- 1Key Laboratory of Microecological Resources and Utilization in Breeding Industry, Ministry of Agriculture and Rural Affairs, Guangdong Haid Group Co., Ltd., Guangzhou, Guangdong, China
- 2College of Fisheries and Life Science, Shanghai Ocean University, Shanghai, China
Mandarin fish (Siniperca chuatsi) can adapt to artificial diets, with the improvement of domestication level. However, the effects of artificial diets on the muscle health of fish are unclear. In this study, 480 homogenous-sized mandarin fish (initial weight of 25.1 ± 0.1 g) were randomly divided into two groups and fed with artificial diets or live prey fish for eight weeks. The transcriptome sequencing analysis identified that artificial diets primarily affected glucose metabolism, lipid metabolism, and immune system in the muscle. Furthermore, artificial diets induced excessive glycogen accumulation in the muscle by increasing the mRNA expression of gluconeogenesis-related genes and decreasing the mRNA expression of glycolysis-related genes. Meanwhile, artificial diets significantly increased triglyceride accumulation in the muscle by upregulating the activity of fatty acid synthetase and the mRNA expression of lipid synthesis-related genes, including srebp1, fas, and plin2. Artificial diets significantly increased the level of malondialdehyde, leading to oxidative stress in the muscle. Besides, artificial diets also upregulated the mRNA expression of pro-inflammation cytokines, including il-1β, ifn-γ, and tnfα. In conclusion, artificial diets disrupted glucose and lipid metabolism and induced oxidative stress and inflammation in the muscle of mandarin fish.
Introduction
The mandarin fish is an economically valued fish species which is widely cultured in China (Liang et al., 2001; Peng et al., 2022). In 2022, the production of mandarin fish is about 400,000 tonnes in China. Mandarin fish is a typical carnivorous, which have been fed with live bait fish (Liang et al., 2008). However, live bait fish is expensive and unstable. Moreover, live prey fish may carry pathogens and parasites (Li et al., 2017). These seriously restrict the sustainable development of mandarin fish aquaculture. In recent years, with the development of selective breeding and domestication, live bait fish can be replaced entirely by artificial diets for mandarin fish (Liang, 2002; Yi et al., 2013; He et al., 2021). However, mandarin fish cannot efficiently utilize artificial diets, which may disrupt metabolism homeostasis and influence fish health (Li et al., 2015).
Previous studies have suggested that compared with live bait fish, artificial diets decrease the growth performance of mandarin fish, which may be the reduced digestibility in the intestine, especially protein digestibility (Li et al., 2015; Li et al., 2017). Similarly, Siamese fighting fish (Betta splendens) where live prey increased their weight gain rate than that of fish fed artificial diets (Mandal et al., 2010). Desai et al. have proved that unsuitable artificial diets contributed to negative impact on growth and health in rainbow trout (Desai et al., 2012). Anti-nutrient factors in plants can alter the structure and function of the intestinal microbiota of carnivorous fish and cause histological and functional changes in the gastrointestinal tract (Miao et al., 2018). In addition, unsuitable artificial diets induce oxidative stress and endoplasmic reticulum stress in mandarin fish, while supplement the artificial diets with betaine can alleviate the negative influence to some extent (Li et al., 2023). In largemouth bass (Micropterus salmoides), artificial diets inhibit the antioxidant enzyme activities and increase malonaldehyde (MDA) content, leading to cell structure and function damage (Mai et al., 2023). Activities of antioxidant enzymes correlate with health and metabolic homeostasis in aquatic animals (Wang et al., 2009). A high-level rapeseed meal diet affect triglyceride accumulation in the liver by inhibiting fatty acid synthesis and enhancing lipolysis (Li et al., 2021). However, the effects of artificial diets on the muscle of mandarin fish remain unclear.
Therefore, this study aimed to explore the effects of live prey fish replaced entirely by artificial diets on the muscle of mandarin fish through transcriptomic analysis, biochemical analysis, and quantitative real-time polymerase chain reaction (RT-qPCR) analysis. This study provided a theoretical guidance for feed optimization of mandarin fish in the future and promoted the healthy development of mandarin fish aquaculture.
Materials and methods
Animal feeding experiment
The animal feeding experiment was strictly implemented in accordance with the provisions of the experimental animal ethics committee of Shanghai Ocean University. Before the actual feeding experiment, mandarin fish were raised for two weeks to adapt to the experimental environment. 480 homogenous-sized mandarin fish (25.1 ± 0.1 g) were randomly stocked into 12 tanks in sextuplicate (40 fish per tank). The mandarin fish were purchased from Guangdong Bairong Aquatic Seed Group Co., Ltd (Guangdong, China). The fish in the control group (CON) were fed with live prey fish (India mrigal (Cirrhinus mrigala) with 17.2% wet-weight protein and 4.3% wet-weight lipid), whereas the fish in the AF group were fed with artificial feed (Table 1). All raw materials were crushed by ultra-fine pulverizer and sieved through 100 μm mesh. Next, raw materials were weighed according to feed composition and mixed by step-by-step expansion method. Then, raw materials were fully conditioned and floating pellets of 5 mm sizes was produced by a twin-screw extruder machine. The pellets were dried at 45°C for 10 h. Finally, the pellets were stored at -20°C until use and analysis. The fish were fed to apparent satiation twice daily for eight weeks. During the experiment, water temperature was 28 ± 1°C, dissolved oxygen was > 5.0 mg/L, nitrite was < 0.1 mg/L, and ammonia nitrogen was < 0.1 mg/L.
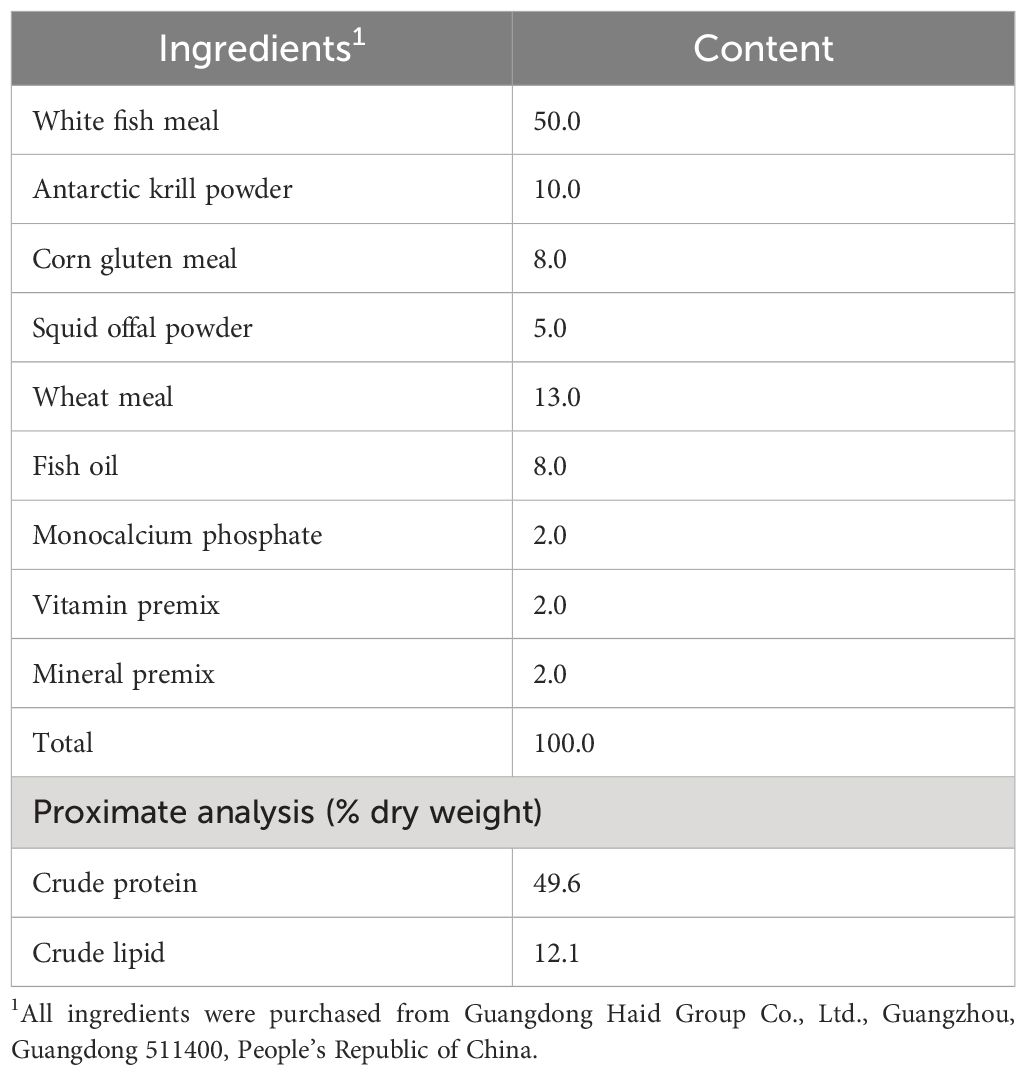
Table 1 Formulation and chemical proximate analysis of the experimental artificial feed (% dry weight).
Sample collection
At the end of the culture experiment, the experimental fish were starved for 24 hours and anesthetized with MS-222 (1:10 000; Sigma, USA). Three fish per tank were randomly sampled to analyze. The muscle above the lateral line of the fish was collected and stored at -80°C for biochemical analysis, gene expression analysis and transcriptome analysis.
Biochemical analysis
The muscle sample was placed into precooled normal saline, homogenized, and centrifuged at 4°C for 20 min (3000 r/min). The supernatant was collected and stored at -20°C for biochemical analysis. The levels of triglyceride (TG) and glycogen in the muscle were detected using commercial reagent kits (Applygen Technologies, China) based on the manufacturer’s instructions. To uncover the effects of artificial diets on the glucose and lipid metabolism in the muscle, the activities of fatty acid synthetase (FAS), pyruvate kinase (PK) and Glucose-6-phosphatase (G6Pase) in the muscle were detected using commercial reagent kits (Solarbio, China). To uncover the effects of artificial diets on the antioxidant capacity in the muscle, the levels of total antioxidant capacity (T-AOC), malonaldehyde (MDA) and reduced glutathione (GSH), and the activities of superoxide dismutase (SOD) and catalase (CAT) in the muscle were detected using commercial reagent kits (Nanjing Jiancheng Bio-engineering Institute, China).
Quantitative real-time PCR
The mRNA level was detected by quantitative real-time PCR, according to our previous studies (Fang et al., 2021; Fang et al., 2022). Primers of each target gene were designed and listed in Table 2. The primers were purchased from Sangon Biotech Co., Ltd (Shanghai, China), including glucose metabolism-related genes (glucose-6-phosphatase (g6pase), pyruvate carboxylase (pc), phosphofructokinase (pfk), pyruvate kinase (pk)), lipid metabolism-related genes (sterol regulatory element binding protein 1 (srebp1), fatty acid synthase (fas), diacylglycerol O-acyltransferase 1 (dgat1), diacylglycerol O-acyltransferase 2 (dgat2), perilipin 2 (plin2), peroxisome proliferation-activated receptor alpha (pparα), carnitine palmitoyl transferase 1 alpha (cpt1α)), antioxidative system-related genes (nuclear factor erythroid2‐related factor 2 (nrf2), Kelch-like ECH-associated protein 1 (keap1), superoxide dismutase (sod), catalase (cat), glutathione peroxidase (gpx)), proinflammation cytokines-related genes (interleukin 1 beta (il-1β), interferon gamma (ifn-γ), tumor necrosis factor alpha (tnfα)), and beta-actin (β-actin). β-actin was used as the housekeeping reference gene. The gene expression levels were analyzed using the 2-△△ct method (Livak and Schmittgen, 2001).
Transcriptome analysis
Muscle tissue samples of mandarin fish in CON and AF groups were collected. Subsequent steps, including RNA extraction, library construction, and transcriptome sequencing were performed by Shanghai Majorbio Bio-pharm Biotechnology using Illumina MiSeq platform (Illumina, USA). Further analyses, including Gene ontology (GO) enrichment and Kyoto Encyclopedia of Genes and Genomes (KEGG) pathway, were analyzed by using the online platform (Majorbio Cloud Platform, China).
Statistical analysis
Results were presented as means ± S.E.M (standard error) and analyzed using SPSS 19.0 software (IBM, USA). The independent t-test was used to inspect the differences between the two groups. P < 0.05 was regarded to be statistically significant.
Results
RNA-seq analysis in the muscle of mandarin fish fed with different diets
RNA sequencing was used to examine the transcriptomic changes in the muscle of mandarin fish fed with different diets. The scatter plot showed that the transcription of 258 genes was upregulated, and the transcription of 243 genes was downregulated in the AF group (Figure 1A). The heatmap illustrated the differentially expressed genes (DEGs) between the two groups (Figure 1B). The DEGs of the two groups were further analyzed by GO and KEGG enrichment. GO enrichment analysis of DEGs revealed that artificial diets primarily affected the processes such as glycolytic process, ATP generation, and nucleotide phosphorylation (Figure 1C). KEGG enrichment analysis of DEGs suggested that significant alterations in glycolysis/gluconeogenesis, glucagon signaling pathway, antigen processing and presentation, lipid and atherosclerosis, and MAPK signaling pathway in the muscle of mandarin fish fed artificial diets (Figure 1D). RNA-seq analysis indicated that artificial diets primary affected glucose and lipid metabolism and immune system.
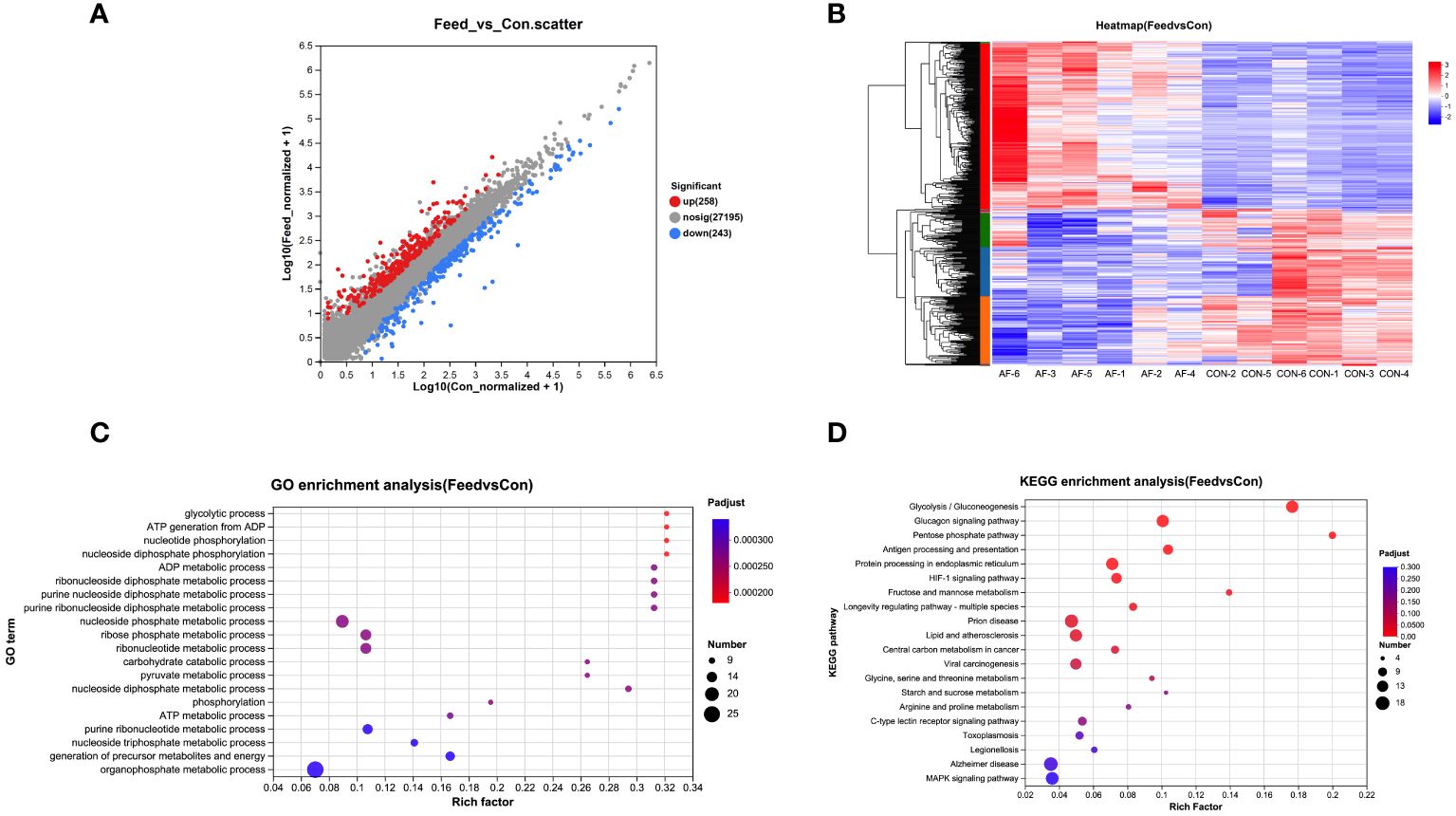
Figure 1 RNA-seq analysis in the muscle of mandarin fish fed with different diets. Scatter plot (A), heatmap (B), GO enrichment analysis (C), and KEGG enrichment analysis (D) in the muscle of mandarin fish fed with the natural or artificial diets. CON, feeding with live prey fish; AF, feeding with artificial diets; GO, Gene ontology; KEGG, Kyoto Encyclopedia of Genes and Genomes (n=6).
Artificial diets disrupted glucose metabolism in the muscle of mandarin fish
The level of muscle glycogen was significantly higher in the AF group compared with the CON group (P < 0.05) (Figure 2A). The activity of G6Pase in the muscle was significantly higher in the AF group than in the CON group (P < 0.05) (Figure 2B). However, the activity of PK was not significantly changed between the two groups (P > 0.05) (Figure 2C). Artificial diets significantly upregulated the mRNA levels of gluconeogenesis-related genes, including g6pase and pc (P < 0.05) (Figure 2D) and downregulated the mRNA levels of glycolysis-related genes, including pfk and pk (P < 0.05) (Figure 2E). These results demonstrated that artificial diets induced excessive muscle glycogen accumulation by disrupting glucose metabolism in the muscle of mandarin fish.
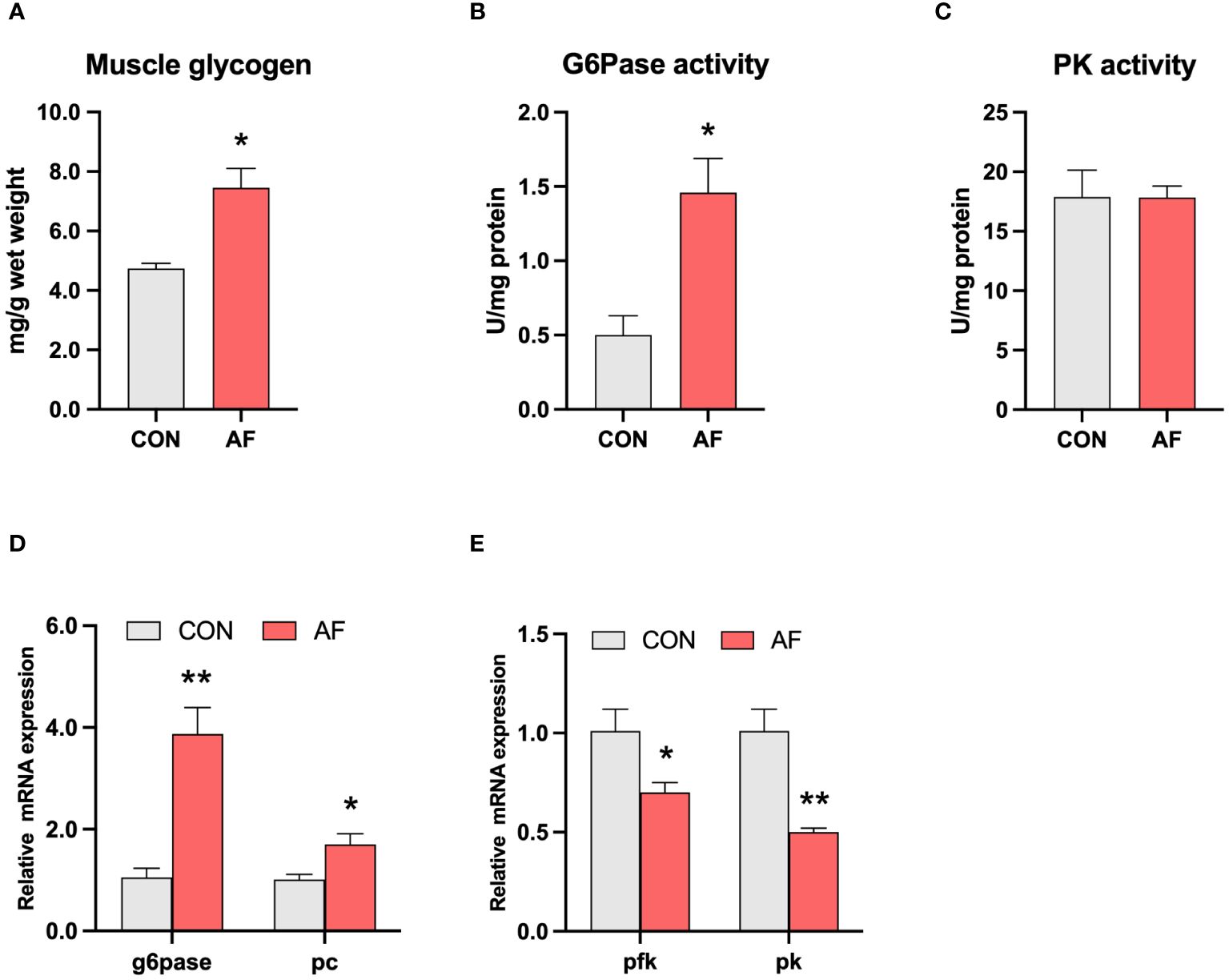
Figure 2 Artificial diets disrupted glucose metabolism in the muscle of mandarin fish. Muscle glycogen (A), G6pase activity (B), and PK activity (C) in the muscle of mandarin fish fed with the natural or artificial diets. Relative mRNA levels of genes related to gluconeogenesis (D) and glycolysis (E) in the muscle of mandarin fish fed with the natural or artificial diets. Data are presented as means ± S.E.M., n=4. Significance was evaluated by independent t-test (*P < 0.05, **P < 0.01). CON, feeding with live prey fish; AF, feeding with artificial diets; G6Pase, glucose-6-phosphatase; PK, pyruvate kinase; pc, pyruvate carboxylase; pfk, phosphofructokinase.
Artificial diets disrupted lipid metabolism in the muscle of mandarin fish
The content of TG in the muscle of mandarin fish fed with artificial diets was remarkably increased than that of live bait fish (P < 0.05) (Figure 3A). Similarly, the activity of FAS was significantly higher in the AF group compared with the CON group (P < 0.05) (Figure 3B). The mRNA expression of lipid synthesis-related genes, including srebp1, fas, and plin2, was significantly higher in the AF group compared with the CON group (P < 0.05) (Figure 3C). However, the mRNA expression of fatty acid β-oxidation-related genes, including pparα and cpt1, was significantly higher in the AF group (P < 0.05) (Figure 3D). These results suggested that artificial diets induced excessive muscle TG accumulation by disrupting lipid metabolism in the muscle of mandarin fish.
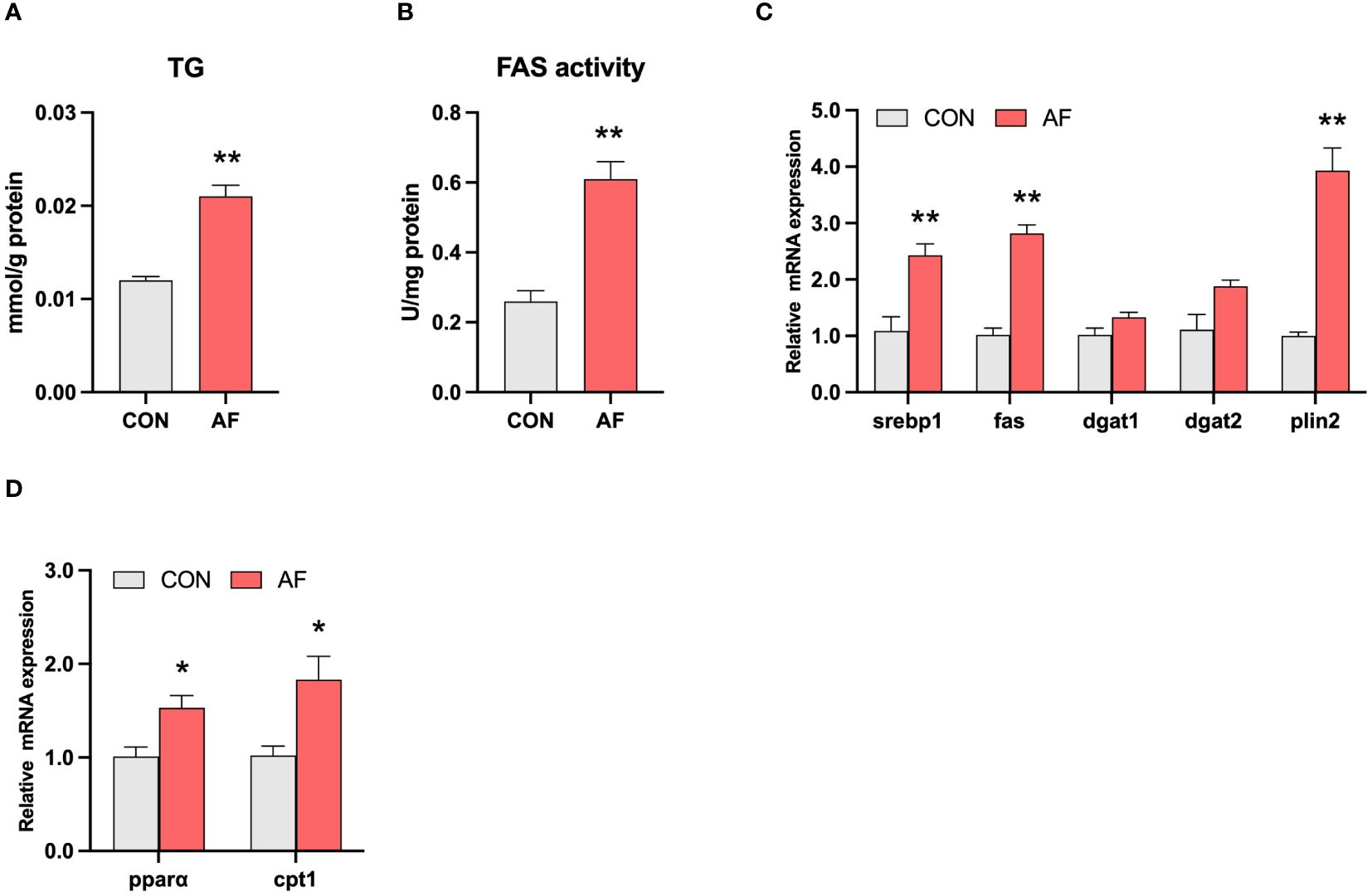
Figure 3 Artificial diets disrupted lipid metabolism in the muscle of mandarin fish. The level of TG (A) and the activity of FAS (B) in the muscle of mandarin fish fed with the natural or artificial diets. Relative mRNA levels of genes related to lipid synthesis (C) and fatty acid β-oxidation (D) in the muscle of mandarin fish fed with the natural or artificial diets. Data are presented as means ± S.E.M., n=4. Significance was evaluated by independent t-test (*P < 0.05, **P < 0.01). CON, feeding with live prey fish; AF, feeding with artificial diets; TG, triglyceride; FAS, fatty acid synthetase; srebp1, sterol regulatory element binding protein 1; dgat1, diacylglycerol O-acyltransferase 1; dgat2, diacylglycerol O-acyltransferase 2; plin2, perilipin 2; pparα, peroxisome proliferation-activated receptor alpha; cpt1α, carnitine palmitoyl transferase 1 alpha.
Artificial diets induced oxidative stress and inflammation in the muscle of mandarin fish
To investigate the effects of artificial diets on the health of mandarin fish, the markers of oxidative stress and inflammation were detected. Results showed that the level of MDA in the muscle of mandarin fish fed with artificial diets was significantly increased compared with the CON group (P < 0.05) (Figure 4A). However, the levels of T-AOC and reduced GSH were significantly higher in the AF group (P < 0.05) (Figures 4B, C). There were no significant differences in activities of SOD and CAT between the two groups (P > 0.05) (Figures 4D, E). Furthermore, artificial diets significantly increased the mRNA expression of nrf2, sod, cat, and gpx in the muscle (P < 0.05) (Figure 4F). Regarding inflammation, artificial diets significantly upregulated the mRNA expression of pro-inflammation cytokines, including il-1β, ifn-γ, and tnfα (P < 0.05) (Figure 4G). These results indicated that artificial diets induced oxidative stress and inflammation in the muscle of mandarin fish.
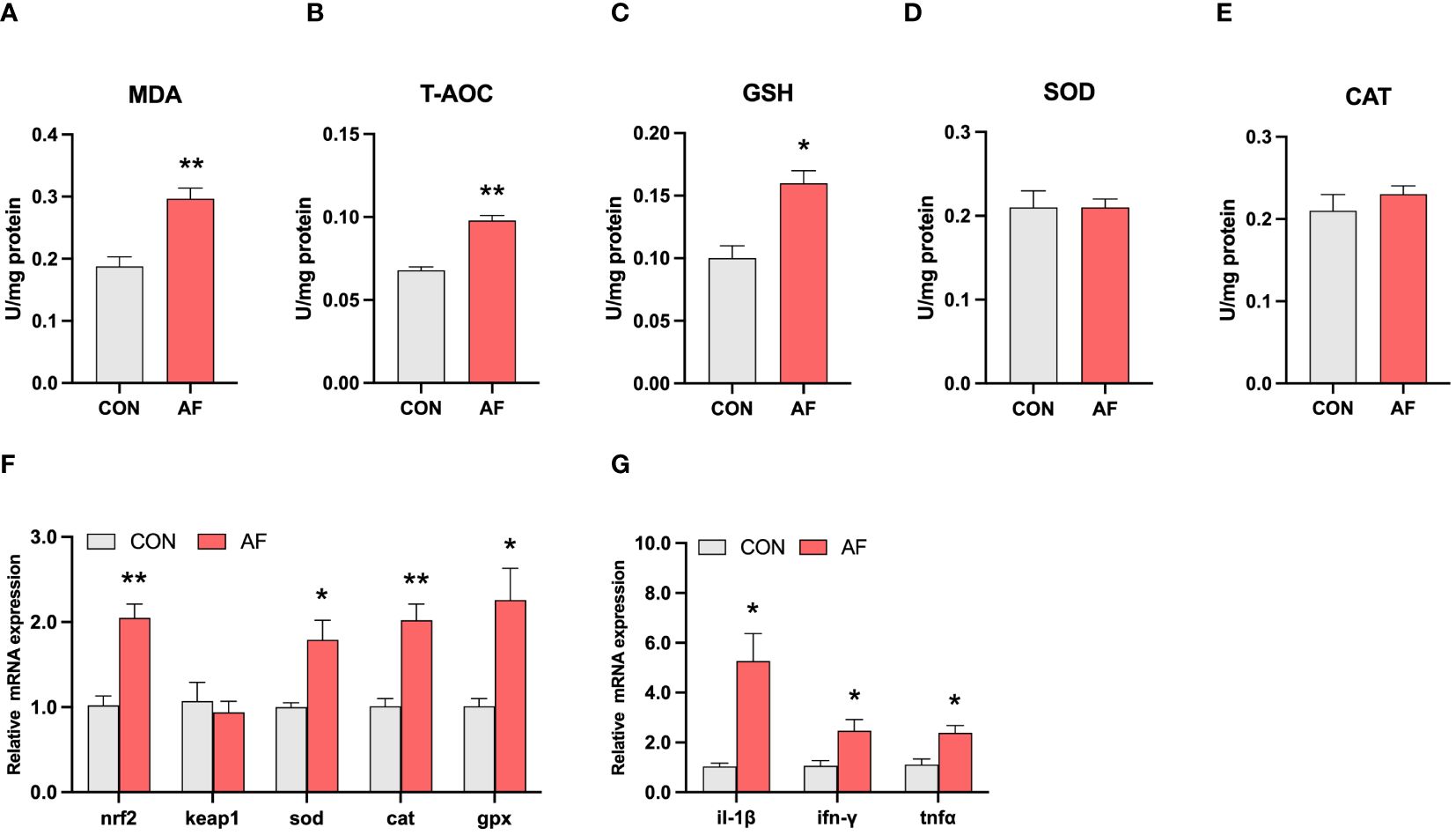
Figure 4 Artificial diets induced oxidative stress and inflammation in the muscle of mandarin fish. The levels of MDA (A), T-AOC (B), and reduced GSH (C) in the muscle of mandarin fish fed with the natural or artificial diets. The activities of SOD (D) and CAT (E) in the muscle of mandarin fish fed with the natural or artificial diets. Relative mRNA levels of genes related to antioxidative stress (F) and proinflammation cytokines (G) in the muscle of mandarin fish fed with the natural or artificial diets. Data are presented as means ± S.E.M., n=4. Significance was evaluated by independent t-test (*P < 0.05, **P < 0.01). CON, feeding with live prey fish; AF, feeding with artificial diets; MDA, malondialdehyde; T-AOC, total antioxidant capacity; GSH, reduced glutathione; SOD, superoxide dismutase; CAT, catalase; nrf2, nuclear factor erythroid2‐related factor 2; keap1, Kelch-like ECH-associated protein 1; sod, superoxide dismutase; cat, catalase; gpx, glutathione peroxidase. il-1β, interleukin 1 beta; ifn-γ, interferon gamma; tnfα, tumor necrosis factor alpha.
Discussion
In this study, to uncover the effects of artificial diets on the muscle of mandarin fish, we first conducted the transcriptome sequencing analysis. The results showed that the different expressed genes between the two groups were enriched in metabolism, especially glucose and lipid metabolism. The results were consistent with previous studies that steroid biosynthesis and glycerolipid metabolism were mostly enriched after the domestication of artificial diets (He et al., 2021). These results suggested that the glucose and lipid metabolism in the muscle were remarkably changed after feeding artificial diets, as the different constituents between artificial diets and live prey fish.
It is well known that carnivorous fish have a high carbohydrate intolerance in diets (Kamalam et al., 2012; Liu et al., 2017). Similarly, mandarin fish exhibited anorexia and hepatic steatosis after feeding a high-carbohydrate diet (You et al., 2020; Zhang et al., 2021). Muscle metabolic response is complex, including the regulation of metabolites and physiological processes (Guderley, 2004). In this study, artificial diets significantly increased glycogen content in the muscle. As expected, the transcriptional levels of gluconeogenesis-related genes were upregulated, and transcriptional levels of gluconeogenesis-related genes were downregulated. Previous studies indicated that muscle glycogen increased significantly after feeding fish with high-carbohydrate diets (Moon, 2001; Suárez et al., 2002). Tian et al. have proved that high-macronutrients (protein, fat and carbohydrate) increased muscular glycogen contents by up-regulation of pc expressions in grass carp (Ctenopharyngodon idella) (Tian et al., 2020). Overall, these results suggested that artificial diets induced glycogen accumulation in the muscle of mandarin fish by disrupting glucose metabolism.
In this study, artificial diets induced lipid deposition in the muscle of mandarin fish. To explore the mechanism for changes, the transcriptional levels of genes related to lipid metabolism were further detected. The lipid metabolism in the muscle of fish consists of lipid uptake, lipid synthesis, and fatty acid β-oxidation (Chen et al., 2023). Regarding lipid synthesis, the gene levels of srebp1, fas and plin2 were significantly increased after feeding artificial diets. These may be the major reason for artificial diets-induced lipid accumulation in the muscle. However, the genes levels of fatty acid β-oxidation were also increased after feeding artificial diets, which may be an adaptive mechanism to alleviate excessive lipid accumulation. Overall, these results suggested that artificial diets promoted lipid synthesis, resulting in excessive lipid accumulation in the muscle of mandarin fish.
Oxidative stress is involved in the pathogenesis of metabolic disturbances (Silhavy et al., 2014). Fish have an antioxidant defense system (antioxidants such as GSH, thioredoxin, and ascorbic acid; antioxidant enzymes such as SOD, GPx, and CAT) to prevent oxidative damage caused by reactive oxygen species (ROS) (Hoseinifar et al., 2020). The lack of balance of the antioxidant defense system and production of ROS causes oxidative stress (Meng et al., 2017; Guo et al., 2023). MDA is a biomarker of oxidative stress (Ali et al., 2020). In this study, artificial diets significantly increased the level of MDA in the muscle of mandarin fish. However, the levels of T-AOC and reduced GSH were also upregulated in fish fed with artificial diets, which may be an adaptive mechanism to relief oxidative stress in the muscle. These results were consistent with a previous study that artificial diets increased the level of MDA in the serum of mandarin fish hybrid (Siniperca chuatsi ♀ x Siniperca scherzeri ♂) (Li et al., 2017). Overall, these results suggested that artificial diets induced oxidative stress in the muscle of mandarin fish.
Prolonged metabolic disturbance and oxidative stress cause inflammation by activating transcription factors such as NF-κB and p53, which enhance the transcriptional level of pro-inflammatory cytokines (Tan et al., 2017; Chen et al., 2022; Dharshini et al., 2023). In this study, the gene expression of pro-inflammatory cytokines was significantly increased in mandarin fish fed with artificial diets. These results were consistent with previous studies that the imbalance of nutrition in artificial diets caused inflammation, which was harmful to the health of fish (Li et al., 2015; Li et al., 2017). Overall, these results suggested that artificial diets induced inflammation in the muscle of mandarin fish.
In conclusion, for the first time, this study systematically revealed that artificial diets disrupted glucose and lipid metabolism, leading to excessive glycogen and TG accumulation in the muscle of mandarin fish. Furthermore, artificial diets induced oxidative stress and inflammation in the muscle, which was harmful to the health of mandarin fish.
Data availability statement
The original contributions presented in the study are included in the article/supplementary material. Further inquiries can be directed to the corresponding authors.
Ethics statement
The animal study was approved by The animal feeding experiment was strictly implemented in accordance with the provisions of the experimental animal ethics committee of Shanghai Ocean University. The study was conducted in accordance with the local legislation and institutional requirements.
Author contributions
WF: Conceptualization, Investigation, Writing – original draft, Writing – review & editing. XL: Conceptualization, Writing – review & editing. BY: Conceptualization, Writing – review & editing. LW: Conceptualization, Writing – review & editing. XQ: Conceptualization, Writing – review & editing.
Funding
The author(s) declare financial support was received for the research, authorship, and/or publication of this article. This research was supported by Panyu Innovation and Entrepreneurship Leading Team Project (2021-R01-4).
Acknowledgments
We thank Youxia Wang, Qiang Chen, Kun Cui, Qiuchi, Chen, and Yongtao Liu for their help during the experiment.
Conflict of interest
Authors WF, BY, LW, and XQ were employed by the company Guangdong Haid Group Co., Ltd.
The remaining author declares that the research was conducted in the absence of any commercial or financial relationships that could be construed as a potential conflict of interest.
Publisher’s note
All claims expressed in this article are solely those of the authors and do not necessarily represent those of their affiliated organizations, or those of the publisher, the editors and the reviewers. Any product that may be evaluated in this article, or claim that may be made by its manufacturer, is not guaranteed or endorsed by the publisher.
References
Ali S. S., Ahsan H., Zia M. K., Siddiqui T., Khan F. H. (2020). Understanding oxidants and antioxidants: Classical team with new players. J. Food Biochem. 44, e13145. doi: 10.1111/jfbc.13145
Chen J., Zhuo M. Q., Jiang J. L., Yu A. G., Yu D. H., Huang F. (2023). Effects of dietary lipid levels on fiber quality, lipidomic profiles, antioxidant and inflammation responses in muscle of yellow catfish Pelteobagrus fulvidraco. Aquacult. Rep. 33, 101855. doi: 10.1016/j.aqrep.2023.101855
Chen M. Y., Meng X. F., Han Y. P., Yan J. L., Xiao C., Qian L. B. (2022). Profile of crosstalk between glucose and lipid metabolic disturbance and diabetic cardiomyopathy: Inflammation and oxidative stress. Front. Endocrinol. 13, 983713. doi: 10.3389/fendo.2022.983713
Desai A. R., Links M. G., Collins S. A., Mansfield G. S., Drew M. D., Van Kessel A. G., et al. (2012). Effects of plant-based diets on the distal gut microbiome of rainbow trout (Oncorhynchus mykiss). Aquaculture 350, 134–142. doi: 10.1016/j.aquaculture.2012.04.005
Dharshini L. C. P., Rasmi R. R., Kathirvelan C., Kumar K. M., Saradhadevi K. M., Sakthivel K. M. (2023). Regulatory components of oxidative stress and inflammation and their complex interplay in carcinogenesis. Appl. Biochem. Biotechnol. 195, 2893–2916. doi: 10.1007/s12010-022-04266-z
Fang W., Chen Q. C., Cui K., Chen Q., Li X. S., Xu N., et al. (2021). Lipid overload impairs hepatic VLDL secretion via oxidative stress-mediated PKCS-HNF4α-MTP pathway in large yellow croaker (Larimichthys crocea). Free Radical Biol. Med. 172, 213–225. doi: 10.1016/j.freeradbiomed.2021.06.001
Fang W., Liu Y. T., Chen Q. C., Xu D., Liu Q. D., Cao X. F., et al. (2022). Palmitic acid induces intestinal lipid metabolism disorder, endoplasmic reticulum stress and inflammation by affecting phosphatidylethanolamine content in large yellow croaker Larimichthys crocea. Front. Immunol. 13, 984508. doi: 10.3389/fimmu.2022.984508
Guderley H. (2004). Metabolic responses to low temperature in fish muscle. Biol. Rev. 79, 409–427. doi: 10.1017/S1464793103006328
Guo J. R., Wang L., Song K., Lu K. L., Li X. S., Zhang C. X. (2023). Physiological response of spotted seabass (Lateolabrax maculatus) to different dietary available phosphorus levels and water temperature: changes in growth, lipid metabolism, antioxidant status and intestinal microbiota. Antioxidants 12, 2128. doi: 10.3390/antiox12122128
He S., You J. J., Liang X. F., Zhang Z. L., Zhang Y. P. (2021). Transcriptome sequencing and metabolome analysis of food habits domestication from live prey fish to artificial diets in mandarin fish (Siniperca chuatsi). BMC Genomics 22, 129. doi: 10.1186/s12864-021-07403-w
Hoseinifar S. H., Yousefi S., Van Doan H., Ashouri G., Gioacchini G., Maradonna F., et al. (2020). Oxidative stress and antioxidant defense in fish: the implications of probiotic, prebiotic, and synbiotics. Rev. Fisheries. Sci. Aquacult. 29, 198–217. doi: 10.1080/23308249.2020.1795616
Kamalam B. S., Medale F., Kaushik S., Polakof S., Skiba-Cassy S., Panserat S. (2012). Regulation of metabolism by dietary carbohydrates in two lines of rainbow trout divergently selected for muscle fat content. J. Exp. Biol. 215, 2567–2578. doi: 10.1242/jeb.070581
Li Y., Li J. Z., Lu J. T., Li Z., Shi S. C., Liu Z. J. (2017). Effects of live and artificial feeds on the growth, digestion, immunity and intestinal microflora of mandarin fish hybrid (Siniperca chuatsi ♀ × Siniperca scherzeri ♂). Aquacult. Res. 48, 4479–4485. doi: 10.1111/are.2017.48.issue-8
Li J., Liang X. F., Alam M. S., Luo H. C., Zhang Y. P., Peng B. B., et al. (2021). Adaptation of AMPK-mTOR-signal pathways and lipid metabolism in response to low- and high-level rapeseed meal diet in Chinese perch (Siniperca chuatsi). J. Comp. Physiol. B-Biochem. Syst. Environ. Physiol. 191, 881–894. doi: 10.1007/s00360-021-01393-7
Li Y., Shi J. H., Shi S. C., Liu Z. J., Li Z., Li J. Z. (2015). Effect of Live, Frozen and Artificial Feeds on Digestive Enzymes, Aminotransferase, Histology of Liver and Intestine in Mandarin Fish Hybrid (Siniperca chuatsi ♀ x Siniperca scherzeri ♂). Israeli. J. Aquaculture-Bamidgeh. 67, 1185. doi: 10.46989/001c.20701
Li H. Y., Zeng Y. Z., Zheng X. Y., Wang G. J., Tian J. J., Gong W. B., et al. (2023). Dietary betaine attenuates high-carbohydrate-diet-induced oxidative stress, endoplasmic reticulum stress, and apoptosis in mandarin fish (Siniperca chuatsi). Antioxidants 12, 1860. doi: 10.3390/antiox12101860
Liang X. F. (2002). Study on Chinese perch (Siniperca chuatsi) artificial diets. Fisheries. Sci. Technol. Inf. 29, 64–67.
Liang X. F., Lin X. T., Li S. Q., Liu J. K. (2008). Impact of environmental and innate factors on the food habit of Chinese perch Siniperca chuatsi (Basilewsky) (Percichthyidae). Aquacult. Res. 39, 150–157. doi: 10.1111/j.1365-2109.2007.01870.x
Liang X. F., Oku H., Ogata H. Y., Liu J., He X. (2001). Weaning Chinese perch Siniperca chuatsi (Basilewsky) onto artificial diets based upon its specific sensory modality in feeding. Aquacult. Res. 32, 76–82. doi: 10.1046/j.1355-557x.2001.00006.x
Liu Z., Chen N., Wang M., Lian X., Yan C., Yin J. (2017). Suitable dietary starch source and supplementation level for largemouth bass (Micropterus salmoides). J. Fishery. Sci. China 24, 317–331. doi: 10.3724/SP.J.1118.2017.16111
Livak K. J., Schmittgen T. D. (2001). Analysis of relative gene expression data using real-time quantitative PCR and the 2-ΔΔCT method. Methods 25, 402–408. doi: 10.1006/meth.2001.1262
Mai Q. Y., Jin Y. Q., Chen Y. F., Dong H. X., Wu Y. L., Sun D. L., et al. (2023). Assessing the effects of dietary live prey versus an artificial compound feed on growth performance, immune response, and intestinal microflora of largemouth bass Micropterus salmoides. Aquacult. Int. 31, 1213–1230. doi: 10.1007/s10499-022-01021-3
Mandal S. C., Sahu N. P., Kohli M. P. S., Das P., Gupta S. K., Munilkumar S. (2010). Replacement of live feed by formulated feed: effect on the growth and spawning performance of Siamese fighting fish (Betta splendens, Regan, 1910). Aquacult. Res. 41, 1707–1716. doi: 10.1111/j.1365-2109.2010.02564.x
Meng D., Zhang P., Zhang L. L., Wang H., Ho C. T., Li S. M., et al. (2017). Detection of cellular redox reactions and antioxidant activity assays. J. Funct. Foods. 37, 467–479. doi: 10.1016/j.jff.2017.08.008
Miao S. Y., Zhao C. Z., Zhu J. Y., Hu J. T., Dong X. J., Sun L. S. (2018). Dietary soybean meal affects intestinal homoeostasis by altering the microbiota, morphology and inflammatory cytokine gene expression in northern snakehead. Sci. Rep. 8, 113. doi: 10.1038/s41598-017-18430-7
Moon T. W. (2001). Glucose intolerance in teleost fish: face or fiction? Comp. Biochem. Physiol. B-Biochem. Mol. Biol. 129, 243–249. doi: 10.1016/S1096-4959(01)00316-5
Peng D., Liang X. F., Chai F. R., Feng H. X., Li J., Tang S. L., et al. (2022). Effects of dietary carbohydrate to lipid ratios on growth, biochemical indicators, lipid metabolism, and appetite in Chinese perch (Siniperca chuatsi). Fish. Physiol. Biochem. 48, 101–116. doi: 10.1007/s10695-021-01043-3
Silhavy J., Zídek V., Mlejnek P., Landa V., Simáková M., Strnad H., et al. (2014). Fumaric acid esters can block pro-inflammatory actions of human CRP and ameliorate metabolic disturbances in transgenic spontaneously hypertensive rats. PloS One 9, E268. doi: 10.1016/j.atherosclerosis.2014.05.802
Suárez M. D., Sanz A., Bazoco J., García-Gallego M. (2002). Metabolic effects of changes in the dietary protein:: carbohydrate ratio in eel (Angilla Anguilla) and trout (Oncorhynchus mykiss). Aquacult. Int. 10, 143–156. doi: 10.1023/A:1021371104839
Tan P., Dong X. J., Xu H. L., Mai K. S., Ai Q. H. (2017). Dietary vegetable oil suppressed non-specific immunity and liver antioxidant capacity but induced inflammatory response in Japanese sea bass (Lateolabrax japonicus). Fish. Shellfish. Immunol. 63, 139–146. doi: 10.1016/j.fsi.2017.02.006
Tian J., Wu F., Yu L. J., Lu X., Jiang M., Liu W., et al. (2020). The effects of high-macronutrient (protein, fat and carbohydrate) diets on growth performance and muscular metabolic responses in grass carp. Aquacult. Nutr. 26, 2135–2146. doi: 10.1111/anu.13152
Wang W. N., Zhou J., Wang P., Tian T. T., Zheng Y., Liu Y., et al. (2009). Oxidative stress, DNA damage and antioxidant enzyme gene expression in the Pacific white shrimp, Litopenaeus vannamei when exposed to acute pH stress. Comp. Biochem. Physiol. C-Toxicol. Pharmacol. 150, 428–435. doi: 10.1016/j.cbpc.2009.06.010
Yi T. L., Sun J., Liang X. F., He S., Li L., Wen Z. Y., et al. (2013). Effects of polymorphisms in pepsinogen (PEP), amylase (AMY) and trypsin (TRY) genes on food habit domestication traits in mandarin fish. Int. J. Mol. Sci. 14, 21504–21512. doi: 10.3390/ijms141121504
You J. J., Ren P., He S., Liang X. F., Xiao Q. Q., Zhang Y. P. (2020). Histone methylation of H3K4 involved in the anorexia of carnivorous mandarin fish (Siniperca chuatsi) after feeding on a carbohydrate-rich diet. Front. Endocrinol. 11, 323. doi: 10.3389/fendo.2020.00323
Keywords: mandarin fish, glucose and lipid metabolism, oxidative stress, inflammation, transcriptome sequencing analysis
Citation: Fang W, Leng X, Yun B, Wang L and Qian X (2024) Artificial diets affect glucose and lipid metabolism, antioxidant capacity, and inflammatory response in the muscle of mandarin fish (Siniperca chuatsi). Front. Mar. Sci. 11:1445902. doi: 10.3389/fmars.2024.1445902
Received: 08 June 2024; Accepted: 19 June 2024;
Published: 05 July 2024.
Edited by:
Jiamin Li, Jiangxi Agricultural University, ChinaCopyright © 2024 Fang, Leng, Yun, Wang and Qian. This is an open-access article distributed under the terms of the Creative Commons Attribution License (CC BY). The use, distribution or reproduction in other forums is permitted, provided the original author(s) and the copyright owner(s) are credited and that the original publication in this journal is cited, in accordance with accepted academic practice. No use, distribution or reproduction is permitted which does not comply with these terms.
*Correspondence: Xueqiao Qian, cXhxQGhhaWQuY29tLmNu