- 1Ecology and Evolutionary Biology, University of Reading, Reading, United Kingdom
- 2Centre for Research into Ecological Environmental Modelling, University of St Andrews, Scotland, United Kingdom
- 3Molecular Biosciences, Northwestern University, Evanston, IL, United States
- 4Earth Sciences, Field Museum of Natural History, Chicago, IL, United States
Population abundance and density estimates provide key information for conservation assessment and prioritization of efforts and management. However, data are still largely unavailable for many taxa, including sea snakes, which appear to be facing global declines. Here, we present the first quantitative abundance and density estimates for the geographically isolated sea snake Hydrophis platurus xanthos endemic to the inner basin of Golfo Dulce, Costa Rica. Using systematic distance sampling methods, we obtained and analyzed 199 snake detections from 46 transect lines covering the entire known distribution (totaling nine days and 469 km of effort). Our modeling methods accounted for (i) the probability of detecting a snake given it was available to be detected (ii) the average availability of snakes at the water surface during a 24-hr cycle, and (iii) the by-hour variance in the taxon’s activity pattern. The best estimate of population abundance was 29781 individuals (95% CI=20104–44115) with an estimated density of 76 snakes/km2. Without historical abundance estimates or minimum viable population size, it is unknown whether this number represents a healthy population. However, with all individuals inhabiting a relatively small inlet increasingly exposed to anthropogenic impacts, the long-term persistence of H. p. xanthos may be threatened by ongoing impacts (boat propeller strikes, agricultural runoff, and climate change), as well as unforeseen events in the future.
Introduction
The late Holocene, frequently referred to as the Anthropocene, is marked by destabilizing changes in nature (Steffen et al., 2007), eliciting concern for ecosystem functioning and the future of biodiversity (Dulvy et al., 2003; Hector and Bagchi, 2007; Cardinale et al., 2012). Supporting and protecting biodiversity requires ecological knowledge including reliable population estimates from which abundance trends can be measured (Dornelas et al., 2018). Securing abundance data, however, is exceedingly difficult. Accurate estimates are available for few species and filling the gaps presents challenges associated with the diversity of habitats and natural histories that need to be studied with limited resources. To date, only 5% of all described species worldwide have been evaluated for risk of extinction (IUCN, 2022) ever as thousands are disappearing, often without ever being identified (Pimm et al., 1995; IPBES, 2019; WWF, 2020).
Among at-risk fauna, a fifth of all reptile species may be threatened with extinction (Böhm et al., 2013), and snakes are declining at an alarming rate (Gibbons et al., 2000; Reading et al., 2010). Marine populations may be increasingly susceptible to pathogens, predators, boat strikes, environmental changes (Somaweera et al., 2021), and trawlers (Fry et al., 2001), but numbers may also be reduced by inexplicable causes (Elfes et al., 2013; Goiran and Shine, 2013; Lukoschek et al., 2013; Udyawer et al., 2018). Reading et al. (2010) identified three general characteristics among snake species experiencing sharp decreases: they inhabit narrow ranges, tend to be sedentary (minimal travel), and display ambush-style foraging. It is hypothesized that these traits make them more vulnerable to anthropogenic impacts and/or habitat degradation. All three of these traits occur in a recently described sea snake: Hydrophis platurus xanthos (Bessesen and Galbreath, 2017). This taxon exhibits geographic rarity occupying a relatively small area of aquatic habitat within the semi-closed embayment of Golfo Dulce, Costa Rica (Bessesen, 2012), it rarely swims across the ocean surface, and uses a floating ambush posture to opportunistically capture tiny fish (Kropach, 1973; Brischoux and Lillywhite, 2011; Bessesen and Galbreath, 2017). Moreover, confined to an area of active human use, there are already several potential threats to the population, including boat traffic (Bessesen and González-Suárez, 2021) and water pollutants (Spongberg, 2004; Fournier et al., 2019). Climate change poses additional risks (Lillywhite et al., 2018; Udyawer et al., 2018). Sensitive to thermal shifts (Heatwole et al., 2012) already reported in the region (Morales-Ramírez et al., 2015; Murayama et al., 2018) and bound by rainforest and mountains to the north, H. p. xanthos is unable to migrate into higher latitudes to escape warming waters (Poloczanska et al., 2016). Despite its likely vulnerability and ongoing threats in its habitat, quantitative data on population abundance and density have to date been unavailable. Such data are key to assessing this taxon’s conservation status and detecting potential declines in the future.
Distance sampling is a long-established method that uses line transect data to model population abundance and density (Eberhardt, 1978; Buckland et al., 1993; Williams et al., 2017). This method has been used with a variety of cetaceans (Jaramillo-Legorreta et al., 1999; Miller et al., 2013; Doniol-Valcroze et al., 2015; Kreb et al., 2020), but has not been applied to sea snakes. Distance sampling has been considered for terrestrial snakes (Dorcas and Willson, 2009) but often failed to return reliable results (Rodda and Campbell, 2002; Stellati et al., 2019), largely because of difficulties in achieving the required minimal number of detections (60–80 per Buckland et al., 2001; Buckland et al., 2015). For terrestrial snakes, abundance studies have historically used mark-recapture techniques when possible; otherwise, occupancy monitoring or relative abundancy indices have been employed (Dorcas and Willson, 2009). Abundance estimates for sea snakes are exceedingly rare (IUCN, 2022) and usually provide single-site data for broader-ranging species (Saint Girons, 1964; Voris, 1985; Lading et al., 1991; Shine et al., 2021). Mark-recapture was attempted with the related pelagic sea snake, H. p. platurus, but proved unsuccessful (Kropach, 1973). Because H. p. xanthos is limited to a narrow range, however, and much like a cetacean is detectable at the ocean surface between dives, distance sampling is a viable approach for this taxon.
Baseline occupancy and relative abundance data for H. p. xanthos have been previously published (Bessesen, 2012; Bessesen, 2015), and two questionnaire surveys with local fishermen and tour boat guides offered insight into perceived abundance and trends (Bessesen and González-Suárez, 2021). Lillywhite et al. (2015) reported an encounter rate of 21.4 ± 4.4 snakes/h (mean ± SD) based on two-nights of data. By completing 45.9 survey hours and applying robust statistical methods we offer the first total abundance and density estimates for this rare, likely vulnerable marine snake. We hope this work can serve to inform stakeholders in the conservation of H. p. xanthos, and more broadly contribute to the knowledge base for marine reptiles and biodiversity in the American tropics. Our methods may prove informative to those working to estimate sea snake population abundance and density estimates elsewhere in the world.
Materials and Methods
Study Area and Survey Design
Golfo Dulce is an inlet estimated to be 725–750 km2 in the Puntarenas province of southwestern Costa Rica (Wolff et al., 1996; Bessesen, 2015). Formed by shifting tectonic blocks, the marine embayment’s fiord-like morphology is considered rare to the tropics (Berrangé, 1989; Hebbeln and Cortés, 2001; Malzer and Fiebig, 2008). Golfo Dulce is characterized by a > 200 m-deep inner basin (Wolff et al., 1996; Svendsen et al., 2006) sheltered from the broader Pacific masses by a 60-m sill at latitude 8° 30’ N (Hebbeln et al., 1996). Our ~400 km2 study area encapsulates the full range and only known habitat of H. p. xanthos (Bessesen, 2012; Bessesen, 2015; Bessesen and Galbreath, 2017). A systematic distance sampling survey with a random starting point was designed in the software program Distance (version 7.3; Thomas et al., 2010) and resulted in 46 parallel transect lines spaced 0.75 km apart. Most transects were shore-to-shore (n=38), while the eight most south-easterly transects provided survey coverage only across the inner basin (Figure 1).
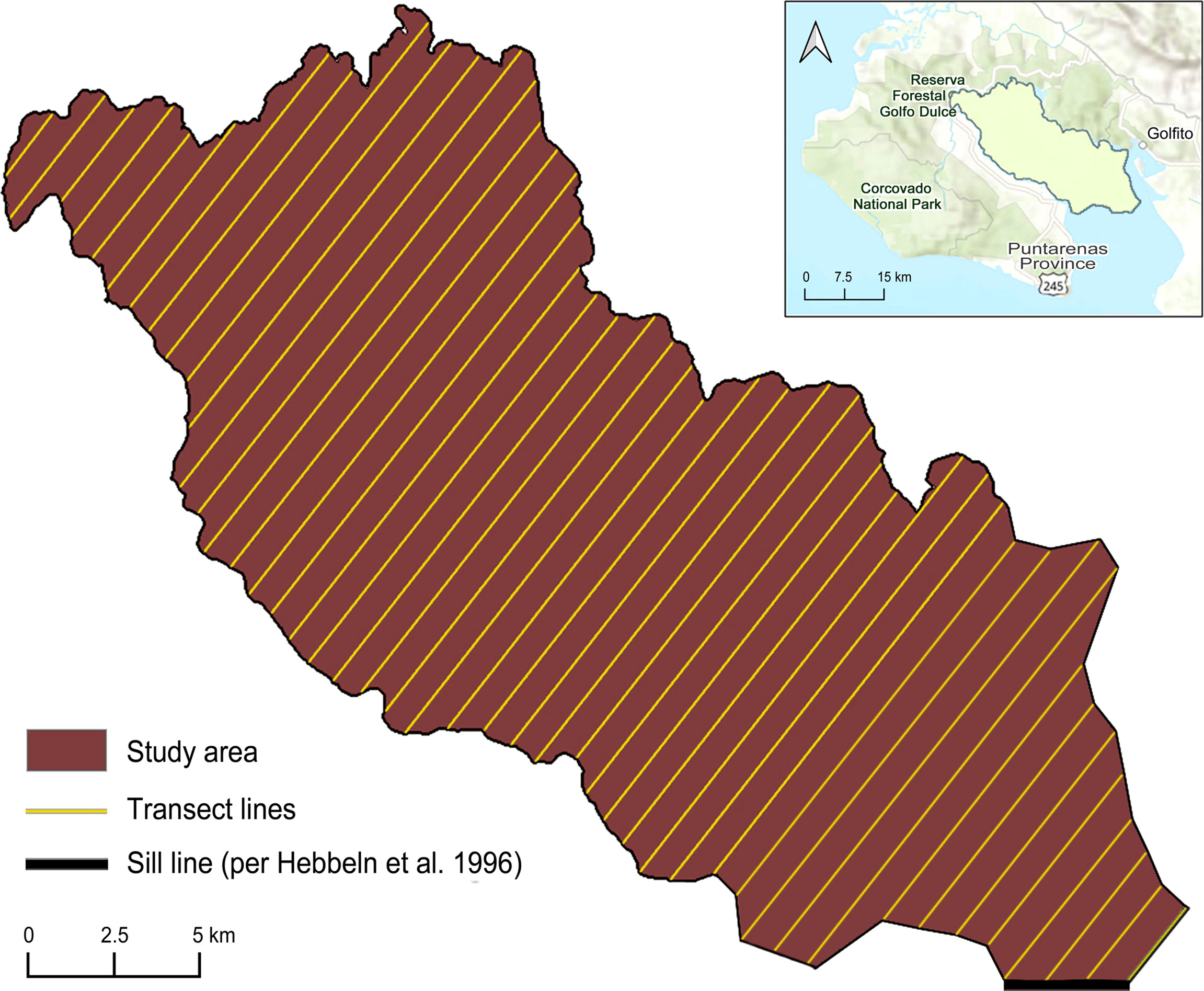
Figure 1 Study area with 46 transect lines spanning the inner basin of Golfo Dulce, Costa Rica; transects were generated in Distance 7.3 using a systematic design with a random starting point (Thomas et al., 2010), inset map created in ArcGIS (ESRI, 2011).
Distance Sampling
Both observers, the first author (BB) and research assistant Jorge Largaespada (JL), had previous experience detecting xanthic sea snakes, and conducted distance-estimation training prior to and during the survey. We completed 12 training sessions totaling 5 hours and 23 minutes with 165 trials (estimation attempts) following recommendations in Williams et al. (2017). Accuracy of visual estimates during training sessions was tested using an Aofar HX-700N/800H laser rangefinder (accurate to +/- 1m) and/or a small yellow buoy attached to a marked fishing line. Accuracy to +/- 1m was comparable between both observers with a combined precision rate of 98% achieved in the last 64 trials.
Transects were surveyed from 5–13 February 2020 following protocols in Buckland et al., 1993; Buckland et al., 2001; Buckland et al., 2015). As H. p. xanthos is nocturnal (Bessesen and González-Suárez, 2022), surveys were completed at night with observation hours typically beginning just after sunset and ending between 2200–0200 h. An expert local boat captain navigated our 7-m research vessel at 8–13 km per hour (generally 12 km/h) using a handheld Garmin GPSMAP 64 to maintain course following the transects. Three LED lamps were attached to the bow of the boat to illuminate forward waters from 90 degrees port (left) to 90 degrees starboard (right) and extending out to approximately 20 m distance. During the surveys, the observers remained at the bow (BB sitting and JL standing immediately behind) and continuously searched the area for snakes using additional handheld torches and headlamps as needed; visual coverage was not divided, but rather, both observers scanned the same span of water across the front and forward sides of the boat. Perception bias (the inability to detect snakes despite their presence) naturally occurs with increasing distance, a tenet of distance sampling, but neither darkness nor wave height caused additional perception bias because our lights shined through the water, eliminating most wave effect on visibility, and the reflectiveness of the yellow skin allowed ready detection of snakes within the defined observation area (Figure 2). When a detection occurred, the boat was immediately slowed, and the perpendicular distance of the snake to the centerline was visually estimated to the agreement of both observers. Full-time observational coverage was maintained by JL during data recording, and all details were logged before the boat resumed speed.
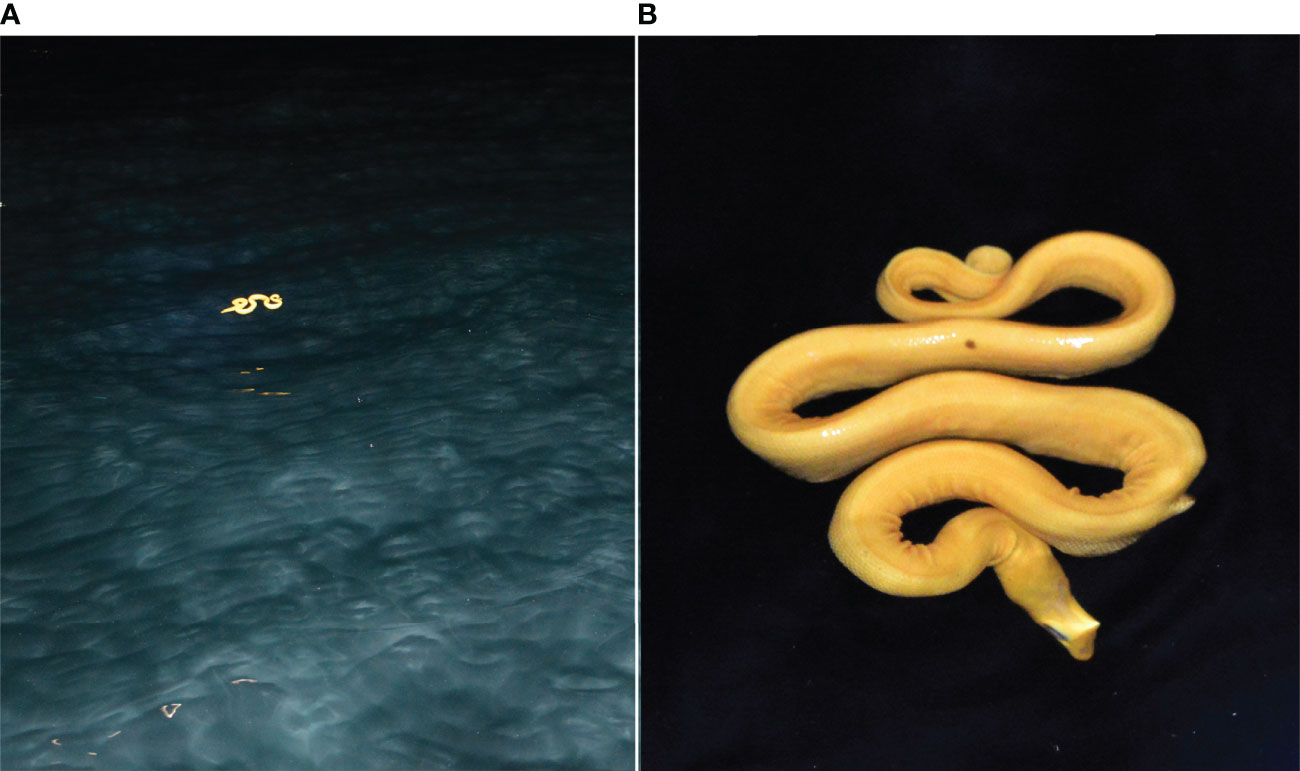
Figure 2 (H) p. xanthos floating at the sea surface at night: (A) seen at ~9 m distance; photography rendered a background darker than it appeared in situ; and (B) photographed at closer range; the light-induced distortions are artifacts; photo credit B. Bessesen.
Efforts were made to ensure detection of all snakes at the sea surface. To accurately utilize distance sampling methods, it is essential to obtain accurate perpendicular distances between the line and the detected snake before the snake changes its position in response to human presence. We first anticipated this would require us to obtain radial distance from the boat and the angle between the line and the snake at the time of first detection, which would then be converted into perpendicular distances; however, the snakes showed no reaction to our presence, allowing us to directly obtain perpendicular distances as we passed. In addition, the side of the boat (port or starboard) was recorded along with the date, time, GPS coordinates for boat location at detection, visibility, prevailing weather, and Beaufort wind force (BWF). Environmental variables were also recorded at the start and end of each track line and at 30-minute intervals during the search if no sightings were logged within the period.
Statistical Modeling
We used the program Distance (Thomas et al., 2010) and the package ‘Distance’ (Miller et al., 2019) in R (version 4.0.3; R Core Team, 2020) to model our line transect data. Three transect lines were repeated during the survey, and from each of these we analyzed the track with the highest recorded detections. Several conventional distance sampling and multiple covariate distance sampling models were explored. These included three key functions (half-normal, hazard rate, and uniform), with various adjustment terms (cosine or simple polynomial) and hour and BWF as covariates (Table 1). Hour and BWF were also tested for collinearity using a Kendall’s Tau coefficient test (cor function in R). Models were compared using the Akaike information criterion (AIC; Akaike, 1979) and the model with the lowest AIC score was deemed as the best fitting model. The input data for the models included the study area (A=394 km2), number of transect lines (k=46), survey effort by line length (L=469 km), visibility-based truncation width (w=20 m), coverage area (2wL=18.76 km2), and perpendicular distance of snake-centerline for each detection.
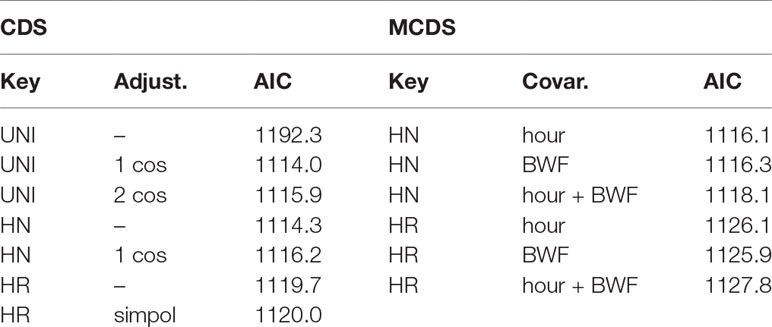
Table 1 AIC values for conventional distance sampling (CDS) and multiple covariate distance sampling (MCDS; with covariates hour and BWF) models, using the key functions of uniform (UNI), half-normal (HN) or hazard rate (HR), and adjustment terms of 1–2 cosines (cos) or simple polynomial (simpol); covariates cannot be used with the uniform key.
Because H. p. xanthos spends most of its time below the water surface, snakes at depth in the water column passed undetected violating the key assumption of distance sampling that detectability at the centerline is complete, often referred to as g(0)=1. As this is a common violation among diving marine animals, an availability bias correction has been proposed using dive data (Laake et al., 1997; Laake et al., 2004). We used the R package ‘hmltm’ (Borchers et al., 2013) to compute a correction factor based on the probability of an animal being at depth (unavailable for detection) or near the surface (available for detection). Specifically, we chose the ‘laake’ model (Laake et al., 1997) as it considers boat speed and farthest visible distance during the distance sampling survey. Because dive data are not available for H. p. xanthos we relied on data from Rubinoff et al. (1986) who used ultrasonic pressure transmitters with boat-based hydrophones and receivers to track 15 H. p. platurus off the coast of Panama for 3.8 to 31.2 hours. The published data included track time, dive count and average dive duration for each specimen. To focus only on full dive cycles, we first adjusted the published track time by removing any initial surface time prior to the first dive (incomplete dives at the end of the tracking period were already truncated). We then calculated the average dive cycle for each individual by dividing the adjusted track time by dive count. Average dive duration served as ‘unavailable’ time per cycle and was subtracted from the dive cycle to obtain average time ‘available’ (Table 2). Finally, we averaged the unavailable and available times across individuals, weighted by the adjusted track time (as we expected individuals tracked for longer to provide more reliable data). We acknowledge that using Rubinoff et al. (1986) dive data assumes time-at-the-surface and time-below-the-surface of H. p. platurus is similar to that of H. p. xanthos and that the reported diving-surfacing behavior was ‘normal’ and not affected by capture and anesthesia or device attachment (all specimens reportedly swam normally in the lab prior to their release).
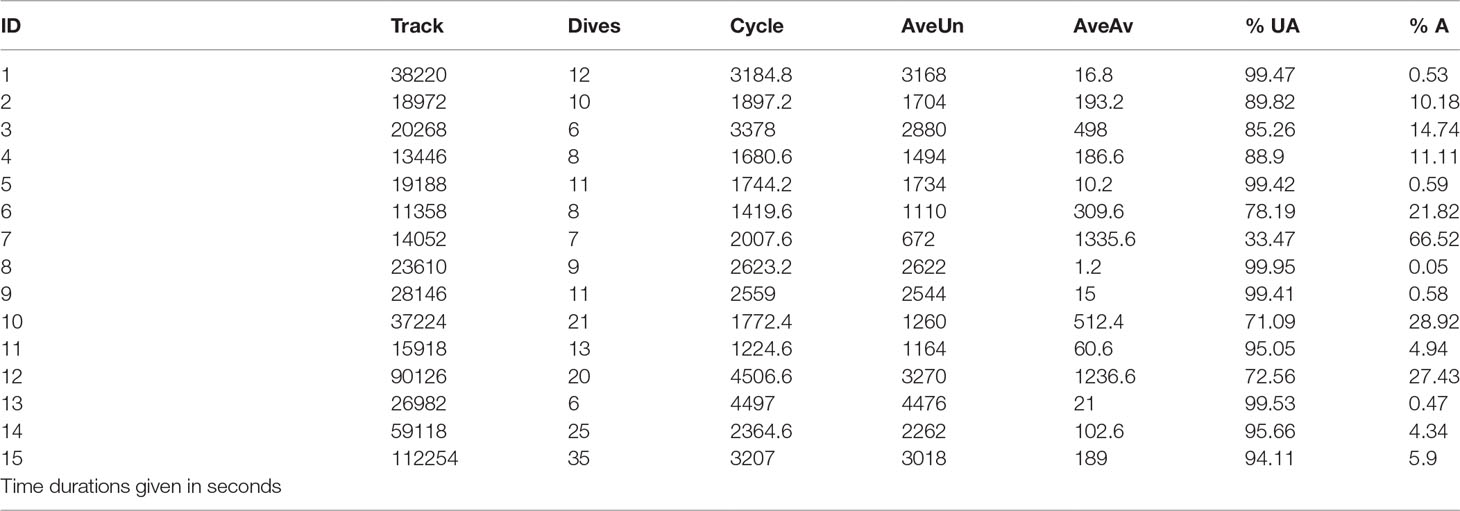
Table 2 Summary of availability data used for ‘laake’ correction, including specimen identification, adjusted time tracked, dive count, means for dive cycle, dive duration per cycle (AveUn), and surface duration per cycle (AveAv), with percentage of time unavailable and available, respectively; these data were calculated from dive data for Hydrophis [Pelamis] platurus (see Rubinoff et al., 1986).
Diurnal sightings of H. p. xanthos are rare, so we conducted night surveys to maximize detection probability, but in doing so we violated the assumption that distance sampling data are collected at random times in relation to the organism’s activity patterns. Also, the correction factor for availability, as described above, constitutes an average across a 24-hr period. To address potential biases resulting from this, we developed an activity bias correction method. We estimated surface activity rates using sighting data from a diel study with constant hourly survey effort across multiple 24-hour periods (Bessesen and González-Suárez, 2022). This was done by fitting a kernel density in the R package ‘activity’ (Rowcliffe, 2021), with a 1.5 kernel bandwidth multiplier as recommended by Rowcliffe et al. (2014), to calculate surface activity rates for each clock hour. We then compared the overall average for the complete 24-hour period with the narrower night-time period weighted by time spent in each hour during the distance sampling survey (to account for variable effort within the survey interval). The activity bias correction factor was defined as the ratio between these two rates (24-hrs rate/night-time rate).
The best estimate and 95% confidence intervals of abundance from the best-fitting conventional distance sampling model were multiplied by both correction factors to obtain a final estimate of abundance with uncertainty. We note that we could not account for uncertainty in the correction factors, so the confidence intervals may be too narrow. Lastly, population density was calculated by dividing the final corrected abundance estimate by the range of the study area (in km2). For comparison with pelagic sea snake studies that have reported an encounter-rate ‘density’ as snakes-per-hour (Kropach, 1971a; Kropach, 1973; Rubinoff et al., 1986; Lillywhite et al., 2015), we also report the average rate of snakes/hr during the distance-sampling survey period.
Results
During 45.9 observation hours over 9 nights of sampling, 203 H. p. xanthos sightings were recorded in sea states of BWF 2–5; no detections were made on the eight most south-easterly transect lines. After removal of data from repeated transects we analyzed 199 detections, all were estimated to be within 20 m from the centerline, a natural truncation of distance created by the limitations of our lights. The best conventional distance sampling model (lowest AIC; Table 1) fitted a uniform + cosine detection function, with the data grouped in 0.95-m intervals for an χ2 goodness-of-fit statistic of p=0.997 (Figure 3), although estimates from all models were comparable. Multiple covariate distance sampling models showed no effect of hour and/or BWF on detection by distance with the best model (lowest Delta AIC; Table 2) having no covariates. Likewise, we found no collinearity between hour and BWF [corr (Kendall)=-0.29; χ2 = 8.7, p<0.0001].
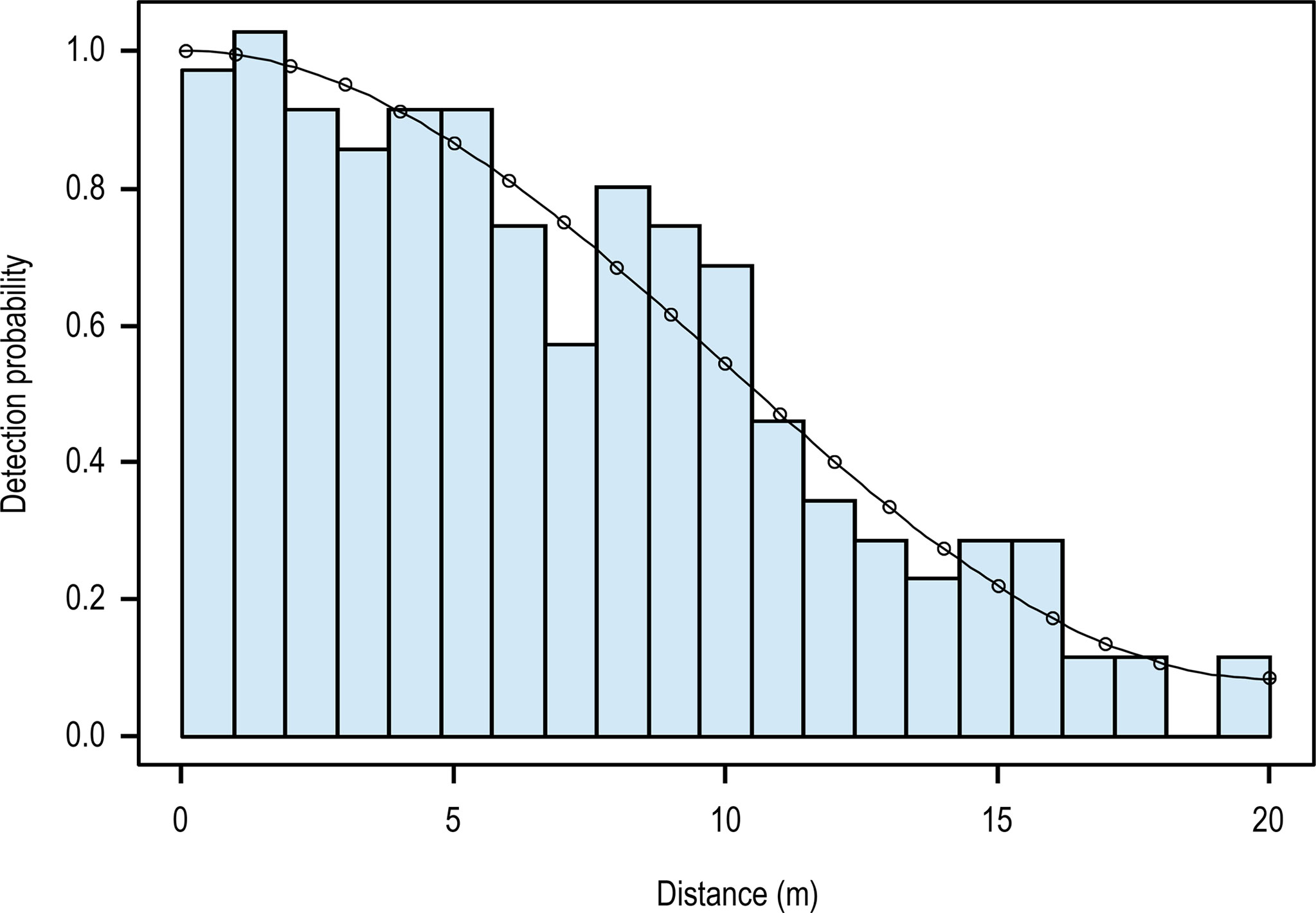
Figure 3 Detection function (uniform + cosine) fitted to 199 detections of xanthic sea snakes by distance with histogram of observed distances between the transect line and detected snakes; line indicates the estimated detection probabilities.
Using the uniform + cosine model, we estimated a baseline of 7705 individuals (95% CI =5202–11414). This value was then corrected applying the availability bias correction (=0.1287575) and the activity bias correction (=0.4976385) to return a total abundance of H. p. xanthos at 29781 individuals (95% CI=20104–44115). Spatial density (abundance/area) was calculated at 76 snakes/km2. Over the course of the distance-sampling survey, our encounter rate averaged 4.8 ± 5.5 snakes/h (range: 0–23).
Discussion
Hydrophis platurus xanthos is one of only two sea snake taxa in the Americas. Unlike transitory pelagic sea snakes, H. p. platurus, in the Pacific Ocean, the yellow sea snake is an obligate resident of the inner basin of Golfo Dulce, Costa Rica. We estimate its total population size as fewer than 30,000 individuals. For comparison, Kropach (1973) estimated the local population of pelagic sea snakes in just the Gulf of Panama to be up to a million individuals. Unlike the sizeable aggregations of H. p. platurus (100s or 1000s at a time) reported off Central America (Kropach, 1971a; Dunson and Ehlert, 1971; Tu, 1976; Vallarino and Weldon, 1996), no aggregations of H. p. xanthos have been observed in Golfo Dulce, and the lead author (BB) has recorded only 765 individual sightings working in the embayment since 2010 (> 880 observation hours). To our knowledge this study is the first quantitative abundance estimate for the global population of any sea snake taxon. Of the 47 species in the genus Hydrophis listed on The IUCN Red List (2022), abundance trends are unknown for 40 of them, and no global population estimates are provided. Obtaining abundance estimates on the species level is challenging because most sea snakes have wide and/or fragmented ranges and studies are typically site-specific with animals potentially leaving or arriving to the study area (Kropach, 1973; Bonnet et al., 2014). Studying a geographically bound population provided a substantial and uncommon advantage; still, we hope our methods may prove of some value to future studies of sea snake abundance in other areas.
Although distance sampling has been described as a viable method for snakes (Dorcas and Willson, 2009), studies of terrestrial species reported underestimations of population size due to small samples and low or inconsistent detections (Rodda and Campbell, 2002; Stellati et al., 2019). We obtained a sample size greater than three times the 60–80 detections recommended by Buckland et al. (2001) and Buckland et al. (2015). Also, given the snakes were at the surface, they were readily observable and did not react to our presence, hence, potential biases including perception bias and bias due to distance-errors were effectively eliminated. We still had to address some limitations in the data, as our observed detections could not account for the number of snakes below the sea surface. Additional steps included the novel application of a known availability bias correction (Laake et al., 1997) and the proposal of a new correction for activity bias. These corrections required making some assumptions, especially using dive data from a related species when diving behaviors may differ. For example, pelagic sea snakes dive to avoid turbulent waters (Rubinoff et al., 1986) while xanthic sea snakes regularly surface in higher sea states (BWF 3–5; Bessesen and González-Suárez, 2022). If having more flexibility in surfacing conditions and/or a steady diel pattern results in H. p. xanthos spending more time at the surface, our corrections could have overestimated the population size. Greater accuracy may be derived with taxon-specific dive data, but our estimates provide a first approximation to the total abundance of this unique yellow sea snake and serve as markers for future trend studies.
Density provides another gauge by which change can be assessed. We estimated a considerably lower spatial density than reported for other marine snake taxa. Judging density by square meter (0.000076 snakes/m2 for H. p. xanthos in our study area) allows comparison with marine snake studies more commonly conducted in small, designated areas. Saint Giron (1964), for example, estimated Laticauda colubrina in just one small inlet of New Caledonia at a density of 0.20/m2. Lading et al. (1991) recorded 721 L. colubrina on Kalampunian Damit Island (a study area of <0.5 km2), indicating a density estimate of 0.0014/m2. In a stretch of 3000 m along the edge of the Great Barrier Reef, Burns and Heatwole (Heatwole, 1999) estimated Aipysurus laevis density to be 0.70–0.94/m2. Shine et al. (2021) studied Emydocephalus annulatus at three 20 x 350 m sites in New Caledonia and found annual densities of 0.005–0.036/m2 (estimates based on published data).
For herpetofauna, an encounter rate is sometimes used as a relative ‘density’ measurement. In the Pacific Ocean, Rubinoff et al. (1986) reported up to 165 pelagic sea snakes per hour, and Kropach (1971a) recorded 4–180 pelagic sea snakes per hour in Panama. In northern Costa Rica, Lillywhite et al. (2015) once counted 1029 pelagic sea snakes in a single hour, though their average was only ~19 snakes/hr. Inside Golfo Dulce, that team published an encounter rate of ~21 xanthic sea snakes per hour during two nights of collection (17/h the first night and 25.7/h the second night). This is comparable to the highest encounter rate for xanthic sea snakes ever recorded by the lead author (29/hr during an around-the-clock diel study; Bessesen & González-Suárez, 2022); however, across all studies, variance between nightly counts was apparent, likely associated to environmental conditions that are more or less suitable for surfacing. Hence, Lillywhite’s team may have benefitted from a small sample collected under ideal conditions. Multiple environmental variables are likely to influence surfacing behavior, including the photoperiod, wind force, red tide (which does occur in Golfo Dulce; pers. obs.), weather, lunar phase, productively, and other factors that have not yet been identified (Lillywhite et al., 2015; Bessesen & González-Suárez, 2022). Precipitation, for example, could draw more individuals to the surface and/or extend the surfacing duration at a particular moment since sea snakes are known to drink from fresh-water lenses formed by rain (Lillywhite et al., 2014, Lillywhite et al., 2019). Relatedly, seasonality could also contribute. Counts of xanthic sea snakes were generally the same during dry- and rain-season sighting surveys (Bessesen, 2015), though the data were too limited for robust comparisons. When searching for pelagic sea snakes in the northern Pacific waters off Costa Rica, Lillywhite et al. (2015) reported similar numbers year-round but with a tendency for more sightings in the dry season. If the same is true for H. p. xanthos in Golfo Dulce, then our February distance-sampling detections could have overrepresented the number of surfacing snakes, leading to an overestimation of the population.
Our findings suggest the H. p. xanthos population is quite small and because sea snakes are known to exhibit k-selected traits, including low fecundity (clutch size of H. p. platurus is 1-6: Visser, 1967), which can reduce their resistance to negative impacts (Heatwole, 1999; Rao et al., 2021), Golfo Dulce’s endemic sea snake may be particularly vulnerable to threats. Current anthropogenic hazards include boat strikes and water contamination. There has been a substantial increase in boat traffic over the last decade (Bessesen and González-Suárez, 2021), and snakes can be injured in collisions (pers. obs.), plus water quality in the gulf is declining (Spongberg, 2004; Fournier et al., 2019). The destabilizing effects of climate change may be creating additional challenges. Sea snakes exhibit thermal sensitivity (Heatwole et al., 2012) and surface waters in Golfo Dulce appear to be rising (Murayama et al., 2018). To presumably help H. p. xanthos avoid overheating in the relatively warm waters of their habitat, these snakes have already lost the melanistic dorsum of their ancestors (Solórzano, 2011) and switched to a nocturnal diel cycle (Bessesen, 2012; Lillywhite et al., 2015). During the day, light skin and eyes risk damage from solar radiation and it is possible that conspicuous yellow coloring exposes the snakes to predation, especially by avian hunters (Bessesen and González-Suárez, 2022), as well as harassment by dolphins (Bessesen et al., 2021); because they must regularly surface to ventilate, nocturnal feeding mitigates but does not eliminate those risks. For sea snakes, access to fresh drinking water is limited in the marine environment (Rash and Lillywhite, 2019), making rainwater lenses critical for hydration (Lillywhite et al., 2019). Regional precipitation is naturally reduced during the dry season, and. climate-induced environmental changes, including stochastic periods of drought, could reduce long-term sustainability of the xanthic population (Lillywhite et al., 2018; Udyawer et al., 2018). If H. p. xanthos has existed as a small population since its origin, selection may have naturally purged deleterious alleles and thereby mitigated potential genetic decline, providing some resistance to bottlenecking (Morin et al., 2021). Nevertheless, because this unique and geographically rare population occurs in a single location with small area of occupancy, it is inherently vulnerable.
Importantly, H. p. xanthos is not just a residential colony of H. p. platurus. Although a few all-yellow specimens have been found outside Golfo Dulce (Kropach, 1971b; Bolaños et al., 1974), suggesting individuals may occasionally escape (Bessesen, 2015) and rarely a pelagic snake may be carried into the embayment (Bessesen and Galbreath, 2017), interbreeding is unlikely (unpub. data). The population appears allopatric (Sheehy et al., 2012) and has been isolated from its yellow-bellied conspecifics sufficiently long to show morphological and behavioral divergence (Bessesen and Galbreath, 2017; Bessesen and González-Suárez, 2022). Indeed, lacking genetic exchange with sea snakes from the broader Indo-Pacific, H. p. xanthos has become a highly discrete organism, an ‘evolutionarily significant unit’ (ESU; per Ryder, 1986; Casacci et al., 2014), which may ultimately be considered for full species status (Bessesen and Galbreath, 2017). Our first population and density estimates of H. p. xanthos fill an important knowledge gap, but future work will be necessary to evaluate change in abundance, range size, and extinction risk. Count comparisons from three sighting surveys conducted over a ten-year period encouragingly suggest a stable or possibly growing population (unpubl. data), but caution is needed as we lack historical estimates or true time series of abundance. In the meantime, a conservative approach is recommended.
Since H. p. xanthos is part of a larger biotic community, and Rasmussen et al. (2021) demonstrated sea snakes to be bioindicators, monitoring this population could provide insights into the status of other marine fauna in its habitat. Costa Rica’s Ministry of Environment (MINAE) and National System of Conservation Areas (SINAC) have made considerable efforts to preserve their country’s rich biodiversity with increasing focus on marine resources (Alvarado et al., 2012; Palacios Martinez, 2016). Our study highlights the importance of additional protections and regulatory enforcement in Golfo Dulce, which could serve to ensure the future of their unique endemic sea snake, H. p. xanthos.
Data Availability Statement
The raw data supporting the conclusions of this article will be made available by the authors, without undue reservation.
Ethics Statement
Ethical review and approval was not required for the animal study because there was no contact with or disturbance of any study animals.
Author Contributions
BB conceived and designed the study, collected, and analyzed the data, and led the writing of the manuscript. CO guided and inspected the statistical analyses and reviewed the manuscript. GG contributed to interpretation of the results and preparation of the manuscript. MG-S participated in the methodology, data analyses, and manuscript writing. All authors contributed to the article and approved the submitted version.
Funding
This work was not supported by any funding organizations; open access publication fees were provided by the University of Reading.
Conflict of Interest
The authors declare that the research was conducted in the absence of any commercial or financial relationships that could be construed as a potential conflict of interest.
Publisher’s Note
All claims expressed in this article are solely those of the authors and do not necessarily represent those of their affiliated organizations, or those of the publisher, the editors and the reviewers. Any product that may be evaluated in this article, or claim that may be made by its manufacturer, is not guaranteed or endorsed by the publisher.
Acknowledgments
This research was supported by the University of Reading School of Biological Sciences with tremendous thanks to Len Thomas at the University of St. Andrews for instruction on distance sampling when animals are missed at zero distance and suggestions for how to address activity bias. Fieldwork was conducted under Costa Rican research permit N°: SINAC-ACOSA-DT-PI-R-010-2019 issued by the Ministry of Environment and Energy (MINAE) and National System of Conservation Areas (SINAC) with the institutional sponsorship of Osa Conservation. We sincerely thank our field team: observer Jorge Largaespada and boat captains Luis Nieto Vásquez and Marisol Jose Eladio. Thanks to Harvey Woodard and Susan Rogers for providing superb food and lodging at Saladero Eco Lodge. Additional support was provided by Mike Boston, Julio Ranalli, Guido Saborío Rodriguez, and Elizabeth Makings.
References
Akaike H. (1979). A Bayesian Extension of the Minimum AIC Procedure of Autoregressive Model Fitting. Biometrika 66 (2), 237–242. doi: 10.1093/biomet/66.2.237
Alvarado J. J., Cortés J., Esquivel M. F., Salas E. (2012). Costa Rica’s Marine Protected Areas: Status and Perspectives. Rev. Biología Trop. 60 (1), 129–142. doi: 10.15517/rbt.v60i1.2657
Berrangé J. P. (1989). The Osa Group: An Auriferous Pliocene Sedimentary Unit From the Osa Peninsula, Southern Costa Rica. RGAC 10, 67–93. doi: 10.15517/rgac.v0i10.13002
Bessesen B. L. (2012). Geospatial and Behavioral Observations of a Unique Xanthic Colony of Pelagic Sea Snakes, Pelamis Platurus, Residing in Golfo Dulce, Costa Rica. Herpetological Rev. 43, 22–22.
Bessesen B. L. (2015). Occurrence and Distribution Patterns of Several Marine Vertebrates in Golfo Dulce, Costa Rica. Rev. Biología Trop. 63 (Supl.1), 261–272. doi: 10.15517/rbt.v63i1.23106
Bessesen B. L., Galbreath G. J. (2017). A New Subspecies of Sea Snake, Hydrophis Platurus Xanthos, From Golfo Dulce, Costa Rica. ZooKeys 686, 109–123. doi: 10.3897/zookeys.686.12682
Bessesen B. L., González-Suárez M. (2021). The Value and Limitations of Local Ecological Knowledge: Longitudinal and Retrospective Assessment of Flagship Species in Golfo Dulce, Costa Rica. People Nat. 3, 627–638. doi: 10.1002/pan3.10219
Bessesen B. L., González-Suárez M. (2022). Safe From Sunburn: The Divergent Diel Pattern of a Hydrophis Sea Snake. Ecol. Evol. 12 (1), e8436. doi: 10.1002/ece3.8436
Bessesen B. L., González-Suárez M., Herra-Miranda D., Oviedo L. (2021). Hydrophis Platurus Xanthos (Golfo Dulce Yellow Seasnake): Harassment by Dolphins. Natural History Note. Herpetological Rev. 52 (2), 425–426.
Böhm M., Collen B., Baillie J. E. M., Bowles P., Chanson J., Cox N., et al. (2013). The Conservation Status of the World’s Reptiles. Biol. Conserv. 157, 372–385. doi: 10.1016/j.biocon.2012.07.015
Bolaños R., Flores A., Taylor R., Cerdas L. (1974). Color Patterns and Venom Characteristics in Pelamis Platurus. Copeia 1974 (4), 909–912. doi: 10.2307/1442589
Bonnet X., Brischoux F., Bonnet C., Plichon P., Fauvel T. (2014). Coastal Nurseries and Their Importance for Conservation of Sea Kraits. PLoS One 9 (3), e90246. doi: 10.1371/journal.pone.0090246
Borchers D. L., Zucchini W., Heide-Jørgensen M. P., Cañadas A., Langrock R. (2013). Using Hidden Markov Models to Deal With Availability Bias on Line Transect Surveys. Biometrics 69, 703–713. doi: 10.1111/biom.12049
Brischoux F., Lillywhite H. B. (2011). Light- and Flotsam-Dependent ‘Float-and-Wait’ Foraging by Pelagic Sea Snakes (Pelamis Platurus). Mar. Biol. 158, 2343–2347. doi: 10.1007/s00227-011-1738-z
Buckland S. T., Anderson D. R., Burnham K. P., Laake J. L. (1993). Distance Sampling: Estimating Abundance of Biological Populations (New York: Springer), 446 pp.
Buckland S. T., Anderson D. R., Burnham K. P., Laake J., Borchers D. L., Thomas L. (2001). Introduction to Distance Sampling: Estimating Abundance of Biological Populations (Oxford: Oxford University Press), 448 pp.
Buckland S. T., Rexstad E. A., Marques T. A., Oedekoven C. S. (2015). Distance Sampling: Methods and Applications (New York: Springer), 277 pp.
Cardinale B. J., Duffy J. M., Gonzalez A., Hooper D. U., Perrings C., Venail P., et al. (2012). Biodiversity Loss and its Impact on Humanity. Nature 486, 59–67. doi: 10.1038/nature11148
Casacci L. P., Barbero F., Balletto E. (2014). The “Evolutionarily Significant Unit” Concept and its Applicability in Biological Conservation. Ital. J. Zoology 81 (2), 182–193. doi: 10.1080/11250003.2013.870240
Doniol-Valcroze T., Gosselin J.-F., Pike D., Lawson J., Asselin N., Hedges K., et al. (2015). Abundance Estimates of Narwhal Stocks in the Canadian High Arctic in 2013 (Ottawa, Canada: Canadian Science Advisory Secretariat (CSAS) Research Document) Available at: https://waves-vagues.dfo-mpo.gc.ca/Library/365100.pdf.
Dorcas M. E., Willson J. D. (2009). “Innovative Methods for Studies of Snake Ecology and Conservation,” in Snakes: Ecology and Conservation. Eds. Mullin S. J., Seigel R. A. (Ithaca NY: Comstock Publishing Associates), 365 pp.
Dornelas M., Antão L. H., Moyes F., Bates A. E., Magurran A. E., Adam D., et al. (2018). BioTIME: A Database of Biodiversity Time Series for the Anthropocene. Global Ecol. Biogeography 27, 760–786. doi: 10.1111/geb.12729
Dulvy N. K., Sadovy Y., Reynolds J. D. (2003). Extinction Vulnerability in Marine Populations. Fish Fish 4, 25–64. doi: 10.1046/j.1467-2979.2003.00105.x
Dunson W. A., Ehlert G. W. (1971). Effects of Temperature, Salinity, and Surface Water Flow on Distribution of the Sea Snake Pelamis. Limnol Oceanogr 16 (6), 845–853. doi: 10.4319/lo.1971.16.6.0845
Eberhardt L. L. (1978). Transect Methods for Population Studies. J. Wildlife Manage. 42 (1), 1–31. doi: 10.2307/3800685
Elfes C. T., Livingstone S. R., Lane A., Lukoschek V., Sanders K. L., Courney A. J., et al. (2013). Fascinating and Forgotten: The Conservation Status of Marine Elapid Snakes. Herpetological Conserv. Biol. 8 (1), 37–52.
Fournier M. L., Castillo L. E., Ramírez F., Moraga G., Ruepert C. (2019). Evaluación Preliminar Del Área Agrícola Y Su Influencia Sobre La Calidad Del Agua En El Golfo Dulce, Costa Rica. RCA 53 (1), 92–112. doi: 10.15359/rca.53-1.5
Fry G., Milton D., Wassenberg T. (2001). The Reproductive Biology and Diet of Sea Snake Bycatch of Prawn Trawling in Northern Australia: Characteristics Important for Assessing the Impacts on Populations. Pacific Conserv. Biol. 7, 55–73. doi: 10.1071/PC010055
Gibbons J. W., Scott D. E., Ryan T. J., Buhlmann K. A., Tuberville T. D., Metts B. S., et al. (2000). The Global Decline of Reptiles, Déjà Vu Amphibians. BioScience 50, 653–666. doi: 10.1641/0006-3568(2000)050[0653:TGDORD]2.0.CO;2
Goiran C., Shine R. (2013). Decline in Sea Snake Abundance on a Protected Coral Reef System in the New Caledonian Lagoon. Coral Reefs 32, 281–284. doi: 10.1007/s00338-012-0977-x
Heatwole H., Grech A., Monahan J. F., King S., Marsh H. (2012). Thermal Biology of Sea Snakes and Sea Kraits. Integr. Comp. Biol. 52, 257–273. doi: 10.1093/icb/ics080
Hebbeln D., Beese D., Cortes J. (1996). Morphology and Sediment Structures in Golfo Dulce, Costa Rica. Rev. Biología Trop. 44 (3), 1–10. doi: 10.1007/s003670000047
Hebbeln D., Cortés J. (2001). Sedimentation in a Tropical Fiord: Golfo Dulce, Costa Rica. Geo-Marine Lett. 20 (3), 142–148. doi: 10.1007/s003670000047
Hector A., Bagchi R. (2007). Biodiversity and Ecosystem Multifunctionality. Nature 448, 188–191. doi: 10.1038/nature05947
IPBES (2019). “Draft Chapter 2.2 Status and Trends – Nature,” in The Global Assessment Report on Biodiversity and Ecosystem Services. Eds. Brondizio E. S., Settele J., Díaz S., Ngo H. T. (France: IPBES Plenary at its 7th session in May 2019 in Paris).
Jaramillo-Legorreta A. M., Rojas-Bracho L., Gerrodette T. (1999). A New Abundance Estimate for Vaquitas, First Step for Recovery. Mar. Mammal Sci. 15, 957–973. doi: 10.1111/j.1748-7692.1999.tb00872.x
Kreb D., Lhota S., Porter L., Redman A., Susanti I., Lazecky M. (2020). Long-Term Population and Distribution Dynamics of an Endangered Irrawaddy Dolphin Population in Balikpapan Bay, Indonesia in Response to Coastal Development. Front. Mar. Sci. 7, 533197. doi: 10.3389/fmars.2020.533197
Kropach C. (1971a). Sea Snake (Pelamis Platurus) Aggregations on Slicks in Panama. Herpetologica 27, 131–135.
Kropach C. (1971b). Another Color Variety of the Sea-Snake Pelamis Platurus From Panama Bay. Herpetologica 27 (3), 326–327.
Kropach C. (1973). A Field Study of the Sea Snake Pelamis Platurus (Linnaeus) in the Gulf of Panama. Ph.D. Thesis (Flushing, New York: City University of New York, Flushing), 200 pp. Available at: https://www.worldcat.org/search?qt=hotseries&q=se%3A%22%2873-11%2C357%29.%22
Laake J., Borchers L., Borchers D. L. (2004). “Methods for Incomplete Detection at Distance Zero. Pp. 108–189,” in Advanced Distance Sampling. Eds. Buckland S. T., Anderson D. R., Burnham K. P., Laake J. L., Borchers D. L., Thomas L. (Oxford: Oxford University Press).
Laake J. L., Calambokidis J., Osmek S. D., Rugh D. J. (1997). Probability of Detecting Harbor Porpoise From Aerial Surveys: Estimating G(0). J. Wildlife Manage. 61 (1), 63–75. doi: 10.2307/3802415
Lading E. A., Stuebing R. B., Voris H. K. (1991). A Population Size Estimate of the Yellow-Lipped Sea Krait, Laticauda Colubrina, on Kalampunian Damit Island, Sabah, Malaysia. Copeia 1991 (4), 1139–1142. doi: 10.2307/1446117
Lillywhite HB. 2014. How Snakes Work: Structure, Function, and Behavior of the World’s Snakes. New York: Oxford University Press. 256 pp.
Lillywhite H. B., Sheehy III CM, Brischoux F., Pfaller J. B. (2015). On the Abundance of a Pelagic Sea Snake. J. Herpetology 49 (2), 184–189. doi: 10.1670/14-004
Lillywhite H. B., Sheehy III CM, Heatwole H., Brischoux F., Steadman D. W. (2018). Why are There No Sea Snakes in the Atlantic? BioScience. 68, 15–24. doi: 10.1093/biosci/bix132
Lillywhite H. B., Sheehy III CM, Sandfoss M. R., Crowe-Riddell J., Grech A. (2019). Drinking by Sea Snakes From Oceanic Freshwater Lenses at First Rainfall Ending Seasonal Drought. PLoS One 14 (2), e0212099. doi: 10.1371/journal.pone.0212099
Lukoschek V., Berger M., Ceccarelli D., Richards Z., Pratchett M. (2013). Enigmatic Declines of Australia’s Sea Snakes From a Biodiversity Hotspot. Biol. Conserv. 166, 191–202. doi: 10.1016/j.biocon.2013.07.004
Malzer O., Fiebig M. (2008). Outline of the Geology of the Golfo Dulce Region (Costa Rica) and its Surroundings in Central America. Stapfia 88, 23–30.
Miller L. J., Mackey A. D., Solangi M., Kuczaj S. A. (2013). Population Abundance and Habitat Utilization of Bottlenose Dolphins in the Mississippi Sound. Aquat. Conservation: Mar. Freshw. Ecosyst. 23, 145–151. doi: 10.1002/aqc.2278
Miller D. L., Rexstad E., Thomas L., Marshall L., Laake J. L. (2019). Distance Sampling in R. J. Stat. Software 89 (1), 1–28. doi: 10.18637/jss.v089.i01
Morales-Ramírez A., Acuña-González J., Lizano O., Alfaro E., Gómez E. (2015). Rasgos Oceanográficos En El Golfo Dulce, Pacífico De Costa Rica: Una Revisión Para La Toma De Decisiones En Conservación Marina. Rev. Biología Trop. 63 (Supl.1), 131–160. doi: 10.15517/rbt.v63i1.23100
Morin P. A., Archer F. I., Avila C. D., Balacco J. R., Bukhman Y. V., Chouw W., et al. (2021). Reference Genome and Demographic History of the Most Endangered Marine Mammal, the Vaquita. Mol. Ecol. Resour. 21, 1008–1020. doi: 10.1111/1755-0998.13284
Murayama H., Barney M., Esmaeili Neyestani S., Furey S. (2018). Evaluating Potential Sites for Coral Reef Rehabilitation in the Golfo Dulce, Costa Rica Based on Turbidity and Sea Surface Temperature (Georgia: NASA DEVELOP National Program technical report), 26 pp.
Palacios Martinez G. E. (2016). Justificación Técnica Para La Creación De La Reserva Marina “Álvaro Ugalde Víquez” (Puntarenas Province, Costa Rica: Reporte técnico de Centro Cientifico Tropical), 56 pp.
Pimm S. L., Russell G. J., Gittleman J. L., Brooks T. M. (1995). The Future of Biodiversity. Science 269, 347–350. doi: 10.1126/science.269.5222.347
Poloczanska E. S., Burrows M. T., Brown C. J., García Molinos J., Halpern B. S., Hoegh-Guldberg O., et al. (2016). Responses of Marine Organisms to Climate Change Across Oceans. Front. Mar. Sci. 3, 62. doi: 10.3389/fmars.2016.00062
Rao C., Dsauza S., Gupta T., Manoharakrishnan M., Lobo A. S. (2021). Fisheries Induced Shift in Sea Snake Community Assemblages Along the Konkan Coast, India. Aquat. Conservation: Mar. Freshw. Ecosyst. 31, 2402–2411. doi: 10.1002/aqc.3658
Rash R., Lillywhite H. B. (2019). Drinking Behaviors and Water Balance in Marine Vertebrates. Mar. Biol. 166, 122. doi: 10.1007/s00227-019-3567-4
Rasmussen A. R., Hay-Schmidt A., Boneka F., Allentoft M. E., Sanders K. L., Elberg J. (2021). Viviparous Sea Snakes Can be Used as Bioindicators for Diverse Marine Environments. Philippine J. Systematic Biol. 14 (2), 1–16. doi: 10.26757/pjsb2020b14013
R Core Team (2020) R: A Language and Environment for Statistical Computing (Vienna, Austria: R Foundation for Statistical Computing) (Accessed 9 November 2020).
Reading C. J., Luiselli L. M., Akani G. C., Bonnet X., Amori G., Ballouard J. M., et al. (2010). Are Snake Populations in Widespread Decline? Biol. Lett. 6, 777–780. doi: 10.1098/rsbl.2010.0373
Rodda G. H., Campbell E. W. (2002). Distance Sampling of Forest Snakes and Lizards. Herpetological Rev. 33, 271–274.
Rowcliffe J. M., Kays R., Kranstauber B., Carbone C., Jansen P. A. (2014). Quantifying Levels of Animal Activity Using Camera Trap Data. Methods Ecol. Evol. 5, 1170–1179. doi: 10.1111/2041-210X.12278
Rubinoff I., Graham J. B., Motta J. (1986). Diving of the Sea Snake Pelamis Platurus in the Gulf of Panama I. Dive Depth and Duration. Mar. Biol. 91, 181–191. doi: 10.1007/bf00569434
Ryder O. A. (1986). Species Conservation and Systematics: The Dilemma of Subspecies. Tree 1 (1), 9–10. doi: 10.1016/0169-5347(86)90059-5
Saint Girons H. (1964). Notes Sur L’ecologie Et La Structure Des Populations Des Laticaudinae (Serpentes, Hydrophiidae) En Novelle Caledonie. Terre Vie 111, 185–214.
Sheehy III CM, Solórzano A., Pfaller J. B., Lillywhite H. B. (2012). Preliminary Insights Into the Phylogeography of the Yellow-Bellied Sea Snake, Pelamis Platurus. Integr. Comp. Biol. 52, 321–330. doi: 10.1093/icb/ics088
Shine R., Brown G. P., Goiran C. (2021). Population Dynamics of the Sea Snake Emydocephalus Annulatus (Elapidae, Hydrophiinae). Sci. Rep. 11, 20701. doi: 10.1038/s41598-021-00245-2
Solórzano A. (2011). Variación De Color De La Serpiente Marina Pelamis Platura (Serpentes: Elapidae) En El Golfo Dulce, Puntarenas, Costa Rica. Cuadernos Investigación UNED 3, 89–96. doi: 10.22458/urj.v3i1.211
Somaweera R., Udyawer V., Guinea M. L., Ceccarelli D. M., Clark R. H., Glover M., et al. (2021). Pinpointing Drivers of Extirpation in Sea Snakes: A Synthesis of Evidence From Ashmore Reef. Front. Mar. Sci. 8, 658756. doi: 10.3389/fmars.2021.658756
Spongberg A. (2004). PCB Contamination in Marine Sediments From Golfo Dulce, Pacific Coast of Costa Rica. Rev. Biología Trop. 52, 23–32.
Steffen W., Crutzen P. J., McNeill J. R. (2007). The Anthropocene: Are Humans Now Overwhelming the Great Forces of Nature. AMBIO: A J. Hum. Environ. 36 (8), 614–621. doi: 10.1579/0044-7447(2007)36[614:TAAHNO]2.0.CO;2
Stellati L., Borgianni N., Bissattini A. M., Buono V., Haubrock P. J., Balzani P., et al. (2019). Living With Aliens: Suboptimal Ecological Condition in Semiaquatic Snakes Inhabiting a Hot Spot of Allodiversity. Acta Oecologica 100, 103466. doi: 10.1016/j.actao.2019.103466
Svendsen H., Rosland R., Myking S., Vargas J. A., Lizano O. G., Alfaro E. J. (2006). A Physical Oceanographic Study of Golfo Dulce, Costa Rica. Rev. Biología Trop. 54, 147–170.
Thomas L., Buckland S. T., Rexstad E. A., Laake J. L., Strindberg S., Hedley S. L., et al. (2010). Distance Software: Design and Analysis of Distance Sampling Surveys for Estimating Population Size. J. Appl. Ecol. 47, 5–14. doi: 10.1111/j.1365-2664.2009.01737.x
Tu A. T. (1976). Investigation of the Sea Snake, Pelamis Platurus (Reptilia, Serpentes, Hydrophiidae), on the Pacific Coast of Costa Rica, Central America. J. Herpetology 10 (1), 13–18. doi: 10.2307/1562922
Udyawer V., Barnes P., Bonnet X., Brischoux F., Crowe-Riddell J. M., D’Anastasi B., et al. (2018). Future Directions in the Research and Management of Marine Snakes. Front. Mar. Sci. 5 Article 399. doi: 10.3389/fmars.2018.00399
Vallarino O., Weldon P. J. (1996). Reproduction in the Yellow-Bellied Sea Snake (Pelamis Platurus) From Panama: Field and Laboratory Observations. Zoo Biol. 15, 309–314. doi: 10.1002/(SICI)1098-2361(1996)15:3<309::AID-ZOO9>3.0.CO;2-F
Visser J. (1967). Color Varieties, Brood Size, and Food of South African Pelamis Platurus (Ophidia: Hydrophiidae). Copeia 1967 (1), 219. doi: 10.2307/1442197
Voris H. K. (1985). Population Size Estimates for a Marine Snake (Enhydrina Schistosa) in Malaysia. Copeia 1985 (4), 955–961. doi: 10.2307/1445247
Williams R., Ashe E., Gaut K., Gryba R., Moore J. E., Rexstad E., et al. (2017). Animal Counting Toolkit: A Practical Guide to Small-Boat Surveys for Estimating Abundance of Coastal Marine Mammals. Endangered Species Res. 34, 149–165. doi: 10.3354/esr00845
Wolff M., Hartman H. J., Koch V. (1996). A Pilot Trophic Model for Golfo Dulce, A Tropical Fjord-Like Embayment, Costa Rica. Rev. Biología Trop. 44, 215–231.
Keywords: abundance estimation models, activity bias, availability bias, distance sampling, Golfo Dulce yellow sea snake, Hydrophis platurus xanthos, line transect survey, marine reptile
Citation: Bessesen BL, Oedekoven CS, Galbreath GJ and González-Suárez M (2022) Population Abundance and Density Estimates for Costa Rica’s Endemic Sea Snake, Hydrophis platurus xanthos. Front. Mar. Sci. 9:924966. doi: 10.3389/fmars.2022.924966
Received: 21 April 2022; Accepted: 02 June 2022;
Published: 07 July 2022.
Edited by:
Salvatore Siciliano, Fundação Oswaldo Cruz (Fiocruz), BrazilReviewed by:
Harvey B. Lillywhite, University of Florida, United StatesColeman Sheehy, Florida Museum of Natural History, United States
Copyright © 2022 Bessesen, Oedekoven, Galbreath and González-Suárez. This is an open-access article distributed under the terms of the Creative Commons Attribution License (CC BY). The use, distribution or reproduction in other forums is permitted, provided the original author(s) and the copyright owner(s) are credited and that the original publication in this journal is cited, in accordance with accepted academic practice. No use, distribution or reproduction is permitted which does not comply with these terms.
*Correspondence: Brooke L. Bessesen, Yi5sLmJlc3Nlc2VuQHBnci5yZWFkaW5nLmFjLnVr