- 1Instituto Politécnico Nacional, Centro Interdisciplinario de Ciencias Marinas, La Paz, BCS, Mexico
- 2Mobula Conservation, La Paz, BCS, Mexico
- 3Pelagios Kakunja A.C, La Paz, BCS, Mexico
- 4The Manta Trust, Corscombe, Dorset, United Kingdom
- 5Ocean Ecology Lab, Marine Mammal Institute, Department of Fisheries, Wildlife & Conservation Sciences, Oregon State University, Newport, OR, United States
- 6Department of Ecology and Evolutionary Biology, University of California Santa Cruz, Santa Cruz, CA, United States
- 7Nicholas School of the Environment, Duke University, Durham, NC, United States
- 8Institute of Marine Science, University of California Santa Cruz, Santa Cruz, CA, United States
- 9Tethys Research Institute, Milan, Italy
Manta and devil rays (mobulids) are filter feeding elasmobranchs with extreme K-selective life histories found circumglobally from temperate to tropical waters. Their vulnerability to fisheries exploitation, bycatch, boat collisions, entanglement and unregulated tourism is exacerbated by their aggregative behavior. Studies have identified aggregation sites around the world for all nine mobulid species, with these groupings varying from a few individuals to thousands. However, the terminology used to define these aggregations and the drivers underpinning them remain unclear, hindering the development of effective management and conservation strategies. Here, we analyze aggregation behavior for mobulid species, providing consistent definitions for grouping events and summarizing the existing research on drivers and environmental factors triggering these events. We find that aggregation behaviors facilitate socializing and key life history functions in mobulids, including feeding, courtship and mating, predation avoidance, cleaning, and thermoregulation. Conservation threats and management mitigation opportunities associated with aggregations sites include fisheries, tourism, spatial protection, and climate change. Finally, we highlight knowledge gaps for future research prioritization and developments in the field for the identification of aggregation sites, the study of aggregation size and demographics and the functions and timing of aggregations.
1 Introduction
It is well documented that many elasmobranchs form large aggregations, varying from temporary groups to structured, long-term associations (e.g., hammerhead sharks, basking sharks, blacktip sharks, and common stingrays) (Klimley, 1987; Ward et al., 2004; Croft et al., 2006; Ward et al., 2007; Croft et al., 2011; Crowe et al., 2018; Chaikin et al., 2020; Ayres et al., 2021; Sims et al., 2022). The functions of such aggregation behaviors have been hypothesized to include reproduction (Klimley, 1987; Chaikin et al., 2020; Sims et al., 2022), feeding (De-la-Parra-Venegas et al., 2011), energy conservation, (Klimley and Nelson, 1984; Economakis and Lobel, 1998; Hight and Lowe, 2007), refuge from predators (Heupel et al., 2007; McAllister et al., 2017) and social learning (Sih et al., 2009; Brown and Laland, 2011). The formation of these aggregations can be seasonal and is often linked to environmental factors and life history stages (Rohner et al., 2013; Kajiura and Tellman, 2016), food abundance (Clua et al., 2013; Hacohen-Domené et al., 2015), critical habitats (Oh et al., 2017; Chiriboga-Paredes et al., 2022) and reproduction (Heupel and Simpfendorfer, 2005; Reyier et al., 2008). The seasonality of these aggregation events, along with the large number of individuals at specific areas (i.e., coastal areas, productive systems) can also increase the likelihood and intensity of exposure to anthropogenic threats such as targeted fishing (Litvinov, 2006; Croll et al., 2016), bycatch (Watson et al., 2009; Hall and Roman, 2013), habitat degradation (Cattano et al., 2021), irresponsible tourism (Venables et al., 2016; Zemah-Shamir et al., 2019), boat strikes (Lester et al., 2020; Allen et al., 2021; Womersley et al., 2022) or climate change (particularly if mismatches in the timing of migration, reproduction or foraging interactions occur).
Among elasmobranchs, the nine manta and devil ray species (collectively referred to as mobulids) inhabit tropical to temperate waters circumglobally (Couturier et al., 2012; Stevens et al., 2018a). Mobulids seasonally form aggregations ranging from a few to thousands of individuals (Anderson et al., 2011; Couturier et al., 2012; De-Boer et al., 2015; Stevens, 2016; Harris and Stevens, 2021; Palacios et al., 2021). These aggregations often occur in habitats or locations associated with concentrated food resources (Couturier et al., 2011; Armstrong et al., 2016; Harris et al., 2020; Harris and Stevens, 2021), parasite removal (O’Shea et al., 2010; Jaine et al., 2012), thermal refugia (Stevens, 2016; Palacios et al., 2021), reproductive behavior (Mendonça et al., 2020), and predator avoidance (Germanov et al., 2019; Pate and Marshall, 2020). Mobulids are also highly vulnerable to overexploitation due to their low fecundity (one pup per pregnancy, low birth rate, and delayed reproduction), leading to slow population growth rates (Stevens et al., 2000; Dulvy et al., 2014). The primary anthropogenic threat to mobulids is fisheries exploitation from both targeted fisheries and bycatch (Croll et al., 2016). Mobulid rays’ tendency to aggregate in productive pelagic areas, where commercially valuable species are targeted by both artisanal and industrial fisheries using a variety of gears (e.g. gillnets, purse-seines, and longlines) results in bycatch being a primary impact for most species of mobulid rays (Hall and Roman, 2013; Rohner et al., 2013; Croll et al., 2016; Lezama-Ochoa et al., 2019a) and contributes to declines in mobulid populations globally (Couturier et al., 2013; Lawson et al., 2017; Rohner et al., 2017). As a result, all mobulid species are listed as Endangered or Vulnerable on the IUCN’s Red List of Threatened Species (IUCN, 2020). Furthermore, mobulid aggregations in coastal areas can lead to exposure to boat collisions (Germanov et al., 2019; Pate and Marshall, 2020; Strike et al., 2022), habitat degradation (Stewart et al., 2018a) and unregulated tourism impacts (Venables, 2013; Murray et al., 2020; Gómez-García et al., 2021). The identification of aggregation sites for some species has led to the creation of Marine Protected Areas (MPAs) (e.g., UNESECO World Heritage Sites Revillagigedo Archipelago in Mexico, and Hanifaru Bay MPA in Maldives) and the establishment of management measures, such as best practices to reduce the mortality of mobulids caught incidentally by tuna purse seiners, or codes of conducts to ensure responsible tourism practices (Poisson et al., 2012; Lezama-Ochoa et al., 2019b; Murray et al., 2020; Cronin et al., 2022).
The aim of this review is to: 1) provide consistent definitions and terminology for mobulid aggregation and grouping events; 2) summarize existing research on the drivers and environmental factors underpinning aggregation events in mobulids; 3) identify conservation threats, management strategies, and mitigation opportunities associated with aggregation sites; and 4) identify knowledge gaps for future research prioritization.
2 Aggregation and social grouping in mobulids
The terminology used in describing aggregation behavior in elasmobranchs is often inconsistent and confusing (McInturf et al. in review). Here we present a conceptual framework (Figure 1) to classify the different types of aggregation behaviors that have been described in the elasmobranch literature. We propose aggregation as the most general term, which can be used to describe any group of individuals that forms for any purpose (Johnson et al., 2002; Guttridge et al., 2009; Guttal and Couzin, 2010; Jacoby et al., 2012). Some aggregations can be social groups, within which individuals exhibit interactive behaviors such as courtship, mating (Sims et al., 2022), or cooperative feeding (Mourier et al., 2012), and dynamic associative relationships among individuals may be present (Guttridge et al., 2009; Jacoby et al., 2012; Perryman et al., 2022). In several marine species including elasmobranchs, these associations have been described as fission-fusion dynamics, where groups merge (fusion) or split (fission) depending on various factors including the costs and benefits of the size of the group (e.g., increased competition for food versus reduced predation risk) (Haulsee et al., 2016; Perryman et al., 2019).
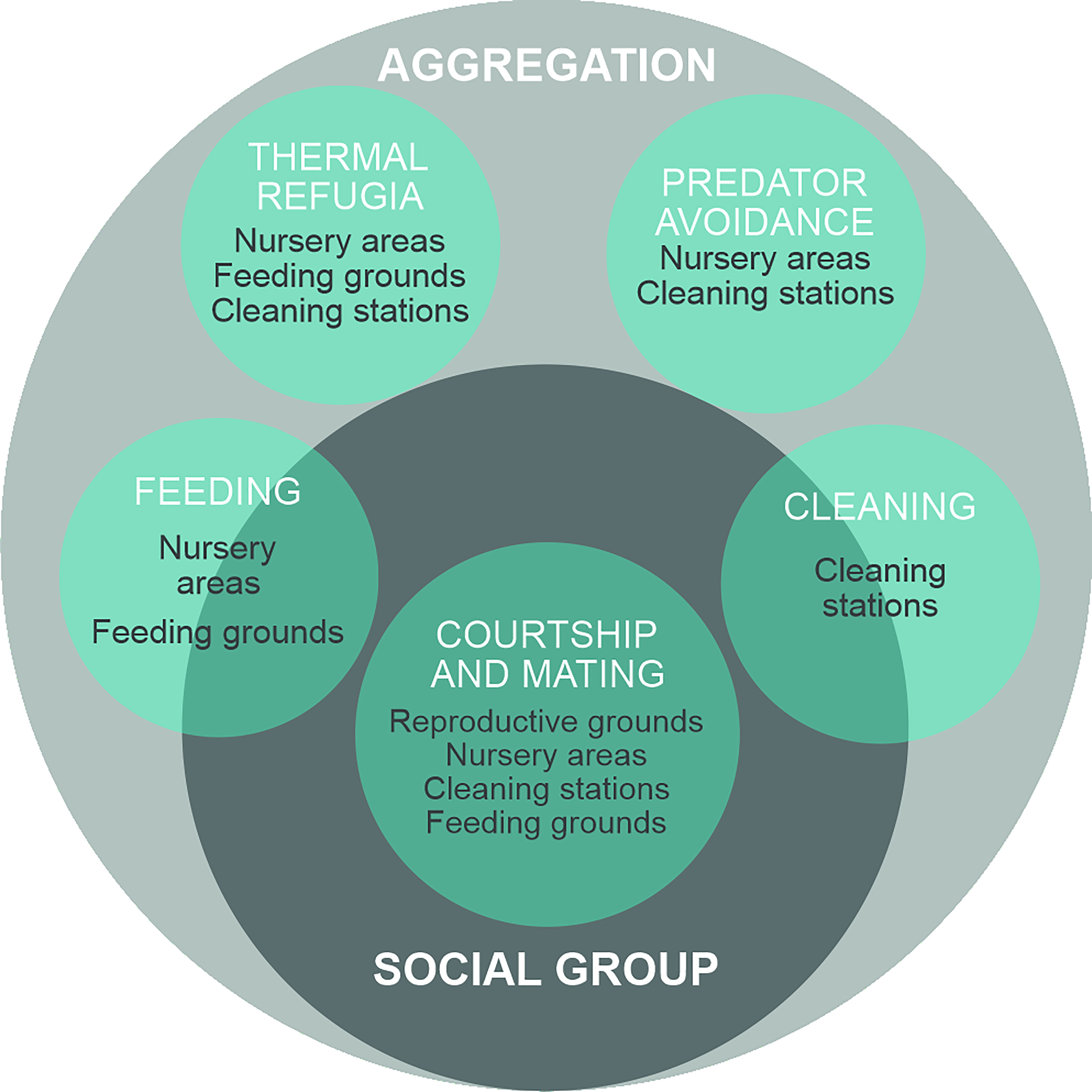
Figure 1 Conceptual framework of grouping behaviors in elasmobranchs. Aggregation (individuals are passively drawn together) is the general term to describe any group and the first prerequisite for the formation of social groups (individuals are drawn to one another). Life history functions (in white italics) acting as drivers of manta and devil ray aggregations. In grey are the key aggregation sites where these life history fuctions occurs.
Social grouping can facilitate collective behaviors like cooperative or optimal feeding (Sih et al., 2009; Villegas-Ríos et al., 2022), predator avoidance (Chivers et al., 1995; Ward et al., 2011), and social learning (Lachlan et al., 1998; Brown and Laland, 2011). Aggregations are likely the first prerequisite for the establishment of social grouping because they facilitate the establishment of social interactions among aggregating individuals (Sims et al., 2000; Jacoby et al., 2012).
Aggregation behavior has been documented in all mobulid species (Table 1) (Childs, 2001; Weeks et al., 2015; Stevens, 2016; Couturier et al., 2018; Lezama-Ochoa et al., 2019a; Solleliet-Ferreira et al., 2020; Harris and Stevens, 2021; McCann et al., 2021; Palacios et al., 2021). However, less attention has been paid to the social interactions within aggregations that may be indicative of more complex social grouping (Marshall and Bennett, 2010a; Stewart et al., 2017a; Stevens et al., 2018b; Murray, 2019; Perryman et al., 2019; Perryman et al., 2022). Nonetheless, some mobulid aggregations have been described as social groups (Marshall and Bennett, 2010a; Stevens, 2016; Stevens et al., 2018b; Perryman et al., 2019; Perryman et al., 2022). Within aggregations and social groups, segregation by size and sex has been observed (Notarbartolo-di-Sciara, 1988; Cerutti, 2005; Stevens, 2016; Stewart et al., 2018a; Germanov et al., 2019; Perryman et al., 2019; Palacios et al., 2021) (Table 1 Supplementary material).
Mobulids possess the highest brain: body weight ratios of all fishes, with enlarged telencephalon regions (Ari, 2011). The telencephalon region drives complex social behaviors in other animals, including for the establishment of dominance hierarchies and social bonds (Dunbar and Shultz, 2007; Ari, 2011). The few studies analyzing the social structure of mobulids have focused only on reef manta rays (Mobula alfredi) and have found social groups that last for weeks or months where interactions are open with fluid hierarchical social structures among females and juveniles (Perryman et al., 2019; Perryman et al., 2022) or unstructured aggregations with associations based on spatiotemporal overlap among individuals driven by food availability (Murray, 2019).
2.1 Drivers of mobulid aggregations
Aggregation behaviors facilitate key life history functions in mobulids, including feeding, courtship and mating, predation avoidance, cleaning, and thermoregulation (Figure 1). Locations where one or more aggregation drivers are present and predictable can become key aggregation sites that are used repeatedly by mobulids, such as cleaning stations and feeding sites, or sites that facilitate long-term stable aggregations such as nurseries (Figure 2). Overlap of key life history functions occur at several aggregation sites (Table 1 Supplementary material) (Childs, 2001; Stewart et al., 2018b; Fonseca-Ponce et al., 2022) where different social behaviors may occur (Perryman et al., 2019). Below, we describe the importance of aggregation behavior in each of these life history functions and at these key sites.
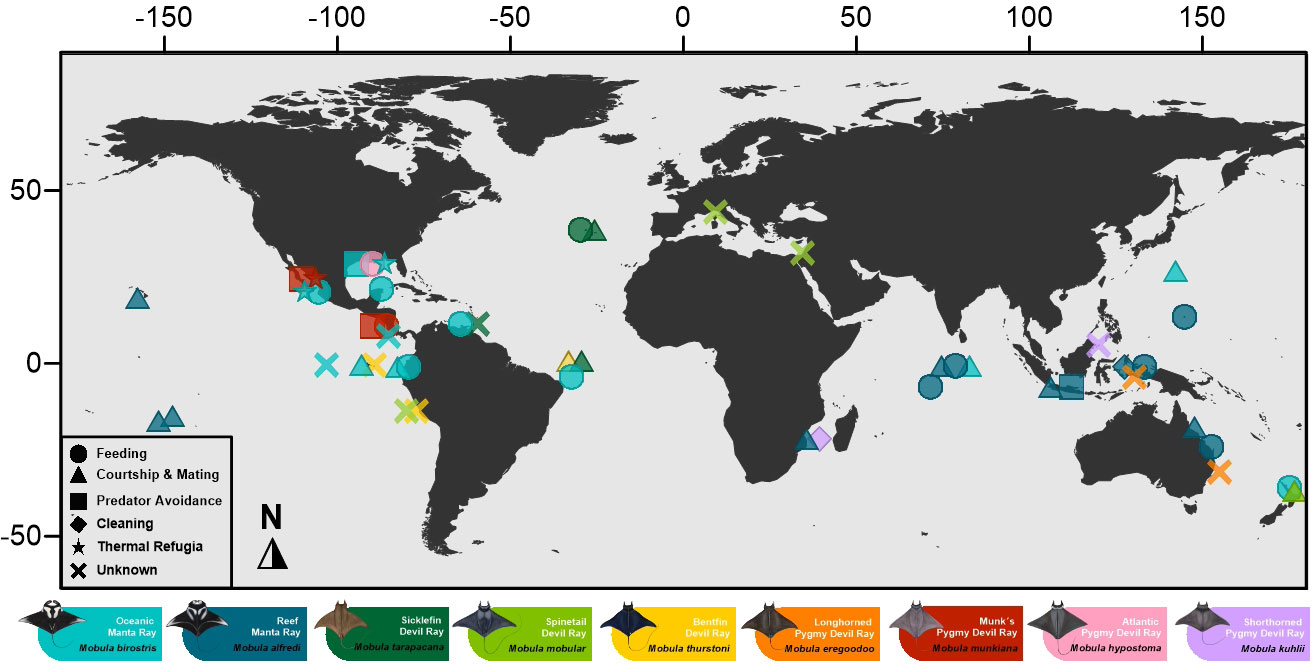
Figure 2 Manta and devil rays aggregation sites worldwide by species (colors) and drivers of the aggregation or social group (geometric figure). Aggregations illustrated with an X have no information related to the purpose of the group.
2.1.1 Feeding aggregations
Large aggregations are often driven by feeding behavior associated with mobulids’ reliance on dense prey assemblages that may correspond to seasons and locations with higher productivity and prey abundance (Notarbartolo-di-Sciara, 1988; Anderson et al., 2011; Jaine et al., 2012; Stevens, 2016; Hacohen-Domené et al., 2017; Stewart et al., 2017b; Lezama-Ochoa et al., 2019b; Lezama-Ochoa et al., 2020; Harris and Stevens, 2021). In the most closely studied example of large feeding aggregations (M. alfredi), no cooperative grouping behavior was observed (Stevens, 2016; Murray, 2019; Armstrong et al., 2021), indicating that these feeding aggregations are likely site-specific behaviors in which prey availability and site characteristics lead to local enhancement of prey (Silverman et al., 2004; Jourdain and Vongraven, 2017). The co-occurrence of multiple individuals, with prey availability likely also determining the group size (Murray, 2019). These aggregations in areas of local enhancement may also be easier to find because of the presence of conspecifics feeding on them, further increasing aggregation size and improving foraging efficiency by reducing search time (Thorpe, 1963; Galef, 2013). In some cases, the coordination of several feeding individuals may also facilitate prey concentration, enhance prey capture, and increase foraging efficiency (Lett et al., 2014). In mobulids, this coordinated behavior occurs during several feeding strategies, such as piggyback, chain, lunge, or cyclone feeding (Stevens, 2016; Stevens et al., 2018b; Bucair et al., 2021) described below.
Large-scale oceanographic processes and regimes such as seasonal upwelling systems (La Niña in the Eastern Tropical Pacific, and the South Asian Monsoon in the Indian Ocean) have a strong influence on the occurrence of mobulid aggregations (Anderson et al., 2011; Stevens, 2016; Burgess, 2017; Beale et al., 2019; Lezama-Ochoa et al., 2019a; Lezama-Ochoa et al., 2019b; Harris et al., 2020; Fonseca-Ponce et al., 2022; Harty et al., 2022). At fine scales, the bathymetry of aggregation sites, combined with tidal cycles and tidal currents, helps accumulate and condense zooplankton at densities up to 40 times higher than in surrounding waters (Armstrong et al., 2016), triggering feeding aggregations such as those described in the Maldives (Stevens, 2016; Harris and Stevens, 2021), eastern Australia (Armstrong et al., 2016), and the Chagos Archipelago (Harris et al., 2021). In conjunction with these environmental factors, moon phases have also been shown to influence the presence of M. alfredi at several aggregation sites (Dewar et al., 2008; Anderson et al., 2011; Jaine et al., 2012; Harris and Stevens, 2021). These mobulid feeding aggregations can be influenced by zooplankton size, species, and concentration. For example, in Hanifaru Bay, Maldives, Armstrong et al. (2021) found that M. alfredi formed feeding aggregations when the zooplankton community was dominated by large crustaceans (copepods) and total biomass was greater than a theoretical prey density threshold for net energy gain (25.2 mg m3). At this feeding aggregation site, the foraging opportunities appeared to occur when strong lunar tides overcame the force of the prevailing monsoonal current, drawing plankton-rich water from deep waters outside the atoll into the shallow reef inlet (Harris and Stevens, 2021) attracting up to 250 M. alfredi individuals (Stevens, 2016; Harris et al., 2020) (Table 2). Interactions between lunar tides and bathymetry may be a coherent explanation for why moon phase and tidal range have been identified as important predictors of mobulid presence at many aggregation sites (Dewar et al., 2008; Anderson et al., 2011; Jaine et al., 2012; Harris and Stevens, 2021; Fonseca-Ponce et al., 2022). In contrast, at Lady Elliot Island, Australia, M. alfredi began feeding in aggregations at lower prey densities than this theoretical density threshold (Armstrong et al., 2016). Here, river outflow, dynamic eddy activity, convergent fronts and ebb tides triggered large M. alfredi feeding aggregations of more than 150 individuals (Weeks et al., 2015).
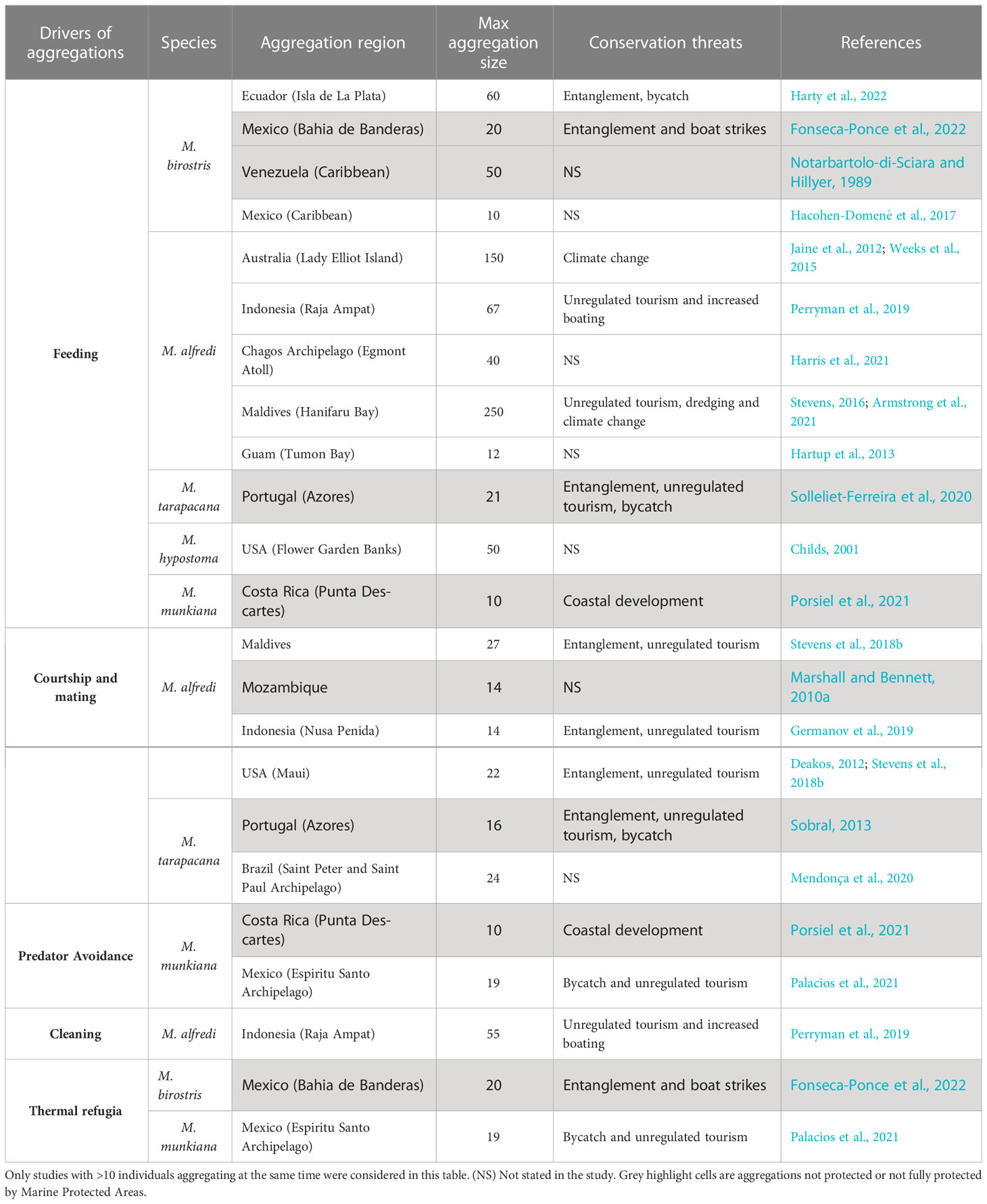
Table 2 Types of aggregations and social groups based on drivers of the groups for manta and devil rays.
During feeding aggregations, social groups can be formed as coordinated feeding strategies emerge (e.g., chain, piggyback and lunge feeding). Here, mobulids use the position of a conspecific to improve their chances of successful feeding, enhancing prey capture, hydrodynamic efficiency, and collision avoidance between individuals (Stevens, 2016; Stevens et al., 2018a; Solleliet-Ferreira et al., 2020; Armstrong et al., 2021; Harris and Stevens, 2021). Such coordinated feeding has been described in M. alfredi, M. birostris, M. kuhlii, M. munkiana, M. hypostoma, M. eregoodoo and M. tarapacana while feeding on zooplankton and shoals of anchovies or mesopelagic lanternfish (Stevens, 2016; Stevens et al., 2018a; Stewart et al., 2018c; Solleliet-Ferreira et al., 2020; Bucair et al., 2021) (Figure 3). An additional type of coordinated feeding, cyclone feeding, has been described for M. alfredi in the Maldives (Stevens, 2016; Stevens et al., 2018a) involving up to 150 individuals circling in an anticlockwise direction in the water column, resembling a 15 m diameter cyclone, for as long as 60 minutes (Stevens, 2016). This behavior presumably creates hydrodynamic conditions favorable to foraging success and is correlated with high zooplankton biomass values over 200 mg m-3 (Armstrong et al., 2021) (Figure 3).
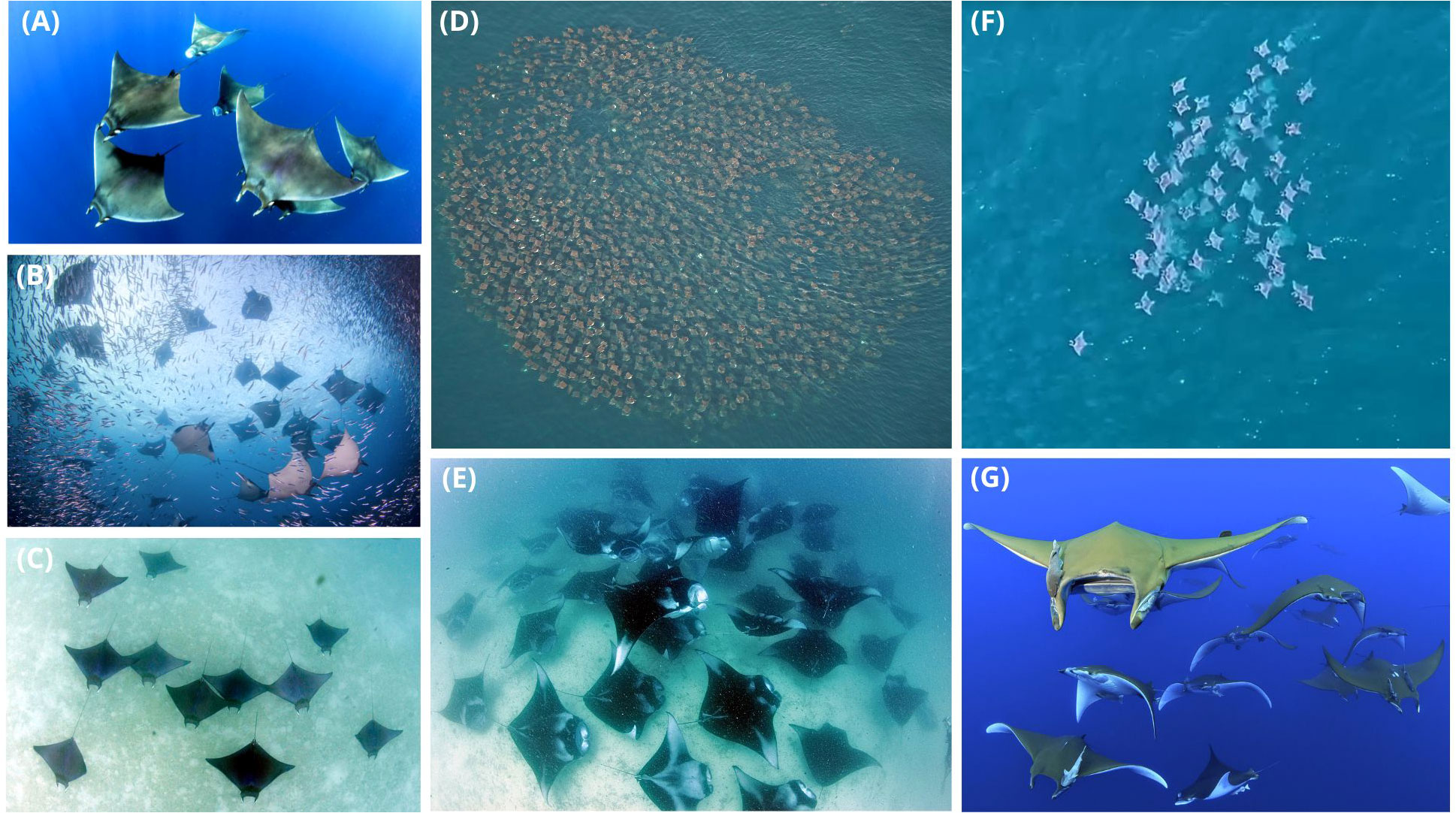
Figure 3 Mobulid aggregations. (A) M. hypostoma in the Mexican Caribbean. Photo © Guy Stevens. (B) A feeding aggregation of M. eregoodoo at Raja Ampat, Indonesia. Photo © Jeff Lemelin. (C) M. kuhlii aggregation in the Maldives. Photo © Guy Stevens. (D) M. munkiana aggregating in the Gulf of California, Mexico. Photo © Sidharta Velázquez-Hernández, Ocean Life Flights. (E) M. alfredi during a cooperative feeding aggregation in Hanifaru Bay, Maldives. Photo © Guy Stevens. (F) Aggregation of M. mobular in the Gulf of California, Mexico. Photo © Sidharta Velázquez-Hernández, Ocean Life Flights. (G) M. tarapacana at Princess Alice Banks, Azores. Photo © Tane Sinclair-Taylor.
2.1.2 Courtship and mating aggregations
Courtship and mating in elasmobranchs are often complex and variable across species (Carrier et al., 1994; Pratt and Carrier, 2001). Under our conceptual framework, all reproductive aggregations would be considered social groups. Some elasmobranch species form reproductive social groups via active partner preferences that can be sporadic and short (Jacoby et al., 2012). In M. alfredi, reproductive social groups seem to be initiated by males (Stevens et al., 2018b), although females triggering courtship with olfactory pheromonal signals have been also hypothesized (Stevens, 2016). The duration of reproductive social groups may be related to the number of males involved in the courtship of the female, with more extended periods when more males are involved (Marshall and Bennett, 2010a). This reproductive behavior has been described for five mobulid species (Table 2) at oceanic islands, seamounts, ridge systems, coral reefs, feeding aggregation sites, cleaning stations, and within the thermocline at depths between 50 to 80 m (Yano et al., 1999; Marshall and Bennett, 2010a; Sobral, 2013; Duffy and Tindale, 2018; Stevens et al., 2018b; Germanov et al., 2019; Stewart et al., 2019; McCallister et al., 2020; Mendonça et al., 2020). During courtship events, several individuals are generally involved, with one or two females chased by as many as 26 males (Stevens et al., 2018b).
Reproductive social groups are often seasonal. In M. alfredi in Mozambique, fresh mating wounds and mating events were observed during the austral summers from October to January (Marshall and Bennett, 2010a). While M. tarapacana (Saint Peter and Saint Paul Archipelago, Brazil) and M. munkiana (Gulf of California, Mexico) are present year-round, social groups displaying courtship and mating behaviors occur only during spring and summer months. Mobulids are believed to give birth shortly before mating (Uchida et al., 2008; Stevens et al., 2018a) and the seasonality of courtship and mating groups may therefore be linked to seasonal food availability and higher temperatures that would benefit the development of neonates (Notarbartolo-di-Sciara, 1988; Mendonça et al., 2020; Palacios et al., 2021). The reported aggregations of M. mobular in the southeast corner of the Mediterranean Sea (off the coasts of Palestine and Israel) during the coldest time of year could be related to the rays accessing the portion of the Mediterranean where sea temperatures are mildest. Evidence of mating was reported in this region based on the presence of oozing sperm in males caught in the fishery (Abudaya et al., 2017).
2.1.3 Predator avoidance aggregations
Forming aggregations may help diminish the risk of predation in elasmobranchs (Heupel and Simpfendorfer, 2005). Mobulids have several known predators, mainly sharks and killer whales (Alava and Merlen, 2009; Marshall and Bennett, 2010a; Stevens et al., 2018a). Larger species of mobulids (M. birostris and M. alfredi) tend to form smaller aggregations than smaller species, such as the pygmy devil rays (M. munkiana, M. eregoodoo, M. hypostoma, and M. kuhlii; Table 1) and are more likely to survive attacks with different degrees of sublethal injuries (Marshall and Bennett, 2010b; Deakos et al., 2011; Strike et al., 2022). However, survival from predation on pygmy devil rays is less likely to occur, although no studies have formally addressed sub-lethal injuries or natural mortality of pygmy devil rays. The larger aggregations formed by pygmy devil rays may have evolved as a predator-avoidance strategy for these smaller species (Broadhurst et al., 2018; Notarbartolo-di-Sciara et al., 2019).
Aggregating at protected or sheltered habitats during vulnerable periods, such as early life stages, to reduce predation risk has been observed in several elasmobranch species (Heupel et al., 2007; Heupel and Simpfendorfer, 2011). Aggregations at cleaning stations may also help reduce predation risk because of the shallow bottom habitats that help protect mobulids (M. alfredi) from shark attacks from below (Stevens, 2016; Stewart et al., 2018b).
Mobulids exhibit substantial maternal investment in a single offspring, and individuals may take 5-15 years to reach sexual maturity, depending on the species (Couturier et al., 2012; Stevens, 2016; Stewart et al., 2018a). Mobulids appear to utilize nursery areas, where neonates or juveniles aggregate in a similar manner to many other elasmobranchs (Heupel et al., 2007; Martins et al., 2018). This aggregation behavior results in higher residency in habitats that appear to enhance the survival of neonates and juveniles (Heupel and Simpfendorfer, 2011). During early life stages, social behaviors (Reyier et al., 2008), active association with conspecifics (Guttridge et al., 2009), and even individual personalities (Finger et al., 2018) have been described for some shark species (e.g., lemon sharks). To date, there is no evidence of similar social behaviors in mobulid nurseries. However, aggregating in nursery areas at vulnerable life history stages may be critical to reduce predation in early life stage mobulids. Mobulid nurseries have been identified at reef lagoons (Setyawan et al., 2022a), along the coast (Pate and Marshall, 2020; Knochel et al., 2022), estuarine systems (Medeiros et al., 2015), and at shallow bays (Germanov et al., 2019; Palacios et al., 2021). These nearshore areas likely provide refuge from predators (Stevens, 2016; Stewart et al., 2018a; Stewart et al., 2018b; Palacios et al., 2021; Setyawan et al., 2022a) as well as foraging opportunities (Setyawan et al., 2022a). Nursery areas have been described for juvenile M. birostris, (Childs, 2001; Medeiros et al., 2015; Stewart et al., 2018b; Pate and Marshall, 2020; Knochel et al., 2022), M. alfredi (Germanov et al., 2019; Setyawan et al., 2022a), and M. munkiana (Palacios et al., 2021). Neonates and juveniles have been shown to use and aggregate in these nursery areas with the occasional presence of adult individuals (Germanov et al., 2019; Palacios et al., 2021). Group sizes within mobulid nurseries tend to be smaller than adult aggregations (e.g., maximum of 19 juvenile individuals in M. munkiana compared with adult aggregations of thousands of individuals) (Palacios et al., 2021). In some of these nursery areas there is segregation by size (Palacios et al., 2021), similar to descriptions in other juvenile elasmobranchs (Guttridge et al., 2011), which could be the result of differences in swimming capabilities or habitat preferences at different size/age stages (Jacoby et al., 2012).
2.1.4 Cleaning aggregations
Cleaning stations play a vital role in the ecology and health of several mobulid species by providing an opportunity for them to rid themselves of harmful parasites by being cleaned by small fishes (O’Shea et al., 2010). Cleaning stations are well-defined areas, typically on inshore reefs or around seamounts (Jaine et al., 2012) where small ‘cleaner’ fishes (primarily Labridae) feed on the ectoparasites, mucus, dead or diseased tissue and scales of their larger ‘clients’ (Grutter, 1996; O’Shea et al., 2010) creating a mutually symbiotic interaction (Hay et al., 2004). Aggregations at cleaning stations have been documented in M. kuhlii (Murie and Marshall, 2016), M. birostris and M. alfredi (which in many cases exhibit strong site fidelity to the station) (Table 2) (Dewar et al., 2008; O’Shea et al., 2010; Marshall and Bennett, 2010b; Stewart et al., 2016a; Setyawan et al., 2018).
In addition to providing health benefits, these sites also provide opportunities where social interactions among individuals can occur (O’Shea et al., 2010; Stevens, 2016; Stevens et al., 2018a; Stewart et al., 2018b; Perryman et al., 2019). The aggregation of mobulids at cleaning stations also has the potential to facilitate reproductive social behaviors, acting as lek sites for these species (Stevens, 2016). For example, courtship and mating behavior in M. alfredi are frequently observed at cleaning stations in the Maldives (Stevens, 2016; Stevens et al., 2018b) and Indonesia (Dewar et al., 2008; Germanov et al., 2019; Perryman et al., 2019). In Australia, M. alfredi visits at cleaning stations last for an average of 30 minutes per visit and up to five hours at the same site, facilitating frequent social interactions among individuals before, during, or after cleaning (O’Shea et al., 2010). In Raja Ampat, Indonesia, cleaning sites with as many as of 55 mobulids present at the same time have been described (Perryman et al., 2019). At the Revillagigedo Archipelago, Mexico, M. birostris have been observed socializing at different cleaning stations, including repetitive movements of the cephalic lobes and half-somersault maneuvers between individuals (Stewart et al., 2017a; Stewart et al., 2019; Perryman et al., 2021).
2.1.5 Thermal refugia aggregations
Water temperature is a major driver of changes in movement and habitat use in elasmobranchs (Schlaff et al., 2014), and may affect metabolic and physiological functions such as digestion, reproduction, and somatic growth (Wallman and Bennett, 2006; Hight and Lowe, 2007; Tenzing, 2014). Mobulids are found in tropical and temperate seas with an optimal thermal range from 20 to 26°C in the case of M. alfredi (Couturier et al., 2012; Lassauce et al., 2022). Despite this, several species (M. birostris, M. alfredi, M. tarapacana, M. mobular and M. munkiana) preform deep dives, possibly to feed on zooplankton and other prey concentrated in the mesopelagic and bathypelagic zones (Stewart et al., 2016b; Andrzejaczek et al., 2022; Lassauce et al., 2022) where water temperatures can reach < 4°C (Thorrold et al., 2014). After deep dives in cold water, mobulids bask at the surface as a behavioral thermoregulation mechanism to warm up their body temperatures (Canese et al., 2011; Thorrold et al., 2014; Stewart et al., 2016b; Lassauce et al., 2022). Aggregations during basking behavior at shallow coastal waters have been observed in M. birostris in Bahia de Banderas (Mexico), presumably after mesopelagic and thermocline associated foraging (Fonseca-Ponce et al., 2022). Coastal nursery areas for M. birostris in the Gulf of Mexico and enclosure estuaries in Brazil and for M. munkiana in the Gulf of California (where higher residency occurred during warmer temperatures) could also provide thermal refugia that accelerate the metabolic rates and growth of juveniles and thereby reduce the duration of these vulnerable life history stages (Tenzing, 2014; Stewart et al., 2018b; Palacios et al., 2021) (Table 2). In Maldives, individuals aggregating at warmer inshore reefs around cleaning stations and staying for longer periods than the cleaning activity, may be augmenting metabolic and physiological functions after deep water feeding forays (Stevens, 2016). Surface aggregations of M. mobular and M. tarapacana in the Mediterranean Sea and the Azores, respectively, have been repeatedly observed (Celona, 2004; Sobral, 2013; Notarbartolo-di-Sciara et al., 2015; Solleliet-Ferreira et al., 2020), coinciding with diving data in the area, suggesting that basking behavior could be a thermal recovery strategy after deep dives (Canese et al., 2011; Thorrold et al., 2014) (Figure 3). Aggregations at the surface during basking behavior may exacerbate the vulnerability of these species to anthropogenic threats, such as boat strikes (Stevens, 2016; Fonseca-Ponce et al., 2022; Strike et al., 2022) and bycatch (Canese et al., 2011).
3 Conservation threats and management mitigation opportunities
As a group with extreme K-selective life history strategies, mobulids are vulnerable to overexploitation, and as a result are suffering from large population declines worldwide (Dulvy et al., 2014; Pardo et al., 2016; Rohner et al., 2017; Dulvy et al., 2021; Fernando and Stewart, 2021). Mobulid aggregations occur at sites that serve important functions (e.g., feeding, reproduction) for the survival and recovery of threatened populations (Stevens et al., 2018b; Germanov et al., 2019; Setyawan et al., 2022a). The tendency of mobulids to aggregate in these areas can increase their vulnerability to anthropogenic activities by increasing the likelihood that multiple individuals will be impacted by any single threat (e.g., a single gill net or purse seine) (Croll et al., 2016; Rohner et al., 2017; Stewart et al., 2018a). Effective conservation and management for mobulids will benefit from the identification of mobulid aggregation sites, knowledge of the geographical extent from which the aggregation sites draw individuals, their seasonality, and the various environmental components influencing them to develop effective threat mitigation strategies through conservation measures at aggregations sites.
3.1 Fisheries
Targeted fishery exploitation and bycatch of mobulid aggregations is leading to major populations declines (e.g., M. mobular, M. tarapacana. M. birostris and M. thurstoni in Sri Lanka; Fernando and Stewart, 2021) and even local extinctions (e.g., M. birostris in the Gulf of California and Mobula spp. in several regions in Indonesia; Lewis et al., 2015; Stewart et al., 2016a). Fisheries targeting mobulids still occur throughout the ranges of most species, including areas where aggregations and social groups occur (e.g., Sri Lanka, Indonesia, India, Peru, Philippines, Palestine, West Africa; Couturier et al., 2013; Acebes and Tull, 2016; Setyawan et al., 2020; Fernando and Stewart, 2021; Guirkinger et al., 2021). In industrial fisheries, tuna purse seine vessels in all tropical oceans of the world have relatively high bycatch of mobulids due to the overlap between tuna and mobulid species distributions within productive regions (Croll et al., 2012; Lezama-Ochoa et al., 2019a; Lezama-Ochoa et al., 2019b; Grande et al., 2020; Lezama-Ochoa et al., 2020; Guirhem et al., 2021). Captures of up to 220 individuals (M. thurstoni) from a single purse seine set have been reported (Lezama-Ochoa et al., 2019a), indicating the potential for incidental captures of aggregations to have a major impact on mobulid populations. Records of >50 bycaught mobulids within a single purse seine set normally occur at productive oceanographic fronts (e.g., off Peru in March for M. mobular; the Galapagos islands in December for M. thurstoni) (Lezama-Ochoa et al., 2019a) (Table 3). Though four of the five large tuna Regional Fisheries Organizations (RFMOs) ban the retention and sale of mobulids, these measures do not influence the likelihood or rate of bycatch, meaning that they cannot prevent bycatch from impacting aggregations (Cronin et al., 2023). The spatial and temporal distribution data of mobulid bycatch collected by scientific observer programs, stablished by RFMOs, are a powerful tool for identifying where and when large mobulid aggregations may occur and where they overlap with tuna purse seine fisheries. These datasets have been used in the creation of species distribution models for several mobulid species (Lezama-Ochoa et al., 2019b; Lezama-Ochoa et al., 2020). The use of tracking data to validate or complete fisheries datasets are essential to support the effective development of spatially restricted seasonal areas as management strategies (Lezama-Ochoa et al., 2019a). Understanding the oceanographic preferences of these species may help to reduce their interactions with fisheries if dynamic spatial management approaches are implemented, as has already successful applied to other fisheries to reduce bycatch (Hazen et al., 2018).
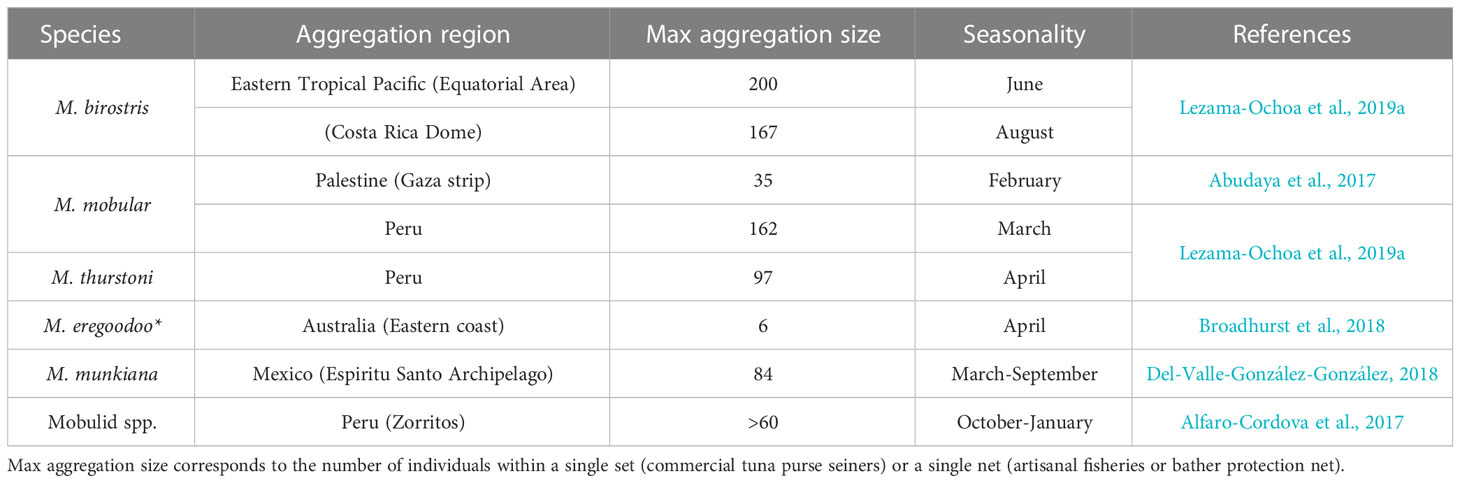
Table 3 Manta and devil ray aggregations obtained from artisanal fisheries, industrial fisheries, and bather protection nets* data.
Mobulid bycatch in artisanal fisheries is poorly documented worldwide, but it exists in at least 21 small scale fisheries (Lewis et al., 2015; Croll et al., 2016; Alfaro-Cordova et al., 2017). In a coastal stingray fishery in the Gulf of California, up to 84 M. munkiana have been bycaught in a single net at an aggregation area described as a nursery ground for this species (Del-Valle-González-González, 2018; Palacios et al., 2021) (Table 3). In coastal Peru, an artisanal pelagic gillnet fishery using surface driftnet to target pelagic sharks and yellowfin tuna, reported several sets with more than 60 mobulid individuals per set in the coastal area in front of the Zorritos locality (Alfaro-Cordova et al., 2017). There is an urgent need for regulations in artisanal fisheries to monitor and mitigate the bycatch rates of mobulids, as well as the quantification of post-release survival of bycaught mobulid species in these fisheries. Furthermore, bather-protection nets (otherwise known as “shark nets”) aimed to reduce shark attack risk in Australia have also been shown to affect mobulid ray species including M. kuhlii, M. eregoodoo and M. alfredi (Broadhurst et al., 2018; Broadhurst and Cullis, 2020). Bycatch mitigation strategies for bather-protection nets include extended soak times (up to four days) to reduce mortalities and the locating nets in areas and seasons when unwanted species are less common (Broadhurst and Cullis, 2020).
Mitigation of bycatch at aggregation sites could be achieved with spatio-temporal closures for gillnets at critical habitats, such as nursery areas or feeding aggregations. Fishing bans of mobulids should also be considered throughout their range, while identifying mobulid aggregations at specific times and locations can offer important management and conservation opportunities (Clark et al., 2014) such as the designation of spatial protection areas. Such an approach is being included in the identification of Important Shark and Ray Areas (ISRAs) for mobulids (Hyde et al., 2022). Furthermore, socio-economic surveys among fishermen communities and associated stakeholders should also be implemented to understand the economic and social impacts that can arise from such management designations (e.g., Peru; Guirkinger et al., 2021 and Indonesia; Booth et al., 2020; Booth et al., 2021).
3.2 Tourism
Predictable aggregations of mobulids at specific locations offer opportunities to develop non-consumptive uses such as ecotourism focused on observing and swimming with mobulids. Tourist activities with mobulids at aggregation sites have been reported for several species worldwide: M. alfredi in Australia, Indonesia, and Maldives (Venables, 2013; Germanov et al., 2019; Murray et al., 2020), M. birostris in Mexico and Ecuador (Kumli and Rubin, 2010; Burgess, 2017; Gómez-García et al., 2021; Harty et al., 2022), M. tarapacana in the Azores (Sobral, 2013; Solleliet-Ferreira et al., 2020), and M. munkiana in the Gulf of California (Palacios et al., 2021). Manta and devil ray tourism not associated with aggregation sites is also widespread globally (O’Malley et al., 2013). Mobulid tourism can contribute significantly to local economies (Gallagher and Hammerschlag, 2011; O’Malley et al., 2013), with direct economic benefits of manta ray tourism to the global economy estimated to be at least $140 USD million annually (O’Malley et al., 2013). While ecotourism is a potential economic opportunity, the large number of individuals present at mobulid aggregations can increase their vulnerability to unregulated or poorly implemented tourism activities (Harris et al., 2020).
Tourist disruption at feeding aggregation sites, where mobulids benefit from temporally high-density prey patches, can reduce food intake, potentially diminishing fitness (Venables, 2013; Murray et al., 2020). Similarly, tourism at mobulid cleaning aggregation sites can result in anthropogenic impacts from SCUBA divers with poor buoyancy generating physical damage to the cleaning site substrate, which is often coral and may also impact the cleaner fish community (Toyoshima and Nadaoka, 2015). Mobulids may be disrupted during their cleaning activities or when engaging in social behaviors such as courtship or mating (Perryman et al., 2019; Murray et al., 2020).
Anthropogenic disturbance to M. alfredi by boats accessing nursery and courtship areas for tourism was observed in Indonesia (Germanov et al., 2019). In Ningaloo Reef, Australia, feeding M. alfredi were disturbed or showed a behavioral response in 34% of tourism interactions (Venables, 2013). In the Maldives, Hanifaru Bay is considered the most important feeding site for M. alfredi in terms of the number of individuals aggregating at the same time (Harris et al., 2020; Armstrong et al., 2021). Due to the predictability and size of the groups at this site, a large tourist industry around manta rays has been established at the site. In a recent study, avoidance responses by M. alfredi at Hanifaru were reported in 37% of the observations, and the animals’ natural behavior was stopped or changed during in-water interactions with humans (Murray et al., 2020). In Mexico, M. birostris at aggregation sites showed higher probabilities of evasive behavior when interacting with divers actively chasing individuals (Gómez-García et al., 2021). While several studies have described anthropogenic disturbances for manta rays at aggregation sites (Venables, 2013; Germanov et al., 2019; Murray et al., 2020; Gómez-García et al., 2021), and addressed potentially problematic human-manta interactions by developing science-based best practices guidelines or codes of conduct (e.g. How to Swim with Manta Rays; swimwithmantas.org), these types of studies and guidelines are largely non-existent for the smaller devil rays. Finally, increased tourism at mobulid aggregations may increase the risk of other lethal and sublethal impacts such as boat strikes and entanglements in mooring lines (Lester et al., 2020; Allen et al., 2021; Strike et al., 2022; Womersley et al., 2022).
To address these problems, greater effort in educating guides, boat captains, and tourists should be put in place at local communities and businesses that benefit from tourist activities with mobulids. This can be achieved by guides who give educational briefings before in-water activities and then enforce these recommendations throughout the encounter (Murray et al., 2020). MPAs can be established to provide regulations and enforcement of boat traffic limits, boat speeds, and snorkeler or diver capacities in such critical aggregation sites, helping to reduce anthropogenic threats to mobulids.
3.3 Spatial protection and important shark and ray areas
Spatial protection, particularly if based on ISRAs, can play an important role in protecting populations from anthropogenic impacts at aggregation sites (Germanov et al., 2019; Murray et al., 2020; Setyawan et al., 2022b). ISRAs provide refereed, actionable information on sites that are critical to mobulid survival, such as aggregations (Hyde et al., 2022). While it is likely not feasible to protect the entire home ranges of most mobulid species, aggregation sites for mobulids may encompass important areas for critical life history stages (Marshall and Bennett, 2010a; Stevens, 2016; Germanov et al., 2019; Setyawan et al., 2022b). Indeed, the establishment of spatial protections for megafauna at aggregation sites has proven to be extremely successful when paired with adequate enforcement and surveillance and when local communities directly benefit from the protection of their surrounding areas (e.g., Cabo Pulmo, El Vizcaíno Biosphere Reserve and Bahía de Loreto National Park; Aburto-Oropeza et al., 2011; Urbán and Viloria-Gómora, 2021).
Many mobulid aggregation sites with established spatial protection are primarily focused on the larger-bodied mobulid species, especially manta rays (Weeks et al., 2015; Germanov et al., 2019; Armstrong et al., 2021; Harris et al., 2021). Important aggregation areas are largely unknown for pygmy devil rays, which are smaller in size but with a greater tendency for aggregation behavior and a more coastal distribution (Murie and Marshall, 2016; Stevens et al., 2018a; Notarbartolo-di-Sciara et al., 2019; Palacios et al., 2021). These species are likely to be threatened by coastal and artisanal fisheries (Smith et al., 2009; Rojas Perea, 2016; Del-Valle-González-González, 2018), where very little information exists on bycatch rates (Fernando and Stewart, 2021; Mustika et al., 2021) making it challenging to use fishery reports to identify aggregation areas or to establish bycatch mitigation strategies.
Despite protection of mobulid species in most of their distributional range and their inclusion in international trade management measures such as the Convention on International Trade in Endangered Species of Wild Fauna and Flora (CITES) and the Convention on the Conservation of Migratory Species of Wild Animals (CMS), targeted fisheries and opportunistic bycatch retention persists in some countries (Akyol et al., 2005; Acebes and Tull, 2016; Alfaro-Cordova et al., 2017; Fernando and Stewart, 2021; Guirkinger et al., 2021). Without specific action to understand the occurrence of mobulid aggregations and their overlap with and susceptibility to human impacts, a major biological and ecological vulnerability for these species will remain unaddressed.
3.4 Climate change
Climate change is one of the most important anthropogenic threats to vulnerable marine megafauna such as mobulid rays and is predicted to increase the intensity and frequency of changes in environmental conditions (Cheung et al., 2009; Stewart et al., 2018a). These environmental changes may cause mobulid aggregation sites to shift to new locations in search of more favorable conditions or to follow new prey distributions. As a result, predator and prey shifts may occur at different magnitudes and directions (Hazen et al., 2013), potentially impacting specialized predators like mobulid rays. Phenological changes, including changes to reproductive periodicity, foraging, or migration may affect their populations. Current protected areas at aggregation sites may not be efficient if mobulids’ new distributions do not match previously established boundaries. In these cases, more dynamic management approaches may be preferential (Lewison et al., 2015; Dunn et al., 2016). Understanding long-term changes in the distribution of mobulid rays is essential to identify species most at risk and anticipate management options. Species distribution model outputs combined with global climate models are an important tool for projecting species’ shifts and future habitat.
4 Key knowledge gaps and developments in the field
4.1 Identification of aggregation sites
Most mobulid aggregation sites have been identified by opportunistic observations from divers or fishers using Traditional Ecological Knowledge surveys (Anderson et al., 2011; Sobral, 2013; Burgess, 2017; Palacios et al., 2021). Once aggregation sites are proposed or identified through initial observations, they can be further studied using tracking technology (such as active and passive acoustic telemetry, as well as satellite tagging studies) to determine when and how often mobulid aggregations occur in these locations in the absence of active observation effort (Figure 4) (Dewar et al., 2008; Andrzejaczek et al., 2020; Peel et al., 2020; Setyawan et al., 2020; Harris and Stevens, 2021; Palacios et al., 2021). For the identification of aggregation sites in pelagic habitats, fishery-dependent data offer a unique opportunity to obtain large-scale information on species distribution, group sizes, and seasonality over long periods of time and with wide spatiotemporal coverage (Notarbartolo-di-Sciara, 1988; White et al., 2006; Croll et al., 2016; Abudaya et al., 2017; Lezama-Ochoa et al., 2019a; Fernando and Stewart, 2021). Scientific observer programs implemented in some industrial fisheries can facilitate the collection of information on environmental parameters from remote sensing databases, bycatch species, abundance, and bycatch size frequencies (Lezama-Ochoa et al., 2019a; Lezama-Ochoa et al., 2019b; Lezama-Ochoa et al., 2020). Remote aggregation sites can also be identified by sporadic or standardized aerial surveys, as demonstrated through the identification of aggregation sites of M. mobular in the Mediterranean and M. birostris in the Caribbean Seas (Notarbartolo-di-Sciara and Hillyer, 1989; Duffy and Abbott, 2003; Notarbartolo-di-Sciara et al., 2015). Further, the use of aerial surveys from seaplanes or helicopters assisting industrial fisheries to locate their target catch could potentially aid real-time identification and bycatch avoidance of mobulid grouping events (Cronin et al., 2022).
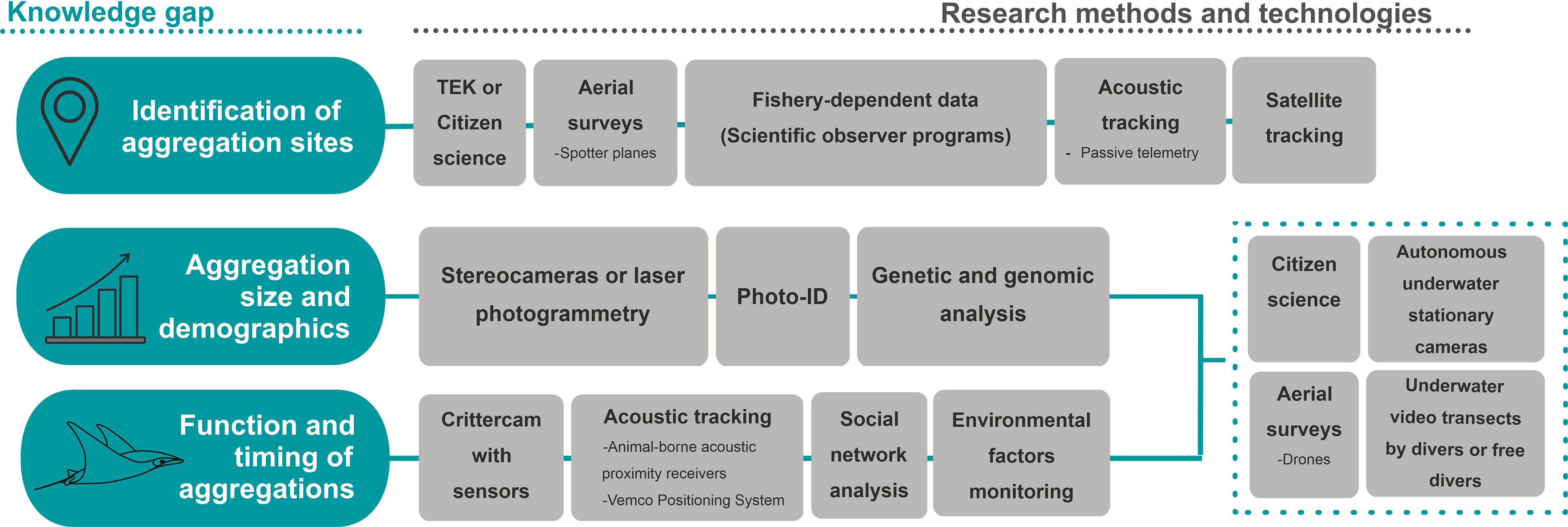
Figure 4 Illustration of key knowledge gaps in manta and devil ray aggregation research, and proposed methods and technologies for developments in the field. In the dotted square are common methods for key knowledge gaps.
4.2 Aggregation size and demographics
Sorting of aggregations by size and sex have been observed for several species of mobulids (Stevens, 2016; Germanov et al., 2019; Perryman et al., 2019; Mendonça et al., 2020; Palacios et al., 2021). For manta rays, photo identification using distinctive ventral markings has been used for decades to produce comprehensive databases of dozens of manta ray populations globally and estimate their population size and structure (Marshall et al., 2011; Germanov and Marshall, 2014; Stevens et al., 2018b; Harty et al., 2022; Cabral et al., 2023). Nevertheless, information on the demographic composition of aggregations and how it relates to aggregation size and function is lacking for most species, especially the smaller devil rays.
Emerging technologies can facilitate the collection of more detailed demographic data in aggregations. To investigate individual size distributions in aggregations, the use of photogrammetry (both in-water and via aerial drones) is an affordable and non-invasive option for the analysis of the size and sex structures of aggregations (Deakos, 2010; Setyawan et al., 2020; Setyawan et al., 2022c). Body size of individuals, which is a proxy for maturity stage in mobulids (Stevens, 2016; Rambahiniarison et al., 2018), can be measured using calibrated underwater stereo camera systems and paired-laser photogrammetry, enabling accurate estimates of ray disc width and other body measures (Deakos, 2010; Langlois et al., 2012). Furthermore, the use of calibrated stereo camera systems and R software packages such as StereoMorph (Olsen and Westneat, 2015) allow for accurate measurement of several individuals in the same frame. The use of drones for mobulid research has not been widely applied, but the few studies using this technology have shown that they can be a useful tool to determine morphometric measurements, such as disc length, width, and cranial width using the known length of a floating object as a reference scale (Setyawan et al., 2022c). Furthermore, photo identification of somersaulting manta rays, determination of female maturity based on the presence of mating scars, and male maturity by the extension and calcification of claspers is also possible to collect at aggregation and social grouping sites using drones (Pate and Marshall, 2020; Setyawan et al., 2020; Setyawan et al., 2022c). Autonomous underwater cameras placed in strategic sites such as cleaning stations can provide continuous coverage, allowing presence/absence analysis and eliminating the potential deterrent effect of diver presence (Barr and Abelson, 2019). From underwater video transects operated by SCUBA divers or free divers, to stationary underwater video stations at grouping sites, these methods have proven valuable in collecting data on abundance, body size, sex ratios, maturity status and even behavior of mobulids at grouping sites (O’Shea et al., 2010; Deakos, 2012; Stewart et al., 2018a; Stevens et al., 2018b;Barr and Abelson, 2019). Beyond observational technology, emerging population genetic and genomic techniques (e.g., RAD sequencing and/or full genome sequencing) can allow for assessments of relatedness within aggregations, as well as calculations of population size estimates that can be used to contextualize aggregations within the larger population (Sigsgaard et al., 2016; Lieber et al., 2020). Additionally, genetic studies could test whether kin aggregation occurs in mobulids, whether aggregating individuals originate from shared or differing original populations, and broadly the extent to which genetic factors shape aggregation behavior (Selwyn et al., 2016). Recent research using population genomic methods for mobulids (e.g., Hosegood et al., 2020; Lassauce et al., 2022; López et al., 2022) has laid the foundation for more fine-scale investigations of genomics within and between aggregating groups.
4.3 Function and timing of aggregations
Understanding function, structure, timing, frequency, and size of mobulids aggregations is critical for mobulid conservation, as it will improve predictions of where and when large aggregations are most vulnerable to human impacts, and what life stages or demographic units are represented. To address these gaps, habitat use and behaviors at aggregation sites can be studied using technologies such as drones (Setyawan et al., 2020; Setyawan et al., 2022c), acoustic telemetry (Dewar et al., 2008; Harris and Stevens, 2021; Palacios et al., 2021), crittercams with sensors (Stewart et al., 2019), in-person observations from citizen science (Sobral, 2013; Germanov et al., 2019) and underwater visual census with fixed cameras or operated by divers or snorkelers (Germanov et al., 2019) (Figure 4). Drones can also collect high-resolution aerial images of marine megafauna, documenting grouping behavior, abundance, and distribution, in a replicable manner over time and space (Johnston, 2019; Schofield et al., 2019) with low disturbance towards wildlife and habitats when appropriately used (Kiszka et al., 2016; Colefax et al., 2018). Detectability issues can be challenging for submerged fauna like sharks and rays (Brack et al., 2018). However, mobulids often have dark dorsal coloration, large body sizes (Stevens et al., 2018a), a tendency to form aggregations or social groups (Stevens, 2016; Perryman et al., 2019; Lezama-Ochoa et al., 2019a) and a preference to swim near the surface in the water column (Croll et al., 2012; Thorrold et al., 2014; Stevens et al., 2018a; Lezama-Ochoa et al., 2019a) making them good candidates for study using drone technology. Using fixed transects with drones can be an efficient strategy to monitor seasonal changes in abundance, behavior, and population demographics, while also providing quantitative data on habitat use that can be replicated over years (Perryman et al., 2019; Setyawan et al., 2020). In addition to aerial observation, visitation patterns obtained through acoustic telemetry or visual data collected from underwater surveys can inform habitat use and aggregation behavior, especially when correlated with environmental factors such as temperature, conductivity, chlorophyll-a concentrations, zooplankton abundance and tides (Dewar et al., 2008; Jaine et al., 2012; Barr and Abelson, 2019; Harris et al., 2020; Setyawan et al., 2020; Armstrong et al., 2021; Harris and Stevens, 2021; Palacios et al., 2021). These data can help conservation and management efforts to correctly identify habitat drivers of aggregations and therefore implement more informed spatial-temporal measures for conservation.
Social dynamics can also be studied with acoustic telemetry (e.g., Vemco Positioning System), allowing for the calculation of near-continuous fine-scale animal locations with overlapping receiver arrays (Espinoza et al., 2011) to elucidate association patterns among tagged individuals (Armansin et al., 2016; Perryman et al., 2022). Crittercams with sensors (Stewart et al., 2019) or animal-borne acoustic proximity receivers, where the individual tagged can transmit their own code and store signals from other tagged animals (Holland et al., 2009) are other options to quantify interactions between individuals. Though this method is limited by the need to recover the instruments from animal (Stewart et al., 2019) via passive release or the recapture of the tagged animal to retrieve the data (Guttridge et al., 2010).
5 Conclusions
Aggregative behavior facilitates multiple life history functions, providing benefits for the intake of food as well as for the survival of the species through mating and protection of early life stages. However, aggregations may also exacerbate the risk of major impacts to mobulid populations if threats occur at key aggregations sites. Similar to fisheries targeting spawning aggregations of bony fishes, targeted fisheries or bycatch at mobulid courtship and mating aggregation sites can affect the viability of the next cohort.
With emerging technologies, the study of aggregations and social behaviors are becoming more accessible and affordable. Yet, the nature and location of mobulid aggregation sites are sometimes difficult to identify because of a lack of consistency in which the term ‘aggregation’ is used. Often, aggregation sites are described without giving information on the details of the behavior and the number of individuals present rather, they are described loosely as ‘an area where mobulids are frequently sighted.’ This terminology makes it difficult to assess the importance of the site for aggregation behavior.
While aggregative behavior is a characteristic of all mobulid species, smaller species often form larger aggregations, potentially leading to higher vulnerability given heightened exposure to anthropogenic risks associated with aggregations. Paradoxically, these smaller devil rays are less studied and enjoy less legal protection in comparison to the larger manta rays. Therefore, it is important than in future research and studies the pygmy devil rays are prioritized. Otherwise, the partial disappearance of pygmy devil rays from some of their range is likely to occur, as has already occurred in the eastern Atlantic for M. hypostoma cf. rochebrunei. Furthermore, many of the pygmy devil rays are still not protected from fisheries in most parts of their range, which exacerbates the urgency to study their aggregation sites and behaviors.
Author contributions
MP, JS, DC, GS, GN, MC, NL-O, KZ, RG-A, and FG-M conceived of the paper and figure concepts. MP wrote the main manuscript text. MDP and AR designed and elaborated the figures. All authors contributed to writing and editing the manuscript. All authors contributed to the article and approved the submitted version.
Funding
MP was supported by Consejo Nacional de Ciencia y Tecnología, BEIFI, Walter Munk Foundation for the Oceans and Dive Ninjas Expeditions and Fundación México Azul. RG-A and FG-M thanks to Instituto Politecnico Nacional for fellowships (COFAA and EDI).
Conflict of interest
The authors declare that the research was conducted in the absence of any commercial or financial relationships that could be construed as a potential conflict of interest.
Publisher’s note
All claims expressed in this article are solely those of the authors and do not necessarily represent those of their affiliated organizations, or those of the publisher, the editors and the reviewers. Any product that may be evaluated in this article, or claim that may be made by its manufacturer, is not guaranteed or endorsed by the publisher.
Supplementary material
The Supplementary Material for this article can be found online at: https://www.frontiersin.org/articles/10.3389/fmars.2023.1148234/full#supplementary-material
Supplementary Table 1 | Summary of published literature on mobulid aggregations, where the numbers of individuals of manta or devil rays aggregating at a given time were stated. (NS) Not stated in the study.
References
Abudaya M., Ulman A., Salah J., Fernando D., Wor C., Notarbartolo di Sciara G. (2017). Speak of the devil ray (Mobula mobular) fishery in Gaza. Rev. Fish Biol. Fish. 28, 229–239. doi: 10.1007/s11160-017-9491-0
Aburto-Oropeza O., Erisman B., Galland G. R., Mascareñas-Osorio I., Sala E., Ezcurra E. (2011). Large Recovery of fish biomass in a no-take marine reserve. PloS One 6 (8), e23601. doi: 10.1371/journal.pone.0023601
Acebes J. M. V., Tull M. (2016). The history and characteristics of the mobulid ray fishery in the bohol Sea, Philippines. PloS One 11 (8), e0161444. doi: 10.1371/journal.pone.0161444
Akyol O., Erdem M., Unal V., Ceyhan T. (2005). Investigations on drift-net fishery for swordfish (Xiphias gladius l.) in the Aegean Sea. Turk. J. Vet. Anim. Sci. 29, 1225–1231.
Alava J. J., Merlen G. (2009). Video-documentation of a killer whale (Orcinus orca) predatory attack on a giant manta (Manta birostris) in the Galapagos islands. Lat. Am. J. Aquat. Mamm. 7, 81–84. doi: 10.5597/lajam00139
Alfaro-Cordova E., Del Solar A., Alfaro-Shigueto J., Mangel J. C., Diaz B., Carrillo O., et al. (2017). Captures of manta and devil rays by small-scale gillnet fisheries in northern Peru. Fish. Res. 195, 28–36. doi: 10.1016/j.fishres.2017.06.012
Allen H. L., Stewart B. D., McClean C. J., Hancock J., Rees R. (2021). Anthropogenic injury and site fidelity in maldivian whale sharks (Rhincodon typus). Aquat. Conserv. Mar. Freshw. Ecosyst. 31, 1429–1442. doi: 10.1002/aqc.3524
Anderson R. C., Adam M. S., Goes J. I. (2011). From monsoons to mantas: seasonal distribution of Manta alfredi in the Maldives. Fish. Oceanog. 20, 104–113. doi: 10.1111/j.1365-2419.2011.00571.x
Andrzejaczek S., Chapple T. K., Curnick D. J., Carlisle A. B., Castleton M., Jacoby D. M. P., et al. (2020). Individual variation in residency and regional movements of reef manta rays Mobula alfredi in a large marine protected area. Mar. Ecol. Prog. Ser. 639, 137–153. doi: 10.3354/meps13270
Andrzejaczek S., Lucas T. C., Goodman M. C., Hussey N. E., Armstrong A. J., Carlisle A., et al. (2022). Diving into the vertical dimension of elasmobranch movement ecology. Sci. Adv. 8, eabo1754. doi: 10.1126/sciadv.abo1754
Ari C. (2011). Encephalization and brain organization of mobulid rays (Myliobatiformes, elasmobranchii) with ecological perspectives. Open Anat. J. 3, 1. doi: 10.2174/1877609401103010001
Armansin N. C., Lee K. A., Huveneers C., Harcourt R. G. (2016). Integrating social network analysis and fine-scale positioning to characterize the associations of a benthic shark. Anim. Behav. 115, 245–258. doi: 10.1016/j.anbehav.2016.02.014
Armstrong A. O., Armstrong A. J., Jaine F. R. A., Couturier L. I. E., Fiora K., Uribe-Palomino J., et al. (2016). Prey density threshold and tidal influence on reef manta ray foraging at an aggregation site on the great barrier reef. PloS One 11, e0153393. doi: 10.1371/journal.pone.0153393
Armstrong A. O., Stevens G. M. W., Townsend K. A., Murray A., Bennett M. B., Armstrong A. J., et al. (2021). Reef manta rays forage on tidally driven, high density zooplankton patches in hanifaru bay, Maldives. PeerJ 9, e11992. doi: 10.7717/peerj.11992
Ayres K. A., Ketchum J. T., González-Armas R., Galván-Magaña F., Hearn A., Elorriaga-Verplancken F. R., et al. (2021). Seasonal aggregations of blacktip sharks Carcharhinus limbatus at a marine protected area in the gulf of California, assessed by unoccupied aerial vehicle surveys. Mar. Ecol. Prog. Ser. 678, 95–107. doi: 10.3354/meps13897
Barr Y., Abelson A. (2019). Feeding – cleaning trade-off: Manta ray “Decision-making” as a conservation tool. Front. Mar. Sci. 6. doi: 10.3389/fmars.2019.00088
Beale C. S., Stewart J. D., Setyawan E., Sianipar A. B., Erdmann M. V. (2019). Population dynamics of oceanic manta rays (Mobula birostris) in the raja ampat archipelago, West Papua, Indonesia, and the impacts of the El niño–southern oscillation on their movement ecology. Divers. Distrib. 25, 1472–1487. doi: 10.1111/ddi.12962
Booth H., Mardhiah U., Siregar H., Hunter J., Putra M. I. H., Marlow J., et al. (2021). An integrated approach to tackling wildlife crime: Impact and lessons learned from the world's largest targeted manta ray fishery. Conserv. Sci. Pract. 3 (2), e314. doi: 10.1111/csp2.314
Booth H., Pooley S., Clements T., Putra M. I. H., Lestari W. P., Lewis S., et al. (2020). Assessing the impact of regulations on the use and trade of wildlife: An operational framework, with a case study on manta rays. Global Ecol. Conserv. 22, e00953. doi: 10.1016/j.gecco.2020.e00953
Brack I. V., Kindel A., Oliveira L. F. B. (2018). Detection errors in wildlife abundance estimates from unmanned aerial systems (UAS) surveys: synthesis, solutions and challenges. Meth. Ecol. Evol. 9, 1864–1873. doi: 10.1111/2041-210X.13026
Broadhurst M. K., Cullis B. R. (2020). Mitigating the discard mortality of non-target, threatened elasmobranchs in bather-protection gillnets. Fish. Res. 222, 105435. doi: 10.1016/j.fishres.2019.105435
Broadhurst M. K., Laglbauer B. J. L., Carrier K. B., Coleman M. A. (2018). Reproductive biology and range extension for Mobula kuhlii cf. eregoodootenkee. Endang. Species Res. 35, 71–80. doi: 10.3354/esr00876
Brown C., Laland K. (2011). “Social learning in fishes,” in Fish cognition and behavior. Eds. Pitcher T. J., Brown C., K. Laland, Krause J. (West Sussex, UK: Blackwell Publishing Ldt), 240–252. doi: 10.1002/9781444342536.ch11
Bucair N., Venables S. K., Balboni A. P., Marshall A. D. (2021). Sightings trends and behaviour of manta rays in Fernando de noronha archipelago, Brazil. Mar. Biodivers. Rec. 14, 1–11. doi: 10.1186/s41200-021-00204-w
Burgess K. B. (2017). Feeding ecology and habitat use of the giant manta ray manta birostris at a key aggregation site off mainland ecuador. (PhD thesis) (Queesnland, AUS: The University of Queensland). doi: 10.14264/uql.2018.157
Cabral M. M. P., Stewart J. D., Marques T. A., Ketchum J. T., Ayala-Bocos A., Hoyos-Padilla E. M., et al. (2023). The influence of El niño southern oscillation on the population dynamics of oceanic manta rays in the Mexican pacific. Hydrobiologia 850, 257–267. doi: 10.1007/s10750-022-05047-9
Canese S., Cardinali A., Romeo T., Giusti M., Salvati E., Angiolillo M., et al. (2011). Diving behavior of the giant devil ray in the Mediterranean Sea. Endang. Species Res. 14, 171–176. doi: 10.3354/esr00349
Carrier J. C., Pratt J. H. L., Martin L. K. (1994). Group reproductive behaviors in free-living nurse sharks, Ginglymostoma cirratum. Copeia, 3, 646–656. doi: 10.2307/1447180
Cattano C., Turco G., Di Lorenzo M., Gristina M., Visconti G., Milazzo M. (2021). Sandbar shark aggregation in the central Mediterranean Sea and potential effects of tourism. Aquat. Conserv. Mar. Freshw. Ecosyst. 31, 1420–1428. doi: 10.1002/aqc.3517
Celona A. (2004). Caught and observed giant devil rays Mobula mobular (Bonnaterre 1788) in the strait of Messina. Ann. Ser. Hist. Nat. 14, 11–18.
Cerutti F. (2005). Isótopos estables de carbono y nitrógeno en mantas (Batoidea: Mobulidae) como indicadores tróficos (Universidad Autónoma De Baja California Sur: Bachelor tesis) La Paz, MX).
Chaikin S., Belmaker J., Barash A. (2020). Coastal breeding aggregations of threatened stingrays and guitarfish in the Levant. Aquat. Conserv: Mar. Freshw. Ecosyst. 30, 1160–1171. doi: 10.1002/aqc.3305
Cheung W. W., Lam V. W., Sarmiento J. L., Kearney K., Watson R., Pauly D. (2009). Projecting global marine biodiversity impacts under climate change scenarios. Fish. Fish. 10, 235–251. doi: 10.1111/j.1467-2979.2008.00315.x
Childs J. N. (2001). The occurrence, habitat use and behavior of sharks and rays associating with topographic highs in the northwestern gulf of mexico. (Master’s thesis) (Texas, USA: Texas A&M University).
Chiriboga-Paredes Y., Palomino Á., Goodman L., Córdova F., Páez V., Yépez M., et al. (2022). Discovery of a putative scalloped hammerhead shark Sphyrna lewini (Carcharhiniformes: Sphyrnidae) nursery site at the Galapagos islands, Eastern tropical pacific. Environ. Biol. Fish. 105, 181–192. doi: 10.1007/s10641-021-01207-3
Chivers D. P., Brown G. E., Smith R. J. F. (1995). Familiarity and shoal cohesion in fathead minnows (Pimephales promelas): implications for antipredator behaviour. Can. J. Zool. 73, 955–960. doi: 10.1139/z95-111
Clark M. R., Rowden A. A., Schlacher T. A., Guinotte J., Dunstan P. K., Williams A., et al. (2014). Identifying ecologically or biologically significant areas (EBSA): a systematic method and its application to seamounts in the south pacific ocean. Ocean Coast. Manage. 91, 65–79. doi: 10.1016/j.ocecoaman.2014.01.016
Clua E., Chauvet C., Read T., Werry J. M., Lee S. Y. (2013). Behavioral patterns of a tiger shark (Galeocerdo cuvier) feeding aggregation at a blue whale carcass in prony bay, new Caledonia. Mar. Freshw. Behav. Physiol. 46, 1–20. doi: 10.1080/10236244.2013.773127
Colefax A. P., Butcher P. A., Kelaher B. P. (2018). The potential for unmanned aerial vehicles (UAVs) to conduct marine fauna surveys in place of manned aircraft. ICES J. Mar. Sci. 75, 1–8. doi: 10.1093/icesjms/fsx100
Couturier L. I. E., Bennett M. B., Richardson A. J. (2013). Mystery of giant rays off the Gaza strip solved. Oryx 47, 480. doi: 10.1017/S003605313000793
Couturier L. I. E., Jaine F. R. A., Townsend K. A., Weeks S. J., Richardson A. J., Bennett M. B. (2011). Distribution, site affinity and regional movements of the manta ray, Manta alfredi (Krefft 1868), along the east coast of Australia. Mar. Freshw. Res. 62, 628–637. doi: 10.1071/MF10148
Couturier L. I. E., Marshall A. D., Jaine F. R. A., Kashiwagi T., Pierce S. J., Townsend K. A., et al. (2012). Biology, ecology and conservation of the mobulidae. J. Fish Biol. 80, 1075–1119. doi: 10.1111/j.1095-8649.2012.03264.x
Couturier L. I. E., Newman P., Jaine F. R. A., Bennett M. B., Venables W. N., Cagua E. F., et al. (2018). Variation in occupancy and habitat use of Mobula alfredi at a major aggregation site. Mar. Ecol. Prog. Series. 599, 125–145. doi: 10.3354/meps12610
Croft D. P., James R., Thomas P. O. R., Hathaway C., Mawdsley D., Laland K. N., et al. (2006). Social structure and co-operative interactions in a wild population of guppies (Poecilia reticulata). Behav. Ecol. Sociobiol 59, 644–650. doi: 10.1007/s00265-005-0091-y
Croft D. P., Madden J. R., Franks D. W., James R. (2011). Hypothesis testing in animal social networks. Trends. Ecol. Evol. 26, 502–507. doi: 10.1016/j.tree.2011.05.012
Croll D. A., Dewar H., Dulvy N. K., Fernando D., Francis M. P., Galván-Magaña F., et al. (2016). Vulnerabilities and fisheries impacts: the uncertain future of manta and devil rays. Aqua. Conserv. Mar. Freshw. Ecosyst. 26, 562–575. doi: 10.1002/aqc.2591
Croll D. A., Newton K. M., Weng K., Galván-Magaña F., O’Sullivan J., Dewar H. (2012). Movement and habitat use by the spine-tail devil ray in the Eastern pacific ocean. Mar. Ecol. Prog. Series. 465, 193–200. doi: 10.3354/meps09900
Cronin M. R., Amaral J. E., Jackson A. M., Jacquet J., Seto K. L., Croll D. A. (2023). Policy and transparency gaps for oceanic shark and rays in high seas tuna fisheries. Fish Fish. 24, 56–70. doi: 10.1111/faf.12710
Cronin M. R., Croll D. A., Hall M. A., Lezama-Ochoa N., Lopez J., Murua H., et al. (2022). Harnessing stakeholder knowledge for the collaborative development of mobulid bycatch mitigation strategies in tuna fisheries. ICES J. Mar. Sci. 0, 1–15. doi: 10.1093/icesjms/fsac093
Crowe L. M., O'Brien O., Curtis T. H., Leiter S. M., Kenney R. D., Duley P., et al. (2018). Characterization of large basking shark Cetorhinus maximus aggregations in the western north Atlantic ocean. J. Fish. Biol. 92, 1371–1384. doi: 10.1111/jfb.13592
Deakos M. H. (2010). Paired-laser photogrammetry as a simple and accurate system for measuring the body size of free-ranging manta rays Manta alfredi. Aquat. Biol. 10, 1–10. doi: 10.3354/ab00258
Deakos M. H. (2012). The reproductive ecology of resident manta rays (Manta alfredi) off Maui, Hawaii, with an emphasis on body size. Environ. Biol. Fishes. 94, 443–456. doi: 10.1007/s10641-011-9953-5
Deakos M. H., Baker J. D., Bejder L. (2011). Characteristics of a manta ray Manta alfredi population off Maui, Hawaii, and implications for management. Mar. Ecol. Prog. Ser. 429, 245–260. doi: 10.3354/meps09085
De-Boer M. N., Saulino J. T., Lewis T. P., Notarbartolo-Di-Sciara G. (2015). New records of whale shark (Rhincodon typus), giant manta ray (Manta birostris) and Chilean devil ray (Mobula tarapacana) for Suriname. Mar. Biodivers. Rec. 8, e10. doi: 10.1017/S1755267214001432
De-la-Parra-Venegas R., Hueter R., Gonzalez-Cano J., Tyminski J., Gregorio-Remolina J., Maslanka M., et al. (2011). An unprecedented aggregation of whale sharks, Rhincodon typus, in Mexican coastal waters of the Caribbean Sea. PloS One 6 (4), e18994. doi: 10.1371/journal.pone.0018994
Del-Valle-González-González (2018). Diversidad de los peces batoideos de la zona sur de la isla espíritu Santo, BCS, méxico. (Masters tesis) (La Paz, MX: Instituto Politécnico Nacional, Centro Interdisciplinario de Ciencias Marinas).
Dewar H., Mous P., Domeier M., Muljadi A., Pet J., Whitty J. (2008). Movements and site fidelity of the giant manta ray, Manta birostris, in the komodo marine park, Indonesia. Mar. Biol. 155, 121–133. doi: 10.1007/s00227-008-0988-x
Duffy C. A. J., Abbott D. (2003). Sightings of mobulid rays from northern new Zealand, with confirmation of the occurrence of Manta birostris in new Zealand waters. NZ. J. Mar. Fresh. Res. 37, 715–721. doi: 10.1080/00288330.2003.9517201
Duffy C. A., Tindale S. C. (2018). First observation of the courtship behaviour of the giant devil ray Mobula mobular (Myliobatiformes: Mobulidae). NZ. J. Zool. 45, 387–394. doi: 10.1080/03014223.2017.1410850
Dulvy N. K., Pacoureau N., Rigby C. L., Pollom R. A., Jabado R. W., Ebert D. A., et al. (2021). Overfishing drives over one-third of all sharks and rays toward a global extinction crisis. Curr. Biol. 31, 4773–4787. doi: 10.1016/j.cub.2021.08.062
Dulvy N. K., Pardo S. A., Simpfendorfer C. A., Carlson J. K. (2014). Diagnosing the dangerous demography of manta rays using life history theory. PeerJ 2, e400. doi: 10.7717/peerj.400
Dunbar R. I. M., Shultz S. (2007). Evolution in the social brain. Sci 317, 1344–1347. doi: 10.1126/science.1145463
Dunn D. C., Maxwell S. M., Boustany A. M., Halpin P. N. (2016). Dynamic ocean management increases the efficiency and efficacy of fisheries management. Proc. Natl. Acad. Sci. 113, 668–673. doi: 10.1073/pnas.1513626113
Economakis A. E., Lobel P. S. (1998). Aggregation behavior of the grey reef shark, Carcharhinus amblyrhynchos, at Johnston atoll, central pacific ocean. Environ. Biol. Fishes. 51, 129–139. doi: 10.1023/A:1007416813214
Espinoza M., Farrugia T. J., Webber D. M., Smith F., Lowe C. G. (2011). Testing a new acoustic telemetry technique to quantify long-term, fine-scale movements of aquatic animals. Fish Res. 108 (2), 364e371. doi: 10.1016/j.fishres.2011.01.011
Fernando D., Stewart J. D. (2021). High bycatch rates of manta and devil rays in the “small-scale'' artisanal fisheries of Sri Lanka. PeerJ 9, e11994. doi: 10.7717/peerj.11994
Finger J. S., Guttridge T. L., Wilson A. D. M., Gruber S. H., Krause J. (2018). Are some sharks more social than others? short-and long-term consistencies in the social behavior of juvenile lemon sharks. Behav. Ecol. Sociobiol. 72, 1–10. doi: 10.1007/s00265-017-2431-0
Fonseca-Ponce I. A., Zavala-Jiménez A. A., Aburto-Oropeza O., Maldonado-Gasca A., Galván-Magaña F., González-Armas R., et al. (2022). Physical and environmental drivers of oceanic manta ray mobula birostris sightings at an aggregation site in bahía de banderas, Mexico. Mar. Ecol. Prog. Series. 694, 133–148. doi: 10.3354/meps14106
Galef B. G. (2013). Imitation and local enhancement: detrimental effects of consensus definitions on analyses of social learning in animals. Behav. Process. 100, 123–130. doi: 10.1016/j.beproc.2013.07.026
Gallagher A. J., Hammerschlag N. (2011). Global shark currency: the distribution, frequency, and economic value of shark ecotourism. Curr. Issues Tourism. 14, 797–812. doi: 10.1080/13683500.2011.585227
Germanov E. S., Bejder L., Chabanne D. B. H., Dharmadi D., Hendrawan I. G., Marshall A. D., et al. (2019). Contrasting habitat use and population dynamics of reef manta rays within the nusa penida marine protected area, Indonesia. Front. Mar. Sci. 6. doi: 10.3389/fmars.2019.00215
Germanov E. S., Marshall A. D. (2014). Running the gauntlet: regional movement patterns of Manta alfredi through a complex of parks and fisheries. PloS One 9, e110071. doi: 10.1371/journal.pone.0110071
Gómez-García M. J., Blázquez-Moreno M. C., Stewart J. D., Leos-Barajas V., Fonseca-Ponce I. A., Zavala-Jiménez A. A., et al. (2021). Quantifying the effects of diver interactions on manta ray behavior at their aggregation sites. Front. Mar. Sci. 8. doi: 10.3389/fmars.2021.639772
Grande M., Ruiz J., Murua H., Murua J., Goñi N., Arregi I. K. I., et al. (2020). Progress on the code of good practices on the tropical tuna purse seine fishery in the Atlantic ocean. Collect. Vol. Sci. Pap. ICCAT. 76, 193–234.
Grutter A. S. (1996). Experimental demonstration of no effect by the cleaner fish Labroides dimidiatus (Cuvier and valenciennes) on the host fish Pomacentrus moluccencis (Bleeker). J. Exp. Mar. Biol.Ecol. 196, 285–298. doi: 10.1016/0022-0981(95)00135-2
Guirhem G., Arrizabalaga H., Lopetegui L., Murua H., Ochoa N. L., Griffiths S., et al. (2021). Preliminary habitat suitability model for devil rays in the Western Indian ocean. IOTC-2021-WPEB17(AS)-27_rev1. Available at: https://www.researchgate.net/publication/355397345_A_Preliminary_Habitat_Suitability_Model_for_Devil_Rays_in_the_Western_Indian_Ocean.
Guirkinger L., Rojas-Perea S., Ender I., Ramsden M., Lenton-Lyons C., Geldmann J. (2021). Motivations for compliance in Peruvian manta ray fisheries. Mar. Policy. 124, 104315. doi: 10.1016/j.marpol.2020.104315
Guttal V., Couzin I. D. (2010). Social interactions, information use, and the evolution of collective migration. Proc. Natl. Acad. Sci. 107, 16172–16177. doi: 10.1073/pnas.1006874107
Guttridge T. L., Gruber S. H., DiBattista J. D., Feldheim K. A., Croft D. P., Krause S., et al. (2011). Assortative interactions and leadership in a free-ranging population of juvenile lemon shark Negaprion brevirostris. Mar. Ecol. Prog. Series. 423, 235–245. doi: 10.3354/meps08929
Guttridge T. L., Gruber S. H., Gledhill K. S., Croft D. P., Sims D. W., Krause J. (2009). Social preferences of juvenile lemon sharks, Negaprion brevirostris. Anim. Behav. 78, 543–548. doi: 10.1016/j.anbehav.2009.06.009
Guttridge T. L., Gruber S. H., Krause J., Sims D. W. (2010). Novel acoustic technology for studying free-ranging shark social behaviour by recording individuals’ interactions. PloS One 5 (2), e9324. doi: 10.1371/journal.pone.0009324
Hacohen-Domené A., Martínez-Rincón R. O., Galván-Magaña F., Cárdenas-Palomo N., de la Parra-Venegas R., Galván-Pastoriza B., et al. (2015). Habitat suitability and environmental factors affecting whale shark (Rhincodon typus) aggregations in the Mexican Caribbean. Enviro. Biol. Fish. 98, 1953–1964. doi: 10.1007/s10641-015-0413-5
Hacohen-Domené A., Martinez-Rincon R. O., Galvan-Magaña F., Cardenas-Palomo N., Herrera-Silveira J. (2017). Environmental factors influencing aggregation of manta rays (Manta birostris) off the northeastern coast of the Yucatan peninsula. Mar. Ecol. 38 (3), e12432. doi: 10.1111/maec.12432
Hall M., Roman M. (2013). “Bycatches and non-tuna catches in the tropical tuna purse seine fisheries of the world,” in FAO fisheries and aquaculture technical paper no. 568 (Rome, Italy: Food and Agriculture Organization of the United Nations).
Harris J. L., Hosegood P., Robinson E., Embling C. B., Hilbourne S., Stevens G. M. W. (2021). Fine-scale oceanographic drivers of reef manta ray (Mobula alfredi) visitation patterns at a feeding aggregation site. Ecol. Evol. 11, 4588–4604. doi: 10.1002/ece3.7357
Harris J. L., McGregor P., Oates Y., Stevens G. (2020). Gone with the wind: seasonal distribution and habitat use by the reef manta ray (Mobula alfredi) in the Maldives, implications for conservation. Aquat. Conserv. Mar. Freshw. Ecosyst. 30, 1649–1664. doi: 10.1002/aqc.3350
Harris J. L., Stevens G. M. W. (2021). Environmental drivers of reef manta ray (Mobula alfredi) visitation patterns to key aggregation habitats in the Maldives. PloS One 16 (6), e0252470. doi: 10.1371/journal.pone.0252470
Hartup J. A., Marshell A., Stevens G., Kottermair M., Carlson P. (2013). Manta alfredi target multispecies surgeonfish spawning aggregations. Coral Reefs. 32, 367–367. doi: 10.1007/s00338-013-1022-4
Harty K., Guerrero M., Knochel A. M., Stevens G. M. W., Marshall A., Burgess K., et al. (2022). Demographics and dynamics of the world’s largest known population of oceanic manta rays Mobula birostris in coastal Ecuador. Mar. Ecol. Prog. Ser. 700, 145–159. doi: 10.3354/meps14189
Haulsee D. E., Fox D. A., Breece M. W., Brown L. M., Kneebone J., Skomal G. B., et al. (2016). Social network analysis reveals potential fission-fusion behavior in a shark. Sci. Rep. 6, 1–9. doi: 10.1038/srep34087
Hay M. E., Parker J. D., Burkepile D. E., Caudill C. C., Wilson A. E., Hallinan Z. P., et al. (2004). Mutualisms and aquatic community structure: The enemy of my enemy is my friend. Annu. Rev. Ecol. Evol. Syst. 34, 175–197. doi: 10.1146/ANNUREV.ECOLSYS.34.011802.132357
Hazen E. L., Jorgensen S., Rykaczewski R. R., Bograd S. J., Foley D. G., Jonsen I. D., et al. (2013). Predicted habitat shifts of pacific top predators in a changing climate. Nat. Clim. Change. 3, 234–238. doi: 10.1038/nclimate1686
Hazen E. L., Scales K. L., Maxwell S. M., Briscoe D. K., Welch H., Bograd S. J., et al. (2018). A dynamic ocean management tool to reduce bycatch and support sustainable fisheries. Sci. Adv. 4 (5), eaar3001. doi: 10.1126/sciadv.aar3001
Heupel M. R., Carlson J. K., Simpfendorfer C. A. (2007). Shark nursery areas: concepts, definition, characterization and assumptions. Mar. Ecol. Prog. Series. 337, 287–297. doi: 10.3354/meps337287
Heupel M. R., Simpfendorfer C. A. (2005). Quantitative analysis of aggregation behavior in juvenile blacktip sharks. Mar. Biol. 147, 1239–1249. doi: 10.1007/s00227-005-0004-7
Heupel M. R., Simpfendorfer C. A. (2011). Estuarine nursery areas provide a low mortality environment for young bull sharks Carcharhinus leucas. Mar. Ecol. Prog. Ser. 433, 237–244. doi: 10.3354/meps09191
Hight B. V., Lowe C. G. (2007). Elevated body temperatures of adult female leopard sharks, Triakis semifasciata, while aggregating in shallow nearshore embayments: evidence for behavioral thermoregulation? J. Exp. Mar. Bio.l Ecol. 3521, 114–128. doi: 10.1016/j.jembe.2007.07.021
Holland K. N., Meyer C. G., Dagorn L. C. (2009). Inter-animal telemetry: results from first deployment of acoustic ‘business card’ tags. Endang. Species. Res. 10, 287–293. doi: 10.3354/esr00226
Hosegood J., Humble E., Ogden R., De Bruyn M., Creer S., Stevens G. M., et al. (2020). Phylogenomics and species delimitation for effective conservation of manta and devil rays. Mol. Ecol. 29, 4783–4796. doi: 10.1111/mec.15683
Hyde C. A., Notarbartolo-di-Sciara G., Sorrentino L., Boyd C., Finucci B., Fowler S. L., et al. (2022). Putting sharks on the map: a global standard for improving shark area-based conservation. Front. Mar. Sci. 1660. doi: 10.3389/fmars.2022.968853
IUCN. (2020). The IUCN red list of threatened species. version 2020-2. Available at: https://www.iucnredlist.org/ (Accessed 24 October 2021).
Jacoby D. M. P., Croft D. P., Sims D. W. (2012). Social behaviour in sharks and rays: analysis, patterns and implications for conservation. Fish Fish. 13, 399–417. doi: 10.1111/j.1467-2979.2011.00436.x
Jaine F. R. A., Couturier L. I. E., Weeks S. J., Townsend K. A., Bennett M. B., Fiora K., et al. (2012). When giants turn up: sighting trends, environmental influences and habitat use of the manta ray Manta alfredi at a coral reef. PloS One 7, e46170. doi: 10.1371/journal.pone.0046170
Johnson D. D. P., Kays R., Blackwell P. G., Macdonald D. W. (2002). Does the resource dispersion hypothesis explain group living? Trends Ecol. Evol. 17, 563–570. doi: 10.1016/S0169-5347(02)02619-8
Johnston D. W. (2019). Unoccupied aircraft systems in marine science and conservation. Annu. Rev. Mar. Sci. 11, 439–463. doi: 10.1146/annurev-marine-010318-09532
Jourdain E., Vongraven D. (2017). Humpback whale (Megaptera novaeangliae) and killer whale (Orcinus orca) feeding aggregations for foraging on herring (Clupea harengus) in northern Norway. Mamm Biol. 86, 27–32. doi: 10.1016/j.mambio.2017.03.006
Kajiura S. M., Tellman S. L. (2016). Quantification of massive seasonal aggregations of blacktip sharks (Carcharhinus limbatus) in southeast Florida. PloS One 11, e0150911. doi: 10.1371/journal.pone.0150911
Kiszka J. J., Mourier J., Gastrich K., Heithaus M. R. (2016). Using unmanned aerial vehicles (UAVs) to investigate shark and ray densities in a shallow coral lagoon. Mar. Ecol. Prog. Ser. 560, 237–242. doi: 10.3354/meps11945
Klimley A. P. (1987). The determinants of sexual segregation in the scalloped hammerhead shark. Sphyrna lewini. Env. Biol. Fish. 18, 27–40. doi: 10.1007/BF00002325
Klimley A. P., Nelson D. R. (1984). Diel movement patterns of the scalloped hammerhead shark (Sphyrna lewini) in relation to El bajo espiritu Santo: a refuging central-position social system. Behav. Ecol. Sociobiol. 15, 45–54. doi: 10.1007/BF00310214
Knochel A. M., Cochran J. E., Kattan A., Stevens G. M., Bojanowksi E., Berumen M. L. (2022). Crowdsourced data reveal multinational connectivity, population demographics, and possible nursery ground of endangered oceanic manta rays in the red Sea. Aquat. Conserv. Mar. Freshw. Ecosyst. 32, 1774–1786. doi: 10.1002/aqc.3883
Kumli K. R., Rubin R. D. (2010). Photo-identification of the manta ray, manta birostris, in the revillagigedos islands (Quebec: Page Joint Meeting of Ichthyologists and Herpetologists).
Lachlan R. F., Crooks L., Laland K. N. (1998). Who follows whom? shoaling preferences and social learning of foraging information in guppies. Anim. Behav. 56, 181–190. doi: 10.1006/anbe.1998.0760
Langlois T. J., Fitzpatrick B. R., Fairclough D. V., Wakefield C. B., Hesp S. A., McLean D. L., et al. (2012). Similarities between line fishing and baited stereo-video estimations of length-frequency: novel application of kernel density estimates. PloS One 7, e45973. doi: 10.1371/journal.pone.0045973
Lassauce H., Dudgeon C. L., Armstrong A. J., Wantiez L., Carroll E. L. (2022). Evidence of fine-scale genetic structure for reef manta rays Mobula alfredi in new Caledonia. Endang. Species Res. 47, 249–264. doi: 10.3354/esr01178
Lawson J. M., Fordham S. V., O’Malley M. P., Davidson L. N. K., Walls R. H. L., Heupel M. R., et al. (2017). Sympathy for the devil: A conservation strategy for devil and manta rays. PeerJ 5, e3027. doi: 10.7717/peerj.3027
Lester E., Meekan M. G., Barnes P., Raudino H., Rob D., Waples K., et al. (2020). Multi-year patterns in scarring, survival and residency of whale sharks in ningaloo marine park, Western Australia. Mar. Ecol. Prog. Ser. 634, 115–125. doi: 10.3354/meps13173
Lett C., Semeria M., Thiebault A., Tremblay Y. (2014). Effects of successive predator attacks on prey aggregations. Theor. Ecol. 7, 239–252. doi: 10.1007/s12080-014-0213-0
Lewis S. A., Setiasih N., Dharmadi D., O'Malley M. P., Campbell S. J., Yusuf M., et al. (2015). Assessing Indonesian manta and devil ray populations through historical landings and fishing community interviews. PeerJ PrePrints 6, e1334v1. doi: 10.7287/peerj.preprints.1334v1
Lewison R., Hobday A. J., Maxwell S., Hazen E., Hartog J. R., Dunn D. C., et al. (2015). Dynamic ocean management: identifying the critical ingredients of dynamic approaches to ocean resource management. BioScience 65, 486–498. doi: 10.1093/biosci/biv018
Lezama-Ochoa N., Hall M. A., Pennino M. G., Stewart J. D., López J., Murua H. (2019b). Environmental characteristics associated with the presence of the spinetail devil ray (Mobula mobular) in the eastern tropical pacific. PloS One 14(8), e0220854. doi: 10.1371/journal.pone.0220854
Lezama-Ochoa N., Hall M., Román M., Vogel N. (2019a). Spatial and temporal distribution of mobulid ray species in the eastern pacific ocean ascertained from observer data from the tropical tuna purse-seine fishery. Environ. Biol. Fish. 102, 1–17. doi: 10.1007/s10641-018-0832-1
Lezama-Ochoa N., Lopez J., Hall M., Bach P., Abascal F., Murua H. (2020). Spatio-temporal distribution of the spinetail devil ray Mobula mobular in the eastern tropical Atlantic ocean. Endang. Species. Res. 43, 447–460. doi: 10.3354/esr01082
Lieber L., Hall G., Hall J., Berrow S., Johnston E., Gubili C., et al. (2020). Spatio-temporal genetic tagging of a cosmopolitan planktivorous shark provides insight to gene flow, temporal variation and site-specific re-encounters. Sci. Rep. 10, 1–17. doi: 10.1038/s41598-020-58086-4
Litvinov F. F. (2006). On the role of dense aggregations of males and juveniles in the functional structure of the range of the blue shark Prionace glauca. J. Ichthyol. 46, 613–624. doi: 10.1134/S0032945206080091
López K. E. R., Guadalupe J. J., Gordillo-Romero M., Montero-Oleas A., Pazmiño D. A., Guerrero M., et al. (2022). Exploring the genetic diversity and population structure of mobula birostris in two key aggregation zones in the Eastern tropical pacific. Mar. Ecol. Prog. Ser. 699, 75–89. doi: 10.3354/meps14171
Marshall A. D., Bennett M. B. (2010a). Reproductive ecology of the reef manta ray manta alfredi in southern Mozambique. J. Fish Biol. 77, 169–190. doi: 10.1111/j.1095-8649.2010.02669.x
Marshall A. D., Bennett M. B. (2010b). The frequency and effect of shark-inflicted bite injuries to the reef manta ray Manta alfredi. Afr. J. Mar. Sci. 32, 573–580. doi: 10.2989/1814232X.2010.538152
Marshall A. D., Dudgeon C. L., Bennett M. B. (2011). Size and structure of a photographically identified population of manta rays Manta alfredi in southern Mozambique. Mar. Biol. 158, 1111–1124. doi: 10.1007/s00227-011-1634-6
Martins A. P. B., Heupel M. R., Chin A., Simpfendorfer C. A. (2018). Batoid nurseries: definition, use and importance. Mar. Ecol. Prog. Series. 595, 253–267. doi: 10.3354/meps12545
McAllister J. D., Barnett A., Lyle J. M., Stehfest K. M., Semmens J. M. (2017). Examining trends in abundance of an overexploited elasmobranch species in a nursery area closure. Mar. Freshw. Res. 69, 376–384. doi: 10.1071/MF17130
McCallister M., Mandelman J., Bonfil R., Danylchuk A., Sales M., Ajemian M. (2020). First observation of mating behavior in three species of pelagic myliobatiform rays in the wild. Enviro. Biol. Fish. 103, 163–173. doi: 10.1007/s10641-019-00943-x
McCann D., McCann C., Yew C. M., Araujo G., Manjaji-Matsumoto B. M. (2021). The shortfin devilray (Mobula kuhlii) aggregates at pulau Si amil, sabah, Malaysia. Pac. Conserv. Biol. 28, 532–537. doi: 10.1071/PC21017
Medeiros A. M., Luiz O. J., Domit C. (2015). Occurrence and use of an estuarine habitat by giant manta ray Manta birostris. J. Fish Biol. 86, 1830–1838. doi: 10.1111/jfb.12667
Mendonça S. A., Macena B. C. L., Araújo C. B. B., Bezerra N. P. A., Hazin F. H. V. (2020). Dancing with the devil: courtship behaviour, mating evidence and population structure of the Mobula tarapacana (Myliobatiformes: Mobulidae) in a remote archipelago in the equatorial mid-Atlantic Ocean. Neotrop. Ichthyol. 18 (3), e200008. doi: 10.1590/1982-0224-2020-0008
Mourier J., Vercelloni J., Planes S. (2012). Evidence of social communities in a spatially structured network of a free-ranging shark species. Anim. Behav. 83, 389–401. doi: 10.1016/j.anbehav.2011.11.008
Murie C. J., Marshall A. D. (2016). Mobula kuhlii cleaning station identified at an inshore reef in southern Mozambique. Peer J. PrePrints. 4, el724vl. doi: 10.7287/peerj.preprints.1724v1
Murray A., Garrud E., Ender I., Lee-Brooks K., Atkins R., Lynam R., et al. (2020). Protecting the million-dollar mantas; creating an evidence-based code of conduct for manta ray tourism interactions. J. Ecot. 19, 132–147. doi: 10.1080/14724049.2019.1659802
Mustika P. L. K., Wonneberger E., Erzini K., Pasisingi N. (2021). Marine megafauna bycatch in artisanal fisheries in gorontalo, northern sulawesi (Indonesia): An assessment based on fisher interviews. Ocean Coast. Manage. 208, 105606. doi: 10.1016/j.ocecoaman.2021.105606
Notarbartolo-di-Sciara G. (1988). Natural history of the rays of the genus mobula in the gulf of California. Fish. Bul. 86, 45–66.
Notarbartolo-di-Sciara G., Adnet S., Bennett M., Broadhurst M. K., Fernando D., Jabado R. W., et al. (2019). Taxonomic status, biological notes, and conservation of the longhorned pygmy devil ray Mobula eregoodoo (Cantor 1849). Aquat. Conserv. Mar. Freshw. Ecosyst. 30, 104–122. doi: 10.1002/aqc.3230
Notarbartolo-di-Sciara G., Hillyer E. V. (1989). Mobulid rays of eastern Venezuela (Chondrichthyes, mobulidae). Copeia 3, 607–614. doi: 10.2307/1445487
Notarbartolo-di-Sciara G., Lauriano G., Pierantonio N., Cañadas A., Donovan G., Panigada S. (2015). The devil we don’t know: investigating habitat and abundance of endangered giant devil rays in the north-Western Mediterranean Sea. PloS One 10, e0141189. doi: 10.1371/journal.pone.0141189
Oh B. Z., Thums M., Babcock R. C., Meeuwig J. J., Pillans R. D., Speed C., et al. (2017). Contrasting patterns of residency and space use of coastal sharks within a communal shark nursery. Mar. Freshw. Res. 68, 1501–1517. doi: 10.1071/MF16131
Olsen A. M., Westneat M. W. (2015). StereoMorph: an r package for the collection of 3D landmarks and curves using a stereo camera set-up. Methods Ecol. Evol. 6, 351–356. doi: 10.1111/2041-210X.12326
O’Malley M. P., Lee-Brooks K., Medd H. B. (2013). The global economic impact of manta ray watching tourism. PloS One 8, e65051. doi: 10.1371/journal.pone.0065051
O’Shea O. R., Kingsford M. J., Seymour J. (2010). Tide-related periodicity of manta rays and sharks to cleaning stations on a coral reef. Mar. Freshw. Res. 61, 65–73. doi: 10.1071/MF08301
Palacios M. D., Hoyos-Padilla E. M., Trejo-Ramírez A., Croll D. A., Galván-Magaña F., Zilliacus K. M., et al. (2021). Description of first nursery area for a pygmy devil ray species (Mobula munkiana) in the gulf of California, Mexico. Sci. Rep. 11, 1–11. doi: 10.1038/s41598-020-80506-8
Pardo S. A., Kindsvater H. K., Cuevas-Zimbrón E., Sosa-Nishizaki O., Pérez-Jiménez J. C., Dulvy N. K. (2016). Growth, productivity and relative extinction risk of a data-sparse devil ray. Sci. Rep. 6, 1–10. doi: 10.1038/srep33745
Pate J. H., Marshall A. D. (2020). Urban manta rays: potential manta ray nursery habitat along a highly developed Florida coastline. Endang. Species. Res. 43, 51–64. doi: 10.3354/esr01054
Peel L. R., Stevens G. M. W., Daly R., Keating-Daly C. A., Collin S. P., Nogués J., et al. (2020). Regional movements of reef manta rays (Mobula alfredi) in Seychelles waters. Front. Mar. Sci. 7. doi: 10.3389/fmars.2020.00558
Perryman R. J. Y., Carpenter M., Lie E., Sofronov G., Marshall A. D., Brown C. (2021). Reef manta ray cephalic lobe movements are modulated during social interactions. Behav. Ecol. Sociobiol. 75, 1–15. doi: 10.1007/s00265-021-02973-x
Perryman R. J. Y., Mourier J., Venables S. K., Tapilatu R. F., Setyawan E., Brown C. (2022). Reef manta ray social dynamics depend on individual differences in behaviour. Anim. Behav. 191, 43–55. doi: 10.1016/j.anbehav.2022.06.010Poisson
Perryman R. J. Y., Venables S. K., Tapilatu R. F., Marshall A. D., Brown C., Franks D. W. (2019). Social preferences and network structure in a population of reef manta rays. Behav. Ecol. Sociobiol. 73, 1–18. doi: 10.1007/s00265-019-2720-x
Poisson F., Vernet A. L., Séret B., Dagorn L. (2012). Good practices to reduce the mortality of sharks and rays caught incidentally by the tropical tuna purse seiners. EU FP7 project, 210496.
Porsiel N., Hernández S., Cordier D., Heidemeyer M. (2021). The devil is coming: Feeding behavior of juvenile munk’s devil rays (Mobula munkiana) in very shallow waters of punta Descartes, Costa Rica. Rev. Biol. Trop. 69, 256–266. doi: 10.15517/rbt.v69iS2.48744
Pratt H. L., Carrier J. C. (2001). A review of elasmobranch reproductive behavior with a case study on the nurse shark, Ginglymostoma cirratum. Environ. Biol. Fishes. 60, 157–188. doi: 10.1023/A:1007656126281
Rambahiniarison J. M., Lamoste M. J., Rohner C. A., Murray R., Snow S., Labaja J., et al. (2018). Life history, growth, and reproductive biology of four mobulid species in the bohol Sea, Philippines. Front. Mar. Sci. 5, 269. doi: 10.3389/fmars.2018.00269
Reyier E. A., Adams D. H., Lowers R. H. (2008). First evidence of a high-density nursery ground for the lemon shark, Negaprion brevirostris, near cape Canaveral, Florida. Florida Scientist. 71, 134–148.
Rohner C. A., Flam A. L., Pierce S. J., Marshall A. D. (2017). Steep declines in sightings of manta rays and devilrays (Mobulidae) in southern Mozambique. PeerJ 5, e3051v1. doi: 10.7287/peerj.preprints.3051v1
Rohner C. A., Pierce S. J., Marshall A. D., Weeks S. J., Bennett M. B., Richardson A. J. (2013). Trends in sightings and environmental influences on a coastal aggregation of manta rays and whale sharks. Mar. Ecol. Prog. Series. 482, 153–168. doi: 10.3354/meps10290
Rojas Perea S. L. (2016). Estudio morfológico de batoideos del género mobula (Myliobatiformes: Myliobatidae) del mar peruano. (Master’s thesis) (Lima, PE: Universidad Ricardo Palma).
Schlaff A. M., Heupel M. R., Simpfendorfer C. A. (2014). Influence of environmental factors on shark and ray movement, behaviour and habitat use: a review. Rev. Fish Biol. Fish. 24, 1089–1103. doi: 10.1007/s11160-014-9364-8
Schofield G., Esteban N., Katselidis K. A., Hays G. C. (2019). Drones for research on sea turtles and other marine vertebrates–a review. Biol. Cons. 238, 108214. doi: 10.1016/j.biocon.2019.108214
Selwyn J. D., Hogan J. D., Downey-Wall A. M., Gurski L. M., Portnoy D. S., Heath D. D. (2016). Kin-aggregations explain chaotic genetic patchiness, a commonly observed genetic pattern, in a marine fish. PloS One 11, e0153381. doi: 10.1371/journal.pone.0153381
Setyawan E., Erdmann M., Gunadharma N., Gunawan T., Hasan A., Izuan M., et al. (2022b). A holistic approach to manta ray conservation in the papuan bird’s head seascape: Resounding success, ongoing challenges. Mar. Policy. 137, 104953. doi: 10.1016/j.marpol.2021.104953
Setyawan E., Erdmann M. V., Lewis S. A., Mambrasar R., Hasan A. W., Templeton S., et al. (2020). Natural history of manta rays in the bird’s head seascape, Indonesia, with an analysis of the demography and spatial ecology of Mobula alfredi (Elasmobranchii: Mobulidae). J. Ocean Sci. Found. 36, 49–83. doi: 10.5281/zenodo.4396260
Setyawan E., Erdmann M. V., Mambrasar R., Hasan A. W., Sianipar A. B., Constantine R., et al. (2022a). Residency and use of an important nursery habitat, raja ampat’s wayag lagoon, by juvenile reef manta rays (Mobula alfredi). Front. Mar. Sci. 9. doi: 10.3389/fmars.2022.815094
Setyawan E., Sianipar A. B., Erdmann M. V., Fischer A. M., Haddy J. A., Beale C. S., et al. (2018). Site fidelity and movement patterns of reef manta rays (Mobula alfredi: Mobulidae) using passive acoustic telemetry in northern raja ampat, Indonesia. Nat. Conserv. Res. 3, 1–15. doi: 10.24189/ncr.2018.043
Setyawan E., Stevenson B. C., Izuan M., Constantine R., Erdmann M. V. (2022c). How big is that manta ray? a novel and non-invasive method for measuring reef manta rays using small drones. Drones 6 (3), 63. doi: 10.3390/drones6030063
Sigsgaard E. E., Nielsen I. B., Bach S. S., Lorenzen E. D., Robinson D. P., Knudsen S. W., et al. (2016). Population characteristics of a large whale shark aggregation inferred from seawater environmental DNA. Nat. Ecol. Evol. 1, 1–5. doi: 10.1038/s41559-016-0004
Sih A., Hanser S. F., McHugh K. A. (2009). Social network theory: new insights and issues for behavioral ecologists. Behav. Ecol. Sociobiol. 63, 975–988. doi: 10.1007/s00265-009-0725-6
Silverman E. D., Veit R. R., Nevitt G. A. (2004). Nearest neighbors as foraging cues: information transfer in a patchy environment. Mar. Ecol. Prog. Ser. 277, 25–36. doi: 10.3354/meps277025
Sims D. W., Berrow S. D., O'Sullivan K. M., Pfeiffer N. J., Collins R., Smith K. L., et al. (2022). Circles in the sea: Annual courtship ‘torus’ behaviour of basking sharks Cetorhinus maximus identified in the eastern north Atlantic ocean. J. Fish. Biol. 101, 1160–1181. doi: 10.1111/jfb.15187
Sims D. W., Southall E. J., Quayle V. A., Fox A. M. (2000). Annual social behaviour of basking sharks associated with coastal front areas. Proc. R. Soc Lond B. 267, 1897–1904. doi: 10.1098/rspb.2000.1227
Smith W. D., Bizzarro J. J., Cailliet G. M. (2009). The artisanal elasmobranch fishery on the east coast of Baja California, Mexico: Characteristics and management considerations. Cienc. Mar. 35, 209–236. doi: 10.7773/cm.v35i2.1534
Sobral A. F. L. (2013). Biology, ecology, and conservation of mobulid rays in the azores. (Master’s thesis) (Azores, Por: Universidade dos Açores).
Solleliet-Ferreira S., Macena B. C. L., Laglbauer B. J. L., Sobral A. F., Afonso P., Fontes J. (2020). Sicklefin devilray and common remora prey jointly on baitfish. Environ. Biol. Fish. 103, 993–1000. doi: 10.1007/s10641-020-00990-9
Stevens G. M. W. (2016). Conservation and population ecology of manta rays in the maldives. (PhD thesis) (York, UK: University of York).
Stevens J. D., Bonfil R., Dulvy N. K., Walker P. A. (2000). The effects of fishing on sharks, rays, and chimaeras (chondrichthyans), and the implications for marine ecosystems. ICES J. Mar. Sci. 57, 476–494. doi: 10.1006/jmsc.2000.0724
Stevens G. M. W., Fernando D., Dando M., Notarbartolo di Sciara G. (2018a). Guide to the Manta and Devil Rays of the World (UK, Wild Nature Press).
Stevens G. M. W., Hawkins J. P., Roberts C. M. (2018b). Courtship and mating behavior of manta rays mobula alfredi and M. birostris in the Maldives. J. Fish Biol. 93, 344–359. doi: 10.1111/jfb.13768
Stewart J. D., Barroso A., Butler R. H., Munns R. J. (2018c). Caught at the surface: myctophids make easy prey for dolphins and devil rays. Ecol 99, 1894–1896. doi: 10.1002/ecy.2348
Stewart J. D., Beale C. S., Fernando D., Sianipar A. B., Burton R. S., Semmens B. X., et al. (2016a). Spatial ecology and conservation of Manta birostris in the indo-pacific. Biol. Conserv. 200, 178–183. doi: 10.1016/j.biocon.2016.05.016
Stewart J. D., Hoyos-Padilla E. M., Kumli K. R., Rubin R. D. (2016b). Deep-water feeding and behavioral plasticity in Manta birostris revealed by archival tags and submersible observations. Zoology 119, 406–413. doi: 10.1016/j.zool.2016.05.010
Stewart J. D., Jaine F. R., Armstrong A. J., Armstrong A. O., Bennett M. B., Burgess K. B., et al. (2018a). Research priorities to support effective manta and devil ray conservation. Front. Mar. Sci. 5. doi: 10.3389/fmars.2018.00314
Stewart J. D., Nuttall M., Hickerson E. L., Johnston M. A. (2018b). Important juvenile manta ray habitat at flower garden banks national marine sanctuary in the northwestern gulf of Mexico. Mar. Biol. 165, 111. doi: 10.1007/s00227-018-3364-5
Stewart J. D., Rohner C. A., Araujo G., Avila. J., Fernando D., Forsberg K., et al. (2017b). Trophic overlap in mobulid rays: insights from stable isotope analysis. Mar. Ecol. Prog. Ser. 580, 131–151. doi: 10.3354/meps12304
Stewart J. D., Smith T., Marshall G., Abernathy K., Fonseca-Ponce I. A., Froman N., et al. (2019). Novel applications of animal-borne crittercams reveal thermocline feeding in two species of manta ray. Mar. Ecol. Prog. Ser. 632, 145–158. doi: 10.3354/meps13148
Stewart J. D., Stevens G. M., Marshall G. J., Abernathy K. (2017a). Are mantas self aware or simply social? a response to ari and D’Agostino 2016. J. Ethol. 35, 145–147. doi: 10.1007/s10164-016-0491-7
Strike E. M., Harris J. L., Ballard K. L., Hawkins J. P., Crockett J., Stevens G. M. W. (2022). Sublethal injuries and physical abnormalities in Maldives manta rays, Mobula alfredi and. Mobula birostris. Front. Mar. Sci. 9. doi: 10.3389/fmars.2022.773897
Tenzing P. (2014). The eco-physiology of two species of tropical stingrays in an era of climate change (Townsville, AUS: James Cook University).
Thorpe W. H. (1963). Learning and instinct in animals (Methuen and Co, London: Harvard Univ. Press).
Thorrold S. R., Afonso P., Fontes J., Braun C. D., Santos R. S., Skomal G. B., et al. (2014). Extreme diving behaviour in devil rays links surface waters and the deep ocean. Nat. Commun. 5, 4274. doi: 10.1038/ncomms5274
Toyoshima J., Nadaoka K. (2015). Importance of environmental briefing and buoyancy control on reducing negative impacts of SCUBA diving on coral reefs. Ocean Coast. Manage. 116, 20–26. doi: 10.1016/j.ocecoaman.2015.06.018
Uchida S., Toda M., Matsumoto Y. (2008). “Captive records of manta rays in Okinawa churaumi aquarium,” in Joint meeting of ichthyologists and herpetologists(Montreal, QC), 23–28.
Urbán R. J., Viloria-Gómora L. (2021). Challenges of whale watching and swim with dolphins in Mexico. Front. Mar. Sci. 8. doi: 10.3389/fmars.2021.624596
Venables S. (2013). Short term behavioral responses of manta rays, manta alfredi, to tourism interactions in coral bay, Western australia. (PhD thesis) (Perth, AUS: Murdoch University).
Venables S., McGregor F., Brain L., van Keulen M. (2016). Manta ray tourism management, precautionary strategies for a growing industry: a case study from the ningaloo marine park, Western Australia. Pac. Conserv. Biol. 22, 295–300. doi: 10.1071/PC16003
Villegas-Ríos D., Jacoby D. M. P., Mourier J. (2022). Social networks and the conservation of fish. Commun. Biol. 5, 178. doi: 10.1038/s42003-022-03138-w
Wallman H. L., Bennett W. A. (2006). Effects of parturition and feeding on thermal preference of Atlantic stingray, Dasyatis sabina (Lesueur). Env. Biol. Fish. 75, 259–267. doi: 10.1007/s10641-006-0025-1
Ward A. J. W., Hart P. J. B., Krause J. (2004). Assessment and assortment: how fishes use local and global cues to choose which school to go to. Proc. R. Soc Lond B. 271, 328–330. doi: 10.1098/rsbl.2004.0178
Ward A. J., Herbert-Read J. E., Sumpter D. J., Krause J. (2011). Fast and accurate decisions through collective vigilance in fish shoals. Proc. Natl. Acad. Sci. 108, 2312–2315. doi: 10.1073/pnas.1007102108
Ward A. J. W., Webster M. M., Hart P. J. B. (2007). Social recognition in wild fish populations. Proc. R. Soc Lond B. 274, 1071–1077. doi: 10.1098/rspb.2006.0231
Watson J. T., Essington T. E., Lennert-Cody C. E., Hall M. A. (2009). Trade-offs in the design of fishery closures: management of silky shark bycatch in the Eastern pacific ocean tuna fishery. Conserv. Biol. 23, 626–635. doi: 10.1111/j.1523-1739.2008.01121.x
Weeks S. J., Magno-Canto M. M., Jaine F. R. A., Brodie J., Richardson A. J. (2015). Unique sequence of events triggers manta ray feeding frenzy in the southern great barrier reef, Australia. Remote Sens. 7, 3138–3152. doi: 10.3390/rs70303138
White W. T., Giles J., Dharmadi P. I. (2006). Data on the bycatch fishery and reproductive biology of mobulid rays (Myliobatiformes) in Indonesia. Fish. Res. 82, 65–73. doi: 10.1016/j.fishres.2006.08.008
Womersley F. C., Humphries N. E., Queiroz N., Vedor M., da-Costa I., Furtado M., et al. (2022). Global collision-risk hotspots of marine traffic and the world’s largest fish, the whale shark. Proc. Natl. Acad. Sci. 119, e2117440119. doi: 10.1073/pnas.2117440119
Yano K., Sato F., Takahashi T. (1999). Observations of mating behavior of the manta ray, Manta birostris, at the ogasawara islands, Japan. Ichthyol. Res. 46, 289–296. doi: 10.1007/BF02678515
Keywords: mobula, grouping behavior, elasmobranch, social group, management, feeding, courtship and mating, cleaning
Citation: Palacios MD, Stewart JD, Croll DA, Cronin MR, Trejo-Ramírez A, Stevens GMW, Lezama-Ochoa N, Zilliacus KM, González−Armas R, Notarbartolo di Sciara G and Galván−Magaña F (2023) Manta and devil ray aggregations: conservation challenges and developments in the field. Front. Mar. Sci. 10:1148234. doi: 10.3389/fmars.2023.1148234
Received: 19 January 2023; Accepted: 31 March 2023;
Published: 17 April 2023.
Edited by:
David M. P. Jacoby, Lancaster University, United KingdomReviewed by:
Robert Perryman, Macquarie University, AustraliaNatascha Wosnick, Federal University of Paraná, Brazil
Copyright © 2023 Palacios, Stewart, Croll, Cronin, Trejo-Ramírez, Stevens, Lezama-Ochoa, Zilliacus, González−Armas, Notarbartolo di Sciara and Galván−Magaña. This is an open-access article distributed under the terms of the Creative Commons Attribution License (CC BY). The use, distribution or reproduction in other forums is permitted, provided the original author(s) and the copyright owner(s) are credited and that the original publication in this journal is cited, in accordance with accepted academic practice. No use, distribution or reproduction is permitted which does not comply with these terms.
*Correspondence: Marta D. Palacios, bWFydGFkLnBhbGFjaW9zQGdtYWlsLmNvbQ==