- 1Cooperative Institute for Climate, Ocean, and Ecosystem Studies, University of Washington, Seattle, WA, United States
- 2Marine Mammal Laboratory, Alaska Fisheries Science Center, NOAA Fisheries, Seattle, WA, United States
- 3Protected Resources Division, NOAA, National Marine Fisheries Service, Anchorage, AK, United States
- 4Joint Base Elmendorf Richardson, US Air Force Conservation Department, Anchorage, AK, United States
- 5College of Business and Information Systems, Dakota State University, Madison, SD, United States
- 6School of Aquatic and Fishery Sciences, University of Washington, Seattle, WA, United States
Cook Inlet beluga (CIB), Delphinapterus leucas, have not recovered from subsistence overharvest despite conservation efforts initiated in 2000. Reasons for this lack of recovery are still unclear with anthropogenic noise identified as a high threat in this population’s recovery plan. Baseline information on CIB habitat use and soundscape characterization is crucial in evaluating impacts from anthropogenic activities. Using passive acoustic monitoring, we documented the seasonality and foraging occurrence of CIB, together with killer whale (Orcinus orca), harbor porpoise (Phocoena phocoena) and humpback whale (Megaptera novaeangliae), for one year in Chinitna and Tuxedni bays and rivers, two historically important but recently overlooked areas of their critical habitat in western lower Cook Inlet, Alaska. This area’s ecological interest converges with planned mining, marine renewable energy, offshore oil and gas production, and commercial shipping. Results revealed higher presence of harbor porpoise, killer whale, and humpback whale in Chinitna compared to Tuxedni but much lower CIB presence. CIB were not detected in Chinitna Bay but were in the river on 21 days September-February and one day in June. However, CIB were never detected feeding in Chinitna River. CIB acoustic crypsis and preferential use of very shallow habitat in Chinitna revealed a perceived predation risk from killer whales. In contrast, CIB were recorded foraging in both Tuxedni Bay and River and were detected September-April on 127 days making it an important winter foraging ground. Low levels of anthropogenic noise disturbance were quantified in both bays making them some of the most undisturbed sections of their critical habitat, but at the same time highly sensitive to further noise disturbance. Commercial shipping was the main noise source likely causing acoustic disturbance to CIB due to communication masking despite current low levels of temporal overlap with beluga presence. We recommend that applicable regulators consider restricting high sound producing anthropogenic activities in and adjacent to Tuxedni Bay from September 1st to May 15th when CIB are actively using this area. Managing future anthropogenic activities to minimize habitat degradation and acoustic disturbance in this winter foraging refuge will be a key component to support the recovery of this endangered population.
1 Introduction
Cook Inlet beluga whales (CIB, Delphinapterus leucas), which are year-round residents of Cook Inlet, Alaska, were listed as endangered in 2008 under the Endangered Species Act (ESA) (USFWS, 1973) following a major decline in abundance (~50%) in the 1990s associated with overhunting (NMFS, 2016). The best historical abundance estimate of the CIB population is 1,293 belugas from a survey in 1979 (Calkins, 1989). Subsistence hunting in Cook Inlet completely ceased in 2005 (Mahoney and Shelden, 2000; Shelden et al., 2021) and the assumption was the population would start recovering, but CIB numbers have continued to decrease until very recently. The latest population estimate from 2022 indicates there are only 331 animals left, but the 10-year trend suggests the population is stable at these low levels (Goetz et al., 2023).
Following the ESA listing, critical habitat was designated for the CIB population in 2011 (NMFS, 2011). In 2016, NMFS released a Recovery Plan with 64 recovery actions (NMFS, 2016) but despite over a decade of dedicated conservation and research efforts, the CIB population has failed to recover and the reason(s) why remain unknown. The NMFS Recovery Plan identifies ten potential threats to recovery, with three categorized as high relative concern threats: catastrophic events, cumulative effects of multiple stressors, and noise.
From late spring through early fall, nearly the entire CIB population is now found predominantly in upper Cook Inlet (UCI) with their historic range contracting north from lower Cook Inlet (LCI) as their numbers declined (Shelden et al., 2018). Most of the recent work on CIB has thus focused in UCI with much less known about the contemporary use of LCI by CIB in the winter. This is compounded by limited knowledge of the whales’ distribution outside of summer because of the combination of poor sighting conditions (e.g., sea ice, minimal daylight hours) and whale behavior (e.g., offshore, more dispersal, longer dives) making visual detection difficult (Shelden and Hobbs, 2015). Limited satellite telemetry data from 1999–2003 showed winter use of the mid and LCI (Hobbs et al., 2005; Shelden et al., 2018). This was also the case from 2018–2023 when NMFS flew aerial distribution surveys for CIB in the winter (V. Gill, unpublished) and found belugas utilizing LCI from Tuxedni Bay and the Kasilof River north to the UCI. The only consistent winter sampling effort in LCI in the recent past has been passive acoustic monitoring efforts (Castellote et al., 2020) demonstrating seasonal presence of CIB in areas of mid and LCI.
The west coast of LCI is remote, roadless, and relatively pristine compared to the east coast of LCI. This region supports a rich marine ecosystem (Abookire and Piatt, 2005; Arimitsu et al., 2021) with large salmon runs (Hollowell et al., 2015), important seabird colonies and shorebird roosts (Speckman et al., 2005; Ruthrauff et al., 2013), and many species of baleen and toothed cetaceans (Young et al., 2023). Two of the most productive bays along the west coast of LCI are Tuxedni and Chinitna along with the salmon-producing rivers that flow into them. Prior to the early 1990s, both Chinitna and Tuxedni Bays were important habitat for CIB (Rugh et al., 2000) but sightings have been uncommon in these areas as the population declined and contracted its distribution into UCI (Rugh et al., 2010; Shelden et al., 2016). Consequently, research focus moved to UCI.
Although the west side of LCI is relatively undisturbed, there are still threats from large-scale exploration and development. Big mining projects are proposed in this area that could have impacts in marine waters (USACE, 2020; Brown et al., 2022). Both Tuxedni and Chinitna bays directly adjoin the Federal Outer Continental Shelf (OCS) waters of the Bureau of Ocean Energy Management (BOEM) Cook Inlet Planning Area (CIPL). As a result, offshore oil & gas exploratory surveys have been completed in recent years (Hilcorp Alaska, 2018; NOAA, 2019) and, despite mitigation measures for the near-distance take of marine mammals, caused unavoidable disturbance (Gordon et al., 2003; Kavanagh et al., 2019). Further, BOEM is now also exploring the possibility of renewable energy in the OCS waters of LCI which represents the primary option for offshore wind and tidal energy development in Alaska due to its proximity to the Alaska’s Railbelt Grid (Meadows et al., 2023). Whether renewable or non-renewable energy, further exploration and development is expected to occur in LCI that may adversely impact CIB habitat.
Securing year-round baseline information on soundscape, marine mammal occurrence, and habitat use is crucial in evaluating the level of impacts new developments will have on cetaceans in LCI. Using passive acoustic monitoring methods, we documented the seasonal distribution and foraging occurrence of CIB, and the seasonality of harbor porpoise (Phocoena phocoena), killer whale (Orcinus orca), and humpback whale (Megaptera novaeangliae) in two main bays and rivers (Tuxedni and Chinitna) on the west coast of LCI for one year (September 2021–2022). We also characterized the occurrence and levels of anthropogenic noise sources that have the potential to disturb CIB in these areas.
2 Methodology
2.1 Study area
Tuxedni Bay and Chinitna Bay are fjord estuaries located on the west coast of LCI (Figure 1) in CIB critical habitat, and they are characterized by brackish waters, high turbidity, ice presence in winter, and shallow depths (<60m). Both estuaries have several rivers flowing into them, but their main fresh water inflow is generated at the bay-head by a glacial river under the same name as each of the bays. Both bays are surrounded by Lake Clark National Park and Preserve and the Chigmit Mountains of the Alaska Range. Two islands, Chisik and Duck, at the mouth of Tuxedni Bay, are part of the Alaska Maritime National Wildlife Refuge and have been afforded protection in some form since 1909.
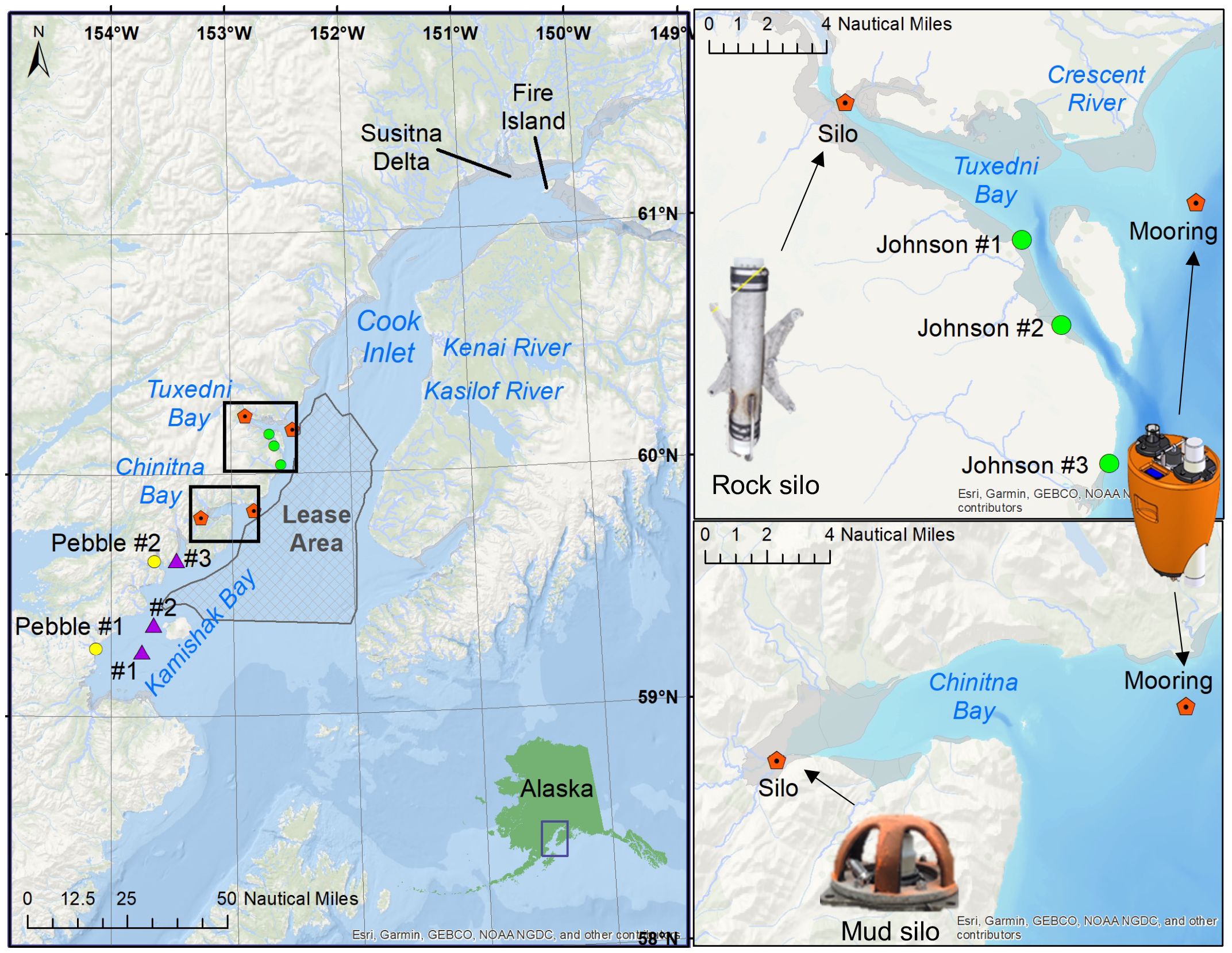
Figure 1 Left panel – Map of Cook Inlet, AK, depicting the locations of the different industrial activities around Chinitna Bay and Tuxedni Bay: BOEM Cook Inlet Planning Area (grey hatched polygon), Johnson Tract Mine proposed ore terminals (green circles) in Tuxedni Bay, and Pebble Mine proposed port facilities (yellow circles) and lightering stations (purple triangles) in Kamishak Bay. Right panels – Location of the mooring and silos in Tuxedni Bay (upper panel), and Chinitna Bay (lower panel), in lower Cook Inlet, AK.
2.2 Instrument deployment
Two types of instrument deployment methods were used in this study: low-profile moorings (Castellote et al., 2016, Castellote et al., 2020) and silos (Polasek et al., 2021). Moorings include two co-located acoustic instruments: an acoustic recorder (ST-600, Ocean Acoustics NZ) to sample the ambient noise and record marine mammal social signals, and an echolocation logger (F-POD, Chelonia ltd.) to detect odontocete echolocation, including feeding buzzes. Both instruments are contained in a syntactic foam mooring package with an acoustical release (Vemco AR-2, Innovasea Systems Inc.) for instrument recovery. Silos are single or dual instrument housings (for ST-600, F-POD, or both) made of stainless steel and a low acoustic impedance plastic (ABS) guard to protect the instrument and hydrophone from ice and vegetation debris. Silos are inserted in a pre-drilled slot in hard sediment, or bolted to rocks, at the lower end of the inter-tidal zone during a strong low tide. Silo stations also included co-located ST-600 and F-PODs. This approach allowed sampling extremely shallow areas near the shoreline or riverbanks typically visited by CIB. All moorings and silos included a temperature and pressure sensor (HOBO U-20, Onset Corp.) to investigate the influence of tidal depth on CIB presence. One temperature and pressure sensor was also attached to a tree next to the silo locations to sample the barometric pressure and was used to calculate water depth. For this study, one mooring was deployed at the entrance of each bay along with a silo station at the end of the bay within the tidally influenced reaches of each river. The moorings were deployed at a depth of 18 m at mean lower low water to prevent interaction with sea ice and toward the north side of the bay entrances because CIB access was expected to occur from this direction. River bank mud-drilled silos where used in Chinitna River and a dual rock silo was used in Tuxedni River. For the winter period (September 2021 to May 2022), an additional mud silo and single rock silo were deployed in these stations respectively, each housing a second F-POD programmed with a 4-month delayed start. This second set of instrumentation enabled continuous sampling throughout the entire deployment period as they were configured to begin sampling when the initial set of instruments exhausted their power supply or memory storage capacity.
The ST-600 sound recorder was programed to sample on a duty cycle of 5 minutes on and 10 minutes off with a sampling rate of 48 kHz, which encompasses beluga social signals (i.e., calls and whistles), other marine mammal signals, and environmental noise of both natural and anthropogenic origin. The F-POD echolocation logger records snippets of sound and acoustic parameters of detections while processing the incoming signal in real time on a continuous basis to log the occurrence of high frequency short impulsive signals in the range 40 to 160 kHz, typically used by odontocetes for echolocation purposes. Moorings and silos were serviced in the spring and fall by boat and helicopter to maintain a continued sampling for the period of September 2021 to September 2022.
2.3 Data analysis
2.3.1 Echolocation detection
Echolocation data were analyzed with the dedicated software F-POD.exe (v 4.5.2023), following Castellote et al. (2016), where all algorithm-based (KERNO-F classifier v1.0, see Ivanchikova and Tregenza (2023)) echolocation detections are manually validated to exclude false detections or correct misclassified detections. The current F-POD algorithms only classify delphinid vs. narrowband high frequency (NBHF) click trains. In this study area, delphinid detections were assigned to beluga or killer whale click trains, and NBHF to harbor porpoise click trains as no other odontocete species are present. Even though Dall’s porpoises (Phocoenoides dalli) are observed in LCI and Kachemak Bay, they tend to prefer waters greater than 180 m in depth, and only harbor porpoises have been reported in Chinitna Bay and Tuxedni Bay from decades of aerial surveys (Shelden et al., 2014).
2.3.2 Beluga feeding behavior detection
F-POD full-train detail exports were used to identify beluga feeding events based on a combination of the inter-click interval (ICI) of click trains and the increment range of these intervals throughout the click train, as described in Castellote et al. (2020). Specifically, buzzes with minimum ICI <8.98 ms and consistently decreasing ICI trend (ICI increment range <1.49 ms) were classed as terminal buzzes related to prey capture. CIB feeding events were summarized in positive minutes/day (any minute with at least one terminal buzz).
2.3.3 Beluga social signals detection
We implemented a machine learning ensemble model as proposed by Zhong et al. (2020) for CIB vocalizations. The ensemble combines the results of four pre-trained convolutional neural networks (CNN): AlexNet, VGG16, ResNet50, and DenseNet, each generating a predictive numeric score for each 2 s of data. The scoring ranged from 0 (no resemblance to pre-trained beluga social signals) to 1 (full resemblance). For each of the four datasets obtained in this study (mooring or silo from Tuxedni or Chinitna), a manual scoring verification of a subsample of the 2 s scorings used a custom built application that allowed us to visualize each 2 s window in a spectrogram and aurally review the corresponding 2 s audio clip, as well as a 10 s window to assess context, and assign a label or make corrections to the classification as needed. The subsample consisted of a selection of 500 events (or as many as available if less than 500) for each scoring from 0.5 to 1 on a one decimal step. The verification aimed at identifying a threshold score that would keep beluga false positive rate at or below 2.5%. All scorings at or above the selected threshold were manually validated.
2.3.4 Anthropogenic noise analysis
Ambient noise was analyzed following Castellote et al. (2019) to characterize the occurrence and contribution of the different identifiable anthropogenic sources. Recordings were processed using the Triton package v. 2021 (https://www.cetus.ucsd.edu/technologies_triton.html) within the MATLAB software (MathWorks Inc., Natick, MA), generating long-term spectral averages (LTSA) with an averaging scale of 5 s and 10 Hz. LTSAs are spectrograms with each time slice typically covering a window of several seconds (5 s for analysis here) that consist of an average of many, non-overlapping, fast Fourier transforms (FFTs). FFTs were calculated using Welch’s method (Welch, 1967) with Hanning windows. This approach allows highlighting periods and frequencies when noise departs from the average values, typically caused by transient signals (Wiggins and Hildebrand, 2007). These signals were evaluated visually and aurally by generating non-averaged spectrograms to identify the source. The Triton module (remora) Logger (Wiggins et al., 2007) was used to log the start and end date and times of each classed event. The remora Soundscape-Metrics (https://github.com/MarineBioAcousticsRC/Triton/tree/master/Remoras/Soundscape-Metrics) was used to obtain one-minute average broadband (20 Hz–24 kHz) sound pressure levels (SPL) and one-third octave levels (TOL) ranging from the nominal center frequencies of 25 Hz to 20 kHz. SPLs and TOLs were computed for all anthropogenic noise events that lasted at least one minute, and results were summarized by noise class and study site for number of events, events per day, duration, and percent of time. In this study, noise from commercial shipping is distinguished from service diesel generator noise, even if generators are within commercial vessels, these two noise classes can be distinguished by the spectral characteristics of their sound (Arveson and Vendittis, 2000). Generator noise is masked by other more predominant sources of noise in modern commercial vessels at typical transit speeds, therefore, generator noise is typically detected when vessels are moving slowly, in idle mode, or anchored. It should also be noted that smaller, non-commercial vessels might also radiate noise from generators (Malinowski and Gloza, 2002).
2.3.5 Consolidating cetacean detections
The anthropogenic noise analysis via LTSA allowed a thorough review of all the sampled periods for cetacean social signals including beluga, humpback whale, and killer whale. All species detections were labeled during that analysis process. Calls from these species were also identified during the manual validation of the CNN ensemble output. Therefore, results from all methods (LTSA and CNN ensemble for beluga, humpback whale, and killer whale, and also F-POD for beluga) were combined into a database framework to summarize the presence and absence of each species in non-duplicated detection positive hours per day (DPH, any hour with at least one detection from either analysis method).
2.3.6 Anthropogenic noise disturbance analysis
In order to evaluate whether the identified anthropogenic noise sources have the potential to interfere with beluga communication, three TOL metrics were calculated from the anthropogenic noise events per location to compare to beluga hearing thresholds: the median (50th percentile), the maximum (event of highest SPL), and the maximum composite (maximum level for each third octave band from all events of each class). Following Brewer et al. (2023), results were graphically represented to evaluate the level of noise exceeding hearing thresholds, (i.e., the level of beluga auditory masking by anthropogenic noise). TOL from background noise in quiet conditions were also represented graphically to provide context for the evaluation of anthropogenic noise contribution to the soundscape of each bay, as well as define when beluga hearing is limited by the natural background noise within its habitat. Beluga hearing data consists of minimum TOL thresholds over all individuals tested both with in-water auditory evoked potentials (AEP) and behavioral methods (Castellote et al., 2014; Erbe et al., 2016; Mooney et al., 2018) including a CIB calf (Mooney et al., 2020). A total of 50 one-minute periods of quiet conditions were arbitrarily selected through all months of the sampling period for each bay based on the characteristics observed in the LTSAs. In particular, the selection criteria considered: no anthropogenic sources discerned in the 20 Hz – 24 kHz spectrogram, low sea state (i.e., no discernable breaking wave noise), absence of rain, ice, or biological transient signals, and no flow noise caused by high current periods. The same methods used in anthropogenic noise events were applied here to compute one-minute average TOLs and SPLs representing these quiet periods.
A second approach to assess the potential for noise disturbance was by quantifying the amount of temporal overlap between CIB acoustic presence and anthropogenic noise occurrence. CIB detections were grouped into acoustic encounters (i.e., Lammers et al., 2013). CIB detections separated by 60 minutes or more were logged as separate encounters. The rational for this grouping is to account for the time period when belugas are present but in silent mode in between detections. Encounter start and end periods were compared to the start and end times of anthropogenic noise events, and the level of temporal overlap was quantified for each CIB encounter.
2.3.7 Beluga relationship with tides
Data from the temperature and pressure sensors was processed with HOBOware Pro software (v. 3.7.25 Onset Computer Corp.) using the Barometric Compensation Assistant. This module uses water pressure data and compensates it with barometric pressure to create a water depth series.
Because pressure sampling was conducted at intervals between 10 and 20 minutes to ensure sampling throughout the deployment period at silos and moorings, data were linearly interpolated to approximate a depth value every minute. A rolling average of 30 minutes of data was used to assign an increasing (flooding) or decreasing (ebbing) tidal trend for each minute. High and low tidal states were identified by inflections in the trend. Slack periods were defined as the 22 minutes before and after these inflection points. The 45-minute slack period duration was chosen based on visual inspection of depth data from the sampled sites.
Minute-level CIB acoustic detections were associated with the corresponding depth and tidal trend (flooding, ebbing, high, low) and summarized graphically. A contingency table and χ2 test for independence was applied to evaluate whether CIB acoustic detections were randomly distributed across the 4 tidal periods for each sampled site.
3 Results
3.1 Gaps in sampled periods
Initial instrument deployments were completed in August and September 2021 for silos and moorings, respectively. Servicing of all instruments was completed in May 2022, and a final recovery in September and October 2022 for moorings and silos, respectively. The project suffered considerable data gaps due to damaged instruments, ice entrapment, or F-POD malfunction (Figure 2). The sound recorder deployed in Chinitna River within a mud silo in August 2021 stopped recording on October 4th during a strong low tide that exposed the instrument to the air, allowing a brown bear (Ursus arctos) to likely jar (i.e. pouncing with forelegs on the silo guard) the silo enough to impact the electronics based on the markings and the high presence of this species in the area (Schmidt et al., 2022). The overwinter period at Chinitna River was therefore only sampled with the F-PODs prior to the bear interaction. A second recorder leaked and was water damaged in the mooring from Chinitna Bay merely 8 hours after re-deployment during May 2022, caused by a sea otter (Enhydra lutris), another highly abundant species in the area (Gerlach-Miller et al., 2018), chewing on the hydrophone connector. The 2022 ice-free season was only sampled with the F-POD for this location. The rock silos installed in Tuxedni River were very likely entrapped in ice from December 12, 2021 until April 2, 2022 based on the low signal in both the sound recordings and F-POD data. We considered this period as off-effort. For both the Chinitna and Tuxedni Rivers, the F-PODs deployed in fall 2021 failed to save power during the 4-month delay period due to defective firmware, and instrument power was partially depleted when they started their sampling cycle on January 1, 2022. Consequently, these F-PODs only logged for ~ 2 months instead of the 4 months expected. For Tuxedni River, the recorder sampled the rest of the 2021 winter until servicing in May 2022. For the Chinitna River, because the recorder was impacted by a bear earlier in October 2021, sampling effort ended on February 21, 2022 when the delayed start F-POD depleted its power, and sampling effort in this location was not resumed until the May 2022 servicing. The Hobo pressure/temperature sensor in the Chinitna Bay mooring leaked and data were lost, impeding any analysis of CIB relationship to tide in this study site. These instruments are factory sealed and data are offloaded via an optical port so we are unaware of the cause of the leak. Lastly, memory cards containing data from the sound recorders from both Chinitna River and Tuxedni River for the period May-October 2022, as well as their backup contained in an external drive, were stolen during field work travel but fortunately F-POD data covered those periods.
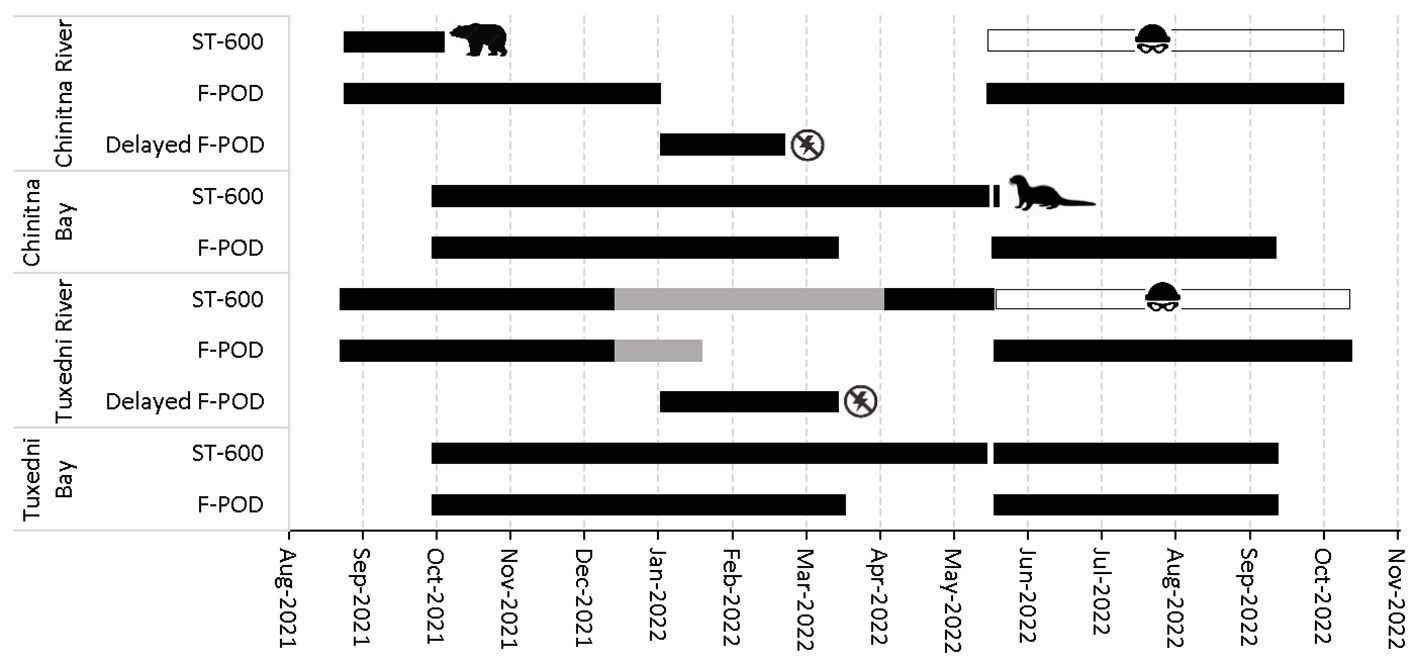
Figure 2 Passive acoustic monitoring effort (black bars) in Tuxedni and Chinitna (river and bay of each). Ice entrapment (off-effort) of Tuxedni River instruments is depicted in gray. Animal symbols depict the interaction that caused the end of sampling. No power symbols depict the delayed start F-PODs that failed to sample the expected 4 months. The thief symbols represent the stolen datasets from Tuxedni and Chinitna River ST-600’s.
3.2 Chinitna cetacean detections
Chinitna datasets yielded very low CIB presence (Figure 3). CIB were never detected in the Bay despite nearly continuous sampling effort from September 2021 until September 2022, though the period of May 20 through September 13, 2022 was sampled only with F-PODs. CIB were detected in Chinitna River on 21 days total across the months of September, October, December, February, and June, with detection periods reaching a maximum of four hours/day of presence. March and April 2022 were not sampled for this river location. Feeding behavior, however, was never detected in Chinitna River. Humpback whales were detected in the bay for 10 days between mid-December and mid-January with up to 10 hours/day on December 20 and 22, and for seven days between April 25 and May 10. Killer whales were detected in the bay on multiple days in December and February through May, with a maximum of 7 hours/day. Killer whales were also detected once in the river on September 20, 2021 for just 1 hour. Harbor porpoise were detected on a daily basis except for 19 days in January, 2 days in February and 2 days in March with a maximum of 22 hours/day. Harbor porpoise were also detected in the river on August 25 - 28, September 1 and 8, 2021, and June 7, 2022 with a maximum of 4 hours/day.
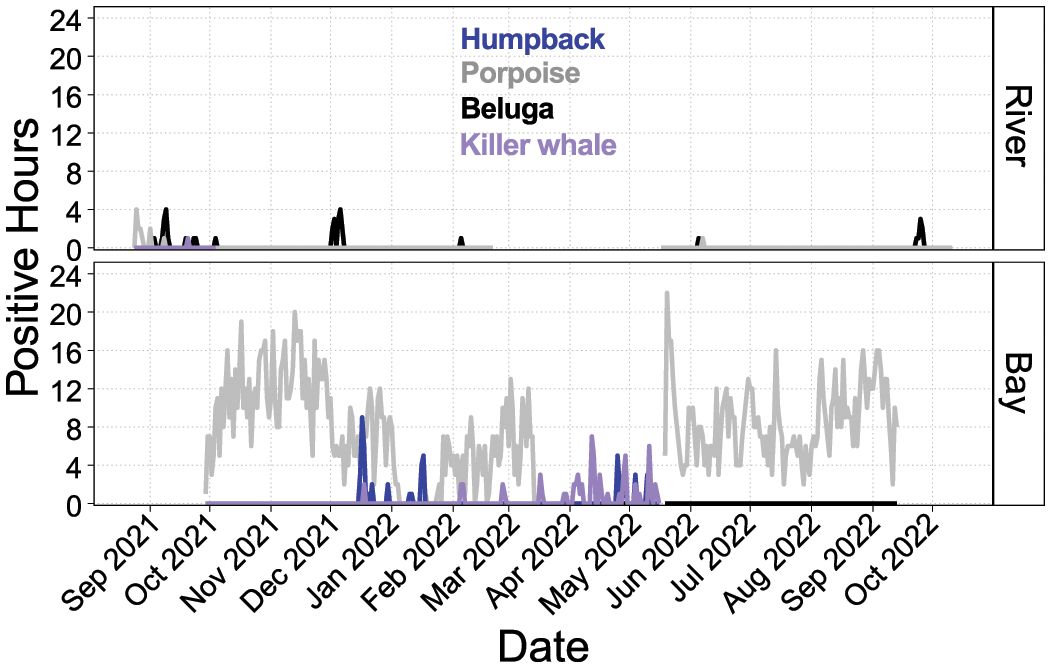
Figure 3 Detection positive hours for humpback whale, harbor porpoise, beluga and killer whale at Chinitna Bay and river. Gaps in the line series denote no sampling effort.
3.3 Chinitna anthropogenic noise
A total of 140 anthropogenic noise events were identified in Chinitna Bay for the period sampled with the recorder (229 days, September 29, 2021 to May 16, 2022), with four sources of noise plus one unknown class (later identified as impact hammer pile driving but of unknown origin, see discussion) (Table 1). Commercial ship noise was the most prevalent noise contributor, but overall anthropogenic noise was present only 6.5% of the time (Figure 4).
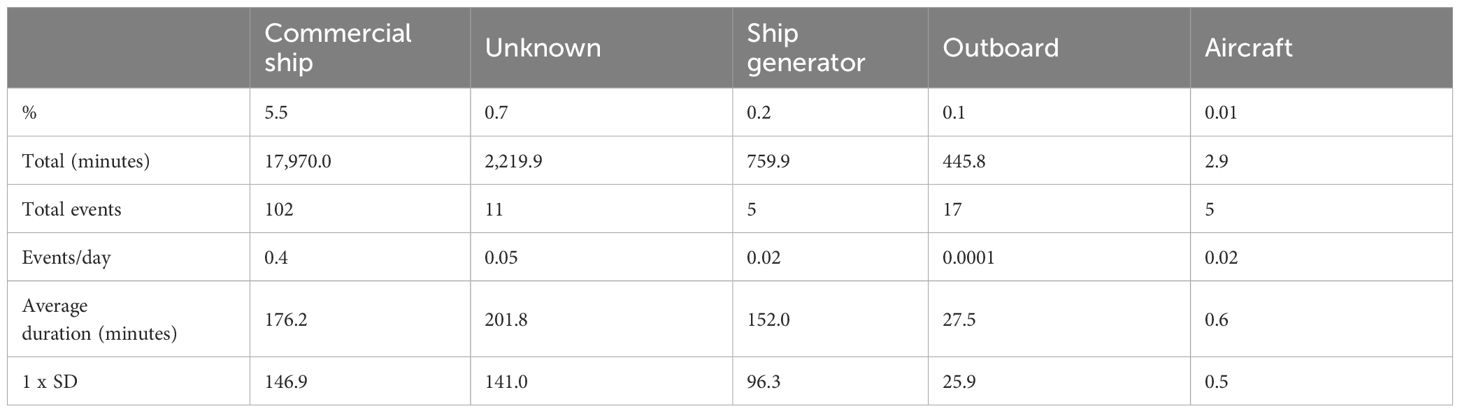
Table 1 Descriptive results for anthropogenic noise events (20 Hz to 24 kHz) identified in Chinitna Bay, Cook Inlet, Alaska, from September 2021 to May 2022.
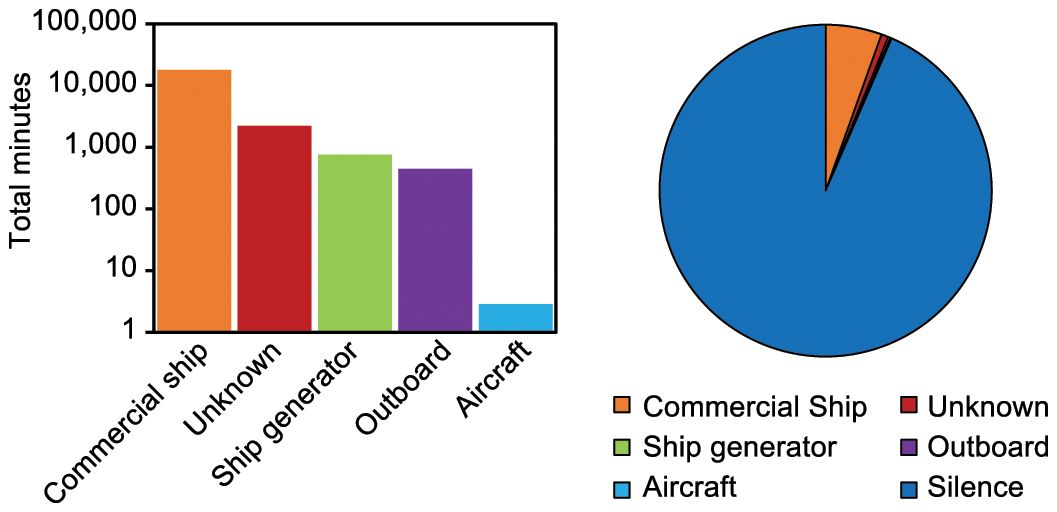
Figure 4 Left – Bar diagram with total number of minutes per noise class, and right – pie chart with percent of time for each noise class and silence (period with no evident contributions from anthropogenic sources) for Chinitna Bay, Cook Inlet, Alaska, from September 2021 to May 2022.
Received levels of anthropogenic sources in Chinitna ranged from 93 to 132 dB SPL (re 1 µPa for all cases), with commercial ship and ship generator noise as the highest amplitude classes (Figure 5). The SPL for quiet periods ranged from 91 to 106 dB, with a mean of 97 dB (1 × SD of 4 dB).
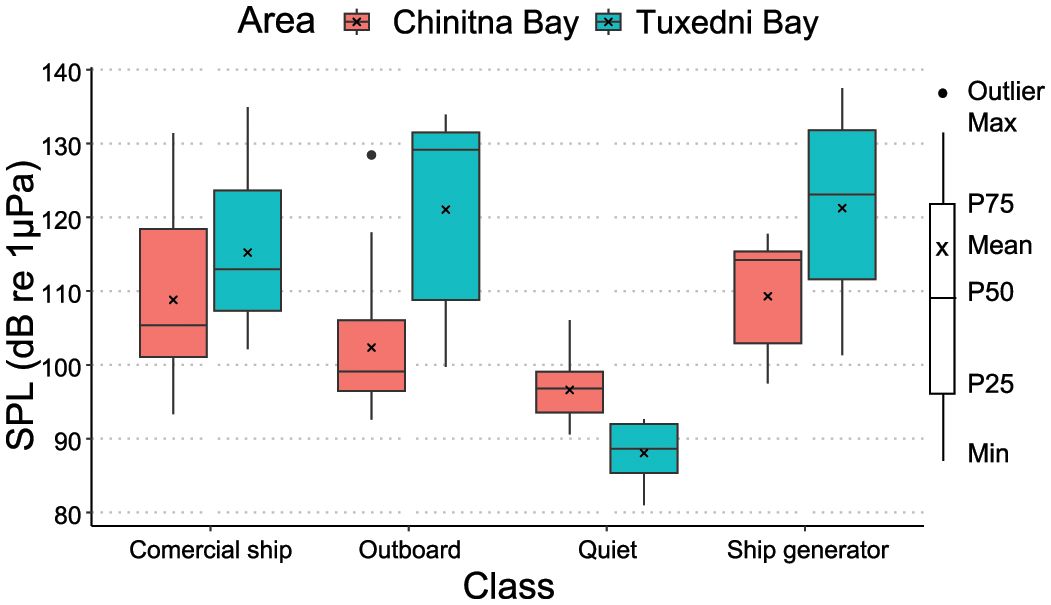
Figure 5 SPL values (20 Hz to 24 kHz) for identified anthropogenic noise classes in both Chinitna Bay (red), and Tuxedni Bay (blue), Cook Inlet, Alaska. Horizontal bars give median SPL, crosses give mean SPL, boxes show the interquartile range and whiskers give the full range of SPL.
Chinitna River was very quiet, with only 3 outboard motor events in the sampled period but it should be noted the sampled period was very limited (41 days, August 24, to October 4, 2021) due to the ST-600 instrument being damaged by a bear. Outboard motor noise events lasted on average 2.2 minutes (SD 0.3) and their SPL ranged from 109 to 128 dB.
3.4 Tuxedni cetacean detections
Tuxedni datasets yielded CIB presence in both the bay and the river on multiple months, primarily from September to April (Figure 6). CIB were detected in the river on September 8, 2021 prior to being detected in the bay. The final day of detections in 2021 in the river was November 13, CIB were then detected only in the bay from November 18, 2021 to April 1, 2022. On April 3, CIB were detected in both sites and continued to be present until April 12 in the bay and April 17 in the river. Detection periods in the bay reached the maxima of 24 hours/day, and 13 hours/day in the river. Periods not sampled due to issues described in previous sections were September 2021 and October 2022 in the bay, and mid-January 2021 to the end of March 2022 in the river. Feeding behavior was detected multiple times in both the bay and river, with maximum occurrence in September and October of both years, and low but consistent occurrence through the month of November 2021 and January 2022. Humpback whales were detected only on May 25 in Tuxedni Bay. Killer whales were also detected but only in the bay on 2 days each in January, May, and June, and 1 day in July, with a maximum of 5 hours/day. Harbor porpoise were detected in the bay on a nearly daily basis from September 30, 2021 until January 3, 2022 and from February 12 until May 24, with up to 18 hours/day. Harbor porpoise were also detected in the river on August and September 2021 for no more than 1 hour/day, and in June, August and September 2022 for no more than 2 hours/day.
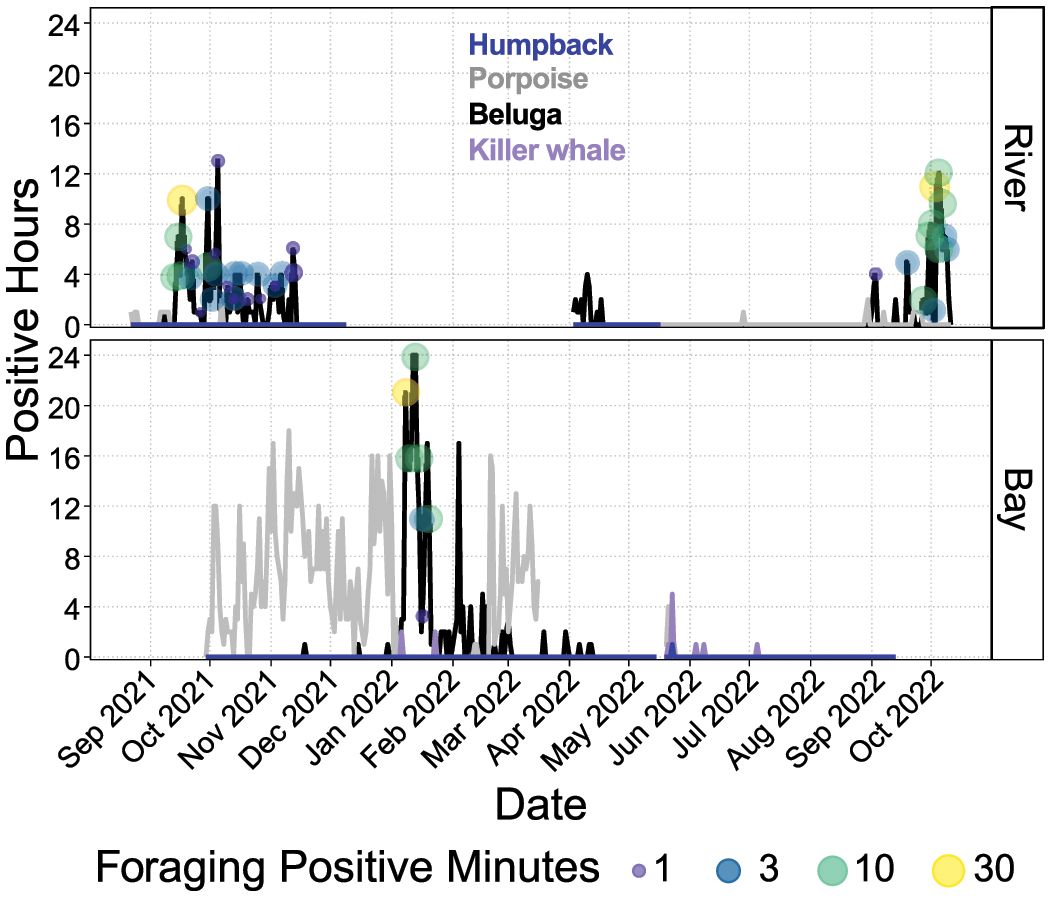
Figure 6 Detection positive hours for humpback whale, harbor porpoise, beluga and killer whale at Tuxedni Bay and river. Gaps in the line series denote no sampling effort. Beluga feeding behavior as positive minutes per day are denoted as circles in the beluga line series.
When comparing positive hours by species and location, Chinitna Bay yielded the highest number of positive hours (n = 2368) primarily driven by harbor porpoise, humpback, and killer whale presence, followed by Tuxedni Bay (n = 1158) driven by harbor porpoise and CIB presence, followed by Tuxedni River (n = 290) driven by CIB presence, and finally Chinitna River (n = 52) driven by CIB and harbor porpoise presence (Figure 7).
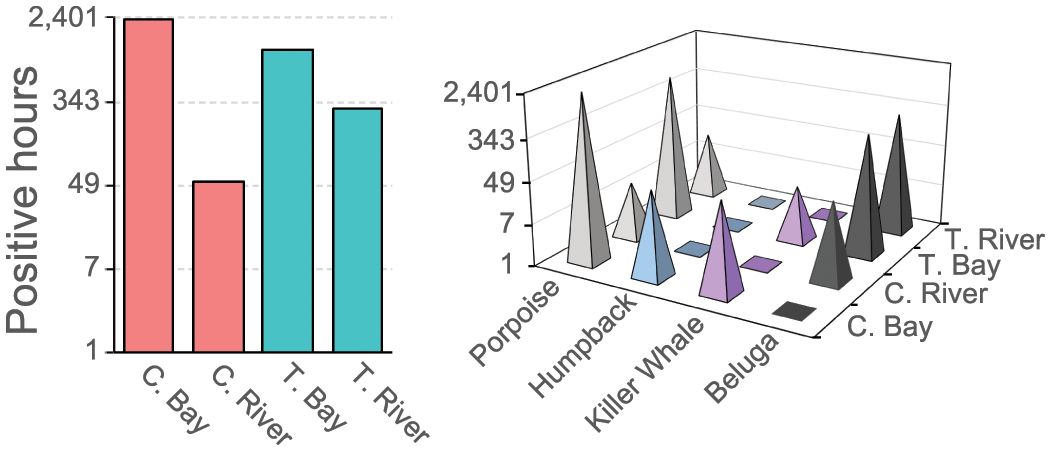
Figure 7 Left panel – total number of detection positive hours per study site, and right panel – distribution of detection positive hours per species and site.
3.5 Tuxedni anthropogenic noise
A total of 505 anthropogenic noise events were identified in Tuxedni Bay for the period sampled with the recorder (345 days, September 29, 2021 to May 15, 2022, and May 19, 2022 to September 13th 2022), with 4 sources of noise (Table 2). Commercial ship noise was the most prevalent anthropogenic contributor, and overall anthropogenic noise was present 14.2% of the time (Figure 8).
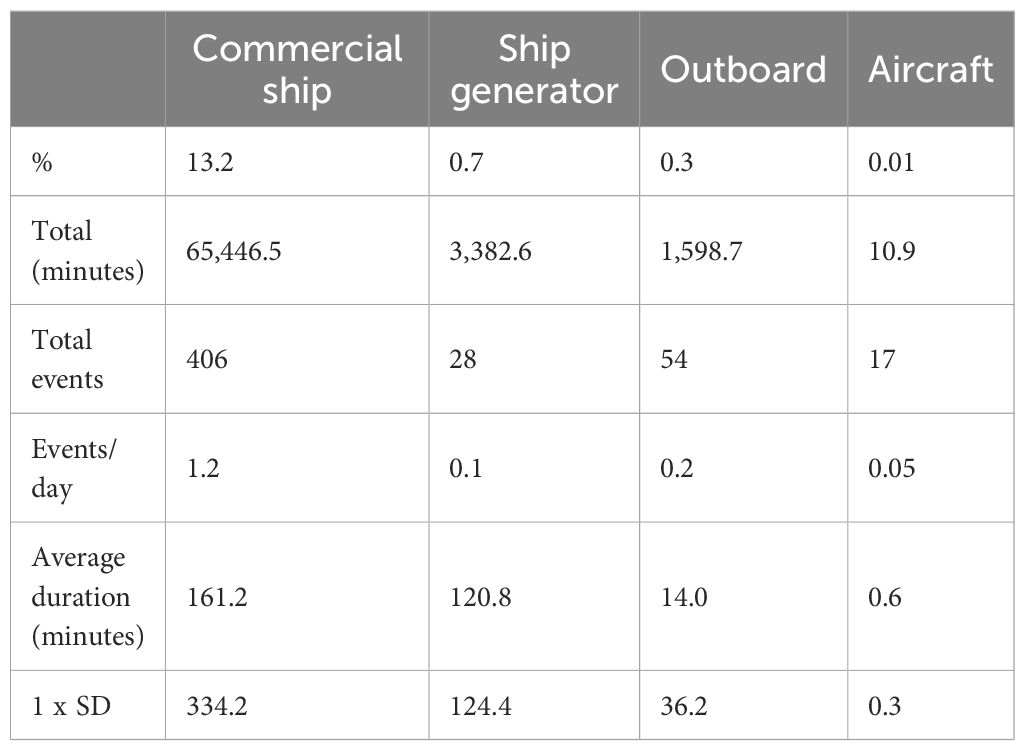
Table 2 Descriptive results for anthropogenic noise events (20 Hz to 24 kHz) identified in Tuxedni Bay, Cook Inlet, Alaska, from September 2021 to September 2022.
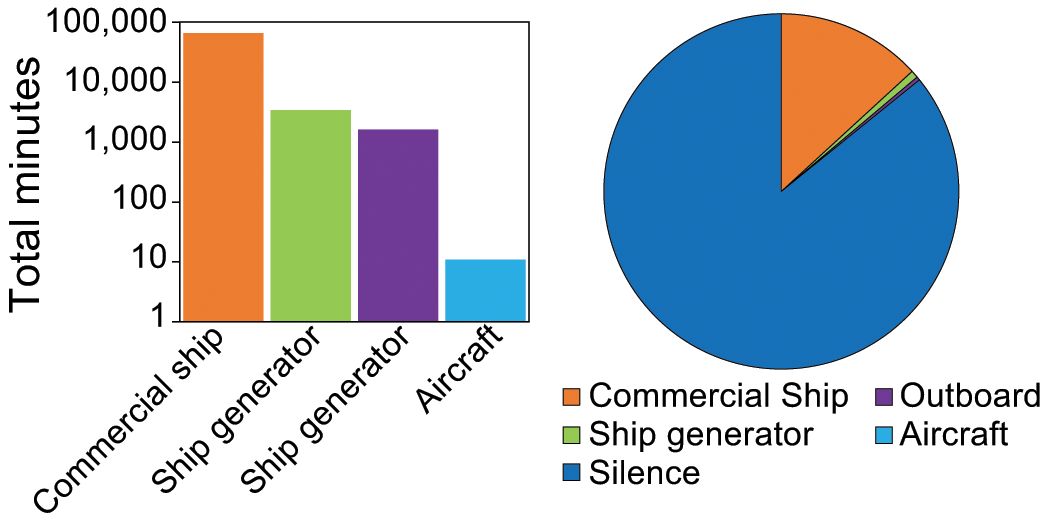
Figure 8 Left – Bar diagram with total number of minutes per noise class, and right – pie chart with percent of time for each noise class and silence (period with no evident contribution form anthropogenic sources) for Tuxedni Bay, Cook Inlet, Alaska, from September 2021 to September 2022.
Received levels of anthropogenic sources ranged from 102 to 138 dB, with outboard motor and ship generator as the highest amplitude classes (Figure 5). The SPL for quiet periods ranged from 85 to 93 dB with a mean of 88 dB (1 × SD 3.2 dB), which is 9 dB lower than Chinitna Bay. Tuxedni River was very quiet, with only one aircraft event identified in the sampled period (268 days, August 22nd 2021 to May 5th 2022) which lasted 53 s with a received level of 116 dB.
3.6 Relationship between CIB acoustic presence and tide period
CIB acoustic detections in both river locations occurred mostly during the ebbing tidal period, however the detections in Tuxedni Bay were distributed between the ebbing and flooding periods (Figure 9).
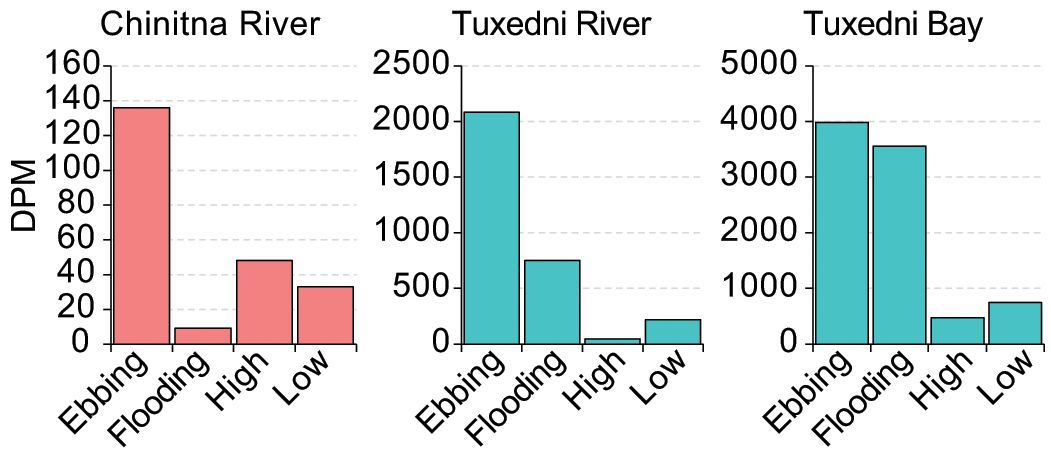
Figure 9 Tidal period distribution of beluga acoustic detections in DPM for the three sites where depth data were available, Chinitna River, Tuxedni River and Tuxedni Bay, Cook Inlet, AK.
The contingency table and χ2 test results yielded a highly significant relationship between acoustic CIB presence and tidal period both for the overall results, χ2 (6, N =12,097, p <.001), and independently for each of the 3 study sites where depth data were available (Table 3). CIB DPM were not randomly distributed across the four tidal periods in any of the 3 study sites where depth data were available.
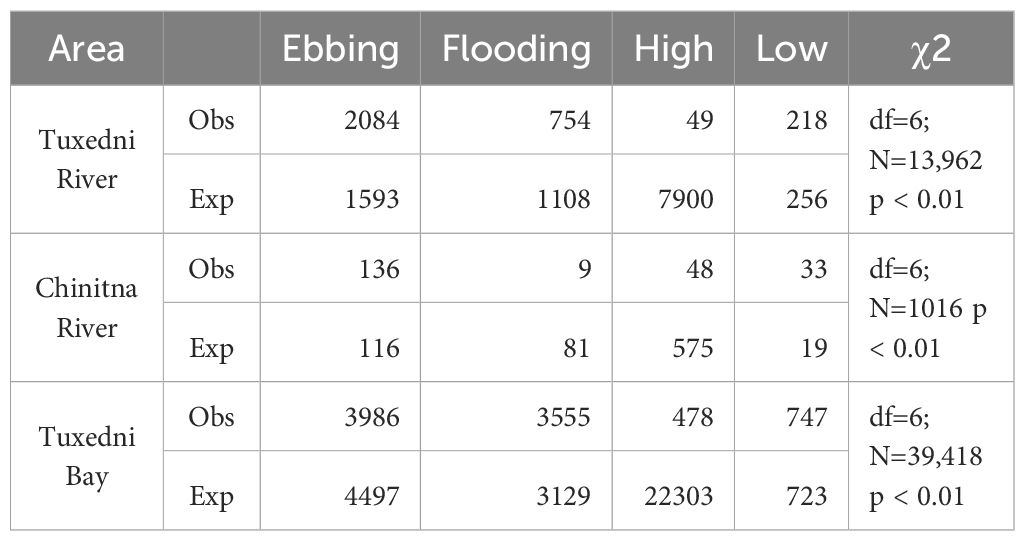
Table 3 Contingency table and χ2 results for beluga acoustic detections (in DPM) and the 4 tidal periods for each study site where depth was available.
The relationship of CIB DPM with depth was very different between the three sites. For the river sites, the majority of the CIB detections occurred at the shallowest end of the depth range (Figure 10). For Chinitna River this relationship was extreme, with most detections falling in the 0–0.5 m depth range (note these are meters of water over the sensors, which were placed in the river bank at unknown elevation from the actual bottom of the river channel). For Tuxedni River, detections were spread in the 0–2.5 m range, although the higher proportion of detections fell into the 0–0.5 m depth. For Tuxedni Bay, CIB detections were distributed along a wider range of depths, with the majority between 12 and 16.5 m depth.
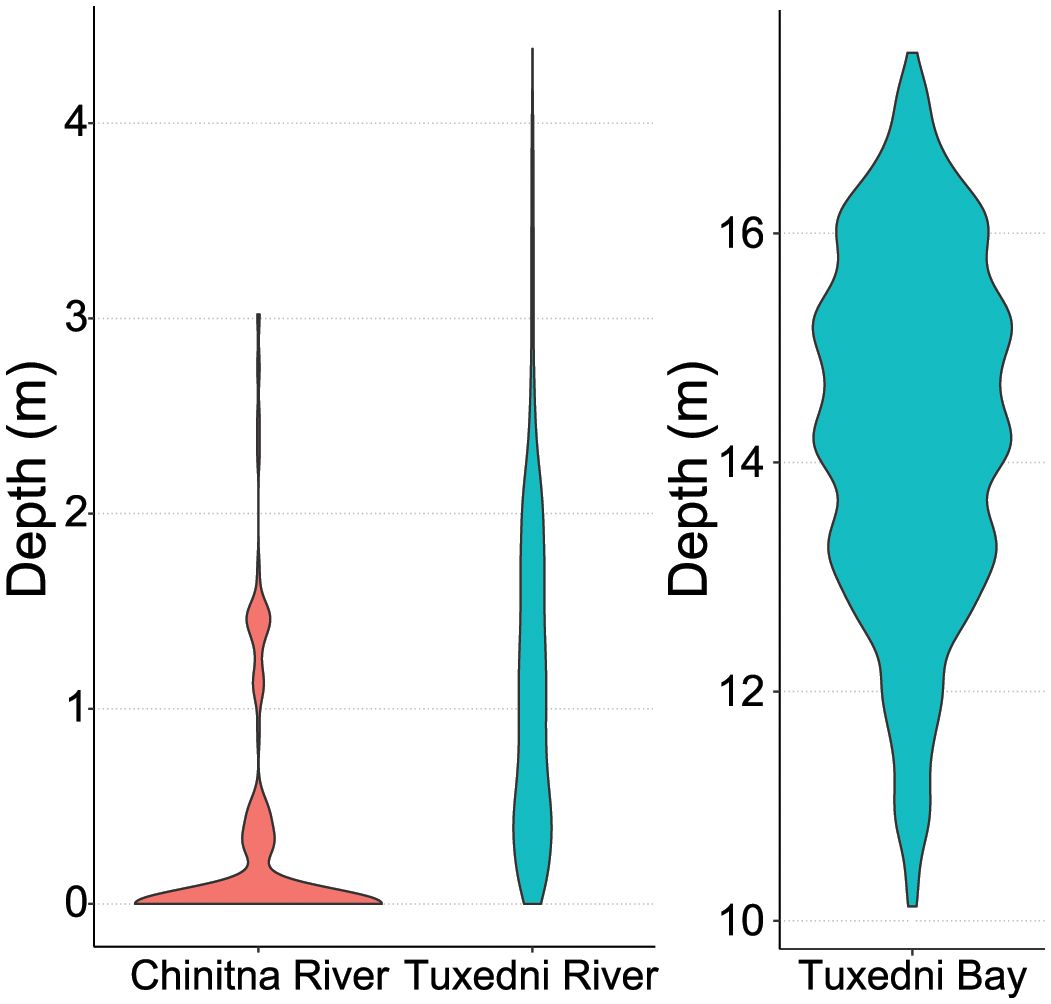
Figure 10 Violin plot outlines illustrating kernel probability density (i.e. the width of the color area represents the proportion of the data located there) of beluga acoustic detections in DPM and depth at the time of each detection, for the three areas where depth data were available, Chinitna River, Tuxedni River and Tuxedni Bay, Cook Inlet, AK. Note Tuxedni Bay data ranged deeper in depth and therefore are plotted independently of the river data.
3.7 Anthropogenic noise disturbance
The predominant anthropogenic noise sources identified in the data are commercial ship, ship generator, outboard motor, and for Chinitna Bay, class unknown (Figures 4, 8). The aircraft class, although identified in three of the four areas, was less prevalent and occurred in small numbers, and therefore is not included in this analysis. Because the river data from both bays yielded very few anthropogenic noise events, only the bay data are considered in this analysis.
All three anthropogenic noise sources evaluated for the potential to mask CIB hearing exceeded hearing thresholds at or below the third octave band centered at 500 Hz (Figure 11). Maximum composite, maximum and median TOLs exceeded CIB hearing thresholds in all cases except the median for outboard noise in Chinitna Bay, which only partially exceeded hearing thresholds for some frequency bands above 600 Hz. In the absence of anthropogenic noise, CIB hearing is limited by background noise in Chinitna Bay at frequency bands above 400 Hz, and in Tuxedni Bay at bands above 800 Hz (quiet TOLs exceed hearing thresholds at and beyond these frequencies, therefore, CIB cannot hear signals within that frequency range unless they are of higher amplitude than the background noise in quiet conditions). However, anthropogenic noise can further limit beluga hearing in lower frequency bands and for a much higher magnitude. For example, commercial ship noise in Tuxedni Bay will limit CIB hearing at any frequencies above 250 Hz for median levels, and 170 Hz for the maximum composite level, and median levels for commercial ship noise will increase hearing thresholds by 10–20 dB, and the maximum composite level by 20–43 dB.
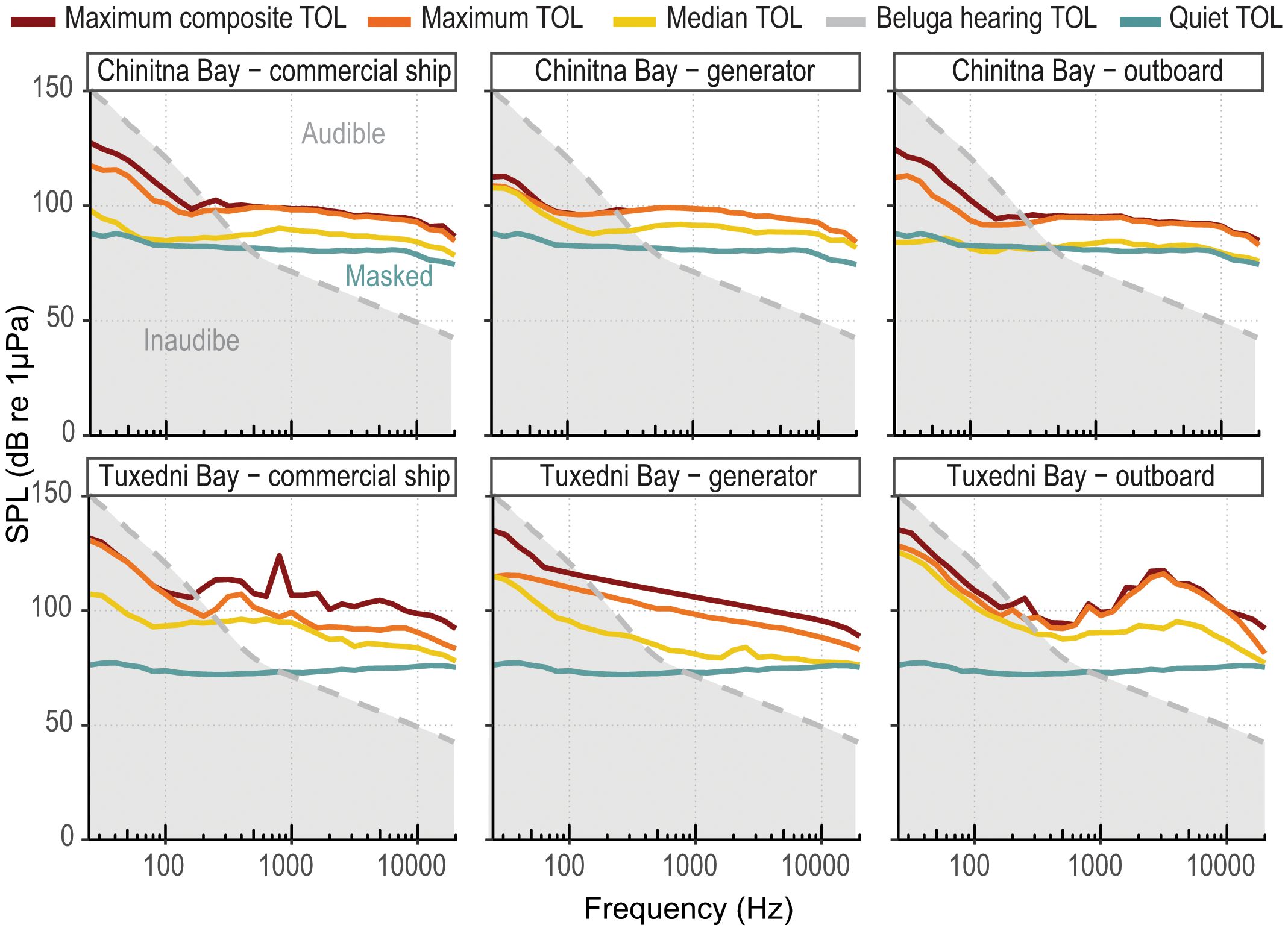
Figure 11 TOL for the maximum composite, maximum event, median, and quiet conditions grouped by area and anthropogenic noise class, as well as minimum beluga hearing thresholds. Grey area will be inaudible for beluga, white area will be audible, and light blue will be the naturally masked area in quiet conditions for each bay. The respective anthropogenic noise source and levels will mask any white area below the red, orange and yellow lines.
The TOLs from commercial ship and generator noise were relatively similar within the bays, and of higher general amplitude in Tuxedni than Chinitna (Figure 5). Outboard motor noise band levels were also higher in Tuxedni Bay, in particular for the range 1 to 20 kHz, and while all three TOL metrics were above quiet levels in Tuxedni Bay, the median levels in Chinitna Bay were barely discernable from quiet levels.
Results for evaluating the amount of temporal overlap between CIB acoustic encounters and anthropogenic noise occurrence was limited to the periods when sound recordings were available at each location. A total of 239 CIB encounters were obtained, of which 37 (15.5%) were overlapped by anthropogenic noise. The overlap in all 37 encounters was caused by commercial ship noise, and one encounter also included aircraft noise. This temporal overlap ranged from several minutes to the entire duration of the encounter. The distribution of temporal overlap by anthropogenic noise for all encounters is shown in Figure 12.
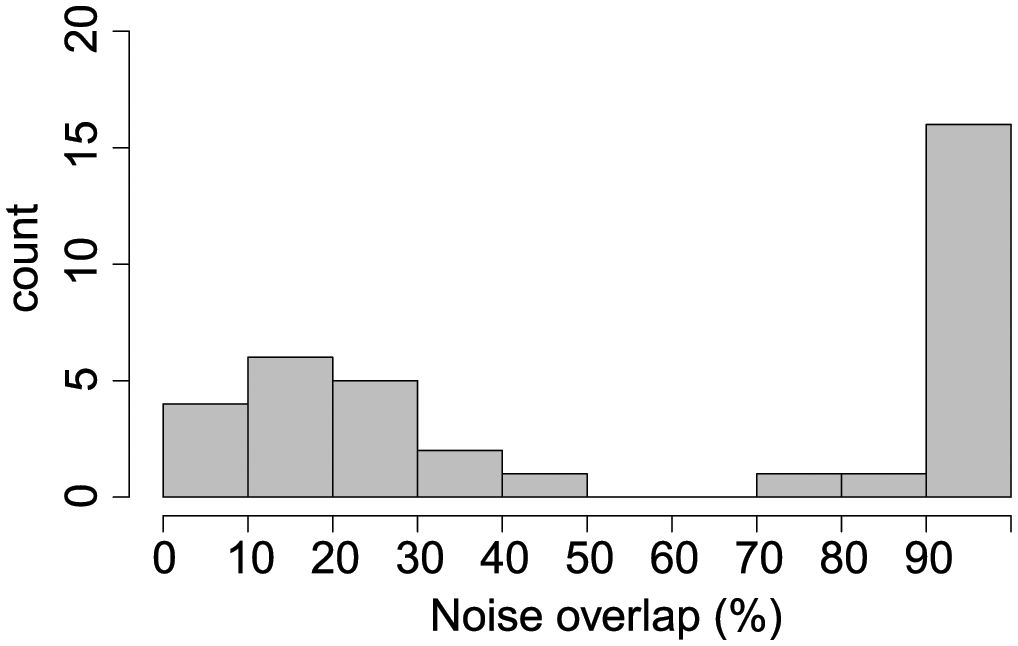
Figure 12 Histogram of the percent of temporal overlap between co-occurring beluga acoustic encounters and anthropogenic noise events for Tuxedni Bay, Cook Inlet, from September 2021 to May 2022.
4 Discussion
4.1 Chinitna bay
Differences in cetacean presence between the two study areas are evident (Figure 7). Chinitna (bay and river) hosted higher cetacean presence overall than Tuxedni (bay and river). Harbor porpoise presence was 2.5 times higher in Chinitna than in Tuxedni. Humpback whale presence was almost exclusive to Chinitna Bay, and killer whales were predominant in Chinitna Bay. However, CIB presence was 14 times higher in Tuxedni than in Chinitna. Predation pressure likely played a role in the differences observed for CIB presence between the two areas. In particular, CIB were never detected in Chinitna Bay, but 38 positive hours were obtained in Chinitna River distributed over 21 different days (Figure 3). CIB most likely moved cryptically in and out of the river near shore undetected by the mooring located 2.4 km from shore at the north end of the bay entrance, a distance at which CIB calls would have been detected (Lammers et al., 2013). This quiet condition has been described as anti-predation behavior reflecting an awareness of predation risk in their habitat (Castellote et al., 2022). Access in and out of the river via deeper waters of the central bay, south of the mooring site and outside the detection range, is unlikely as it is known that beluga exhibit a strong antipredator response by fleeing to shallow coastal waters when predation risk is perceived (Huntington, 2000; Lydersen et al., 2001; Westdal et al., 2016) and the track of a satellite tagged beluga that visited this area in October 2002 almost matched the shoreline (Shelden et al., 2018). This acoustically elusive behavior was also apparent in September 2022 when CIB accessed Tuxedni River without detection in the bay (Figure 6). These results suggest that, at least for areas where killer whale presence is common, acoustic monitoring results should not be extrapolated to wider general areas, and fine scale spatial sampling should be implemented. For example, if we had only deployed moorings in Chinitna Bay we would have concluded that CIB did not use the Chinitna Bay or River when in reality they were silently moving through the bay to get to the river (Figure 3). Similarly, if we had only deployed moorings in Tuxedni Bay we would have concluded CIB only used the Tuxedni area from December to April, missing their use of the river from September to November (Figure 6).
CIB detection periods in Chinitna River were shorter than in Tuxedni River, and feeding was not detected. This suggests CIB might use that area of the river as a corridor to access prey further up river.
Additionally, we presume a possible effect from deploying the silos several feet above the ambient river level. We believe this caused CIB to be missed when venturing into these rivers, during the early flood and late ebb because the tide was too low for our instruments to be in the water. Indeed, most detections occurred when CIB were returning to the bay during the ebbing tidal period before the instruments got exposed to the air (Figures 9, 10).
It was surprising that a short CIB detection was obtained in this river section on June 5th 2022. This matches the sporadic sightings of single CIB or small groups in this region of LCI from surveys 30+ years ago prior to their decline (Shelden et al., 2016). Even though this was a single event, it is significant as it suggests there might still be a wider spatial distribution in early summer than recent accounts, and highlights the practicality of acoustic monitoring.
Killer whales were detected in the Chinitna area from February to May and in September and December. Killer whales were detected once in the river on September 20, 2021 within 17 hours of CIB detections. On February 5, 2022, killer whales were detected in the bay and CIB in the river. Although not all killer whale detections could be assigned to a specific ecotype, the majority were determined to be marine mammal-eating (transients), including the two encounters described above. CIB predation by transient killer whale has been reported previously in Chinitna Bay (Shelden et al., 2003). Our data suggests CIB are using Chinitna River to avoid killer whale predation. First, it is surprising that none of the CIB detections in the river were associated with feeding behavior. While this could indicate that this reach of the river is used primarily to access upstream foraging grounds, a more parsimonious explanation could be that CIB use the river to escape predation, potentially by maintaining a depth too shallow for any killer whale that might be present in the area. Second, the data indicate that CIB were aware of the predation risk in Chinitna, given their acoustically cryptic behavior and presumed preference for near-shore habitat. And third, killer whale detections within the river itself suggest their awareness of and potential search for CIB within the river.
Transient killer whales are notoriously cryptic when in hunting mode, emitting few, very brief echolocation emissions, to avoid detection (Guinet, 1992; Barrett-Lennard et al., 1996; Castellote et al., 2022). For this reason, acoustic presence of transient killer whales in Chinitna and Tuxedni areas is likely underestimated.
Humpback whales were detected on 17 days in Chinitna Bay up to 9 hours/day in December, January, April and May. Song (January), feeding (April), and social (April-May), signals were identified. Six of the 17 days of humpback presence overlapped with killer whale detections. Accounts of transient killer whale predation on humpback whales in Alaska are rare but there have been observed predation events in LCI during summer on sub-adults and cow/calf pairs, as well as carcasses of sub-adults that have been found with evidence of killer whale predation and consumption (Shelden et al., 2003; Saulitis et al., 2015).
Harbor porpoise were present in Chinitna Bay almost daily except for the period January 4, until the 24, which corresponded with a period of 8/10th to 10/10th sea ice concentration levels, the highest of all winter for Chinitna Bay (National Weather Service Alaska Sea Ice Program). Ice displacement has been described for this species in the UCI (Castellote et al., 2016), therefore, we assume this was also the case in Chinitna Bay.
4.2 Tuxedni bay
CIB foraging behavior was identified in Tuxedni primarily in two periods, September-October in the river, and January in the bay. Interestingly, CIB presence was still very high in February and, to a lesser extent, in March and April but foraging behavior was not identified. For both fall and winter, feeding events reached up to 37 minutes/day, which is a high value compared to other foraging areas monitored acoustically with the same methodology (Castellote et al., 2020, Castellote et al., 2021). CIB presence in winter in the bay reached 24 hours for two consecutive days. This level of concentrated acoustic presence has only been observed in the Susitna delta (UCI) in June when CIB concentrate in this area preying on salmon (Castellote et al., 2021). Very limited knowledge is available on CIB prey in the Tuxedni area in fall and winter. Coho salmon (Oncorhynchus kisutch) have been documented in Tuxedni River but no information is available on the timing of that run (Barclay and Habicht, 2019). However, a coho salmon spawning run is known to occur in the nearby Crescent River (Figure 1) from mid-August extending into at least October (Tarbox, 1988). Similar timing of coho in Tuxedni River could partially explain the presence of feeding CIB in fall. Summer surveys for fish in the Tuxedni area have identified a rich diversity of forage fish, in particular herring (Clupea pallasii), capelin (Mallotus villosus), which spawn in April-May and are believed to migrate offshore in winter, Pacific sand lance (Ammodytes hexapterus), which spawn in fall and winter, and longfin smelt (Spirinchus thaleichthys), which have been observed returning to Kenai River in late November through early December (Marston and Frothingham, 2022). Other species include Pacific sandfish (Trichodon trichodon), Pacific lamprey (Lampetra tridentata), snake prickleback (Lumpenus sagitta), Pacific cod (Gadus macrocephalus), Alaska Pollock (Gadus chalcogrammussculpins), dolly varden (Salvelinus malma), sculpins (Cottoidea), flat-fishes, and crangonid and pandalid shrimp (Fechhelm et al., 1999; Robards et al., 1999; Speckman et al., 2005; Arimitsu et al., 2021). Many of these species have been identified as part of the diet of CIB (Quakenbush et al., 2015); thus, the Tuxedni area should be considered an important fall/winter foraging ground. No other winter foraging ground has been described yet and further research in identifying fall and winter prey communities in this area should be prioritized. Population modeling efforts on CIB highlight how survival and resilience against disturbed environments are influenced by winter energy intake (McHuron et al., 2023). Therefore, understanding what prey is targeted by CIB in this area, and evaluating the trend of these prey assemblages are critical actions to facilitate proper management for the recovery of this endangered population.
Similar to Chinitna River, in Tuxedni River CIB were detected primarily during the ebbing tidal period (Figure 9). Therefore, we can also assume that CIB detected here were on their way back to the bay after their incursion into the river with the flooding tidal period. The proportion of detections during the flooding period was slightly greater than in Chinitna River, and the distribution of these detections was more spread across depth (Figure 10). This might be due to the silos in this study site being placed a bit lower in the intertidal zone, which in turn allowed a more complete capture of CIB presence when transiting this part of the river. The Tuxedni Bay depth data show a more balanced proportion of CIB detections between the ebbing and the flooding tidal periods (Figure 9), and detections are well distributed across the range of depths reported (Figure 10). The χ2 test results in Table 3 confirm depth is not independent of CIB presence in the bay, but that may be due to the low number of CIB detections occurring during the low and high slack periods.
Our Tuxedni River instruments were entrapped in ice from December 12 until April 2, drastically attenuating sound sensing, therefore absence of CIB in the river within this period cannot be confirmed. However, some interesting observations can still be described. CIB use of Tuxedni River ended on November 13, 2021 (30 days prior to instruments icing) and sea ice formation in the bay-head and river began on November 17, 2021 (2/10th to 4/10th sea ice concentration, National Weather Service Alaska Sea Ice Program). First CIB detections in the bay occurred on November 18, 2021, highlighting how CIB apparently ceased use of the river but instead focused on the bay at the onset of sea ice formation. However, first spring detections in the river occurred on April 3, 2022 (one day after instruments thawed) when sea ice concentration in the river and bay-head was at 7/10th to 9/10th (National Weather Service Alaska Sea Ice Program). CIB detections continued to occur almost on a daily basis until April 17, the last day of spring detections in this site. Sea ice concentration remained at 7/10th to 9/10th until break up on April 20 (National Weather Service Alaska Sea Ice Program), suggesting CIB used the river and bay-head under heavy sea ice coverage. This differing relationship with ice in fall and spring suggests CIB movements in and out of the river may be driven by prey preferences rather than just ice presence.
Results from this study increase our contemporary knowledge on the seasonal occurrence of CIB in LCI which had been a data gap. Until now, based on aerial surveys in 2018 - 2023, CIB occurrence was documented in Tuxedni in September, March, and April (V. Gill, unpublished), and based on previous acoustic monitoring (2009–2011), CIB occurrence in Tuxedni Bay was limited to January-April with significant inter-annual variation (Castellote et al., 2020). These new acoustic results, incorporating sampling in the bay-head through the use of silos, strengthen our knowledge of CIB use of this habitat, and further support the importance of Tuxedni area as CIB foraging ground from September to April.
Humpback whales were only detected in Tuxedni Bay on May 23, 2022 for a period of 5 hours, consisting of social signals. This species is seasonally observed in the lower inlet, and not commonly sighted during aerial surveys further north than the latitude of Chinitna Bay (Shelden et al., 2013). Rip tides, higher turbidity, and more estuarine conditions further north in the inlet may not be preferred by this species, and opportunistic sightings in UCI are often associated to strandings or carcasses (NMFS unpublished).
Killer whales were detected on seven days in Tuxedni Bay in January, and May to July, with up to 5 hours/day. Although CIB were not detected when killer whales calls were identified from May to July, spatio-temporal overlap of these two species occurred in January, when CIB presence was at its highest. On January 6, 2022, a spike of transient killer whale calls, often overlapping thus indicating vocalization by multiple individuals, coincided within a 29 minute window with CIB calls, and on January 23, 2022 another spike in transient killer whale calls were detected 31 minutes after CIB calls. Although successful predation events cannot be confirmed with these data, it has been documented that transient killer whales only show significant amounts of vocal behavior after a marine mammal kill (Guinet, 1992; Deecke et al., 2005; Guinet et al., 2006). A review of killer whale and CIB interactions by Shelden et al. (2003) estimated one CIB predation per year from accounts reported between 1985 and 2002. The authors discuss how this is probably a function of observational effort and likely underrepresents predation rates. The NMFS CIB Recovery Plan (NMFS, 2016) states that the loss of more than one CIB whale annually due to predation could impede recovery. Our results with killer whale visiting this CIB hotspot area in winter at least two times, and both overlapping spatio-temporally with CIB, indicate predation might be occurring more often than previously determined.
4.3 Anthropogenic noise
Commercial ships were the dominant anthropogenic noise source in both Tuxedni Bay and Chinitna Bay, similar to areas of UCI that have been measured (Castellote et al., 2019), however the levels reported in our LCI sites were much lower than the measured UCI sites both in terms of prevalence and SPLs. (Figure 1). Ship generator was the second most common noise source for both bays in our study, and it was likely caused by vessels anchored or idling within acoustic range of the moored instruments. Commercial shipping, and its associated generator noise, dominated the anthropogenic soundscape accounting for 99% of all noise in Tuxedni Bay and 88% for Chinitna Bay (Figures 4, 6). An ‘unknown’ class of noise was recorded in Chinitna Bay which encompassed low frequency impulsive sounds in sequences of approximately 3.4 hours in duration (Figure 4), with impulses at 1 s intervals and mean SPL of 151 dB. This precisely matches what has been described acoustically for in-water impact pile driving operations (Dahl, 2015). Neither NMFS nor the U.S. Army Corps of Engineers were aware of any permitted piling activity in Chinitna Bay, therefore if this was pile driving, it occurred with no mitigations in place to avoid impact to CIB or other protected species. It is highly concerning that a potentially harmful (Thompson et al., 2020), and unregulated pile driving operation occurred in Chinitna Bay in the fall when CIB are using this area.
Commercial ships are transiting Cook Inlet year-round entering and exiting the Port of Alaska (Anchorage), Port of Nikiski, and Port of Homer, with shipping lanes roughly 18 nautical miles from the Tuxedni Bay mooring and 20 nautical miles from the Chinitna mooring. Seasonally, commercial fisheries vessels operate closer to the sampled areas out of the ports of Kenai and Homer. By August 15, the drift gillnet fleet is restricted to fishing in areas on the west side of LCI adjacent to Chinitna Bay (NMFS, 2023). Some vessel and generator noise might be related to this fleet using the bays as protected overnight anchoring. Interestingly, the prevalence of commercial ship noise in Tuxedni Bay was more than twice that of Chinitna Bay (Figures 4, 8) and with higher SPLs (Figure 5). Sport fishing and ecotourism boat-based tours are offered seasonally to visit Chinitna and Tuxedni from Homer, and remote lodges and private cabins also bring small vessel traffic. But likely the difference between both bays might have been driven by wildlife research-based vessel activity in Tuxedni Bay and around Chisik and Duck Islands (i.e., Arimitsu et al., 2021).
Other identified sources of anthropogenic noise for both areas were outboard and aircraft, but the prevalence of these sources was minimal (Figures 4, 8). Despite the higher prevalence for commercial ship and generator noise, Tuxedni and Chinitna remain among the quietest and most pristine soundscapes of all the quantified locations in Cook Inlet, with Tuxedni Bay sustaining 85.8% of time with no evident sources of anthropogenic noise, and Chinitna 93.5%. Their respective mean quiet SPL values of 96.6 dB for Chinitna and 88 dB for Tuxedni (Figure 5) were below the mean values from all other reported sites in Cook Inlet to date (Castellote et al., 2019).
A key question to understand the potential for CIB disturbance by anthropogenic sources of noise detected in the study areas was to assess if these were heard by CIB at the levels reported from our data. The three main sources of noise (commercial ship, generator, and outboard motor) exceeded beluga hearing thresholds in both bays for median and maximum TOLs, and in some instances by large differences (Figure 11). Thus, it must be assumed that CIB were impacted by masked hearing and reduced communication space during the majority of the noise events identified in our data. Masking was evaluated up to 24 kHz, the limit imposed by the sampling rate used in our instruments, and while these sources of noise can extend beyond this limit, all the key acoustic components of beluga sound emissions are occurring within that frequency limit, except for echolocation signals (Brewer et al., 2023). Based on our results, we believe that CIB in our study area are negatively affected by the current level and type of anthropogenic noise in that their communication and passive listening for acoustic cues (i.e., prey or predator signals) are disrupted. While the magnitude of the masking evaluated in our comparison was very elevated in terms of frequency range and excess dB, only a small percent (15.5%) of CIB encounters were masked by noise, and only by the commercial ship noise class (except one encounter which also included aircraft noise). This was due to the relatively low prevalence of anthropogenic noise in both areas compared to other parts of Cook Inlet. This low level of overlap could reduce the concern for potential negative effects of acoustic disturbance. However, it is interesting to note that half of the CIB encounters masked by noise were affected in its totality (100% noise overlap, Figure 12), suggesting that any future increase in shipping activity in the area could quickly escalate the potential negative effects of masking, unless restricted to the season when CIB presence is minimal or absent (April to August). It is currently very difficult to evaluate population consequences for this or any other level of acoustic masking in marine mammals (Erbe et al., 2016). However, recent work has demonstrated how cetacean species exhibiting costly antipredator responses (i.e., fleeing and cessation of feeding) also have stronger behavioral reactions to anthropogenic noise (Miller et al., 2022), particularly for belugas, which have been observed to react to ship noise at very long distances, up to 79 km (Martin et al., 2022). Similar to predation risk, disturbance stimuli can indirectly affect fitness and population dynamics via the energetic and lost opportunity costs of risk avoidance (Frid and Dill, 2002). Cetacean species that rely upon crypsis and escape behaviors, such as beluga, will be most sensitive to disturbance by anthropogenic noise, especially when in areas with increased predation (Miller et al., 2022). Therefore, if these adaptive responses known to occur in CIB are also triggered by the exposure of anthropogenic noise sources, even if in this case for a relatively low amount of time, there is potential for negative effects beyond acoustic masking. Additionally, the quiet environments of Tuxedni and Chinitna may in fact mean CIB, and other cetaceans, may be more behaviorally responsive to anthropogenic noise in these pristine areas than in more industrialized areas due to the novelty of the stimuli (Sih, 2013; Wensveen et al., 2019), paired with the larger noise footprints in quieter environments.
Tuxedni Bay, from fall to spring, qualifies for what has been termed as an “opportunity site” — important habitat that experiences low ship noise (Williams et al., 2015). This term derives from incorporating ocean noise into spatial planning and swapping the aim of the analysis: rather than identifying areas of important habitat with high ship noise requiring mitigation, here conservation gain is facilitated by identifying opportunities to protect important habitat that happen to be currently quiet. The conservation task may simply involve maintaining the acoustic status quo of this habitat. Few other areas of CIB critical habitat can be designated this way due to the spatial overlap between anthropogenic activities (or the noise generated by these) and the habitat importance to CIB. And, while important foraging grounds are recognized in UCI during the ice-free season (NMFS, 2008), no winter foraging grounds had been conclusively confirmed in LCI until now, but results from this study, combined with observations from multiple years of visual and acoustic efforts, allow us to propose Tuxedni Bay as the first one.
Spatio-temporal restrictions on noise generating activities offer one of the most effective means of protecting cetaceans and their habitats from the cumulative and synergistic effects of noise as well as from other anthropogenic stressors (Castellote, 2007; Dolman, 2007; Nowacek, 2013; Chou et al., 2021). For this, and the above discussed reasons, we suggest that applicable regulators consider a seasonal restriction for high noise-producing anthropogenic activities, such as seismic surveys or in-water pile driving operations, in and adjacent to Tuxedni Bay from September 1 to May 15 when CIB are present in this habitat.
This is the first time a winter foraging area (Tuxedni) has been identified for CIB. The importance of this area is further accentuated by its pristine soundscape and the bay and river should be viewed as a noise refuge for this endangered whale. In view of the converging interests by industry in this area of Cook Inlet, managing anthropogenic activities to minimize habitat degradation and acoustic disturbance will be a key component to support the recovery of this endangered population.
Data availability statement
The datasets presented in this study can be found in online repositories. The names of the repository/repositories and accession number(s) can be found below: https://www.fisheries.noaa.gov/inport/item/72248.
Author contributions
MC: Conceptualization, Formal analysis, Funding acquisition, Methodology, Project administration, Visualization, Writing – original draft. VG: Conceptualization, Funding acquisition, Project administration, Writing – original draft, Writing – review & editing. CG: Conceptualization, Methodology, Resources, Writing – review & editing. AG: Methodology, Resources, Writing – review & editing. BH: Data curation, Formal analysis, Methodology, Visualization, Writing – review & editing. AB: Formal analysis, Visualization, Writing – review & editing. JK: Formal analysis, Writing – review & editing.
Funding
The author(s) declare financial support was received for the research, authorship, and/or publication of this article. We acknowledge the Alaska Region of the Bureau of Ocean Energy Management (BOEM), U.S. Department of the Interior, and the Alaska Region of NOAA Fisheries (NOAA) for funding this study. We are indebted to Sean Burrill and Cathy Coon (BOEM) for partnering with us and championing this project for funding. This publication was partially funded by the Cooperative Institute for Climate, Ocean, and Ecosystem Studies (CIOCES) under NOAA Cooperative Agreement NA20OAR4320271, Contribution No. 2024–1338.
Acknowledgments
We appreciate the help of Barbara Lake and Julie Scheurer (NOAA) with equipment logistics and Dana Whitley (NOAA) with contracts. Logistical support was received by the Silver Salmon Creek Lodge (David and Oliver Coray), Coldwater AK (Brad and Lisa Conely), Soloy Helicopters, and Maritime Helicopters. The Alaska Department of Fish and Game Marine Mammal Program provided warehouse space in Anchorage to store and work on acoustic equipment, we are indebted to Lori Polasek, Michael Rehberg, and Tom Gage. Kim Shelden (NOAA) produced Figure 1. Bonnie Easley-Appleyard (NOAA) and Kim Shelden reviewed an earlier draft version and provided useful comments and edits. All work was conducted under National Marine Fisheries Service (NMFS) permit no. 25563.
Conflict of interest
The authors declare that the research was conducted in the absence of any commercial or financial relationships that could be construed as a potential conflict of interest.
The reviewer CHR declared a past co-authorship with the author VG to the handling editor.
Publisher’s note
All claims expressed in this article are solely those of the authors and do not necessarily represent those of their affiliated organizations, or those of the publisher, the editors and the reviewers. Any product that may be evaluated in this article, or claim that may be made by its manufacturer, is not guaranteed or endorsed by the publisher.
Author disclaimer
The findings and conclusions in this article are those of the authors and do not necessarily represent the views of NOAA Fisheries.
References
Abookire A. A., Piatt J. F. (2005). Oceanographic conditions structure forage fishes into lipid-rich and lipid-poor communities in lower Cook Inlet, Alaska, USA. Mar. Ecol. Prog. Ser. 287, 229–240. doi: 10.3354/meps287229
Arimitsu M., Schoen S., Piatt J., Marsteller C., Drew G. (2021). Monitoring the recovery of seabirds and forage fish following a major ecosystem disruption in Lower Cook Inlet (Washington, D.C.: BOEM. U.S. Department of the Interior). Available at: https://espis.boem.gov/final%20reports/BOEM_2021-031.pdf
Arveson P. T., Vendittis D. J. (2000). Radiated noise characteristics of a modern cargo ship. J. Acoustical Soc. America 107, 118–129. doi: 10.1121/1.428344
Barclay A. W., Habicht C. (2019). Genetic baseline for Cook Inlet coho salmon and evaluations for mixed stock analysis (Anchorage: Alaska Department of Fish and Game).
Barrett-Lennard L. G., Ford J. K. B., Heise K. A. (1996). The mixed blessing of echolocation: differences in sonar use by fish-eating and mammal-eating killer whales. Anim. Behav. 51, 553–565. doi: 10.1006/anbe.1996.0059
Brewer A. M., Castellote M., Van Cise A. M., Gage T., Berdahl A. M. (2023). Communication in Cook Inlet beluga whales: Describing the vocal repertoire and masking of calls by commercial ship noise. J. Acoustical Soc. America 154, 3487–3505. doi: 10.1121/10.0022516
Brown R. C., Gray J. N., Jones L. (2022). Updated Mineral Resource Estimate and NI 43–101 Technical Report for the Johnson Tract Project, Alaska (Vancouver, BC: HighGold Mining Inc).
Calkins D. G. (1989). “Status of belukha whales in Cook Inle,” in Cook Inlet, and North Aleutian Basin information update meeting ed (Department of Commerce, Anchorage).
Castellote M. (2007). General review of protocols and guidelines for minimizing acoustic disturbance to marine mammals from seismic surveys. J. Int. Wildlife Law Policy 10, 273–288. doi: 10.1080/13880290701769262
Castellote M., Mooney A., Andrews R., Deruiter S., Lee W. J., Ferguson M., et al. (2021). Beluga whale (Delphinapterus leucas) acoustic foraging behavior and applications for long term monitoring. PloS One 16, e0260485. doi: 10.1371/journal.pone.0260485
Castellote M., Mooney T. A., Quakenbush L., Hobbs R., Goertz C., Gaglione E. (2014). Baseline hearing abilities and variability in wild beluga whales (Delphinapterus leucas). J. Exp. Biol. 217, 1682–1691. doi: 10.1242/jeb.093252
Castellote M., Small R. J., Lammers M. O., Jenniges J. J., Mondragon J., Atkinson S. (2016). Dual instrument passive acoustic monitoring of belugas in Cook Inlet, Alaska. J. Acoustical Soc. America 139, 2697–2707. doi: 10.1121/1.4947427
Castellote M., Small R. J., Lammers M. O., Jenniges J., Mondragon J., Garner C. D., et al. (2020). Seasonal distribution and foraging occurrence of Cook Inlet beluga whales based on passive acoustic monitoring. Endangered Species Res. 41, 225–243. doi: 10.3354/esr01023
Castellote M., Small R. J., Stafford K. M., Whiting A., Frost K. J. (2022). Beluga (D. leucas), harbor porpoise (P. phocoena), and killer whale (O. orca) acoustic presence in kotzebue sound, alaska: Silence speaks volumes. Front. Remote Sens. 3. doi: 10.3389/frsen.2022.940247
Castellote M., Thayre B., Mahoney M., Mondragon J., Lammers M. O., Small R. J. (2019). Anthropogenic noise and the endangered cook inlet beluga whale, delphinapterus leucas: acoustic considerations for management. Mar. Fisheries Rev. 80, 63–88. doi: 10.7755/MFR
Chou E., Southall B. L., Robards M., Rosenbaum H. C. (2021). International policy, recommendations, actions and mitigation efforts of anthropogenic underwater noise. Ocean Coast. Manage. 202, 105427. doi: 10.1016/j.ocecoaman.2020.105427
Dahl P. H. (2015). The Underwater sound field from Impact Pile Driving and Its Potential effects on Marine life. Acoustics Today 11, 18–25.
Deecke V. B., Ford J. K. B., Slater P. J. B. (2005). The vocal behaviour of mammal-eating killer whales: communicating with costly calls. Anim. Behav. 69, 395–405. doi: 10.1016/j.anbehav.2004.04.014
Dolman S. (2007). Spatio-temporal restrictions as best practice precautionary response to ocean noise. J. Int. Wildlife Law Policy 10, 219–224. doi: 10.1080/13880290701769270
Erbe C., Reichmuth C., Cunningham K., Lucke K., Dooling R. (2016). Communication masking in marine mammals: A review and research strategy. Mar. pollut. Bull. 103, 15–38. doi: 10.1016/j.marpolbul.2015.12.007
Fechhelm R. G., Wilson W. J., Griffiths W. B., Stables T. B., Marino D. A. (1999). Forage fish assessment in cook inlet oil and gas development areas 1997–1998 (Anchorage, Alaska: U.S. Department of the Interior Minerals Management Service, Alaska Outer Continental Shelf Region). Available at: https://www.govinfo.gov/content/pkg/GOVPUB-I-e3037232f8419d88f3d2aaba6e2b6144/pdf/GOVPUB-I-e3037232f8419d88f3d2aaba6e2b6144.pdf.
Frid A., Dill L. (2002). Human-caused disturbance stimuli as a form of predation risk. Conserv. Ecol. 6, 11–27. doi: 10.5751/ES-00404-060111
Gerlach-Miller J., Esslinger G. G., Weitzman B. (2018). Aerial surveys of sea otters (Enhydra lutris) in Lower Cook Inlet, Alaska, May 2017 (Anchorage, AK: U.S. Geological Survey. Alaska Science Center). Available at: https://www.usgs.gov/data/sea-otter-aerial-survey-data-lower-cook-inlet-alaska-2017.
Goetz K. T., Shelden K. E. W., Sims C. L., Waite J. M., Wade P. R. (2023). “Abundance and Trend of Belugas (Delphinapterus leucas) in Cook Inlet, Alaska, June 2021 and June 2022” (Seattle WA: Alaska Fisheries Science Center, NOAA, National Marine Fisheries Service). Available at: https://www.fisheries.noaa.gov/s3//2023-06/PR2023-03.pdf.
Gordon J., Gillespie D., Potter J., Frantzis A., Simmonds M. P., Swift R., et al. (2003). A review of the effects of seismic surveys on marine mammals. Mar. Technol. Soc. J. 37, 16–34. doi: 10.4031/002533203787536998
Guinet C. (1992). Comportement de chasse des orques (Orcinus orca) autour des Iles Crozet. Can. J. Zoology 70, 1656–1667. doi: 10.1139/z92-231
Guinet C., Barrett-Lennard L. G., Loyer B. (2006). Co-ordinated attack behavior and prey sharing by killer whales at crozet archipelago: strategies for feeding on negatively-buoyant prey. Mar. Mammal Sci. 16, 829–834. doi: 10.1111/j.1748–7692.2000.tb00976.x
Hilcorp Alaska L. (2018). Lower Cook Inlet 2018 airborne gravity and magnetic data survey environmental evaluation document (Anchorage, AK: Hilcorp Alaska, LLC).
Hobbs R. C., Laidre K. L., Mahoney B. A., Eagleton M. (2005). Movements and area use of belugas, delphinapterus leucas, in a Subarctic Alaskan estuary. Arctic 58, 331–340. doi: 10.14430/arctic447
Hollowell G., Otis E. O., Ford E. (2015). 2014 Lower Cook Inlet Area Finfish Management Report (Homer, AK: Alaska Deparmtment of Fish and Game).
Huntington H. P. (2000). Traditional knowledge of the ecology of belugas, Delphinapterus leucas, in Cook Inlet, Alaska. Mar. Fisheries Rev. 62, 134–140.
Ivanchikova J., Tregenza N. (2023). Validation of the F-POD-A fully automated cetacean monitoring system. PloS One 18, e0293402. doi: 10.1371/journal.pone.0293402
Kavanagh A. S., Nykanen M., Hunt W., Richardson N., Jessopp M. J. (2019). Seismic surveys reduce cetacean sightings across a large marine ecosystem. Sci. Rep. 9, 19164. doi: 10.1038/s41598–019-55500–4
Lammers M. O., Castellote M., Small R. J., Atkinson S., Jenniges J., Rosinski A., et al. (2013). Passive acoustic monitoring of Cook Inlet beluga whales (Delphinapterus leucas). J. Acoustical Soc. America 134, 2497–2504. doi: 10.1121/1.4816575
Lydersen C., R. M. A., M. K. K., I. G. (2001). Summer and autumn movements of white whales Delphinapterus leucas in Svalbard, Norway. Mar. Ecol. Prog. Ser. Mar. Ecol. Prog. Ser. 219, 265–274. doi: 10.3354/meps219265
Mahoney B. A., Shelden K. E. W. (2000). Harvest history of belugas, Delphinapterus leucas, in Cook Inlet, Alaska. Mar. Fisheries Rev. 62, 124–133.
Malinowski S. J., Gloza I. (2002). Underwater noise characteristics of small ships. Acta Acustica United Acustica 88, 718–721.
Marston B., Frothingham A. (2022). Upper Cook Inlet Commercial Fisheries Annual Management Report 2020 (Anchorage: Alaska Department of Fish and Game).
Martin M. J., Halliday W. D., Storrie L., Citta J. J., Dawson J., Hussey N. E., et al. (2022). Exposure and behavioral responses of tagged beluga whales (Delphinapterus leucas) to ships in the Pacific Arctic. Mar. Mammal Sci. 39, 387–421. doi: 10.1111/mms.12978
McHuron E. A., Castellote M., Himes Boor G. K., Shelden K. E. W., Warlick A. J., McGuire T. L., et al. (2023). Modeling the impacts of a changing and disturbed environment on an endangered beluga whale population. Ecol. Model. 483, 110417. doi: 10.1016/j.ecolmodel.2023.110417
Meadows R., Cooperman A., Koleva M., Draxl C., Kilcher L., Baca E., et al. (2023). Feasibility Study for Renewable Energy Technologies in Alaska Offshore Waters. Ed. B.o.O.E. Management (Anchorage, AK: U.S. Department of the Interior).
Miller P. J. O., Isojunno S., Siegal E., Lam F. A., Kvadsheim P. H., Cure C. (2022). Behavioral responses to predatory sounds predict sensitivity of cetaceans to anthropogenic noise within a soundscape of fear. Proc. Natl. Acad. Sci. 119, e2114932119. doi: 10.1073/pnas.2114932119
Mooney T. A., Castellote M., Jones I., Rouse N., Rowles T., Mahoney B., et al. (2020). Audiogram of a Cook Inlet beluga whale (Delphinapterus leucas). J. Acoustical Soc. America 148, 3141. doi: 10.1121/10.0002351
Mooney T. A., Castellote M., Quakenbush L., Hobbs R., Gaglione E., Goertz C. (2018). Variation in hearing within a wild population of beluga whales (Delphinapterus leucas). J. Exp. Biol. 221, 171959. doi: 10.1242/jeb.171959
NMFS (2008). Conservation Plan for the Cook Inlet beluga whale (Delphinapterus leucas) (Juneau, Alaska: National Marine Fisheries Service). Available at: https://www.federalregister.gov/documents/2011/04/11/2011-8361/endangered-and-threatened-species-designation-of-critical-habitat-for-cook-inlet-beluga-whale.
NMFS (2011). Endangered and Threatened Species: Designation of Critical Habitat for Cook Inlet Beluga Whale. Ed. N.O.a.A. Administration (Washington, DC: National Oceanic and Atmospheric Administration). Available at: https://www.federalregister.gov/documents/2011/04/11/2011-8361/endangered-and-threatened-species-designation-of-critical-habitat-for-cook-inlet-beluga-whale.
NMFS (2016). Recovery Plan for the Cook Inlet Beluga Whale (Delphinapterus leucas) (Juneau: National Marine Fisheries Service Alaska Region).
NMFS (2023). Effects of Proposed Amendment 16 to the Fishery Management Plan for the Salmon Fisheries in the EEZ off Alaska on ESA-Listed Species (Washington, DC: National Oceanic and Atmospheric Administration). Available at: https://www.fisheries.noaa.gov/action/amendment-16-fmp-salmon-fisheries-alaska add color with link.
NOAA (2019). Takes of Marine Mammals Incidental to Specified Activities; Taking Marine Mammals Incidental to Oil and Gas Activities in Cook Inlet, Alaska (Washington, DC: National Oceanic and Atmospheric Administration). Available at: https://www.federalregister.gov/documents/2021/01/25/2021-01426/takes-of-marine-mammals-incidental-to-specified-activities-taking-marine-mammals-incidental-to-oil.
Nowacek D. (2013). Responsible practices for minimizing and monitoring environmental impacts of marine seismic surveys with an emphasis on marine mammals. Aquat. Mammals 39, 356–377. doi: 10.1578/AM.39.4.2013.356
Polasek L., Castellote M., Nelson M., Wooler M. J. (2021). Foraging Ecology and Habitat Use of Cook Inlet Beluga Whales (Delphinapterus leucas) - Final Report (Anchorage, AK: Alaska Department of Fish and Game).
Quakenbush L. T., Suydam R. S., Brown A. L., Lowry L. F., Frost K. J., Mahoney B. A. (2015). Diet of beluga whales, Delphinapterus leucas, in Alaska from stomach contents, March-November. Mar. Fisheries Rev. 77, 70–84. doi: 10.7755/MFR
Robards M. D., Piatt J. F., Ketle A. B., Abookire A. A. (1999). Temporal and geographic variation in fish communities of lower Cook Inlet, Alaska. Fisheries Bull. 97, 962–977.
Rugh D. J., Shelden K. E. W., Hobbs R. C. (2010). Range contraction in a beluga whale population. Endangered Species Res. 12, 69–75. doi: 10.3354/esr00293
Rugh D. J., Shelden K. E. W., Mahoney B. A. (2000). Distribution of belugas, Delphinapterus leucas, in Cook Inlet, Alaska, during June/July 1993–2000. Mar. Fisheries Rev. 62, 6–21.
Ruthrauff D. R., Gill R. E. J., Tibbitts T. L. (2013). Coping with the cold: an ecological context for the abundance and distribution of rock sandpipers during winter in upper Cook Inlet, Alaska. Arctic 66, 269–278. doi: 10.14430/arctic4306
Saulitis E., Holmes L. A., Matkin C., Wynne K., Ellifrit D., St-Amand C. (2015). Short Note: Biggs Killer Whale (Orcinus orca) Predation on Subadult Humpback Whales (Megaptera novaeangliae) in Lower Cook Inlet and Kodiak, Alaska. Aquat. Mammals 41, 341–344. doi: 10.1578/AM.41.3.2015.341
Schmidt J. H., Wilson T. L., Thompson W. L., Mangipane B. A. (2022). Integrating distance sampling survey data with population indices to separate trends in abundance and temporary immigration. J. Wildlife Manage. 86, 22185. doi: 10.1002/jwmg.22185
Shelden K. E. W., Agler B. A., Brueggeman J. J., Cornick L. A., Speckman S. G., Prevel-Ramos A. (2014). Harbor Porpoise, Phocoena phocoena vomerina, in Cook Inlet, Alaska. Mar. Fisheries Rev. 76, 22–50. doi: 10.7755/MFR.76.1–2.2
Shelden K. E. W., Goetz K. T., Hobbs R. C., Hoberecht L. K., Laidre K. L., Mahoney B. A., et al. (2018). Beluga Whale, Delphinapterus leucas, Satellite-Tagging and Health Assessments in Cook Inlet, Alaska 1999 to 2002. (Seattle WA: Alaska Fisheries Science Center, NOAA, National Marine Fisheries Service).
Shelden K. E. W., Goetz K. T., Rugh D. J., Calkins D. G., Mahoney B. A., Hobbs R. (2016). Spatio-temporal changes in beluga whale, Delphinapterus leucas, distribution: results from aerial surveys, (1977–2014), opportunistic sightings, (1975–2014), and satellite tagging, (1999–2003) in Cook Inlet, Alaska. Mar. Fisheries Rev. 77, 1–31. doi: 10.7755/MFR
Shelden K., Hobbs R. (2015). The status of beluga whales, Delphinapterus leucas, in Cook Inlet, Alaska, and surrounding waters: part I: preface. MFR Mar. Fisheries Rev. 77, i. doi: 10.7755/MFR
Shelden K. E. W., Mahoney B. A., O’Corry-Crowe G., Stanek R. T., Frost K. J. (2021). Beluga, Delphinapterus leucas, harvest in Cook Inlet, Alaska 1987 to 2022. Ed. D. Commerce Seattle WA: Alaska Fisheries Science Center, NOAA, National Marine Fisheries Service.
Shelden K. E. W., Rugh D. J., Goetz K. T., Sims C. L., Brattström V., Mocklin J. A., et al. (2013). “Aerial surveys of Beluga whales, Delphinapterus leucas,” in Cook Inlet, Alaska, June 2005 to 2012. Ed. C. Alaska Fisheries Science Seattle WA: Alaska Fisheries Science Center, NOAA, National Marine Fisheries Service.
Shelden K. E. W., Rugh D. J., Mahoney B. A., E. D. M. (2003). Killer whale predation on belugas in Cook Inlet, Alaska: implications for a depleted population. Mar. Mammal Sci. 19, 529–544. doi: 10.1111/j.1748-7692.2003.tb01319.x
Sih A. (2013). Understanding variation in behavioural responses to human-induced rapid environmental change: a conceptual overview. Anim. Behav. 85, 1077–1088. doi: 10.1016/j.anbehav.2013.02.017
Speckman S. G., Piatt J. F., Minte-Vera C. V., Parrish J. K. (2005). Parallel structure among environmental gradients and three trophic levels in a subarctic estuary. Prog. Oceanography 66, 25–65. doi: 10.1016/j.pocean.2005.04.001
Tarbox K. E. (1988). Migratory rate and behavior of salmon in upper Cook Inlet, Alaska 1983–1984. Fishery Res. Bull. 88, 1–67.
Thompson P. M., Graham I. M., Cheney B., Barton T. R., Farcas A., Merchant N. D. (2020). Balancing risks of injury and disturbance to marine mammals when pile driving at offshore windfarms. Ecol. Solutions Evidence 1, 12034. doi: 10.1002/2688-8319.12034
USACE (2020). Pebble Project. Environmental Impact Statemement. Executive Summary (Anchorage, AK: U.S. Army Corps of Engineers).
USFWS (1973). Endangered Species Act of 1973 As Amended through the 108th Congress. Ed. D.o.t. Interior (Washington DC: U.S. Fish and Wildlife Service).
Welch P. (1967). The use of fast fourier transform for the estimation of power spectra: a method based on time averaging over short, modified periodograms. IEEE Trans on Audio and Electroacoustics. 15, 70–73. doi: 10.1109/TAU.1967.1161901
Wensveen P. J., Isojunno S., Hansen R. R., von Benda-Beckmann A. M., Kleivane L., van I. S., et al. (2019). Northern bottlenose whales in a pristine environment respond strongly to close and distant navy sonar signals. Proceedings: Biol. Sci. 286, 20182592. doi: 10.1098/rspb.2018.2592
Westdal K. H., Davies J., McPherson A., Orr J., Ferguson S. H. (2016). Behavioural changes in Belugas (Delphinapterus leucas) during a Killer Whale (Orcinus orca) attack in southwest Hudson Bay. Can. field-naturalist 130, 315–319. doi: 10.22621/cfn.v130i4.1925
Wiggins S. M., Hildebrand J. A. (2007). “High-frequency acoustic recording package (HARP) for broad-band, long-term marine mammal monitoring,” in International symposium on underwater technology and international workshop on scientific use of submarine cables & related technologies: IEEE. (Tokyo: Institute of Electrical and Electronics Engineers (IEEE)). 551–557.
Wiggins S. M., Soldevilla M. S., Roch M. A., Hoenigman R. (2007). Triton User Manual: A software package for evaluating acoustic data sets (San Diego: Scripps Institution of Oceanography).
Williams R., Erbe C., Ashe E., Clark C. W. (2015). Quiet(er) marine protected areas. Mar. pollut. Bull. 100, 154–161. doi: 10.1016/j.marpolbul.2015.09.012
Young N. C., Brower A. A., Muto M. M., Freed J. C., Angliss R. P., Friday N. A., et al. (2023). Alaska marine mammal stock assessment (Seattle, WA: Marine Mammal Laboratory. Alaska Fisheries Science Center). Available at: https://www.fisheries.noaa.gov/national/marine-mammal-protection/marine-mammal-stock-assessment-reports.
Keywords: white whale, F-POD, endangered, acoustics, anthropogenic noise, masking, predation pressure, ship noise
Citation: Castellote M, Gill VA, Garner CD, Gilstad AJ, Hou BX, Brewer AM and Knoth JM (2024) Using passive acoustics to identify a quiet winter foraging refuge for an endangered beluga whale population in Alaska. Front. Mar. Sci. 11:1393380. doi: 10.3389/fmars.2024.1393380
Received: 08 March 2024; Accepted: 21 May 2024;
Published: 15 July 2024.
Edited by:
Todd Atwood, U.S. Geological Survey, United StatesReviewed by:
William Halliday, Wildlife Conservation Society, CanadaCarlos Hipolito Romero, University of Florida, United States
Copyright © 2024 Castellote, Gill, Garner, Gilstad, Hou, Brewer and Knoth. This is an open-access article distributed under the terms of the Creative Commons Attribution License (CC BY). The use, distribution or reproduction in other forums is permitted, provided the original author(s) and the copyright owner(s) are credited and that the original publication in this journal is cited, in accordance with accepted academic practice. No use, distribution or reproduction is permitted which does not comply with these terms.
*Correspondence: Manuel Castellote, bWFudWVsLmNhc3RlbGxvdGVAbm9hYS5nb3Y=