- 1Laboratory of Zoology, University of Yaoundé I, Yaoundé, Cameroon
- 2African Marine Mammal Conservation Organization, Dizangue, Cameroon
- 3Division of Natural Sciences, New College of Florida, Sarasota, FL, United States
- 4African Manatee Program, African Aquatic Conservation Fund, Joal, Senegal
- 5Fundación Internacional para la Naturaleza y la Sustentabilidad, Chetumal, Quintana Roo, Mexico
- 6Japan manatee Education and Study Lab, Tokyo, Japan
The African manatee (Trichechus senegalensis) is an elusive, data-deficient, and endangered species which inhabits marine and freshwater systems throughout Western and Central Africa. A major challenge in understanding the species ecology and distribution is the difficulty in detecting it using traditional visual surveys. The recent invasion of Giant Salvinia (Salvinia molesta) at the most important site for the species in Cameroon further limits their detectability and may restrict their movements and habitat use. To investigate methods’ effectiveness in detecting African manatees, we conducted monthly vessel surveys from which visual point scans, 360° sonar scans, and passive acoustic monitoring were conducted simultaneously at ten locations and over 12 months in Lake Ossa, Cameroon. Manatee detection frequency was calculated for each method and the influence of some environmental conditions on the methods’ effectiveness and manatee detection likelihood was assessed by fitting a binary logistic regression to our data. Detection frequencies were significantly different between methods (p < 0.01) with passive acoustics being the most successful (24.17%; n = 120), followed by the 360° sonar scan (11.67%; n = 120), and the visual point scan (3.33%; n = 120). The likelihood of detecting manatees in Lake Ossa was significantly influenced by water depth (p = 0.02) and transparency (p < 0.01). It was more likely to detect manatees in shallower water depths and higher water transparency. Passive acoustic detections were more effective in uninvaded areas of the Lake. We recommend using passive acoustics to enhance African manatee detections in future surveys.
1 Introduction
Sirenians (Order Sirenia) include four species now living, which are distributed within two families: the Dugongid (Dugongs - Dugong dugon) and the Trichechids (the West Indian manatee - Trichechus manatus; the Amazonian manatee - Trichechus inunguis and the African manatee - Trichechus senegalensis). Among sirenians, the African manatee is the least studied species and moreover, the least studied large mammal in Africa (Trimble and Van Aarde, 2010). Similar to the other sirenians, African manatees are classified as Vulnerable in the IUCN Red-List and in Appendix I of both the Convention on the International Trade of Endangered Species of Wild Fauna and Flora and the Convention on Migratory Species (Convention on the Conservation of Migratory Species, 2009; Convention on International Trade of Endangered Species, 2013; Keith Diagne, 2015). Their distribution spans 21 countries, including 18 along the African Atlantic coast (from southern Mauritania to central Angola) and three inland countries (Chad, Niger, and Mali). In Cameroon, manatees are found throughout the coastal areas of the country, including in numerous rivers (Ndian, Wouri, Sanaga, Nyong, Cross, Benue, Nkam, and Ntem Rivers), and in Lake Ossa and Lake Tissongo (Nishiwaki et al., 1982; Powell, 1996; Keith Diagne, 2015; Takoukam Kamla, 2019). The lower Sanaga River Watershed is the most monitored manatee habitat in Cameroon, home to approximately 250 manatees, including a minimum of 49 manatees in Lake Ossa (Takoukam Kamla, 2019). Lake Ossa is considered a manatee sanctuary, as it is protected and hosts a year-round, relatively abundant manatee population when compared to other habitats (Mayaka et al., 2013; Keith Diagne, 2015; Takoukam Kamla, 2019). However, Lake Ossa was recently invaded by Giant Salvinia (Salvinia molesta), a free-floating aquatic fern native to Brazil and known to have infested many countries and caused major harmful environmental impacts on native ecosystems in tropical and subtropical regions (Oliver, 1993; McFarland et al., 2004). This invasive plant has covered approximately 50% of Lake Ossa’s surface (4,000 ha) in less than five years. The plant seems to outcompete the antelope grass (Echinochloa pyramidalis), which is the major manatee local food, representing on average 63% of their diet composition (Takoukam Kamla, 2019). Also, the dense and widespread mats formed by Giant Salvinia on the surface of Lake Ossa may influence their habitat use and/or making their detection more challenging.
In addition to habitat degradation like the current situation of Lake Ossa, African manatees face other major threats throughout their range including poaching, bycatch in fishing gear, and habitat fragmentation by dams (Keith Diagne, 2015). Fewer than 10,000 individuals of the species are estimated to remain, and it is estimated that 30% or more are highly likely to perish within 90 years due to threats (Keith Diagne, 2015). A major challenge to the conservation of African manatees is the limited available data due in part to the difficulty in detecting and gathering information on them in the wild. African manatees are naturally cryptic, elusive, and only mildly social; due to poaching these traits seem to have been exacerbated. As in most of their range, previous African manatee ecological surveys in Cameroon were mostly based on visual detections (Grigione, 1996; Takoukam Kamla, 2012; Ngafack, 2014) and/or interviews of local communities (Nishiwaki et al., 1982; Powell, 1996; Mayaka et al., 2013; Mayaka et al., 2015; Mayaka et al., 2019). A few studies used DNA to detect manatees (e-DNA, Hunter et al., 2018) and to estimate their population numbers (Takoukam Kamla, 2019). However, genetic analyses are expensive, visual survey methods are limited by the low detectability of manatees and the highly turbid and tannic waters they inhabit, whereas citizen data are limited by the unreliability of public reports of bycatch and poaching due to the fear of being prosecuted.
Active and passive acoustics are used extensively in the field to detect marine mammals and monitor their behavior. Many studies have established sound navigation and ranging (SONAR) scans as a valuable method of underwater detection of Antillean manatees (T. m. manatus) in their turbid waters of Mexico and Central America, with manatee detection frequencies of up to 93% (Gonzalez-Socoloske et al., 2009; Gonzalez-Socoloske and Olivera-Gomez, 2012; Guzman and Condit, 2017; Puc-Carrasco et al., 2017; Castelblanco-Martínez et al., 2018). In addition, manatees produce a variety of vocalizations including squeaks, screeches, whines, and trills (Umeed et al., 2018; Brady et al., 2020; Baotic et al., 2022) shown to be important for their underwater communication and emitted across various behavioral states (Bengtson and Fitzgerald, 1985; O’Shea and Poché, 2006; Brady et al., 2021). Passive acoustics have been used in many studies to monitor manatees and have yielded up to 100% detection frequencies (LaCommare et al., 2008; Kikuchi et al., 2013; Rivera Chavarria et al., 2015; Rycyk et al., 2021; Rycyk et al., 2022). While the above methodologies have been used to monitor other species of manatee (Florida manatees: Rycyk et al., 2022; Antillean manatees: Ramos et al., 2020), they have been rarely implemented with African manatees. Rycyk et al. (2021) deployed passive acoustic recorders in Lake Ossa in 2020 for two, 3-day periods, resulting in the detection of thousands of vocalizations and enabling the first characterization of African manatee calls; the authors found that African manatee vocalizations are similar to those of other manatee species, with mean fundamental frequency of 4.65 kHz and average duration of 0.181s. Finally, Takoukam Kamla (personal communication) conducted sporadic sonar detections.
In this study, we compared three methods of monitoring African manatees in Lake Ossa, Cameroon: visual point scans by boat-based observers, passive acoustic monitoring for manatee sounds, and 360° sonar scans. Our goals were to: 1) determine and compare the manatee detection frequency of each of the three methods; 2) determine which environmental conditions influence the likelihood of detecting manatees; and 3) make recommendations for future manatee surveys based on the combination of survey methods and environmental conditions evaluated.
2 Material and methods
2.1 Study site
This study was conducted in Lake Ossa Wildlife Reserve (LOWR, Figure 1), an IUCN category IV protected area, situated in the Littoral region of Cameroon between the 3.75 N and 3.87 N (latitude), and 9.75 E and 10.07 E (longitude) and at an average elevation of approximately 45 m (Wirrmann and Elouga, 1998). Situated approximately 40 km inland, Lake Ossa is a fully freshwater system, which is connected to the country’s major river, the Sanaga River, through a narrow and comparatively deep (5-9 m) channel. Through this channel, the depth of Lake Ossa is seasonally influenced by the incursion of water from the Sanaga River (Takoukam Kamla, 2019) which also influences the lake’s water transparency. Lake Ossa is a shallow lacustrine complex, with a maximum depth of 7 m measured during the rainy season (March-November) (Giresse et al., 2005). The Lake Ossa complex encompasses three connected lakes: Lake Mévia (700 ha) in the north, Lake Mwembè (300 ha) in the south, and Lake Ossa (3000 ha)—also known as Big Lake—situated between the two. The waters of Lake Ossa are relatively calm, with Beaufort Sea state typically fluctuating between 0 and 2, and water transparency is higher during the rainy season than the dry season (Takoukam Kamla, 2019). However, since 2016 the lake is being invaded by Giant Salvinia, a free-floating aquatic fern (Figure 2) originating from Southern Brazil which has the potential to choke streams and lakes (Westbrook, 1984) and to kill aquatic wildlife such as fish (Barrett, 1989). To date, Giant Salvinia covers over 50% of the lake’s surface with Malongue, Koungue, and Mevia being the most invaded areas.
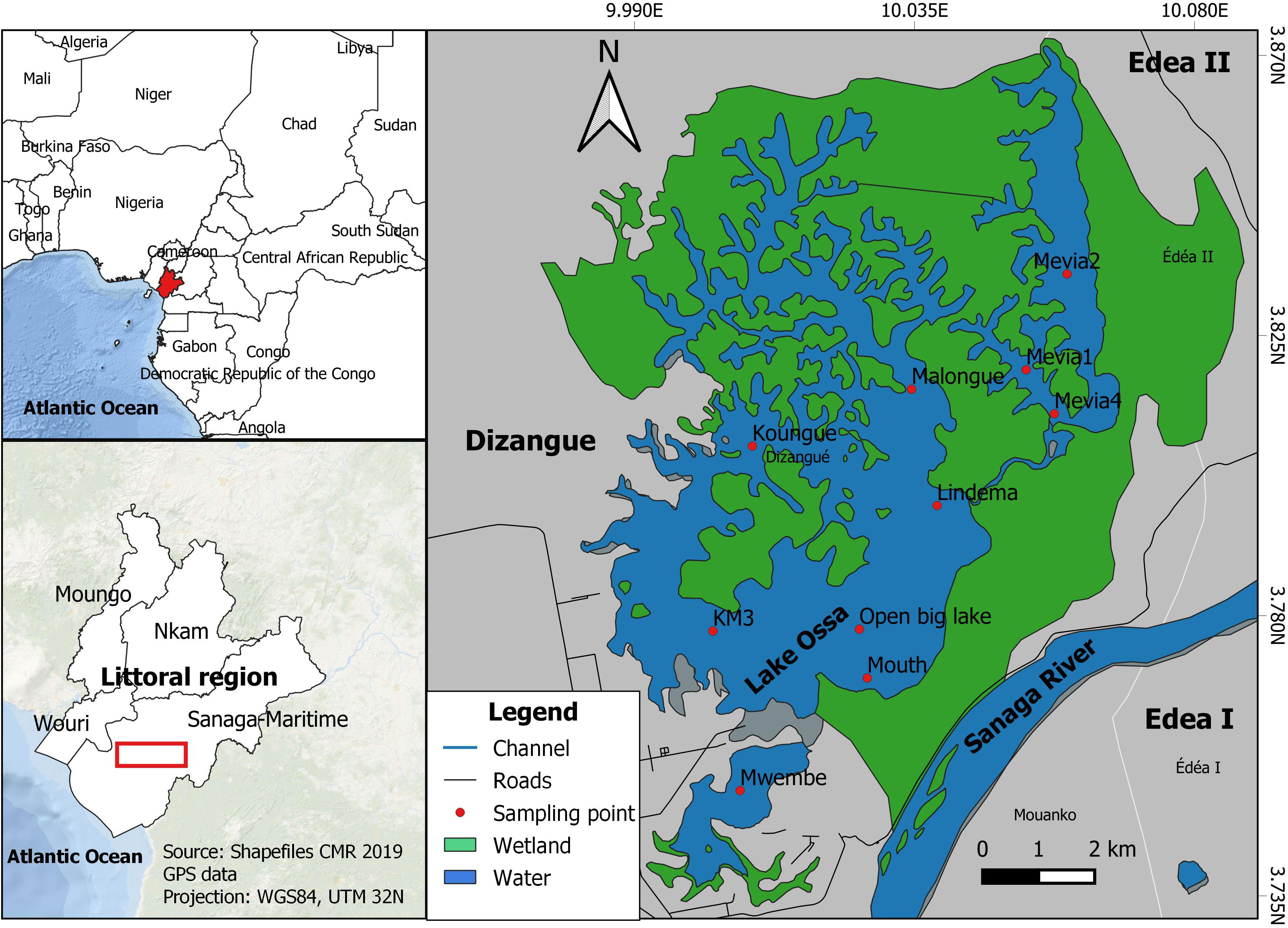
Figure 1 Map of Lake Ossa highlighting sampling sites with red circles. Koungue, Malongue, Mevia 1, Mevia 2 and Mevia 4 are sites situated in the salvinia-invaded area of the lake, whereas the other sites are in the uninvaded area.
2.2 Experimental design
On a monthly basis, between November 2020 and October 2021 (12 months), we simultaneously implemented one-hour visual point scans, 360° sonar scans, and passive acoustic monitoring (PAM) of the African manatee at ten sites in Lake Ossa (Figure 1). To investigate the influence of Giant Salvinia on manatee detection, we included five sites within the Salvinia-invaded area and five within the uninvaded area. A total of 120 surveys (1 survey/site/month = 1*10*12 = 120 surveys) were conducted with each monitoring method. The boat was anchored during the implementation of all the survey methods.
A visual point scan survey consisted of two experienced manatee observers sitting at the two ends of an anchored boat. The survey boat was in fiberglass and approximately 3 m long. Each observer watched the water’s surface at a height of approximately 1.8 m. Observers searched for signs of manatee presence by continuously scanning a 180° area, i.e., one side of the vessel up to approximately 100 m away (Figure 3) (Self-Sullivan et al., 2003; LaCommare et al., 2008; Takoukam Kamla, 2012). Signs of manatee presence include direct signs (e.g., physical presence of manatees, air bubbles, and mud plumes created by their passage) and indirect signs (e.g., feces, feeding sign marks on vegetation) (Takoukam Kamla, 2012). To reduce potential bias, team members did not communicate during scans and only shared their detections after the visual point scan ended.
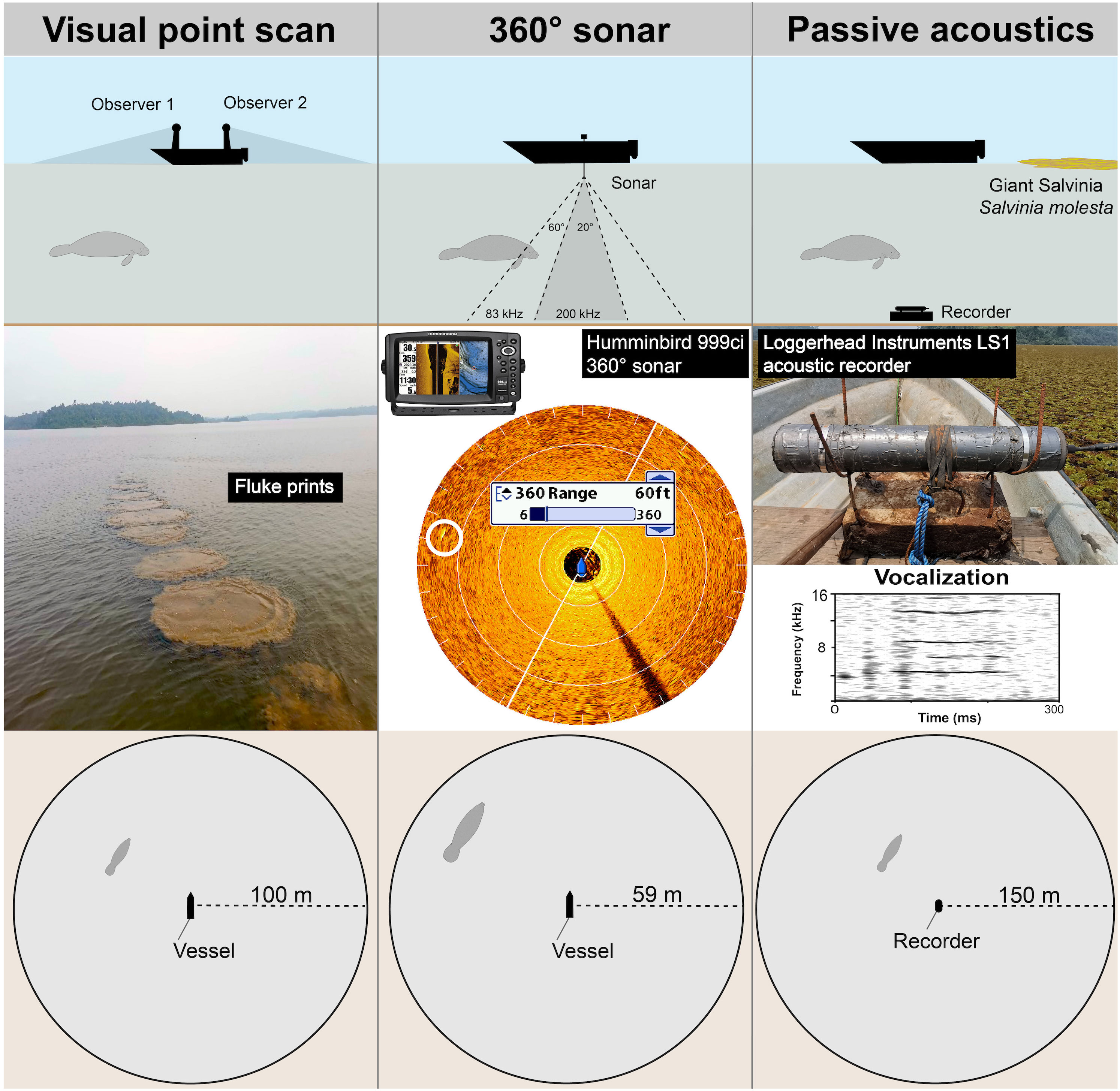
Figure 3 Schematic comparison of the procedures involved in each of the three survey methods for detecting African manatees. The three top images illustrate in-situ experimental design for each method. The three center images show some cues for each method, e.g., manatee footprint for visual point scan (center left image), manatee sonar image for 360° sonar scan (center middle image; manatee in the white circle) and a spectrogram of a manatee vocalization for passive acoustics (center right image). The three bottom images illustrate detection ranges for the three methods.
For the 360° sonar survey of manatees, we used a transom-mounted Humminbird sonar (Humminbird 999CI HD SI) equipped with a spinning (rotating) transducer (i.e., 360° sonar) and powered by a 12V Bluetop marine battery. The dual-beam system produced sound waves at 83 and 200 kHz (Figure 3). Sonar images were displayed on an 8” HD screen and were captured by pressing the screen-capture button on the device. Screenshots were saved in jpeg format on a SD (secure digital) memory card for later confirmation of manatee presence after inspection of sonar images. Here, manatee detection consisted of an experienced sonar image researcher watching and focusing only on the screen of the sonar device. The device was set to scan the water column to a radius of up to 59 m. This distance was occasionally reduced when a target object was closer to the boat to obtain a better image then set back to 59 m after verification. The transducer was set to rotate at the device’s default average speed, and the sonar image on the screen was refreshed thrice per minute. Morphologically (body shape and length), no species is similar to the African manatee in Lake Ossa. Thus, four elements were taken into account when determining whether or not a target object on a sonar image was a manatee: the relative size of the object, its shape, strength of acoustic reflectivity, and mobility. It is not clear if and how sound waves of the sonar would interfere with manatee hearing sensitivity as little is known about the ultrasonic content of African manatee vocalizations. Manatees can produce vocalizations at up to 83.1 kHz (Antillean manatee Trichechus manatus manatus; Ramos et al., 2020) and 90.5 kHz (Florida manatees - Trichechus manatus latirostris; Gaspard et al., 2012). However, studies based on Florida manatees showed that their hearing sensitivity may be best between 6 and 32 kHz (Gerstein et al., 1999; Gaspard et al., 2012). The threshold dB levels for the Florida manatee at 90.5 kHz and 76.1 kHz are very high (141.1 dB and 128.4 dB respectively; Gaspard et al., 2012) and manatees rarely vocalize above those thresholds. Thus, we suggest that the sonar sound waves did not significantly influence manatees in this study.
Passive acoustic monitoring (PAM) of manatees was undertaken using an LS1 remote acoustic recorder (LS1, Loggerhead Instruments, HTI-96-min hydrophone with a sensitivity of -180.2 dB re 1 V/µPa and frequency response of 2 Hz to 30 kHz) deployed underwater at multiple sites (Figure 3). The device was set to record continuously (sample rate: 44.1 kHz; 16-bit) for an hour and store recordings in 5-min sound clips in wav format. The recorder was attached horizontally to a concrete weight of approximately 25 kg with a rope attached to facilitate deployment and retrieval, set approximately 50 cm off the bottom of the lake. The recorder was retrieved after one-hour deployments (end of survey), and the recordings downloaded for later analysis of the presence of manatee vocalizations (see below for methods). Based on an estimate of manatee vocalization detection range using similar equipment and in a similar environment (Rycyk et al., 2022), manatees might be detected using the PAM method at up to 148 m. A schematic illustration of the implementation of all three methodologies appears in Figure 3.
All surveys were conducted between 06:00 and 10:00 AM, a timeframe during which manatees are more likely to be visually spotted in Lake Ossa (Takoukam Kamla, 2012). According to Rycyk et al. (2021), African manatees in Lake Ossa seem to be more active out of daylight hours, presumably in avoidance of human presence. For all survey methods, detections were scored as presence/absence for each one-hour survey. Prior to the beginning of each survey, Beaufort Sea state and Giant Salvinia coverage (percentage of the water surface covered by Giant Salvinia approximately 500 m around the boat) were visually estimated. Water depth (m) and temperature (°C) were measured using a DepthTrax 1H sonar depth finder. Transparency was measured with a Secchi disk to the nearest cm.
2.3 Data analysis
2.3.1 Analysis of acoustic recordings
Each 5-minute audio file was aurally and visually inspected in small sections (~10 s) by an experienced researcher using Raven Pro 1.6 (512-point DFT, Hann window, 50% overlap) (Center for Conservation Bioacoustics, 2014). Presence of African manatee vocalizations were determined for each 1-hour passive acoustic monitoring period. Regardless of the number of vocalizations, a manatee was considered present when at least one manatee vocalization was noted in the recordings. Examples of spectrograms of African manatee vocalizations can be found in Rycyk et al. (2021).
2.3.2 Statistical analyses
We used software R, version 4.0.5 (R Core Team, 2021) to plot figures and to run all statistical tests and modelling. Manatee detection frequency was reported for each survey method as the percentage of surveys in which manatee presence was recorded. We ran a 3-sample (visual point scan, 360° sonar scan, and PAM) test for equality of proportions to ascertain statistically significant differences between the manatee detection frequencies of the three survey methods and subsequently ran post-hoc comparisons (visual point scan and 360° sonar scan, visual point scan and PAM, 360° sonar scan and PAM) with a Bonferroni correction using respectively the “prop_test” and “pairwise_prop_test” functions of package “rstatix” (Kassambara, 2022). To determine if and how some environmental conditions and their interaction with the survey methods influence the likelihood of manatee detection, we built and fitted a Binomial Generalized Linear Mixed Effects Model (GLMM) to our data. Manatee detection was predicted from environmental variables, namely Giant Salvinia invasion (invaded vs uninvaded by Giant Salvinia), Giant Salvinia coverage, Beaufort Sea state, water depth, temperature, and transparency – and their interaction with the survey methods. Environmental variables were set to be fixed effects in the model. As each site was monitored 12 times (once per month), and there were 30 surveys per month (1 survey * 3 methods * 10 sites = 30) we accounted for spatial and temporal autocorrelation in the model by adding the variables “site” and “month” as random effects. To test the relevance of including “Site” and “Month” as random variables, we first did a likelihood ratio test between a fixed-effects minimal baseline model (model1 = glm(Detection ~ 1)) and a baseline mixed-model with only the random effects (model2 = glmer(Detection ~ (1|Site) + (1|Month))) using the “lrtest” function from the “lmtest” package (Zeileis and Hothorn, 2002). The latter model (model2) yielded a better fit than the first (model1) (p < 0.01). We then compared model2 to two mixed-models each containing only one of the two random effects: model3 = glmer(Detection ~ (1|Site)) and model4 = glmer(Detection ~ (1|Month)). Model2 still yielded a better fit than model3 (p < 0.01) and model4 (p = 0.03). It was therefore relevant to include both site and month as random effects in our model. Detection (i.e. manatee detection) was a binary response variable with values 0 and 1, hence obeying a Bernoulli distribution (Christensen, 1990; Agresti, 2002). Thus, the full/saturated model predicted manatee detection as a function of the above-cited environmental variables and their interaction with the variable “methods” (survey methods) and in the condition where “site” and “month” were set to be random effects.
To obtain the minimal adequate model, we parsimoniously simplified the full model following the Occam’s razor principle. We used a manual step-wise step-up procedure during which the full model’s predictors (fixed effects) were removed one after another and then the resulting model was compared to the previous candidate model. To remove a predictor (environmental condition) from the saturated model, we would first remove only its interaction with the variable “Methods”, and if this does not significantly influence the model, we would then take it off. If taking the predictor off significantly influence the model, then we put it back without the interaction term. If this does not influence the model, we then progress to the next predictor. Model discrimination/selection was done on the basis of an analysis of variance using the function “anova” from the “stats” package in R (R Core Team, 2021). We used function “glmer” of package “lme4” (Bates et al., 2015) to run the models, using the “binomial” family and the “logit” link function.
The minimal adequate model obtained was as follows: Detection ~ Methods*Invasion + Depth + Transp + (1|Site) + (1|Month), where “Detection” is the binary response variable (1 for manatee detection and 0 for non-detection), “Methods” are the survey methods (coded as PAM, Sonar.scan and Visual.point.scan). “Invasion” represents whether the survey area is invaded or not (uninvaded) by Giant Salvinia and “Depth” and “Transp” represent water depth and transparency respectively. The asterisk indicates an interaction between at least one survey method and Giant Salvinia invasion. Aside from water depth and transparency (numeric variables), all the other variables in the model were set as factors. To validate the final model, we verified the level of collinearity between the fixed effects and the level of residuals’ autocorrelation using respectively the “check_collinearity” function of package “performance” (Lüdecke et al., 2021) and the “acf” function of package “stats”. Model’s autocorrelation structure (AR1) was inspected and its fit parameters were summarized in a table using the “tab_model” function of package “sjPlot” (Lüdecke, 2022). We further inspected the model’s fit by generating the Somers’ Dxy rank correlation (and the associated C-value) between the predicted probabilities and the observed values using the “somers2” function of package “Hmisc” (Harrell, 2022). Post-hoc analyses of the interaction in our model were done using the “emmeans” package (Lenth, 2022). Finally, we graphically plotted how the explanatory variables predict the probability of detecting manatees using the “effects” package (Fox and Weisberg, 2019).
3 Results
No African manatee chewing sounds were recorded, so passive acoustic detections of manatees in this study only referred to the detection of their vocalizations. PAM resulted in the highest manatee detection frequency (24.17%; n = 120), followed by the 360° sonar scan (11.67%; n = 120) and the visual point scan (3.33%; n = 120) (Table 1; Figure 4). The test for equality of proportions (based on Pearson’s chi-squared test statistic) revealed statistically significant differences in manatee detection frequencies between visual point scan, 360° sonar scan, and PAM (p < 0.01; Table 2). Post-hoc comparisons showed a significant difference only between PAM and visual point scan (p < 0.01; Table 2). The detection frequency through PAM was either equal (at 2 over 10 sites) or greater than those of the two other survey methods (at 7 over 10 sites) at all the monitored sites (Figure 5).

Table 1 Detection frequency of African manatees using visual point scans, 360° sonar and Passive Acoustics Monitoring (PAM) (n = 120 surveys for each method).
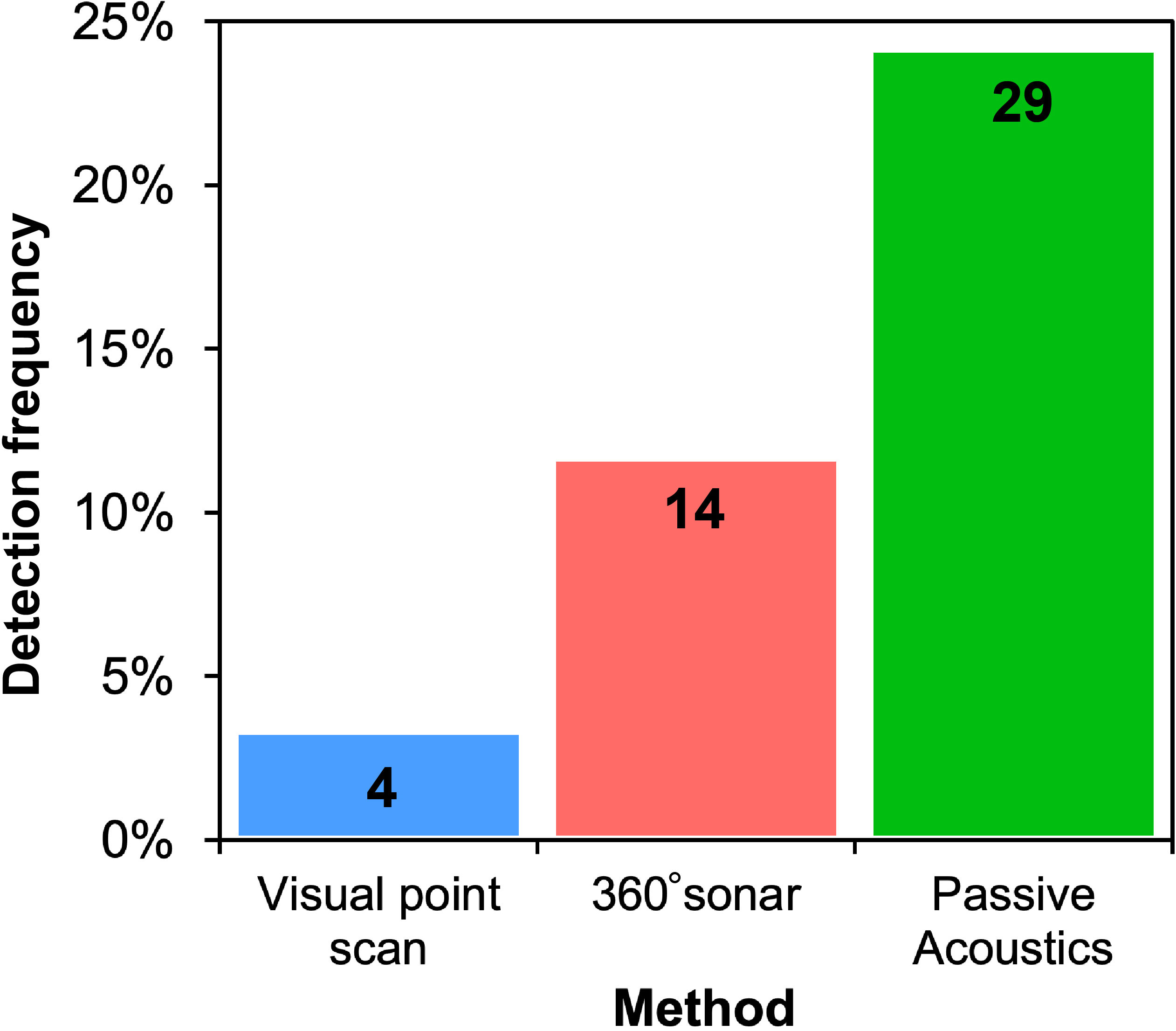
Figure 4 The number of manatee detections and detection frequency (%) across three survey methods (visual point scan, 360° sonar, and passive acoustics). Data are pooled across all ten sampling sites and include all 12 monthly samples (n= 120 surveys for each method).
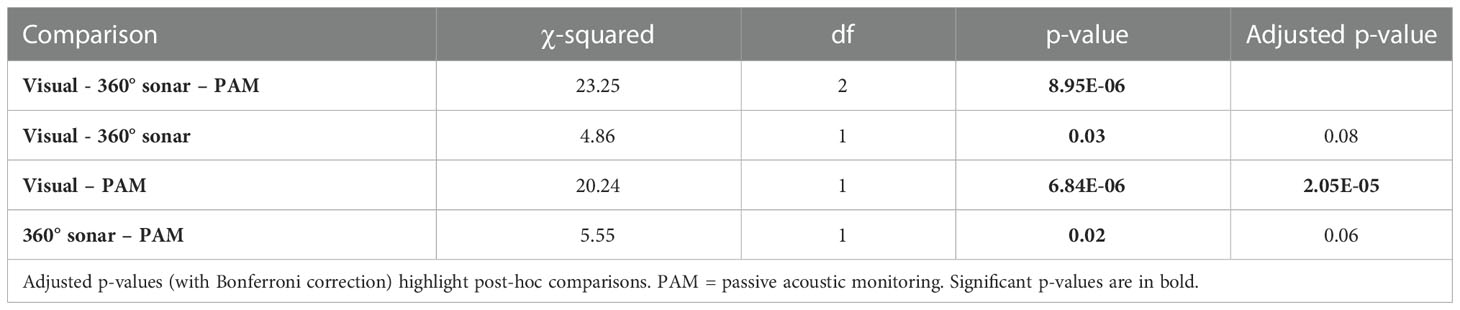
Table 2 Output of 3-sample tests for equality of proportions based on Pearson’s chi-squared test statistic, comparing methods detection frequencies.
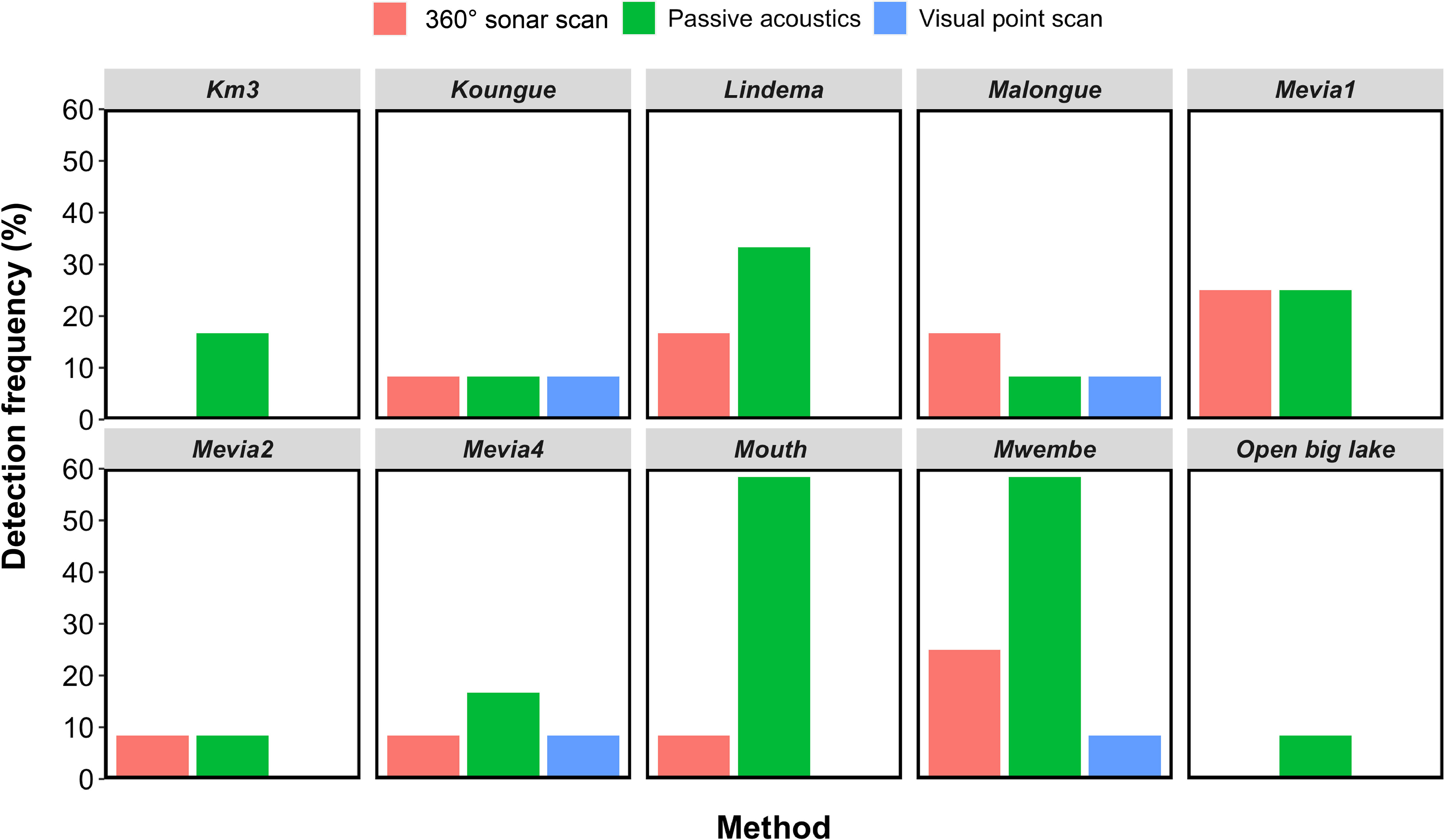
Figure 5 African manatee detection frequency (%) of visual point scan, 360° sonar scan and passive acoustics at 10 sites of Lake Ossa. Each site was sampled monthly for 12 months (n = 12 surveys per site).
When all three methods were combined, manatees were detected in 38 of the 120 surveys. In more than half of the surveys with positive detections (57.9%, n = 22), manatees were only detected by passive acoustics. Similarly, 21.1% of surveys with positive detections were specific to the 360° sonar scan. There was no survey where manatees were detected only by the visual point scan method.
The output of the minimal adequate model is summarized in Table 3. There was low collinearity (variance inflation factor < 4 for all the predictors) between the explanatory variables and no residuals’ autocorrelation. Thus, our model did not violate important assumptions. The total variance explained by the model (conditional R2) was 0.52 (Table 3). Somers’s Dxy and its associated C-value were respectively 0.78 and 0.89 (Table 3).
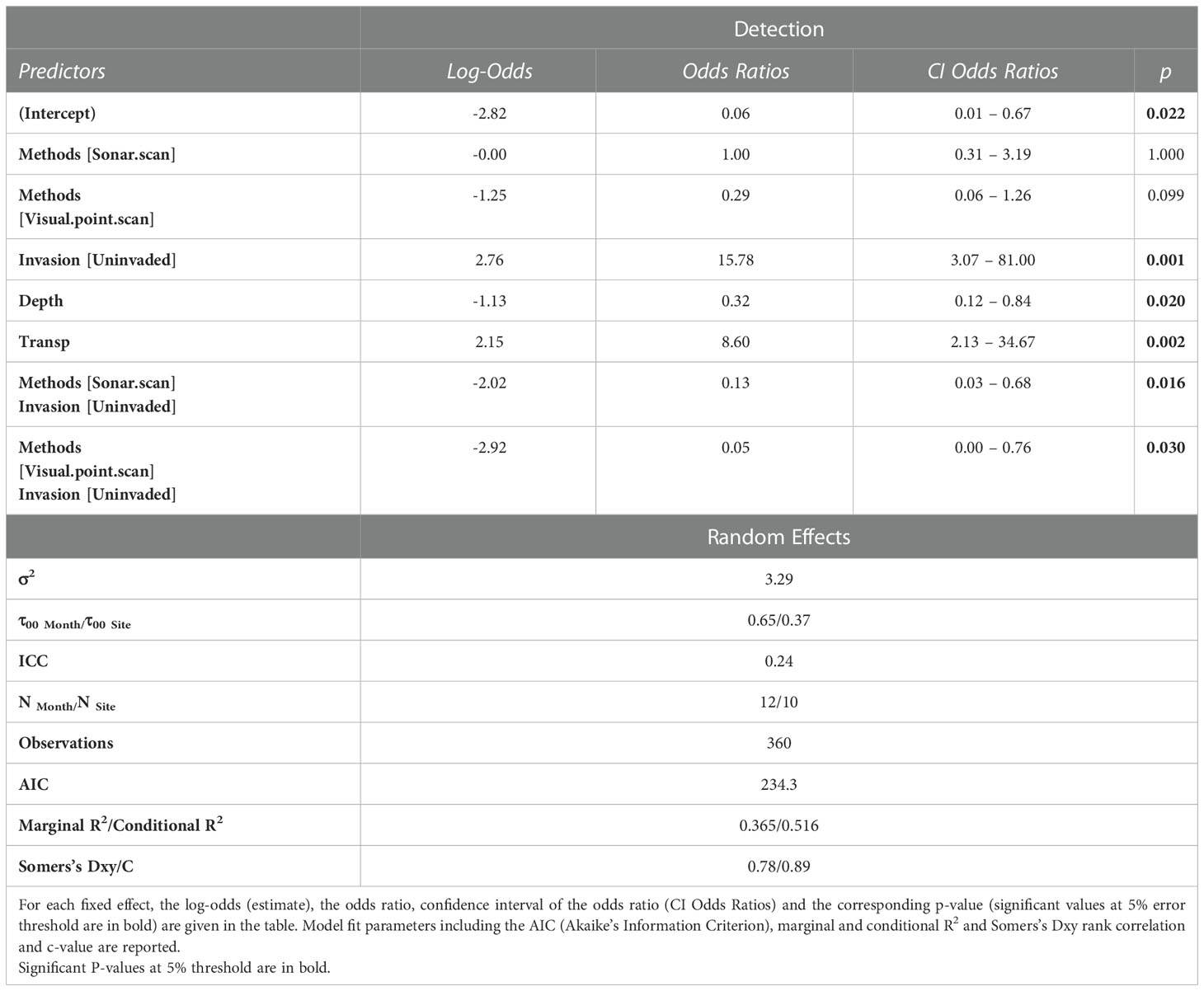
Table 3 Output of the binary logistic regression predicting African manatee detection in Lake Ossa as a function of water depth and transparency and an interaction between the survey methods and Giant Salvinia invasion.
Water depth (p < 0.01) and transparency (p = 0.02) were significant predictors of positive manatee detections (Table 3). The likelihood of detecting African manatees increases with lower water depth and higher water transparency (Figure 6). Also, the interaction between the survey methods and Giant Salvinia invasion had a significant predictive effect on the model outcome (Table 3). Post-hoc analyses of this interaction revealed that unlike the 360° sonar and the visual point scan methods, the likelihood of detecting African manatees using PAM was significantly different between the invaded and the uninvaded areas of Lake Ossa (p < 0.01; Table 4). Passive acoustic detections of manatees were more likely to occur in the uninvaded areas than areas invaded by Giant Salvinia (Figure 6). In addition, within the uninvaded area of the Lake, the likelihood of detecting the African manatee was significantly higher when using PAM than the 360° sonar (p < 0.01) or the visual point scan methods (p < 0.01) (Table 4; Figure 6). The likelihood of detecting manatees in the invaded area of Lake Ossa was not significantly different between survey methods (Table 4). The fixed effects accounted for about 37% of the variance in our model whereas all the predictors (including the random effects) accounted for about 52% of the variance (Table 3).
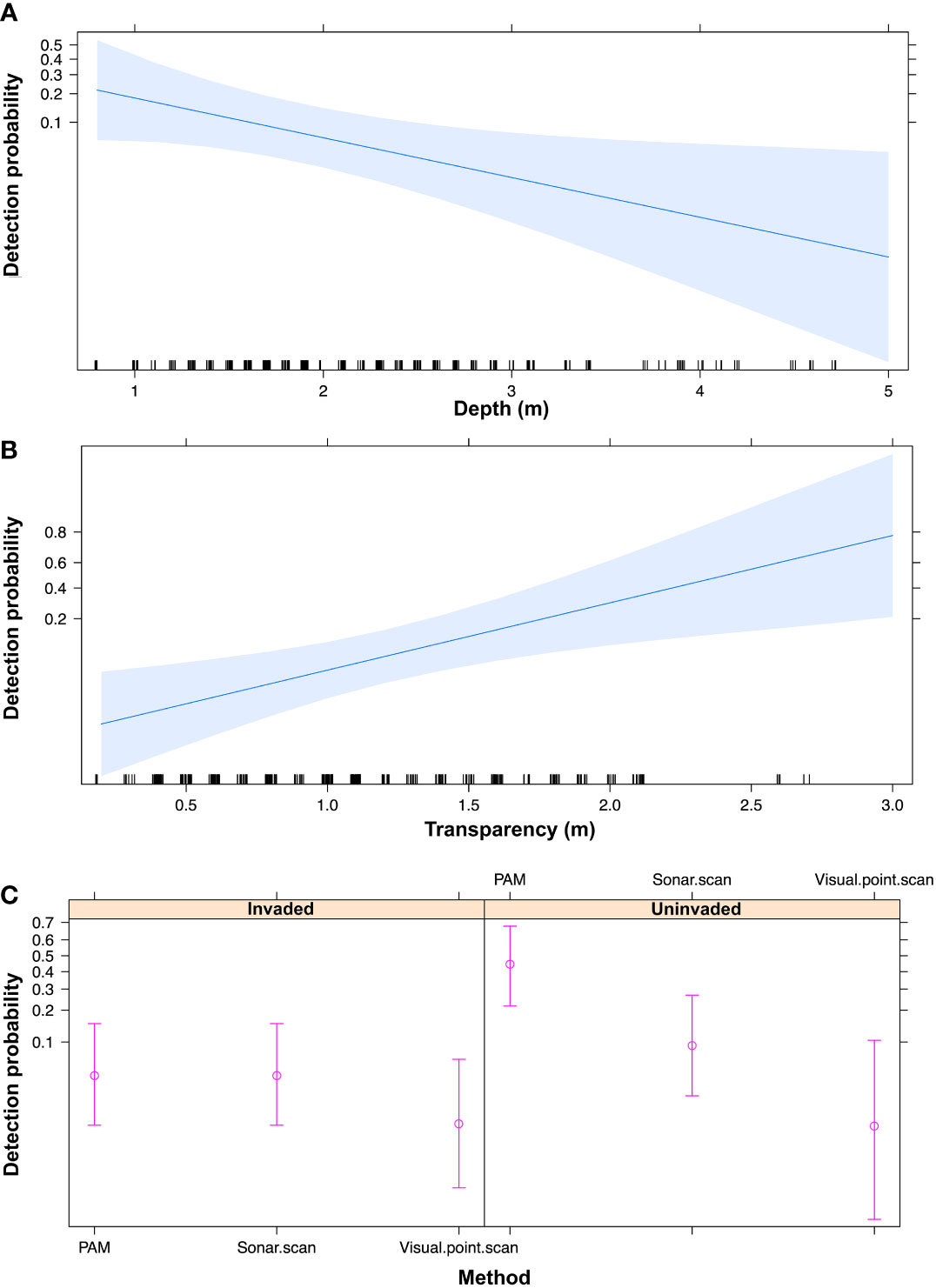
Figure 6 Plots illustrating the effects of water depth (A), transparency (B), and the interaction between survey methods and Giant Salvinia invasion (C) on the probability of detecting manatees in Lake Ossa. PAM = passive acoustic monitoring. Error bars in plot C indicate 95% confidence intervals.
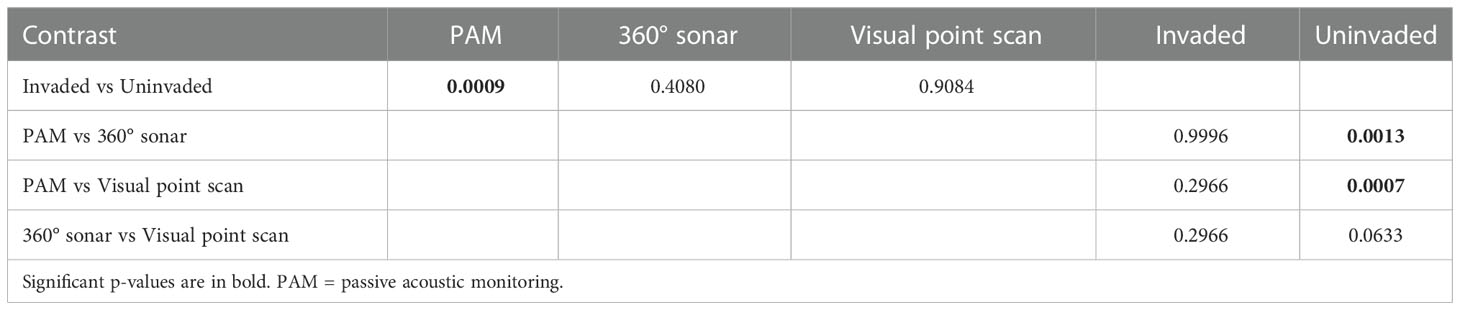
Table 4 P-values obtained after post-hoc inspection of the interaction between the survey methods and Giant Salvinia invasion (significant in the model) using the “emmeans” package in R.
4 Discussion
Developing improved methods for detecting endangered species where their populations are in decline is crucial to their preservation. Our previous work illustrated the strength of passive acoustics for detecting manatees, and similar methods have been successful in habitats of the West Indian manatee (LaCommare et al., 2008; Gonzalez-Socoloske et al., 2009; Gonzalez-Socoloske and Olivera-Gomez, 2012; Kikuchi et al., 2013; Rivera Chavarria et al., 2015; Guzman and Condit, 2017; Puc-Carrasco et al., 2017; Castelblanco-Martínez et al., 2018; Rycyk et al., 2021; Rycyk et al., 2022).
In this study, we implemented three methods of monitoring African manatees, including one classic method (visual point scan) and two novel methods (360° sonar and PAM). We also investigated which survey method, environmental conditions, or interaction between these latter influenced manatee detection by fitting a parsimoniously selected binary logistic regression model (GLMM) to our data. Our findings highlighted that passive acoustics exhibited the greatest detection frequency, followed by 360° sonar and then visual point scan. Water depth and transparency significantly predicted manatee detection. When using PAM, manatees were more likely to be detected in the uninvaded area than in areas invaded by Giant Salvinia. Within the uninvaded area, it was more likely to detect manatees through PAM than the 360° sonar and the visual point scan methods.
4.1 Detection frequencies
The detection frequency of passive acoustics was two-fold greater than that of the 360° sonar, the latter being four times greater than that of visual point scan. These differences in detection frequencies were statistically significant (p < 0.01) and occurred between PAM and the visual point scan (p < 0.01). The superiority of passive acoustics in detecting manatees can be explained by several factors. In turbid environments like Lake Ossa where visual cues are limited, sound is the most efficient communication modality (Bauer et al., 2003). While the vocal behavior of African manatees remains largely unexplored, other manatee species are known to produce sounds in most behavioral contexts, including milling, cavorting, resting, and feeding (Bengtson and Fitzgerald, 1985; Sousa-Lima et al., 2002; Nowacek et al., 2003; Sousa-Lima et al., 2008; Miksis-Olds and Tyack, 2009). Manatee vocalization rate is highest during milling and cavorting, and lowest during feeding and resting (Bengtson and Fitzgerald, 1985). However, most vocalizations are between mothers and their calves to maintain contact especially when feeding or traveling in murky waters. Florida manatees have an estimated vocalization rate of 12.7 vocalizations/manatee/60-min (Rycyk et al., 2022). It is likely that PAM would be more effective when manatee abundance within the acoustic range increases, as the number of vocalizations would likely increase. Conversely, PAM might be less effective when only a single manatee is within acoustic range. We did one-hour scans, thus had several opportunities to record a manatee vocalization when it was within acoustic range and if it stayed in the area. Although we did not record manatee feeding sounds in this study, it would be worth looking for them in future manatee passive acoustic surveys as they could improve the detection probability.
The superiority of passive acoustics could also be due to the many limits of the two other survey methods. We used a 360° Humminbird sonar for the active acoustic survey of manatees. Animal sonar images are of better quality when the boat is moving slowly along a transect (side scan images) than when staying stationary (Gonzalez-Socoloske et al., 2009). Stationary active acoustic monitoring (360° sonar imaging) was chosen in our study design to mitigate biases; if moving, engine noise would alert manatees, which could flee and thus influence visual point scan and passive acoustic detections. Also, sonar detection probability is negatively correlated with the distance of the target (manatees in our case) from the sonar and is estimated to be 0.5 at 33 m and drops below 0.05 at ranges over 59 m (based on grey seals (Halichoerus grypus), Hastie et al., 2019). Moreover, the study range of the 360° sonar (59 m radius around the boat) in this study was much smaller compared to our passive acoustic system which could likely detect manatees up to approximately 148 m around the boat (based on an estimate of manatee vocalization detection range using similar equipment and in a similar environment, Rycyk et al., 2022). The 360° sonar and visual point scan methods could be subjected to many perception and availability biases. The 360° sonar could be subjected to perception bias when manatees were 40 m and above (40-59m) as they would be less distinguishable on sonar images. Perception bias occurs when an animal is potentially detectable but not detected; this includes perception bias (stricto sensu) whereby an animal is available for detection, but the detection system fails to detect the available cue (Marsh and Sinclair, 1989) and detection bias whereby an animal is available in the study area but is not detected because for example the detection probability drops with distance (Buckland et al., 2015). Also, availability bias occurs when an animal is within the study area but unavailable for detection because the cue is canceled by other animals, turbid waters, ambient noise, etc. (Marsh and Sinclair, 1989; Buckland et al., 2015; Verfuss et al., 2018); e.g., the manatee is within the acoustic range but does not vocalize or its vocalizations are masked by ambient noise causing an availability bias for passive acoustics.
Visual point scan was the method with the least manatee detection frequency. Given the very low water transparency, visual detection of manatees in Lake Ossa necessitates that the manatee surfaces to breathe or create water bubbles and/or mud at the water surface during their movements. Even though surveys lasted one hour each and were done in silence, manatees seem to prefer slow, cryptic movements that are difficult to detect by humans from the surface. This behavior is likely an adaptation to poaching as cryptic movements limit their visual detectability and thus the likelihood of being spotted by poachers. Most manatee visual detections during our survey were opportunistic sightings (occurred when moving from one area to another), whereby manatees fled when hearing the noise of our boat engine, thus creating water bubbles and mud at the water surface. Line transect surveys might be a more suitable visual survey method in shallow water systems like Lake Ossa as they cover a wider study area than point scans. When water depth is low, abrupt manatee movements stir up bottom sediments which change water color at the surface, thus indicating manatee presence (mud plumes). Also, manatee “footprints” created by tail movement during swimming can be easily detected in Lake Ossa as the Lake is mostly calm (Beaufort Sea state mostly between 0 and 2). However, all these visual cues are less likely detectable in deeper and murkier water systems. In this study, 50% of the monitored sites were invaded by Giant Salvinia. This plant can completely cover the water surface, therefore hiding any visible sign of manatees (Figure 2) and potentially causing availability bias for this method.
One limit of our surveys was that we do not know the detection probability of each method as we were not sure whether surveys with no positive detections of manatees were due to the absence of manatees during surveys or to the failure of the survey methods. In Lake Ossa, no animal is morphologically similar to manatees nor produces similar visual cues (“footprints”, air bubbles, mud plumes). Also, no animal produces vocalizations that are similar to those of manatees. Thus, the probability of false positive detection of manatees was very limited for both survey methods. However, during this study, a survey served as a positive control whereby detection could be compared between methods as a manatee was known to be present. Therefore, our study highlights that PAM is a promising method of detecting African manatees, especially in turbid habitats. PAM could be very useful in filling the many gaps in African manatee ecology and lends itself to occupancy modeling to understand the distribution and habitat use. There is little literature on the influence of age and sex classes on manatee vocalization rate but it known that this varies according to the behavioral state, with milling and cavorting having the highest (Bengtson and Fitzgerald, 1985). However, is it less likely that the vocalization rate significantly influences occupancy modelling as this type of models deals with presence/absence rather than abundance data. Even in a context of low vocalization rate, a single vocalization is enough to determine presence. The value of passive acoustics can be further strengthened if vocalizations are individually distinct, so that the minimum number of manatees present could be calculated and population size estimated using mark-recapture analysis techniques (Longden et al., 2020) and/or vocalization clustering (Merchan et al., 2019). Longden et al. (2020) used mark-recapture techniques of individually distinctive signature whistles of common bottlenose dolphins (Tursiops truncatus) to estimate their population size in Walvis Bay, Namibia. The acoustically estimated population size was comparable to the known population size in the area. Manatee vocalizations can contain individually-distinctive parameters (Sousa-Lima et al., 2002) that could serve in similar studies. Indeed, Merchan et al. (2019) successfully used vocalization clustering to identify and count Antillean manatees in Panamanian wetlands.
In addition to being the method with the highest detection frequency, more than half (57.9%) of the overall manatee detections were specific to passive acoustics. Thus, our overall manatee detection frequency would have reduced by more than half if we had used only 360° sonar and visual point scan methods. One-fifth of manatee detections were specific to the 360° sonar scan and none were specific to visual point scans. The high ratio of specific passive acoustic detections could be because PAM covered a wider detection range and that verified manatee sounds are less subject to perception biases compared to the 360° sonar and the visual point scans. Perception biases can be human-based or can originate from environmental conditions. Below we discuss which and how environmental conditions and their interaction with the survey methods influenced manatee detection.
4.2 Environmental conditions influencing manatee detection
We built and fitted a binary logistic regression model (GLMM) to our data to determine which and how environmental conditions and their interaction with the survey methods influenced manatee detection. Our model’s total explanatory power was substantial (conditional R2 = 0.52; Table 3) (Nakagawa et al., 2017). Moreover, the C-value and Somers’s Dxy were respectively 0.89 and 0.78. C-values above 0.8 indicate strong predictive capacity (Baayen, 2008). Somers’s Dxy ranges between 0 (complete randomness) and 1 (perfect prediction) (Baayen, 2008). Thus, our model had a substantial predictive power. Water depth and transparency, and an interaction between Giant Salvinia invasion (invaded vs. uninvaded) and the survey methods had significant predictive effects on the likelihood of detecting African manatees in Lake Ossa (Table 3).
The likelihood of detecting manatees decreased with increasing water depth (Figure 6). The odds of detecting manatees when water depth increases by one unit (1 m) is 0.32 (Table 3), meaning there was 68% less chance of detecting a manatee when the water level increased by one meter. The greater manatee detection probability in shallower water depths could be the result of the fact that in low water season, manatees are constrained to converge in the lake basin rather than staying in inaccessible areas, therefore increasing their detection probability. Unfortunately, there is no available data regarding the difference in the surface area of Lake Ossa between low and high-water seasons. Inspecting this would help to better understand manatee habitat use and better analyze the effect of water depth on manatee detectability in Lake Ossa.
Conversely, the likelihood of detecting manatees increased with higher water transparency (Figure 6). The odds of detecting manatees in Lake Ossa was more than eight times higher when water transparency increased by one meter (Table 3). Even though our model did not highlight this, it is more likely to visually spot manatees in higher water transparency (Takoukam Kamla, 2012). Also, sonar images are of better water quality (and thus increase chance of detection) when turbidity is low i.e., high water transparency (Flowers and Hightower, 2013).
Within the uninvaded area of Lake Ossa, the likelihood of detecting manatees was significantly higher when using PAM than the 360° sonar or the visual point scan methods (Table 4; Figure 6). Unlike the two other methods, the probability of detecting manatees through PAM was significantly higher within the uninvaded areas than in areas invaded by Giant Salvinia. For all the survey methods, the likelihood of detecting manatees was greater within the uninvaded area. Manatees may find it difficult to surface and breathe in invaded areas as Giant Salvinia forms dense and thick carpets on the water surface. Also, manatees in Lake Ossa are not known to consume Giant Salvinia (Takoukam Kamla, 2019), and this invasive plant competes with the major manatee local food plant (Antelope grass). Thus, it is very likely that manatees prefer uninvaded areas of the lake.
4.3 Recommendations for future manatee surveys based on survey methods and environmental conditions
In this study, we compared three methods in stationary positions, PAM, 360° sonar, and visual point scan, to monitor African manatees. One of the survey methods, PAM, accounted for more than half of all positive detections. We recommend, in the scenario of choosing only one of these methods, using PAM in future manatee surveys. However, combining both PAM and 360° sonar is ideal, as it would increase detection probabilities. PAM has the advantage of being non-invasive and passive (does not require human control in-situ) and can cost-effectively cover longer time-scales including night sampling. Indeed, deploying acoustic devices over longer periods can improve the likelihood of detecting target animals. This survey method would particularly be useful in highly turbid water systems where cues of a target species are difficult to visually detect. However, underwater acoustic devices may be expensive to get (particularly in low-income countries) and can only cover limited survey areas, especially when the number of devices is very limited. In shallow and calm water systems like Lake Ossa, visual surveys would be more effective when water transparency is high. Line transects surveys could also be preferred to PAM when the study aims to cover large survey areas in a time-effective way. Similarly, when available, side-scan imaging would be preferable to 360° imaging as the first allows covering larger areas and can be coupled with line transects surveys to enhance detectability. African, Amazonian, and Antillean manatees share some common habitat characteristics (habitat turbidity and depth). These recommendations could be applicable to other manatee species and other species inhabiting similar habitats.
5 Conclusions
Passive acoustics clearly was more successful in detecting African manatees than 360° sonar and visual point scans. The visual point scan had a very low detection rate and was four times less successful than the 360° sonar scan. When combined, the 360° sonar and passive acoustics accounted for 100% of all surveys with positive detections of manatees. In Lake Ossa the likelihood of detecting manatees increases with higher water transparency and shallower water depths and when moving from areas invaded by Giant Salvinia to uninvaded areas. The invasion of Giant Salvinia is likely influencing manatee distribution in Lake Ossa. We recommend using passive acoustics, or even better, the combination of passive acoustics and 360° sonar to enhance manatee detection and by extension, enhance our understanding of the very elusive African manatee. Passive acoustics could further provide in-depth information on African manatees and enhance their detection when acoustic recorders are deployed over longer time periods. Visual surveys may be more effective in clearer water systems and would be preferable to PAM when aiming to maximise coverage in studies with limited acoustic receivers and where limited sonar detections can be expected.
Data availability statement
The raw data supporting the conclusions of this article will be made available by the authors, without undue reservation.
Ethics statement
Ethical review and approval was not required for the animal study because we did not directly handle or interact with the study animal.
Author contributions
CF conceptualized the study, collected and analysed the data, wrote the main draft of the manuscript and participated in editing the manuscript. AR contributed with resources, planning, guidance, and review/editing of the manuscript. ATK conceptualized the study, contributed with guidance, and review/editing of the manuscript. ER contributed with data analyses, and review/editing of the manuscript. SK and LK-D contributed with guidance, review/editing of the manuscript. MK contributed with review/editing of the manuscript. All authors contributed to the article and approved the submitted version.
Funding
This study was funded by a CARN Aspire Grant, a Small Grant in-Aid of Research from the Society for Marine Mammalogy, African Graduate Student Research Funds from the American Society of Mammologists and the Africa section of the Society for Conservation Biology.
Acknowledgments
We thank the African Marine Mammal Conservation Organization (AMMCO) for their logistics support. The memorandum of understanding between AMMCO and the Cameroonian Ministry of Forests and Wildlife permitted us to work within the Lake Ossa wildlife reserve.
Conflict of interest
The authors declare that the research was conducted in the absence of any commercial or financial relationships that could be construed as a potential conflict of interest.
Publisher’s note
All claims expressed in this article are solely those of the authors and do not necessarily represent those of their affiliated organizations, or those of the publisher, the editors and the reviewers. Any product that may be evaluated in this article, or claim that may be made by its manufacturer, is not guaranteed or endorsed by the publisher.
References
Baayen R. H. (2008). Analyzing linguistic data. a practical introduction to statistics using r (Cambridge: Cambridge University press), 368.
Baotic A., Brady B., Ramos E. A., Stoeger A. (2022). Elephants and sirenian: a comparative review across related taxa in regard to learned vocal behavior. Comp. Cog. Beh. Rev. 17, 89–108. doi: 10.3819/CCBR.2022.170004
Barrett S. C. H. (1989). Waterweed invasions. Sci. Am. 261, 90–97. doi: 10.1038/scientificamerican1089-90
Barton K. (2022) MuMIn: Multi-model inference. r package version 1.46.0. Available at: https://CRAN.R-project.org/package=MuMIn.
Bates D., Maechler M., Bolker B., Walker S. (2015). Fitting linear mixed-effects models using lme4. J. Stat. Soft. 67, 1–48. doi: 10.18637/jss.v067.i01
Bauer G. B., Colbert D. E., Gaspard J. C. III, Littlefield B., Fellner W. (2003). Underwater visual acuity of Florida manatees (Trichechus manatus latirostris). Int. J. Comp. Psychol. 16, 130–142. doi: 10.46867/C43P4V
Bengtson J. L., Fitzgerald S. M. (1985). Potential role of vocalizations in West Indian manatees. J. Mammalogy 66, 816–819. doi: 10.2307/1380821
Brady B., Hedwig D., Trygonis V., Gerstein E. (2020). Classification of Florida manatee (Trichechus manatus latirostris) vocalizations. J. Acoust. Soc Am. 147, 1597–1606. doi: 10.1121/10.0000849
Brady B., Moore J., Love K. (2021). Behavior related vocalizations of the Florida manatee (Trichechus manatus latirostris). Mar. Mamm. Sci. 38, 975–989. doi: 10.1111/mms.12904
Buckland S. T., Rexstad E. A., Marques T. A., Oedekoven C. S. (2015). Distance sampling: Methods and applications. ISBN. doi: 10.1007/978-3-319-19219-2
Castelblanco-Martínez D. N., dos Reis V., de Thoisy B. (2018). How to detect an elusive aquatic mammal in complex environments? a study of the endangered antillean manatee Trichechus manatus manatus in French Guiana. Oryx 52, 382–392. doi: 10.1017/S0030605316000922
Center for Conservation Bioacoustics (2014). Raven pro: Interactive sound analysis software (Version 1.5). [Computer software] (Ithaca, NY: The Cornell Lab of Ornithology).
Convention on the Conservation of Migratory Species (CMS) (2009). West African Manatee—Trichechus senegalensis. https://www.cms.int/en/document/west-african-manatee-trichechus-senegalensis-0. (Last viewed 05/18/2021).
Convention on International Trade of Endangered Species (CITES) (2013). Trichechus senegalensis Link, 1795. Available: https://www.speciesplus.net/species#/taxon_concepts/4306/legal. (Last viewed 05/18/2021)
Flowers H. J., Hightower J. E. (2013). A novel approach to surveying sturgeon using side-scan sonar and occupancy modeling. Mar. Coast. Fisheries 5, 211–223. doi: 10.1080/19425120.2013.816396
Fox J., Weisberg S. (2019). An r companion to applied regression, 3rd edition (Thousand Oaks, CA) SAGE Publications, Inc. Available at: https://socialsciences.mcmaster.ca/jfox/Books/Companion/index.html.
Gaspard J. C., Bauer G. B., Reep R. L., Dziuk K., Cardwell A., Read L., et al. (2012). Audiogram and auditory critical ratios of two Florida manatees (Trichechus manatus latirostris). J. Exp. Biol. 215, 1442–1447. doi: 10.1242/jeb.065649
Gerstein E. R., Gerstein L., Forsythe S. E., Blue J. E. (1999). The underwater audiogram of the West Indian manatee trichechus manatus. J. Acoust. Soc Am. 105, 3575–3583. doi: 10.1121/1.424681
Giresse P., Maley J., Kossoni A. (2005). Sedimentary environmental changes and millennial climatic variability in a tropical shallow lake (Lake ossa, Cameroon) during the Holocene. Paleogeogr. Paleoclimatol. Paleoecol. 218, 257–285. doi: 10.1016/j.palaeo.2004.12.018
Gonzalez-Socoloske D., Olivera-Gomez L. D. (2012). Gentle giants in dark waters: Using side-scan sonar for manatee research. Open Rem. Sens. J. 5, 1–14. doi: 10.2174/1875413901205010001
Gonzalez-Socoloske D., Olivera-Gomez L. D., Ford R. E. (2009). Detection of free-ranging West Indian manatees Trichechus manatus using side-scan sonar. Endang. Spec. Res. 8, 249–257. doi: 10.3354/esr00232
Grigione M. M. (1996). Observations on the status and distribution of the West African manatee in Cameroon. Afri. J. Ecol. 34, 189–195. doi: 10.1111/j.1365-2028.1996.021-89021.x
Guzman H. M., Condit R. (2017). Abundance of manatees in Panama estimated from side-scan sonar. Wildl. Soc Bull. 41, 556–565. doi: 10.1002/wsb.793
Harrell Jr F.E. (2022) Hmisc: Harrell miscellaneous. r package version 4.7-0. Available at: https://CRAN.R-project.org/package=Hmisc.
Hastie G. D., Wu G., Moss S., Jepp P., MacAulay J., Lee A., et al. (2019). Automated detection and tracking of marine mammals: A novel sonar tool for monitoring effects of marine industry. Aquat. Conserv. Mar. Freshw. Ecosyst. 29, 119–130. doi: 10.1002/aqc.3103
Hunter M. E., Meigs-Friend G., Ferrante J. A., Takoukam Kamla A., Dorazio R. M., Keith-Diagne L., et al. (2018). Surveys of environmental DNA (eDNA): a new approach to estimate occurrence in vulnerable manatee populations. Endan. Spec. Res. 35, 101–111. doi: 10.3354/esr00880
Kassambara A. (2022) Rstatix: Pipe-friendly framework for basic statistical tests. r package version 0.7.1. Available at: https://CRAN.R-project.org/package=rstatix.
Keith Diagne L. (2015). Trichechus senegalensis. IUCN Red List Threatened Species. doi: 10.2305/IUCN.UK.2015-4.RLTS.T22104A81904980.en
Kikuchi M., Akamatsu T., Gonzalez-Socoloske D., de Souza D. A., Olivera-Gomez L. D., da Silva V. M. F. (2013). Detection of manatee feeding events by animal-borne underwater sound recorders. J. Mar. Biol. Assoc. UK 94, 1139–1146. doi: 10.1017/S0025315413001343
LaCommare K. S., Self-Sullivan C., Brault S. (2008). Distribution and habitat use of antillean manatees (Trichechus manatus manatus) in the drowned cayes area of Belize, central America. Aquat. Mammals 34, 35–43. doi: 10.1578/AM.34.1.2008.35
Lenth R. V. (2022) Emmeans: Estimated marginal means, aka least-squares means. r package version 1.7.5. Available at: https://CRAN.R-project.org/package=emmeans.
Longden E. D., Elwen H. S., McGovern B., James B. S., Embling C. B., Gridley T. (2020). Mark–recapture of individually distinctive calls–a case study with signature whistles of bottlenose dolphins (Tursiops truncatus). J. Mammalogy 101, 1289–1301. doi: 10.1093/jmammal/gyaa081
Lüdecke D. (2022) sjPlot: Data visualization for statistics in social science_. r package version 2.8.11. Available at: https://CRAN.R-project.org/package=sjPlot.
Lüdecke D., Ben-Shachar M. S., Patil I., Waggoner P., Makowski D. (2021). Performance: An r package for assessment, comparison and testing of statistical models. J. Open Source Softw. 6, 3139. doi: 10.21105/joss.03139
Marsh H., Sinclair D. F. (1989). Correcting for visibility bias in strip transect aerial surveys of aquatic fauna. J. Wildl. Manage. 53, 1017–1024. doi: 10.2307/3809604
Mayaka T. B., Awah H. C., Ajonina G. (2013). Conservation status of manatee (Trichechus senegalensis link 1795) in lower sanaga basin, Cameroon: An ethnobiological assessment. Trop. Conserv. Sci. 6, 521–538. doi: 10.1177/194008291300600406
Mayaka T. B., Koh-Dimbot J. P., Keith-Diagne L. W. (2019). Occurrence patterns of African manatees, conflicts with humans, and local perception in the southern korup area, Cameroon. Aquat. Conservation: Mar. Freshw. Ecosyst. 29, 1801–1813. doi: 10.1002/aqc.3210
Mayaka T. B., Takoukam Kamla A., Self-Sullivan C. (2015). Using pooled local expert opinions (PLEO) to discern patterns in sightings of live and dead manatees (Trichechus senegalensis, link 1785) in lower sanaga basin, Cameroon. PloS One 10, e0128579. doi: 10.1371/journal.pone.0128579
McFarland D. G., Nelson L. S., Grodowitz M. J., Smart R. M., Owens C. S. (2004). Salvinia molesta d. Mitchell (Giant salvinia) in the united states: A review of species ecology and approaches to management. final report. US (Vicksburg, Mississippi: Army Corps of Engineers - Engineer Research and Development Center), 35 pp.
Merchan F., Echevers G., Poveda H., Sanchez-Galan J. E., Guzman H. M. (2019). Detection and identification of manatee individual vocalizations in Panamanian wetlands using spectrogram clustering. J. Acoust. Soc Am. 146, 1745–1757. doi: 10.1121/1.5126504
Miksis-Olds J. L., Tyack P. L. (2009). Manatee (Trichechus manatus) vocalization usage in relation to environmental noise levels. J. Acoust. Soc Am. 125, 1806–1815. doi: 10.1121/1.3068455
Nakagawa S., Johnson P. C. D., Schielzeth H. (2017). The coefficient of determination R2 and intra-class correlation coefficient from generalized linear mixed-effects models revisited and expanded. J. R. Soc Interf. 14, 20170213. doi: 10.1098/rsif.2017.0213
Ngafack P. R. (2014). L’effet combiné de sites, de périodes et de saisons sur l’indice de présence du lamantin (Trichechus senegalensis link 1795) et les caractéristiques physiques de l’eau dans la reserve de faune du lac ossa, région du littoral, cameroun. [master’s thesis] (Dschang: University of Dschang).
Nishiwaki M., Yamaguchi M., Shokita S., Uchida S., Kataoka T. (1982). Recent survey on the distribution of the African manatee. Scient. Rep. Whale Res. Inst. 34, 137–147.
Nowacek D. P., Casper B. M., Wells R. S., Nowacek S. M., Mann D. A. (2003). Intraspecific and geographic variation of West Indian manatee (Trichechus manatus spp.) vocalizations. J. Acoust. Soc Am. 114, 66–69. doi: 10.1121/1.1582862
O’Shea T. J., Poche L. B. (2006). Aspects of underwater sound communication in Florida manatees (Trichechus manatus latirostris). J. Mammalogy 87, 1061–1071.
Powell J. A. (1996). “The distribution and biology of the West African manatee (Trichechus senegalensis link 1795),” in United Nations Environmental Program, Regional Seas Program, Oceans and Coastal Areas, Nairobi, Kenya. 68.
Puc-Carrasco G., Morales-Vela B., Olivera-Gómez L. D., González-Solís D. (2017). First field-based estimate of antillean manatee abundance in the San Pedro river system suggests large errors in current estimates for Mexico. Cienc. Marinas 43, 285–299. doi: 10.7773/cm.v43i4.2704
R Core Team. (2021). R: A language and environment for statistical computing. Vienna, Austria: R Foundation for Statistical Computing. URL https://www.R-project.org/.
Ramos E. A., Maust-Mohl M., Collom K. A., Brady B., Gerstein E. R., Magnasco M. O., et al. (2020). The antillean manatee produces broadband ultrasonic vocalizations in Belize. J. Acoust. Soc Am. Exp. Let. 147, EL80. doi: 10.1121/10.0000602
Rivera Chavarria M., Castro J., Camacho A. (2015). The relationship between acoustic habitat, hearing and tonal vocalizations in the antillean manatee (Trichechus manatus manatus, Linnaeus 1758). Biol. Open 4, 1237–1242. doi: 10.1242/bio.013631
Rycyk A. M., Berchem C., Marques T. A. (2022). Estimating Florida manatee (Trichechus manatus latirostris) abundance using passive acoustic methods. J. Acoust. Soc Am. Exp. Let. 2, 051202. doi: 10.1121/10.0010495
Rycyk A. M., Factheu C., Ramos E. A., Brady B. A., Kikuchi M., Nations H. F., et al. (2021). First characterization of vocalizations and passive acoustic monitoring of the vulnerable African manatee (Trichechus senegalensis). J. Acoust. Soc Am. 150, 3028–3037. doi: 10.1121/10.0006734
Self-Sullivan C., Smith G. W., Packard J. M., LaCommare K. S. (2003). Seasonal occurrence of male antillean manatees (Trichechus manatus manatus) on the Belize barrier reef. Aquat. Mammals. 29, 342–354. doi: 10.1578/01675420360736514
Sousa-Lima R. S., Paglia A. P., Da Fonseca G. A. B. (2002). Signature information and individual recognition in the isolation calls of Amazonian manatees, Trichechus inunguis (Mammalia: Sirenia). Anim. Behav. 63, 301–310. doi: 10.1006/anbe.2001.1873
Sousa-Lima R. S., Paglia A. P., da Fonseca G. A. B. (2008). Gender, age, and identity in the isolation calls of antillean manatees (Trichechus manatus manatus). Aquat. Mammals 34, 109–122. doi: 10.1578/AM.34.1.2008.109
Takoukam Kamla A. (2012). “Activity center, habitat use and conservation of the west African manatee (Trichechus senegalensis Link 1795),” in The douala-edea and lake ossa wildlife reserves (Dschang: University of Dschang).
Takoukam Kamla A. (2019). “Genetic diversity, diet, and habitat quality of the African manatee (Trichechus senegalensis,” in The downstream of the sanaga river watershed, Cameroon (Florida: University of Florida).
Trimble M. J., Van Aarde R. J. (2010). Species inequality in scientific study: Species inequality in science. Conserv. Biol. 24, 886–890. doi: 10.1111/j.1523-1739.2010.01453.x
Umeed R., Attademo F. L. N., Bezerra B. (2018). The influence of age and sex on the vocal repertoire of the antillean manatee (Trichechus manatus manatus) and their responses to call playback. Mar. Mammal Sci. 34, 577–594. doi: 10.1111/mms.12467
Verfuss U. K., Gillespie D., Gordon J., Marques A. T., Miller B., Plunkett R., et al. (2018). Comparing methods suitable for monitoring marine mammals in low visibility conditions during seismic surveys. Mari. Pol. Bul. 126, 1–18. doi: 10.1016/j.marpolbul.2017.10.034
Westbrook R. (1984). Federal noxious weeds: Kariba weed. Weeds Today 15, 8–9. doi: 10.1079/cabicompendium.48447
Wirrmann D., Elouga M. (1998). Discovery of an iron age site in lake ossa, cameroonian littoral province. Earth Planet. Sci. 326, 383–389.
Keywords: Trichechus senegalensis, Salvinia molesta, passive acoustics, 360° sonar, Cameroon
Citation: Factheu C, Rycyk AM, Kekeunou S, Keith-Diagne LW, Ramos EA, Kikuchi M and Takoukam Kamla A (2023) Acoustic methods improve the detection of the endangered African manatee. Front. Mar. Sci. 9:1032464. doi: 10.3389/fmars.2022.1032464
Received: 30 August 2022; Accepted: 13 December 2022;
Published: 06 January 2023.
Edited by:
Salvatore Siciliano, Escola Nacional de Saúde Pública Sergio Arouca (Fiocruz), BrazilReviewed by:
Chandra Paulina Salgado Kent, Edith Cowan University, AustraliaSergio Carvalho Moreira, Federal University of Rio de Janeiro, Brazil
Copyright © 2023 Factheu, Rycyk, Kekeunou, Keith-Diagne, Ramos, Kikuchi and Takoukam Kamla. This is an open-access article distributed under the terms of the Creative Commons Attribution License (CC BY). The use, distribution or reproduction in other forums is permitted, provided the original author(s) and the copyright owner(s) are credited and that the original publication in this journal is cited, in accordance with accepted academic practice. No use, distribution or reproduction is permitted which does not comply with these terms.
*Correspondence: Clinton Factheu, Y2xpbnRvbmZhY3RoZXVAZ21haWwuY29t