- 1Chesapeake Biological Laboratory, University of Maryland Center for Environmental Science, Solomons, MD, United States
- 2Marine Invasive Species Program, California State Lands Commission, Long Beach, CA, United States
- 3Jotun A/S, Sandefjord, Norway
- 4AkzoNobel/International Paint Ltd., Gateshead, United Kingdom
- 5HullWiper, Ltd., Dubai, United Arab Emirates
- 6SGS Marine Field Services & Monitoring, Wilmington, NC, United States
Microplastic pollution in the ocean is a critical, global environmental concern. Although believed to be a comparatively small contributor, the paints applied to commercial ships have been identified as a source of microplastics because polymers are used as binding agents in all anticorrosive and antifouling marine coatings. Furthermore, the release of microplastics from coatings may be amplified by in-water cleaning operations to remove biofouling. A comprehensive, detailed assessment and review of the array of commercial ships’ coatings, specifically characteristics, behavior, and fate over time, has not been conducted in the context of ocean microplastic pollution. This Policy Brief identifies gaps and proposes actions to better understand the fate of released particles with the goal to inform appropriate and effective solutions.
Introduction
Plastics (i.e., solid materials made from polymers) have become indispensable in our daily lives, owing to their adaptability, versatility, and relatively low cost. In the late 1960s, due to their widespread use around the world, plastics were first reported as marine pollutants with the discovery of polymers in the remains of birds, specifically, Laysan albatrosses (Phoebastria immutabilis) (Kenyon and Kridler, 1969). Instead of harmlessly degrading, it was discovered that many plastics often fragment into smaller, and very long-lived, particles in the ocean (Carpenter and Smith, 1972).
Recently, there has been intense global interest in plastic pollution in the oceans, particularly regarding the “garbage patch” of marine debris in the North Pacific Ocean (e.g., Lebreton et al., 2018). On a smaller scale, high concentrations of microplastics have also been found within the water column in various locations (e.g., Coyle et al., 2020). An estimated 14.4 M tons of microplastics have sunk to the ocean floor since polymers were first used (Barrett et al., 2020). Additionally, microplastics physical and chemical properties may allow them to adsorb toxic chemicals (e.g., heavy metals and organic contaminants) from the environment (Holmes et al., 2012; Godoy et al., 2019). If consumed by marine organisms, toxins can be introduced into trophic networks and food webs (Verla et al., 2019; Costa et al., 2020; Zhu et al., 2021).
At a coarse level, microplastics can be binned into primary microplastics (directly released into marine systems as small particulates) and secondary microplastics (fragments from the degradation of larger plastic items). The sources of ocean microplastics can be further classified into seven major categories (Boucher and Friot, 2017):
● plastic pellets (from manufacturing, transport, and recycling);
● synthetic textiles (abrasion during laundry);
● tires (abrasion while driving);
● road markings (weathering and abrasion by vehicles);
● marine ship/boat coatings (weathering and incidents during application, maintenance, and disposal);
● personal care products (pouring during product use); and
● city dust (weathering, abrasion, and/or pouring of building coatings, synthetic soles of footwear, synthetic cooking utensils, detergents, artificial turfs, etc.).
Multiple studies have attempted to estimate the contribution of these different sources of microplastics towards overall ocean pollution (e.g., Pruter, 1987; Andrady, 2017; Su et al., 2022), but uncertainties remain in both the many possible sources and each of their relative impacts.
Although terrestrial sources are considered the major inputs of plastic pollution, direct ocean-based sources, such as plastics released during commercial shipping activities, aquaculture operations, and fishing, are also recognized contributors (Peng et al., 2022). A review by the International Maritime Organization (IMO) concluded that commercial shipping operations may be a notable source of marine plastic pollution through the release of waste, marine coatings, and gray water (e.g., discharges from galleys, sinks, shower, laundries) (GESAMP, 2021). For example, cruise ships’ gray water can contain 2,000 to 50,000 microplastic particles per liter (Mikkola, 2020). Further, several studies describe debris collected on beaches as likely connected directly to shipping activities (e.g., Santos et al., 2005; Smith et al., 2018; Ryan et al., 2019). Indeed, preventative regulations promulgated by the IMO (e.g., Annex V of MARPOL) are intended to improve ship waste (including plastics) management systems.
Recently, attention has turned to the potential for release of microplastics from commercial ships’ coatings. Herein, “marine coatings” means any coating or coating system (a.k.a. paint) that is used on the outside surfaces of ships (above or below the waterline), including antifouling coatings, anticorrosion coatings, cosmetic finishes, and tie-coats (used to join successive coating layers together). High performing biocidal and non-biocidal antifouling coatings are essential in minimizing the transfer of non-indigenous species (NIS) in biofouling, reducing the need for underwater cleaning of biofouling, and reducing ship drag and fuel consumption (thereby decreasing operational costs and greenhouse gas emissions). However, because marine coatings use polymers as binding agents, there is also a potential for the release of microplastics (e.g., Gaylarde et al., 2021; Turner, 2021).
The liberation of microplastics associated with marine coatings (during application, throughout the coating’s approximate five-year service life, and during removal and disposal) has been suggested to be an important contributor to ocean plastic pollution (e.g., Dibke et al., 2021). For example, Boucher and Friot (2017) predict that perhaps 3.7% (~55,000 tons/year) of the 1.5 M tons/year of primary ocean microplastics are from ship and yacht coatings. However, given the limited available data, more comprehensive and careful estimates are critically needed to fully understand the scope and scale of microplastics release from ships coatings and to identify possible solutions.
The main release of microplastics associated with a ship’s coating is thought to be a direct result of ultraviolet (UV) radiation, heat, microbial activity, and mechanical stress from wave and wind energy, all of which are known to degrade marine coatings (Min et al., 2020). These processes may be further exacerbated and accelerated by in-water cleaning (IWC) of biofouling (the physical removal of biofilms, macrofouling, or both from submerged ship surfaces) (Scianni and Georgiades, 2019; Tamburri et al., 2020; Tamburri et al., 2021).
While estimates of microplastic release from ships’ coatings have raised environmental concerns (e.g., conveying toxicity to non-targeted organisms and biocide leaching from microplastics), this complicated issue has yet to be thoroughly investigated. Many facets of this issue are largely unknown, including:
● the concentrations and types of polymers used as binding agents in the many different marine coatings currently in use;
● the variable behavior and fate of the different polymers in different coatings over time; and
● the concentrations, biological availability, and environmental impacts of these diverse polymers (and their by-products).
This Policy Brief discusses relevant existing and emerging regulations, proposes standardized terminology, and describes knowledge gaps that need to be addressed before meaningful steps can be taken to minimize the potentially harmful release of microplastics from commercial ship coatings.
Existing policy
The overarching and global instrument addressing the release of plastics from the shipping industry is the International Convention for the Prevention of Pollution from Ships, which was adopted in 1973 by the IMO and modified in 1978 (MARPOL 73/78; IMO, 1978). Annexes I and II entered into force in 1983. Annex V (Regulations for the Prevention of Pollution by Garbage from Ships) took the step to ban the discharge of all plastic from ships. It entered into force in 1988 and is nearly universally applied: at present, 155 contracting States representing 98.49% of the world’s shipping tonnage have ratified it (IMO, 2022b). More broadly, the Convention on the Prevention of Marine Pollution by Dumping of Wastes and other Matter, 1972 (London Convention) prevented dumping at sea. It was modernized by the 1996 Protocol (London Protocol), which entered into force in 2006. To specifically address the fraction of marine plastic litter that emanates from ships, the IMO developed an Action Plan in 2018 (MEPC.310[73]; IMO, 2018).
Microplastic regulations at international, national, regional (e.g., state or territory), and local levels are relatively numerous, but they are narrowly targeted toward individual sources, such as microbeads in cosmetics and other personal care products (e.g., U.S. Microbead-Free Waters Act of 2015, H.R. 1321). Currently, no policy or regulation exists that addresses the release of microplastics from maritime shipping activities. One proposal that would directly affect the use of microplastics in coatings is the European Union’s Registration, Evaluation, Authorization, and Restriction of Chemicals Act (REACH). The legislation would restrict the intentional addition of microplastic particles in everyday products, including paints and coatings, although the official proposal has been delayed and is not yet in force (Faber et al., 2021; ECHA, 2022). However, given the global nature of commercial shipping, effective regulations will be challenging unless implemented at an international level. Furthermore, regulations to limit/prohibit the use of polymers in marine coatings should only be considered after there is a much more holistic understanding of potential environmental concerns.
Terminology
Microplastics were first described by Thompson et al. (2004), and they subsequently have been categorized by different organizations into groups based on size. At present, no size distribution scheme is used in a globally consistent way (ISO, 2020). For comparison across research studies and for clarity as regulations are considered and implemented, a universally accepted definition is critical. We propose combining two of the most-often used classifications, GESAMP (2019) and ISO (2020) (Table 1). In this manner, marine plastic “litter” is binned into various size classes (Table 1), with microplastics from ships’ coatings defined as particles ≥1 µm and <5 mm.
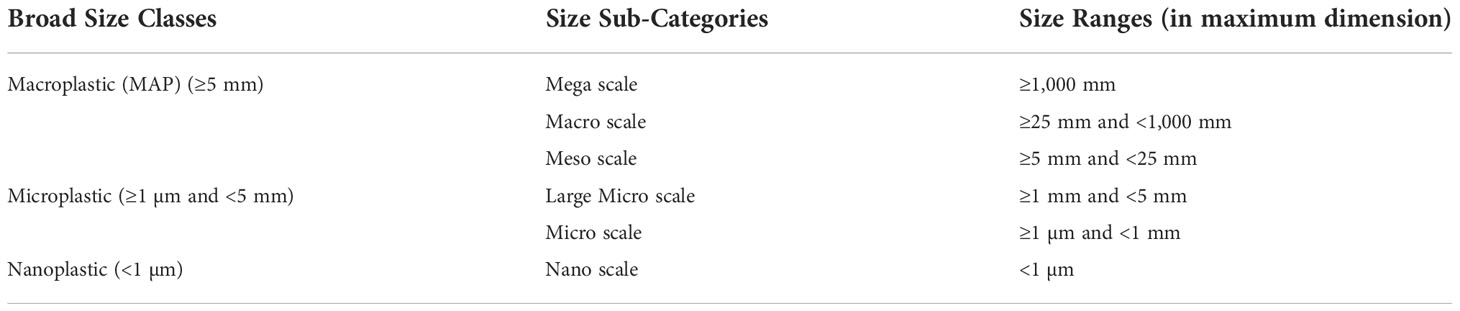
Table 1 Proposed classification of ocean plastics with respect to marine coatings, which combine definitions in ISO/TR 21960:2020 and GESAMP 2019.
Gaps and recommendations
While no existing regulations address microplastic release from ship coatings, growing awareness of this issue has begun to stimulate interest in the possible development of future guidelines, best-practices, and policies. However, the success of any approach to minimize the impacts of microplastic release from ship coatings is dependent on sound, scientific understanding of the:
● variability in composition of marine coatings;
● behavior and fate of polymers in different coatings, in different environments, over the lifetime of the coatings (e.g., mechanisms of possible release, the chemical and physical properties, movement, and degradation); and
● the concentrations, biological availability, and environmental impacts of any released microplastics and their by-products.
This section discusses the main uncertainties and proposes actions to address them.
Composition – Polymer binders are the primary contributor to most of the functional performance of coatings and the main potential source of plastic materials (OECD, 2009). Minor contents of other organic polymers are used in most coatings as functional additive ingredients. The total percentage of polymer binders can vary widely, depending on the type of coating and the specific performance requirements. This range is found because coatings’ functional features—such as barrier properties, protection, gloss, hardness, weathering resistance – are all influenced by the amount and type of binder used. Indeed, the binder content (percentage of the total solid mass) typically varies from 10-90% (~40% for many commonly used coatings) (Tasche et al., 2020). However, it must be noted that the polymers used in coatings may range from low-molecular weight organic oligomers to high-molecular weight and non-degradable polymers, and mixing different polymers within a single coating is not uncommon (Tasche et al., 2020). A further complicating factor is that the relative concentration of polymers in any specific coating type will often change throughout its service life (i.e., as the coating interacts with the environment over time, including the normal leaching of biocides and pigments) (Kiil et al., 2001; Yebra et al., 2004; Yebra et al., 2006).
Plastic bottles and bags, which often end up as ocean plastic pollution, are nearly 100% polymers, with a relatively small amount of non-polymeric additives (e.g., Siddiqui et al., 2008; Hansen et al., 2013). Conversely, standard coating particles are irregular composite materials containing a greater proportion of additives and, consequently, tend to be more brittle, angular, opaque, dense, heterogeneous, and layered than other microplastics of equivalent dimensions (Turner, 2021). All of this variability begs the question: How much polymer is needed for a particle to be considered a “microplastic”? We propose to define ships’ microplastics as any particle containing ≥10% polymer content, with the entire mass of the particle considered a microplastic. This conservative approach is based on the lower end of polymer content found in marine coatings and is, therefore, environmentally protective.
Behavior and fate of microparticles – Marine coatings vary widely in their intended function and lifetime, and they are designed to fit individual needs and performance criteria (Turner, 2021). Additives such as metal–containing and organic biocides, pigments, and corrosion inhibitors are typically included in specific marine coating applications (Singh and Turner, 2009; Gade et al., 2012; Soroldoni et al., 2017). Not surprisingly, these components can increase environmental concerns, including producing potentially toxic conditions for aquatic organisms (Soon et al., 2021).
Many coatings have weathering resistance or corrosion protection as their primary function. Here, the binders used are specifically chosen for their durability and resilience to chemical, physical, or mechanical degradation. Alternatively, “self-polishing” antifouling coatings are specifically designed to be consumed (sloughed off or removed) during their service life. This action occurs through controlled chemical processes such as hydrolysis or ion exchange, thereby providing optimal resistance to biofouling on the ship’s submerged surfaces (e.g., Bressy et al., 2009; Kwon et al., 2020). Through self-polishing mechanisms, these polymers are generally understood to be released as hydrophilic, water-soluble polymers rather than released as solid, particulate materials (Zhang and Chiao, 2015). Such marine coating systems themselves could still be a source of microplastics (e.g., when the coating is damaged or abraded, including on ice-breaking vessels; Leistenschneider et al., 2021).
Marine coatings on the outside hulls of commercial ships are generally applied as part of a coating system or scheme. They often comprise several layers of topcoat (e.g., antifouling paint or cosmetic finish) over an anticorrosive coating, “tie-coats” (which act as bridges between layers), or both. The diversity of chemistries and properties of binders used in a coating will ultimately be an important factor in determining its risk of releasing microplastics throughout its in-service period (i.e., the typical five-year interval between successive dry dockings). We recommend a comprehensive evaluation of the intended use of commonly used coatings and their inherent features, combined with situations/scenarios that can cause particles to be released. We also recommend empirical assessments of marine coating associate particle movement (once released from a ship), and particle physical and chemical changes over time and under diverse environmental conditions.
Concentrations, biological availability, and environmental impacts – Microplastic particles can be released from ships to the environment through five scenarios, during: (a) coating application, (b) in-service use, (c) IWC of biofouling, (d) routine ship maintenance (e.g., submerged surface and equipment inspections and testing; replacing anodes; pipe and valve maintenance, repair, replacement and blanking; repair and replacing suction and vent grates), and (e) end-of-life (i.e., coating removal and disposal or ship decommissioning/scrapping) (Figure 1).

Figure 1 Representation (not to scale) of the five scenarios for microplastic release from a ship’s coating throughout its typical five-year, in-service period: (A) shipyard/drydocking application; (B) in-service use, including normal wear and tear; (C) in-water cleaning of biofouling on submerged ship surfaces, which can be proactive (using remotely operated vehicles [red circle]) or reactive (using diver- or remotely operated cleaning [yellow rectangle]); (D) routine/required ship and coating maintenance, such as replacing anodes or maintaining pipes and valves; and (E) shipyard/drydocking end-of-life coating system removal and disposal and/or surface preparation for re-coating.
Four of the five scenarios (excluding in-service wear) can be managed or controlled because human involvement is inherent in their operations. In each scenario, preventive measures (e.g., using closed systems, collecting debris, or applying specialized techniques) can be implemented to mitigate or avoid/minimize releasing coating particles. Certainly, these activities will be most effective if best practices are universally identified and adopted (e.g., EU IPPC legislation covering shipyards).
In-water cleaning systems that: (a) minimize the release of coating particles during cleaning, (b) effectively capture particulate material removed from ships surfaces, or (c) both, will limit the amount of microplastics emitted during operations (e.g., Scianni and Georgiades, 2019; Tamburri et al., 2021). The IWC system itself may also be a source of microplastics if, for example, cleaning brushes wear and produce microplastics. However, not all IWC use plastic brushes and, when they do, the amount of brush wear/loss can be quantified (e.g., before- and after- measures). Brush-associated plastics can also be captured and disposed of, like other cleaning debris. Regardless, if the use and frequency of IWC increases across the global fleet of commercial ships, ensuring effective capture and disposal of coating particles, and cleaning debris more broadly, will become critical. It should be noted that the frequency of IWC will also be influenced by the choice of coating system and the ship’s operational profile and environmental conditions (although some ships are not cleaned during their specified in-service period).
During the marine coating’s normal in-service use stage, there is little direct, targeted human influence on the coating. The majority of particles release will take place through adventitious processes, such as weathering, corrosion, degradation, erosion or mechanical damages from unintended operational incidents (e.g., groundings, fender damage, anchor chain damage, or collisions). Microplastic release from such processes is generally relevant to coatings that are directly exposed to the environment during the in-service use stage (generally topcoats), as other coatings that are part of a coating system (e.g., primers, tie-coats, middle coats) will not be exposed to natural conditions that may cause particle release. Release from mechanical damage during the use stage is more likely to influence all coating types but typically in relatively small affected areas.
It is expected that there is a significant difference in the morphology of particles formed during the in-service stage, depending on their mode of generation. Particles from the normal wearing processes might be expected to be significantly smaller in size and of a relatively uniform nature. It is also possible that the binder polymers may be partially degraded from such processes, so that further degradation and decomposition may happen more rapidly. On the other hand, particles from mechanical damage are more likely to be larger, denser than seawater, and less uniform in size and can generally be expected to have the same properties as the intact coating. For these types of sinking particles, further degradation and decomposition may take longer, causing the material to persist for a long period. We recommend determining coatings’ degradation and decomposition rates, as well as the bioavailability (i.e., the proportion of the total quantity of particles present in the environment that is available for uptake/ingestion by various marine organisms; see Botterell et al., 2019) of microplastics released from ships’ coatings.
Conclusions
While the scenarios for the release of microplastics from ships’ coatings can be categorized (Figure 1), the types, amounts, fates, and environmental effects of the coating polymers—under each pathway and over time—are highly variable and poorly understood. Focused, interdisciplinary research efforts are needed to collect, aggregate, and interpret data to better understand the relative risks of release. In turn, this information will be used to develop or improve environmentally protective best-practices and regulations. Because different sectors (coating manufacturers, ship owners/operators, IWC service providers, and in-water maintenance and repair service providers) of the maritime value chain represent possible pathways for the release of microplastics, it is necessary that each sector investigate their respective areas, and they coordinate efforts between sectors and with academic and agency researchers. In doing so, a holistic view of ships’ microplastics will emerge, allowing for successful environmental stewardship.
Author contributions
MT led the overall project design, execution, and drafting of this manuscript. LD, CS, ZS, CØ, NO, and SD contributed information, expertise, and insight, and they assisted in drafting various sections of the publication. All authors contributed to the article and approved the submitted version.
Funding
This effort was funded by the U.S. Department of Transportation Maritime Administration and Maryland Department of Transportation’s Port Administration, through the University of Maryland Center for Environmental Science.
Conflict of interest
No potential conflict of interest was reported by the authors. Author LD is employed by SGS Marine Field Services & Monitoring, author CØ is employed by Jotun A/S, author NO is employed by Akzo Nobel/International Paint Ltd., and author SD is employed by HullWiper Ltd.
The remaining authors declare that the research was conducted in the absence of any commercial or financial relationships that could be construed as a potential conflict of interest.
Publisher’s note
All claims expressed in this article are solely those of the authors and do not necessarily represent those of their affiliated organizations, or those of the publisher, the editors and the reviewers. Any product that may be evaluated in this article, or claim that may be made by its manufacturer, is not guaranteed or endorsed by the publisher.
Disclaimer
The information contained within the manuscript reflects the views of the authors only, and not the views of their institution, agency, organization, or company.
References
Andrady A. L. (2017). The plastic in microplastics: A review. Mar. pollut. Bull. 119, 12–22. doi: 10.1016/j.marpolbul.2017.01.082
Barrett J., Chase Z., Zhang J., Holl M. M. B., Willis K., Williams A., et al. (2020). Microplastic pollution in deep-sea sediments from the great Australian bight. Front. Mar. Sci. 7, 1–10. doi: 10.3389/fmars.2020.576170
Botterell Z. L. R., Beaumont N., Dorrington T., Steinke M., Thompson R. C., Lindeque P. K. (2019). Bioavailability and effects of microplastics on marine zooplankton: a review. Environ. pollut. 245, 98–110. doi: 10.1016/j.envpol.2018.10.065
Boucher J., Friot D. (2017). Primary microplastics in the oceans: A global evaluation of sources (Gland, Switzerland: IUCN).
Bressy C., Margaillan A., Faÿ F., Linossier I., Réhel K. (2009). “18 - tin-free self-polishing marine antifouling coatings,” in Woodhead publishing series in metals and surface engineering, advances in marine antifouling coatings and technologies. Eds. Hellio C., Yebra D. (Cambridge, UK: Woodhead Publishing Limited), 445–491.
Carpenter E. J., Smith J. K. L. (1972). Plastics on the Sargasso sea surface. Sci. 175 4027, 1240–1241. doi: 10.1126/science.175.4027.1240
Costa E., Piazza V., Lavorano S., Faimali M., Garaventa F., Gambardella C. (2020). Trophic transfer of microplastics from copepods to jellyfish in the marine environment. Front. Environ. Sci. 8, 571732. doi: 10.3389/fenvs.2020.571732
Coyle R., Hardiman G., O’Driscoll K. (2020). Microplastics in the marine environment: A review of their sources, distribution processes, uptake and exchange in ecosystems. Case. Stud. Chem. Environ. Eng. 2, 100146. doi: 10.1016/j.cscee.2020.100010
Dibke C., Fischer M., Scholz-Böttcher B. M. (2021). Microplastic mass concentrations and distribution in German bight waters by pyrolysis–gas chromatography–mass Spectrometry/Thermochemolysis reveal potential impact of marine coatings: Do ships leave skid marks? Environ. Sci. Technol. 55(4), 2285–2295. doi: 10.1021/acs.est.0c04522
ECHA (European Chemicals Agency) (2022) Registry of restrictions until outcome. Available at: https://echa.europa.eu/registry-of-restriction-intentions/-/dislist/details/0b0236e18244cd73 (Accessed 15 July 2022).
Faber M., Marinkovic M., de Valk E., Waaijers-van der Loop. S. L. (2021)Paints and microplastics: exploring recent developments to minimise the use and release of microplastics in the Dutch pain value chain. In: RIVM report 2021-0037. Available at: https://www.rivm.nl/bibliotheek/rapporten/2021-0037.pdf (Accessed 15 July 2022).
Gade A. L., Heiaas H., Lillicrap A., Hylland K. (2012). Ecotoxicity of paint mixtures: Comparison between measured and calculated toxicity. Sci. Total Environ. 435-436, 526–540. doi: 10.1016/j.scitotenv.2012.07.011
Gaylarde C. C., Neto J. A. B., da Fonseca E. M. (2021). Paint fragments as polluting microplastics: A brief review. Mar. pollut. Bull. 162, 111847. doi: 10.1016/j.marpolbul.2020.111847
GESAMP (2019). “Guidelines or the monitoring and assessment of plastic litter and microplastics in the ocean,” in IMO/FAO/UNESCO-IOC/UNIDO/WMO/IAEA/UN/UNEP/UNDP/ISA joint group of experts on the scientific aspects of marine environmental protection, (Nairobi: United Nations Environment Programme).
GESAMP (2021). “Sea-Based sources of marine litter,” in IMO/FAO/UNESCO-IOC/UNIDO/WMO/IAEA/UN/UNEP/UNDP/ISA joint group of experts on the scientific aspects of marine environmental protection. (London: International Maritime Organization).
Godoy V., Blazquezm G., Calero M., Quesada L., Martin-Lara M. A. (2019). The potential of microplastics as carriers of metals. Environ. Pullut. 255, 113363. doi: 10.1016/j.envpol.2019.113363
Hansen E., Nilsson N. H., Lithner D., Lassen C. (2013). “Hazardous substances in plastic materials,” in Report TA-3017 prepared by COWI in cooperation with Danish technological institute. (Vejle: Denmark).
Holmes L. A., Turner A., Thompson R. C. (2012). Adsorption of trace metals to plastic resin pellets in the marine environment. Environ. Pullut. 160, 42–48. doi: 10.1016/j.envpol.2011.08.052
IMO (1978). International convention for the prevention of pollution from ships (MARPOL 73/78). (London: International Maritime Organization).
IMO (2018). Action plan to address marine plastic litter from ships. MEPC.310(73). (London: International Maritime Organization).
IMO (2022b) Status of treaties. Available at: https://wwwcdn.imo.org/localresources/en/About/Conventions/StatusOfConventions/StatusOfTreaties.pdf (Accessed 18 July 2022).
International Organization for Standardization (ISO) (2020). Plastics–environmental aspects–state of knowledge and methodologies. ISO/TR 21960, 2020. (Geneva: International Organization for Standardization).
Kenyon K. W., Kridler E. (1969). Laysan albatrosses swallow indigestible matter. Auk. 86 (2), 339–343. doi: 10.2307/4083505
Kiil S., Weinell C. E., Pedersen M. S., Dam-Johansen K. (2001). Analysis of self-polishing antifouling paints using rotary experiments and mathematical modeling. Ind. Eng. Chem. Res. 40, 3906–3920. doi: 10.1021/ie010242n
Kwon S. H., Lee I., Park H., Lee S. G. (2020). Decomposition mechanisms of self-polishing copolymers for antifouling coating materials through first-principles approach. Prog. Org. Coat. 138, 105406. doi: 10.1016/j.porgcoat.2019.105406
Lebreton L., Slat B., Ferrari F., Sainte-Rose B., Aitken J., Marthouse R. (2018). Evidence that the Great Pacific Garbage Patch is rapidly accumulating plastic. Sci. Rep. 8, 4666.
Leistenschneider C., Burkhardt-Holm P., Mani T., Primpke S., Taubner H., Gerdts G. (2021). Microplastics in the weddell Sea (Antarctica): a forensic approach for discrimination between environmental and vessel-induced microplastics. Environ. Sci. Technol. 55, 15900–15911. doi: 10.1021/acs.est.1c05207
Mikkola O. (2020). Estimating microplastic concentrations and loads in cruise ship grey waters. [dissertation/master’s thesis] (Espoo, Finland: Aalto University).
Min K., Cuiffi J. D., Mathers R. T. (2020). Ranking environmental degradation trends of plastic marine debris based on physical properties and molecular structure. Nat. Commun. 11, 727. doi: 10.1038/s41467-020-14538-z
OECD (2009). “Emission scenario documents on coating industry (paints, lacquers and varnishes),” in OCED series on emission scenario documents, no. 22, ENV/JM/MONO, vol. 2009) (Paris: OECD).
Peng G., Xu B., Li D. (2022). Gray Water from ships: A significant sea-based source of microplastics? Environ. Sci. Technol. 56, 4–7. doi: 10.1021/acs.est.1c05446
Pruter A. T. (1987). Sources, quantities and distribution of persistent plastics in the marine environment. Mar. pollut. Bull. 18, 305–310. doi: 10.1016/S0025-326X(87)80016-4
Ryan P. G., Dilley B. J., Ronconi R. A., Connan M. (2019). Rapid increase in Asian bottles in the south Atlantic ocean indicates major debris inputs from ships. PNAS. 116 (42), 20892–29897. doi: 10.1073/pnas.1909816116
Santos I. R., Friedrich A. C., Barretto F. P. (2005). Overseas garbage pollution on beaches of northeast Brazil. Mar. pollut. Bull. 50, 782–786. doi: 10.1016/j.marpolbul.2005.04.044
Scianni C., Georgiades E. (2019). Vessel in-water cleaning or treatment: Identification of environmental risks and science needs for evidence-based decision making. Front. Mar. Sci. 6, 467. doi: 10.3389/fmars.2019.00467
Siddiqui M. N., Gondal M. A., Redhwi H. H. (2008). Identification of different type of polymers in plastics waste. J. Environ. Sci. Health 43, 1303–1310. doi: 10.1080/10934520802177946
Singh N., Turner A. (2009). Trace metals in antifouling paint particles and their heterogenous contamination of coastal sediments. Mar. pollut. Bull. 58 (4), 559–564. doi: 10.1016/j.marpolbul.2008.11.014
Smith S. D. A., Banister K., Fraser N., Edgar R. J. (2018). Tracing the source of marine debris on the beaches of northern new south Wales, Australia: The bottles on beaches program. Mar. pollut. Bull. 126, 304–307. doi: 10.1016/j.marpolbul.2017.11.022
Soon Z. Y., Jung J.-H., Loh A., Yoon C., Shin D., Kim M. (2021). Seawater contamination associated with in-water cleaning of ship hulls and the potential risk to the marine environment. Mar. pollut. Bull. 171, 112694. doi: 10.1016/j.marpolbul.2021.112694
Soroldoni S., Abreu F., Castro I. B., Duarte F. A., Pinho G. L. L. (2017). Are antifouling paint particles a continuous source of toxic chemicals to the marine environment? J. Hazard. Mater. 330, 76–82. doi: 10.1016/j.jhazmat.2017.02.001
Su L., Xiong X., Zhang Y., Wu C., Xu X., Sun C., et al. (2022). Global transportation of plastics and microplastics: A critical review of pathways and influences. Sci. Total Environ. 831, 154884. doi: 10.1016/j.scitotenv.2022.154884
Tamburri M. N., Davidson I. C., First M. R., Scianni C., Newcomer K. A., Inglis G. J., et al. (2020). In-water cleaning and capture system to remove ship biofouling: An initial evaluation of efficacy and environmental safety. Front. Mar. Sci. 7, 437. doi: 10.3389/fmars.2020.00437
Tamburri M. N., Georgiades E. T., Scianni C., First M. R., Ruiz G. M., Junemann C. E. (2021). Technical considerations for development of policy and approvals for in-water cleaning of ship biofouling. Front. Mar. Sc. 8, 804766. doi: 10.3389/fmars.2021.804766
Tasche J., Sabattie E. F. D., Thompson R. L., Campana M., Wilson M. R. (2020). Oligomer/polymer blend phase diagram and surface concentration profiles for suqalane/polybutadiene: experimental measurements and predictions from SAFT-γ mie and molecular dynamic simulations. Macromolecules 53, 2299–2309. doi: 10.1021/acs.macromol.9b02155
Thompson R. C., Olsen Y., Mitchell R. P., Davis A., Rowland S. J., John A. W. G., et al. (2004). Lost at sea: Where is all the plastic? Sci. 304, 838. doi: 10.1126/science.1094559
Turner A. (2021). Paint particles in the marine environment: An overlooked component of microplastics. Water Res. X. 12, 100110. doi: 10.1016/j.wroa.2021.100110
Verla A. W., Enyoh C. E., Verla E. N., Nwarnorh K. O. (2019). Microplastic-toxic chemical interaction: a review study on quantified levels, mechanism and implication. SN Appl. Sci. 1, 1400. doi: 10.1007/s42452-019-1352-0
Yebra D. M., Kiil S., Dam-Johansen K. (2004). Antifouling technology–past, present and future steps towards efficient and environmentally friendly antifouling coatings. Prog. Org. Coat. 50, 75–104. doi: 10.1016/j.porgcoat.2003.06.001
Yebra D. M., Kiil S., Dam-Johansen K., Weinell C. E. (2006). Mathematical modeling of tin-free chemically-active antifouling paint behavior. AIChE J. 52, 1926–1940. doi: 10.1002/aic.10787
Zhang H., Chiao M. (2015). Anti-fouling coatings of poly(dimethylsiloxane) devices for biological and biomedical applications. J. Med. Biol. Eng. 35, 143–155. doi: 10.1007/s40846-015-0029-4
Keywords: ocean plastics, marine coatings, polymers, ship anti-fouling systems, microplastics
Citation: Tamburri MN, Soon ZY, Scianni C, Øpstad CL, Oxtoby NS, Doran S and Drake LA (2022) Understanding the potential release of microplastics from coatings used on commercial ships. Front. Mar. Sci. 9:1074654. doi: 10.3389/fmars.2022.1074654
Received: 19 October 2022; Accepted: 09 November 2022;
Published: 24 November 2022.
Edited by:
Sonja M. Ehlers, Bundesanstalt für Gewässerkunde (BFG), GermanyReviewed by:
Eoghan Mánus Cunningham, University of Oxford, United KingdomAndrew Turner, University of Plymouth, United Kingdom
Copyright © 2022 Tamburri, Soon, Scianni, Øpstad, Oxtoby, Doran and Drake. This is an open-access article distributed under the terms of the Creative Commons Attribution License (CC BY). The use, distribution or reproduction in other forums is permitted, provided the original author(s) and the copyright owner(s) are credited and that the original publication in this journal is cited, in accordance with accepted academic practice. No use, distribution or reproduction is permitted which does not comply with these terms.
*Correspondence: Mario Tamburri, dGFtYnVycmlAdW1jZXMuZWR1