- 1University of Western Australia (UWA) Oceans Institute and School of Biological Sciences, University of Western Australia, Crawley, WA, Australia
- 2Institute of Marine Research, His, Norway
- 3Department of Primary Industries, Fisheries, Coffs Harbour, NSW, Australia
- 4The School of Biological Sciences, University of Auckland, Auckland, New Zealand
- 5School of Natural Sciences, Massey University, Auckland, New Zealand
- 6School of Biological Sciences, University of Canterbury, Christchurch, New Zealand
- 7Deakin Marine Research and Innovation Centre, School of Life and Environmental Sciences, Deakin University, Queenscliff, VIC, Australia
- 8Department of Ecology & Evolutionary Biology, University of California, Irvine, Irvine, CA, United States
- 9Department of Biology, University of Victoria, Victoria, BC, Canada
- 10The Kelp Rescue Initiative and Bamfield Marine Sciences Centre, Bamfield, BC, Canada
- 11The Dove Marine Laboratory, School of Natural and Environmental Sciences, Newcastle University, Newcastle-upon-Tyne, United Kingdom
- 12Department of Life Sciences, Aberystwyth University, Aberystwyth, United Kingdom
- 13British Columbia Conservation Foundation, Vital Kelp Co., Sunshine Coast, BC, Canada
- 14MARE – Marine and Environmental Sciences Centre & ARNET – Aquatic Research Network, ESTM, Polytechnic of Leiria, Peniche, Portugal
- 15Puget Sound Restoration Fund, Bainbridge Island, WA, United States
- 16Institute for Marine & Antarctic Studies, University of Tasmania, Hobart, TAS, Australia
- 17Centre for Marine Socioecology, University of Tasmania, Hobart, TAS, Australia
- 18Project Baseline, Wellington, New Zealand
- 19Department of Biology, University of British Columbia (Okanagan), Vancouver, BC, Canada
- 20Benthic Ecosystems and Environmental Change, Marine Biological Association of the UK, Plymouth, United Kingdom
- 21Marine Botany, University of Bremen, Bremen, Germany
- 22SeaForester Lda, Cascais, Portugal
- 23School of Biological, Earth & Environmental Sciences, University of New South Wales (UNSW), Sydney, NSW, Australia
Introduction: To counteract the rapid loss of marine forests globally and meet international commitments of the UN Decade on Ecosystem Restoration and the Convention on Biological Diversity ‘30 by 30’ targets, there is an urgent need to enhance our capacity for macroalgal restoration. The Green Gravel Action Group (GGAG) is a global network of 67 members that are working on the restoration of a diverse range of macroalgal forests and it aims to facilitate knowledge exchange to fast-track innovation and implementation of outplanting approaches worldwide.
Methods: Here, we overview 25 projects conducted by members of the group that are focused on testing and developing techniques for macroalgal restoration. Based on these projects, we summarise the major challenges associated with scaling up the area of marine forests restored.
Results: We identify several critical challenges that currently impede more widespread rollout of effective large-scale macroalgal restoration worldwide: 1) funding and capacity limitations, 2) difficulties arising from conditions at restoration sites, 3) technical barriers, and 4) challenges at the restoration-policy interface.
Discussion: Despite these challenges, there has been substantial progress, with an increasing number of efforts, community engagement and momentum towards scaling up activities in recent years. Drawing on the collective expertise of the GGAG, we outline key recommendations for the scaling up of restoration efforts to match the goals of international commitments. These include the establishment of novel pathways to fund macroalgal restoration activities, building skills and capacity, harnessing emerging innovations in mobile hatchery and seeding technologies, and the development of the scientific and governance frameworks necessary to implement and monitor macroalgal restoration projects at scale.
1 Introduction
Habitat loss is one of the largest threats to species and ecosystem functioning globally (Airoldi et al., 2008; Powers and Jetz, 2019). Environmental protection laws and resolutions are increasingly being implemented to mitigate damage to both terrestrial and marine habitats. For example, the United Nations Decade on Ecosystem Restoration (2021-2030; United Nations Environment Agency, 2019) and the Convention on Biological Diversity (CBD)’s recent adoption of the Kunming-Montreal Global Biodiversity Framework, which includes the ‘30-by-30’ target to secure effective conservation of 30% of the world’s habitats by 2030 (Convention on Biological Diversity, 2021). Yet, despite these and other initiatives, habitats continue to decline globally (IPCC 2021). To mitigate loss and meet formal commitments for restoration, there is an urgent need to massively scale up current restoration efforts at both local and global levels (Murcia et al., 2016; Vardi et al., 2021).
Marine forests formed by large, brown macroalgae (orders Laminariales, Fucales and Tilopteriadales; Wernberg et al, 2018) represent the most spatially extensive vegetated nearshore ecosystems on Earth (Duarte et al., 2022), and are of high ecological and socio-economic value globally (Bennett et al., 2015; Feehan et al., 2021; Eger et al, 2023). Over the past 50 years there has been an accelerating loss of macroalgal cover in many regions, with an estimated 60% of long-term records showing decline (Wernberg and Filbee-Dexter, 2019), and over ten thousand square kilometers of macroalgal forests currently considered to be in a degraded state (Rogers-Bennett and Catton, 2019; Filbee-Dexter et al., 2022).
The extent of decline presents a significant challenge for marine managers and practitioners aiming to protect and restore macroalgal ecosystems. Bringing back lost marine forests often requires active interventions, such as transplanting healthy macroalgae to degraded areas using divers (Wood et al., 2019; Eger et al., 2022). Whilst these approaches have been shown to be effective at small scales (i.e. tens of meters, e.g. Campbell et al., 2014), examples of restoration at the scales of original loss (i.e. tens of kilometers) are very rare (Eger et al., 2022b). This is likely because of high upfront restoration costs (Eger et al., 2020a) and that working in temperate/subpolar coastal systems is challenging, involving work in wave-exposed, often remote and harsh environments, where personnel are limited by restricted timeframes and accessibility (Fredriksen et al., 2020; Eger et al., 2022).
In addition to UN and CBD goals, the Kelp Forest Alliance (https://kelpforestalliance.com/) recently launched a macroalgae-specific goal, the Kelp Forest Challenge, which aligns with the Kunming-Montreal Global Biodiversity Framework and aims to actively restore ten thousand square kilometers of macroalgal forests by 2040 (Eger et al., 2023). According to best estimates, meeting these global ambitions will require restoring >60 times the area of macroalgal cover that has been restored over the past half century (Eger et al., 2022). To achieve this goal the restoration sector urgently requires new, financially viable and relatively simple tools suitable for deploying effective restoration actions that target a diversity of environments, are able to reach even remote locations, and cover large areas.
An emerging tool to upscale macroalgal forest restoration is the active ‘outplanting’ of macroalgae that have been cultured in a hatchery or aquarium setting. Perhaps one of the most well-known of these methods is ‘Green Gravel’, a technique initially developed for restoring Saccharina latissima in the fjords of Norway (Fredriksen et al., 2020), but with similar analogues elsewhere (e.g. Falace et al., 2018; Vanderklift et al., 2020). Here, we define ‘outplanting’ as any technique where macroalgal propagules are seeded in a laboratory or nursery environment onto substrates such as gravel, rocks, ceramic tiles or twine, and then outplanted in the field directly or following a period of being reared ex situ. The juvenile macroalgae can then overgrow or disperse from the substrate (e.g. Alsuwaiyan et al., 2022) and attach to the underlying reef, forming the basis of restoration efforts that hopefully can expand naturally over time (Verdura et al., 2018; Tamburello et al., 2019; Layton et al., 2020).
Such outplanting approaches may be particularly valuable or necessary where macroalgae are recruitment-limited and/or must be re-introduced into a system (Layton et al., 2020; Cebrian et al., 2021). Once developed further, these methods may also help minimize some existing challenges of macroalgal forest restoration, because the macroalgae might be deployed with only minimal use of laborious/technical diving based approaches, and can potentially enable the deployment of many (e.g. millions or billions) macroalgae at once. Moreover, synergizing and leveraging the hatchery/aquarium component of restoration outplanting with already established aquaculture knowledge and methods may further minimize costs and labor, and simultaneously drive innovation toward sustainable, large-scale, and cost-effective macroalgal forest restoration (Layton & Johnson, 2021; Filbee-Dexter et al., 2022). Outplanting methods (and collaborations with aquaculture) can also allow a direct mechanism to implement ‘future-proof’ management solutions, such as using selected thermally tolerant macroalgal genotypes to boost the resilience of macroalgal forests that are forecasted to experience an intensification of climate-driven stressors (Coleman et al., 2020; Wood et al., 2021; Layton et al., 2022b).
In 2020, the Green Gravel Action Group (hereafter, GGAG) was established. Named after the Green Gravel method developed in Norway, the GGAG is a global network of macroalgae restoration researchers and practitioners that aims to facilitate the communication of research and protocols for outplanting and upscaling restoration activities across different macroalgal species, locations, and habitats (www.greengravel.org). Since its inception, the GGAG meets online twice yearly to discuss key questions, activities, challenges, solutions and project outcomes.
Here, we build on topics discussed during the 2021-2023 meetings to evaluate challenges and opportunities to upscale macroalgal forest restoration. First, we provide an overview of GGAG members’ projects, including characteristics, objectives and status. We then draw on our recent experiences in developing and applying outplanting and other techniques to highlight the lessons learnt so far and to discuss key challenges in scaling up macroalgal restoration activities. Finally, we present key recommendations towards meeting the CBD challenge of restoring 30% of degraded macroalgal forests by 2030 and the targets in the UN Decade of Restoration.
2 Overview and status of Green Gravel Action Group member projects
2.1 Methods
We surveyed the GGAG to describe projects within the group, including status, objectives and factors affecting restoration design. The survey themes also explored issues of institutional alignment and support (resources, capacity and social support), governance (i.e. legislation and policies) and project leaders’ experiences and perceptions related to these three themes. A copy of the survey questions is provided in the Supplementary Materials.
The survey was sent to the group (67 members) via the Qualtrics online survey platform (Qualtrics, Provo, UT) between August 2022 and March 2023. Respondents were either project primary investigators or on-the-ground leads that responded on behalf of the entire project team, to avoid multiple reporting from individual groups. The survey used a combination of closed and open-ended questions; quantitative elements about each project were measured using closed-ended questions (e.g. “How long has your project been running?”) or multiple choice (e.g. “What stage of restoration (of four stages described) best describes your project?”) which allowed for multiple categories to be selected if multiple answers were applicable (e.g. What is/was the main aim of the project? (Check as many as apply)). There was also an option for additional open-ended text responses (e.g. “Other: please explain”) for qualitative information. Participants’ perceptions of support from local communities, authorities and legislative frameworks were also explored via a series of questions where a 1 to 10 Likert Opinion Scale was used (e.g. “In your opinion, how would you rate the interest of local authorities on the status of kelp (macroalgal) forests in your study area?”; 1 = extremely low, 10 = high).
Standard descriptive statistics and mean comparisons were used to analyze and summarize quantitative responses in R v4.3.1 (R Core Team 2021). When multiple responses were recorded by a project (e.g. those that recorded affiliations with both academic and commercial sectors), each answer was included as one datapoint. We then used the GGAG discussions and open-ended survey responses to compile a list of unknowns and roadblocks pertaining to project planning, initiation, upscaling and monitoring. These provided a framework for the discussion and review of key challenges, solutions and recommendations presented below.
2.2 Survey results
2.2.1 Status and project objectives
The survey received responses from participants representing 25 projects, spanning nine countries and 17 ecoregions (sensu Spalding et al., 2007) around the globe (Figure 1A). We found that the use of culturing and outplanting techniques for restorative purposes has garnered interest for use across a wide diversity of macroalgal species, most of which were from the Laminarian kelps (Order Laminariales, eight species), with a further two projects investigating potential applications for fucoids (Order Fucales, two species).
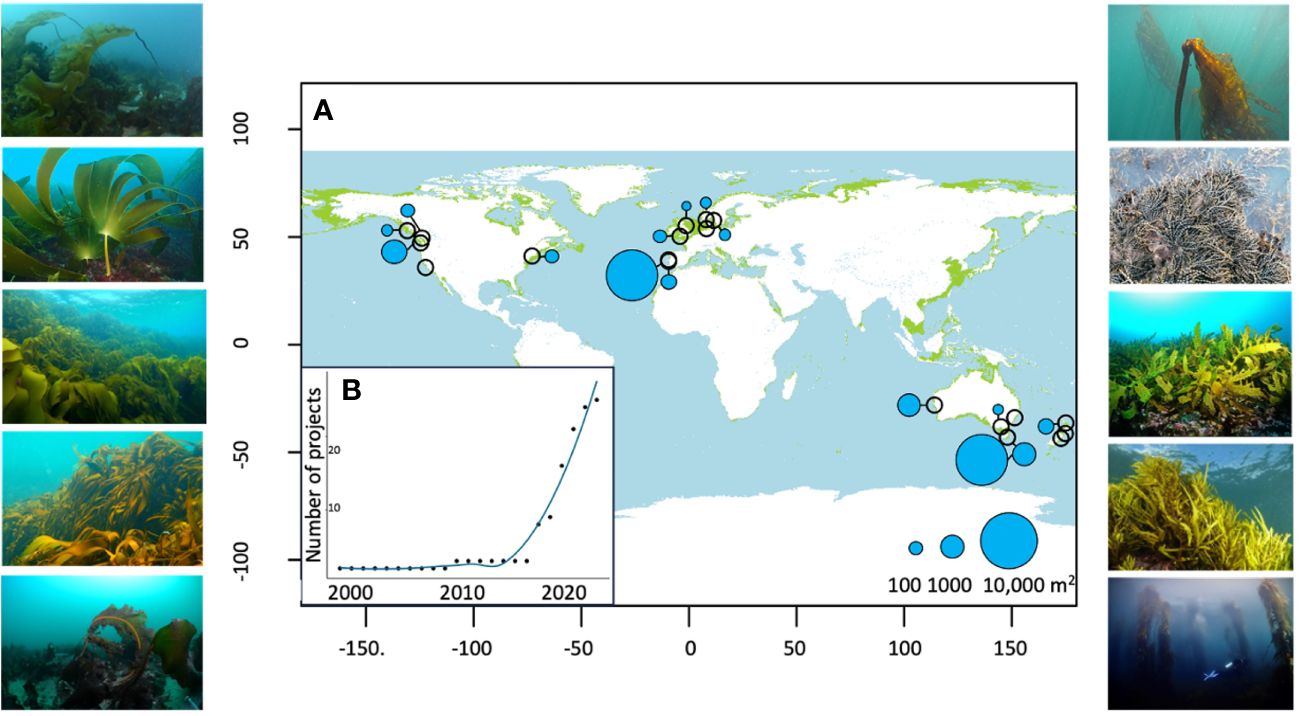
Figure 1 Green Gravel Action Group projects. (A) Map of the locations of GGAG projects; open circles represent projects in planning and scoping stages; blue circles indicate location where field based restoration projects have been initiated. Blue circle size indicates the area over which the project is actively outplanting macroalgae. (B) Cumulative number of projects starting each year for the period of 2000-2023. Side panels: study species being worked on in the GGAG (in clockwise order from the top left): Saccharina latissima, Nereocystis luetkeana, Hormosira banksii, Ecklonia radiata, Phyllospora comosa, Macrocystis pyrifera, Alaria marginata, Laminaria digitata, Laminaria hyperborea and Laminaria ochroleuca (photos by Karen Filbee-Dexter, Kathy Burnham, Paige Bentley, John Turnbull, Scott Ling, Robert Scheibling and João Franco).
Most of the projects in the GGAG were new, with 92% of projects initiated since 2018 (Figure 1B). The greatest share of projects were in early stages of development (Figure 2A), with five (20%) at initiation and stimulation stages (which entailed scoping and organizing permits, securing funding and/or conducting laboratory-based feasibility studies) and fourteen other projects (56%) at the field initiation stage (defined as in-water restoration work at a site-level scale). The remaining respondents (<25%) indicated that their projects were ready to scale up: four (16%) were currently in the scaling up stage (defined as when a project was applying a proven restoration technique to multiple sites), and two (8%) self-identified as being at the final sustenance and monitoring stage (defined as the point at which outplanting was completed and the project was focused on monitoring the next generation and quantifying associated benefits).
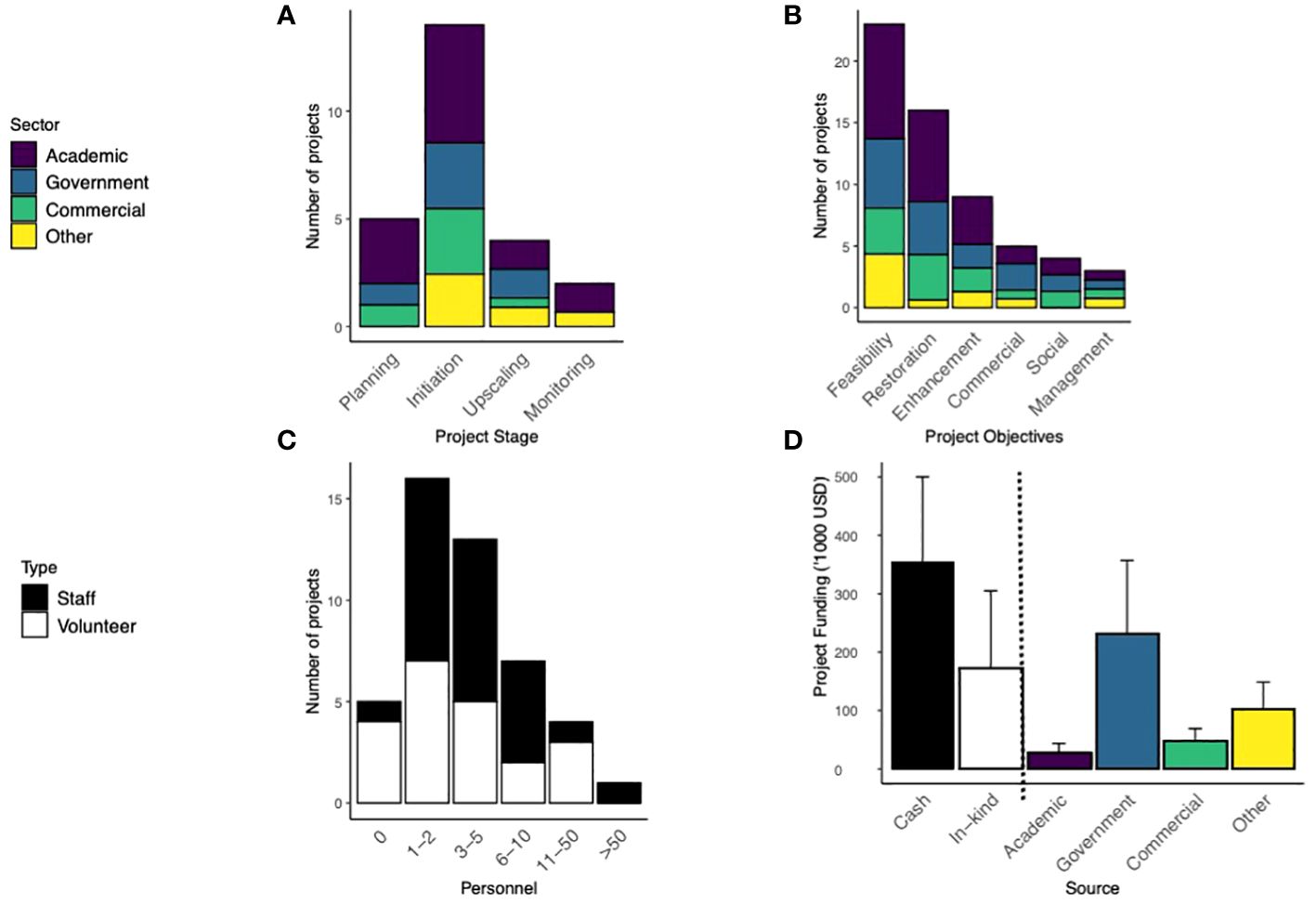
Figure 2 Projects initiated by individual members contributing to the Green Gravel Action Group, based on the 2022-2023 survey. (A) Stage of each project split by sector alignment; projects could align to more than one sector with 50% of the projects reported as multi-sectorial. Stages were defined as Planning: scoping/organising permits, funding and support necessary to conduct restoration, lab-based feasibility studies. Initiation: ocean-based work at site-level scale. Upscaling: applying proven restoration techniques to multiple sites. Monitoring: initial planting completed, focus is on associated benefits or next generation of macroalgae; (B) project objectives split by sector alignment; responses under the ‘Other’ category in the survey consisted of cultural and tourism uses and were subsequently coded here as ‘Social’; (C) number of dedicated personnel (staff and volunteers) affiliated with each project; (D) project funding split by cash and in-kind sources (left); cash funding was further separated into sector sources (right). Mean and standard error of responses across projects are shown, hence sectoral funding does not equal cash on the left.
Due to the nature of the group, most projects (96%) reported using aquarium-based culturing and outplanting restoration techniques (with one well-established transplanting, rather than outplanting, project in Sydney assessing the feasibility of using outplanting techniques in the future). Notably, however, the outplanting of cultivated macroalgae was frequently (54% of projects) combined with other restoration methods including transplants of wild macroalgae (28%), sea urchin removals (12%) and spore bags (4%; defined in Eger et al., 2022). The average total area that projects reported working across was 30 km2, although physical outplanting activities were only being conducted within a much smaller subset of this, from 4 to 8,000 m2 (Figure 1A). The use of multiple, small-scale (i.e. meters across) plots at one or more sites to achieve larger-scale plantings was common. This is likely because most projects were focused on research and method development, with a key aim being to test the feasibility/effectiveness of restoration techniques (88% of projects). Many projects also had additional and multiple aims that were not mutually exclusive, such as restoration (64%), enhancement of the resilience of existing forests (28%) and management of uses of existing forests (harvesting and fisheries; 12%). Cultural and tourism uses (28%) were also listed under the ‘Other’ category (Figure 2B).
Interestingly, whilst all participants reported project goals aligned with the restoration of macroalgal forests that had been lost in their region, the understanding of the extent of this loss was relatively low, with 48% of participants indicating they did not know how much macroalgal forest had been lost in their area. This is reflective of the wider paucity of data on macroalgal forests and the difficulty in surveying and mapping such dynamic subtidal environments. Those participants that did have an understanding of the spatial extent of local losses, provided estimates from 0.1 km2 (North-East New Zealand) to 7800 km2 (Norway): highlighting that affected macroalgal forest ecosystems vary drastically in many characteristics, including regional extent.
2.2.2 Factors affecting project design
Almost all respondents (96%) indicated that multiple factors influenced the choice of restoration site, however the most common response was academic alignment/interest. For example, 72% of respondents indicated that sites were chosen to test a hypothesis, such as whether locations that historically supported macroalgae were suitable for macroalgal survival and growth, or to test how wave exposure and substrate impact restoration success. This was followed in order of commonality by convenience and ease of access (56%) and local community demand (44%), with political will being a relatively minor driver (12%). A quarter (24%) of projects also reported that site selection was also driven by factors not listed in the survey, such as knowledge of where the species used to be present, the presence of Marine Protected Areas, requests by Indigenous groups, and potential integration with marine-based industries.
The sourcing or “provenance” of the donor stock used in habitat restoration is a critical yet complex issue for restoration projects (Breed et al., 2018);. Many projects (68%) reported that their provenancing choices were influenced by multiple parameters, with the most common being convenience (56% projects), the size and health of wild macroalgal populations (48%), and official regulations or guidelines (44%). While traditional guidelines for restoration practitioners strongly emphasize mimicking genetic characteristics of natural populations by sourcing donors from ‘local’ populations (Bischoff et al., 2010; Bucharova et al., 2019), the marine restoration field is increasingly considering more complex approaches, such as the sourcing of more diverse or resilient stock that might aid restoration success under climate-change scenarios (Wood et al., 2021; Bay et al., 2023).
We found that only a third of surveyed projects (36%) considered the inclusion of desirable genetic traits when considering macroalgal provenance, and inclusion of other desirable macroalgal traits (16%) even less so. When projects did use genetic information, provenance generally adhered to traditional ‘local is best’ protocols, despite many restoration projects taking place in areas that were already affected by and vulnerable to climate stress. Six projects (in Western Australia, Tasmania, Sydney, Auckland, British Columbia and California), were however conducting laboratory and/or field-based experiments related to the potential future use of thermally-tolerant macroalgae in assisted evolution strategies. Because there is a lack of policy and consistent definition surrounding population delineation, participants were asked to both define the source of their population and write down the geographic distance between source and restoration sites. Most projects reported actively using seedstock from the same population (60%) or the most similar to that which had been lost (28%); while the definition of the same population was generally limited to a small geographic range (average 7.5 km from restoration sites), projects that chose the next closest or most similar population possible ultimately sourced macroalgae over a wide range of distances (5 to 250 km, with an average of 118 km from donor population-restoration site). One project in Tasmania, Australia, reported using macroalgae that was explicitly distinct from the historical population, due to selection for desirable traits (thermal tolerance for climate-change resilience) and near total local losses of the target species (Layton & Johnson, 2021; Layton et al., 2022a; Box 1). In this case however, the stated distance between donor population and restoration sites (~175 km) was still comparable to other projects that used donor material that was defined as the most similar population possible once the original population had been lost. Very few projects (8%) were unsure of the provenance of their macroalgae because it had been bought commercially and where no information was available.
Box 1. Permitting and outplanting trials of warm-tolerant Macrocystis pyrifera in Tasmania, Australia.
Climate change and ocean warming has led to >95% reductions in giant kelp (Macrocystis pyrifera) forest cover in Tasmania, Australia since mid-last century (Johnson et al., 2011; Butler et al., 2020). Recruitment limitation due to the catastrophic losses and the ongoing stressor of ocean warming led to outplanting trials and the use of local strains of naturally warm-tolerant giant kelp (Layton et al., 2020, Layton & Johnson, 2021; Layton et al, 2022a). Permitting for this quite radical intervention (Coleman and Kelaher, 2009; Morrison et al., 2022) involved detailed discussions and involvement with local regulators along with informal community discussions – most of which focused on addressing initial concerns and questions. Ultimately, risk and evidence-based decision-making allowed the initial trials (~100 m2) to proceed, and revolved around core knowledge of population genetics, natural variation in warm tolerance, and the complete loss of giant kelp at the restoration sites (Layton and Johnson, 2021). Those early trials formed the foundation for ongoing research (e.g. Layton et al., 2022a; Iha et al., 2023). Moreover, some of the original planted kelp still persist in the field and have even resulted in limited local reproduction and recruitment. Photo: Reseeded giant kelp patch; Cayne Layton.
2.2.3 Institutional alignment, resources and capacity
Restoration projects were often multi-sectorial (60% of projects) and were primarily aligned to one or more of academic research (72%), government (36%) or commercial organizations (28%). Seven projects (28%) were aligned or partnered with ‘Other’ organizations that spanned NGOs, community and Indigenous groups. Regardless of affiliation, most projects were being run by small teams of ≦10 staff, with 36% having ≦2 staff and 32% having three-to-five staff (Figure 2C). The largest project in terms of personnel was an urchin-culling project from Tasmania, Australia. This multi-partnered project had significant collaboration with commercial urchin fishers and so had >50 staff affiliated with the project. Projects were often supported by a volunteer base (72% projects; Figure 2C), with the exception of three projects in Norway, the USA and Australia.
On average, the total funding period for projects in the GGAG was 3.1 years, which included 2.1 rounds of funding success that were typically 1-2 years long. Total project funding ranged from zero (i.e. completely unfunded) to >1 million USD, which five projects received over their full duration (four of which were from different states of Australia). The most well-funded projects included both research and conservation aims, and had a mixture of funding sources (Figure 2D). Participants estimated that it took an average total of 28 days full time effort (between 10-1200 hours over the project with an average of ~100 hours per year) to write applications and obtain funding for the work, but several respondents noted that this was a substantial underestimate of time burden, since it did not account for the hours of work required to first understand the system to be able to develop funding applications.
2.2.4 Community support
Many projects participated in some kind of community outreach (72%) such as media releases, educational workshops or community plantings. Perceptions of local community understanding of the state of macroalgal forests and their value was relatively low overall (average 4.95 and 5.95 out of 10 in Likert scale), however these varied widely both between and within regions. For example, projects in most Australian and New Zealand states, Germany, England and Sweden reported local public understanding values of less than five out of ten, while Tasmania Australia, North-East New Zealand and Norway reported values of eight to ten. When asked about perceptions of the local community, authority, government and industry support of each project in terms of (i) overall interest, (ii) legislative frameworks and permits, and (iii) funding, responses were also highly variable (Supplementary Figure S1), but were similar to previous responses about understanding and awareness of the state and value of macroalgal forests overall.
2.2.5 Governance
The legislation and permitting required for macroalgal restoration activities was frequently perceived as complex and confusing by the survey respondents. Bureaucratic and regulatory hurdles, and lack of awareness of legislative processes were common challenges to implementing large-scale (i.e > hundreds of meters) macroalgal restoration projects within existing legal frameworks. An average of 3.5 (but up to ten in one case) different permits were sought per project, often from multiple agencies ranging from federal (48% requiring one or more), to state (60% requiring one or more) and local (40% requiring one or more) levels. Permitting from First Nations governments was also required for some projects, although we did not receive more specific information from those respondents. Most projects operated under multiple permits, including scientific/experimental permits (72%) that required any foreign materials or outplanted macroalgae to be removed at the conclusion of the work unless additional permission was sought. Just over half (52%) of the projects worked under separate permits that allowed for more activities with permanent environmental impacts (e.g. restoration-specific). Each of these were obtained from different (at times, multiple) Government levels in each region. As with funding, the number of hours spent working on permit applications was considerable, with participants reporting having spent an average of 34 hours a year and 207 hours over the whole project lifetime to comply with existing permits and legislations. This represents a significant proportion of potential time allocated to projects where personnel were commonly committed to less than full-time equivalent employment. Around 20% of projects had also experienced a refusal of their permit applications for various reasons, such as a lack of restoration policy to support the activities, or the restriction on commercial partnerships (in this specific case, the establishment of an urchin fishery).
Despite those commonly-cited regulatory hurdles, when asked if legislation/regulation should be changed to facilitate the initiation of projects, 56% of respondents were undecided or did not answer, 28% said no change was needed and 16% said change was needed. When asked the same question but relevant to upscaling activities, 68% were undecided or did not answer, 20% of respondents indicated that a change was needed and 12% respondents indicated that no change in legislation was needed (Supplementary Figure S1). In the open-ended text section, 44% respondents commented on a lack of precedent or specific policy related to macroalgae or macroalgal restoration activities in their respective region, which led to permit processes being time consuming, including extending far beyond project funding cycle timeframes, and not being allowed in marine protected areas (even if the MPAs might be well-suited for restoration in specific cases). Several respondents commented that permits were only granted for small-scale scientific experiments, which risked removal of restored macroalgae at the expiry of the permit, as large-scale restoration activities required lengthy development or aquaculture lease permits that would be both expensive and unobtainable within project timeframes.
3 Discussion of key challenges and recommendations
Interest and demand for scalable restoration technologies are clearly growing, yet our survey and discussions with GGAG participants highlighted several critical challenges that currently impede more widespread rollout of effective large-scale macroalgal restoration worldwide: 1) funding and capacity limitations, 2) difficulties arising from conditions at restoration sites, 3) technical barriers and 4) challenges at the restoration-policy interface. We explore these challenges and provide our recommendations below:
3.1 Challenge: funding and capacity limitations
While recent funding trends for macroalgal restoration have resulted in an increase in the number of projects, we found that almost all of these still face significant economic limitations, restricting their access to essential resources such as personnel, equipment, technology, and continuing technical assistance. The short duration of funding cycles supporting restoration means that project timescales (~1-3 years) are generally significantly mismatched with both the realistic requirements for planning and conducting restoration activities and also the biological/ecological complexities of habitat restoration, leading to fundamental challenges across the long-term. For example, while some macroalgal species are reproductive within a year of recruitment, others require ~1.5 years to achieve a reproductive canopy from ‘seed’ (i.e. young sporophytes large enough for outplanting). With most grants supporting projects for 1-3 years, even the most ‘successful’ projects are rushed and have insufficient support for long-term outcomes, without spending significant resources on further grant applications. Factoring in that (i) start-up time and costs e.g. from purchasing (or developing) equipment are usually lengthy and high and (ii) the need to monitor and assess subsequent, cascading impacts of outplanting activities on the ecosystem, most projects theoretically require funding for at least 5 years. Limited funding can also lead to ineffectiveness and impaired project outcomes in the long-term. For example, small teams of highly trained staff often rely on volunteers to conduct field activities, which necessitates simple, easy-to-use techniques that are achievable by untrained personnel. This reliance on volunteers has assisted funding-restricted projects, but may not be a viable model in every jurisdiction, and can be difficult to upscale because large projects often require more technical methods or industrial working environments. Further, there is a trend of increasing restrictions on volunteer activities due to perceived health, safety, and litigation risk. One of the driving factors behind current funding structures was that the majority of projects still sit within academia (72% projects). These results align with previous findings that scientists who engaged in marine coastal restoration globally were mainly motivated by experimental reasons, compared to terrestrial restoration practitioners, who were motivated by broader ecosystem services improvement (Bayraktarov et al., 2020), and may be in a better position to leverage funding for restoration activities from various funding sources.
3.2 Recommendations: explore new funding strategies and skills development
As has occurred in terrestrial systems for some time (BenDor et al., 2014), existing and emerging funding strategies (e.g. research grants, government investment, blue economy startups, philanthropy, carbon and nutrient credit schemes) are helping accelerate growth in macroalgae restoration projects (Figure 1B). New markets and models pose significant opportunities for improving access to long-term funding for restoration projects. Multiple groups within the GGAG are funding research and technology developments through a mixture of grants from government and NGOs/foundations and philanthropy. Commercial enterprises such as the environmental impact company SeaForester (Box 2) have built business support through both these pathways and corporate sponsorships. Whilst several projects were involved in industry-research agreements, however, restoration is still not seen as a profitable enterprise. Importantly, our results suggest a clear distinction in suitable funding models for restoration projects in different stages, with cost per area metrics only appropriate for use with the minority of projects that are in the scaling and monitoring stages.
Box 2. SeaForester’s mobile nurseries.
Portuguese environmental impact company SeaForester is developing mobile macroalgae nurseries to provide an innovative technological solution to overcome the current challenges with upscaling macroalgal forest restoration. The mobile nurseries provide easy-to-use commercial macroalgae culturing facilities with remote monitoring solutions and high-quality water filtration systems, enabling the local production of high volumes of seeded materials. The aim is to implement a network of ‘seaforestation’ units across the world together with local partners, generating impact at a global scale. Embedded within its nursery and field operations, SeaForester is conducting experimental research through partnerships with scientific institutions, such as MARE-IPLeiria in Portugal, to drive innovations in marine forest restoration. These partnerships will also ensure that restoration activities are implemented considering ecological drivers and conditions of the local marine environment. Photo: Mobile macroalgae nursery; Jan Verbeek.
To make the most of these opportunities will also require expanding team skills to include expertise in financing, engineering, and team management. As an active area of research and development, few projects can guarantee the real-world returns on investment at this stage (e.g. $/m2 of macroalgae restored) and most are far away from being commercially viable activities. This inherent risk can produce a conflict that can only be resolved with transparency among project partners and investors about the uncertainty involved in research and development. Further investment towards increasing local skills pathways, policy and funding mechanisms, and the development of research and innovation in restoration methods is required before macroalgal restoration can be fully commercialized.
Canvassing a broader selection of stakeholders (e.g. research institutions, NGOs and charities, Indigenous groups, local governments and commercial industry) can also help restoration projects identify beneficial partners or innovative programs (McHugh et al., 2022). For example, projects might participate in the blue economy, develop local environmental remediation programs using macroalgal restoration, or engage with local or Indigenous authorities to leverage tax credits or funding streams toward restoration (Gosnell & Kelly, 2010; Kern et al., 2016). Engaging the broader public offers various benefits, including improved funding, governance support (see section Engage with local authorities and stakeholders from the outset below), and increased local stewardship. Public discussions are also useful for addressing ethics, standards, and cost/benefit considerations. Most GGAG projects are doing well at this, although the generally low perceptions of public awareness suggest that the benefits of public discussions, outreach and engagement (similar to funding) require longer than the average project duration to take effect.
3.3 Challenge: dealing with sources of environmental stress at restoration sites
Restoration success across the GGAG has been highly site- and context- dependent, highlighting the need to understand the complex biotic and abiotic drivers of outplanting (and more broadly, restoration) effectiveness on both local and regional scales. For example, poor water quality and associated pollution have been significant drivers of macroalgal forest decline over the past century and continue to threaten coastal ecosystems as coasts become more heavily urbanized (Airoldi & Beck, 2007; Burkholder et al., 2007; Lotze et al., 2011). In areas where substantial efforts have been made to first ameliorate stressful conditions prior to restoration trials, projects may prove successful from some efforts (e.g. Sydney, Australia; Campbell et al., 2014), but not all (e.g. Wood et al., 2020). This is not a challenge that is unique to marine environments, indeed restoration attempts in terrestrial systems are also highly variable (Suding, 2011). In such cases, a deeper understanding of the causes of restoration failure is needed and subsequent activities require careful evaluation and targeted planning (Copeland et al., 2021).
In some settings, biotic drivers are the dominant cause of macroalgal mortality and represent a major challenge for restoration, where reef ecosystems may be locked into alternative states such as rocky barrens maintained by sea urchin grazing (Filbee-Dexter & Scheibling, 2014; Ling et al., 2015) or dominated by small turf-forming macroalgae (Filbee-Dexter & Wernberg, 2018). Grazing pressure by highly mobile grazers such as herbivorous fishes can also play a significant role in some systems, limiting survival of early stages of macroalgal development (Franco et al., 2015; Savonitto et al., 2021). While experiments (16% of projects we surveyed) have paired seeding with some method of grazer control, only a modest amount of these have trialled such activities at scale (Norway and Tasmania). Critically, as with the active outplanting of propagules, grazer control activities can also be expensive (Tracey et al., 2014), and so unless natural top-down controls are reinstated, they must be continually maintained (i.e. beyond the 1-3 year project timescales) to prevent ecosystem regression (Layton et al., 2020; Keane & Ling, 2022).
Climate change now represents one of the most significant challenges for macroalgal dominated ecosystems (Wernberg et al., 2024) and therefore macroalgal restoration, due to long-term ocean warming and increased severity and frequency of extreme climate events threatening natural and even ‘successfully’ restored systems (Wood et al., 2019; Coleman et al., 2020; Layton et al., 2022b). Many GGAG projects are being conducted in areas where severe events like marine heatwaves or flooding have caused widespread mortality (e.g. macroalgal losses in Western Australia, southern Norway, eastern USA and western Canada; Wernberg et al., 2016; Feehan et al., 2021; Starko et al., 2022), or where climate-induced warming is predicted to exceed local physiological thresholds in the future (e.g. Vranken et al., 2021; Wood et al., 2021). Despite this, mitigative techniques such as the deployment of more resilient or stress-tolerant macroalgal strains that might aid restoration success (Coleman and Kelaher, 2009; Wood et al., 2019; Coleman & Wernberg, 2021) remain largely underexplored in these projects, with only one project reporting use of such techniques (Box 1). In contrast, climate-change planning is rapidly becoming a critical component of restoration in other systems (Rossetto et al., 2019; Bay et al., 2023). We believe that macroalgal restoration lags in this respect due to (i) a general lack of scientific understanding of potential consequences, which require thorough testing both in controlled and field settings, (ii) restrictive policy and regulations prohibiting translocation of foreign genotypes and (iii) no overarching government stance or policy on these types of future-proofing activities.
3.4 Recommendations: account for changing ecosystem states
Controlling CO2 emissions to reduce further climate change impacts remains the single primary issue for both the restoration and persistence of macroalgal ecosystems. There remain many uncertainties around the long-term success of restoration initiatives, even for those that have reported success in their initial stages. As relationships between drivers and decline are better understood, researchers should develop further recommendations, for example in the form of habitat suitability indices for restoration site selection, which can be updated to reflect predicted environments and outcomes. In addition to this, a range of additional strategies to mitigate existing climate change threats should also be explored. In systems with persistent and forecast changes such as topicalization and influx of herbivores (Vergés et al., 2019), the development of new fisheries focused on key grazers can significantly reduce pressure on macroalgal forests, aiding re-establishment (e.g. Keane & Ling, 2022). In cases where the harvesting of grazers is not commercially profitable or incentivized at the outset, such activities might rely on government subsidization and partnerships with industry to create new commercial products (Cresswell et al., 2022; Zilia et al., 2023).
Macroalgal culturing also presents opportunities for selection and/or priming and propagation of stress-tolerant individuals during the hatchery phase (Coleman and Wernberg, 2021; Jueterbock et al., 2021; Clausing et al., 2022), but such activities must be well-designed and monitored to minimize harmful effects on the environment. This is an emerging area of research, for example the University of Western Australia and New South Wales Fisheries in Australia are developing an interactive web platform (www.reefadapt.org) that operationalizes existing genomic data to allow marine managers and restoration practitioners to rapidly and easily make appropriate provenance decisions for their restoration activities, including local and climate-adjusted provenancing. Alongside these emerging tools however, we urgently need to start discussions among government, community and stakeholders to decide what we are trying to achieve in marine restoration and what we are willing to let go of, particularly in a future of increasing environmental change, and to develop suitable policy and regulations to support this.
3.5 Challenge: technical barriers and narrow focus
Methods for restoring macroalgal systems with outplanting approaches are still highly experimental, with best practice techniques still being developed for different species and systems. As a result, guidelines for genetic diversity, strain-selection, deployment and monitoring are emerging topics, whilst questions around what constitutes restoration ‘success’ remain overly simplistic - such as the area or number of macroalgae planted or surviving after several months. Across the GGAG, outplanting success has been dependent on a range of local factors and conditions. For example, although ‘Green Gravel’ deployment of macroalgae attached to smaller rocks may be suitable for fjords or other sheltered locations such as Norway, larger rocks or boulders are proving more successful in shallow and wave-exposed areas such as those found along the coasts Western Australia (Wood et al., pers obs). Moreover, selecting appropriately-sized substrates for very large and buoyant macroalgal species, like Macrocystis pyrifera and Nereocystis luetkeana, pose development challenges, as rapid holdfast expansion onto larger substrate is critical to secure the macroalgae before their buoyancy carries them away (Scott Ling pers. experimental obs. 2020). Gravel and rocks, while cheap, are also susceptible to rolling (leading to transplant failure) on steep, shallow, exposed coastlines. In these habitats, seeding macroalgae onto other substrates such as aquaculture lines, infrastructure or adhesives may provide alternative opportunities for upscaling. The few projects targeting large scale restoration identified many logistical challenges associated with upscaling outplanting methods from plot-based trials to kilometers of macroalgal forests. For example, how to seed thousands/millions of juvenile macroalgae in conditions that are similar to restoration sites but that avoid contamination issues (i.e. by diatoms, red algae, fungi, and ciliates) (Box 2); how to transport and deploy them in the field with minimal damage and stress to both the macroalgae themselves and the rest of the ecosystem; and, how to monitor them (and any cascading ecosystem impacts) over long time scales and across expansive, often difficult to access areas. We consider that synergies with commercial macroalgae nurseries and aquaculture operations are therefore of key and immediate relevance.
3.6 Recommendations: embrace commercial partnerships and embed experiments as projects scale
All projects should continue to incorporate rigorous scientific trials while beginning to scale up and make both successes and failures transparently available for other practitioners to learn from. Industry partnerships may provide opportunities for both funding and technical expertise as projects scale up. Since macroalgal restoration activities are funding-limited, the methodological trials conducted by new projects are of high value for the ongoing progress of the field. While not included in the survey, we have developed an overview of the technical questions many projects are working on to aid prospective practitioners and entities interested in pursuing this work (Figure 3).
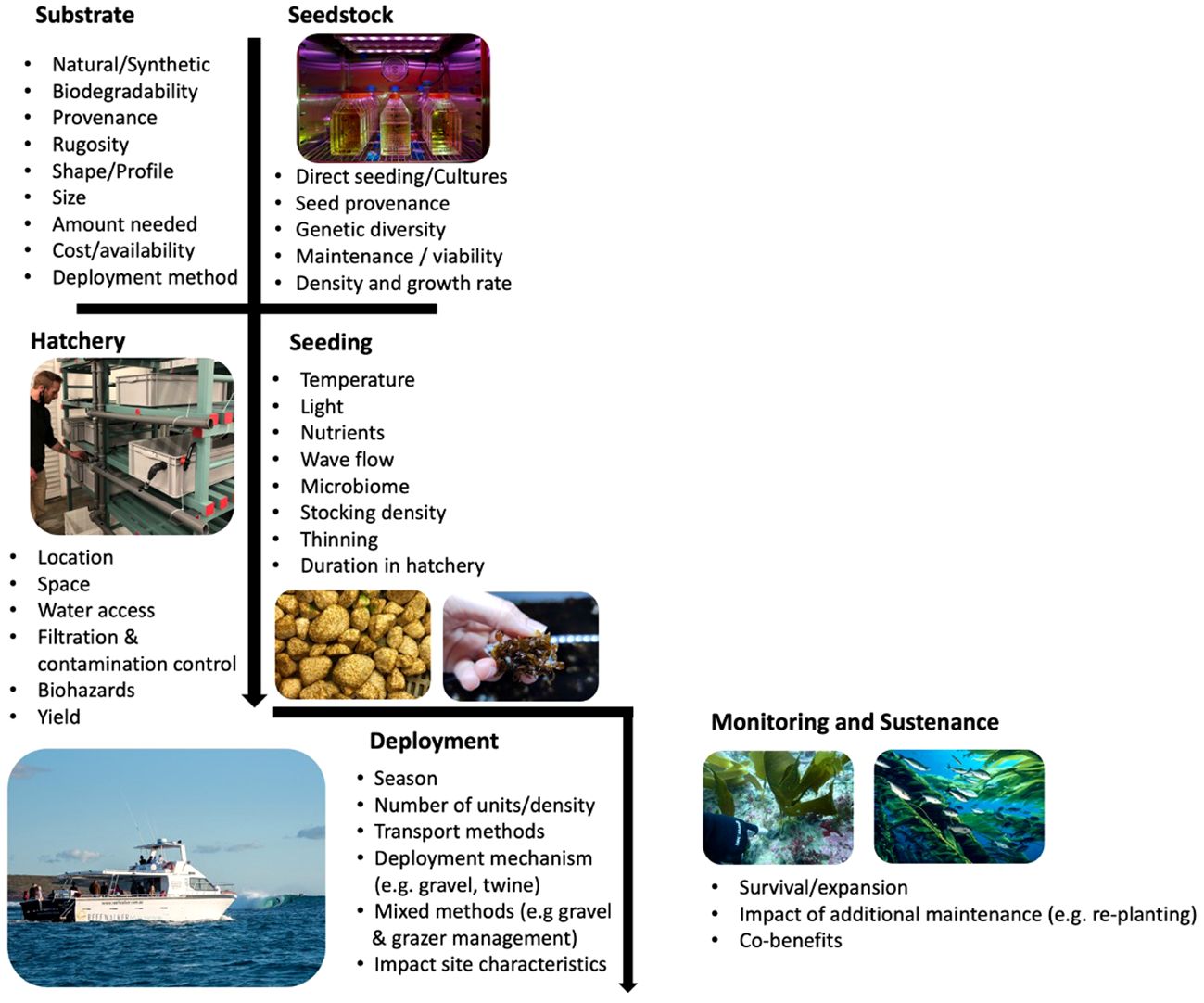
Figure 3 Key unknowns/research questions being worked on by GGAG members during development of macroalgal outplanting protocols. Best practice protocols vary depending on the target species, local conditions, available skills, infrastructure and scale of activities being conducted.
The development of cost-effective tools, resources and monitoring initiatives to assist restoration projects in identifying areas suitable for restoration is a core requirement for both site selection and ongoing monitoring of success (Smith et al., 2023). While many current projects rely on SCUBA-based surveys at candidate sites, moving towards remote-sensing monitoring programs (e.g. using AUVs and machine-learning programs; Bell et al., 2023) might help to understand broader ecosystem changes and long-term restoration success. Finally, ecological systems are complex and highly variable, such that restoration success can be a combination of both conducting ‘suitable’ activities and also a matter of outplanting in high numbers. Several GGAG projects reported site by site differences in success that were difficult to explain, therefore deployments across multiple sites and times is recommended.
3.7 Challenge: hurdles at the restoration-policy interface
Domestic laws and policies for marine management differ considerably between and within countries, however restoration policies and regulations are critically underdeveloped in nearly all regions where GGAG projects are active. As projects move beyond pure research activities, many people reported challenges in gaining permissions. Further, more than half of participants in the survey did not respond as to whether governance arrangements were adequate for them to complete their work, suggesting a lack of knowledge on this particular topic (Morrison et al., 2022). This is likely reflective of projects in nascent stages, and/or conducted by academic institutions where experimental or research permits are easier to obtain than authority to conduct restoration at scale.
The rapid acceleration of marine restoration activities has led to a lag in development of new policies to support these efforts, with governments only just beginning to recognize and address the need for guidelines in this area. Compared to the long history of conservation intervention and farming on land, marine benthic restoration is an emerging area, with governing agencies also grappling with how the activities fit within legislation and what is appropriate. For example, in several regions, marine environment legislation covers fisheries, marine protected areas, water quality, sea dumping and disturbance of the seabed. Restoration activities can intersect with many of these, yet do not neatly fit into any, leading to them being granted under permits designed for research activities, aquaculture, or even sea dumping (McLeod et al., 2019; Shumway et al., 2021). Legislation can also be competing, for example maintaining rights of local urchin fishery compared to culling activities to aid restoration of marine forests that underpin alternative fisheries and/or biodiversity. In the case of protected areas, reducing populations of native species such as urchins can only be granted case by case under stringent requirements to meet legislation actions (e.g. strong evidence needed to have a successful case for intervention). Lack of marine restoration-specific policies can slow down project progress and may lead to action being taken too late.
3.8 Recommendation: engagement with local authorities and rights-holders from the outset
Early engagement and development of working relationships between restoration practitioners and local authorities will be important for the successful development of restoration projects at scale. These relationships are crucial for the rapid co-development of locally relevant guidelines, policies, and permits, which could otherwise become significant barriers for moving beyond research scale permits to industrial-scale restoration projects. This may require restoration projects to use policy and industry specialists that understand legislative settings as well as restoration research, to liaise and begin discussions with governments. An example of restoration policy that may act as a guide for development of new marine restoration policies is the Joint Aquatic Resource Permit (JARPA), which consolidates marine development and restoration permits from Federal, State and local levels of government, reducing complexity and processing times (Manning, 2001; Shumway et al., 2021), and can be used as a guide for development of new marine restoration policies. Given the majority of restoration projects are working in areas expected to warm significantly over the next half-century, the use of future-proofing strategies (cf. Coleman et al., 2020) must also be considered in legislation and associated policy and regulations (Box 1).
While permission or involvement from local Indigenous rights holders is not always a formal requirement, the opportunities for shared benefits from genuine partnership and co-ownership of research and restoration programs should not be overlooked (Trisos et al., 2021; Fischer et al., 2022). There is now a growing appreciation of benefits from genuine partnership between Indigenous and non-Indigenous peoples and the incorporation or acknowledgement of Indigenous ways of knowing and doing in both research and coastal management. This cohesive approach can also help to motivate changes to legislation stymying rapid responses to environmental challenges such as the loss of marine forests (Box 3).
Box 3. Co-development of Green Gravel projects with Māori communities in Aotearoa/New Zealand.
The health of the environment is crucial to the identity, sense of culture and ongoing ability of indigenous people of Aotearoa/New Zealand to keep customary values and food gathering practices alive. Coastal kinship groups (hapū) and tribes (iwi) have witnessed dramatic changes in their marine ecosystems as a result of land-use change and overfishing. Passive ecosystem restoration approaches such as marine protected areas have proven successful in restoring kelp forests in New Zealand where overfishing was a primary driver of trophic cascades and kelp decline (Shears & Babcock, 2003). However, MPAs are frequently challenged by hapū and iwi because they prevent access in perpetuity, including food gathering practices, which are used to maintain rights of occupation and custodianship for hapū. Conversely, active ecosystem restoration approaches such as green gravel that place the responsibility for nurturing the environment on the community are in keeping with the Māori world view. This world view considers the environment holistically, thus when working with hapū there is no change in mindset required to transition between gardening and conservation practices on land such as tree planting and the planting of kelp seedlings on green gravel in the sea. Greater emphasis by the New Zealand government and local councils to uplift self-determination within Māori communities means that iwi-led, active restoration activities such as green gravel are encouraged, thereby eroding some legislative challenges. The challenge then becomes nurturing a genuine partnership between researchers and indigenous people that allows the co-development of the principles underpinning restoration as well as the research and techniques required to make these endeavors successful. There are numerous examples of green gravel approaches throughout New Zealand ranging from initial scoping to implementation and scale-up, but a common theme in many of these programs is a strong indigenous involvement in the program. Photo: Kaitiaki preparing to deploy green gravel at Tutukaka Harbour; Seacologynz.
4 Conclusion
Over the past five years, macroalgal restoration initiatives have rapidly expanded and gained momentum. Yet, to meet international commitments and successfully restore >60 times the current amount of restored macroalgal habitat clearly requires unprecedented investment. Here, we have outlined the current status of 25 projects considered to be at the forefront of the macroalgal restoration field. Despite a recent surge in interest globally, macroalgal restoration activities remain largely hindered by limitations in long-term funding for projects, personnel, technical capacity, and the policy required to conduct activities on the ground. Sharing initiatives such as the Green Gravel Action Group are useful to create space and opportunities to compare and generate knowledge to develop successful projects. Further, by communicating successes and learning from our failures we hope to fast-track restoration scope across a wide range of macroalgal systems globally.
Data availability statement
The de-identified raw data supporting the conclusions of this article will be made available by the authors, without undue reservation.
Ethics statement
The studies involving humans were approved by the University of Western Australia Human Ethics Board (ET001074). The studies were conducted in accordance with the local legislation and institutional requirements. The participants provided their written informed consent to participate in this study. Written informed consent was obtained from the individual(s) for the publication of any potentially identifiable images or data included in this article.
Author contributions
GW: Conceptualization, Data curation, Formal analysis, Investigation, Methodology, Project administration, Visualization, Writing – original draft, Writing – review & editing. KF-D: Conceptualization, Funding acquisition, Investigation, Methodology, Writing – original draft, Writing – review & editing, Resources. MC: Conceptualization, Funding acquisition, Investigation, Writing – original draft, Writing – review & editing, Methodology. JVa: Investigation, Writing – original draft, Writing – review & editing, Conceptualization, Methodology. JA: Investigation, Writing – original draft, Writing – review & editing. PB: Investigation, Writing – original draft, Writing – review & editing. PC: Investigation, Writing – original draft, Writing – review & editing. PD: Investigation, Writing – original draft, Writing – review & editing. LD: Investigation, Writing – original draft, Writing – review & editing. HE: Investigation, Writing – original draft, Writing – review & editing. LE: Investigation, Writing – original draft, Writing – review & editing. PF: Investigation, Writing – original draft, Writing – review & editing. JF: Investigation, Writing – original draft, Writing – review & editing. HH: Investigation, Writing – original draft, Writing – review & editing. SL: Investigation, Writing – original draft, Writing – review & editing. JL: Investigation, Writing – original draft, Writing – review & editing. CL: Investigation, Writing – original draft, Writing – review & editing. EL: Investigation, Writing – original draft, Writing – review & editing. BM: Investigation, Writing – original draft, Writing – review & editing. NM: Investigation, Writing – original draft, Writing – review & editing. PM: Investigation, Writing – original draft, Writing – review & editing. CN: Investigation, Writing – original draft, Writing – review & editing. JP: Investigation, Writing – original draft, Writing – review & editing. DS: Investigation, Writing – original draft, Writing – review & editing. FS: Investigation, Writing – original draft, Writing – review & editing. SS: Investigation, Writing – original draft, Writing – review & editing. SCS: Investigation, Writing – original draft, Writing – review & editing. JVe: Investigation, Writing – original draft, Writing – review & editing, Conceptualization, Funding acquisition, Methodology. AV: Investigation, Writing – original draft, Writing – review & editing. CW: Investigation, Writing – original draft, Writing – review & editing. TW: Conceptualization, Funding acquisition, Investigation, Methodology, Resources, Writing – original draft, Writing – review & editing.
Funding
The author(s) declare financial support was received for the research, authorship, and/or publication of this article. KF-D, MC and TW were supported by the Australian Research Council (LP190100346, DP190100058). KF-D and TW were also supported by the Schmidt Marine Technology Partners. DS was supported by a UKRI Future Leaders Fellowship (MR/S032827/1). JNF was funded by the European Economic Area Grants Fund (PT-INNOVATION-0081 and PT-INNOVATION-0077). JL and PD were supported by the California Sea Grant Kelp Recovery Research Program (R/HCE-17). SL supported by the Australian Research Council (FT200100949). SS and LD were supported by Mitacs and Pacific Salmon Foundation. SS was funded by the Forrest Research Foundation.
Acknowledgments
We would like to thank Mason Sullivan for assistance in coordinating the GGAG online meetings.
Conflict of interest
Author LE was employed by the company Vital Kelp Co. Author JV works for Seaforester, a for profit seaweed restoration company. www.seaforester.org
The remaining authors declare that the research was conducted in the absence of any commercial or financial relationships that could be construed as a potential conflict of interest.
The author(s) declared that they were an editorial board member of Frontiers, at the time of submission. This had no impact on the peer review process and the final decision.
Publisher’s note
All claims expressed in this article are solely those of the authors and do not necessarily represent those of their affiliated organizations, or those of the publisher, the editors and the reviewers. Any product that may be evaluated in this article, or claim that may be made by its manufacturer, is not guaranteed or endorsed by the publisher.
Supplementary material
The Supplementary Material for this article can be found online at: https://www.frontiersin.org/articles/10.3389/fmars.2024.1364263/full#supplementary-material
References
Airoldi L., Balata D., Beck M. W. (2008). The gray zone: relationships between habitat loss and marine diversity and their applications in conservation. J. Exp. Mar. Biol. Ecol. 366, 8–15. doi: 10.1016/j.jembe.2008.07.034
Airoldi L., Beck M. (2007). “Loss, status and trends for coastal marine habitats of Europe,” in Oceanography and marine biology: an annual review, vol. 20074975 . Eds. Gibson R., Atkinson R., Gordon J. (CRC Press, Boca Raton), 345–405.
Alsuwaiyan N. A., Filbee-Dexter K., Vranken S., Burkholz C., Cambridge M., Coleman M. A., et al. (2022). Green gravel as a vector of dispersal for kelp restoration. Front. Mar. Sci. 9, 910417. doi: 10.3389/fmars.2022.910417
Bay L. K., Ortiz J. C., Humanes A., Riginos C., Baums I. B., Aranda M., et al. (2023). CORDAP R&D technology roadmap for understanding the natural adaptation and assisted evolution of corals to climate change. CORDAP. Available at: www.cordap.org.
Bayraktarov E., Brisbane S., Hagger V., Smith C. S., Wilson K. A., Lovelock C. E., et al. (2020). Priorities and motivations of marine coastal restoration research. Front. Mar. Sci. 7, 484. doi: 10.3389/fmars.2020.00484
Bell T. W., Cavanaugh K. C., Saccomanno V. R., Cavanaugh K. C., Houskeeper H. F., Eddy N., et al. (2023). Kelpwatch: A new visualization and analysis tool to explore kelp canopy dynamics reveals variable response to and recovery from marine heatwaves. PloS One 18, e0271477. doi: 10.1371/journal.pone.0271477
BenDor T. K., Lester T. W., Livengood A., Davis A., Yonavjak L. (2014). “Exploring and understanding the restoration economy,” in Final report to Walton Family Fund. Chapel Hill, USA: Center for Urban and Regional Studies, The University of North Carolina. Available at: https://curs.unc.edu/files/2014/01/RestorationEconomy.pdf.
Bennett S., Wernberg T., Connell S. D., Hobday A. J., Johnson C. R., Poloczanska E. S. (2015). The ‘Great Southern Reef’: social, ecological and economic value of Australia’s neglected kelp forests. Mar. Freshw. Res. 67, 47–56. doi: 10.1071/MF15232
Bischoff A., Steinger T., Müller-Schärer H. (2010). The importance of plant provenance and genotypic diversity of seed material used for ecological restoration. Restor. Ecol. 18, 338–348. doi: 10.1111/rec.2010.18.issue-3
Breed M. F., Harrison P. A., Bischoff A., Durruty P., Gellie N. J., Gonzales E. K., et al. (2018). Priority actions to improve provenance decision-making. BioScience 68, 510–516. doi: 10.1093/biosci/biy050
Bucharova A., Bossdorf O., Hölzel N., Kollmann J., Prasse R., Durka W. (2019). Mix and match: regional admixture provenancing strikes a balance among different seed-sourcing strategies for ecological restoration. Conserv. Genet. 20, 7–17. doi: 10.1007/s10592-018-1067-6
Burkholder J. M., Tomasko D. A., Touchette B. W. (2007). Seagrasses and eutrophication. J. Exp. Mar. Biol. Ecol. 350, 46–72. doi: 10.1016/j.jembe.2007.06.024
Butler C. L., Lucieer V. L., Wotherspoon S. J., Johnson C. R. (2020). Multi-decadal decline in cover of giant kelp Macrocystis pyrifera at the southern limit of its Australian range. Mar. Ecol. Prog. Ser. 653, 1–18. doi: 10.3354/meps13510
Campbell A. H., Marzinelli E. M., Verges A., Coleman M. A., Steinberg P. D. (2014). Towards restoration of missing underwater forests. PloS One 9, e84106. doi: 10.1371/journal.pone.0084106
Cebrian E., Tamburello L., Verdura J., Guarnieri G., Medrano A., Linares C., et al. (2021). Galobart C and fraschetti S A roadmap for the restoration of mediterranean macroalgal forests. Front. Mar. Sci. 8. doi: 10.3389/fmars.2021.709219
Clausing R., de la Fuente G., Falace A., Chiantore M. (2022). Accounting for environmental stress in restoration of intertidal foundation species. J. Appl. Ecol. 00, 1–14. doi: 10.1111/1365-2664.14334
Coleman M. A., Kelaher B. P. (2009). Connectivity among fragmented populations of a habitat-forming alga, Phyllospora comosa (Phaeophyceae, Fucales) on an urbanised coast. Mar. Ecol. Prog. Ser. 381, 63–70. doi: 10.3354/meps07977
Coleman M. A., Wernberg T. (2021). A glass half full: Solutions-oriented management under climate change. Trends Ecol. Evol. 36, 385–386. doi: 10.1016/j.tree.2021.02.009
Coleman M. A., Wood G., Filbee-Dexter K., Minne A. J. P., Goold H. D., Vergés A., et al. (2020). Restore or redefine: future trajectories for restoration. Front. Mar. Sci. 7. doi: 10.3389/fmars.2020.00237
Convention on Biological Diversity (2021) First draft of the post 2020 global biodiversity framework (Accessed 5 July 2021).
Copeland S. M., Baughman O. W., Boyd C. S., Davies K. W., Kerby J., Kildisheva O. A., et al. (2021). Improving restoration success through a precision restoration framework. Restor. Ecol. 29, e13348. doi: 10.1111/rec.13348
Cresswell K., Hartmann K., Gardner C., Keane J. (2022). Tasmanian longspined sea urchin fishery assessment 2020/21. Hobart Australia: University of Tasmania and Institute of Marine and Antarctic Studies.
Duarte C. M., Gattuso J. P., Hancke K., Gundersen H., Filbee-Dexter K., Pedersen M. F., et al. (2022). Global estimates of the extent and production of macroalgal forests. Global Ecol. Biogeogr. 31, 1422–1439. doi: 10.1111/geb.13515
Eger A. M., Aguirre J. D., Altamirano M., Arafeh-Dalmau N., Arroyo N. L., Bauer-Civiello A. M., et al. (2023). The Kelp Forest Challenge: A collaborative global movement to protect and restore 4 million hectares of kelp forests. J. Appl. Phycology, 1–14. doi: 10.1007/s10811-023-03103-y
Eger A. M., Ling. S. D., McHugh T. A., Filbee-Dexter K. A., Kim J. H., Marzinelli E., et al. (2022b). “Kelp forest restoration in action (methods),” in Kelp restoration guidebook: lessons learned from kelp projects around the world. Eds. Eger A. M., Layton C., McHugh T. A., Gleason M., Eddy N. (The Nature Conservancy, Sacramento, CA, USA), 44–46.
Eger A. M., Marzinelli E. M., Christie H., Fagerli C. W., Fujita D., Gonzalez A. P., et al. (2022). Global kelp forest restoration: Past lessons, present status, and future directions. Biol. Rev. 97, 1449–1475. doi: 10.1111/brv.12850
Eger A. M., Vergés A., Choi C. G., Christie H., Coleman M. A., Fagerli C. W., et al. (2020a). Financial and institutional support are important for large-scale kelp forest restoration. Front. Mar. Sci. 7, 535277. doi: 10.3389/fmars.2020.535277
Falace A., Kaleb S., de la Fuente G., Asnaghi V., Chiantore M. (2018). Ex situ cultivation protocol for Cystoseira amentacea var. stricta (Fucales, Phaeophyceae) from a restoration perspective. PloS One 13, e0193011. doi: 10.1371/journal.pone.0193011
Feehan C. J., Filbee-Dexter K., Wernberg T. (2021). Embrace kelp forests in the coming decade. Science 373, 863. doi: 10.1126/science.abl3984
Filbee-Dexter K., Scheibling R. E. (2014). Sea urchin barrens as alternative stable states of collapsed kelp ecosystems. Mar. Ecol. Prog. Ser. 495, 1–25. doi: 10.3354/meps10573
Filbee-Dexter K., Wernberg T. (2018). Rise of turfs: a new battlefront for globally declining kelp forests. Bioscience 68, 64–76. doi: 10.1093/biosci/bix147
Filbee-Dexter K., Wernberg T., Barreiro R., Coleman M. A., de Bettignies T., Feehan C. J., et al. (2022). Leveraging the blue economy to transform marine forest restoration. J. phycology 58, 198–207. doi: 10.1111/jpy.13239
Fischer M., Maxwell K., Pedersen H., Greeno D., Jingwas N., Graham Blair J., et al. (2022). Empowering her guardians to nurture our Ocean’s future. Rev. Fish Biol. Fisheries 32, pp.271–pp.296. doi: 10.1007/s11160-021-09679-3
Franco J. N., Wernberg T., Bertocci I., Duarte P., Jacinto D., Vasco-Rodrigues N., et al. (2015). Herbivory drives kelp recruits into ‘hiding’in a warm ocean climate. Mar. Ecol. Prog. Ser. 536, 1–9. doi: 10.3354/meps11445
Fredriksen S., Filbee-Dexter K., Norderhaug K. M., Steen H., Bodvin T., Coleman M. A., et al. (2020). Green gravel: a novel restoration tool to combat kelp forest decline. Sci. Rep. 10, 3983. doi: 10.1038/s41598-020-60553-x
Gosnell H., Kelly E. C. (2010). Peace on the river? Social-ecological restoration and large dam removal in the Klamath basin, USA. Water Alternatives, 3 (2), 361–383.
Iha C., Layton C., Flentje W., Lenton A., Johnson C., Fraser C. I., et al. (2023). Organellar genomes of giant kelp from the southern hemisphere. Appl. Phycology 4, pp.78–pp.86. doi: 10.1080/26388081.2023.2193619
Johnson C. R., Banks S. C., Barrett N. S., Cazassus F., Dunstan P. K., Edgar G. J., et al. (2011). Climate change cascades: shifts in oceanography, species’ ranges and subtidal marine community dynamics in eastern Tasmania. J. Exp. Mar. Biol. Ecol. 400, 17–32. doi: 10.1016/j.jembe.2011.02.032
Jueterbock A., Minne A. J., Cock J. M., Coleman M. A., Wernberg T., Scheschonk L., et al. (2021). Priming of marine macrophytes for enhanced restoration success and food security in future oceans. Front. Mar. Sci. 8, 658485. doi: 10.3389/fmars.2021.658485
Keane J., Ling S. (2022). Diver control of long-spined sea urchin in tasmania (Hobart, Tasmania, Australia: University Of Tasmania). Available at: https://figshare.utas.edu.au/articles/chapter/Diver_Control_of_Long-spined_Sea_Urchin_in_Tasmania/23070329.
Kern J., Jennings L., Senkyr L., Center N. R., Hoff R. (2016). Habitat restoration in an urban waterway: lessons learned from the Lower Duwamish River, in "2015 Workshop Report". U.S. Department of Commerce, National Oceanic and Atmospheric Divison, NOAA Fisheries, USA.
Layton C., Coleman M. A., Marzinelli E. M., Steinberg P. D., Swearer S. E., Vergés A., et al. (2020). Kelp forest restoration in Australia. Front. Mar. Sci. 7, 74. doi: 10.3389/fmars.2020.00074
Layton C., Johnson C. R. (2021). “Assessing the feasibility of restoring giant kelp forests in Tasmania,” in Report to the national environmental science program (Hobart, Tasmania, Australia: Marine Biodiversity Hub). doi: 10.13140/RG.2.2.14537.06244
Layton C., Perez Matus A., Gonzalez A. V., Coleman M. A. (2022b). “Future-proofing kelp forest restoration for climate change,” in Kelp restoration guidebook: lessons learned from kelp projects around the world. Eds. Eger A. M., Layton C., McHugh T. A., Gleason M., Eddy N. (The Nature Conservancy, Sacramento, CA, USA), 44–46.
Layton C., Smid E., Johnson C. R. (2022a). “A physiological assessment of giant kelp (Macrocystis pyrifera) strains that exhibit warm tolerance,” in Report to the national environmental science program (Hobart, Tasmania, Australia: University of Tasmania).
Ling S. D., Scheibling R. E., Rassweiler A., Johnson C. R., Shears N., Connell S. D., et al. (2015). Global regime shift dynamics of catastrophic sea urchin overgrazing. Philos. Trans. R. Soc. B: Biol. Sci. 370, 20130269. doi: 10.1098/rstb.2013.0269
Lotze H. K., Coll M., Magera A. M., Ward-Paige C., Airoldi L. (2011). Recovery of marine animal populations and ecosystems. Trends Ecol. Evol. 26, 595–605. doi: 10.1016/j.tree.2011.07.008
Manning S. (2001). State of washington joint aquatic resource permit application (JARPA) (UC Davis: Road Ecology Center). Available at: https://escholarship.org/uc/item/0cr570jt.
McHugh T. A., Lee L. C., Verges A. (2022). “The human elements: communities and partners in kelp restoration,” in Kelp restoration guidebook: lessons learned from kelp projects around the world. Eds. Eger A. M., Layton C., McHugh T. A., Gleason M., Eddy N. (The Nature Conservancy, Sacramento, CA, USA), 44–46.
McLeod I. M., Boström-Einarsson L., Johnson C., Kendrick G., Layton C., Rogers A. A., et al. (2019). The role of restoration for conserving Matters of National Environmental Significance. Report to the National Environmental Science Programme, Marine Biodiversity Hub. 186.
Morrison T. H., Adger W. N., Agrawal A., Brown K., Hornsey M. J., Hughes T. P., et al. (2022). Radical interventions for climate-impacted systems. Nat. Climate Change 12, 1100–1106. doi: 10.1038/s41558-022-01542-y
Murcia C., Guariguata M. R., Andrade Á., Andrade G. I., Aronson J., Escobar E. M., et al. (2016). Challenges and prospects for scaling-up ecological restoration to meet international commitments: Colombia as a case study. Conserv. Lett. 9, pp.213–pp.220. doi: 10.1111/conl.12199
Powers R. P., Jetz W. (2019). Global habitat loss and extinction risk of terrestrial vertebrates under future land-use-change scenarios. Nat. Climate Change 9, 323–329. doi: 10.1038/s41558-019-0406-z
Rogers-Bennett L., Catton C. A. (2019). Marine heat wave and multiple stressors tip bull kelp forest to sea urchin barrens. Sci. Rep. 9, 15050. doi: 10.1038/s41598-019-51114-y
Rossetto M., Bragg J., Kilian A., McPherson H., van der Merwe M., Wilson P. D. (2019). Restore and Renew: a genomics-era framework for species provenance delimitation. Restor. Ecol. 27, pp.538–pp.548. doi: 10.1111/rec.12898
Savonitto G., de la Fuente G., Tordoni E., Ciriaco S., Srijemsi M., Bacaro G., et al. (2021). Addressing reproductive stochasticity and grazing impacts in the restoration of a canopy- forming brown alga by implementing mitigation solutions. Aquat. Conservation Mar. Freshw. Ecosyst. 31, 1–13. doi: 10.1002/aqc.3555
Shears N. T., Babcock R. C. (2003). Continuing trophic cascade effects after 25 years of no-take marine reserve protection. Mar. Ecol. Prog. Ser. 246, 1–16. doi: 10.3354/meps246001
Shumway N., Bell-James J., Fitzsimons J. A., Foster R., Gillies C., Lovelock C. E. (2021). Policy solutions to facilitate restoration in coastal marine environments. Mar. Policy 134, 104789. doi: 10.1016/j.marpol.2021.104789
Smith C. J., Verdura J., Papadopoulou N., Fraschetti S., Cebrian E., Fabbrizzi E., et al. (2023). A decision-support framework for the restoration of Cystoseira sensu lato forests. Front. Mar. Sci. 10, 1159262. doi: 10.3389/fmars.2023.1159262
Spalding M. D., Fox H. E., Allen G. R., Davidson N., Ferdaña Z. A., Finlayson M. A. X., et al. (2007). Marine ecoregions of the world: a bioregionalization of coastal and shelf areas. BioScience 57, 573–583. doi: 10.1641/B570707
Starko S., Neufeld C. J., Gendall L., Timmer B., Campbell L., Yakimishyn J., et al. (2022). Microclimate predicts kelp forest extinction in the face of direct and indirect marine heatwave effects. Ecol. Appl. 32, e2673. doi: 10.1002/eap.2673
Suding K. N. (2011). Toward an era of restoration in ecology: successes, failures, and opportunities ahead. Ann,. Rev. Ecol. Evol. Syst. 42, 465–487. doi: 10.1146/annurev-ecolsys-102710-145115
Tamburello L., Papa L., Guarnieri G., Basconi L., Zampardi S., Scipione M. B., et al. (2019). Are we ready for scaling up restoration actions? An insight from Mediterranean macroalgal canopies. PloS One 14, e0224477. doi: 10.1371/journal.pone.0224477
Tracey S. R., Mundy C., Baulch T., Marzloff M., Hartmann K., Ling S., et al. (2014). “Trial of an industry implemented, spatially discrete eradication/control program for Centrostephanus rodgersii in Tasmania,” in FRDC project no. 2011/087 (Institute for Marine and Antarctic Studies, Tasmania).
Trisos C. H., Auerbach J., Katti M. (2021). Decoloniality and anti-oppressive practices for a more ethical ecology. Nat. Ecol. Evol. 5, 1205–1212. doi: 10.1038/s41559-021-01460-w
United Nations Environment Agency (2019) Resolution 73/284: united nations decade on ecosystem restoratio –2030). Available online at: https://undocs.org/A/RES/73/284 (Accessed 22 Dec 2023).
Vanderklift M. A., Doropoulos C., Gorman D., Leal I., Minne A. J., Statton J., et al. (2020). Using propagules to restore coastal marine ecosystems. Front. Mar. Sci. 7, 724. doi: 10.3389/fmars.2020.00724
Vardi T., Hoot W. C., Levy J., Shaver E., Winters R. S., Banaszak A. T., et al. (2021). Six priorities to advance the science and practice of coral reef restoration worldwide. Restor. Ecol. 29, e13498. doi: 10.1111/rec.13498
Verdura J., Sales M., Ballesteros E., Cefalì M. E., Cebrian E. (2018). Restoration of a canopy-forming alga based on recruitment enhancement: methods and long-term success assessment. Front. Plant Sci. 9, 1832. doi: 10.3389/fpls.2018.01832
Vergés A., McCosker E., Mayer-Pinto M., Coleman M. A., Wernberg T., Ainsworth T., et al. (2019). Tropicalisation of temperate reefs: Implications for ecosystem functions and management actions. Funct. Ecol. 33, 1000–1013. doi: 10.1111/1365-2435.13310
Vranken S., Wernberg T., Scheben A., Severn-Ellis A. A., Batley J., Bayer P. E., et al. (2021). Genotype–Environment mismatch of kelp forests under climate change. Mol. Ecol. 30, 3730–3746. doi: 10.1111/mec.15993
Wernberg T., Bennett S., Babcock R. C., De Bettignies T., Cure K., Depczynski M., et al. (2016). Climate-driven regime shift of a temperate marine ecosystem. Science 353, 169–172. doi: 10.1126/science.aad8745
Wernberg T., Coleman M. A., Bennett S., Thomsen M. S., Tuya F., Kelaher B. P. (2018). Genetic diversity and kelp forest vulnerability to climatic stress. Sci. Rep. 8, p.1851.
Wernberg T., Filbee-Dexter K. (2019). Missing the marine forests for the trees. Mar. Ecol. Prog. Ser. 612, 209–215. doi: 10.3354/meps12867
Wernberg T., Thomsen M. S., Baum J. K., Bishop M. J., Bruno J., Coleman M. A., et al. (2024)Impacts of climate change on marine foundation species. In: Annual review of marine science (Accessed 7/6/23).
Wood G., Marzinelli E. M., Campbell A. H., Steinberg P. D., Vergés A., Coleman M. A. (2021). Genomic vulnerability of a dominant seaweed points to future-proofing pathways for Australia’s underwater forests. Global Change Biol. 27, 2200–2212. doi: 10.1111/gcb.15534
Wood G., Marzinelli E. M., Coleman M. A., Campbell A. H., Santini N. S., Kajlich L., et al. (2019). Restoring subtidal marine macrophytes in the Anthropocene: trajectories and future-proofing. Mar. Freshw. Res. 70, 936–951. doi: 10.1071/MF18226
Wood G., Marzinelli E. M., Vergés A., Campbell A. H., Steinberg P. D., Coleman M. A. (2020). Using genomics to design and evaluate the performance of underwater forest restoration. J. Appl. Ecol. 57, 1988–1998. doi: 10.1111/1365-2664.13707
Keywords: macroalgae, restoration, upscaling, green gravel, kelp, seaweed, ecology, future-proof
Citation: Wood GV, Filbee-Dexter K, Coleman MA, Valckenaere J, Aguirre JD, Bentley PM, Carnell P, Dawkins PD, Dykman LN, Earp HS, Ennis LB, Francis P, Franco JN, Hayford H, Lamb JB, Ling SD, Layton C, Lis E, Masters B, Miller N, Moore PJ, Neufeld C, Pocklington JB, Smale D, Stahl F, Starko S, Steel SC, Verbeek J, Vergés A, Wilding CM and Wernberg T (2024) Upscaling marine forest restoration: challenges, solutions and recommendations from the Green Gravel Action Group. Front. Mar. Sci. 11:1364263. doi: 10.3389/fmars.2024.1364263
Received: 02 January 2024; Accepted: 11 March 2024;
Published: 09 April 2024.
Edited by:
Oscar Serrano, Edith Cowan University, AustraliaReviewed by:
José M. Rico, University of Oviedo, SpainMariachiara Chiantore, University of Genoa, Italy
Copyright © 2024 Wood, Filbee-Dexter, Coleman, Valckenaere, Aguirre, Bentley, Carnell, Dawkins, Dykman, Earp, Ennis, Francis, Franco, Hayford, Lamb, Ling, Layton, Lis, Masters, Miller, Moore, Neufeld, Pocklington, Smale, Stahl, Starko, Steel, Verbeek, Vergés, Wilding and Wernberg. This is an open-access article distributed under the terms of the Creative Commons Attribution License (CC BY). The use, distribution or reproduction in other forums is permitted, provided the original author(s) and the copyright owner(s) are credited and that the original publication in this journal is cited, in accordance with accepted academic practice. No use, distribution or reproduction is permitted which does not comply with these terms.
*Correspondence: Georgina Valentine Wood, george.wood@uwa.edu.au