglise, and T. C. L. Bridge (Cham: Springer International Publishing), 779–800.
Weil, E., and Rogers, C. S. (2011). “Coral reef diseases in the Atlantic-Caribbean,” in Coral Reefs: An Ecosystem in Transition, eds Z. Dubinsky and N. Stambler (Dordrecht: Springer Netherlands), 465–491. doi: 10.1007/978-94-007-0114-4_27
Weil, E., Croquer, A., and Urreiztieta, I. (2009). Temporal variability and impact of coral diseases and bleaching in La Parguera, Puerto Rico from 2003–2007. Caribb. J. Sci. 45, 221–246. doi: 10.18475/cjos.v45i2.a10
Keywords: tissue loss disease, white plague, white syndrome, coral disease intervention, amoxicillin, coral antibiotics, florida’s coral reef, southeast florida coral reef ecosystem conservation area
Citation: Walker BK, Turner NR, Noren HKG, Buckley SF and Pitts KA (2021) Optimizing Stony Coral Tissue Loss Disease (SCTLD) Intervention Treatments on Montastraea cavernosa in an Endemic Zone. Front. Mar. Sci. 8:666224. doi: 10.3389/fmars.2021.666224
Received: 09 February 2021; Accepted: 27 May 2021;
Published: 02 July 2021.
Edited by:
Lorenzo Alvarez-Filip, National Autonomous University of Mexico, MexicoReviewed by:
Aldo Cróquer, The Nature Conservancy, Dominican RepublicMichael S. Studivan, University of Miami, United States
Ernesto Weil, University of Puerto Rico at Mayagüez, Puerto Rico
Copyright © 2021 Walker, Turner, Noren, Buckley and Pitts. This is an open-access article distributed under the terms of the Creative Commons Attribution License (CC BY). The use, distribution or reproduction in other forums is permitted, provided the original author(s) and the copyright owner(s) are credited and that the original publication in this journal is cited, in accordance with accepted academic practice. No use, distribution or reproduction is permitted which does not comply with these terms.
*Correspondence: Brian K. Walker, d2Fsa2VyYkBub3ZhLmVkdQ==