- 1Norwegian College of Fishery Science, Faculty of Bioscience, Fisheries and Economics, UiT The Arctic University of Norway, Tromsø, Norway
- 2Key Laboratory of Freshwater Biodiversity Conservation, Ministry of Agriculture of China, Yangtze River Fisheries Research Institute, Chinese Academy of Fishery Sciences, Wuhan, China
- 3Department of Animal and Aquatic Sciences, Faculty of Agriculture, Chiang Mai University, Chiang Mai, Thailand
- 4Aquaculture Laboratory, Department of Zoology, The University of Burdwan, Burdwan, India
Growing demands stimulate the intensification of production and create the need for practices that are both economically viable and environmentally sustainable. As European Union banned the use of antibiotics in production in 2003, several alternative treatments have been suggested, including probiotics. The first probiotic study in aquaculture was published in 1986, and since then probiotics have been considered as a beneficial tool in this industry. Today current evidence suggests that administration of certain probiotic strains might be able to enhance growth rate, improve the welfare of different fish species by modulating gut microbiota, improve physiological functions, such as metabolism, digestion, immunity, stress tolerance, intestinal histology, and disease resistance. Even though lactic acid bacteria and Bacillus spp. are the most frequently used probiotics in aquaculture, numerous studies have been published on other interesting probiotics. Therefore, the purpose of this paper is to summarize, comment, and discuss the current knowledge related to the effects of Aeromonas, Aliivibrio, Alteromonas, Arthrobacter, Bifidobacterium, Brochothrix, Clostridium, Enterovibrio, Kocuria, Microbacterium, Micrococcus, Paenibacillus, Phaeobacter, Pseudoalteromonas, Pseudomonas, Rhodococcus, Rhodopseudomonas, Rhodosporidium, Roseobacter, Shewanella and Vibrio as probiotics in finfish aquaculture, and present general information on their presence in the gastrointestinal tract of finfish. Moreover, some considerations for future studies are also indicated.
Introduction
By 2025, aquaculture is expected to play a leading role in the global supply of fish. However, the growth of this industry could be considerably hampered by failures to predict, avoid, and contain infections. Unsurprisingly, the intensification of aquatic production has led to a significant increase in the frequency of disease and a growing inefficiency among the antibiotics used to treat these.
Antibiotics have been used in aquaculture for more than 50 years (Shamsuzzaman and Kumar, 2012), and previously the most common method for dealing with the occurrence of bacterial infections was the administration of antibiotics (Cabello, 2006; Cabello et al., 2020; Lulijwa et al., 2020), and the rapid growth of for example the Chilean salmon industry was accompanied by intensive use of antibiotics. However, as antibiotic administrations became the target of increasing public criticism and political controversy, Sweden was the first country in Europe, to ban the use of antimicrobial growth promoters as early as 1986. In 2003, European Union stated in Regulation (EC) No. 1831/2003; “Antibiotics, other than coccidiostats or histomonostats, shall not be authorized as feed additives”. However, recent findings have revealed that mono- (e.g., Gudmundsdottir and Bjornsdottir, 2007) and polyvalent vaccines (e.g., Tobar et al., 2015) are effective for disease control in aquaculture, especially for salmonids. However, for many Chinese and Indian finfish-, shellfish- and cucumber species, vaccines are not available, and will hardly be available soon. However, there are several alternative treatment options available; phytobiotics, phage therapy, bacterial membrane vesicles (e.g., Jan, 2017; Tandberg et al., 2019; Mertes et al., 2021), quorum sensing interference, postbiotics (secreted by live bacteria, or released after bacterial lysis; Ang et al., 2020; Cuevas-González et al., 2020; Teame et al., 2020), postbiotics in combination with prebiotics, paraprobiotics (cell wall components; Taverniti and Guglielmetti, 2011; Choudhury and Kamilya, 2019; Nataraj et al., 2020), pro-, pre- and synbiotics. However, Cheng et al. (2014) argue that these “so-called alternatives” are not ideal antibiotics replacers.
According to Bermudez-Brito et al. (2012), “Probiotics are live microorganisms that provide health benefits to the host when ingested in adequate amounts”. The probiotic concept is primarily based on the assumption that direct feeding of microbial cultures possesses beneficial effects on growth performance, digestive processes, the immune system and animal health. The use of probiotics gained attention within aquaculture in the mid 1980s (Kozasa, 1986), and since then numerous reviews papers have been published (e.g., Gatesoupe, 1999; Irianto and Austin, 2002a; Merrifield et al., 2010; Dimitroglou et al., 2011; Hoseinifar et al., 2018; Ringø et al., 2018; Wang et al., 2019a; Hoseinifar et al., 2020; Hu et al., 2020; Ringø et al., 2020a; Ringø et al., 2020b; Yao et al., 2020; Nayak, 2021; van Doan et al., 2021). In this context, it is also worth mention that administration of antibiotics lead to their accumulation in the tissues (Chen et al., 2020a), emergence of antimicrobial resistant bacteria in the environment (e.g., Marti et al., 2014; Chen et al., 2020a; Lulijwa et al., 2020), modulation of the gut microbiota (dysbiosis) (e.g., Ringø et al., 2016; Kim et al., 2019; Legrand et al., 2020), suppression of certain gut bacteria (Saettone et al., 2020), and increased abundance of intestinal bacteria that act as reservoirs for antibiotic resistance genes (Salyers et al., 2004; Saenz et al., 2019). In addition, modulation of the gut microbiota to an undesirable community can induce mucosal inflammation (Tamboli et al., 2004; Turroni et al., 2014). Furthermore, many antibiotics currently used in aquaculture are, or are closely related to agents used to treat bacterial diseases in humans, which makes their uncontrolled application in animal production an enormous risk to host health. It is thus clear that new methods to control aquatic infections is required.
Among the probiotic bacteria used in aquaculture are lactic acid bacteria (LAB) and Bacillus most frequently used (e.g., Ringø et al., 2018a; Kuebutornye et al., 2019; Soltani et al., 2019; Ringø et al., 2020a; Nayak, 2021; James et al., 2021; van Doan et al., 2021). However, in addition to LAB and bacilli are numerous probiotics such as Aeromonas, Alteromonas, Arthrobacter, Bifidobacterium, Brochothrix, Clostridium, Kocuria, Microbacterium, Micrococcus, Paenibacillus, Phaeobacter, Pseudoalteromonas, Pseudomonas, Rhodococcus, Rhodopseudomonas, Rhodosporidium, Roseobacter, Shewanella and Vibrio used.
The use of probiotics is an alternative approach to reduce pathogen adherence and colonization in larval-, fry- and juvenile intestines by modulating the intestinal microbiota with beneficial bacteria. They can be added to the diet or water in order to enhance the proportion of health-promoting bacteria in the gut. An advantage of this method is that it can be implemented during the early stages of development when vaccination by injection is impractical.
The review of Irianto and Austin (2002a); Tapia-Paniagua et al. (2012); De et al. (2014); Newaj-Fyzul et al. (2014), Tan et al. 2020), Cámara-Ruiz et al. (2020); Hayatgheib et al. (2020), and van Doan et al. (2021) presented some information on administrations of the probiotic bacteria discussed in the present study, and to avoid duplication with that presented in the above mention reviews, these studies are only briefly presented in Tables.
The present review address to present an overview of interesting probiotic bacteria, not LAB and bacilli, with focus on growth performance, modulation of the gut microbiota, gut histology, effect on immunesystem, and disease resistance in finfish. Furthermore, some general information is presented on the probiotics discussed, and their presence in the GI tract of finfish.
Administration and Mode of Actions
Probiotic administration has been described in several reviews (e.g., Verschuere et al., 2000; Irianto and Austin, 2002a; Villamil et al., 2010; Dawood and Koshio, 2016; Kumar et al., 2016; Hoseinifar et al., 2018; Jahangiri and Esteban, 2018; Ringø et al., 2020a; Vargas-Albores et al., 2021), and in order to avoid overlaps, the administration methods are only briefly presented.
i) Oral administration via diet or water/bath, ii) Administration of several probiotics in combination (Fuller, 1989; Kesarcodi-Watson et al., 2012; Melo-Bolivar et al., 2021), iii) Inactivated bacteria, iv) Spores, v) Culturing, and added to feed as freeze-dried cultures, which sometimes are coated with lipids, vi) Encapsulation e.g., by calcium alginate beads (e.g., Rosas-Ledesma et al., 2012; Cordero et al., 2015; Prado et al., 2020), vii) Lyophilization, viii) Administration – continuously or regular intervals, but are the probiotics permanently colonisers in the GI tract, ix) Co-administration of probiotics with prebiotics or plant products, and x) Host specificity, or strains from other species or commercial probiotics (Lazado et al., 2015; van Doan et al., 2020).
The modes of action of probiotics are well discussed, and several hypotheses have been suggested (e.g., Irianto and Austin, 2002a; Prado et al., 2010; Kumar et al., 2016; Zorriehzahra et al., 2016; Wang et al., 2017; de Melo Pereira et al., 2018; Hoseinifar et al., 2018; Chauhan and Singh, 2019; Ran et al., 2021), and according to these reviews the modes of action are: a) competitive adhesion of probiotic microorganisms to epithelial receptors may prevent the attachment of pathogenic bacteria (rational behind “competitive exclusion”), b) aggregation of probiotics and pathogenic bacteria, c) competition for nutrients between probiotic and undesired bacteria, d) increased synthesis of lactic acid and reduction of intestinal pH, e) production of specific antibacterial substances, f) reduced production of toxic amines and decrease of ammonia level in the GI tract, g) beneficial effects on the intestinal immune system, h) interference with quorum sensing, i) bioremediator, j) improved defense against bacterial and viral infections, k) alleviate negative effects induced by crowding stress, and l) antioxidant properties.
Gram-Negatives
In Table 1 are the beneficial effects of Gram-negative probiotic bacteria used in finfish aquaculture revealed. In addition, some vital information is presented from in vitro studies.

Table 1 Effect of Gram-negative bacteria on growth performance, gut health, immune system and disease resistance in finfish, and some in vitro studies.
Acinetobacter
Acinetobacter belong to Gammaproteobacteria, is oxidase-negative, aerobic coccobacilli with twitching motility. They are isolated from the GI tract of finfish (e.g., Ringø et al., 2006a; Navarrete et al., 2013; Liu et al., 2019; Wang et al., 2020a), but their use as probiotics in finfish are less investigated (Bunnoy et al., 2019a), and as a part in multi-strain probiotic supplementation (Li et al., 2019a).
In a study with bighead catfish (Clarias macrocephalus; 150g), Bunnoy et al. (2019a) used an Acinetobacter originally isolated from skin mucus of bighead catfish revealing strong antibacterial activity against several freshwater pathogens in vitro (Bunnoy et al., 2019b). After 15- and 30-days administration, phagocytic index, phagocytic-, lysozyme-, and respiratory burst activity, and alternative complement pathway significantly enhanced, and upregulation of immune-related genes was observed. After 30 days administration, increased resistance was observed following intraperitoneal injection with Aeromonas hydrophila. The main reason why Acinetobacter species have little been used as probiotics in finfish, may be due to reports on opportunistic fish pathogenic agents within the genus.
Aeromonas
Aeromonas is facultative anaerobic, rod-shaped bacteria. Even though Aeromonas are mainly associated with diseases (Feckaninova et al., 2017), there are present in the GI tract of healthy finfish (e.g., Ringø et al., 1997; Navarrete et al., 2013; Chen et al., 2014; Abdelhamed et al., 2019). In finfish, information is available on the use of Aeromonas as probiotics (Irianto and Austin, 2002b; Lategan et al., 2004; Brunt and Austin, 2005; Makridis et al., 2005; Brunt et al., 2007; Brunt et al., 2008; Makridis et al., 2008; Pieters et al., 2008; Abbass et al., 2010; Wu et al., 2015; Hao et al., 2017), as well as a part of multi strains probiotics supplementation; Aeromonas veronii in combination with Flavobacterium sasangense (Chi et al., 2014).
Irianto and Austin (2002b) indicated that feed supplemented with A. hydrophila for 7 and 14 days led to better survival rate of rainbow trout (Oncorhynchus mykiss) following challenge with Aeromonas salmonicida. In contrast, Makridis et al. (2005) revealed no clear effect on survival of gilthead sea bream (Sparus aurata) larvae exposed to 6 x 105 Aeromonas mL-1.
Saprolegina paracitica (saprolegniosis; caused by fungal infections) is reported in silver perch (Bidyanus bidyanus), and Lategan et al. (2004) revealed that administration of Aeromonas media strain A199 to the tank water of silver perch halted the outbreak. In three later studies using Aeromonas sobria GC2 as probiont to rainbow trout, Brunt and Austin (2005) and (2008); Brunt et al. (2007) revealed enhanced survival after challenge with a range of pathogens. Similarly, Pieters et al. (2008) displayed that dietary inclusion of A. sobria protected rainbow trout against surface infections and against a eukaryotic pathogen, Ichthyophthirius multifiliis. Abbass et al. (2010) evaluated intraperitoneal and intramuscular injections of subcellular component of A. sobria GC2 to rainbow trout and revealed protection against Yersinia ruckeri.
Even though, diseases caused by A. veronii in freshwater fish are reported (e.g., Liu et al., 2018), the bacterium has been used as probiotic supplement. A dietary supplementation of A. veronii isolated from grass carp (Ctenopharyngodon idella) was administrated to grass carp, 108 CFU g-1, for 28 days and challenged with A. hydrophila (Wu et al., 2015). A significant increase of respiratory burst, phagocytic and lysozyme activities, and upregulation of immune related genes (IL-8, IL-1β, lysozyme-C and TNF-α), and resistance against A. hydrophila were observed. Modulation of the gut microbiota of grass carp by A. veronii administration was revealed by Hao et al. (2017), as Brevundimonas was the abundant genus in the GI tract, while Lactococcus, Pseudomonas and Vibrio decreased, and Flavobacterium and Lactococcus was not detected in probiotic administrated fish compared to control fed fish.
Alcaligenes
A genus of rod-shaped, motile, aerobic bacteria, and some strains of Alcaligenes are capable of anaerobic respiration in the presence of nitrate or nitrite. The genus does not use carbohydrates. Strains of Alcaligenes are reported in the intestinal tracts of vertebrates as well as finfish (e.g., Ringø, 1993; Navarrete et al., 2013; Sedlacek et al., 2016; Karlsen et al., 2017).
A decapeptide (cyclo‐(l‐Pro‐Gly)5) from Alcaligenes faecalis revealed immunostimulatory activities in a study with crucian carp (Carassius carassius) (Wang et al., 2011), and a challenge experiment displayed that fish injected with the decapeptide significantly improved survival (87.0%) compared with the control (54.6%) after infection with live A. hydrophila. An Alcaligenes sp. at inclusion level of 108 CFU g-1 feed, was fed to Malaysian Mahseer (Tor tambroides) for 90 days (Asaduzzaman et al., 2018). Weight gain, gut histology (villi height, villi with and villi area), and production of short chain fatty acids (SCFAs) significantly improved, and modulation of the gut microbiota Wang et al. (2020a) conducted a three-month feeding trial with Nile tilapia (Oreochromis niloticus) to determine the effect of Alcaligenes faecalis Y311 supplementation and revealed increase of alkaline phosphatase activities in gill and intestine. Probiotic administration did not affect the dominant bacteria but affected the relative abundance of some low abundance bacteria, Methyloparacoccus, Enterococcus, Limnohabitans, Tepidimonas and Cetobacterium in skin, gill and intestine. One interesting finding was that the relative abundance of Acinetobacter, potential pathogen, decreased in the gut by A. faecalis Y311 administration.
Alteromonas
Genus Alteromonas are facultative anaerobic bacteria, and in the absence of oxygen, the genus possess capabilities to use of a variety of other electron acceptors for respiration, for example trimethylamineoxide (Ringø et al., 1984). The genus is isolated from the GI tract of finfish (e.g., Akimoto et al., 1990; Jiang et al., 2018; Fonseca et al., 2019), and some information is available on the use of Alteromonas as probiotic supplement in shellfish (Ringø, 2020), but less information is available on their use as probiotics in finfish aquaculture (Mladineo et al., 2016). Mladineo et al. (2016) revealed improved phagocytic activity, respiratory burst, and gene expression of lysozyme, Mx protein, caspase 3, TNF-a, and IL-10, by Alteromonas sp. administrated to European sea bass (Dicentrarchus labrax).
Chromobacterium
Chromobacterium is a facultative anaerobic, motile and non-sporing coccobacillus, present in the GI tract of finfish (Ziólkowska et al., 2009; Zhou et al., 2016).
In a recent study, Yi et al. (2019) evaluated the effect of administration of a Chromobacterium aquaticum isolated from lake water with bacteriocin-like activity on zebrafish (Danio rerio) and revealed improved hepatic mRNA expression of carbohydrate metabolism-related genes, including glucokinase, hexokinase, glucose-6-phosphatase and pyruvate kinase, and growth-related genes, induced effect on innate immune-related genes and enhanced resistance against A. hydrophila and Streptococcus iniae by probiotic administration.
Enterobacter
Genus Enterobacter is rod shaped, facultative anaerobic, non-spore-forming bacteria and belong to family Enterobacteriaceae. During the last years, information has become available showing the presence of Enterobacter in intestine of finfish (e.g., Tapia-Paniagua et al., 2014a; Tapia-Paniagua et al., 2019; Terova et al., 2019; Nguyen et al., 2020).
Burbank et al. (2011) reported administration of Enterobacter strain PIC15 and Enterobacter amnigenus to rainbow trout for 7 days and revealed significant improved resistance against Flavobacterium psychrophilum. Furthermore, as both probiotic strains were isolated from the GI tract, this finding may indicate their ability to colonize the GI tract. Kenyi cichlid (Maylandia lombardoi) fed 60 days on an Enterobacter cloacae isolated from curd revealed enhanced growth, respiratory burst activity, and modulated the gut microbiota (Girijakumari et al., 2018). Moreover, it is of interest to notice that dietary administration of Enterobacter cloacae in combination with Bacillus mojavensis at 108 CFU g−1 to rainbow trout for 60 days improved protection against Y. ruckeri, as survival rate increased to 99.2% vs. 35% in the control group (Capkin and Altinok, 2009).
Phaeobacter
Genus Phaeobacter belongs to family Rhodobacteraceae and was first suggested by Martens et al. (2006). Phaeobacter is important as a carbon and sulfur metabolizer, and a biofilm former and antibiotic tropodithietic acid producer (TDA) a sulfur-containing compound (Porsby et al., 2008; Dittmann et al., 2020). Most of the cultured strains of Phaeobacter are isolated from aquatic environments, and from the intestine of finfish (Hjelm et al., 2004; Planas et al., 2004; Terova et al., 2019). Information is available on its use as probiotic, as well as a part of multi strains probiotics supplementation with Bacillus pumilus (Schmidt et al., 2017), and Phaeobacter inhibens in combination with vibriophage KVP40 (Rasmussen et al., 2019).
In a previous review, Dimitroglou et al. (2011) discussed probiotic administration of Phaeobacter in Mediterranean finfish, and in order to avoid overlaps we recommend readers with interest to have a closer look at the papers discussed in the above mention review.
Phaeobacter gallaeciensis isolated from seawater of scallop cultures and administrated to Atlantic cod (Gadus morhua) larvae enhanced survival towards Vibrio anguillarum serotype 01 (D’Alvise et al., 2012). In a later study with Atlantic cod larvae, improved resistance against V. anguillarum 02α was noticed when Phaeobacter sp. isolated from turbot hatchery was added into well dishes at 107 CFU mL-1 (D’Alvise et al., 2013). Based on their results, the author suggested that Phaeobacter was a promising probiont in marine larval culture, and that TDA contribute to its probiotic effect as a mutant of P. gallaeciensis did not reduce V. anguillarum numbers.
Planas et al. (2004) revealed that Phaeobacter 27-4 administration improved survival against V. anguillarum and detected 27-4 in GI lumen of turbot (Scophthalmus maximus) when the probiont was administrated to the larvae incorporated in rotifers, but 27-4 did not colonize the larval gut and intestinal epithelium.
A Phaeobacter strain isolated from turbot hatchery, grown on ceramic biofilter (probiofilter) revealed resistance against V. anguillarum, improved seawater quality by decreasing turbidity, but the bacterial diversity in larval turbot (Psetta maxima) gut was unchanged (Prol-García and Pintado, 2013). In a recent study, Dittmann et al. (2020) studied administration of a TDA producing P. inhibens strain DSM17395 isolated from mariculture environment to turbot larvae, and observed no effect on gut community structure, even though the relative abundance of Rhodobacterales in the GI tract decreased.
Pseudoalteromonas
Gauthier et al. (1995) proposed that genus Pseudoalteromonas, aerobic, non-spore forming rods was split from Alteromonas. They produce a broad range of anti-bacterial products (Jin et al., 2010; Offret et al., 2016; Richards et al., 2017), and are reported in finfish intestine (e.g., Fjellheim et al., 2007; Ringø et al., 2008; Jiang et al., 2018), as probiotics (Mladineo et al., 2016), and as a part of a multi-strain probiotic preparations with Microbacterium, Ruegeria and Vibrio fed to Atlantic cod larvae (Skjermo et al., 2015).
In a study using Pseudoalteromonas sp. Administrated to European sea bass (Dicentrarchus labrax), Mladineo et al. (2016) documented best stimulation of phagocytic activity, respiratory burst, and gene expression of lysozyme, Mx protein, caspase 3, TNF-a, and IL-10, by Pseudoalteromonas compared to Alteromonas sp. And Enterovibrio coralii administration.
Pseudomonas
Genus Pseudomonas belong to family Pseudomonadaceae, are rod-shaped, aerobic, catalase- and oxidase positive and contain approximately 200 species. The genus has a great metabolic diversity (Palleroni, 1992; Silby et al., 2011), and produce exopolysaccharides, which could make it difficult for pseudomonads to be phagocytosed by mammalian white blood cells and contribute to surface-colonizing biofilms that are difficult to remove from food preparation surfaces (Royan et al., 1999).
Several studies have revealed the probiotic potential of Pseudomonas (e.g., Giri et al., 2011; Giri et al., 2015; Giri et al., 2016), and they are able to colonize a wide range of niches, including the GI tract of finfish (e.g., Ringø et al., 1997; Ringø et al., 2006a; Navarrete et al., 2013; Wang et al., 2020a).
Genus Pseudomonas is frequently used in finfish aquaculture, and in order to avoid overlaps, previous studies discussed in the review of De et al. (2014); Hayatgheib et al. (2020) and Irianto and Austin (2002a) are only briefly presented in Table 1. Siderophore-producing Pseudomonads strains have successfully been applied as biocontrol agens to finfish. In an early study, Smith and Davey (1993) showed that the fluorescent pseudomonad F19/3 isolated from Atlantic salmonatlantic salmon (Salmo salar) with furunculosis inhibitied in vitro growth of A. salmonicida in culture media, and improved resistance of presmolts of brown trout (Salmo trutta) against A. salmonicida and the authors suggested that this finding was due to that the strain inhibited the pathogen by competing for free iron. Similarly, Pseudomonas fuorecens was regarded as effective probiont for rainbow trout conferring protection against V. anguillarum (Gram et al., 1999), but the strain did not protect Atlantic salmon against A. salmonicida despite that in vitro inhibition was revealed (Gram et al., 2001). (Korkea-aho et al. (2012) revealed that Pseudomonas sp. M162 administrated to rainbow trout fry colonised the GI tract, improved immunity and protection against F. psychrophilum, while administration of P. fluorescens at a inclusion level of 107 displayed enhanced growth rate, and feed conversion ratio of African catfish (Clarias gariepinus) (Osungbemiro et al., 2018). Haematological analyses of African catfish fed the P. fluorescens diets had significantly higher white blood cell than control diet. In addition, lower mortality rate and several intestine histopathological alterations were revealed in catfish fed diets supplemented with P. fluorescens. In two recent studies, González-Palacios et al. (2019; 2020) revealed that two P. fluorescens isolated from skin of trout could adhere to mucus, reduced adhesion of zoospores and cysts of S. paracitica in rainbow trout, stimulated phagocytic activity of macrophages, serum lysozyme activity and serum protein concentration, and might be promising for biocontrol of saprolegninosis. Giri et al. (2020) conducted a 8 weeks feeding trial with juvenile common carp to determine the effect of heat-killed Pseudomonas aeruginosa strain VSG2, and revealed enhanced lysozyme, protein level, and alkaline phosphatase. In serum and skin mucus, superoxide dismutase, glutathione, glutathione peroxidase, and myeloperoxidase levels significantly enhanced. Furthermore, mRNA expression of antioxidant genes significantly improved in liver. These positive effects were also noticed by improved resistance against A. hydrophila. Qi et al. (2020) displayed that Pseudomonas monteilii JK-1 significantly inhibited in vitro growth of A. hydrophila, and based on this criterion administrated the bacteria to grass carp, and showed that the bacteria was not toxic to the fish, and improved resistance against A. hydrophila. According to Fu et al. (2017), administration of Pseudomonas stutzeri F11 to grass carp reduced the levels of ammonia-N, nitrite-N, and total N in the water over an extended range, but administration did not had any effect on nitrate-N level. Modulation of the water microbial community was observed, by increasing the relative abundance of Bacteroidetes and Firmicutes, in contrast to Proteobacteria, Actinobacteria and Verrucomicrobia which decreased.
P. aeruginosa strain VSG2 has also been used in a multi-strain probiotic preparation with Bacillus subtilis and Lactobacillus plantarum (Giri et al., 2015), revealing improved growth performance, immunity and disease resistance in rohu.
Psychrobacter
Genus Psychrobacter belongs to the family Moraxellaceae, are Gram-negative aerobic, oxidase-negative, catalase-positive, non-pigmented and non-motile coccoid bacteria. Information is available showing the presence of Psychrobacter in intestine of finfish (e.g., Bakke-McKellep et al., 2007; Ringø et al., 2006a; Ringø et al., 2016b), and some Psychrobacter strains have successfully been used as probiotics to finfish (Sun et al., 2011; Sun et al., 2014; Makled et al., 2017; Makled et al., 2020).
Sun et al. (2011) administrated Psychrobacter sp. SE6 to grouper (Epinephelus coioides) and revealed only improvement in feed conversion ratio and serum component 4, while no effect was noticed on weight gain, specific growth rate (SGR), hepatopancreatic protease and lipase activity, intestinal amylase activity, and serum lysozyme - and superoxide dismutase activity, and serum component 3. In a later study, Sun et al. (2014) revealed that viable SE6 administration upregulated expression of TRL2 and TRL5 and cytokines, while results of heat-inactivated SE6 administration revealed that the MyD88-independent TLR2 signaling pathway was involved in the recognition of SE6. Use of Psychrobacter namhaensis administrated to Nile tilapia for 50 days, showed improved growth rate and feed utilisation ratio, haematocrit-, haemoglobin-, erythrocytes- and total leucocytes values by 2.8 x 107 CFU mL-1 supplementation vs. control fish (Makled et al., 2017). Moreover, immunoglobulin, alternative hemolysis, phagocytic and lysozyme activities significantly increased by feeding similar administration level. In a later study by the same authors, Makled et al. (2020) used Psychrobacter maritimus S, isolated from sediment as probiont to Nile tilapia. Growth rates, digestive enzymes (protease, lipase and amylase), phagocytic- and lysozyme activity, alternative complement hemolysis, hematological parameters significantly increased by 3.3 x 108 CFU mL-1 feeding, but slightly decreased at the highest inclusion level. Similarly, expression of interleukin-4 and 12 genes was significantly up-regulated by 3.3 x 108 CFU mL-1 feeding, while heat shock protein gene was down-regulated. Based on their results, the authors concluded that P. maritimus S at inclusion level of 3.3 x 108 CFU mL-1 is a promising probiont for Nile tilapia fingerlings.
Even though some information is available on Psychrobacter as probiotic in finfish, the genus deserves more attention as findings indicate that Psychrobacter might be capable of producing and secrete antimicrobial compounds (Wanka et al., 2018).
Rhodopseudomonas palustris
Rhodopseudomonas palustris is a rod-shaped photosynthetic bacterium, with an ability to switch between four different modes of metabolism. The bacterium has been isolated from swine waste lagoons, earthworm droppings, marine coastal sediments, sludge for use in a recirculating aquaculture system (Kim et al., 1999), pond water (Wang, 2011; Zhang et al., 2014), used in fluidizes bed biofilters (Zhan and Liu, 2013), and in some probiotic studies (Zhou et al., 2010; Wang, 2011; Zhang et al., 2014; Liu et al., 2020).
Zhou et al. (2010) revealed that administration during 40 days of a diet supplemented with R. palustris GO6 increased significantly the tilapia growth performance, respiratory burst-, superoxide dismutase-, catalase- and myeloperoxidase activities, while no effect was revealed regarding total serum protein, albumin, globulin, serum lysozyme content. In this study, administration of two bacilli species were included, and the author’s conclusion was Bacillus coagulans, followed by G06 were better water additives than B. subtilis to tilapia. In a 60-day study with grass carp, Wang (2011) reported that R. palustris administration enhanced growth performance and amylase activity in proximal intestine (PI) and distal intestine (DI), while protease- and cellulase activities in PI and DI were not affected. Although R. palustris supplementation revealed some positive effect, the best results were revealed by Bacillus coagulans. Zhang et al. (2014) demonstrated that R. palustris administration to grass carp culture significantly decreased ammonia-N, total inorganic-N and total-N in water, and modulated the water microbiota, by affecting the relative abundance of Proteobacteria, Bacteroides and Actinobacteria. In a study with yellow catfish (Pelteobagrus vachelli), Liu et al. (2020) revealed that R. palustris in effluent increased protease-, amylase-, and lipase activities, and alkaline phosphatase, acid phosphatase, superoxide dismutase and catalase by up-regulating gene expression. Furthermore, disease resistance towards A. hydrophila, and modulation of the gut microbiota was observed by significantly increased the relative abundance of bifidobacteria and lactobacilli.
Roseobacter
Roseobacter species have been identified as both oval and rod-like shaped motile cells, are marine species and have a major role in oceanic sulfur cycling (Buchan and Moran, 2005; Wagner-Döbler and Biebl, 2006). They are heterotrophs, anaerobic and possess N-acyl homoserine lactones (AHLs) based quorum sensing systems (Tang et al., 2010; Cude and Buchan, 2013). During the last years, information has become available showing the presence of Roseobacter in intestine of finfish (e.g., Hjelm et al., 2004; Fjellheim et al., 2007).
Roseobacter species as candidate probiotic bacteria of the fish could antagonize fish-pathogenic bacteria without harming the fish or their live feed. Makridis et al. (2005; 2008) revealed similar survival of gilthead sea bream larvae exposed to 6 x 105 Roseobacter mL-1 when larvae were reared in sterile seawater, while lower survival was noticed when larvae were held in filtered seawater. spp. sp.
Roseobacter species revealing antagonism against Vibrio species in combination with algae could be a possible probiotic organism in larval rearing.
Shewanella
The genus is included in family Shewanellaceae, is facultative anaerobic in the absence of oxygen, and members of the genus have capabilities to use of a variety of electron acceptors for respiration. Most of the bacteria in the genus are revealed in extreme aquatic habitats, at low temperature, and at high pressure. Shewanella are a normal component of the surface microbiota of several finfish species (e.g., Satomi et al., 2006; Fjellheim et al., 2007; Satomi et al., 2007; Navarrete et al., 2013; Egerton et al., 2018).
Genus Shewanella is one of the most frequently used Gram-negative probiotics in finfish aquaculture, as the bacterium inhibit in vitro growth of Photobacterium damselae subsp. piscicida, Vibrio harveyi, Vibrio alginolyticus and V. anguillarum (Chabrillón et al., 2005a; Chabrillón et al., 2005b; Chabrillón et al., 2006).
Within genus Shewanella, is Shewanella putrefaciens Pdp11 most frequently used. Guzmán-Villanueva et al. (2014) used Pdp11 as a probiotic supplementation to gilthead seabream (14.5 g) in a 4-week study, and revealed significant lower serum IgM levels, and serum peroxidase activity after 4 weeks, while growth performance (SGR and condition factor), serum antiprotease -, leucocyte peroxidase- serum antiprotease and leucocyte peroxidase activities were unaffected by probiotic feeding compared to control fed fish. In two studies using enriched of Artemia by S. putrefaciens Pdp11 to Senegalese sole (Solea senegalensis) larvae, Lobo et al. (2014a; 2014b), revealed improved growth and modulation of the gut microbiota. In a later study, Lobo et al. (2016) showed that Artemia metanauplii used as live vector for Pdp11 administration, improved growth of the sole and affected lipid profile.
In a recent study, Chen et al. (2020b) revealed that administration of Pdp11 facilitated wound closure, and increased the albumin/globulin ratio, protease and peroxidase activities in skin mucus, 7 days after post-wounding, but decreased serum aspartate aminotransferase. In addition, probiotic administration up-regulated gene expression of antioxidant enzymes and anti-inflammatory cytokines (il-10 and tgf- β) but decreases pro-inflammatory cytokines (il-1 β, il-6, il-8 and tnf-α). Based on their results, the authors concluded that Pdp11 had a positive effect on wound healing and skin damage. This conclusion was strengthened by Chen et al. (2020c) evaluating administration of Pdp11 on gene expression of the intestinal inflammatory response and barrier function of gilthead seabream.
An interesting approach regarding probiotics, using Shewanella sp. MR-7 isolated from turbot intestine that could utilize soybean meal (SBM) in turbot intestine was evaluated by Li et al. (2019b). SBM fermented by the bacterium and fed to turbot counteracted inflammatory response and modulated mucosal microbiota at both phylum and genus level, but no significant effect was noticed on trypsin, diastase (catalyze the breakdown of starch into maltose) and lipase activities. These interesting findings merit further investigations by including disease-, immunological- and gene expression studies.
S. putrefaciens Pdp11 is also used in combination with Bacillus sp. and palm fruits extracts in a study evaluated antioxidant enzyme gene expression in the mucus of gilthead seabream (Sparus aurata L.) (Esteban et al., 2014).
According to Seoane et al. (2019), S. putrefaciens Pdp11, presents features that can explain its probiotic benefits; specific proteins for adhesion and colonization of the GI tract, resistance to bile salt, and inhibition of pathogen adhesion in the gut.
A dietary supplementation of Shewanella xiamenensis A-1 and A-2 isolated from grass carp was administrated to grass carp for 28 days and thereafter challenged with A. hydrophila (Wu et al., 2015). A significant enhancement of respiratory burst, and phagocytic and lysozyme activities, and upregulation of immune related genes (IL-8, IL-1β, lysozyme-C and TNF-α), and resistance against A. hydrophila were revealed. In later study, Hao et al. (2017) supplemented grass carp diet with a dose of 108 CFU g−1 of S. xiamenensis for 28 days, and at the end of feeding modulation of the gut microbiota was noticed. The relative abundance of Meganema and Rubellimicrobium increased, Lactococcus, Pseudomonas and Citrobacter (cellulose degrading bacteria) decreased, while Flavobacterium was not detected compared to control fed fish.
Asaduzzaman et al. (2018) revealed that administration of Shewanella sp. to Malaysian Mahseer for 90 days, improved gut histology (villi height, villi with and villi area), gut production of SCFAs, and modulated the gut microbiota. In a more recent study, administration of Shewanella sp. MR-7 to turbot, ameliorate lipopolysaccharide induced intestinal dysfunction (villus and microvilli height), and modulated the gut microbiota by enhancing the relative abundance of Lactobacillus, and reducing the relative abundance of Pseudomonas (Zhang et al., 2020).
Aliivibrio
The taxonomy and phylogeny of genus Photobacterium is revised, for example, Photobacterium logei and Photobacterium fischeri are now considered members of genus Aliivibrio (Labella et al., 2017). Aliivibrio is reported in the intestinal tracts of vertebrates as well as finfish (Green et al., 2013; Karlsen et al., 2017; Rud et al., 2017; Hamilton et al., 2019), and some information is available on their use as probiotics in finfish aquaculture.
Vibrio viscosus reclassified as Moritella viscosa (Benediktsdottir et al., 2000) is a bacteria species associated with “winter ulcer”, affecting salmonids reared in seawater. In two recent studies, Klakegg et al. (2020a; 2020b) investigated the effect of Aliivibrio strains isolated from the mandibulum of farmed Atlantic salmon on growth performance and ulcer prevalence of Atlantic salmon, and ulcer prevalence of lumpfish (Cyclopterus lumpus), respectively. Both studies, revealed improved growth performance and ulcer prevalence after adding Aliivibrio into the tank water. Based on their results, the author suggested that Aliivibrio administration may have impact on welfare, economy and sustainability in aquaculture as fewer ulcer outbreak caused by M. viscosa was noticed.
Enterovibrio
In a study evaluating Enterovibrio coralii administration to European sea bass (Dicentrarchus labrax), Mladineo et al. (2016) revealed no significant stimulation of phagocytic activity, respiratory burst, and gene expression of lysozyme, Mx protein, caspase 3, TNF-a, and IL-10.
Vibrio
Genus Vibrio has a curved-rod (comma) shape, are reported in salt water, and are facultative anaerobe and oxidase positive bacteria. Even through several species of Vibrio are among the most common bacteria leading to massive mortality of cultured fish, and shellfish (Ina-Salwany et al., 2019), several studies have reported that Vibrio is dominant in the GI tract of fish (e.g., Eddy and Jones, 2002; Fonseca et al., 2019). Even though genus Vibrio are pathogenic, several studies have used apathogenic Vibrio as probiotic in finfish aquaculture (Austin et al., 1995; Gatesoupe, 1997; Ringø and Vadstein, 1998; DeSchrijver and Ollevier, 2000; Ottesen and Olafsen, 2000; Makridis et al., 2001; Aerts et al., 2018; Schaeck et al., 2016), as well as a part in a multi-strain probiotic supplementation with Microbacterium, Ruegeria and Pseudoalteromonas (Skjermo et al., 2015), and in combination with A. veronii and Flavobacterium sasangense (Chi et al., 2014).
In an early study, Ottesen and Olafsen (2000) evaluated the effect of water administration of Vibrio iliopiscarius and apathogenic Vibrio salmonicida on Atlantic halibut (Hippoglossus hippoglossus) larval survival. Pre-incubation of larvae with apathogenic V. salmonicida improved survival to 94.4%, whereas V. iliopiscarius administration reduced survival to 63% compared to 81% survival in the control group.
Makridis et al. (2001) reported the use of Vibrio strain PB 1-11 and strain PB 6-1 encapsulated in Artemia franciscana and revealed that total CFU in water was lower by encapsulation, while larval gut microbiota was not significantly affected by encapsulation. Schaeck et al. (2016) used a Vibrio lentus as probiotic supplement in a study with gnotobiotic European sea bass (Dicentrarchus labrax) larvae, and displayed improved resistance against V. harveyi, but administration of Vibrio proteolyticus did not revealed any effect on larval survival. In a following study, Schaeck et al. (2017) revealed that V. lentus administration to gnotobiotic European sea bass larvae significantly modified gene expression did not affect apoptotic and cell proliferative indexes. Aerts et al. (2018) evaluate the probiotic potential of V. lentus, as inoculum into well water containing gnotobiotic European sea bass larvae at day 8 post hatching, and revealed significantly decreased glucocorticoid baseline levels in larvae, and the authors suggested that their findings provided a better insight into the hypothalamic-pituitary-interrenal axis. Furthermore, Vibrio species, such as Vibrio natriegens which show high capacity to hydrolyze casein, could increase feed efficiency and improve the growth rate of fish (Rahman et al., 2016).
Gram-Positives
The beneficial effects of Gram-positive probiotic bacteria used in finfish aquaculture, and studies discussed in the review of De et al. (2014); Hayatgheib et al. (2020); Tran et al. (2020) and van Doan et al. (2021) are only briefly presented in Table 2.
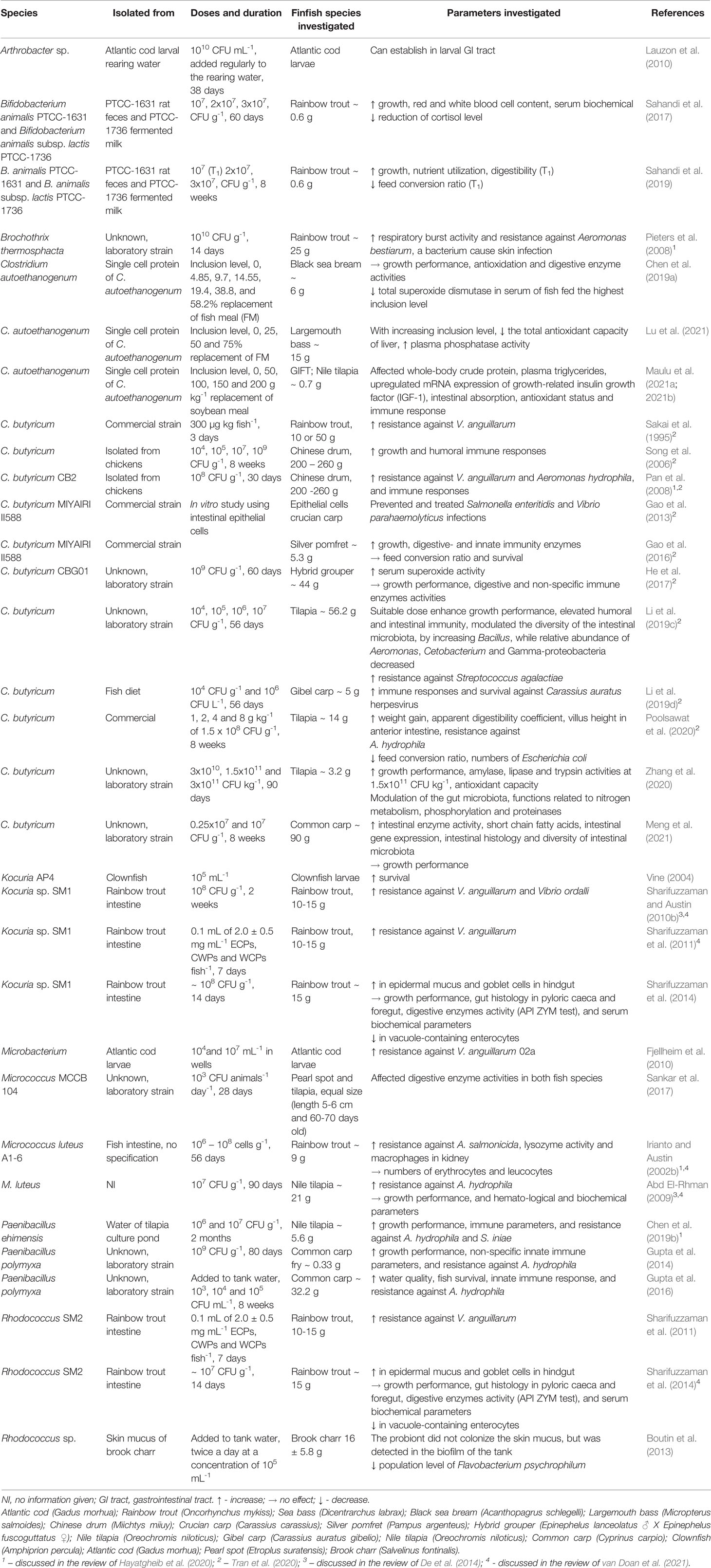
Table 2 Effect of Gram-positive bacteria on growth performance, gut health, immune system and disease resistance in finfish, and an in vitro study.
Arthrobacter
Genus Arthrobacter, has no spores and capsule, utilizes a wide and diverse range of organic substances, and has ability to produce antimicrobial compounds (O’Brien et al., 2004; Papaleo et al., 2012). They are reported in finfish intestine (e.g., Ringø et al., 2006a; Ringø et al., 2008; Nayak, 2010; Wang et al., 2019b), but less information is available on their use as probiotic to finfish (Lauzon et al., 2010). Some information is available on its use in a multi-strain probiotic mixture (Geng et al., 2012a; Peixoto et al., 2018).
An Arthrobacter sp. strain that showed inhibitory potential against fish pathogens in vitro was used in a bath treatment of Atlantic cod larvae (Lauzon et al., 2010), and regularly administration to the rearing water, revealed that the bacterium could establish in the larval intestine.
Bifidobacterium
Numerous health benefits have been claimed for genus Bifidobacterium, the “good bacteria”. Compared to Lactobacillus acidophilus, are bifidobacteria less acid tolerant as they do not grow below pH 5.0 (Shah, 1997), while Lb. acidophilus grow below 4.0. Bifidobacterium are reported in the GI tract of finfish (Vlkova et al., 2012; Piazzon et al., 2019; Wang et al., 2020b), but Bifidobacterium is less incorporated into diets or added to the rearing water in finfish aquaculture (Sahandi et al., 2017; Sahandi et al., 2019). In addition, Bifidobacterium bifidium was administrated to Siberian sturgeon (Acipenser baerii) in combination with Lactobacillus spp. and B. subtilis, and the results revealed improved growth performance, hematological and immune parameters (Hassani et al., 2020).
In two studies with rainbow trout, Sahandi et al. (2017; 2019) used two bifidobacteria, Bifidobacterium animalis PTCC-1631 and Bifidobacterium animalis subsp. lactis PTCC-1736, isolated from rat feces and fermented milk, respectively, and administration of 107 CFU g-1, revealed positive effect on growth, nutrient utilization, digestibility, feed conversion ratio, red and white blood cell content, serum biochemical and reduction of cortisol level of rainbow trout.
in vitro Brochothrix
Genus Brochothrix is non-spore-forming, non-motile catalase-positive, facultative anaerobic, rod-shaped bacteria that show characteristic changes in cell morphology during growth. Sneath and Jones (1976) proposed the genus for some meat spoilage bacteria, previously designated as Microbacterium thermosphactum. The scientific interests have mostly focused on Brochothrix thermosphacta as the bacterium is associated with off-odour development in meats, especially in prepacked products held at refrigeration temperatures. They are isolated from the GI tract of finfish (Ringø et al., 2006a; Ringø et al., 2008; Higuera-Llanten et al., 2018), but less information is available on their use as probiotics in aquaculture (Pieters et al., 2008).
Administration of B. thermosphacta BA211 at 1010 g-1 in rainbow trout diet for 2 weeks increased fish survival against challenge with Aeromonas bestiarum (Pieters et al., 2008).
Clostridium
Genus Clostridium is a butyric‐acid producer, and butyrate is the preferred energy source for the colon epithelial cells, contributes to the maintenance of the gut barrier functions, and has immunomodulatory and anti-inflammatory properties (Riviere et al., 2016; Guo et al., 2020). Clostridium are isolated from finfish intestine (e.g., Wu et al., 2012; Abdelhamed et al., 2019; Pérez-Pascual et al., 2020; Rimoldi et al., 2020), and some strains are frequently used as probiotics to enhance growth and immune response in finfish (for review see Tran et al., 2020; Table 2) as well as in shellfish aquaculture (Ringø, 2020; Tran et al., 2020).
Single cell protein of Clostridium autoethanogenum has been evaluated in four recent studies (Table 2). Chen et al. (2019a) evaluated the effect of partial replacement of fish meal with C. autoethanogenum single-cell protein (CAP) fed to juvenile black sea bream (Acanthopagrus schlegelli) and revealed that dietary treatments did not significantly affect malondialdehyde, catalase, total antioxidant capacity and digestive protease, lipase and amylase activities. On the other hand, total superoxide dismutase in serum of fish fed the highest inclusion CAP level, 58.2%, was significantly lower than that of control fed fish.
An overview on the use of C. butyricum as probiotics was presented by Tran et al. (2020) and to avoid overlaps, the results discussed in the abovementioned review are only summarized in Table 2. He et al. (2017) noted no effect on growth performance, digestive enzyme activities, serum- lysozyme, catalase and glutathione peroxidase activities, but only improved serum superoxide activity by feeding hybrid grouper (Epinephelus lanceolatus ♂ x Epinephelus fuscoguttatus ♀) C. butyricum, at inclusion level of 109 CFU kg-1, compared to fish fed only Lb. acidophilus or a combination with Lb. acidophilus, Bacillus cereus and C. butyricum. In a recent study, Meng et al. (2021) administrated a commercial C. butyricum at two inclusion levels; 0.25 x 107 (LVB) and 107 (HCB) CFU g−1, for 8 weeks to address the effect on intestinal enzyme activity, SCFAs, intestinal gene expression and diversity of intestinal microbiota of common carp (Cyprinus carpio). A substantial beneficial effect was noticed, probiotic administration by HCB significantly enhanced intestinal catalase and lysozyme, positively affected mucin secretion and the height of microvilli, and intestinal gene expression of IL-10, TLR-2, MyD-88, ZO-1 and Occludin. Butyric- and propionic acid content were elevated in both clostridia treatments. Furthermore, the intestinal content microbiota was affected, with improved abundance of Bacteroides and a significant decrease in Fusobacteria and Proteobacteria. Zhang et al. (2020) used a commercial C. butyricum strain, previously used in shellfish studies at three administration levels. The authors revealed that inclusion level of 1.5x1011 CFU kg-1, improved growth performance, digestive enzymes (amylase, lipase and trypsin), and antioxidant capacity in spleen, head kidney and liver of tilapia. Furthermore, modulation of the gut microbiota, functions related to phosphorylation, proteinases, and nitrogen metabolism were noticed.
Kocuria
Kocuria was first reported by Kocuria is coccus shaped, and have rigid cell walls and are either aerobic or facultative anaerobic bacteria (Venkataramana et al., 2016), and has been isolated from the GI tract of finfish (e.g., Bakke-McKellep et al., 2007; Linh et al., 2018; Sharifuzzaman et al., 2018).
According to Vine (2004) Kocuria AP4 administrated to clownfish larvae, improved survival. Rainbow trout orally fed Kocuria SM1 (108 cells g-1) originally isolated from GI tract of rainbow trout, has been administrated to rainbow trout in three studies (Sharifuzzaman and Austin, 2010a; Sharifuzzaman and Austin, 2010b; Sharifuzzaman et al., 2011). In a later study using Kocuria SM1, Sharifuzzaman et al. (2014) revealed increase in in epidermal mucus and goblet cells in hindgut, but growth performance, gut histology in pyloric caeca and foregut, serum biochemical parameters (hemoglobin, urea, creatinine and glucose), and digestive enzymes activity (API ZYM test) were not affected by probiotic feeding, while a notable decrease in vacuole-containing enterocytes was noticed. Furthermore, an interesting finding was that inflammation was not observed in fish fed Kocuria SM1. In most probiotic studies, the frequency of the probiont is evaluated after continuous feeding, but in the study of Sharifuzzaman et al. (2014) probiotic feeding by Kocuria SM1or Rhodococcus SM2 was stopped after 14 days and reverted back to the control diet for 14 days. The results of both probiotic bacteria revealed that percentage of the probiont in digesta reached maximum, at the end of day 14, but disappear upon switching to the control diet after 28 days. The authors concluded that this observation indicate no primary colonization in the GI tract, but to fully conclude the autochthonous microbiota should be analyzed.
Microbacterium
Genus Microbacterium belongs to the family Microbacteriaceae within suborder Micrococcineae. Microbacterium are non-spore-forming, rod-shaped bacteria, and was classified according to the amended genus description by Collins et al. (1983) and redefinition of Takeuchi and Hatano (1998). They are isolated from the GI tract of finfish (e.g., Ringø et al., 2006a; Ringø et al., 2006b; Hu et al., 2015), used as probiotics (Fjellheim et al. (2010), and in a multi-strain probiotic mixture with Pseudoalteromonas, Ruegeria and Vibrio fed to Atlantic cod larvae (Skjermo et al., 2015).
In a study to evaluate the selection of candidate probiotics, Fjellheim et al. (2010) revealed that Microbacterium sp. ID-3-10 improved survival of Atlantic cod larvae exposed to V. anguillarum 02a.
Micrococcus
Genus Micrococcus was divided into Micrococcus, Kocuria, Nesterenkonia, Kytococcus and Dermacoccus based on phylogenetic and chemotaxonomic studies by Stackebrandt et al. (1995). Information is available on their presence in finfish intestine (e.g., Ringø, 1993; Bakke-McKellep et al., 2007; Hu et al., 2015), and furthermore information is available on the potential of gut Micrococcus isolates as probiotics (e.g., Nurhidayu et al., 2012; Akayli and Urku, 2014; Akayli et al., 2016), and their use as probiotics in finfish aquaculture (Irianto and Austin, 2002b; Abd El-Rhman et al., 2009; Sankar et al., 2017).
Feeding Micrococcus luteus to rainbow trout and Nile tilapia, reduced mortality after challenged with A. salmonicida (Irianto and Austin, 2002b) and A. hydrophila Abd El-Rhman et al. (2009). In a later study, Sankar et al. (2017) evaluated the effect of Micrococcus administration on digestive enzymes (pepsin, α-amylase, protease and lipase) activities, and revealed differences in pearl spot (Etroplus suratensis) and tilapia after 60 days feeding.
Paenibacillus
Previously, Paenibacillus species were included in genus Bacillus due to their common morphological and physiological characteristics. However, based on 16S rRNA gene sequences in 1993, Paenibacillus was reassigned as a new genus. Genus Paenibacillus has been isolated from humans, animals, plants as well as fish (Midhun et al., 2017; Ma et al., 2018; Wang et al., 2019c), but their use as probiotics in finfish aquaculture is less investigated.
Common carp fry administrated with Paenibacillus polymyxa improved growth performance, non-specific immune (lysozyme, respiratory burst and myeloperoxidase activities), and resistance against A. hydrophila (Gupta et al., 2014). Later the same authors (Gupta et al., 2016) revealed that P. polymyxa supplemented to the water at three concentrations, improved water quality, common carp survival, innate immune response (lysozyme, respiratory burst, myeloperoxidase, catalase and superoxide dismutase activities), and resistance against A. hydrophila, at 103 and 104 CFU mL-1 supplementation. In a recent study, Chen et al. (2019b) demonstrated that administration of Paenibacillus ehimensis enhanced growth performance, immune parameters, and resistance against A. hydrophila and S. iniae.
Rhodococcus
Genus Rhodococcus is aerobic, nonsporulating, non-motile bacteria closely related to Mycobacterium and Corynebacterium. Few species are pathogenic and Rhodococcus have been revealed in a broad range of environments, including soil and water, as well as fish intestine (Tapia-Paniagua et al., 2014a; Song et al., 2016; Sharifuzzaman et al., 2018). Strains of Rhodococcus is experimentally advantageous due to its relatively fast growth rate and simple developmental cycle.
In a study by Sharifuzzaman et al. (2011), the authors used paraprobiotic (cellular components) of Rhodococcus SM2 isolated from the intestine of rainbow trout and displayed enhanced trout immune response and a significant resistance to V. anguillarum challenge. In a subsequent study, Sharifuzzaman et al. (2014) revealed that administration with Rhodococcus SM2, ~ 107 CFU g-1 for 14 days, epidermal mucus and goblet cells in hindgut increased, while no significant effect was noticed on growth performance, gut histology in pyloric caeca and foregut, digestive enzymes activity, and serum biochemical parameters. Inflammation was not observed in fish fed Rhodococcus SM2.
In a study with brook charr (Salvelinus fontinalis), Rhodococcus sp. originally isolated from skin mucus of brook charr and added to the tank water twice a day at a concentration of 105 mL−1, Boutin et al. (2013) observed that the bacteria did not colonize the skin mucus, but was detected in the biofilm of the tank. An interesting beneficial effect was noticed, the population of the pathogen F. psychrophilum decreased in water by modulating the water microbiota. Furthermore, the bacterial communities in water samples were more diverse than the skin mucus microbiota.
An interesting aspect of Rhodococcus was recently evaluated by Garai et al. (2021), as they investigated degradation of mycotoxin. This is highly relevant as mycotoxins are secondary metabolites of fungi, which are common in food, and from time to time also present in aquafeed (Pietsch, 2020).
Multi-Strain Probiotics
In aquaculture, multi-strain probiotics have been considered to be more effective than a single strain in aquaculture, and readers with special interest in this topic, are referred to the recent reviews of Melo-Bolivar et al. (2021).
Conclusions and Further Directions
Nearly 90% of the global aquaculture production is carried out in countries in Asia, and the development is so fast that infectious disease outbreak happens regularly, and to solve this problem antibiotics are used with few regulations. However, the abuse of antibiotic treatment in aquaculture with tetracycline, β-lactams, sulfonamides, quinolones etc. results in development of antibiotic resistance in the pathogens, accumulation of residual in finfish products, depression of immune system, and translation of resistant genes to terrestrial animals and humans. Therefore action for alternative treatment methods in aquaculture are needed. In addition to the concept of probiotics, paraprobiotic is relatively established in higher vertebrate models and related food production sectors, but its application in aquaculture is still in its early stage (Choudhury and Kamilya, 2019; Teame et al., 2020), and merits investigations. Another alternative method is postbiotic that may be useful in aquaculture (Ang et al., 2020; Cuevas-González et al., 2020; Teame et al., 2020), a topic that merits further studies.
Moreover, peptides and exopolysaccharides revealed antimicrobial properties against bacterial pathogens, and SCFAs display both antimicrobial activities against bacterial pathogens and immune stimulating effects to aquatic organism, and cell surface proteins and teichoic acid can act as vaccine. Furthermore, it is well known that dietary manipulation affect the gut microbiota and improve fish health (e.g., Ringø et al., 2016; Turchini et al., 2022).
Dose as defined as the concentration (number of probiotic cells) must be carefully determined, as overdosing may result in lower efficacy with increasing costs, and under dosing could reduce the efficacy of the probiont. Previously, administration doses between 104 and 106 cells mL-1 to the total culture volume was suggested to be sufficient in introducing a probiotic capable of dominating the intestinal microbiota (Vine et al., 2006), but nowadays doses between 107 and 109 cells mL-1 are used. However, in order to maintain the desired probiotic concentration in the culture water, additional doses may be required, and its frequency may depend on the probiotic species, stage of fish development, diet, culture conditions (Verschuere et al., 2000).
Even though focus has been directed towards LAB and bacilli within probiotics in aquaculture (e.g., Ringø et al., 2018; Ringø et al., 2020a; James et al., 2021; Nayak, 2021), we strongly recommend the scientific community to focus on other interesting probionts. They play important roles in mediating and stimulating GI development, aiding digestive function, maintaining mucosal tolerance, enhance the immune response, and provide protection against diseases, development of metabolic syndrome, vitamin synthesis, modulation of the gut microbiota, and interactions on the gut-brain axis and gut-kidney axis. In addition, multistrain probiotic administration increased the gut microbiota diversity (Halkjær et al., 2020) illustrating that the gut microbiota merits further investigation in finfish aquaculture.
Probiotic applications may be extended in aquaculture with the use of exo-enzymes producing strains in bioprocessing of the complex feed ingredients and diverse microbial bio-active compounds and/or metabolites (e.g., antimicrobial compounds, quorum quenching enzymes, SCFA as functional feed additives. Along with probiotic potential, studies should be directed to develop the probiotic-products as synbiotics or postbiotics and their efficacy in culture condition are required to be evaluated.
The beneficial effects of LAB and Bacillus and their bacteriocins as alternatives to antibiotic growth promoters in animal production is well known (e.g., Caulier et al., 2019; Vieco-Saiz et al., 2019). However, as no information is available on bacteriocins from other promising probiotics in aquaculture, we highly recommend that this topic receive more attention.
Bacteria communicate with one another using chemical signal molecules, a process, and termed quorum sensing (QS), the enzymatic degradation of AHLs, has been suggested as a promising strategy to control bacterial diseases (e.g., Defoirdt, 2018; Ghanei-Motlagh et al., 2020). For example, Ghanei-Motlagh et al. (2020) revealed that Shewanella isolated from Asian sea bass showed high ability to degrade synthetic- and natural AHLs produced by V. harveyi and V. alginolyticus.
Within the probiotic bacteria discussed in the present study, a topic that merits investigation is the interactions between probiotics and antioxidant properties, a topic reviewed by Wang et al. (2017).
Even though probiotic inclusion in the diet is the most frequently used administration method, several studies have administrated probiotics in the water (Jahangiri and Esteban, 2018; the present study). However, to fully conclude that water administration is a suitable method, further studies need to be conducted in intensive production.
In the conclusion, application of beneficial microbes is a sustainable approach.
Author Contributions
ER: Introduction, Acinetobacter, Alcaligenes, Aliivibrio, Arthrobacter, Bifidobacterium, Brochothrix, Chromobacterium, Clostridium, Enterovibrio, Kocuria, Microbacterium, Micrococcus, Paenibacillus, Phaeobacter, Pseudoalteromonas, Rhodococcus, Rhodopseudomonas, Shewanella and editorial. XL: Pseudomonas, Roseobacter, Vibrio and Rhodosporidium. HD: Aeromonas, and Alteromonas. KG: proofreading, editing and provided critical feedback in revision. All authors contributed to the article and approved the submitted version.
Funding
The publication charges for this article have been funded by a grant from the publication fund of UiT The Arctic University of Norway.
Conflict of Interest
The authors declare that the research was conducted in the absence of any commercial or financial relationships that could be construed as a potential conflict of interest.
Publisher’s Note
All claims expressed in this article are solely those of the authors and do not necessarily represent those of their affiliated organizations, or those of the publisher, the editors and the reviewers. Any product that may be evaluated in this article, or claim that may be made by its manufacturer, is not guaranteed or endorsed by the publisher.
References
Abbass A., Sharifuzzaman S. M., Austin B. (2010). Cellular Components of Probiotics Control Yersinia Ruckeri Infection in Rainbow Trout, Oncorhynchus Mykiss (Walbaum). J. Fish Dis. 33, 31–37. doi: 10.1111/j.1365-2761.2009.0186.x
Abdelhamed H., Ozdemir O., Waldbieser G., Perkins A. D., Lawrence M. L., Karsi A. (2019). Effects of Florfenicol Feeding on Diversity and Composition of the Intestinal Microbiota of Channel Catfish (Ictalurus Punctatus. Aquacult. Res. 50, 3663–3672. doi: 10.1111/are.14325
Abd El-Rhman A. M., Khattab Y. A. E., Shalaby A. M. E. (2009). Micrococcus Luteus and Pseudomonas Species as Probiotics for Promoting the Growth Performance and Health of Nile Tilapia, Oreochromis Niloticus. Fish Shellfish Immunol. 27, 175–180. doi: 10.1016/j.fsi.2009.03.020
Aerts J., Schaeck M., De Swaef E., Ampe B., Decostere A. (2018). Vibrio Lentus as a Probiotic Candidate Lowers Glucocorticoid Levels in Gnotobiotic Sea Bass Larvae. Aquaculture 492, 40–45. doi: 10.1016/j.aquaculture.2018.03.059
Akayli T., Albayrak G., Urku C., Canak O., Yoruk E. (2016). Characterization of Micrococcus Luteus and Bacillus Marisflavi Recovered From Common Dentex (Dentex Dentex) Larviculture System. Med. Mar. Sci. 17 (1), 163–169. doi: 10.12681/mms.1322
Akayli T., Urku C. (2014). A Study on Probiotic Bacteria Isolated From Common Dentex (Dentex Dentex) Larvae and Their Antagonistic Effect on Photobacterium Damselae Subsp Damselae. Israeli J. Aquacult. Bamidgeh 66, 100. doi: 10.46989/001c.20783
Akimoto M., Ishii T., Yamagaki K., Ohtaguchi K., Koide K., Yazawa K. (1990). Production of Eicosapentaenoic Acid by a Bacterium Isolated From Mackerel Intestines. J. Am. Oil Chem. Soc 67 (12), 911–915. doi: 10.1007/BF02541846
Ang C. Y., Sano M., Dan S., Leelakriangsak M., Lal T. (2020). Postbiotics Applications as Infectious Disease Control Agent in Aquaculture. Biocontrol Sci. 25 (1), 1–7. doi: 10.4265/bio.25.1
Asaduzzaman M., Ie.hata S., Akter S., Kader M. A., Ghosh S. K., Khan M. N. A., et al. (2018). Effects of Host Gut-Derived Probiotic Bacteria on Gut Morphology, Microbiota Composition and Volatile Short Chain Fatty Acids Production of Malaysian Mahseer Tor Tambroides. Aquacult. Rep. 9, 53–61. doi: 10.1016/j.aqrep.2017.12.003
Austin B., Stuckey L., Robertson P., Effendi I., Griffith D. (1995). A Probiotic Strain of Vibrio Alginolyticus Effective in Reducing Diseases Caused by Aeromonas Salmonicida, Vibrio Anguillarum and Vibrio Ordalii. J. Fish Dis. 18, 93–96. doi: 10.1111/j.1365-2761.1995.tb01271.x
Bakke-McKellep A. M., Penn M. H., Salas P. M., Refstie S., Sperstad S., Landsverk T., et al. (2007). Effects of Dietary Soybean Meal, Inulin and Oxytetracycline on Gastrointestinal Histological Characteristics, Distal Intestine Cell Proliferation and Intestinal Microbiota in Atlantic Salmon (Salmo Salar L.). Brit. J. Nutr. 97, 699–713. doi: 10.1017/S0007114507381397
Benediktsdottir E., Verdonck L., Sproer C., Helgason S., Swings J. (2000). Characterization of Vibrio Viscosus and Vibrio Wodanis Isolated at Different Geographical Locations: A Proposal for Reclassification of Vibrio Viscosus as Moritella Viscosa Comb. Nov. Inter. J. Sys. Evol. Microbiol. 50, 479–488. doi: 10.1099/00207713-50-2-479
Bermudez-Brito M., Plaza-Diaz J., Munoz-Quezada S., Gomez-Llorente C., Gil A. (2012). Probiotic Mechanisms of Action. Ann. Nutr. Metab. 61, 160–174. doi: 10.1159/000342079
Boutin S., Audet C., Derome N. (2013). Probiotic Treatment by Indigenous Bacteria Decreases Mortality Without Disturbing the Natural Microbiota of Salvelinus Fontinalis. Can. J. Microbiol. 59 (10), 662–670. doi: 10.1139/cjm-2013-0443
Brunt J., Austin B. (2005). Use of a Probiotic to Control Lactococcosis and Streptococcosis in Rainbow Trout, Oncorhynchus Mykiss (Walbaum). J. Fish Dis. 28 (12), 693–701. doi: 10.1111/j.1365-2761.2005.00672.x
Brunt J., Hansen R., Jamieson D. J., Austin B. (2008). Proteomic Analysis of Rainbow Trout (Oncorhynchus Mykiss, Walbaum) Serum After Administration of Probiotics in Diets. Vet. Immunol. Immunophathol. 121 (3-4), 199–205. doi: 10.1016/j.vetimm.2007.09.010
Brunt J., Newaj-Fyzul A., Austin B. (2007). The Development of Probiotics for the Control of Multiple Bacterial Diseases of Rainbow Trout, Oncorhynchus Mykiss (Walbaum). J. Fish Dis. 30 (10), 573–579. doi: 10.1111/j.1365-2761.2007.00836.x
Buchan A., Moran M. A. (2005). Overview of the Marine Roseobacter Lineage. Appl. Environ. Microbiol. 71, 5665–5677. doi: 10.1128/AEM.71.10.5665-5677.2005
Bunnoy A., Na-Nakorn U., Kayansamruaj P., Srisapoome P. (2019b). Acinetobacter Strain KU011TH, a Unique Organism Related to Acinetobacter Pittii and Isolated From the Skin Mucus of Healthy Bighead Catfish and its Efficacy Against Several Fish Pathogens. Microorganisms 7, 549. doi: 10.3390/microorganisms7110549
Bunnoy A., Na-Nakorn U., Srisapoome P. (2019a). Probiotic Effects of a Novel Strain, Acinetobacter KU011TH, on the Growth Performance, Immune Responses, and Resistance Against Aeromonas Hydrophila of Bighead Catfish (Clarias Macrocephalus Günther 1864). Microorganisms 7, 613. doi: 10.3390/microorganisms7120613
Burbank D. R., Shah D. H., LaPatra S. E., Fornshell G., Cain K. D. (2011). Enhanced Resistance to Coldwater Disease Following Feeding of Probiotic Bacterial Strains to Rainbow Trout (Oncorhynchus Mykiss). Aquaculture 321, 185–190. doi: 10.1016/j.aquaculture.2011.09.004
Cabello F. C. (2006). Heavy Use of Prophylactic Antibiotics in Aquaculture: A Growing Problem for Human and Animal Health and for the Environment. Environ. Microbiol. 8, 1137–1144. doi: 10.1111/j.1462-2920.2006.01054.x
Cabello F. C., Godfrey H. P., Ivanova L., Shah S. Q. A., Sørum H., Tomova A. (2020). Freshwater Salmon Aquaculture in Chile and Transferable Antimicrobial Resistance. Environ. Microbiol. 22 (2), 559–563. doi: 10.1111/j.1462-2920.14891
Cámara-Ruiz M., Balebona M. C., Morinigo M.Á., Esteban M.Á. (2020). Probiotic Shewanella Putrefaciens (SpPdp11) as Fish Health Modulator: A Review. Microorganisms 8, 1990. doi: 10.3390/microorganisms8121990
Capkin E., Altinok I. (2009). Effects of Dietary Probiotic Supplementations on Prevention/Treatment of Yersiniosis Disease. J. Appl. Microbiol. 106, 1147–1153. doi: 10.1111/j.1365-2672.2008.04080.x
Caulier S., Nannan C., Gillis A., Licciardi F., Bragard C., Mahillon J. (2019). Overview of the Antimicrobial Compounds Produced by Members of the Bacillus Subtilis Group. Front. Microbiol. 10. doi: 10.3389/fmicb.2019.00302
Cerezuela R., Guardiola F. A., Cuesta A., Esteban M. A. (2016). Enrichment of Gilthead Seabream (Sparus Aurata L.) Diet With Palm Fruit Extracts and Probiotics: Effects on Skin Mucosal Immunity. Fish Shellfish Immunol. 49, 100–109. doi: 10.1016/j.fsi.2015.12.028
Chabrillón M., Arijo S., Díaz-Rosales P., Balebona M. C., Morinigo M. A. (2006). Interference of Listonella Anguillarum With Potential Probiotic Microorganisms Isolated From Farmed Gilthead Seabream (Sparus Aurata, L.). Aquacult. Res. 37, 78–86. doi: 10.1111/j.1365-2109.2005.01400.x
Chabrillón M., Rico R. M., Arijo S., Diaz-Rosales P., Balebona M. C., Morinigo M. A. (2005b). Interactions of Microorganisms Isolated From Gilthead Seabream, Sparus Aurata L., on Vibrio Harveyi, a Pathogen of Farmed Senegalese Sole, Solea Senegalensis (Kaup). J. Fish Dis. 28, 531–537. doi: 10.1111/j.1365-2761.2005.00657.x
Chabrillón M., Rico S., Balebona M. C., Morinigo M. A. (2005a). Adhesion to Sole, Solea Senegalensis Kaup, Mucus of Microorganisms Isolated From Farmed Fish, and Their Interaction With Photobacterium Damselae Subsp. Piscicida. J. Fish Dis. 28, 229–237. doi: 10.1111/j.1365-2761.2005.00623.x
Chauhan A., Singh R. (2019). Probiotics in Aquaculture: A Promising Emerging Alternative Approach. Symbiosis 77, 99–113. doi: 10.1007/s13299-018-0580-1
Chen Z., Ceballos-Francisco D., Guardiola F. A., Esteban M.Á. (2020b). Dietary Administration of the Probiotic Shewanella Putrefaciens to Axperimentally Wounded Gilthead Seabream (Sparus Aurata L.) Facilitates the Skin Wound Healing. Sci. Rep. 10, 11029. doi: 10.1038/s41598-020-68024-z
Chen Z., Ceballos-Francisco D., Guardiola F. A., Esteban M.Á. (2020c). Influence of Skin Wounds on the Intestinal Inflammatory Response and Barrier Function. Protective Role of Dietary Shewanella Putrefaciens SpPdp11 Administration to Gilthead Seabream (Sparus Aurata L.). Fish Shellfish Immunol. 99, 414–423. doi: 10.1016/j.fsi.2020.02.022
Chen S., Liu C., Hu S. (2019b). Dietary Administration of Probiotic Paenibacillus Ehimensis NPUST1 With Bacteriocin-Like Activity Improves Growth Performance and Immunity Against Aeromonas Hydrophila and Streptococcus Iniae in Nile Tilapia (Oreochromis Niloticus). Fish Shellfish Immunol. 84, 695–703. doi: 10.1016/j.fsi.2028.10.059
Chen Y., Sagada G., Xu B., Chao W., Zou F., Ng W.-K., et al. (2019a). Partial Replacement of Fishmeal With Clostridium Autoethanogenum Single-Cell Protein in the Diet for Juvenile Black Sea Bream (Acanthopagrus Schlegelli). Aquacult. Res 51 (3), 1000–1011. doi: 10.1111/are.14446
Chen J., Sun R., Pan C., Sun Y., Mai B., Li Q. X. (2020a). Antibiotics and Food Safety in Aquaculture. J. Agricult. Food Chem. 68, 11908–11919. doi: 10.1021/acs.jafc.0c03996
Chen Y., Zhu X., Yang Y., Han D., Jin J., Xie S. (2014). Effect of Dietary Chitosan on Growth Performance, Haematology, Immune Response, Intestinal Morphology, Intestine Microbiota and Disease Resistance in Gibel Carp (Carassius Auratus Gibelio). Aquacult. Nutr. 20, 532–546. doi: 10.1111/anu.12106
Cheng G., Hao H., Xie S., Wang X., Dai M., Huang L., et al. (2014). Antibiotic Alternatives: The Substitution of Antibiotics in Animal Husbandry? Front. Microbiol. 5. doi: 10.3389/fmicb.2014.00217
Chi C., Jiang B., Yu X.-B., Liu T.-Q., Xia L., Wang G.-X. (2014). Effects of Three Strains of Intestinal Autochthonous Bacteria and Their Extracellular Products on the Immune Response and Disease Resistance of Common Carp, Cyprinus Carpio. Fish Shellfish Immunol. 36 (1), 9–18. doi: 10.1016/j.fsi.2013.10.003
Choudhury T. G., Kamilya D. (2019). Paraprobiotics: An Aquaculture Perspective. Rev. Aquacult. 11, 1258–1270. doi: 10.1111/raq.12290
Collins M. D., Jones D., Kroppenstedt R. M. (1983). Reclassification of Brevibacterium-Imperiale (Steinhaus) and Corynebacterium-Laevaniformans (Dias and Bhat) in a Redefined Genus Microbacterium (Orla-Jensen), As Microbacterium-Imperiale Comb Nov and Microbacterium-Laevaniformans Nom Rev - Comb Nov. Syst. Appl. Microbiol. 4 (1), 65–78.
Cordero H., Guardiola F. A., Tapia-Paniagua S. T., Cuesta A., Meseguer J., Balebona M. C., et al. (2015). Modulation of Immunity and Gut Microbiota After Dietary Administration of Alginate Encapsulated Shewanella Putrefaciens Pdp11 to Gilthead Seabream (Sparus Aurata L.). Fish Shellfish Immunol. 45 (2), 608–618. doi: 10.1016/j.fsi.2015.05.010
Cordero H., Mauro M., Cuesta A., Cammarata M., Esteban M. A. (2016b). In Vitro Cytokine Profile Revealed Differences From Dorsal and Ventral Skin Susceptibility to Pathogen-Probiotic Interaction in Gilthead Seabream. Fish Shellfish Immunol. 56, 188–191. doi: 10.1016/j.fsi.2016.07.018
Cordero H., Morcillo P., Cuesta A., Brinchmann M. F., Esteban M. A. (2016c). Differential Proteome Profile of Skin Mucus of Gilthead Seabream (Sparus Aurata) After Probiotic Intake and/or Overcrowding Stress. J. Proteo. 132, 41–50. doi: 10.1016/j.jprot.2015.11.017
Cordero H., Morcillo P., Meseguer J., Cuesta A., Esteban M. A. (2016a). Effects of Shewanella Putrefaciens on Innate Immunity and Cytokine Expression Profile Upon High Stocking Density of Gilthead Seabream Specimens. Fish Shellfish Immunol. 51, 33–40. doi: 10.1016/j.fsi.2016.02.008
Cude W. N., Buchan A. (2013). Acyl-Honnoserine Lactone-Based Quorum Sensing in the Roseobacter Clade: Complex Cell-to-Cell Communication Controls Multiple Physiologies. Front. Microbiol. 4. doi: 10.3389/fmicb.2013.00336
Cuevas-González P. F., Licega A. M., Aguilar-Toalá J. E. (2020). Postbiotics and Parabiotics: From Concepts to Application. Food Res. Inter. 136, 109502. doi: 10.1016/j.foodre.2020.109502
D’Alvise P. W., Lillebø S., Prol-Garcia M. J., Wergeland H. I., Nielsen K. F., Bergh Ø., et al. (2012). Phaeobacter Gallaeciensis Reduces Vibrio Anguillarum in Cultures of Microalgae and Rotifers, and Prevents Vibriosis in Cod Larvae. PlosOne 7 (8), e43996. doi: 10.1371/journalpone.0043996
D’Alvise P. W., Lillebø S., Wergeland H. I., Gram L., Bergh Ø. (2013). Protection of Codlarvae From Vibriosis by Phaeobacter Spp.: A Comparison of Strains and Introduction Times. Aquaculture 384-387, 82–85. doi: 10.1016/j.aquaculture.2012.12.013
Dawood M. A. O., Koshio S. (2016). Recent Advances in the Role of Probiotics and Prebiotics in Carp Aquaculture: A Review. Aquaculture 454, 243–251. doi: 10.1016/j.aquaculture.2015.12.033
Defoirdt T. (2018). Quorum-Sensing Systems as Targets for Antivirulence Therapy. Trend. Microbiol. 26, 4. doi: 10.1016/j.tim.2017.10.005
De B. C., Meena D. K., Behera B. K., Das P., Das Mohapatra P. K., Sharma A. P. (2014). Probiotics in Fish and Shellfish Culture: Immunomodulatory and Ecophysiological Responses. Fish Physiol. Biochem. 40, 921–971. doi: 10.1007/s10695-013-9897-0
de Melo Pereira G. V., de Oliveira Coelho B., Magalhães Jùnior A. I., Thomaz-Soccol V., Soccol C. R. (2018). How to Select a Probiotic? A Review and Update of Methods and Criteria. Biotechnol. Adv. 36, 2060–2076. doi: 10.1016/j.biotechadv.2018.09.003
DeSchrijver R., Ollevier F. (2000). Protein Digestion in Juvenile Turbot (Scophthalmus Maximus) and Effects of Dietary Administration of Vibrio Proteolyticus. Aquaculture 186, 107–116. doi: 10.1016/S0044-8486(99)00372-5
Díaz-Rosales P., Arijo S., Chabrillón M., Alarcon F. J., Tapia-Paniagua S. T., Martinez-Manzanares E., et al. (2009). Effects of Two Closely Related Probiotics on Respiratory Burst Activity of Senegalese Sole (Solea Senegalensis, Kaup) Phagocytes, and Protection Against Photobacterium Damselae Subsp. Piscicida. Aquaculture 293, 16–21. doi: 10.1016/j.aquaculture.2009.03.050
Diaz-Rosales P., Salinas I., Rodríguez A., Cuesta A., Chabrillón M., Balebona M. C., et al. (2006). Gilthead Seabream (Sparus Aurata L.) Innate Immune Response After Dietary Administration of Heat-Inactivated Potential Probiotics. Fish Shellfish Immunol. 20, 482–492. doi: 10.1016/j.fsi.2005.06.007
Dimitroglou A., Merrifield D. L., Carnevali O., Picchietti S., Avella M., Daniels C., et al. (2011). Microbial Manipulations to Improve Fish Health and Production - A Mediterranean Perspective. Fish Shellfish Immunol. 30 (1), 1–16. doi: 10.1016/j.fsi.2010.08.009
Dittmann K. K., Rasmussen B. B., Melchiorsen J., Sonnenschein E. C., Gram L., Bentzon-Tilia M. (2020). Changes in the Microbiome of Mariculture Feed Organisms After Treatment With a Potentially Probiotic Strain of Phaeobacter Inhibens. Appl. Environ. Microbiol. 86 (14), e00499–e00420. doi: 10.1128/AEM.00499-20
Eddy S. D., Jones S. H. (2002). Microbiology of Summer Flounder Paralichthys Dentatus Fingerling Production at a Marine Fish Hatchery. Aquaculture 211 (1-4), 9–28. doi: 10.1016/j.fsi.2005.06.007
Egerton S., Culloty S., Whooley J., Stanton C., Ross R. P. (2018). The Gut Microbiota of Marine Fish. Front. Microbiol. 9. doi: 10.3389/fmicb.2018.00873
Esteban M. A., Cordero H., Martinez-Tome M., Jimenez-Monreal A. M., Bakhrouf A., Mahdhi A. (2014). Effect of Dietary Supplementation of Probiotics and Palm Fruits Extracts on the Antioxidant Enzyme Gene Expression in the Mucosae of Gilthead Seabream (Sparus Aurata L.). Fish Shellfish Immunol. 39 (2), 532–540. doi: 10.1016/j.fsi.2014.06.012
Feckaninova A., Koscova J., Mudronova D., Popelka P., Toropilova J. (2017). The Use of Probiotic Bacteria Against Aeromonas Infections in Salmonid Aquaculture. Aquaculture 469, 1–8. doi: 10.1016/j.aquaculture.2016.11.042
Fjellheim A. J., Klinkenberg G., Skjermo J., Aasen I. M., Vadstein O. (2010). Selection of Candidate Probionts by Two Different Strategies From Atlantic Cod (Gadus Morhua L.) Larvae. Vet. Microbiol. 144, 153–159. doi: 10.1016/j.vetmic.2009.12.032
Fjellheim A. J., Playfoot K. J., Skjermo J., Vadstein V. (2007). Vibrionaceae Dominates the Microflora Antagonistic Towards Listonella Anguillarum in the Intestine of Cultured Atlantic Cod (Gadus Morhua L.) Larvae. Aquaculture 269 (1-4), 98–106. doi: 10.1016/j.aquaculture.2007.04.021
Fonseca F., Cerqueira R., Fuentes J. (2019). Impact of Ocean Acidification on the Intestinal Microbiota of the Marine Sea Bream (Sparus Aurata L.). Front. Physiol. 10. doi: 10.3389/fphys.2019.01446
Fu L., Zhang X., Wang Y., Peng L., Li W. (2017). Nitrogen Removal Characteristics of Pseudomonas Stutzeri F11 and its Application in Grass Carp Culture. Fish. Sci. 83, 89–98. doi: 10.1007/s12562-016-1038-0
Gao Q., Xiao C., Min M., Zhang C., Peng S., Shi Z. (2016). Effects of Probiotics Dietary Supplementation on Growth Performance, Innate Immunity and Digestive Enzymes of Silver Pomfret, Pampus Argenteus. Indian J. Anim. Res. 50 (6), 936–941. doi: 10.18805/ijar.9640
Gao Q., Xiao Y., Sun P., Peng S., Yin F., Ma X., et al. (2013). In Vitro Protective Efficacy of Clostridium Butyricum Against Fish Pathogen Infections. Indian J. Microbiol. 53 (4), 453–459. doi: 10.1007/s12088-013-0394-z
Garai E., Risa A., Varga E., Cserháti M., Kriszt B., Urbányi B., et al. (2021). Evaluation of the Multimycotoxin-Degrading Efficiency of Rhodococcus Erythropolis NI1 Strain With the Three-Step Zebrafish Microinjection Method. Intern. J. Mol. Sci. 22, 724. doi: 10.3390/ijms22020724
Garcia de la Banda I., Lobo C., León-Rubio J. M., Tapia-Paniagua S., Balebona M. C., Morinigo M. A., et al. (2010). Influence of Two Closely Related Probiotics on Juvenile Senegalese Sole (Solea Senegalensis, Kaup 1858) Performance and Protection Against Photobacterium Damselae Subsp. Piscicida. Aquaculture 306, 281–288. doi: 10.1016/j.aquaculture.2010.05.008
Garcia de la Banda I., Lobo C., Mariana C., Leon-Rubio J. M., Salvador A., GemaPazos L. M. L., et al. (2012). Influence of Dietary Administration of a Probiotic Strain Shewanella Putrefaciens on Senegalese Sole (Solea Senegalensis, Kaup 1858) Growth, Body Composition and Resistance to Photobacterium Damselae Subsp. Piscicida. Aquacult. Res. 43 (5), 662–669. doi: 10.1111/j.1365-2109.2011.02871.x
Gatesoupe F. J. (1997). Siderophore Production and Probiotic Effect of Vibrio Sp. Associated With Turbot Larvae, Scophthalmus Maximus. Aqua. Living Res. 10, 239–246. doi: 10.1051/alr:1997026
Gatesoupe F. J. (1999). The Use of Probiotics in Aquaculture. Aquaculture 180, 147–165. doi: 10.1016/S0044-8486(99)00187-8
Gauthier G., Gauthier M., Christen R. (1995). Phylogenetic Analysis of the Genera Alteromonas, Shewanella, and Moritella Using Genes Coding for Small-Subunit rRNA Sequences and Division of the Genus Alteromonas Into Two Genera, Alteromonas (Emended) and Pseudoalteromonas Gen. Nov., and Proposal of Twelve New Species Combinations. Inter. J. Sys. Bacteriol. 45 (4), 755–761. doi: 10.1099/00207713-45-4-755
Geng X., Dong X. H., Tan B. P., Yang Q. H., Chi S. Y. (2012a). Effects of Dietary Chitosan and Bacillus Subtilis on the Growth Performance, non-Specific Immunity and Disease Resistance of Cobia, (Rachycentron Canadum). Fish Shellfish Immunol. 31, 400–406. doi: 10.1016/j.fsi.2011.06.006
Ghanei-Motlagh R., Mohammadian T., Gharibi D., Menanteau-Ledouble S., Mahmoudi E., Khosravi M., et al. (2020). Quorum Quenchingpproperties and Probiotic Potentials of Intestinal Associated Bacteria in Asian Sea Bass Lates Calcarifer. Mar. Drugs 18, 23. doi: 10.3390/md18010023
Girijakumari N. R., Ethiraja K., Marimuthu P. ,. N. (2018). In Vitro and In Vivo Evaluation of Probiotic Properties of Enterobacter Cloacae in Kenyi Cichlid, Maylandia Lombardoi. Aquacult. Inter. 26, 959–980. doi: 10.1007/s10499-018-0262-2
Giri S. S., Jun J. W., Yun S., Kim H. J., Kim S. G., Kim S. W., et al. (2020). Effects of Dietary Heat-Killed Pseudomonas Aeruginosa Strain VSG2 on Immune Functions, Antioxidant Efficacy, and Disease Resistance in Cyprinus Carpio. Aquaculture 514, 734489. doi: 10.1016/j.aquaculture.2019.734489
Giri S. S., Sen S. S., Chi C., Kim H. J., Yun S., Park S. C. (2015). Effects of Cellular Products of Potential Bacteria on the Immune Response of Labeo Rohita and Susceptibility to Aeromonas Hydrophila Infection. Fish Shellfish Immunol. 46, 716–722. doi: 10.1016/j.fsi.2015.08.012
Giri S. S., Sen S. S., Jun J. W., Park S. C., Sukumara V. (2016). Heat-Killed Whole-Cell Products of the Probiotic Pseudomonas Aeruginosa VSG2 Strain Affect In Vitro Cytokine Expression in Head Kidney Macrophages of Labeo Rohita. Fish Shellfish Immunol. 50, 310–316. doi: 10.1016/j.fsi.2016.02.007
Giri S. S., Sen S. S., Sukumaran V. (2012). Effects of Dietary Supplementation of Potential Probiotic Pseudomonas Aeruginosa VSG-2 on the Innate Immunity and Disease Resistance of Tropical Freshwater Fish, Labeo Rohita. Fish Shellfish Immunol. 32, 1135–1140. doi: 10.1016/j.fsi.2012.03.019
Giri S. S., Sukumaran V., Sen S. S., Vinumonia J., Banu B. N., Jena P. K. (2011). Antagonistic Activity of Cellular Components of Potential Probiotic Bacteria, Isolated From the Gut of Labeo Rohita, Against Aeromonas Hydrophila. Prob. Antimicro. Prot. 3 (3-4), 214–222. doi: 10.1007/s12602-011-9078-3
González-Palacios C., Fregeneda-Grandes J.-M., Aller-Gancedo J.-M. (2019). Biocontrol of Saprolegniosis in Rainbow Trout (Oncorhynchus Mykiss Walbaum) Using Two Bacterial Isolates (LE89 and LE141) of Pseudomonas Fluorescens. J. Fish Dis. 42, 269–275. doi: 10.1111/jfd.12928
González-Palacios C., Fregeneda-Grandes J.-M., Aller-Gancedo J.-M. (2020). Possible Mechanisms of Action of Two Pseudomonas Fluorescens Isolates as Probiotics on Saprolegniosis Control in Rainbow Trout (Oncorhynchus Mykiss Walbaum). Animals 10, 1507. doi: 10.3390/ani10091507
Gram L., Lovold T., Nielsen J., Melchiorsen J., Spangaard B. (2001). In Virto Antagonism of the Probiont Pseudomonas Fluorecens AH2 Against Aeromonas Salmonicida Does Not Confer Protection of Salmon Against Furunculosis. Aquaculture 199 (1-2), 1–11. doi: 10.1016/S0044-8486(01)00565-8
Gram L., Melchiorsen J., Spanggaard B., Huber I., Nielsen T. (1999). Inhibition of Vibrio Anguillarum by Pseudomonas Fluorescens Strain AH2 a Possible Probiotic Treatment of Fish. Appl. Environ. Microbiol. 65 (3), 969–973. doi: 10.1128/AEM.65.3.969-973.1999
Green T. J., Smullen R., Barnes A. C. (2013). Dietary Soybean Protein Concentrate-Induced Intestinal Disorder in Marine Farmed Atlantic Salmon, Salmo Salar is Associated With Alterations in Gut Microbiota. Vet. Microbiol. 166 (1-2), 286–292. doi: 10.1016/j.vetmic.2013.05.009
Gudmundsdottir B. K., Bjornsdottir B. (2007). Vaccination Against Atypical Furunculosis and Winter Ulcer Disease of Fish. Vaccine 25 (30), 5512–5523. doi: 10.1016/j.vaccine.2007.02.009
Guo P., Zhang K., Ma X., He P. (2020). Clostridium Species as Probiotics: Potential and Challenges. J. Anim. Sci. Biotechnol. 11, 24. doi: 10.1186/s40104-019-0402-1
Gupta A., Gupta P., Dhawan A. (2014). Dietary Supplementation of Probiotics Affects Growth, Immune Response and Disease Resistance of Cyprinus Carpio Fry. Fish Shellfish Immunol. 41 (2), 113–119. doi: 10.1016/j.fsi.2014.08.023
Gupta A., Gupta P., Dhawan A. (2016). Paenibacillus Polymyxa as a Water Additive Improved Immune Response of Cyprinus Carpio and Disease Resistance Against Aeromonas Hydrophila. Aquacult. Rep. 4, 86–92. doi: 10.1016/j.aqrep.2016.07.002
Guzmán-Villanueva L. T., Tovar-Ramírez D., Gisbert E., Cordero H., Guardiola F. ,. A., Cuesta A., et al. (2014). Dietary Administration of β-1,3/1,6-Glucan and Probiotic Strain Shewanella Putrefaciens, Single or Combined, on Gilthead Seabream Growth, Immune Responses and Gene Expression. Fish Shellfish Immunol. 39, 34–41. doi: 10.1016/j.fsi.2014.04.024
Halkjær S. I., de Knegt V. E., Lo B., Nilas L., Cortes D., Pedersen A. E., et al. (2020). Multistrain Probiotic Increases the Gut Microbiota Diversity in Obese Pregnant Women: Results From a Randomized, Double-Blind Placebo-Controlled Study. Curr. Dev. Nutr. 4 (7), nzaa095. doi: 10.1093/cdn/nzaa095
Hamilton E. F., Element G., van Coeverden de Groot P., Engel K., Neufeld J. D., Shah V., et al. (2019). Anadromous Arctic Char Microbiomes: Bioprospecting in the High Arctic. Front. Bioeng. Biotechnol. 7. doi: 10.3389/fbioe.2019.00032
Hao K., Wu Z. Q., Li D. L., Yu X. B., Wang G. X., Ling F. (2017). Effects of Dietary Administration of Shewanella Xiamenensis A-1, Aeromonas Veronii A-7, and Bacillus Subtilis, Single or Combined, on the Grass Carp (Ctenopharyngodon Idella) Intestinal Microbiota. Prob. Antimicro. Prot. 9 (4), 386–396. doi: 10.1007/s12602-017-9269-7
Hassani M. H. S., Jourdehi A. Y., Zelti A. H., Masouleh A. S., Lakani F. B. (2020). Effects of Commercial Superzist Probiotic on Growth Performance and Hematological and Immune Indices in Fingerlings Acipenser Baerii. Aquacult. Inter. 28 (1), 377–387. doi: 10.1007/s10499-019-00468-1
Hayatgheib N., Moreau E., Calvez S., Lepelletier D., Pouliquen H. (2020). A Review of Functional Feeds and the Control of Aeromonas Infections in Freshwater Fish. Aquacult. Inter. 28 (3), 1083–1123. doi: 10.1007/s10499-020-00514-3
He R.-P., Feng J., Tian X.-L., Dong S.-L., Wen B. (2017). Effects of Dietary Supplementation of Probiotics on the Growth, Activities of Digestive and non-Specific Immune Enzymes in Hybrid Grouper (Epinephelus Lanceolatus ♂ X Epinephelus Fuscoguttatus ♀). Aquacult. Res. 48, 5782–5790. doi: 10.1111/are.13401
Higuera-Llanten S., Vasquez-Ponce F., Barrientos-Espinoza B., Mardones F., Marshall S., Olivares-Pacheco J. (2018). Extended Antibiotic Treatment in Salmon Farms Select Multiresistant Gut Bacteria With a High Prevalence of Antibiotic Resistance Genes. PLoSOne 13 (9), e0203641. doi: 10.1371/journal.pone.0202641
Hjelm M., Bergh Ø., Riaza A., Nielsen J., Melchiorsen J., Jensen S., et al. (2004). Selection and Identification of Autochtonous Potential Probiotic Bacteria From Turbot Larvae (Scophthalmus Maximus) Rearing Units. Sys. Appl. Microbiol. 27, 360–371. doi: 10.1078/0723-2020-00256
Hoque F., Jawahar, Abraham T., Nagesh T. S., Kamilya D. (2019). Pseudomonas Aeruginosa FARP72 Offers Protection Against Aeromonas Hydrophila Infection in Labeo Rohita. Probio. Anti Prot. 11 (3), 973–980. doi: 10.1007/s12602-018-9456-1
Hoseinifar S. H., Sun Y.-Z., Wang A., Zhou Z. (2018). Probiotic as Means of Diseases Control in Aquaculture, a Review of Current Knowledge and Future Perspectives. Front. Microbiol. 9. doi: 10.3389/fmicb.2018.02429
Hoseinifar S. H., Yousefi S., van Doan H., Ashouri G., Gioacchini G., Maradonna F., et al. (2020). Oxidative stress and antioxidant defense in fish: The implications of probiotic, prebiotic, and synbiotics. Rev. Fish. Sci. Aquacult 29 (2), 198–217. doi: 10.1080/23308249.2020.1795616
Hu H., Mai K. S., Zhang Y. J., Ai Q. H., Xu W., Zhang W. B., et al. (2015). Effects of Dietary Xylan on Growth Performance, Digestive Enzyme Activity and Intestinal Morphology of Juvenile Turbot (Scophthalmus Maximus L.). Israeli J. Aquacult. Bamidgeh 67, 115. doi: 10.46989/001c.20730
Hu J., Yao Y., Yang Y., Gao C., Zhang F., Xia R., et al. (2021). Advances in Intra-Species Molecular Typing Analysis of Aquatic Probiotics Approved by the Chinese Ministry of Agriculture. Rev. Aquacult 13 (1), 178–188. doi: 10.1111/raq.12469
Ina-Salwany M. Y., Al-saari N., Mohamad A., Mursidi F. A., Mohd-Aris A., Amal M. N. A., et al. (2019). Vibriosis in Fish: A Review on Disease Development and Prevention. J. Aqua. Anim. Health 31 (1), 2–22. doi: 10.1002/aah.10045
Irianto A., Austin B. (2002a). Probiotics in Aquaculture. J. Fish Dis. 25 (11), 633–642. doi: 10.1046/j.1365-2761.2002.00422.x
Irianto A., Austin B. (2002b). Use of Probiotics to Control Furunculosis in Rainbow Trout, Oncorhynchus Mykiss (Walbaum). J. Fish Dis. 25 (6), 333–342. doi: 10.1046/j.1365-2761.2002.00375.x
Irianto A., Austin B. (2003a). Use of Dead Probiotic Cells to Control Furunculosis in Rainbow Trout, Oncorhynchus Mykiss (Walbaum). J. Fish Dis. 26, 59–62. doi: 10.1046/j.1365-2761.2003.00414.x
Irianto A., Austin B. (2003b). Oral Administration of Formalin-Inactivated Cells of Aeromonas Hydrophila A3-51 Controls Infection by Atypical A. Salmonicida in Goldfish, Carassius Auratus (L.). J. Fish Dis. 26, 117–120. doi: 10.1046/j.1365-2761.2003.00439.x
Jahangiri L., Esteban M. A. (2018). Administration of Probiotics in the Water in Finfish Aquaculture Systems: A Review. Fishes 3, 33. doi: 10.3390/fishes3030033
James G., Das B. C., Jose S., Kuma R. V. J. (2021). Bacillus as an Aquaculture Friendly Microbe. Aquacult. Intern. 29, 323–353. doi: 10.1007/s10499-020-00630-0
Jan A. T. (2017). Outer Membrane Vesicles (OMVs) of Gram-Negative Bacteria: A Perspective Update. Front. Microbiol. 8. doi: 10.3389/fmicb.2017.01043
Jiang Y., Zhang Z., Wang Y. G., Jing Y. Y., Liao M. J., Rong X. J., et al. (2018). Effects of Probiotic on Microfloral Structure of Live Feed Used in Larval Breeding of Turbot Scophthalmus Maximus. J. Ocenol. Limnol. 36 (3), 1002–1012. doi: 10.1007/s00343-018-7049-1
Jin G. A., Wang S. S., Yu M., Yan S. L., Zhang X. H. (2010). Identification of a Marine Antagonistic Strain JG1 and Establishment of a Polymerase Chain Reaction Detection Technique Based on the gyrB Gene. Aquacult. Res. 41 (12), 1867–1874. doi: 10.1111/j.1365-2109.2010.02591.x
Jurado J., Villasanta-Gonzalez A., Tapia-Paniagua S. T., Balebona M. C., de la Banda I. G., Morinigo M. A., et al. (2018). Dietary Administration of the Probiotic Shewanella Putrefaciens Pdp11 Promotes Transcriptional Changes of Genes Involved in Growth and Immunity in Solea Senegalensis Larvae. Fish Shellfish Immunol. 77, 350–363. doi: 10.1016/j.fsi.2018.04.018
Karlsen C., Ottem K. F., Brevik O. J., Davey M., Sørum H., Winther-Larsen H. C. (2017). The Environmental and Host-Associated Bacterial Microbiota of Arctic Sea-Water-Farmed Atlantic Salmon With Ulcerative Disorders. J. Fish Dis. 40 (11), 1645–1663. doi: 10.1111/jfd.12632
Kesarcodi-Watson A., Kaspar H., Lategan M. J., Gibson L. (2012). Performance of Single and Multi-Strain Probiotics During Hatchery Production of Greenshell (TM) Mussel Larvae, Perna Canaliculus. Aquaculture 354, 56–53. doi: 10.1016/j.aquaculture.2012.04.026
Kim A., Kim N., Roh H. J., Chun W.-K., Ho D. T., Lee Y., et al. (2019). Administration of Antibiotics can Cause Dysbiosis in Fish Gut. Aquaculture 512, 734330. doi: 10.1016/j.aquaculture.2019.734330
Kim J. K., Lee B. K., Kim S. H., Moon J. H. (1999). Characterization of Denitrifying Photosynthetic Bacteria Isolated From Photosynthetic Sludge. Aquacult. Eng. 19 (3), 179–193. doi: 10.1016/S0144-8609(98)00050-8
Klakegg Ø., Salonius K., Nilsen A., Fülberth M., Sørum H. (2020a). Enhanched Growth and Decreased Mortality in Atlantic Salmon (Salmo Salar) After Probiotic Bath. J. Appl. Microbiol. 129, 146–160. doi: 10.1111/jam.14649
Klakegg Ø., Salonius K., Nilsen A., Fülberth M., Sørum H. (2020b). Improved Health and Better Survival of Farmed Lumpfish (Cyclopterus Lumpus) After a Probiotic Bath With Two Strains of Aliivibrio. Aquaculture 518, 734810. doi: 10.1016/aquaculture.2019.734810
Korkea-aho T. L., Papadoupoulou A., Heikkinen J., von Wright A., Adams A., Austin B., et al. (2012). Pseudomonas Sp. M162 Confers Protection Against Rainbow Trout Fry Syndrome by Stimulating Immunity. J. Appl. Microbiol. 113, 24–35. doi: 10.1111/j.1365-2672.2012.05325.x
Kozasa M. (1986). Toyocerin _Bacillus Toyoi as Growth Promotor for Animal Feeding. Microbiol. Aliment. Nutr. 4, 121–135.
Kuebutornye F. K. A., Abarike E. D., Lu Y. (2019). A Review on the Application of Bacillus as Probiotics in Aquaculture. Fish Shellfish Immunol. 87, 820–828. doi: 10.1016/j.fsi.2019.02.010
Kumar V., Roy S., Kumar Meena D., Kumar Sarkar U. (2016). Application of Probiotics in Shrimp Aquaculture: Importance, Mechanisms of Action, and Methods of Administration. Rev. Fish. Sci. Aquacult. 24 (4), 342–358. doi: 10.1080/23308249.2016.119341
Labella A. M., Arahal D. R., Castro D., Lemos M. L., Borrego J. J. (2017). Revisiting the Genus Photobacterium: Taxonomy, Ecology and Pathogenesis. Int. Microbiol. 20 (1), 1–10. doi: 10.2436/20.1501.280
Lategan M. J., Torpy F. R., Gibson L. F. (2004). Biocontrol of Saprolegniosis in Silver Perch Bidyanus Bidyanus (Mitchell) by Aeromonas Media Strain A199. Aquaculture 235, 77–88. doi: 10.1016/j.aquaculture.2003.09.014
Lauzon H. L., Gudmundsdottir S., Steinarsson A., Oddgeirsson M., Petursdottir S. K., Reynisson E., et al. (2010). Effects of Bacterial Treatment at Early Stages of Atlantic Cod (Gadus Morhua L.) on Larval Survival and Development. J. Appl. Microbiol. 108, 624–632. doi: 10.1111/j.1365-2672.2009.04454.x
Lazado C. C., Caipang C. M. A., Estante E. G. (2015). Prospects of Host-Associated Microorganisms in Fish and Penaeids as Probiotics With Immunomodulatory Functions. Fish Shellfish Immunol. 45, 2–12. doi: 10.1016/j.fsi.2015.02.023
Legrand T. P. R. A., Catalano S. R., Wos-Oxley M. L., Wynne J. W., Weyrich L. S., Oxley A. P. A. (2020). Antibiotic-Induced Alterations and Repopulation Dynamics of Yellowtail Microbiota. Anim. Microb. 2, 26. doi: 10.1186/s42523-020-00046-4
Li Z., Bao N., Ren T., Han Y., Jiang Z., Bai Z., et al. (2019a). The Effect of Multi-Strain Probiotic on Growth Performance, non-Specific Immune Response, and Intestinal Health of Juvenile Turbot, Scophthalmus Maximus L. Fish Physiol. Biochem. 15, 1393–1407. doi: 10.1007/s10695-019-00635-4
Li T., Ke F., Gui J.-F., Zhou L., Zhang X.-J., Zhang Q.-Y. (2019d). Protective Effect of Clostridium Butyricum Against Carassius Auratus Herpesvirus in Gibel Carp. Aquacult. Inter. 27 (3), 905–914. doi: 10.1007/s10499-019-00377-3
Linh N. T. H., Nagai S., Nagasaka N., Okane S., Taoka Y. (2018). Effect of Lactococcus Lactis K-C2 on the Growth Performance, Amino Acid Content and Gut Microflora of Amberjack Seriola Dumerili. Fish. Sci. 84 (6), 1051–1062. doi: 10.1007/s12562-018-1243-0
Liu Y., Chen Z., Dai J., Yang P., Xu W., Ai Q., et al. (2019). Sodium Butyrate Supplementation in High-Soybean Meal Diets for Turbot (Scophthalmus Maximus): Effects on Inflammatory Status, Mucosal Barriers and Microbiota in the Intestine. Fish Shellfish Immunol. 88, 65–75. doi: 10.1016/j.fsi.2019.02.064
Liu R. J., Wu W. S., Xu X. H., Wang Y. L., Yu T., Wang J. H., et al. (2020). Rhodopseudomonas Palustris in Effluent Enhances the Disease Resistance, TOR and NF-Kappa B Signalling Pathway, Intestinal Microbiota and Aquaculture Water Quality of Pelteobagrus Vachelli. Aquacult. Res. 51 (10), 3959–3971. doi: 10.1111/are.14736
Liu Z. G., Zheng A. F., Chen M. M., Lian Y. X., Zhang X. K., Zhang S. Z., et al. (2018). Isolation and Identification of Pathogenic Aeromonas Veronii From a Dead Yangtze Finless Porpoise. Dis. Aquat. Org. 132 (1), 13–22. doi: 10.3354/dao03288
Li C., Zhang B., Wang X., Pi X., Wang X., Zhou H., et al. (2019b). Improved Utilization of Soybean Meal Through Fermentation With Commensal Shewanella Sp. MR-7 in Turbot (Scophthalmus Maximus L.). Micro. Cell Fact 18, 214. doi: 10.1186/s12934-019-1265-z
Li H., Zhou Y., Ling H., Luo L., Qi D., Feng L. (2019c). The Effect of Dietary Supplementation With Clostridium Butyricum on the Growth Performance, Immunity, Intestinal Microbiota and Disease Resistance of Tilapia (Oreochromis Niloticus). PLoSOne 14 (12), e0223428. doi: 10.1371/journal.pone.0223428
Lobo C., Martin M. V., Moreno-Ventas X., Tapia-Paniagua S. T., Rodriguez C., Morinigo M. A., et al. (2016). Shewanella Putrefaciens PdP11 Probiotic Supplementation as Enhancer of Artemia N-3 HUFA Contents and Growth Performance in Senegalese Sole Larviculture. Aquacult. Nutr. 24 (1), 548–561. doi: 10.1111/anu.12587
Lobo C., Moreno-Ventas X., Tapia-Paniagua S., Rodriguez C., Morinigo M. A., de la Banda I. G. (2014a). Dietary Probiotic Supplementation (Shewanella Putrefaciens Pdp11) Modulates Gut Microbiota and Promotes Growth and Condition in Senegalese Sole Larviculture. Fish Physiol. Biochem. 40 (1), 295–309. doi: 10.1007/s10695-013-9844-0
Lobo C., Tapia-Paniagua S., Moreno-Ventas X., Alarcon F. J., Rodriguez C., Balebona M. ,. C., et al. (2014b). Benefit of Probiotic Administration on Growth and Performance Along Metamorphosis and Weaning of Senegalese Sole (Solea Senegalensis). Aquaculture 40 (1), 295–309. doi: 10.1016/j.aquaculture.2014.06.011
Lulijwa R., Rupia E. J., Alfaro A. C. (2020). Antibiotic Use in Aquaculture, Policies and Regulation, Health and Environmental Risks: A Review of the Top 15 Major Producers. Rev. Aquacult. 12, 640–663. doi: 10.1111/raq.12344
Lu Q., Xi L., Liu Y., Gong Y., Su J., Han D., et al. (2021). Effects of Dietary Inclusion of Clostridium Autoethanogenum Protein on the Growth Performance and Liver Health of Largemouth Bass (Micropterus Salmoides). Front. Mar. Sci. 8. doi: 10.3389/fmars.2021.764964
Makled S. O., Hamdan A. M., El-Sayed A. F. M. (2020). Growth Promotion and Immune Stimulation in Nile Tilapia, Oreochromis Niloticus, Fingerlings Following Dietary Administration of a Novel Marine Probiotic, Psychrobacter Maritimus S. Prob. Antimicrob. Prot. 12 (2), 365–374. doi: 10.1007/s12602-019-09575-0
Makled S. O., Hamdan A. M., El-Sayed A. F. M., Hafez E. E. (2017). Evaluation of Marine Psychrophile, Psychrobacter Namhaensis SO89, as a Probiotic in Nile Tilapia (Oreochromis Niloticus) Diets. Fish Shellfish Immunol. 61, 194–200. doi: 10.1016/j.fsi.2017.01.001
Makridis P., Bergh Ø., Skjermo J., Vadstein O. (2001). Addition of Bacteria Bioencapsulated in Artemia Metanauplii to a Rearing System for Halibut Larvae. Aquacult. Inter. 9, 225–235. doi: 10.1023/A:1016815929846
Makridis P., Martins S., Reis J., Dinis M. T. (2008). Use of Probiotic Bacteria in the Rearing of Senegalese Sole (Solea Senegalensis) Larvae. Aquacult. Res. 39, 627–634. doi: 10.1111/j.1365-2109.2008.01933.x
Makridis P., Martins S., Vercauteren T., Driessche K., Decamp O., Dinis M. T. (2005). Evaluation of Candidate Probiotic Strains for Gilthead Seabream Larvae (Sparus Aurata) Using an In Vivo Approach. Lett. Appl. Microbiol. 40, 274–277. doi: 10.1111/j.1472-765X.2005.01676.x
Ma Q., Li L. Y., Le J. Y., Lu D. L., Qiao F., Zhang M. L., et al. (2018). Dietary Microencapsulated Oil Improves Immune Function and Intestinal Health in Nile Tilapia Fed With High-Fat Diet. Aquaculture 496, 19–29. doi: 10.1016/j.aquaculture.2018.06.080
Martens T., Heidorn T., Pukall R., Simon M., Tindall B. J., Brinkhoff T. (2006). Reclassification of Roseobacter Gallaeciensis Ruiz-Ponte Et Al. 1998 as Phaeobacter Gallaeciensis Gen. Nov., Comb. Nov., Description of Phaeobacter Inhibens Sp Nov., Reclassification of Ruegeria Algicola (Lafay Et Al. 1995) Uchino Et Al. 1999 as Marinovum Algicola Gen. Nov., Comb. Nov., and Emended Descriptions of the Genera Roseobacter, Ruegeria and Leisingera. Int. J. Sys. Evol. Microbiol. 56, 1293–1304. doi: 10.1099/ijs.0.63724-0
Marti E., Variatza E., Balcázar J. L. (2014). The Role of Aquatic Ecosystems as Reservoirs of Antibiotic Resistance. Trends Microbiol. 22, 1. doi: 10.1016/j.tim.2013.11.001
Maulu S., Liang H., Ge X., Yu H., Huang D., Ke J., et al. (2021a). Effect of Dietary Clostridium Autoethanogenum Protein on Growth, Body Composition, Plasma Parameters and Hepatic Genes Expression Related to Growth and AMP/TOR/PI3K Signaling Pathway of the Genetically Improved Farmed Tilapia (GIFT: Oreochromis Niloticus) Juveniles. Anim. Feed Sci. Technol. 276, 114914. doi: 10.1016/j.anifeedsci.2021.114914
Maulu S., Liang H., Ge X., Yu H., Huang D., Ke J., et al. (2021b). Dietary Clostridium Autoethanogenum Protein Modulates Intestinal Absorption, Antioxidant Status, and Immune Response in GIFT (Oreochromis Niloticus) Juveniles. Aquacult. Res. 52, 5787–5799. doi: 10.1111/are.15454
Melo-Bolivar J. F., Ruiz Pardo R. Y., Hume M. E., Villamil Diaz L. ,. M. (2021). Multistrain Probiotics Use in Main Commercially Cultured Freshwater Fish: A Systematic Review of Evidence. Rev. Aquacult 13 (4), 1758–1780. doi: 10.1111/raq.12543
Meng X., Wu S., Hu W., Zhu Z., Yang G., Zhang Y., et al. (2021). Clostridium Butyricum Improves Immune Responses and Remodels the Intestinal Microbiota of Common Carp (Cyprinus Carpio L.). Aquaculture 530, 735753. doi: 10.1016/j.aquaculture.2020.735753
Merrifield D. L., Dimitroglou A., Foey A., Davies S. J., Baker R. R., Bøgwald J., et al. (2010). The Current Status and Future Focus of Probiotic and Prebiotic Applications for Salmonids. Aquaculture 302, 1–18. doi: 10.1016/j.aquaculture.2010.02.007
Mertes V., Bekkelund A. K., Lagos L., Ciani E., Colquhoun D., Hallene-Hox H., et al. (2021). The Use of Extracellular Membrane Vesicles for Immunization Against Francisellosis in Nile Tilapia (Oreochromis Niloticus) and Atlantic Cod (Gadus Morhua L.). Vaccines 9, 34. doi: 10.3390/vaccines9010034
Midhun S. J., Neethu S., Vysakh A., Arun D., Radhakrishnan E. K., Jyothis M. (2017). Antibacterial Activity and Probiotic Characterization of Autochthonous Paenibacillus Polymyxa Isolated From Anabas Testudineus (Bloc, 1792). Microb. Patho. 113, 403–411. doi: 10.1016/j.micpath.2017.11.019
Mladineo I., Buselic I., Hrabar J., Radonic I., Vrbatovic A., Jozic S., et al. (2016). Autochthonous Bacterial Isolates Successfully Stimulate In Vitro Peripheral Blood Leukocytes of the European Sea Bass (Dicentrarchus Labrax). Front. Microbiol. 7. doi: 10.3389/fmic.2018.01244
Nataraj B. H., Ali S. A., Behare P. V., Yadav H. (2020). Postbiotics-Parabiotics; New Horizons in Microbial Biotherapy and Functional Foods. Microb. Cell Fact. 19, 168. doi: 10.1186/s2934-020-01426-w
Navarrete P., Fuentes P., de la Fuente L., Barros L., Magne F., Opazo R., et al. (2013). Short-Time Effects of Dietary Soybean Meal and Lactic Acid Bacteria on the Intestinal Morphology and Microbiota of Atlantic Salmon (Salmo Salar). Aquacult. Nutr. 19 (5), 827–836. doi: 10.1111/anu.12047
Nayak S. K. (2010). Probiotics and Immunity: A Fish Perspective. Fish Shellfish Immunol. 29 (1), 2–14. doi: 10.1016/j.fsi.2010.02.017
Nayak S. K. (2021). Multifaceted Applications of Probiotic Bacillus Species in Aquaculture With Special Reference to Bacillus Subtilis. Rev. Aquacult. 13, 862–906. doi: 10.1111/raq.12503
Newaj-Fyzul A., Al-Harbi A. H., Austin B. (2014). Developments in the Use of Probiotics for Disease Control in Aquaculture. Aquaculture 431, 1–11. doi: 10.1016/j.aquaculture.2013.08.026
Nguyen C. D. H., Amoroso G., Ventura T., Elizur A. (2020). Assessing the Pyloric Caeca and Distal Gut Microbiota Correlation With Flesh Color in Atlantic Salmon (Salmo Salar L. 1758). Microorganisms 8, 1244. doi: 10.3390/microorganisms8081244
Nurhidayu A., Ina-Salwany M. Y., Daud H. M., Harmin S. A. (2012). Isolation, Screening and Characterization of Potential Probiotics From Farmed Tiger Grouper (Epinephelus Fuscoguttatus). Afr. J. Microbiol. Res. 6 (9), 1924–1933. doi: 10.5897/AJMR11.913
O’Brien A., Sharp R., Russell N. J., Roller S. (2004). Antarctic Bacteria Inhibit Growth of Food-Borne Microorganisms at Low Temperatures. FEMS Microbiol. Ecol. 48 (2), 157–167. doi: 10.1016/j.femsec.2004.01.001
Offret C., Desriac F., Le Chevalier P., Mounier J., Jegou C., Fleury Y. (2016). Spotlight on Antimicrobial Metabolites From the Marine Bacteria Pseudoalteromonas: Chemodiversity and Ecological Significance. Mar. Drugs 14 (7), 26. doi: 10.3390/md14070129
Osungbemiro N. R., Belloolusoji O. A., Oladipupo M. (2018). “Effects of Probiotic Pseudomonas Fluorescens on the Growth Performance, Immune Modulation, and Histopathology of African Catfish (Clarias Gariepinus). ICAND 2018,” in International Conference on Animal Diseases and Nutrition(New York, USA).
Ottesen O. H., Olafsen J. A. (2000). Effects on Survival and Mucous Cell Proliferation of Atlantic Halibut, Hippoglossus Hippoglossus L., Larvae Following Microflora Manipulation. Aquaculture 187, 225–238. doi: 10.1016/S0044-8486(00)00314-8
Palleroni N. J. (1992). “Introduction to the Family Pseudomonadaceae,” in The Prokaryotes, 2nd ed (New York Dordrecht London: Springer Heidelberg), 3071–3085. doi: 10.1007/978-3-642-30194-0
Pan X., Wu T., Song Z., Tang H., Zhao Z. (2008). Immune Responses and Enhanced Disease Resistance in Chinese Drum, Miichthys Miiuy (Basilewsky), After Oral Administration of Live or Dead Cells of Clostridium Butyricum CB2. J. Fish Dis. 31, 679–686. doi: 10.1111/j.1365-2761.2008.00955.x
Papaleo M. C., Fondi M., Maida I., Perrin E., Lo Giudice A., Michaud L., et al. (2012). Sponge-Associated Microbial Antarctic Communities Exhibiting Antimicrobial Activity Against Burkholderia Cepacia Complex Bacteria. Biotechnol. Adv. 30 (1), 272–293. doi: 10.1016/j-biotechadv.2011.06.011
Peixoto M. J., Domingues A., Batista S., Goncalves J. F. M., Gomes A. M., Cunha S., et al. (2018). Physiopathological Responses of Sole (Solea Senegalensis) Subjected to Bacterial Infection and Handling Stress After Probiotic Treatment With Autochthonous Bacteria. Fish Shellfish Immunol. 83, 348–358. doi: 10.1016/j.fsi.2018.09.045
Pérez-Pascual D., Estellé J., Dutto G., Rodde C., Bernardet J.-F., Marchand Y., et al. (2020). Growth Performance and Adaptability of European Sea Bass (Dicentrarchus Labrax) Gut Microbiota to Alternative Diets Free of Fish Products. Microorganisms 8, 18. doi: 10.3390/microorganisms8091346
Piazzon M. C., Naya-Catala F., Simo-Mirabet P., Picard-Sanchez A., Roig F. J., Calduch-Giner J. A., et al. (2019). Sex, Age, and Bacteria: How the Intestinal Microbiota is Modulated in a Protandrous Hermaphrodite Fish. Front. Microbiol. 10. doi: 10.3389/fmicb.2019.02512
Pieters N., Brunt J., Austin B., Lyndon A. R. (2008). Efficacy of in-Feed Probiotics Against Aeromonas Bestiarum and Ichthyophthirius Multifiliis Skin Infections in Rainbow Trout (Oncorhynchus Mykiss, Walbaum). J. Appl. Microbiol. 105, 723–732. doi: 10.1111/j.1365-2672.2008.03817.x
Pietsch C. (2020). Risk Assessment for Mycotoxin Contamination in Fish Feeds in Europe. Mycotoxin Res. 36, 41–62. doi: 10.1007/s12550-019-00368-6
Planas M., Pérez-Lorenzo M., Hjelm M., Gram L., Uglenes Fiksdal I., Bergh Ø., et al. (2004). Probiotic Effect In Vivo of Roseobacter Strain 27-4 Against Vibrio (Listonella) Anguillarum Infections in Turbot (Scophthalmus Maximus L.) Larvae). Aquaculture 255, 323–333. doi: 10.1016/j.aquaculture.2005.11.039
Poolsawat L., Li X., He M., Ji D., Leng X. (2020). Clostridium Butyricum as Probiotic for Promoting Growth Performance, Feed Utilization, Gut Health and Microbiota Community of Tilapia (Oreochromis Niloticus X O. Aureus). Aquacult. Nutr. 26, 657–670. doi: 10.1111/anu.13025
Porsby C. H., Nielsen K. F., Gram L. (2008). Phaeobacter and Ruegeria Species of the Roseobacter Clade Colonize Separate Niches in a Danish Turbot (Scophthalmus Maximus)-Rearing Farm and Antagonize Vibrio Anguillarum Under Different Growth Conditions. Appl. Environ. Microbiol. 74 (23), 7356–7364. doi: 10.1128/AEM.01738-08
Prado S., Barja J. L., Luzardo A., Dubert J., Blanco J. (2020). Encapsulation of Live Marine Bacteria for Use in Aquaculture Facilities and Process Evaluation Using Response Surface Methodology. Appl. Microbiol. Biotechnol. 104 (5), 1993–2006. doi: 10.1007/s00253-019-10332-0
Prado S., Romalde J. L., Barja J. L. (2010). Review of Probiotics for Use in Bivalve Hatcheries. Vet. Microbiol. 145, 187–197. doi: 10.1016/j.vetmic.2010.08.021
Prol M. J., Bruhn J. B., Pintado J., Gram L. (2009). Real-Time PCR Detection and Quantification of Fish Probiotic Phaeobacter Strain 27-4 and Fish Pathogenic Vibrio in Microalgae, Rotifer, Artemia and First Feeding Turbot (Psetta Maxima) Larvae. J. Appl. Microbiol. 106 (4), 1292–1303. doi: 10.1111/j.1365-2672.2008.04096.x
Prol-García M. J., Pintado J. (2013). Effectiveness of Probiotic Phaeobacter Bacteria Grown in Biofilters Against Vibrio Anguillarum Infections in the Rearing of Turbot (Psetta Maxima) Larvae. Mar. Biotechnol. 15, 726–738. doi: 10.1007/s1026-013-9521-4
Qi X., Xue M. Y., Cui H. B., Yang K. C., Song K. G., Zha J. W., et al. (2020). Antimicrobial Activity of Pseudomonas Monteilii JK-1 Isolated From Fish Gut and its Major Metabolite, 1-Hydroxyphenazine, Against Aeromonas Hydrophila. Aquaculture 526, 735366. doi: 10.1016/j.aquaculture.2020.735366
Rahman F., Meryandini A., Rusmana I. (2016). Substrates Break Down and Agarase Activity of Vibrio Spp. As Probiotics Candidate for Abalon. Eur. J. Adv. Res. Biol. Life Sci. 4 (3), 14–22.
Ran C., Li Y., Ma X., Xie Y., Xie M., Zhang Y., et al. (2021). Interactions Between Commensal Bacteria and Viral Infection: Insight for Viral Disease Control in Farmed Animals. Sci. China Life Sci. 64, 1437–1448. doi: 10.1007/s1111427-020-1721-5
Rasmussen B. B., Klatzis P. G., Middelboe M., Gram L. (2019). Combining Probiotic Phaeobacter Inhibens DSM and Broad-Host-Range Vibriophage KVP40 Against Fish Pathogenic Vibrios. Aquaculture 513, 734415. doi: 10.1016/aquaculture.2019.734415
Richards G. P., Watson M. A., Needleman D. S., Uknalis J., Boyd E. F., Fay J. P. (2017). Mechanisms for Pseudoalteromonas Piscicida-Induced Killing of Vibrios and Other Bacterial Pathogens. Appl. Environ. Microbiol. 83 (11), e00175–e00117. doi: 10.1128/AEM.00175-17
Rimoldi S., Torrecillas S., Montero D., Gini E., Makol A., Valdenegro V., et al. (2020). Assessment of Dietary Supplementation With Galactomannan Oligosaccharides and Phytogenics on Gut Microbiota of European Sea Bass (Dicentrarchus Labrax) Fed Low Fishmeal and Fish Oil Based Diet. Pos One 15 (4), e0231494. doi: 10.1371/journal.pone.0231494
Ringø E. (1993). The Effect of Chromic Oxide (Cr2O3) on Aerobic Bacterial Populations Associated With the Intestinal Mucosa of Arctic Charr, Salvelinus Alpinus (L). Can. J. Microbiol. 39 (12), 1169–1173. doi: 10.1139/m93-177
Ringø E. (1999). Does Carnobacterium Divergens Isolated From Atlantic Salmon (Salmo Salar L.) Colonise the Gut of Early Developing Turbot (Scophthalmus Maximus L.) Larvae? Aquacult. Res. 30 (3), 229–232. doi: 10.1046/j.1365-2109.1999.00269.x
Ringø E. (2020). Probiotics in Shellfish Aquaculture. Aquacult. Fish. 5 (1), 1–27. doi: 10.1016/j.aaf.2019.12.001
Ringø E., Birkbeck T. H., Munro P. D., Vadstein O., Hjelmeland K. (1996). The Effect of Early Exposure to Vibrio Pelagius on the Aerobic Bacterial Flora of Turbot, Scophthalmus Maximus (L.) Larvae. J. Appl. Bacteriol. 81 (2), 207–211. doi: 10.1111/j.1365-2672.1996.tb04502.x
Ringø E., Hoseinifar S. H., Ghosh K., van Doan H., Beck B. R., Song S. K. (2018). Lactic Acid Bacteria in Finfish – an Update. Front. Microbiol. 9. doi: 10.3389/fmicb.2018.01818
Ringø E., Olsen R. E., Øverli Ø., Løvik F. (1997). Effect of Dominance Hierarchy Formation on Aerobic Microbiota Associated With Epithelial Mucosa of Subordinate and Dominant Individuals of Arctic Charr, Salvelinus Alpinus (L.). Aquacult. Res. 28, 901–904. doi: 10.1111/j.1365-2109.1997.tb01014.x
Ringø E., Sperstad S., Kraugerud O. F., Krogdahl Å. (2008). Use of 16S rRNA Gene Sequencing Analysis to Characterise Culturable Intestinal Bacteria in Atlantic Salmon (Salmo Salar L.) Fed Diets With non-Starch Polysaccharides From Soy and Cellulose. Aquacult. Res. 39, 1087–1100. doi: 10.1111/j.1365-2109.2008.01972.x
Ringø E., Sperstad S., Myklebust R., Mayhew T. M., Mjelde A., Melle W., et al. (2006b). The Effect of Dietary Krill Supplementation on Epithelium-Associated Bacteria in the Hindgut of Atlantic Salmon (Salmo Salar L.). A Microbial and Electron Microscopical Study. Aquacult. Res. 37, 1644–1653. doi: 10.1111/j.1365-2109.2006.01611.x
Ringø E., Sperstad S., Myklebust R., Refstie S., Krogdahl Å. (2006a). Characterisation of the Microbiota Associated With the Intestine of Atlantic Cod (Gadus Morhua L.). The Effect of Fish Meal, Soybean Meal and a Bioprocessed Soybean Meal. Aquaculture 261, 829–841. doi: 10.1016/j.aquaculture.2006.06.030
Ringø E., Stenberg E., Strøm A. R. (1984). Amino Acid and Lactate Catabolism in Trimethylamine-Oxide Respiration of Alteromonas Putrefaciens NCMB 1735. Appl. Environ. Microbiol. 47, 1084–1089. doi: 10.1128/aem.47.5.1084-1089.1984
Ringø E., Vadstein O. (1998). Colonization of Vibrio Pelagius and Aeromonas Caviae in Early Developing Turbot, Scophthalmus Maximus (L.) Larvae. J. Appl. Microbiol. 84, 227–233. doi: 10.1046/j.1365-2672.1998.00333.x
Ringø E., van Doan H., Lee S. H., Soltani M., Hoseinifar S. H., Ramasamy H., et al. (2020a). Probiotics, Lactic Acid Bacteria and Bacilli: Interesting Supplementation for Aquaculture. J. Appl. Microbiol. 129, 116–136. doi: 10.1111/jam.14628
Ringø E., van Doan H., Lee S., Song S. K. (2020b). Lactic Acid Bacteria in Shellfish: Possibilities and Challenges. Rev. Fish. Sci. Aquacult. 28 (2), 139–169. doi: 10.1080/23308249.2019.1683151
Ringø E., Zhou Z., Gonzalez Vecino J. L., Wadsworth S., Romero J., Krogdahl Å., et al. (2016). Effects of Dietary Components on the Gut Microbiota of Aquatic Animals: A Never-Ending Story? Aquacult. Nutr. 22, 219–282. doi: 10.1111/anu12346
Riviere A., Selak M., Lantin D., Leroy F., De Vuyst L. (2016). Bifidobacteria and Butyrate-Producing Colon Bacteria: Importance and Strategies for Their Stimulation in the Human Gut. Front. Microbiol. 7. doi: 10.3389/fmicb.2016.00979
Rosas-Ledesma P., Leon-Rubio J. M., Alarcon F. J., Morinigo M. A., Balebona M. C. (2012). Calcium Alginate Capsules for Oral Administration of Fish Probiotic Bacteria: Assessment of Optimal Conditions for Encapsulation. Aquacult. Res. 43 (1), 106–116. doi: 10.1111/j.1365-2109.2011.02809.x
Royan S., Parulekar C., Mavinkurve S. (1999). Exopolysaccharides of Pseudomonas Mendocina P2d. Lett. Appl. Microbiol. 29 (5), 342–346. doi: 10.1046/j.1472-765X.1999.00638
Rud I., Kolarevic J., Holan A. B., Berget I., Calabrese S., Terjesen B. F. (2017). Deep-Sequencing of the Bacterial Microbiota in Commercial-Scale Recirculating and Semi-Closed Aquaculture Systems for Atlantic Salmon Post-Smolt Production. Aquacult. Eng. 78, 50–62. doi: 10.1016/j.aquaeng.2016.10.003
Sáenz de Rodrigáñez M. A., Díaz-Rosales P., Chabrillón M., Smidt H., Arijo S., Leon-Rubio J. M., et al. (2009). Effect of Dietary Administration of Probiotics on Growth and Intestine Functionality of Juvenile Senegalese Sole (Sole Senegalensis, Kaup 1858). Aquacult. Nutr. 15, 177–185. doi: 10.1111/j.1365-2095.2008.00581.x
Saenz J. S., Marques T. V., Barone R. S. C., Cyrino J. E. P., Kublik S., Nesme J., et al. (2019). Oral Administration of Antibiotics Increased the Potential Mobility of Bacterial Resistant Genes in the Gut of Fish Piaractus Mesopotamicus. Microbiome 7, 14. doi: 10.1186/s40168-019-0632-7
Saettone V., Biasato I., Radice E., Schiavone A., Bergero D., Meineri G. (2020). State-Of-the-Art of the Nutritional Alternatives to the Use of Antibiotics in Human and Monogastric Animals. Animals 10, 2199. doi: 10.3390/ani10122199
Sahandi J., Jafaryan H., Soltani M., Ebrahimi P. (2017). Enhancement of Growth Performance and Haematological Changes in Rainbow Trout (Oncorhynchus Mykiss) Alevins Fed With Bifidobacterium Bacteria. J. Fish. 5 (1), 439–444. doi: 10.1017/jfish.v5i1.2017.152
Sahandi J., Jafaryan H., Soltani M., Ebrahimi P. (2019). The Use of Two Bifidobacterium Strains Enhanced Growth Performance and Nutrient Utilisation of Rainbow Trout (Oncorhynchus Mykiss) Fry. Prob. Antimicrob. Prot. 11, 966–972. doi: 10.1007/s12602-018-9455-2
Sakai M., Yoshida T., Atsuta S., Kobayashi M. (1995). Enhancement of Resistance to Vibriosis in Rainbow Trout, Oncorhynchus Mykiss (Walbaum), by Oral Administration of Clostridium Butyricum Bacterin. J. Fish Dis. 18, 187–190. doi: 10.1111/j.1365-2761.1995.tb00276.x
Salinas I., Diaz-Rosales P., Cuesta A., Meseguer J., Chabrillón M., Morinigo M. A., et al. (2006). Effect of Heat-Inactivated Fish and non-Fish Derived Probiotics on the Innate Immune Parameters of a Teleost Fish (Sparus Aurata L.). Vet. Immunol. Immunopathol. 111 (3-4), 279–286. doi: 10.1016/j.vetimm.2006.01.020
Salyers A. A., Gupta A., Wang Y. (2004). Human Intestinal Bacteria as Reservoirs for Antibiotic Resistance Genes. Trends Microbiol. 14 (9), 412–416. doi: 10.1016/j.tim.2004.07.004
Sankar H., Philip B., Philip R., Singh I. S. B. (2017). Effect of Probiotics on Digestive Enzyme Activities and Growth of Cichlids, Etroplus Suratensis (Pearl Spot) and Oreochromis Mossambicus (Tilapia). Aquacult. Nutr. 23 (4), 852–864. doi: 10.1111/anu.12452
Satomi M., Vogel B. F., Gram L., Venkateswaran K. (2006). Shewanella Putrefaciens Sp. Nov and Shewanella Morhuae Sp. Nov., Isolated From Marine Fish of the Baltic Sea. Int. J. Sys. Evol. Microbiol. 56, 243–249. doi: 10.1099/ijs.0.63931-0
Satomi M., Vogel B. F., Venkateswaran K., Gram L. (2007). Description of Shewanella Glacialipiscicola Sp. Nov and Shewanella Algidipiscicola Sp. Nov., Isolated From Marine Fish of the Danish Baltic Sea, and Proposal That Shewanella Affinis is a Later Heterotypic Synonym of Shewanella Colwelliana. Int. J. Sys. Evol. Microbiol. 57, 347–352. doi: 10.1099/ijs.0.64708-0
Schaeck M., Duchateau L., Van den Broeck W., Van Trappen S., De Vos P., Coulombet C., et al. (2016). Vibrio Lentus Protects Gnotobiotic Sea Bass (Dicentrarchus Labrax L.) Larvae Against Challenge With Vibrio Harveyi. Vet. Microbiol. 185, 41–48. doi: 10.1016/j.vetmic.2016.01.024
Schaeck M., Reyes-Lopez F. E., Vallejos-Vidal E., Van Cleemput J., Duchateau L., Van den Broeck W., et al. (2017). Cellular and Transcriptomic Response to Treatment With the Probiotic Candidate Vibrio Lentus in Gnotobiotic Sea Bass (Dicentrarchus Labrax) Larvae. Fish Shellfish Immunol. 63, 147–156. doi: 10.1016/j.fsi.2017.01.028
Schmidt V., Gomez-Chiarri M., Roy C., Smith K., Amaral-Zettler L. (2017). Subtle Microbiome Manipulation Using Probiotics Reduces Antibiotic-Associated Mortality in Fish. MSystems 2 (6), e00133–e00117. doi: 10.1128/mSystems.00133-17
Sedlacek I., Stankova E., Svec P. (2016). Composition of Cultivable Enteric Bacteria From the Intestine of Antarctic Fish (Family Nototheniidae). Czech J. Anim. Sci. 61 (3), 127–132. doi: 10.17221/8785-CJAS
Seoane P., Tapia-Paniagua S. T., Bautista R., Alcaide E., Esteve C., Martinez-Manzanares E., et al. (2019). TarSynFlow, a Workflow for Bacterial Genome Comparisons That Revealed Genes Putatively Involved in the Probiotic Character of Shewanella Putrefaciens Strain Pdp11. PeerJ 7, e6526. doi: 10.7717/peerj.6526
Shah N. P. (1997). Isolation and Enumeration of Bifidobacteria in Fermented Milk Products: A Review. Milchwissenschaft 52, 71–76.
Shamsuzzaman M. M., Kumar B. T. (2012). Aqua Chemicals in Shrimp Farm: A Study From South-West Coast of Bangladesh. Egyptian J. Aquat. Res. 38, 275–285. doi: 10.1016/j.ejar.2012.12.08
Sharifuzzaman S. M., Abbass A., Tinsley J. W., Austin B. (2011). Subcellular Components of Probiotics Kocuria SM1 and Rhodococcus SM2 Induce Protective Immunity in Rainbow Trout (Oncorhynchus Mykiss, Walbaum) Against Vibrio Anguillarum. Fish Shellfish Immunol. 30, 347–353. doi: 10.1016/j.fsi.2010.11.005
Sharifuzzaman S. M., Al-Harbi A. H., Austin B. (2014). Characteristics of Growth, Digestive System Functionality, and Stress Factors of Rainbow Trout Fed Probiotics Kocuria SM1 and Rhodococcus Sm2. Aquaculture 418, 55–61. doi: 10.1016/j.aquaculture.2013.10.006
Sharifuzzaman S. M., Austin B. (2010a). Development of Protection in Rainbow Trout (Oncorhynchus Mykiss, Walbaum) to Vibrio Anguillarum Following Use of the Probiotic Kocuria Sm1. Fish Shellfish Immunol. 29, 212–216. doi: 10.1016/j.fsi.2010.03.008
Sharifuzzaman S. M., Austin B. (2010b). Kocuria SM1 Controls Vibriosis in Rainbow Trout (Oncorhynchus Mykiss, Walbaum). J. Appl. Microbiol. 108, 2162–2170. doi: 10.1111/j.1365-2672.2009.04618.x
Sharifuzzaman S. M., Rahman H., Austin D. A., Austin B. (2018). Properties of Probiotics Kocuria SM1 and Rhodococcus SM2 Isolated From Fish Guts. Prob. Antimicrob. Prot. 10 (3), 534–542. doi: 10.1007/s12601-017-9290-x
Silby M. W., Winstanley C., Godfrey S. A. C., Levy S. B., Jackson R. W. (2011). Pseudomonas Genomes: Diverse and Adaptable. FEMS Microbiol. Rev. 35 (4), 652–680. doi: 10.1111/j.1574-6976.2011.00269.x
Skjermo J., Bakke I., Dahle S. W., Vadstein O. (2015). Probiotic Strains Introduced Through Live Feed and Rearing Water Have Low Colonizing Success in Developing Atlantic Cod Larvae. Aquaculture 438, 17–23. doi: 10.1016/j.aquaculture.2014.12.027
Smith P., Davey S. (1993). Evidence for the Competitive Exclusion of Aeromonas Salmonicida From Fish With Stress-Inducible Furunculosis by a Fluorescent Pseudomonad. J. Fish Dis. 16 (5), 521–554. doi: 10.1111/j.1365-2761.1993.tb00888.x
Sneath P. H. A., Jones D. (1976). Brochothrix, a New Genus Tentatively Placed in the Family Lactobacillaceae. J. Sys. Bacteriol. 26, 102–104. doi: 10.1099/00207713-26-2-102
Soltani M., Ghosh K., Hoseinifar S. H., Kumar V., Lymbery A., Roy S., et al. (2019). Genus Bacillus, Promising Probiotics in Aquaculture: Aquatic Animal Origin, Bio-Active Components, Bioremediation in Fish and Shellfish. Rev. Fish. Sci. Aquacult. 27 (3), 331–379. doi: 10.1080/23308249.2019.1597010
Song W., Li L. Z., Huang H. L., Jiang K. J., Zhang F. Y., Chen X. Z., et al. (2016). The Gut Microbial Community of Antarctic Fish Detected by 16S rRNA Gene Sequence Analysis. BioMed. Res. Inter. 2016, 3241529. doi: 10.1155/2016/3241529
Song Z.-F., Wu T.-X., Cai L.-S., Zhang L.-J., Zheng X.-D. (2006). Effects of Dietary Supplementation With Clostridium Butyricum on the Growth Performance and Humoral Immune Response in Miichtys Miiuy. J. Zhejiang Univ. Sci. B 7, 596–602. doi: 10.1631/jzus.2006.B0596
Stackebrandt E., Koch C., Gvozdiak O., Schumann P. (1995). Taxonomic Dissection of the Genus Micrococcus: Kocuria Gen. Nov., Nesterenkonia Gen. Nov., Kytococcus Gen. Nov., Dermacoccus Gen. Nov., and Micrococcus Cohn 1872 Gen. Emend. Int. J. Syst. Bacteriol. 45 (4), 682–693. doi: 10.1099/00207713-45-4-682
Strom-Bestor M., Wiklund T. (2011). Inhibitory Activity of Pseudomonas Sp. On Flavobacterium Psychrophilum In Vitro. J. Fish Dis. 34, 255–264. doi: 10.1111/j.1365-2761.2010.01232.x
Sun Y. Z., Xia H. Q., Yang H. L., Wang Y. L., Zou W. C. (2014). TLR2 Signaling may Play a Key Role in the Probiotic Modulation of Intestinal Microbiota in Grouper Epinephelus Coioides. Aquaculture 430, 50–56. doi: 10.1016/j.aquaculture.2014.03.042
Sun Y. Z., Yang H. L., Ma R. L., Zhang C. X., Lin W. Y. (2011). Effect of Dietary Administration of Psychrobacter sp. on the growth, feed utilization, digestive enzymes and immune responses of grouper Epinephelus coioides. Aquacult. Nutr. 17, e733–e740. doi: 10.1111/j.1365-2095.2010.00837.x
Suryaningsih W., Maulana R., Istiqomah I., Isnansetyo A. (2021). In Vitro Adhesion of Bacillus Sp. And Enterobacter Sp. Probiotics on Intestinal Epithelial Cells of Red Tilapia (Oreochromis Sp.) and the Application Effects on the Fish Growth and Survival Rate. IOP Conf. Ser. Earth Environ. Sci. 919, 12056. doi: 10.1088/1755-1315/919/1/012056
Takeuchi M., Hatano K. (1998). Proposal of Six New Species in the Genus Microbacterium and Transfer of Flavobacterium Marinotypicum ZoBell and Upham to the Genus Microbacterium as Microbacterium Maritypicum Comb. Nov.. Int. J. Syst. Bacteriol. 48, 973–82. doi: 10.1099/00207713-48-973
Tamboli C. P., Neut C., Desreumaux P., Colombel J. F. (2004). Dysbiosis as a Prerequisite for IBD. Gut 53 (7), 1057.
Tandberg J. I., Lagos L., Kashulin A., Sørum H., Winter-Larsen H. C. (2019). Bacterial Membrane Vesicles as Vaccines in Aquaculture. Fish Shellfish Immunol. 91, 425–425 (abstract). doi: 10.1016/j.fsi.2019.04.163
Tang K., Huang H., Jiao N., Wu C. H. (2010). Phylogenomic Analysis of Marine Roseobacters. PloS One 5 (7), e11604. doi: 10.1371/journal.pone.0011604
Tan P., Wu X., Zhu W., Lou B., Chen R., Wang L. (2020). Effect of Tributyrin Supplementation in High-Soya Bean Meal Diet on Growth Performance, Body Composition, Intestine Morphology and Microbiota of Juvenile Yellow Drum (Nibea Albiflora). Aquacult. Res. 51, 2004–2019. doi: 10.1111/are.14552
Tapia-Panagia S. T., Fumanal M., Anguis V., Fernández-Díaz C., Alarcón F. J., Moriñigo M. A., et al. (2019). Modulation of Intestinal Microbiota in Solea Senegalensis Fed Low Dietary Level of Ulva Ohnoi. Front. Microbiol. 10, 171. doi: 10.3389/fmicb.2019.00171
Tapia-Paniagua S. T., Chabrillón M., Diaz-Rosales P., de la Banda I. G., Lobo C., Balebona M. C., et al. (2010). Intestinal Microbiota Diversity of the Flat Fish Solea Senegalensis (Kaup, 1858) Following Probiotic Administration. Microb. Ecol. 60 (2), 310–319. doi: 10.1007/s00248-010-9680-z
Tapia-Paniagua S. T., Díaz-Rosales P., León-Rubio J. M., García de la Banda I., Lobo C., Alarcón F. J., et al. (2012). Use of the Probiotic Shewanella Putrefaciens Pdp11 on the Culture of Senegalese Sole (Solea Senegalensis Kaup 1858) and Gilthead Seabream (Sparus Aurata L.). Aquacult. Int. 20, 1025–1039. doi: 10.1007/s10499-012-9509-5
Tapia-Paniagua S. T., Lobo C., Moreno-Ventas X., de la Banda I. G., Moriñigo M. A., Balebona M. C. (2014a). Probiotic Supplementation Influences the Diversity of the Intestinal Microbiota During Early Stages of Farmed Senegalese Sole (Solea Senegalensis, Kaup 1858). Mar. Biotechnol. 16, 716–728. doi: 10.1007/s10126-014-9588-6
Tapia-Paniagua S. T., Vidal S., Lobo C., Prieto-Álamo M. J., Jurado J., Cordero H., et al. (2014b). The Treatment With the Probiotic Shewanella Putrefaciens Pdp11 of Specimens of Solea Senegalensis Exposed to High Stocking Densities to Enhance Their Resistance to Disease. Fish Shellfish Immunol. 41 (2), 209–221. doi: 10.1016/j.fsi.2014.08.019
Taverniti V., Guglielmetti S. (2011). The Immunomodulatory Properties of Probiotic Microorganism Beyond Their Viability (Ghost Probiotics: Proposal of Paraprobiotic Concept). Genes Nutr. 6 (3), 261–271. doi: 10.1007/s12263-011-0218-x
Teame T., Wang A., Xie M., Zhang Z., Yang Y., Ding Q., et al. (2020). Paraprobiotics and Postbiotics of Probiotic Lactobacilli, Their Positive Effects on the Host and Action Mechanisms: A Review. Front. Nutr. 7. doi: 10.3389/fnut.2020.570144
Terova G., Rimoldi S., Ascione C., Gini E., Ceccotti C., Gasco L. (2019). Rainbow Trout (Oncorhynchus Mykiss) Gut Microbiota is Modulated by Insect Meal From Hermetia Illucens Prepupae in the Diet. Rev. Fish Biol. Fish. 29, 465–486. doi: 10.1007/s11160-019-09558-y
Tobar I., Arancibia S., Torres C., Vera V., Soto P., Carrasco C., et al. (2015). Successive Oral Immunizations Against Piscirickettsia Salmonis and Infectious Salmon Anemia Virus are Required to Maintain a Long-Term Protection in Farmed Salmonids. Front. Immunol. 6. doi: 10.3389/fimmu.2015.00244
Tran N. T., Li Z., Ma H., Zhang Y., Zheng H., Gong Y., et al. (2020). Clostridium Butyricum: A Promising Probiotic Confers Positive Health Benefits in Aquatic Animals. Rev. Aquacult. 12, 2573–2589. doi: 10.1111/raq.12459
Turchini G. M., Francis D. S., Du Z.-Y., Olsen R. E., Ringø E., Tocher D. R. (2022). ““Chapter 5. The Lipids”,” in Fish Nutrition, 4th Edition. Ed. Hardy R. (125 London Wall, London EC2Y 5AS, United Kingdom: Elsevier), 244. ISBN: 978-0-12-819587-1
Turroni F., Ventura M., Buttó L. F., Duranti S., O`Toole R. W., O`Connel Motherway M., et al. (2014). Molecular Dialogue Between the Human Gut Microbiota and the Host: A Lactobacillus and Bifidobacterium Perspective. Cell. Mol. Life Sci. 71, 183–203. doi: 10.1007/s00018-013-1318-0
Van Doan H., Hoseinifar S. H., Ringø E., Esteban M.Á., Dadar M., Dawood M. A. O., et al. (2020). Host-Associated Probiotics: A Key Factor in Sustainable Aquaculture. Rev. Fish. Sci. Aquacult. 28 (1), 16–42. doi: 10.1080/23308249.2019.1643288
van Doan H., Soltani M., Ringø E. (2021). In Vitro Antagonistic Effect and In Vivo Protective Efficacy of Gram-Positive Probiotics Versus Gram-Negative Bacterial Pathogens in Finfish and Shellfish. Aquaculture 538, 736581. doi: 10.1016/j.aquaculture.2021.736581
Varela J. L., Ruíz-Jarabo I., Vargas-Chacoff L., Arijo S., Leon-Rubio J. M., Garcia-Millan I., et al. (2010). Dietary Administration of Probiotic Pdp11 Promotes Growth and Improves Stress Tolerance to High Stocking Density in Gilthead Seabream Sparus Auratus. Aquaculture 309, 265–271. doi: 10.1016/j.aquaculture.2010.09.029
Vargas-Albores F., Martíenez-Córdova L. R., Herández-Mendoza A., Cicala F., Lago-Lestón A., Martínez-Porchas M. (2021). Therapeutic Modulation of Fish Gut Microbiota, a Feasible Strategy for Aquaculture? Aquaculture 544, 737050. doi: 10.1016/j.aquaculture.2021.737050
Venkataramana K., Padmavali P., Ritu V., Adnan B. B., Vinod K., Maheshwar R. K., et al. (2016). Emerging Bacterial Infection: Identification and Clinical Significance of Kocuria Species. Cureus 8 (8), e731. doi: 10.7759/cureus.731
Verschuere L., Rombaut G., Sorgeloos P., Verstraete W. (2000). Probiotic Bacteria as Biological Control Agents in Aquaculture. Microbiol. Mol. Biol. Rev. 64 (4), 655–671. doi: 10.1128/MMBR.64.4.655-671.2000
Vidal S., Tapia-Paniagua S. T., Morinigo J. M., Lobo C., de la Banda I. G., Balebona M. D., et al. (2016). Effects on Intestinal Microbiota and Immune Genes of Solea Senegalensis After Suspension of the Administration of Shewanella Putrefaciens Pdp11. Fish Shellfish Immunol. 58, 274–283. doi: 10.1016/j.fsi.2016.09.010
Vieco-Saiz N., Belguesmia Y., Raspoet R., Auclair E., Gancel F., Kempf I., et al. (2019). Benefits and Inputs From Lactic Acid Bacteria and Their Bacteriocins as Alternatives to Antibiotic Growth Promoters During Food-Animal Production. Front. Microbiol. 10. doi: 10.3389/fmicb.2019.00057
Villamil L., Figueras A., Planas M., Novoa B. (2010). Pediococcus Acidilactici in the Culture of Turbot (Psetta Maxima) Larvae, Administration Pathways. Aquaculture 307, 83–88. doi: 10.1016/j.aquaculture.2010.07.004
Vine N. G. (2004). Towards the Development of a Protocol for the Selection of Probiotics in Marine Fish Larviculture. PhD Thesis (South Africa: Rhodes University, Grahamstown), 222.
Vine N. G., Leukes W. D., Kaiser H. (2006). Probiotics in Marine Larviculture. FEMS Microbiol. Rev. 30, 404–427. doi: 10.1111/j.1574-6976.2006.00017.x
Vlkova E., Kalous L., Bunesova V., Rylkova K., Svetlikova R., Rada V. (2012). Occurrence of Bifidobacteria and Lactobacilli in Digestive Tract of Some Freshwater Fishes. Biologia 67 (2), 411–416. doi: 10.2478/s11756-012-0017-x
Wagner-Döbler I., Biebl H. (2006). Environmental Biology of the Marine Roseobacter Lineage. Ann. Rev. Microbiol. 60, 255–280. doi: 10.1146/annurev.micro.60.080805.142115
Wang Y. (2011). Use of Probiotics Bacillus Coagulans, Rhodopseudomonas Palustris and Lactobacillus Acidophilus as Growth Promoters in Grass Carp (Ctenopharyngodon Idella) Fingerlings. Aquacult. Nutr. 17 (2), e372–e378. doi: 10.1111/j.1365-2095.2010.00771.x
Wang R.-F., An X.-P., Wang Y., Qi J.-W., Zhang J., Liu Y.-H., et al. (2020b). Effects of Polysaccharide From Fermented Wheat Bran on Growth Performance, Muscle Composition, Digestive Enzyme Activities and Intestinal Microbiota in Juvenile Common Carp. Aquacult. Nutr. 26 (4), 1096–1107. doi: 10.1111/anu.13067
Wang C., Chuprom J., Wang Y., Fu L. (2019a). Beneficial Bacteria for Aquaculture: Nutrition, Bacteriostasis and Immunoregulation. J. Appl. Microbiol. 128, 28–40. doi: 10.1111/jam.14383
Wang G. X., Li F.-Y., Cui J., Wang Y., Liu T.-Y., Han J., et al. (2011). Immunostimulatory Activities of a Decapeptide Derived From Alcaligenes Faecalis FY-3 to Crucian Carp. Scan. J. Immunol. 74 (1), 14–22. doi: 10.1111/j.1365-3083.2011.02533.x
Wang C. A., Liu H. B., Mu G. Q., Lu S. X., Wang D., Jiang H. B., et al. (2019b). Effects of Traditional Chinese Medicines on Immunity and Culturable Gut Microflora to Oncorhynchus Masou. Fish Shellfish Immunol. 93, 322–327. doi: 10.1016/j.fsi.2019.07.071
Wang Y., Wu Y., Wang Y., Xu H., Mei X., Yu D., et al. (2017). Antioxidant Properties of Probiotic Bacteria. Nutrients 9, 521. doi: 10.3390/nu9050521
Wang M., Yi M., Lu M., Gao F., Liu Z., Huang Q., et al. (2020a). Effects of Probiotics Bacillus Cereus NY5 and Alcaligenes Faecalis Y311) Used as Water Additives on the Microbiota and Immune Enzyme Activities in Three Mucosal Tissues in Nile Tilapia Oreochromis Niloticus Reared in Outdoor Tanks. Aquacult. Rep. 17, 9. doi: 10.1016/j.aqrep.2020.100309
Wang P., Zhou Q. C., Feng J., He J. J., Lou Y. D., Zhu J. Q. (2019c). Effect of Dietary Fermented Soybean Meal on Growth, Intestinal Morphology and Microbiota in Juvenile Large Yellow Croaker, Larimichthys Crocea. Aquacult. Res. 50 (3), 748–757. doi: 10.1111/are.13929
Wanka K. M., Damerau T., Costas B., Krueger A., Schulz C., Wuertz S. (2018). Isolation and Characterization of Native Probiotics for Fish Farming. BMC Microbiol. 18, 119. doi: 10.1186/s2866-018-1260-2
Wu S., Wang G., Angert E. R., Wang W., Li W., Zou H. (2012). Composition, Diversity, and Origin of the Bacterial Community in Grass Carp Intestine. PLoSOne 7 (2), e30440. doi: 10.1371/journal.pone.0030440
Wu Z.-Q., Jiang C., Ling F., Wang G.-X. (2015). Effects of Dietary Supplementation of Intestinal Autochthonous Bacteria on the Innate Immunity and Disease Resistance of Grass Carp (Ctenopharyngodon Idellus). Aquaculture 438, 105–114. doi: 10.1016/j.aquaculture.2014.12.041
Yao Y.-Y., Yang Y.-L., Gao C.-C., Zhang F.-L., Xia R., Li G., et al. (2020). Surface Display System for Probiotics and its Application in Aquaculture. Rev. Aquacult 12 (4), 2333–2350. doi: 10.1111/raq.12437
Yi C.-C., Liu C.-H., Chuang K.-P., Chang Y. T., Hu S.-Y. (2019). A Potential Probiotic Chromobacterium Aquaticum With Bacteriocin-Like Activity Enhances the Expression of Indicator Genes Associated With Nutrient Metabolism, Growth Performance and Innate Immunity Against Pathogen Infections in Zebrafish (Danio Rerio). Fish Shellfish Immunol. 93, 124–134. doi: 10.1016/j.fsi.2019.07.042
Zhang B., Li C., Wang X., Liu C., Zhou H., Mai K., et al. (2020). Administration of Commensal Shewanella Sp. MR-7 Ameliorates Lipopolysaccharide-Induced Intestine Dysfunction in Turbot (Scophthalmus Maximus L.). Fish Shellfish Immunol. 102, 460–468. doi: 10.1016/j.fsi.2020.04.068
Zhang X., Shu M. A., Wang Y. B., Fu L. Q., Li W. F., Deng B., et al. (2014). Effect of Photosynthetic Bacteria on Water Quality and Microbiota in Grass Carp Culture. World J. Microbiol. Biotechnol. 30 (9), 2523–2531. doi: 10.1007/s11274-014-1677-1
Zhan P. R., Liu W. (2013). Use of Fluidized Bed Biofilter and Immobilized Rhodopseudomonas Palustris for Ammonia Removal and Fish Health Maintenance in a Recirculation Aquaculture System. Aquacult. Res. 44 (3), 327–334. doi: 10.1111/j.1365-2109.2011.03038.x
Zhou X. X., Tian Z. Q., Wang Y. B., Li W. F. (2010). Effect of Treatment With Probiotics as Water Additives on Tilapia (Oreochromis Niloticus) Growth Performance and Immune Response. Fish Physiol. Biochem. 36 (3), 501–509. doi: 10.1007/s10695-009-9320-z
Zhou S., Zhang A., Yin H. P., Chu W. H. (2016). Bacillus Sp. QSI-1 Modulate Quorum Sensing Signals Reduce Aeromonas Hydrophila Level and Alter Gut Microbial Community Structure in Fish. Front. Cell. Infect. Microbiol. 6. doi: 10.3389/fcimb.2016.00184
Ziólkowska M., Rokicki J., Kedzia A. (2009). The Effect of Infection by Pomphorhynchus Laevis (Acanthocephala) on the Composition of the Intestinal Bacterial Flora in Flounder, Platichthys Flesus. J. Mar. Bio. Ass. United Kingdom 89 (6), 1177–1179. doi: 10.1017/S0025315409000447
Keywords: probiotic bacteria, non-LAB, bacilli, finfish, aquaculture
Citation: Ringø E, Li X, Doan Hv and Ghosh K (2022) Interesting Probiotic Bacteria Other Than the More Widely Used Lactic Acid Bacteria and Bacilli in Finfish. Front. Mar. Sci. 9:848037. doi: 10.3389/fmars.2022.848037
Received: 03 January 2022; Accepted: 29 April 2022;
Published: 02 June 2022.
Edited by:
Benjamin Costas, University of Porto, PortugalReviewed by:
Ana Teresa Gonçalves, GreenCoLAB, PortugalFrancisco Vargas-Albores, Consejo Nacional de Ciencia y Tecnología (CONACYT), Mexico
Viswanath Kiron, Nord University, Norway
Copyright © 2022 Ringø, Li, Doan and Ghosh. This is an open-access article distributed under the terms of the Creative Commons Attribution License (CC BY). The use, distribution or reproduction in other forums is permitted, provided the original author(s) and the copyright owner(s) are credited and that the original publication in this journal is cited, in accordance with accepted academic practice. No use, distribution or reproduction is permitted which does not comply with these terms.
*Correspondence: Einar Ringø, RWluYXIuUmluZ29AdWl0Lm5v; orcid.org/0000-0002-4940-5688