- 1Cell, Molecular Biology and Genomics Group, Department of Biology, Norwegian University of Science and Technology, Trondheim, Norway
- 2Taskforce Salmon Lice, Department of Biology, Norwegian University of Science and Technology, Trondheim, Norway
The arthropod salmon louse (Lepeophtheirus salmonis) is a major threat to Atlantic salmon aquaculture and wild salmonids. Essentially like in monoculture, very high concentrations of susceptible hosts may result in high reproduction and severe production of waves of pests. Pest management is crucial both for fish health and protection of wild fish populations from aquaculture influence. Various methods have been utilized to control salmon lice infestations, such as pesticide use, physical treatments, construction modifications, fallowing, breeding, vaccination, and biological control. Most of the methods are partially successful, but none completely fulfills the necessary pest control strategy. Like in agriculture, lice/pest management is an arms race, but the marine environment makes it even more difficult to precisely hit the target pest and avoid unintended negative effects on general wildlife. In this study, we provide an overview of the methods and principles of salmon lice management and address current possibilities and limitations. We also highlight the potential of emerging strategies and enabling technologies, like genome editing, RNA interference, and machine learning, in arthropod management in aquaculture.
Introduction
Human population is projected to increase to 11.2 billion by the end of the century, and the buying power is expected to continue to increase (UN Economic & Social Affairs, 2019). These increases will drive the need for human food, and scarcity is expected to be a great challenge for humans in the coming century (FAO, 2020a). The cultivable land needed for agriculture is decreasing and the future of food production is likely in the marine environment. At least one-third of agricultural output is currently lost due to damage caused by pests and diseases (Oerke et al., 2012). Arthropods, by far the largest invertebrate phylum that include approximately eighty percent of all animal species inhabiting the earth (Zhang, 2013; Ghafor, 2020), cause a main problem. Agricultural losses due to arthropods are enormous (Bradshaw et al., 2016), and major attention has been paid to the study of terrestrial arthropods. Comparatively, the marine arthropods and parasites have received less attention.
Over the last 70 years, global fish and seafood production has been a success story and the production has increased sevenfold (FAO, 2020b). The production value of the Atlantic salmon and other salmonids added up to 13.1 billion euros in 2018. Vaccination programs and best practice in cultivation have resulted in a drastic reduction in disease outbreaks as well as in medical treatments. The use of antibiotics in salmon farming in Norway alone has, for example, been reduced from approximately 2.13 μg/kg fish in 2006 to 0.14 μg/kg fish in 2019 (Directorate of Fisheries, 2020; Norwegian Institute of Public Health, 2020) mainly due to mass vaccination of juvenile salmonids. During these years, the use of antibiotics has also been drastically reduced in Scotland and Canada (Love et al., 2020). Pest control still represents, however, a major challenge and limits the growth potential of the salmon industry. As in terrestrial agriculture, the cost of arthropods to the salmon industry is very high. Salmon lice (Lepeophtheirus salmonis) are a major threat to both farmed and wild Atlantic salmon in the North Atlantic region (Costello, 2006; Torrissen et al., 2013), and Caligus rogercresseyi is considered a major threat to salmon in Chile (Gonzalez et al., 2000; González et al., 2015). L. salmonis is known to have two allopatric subspecies, L. salmonis salmonis from the Atlantic and L. salmonis oncorhynchi from the pacific, each having biological, morphological, and genetic differences (Skern-Mauritzen et al., 2014). The financial losses caused by lice infestation amount to about 9% of overall production revenue (Abolofia et al., 2017). The first-hand value of salmon in 2019 was approx. 6 billion euro in Norway (SSB, 2020), whereas in Scotland it was valued at approx. 1.2 billion euro (Munro, 2020). The expenses for combating salmon lice in the Norwegian salmon industry was estimated to be around 500 million euro (Iversen et al., 2019), 17 million euro in Canada, 304 million euro in Chile, and 78 million euro in Scotland in 2019 (Just Economics, 2021). Salmon lice limit the further growth of the salmon aquaculture industry.
In this study, we present a broad overview of current scientific advances as well as methods and principles employed to combat this significant marine parasite (Figure 1).
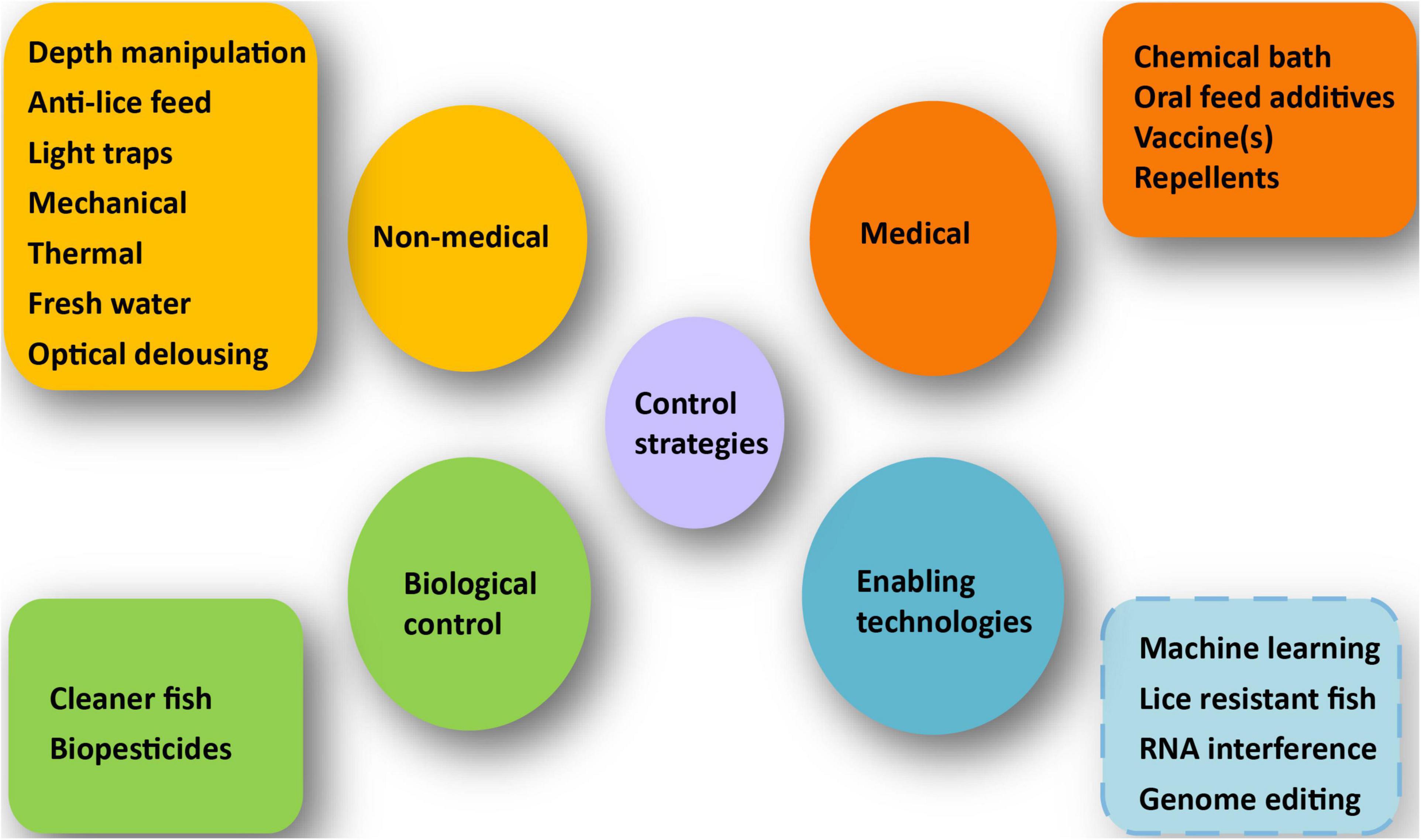
Figure 1. Various control strategies for salmon lice management. The figure displays an overview of recent scientific advances, as well as methods and strategies for combating salmon lice, discussed in the text. The dotted blue box depicts the technologies that are still in the developing phase but might have a big influence on salmon lice management in the future.
History
The Norwegian-Danish Bishop Erik L. Pontoppidan first described the salmon lice in a text, stating that the salmon had partly returned from the sea to freshwater to get rid of greenish vermin by rubbing and washing in the rapid stream of waterfalls (Pontoppidan, 1755; see Torrissen et al., 2013). Salmon louse was later classified by the Danish zoologist Henrik Nikolai Krøyer as Lepeophtheirus salmonis in 1837 (Krøyer, 1837; IMR, 2009). Severe infestation of sea lice on fish, causing fish deaths in Canada, was described in a 1940 report (White, 1940; see Torrissen et al., 2013). In the early 1970s, Harald Skjervold and Trygve Gjedrem developed modern principles for the breeding of the Atlantic salmon and pioneered an important industry in Norway (Syrstad, 2009). The first outbreak of sea lice in aquaculture facilities was reported in the 1960s (Misund, 2018). It is therefore worth noting that salmon lice were present in salmon populations as a natural parasite long before aquaculture. Adverse reactions of salmon lice to other salmonids and to wild salmon were recorded for the first time in 1992. The later industrialized farming of salmon increased the number of hosts, which in turn increased the number of lice and, subsequently, the infection rate of both farmed salmon and wild salmonids (Heuch and Mo, 2001). The number of salmonids in fish pens in Norway has risen from approximately 9.5 million in 1994 to 445 million in 2019, according to data from the Norwegian Directorate of Fisheries. Chile is the world’s second largest producer of salmon after Norway, accounting for 30% of total farmed salmon production (FAO, 2019). Salmonid industry is also booming in the United Kingdom and Canada, and the number is rapidly increasing.
Impact of Salmon Lice
The salmon louse is a salmonid parasite specialized on host fish of the three genera Salmo, Salvelinus, and Oncorhynchus (Wootten et al., 1982). Salmon lice have a significant effect on salmonids during the marine part of their life cycle since they feed on fish mucus, blood, and epidermal tissues (Costello, 2006). The effects of these parasites on fish include physiological stress, growth reduction, immune system suppression, and osmoregulation imbalance (Johnson et al., 2004; Costello, 2006). Higher rates of infestation lead to skin lesions, secondary microbial and viral infections, and, ultimately, increased mortality in the absence of treatment (Grimnes and Jakobsen, 1996; Pike and Wadsworth, 1999). The infestation of salmon lice has a huge economic impact on the aquaculture industry and is projected to escalate further in the coming years (Liu and Bjelland, 2014). In Norway, salmon lice levels are regulated by law in order to protect wild salmon (traffic light system) (Fiskeridepartementet, 2015), and this increases the intensity of treatment and handling, which drives up costs and causes fish welfare issues. Increased cost of production due to salmon lice come from medical and non-medical treatments, cleaner fish, net cleaning, stress and increased mortality, reduced weight gain, fish handling costs, and higher ratios of feed consumption (Iversen et al., 2018, 2019). Salmon lice may also be reservoirs of fish pathogens (Gonçalves et al., 2020).
Control of Salmon Lice
Control of salmon lice includes non-medical approaches and medical delousing. Non-medical approaches are divided into preventive and physical delousing methods. Due to its consistency and efficacy, the most common delousing method is medical treatments (Aaen et al., 2015). Attempts to develop resistant fish and vaccines against salmon lice have also been reported (Kaur et al., 2015; Contreras et al., 2020; Swain et al., 2020). Regulatory authorities in salmon-producing nations have established mandatory and maximum thresholds on the number of mature or motile lice per farmed fish at production sites to limit the transmission of sea lice and sea-lice larvae from farming facilities to wild salmon smolts. There is a legal limit in Norway for the maximum mean number of lice per farmed salmon, as well as obligatory reporting of lice numbers to authorities and delousing if the limit is exceeded (Heuch et al., 2005). The regulatory requirement of lice abundance threshold in various locations is summarized in Table 1.
Medical Methods
Medical Treatment and Resistance
Medical treatment has been widely used to combat the problem of sea lice, as it is the most productive and predictable measure (Aaen et al., 2015). In 1974, Norway began treatment of sea lice with the use of organophosphate metrifonate, followed by Scotland in 1979, Chile in 1981, and Canada and Faroe Islands in the mid to late 1990s (Grave et al., 2004; Aaen et al., 2015). Since then, different forms of chemicals have been used as bath treatments, e.g., organophosphates, pyrethroids and hydrogen peroxide, and as feed additives, e.g., emamectin benzoate and benzoyl urea (Table 2) (Burridge et al., 2010; Aaen et al., 2015). The cost of medication is relatively high and was estimated to be in the range of 1–2 billion NOK (100–200 million euro) in 2014 (Iversen et al., 2018), excluding the costs of Chile, the United Kingdom, Canada, Ireland, Faroe Islands, and the United States.
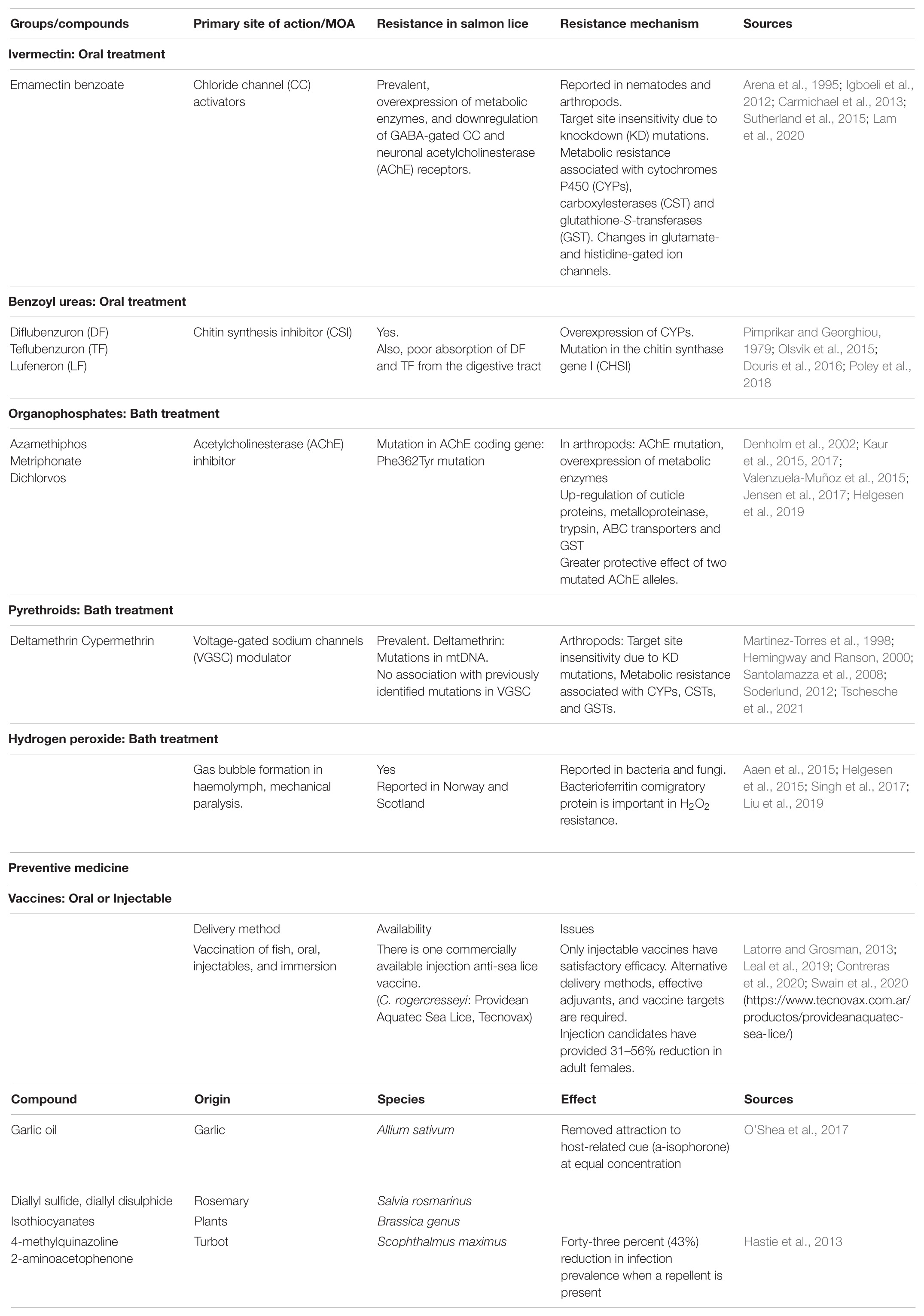
Table 2. Chemicals, Vaccines, and Repellents used in salmon lice control, as well as the concerns associated with them.
The extensive use of chemical agents to combat sea lice has led to an inevitable drift toward drug-resistant parasites (Aaen et al., 2015). Successful treatment of the pest depends on the doses administered. For parasites like sea lice, the dosage is also determined by the host’s ability to tolerate the toxicity of the agent. A smaller reduction in dosage may reduce the effect on parasites and generate resistance driven by natural selection in pests (Kunz and Kemp, 1994). Various genetic resistance mechanisms, such as point mutation in a target gene, upregulation of detoxification metabolism and efflux pumps in the intestines of parasites, changes in the thickness of the cuticle and other mechanisms to reduce chemical penetration, have been documented in arthropods (Brattsten et al., 1986; Aaen et al., 2015).
Resistance to a number of commonly used delousing chemicals has been registered, as well as ineffective treatment with emamectin benzoate and pyrethroids since 2008 (Aaen et al., 2015; Grøntvedt et al., 2016; Fjørtoft et al., 2020). Treatment failure and low treatment efficacy with azamethiphos, first noted in 2009 after re-introduction, is widespread along the coast (Kaur et al., 2015; Grøntvedt et al., 2016; Jensen et al., 2017). Losses of sensitivity to hydrogen peroxide is also reported (Helgesen et al., 2017). The number of prescriptions for medical treatment of sea lice is decreasing in recent years. Chemical agents are not prescribed when the efficacy of the treatment is low due to resistance. This could have contributed significantly to the decrease in prescription rates (Helgesen et al., 2017).
Chemical agents used against salmon lice might have negative effects on non-target species. Spillover from treatment around the fish farm could pose a significant risk to non-target organisms such as lobster (Olsvik et al., 2015), shrimp, and other crustaceans and bivalves. Local treatments in the marine environment have limitations due to, for example, changing volume of water, addition of solvent to large volumes, and water currents, making precision difficult. Negative effects on many non-target species studied have been observed at lower concentration levels of chemotherapeutic agents than those used for salmon lice treatment (Macken et al., 2015; Urbina et al., 2019). This may have an undesirable effect on the food web in coastal ecosystems of surrounding waters. Medical use has its limitations and alternative methods like vaccine development has gained scientific interest and financial support.
Vaccines
In the face of the current COVID-19 pandemic, research on vaccine development has received significant financial and political support. In the year 2020, a substantial number of studies using different approaches to vaccine development were tested by commercial and academic actors. Special attention has been paid to mRNA-based vaccines due to the high potency, rapid development, low-cost manufacturing, and high efficacy demonstrated in studies based on animal models (Sandbrink and Shattock, 2020). In vitro-synthesized mRNA-based vaccines combined with lipid nanoparticles have been the first to be approved against SARS-CoV-2 outbreak (Anderson et al., 2020; Mulligan et al., 2020). Although mRNA vaccines are not currently available in aquaculture, this brings hope for successful research and potential application in other areas where vaccines are needed, as well as vaccine research in general.
In aquaculture, the introduction of vaccines as a preventive method is a necessity for sustainable fish farming (Adams, 2019). From the experience of the viral and bacterial vaccines produced for salmon, we see how effective vaccines can be in aquaculture (Sommerset et al., 2005). However, cost-efficient vaccination against salmon lice remains an unsolved challenge. Vaccines against ectoparasites are still in their infancy, with a few successful commercial products providing reliable protection, such as against cattle tick (Willadsen et al., 1995). The complexity of interactions between vertebrate host and arthropod parasites is yet to be explored for potential vaccine targets (Raynard et al., 2002; Fuente et al., 2016). Translated research from advances in the tick vaccine have already provided promising results in peptide vaccines against salmon lice. Peptides from salmon lice were conjugated with T-cell epitopes (TCEs), that are universally immunogenic in mammalian systems (Panina-Bordignon et al., 1989; Leal et al., 2019). TCEs from tetanus toxin and measles virus are known to increase the immunogenicity in salmonid immune system (Leal et al., 2019; Contreras et al., 2020; Swain et al., 2020). Moreover, technologies, such as adjuvants and nanoparticles, in combination with conventional approaches for enhanced immunogenicity are additional perspectives for the future (Dadar et al., 2017; Angulo et al., 2020).
So far, there is only one commercial injectable vaccine available for sea lice, C. rogercresseyi namely Tecnovax’s Providean Aquatec Sea Lice. The vaccine was reported to have efficacy of 61–86% reduction of the number of post-challenge parasitic stages of lice in immunized trout (Latorre and Grosman, 2013). Only injectable vaccines have provided positive results, but they are far from optimal. While protection is generally high, application is labor-intensive and stressful for the fish (Angulo et al., 2020). However, with the introduction of high-throughput injectable devices capable of injecting thousands of fishes under anesthesia every hour, application time has been greatly reduced. Furthermore, additional efforts in salmon lice vaccine research could also be directed toward alternative routes of administration. One of the preferred methods is oral administration, as it can be easily supplied as a component of the fish feed (Mutoloki et al., 2015), having the potential to be adopted by aquaculture industries. There is so far no oral vaccine available for salmon lice, but the challenge is addressed in the research project Mucoprotect (NFR, 2021). Oral vaccines, however, need to confer immunity at clinically relevant levels while being affordable for usage in the pre-smolt stage. Fish immunology is a rising field of bewildering complexity just on the verge of inception. It will require years of research to achieve cost-efficient and sustainable vaccination against salmon lice.
Repellents
Another class of compounds, repellents, which are a subclass of semiochemicals, act on parasite olfactory systems, interfering with the settlement on the host. These chemicals act as masking compounds for salmon-derived kairomones, a sensory cue for salmon lice. Some natural compounds derived from plants have been tested as novel feed-through repellents and shown positive results by masking host-related compounds in Y-tube behavioral bioassays (O’Shea et al., 2017). Interestingly, even compounds derived from other fish have been shown to act as repellents, significantly reducing the infection rate of L. salmonis (Hastie et al., 2013). Despite showing promising results in laboratory conditions, these repellants require further validation of their efficacy and cost-efficiency in open farms. It will likely be challenging to solve the problem of salmon lice infestation solely by introducing repellants due to the rapid dilution of chemical substances in open sea, but they may be employed in combination with other treatments.
Non-Medical Methods
There is a shift toward the use of non-medical solutions to control salmon lice infestation levels (Overton et al., 2019). This is, among others, as a result of the broad resistance developed against all available chemical agents (Aaen et al., 2015). Preventive measures such as cage depth manipulations, anti-lice functional feeds, lice skirts, and lice traps are part of salmon louse management plans. The use of direct physical and mechanical removal of lice and cleaner fish is growing. Furthermore, the relevance of environmental effects, such as temperature and local wind, and current trends in salmon louse management strategies with antagonistic selective effects that delay louse development should be considered when choosing different approaches (Jevne and Reitan, 2019; Coates et al., 2021). Current and novel innovations used to pursue non-medical alternatives are summarized in Table 3.
Preventive Methods
Cage Depth Manipulations
Salmon lice larvae have evolutionary preference for residing in the upper part of the water column, a strategy to increase the likelihood of finding potential hosts (Heuch et al., 1995). This phototropic behavior has been explored in countermeasures taken to prevent lice contact with fish. Lice skirts, where tarpaulin is wrapped 5–10 m around the upper part of salmon pens, may act as a shield to prevent infective stages of lice from entering the pens from outside the surface waters (Frank et al., 2014). The skirt may decrease the infestation levels, but it may also cause a serious decrease in oxygen levels available for fish inside the skirt (Stien et al., 2012).
Similarly, with deep lighting and submerged feeding, fish change their swimming behavior during the feeding process and are attracted to larger depths assumed to have lower lice larval concentrations. The lice population present at any given time on salmon was found to be lower with deep lighting alone than with submerged feeding alone (Frenzl et al., 2014). During the day, however, natural light may have an impact on the efficacy of deep lighting due to the preference of salmon for natural light and their migration to the upper part of the water column (Frenzl et al., 2014). Furthermore, submerged lights can have a negative influence because certain wavebands of light tend to attract lice and may therefore raise infection pressure (Alsvik, 2019; Bjørnstad and Solstad, 2019; Børset, 2019; Vatn, 2019; Andersen, 2020; Nordtug et al., 2021).
Snorkel cages create a barrier between salmon and parasites, where a net roof at a depth of 10–20 m in the salmon pen, with a snorkel at the center, keeps salmon in deep water. The purpose of the snorkel is to provide a protection zone with low lice larval concentrations that allows the fish to reach the surface to take air into their swim bladders while reducing contact with the infective stages of lice (Stien et al., 2016). In one commercial study, the use of snorkel-based technologies was reported to result in a substantial reduction of salmon lice (Geitung et al., 2019). Finally, when different strategies are integrated, they are more successful than if used independently (Jackson et al., 2018).
Anti-lice Functional Feeds
The stimulus substances in functional feeds are composed of pathogen-associated molecular patterns (PAMPs) that are recognized by the immune system as foreign using pattern-recognition receptors, initiating signaling that leads to immune response (Mogensen, 2009). Immunostimulants, which are feed additives that protect against salmon lice and secondary infections, have been developed and widely used in aquaculture. Available functional feeds in the market include exogenous nucleotides and yeast components that have given 11–41% reduction in L. salmonis infestation levels (Yossa and Dumas, 2016). In addition, feeds containing plant-based glucosinolates have been reported to reduce lice counts up to 25% in fish compared to control diets without glucosinolates (Holm et al., 2016; Skugor et al., 2016). These feed additives have to be applied for a prolonged period (3–10 weeks) and apparently do not provide long-term protection. Therefore, these methods should be regarded as a temporary supplement with the potential to be efficient, especially when combined with other methods.
Lice Traps
Salmon louse has several sensory systems that are involved in host location during the infective stages. These systems can be used as bait to attract lice in underwater traps (Nordtug et al., 2021). One such sensory system has to do with vision, which guides parasites to either a flickering light that resembles fish swimming through sunrays or a continuous light source. It has a longer distance range than mechanosensory and olfactory systems and is likely to be shared across lice species (Fields et al., 2018; Bjørnstad and Solstad, 2019). Furthermore, lice are capable of changing hosts during their adult life stage, thus making it possible to capture them in traps when changing hosts (Ritchie, 1997). These factors have contributed to the development of light-emitting traps (LET) with a light source and an air lift system to capture parasites (Pahl et al., 1999). LET represent an attempt to reduce sea lice infestation and risks of potential infection with smaller ecological impact and at a reduced cost when compared to the chemical treatments (Flamarique et al., 2009; Burridge et al., 2010). It has been shown that alternating light attracts adult salmon lice, whereas the larvae are attracted to continuous light (Flamarique et al., 2000). Thus, future prototypes of LET should have several light sources in order to efficiently capture lice at different life stages, and possibly be combined with salmon-related olfactory cues to enhance efficiency. Current LET based on the light-emitting diode have been tested under laboratory conditions and found to catch 70% of larvae and 8% of female adults, suggesting that females are probably also attracted to continuous light, although at a lower efficiency (Flamarique et al., 2009). Another prototype, by the Chilean company Indesol1, is in the process of being patented. LET often require additional testing in the open sea, where they are exposed to sea currents which transport all stages of lice to downstream waters. The velocity of sea currents is typically higher than the swimming speed of lice, which may reduce the efficiency of LET. Lice traps have the potential to be an environmentally friendly form of lice management, but concrete research on their effectiveness and shortcomings in turbulent water and sea cages, and their effect on other phototactic organisms, is necessary.
Physical Methods
Thermal Treatments
Salmonids can tolerate a temperature of 20–34°C for a limited period of time (Elliot, 1981; Elliott and Elliott, 1995). In thermal treatments, the lice’s susceptibility to abrupt increase in temperature is exploited for delousing. The salmon are crowded in grow-out cages and pumped into a thermal treatment system installed in a ship before being bathed in hot seawater for 30 s on board and allowed to return to sea cages (Noble et al., 2018). While thermal treatments are an effective approach for delousing, some reports have revealed that these treatments may cause tissue injury and severe welfare issues for the salmon (Gismervik et al., 2017, 2019). Other reports, however, conclude differently. Exposure of fish to a temperature of 34°C for 30 s in a recent study did not result in a significant increase in acute lesions apart from fin lesions (Moltumyr et al., 2021).
Flushers and Hydrolicer
Sea lice can be dislodged by water jets by passing salmon into a water jet system to flush out lice. Three companies currently use mechanical lice removal systems: Flatsetsund (FLS) Engineering AS, SkaMik AS, and the Hydrolicer®. The fish is injected into the flushing system for lice removal during treatment. FLS and SkaMik use similar systems, with the former using a flush/spray to remove lice and the latter including a brush system (Gismervik et al., 2017). Hydrolicer® pumps fish into a confined piping system and vacuums out lice using inverse water turbulence (Erikson et al., 2018; Overton et al., 2019). The flushers are attached to a boat or barge near the salmon pens. Fish are briefly pushed into the flusher where detached lice are removed, and the salmon thereafter are returned to the pen. Significant removal of mobile lice using these systems have been reported. There are no published studies on fish welfare and mortality, but losses of scale, gill bleeding, wounds and salmon mortality have been recorded (Gismervik et al., 2017; Holan et al., 2017; Hjeltnes et al., 2019; Westgård, 2020). A recent study has assessed the mortality of various delousing methods and concluded that thermal and mechanical delousing have the highest overall median mortality (Walde et al., 2021).
Freshwater Treatments
Salmon lice are more sensitive to freshwater (Wright et al., 2016) and this vulnerability is targeted in the delousing process of freshwater exposure. Freshwater treatment has historically been used to treat amoebic gill disease, but the use for salmon lice treatment is novel (Powell et al., 2015). Historically, it has been observed that wild salmon may migrate up the river to get rid of lice (Pontoppidan, 1755). A recent study of freshwater treatments in Central Norway showed a high reduction rate of salmon lice in all stages, as well as a very high reduction rate of Caligus elongatus (Gaasø, 2019). However, some reports have claimed that some attached lice can tolerate transfer into freshwater for 24 h. Transcriptome data shows upregulation of the proline synthesis pathway, which is used to offset cellular stress due to environmental effects (Liang et al., 2013; Borchel et al., 2021). Freshwater immobilizes the lice, but they might revive when they return to seawater (Andrews and Horsberg, 2020). To avoid the revival of lice in seawater, it may be necessary to filter the water before disposal. Adult stages of L. salmonis can live in low-salinity waters through a host–dependent interaction, where the lice absorb ions from the host to replace ions lost to the environment. This mechanism increases their tolerance to freshwater (Hahnenkamp and Fyhn, 1985; Johnson and Albright, 1991). The lice also detach as a result of the mechanical effects of crowding and handling before freshwater treatment, but most lice detach during the freshwater exposure (Gaasø, 2019). These detached lice are affected by freshwater exposure and die due to the changes in osmolarity compared to the few attached lice (Wright et al., 2016; Ljungfeldt et al., 2017). So far, freshwater treatment is considered one of the environmentally sustainable methods for delousing with less strain on fish welfare, but it is more time-consuming and expensive than thermal and mechanical methods. Some concerns have been expressed about the possibility that the lice may develop freshwater resistance.
Optical Delousing
Optical or laser delousing is achieved using a system composed of machine vision and targeted laser pulse removal. The laser pulses are directed to a single parasite detected by a submerged automated camera unit scanning the fish. The data generated by the computer vision is continuously transmitted to the database to further train the machine learning system. The laser pulses do not seem to damage the skin of salmon, and the system is able to recognize the fish’s eyes and thereby prevent harm to them (Frenzl, 2017; Bui et al., 2020). Recent research suggests the lack of lethality of lasers rather than the lack of target detection requiring alternative delousing approaches (Bui et al., 2020). Optical delousing is a promising approach where physics meets informatics and pattern recognition in a multidisciplinary environment. New delousing methods currently under development are exploiting the possibilities of optical delousing. However, proper evaluation of the procedure and increasing the laser effectiveness are critical.
Use of Biological Controls
Cleaner Fish
Cleaner fish live in symbiosis with other fish species and feed on dead skin, ectoparasites and zooplanktons (Morado et al., 2019). Since the 1980s, cleaner fish have been used in preventative measures against lice (Bjordal, 1991; Torrissen et al., 2013). The use of cleaner fish as defense against salmon lice has increased steadily since 2008, with about 60 million cleaner fish used for the purpose in 2019 (Directorate of Fisheries, 2019). Biological control using cleaner fish has become a strong favorite among salmon producers, as new approaches that are less strenuous for salmon are deemed necessary (Barrett et al., 2020). Nowadays, cleaner fish, which include lumpfish (Cyclopterus lumpus), corkwing wrasse (Symphodus melops), ballan wrasse (Labrus bergylta), goldsinny wrasse (Ctenolabrus rupestris), and cuckoo wrasse (Labrus mixtus), are a prevalent alternative to current delousing control methods (Overton et al., 2020). This method is considered to be economical and less strenuous to salmon than physical and medical treatments, and the general public accepts the use of cleaner fish more readily than chemical treatments (Groner et al., 2013; Imsland et al., 2018; Powell et al., 2018; Overton et al., 2020).
Despite the wide acceptance and immense benefits, mixed results of the commercial success of the method have been reported. Easily accessible feed pellets, zooplankton abundance in the water, and prey located in biofouling may reduce the need for cleaner fish to feed on lice (Imsland et al., 2015). Concerns have also been raised about unsuitable environmental conditions that may reduce the efficacy and increase mortality among species of cleaner fish. Reports of diseases and high mortality of the cleaner fish deployed in salmon farms and ethical consideration of using vertebrates listed in animal welfare legislation are frequent (Nilsen and Colquhoun, 2014; Treasurer and Feledi, 2014). In addition, most wrasses are wild-caught, which can affect the abundance and population dynamics of the wild stocks negatively, and the sustainability of the use must be justified (Skiftesvik et al., 2014; Halvorsen et al., 2017). More focused, evidentiary use of cleaner fish could increase their effectiveness and help ease economic, environmental and ethical issues.
Biopesticides
Biopesticides are compounds used, instead of broad chemical pesticides, to manage agricultural pests through specific biological effects. They include biocontrol products like natural organisms or substances derived from natural products, such as genes or metabolites from animals, plants, bacteria, or certain minerals, that are used to manage pests (Sporleder and Lacey, 2013). Additionally, there are several reports of parasites that can manipulate and control arthropod behavior. The diversity of arthropod-manipulatory parasites varies from viruses to worms (Sporleder and Lacey, 2013). Viruses have been produced as biological insecticides for the control of pests in forests and agricultural crops. Baculoviruses are large, occlusive dsDNA viruses that infect arthropods (Anderson and May, 1980; Cory and Myers, 2003; Elderd et al., 2013). Many studies have shown substantial and important benefits for crop protection with both natural and genetically modified (GM) baculoviruses (Kamita et al., 2017). Further research on the use of salmon lice parasites, such as viruses or fungi, as a substitute for conventional chemical treatments opens the door to smart biological control of salmon lice in aquaculture.
Enabling Technologies
The 20-first century is the era of biotechnology and information technology. Data availability and technological advancements have aided humanity in a variety of ways. We address some of the technologies that are or could be useful in counteracting salmon lice.
Machine Learning
Machine learning (ML) uses computer algorithms that are improved automatically by experience, which can create models based on sampled data to make predictions or decisions without being explicitly programmed to do so. Computer vision represents an ML environment where it is impossible for traditional algorithms to execute specific tasks (Szeliski, 2011). For many years, computer vision has been used to identify and measure planktonic individuals (Kocak et al., 1999; Zheng et al., 2017; Luo et al., 2018; Li et al., 2020), making the operation more powerful than manual detection. The effects of the use of machine vision can be as strong as those of human experts.
Computer vision is utilized in pattern recognition and followed by laser shooting during optical delousing. It could also be developed to monitor the infestation levels of lice. In addition, studies of the behavior of planktonic lice, such as their responses to light stimulus, are carried out (Kvæstad et al., 2020). Besides, ML has been applied in the identification of diseased fish suffering from secondary infections based on mass spectrometry data (López-Cortés et al., 2017) and in a random forest-based approach for genome-wide SNP analysis (Jacobs et al., 2018). In future, ML has the potential to enable smart and autonomous control and monitoring of salmon lice infestations.
Monitoring of Salmon Lice
Computer vision may also be applied to monitor salmon lice infestations in salmon farms. This may imply the development of a fully automatic sea lice counter for continuous counting and sorting of sea lice on farmed salmon without the need for handling. The method provides a more comprehensive, impartial, and precise assessment of the lice situation in a fish cage. Since there is a limit on the maximum mean numbers of average female salmon lice per fish (Heuch et al., 2005), it is beneficial to have a system that provides real-time data for decision-making in intervention selection. Ecotone AS have developed a system called SpectraLice for this purpose, which can distinguish various stages of salmon lice using their proprietary hyperspectral imaging technology to detect light through the entire electromagnetic spectrum (Spectralice, 2021). Recently, the Norwegian Food Safety Authority (NFSA) approved the use of another louse counting technology, this time from Aquabyte, adopting their robust camera setup and patented optical architecture in 56 cages (Redaksjonen, 2021). NFSA have also given Stingray permission to use their image-based lice count system in 15 more cages (Drønen, 2021). One significant benefit of enabling technologies in lice monitoring is that they reduce costs in the long term and eliminate the need for fish handling and manual counting, which improves fish welfare.
Breeding and Use of Lice Resistant Fish
Selective breeding based on phenotypic values of a phenotype is typically used to generate genetic improvement of a quantitative trait in a population (Narain and Mishra, 1975). Using the same concept, salmon lice resistance can be improved by breeding new generations of farmed salmon using fish from families with low susceptibility to lice infestation. It has been verified that some select populations exhibit significantly reduced needs for chemical treatment to control lice outbreaks (Gharbi et al., 2015). QTL (quantitative trait locus) analysis for the evaluation of gene variation in the breeding candidate for genomic selection has been used to select lines that are resistant or have better tolerance to salmon lice (Aquagen, 2017). This method has been successfully used in the selection of salmon with higher degree of resistance against Infectious Pancreatic Necrosis (IPN), with fewer outbreaks of IPN in the short term (Houston et al., 2008; Moen et al., 2009). A crucial step to counteract the lice problem can be achieved through a breeding process, but it will likely take several generations of salmon to see the effect. A more direct approach will be to find the genetic differences between the Atlantic and Pacific salmon, since the latter is less attractive as hosts for sea lice, and use this genetic information in breeding or genetic modification of the Atlantic salmon (Moore, 2020; Robinson, 2021). This methodology is currently being developed as part of the CrispResist project sponsored by the Norwegian Seafood Research Fund (FHF).
RNA Interference
Technological advances in molecular biology approaches to salmon lice are an integral aspect of the study of gene function and identifying breeding targets. The key to salmon louse management could be found in the study of genes, and technical advancement in this area is warranted. RNA interference (RNAi) is a form of gene knockdown that targets mRNA (not necessarily only mRNA). For many years, RNAi technology has been applied in aquaculture research, contributing to our understanding of functional genomics and aquatic biology (Abo-Al-Ela, 2021). A schematic diagram is shown comparing RNAi to genome editing (Figure 2). The method of post-transcriptional silencing of genes using RNAi has been developed for salmon lice (Campbell et al., 2009; Eichner et al., 2014) and several important genes have been functionally characterized. RNAi has facilitated in the study of the molecular biology of the louse, such as the function of specific genes in developmental processes.
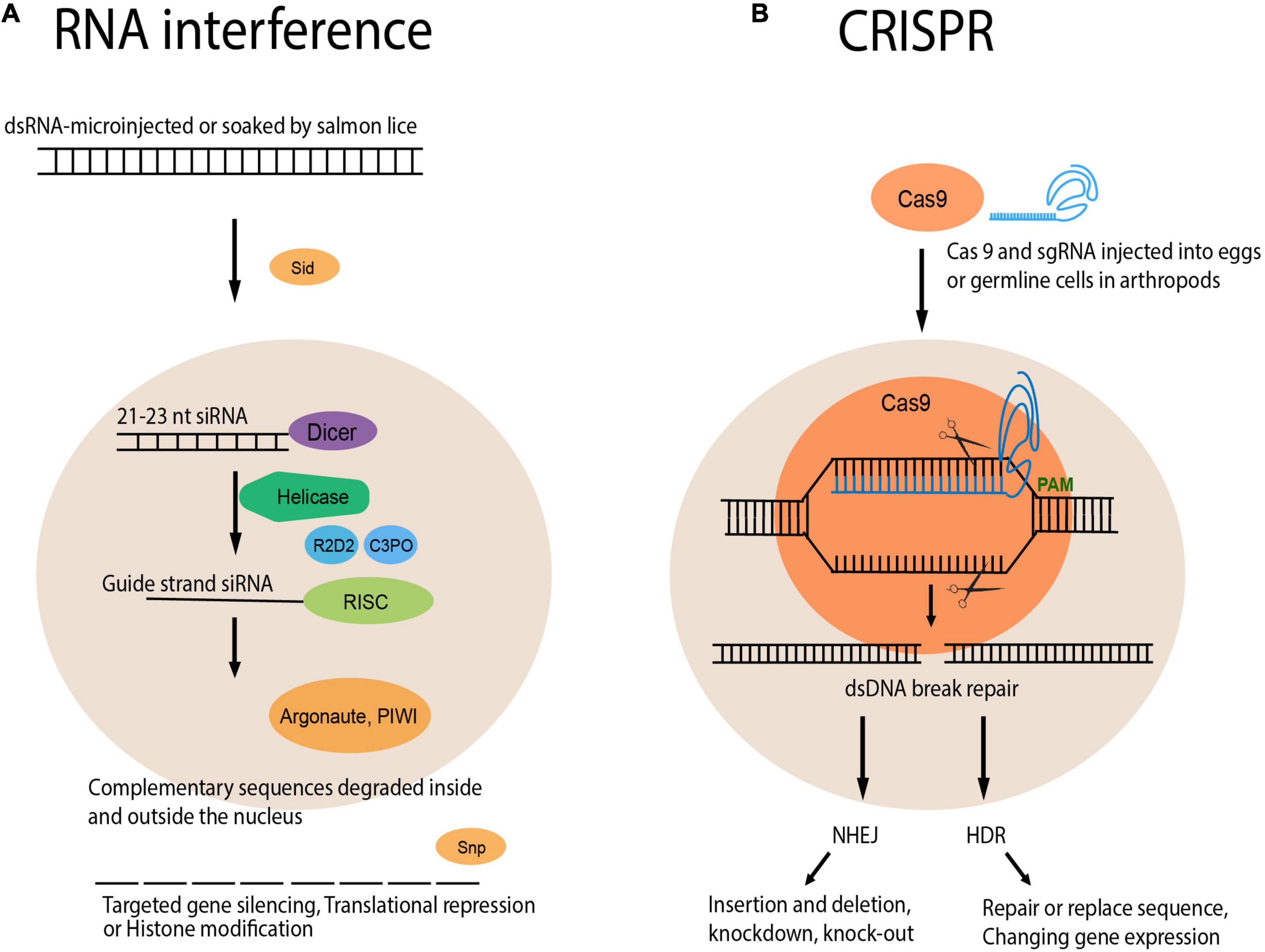
Figure 2. Schematic representation of RNA interference and CRISPR/Cas9 technology. Two methods to target the activity of specific genes in the organism that are valuable for the functional study of genes and genetic elements. (A) RNA interference is a reverse genetics approach to study genes by knocking them down and then evaluating the effects. RNAi can be achieved in salmon lice by microinjecting dsRNA into pre-adult/adult lice or by immersing nauplii in dsRNA solution. dsRNA is incorporated in the cell with Sid, cleaved by Dicer into 21 nt small interfering RNA (siRNA), loaded into RNA-induced silencing complex (RISC) by R2D2 and C3PO. Argonaute and PIWI endonucleases degrade complementary RNA inside the nucleus, whereas Snp exonucleases degrades RNA outside. (B) CRISPR/Cas9 has the precision to target and knock out a single gene or genes in a gene family. Although the CRISPR/Cas9 is yet available for salmon lice, Cas9 and single guide RNA (sgRNA) can be injected into the eggs or germline cells similar to other arthropods. sgRNA forms a complex with Cas9 endonuclease, producing a dsDNA break. The dsDNA break is repaired by Non-homologous end joining (NHEJ) or homology directed repair (HDR). As a consequence, genes would be knocked out, knocked down, upregulated, or silenced. Adapted from Perkin et al. (2016).
RNA interference screens have aided the study of host–parasite interactions, host recognition (Komisarczuk et al., 2017; Núñez-Acuña et al., 2019), and physiology at various life stages of lice (Sandlund et al., 2016, 2018; Komisarczuk et al., 2018, 2020; Braden et al., 2020; Guragain et al., 2020). RNAi has also facilitated in the functional studies of key genes involved in lice reproduction (Dalvin et al., 2009; Tröße et al., 2014; Eichner et al., 2015a; Khan et al., 2017; Borchel and Nilsen, 2018; Borchel et al., 2019; Heggland et al., 2019a,b). In addition, the method is used to identify the potential vaccine targets in C. rogercresseyi (Carpio et al., 2011; Maldonado-Aguayo and Gallardo-Escárate, 2014). The genes and pathways in salmon louse studied using RNAi are summarized in Table 4, which offers a broad range of information on gene function and various phenotypes resulting from gene knockdown.
Genome Editing
Genome editing, which includes precise manipulation of DNA sequences to alter the cell fate and organism traits, offers the potential to broaden our understanding of genetics and biology (Doudna, 2020). An attractive genome editing tool used in multiple organisms and cells, including arthropods, is CRISPR/Cas9 (Clustered, Regularly Interspersed, Short Palindromic Repeats/Cas9), which was discovered as a bacterial adaptive immune system (Ran et al., 2013). CRISPR/Cas9 genome editing can be utilized to target specific sequences in a genome and to destroy a specific gene activity or to modify the activity of the encoded protein (Anzalone et al., 2020). Furthermore, advancements in the Cas12 and Cas13 systems may open up new avenues for genome editing and RNA editing, respectively (Yan et al., 2019). Genome editing has resulted in a revolution in functional studies of genes by providing a tool for down to single nucleotide editing. This is done without leaving any foreign DNA in the edited organism/individual. Genome editing is already used to improve plant and animal traits and has a big potential both for targeted improvements and identification of traditional breeding targets. An international project that has as its goal to identify genetic salmon lice resistance markers in the Pacific salmon and use this information to modify the Atlantic salmon using the CRISPR/Cas9 technology has already been initiated. In contrast to salmon, where gene editing technology is well established, the technological development of CRISPR/Cas9 in salmon lice is more challenging because of problems associated with targeting of germline cells, the method of delivery, and the absence of a visible marker for mutant screening. When developed, genome editing methods for salmon lice will open a completely new toolbox and avenue for studies of salmon lice and likely offer great potential for the development of principles of salmon lice control. The genome sequence of salmon lice was reported in 2012 and provides a fundamental aid in identifying the targets2.
Future Perspectives
Over the last decades, numerous preventive methods and treatments have been developed, and scientific studies undertaken, to combat salmon lice. As we have outlined here, none of these methods have been highly successful in counteracting salmon lice infestations. Most preventive methods have shown some efficiency, and others have yet to be tested commercially. We foresee that a key to future solutions could be unlocked through genetic studies of both sea lice and the Atlantic salmon, as well as of their physiological and ecological interactions. In addition, vaccine research, sophisticated engineering devices, and improvement in non-medical methods can provide us with the necessary tools to reduce the damage inflicted by salmon lice infestations. The ultimate biological goal in the quest to control salmon lice might be to breed super-resistant salmon, but there would likely still be a constant fight or arms race between lice and lice management strategies, comparable to the situation for terrestrial arthropods in agriculture. Cleaner fish provides good protection but have limitations of fish welfare and high cost. Another option might be to breed salmon that has been genetically modified to produce compounds that repel salmon lice without harming humans or fish. Natural enemies, such as biocontrol agents, could be another strategy for louse control that is less harmful to humans and the environment. The industry is using a wide range of counteracting measures to treat salmon lice in aquaculture. We have pointed to different strategies used, and the rapid development trend within the non-medical treatments.
We believe that a combination of multiple strategies is preferred to combat salmon lice until novel and better solutions become available in the future. The agriculture like approach, such as crop rotation or leaving fallow for greater protection, could be developed. Identifying key biological mechanisms in host–parasite interactions, metabolic profiling, and omics studies are critical. Methods such as RNAi and genome editing will play a central role in these types of investigations. The quest for better means of controlling salmon lice should not be stopped, and research activity should continue.
Author Contributions
AMB and PG conceived and designed the study. PG, AMB and MT wrote the manuscript, with intellectual inputs from YO, PW, and ASB. All authors have reviewed and approved the manuscript.
Funding
This study was part of the project “Taskforce Salmon Lice” at the Norwegian University of Science and Technology (NTNU) funded by the salmon industry in Mid-Norway, the Norwegian Seafood Research Fund (project number 901241), and the NTNU (https://www.ntnu.edu/oceans/taskforce).
Conflict of Interest
The authors declare that the research was conducted in the absence of any commercial or financial relationships that could be construed as a potential conflict of interest.
Acknowledgments
The authors would like to thank their industry partners in the Taskforce Salmon Lice project for providing the resources for the study.
Footnotes
- ^ https://www.fishfarmingexpert.com/article/usinglights-to-lure-lice-to-their-deaths/
- ^ https://metazoa.ensembl.org/Lepeophtheirus_salmonis/Info/Index
References
Aaen, S. M., Helgesen, K. O., Bakke, M. J., Kaur, K., and Horsberg, T. E. (2015). Drug resistance in sea lice: A threat to salmonid aquaculture. Trends Parasitol. 31, 72–81. doi: 10.1016/j.pt.2014.12.006
Abo-Al-Ela, H. G. (2021). RNA interference in aquaculture: a small tool for big potential. J. Agric. Food Chem. 69, 4343–4355. doi: 10.1021/acs.jafc.1c00268
Abolofia, J., Asche, F., and Wilen, J. E. (2017). The cost of lice: quantifying the impacts of parasitic sea lice on farmed salmon. Mar. Resour. Econ. 32, 329–349. doi: 10.1086/691981
Adams, A. (2019). Progress, challenges and opportunities in fish vaccine development. Fish Shellfish Immunol. 90, 210–214. doi: 10.1016/j.fsi.2019.04.066
Alsvik, M. (2019). The Response of Salmon Lice Nauplii and Copepodids (Lepeophtheirus Salmonis) to Artificial Light Stimuli. Available online at: https://ntnuopen.ntnu.no/ntnu-xmlui/handle/11250/2656636 (accessed July 7, 2021).
Andersen, R. (2020). The Effect of Natural and Artificial Light on in situ Vertical Migration of Salmon Lice (Lepeophtheirus Salmonis) Copepodids. Trondheim: NTNU.
Anderson, E. J., Rouphael, N. G., Widge, A. T., Jackson, L. A., Roberts, P. C., Makhene, M., et al. (2020). Safety and immunogenicity of SARS-CoV-2 mRNA-1273 vaccine in older adults. N. Engl. J. Med. 383, 2427–2438. doi: 10.1056/NEJMoa2028436
Anderson, R. M., and May, R. M. (1980). Infectious diseases and population cycles of forest insects. Science 210:658. doi: 10.1126/science.210.4470.658
Andrews, M., and Horsberg, T. E. (2020). Sensitivity towards low salinity determined by bioassay in the salmon louse, Lepeophtheirus salmonis (Copepoda: Caligidae). Aquaculture 514:734511. doi: 10.1016/j.aquaculture.2019.734511
Angulo, C., Tello−Olea, M., Reyes−Becerril, M., Monreal−Escalante, E., Hernández−Adame, L., Angulo, M., et al. (2020). Developing oral nanovaccines for fish: a modern trend to fight infectious diseases. Rev. Aquac. 2020:12518. doi: 10.1111/raq.12518
Anzalone, A. V., Koblan, L. W., and Liu, D. R. (2020). Genome editing with CRISPR–Cas nucleases, base editors, transposases and prime editors. Nat. Biotechnol. 38, 824–844. doi: 10.1038/s41587-020-0561-9
Aquagen. (2017). gen-innova-gain-2016-2017-eng.pdf. Gen-Innova-Gain. Available Online at: https://aquagen.no/wp-content/uploads/2016/10/gen-innova-gain-2016-2017-eng.pdf (Accessed April 14, 2021).
Arena, J. P., Liu, K. K., Paress, P. S., Frazier, E. G., Cully, D. F., Mrozik, H., et al. (1995). The mechanism of action of avermectins in caenorhabditis elegans: correlation between activation of glutamate-sensitive chloride current, membrane binding, and biological activity. J. Parasitol. 81, 286–294. doi: 10.2307/3283936
Barrett, L. T., Overton, K., Stien, L. H., Oppedal, F., and Dempster, T. (2020). Effect of cleaner fish on sea lice in Norwegian salmon aquaculture: a national scale data analysis. Int. J. Parasitol. 50, 787–796. doi: 10.1016/j.ijpara.2019.12.005
Bjordal, Å (1991). Wrasse as cleaner-fish for farmed salmon. 17–28. Available Online at: https://imr.brage.unit.no/imr-xmlui/handle/11250/109019 (Accessed March 8, 2021).
Bjørnstad, L. F., and Solstad, M. A. (2019). Investigation of Light Response and Swimming Behavior of Salmon Lice (Lepeophtheirus Salmonis) Using Feature Detection and Object Tracking. Available online at: https://ntnuopen.ntnu.no/ntnu-xmlui/handle/11250/2622957 (accessed April 6, 2021).
Borchel, A., Heggland, E. I., and Nilsen, F. (2021). The transcriptomic response of adult salmon lice (Lepeophtheirus salmonis) to reduced salinity. Comp. Biochem. Physiol. Part D Genomics Proteomics 37:100778. doi: 10.1016/j.cbd.2020.100778
Borchel, A., Kongshaug, H., and Nilsen, F. (2019). Identification and description of the key molecular components of the egg strings of the salmon louse (Lepeophtheirus salmonis). Genes 10:1004. doi: 10.3390/genes10121004
Borchel, A., and Nilsen, F. (2018). A novel gene-family involved in spermatophore generation in the economically important salmon louse Lepeophtheirus salmonis. Mol. Reprod. Dev. 85, 478–489. doi: 10.1002/mrd.22984
Børset, E. (2019). Investigating the Phototactic Response of Salmon Lice. Available online at: https://ntnuopen.ntnu.no/ntnu-xmlui/handle/11250/2617257 (accessed March 7, 2021).
Braden, L., Michaud, D., Igboeli, O. O., Dondrup, M., Hamre, L., Dalvin, S., et al. (2020). Identification of critical enzymes in the salmon louse chitin synthesis pathway as revealed by RNA interference-mediated abrogation of infectivity. Int. J. Parasitol. 50, 873–889. doi: 10.1016/j.ijpara.2020.06.007
Bradshaw, C. J. A., Leroy, B., Bellard, C., Roiz, D., Albert, C., Fournier, A., et al. (2016). Massive yet grossly underestimated global costs of invasive insects. Nat. Commun. 7:12986. doi: 10.1038/ncomms12986
Brattsten, L. B., Holyoke, C. W., Leeper, J. R., and Raffa, K. F. (1986). Insecticide resistance: challenge to pest management and basic research. Science 231, 1255–1260. doi: 10.1126/science.231.4743.1255
Bui, S., Geitung, L., Oppedal, F., and Barrett, L. T. (2020). Salmon lice survive the straight shooter: A commercial scale sea cage trial of laser delousing. Prev. Vet. Med. 181:105063. doi: 10.1016/j.prevetmed.2020.105063
Burrells, C., Williams, P. D., and Forno, P. F. (2001). Dietary nucleotides: a novel supplement in fish feeds: 1. Effects on resistance to disease in salmonids. Aquaculture 199, 159–169. doi: 10.1016/S0044-8486(01)00577-4
Burridge, L., Weis, J. S., Cabello, F., Pizarro, J., and Bostick, K. (2010). Chemical use in salmon aquaculture: A review of current practices and possible environmental effects. Aquaculture 306, 7–23. doi: 10.1016/j.aquaculture.2010.05.020
Campbell, E., Pert, C., and Bowman, A. (2009). RNA-interference methods for gene-knockdown in the sea louse, Lepeophtheirus salmonis: Studies on a putative prostaglandin E synthase. Parasitology 136, 867–874. doi: 10.1017/S0031182009990357
Carmichael, S. N., Bron, J. E., Taggart, J. B., Ireland, J. H., Bekaert, M., Burgess, S. T. G., et al. (2013). Salmon lice (Lepeophtheirus salmonis) showing varying emamectin benzoate susceptibilities differ in neuronal acetylcholine receptor and GABA-gated chloride channel mRNA expression. BMC Genom. 14:408. doi: 10.1186/1471-2164-14-408
Carpio, Y., Basabe, L., Acosta, J., Rodríguez, A., Mendoza, A., Lisperger, A., et al. (2011). Novel gene isolated from Caligus rogercresseyi: A promising target for vaccine development against sea lice. Vaccine 29, 2810–2820. doi: 10.1016/j.vaccine.2011.01.109
Coates, A., Phillips, B. L., Bui, S., Oppedal, F., Robinson, N. A., and Dempster, T. (2021). Evolution of salmon lice in response to management strategies: a review. Rev. Aquac. 2021, 1–26. doi: 10.1111/raq.12528
Contreras, M., Karlsen, M., Villar, M., Olsen, R. H., Leknes, L. M., Furevik, A., et al. (2020). Vaccination with ectoparasite proteins involved in Midgut function and blood digestion reduces salmon louse infestations. Vaccines 8:32. doi: 10.3390/vaccines8010032
Cory, J. S., and Myers, J. H. (2003). The ecology and evolution of insect baculoviruses. Annu. Rev. Ecol. Evol. Syst. 34, 239–272. doi: 10.1146/annurev.ecolsys.34.011802.132402
Costello, M. J. (2006). Ecology of sea lice parasitic on farmed and wild fish. Trends Parasitol. 22, 475–483. doi: 10.1016/j.pt.2006.08.006
Covello, J. M., Friend, S. E., Purcell, S. L., Burka, J. F., Markham, R. J. F., Donkin, A. W., et al. (2012). Effects of orally administered immunostimulants on inflammatory gene expression and sea lice (Lepeophtheirus salmonis) burdens on Atlantic salmon (Salmo salar). Aquaculture 367, 9–16. doi: 10.1016/j.aquaculture.2012.08.051
Dadar, M., Dhama, K., Vakharia, V. N., Hoseinifar, S. H., Karthik, K., Tiwari, R., et al. (2017). Advances in aquaculture vaccines against fish pathogens: global status and current trends. Rev. Fish. Sci. Aquac. 25, 184–217. doi: 10.1080/23308249.2016.1261277
Dalvin, S., Frost, P., Biering, E., Hamre, L. A., Eichner, C., Krossøy, B., et al. (2009). Functional characterisation of the maternal yolk-associated protein (LsYAP) utilising systemic RNA interference in the salmon louse (Lepeophtheirus salmonis) (Crustacea: Copepoda). Int. J. Parasitol. 39, 1407–1415. doi: 10.1016/j.ijpara.2009.04.004
Denholm, I., Devine, G. J., Horsberg, T. E., Sevatdal, S., Fallang, A., Nolan, D. V., et al. (2002). Analysis and management of resistance to chemotherapeutants in salmon lice, Lepeophtheirus salmonis (Copepoda: Caligidae). Pest Manag. Sci. 58, 528–536. doi: 10.1002/ps.482
Directorate of Fisheries. (2019). Use of cleanerfish 1998-2019. Available Online at: https://www.fiskeridir.no/English/Aquaculture/Statistics/Cleanerfish-Lumpfish-and-Wrasse/_/attachment/download/d70cefea-4c9a-4537-8250-6f1ef7e6827e:80a559bdde3404f6a058e94013c2455dfdbe2b34/sta-laks-mat-10-rensefisk.xlsx (Accessed March 8, 2021)
Directorate of Fisheries. (2020). Atlantic salmon and rainbow trout: Sale 1994-2019. English. Available Online at: https://www.fiskeridir.no/English/Aquaculture/Statistics/Atlantic-salmon-and-rainbow-trout/_/attachment/download/47d5b9f9-6a7e-4594-a394-8ab9eadf59d3:6eb0635c1bb4363154cf0cde0f50a734d656182a/sta-laks-mat-06-salg.xlsx (Accessed March 17, 2021)
Doudna, J. A. (2020). The promise and challenge of therapeutic genome editing. Nature 578, 229–236. doi: 10.1038/s41586-020-1978-5
Douris, V., Steinbach, D., Panteleri, R., Livadaras, I., Pickett, J. A., Leeuwen, T. V., et al. (2016). Resistance mutation conserved between insects and mites unravels the benzoylurea insecticide mode of action on chitin biosynthesis. Proc. Natl. Acad. Sci. 113, 14692–14697. doi: 10.1073/pnas.1618258113
Drønen, O. A. (2021). Så mange har søkt om dispensasjon med Stingray sitt system - Kyst.no. Available Online at: https://www.kyst.no/article/saa-mange-har-soekt-om-dispensasjon-med-stingray-sitt-system/ (Accessed April 14, 2021)
Eichner, C., Dalvin, S., Skern-Mauritzen, R., Malde, K., Kongshaug, H., and Nilsen, F. (2015a). Characterization of a novel RXR receptor in the salmon louse (Lepeophtheirus salmonis, Copepoda) regulating growth and female reproduction. BMC Genomics 16:81. doi: 10.1186/s12864-015-1277-y
Eichner, C., Harasimczuk, E., Nilsen, F., Grotmol, S., and Dalvin, S. (2015b). Molecular characterisation and functional analysis of LsChi2, a chitinase found in the salmon louse (Lepeophtheirus salmonis salmonis, Krøyer 1838). Exp. Parasitol. 151–152, 39–48. doi: 10.1016/j.exppara.2015.01.011
Eichner, C., Nilsen, F., Grotmol, S., and Dalvin, S. (2014). A method for stable gene knock-down by RNA interference in larvae of the salmon louse (Lepeophtheirus salmonis). Exp. Parasitol. 140, 44–51. doi: 10.1016/j.exppara.2014.03.014
Elderd, B. D., Rehill, B. J., Haynes, K. J., and Dwyer, G. (2013). Induced plant defenses, host–pathogen interactions, and forest insect outbreaks. Proc. Natl. Acad. Sci. 110, 14978–14983. doi: 10.1073/pnas.1300759110
Elliot, J. M. (1981). “Some aspects of thermal stress on freshwater teleosts,” in Stress and Fish, ed. A. D. Pickering (New York: Academic press), 209.
Elliott, J. M., and Elliott, J. A. (1995). The effect of the rate of temperature increase on the critical thermal maximum for parr of Atlantic salmon and brown trout. J. Fish Biol. 47, 917–919. doi: 10.1111/j.1095-8649.1995.tb06014.x
Erikson, U., Solvang, T., Schei, M., Ag, S., Strand, A., and Aalberg, K. (2018). Hydrolicer - Utredning av system, stress og velferd ved avlusing. Norway: Sintef Ocean, 95.
FAO. (2019). FAO Fisheries & Aquaculture - Statistics - FIGIS - Time-series query on: Production. Rome, Italy: FAO.
FAO. (2020a). The State of Food Security and Nutrition in the World 2020: Transforming food systems for affordable healthy diets. Rome, Italy: FAO, IFAD, UNICEF, WFP and WHO.
Fields, D. M., Skiftesvik, A. B., and Browman, H. I. (2018). Behavioural responses of infective-stage copepodids of the salmon louse (Lepeophtheirus salmonis, Copepoda:Caligidae) to host-related sensory cues. J. Fish Dis. 41, 875–884. doi: 10.1111/jfd.12690
Fiskeridepartementet, N. (2015). Meld. St. 16 (2014-2015). Regjeringen.no. Available Online at: https://www.regjeringen.no/no/dokumenter/meld.-st.-16-2014-2015/id2401865/ (Accessed April 14, 2021)
Fjørtoft, H. B., Nilsen, F., Besnier, F., Espedal, P. G., Stene, A., Tveten, A.-K., et al. (2020). Aquaculture-driven evolution: distribution of pyrethroid resistance in the salmon louse throughout the North Atlantic in the years 2000–2017. ICES J. Mar. Sci. 77, 1806–1815.
Flamarique, I. N., Browman, H. I., Belanger, M., and Boxaspen, K. (2000). Ontogenetic changes in visual sensitivity of the parasitic salmon louse Lepeophtheirus salmonis. J. Exp. Biol. 203, 1649–1657.
Flamarique, I. N., Gulbransen, C., Galbraith, M., and Stucchi, D. (2009). Monitoring and potential control of sea lice using an LED-based light trap. Can. J. Fish. Aquat. Sci. 66, 1371–1382. doi: 10.1139/F09-094
Frank, K., Gansel, L. C., Lien, A. M., and Birkevold, J. (2014). Effects of a shielding skirt for prevention of sea lice on the flow past stocked salmon fish cages. J. Offshore Mech. Arct. Eng. 137:4028260. doi: 10.1115/1.4028260
Frenzl, B., Stien, L. H., Cockerill, D., Oppedal, F., Richards, R. H., Shinn, A. P., et al. (2014). Manipulation of farmed Atlantic salmon swimming behaviour through the adjustment of lighting and feeding regimes as a tool for salmon lice control. Aquaculture 42, 183–188. doi: 10.1016/j.aquaculture.2013.12.012
Fuente, J., de la Kopáček, P., Lew−Tabor, A., and Maritz−Olivier, C. (2016). Strategies for new and improved vaccines against ticks and tick-borne diseases. Parasite Immunol. 38, 754–769. doi: 10.1111/pim.12339
Gaasø, M. (2019). Sea lice (Lepeophtheirus salmonis and Caligus elongatus) during freshwater treatment. Available Online at: https://ntnuopen.ntnu.no/ntnu-xmlui/handle/11250/2656633 (Accessed April 7, 2021).
Geitung, L., Oppedal, F., Stien, L. H., Dempster, T., Karlsbakk, E., Nola, V., et al. (2019). Snorkel sea-cage technology decreases salmon louse infestation by 75% in a full-cycle commercial test. Int. J. Parasitol. 49, 843–846. doi: 10.1016/j.ijpara.2019.06.003
Ghafor, I. M. (2020). Crustacean, Crustacea, Genaro Diarte-Plata and Ruth Escamilla-Montes. London: IntechOpen.
Gharbi, K., Matthews, L., Bron, J., Roberts, R., Tinch, A., and Stear, M. (2015). The control of sea lice in Atlantic salmon by selective breeding. J. R. Soc. Interface 12:20150574. doi: 10.1098/rsif.2015.0574
Gislason, H. (2018). Statistical modelling of sea lice count data from salmon farms in the Faroe Islands. J. Fish Dis. 41, 973–993. doi: 10.1111/jfd.12742
Gismervik, K., Gåsnes, S. K., Gu, J., Stien, L. H., Madaro, A., and Nilsson, J. (2019). Thermal injuries in Atlantic salmon in a pilot laboratory trial. Vet. Anim. Sci. 8:100081. doi: 10.1016/j.vas.2019.100081
Gismervik, K., Nielsen, K. V., Lind, M. B., and Viljugrein, H. (2017). Mekanisk avlusing med FLS-avlusersystem - dokumentasjon av fiskevelferd og effekt mot lus. Norway: Norwegian. Veterinary. Institute, 43.
Glaropoulos, A., Stien, L. H., Folkedal, O., Dempster, T., and Oppedal, F. (2019). Welfare, behaviour and feasibility of farming Atlantic salmon in submerged cages with weekly surface access to refill their swim bladders. Aquaculture 502, 332–337. doi: 10.1016/j.aquaculture.2018.12.065
Gonçalves, A. T., Collipal-Matamal, R., Valenzuela-Muñoz, V., Nuñez-Acuña, G., Valenzuela-Miranda, D., and Gallardo-Escárate, C. (2020). Nanopore sequencing of microbial communities reveals the potential role of sea lice as a reservoir for fish pathogens. Sci. Rep. 10:2895. doi: 10.1038/s41598-020-59747-0
Gonzalez, L., Carvajal, J., and George-Nascimento, M. (2000). Differential infectivity of Caligus flexispina (Copepoda, Caligidae) in three farmed salmonids in Chile. Aquaculture 183, 13–23.
González, M. P., Marín, S. L., and Vargas-Chacoff, L. (2015). Effects of Caligus rogercresseyi (Boxshall and Bravo, 2000) infestation on physiological response of host Salmo salar (Linnaeus 1758): Establishing physiological thresholds. Aquaculture 438, 47–54.
Grave, K., Horsberg, T., Lunestad, B., and Litleskare, I. (2004). Consumption of drugs for sea lice infestations in Norwegian fish farms: methods for assessment of treatment patterns and treatment rate. Dis. Aquat. Organ. 60, 123–131. doi: 10.3354/dao060123
Grimnes, A., and Jakobsen, P. J. (1996). The physiological effects of salmon lice infection on post- smolt of Atlantic salmon. J. Fish Biol. 48, 1179–1194. doi: 10.1006/jfbi.1996.0119
Groner, M. L., Cox, R., Gettinby, G., and Revie, C. W. (2013). Use of agent-based modelling to predict benefits of cleaner fish in controlling sea lice, Lepeophtheirus salmonis, infestations on farmed Atlantic salmon, Salmo salar L. J. Fish Dis. 36, 195–208. doi: 10.1111/jfd.12017
Grøntvedt, R. N., Jansen, P. A., Horsberg, T. E., Helgesen, K., and Tarpai, A. (2016). The surveillance programme for resistance to chemotherapeutants in salmon lice (Lepeophtheirus salmonis) in Norway 2015 Surveillance programs for terrestrial and aquatic animals in Norway. Nor. Vet. Inst. Oslo Nor. Available online at: https://www.vetinst.no/rapporter-og-publikasjoner/rapporter/2015/thermal-de-licing-of-salmonid-fish-documentation-of-fish-welfare-and-effect
Guragain, P., Sporsheim, B., Skjesol, A., Baatnes, A. S., Olsen, Y., Bones, A. M., et al. (2020). Phenylalanine Hydroxylase RNAi Knockdown negatively affects larval development, molting and swimming performance of salmon lice. Front. Mar. Sci. 7:8463. doi: 10.3389/fmars.2020.608463
Hahnenkamp, L., and Fyhn, H. J. (1985). The osmotic response of salmon louse, Lepeophtheirus salmonis (Copepoda: Caligidae), during the transition from sea water to fresh water. J. Comp. Physiol. B 155, 357–365. doi: 10.1007/BF00687479
Halvorsen, K. T., Larsen, T., Sørdalen, T. K., Vøllestad, L. A., Knutsen, H., and Olsen, E. M. (2017). Impact of harvesting cleaner fish for salmonid aquaculture assessed from replicated coastal marine protected areas. Mar. Biol. Res. 13, 359–369. doi: 10.1080/17451000.2016.1262042
Harasimczuk, E., Overgard, A.-C., Grotmol, S., Nilsen, F., and Dalvin, S. (2018). Characterization of three salmon louse (Lepeophtheirus salmonis) genes with fibronectin II domains expressed by tegumental type 1 glands. Mol. Biochem. Parasitol. 219, 1–9. doi: 10.1016/j.molbiopara.2017.12.002
Hastie, L. C., Wallace, C., Birkett, M. A., Douglas, A., Jones, O., Mordue Luntz, A. J., et al. (2013). Prevalence and infection intensity of sea lice (Lepeophtheirus salmonis) on Atlantic salmon (Salmo salar) host is reduced by the non-host compound 2-aminoacetophenone. Aquaculture 410–411, 179–183. doi: 10.1016/j.aquaculture.2013.06.035
Heggland, E. I., Eichner, C., Støve, S. I., Martinez, A., Nilsen, F., and Dondrup, M. (2019a). A scavenger receptor B (CD36)-like protein is a potential mediator of intestinal heme absorption in the hematophagous ectoparasite Lepeophtheirus salmonis. Sci. Rep. 9, 1–14. doi: 10.1038/s41598-019-40590-x
Heggland, E. I., Tröße, C., Eichner, C., and Nilsen, F. (2019b). Heavy and light chain homologs of ferritin are essential for blood-feeding and egg production of the ectoparasitic copepod Lepeophtheirus salmonis. Mol. Biochem. Parasitol. 232:111197. doi: 10.1016/j.molbiopara.2019.111197
Helgesen, K. O., Jansen, P. A., Horsberg, T. E., and Tarpai, A. (2017). The surveillance programme for resistance to chemotherapeutants in salmon lice (Lepeophtheirus salmonis) in Norway 2016. Nor. Vet. Inst. 2–16. Available online at: https://www.vetinst.no/en/surveillance-programmes/salmon-lice-resistance-to-chemotherapeutants/_/attachment/download/66d8a702-4e8e-4933-9e7c-0a7fbaa627a8:823d6fb4028fe54d41f5e028ae91d6d167f142bf/OK%20Resistance%20in%20salmon%20lice%202017.pdf
Helgesen, K. O., Romstad, H., Aaen, S. M., and Horsberg, T. E. (2015). First report of reduced sensitivity towards hydrogen peroxide found in the salmon louse Lepeophtheirus salmonis in Norway. Aquac. Rep. 1, 37–42. doi: 10.1016/j.aqrep.2015.01.001
Helgesen, K. O., Røyset, K., Aspehaug, V., and Jansen, P. A. (2019). The protective effect of the Phe362Tyr mutation in salmon lice’ (Lepeophtheirus salmonis) AChE when exposed to full-scale azamethiphos bath treatments. Aquaculture 505, 517–522. doi: 10.1016/j.aquaculture.2019.03.016
Hemingway, J., and Ranson, H. (2000). Insecticide resistance in insect vectors of human disease. Annu. Rev. Entomol. 45, 371–391. doi: 10.1146/annurev.ento.45.1.371
Heuch, P. A., Bjørn, P. A., Finstad, B., Holst, J. C., Asplin, L., and Nilsen, F. (2005). A review of the norwegian ‘National action plan against salmon lice on salmonids’: The effect on wild salmonids. Aquaculture 246, 79–92. doi: 10.1016/j.aquaculture.2004.12.027
Heuch, P. A., and Mo, T. A. (2001). A model of salmon louse production in Norway: Effects of increasing salmon production and public management measures. Dis. Aquat. Organ. 45, 145–152. doi: 10.3354/dao045145
Heuch, P. A., Parsons, A., and Boxaspen, K. (1995). Diel vertical migration: A possible host-finding mechanism in salmon louse (Lepeophtheirus salmonis) copepodids? Can. J. Fish. Aquat. Sci. 52, 681–689. doi: 10.1139/f95-069
Hevrøy, E. M., Boxaspen, K., Oppedal, F., Taranger, G. L., and Holm, J. C. (2003). The effect of artificial light treatment and depth on the infestation of the sea louse Lepeophtheirus salmonis on Atlantic salmon (Salmo salar L.) culture. Aquaculture 220, 1–14. doi: 10.1016/S0044-8486(02)00189-8
Hjeltnes, B., Britt, B.-J., Geir, B., Asle, H., and Cecilie, S. W. (2019). The health situation in norwegian aquaculture. Nor. Vet. Inst. Oslo Nor. 37–41. Available online at: https://www.vetinst.no/rapporter-og-publikasjoner/rapporter/2019/fish-health-report-2018
Holan, A. B., Roth, B., Breiland, M. S. W., Kolarevic, J., Hansen, ØJ., and Hermansen, Ø, et al. (2017). Beste praksis for medikamentfrie metoder for lakseluskontroll (MEDFRI). Available Online at: https://nofima.no/publikasjon/1471259/.
Holm, H. J., Wadsworth, S., Bjelland, A. K., Krasnov, A., and Evensen, Ø, and Skugor, S. (2016). Dietary phytochemicals modulate skin gene expression profiles and result in reduced lice counts after experimental infection in Atlantic salmon. Parasit. Vectors 9, 1–14. doi: 10.1186/s13071-016-1537-y
Houston, R. D., Haley, C. S., Hamilton, A., Guy, D. R., Tinch, A. E., Taggart, J. B., et al. (2008). Major quantitative trait loci affect resistance to infectious pancreatic Necrosis in Atlantic Salmon (Salmo salar). Genetics 178, 1109–1115. doi: 10.1534/genetics.107.082974
Igboeli, O. O., Fast, M. D., Heumann, J., and Burka, J. F. (2012). Role of P-glycoprotein in emamectin benzoate (SLICE®) resistance in sea lice, Lepeophtheirus salmonis. Aquaculture 34, 40–47. doi: 10.1016/j.aquaculture.2012.03.026
IMR. (2009). Lakselus. Available Online at: http://www.imr.no/temasider/parasitter/lus/lakselus/nb-no (Accessed February 11, 2019)
Imsland, A. K. D., Hanssen, A., Nytrø, A. V., Reynolds, P., Jonassen, T. M., Hangstad, T. A., et al. (2018). It works! Lumpfish can significantly lower sea lice infestation in large-scale salmon farming. Biol. Open 7:bio036301. doi: 10.1242/bio.036301
Imsland, A. K., Reynolds, P., Eliassen, G., Hangstad, T. A., Nytrø, A. V., Foss, A., et al. (2015). Feeding preferences of lumpfish (Cyclopterus lumpus L.) maintained in open net-pens with Atlantic salmon (Salmo salar L.). Aquaculture 436, 47–51. doi: 10.1016/j.aquaculture.2014.10.048
Iversen, A., Hermansen, Ø, Nystøyl, R., Hess, E. J., Rolland, K. H., Garshol, L. D., et al. (2019). Kostnadsutvikling og forståelse av drivkrefter i norsk lakseoppdrett Faglig sluttrapport. Tromsø, Norway: Nofima.
Iversen, A., Hermansen, Ø, Nystøyl, R., Marthinussen, A., and Garshol, D. (2018). Kostnadsdrivere i Lakseoppdrett 2018, Fokus på Smolt og Kapitalbinding. Tromsø, Norway, Nofima. Available online at: https://nofima.no/publikasjon/1642304/
Jackson, D., Moberg, O., Djupevåg, E. M. S., Kane, F., and Hareide, H. (2018). The drivers of sea lice management policies and how best to integrate them into a risk management strategy: An ecosystem approach to sea lice management. J. Fish Dis. 41, 927–933. doi: 10.1111/jfd.12705
Jackson, D., O’Donohoe, P., McDermott, T., Kane, F., Kelly, S., and Drumm, A. (2013). Report on Sea Lice Epidemiology and Management in Ireland with Particular Reference to Potential Interactions with Wild Salmon (Salmo salar) and Freshwater Pearl Mussel (Margaritifera margaritifera) Populations. Marine Institute. Available Online at at: https://oar.marine.ie/handle/10793/893 (Accessed June 4, 2021).
Jacobs, A., De Noia, M., Praebel, K., Kanstad-Hanssen, Ø, Paterno, M., Jackson, D., et al. (2018). Genetic fingerprinting of salmon louse (Lepeophtheirus salmonis) populations in the North-East Atlantic using a random forest classification approach. Sci. Rep. 8:1203. doi: 10.1038/s41598-018-19323-z
Jensen, E. M., Sevatdal, S., Bakke, M. J., Kaur, K., and Horsberg, T. E. (2017). A selection study on a laboratory-designed population of salmon lice (Lepeophtheirus salmonis) using organophosphate and pyrethroid pesticides. PLoS One 12:e0178068. doi: 10.1371/journal.pone.0178068
Jevne, L. S., and Reitan, K. I. (2019). How are the salmon lice (Lepeophtheirus salmonis Krøyer, 1837) in Atlantic salmon farming affected by different control efforts: A case study of an intensive production area with coordinated production cycles and changing delousing practices in 2013–2018. J. Fish Dis. 42, 1573–1586. doi: 10.1111/jfd.13080
Johnson, S. C., and Albright, L. J. (1991). Development, growth, and survival of Lepeophtheirus Salmonis (Copepoda: Caligidae) under laboratory conditions. J. Mar. Biol. Assoc. U. K. 71, 425–436. doi: 10.1017/S0025315400051687
Johnson, S. C., Bravo, S., Nagasawa, K., Kabata, Z., Hwang, J., Ho, J., et al. (2004). A review of the impact of parasitic copepods on marine aquaculture. Zool. Stud. 43, 229–243.
Just Economics. (2021). Dead Loss: the high cost of poor salmon farming Just Econ. Available Online at: https://www.justeconomics.co.uk/health-and-well-being/dead-loss (Accessed June 4, 2021)
Kamita, S. G., Kang, K.-D., Inceoglu, A. B., and Hammock, B. D. (2017). “Genetically Modified Baculoviruses for Pest Insect Control⋆,” in Reference Module in Life Sciences. Amsterdam: Elsevier.
Kaur, K., Besnier, F., Glover, K. A., Nilsen, F., Aspehaug, V. T., Fjørtoft, H. B., et al. (2017). The mechanism (Phe362Tyr mutation) behind resistance in Lepeophtheirus salmonis pre-dates organophosphate use in salmon farming. Sci. Rep. 7, 1–9. doi: 10.1038/s41598-017-12384-6
Kaur, K., Helgesen, K. O., Bakke, M. J., and Horsberg, T. E. (2015). Mechanism behind Resistance against the Organophosphate Azamethiphos in Salmon Lice (Lepeophtheirus salmonis). PLoS One 10:e0124220. doi: 10.1371/journal.pone.0124220
Khan, M. T., Dalvin, S., Nilsen, F., and Male, R. (2017). Microsomal triglyceride transfer protein in the ectoparasitic crustacean salmon louse (Lepeophtheirus salmonis). J. Lipid Res. 58, 1613–1623. doi: 10.1194/jlr.M076430
Khan, M. T., Dalvin, S., Waheed, Q., Nilsen, F., and Male, R. (2018). Molecular characterization of the lipophorin receptor in the crustacean ectoparasite Lepeophtheirus salmonis. PLoS One 13:e0195783. doi: 10.1371/journal.pone.0195783
Kocak, D. M., da Vitoria, Lobo, N., and Widder, E. A. (1999). Computer vision techniques for quantifying, tracking, and identifying bioluminescent plankton. IEEE J. Ocean. Eng. 24, 81–95. doi: 10.1109/48.740157
Komisarczuk, A. Z., Grotmol, S., and Nilsen, F. (2017). Ionotropic receptors signal host recognition in the salmon louse (Lepeophtheirus salmonis, Copepoda). PLoS One 12:e0178812. doi: 10.1371/journal.pone.0178812
Komisarczuk, A. Z., Kongshaug, H., Li, M., and Nilsen, F. (2020). RNAi mediated myosuppressin deficiency affects muscle development and survival in the salmon louse (Lepeophtheirus salmonis). Sci. Rep. 10:15849. doi: 10.1038/s41598-020-69891-2
Komisarczuk, A. Z., Kongshaug, H., and Nilsen, F. (2018). Gene silencing reveals multiple functions of Na+/K+-ATPase in the salmon louse (Lepeophtheirus salmonis). Exp. Parasitol. 185, 79–91. doi: 10.1016/j.exppara.2018.01.005
Krøyer, H. N. (1837). De Danske Östersbanker, et Bidrag til Kundskab om Danmarks Fiskerier. Kjøbenhavn, Denmark: Trykt i S. Triers officin.
Kunz, S., and Kemp, D. (1994). Insecticides and acaricides: resistance and environmental impact. Rev. Sci. Tech. Int. Off. Epizoot. 13, 1249–1286.
Kvæstad, B., Nordtug, T., and Hagemann, A. (2020). A machine vision system for tracking population behavior of zooplankton in small-scale experiments: a case study on salmon lice (Lepeophtheirus salmonis Krøyer, 1838) copepodite population responses to different light stimuli. Biol. Open 9:bio050724. doi: 10.1242/bio.050724
Lam, C. T., Rosanowski, S. M., Walker, M., and St-Hilaire, S. (2020). Sea lice exposure to non-lethal levels of emamectin benzoate after treatments: a potential risk factor for drug resistance. Sci. Rep. 10, 1–8. doi: 10.1038/s41598-020-57594-7
Latorre, D., and Grosman, M. (2013). Peptides inducing an immune response against copepods and/or the development of a mucous shield in fish; vaccines, uses and methods for modulating the fish immune response and/or for inducing development of a mucous shield in fish. Available Online at: https://patents.google.com/patent/CA2873599A1/en?oq=CA2873599 (Accessed June 3, 2021).
Leal, Y., Velazquez, J., Hernandez, L., Swain, J. K., Rodríguez, A. R., Martínez, R., et al. (2019). Promiscuous T cell epitopes boosts specific IgM immune response against a P0 peptide antigen from sea lice in different teleost species. Fish Shellfish Immunol. 92, 322–330. doi: 10.1016/j.fsi.2019.06.018
Li, Q., Sun, X., Dong, J., Song, S., Zhang, T., Liu, D., et al. (2020). Developing a microscopic image dataset in support of intelligent phytoplankton detection using deep learning. ICES J. Mar. Sci. 77, 1427–1439. doi: 10.1093/icesjms/fsz171
Liang, X., Zhang, L., Natarajan, S. K., and Becker, D. F. (2013). Proline mechanisms of stress survival. Antioxid. Redox Signal. 19, 998–1011. doi: 10.1089/ars.2012.5074
Liu, X., Qiu, W., Rao, B., Cao, Y., Fang, X., Yang, J., et al. (2019). Bacterioferritin comigratory protein is important in hydrogen peroxide resistance, nodulation, and nitrogen fixation in Azorhizobium caulinodans. Arch. Microbiol. 201, 823–831. doi: 10.1007/s00203-019-01654-8
Liu, Y., and Bjelland, H. V. (2014). Estimating costs of sea lice control strategy in Norway. Prev. Vet. Med. 117, 469–477. doi: 10.1016/j.prevetmed.2014.08.018
Ljungfeldt, L. E. R., Quintela, M., Besnier, F., Nilsen, F., and Glover, K. A. (2017). A pedigree-based experiment reveals variation in salinity and thermal tolerance in the salmon louse, Lepeophtheirus salmonis. Evol. Appl. 10, 1007–1019. doi: 10.1111/eva.12505
López-Cortés, X. A., Nachtigall, F. M., Olate, V. R., Araya, M., Oyanedel, S., Diaz, V., et al. (2017). Fast detection of pathogens in salmon farming industry. Aquaculture 470, 17–24. doi: 10.1016/j.aquaculture.2016.12.008
Love, D. C., Fry, J. P., Cabello, F., Good, C. M., and Lunestad, B. T. (2020). Veterinary drug use in United States net pen Salmon aquaculture: Implications for drug use policy. Aquaculture 518:734820. doi: 10.1016/j.aquaculture.2019.734820
Luo, J. Y., Irisson, J.-O., Graham, B., Guigand, C., Sarafraz, A., Mader, C., et al. (2018). Automated plankton image analysis using convolutional neural networks. Limnol. Oceanogr. Methods 16, 814–827. doi: 10.1002/lom3.10285
Luthman, O., Jonell, M., and Troell, M. (2019). Governing the salmon farming industry: Comparison between national regulations and the ASC salmon standard. Mar. Policy 106:103534. doi: 10.1016/j.marpol.2019.103534
Macken, A., Lillicrap, A., and Langford, K. (2015). Benzoylurea pesticides used as veterinary medicines in aquaculture: Risks and developmental effects on nontarget crustaceans. Environ. Toxicol. Chem. 34, 1533–1542. doi: 10.1002/etc.2920
Maldonado-Aguayo, W., and Gallardo-Escárate, C. (2014). Increasing transcriptome response of serpins during the ontogenetic stages in the salmon louse Caligus rogercresseyi (Copepoda: Caligidae). Mar. Genom. 15, 55–64. doi: 10.1016/j.margen.2014.04.006
Martinez-Torres, D., Chandre, F., Williamson, M. S., Darriet, F., Bergé, J. B., Devonshire, A. L., et al. (1998). Molecular characterization of pyrethroid knockdown resistance (kdr) in the major malaria vector Anopheles gambiae s.s. Insect Mol. Biol. 7, 179–184. doi: 10.1046/j.1365-2583.1998.72062.x
Ministry of Trade, Industry and Fisheries. (2013). Forskrift om bekjempelse av lakselus i akvakulturanlegg - Lovdata. Available Online at: https://lovdata.no/dokument/SF/forskrift/2012-12-05-1140 (Accessed June 4, 2021).
Ministry of Trade, Industry and Fisheries. (2017). Forskrift om endring i forskrift om bekjempelse av lakselus i akvakulturanlegg - Lovdata. Available Online at: https://lovdata.no/dokument/LTI/forskrift/2017-03-06-275 (Accessed June 4, 2021).
Misund, A. U. (2018). From a natural occurring parasitic organism to a management object: Historical perceptions and discourses related to salmon lice in Norway. Mar. Policy 99, 400–406. doi: 10.1016/j.marpol.2018.10.037
Moen, T., Baranski, M., Sonesson, A. K., and Kjøglum, S. (2009). Confirmation and fine-mapping of a major QTL for resistance to infectious pancreatic necrosis in Atlantic salmon (Salmo salar): population-level associations between markers and trait. BMC Genom. 10:368. doi: 10.1186/1471-2164-10-368
Mogensen, T. H. (2009). Pathogen recognition and inflammatory signaling in innate immune defenses. Clin. Microbiol. Rev. 22, 240–273. doi: 10.1128/CMR.00046-08
Moltumyr, L., Gismervik, K., Gu, J., Gåsnes, S. K., Kristiansen, T. S., Rønnestad, I., et al. (2021). Does the thermal component of warm water treatment inflict acute lesions on Atlantic salmon (Salmo salar)? Aquaculture 532:736048. doi: 10.1016/j.aquaculture.2020.736048
Moore, G. (2020). Cutting the lice problem with ‘genetic scissors’ - FishFarmingExpert.com. Available Online at: https://www.fishfarmingexpert.com/article/cutting-the-lice-problem-with-genetic-scissors/ (Accessed April 13, 2021)
Morado, N., Mota, P. G., and Soares, M. C. (2019). The rock cook wrasse centrolabrus exoletus aims to clean. Front. Ecol. Evol. 7:182. doi: 10.3389/fevo.2019.00182
Mulligan, M. J., Lyke, K. E., Kitchin, N., Absalon, J., Gurtman, A., Lockhart, S., et al. (2020). Phase I/II study of COVID-19 RNA vaccine BNT162b1 in adults. Nature 586, 589–593. doi: 10.1038/s41586-020-2639-4
Munro, L. A. (2020). Scottish Fish Farm Production Survey 2019 - gov.scot. Available Online at: https://www.gov.scot/publications/scottish-fish-farm-production-survey-2019/pages/12/ (Accessed June 8, 2021)
Mutoloki, S., Munang’andu, H. M., and Evensen, Ø (2015). Oral vaccination of fish – antigen preparations, uptake, and immune induction. Front. Immunol. 6:519. doi: 10.3389/fimmu.2015.00519
Narain, P., and Mishra, A. K. (1975). Efficiency of selective breeding based on a phenotypic index. J. Genet. 62, 69–76. doi: 10.1007/BF02985168
NFR, P. B. (2021). MucoProtect. Novel bio-mimicking nanocarriers for oral delivery of mucosal vaccines - protection at the first line of defence. - Prosjektbanken. Prosjektbanken - Forskningsrådet. Available Online at: https://prosjektbanken.forskningsradet.no/project/FORISS/280520?Kilde=FORISS&distribution=Ar&chart=bar&calcType=funding&Sprak=no&sortBy=score&sortOrder=desc&resultCount=30&offset=0&Fritekst=mucoprotect&source=FORISS&projectId=280520 (Accessed March 17, 2021).
Nilsen, A., and Colquhoun, D. (2014). Nilsen A, Viljugrein H, Røsæg MV, Colquhoun D. Rensefiskhelse – kartlegging av dødelighet og dødelighetsårsaker. Veterinærinstituttets rapportserie 12-2014. Oslo: Veterinærinstituttet.
Nilsen, A., Erikson, U., Aunsmo, A., Østvik, A., and Heuch, P. A. (2010). Mekanisk Fjerning av Lakselus “FLS avlusersystem”–Test av Ejektor- Pumpe fra Flatsetsund Engineering AS. Veterinærinstituttets Rapportserie 11-2010. Oslo: Veterinærinstituttet.
Noble, C., Gismervik, K., Iversen, M. H., Kolarevic, J., Nilsson, J., Stien, L. H., et al. (2018). Welfare Indicators for farmed Atlantic salmon?: tools for assessing fish welfare. Tromsø, Norway: Nofima, 351.
Nordtug, T., Kvæstad, B., and Hagemann, A. (2021). Responses and preferences of salmon louse (Lepeophtheirus salmonis Krøyer 1836) copepodids to underwater artificial light sources. Aquaculture 532:736036. doi: 10.1016/j.aquaculture.2020.736036
Norwegian Institute of Public Health. (2020). 2020: Bruk av legemidler i fiskeoppdrett. 2020 Bruk Av Legemidler Fiskeoppdrett. Available Online at: https://www.fhi.no/hn/legemiddelbruk/fisk/2020-bruk-av-legemidler-i-fiskeoppdrett/ (Accessed March 17, 2021)
Núñez-Acuña, G., Gallardo-Escárate, C., Skiftesvik, A. B., Fields, D. M., and Browman, H. I. (2019). Silencing of ionotropic receptor 25a decreases chemosensory activity in the salmon louse Lepeophtheirus salmonis during the infective stage. Gene 697, 35–39. doi: 10.1016/j.gene.2019.02.012
Oerke, E.-C., Dehne, H.-W., Schönbeck, F., and Weber, A. (2012). Crop Production and Crop Protection: Estimated Losses in Major Food and Cash Crops. Amsterdam: Elsevier.
Olsvik, P. A., Samuelsen, O. B., Agnalt, A.-L., and Lunestad, B. T. (2015). Transcriptional responses to teflubenzuron exposure in European lobster (Homarus gammarus). Aquat. Toxicol. 167, 143–156. doi: 10.1016/j.aquatox.2015.07.008
Oppedal, F., Folkedal, O., Stien, L. H., Vågseth, T., Fosse, J. O., Dempster, T., et al. (2020). Atlantic salmon cope in submerged cages when given access to an air dome that enables fish to maintain neutral buoyancy. Aquaculture 525:735286. doi: 10.1016/j.aquaculture.2020.735286
O’Shea, B., Wadsworth, S., Marambio, J. P., Birkett, M. A., Pickett, J. A., and Mordue Luntz, A. J. (2017). Disruption of host-seeking behaviour by the salmon louse, Lepeophtheirus salmonis, using botanically derived repellents. J. Fish Dis. 40, 495–505. doi: 10.1111/jfd.12526
Øvergård, A. C., Eichner, C., Nilsen, F., and Dalvin, S. (2017). Molecular characterization and functional analysis of a salmon louse (Lepeophtheirus salmonis, Krøyer 1838) heme peroxidase with a potential role in extracellular matrixes. Comp. Biochem. Physiol. Part Mol. Integr. Physiol. 206, 1–10. doi: 10.1016/j.cbpa.2017.01.004
Overton, K., Barrett, L., Oppedal, F., Kristiansen, T., and Dempster, T. (2020). Sea lice removal by cleaner fish in salmon aquaculture: a review of the evidence base. Aquac. Environ. Interact. 12, 31–44. doi: 10.3354/aei00345
Overton, K., Dempster, T., Oppedal, F., Kristiansen, T. S., Gismervik, K., and Stien, L. H. (2019). Salmon lice treatments and salmon mortality in Norwegian aquaculture: a review. Rev. Aquac. 11, 1398–1417. doi: 10.1111/raq.12299
Pahl, B. C., Cole, D. G., and Bayer, R. C. (1999). Sea lice control I. J. Appl. Aquac. 9, 85–96. doi: 10.1300/J028v09n01_08
Panina-Bordignon, P., Tan, A., Termijtelen, A., Demotz, S., Corradin, G., and Lanzavecchia, A. (1989). Universally immunogenic T cell epitopes: promiscuous binding to human MHC class II and promiscuous recognition by T cells. Eur. J. Immunol. 19, 2237–2242. doi: 10.1002/eji.1830191209
Perkin, L. C., Adrianos, S. L., and Oppert, B. (2016). Gene disruption technologies have the potential to transform stored product insect pest control. Insects 7:46. doi: 10.3390/insects7030046
Pike, A. W., and Wadsworth, S. L. (1999). “Sealice on Salmonids: Their Biology and Control,” in Advances in Parasitology, eds J. R. Baker, R. Muller, and D. Rollinson (San Diego: Academic Press), 233–337.
Pimprikar, G. D., and Georghiou, G. P. (1979). Mechanisms of resistance to diflubenzuron in the house fly, Musca domestica (L.). Pestic. Biochem. Physiol. 12, 10–22. doi: 10.1016/0048-3575(79)90089-0
Poley, J. D., Braden, L. M., Messmer, A. M., Igboeli, O. O., Whyte, S. K., Macdonald, A., et al. (2018). High level efficacy of lufenuron against sea lice (Lepeophtheirus salmonis) linked to rapid impact on moulting processes. Int. J. Parasitol. Drugs Drug Resist. 8, 174–188. doi: 10.1016/j.ijpddr.2018.02.007
Pontoppidan, E. (1755). The Natural History of Norway: Translated from the Danish Original, Page 132. London: A Linde. Available online at: https://www.biodiversitylibrary.org/item/131226#page/11/mode/1up
Powell, A., Treasurer, J. W., Pooley, C. L., Keay, A. J., Lloyd, R., Imsland, A., et al. (2018). Use of lumpfish for sea-lice control in salmon farming: challenges and opportunities. Rev. Aquac. 10, 683–702. doi: 10.1111/raq.12194
Powell, M. D., Reynolds, P., and Kristensen, T. (2015). Freshwater treatment of amoebic gill disease and sea-lice in seawater salmon production: Considerations of water chemistry and fish welfare in Norway. Aquaculture 448, 18–28. doi: 10.1016/j.aquaculture.2015.05.027
Ran, F. A., Hsu, P. D., Wright, J., Agarwala, V., Scott, D. A., and Zhang, F. (2013). Genome engineering using the CRISPR-Cas9 system. Nat. Protoc. 8, 2281–2308. doi: 10.1038/nprot.2013.143
Raynard, R. S., Bricknell, I. R., Billingsley, P. F., Nisbet, A. J., Vigneau, A., and Sommerville, C. (2002). Development of vaccines against sea lice. Pest Manag. Sci. 58, 569–575. doi: 10.1002/ps.474
Redaksjonen. (2021). Antallet merder med dispensasjon fra Mattilsynet tredoblet - Kyst.no. Available Online at: https://www.kyst.no/article/antallet-merder-med-dispensasjon-fra-mattilsynet-tredoblet/ (Accessed April 14, 2021)
Refstie, S., Baeverfjord, G., Seim, R. R., and Elvebø, O. (2010). Effects of dietary yeast cell wall β-glucans and MOS on performance, gut health, and salmon lice resistance in Atlantic salmon (Salmo salar) fed sunflower and soybean meal. Aquaculture 305, 109–116. doi: 10.1016/j.aquaculture.2010.04.005
Ritchie, G. (1997). The host transfer ability of Lepeophtheirus salmonis (Copepoda: Caligidae) from farmed Atlantic salmon, Salmo salar L. J. Fish Dis. 20, 153–157. doi: 10.1046/j.1365-2761.1997.00285.x
Robinson, N. A. (2021). Drawing on the natural abilities of Pacific salmon to fight sea lice. Draw. Nat. Abil. Pac. Salmon Fight Sea Lice Nofima. Available Online at: https://nofima.no/en/nyhet/2020/10/drawing-on-the-natural-abilities-of-pacific-salmon-to-fight-sea-lice/ (Accessed April 13, 2021).
Sandbrink, J. B., and Shattock, R. J. (2020). RNA Vaccines: A suitable platform for tackling emerging pandemics? Front. Immunol. 11:8460. doi: 10.3389/fimmu.2020.608460
Sandlund, L., Kongshaug, H., Nilsen, F., and Dalvin, S. (2018). Molecular characterization and functional analysis of components of the TOR pathway of the salmon louse, Lepeophtheirus salmonis (Krøyer, 1838). Exp. Parasitol. 188, 83–92. doi: 10.1016/j.exppara.2018.04.004
Sandlund, L., Nilsen, F., Male, R., and Dalvin, S. (2016). The ecdysone receptor (EcR) is a major regulator of tissue development and growth in the marine salmonid ectoparasite, Lepeophtheirus salmonis (Copepoda, Caligidae). Mol. Biochem. Parasitol. 208, 65–73. doi: 10.1016/j.molbiopara.2016.06.007
Santolamazza, F., Calzetta, M., Etang, J., Barrese, E., Dia, I., Caccone, A., et al. (2008). Distribution of knock-down resistance mutations in Anopheles gambiae molecular forms in west and west-central Africa. Malar. J. 7, 1–8. doi: 10.1186/1475-2875-7-74
Scottish Statutory Instruments. (2021). The Fish Farming Businesses (Reporting) (Scotland) Order 2020. Available Online at: https://www.legislation.gov.uk/ssi/2020/447/contents/made (Accessed June 4, 2021)
Singh, A., Kumar, N., Tomar, P. P. S., Bhose, S., Ghosh, D. K., Roy, P., et al. (2017). Characterization of a bacterioferritin comigratory protein family 1-Cys peroxiredoxin from Candidatus Liberibacter asiaticus. Protoplasma 254, 1675–1691. doi: 10.1007/s00709-016-1062-z
Skern-Mauritzen, R., Torrissen, O., and Glover, K. A. (2014). Pacific and Atlantic Lepeophtheirus salmonis (Krøyer, 1838) are allopatric subspecies: Lepeophtheirus salmonis salmonis and L. salmonis oncorhynchi subspecies novo. BMC Genet. 15:32. doi: 10.1186/1471-2156-15-32
Skiftesvik, A. B., Blom, G., Agnalt, A.-L., Durif, C. M. F., Browman, H. I., Bjelland, R. M., et al. (2014). Wrasse (Labridae) as cleaner fish in salmonid aquaculture – The Hardangerfjord as a case study. Mar. Biol. Res. 10, 289–300. doi: 10.1080/17451000.2013.810760
Skugor, S., Jodaa Holm, H., Bjelland, A. K., Pino, J., Evensen, Ø, Krasnov, A., et al. (2016). Nutrigenomic effects of glucosinolates on liver, muscle and distal kidney in parasite-free and salmon louse infected Atlantic salmon. Parasit. Vect. 9, 1–17. doi: 10.1186/s13071-016-1921-7
Soderlund, D. M. (2012). Molecular mechanisms of pyrethroid insecticide neurotoxicity: Recent advances. Arch. Toxicol. 86, 165–181. doi: 10.1007/s00204-011-0726-x
Sommerset, I., Krossøy, B., Biering, E., and Frost, P. (2005). Vaccines for fish in aquaculture. Expert Rev. Vacc. 4, 89–101. doi: 10.1586/14760584.4.1.89
Sparks, W. O., Bartholomay, L. C., and Bonning, B. C. (2008). “Insect Immunity to Viruses,” in Insect Immunology, ed. N. E. Beckage (San Diego: Academic Press), 209–242.
Spectralice. (2021). SpectraLice. Ecotone. Available Online at: https://ecotone.com/automatisk-luseteller-fra-ecotone/?lang=en (Accessed April 14, 2021).
Sporleder, M., and Lacey, L. A. (2013). “Chapter 16 - Biopesticides,” in Insect Pests of Potato, eds A. Alyokhin, C. Vincent, and P. Giordanengo (San Diego: Academic Press), 463–497.
SSB. (2020). Akvakultur (avsluttet i Statistisk sentralbyrå). ssb.no. Available Online at: https://www.ssb.no/jord-skog-jakt-og-fiskeri/statistikker/fiskeoppdrett/aar/2020-10-29 (Accessed March 12, 2021)
Stien, L. H., Dempster, T., Bui, S., Glaropoulos, A., Fosseidengen, J. E., Wright, D. W., et al. (2016). “Snorkel” sea lice barrier technology reduces sea lice loads on harvest-sized Atlantic salmon with minimal welfare impacts. Aquaculture 458, 29–37. doi: 10.1016/j.aquaculture.2016.02.014
Stien, L. H., Nilsson, J., Hevrøy, E. M., Oppedal, F., Kristiansen, T. S., Lien, A. M., et al. (2012). Skirt around a salmon sea cage to reduce infestation of salmon lice resulted in low oxygen levels. Aquac. Eng. 51, 21–25. doi: 10.1016/j.aquaeng.2012.06.002
Sutherland, B. J. G., Poley, J. D., Igboeli, O. O., Jantzen, J. R., Fast, M. D., Koop, B. F., et al. (2015). Transcriptomic responses to emamectin benzoate in Pacific and Atlantic Canada salmon lice Lepeophtheirus salmonis with differing levels of drug resistance. Evol. Appl. 8, 133–148. doi: 10.1111/eva.12237
Swain, J. K., Carpio, Y., Johansen, L.-H., Velazquez, J., Hernandez, L., Leal, Y., et al. (2020). Impact of a candidate vaccine on the dynamics of salmon lice (Lepeophtheirus salmonis) infestation and immune response in Atlantic salmon (Salmo salar L.). PLoS One 15:e0239827. doi: 10.1371/journal.pone.0239827
Syrstad, O. (2009). Harald Skjervold. Nor. Biogr. Leks. Available Online at: http://nbl.snl.no/Harald_Skjervold (Accessed March 12, 2021)
Torrissen, O., Jones, S., Asche, F., Guttormsen, A., Skilbrei, O. T., Nilsen, F., et al. (2013). Salmon lice - impact on wild salmonids and salmon aquaculture. J. Fish Dis. 36, 171–194. doi: 10.1111/jfd.12061
Treasurer, J., and Feledi, T. (2014). The physical condition and welfare of five species of wild-caught wrasse stocked under aquaculture conditions and when stocked in atlantic salmon, salmo salar, production cages. J. World Aquac. Soc. 45, 213–219. doi: 10.1111/jwas.12099
Tröße, C., Kongshaug, H., Dondrup, M., and Nilsen, F. (2015). Characterisation of iron regulatory protein 1A and 1B in the blood-feeding copepod Lepeophtheirus salmonis. Exp. Parasitol. 157, 1–11. doi: 10.1016/j.exppara.2015.06.010
Tröße, C., Nilsen, F., and Dalvin, S. (2014). RNA interference mediated knockdown of the KDEL receptor and COPB2 inhibits digestion and reproduction in the parasitic copepod Lepeophtheirus salmonis. Comp. Biochem. Physiol. B Biochem. Mol. Biol. 170, 1–9. doi: 10.1016/j.cbpb.2013.12.006
Tschesche, C., Bekaert, M., Bassett, D. I., Mitchell, C., North, B., Boyd, S., et al. (2021). Investigation of deltamethrin resistance in salmon lice (Lepeophtheirus salmonis) provides no evidence for roles of mutations in voltage-gated sodium channels. Pest Manag. Sci. 77, 1052–1060. doi: 10.1002/ps.6120
UN Economic & Social Affairs. (2019). World population prospects 2019. Available Online at: https://population.un.org/wpp/Download/Standard/Population/.
Urbina, M. A., Cumillaf, J. P., Paschke, K., and Gebauer, P. (2019). Effects of pharmaceuticals used to treat salmon lice on non-target species: Evidence from a systematic review. Sci. Total Environ. 649, 1124–1136. doi: 10.1016/j.scitotenv.2018.08.334
Valenzuela-Muñoz, V., Chavez-Mardones, J., and Gallardo-Escárate, C. (2015). RNA-seq analysis evidences multiple gene responses in Caligus rogercresseyi exposed to the anti-salmon lice drug azamethiphos. Aquaculture 446, 156–166. doi: 10.1016/j.aquaculture.2015.05.011
Vatn, J. A. Å (2019). Metode for Kartlegging av Den Fototaktiske Svømmeresponsen til Lepeophtheirus Salmonis. Available online at: https://nmbu.brage.unit.no/nmbu-xmlui/handle/11250/2600181 (accessed July 7, 2021).
Walde, C. S., Jensen, B. B., Pettersen, J. M., and Stormoen, M. (2021). Estimating cage-level mortality distributions following different delousing treatments of Atlantic salmon (salmo salar) in Norway. J. Fish Dis. 44, 899–912. doi: 10.1111/jfd.13348
Westgård, A. K. M. (2020). Statement SkaMik. Aqua Kompetanse AS, Rørvik, Norway. Available online at: https://skamik.no/wp-content/uploads/2020/08/statement-skamik_21-07-20.pdf (accessed July 7, 2021).
White, H. C. (1940). “Sea Lice” (Lepeophtheirus) and death of salmon. J. Fish. Res. Board Can. 5a, 172–175. doi: 10.1139/f40-017
Willadsen, P., Bird, P., Cobon, G. S., and Hungerford, J. (1995). Commercialisation of a recombinant vaccine against Boophilus microplus. Parasitology 110, S43–S50. doi: 10.1017/s0031182000001487
Williams, T., Virto, C., Murillo, R., and Caballero, P. (2017). Covert infection of insects by baculoviruses. Front. Microbiol. 8:1337. doi: 10.3389/fmicb.2017.01337
Wootten, R., Smith, J. W., and Needham, E. A. (1982). Aspects of the biology of the parasitic copepods Lepeophtheirus salmonis and Caligus elongatus on farmed salmonids, and their treatment. Proc. R. Soc. Edinb. Sect. B Biol. Sci. 81, 185–197. doi: 10.1017/S0269727000003389
Wright, D. W., Geitung, L., Karlsbakk, E., Stien, L. H., Dempster, T., Oldham, T., et al. (2018). Surface environment modification in Atlantic salmon sea-cages: effects on amoebic gill disease, salmon lice, growth and welfare. Aquac. Environ. Interact. 10, 255–265. doi: 10.3354/aei00269
Wright, D. W., Oppedal, F., and Dempster, T. (2016). Early-stage sea lice recruits on Atlantic salmon are freshwater sensitive. J. Fish Dis. 39, 1179–1186. doi: 10.1111/jfd.12452
Yan, F., Wang, W., and Zhang, J. (2019). CRISPR-Cas12 and Cas13: the lesser known siblings of CRISPR-Cas9. Cell Biol. Toxicol. 35, 489–492. doi: 10.1007/s10565-019-09489-1
Yossa, R., and Dumas, A. (2016). Approaches for controlling sea lice infestation in global salmon farming: What is applicable in Canadian aquaculture industry. J. Appl. Aquac. 28, 314–329. doi: 10.1080/10454438.2016.1234877
Zhang, Z.-Q. (2013). Animal biodiversity: An outline of higher-level classification and survey of taxonomic richness (Addenda 2013). Zootaxa 3703, 1–82.
Keywords: enabling technologies, genome editing, marine arthropod, salmon lice, delousing, medical treatments, non-medical treatments
Citation: Guragain P, Tkachov M, Båtnes AS, Olsen Y, Winge P and Bones AM (2021) Principles and Methods of Counteracting Harmful Salmon–Arthropod Interactions in Salmon Farming: Addressing Possibilities, Limitations, and Future Options. Front. Mar. Sci. 8:701793. doi: 10.3389/fmars.2021.701793
Received: 28 April 2021; Accepted: 28 June 2021;
Published: 20 July 2021.
Edited by:
Benjamin Costas, University of Porto, PortugalReviewed by:
Laura Braden, AquaBounty, CanadaOystein Evensen, Norwegian University of Life Sciences, Norway
Copyright © 2021 Guragain, Tkachov, Båtnes, Olsen, Winge and Bones. This is an open-access article distributed under the terms of the Creative Commons Attribution License (CC BY). The use, distribution or reproduction in other forums is permitted, provided the original author(s) and the copyright owner(s) are credited and that the original publication in this journal is cited, in accordance with accepted academic practice. No use, distribution or reproduction is permitted which does not comply with these terms.
*Correspondence: Atle M. Bones, YXRsZS5tLmJvbmVzQG50bnUubm8=