- 1Fisheries Resources Institute, Japan Fisheries Research and Education Agency, Yokohama, Japan
- 2Specified Nonprofit Corporation Marine Wildlife Center of Japan, Abashiri, Japan
- 3Department of Ocean and Fisheries Sciences, Faculty of Bioindustry, Tokyo University of Agriculture, Abashiri, Japan
Under global warming, impacts on animals’ spatial distribution in response to ocean warming have been anticipated for marine endotherms like cetaceans. Therefore, determining the distribution patterns of small cetaceans is key to understanding how their distributional patterns are susceptible to changing oceanic environments. To investigate the effect of environmental variations on distributional patterns of small cetaceans on a fine scale, we attached eight satellite tags to Dall’s porpoises bycaught in Eastern Hokkaido. After release, the tagged porpoises moved into the western North Pacific and its marginal sea, the Sea of Okhotsk. Our tagging results showed that the habitat ranges of Dall’s porpoises were restricted by water temperature. To avoid warm water, tagged porpoises changed their directions when they faced surface thermocline of 17°C. Dall’s porpoise tracked for the longest periods (116 days) showed southwestward movement after entering the Sea of Japan through the Soya Strait, while the other seven tagged porpoises stayed in the Sea of Okhotsk and off the Pacific coast around Hokkaido and Chishima islands for the entire tracking periods. Vertical movements were also recorded by these tags. Long-term tracking of Dall’s porpoises’ movement provides important insights into seasonal migration patterns, particularly for fall southward migration, which has not been well investigated compared with spring northward migration. Information on seasonal migration, distribution and percentage of time spent at the surface will improve our approach to monitoring Dall’s porpoise populations and discover important knowledge for improving the conservation and management of the population.
1 Introduction
What determines distribution patterns of small cetaceans is a key question to understanding how their distributional patterns are susceptible to changing oceanic environments under the long-term global warming. Since industrialization, we have experienced increasing seawater temperatures worldwide, particularly in the waters off Japan’s coast. The number of extreme ocean-warming events such as ocean heatwaves, has gradually increased since the 1980s and became frequent after the mid-2010s (Hayashi et al., 2022). Effects on animal growth, reproduction, mortality, and spatial distribution in response to ocean warming have been anticipated, even for marine endotherms like cetaceans (Clarke et al., 2013; Thorne et al., 2017; Stewart et al., 2023).
The mechanism of habitat selection by small cetaceans is often complex, and a single parameter, such as water temperature, might not sufficiently to explain such complex mechanisms. For example, small cetaceans can swim fast, with up to 3m/second of routine speeds (Fish and Hui, 1991) and have the ability to travel for long distances of over 100km/day (Hui, 1987; Scott and Chivers, 2009; Rasmussen et al., 2013). They feed on a wide variety of species, from pelagic to deepwater fishes and squids (Blanco et al., 2001; Ohizumi, 2002; Ohizumi et al., 2003; Wang et al., 2012). The nature of opportunistic feeding allows them to switch feeding habits depending on prey availability and environmental conditions (Ohizumi et al., 2000). Marine mammals are endotherms with thermal regulatory mechanisms, such as metabolic heat production (Levesque and Marshall, 2021). Thus, they can maintain their internal body temperature and endure changes in the ambient environment (Buckley et al., 2012). These biological traits allow them to disperse widely and tolerate various environmental conditions, which could affect their adaptation to the wide habitat ranges of many small cetaceans. However, there has been sufficient evidence that temperature distributions determine the habitat selection of small cetacean populations. For example, species compositions of small cetaceans change latitudinally, which can be explained by the frontal structures in the North Pacific (Kanaji et al., 2016). The distribution ranges of four pelagic species, Dall’s porpoise, Pacific white-sided dolphins, common dolphins, and pantropical spotted dolphins, are separated by the subarctic front, subarctic boundary, and Kuroshio extension, respectively (Kanaji et al., 2016). In habitat modeling, seawater temperature is often selected as an important parameter to predict spatial distribution and abundance (Becker et al., 2014; Kanaji and Gerrodette, 2020). These results were derived from long-term sighting records, representing the snapshot status of animal positions and ambient environments. The truth is that animals are constantly moving, and oceanographic conditions change dynamically. To analyze such dynamic nature in animal habitats, fine-scale satellite tracking is a powerful tool that enables us to directly analyze animal movements in ocean environments. In fact, satellite-tracked data clearly showed that bottlenose dolphins mainly inhabit colder inshore waters inside Kuroshio main streams in the coastal waters off Japan (Kanaji et al., 2022).
Dall’s porpoise, Phocoenoides dall, is a species of phocoenid cetaceans endemic to the North Pacific and adjacent waters (Jefferson, 2018). At least 11 subpopulations have been recognized for the species (International Whaling Commission, 2002). Among them, two populations inhabit the coastal waters of Japan, and these were easily distinguished by their color morphs (Kasuya, 1978; Amano and Marui, 2000; Amano and Hayano, 2007). The Sea of Japan–Sea of Okhotsk (JO) population is one of the populations that have a dalli-type color morph, while a truei-type morph is observed in a single population, the so-called truei-type (TT) population (Miyashita, 1991). JO population migrates northward to the southern Sea of Okhotsk from spring to summer for breeding and feeding, and southward to the southern Sea of Japan for wintering (Amano and Kuramochi, 1992; Amano and Hayano, 2007; Kasuya, 2011). The TT population migrates to the central Sea of Okhotsk from spring to summer and the Pacific coast of northern Japan for winter (Amano and Kuramochi, 1992; Amano and Hayano, 2007; Kasuya, 2011). In the broad term, Dall’s porpoises begin moving northward with increasing temperature and southward with decreasing temperature (Miyashita and Kasuya, 1988). The Dall’s porpoise is one of the small cetacean species showing the most prominent north-south migration patterns in the western North Pacific, but details of the movement mechanism are still unclear. Elucidating the porpoises’ habitat utilization patterns and their relationship with ambient environments would answer our key questions: how does ambient temperature shape the habitat range and trigger their movements? This challenging question can be answered by tracking Dall’s porpoise movements for as long as possible, hopefully across seasons. Long retention of satellite tags depends on the possibility of attaching tags safely and precisely to non-harmful body positions. Dall’s porpoises are sometimes bycaught by salmon set nets in the coastal waters off Hokkaido in early summer, which provides a rare opportunity to track porpoise movements from summer to fall.
Here, we attached eight tags to Dall’s porpoises to monitor their movements. The data retrieved from the tags were processed using a statistical model to determine the most reliable animal positions. Their movement trajectories were overlayed with spatial maps of several environmental variables. Additionally, we retrieved the data of vertical habitat utilization. Based on these datasets, three-dimensional habitat utilization patterns, including temperature variables and other environmental factors, were analyzed.
2 Materials and methods
2.1 Tagging
We were immediately informed by set net fishers when Dall’s porpoises were bycaught; they then rushed to the location attach tags. When porpoises were alive in a set net, we carefully hauled in the net and brought the porpoise alongside the ship after taking other fish catches onto the boat. We attached two types of satellite tags: SPLASH 10 and miniPAT (Wildlife Computers, Inc., Redmond, WA, USA). Two Dall’s porpoises were tagged with SPLASH 10, and six were tagged with miniPAT (Table 1). SPLASH 10 tag provides location data with higher resolution and credibility, while miniPAT tag is much easier to attach using a pole and plastic anchor. Only when there was a chance (i.e. a porpoise stayed calm, an animal body was able to be retained at appropriate position, and fishers ware available to help entire process), we tried to attach SPLASH 10 tag. Body size was measured, and skin biopsy was collected from Dall’s porpoises’ dorsal area if possible. Biopsy samples were used for molecular-based sex determination. A sex determining region of the Y chromosome (SRY) was amplified using specific PCR primers (Jayasankar et al., 2008).
The SPLASH 10 is a fin-mounted ARGOS satellite tag that transmits animal locations and time spent in each depth layer in quasi-real time. The tags were attached to the trailing edge of the dorsal fin using Delrin tubes (DuPont de Nemours, Inc, Wilmington, DE, USA), stainless steel bolts, and nuts in accordance with the manufacturer’s protocols (Figure 1A). This tagging approach is commonly used for many species in several study fields, and successfully tracked animal movements for several months (Wells et al., 2009; Balmer et al., 2010, 2014; Mullin et al., 2017; Deming et al., 2020; Heide-Jørgensen et al., 2020; Kanaji et al., 2022). Data transmission continued for up to 300 uplinks every day. Depth bins were set to 0–10 m, 10–20 m, 20–50 m, 50–100 m, 100–150 m, 150–200 m, 200–300 m, 300–400 m, 400–500 m and > 500 m. Diving profiles were summarized every hour and transmitted together with location information.
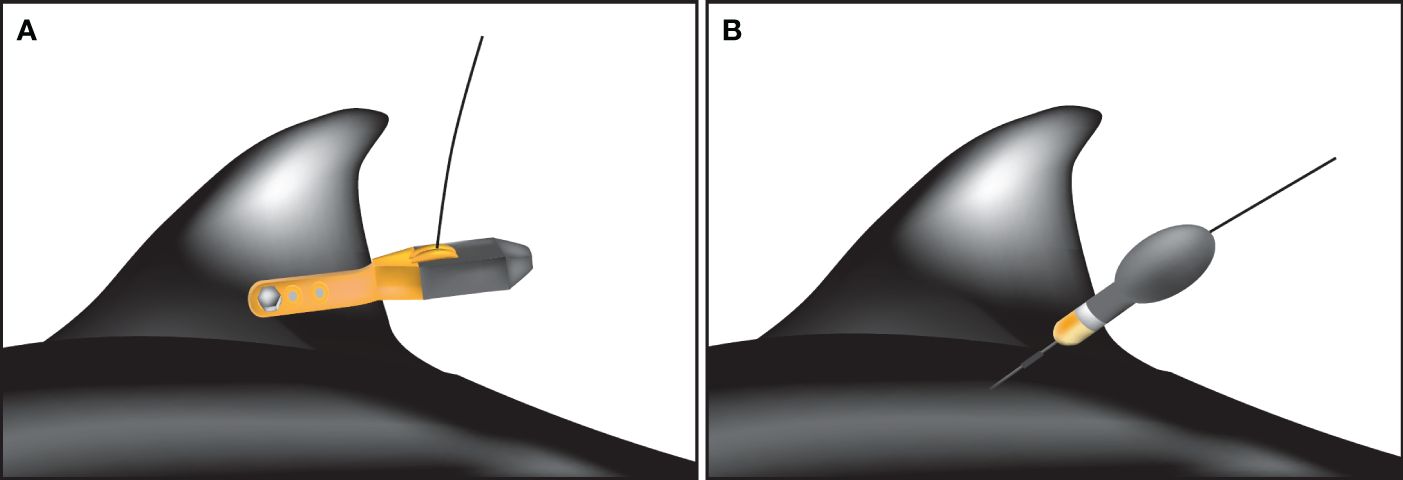
Figure 1 Illustration of tag attachment on Dall’s porpoise body. SPLASH 10 tag was attached to the trailing edge of the dorsal fin (A), while miniPAT tag was attached to an area near the dorsal fin base (B).
The miniPAT is a pop-up archival transmitting tag that archives summarized datasets of depth, temperature, and light level, and transmits all archived data once a tag is released from the animal body. Tag release was programmed to 100 days after deployment. Using a plastic anchor (Wilton Dart, Wildlife Computers, Inc.), the tag was attached to an area near the dorsal fin base (Figure 1B). This tagging approach does not require retaining an animal for long. Thus, it is particularly useful for free-ranging animals (Minamikawa et al., 2013). The Wilton Dart is only 38 mm long and 11 mm in diameter, which prevent deep penetration into the body muscle. Setting to depth bins was the same as for SPLASH 10, but diving profiles were set to be summarized every six hours to reduce transmitting burden after release from an animal.
A researcher with a veterinarian’s license conducted the entire tagging process. After releasing it from the set net, we carefully watched the animal’s behavior and confirmed that the porpoise did not display any unusual behavior. These field protocols followed domestic (The Committee on Taxonomical Names and Collections of the Mammalogical Society of Japan, 2001) and international guidelines for wild animal treatments (The Society for Marine Mammalogy, 2024). In accordance with those guidelines, tagging procedures used in this study were reviewed and authorized by the internal committee at the Japan Fisheries Research and Education Agency (authorization permit #44) and the Tokyo University of Agriculture (authorization permit #2019115).
2.2 Data processing
ARGOS location data obtained from SPLASH 10 were processed using a switching state-space model (SSSM) (Jonsen et al., 2005, 2013). SSSM provides location estimates by filtering data based on location classes (LCs 3, 2, 1, 0, A, and B) and random-walk processes. LCs were given by satellite and transmitter geometry and the number of messages received during each pass of ARGOS (CLS, 2016). SSSM was fitted to those ARGOS data using package “bsam” (Jonsen et al., 2005) in R (R Core Team, 2023). Through this processing, unrealistic location data (e.g., outliers) were modified and sorted at fixed time intervals. Here, we estimated animal location every 12 hours.
The miniPAT archived depth, temperature, and light level, and those summaries were transmitted via ARGOS after releasing the tag from the animal body. The tag never provides real-time location information. Instead, archived data can be used to estimate locations of animal movement trajectories (Pedersen et al., 2011). Among them, daily light level enables us to infer longitudinal coordinates, and depth and temperature values experienced by the animal will be used to identify spatial areas with similar environmental conditions. GPE-3 (Wildlife Computers, Inc. Redmond, WA, USA) is an online-based modeling tool to fit state-space models to those data archived in a tag and provides location estimates. A swim-speed parameter is required for the model, which controls how far animals can disperse from their original position. Here, we set a default parameter to 3 m/s based on the maximum reported routine speeds (Fish and Hui, 1991) and additionally used 2 m/s and 4 m/s to test the model’s sensitivity.
2.3 Environmental variables
The estimated animal movement trajectories were overlayed on environmental variable surfaces. Depth data were obtained from ETOPO1, a 1-arc-minute global relief model (Amante and Eakins, 2009). Sea surface temperature, temperature at a depth of 100 m, and surface water velocity data were obtained daily from the Japan Fisheries Research and Education Agency Regional Ocean Modeling System II (FRA-ROMS II) (Kuroda et al., 2017). The concentration of chlorophyll-a was obtained from ocean color images (https://oceancolor.gsfc.nasa.gov/; accessed January 19, 2024). Monthly averaged chlorophyll-a concentration was used here because daily base data often includes missing data due to cloud cover. Sea surface temperatures experienced by tagged porpoises were also measured by a sensor equipped on each tag.
3 Results
3.1 Horizontal movements
All six Dall’s porpoises tagged in 2019 had their body size measured and skin biopsy samples taken. Their body size ranged from 182 to 217 cm, and all were determined to be male (Table 1).
Raw location data received from two SPLASH 10 tags are shown in Figure 2A. Those raw data exhibited a few unreasonable location data, such as points on land. Such outlier data were filtered and sorted by an interval of 12 hours through modeling of SSSM (Figure 2B). The Dall’s porpoise tagged on July 4, 2019 (#180403) moved along the southern Chishima islands, while the porpoise tagged on July 25, 2019 (#180404) moved near the southern Chishima islands within the first seven weeks and then moved into the Pacific side. This individual returned to the Sea of Okhotsk 12 weeks after the attachment of the tag and further north to the coastal waters off the Russian Far East (Figure 2; Appendix Figure A1).
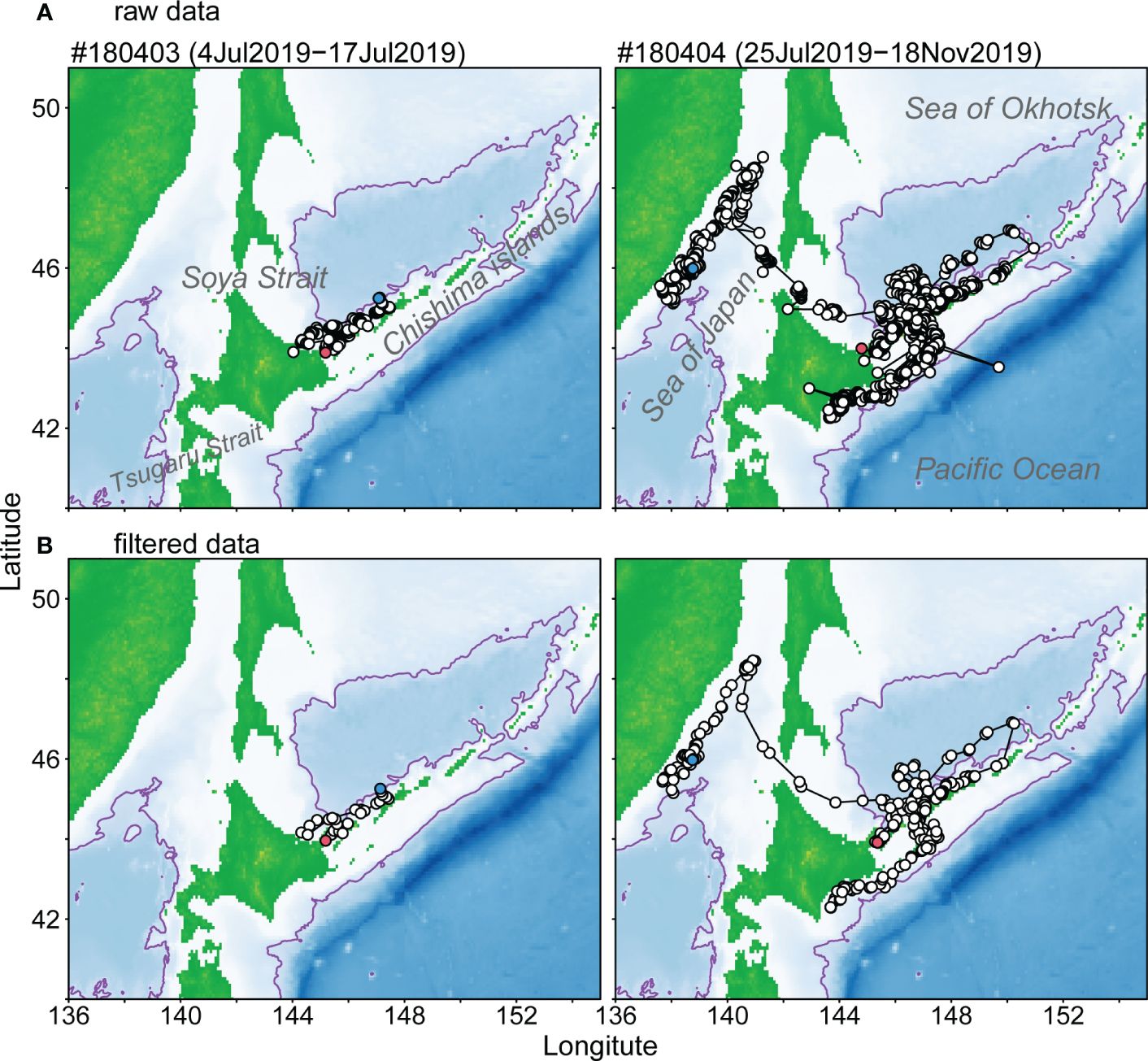
Figure 2 Locations and tracking of two Dall’s porpoises tagged with SPOT10 tags from raw satellite data obtained from Advanced Research and Global Observation Satellite (ARGOS) (A) and data filtered using a switching state-space model (SSSM) (B). Location to start and end tracking animals were shown red and blue plots. Purple lines represent 2,000 m isobath.
For each porpoise tagged with miniPAT, three movement trajectories were estimated with different swimming speed parameters (Figure 3). The estimated range of animal movements tended to be larger when higher swimming speed was assumed (e.g., 4 m/s) than when using a slower swimming speed (e.g., 2 m/s), but their movement patterns were not largely affected by parameter settings (Figure 3). Among six Dall’s porpoises, three individuals (#151171, #181205, and #181208) spent almost all tracking periods within the Sea of Okhotsk. They moved widely in the southern part of the sea, but most of the estimated locations were in the waters within 2,000 m isobath (Figure 3). The other three Dall’s porpoises (#151172, #181204, and #181206) showed similar movement to one of the individuals tagged with SPLASH 10; the porpoises moved into the Pacific Ocean through the straits among Chishima islands but then returned to the Sea of Okhotsk (Figure 3).
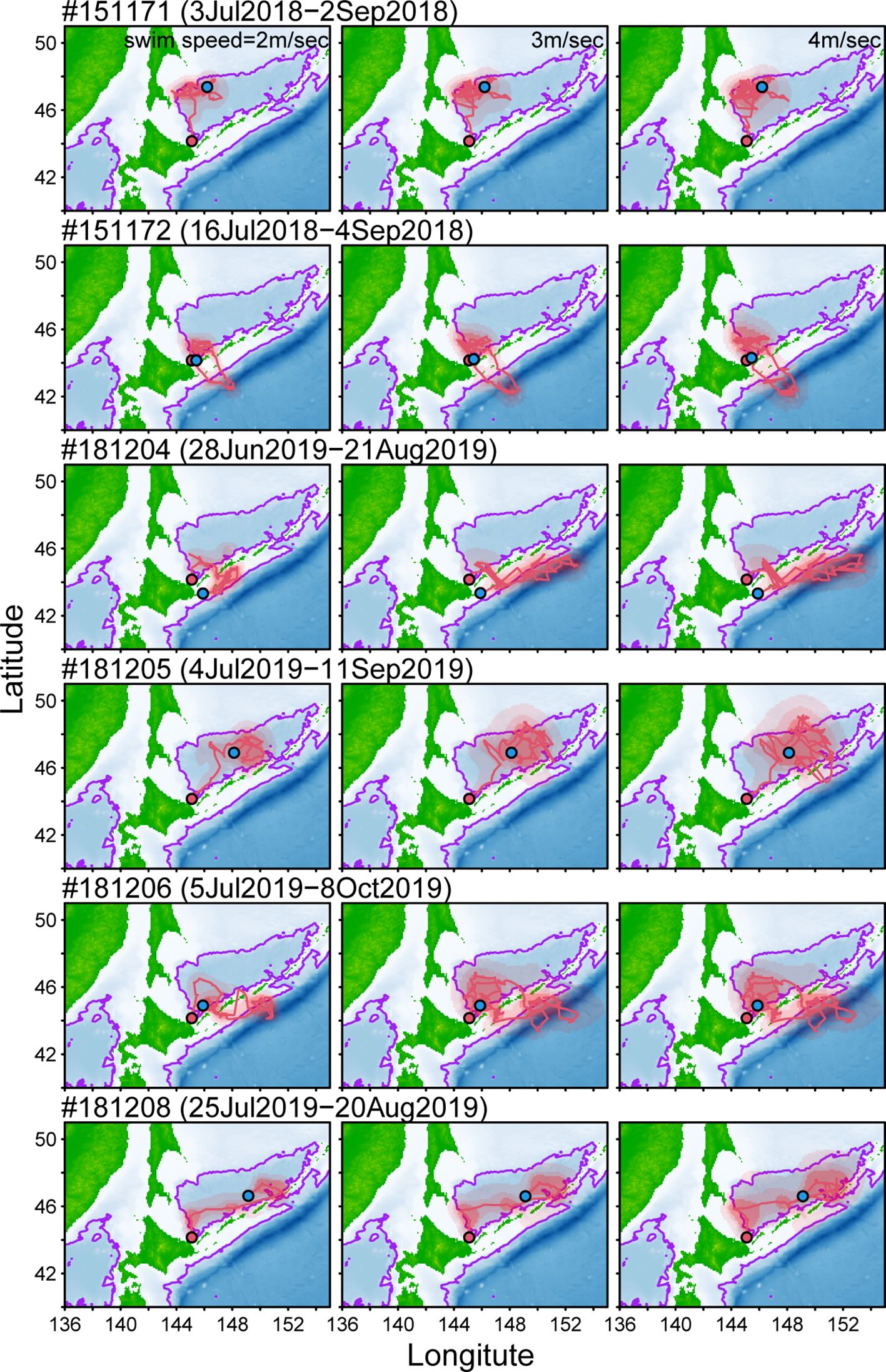
Figure 3 Estimated locations and tracking of six Dall’s porpoises tagged with miniPAT tags. Estimation was conducted using an online-based modeling tool (GPE-3) with three swim speed parameters (left: 2m/s, middle: 3m/s, and right: 4m/s). Location to start and end tracking animals were shown red and blue plots. Red shaded areas represent 95, 75, and 50% probability surfaces. Purple lines represent 2,000 m isobath.
As a result, we found two distinct movement patterns; one is that porpoises stayed in the Sea of Okhotsk during the tracking periods (Pattern A), and another is that porpoises moved into the Pacific Ocean but then returned to the Sea of Okhotsk again (Pattern B). Those movement trajectories were overlayed on four environmental variable surfaces: sea surface temperature, the temperature at a depth of 100 m, chlorophyll-a concentration, and surface velocity (Figure 4). Temperature and chlorophyll-a concentration were relatively lower, and velocity was lower in the Sea of Okhotsk than in the Pacific Ocean. Sea surface temperature was highly correlated with movement trajectories, particularly for Dall’s porpoises of movement Pattern B (Figure 4; Appendix Figure A1). For example, porpoise #180404 moved along the coast of Hokkaido after entering the Pacific Ocean, but changed direction soon after facing warm waters (isotherms of 17°C). It returned to the Sea of Okhotsk in the next two weeks (Appendix Figure A1). Similar patterns were observed for porpoises #151172, #181204, and #181206, which changed direction when they approached 17°C of isotherms (Appendix Figure A1). Such correlation with temperature distribution was also observed for the porpoises of movement Pattern B. For example, porpoise #151171 moved northward after releasing, but changed direction eastward when 17°C of isotherm came close to the porpoise (four weeks after releasing) (Appendix Figure A1). Similarly, porpoise #181208 changed direction eastward as 17°C of isotherms moved eastward a week after release (Appendix Figure A1).
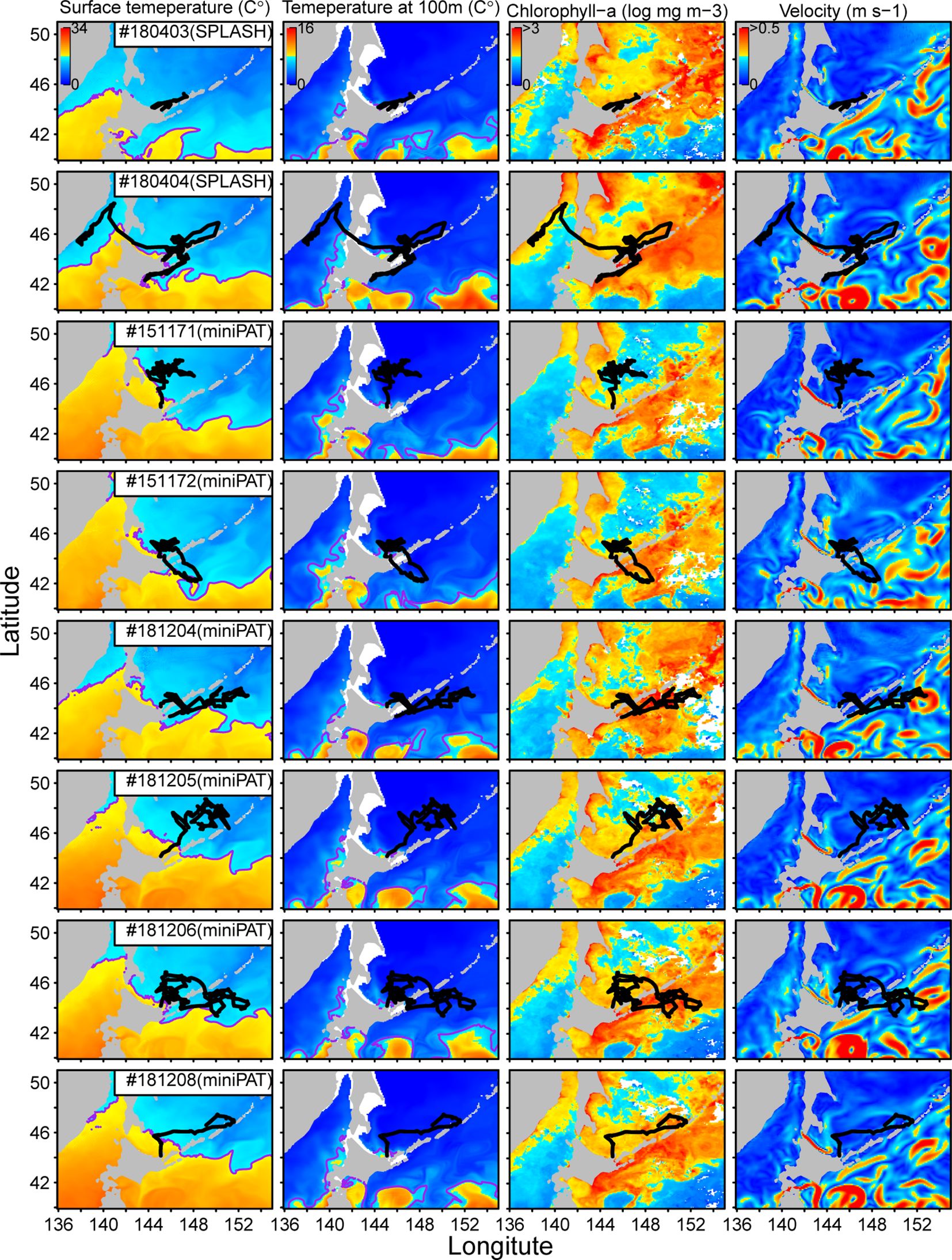
Figure 4 Movement trajectories of eight Dall’s porpoises overlayed on the four environmental variables surfaces (sea surface temperature, temperature at a depth of 100m, chlorophyll-a concentration, and surface velocity). Trajectories of two porpoises tagged with SPOT10 tags were from model filtered data and those of six tagged with miniPAT tags were from estimation with swim speed parameter of 3m/s. Purple lines on sea the surface temperature maps represent 17°C isotherms.
Spatial patterns of surface velocity resembled those of sea surface temperature (Figure 4). In the Pacific Ocean, the warm waters with >17°C of sea surface temperature were accompanied by eddies with complex flows and relatively higher velocity. The porpoises tended to avoid such surface currents and used calm waters as their habitats (Figure 4). Conversely, we could not identify clear relationships between the movement patterns of the porpoises with a temperature at a depth of 100 m and chlorophyll-a concentration (Figure 4). The thermocline of 6°C at a depth of 100 m is often used as an index of the front of cold Oyahshio waters (Kawai, 1972). This front was found further south of the movement trajectories of Dall’s porpoises observed in the study (Figure 4).
Time-series of sea surface temperature experienced by tagged Dall’s porpoises was obtained by associating ocean model data (FRA-ROMS II) to estimated locations and directly obtained from a temperature sensor equipped on a satellite tag (Figure 5). Overall, these time-series were similar between different data sources. However, only porpoise #181205 showed large discrepancy between ocean model data and sensor data for certain periods (Figure 5). Considering that temperature data by a tag sensor was unreasonably lower during those periods, those discrepancies would be caused by technical error in the sensor. In these time-series, we also found that Dall’s porpoise frequently approached or passed 17°C of isotherms but did not stay longer in warmer water and returned to colder area soon after facing 17°C of isotherms (Figure 5).
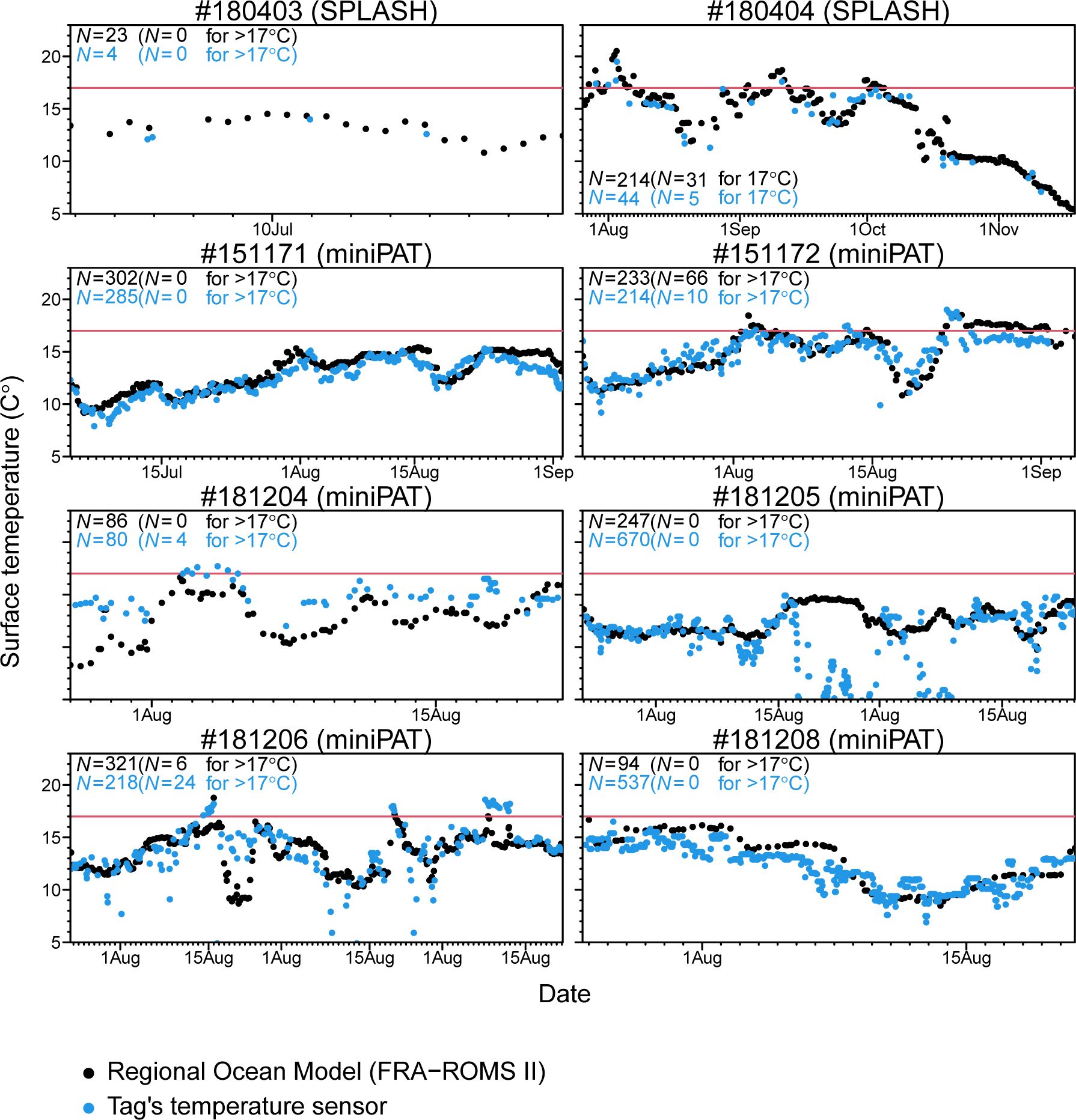
Figure 5 Time-series of sea surface temperature experienced by tagged Dall’s porpoises. Temperature values was obtained by associating ocean model data (FRA-ROMS II) to estimated locations (black plots) and directly obtained from a temperature sensor equipped on a satellite tag (blue plots). Location information was based on model filtered data for two porpoises tagged with SPOT10 tags and estimated by using a swim speed parameter of 3m/s for six porpoises tagged with miniPAT tags. Red horizontal lines represent sea surface temperature of 17°C.
3.2 Vertical movements
The percentage of time spent in each depth layer was summarized within hourly bins for two porpoises attached with SPOT 10, and by 6-hour bins for six porpoises attached with miniPAT. Those time series showed that Dall’s porpoises spent more time near the surface (0–10 m) while frequently diving up to 100 m (Figure 6). Diving deeper than 300 m was not frequent (Figure 6). A clear correlation between dive depth and habitat depth was not observed (Figure 6). In fact, the porpoises of movement Pattern B also mainly used near-surface layers shallower than 100 m, although they spent most time in waters with bottom a depth exceeding 2,000 m. Additionally, dive depth profiles were summarized by time ranges of an hour and six hours for the porpoises attached with SPOT 10 and miniPAT, respectively (Figure 7). A weak tendency for inhabiting a layer shallower than 50 m during mornings (3:00–9:00 Japan Standard Time) was observed for some individuals (Figure 7).
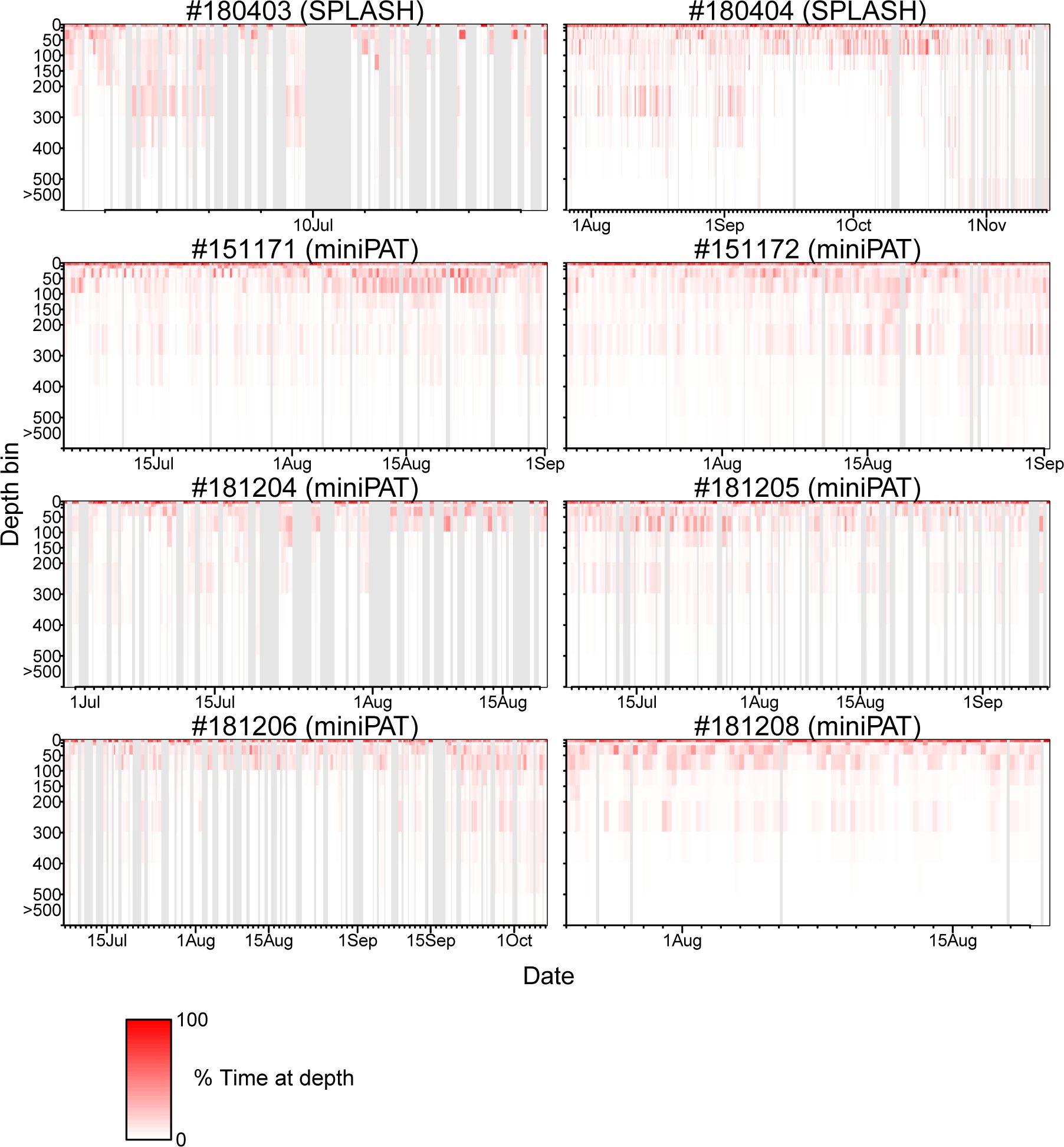
Figure 6 Dive profile time-series of eight Dall’s porpoises. The percentage of time spent in each depth layer was summarized by an hour for two porpoises attached with SPOT 10 tags (top two panels), and by six hours for six porpoises attached with miniPAT tags (bottom six panels). Grey shaded area represents missing data.
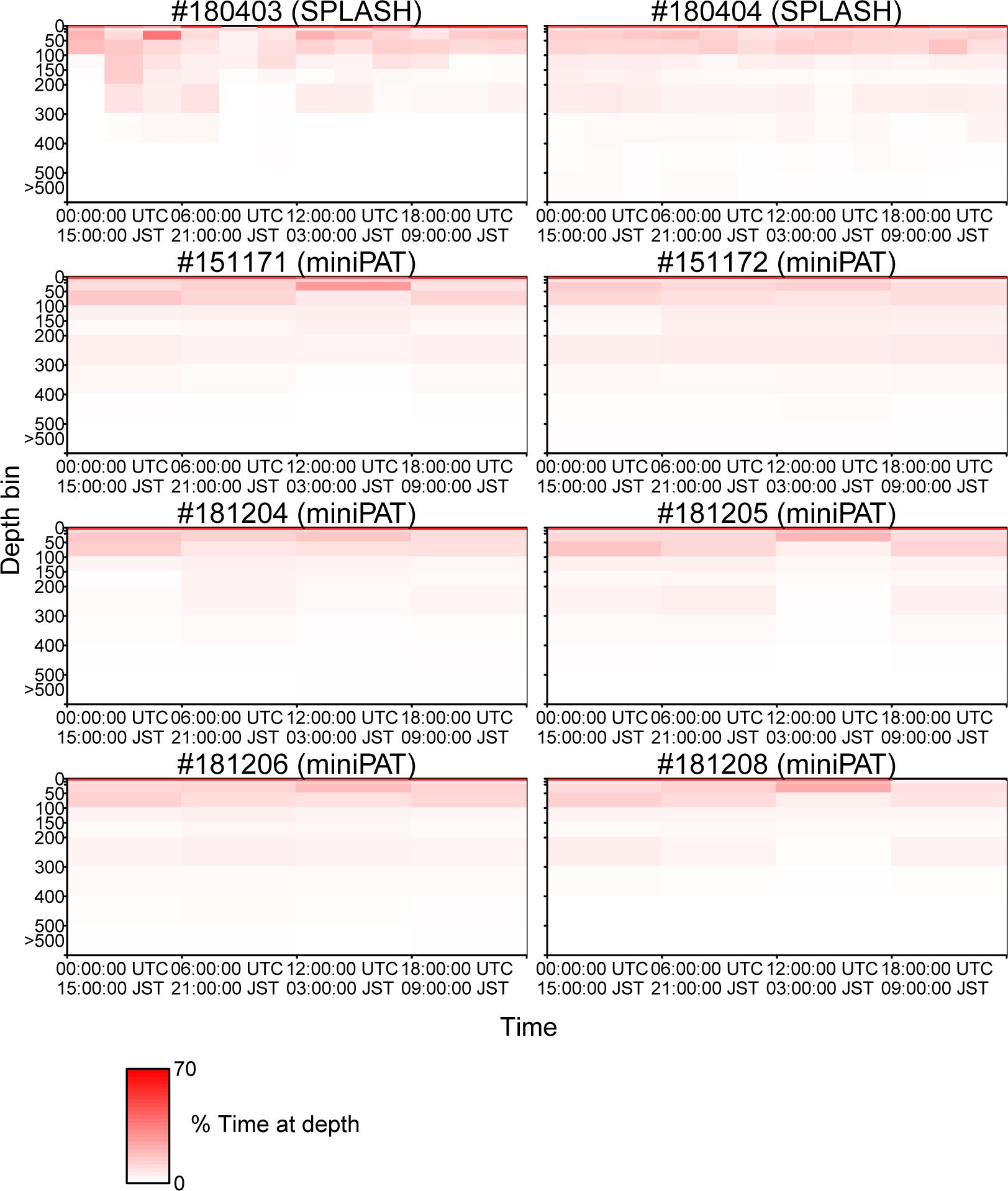
Figure 7 Dive profiles (the percentage of time spent in each depth layer) summarized by 6 hours of time intervals (0:00–6:00, 6:00–12:00, 12:00–18:00, and 18:00–24:00 UTC).
4 Discussion
4.1 Temperature related distribution patterns
Our tagging results revealed that the habitat ranges of Dall’s porpoises showing the movement Pattern B were restricted by water temperature, particularly by a thermocline of 17°C. Thus, to avoid warm water, tagged porpoises changed direction when they faced a surface thermocline of 17°C (Figures 4, 5; Appendix Figure A1). The time-series temperatures data showed that three porpoises frequently entered the waters with >17°C but did not stay long and soon returned to colder waters (Figure 5). Dall’s porpoise #15117 showed moving along thermocline of 17°C (Figure 5). The movement patterns of Dall’s porpoises were more clearly correlated with surface temperature than with temperature at a depth of 100 m (Figure 4), which is consistent with the data showing that porpoises spent more times near the surface (Figure 6). On the velocity maps, Dall’s porpoises tended to avoid waters with higher velocity caused by the Oyashio mainstream and its bifurcations and eddies in the Pacific Ocean. These results suggested that Oyashio current forms the habitat boundary of the JO population of Dall’s porpoises. The Oyashio current flows further southwestward along the Chishima islands and the eastern coast of Japan. Then, it turns eastward at latitudes of approximately 38°–40°N, which shapes cold Oyahsio waters in the subarctic western North Pacific (Nishikawa et al., 2020). The Oyashio front water, represented by a thermocline of 6°C at a depth of 100 m, was located further south of the observed habitats of the JO population (Figure 4). According to previous sighting records, immature Dall’s porpoises of the TT population were distributed south of JO population (Miyashita, 1991). It is plausible that the Oyashio current spatially separated the two Dall’s porpoise populations.
Ocean currents often works as a species distribution boundary through discontinuity in physical and biological environments. Therefore, these distribution patterns might be related to prey distribution and abundance. However, even if the Oyashio current separated the habitats of two populations, such a mechanism could not explain distribution patterns in the Sea of Okhotsk (movement Pattern A). Two Dall’s porpoises demonstrated a tendency to avoid the thermocline of 17°C in the Sea of Okhotsk. It is difficult to assume that prey compositions are the same between the Pacific Ocean and the Sea of Okhotsk. These observations imply that temperature distribution affects the habitat selection of Dall’s porpoises more directly than believed previously. However, there may be a demographic component to this temperature preference. Using the molecular sexing method, we determined that all six Dall’s porpoises tagged in 2019 were males (Table 1). Body length was measured for these six Dall’s porpoises, which ranged from 182 to 223 cm (Table 1). According to Amano and Kuramochi (1992), the smallest and largest body length of immature Dall’s porpoise was 181 cm, which suggested that the Dall’s porpoises tagged in this study were at least at the early maturing stage. According to the stranding database, 76 Dall’s porpoises of the JO population were bycaught or stranded in Rausu town, our tagging site, in 2010–2023 (National Museum of Nature and Science, 2024). Among animals whose sex was identified, 53 Dall’s porpoises were males, and only 10 were female. Generally, larger body sizes have greater energy requirements (Perez and Mooney, 1990). Migrating northward would be beneficial, particularly for larger mature porpoises, because primary productivity is much greater at high latitudes where the pronounced vertical mixing supplies nutrients to the photic zone (Lalli and Parsons, 1997). Conversely, endotherms, such as porpoises, maintain constant body temperatures even in cold waters at higher latitudes. Endotherms can maintain their activity in cold environments if they can obtain sufficient energy to meet their elevated metabolic needs (Porter and Gates, 1969). In this regard, a larger body is beneficial in northern colder habitats because adult animals can generally feed more efficiently than young and reduce body surface relative to body volumes. Remaining in relatively warmer waters might be beneficial for young immature animals. In the previous sighting survey, a small number of Dall’s porpoises of JO population was recorded in the waters warmer than 17°C (Kasuya and Jones, 1984). These sighting positions were located in the Pacific Ocean, where immature animals have been observed (Amano and Kuramochi, 1992).
4.2 Migration patterns
The JO population of Dall’s porpoise has been known to migrate into the Sea of Okhotsk during summer for breeding and feeding (Amano and Kuramochi, 1992; Amano and Hayano, 2007; Kasuya, 2011). There are two estimated migration routes; the eastward route enters into the Pacific Ocean via Tsugaru Strait and then into the Sea of Okhotsk, and the northward route enters into the Sea of Okhotsk via Soya Strait (Figure 2). Based on the absence of pregnant females and mother-calf pairs, the male’s smaller body length, and the relatively lower proportions of mature males, immature porpoises have been considered to mainly use the eastward route (Amano and Kuramochi, 1992). Many mother-calf pairs have been observed on the northward route, which is considered to provide calving ground for the JO population from May to June (Amano and Kuramochi, 1992). All sexed Dall’s porpoises were males (Table 1), and no mother-calf pairs were observed in this sampling site (Kobayashi, M., personal communication). Considering our sampling site was close to the southern edge of the Sea of Okhotsk and Dall’s porpoises were bycaught in early summer, the tagged Dall’s porpoises were possibly migrating from the eastward route.
Conversely, our tagged Dall’s porpoises did not move straight toward breeding areas in the Sea of Okhotsk. Rather, some went back and forth between the Sea of Okhotsk and the Pacific Ocean (Figure 4). Amano and Kuramochi (1992) reported that the maturation rate was significantly higher for both sexes in the Sea of Okhotsk than in the other areas (e.g. northern Sea of Japan and the Pacific Ocean). The sex ratio was also biased to females (70.8%) in the Sea of Okhotsk compared with the areas on eastward (south of Chishima islands) (16.2–30.0%) and northward routes (west of Soya Strait) (40.0–40.3%). Mature males might enter the Sea of Okhotsk to mate with mature females, while immature and early mature males might stay in the Pacific Ocean or around Chishima islands for feeding. In fact, the latter waters were more productive regarding chlorophyll-a concentration (Figure 4), which would provide preferable feeding grounds. Similar habitat separation was also observed for Dall’s porpoises of the TT population (Miyashita, 1991), in which mother-calf pairs were only observed in the central Sea of Okhotsk, but many Dall’s porpoises of the TT population were also observed in the waters off the southern Chishima islands. These two habitats were spatially separated, so Kasuya (2011) considered that such distributional patters represented a separation of habitats between mature and immature porpoises.
Among the eight tagged Dall’s porpoises, only one porpoise (#180404) moved into the Sea of Japan through Soya Strait (Figure 4). Dall’s porpoises were likely prohibited from moving further south by warm waters; thus, no tagged porpoises moved into the sea through Tsugaru Strait at least during the tracking periods. Compared with spring migration, knowledge of migration routes from the Sea of Okhotsk to the Sea of Japan during fall to winter has been limited. In the Tsugaru Strait, Dall’s porpoises of the JO population were mainly observed from April to June and November (Ichimori et al., 2013). Sightings of Dall’s porpoises lasted three months in the spring season, while the fall sighting season was limited to November (Ichimori et al., 2013). Sightings were also observed in January and February, but encounter rates were 10 times smaller than in April to June and November. It is possible that most of Dall’s porpoises of the JO population used the southward (northward in spring–winter) route through Soya Strait during fall and winter, rather than the westward (eastward in spring–winter) route through Tsugaru Strait. However, another scenario is possible; as temperature decreases from fall to winter, the thermocline of 17°C moves southward (Appendix Figure A1), which could trigger westward migration toward Tsugaru Strait. To test these hypotheses, we need to track the movements of Dall’s porpoise for longer periods. This study was the first attempt at satellite-based tracking of Dall’s porpoise in the waters off Japan. The maximum tracking period was 116 days (Table 1). These tracking records were much longer than those of other small cetacean species tagged around Japan (Minamikawa et al., 2007, 2013; Kanaji et al., 2022). Further accumulation of tracking data will facilitate our knowledge about migration and habitat utilization patterns in future.
4.3 Vertical movements
According to the diving profiles retrieved from the eight tags, Dall’s porpoises spent more time near the surface while frequently diving up to 100 m deep (Figure 6). Diving deeper than 300 m was not frequent. Particularly, Dall’s porpoise #180404 rarely dived deeper than 100 m when the porpoise moved along shallow coastal waters from mid-September to early October (Figure 6; Appendix Figure A1). Other than this, the diving profiles did not changed among individuals and seasons. Cetacean’s diving profiles are often related to their feeding behaviors (Aoki et al., 2007; Minamikawa et al., 2007; Aguilar Soto et al., 2008; Minamikawa et al., 2013); vertically wide habitat use observed in the diving profiles corresponded well with a wide variety of prey species of Dall’s porpoise ranging from pelagic to deepwater fishes and squids (Ohizumi et al., 2000, 2003; Okamoto et al., 2010). Ohizumi et al. (2000) pointed out that Dall’s porpoises would not dive deeper than 500 m because the habitat layer for important prey species is 300–400 m deep. Except for #180404, Dall’s porpoises tended to use shallow layers in the morning (3:00–9:00 Japan Standard Time) (Figure 7). According to the visual observation of feeding groups, feeding activities of Dall’s porpoises were more frequently observed in the morning in the North Pacific, though clear diurnal patterns were not observed in the Sea of Okhotsk (Amano et al., 1998). However, Ohizumi et al. (2000) asserted that Dall’s porpoises can change their feeding time based on preferred prey availability. Our results might suggest that Dall’s porpoises forage pelagic species in the morning or shallow migrating deepwater species in the dawn, but we could not conclude that because the six hour (an hour for two tags) time resolution of our diving profiles was not adequate to identify the diurnal pattern of their diving behaviors.
4.4 Conservation implications
It has been widely alerted that global warming seriously impacts marine environments and ecosystems worldwide (Johnson and Lyman, 2020; Venegas et al., 2023). Global warming significantly affects animals inhabiting cold, higher latitudes (van Weelden et al., 2021). Indeed, our tagged porpoises avoided warm waters, which provided direct evidence that Dall’s porpoises could change their distribution ranges in response to ocean warming. The distribution ranges of Dall’s porpoises were related to the front of Oyashio current, and a northward shift of the fronts up to latitudes of 2° is predicted by the late twenty-first century in an extreme simulation scenario (Nishikawa et al., 2020).
In addition, Dall’s porpoises of the JO population have been targeted by hand-harpoon fisheries and sometimes incidentally killed by set nets (National Museum of Nature and Science, 2024). After the great earthquake in 2011, many licensed boats quit taking Dall’s porpoises, and currently, few Dall’s porpoises are harvested from the JO population. The estimated abundance was at least 100,000 individuals (Kanaji et al., 2015), which is sufficiently large to sustain the population given the known mortality rate. However, past large-scale direct catches for the porpoises could have a substantial impact on the population.
The refined understanding of habitat selection provided by the present study would also improve monitoring frameworks for Dall’s porpoise abundances. Monitoring the abundance of the fishery-targeted population is undoubtedly important to assess these effects and prevent severe depletion and extinction. Abundance estimation has been conducted based on the assumption of distribution ranges of three distinct populations in the Sea of Okhotsk (Miyashita, 1991). The assumed geographical range for the JO population corresponded with movement trajectories observed in the study (Figures 2, 3). Northern limits of movement trajectories roughly corresponded with an isobath of 2,000 m (Figures 2, 3), which provided more direct knowledge of the distribution range of the JO population in the Sea of Okhotsk. The correction of bias due to animal diving is also a key component of abundance estimation (Barlow, 2021). The diving profile data provide important information for more precise abundance estimation (Kanaji et al., 2020). This study could answer our primary biological question, and contribute important knowledge for improving the conservation and management of the population.
Data availability statement
The datasets presented in this study can be found in online repositories. The names of the repository/repositories and accession number(s) can be found in the article/Supplementary Material.
Ethics statement
The animal study was approved by Internal committee at the Japan Fisheries Research and Education Agency (authorization permit #44) and the Tokyo University of Agriculture (authorization permit #2019115). The study was conducted in accordance with the local legislation and institutional requirements.
Author contributions
YK: Conceptualization, Formal analysis, Methodology, Software, Writing – original draft. HS: Data curation, Formal analysis, Investigation, Methodology, Software, Writing – review & editing. SI: Investigation, Methodology, Writing – review & editing. NA: Investigation, Writing – review & editing. MK: Investigation, Methodology, Writing – review & editing.
Funding
The author(s) declare financial support was received for the research, authorship, and/or publication of this article. The field survey was conducted as a part of the research and assessment programme for fisheries resources, the Fisheries Agency of Japan.
Acknowledgments
We express our sincere thanks to the members of Minehama Suisan, Co., Ltd. We also thank Dr. Shingo Minamikawa for his advice for tagging methods. This manuscript greatly benefited from the comments of the editor, xxx reviewers.
Conflict of interest
The authors declare that the research was conducted in the absence of any commercial or financial relationships that could be construed as a potential conflict of interest.
Publisher’s note
All claims expressed in this article are solely those of the authors and do not necessarily represent those of their affiliated organizations, or those of the publisher, the editors and the reviewers. Any product that may be evaluated in this article, or claim that may be made by its manufacturer, is not guaranteed or endorsed by the publisher.
Supplementary material
The Supplementary Material for this article can be found online at: https://www.frontiersin.org/articles/10.3389/fmars.2024.1429358/full#supplementary-material
References
Aguilar Soto N., Johnson M. P., Madsen P. T., Díaz F., Domínguez I., Brito A., et al. (2008). Cheetahs of the deep sea: Deep foraging sprints in short-finned pilot whales off Tenerife (Canary Islands). J. Anim. Ecol. 77, 936–947. doi: 10.1111/j.1365-2656.2008.01393.x
Amano M., Hayano A. (2007). Intermingling of dalli-type Dall’s porpoises into a wintering truei-type population off Japan: Implications from color patterns. Mar. Mammal Sci. 23, 1–14. doi: 10.1111/j.1748-7692.2006.00077.x
Amano M., Kuramochi T. (1992). Segregative migration of Dall’s porpoise (Phocoenoides dalli) in the sea of Japan and sea of Okhotsk. Mar. Mammal Sci. 8, 143–151. doi: 10.1111/j.1748-7692.1992.tb00373.x
Amano M., Marui M. (2000). Re-evaluation of geographic variation in the white fluke patch of dalli-type Dall’s porpoises. Mar. Mammal Sci. 16, 631–636. doi: 10.1111/j.1748-7692.2000.tb00956.x
Amano M., Yoshioka M., Kuramochi T., Mori K. (1998). Diurnal feeding by Dall’s porpoise, phocoenoides dalli. Mar. Mammal Sci. 14, 130–135. doi: 10.1111/j.1748-7692.1998.tb00695.x
Amante C., Eakins B. W. (2009). ETOPO1 1 arc-minute global relief model: procedures, data sources and analysis. NOAA Tech. Memo. NESDIS NGDC-24, 1–19.
Aoki K., Amano M., Yoshioka M., Mori K., Tokuda D., Miyazaki N. (2007). Diel diving behavior of sperm whales off Japan. Mar. Ecol. Prog. Ser. 349, 277–287. doi: 10.3354/meps07068
Balmer B. C., Schwacke L. H., Wells R. S. (2010). Linking dive behavior to satellite-linked tag condition for a bottlenose dolphin (Tursiops truncatus) along florida’s Northern gulf of Mexico coast. Aquat. Mamm. 36, 1–8. doi: 10.1578/AM.36.1.2010.1
Balmer B. C., Wells R. S., Howle L. E., Barleycorn A. A., McLellan W. A., Ann Pabst D., et al. (2014). Advances in cetacean telemetry: A review of single-pin transmitter attachment techniques on small cetaceans and development of a new satellite-linked transmitter design. Mar. Mammal Sci. 30, 656–673. doi: 10.1111/mms.12072
Barlow J. (2021). “Trackline detection probability for long-diving whales,” in Marine Mammal Survey and Assessment Methods, eds. Laake J. L., Robertson D. G., Amstrup S. C., Manly B. F. J. (London: CRC Press), 209–221. doi: 10.1201/9781003211167-19
Becker E. A., Forney K. A., Foley D. G., Smith R. C., Moore T. J., Barlow J. (2014). Predicting seasonal density patterns of California cetaceans based on habitat models. Endanger. Species Res. 23, 1–22. doi: 10.3354/esr00548
Blanco C., Salomón O., Raga J. A. (2001). Diet of the bottlenose dolphin (Tursiops truncatus) in the western Mediterranean Sea. J. Mar. Biol. Assoc. United Kingdom 81, 1053–1058. doi: 10.1017/S0025315401005057
Buckley L. B., Hurlbert A. H., Jetz W. (2012). Broad-scale ecological implications of ectothermy and endothermy in changing environments. Glob. Ecol. Biogeogr. 21, 873–885. doi: 10.1111/j.1466-8238.2011.00737.x
Clarke J. K., Stafford S. E., Moore B., Rone L., Aerts L., Crance J. (2013). Subarctic cetaceans in the southern Chukchi Sea: Evidence of recovery or response to a changing ecosystem. Oceanography 26, 136–149. doi: 10.5670/oceanog.2013.81
CLS (Collecte LocalisationSatellites). (2016). Argos user’s manual, CLS (Ramonville-Saint-Agne, France).
Deming A. C., Wingers N. L., Moore D. P., Rotstein D., Wells R. S., Ewing R., et al. (2020). Health impacts and recovery from prolonged freshwater exposure in a common bottlenose dolphin (Tursiops truncatus). Front. Vet. Sci. 7, 5759–5784. doi: 10.1111/j.1748-7692.1998.tb00745.x
Fish F. E., Hui C. A. (1991). Dolphin swimming–a review. Mamm. Rev. 21, 181–195. doi: 10.1111/j.1365-2907.1991.tb00292.x
Hayashi M., Shiogama H., Ogura T. (2022). The contribution of climate change to increasing extreme ocean warming around Japan. Geophys. Res. Lett. 49, 1–10. doi: 10.1029/2022GL100785
Heide-Jørgensen M. P., Blackwell S. B., Williams T. M., Sinding M. H. S., Skovrind M., Tervo O. M., et al. (2020). Some like it cold: Temperature-dependent habitat selection by narwhals. Ecol. Evol. 10, 8073–8090. doi: 10.1002/ece3.6464
Hui C. A. (1987). Power and speed of swimming dolphins. J. Mammal. 68, 126–132. doi: 10.2307/1381055
Ichimori T., Suzuki S., Kojima S., Ishimori K., Sugitani M., Matsuda A., et al. (2013). Seasonal variability and yearly trend of cetacean encounters in decadal sighting survey in the Tsugaru Strait, Japan. Japan Cetol. 23, 29–33. doi: 10.5181/cetology.0.23_29
International Whaling Commission (2002). Report of the standing sub-committee on small cetaceans. J. Cetacean Res. Manage. 4, 325–338.
Jayasankar P., Anoop B., Rajagopalan M. (2008). PCR-based sex determination of cetaceans and dugong from the Indian seas. Curr. Sci. 94, 1513–1516.
Jefferson T. A. (2018). “Dall’s Porpoise Phocoenoides dalli,” in The Encyclopedia of marine mammals, 3rd edn, eds. Würsig B., Thewissen J. G. M., Kovacs K. M., (London: Academic Press), 239–242.
Johnson C. G., Lyman M. J. (2020). Warming trends increasingly dominate global ocean. Nat. Clim. Change 10, 757–761. doi: 10.1038/s41558-020-0822-0
Jonsen I. D., Basson M., Bestley S., Bravington M. V., Patterson T. A., Pedersen M. W., et al. (2013). State-space models for bio-loggers: A methodological road map. Deep. Res. Part II Top. Stud. Oceanogr. 88–89, 34–46. doi: 10.1016/j.dsr2.2012.07.008
Jonsen I. D., Flemming J. M., Myers R. A. (2005). Robust state-space modeling of animal movement data. Ecology 86, 2874–2880. doi: 10.1890/04-1852
Kanaji Y., Funasaka N., Sasaki H. (2022). Mesoscale movement patterns of common bottlenose dolphins along Kuroshio Current and its bifurcation. Fish. Oceanogr. 31, 429–442. doi: 10.1111/fog.12593
Kanaji Y., Gerrodette T. (2020). Estimating abundance of Risso’s dolphins using a hierarchical Bayesian habitat model: A framework for monitoring stocks of animals inhabiting a dynamic ocean environment. Deep. Res. Part II Top. Stud. Oceanogr. 175, 104699. doi: 10.1016/j.dsr2.2019.104699
Kanaji Y., Miyashita T., Yoshida H., Okazaki M., Kishiro T. (2015). Abundance estimates of dalli-type and truei-type of Dall’s porpoise Phocoenoides dalli in the western central part of the Sea of Okhotsk, July–September between 1990 and 2010. Fish. Sci. 81, 611–619. doi: 10.1007/s12562-015-0887-2
Kanaji Y., Okazaki M., Watanabe H., Miyashita T. (2016). Biogeography of small odontocetes in relation to wide-scale oceanographic structure in the North Pacific Ocean. Fish. Oceanogr. 25, 119–132. doi: 10.1111/fog.12140
Kanaji Y., Yoshida H., Sasaki H., Okazaki M., Kobayashi M. (2020). Distribution and abundance of dalli-type Dall’s porpoises Phocoenoides dalli migrating into waters off southeastern Hokkaido, Japan, during summer: results of 2014–2016 aerial surveys. Fish. Sci. 86, 287–298. doi: 10.1007/s12562-019-01382-4
Kasuya T. (1978). The life history of Dall’s porpoises with special reference to the stock off the Pacific coast of Japan. Sci. Rep. Whales Res. Inst. 30, 1–63.
Kasuya T. (2011). Conservation Biology of Small Cetaceans around Japan (Tokyo: University of Tokyo Press).
Kasuya T., Jones L. L. (1984). Behavior and segregation of the Dall’s porpoise in the northwestern North Pacific ocean. Sci. Rep. Whales Res. Inst. 35, 107–128.
Kawai H. (1972). “Hydorography of the Kuroshio and the Oyashio,” in Physical oceanography II, Fundamental Lectures of Oceanography. Eds. Iwashita M., Komaki Y., Hoshino M., Horibe S., Masuzawa J. (Tokai University Press, Tokyo), 129–320.
Kuroda H., Setou T., Kakehi S., Ito S., Taneda T., Azumaya T., et al. (2017). Recent advances in Japanese fisheries science in the Kuroshio-Oyashio region through development of the FRA-ROMS ocean forecast system: overview of the reproducibility of reanalysis products. Open J. Mar. Sci. 07, 62–90. doi: 10.4236/ojms.2017.71006
Lalli C. M., Parsons T. R. (1997). Biological Oceanography: An Introduction. 2nd ed (Oxford: Butterworth-Heinema).
Levesque D. L., Marshall K. E. (2021). Do endotherms have thermal performance curves? J. Exp. Biol. 224, jeb141309. doi: 10.1242/jeb.141309
Minamikawa S., Iwasaki T., Kishiro T. (2007). Diving behaviour of a Baird’s beaked whale, Berardius bairdii, in the slope water region of the western North Pacific: First dive records using a data logger. Fish. Oceanogr. 16, 573–577. doi: 10.1111/j.1365-2419.2007.00456.x
Minamikawa S., Watanabe H., Iwasaki T. (2013). Diving behavior of a false killer whale, Pseudorca crassidens, in the Kuroshio-Oyashio transition region and the Kuroshio front region of the western North Pacific. Mar. Mammal Sci. 29, 177–185. doi: 10.1111/j.1748-7692.2011.00532.x
Miyashita T., Kasuya T. (1988). Distribution and abundance of Dall’s porpoises off Japan. Sci. Rep. Whales Res. Inst. 39, 121–150.
Miyashita T. (1991). “Stocks and abundance of Dall’s porpoises in the Okhotsk Sea and adjacent waters.” in Paper SC/43/SM7 presented to International Whaling Commission Scientific Committee, 1–24.
Mullin K. D., McDonald T., Wells R. S., Balmer B. C., Speakman T., Sinclair C., et al. (2017). Density, abundance, survival, and ranging patterns of common bottlenose dolphins (Tursiops truncatus) in Mississippi Sound following the Deepwater Horizon oil spill. PloS One 12, 1–30. doi: 10.1371/journal.pone.0186265
National Museum of Nature and Science. (2024). Marine mammals stranding database. Available online at: https://www.kahaku.go.jp/research/db/zoology/marmam/drift/index.php (Accessed January 30, 2024).
Nishikawa H., Nishikawa S., Ishizaki H., Wakamatsu T., Ishikawa Y. (2020). Detection of the Oyashio and Kuroshio fronts under the projected climate change in the 21st century. Prog. Earth Planet. Sci. 7, 29. doi: 10.1186/s40645-020-00342-2
Ohizumi H. (2002). Dietary studies of toothed whales: A review of technical issues and new topics. Fish. Sci. 68, 264–267. doi: 10.2331/fishsci.68.sup1_264
Ohizumi H., Kuramochi T., Amano M., Miyazaki N. (2000). Prey switching of Dall’s porpoise Phocoenoides dalli with population decline of Japanese pilchard Sardinops melanostictus around Hokkaido, Japan. Mar. Ecol. Prog. Ser. 200, 265–275. doi: 10.3354/meps200265
Ohizumi H., Kuramochi T., Kubodera T., Yoshioka M., Miyazaki N. (2003). Feeding habits of Dall’s porpoises (Phocoenoides dalli) in the subarctic North Pacific and the Bering Sea basin and the impact of predation on mesopelagic micronekton. Deep. Res. Part I Oceanogr. Res. Pap. 50, 593–610. doi: 10.1016/S0967-0637(03)00033-5
Okamoto R., Ohizumi H., Uchikawa K., Ito M., Iwasaki T., Kato H. (2010). Prey selection of Dali’s porpoise Phocoenoides dalli on the continental slope off the Pacific coast of Sanriku in winter. Nippon Suisan Gakkaishi 76, 54–61. doi: 10.2331/suisan.76.54
Pedersen M. W., Patterson T. A., Thygesen U. H., Madsen H. (2011). Estimating animal behavior and residency from movement data. Oikos 120, 1281–1290. doi: 10.1111/j.1600-0706.2011.19044.x
Perez M. a., Mooney W. B. M. (1990). Estimated feeding rate relationship for marine mammals based on captive animal data. NOAA Tech. Memo. NMFS-F/NWC 184, 1–30.
Porter W. P., Gates D. M. (1969). Thermodynamic equilibria of animals with environment. Ecol. Monogr. 39, 227–244. doi: 10.2307/1948545
Rasmussen M. H., Akamatsu T., Teilmann J., Vikingsson G., Miller L. A. (2013). Biosonar, diving and movements of two tagged white-beaked dolphin in Icelandic waters. Deep. Res. Part II Top. Stud. Oceanogr. 88–89, 97–105. doi: 10.1016/j.dsr2.2012.07.011
R Core Team. (2023). R: A language and environment for statistical computing (Vienna, Austria). Available at: https://www.r-project.org/.
Scott M. D., Chivers S. J. (2009). Movements and diving behavior of pelagic spotted dolphins. Mar. Mammal Sci. 25, 137–160. doi: 10.1111/j.1748-7692.2008.00241.x
Stewart J. D., Joyce T. W., Durban J. W., Calambokidis J., Fauquier D., Fearnbach H., et al. (2023). Boom-bust cycles in gray whales associated with dynamic and changing Arctic conditions. Science 382, 207–211. doi: 10.1126/science.adi1847
The Committee on Taxonomical Names and Collections of the Mammalogical Society of Japan (2001). Guidelines for the procedure of obtaining mammals specimens as approved by the Mammalogical Society of Japan. Honyurui Kagaku 41, 215–233.
The Society for Marine Mammalogy. (2024). Guideline for treatment of marine mammals. Available online at: https://marinemammalscience.org/about-us/ethics/marine-mammal-treatment-guidelines/ (Accessed April 23, 2024).
Thorne L. H., Foley H. J., Baird R. W., Webster D. L., Swaim Z. T., Read A. J. (2017). Movement and foraging behavior of short-finned pilot whales in the Mid-Atlantic Bight: Importance of bathymetric features and implications for management. Mar. Ecol. Prog. Ser. 584, 245–257. doi: 10.3354/meps12371
van Weelden C., Towers J. R., Bosker T. (2021). Impacts of climate change on cetacean distribution, habitat and migration. Clim. Change Ecol. 1, 100009. doi: 10.1016/j.ecochg.2021.100009
Venegas R. M., Acevedo J., Treml E. A. (2023). Three decades of ocean warming impacts on marine ecosystems: A review and perspective. Deep. Res. Part II Top. Stud. Oceanogr. 212, 105318. doi: 10.1016/j.dsr2.2023.105318
Wang M. C., Shao K. T., Huang S. L., Chou L. S. (2012). Food partitioning among three sympatric odontocetes (Grampus griseus, Lagenodelphis hosei, and Stenella attenuata). Mar. Mammal Sci. 28, 143–157. doi: 10.1111/j.1748-7692.2011.00501.x
Keywords: global warming, satellite tracking, MiniPAT, ARGOS, Oyashio, dalli-type population, Phocoenidae, bycatch
Citation: Kanaji Y, Sasaki H, Ikuta S, Azuma N and Kobayashi M (2024) Temperature-related movement and habitat utilization patterns of Dall’s porpoises, small cetaceans seasonally migrating into the subarctic Pacific. Front. Mar. Sci. 11:1429358. doi: 10.3389/fmars.2024.1429358
Received: 08 May 2024; Accepted: 18 June 2024;
Published: 02 July 2024.
Edited by:
K. David Hyrenbach, Hawaii Pacific University, United StatesReviewed by:
Thomas A. Jefferson, Clymene Enterprises, United StatesAlyson H. Fleming, Smithsonian Institution, United States
Copyright © 2024 Kanaji, Sasaki, Ikuta, Azuma and Kobayashi. This is an open-access article distributed under the terms of the Creative Commons Attribution License (CC BY). The use, distribution or reproduction in other forums is permitted, provided the original author(s) and the copyright owner(s) are credited and that the original publication in this journal is cited, in accordance with accepted academic practice. No use, distribution or reproduction is permitted which does not comply with these terms.
*Correspondence: Yu Kanaji, a2FuYWppX3l1OTZAZnJhLmdvLmpw