- 1Posgrado en Biología, Sistema de Estudios de Posgrado, Universidad de Costa Rica, San José, Costa Rica
- 2Centro de Investigación en Ciencias del Mar y Limnología (CIMAR), Universidad de Costa Rica, San José, Costa Rica
- 3Centro de Investigación en Biodiversidad y Ecología Tropical (CIBET), Escuela de Biología, Universidad de Costa Rica, San José, Costa Rica
- 4Escuela de Biología, Universidad de Costa Rica, San José, Costa Rica
The decline of coral reefs has increased interest in ecological restoration. Due to the scarcity of coral gardening projects in the Eastern Tropical Pacific, improving our understanding of such techniques is key. We report the results of coral gardening using the branching Pocillopora spp. and massive coral species (Pavona gigantea, Pavona clavus and Porites lobata) in an upwelling area in Costa Rica. We examined whether nursery type influenced Pocillopora spp. survival and growth, and how environmental conditions shaped restoration. We monitored the survival and growth of Pocillopora spp. fragments (n = 334) and microfragments of massive species (P. gigantea [n = 148], P. clavus [n = 37], P. lobata [n = 66]) over 11 months. Survival at the end of the gardening period was 51% for Pocillopora spp., 59% for P. clavus, 55% for P. gigantea, and 17% for P. lobata, with a decline after a cease in maintenance caused by the COVID-19 lockdown. Pocillopora spp. fragments in the floating nurseries exhibited higher growth (7.52 ± 1.98 and 6.64 ± 2.91 cm yr-1) than in the A-frame (4.16 ± 2.35 cm yr-1), which suggests the benefits of suspending fragments. For massive microfragments coral growth was 1.92-4.66 cm2 yr-1 and were affected by pigmentation loss, causing partial tissue loss and mortality. Our results point towards acclimation to local conditions, and show the need to develop site-specific cost-efficient gardening techniques for massive species, allowing for a multi-species approach to ensure long-term ecosystem recovery.
1 Introduction
Coral reefs are facing a wide array of local and global threats (Hoegh-Guldberg et al., 2007; Glynn et al., 2017; Hughes et al., 2017) that put them at risk (Dixon et al., 2022). Since traditional conservation efforts (e.g., marine protected areas) have not always ensured meeting conservation goals (Possingham et al., 2015; Pendleton et al., 2018), and the ability of corals to acclimatize to changing conditions rapidly enough is highly dubious (Torda et al., 2017), there is an increasing interest in active conservation approaches, such as coral gardening and ecological restoration (Rinkevich, 2014).
Several approaches are used for coral reef restoration. Asexual methods, such as the direct transplantation of coral fragments or coral gardening, are the most widespread (Bayraktarov et al., 2019). Coral gardening incorporates an initial-phase during which coral fragments grow in ex situ or in situ nurseries, protected from sedimentation, predation and competition; where coral growth and survival increases (Shafir and Rinkevich, 2010; Lirman et al., 2014; Rinkevich, 2014; Afiq-Rosli et al., 2017). Different types of structures can serve as in situ coral nurseries, such as mid-water floating structures (e.g., coral trees, rope nurseries, platforms) or fixed on the seafloor (e.g., tables, coral spiders, A-frames) (Rinkevich, 2014). The type of nursery used will depend on the local oceanographic conditions and coral growth strategies form (Young et al., 2012), and it has been seen to influence coral performance (Hernández-Delgado et al., 2014; O’Donnell et al., 2017; Kuffner et al., 2017; Schopmeyer et al., 2017). Therefore, it is vital to determine the adequate structures for each specific site and species.
Throughout the Eastern Tropical Pacific (ETP), the number of coral restoration projects is increasing, only few have use coral gardening approach (Bayraktarov et al., 2020; Ishida-Castañeda et al., 2020). The ETP is characterized by suboptimal conditions for reef development (Glynn and Ault, 2000): the region is affected by the El Niño-Southern Oscillation (ENSO), which can lead to coral bleaching and mortality (Glynn, 1984), presents low aragonite saturation (Rixen et al., 2012; Sánchez-Noguera et al., 2018b), and includes areas influenced by seasonal upwelling (Cortés, 1997). Reefs in the ETP are discontinuous, narrow, and composed by few coral species (Glynn et al., 2017). As most reef sites in the ETP present high abundance and dominance of the branching Pocillopora corals, restoration efforts mainly focus on this genus (Liñán-Cabello et al., 2011; Tortolero-Langarica et al., 2014; Ishida-Castañeda et al., 2020; Combillet et al., 2022), and limited work exists on the so-far challenging massive species (Tortolero-Langarica et al., 2020; Vargas-Ugalde et al., 2020). Furthermore, the influence of nursery design, microfragmentation, life history of donor colonies, and local environmental variables on the variation of coral fragment growth remain poorly studied (Schopmeyer et al., 2017; Knapp et al., 2022).
Bahía Culebra, located in the seasonal upwelling North Pacific of Costa Rica, historically harbored some of the most extensive coral reefs in the area, built mainly by the genera Pocillopora (Jiménez, 2001; Cortés and Jiménez, 2003). Mean live coral cover has drastically collapsed in the last two decades, from 44.0 ± 0.3% (>90% in some sites) in the 1990s (Jiménez, 2001) to 1-4% in 2011, due to several disturbances (Sánchez-Noguera et al., 2018a), such as ENSO, anthropic eutrophication, harmful algal blooms, the proliferation of the macroalgae Caulerpa sertularioides, and a population outbreak of the sea urchin Diadema mexicanum (Alvarado et al., 2012; Fernández-García et al., 2012). This resulted in widespread coral bleaching and mortality (Sánchez-Noguera, 2012), and as consequence, coral framework rapidly weakened (Alvarado et al., 2012, 2016), and some reefs completely depleted (Sánchez-Noguera, 2012; Arias-Godínez et al., 2019).
Despite these adverse circumstances, reefs in Bahía Culebra are still of socioeconomic importance (Sánchez-Noguera, 2012). Therefore, efforts to restore them are needed to ensure their long-term maintenance, ecological functionality, and integrity. The semi-enclosed nature of the bay, protected from strong wave action (Sánchez-Noguera, 2012), makes it a uniquely suitable site for the establishment of coral nurseries and the development of a coral gardening project. Given the conditions that seasonal upwelling brings (Rixen et al., 2012; Stuhldreier et al., 2015), the lack of previous restoration experiences, it is necessary to improve our understanding of coral gardening techniques and their potential to determine the most effective approach for these conditions.
This study represents the assessment of the first restoration project in the area, in order to evaluate the success of different coral gardening techniques. The goals of this study are to (1) monitor the health and survival of fragments of the branching Pocillopora spp. and massive Pavona gigantea, Pavona clavus and Porites lobata corals during nursery stage, (2) quantify the growth rate of arranged coral species, and (3) determine the effect of nursery type and environmental conditions on coral fragment growth and survival.
2 Materials and methods
2.1 Study area
Bahía Culebra (10°37’N, 85°39’W) is a semi-enclosed bay in the Gulf of Papagayo (Figure 1), which extends for more than 20 km2 and reaches 42 m in depth (Rodríguez-Sáenz and Rodríguez-Fonseca, 2004). It is in one of the three seasonal upwelling areas in the ETP, which affects the region from December to April (Jiménez et al., 2010; Alfaro and Cortés, 2011), and bring up more acidic (pH 7.8) and nutrient-rich waters (Rixen et al., 2012; Stuhldreier et al., 2015; Sánchez-Noguera et al., 2018b).
2.2 Experimental design
Three structures types were used as in situ coral nurseries, which differ in terms of their position in the water column, design, and materials. Coral trees (Figure 2A) consist of a central PVC column and fiberglass rods, in which coral fragments are hung using monofilament lines. Rope line nurseries (Figure 2B) are constructed with a series of ropes attached to two PVC tubes at each end, and the small coral fragments are inserted into the coils of the ropes. While these two nurseries are suspended in the water column, A-frames (Figure 2C) are benthic-attached structures built out of electro-welded wire mesh bent to form an “A” shape, with coral fragments attached using plastic cable ties. All coral nurseries were placed at a depth of 5 m.
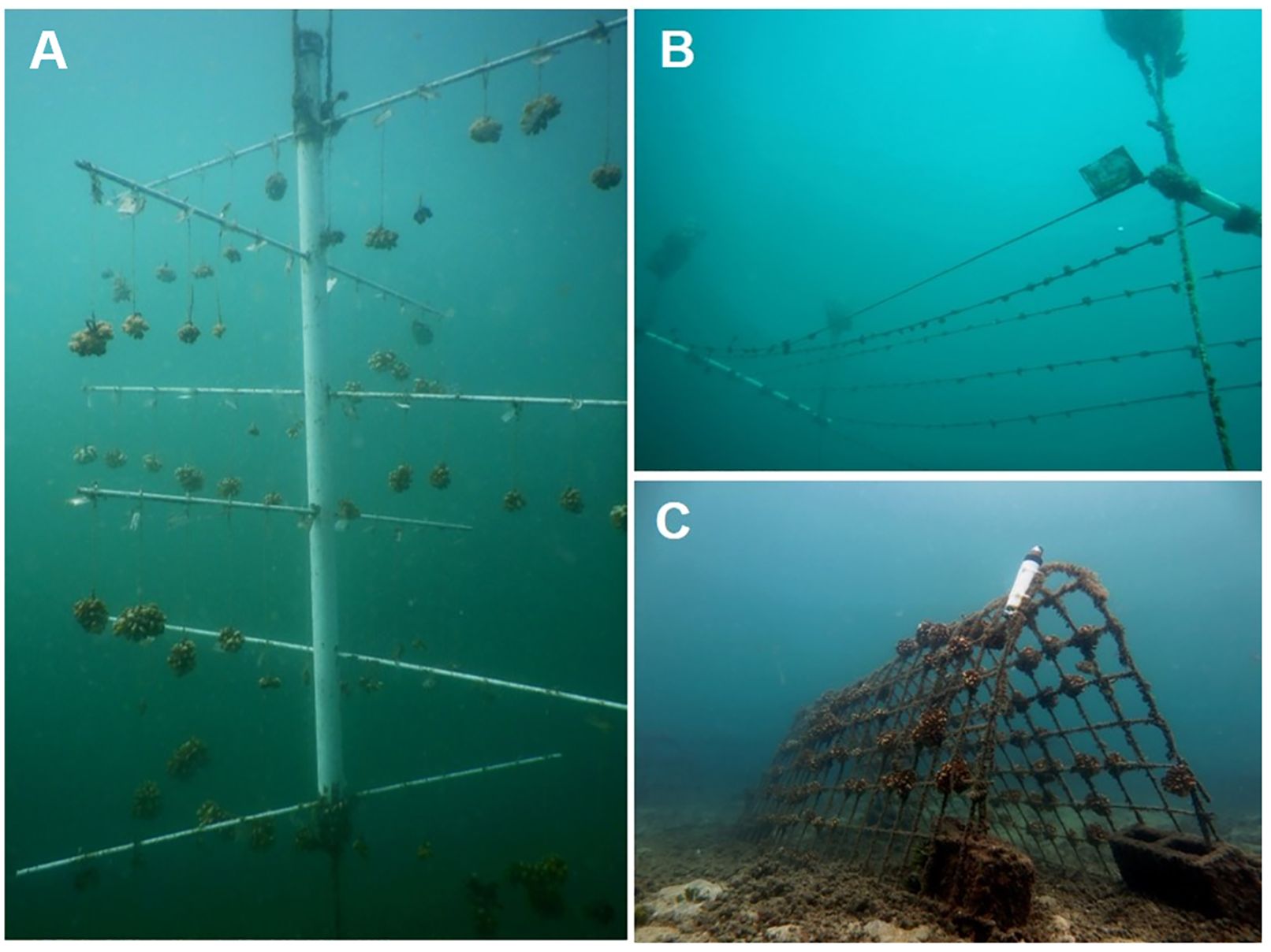
Figure 2. Nurseries used for cultivation of Pocillopora spp. fragments in Playa Jícaro, Costa Rica. (A) Coral tree, (B) rope line nursery, (C) A-frame structure.
Coral fragments (n = 585) from four species (Pocillopora spp., n = 334; P. gigantea, n = 148; P. clavus, n = 37; and P. lobata, n = 66) were obtained from donor colonies on eight different reefs and coral communities around Bahía Culebra (Figure 1). Donor colonies were randomly selected at depths between 2-7 m, growing at least 5 m from each other to maximize the chance of sampling distinct genets. Five fragments of 2-5 cm were extracted from each donor colony in the case of Pocillopora spp. colonies. In this study P. damicornis and P. elegans are grouped as “Pocillopora spp.” This categorization is employed due to the inherent difficulty in discriminating between individual species in the field based solely on their morphology (Pinzón et al., 2013). For massive species microfragments 1.5-2 cm2 were obtained from donor colonies, using a diamond band saw (Gryphon®, Model C-40), and then were glued to a ceramic disk (Figure 3). Fragments were tagged individually and placed only in coral trees nurseries at Playa Jícaro (10°37’11.388”N, 85°40’32.916”W) (Figures 2, 3A), whereas Pocillopora spp. fragments were arrayed in the three different nurseries: coral tree (n = 72), rope line nursery (n = 150), and A-frame (n = 112).
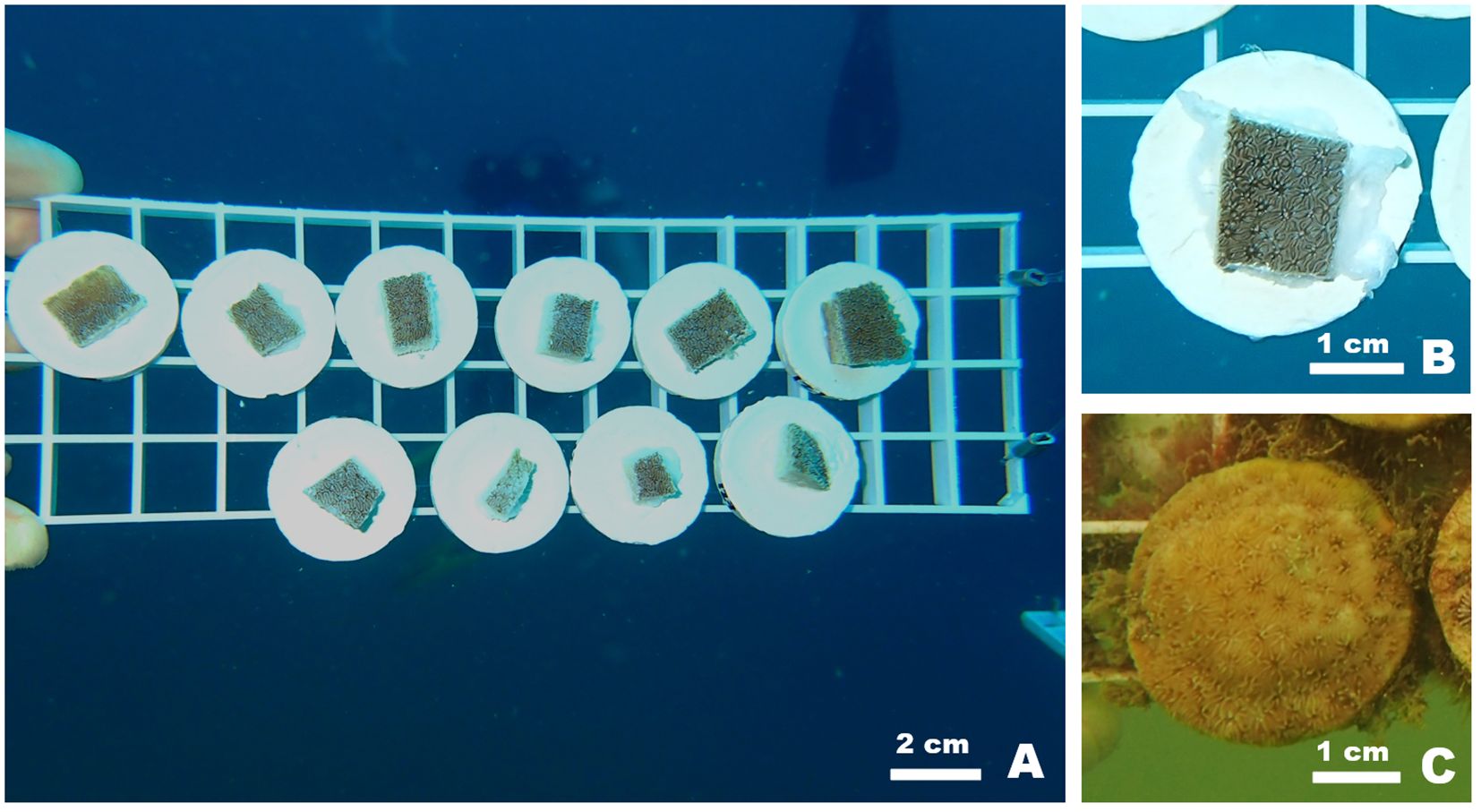
Figure 3. Microfragments of massive species cultivated in the coral tree nursery (A), and growth of the same Pavona gigantea microfragment at the start of the nursery stage in September 2019 (B) and by the end of its cultivation, in August 2020 (C), in the nursery site in Playa Jícaro, Costa Rica.
The experiment was conducted between September 2019 and August 2020, over a period of 330 days for mid-water floating nurseries. For A-frames, the experiment extended from August 2020 to August 2021, covering a period of 365 days. Maintenance, carried out twice a month when conditions allowed, involved using brushes to remove fouling organisms from all nursery structures.
In addition, costs (in USD) of nursery building and installation, and fragment production were calculated, excluding indirect expenses (i.e., travel, accommodation, scuba gear, maintenance, and monitoring). For each nursery, we calculated the cost per fragment considering their capacity and the final cost per fragment obtained, considering final survivorship by the end of their nursery stage (Supplementary Table S1).
2.3 Data collection
To evaluated coral growth, in situ photographs of each fragment were taken monthly, which were later analyzed using ImageJ software to measure the length (cm), and area (cm2) for Pocillopora spp. fragments and the area (cm2) of each massive microfragment. As for Pocillopora spp., despite their branching growth, there is a greater vertical extension upwards, which we aimed to measure using the length of each fragment. Photographs were taken from the same angle and direction, with a plastic caliper as reference, using an Olympus Tough TG-6 underwater camera. The survival and health condition of each individual coral fragment (alive, pale/bleached, tissue lost, dead or lost) was recorded. Lost fragment and those with <10% of live tissue were considered as dead, as probability of recovery was considered extremely low. No data were obtained during March 2020 due to the COVID-19 pandemic national lockdown.
To characterize environmental conditions, water samples were collected each month to determine salinity (PSU) and nutrient concentration (NO3-, NO2-, PO43-, NH4+, and SiO4), using a continuous flow autoanalyzer (QuikChem 8500, Lachat Instruments). Because of the COVID-19 pandemic national lockdown, water samples for nutrient concentration and salinity were not collected between March and June 2020. Seawater temperature was recorded every 30 min using in situ HOBO® data loggers.
2.4 Data analysis
Kaplan-Meier tests were used to determine whether the survival curves of Pocillopora spp. fragments differed significantly between nursery types and donor colony sites. Only donor colony sites that were shared between the A-frame, rope line, and coral tree nurseries were included in the comparison between nursery types. Comparisons between donor colony sites were evaluated separately for each nursery type. A Kaplan-Meier test was also used to determine whether the survival curves of microfragments of P. clavus, P. gigantea, and P. lobata differed significantly. Mean annual coral growth in length (cm yr-1) and area (cm2 yr-1) were estimated. Only those fragments that had survived throughout the experimental period were considered for calculation of growth rates. For Pocillopora spp., the length and area growth of each fragment were subtracted from the measurement of the following month, whereas the growth rate of massive microfragments was only calculated in terms of area increase. Data were tested for normality and equality of variances using the Shapiro-Wilk test and Bartlett test to ensure they met model assumptions. Differences in Pocillopora spp. fragment growth rates between nurseries were analyzed using a one-way ANOVA and post-hoc Tukey tests. Massive species growth rates were compared between species using a one-way ANOVA. All statistical analysis was performed in R (R Development Core Team, 2021).
3 Results
3.1 Coral fragment survival
After 10 months, 51% of Pocillopora spp. fragments had survived. The survival curves of Pocillopora spp. fragments differed between the nurseries (χ2 = 109, p < 0.001) (Figure 4A). Fragments in the A-frame and the coral tree had similar survival curves, while the survival was significantly lower in the rope line, where coral fragments suffered a marked decrease in their survival after five months (Figure 4A). After ten months, the probability of survival was about 26% lower in the rope line than in the A-frame or the coral tree. Paleness was observed in Pocillopora spp. fragments between June and August 2021, with a peak of 21% of the remaining live fragments affected in July.
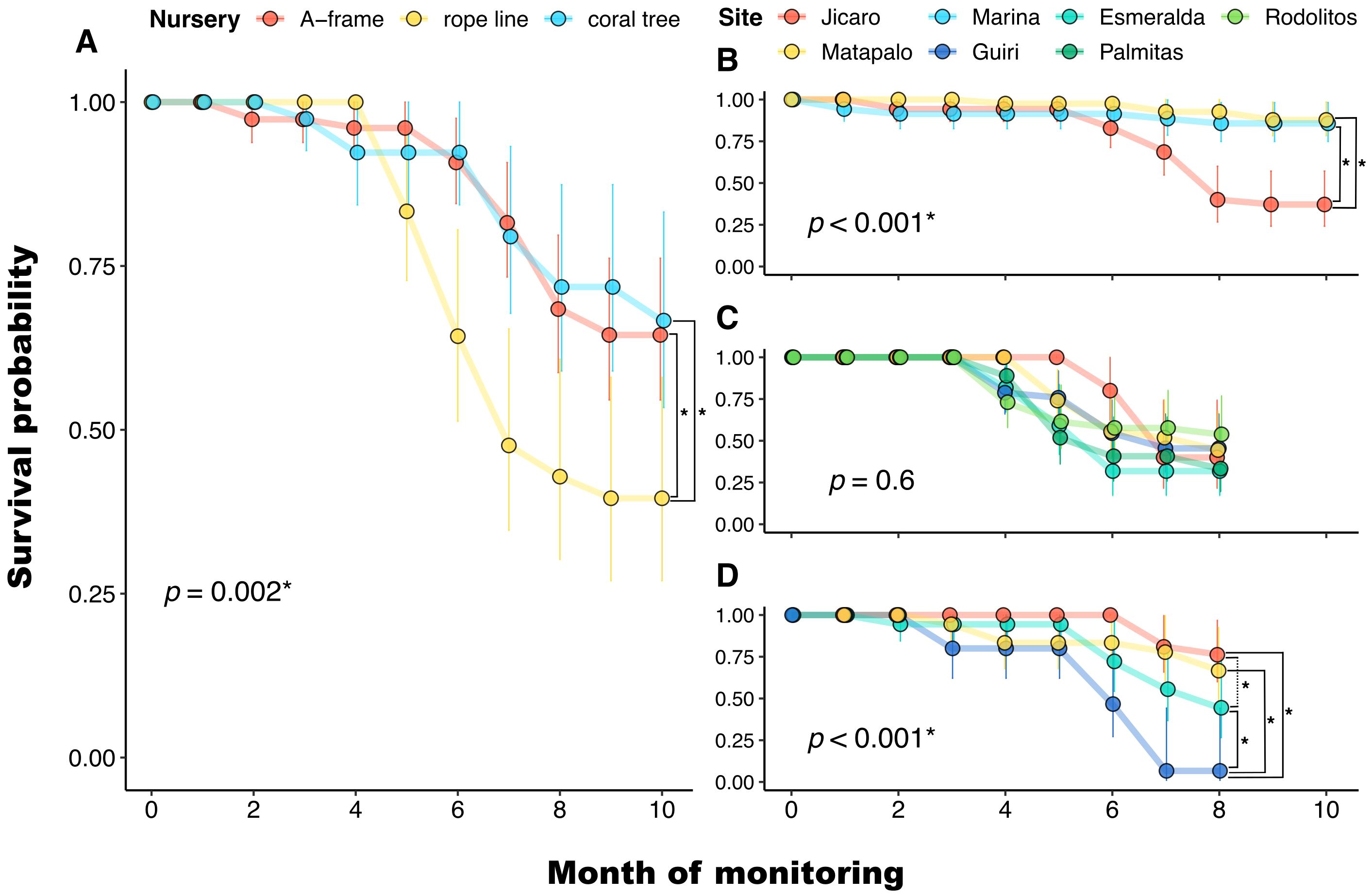
Figure 4. Kaplan-Meier survival curves of Pocillopora spp. fragments after ten months of growth in nurseries in Playa Jícaro (Bahía Culebra, Costa Rica). (A) Comparison between nurseries including only shared donor sites (Jícaro and Matapalo) (A-frames [n = 77 ], rope line [n = 42
], and coral tree [n = 39
]). Comparison between donor sites for the (B) A-frame, (C) rope line, and (D) coral tree nurseries. Error bars indicate the 95% confidence intervals. The p-value of the Kaplan-Meier test comparing the survival curves is shown in each panel. Asterisks (*) indicate significant differences (p < 0.05). Square brackets indicate a significant difference between two specific survival curves.
The survival curves of Pocillopora spp. fragments from different donor sites differed significantly for fragments growing in the A-frame (χ2 = 29.4, p < 0.001) and coral tree (χ2 = 26.8, p < 0.001) nurseries. In the case of the A-frame, the survival curves of corals from Jícaro differed from the other two sites, but no differences were detected between fragments from Matapalo and Marina, which had a higher survival than fragments from Jícaro (Figure 4B). Corals from Guiri growing in the coral tree nursery had significantly lower survival than corals from the other three sites (Figure 4D). The survival curves of corals from Jícaro and Esmeralda growing in the coral tree were also significantly different (Figure 4D). For the rope line, no significant differences were found in the survival curves of fragments from different donor sites (χ2 = 4, p = 0.6) (Figure 4C).
For massive species, final survival after nine months was 59% for P. clavus, 55% for P. gigantea, and 17% for P. lobata. Survival curves differed significantly between P. lobata and the two coral species (χ2 = 44.6, p < 0.001) (Figure 5). Microfragments experienced two episodes of bleaching/paleness in the nursery: the first, between December 2019 and February 2020 (affecting 90.4% of live fragments in January 2020) and the second, between June and August 2020. After the first event, 70.5% of microfragments recovered their pigmentation after two months. However, many fragments died or lost part of their live tissue following the second event, which was the most intense in terms of loss of pigmentation and affected 92.3% of live fragments at its peak in June 2020 (Figure 6). Fragments of massive colonies were the most susceptible to bleaching.
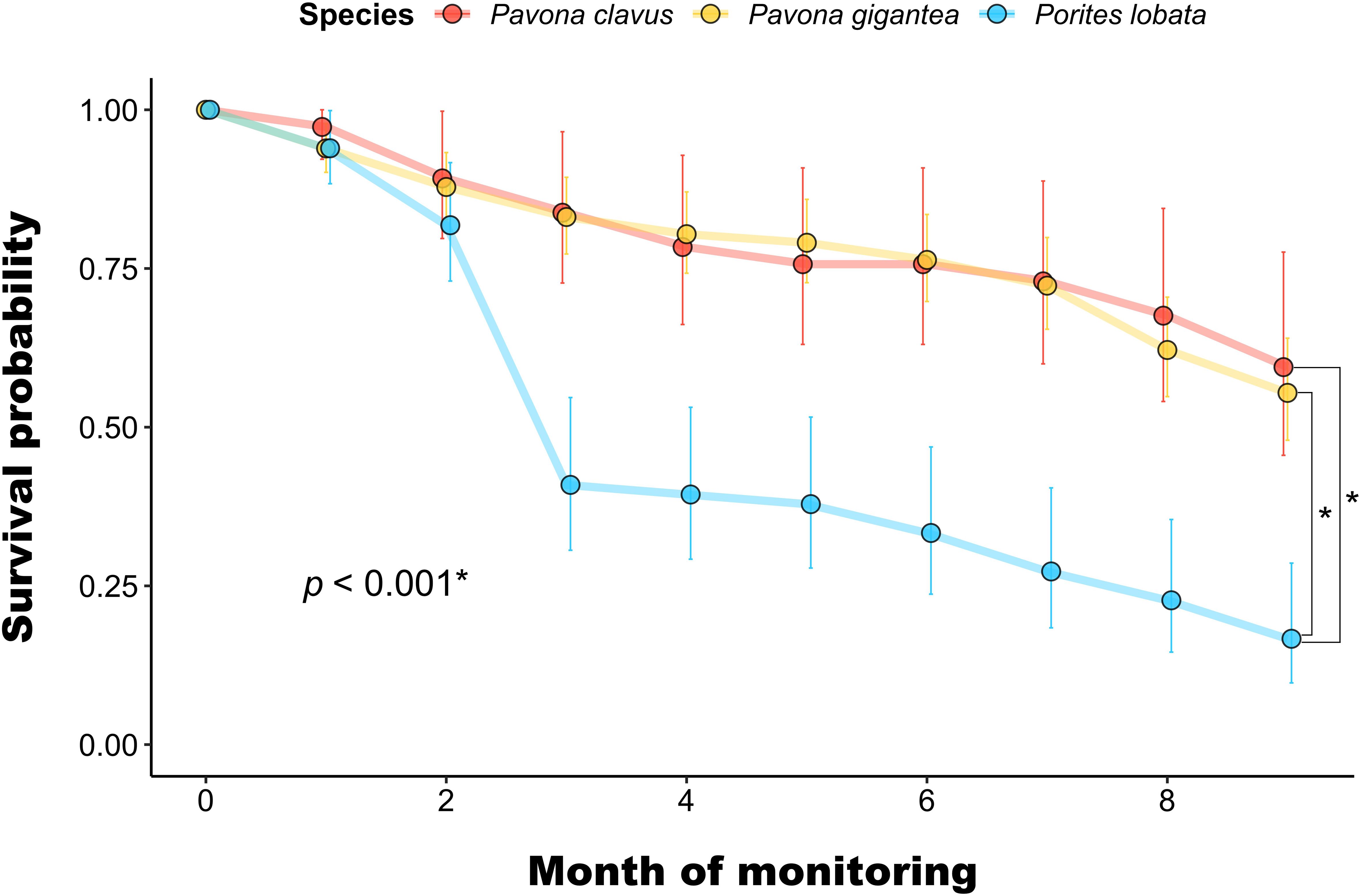
Figure 5. Kaplan-Meier survival curves of microfragments of massive Pavona clavus (n = 37 ), Pavona gigantea (n = 148
), and Porites lobata (n = 66
) after nine months of growth in the nursery site in Playa Jícaro (Bahía Culebra, Costa Rica). Error bars indicate the 95% confidence intervals. The p-value of the Kaplan-Meier test comparing the survival curves is shown. Asterisks (*) indicate significant differences (p < 0.05). Square brackets indicate a significant difference between two specific survival curves.
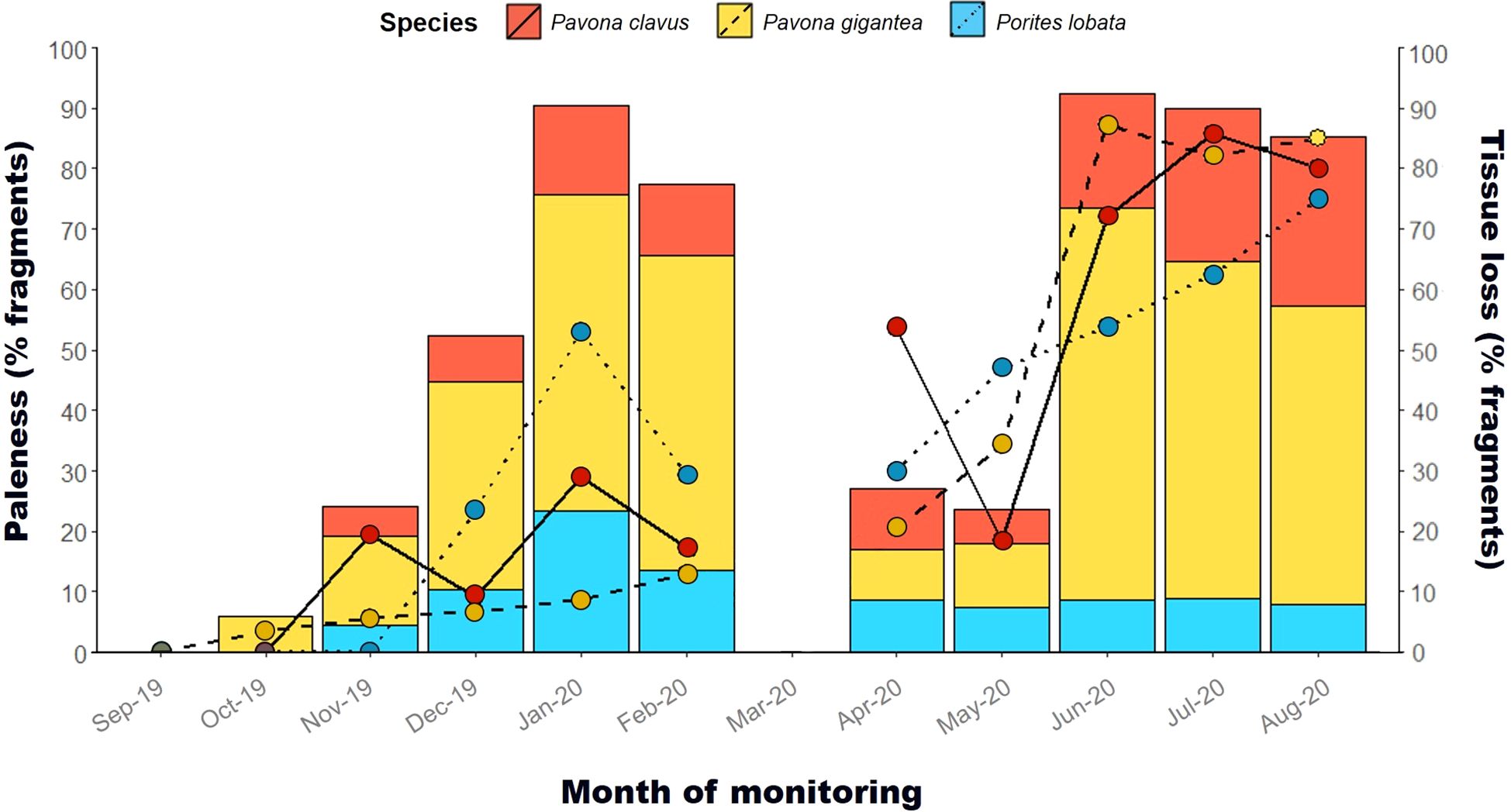
Figure 6. Proportion (% of fragments affected) of bleaching or paleness (bars), and partial tissue loss (lines) in microfragments of massive species (Pavona clavus , Pavona gigantea
, and Porites lobata
) during nursery stage (September 2019-August 2020) in Playa Jícaro (Bahía Culebra, Costa Rica). No data is available for March 2020 due to the COVID-19 pandemic national lockdown.
3.2 Coral fragment growth
Pocillopora spp. fragments placed in the nurseries were initially 3.99 ± 1.70 cm long and 6.67 ± 5.93 cm2 in area. The size of live Pocillopora spp. fragments increased to a mean of 9.04 ± 2.89 cm in length, and 45.21 ± 28.47 cm2 in area by the end of the nursery stage (t160 = -17.53, p < 0.005), which represent a 126.6% mean increase in length and a 577.8% in area from initial size. Mean growth rate of Pocillopora spp. fragments was 5.71 ± 2.88 cm yr-1 (45.03 ± 30.84 cm2 yr-1), and it was lower in A-frames than in the other two nurseries (F2,155 = 24.37, p < 0.005) (Table 1). Pocillopora spp. growth rate through time did not show any pattern, although significant differences were found among months (p < 0.05 in all cases).
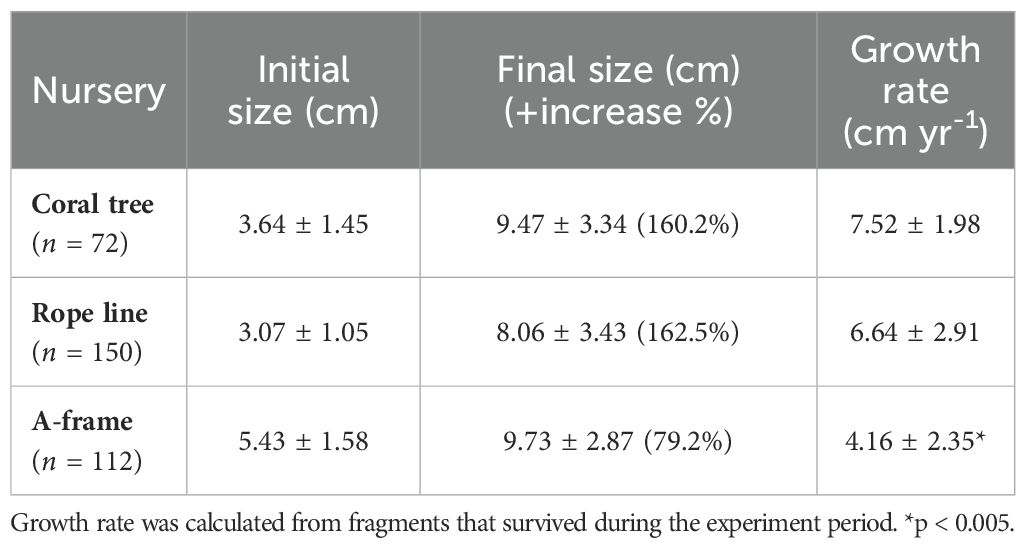
Table 1. Initial and final sizes (mean length ± SD), and mean growth rate (± SD) of Pocillopora spp. fragments cultivated in the different in situ coral nurseries in Playa Jícaro (Bahía Culebra, Costa Rica).
Pocillopora spp. annual growth rate also differed among donor sites. In the coral tree, Pocillopora spp. fragments from Esmeralda grew at a significantly higher rate than the other donor sites (F2,24 = 9.09, p < 0.005). For fragments in the rope line nursery, growth rates from Jícaro fragments were significantly lower from all other sites except for Palmitas (F5,55 = 4.27, p < 0.005). In the A-frames, fragments from Marina grew at a significantly lower rate than the site with the highest rate (F2,66 = 4.57, p < 0.05).
When comparing annual growth rates of shared donor sites between nurseries, fragments from Jícaro grew faster in coral trees (F2,22 = 16.07, p < 0.005), while fragments from Matapalo did so in the rope line nursery, statistically differing from fragments growing in the A-frame (F2,50 = 4.41, p < 0.05) (Figure 7).
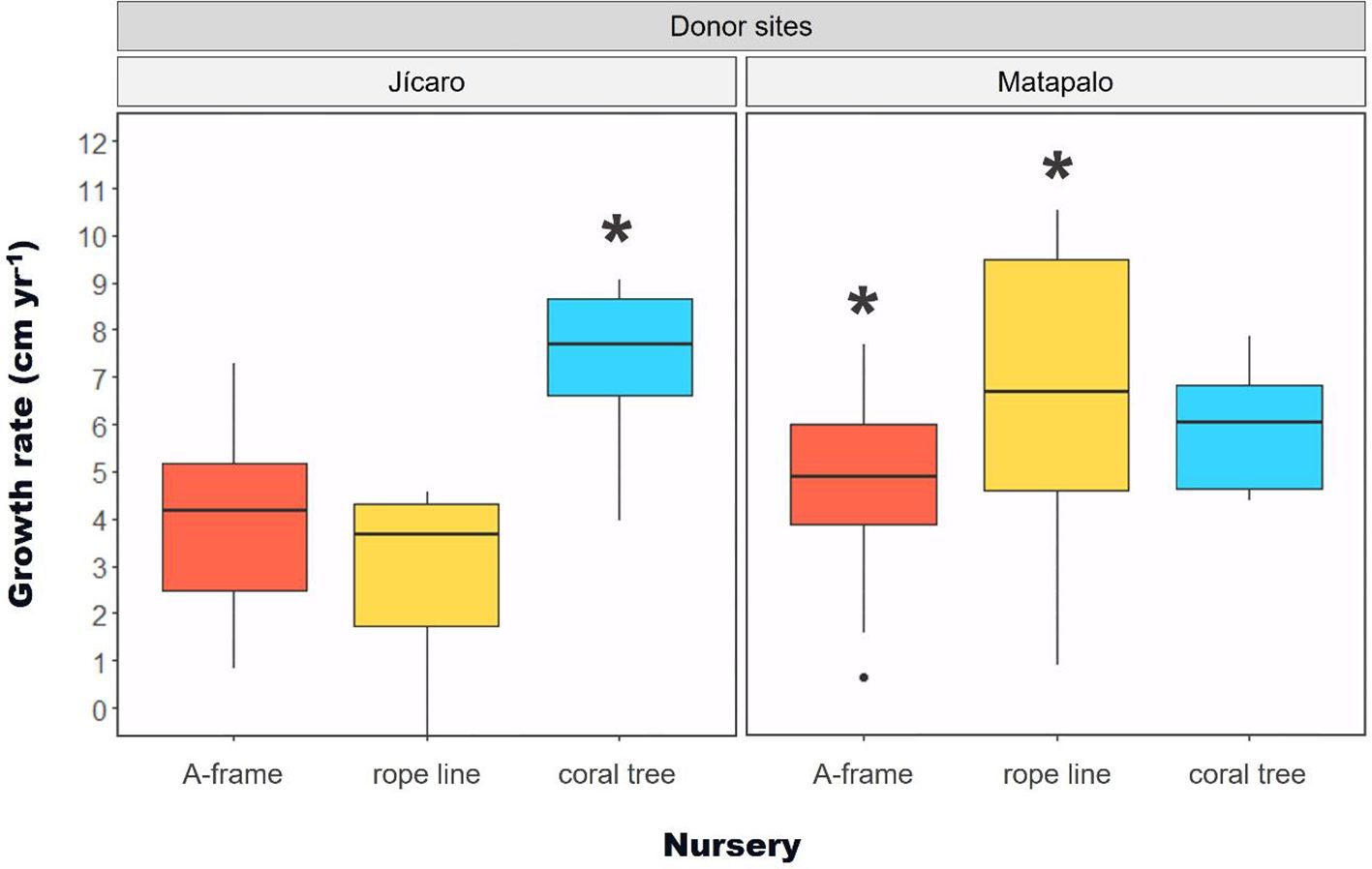
Figure 7. Box plot depicting the growth rate (cm yr-1) of Pocillopora spp. fragments from shared donor sites (Jícaro and Matapalo) between nurseries. Significance denoted by * indicates p < 0.05. Black inner lines represent mean values.
Massive species significantly increased their area from initial size 291.2% for P. gigantea fragments (R2= 0.58, F1,493 = 692.3, p < 0.005), 94.0% for P. clavus (R2 = 0.25, F1,198 = 67.57, p < 0.005), and 187.6% in P. lobata (R2 =0.54, F1,68 = 81.04, p < 0.005). Growth rates for massive species (Table 2) differed between P. gigantea and P. clavus (F2,69 = 20.98, p < 0.005), but not with P. lobata (p > 0.05).
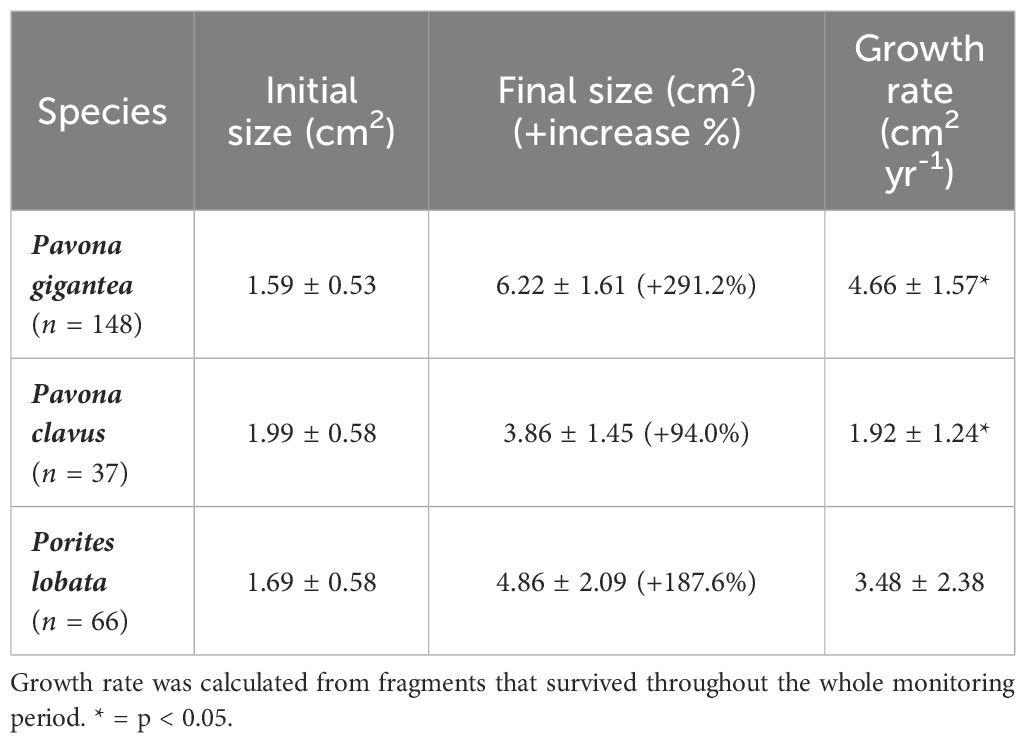
Table 2. Initial and final sizes (mean area ± SD), and mean growth rate (± SD) of microfragments of massive species cultivated in in situ coral nurseries in Playa Jícaro (Bahía Culebra, Costa Rica).
Mean seawater temperature in the nursery site was 27.47 ± 1.90°C, with a minimum of 18.53°C (March 2020) and a maximum of 31.08°C (May 2020) (Supplementary Figure S2). Significant differences in seawater temperature between upwelling (26.37 ± 1.89°C) and non-upwelling period (28.46 ± 1.27°C) were detected (t31584 = 126.14, p < 0.0005). Nonetheless, mean monthly Pocillopora spp. growth rates did not differ between seasons (coral tree: t77.998 = -0.399, p = 0.691; rope line: t263.99 = 1.531, p = 0.127; A-frame: t748.69 = 0.365, p = 0.715). For massive species, differences were only detected in P. gigantea, with a higher mean rate during the dry upwelling season (0.52 ± 0.48 cm2 mo-1) than in the rainy non-upwelling season (0.33 ± 0.50 cm2 mo-1) (t446.93 = -4.123, p < 0.005).
Mean seawater salinity was 33.37 ± 1.08 PSU and showed differences between dry upwelling (33.83 ± 0.99 PSU) and rainy non-upwelling season (32.85 ± 0.95 PSU) (t54.736 = -3.83, p < 0.0005). Nutrient concentration did not show any pattern and did not differ between seasons (p > 0.05 in all cases) (Supplementary Table S2).
4 Discussion
Coral reef restoration must optimize its techniques to be as efficient and practical as possible to ensure long-term feasibility and success. Thus, it is key that restoration efforts are adapted to regional and local conditions. We found that nursery type influences coral survival and growth, with fragments growing faster in mid-water floating nurseries. Furthermore, we found that branching species responded differently to coral gardening techniques and environmental conditions during their cultivation period, which aligns with the results found in other studies in the Philippines (Shaish et al., 2008), and different sites in the Caribbean, such as the Bahamas (Maurer et al., 2022), Florida (Goergen et al., 2018), and Puerto Rico (Aponte-Marcano et al., 2023). Fragment survival was 51% for Pocillopora spp. after 10 months and 59% for P. clavus, 55% for P. gigantea, and 17% for P. lobata after 9 months. The steep decline in survival after the fifth and sixth month in the nurseries could be partially explained by the proliferation of highly competitive and aggressive coral competitors such as algae, ascidians, barnacles, bivalves, and sponges caused by the temporary interruption (February to May 2020) in maintenance as a consequence of the COVID-19 pandemic national lockdown. This has been experienced in several projects worldwide, with some effect on coral cultivation success (Montano et al., 2022). Periodic maintenance is key to reduce competition (Edwards, 2010; Goergen et al., 2018), Montano et al., 2022), and even more so in the North Pacific of Costa Rica, where productivity is high and hence, so is competitor recruitment, particularly during upwelling season (Stuhldreier et al., 2015).
Survival is also affected by coral growth form and other species traits like colony size, as they influence the coral’s response to environmental stressors (Loya et al., 2001; Hoogenboom et al., 2017). Low survival is common for species with massive growth, which have proven to be challenging to work with in restoration efforts (Rivas et al., 2021; Knapp et al., 2022). In the Philippines, branched corals such as Pocillopora also had greater survival than massive corals (genus Porites) (Shaish et al., 2008). Microfragments of massive species underwent two bleaching/paleness episodes, the first one occurring between December 2019 and February 2020. During this period, Bahía Culebra was influenced by seasonal upwelling, with abrupt drops in seawater temperature down to 18.5°C. Even though low temperatures may elicit coral bleaching (Lirman et al., 2011), corals in the bay are periodically subject this thermal regime and are thus expected to be acclimated to these local conditions (Liñán-Cabello et al., 2011; Rodríguez-Troncoso et al., 2014). However, the process of microfragmentation may damage healthy tissue, and it drastically reduces their size; therefore, their vulnerability to stressors such as competition, particularly if it is as intense as in Bahía Culebra, increases (Ferrari et al., 2012; Forsman et al., 2015; Marín-Moraga et al., 2023), which may have resulted in the observed paleness, partial tissue loss, and mortality. This was the case in our massive coral nurseries, where algae, hydroids, barnacles, ascidians, and bivalves, rapidly and aggressively grew on the structure, as well as on and around the coral microfragments. Hence, given these particular conditions, we suggest minimizing the available surface for the potential recruitment and growth of coral competitors.
Although the first episode of loss of pigmentation affected over 90% of microfragments, most recovered their pigmentation in the following months; yet the second episode (June-August 2020) did cause widespread mortality on microfragments. The highest temperatures during nursery stages were recorded in May 2020, with maximums of 31.1°C and exceeding 30°C for 12 consecutive days, which could have caused the bleaching and paleness reported in the following month (Glynn and D’Croz, 1990). Pavonid corals have also shown little tolerance to repeated bleaching, as it reduces their resilience (Manzello, 2010). Thus, the coupled effects of a repeated loss of pigmentation episode and the simultaneous temporary cease in maintenance led to the reduced survival of massive species. This situation contrasts with the branching Pocillopora spp., in which no bleaching or loss of pigmentation was observed during upwelling season and with very limited prevalence in August 2021. This might indicate that Pocillopora, previously considered as a highly sensitive genus to natural stressors (Glynn and Ault, 2000), has a higher tolerance threshold than massive-growing corals like pavonids (Manzello, 2010; Cruz-García et al., 2020; Romero-Torres et al., 2020), and could be less vulnerable due to the larger size of the fragments (Lizcano-Sandoval et al., 2018).
Under nursery conditions, the initially small coral fragments of the fast-growing Pocillopora spp. grew to be colonies in less than a year. In contrast, massive slow-growing species required a much longer time to grow, as by the end of the monitoring period, not all microfragments covered the totality of the ceramic disk. Particularly, the massive species P. gigantea grew 866% slower than the branching Pocillopora spp. These differences are to be expected provided their differential growth form and growth strategies (Rinkevich, 2014; Lirman and Schopmeyer, 2016; Page et al., 2018), since massive species present higher calcium carbonate production and calcification rates; thus, their growth rates are lower than those of branching corals (Wellington, 2004; Tortolero-Langarica et al., 2022).
Nursery design influenced fragment growth, as Pocillopora spp. fragments grew significantly faster in the mid-water floating structures than in the A-frame (81% lower than the coral tree and 60% lower than the rope line nursery). Since all coral nurseries were within a few meters of each other and at the same depth, we expect environmental variables (temperature, salinity, and nutrient concentration) to be the same. Another study using benthic-attached structures (“spiders”) in our nursery site, carried out at the same time as the mid-water floating nurseries, recorded a growth rate of 4.12 ± 2.77 cm yr-1 (Combillet et al., 2022), much alike our results in the A-frame, which also aligns with the reported rates for Pocillopora spp. in the southern Mexican Pacific using similar structures (García-Medrano et al., 2023). Similarly, other studies have reported higher growth rates in mid-water floating nurseries than in benthic-attached structures (Kuffner et al., 2017; O’Donnell et al., 2017; Schopmeyer et al., 2017; Ruiz-Diaz et al., 2022). This could indicate the value of suspending fragments, as it may increase water flow around corals, which improves nutrition and oxygen supply, and reduces sedimentation and debris that might accumulate on the corals (Rinkevich, 2014). By being suspended, fragments can also grow in all directions, which increases their three-dimensional complexity (Ishida-Castañeda et al., 2020). Additionally, area for competitor recruitment directly around the coral is also limited compared to A-frames (pers. Obs.). It is possible that fragments growing closer to the substrate (i.e., in the benthic-attached A-frame) form sturdier and denser skeletons, as they might be exposed to stronger currents (Kuffner et al., 2017; O’Donnell et al., 2017). If proven, this could have potential advantages for restoration of higher-energy environments (Chindapol et al., 2013; Doszpot et al., 2019).
Even when being cultivated in the same nursery, and therefore, subject to the same conditions, survival and growth among fragments can vary according to intrinsic factors like genotype, symbionts, and life history (Lirman et al., 2014; van Oppen et al., 2015; Drury et al., 2017). Despite not possessing information on every individual genotype of the fragmented donor Pocillopora spp. colonies, we hypothesize that donor sites could here be used as a proxy for different environmental tolerances, especially since donor colonies were fragmented from small areas in each site (Ruiz-Diaz et al., 2022). We detected significant growth rate differences among donor sites in the same nursery, and differences in survival among some donor sites. The differential response when being subject to the same rearing conditions could be a result of their life history and acclimation to the conditions of the donor site (Baums et al., 2019), which might differ from one another in temperature, depths, currents, water turbidity, and upwelling intensity. For instance, fragments from Marina (86% survival) were extracted from shallow areas (<2 m), which exposed them to high irradiance and seawater temperatures, possibly originating more resistant and resilient corals (Putnam and Gates, 2015; Hughes et al., 2019; Romero-Torres et al., 2020). Nonetheless, these fragments also presented the lowest growth rates, which might suggest trade-offs between stress resilience and growth (Edmunds, 2017; Ladd et al., 2017). In addition, these differences did not remain consistent among nursery types, but varied between shared donor sites in all nurseries. This might indicate some degree of interaction between life history and form of cultivation. Therefore, it is vital to maintain diversity of donor sites in the different nurseries, as this will ensure diversity of physiological responses when facing disturbances (van Oppen et al., 2015; Drury et al., 2017; Baums et al., 2019).
Through their time in the nurseries, growth rates of each individual fragment may vary as a result of its size or changes in environmental variables (Edmunds and Putnam, 2020; Foo and Asner, 2020; 2021). The presence of upwelling did not affect growth of coral fragments, with the exception of P. gigantea. The demonstrated ability of these fragments to maintain physiological processes like growth under upwelling conditions indicates an acclimation response (Rodríguez-Troncoso et al., 2010, 2014, 2016). Thus, it is likely that the higher growth rates experienced by P. gigantea in the first two months and during upwelling season could be a result of the microfragmentation process, as it has been found to stimulate radial extension of tissue in the short-term (Page and Vaughan, 2014; Forsman et al., 2015). The similar and lower growth of P. clavus and P. lobata through time could be explained by their quick initial loss of pigmentation and partial tissue loss, indicating stress (Manzello, 2010). During the nursery period, mean nutrient concentrations –except phosphates– were higher than those previously reported in Bahía Culebra (Fernández, 2007; Sánchez-Noguera, 2012), which could indicate some degree of eutrophication in the bay’s waters, a known stressor to corals as it promotes algal overgrowth and competition (Fabricius, 2005).
Even though economic costs of restoration are decreasing as new low-cost, low-tech techniques are developed (Bayraktarov et al., 2019; Rinkevich, 2019), they are still a limitation for many projects (Hesley et al., 2017), which in turn hinders their potential effect on ecosystem recovery. Thus, for coral reef restoration to reach meaningful scales, costs need to be optimized (Shaish et al., 2008; Hernández-Delgado et al., 2014; Maurer et al., 2022). We found that the efficiency of monitoring and maintenance were affected by nursery design. Frequent (at least twice a month) and labor-intensive maintenance is needed in Bahía Culebra’s nurseries, due to the high productivity of its waters (Stuhldreier et al., 2015) that promotes the proliferation of highly aggressive coral competitors and fouling organisms. For the coral nurseries used for Pocillopora spp., 10-30 min of two divers were required, while the massive microfragment nursery required extended maintenance (>1.5 h of two divers), as a result of the technique and nursery design. Since labor and maintenance are generally the highest costs of restoration, the type of nursery used and the duration of the nursery stage need to be considered (Knapp et al., 2022; Maurer et al., 2022).
Microfragments of massive species were the least cost-efficient form of cultivation, with each final microfragment costing US$12.76. Due to their slow growth, massive species tend to require prolonged nursery periods (Levy et al., 2010; Shaish et al., 2010), which increases costs. We therefore propose reducing nursery time and producing larger fragments (>15 cm2), which would also be less vulnerable to stressors (Raymundo and Maypa, 2004; Forsman et al., 2015; Marín-Moraga et al., 2023) and would reduce empty space on the ceramic disk, limiting recruitment of coral competitors and increasing fragment survival. Identifying the optimal fragment size that reduces nursery time, ensures high survival, and optimizes growth is an essential next step for improving time-effectiveness and cost-efficiency to scale up efforts with slow-growing massive species (Forsman et al., 2021; Knapp et al., 2022). Alternatively, the direct transplantation of fragments has proven to be an effective low-cost approach for massive species not only in the ETP (Tortolero-Langarica et al., 2020) but also in other regions like the Caribbean (Forrester et al., 2019) and the Indo-Pacific (dela Cruz et al., 2015), bypassing the need and costs of nursery cultivation (Tortolero-Langarica et al., 2020).
The lower installation and labor costs, and higher survival (62.5%) in the A-frame were somewhat offset by their lower growth rates. These differences could be surpassed by identifying which donor sites yield the highest coral growth, and which reef sites in the bay allow for higher growth rates (Alvarado et al. in prep.). Beyond higher survival and less maintenance, A-frames provide practical advantages when several divers are collecting data and cleaning the structures. Furthermore, benthic-attached structures like the A-frames have proven to enhance structural complexity (Yanovski and Abelson, 2019), acting as an aggregation site for many species (Loke et al., 2015; Levy et al., 2022), and as a substrate on which to permanently attach fragments when no natural substrate is available for outplanting (Combillet et al., 2022). This is particularly relevant due to the rapid degradation of the reef framework and loss of structural complexity experienced in Bahía Culebra in the last two decades, which resulted in habitat loss for many species and caused shifts in reef fish communities (Alvarado et al., 2012; Arias-Godínez et al., 2019). Hence, areas where the reef framework has been destroyed, like blast fishing sites in the Indo-Pacific (Williams et al., 2018; Fox et al., 2005) or sites affected by hurricanes in the Caribbean (Hughes, 1994), could greatly benefit from this restoration technique. It is important to consider that these structures also tend to be more durable in strong current sites (Maurer et al., 2022), and are less susceptible to recruitment of fast-growing organisms (pers. obs.). Therefore, as different coral nurseries yield different results and advantages, which could be site-specific, maintaining the diversity of structures used as nurseries could potentially improve restoration benefits.
5 Conclusions
Altogether, our results might indicate that coral gardening is a feasible technique for branching Pocillopora spp. corals, as it promoted their growth before being outplanted. The importance of considering multiple factors during the cultivation of coral fragments is also highlighted. To ensure long-term ecological restoration, the growth rate of nursery-reared corals should not be the focal point of nursery propagation, as it is a poor predictor of performance and trade-offs with survival, resistance, and reproductive capacity may exist (Cunning et al., 2014; Shaw et al., 2016; Edmunds, 2017; Ladd et al., 2017), and it might not consistent over time and across restoration sites and regions (Goergen et al., 2018). Although previous studies in the ETP have used the gardening approach (Ishida-Castañeda et al., 2020; Robles-Payán et al., 2021; García-Medrano et al., 2023), this study is the first to evaluate differences in coral growth and survival using different types of in situ nurseries (mid-water floating vs. benthic-attached). Despite the overall low survivorship and slower growth of massive corals, these species may have advantageous characteristics, such as higher calcification rates and calcium carbonate production (Tortolero-Langarica et al., 2022), and a higher resistance to physical impacts (Loya et al., 2001; Rivas et al., 2021) and changing environmental conditions (Schlöder and D’Croz, 2004). Therefore, it is necessary to improve our understanding of cultivation techniques for massive species. Considering response diversity in the face of disturbance, restoration efforts should shift the focus from the cultivation of fast-growing genera like Pocillopora to a multi-species approach (Lirman et al., 2011; dela Cruz et al., 2015; Baums et al., 2019). This would promote functional diversity and ecosystem resilience (Nyström, 2006; Baskett et al., 2014), and thus increase the chances for the long-term existence of coral reefs at a time when it is urgently needed.
Data availability statement
The raw data supporting the conclusions of this article will be made available by the authors, without undue reservation.
Ethics statement
The manuscript presents research on animals that do not require ethical approval for their study.
Author contributions
SM-G: Investigation, Methodology, Resources, Visualization, Writing – review & editing. SF-M: Data curation, Investigation, Methodology, Resources, Validation, Visualization, Writing – original draft, Writing – review & editing. FQ-P: Data curation, Formal analysis, Validation, Writing – review & editing. JA: Conceptualization, Funding acquisition, Investigation, Methodology, Project administration, Supervision, Writing – original draft, Writing – review & editing.
Funding
The author(s) declare financial support was received for the research, authorship, and/or publication of this article. This project was funded by Vicerrectoría de Investigación of Universidad de Costa Rica through Project B9089, Península Papagayo through their environmental sustainability program, and the German Agency for International Cooperation (GIZ).
Acknowledgments
The present study would not have been possible without the support of Centro de Investigación en Ciencias del Mar y Limnología (CIMAR), from Universidad de Costa Rica. We are especially thankful to José Andrés Marín, Adriana Arce, Cindy Fernández, Gabriela López, Celeste Sánchez-Noguera, Lisa Combillet, Camila Valverde, Ben Chomitz, Sergio Madrigal, Andrea Bogantes, Maricruz Calvo, Carlos Marenco, Mónica Gutierrez, Miguel Sánchez, and the staff of Marina Papagayo for their support during fieldwork. We thank Alma Paola Rodríguez-Troncoso for her comments and improvements of the manuscript.
Conflict of interest
The authors declare that the research was conducted in the absence of any commercial or financial relationships that could be construed as a potential conflict of interest.
The reviewer FAR-Z declared a past co-authorship with the author JA to the handling editor.
Publisher’s note
All claims expressed in this article are solely those of the authors and do not necessarily represent those of their affiliated organizations, or those of the publisher, the editors and the reviewers. Any product that may be evaluated in this article, or claim that may be made by its manufacturer, is not guaranteed or endorsed by the publisher.
Supplementary material
The Supplementary Material for this article can be found online at: https://www.frontiersin.org/articles/10.3389/fmars.2024.1400026/full#supplementary-material
References
Afiq-Rosli L., Taira D., Loke H. X., Toh T. C., Toh K. B., Ng C. S. L., et al. (2017). In situ nurseries enhance coral transplant growth in sedimented waters. Mar. Biol. Res. 13, 878–887. doi: 10.1080/17451000.2017.1307988
Alfaro E., Cortés J. (2011). Atmospheric forcing of cool subsurface water events in Bahía Culebra, Gulf of Papagayo, Costa Rica. Rev. Biol. Trop. 60, 173–186. doi: 10.15517/rbt.v60i2.20001
Alvarado J. J., Cortés J., Guzmán H., Reyes-Bonilla H. (2016). Density, size, and biomass of Diadema mexicanum (Echinoidea) in Eastern Tropical Pacific coral reefs. Aquat. Bio. 24, 151–161. doi: 10.3354/ab00645
Alvarado J. J., Cortés J., Reyes-Bonilla H. (2012). Reconstruction of Diadema mexicanum bioerosion impact on three Costa Rican Pacific coral reefs. Rev. Biol. Trop. 60, 121–132. doi: 10.15517/rbt.v60i2.19975
Aponte-Marcano P. I., Suleimán-Ramos S. E., Mercado-Molina A. E. (2023). Effectiveness of different nursery designs for the restoration of the threatened coral Acropora cervicornis in Culebra, Puerto Rico. Conserv. Evid. J. 20, 30–39. doi: 10.52201/CEJ20/PNCI5011
Arias-Godínez G., Jiménez C., Gamboa C., Cortés J., Espinoza M., Alvarado J. J. (2019). Spatial and temporal changes in reef fish assemblages on disturbed coral reefs, north Pacific coast of Costa Rica. Mar. Ecol. 40, e12532. doi: 10.1111/maec.12532
Baskett M. L., Fabina N. S., Gross K. (2014). Response diversity can increase ecological resilience to disturbance in coral reefs. Am. Nat. 184, 16–31. doi: 10.1086/676643
Baums I. B., Baker A. C., Davies S. W., Grottoli A. G., Kenkel C. D., Kitchen S. A., et al. (2019). Considerations for maximizing the adaptive potential of restored coral populations in the western Atlantic. Ecol. Appl. 29, e01978. doi: 10.1002/eap.1978
Bayraktarov E., Banaszak A. T., Montoya-Maya P., Kleypas J., Arias-González J. E., Blanco M., et al. (2020). Coral reef restoration efforts in Latin American countries and territories. PloS One 15, e0228477. doi: 10.1371/journal.pone.0228477
Bayraktarov E., Stewart-Sinclair P. J., Brisbane S., Boström-Einarsson L., Saunders M. I., Lovelock C. E., et al. (2019). Motivations, success, and cost of coral reef restoration. Rest. Ecol. 27, 981–991. doi: 10.1111/rec.12977
Chindapol N., Kaandorp J. A., Cronemberger C., Mass T., Genin A. (2013). Modelling growth and form of the scleractinian coral Pocillopora verrucosa and the influence of hydrodynamics. PloS Comp. Biol. 9, e1002849. doi: 10.1371/journal.pcbi.1002849
Combillet L., Fabregat-Malé S., Mena S., Marín-Moraga J. A., Gutiérrez M., Alvarado J. J. (2022). Pocillopora spp. growth analysis on restoration structures in an Eastern Tropical Pacific upwelling area. PeerJ 10, e13248. doi: 10.7717/peerj.13248
Cortés J. (1997). Biology and geology of eastern Pacific coral reefs. Coral Reefs 16, S36–S46. doi: 10.1007/s003380050240
Cortés J., Jiménez C. (2003). “Corals and coral reefs of the Pacific of Costa Rica: history, research and status,” in Latin American Coral Reefs. Ed. Cortés J. (Elsevier Science, Amsterdam, Netherlands), 361–367. doi: 10.1016/B978-044451388-5/50017-5
Cruz-García R., Rodríguez-Troncoso A. P., Rodríguez-Zaragoza F. A., Mayfield C. A., Cupul-Magaña A. L. (2020). Ephemeral effects of El Niño–Southern Oscillation events on an eastern tropical Pacific coral community. Mar. Fresh. Res. 71, 1259–1268. doi: 10.1071/MF18481
Cunning R., Gillette P., Capo T., Galvez K., Baker A. C. (2014). Growth tradeoffs associated with thermotolerant symbionts in the coral Pocillopora damicornis are lost in warmer oceans. Coral Reefs 34, 155–160. doi: 10.1007/s00338-014-1216-4
dela Cruz D. W., Rinkevich B., Gomez E. D., Yap H. T. (2015). Assessing an abridged nursery phase for slow growing corals used in coral restoration. Ecol. Eng. 84, 408–415. doi: 10.1016/j.ecoleng.2015.09.042
Dixon A. M., Forster P. M., Heron S. F., Stoner A. M. K., Beger M. (2022). Future loss of local scale thermal refugia in coral reef ecosystems. PloS Climate 1, e0000004. doi: 10.1371/journal.pclm.0000004
Doszpot N. E., McWilliam M. J., Pratchett M. S., Hoey A. S., Figueira W. F. (2019). Plasticity in three-dimensional geometry of branching corals along a cross-shelf gradient. Diversity 11, 44. doi: 10.3390/d11030044
Drury C., Manzello D. P., Lirman D. (2017). Genotype and local environment dynamically influence growth, disturbance response and survivorship in the threatened coral, Acropora cervicornis. PloS One 12, e0174000. doi: 10.1371/journal.pone.0174000
Edmunds P. J. (2017). Intraspecific variation in growth rate is a poor predictor of fitness for reef corals. Ecol. 98, 2191–2200. doi: 10.1002/ecy.1912
Edmunds P. J., Putnam H. M. (2020). Science-based approach to using growth rate to assess coral performance and restoration outcomes. Biol. Let. 16, 20200227. doi: 10.1098/rsbl.2020.0227
Edwards A. J. (2010). Reef rehabilitation manual (St Lucia, Australia: Coral reef targeted research & capacity building for Management Program).
Fabricius K. E. (2005). Effects of terrestrial runoff on the ecology of corals and coral reefs: review and synthesis. Mar. Pol. Bull. 50, 125–146. doi: 10.1016/j.marpolbul.2004.11.028
Fernández C. (2007). Propagación del alga Caulerpa sertularioides (Chlorophyta) en Bahía Culebra, Golfo de Papagayo, Pacífico norte de Costa Rica. M.Sc. San José, Costa Rica: Universidad de Costa Rica, San José, Costa Rica.
Fernández-García C., Cortés J., Alvarado J. J., Nívia-Ruiz J. (2012). Physical factors contributing to the benthic dominance of the algae Caulerpa sertularioides (Caulerpaceae, Clorophyta) in the upwelling Bahía Culebra, North Pacific of Costa Rica. Rev. Biol. Trop. 60, 93–107. doi: 10.15517/rbt.v60i2.19970
Ferrari R., Gonzalez-Rivero M., Mumby P. J. (2012). Size matters in competition between corals and macroalgae. Mar. Ecol. Prog. Ser. 467, 77–88. doi: 10.3354/meps09953
Foo S. A., Asner G. P. (2020). Sea surface temperature in coral reef restoration outcomes. Environ. Res. Let. 15, 074045. doi: 10.1088/1748-9326/ab7dfa
Foo S. A., Asner G. P. (2021). Impacts of remotely sensed environmental drivers on coral outplant survival. Rest. Ecol. 29, e13309. doi: 10.1111/rec.13309
Forrester G., Chan M., Conetta D., Dauksis R., Nickles K., Siravo A. (2019). Comparing the efficiency of nursery and direct transplanting methods for restoring endangered corals. Ecol. Restor. 37, 81–89. doi: 10.3368/ER.37.2.81
Forsman Z. H., Page C. A., Toonen R. J., Vaughan D. (2015). Growing coral larger and faster: micro-colony-fusion as a strategy for accelerating coral cover. PeerJ 3, e1313. doi: 10.7717/peerj.1313
Forsman Z. H., Page C. A., Vaughan D. (2021). “Coral fusion: harnessing coral clonality for reef restoration,” in Active Coral Restoration: Techniques for a Changing Planet. Ed. Vaughan D. (J. Ross Publishing, Plantation, Florida), 172–201.
Fox H., Mous P., Pet J., Muljadi A., Caldwell R. (2005). Experimental assessment of coral reef rehabilitation following blast fishing. Conserv. Biol. 19, 98–107. doi: 10.1111/j.1523-1739.2005.00261.x
García-Medrano D., López-Pérez A., Guendulain-García S., Valencia-Méndez O., Granja-Fernández R., González-Mendoza T., et al. (2023). Gardening Pocillopora spp. fragments and their potential for rebuilding reef systems in the southern Mexican Pacific. Rest. Ecol. 31, e14006. doi: 10.1111/rec.14006
Glynn P. W. (1984). Widespread coral mortality and the 1982-83 El Niño warming event. Environ. Conser. 11, 133–146. doi: 10.1017/S0376892900013825
Glynn P. W., Alvarado J. J., Banks S., Cortés J., Feingold J. S., Jiménez C., et al. (2017). “Eastern Pacific coral reef provinces, coral community structure and composition: an overview,” in oral reefs of the Eastern Pacific: Persistence and loss in a dynamic environment. Eds. Glynn P. W., Manzello D., Enochs I. (Springer Science+Business Media, Dordrecht, the Netherlands), 107–176. doi: 10.1007/978-94-017-7499-4_5
Glynn P. W., Ault J. (2000). A biogeographic analysis and review of the far eastern Pacific coral reef region. Coral Reefs 19, 1–23. doi: 10.1007/s003380050220
Glynn P. W., D’Croz L. (1990). Experimental evidence for high temperature stress as the cause of El Niño-coincident coral mortality. Coral Reefs 8, 181–191. doi: 10.1007/BF00265009
Goergen E., Ostroff Z., Gilliam D. (2018). Genotype and attachment technique influence the growth and survival of line nursery corals. Restor. Ecol. 26, 622–628. doi: 10.1111/rec.12545
Hernández-Delgado E. A., Mercado-Molina A. E., Alejandro-Camis P. J., Candelas-Sánchez F., Fonseca-Miranda J. S., González-Ramos C. M., et al. (2014). Community-based coral rehabilitation in a changing climate: lessons learned from hurricane, extreme rainfall, and changing land use impacts. Open J. Ecol. 4, 918–944. doi: 10.4236/oje.2014.414077
Hesley D., Burdeno D., Drury C., Schopmeyer S., Lirman D. (2017). Citizen science benefits coral reef restoration activities. Journal for Nature Conservation 40, 94–99. doi: 10.1016/j.jnc.2017.09.001
Hoegh-Guldberg O., Mumby P. J., Hooten A. J., Steneck R. S., Greenfield P., Gómez E., et al. (2007). Coral reefs under rapid climate change and ocean acidification. Science 318, 1737–1742. doi: 10.1126/science.1152509
Hoogenboom M. O., Frank G. E., Chase T. J., Jurriaans S., Álvarez-Noriega M., Peterson K., et al. (2017). Environmental drivers of variation in bleaching severity of Acropora species during an extreme thermal anomaly. Front. Mar. Sci. 4. doi: 10.3389/fmars.2017.00376
Hughes T. P. (1994). Catastrophes, phase shifts, and large-scale degradation of a Caribbean coral reef. Science 265, 1547–1551. doi: 10.1126/science.265.5178.1547
Hughes T. P., Kerry J., Álvarez-Noriega M., Álvarez-Romero J. G., Anderson K. D., Baird A. H., et al. (2017). Global warming and recurrent mass bleaching of corals. Nature 543, 373–377. doi: 10.1038/nature21707
Hughes T. P., Kerry J. T., Connolly S. R., Baird A. H., Eakin C. M., Heron S. F., et al. (2019). Ecological memory modifies the cumulative impact of recurrent climate extremes. Nat. Clim. Change 9, 40–43. doi: 10.1038/s41558-018-0351-2
Ishida-Castañeda J., Pizarro V., López-Victoria M., Zapata F. A. (2020). Coral reef restoration in the Eastern Tropical Pacific: feasibility of the coral nursery approach. Rest. Ecol. 28, 22–28. doi: 10.1111/rec.13047
Jiménez C. (2001). Arrecifes y ambientes coralinos de Bahía Culebra, Pacífico de Costa Rica: aspectos biológicos, económico-recreativos y de manejo. Rev. Biol. Trop. 49, 215–231.
Jiménez C., Bassey G., Segura Á., Cortés J. (2010). Characterization of the coral communities and reefs of two previously undescribed locations in the upwelling region of Gulf of Papagayo (Costa Rica). Rev. Cien. Mar. Cost. 2, 95–108. doi: 10.15359/revmar.2.8
Knapp I. S. S., Forsman Z. H., Greene A., Johnston E. C., Bardin C. E., Chan N., et al. (2022). Coral micro-fragmentation assays for optimizing active reef restoration efforts. PeerJ 10, e13653. doi: 10.7717/peerj.13653
Kuffner I. B., Bartels E., Stathakopoulos A., Enochs I. C., Kolodziej G., Toth L. T., et al. (2017). Plasticity in skeletal characteristics of nursery-raised staghorn coral, Acropora cervicornis. Coral Reefs 36, 679–684. doi: 10.1007/s00338-017-1560-2
Ladd M. C., Shantz A. A., Bartels E., Burkepile D. E. (2017). Thermal stress reveals a genotype-specific tradeoff between growth and tissue loss in restored Acropora cervicornis. Mar. Ecol. Prog. Ser. 572, 129–139. doi: 10.3354/meps12169
Levy N., Berman O., Yuval M., Loya Y., Treibitz T., Tarazi E., et al. (2022). Emerging 3D technologies for future reformation of coral reefs: Enhancing biodiversity using biomimetic structures based on designs by nature. Sci. Total Env. 830, 154749. doi: 10.1016/j.scitotenv.2022.154749
Levy G., Shaish L., Haim A., Rinkevich B. (2010). Mid-water rope nursery – testing design and performance of a novel reef restoration instrument. Ecol. Eng. 36, 560–569. doi: 10.1016/j.ecoleng.2009.12.003
Lizcano-Sandoval L. D., Londoño-Cruz E., Zapata F. A. (2018). Coral reef resilience to thermal stress in the Eastern Tropical Pacific. Marine Biology Research 14, 887–897. doi: 10.1080/17451000.2018.1528011
Liñán-Cabello M. A., Flores-Ramírez L. A., Laurel-Sandoval M. A., García-Mendoza E., Soriano-Santiago O., Delgadillo-Nuño M. A. (2011). Acclimation in Pocillopora spp. during a coral restoration program in Carrizales Bay, Colima, Mexico. Mar. Fresh. Behav. Physiol. 44, 61–72. doi: 10.1080/10236244.2010.537440
Lirman D., Schopmeyer S. (2016). Ecological solutions to reef degradation: optimizing coral reef restoration in the Caribbean and Western Atlantic. PeerJ 4, e2597. doi: 10.7717/peerj.2597
Lirman D., Schopmeyer S., Galvan V., Drury C., Baker A. C., Baums I. B. (2014). Growth dynamics of the threatened Caribbean staghorn coral Acropora cervicornis: influence of host genotype, symbiont identity, colony size, and environmental setting. PloS One 9, e107253. doi: 10.1371/journal.pone.0107253
Lirman D., Schopmeyer S., Manzello D., Gramer L. J., Precht W. F., Muller-Karger F., et al. (2011). Severe 2010 cold-water event caused unprecedented mortality to corals of the Florida reef tract and reversed previous survivorship patterns. PloS One 6, e23047. doi: 10.1371/journal.pone.0023047
Loke L. H. L., Ladle R. J., Bouma T. J., Todd P. A. (2015). Creating complex habitats for restoration and reconciliation. Ecol. Eng. 77, 307–313. doi: 10.1016/j.ecoleng.2015.01.037
Loya Y., Sakai K., Yamazato K., Nakano Y., Sambali H., van Woesik R. (2001). Coral bleaching: the winners and the losers. Ecol. Let. 4, 122–131. doi: 10.1046/j.1461-0248.2001.00203.x
Manzello D. P. (2010). Coral growth with thermal stress and ocean acidification: lessons from the eastern tropical Pacific. Coral Reefs 29, 749–758. doi: 10.1007/s00338-010-0623-4
Marín-Moraga J., Chacón-Guzmán J., Méndez-Venegas M., Hernández-Mora R., Cortés J. (2023). Ex situ culture of coral species Porites lobata (Scleractinia: Poritidae) and Pocillopora damicornis (Scleractinia: Pocilloporidae), Costa Rica: first assessment and implications. Rev. Biol. Trop. 71, e54926. doi: 10.15517/rev.biol.trop.v71is1.54926
Maurer L., Puishys L., Pham Ho N. K., Dahlgren C., Kamerman T. Y., Martin S., et al. (2022). Acropora cervicornis and Acropora palmata cultured on a low maintenance line nursery design in the Bahamas. PloS One 17, e0267034. doi: 10.1371/journal.pone.0267034
Montano S., Dehnert I., Seveso D., Maggioni D., Montalbetti E., Strona G., et al. (2022). Effects of the COVID-19 lockdowns on the management of coral restoration projects. Restor. Ecol. 30, e13646. doi: 10.1111/rec.13646
Nyström M. (2006). Redundancy and response diversity of functional groups: implications for the resilience of coral reefs. AMBIO 35, 30–35. doi: 10.1579/0044-7447-35.1.30
O’Donnell K. E., Lohr K. E., Bartels E., Patterson J. T. (2017). Evaluation of staghorn coral (Acropora cervicornis, Lamarck 1816) production techniques in an ocean-based nursery with consideration of coral genotype. J. Exp. Mar. Biol. Ecol. 487, 53–58. doi: 10.1016/j.jembe.2016.11.013
Page C. A., Muller E. M., Vaughan D. E. (2018). Microfragmenting for the successful restoration of slow growing massive corals. Ecol. Eng. 123, 86–94. doi: 10.1016/j.ecoleng.2018.08.017
Page C. A., Vaughan D. E. (2014). “The cultivation of massive corals using “micro-fragmentation” for the “reskinning” of degraded coral reefs,” in Benthic Ecology Meeting (University of North Florida, Jacksonville, Florida: Benthic Ecology MeetingAt).
Pendleton L. H., Ahmadia G. N., Browman H. I., Thurstan R. H., Kaplan D. M., Bartolino V. (2018). Debating the effectiveness of marine protected areas. ICES J. Mar. Sci. 75, 1156–1159. doi: 10.1093/icesjms/fsx154
Pinzón J. H., Sampayo E., Cox E., Chauka L. J., Chen C. A., Voolstra C. R., et al. (2013). Blind to morphology: genetics identifies several widespread ecologically common species and few endemics among Indo-Pacific cauliflower corals (Pocillopora, Scleractinia). J. Biogeogr. 40, 1595–1608. doi: 10.1111/jbi.12110
Possingham H. P., Bode M., Klein C. J. (2015). Optimal conservation outcomes require both restoration and protection. PloS Biol. 13, e1002052. doi: 10.1371/journal.pbio.1002052
Putnam H. M., Gates R. D. (2015). Preconditioning in the reef-building coral Pocillopora damicornis and the potential for trans-generational acclimatization in coral larvae under future climate change conditions. J. Exp. Biol. 218, 2365. doi: 10.1242/jeb.123018
Raymundo L. R., Maypa A. P. (2004). Getting bigger faster: mediation of size-specific mortality via fusion in juvenile coral transplants. Ecol. Appl. 14, 281–295. doi: 10.1890/02-5373
R Development Core Team (2021). R: A language and environment for statistical computing (Vienna, Austria: R Foundation for Statistical Computing).
Rinkevich B. (2014). Rebuilding coral reefs: does active reef restoration lead to sustainable reefs? Cur. Op. Environ. Sust. 7, 28–36. doi: 10.1016/j.cosust.2013.11.018
Rinkevich B. (2019). The active reef restoration toolbox is a vehicle for coral resilience and adaptation in a changing world. J. Mar. Sci. Eng. 7, 201. doi: 10.3390/jmse7070201
Rivas N., Hesley D., Kaufman M., Unsworth J., D’Alessandro M., Lirman D. (2021). Developing best practices for the restoration of massive corals and the mitigation of predation impacts: influences of physical protection, colony size, and genotype on outplant mortality. Coral Reefs 40, 1227–1241. doi: 10.1007/s00338-021-02127-5
Rixen T., Jiménez C., Cortés C. (2012). Impact of upwelling events on the sea water carbonate chemistry and dissolved oxygen concentration in the Gulf of Papagayo (Culebra Bay), Costa Rica: Implications for coral reefs. Rev. Biol. Trop. 60, 187–195. doi: 10.15517/rbt.v60i2.20004
Robles-Payán A., Reyes-Bonilla H., Cáceres-Martínez C. (2021). Crecimiento y supervivencia de corales durante la fase inicial de cultivo en La Paz, Baja California Sur, México. Rev. Mex. Biodiv. 92, e923594. doi: 10.22201/ib.20078706e.2021.92.3594
Rodríguez-Sáenz K., Rodríguez-Fonseca J. (2004). Avistamientos del delfín manChado, Stenella attenuata (Cetacea: Delphinidae) en Bahía Culebra, Costa Rica 1999-2000. Rev. Biol. Trop. 49, 189–193.
Rodríguez-Troncoso A. P., Carpizo-Ituarte E., Cupul-Magaña A. L. (2010). Differential response to cold and warm water conditions in Pocillopora colonies from the Central Mexican Pacific. J. Exp. Mar. Biol. Ecol. 391, 57–64. doi: 10.1016/j.jembe.2010.06.006
Rodríguez-Troncoso A. P., Carpizo-Ituarte E., Cupul-Magaña A. L. (2016). Physiological response to high temperature in the Tropical Eastern Pacific coral Pocillopora verrucosa. Mar. Ecol. 37, 1168–1175. doi: 10.1111/maec.12392
Rodríguez-Troncoso A. P., Carpizo-Ituarte E., Pettay D. T., Warner M. E., Cupul-Magaña A. L. (2014). The effects of an abnormal decrease in temperature on the Eastern Pacific reef−building coral Pocillopora verrucosa. Mar. Biol. 161, 131–139. doi: 10.1007/s00227-013-2322-5
Romero-Torres M., Acosta A., Palacio-Castro A. M., Treml E. A., Zapata F. A., Paz-García D. A., et al. (2020). Coral reef resilience to thermal stress in the Eastern Tropical Pacific. Global Change Biol. 26, 3880–3890. doi: 10.1111/gcb.15126
Ruiz-Diaz C. P., Toledo-Hernández C., Sánchez-González J. L., Betancourt B. (2022). The effects of depth-related environmental factors on traits in acropora cervicornis raised in nurseries. Water 14 (2), 212. doi: 10.3390/w14020212
Sánchez-Noguera C. (2012). Cambios socioeconómicos y ambientales en Bahía Culebra, Guanacaste, Costa Rica: implicaciones para su gestión. San José, Costa Rica: Universidad de Costa Rica, San José, Costa Rica.
Sánchez-Noguera C., Jiménez C., Cortés J. (2018a). Desarrollo costero y ambientes marino-costeros en Bahía Culebra, Guanacaste, Costa Rica. Rev. Biol. Trop. 66, 309–327. doi: 10.15517/rbt.v66i1.33301
Sánchez-Noguera C., Stuhldreier I., Cortés J., Jiménez C., Morales Á., Wild C., et al. (2018b). Natural ocean acidification at Papagayo upwelling system (north Pacific Costa Rica): implications for reef development. Biogeosci. 15, 2349–2360. doi: 10.5194/bg-15-2349-2018
Schlöder C., D’croz L. (2004). Responses of massive and branching coral species to the combined effects of water temperature and nitrate enrichment. J. Exp. Mar. Bio. Ecol. 313, 255–268. doi: 10.1016/J.JEMBE.2004.08.012
Schopmeyer S. A., Lirman D., Bartels E., Gilliam D. S., Goergen E. A., Griffin S. P., et al. (2017). Regional restoration benchmarks for Acropora cervicornis. Coral Reefs 36, 1047–1057. doi: 10.1007/s00338-017-1596-3
Shafir S., Rinkevich B. (2010). Integrated long term mid-water coral nurseries: a management instrument evolving into a floating ecosystem. Mauritus Res. J. 16, 365–379.
Shaish L., Levy G., Gomez E., Rinkevich B. (2008). Fixed and suspended coral nurseries in the Philippines: establishing the first step in the “gardening concept” of reef restoration. J. Exp. Mar. Biol. Ecol. 358, 86–97. doi: 10.1016/j.jembe.2008.01.024
Shaish L., Levy G., Katzir G., Rinkevich B. (2010). Coral reef restoration (Bolinao, Philippines) in the face of frequent natural catastrophes. Rest. Ecol. 18, 285–299. doi: 10.1111/j.1526-100X.2009.00647.x
Shaw E. C., Carpenter R. C., Lantz C. A., Edmunds P. J. (2016). Intraspecific variability in the response to ocean warming and acidification in the scleractinian coral Acropora pulchra. Mar. Biol. 163, 210. doi: 10.1007/s00227-016-2986-8
Stuhldreier I., Sánchez-Noguera C., Roth F., Cortés J., Rixen T., Wild C. (2015). Upwelling increases net primary production of corals and reef-wide gross primary production along the Pacific coast of Costa Rica. Front. Mar. Sci. 2. doi: 10.3389/fmars.2015.00113
Torda G., Donelson J. M., Aranda M., Barshis D. J., Bay L., Berumen M. L., et al. (2017). Rapid adaptive responses to climate change in corals. Nat. Clim. Change 7, 627–636. doi: 10.1038/nclimate3374
Tortolero-Langarica J. J. A., Cupul-Magaña A. L., Rodríguez-Troncoso A. P. (2014). Restoration of a degraded coral reef using a natural remediation process: A case study from a Central Mexican Pacific National Park. Ocean Coast. Manage. 96, 12–19. doi: 10.1016/j.ocecoaman.2014.04.020
Tortolero-Langarica J. J. A., Rodríguez-Troncoso A. P., Cupul-Magaña A. L., Morales-de-Anda D. E., Caselle J. E., Carricart-Ganivet J. P. (2022). Coral calcification and carbonate production in the eastern tropical Pacific: The role of branching and massive corals in the reef maintenance. Geobiol. 20, 533–545. doi: 10.1111/gbi.12491
Tortolero-Langarica J. J. A., Rodríguez-Troncoso A. P., Cupul-Magaña A. L., Rinkevich B. (2020). Micro-fragmentation as an effective and applied tool to restore remote reefs in the Eastern Tropical Pacific. Int. J. Environ. Res. Pub. Health 17, 6574. doi: 10.3390/ijerph17186574
van Oppen M. J. H., Oliver J. K., Putnam H. M., Gates R. D. (2015). Building coral reef resilience through assisted evolution. Proc. Nat. Acad. Sci. 112, 2307–2313. doi: 10.1073/pnas.1422301112
Vargas-Ugalde R., Gómez-Salas C., Pérez-Reyes C., Umaña-Vargas E., Acosta-Nassar M. (2020). Jardinería” para la restauración coralina en el Golfo Dulce, Costa Rica: Una prueba práctica. UNED Res. J. 12, 40–53. doi: 10.22458/urj.v12i1.2809
Wellington G. (2004). An experimental analysis of the effects of light and zooplankton on coral zonation. Oecologia 52, 311–320. doi: 10.1007/BF00367953
Williams S., Sur C., Janetski N., Hollarsmith J., Rapi S., Barron L., et al. (2018). Large-scale coral reef rehabilitation after blast fishing in Indonesia. Restor. Ecol. 27, 447–456. doi: 10.1111/rec.12866
Yanovski R., Abelson A. (2019). Structural complexity enhancement as a potential coral-reef restoration tool. Ecol. Engin. 132, 87–93. doi: 10.1016/j.ecoleng.2019.04.007
Keywords: Bahía Culebra, coral gardening, Eastern Tropical Pacific, ecological restoration, microfragmentation, Pocillopora
Citation: Fabregat-Malé S, Mena-González S, Quesada-Perez F and Alvarado JJ (2024) Testing the feasibility of coral nurseries in an upwelling area in the North Pacific of Costa Rica. Front. Mar. Sci. 11:1400026. doi: 10.3389/fmars.2024.1400026
Received: 12 March 2024; Accepted: 12 August 2024;
Published: 02 September 2024.
Edited by:
Daniel Wangpraseurt, University of California, San Diego, United StatesReviewed by:
Fabian Alejandro Rodriguez-Zaragoza, University of Guadalajara, MexicoAlex E. Mercado-Molina, Sociedad Ambiente Marino, Puerto Rico
Copyright © 2024 Fabregat-Malé, Mena-González, Quesada-Perez and Alvarado. This is an open-access article distributed under the terms of the Creative Commons Attribution License (CC BY). The use, distribution or reproduction in other forums is permitted, provided the original author(s) and the copyright owner(s) are credited and that the original publication in this journal is cited, in accordance with accepted academic practice. No use, distribution or reproduction is permitted which does not comply with these terms.
*Correspondence: Juan José Alvarado, anVhbi5hbHZhcmFkb0B1Y3IuYWMuY3I=