- 1Observatoire PELAGIS, UAR 3462 CNRS, La Rochelle Université, La Rochelle, France
- 2Centre d’Etude Biologique de Chizé, UMR 7372 CNRS, La Rochelle Université, Chizé, France
- 3Marine Conservation Research, Kelvedon, United Kingdom
- 4Share the Ocean, Larmor-Baden, France
Marine organisms continually adapt their physiology and behaviour to temporal variations in their environment, resulting in diurnal rhythmic behaviour, particularly when foraging. In delphinids, these rhythms can be studied by recording echolocation clicks, which can provide indicators of foraging activity. The foraging rhythms of delphinids and their relationship to temporal parameters are poorly documented and most studies so far have used moored passive acoustic systems. The present study provides, for the first time, information on the activity rhythms of delphinids investigated in relation with temporal variables at a basin scale from a moving platform, in the western and central Mediterranean Sea. We used passive acoustic recordings collected by hydrophones towed along transect lines during the ACCOBAMS Survey Initiative in the summer 2018. We extracted variables that may influence daily and monthly rhythms, including time of day, lunar cycle, lunar illumination and sea state and fitted generalised additive models. The nycthemeral and lunar cycles were the two main factors influencing dolphin activity rhythms. Echolocation activity was predominant at night, with a maximum of 0.026 acoustic events per minute at 21:00/22:00 compared to as few as 0.0007 events per minute at 11:00. These events were also more frequent during the third quarter of the moon; 0.033 acoustic events on day 22 of the lunar cycle as opposed to 0.0008 on day 8 of the lunar cycle, corresponding to the first quarter of the moon. Variations in the echolocation activity of delphinids in the Mediterranean Sea could reflect variation in their foraging effort and be related to prey density, composition, accessibility and catchability within dolphin foraging depth range. These results should also improve interpretation of passive acoustic monitoring data.
1 Introduction
Marine animals live in habitats that are subject to temporal variations that can be seasonal, lunar or daily and that shape the adaptive strategies of each species (Häfker et al., 2023). Species adapt their physiology and behaviour to these environmental fluctuations and associated rhythms (Pirotta et al., 2020). These adaptations result in biological activity being in phase with the main natural cycles, which are governed by the Earth’s orbit around the Sun (seasons), the Moon’s orbit around the Earth (tides) and the Earth’s rotation (nycthemeron). Biological rhythms are controlled by endogenous systems, some of which are light sensitive. Changes in light levels can trigger physiological or behavioural responses (Simonis et al., 2017). Therefore, the nycthemeral cycle can be the source of daily changes and adaptations, although the exact nature of these rhythms also depends on other factors such as habitat type, coordinates, and physicochemical properties (Häfker et al., 2023). The aggregation of marine predators for feeding has been shown to be associated with primary production, bathymetry, and sea surface temperature (Hastie et al., 2004; Prieto et al., 2017; Scales et al., 2016).
The best known example of a biological rhythm in the pelagic environment is the Diel Vertical Migration (DVM) of the Deep Scattering Layer (DSL), composed mainly of planktonic and micronectonic organisms such as a variety of crustaceans (euphausiids, mysids, copepods), fish (e.g., myctophids) or cephalopods (e.g., histioteuthids) which generally migrate towards the surface layer at dusk and return to the depths at dawn (Wang et al., 2019). According to Marohn et al. (2021), this phenomenon would reflect a balance between meeting food requirements and avoiding predators. The most plausible hypothesis is that the vertical migrations of marine organisms at the base of the food web lead to similar rhythms in their mesopelagic and epipelagic predators, such as number of fish, squid and sharks (Häfker et al., 2023) which forage when their prey is most efficiently exploited. Similarly, air-breathing diving top predators such as cetaceans, that feed on these meso-and epipelagic species, would develop a strategy in which the optimal decision about where and when to feed would allow them to maximise their energy intake, especially as these predators must hold their breath during dives to access resources (Miller et al., 2010). The challenge for these marine mammals is to find the best compromise between breathing at the surface and feeding at depth. To achieve this, they are likely to adapt their feeding rhythms to the dynamics, availability, or catchability of their prey (Giorli et al., 2016).
In pelagic ecosystems, cetacean activity tends to be nocturnal, with an increase in activity in the evening, followed by a peak at night and a decrease in the early morning (Linnenschmidt et al., 2013). This pattern likely occurs due to the greater availability of prey closer to the sea surface at night. Furthermore, in demersal ecosystems, cetaceans that prey on bottom-dwelling fish, many of which are not particularly active at night, may exhibit different diel activity patterns (Brandt et al., 2014). In the Mediterranean Sea, where neritic habitats are quite limited, Giorli et al. (2016) highlighted that several typically offshore dwelling cetaceans (Risso’s dolphin Grampus griseus, long-finned pilot whale Globicephala melas, Cuvier’s beaked whale Ziphius cavirostris, and sperm whale Physeter macrocephalus) exhibited mostly nocturnal foraging activity. Similarly, Caruso et al. (2017) studied several delphinids (striped dolphin Stenella coeruleoalba, bottlenose dolphin Tursiops truncatus, common dolphin Delphinus delphis, Risso’s dolphin and long-finned pilot whale) in the Ionian Sea and found a daily pattern in foraging activity, with greater activity at night. The explanatory hypothesis put forward in these two studies is that the foraging behaviour of odontocetes would be dictated by the presence and dynamics of their prey, which are more active and putatively easier to capture at night.
There are several approaches to study cetacean activity rhythms. Longitudinal approaches (e.g., biologging techniques and focal tracking) have the advantage of following the activity of known individuals over time (Brauer et al., 2022), although they can be very time-consuming to implement and, in the case of visual tracking, are typically dependent on good weather and daylight conditions. Cross-sectional approaches sample activity data across a population as a function of time, for example during acoustic or visual surveys. They provide rapid results that reflect the general characteristics of a population. However, individual variability is not measurable (Brauer et al., 2022). The distribution, dynamics and activity rhythms of vocalising cetaceans can be studied using this second method, thanks to acoustic surveys (Barlow et al., 2021). Here we used a cross-sectional approach to investigate delphinid foraging activity using a large-scale acoustic line-transect survey.
Sound emission is common to all cetaceans, which have developed highly sophisticated sound production systems. Sound plays an important role in their daily lives, being used for communication, socialisation, navigation, and predation (Hildebrand, 2009). Vocalisations are also used to compensate for poor visibility conditions such as night, depth and turbidity, and are a critical component of the evolutionary success of odontocetes in general (Au, 1993). Odontocetes emit different types of acoustic signals, sometimes near the surface, sometimes at depth (Gillespie et al., 2009), which can travel long distances (probably tens of kilometres in some whale species, Bittle and Duncan, 2013). Acoustic signals emitted by odontocetes can be classified into three categories: tonal signals (or whistles), pulsed calls and echolocation clicks (Azzolin et al., 2014). Whistles and certain pulsed calls are long and complex sounds with intermediate frequencies (often below 10 kHz, González-Hernández et al., 2017), modulated and with a narrow bandwidth. They mainly provide information about the identity of a species, population or individual, as well as on the physiological and behavioural state of the emitter. These signals are essential for maintaining organisation and cohesion within social groups (Azzolin et al., 2014). Clicks, emitted in series (known as click trains), and certain pulsed calls, are short, repetitive sounds that cover a wide range of frequencies (from 20 kHz to over 100 kHz, González-Hernández et al., 2017). They are used for foraging, navigating and detection of predators (Au, 2018). Foraging involves all the activities required to reach, locate, detect and capture prey, with searching generally being the most time-consuming activity and this includes echolocation, which is becoming more important for both navigation and prey detection (Madsen and Wahlberg, 2007; Giorli et al., 2016). As clicks allow the detection of prey in the environment, they are considered to be the most relevant acoustic indicator of foraging activity (Caruso et al., 2017). In this context, we assumed that temporal pattern in foraging activity would strongly affect click production rate. In addition, clicks are the most abundant type of sound emitted, and therefore would provide a larger sample size than the other types of vocalisations. Studying them can therefore help our understanding of how cetacean foraging activity changes over time.
The Mediterranean Sea is home to 7% of the world’s marine biodiversity, of which around a fifth is considered endemic (Pace et al., 2015). Eleven cetacean species are known to permanently occur in the Mediterranean Sea and are regularly observed in the basin: the bottlenose dolphin, the striped dolphin, the common dolphin, the Risso’s dolphin, the rough-toothed dolphin Steno bredanensis, the long-finned pilot whale, the Cuvier’s beaked whale, the sperm whale, the fin whale Balaenoptera physalus, the orca, Orcinus orca and the Black Sea harbour porpoise Phocoena phocoena relicta, which is present in the northern Aegean Sea (Cucknell et al., 2016; Notarbartolo di Sciara and Tonay, 2021). The false killer whale Pseudorca crassidens, the minke whale Balaenoptera acutorostrata, and the humpback whale Megaptera novaeangliae are occasional visitors (Notarbartolo di Sciara and Tonay, 2021).
The main objective of this study was to analyse the rhythm of foraging activity of Mediterranean pelagic delphinids of which the striped, common and bottlenose dolphins are the most abundant (Panigada et al., 2024). Most previous studies of delphinid foraging rhythms in the Mediterranean Sea have used fixed acoustic devices within limited geographic areas (Giorli et al., 2016; Cascão et al., 2020). Here we used acoustic recordings provided by the Agreement on the Conservation of Cetaceans of the Black Sea, Mediterranean Sea and contiguous Atlantic area (ACCOBAMS) and collected during the vessel component of the basin-wide 2018 ACCOBAMS Survey Initiative (ASI), which aimed to assess cetacean abundance and distribution in the Mediterranean Sea. Acoustic recordings were collected day and night using towed hydrophones along predetermined transect lines. Only echolocation clicks were analysed, as they are the most relevant signals to study the foraging activity. Diel rhythms in the number of acoustic events were investigated, as well as their relationship with a selection of temporal parameters. We hypothesised that a strong diel pattern would prevail, with more acoustic events during the night. We first extracted click emissions from acoustic recordings. The number of acoustic events per unit of time was then modelled in a generalised additive model (GAM) framework using a selection of temporal variables thought to be involved in the foraging strategies of delphinids.
2 Materials and methods
2.1 Study area
The study area covered the entire Mediterranean basin, from 34°S to 45°N and from 6°W to 17°E (Figure 1). The Mediterranean Sea is a semi-enclosed mid-latitude sea virtually isolated from the main oceanic systems, stretching east-west over about 3,800 kilometres. The general circulation of the Mediterranean Sea is cyclonic, with Atlantic surface waters flowing along the southern shores of the basin and returning along the northern shores, whose complexity generates many eddies. Winter cooling of the surface waters in the north of the basin is responsible for the formation of rich, deep waters that flow towards the Atlantic Ocean (Robinson et al., 2001; Millot and Taupier-Letage, 2005). Although the Mediterranean Sea is generally considered to range from oligotrophic to ultra-oligotrophic, it is characterised by significant spatial variability in primary productivity, with decreasing productivity observed from west to east (Lazzari et al., 2012). Geomorphological features such as canyons, seamounts and deep trenches also provide a variety of unique habitats (Aïssi et al., 2015) resulting in highly diverse and rich ecosystems.
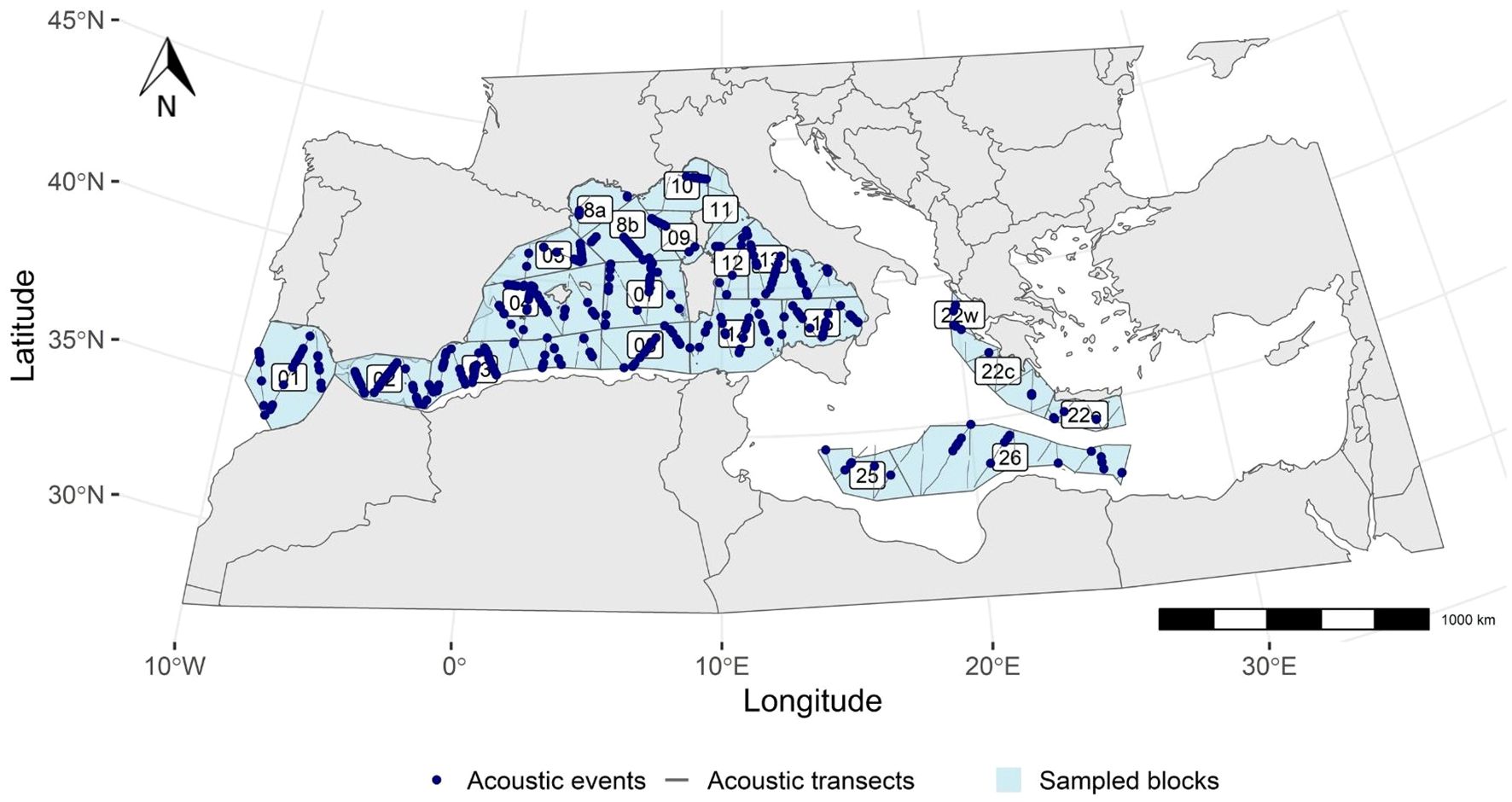
Figure 1 2018 ASI survey area. The blue polygons represent the sampled blocks, the grey lines represent the linear transects followed by the R/V Song of the Whale and the blue dots illustrate the recorded acoustic events.
2.2 Data collection
The acoustic data used in this study were collected from May to September 2018 during the ASI survey, during which two types of platforms were used to collect cetacean data; aircraft conducted visual detection only, and vessels supplemented the visual observations collected by the aerial component with acoustic data, particularly for deep-diving sperm whales and Cuvier’s beaked whales (Boisseau et al., 2024; Panigada et al., 2024). In this study, we focused only on acoustic data from delphinids collected aboard the R/V Song of the Whale. Overall, the visual data collected from the boat indicated that delphinids were represented by three main species: the striped, the common and the bottlenose dolphins. Risso’s dolphin, long-finned pilot whale and rough-toothed dolphin were also present, although less frequently.
The acoustic survey was conducted continuously (24 hours per day) wherever permitted by the riparian states, except where weather conditions, water depth (minimum 50 m) or technical constraints did not allow it. It focused on the Atlantic region near the Strait of Gibraltar, the western Mediterranean basin from the Alborán Sea to the Tyrrhenian Sea, and parts of the central Mediterranean basin (the Ionian waters and the Hellenic Trench; Figure 1). No survey effort was allocated in the Aegean Sea. The study area was divided into 21 blocks, designed to estimate cetacean abundance (ACCOBAMS, 2021).
Sampling was carried out along linear transects, and the hydrophone array was towed 400 m behind the vessel. This sampling method was used to maximise the coverage of the study area and to provide a robust estimate of species density and abundance (Buckland et al., 2004). Transects were defined using the Distance software (version 7.3, ACCOBAMS, 2021) and a zig-zag pattern was used to achieve equal probability coverage. A total of 17,271 km of transects were travelled, representing approximately 74,000 minutes of effort. This total sampling effort was divided into one-minute duration segments. The vessel speed was maintained between 5 and 8 knots to minimise bias due to species movement. The hydrophone array was housed in an oil-filled tube and consisted of two pairs of hydrophones in a linear configuration. The pair of broadband hydrophones, spaced 0.25 m apart, recorded sounds from 1 to 100 kHz with a sensitivity of -204 dB re 1V/µPa, in the frequency band in which most odontocete vocalisations are produced. The outputs of the broadband hydrophones were digitised at a sampling rate of 192 kHz. The audio recordings were stored in 16-bit wav files.
2.3 Acoustic data analysis
Field recordings were compressed into binary storage files using PAMGuard (version 2.2.7.0, Gillespie et al., 2008). These files were manually analysed using the ‘click detector’ module, which allows the user to identify acoustic events, following the methodology of Ollier et al. (2023). Only click series, defined as sequences of clicks produced by the same animal or group of animals that exhibited a consistent change in bearing (i.e., from the bow to the stern of the vessel) were examined. Sequences of clicks that did not show a consistent bearing trajectory or did not cross the 90° line were not considered as acoustic events. To ensure that the series of echolocation clicks identified were indeed from delphinids, the characteristics of the clicks making up the series were examined. The waveform of a click had to cross the horizontal axis several times (known as “zero crossing”). The spectrum of each click should ideally have a frequency peak between 20 kHz and 40 kHz. If this frequency peak was below 10 kHz, it could be a sound signal emitted by a boat (ACCOBAMS, 2021).
2.4 Statistical analysis
2.4.1 Variables
We selected variables relevant to temporal scales ranging from hourly to monthly time resolution such as time of day, lunar cycle, lunar illumination and sea state (Table 1). The time of day of the acoustic events was considered as a circular variable to account for the temporal cyclicity (so that the statistical models considered that 00:00 = 24:00). This improved the accuracy of the model and captured the large cyclical variations in the data. As the study area was relatively large, and to avoid any time zone bias, the times at which these acoustic events were detected, given in Coordinated Universal Time (UTC), were converted to solar time (Waugh, 1973) by using the equation:
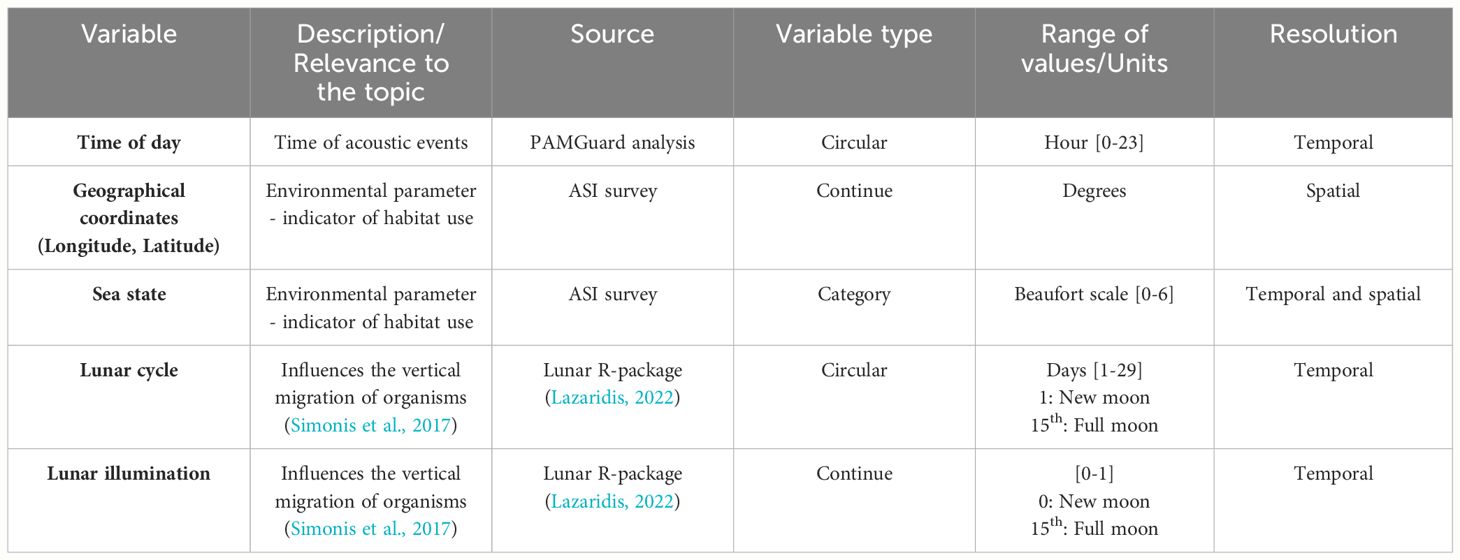
Table 1 Temporal and spatial variables used in the GAMs that potentially influence the cetacean rhythm activity.
The longitude in the equation is expressed in decimal degrees. The value 4 represents minutes and comes from the fact that the Earth rotates in 24 hours (= 1440 minutes), i.e. one degree of rotation every 4 minutes. Depending on whether the longitude is positive or negative (whether the acoustic event was detected east or west of the Greenwich meridian), the duration in minutes obtained is added to or subtracted from Coordinated Universal Time to obtain Solar Time (Waugh, 1973).
Sea state is expected to affect foraging efficiency on epipelagic prey. It was recorded during the ASI survey according to the Beaufort scale. The lunar cycle and lunar illumination are expected to modulate the extent and intensity of the diel vertical migration of planktonic and micronectonic organisms. The lunar cycle lasts 29.53 days, and during this cycle the moon goes through different phases (Wang et al., 2015): new moon (day 1 of the lunar cycle), first quarter (day 8 of the lunar cycle), full moon (day 15 of the lunar cycle), and last quarter (day 22 of the lunar cycle). The lunar cycle is also a circular variable as the lunar phases change from day to day, completing a cycle at the end of a lunation. The phase of the lunar cycle and the lunar illumination of each day were obtained using the ‘lunar’ R-package (Lazaridis 2022). To obtain the day of the lunar cycle, the lunar phase was divided by 0.212769 (the lunar phase increases by this value per day for a complete cycle). Beside nocturnal illumination, the lunar cycle also determines tidal range. Although the tide is limited in the Mediterranean Sea it does exist with an amplitude from 0 around the Balearic Islands to 60 cm in the Gulf of Gabès, Tunisia. Tidal range is greater at full and new moon and is minimal at first and last quarters, following a cyclical pattern with a period of approximately 15 days. Recognising the importance of spatial distribution of delphinids in the Mediterranean Sea (Cañadas et al., 2023), we included longitude and latitude as spatial factors rather than as explanatory variables to focus on temporal patterns.
2.4.2 Generalised additive models
GAMs (Wood, 2006) were fitted to determine how the number of acoustic events could be explained by these different variables. GAMs were chosen because unlike linear models, they offer great flexibility in describing non-linear relationships between predictors and a response variable. They have the advantage that the data dictate how the shape of the response variable is affected by each covariate by fitting non-parametric models (Marian et al., 2021). The explained variable was the number of acoustic events per minute. Correlations between variables were assessed using a correlation matrix. If the Pearson coefficient between two variables was greater than 0.5, then the variables could not be included together in any model selection. Due to the very large number of null values and the overdispersion of the data, a Tweedie distribution was used for the analysis (Foster and Bravington, 2013). For the time of day and lunar cycle variables, the degrees of freedom (k) were set to a maximum of 15 and 7 respectively, to allow the relationships to have multiple inflection points (non-linear responses expected for these two variables). To account for the circularity of these two variables, we have included circular smoothing terms (splines, bs = ‘cc’). This approach allowed the model to better capture temporal variations and effectively model the cyclicality of the data. For the other variables, we set the degrees of freedom to a maximum of 4, as the expected relationships were less complex, and used thin plate regression splines (bs = ‘tp’) as these are considered optimal (Wood, 2017). GAMs were fitted using the ‘gam’ function in the ‘mgcv’ R-package (Wood, 2017). Model selection was performed to determine the best model from all the models tested (combinations of 1 to 6 variables). The best model was selected based on the Akaike information criterion (AIC; Akaike, 1998), the AIC weight (‘akaike.weight’ function in the ‘qpcR’ R-package, Spiess, 2018), and the explained deviance. The importance of each variable was determined by summing the Akaike weights of the models in which the variable was selected (Symonds and Moussalli, 2011). The variables were then ranked according to their individual contribution to the models. All analyses were performed using the R software (version 4.1.2, R Core Team, 2022).
3 Results
Approximately 12,000 km were covered by the R/V Song of the Whale between May and September 2018, which represents a total of 74,000 minutes of sampling effort. Almost 40,000 minutes were sampled during the day while 34,000 were sampled at night. We identified a total of 741 acoustic events in PAMGuard during this sampling period. 41 events were recorded in May, 288 in June, 290 in July, 64 in August and 58 in September. Acoustic events were detected in all blocks sampled (Figure 1). More acoustic events were recorded at night (shaded area, Figure 2) with two peaks, reaching around 0.026 acoustic events per minute at 21:00/22:00 (solar time) and then around 01:00. After 01:00, the number of events decreased considerably; down to 0.0007 events per minute at 11:00 (Figure 2).
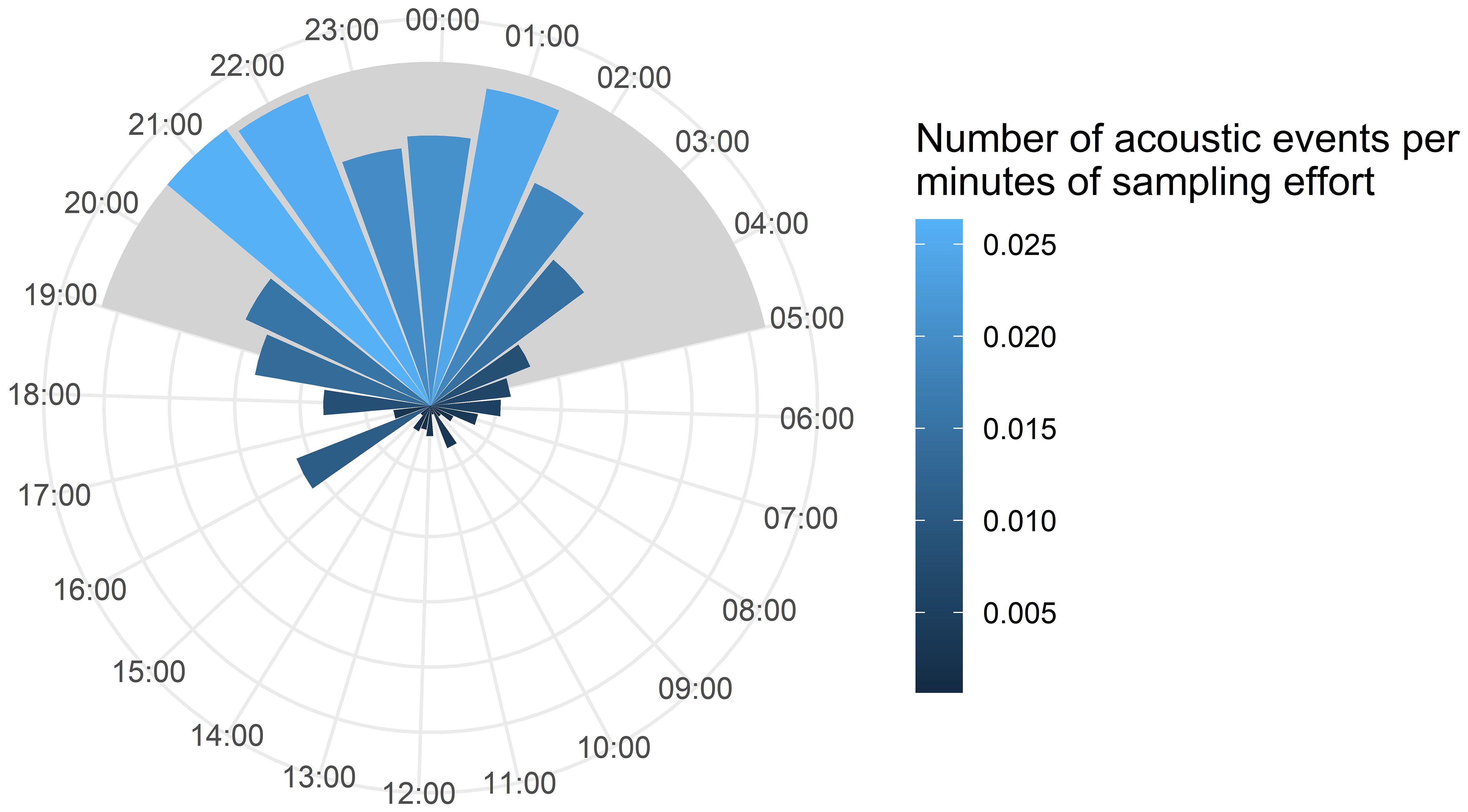
Figure 2 Temporal distribution of acoustic events corrected by sampling effort over the nycthemeral cycle. The shaded area represents the nocturnal phase. The largest radius has a value of 0.026 acoustic events per minute of effort at 21:00.
No correlations were found between the variables in the correlation matrix (Figure 3). Therefore, all the variables could be selected simultaneously in the models tested (Supplementary Table 1). The variables that contributed most to explaining the number of events were the time of day and the sea state, with Akaike weights of 99-100% (Table 2). They were followed by the lunar cycle with an AIC weight of 80%. In contrast, lunar illumination, had a relatively low AIC weight of 60% (Table 2). The best model explained 14.3% of the deviance and included all the variables tested, although lunar illumination was not significant (p = 0.39, Supplementary Table 1). The second model also explained 14.3% of the deviance and did not include lunar illumination. As the top two models were very similar (ΔAIC< 2), the second model was selected as the final model because lunar illumination was not significant (Ssupplementary Table 1). The final model included the spatial effect and the variables time of day, lunar cycle and sea state.
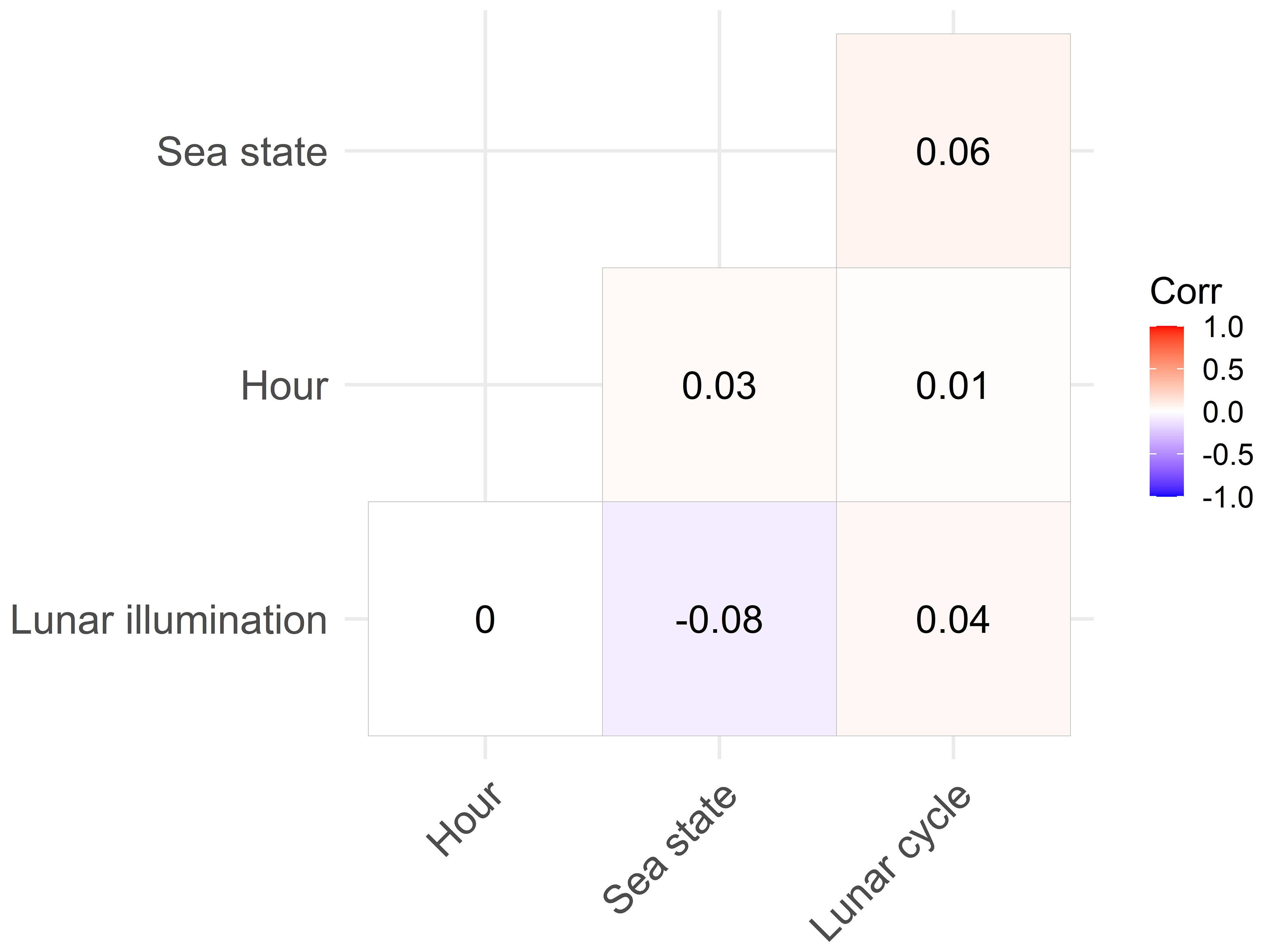
Figure 3 Correlation matrix including all variables tested in model selection. The numbers represent the coefficients of Pearson.
The number of acoustic events increased as night approached, peaking around 21:00/22:00 and at 01:00, with only a slight decrease between these two periods (Figure 4A). The number of acoustic events then decreased to a diurnal minimum around 11:00 (Figure 4A). For the lunar cycle variable, the number of events decreased from day 0, corresponding to the new moon, to a minimum on day 8, corresponding to the first quarter of the moon (Figure 4B). The response variable then increased, reaching a maximum during the third quarter of the moon (day 22 of the lunar cycle) and decreasing again until the end of the lunar cycle (day 29, Figure 4B). The relationship with sea state was unimodal from 1 to 4 and became positive at higher sea states (above 4, Figure 4C). Longitudinal and latitudinal gradients were observed, with more frequent acoustic events in the northern part of the western and central Mediterranean sub-regions (Figure 4D).
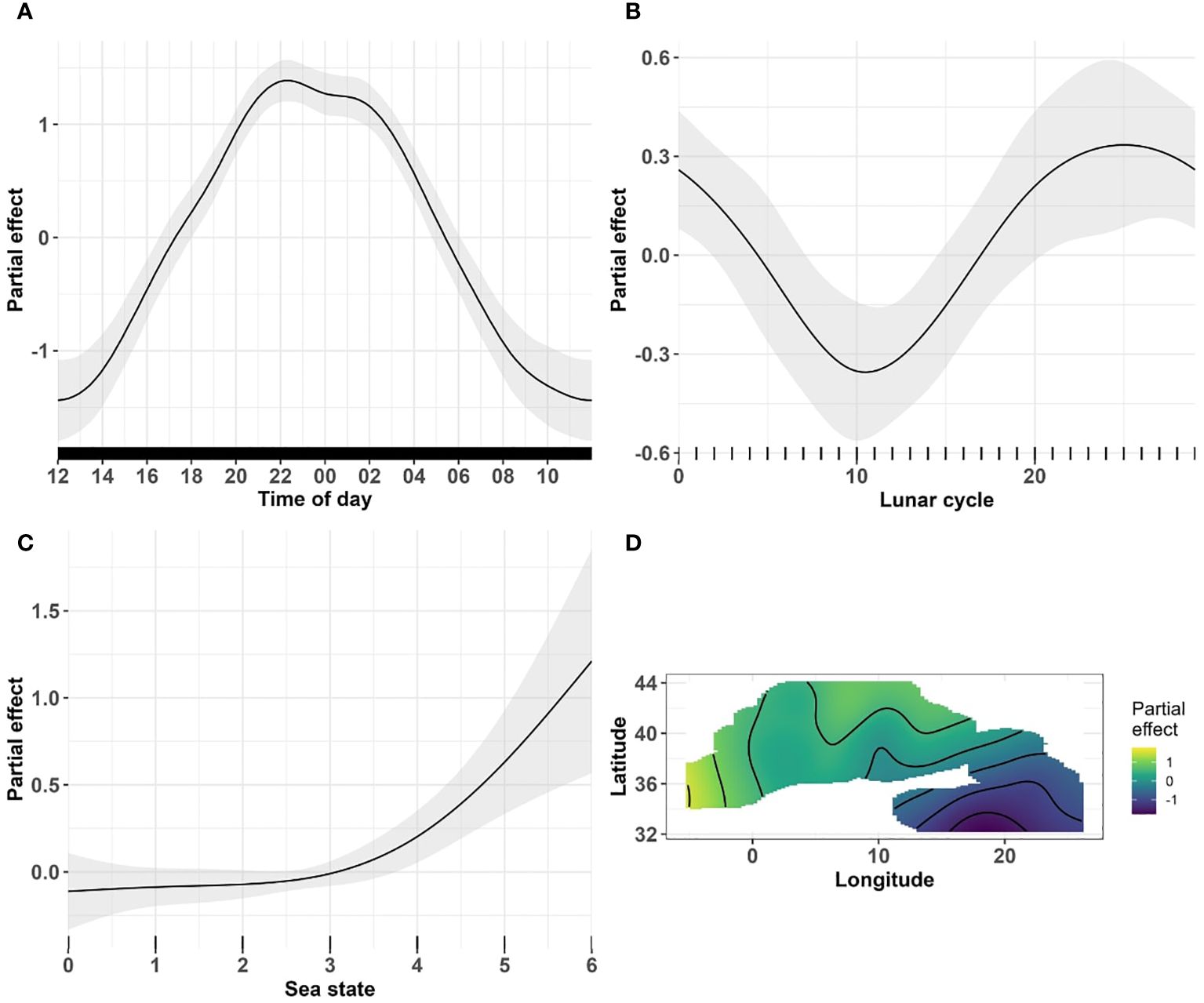
Figure 4 Results of the selected GAM model with a Tweedie distribution for the variables (A) time of day (B) lunar cycle, (C) sea state and (D) for the joint smoothing of longitude and latitude (yellow represent the highest predictions and blue the lowest) and. The black lines on the x-axis represent the distribution of the data. (A) time of day is a continuous variable with a resolution in minutes and labels in hours. (B) lunar cycle and (C) sea state are categorical variables. The shaded area illustrates the confidence interval associated with the curve estimate.
4 Discussion
4.1 General considerations
To date, knowledge of the dynamics of delphinid foraging activity throughout the Mediterranean basin has been relatively patchy. Most studies analysing delphinid foraging rhythms have been carried out using fixed passive acoustics and have focused on specific Mediterranean subregions or even local sites (e.g. Sicily, Caruso et al., 2017; Ligurian Sea, Giorli et al., 2016). These studies showed a diel foraging activity pattern with a peak at night. However, in these settings, it can be challenging to discriminate true patterns in echolocation activity around the recording stations from local movements in and out of the detection radius of these stations. The present study provides, for the first time, information on the activity rhythms of delphinids investigated at a basin scale from a moving platform, in the western and central Mediterranean Sea. At such a large scale, we can assume that the abundance of dolphins in the study area does not change during a diel cycle. Therefore, any change in the detection rate of acoustic events on the hydrophone array is assumed to be primarily due to changes in the dolphin vocal behaviour in relation to their foraging activity rather than changes in their occurrence. This is an inherent benefit of using large spatial datasets to identify activity rhythms. The GAM results suggested that delphinids in the Mediterranean Sea exhibit a strong diel foraging pattern, as evidenced by a nocturnal predominance of acoustic events. The distribution of effort between day and night condition was fairly well balanced and cannot be a source of bias in the number of acoustic events per unit time. The lunar cycle and also had a clear influence on the number of acoustic events, with more events being detected during the third quarter of the moon.
Carrying out this study at the scale of the western and central Mediterranean provided an overall picture of delphinid activity in the area but required spatial variability and time zone to be considered. To do this, we transformed all UTC times into solar time so that the time zone did not bias the results (Carlucci et al., 2016) and we included a spatial effect in the model to smooth out the effect of the distribution of animals in the model. A very clear signal of the influence of selected temporal variables on the number of acoustic events was found as the uncertainties associated with the relationships obtained were very low (narrow confidence intervals, Figure 4), suggesting that the highlighted patterns were quite reliable.
However, the selected model only explained 14.3% of the deviance suggesting that processes other than those considered in this study could also contribute to explain the variation in the number of acoustic events. For example, tidal height or sea surface temperature were considered by Gauger et al. (2022) and found to influence the number of acoustic events. Benoit-Bird and Au (2003) analysed and compared the relative abundance and density of spinner dolphins (Stenella longirostris) and the mesopelagic community off three Hawaiian islands using a modified echo-sounder and showed that the dolphins followed the vertical dynamics of their prey. In this study, we were limited to using temporal variables that were available at all locations in a large study area, i.e. through available remote sensing datasets or oceanographic model outputs. This lack of direct data on prey behaviour throughout the study area necessitated the use of other parameters such as time of day or lunar cycle, thought to influence prey density and catchability at dolphin foraging depths. More detailed information on local delphinid prey activity and their catchability may have allowed for a more precise explanation of delphinid activity rhythms.
Grouping species which have distinct ecological and behavioural requirements (Cipriano et al., 2022) may also contribute to the relatively low deviance explained by the model. The exclusive use of echolocation clicks provides a solid description of delphinids foraging activity but clicks do not allow the precise identification of the species recorded. Here, delphinids were mainly represented by three species, the striped, bottlenose and common dolphins, which may have different diel activity patterns, with other species like the long-finned pilot whale, the Risso’s dolphin and the rough-toothed dolphin being extremely marginal in the data set (ACCOBAMS, 2021; Ollier et al., 2023). Striped dolphins, for example, typically live in productive waters deeper than 350 m off the continental shelf (Carlucci et al., 2016). They exploit mesopelagic fish, cephalopods, and planktonic crustaceans (Würtz and Marrale, 1993) and follow their vertical distribution and dynamic movements. The bottlenose dolphin is quite flexible in its requirements, with populations living in extreme coastal, estuarine or even lagoon habitats, while others live offshore in oceanic waters (Wursig and Wursig, 1979). Its diet is quite eclectic, with combinations of benthic, demersal and pelagic shelf species versus oceanic assemblages according to the habitats where it is found (e.g., Bearzi et al., 2009; Blanco et al., 2001; Neri et al., 2023; Queiros et al., 2018). The common dolphin occurs in both pelagic and neritic environments, often sharing the former with striped dolphins and the latter with bottlenose dolphins (Notarbartolo di Sciara and Tonay, 2021). The temporal patterns presented here result from a combination of the foraging strategies of these three species in approximate proportions of 80% striped dolphins, 10% bottlenose dolphins and 10% common dolphins (proportions derived from table 4 in Panigada et al., 2024). Hence, the present results might be considered as a fair image of striped dolphin behaviour, slightly blurred by limited inputs from the other two species. However, as we were not able to distinguish species from the click trains alone in this study, any assumptions regarding species identity should be treated with caution.
4.2 Activity rhythms of delphinids in the Mediterranean Sea
The results of this study, showing that Mediterranean delphinids have a very distinct diel rhythm of acoustic event production, mainly displayed processes related to the striped dolphins in offshore habitats (Panigada et al., 2024). The other species present did not contribute much to the overall picture and were likely merely contributing to the uncertainty around the observed general patterns. These variations in the number of acoustic events illustrated the activity or foraging strategy of the dolphins, which would vary according to the availability and type of prey (Benoit-Bird and Au, 2003).
The variable that contributed most to our model was the time of day of the nycthemeral cycle. Here, the variation in the number of acoustic events expresses a change in foraging activity, based on the availability of prey. The latter is shaped by depth distribution and density of prey. Dolphins assess the accessibility of prey, the potential encounter rate during foraging dives, and the associated costs in order to maximise their foraging success (MacArthur and Pianka, 1966). When prey is scarce or too deep, dolphins would stop foraging because it would not be profitable (Cascão et al., 2020), hence few acoustic events would be recorded. When prey is more abundant and accessible, dolphins would forage actively (MacArthur and Pianka, 1966). In the latter situation, it is also possible to imagine that when prey is most abundant less foraging effort would be necessary to meet calorific requirements, whereas when there is slightly less prey but still enough for foraging to be profitable, then foraging would be greater, hence acoustic events more numerous.
In our study, dolphins exhibited a higher number of acoustic events emitted during the night, suggesting a nocturnal peak in foraging activity. This is thought to occur in response to nocturnal changes in the dynamics and vertical distribution of cetacean prey (Thompson and Miller, 1990). The latter migrate vertically between the surface to feed at night and deeper layers to escape from predators during the day (Marohn et al., 2021). During the ascent of the DSL to the surface, the defence mechanisms of organisms, particularly small pelagic fish, are thought to be reduced; they would be present in less aggregated schools and would have a reduced individual swimming speeds (Zein et al., 2019). Furthermore, as night-time can lead to a loss of visual information and an increase in backscatter (Mass and Supin, 2018), the nocturnal increase in prey concentration near the surface would be advantageous for nocturnal air-breathing predators; this is the case for odontocetes, whose highly developed sonar allows them to detect prey regardless of light conditions.
To maximise foraging success while minimising dive duration, pelagic delphinids might be expected to concentrate their foraging activity at night, when the DSL get closer to the surface (Giorli et al., 2016). Maximum amounts of prey would then be available, easy to capture and accessible near the surface. Dolphins consume more oxygen per unit time than deep diving odontocetes which may consume less oxygen due to evolutionary adaptations (Tyack et al., 2006). As a result, delphinids deplete their oxygen reserves more quickly before reaching their aerobic diving limit. To maximise hunting time relative to vertical transit time, they would ideally limit their foraging depth and thus foraging at night may be more favourable to them. Simonis et al. (2017) have highlighted a similar diurnal pattern in certain delphinids, stating that foraging activity was mainly nocturnal, and that social behaviours tended to take place during the day.
The number of acoustic events were found to be higher in the north and west of the western and central Mediterranean subregions. According to Goffredo and Dubinsky (2013), the different Mediterranean subregions are characterised by different micro phytoplanktonic compositions, with diatoms dominating in the west, and coccolithophorids and dinoflagellates in the east. The Mediterranean basin would then be characterised by significant spatial variability in terms of primary production, with decreasing levels of primary productivity from west to east, and to a lesser extent from north to south. This gradient could be a cause for a lower availability of cetacean prey, resulting in a lower densities of cetaceans (see Cañadas et al., 2023 for an analysis of gradient in cetacean distribution observed during the ASI) and therefore fewer acoustic events in the eastern and southern parts of the basin.
Other parameters may influence the increase in acoustic events, but more indirectly, as they could affect the migration dynamics of the DSL. This is the case for the lunar cycle. The number of acoustic events was minimal on day 8, corresponding to the first quarter of the moon, and maximal during the third quarter (22st day of the lunar cycle). According to Ochoa and collaborators (2013), the extent of these vertical migrations would depend on the intensity of nocturnal versus diurnal illumination and would only be possible if light intensity fluctuates sufficiently during the diel cycle. During full moon nights, when nocturnal illumination is the highest, vertical migrations would be delayed or attenuated (Ochoa et al., 2013) and fish would therefore be less available near the surface. As a result, small cetaceans may reduce their use of acoustic foraging behaviour or switch to visual predation, resulting in fewer acoustic events. However, the results of this study are inconsistent with previous research that have observed minimal acoustic foraging activity during the full moon. Simonis et al. (2017) found that common dolphin echolocation activity in the Southern California Bight was the lowest during the full moon and the highest during the third quarter phase. They suggested that the brightness of the full moon delayed or shortened vertical migrations to avoid predators, causing predators to reduce foraging or switch to visual predation. During the third quarter, a prolonged period of darkness after sunset allows mesopelagic prey to migrate to the surface before the moon rises, making them more vulnerable and available to predators. In the Mediterranean Sea, Caruso et al. (2017) found no evidence of a relationship between echolocation activity and the lunar cycle in a pelagic area. These mixed results suggest that the response of small cetaceans to the lunar cycle is more complex than previously thought (Benoit-Bird et al., 2009; Caruso et al., 2017; Simonis et al., 2017; Shaff and Baird, 2021; Cohen et al., 2023). Therefore, in our study, lunar illumination alone cannot explain the variation in the rhythm of delphinid activity as maximum acoustic activity was found close to the third quarter of the moon and not at full moon. The mesopelagic community would be affected by the lunar cycle in a more complex way than just a direct effect of nocturnal light that would limit the DSL vertical migration and in cascade reduce nocturnal prey density in the surface layer at full moon. Indeed, detailed analyses of the DSL composition at night suggest that the main phyla constituting the DSL, i.e. fish, squids and crustaceans, and probably the main taxa constituting those, might display more complex variation in relation to the lunar cycle, drastically affecting prey availability for top predators (e.g. Battaglia et al., 2020). For example, blue-finned tuna (Thunnus thynnus) in the Strait of Messina show extensive changes to their prey composition during the lunar cycle, with mesopelagic fish being predominant during first quarter and full moon, mesopelagic squid after first and third quarters and other invertebrates at third quarter and new moon. If different prey types are available at different times of the lunar cycle, dolphins may use different foraging strategies, including variable echolocation rates.
Sea state was also selected in the best model. This variable may have an influence on cetacean sound activity. The number of acoustic events increased from Beaufort 4. However, it is difficult to interpret this trend due to the limited data coverage in these conditions. Changes could appear with significant ambient noise, whether natural or anthropogenic, as an adaptation strategy (Isojunno et al., 2022). For example, cetaceans may compensate for higher ambient noise by increasing the amplitude or shifting the frequency or temporal pattern of their acoustic signals (Isojunno et al., 2022). Elevated ambient noise may also affect the detection range of a hydrophone array. An independent measure of ambient noise may be useful in future surveys to explore whether dolphins adjust their sound activity to ambient noise.
4.3 Monitoring and conservation implications
Our results have important implications for the monitoring of small delphinid populations using passive towed acoustics. Highlighting the strong signal in the intensity of delphinid acoustic activity as a function of time may have implications for cetacean abundance estimation programmes. A uniform acoustic detection probability over the nycthemeral cycle cannot be used because the relationship between the number of acoustic events and the number of individuals would change with the activity of those individuals. Therefore, a variable cue rate should be included when estimating dolphin abundance in order to duly consider variation in acoustic detection probability with the time of day. This would apply to the analysis of data from towed passive acoustic surveys following a linear transect strategy. In contrast to stationary passive acoustic surveys using moored hydrophones, changes in dolphin activity on the one hand and small scale movements affecting local dolphin density within the detection range of the hydrophone on the other hand, determine together the number of acoustic detection per unit time and are hard to disentangle.
The existence of these activity rhythms may also have conservation implications. While fishing may occur day or night (Levy et al., 2015), depending on the diversity of fishing practices, increased foraging activity at night could make dolphins more vulnerable to fisheries bycatch as they would be more focused on foraging and less alert to environmental hazard (Todd et al., 2020). Anthropogenic sound sources in the same frequency bands as dolphins can have a masking effect on these signals (Marian et al., 2021) which can affect foraging success and, indirectly, the survival of the species.
5 Conclusion
This study is the first of its kind to be carried out across a large part of the Mediterranean Sea. It was conducted to explore the activity rhythms of Mediterranean delphinids, mostly represented by the striped dolphin in offshore habitats. A nycthemeral rhythm of echolocation activity was demonstrated. These dolphins showed a predominantly nocturnal foraging pattern, illustrated by much higher echolocation clicks at night. This rhythmicity of foraging activity was shown to be linked to temporal variables such as time of day, sea state and lunar cycle. This result is consistent with available knowledge on the DSL vertical migration. In the context of foraging ecology, the use of echolocation clicks by towed passive acoustics has proved to be highly relevant for identifying diel patterns in delphinid foraging activity.
Data availability statement
The original contributions presented in the study are included in the article/Supplementary Material. Further inquiries can be directed to the corresponding author.
Author contributions
MS: Data curation, Methodology, Formal analysis, Writing – original draft. CO: Data curation, Methodology, Formal analysis, Conceptualization, Writing – review & editing. OB: Writing, review & editing. VR: Conceptualization, Methodology, Writing – review & editing. AV: Conceptualization, Methodology, Formal analysis, Writing – review & editing.
Funding
The author(s) declare financial support was received for the research, authorship, and/or publication of this article. The authors thank ACCOBAMS for covering the publication fees. The authors thank the funding bodies that facilitated ASI: Mava Foundation, Prince Albert II Foundation, the Spanish Ministry of Agriculture, Fisheries, Food and Environmental Affairs, the French Agency for Biodiversity, the Italian Ministry for Environment and Protection of Land and Sea, the Principality of Monaco and the International Fund for Animal Welfare. ASI was implemented with contributions from all ACCOBAMS parties.
Acknowledgments
We would like to thank ACCOBAMS and their technical and financial partners for making this survey possible and providing the dataset collected during the vessel component of the ASI. In addition, we thank the ACCOBAMS Permanent Secretariat, the ASI Steering Committee, National Focal Points and the ASI Contact Group. We are also indebted to the relevant government bodies for providing permissions and/or logistical support in the field. We are extremely pleased to extend our acknowledgements to the team on board R/V Song of the Whale during the ASI, and all the observers who have contributed to collecting the data.
Conflict of interest
The authors declare that the research was conducted in the absence of any commercial or financial relationships that could be construed as a potential conflict of interest.
Publisher’s note
All claims expressed in this article are solely those of the authors and do not necessarily represent those of their affiliated organizations, or those of the publisher, the editors and the reviewers. Any product that may be evaluated in this article, or claim that may be made by its manufacturer, is not guaranteed or endorsed by the publisher.
Supplementary material
The Supplementary Material for this article can be found online at: https://www.frontiersin.org/articles/10.3389/fmars.2024.1378524/full#supplementary-material
References
ACCOBAMS (2021). Estimates of abundance and distribution of cetaceans, marine mega-fauna and marine litter in the Mediterranean Sea from 2018-2019 surveys. Eds. Panigada S., Boisseau O., Cañadas A., Lambert C., Laran S., McLanaghan R., Moscrop A. (Monaco: ACCOBAMS - ACCOBAMS Survey Initiative Project), 177.
Aïssi M., Arcangeli A., Crosti R., Daly Yahia M. N., Loussaief B., Moulins A., et al. (2015). Cetacean occurrence and spatial distribution in the central Mediterranean Sea using ferries as platform of observation. Russian J. Mar. Biol. 41, 343–350. doi: 10.1134/S1063074015050028
Akaike H. (1998). “Information theory and an extension of the maximum likelihood principle,” in Selected Papers of Hirotugu Akaike. Springer Series in Statistics. Eds. Parzen E., Tanabe K., Kitagawa G. (Springer, New York, NY), 199–213. doi: 10.1007/978-1-4612-1694-0_15
Au W. W. L. (1993). The Sonar of Dolphins (New York, NY: Springer Science & Business Media). Springer.
Au W. W. L. (2018). “Echolocation,” in Encyclopedia of Marine Mammals, 3rd ed. Eds. Wursig B., Thewissen J. G. M., Kovacs K. M. (Academic Press, London), 289–299. doi: 10.1016/C2015-0-00820-6
Azzolin M., Gannier A., Lammers M. O., Oswald J. N., Papale E., Buscaino G., et al. (2014). Combining whistle acoustic parameters to discriminate Mediterranean odontocetes during passive acoustic monitoring. J. Acoustical Soc. America 135, 502–512. doi: 10.1121/1.4845275
Barlow J., Cheeseman T., Trickey J. S. (2021). Acoustic detections of beaked whales, narrow-band high-frequency pulses and other odontocete cetaceans in the Southern Ocean using an autonomous towed hydrophone recorder. Deep Sea Res. Part II: Topical Stud. Oceanography 193, 104973. doi: 10.1016/j.dsr2.2021.104973
Bearzi G., Fortuna C., Reeves R. (2009). Ecology and conservation of common bottlenose dolphins Tursiops truncatus in the Mediterranean Sea. Mammal Rev. 39, 92. doi: 10.1111/j.1365-2907.2008.00133.x
Benoit-Bird K. J., Au W. W. (2003). Prey dynamics affect foraging by a pelagic predator (Stenella longirostris) over a range of spatial and temporal scales. Behav. Ecol. Sociobiology 53, 364–373. doi: 10.1007/s00265-003-0585-4
Benoit-Bird K. J., Dahood A. D., Würsig B. (2009). Using active acoustics to compare lunar effects on predator–prey behavior in two marine mammal species. Mar. Ecol. Prog. Ser. 395, 119–135. doi: 10.3354/meps07793
Bittle M., Duncan A. (2013). A review of current marine mammal detection and classification algorithms for use in automated passive acoustic monitoring. Proc. Acoustics 8, 1–8.
Boisseau O., Reid J., Ryan C., Moscrop A., McLanaghan R., Panigada S. (2024). Acoustic estimates of sperm whale abundance in the Mediterranean Sea as part of the ACCOBAMS Survey Initiative. Front. Mar. Sci. 11, 1164026. doi: 10.3389/fmars.2024.1164026
Brandt M. J., Hansen S., Diederichs A., Nehls G. (2014). Do man-made structures and water depth affect the diel rhythms in click recordings of harbor porpoises (Phocoena phocoena)? Mar. Mammal Sci. 30, 1109–1121. doi: 10.1111/mms.12112
Brauer K., Sendatzki R., Proyer R. T. (2022). A primer on studying effects of relationship duration in dyadic research: Contrasting cross-sectional and longitudinal approaches. J. Soc. Pers. Relat. 39, 2117–2133. doi: 10.1177/02654075221074677
Buckland S. T., Anderson D. R., Burnham K. P., Laake J. L., Borchers D. L., Thomas L. (2004). Advanced distance sampling: estimating abundance of biological populations (Oxford: Oxford University Press). doi: 10.1093/oso/9780198507833.001.0001
Cañadas A., Pierantonio N., Araújo H., David L., Di Meglio N., Dorémus G., et al. (2023). Distribution patterns of marine megafauna density in the Mediterranean Sea assessed through the ACCOBAMS Survey Initiative (ASI). Front. Mar. Sci. 10. doi: 10.3389/fmars.2023.1270917
Carlucci R., Fanizza C., Cipriano G., Paoli C., Russo T., Vassallo P. (2016). Modeling the spatial distribution of the striped dolphin (Stenella coeruleoalba) and common bottlenose dolphin (Tursiops truncatus) in the Gulf of Taranto (Northern Ionian Sea, Central-eastern Mediterranean Sea). Ecol. Indic. 69, 707–721. doi: 10.1016/j.ecolind.2016.05.035
Caruso F., Alonge G., Bellia G., De Domenico E., Grammauta R., Larosa G., et al. (2017). Long-term monitoring of dolphin biosonar activity in deep pelagic waters of the Mediterranean Sea. Sci. Rep. 7, 4321. doi: 10.1038/s41598-017-04608-6
Cascão I., Lammers M. O., Prieto R., Santos R. S., Silva M. A. (2020). Temporal patterns in acoustic presence and foraging activity of oceanic dolphins at seamounts in the Azores. Sci. Rep. 10, 3610. doi: 10.1038/s41598-020-60441-4
Cipriano G., Carlucci R., Bellomo S., Santacesaria F. C., Fanizza C., Ricci P., et al. (2022). Behavioral pattern of Risso’s dolphin (Grampus griseus) in the gulf of Taranto (Northern Ionian Sea, central-eastern Mediterranean sea). J. Mar. Sci. Eng. 10, 175. doi: 10.3390/jmse10020175
Cohen R. E., Frasier K. E., Baumann-Pickering S., Hildebrand J. A. (2023). Spatial and temporal separation of toothed whales in the western North Atlantic. Mar. Ecol. Prog. Ser. 720, 1–24. doi: 10.3354/meps14396
Cucknell A. C., Frantzis A., Boisseau O., Romagosa M., Ryan C., Tonay A. M., et al. (2016). Harbour porpoises in the Aegean Sea, Eastern Mediterranean: the species’ presence is confirmed. Mar. Biodiversity Records 9, 1–13. doi: 10.1186/s41200-016-0050-5
Foster S. D., Bravington M. V. (2013). A poisson-gamm model for analysis of ecological non-negative continuous data. Environ. Ecol. Stat 20, 533–552. doi: 10.1007/s10651-012-0233-0
Gauger M. F., Romero-Vivas E., Peck M. A., Balart E. F., Caraveo-Patiño J. (2022). Seasonal and diel influences on bottlenose dolphin acoustic detection determined by whistles in a coastal lagoon in the southwestern Gulf of California. PeerJ 10, e13246. doi: 10.7717/peerj.13246
Gillespie D., Mellinger D. K., Gordon J., Mclaren D., Redmond P. A. U. L., McHugh R., et al. (2008). PAMGuard: Semiautomated, open source software for real-time acoustic detection and localisation of cetaceans. J. Acoustical Soc. America 30, 54–62. doi: 10.1121/1.4808713
Giorli G., Au W. W., Neuheimer A. (2016). Differences in foraging activity of deep sea diving odontocetes in the Ligurian Sea as determined by passive acoustic recorders. Deep Sea Res. Part I: Oceanographic Res. Papers 107, 1–8. doi: 10.1016/j.dsr.2015.10.002
Goffredo S., Dubinsky Z. (2013). The Mediterranean Sea: Its history and present challenges. Springer Sci. Business Media. doi: 10.1007/978-94-007-6704-1_12
González-Hernández F. R., Sánchez-Fernández L. P., Suárez-Guerra S., Sánchez-Pérez L. A. (2017). Marine mammal sound classification based on a parallel recognition model and octave analysis. Appl. Acoustics 119, 17–28. doi: 10.1016/j.apacoust.2016.11.016
Häfker N. S., Andreatta G., Manzotti A., Falciatore A., Raible F., Tessmar-Raible K. (2023). Rhythms and clocks in marine organisms. Annu. Rev. Mar. Sci. 15, 509–538. doi: 10.1146/annurev-marine-030422-113038
Hastie G., Wilson B., Thompson P. (2004). Functional mechanisms underlying cetacean distribution patterns: hotspots for bottlenose dolphins are linked to foraging. Mar. Biol. 144, 397–403. doi: 10.1007/s00227-003-1195-4
Hildebrand J. A. (2009). Anthropogenic and natural sources of ambient noise in the ocean. Mar. Ecol. Prog. Ser. 395, 5–20. doi: 10.3354/meps08353
Isojunno S., von Benda-Beckmann A. M., Wensveen P. J., Kvadsheim P. H., Lam F. P. A., Gkikopoulou K. C., et al. (2022). Sperm whales exhibit variation in echolocation tactics with depth and sea state but not naval sonar exposures. Mar. Mammal Sci. 38, 682–704. doi: 10.1111/mms.12890
Lazaridis E.. (2022). lunar: Lunar Phase & Distance, Seasons and Other Environmental Factors. R package version 0.2-01.
Lazzari P., Solidoro C., Ibello V., Salon S., Teruzzi A., Béranger K., et al. (2012). Seasonal and inter-annual variability of plankton chlorophyll and primary production in the Mediterranean Sea: a modelling approach. Biogeosciences 9, 217–233. doi: 10.5194/bg-9-217-2012
Levy Y., Frid O., Weinberger A., Sade R., Adam Y., Kandanyan U., et al. (2015). A small fishery with a high impact on sea turtle populations in the eastern Mediterranean. Zoology Middle East 61, 300–317. doi: 10.1080/09397140.2015.1101906
Linnenschmidt M., Teilmann J., Akamatsu T., Dietz R., Miller L. A. (2013). Biosonar, dive, and foraging activity of satellite tracked harbor porpoises (Phocoena phocoena). Mar. Mammal Sci. 29, E77–E97. doi: 10.1111/j.1748-7692.2012.00592.x
MacArthur R. H., Pianka E. R. (1966). On optimal use of a patchy environment. Am. Nat. 100, 603–609. doi: 10.1086/282454
Madsen P. T., Wahlberg M. (2007). Recording and quantification of ultrasonic echolocation clicks from free-ranging toothed whales. Deep sea Res. Part I: oceanographic Res. papers 54, 1421–1444. doi: 10.1016/j.dsr.2007.04.020
Marian A. D., Monczak A., Balmer B. C., Hart L. B., Soueidan J., Montie E. W. (2021). Long- term passive acoustics to assess spatial and temporal vocalization patterns of Atlantic common bottlenose dolphins (Tursiops truncatus) in the May River estuary, South Carolina. Mar. Mammal Sci. 37, 1060–1084. doi: 10.1111/mms.12800
Marohn L., Schaber M., Freese M., Pohlmann J. D., Wysujack K., Czudaj S., et al. (2021). Distribution and diel vertical migration of mesopelagic fishes in the Southern Sargasso Sea— observations through hydroacoustics and stratified catches. Mar. Biodiversity 51, 1–24. doi: 10.1007/s12526-021-01216-6
Mass A. L., Supin A. Y. (2018). “Vision,” in Encyclopedia of Marine Mammals, 3rd ed. Eds. Wursig B., Thewissen J. G. M., Kovacs K. M. (Academic Press, London), 1035–1044. doi: 10.1016/C2015-0-00820-6
Miller P. J. O. M., Shapiro A. D., Deecke V. B. (2010). The diving behaviour of mammal-eating killer whales (Orcinus orca): variations with ecological not physiological factors. Can. J. Zoology 88, 1103–1112. doi: 10.1139/Z10-080
Millot C., Taupier-Letage I. (2005). Circulation in the Mediterranean sea. Mediterr. Sea 5K, 29–66. doi: 10.1007/b107143
Notarbartolo di Sciara G., Tonay A. (2021). Conserving whales, dolphins and porpoises in the 891 Mediterranean and Black Seas (Monaco: An ACCOBAMS status report-ACCOBAMS).
Ochoa J., Maske H., Sheinbaumc J., Candela J. (2013). Diel and lunar cycles of vertical migration extending to below 1000 m in the ocean and the vertical connectivity of depth-tiered populations. Limnology Oceanography 58, 1207–1214. doi: 10.4319/lo.2013.58.4.1207
Ollier C., Sinn I., Boisseau O., Ridoux V., Virgili A. (2023). Matching visual and acoustic events to estimate detection probability for small cetaceans in the ACCOBAMS Survey Initiative. Front. Mar. Sci. 10. doi: 10.3389/fmars.2023.1244474
Pace D. S., Tizzi R., Mussi B. (2015). Cetaceans value and conservation in the Mediterranean Sea. J. Biodivers. Endanger. Species S1, S1.004. doi: 10.4172/2332-2543.S1.004
Panigada S., Pierantonio N., Araújo H., David L., Di Meglio N., Dorémus G., et al. (2024). The ACCOBAMS Survey Initiative: the first synoptic assessment of cetacean abundance in the Mediterranean Sea through aerial surveys. Front. Mar. Sci. 10, 1270513. doi: 10.3389/fmars.2023.1270513
Pirotta E., Brotons J. M., Cerdà M., Bakkers S., Rendell L. E. (2020). Multi-scale analysis reveals changing distribution patterns and the influence of social structure on the habitat use of an endangered marine predator, the sperm whale Physeter macrocephalus in the Western Mediterranean Sea. Deep Sea Res. Part I: Oceanographic Res. Papers 155, 103169. doi: 10.1016/j.dsr.2019.103169
Prieto R., Tobeña M., Silva M. A. (2017). Habitat preferences of baleen whales in a mid-latitude habitat. Deep Sea Res. Part II: Topical Stud. Oceanography 141, 155–167. doi: 10.1016/j.dsr2.2016.07.015
R Core Team. (2022). R: A language and environment for statistical computing (Vienna: R Foundation for Statistical Computing). Available at: https://www.R-project.org/.
Robinson A. R., Leslie W. G., Theocharis A., Lascaratos A. (2001). Mediterranean sea circulation. Ocean currents 1, 710–725. doi: 10.1006/rwos.2001.0376
Scales K. L., Miller P., Ingram S. N., Hazen E. L., Bograd S. J., Richard A. (2016). Identifying predictable foraging habitats for a wide-ranging marine predator using ensemble ecological niche models. Diversity Distributions 22, 212–224. doi: 10.1111/ddi.12389
Shaff J. F., Baird R. W. (2021). Diel and lunar variation in diving behavior of rough-toothed dolphins (Steno bredanensis) off Kauaʻi, Hawaiʻi. Mar. Mammal Sci. 37, 1261–1276. doi: 10.1111/mms.12811
Simonis A. E., Roch M. A., Bailey B., Barlow J., Clemesha R. E., Iacobellis S., et al. (2017). Lunar cycles affect common dolphin Delphinus delphis foraging in the Southern California Bight. Mar. Ecol. Prog. Ser. 577, 221–235. doi: 10.3354/meps12247
Symonds M. R., Moussalli A. (2011). A brief guide to model selection, multimodel inference and model averaging in behavioural ecology using Akaike’s information criterion. Behav. Ecol. sociobiology 65, 13–21. doi: 10.1007/s00265-010-1037-6
Thompson P. M., Miller D. (1990). Summer foraging activity and movements of radio-tagged common seals (Phoca vitulina) in the Moray Firth, Scotland. J. Appl. Ecol. 27, 492–501. doi: 10.2307/2404296
Todd N. R., Cronin M., Luck C., Bennison A., Jessopp M., Kavanagh A. S. (2020). Using passive acoustic monitoring to investigate the occurrence of cetaceans in a protected marine area in northwest Ireland. Estuarine Coast. Shelf Sci. 232, 106509. doi: 10.1016/j.ecss.2019.106509
Tyack P. L., Johnson M., Soto N. A., Sturlese A., Madsen P. T. (2006). Extreme diving of beaked whales. J. Exp. Biol. 209, 4238–4253. doi: 10.1242/jeb.02505
Wang Z. T., Nachtigall P. E., Akamatsu T., Wang K. X., Wu Y. P., Liu J. C., et al. (2015). Passive acoustic monitoring the diel, lunar, seasonal and tidal patterns in the biosonar activity of the Indo-Pacific humpback dolphins (Sousa chinensis) in the Pearl River Estuary, China. PloS One 10, e0141807. doi: 10.1371/journal.pone.0141807
Wang X., Zhang J., Zhao X., Chen Z., Ying Y., Li Z., et al. (2019). Vertical distribution and diel migration of mesopelagic fishes on the northern slope of the South China Sea. Deep Sea Res. Part II: Topical Stud. Oceanography 167, 128–141. doi: 10.1016/j.dsr2.2019.05.009
Wood S. N. (2006). On confidence intervals for generalized additive models based on penalized regression splines. Aust. New Z. J. Stat 48, 445–464. doi: 10.1111/j.1467-842X.2006.00450.x
Wood S. N. (2017). Generalized Additive Models: An Introduction with R. 2nd edition (Chapman and Hall/CRC). doi: 10.1201/9781315370279
Wursig B., Wursig M. (1979). Behavior and ecology of the bottlenose dolphin (Tursiops truncatus) in the South Atlantic. Fishery Bull. 77, 399–412.
Würtz M., Marrale D. (1993). Food of striped dolphin, Stenella coeruleoalba, in the Ligurian Sea. J. Mar. Biol. Assoc. United Kingdom 73, 571–578. doi: 10.1017/S0025315400033117
Keywords: foraging activity rhythms, Generalised Additive Models (GAMs), delphinids, echolocation clicks, Mediterranean Sea
Citation: Sol M, Ollier C, Boisseau O, Ridoux V and Virgili A (2024) Temporal patterns in dolphin foraging activity in the Mediterranean Sea: insights from vocalisations recorded during the ACCOBAMS Survey Initiative. Front. Mar. Sci. 11:1378524. doi: 10.3389/fmars.2024.1378524
Received: 29 January 2024; Accepted: 11 July 2024;
Published: 06 August 2024.
Edited by:
Xuelei Zhang, Ministry of Natural Resources, ChinaReviewed by:
Franciele Rezende De Castro, Instituto Aqualie, BrazilLiangliang Yang, Shantou University, China
Copyright © 2024 Sol, Ollier, Boisseau, Ridoux and Virgili. This is an open-access article distributed under the terms of the Creative Commons Attribution License (CC BY). The use, distribution or reproduction in other forums is permitted, provided the original author(s) and the copyright owner(s) are credited and that the original publication in this journal is cited, in accordance with accepted academic practice. No use, distribution or reproduction is permitted which does not comply with these terms.
*Correspondence: Morgane Sol, bS5zb2wxM0Bob3RtYWlsLmZy