- 1Ministry of Education Key Laboratory of Water and Sediment Science, School of Environment, Beijing Normal University, Beijing, China
- 2State Key Laboratory of Water Environment Simulation, School of Environment, Beijing Normal University, Beijing, China
- 3School of Government, Beijing Normal University, Beijing, China
- 4College of Art and Science, Lehigh University, Bethlehem, PA, United States
Zostera japonica, as one of the major seagrasses in the Yellow River Estuary, plays a critical ecological role, particularly in providing habitat for marine organisms, stabilizing sediment, and contributing significantly to carbon sequestration. In recent years, Zostera japonica seagrass beds have receded extensively due to multiple impacts of natural factors and human activities. This study investigates the complex effects of extreme climate events and human activities on the growth mechanisms of Zostera japonica in the Yellow River Estuary using a combination of field sampling, laboratory analysis, and quantitative calculations. The result shows that there are significant differences in sediment characteristics between the north and south shores, with the south shore having finer sediments and higher nutrient content, which support more robust seagrass growth. The Water and Sediment Regulation Scheme (WSRS) dramatically alters water quality by reducing salinity and increasing turbidity, thus inhibiting photosynthesis and disrupting the physiological functions of Zostera japonica. Additionally, WSRS introduces an increase in heavy metals, which could potentially impact plant health and stress levels. Extreme weather events, particularly Super Typhoon Lekima, further compound these impacts by causing soil erosion, uprooting seagrass beds, and reducing biomass and seed production. The interplay of WSRS, climate change, and anthropogenic activities necessitates integrated management strategies to mitigate adverse effects and enhance habitat resilience. This study underscores the need for specific management strategies, such as controlling heavy metal inflows, implementing sediment stabilization techniques, and regulating freshwater discharge during key growth periods, to mitigate adverse effects and enhance habitat resilience for Zostera japonica in the Yellow River Estuary.
1 Introduction
Seagrasses are vital components of coastal ecosystems worldwide, known for their high primary productivity and essential roles in carbon sequestration, habitat provision, and shoreline protection. Seagrasses are submerged angiosperm that grows widely in intertidal to subtidal zones in shallow waters of tropical and temperate seas, and can cover thousands of kilometers of coastline, adapting to the dramatically changing environmental conditions at the ocean margins (Peng, 2007; Zhang et al., 2016). Seagrass ecosystems are among the most productive aquatic ecosystems in the biosphere, rivalling mangroves and coral reefs in their carbon sequestration capacity and provision of critical ecological services (Peng, 2007; Garcia-Marin et al., 2013; Wang et al., 2016). Large areas of continuous seagrass form seagrass beds, which have high ecological and economic value. Zostera japonica, in particular, plays a crucial role in these ecosystems by providing habitat and food for a variety of marine organisms, participating in nutrient cycling, and stabilizing sediment (Les and Waycott, 1997; Mao et al., 2022).
The Yellow River estuary is a typical and unique ecological environment that significantly influences the growth of Zostera japonica. Its distinctive geographical and ecological characteristics, including high sediment loads and variable salinity, create a dynamic environment for seagrass growth. The Yellow River, being the most sediment-laden river in the world, delivers substantial sediment to the estuary, profoundly shaping the estuarine ecosystem. High sediment loads increase turbidity, which limits light penetration essential for photosynthesis in seagrasses. Additionally, the constant deposition of sediments alters the physical landscape, influencing nutrient availability and habitat structure. These sediment dynamics create a highly variable environment that impacts the growth and survival of species like Zostera japonica, challenging their adaptation to frequent changes in water quality, sediment texture, and nutrient concentrations (Han et al., 2017; Hou et al., 2020; Yi et al., 2024).
Global climate change has led to an increase in the frequency and intensity of extreme weather events such as heatwaves, cold spells, and storm surges. These events pose significant threats to seagrass beds particularly to Zostera japonica, by inducing temperature stress during heatwaves, causing cellular damage during cold spells, and resulting in mechanical damage from storm surges, which can uproot plants and disrupt sediment stability (Gao et al., 2023). For instance, heatwaves can cause thermal stress, leading to decreased photosynthetic efficiency and increased respiration rates in seagrasses. Cold spells can result in cellular damage and reduced growth rates. Storm surges can lead to physical damage to seagrass beds, including sediment displacement, which buries or erodes seagrass shoots, and root system damage, which weakens plant anchorage and reduces nutrient uptake. These disturbances further destabilize the habitat, making recovery for species like Zostera japonica more challenging (Bradley and Stolt, 2006; Hou et al., 2020). These climatic events disrupt the delicate balance of the seagrass ecosystem, leading to long-term degradation and loss of habitat.
Human activities such as coastal development, pollutant discharge, and overfishing have significantly disturbed seagrass ecosystems. Coastal development often leads to habitat destruction and increased sedimentation, which smothers seagrass beds and reduces light availability for photosynthesis. Pollutant discharge, including heavy metals and pesticides, directly affects the health and survival of seagrass plants. Overfishing disrupts the food chain and ecological balance within seagrass ecosystems by removing key species, such as fish and invertebrates, that play vital roles in nutrient cycling and sediment aeration (Xu et al., 2009; Marb and Duarte, 2010; Wang et al., 2016). In the Yellow River estuary, specific human activities such as the Xiaolangdi water and sediment regulation scheme (WSRS) have exacerbated these issues. WSRS, implemented in 2002, has altered the natural flow of the Yellow River, resulting in significant sediment discharge during the Zostera japonica growth period. This leads to increased turbidity and nutrient loading, which significantly impact Zostera japonica. The heightened turbidity reduces light penetration, limiting photosynthesis and weakening the plant’s energy production. Excess nutrient loading can also stimulate algal blooms, which further decrease light availability and compete with Zostera japonica for resources, ultimately hindering its growth and reproduction. The combined effects of waves, tides, and winds create a constantly changing environment that further stresses seagrass growth (Yang et al., 2011; Zhang et al., 2021).
Current research has provided insights into the impacts of extreme climate events and human activities on seagrass beds. However, there is a lack of comprehensive studies that simultaneously examine the synergistic effects of extreme climatic events and human activities on Zostera japonica, particularly in the unique conditions of the Yellow River estuary. Existing studies have primarily focused on either climatic impacts or human activities in isolation, without addressing their synergistic effects on Zostera japonica growth mechanisms. In particular, there is a lack of systematic research on how the estuary’s extreme sedimentation dynamics and heavy metal pollution collectively affect the physiological and biochemical responses of Zostera japonica (Ma et al., 2020; Yue et al., 2021; Zhang et al., 2021). Furthermore, research specific to the Yellow River estuary is limited, especially in understanding the detailed physiological and biochemical responses of Zostera japonica to the dynamic and challenging conditions of this environment. The catastrophic impact of events such as the 2019 Super Typhoon Lekima, which caused extensive damage to seagrass beds and significant reductions in soil organic carbon and nitrogen pools, highlights the need for focused research in this area (Yue et al., 2021).
This study aims to comprehensively analyze the effects of extreme climatic events and human activities on the growth mechanisms of Zostera japonica in the Yellow River estuary. By conducting long-term monitoring and detailed analysis of plant growth conditions and environmental factors, this research seeks to fill existing knowledge gaps and provide scientific evidence to support the conservation and management of seagrass habitats in this region. The findings of this study have significant implications for understanding and mitigating the effects of global climate change on marine ecosystems. By elucidating the growth mechanisms of Zostera japonica under the combined influence of extreme climatic events and human activities, this research will contribute to the development of effective conservation and restoration strategies for seagrass beds. Additionally, the study will offer valuable insights for the management of the Yellow River estuary, promoting sustainable practices that protect and enhance the ecological health of this critical habitat.
2 Method
2.1 Study area and sampling locations
The Yellow River estuary is located in eastern China, in the Bohai Bay in northeastern Shandong Province, and is one of the most representative sediment deposition deltas in the world. The Yellow River, with a total length of about 5,464 kilometers, as one of the rivers with the highest sand content in the world and the second largest river in China, inputs large amounts of sediment and freshwater into the Bohai Sea every year, creating a typical estuarine environment. The Yellow River estuary region thus exhibits a highly dynamic ecological environment, including dramatic hydrological and sedimentary changes. These sediment deposits are constantly modifying the morphology of the estuary, creating wide shallows and wetlands that provide unique habitats for many plants and animals.
Zostera japonica in the Yellow River estuary grows in the shallow subtidal zone of the estuary, with a suitable water depth of 1-2 m, characterized by moderate salinity levels (typically ranging from 20 to 40 psu) and sediment composed primarily of fine sand and silt. These sediments support nutrient retention and provide growth stability, yet seasonal river discharge and tidal currents introduce significant fluctuations in salinity and turbidity. Such variability affects light penetration, impacting photosynthesis and overall growth. High temperatures and low oxygen levels further constrain its growth, and when salinity levels rise above 40 psu, germination rates significantly decrease. These dynamic environmental conditions pose challenges for the reproduction and stability of Zostera japonica populations. It is distributed on both the north and south sides, and its growth period is from April to October each year. The distribution area of Zostera japonica in the Yellow River Delta was reduced by more than 100-fold after Super Typhoon Lekima, and the loss of soil organic carbon was greater than 35%, and the loss of total soil nitrogen in the top 35 cm sediment was greater than 65% (Yue et al., 2021).
According to the different sediment deposition types, two study sites on the north (119°11’E, 37°86’N) and south (119°27’E, 37°74’N) shores of the Yellow River Estuary were selected with reference to the study of Zhang et al. (2021) (Figure 1). The north shore site, characterized by erosion and coarser sediment, reflects the dynamic, nutrient-limited conditions of areas affected by strong hydrodynamic forces. In contrast, the south shore site, dominated by finer sediment deposition, represents more stable conditions with higher nutrient retention, conducive to seagrass growth. These sites collectively capture the diversity of sedimentary and hydrodynamic conditions found across the broader estuarine environment. A square sample area of approximately 100 m x100 m was selected at each study site, with nine 1 m x 1 m sample plots uniformly designed.
2.2 Survey on the growth of Zostera japonica
Zostera japonica was selected to monitor its growth from April to September of the growth cycle, and five sampling activities were carried out at two sampling sites in April, May, June, July and September 2021, including one additional sampling in July due to WSRS at Xiaolangdi and one stop sampling in August due to bad weather. The collected samples included Zostera japonica plants (including roots), surface water, surface soil, and rhizosphere soil. These samples were transported to the laboratory under dark conditions and maintained at a low temperature of approximately 4°C to prevent degradation. Samples were stored in insulated containers with ice packs to ensure a stable temperature throughout the transportation process, minimizing any potential alterations in their chemical and biological properties. And sample collection activities were conducted following approval from the Shandong Yellow River Delta National Nature Reserve.
2.3 Indoor experimental design and index determination
2.3.1 Plant analysis
Zostera japonica plants were carefully washed with distilled water to avoid contamination from external substances, and the plant height of each sample was measured using a precise digital caliper to ensure accuracy. The plants were then processed by killing at 105°C for 12 minutes and drying at 70°C for 24 hours. The dry weight of the plants, including both above-ground and below-ground parts, was measured using an analytical balance. The dried plant material was ground, passed through a 150 μm nylon mesh, and frozen for subsequent carbon and nutrient analysis. Soil total carbon (TC) and total nitrogen (TN) concentrations were determined using an Elementar Vario Macro Cube elemental analyzer, calibrated with certified standard materials to ensure accuracy. The analyzer was set to detect TC and TN with a precision of ±0.1% and accuracy within ±0.5%. Regular calibration checks were conducted before each batch of samples to maintain data reliability and to minimize measurement drift. Total phosphorus (TP) was measured using a high-resolution inductively coupled plasma emission spectrometer (ICP-OES, Thermo Fisher), and the concentrations of various metal elements were determined using an inductively coupled plasma mass spectrometer (ICP-MS, Thermo Fisher). All sample processing and analyses were conducted at the State Key Laboratory of Water Environment Simulation, School of Environment, Beijing Normal University (Beijing, China).
2.3.2 Sediment and water quality analysis
In the sampling point site using YSI (EXO multi-parameter water quality monitor, U.S.) to monitor water temperature (T), conductivity (cond.), dissolved oxygen (DO), pH, total soluble solids (TDS), turbidity (NTU) and chlorophyll (Chla) and other water quality indicators. The analyzer’s sensitivity allows detection of nutrient concentrations down to 0.01 mg/L, with an accuracy of ±0.2%. Regular calibration was performed using certified standards to ensure data accuracy and consistency across all measurements. And each sampling point of the collected water samples into acid-washed polyethylene plastic bottles, cryopreservation, brought back to the laboratory. Water quality indices, including TN, TC, and TP, were measured using a water quality analyzer. Sediment samples were dried naturally in the laboratory and the particle size was determined using a particle size analysis laser particle size analyzer (Horiba, France). The remaining sediment samples were ground and passed through a 100-mesh nylon sieve. TC and TN contents in the sediment were measured using an elemental analyzer (VARIOEL Elemental Analyzer, ELEMENTAR, Germany), while TP content was determined using high-resolution inductively coupled plasma atomic emission spectrometry (HR-ICP-AES, SPECTRO, Germany). The samples were processed following standard protocols, and the concentrations of TP, Fe, Mn, Cu, Zn, Pb, Cr, and Cd were determined using ICP-MS and ICP-OES.
2.3.3 Heavy metal risk assessment
An ecological risk index (RI) was used to evaluate the potential risk to Zostera japonica from metals in sediments in the study area. The RI values were used to estimate the cumulative ecological risk based on the concentration of known metals in the sediment and the toxicity response coefficient of each metal (Hakanson, 1980), where RI < 150 was considered low risk, 150 - 300 was considered medium risk, and RI > 300 was considered high risk (Liu et al., 2020; Wang et al., 2021a). The calculation formula is as follows.
where Tr is the toxicity response factor of metal i, RI is the cumulative impact of metals in sediment, Er is the single metal ecological risk index, Csediment is the concentration of metal i in sediment, and Cbackgroud is the concentration of background concentration of metal i in the study area (μg-1), data from Zhao and Yan (1994) and Wang et al. (2021b).
The degree of accumulation of each metal element in Zostera japonica was evaluated by measuring the bioconcentration factor (BCF), which reflects the ability of organisms to absorb metal elements from the sediment, and was calculated as follows.
where CZ, japonica tissue and Csediment are the metal content (μg-1) in Zostera japonica tissue and sediment, respectively.
3 Results
3.1 Sediment and water quality indexes
3.1.1 Physicochemical indicators of surface water
DO and pH values in the surface waters of the study area during the sampling period were not significantly different between the north and south shores, while conductivity, total suspended solids and turbidity were significantly higher on the north shore than on the south shore, and all showed a significant decrease in mid-July. The concentrations of TN and TP in the north shore were significantly lower than those in the south shore (Table 1), and the concentrations of TN and TP in the water in the south shore were 2.96~ 3.19 mg/L and 0.09 ~0.45 mg/L, respectively, which were higher than those in the north shore of 1.79~ 2.66 mg/L and 0.02~0. 25 mg/L (Table 2). During the period of WSRS, the physical and chemical indexes of surface water changed significantly. The salinity, conductivity, and total suspended solids of both north and south shores showed a significant decrease due to the large increase of diluted water, and gradually increased after WSRS; Turbidity, TN, TP, and DO levels increased noticeably during the period of WSRS due to the inflow of large amounts of sediment and decreased after the end of WSRS. Comparing these findings with the optimal habitat thresholds for Zostera japonica (Yi et al., 2023), which thrives in turbidity levels below 150 NTU and prefers TN and TP concentrations conducive to its nutrient needs, it is evident that turbidity levels exceeded the ideal range during peak WSRS sediment inflow, potentially limiting photosynthesis. Additionally, the salinity levels dropped below the preferred 20-25 ppt range during WSRS, potentially stressing Zostera japonica due to osmotic imbalance.
On the south shore, Chla concentrations were higher in the early stage of WSRS, ranging from 5.0 to 9.1 μg/L, indicating higher photosynthetic activity. As the WSRS progressed, Chla concentrations significantly decreased to 3.8~4.4 μg/L and 1.6~3.7 μg/L in the middle and late stages of the WSRS. In contrast, Chla concentrations on the north shore were significantly lower than those on the south shore at all WSRS stages. In the early stage, Chla concentration was only 1.7~2.1 μg/L, while in the middle and late stages, Chla further decreased to 1.2~1.7 μg/L and 0.06~0.14 μg/L.
3.1.2 Physicochemical indicators of sediments
The surface sediments of the seagrass beds in the sampling areas of the north and south shores were classified according to the Folk’s classification system. On the north shore, sediments were initially classified as Silty Sand before WSRS, characterized by a mixture of fine sand and silt with low clay content. During WSRS, these sediments transitioned to Sandy Silt, with a noticeable decrease in sandy gravel and an increase in silt and clay content, indicating enhanced deposition of finer particles. On the south shore, both surface and root sediments were primarily classified as Sandy Silt throughout the study period, except for the initial sample, which was Silty Sand. Similar to the north shore, the south shore sediments also exhibited a trend of decreasing sandy gravel and increasing silt and clay proportions during WSRS, reflecting changes in sediment dynamics influenced by the water and sediment regulation scheme (Figure 2).
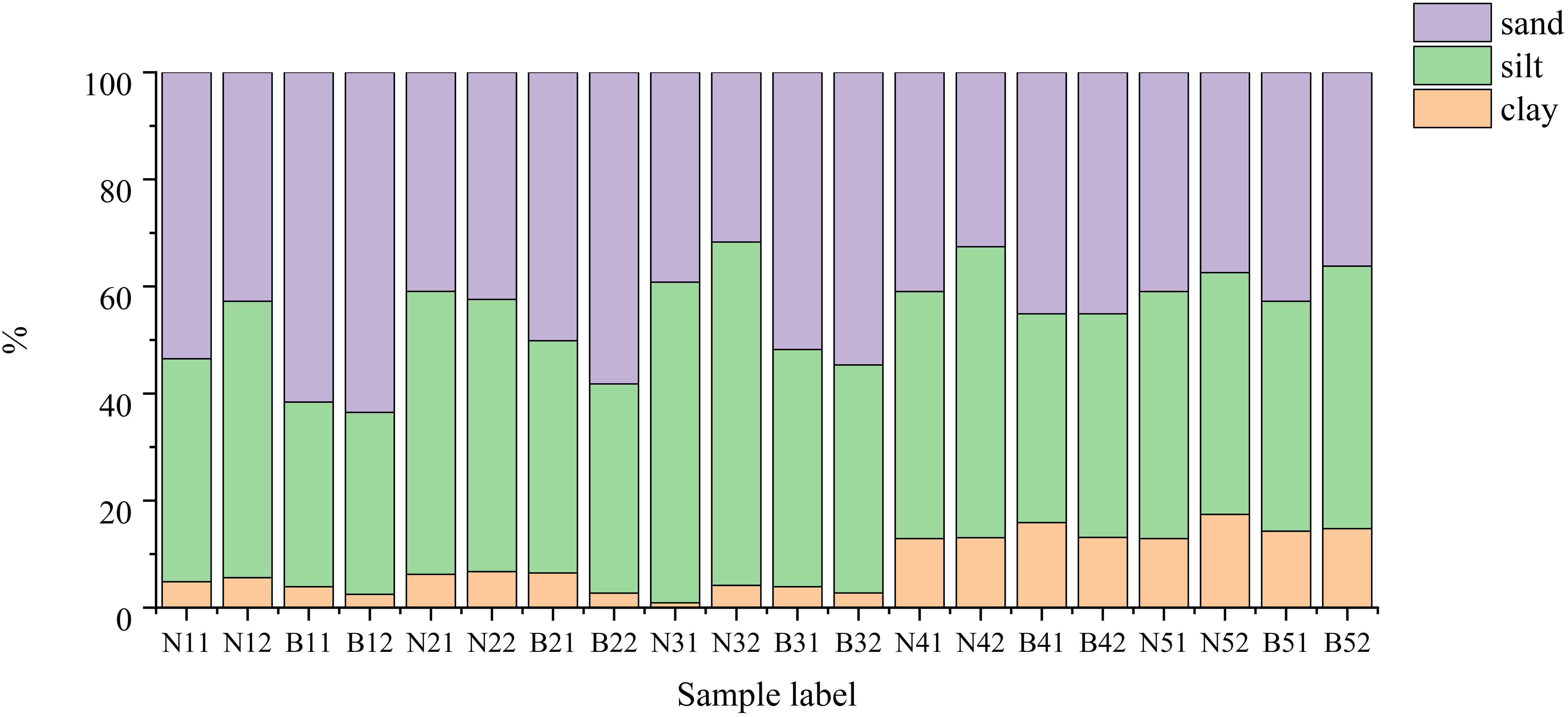
Figure 2. Sediment particle size distribution ratio, where N indicates the south shore B indicates the north shore, 11 indicates the first sampled surface sediment and 12 indicates the first sampled root sediment.
The median particle size (D50) of the surface sediments on the north shore showed a general decreasing trend during the sampling period, with a small increase at the end of June and then a continuous decrease, fluctuating from 50.55 to 70.87 μm. The surface sediments on the south shore showed a decreasing and then increasing trend, reaching a minimum at the end of June, fluctuating from 51.02 to 59.82 μm (Figure 3A). The D50 of the root sediments on the north shore showed a linear decrease during the sampling period, with D50 decreasing significantly from 72.79 μm to 51.72 μm, while the south shore root sediment fluctuated from 49.49 to 56.30 μm, consistently smaller than the particle size on the north shore (Figure 3B).
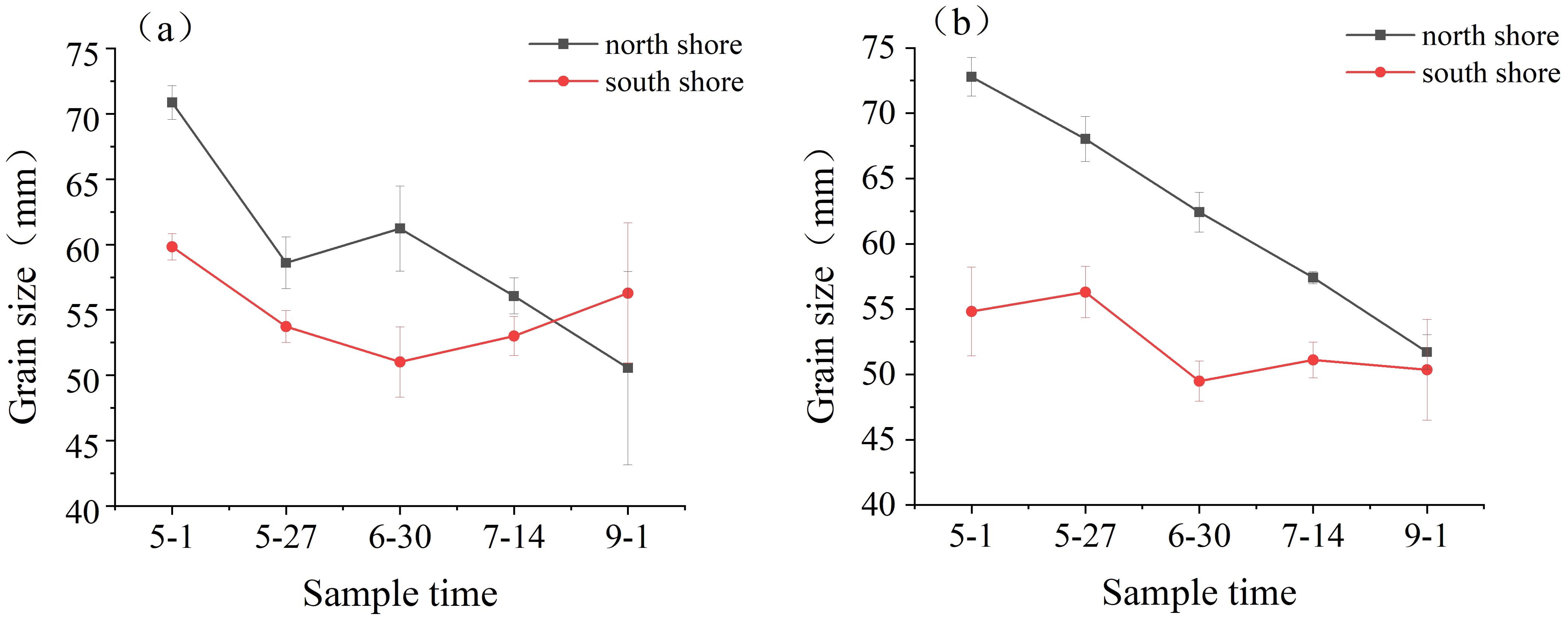
Figure 3. Sediment grain size in the sample area, where (A) is the median grain size of surface sediment and (B) is the median grain size of root sediment.
The temporal and spatial trends of TC, TN and TP contents in seagrass bed sediments at the sampling sites on the north and south shores of the Yellow River estuary are shown in Figure 4. Except for TN in surface sediments on the north shore, the contents of other substances generally decreased in the early stage, increased at the end of June due to WSRS, and then continue to decrease; TN contents in surface sediments on the north shore continued to increase after a small decrease at the end of May, showing an overall increasing trend. With the continuous deposition of sediment and nutrients in the estuary, the content of TC, TN and TP in the root sediment on the north shore increased by 6.0%, 21.5% and 40.9%, respectively, at the end of June compared to the first sampling, while the surface sediment of the south shore increased by 94.5%, 99.2% and 9.8%. The root sediment on the south shore decreased by 7.2%, 32.3% and increased by 2.3%, respectively. The mean concentrations of TC, TN and TP in the sediments of the north and south shores were not significantly different.
3.2 Growth dynamics of Zostera japonica
3.2.1 Growth status of Zostera japonica
There are obvious differences in the growth of Zostera japonica between the north and south shores of the Yellow River Estuary (Figure 5). On the south shore, the biomass of Zostera japonica first increased and then decreased, reaching a maximum biomass of about 53g/m2 at the end of June, with a continuous increase in plant height, reaching a maximum average of 25.85 ± 7.9 cm in September. On the north shore, Zostera japonica was sparsely distributed, reaching a maximum biomass of about 11g/m2 at the end of May and a maximum plant height of 9.3 ± 2.1 cm at the end of June, with incomplete morphology and broken leaves. The biomass and plant height were significantly lower than those of the south shore, and no surviving Zostera japonica was found during the sampling in September, and Zostera japonica disappeared from the north shore.
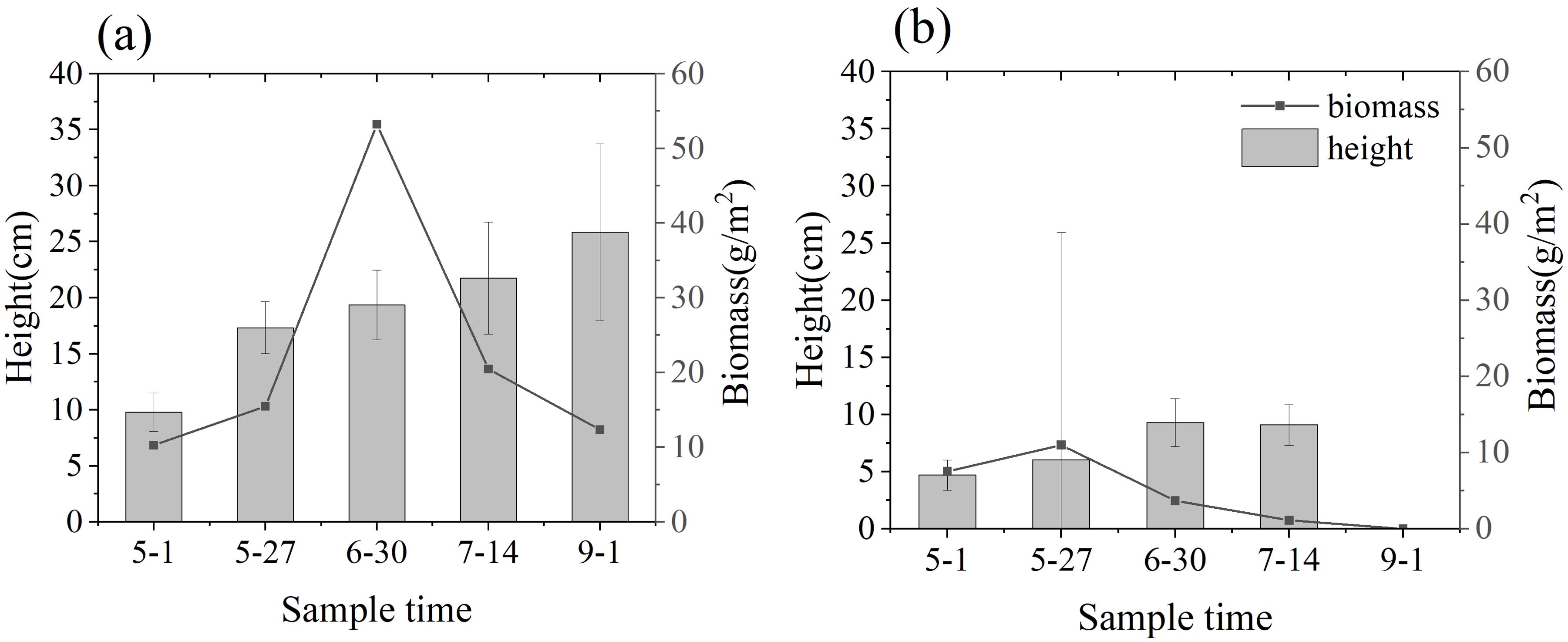
Figure 5. Plant height and biomass of Zostera japonica in the Yellow River estuary (A) south shore; (B) north shore.
3.2.2 Changes in nutrients of Zostera japonica
Due to the limited number of Zostera japonica on the north shore and insufficient plant quality in the later stages to meet the minimum mass required for elemental analysis, TC and TN of Zostera japonica on the north shore were only measured for the first three samples. The concentrations of TC and TN in Zostera japonica on the south shore of the Yellow River Estuary showed a decreasing trend from April to late May, followed by a significant increase, and then kept decreasing. Total phosphorus showed an increasing trend from April to the end of June, followed by a decreasing trend. The contents of TC, TN, and TP in Zostera japonica ranged from 18.06% to 31.43%, 1.34% to 2.32%, and 0.20% to 1.36%, respectively (Figure 6). On the north shore, the concentrations of TN in Zostera japonica showed a decreasing trend from May to the end of June, while TP did not fluctuate much except for a low value in mid-July. The contents of TC, TN, and TP in Zostera japonica on the north shore ranged from 9.41% to 28.16%, 0.87% to 2.53%, and 0.06% to 0.49%, respectively (Figure 6). Overall, the concentrations of TN and TC in Zostera japonica on the north shore were higher than those on the south shore in the early period, while TP was generally lower than on the south shore.
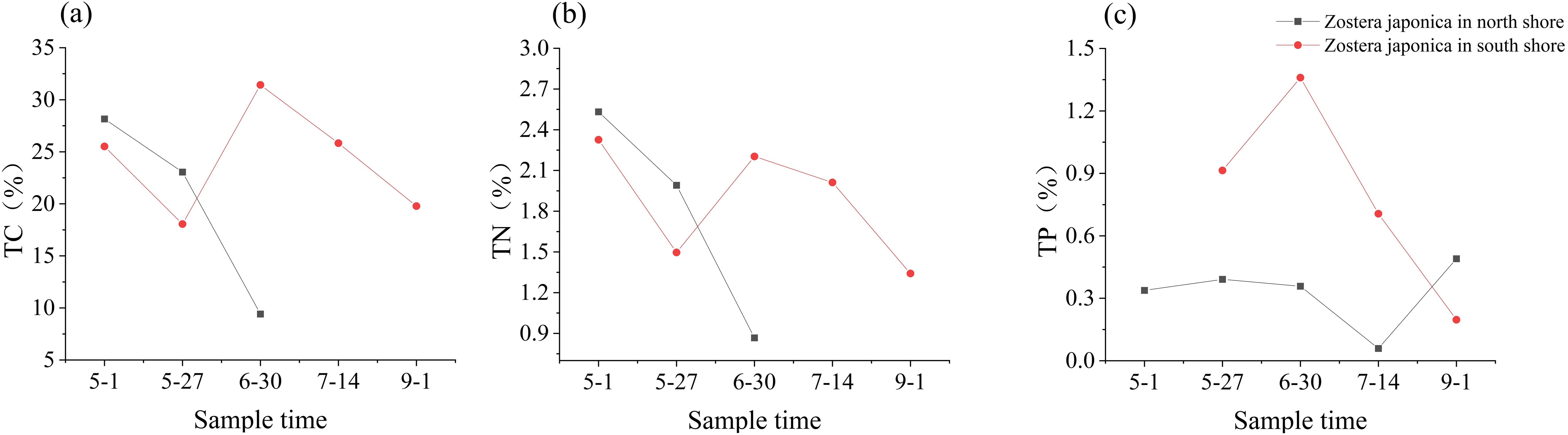
Figure 6. Changes in TC, TN and TP contents of Zostera japonica plants in the Yellow River estuary (A) TC content; (B) TN content; (C) TP content.
3.3 Heavy metal analysis of sediment and Zostera japonica
3.3.1 Heavy metal content determination
As shown in Figure 7, the metal contents of sediments on the north and south shores of the Yellow River estuary did not differ much, and the metal concentrations at the two sites remained relatively stable throughout the sampling period. The metal content of Zostera japonica fluctuated significantly throughout the sampling period. The Fe content was lower than the elemental content of sediments on both shores, while Cd, Mn, Pb, and Zn were higher than the elemental content of sediments on both shores, and the trend of Cr and Cu was not obvious. The average concentrations of Cd, Cr, Cu, Fe, Mn, Pb, and Zn in the sediments of the Zostera japonica growth area on the north shore were 0.46 μg/g, 17.47μg/g, 11.66μg/g, 17.13μg/g, 0.74μg/g, 26.45μg/g, and 3.35μg/g, respectively, and 0.47μg/g, 16.0μg/g, 10.4μg/g, and 10.4μg/g, respectively, on the south shore. The average concentrations of Cd, Cr, Cu, Fe, Mn, Pb and Zn in Zostera japonica on the north shore were 2.93μg/g, 27.78μg/g, 13.94μg/g, 10.02μg/g, 1.81μg/g, 190.56μg/g and 8.40μg/g, respectively, and 3.53μg/g, 12.34μg/g, 14.64μg/g, 7.93μg/g, 2.17μg/g, 72.65μg/g and 4.46μg/g on the south shore, and the concentrations of Cr, Pb and Zn were significantly higher than those on the north shore. In addition, the concentrations of all these metals in Zostera japonica decreased slightly with the growth period and then increased with the maturation of Zostera japonica.
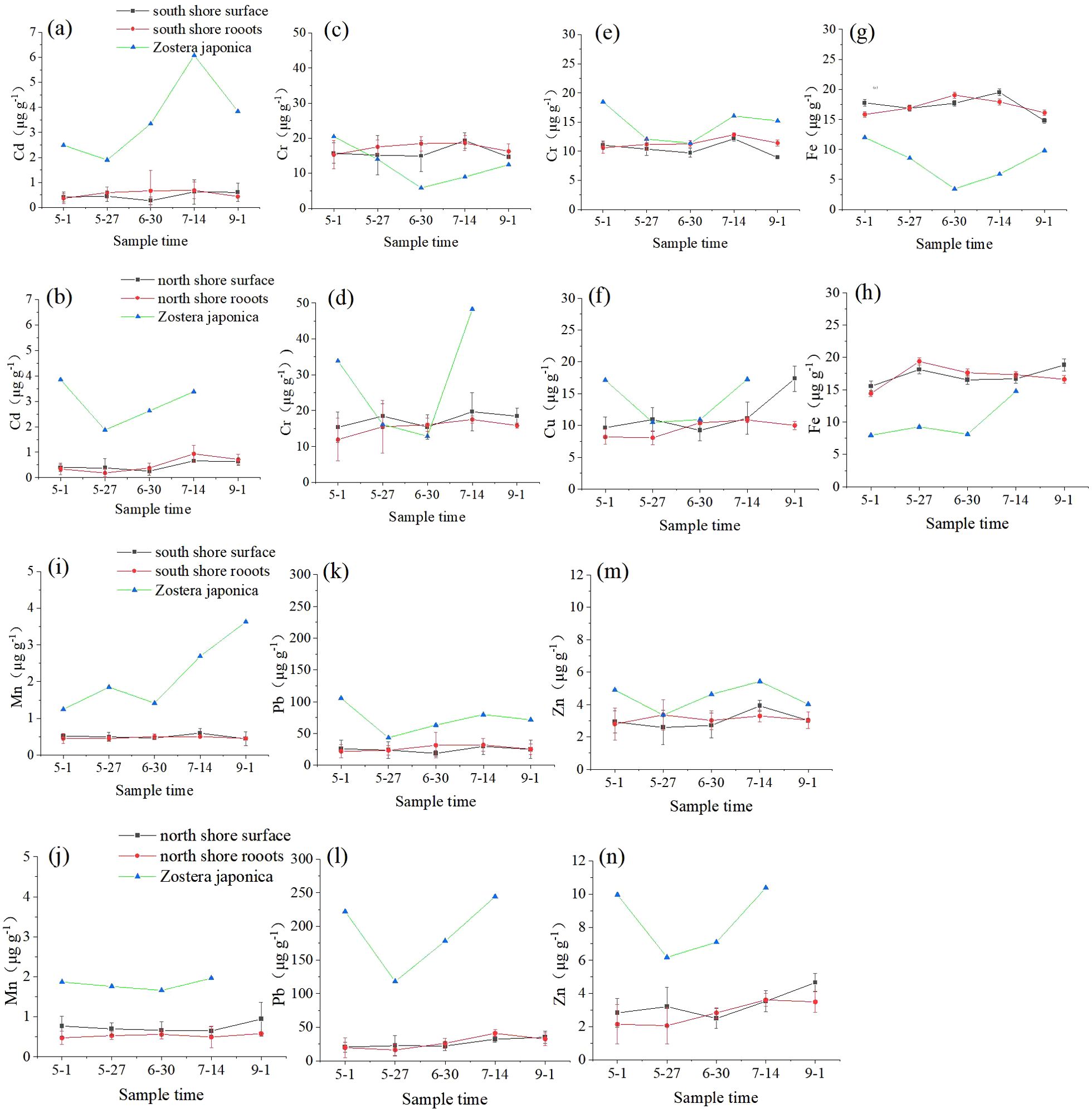
Figure 7. Changes in Cd (A, B), Cr (C, D), Cu (E, F), Fe (G, H), Mn (I, J), Pb (K, L) and Zn (M, N) contents in sediments and Zostera japonica plants in the Yellow River estuary on the north and south shores.
3.3.2 Heavy metal risk assessment
The RI value estimates the cumulative ecological risk based on the concentrations of all metals in the sediment and the toxic response coefficient of each metal (Hakanson, 1980). As shown in Tables 3, 4, the cumulative ecological risk of heavy metals in sediments on both shores was low in the early stage, increased in the later stage with the beginning of WSRS, and finally decreased with the end of WSRS. Cd was identified as the main risk metal. The south shore remained at a medium risk level, while the north shore was at a low risk level until the end of WSRS, when it transitioned to a high risk level before decreasing to a medium risk level.
The bioconcentration factor (BCF) was used to evaluate the enrichment capacity of Zostera japonica for heavy metals in sediments. Among these metals, Cd, Mn, Pb, and Zn were heavily enriched in Zostera japonica tissues, with mean BCF values of 7.0 and 6.0 for Cd on the south and north shores, respectively; 4.4 and 2.3 for Mn on the south and north shores, respectively; 2.8 and 7.1 for Pb on the south and north shores, respectively; and 1.5 and 2.7 for Zn on the south and north shores, respectively (Figure 8).
Except for Cd and Mn, the enrichment capacity of Zostera japonica on the north shore was higher than that of Zostera japonica on the south shore. The accumulation of a large number of heavy metals poses a certain threat to the growth of Zostera japonica and the survival of other aquatic organisms, with Cd and Pb being the main enrichment of Zostera japonica on the north shore and Cd and Mn being the main enrichment metals on the south shore.
3.4 Analysis of the relationship between Zostera japonica growth and environmental factors
The Pearson correlation coefficients between the height and biomass of Zostera japonica plants and various environmental factors are shown in Figure 9. In the south shore sample area, significant correlations (P < 0.05) were found between the height and biomass of Zostera japonica and water salinity, total suspended solids, and chlorophyll content, with correlation coefficients around 0.9. Additionally, strong correlations were observed with plant and sediment nutrient contents, with coefficients close to 0.8. In the north shore sample area, the correlation coefficients between the height and biomass of Zostera japonica and water salinity, total suspended solids, cumulative plant nutrients C and N, and nutrient C content in sediment exceeded 0.9, indicating significant relation. These results indicate that the growth of Zostera japonica in both sample areas is influenced by water salinity, total suspended solids, and sediment nutrient content. The growth of Zostera japonica on the south shore is more susceptible to hydrodynamic effects, as indicated by the significant correlations with water quality factors. During the flood season and WSRS, the increased flow of the Yellow River into the sea altered water quality, increased turbidity, and affected the photosynthesis and oxygen conditions of Zostera japonica. The increased inundation depth and prolonged inundation time also impacted the growth of Zostera japonica.
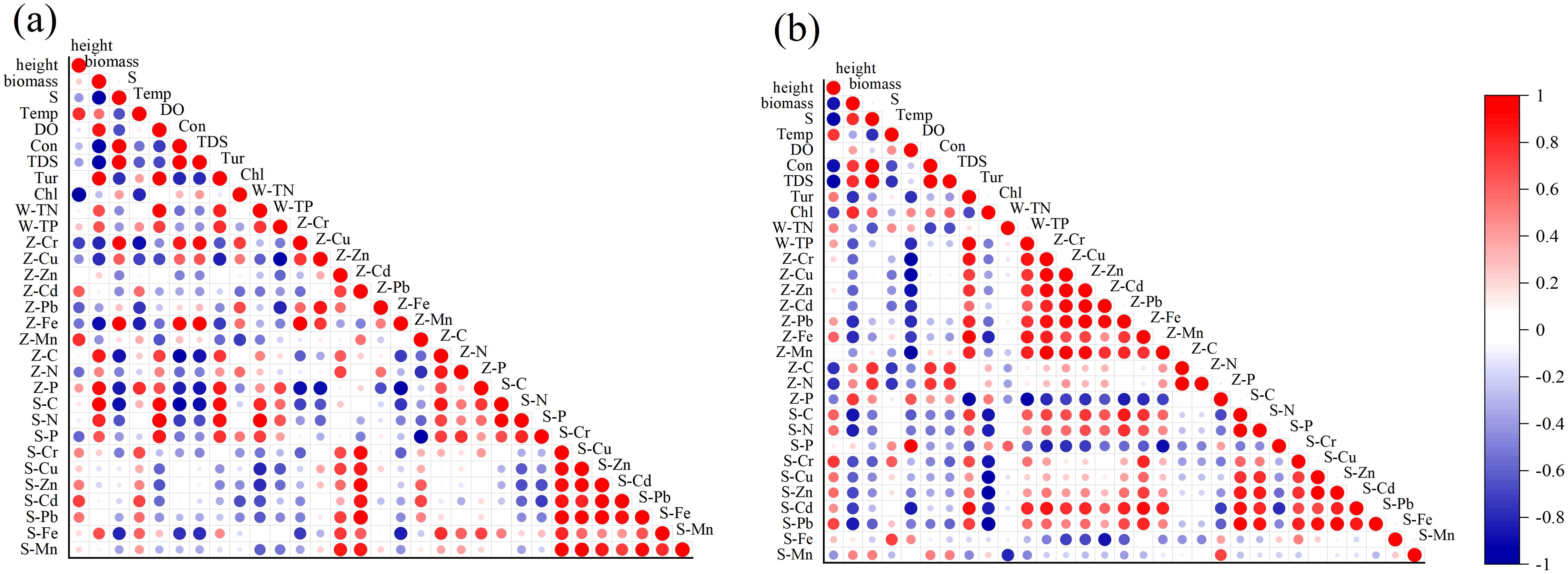
Figure 9. Results of Pearson’s correlation coefficient test between the growth of Zostera japonica and environmental factors on the south shore (A) and north shore (B).
Correlation analysis of nutrients and heavy metals in plants and sediments at the two sample sites showed that Zostera japonica at both sites had some correlation with the corresponding nutrient and metal content in sediments. On the south shore, strong correlations were found between C, N, Cd, and Zn in Zostera japonica and the corresponding elements in sediments. On the north shore, significant correlations were observed between Cd, Pb, and P in Zostera japonica and the corresponding elements in sediments. The weaker correlations for other elements may be related to the continuous scouring of the sediment by seawater during the sampling period and the small sample size of Zostera japonica on the north shore. Additionally, some elements in plants showed significant correlations (P < 0.05) with other elements in plants or sediments, such as Fe and Cr in Zostera japonica on the south shore and Pb in plants and P in sediments. These findings highlight the complex interactions between environmental factors and the growth of Zostera japonica in the Yellow River estuary, emphasizing the importance of considering multiple factors in the conservation and management of seagrass beds.
4 Discussion
The WSRS significantly affects the Yellow River estuary, particularly concerning water quality, sediment dynamics, and the health of Zostera japonica seagrass beds. WSRS induced significant fluctuations in water quality parameters (Yi et al., 2023). Before WSRS, the estuary maintained relatively stable salinity levels, supporting optimal growth conditions for Zostera japonica. However, during WSRS, the influx of freshwater drastically reduced salinity and increased turbidity. These conditions inhibited light penetration, which is essential for photosynthesis, thereby stressing the seagrass. The reduction in salinity disrupted the osmotic balance of Zostera japonica, affecting its physiological functions and overall health. The south shore, closer to the estuary, is more influenced by freshwater inflow, which carries finer sediments and organic-rich particles. These finer particles have a higher surface area and better adsorption capacity, which enhances the retention of nutrients in the sediment (Yang et al., 2011). Consequently, this nutrient-rich environment provides Zostera japonica with a readily available nutrient supply, likely leading to the observed significant increases in TC and TN concentrations within the seagrass tissue. The stable, fine-grained sediments not only reduce nutrient leaching but also improve root anchorage, allowing Zostera japonica to effectively absorb and accumulate these nutrients for growth (Zhang et al., 2021). On the north shore, although sediment composition changed less dramatically, the existing coarser sediments continued to pose challenges for nutrient retention and root stability, leading to poorer growth conditions for the seagrass. Moreover, the water carried by WSRS is not only fresh but also contains high levels of heavy metals. This influx significantly altered the distribution and concentration of heavy metals in the estuarine sediments and in Zostera japonica.
The changes in sediment input and particle composition brought about by the WSRS elevated the heavy metal loads in sediments in the short term. However, this phenomenon was a short-term fluctuation, and the heavy metal concentrations and their risks in the sediments subsequently receded after the end of WSRS (Liu et al., 2019; Chen et al., 2021; Wang et al., 2021b). The relatively low Fe content in Zostera japonica is primarily due to Fe in the sediment being present in an oxidized form that is not easily absorbed, resulting in low bioavailability. Cd, Mn, Pb, and Zn exhibit higher mobility and bioavailability, making them more readily absorbed and accumulated in plant tissues (Lin et al., 2016; Hu et al., 2019). Nevertheless, to alleviate the adverse physiological effects of heavy metals, seagrasses may utilize two primary tolerance strategies: an exclusion or compartmentalization approach, where the highest concentrations of metals are sequestered in belowground structures (such as roots), and a translocation strategy, which results in the majority of metals accumulating in aboveground parts (like leaves). By routinely regenerating their leaves, seagrasses can effectively reduce toxic metal accumulation (Bonanno and Raccuia, 2018; Hu et al., 2019). Thus, the threat to the ecosystem from heavy metal pollution in the region is relatively limited.
The main way to maintain stable reproduction of Zostera japonica populations is through targeted management strategies, such as sediment stabilization to prevent erosion, controlled seeding from healthy populations, and regulating water flow to reduce stress from salinity and turbidity changes. Establishing protected zones can also limit human disturbances, supporting natural regeneration (Yue et al., 2020). Yue et al. (2021) found that Super Typhoon Lekima in August 2019 caused severe damage to Zostera japonica seagrass beds in the Yellow River Estuary, and the main cause of Zostera japonica mortality was soil erosion caused by the typhoon, when Zostera japonica was flowering and seed production was severely reduced, and the lack of seeds and overwintering shoots was the main reason for the disappearance of Zostera japonica seagrass beds in the field study in 2020 (Yue et al., 2020), and also the main reason for the disappearance of Zostera japonica seagrass beds and the decline of biomass in the north shore and the decline of biomass in the south shore found in this study. In 2019, the south shore’s Zostera japonica exhibited greater biomass and plant height, with a maximum of 142 g/m² and 23.78 cm, respectively, compared to the north shore’s 80 g/m² and 18.41 cm. However, both areas saw declines in 2021, with the north shore experiencing an eightfold biomass reduction and near disappearance of seagrass by September, while the south shore’s biomass decreased threefold without significant changes in plant height (Hou, 2020).
In addition, large areas and numbers of Zostera japonica disappeared with incomplete growth patterns on the north shore in 2020 and 2021. Large numbers of snails were found to have been densely distributed on the surface of the north shore from April-September during field sampling in 2021 (Figure 10A), while this phenomenon was not found during field sampling in 2019. Since snails mainly feed on young aquatic plants, aquatic phytoplankton, moss, microorganisms, and humus, the large decrease in Zostera japonica on the north shore may be related to the presence of large numbers of snails and other benthic animals. According to the normal growth pattern of plants, it is not valid that the height and biomass of north shore Zostera japonica plants have been reduced since the end of June. It was observed that the roots of north shore Zostera japonica plants were not destroyed, and only the middle leaves and stems had more neat serrated breaks at them (Figure 10B), resulting in a significant reduction in plant length. Snails feed at night, and at night high tide Zostera japonica leaves are close to the ground under the action of the bottom current and can be ingested by snails, crabs, and clams. The reason for the appearance of large numbers of snails in 2021 is unclear. It may be that the snails were transported to the north shore by tidal currents, or the habitat may have changed due to natural phenomena such as typhoons, which adversely affected the growth of Zostera japonica and may even be the direct cause of the disappearance of Zostera japonica from the north shore. In addition, since the south shore is located in the Yellow River Estuary National Ecological Marine Special Protection Zone, which is free from human activities, while the north shore is located in the Gudong Seawall, where oil field exploration projects, seawall projects and tourist attractions have been built, human activities are inevitable and are one of the factors affecting Zostera japonica seagrass beds. Potential management interventions include introducing natural predators to control snail populations, installing physical barriers around vulnerable seagrass beds, or selectively removing snails during critical growth periods.
The comprehensive impact on Zostera japonica seagrass beds in the Yellow River estuary results from a confluence of natural and anthropogenic factors. WSRS significantly alters water quality and sediment dynamics, reducing salinity and increasing turbidity, which impede photosynthesis and disrupt physiological functions essential for seagrass health. The influx of sediments, including heavy metals like Cd, further exacerbates these stressors by contaminating the habitat and increasing ecological risks. Extreme weather events, such as Super Typhoon Lekima, compound these impacts by causing severe physical disturbances, such as soil erosion and uprooting of seagrass beds, leading to substantial biomass loss and reduced seed production. These events also alter sediment composition and nutrient distribution, hindering the natural regeneration of seagrass. The presence of benthic organisms, like snails, and human activities, including industrial pollution and coastal development, further stress the seagrass beds, particularly on the north shore. WSRS had short-term adverse effects on seagrass growth, compounded by heavy metal contamination, climate change, and benthic organisms, highlighting the complex interplay of natural and anthropogenic factors affecting these habitats (Yue et al., 2021; Song et al., 2021; Wang et al., 2021a, b).This complex interplay of factors necessitates integrated management strategies to mitigate adverse effects, enhance habitat resilience, and ensure the long-term sustainability of Zostera japonica in the Yellow River estuary.
5 Conclusion
This comprehensive study elucidates the multifaceted impacts of extreme climate events and human activities on the growth mechanisms of Zostera japonica in the Yellow River estuary. The findings highlight significant differences in sediment habitats between the north and south shores, influenced by WSRS and other environmental factors. The south shore, with its finer sediments and higher nutrient content, generally supports more robust seagrass growth compared to the north shore, which is characterized by coarser sediments and lower nutrient levels.
WSRS affects water quality and sediment dynamics, leading to reduced salinity and increased turbidity, which impair photosynthesis and disrupt the physiological functions of Zostera japonica. Additionally, the influx of heavy metals during WSRS may pose some ecological risks, further stressing the seagrass beds. Extreme weather events, such as Super Typhoon Lekima, exacerbate these impacts by causing physical disturbances, such as soil erosion and uprooting of seagrass beds, which result in substantial biomass loss and reduced seed production. The combined effects of WSRS, climate change, and anthropogenic activities underscore the complexity of managing and conserving Zostera japonica habitats. Effective management strategies should integrate efforts to mitigate the negative impacts of freshwater influx, control pollutant loads, and maintain optimal water quality. Restoration efforts should focus on stabilizing sediments, enhancing nutrient retention, and supporting the natural regeneration of seagrass beds.
Future research should emphasize long-term monitoring to understand the cumulative impacts of these stressors and explore the potential for natural recovery. By developing targeted conservation efforts and adaptive management strategies, it is possible to promote the resilience and sustainability of Zostera japonica in the Yellow River estuary.
Data availability statement
The raw data supporting the conclusions of this article will be made available by the authors, without undue reservation.
Author contributions
YG: Data curation, Investigation, Software, Visualization, Writing – original draft. KC: Software, Writing – original draft, Resources. ZM: Resources, Investigation, Writing – original draft. YY: Conceptualization, Funding acquisition, Methodology, Supervision, Validation, Writing – review & editing.
Funding
The author(s) declare financial support was received for the research, authorship, and/or publication of this article. This work is supported by the Joint Funds of the National Natural Science Foundation of China Grant No.(U2243236), the Innovative Research Group of the National Natural Science Foundation of China (52221003), and the National Science Fund for Distinguished Young Scholars Grant No.(52025092).
Conflict of interest
The authors declare that the research was conducted in the absence of any commercial or financial relationships that could be construed as a potential conflict of interest.
Publisher’s note
All claims expressed in this article are solely those of the authors and do not necessarily represent those of their affiliated organizations, or those of the publisher, the editors and the reviewers. Any product that may be evaluated in this article, or claim that may be made by its manufacturer, is not guaranteed or endorsed by the publisher.
References
Bonanno G., Raccuia S. A. (2018). Comparative assessment of trace element accumulation and bioindication in seagrasses Posidonia oceanica, Cymodocea nodosa and Halophila stipulacea. Mar. pollut. Bull. 131, 260–266. doi: 10.1016/j.marpolbul.2018.04.039
Bradley M. P., Stolt M. H. (2006). Landscape-level seagrass–sediment relations in a coastal lagoon. Aquat. Bot. 84, 121–128. doi: 10.1016/j.aquabot.2005.08.003
Chen Y., Liu M., Zhang X., Bu X., Chen J., Wu X. (2021). Metals in Yellow River estuary sediments during the 2018 water-sediment regulation scheme period. Mar. pollut. Bull. 166, 112177. doi: 10.1016/j.marpolbul.2021.112177
Gao Y., Yi Y., Chen K., Xie H. (2023). Simulation of suitable habitats for typical vegetation in the Yellow River Estuary based on complex hydrodynamic processes. Ecol. Indic. 154, 110623. doi: 10.1016/j.ecolind.2023.110623
Garcia-Marin P., Cabaco S., Hernandez I., Vergara J. J., Silva J., Santos R. (2013). Multi-metric index based on the seagrass Zostera noltii (ZoNI) for ecological quality assessment of coastal and estuarine systems in SW Iberian Peninsula. Mar. pollut. Bull. 68, 46–54. doi: 10.1016/j.marpolbul.2012.12.025
Hakanson L. (1980). An ecological risk index for aquatic pollution control. A sedimentological approach. Water Res. 14, 975–1001. doi: 10.1016/0043-1354(80)90143-8
Han Q., Soissons L., Liu D., van Katwijk M., Bouma T. (2017). Individual and population indicators of Zostera japonica respond quickly to experimental addition of sediment-nutrient and organic matter. Mar. pollut. Bull. 114, 201–209. doi: 10.1016/j.marpolbul.2016.08.084
Hou C., Song J., Yan J., Wang K., Li C., Yi Y. (2020). Growth indicator response of Zostera japonica under different salinity and turbidity stresses in the Yellow River Estuary, China. Mar. Geology 424, 106169. doi: 10.1016/j.margeo.2020.106169
Hou C. Y. (2020). Response of Zostera japonica to environmental conditions of water and sand in the Yellow River Estuary. Beijing Normal Univ.
Hu C., Yang X., Gao L., Zhang P., Li W., Dong J., et al. (2019). Comparative analysis of heavy metal accumulation and bioindication in three seagrasses: which species is more suitable as a bioindicator? Sci. Total Environ. 669, 41–48. doi: 10.1016/j.scitotenv.2019.02.425
Les D., Waycott C. (1997). Phylogenetic studies in Alismatidae, II: evolution of marine angiosperms (Seagrasses) and hydrophily. Systematic Bot. 22, 443–463. doi: 10.2307/2419820
Lin H., Sun T., Xue S., Jiang X. (2016). Heavy metal spatial variation, bioaccumulation, and risk assessment of Zostera japonica habitat in the Yellow River Estuary, China. Sci. Total Environ. 541, 435–443. doi: 10.1016/j.scitotenv.2015.09.050
Liu M., Fan D., Bi N., Sun X., Tian Y. (2019). Impact of water-sediment regulation on the transport of heavy metals from the Yellow River to the sea in 2015. Sci. Total Environ. 658, 268–279. doi: 10.1016/j.scitotenv.2018.12.170
Liu W., Meng Y., Jin B., Jiang M., Lin Z., Hu L., et al. (2020). Spatial distribution characteristics of heavy metals in surface sediments of the North Canal and ecological risk evaluation by ICP-OES. Spectrosc. Spectral Anal. 40, 3912.
Ma X., Wang A., Fu S., Yue X., Qiu D., Sun L., et al. (2020). Ecological effects of invasion of Zostera japonica Zostera japonica by yellow estuary intergrass. Environ. Ecol. 04), 65–71.
Mao W., Zhao Y., He B., Jia B., Li W. (2022). A review of degradation mechanisms and restoration measures of seagrass ecosystems. J. Desert Res. 01), 87–95.
Marb N., Duarte C. (2010). Mediterranean warming triggers seagrass (Posidonia oceanica) shoot mortality. Global Change Biol. 16(8), 2366-2375. doi: 10.1111/j.1365-2486.2009.02130
Peng S. (2007). The dynamics of biomass and caloric value of seagrass dwarf macroalgae. Guangxi Univ.
Song X., Zhou Y., Zeng J., Shou L., Zhang X., Yue S., et al. (2021). Distinct root system acclimation patterns of seagrass Zostera japonica in sediments of different trophic status: a research by X-ray computed tomography. J. Oceanology Limnology 39, 2267–2280. doi: 10.1007/s00343-020-0421-y
Wang K., Zhang J., Yi Q., Wang X., Yi Y. (2021b). Metals in sediments and their accumulation in Zostera japonica in different sediment habitats of the Yellow River Estuary. J. Soils Sediments 21, 1539–1549. doi: 10.1007/s11368-021-02875-5
Wang S., Cui Y., Liu J., Xia Z. (2016). Advances in research on seagrass and seagrass field ecosystems. Acta Prataculturae Sin. 25, 149–159.
Wang X., Yan J., Bai J., Shao D., Cui B. (2021a). Effects of interactions between macroalgae and seagrass on the distribution of macrobenthic invertebrate communities at the Yellow River Estuary, China. Mar. pollut. Bull. 164, 112057. doi: 10.1016/j.marpolbul.2021.112057
Xu Z., Luo Y., Zhu A., Cai W. (2009). Degradation and restoration of seagrass bed ecosystems. Chin. J. Ecol. 28, 2613–2618.
Yang Z., Ji Y., Bi N., Lei K., Wang H. (2011). Sediment transport off the Huanghe (Yellow River) delta and in the adjacent Bohai Sea in winter and seasonal comparison. Estuar. Coast. Shelf Sci. 93, 173–181. doi: 10.1016/j.ecss.2010.06.005
Yi Y., Gao Y., Wu X., Jia W., Liu Q. (2023). Modeling the effect of artificial flow and sediment flux on the environment and plankton of an estuary. Int. J. Sediment Res. 38, 335–348. doi: 10.1016/j.ijsrc.2023.02.001
Yi Y., Zhao F., Hou C., Zhang C., Tang C. (2024). Mechanism and threshold of environmental stressors on seagrass in high-turbidity estuary: case of Zostera japonica in Yellow River Estuary, China. Front. Mar. Sci. 11, 1432106. doi: 10.3389/fmars.2024.1432106
Yue S., Zhang X., Xu S., Liu M., Qiao Y., Zhang Y., et al. (2021). The super typhoon Lekima, (2019) resulted in massive losses in large seagrass (Zostera japonica) meadows, soil organic carbon and nitrogen pools in the intertidal Yellow River Delta, China. Sci. Total Environ. 793, 148398. doi: 10.1016/j.scitotenv.2021.148398
Yue S., Zhang X., Xu S., Zhang Y., Zhao P., Wang X., et al. (2020). Reproductive strategies of the seagrass Zostera japonica under different geographic conditions in northern China. Front. Mar. Sci. 7, 574790. doi: 10.3389/fmars.2020.574790
Zhang J., Wang K., Yi Q., Pei Y., Hou. C., Yi Y. (2021). Growth of Zostera japonica in different sediment habitats of the Yellow River Estuary in China. Environ. Sci. pollut. Res. 28, 31151–31162. doi: 10.1007/s11356-021-12925-7
Zhang P. D., Liu Y. S., Guo D., Li W. T., Zhang Q. (2016). Seasonal variation in growth, morphology, and reproduction of Eelgrass Zostera marina on the eastern coast of the Shandong Peninsula, China. J. Coast. Res. 32(2), 315–322.
Keywords: Zostera japonica, Yellow River estuary, WSRS, extreme climate, habitat, heavy metal contamination
Citation: Gao Y, Chen K, Ma Z and Yi Y (2024) Study on the effects of extreme climate and human activities on the growth mechanisms of Zostera japonica in the Yellow River estuary. Front. Mar. Sci. 11:1470151. doi: 10.3389/fmars.2024.1470151
Received: 25 July 2024; Accepted: 11 November 2024;
Published: 27 November 2024.
Edited by:
Kejun Yang, Sichuan University, ChinaReviewed by:
Zhenchang Zhu, Guangdong University of Technology, ChinaNa Zhao, Henan University of Science and Technology, China
Copyright © 2024 Gao, Chen, Ma and Yi. This is an open-access article distributed under the terms of the Creative Commons Attribution License (CC BY). The use, distribution or reproduction in other forums is permitted, provided the original author(s) and the copyright owner(s) are credited and that the original publication in this journal is cited, in accordance with accepted academic practice. No use, distribution or reproduction is permitted which does not comply with these terms.
*Correspondence: Yujun Yi, eWl5dWp1bkBibnUuZWR1LmNu